- 1Department of Research Infrastructures for Marine Biological Resources, Stazione Zoologica Anton Dohrn, Naples, Italy
- 2Department of Biology, University of Naples Federico II, Naples, Italy
- 3Department of Integrative Marine Ecology, Stazione Zoologica Anton Dohrn, Naples, Italy
- 4Department of Life and Environmental Sciences, Polytechnic University of Marche, Ancona, Italy
- 5Department of Sciences and Engineering of Materials, Environment and Urbanistic, Polytechnic University of Marche, Ancona, Italy
- 6Department of Sciences and Department of Biological and Environmental Sciences and Technologies, University of Salento, Lecce, Italy
Polychaetes of the genus Sabellaria (Annelida, Sabellariidae) are gregarious bioconstructors that build reefs by assembling rigid tubes with sand grains in shallow waters. Sabellarid bioconstructions provide important ecosystem services such as sediment stabilization, water filtration and the mitigation of coastal erosion as well as nursery areas, shelter and feeding grounds for several marine species. Moreover, sabellarid reefs are exposed to both natural and anthropogenic disturbance and are therefore listed by international directives among the marine habitats deserving protection. We conducted a pilot study to assess the feasibility of habitat restoration with the sabellarid reef through a novel transplantation method. Fragments of S. spinulosa reef were collected at 1 m depth, fixed using epoxy putty into terracotta vases and then attached on the landward side of the two breakwaters in a coastal marine area enclosed in a Site of National Interest (SNI) of the central-western Adriatic (Mediterranean Sea). Overall, 14 of the 24 transplanted fragments (54.2%) survived during the study period (17 months). The total area of the transplanted reef fragments reduced during the early phase, appearing stable toward the end of the experiment. The transplantation method resulted effective given the survival rate observed, however, we did not observe the expected increase in the reef surface. Small-scale variation in environmental conditions such as organic load, sediment granulometry and hydrodynamics might have affected the growth capacity of the transplanted reef fragments. Further studies considering the microscale environmental requirements of this species are needed to better understand the feasibility of sabellarid reef restoration and its large-scale implementation.
Introduction
Marine biogenic reefs are bioconstructions typically built up by calcifying benthic organisms with calcareous structures (e.g., calcareous algae, oysters, corals, gastropods, and serpulid polychaetes), and resulting in complex tridimensional formations. Bioconstructions, however, also originate from organisms with sand-binding and cementing capacities, such as the sabellarid polychaetes, that can actively build up reefs (Ingrosso et al., 2018). In fact, polychaetes of the genus Sabellaria (Annelida, Sabellariidae) are gregarious bioconstructors widely distributed worldwide (Kirtley, 1994). They build reefs in shallow waters of various morphologies (mushrooms, pillows, barriers, and platforms) by assembling rigid tubes using sand grains (Dubois et al., 2002; Pearce, 2014). After the pelagic phase, larvae settle and metamorphosize on hard substrates (e.g., shells or rocks). Provided the availability of sand in the surroundings, the worm collects sediment particles with its tentacles. The building organ, a horseshoe-shaped structure that sorts sediment grains by size, uses these grains to create a rigid tube. The sand grains are in fact cemented by proteinaceous adhesives and placed on the outer edge of the tube (Vovelle, 1965).
Sabellarid bioconstructions provide important ecosystem services such as sediment stabilization, water filtration and the mitigation of coastal erosion (Naylor and Viles, 2000; Hendrick and Foster-Smith, 2006; Dubois et al., 2009; Desroy et al., 2011; Jones et al., 2020). Moreover, these complex three-dimensional structures provide nursery and feeding grounds for several invertebrate and fish species as well as a variety of microhabitats for benthic invertebrates, thus supporting coastal biodiversity (Dubois et al., 2002; Pearce et al., 2007; Plicanti et al., 2016; Schimmenti et al., 2016; Bertocci et al., 2017a; Gravina et al., 2018; Bonifazi et al., 2019) and ecosystem functioning (Jones et al., 2020; Muller et al., 2021).
Sabellarid reefs can persist for many years although they are highly dynamic structures with cyclical phases of construction and destruction (Gruet, 1986; Porras et al., 1996; Lisco et al., 2017; Gravina et al., 2018). Reef’s growth occurs both for the increase in the worm size and for the recruitment of planktonic larvae that settle preferentially on tubes of conspecific adults (Dubois et al., 2002; Ingrosso et al., 2018).
Reefs are exposed to both natural and anthropogenic disturbance. Storms and extreme fluctuation of environmental variables (i.e., salinity, temperature, hydrodynamics) may affect these biogenic structures (Tillin and Jackson, 2018). However, the main threats for sabellarid reef conservation are human activities, especially those that cause mechanical disturbance. Reefs can be significantly damaged by trampling, the use of fishing gears (beam-trawlers), the collection of sabellarid worms used as fishing bait, and harvesting of commercial species associated to the reef (e.g., mussels and oysters) (Dubois et al., 2002; Plicanti et al., 2016; Van der Reijden et al., 2019). Moreover, the combined effects of natural and anthropogenic stressors, may determine detrimental effects on the dynamics of these biogenic structures (Bertocci et al., 2017b). After disturbance, the natural recovery of damaged reefs may occur (Vorberg, 2000; Pearce et al., 2007; Plicanti et al., 2016), albeit extreme climate events may determine long term effects on the reef persistence (Firth et al., 2015).
Because of their vulnerability, Sabellaria reefs are designated as “Special Area of Conservation” in the Annex I of the EC Habitats Directive (Council Directive EEC/92/43 on the Conservation of Natural Habitats and of Wild Fauna and Flora). Despite their fundamental ecological role, however, sabellarid reefs are listed as “Data Deficient” in the European Red List of Habitats by the IUCN (Gubbay et al., 2016). In the Mediterranean Sea the genus Sabellaria is represented by the honeycomb worm S. alveolata (Linnaeus, 1767) and the ross worm S. spinulosa (Leuckart,1849). Along the Italian Peninsula biogenic reefs formed by S. alveolata are reported along the western coast including Liguria (Delbono et al., 2003), Latium (La Porta and Nicoletti, 2009), Tuscany (Casoli et al., 2019), Campania (Sanfilippo et al., 2020) and around Sicily (Bertocci et al., 2017a; Sanfilippo et al., 2020), while S. spinulosa reefs are exclusively reported in the Adriatic Sea along the coast of Apulia (Lezzi et al., 2015; Lisco et al., 2017; Gravina et al., 2018), Abruzzo, Marche and Emilia Romagna (Ingrosso et al., 2018).
