- 1Faculty of Biosciences and Aquaculture, Nord University, Bodø, Norway
- 2Aquaculture Program, Institut de Recerca i Tecnologia Agroalimentàries (IRTA), Centre de la Ràpita (IRTA-SCR), La Ràpita, Spain
- 3AZTI, Food Research, Basque Research and Technology Alliance (BRTA), Bizkaia, Spain
In this study, we evaluated the replacement of dietary protein sources like fishmeal (FM) and plant proteins (PP) by Brewer’s spent dry yeast (SDY) on the transcriptomic response (RNA-seq, NextSeq500 platform Illumina) in the liver, anterior-mid intestine, and head kidney in juveniles of gilthead sea bream (Sparus aurata). The inclusion of SDY at 30% in the experimental diet (40% crude protein, 16% crude lipid) resulted in a reduction in FM (10%) and PP (31.4%) contents. Using RNA-seq, a total of 19.4 million raw reads per library were obtained, from whose 99.8% of the sequenced data were retained. The alignment efficiency of uniquely mapped reads was 90.3, 89.5, and 89.8% for head kidney, liver, and anterior-mid intestine, respectively. In total, 218 differentially expressed genes (DEGs) were identified among all tissues, out of which, 141 were up- and 77 down-regluated. The enrichment analysis of DEGs revealed that SDY had a modulatory effect on several processes related to host’s immunity, oxygen’s carrier capacity, steroidogenesis, metabolism, and digestion. It is of special relevance the immunomodulatory effects of the tested ingredient as data from RNA-seq showed from the three target tissues analyzed. These results indicated that this ingredient in addition to being considered as a sustainable raw material for replacing conventional protein sources in aquafeeds may also be considered as a functional ingredient due to its content in β-glucans. The overall results of this study coupled with previous nutritional studies on this ingredient indicated the suitability of brewery’s by-products like SDY in aquafeeds for carnivorous species like gilthead seabream, as well as supporting a circular bioeconomy model that reuses, recovers, and recycles resources instead of producing wastes.
Introduction
Although it is generally accepted that aquaculture can relieve pressure on wild fisheries by producing alternative fish for human consumption, the production of those fish often requires inputs from wild fish stocks in the form of feed ingredients. The paradox stems from the diversity of farmed species and husbandry systems (Stevens et al., 2018). Regardless of the remarkable advances in aquafeed formulation, most feeds used in aquaculture still rely on fishmeal (FM) and fish oil derived from fisheries. However, there is increasing pressure on these raw materials and conventional alternative plant protein (PP) sources due to growing demands from a variety of users. For instance, the use of soy protein and other plant products in aquaculture reduces their availability for direct human consumption (Ytrestøyl et al., 2015). Under this scenario, the sustainability and competitiveness of aquaculture may depend on their replacement with alternative ingredients ranging from plant-based meal and plant oil to terrestrial by-products and microbial ingredients (Naylor et al., 2021). Thus, the mantra about the economic and environmental sustainability of the aquaculture industry based on the identification and use of alternative raw ingredients to the classical ones is still valid.
Microbial products, particularly yeast, are potential sustainable ingredients in aquafeeds due to the ability to convert low-value non-food biomass from forestry, and agricultural and food technology industries into high-value feed ingredients with limited dependence on arable land, water, and changing climatic conditions (Øverland and Skrede, 2017; Agboola et al., 2021). Among them, Brewer’s spent yeast (SDY), a by-product of the brewing industry, is a rich source of B vitamins, proteins (45–60%) and other compounds like β-glucans and mannoproteins (Jaeger et al., 2020; Agboola et al., 2021). Therefore, BSY is mainly utilized in animal feed formulations as a low-cost source of protein (Agboola et al., 2021; Estévez et al., 2021; Nazzaro et al., 2021) even though it may be also used as an ingredient in functional feeds due to its potential health benefits to the host (Jaeger et al., 2020). Under this scenario, Estévez et al. (2021) have shown that the replacement of FM and plant protein sources by SDY in the feed of a carnivorous marine teleost fish species, the gilthead sea bream (Sparus aurata), resulted in a better growth, as well as a higher protein digestibility and feed efficiency indexes than in their congeners fed a reference diet. The above-mentioned results showed that this by-product from the brewing industry is a promising feed ingredient in aquafeeds, especially when considering its use in terms of an alternative plant protein source, but also when considering its associated sustainable and environmental benefits. Those benefits can be achieved by means of a circular economy strategy, which helps in providing added value to the brewing industry through wastes’ reuse and, consequently, reducing the industry carbon footprint (Kerby and Vriesekoop, 2017; Jaeger et al., 2020).
Once validated the use of SDY as an alternative protein source in aquafeeds (Estévez et al., 2021), the present study aimed to evaluate at the transcriptomic level the impact of SDY in the head kidney, intestine, and liver of S. aurata. This marine species is the most important Mediterranean aquaculture fish species in terms of volume and economic value (FAO, 2020). Regarding the selected target tissues, the head kidney was chosen as it is one of the most important organs in fish due to its role in endocrine and hematopoietic functions, as well as a major secondary lymphoid organ (Tort, 2011). Furthermore, the liver was selected as the central metabolic organ of the body with a predominant role in the intermediary metabolism, with important functions in lipid storage and digestive and detoxification functions (Bruslé and González, 1996). Finally, the intestine was chosen due to its involvement in important physiological digestive functions, being the primary site of food digestion and nutrient uptake, whereas it is also reputed for providing support to gut microbiota and maintaining an effective barrier defense against pathogens and tolerance toward dietary antigens (Salinas and Parra, 2015; Wang et al., 2018). Thus, a proper understanding of the effects of this new alternative ingredient at systemic and local levels as well as its mode of action upon the selected target tissues would support its proper use as an ingredient for sustainable aquafeeds.
Materials and Methods
Experimental Diets
Two experimental diets (Table 1) were designed in order to evaluate the impact of brewer’s SDY on gilthead sea bream growth and feed efficiency performance (Estévez et al., 2021), as well as evaluate global transcriptome changes in selected tissues (head kidney, anterior-mid intestine, and liver). A control diet was formulated containing 15% of FM and 46.7% of a blend of PP sources, whereas lipids were mainly provided by fish oil (7.9%), soybean oil (6.7%), and lecithin (2.0%). The inclusion of Brewer’s SDY in the experimental diet resulted in a reduction in FM (10%) and PP (31.4%) contents (Table 1). This alternative protein source and level of dietary inclusion were selected according to previous results (Estévez et al., 2021).
