- 1Key Laboratory of Mariculture, Ministry of Education, Ocean University of China, Qingdao, China
- 2College of Information and Electrical Engineering, China Agricultural University, Beijing, China
- 3Function Laboratory for Marine Fisheries Science and Food Production Processes, Qingdao National Laboratory for Marine Science and Technology, Qingdao, China
The light spectrum varies with the altitude of the sun and shows different light colors in clear water. In this study, we aimed to investigate the response of juvenile steelhead trout Oncorhynchus mykiss (34.67 ± 2.69 g initial weight) under different light color conditions. The effects of different blue and red light combinations on plasma biochemical parameters, digestive enzyme activity, and RNA/DNA ratio were assessed in trout over 16 weeks. Six treatments were randomly assigned to 24 tanks with four replicates per treatment: a constant light intensity of 150 lx: 12 h white light then 12 h dark (12W); 12 h blue light then 12 h dark (12B); 12 h red light then 12 h dark (12R); 1.5 h blue light, 9 h red light, 1.5 h blue light, then 12 h dark (3B9R); 3 h blue light, 6 h red light, 3 h blue light, then 12 h dark (6B6R); and 12 h of both blue and red light then 12 h dark (T12BR). Fish exposed to the 3B9R light environment showed significantly increased plasma levels of total protein (TP), enhanced activities of midgut lipase, trypsin, and gastric lipase; and increased RNA content in the liver and muscle tissue to promote protein synthesis efficiency, thereby improving digestive and anabolic performance compared to fish in the other treatments. This indicates that steelhead trout have adapted well to such variable light conditions during long-term evolution. In contrast, trout exposed to the 6B6R light environment showed significant reductions in plasma glucose, TP, and triglyceride levels, decreased activity of gastrointestinal digestive enzymes, and reduced protein synthesis capacity in the muscle and liver, resulting in weakened digestive and anabolic performance. Furthermore, despite the high RNA content and RNA/DNA ratio in fish exposed to a 12R light environment, relatively high plasma cholesterol and triglycerides levels were observed, which might indicate oxidative stress. Therefore, this light is not considered suitable for long-term cultivation. In conclusion, the 3B9R treatment was the optimal light condition tested and can be used to improve the digestive and anabolic performance of steelhead trout.
Introduction
Fish growth is influenced by a combination of exogenous environmental factors and genetic background (Taylor et al., 2006). Light is a vital environmental factor that plays a central role, with light intensity (quantity), photoperiod (duration), and light color (spectrum) affecting the physiological indicators of digestion and metabolism in fish (Villamizar et al., 2011; Zhang et al., 2020a). Light can stimulate the visual organs of fish to produce certain biological information and can influence their physiological and biochemical characteristics by regulating the central nervous and endocrine systems (Ruchin, 2021).
Previous studies have shown that light intensity and photoperiod have significant effects on the digestive and anabolic performance of fish. For example, in groupers (Epinephelus coioides), a light intensity of 320–1150 lx significantly increased total protease, amylase, and lipase activities in the liver, stomach, and intestine, and decreased plasma cortisol levels (Wang et al., 2013; Wang et al., 2015). In contrast, Tian et al. (2015) reported that a light intensity above 400 lx could significantly increase plasma cortisol levels in blunt snout bream (Megalobrama amblycephala). Shan et al. (2008) found that an 18L:6D photoperiod could enhance the lipase activity of miiuy croaker (Miichthys miiuy). Similarly, Wei et al. (2019) showed that long photoperiods (> 16 h/day) could improve lipometabolism as well as increase plasma triglyceride and non-esterified fatty acid content in gibel carp (Carassius auratus). However, Li et al. (2021) found that the 24L:0D condition could significantly inhibit digestive enzyme activity in European sea bass (Dicentrarchus labrax L.).
Light color also has a significant effect on the digestive and anabolic performance of fish. Under blue and green light environments, accelerated digestive and anabolic performance has been reported for several species, including goldfish (Carassius auratus) (Noureldin et al., 2021), turbot (Scophthalmus maximus) (Wu et al., 2021), spotted halibut (Trachinotus blochii) (Mapunda et al., 2021), and olive flounder (Paralichthys olivaceus) (Zou et al., 2022). Conversely, red light environments can enhance the anabolic performance of fish, such as pikeperch (Sander lucioperca) (Baekelandt et al., 2019) and yellow perch (Perca flavescens) (Head and Malison, 2000). However, most studies have focused on the effect of a single light color on fish physiological and biochemical characteristics, whereas limited information is available regarding the influences of light color combinations on fish digestive and metabolic performance.
Based on our unpublished data, we found that red light color can be beneficial in improving the growth performance of steelhead trout, while blue light color can improve their immune performance. Generally, the growth and immune performance of fish may be cooperative. This phenomenon indicated that single light color may not be suitable for rearing steelhead trout. The altitude of the sun changes throughout the day, and the path length and spectral composition (light color) of light reaching a particular water layer vary with time (Wetzel, 1983; Ruchin, 2021). The light color within a given water layer tends to change from blue to red to blue over time. However, the effects of changes in underwater light color on the physiological and biochemical characteristics of fish remain unclear. Presumably, fish have acclimatized to changing light color environments as a result of long-term evolution. Providing farmed fish with a changing light color environment similar to that experienced in nature may be more conducive to their digestive and anabolic performance. To explore the physiological and ecological responses of juvenile steelhead trout to light color variation, we investigated the influence of various blue and red light combinations on plasma biochemical parameters, digestive enzyme activity, and muscle and liver RNA and DNA content to identify suitable light color environments.
Materials and Methods
Source and Acclimation of Fish
Triploid steelhead trout eyed eggs were purchased from Troutlodge, Inc. (Washington, USA). Prior to the experiments, the juvenile trout were acclimatized to a brackish saltwater environment (salinity: 14.2 ± 0.7) for 2 weeks. Fish were fed to apparent satiation twice daily at 08:00 and 18:30 with a commercial trout feed (Greatseven Inc., Qingdao, China) and maintained on 24 h oxygen supply and 12L:12D photoperiod.
Trial Design
For light evaluation, we utilized the mean light intensity values measured at the surface, middle, and bottom water layers as a baseline and established six light color treatments for 150 lx light intensity (12R, 12B, 12W, 3B9R, 6B6R, and T12BR). The light intensity was changed by adjusting the height of the light to the water surface. The light color regimes for the six treatments are listed in Table 1. The spectra of white light (full spectrum), blue light (peak at 454.9 nm), red light (peak at 614.8 nm), and mixed blue and red light (peaks at 454.9 nm and 614.8 nm, respectively) are shown in Figure 1. For all treatments, the photoperiod was a 12L:12D cycle (light period: 07:30 to 19:30). The light intensity and spectra were determined using a handheld illuminometer (PLA-300; Everfine Inc., Hangzhou, China). A packaged LED (COB) was used, which was designed and produced by Qingdao Lanchi Technology Company. The 16-week trial was based on a completely randomized block design with four replicates per treatment and with each replicate group comprising 20 fish per tank (380 L volume, 0.72 m height × 0.95 m diameter; white background color). Each tank was covered with a black light-absorbing cloth to prevent light contamination between treatments.
