- 1Institut Maurice-Lamontagne, Fisheries and Oceans Canada, Mont-Joli, QC, Canada
- 2Institut des Sciences de la Mer de Rimouski (ISMER), Université du Québec à Rimouski (UQAR), Rimouski, QC, Canada
- 3Ministère des Forêts, de la Faune et des Parcs, Direction de l’Expertise sur la Faune Aquatique, Québec, QC, Canada
- 4Département de Biologie, Université Laval, Québec, QC, Canada
Mussel farming influences benthic environments by organic loading and the addition of physical structure within aquaculture leases. This study evaluated near-field (distance to mussel aquaculture structures, line-scale) and bay-scale (inside vs. outside a blue mussel, Mytilius edulis, farm) effects of an offshore mussel farm in Îles de la Madeleine (Canada) on epibenthic macrofaunal communities. Benthic communities were evaluated by underwater visual counts using SCUBA in June and July 2014. The mussel farm influenced benthic communities at line- and bay-scales. Overall, crabs (Cancer irroratus, Pagarus pubecsens, Pagarus acadianus), sea stars (Asterias rubens), Northern moon snails (Polinices heros), and American lobsters (Homarus americanus) were more abundant in farm sites than outside of farm sites and ocean quahogs (Arctica islandica) were more abundant outside. No clear spatial trend was observed for winter flounder (Pseudopleuronectes americanus) and the sand dollar (Echinarachnius parma) but both species differed (flounder) or showed a trend to differ (sand dollar) between sampling dates. Spatial structure in the distribution of macrofauna was evident within the aquaculture lease as most species were more abundant directly below and close to mussel lines and anchor blocks. There was no spatial structure in non-farm sites. Further investigation is needed to evaluate if mussel farms serve as ecological traps for the species that congregate within them. The long-term effects of the observed spatial effects of offshore mussel culture on macrofauna fitness remains unknown.
Introduction
Declining wild stocks and increasing demand for seafood have led to a rapid expansion of the aquaculture industry and concerns of its potential impact on the environment. Bivalve aquaculture has various near- and far-field effects on marine ecosystems. Far-field effects are mostly limited to effects on water column processes, such as alteration of plankton communities (Prins et al., 1997) and hydrodynamic processes (Plew et al., 2005). Although near-field effects (i.e., within-and immediately surrounding farms) may occur in the water column due to hydrodynamic modifications and farmed bivalves grazing on plankton (see review in Weitzman et al., 2019), most research on near-field effects of bivalve culture has focused on the benthic environment with an emphasis on infaunal and epibenthic macrofaunal communities, sediment structure, and nutrient fluxes and benthic respiration (Souchu et al., 2001; Giles et al., 2006; McKindsey et al., 2011).
The effects of bivalve culture on benthic macrofauna may be evident at several spatial scales. At a bay-scale, culture sites may differ from non-culture sites in the same general area and, at the farm-scale, communities may differ between farm areas at different stages of production (e.g., Toupoint et al., 2008; Drouin et al., 2015). At a smaller spatial scale (line-scale), communities close to or directly below culture gear may differ from those further from such structures (McKindsey et al., 2012; Drouin et al., 2015). In all cases, variations in benthic communities among locations are largely due to two main mechanisms: the addition of physical structure to the environment and organic loading effects.
As suspended bivalve culture is commonly done above unvegetated soft-bottom habitats, culture gear adds considerable physical structure and complexity to an environment that is largely devoid of three-dimensional surfaces (Dumbauld et al., 2009; Forrest et al., 2009; McKindsey et al., 2011). The introduction of aquaculture gear (e.g., ropes and anchor blocks) modifies habitat characteristics and may attract vagile organisms directly (as shelter) or indirectly (as substrate for sessile hard-bottom prey) (Drouin et al., 2015). When fouled, aquaculture structures may increase the abundance and diversity of fauna in farm sites relative to non-farm sites (Saranchova and Flyachinskaya, 2001; Miron et al., 2002; D’Amours et al., 2008) but not necessarily the productivity of these species (Clynick et al., 2008). Bivalve fall-off also alters the habitat of otherwise flat, soft bottoms, adding physical structure through the addition of living hard-bodied organisms and shell debris, although this effect is most likely to occur in mussel farms as other bivalves (e.g., oysters and scallops) are most typically grown in baskets or other structures and do not fall from culture structures to the extent that mussels do.
Many studies of the effects of bivalve culture on the benthic environment have focused on the accumulation of biodeposits (organic material in feces and pseudofeces) from farmed bivalves (Matisson and Lindén, 1983; Callier et al., 2008; Weise et al., 2009). In general, this type of organic loading may alter benthic infaunal and epifaunal communities. This, in turn, may influence the types of larger animals that may feed on these communities (McKindsey et al., 2011; Froehlich et al., 2017). However, as noted by Cranford et al. (2006) and Fréchette (2012), organic loading also occurs in the form of fallen mussels (and associated fauna). Casual observations (authors’ pers. obs.) show that there are often notable quantities of fallen mussels on the seabed within mussel farm sites, particularly directly below and close to culture structures, in eastern Canada. This has also been reported elsewhere around the world where mussels are farmed (e.g., Kaspar et al., 1985; Freire and González-Gurriarán, 1995; Inglis and Gust, 2003; Wilding and Nickell, 2013). Fallen mussels represent a direct input of food and may act as a trophic subsidy for the animals that live in and around culture sites with scavengers and opportunistic predators likely benefiting from this novel prey source. Evidence of this trophic link was provided by Freire and González-Gurriarán (1995), who documented a greater proportion of mussels in the diet of crabs located in a Spanish mussel farm area than comparable areas outside of it. More recently, Sardenne et al. (2019) used lab and field studies to determine that large lobsters fed mainly on mussels from a mussel farm.
At the bay-scale, predators and scavengers are commonly more abundant within mussel farms than outside of them because of the greater abundance of physical structure and trophic interactions (i.e., modified benthic communities and the addition of prey through fall-off of farmed and associated species). At the farm-scale, predators and scavengers may be more abundant in some areas than others as the level of fall-off and physical structure in areas is a function of the stage of production (e.g., fall-off is greater and more anchor blocks are deployed to maintain culture structures in place for larger mussels). Farm-related physical structures, such as anchor blocks, ropes, associated farmed animals, and fall-off are not randomly distributed in farm sites – they occur in well-defined rows. Accordingly, vagile macrofauna have been observed to be more abundant in the vicinity of culture structures and fallen mussels than in areas between mussel lines, thus accounting for line-scale variation in species abundances (Inglis and Gust, 2003; D’Amours et al., 2008; Wilding and Nickell, 2013; Drouin et al., 2015).
Numerous studies have documented that the abundance of vagile predators and scavengers (e.g., crustaceans, sea stars, and gastropods) is increased within bivalve culture sites, particularly mussel farms (Romero et al., 1982; Inglis and Gust, 2003; D’Amours et al., 2008; Callier et al., 2018; Barrett et al., 2019). These studies have mostly focused on mussel farms in shallow, protected embayments. However, the expansion of the aquaculture industry is increasingly leading to the development of offshore farm sites as the inshore industry is becoming saturated in some areas and conflicts with other users increase (Jansen et al., 2016; Mascorda Cabre et al., 2021). According to Jansen et al. (2016) and Mascorda Cabre et al. (2021), cultivation of lower trophic species, such as bivalves and specifically mussels, has a high biological and economic potential for offshore production and this type of aquaculture will most likely expand in the future. Offshore sites have a different architecture (e.g., cultured bivalves being suspended higher off the bottom, longlines with greater spacing between them) and the general environment is different from that of enclosed embayments (e.g., commonly stronger currents, colder waters, deeper areas, different species). Such differences may affect interactions between bivalve culture and benthic communities. Few studies have concentrated on the distribution of vagile epibenthic macrofauna in offshore sites, including those for mussel farms (Froehlich et al., 2017). In the context of marine spatial planning, it is important to quantify the effects of offshore aquaculture on capture fisheries (and ecologically important) species to better predict if farms result in net gains or losses for impacted populations (Clavelle et al., 2019). Indeed, Barrett et al. (2022) recently suggested that non-fed aquaculture may increase productivity of associated species, providing a more holistic view of the costs and benefits of aquaculture production in support of decision-making and the development of sustainable aquaculture (Weitzman, 2019).
The general objective of this study was to evaluate the effects of offshore suspended mussel Mytilus edulis aquaculture on the spatial distribution of vagile epibenthic macrofauna. Two hypotheses were evaluated: (1) that the abundance of epibenthic macrofauna is greater within farm sites relative to non-farm sites (hereafter, reference sites—bay-scale variation), and (2) that the abundance of epibenthic macrofauna within farm sites increases with proximity to farm infrastructure (e.g., mussel longlines and anchor blocks—line-scale variation). Sampling was done twice to evaluate if the benthic communities display temporal variation.
