- 1Guangdong Provincial Key Laboratory of Improved Variety Reproduction in Aquatic Economic Animals, Institute of Aquatic Economic Animals, School of Life Sciences, Sun Yat-sen University, Guangzhou, China
- 2Zhejiang NHU Company Ltd., Shaoxing, China
A 56-day culture experiment followed by an acute low salinity challenge was conducted to evaluate the effects of Rhodobacter sphaeroides protein (RSP) substitution of fishmeal and Coenzyme Q10 (CoQ10) supplementation on growth performance, intestinal microbiota and compressive capacity of Litopenaeus vannamei (L. vannamei). Four experimental diets were formulated: FM diet (20% fishmeal), RSP diet (20% RSP instead of 20% fishmeal), CoQ10 diet (20% fishmeal supplemented with CoQ10 at 0.08 g kg–1), RSP + CoQ10 diet (20% RSP supplemented with CoQ10 at 0.08 g kg–1). The obtained results were denoted that L. vannamei fed CoQ10 diet could improve growth performance (weigh gain and specific growth rate), condition factor and crude lipid, and decrease hepatosomatic index, but no differences were found in survival rates. High throughput sequencing on intestinal microbiota indicated that intestinal microbiota of L. vannamei consisted mainly of Proteobacteria and Firmicutes. The species richness of the RSP diet was remarkably higher than that of the other diets. Moreover, the presence of RSP and CoQ10 may improve intestinal homeostasis by inhibiting the propagation of Vibrio. Compared to FM diet and RSP diet, supplementation of CoQ10 significantly improved the compressive capacity of L. vannamei against the acute low salinity challenge, as indicated by higher survival rates as well as higher activities of T-AOC and higher transcript levels of SOD, HSP70, and Relish gene. Our findings demonstrated that RSP could serve as a novel FM and CoQ10 could serve as a prospective feed additive to help L. vannamei to overcome environmental stresses.
Introduction
Although as a highly efficient osmoregulator, the salinity of L. vannamei’ live was wide-ranging, from 0.5 to 50 ppt (Xu et al., 2018). More and more studies have shown that low salinity can increase ammonia excretion and oxygen consumption, and decrease ammonia or nitrite tolerant in L. vannamei (Jiang et al., 2000; Shinji et al., 2012; Wang et al., 2015). Besides, 5 and 54 ppt salinities increase the susceptibility of L. vannamei to White Spot Syndrome Virus (WSSV) (Ramos-Carreno et al., 2014). Meanwhile, L. vannamei juveniles exhibited an impaired resistance to pathogens due to long-term low salinity (2.5–5 ppt) (Lin et al., 2012). On the other hand, the growth performance of juvenile L. vannamei would be significantly suppressed when exposure to salinity 3 ppt (Huang et al., 2019). All these data indicate that the tolerance to stress, the resistance to pathogens and the poor survival rates have become restrictive factors for inland low salinity L. vannamei farming (Li et al., 2008). And so far, a few studies have started to address the effects of low salinity on survival and growth of L. vannamei (Zheng et al., 2017; Li et al., 2019). In the long-term feeding experiment, there is a need to further explore some new fishmeal substitutions and dietary supplementations to enhance nutrient uptake and increase host defense against acute salinity challenge.
Coenzyme Q10 (CoQ10; also called ubiquinone) is naturally occurring endogenous lipophilic antioxidant, the main function of which was to produce the ATP by the mitochondrial Electron Transport Chain (ETC). CoQ10 has been reported to contribute a significantly improvement to human and animal health in multiple diseases, like cancer, obesity, diabetes, etc. (Roffe et al., 2004; Adarsh et al., 2008; Sohet et al., 2009; Prakash et al., 2010; Varela-López et al., 2016). In addition, CoQ10 has an anti-inflammatory potency in THP-1 cells (Schmelzer et al., 2008, 2009). It has been suggested that there was a markedly higher weigh gain when CoQ10 was supplemented at 0.04 g kg–1 in broiler diet (Geng et al., 2007), a few studies also using CoQ10 to reducing the mortality of broiler in a stress environment (Gopi et al., 2014). El et al. observed that inclusion of CoQ10 has improved the growth performance, health being and antioxidant capacity in Nile tilapia (El et al., 2020). Therefore, CoQ10 was regarded as an ideal supplement to increase the antioxidative capacity to combat oxidative stress, while related studies on its effect on farmed shrimp were lacked. As known as a high potential as nutritious feed, Rhodobacter sphaeroides is a purple non-sulfur bacterium that contains essential vitamins and carotenoid pigments (Sasaki et al., 1998). Supplements of Rhodobacter sphaeroides improved water quality, reduced the mortality rates, and stimulated immune system and the growth of seawater red tilapia (Chiu and Liu, 2014). L. vannamei fed with Rhodopseudomonas addition diets were more resistant against ammonia stress and Vibrio pathogens (Alloula et al., 2020). Studies have found the protein content of Rhodobacter sphaeroides protein was up to 63%, which can replace FM up to 100% with no negative effects on survival rate, feed intake, growth performance, antioxidant and intestinal tissue health (Liao et al., 2021). On the other hand, Rhodobacter sphaeroides had been established as a strong candidate for CoQ10 production (Choi et al., 2005). Although Rhodobacter sphaeroides and CoQ10 have been researched widely in aquaculture, the resistance to acute low salinity of which remained to be further investigated.
As a burgeoning area, the metagenomics approach was extensively used in the research of mammalian intestinal microbiota (Maccaferri et al., 2011; Zhu et al., 2011; Song et al., 2017). By contrast, metagenomics is seldom used in aquaculture (Martínez-Porchas and Vargas-Albores, 2017). Recently, researches on the intestinal microbiota in L. vannamei have been carried out successively (Chen et al., 2017; Gainza et al., 2018; Hou et al., 2018; Huang et al., 2018). As the main constituents of the shrimp digestive system, it has been recognized now that intestine was an extremely intricate ecosystem, which contains a variety of active microorganisms (Fan and Pedersen, 2021). What’s more, regarded as the “second genome” or “additional organ” (Bäckhed et al., 2007), intestinal microbiota has significantly influenced host metabolism, body composition and immune capacity (Clarke et al., 2014). Recent study has indicated that the composition of intestinal microbiota was more influenced by host diets than the water environment (Li et al., 2017). Interestingly, the addition of probiotics in shrimp commercial dietary could remarkably modify the intestinal microflora (Vargas-Albores et al., 2017). To further evaluate whether CoQ10 can be safe additives modulating microbial community structure in L. vannamei, the intestines of shrimp fed with different diets were chosen for 16S rRNA gene sequencing analysis, which may provide a solid theoretical basis for fishmeal substitutions or dietary supplementations. In a word, the experiment was aimed at elucidation of the impacts of RSP substitution of fishmeal and CoQ10 supplementation on growth performance, intestinal microbiota and stress tolerance against acute low salinity challenge.
Materials and Methods
Cultured Environmental Conditions
For this research, the cultured Pacific White Shrimp (L. vannamei) were afforded by Evergreen South Ocean Tech Co., Ltd, Zhanjiang, China. 16 aquaria (300 L, 0.6 m2 bottom, 4 aquaria per dietary treatment) with 40 shrimp/aquaria were used for four experimental diets. The shrimp weight 0.85 g were selected and adapted for a week with basic feed prior to the experiments. For the evaluation of the impacts of RSP (All the main raw materials (include: Rhodobacter sphaeroides and glucose, corn steep liquor, inorganic salts, etc.) were fermented, then inactivated and dried to gain the Rhodobacter sphaeroides protein product; Zhejiang NHU Company Ltd., Xinchang, Zhejiang) and CoQ10 (Zhejiang NHU Company Ltd., Xinchang, Zhejiang) on L. vannamei, four experimental diets were designed as follows: FM diet contains 20% fishmeal; RSP diet contains 20% RSP instead of 20% fishmeal; CoQ10 diet contains 20% fishmeal supplemented with CoQ10 at 0.08 g kg–1; RSP + CoQ10 diet contains 20% RSP supplemented with CoQ10 at 0.08 g kg–1 (Table 1). The composition of basal feed was as follows: crude protein 38%, crude fat 6% and moisture 9%. The method of diet preparation was the same as described by Niu et al. (2010). In short, all dry ingredients of four diets were weighed and mixed fully to homogeneity in a Hobart-type mixer (A-200T Mixer Bench Model unit, Resell Food Equipment Ltd., Ottawa, Canada). After that soy oil, fish oil and soybean lecithin were then added and mixed well for 5 min, and the mixed components were added and mixed to deionized water for 10 min. Post conditioning, the wet mixture were randomly extruded using monoscrew extruder (Institute of Chemical Engineering, South China University of Technology, Guangzhou, P.R. China) having barrel inner diameter of 1.2 mm. The four diets were stored at −20°C before use. All shrimp were fed to apparent satiation three cycles per day at 8:00, 12:00, and 18:00 by hand with a total amount of approximately 6% of its body weight, and maintained in fresh seawater with the temperature ranged from 27 to 29°C, pH was 8.0–8.2, salinity was approximately 30 ppt. The aquaria conditions were kept identical during feeding experiment.
