- 1Key Laboratory of Marine Biotechnology of Fujian Province, Institute of Oceanology, Fujian Agriculture and Forestry University, Fuzhou, China
- 2Xiamen Key Laboratory for Feed Quality Testing and Safety Evaluation, Fisheries College of Jimei University, Xiamen, China
An 8-week feeding trial was conducted to investigate how dietary taurine supplementation attenuates the lipid deposition induced by dietary high lipid in juvenile orange-spotted grouper (Epinephelus coioides). Three isonitrogenous (47% crude protein) semipurified diets were formulated to contain two levels of lipid and termed as 10% lipid diet, 15% lipid diet, and 15% lipid with 1% taurine (namely, diet 10L, diet 15L, and diet 15L + T, respectively). Groupers fed diet 15L + T showed higher weight gain and feed efficiency compared with diet 15L. Groupers fed diet 15L showed higher liver lipid contents, plasma total cholesterol (TC), and leptin contents vs. those fed diet 10L. Diet 15L + T decreased hepatosomatic index, liver lipid content, and plasma TC and adiponectin contents, and increased liver 3-hydroxy-3-methy1 glutary1 coenzyme A reductase content compared with diet 15L. Fish fed diet 15L had higher contents of chenodeoxycholic acid, deoxycholic acid, and lithocholic acid, and lower contents of glycodeoxycholic acid (GDCA), glycolithocholic acid, glycoursodeoxycholic acid, taurodeoxycholic acid (TDCA), and β-, γ-, and ω-muricholic acid (MCA) when compared with fish fed diet 10L. Diet 15L + T downregulated the contents of glycocholic acid, glycochenodeoxycholic acid, taurohyodeoxycholic acid, tauromuricholic acid, TDCA, ursodeoxycholic acid, GDCA, and β-MCA vs. diet 15L. Diet 15L upregulated expression of peroxisome proliferator-activated receptor α (pparα) gene but downregulated expression of acyl-CoA carboxylase (acc), fatty acid synthase (fas), and glucose-6-phosphate dehydrogenase (g6pd) genes in comparison with diet 10L. The gene expression level of fas and 6pgd was downregulated and the pparα gene expression level was upregulated in fish fed diet 15L + T compared with those in fish fed diet 15L. Overall, this study indicated that dietary taurine supplementation can attenuate the liver lipid deposition of groupers caused by feeding 15% lipid through accelerating lipid absorption of taurine-conjugated bile acids and fatty acid β-oxidation and inhibiting lipogenesis.
Introduction
Taurine (2-aminoethanesulfonic acid), as a free amino acid, does not participate in protein synthesis but is known to play a wide range of key roles in animal physiology, such as immune regulation, osmoregulation, antioxidation, nervous system development and regeneration, and bile acid (BA) conjugation (Huxtable, 1992; Salze and Davis, 2015; Wu, 2020; Xu et al., 2020). BAs are regarded as biosurfactants, which emulsify lipid into micelles to facilitate its digestion and absorption by allowing for more cleavage sites for lipase (Suga et al., 2019; Romano et al., 2020). However, the emulsification of long-chain triglycerides (TGs) is achieved by conjugated BAs, which are better emulsifiers than BAs due to their hydrophilic and lipophilic properties (Gupta and Kim, 2003). It was reported that most primary BAs are linked with taurine or glycine to form conjugated BAs (Chiang and Ferrell, 2020), and taurocholic acid (TCA) or taurodeoxycholic acid (TDCA) formed by taurine conjugating with BAs is predominant in BAs for most teleost fish except common carp (Cyprinus carpio) (Kim et al., 2007, 2015). Previous studies demonstrated that dietary taurine addition increased taurine-conjugated BAs contents in rockfish (Sebastes schlegeli) and tiger puffer (Takifugu rubripes) (Kim et al., 2015; Xu et al., 2020). Thus, taurine may regulate lipid metabolism through accelerating BAs to form taurine-conjugated BAs.
It is clear that dietary taurine supplementation can reduce peripheral cholesterol and visceral fat contents of diabetic rats (Tsuboyama-Kasaoka et al., 2006) and obese humans (Brons et al., 2004; Zhang et al., 2004). The lipid-lowering effect of taurine was also observed in several farmed fish species. For example, dietary taurine addition resulted in a reduction of whole-body lipid content in European seabass (Dicentrarchus labrax) (Martins et al., 2021), serum total cholesterol (TC), and TG contents in yellow catfish (Pelteobagrus fulvidraco) (Li et al., 2016), and liver lipid contents in California yellowtails (Seriola dorsalis) (Garcia-Organista et al., 2019) and rice field eels (Monopterus albus) (Hu et al., 2018). Of particular concern are the liver lipid deposition and even fatty liver syndrome caused by the widespread use of high-lipid feed in intensive aquaculture for protein-sparing and growth-promoting (Du et al., 2005; Li et al., 2012). Moreover, the excessive dietary lipid can also cause poor appetite, growth retardation (Dai et al., 2018, 2019; Nath et al., 2019). It was well-reported that taurine could act as an attractant to increase the feed intake (FI) of fish (Matsunari et al., 2008; Takagi et al., 2008; Qi et al., 2012). In view of the aforementioned lipid-lowering effect of taurine, dietary taurine supplementation may therefore be an effective way to eliminate the negative effects caused by high-lipid feeding. However, it is unclear whether the lipid-lowering effect of taurine is involved in taurine-mediated BA metabolism.
The orange-spotted grouper, Epinephelus coioides, is a commercially important farmed carnivorous fish species in Southeast Asian countries, including China (Shapawi et al., 2019; Feng et al., 2020). The optimal lipid level in feed was reported to be 10% for juvenile E. coioides, and the fish showed a downward growth trend, accompanied by an increasing trend of liver lipid accumulation when the dietary lipid level was increased from 12 to 14% (Luo et al., 2005). However, the visible fatty liver symptom usually occurs in different grouper species such as E. akaara (Ma, 1996), E. awoara (Zhou et al., 1995), E. malabaricus (Tuan and Williams, 2007), and E. bruneus (Yoshii et al., 2010) when they were fed with high-lipid diets, though varied lipid requirements for the fish species. Our latest research results showed that 1% dietary taurine was optimal for the growth of E. coioides and the dietary taurine supplementation can reduce lipid accumulation by decreasing the contents of TGs containing 18:2n-6 at the sn-2 and sn-3 positions at 15% lipid (Bai et al., 2021a,b). In this study, three diets containing 10% lipid, 15% lipid, and 15% lipid with 1% taurine were formulated to investigate how a high lipid diet does affect liver lipid deposition and to explore the lipid-lowering effect of dietary taurine supplementation concerning taurine-mediated changes of conjugated BAs.
Materials and Methods
Diets, Feeding Trial, and Sampling
Three isonitrogenous (47% crude protein) semipurified diets were formulated to contain two levels of lipid and termed as 10% lipid diet, 15% lipid diet, and 15% lipid with 1% taurine supplement (namely, diets 10L, 15L, and 15L + T, respectively) (Table 1). The experimental diets were produced according to the method described in detail previously (Bai et al., 2021a). Finally, the pellets were stored in a refrigerator at −20°C.
Juvenile groupers were obtained from a local hatchery (Zhangpu county, Fujian, China) and, before the experiment, were acclimated to the experiment condition for 3 weeks. A total of 270 juvenile groupers (36.30 ± 0.09 g/fish) were randomly allocated into nine fiberglass tanks of recirculating system, and three replicates (30 fish per replicate) were assigned to each treatment. Groupers were hand-fed to apparent satiation two times daily at 8:30 and 18:00 over 8 weeks. Feces and excess feed were removed by siphoning 30 min after each meal. Excess feed was collected, dried at 65°C, and weighed for use in the calculation of FI. During the experimental period, the water temperature was 28.0 ± 0.5°C, dissolved oxygen level was 6.1 ± 0.2 mg/l, and ammonia nitrogen level was 0.21 ± 0.03 mg/l.
At the end of the feeding trial, groupers in each tank were batch-weighed and recorded on a wet weight basis after fasting for 24 h to determine the weight gain (WG) and feed efficiency (FE). Blood samples were taken from the caudal vasculature of 10 fish per tank by a sterile 2 ml heparinized syringe and centrifuged at 1,027 g for 10 min at 4°C to obtain plasma. The plasma samples in each tank were pooled and stored at −80°C for biochemical indices. After drawing blood, the bile of gallbladders was then collected and pooled by tank, and frozen immediately in liquid N2 for BA profiles analysis. Livers were collected from the same fish and weighed to calculate hepatosomatic index (HSI), pooled by tank and frozen immediately in liquid N2, and stored at −80°C for the analysis of lipid and enzyme contents, and the expression of genes related to lipid metabolism.
Bile Acid Profile Analysis
An aliquot of 500 μl precooled methanol and 20 μl internal standard solution (Sigma-Aldrich, Mosby, MO, United States) were added into the mixed solution of 10 μl aliquot of BAs sample and 90 μl precooled water and then mixed homogeneously via the vortex. After centrifugation (14,000 g, 4°C, 15 min), the supernatant was collected and concentrated by vacuum drying. The concentrates were re-dissolved in 50% methanol-water solution, and resultant supernatants were collected after centrifugation (14,000 g, 4°C, 15 min) for subsequent analysis of BAs.
Waters ACQUITY UPLC I-Class (Waters Corporation, Milford, Massachusetts, United States) and 5500 QTRAP mass spectrometer (SCIEX Corporation, Foster City, California, United States) with an electrospray ion source (ESI) source were used in the BA profile analysis. Briefly, the supernatants were separated using an ACQUITY UPLC BEH C18 (1.7 μm, 2.1 mm × 100 mm column), and the samples were placed in an 8°C automatic sampler with column temperature of 45°C, and the flow rate was 300 μl/min and the injection volume was 2 μl. The 0.1% formic acid-water solution and methanol were used as mobile phases A and B, respectively. The correlation gradient was programed as follows: 0–6 min from 60% B to 65% B, 6–13 min from 65% B to 80% B, 13–13.5 min from 80% B to 90% B, and 13.5–15 min, B phase maintained at 90%. The mass spectrometer was operated in the ESI negative mode under the following conditions: source temperature: 550°C; ion source gas1 (Gas1): 55; ion source gas2 (Gas2): 55; curtain gas (CUR): 40; Ion Sapary Voltage Floating (ISVF): −4,500 V. The ion pair was detected by the multiple reaction monitoring mode. The chromatographic peak area and retention time were extracted using multquant 3.02 (SCIEX Corporation, Foster City, California, United States). To determine the stability of data, a QC sample was run and analyzed every ten samples. A standard substance of BAs was used to correct retention time and identify metabolites. The stability of the system and reliability of data was proved by extracted-ion chromatogram spectra of the standard mixture and relative SD distribution of quality control (QC) samples (Supplementary Figures 1, 2). The contents of BAs were determined by a standard curve (Supplementary Figure 3).