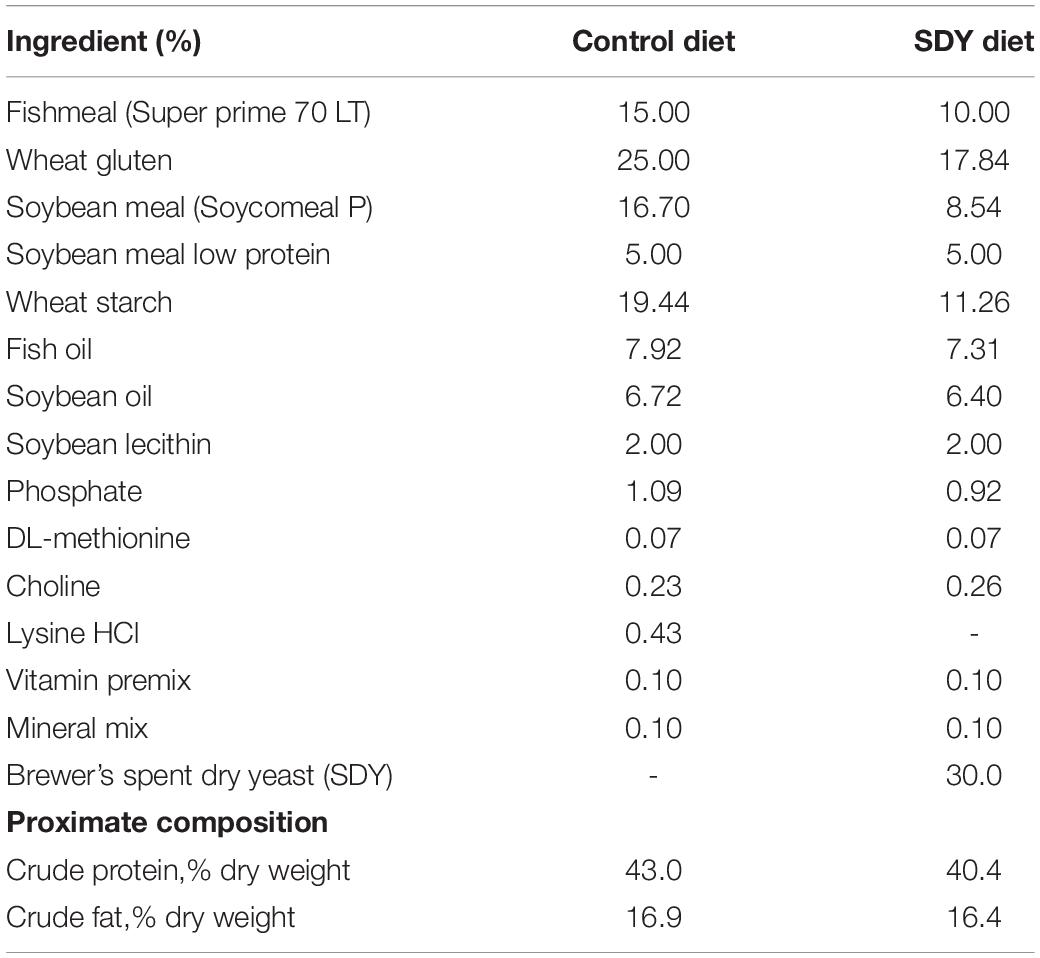
Table 1. List of ingredients and proximal composition of experimental diets used for evaluating the inclusion of Brewer’s spent dry yeast (SDY) in compound diets for gilthead sea bream (Sparus aurata).
Experimental diets (pellet size: 4 mm) were manufactured by IRTA (Mas de Bover, El Morell, Spain). The main ingredients were ground in a hammer mill (Rosal VRE-40, Barcelona, Spain). Powder ingredients and oils were then mixed according to the target formulation in a 500 L Rosal Mixer, with a double horizontal ribbon, for 6 min. Both diets were manufactured by temperature-controlled extrusion (90°C at the conditioner and 120°C at the screw) by means of an extruder press (Rosal RS-50). Upon extrusion, feed batches were dried and cooled by a hot air aspiration system (MABRIK S.A., Barbera del Vallés, Spain).
Fish and Experimental Design
Samples of target tissues used for transcriptomic analyses were taken from a previous trial in which we evaluated different by-products from the brewer’s industry as potencial feed ingredients in gilthead sea bream (Estévez et al., 2021). In brief, juveniles (age1 +) were obtained from a commercial fish farm (Albadalejo, San Pedro del Pinatar, Murcia, Spain) and transported by road to IRTA-Sant Carles de la Ràpita research facilities (REGA number: ES431360036277; Sant Carles de la Ràpita, Tarragona, Spain). Fish were kept under quarantine conditions and fed a commercial diet for 2 weeks. Then, fish (N = 90) were gently anesthetized (tricaine methanesulfonate, MS-222, 150 mg/L), individually weighed for initial body weight (BWi) and distributed among 6 experimental 200 L tanks (3 replicate tanks per dietary group; n = 15 fish per tank; initial stocking density = 13–14 kg m–3) as described in Estévez et al. (2021). The mean BWi values for fish from the control and SDY diets were 114.8 ± 16.7 g and 112.7 ± 14.8 g (mean ± standard deviation), respectively. Both diets were tested in triplicate for 60 days. Fish were fed at an initial feeding ration of 1.8% of the stocked biomass by means of two daily meals (08:00 and 14:00 h). During the nutritional trial, water temperature (pH meter 507; Crison Instruments, Barcelona, Spain), salinity (MASTER-20T; ATAGO Co., Ltd., Tokyo, Japan), and dissolved oxygen (OXI330; Crison Instruments) were 20.1 ± 3.0°C, 35.1 ± 0.1 ‰, and 6.6 ± 0.6 mg L–1 (mean ± SD), respectively. Water flow rate in experimental tanks was maintained at approximately 9.0 L min–1 (open-flow system), which guaranteed one full tank’s water renewal per hour. Photoperiod followed natural changes according to the season of the year (September –November; 40°37′41″ N).
At the end of the trial, all fish were netted, anaesthetized with MS-222 (150 mg/L), and individually weighted for their final body weight (BWf). In addition, three fish per tank (9 fish per dietary condition) were sacrificed with an overdose of MS222, and their head kidney, liver, and mid-anterior-mid dissected for further transcriptomic analyses. In particular, sampled tissues (200 mg) were preserved in RNAlater® (Sigma-Aldrich, Spain) overnight, and then stored at −80°C until further RNA extraction.
RNA Sequencing and Data Analysis
Total RNA from each tissue was extracted from five randomly chosen specimens using the TRIzol reagent (Invitrogen, San Diego, CA, United States) following the manufacturer’s instructions; the other samples from the remaining three fish were kept as backup. Then, total RNA concentration was quantified using a NanoDrop ND-2000 (Thermo Fisher Scientific, Waltham, United States), and RNA integrity and quality checked with Agilent 2100 Bioanalyzer (Agilent Technologies, Santa Clara, United States). Samples were shipped in dry ice to Nord University (Bodø, Norway) for further RNA sequencing. At their arrival, RNA integrity was checked again on an Agilent 4150 TapeStation system (Agilent Technologies) in order to confirm that the RNA quality of the samples did not deteriorate during shipment.