Sampling
The feeding experiment was carried out for 8 weeks. At the start and the end of the feeding experiment, all shrimp were weighed. The routine nutritional compositions of the body samples were then analyzed (n = 5), meanwhile, intestine samples were obtained for the analysis of intestinal microbiota (n = 5). All samples were frozen in liquid nitrogen immediately and then stored in −80°C until measurement.
Survival Rates and Growth Performance
During the feeding experiment (8 weeks), the number of dead individuals were counted from beginning to end for measurement of the survival rates. In this study, weigh gain (WG), feed conversion ratio (FCR), specific growth rate (SGR), condition factor (CF), and hepatosomatic index (HSI) were used to evaluate the growth performance in L. vannamei. The parameters were calculated as follows: WG = 100 × (final body weight - initial body weight)/initial body weight; SGR = 100 × (Ln final mean weight—Ln initial mean weight)/number of days; FCR = dry diet fed/wet weight gain; CF = 100 × (body weight)/(body length)3; HSI = 100 × hepatopancreas weight/whole body weight.
16S rRNA Gene Sequence Analysis
Genome DNA of the intestinal contents was extracted with a DNA microbiome kit (Qiagen, Germany). The integrality of total DNA was assessed with 1% agarose gel electrophoresis, DNA purity and concentration were quantified using a spectrophotometer (Thermo Fisher Scientific Inc., United States). Purified genomic DNA was then sent for sequencing at the Novogene Biological Information Technology Co. (Tianjin, China). Quality filtering and splicing on raw data were performed using QIIME procedures (Caporaso et al., 2010). The retained clean data was performed Operational Taxonomic Unit (OTU) cluster analysis. Clustering of OTUs (at 97% similarity) were performed by VSEARCH for further annotation. The further diversity difference analysis was conducted according to the results of the relative OTU abundance. Alpha diversity measurements were evaluated with ACE, Chao1 and Rank-Abundance curves (richness), Shannon and Simpson (diversity) (Schloss et al., 2011). Beta diversity measurements were manifested as principal component analysis (PCA) and principal coordinates analysis (PCoA). Finally, the microbial communities’ differences between four diet treatments were evaluated using Linear Discriminant Analysis Effect Size (LEfSe) method.
Challenge Tests
At the end of the 8-week feeding trial, 10 shrimp from each experimental aquarium were transferred randomly into the desalinated seawater (salinity, 2 ppt). The mortality was monitored for 4.5 h post- attack, and the relative percentage survival rates were calculated. In addition, hepatopancreas samples were collected for further analysis.
Biochemical Analysis
The frozen hepatopancreas were weighed and homogenized by adding ice-cold PBS (1:10 dilution) until the hepatopancreas were completely broken. The homogenate tissues were centrifuged for 20 min (3,000 × g at 4°C). T-AOC, GSH-PX and MDA were detected by commercial assay kits (cat. nos. A015, A005, and A003, respectively; Nanjing Jiancheng Bioengineering Institute, China) following the manufacturers’ instructions. All assays were completed within a week after preparation.
qPCR Analysis of Immune- Related and Oxidative Stress Damage Related Genes
For the purposes of investigating the effects of RSP and CoQ10 on the immunity and antioxidative capacity of L. vannamei, the hepatopancreas samples from 4 shrimp per each dietary were collected after low salinity challenge. The total RNA samples were extracted from hepatopancreas by RNeasy™ animal RNA extraction kit (Beyotime, Shanghai, China) according to the protocol, and then transcribed to complementary DNA (cDNA) with a PrimeScript RT Reagent kit (Takara Bio, Inc.). The gene expression levels of four immune- related and oxidative stress damage related genes HSP70, Caspase-3, SOD and Relish were investigated in the hepatopancreas by the Light Cycler 480 (Roche Applied Science, Basel Switzerland). The qRT-PCR reaction program was performed as the following thermocycler condition: 95°C for 10 min, followed by 40 cycles of 95°C for 5 s, 60°C for 30 s and 72°C for 30 s, finally at 4°C for 5 min. Specific shrimp primers of related genes used in this study were shown in Table 2, the expression levels of related genes were quantified using the 2–ΔΔCt method.
Statistical Analysis
All results were presented as the mean ± standard error (SE). One-way ANOVA was performed to determine statistically significant differences of the different treatment using SPSS software. Multiple comparisons were made using Duncan’s multiple tests. Other statistical analyses were performed with the R or VSEARCH software environment. A P-value < 0.05 was considered significant.
Results
Growth Performance
The growth performance and survival rates of L. vannamei were shown in Figure 1. In the whole feeding period, shrimp fed with RSP diet had remarkably higher WG and FCR than shrimp fed with FM diet (P < 0.05; Figures 1A,C). However, survival rates were similar among all diets (P > 0.05; Figure 1B). A higher SGR was found in shrimp fed with CoQ10 diet than that in shrimp fed those diets and no significant differences were found among other diets (P > 0.05; Figure 1D). CF of shrimp fed with FM diet was remarkably lower than those of shrimp fed with RSP diet, CoQ10 diet and RSP + CoQ10 diets, while no significant differences were found among RSP diet, CoQ10 diet and RSP + CoQ10 diets (P > 0.05; Figure 1E). HSI of shrimp fed with FM diet was significantly higher than those of shrimp fed RSP diet and CoQ10 diets (P < 0.05), while no significant differences were found in HSI between FM diet and RSP + CoQ10 diets (P > 0.05; Figure 1F).
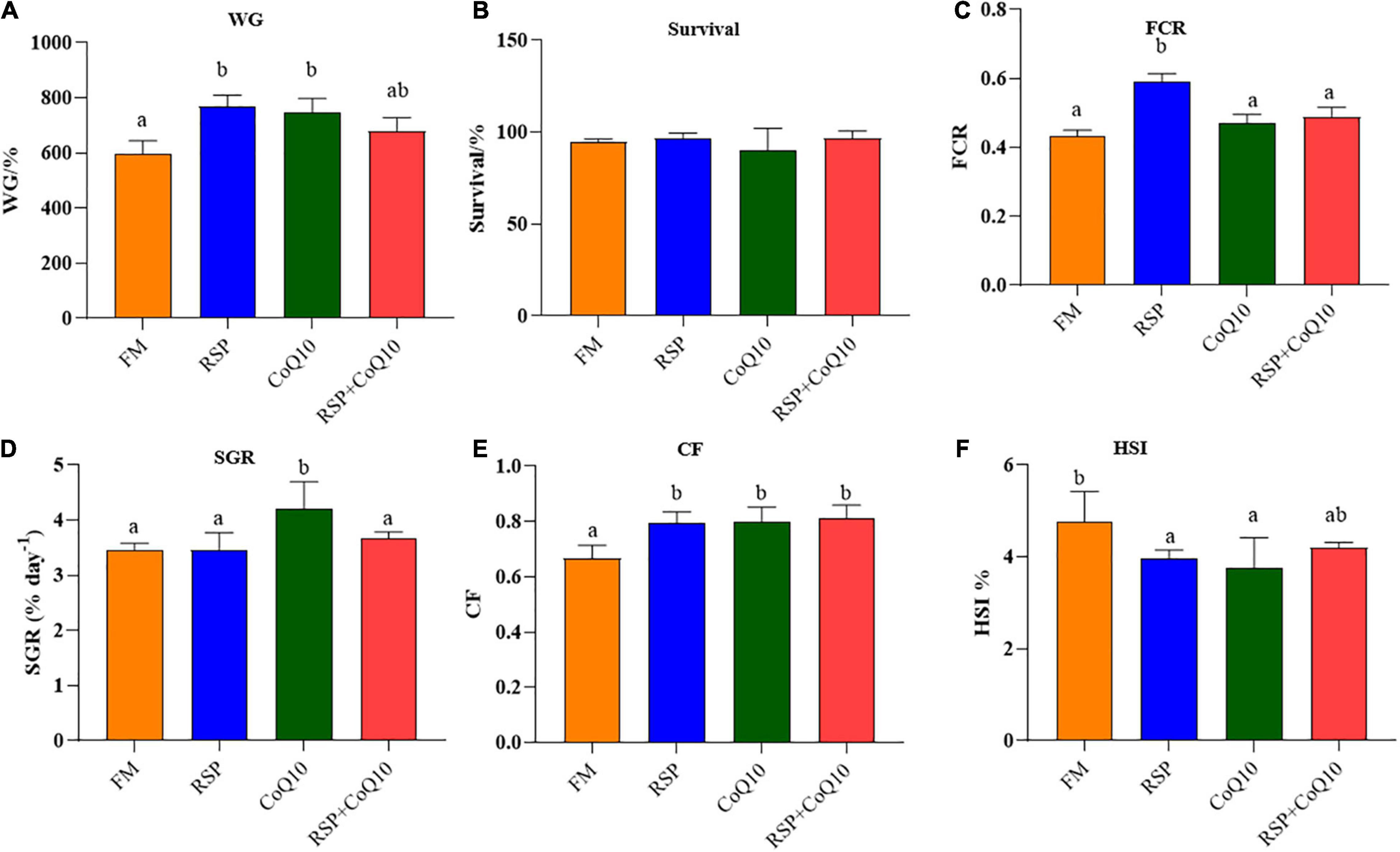
Figure 1. The effect of four different diet treatments on growth performance of shrimp. (A) WG of shrimp in different diet treatments. (B) Survival rates of shrimp in different diet treatments. (C) FCR of shrimp in different diet treatments. (D) SGR of shrimp in different diet treatments. (E) CF of shrimp in different diet treatments. (F) HSI of shrimp in different diet treatments. WG, weigh gain; FCR, feed conversion ratio; SGR, specific growth rate; CF, condition factor; HSI, hepatosomatic index.