Principal component analysis (PCA) and orthogonal partial least squares discriminant analysis (OPLS-DA) were performed after all BAs were scaled with unit variance (UV). PCA was performed to examine the intrinsic variation in the data set. OPLS-DA was applied to maximize the separation between samples. The confidence level for membership probability was considered to be 95% (observations at < 5% were considered outliers). The farther the distance between components, the greater the difference. The potential overfitting of the OPLS-DA model was checked by performing 200 permutation tests and visualization using a validation plot (Supplementary Figure 4). The biplots were used to present the correlation between BA molecule species and different dietary treatments. A threshold of corrected p-value < 0.05 and variable important in projection value > 1 were used to determine the differential BAs.
Proximate Composition Determination
The moisture, ash, crude protein, and lipid contents of diets were determined according to the method of the Association of Official Analytical Chemists (AOAC, 2006). The moisture content was determined by drying the samples to a constant weight at 105°C. The crude protein contents (N × 6.25) were assayed by the Kjeldahl method using Kjeltec™ 8400 Auto Sample Systems (Foss Tecator AB, Hoganas, Sweden). Crude lipid was measured via the Soxtec extraction method using a solvent extraction system (Soxtec Avanti 2050 Auto System, Foss Tecator AB, Hoganas, Sweden), and the ash content was determined after incineration in a muffle furnace at 550°C for 8 h. The liver lipid was extracted by chloroform-methanol (2:1, vol/vol) solution and determined gravimetrically after drying a 5 ml aliquot under nitrogen (Folch et al., 1957).
Plasma and Liver Biochemical Indices Analysis
High-density lipoprotein cholesterol (HDL-C), low-density lipoprotein cholesterol (LDL-C), TG, and TC of plasma were measured using commercial assay kits. Leptin (LEP) and adiponectin (ADPN) contents in plasma and 3-hydroxy-3-methy1 glutary1 coenzyme A reductase (HMGR) content in the liver were determined by using ELISA kits according to the manufacturer’s instructions. All the commercial kits were purchased from Jiancheng Bioengineering Institute (Nanjing, China).
Real-Time Quantitative PCR Analysis of Lipid Metabolism Genes in the Liver
Total RNA was extracted using Trizol reagent (Invitrogen, Carlsbad, United States) with quantity and quality assessed via NanoDrop ND-2000 spectrophotometer (NanoDrop Technologies, Waltham, United States) and 1.2% denaturing agarose gel electrophoresis. RNA samples with 260/280 absorbance ratio of 1.86–2.00 were treated with 30 μl recombinant DNase I (RNase-free) (Takara, Tokyo, Japan), after which reverse transcription was conducted immediately on 1,000 ng RNA sample to synthesize cDNA using the PrimeScript™ RT Reagent Kit (Takara, Tokyo, Japan) following the manufacturer’s instructions. The cDNAs were stored at −80°C until quantitative real-time (qRT)-PCR analysis.
Real-time quantitative-PCR was performed to determine relative mRNA levels of four genes related to lipid metabolism, namely, fatty acid synthase (fas), acyl-CoA carboxylase (acc), glucose-6-phosphate dehydrogenase (g6pd), and peroxisome proliferator-activated receptor α (pparα) with primers designed by Primer Premier 5.0 (Supplementary Table 1). β-actin was used as a house-keeping gene after confirming its stability across the experimental treatments according to the previous study (Pfaffl et al., 2004). The amplification efficiency of primers was approximately 100% according to the specific gens standard curves generated from serial dilutions (Hanaki et al., 2014). The qRT-PCR was performed using a Light Cycler®96 (Roche, Rotkreuz, Switzerland) with a reaction system containing 10 μl TB Green premix Ex Taq™ (Tli RNaseH Plus) (2×), 2 μl cDNA template, 0.8 μl forward and reverse primers (10 μM), and 6.0 μl diethyl pyrocarbonate-treated water. The program parameters were set as follows: 30 s at 95°C, 40 cycles at 95°C for 10 s, and 60°C for 30 s. The data were optimized using the comparative Ct (2–ΔΔCt) value method as described previously (Livak and Schmittgen, 2001).
Calculations and Statistical Analysis
Data transformation was performed the prior statistical analysis. Student’s t-test was applied for comparison of diet 10L vs. diet 15L and diet 15L vs. diet 15L + T. PCA and OPLS-DA were used to analyze the data and find the changes of metabolites using SIMCA-P + 14.1 software package. The plot of correlation analysis was drawn by EXCEL 2019. All the results are presented as means ± SEM (n = 3).
Results
Growth Performance, Feed Utilization, Lipid Content, and Biometric Indices
As shown in Figure 1, FI was not affected by dietary treatments. There was no difference in WG, FE, and HSI between groupers fed diet 10L and diet 15L. Groupers fed diet 15L + T had significantly higher WG and FE, and lower HSI and liver lipid content when compared with those fed diet 15L. The liver lipid content was significantly higher in fish fed diet 15L than in those fed diet 10L.
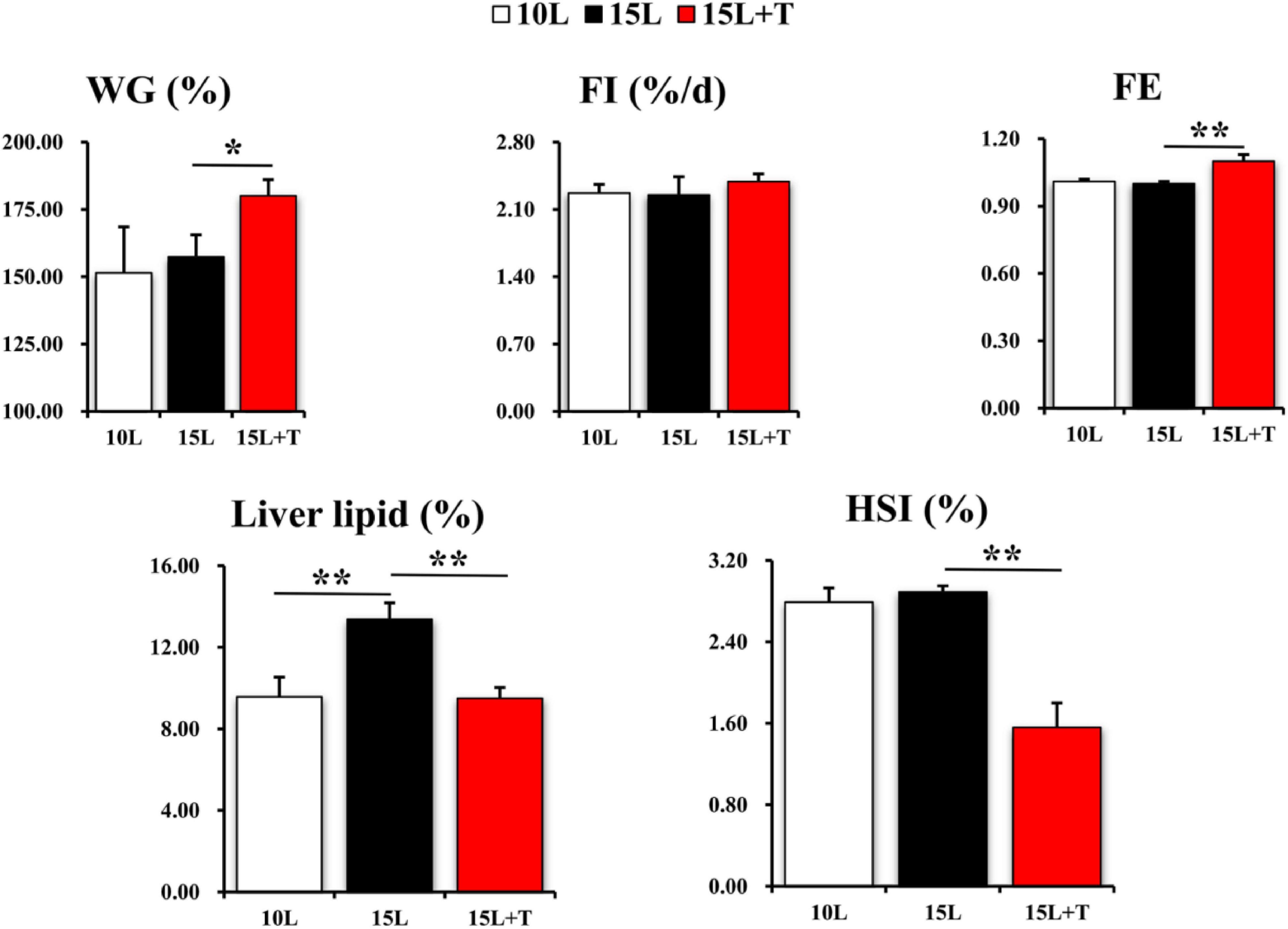
Figure 1. Growth performance, feed utilization, lipid content, and biometric indices of grouper fed the experimental diets. Values are means ± SEM (n = 3). The Student’s t-test was applied for comparison of diet 10L vs. diet 15L and diet 15L vs. diet 15L + T. * and ** represent significant differences with P < 0.05 and P < 0.01, respectively. Parameters were calculated as follows: Weight gain (WG, %) = 100 × {[final body weight (g, wet weight) − initial body weight (g, wet weight)]/initial body weight (g, wet weight)}; Feed intake (FI, %/d) = 100 × {feed consumed (g, dry weight)/[final body weight (g, wet weight) + initial body weight (g, wet weight)]/2 × days}. Feed efficiency (FE) = weight gain (g, wet weight)/feed consumed (g, dry weight); Hepatosomatic index (HSI, %) = 100 × (liver weight/wet body weight).
