- 1Department of Natural Resources Science, University of Rhode Island, Kingston, RI, United States
- 2Beyond the Reef, Road Town, British Virgin Islands
- 3Ministry of Natural Resources, Labour and Immigration, Road Town, British Virgin Islands
- 4Department of Biological Sciences, Wellesley College, Wellesley, MA, United States
- 5Department of Biological Sciences, University of Rhode Island, Kingston, RI, United States
Stony coral tissue loss disease has spread widely in the Caribbean and causes substantial changes to coral community composition because of its broad host range and high fatality rate. To reduce SCTLD impacts, intervention programs throughout the region have divers treating corals with antibiotics. We assessed the effect of antibiotic treatment in the British Virgin Islands by comparing coral communities at 13 treated sites to those at 13 untreated sites. The prevalence of white syndromes (assumed to be primarily SCTLD), the severity of white syndrome lesions, partial colony mortality and complete colony mortality all showed a qualitative pattern consistent with benefits of treatment: they were reduced at treated sites for species highly susceptible to SCTLD. In contrast, the prevalence and severity of lesions from other diseases, and other causes of tissue loss, were all unrelated to treatment. Re-ordering of rank abundance at the community-level was also consistent with a positive effect of treatment because rare, highly SCTLD-susceptible species increased slightly in relative cover at treated sites. Although there was limited statistical support for these responses individually, collectively the overall pattern of results indicates a modest beneficial effect of the intervention program for highly susceptible species. Diver-based intervention programs using antibiotics may thus be a viable part of management plans for STLD at the epidemic stage.
Introduction
Outbreaks of disease are a significant cause of coral mortality that can alter the composition of coral communities (Rogers and Miller, 2013). Increasing disease impacts over the past four decades are associated with the emergence of new diseases and exacerbated by synergisms with increasing water temperature and localized pollution (Ward and Lafferty, 2004; Harvell et al., 2007; Bourne et al., 2009; Ruiz-Moreno et al., 2012).
A recently emergent disease, of particular concern in the Caribbean, is Stony Coral Tissue Loss Disease (SCTLD). The disease was first documented on reefs in Florida, USA (Precht et al., 2016) and has subsequently spread to other parts of the Caribbean (Kramer et al., 2019). The cause of the disease remains uncertain, but appears to be an infectious agent (Muller et al., 2020) that can be transmitted by physical contact and in seawater (Aeby et al., 2019; Sharp et al., 2020). Outbreaks are identified using epidemiological criteria based on which species show signs and on the spatial and temporal pattern of mortality at a site (FKNMS/DEP, 2018). Lesions have a distinctive pathology under the microscope (Landsberg et al., 2020) and can appear at the edge of a coral colony, or within areas of live tissue, and multiple areas of tissue of loss often appear in the same colony. Disease progression differs from other syndromes in being extremely rapid and almost always kills the colony (Precht et al., 2016; Walton et al., 2018). SCTLD affects at least 20 coral species, including several that are abundant reef-builders in the region and others that form massive slow-growing colonies and so have long recovery times (FKNMS/DEP, 2018). These features of the disease combine to generate severe impacts of SCTLD outbreaks on coral community composition (Precht et al., 2016; Walton et al., 2018; Brandt et al., 2021; Estrada-Saldívar et al., 2021).
Acute impacts of SCTLD on coral communities created an urgent need to develop management actions that can either reduce pathogen abundance, abate the local stressors that intensify impacts, or promote recovery after an outbreak (Beeden et al., 2011). Diseases of terrestrial wildlife have been managed by treating hosts to reduce pathogen abundance (Wobeser, 2002), but there have been few attempts to use this strategy on reef corals (Gil-Agudelo et al., 2004; Atad et al., 2012). Although ongoing research into SCTLD etiology may not identify a single pathogen that reproduces signs of the disease (Vega Thurber et al., 2020), several lines of evidence indicate bacterial involvement in the infection (Meyer et al., 2019; Rosales et al., 2020), which suggests the potential for management actions that use antibiotics to reduce pathogen abundance.
Intervention plans using divers have been successful at treating corals infected with SCTLD. Waterborne antibiotics stop the spread of SCTLD lesions in the laboratory (Aeby et al., 2019; Miller et al., 2020), which spurred the development of topical antibiotic pastes that could be used by divers to treat wild corals (Neely et al., 2020). Controlled field tests in Florida indicate that 67-95% of lesions either healed or stopped spreading after antibiotic paste was applied around their perimeter. The protective effect on treated lesions lasted months, but did not always prevent new lesions from appearing on treated colonies or untreated colonies nearby (Neely et al., 2020; Shilling et al., 2021; Walker et al., 2021). A formal intervention program based on these findings was established in Florida (Neely, 2020; Florida DEP, 2021) and a more comprehensive analysis confirmed long-term benefits of treatment for five heavily impacted species at multiple sites (Neely et al., 2021). Based on this success, in-water treatment programs are now reportedly active in at least 11 Caribbean countries/territories (Roth et al., 2020).
While benefits of antibiotic application for treated colonies are well-established, we are not aware of any studies testing the effects of treatment programs on the entire coral community. Our objective was thus to perform a preliminary test for effects of antibiotic treatment at the community-level. We studied a treatment program in the British Virgin Islands (BVI), where SCTLD outbreaks began in 2020. The rapidity and large-scale of the outbreak required selecting a set of priority sites for treatment and meant that only a fraction of corals at treated sites received antibiotic applications. We tested whether the positive effects of antibiotic application would extend beyond treated corals and confer community-wide benefits at treated sites. We explored if the BVI treatment program reduced the overall prevalence of SCTLD at treated sites, the severity of lesions on infected corals, and whether populations at treated sites showed reduced colony mortality. Finally, at the community level, we checked for shifts in community composition at treated sites that could be attributed to the treatment program.
Materials and Methods
BVI SCTLD Outbreak and Treatment Program
SCTLD was first reported in the BVI in May 2020 and, using established epidemiological criteria (FKNMS/DEP, 2018), outbreaks have been identified at over 50 locations in the territory since then. A treatment program was developed as part of the BVI Government’s response plan for SCTLD. The program is a partnership between the BVI Ministry of Natural Resources, Labour and Immigration (BVI- MNRLI), the Governor’s Office, the National Parks of the Virgin Islands (BVI-NPT), UNITE BVI, Non-Profit Organizations (NPOs), and interested private groups and individuals. Forty two sites were initially selected for treatment based on a combination of factors (following Neely, 2018). Ecological factors included coral community composition, demographic structure, and site isolation. To maximize the total reef area that could be treated with available resources, the treatability of sites was also considered based on their size and accessibility to divers. Lastly, priority was given to sites in the BVI network of marine protected areas and parks.
Experienced local divers were trained by BVI-MNRLI staff to identify corals and apply amoxicillin paste (Coral Cure Base2b, Ocean Alchemists LLC) to infected colonies using procedures adapted from established protocols (e.g., Neely, 2018). Groups of trained divers called strike teams were each assigned a subset of the treatment sites to which they made regular visits; the target was every 2-4 weeks (Supplemental Figure 1). Coral species prioritized for treatment were those of medium and high susceptibility to SCTLD (FKNMS/DEP, 2018), particularly abundant reef-builders in the territory such as Orbicella spp., Montastraea cavernosa and Colpophyllia natans (Keefner, 2019). Individual coral colonies were prioritized if they were large, relatively free of lesions and surrounded by unaffected colonies.
Strike team divers kept detailed records of their activity and we assembled a subset of their records for the 13 treatment sites in our study. Data compiled included the species or genus of each treated colony (Supplementary Table 1), colony size (length/width in cm), the number of active lesions considered to be SCTLD, the amount of antibiotic paste applied (ml) and whether there were signs of previous treatment.
Study Design
Quantitative monitoring data for coral diseases prior to SCTLD arrival are limited, so we evaluated treatment effects using an after-control-impact (ACI) design that involved a spatial comparison of 13 treated sites and 13 untreated reference sites (Underwood, 1997; Smith, 1998). Sites were used as replicates in our analyses because our goal was to compare treated and reference sites, rather than compare treated and untreated corals at the same site.
Each treated site was paired with a nearby reference site that was similar in depth, wave exposure and reef topography. Proximity of paired sites also increased the chance that SCTLD reached them at similar times (Figure 1 and Table 1). Using this ACI design to isolate treatment effects assumes that, on average, the difference between paired sites is attributable to antibiotic treatment (i.e., a space-for-time substitution). For practical reasons, there are, however, other differences between treated and reference sites to address when assessing potential treatment effects. All 13 treated sites are established recreational dive sites with permanent moorings for the specific use of dive boats, whereas none of the reference sites have moorings and are rarely visited by divers. SCTLD treatment is thus confounded with boat and diver visitation. Divers and boats can both negatively impact reef corals, but the symptoms and the species most susceptible are different from those impacted by SCTLD (Hawkins et al., 1999; Forrester et al., 2015; Lyons et al., 2015; Flynn and Forrester, 2019). We used these expected differences to differentiate these potentially confounding effects from those of SCTLD treatment. Because SCTLD can be transmitted by direct contact (Aeby et al., 2019), regular recreational diving activity at the treated sites might also enhance the spread of disease and counteract any benefits of antibiotic treatment.