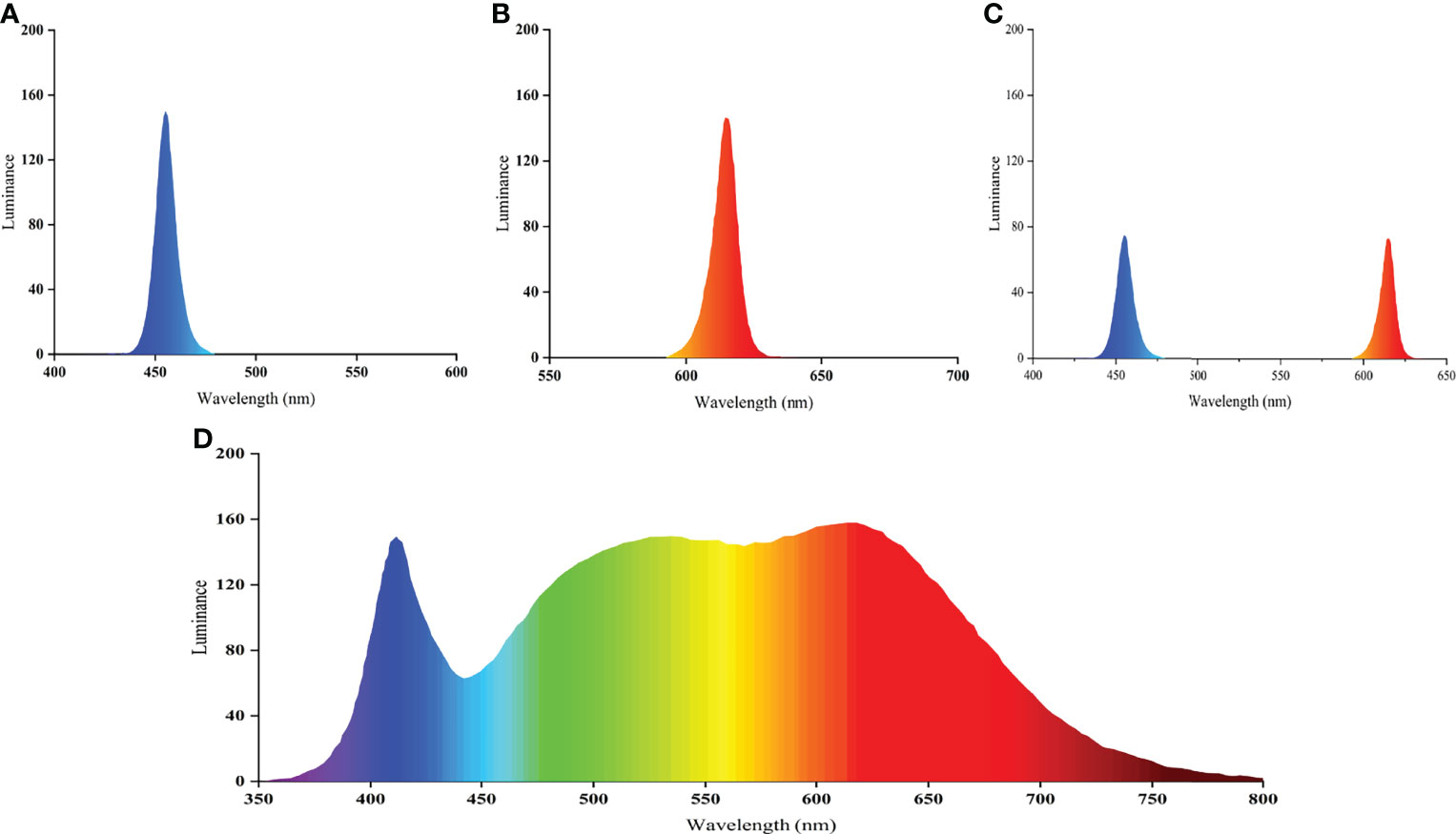
Figure 1 Relative spectral composition of blue light (A), red light (B), mixed blue and red light (C), and white light (D).
At the beginning and end of the trial, feeding was suspended for 36 h to ensure that the digestive tracts of the fish were empty. Prior to commencing the trial, the fish were anesthetized by immersion in a 30 mg/L tricaine methanesulfonate (MS-222; Sigma-Aldrich, USA) solution, after which they were gently blotted dry with tissue and weighed. During the culture period, all trout (initial weight, 34.67 ± 2.69 g) were fed twice daily (at 08:00 and 18:30) with commercial trout feed. Residual unconsumed feed was collected after feeding for 30 min, and the daily feed intake was calculated by measuring the feed moisture content and correcting the feed intake for leaching. Water was renewed using a single-flow system at a water flow rate of 1.15 L/min. Water temperature, salinity, dissolved oxygen content, and pH were monitored three times daily using a YSI ProPlus handheld multiparameter meter (YSI Inc., Ohio, USA). Water samples were collected at 3-day intervals, and the total ammonia nitrogen (TAN), phosphate, nitrite nitrogen, and nitrate nitrogen concentrations were analyzed using a Cleverchem 380 automatic chemical analyzer (DeChem-Tech Inc., Hamburg, Germany).
During the culture period, values for the water quality parameters (mean ± standard deviation), temperature, salinity, dissolved oxygen, pH, TAN, phosphate, nitrite nitrogen, and nitrate nitrogen were maintained at 16.5 ± 0.2°C, 14.2 ± 0.7, 8.7 ± 0.3 mg/L, 7.3 ± 0.1, at 0.03 ± 0.03 mg N/L, 0.11 ± 0.08 mg P/L, 0.09 ± 0.05 mg N/L, and 3.43 ± 1.9 mg N/L, respectively.
At 4-week intervals, the fish in each tank were treated with 30 mg/L tricaine MS-222, counted, and weighed. At the end of the trial, the final weights of steelhead trout in the 12W, 12R, 12B, 3B9R, 6B6R, and T12BR groups were 277.32 ± 6.23, 278.24 ± 4.89, 294.13 ± 13.20, 295.74 ± 5.61, 264.78 ± 4.86, and 283.10 ± 16.90 g, respectively. The 3B9R group had the highest final weight, which was significantly higher than that of the 6B6R group. The average daily intake of all groups was 2.26 ± 0.09 g, and no significant differences were found among the groups. At the time of sampling, three fish were randomly selected from each tank and immediately anesthetized with 100 mg/L MS-222. The midgut, stomach, liver, and muscle tissues were immediately collected and frozen in liquid nitrogen. Blood samples were collected in heparin sodium anticoagulant EP tubes, centrifuged at 10,000 r/min (4°C) for 10 min to separate plasma, and immediately placed in liquid nitrogen. All samples were then transferred to a −80°C ultra-low temperature freezer for preservation.
Digestive Enzyme Activity Analyses
After being accurately weighed, midgut and stomach tissues were placed in pre-chilled saline at a weight (g): volume (mL) ratio of 1:9 and homogenized using an automatic freezer mill grinder (Jingxin Industrial Development Inc., Shanghai, China). The tissue homogenate solutions were centrifuged at 3,500 r/min and 4°C for 10 min, and the resulting supernatants were collected for analysis. The commercially available kits (Nanjing Jiancheng Bioengineering Institute, Nanjing, China; A045-4-2, A054-2-1, C016-1-1, A080-2-2, and A080-1-1) were used to determine the total protein content and the activities of lipase, amylase, trypsin, and pepsin in tissues. The total protein content, lipase and amylase activity, and trypsin and pepsin activity were determined as described by Wang et al. (2021b), Lu et al. (2020), and Zhang et al. (2020b), respectively. The determination process was strictly based on the instructions, and absorbance values of total protein content, lipase, amylase, trypsin, and pepsin were measured at 562, 580, 660, 253, and 660 nm, respectively, using a microplate reader (Synergy2; BioTek Inc., USA) and a UV spectrophotometer (UV-5100; Shanghai Metash Instruments Co., Ltd, Shanghai, China). The lipase activity was presented as units per gram of protein; the activities of amylase, trypsin, and pepsin were presented as units per milligram of protein.
Plasma Biochemical Analyses
Plasma levels of glucose (Glu), total protein (TP), triglyceride (TG), and total cholesterol (TC) were determined using a Cobas C-311 automatic biochemical analyzer (Roche/Hitachi Inc., Basel, Switzerland). All commercial kits were obtained from Roche, Inc. (Basel, Switzerland).