Muscle Proximate Composition
SA The crude lipid content in muscle was significantly lower in shrimp fed FM diet than from the other three diets (P < 0.05; Table 3). Meanwhile, no considerable differences were found in crude protein and moisture contents of muscle in all diets (P > 0.05).
Alpha Diversity Index Statistics and Beta Diversity Analysis of Microbial Communities
The alpha diversity parameters include ACE, Chao1, Shannon and Simpson index, which represent the extents of microbial communities’ richness and diversities. Clearly, the microbial community richness from RSP diet was significantly higher than that from other diets, as supported by ACE (P < 0.05) and Chao1 (P < 0.05; Figures 2A,B). The Shannon index was highest in RSP diet, following in RSP + CoQ10 diet, and lowest in FM diet (Figure 2C). What’s more, Simpson’s index was lower in RSP diet than that in FM diet and CoQ10 diets (Figure 2D), microbial diversity in FM diet was nearly identical with CoQ10 diet (Shannon index 4.5, Simpson index 0.90). All the results manifested that 100% of fishmeal replaced by RSP can increase intestinal microbial diversity.
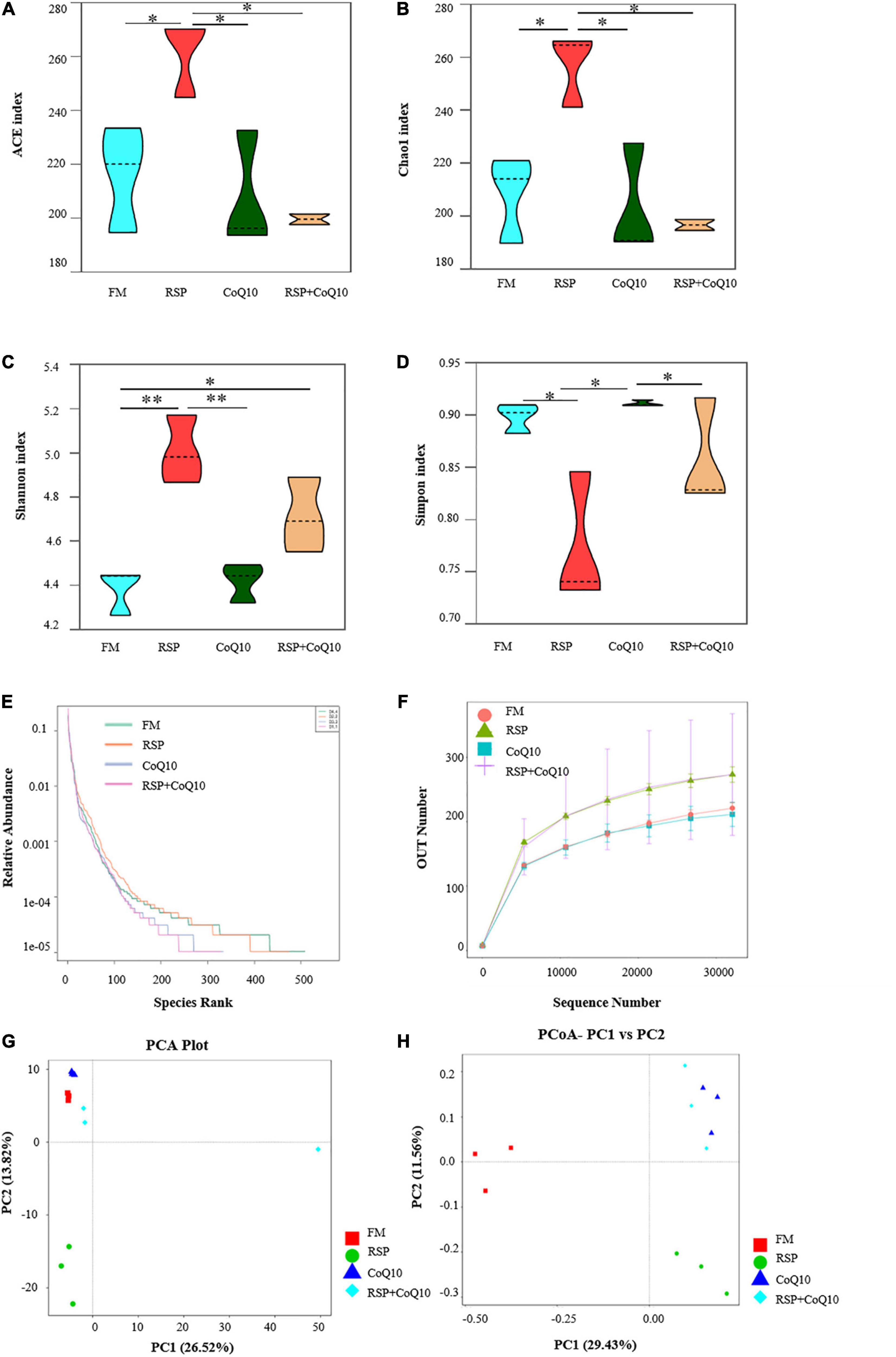
Figure 2. Alpha diversity index statistics and beta diversity analysis of intestinal microbiota in L. vannamei fed four different diet treatments. (A–D) Were the Ace, Chao1, Shannon and Simpson index of OTU level separately. Statistical differences were determined using two-way ANOVA. *P < 0.05; **P < 0.01. (E,F) Dilution curve and grade abundance curves based on OTU analysis of L. vannamei intestines. (G) Principal co-ordinates analysis (PCoA) distribution plot. (H) Principal component analysis (PCoA) normalized distribution plot. PC1, the first principle component; PC2, the second principle component.
OTU analysis-based dilution and grade abundance curves from different treatments were given in Figures 2E,F. The horizontal axis of FM diet and RSP diets were broader than that of CoQ10 diet and RSP + CoQ10 diets, manifesting that the species composition from FM diet and RSP diets were more specific and richer compared with CoQ10 diet and RSP + CoQ10 diets.
Beta diversity analysis was to show the similarities and differences of microbial communities from different treatments. According to PCA analysis, a similar microbial community structure was observed among the basic diet, CoQ10 diet and RSP + CoQ10 diet (Figure 2G), which showed that RSP substitution of fishmeal had a bigger effect on microbial community structure. A principal coordinate analysis (PCoA) showed that the four diet treatments were divided into three parts that manifested the effects of RSP and CoQ10 on the similarity of intestinal microbial composition in L. vannamei (Figure 2H), indicating that RSP substitution had impacted L. vannamei microbiota significantly (P < 0.05) but not CoQ10 addition.
Taxonomic Compositions and Changes of Intestinal Microbiota
An OTU was defined by sequences were clustered at the 97% similarity, each OTU represented a corresponding sequence. Observed numbers of OTUs have shown that the highest OTU number of 530 was with RSP + CoQ10 diet and the lowest of 218 with CoQ10 diet with the remaining OUT numbers were in between (222–460) (Figure 3A). A Venn map was constructed to identify dominant OTUs presented in all diet treatments, indicating that there were 50, 141, 56, and 221 unique OTUs in FM diet, RSP diet, CoQ10 diet and RSP + CoQ10 diets, respectively, which means the dietary fishmeal replaced with RSP and the dietary CoQ10 addition can influence intestinal microbial and generate corresponding unique microbiota (Figure 3B).