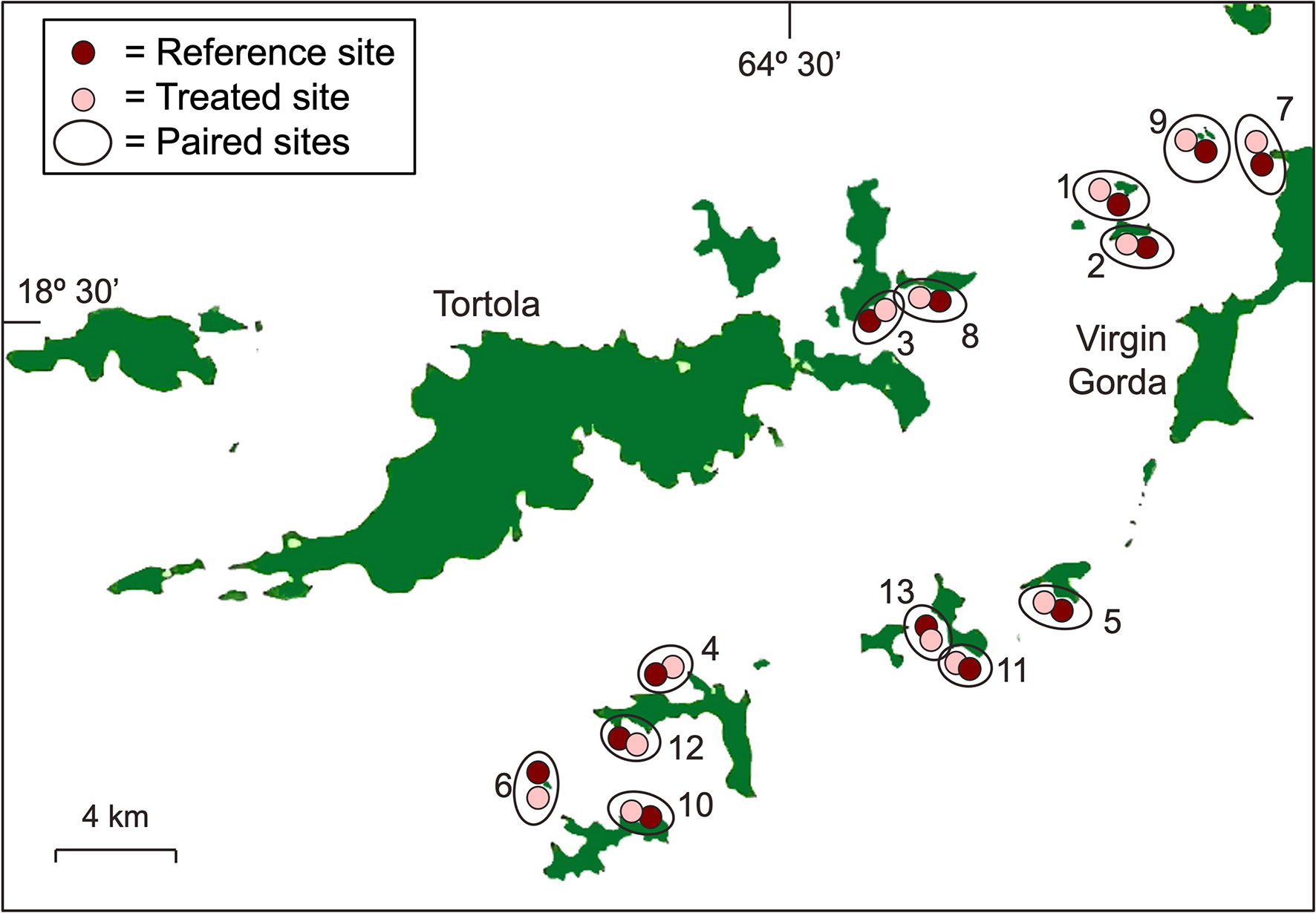
Figure 1 Map of the thirteen pairs of treated and reference sites that were studied. Numbering of the site-pairs correspond to the numbers in Table 1.
Survey Methods
Eleven site-pairs were sampled in July 2021 and the remaining two in October 2021 (Thumb Rock and Indians). We surveyed each site on SCUBA using haphazardly placed 30-m transects. The areas typically visited by strike-team divers were known to the local coordinators, so the tapes were placed within these areas, but no strict boundaries were imposed on strike team divers so they may have treated some corals outside of the surveyed area. Within each site-pair, we surveyed the same number of transects at the treated and reference site, with minor exceptions due to time constraints. However, we varied the number of transects among site-pairs (from 4-7 transects per site); sampling more transects where corals were sparser. This strategy ensured that sampling effort (metres of transect surveyed per site) was similar at treated (mean ± 95% CI = 152 ± 17) and reference (mean ± 95% CI = 146 ± 22) sites (paired t-test, df = 12, t = -0.82, p = 0.43). All scleractinian coral colonies intersected by the transect tapes that were > 10 cm in maximum linear dimension were identified to species and recorded (Supplemental Table 1).
Colony-Level Indicators
We estimated lesion severity as a colony-level indicator of treatment effect (Caldwell et al., 2018). Each colony was visually assessed for signs of disease, bleaching, and other agents of tissue loss. Lesion severity was estimated as the proportion of the colony affected by each agent of tissue loss, including areas of active disease and recently dead tissue associated with the lesions (Supplemental Figure 2). For individual colonies, SCTLD cannot be distinguished from other diseases with similar signs (white plague, white pox and other white syndromes) and so we classified all colonies with focal or multi-focal lesions consistent with SCTLD as white syndrome (WS) (FKNMS/DEP, 2018). Other visually distinctive diseases (black band disease, red band disease, yellow band disease, yellow blotch disease, dark spot disease, ciliate infection, and growth anomalies) were grouped as non-white syndromes (NWS). Other agents of tissue loss such as predation, abrasion, and competitive overgrowth were grouped as non-disease (ND). Recently dead tissue associated with a lesion was defined as dead tissue with white corallites still identifiable to species, plus limited sedimentation or overgrowth by turf algae organisms, suggesting that tissue loss occurred within the preceding few months (for details see Lang et al., 2013). To assess older tissue loss that was not always readily associated with active lesions, we also visually estimated the proportion of long-dead tissue on each colony. Areas of tissue loss that occurred from a few months to a few years in the past have eroded corallite structure, and may have substantial overgrowth with algae or other organisms (for details see Lang et al., 2013).
Population-Level Indicators
To assess treatment effects at the population level, we estimated disease prevalence (Begon et al., 2002) and colony mortality. For colonies with some live tissue, disease prevalence was estimated as the proportion of colonies at a site infected by each syndrome (WS, NWS and ND). Colony mortality at each site was estimated as the proportion of colonies sampled that were completely dead.
Community-Level Indicators
We used coral cover as our primary community-level indicator because cover is the most appropriate unit of abundance for colonial organisms (Hughes, 1984). Each coral intercepted by the transect tapes was measured in maximum length (L) parallel to the tape, width (W) orthogonal to the tape and height (H). Only coral colonies > 10 cm in maximum linear dimension were sampled. Simple geometric approximations were used to estimate colony surface area (CSA), assuming colonies were either hemispherical in shape (for massive colonies) or cylindrical (for branching, foliose, tabulate and columnar colonies) (Fisher et al., 2008). CSA for hemispheres was
where
and for cylinders was
where
We calculated coral colony density (D) at each site using the Strong Method (Bakus, 2006). Density (colonies per 100 m2) is calculated as
where t = distance covered by transects at a site in m. We calculated live coral cover (CC) at each site as
where meanPL is the mean % live tissue (Fisher et al., 2008). Coral cover had units of m2 coral per 100m2 seabed and so is analogous to percent cover.
Analysis
Colony-and Population-Level Indicators
For colony- and population-level indicators, we predicted that the treatment program would reduce the severity of WS lesions, WS prevalence, older tissue loss, and colony mortality. These predicted differences between treated and reference sites should increase with species susceptibility to SCTLD (Figure 2). To test these predictions, we divided species into groups based on their SCTLD susceptibility [following FKNMS/DEP, 2018 (Supplemental Table 1)] and modelled each indicator as a function of SCTLD-susceptibility and treatment (treated and reference), the interaction SCTLD-susceptibility x treatment and site-pair (a random blocking factor). A significant SCTLD-susceptibility x treatment interaction provides statistical support for our prediction. In contrast, the severity of NWS and ND lesions and the prevalence of these syndromes were not expected to vary with treatment or SCTLD-susceptibility.
