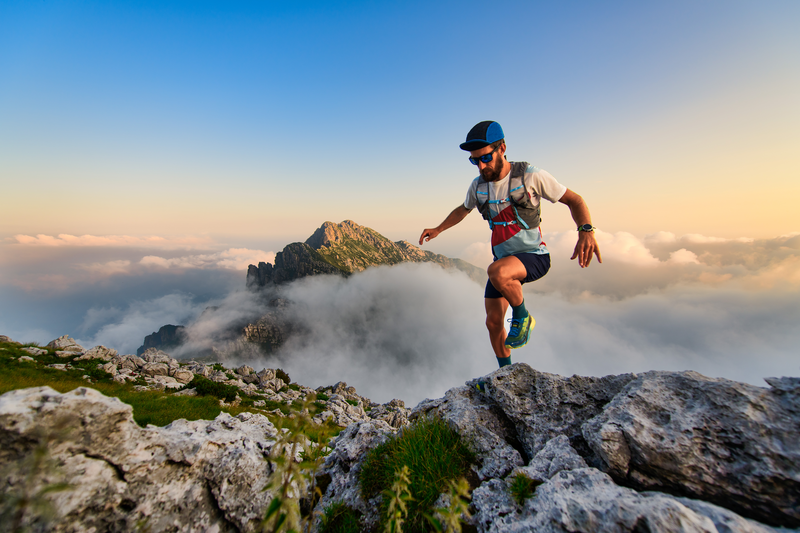
94% of researchers rate our articles as excellent or good
Learn more about the work of our research integrity team to safeguard the quality of each article we publish.
Find out more
REVIEW article
Front. Mar. Sci. , 09 March 2022
Sec. Marine Pollution
Volume 9 - 2022 | https://doi.org/10.3389/fmars.2022.851281
This article is part of the Research Topic Plastics in Aquatic Systems: from Transport and Fate to Impacts and Management Perspectives View all 12 articles
Plastic pollution is a fast-rising environmental catastrophe. Microplastics and nanoplastics (MNPs) are ubiquitous components of most aquatic environments, and their burgeoning prevalence is endangering aquatic organisms. Recent studies have documented the entanglement of marine and freshwater biota by plastic litters, particularly ghost fishing gear, resulting in suffocation, drowning, or starving to death. Numerous reports have shown that aquatic organisms readily ingest and accumulate these emerging contaminants in their digestive systems. Given experimental evidence that contaminants-laden MNPs can persist in the gastrointestinal tract for considerable durations, investigations have documented a high probability of lethal and sublethal toxicological effects associated with direct and indirect MNPs ingestions. These include chronic protein modulation, DNA damage, embryotoxicity, gastrointestinal toxicity, genotoxicity, growth inhibition toxicity, histopathotoxicity, liver toxicity, neurotoxicity, oxidative stress, reproductive toxicity, and tissue damage. Today, reports have proven the transfer of MNPs across the aquatic food web to humans. However, the mechanisms of multiple contaminants-laden MNPs-induced toxicities, size-dependent toxicity, and the comprehensive mode-of-action and alterations of digestive, reproductive, and neurological systems’ functionality in marine organisms are still unclear. Thus, this review mainly addresses the prevalence, food web interactions, and toxicity assessment of micro(nano) plastics in marine and freshwater organisms. It summarizes documented studies based on the following broad objectives: (1) the occurrence and prevalence of micro(nano) plastic particles in marine and freshwater environments; (2) the ingestion of MNPs by aquatic biota and the food web exposure routes and bioaccumulation of contaminated MNPs by higher trophic entities; (3) the adsorption and desorption of persistent organic pollutants, metals, and chemical additives on/from micro(nano)plastics; and (4) the probable ecotoxicological effects of micro(nano)plastics ingestion on aquatic biota.
Our oceans are littered with plastics originating from both terrestrial and marine sources. It is estimated that about 80% of ocean plastics originate from land-based emissions, while the rest comes from marine sources (Li et al., 2016). Plastics are synthetic organic polymers made through addition or condensation reactions of substituted or unsubstituted hydrocarbons, which possess stable, lipophilic, and water-resistant structures. They are used in many commercially available products due to low cost, durability, and flexibility leading to a geometric increase in production. Numerous single-use plastics, particularly those used in beverage, food, and consumer product packaging, have a short useful life and are readily discarded into the environment. Millions of single-use COVID-generated plastics, including face masks, protective medical aprons, gloves, medical test kits, hand-sanitizer bottles, and takeout plastics, and medical test kits have been improperly discarded into the terrestrial environment, potentially increasing the amount of plastic washing up on ocean shorelines, beach resorts, and exacerbating the plastic pollution problems (Benson et al., 2021a,b). Particulate plastics generated on land are mostly carried by run-off, soil erosion, and stormwater through drainages into rivers, lagoons, and eventually to the sea and ocean. Additionally, fine plastics particles may reach freshwater and marine ecosystems via other land-based processes such as wastewater treatment plant effluent as well as shipping operations (Li et al., 2018; Bradney et al., 2019). Mismanaged plastics originating primarily from land-based sources continue to pervade the environment, and up to 12.7 million metric tons are estimated to enter the ocean annually (Jambeck et al., 2015). Additionally, plastic debris from anthropogenic sources into the marine and freshwater ecosystems could come from commercial fisheries that abandon fishing gears and equipment including lines, nets, lines, plastics lures, ropes, and occasionally abandoned trawlers (Macfadyen et al., 2009). The vast majority of these ocean plastics are in the form of macroplastics, mesoplastics, microplastics, and nanoplastics which have washed ashore, been buried or littered along the coastlines, or been transported to offshore environments via hydrological influences.
Particles of plastics are often categorized according to their size. Despite the absence of agreement on how to describe and categorize plastic particles, this report considered the following classifications. Macroplastics, mesoplastics, microplastics, and nanoplastics are plastic particles with size diameter range that are >200 mm, 4.76–200 mm, 0.01 μm–1 mm, and less than 0.1 μm, respectively (Eriksen et al., 2014). However, Hartmann et al. (2019) categorize plastic particles as macroplastics (>1 cm), mesoplastics (1–<10 mm), microplastics (1–<1,000 μm), and nanoplastics (1–<1,000 nm). Although there is no consensus on categorizing plastic particles, in this manuscript, microplastics (MPs) are regarded as the category of plastics with a diameter of 1 nm–<5 mm, and nanoplastics (NPs) are characterized as plastic particles having a lower size less than 1 nm. MPs which are classified into primary and secondary based on their sources are considered as an emerging persistent micropollutant threatening our global aquatic and terrestrial environments. While primary microplastics are originally manufactured for use in cosmetics, toothpaste, or pharmaceutical drugs (Mintenig et al., 2017), secondary microplastics, on the other hand, exist from the degradation of larger plastics into smaller pieces under different chemical, physical, and biological conditions (Horton et al., 2017a; Padervand et al., 2020; Agboola and Benson, 2021). The steady rise in the production and use of plastics led to an increase in presence of microplastics in the environment (Cole et al., 2011). MPs are found in a variety of everyday products, including facial scrub cleansers, sea salts, and toothpastes (Chang, 2015; Zhang et al., 2020a; Agboola and Benson, 2021). MPs enter aquatic ecosystems by a variety of routes, the most common of which being surface runoff, air currents, and wastewater treatment plants (WWTPs) effluent (Dris et al., 2015; Turan et al., 2021). The most common types of microplastics detected in WWTPs are fibers and microbeads. Wastewaters from domestic, commercial, and industrial sources are usually treated at municipal WWTPs and their effluents constitute a major source of MPs entering freshwater systems, most commonly rivers, from which they are transported to the ocean (Iyare et al., 2020; Zhang et al., 2020a). However, the introduction of microplastics and nanoplastics (MNPs) into the terrestrial and aquatic environments through WWTPs processes could potentially exacerbate the plastic pollution problem. Inappropriate management and disposal have led to a higher concentration of microplastics in freshwaters including lakes (Eriksen et al., 2013; Lenaker et al., 2019; Pico et al., 2020; Felismino et al., 2021) or rivers (Leslie et al., 2017; Rodrigues et al., 2018), and sand beaches (Benson and Fred-Ahmadu, 2020; De-la-Torre et al., 2020a). Researchers have reported an abundance of microplastics in the ocean which is both an aesthetical eyesore and an endangerment to marine life. According to estimates using different datasets, the amount of plastics in the aquatic ecosystems has increased by 7,000 (Cózar et al., 2014) and 250,000 tonnes (Eriksen et al., 2014) in the last few years, and is projected to reach 270 million tonnes by 2060 (Lebreton and Andrady, 2019). They are also reported to be enormously present in seawater including the Arctic (Morgana et al., 2018) and the Antarctic (Waller et al., 2017). Nevertheless, the precise volume of plastic waste particles floating around the surface of global freshwater and marine ecosystems remains unknown.
To the best of our knowledge, several landmark ecotoxicological investigations on the occurrence, ubiquity, and toxicity of micro (nano) plastics in marine and freshwater organisms have been conducted. In this manuscript, we conducted a meticulous review of peer-reviewed publications on the prevalence, food web interactions, and toxicity investigations of micro(nano)plastics in aquatic animals, and also identified future research priorities and constraints. The objectives of this review are to (1) highlight the sources, prevalence, and fate of micro(nano)plastics in aquatic ecosystems, (2) explicate food web interactions between micro(nano)plastics and aquatic organisms, and (3) review the toxicity and respective health risks associated with ingestion of micro(nano)plastics by marine and freshwater animals. The main aim of this review is to present relevant research findings and effects regarding the prevalence, food web interactions, and toxicity assessments of micro(nano)plastic particles on aquatic species.
The aim of the literature search was to compile a list of core studies that addressed the research questions for the present review. Relevant databases including Elsevier’s ScienceDirect1 and Clarivate’s Web of Science2 were used to select peer-reviewed papers for the literature review conducted between 8th and 24th June of 2021, with an updated search on October 23rd, 2021. Advanced and basic selection techniques were utilized for the literature search, which involved keywords combination in forming queries for keywords and title elements. The following steps were used to narrow the search: “microplastics” OR “nanoplastics” AND “marine” OR “freshwater” were consistently retained as the basis in all bibliographic searches with keywords incorporated with the Boolean connector AND. Keywords used in this literature review were: “trophic transfer,” “trophic interactions,” “trophic web,” trophic chain,” “toxicity,” “abundance,” and “impact” to expand the search by using Boolean connector AND, OR. Afterward, each query was further expanded with word combinations including “microplastics in marine ecosystems,” “microplastics in freshwater ecosystems,” “nanoplastics in marine ecosystems,” and “nanoplastics in freshwater ecosystems.” The review was limited to research papers published in any year and on the research subject matter. The literature review identified 932 and 484 scholarly journal articles for MPs studies in marine and freshwater, respectively, published between 2011 and 2021 (as of October 23rd). Figure 1 illustrates a progressive increase in studies conducted for marine ecosystems MPs (R2 = 98%), as well as those carried out for freshwater ecosystems MPs (R2 = 96%) since 2011 when the first original research article meeting the selected search criteria was identified in the two databases used. The significant increase in research papers throughout the reviewed period reflects the scientific community’s growing interest in the occurrence, trophic food web interactions, and toxicity of MPs and NPs in marine and freshwater ecosystems.