Materials and Methods
Study Sites
The study was done in Baie de Plaisance (N 47°21′, W 61° 44′), îles de la Madeleine, Québec, Canada (Figure 1). The mussel lease covers an area of approximately 2.5 km2, 4 km offshore, where 187 longlines are deployed primarily for mussel aquaculture but the area also supported limited oyster aquaculture. Longlines are 100 m long and are anchored at each end by cement blocks or screw anchors, suspended off-bottom by variable numbers (depending on grow-out stage) of 40 cm spherical buoys and ballasted by ca. 10 concrete anchor blocks (typically about 30 × 30 × 40 cm high) spaced at ca. 10 m. Rows of longlines are spaced 50 m from adjacent ones and individual longlines within rows are separated by 70 m. The site is about 20 m deep and 2 m-long mussel socks are suspended from longlines ca. 10 m above the bottom. The studied area experiences a tidal range of 0.60 m and water temperature can reach 20°C in summer and drop below 0°C during the winter (December to April). The bottom is characterized by a very flat sandy substratum with little physical heterogeneity. In all sites, underwater visibility was typically >5 m. Up to 85% of the mussel lease is used at a time and contains mussels at different stages of production.
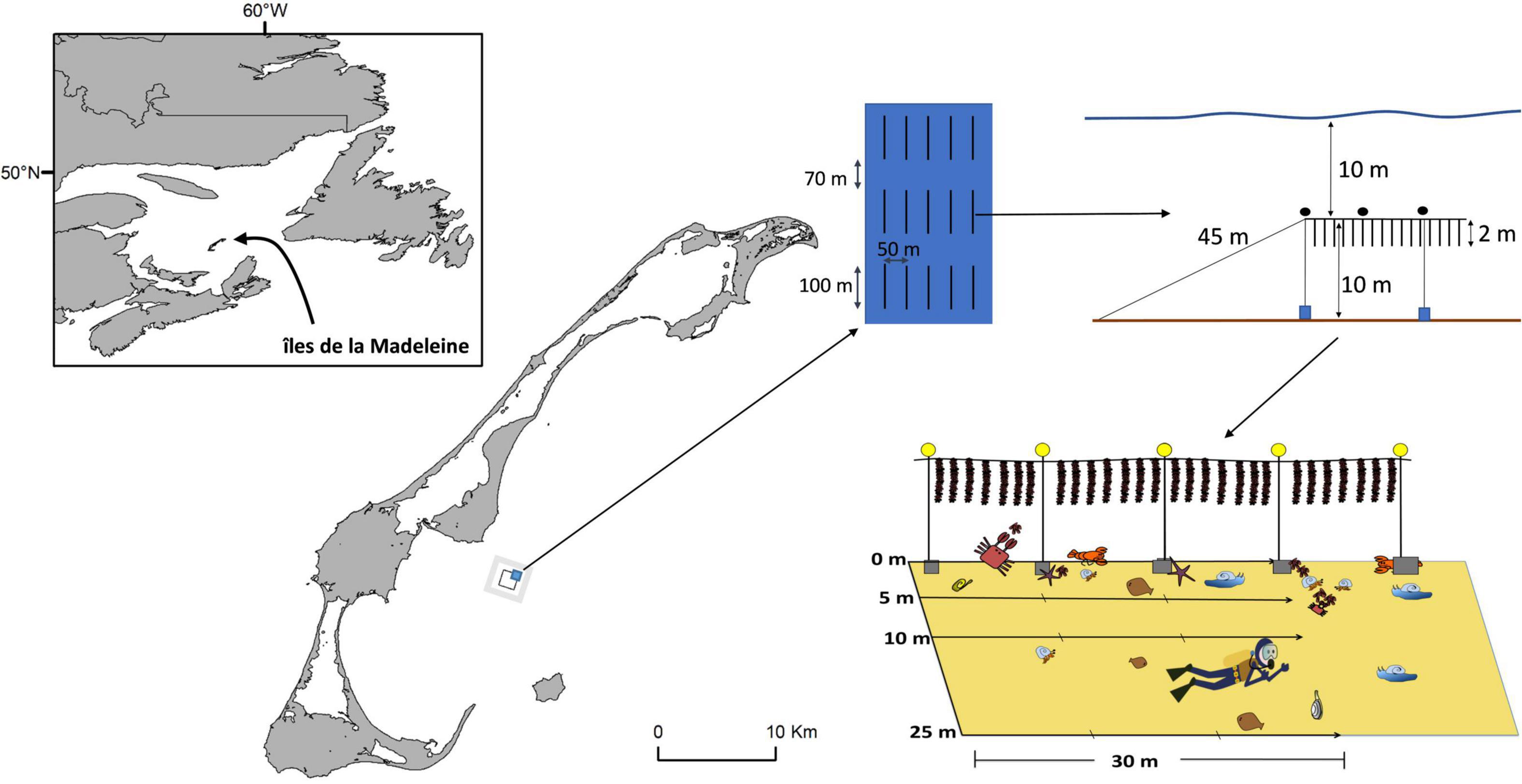
Figure 1. Location of the mussel lease site in Baie de Plaisance, îles de la Madeleine, eastern Canada. The black polygon indicates the mussel lease (Farm area) and the gray area surrounding it the Reference area. Details of farm layout and sampling are also provided.
Sampling Protocol
Sampling was done at two different periods, June 8–14 and July 19–23, 2014. Two areas were studied, one inside (Farm area) and one outside (Reference area, encompassing all areas surrounding the farm and > 500 m to about 1 km distant from it) the offshore mussel farm site. At each area and each period, 8 stations were chosen haphazardly to cover the surface of the mussel farm (concentrating on longlines with 2-year-old mussels) and non-farm areas, for a total of 32 sampling stations. As described by D’Amours et al. (2008), two SCUBA divers made underwater visual counts along transects. The first diver swam near the bottom holding a 2 m pole equipped with a compass and swam transects along the same bearing that mussel lines were oriented while deploying a measuring tape. The second diver identified, counted, and noted epibenthic macroinvertebrates and fish by swimming directly above the bar guided by the first diver. All taxa were distinct and a clear distinction between hermit crabs was made; large hermit crabs were identified as Pagarus pubecsens and small hermit crabs as Pagarus acadianus. Inside the farm, benthic macrofauna were noted along 30 m transects, divided into contiguous (n = 3) 10 m segments, parallel to mussel lines at each of 4 distances from them: 0 m (directly under mussel lines), and at 5, 10, and 25 m away from them (i.e., directly between two adjacent longlines). Transects evaluated in non-farm stations were done following the same pattern and orientation of four transects, with the exception that there was no mussel line, to evaluate if spatial structure of benthic communities differed between farm and reference areas.
Data Analysis
Temporal, bay- and line-scale variation in abundances and taxonomic richness were evaluated using 4-way mixed effect models with the factors Farm (fixed; farm area or reference area), Period (random; June or July), Distance (fixed; from mussel line: 0, 5, 10, and 25 m) and Site (random; nested in Farm and Period) and the interactions among these factors. Samples from contiguous transect are considered as replicates nested within each Distance in a given Site. The assumptions of normality and homoscedasticity for parametric analyses were verified by visual evaluation of residuals and Cochran’s test and were never met, despite trying various data transformations. Thus, all univariate models (i.e., for abundances of each species, total abundance, and richness) were evaluated using PERMANOVA with PRIMER 7 + PERMANOVA based on Euclidean distances (n = 4,999 unrestricted permutations) as suggested by Anderson (2001). All univariate analyses (with the exception of winter flounder and small hermit crabs) showed that the factor Period and its interactions with other factors were not significant (P > 0.2) and thus this factor was removed from the analyses and the reduced model rerun for all species other than winter flounder and small hermit crabs. Significant factors were further examined using appropriate pairwise comparisons. Variation in multivariate community structure was visualized using non-metric multidimensional scaling (MDS) and evaluated using PERMANOVA (fourth-root transformation of abundance data and based on Bray Curtis similarity distances, n = 4,999 unrestricted permutations). PERMDISP was performed on the same Bray–Curtis matrix to evaluate variation in multivariate dispersion among a priori groups (i.e., contrasting variation in multivariate community structure between outside and inside of the farm and among distance classes within the farm). Likewise, species accounting for differences between Farm and Reference areas and between distances within the farm were identified using SIMPER.
Results
Epibenthic macrofaunal communities were dominated by sand dollars Echinarachnius parma (70.5%), small hermit crabs Pagarus acadianus (9.59%), large hermit crabs Pagarus pubecsens (5.2%), sea stars Asterias rubens (4.3%), Atlantic rock crabs Cancer irroratus (3.8%), ocean quahogs Arctica islandica (2.9%), winter flounders Pseudopleuronectes americanus (2.9%), Northern moon snails Polinices heros (0.6%), and American lobsters Homarus americanus (0.3%). Other vagile organisms were occasionally observed, mostly within mussel farms and directly on anchors blocks (data not shown), such as sea ravens Hemitripteridae sp. and sculpins Myoxocephalus sp., although they were not considered in the present study due to their rarity.