Bile Acid Profile
Figure 2A showed that the TCA, TDCA, and taurochenodeoxycholic acid (TCDCA) were the predominant BAs among groupers fed diet 10L, 15L, and 15L + T. The PCA score plot (Figure 2B) showed that components of BA molecule species in the bile were clustered as three groups and separated from each other with no overlap. The OPLS-DA score plots and biplots showed that the models of comparison of diet 10L vs. diet 15L and diet 15L vs. diet 15L + T were well-established (Figures 3A,D). Biplot showed the best contributor to different treatments (Figures 3B,E). All differential BAs in diet 10L vs. diet 15L and diet 15L vs. diet 15L + T are presented in Figure 3C. The contents of chenodeoxycholic acid (CDCA), deoxycholic acid (DCA), and lithocholic acid (LCA) significantly increased with dietary lipid increasing from 10 to 15%, and the glycodeoxycholic acid (GDCA), glycolithocholic acid (GLCA), glycoursodeoxycholic acid (GUDCA), TDCA, and β-, γ-, and ω-muricholic acid (MCA) contents were totally opposite. The diet taurine downregulated the contents of all differential BAs, namely, GDCA, glycocholic acid (GCA), glycochenodeoxycholic acid (GCDCA), taurohyodeoxycholic acid (THDCA), tauromuricholic acid (TMCA), tauroursodeoxycholic acid (TUDCA), ursodeoxycholic acid (TUDCA), and β-MCA in comparison with taurine free diet at 15% lipid (Figure 3F). The GDCA and β-MCA contents were downregulated by increasing dietary lipid and taurine levels.
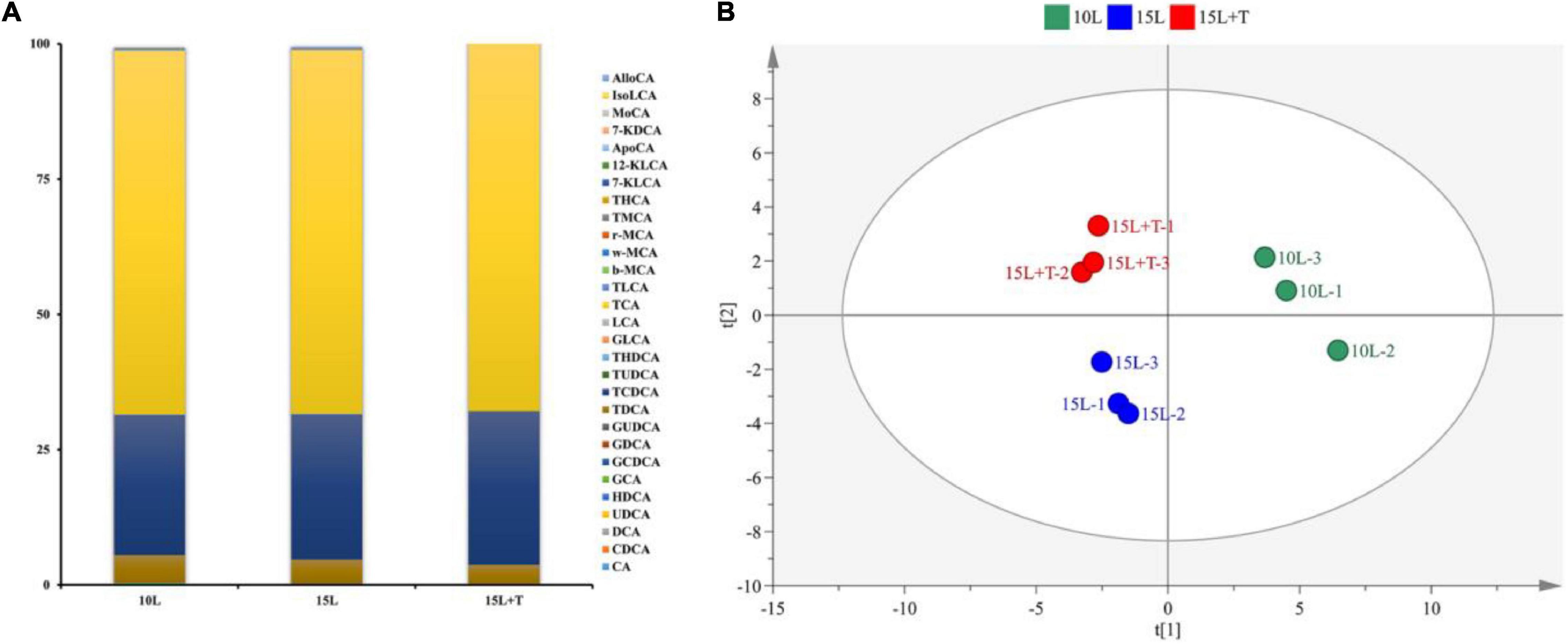
Figure 2. The contents (A) and principal component analysis score plot (B) of bile acids in groupers fed the diets 10L, 15L and 15L+T. AlloCA, allolithocholic acid; IsoLCA, isolithocholic acid; MoCA, murocholic acid; KDCA, ketodeoxycholic acid; ApoCA, apocholic acid; KLCA, ketolithocholic acid; THCA, taurohyomuricholic acid; TMCA, tauromuricholic acid; MCA, muricholic acid; TLCA, taurolithocholic acid; TCA, taurocholic acid; LCA, lithocholic acid; GLCA, glycolithocholic acid; THDCA, taurohyodeoxycholic acid; TUDCA, tauroursodeoxycholic acid; TCDCA, taurochenodeoxycholic acid; TDCA, taurodeoxycholic acid; GUDCA, glycoursodeoxycholic acid; GDCA, glycodeoxycholic acid; GCDCA, glycochenodeoxycholic acid; GCA, glycocholic acid; HDCA, hyodeoxycholic acid; UDCA, ursodeoxycholic acid; DCA, deoxycholic acid; CDCA, chenodeoxycholic acid; CA, cholic acid.
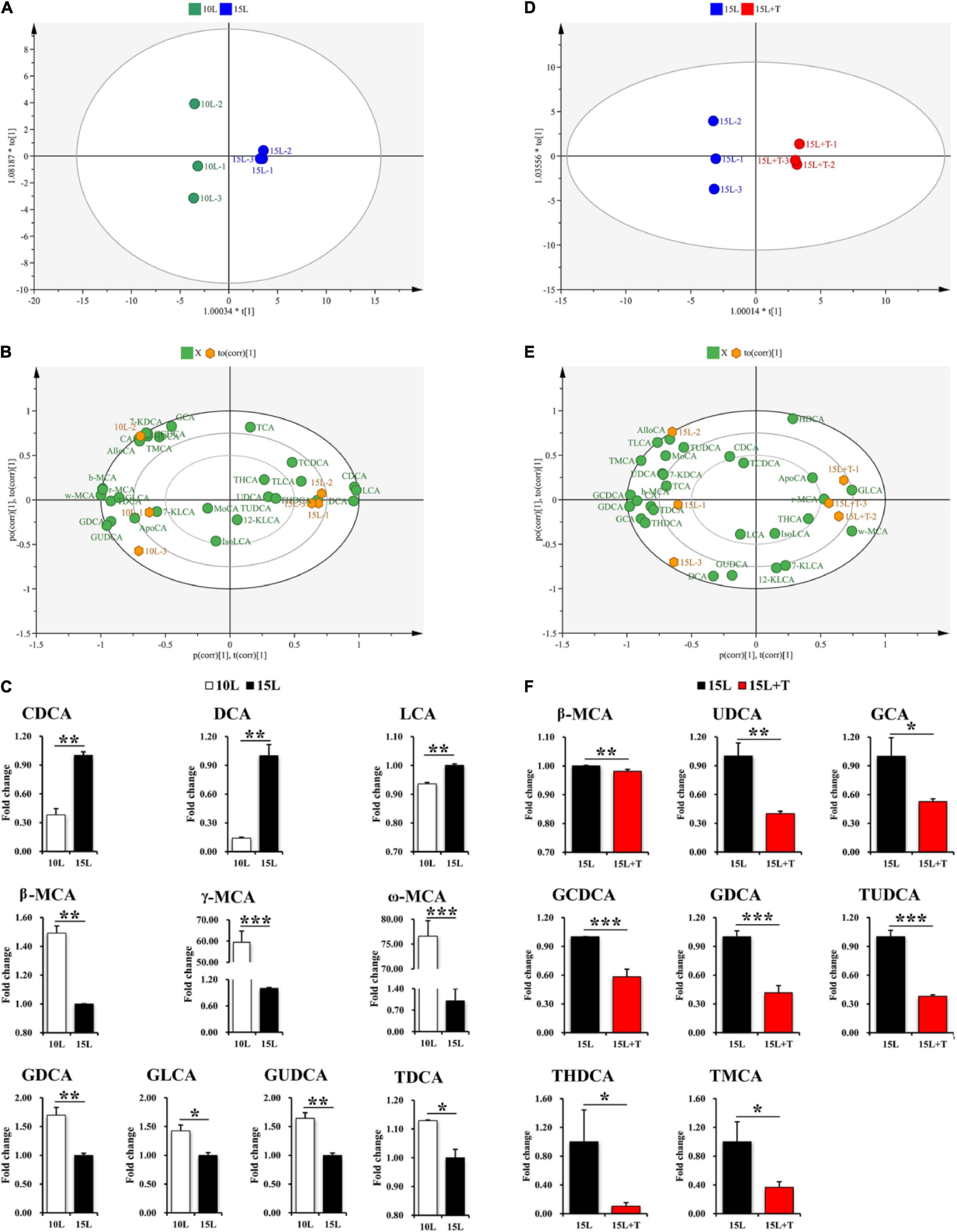
Figure 3. Orthogonal partial least squares discriminant analysis (OPLS-DA) score plots (A,D), biplot (B,E), and significantly different bile acids (C,F) of juvenile grouper fed the experimental diets. Values are means ± SEM (n = 3). Student’s t-test was applied for comparison of diet 10L vs. diet 15L and diet 15L vs. diet 15L + T. *, **, and *** represent significant differences with P < 0.05, P < 0.01, and P < 0.001.
Plasma and Hepatic Biochemical Components
The plasma contents of TG, HDL-C, and LDL-C were not affected by dietary treatments (Figure 4). However, the plasma TC content was increased when the dietary lipid level was increased from 10 to 15%, and the value was decreased when dietary taurine was increased from 0 to 1% in the case of dietary 15% lipid level. About 1% dietary taurine significantly decreased plasma ADPN content and increased liver HMGR content at 15% lipid diet. There was no difference in plasma ADPN and liver HMGR contents between diet 10L and diet 15L. The plasma LEP content was increased by increasing dietary lipid from 10 to 15%, but not affected by dietary taurine levels.