Figure 1. Yearly published research articles on micro- and nano-plastic particles in marine and freshwater ecosystems. The total for 2021 represents the research articles as at October 23, 2021.
According to van Sebille et al. (2015) there are an estimated 51 trillion particles of microplastics floating on the surface of the ocean, and have been established as vectors for the transfer of persistent organic and inorganic contaminants including plastic additives (Benson and Fred-Ahmadu, 2020; Torres et al., 2021), organochlorine pesticides (O) (Shi et al., 2020), polychlorinated biphenyls (PCBs), polycyclic aromatic hydrocarbons (PAHs) (Frias et al., 2010; Hirai et al., 2011; Fisner et al., 2013; Tan et al., 2019), and heavy metals (Turner and Holmes, 2015; Brennecke et al., 2016; Fred-Ahmadu et al., 2020a; Xiang et al., 2021). Microplastics and nanoplastics are composed of a variety of polymers that are hydrophobic, having varied molecular structures and characterized with the capability to adsorb organic pollutants and metals on interaction with them.
Microplastics are remarkably variable in terms of particle size, morphology, color, material type, and density, and are regarded as a complex aggregation of emerging micropollutants of concern (Rochman et al., 2019). As a result of these unique particle characteristics, MPs can enter aquatic food webs at a variety of trophic levels and eco-niches with greater ease. However, in aquatic ecosystems, the distribution, fate and transport mechanisms, bioavailability, and trophic transfer among primary, secondary, and tertiary aquatic biotas are all influenced by these unique characteristics of microplastics (Sharma and Chatterjee, 2017; Bank and Hansson, 2022). Currently, there is a dearth of study on plastic particles transfer through freshwater food webs, and impacts of MPs exposure to freshwater organisms is limited. Thus far, our understanding of MPs uptake and potential toxicity has come mostly from laboratory studies that employ uncomplicated contact regimes with minimal environmental applicability. In particular, MPs in the marine environment can be transported from the top epipelagic and mesopelagic zones to mixed and deep layer depths by the continual vertical sinking of suspended organic matter, which may increase MPs bioavailability to bottom-dwelling organisms (Porter et al., 2018). It has been reported that 99.8% of plastic contamination in aquatic ecosystems since the 1950s has sunk to the seafloor in 2016, with an incremental 9.4 million tonnes accumulating yearly, according to modeling estimates by Koelmans et al. (2017).
Furthermore, due to MNPs small sizes and varied particle characteristics, those that are in suspension in the water column, floating on water surfaces (buoyant particles, <1.0 g cm–3), and sedimenting plastic particles (benthic or seafloor materials, density >1 g cm–3) are often mistaken for food by aquatic organisms, especially fishes (Galloway et al., 2017; Steer et al., 2017), or ingested by filter-feeding organisms, like bivalves (Scherer et al., 2018). Uptake of microplastics by fishes block their guts and intestines – depending on their size (Savoca et al., 2019), and increases the chance of organic pollutants sorbed onto microplastics to be leached into fish organs, thus causing health defects. The process of ingestion of microplastics and leaching of pollutants influences the transport of both through the food web and poses a threat to aquatic life (Betts, 2008; Wright et al., 2013a). A study testing the hypothesis of accumulation of polyethylene (PE) microplastics in animals, their possible cytotoxicity and effects on behavior and mutagens at upper trophic levels, recorded that accumulation of PE in Danio rerio was associated with behavioral disorders observed at upper trophic levels. The study showed the adherence, absorption and translocation of MPs through the aquatic food chain (da Costa Araújo et al., 2020). Direct and indirect ingestions of organic pollutants interfere with growth, fertility, and lifespan while dosage could alter metabolism (Jovanović, 2017), immunity (Veneman et al., 2017), behavioral pattern (Gambardella et al., 2017), and energy budgeting (Wright et al., 2013b).
According to contemporary ecotoxicological investigations, the ingestion of microplastic particles by marine animals could culminate in both sublethal and lethal effects such as toxicosis in freshwater and marine fish species (Veneman et al., 2017; Jin et al., 2018; Lei et al., 2018; LeMoine et al., 2018; Hamed et al., 2020; Malafaia et al., 2020; van der Hal et al., 2020; Zhang et al., 2020b; Tongo and Erhunmwunse, 2022), oysters (Green, 2016; Sussarellu et al., 2016), mussels (Browne et al., 2008; von Moos et al., 2012; Jang et al., 2016; Paul-Pont et al., 2016; Ribeiro et al., 2017; Faggio et al., 2018; Pittura et al., 2018; Magni et al., 2019; Revel et al., 2019), clams (Ribeiro et al., 2017; Luan et al., 2019), and sea urchins (Della Torre et al., 2014). In the same vein, the ingestion toxicity effects of nanoplastics on fish (Greven et al., 2016; Brandts et al., 2018a; Pitt et al., 2018a; Sökmen et al., 2020), shrimps (Chae et al., 2019), and mussels (Brandts et al., 2018b; De-la-Torre et al., 2020b) have also been reported.
Multiple adverse effects (Figure 2), on marine and freshwater animals have been reported by several researchers, including changes in fertility (Sussarellu et al., 2016), reproduction abnormalities (Della Torre et al., 2014; Sussarellu et al., 2016), inflammatory responses (von Moos et al., 2012), gut inflammation (Jin et al., 2018), metabolism disorder (LeMoine et al., 2018; Yu et al., 2018; Banaee et al., 2019; Magni et al., 2019), immune system disabilities (Jang et al., 2016), endocrine disruption (Rochman et al., 2014), reduction in mortality rate (Green, 2016; Rist et al., 2016), hepatic stress (Greven et al., 2016; Brandts et al., 2018a,b), cytotoxicity (von Moos et al., 2012; Ribeiro et al., 2017; Hamed et al., 2020; Xia et al., 2020; Ali et al., 2021), particle transfer into the cardiovascular system (Browne et al., 2008; Ribeiro et al., 2017), and pathological impairment of respiratory and gastrointestinal tracts (Hämer et al., 2014; Mattsson et al., 2014; Jeong et al., 2016; Lu et al., 2016). Other effects of plastic ingestion by aquatic animals include the obstruction and damage of the digestive system (Camedda et al., 2014), gastrointestinal blockage, constriction, and injuries (Parga, 2012; Di Bello et al., 2013), immune system depression (Limonta et al., 2019; Huang et al., 2020; Hu et al., 2021), reduced growth rates, fecundity, reproductive success, and late sexual maturation (Hoarau et al., 2014; Vegter et al., 2014; Sussarellu et al., 2016; Liu et al., 2022), growth and reproductive abnormalities (Naidoo and Glassom, 2019; Pannetier et al., 2020; Liu et al., 2022), and metabolism, oxidative stress, and gastrointestinal dysfunction (Qiao et al., 2019a; Li et al., 2022).
The food web is a complex feeding relationship among species within an ecosystem. It is a collection of interconnected food chains that illustrates the transfer of energy and nutrients from plant sources through herbivores to carnivores (Hui, 2012). Organisms in the aquatic ecosystem food web belong to different trophic levels including the (i) producers, (ii) primary consumers, (iii) secondary consumers, (iv) tertiary consumers, and (v) decomposers. Producers are autotrophs that create their food through photosynthesis utilizing sunlight, carbon dioxide, and water. Autotrophs such as algae and plankton are eaten by primary consumers like zooplankton, small fishes, and crustaceans. Turtles and sea urchins are also primary consumers. Secondary consumers such as sea otters feed on the herbivorous primary consumers and tertiary consumers feed on the secondary. The apex predators like large sharks, dolphins, and whales are top predators. The last trophic level in the food web comprises the decomposers (fungi and bacteria). They turn organic wastes, such as decaying plants and animals, into nutrients. They complete the cycle of life by returning nutrients to the soil or oceans for use by autotrophs (National Geographic Society, 2021).
In a healthy food web, autotrophs are present in higher abundance than herbivores therefore, biomass and energy production decrease with each trophic level. Conversely, toxic chemicals such as persistent organic pollutants and microplastics increase with each trophic level because organic chemicals can store in the fatty tissues of animals while microplastics have been shown to be stored in the guts, gills, liver, and brain of fish (Ding et al., 2018). The toxic chemicals and microplastics can be transferred from one trophic level to another upon ingestion leading to bioaccumulation in the food web.
Figure 3 shows how microplastics attached to phytoplankton and zooplanktons are ingested by small fishes, which in turn are fed upon by other primary consumers that are prey for secondary consumers at the higher trophic levels. Subsequently, microplastics spread through the aquatic food web. Microplastics discovered in fur seals were suspected to have been present due to the consumption of a pelagic fish Electrona subaspera that ingested microplastics (Eriksson and Burton, 2003). Microplastics are not biodegradable, thus remain in the digestive tracts of marine organisms across the food web, having biological and physical impacts on marine lives. The effect of chemically-laden contaminated microplastic ingestion might not be obvious immediately in big fishes, but the continuous accumulation of these microplastics could eventually be lethal.
Figure 3. A pictorial representation of the transport of microplastics across the marine aquatic food web.
Various researches on the prevalence and suffusive contamination of microplastic particles in the aquatic environment have concentrated on marine systems more than freshwater ecosystems (Isobe et al., 2017, 2019; Blettler et al., 2018; Zheng et al., 2019; Mataji et al., 2020; Nematollahi et al., 2020; Saeed et al., 2020; Ain Bhutto and You, 2022). Microplastics enter freshwater ecosystems (rivers, lakes, and streams) through a variety of ways, including sewage and landfill effluents, urban run-off, atmospheric deposition, wastewater treatment plants, and improper waste disposal (Horton et al., 2017b; Wu et al., 2019; Müller et al., 2020; Castro-Castellon et al., 2021). Rivers have nonetheless been identified as the primary route through which plastic waste enters the marine environment (Li et al., 2020). Climatological conditions and hydrodynamic processes in rivers, lakes, and streams such as current, waves, wind, river discharge rate, and geographical location have been identified as the primary factors that influence the occurrence and distribution (sinking and resuspension rates) of microplastic particles in freshwater ecosystems (Rodrigues et al., 2018; Bellasi et al., 2020; Dahms et al., 2020). Furthermore, microplastic particles concentration and bioavailability to planktonic, nektonic, and bottom-dwelling freshwater biotas (Meng et al., 2020; Krause et al., 2021), as well as their widespread relative abundance in freshwater compartments, can inevitably lead to ingestion by multiple biological organisms including diatoms, planktonic crustaceans, fish, mussels, and zooplankton.