Univariate Analyses
Variation in abundance was observed between farm and reference sites for seven of the nine epibenthic species evaluated. The abundance of large hermit crabs, Northern moon snails, and ocean quahogs varied significantly between farm and reference areas, being more abundant in farm than reference sites, with the exception of ocean quahog, which was more abundant in reference than in farm sites (Figure 2 and Table 1). The abundance of American lobster, Atlantic rock crab and common sea star showed significant 2-way interactions between Farm and Distance. Atlantic rock crab and sea star abundance increased significantly with proximity to longlines, whereas American lobster abundance was greater at 0 m than at other distances from the longlines (Figure 2 and Table 1). Winter flounder was significantly more abundant in June than July but did not show any spatial variation in abundance (Figure 2 and Table 2). The abundance of small hermit crabs varied as a function of the Period × Distance interaction (Figure 2 and Table 2) such that its abundance decreased with proximity to longlines—being least abundant at 0 m in June—but no trend was observed in July. Despite a fairly clear trend in the abundance of sand dollars (Figure 2 shows that sand dollar abundance in reference sites in June to be about twice that observed in farm sites and of reference sites in July), this effect was not statistically significant (Table 1), likely due to very high variability among sites. Overall, taxonomic richness was significantly greater inside the mussel farm than in the reference sites (Figure 3 and Table 1). There were no statistically significant patterns for total abundance, again most likely due to the abundance of sand dollars among sites. When the abundance of this species is removed from the calculation of “total abundance,” the abundance of all other species combined is greater in Farm sites than Reference sites (Figure 3 and Table 2), mirroring the overall trends observed for most individual species.
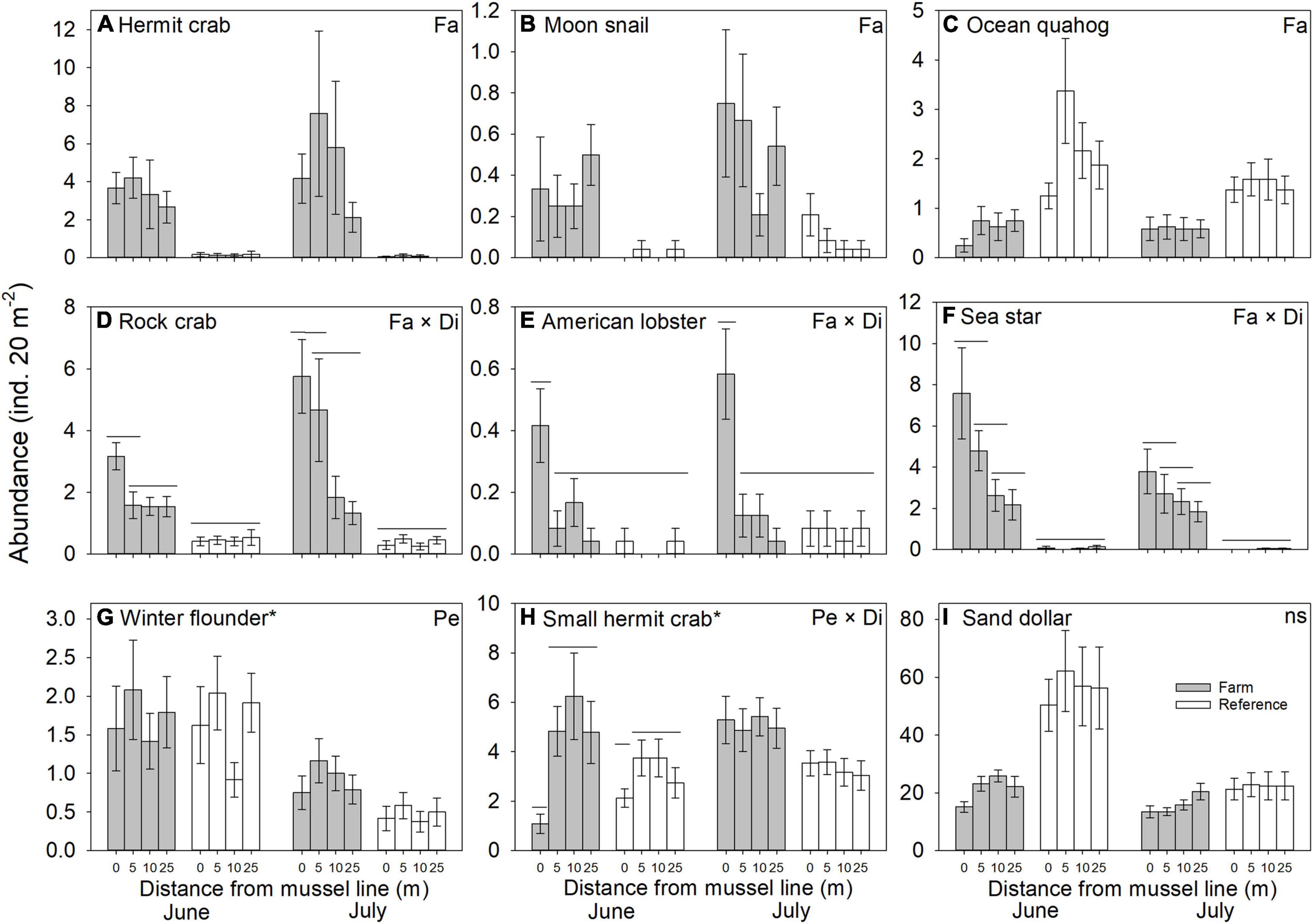
Figure 2. Abundance (mean ± SE, n = 24) of macrofauna recorded along transects in Farm (gray) and Reference (white) areas at two sampling Periods (June and July) with Distance to mussel lines (0, 5, 10 and 25 m) in an offshore blue mussel (Mytilus edulis) culture site. Significant effects on macrofauna abundance among different treatments are specified in the upper right-hand corner; only significant differences between distances where interactions are significant are indicated (by non-adjoining bars); effects of Farm are evident. All pair-wise comparisons were made with reduced model (i.e., without the factor Period) except for species indicated by an asterisk; ns = not significant.
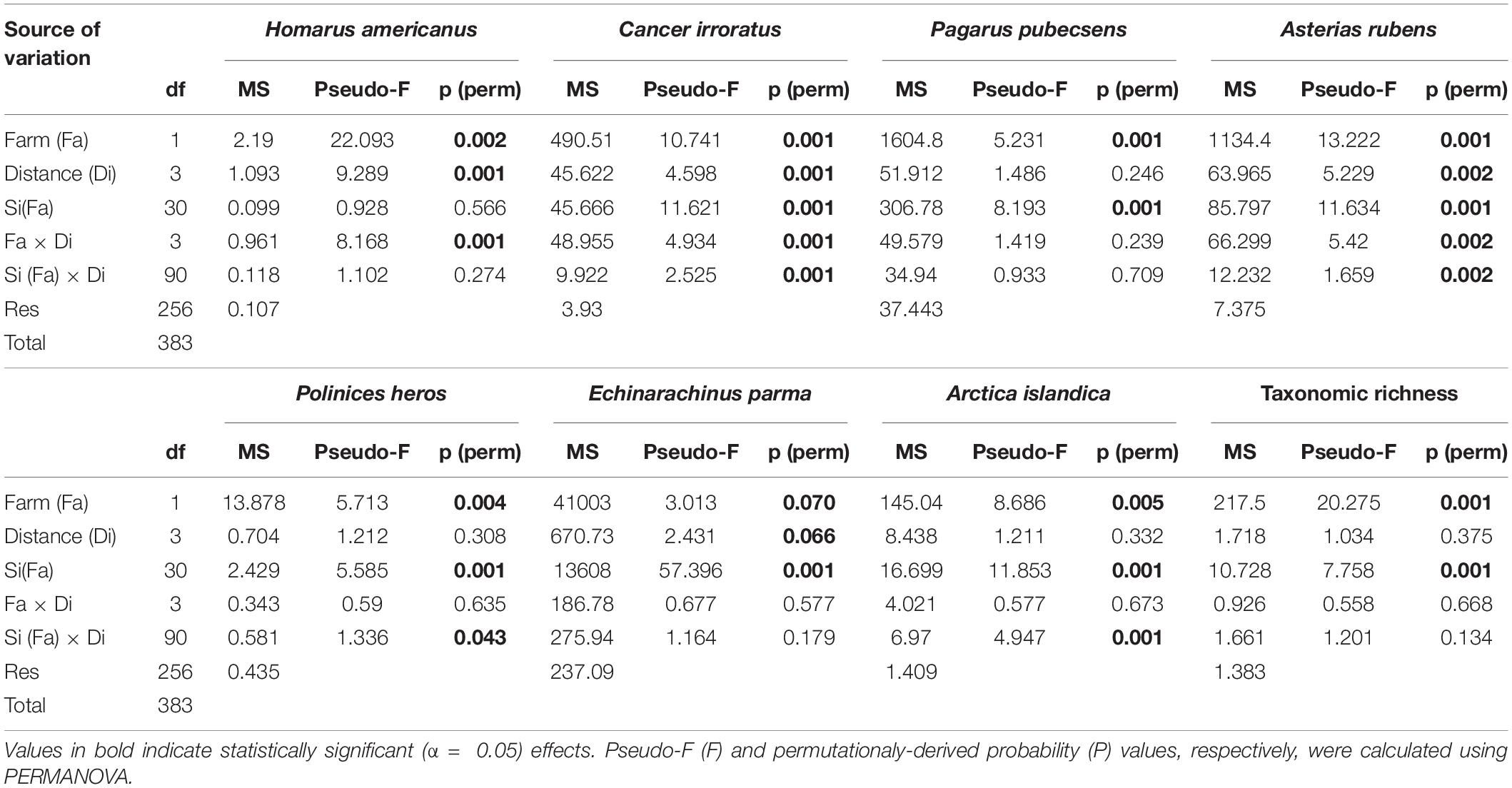
Table 1. Results of PERMANOVA analysis (reduced model; untransformed data) for variation in the abundance of American lobster Homarus americanus, Atlantic rock crab Cancer irroratus, hermit crab Pagarus pubecsens, common sea star Asterias rubens, northern moon snail Polinices heros, sand dollar Echinarachnis parma and ocean quahog Arctica islandica, total abundance, taxonomic richness, and multivariate community structure to test the blue mussel (Mytilus edulis) culture lease effects of Farm (Farm vs. reference), Distance (from mussel line: 0, 5, 10, and 25 m) and Site (1–32) and their interactions.