RNA and DNA Extractions
Total RNA was extracted from muscle and liver tissue samples using a UNIQ-10 TRIzol Total RNA Extraction kit (Sangon Inc., Shanghai, China), and DNA was extracted using a marine animal tissue genomic DNA extraction kit (Tiangen Inc., Beijing, China). RNA and DNA concentrations were measured at 260 nm using a microspectrophotometer (Nano-300, Allsheng Inc., Hangzhou, China). Purity of the sample was confirmed by determining the OD260/280 nm absorption ratio. The OD260/280 values for muscle tissue RNA and DNA were 1.96 ± 0.03 and 1.84 ± 0.02, respectively. The OD260/280 values for liver tissue RNA and DNA were 1.96 ± 0.03 and 1.83 ± 0.02, respectively. All samples showed OD260/280 readings between 1.8 and 2.0 for both RNA and DNA.
Statistical Analysis
All data were analyzed using SAS version 9.4 (SAS Institute Inc., Cary, North Carolina, USA). The normal distribution and homogeneity of variance of the data were initially assessed using the Shapiro–Wilk and Levene tests, respectively. Normally distributed homogeneous data were analyzed using one-way analysis of variance (ANOVA), with mean values compared using Tukey’s multiple comparison tests. For analysis of non-normally distributed data, we applied the non-parametric Mann–Whitney rank sum test and Kruskal–Wallis ANOVA of ranks. The results are expressed as the mean ± standard deviation, with differences considered significant at P < 0.05.
Ethics Approval Statement
All procedures were performed under the Regulations of the Administration of Affairs Concerning Experimental Animals of China, as well as the Regulations of the Administration of Affairs Concerning Experimental Animals of Shandong Province.
Results
Digestive Enzyme Activity
Digestive enzyme activity data obtained in juvenile steelhead trout are summarized in Figure 2. Midgut lipase and trypsin levels were significantly higher in the 3B9R and 12R groups than in the 6B6R group (P < 0.05). Furthermore, the midgut and gastric lipase activities showed similar trends. The 3B9R and 12R groups had significantly higher lipase activities than the other groups (P < 0.05). However, no significant differences were observed in the amylase (midgut and gastric) or pepsin levels among the groups (P > 0.05).
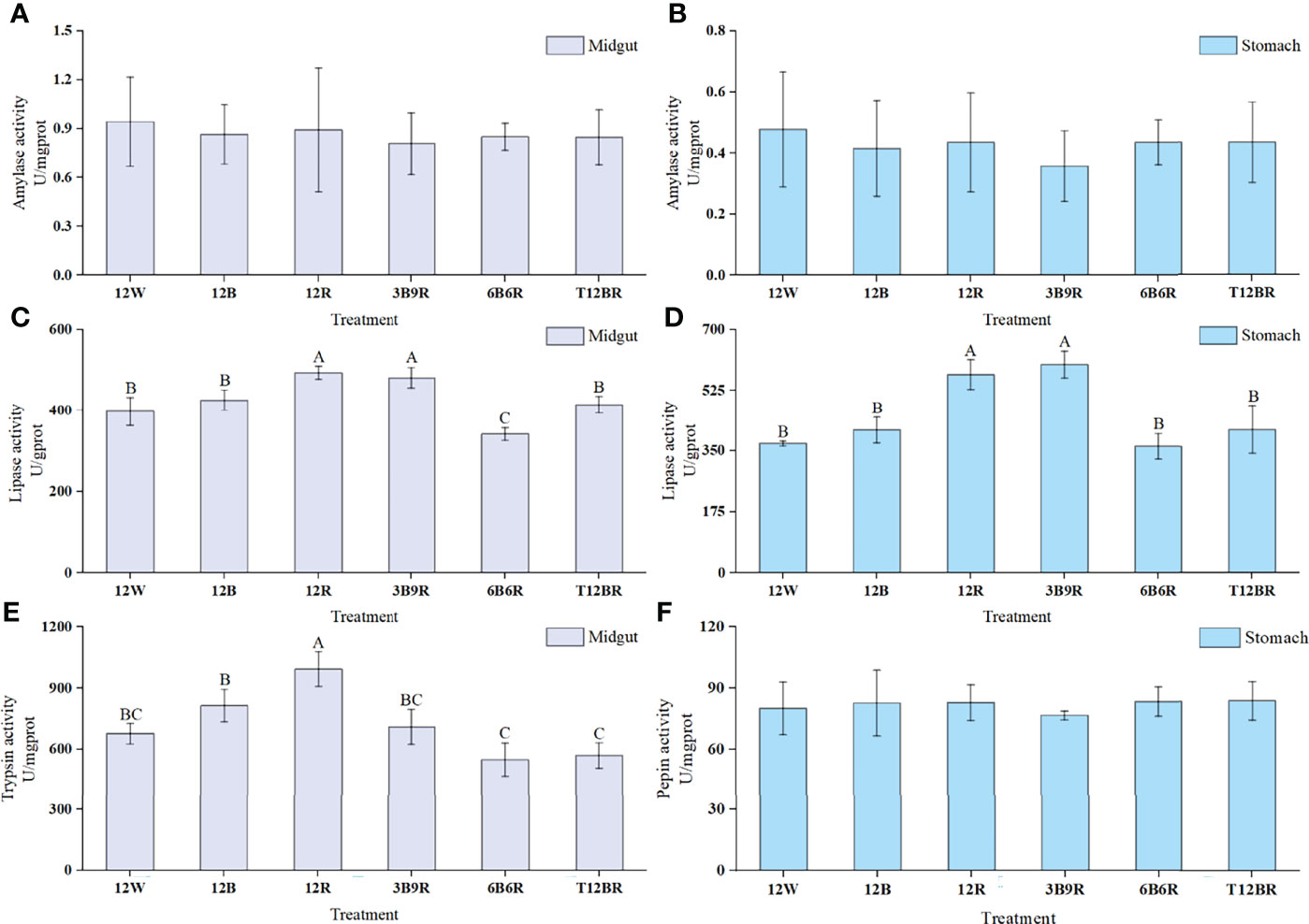
Figure 2 Digestive enzyme activities of the midgut and stomach in juvenile steelhead trout reared under different light color conditions. (A) Amylase activity in the midgut; (B) amylase activity in the stomach; (C) lipase activity in the midgut; (D) lipase activity in the stomach; (E) trypsin activity in the midgut; (F) pepsin activity in the stomach. Different capital letters denote significant differences among the steelhead trout treatments. Results are expressed as mean ± standard deviation (n=12).
Plasma Biochemistry
Results obtained for the plasma Glu, TP, TG, and TC levels are presented in Figure 3. Glu levels in trout in the 6B6R group were significantly lower than those in the other groups (P < 0.05). The highest levels of TP were detected in the 3B9R group, which were significantly higher than the levels recorded in the 12W and 6B6R groups (P < 0.05). The TG levels in the 12R group were significantly higher than those in the 12W group (P < 0.05). The highest levels of TC were detected in the 12R group, which were significantly higher than those in the other groups (P < 0.05), whereas the levels of TC in the 12W and 6B6R groups were significantly lower than those in the other groups (P < 0.05).