Lesion severity and old tissue loss are measured percentages and so were analysed using linear models with normally distributed errors and constant variance after arcsine transformation (Crawley, 2012). Prevalence and colony mortality are proportions derived from binomial processes and so were modelled with a 2-vector response variable (infected:not infected and dead:alive respectively) using binomial generalized linear mixed models (following Crawley, 2012; Kosmidis and Firth, 2021). Because the binomial model includes a random effect, it was fitted using a Bayesian method with a zero-mean normally distributed prior on the fixed effects. Statistical modelling was done in the R programming environment (R Core Team, 2021), using the package blme (Chung et al., 2013).
Community-Level Indicators
At the community-level, we used recently-developed indices based on rank-abundance curves (RACs) to assess treatment effects (Avolio et al., 2015; Avolio et al., 2019). RACs are plots of abundance in which species are arranged from most common to rarest (lowest to highest rank, Whittaker, 1965). They provide a flexible approach to describe community composition that, importantly for our purposes, retains information on species identities (Avolio et al., 2019).
Treatment programs might ameliorate the effects of SCTLD in many possible ways, but we focused on two possibilities. First, successful treatment might cause re-ordering of relative abundances if highly susceptible species become more abundant (lower in relative rank) at treated sites, while non-susceptible species become rarer (higher in relative rank) (Figure 3). We tested this prediction using a rank-difference index, which compares the difference in rank of each species between paired sites and ranges from 0 to 0.5, where 0.5 is the maximum rank change (Avolio et al., 2015; Avolio et al., 2019). Species were grouped by SCTLD-susceptibility to assess re-ordering based on susceptibility. Second, when SCTLD impacts are severe, antibiotic applications might lead to higher species richness at treated sites by preventing the extirpation of rare SCTLD-susceptible species (Figure 3). We tested this prediction using a richness-difference index, which quantifies the proportional difference in species richness between paired sites (Avolio et al., 2019), noting the SCTLD-susceptibility of any species lost.
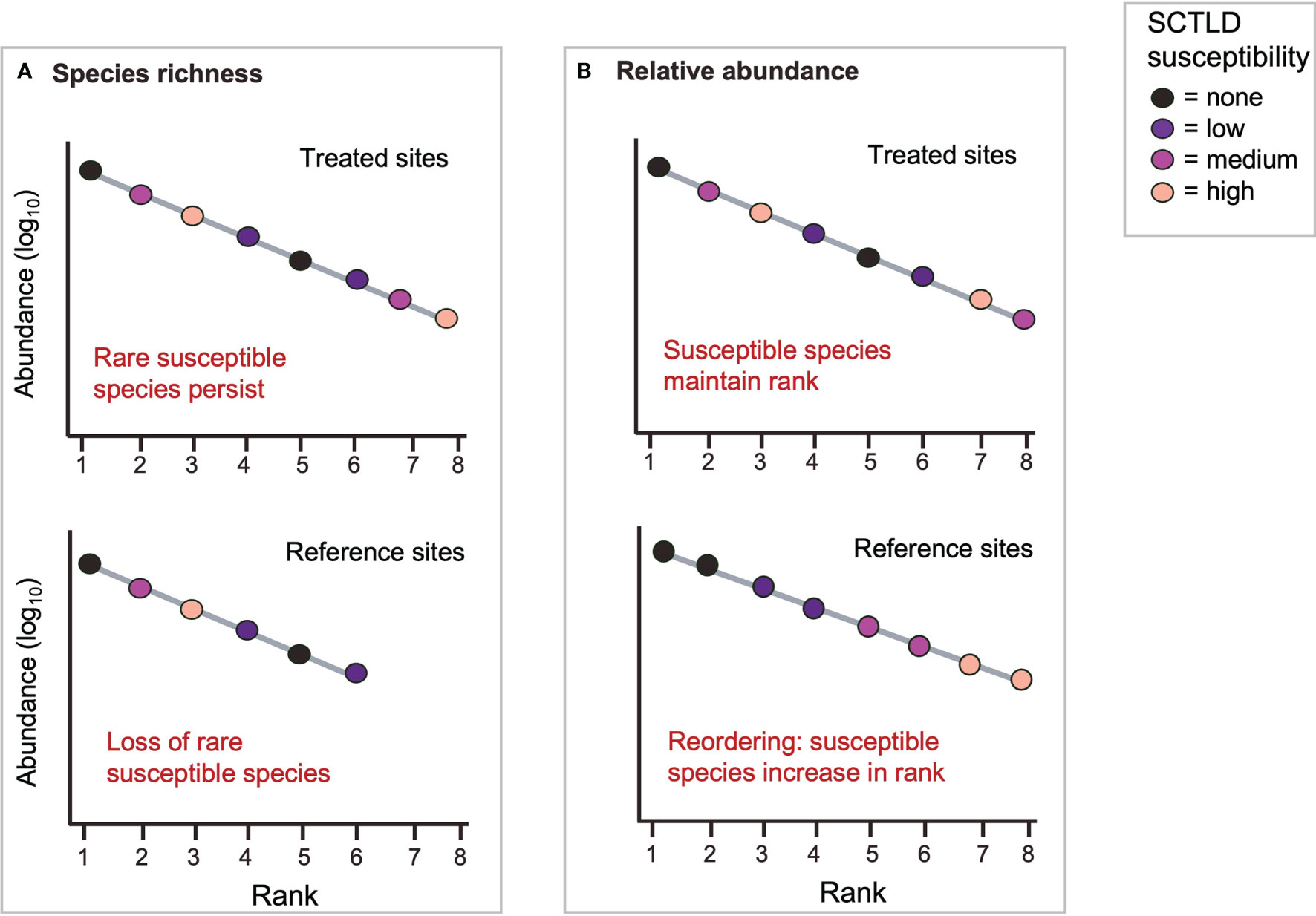
Figure 3 Two predicted effects of treatment on community-level patterns of rank abundance. (A) Treatment prevents the extirpation of rare species highly susceptible to SCTLD. (B) Treatment causes re-ordering of relative rank: susceptible species increase in abundance relative to non-susceptible ones.
To assess other possible differences between treated and reference sites, we used other indices calculated from the RAC curves to compare: (1) curve-differences – quantified based on differences in relative rank and cumulative abundance, (2) species-differences – quantified as the proportion of species not shared between paired sites, (3) evenness-differences – the proportional difference in evenness of relative abundance, (4) abundance-differences – the difference in cover between paired sites and (5) multivariate-differences – the proportional difference between treatments in group centroids and in the dispersion of replicates within treatments based on Bray-Curtis dissimilarity (following methods described by Avolio et al., 2019). For all RAC indices, we calculated mean differences between RAC curves as a function of treatment (treated and reference) and site-pair (a blocking factor). Replicates were the RAC curves for each site and t-tests were used to test the null hypothesis that mean differences were zero. Statistical modelling was done in R, using the packages BiodiversityR (Kindt and Coe, 2005) and codyn (Hallett et al., 2016).
Results
Corals Treated by the Strike Teams
Divers treated 3623 coral colonies at the 13 treatment sites and there were 1070 repeat treatments. A total of 38.2 L of antibiotic paste was applied, averaging 8.1 mL per treatment. For practical reasons, the sites differed in when treatment dives began, the number of visits, and the total number of colonies treated (Table 1).
The coral taxa treated matched program priorities. Corals most frequently treated were the medium-susceptibility reef-builders Orbicella spp. and Monastraea cavernosa that dominate mid-depth BVI reefs (Keefner, 2019). Other frequently treated taxa were those forming massive or meandroid colonies that are highly susceptible to SCTLD (Figure 4). The pattern of treatment by taxa was fairly consistent among sites (Supplemental Figure 3). The mean size of treated colonies was greater than those surveyed at the sites, as expected because divers prioritized larger colonies for treatment (Supplemental Figure 4).