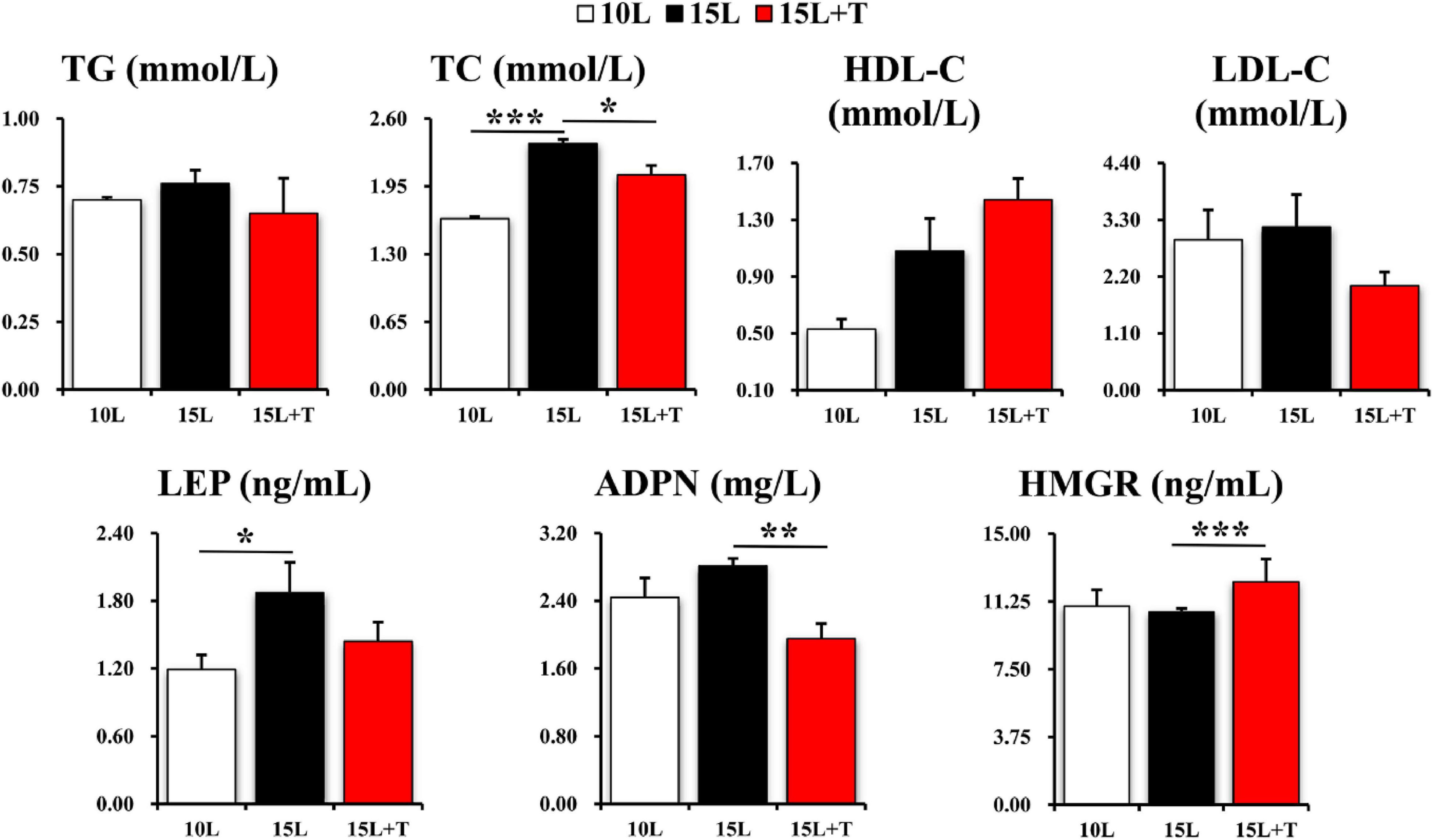
Figure 4. Plasma and liver biochemical indices in juvenile grouper fed the experimental diets. Values are means ± SEM (n = 3). Student’s t-test was applied for comparison of diet 10L vs. diet 15L and diet 15L vs. diet 15L + T. *, **, and *** represent significant differences with P < 0.05, P < 0.01, and P < 0.001.
Expression of Genes Related to Liver Lipid Metabolism
Groupers fed diet 15L showed a significantly lower expression level of acc when compared to those fed diet 10L (Figure 5). The expression levels of fas and g6pd genes were lower in groupers fed diet 15L than in those fed diet 10L, and higher than in those fed diet 15L + T. However, the pparα gene showed the opposite expression change. The dietary taurine addition in 15% lipid feed did not affect acc gene expression.
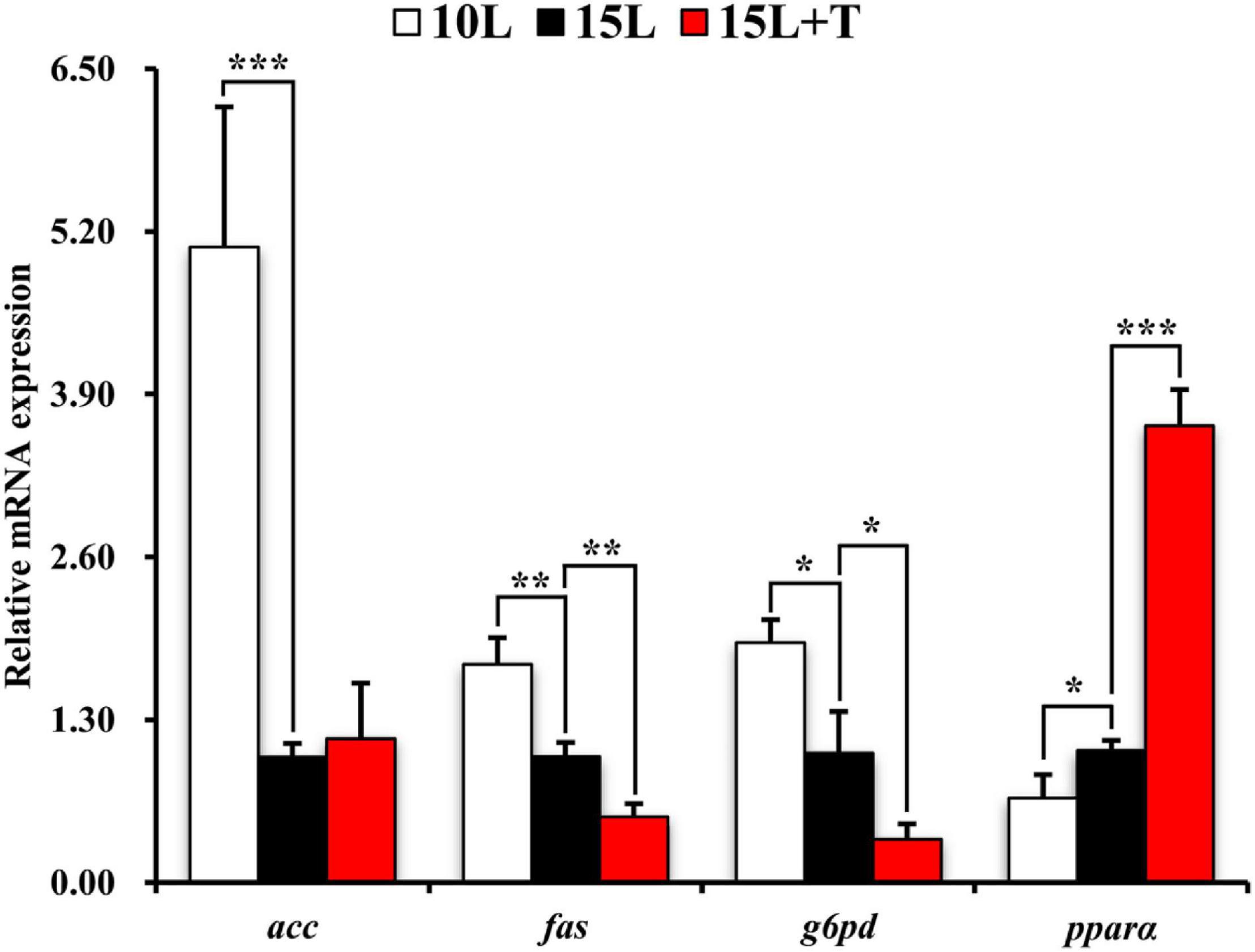
Figure 5. Expression levels of genes involved in lipid anabolism and catabolism in the liver of juvenile grouper fed the experimental diets. Values are means ± SEM (n = 3). Student’s t-test was applied for comparison of diet 10 L vs. diet 15 L and diet 15 L vs. diet 15 L + T. *, **, and *** represent significant differences with P < 0.05, P < 0.01, and P < 0.001.
Discussion
Many studies have shown that dietary taurine has a positive effect on the growth performance of fish (de Moura et al., 2018; Liu et al., 2018; Candebat et al., 2020). In this study, compared to groupers fed taurine free diet (15L), fish fed diet containing 1% taurine (diet 15L + T) showed a significantly higher growth rate, as observed in juvenile Cobia (Rachycentron canadum), European sea bass (Dicentrarchus labrax), and parrot fish (Oplegnathus fasciatus) (Lunger et al., 2007; Lim et al., 2013; Martins et al., 2018). Results in yellowtail (Seriola quinqueradiata) and red sea bream (Pagrus major) showed that dietary taurine addition improved the FI (Matsunari et al., 2005; Matsunari et al., 2008). Although FI was not affected by dietary taurine levels at 15% lipid in this study, the trend of FE in response to dietary taurine addition was similar to WG. This finding indicated that taurine promotes the growth rate of grouper via improving feed utilization. In addition, the growth rate and feed utilization were not affected by dietary lipid levels in the absence of taurine. These results disagreed with those that occurred in meager (Argyrosomus regius) and silver barb (Puntius gonionotous), i.e., high lipid diet led to a reduced growth rate (Chatzifotis et al., 2010; Nayak et al., 2018). The contradictory result may be due to different fish species and rearing environments, etc.