Albeit the natural recovery of damaged marine ecosystems following management and protection actions is desirable, it may not occur, or it may be a long-lasting process (Montefalcone et al., 2009; Possingham et al., 2015; Colletti et al., 2020). Direct interventions like restoration actions are thus necessary when the resilience of natural systems is seriously compromised (Lotze et al., 2006). Ecological restoration is the process of assisting the recovery of an ecosystem that has been degraded, damaged, or destroyed by human activities, bringing it back as close as possible to its undisturbed state (Society for Ecological Restoration International Science, and Policy Working Group, 2004). Recently, ecological restoration of marine habitats and ecosystems is receiving greater attention as a tool for preserving marine biodiversity and rehabilitating ecosystem functioning (Merces-project.eu, 2021).1 It, indeed, is a global project launched by the United Nations for the decade 2021–2030 (Decade on Ecosystem Restoration, 2021)2 and is an integral part of the European Green Deal and the Mission Starfish 2030.3 So far, some efforts have been made to restore degraded and threatened marine habitats (e.g., Boström-Einarsson et al., 2020; Cebrian et al., 2021; Montseny et al., 2021). In the Mediterranean Sea, several studies focused on the restoration of coralligenous formations (Fava et al., 2010; Forcioli et al., 2011; Montero-Serra et al., 2017; Topçu et al., 2018; Montseny et al., 2019; Pagès Escolà et al., 2020; Casoli et al., 2021), seagrass meadows (Pirrotta et al., 2015; Alagna et al., 2019; Da Ros et al., 2021; Mancini et al., 2021; Piazzi et al., 2021; Ventura et al., 2021), macroalgal forests (De La Fuente et al., 2019; Tamburello et al., 2019), and other vulnerable habitat-forming species (Baldacconi et al., 2010; Musco et al., 2017). Despite their ecological role and fragility, sabellarid reef habitats have been neglected in restoration studies so far. In addition, due to its brittleness, the biogenic structure produced by sabellarid worms appears hardly transplantable by direct translocation and fixation as for other bioconstructions such as corals (e.g., Musco et al., 2017). Therefore, an ad hoc method to avoid loss or disruption of the transplanting is required.
By this pilot study, we aim to test the feasibility of restoration of sabellarid reefs in a Site of National Interest (SNI) of the central-western Adriatic (Mediterranean Sea), off-limits to any human activity since 2002 and where priority actions of environmental remediation are required by governmental laws due the high level of degradation of the area (Corinaldesi et al., 2022). We aim to test if transplantation is a feasible restoration method for sabellarid bioconstructions. Provided the effectiveness of the transplantation technique herein developed, we hypothesized that transplanted fragments could represent cores for the formation of new reefs.
Materials and Methods
Study Area
The study area is located at Falconara Marittima (43° 39′ 02.5″ N, 13° 21′ 11.1″ E) along the western coast of the central Adriatic Sea in the Marche region (Italy, Mediterranean Sea). From 1919 to 1990 the area suffered from anthropogenic impact caused by several industrial activities that contaminated the soil and the groundwater with pyrite, phosphorus, heavy metals, fluorides, hydrocarbons and PAHs. At present, no evident effects of pollution on the native marine benthic biota are reported (see Corinaldesi et al., 2022 for further details). The area is mainly sandy, and several breakwaters protect the coast. The area is fed by fluvial sediments from the mouth of the Esino river and is subject to heavy storms, especially in winter, with a prevalence of turbulence from southeast. The breakwater barriers were built to counteract coastal erosion due to the extraction of aggregates in the river bed and for the construction of the embankment serving the refinery plant on the right bank of the mouth (Mancinelli et al., 2005). The transplant experiment was set up in September 2019 and monitored until February 2021 in two breakwaters (hereafter site A and site B), approximately 1.25 km away from each other. The two breakwaters are about 80 m long and are made of carbonate boulders, lasting about 100 m away from the coast (Figure 1). The benthic assemblage associated with hard substrates in the area, including the considered breakwaters, are mainly represented by mussel and oyster beds mixed with algae and small sparse sabellarid formations.
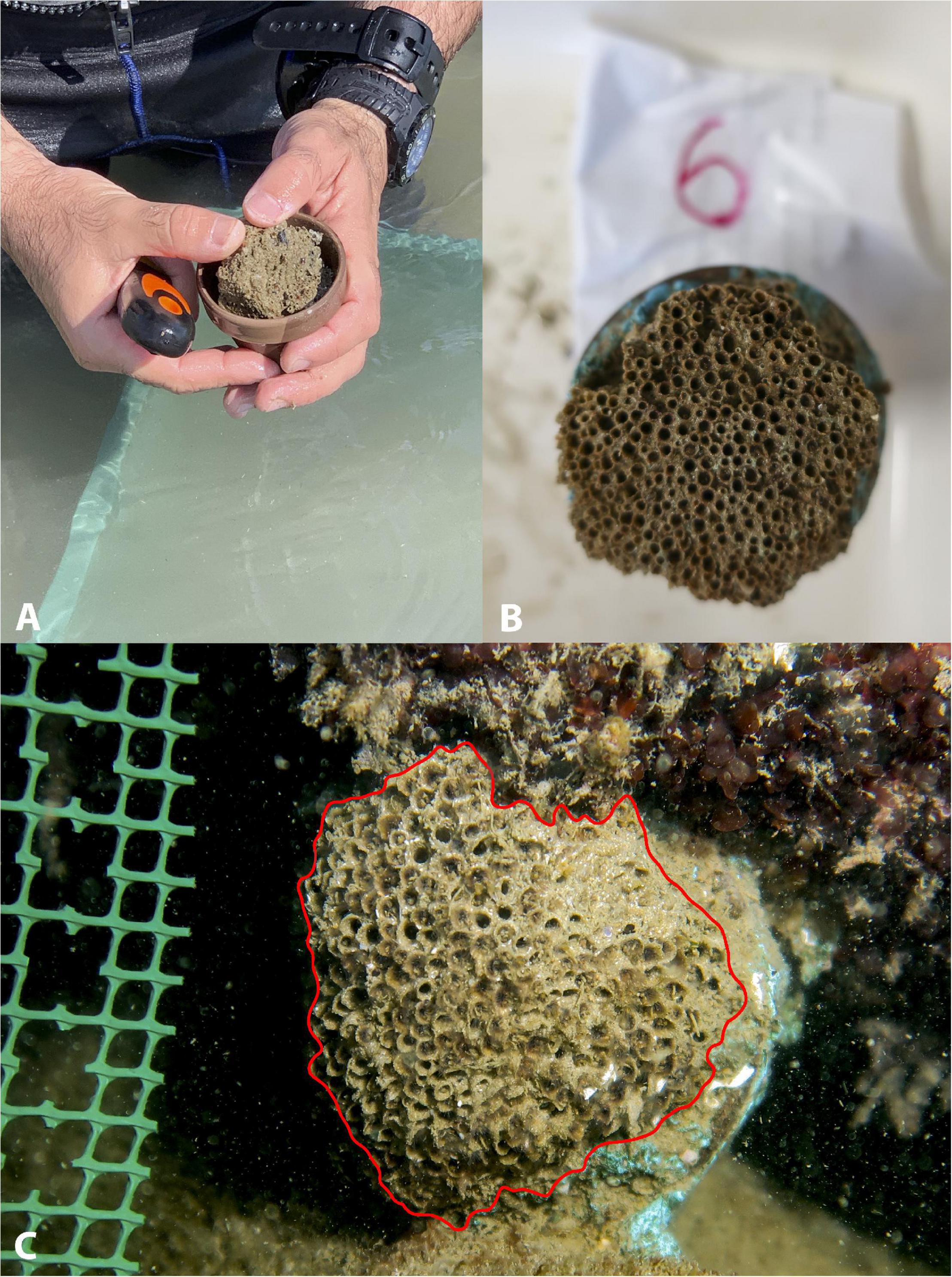
Figure 1. Fixation of a sabellarid reef fragment inside a vase (A). Translocation unit (B). Method used to calculate the sabellarid fragment surface by ImageJ using a reference greed (C).