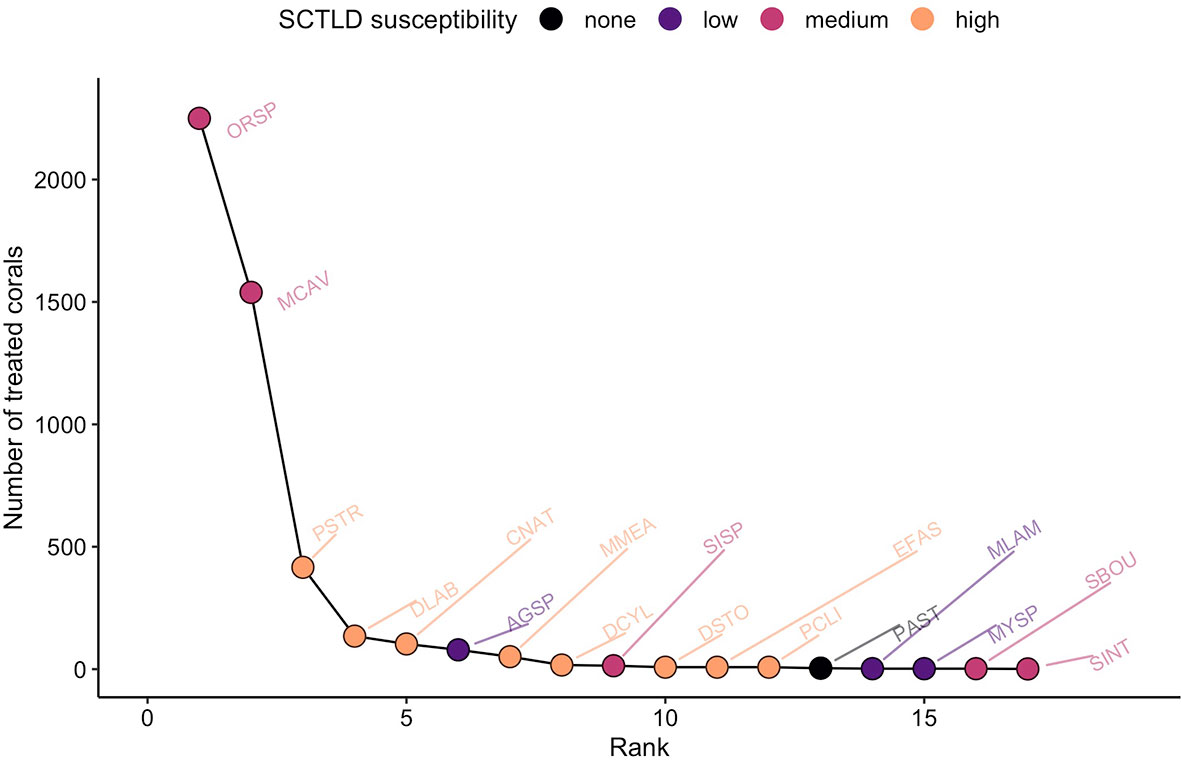
Figure 4 Rank-abundance curve for corals treated with antibiotics. Data from the 13 treated sites are pooled. Coral taxa are labelled by 4-letter codes listed in Supplemental Table 1.
Colony-Level Indicators
WS lesions were only observed on species susceptible to SCTLD, suggesting that SCTLD was their primary cause (Figure 5). Mean severity was significantly reduced at treated sites for high-susceptibility species and, in that sense, the pattern of results conformed to that predicted in Figure 2, but there was no significant SCTLD-susceptibility x treatment interaction (Table 2). Older tissue loss also conformed qualitatively to the pattern predicted by a positive treatment effect (Figure 5) but the SCTLD-susceptibility x treatment interaction was not significant (Table 2).
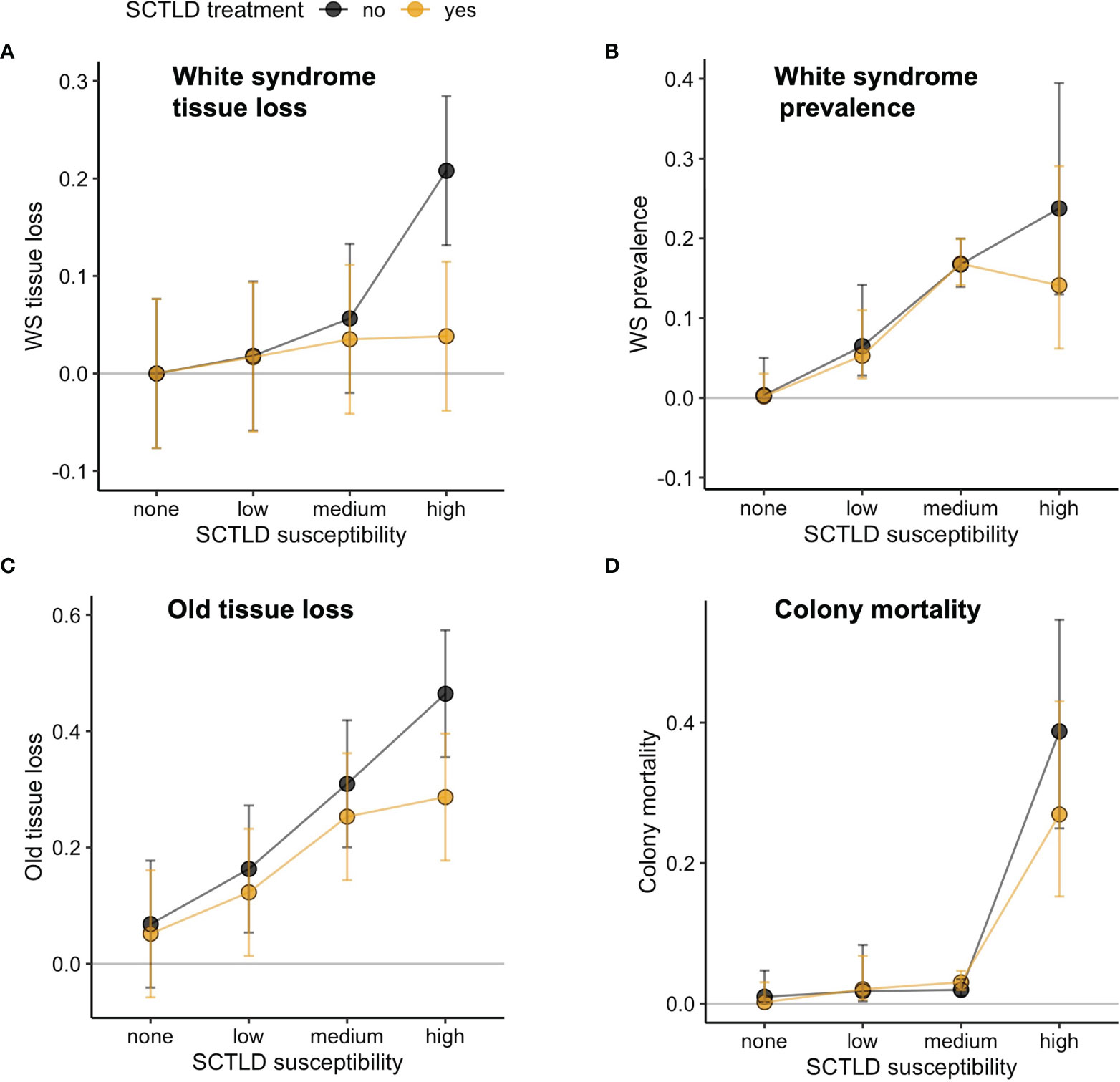
Figure 5 Effects of antibiotic treatment for SCTLD (yes = treated sites, no = reference sites) on (A) WS tissue loss (proportion of colony affected), (B) WS prevalence (proportion of population infected), (C) Older tissue loss (proportion of dead tissue), and (D) colony mortality (proportion of population dead). Plotted are means ± 95% CI.
As expected, NWS and ND lesions caused much less tissue loss than WS lesions, did not increase with SCTLD susceptibility, nor differ between treated and untreated sites (Supplemental Figure 5; Supplemental Table 2). Abrasions caused by boat anchors, anchor chains and diver contact would all have been scored as ND lesions. Because lesions caused by boat anchors or anchor chains are visually distinctive (see Forrester et al., 2015; Flynn and Forrester, 2019), and are often seen in combination with other signs that cannot be confused with disease, their absence allows us to exclude this possible source of confounding. We also saw few abrasions clearly related to diver contact (see Hawkins et al., 1999; Zakai and Chadwick-Furman, 2002), but are less confident of our ability to recognize this source of tissue loss. Nonetheless, any unrecognized damage from diver contact at treated sites was insufficient to increase overall ND lesion severity.
Population-Level Indicators
White syndrome prevalence was zero for non-susceptible species and highest for medium- and high-susceptibility species, further suggesting that SCTLD was the primary cause of WS lesions in the study area (Figure 5). Mean WS prevalence of highly susceptible species was slightly reduced at treatment sites, as predicted with a beneficial treatment effect, but statistical support for a SCTLD-susceptibility x treatment interaction was lacking (Table 2).
Any spreading of SCTLD by the recreational divers who frequently visit treated sites should increase WS prevalence at treated sites and so tend to counteract any reduction due to successful antibiotic treatments. We cannot separate these countervailing influences on WS prevalence, but the results suggest that the net effect is neutral or very slightly positive (Figure 5).
The overall prevalence of WS was more than double that of NWS and ND (Supplemental Figure 5), suggesting that SCTLD was the most frequent agent of tissue loss at the studied sites. As expected, the prevalence of NWS and ND lesions appeared unrelated to SCTLD susceptibility and did not differ between treated and untreated sites (Supplemental Figure 5). No treatment effects on ND prevalence also suggests the lack of confounding from boat and diver damage, as argued above.
Colony mortality was zero for non-susceptible species and negligible for low and medium susceptibility species (Figure 5). Mortality of species highly susceptible to SCTLD was slightly reduced at treated sites, as predicted if treatment is beneficial, but the SCTLD-susceptibility x treatment interaction term was not significant (Table 2).