Previous studies showed that liver lipid content in Nile tilapia (Oreochromis niloticus) increased with increasing dietary lipid levels (He et al., 2015; Zhou et al., 2019), which was consistent with our current study results. We observed no variations in HSI and plasma TG, HDL-C, and LDL-C contents between groupers fed diet 10L and diet 15L. Plasma components could reflect the absorption, transportation, utilization, and deposition of lipids (Xu et al., 2016). Thus, maybe the grouper has a relatively high tolerance to dietary high lipid under the conditions of this experiment, which supported our result of growth performance. However, decreased HSI and liver lipid content were observed in 15% lipid feed with 1% taurine addition in comparison with 15% lipid feed without taurine addition. This finding supported previous observations on turbot (Scophthalmus maximus), Nile tilapia, and yellowfin seabream (Acanthopagrus latus) (Yun et al., 2012; Michelato et al., 2018; Dehghani et al., 2020), indicating a lipid-lowering effect of taurine in grouper. Furthermore, we also determined plasma hormones contents to find clues to the change of liver lipid content in the presence of taurine in high lipid feeds. Both ADPN and LEP are hormones secreted by adipose tissue and released into the bloodstream. LEP can reduce lipid accumulation by directly inhibiting lipid synthesis in adipocytes (de Gortari et al., 2020). ADPN can enhance the decomposition and energy consumption of fatty acids, and reduce the TG contents in the muscle and liver of large yellow croaker (Larimichthys crocea) (Ji et al., 2020). However, it was also reported that ADPN acts as the feedback signaling regulator of energy expenditure and metabolism (O’Brien et al., 2018). In the current study, groupers fed diet 15L + T showed lower plasma ADPN content than those fed diet 15L, indicating a decrease in liver lipid deposition in groupers. Meanwhile, consistent with our result, the increased plasma LEP content of mice was found to be accompanied by HSI, as a result of the high-lipid FI (Zhao et al., 2020). Dietary taurine reduced the secretion of LEP in rats and plasma LEP content in M. albus (Kim et al., 2012; Li, 2016), which was not observed in this study. However, the associations of dietary taurine with hormone secret and transport were not well-clarified so far, which needs further study.
Cholesterol is an important component of blood lipids. In this study, plasma TC content was upregulated by a 15% lipid diet without taurine addition but downregulated by a 15% lipid diet with taurine addition, as evidenced with increased liver lipid content in this study and previous studies in tiger puffer and European sea bass (Xu et al., 2020; Martins et al., 2021). Thus, taurine could reduce the risk of atherosclerosis by removing cholesterol (Gupta and Kim, 2003). We also observed an upregulation of liver HMGR content of groupers fed a 15% lipid diet with taurine in comparison with a 15% lipid diet without taurine. It was demonstrated that HMGR is the key rate-limiting enzyme that catalyzes the synthesis of a precursor of cholesterol in the regulation of cholesterol synthesis (Mahboobi et al., 2018). Our results may indicate the promotion of cholesterol biosynthesis by taurine via HMGR (Xu et al., 2020). The liver lipid content was found to have a positive association with the plasma content of TC, HMGR, LEP, and ADPN (Figure 6), indicating lipid accumulation in the liver was the result of the combined action of enzymes, hormones, and plasma lipids (Zhao et al., 2020). Furthermore, cholesterol is an integral component of cell membranes and acts as precursors of some hormones and BAs (Abramo, 1989; Suga et al., 2019), which combines with taurine to form taurine-conjugated BAs, the major BAs in most teleost fish (Kim et al., 2015). Thus, The BA profile of groupers was determined in this study.
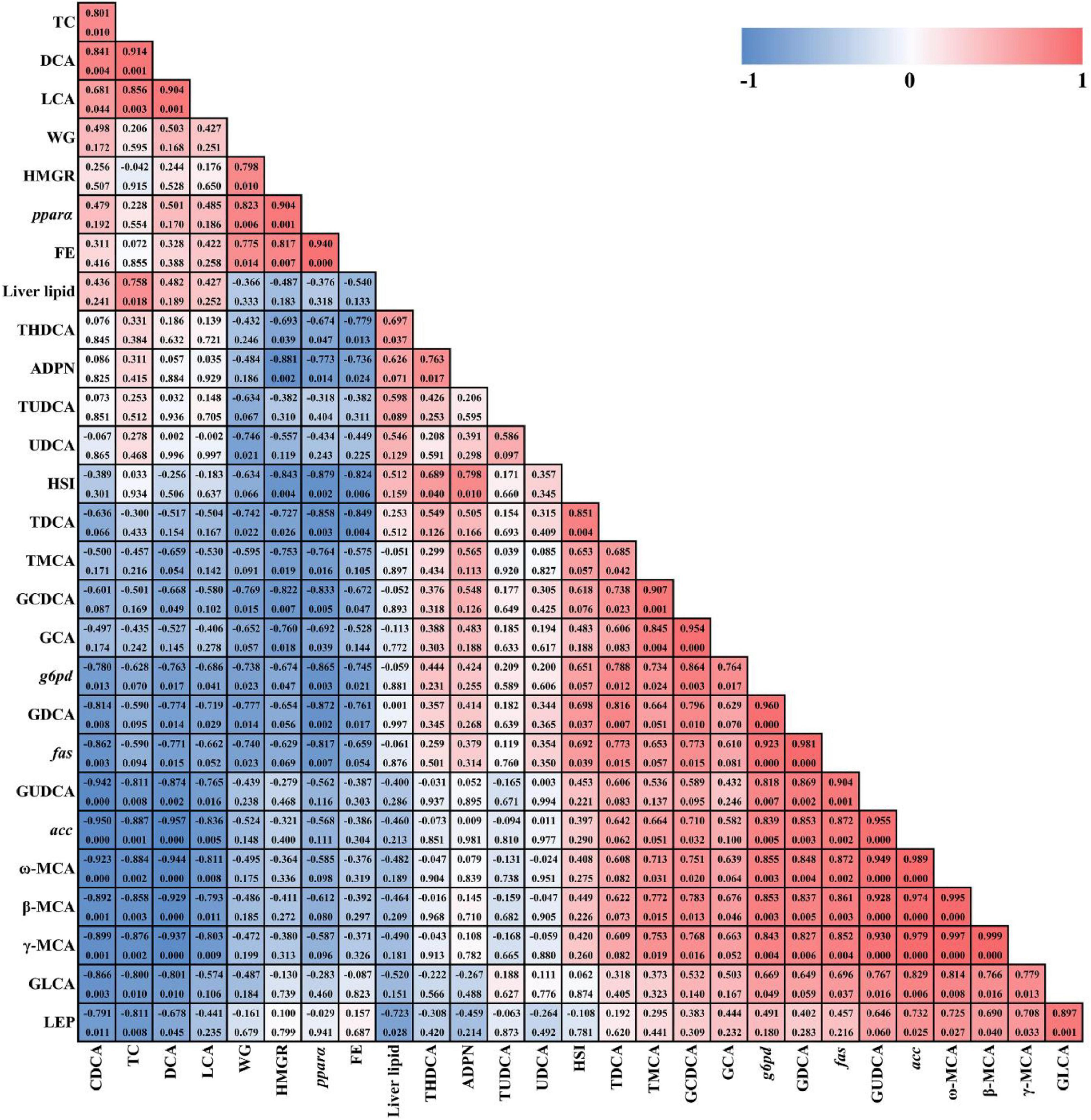
Figure 6. The Pearson’s correlation analysis of the correlation matrix of the main detection parameters. Positive correlations are shown in pink, negative correlations in blue. The numbers from top to bottom were correlation coefficients and P-value in each color block, respectively.
Bile acids (BAs), amphiphilic (hydrophilic and lipophilic) molecules, are steroids biosynthesized from cholesterol in the liver and stored in the gallbladder, and released into the intestinal lumen after food ingestion (Nguyen et al., 2018; Suga et al., 2019). BAs can effectively emulsify lipids to form small chylous particles, which increase the contact surface between lipid molecules and lipase to accelerate the hydrolysis of lipid molecules in vivo (Ding et al., 2020). The BAs synthesis and its composition vary among animal species (Chiang and Ferrell, 2020). For example, 25 and 75% of primary BAs were conjugated with taurine and glycine in humans, respectively; while 95 and 5% of primary BAs are taurine-conjugated and glycine-conjugated in mice, respectively (Hofmann et al., 2010). However, it was previously believed that almost all BAs secreted by finfish were taurine-conjugated BAs (Kim et al., 2007; Kim et al., 2015). Here, a total of 29 BAs molecules in the bile of the grouper were detected and identified. The majority of them were taurine-conjugated BAs, but a small part of BAs are glycine-conjugated which was consistent with previous studies in other fish species (Garcia-Organista et al., 2019; Xu et al., 2020). It was reported that taurine-conjugated BAs have a high ability to remove cholesterol by enhancing serum HDL and suppressing LDL and very low-density lipoprotein concentration (Gupta et al., 2009). In this study, the three taurine-conjugated BAs such as TCA, TCDCA, and TDCA were the main components of BAs, and the contents of TDCA, GDCA, GLCA, and GUDCA were downregulated by the dietary lipid increasing from 10 to 15%, indicating an inhibiting of cholesterol metabolism, which agreed with plasma TC content discussed before. Interestingly, we also observed downregulation of GDCA, GCA, and GCDCA contents by dietary taurine addition at 15% lipid, which may indicate the higher competition of taurine combining with BAs than glycine in grouper and mice (Hofmann et al., 2010). In a study with Swiss female mice, CA promotes cholesterol absorption, but CDCA does not (Reynier et al., 1981). In our current study, CA content was not affected by dietary lipid level, but CDCA content was promoted by high lipid feed. Similar results were also observed in a recent study (Lu et al., 2018). The findings indicated that cholesterol absorption was limited. Moreover, CA and CDCA are efficacious endogenous ligands, whereas UDCA, MCA, and TMCA are antagonists of farnesoid X receptor (FXR) (Sayin et al., 2013; Su et al., 2019), which could inhibit TG synthesis by inducing small heterodimer partner in mice and grass carp (Ctenopharyngodon idella) (Li et al., 2013; Tian et al., 2021). In this study, β-MCA, γ- and ω-MCA contents were lower in groupers fed diet 15L than that in those fed diet 10L. Moreover, UDCA, β-MCA, and TMCA contents continued to decline when taurine content was increased from 0 to 1% in a 15% lipid diet. This means the activation of FXR via reducing their antagonists could be triggered by taurine too. Notably, compared to taurine free diet at a 15% lipid level, taurine supplementation reduced the contents of THDCA and TUDCA. A previous study in humans demonstrated that the ratio of taurine to glycine-conjugated BAs is about 3:1 and this could be reduced by low taurine supply (Haslewood, 1978). Our results suggested that taurine addition at a 15% lipid diet could promote the taurine-conjugated BAs forming and play more important roles in lipid-lowering effects. Study results showed that most BAs through re-absorption in the intestinal lumen was routed to the liver where they were recycled to the gallbladder (Dawson, 2018). However, the re-absorption of BAs in the intestinal lumen was inhibited by dietary taurine in rats (Chen et al., 2003; Lam et al., 2006; Nishimura et al., 2009). This finding further supported the reduction of BAs caused by dietary taurine addition in our current study. Thus, taking liver lipid content and BAs profile into consideration (Figure 6), we speculate that taurine-conjugated BAs have a stronger ability to emulsify and absorb lipids than glycine-conjugated and other BAs in the fish species, which might be one of the reasons for the growth improvement. Interestingly, DCA and LCA, are hydrophobic cholic acids that have strong toxicity to colonic cells, they induce cell death involving either death receptor or mitochondrial (Rodrigues et al., 2004; Nguyen et al., 2018). The DCA and LCA contents were higher in groupers fed diet 15L than that in those fed diet 10L in this study and were found a positive correlation with liver lipid content (Figure 6), indicating the negative effect of high dietary lipid intake on the lipid metabolism of fish.