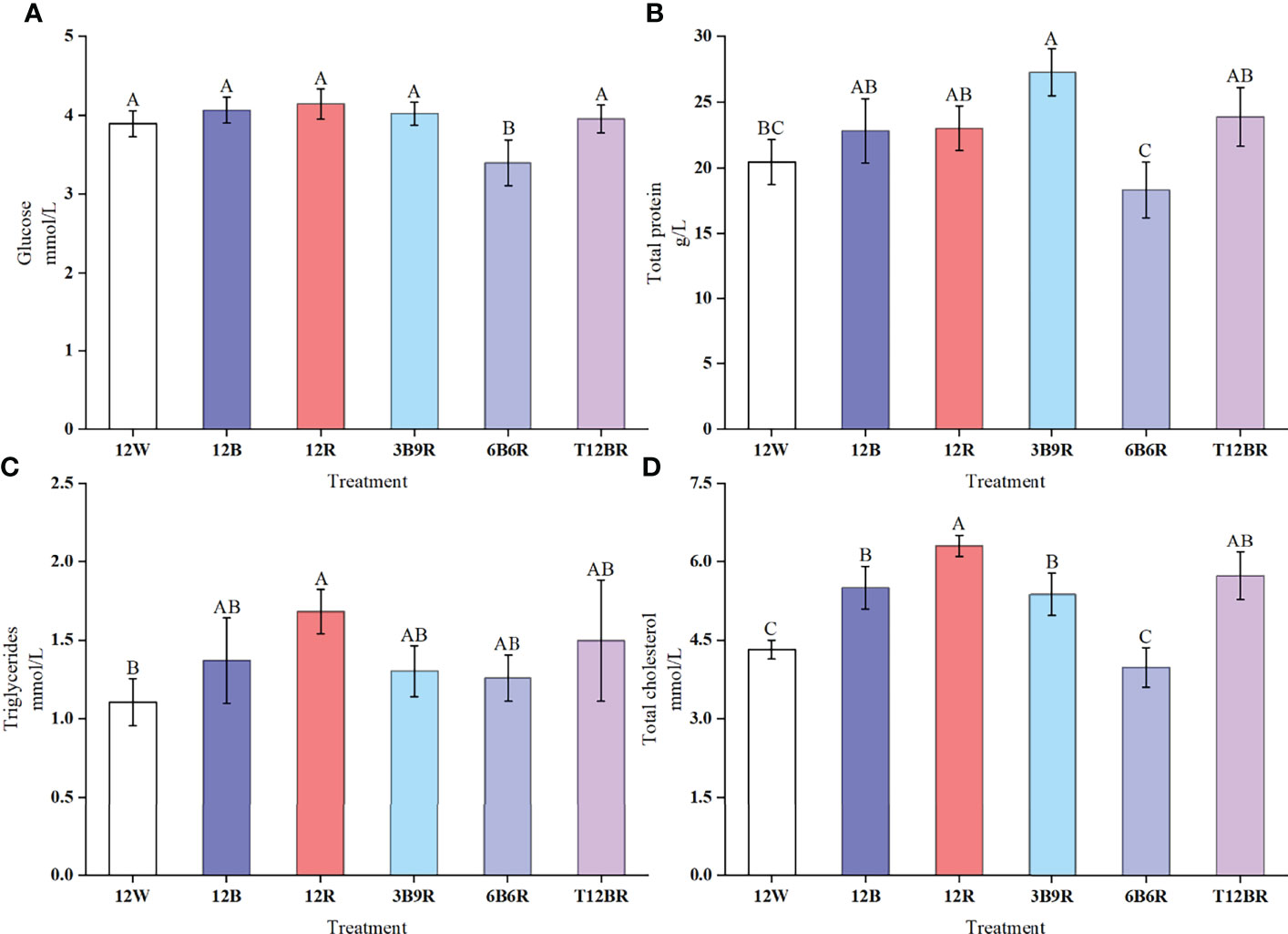
Figure 3 Plasma enzyme levels of Glu (glucose, A), TP (total protein, B), TG (triglycerides, C), and TC (total cholesterol, D) in juvenile steelhead trout reared under different light color conditions. Different capital letters denote significant differences among the steelhead trout treatments. Results are expressed as the mean ± standard deviation (n = 12).
RNA/DNA Content and Ratio
Data relating to the muscle and liver RNA/DNA content and ratios of juvenile steelhead trout are presented in Table 2. The muscle RNA content in the 3B9R group was significantly higher than that in the 12W, 12B, and 6B6R groups (P < 0.05). Interestingly, the muscle RNA/DNA ratio showed a similar trend to the muscle RNA content, with the 3B9R group having significantly higher values than the T12BR, 12W, 12B, and 6B6R groups (P < 0.05). The lowest liver RNA content was detected in the 6B6R group, which was significantly lower than that recorded for the 12R, 3B9R, T12BR, and 12B groups (P < 0.05). In addition, the liver RNA/DNA ratio was significantly higher in the 12R and 3B9R groups than that in the 12B, 12W, and 6B6R groups (P < 0.05). However, no significant differences in muscle and liver DNA content were observed among the groups (P > 0.05).
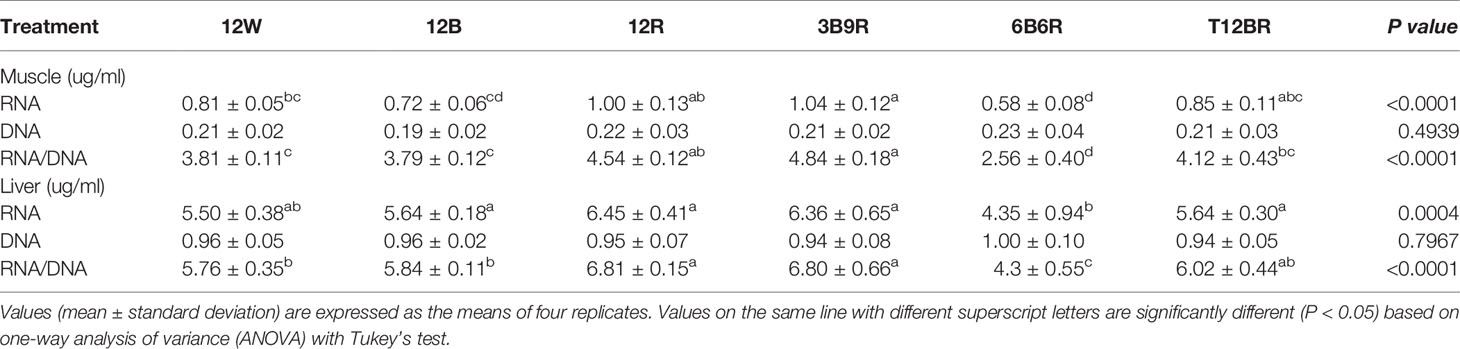
Table 2 RNA, DNA, and RNA/DNA ratio in the muscle and liver of steelhead trout reared under different light color conditions.
Discussion
The altitude of the sun considerably influences the light color composition in the water. In the morning and evening, because of the low altitude of the sun and the long path length of the incident light, red light is quickly absorbed after entering the water, thus increasing the proportion of blue light in the water. At noon, the incident light path is shorter, increasing the proportion of red light in the water. Therefore, the light color tends to exhibit a blue-red-blue variation in the water during the daytime (Wetzel, 1983; Ruchin, 2021). This light color variation in the water is similar to that observed in the 3B9R light environment in the current study. In the 3B9R light environment, TP levels, digestive enzyme activity, muscle/liver RNA content, and RNA/DNA ratio in trout significantly increased compared other light environments, implying that the digestive and anabolic performance was significantly improved under 3B9R light environment. These findings indicate that trout can adapt to variations in the daily rhythm of light conditions during their long-term natural evolution.
Our results showed that the combination of blue and red light was more effective than single light in improving the digestive and anabolic performance in steelhead trout. However, information on the effects of the combination of blue and red light colors in trout is limited. Our findings are similar to those reported by Karakatsouli et al. (2008), who found that red light was beneficial in increasing the anabolic level of rainbow trout (Oncorhynchus mykiss), and Guller et al. (2020), who found that blue light can enhance the antioxidant enzyme synthesis of rainbow trout. In contrast, Karakatsouli et al. (2007) documented that blue light can inhibit anabolism and reduce the stress response. Therefore, responses to colored light may vary depending on the species, life stage, and size-class.
Digestive enzyme activity is a vital indicator of the maturity and function of the digestive system in fish (Fang et al., 2019; Pérez-Sirkin et al., 2020). Digestive enzyme activity is closely related to fish feeding habits and is influenced by environmental factors (Castro-Ruiz et al., 2019; Yu et al., 2020; Yan et al., 2021). In the present study, the lipase activity of the 3B9R group was significantly higher than that of the 6B6R group (Figure 2), whereas no significant difference in amylase activity was observed. This difference may be explained by the fact that steelhead trout is a carnivorous fish and its lipase activity is susceptible to light color. Furné et al. (2005) reported higher lipase activity in carnivorous fish, whereas higher amylase activity was observed in herbivorous and omnivorous fish. In a recent study on spotted sea bass (Lateolabrax maculatus), Hou et al. (2019) found that exposure to blue light could significantly increase digestive enzyme activity. Our results indicate that trout can adapt to variable blue-red-blue light environments during long-term natural evolution. Hence, a suitable light environment can promote the development of the digestive system, as well as the gastrointestinal digestion and absorption capacity of trout, ultimately improving the digestive performance.