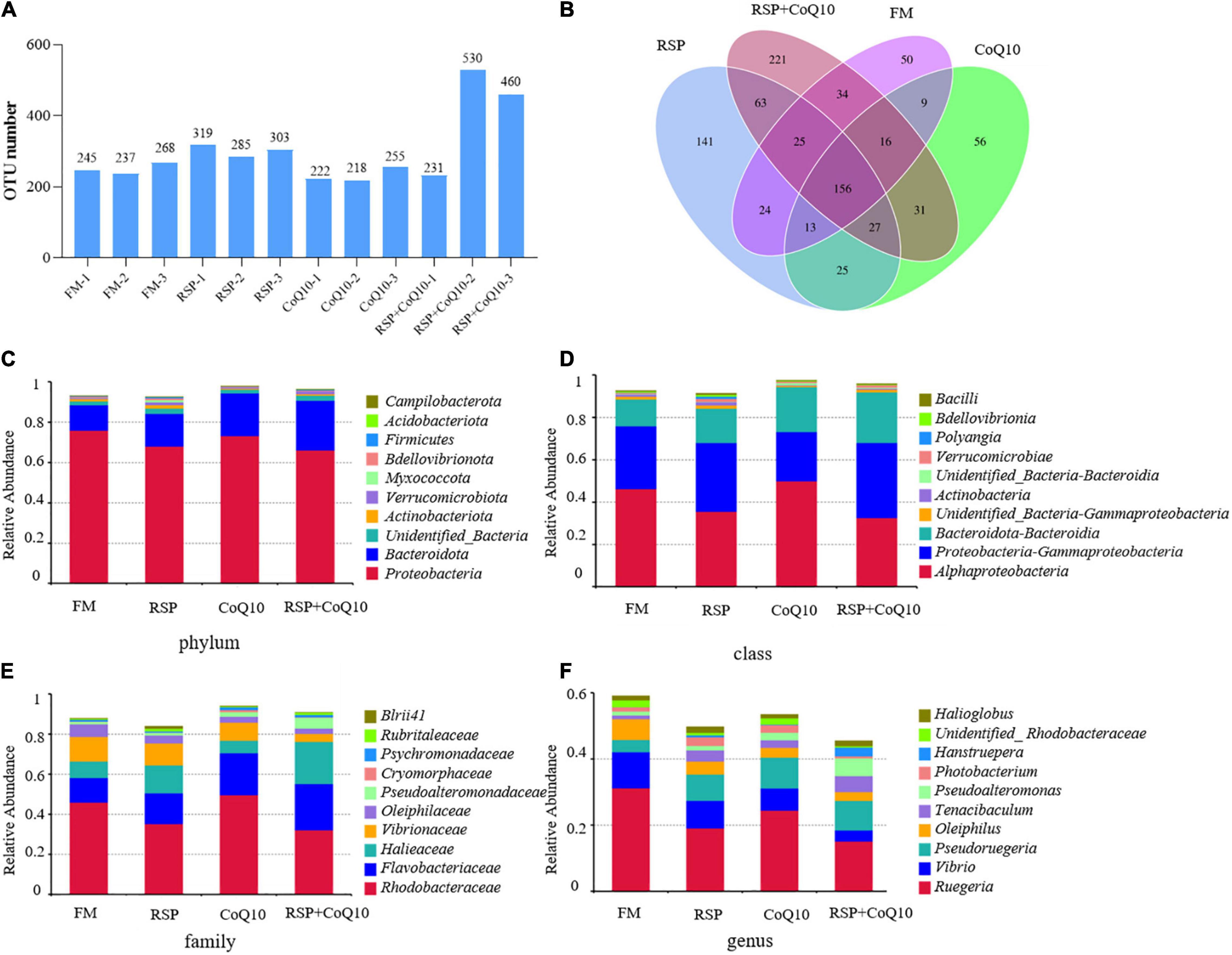
Figure 3. Taxonomic analysis of intestinal microbiota in L. vannamei fed four different diet treatments. (A) Distribution map of OTU number in samples from different diet treatments. (B) Comparison of OTUs in the four diet treatments by Venn diagram. Relative abundance of bacterial community in all diet treatments at (C) phylum, (D) class, (E) family and (F) genus levels in different diet treatments. AL, ageratum-liquid; OTU, operational taxonomic unit (OTU).
Compared with the OTU sequences with related database, Proteobacteria still the only predominant phylum in all diet treatments with the abundance of 75.9, 68.4, 73.2, and 66.1% in FM diet, RSP diet, CoQ10 diet and RSP + CoQ10 diets, correspondingly, the second dominant phylum was Bacteroidota with an abundance of 12.6, 16.1, 21.1, and 24.6% in FM diet, RSP diet, CoQ10 diet and RSP + CoQ10 diets (Figure 3C). Clearly, more diverse phyla were found in RSP + CoQ10 diet, including Thermotogae, Caldatribacteriota, Gemmatimonadota, and Parcubacteria. The above results revealed that the amount of Proteobacteria was changed not due to RSP replacement for fishmeal, but as a result of the addition of CoQ10. On the other hand, both dietary fishmeal replaced with RSP and dietary CoQ10 addition have promoted the proliferation of Bacteroidota, manifesting that RSP substitution and CoQ10 supplementation could improve the intestinal microecological environment.
At bacterial class level, Alphaproteobacteria, Gammaproteobacteria, and Bacteroidia were confirmed to be abundant in all diet treatments. The relative abundance of Alphaproteobacteria revealed that a highest relative abundance of 49.9% in CoQ10 diet and a lowest of 32.6% in RSP + CoQ10 diet. Whereas the relative abundance of Gammaproteobacteria displayed the opposite trend that shrimp fed with RSP + CoQ10 diet has the highest abundance of 35.4% and fed with CoQ10 diet has the lowest abundance of 23.2%. The third dominant class was Bacteroidia with an abundance of 12.6, 16.1, 21.1, and 23.8% in FM diet, RSP diet, CoQ10 diet and RSP + CoQ10 diets (Figure 3D). At the family level (Figure 3E), Rhodobacteraceae was the dominant family in all diets with its relative abundance at 45.9, 35.2, 49.6, and 32.1% in FM diet, RSP diet, CoQ10 diet and RSP + CoQ10 diets, separately, indicating its predominance was weakened in RSP diet and RSP + CoQ10 diets independent of the presence of absence of CoQ10. At the genus level (Figure 3F), the number and abundance of predominant genus in different diets varied considerably. Ruegeria was the predominant genus in all diets, the relative abundance of Ruegeria manifested that the highest relative abundance of 31.2% was with FM diet and a lowest of 15.1% with RSP + CoQ10 diet with the rest in between (19.1–22.4%). At the end of the test, compared with FM diet, the relative abundance of Vibrio was decreased significantly in RSP diet, CoQ10 diet and RSP + CoQ10 diets, however, in RSP diet, CoQ10 diet and RSP + CoQ10 diets, almost an addition of Pseudoruegeria was observed. Altogether, the microbial compositions of predominant phyla were obviously similar among these four diet treatments. All these data indicated that RSP substitution and CoQ10 supplementation may maintain intestinal homeostasis and decrease the colonization by intestinal opportunistic pathogens, like Vibrio.
LefSe Analysis of Gut Microbiota
LefSe analysis was carried out to further investigate the changes of L. vannamei’ intestinal microbial biomarkers caused by RSP substitution and CoQ10 supplementation (Figure 4). Based on the threshold that a Linear Discriminant Analysis (LDA) score of > 4.0, the dominant phylum, order, class, family and genus were screened. The biomarkers at different levels associated with FM diet were, in descending, Cellvibrionales, Halieaceae, and Bacteroidia. In RSP diet, the specific biomarkers were, Rhodobacteraceae, Rhodobacterales, and Alphaproteobacteria. Moreover, in CoQ10 diet, the specific biomarkers were, Photobacterium_damselae, Photobacterium, and Actinobacteriota, by contrast, the specific biomarkers were, Bacteria, Protebacteria, and Oceanospirillales in RSP + CoQ10 diet.
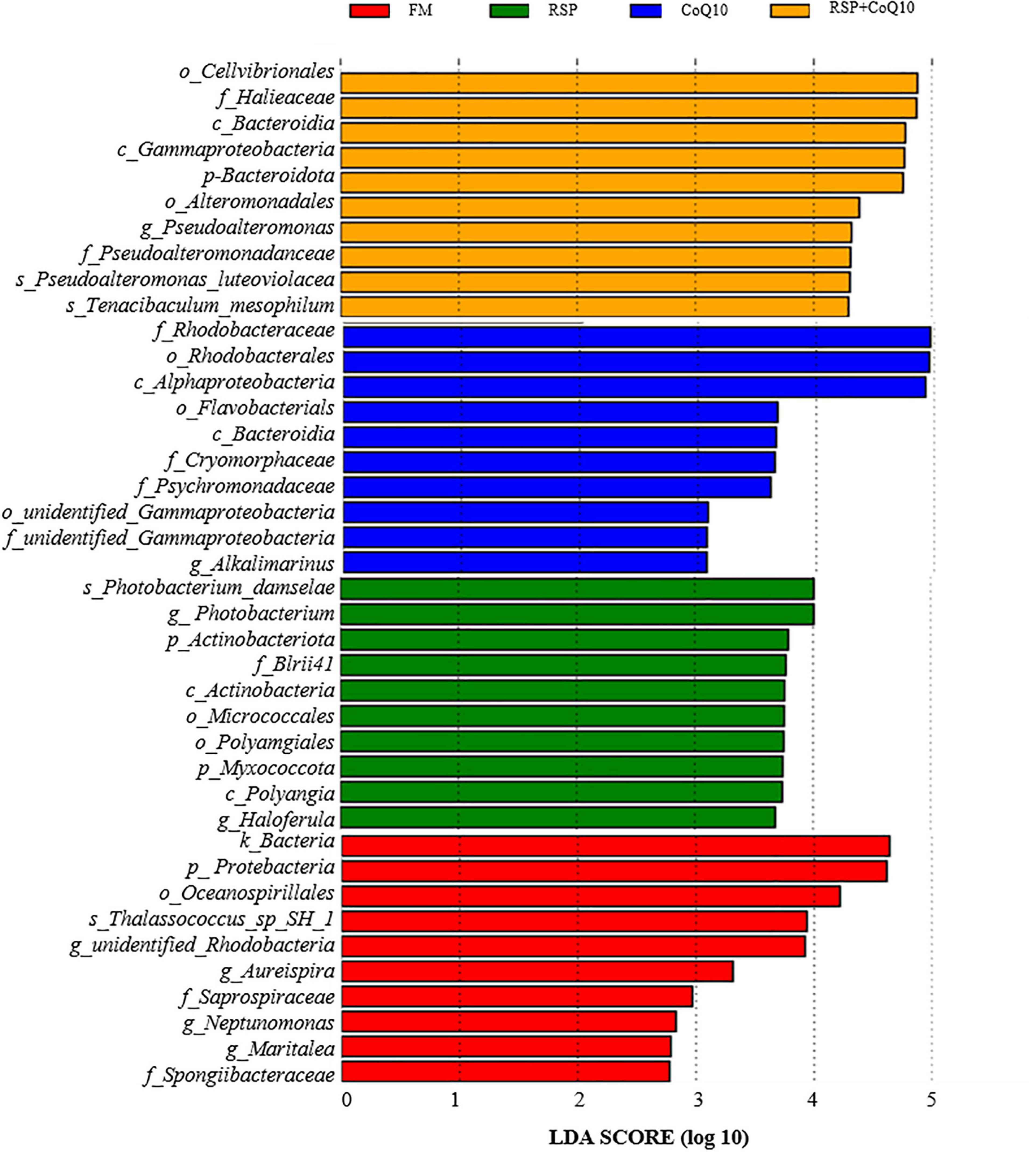
Figure 4. Identified differentially abundant taxa between four diet treatments from by linear discriminant analysis coupled with LEfSe (LDA > 2.5, P < 0.05). Yellow box: enriched in FM diet, blue box: enriched in RSP diet, green box: enriched in CoQ10 diet, red box: enriched in RSP + CoQ10 diet. LDA, linear discriminant analysis; LefSe, LDA effect size.