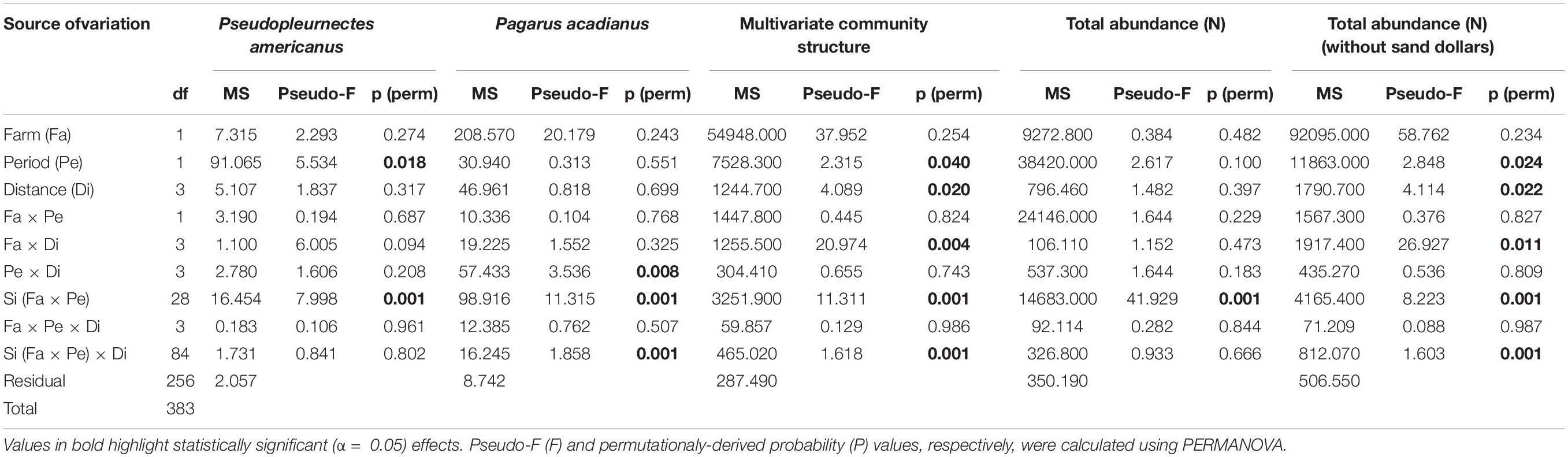
Table 2. Results of PERMANOVA analysis (full model; untransformed data for univariate metrics but fourth-root transformed data for multivariate community structure) for variation in the abundance of winter flounder Pseudopleuronectes americanus and small hermit crab Pagarus acadianus, multivariate community structure, total abundance and total abundance without sand dollar Echinarachnius parma to test the effects of Farm, Period, Distance, Site and their interactions.
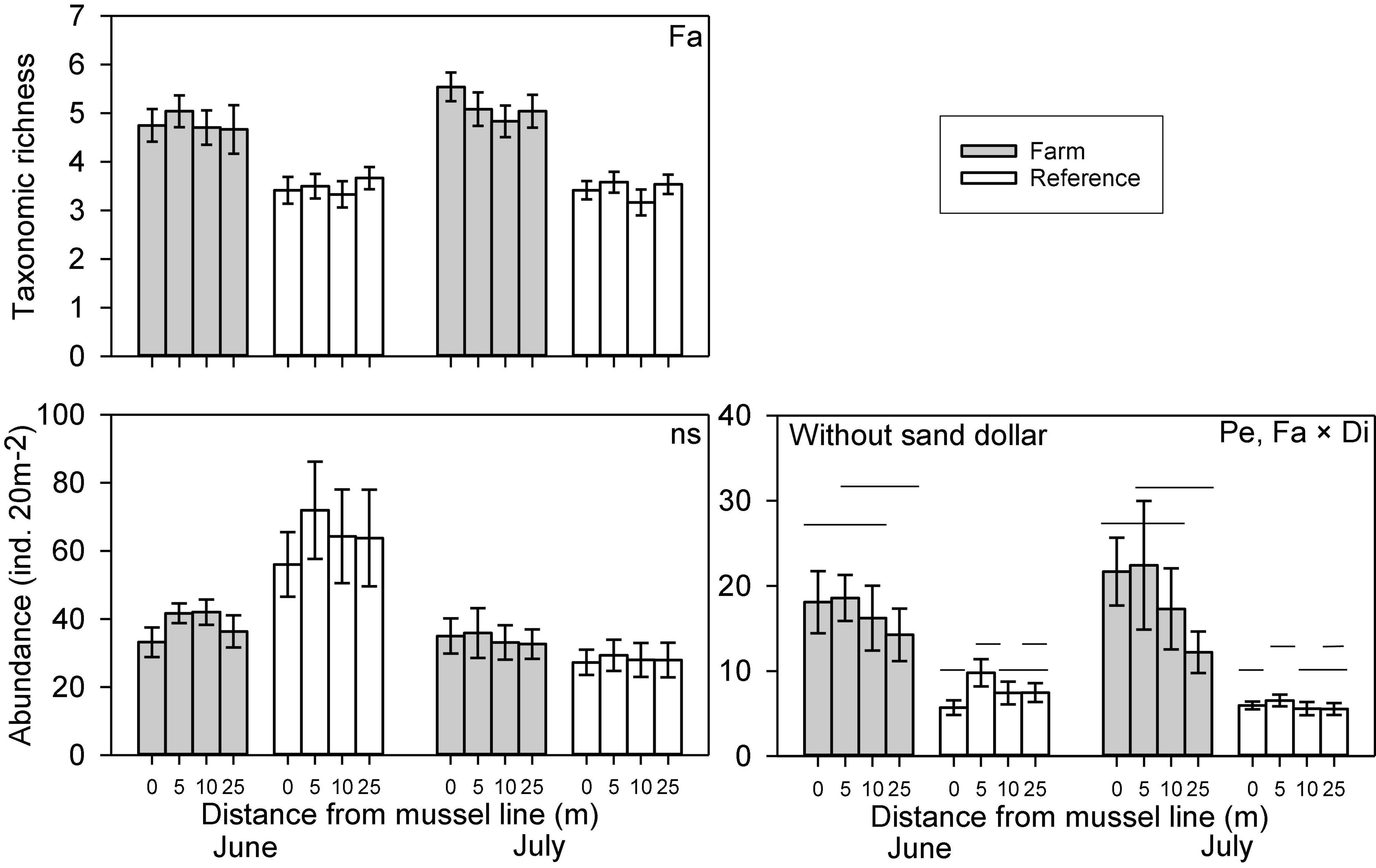
Figure 3. Abundance (with and without sand dollars) and taxonomic richness (mean ± SE, n = 24) of macrofauna recorded along transects in Farm (gray) and Reference (white) areas at two sampling Periods (June and July) with Distance to mussel lines (0, 5, 10, and 25m) in an offshore blue mussel (Mytilus edulis) culture site. Significant differences in macrofauna abundances and taxonomic richness among different treatments are specified in the upper right-hand corner; only significant differences between distances where interactions are significant are indicated (by lines on different planes); effects of Farm are evident. The pair-wise comparison was made with full model (i.e., with the factor Period).
Multivariate Analyses
Macrofaunal community structure varied as a function of the Farm × Distance interaction (Table 2), with communities from farm and reference areas differing. Within the farm, communities directly under the mussel lines differed from those at other distances (i.e., 0 m ≠ 5 m = 10 m = 25 m) and were most different from those in reference sites. In both periods, MDS plots (Figure 4) show clear farm-scale effects where Farm sites differ from Reference sites with little overlap between site points. Multivariate dispersion differed between farm and reference sites, with variation among replicate contiguous transects being greater inside of the farm than outside of it [ = 25.026 and 20.642 for dissimilarity outside and inside of the farm, respectively, F(1,382) = 21.225, p = 0.001]. In contrast, multivariate dispersion within the farm did not differ among distances [F(3, 39) = 0.128, p = 0.961]. In all contrasts among distance classes within farms, sea stars, small and large hermit crabs, flounders, and rock crabs, together, accounted for between 71 and 77% of the observed dissimilarity in community structure among distances, with the contribution of the various species differing depending on the contrast.