The mRNA expression levels of several genes related to both lipid metabolism and lipid accumulation to elucidate the molecular mechanisms underlying the taurine-mediated lipid-lowering effect on groupers. Acyl-CoA carboxylase is a cytosolic enzyme-producing malonyl-CoA, the first step in the biosynthesis of long-chain fatty acids (Qian et al., 2015). G6PD is a key enzyme related to the production of NADPH, and essential for de novo fatty acid biosynthesis catalyzed by FAS (Chen et al., 2013; Zheng et al., 2013). PPARα can modulate the expression of genes encoding several mitochondrial fatty acid-catabolizing enzymes in addition to mediating inducible mitochondrial and peroxisomal fatty acid β-oxidation (Aoyama et al., 1998). In this study, diet 15L upregulated the expression of the pparα gene but downregulated the expression of acc, fas, and 6pgd genes compared with diet 10L. The findings were consistent with previous results in blunt snout bream (Megalobrama amblycephala), hybrid grouper (Epinephelus lanceolatus♀ × Epinephelus fuscoguttatus♂), and large yellow croaker (Yan et al., 2015; Cao et al., 2019; Tan et al., 2019). Moreover, the expression levels of fas and 6pgd genes were decreased, and the expression level of the pparα gene was promoted with the dietary taurine increase. This indicated that liver lipid deposition reduction caused by dietary taurine addition may be the result of lipogenesis inhibition and fatty acid β-oxidation acceleration.
Conclusion
Under the present experimental conditions, the growth performance of the grouper was not affected by dietary lipid level increased from 10 to 15%, but significantly increased by dietary taurine addition at 15% lipid. High lipid diet increased the liver lipid content and decreased the contents of glycine-conjugated BAs and TDCA. Dietary taurine supplementation in a 15% lipid diet could decrease the lipid deposition in the liver of groupers through forming more taurine-conjugated BAs and triggering the activation of FXR, and inhibiting the expression of genes involved in lipid anabolism and accelerating fatty acid β-oxidation (Figure 6). Taurine-conjugated BAs have a higher ability to accelerate lipids emulsification and absorption than glycine-conjugated and other BAs in the fish species.
Data Availability Statement
The datasets presented in this study can be found in online repositories. The names of the repository/repositories and accession number(s) can be found in the article/Supplementary Material.
Ethics Statement
The animal study was reviewed and approved by Animal Care and Use Committee of Fujian Agriculture and Forestry University.
Author Contributions
XW: conceptualization, data curation, formal analysis, investigation, methodology, project administration, validation, visualization, and writing-original draft. FB: data curation, formal analysis, investigation, validation, and methodology. XN: investigation and data curation. YS: supervision and writing-review. JY: conceptualization, formal analysis, funding acquisition, resources, supervision, and writing-review. All authors contributed to the article and approved the submitted version.
Funding
This study was supported by funding from the National Natural Science Foundation of China (Grant Nos. 31772861 and 31372546) and the Science and Technology Project of Fujian Province of China (Grant No. 2020N0012).
Conflict of Interest
The authors declare that the research was conducted in the absence of any commercial or financial relationships that could be construed as a potential conflict of interest.
Publisher’s Note
All claims expressed in this article are solely those of the authors and do not necessarily represent those of their affiliated organizations, or those of the publisher, the editors and the reviewers. Any product that may be evaluated in this article, or claim that may be made by its manufacturer, is not guaranteed or endorsed by the publisher.
Supplementary Material
The Supplementary Material for this article can be found online at: https://www.frontiersin.org/articles/10.3389/fmars.2022.859428/full#supplementary-material
References
Abramo, D. (1989). “Lipid requirements of shrimp,” in Advances in Tropical Aquaculture, Workshop at Tahiti, French Polynesia (Nantes: IFREMER).
AOAC (2006). Official Methods of Analysis, 15th Edn. Washington: Association of Official Analytical Chemists.
Aoyama, T., Peters, J. M., Iritani, N., Nakajima, T., Furihata, K., Hashimoto, T., et al. (1998). Altered constitutive expression of fatty acid-metabolizing enzymes in mice lacking the peroxisome proliferator-activated receptor alpha (PPARalpha). J. Biol. Chem. 273, 5678–5684. doi: 10.1074/jbc.273.10.5678
Bai, F., Niu, X., Wang, X., and Ye, J. (2021a). Growth performance, biochemical composition and expression of lipid metabolism related genes in groupers (Epinephelus coioides) are altered by dietary taurine. Aquacult. Nutr. 27, 2690–2702. doi: 10.1111/anu.13395
Bai, F., Wang, X., Niu, X., Shen, G., and Ye, J. (2021b). Lipidomic profiling reveals the reducing lipid accumulation effect of dietary taurine in groupers (Epinephelus coioides). Front. Mol. Biosci. 8:814318. doi: 10.3389/fmolb.2021.814318
Brons, C., Spohr, C., Storgaard, H., Dyerberg, J., and Vaag, A. (2004). Effect of taurine treatment on insulin secretion and action, and on serum lipid levels in overweight men with a genetic predisposition for type II diabetes mellitus. Eur. J. Clin. Nutr. 58, 1239–1247. doi: 10.1038/sj.ejcn.1601955
Candebat, C. L., Booth, M., Codabaccus, M. B., and Pirozzi, I. (2020). Dietary methionine spares the requirement for taurine in juvenile Yellowtail Kingfish (Seriola lalandi). Aquaculture 522:735090. doi: 10.1016/j.aquaculture.2020.735090
Cao, X. F., Liu, W. B., Zheng, X. C., Yuan, X. Y., Wang, C. C., and Jiang, G. Z. (2019). Effects of high-fat diets on growth performance, endoplasmic reticulum stress and mitochondrial damage in blunt snout bream Megalobrama amblycephala. Aquacult. Nutr. 25, 97–109. doi: 10.1111/anu.12834
Chatzifotis, S., Panagiotidou, M., Papaioannou, N., Pavlidis, M., Nengas, I., and Mylonas, C. C. (2010). Effect of dietary lipid levels on growth, feed utilization, body composition and serum metabolites of meagre (Argyrosomus regius) juveniles. Aquaculture 307, 65–70. doi: 10.1016/j.aquaculture.2010.07.002
Chen, Q.-L., Luo, Z., Liu, X., Song, Y.-F., Liu, C.-X., Zheng, J.-L., et al. (2013). Effects of waterborne chronic copper exposure on hepatic lipid metabolism and metal-element composition in Synechogobius hasta. Arch. Environ. Contam. Toxicol. 64, 301–315. doi: 10.1007/s00244-012-9835-7
Chen, W., Nishimura, N., Oda, H., and Yokogoshi, H. (2003). Effect of taurine on cholesterol degradation and bile acid pool in rats fed a high-cholesterol diet. Adv. Exp. Med. Biol. 526, 261–267. doi: 10.1007/978-1-4615-0077-3_33
Chiang, J. Y., and Ferrell, J. M. (2020). Up to date on cholesterol 7 alpha-hydroxylase (CYP7A1) in bile acid synthesis. Liver Res. 4, 47–63. doi: 10.1016/j.livres.2020.05.001
Dai, Y.-J., Cao, X.-F., Zhang, D.-D., Li, X.-F., Liu, W.-B., and Jiang, G.-Z. (2019). Chronic inflammation is a key to inducing liver injury in blunt snout bream (Megalobrama amblycephala) fed with high-fat diet. Dev. Comp. Immunol. Microbiol. Infect Dis. 97, 28–37. doi: 10.1016/j.dci.2019.03.009
Dai, Y.-J., Jiang, G.-Z., Yuan, X.-Y., and Liu, W.-B. (2018). High-fat-diet-induced inflammation depresses the appetite of blunt snout bream (Megalobrama amblycephala) through the transcriptional regulation of leptin/mammalian target of rapamycin. Br. J. Nutr. 120, 1422–1431. doi: 10.1017/s000711451800288x
Dawson, P. A. (2018). “Bile formation and the enterohepatic circulation,” in Hysiology of the Gastrointestinal Tract, 6th ed., ed. H. M. Said (Cambridge: Academic Press), 931–956. doi: 10.1016/b978-0-12-809954-4.00041-4
de Gortari, P., Alcántara-Alonso, V., Matamoros-Trejo, G., Amaya, M., and Alvarez-Salas, E. (2020). Differential effects of leptin administration on feeding and HPT axis function in early-life overfed adult rats. Peptides 127:170285. doi: 10.1016/j.peptides.2020.170285
de Moura, L. B., Diógenes, A. F., Campelo, D. A., de Almeida, F. L., Pousão-Ferreira, P. M., Furuya, W. M., et al. (2018). Taurine and methionine supplementation as a nutritional strategy for growth promotion of meagre (Argyrosomus regius) fed high plant protein diets. Aquaculture 497, 389–395. doi: 10.1016/j.aquaculture.2018.07.038
Dehghani, R., Oujifard, A., Mozanzadeh, M. T., Morshedi, V., and Bagheri, D. (2020). Effects of dietary taurine on growth performance, antioxidant status, digestive enzymes activities and skin mucosal immune responses in yellowfin seabream, Acanthopagrus latus. Aquaculture 517:734795. doi: 10.1016/j.aquaculture.2019.734795
Ding, T., Xu, N., Liu, Y., Du, J., Xiang, X., Xu, D., et al. (2020). Effect of dietary bile acid (BA) on the growth performance, body composition, antioxidant responses and expression of lipid metabolism-related genes of juvenile large yellow croaker (Larimichthys crocea) fed high-lipid diets. Aquaculture 518:734768. doi: 10.1016/j.aquaculture.2019.734768
Du, Z. Y., Liu, Y. J., Tian, L. X., Wang, J. T., Wang, Y., and Liang, G. Y. (2005). Effect of dietary lipid level on growth, feed utilization and body composition by juvenile grass carp (Ctenopharyngodon idella). Aquacult. Nutr. 11, 139–146. doi: 10.1111/j.1365-2095.2004.00333.x
Feng, H., Yi, K., Qian, X., Niu, X., Sun, Y., and Ye, J. (2020). Growth and metabolic responses of juvenile grouper (Epinephelus coioides) to dietary methionine/cystine ratio at constant sulfur amino acid levels. Aquaculture 518:734869. doi: 10.1016/j.aquaculture.2019.734869
Folch, J., Lees, M., and Stanley, G. S. (1957). A simple method for the isolation and purification of total lipides from animal tissues. J. Biol. Chem. 226, 497–509. doi: 10.1016/s0021-9258(18)64849-5
Garcia-Organista, A. A., Mata-Sotres, J. A., Viana, M. T., and Rombenso, A. N. (2019). The effects of high dietary methionine and taurine are not equal in terms of growth and lipid metabolism of juvenile California Yellowtail (Seriola dorsalis). Aquaculture 512:734304. doi: 10.1016/j.aquaculture.2019.734304
Gupta, R. C., and Kim, S. J. (2003). Role of taurine in organs’ dysfunction and in their alleviation. Crit. Care Shock 6, 191–197.