Microplastics ingestion by biotas in the freshwater ecosystems are largely influenced by multiple interactions involving abiotic (e.g., temperature) and biotic factors (e.g., feed abundance, type of diet, size of feeding substance, availability of competitors, and physiological condition) (Scherer et al., 2018), as well as feed behavior, morphology of MPs, and exposure route (Lambert and Wagner, 2018; Elizalde-Velázquez et al., 2020; Filgueiras et al., 2020; Garcia et al., 2020). It is well known that organisms in freshwater ecosystems are constituents of complex trophic food chain, feeding on a plethora of different types of food items, using a variety of distinct feeding methods including filter, fluid, suspension, sediment, and suction feeding. Filter and suspension feeders including planktonic crustaceans (e.g., Daphnids), mussels, protozoans, and rotifers are particularly susceptible to microplastics intake due to their frequent ingestion of living (bioseston e.g., nekton and plankton) and non-living (tripton e.g., plant debris) particulate matter suspended in freshwater bodies (Thouvenot et al., 1999; Browne et al., 2008; Scherer et al., 2018; Koelmans et al., 2022). These interactions and trophic processes are poorly understood and further complicate our understanding of their MPs impacts and toxicosis to freshwater biota (Castro-Castellon et al., 2021; Ma and You, 2021; Ain Bhutto and You, 2022).
Furthermore, a couple of studies have reported on the spatial distribution of MPs and their impacts on freshwater food webs. However, the mechanistic routes of MPs and sorbed additives exposure, bioaccumulation, and biomagnification through food chains and the associated toxicities on higher trophic level biota in freshwater ecosystems are still unknown. On the other hand, scanty studies have been conducted on the fate of nanoplastic particles in freshwater environments, owing to a lack of standardized analytical and reliable methods for sampling, detection, and characterization of NPs. Little is known about the toxicity of NPs in freshwater ecosystems. However, the few documented studies are largely lab-based reports that might not reflect similar biological toxicity if replicated in the field (Zhang et al., 2022). These laboratory studies employed polystyrene nanoplastics, and the toxicological effects reported include reproductive abnormalities (Li et al., 2020, 2020a,b), oxidative stress and gastrointestinal dysfunction (Chae et al., 2018; Pitt et al., 2018b; Huang et al., 2020; Li et al., 2020, 2020a), increased mortality (Liu et al., 2019; Liu et al., 2020; Zhang et al., 2020c), growth inhibition and disorder (Liu et al., 2019; Lin et al., 2020; Liu et al., 2020; Zhang et al., 2020c), and cardiovascular dysfunction and neurotoxicity (Chen et al., 2017a,b).
In aquatic ecosystems, microplastics are known to disintegrate further into nanoplastics when exposed to ultraviolet radiation, facilitated by shear forces of tidal currents and chemically or biologically driven degradation mechanisms, thus resulting in deleterious endpoints for freshwater and marine food webs. When micro(nano)plastics reach the aquatic environment, they may be directly ingested by zooplankton, planktivorous, and piscivorous fish in the aquatic food web (Abbasi et al., 2018; Ain Bhutto and You, 2022), and then transferred through the food chain, where they are finally consumed by humans. The ingestion of particulate plastics by humans is largely from consuming contaminated seafood, sea salts, and water (Kim et al., 2018; Schymanski et al., 2018; Bradney et al., 2019; Wong et al., 2020; Selvam et al., 2020). Toxicological studies have shown that plastic particles in the gastrointestinal systems of humans could pose severe biological effects to the digestive systems and impair immune functioning (Chang et al., 2020).
Studies on the prevalence, exposure, and transfer of micro(nano)plastics from the lower trophic levels across the food web to humans are emerging. Almost all of these investigations are concerned with the plastics consumed by phytoplankton, zooplankton, oysters, crustaceans, and fish. A few studies have also reported the transfer of MNPs across different trophic levels (Nelms et al., 2018; Ribeiro et al., 2019). Furthermore, Farrell and Nelson (2013) reported the trophic level transfer of microplastics in Mytilus edulis (L.) to Carcinus maenas (L.), while the ingestion and biotransfer of MPs in the planktonic food web has been documented (Setälä et al., 2014). However, the bulk of food web dynamics comprises multiple organisms with modest and significant interactions among organisms and animals in other food webs, thus complicating risk and ecotoxicological assessments (Bellas et al., 2016; Walkinshaw et al., 2020). An in-depth investigation of the transfer of MNPs and sorbed organic and inorganic pollutants through aquatic food webs to humans has been reported (Bradney et al., 2019). According to documented toxicological studies, microplastics and nanoplastics are readily absorbed by human epithelial (Magri et al., 2018) and cutaneous (Rubio et al., 2020) tissues when exposed to particulate plastics. Bioaccumulation of polymeric particles, as well as absorption/desorption of MNP-bound hydrophobic organic compounds and trace elements when they reach the gastrointestinal and respiratory systems, could have long-term negative impacts on human health (Cole et al., 2015; Pozo et al., 2019; Rubio et al., 2020; Zazouli et al., 2022).
In recent times, food web interactions of ingested microplastics and nanoplastics by aquatic organisms are the subject of laboratory and field comparative studies. MPs are ingested by a number of aquatic organisms, and these particulate plastics have been found to be transferred through the food chain in several laboratory studies. The majority of published studies indicate the presence of microplastics in organisms’ gastrointestinal and respiratory systems (Chua et al., 2014; Kaposi et al., 2014). The transfer of ingested microplastics from aquatic organisms’ gut to the adjoining tissues, as well as excretion and trophic transfer, have largely been investigated in controlled laboratory experiments (Browne et al., 2008; Hesler et al., 2019; Cousin et al., 2020; Kong et al., 2020). Several laboratory studies have reported the ingestion of primary microplastics by organisms across the aquatic food web like zooplankton (Cole et al., 2013; Setälä et al., 2014) and other macro-sized invertebrates (Setälä et al., 2016; Gray and Weinstein, 2017), as well as fishes (Rochman et al., 2013; Batel et al., 2016). Setälä et al. (2014) reported the ingestion and planktonic trophic transmission of fluorescent polystyrene (PS) microspheres (10 μm) from zooplankton to the mysid shrimp Mysis relicta. Furthermore, the food chain interspecies transfer of fluorescently labeled MPs between mussels (M. edulis) and shore crabs (C. maenas) has been reported (Farrell and Nelson, 2013; Watts et al., 2014). Microplastic intake has also been studied in various field investigations, primarily using marine organisms. However, no distinction between direct ingestion of MPs and trophic transfer is apparent considering such field investigations (Duis and Coors, 2016). On the other hand, limited data exists on freshwater organisms’ ingestion of microplastic particles in field research studies (Sanchez et al., 2014; Hurley et al., 2017; Nel et al., 2018; Bertoli et al., 2022; Buwono et al., 2022). Furthermore, nanoplastic particles could be transferred trophically up the planktonic food chain through multiple secondary aquatic organisms at the higher trophic level. Moreover, our contemporary knowledge of the interactions between lower trophic level species and nanoplastic particulates remains relatively sparse (Latchere et al., 2021; Zhu et al., 2021; Sendra et al., 2020; Haegerbaeumer et al., 2019).
Plastic particles in the aquatic environment experience variations in physical properties which in turn influence their deposition, transport, retention dynamics, and bioavailability (Krause et al., 2021). The bioavailability and uptake of microplastics in the aquatic food web can occur at all trophic levels as evidenced in the detection of microplastics in organisms ranging from benthic planktons to large fishes in the water column (Lima et al., 2014; Digka et al., 2018). The bioavailability and uptake are predicated on several factors such as density, color, size, and the abundance of the polymer particles and hydrodynamic conditions such as flow dynamics of water.
The density of plastics is an important characteristic that determines whether the microplastic particles will be suspended in the water column or sink to the benthic sediment. This will subsequently determine the type of organism that might ingest such plastic particles. Low-density plastics such as polyethylene with specific gravity 0.91–0.94 are likely to be ingested by filter feeders and suspension feeders which reside in the upper column of seawater. Annelids feed on microplastics in intertidal beach sediment (van Cauwenberghe et al., 2015) and seabirds pick up floating particles on seawater. Biofouling of MPs changes the buoyancy of plastics by increasing their mass and density causing the low-density plastics to sink and become available to benthic organisms for ingestion (Long et al., 2015). Other factors that affect the buoyancy of plastics include adsorption of minerals to plastic surfaces (Corcoran et al., 2015), microplastics ability to combine with fecal pellets (Cole et al., 2016), and the types and amount of additives included during the manufacture of plastics. Polymers with density lower than that of seawater (∼1.02 g/cm3) are expected to float.
For visual predators, the color and sometimes the shape of prey is an important characteristic. A study by Shaw and Day (1994) reported that some commercially important fish and their larvae fed on small zooplankton which is usually white, tan, or yellow in color; the chances of mistaking these classes of plastic particles as prey and ingesting them selectively are high. The researchers found a consistent decrease in the number of white plastic particles in fish sampled from the North Pacific and concluded that marine organisms showed a preference for certain colors, sizes, and shapes of plastic particles (Shaw and Day, 1994). Similarly, Ory et al. (2017) investigated the ingestion of blue microplastics by Amberstripe scad (Decapterus muroadsi) in the South Pacific. According to their findings, Amberstripe scads unintentionally ingested microplastics that resembled their natural prey (a blue copepod). Other than fish, holothurians (sea cucumbers) are another class of sea organisms that ingest microplastics of specific colors and shapes (Ivar and Costa, 2014).