Field Activities and Transplantation Method
In September 2019, fragments of S. spinulosa reef were collected at 1 m depth from sabellarid formations growing associated with coastal-defense carbonate boulders located alongshore in the study area. The fragments were transported in aerated seawater tanks in the facilities of the Polytechnic University of Marche. Once in the laboratory, fragments were fixed using bicomponent epoxy putty (Subcoat S, Veneziani Yachting Italy) into terracotta vases (diameter 7 cm, height 6 cm). A total of 24 translocation units (i.e., vase plus sabellarid fragment) were set up. After 24 h, once the fragments appeared well fixed into the vases, the translocation units were transported in aerated tanks to the study area and attached with epoxy putty on the landward side of the two selected breakwaters, named site A and site B (Figure 2). Site A and site B were respectively located outside and within the SNI area. At both sites, 12 translocation units were fixed by SCUBA divers, at 1–2 m depth, laying approximately 1 m from the sandy bottom (Figure 1).
A crucial issue for the success of this transplant experiment is the effective anchoring of the translocation units able to persist to local hydrodynamic conditions and avoid detachment. The underwater fixation of transplants using epoxy putty is a critical phase in transplantation experiments since transplants may be lost due to non-effective hardening of the bicomponent mixture and the relatively long time needed for its complete hardening (Casoli et al., 2021). This is particularly critical in shallow waters as in the present case study. We did not attach the species directly to the substrate because of the brittleness of the biogenic structure that prevented the bicomponent putty to effectively adhere, especially under the considered experimental constraints. Since our experiment required the use of epoxy putty during two phases (i.e., the fixation within the vase and the fixation of the transplant unit to the boulders) we followed a protocol similar to the one used by Musco et al. (2017) for the transplant of coral nubbins, in order to limit the risk of fragment loss in the first of the two critical phases. Moreover, to further reduce the risk of loss, each terracotta vase was fixed in the crevice between two boulders. To calculate the sabellarid fragment surface, the translocation units were photographed frontally together with a piece of rigid plastic greed (mesh size 1 cm) deployed close to the units in order to obtain the metric reference for the subsequent analyses. Pictures were taken using a Canon G7XII in Nauticam housing equipped with Inon S-2000 strobe soon after the transplant units were fixed to the substrate in September 2019 (T0) and, subsequently, in February 2020 (T1), July 2020 (T2), October 2020 (T3), and February 2021 (T4). Despite photogrammetric methods resulted effective to evaluate the three-dimensional dynamics of sabellarid reefs (Ventura et al., 2020), the experimental constraints of the present study (i.e., the lack of space between boulders, and necessary for circular shooting), led us to use the fragment surface as a proxy of the transplant growth instead of its three-dimensional shape.
During each sampling occasion the number of vases left counted to check for the effectiveness of the anchoring method. The number of alive sabellarid fragments (i.e., hosting alive sabellarid worms) was also registered at each sampling time. Per each sampling time, the surface area of the transplanted reef fragments was calculated using the Polygon Selection function of the ImageJ software to draw a polygon around the area including all the tubes hosting alive sabellarid worms and considering the metric reference used during shooting for calculating the fragment surface (Figure 1; Abramoff et al., 2004).
Data Analysis
To check for the overall efficacy of the anchoring method, the persistence of the vases was calculated as the ratio between the number of vases found at T1, T2, T3, and T4, and the original number of vases deployed at T0 (Figure 3 and Table 1). The survival of the reef fragments was calculated as the ratio between the number of transplanted fragments found alive at T1, T2, T3, and T4, and the original number of transplanted fragments (Table 1). Differences in the surface area of fragments among sampling times and between the two sites were tested using a 2-way ANOVA. The analyses were carried out using the software PRIMER 7.0.20 with the add-on PERMANOVA + (Anderson et al., 2008).
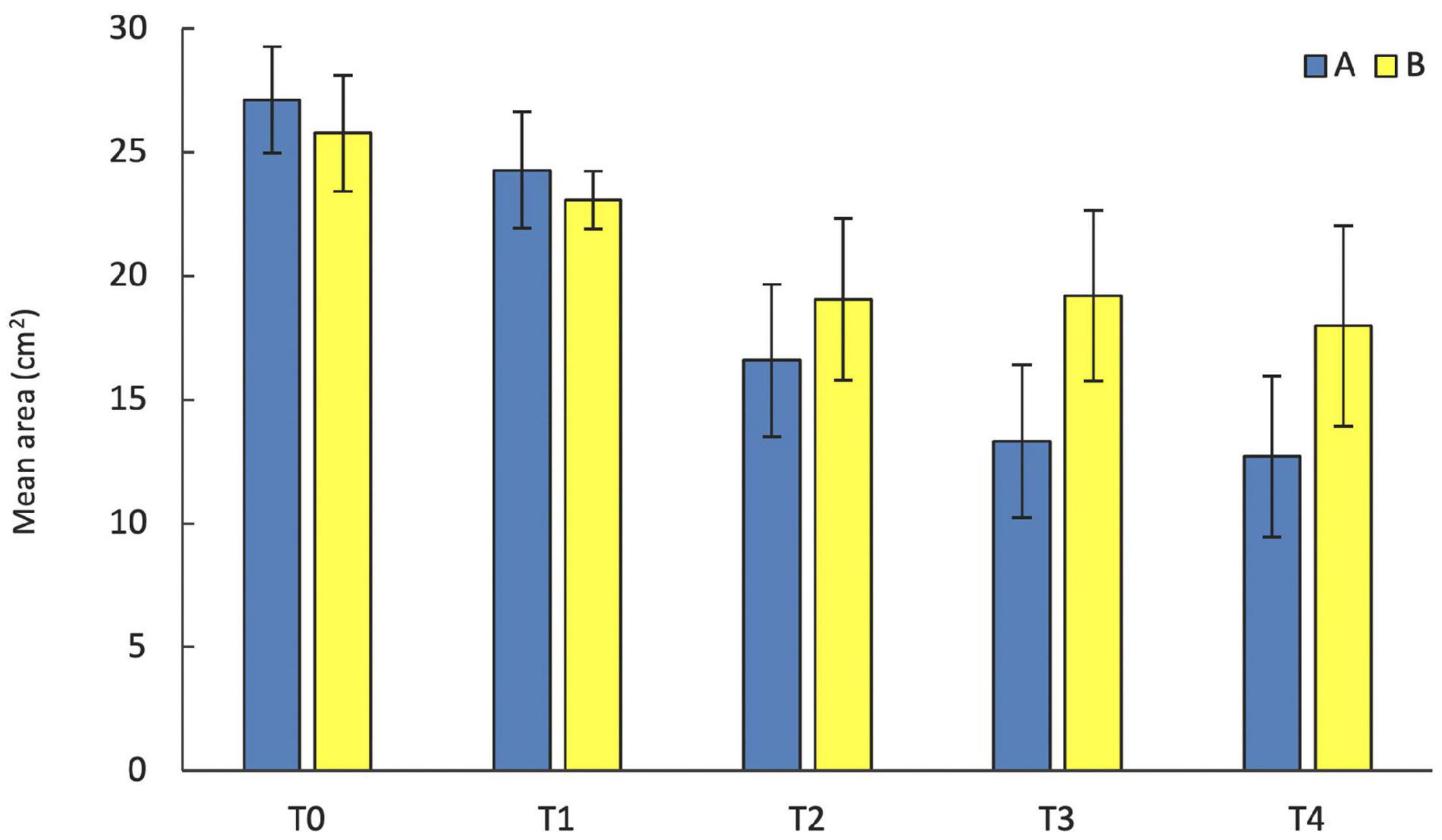
Figure 3. Mean area (± standard error) of S. spinulosa reef fragments sampled at the two analyzed sites during five sampling times (T0 = September, T1 = February, T2 = July, T3 = October, and T4 = February).