Functional Prediction of the Microbiota
Based on PICRUSt, the functional capacity of the intestinal microbiota was predicted. The detailed results were given in Figure 5, evidently, the most abundant function was related to environmental information processing in FM diet. In RSP + CoQ10 diet, genetic information processing functions were doubled when compared to FM diet. Correspondingly, human diseases were enriched in CoQ10 diet. In combination, these COG function classification results showed that the L. vannamei intestinal microbial taxa presented the distinct biological functions with the presence of absence of RSP substitution and CoQ10 supplementation.
Survival Rates of After Acute Low Salinity Challenge
The survival rates of four experimental diet treatments were shown in Table 4 after acute low salinity challenge. The survival rate in shrimp fed with RSP diet was considerably lower than those in shrimp fed with CoQ10 diet and RSP + CoQ10 diets (P < 0.05). No statistically appreciable differences were observed in shrimp fed with FM diet and RSP diets (P > 0.05).
Biochemical Parameters of Hepatopancreas After Acute Low Salinity Challenge
The hepatopancreas content of MDA and activities of T-AOC and GSH-PX were illustrated in Table 5. The hepatopancreas activities of T-AOC in shrimp fed CoQ10 diet and RSP + CoQ10 diets were remarkably higher than that in shrimp fed FM diet and RSP diets (P < 0.05), while no significant differences were presented in T-AOC activity between FM diet and RSP diets (P > 0.05). In addition, no considerable differences were found in hepatopancreas MDA contents and GSH-PX activities among all diets (P > 0.05).
Relative Expression of Immune-Related and Antioxidant-Related Genes in Hepatopancreas of Shrimp After Low Salinity Challenge
As showed in Figure 6, after 4.5 h acute low salinity challenge, the expression level of SOD in hepatopancreas of shrimp fed CoQ10 diet and RSP + CoQ10 diets were remarkably higher than those of shrimp fed FM diet and RSP diets (P < 0.05). Relative expression of HSP70 for shrimp fed CoQ10 diet and RSP + CoQ10 diets were higher than that for shrimp fed FM diet and RSP diets (P < 0.05). However, no significant differences were found in Caspase-3 expression among all diets (P > 0.05). The lowest Relish expression was found in shrimp fed FM diet (P < 0.05).
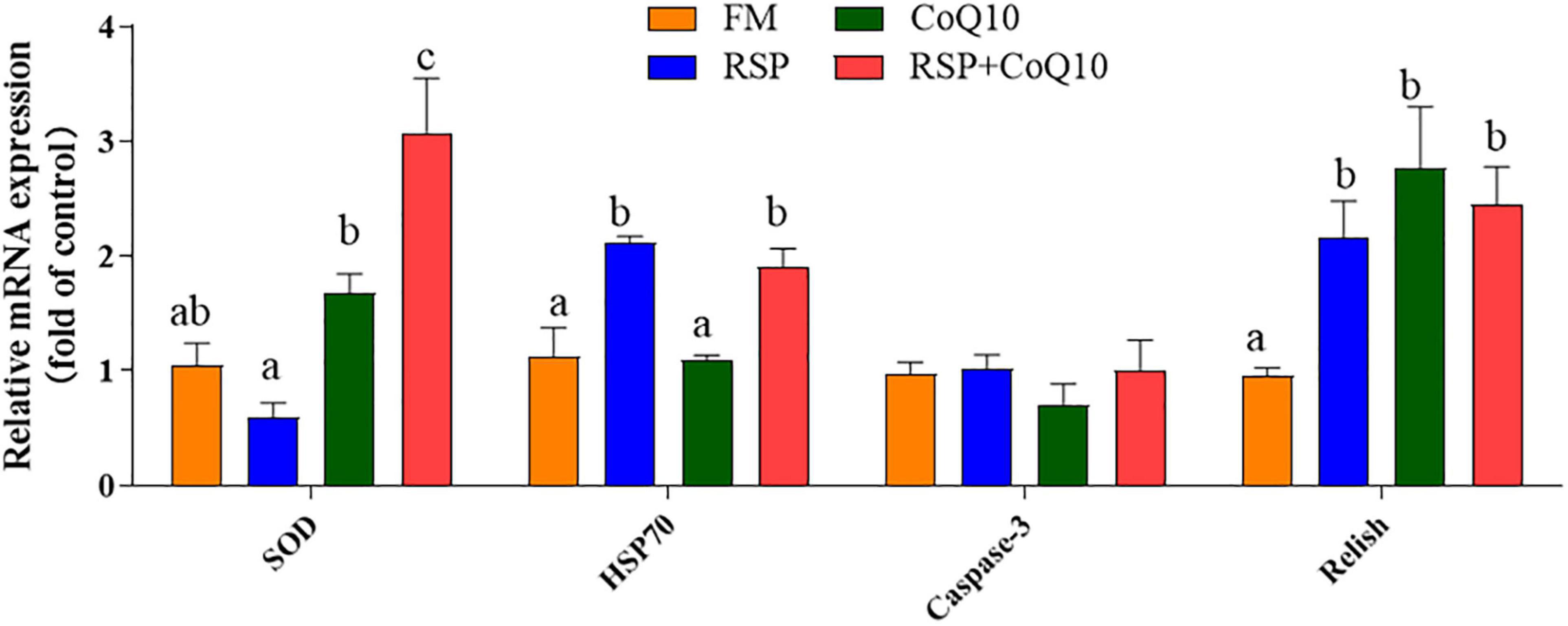
Figure 6. Relative expression of immune-related and antioxidant-related genes in hepatopancreas of shrimp fed with four diet treatments after low salinity stress. Results are mean ± SEM (n = 3). The column with different superscripts manifests significant differences (P < 0.05). HSP70, heat shock protein 70; SOD, superoxide dismutase.
Discussion
The current studies of RSP as an alternative fishmeal source in L. vannamei feed were limited. The PNSB extracted from Rhodobacter sphaeroides was proved to benefit for L. vannamei (Chumpol et al., 2018). Our previous study has proved that RSP played a significant role in enhancing growth performance, increasing survival rates, reinforcing immune response, facilitating resistance and oxidative capacity against low salt challenge in L. vannamei (Liao et al., 2021). Meanwhile, CoQ10 was recognized as a natural substance with a formidable antioxidant capacity, whose natural sources varied from prokaryotes organisms to eukaryotes, comprising an abundance of bacteria (Agrobacterium tumefaciens, Rhodobacter sphaeroides, and Paracoccus denitrificans) (Cluis et al., 2007). With the exception of Agrobacterium, the great potential of a facultative photosynthetic bacteria-Rhodobacter sphaeroides for yielding CoQ10 had been confirmed (Choi et al., 2005). In this research, the growth performance (WG and SGR) of L. vannamei showed a significantly growth with CoQ10 supplementation in FM diet. Correspondingly, there were no significant differences between RSP diet and RSP + CoQ10 diets in WG and SGR. To a certain extent, RSP could serve as a complete fishmeal substitution in L. vannamei without compromising growth performance. Gopi et al. (2014) reported an enhancement in WG at 0.02 and 0.04 g kg–1 CoQ10 diet in broiler. Moreover, El et al. (2020) observed that WG and SGR of Nile tilapia showed no remarkable differences at the level of 0.04 g kg–1 CoQ10 dietary. While, 0.08 g kg–1 CoQ10 supplementation could significantly ameliorate growth performance of L. vannamei in this study.
It is well known that the intestinal microbiota has many roles in the health of aquatic animal, the majority of which were benign or neutral, including in immune response, nutrient absorption and intestinal morphology (Fujimura et al., 2010; Huang et al., 2015). Indeed, the intestinal microbiota would be susceptible to the food and aqueous environment (Gao et al., 2014). As an important determinant of ecosystem functioning (Johnke et al., 2020), biodiversity is generally quantified by richness, evenness or diversity (McArt et al., 2012). Balanced intestinal microbiota are important for the growth and health of L. vannamei. In this study, the value of Shannon index increased significantly in L. vannamei fed RSP diet treatment, which indicated that shrimp fed RSP diet had greater community diversity than other three diets. Meanwhile, the trend of ACE index and Chao1 index were consistent with Shannon index, while Simpson index was just opposite, all these data manifested that it was dietary RSP substitution of fishmeal that enriched the intestinal microbial community of L. vannamei. The PCoA score plot statistics explained that the intestinal samples from CoQ10 addition did cluster in the same district, while FM diet and RSP diets did not, indicating fluctuations in the intestinal microbial compositions of L. vannamei with the addition of CoQ10, which may be caused by dietary CoQ10 supplementation.