For library preparation, RNA concentration was calculated using the Qubit RNA high-sensitivity assay on a Qubit 3.0 fluorometer (Thermo Fisher Scientific, Waltman, United States). RNA-Seq libraries were prepared using the NEBNext ultra II directional RNA library preparation kit with a poly(A) mRNA magnetic isolation module (NEB #E7490) in accordance with the manufacturer’s protocol (New England BioLabs Inc., Ipswich, United Kingdom). Briefly, after poly(A) enrichment of 1 μg total RNA, mRNA was fragmented to about 100–200 nt in length and used for 1st and 2nd strand cDNA synthesis. After A-tailing, end repair, and adaptor ligation, the second strand was selectively removed using uracil-specific excision reagent (USER® II Enzyme; New England BioLabs Inc.). The resulting directional RNA-seq libraries were amplified with 8 PCR cycles and posteriorly purified using AMPure XP beads (Beckman Coulter, Inc., Brea, United States). Quality and quantity of the RNA-seq libraries were assessed using the Agilent 2200 TapeStation. Unique indices were assigned to each sample and libraries were pooled at equimolar concentrations. Sequencing was performed at Nord University (Norway) using two high output flowcells (75 cycles) on the NextSeq500 (Illumina, San Diego United States) with 4% PhiX. Library sequencing yielded 764 million 75 bp single-end reads RNA-Seq libraries.
Raw sequences were adapter-trimmed and quality checked using Trim galore v0.4.4 (Babraham Bioinformatics, United Kingdom) with the following trimming parameters: -phred33, -fastqc, and -q20. Alignments were performed using the hisat2 v2.1.0 platform with default parameters and the latest S. aurata genome assembly (GCA_900880675.2; NCBI, Bethesda, United States). Count-based matrices were created for each sample using featureCounts v1.5.3 and they were then combined in R using the plyr and dplyr packages. Differential expression analysis was performed using DESeq2 (Anders and Huber, 2010) and the final list of differentially expressed genes (DEGs) was filtered accordingly (adjusted P-value < 0.05) and absolute log2 fold change (FC) of 1.0. Gene set enrichment analysis was performed for down- and up-regulated DEGs separately, using g:GOSt functional profiling on gProfiler (version e104_eg51_p15_3922dba) with default parameters (Raudvere et al., 2019). The selected organism was gilthead sea bream (Sparus aurata) and the tailor-made algorithm g:SCS was used for multiple testing correction. Heatmaps were prepared using the R package “pheatmap.” Transcriptomic raw data for samples analyzed in the current study are available through the public repository Gene Expression Omnibus (GEO) at the US National Centre for Biotechnology Information (NCBI) (accession number GSE194206).
Ethics Statement
All animal experimental procedures complied with the Guiding Principles for Biomedical Research Involving Animals (EU2010/63), the guidelines of the Spanish laws (law 32/2007 and RD 1201/2015) and were authorized by the Ethical Committee of the Institute for Research and Technology in Food and Agriculture (IRTA, Spain) for the use of laboratory animals (E-10/2020).
Results
Results on growth performance, feed efficiency, and diet digestibility are presented elsewhere (Estévez et al., 2021). In brief, no differences in growth performance were found among both experimental groups with BWf average values comprised between 176.1 and 179.0 g. Regarding feed efficiency variables, gilthead sea bream fed the SDY-supplemented diet showed better feed conversion ratio (FCR = 2.0 ± 0.05) and protein efficiency rate (PER = 1.1 ± 0.03) than their congeners fed the control diet (FCR = 2.1 ± 0.29; PER = 1.0 ± 0.11) (P < 0.05).
RNA Sequencing
On average, we obtained 19.4 million raw reads per library. After quality and adapter trimming, 99.8% of the sequenced data were retained. The alignment efficiency of uniquely mapped reads was 90.3, 89.5, and 89.8% for head kidney, liver, and intestine, respectively. The rest of the reads (∼10%) were excluded from further analysis, either as aligned reads with multiple matches or reads that failed to align to the reference transcriptome (Figure 1 and Supplementary Table 1). In total, 218 DEGs were identified among all tissues, out of which, 141 were up- and 77 down-regulated (q < 0.05; −1 > log2 (FC) > 1; Supplementary Table 2). These DEGs had a tissue-specific profile and, therefore, we investigated their functionality for each tissue separately.
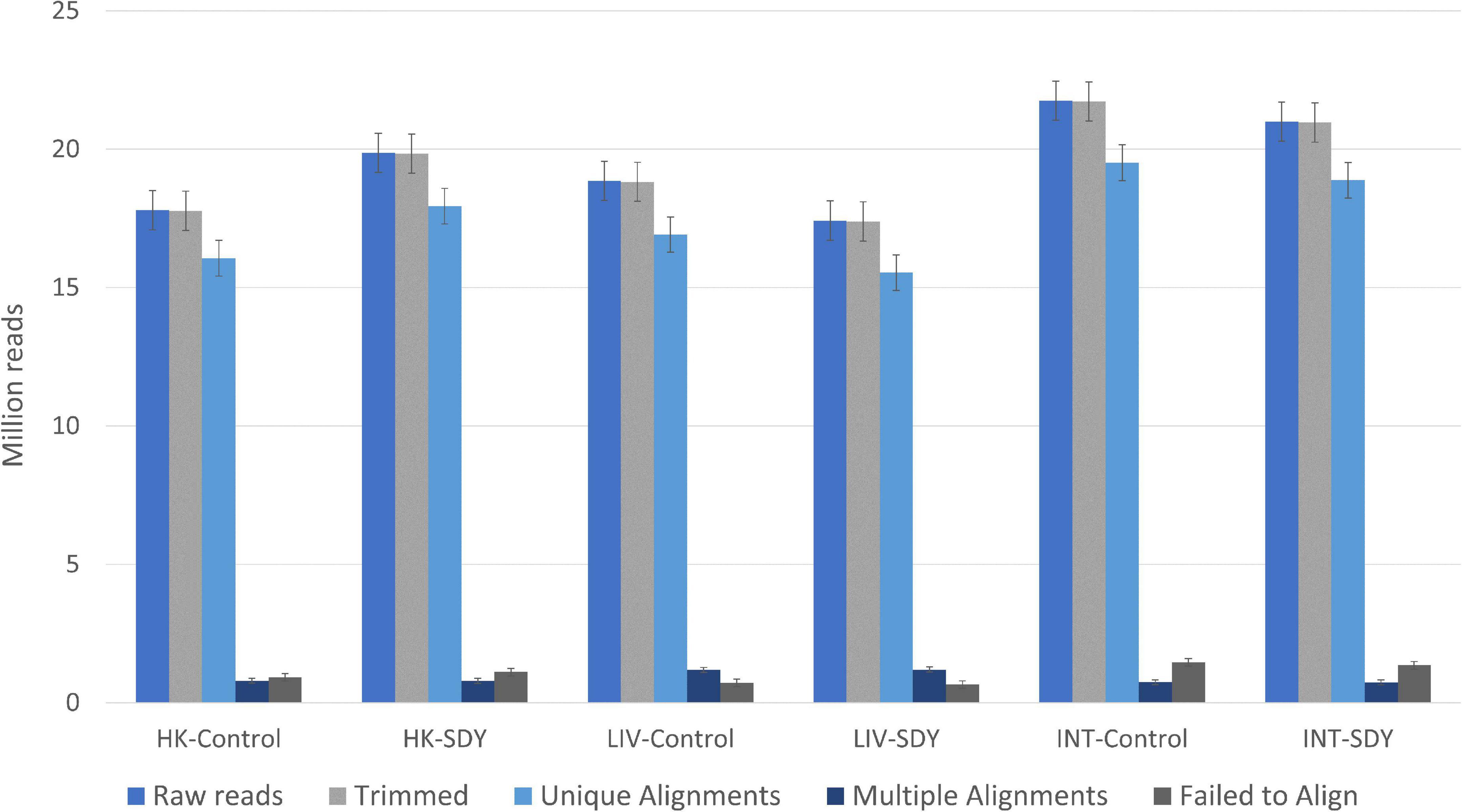
Figure 1. Number of reads obtained by RNA-seq (raw reads, trimmed reads, unique alignment reads, multiple alignment reads, and reads that failed to align) for each of the targeted tissues (head kidney, liver, and anterior-mid intestine) of gilthead sea bream (Sparus aurata) in response to the control (Control) and the Brewer’s SDY diets. The error bar represents the standard deviation of the mean values obtained for each tissue (n = 5 replicates). HK, head kidney; LIV, liver; INT, anterior-mid intestine.