Gupta, R. C., D’Archivio, M., and Masella, R. (2009). “Taurine as drug and functional food component,” in Glutathione and Sulfur Amino Acids in Human Health and Disease, eds R. Masella and G. Mazza (Hoboken: John Willey and Sons), 543–579.
Hanaki, K.-I., Ike, F., Kajita, A., Yasuno, W., Yanagiba, M., Goto, M., et al. (2014). A broadly reactive one-step SYBR Green I real-time RT-PCR assay for rapid detection of murine norovirus. PLoS One 9:e98108. doi: 10.1371/journal.pone.0098108
Haslewood, G. A. D. (1978). The Biological Importance of Bile Salts. New York: North-Holland Publishing Company.
He, A. Y., Ning, L. J., Chen, L. Q., Chen, Y. L., Xing, Q., Li, J. M., et al. (2015). Systemic adaptation of lipid metabolism in response to low-and high-fat diet in Nile tilapia (Oreochromis niloticus). Physiol. Rep. 3:e12485. doi: 10.14814/phy2.12485
Hofmann, A. F., Hagey, L. R., and Krasowski, M. D. (2010). Bile salts of vertebrates: structural variation and possible evolutionary significance. J. Lipid Res. 51, 226–246. doi: 10.1194/jlr.r000042
Hu, Y., Yang, G., Li, Z., Hu, Y., Zhong, L., Zhou, Q., et al. (2018). Effect of dietary taurine supplementation on growth, digestive enzyme, immunity and resistant to dry stress of rice field eel (Monopterus albus) fed low fish meal diets. Aquacult. Res. 49, 2108–2118. doi: 10.1111/are.13665
Huxtable, R. (1992). Physiological actions of taurine. Physiol. Rev. 72, 101–163. doi: 10.1152/physrev.1992.72.1.101
Ji, R., Xu, X., Xiang, X., Zhu, S., Li, Y., Mai, K., et al. (2020). Regulation of adiponectin on lipid metabolism in large yellow croaker (Larimichthys crocea). Biochim. Biophys. Acta Mol. Cell Biol. Lipids 1865:158711. doi: 10.1016/j.bbalip.2020.158711
Kim, K. S., Oh, D. H., Kim, J. Y., Lee, B. G., You, J. S., Chang, K. J., et al. (2012). Taurine ameliorates hyperglycemia and dyslipidemia by reducing insulin resistance and leptin level in Otsuka Long-Evans Tokushima fatty (OLETF) rats with long-term diabetes. Exp. Mol. Med. Rep. 44, 665–673. doi: 10.3858/emm.2012.44.11.075
Kim, S. K., Kim, K. G., Kim, K. D., Kim, K. W., Son, M. H., Rust, M., et al. (2015). Effect of dietary taurine levels on the conjugated bile acid composition and growth of juvenile Korean rockfish Sebastes schlegeli (Hilgendorf). Aquacult. Res. 46, 2768–2775. doi: 10.1111/are.12431
Kim, S.-K., Matsunari, H., Takeuchi, T., Yokoyama, M., Murata, Y., and Ishihara, K. (2007). Effect of different dietary taurine levels on the conjugated bile acid composition and growth performance of juvenile and fingerling Japanese flounder Paralichthys olivaceus. Aquaculture 273, 595–601. doi: 10.1016/j.aquaculture.2007.10.031
Lam, N. V., Chen, W., Suruga, K., Nishimura, N., Goda, T., Oda, H., et al. (2006). Effects of taurine on mRNA levels of nuclear receptors and factors involved in cholesterol and bile acid homeostasis in mice. Adv. Exp. Med. Biol. 583, 193–202. doi: 10.1007/978-0-387-33504-9_20
Li, G., Kong, B., Zhu, Y., Zhan, L., Williams, J. A., Tawfik, O., et al. (2013). Small heterodimer partner overexpression partially protects against liver tumor development in farnesoid X receptor knockout mice. Toxicol. Appl. Pharmacol. 272, 299–305. doi: 10.1016/j.taap.2013.06.016
Li, M., Lai, H., Li, Q., Gong, S., and Wang, R. (2016). Effects of dietary taurine on growth, immunity and hyperammonemia in juvenile yellow catfish Pelteobagrus fulvidraco fed all-plant protein diets. Aquaculture 450, 349–355. doi: 10.1016/j.aquaculture.2015.08.013
Li, X., Jiang, Y., Liu, W., and Ge, X. (2012). Protein-sparing effect of dietary lipid in practical diets for blunt snout bream (Megalobrama amblycephala) fingerlings: effects on digestive and metabolic responses. Fish Physiol. Biochem. 38, 529–541. doi: 10.1007/s10695-011-9533-9
Li, Z. (2016). Effect of Taurine on Growth, Immune and Metabolism in Rice Filed Eel (Monopterus albus) Fed Low Fish Meal. Hunan: Hunan Agricultural University.
Lim, S.-J., Oh, D.-H., Khosravi, S., Cha, J.-H., Park, S.-H., Kim, K.-W., et al. (2013). Taurine is an essential nutrient for juvenile parrot fish Oplegnathus fasciatus. Aquaculture 414, 274–279. doi: 10.1016/j.aquaculture.2013.08.013
Liu, Y., Yang, P., Hu, H., Li, Y., Dai, J., Zhang, Y., et al. (2018). The tolerance and safety assessment of taurine as additive in a marine carnivorous fish, Scophthalmus maximus L. Aquacult. Nutr. 24, 461–471. doi: 10.1111/anu.12579
Livak, K. J., and Schmittgen, T. D. (2001). Analysis of relative gene expression data using real-time quantitative PCR and the 2–ΔΔCT method. Methods 25, 402–408. doi: 10.1006/meth.2001.1262
Lu, Y., Du, Y., Qin, L., Wu, D., Wang, W., Ling, L., et al. (2018). Gypenosides altered hepatic bile acids homeostasis in mice treated with high fat diet. Evid. Based Complementary Altern. Med. 2018, 1–10. doi: 10.1155/2018/8098059
Lunger, A. N., McLean, E., Gaylord, T., Kuhn, D., and Craig, S. (2007). Taurine supplementation to alternative dietary proteins used in fish meal replacement enhances growth of juvenile cobia (Rachycentron canadum). Aquaculture 271, 401–410. doi: 10.1016/j.aquaculture.2007.07.006
Luo, Z., Liu, Y.-J., Mai, K.-S., Tian, L.-X., Liu, D.-H., Tan, X.-Y., et al. (2005). Effect of dietary lipid level on growth performance, feed utilization and body composition of grouper Epinephelus coioides juveniles fed isonitrogenous diets in floating netcages. Aquacult. Int. 13, 257–269. doi: 10.1007/s10499-004-2478-6
Ma, P. (1996). Influence of lipid diet on fat accumulation in gut of juvenile red-spotted grouper. J. Oceanog. Taiwan Str. 15, 55–57.