Community-Level Indicators
There was no overall difference in % coral cover between treated and reference sites (mean abundance-difference = 0.04%, 95% CI = -0.04% to 0.12%, df = 201, t = 0.94, p = 0.35), but there was evidence of a difference in community composition. The proportional multivariate difference between treatments was 0.12, far greater than dispersion within the centroids (0.0009) and rank-abundance curves for paired sites differed in overall shape (mean curve-difference = 102,842, 95% CI = 41,525 to 164,160, df = 12, t = 3.65, p = 0.003). Shape differences reflect the combined influence of shifts in cumulative abundance and relative rank (Figure 6). Paired sites had similar evenness in relative abundance (mean proportional difference = -0.046, 95% CI = -0.154 to 0.210, df = 12, t = -0.945, p = 0.363), but of most relevance to our objectives was that treated and untreated sites displayed shifts in relative rank (mean rank-difference = 0.19, 95% CI = 0.17 to 0.21, df = 12, t = 21.9, p <0.0001).
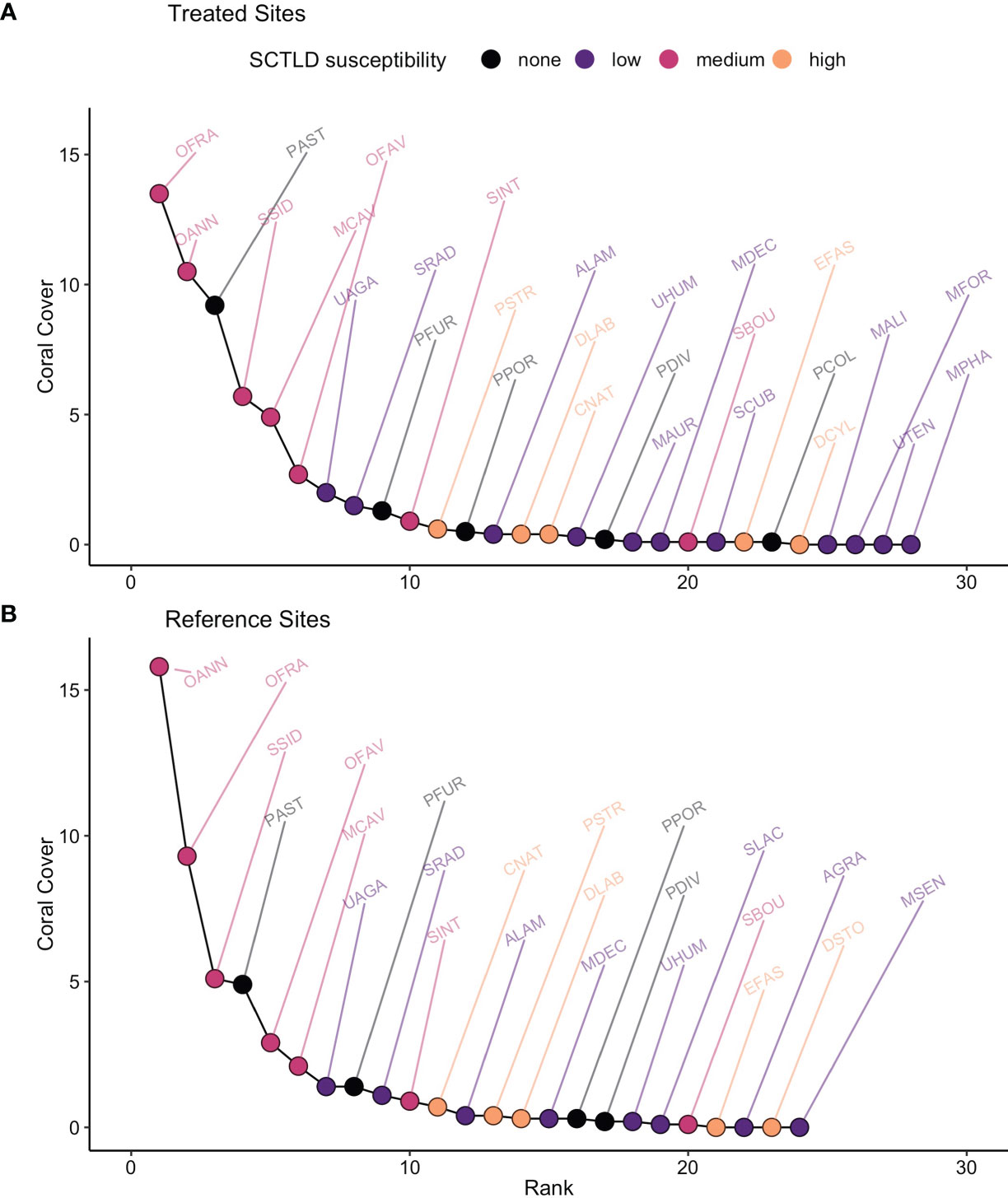
Figure 6 Pooled rank-abundance curves for (A) treated and (B) reference sites. Coral taxa are labelled by 4-letter codes listed in Supplemental Table 1.
There was some support for our prediction that treatment would cause a re-ordering of relative rank based on species’ susceptibility to SCTLD (Figure 3). Species of medium susceptibility to SCTLD were relatively common (all but one ranked < 10, Figure 6). Highly susceptible species, in contrast, tended to be rare (always ranked > 10, Figure 6) and their rarity makes them more susceptible to shifts in relative abundance. When species were grouped by susceptibility, the pattern of changes in mean relative ranks was consistent with a positive effect of antibiotic treatment (Figure 7). High susceptibility species tended to increase in relative abundance (became roughly 10% lower in rank) at treated sites, non-susceptible species tended to decrease in relative abundance (became roughly 5% higher in rank), and species of low- and medium-susceptibility were relatively unchanged (Figure 7).
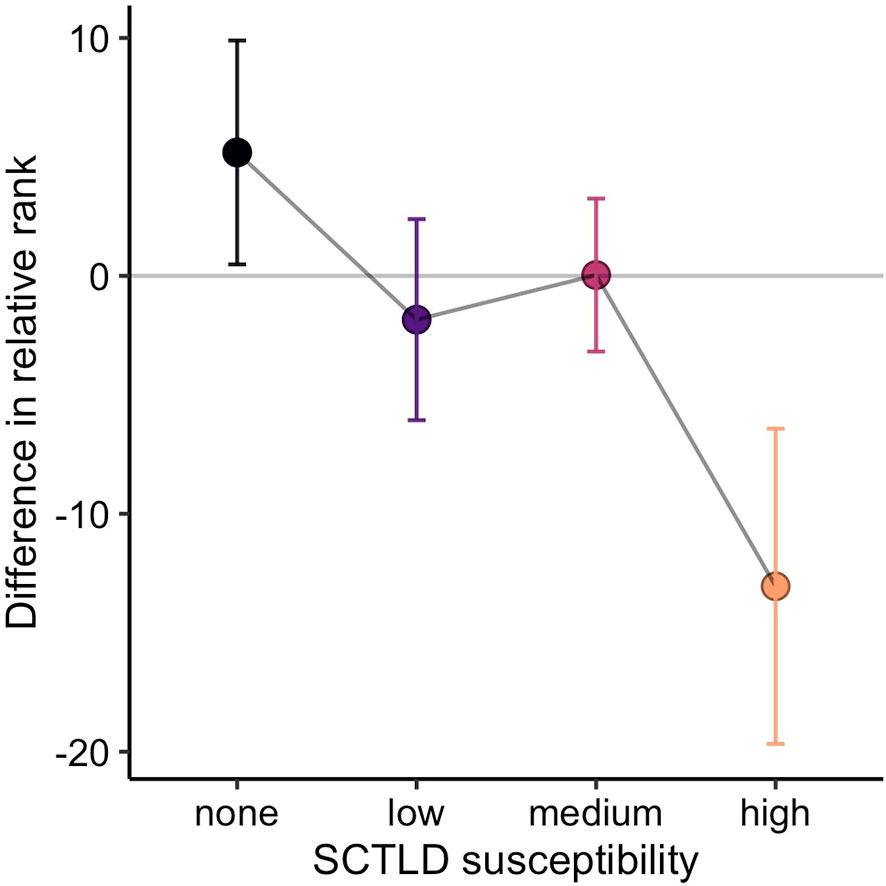
Figure 7 Mean change in relative rank (± 95% CI) of corals at paired treated and reference sites. Positive values indicate higher rank (i.e., lower relative abundance) at treated sites and negative values indicate lower rank (i.e., higher relative abundance) at treated sites.
Treated and reference sites also differed in overall species richness; 28 species were detected at treated sites and 24 at reference sites (Figure 6). The richness-difference index was roughly 10% higher at treated sites relative to nearby reference sites (mean proportional difference = 0.11, 95% CI = 0.06 to 0.21, df = 12, t = 2.31, p = 0.038). There was, however, no support for our prediction that the species missing from reference sites would be those highly susceptible to SCTLD (Figure 3). Three of the missing species were of low-susceptibility and one was not-susceptible (Figure 6). Similarly, treated and reference sites also differed in species composition (mean proportional difference in species = 0.23, 95% CI = 0.17 to 0.22, df = 12, t = 8.66, p < 0.001), but we saw no evidence that this was related to SCTLD. Species unique to treated or reference sites were simply rare species whose detection is likely to be a function of scarcity, and there was no tendency for them to be high or low in susceptibility to SCTLD (Figure 6).