The Immunomodulatory and Steroidogenic Effects of Spent Dry Yeast on the Head Kidney
Head kidney was the tissue with the highest number of DEGs (99; q < 0.05; −1 > log2 (FC) > 1) when compared to the other target tissues analyzed (Supplementary Table 2). In particular, 62 DEGs (62.6%) were up-regulated and 37 DEGs (37.4%) were down-regulated in the head kidney of fish fed the SDY diet compared to the control group (Figure 2A). The principal component analysis (PCA) showed no distinction between groups in the first component (PC1), which explained 90% of the variation, although a slight distinction was present in the second component (PC2) that explained a rather small fraction of the variation (4%) (Supplementary Figure 1). Among the top 10 up-regulated genes in head kidney (log2 (FC) > 6.7), we identified 6 genes associated with steroidogenesis (nr5a1a, bicc2, hsd3b1, cyp11b1, cyp21a2, cyp17a1), and others related to chemokine activity (ccl17, cxc11, and cxc6). These findings were also supported by the functional enrichment analysis (Supplementary Table 3; q < 0.05). Furthermore, the gene ontology annotation of all up-regulated genes showed an enrichment of molecular functions related to chemokine activity (GO:0008009) and chemokine receptor binding (GO:0042379), as well as to biological processes linked to steroid metabolic (GO:0008202) and biosynthetic (GO:0006694) processes, and glucocorticoid metabolism (GO:0008211). Additionally, the oxidoreductase (GO:0016705) and monooxygenase activity (GO:0004497) processes were also identified.
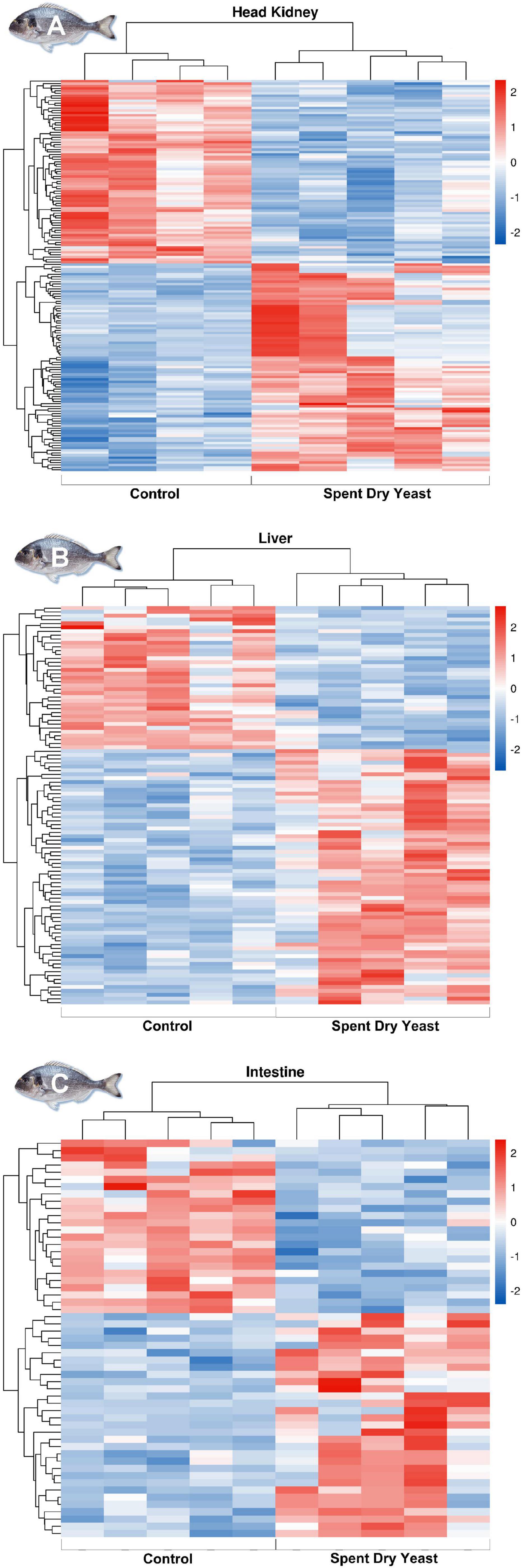
Figure 2. Hierarchical clustering of transcriptomic response from the head kidney (A), liver (B), and anterior-mid intestine (C) of gilthead seabream (Sparus aurata) fed the control and the Brewer’s SDY diets. This heat map is based on similitude patterns of the differentially expressed genes from five replicates per tissue both dietary groups with the only exception of the head kidney where one replicate from the control group was excluded from the analysis as shown in Supplementary Figure 1 (results from the Principal Component Analysis).
Down-regulated genes showed an enrichment in oxygen carrier activity and at the cellular component level an enrichment in hemoglobin complex (Supplementary Table 3, q < 0.05). Genes associated with these functions coded for hemoglobin subunits alpha-A and beta (hbaa and hbb), which are involved in oxygen transport, with the latter being a potential regulator of inflammation. In addition, one of the most down-regulated genes was the lipoxygenase homology PLAT domains 1b (loxhd1b), which is also involved in heme binding and oxygen binding affinity. Among the down-regulated genes (log2(FC) < −5.24), we identified the carcinoembryonic antigen-related cell adhesion molecule 6 (ceacam6) responsible for cell–cell adhesion and neutrophil adhesion to cytokine-activated endothelial cells, as well as the ceacam2 gene, which is involved in energy homeostasis and negative regulation of feeding behavior.
Spent Dry Yeast Affects Genes Involved in Liver Metabolism, Growth and Immunity
In the liver, we identified 75 DEGs, of which 52 were up-regulated (69.3%) and 23 down-regulated (30.7%) in fish fed the SDY diet (Figure 2B; q < 0.05; −1 < log2 (FC) < 1). The PCA revealed a distinction of the two diets within the PC1, which explained 22% of the total variance (Supplementary Figure 2). Up-regulated genes (psat1, phgdh, wars1, hars1; Supplementary Table 2, q < 0.05) were primarily associated with metabolic and biosynthetic processes of L-serine and cellular amino acids, as well as organic carboxylic and oxoacids. Among up-regulated genes, we also identified socs3 and cish that are involved in the regulation of inflammatory responses and growth via the regulation of the JAK-STAT receptor signaling pathway (Supplementary Table 4, q < 0.05).