Mahboobi, S., Rahimi, F., and Jafarnejad, S. (2018). Effects of prebiotic and synbiotic supplementation on glycaemia and lipid profile in type 2 diabetes: a meta-analysis of randomized controlled trials. Adv. Pharm. Bull. 8, 565–574. doi: 10.15171/apb.2018.065
Martins, N., Diogenes, A. F., Magalhães, R., Matas, I., Oliva-Teles, A., and Peres, H. (2021). Dietary taurine supplementation affects lipid metabolism and improves the oxidative status of European seabass (Dicentrarchus labrax) juveniles. Aquaculture 531:735820. doi: 10.1016/j.aquaculture.2020.735820
Martins, N., Estevão-Rodrigues, T., Diógenes, A., Diaz-Rosales, P., Oliva-Teles, A., and Peres, H. (2018). Taurine requirement for growth and nitrogen accretion of European sea bass (Dicentrarchus labrax, L.) juveniles. Aquaculture 494, 19–25. doi: 10.1016/j.aquaculture.2018.05.007
Matsunari, H., Furuita, H., Yamamoto, T., Kim, S.-K., Sakakura, Y., and Takeuchi, T. (2008). Effect of dietary taurine and cystine on growth performance of juvenile red sea bream Pagrus major. Aquaculture 274, 142–147. doi: 10.1016/j.aquaculture.2007.11.002
Matsunari, H., Takeuchi, T., Takahashi, M., and Mushiake, K. (2005). Effect of dietary taurine supplementation on growth performance of yellowtail juveniles Seriola quinqueradiata. Fish. Sci. 71, 1131–1135. doi: 10.1111/j.1444-2906.2005.01072.x
Michelato, M., Furuya, W. M., and Gatlin, D. M. III. (2018). Metabolic responses of Nile tilapia Oreochromis niloticus to methionine and taurine supplementation. Aquaculture 485, 66–72. doi: 10.1016/j.aquaculture.2017.11.003
Nath, S., Matozzo, V., Bhandari, D., and Faggio, C. (2019). Growth and liver histology of Channa punctatus exposed to a common biofertilizer. Nat. Prod. Res. 33, 1591–1598. doi: 10.1080/14786419.2018.1428586
Nayak, M., Saha, A., Pradhan, A., Samanta, M., Mohanty, T. K., and Giri, S. S. (2018). Influence of dietary lipid levels on growth, nutrient utilization, tissue fatty acid composition and desaturase gene expression in silver barb (Puntius gonionotous) fingerlings. Comp. Biochem. Physiol. B Biochem. Mol. Biol. Evol. 226, 18–25. doi: 10.1016/j.cbpb.2018.08.005
Nguyen, T. T., Ung, T. T., Kim, N. H., and Do Jung, Y. (2018). Role of bile acids in colon carcinogenesis. World J. Clin. Cases 6, 577–588. doi: 10.12998/wjcc.v6.i13.577
Nishimura, N., Yamamoto, T., and Ota, T. (2009). Taurine feeding inhibits bile acid absorption from the ileum in rats fed a high cholesterol and high fat diet. Adv. Exp. Med. Biol. 643, 285–291. doi: 10.1007/978-0-387-75681-3_29
O’Brien, L. C., Graham, Z. A., Chen, Q., Lesnefsky, E. J., Cardozo, C., and Gorgey, A. S. (2018). Plasma adiponectin levels are correlated with body composition, metabolic profiles, and mitochondrial markers in individuals with chronic spinal cord injury. Spinal Cord 56, 863–872. doi: 10.1038/s41393-018-0089-8
Pfaffl, M. W., Tichopad, A., Prgomet, C., and Neuvians, T. P. (2004). Determination of stable housekeeping genes, differentially regulated target genes and sample integrity: bestKeeper–Excel-based tool using pair-wise correlations. Biotechnol. Lett. 26, 509–515. doi: 10.1023/b:bile.0000019559.84305.47
Qi, G., Ai, Q., Mai, K., Xu, W., Liufu, Z., Yun, B., et al. (2012). Effects of dietary taurine supplementation to a casein-based diet on growth performance and taurine distribution in two sizes of juvenile turbot (Scophthalmus maximus L.). Aquaculture 358, 122–128. doi: 10.1016/j.aquaculture.2012.06.018
Qian, Y., Li, X. F., Zhang, D. D., Cai, D. S., Tian, H. Y., Liu, W. B., et al. (2015). Effects of dietary pantothenic acid on growth, intestinal function, anti-oxidative status and fatty acids synthesis of juvenile blunt snout bream Megalobrama amblycephala. PLoS One 10:e0119518. doi: 10.1371/journal.pone.0119518
Reynier, M. O., Montet, J. C., Gerolami, A., Marteau, C., Crotte, C., Montet, A. M., et al. (1981). Comparative effects of cholic, chenodeoxycholic, and ursodeoxycholic acids on micellar solubilization and intestinal absorption of cholesterol. J. Lipid Res. 22, 467–473. doi: 10.1016/s0022-2275(20)34961-0
Rodrigues, C. M., Castro, R. E., and Steer, C. J. (2004). The role of bile acids in the modulation of apoptosis. Prin. Med. Biol. 15, 119–145. doi: 10.1016/S1569-2582(04)15005-8
Romano, N., Kumar, V., Yang, G., Kajbaf, K., Rubio, M. B., Overturf, K., et al. (2020). Bile acid metabolism in fish: disturbances caused by fishmeal alternatives and some mitigating effects from dietary bile inclusions. Rev. Aquac. 12, 1792–1817. doi: 10.1111/raq.12410
Salze, G. P., and Davis, D. A. (2015). Taurine: a critical nutrient for future fish feeds. Aquaculture 437, 215–229. doi: 10.1016/j.aquaculture.2014.12.006
Sayin, S. I., Wahlström, A., Felin, J., Jäntti, S., Marschall, H.-U., Bamberg, K., et al. (2013). Gut microbiota regulates bile acid metabolism by reducing the levels of tauro-beta-muricholic acid, a naturally occurring FXR antagonist. Cell Metab. 17, 225–235. doi: 10.1016/j.cmet.2013.01.003
Shapawi, R., Abdullah, F. C., Senoo, S., and Mustafa, S. (2019). Nutrition, growth and resilience of tiger grouper (Epinephelus fuscoguttatus)× giant Grouper (Epinephelus lanceolatus) hybrid-a review. Rev. Aquac. 11, 1285–1296. doi: 10.1111/raq.12292
Su, G., Sun, H., Wu, G., and Hu, J. (2019). Enterohepatic circulation of bile acids and regulation roles of bile acid receptors in glycolipid metabolism. Chin. J. Anim. Nutr. 31, 3511–3521. doi: 10.1126/scitranslmed.aaf4823
Suga, T., Yamaguchi, H., Ogura, J., Shoji, S., Maekawa, M., and Mano, N. (2019). Altered bile acid composition and disposition in a mouse model of non-alcoholic steatohepatitis. Toxicol. Appl. Pharmacol. 379:114664. doi: 10.1016/j.taap.2019.114664
Takagi, S., Murata, H., Goto, T., Endo, M., Yamashita, H., and Ukawa, M. (2008). Taurine is an essential nutrient for yellowtail Seriola quinqueradiata fed non-fish meal diets based on soy protein concentrate. Aquaculture 280, 198–205. doi: 10.1016/j.aquaculture.2008.05.012
Tan, X., Sun, Z., and Ye, C. (2019). Dietary Lycium barbarum extract administration improved growth, meat quality and lipid metabolism in hybrid grouper (Epinephelus lanceolatus♂ × E. fuscoguttatus♀) fed high lipid diets. Aquaculture 504, 190–198. doi: 10.1016/j.aquaculture.2019.01.044
Tian, J.-J., Jin, Y.-Q., Yu, E.-M., Sun, J.-H., Xia, Y., Zhang, K., et al. (2021). Farnesoid X receptor is an effective target for modulating lipid accumulation in grass carp, Ctenopharyngodon idella. Aquaculture 534:736248. doi: 10.1016/j.aquaculture.2020.736248
Tsuboyama-Kasaoka, N., Shozawa, C., Sano, K., Kamei, Y., Kasaoka, S., Hosokawa, Y., et al. (2006). Taurine (2-aminoethanesulfonic acid) deficiency creates a vicious circle promoting obesity. Endocrinology 147, 3276–3284. doi: 10.1210/en.2005-1007
Tuan, L. A., and Williams, K. C. (2007). Optimum dietary protein and lipid specifications for juvenile Malabar grouper (Epinephelus malabaricus). Aquaculture 2007, 129–138. doi: 10.1016/j.aquaculture.2007.03.007
Wu, G. (2020). Important roles of dietary taurine, creatine, carnosine, anserine and 4-hydroxyproline in human nutrition and health. Amino Acids 52, 329–360. doi: 10.1007/s00726-020-02823-6
Xu, C., Li, X., Tian, H., Zhou, M., Cai, W., and Liu, W. (2016). Effects of feeding rates on plasma lipid profiles and the mRNA expressions of genes related to lipid metabolism in juvenile blunt snout bream (Megalobrama amblycephala). J. Fish. China 40, 1732–1741.
Xu, H., Zhang, Q., Kim, S.-K., Liao, Z., Wei, Y., Sun, B., et al. (2020). Dietary taurine stimulates the hepatic biosynthesis of both bile acids and cholesterol in the marine teleost, tiger puffer (Takifugu rubripes). Br. J. Nutr. 123, 1345–1356. doi: 10.1017/S0007114520000161
Yan, J., Liao, K., Wang, T., Mai, K., Xu, W., and Ai, Q. (2015). Dietary lipid levels influence lipid deposition in the liver of large yellow croaker (Larimichthys crocea) by regulating lipoprotein receptors, fatty acid uptake and triacylglycerol synthesis and catabolism at the transcriptional level. PLoS One 10:e0129937. doi: 10.1371/journal.pone.0129937
Yoshii, K., Takakuwa, F., Nguyen, H. P., Masumoto, T., and Fukada, H. (2010). Effect of dietary lipid level on growth performance and feed utilization of juvenile kelp grouper Epinephelus bruneus. Fish. Sci. 76, 139–145. doi: 10.1007/s12562-009-0191-0
Yun, B., Ai, Q., Mai, K., Xu, W., Qi, G., and Luo, Y. (2012). Synergistic effects of dietary cholesterol and taurine on growth performance and cholesterol metabolism in juvenile turbot (Scophthalmus maximus L.) fed high plant protein diets. Aquaculture 324, 85–91. doi: 10.1016/j.aquaculture.2011.10.012
Zhang, M., Bi, L. F., Fang, J. H., Su, X. L., Da, G. L., Kuwamori, T., et al. (2004). Beneficial effects of taurine on serum lipids in overweight or obese non-diabetic subjects. Amino Acids 26, 267–271. doi: 10.1007/s00726-003-0059-z
Zhao, D. P., Hou, Y., Yue, N., Liu, J. L., Gao, M. W., Zhang, J. J., et al. (2020). Antiobesity function of the chitosan compounds on fatty rats established by high fat diet. Sci. Technol. Food Ind. 41, 322–325.
Zheng, J.-L., Luo, Z., Zhu, Q.-L., Tan, X.-Y., Chen, Q.-L., Sun, L.-D., et al. (2013). Molecular cloning and expression pattern of 11 genes involved in lipid metabolism in yellow catfish Pelteobagrus fulvidraco. Gene 531, 53–63. doi: 10.1016/j.gene.2013.08.028
Zhou, L., Hu, J., and Chen, X. (1995). The most suitable content of fat in the artificial diets to culture Epinephelus awoara. J. Xiamen Fish. Coll. 17, 13–16.
Keywords: taurine, high lipid diet, bile acid profile, lipid metabolism, liver lipid deposition, Epinephelus coioides
Citation: Wang X, Bai F, Niu X, Sun Y and Ye J (2022) The Lipid-Lowering Effect of Dietary Taurine in Orange-Spotted Groupers (Epinephelus coioides) Involves Both Bile Acids and Lipid Metabolism. Front. Mar. Sci. 9:859428. doi: 10.3389/fmars.2022.859428
Received: 21 January 2022; Accepted: 10 February 2022;
Published: 09 March 2022.
Edited by:
Samad Rahimnejad, University of South Bohemia in České Budějovice, CzechiaReviewed by:
Shiwei Xie, Guangdong Ocean University, ChinaHouguo Xu, Chinese Academy of Fishery Sciences (CAFS), China
Copyright © 2022 Wang, Bai, Niu, Sun and Ye. This is an open-access article distributed under the terms of the Creative Commons Attribution License (CC BY). The use, distribution or reproduction in other forums is permitted, provided the original author(s) and the copyright owner(s) are credited and that the original publication in this journal is cited, in accordance with accepted academic practice. No use, distribution or reproduction is permitted which does not comply with these terms.
*Correspondence: Jidan Ye, yjyjd@jmu.edu.cn