Discussion
Collectively, we suggest that the results indicate slight beneficial effects of the intervention program, primarily for rare, highly susceptible species. All four indicators predicted to reflect SCTLD impacts (WS lesion severity, WS prevalence, tissue loss, and colony mortality) varied with treatment and SCTLD-susceptibility in a pattern qualitatively consistent with benefits of antibiotic application, whereas all four indicators unrelated to SCTLD (lesion severity and prevalence of NWS and ND syndromes) showed different patterns (compare Figure 5 and Supplemental Figure 4). Although there was little statistical support for these responses individually, the eight responses display a collective pattern suggestive of a subtle beneficial effect of antibiotic applications at the colony- and population-level. The re-ordering of rank abundance at the community-level, whereby rare highly SCTLD-susceptible species showed modest increases in cover in response to treatment, further reinforces this interpretation.
This signal indicative of a beneficial treatment effect was observed despite noise in the data created by variation in SCTLD-susceptibility and responsiveness to antibiotics. We predicted that coral species would respond to treatment according to their sensitivity to SCTLD, and used susceptibility groupings developed in Florida where SCTLD was first reported and has been most extensively studied (FKNMS/DEP, 2018). Subsequent work in other areas suggests variation in susceptibility (Alvarez-Filip et al., 2019; Brandt et al., 2021), and BVI strike team divers observed lesions consistent with SCTLD on species previously classified as unaffected or data-deficient (including Porites astreoides, Agaricia spp. and Undaria spp.). Our analysis also assumes that species respond equally to treatment and, although evidence from Florida supports this assumption for five species treated frequently in the BVI (Neely et al., 2021), strike team divers noted that two other highly susceptible species (Dendrogyra cylindrus and Meandrina meandrites) responded poorly to treatment in the BVI and were extirpated at several sites prior to the surveys.
Our argument for benefits of the intervention also comes with the caveat that we cannot rule out all other possible reasons for differences between treated and reference sites. We were able to rule out confounding effects of coral damage from boats or diver contact but, although there was no evidence that divers damage corals, we cannot rule out the possibility that recreational divers touching corals are spreading SCTLD at treated sites. The results do, however, suggest that benefits of treatment either counteract or override any such effect. Other differences stem from the fact that treated and reference sites differed in species richness and composition in ways not obviously linked to SCTLD susceptibility, and so may have preceded SCTLD arrival. This was likely a result of site-selection priorities for the intervention because ease of diver access and high species diversity were both factors weighed when selecting sites for treatment. Interpreting our results is, however, complicated by the fact that both species richness and composition can affect community disease susceptibility (Rohr et al., 2020). The effect can be either positive (the dilution effect, Keesing et al., 2010) or negative (the amplification effect, Keesing et al., 2006) and is not simple to predict a-priori (Rohr et al., 2020). In other studies, SCTLD prevalence was unrelated to coral cover (Muller et al., 2020; Sharp et al., 2020) but was positively correlated with species richness in Florida and the US Virgin Islands, suggesting a possible amplification effect for SCTLD (Muller et al., 2020; Costa et al., 2021). Any similar amplification effect at BVI treated sites due to their higher species richness was apparently counteracted by a beneficial effect of treatment.
With these caveats in mind, it was encouraging that we detected benefits while studying the entire community, rather than just those colonies that received treatment. There are two possible mechanisms for site-wide effects: (1) only treated corals benefited from antibiotic application, but treated corals were a sufficiently large fraction of the total to make benefits detectable using a community-wide sample, or (2) treating some infected corals indirectly benefits untreated corals at the site by slowing the spread of SCTLD. The latter mechanism makes intervention programs more valuable, and the contagious spread of SCTLD among neighbouring colonies (Williams et al., 2021) makes it plausible, but interventions in other systems provide examples of both scenarios. Vaccinating wild mammals, for example, has reduced the prevalence of contagious diseases (Brochier et al., 1991), the severity of infections (Chambers et al., 2011), and triggered host population increases (Goszczyński et al., 2008). In some situations, vaccination reduced the spread of disease to untreated hosts (Carter et al., 2012) and to other susceptible species (Brochier et al., 1991), but other treatment programs appeared to benefit only vaccinated individuals (White et al., 2011). In our study, because the sites treated with antibiotics did not have precisely defined boundaries and we did not count every coral at each site, we can make only a very rough estimate of the fraction of corals that were treated approximately (mean % treated ± 95% CI for high-susceptibility species = 5.8% ± 5.2% and for medium susceptibility species = 1.4% ± 1.8%, see Supplemental Table 3). Even these crude estimates suggest that too few corals were treated for mechanism (1) to be plausible. Treating a small fraction of the corals at a site thus appears to indirectly benefit untreated corals and confer a subtle benefit at the community-level.
Conclusion
Successful management plans for SCTLD are likely to contain several components that span the pre-arrival phase and invasion-front, as well as the epidemic and established phases (sensu Langwig et al., 2015). We showed that intervention programs using antibiotics may be a viable part of management plans for SCTLD at the epidemic stage, which complements work in Florida showing their value once SCTLD is well-established (Neely et al., 2021). Because diver-based interventions are expensive, time-consuming, and limited in scale to relatively small areas, future priorities should also include evaluations of how to optimize the impact of these treatment programs. Our study was of limited scope and so used a simple comparison of treated and untreated areas. With more detailed information on the fraction of corals treated at each site and the temporal progression of the outbreak, it would be valuable in future to quantify benefits as a function of treatment effort and outbreak-stage. Studies tracking possible changes in the effectiveness of antibiotic treatment over time will also be extremely important because of the potential development of antibiotic resistance and other unanticipated alternations to coral-microbe interactions (Sweet et al., 2011; Sweet et al., 2014). Where SCTLD has yet to invade, we hypothesize that the benefits of treatment may be greatest for highly susceptible species at the invasion front due to the potential reduction of disease transmission among neighbouring corals.
Data Availability Statement
The datasets presented in this study can be found in online repositories. The names of the repository/repositories and accession number(s) can be found below: https://doi.org/10.5061/dryad.4qrfj6qbw.
Author Contributions
GF and LA acquired funding for the study. GF designed the study, performed the statistical analyses, and drafted the manuscript. LA, GF, LF and KN performed the field research and compiled the survey data. LA and AH compiled strike team data. All authors contributed to the final article and approved the submitted version.
Funding
Funding for the study was provided by the Falconwood Foundation and Beyond the Reef. Field surveys for the project were conducted under BVI research permit ENV E1/17. Funding for the treatment program was provided by Unite BVI.
Conflict of Interest
The authors declare that the research was conducted in the absence of any commercial or financial relationships that could be construed as a potential conflict of interest.
Publisher’s Note
All claims expressed in this article are solely those of the authors and do not necessarily represent those of their affiliated organizations, or those of the publisher, the editors and the reviewers. Any product that may be evaluated in this article, or claim that may be made by its manufacturer, is not guaranteed or endorsed by the publisher.
Acknowledgments
We thank Lianna Jarecki, plus staff from Commercial Dive Services, Dive BVI and Guana Island for their logistical support of the field research. We thank Elisabeth Carpinelli, Michael Caron, Kaitlyn Eriksson, Maddie Reagan, Hazelynn Rios and Talia Scott for help with data compilation. We are grateful to the BVI National Parks Trust and Governor’s Office for permission to use the strike team data, and special thanks to all the strike team divers for their efforts as part of the intervention program.
Supplementary Material
The Supplementary Material for this article can be found online at: https://www.frontiersin.org/articles/10.3389/fmars.2022.859740/full#supplementary-material
References
Aeby G. S., Ushijima B., Campbell J. E., Jones S., Williams G. J., Meyer J. L., et al. (2019). Pathogenesis of a Tissue Loss Disease Affecting Multiple Species of Corals Along the Florida Reef Tract. Front. Marine Sci. 6, 678. doi: 10.3389/fmars.2019.00678
Alvarez-Filip L., Estrada-Saldívar N., Pérez-Cervantes E., Molina-Hernández A., González-Barrios F. J. (2019). A Rapid Spread of the Stony Coral Tissue Loss Disease Outbreak in the Mexican Caribbean. PeerJ 7, e8069. doi: 10.7717/peerj.8069
Atad I., Zvuloni A., Loya Y., Rosenberg E. (2012). Phage Therapy of the White Plague-Like Disease of Favia Favus in the Red Sea. Coral Reefs 31, 665–670. doi: 10.1007/s00338-012-0900-5
Avolio M. L., Carroll I. T., Collins S. L., Houseman G. R., Hallett L. M., Isbell F., et al. (2019). A Comprehensive Approach to Analyzing Community Dynamics Using Rank Abundance Curves. Ecosphere 10, e02881. doi: 10.1002/ecs2.2881
Avolio M. L., Pierre K. J. L., Houseman G. R., Koerner S. E., Grman E., Isbell F., et al. (2015). A Framework for Quantifying the Magnitude and Variability of Community Responses to Global Change Drivers. Ecosphere 6, 1–14. doi: 10.1890/ES15-00317.1
Bakus G. J. (2006). Quantitative Analysis of Marine Biological Communities: Field Biology and Environment (Hoboken, New Jersey, USA: John Wiley & Sons).