Down-regulated genes were enriched for metal ion, cation, and zinc ion binding molecular functions (Supplementary Table 4, q < 0.05). The E3 ubiquitin-protein ligase TRIM21-like gene (trim21) was down-regulated in fish fed the SDY diet compared to the control group [log2(FC) = −3.9]. Similarly, among the most down-regulated genes, we identified the NACHT, LRR, and PYD domains-containing protein 12-like (nlrp12) and protein mono-ADP-ribosyltransferase PARP14-like (parp14), which are also involved in immunity through the regulation of NF-kappaβ signaling and tyrosine phosphorylation of STAT proteins, respectively.
Intestinal Host Immunity and Digestion Are Affected by the Spent Dry Yeast Diet at a Molecular Level
The tissue with the least DEGs, among the studied ones, was the anterior-mid intestine. Out of 44 DEGs, 27 were up- and 17 down-regulated in fish fed the SDY diet compared to the control group, respectively (Figure 2C; q < 0.05; −1 > log2 (FC) > 1). PCA did not reveal any distinct groupings between groups across both components, which only explained 54% of the total variation in the intestine (Supplementary Figure 3). Interestingly, up-regulated genes were enriched for lysozyme (GO:0003796), peptidoglycan muralytic (GO:0061783), and hydrolase activities (GO:0004553; GO:0016798), all of them being highly relevant to tissue-specific functions such as intestinal host defense (Supplementary Table 5). Similarly, among the most down-regulated DEGs, we identified a gene linked to fatty acid oxidation (hao2) as well as the retinoic acid receptor beta (rarb) and the major histocompatibility complex class I-related gene protein (hla), which are involved in intestinal immunity (Supplementary Table 5).
Discussion
In this study, we decided to explore the dietary effects of Brewer’s SDY at transcriptomic level by means of RNA-seq on three different tissues selected by their relevant body functions. Regarding the head kidney, RNA-seq results showed that most of DEGs in this hematopoietic and lymphoid tissue of gilthead sea bream fed the SDY were related to increased transcription levels of genes related to chemokine activity (GO:0008009) and chemokine receptor binding (GO:0042379). This is of special relevance since chemokines are involved in the initiation and regulation of the inflammatory response. In particular, the production of pro-inflammatory chemokines (i.e., CCL2, CCL3, CCL5, CXCL1, CXCL2, and CXCL8) is generally induced to recruit immune cells to the infection site, while chemokines considered as homeostatic (i.e., CCL19 and CCL21) are involved in controlling the migration of cells during normal processes of tissue maintenance or development; thus, initiating and regulating the inflammatory response (Laing and Secombes, 2004; Sakai et al., 2021). Under present experimental conditions, we found a transcriptional regulation of ccl17, cxc6, cxc11 in the head kidney of fish fed the SDY-supplemented diet. The above-mentioned chemokines are reputed for being involved in the activation (cxc6) and recruitment (ccl17, cxc11) of T lymphocytes. These results are in agreement with the lymphoid function of this tissue, which may be translated into an enhanced host’s immune response as similar results have been observed in fish fed functional diets supplemented with zootechnical feed additives with immunomodulatory properties (Martin and Król, 2017; Hoseinifar et al., 2019; Salomón et al., 2021). The potential immunomodulatory function of the SDY diet may be attributed to its content in β-glucan (81 g/kg feed). In this sense, β-glucans are well-known in aquaculture because they promote hematopoiesis and enhance immunity (Meena et al., 2013).
Under the present experimental conditions, we also found changes in expression of genes involved in steroid synthesis and secretion (GO:0008202, GO:0006694). Among DEGs involved in steroidogenesis, nr5a1a was found to be up-regulated in the head kidney of fish fed the SDY-supplemented diet. This gene is expressed in the interrenal gland, gonads, and hypothalamus (Chai et al., 2003). In teleosts, the interrenal gland is embedded in the head kidney and is the major site of steroid synthesis in response to signals from the pituitary and hypothalamus (Milano et al., 1997). Similarly, cytochrome P450 11b (cyp11b), steroid 21-hydroxylase (cyp21a2), hydroxy-delta-5-steroid dehydrogenase, 3 beta- and steroid delta-isomerase (hsd3b1), and steroid 17-alpha-hydroxylase/17,20 lyase-like (LOC115589985) were also differentially expressed, which is of special relevance since those genes are involved in the biosynthesis of steroid hormones like cortisol through their precursors (Mommsen et al., 1999; Li et al., 2003). These results are of special importance, since glucocorticoids are synthesized by interrenal cells of the head kidney and regulate osmolarity, metabolism, and immune responses (Vazzana et al., 2010). Furthermore, the up-regulation of bicaudal C homolog 2 (bicc2) that is involved in renal gluconeogenesis might show that gilthead sea bream fed the SDY-supplemented diet may have a more effective stress response, as data from genes involved in steroidogenesis also indicated.
Surprisingly, the head kidney of gilthead sea bream fed the SDY-supplemented diet showed a down-regulation of hemoglobin subunits alpha-A and beta genes (hbaa and hbb), which encoded for α and β subunits of hemoglobin. In addition, we also found a down-regulation of the lipoxygenase homology PLAT domains 1b (loxhd1b—LOC115592348), which is also involved in heme binding and oxygen binding affinity. Under hypoxic conditions, changes in transcription levels of hbaa and hbb in the head kidney of fish have been associated to changes in erythropoiesis and hemoglobin synthesis (Xia et al., 2016). Furthermore, changes in gene expression of hbaa and hbb are responsible for hemoglobinopathies in humans (Sabath, 2017). We found these results puzzling, since the nutritional trial was conducted under normoxic conditions (> 85% O2 saturation) and we did not find any sign of distress in gilthead sea bream fed the SDY-supplemented diet caused by a putative anemia. Further research is needed to clarify the physiological effect of SDY on hemoglobin homeostasis and erythropoiesis in order to clarify current results.
Among the three studied tissues, the liver was the second one with a larger number of DEGs. In particular, DEGs were involved metabolic and biosynthetic processes (psat1, phgdh, wars1, hars1). These findings are in agreement with those found in the head kidney, which indicated that fish fed the SDY diet had a more biologically active organ, since the above-mentioned DEGs are involved in protein transcription and biosynthesis (Nguyen et al., 2020; Yu et al., 2021). In addition to the classical role of wars1 in protein synthesis, this gene has been recently described in mammals to be activated upon infection, whereas its protein acts as an endogenous ligand of toll-like receptors (TLR) 2 and TLR4 (Nguyen et al., 2020).