A variety of fish larvae (meroplankton) have been reported to have ingested microplastic particles in the western English Channel, with blue fibers accounting for 66% of the total ingested microfibres (Steer et al., 2017). Desforges et al. (2015) also reported that two foundational zooplankton species Neocalanus cristatus and the euphausiid Euphausia pacifia showed selective ingestion for black, blue, and red microplastic particles. A recent study by Gurjar et al. (2022) indicates that different pelagic and demersal fish species showed selective preference for black [Bombay duck (Harpodon nehereus), Malabar sole fish (Cynoglossus macrostomus), and shrimps (Metapenaeus dobsoni)], blue [Belanger croakers (Johnius belangerii) and white sardine (Escualosa thoracata)], and green and yellow (Malabar sole fish, Belanger croaker, and Bombay duck). Further, a documented study by Renzi et al. (2018) revealed that the European anchovy’s feeding habit of preferentially swallowing dark prey materials could be responsible for the mostly black and blue colored microplastics identified in their digestive systems. Also, a penaeid shrimp (Penaeus monodon) has been reported to dominantly ingest black colored microplastic particles while also swallowing four other different colors (blue, green, red, and white) of plastic particles found in its gastrointestinal tracts (Hossain et al., 2020). However, more research is needed to establish whether freshwater and marine organisms exhibit preferential behavior toward ingestion of microplastic particles of varying color.
The small size of microplastics increases the likelihood of their ingestion by lower trophic organisms which are less selective in their feeding behavior as they take up anything of the appropriate size (Moore, 2008). Higher trophic organisms, on the other hand, may mistakenly ingest microplastic particles as food (Fossi et al., 2012; Hartmann et al., 2017). Quinn et al. (2017) reported the ingestion of both micro-and macro-plastics in demersal flatfish and pelagic fish species harvested from the East and West coasts of Scotland. Similarly, turtles have been reported to ingest micro-, meso-, and macroplastics in several studies due to their size and feeding habits (Clause et al., 2021), while small invertebrates, such as annelids, are prone to ingest microfibres a few hundreds of micrometers in size (Gusmão et al., 2016).
Enhanced quantity of plastic particles in the marine and freshwater environments increases the likelihood of organisms to encounter them, making them available for ingestion. The continuous introduction from land-based sources and subsequent fragmentation under environmental conditions in aquatic ecosystems will ensure a steady supply of MPs and NPs to marine and freshwater organisms (Browne, 2015). Due to their ubiquity, microplastics in particular are unequivocally interacting with the marine food web as indicated by several scientific evidence showing the detection of microplastics in many environmental matrices and biota. According to Gurjar et al. (2022), the mean abundance of microplastics in demersal fish species varied between 5.62 ± 2.27 and 6.6 ± 2.98 items/species, whereas in pelagic species the results ranged from 6.74 ± 2.74 to 9.12 ± 3.57 items/species. As a result, the study concluded that microplastic abundance was higher in pelagic flesh-eating types of fishes than in bottom-feeding fish species. Additionally, James et al. (2020) showed that microplastics were more abundant in pelagic fish species than in benthic species. In general, aquatic fishes existing and feeding in shallow coastal waters have a greater probability of ingesting microplastic particles directly or accidentally through their feeding behaviors than bottom-dwelling species (Pozo et al., 2019; James et al., 2020). This might likely be attributed to the increased introduction of macro-, meso-, and microplastics from land-based anthropogenic activities into the aquatic coastlines.
On the other hand, relatively low ingestion of microplastic particles by aquatic species has been reported in various regions of the world, including south-east Bay of Bengal (Karuppasamy et al., 2020), southwest coast of India (Robin et al., 2020), Mediterranean Sea (Fossi et al., 2018; Giani et al., 2019), and Adriatic Sea (Pellini et al., 2018), all of which showed a reduced abundance of MPs. Moreover, Davison and Asch (2011) estimated that the annual ingestion rate of plastic debris by mesopelagic fishes in the North Pacific Ocean ranged between 12,000 and 24,000 tonnes. Generally, the relative abundance of MNPs in freshwater and marine organisms may be a function of many factors including trophic level, habitat, feeding habits, type and size of species, size of microplastic, type of season, and anthropogenic emissions.
The influence of local hydrodynamic conditions such as current intensity, seawater influx and velocity, tidal currents induced by winds (Sadri and Thompson, 2014; Tan et al., 2019; Fred-Ahmadu et al., 2020b), and depositional environment (Wilson et al., 2021) on the abundance, distribution and dispersion of microplastics have been reported. Beaches with low current intensity or low energy were found to accumulate more microplastic particles on their coastlines. This accumulation allows MPs to enter the food web through uptake by filter-feeding crustaceans such as crabs on shoreline sediments (Horn et al., 2019). In freshwater ecosystems, models have been developed for MPs transport and retention using Stoke’s settling velocity which is a function of particle density, size, and shape (Krause et al., 2021). Hyporheic exchange caused by flow interactions with sediment and benthic algal beds results in the availability of MPs to benthic organisms (Drummond et al., 2020). The remobilization of microplastic particles into the sediment-water interface during base flow and storm flow releases MPs into the water column. However, the dynamics of remobilization of sediment-sorbed MPs due to disturbances and turbulent mixing in the marine environment is yet to be fully understood. Further research is required in this aspect.
Microplastics have been found to be sources of organic pollutants like polycyclic aromatic hydrocarbons (PAHs) which are present in petroleum; a primary ingredient of synthetic plastic. Plastics also include chemical additives like bisphenol-A (BPA), heavymetals, phthalate esters (PAEs), alkylphenols, and polybrominated diphenyl ethers added to them to enhance flexibility and durability (Barnes et al., 2009; Benson and Fred-Ahmadu, 2020; Hajiouni et al., 2022). These chemical additives are not strongly bonded to polymers and could eventually desorb and get leached into tissues of marine organisms upon ingestion (Koelmans, 2015). A study by Collard et al. (2017) reported the leaching of additive chemicals from microplastics into the blood and other organs of European anchovies (Engraulis encrasicolus L.). Likewise, the study by Tanaka et al. (2013) showed the possibility of leaching of chemical additives from plastics into biological tissues of African seabirds and North Pacific seabirds. The release of chemicals from microplastics occurs in a process called desorption. Studies have reported desorption to occur in tissues and organs of marine organisms after ingestion. PAHs sorbed on fluorescent microplastic particles were found to have desorbed in the intestine of Zebrafish before being transferred to the intestinal epithelium and liver (Batel et al., 2016). The ability of microplastics to release sorbed Persistent organic pollutants (POPs) and potentially toxic trace metals into various environmental media and organisms’ tissues have been shown to have detrimental effects.
While microplastics are sources of organic pollutants through the chemical additives incorporated in them during production, they also act as sinks for organic pollutants and metals through adsorption process. The sorption process depends on the physicochemical characteristics of both the POPs and the microplastics (Fred-Ahmadu et al., 2020c). Generally, the hydrophobicity of POPs and microplastics is the major factor that contributes to the interaction between them as well as sorption. Rubber-like feature of polypropylene (PP) and polyethylene (PE) (Bakir et al., 2012), glass-like property of polyvinylchloride (PVC) (Rodrigues et al., 2019), π-π electron system (Nakano, 2010), and amorphous structure of PS, the crystalline structure of polyethylene terephthalate (PET) (Miandad et al., 2018) are all properties that govern their sorption capacity. Aging influences the sorption capacity of microplastics and surface area to volume ratio is another property of microplastics that influence the sorption of organic pollutants and metals to them.
A report by Bakir et al. (2012) showed that microplastics made of polymers like PE, PVC, PP, and PS have a high sorption capacity for polycyclic aromatic hydrocarbons, hexachlorocyclohexanes, polychlorinated benzenes, and dichlorodiphenyltrichloroethane. A study by Turner and Holmes (2015) showed the adsorption of trace metals; silver (Ag), cadmium (Cd), chromium (Cr), cobalt (Co), copper (Cu), mercury (Hg), nickel (Ni), lead (Pb), and zinc (Zn) onto both virgin and aged microplastics with the aged plastic having higher sorption capacity. This sorption increases the long-distance transport of toxic chemicals in the marine environment. Due to electrostatic interactions and complexation on the surface of plastics such as PVC, preferential adsorption of bivalent metals (Pb2+, Cu2+, and Cd2+) was observed by Zou et al. (2020). Furthermore, adsorption of metals on MPs surfaces also occurs via surface charge created through weathering and organic matter precipitation which allows the binding of metal ions to active sites on the microplastics (Maršić-Lučić et al., 2018). The capacity of microplastics to act as adsorbents for metals and POPs requires further research in order to maximize this property for the remediation of wastewater and contaminated soils and sediment.
The impacts of microplastics on aquatic organisms could be physical, biological, or chemical. Physical impacts include entanglement, blockage of sunlight, and ingestion. There are recorded cases where marine animals like seabirds, sea turtles, and marine mammals have been trapped by plastic debris (Nelms et al., 2016; Hiemstra et al., 2021). Ghost nets, which are usually made of synthetic, non-biodegradable fibers like nylon, can persist for several years in the aquatic ecosystems, entangling, and in most cases killing an uncountable number of marine species (Stelfox et al., 2014; Wilcox et al., 2014; Nelms et al., 2016). The entrapment limits mobility for marine life which could eventually lead to discomfort that later results in death by starvation of marine animals caught in such situations (Schuyler et al., 2014). An increase in cases of entrapment has led to movements like “Skip the straw” and “Save the turtles Campaign,” as consequences of entrapment have included a decline in species of sea turtles around the globe (Friends of the Sea, 2009). Gall and Thompson (2015) study showed that almost 700 species interact with microplastics and an increase in this estimation is reported every year.
Microplastics are light weighted enough to float on water bodies, thus hindering the passage of adequate sunlight when excessively present. The hindrance of sunlight has been shown to alter photosynthetic activity (Zhang et al., 2017; Mao et al., 2018) which influences a decrease in chlorophyll content (Zhang et al., 2017; Prata et al., 2018). The altered photosynthetic activity and chlorophyll content could stunt the growth of phytoplankton and algae which form the bases of aquatic food webs, thus resulting in shortage of food for primary and then secondary consumers in aquatic biota. These scenarios are likely to occur in areas where microplastics pollution is severe enough to limit sunlight penetration. Figure 4 shows an example of an extremely polluted site, possibly hindering sunlight penetration.
Figure 4. Example of thousands of floating macro(meso)plastic (A,B) (Nigeria) and micro(nano)plastic (C) (Lima Peru) particles possibly blocking sunlight penetration at extremely polluted coastal sites.
Physical impacts of microplastic that arise from ingestion include malnutrition, intestinal blockage and internal injury, increased buoyancy, and dietary dilution (Nelms et al., 2016). More so, a study by Lei et al. (2018) showed that ingestion of microplastic particles by Zebrafish led to intestinal damage. Inflammation, malnutrition, depleted energy reserves as well as prolonged stay of MPs in the guts of Arenicola marina on exposure to PVC were some of the negative effects recorded (Wright et al., 2013b). Reduced feeding activity leading to a decrease in energy available for growth was detected in Carcinus maenas on exposure to polypropylene fibers (Watts et al., 2014). However, Chen et al. (2020a) in their study observed hyperactivity in adult zebrafish which was evident in an increase in their swimming distance and suggested it was probably as a result of up-regulation of estrogen contents in the fish on exposure to PS. However, physical injuries and adverse impacts of plastic debris to marine organisms can be avoided by regular beach cleaning, public awareness, and proper regulation of activities on and around water bodies.