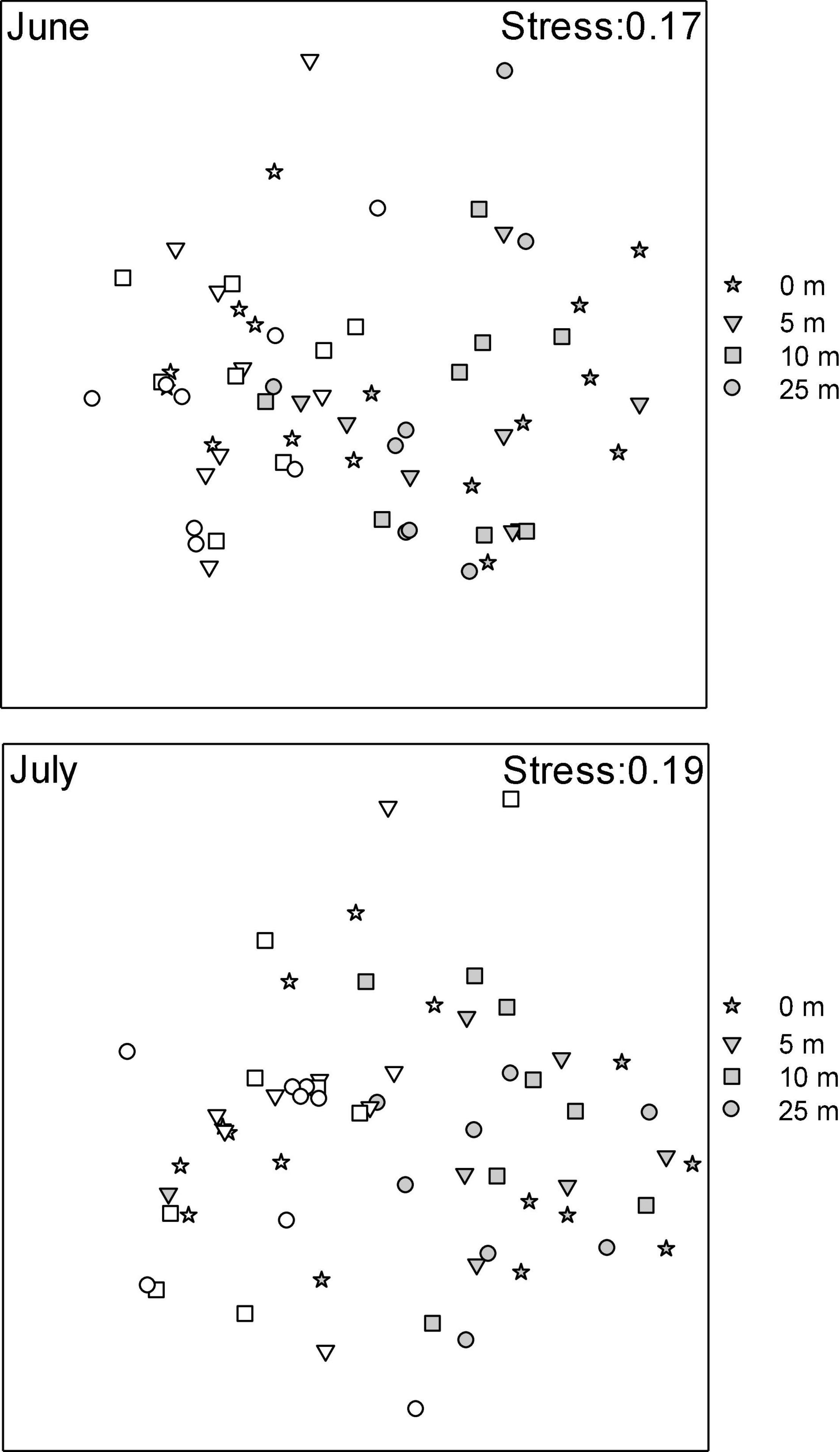
Figure 4. MDS plots of macrofauna (√√) community structure at two sampling Periods (June and July 2014) in mussel farms (gray) with distance to mussel lines (0 m through 25 m) and reference areas (open circles).
Discussion
This study shows that offshore suspended mussel aquaculture in Îles de la Madeleine influences the distribution and abundance of epibenthic macrofauna at both the bay- and line-scale, although the effects varied among species and, at times, between sampling periods. Community structure analyses highlighted three distinct species assemblages: outside the farm sites, within the farm sites but not below mussel lines, and directly below mussel lines. This was reflected by the distribution of most species, except for winter flounder and sand dollars, whose abundance did not vary between farm and reference sites, most of which (except for ocean quahogs, which is actually an infaunal species) were most abundant in farm sites and many of which were more abundant directly below mussel lines than between them. Taxonomic richness and total abundance (less sand dollars) were also greater in farm areas than non-farm areas. This increased taxonomic richness and total abundance likely homogenized benthic communities within the farm relative to those outside of the farm, as evidenced by the decreased multivariate dispersion of samples from farm sites relative to those from reference sites.
Two main mechanisms likely account for the spatial distribution of the vagile macrobenthic organisms observed in the present study (see review in McKindsey et al., 2011). First, effects on trophic structure (increased input of prey resulting from fallen mussels and associated fouling organisms may attract predators, as may biodeposit-enriched and modified infaunal communities). Second, the addition of physical structure to the benthic environment in the form of anchor blocks and fallen mussels alters the seabed by creating colonization surfaces and increasing possible shelter or refuges for a variety of organisms. While other studies have focused on a few species (e.g., Freire and González-Gurriarán, 1995; Inglis and Gust, 2003; Wilding and Nickell, 2013), we focused on entire local macrofaunal communities to allow more general conclusions to be drawn. Given that the impact of this offshore mussel farm on benthic infauna is quite limited (Lacoste et al., 2018) and that lobster, but not crabs, sea stars, or flounders are impacted by the presence of anchor blocks alone without the presence of mussels (Drouin et al., 2015), we assume that observed impacts on species distributions of most species are likely mostly attributable to the presence of fallen mussels as a food source. That is, a simple trophic effect (i.e., the supply of food in the form of fallen mussels to scavengers or predators; Forget et al., 2020) is likely the main reason for the increased abundance of several taxa in farm sites, particularly close to mussel lines within the farm. Mussels are considered the main prey of rock crabs in coastal areas (Drummond-Davis et al., 1982). Given that many mussels fall from culture structures during grow-out, particularly more or less directly below mussel longlines (Wilding and Nickell, 2013), this provides a great trophic advantage to crabs in the area, logically concentrating them where the mussels have fallen. Romero et al. (1982) noted a greater abundance of various crabs in areas with mussel rafts relative to areas without them, suggesting that they are attracted to aquaculture sites where they feed on fallen cultured mussels and associated epifauna. As noted by Hudon and Lamarche (1989), mussels may account for an important fraction of American lobster diet due to their high calorific value. Moreover, a great proportion of the diet of large lobsters is mussels in an area with available farmed, but few wild, mussels (Sardenne et al., 2019). However, rock crabs play a key role in the growth, condition and ovary development of American lobsters of all sizes due to their high protein content and ratio of amino acids (Gendron et al., 2001), likely accounting for the finding that smaller lobsters feed mostly on rock crabs associated with mussel farms (Sardenne et al., 2019). In contrast, Sainte-Marie and Chabot (2002) found that larger lobsters preyed on large, vagile, nutritious prey, such as crustaceans, although this latter work was done in an area that lacked nearby mussel aquaculture sites. Together, this suggests that lobsters are, in part, more abundant in farm sites, particularly close to mussel lines, because of both the abundant prey and the addition of physical structure in the form of anchor blocks.
Sea stars were orders of magnitude more abundant in farm sites than in reference sites, showing the great attractiveness of the farm for these animals. Increased abundance of sea stars within mussel sites associated with mussel lines was also reported by Inglis and Gust (2003) and Barrett et al. (2020) for Coscinasterias muricata and D’Amours et al. (2008) for Asterias sp. Gaymer et al. (2004) showed that mussels (M. edulis) are the preferred prey of Asterias sp., again suggesting a trophic link. Similarly, Wilding and Nickell (2013) reported a negative correlation between sea star abundance and distance to mussel lines and related this to mussel fall-off. However, sea stars may also recruit in large quantities to mussel lines (Barkhouse et al., 2007) and this too could, in part, account for the greater abundance of these animals in the farm relative outside of it.
Large hermit crabs and Northern moon snails were more abundant in mussel farm sites relative to reference sites. Hermit crabs are omnivorous detritivores (Hazlett, 1981) and thus likely able to take advantage of the general increase of detritus in farm areas due to incomplete consumption of fallen mussels and associated organisms by other macropredators in the site. In contrast, Northern moon snails are active foraging predators of molluscs (Commito, 1982) that readily feed on mussels if found in their environment (Kenchington et al., 1998) and thus were likely more abundant in the farm site because of the great concentration of fallen mussels in the area. Other authors reported similar findings of increased abundance of predatory gastropods in Eastern Canada (Grant et al., 1995; D’Amours et al., 2008).
Although small hermit crabs were, overall, more abundant in mussel farm sites than reference sites, they displayed line-scale variation in abundance in June, when they were less abundant directly below mussel lines than at other distances and locations. Small hermit crabs are vulnerable to dexterous predators, including larger hermit crabs, brachyuran crabs (e.g., rock crabs) and lobsters (Angel, 2000) and these predators were particularly more abundant in farm sites close to mussel longlines, potentially explaining their line-scale variation in abundance. Similarly, Drummond-Davis et al. (1982) found that P. acadianus is the most frequently consumed prey by rock crabs in kelp beds in Nova Scotia. Although more food is available in farm sites (e.g., fallen mussels, associated organisms), small hermit crabs may avoid such areas since predation risks are elevated, illustrating a behavior defined by Kerfoot and Sih (1987) whereby aquatic prey adjust to spatial and temporal variation in predation risk.