The potential immunomodulatory properties of the SDY found in the head kidney seemed to be also supported by the up-regulation of genes involved in modulating the inflammatory responses in the liver via the regulation of the JAK-STAT receptor signaling pathway as transcriptomic data suggested. Specifically, by the up-regulation in the liver of cytokine-inducible SH containing protein (cish) and the suppressor of cytokine signaling-like (socs) in comparison to the control group. These genes are members of the suppressor cytokine signaling family whose proteins are involved in the control and attenuation of cytokine-induced responses through the JAK/STAT signal transduction pathway, playing an important role in the regulation of host’s immunity and the inflammatory response, as well as in cell proliferation, migration and survival (Yoshimura, 2009; Yoshimura et al., 2012; Maehr et al., 2014). Furthermore, the down-regulation of the NACHT, LRR, and PYD domains-containing protein 12-like (nlrp12) might suggest an enhancement of the NF-κB pathway, since these proteins have a modulatory role on the NF-κB pathway (Normand et al., 2018). This is of special relevance since the NF-kB pathway plays an important role in the regulation of several cytokines, chemokines, antimicrobial peptides, and interferon-stimulated genes, as well as being crucial in modulating the survival, activation, and differentiation of innate and adaptive immune cells (Sun, 2017). Although these results might be attributed to a potential inflammatory response derived from the inclusion of SDY in the diet, we did not find inflammatory signs in the intestinal mucosa of fish fed the SDY diet (Supplementary Figure 4); thus, the transcriptional regulation of the NF-kB pathway may be attributed to the immunomodulatory properties of the SDY. Furthermore, the E3 ubiquitin-protein ligase TRIM21-like gene (trim21) was also down-regulated in the liver of fish fed the SDY diet. This gene is generally over-expressed under prolonged activation of the immune system, being also described as a negative regulator of antioxidative stress (Lee, 2017). Similarly, protein mono-ADP-ribosyltransferase PARP14-like (parp14) was also among the most down-regulated genes. This gene modulates the humoral immune response through the activation of the JAK/STAT pathway (Schweiker et al., 2018), which suggests a balanced immune response in gilthead sea bream fed the SDY diet as other authors have reported when there is a sort of immune homeostasis based on an equilibrium between pro- and anti-inflammatory processes (Salomón et al., 2020, 2021; Firmino et al., 2021).
The transcriptomic response evaluated in the anterior-mid intestine was in agreement with results found in the two former tissues from gilthead sea bream fed the SDY diet. In particular, four g-type lysozyme genes were up-regulated (LOC115585860, LOC115582400, LOC115582423, and LOC115585885), which were related to the biological process linked to peptidoglycan and aminoglycan metabolic, and catabolic processes in which g lysozyme is involved (Jiménez-Cantizano et al., 2008). In this sense, lysozyme cleaves peptidoglycans of the bacterial cell wall and participates in the activation of the complement system and phagocytes. In addition, lysozymes have also been reported to have antiviral and anti-inflammatory functions, thus playing an important function at both systemic and local immune responses (Saurabh and Sahoo, 2008). Changes in the number of transcripts of the retinoic acid receptor beta (rarb) were not attributed to different dietary vitamin A contents between both experimental diets, since yeast does not contain this lipophilic vitamin. Thus, changes in rarb expression might be associated to the regulation of the intestinal immune condition in fish fed the SDY diet (Gattu et al., 2019). Similarly, we also found the major histocompatibility complex class I-related gene protein (hla) to be down-regulated, which may be associated with a reduction in the immunogenicity of dietary SDY and modulation of the immune response in the intestine (Groh et al., 1996). Although we did not find inflammatory signs in the intestinal mucosa of fish fed the SDY diet (Supplementary Figure 4), the expression profile of immune-related genes in fish fed the SDY diet indicated that further research may be focused on evaluating the effect of SDY on the mucosal-associated lymphoid tissue in the intestine and its immunocompetence under disease conditions.
Furthermore, the inclusion of SDY in the diet down-regulated the expression of hydroxyacid oxidase 2 (hao2) in the anterior-mid intestine of gilthead sea bream, which might be attributed to a dietary regulation of this gene. Hydroxyacid oxidase 2 is a peroxisomal 2-hydroxy acid oxidase responsible for the oxidation of 2-hydroxy fatty acids like 2-hydroxypalmitic acid, which is an intermediate in phytosphingosine metabolic pathway, an important component of sphingolipids found in yeasts and plants (Pruett et al., 2008).
Conclusion
Under present experimental conditions, the partial replacement of conventional dietary protein sources by spent dry yeast (SDY) obtained as a by-product of the brewer’s industry had a modulatory effect on several processes related to host’s immunity, oxygen’s carrier capacity, steroidogenesis, metabolism, and digestion. Results related to the enhancement of the immune condition at systemic and local levels in fish fed the SDY diet are of special relevance, since they indicated that this ingredient in addition to being considered as a sustainable ingredient for replacing conventional marine and terrestrial protein sources in aquafeeds, it may also be considered as a functional one due to its immunomodulatory properties derived from its content in β-glucans. Although the results found in the present study seemed really promising in terms of the functional properties of the tested SDY, further research needs to be focused on clarifying the effect of this ingredient on the biological processes involved in oxygen transport in order to guarantee the safety of this ingredient for fish. The overall results of this study coupled with nutritional studies on this ingredient (Estévez et al., 2021; Nazzaro et al., 2021) indicated that the use of brewery’s by-products like SDY in aquafeeds supports a circular bioeconomy model that reuses, recovers, and recycles resources instead of producing wastes.
Data Availability Statement
The datasets presented in this study can be found in online repositories. The names of the repository/repositories and accession number(s) can be found below: https://www.ncbi.nlm.nih.gov/geo/, accession ID: GSE194206.
Ethics Statement
This animal study was reviewed and approved by the Ethical Committee of the Institute for Research and Technology in Food and Agriculture (IRTA, Spain) for the use of laboratory animals (E-10/2020).
Author Contributions
AE, DS, and BI: conceptualization. EG, JPF, and JMOF: methodology. JPF, IK, and AE: sample processing. IK, JPF, and JMOF: formal analysis. EG and JMOF: resources. IK and EG: writing the original draft. JPF, IK, and JMOF: data visualization and curation. EG and JMOF: supervision. EG, AE, and DS: project administration. AE and DS: funding acquisition. All authors contributed to the article and approved the submitted version.
Funding
This study has been conducted under the Life BREWERY project (LIFE16ENV/ES/000160) that was funded by LIFE European Environment and Resource Efficiency Program, which was the EU’s financial instrument supporting environmental, nature conservation, and climate action projects throughout the EU, and partially funded by the Ministerio de Economía, Industria y Competitividad (Government of Spain) through the grant AGL2018-095653-R-I00 to IRTA. The implementation, updating, and development of EU environmental and climate policy and legislation by co-financing projects with European added value are among its main priorities. JPF has received funding from the Erasmus + Programme of the European Union, and the Autonomous University of Barcelona (UAB) Exchange Programme Traineeship 2020–2021 for conducting a research stage at Nord University (Bodø, Norway). AR was supported by a predoctoral grant (reference number: PRE2019-091259) linked to the project RTI2018-095653-RI00 (Ministerio de Ciencia e Innovación, Spain).
Conflict of Interest
The authors declare that the research was conducted in the absence of any commercial or financial relationships that could be construed as a potential conflict of interest.