Results
The persistence percentage of the vases was 83.33% both at site A and B since 10 of the 12 deployed vases were found attached to the substrate at both sites (Table 1).
Overall, 14 of the 24 S. spinulosa reef transplanted fragments (54.2%) survived during the study period (17 months). At site A, the percentage of alive fragments that lasted for the whole study period was 75%, while at site B it was 41.67%. Of the 10 lost reef fragments, 6 were not found inside the vases and 4 were lost with their vases.
The temporal variation in the area of the transplanted fragments between the two sites was analyzed considering the 14 fragments that survived for the whole study period. Overall, the general pattern reveals a progressive reduction of the area of the transplanted reef fragments. In fact, at T0 the average area was 26.65 ± 1.57 cm2, (mean ± SE). It decreased to 23.86 ± 1.53 cm2 at T1, 17.46 ± 2.24 cm2 at T2, 15.42 ± 2.39 cm2 at T3 and 15.59 ± 2.54 at T4. The pattern was similar at the two sites, albeit the reduction of the fragment area appeared larger at site A than at site B (Figure 3). At the end of the experiment 5 of the 14 fragments (3 in site A, 1 in site B), although evidently including alive worms, showed less than 20% of the initial surface. The ANOVA revealed no significant difference in the reef fragment area between the two considered sites, while significant differences among times were detected (Table 2). The pairwise comparison between sampling times at each sampled site revealed no significant difference in the fragment area between T0 and T1 at site A, whilst significant differences were detected when these two sampling times were pairwise compared with T2, T3, and T4. No significant differences in the fragment area were detected at site B in the pairwise comparison of the 5 sampling times (Table 3).
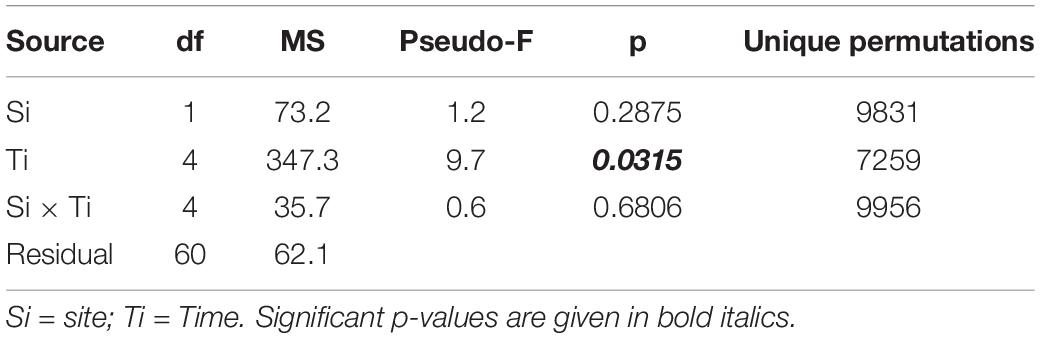
Table 2. Results of 2-way ANOVA testing for difference in fragments mean area (cm2) among location and time of the year.
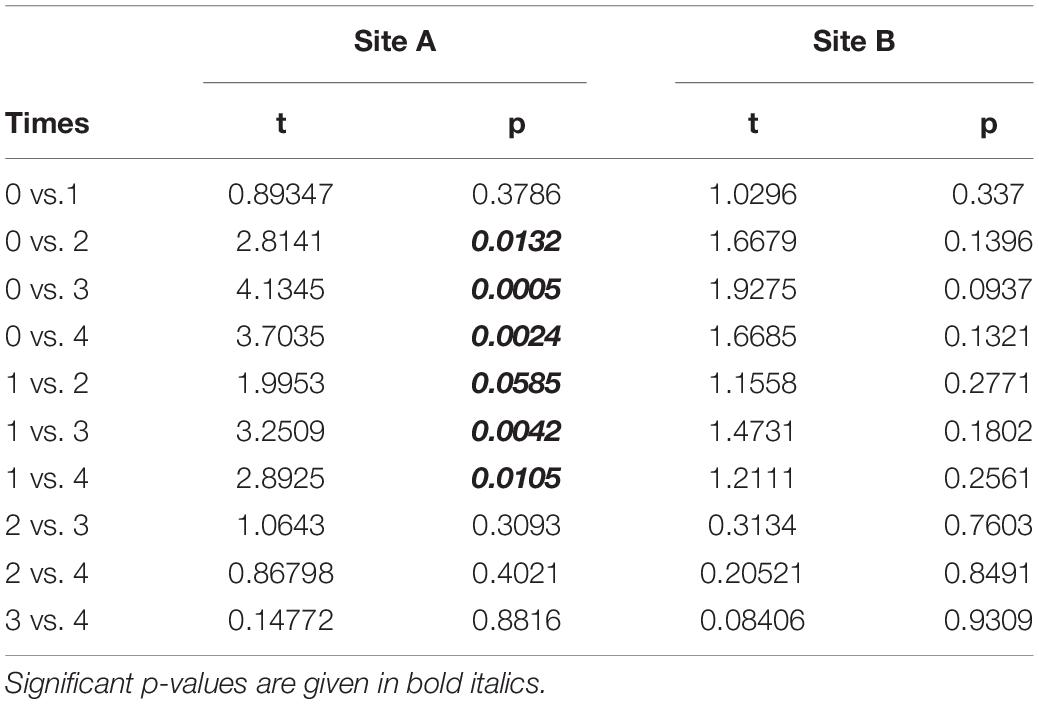
Table 3. Pairwise comparison testing differences in fragments mean area (cm2) between sampling times at the two analyzed sites.
Discussion
To the best of our knowledge, this study represents the first restoration attempt of the sabellarid reef habitat. This study had the double aim to test a suitable method for the translocation of worm reef portions as well as to verify if sabellarid reef restoration represents a feasible option to restore degraded habitats and subject to anthropogenic impacts. As far as the proposed method is concerned, the use of terracotta vases involves two critical steps: (1) the fixation of the fragment into the vase and (2) the fixation of the translocation unit (vase plus fragment) to the rocky substrate in the field. In both cases the method appeared effective. As regards the persistence of the translocation units, twenty vases (83.33%) were found anchored to the breakwater boulders at the end of the experiment, while as regards the persistence of the fragments within the vases, only 2 fragments (9.1%) were lost at T1. The subsequent loss of other fragments was probably due to erosion rather than to the effectiveness of the fixing method, since the total surface area of the remnant reef fragments showed a gradual reduction, especially from T0 to T2. In fact, during the period between T1 and T2 (from February to July 2020) the reduction of the surface area was higher possibly due to seasonal dynamics of the sabellarid reef that usually undergoes erosion during winter also because of adverse climatic conditions (Lisco et al., 2021). The fact that the transplant units persisted after winter suggests that the translocation method herein used may be effective for restoration purposes. It should also be noted that variation in the erosion rate appeared site dependent. After July (T2) translocation units at site B appeared more stable than at site A, where the reduction of the surface area of the reef fragments appeared higher. Since the two sites apparently differed only for their position with respect to the SNI area, while environmental conditions such as depth and exposition were similar and there was no difference in the way the units were fixed, the observed variation could be due to small scale alongshore variation in environmental conditions (e.g., sediment availability, hydrodynamics, and wave action) which may have differently influenced the growth and erosion rates of the fragments at the two sites. In fact, factors such as hydrodynamics and sedimentation rate play a dual role in the reef formation that is inhibited by intense waves and currents that cause erosion, albeit water movement is necessary to keep the sediment in suspension and make it available for the reef growth (Jackson and Hiscock, 2008; Davies et al., 2009).