Beeden R., Maynard J. A., Marshall P. A., Heron S. F., Willis B. L. (2011). A Framework for Responding to Coral Disease Outbreaks That Facilitates Adaptive Management. Environ. Manage. 49, 1–13. doi: 10.1007/s00267-011-9770-9
Begon M., Bennett M., Bowers R. G., French N. P., Hazel S. M., Turner J. (2002). A Clarification of Transmission Terms in Host-Microparasite Models: Numbers, Densities and Areas. Epidemiol. Infect. 129, 147–153. doi: 10.1017/S0950268802007148
Bourne D. G., Garren M., Work T. M., Rosenberg E., Smith G. W., Harvell C. D. (2009). Microbial Disease and the Coral Holobiont. Trends Microbiol. 17, 554–562. doi: 10.1016/j.tim.2009.09.004
Brandt M. E., Ennis R. S., Meiling S. S., Townsend J., Cobleigh K., Glahn A., et al. (2021). The Emergence and Initial Impact of Stony Coral Tissue Loss Disease (SCTLD) in the United States Virgin Islands. Front. Marine Sci. 8, 715329. doi: 10.3389/fmars.2021.715329
Brochier B., Kieny M. P., Costy F., Coppens P., Bauduin B., Lecocq J. P., et al. (1991). Large-Scale Eradication of Rabies Using Recombinant Vaccinia-Rabies Vaccine. Nature 354, 520–522. doi: 10.1038/354520a0
Caldwell J. M., Donahue M. J., Harvell C. D. (2018). Host Size and Proximity to Diseased Neighbours Drive the Spread of a Coral Disease Outbreak in Hawai’i. Proc. R. Soc. B: Biol. Sci. 285, 20172265. doi: 10.1098/rspb.2017.2265
Carter S. P., Chambers M. A., Rushton S. P., Shirley M. D. F., Schuchert P., Pietravalle S., et al. (2012). BCG Vaccination Reduces Risk of Tuberculosis Infection in Vaccinated Badgers and Unvaccinated Badger Cubs. PloS One 7, e49833. doi: 10.1371/journal.pone.0049833
Chambers M. A., Rogers F., Delahay R. J., Lesellier S., Ashford R., Dalley D., et al. (2011). Bacillus Calmette-Guérin Vaccination Reduces the Severity and Progression of Tuberculosis in Badgers. Proc. R. Soc. B: Biol. Sci. 278, 1913–1920. doi: 10.1098/rspb.2010.1953
Chung Y., Rabe-Hesketh S., Dorie V., Gelman A., Liu J. (2013). A Nondegenerate Penalized Likelihood Estimator for Variance Parameters in Multilevel Models. Psychometrika 78, 685–709. doi: 10.1007/s11336-013-9328-2
Costa S. V., Hibberts S. J., Olive D. A., Budd K. A., Long A. E., Meiling S. S., et al. (2021). Diversity and Disease: The Effects of Coral Diversity on Prevalence and Impacts of Stony Coral Tissue Loss Disease in Saint Thomas, U.S. Virgin Islands. Front. Mar. Sci. 8, 682688. doi: 10.3389/fmars.2021.682688
Estrada-Saldívar N., Quiroga-García B. A., Pérez-Cervantes E., Rivera-Garibay O. O., Alvarez-Filip L. (2021). Effects of the Stony Coral Tissue Loss Disease Outbreak on Coral Communities and the Benthic Composition of Cozumel Reefs. Front. Marine Sci. 8, 306. doi: 10.3389/fmars.2021.632777
Fisher W. S., Fore L. S., Hutchins A., Quarles R. L., Campbell J. G., LoBue C., et al. (2008). Evaluation of Stony Coral Indicators for Coral Reef Management. Marine Pollution Bull. 56, 1737–1745. doi: 10.1016/j.marpolbul.2008.07.002
FKNMS/DEP (2018) Stony Coral Tissue Loss Disease Case Definition. Available at: https://floridadep.gov/sites/default/files/Copy%20of%20StonyCoralTissueLossDisease_CaseDefinition%20final%2010022018.pdf (Accessed September 15, 2020).
Florida DEP. (2021). Reconnaissance and Intervention - Coral Disease Response Team | Florida Department of Environmental Protection (Florida DEP). Available at: https://floridadep.gov/rcp/coral/content/reconnaissance-and-intervention-coral-disease-response-team (Accessed January 4, 2022).
Flynn R. L., Forrester G. E. (2019). Boat Anchoring Contributes Substantially to Coral Reef Degradation in the British Virgin Islands. PeerJ 7, e7010. doi: 10.7717/peerj.7010
Forrester G. E., Flynn R. L., Forrester L. M., Jarecki L. L. (2015). Episodic Disturbance From Boat Anchoring Is a Major Contributor to, But Does Not Alter the Trajectory of, Long-Term Coral Reef Decline. PloS One 10, e0144498. doi: 10.1371/journal.pone.0144498
Gil-Agudelo D. L., Smith G. W., Garzón-Ferreira J., Weil E., Petersen D. (2004). “Dark Spots Disease and Yellow Band Disease, Two Poorly Known Coral Diseases With High Incidence in Caribbean Reefs,” in Coral Health and Disease. Eds. Rosenberg E., Loya Y. (Berlin, Heidelberg: Springer), 337–349. doi: 10.1007/978-3-662-06414-6_19
Goszczyński J., Misiorowska M., Juszko S. (2008). Changes in the Density and Spatial Distribution of Red Fox Dens and Cub Numbers in Central Poland Following Rabies Vaccination. Acta Theriol. 53, 121–127. doi: 10.1007/BF03194245
Hallett L. M., Jones S. K., MacDonald A. A. M., Jones M. B., Flynn D. F. B., Ripplinger J., et al. (2016). Codyn: An R Package of Community Dynamics Metrics. Methods Ecol. Evol. 7, 1146–1151. doi: 10.1111/2041-210X.12569
Harvell D., Jordán-Dahlgren E., Merkel S., Rosenberg E., Raymundo L., Smith G., et al. (2007). Coral Disease, Environmental Drivers, and the Balance Between Coral and Microbial Associates. Oceanog. 20, 172–195. doi: 10.5670/oceanog.2007.91
Hawkins J. P., Roberts C. M., Van’T Hof T., De Meyer K., Tratalos J., Aldam C. (1999). Effects of Recreational Scuba Diving on Caribbean Coral and Fish Communities. Conserv. Biol. 13, 888–897. doi: 10.1046/j.1523-1739.1999.97447.x
Hughes T. P. (1984). Population Dynamics Based on Individual Size Rather Than Age: A General Model With a Reef Coral Example. Am. Nat. 123, 778–795. doi: 10.1086/284239
Keefner N. B. (2019) Temporal Effectiveness of Biodiversity Surrogates in Coral Reefs in the British Virgin Islands. Available at: http://digitalcommons.uri.edu/theses/1752 (Accessed January 7, 2022).
Keesing F., Belden L. K., Daszak P., Dobson A., Harvell C. D., Holt R. D., et al. (2010). Impacts of Biodiversity on the Emergence and Transmission of Infectious Diseases. Nature 468, 647–652. doi: 10.1038/nature09575
Keesing F., Holt R. D., Ostfeld R. S. (2006). Effects of Species Diversity on Disease Risk. Ecol. Lett. 9, 485–498. doi: 10.1111/j.1461-0248.2006.00885.x
Kindt R., Coe R. (2005). Tree Diversity Analysis: A Manual and Software for Common Statistical Methods for Ecological and Biodiversity Studies (Nairobi, Kenya: World Agroforestry Centre (ICRAF).
Kosmidis I., Firth D. (2021). Jeffreys-Prior Penalty, Finiteness and Shrinkage in Binomial-Response Generalized Linear Models. Biometrika 108, 71–82. doi: 10.1093/biomet/asaa052
Kramer P. R., Roth L., Lang J. C. (2019) Map of Stony Coral Tissue Loss Disease Outbreak in the Caribbean (AGRRA). Available at: www.agrra.org/coral-disease-outbreak (Accessed January 4, 2021).
Landsberg J. H., Kiryu Y., Peters E. C., Wilson P. W., Perry N., Waters Y., et al. (2020). Stony Coral Tissue Loss Disease in Florida Is Associated With Disruption of Host–Zooxanthellae Physiology. Front. Marine Sci. 7, 576013. doi: 10.3389/fmars.2020.576013
Lang J. C., Marks K. W., Kramer P. A., Kramer P. R., Ginsburg R. N. (2013). AGRRA Protocols Version 5.5 (Florida, USA: Atlantic and Gulf Rapid Reef Assessment Program).