Publisher’s Note
All claims expressed in this article are solely those of the authors and do not necessarily represent those of their affiliated organizations, or those of the publisher, the editors and the reviewers. Any product that may be evaluated in this article, or claim that may be made by its manufacturer, is not guaranteed or endorsed by the publisher.
Supplementary Material
The Supplementary Material for this article can be found online at: https://www.frontiersin.org/articles/10.3389/fmars.2022.857592/full#supplementary-material
References
Agboola, J. O., Øverland, M., Skrede, A., and Hansen, J. Ø. (2021). Yeast as major protein-rich ingredient in aquafeeds: a review of the implications for aquaculture production. Rev. Aquac. 13, 949–970. doi: 10.1111/raq.12507
Anders, S., and Huber, W. (2010). Differential expression analysis for sequence count data. Gen. Biol. 10:R106. doi: 10.1186/gb-2010-11-10-r106
Bruslé, J., and González, G. (1996). “The structure and function of fish liver,” in Fish Morphology, eds J. S. D. Munshi and H. M. Dutta (New Delhi: Science Publishers Inc).
Chai, C., Liu, Y. W., and Chan, W. K. (2003). Ff1b is required for the development of steroidogenic component of the zebrafish interrenal organ. Dev. Biol. 260, 226–244. doi: 10.1016/S0012-1606(03)00219-7
Estévez, A., Padrell, L., Iñarra, B., Orive, M., and Martin, D. S. (2021). Brewery by-products (yeast and spent grain) as protein sources in gilthead sea bream (Sparus aurata) feeds. Aquaculture 543:736921. doi: 10.1016/j.aquaculture.2021.736921
FAO (2020). The State of World Fisheries and Aquaculture 2020. FAO Fisheries and Aquaculture Department. Rome: The Food and Agriculture Organization of the United Nations.
Firmino, J. P., Vallejos-Vidal, E., Balebona, M. C., Ramayo-Caldas, Y., Cerezo, I., Salomón, R., et al. (2021). Diet, immunity and microbiota interactions: an integrative analysis of the intestine transcriptional response and microbiota modulation in gilthead seabream (Sparus aurata) fed an essential oils-based functional diet. Front. Immunol. 12:625297. doi: 10.3389/fimmu.2021.625297
Gattu, S., Bang, Y. J., Pendse, M., Dende, C., Chara, A. L., Harris, T. A., et al. (2019). Epithelial retinoic acid receptor β regulates serum amyloid a expression and vitamin a-dependent intestinal immunity. Proc. Natl. Acad. Sci. U.S.A. 116, 10911–10916. doi: 10.1073/pnas.1812069116
Groh, V., Bahram, S., Bauer, S., Herman, A., Beauchamp, M., and Spies, T. (1996). Cell stress-regulated human major histocompatibility complex class I gene expressed in gastrointestinal epithelium. Proc. Natl. Acad. Sci. U.S.A. 93, 12445–12450. doi: 10.1073/pnas.93.22.12445
Hoseinifar, S. H., Sohrabi, A., Paknejad, H., Jafari, V., Paolucci, M., and Van Doan, H. (2019). Enrichment of common carp (Cyprinus carpio) fingerlings diet with Psidium guajava: the effects on cutaneous mucosal and serum immune parameters and immune related genes expression. Fish Shellfish Immunol. 86, 688–694. doi: 10.1016/j.fsi.2018.12.001
Jaeger, A., Arendt, E. K., Zannini, E., and Sahin, A. W. (2020). Brewer’s Spent Yeast (BSY), an underutilized brewing by-product. Fermentation 6:123. doi: 10.3390/fermentation6040123
Jiménez-Cantizano, R. M., Infante, C., Martin-Antonio, B., Ponce, M., Hachero, I., Navas, J. I., et al. (2008). Molecular characterization, phylogeny, and expression of c-type and g-type lysozymes in brill (Scophthalmus rhombus). Fish Shellfish Immunol. 25, 57–65. doi: 10.1016/j.fsi.2007.12.009
Kerby, C., and Vriesekoop, F. (2017). An overview of the utilisation of brewery by-products as generated by British craft breweries. Beverages 3:24. doi: 10.3390/beverages3020024
Laing, K. J., and Secombes, C. J. (2004). Chemokines. Dev. Comp. Immunol. 28, 443–460. doi: 10.1016/j.dci.2003.09.006
Lee, A. Y. (2017). A review of the role and clinical utility of anti-Ro52/TRIM21 in systemic autoimmunity. Rheumatol. Int. 37, 1323–1333. doi: 10.1007/s00296-017-3718-1
Li, Y. Y., Inoue, K., and Takei, Y. (2003). Interrenal steroid 21-hydroxylase in eels: primary structure, progesterone-specific activity and enhanced expression by ACTH. J. Mol. Endocrinol. 31, 327–340. doi: 10.1677/jme.0.0310327
Maehr, T., Vecino, J. L. G., Wadsworth, S., Wang, T., and Secombes, C. J. (2014). Four CISH paralogues are present in rainbow trout Oncorhynchus mykiss: differential expression and modulation during immune responses and development. Mol. Immunol. 62, 186–198. doi: 10.1016/j.molimm.2014.06.021
Martin, S. A., and Król, E. (2017). Nutrigenomics and immune function in fish: new insights from omics technologies. Dev. Comp. Immunol. 75, 86–98. doi: 10.1016/j.dci.2017.02.024
Meena, D. K., Das, P., Kumar, S., Mandal, S. C., Prusty, A. K., Singh, S. K., et al. (2013). Beta-glucan: an ideal immunostimulant in aquaculture (a review). Fish Physiol. Biochem. 39, 431–457. doi: 10.1007/s10695-012-9710-5
Milano, E. G., Basari, F., and Chimenti, C. (1997). Adrenocortical and adrenomedullary homologs in eight species of adult and developing teleosts: morphology, histology, and immunohistochemistry. Gen. Comp. Endocrinol. 108, 483–496. doi: 10.1006/gcen.1997.7005
Mommsen, T. P., Vijayan, M. M., and Moon, T. W. (1999). Cortisol in teleosts: dynamics, mechanisms of action, and metabolic regulation. Rev. Fish Biol. Fish. 9, 211–268. doi: 10.1210/en.2004-0644
Naylor, R. L., Hardy, R. W., Buschmann, A. H., Bush, S. R., Cao, L., Klinger, D. H., et al. (2021). A 20-year retrospective review of global aquaculture. Nature 591, 551–563. doi: 10.1038/s41586-021-03308-6
Nazzaro, J., San Martin, D., Perez-Vendrell, A. M., Padrell, L., Iñarra, B., Orive, M., et al. (2021). Apparent digestibility coefficients of brewer’s by-products used in feeds for rainbow trout (Oncorhynchus mykiss) and gilthead sea bream (Sparus aurata). Aquaculture 530:735796. doi: 10.1016/j.aquaculture.2020.735796