In contrast to the positive association of animals to the mussel culture site, both ocean quahogs and sand dollars tended to be less abundant in the farm than outside of it. In both cases, this may be due to the increased abundance of predators in the farm, which may also prey on these two species. Predators of ocean quahogs include invertebrates such as brachyuran crabs (e.g., C. irroratus) (Stehlik, 1993), crustaceans (Kraus et al., 1992), seas stars (Kennish et al., 1994), and teleost predators such as sculpins (Myxocephaus sp.) (Langton and Bowman, 1980), all which were most abundant in farm sites. Likewise, predators of sand dollars include sea stars, common rock crabs, and benthic fish (i.e., winter flounder) (Jalbert et al., 1989; Himmelman and Dutil, 1991; Gaymer et al., 2004). Most of these predators were more abundant in farm sites than reference sites. In addition to predation, mortality of ocean quahogs may also occur due to increased sedimentation (Anger et al., 1977). Thus, organic loading from biodeposition below mussel lines may, in part, explain the decreased abundance of these bivalves in farm sites. Sand dollars are more mobile than ocean quahogs and commonly burrow in sediments (Cabanac and Himmelman, 1996), suggesting that increased sedimentation may not account for the observed trends in sand dollar abundance.
The presence of physical structure in marine habitats allows for a greater abundance of organisms and species richness compared to less complex habitats (Gratwicke and Speight, 2005). Anchor blocks, ropes and other aquaculture items, including fallen mussels and shell debris, may create a more heterogeneous benthic environment and modify community richness and biomass (Chesney and Iglesias, 1979). It may also decrease the abundance of species that require flat homogenous substrata, such as sand dollars and ocean quahogs. Significant variation at the line-scale was observed for many taxa. American lobsters were most abundant directly under the mussel line, most often associated with anchor blocks, whereas the abundance of rock crabs and sea stars increased with proximity to mussel lines and Northern moon snails and large hermit crabs showed the same trend. However, the relative importance of trophic and physical structure effects on the distribution of taxa within farms is difficult to resolve. Drouin et al. (2015) did an experiment to separate these factors and found that the association of American lobster with mussel lines is likely primarily due to the presence of anchor blocks but suggested that the abundance of food in mussel farm areas further increased their abundance. As American lobsters cannot quickly bury themselves in sand, they seek protection from other shelters in their environment (Hudon and Lamarche, 1989). In contrast, Drouin et al. (2015) also suggested that rock crabs, which are less dependent on shelter and physical structure (Fogarty, 1976; Cobb et al., 1986; Gendron and Fradette, 1995), likely benefit from fallen mussels, thus explaining the line-scale distribution of this species.
Overall, the distribution of winter flounder does not appear to be influenced by mussel leases as no clear trend was observed except for a temporal variation. Similarly, Clynick et al. (2008) found no difference in the abundance of winter flounder in farm sites relative to reference sites. Winter flounder is a habitat generalist that occurs on a variety of shallow substrates and sediment types (Sogard and Able, 1991). According to Worobec (1984), juveniles prey opportunistically on small infaunal organisms, crustaceans, and other appropriate food as they grow. Only winter flounder was observed to vary temporally in the present study. However, the abundance of other taxa also likely vary temporally but the sampling regime employed (sampling twice at the beginning of summer) was not appropriate to detect such temporal variation.
The present study suggests that most epibenthic macrofauna taxa have a positive response to suspended mussel aquaculture in the studied offshore area. By itself, the addition of aquaculture structural features likely increase local productivity, diversity, and biomass, which are typically greater on hard-substrates (Cowles et al., 2009). Mussel fall-off from longlines likely increases the abundance of many predators in the farm site. Beyond the simple aggregative effect of predators due to fall-off, the impact of such a transfer of trophic energy from the pelagic environment (plankton) and intermediary (mussel filtration and fall-off) to the benthic environment on the animals that live there is poorly understood. Observational and modeling work on benthic scallop culture (Kluger et al., 2016a,b) suggests that the addition of farmed bivalves to the bottom increases the abundance of their predators at the bay-scale, thus benefiting fisheries of any such species, whereas this increased abundance of predators also negatively impacts non-predatory species that are normally present. However, the increased abundance of predators in farm sites may also cause farms to act as ecological traps for these animals if fishing effort is directed in or around them (Fernandez-Jover et al., 2008; Dempster et al., 2009). Although a recent review (Barrett et al., 2022) suggests that such aggregations of wild animals may enhance the productivity of farm environments relative to otherwise equivalent environments, the impact of mussel farms on the fitness of these animals is unknown. Wang and McGaw (2016) suggest that a mussel-only diet is not suitable for lobsters because of low levels of amino acids such as asparagine, alanine and glutamic acid and the carotenoid astaxanthin in mussel flesh. Further studies are needed to obtain a more holistic understanding of the interactions between mussel aquaculture and associated macrofauna to ensure the ecological sustainability of the industry and better understand its effects on the fishing industry and the fisheries species themselves.
Conclusion
Marine aquaculture of many types is expanding into offshore areas to provide seafood products while limiting environmental impacts and conflict between users of the limited space in coastal zones (Froehlich et al., 2017). In the context of marine spatial planning, a better understanding of the interactions between aquaculture and fisheries is of great importance (Clavelle et al., 2019) and may be used to limit the impacts of these two activities on one another (Gentry et al., 2017; Lester et al., 2018). It is hoped that the clear evidence of increased abundance of many macrobenthic species in the examined îles-de-la-Madeleine offshore mussel farm will contribute to a more holistic (Weitzman, 2019) understanding of offshore mussel farm effects to support logical marine spatial planning.
Data Availability Statement
The raw data supporting the conclusions of this article will be made available by the authors, without undue reservation.
Author Contributions
A-SS, AD, PA, and CM contributed to the design and concept of the study. A-SS, AD, and CM contributed to field missions, statistical analysis of the data, and writing the manuscript. AD, PA, and CM obtained funding to complete the work. All authors reviewed and edited the manuscript contributing to the final version.
Funding
This research was funded by Fisheries and Oceans Canada through an Aquaculture Collaborative Research and Development Program (ACRDP) grant to CM (Q-13-01-001), the Société de Développement de l’Industrie Maricole (SODIM), a UQAR-MERINOV grant, and Ressources Aquatiques Québec (RAQ).
Conflict of Interest
The authors declare that the research was conducted in the absence of any commercial or financial relationships that could be construed as a potential conflict of interest.
Publisher’s Note
All claims expressed in this article are solely those of the authors and do not necessarily represent those of their affiliated organizations, or those of the publisher, the editors and the reviewers. Any product that may be evaluated in this article, or claim that may be made by its manufacturer, is not guaranteed or endorsed by the publisher.
Acknowledgments
We thank F. Roy, P. Robichaud, and E. Simard for help in the field, La Moule du Large (C. Vigneau and D. Hébert) for use of their mussel site, and MERINOV (M. Nadeau) for discussions about the work. We also thank D. Drolet and A. Weise for suggestions on analyses and revisions of early drafts of the manuscript.
References
Anderson, M. J. (2001). A new method for non-parametric multivariate analysis of variance. Aust. Ecol. 26, 32–46. doi: 10.1111/j.1442-9993.2001.01070.pp.x
Angel, J. E. (2000). Effects of shell fit on the biology of the hermit crab Pagurus longicarpus. J. Exp. Mar. Biol. Ecol. 243, 169–184. doi: 10.1016/S0022-0981(99)00119-7
Anger, K., Rogal, U., Schriever, G., and Valentin, C. (1977). In-situ investigations on the echinoderm Asterias rubens as a predator of soft-bottom communities in the western Baltic Sea. Helgoland. Wiss. Meer. 29:439. doi: 10.1007/BF01609982
Barkhouse, C., Niles, M., and Davidson, L.-A. (2007). A literature review of sea star control methods for bottom and off bottom shellfish cultures. Can. Ind. Rep. Fish. Aquat. Sci. 279, vii, + 38.