No significant increase nor reduction of the reef surface area was observed throughout the rest of the experiment, although the growth phase of Sabellaria reef in the Mediterranean Sea is reported to occur during spring-summer both due to somatic growth and recruitment (Lisco et al., 2017, 2021; Gravina et al., 2018). This may suggest that the erosion process, initially attributable to the effect of winter climatic conditions stopped after winter but it was not counteracted by spring growth possibly due to the natural rearrangement of the biogenic structures adapting to the local environmental conditions at the transplant sites. Nonetheless, the reduction of the average fragment area appeared to stop at the end of the experiment (T3, T4) at both sites, suggesting that the remnant transplanted fragments reached an equilibrium with the surrounding system. Moreover, it should be considered that the method herein used for the growth rate assessment, specifically the measurement of the area by frontal photographic sampling, may have underestimated the actual three-dimensional growth, at least for part of the transplanted fragments (see Figure 4), since it was not possible to apply photogrammetric methods that resulted effective in the analysis of sabellarid reefs (Ventura et al., 2020). Although the experiment allowed us to record a good survival rate of the transplanted fragments (54% including all fragments containing living worms, 37.5% if fragments having less than 20% of the initial surface are excluded from the count), it is a matter of fact that we did not observe the expected increase in the reef surface, and in some cases the reduction of the surface was particularly relevant.
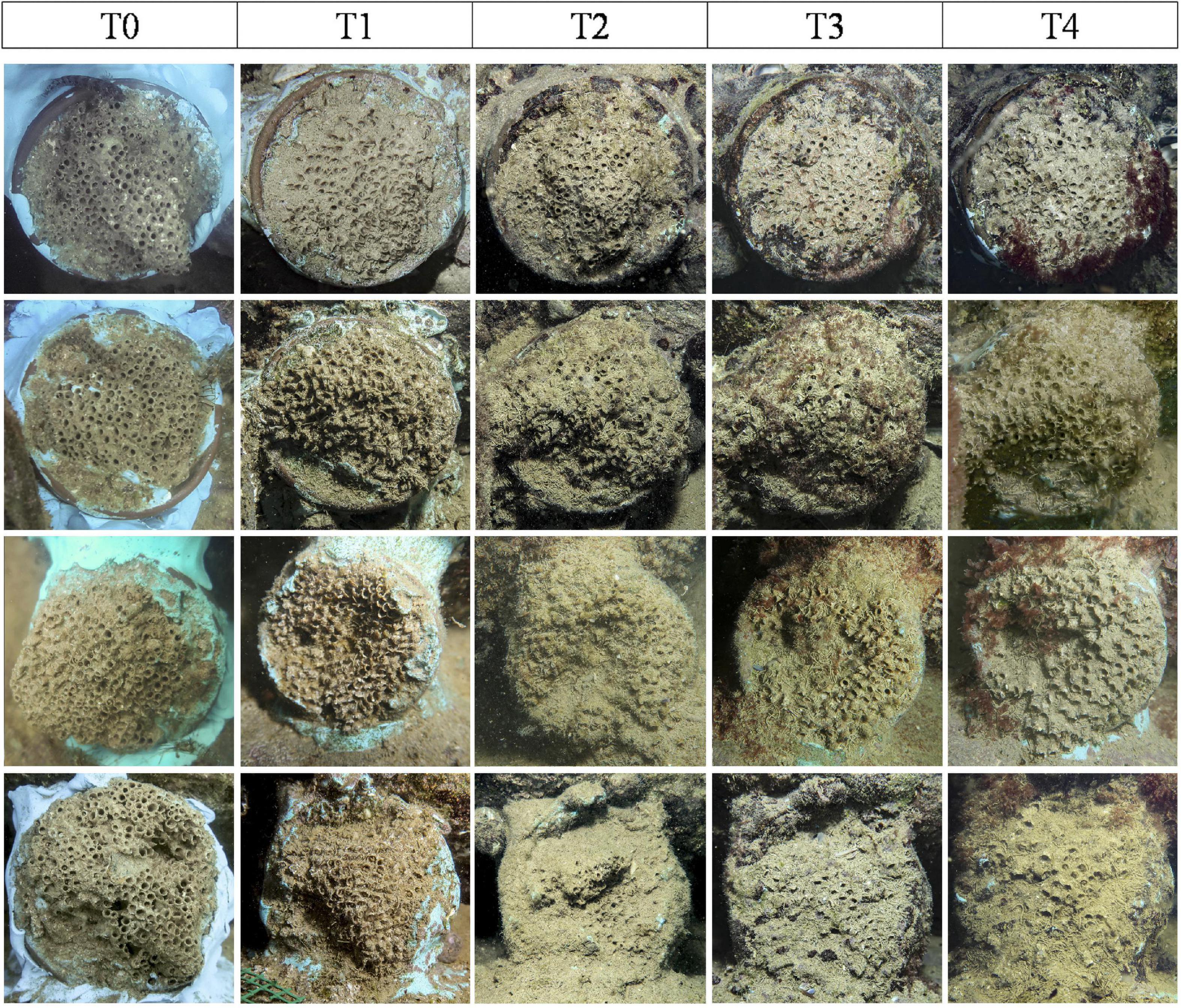
Figure 4. Example of four translocation units monitored during the five experimental times (T0 = September, T1 = February, T2 = July, T3 = October, and T4 = February).
Several factors may have determined the observed lack of growth. It is well known that sabellarid reefs may suffer from the effect of stressors and their combination (Gibb et al., 2014; Bertocci et al., 2017b). In this experiment, the sabellarid reef fragment may have suffered from manipulation as well as from translocation from the donor to the recipient sites which might have had a combined effect on the growth capacity of the fragments. We can exclude an eventual effect of the recipient rocky substrate since it is similar to the carbonatic rock of the donor site, whilst the epoxy putty might have had a role, albeit it was rapidly colonized by encrusting organisms and tubes of S. spinulosa were observed to grow on it (see Figure 4). We exclude that the sabellarid reef growth was affected by anthropogenic contamination of the SNI area as this has been reported to be generally lower than that expected to induce harmful biological effects (Corinaldesi et al., 2022). On the contrary, the fixation of the transplant units in a sheltered microenvironment, such as the crevice between two close boulders, might have influenced the results. In fact, we hypothesize that small scale variation in environmental conditions may influence the growth capacity of the sabellarid reefs although we did not measure abiotic variables such as organic load, sediment granulometry, hydrodynamics. It appears therefore crucial for future experiments of sabellarid reef restoration to consider the microscale environmental features of the recipient sites to provide the most suitable conditions for the development of the restored reefs.
Conclusion
This study provides a translocation method potentially suitable for the restoration of the sabellarid reef habitats. Sabellaria spinulosa appeared tolerant to stress due to manipulation and translocation phases, appearing therefore potentially eligible for restoration actions. The species has shown a good survival rate in respect to the transplant phases, although we did not register the expected growth. Further studies considering the microscale environmental requirements of this species are necessary to better understand the feasibility of reef restoration.