Nguyen, T. T., Yoon, H. K., Kim, Y. T., Choi, Y. H., Lee, W. K., and Jin, M. (2020). Tryptophanyl-tRNA synthetase 1 signals activate TREM-1 via TLR2 and TLR4. Biomolecules 10:1283. doi: 10.3390/biom10091283
Normand, S., Waldschmitt, N., Neerincx, A., Martinez-Torres, R. J., Chauvin, C., Couturier-Maillard, A., et al. (2018). Proteasomal degradation of NOD2 by NLRP12 in monocytes promotes bacterial tolerance and colonization by enteropathogens. Nat. Commun. 9:5338. doi: 10.1038/s41467-018-07750-5
Øverland, M., and Skrede, A. (2017). Yeast derived from lignocellulosic biomass as a sustainable feed resource for use in aquaculture. J. Sci. Food Agric. 97, 733–742. doi: 10.1002/jsfa.8007
Pruett, S. T., Bushnewv, A., Hagedorn, K., Adiga, M., Haynes, C. A., Sullards, M. C., et al. (2008). Biodiversity of sphingoid bases (‘sphingosines’) and related amino alcohols. J. Lipid Res. 49, 1621–1639. doi: 10.1194/jlr.R800012-JLR200
Raudvere, U., Kolberg, L., Kuzmin, I., Arak, T., Adler, P., Peterson, H., et al. (2019). g:Profiler: a web server for functional enrichment analysis and conversions of gene lists (2019 update). Nucleic Acids Res. 47, W191–W198. doi: 10.1093/nar/gkz369
Sabath, D. E. (2017). Molecular diagnosis of thalassemias and hemoglobinopathies: an ACLPS critical review. Am. J. Clin. Pathol. 148, 6–15. doi: 10.1093/ajcp/aqx047
Sakai, M., Hikima, J. I., and Kono, T. (2021). Fish cytokines: current research and applications. Fish. Sci. 87, 1–9. doi: 10.1007/s12562-020-01476-4
Salinas, I., and Parra, D. (2015). “Fish mucosal immunity: intestine,” in Mucosal Health in Aquaculture, eds B. J. Beck and E. Peatman (Cambridge, MA: Academic Press), 135–170. doi: 10.1016/b978-0-12-417186-2.00006-6
Salomón, R., Firmino, J. P., Reyes-López, F. E., Andree, K. B., González-Silvera, D., Esteban, M. A., et al. (2020). The growth promoting and immunomodulatory effects of a medicinal plant leaf extract obtained from Salvia officinalis and Lippia citriodora in gilthead seabream (Sparus aurata). Aquaculture 524:735291. doi: 10.1016/j.aquaculture.2020.735291
Salomón, R., Furones, M. D., Reyes-López, F. E., Tort, L., Firmino, J. P., Esteban, M. A., et al. (2021). A bioactive extract rich in triterpenic acid and polyphenols from Olea europaea promotes systemic immunity and protects Atlantic salmon smolts against furunculosis. Front. Immunol. 12:737601. doi: 10.3389/fimmu.2021.737601
Saurabh, S., and Sahoo, P. K. (2008). Lysozyme: an important defence molecule of fish innate immune system. Aquac. Res. 39, 223–239. doi: 10.1111/j.1365-2109.2007.01883.x
Schweiker, S. S., Tauber, A. L., Sherry, M. E., and Levonis, S. M. (2018). Structure, function and inhibition of poly (ADP-ribose) polymerase, member 14 (PARP14). Mini Rev. Med. Chem. 18, 1659–1669. doi: 10.2174/1389557518666180816111749
Stevens, J. R., Newton, R. W., Tlusty, M., and Little, D. C. (2018). The rise of aquaculture by-products: increasing food production, value, and sustainability through strategic utilisation. Mar. Policy 90, 115–124. doi: 10.1016/j.marpol.2017.12.027
Sun, S. C. (2017). The non-canonical NF-kappaB pathway in immunity and inflammation. Nat. Rev. Immunol. 17, 545–558. doi: 10.1038/nri.2017.52
Tort, L. (2011). Stress and immune modulation in fish. Dev. Comp. Immunol. 35, 1366–1375. doi: 10.1016/j.dci.2011.07.002
Vazzana, M., Vizzini, A., Sanfratello, M. A., Celi, M., Salerno, G., and Parrinello, N. (2010). Differential expression of two glucocorticoid receptors in seabass (teleost fish) head kidney after exogeneous cortisol inoculation. Comp. Biochem. Physiol. 157A, 49–54. doi: 10.1016/j.cbpa.2010.05.003
Wang, A. R., Ran, C., Ringø, E., and Zhou, Z. G. (2018). Progress in fish gastrointestinal microbiota research. Rev. Aquac. 10, 626–640. doi: 10.1111/raq.12191
Xia, M., Chao, Y., Jia, J., Li, C., Kong, Q., Zhao, Y., et al. (2016). Changes of hemoglobin expression in response to hypoxia in a Tibetan schizothoracine fish, Schizopygopsis pylzovi. J. Comp. Physiol. 186B, 1033–1043. doi: 10.1007/s00360-016-1013-1
Yoshimura, A. (2009). Regulation of cytokine signalling by the SOCS and Spred family proteins. Keio J. Med. 58, 73–83. doi: 10.2302/kjm.58.73
Yoshimura, M., Suzuki, R., Sakaguchi, T., Hanada, H., and Yasukawa, H. (2012). SOCS, inflammation, and autoimmunity. Front. Immunol. 3:20. doi: 10.3389/fimmu.2012.00020
Ytrestøyl, T., Aas, T. S., and Asgard, T. (2015). Utilisation of feed resources in production of Atlantic salmon (Salmo salar) in Norway. Aquaculture 448, 365–374. doi: 10.1016/j.aqrep.2019.100216
Keywords: gilthead sea bream, functional feed, alternative dietary protein, RNA-seq, immunity, circular feeds, aquafeed
Citation: Konstantinidis I, Firmino JP, Ruiz A, Iñarra B, San Martin D, Estévez A, Fernandes JMO and Gisbert E (2022) Brewer’s Spent Dry Yeast Modulates Immunity in Gilthead Sea Bream (Sparus aurata). Front. Mar. Sci. 9:857592. doi: 10.3389/fmars.2022.857592
Received: 18 January 2022; Accepted: 28 February 2022;
Published: 07 April 2022.
Edited by:
Ikram Belghit, Norwegian Institute of Marine Research (IMR), NorwayReviewed by:
Gonzalo Martinez-Rodriguez, Department of Marine Biology and Aquacuture, Institute of Marine Sciences of Andalusia (CSIC), SpainYulema Valero Valero, University of Murcia, Spain
Copyright © 2022 Konstantinidis, Firmino, Ruiz, Iñarra, San Martin, Estévez, Fernandes and Gisbert. This is an open-access article distributed under the terms of the Creative Commons Attribution License (CC BY). The use, distribution or reproduction in other forums is permitted, provided the original author(s) and the copyright owner(s) are credited and that the original publication in this journal is cited, in accordance with accepted academic practice. No use, distribution or reproduction is permitted which does not comply with these terms.
*Correspondence: Enric Gisbert, ZW5yaWMuZ2lzYmVydEBpcnRhLmVz