Barrett, L. T., Swearer, S. E., and Dempster, T. (2019). Impacts of marine and freshwater aquaculture on wildlife: a global meta-analysis. Rev. Aquac. 11, 1022–1044. doi: 10.1111/raq.12277
Barrett, L. T., Swearer, S. E., and Dempster, T. (2020). Native predator limits the capacity of an invasive seastar to exploit a food-rich habitat. Mar. Env. Res. 162:105152. doi: 10.1016/j.marenvres.2020.105152
Barrett, L. T., Theuerkauf, S. J., Rose, J. M., Alleway, H. K., Bricker, S. B., Parker, M., et al. (2022). Sustainable growth of non-fed aquaculture can generate valuable ecosystem benefits. Ecosyst. Serv. 53:101396. doi: 10.1016/j.ecoser.2021.101396
Cabanac, A., and Himmelman, J. (1996). Population structure of the sand dollar Echinarachnius parma in the subtidal zone of the northern Gulf of St. Lawrence, eastern Canada. Can. J. Zool. 74, 698–709. doi: 10.1139/z96-079
Callier, M. D., Byron, C. J., Bengtson, D. A., Cranford, P. J., Cross, S. F., Focken, U., et al. (2018). Attraction and repulsion of mobile wild organisms to finfish and shellfish aquaculture: a review. Rev. Aquac. 10, 924–949. doi: 10.1111/raq.12208
Callier, M. D., McKindsey, C. W., and Desrosiers, G. (2008). Evaluation of indicators used to detect mussel farm influence on the benthos: two case studies in the Magdalen Islands, Eastern Canada. Aquaculture 278, 77–88. doi: 10.1016/j.aquaculture.2008.03.026
Chesney, E. J., and Iglesias, J. (1979). Seasonal distribution, abundance and diversity of demersal fishes in the inner Ria de Arosa, northwest Spain. Estuar. Coast. Mar. Sci. 8, 227–239. doi: 10.1016/0302-3524(79)90093-8
Clavelle, T., Lester, S. E., Gentry, R., and Froehlich, H. E. (2019). Interactions and management for the future of marine aquaculture and capture fisheries. Fish Fisher. 20, 368–388. doi: 10.1111/faf.12351
Clynick, B. G., Archambault, P., and McKindsey, C. W. (2008). Distribution and productivity of fish and macroinvertebrates in aquaculture sites in the Magdalen islands (Québec, Canada). Aquaculture 283, 203–210. doi: 10.1016/j.aquaculture.2008.06.009
Cobb, J., Wang, D., Richards, R., and Fogarty, M. (1986). Competition among lobsters and crabs and its possible effects in Narragansett Bay, Rhode Island. Can. Spec. Pub. Fish. Aquat. Sci. 92, 282–290.
Commito, J. A. (1982). Effects of Lunatia heros predation on the population dynamics of Mya arenaria and Macoma balthica in Maine, USA. Mar. Biol. 69, 187–193. doi: 10.1007/BF00396898
Cowles, A., Hewitt, J. E., and Taylor, R. B. (2009). Density, biomass and productivity of small mobile invertebrates in a wide range of coastal habitats. Mar. Ecol. Prog. Ser. 384, 175–185. doi: 10.3354/meps08038
Cranford, P., Anderson, R., Archambault, P., Balch, T., Bates, S., Bugden, G., et al. (2006). Indicators and Thresholds for Use in Assessing Shellfish Aquaculture Impacts on Fish Habitat. DFO Can. Sci. Advis. Sec. Res. Doc. 2006/034. Ottawa, ON: Fisheries and Oceans Canada, Ottawa, viii, + 116.
D’Amours, O., Archambault, P., McKindsey, C. W., and Johnson, L. E. (2008). Local enhancement of epibenthic macrofauna by aquaculture activities. Mar. Ecol. Prog. Ser. 371, 73–84. doi: 10.3354/meps07672
Dempster, T., Uglem, I., Sanchez-Jerez, P., Fernandez-Jover, D., Bayle-Sempere, J., Nilsen, R., et al. (2009). Coastal salmon farms attract large and persistent aggregations of wild fish: an ecosystem effect. Mar. Ecol. Prog. Ser. 385, 1–14. doi: 10.3354/meps08050
Drouin, A., Archambault, P., Clynick, B. G., Richer, K., and McKindsey, C. W. (2015). Influence of mussel aquaculture on the distribution of vagile benthic macrofauna in îles de la Madeleine, eastern Canada. Aquac. Environ. Interact. 6, 175–183. doi: 10.3354/aei00123
Drummond-Davis, N. C., Mann, K. H., and Pottle, R. A. (1982). Some estimates of population density and feeding habits of the rock crab, Cancer irroratus, in a kelp bed in Nova Scotia. Can. J. Fish. Aquat. Sci. 39, 636–639. doi: 10.1139/f82-090
Dumbauld, B. R., Ruesink, J. L., and Rumrill, S. S. (2009). The ecological role of bivalve shellfish aquaculture in the estuarine environment: a review with application to oyster and clam culture in West Coast (USA) estuaries. Aquaculture 290, 196–223. doi: 10.1016/j.aquaculture.2009.02.033
Fernandez-Jover, D., Sanchez-Jerez, P., Bayle-Sempere, J. T., Valle, C., and Dempster, T. (2008). Seasonal patterns and diets of wild fish assemblages associated with Mediterranean coastal fish farms. ICES J. Mar. Sci. 65, 1153–1160. doi: 10.1093/icesjms/fsn091
Fogarty, M. J. (1976). Competition and Resource Partitioning in Two Species of Cancer (Crustacea, Brachyura). Ph.D. thesis. Kingston, RI: University of Rhode Island.
Forget, N., Duplisea, D. E., Sardenne, F., and McKindsey, C. W. (2020). Using qualitative network models to assess the influence of mussel culture on benthic ecosystem dynamics. Ecol. Model. 430:109070. doi: 10.1016/j.ecolmodel.2020.109070
Forrest, B. M., Keeley, N. B., Hopkins, G. A., Webb, S. C., and Clement, D. M. (2009). Bivalve aquaculture in estuaries: review and synthesis of oyster cultivation effects. Aquaculture 298, 1–15. doi: 10.1016/j.aquaculture.2009.09.032
Fréchette, M. (2012). Self-thinning, biodeposit production, and organic matter input to the bottom in mussel suspension culture. J. Sea Res. 67, 10–20. doi: 10.1016/j.seares.2011.08.006
Freire, J., and González-Gurriarán, E. (1995). Feeding ecology of the velvet swimming crab Necora puber in mussel raft areas of the Ría de Arousa (Galicia, NW Spain). Mar. Ecol. Prog. Ser. 119, 139–154. doi: 10.3354/meps119139
Froehlich, H. E., Smith, A., Gentry, R. R., and Halpern, B. S. (2017). Offshore aquaculture: i know it when i see it. Front. Mar. Sci. 4:154. doi: 10.3389/fmars.2017.00154
Gaymer, C. F., Dutil, C., and Himmelman, J. H. (2004). Prey selection and predatory impact of four major sea stars on a soft bottom subtidal community. J. Exp. Mar. Biol. Ecol. 313, 353–374. doi: 10.1016/j.jembe.2004.08.022
Gendron, L., and Fradette, P. (1995). Revue des interactions entre le crabe commun (Cancer irroratus) et le homard américain (Homarus americanus), dans le contexte du développement d’une pêche au crabe commun au Québec. Can. Man. Rep. Fish. Aquat. Sci. 2306, vii, + 47.
Gendron, L., Fradette, P., and Godbout, G. (2001). The importance of rock crab (Cancer irroratus) for growth, condition and ovary development of adult American lobster (Homarus americanus). J. Exp. Mar. Biol. Ecol. 262, 221–241. doi: 10.1016/S0022-0981(01)00297-0
Gentry, R. R., Lester, S. E., Kappel, C. V., White, C., Bell, T. W., Stevens, J., et al. (2017). Offshore aquaculture: spatial planning principles for sustainable development. Ecol. Evol. 7, 733–743. doi: 10.1002/ece3.2637
Giles, H., Pilditch, C. A., and Bell, D. G. (2006). Sedimentation from mussel (Perna canaliculus) culture in the Firth of Thames, New Zealand: impacts on sediment oxygen and nutrient fluxes. Aquaculture 261, 125–140. doi: 10.1016/j.aquaculture.2006.06.048
Grant, J., Hatcher, A., Scott, D. B., Pocklington, P., Schafer, C. T., and Winters, G. V. (1995). A multidisciplinary approach to evaluating impacts of shellfish aquaculture on benthic communities. Estuaries 18, 124–144. doi: 10.2307/1352288
Gratwicke, B., and Speight, M. R. (2005). The relationship between fish species richness, abundance and habitat complexity in a range of shallow tropical marine habitats. J. Fish Biol. 66, 650–667. doi: 10.1111/j.0022-1112.2005.00629.x
Hazlett, B. A. (1981). The behavioral ecology of hermit crabs. Ann. Rev. Ecol. Syst. 12, 1–22. doi: 10.1146/annurev.es.12.110181.000245
Himmelman, J. H., and Dutil, C. (1991). Distribution, population structure and feeding of subtidal seastars in the northern Gulf of St. Lawrence. Mar. Ecol. Prog. Ser. 76, 61–72.
Hudon, C., and Lamarche, G. (1989). Niche segregation between American lobster Homarus americanus and rock crab Cancer irroratus. Mar. Ecol. Prog. Ser. 52, 155–168.
Inglis, G. J., and Gust, N. (2003). Potential indirect effects of shellfish culture on the reproductive success of benthic predators. J. Appl. Ecol. 40, 1077–1089. doi: 10.1111/j.1365-2664.2003.00860.x
Jalbert, P., Himmelman, J., Beland, P., and Thomas, B. (1989). Whelks (Buccinum undatum) and other subtidal invertebrate predators in the northern Gulf of St. Lawrence. Can. Field Nat. 116, 1–15.