The chemical impacts of microplastics usually originate from the ingestion of microplastics contaminated with heavy metals and organic pollutants. It is well established that microplastics can act both as sources and sink for organic pollutants and metals. These pollutants upon ingestion can pose threats to the health of marine organisms that consume them and can as well be transported through the aquatic food web thus posing a direct and/or indirect threat to all entities across the trophic level of the food web. The toxicity of PAH on ingestion by humans include nausea, diarrhea, confusion, kidney, and liver damage. Organohalogenated compounds cause reproductive problems (Sweeney et al., 2015) and phthalate esters (PAEs) disrupt metabolic systems (Diamanti-Kandarakis et al., 2009). The acute effects of POPs and metals on marine organisms have not been widely investigated. However, some studies have given an insight into the accumulation and toxic effect of contaminated microplastic on marine organisms. Nickel (Ni) contaminated polystyrene led to abnormalities like immobilization and changes in the morphology of Daphnia Magna (Kim et al., 2017). Copper-contaminated polystyrene accumulated in the tissue of Zebrafish and was found to be toxic to guts and the liver (Qiao et al., 2019b). The feeding activity of Lugworm was disrupted after it ingested polychlorinated biphenyl-contaminated polyethylene (Besseling et al., 2017). Mercury (Hg) adsorbed on fluorescence red polymer microsphere was found to have accumulated in the brain and muscles of European seabass (Dicentrarchus labrax), resulting in neurotoxicity, oxidative stress and damage, and causing changes in the activities of energy-related enzymes in juveniles of the species (Antao Barboza et al., 2018). The leaching of chemical additives and pollutants from microplastics into the blood and other organs of European anchovies (Engraulis encrasicolus, L.) enhanced vascular thrombosis (Collard et al., 2017).
Few studies have been carried out to investigate the biological impact of microplastics on the marine ecosystem. Geographical transfer of microorganisms is one of the biological roles that microplastics play in the marine environment, due to microorganisms’ ability to inhabit the surface of microplastics and be transported around with them (Oberbeckmann et al., 2015; De-la-Torre et al., 2021). The colonization of microplastics by microorganisms (biofouling) leads to changes in the physical properties of microplastics. Some of these changes include an increase in the density of MPs which causes them to sink to the bottom of the sea making them available to benthic organisms for ingestion and a decrease in hydrophobicity rendering them less accessible for contamination by organic pollutants (Fred-Ahmadu et al., 2020c). While the colonization of microplastics by microorganisms reduces chances of contamination by metals or organic pollutants, it increases their chances of being mistaken for food by other marine life in higher trophic levels, which primarily feed on microorganisms attached to the microplastics. Fractions of microplastics that adhere to planktons and algae can be ingested by other aquatic organisms like fish, shrimp, invertebrates and bivalves as reported by De-la-Torre et al. (2021), Kasamesiri and Thaimuangphol (2020), Jabeen et al. (2017), Rehse et al. (2016).
Biological effects of microplastic ingestion by marine organisms include disruption of oxidative balance, energy metabolism, antioxidant capacity, DNA makeup, immunological, neurological and histological impairment (Prokić et al., 2019). Direct and indirect consumption of contaminated microplastics could result in biological responses like inflammation, reduced feeding and body weight, and mortality stemming from bioaccumulation (Wright et al., 2013b). The study by Chen et al. (2020b) showed that interaction of marine model fish Oryzias melastigma with PS led to alteration of heartbeat, delay in hatching time, and decrease in hatching rate of embryos. According to the transcriptome result, exposure of these embryos to PS led to an increase in diseases as immune responses, genetic formation processing, and metabolism pathway were negatively impacted. Reproductive disruption was detected in Crassostrea gigas on exposure to polystyrene and significant impacts were also observed in offspring (Sussarellu et al., 2016). Polystyrenes were found in the circulatory system of mussels 3 days after they were first found in the guts (Browne et al., 2008; Carbery et al., 2018). A reduction in isocitrate dehydrogenase-IDH, a metabolic biomarker was observed in the gall bladder of Juvenile goby, Pomatoschistus microps fish after exposure to polyethylene (Oliveira et al., 2013). Mortality of brine shrimps was observed after they were exposed to polystyrene microplastics (Suman et al., 2020). Recently, Buwono et al. (2022) demonstrated that MPs (0.0001–1.0 mm) abundance has both direct and indirect biological impacts on oxidative damage on the gills and digestive tract of Gambusia affinis.
There is a wide range of biological, chemical, and physical effects resulting from direct or indirect ingestion of microplastic and nanoplastic particles by aquatic biota because of the relatively diminutive size of these emerging contaminants. The effects are variable and impact critical organs of aquatic organisms leading sometimes to increased morbidity and mortality. Small fishes and lower trophic marine organisms are the most impacted by contaminated micro(nano)plastics because they have the most direct contact with them. Biological impacts of microplastics on marine organisms influence the marine food web the most. This is due to the adherence of primary microorganisms on micro(nano)plastics, which are then fed upon by higher marine animals. Physical impacts include intestinal blockage, internal injury which could result in disturbed mobility, stunted growth, and death. The presence of microplastics that persist in the digestive tracts results in starvation which eventually could lead to death. The chemical effects of ingested microplastics on marine organisms have not been fully understood, because only accumulation has been studied the most. Most of the studies conducted to date have reported mainly on microplastic particles occurrence and prevalence in marine biota. The toxicity and mechanistic interactions of organic chemicals and metals on getting into the marine organisms is not fully understood. Thus, we recommend the following:
i There are currently limited studies on the prevalence and toxicity of MNPs on freshwater organisms, as well as their occurrence, fate, and transport in freshwater ecosystems. More research is needed to clearly understand the gastrointestinal-neuro-endocrine mechanisms of micro-and nanoplastic-induced adverse effects associated with
MNPs ingestion, absorption, metabolism, and excretion by freshwater organisms.
ii Physiological responses and broad toxicological effects in marine organisms, particularly adult marine species, are the focus of the majority of published studies. Furthermore, a relatively limited study has been conducted on the bioaccumulation and bioavailability of micro(nano)plastics. Understanding how microplastics are transferred from one trophic level to the next is essential in determining how long they remain in the digestive tract and how much they accumulate. Therefore, accurate, open and reliable data on the occurrence and ingestion/egestion rate of micro(nano)plastics, as well as the intake of hydrophobic micropollutants by freshwater and marine organisms, are required across a broader variety of intermediate and higher-trophic animals. This will provide a framework for establishing sound and effective risk assessment across a wider population and ecosystem scales.
iii Further studies should be focused on nanoplastics to enhance our understanding of size-dependent toxicity mechanisms.
iv In-depth investigations on the modes-of-action of microplastic and nanoplastic particles ingestions by marine animals, and the toxicological effects of these emerging contaminants’ sizes on the oxidative status, digestive systems changes, neurological system damage, and reproductive alterations should be carried out to gain explicit understanding.
v It would be imperative to conduct further research to understand the pathways of chemicals leached from microplastics and nanoplastics into marine organisms upon exposures and the associated effects.
vi More research is required to explore the metabolic pathways in marine biota after ingestion of MNPs/plastic debris taking into consideration the chemical and physical properties of the MNPs, exposure time, and concentration of MNPs.
vii Finally, the biological mechanisms by which microplastic and nanoplastic particles affect marine and freshwater organisms are still poorly known, and therefore necessitate extensive research.
NB: conceptualization, investigation, supervision, project administration, funding acquisition, and writing−original draft, review, and editing. OA: conceptualization, investigation, and writing−original draft, review, and editing. OF-A: conceptualization and writing−original draft, review, and editing. GD-l-T, AO, and AW: conceptualization and writing−review and editing. All authors contributed to the article and approved the submitted version.
This research was supported by the Covenant University Centre for Research, Innovation, and Discovery (CUCRID), through the Covenant University Seed Grant.
The authors declare that the research was conducted in the absence of any commercial or financial relationships that could be construed as a potential conflict of interest.
All claims expressed in this article are solely those of the authors and do not necessarily represent those of their affiliated organizations, or those of the publisher, the editors and the reviewers. Any product that may be evaluated in this article, or claim that may be made by its manufacturer, is not guaranteed or endorsed by the publisher.
We are grateful to Covenant University for providing publication assistance. We wish to thank Eric Akhiankore for providing graphical support.