Blood functions as a mediator of metabolism and nutrient transport in fish and is widely used to evaluate physiological health, digestion capacity, and anabolic performance (Giri et al., 2018; Chen et al., 2021; Huang et al., 2021). The biochemical characteristics of fish blood can exhibit marked variations in response to environmental changes (Wang et al., 2021a).
In the current study, plasma Glu levels were significantly lower in steelhead trout exposed to the 6B6R light environment than in those exposed to other light environments (Figure 3). We suspect that this can represent oxidative stress in the liver of trout caused by the 6B6R light environment, which influences anabolic performance. Glu is mainly synthesized in the liver. When the liver is exposed to oxidative stress, its synthetic capacity is diminished, resulting in decreased Glu levels (Ogueji et al., 2020). Glu is produced by ATP through aerobic oxidation or glycolysis pathways, providing energy resources for tissues such as the liver, muscle, and gastrointestinal tract. Lower levels of Glu can result in a decreased availability of energy resources for the organism, affecting the anabolic efficiency of other tissues in fish (Feng et al., 2022).
Our findings revealed that the TP levels in the plasma of steelhead trout in the 3B9R group were significantly higher than those in the 6B6R group (Figure 3). This may be because the 6B6R light environment caused oxidative stress in the liver, leading to lower plasma TP levels. TP is synthesized in the liver and plasma cells of the reticuloendothelial system and its protein synthesis capacity decreases when the liver is exposed to oxidative stress (Afshari et al., 2021). Similarly, Refaey and Li (2018) observed a reduced TP synthesis capacity in the liver of channel catfish (Ictalurus punctatus) when subjected to oxidative stress. TP can supply energy to the body via gluconeogenesis (Yang et al., 2020) and can indirectly reflect the anabolic performance of the body (Afshari et al., 2021). Our findings showed that the anabolic performance of the 6B6R group was weakened because of a decrease in the efficiency of nutrient transport and metabolism caused by a reduction in TP levels.
Our results showed that TG and TC levels were significantly higher in the 12R group of steelhead trout than in other groups (Figure 3), possibly because of the long-term participation in high-intensity anabolism of the liver, leading to overload or oxidative stress caused by this light environment. TG and TC are important components of blood lipids and are mainly synthesized in the liver (Su et al., 2022). Variations in blood lipid levels are closely related to the metabolic and physiological status of fish, and changes in their content can reflect the level of lipid metabolism (Qiang et al., 2018; Jia et al., 2021). As an important biomarker, TC levels reflect liver health (Farag et al., 2022). During oxidative stress, the digestive performance and cell membrane function are inhibited (Narra et al., 2017). Owing to the reduced efficiency of TG reabsorption, a large amount of TG accumulates in the blood, causing increased plasma TG levels. TC is transported by high-density lipoproteins to the liver and synthesized by bile acids. When the liver is under high load or oxidative stress, its capacity to synthesize bile acids by TC is reduced, which leads to elevated levels (Mertens et al., 2017; Xu et al., 2020). Our data showed that the plasma TG and TC levels were significantly lower in the 6B6R group than in the other groups. This difference in the response indicates that the liver and intestine of the 6B6R group were affected by light color, and the liver synthesis of lipids and intestinal absorption of lipids were inhibited, resulting in lower levels of TG and TC. TC is a carrier of nutrients for the body; when its levels decrease, the metabolic capacity of steelhead trout decreases. Thus, nutrients cannot be efficiently transformed and utilized, which affects anabolic performance.
RNA directly participates in protein synthesis, and the metabolic rate of the body is positively correlated with the efficiency of RNA synthesis (Kumar et al., 2018). Conversely, the DNA content of genetic information carrier molecules remains stable (Zehra and Khan, 2017). Consequently, the RNA/DNA ratio, a physiological indicator of the body’s protein synthesis capacity, can accurately reflect cell metabolism (Sterzelecki et al., 2021). Our data showed that the DNA content in the muscle and liver of steelhead trout was not significantly different among treatment groups, whereas the RNA content and the RNA/DNA ratio were significantly higher in the 3B9R group than those in the 6B6R group (Table 2). The RNA content and RNA/DNA ratio of different tissues in each group revealed similar trends—which may reflect differences in anabolic performance of steelhead trout under different light color conditions—and were positively correlated with the growth trend of trout in our laboratory (Chen et al., unpublished data). Similarly, a positive correlation was found between the RNA/DNA ratio and growth trend in pearl oysters (Pinctada fucata) (Chang et al., 2021). Studies have revealed that factors such as feeding, temperature, and light can influence the RNA/DNA ratio in the body (Grimm et al., 2015; Angelo et al., 2021; Quintanilla-Ahumada et al., 2021). Kawamura et al. (2017) reported that exposure to red light could significantly increase the muscle RNA/DNA ratio in red sea bream (Pagrus major). Similarly, we found that the 3B9R light environment could enhance anabolic performance by improving the rate of RNA synthesis in steelhead trout.
Light is a vital factor in regulating the physiological characteristics of fish, and an optimal light environment can contribute positively to fish welfare (Owen et al., 2009; Stien et al., 2013; Ruchin, 2021). Our findings on digestive and anabolic performance of trout can partially reflect fish welfare status; however, investigating different aspects of stress response (e.g. oxidative stress process) is important as the welfare index has some limitations (Stien et al., 2013; Parma et al., 2020). Our unpublished data (Chen et al.) showed that blue-red light variation can affect trout growth. This is consistent with the findings of previous studies, which demonstrated that fish growth can be improved by altering the background color of the culture tank and the light color to yield interesting results (Papoutsoglou et al., 2000; Rotllant et al., 2003; Sotoudeh et al., 2019; Kasagi et al., 2020; McLean, 2021; Ninwichian et al., 2022). Overall, our study failed to comprehensively explain fish welfare, which will need to be explored further using an overall model for welfare indicators such as oxidative stress process, behavior, and other aspects.
Conclusion
In the present study, the 3B9R light environment was beneficial for increasing plasma TP levels and enhancing gastrointestinal digestive enzyme activity in steelhead trout. This environment also improved the liver and muscle protein synthesis capacity. We conclude that 3B9R is the most favorable light environment for improving the digestive and anabolic performance of trout. Our results indicate that steelhead trout acclimated to variable light conditions during long-term natural evolution. The 3B9R group of steelhead trout had enhanced secretory synthesis capacity through an increase in TP levels. This also improved RNA synthesis, protein capacity, and digestive capacity. This is the primary reason for the relatively high digestive and anabolic performance of fish exposed to the 3B9R light environment compared to that of fish in other light environments. In contrast, the 6B6R light environment significantly reduced plasma Glu, TP, and TC levels. Additionally, the gastrointestinal digestive enzyme activity was inhibited, and the ability of the muscle and liver to synthesize protein using RNA was reduced, resulting in decreased digestive and anabolic performance. Despite the higher RNA content and RNA/DNA ratio, exposure to the 12R light environment caused relatively high levels of TG and TC in plasma, which indicates the occurrence of oxidative stress. In summary, the 12R light environment is considered unsuitable for long-term cultivation, and the 3B9R light environment may be more appropriate for rearing steelhead trout.
Data Availability Statement
The original contributions presented in the study are included in the article/supplementary material. Further inquiries can be directed to the corresponding authors.
Ethics Statement
All procedures were performed under the Regulations of the Administration of Affairs Concerning Experimental Animals of China, as well as the Regulations of the Administration of Affairs Concerning Experimental Animals of Shandong Province.