Many previous studies have proved that Proteobacteria was the predominant colonizer in L. vannamei’ intestine (Xiong et al., 2015; Rungrassamee et al., 2016), the enrichment of which reflected the dysregulation of microbiota and the unstable microbial community structure (Shin et al., 2015). In this research, the abundance of Proteobacteria in all diets was more than 60%, and Proteobacteria was abundant in FM diet compared to other three dies. Accordingly, the outgrowth of Proteobacteria may trigger the dysbiosis, and diets of RSP substitution and CoQ10 supplementation maintain the intestinal homeostasis compared to FM diet. Rhodobacteraceae has been applied in shrimp aquaculture for its latent talent to promote host growth (Yamazaki et al., 2016) and ability to degrade organic compounds in aquarium (Huang et al., 2018). As beneficial bacteria, Rhodobacteraceae was abundant in CoQ10 diet in L. vannamei’ intestine compared to other three diets. Correspondingly, the growth performance of CoQ10 diet was significantly higher other three diets, and the higher abundance of Rhodobacteraceae might be the major contributor to this difference, therefore, L. vannamei fed CoQ10 diet had better growth performance. In the genus levels, Ruegeria and Vibrio were the most dominant genus in L. vannamei’ intestine fed with four diet treatments. Similarly, a significant amount of Vibrio was also found in some marine organisms (Guerreiro et al., 2018). Vibrio was affiliated to the Vibrionaceae (family), which was probably the most commonly bacterial pathogens (Liu et al., 2016), and excessive of Vibrio could impair the health status of L. vannamei (Xiong et al., 2017). Compared with FM diet, the relative abundance of Vibrio was remarkably decreased after the shrimp fed CoQ10 diet and RSP + CoQ10 diets, whereas the shrimp fed with RSP diet didn’t significantly decrease, which indicated that CoQ10 addition could serve to augment the immune response of L. vannamei and avoid the risk of Vibrio infection.
Different diet treatments could shape different functional predictions of the intestinal microbiota. Function of environmental information processing, cellular processes and human diseases were more plentiful in FM diet. On the contrary, except for above functions, genetic information processing functions, metabolism and organismal systems were more enriched in RSP + CoQ10 diet. These differences in functions of intestinal microbiota would be related to L. vannamei’ dietary supplementations. Due to the capability of microbiota (Firmicutes and Bacteroidetes) to degrade complex polysaccharide (Dudek et al., 2014), taken the abundance of intestinal microbiota into account, more abundant Bacteroidota in L. vannamei fed RSP + CoQ10 diet might be the reason of higher level of metabolism function. In addition, the higher species richness and diversity in RSP diet demonstrated that the complexity and instability of microbiota might lead to more functional categories.
As one of the most significant environmental factors in aquaculture, salinity changes have influenced the growth performance and survival rates of the shrimp (Esparza-Leal et al., 2010). The previous research has proved that no big differences were observed between FM diet and diet treatment of fishmeal replaced with different levels of RSP in survival rates after acute low salinity challenge (Liao et al., 2021). As shown in this research, after acute low salinity challenge, the supplementation of CoQ10 to diets have significantly increased the survival rates of L. vannamei whether exist fishmeal substitution or not. On the other hand, antioxidant capacity of shrimp was the important defense mechanism to withstand environmental extremes. As an endogenous lipophilic antioxidant, CoQ10 plays an important role in preventing the lipid peroxidation level (Navas et al., 2007). During this research, L. vannamei fed with CoQ10 diet had significantly upregulated SOD expression in hepatopancreas after 4.5 h acute low salinity challenge. The higher expression of SOD could be beneficial to increase the resistance of L. vannamei against acute low salinity challenge (Zhang et al., 2012), which indicated that CoQ10 supplementation may increase the antioxidant capability by enhancing the release of superoxide anion. In addition, T-AOC activity in the hepatopancreas was highest in CoQ10 diet treatment after acute low salinity challenge. Low salinity had stimulated the accumulation of ROS (Rosas et al., 2001; Yeh et al., 2010), and the increase of T-AOC activity ameliorated the health status of L. vannamei to some extent. The improvement of health status and antioxidant capacity may directly enhance the survival rates of L. vannamei against acute low salinity challenge, which means that dietary CoQ10 could be used to promote resistance to environmental stress in L. vannamei, however, RSP replacement of FM does not influence the antioxidant ability of L. vannamei against acute low salinity challenge.
As an important regulator of activating the immune signaling pathway, HSP70 can induce the production of immune proteins to reinforce L. vannamei’ resistance against a pathogen (Yik Sung and MacRae, 2013). In this study, significantly higher expression of HSP70 were found in L. vannamei fed RSP diet and RSP + CoQ10 diets under low salt stress. Previous research also demonstrated that chronic exposure in the low salinity environment can decrease the immune parameters in L. vannamei (Lin et al., 2012). Meanwhile, CoQ10 supplementation can improve immune parameters by reducing stresses and promoting mitochondrial respiration (Feher et al., 2007). These results demonstrated that RSP substitution of fishmeal and CoQ10 supplementation enhanced resistance against low salt stress in L. vannamei as an immunostimulant.
Conclusion
In this study, the impacts of dietary RSP substitution of fishmeal and CoQ10 supplementation on the growth performance, muscle composition, intestinal microbiota, antioxidation effects and immunity capacity of L. vannamei after acute low salinity challenge were investigated. These beneficial effects can provide a new perspective that RSP substitution of fishmeal and CoQ10 addition may be enhance resistance at low salinity or other extreme environments.
Data Availability Statement
The datasets presented in this study can be found in online repositories. The names of the repository/repositories and accession number(s) can be found in the article/supplementary material.
Ethics Statement
The animal study was reviewed and approved by the Care and Use of Laboratory Animals of the National Institutes of Health (NIH) and approved by Experimental Animal Ethics Committee of Sun Yat-sen University.
Author Contributions
JN and YG designed the study. ZW, YW, WZ, and XH analyzed parts of results. DW carried out the rearing trial. RY and MC analyzed the data. ZL analyzed results and wrote this manuscript with suggestions from JN and YG. All authors contributed to the article and approved the submitted version.
Funding
This work was supported by the Fund of China Agriculture Research System of MOF and MARA 48 (CARS 48), and Fund of Zhejiang NHU Company Ltd. (HT-99982019-0249), and Project of Science and Technology of Guangdong Province (2019B110209005), and Project of Science and Technology of Guangdong Province (2021B0202050002), and Youth Science and Technology Innovation Talent of Guangdong TeZhi Plan Talent (2019TQ05N129).
Conflict of Interest
YG was employed by Zhejiang Xinhecheng Co., Ltd.
The remaining authors declare that the research was conducted in the absence of any commercial or financial relationships that could be construed as a potential conflict of interest.
Publisher’s Note
All claims expressed in this article are solely those of the authors and do not necessarily represent those of their affiliated organizations, or those of the publisher, the editors and the reviewers. Any product that may be evaluated in this article, or claim that may be made by its manufacturer, is not guaranteed or endorsed by the publisher.
Acknowledgments
We thank the participants who gave their time to the trial.