Abbasi, S., Soltani, N., Keshavarzi, B., Moore, F., Turner, A., and Hassanaghaei, M. (2018). Microplastics in different tissues of fish and prawn from the Musa Estuary, Persian Gulf. Chemosphere 205, 80–87. doi: 10.1016/j.chemosphere.2018.04.076
Agboola, O. D., and Benson, N. U. (2021). Physisorption and chemisorption mechanisms influencing micro(nano)plastics-organic chemical contaminants interactions: a review. Front. Environ. Sci. 9:167. doi: 10.3389/fenvs.2021.678574
Ain Bhutto, S. U., and You, X. (2022). Spatial distribution of microplastics in Chinese freshwater ecosystem and impacts on food webs. Environ. Pollut. 293:118494. doi: 10.1016/j.envpol.2021.118494
Ali, I., Cheng, Q., Ding, T., Yiguang, Q., Yuechao, Z., Sun, H., et al. (2021). Micro- and nanoplastics in the environment: occurrence, detection, characterization and toxicity - a critical review. J. Clean. Prod. 313:127863. doi: 10.1016/j.jclepro.2021.127863
Antao Barboza, L. G., Vieira, L. R., Branco, V., Figueiredo, N., Carvalho, F., Carvalho, C., et al. (2018). Microplastics cause neurotoxicity, oxidative damage and energy-related changes and interact with the bioaccumulation of mercury in the European seabass, Dicentrarchus labrax (Linnaeus, 1758). Aquat. Toxicol. 195, 49–57. doi: 10.1016/j.aquatox.2017.12.008
Bakir, A., Rowland, S. J., and Thompson, R. C. (2012). Competitive sorption of persistent organic pollutants onto microplastics in the marine environment. Mar. Pollut. Bull. 64, 2782–2789. doi: 10.1016/j.marpolbul.2012.09.010
Banaee, M., Soltanian, S., Sureda, A., Gholamhosseini, A., Haghi, B. N., Akhlaghi, M., et al. (2019). Evaluation of single and combined effects of cadmium and micro-plastic particles on biochemical and immunological parameters of common carp (Cyprinus carpio). Chemosphere 236:124335. doi: 10.1016/j.chemosphere.2019.07.066
Bank, M. S., and Hansson, S. V. (2022). “The microplastic cycle: an introduction to a complex issue,” in Microplastic in the Environment: Pattern and Process. Environmental Contamination Remediation and Management, ed. M. S. Bank (Cham: Springer),Google Scholar
Barnes, D. K. A., Galgani, F., Thompson, R. C., and Barlaz, M. (2009). Accumulation and fragmentation of plastic debris in global environments. Philos. Trans. R. Soc. Lond. B Biol. Sci. 364, 1985–1998. doi: 10.1098/rstb.2008.0205
Batel, A., Linti, F., Scherer, M., Erdinger, L., and Braunbeck, T. (2016). The transfer of benzo(a)pyrene from microplastics to Artemia nauplii and further to zebrafish via a trophic food web experiment–CYP1A induction and visual tracking of persistent organic pollutants. Environ. Toxicol. Chem. 35, 1656–1666. doi: 10.1002/etc.3361
Bellas, J., Martínez-Armental, J., Martínez-Cámara, A., Besada, V., and Martínez-Gómez, C. (2016). Ingestion of microplastics by demersal fish from the Spanish Atlantic and Mediterranean coasts. Mar. Pollut. Bull. 109, 55–60. doi: 10.1016/j.marpolbul.2016.06.026
Bellasi, A., Binda, G., Pozzi, A., Galafassi, S., Volta, P., and Bettinetti, R. (2020). Microplastic contamination in freshwater environments: a review, focusing on interactions with sediments and benthic organisms. Environments 7:30. doi: 10.3390/environments7040030
Bertoli, M., Pastorino, P., Lesa, D., Renzi, M., Anselmi, S., Prearo, M., et al. (2022). Microplastics accumulation in functional feeding guilds and functional habit groups of freshwater macrobenthic invertebrates: novel insights in a riverine ecosystem. Sci. Total Environ. 804:150207. doi: 10.1016/j.scitotenv.2021.150207
Benson, N. U., Bassey, D. E., and Palanisami, T. (2021a). COVID pollution: impact of COVID-19 pandemic on global plastic waste footprint. Heliyon 7:e06343. doi: 10.1016/j.heliyon.2021.e06343
Benson, N. U., Fred-Ahmadu, O. H., Bassey, D. E., and Atayero, A. A. (2021b). COVID-19 pandemic and emerging plastic-based personal protective equipment waste pollution and management in Africa. J. Environ. Chem. Eng. 9:105222. doi: 10.1016/j.jece.2021.105222
Benson, N. U., and Fred-Ahmadu, O. H. (2020). Occurrence and distribution of microplastics-sorbed phthalic acid esters (PAEs) in coastal psammitic sediments of tropical Atlantic Ocean, Gulf of Guinea. Sci. Total Environ. 730:139013. doi: 10.1016/j.scitotenv.2020.139013
Besseling, E., Foekema, E. M., Van Den Heuvel-Greve, M. J., and Koelmans, A. A. (2017). The effect of microplastic on the uptake of chemicals by the lugworm Arenicola marina (L.) under environmentally relevant exposure conditions. Environ. Sci. Technol. 51, 8795–8804. doi: 10.1021/acs.est.7b02286
Betts, K. (2008). Why small plastic particles may pose a big problem in the oceans. Environ. Sci. Technol. 42:8995. doi: 10.1021/es802970v
Blettler, M. C. M., Abrial, E., Khan, F. R., Sivri, N., and Espinola, L. A. (2018). Freshwater plastic pollution: recognizing research biases and identifying knowledge gaps. Water Res. 143, 416–424. doi: 10.1016/j.watres.2018.06.015
Brandts, I., Teles, M., Tvarijonaviciute, A., Pereira, M., Martins, M., Tort, L., et al. (2018a). Effects of polymethylmethacrylate nanoplastics on Dicentrarchus labrax. Genomics 110, 435–441. doi: 10.1016/j.ygeno.2018.10.006
Brandts, I., Teles, M., Gonçalves, A., Barreto, A., Franco-Martinez, L., Tvarijonaviciute, A., et al. (2018b). Effects of nanoplastics on Mytilus galloprovincialis after individual and combined exposure with carbamazepine. Sci. Total Environ. 643, 775–784. doi: 10.1016/j.scitotenv.2018.06.257
Bradney, L., Wijesekara, H., Palansooriya, K. N., Obadamudalige, N., Bolan, N. S., Ok, Y. S., et al. (2019). Particulate plastics as a vector for toxic trace-element uptake by aquatic and terrestrial organisms and human health risk. Environ. Int. 131:104937. doi: 10.1016/j.envint.2019.104937
Brennecke, D., Duarte, B., Paiva, F., Caçador, I., and Canning-Clode, J. (2016). Microplastics as vector for heavy metal contamination from the marine environment. Estuar. Coast. Shelf Sci. 178, 189–195. doi: 10.1016/j.ecss.2015.12.003
Browne, M. A. (2015). “Sources and pathways of microplastics to habitats,” in Marine Anthropogenic Litter, eds M. Bergmann, L. Gutow, and M. Klages (Cham: Springer), 229–244. doi: 10.1007/978-3-319-16510-3_9
Browne, M. A., Dissanayake, A., Galloway, T. S., Lowe, D. M., and Thompson, R. C. (2008). Ingested microscopic plastic translocates to the circulatory system of the mussel, Mytilus edulis (L.). Environ. Sci. Technol. 42, 5026–5031. doi: 10.1021/es800249a
Buwono, N. R., Risjani, Y., and Soegianto, A. (2022). Oxidative stress responses of microplastic-contaminated Gambusia affinis obtained from the Brantas River in East Java, Indonesia. Chemosphere 293:133543. doi: 10.1016/j.chemosphere.2022.133543
Camedda, A., Marra, S., Matiddi, M., Massaro, G., Coppa, S., Perilli, A., et al. (2014). Interaction between loggerhead sea turtles (Caretta caretta) and marine litter in Sardinia (Western Mediterranean Sea). Mar. Environ. Res. 100, 25–32. doi: 10.1016/j.marenvres.2013.12.004
Carbery, M., O’Connor, W., and Palanisami, T. (2018). Trophic transfer of microplastics and mixed contaminants in the marine food web and implications for human health. Environ. Int. 115, 400–409. doi: 10.1016/j.envint.2018.03.007
Castro-Castellon, A. T., Horton, A. A., Hughes, J. M. R., Rampley, C., Jeffers, E. S., Bussi, G., et al. (2021). Ecotoxicity of microplastics to freshwater biota: considering exposure and hazard across trophic levels. Sci. Total Environ. 816:151638. doi: 10.1016/j.scitotenv.2021.151638
Chae, Y., Kim, D., Choi, M.-J., Cho, Y., and An, Y.-J. (2019). Impact of nano-sized plastic on the nutritional value and gut microbiota of whiteleg shrimp Litopenaeus vannamei via dietary exposure. Environ. Int. 130:104848. doi: 10.1016/j.envint.2019.05.042
Chae, Y., Kim, D., Kim, S. W., and An, Y. J. (2018). Trophic transfer and individual impact of nano- sized polystyrene in a four-species freshwater food chain. Sci. Rep. 8:284. doi: 10.1038/s41598-017-18849-y
Chang, M. (2015). Reducing microplastics from facial exfoliating cleansers in wastewater through treatment versus consumer product decisions. Mar. Pollut. Bull. 101, 330–333. doi: 10.1016/j.marpolbul.2015.10.074
Chang, X., Xue, Y., Li, J., Zou, L., and Tang, M. (2020). Potential health impact of environmental micro- and nanoplastics pollution. J. Appl. Toxicol. 40, 4–15. doi: 10.1002/jat.3915
Chen, Q., Lackmann, C., Wang, W., Seiler, T. B., Hollert, H., and Shi, H. (2020a). Microplastics lead to hyperactive swimming behaviour in adult zebrafish. Aquat. Toxicol. 224:105521. doi: 10.1016/j.aquatox.2020.105521
Chen, J. C., Chen, M. Y., Fang, C., Zheng, R. H., Jiang, Y. L., Zhang, Y., et al. (2020b). Microplastics negatively impact embryogenesis and modulate the immune response of the marine medaka Oryzias melastigma. Mar. Pollut. Bull. 158:111349. doi: 10.1016/j.marpolbul.2020.111349
Chen, Q., Gundlach, M., Yang, S., Jiang, J., Velki, M., Yin, D., et al. (2017a). Quantitative investigation of the mechanisms of microplastics and nanoplastics toward zebrafish larvae locomotor activity. Sci. Total Environ. 584–585, 1022–1031. doi: 10.1016/j.scitotenv.2017.01.156
Chen, Q., Yin, D., Jia, Y., Schiwy, S., Legradi, J., Yang, S., et al. (2017b). Enhanced uptake of BPA in the presence of nanoplastics can lead to neurotoxic effects in adult zebrafish. Sci. Total Environ. 609, 1312–1321. doi: 10.1016/j.scitotenv.2017.07.144
Chua, E. M., Shimeta, J., Nugegoda, D., Morrison, P. D., and Clarke, B. O. (2014). Assimilation of polybrominated diphenyl ethers from microplastics by the marine amphipod, Allorchestes compressa. Environ. Sci. Technol. 48, 8127–8134. doi: 10.1021/es405717z
Clause, A. G., Celestian, A. J., and Pauly, G. B. (2021). Plastic ingestion by freshwater turtles: a review and call to action. Sci. Rep. 11:5672. doi: 10.1038/s41598-021-84846-x
Cole, M., Lindeque, P. K., Fileman, E., Clark, J., Lewis, C., Halsband, C., et al. (2016). Microplastics alter the properties and sinking rates of Zooplankton faecal pellets. Environ. Sci. Technol. 50, 3239–3246. doi: 10.1021/acs.est.5b05905
Cole, M., Lindeque, P., Fileman, E., Halsband, C., and Galloway, T. S. (2015). The impact of polystyrene microplastics on feeding, function and fecundity in the marine copepod Calanus helgolandicus. Environ. Sci. Technol. 49, 1130–1137. doi: 10.1021/es504525u
Cole, M., Lindeque, P., Fileman, E., Halsband, C., Goodhead, R., Moger, J., et al. (2013). Microplastic ingestion by zooplankton. Environ. Sci. Technol. 47, 6646–6655.