Author Contributions
XC performed the trial and data analysis, wrote the original draft, and proposed the research methods and ideas; YZ and SD supervised research progress, proposed research methods and research ideas, and edited the manuscript. JH performed the data analysis and wrote the original draft; DA, LL, YD, and QG reviewed and edited the original draft. All authors contributed to the article and approved the submitted version.
Funding
This publication was supported by the National Natural Science Foundation of China (NSFC) (Nos. U1906206 and 31872575), the National Key Research and Development Program of China (No. 2019YFD0901000), and the Scientific and Technological Demonstration for Offshore Facilities Fisheries of Shandong Province (2021SFGC0701).
Conflict of Interest
The authors declare that the research was conducted in the absence of any commercial or financial relationships that could be construed as a potential conflict of interest.
Publisher’s Note
All claims expressed in this article are solely those of the authors and do not necessarily represent those of their affiliated organizations, or those of the publisher, the editors and the reviewers. Any product that may be evaluated in this article, or claim that may be made by its manufacturer, is not guaranteed or endorsed by the publisher.
References
Afshari A., Sourinejad I., Gharaei A., Johari S. A., Ghasemi Z. (2021). The Effects of Diet Supplementation With Inorganic and Nanoparticulate Iron and Copper on Growth Performance, Blood Biochemical Parameters, Antioxidant Response and Immune Function of Snow Trout Schizothorax Zarudnyi (Nikolskii 1897). Aquaculture 539, 736638. doi: 10.1016/j.aquaculture.2021.736638
Angelo M., Lisboa M. K., Magnotti C., Pilotto M. R., Mattos J. J., Cerqueira V. R. (2021). Temperature Influence on the Initial Development of Sardinella Brasiliensis Larvae. Aquac. Res. 52, 6497–6503. doi: 10.1111/are.15517
Baekelandt S., Mandiki S. N. M., Schmitz M., Kestemont P. (2019). Influence of the Light Spectrum on the Daily Rhythms of Stress and Humoral Innate Immune Markers in Pikeperch Sander Lucioperca. Aquaculture. 499, 358–363. doi: 10.1016/j.aquaculture.2018.09.046
Castro-Ruiz D., Mozanzadeh M. T., Fernández-Méndez C., Andree K. B., García-Dávila C., Cahu C., et al. (2019). Ontogeny of the Digestive Enzyme Activity of the Amazonian Pimelodid Catfish Pseudoplatystoma Punctifer (Castelnau 1855). Aquaculture. 504, 210–218. doi: 10.1016/j.aquaculture.2019.01.059
Chang G., Wang H., Wang L., Xue C., Zhu C., Zhang J., et al. (2021). RNA/DNA Ratio to Assess the Growth Performance in Postlarvae of the Pearl Oyster Pinctada Fucata (Gould 1850). Aquac. Rep. 19, 100600. doi: 10.1016/j.aqrep.2021.100600
Chen H., Liu H., Li R., Lin X., Luo H., Ji S., et al. (2021). Blood Cell Identification and Hematological Analysis During Natural Sex Reversal in Rice Field Eel (Monopterus Albus). Aquaculture 538, 736543. doi: 10.1016/j.aquaculture.2021.736543
Fang H., Xie J., Liao S., Guo T., Xie S., Liu Y., et al. (2019). Effects of Dietary Inclusion of Shrimp Paste on Growth Performance, Digestive Enzymes Activities, Antioxidant and Immunological Status and Intestinal Morphology of Hybrid Snakehead (Channa Maculata ♀ × Channa Argus ♂). Front. Physiol. 10. doi: 10.3389/fphys.2019.01027
Farag M. R., Alagawany M., Khalil S. R., Abd El-Aziz R. M., Zaglool A. W., Moselhy A. A. A., et al. (2022). Effect of Parsley Essential Oil on Digestive Enzymes, Intestinal Morphometry, Blood Chemistry and Stress-Related Genes in Liver of Nile Tilapia Fish Exposed to Bifenthrin. Aquaculture 546, 737322. doi: 10.1016/j.aquaculture.2021.737322
Feng J., Liu S., Zhu C., Cai Z., Cui W., Chang X., et al. (2022). The Effects of Dietary Lactococcus Spp. On Growth Performance, Glucose Absorption and Metabolism of Common Carp, Cyprinus Carpio L. Aquaculture 546, 737394. doi: 10.1016/j.aquaculture.2021.737394
Furné M., Hidalgo M. C., López A., García-Gallego M., Morales A. E., Domezain A., et al. (2005). Digestive Enzyme Activities in Adriatic Sturgeon Acipenser Naccarii and Rainbow Trout Oncorhynchus Mykiss. A Comparative Study. Aquaculture. 250, 391–398. doi: 10.1016/j.aquaculture.2005.05.017
Giri S. S., Yun S., Jun J. W., Kim H. J., Kim S. G., Kang J. W., et al. (2018). Therapeutic Effect of Intestinal Autochthonous Lactobacillus Reuteri P16 Against Waterborne Lead Toxicity in Cyprinus Carpio. Front. Immunol. 9. doi: 10.3389/fimmu.2018.01824
Grimm C., Lehmann K., Clemmesen C., Brendelberger H. (2015). RNA/DNA Ratio is an Early Responding, Accurate Performance Parameter in Growth Experiments of Noble Crayfish Astacus Astacus(L.). Aquac. Res. 46, 1937–1945. doi: 10.1111/are.12348
Guller U., Onalan S., Arabaci M., Karatas B., Yasar M., Kufrevioglu O. I. (2020). Effects of Different LED Light Spectra on Rainbow Trout (Oncorhynchus Mykiss): In Vivo Evaluation of the Antioxidant Status. Fish Physiol. Biochem. 46 (6), 2169–2180. doi: 10.1007/s10695-020-00865-x
Head A. B., Malison J. A. (2000). Effects of Lighting Spectrum and Disturbance Level on the Growth and Stress Responses of Yellow Perch Perca Flavescens. J. World Aquac. Soc 31, 73–80. doi: 10.1111/j.1749-7345.2000.tb00700.x
Hou Z. S., Wen H. S., Li J. F., He F., Li Y., Qi X., et al. (2019). Effects of Photoperiod and Light Spectrum on Growth Performance, Digestive Enzymes, Hepatic Biochemistry and Peripheral Hormones in Spotted Sea Bass (Lateolabrax Maculatus). Aquaculture. 507, 419–427. doi: 10.1016/j.aquaculture.2019.04.029
Huang M., Yang X., Zhou Y., Ge J., Davis D. A., Dong Y., et al. (2021). Growth, Serum Biochemical Parameters, Salinity Tolerance and Antioxidant Enzyme Activity of Rainbow Trout (Oncorhynchus Mykiss) in Response to Dietary Taurine Levels. Mar Life Sci. Technol. 3, 449–462. doi: 10.1007/s42995-020-00088-2
Jia Y., Gao Y., Gao Y., Li W., Guan C. (2021). Growth Performance, Hematological and Biochemical Parameters, and Hepatic Antioxidant Status of Spotted Knifejaw Oplegnathus Punctatus in an Offshore Aquaculture Net Pen. Aquaculture 541, 736761. doi: 10.1016/j.aquaculture.2021.736761
Karakatsouli N., Papoutsoglou S. E., Panopoulos G., Papoutsoglou E. S., Chadio S., Kalogiannis D. (2008). Effects of Light Spectrum on Growth and Stress Response of Rainbow Trout Oncorhynchus Mykiss Reared Under Recirculating System Conditions. Aquac. Eng. 38 (1), 36–42. doi: 10.1016/j.aquaeng.2007.10.006