Jansen, H. M., Burg, S., Bolman, B., Jak, R. G., Kamermans, P., Poelman, M., et al. (2016). The feasibility of offshore aquaculture and its potential for multi-use in the North Sea. Aquac. Int. 24, 735–756. doi: 10.1007/s10499-016-9987-y
Kaspar, H. F., Gillespie, P., Boyer, L. F., and Mackenzie, A. L. (1985). Effects of mussel aquaculture on the nitrogen cycle of benthic communities in Kenepuru Sound, Marlborough Sound, New Zealand. Mar. Biol. 85, 127–136. doi: 10.1007/BF00397431
Kenchington, E., Duggan, R., and Riddell, T. (1998). Early life history characteristics of the razor clam, Ensis directus, and the Moonsnails, Euspira spp., with applications to fisheries and aquaculture. Can. Tech. Rep. Fish. Aquat. Sci. 2223, vii, + 32.
Kennish, M. J., Lutz, R. A., Dobarro, J. A., and Fritz, L. W. (1994). In situ growth rates of the ocean quahog, Arctica islandica (Linnaeus, 1767) in the Middle Atlantic Bight. J. Shellfish Res. 13, 473–478.
Kerfoot, W. C., and Sih, A. (1987). Predation: Direct and Indirect Impacts on Aquatic Communities. Hanover, NH: University Press of New England.
Kluger, L. C., Taylor, M. H., Mendo, J., Tam, J., and Wolff, M. (2016b). Carrying capacity simulations as a tool for ecosystem-based management of a scallop aquaculture system. Ecol. Model. 331, 44–55. doi: 10.1016/j.ecolmodel.2015.09.002
Kluger, L. C., Taylor, M. H., Rivera, E. B., Silva, E. T., and Wolff, M. (2016a). Assessing the ecosystem impact of scallop bottom culture through a community analysis and trophic modelling approach. Mar. Ecol. Prog. Ser. 547, 121–135. doi: 10.3354/meps11652
Kraus, M., Beal, B., Chapman, S., and McMartin, L. (1992). A comparison of growth rates in Arctica islandica (Linnaeus, 1767) between field and laboratory populations. J. Shellfish Res. 11, 289–289.
Lacoste, É, Drouin, A., Weise, A. M., Archambault, P., and McKindsey, C. W. (2018). Low benthic impact of an offshore mussel farm in Îles-de-la-Madeleine, eastern Canada. Aquac. Environ. Interact. 10, 473–485. doi: 10.3354/aei00283
Langton, R. W., and Bowman, R. E. (1980). Food of Fifteen Northwest Atlantic Gadiform Fishes. NOAA Tech. Rep. NMFS SSRF-740. Washington, DC: U.S. Department of Commerce. iv, + 23.
Lester, S. E., Stevens, J. M., Gentry, R. R., Kappel, C. V., Bell, T. W., Costello, C. J., et al. (2018). Marine spatial planning makes room for offshore aquaculture in crowded coastal waters. Nat. Commun. 9:945. doi: 10.1038/s41467-018-03249-1
Mascorda Cabre, L., Hosegood, P., Attrill, M. J., Bridger, D., and Sheehan, E. V. (2021). Offshore longline mussel farms: a review of oceanographic and ecological interactions to inform future research needs, policy and management. Rev. Aquac. 13, 1864–1887. doi: 10.1111/raq.12549
Matisson, J., and Lindén, O. (1983). Benthic macrofauna succession under mussels, Mytilus edulis L. (Bivalvia), cultured on hanging long-lines. Sarsia 68, 97–102. doi: 10.1080/00364827.1983.10420561
McKindsey, C. W., Archambault, P., and Simard, N. (2012). Spatial variation of benthic infaunal communities in baie de Gaspé (eastern Canada) – influence of mussel aquaculture. Aquaculture 356–357, 48–54. doi: 10.1016/j.aquaculture.2012.05.037
McKindsey, C. W., Archambault, P., Callier, M. D., and Olivier, F. (2011). Influence of suspended and off-bottom mussel culture on the sea bottom and benthic habitats: a review. Can. J. Zool. 89, 622–646. doi: 10.1139/z11-037
Miron, G., Landry, T., and MacNair, N. (2002). Predation potential by various epibenthic organisms on commercial bivalve species in Prince Edward Island: preliminary results. Can. Tech. Rep. Fish. Aquat. Sci. 2392, ix, + 32.
Plew, D. R., Stevens, C. L., Spigel, R. H., and Hartstein, N. D. (2005). Hydrodynamic implications of large offshore mussel farms. IEEE J. Ocean. Eng. 30, 95–108. doi: 10.1109/JOE.2004.841387
Prins, T. C., Smaal, A. C., and Dame, R. F. (1997). A review of the feedbacks between bivalve grazing and ecosystem processes. Aquat. Ecol. 31, 349–359. doi: 10.1023/A:1009924624259
Romero, P., González-Gurriarán, E., and Penas, E. (1982). Influence of mussel rafts on spatial and seasonal abundance of crabs in the Ría de Arousa, North-West Spain. Mar. Biol. 72, 201–210. doi: 10.1007/BF00396921
Sainte-Marie, B., and Chabot, D. (2002). Ontogenetic shifts in natural diet during benthic stages of American lobster (Homarus americanus), off the Magdalen Islands. Fish. Bull. 100, 106–116.
Saranchova, O. L., and Flyachinskaya, L. P. (2001). The Influence of salinity on early ontogeny of the mussel Mytilus edulis and the starfish Asterias rubens from the White Sea. Russ. J. Mar. Biol. 27, 87–93. doi: 10.1023/A:1016643213691
Sardenne, F., Forget, N., and McKindsey, C. W. (2019). Contribution of mussel fall-off from aquaculture to wild lobster Homarus americanus diets. Mar. Env. Res. 149, 126–136. doi: 10.1016/j.marenvres.2019.06.003
Sogard, S. M., and Able, K. W. (1991). A comparison of eelgrass, sea lettuce macroalgae, and marsh creeks as habitats for epibenthic fishes and decapods. Estuar. Coast. Shelf Sci. 33, 501–519. doi: 10.1016/0272-7714(91)90087-R
Souchu, P., Collos, Y., Landrein, S., Deslous-Paoli, J.-M., Bibent, B., and Vaquer, A. (2001). Influence of shellfish farming activities on the biogeochemical composition of the water column in Thau lagoon. Mar. Ecol. Prog. Ser. 218, 141–152. doi: 10.3354/meps218141
Stehlik, L. L. (1993). Diets of the brachyuran crabs Cancer irroratus, C. borealis, and Ovalipes ocellatus in the New York Bight. J. Crustacean Biol. 13, 723–735. doi: 10.1163/193724093X00291
Toupoint, N., Godet, L., Fournier, J., Retière, C., and Olivier, F. (2008). Does Manila clam cultivation affect habitats of the engineer species Lanice conchilega (Pallas, 1766)? Mar. Pollut. Bull. 56, 1429–1438. doi: 10.1016/j.marpolbul.2008.04.046
Wang, G., and McGaw, I. J. (2016). Potential use of mussel farms as multitrophic on-growth sites for american lobster, Homarus americanus (Milne Edwards). Fish. Aquac. J. 7, 1–11. doi: 10.4172/2150-3508.1000161
Weise, A. M., Cromey, C. J., Callier, M. D., Archambault, P., Chamberlain, J., and McKindsey, C. W. (2009). Shellfish-DEPOMOD: modelling the biodeposition from suspended shellfish aquaculture and assessing benthic effects. Aquaculture 288, 239–253. doi: 10.1016/j.aquaculture.2008.12.001
Weitzman, J. (2019). Applying the ecosystem services concept to aquaculture: a review of approaches, definitions, and uses. Ecosyst. Serv. 35, 194–206. doi: 10.1016/j.ecoser.2018.12.009
Weitzman, J., Steeves, L., Bradford, J., and Filgueira, R. (2019). “Far-field and near-field effects of marine aquaculture,” in World Seas: An Environmental Evaluation,: Ecological Issues and Environmental Impacts, 2nd Edn, Vol. III, ed. C. Sheppard (London: Academic Press), 197–220. doi: 10.1016/b978-0-12-805052-1.00011-5
Wilding, T. A., and Nickell, T. D. (2013). Changes in benthos associated with mussel (Mytilus edulis L.) farms on the west-coast of Scotland. PLoS One 8:e68313. doi: 10.1371/journal.pone.0068313
Keywords: offshore mussel aquaculture, Mytilus edulis, Homarus americanus, Cancer irroratus, benthic macrofauna, spatial variation
Citation: Sean A-S, Drouin A, Archambault P and McKindsey CW (2022) Influence of an Offshore Mussel Aquaculture Site on the Distribution of Epibenthic Macrofauna in Îles de la Madeleine, Eastern Canada. Front. Mar. Sci. 9:859816. doi: 10.3389/fmars.2022.859816
Received: 21 January 2022; Accepted: 28 February 2022;
Published: 07 April 2022.
Edited by:
Øivind Strand, Norwegian Institute of Marine Research (IMR), NorwayReviewed by:
Adriana Giangrande, University of Salento, ItalyPaul South, Cawthron Institute, New Zealand
Copyright © 2022 Sean, Drouin, Archambault and McKindsey. This is an open-access article distributed under the terms of the Creative Commons Attribution License (CC BY). The use, distribution or reproduction in other forums is permitted, provided the original author(s) and the copyright owner(s) are credited and that the original publication in this journal is cited, in accordance with accepted academic practice. No use, distribution or reproduction is permitted which does not comply with these terms.
*Correspondence: Christopher W. McKindsey, Y2hyaXMubWNraW5kc2V5QGRmby1tcG8uZ2MuY2E=