Cole, M., Lindeque, P., Halsband, C., and Galloway, T. S. (2011). Microplastics as contaminants in the marine environment: a review. Mar. Pollut. Bull. 62, 2588–2597. doi: 10.1016/j.marpolbul.2011.09.025
Collard, F., Gilbert, B., Compère, P., Eppe, G., Das, K., Jauniaux, T., et al. (2017). Microplastics in livers of European anchovies (Engraulis encrasicolus, L.). Environ. Pollut. 229, 1000–1005. doi: 10.1016/j.envpol.2017.07.089
Corcoran, P. L., Norris, T., Ceccanese, T., Walzak, M. J., Helm, P. A., and Marvin, C. H. (2015). Hidden plastics of Lake Ontario, Canada and their potential preservation in the sediment record. Environ. Pollut. 204, 17–25. doi: 10.1016/j.envpol.2015.04.009
Cousin, X., Batel, A., Bringer, A., Hess, S., Bégout, M.-L., and Braunbeck, T. (2020). Microplastics and sorbed contaminants – Trophic exposure in fish sensitive early life stages. Mar. Environ. Res. 161:105126. doi: 10.1016/j.marenvres.2020.105126
Cózar, A., Echevarría, F., González-Gordillo, J. I., Irigoien, X., Úbeda, B., Hernández-León, S., et al. (2014). Plastic debris in the open ocean. Proc. Natl. Acad. Sci. U.S.A. 111, 10239–10244.
da Costa Araújo, A. P., de Andrade Vieira, J. E., and Malafaia, G. (2020). Toxicity and trophic transfer of polyethylene microplastics from Poecilia reticulata to Danio rerio. Sci. Total Environ. 742:140217. doi: 10.1016/j.scitotenv.2020.140217
Dahms, H. T., van Rensburg, G. J., and Greenfield, R. (2020). The microplastic profile of an urban African stream. Sci. Total Environ. 731:138893. doi: 10.1016/j.scitotenv.2020.138893
Davison, P., and Asch, R. G. (2011). Plastic ingestion by mesopelagic fishes in the North Pacific Subtropical Gyre. Mar. Ecol. Prog. Ser. 432, 173–180. doi: 10.3354/meps09142
De-la-Torre, G. E., Dioses-Salinas, D. C., Castro, J. M., Antay, R., Fernández, N. Y., Espinoza-Morriberón, D., et al. (2020a). Abundance and distribution of microplastics on sandy beaches of Lima. Peru. Mar. Pollut. Bull. 151:110877. doi: 10.1016/j.marpolbul.2019.110877
De-la-Torre, G. E., Apaza-Vargas, D. M., and Santillán, L. (2020b). Microplastic ingestion and feeding ecology in three intertidal mollusk species from Lima, Peru. Rev. Biol. Mar. Oceanogr. 55, 167–171. doi: 10.22370/rbmo.2020.55.2.2502
De-la-Torre, G. E., Dioses-Salinas, D. C., Pérez-Baca, B. L., Millones Cumpa, L. A., Pizarro-Ortega, C. I., Torres, F. G., et al. (2021). Marine macroinvertebrates inhabiting plastic litter in Peru. Mar. Pollut. Bull. 167:112296. doi: 10.1016/j.marpolbul.2021.112296
Della Torre, C., Bergami, E., Salvati, A., Faleri, C., Cirino, P., Dawson, K. A., et al. (2014). Accumulation and embryotoxicity of polystyrene nanoparticles at early stage of development of sea urchin embryos Paracentrotus lividus. Environ. Sci. Technol. 48, 12302–12311. doi: 10.1021/es502569w
Desforges, J. P. W., Galbraith, M., and Ross, P. S. (2015). Ingestion of microplastics by zooplankton in the Northeast Pacific Ocean. Arch. Environ. Contam. Toxicol. 69, 320–330. doi: 10.1007/s00244-015-0172-5
Diamanti-Kandarakis, E., Bourguignon, J. P., Giudice, L. C., Hauser, R., Prins, G. S., Soto, A. M., et al. (2009). Endocrine-disrupting chemicals: an endocrine society scientific statement. Endocr. Rev. 30, 293–342.
Di Bello, A., Valastro, C., Freggi, D., Lai, O. R., Crescenzo, G., and Franchini, D. (2013). Surgical treatment of injuries caused by fishing gear in the intracoelomic digestive tract of sea turtles. Dis. Aquat. Org. 106, 93–102. doi: 10.3354/dao02641
Digka, N., Tsangaris, C., Torre, M., Anastasopoulou, A., and Zeri, C. (2018). Microplastics in mussels and fish from the Northern Ionian Sea. Mar. Pollut. Bull. 135, 30–40. doi: 10.1016/j.marpolbul.2018.06.063
Ding, J., Zhang, S., Razanajatovo, R. M., Zou, H., and Zhu, W. (2018). Accumulation, tissue distribution, and biochemical effects of polystyrene microplastics in the freshwater fish red tilapia (Oreochromis niloticus). Environ. Pollut. 238, 1–9. doi: 10.1016/j.envpol.2018.03.001
Dris, R., Gasperi, J., Rocher, V., Saad, M., Renault, N., and Tassin, B. (2015). Microplastic contamination in an urban area: a case study in Greater Paris. Environ. Chem. 12, 592–599. doi: 10.1071/en14167
Duis, K., and Coors, A. (2016). Microplastics in the aquatic and terrestrial environment: sources (with a specific focus on personal care products), fate and effects. Environ. Sci. Eur. 28:2. doi: 10.1186/s12302-015-0069-y
Drummond, J., Nel, H. A., Packman, A. I., and Krause, S. (2020). Significance of hyporheic exchange for predicting microplastic fate in rivers. Environ. Sci. Technol. Lett. 7, 727–732. doi: 10.1021/acs.estlett.0c00595
Elizalde-Velázquez, A., Carcano, A. M., Crago, J., Green, M. J., Shah, S. A., and Cañas-Carrell, J. E. (2020). Translocation, trophic transfer, accumulation and depuration of polystyrene microplastics in Daphnia magna and Pimephales promelas. Environ. Pollut. 259:113937. doi: 10.1016/j.envpol.2020.113937
Eriksen, M., Lebreton, L. C., Carson, H. S., Thiel, M., Moore, C. J., Borerro, J. C., et al. (2014). Plastic pollution in the world’s oceans: more than 5 trillion plastic pieces weighing over 250,000 tons afloat at sea. PLoS One 9:e111913. doi: 10.1371/journal.pone.0111913
Eriksen, M., Mason, S., Wilson, S., Box, C., Zellers, A., Edwards, W., et al. (2013). Microplastic pollution in the surface waters of the Laurentian Great Lakes. Mar. Pollut. Bull. 77, 177–182. doi: 10.1016/j.marpolbul.2013.10.007
Eriksson, C., and Burton, H. (2003). Origins and biological accumulation of small plastic particles in fur seals from Macquarie Island. Ambio 32, 380–384. doi: 10.1579/0044-7447-32.6.380
Faggio, C., Tsarpali, V., and Dailianis, S. (2018). Mussel digestive gland as a model for assessing xenobiotics: an overview. Sci. Total Environ. 613, 220–229. doi: 10.1016/j.scitotenv.2018.04.264
Farrell, P., and Nelson, K. (2013). Trophic level transfer of microplastic: Mytilus edulis (L.) to Carcinus maenas (L.). Environ. Pollut. 177, 1–3. doi: 10.1016/j.envpol.2013.01.046
Felismino, M. E. L., Helm, P. A., and Rochman, C. M. (2021). Microplastic and other anthropogenic microparticles in water and sediments of Lake Simcoe. J. Great Lakes Res. 47, 180–189.