Karakatsouli N., Papoutsoglou S. E., Pizzonia G., Tsatsos G., Tsopelakos A., Chadio S., et al. (2007). Effects of Light Spectrum on Growth and Physiological Status of Gilthead Seabream Sparus Aurata and Rainbow Trout Oncorhynchus Mykiss Reared Under Recirculating System Conditions. Aquac. Eng. 36 (3), 302–309. doi: 10.1016/j.aquaeng.2007.01.005
Kasagi S., Miura M., Okazaki T., Mizusawa K., Takahashi A. (2020). Effects of Tank Color Brightness on the Body Color, Somatic Growth, and Endocrine Systems of Rainbow Trout Oncorhynchus Mykiss. Gen. Comp. Endocrinol. 298, 113581. doi: 10.1016/j.ygcen.2020.113581
Kawamura G., Bagarinao T. U., Anraku K., Okamoto M. (2017). Effect of Colored Light Regimes on the Stress Response and RNA/DNA Ratio of Juvenile Red Sea Bream, Pagrus Major. Borneo J. Mar Sci. Aquac. 1, 33–38. doi: 10.51200/bjomsa.v1i0.988
Kumar S., Sahu N. P., Ranjan A. (2018). Feeding De-Oiled Rice Bran (DORB) to Rohu, Labeo Rohita: Effect of Varying Dietary Protein and Lipid Level on Growth, Body Composition, and Insulin Like Growth Factor (IGF) Expression. Aquaculture. 492, 59–66. doi: 10.1016/j.aquaculture.2018.04.001
Li X., Wei P., Liu S., Tian Y., Ma H., Liu Y. (2021). Photoperiods Affect Growth, Food Intake and Physiological Metabolism of Juvenile European Sea Bass (Dicentrachus Labrax L.). Aquac. Rep. 20, 100656. doi: 10.1016/j.aqrep.2021.100656
Lu K. L., Cai L. S., Wang L., Song K., Zhang C. X., Rahimnejad S. (2020). Effects of Dietary Protein/Energy Ratio and Water Temperature on Growth Performance, Digestive Enzymes Activity and non-Specific Immune Response of Spotted Seabass (Lateolabrax Maculatus). Aquac. Nutr. 26 (6), 2023–2031. doi: 10.1111/anu.13143
Mapunda J., Mtolera M. S. P., Yahya S. A. S., Ngo V. M., Golan M. (2021). Light Colour Affect the Survival Rate, Growth Performance, Cortisol Level, Body Composition, and Digestive Enzymes Activities of Different Snubnose Pompano (Trachinotus Blochii (Lacépède 1801) Larval Stages. Aquac. Rep. 21, 100804. doi: 10.1016/j.aqrep.2021.100804
McLean E. (2021). Fish Tank Color: An Overview. Aquaculture 530, 735750. doi: 10.1016/j.aquaculture.2020.735750
Mertens K. L., Kalsbeek A., Soeters M. R., Eggink H. M. (2017). Bile Acid Signaling Pathways From the Enterohepatic Circulation to the Central Nervous System. Front. Neurosci. 11. doi: 10.3389/fnins.2017.00617
Narra M. R., Rajender K., Reddy R. R., Murty U. S., Begum G. (2017). Insecticides Induced Stress Response and Recuperation in Fish: Biomarkers in Blood and Tissues Related to Oxidative Damage. Chemosphere. 168, 350–357. doi: 10.1016/j.chemosphere.2016.10.066
Ninwichian P., Phuwan N., Limlek P. (2022). Effects of Tank Color on the Growth, Survival Rate, Stress Response, and Skin Color of Juvenile Hybrid Catfish (Clarias Macrocephalus × Clarias Gariepinus). Aquaculture 554, 738129. doi: 10.1016/j.aquaculture.2022.738129
Noureldin S. M., Diab A. M., Salah A. S., Mohamed R. A. (2021). Effect of Different Monochromatic LED Light Colors on Growth Performance, Behavior, Immune-Physiological Responses of Gold Fish, Carassius Auratus. Aquaculture 538, 736532. doi: 10.1016/j.aquaculture.2021.736532
Ogueji E., Nwani C., Mbah C., Iheanacho S., Nweke F. (2020). Oxidative Stress, Biochemical, Lipid Peroxidation, and Antioxidant Responses in Clarias Gariepinus Exposed to Acute Concentrations of Ivermectin. Environ. Sci. Pollut. Res. 27, 16806–16815. doi: 10.1007/s11356-019-07035-4
Owen M. A. G., Davies S. J., Sloman K. A. (2009). Light Colour Influences the Behaviour and Stress Physiology of Captive Tench (Tinca Tinca). Rev. Fish. Biol. Fish. 20 (3), 375–380. doi: 10.1007/s11160-009-9150-1
Papoutsoglou S. E., Mylonakis G., Miliou H., Karakatsouli N. P., Chadio S. (2000). Effects of Background Color on Growth Performances and Physiological Responses of Scaled Carp (Cyprinus Carpio L.) Reared in a Closed Circulated System. Aquac. Eng. 22 (4), 309–318. doi: 10.1016/s0144-8609(00)00056-x
Parma L., Pelusio N. F., Gisbert E., Esteban M. A., D’Amico F., Soverini M., et al. (2020). Effects of Rearing Density on Growth, Digestive Conditions, Welfare Indicators and Gut Bacterial Community of Gilthead Sea Bream (Sparus Aurata, L. 1758) Fed Different Fishmeal and Fish Oil Dietary Levels. Aquaculture 518, 734854. doi: 10.1016/j.aquaculture.2019.734854
Pérez-Sirkin D. I., Solovyev M., Delgadin T. H., Herdman J. E., Miranda L. A., Somoza G. M., et al. (2020). Digestive Enzyme Activities During Pejerrey (Odontesthes Bonariensis) Ontogeny. Aquaculture 524, 735151. doi: 10.1016/j.aquaculture.2020.735151
Qiang J., Tao Y. F., Bao J. W., Chen D. J., Li H. X., He J., et al. (2018). High Fat Diet-Induced miR-122 Regulates Lipid Metabolism and Fat Deposition in Genetically Improved Farmed Tilapia (GIFT, Oreochromis Niloticus) Liver. Front. Physiol. 9. doi: 10.3389/fphys.2018.01422
Quintanilla-Ahumada D., Quijon P. A., Pulgar J., Manriquez P. H., Garcia-Huidobro M. R., Duarte C. (2021). Exposure to Artificial Light at Night (ALAN) Alters RNA:DNA Ratios in a Sandy Beach Coleopteran Insect. Mar. Pollut. Bull. 165, 112132. doi: 10.1016/j.marpolbul.2021.112132
Refaey M. M., Li D. (2018). Transport Stress Changes Blood Biochemistry, Antioxidant Defense System, and Hepatic HSPs mRNA Expressions of Channel Catfish Ictalurus Punctatus. Front. Physiol. 9. doi: 10.3389/fphys.2018.01628
Rotllant J., Tort L., Montero D., Pavlidis M., Martinez M., Wendelaar Bonga S. E., et al. (2003). Background Colour Influence on the Stress Response in Cultured Red Porgy Pagrus Pagrus. Aquaculture. 223, 129–139. doi: 10.1016/s0044-8486(03)00157-1
Ruchin A. B. (2021). Effect of Illumination on Fish and Amphibian: Development, Growth, Physiological and Biochemical Processes. Rev. Aquac. 13 (1), 567–600. doi: 10.1111/raq.12487
Shan X., Xiao Z., Huang W., Dou S. (2008). Effects of Photoperiod on Growth, Mortality and Digestive Enzymes in Miiuy Croaker Larvae and Juveniles. Aquaculture. 281, 70–76. doi: 10.1016/j.aquaculture.2008.05.034
Sotoudeh E., Feizollahi S., Banan A., Morshedi V., Hosseini A. (2019). Oxidative Status and Digestive Histology of Barramundi Perch in Response to Different Tank Colors. J. Aquat. Anim. Health 31 (2), 139–146. doi: 10.1002/aah.10060
Sterzelecki F. C., Sugai J. K., Mattos J. J., Derner R. B., Pedrotti F. S., Magnotti C. F., et al. (2021). Live Diet for First Feeding of Brazilian Sardine, Sardinella Brasiliensis (STEINDACHNER 1879), Larvae in Captivity. Aquac. Res. 52(11), 5558–5565. doi: 10.1111/are.15431