Data Availability Statement
The raw data supporting the conclusions of this article will be made available by the authors, without undue reservation.
Author Contributions
CC and LM conceived the study. GF, CC, and LM defined the experimental design and sampling strategy. GF, AC, BS, and ML participated in the sampling activity. GF, AC, BS, LM, and CC contributed to data elaboration and interpretation. LM, GF, AC, and BS drafted the first version of the manuscript. All authors contributed to manuscript preparation and to the final version.
Funding
This study has been supported by the project BIOBLUTECH “Blue Biotechnologies for restoring marine ecosystems of the contaminated Site of National Interest (SIN) ex Montedison (Falconara M.ma), ID 9204 supported by Cariverona Foundation.
Conflict of Interest
The authors declare that the research was conducted in the absence of any commercial or financial relationships that could be construed as a potential conflict of interest.
Publisher’s Note
All claims expressed in this article are solely those of the authors and do not necessarily represent those of their affiliated organizations, or those of the publisher, the editors and the reviewers. Any product that may be evaluated in this article, or claim that may be made by its manufacturer, is not guaranteed or endorsed by the publisher.
Acknowledgments
We thank Fabrizio Torsani, Lisa Pola, and Stefano Varrella for participation in the sampling activities.
Footnotes
- ^ http://www.merces-project.eu/
- ^ https://www.decadeonrestoration.org/
- ^ https://ec.europa.eu/info/publications/mission-starfish-2030-restore-our-ocean-and-waters_en
References
Abramoff, M. D., Magalhaes, P. J., and Ram, S. J. (2004). Image processing with ImageJ. Biophot. Int. 11:7.
Alagna, A., D’Anna, G., Musco, L., Fernández, T. V., Gresta, M., Pierozzi, N., et al. (2019). Taking advantage of seagrass recovery potential to develop novel and effective meadow rehabilitation methods. Mar. Pollut. Bull. 149:110578. doi: 10.1016/j.marpolbul.2019.110578
Anderson, M. J., Gorley, R. N., and Clarke, K. R. (2008). Permanova+ for primer: guide to software and statistical methods. Plymouth: PRIMER-E.
Baldacconi, R., Cardone, F., Longo, C., Mercurio, M., Marzano, C. N., Gaino, E., et al. (2010). Transplantation of Spongia officinalis L. (Porifera, Demospongiae): a technical approach for restocking this endangered species. Mar. Ecol. 31, 309–317. doi: 10.1111/j.1439-0485.2009.00299.x
Bertocci, I., Badalamenti, F., Lo Brutto, S., Mikac, B., Pipitone, C., Schimmenti, E., et al. (2017a). Reducing the data-deficiency of threatened European habitats: Spatial variation of sabellariid worm reefs and associated fauna in the Sicily Channel, Mediterranean Sea. Mar. Environ. Res. 130:8. doi: 10.1016/j.marenvres.2017.08.008
Bertocci, I., Domínguez Godino, J. A., Freitas, C., Bio, A., and Domínguez, R. (2017b). Compounded perturbations in coastal areas: contrasting responses to nutrient enrichment and the regime of storm-related disturbance depend on life-history traits. Funct. Ecol. 31, 1122–1134. doi: 10.1111/1365-2435.12815
Bonifazi, A., Lezzi, M., Ventura, D., Lisco, S., Cardone, F., and Gravina, M. F. (2019). Macrofaunal biodiversity associated with different developmental phases of a threatened Mediterranean Sabellaria alveolata (Linnaeus, 1767) reef. Mar. Environ. Res. 145, 97–111. doi: 10.1016/j.marenvres.2019.02.009
Boström-Einarsson, L., Babcock, R. C., Bayraktarov, E., Ceccarelli, D., Cook, N., Ferse, S. C., et al. (2020). Coral restoration–A systematic review of current methods, successes, failures and future directions. PloS One 15:226631. doi: 10.1371/journal.pone.0226631
Casoli, E., Bonifazi, A., Ardizzone, G., Gravina, M. F., Russo, G., Sandulli, R., et al. (2019). Comparative analysis of mollusc assemblages from different hard bottom habitats in the central tyrrhenian sea. Diversity 11:74. doi: 10.3390/d11050074
Casoli, E., Ventura, D., Mancini, G., Cardone, S., Farina, F., Donnini, L., et al. (2021). Rehabilitation of Mediterranean animal forests using gorgonians from fisheries by-catch. Restor. Ecol. 30:e13465. doi: 10.1111/rec.13465
Cebrian, E., Tamburello, L., Verdura, J., Guarnieri, G., Medrano, A., Linares, C., et al. (2021). A roadmap for the restoration of mediterranean macroalgal forests. Front. Mar. Sci. 1456:9219. doi: 10.3389/fmars.2021.709219
Colletti, A., Savinelli, B., Di Muzio, G., Rizzo, L., Tamburello, L., Fraschetti, S., et al. (2020). The date mussel Lithophaga lithophaga: Biology, ecology and the multiple impacts of its illegal fishery. Sci. Total Environ. 2020:140866. doi: 10.1016/j.scitotenv.2020.140866
Corinaldesi, C., Bianchelli, S., Rastelli, E., Varrella, S., Canensi, S., Gambi, C., et al. (2022). The paradox of an unpolluted coastal site facing a chronically contaminated industrial area. Front. Mar. Sci. 8:813887. doi: 10.3389/fmars.2021.813887
Da Ros, Z., Corinaldesi, C., Dell’Anno, A., Gambi, C., Torsani, F., and Danovaro, R. (2021). Restoration of Cymodocea nodosa seagrass meadows: efficiency and ecological implications. Restor. Ecol. 29:13313. doi: 10.1111/rec.13313
Davies, A. J., Last, K. S., Attard, K., and Hendrick, V. J. (2009). Maintaining turbidity and current flow in laboratory aquarium studies, a case study using Sabellaria spinulosa. J. Exp. Mar. Biol. Ecol. 370, 35–40. doi: 10.1016/j.jembe.2008.11.015
De La Fuente, G., Chiantore, M., Asnaghi, V., Kaleb, S., and Falace, A. (2019). First ex situ outplanting of the habitat-forming seaweed Cystoseira amentacea var. stricta from a restoration perspective. PeerJ 7:e7290. doi: 10.7717/peerj.7290
Decade on Ecosystem Restoration (2021). Available online at: https://www.decadeonrestoration.org/ [Accessed January 12, 2021].
Delbono, I., Bianchi, C. N., and Morri, C. (2003). “Le biocostruzioni di Sabellaria alveolata come indicatori ambientali: area costiera fra Chiavari e Sestri Levante,” in Studi per la creazione di strumenti di gestione costiera: Golfo del Tigullio, ed. O. Ferretti (La Spezia: ENEA, Centro Ricerche Ambiente Marino).