Langwig K. E., Voyles J., Wilber M. Q., Frick W. F., Murray K. A., Bolker B. M., et al. (2015). Context-Dependent Conservation Responses to Emerging Wildlife Diseases. Front. Ecol. Environ. 13, 195–202. doi: 10.1890/140241
Lyons P. J., Arboleda E., Benkwitt C. E., Davis B., Gleason M., Howe C., et al. (2015). The Effect of Recreational SCUBA Divers on the Structural Complexity and Benthic Assemblage of a Caribbean Coral Reef. Biodivers Conserv 24, 3491–3504. doi: 10.1007/s10531-015-1009-2
Meyer J. L., Castellanos-Gell J., Aeby G. S., Häse C. C., Ushijima B., d Paul V. J. (2019). Microbial Community Shifts Associated With the Ongoing Stony Coral Tissue Loss Disease Outbreak on the Florida Reef Tract. Front. Microbiol. 10, 2244. doi: 10.3389/fmicb.2019.02244
Miller C., May L., Moffitt Z., Woodley C. (2020). Exploratory Treatments for Stony Coral Tissue Loss Disease: Pillar Coral (Dendrogyra Cylindrus) (Charleston, South Carolina, USA: NOAA National Centers for Coastal Ocean Science , National Ocean Service).
Muller E. M., Sartor C., Alcaraz N. I., van Woesik R. (2020). Spatial Epidemiology of the Stony-Coral-Tissue-Loss Disease in Florida. Front. Marine Sci. 7, 163. doi: 10.3389/fmars.2020.00163
Neely K. (2020). FLORIDA KEYS Coral Disease Strike Team: FY 2019/2020 FINAL REPORT (Miami, Florida, USA: Florida DEP).
Neely K. L., Macaulay K. A., Hower E. K., Dobler M. A. (2020). Effectiveness of Topical Antibiotics in Treating Corals Affected by Stony Coral Tissue Loss Disease. PeerJ 8, e9289. doi: 10.7717/peerj.9289
Neely K. L., Shea C. P., Macaulay K. A., Hower E. K., Dobler M. A. (2021). Short- and Long-Term Effectiveness of Coral Disease Treatments. Front. Marine Sci. 8, 1031. doi: 10.3389/fmars.2021.675349
Precht W. F., Gintert B. E., Robbart M. L., Fura R., van Woesik R. (2016). Unprecedented Disease-Related Coral Mortality in Southeastern Florida. Sci. Rep. 6, 31374. doi: 10.1038/srep31374
R Core Team. (2021). R: A Language and Environment for Statistical Computing. R Foundation for Statistical Computing (Vienna, Austria). Available at: https://www.R-project.org/.
Rogers C. S., Miller J. (2013). Coral Diseases Cause Reef Decline. Science 340, 1522. doi: 10.1126/science.340.6140.1522-a
Rohr J. R., Civitello D. J., Halliday F. W., Hudson P. J., Lafferty K. D., Wood C. L., et al. (2020). Towards Common Ground in the Biodiversity–Disease Debate. Nat. Ecol. Evol. 4, 24–33. doi: 10.1038/s41559-019-1060-6
Rosales S. M., Clark A. S., Huebner L. K., Ruzicka R. R., Muller E. M. (2020). Rhodobacterales and Rhizobiales Are Associated With Stony Coral Tissue Loss Disease and Its Suspected Sources of Transmission. Front. Microbiol. 11, 681. doi: 10.3389/fmicb.2020.00681
Roth L., Kramer P. A., Doyle E., O’Sullivan C. (2020) Caribbean SCTLD Dashboard (AGRRA). Available at: https://www.agrra.org/coral-disease-outbreak/ (Accessed January 4, 2022).
Ruiz-Moreno D., Willis B. L., Page A. C., Weil E., Cróquer A., Vargas-Angel B., et al. (2012). Global Coral Disease Prevalence Associated With Sea Temperature Anomalies and Local Factors. Dis. Aquat. Organisms 100, 249–261. doi: 10.3354/dao02488
Sharp W. C., Shea C. P., Maxwell K. E., Muller E. M., Hunt J. H. (2020). Evaluating the Small-Scale Epidemiology of the Stony-Coral-Tissue-Loss-Disease in the Middle Florida Keys. PloS One 15, e0241871. doi: 10.1371/journal.pone.0241871
Shilling E. N., Combs I. R., Voss J. D. (2021). Assessing the Effectiveness of Two Intervention Methods for Stony Coral Tissue Loss Disease on Montastraea Cavernosa. Sci. Rep. 11, 8566. doi: 10.1038/s41598-021-86926-4
Smith G. J. (1998)“Retrospective Studies,” In: Statistical Methods for Adaptive Management Studies (Victoria, BC, Canada: British Columbia Ministry of Forests). Available at: https://www.for.gov.bc.ca/hfd/pubs/docs/lmh/lmh42.htm (Accessed September 28, 2021).
Sweet M. J., Croquer A., Bythell J. C. (2011). Dynamics of Bacterial Community Development in the Reef Coral Acropora Muricata Following Experimental Antibiotic Treatment. Coral Reefs 30, 1121. doi: 10.1007/s00338-011-0800-0
Sweet M. J., Croquer A., Bythell J. C. (2014). Experimental Antibiotic Treatment Identifies Potential Pathogens of White Band Disease in the Endangered Caribbean Coral Acropora Cervicornis. Proc. R. Soc. B: Biol. Sci. 281, 20140094. doi: 10.1098/rspb.2014.0094
Underwood A. J. (1997). Experiments in Ecology: Their Logical Design and Interpretation Using Analysis of Variance (Cambridge, United Kingdom: Cambridge University Press).
Vega Thurber R., Mydlarz L. D., Brandt M., Harvell D., Weil E., Raymundo L., et al. (2020). Deciphering Coral Disease Dynamics: Integrating Host, Microbiome, and the Changing Environment. Front. Ecol. Evol. 8, 575927. doi: 10.3389/fevo.2020.575927
Walker B. K., Turner N. R., Noren H. K. G., Buckley S. F., Pitts K. A. (2021). Optimizing Stony Coral Tissue Loss Disease (SCTLD) Intervention Treatments on Montastraea Cavernosa in an Endemic Zone. Front. Marine Sci. 8, 666224. doi: 10.3389/fmars.2021.666224
Walton C. J., Hayes N. K., Gilliam D. S. (2018). Impacts of a Regional, Multi-Year, Multi-Species Coral Disease Outbreak in Southeast Florida. Front. Marine Sci. 5, 323. doi: 10.3389/fmars.2018.00323
Ward J. R., Lafferty K. D. (2004). The Elusive Baseline of Marine Disease: Are Diseases in Ocean Ecosystems Increasing? PloS Biol. 2, e120. doi: 10.1371/journal.pbio.0020120
White P. J., Wallen R. L., Geremia C., Treanor J. J., Blanton D. W. (2011). Management of Yellowstone Bison and Brucellosis Transmission Risk – Implications for Conservation and Restoration. Biol. Conserv. 144, 1322–1334. doi: 10.1016/j.biocon.2011.01.003
Whittaker R. H. (1965). Dominance and Diversity in Land Plant Communities: Numerical Relations of Species Express the Importance of Competition in Community Function and Evolution. Science 147, 250–260. doi: 10.1126/science.147.3655.250
Williams S. D., Walter C. S., Muller E. M. (2021). Fine Scale Temporal and Spatial Dynamics of the Stony Coral Tissue Loss Disease Outbreak Within the Lower Florida Keys. Front. Marine Sci. 8, 631776. doi: 10.3389/fmars.2021.631776
Wobeser G. (2002). Disease Management Strategies for Wildlife. Rev. Sci. Tech. OIE 21, 159–178. doi: 10.20506/rst.21.1.1326
Keywords: Amoxicillin, epidemic, rank-abundance curve, scleractinia, volunteers
Citation: Forrester GE, Arton L, Horton A, Nickles K and Forrester LM (2022) Antibiotic Treatment Ameliorates the Impact of Stony Coral Tissue Loss Disease (SCTLD) on Coral Communities. Front. Mar. Sci. 9:859740. doi: 10.3389/fmars.2022.859740
Received: 21 January 2022; Accepted: 23 March 2022;
Published: 19 April 2022.
Edited by:
Sarah Annalise Gignoux-Wolfsohn, Smithsonian Environmental Research Center (SI), United StatesReviewed by:
Michael Sweet, University of Derby, United KingdomAndrew A Shantz, Florida State University, United States
Copyright © 2022 Forrester, Arton, Horton, Nickles and Forrester. This is an open-access article distributed under the terms of the Creative Commons Attribution License (CC BY). The use, distribution or reproduction in other forums is permitted, provided the original author(s) and the copyright owner(s) are credited and that the original publication in this journal is cited, in accordance with accepted academic practice. No use, distribution or reproduction is permitted which does not comply with these terms.
*Correspondence: Graham E. Forrester, Z2ZvcnJlc3RlckB1cmkuZWR1