References
Adarsh, K., Kaur, H., and Mohan, V. (2008). Coenzyme Q10 (CoQ10) in isolated diastolic heart failure in hypertrophic cardiomyopathy (HCM). Biofactors 32, 145–149. doi: 10.1002/biof.5520320117
Alloula, A., Willeb, M., Lucentia, P., Bossierb, P., Van, G., Siegfried, S., et al. (2020). Purple bacteria as added-value protein ingredient in shrimp feed: penaeus vannamei growth performance, and tolerance against Vibrio and ammonia stress. Aquaculture 530:735788. doi: 10.1016/j.aquaculture.2020.735788
Bäckhed, F., Manchester, J. K., Semenkovich, C. F., and Gordon, J. I. (2007). Mechanisms underlying the resistance to diet-induced obesity in germ-free mice. PNAS 104, 979–984. doi: 10.1073/pnas.0605374104
Caporaso, J. G., Kuczynski, J., Stombaugh, J., Bittinger, K., Bushman, F. D., Costello, E. K., et al. (2010). QIIME allows analysis of high-throughput community sequencing data. Nat. Methods 7, 335–336. doi: 10.1038/nmeth.f.303
Chen, W., Ng, T. H., Wu, J., Chen, J., and Wang, H. (2017). Microbiome dynamics in a shrimp grow-out pond with possible outbreak of acute hepatopancreatic necrosis disease. Sci. Rep. 7, 1–12. doi: 10.1038/s41598-017-09923-6
Chiu, K. H., and Liu, W. S. (2014). Dietary administration of the extract of Rhodobacter sphaeroides WL-APD911 enhances the growth performance and innate immune responses of seawater red tilapia (Oreochromis mossambicus× Oreochromis niloticus). Aquaculture 418, 32–38. doi: 10.1016/j.aquaculture.2013.10.007
Choi, J. H., Ryu, Y. W., and Seo, J. H. (2005). Biotechnological production and applications of coenzyme Q10. Appl. Microbiol. Biotechnol. 68, 9–15. doi: 10.1007/s00253-005-1946-x
Chumpol, S., Kantachote, D., Nitoda, T., and Kanzaki, H. (2018). Administration of purple nonsulfur bacteria as single cell protein by mixing with shrimp feed to enhance growth, immune response and survival in white shrimp (Litopenaeus vannamei) cultivation. Aquaculture 489, 85–95. doi: 10.1016/j.aquaculture.2018.02.009
Clarke, G., Stilling, R. M., Kennedy, P. J., Stanton, C., Cryan, J. F., and Dinan, T. G. (2014). Minireview, gut microbiota, the neglected endocrine organ. Mol. Endo. 28, 1221–1238. doi: 10.1210/me.2014-1108
Cluis, C. P., Burja, A. M., and Martin, V. J. (2007). Current prospects for the production of coenzyme Q10 in microbes. Trends Biotechnol. 25, 514–521. doi: 10.1016/j.tibtech.2007.08.008
Dudek, M., Adams, J., Swain, M., Hegarty, M., Huws, S., and Gallagher, J. (2014). Metaphylogenomic and potential functionality of the limpet Patella pellucida’s gastrointestinal tract microbiome. Int. J. Mol. Sci. 15, 18819–18839. doi: 10.3390/ijms151018819
El, B. M., Teiba, I. I., Zaki, M., Alabssawy, A. N., El-Hais, A. M., Gabr, A. A., et al. (2020). Assessing the effectiveness of CoQ10 dietary supplementation on growth performance, digestive enzymes, blood health, immune response, and oxidative-related genes expression of Nile tilapia (Oreochromis niloticus). Fish Shellfish Immunol. 98, 420–428. doi: 10.1016/j.fsi.2020.010.052
Esparza-Leal, H. M., Ponce-Palafox, J. T., Valenzuela-Quinnez, W., Arredondo-Figueroa, J. L., and Gomez, M. G. (2010). Effects of Density on growth and survival of juvenile Pacific White Shrimp, Penaeus vannamei, reared in low-salinity well water. J. World Aqua. Soc. 41, 648–654. doi: 10.1111/j.1749-7345.2010.00406.x
Fan, Y., and Pedersen, O. (2021). Gut microbiota in human metabolic health and disease. Nat. Rev. Microbiol. 19, 55–71. doi: 10.1038/s41579-020-0433-9
Feher, J., Nemeth, E., Nagy, V., and Lengyel, G. (2007). The preventive role of coenzyme Q10 and other antioxidants in injuries caused by oxidative stress. Arch. Med. Sci. 3:305. doi: 10.1186/1471-2202-12-75
Fujimura, K. E., Slusher, N. A., Cabana, M. D., and Lynch, S. V. (2010). Role of the gut microbiota in defining human health. Exp. Rev. Anti Infect. Ther. 8, 435–454. doi: 10.1586/eri.10.14
Gainza, O., Ramirez, C., Ramos, A. S., and Romero, J. (2018). Intestinal microbiota of White Shrimp Penaeus vannamei under intensive cultivation conditions in ecuador. Microb. Ecol. 75, 562–568. doi: 10.1007/s00248-017-1066-z
Gao, F., Li, F., Tan, J., Yan, J., and Sun, H. (2014). Bacterial community composition in the gut content and ambient sediment of sea cucumber Apostichopus japonicus revealed by 16S rRNA gene pyrosequencing. PLoS One 9:e100092. doi: 10.1371/journal.pone.0100092
Geng, A., Li, B., and Guo, Y. (2007). Effects of dietary L-carnitine and coenzyme Q10 at different supplemental ages on growth performance and some immune response in ascites– susceptible broilers. Arch. Anim. Nutr. 61, 50–60. doi: 10.1080/17450390601117041
Gopi, M., Purushothaman, M. R., and Chandrasekaran, D. (2014). Effect of dietary coenzyme Q10 supplementation on the growth rate, carcass characters and cost effectiveness of broiler fed with three energy levels. Springerplus 3, 518. doi: 10.1186/2193-1801-3-518
Guerreiro, I., Serra, C. R., Oliva-Teles, A., and Enes, P. (2018). Short communication, gut microbiota of European sea bass (Dicentrarchus labrax) is modulated by short-chain fructooligosaccharides and xylooligosaccharides. Aqua. Int. 26, 279–288. doi: 10.1007/s10499-017-0220-4
Hou, D., Huang, Z., Zeng, S., Liu, J., Weng, S., and He, J. (2018). Comparative analysis of the bacterial community compositions of the shrimp intestine, surrounding water and sediment. J. Appl. Microbiol. 125, 792–799. doi: 10.1111/jam.13919
Huang, F., Pan, L., Song, M., Tian, C., and Gao, S. (2018). Microbiota assemblages of water, sediment, and intestine and their associations with environmental factors and shrimp physiological health. Appl. Microbiol. Biotechnol. 102, 8585–8598. doi: 10.1007/s00253-018-9229-5
Huang, L., Ran, C., He, S., Ren, P., Hu, J., Zhao, X., et al. (2015). Effects of dietary Saccharomyces cerevisiae culture or live cells with Bacillus amyloliquefaciens spores on growth performance, gut mucosal morphology, hsp70 gene expression, and disease resistance of juvenile common carp (Cyprinus carpio). Aquaculture 438, 33–38. doi: 10.1016/j.aquaculture.2014.12.029
Huang, M., Dong, Y., Zhang, Y., Chen, Q., Xie, J., Xu, C., et al. (2019). Growth and lipidomic responses of juvenile Pacific White Shrimp Litopenaeus vannamei to low salinity. Front. Physiol. 10:1087. doi: 10.3389/fphys.2019.01087
Jiang, D., Lawrence, A. L., Neill, W. H., and Gong, H. (2000). Effects of temperature and salinity on nitrogenous excretion by Litopenaeus vannamei juveniles. J. Exp. Mar. Biol. Ecol. 253, 193–209. doi: 10.1016/S0022-0981(00)00259-8
Johnke, J., Fraune, S., Bosch, T., Hentschel, U., and Schulenburg, H. (2020). Bdellovibrio and like organisms are predictors of microbiome diversity in distinct host groups. Microb. Ecol. 79, 252–257. doi: 10.1007/s00248-019-01395-7
Li, E., Chen, L., Zeng, C., Yu, N., Xiong, Z., Chen, X., et al. (2008). Comparison of digestive and antioxidant enzymes activities, haemolymph oxyhemocyanin contents and hepatopancreas histology of white shrimp, Litopenaeus vannamei, at various salinities. Aquaculture 274, 80–86. doi: 10.1016/j.aquaculture.2007.11.001
Li, H., Xu, C., Zhou, L., Dong, Y., Su, Y., Wang, X., et al. (2019). Beneficial effects of dietary beta-glucan on growth and health status of Pacific white shrimp Litopenaeus vannamei at low salinity. Fish Shellfish Immunol. 91, 315–324. doi: 10.1016/j.fsi.20190.050.052
Li, X., Zhou, L., Yu, Y., Ni, J., Xu, W., and Yan, Q. (2017). Composition of gut microbiota in the Gibel Carp (Carassius auratus gibelio) varies with host development. Microb. Ecol. 74, 239–249. doi: 10.1007/s00248-016-0924-4
Liao, Z. H., Gong, Y. Y., Zhao, W., He, X. S., Wei, D., and Niu, J. (2021). Comparison effect of Rhodobacter sphaeroides protein replace fishmeal on growth performance, intestinal morphology, hepatic antioxidant capacity and immune gene expression of Litopenaeus vannamei under low salt stress. Aquaculture 547:737488. doi: 10.1016/j.aquaculture.2021.737488
Lin, Y. C., Chen, J. C., Li, C. C., Morni, W. Z., Suhaili, A. S., Kuo, Y. H., et al. (2012). Modulation of the innate immune system in white shrimp Litopenaeus vannamei following long-term low salinity exposure. Fish Shellfish Immunol. 33, 324–331. doi: 10.1016/j.fsi.20120.05.006
Liu, F., Liu, G., and Li, F. (2016). Characterization of two pathogenic Photobacterium strains isolated from Exopalaemon carinicauda causing mortality of shrimp. Aquaculture 464, 129–135. doi: 10.1016/j.aquaculture.2016.06.019