Desroy, N., Dubois, S. F., Fournier, J., Ricquiers, L., Le Mao, P., Guerin, L., et al. (2011). The conservation status of Sabellaria alveolata (L.) (Polychaeta: Sabellariidae) reefs in the bay of mont-saint-michel. Aquat. Conserv. 21, 462–471. doi: 10.1002/aqc.1206
Dubois, S., Laurent, B., and Cognie, B. (2009). Feeding response of the polychaete Sabellaria alveolata (Sabellariidae) to changes in seston concentration. J. Exp. Mar. Biol. Ecol. 376, 94–101. doi: 10.1016/j.jembe.2009.06.017.7
Dubois, S., Retière, C., and Olivier, F. (2002). Biodiversity associated with Sabellaria alveolata (Polychaeta: Sabellariidae) reefs: effects of human disturbances. J. Mar. Biol. Assoc. UK 82:817. doi: 10.1017/s0025315402006185
Fava, F., Bavestrello, G., Valisano, L., and Cerrano, C. (2010). Survival, growth and regeneration in explants of four temperate gorgonian species in the Mediterranean Sea. Ital. J. Zool. 77, 44–52. doi: 10.1080/11250000902769680
Firth, L. B., Mieszkowska, N., Grant, L. M., Bush, L. E., Davies, A. J., Frost, M. T., et al. (2015). Historical comparisons reveal multiple drivers of decadal change of an ecosystem engineer at the range edge. Ecol. Evol. 5, 3210–3222. doi: 10.1002/ece3.1556
Forcioli, D., Merle, P. L., Caligara, C., Ciosi, M., Muti, C., Francour, P., et al. (2011). Symbiont diversity is not involved in depth acclimation in the Mediterranean sea whip Eunicella singularis. Mar. Ecol. Prog. Ser. 439, 57–71. doi: 10.3354/meps09314
Gibb, N., Tillin, H. M., Pearce, B., and Tyler-Walters, H. (2014). Assessing the sensitivity of Sabellaria spinulosa to pressures associated with marine activities. Peterborough: JNCC.
Gravina, M. F., Cardone, F., Bonifazi, A., Bertrandino, M. S., Chimienti, G., Longo, C., et al. (2018). Sabellaria spinulosa (Polychaeta, Annelida) reefs in the Mediterranean Sea: Habitat mapping, dynamics and associated fauna for conservation management. Estuar. Coast. Shelf Sci. 200, 248–257. doi: 10.1016/j.ecss.2017.11.017
Gruet, Y. (1986). Spatio-temporal changes of sabellarian reefs built by the sedentary polychaete Sabellaria alveolata (Linné). Mar. Ecol. 7, 303–319. doi: 10.1111/j.1439-0485.1986.tb00166.x
Gubbay, S., Sanders, N., Haynes, T., Janssen, J. A. M., Rodwell, J. R., Nieto, A., et al. (2016). European Red List of Habitats. Part 1. Marine Habitats. Luxembourg: Publications Office of the European Union.
Hendrick, V. J., and Foster-Smith, R. L. (2006). Sabellaria spinulosa reef: a scoring system for evaluating ‘reefiness’ in the context of the Habitats Directive. J. Mar. Biol. Assoc. UK 86, 665–678. doi: 10.1017/S0025315406013555
Ingrosso, G., Abbiati, M., Badalamenti, F., Bavestrello, G., Belmonte, G., Cannas, R., et al. (2018). Mediterranean bioconstructions along the Italian coast. Adv. Mar. Biol. 79, 61–136. doi: 10.1016/bs.amb.2018.05.001
Jackson, A., and Hiscock, K. (2008). “Sabellaria spinulosa Ross worm,” in Marine Life Information Network: Biology and Sensitivity Key Information Reviews, [on-line], eds H. Tyler-Walters and K. Hiscock (Plymouth: Marine Biological Association of the United Kingdom), doi: 10.17031/marlinsp.1133.1
Jones, A., Denis, L., Fournier, J., Desroy, N., Duong, G., and Dubois, S. (2020). Linking multiple facets of biodiversity and ecosystem functions in a coastal reef habitat. Mar. Environ. Res. 162:5092. doi: 10.1016/j.marenvres.2020.105092
Kirtley, D. W. (1994). A review and taxonomic revision of the family Sabellariidae Johnston, 1865 (Annelida; Polychaeta). Boston: Sabecon Press.
La Porta, B., and Nicoletti, L. (2009). Sabellaria alveolata (Linnaeus) reefs in the central Tyrrhenian Sea (Italy) and associated polychaete fauna. Zoosymposia 2, 527–536. doi: 10.11646/zoosymposia.2.1.36
Lezzi, M., Cardone, F., Mikac, B., and Giangrande, A. (2015). Variation and ontogenetic changes of opercular paleae in a population of Sabellaria spinulosa (Polychaeta: Sabellaridae) from the South Adriatic Sea, with remarks on larval development. Sci. Mar. 79, 137–150. doi: 10.3989/scimar.04127.19A
Lisco, S. N., Pierri, C., Lazic, T., Bonifazi, A., Gravina, M. F., Giangrande, A., et al. (2021). Sabellaria alveolata versus Sabellaria spinulosa reefs along the italiancoasts: a new methodological proposal to compare different growth models. Geosci 11:426. doi: 10.3390/geosciences11100426
Lisco, S., Moretti, M., Moretti, V., Cardone, F., Corriero, G., and Longo, C. (2017). Sedimentological features of Sabellaria spinulosa bioconstructions. Mar. Pet. Geol. 87, 203–212. doi: 10.1016/j.marpetgeo.2017.06.013
Lotze, H. K., Lenihan, H. S., Bourque, B. J., Bradbury, R. H., Cooke, R. G., Kay, M. C., et al. (2006). Depletion, degradation, and recovery potential of estuaries and coastal seas. Science 312, 1806–1809. doi: 10.1126/science.1128035
Mancinelli, A., Aminti, P. L., Archetti, R., Atzeni, A., Canu, V., Cappietti, L., et al. (2005). Opere di difesa realizzate con strutture sommerse ed a cresta bassa ad Alghero (SS), Perd’e Sali (CA), Marina di Massa (MS), Marina di Pisa (PI), Porto Recanati (MC), Marina di Montemarciano (AN), Lido di Dante (RA) e Pellestrina (VE). Studi Costieri, Dinamica e difesa dei litorali - Gestione integrata della fascia costiera 9, 7–50.