Stien L. H., Bracke M. B. M., Folkedal O., Nilsson J., Oppedal F., Torgersen T., et al. (2013). Salmon Welfare Index Model (SWIM 1.0): A Semantic Model for Overall Welfare Assessment of Caged Atlantic Salmon: Review of the Selected Welfare Indicators and Model Presentation. Rev. Aquac. 5 (1), 33–57. doi: 10.1111/j.1753-5131.2012.01083.x
Su C., Li J., Lu Y., Wang Y., Ding Y., Pan L., et al. (2022). Interactive Effects of Dietary Cholesterol and Bile Acids on the Growth, Lipid Metabolism, Immune Response and Intestinal Microbiota of Litopenaeus Vannamei: Sparing Effect of Bile Acids on Cholesterol in Shrimp Diets. Aquaculture 547, 737412. doi: 10.1016/j.aquaculture.2021.737412
Taylor J. F., North B. P., Porter M. J. R., Bromage N. R., Migaud H. (2006). Photoperiod can be Used to Enhance Growth and Improve Feeding Efficiency in Farmed Rainbow Trout, Oncorhynchus Mykiss. Aquaculture. 256, 216–234. doi: 10.1016/j.aquaculture.2006.02.027
Tian H. Y., Zhang D. D., Xu C., Wang F., Liu W. B. (2015). Effects of Light Intensity on Growth, Immune Responses, Antioxidant Capability and Disease Resistance of Juvenile Blunt Snout Bream Megalobrama Amblycephala. Fish Shellfish Immunol. 47, 674–680. doi: 10.1016/j.fsi.2015.08.022
Villamizar N., Blanco-Vives B., Migaud H., Davie A., Carboni S., Sánchez-Vázquez F. J. (2011). Effects of Light During Early Larval Development of Some Aquacultured Teleosts: A Review. Aquaculture. 315, 86–94. doi: 10.1016/j.aquaculture.2010.10.036
Wang T., Cheng Y., Liu Z., Long X. (2015). Effects of Light Intensity on Husbandry Parameters, Digestive Enzymes and Whole-Body Composition of Juvenile Epinephelus Coioidesreared in Artificial Sea Water. Aquac. Res. 46, 884–892. doi: 10.1111/are.12241
Wang T., Cheng Y., Liu Z., Yan S., Long X. (2013). Effects of Light Intensity on Growth, Immune Response, Plasma Cortisol and Fatty Acid Composition of Juvenile Epinephelus Coioides Reared in Artificial Seawater. Aquaculture. 414-415, 135–139. doi: 10.1016/j.aquaculture.2013.08.004
Wang J., Li K., Wang L., Xu Q. (2021a). Effects of Different Levels of Stevioside on Growth Performance, Digestive Enzyme Activity, Antioxidant Capacity and Gene Expression of Juvenile Mirror Carp (Cyprinus Carpio). Aquaculture. 543, 737019. doi: 10.1016/j.aquaculture.2021.737019
Wang Z., Zhang C., Lu K., Song K., Li X., Wang L., et al. (2021b). Effects of Supplementing Intestinal Autochthonous Bacteria in Plant-Based Diets on Growth, Nutrient Digestibility, and Gut Health of Bullfrogs (Lithobates Catesbeianus). Front. Microbiol. 12. doi: 10.3389/fmicb.2021.739572
Wei H., Cai W. J., Liu H. K., Han D., Zhu X. M., Yang Y. X., et al. (2019). Effects of Photoperiod on Growth, Lipid Metabolism and Oxidative Stress of Juvenile Gibel Carp (Carassius Auratus). J. Photochem. Photobiol. B-Biol. 198, 111552. doi: 10.1016/j.jphotobiol.2019.111552
Wu L. L., Wang Y. N., Li J., Song Z. C., Xu S. H., Song C. B., et al. (2021). Influence of Light Spectra on the Performance of Juvenile Turbot (Scophthalmus Maximus). Aquaculture 533, 736191. doi: 10.1016/j.aquaculture.2020.736191
Xu H., Zhang Q., Kim S. K., Liao Z., Wei Y., Sun B., et al. (2020). Dietary Taurine Stimulates the Hepatic Biosynthesis of Both Bile Acids and Cholesterol in the Marine Teleost, Tiger Puffer (Takifugu Rubripes). Br. J. Nutr. 123, 1345–1356. doi: 10.1017/S0007114520000161
Yan X., Chen Y., Dong X., Tan B., Liu H., Zhang S., et al. (2021). Ammonia Toxicity Induces Oxidative Stress, Inflammatory Response and Apoptosis in Hybrid Grouper (♀ Epinephelus Fuscoguttatus × ♂ E. lanceolatu). Front. Mar. Sci. 8. doi: 10.3389/fmars.2021.667432
Yang Q., Guo L., Liu B.-S., Guo H.-Y., Zhu K.-C., Zhang N., et al. (2020). Effects of Stocking Density on the Growth Performance, Serum Biochemistry, Muscle Composition and HSP70 Gene Expression of Juvenile Golden Pompano Trachinotus Ovatus (Linnaeus 1758). Aquaculture 518, 734841. doi: 10.1016/j.aquaculture.2019.734841
Yu Z., Wu X. Q., Zheng L. J., Dai Z. Y., Wu L. F. (2020). Effect of Acute Exposure to Ammonia and BFT Alterations on Rhynchocypris Lagowski: Digestive Enzyme, Inflammation Response, Oxidative Stress and Immunological Parameters. Environ. Toxicol. Pharmacol. 78, 103380. doi: 10.1016/j.etap.2020.103380
Zehra S., Khan M. A. (2017). Dietary Thiamin Requirement of Fingerling Channa Punctatus (Bloch) Based on Growth, Protein Gain, Liver Thiamin Storage, RNA/DNA Ratio and Biochemical Composition. Aquac. Nutr. 24, 1015–1023. doi: 10.1111/anu.12638
Zhang X., Bian Z., Yuan X., Chen X., Lu C. (2020a). A Review on the Effects of Light-Emitting Diode (LED) Light on the Nutrients of Sprouts and Microgreens. Trends Food Sci. Technol. 99, 203–216. doi: 10.1016/j.tifs.2020.02.031
Zhang X., Wang H., Zhang J., Lin B., Chen L., Wang Q., et al. (2020b). Assessment of Rapeseed Meal as Fish Meal Alternative in Diets for Juvenile Asian Red-Tailed Catfish (Hemibagrus Wyckioides). Aquac. Rep. 18, 100497. doi: 10.1016/j.aqrep.2020.100497
Keywords: anabolism, blue and red light color combination, digestive enzyme, RNA/DNA ratio, plasma biochemistry, steelhead trout
Citation: Chen X, Zhou Y, Huang J, An D, Li L, Dong Y, Gao Q and Dong S (2022) Blue and Red Light Color Combinations Can Enhance Certain Aspects of Digestive and Anabolic Performance in Juvenile Steelhead Trout Oncorhynchus mykiss. Front. Mar. Sci. 9:853327. doi: 10.3389/fmars.2022.853327
Received: 17 January 2022; Accepted: 11 April 2022;
Published: 06 May 2022.
Edited by:
Samad Rahimnejad, University of South Bohemia in České Budějovice, CzechiaReviewed by:
Rajeswari M V, Sathyabama Institute of Science and Technology, IndiaAshkan Banan, Lorestan University, Iran
Copyright © 2022 Chen, Zhou, Huang, An, Li, Dong, Gao and Dong. This is an open-access article distributed under the terms of the Creative Commons Attribution License (CC BY). The use, distribution or reproduction in other forums is permitted, provided the original author(s) and the copyright owner(s) are credited and that the original publication in this journal is cited, in accordance with accepted academic practice. No use, distribution or reproduction is permitted which does not comply with these terms.
*Correspondence: Yangen Zhou, zhouyg@ouc.edu.cn; Shuanglin Dong, dongsl@ouc.edu.cn