Maccaferri, S., Biagi, E., and Brigidi, P. (2011). Metagenomics, key to human gut microbiota. Dig. Dis. 29, 525–530. doi: 10.1159/000332966
Martínez-Porchas, M., and Vargas-Albores, F. (2017). Microbial metagenomics in aquaculture, a potential tool for a deeper insight into the activity. Rev. Aqua. 9, 42–56. doi: 10.1111/raq.12102
McArt, S. H., Cook-Patton, S. C., and Thaler, J. S. (2012). Relationships between arthropod richness, evenness, and diversity are altered by complementarity among plant genotypes. Oecologia 168, 1013–1021. doi: 10.1007/s00442-011-2150-6
Navas, P., Villalba, J. M., and Cabo, R. D. (2007). The importance of plasma membrane coenzyme Q in aging and stress responses. Mitochondrion 7S, 34–40. doi: 10.1016/j.mito.2007.02.010
Niu, J., Tian, L. X., Liu, Y. J., Yang, H. J., and Mai, K. S. (2010). Effects of dietary chitosan on growth, survival and stress tolerance of postlarval shrimp, litopenaeus vannamei. Aqua. Nutr. 40, 795–802. doi: 10.1111/j.1749-7345.2009.00300.x
Prakash, S., Sunitha, J., and Hans, M. (2010). Role of coenzyme Q (10) as an antioxidant and bioenergizer in periodontal diseases. Indian J. Pharmacol. 42, 334–337. doi: 10.4103/0253-7613.71884
Ramos-Carreno, S., Valencia-Yanez, R., Correa-Sandoval, F., Ruiz-Garcia, N., Diaz-Herrera, F., and Giffard-Mena, I. (2014). White spot syndrome virus (WSSV) infection in shrimp (Litopenaeus vannamei) exposed to low and high salinity. Arch. Virol. 159, 2213–2222. doi: 10.1007/s00705-014-2052-0
Roffe, L., Schmidt, K., and Ernst, E. (2004). Efficacy of coenzyme Q10 for improved tolerability of cancer treatments, a systematic review. J. Clin. Oncol. 22, 4418–4424. doi: 10.1200/JCO.2004.02.034
Rosas, C., Cuzon, G., Gaxiola, G., Priol, Y. L., Pascual, C., Rossignyol, J., et al. (2001). Metabolism and growth of juveniles of Litopenaeus vannamei, effect of salinity and dietary carbohydrate levels. J. Exp. Mar. Biol. Ecol. 259, 1–22. doi: 10.1016/s0022-0981(01)00222-2
Rungrassamee, W., Klanchui, A., Maibunkaew, S., and Karoonuthaisiri, N. (2016). Bacterial dynamics in intestines of the black tiger shrimp and the Pacific white shrimp during Vibrio harveyi exposure. J. Invertebr. Pathol. 133, 12–19. doi: 10.1016/j.jip.2015.11.004
Sasaki, T. K., Tanaka, S., and Nagai, S. (1998). “Use of photosynthetic bacteria for the production of SCP and chemicals from organic wastes”. in Bioconversion of Waste Materials to Industrial Products, eds A. M. Martin. Boston, MA: Springer, 247–292. doi: 10.1007/978-1-4615-5821-7_6
Schloss, P. D., Gevers, D., and Westcott, S. L. (2011). Reducing the effects of PCR amplification and sequencing artifacts on 16S rRNA-based studies. PLoS One 6:e27310. doi: 10.1371/journal.pone.0027310
Schmelzer, C., Lindner, I., Rimbach, G., Niklowitz, P., Menke, T., and Doring, F. (2008). Functions of coenzyme Q10 in inflammation and gene expression. Biofactors 32, 179–183. doi: 10.1002/biof.5520320121
Schmelzer, C., Lorenz, G., Rimbach, G., and Doring, F. (2009). In vitro effects of the reduced form of Coenzyme Q (10) on secretion levels of TNF-alpha and chemokines in response to LPS in the human monocytic cell line THP-1. J. Clin. Biochem. Nutr. 44, 62–66. doi: 10.3164/jcbn.08-182
Shin, N. R., Whon, T. W., and Bae, J. W. (2015). Proteobacteria: microbial signature of dysbiosis in gut microbiota. Trends Biotechnol. 33, 496–503. doi: 10.1016/j.tibtech.2015.06.011
Shinji, J., Okutsu, T., Jayasankar, V., Jasmani, S., and Wilder, M. N. (2012). Metabolism of amino acids during hyposmotic adaptation in the whiteleg shrimp, Litopenaeus vannamei. Amino. Acids 43, 1945–1954. doi: 10.1007/s00726-012-1266-2
Sohet, F. M., Neyrinck, A. M., Pachikian, B. D., De Backer, F. C., Bindels, L. B., Niklowitz, P., et al. (2009). Coenzyme Q10 supplementation lowers hepatic oxidative stress and inflammation associated with diet-induced obesity in mice. Biochem. Pharmacol. 78, 1391–1400. doi: 10.1016/j.bcp.2009.07.008
Song, C., Wang, B., Tan, J., Zhu, L., and Lou, D. (2017). Discovery of tauroursodeoxycholic acid biotransformation enzymes from the gut microbiome of black bears using metagenomics. Sci. Rep. 7:45495. doi: 10.1038/srep45495
Varela-López, A., Giampieri, F., Battino, M., and Quiles, J. (2016). Coenzyme Q and its role in the dietary therapy against aging. Molecules 21:373. doi: 10.3390/molecules21030373
Vargas-Albores, F., Porchas-Cornejo, M. A., Martínez-Porchas, P., Villalpando-Canchola, E., Gollas-Galván, T., and Martínez-Córdova, L. R. (2017). Bacterial biota of shrimp intestine is significantly modified by the use of a probiotic mixture, a high throughput sequencing approach. Helg Mar. Res. 71, 1–10. doi: 10.1186/s10152-017-0485-z
Wang, Y., Li, Z., Li, J., Duan, Y. F., Niu, J., Wang, J., et al. (2015). Effects of dietary chlorogenic acid on growth performance, antioxidant capacity of white shrimp Litopenaeus vannamei under normal condition and combined stress of low-salinity and nitrite. Fish Shellfish Immunol. 43, 337–345. doi: 10.1016/j.fsi.2015.01.008
Xiong, J., Wang, K., Wu, J., Qiuqian, L., Yang, K., Qian, Y., et al. (2015). Changes in intestinal bacterial communities are closely associated with shrimp disease severity. Appl. Microbiol. Biotechnol. 99, 6911–6919. doi: 10.1007/s00253-015-6632-z
Xiong, J., Zhu, J., Dai, W., Dong, C., Qiu, Q., and Li, C. (2017). Integrating gut microbiota immaturity and disease-discriminatory taxa to diagnose the initiation and severity of shrimp disease. Environ. Microbiol. 19, 1490–1501. doi: 10.1111/1462-2920.13701
Xu, C., Li, E., Masserey, I., Liu, Y., Wang, S., Servin, A., et al. (2018). Effect of dietary lipid level on growth, lipid metabolism and health status of the Pacific white shrimp Litopenaeus vannamei at two salinities. Aqua. Nutr. 24, 204–214. doi: 10.1111/anu.12548
Yamazaki, Y., Meirelles, P. M., Mino, S., Suda, W., Oshima, K., Hattori, M., et al. (2016). Individual Apostichopus japonicus fecal microbiome reveals a link with polyhydroxybutyrate producers in host growth gaps. Sci. Rep. 6:21631. doi: 10.1038/srep21631
Yeh, S. T., Lin, Y. C., Huang, C. L., and Chen, J. C. (2010). White shrimp Litopenaeus vannamei that received the hot-water extract of Gracilaria tenuistipitata showed protective innate immunity and up-regulation of gene expressions after low-salinity stress. Fish Shellfish Immunol. 28, 887–894. doi: 10.1016/j.fsi.2010.02.005
Yik Sung, Y., and MacRae, T. H. (2013). Heat shock proteins and disease control in aquatic organisms. J. Aqua. Res. Dev. S2:006. doi: 10.4172/2155-9546.S2-006
Zhang, J., Liu, Y., Tian, L., Yang, H., Liang, G., and Xu, D. (2012). Effects of dietary mannan oligosaccharide on growth performance, gut morphology and stress tolerance of juvenile Pacific white shrimp, Litopenaeus vannamei. Fish Shellfish Immunol. 33, 1027–1032. doi: 10.1016/j.fsi.20120.05.001
Zheng, X., Duan, Y., Dong, H., and Zhang, J. (2017). Effects of dietary Lactobacillus plantarum in different treatments on growth performance and immune gene expression of white shrimp Litopenaeus vannamei under normal condition and stress of acute low salinity. Fish Shellfish Immunol. 62, 195–201. doi: 10.1016/j.fsi.2017.01.015
Keywords: Litopenaeus vannamei, nutrition, Rhodobacter sphaeroides protein, CoQ10, intestinal microbiota, low salt stress
Citation: Liao Z, Gong Y, Wang Z, Wang Y, Yao R, Chen M, Wei D, Zhao W, He X and Niu J (2022) Effects of Dietary Rhodobacter sphaeroides Protein Substitution of Fishmeal and Coenzyme Q10 Supplementation on Growth Performance, Intestinal Microbiota and Stress Tolerance of Litopenaeus vannamei in Acute Low Salinity. Front. Mar. Sci. 9:851649. doi: 10.3389/fmars.2022.851649
Received: 10 January 2022; Accepted: 07 February 2022;
Published: 11 March 2022.
Edited by:
Jun Wang, Chinese Academy of Fishery Sciences (CAFS), ChinaReviewed by:
Jia-Song Zhang, Chinese Academy of Fishery Sciences (CAFS), ChinaChuanpeng Zhou, Chinese Academy of Fishery Sciences (CAFS), China
Copyright © 2022 Liao, Gong, Wang, Wang, Yao, Chen, Wei, Zhao, He and Niu. This is an open-access article distributed under the terms of the Creative Commons Attribution License (CC BY). The use, distribution or reproduction in other forums is permitted, provided the original author(s) and the copyright owner(s) are credited and that the original publication in this journal is cited, in accordance with accepted academic practice. No use, distribution or reproduction is permitted which does not comply with these terms.
*Correspondence: Jin Niu, bml1ajNAbWFpbC5zeXN1LmVkdS5jbg==