Mancini, G., Casoli, E., Ventura, D., Jona Lasinio, G., Belluscio, A., and Ardizzone, G. D. (2021). An experimental investigation aimed at validating a seagrass restoration protocol based on transplantation. Biol. Conserv. 264:109397. doi: 10.1016/j.biocon.2021.109397
Merces-project.eu (2021). Welcome to Merces | Merces. Available online at: http://www.merces-project.eu [Accessed December 1, 2021]
Montefalcone, M., Albertelli, G., Morri, C., Parravicini, V., and Bianchi, C. N. (2009). Legal protection is not enough: Posidonia oceanica meadows in marine protected areas are not healthier than those in unprotected areas of the northwest Mediterranean Sea. Mar. Pollut. Bull. 58, 515–519. doi: 10.1016/j.marpolbul.2008.12.001
Montero-Serra, I., Garrabou, J., Doak, D. F., Figuerola, L., Hereu, B., Ledoux, J. B., et al. (2017). Accounting for life-history strategies and timescales in marine restoration. Conserv. Lett. 11:e12341. doi: 10.1111/conl.12341
Montseny, M., Linares, C., Carreiro-Silva, M., Henry, L. A., Billett, D., Cordes, E. E., et al. (2021). Active ecological restoration of cold-water corals: Techniques, challenges, costs and future directions. Front. Mar. Sci. 1309:621151. doi: 10.3389/fmars.2021.621151
Montseny, M., Linares, C., Viladrich, N., Olariaga, A., Carreras, M., and Palomeras, N. (2019). First attempts towards the restoration of gorgonian populations on the Mediterranean continental shelf. Aquat. Conserv. 29, 1278–1284. doi: 10.1002/aqc.3118
Muller, A., Poitrimol, C., Nunes, F., Boye, A., Curd, A., Desroy, N., et al. (2021). Musical chairs on temperate reefs: Species turnover and replacement within functional groups explain regional diversity variation in assemblages associated with honeycomb worms. Front. Mar. Sci. 8:654141. doi: 10.3389/fmars.2021.654141
Musco, L., Prada, F., D’Anna, G., Galasso, N. M., Pipitone, C., Fernández, T. V., et al. (2017). Turning casualty into opportunity: fragmenting dislodged colonies is effective for restoring reefs of a Mediterranean endemic coral. Ecol. Eng. 98, 206–212. doi: 10.1016/j.ecoleng.2016.10.075
Naylor, L., and Viles, H. A. (2000). A temperate reef builder: an evaluation of the growth, morphology and composition of Sabellaria alveolata (L.) colonies on carbonate platforms in South Wales. Geol. Soc. Spec. Publ. 178, 9–19. doi: 10.1144/GSL.SP.2000.178.01.02
Pagès Escolà, M., Linares, C., Gómez Gras, D., Medrano, A., and Hereu, B. (2020). Assessing the effectiveness of restoration actions for Bryozoans: The case of the Mediterranean Pentapora fascialis. Aquat. Conserv. 30, 8–19. doi: 10.1002/aqc.3236
Pearce, B., Taylor, J., and Seiderer, L. (2007). Recoverability of sabellaria spinulosa following aggregate extraction. MESL 2007:880. doi: 10.13140/RG.2.2.34738.50880
Piazzi, L., Acunto, S., Frau, F., Atzori, F., Cinti, M. F., Leone, L. M., et al. (2021). Environmental engineering techniques to restore degraded posidonia oceanica meadows. Water 13:661. doi: 10.3390/w13050661
Pirrotta, M., Tomasello, A., Scannavino, A., Di Maida, G., Luzzu, F., Bellissimo, G., et al. (2015). Transplantation assessment of degraded Posidonia oceanica habitats: site selection and long-term monitoring. Mediterr. Mar. Sci. 16, 591–604. doi: 10.12681/mms.1045
Plicanti, A., Domínguez, R., Dubois, S. F., and Bertocci, I. (2016). Human impacts on biogenic habitats: effects of experimental trampling on Sabellaria alveolata (Linnaeus, 1767) reefs. J. Exp. Mar. Biol. Ecol. 478, 34–44. doi: 10.1016/j.jembe.2016.02.001
Porras, R., Bataller, J. V., Murgui, E., and Torregrosa, M. T. (1996). Trophic structure and community composition of polychaetes inhabiting some Sabellaria alveolata (L.) reefs along the Valentia Gulf coast, western Mediterranean. Mar. Ecol. 17, 583–602. doi: 10.1111/j.1439-0485.1996.tb00419.x
Possingham, H. P., Bode, M., and Klein, C. J. (2015). Optimal conservation outcomes require both restoration and protection. PLoS Biol. 13:e1002052. doi: 10.1371/journal.pbio.1002052
Sanfilippo, R., Guido, A., Insacco, G., Deias, C., Catania, G., and Reitano, A. (2020). Distribution of sabellaria alveolata (polychaeta sabellariidae) in the mediterranean sea: update and new findings. Zoosymposia. 19, 128–208. doi: 10.11646/ZOOSYMPOSIA.19.1.20
Schimmenti, E., Musco, L., Lo Brutto, S., Mikac, B., Nygren, A., and Badalamenti, F. (2016). Mediterranean record of Eulalia ornata (Annelida: Phyllodocidae) corroborating its fidelity link with the Sabellaria alveolata reef habitat. Mediterr. Mar. Sci. 17, 359–370. doi: 10.12681/mms.1485
Society for Ecological Restoration International Science, and Policy Working Group (2004). The SER international primer on ecological restoration. Tucson, AZ: Society for Ecological Restoration International.
Tamburello, L., Papa, L., Guarnieri, G., Basconi, L., Zampardi, S., Scipione, M. B., et al. (2019). Are we ready for scaling up restoration actions? An insight from Mediterranean macroalgal canopies. PloS One 14:e0224477. doi: 10.1371/journal.pone.0224477
Tillin, H. M., and Jackson, A. (2018). “Sabellaria alveolata reefs on sand-abraded eulittoral rock,” in Marine Life Information Network: Biology and Sensitivity Key Information Reviews, [on-line], eds H. TylerWalters and K. Hiscock (Plymouth: Marine Biological Association of the United Kingdom), doi: 10.17031/marlinhab.351.1
Topçu, N. E., Yilmaz, I., and ÖztürK, B. (2018). “Transplantation of yellow gorgonian fragments from a highly disturbed area,” in 3rd Mediterranean Symposium on the conservation of Coralligenous & other Calcareous Bio-Concretions. Antalya.
Van der Reijden, K. J., Koop, L., O’Flynn, S., Garcia, S., Bos, O., van der Sluis, C., et al. (2019). Discovery of Sabellaria spinulosa reefs in an intensively shed area of the Dutch Continental Shelf, North Sea. J. Sea Res. 144, 85–94. doi: 10.1016/j.seares.2018.11.008
Ventura, D., Dubois, S. F., Bonifazi, A., Jona Lasinio, G., Seminara, M., Gravina, M. F., et al. (2020). Integration of close-range underwater photogrammetry with inspection and mesh processing software: A novel approach for quantifying ecological dynamics of temperate biogenic reefs. Remote. Sens. Ecol. Conserv. 7, 169–186. doi: 10.1002/rse2.178
Ventura, D., Mancini, G., Casoli, E., Pace, D. S., Lasinio, G. J., Belluscio, A., et al. (2021). Seagrass restoration monitoring and shallow-water benthic habitat mapping through a photogrammetry-based protocol. J. Environ. Manag. 304, 114262. doi: 10.1016/j.jenvman.2021.114262
Vorberg, R. (2000). Effects of shrimp fisheries on reefs of Sabellaria spinulosa (Polychaeta). ICES J. Mar. Sci. 57, 1416–1420. doi: 10.1006/jmsc.2000.0920
Keywords: Sabellaria, habitat restoration, bioconstruction, environmental restoration, ross worm, honeycomb worm, Mediterranean sea
Citation: Franzitta G, Colletti A, Savinelli B, Lo Martire M, Corinaldesi C and Musco L (2022) Feasibility of the Sabellarid Reef Habitat Restoration. Front. Mar. Sci. 9:854986. doi: 10.3389/fmars.2022.854986
Received: 14 January 2022; Accepted: 23 February 2022;
Published: 14 March 2022.
Edited by:
Christina Pavloudi, George Washington University, United StatesReviewed by:
Juan Moreira Da Rocha, Autonomous University of Madrid, SpainDaniele Ventura, Sapienza University of Rome, Italy
Copyright © 2022 Franzitta, Colletti, Savinelli, Lo Martire, Corinaldesi and Musco. This is an open-access article distributed under the terms of the Creative Commons Attribution License (CC BY). The use, distribution or reproduction in other forums is permitted, provided the original author(s) and the copyright owner(s) are credited and that the original publication in this journal is cited, in accordance with accepted academic practice. No use, distribution or reproduction is permitted which does not comply with these terms.
*Correspondence: Luigi Musco, luigi.musco@unisalento.it
†These authors have contributed equally to this work and share last authorship