Filgueiras, A. V., Preciado, I., Cartón, A., and Gago, J. (2020). Microplastic ingestion by pelagic and benthic fish and diet composition: a case study in the NW Iberian shelf. Mar. Pollut. Bull. 160:111623. doi: 10.1016/j.marpolbul.2020.111623
Fisner, M., Taniguchi, S., Moreira, F., Bícego, M. C., and Turra, A. (2013). Polycyclic aromatic hydrocarbons (PAHs) in plastic pellets: variability in the concentration and composition at different sediment depths in a sandy beach. Mar. Pollut. Bull. 70, 219–226. doi: 10.1016/j.marpolbul.2013.03.008
Fossi, M. C., Peda‘, C., Compa, M., Tsangaris, C., Alomar, C., Claro, F., et al. (2018). Bioindicators for monitoring marine litter ingestion and its impacts on Mediterranean biodiversity. Environ. Pollut. 237, 1023–1040. doi: 10.1016/j.envpol.2017.11.019
Fossi, M. C., Panti, C., Guerranti, C., Coppola, D., Giannetti, M., Marsili, L., et al. (2012). Are baleen whales exposed to the threat of microplastics? A case study of the Mediterranean fin whale (Balaenoptera physalus). Mar. Pollut. Bull. 64, 2374–2379. doi: 10.1016/j.marpolbul.2012.08.013
Fred-Ahmadu, O. H., Ayejuyo, O. O., and Benson, N. U. (2020a). Dataset on microplastics and associated trace metals and phthalate esters in sandy beaches of tropical Atlantic ecosystems, Nigeria. Data Brief 31:105755. doi: 10.1016/j.dib.2020.105755
Fred-Ahmadu, O. H., Ayejuyo, O. O., and Benson, N. U. (2020b). Microplastics distribution and characterization in epipsammic sediments of tropical Atlantic Ocean, Nigeria. Reg. Stud. Mar. Sci. 38:101365. doi: 10.1016/j.rsma.2020.101365
Fred-Ahmadu, O. H., Bhagwat, G., Oluyoye, I., Benson, N. U., Ayejuyo, O. O., and Palanisami, T. (2020c). Interaction of chemical contaminants with microplastics: principles and perspectives. Sci. Total Environ. 706:135978. doi: 10.1016/j.scitotenv.2019.135978
Frias, J. P. G. L., Sobral, P., and Ferreira, A. (2010). Organic pollutants in microplastics from two Portuguese beaches. Mar. Pollut. Bull. 60, 1988–1992. doi: 10.1016/j.marpolbul.2010.07.030
Gall, S. C., and Thompson, R. (2015). The impact of debris on marine life. Mar. Pollut. Bull. 92, 170–179. doi: 10.1016/j.marpolbul.2014.12.041
Galloway, T. S., Cole, M., and Lewis, C. (2017). Interactions of microplastic debris throughout the marine ecosystem. Nat. Ecol. Evol. 1:0116. doi: 10.1038/s41559-017-0116
Gambardella, C., Morgana, S., Ferrando, S., Bramini, M., Piazza, V., Costa, E., et al. (2017). Effects of polystyrene microbeads in marine planktonic crustaceans. Ecotoxicol. Environ. Saf. 145, 250–257. doi: 10.1016/j.ecoenv.2017.07.036
Garcia, T. D., Cardozo, A. L. P., Quirino, B. A., Yofukuji, K. Y., Ganassin, M. J. M., dos Santos, N. C. L., et al. (2020). Ingestion of microplastic by fish of different feeding habits in urbanized and non-urbanized streams in Southern Brazil. Water Air Soil Pollut. 231:434. doi: 10.1007/s11270-020-04802-9
Giani, D., Baini, M., Galli, M., Casini, S., and Fossi, M. C. (2019). Microplastics occurrence in edible fish species (Mullus barbatus and Merluccius merluccius) collected in three different geographical sub-areas of the Mediterranean Sea. Mar. Pollut. Bull. 140, 129–137. doi: 10.1016/j.marpolbul.2019.01.005
Gray, A. D., and Weinstein, J. E. (2017). Size- and shape-dependent effects of microplastic particles on adult daggerblade grass shrimp (Palaemonetes pugio). Environ. Toxicol. 36, 3074–3080. doi: 10.1002/etc.3881
Green, D. S. (2016). Effects of microplastics on European flat oysters, Ostrea edulis and their associated benthic communities. Environ. Pollut. 216, 95–103. doi: 10.1016/j.envpol.2016.05.043
Greven, A. C., Merk, T., Karagoöz, F., Mohr, K., Klapper, M., Jovanovi ìc, B., et al. (2016). Polycarbonate and polystyrene nanoplastic particles act as stressors to the innate immune system of fathead minnow (Pimephales promelas). Environ. Toxicol. Chem. 35, 3093–3100. doi: 10.1002/etc.3501
Gurjar, U. R., Xavier, K. A. M., Shukla, S. P., Jaiswar, A. K., Deshmukhe, G., and Nayak, B. B. (2022). Microplastic pollution in coastal ecosystem off Mumbai coast, India. Chemosphere 288:132484. doi: 10.1016/j.chemosphere.2021.132484
Gusmão, F., Di Domenico, M., Amaral, A. C. Z., Martínez, A., Gonzalez, B. C., Worsaae, K., et al. (2016). In situ ingestion of microfibres by meiofauna from sandy beaches. Environ. Pollut. 216, 584–590. doi: 10.1016/j.envpol.2016.06.015
Haegerbaeumer, A., Mueller, M.-T., Fueser, H., and Traunspurger, W. (2019). Impacts of micro- and nano-sized plastic particles on benthic invertebrates: a literature review and gap analysis. Front. Environ. Sci. 7:17. doi: 10.3389/fenvs.2019.00017
Hajiouni, S., Mohammadi, A., Ramavandi, B., Arfaeinia, H., De-la-Torre, G. E., Tekle-Röttering, A., et al. (2022). Occurrence of microplastics and phthalate esters in urban runoff: a focus on the Persian Gulf coastline. Sci. Total Environ. 806:150559. doi: 10.1016/j.scitotenv.2021.150559
Hamed, M., Soliman, H. A., Osman, A. G., and Sayed, A. E.-D. H. (2020). Antioxidants and molecular damage in nile Tilapia (Oreochromis niloticus) after exposure to microplastics. Environ. Sci. Pollut. Res. 27, 14581–14588. doi: 10.1007/s11356-020-07898-y
Hämer, J., Gutow, L., Köhler, A., and Saborowski, R. (2014). Fate of microplastics in the marine isopod Idotea emarginata. Environ. Sci. Technol. 48, 13451–13458. doi: 10.1021/es501385y
Hartmann, N. B., Hüffer, T., Thompson, R. C., Hassellöv, M., Verschoor, A., Daugaard, A. E., et al. (2019). Are we speaking the same language? recommendations for a definition and categorization framework for plastic debris. Environ. Sci. Technol. 53, 1039–1047.
Hartmann, N. B., Rist, S., Bodin, J., Meibom, A., Jensen, L. H., Baun, A., et al. (2017). Microplastics as vectors for environmental contaminants: exploring sorption, desorption, and transfer to biota. Integr. Environ. Assess. Manag. 13, 488–493. doi: 10.1002/ieam.1904
Hesler, M., Aengenheister, L., Ellinger, B., Drexel, R., Straskraba, S., Jost, C., et al. (2019). Multi-endpoint toxicological assessment of polystyrene nano- and microparticles in different biological models in vitro. Toxicol. In Vitro 61:104610. doi: 10.1016/j.tiv.2019.104610
Hiemstra, A. F., Rambonnet, L., Gravendeel, B., and Schilthuizen, M. (2021). The effects of COVID-19 litter on animal life. Anim. Biol. 71, 215–231. doi: 10.1163/15707563-bja10052
Hirai, H., Takada, H., Ogata, Y., Yamashita, R., Mizukawa, K., Saha, M., et al. (2011). Organic micropollutants in marine plastics debris from the open ocean and remote and urban beaches. Mar. Pollut. Bull. 62, 1683–1692. doi: 10.1016/j.marpolbul.2011.06.004
Hoarau, L., Ainley, L., Jean, C., and Ciccione, S. (2014). Ingestion and defecation of marine debris by loggerhead sea turtles, Caretta caretta, from by-catches in the South-West Indian Ocean. Mar. Pollut. Bull. 84, 90–96. doi: 10.1016/j.marpolbul.2014.05.031
Horn, D., Miller, M., Anderson, S., and Steele, C. (2019). Microplastics are ubiquitous on California beaches and enter the coastal food web through consumption by Pacific mole crabs. Mar. Pollut. Bull. 139, 231–237. doi: 10.1016/j.marpolbul.2018.12.039
Horton, A. A., Walton, A., Spurgeon, D. J., Lahive, E., and Svendsen, C. (2017a). Microplastics in freshwater and terrestrial environments: evaluating the current understanding to identify the knowledge gaps and future research priorities. Sci. Total Environ. 586, 127–141. doi: 10.1016/j.scitotenv.2017.01.190
Horton, A. A., Svendsen, C., Williams, R. J., Spurgeon, D. J., and Lahive, E. (2017b). Large microplastic particles in sediments of tributaries of the River Thames, UK – abundance, sources and methods for effective quantification. Mar. Pollut. Bull. 114, 218–226. doi: 10.1016/j.marpolbul.2016.09.004
Hossain, M. S., Rahman, M. S., Uddin, M. N., Sharifuzzaman, S. M., Chowdhury, S. R., Sarker, S., et al. (2020). Microplastic contamination in penaeid shrimp from the northern Bay of Bengal. Chemosphere 238:124688. doi: 10.1016/j.chemosphere.2019.124688
Hu, J., Qin, X., Zhang, J., Zhu, Y., Zeng, W., Lin, Y., et al. (2021). Polystyrene microplastics disturb maternal-fetal immune balance and cause reproductive toxicity in pregnant mice. Reprod. Toxicol. 106, 42–50. doi: 10.1016/j.reprotox.2021.10.002
Huang, J. N., Wen, B., Meng, L. J., Li, X. X., Wang, M. H., Gao, J. Z., et al. (2020). Integrated response of growth, antioxidant defense and isotopic composition to microplastics in juvenile guppy (Poecilia reticulata). J. Hazard. Mater. 399:123044. doi: 10.1016/j.jhazmat.2020.123044
Hui, D. (2012). Food Web: Concept and Applications. Available online at: https://www.nature.com/scitable/knowledge/library/food-web-concept-and-applications-84077181/ (accessed on February 16, 2021)
Hurley, R. R., Woodward, J. C., and Rothwell, J. J. (2017). Ingestion of microplastics by freshwater tubifex worms. Environ. Sci. Technol. 51, 12844–12851. doi: 10.1021/acs.est.7b03567
Isobe, A., Iwasaki, S., Uchida, K., and Tokai, T. (2019). Abundance of non-conservative microplastics in the upper ocean from 1957 to 2066. Nat. Commun. 10:417. doi: 10.1038/s41467-019-08316-9
Isobe, A., Uchiyama-Matsumoto, K., Uchida, K., and Tokai, T. (2017). Microplastics in the Southern Ocean. Mar. Pollut. Bull. 114, 623–626. doi: 10.1016/j.marpolbul.2016.09.037
Ivar, J. A., and Costa, M. F. (2014). The present and future of microplastic pollution in the marine environment. Environ. Pollut. 185, 352–364. doi: 10.1016/j.envpol.2013.10.036
Iyare, P. U., Ouki, S. K., and Bond, T. (2020). Microplastics removal in wastewater treatment plants: a critical review. Environ. Sci. Water Res. Technol. 6, 2664–2675. doi: 10.1039/D0EW00397B
Jabeen, K., Su, L., Li, J., Yang, D., Tong, C., Mu, J., et al. (2017). Microplastics and mesoplastics in fish from coastal and fresh waters of China. Environ. Pollut. 221, 141–149. doi: 10.1016/j.envpol.2016.11.055
Jambeck, J. R., Geyer, R., Wilcox, C., Siegler, T. R., Perryman, M., Andrady, A., et al. (2015). Plastic waste inputs from land into the ocean. Science 347, 768–771. doi: 10.1126/science.1260352
James, K., Vasant, K., Padua, S., Gopinath, V., Abilash, K. S., Jeyabaskaran, R., et al. (2020). An assessment of microplastics in the ecosystem and selected commercially important fishes off Kochi, south eastern Arabian Sea, India. Mar. Pollut. Bull. 154:111027. doi: 10.1016/j.marpolbul.2020.111027
Jang, M., Shim, W. J., Han, G. M., Rani, M., Song, Y. K., and Hong, S. H. (2016). Styrofoam debris as a source of hazardous additives for marine organisms. Environ. Sci. Technol. 50, 4951–4960. doi: 10.1021/acs.est.5b05485
Jeong, C.-B., Won, E.-J., Kang, H.-M., Lee, M.-C., Hwang, D.-S., Hwang, U.-K., et al. (2016). Microplastic size-dependent toxicity, oxidative stress induction, and p-JNK and p-p38 activation in the monogonont rotifer (Brachionus koreanus). Environ. Sci. Technol. 50, 8849–8857. doi: 10.1021/acs.est.6b01441
Jin, Y., Xia, J., Pan, Z., Yang, J., Wang, W., and Fu, Z. (2018). Polystyrene microplastics induce microbiota dysbiosis and inflammation in the gut of adult zebrafish. Environ. Pollut. 235, 322–329. doi: 10.1016/j.envpol.2017.12.088
Jovanović, B. (2017). Ingestion of microplastics by fish and its potential consequences from a physical perspective. Integr. Environ. Assess. Manag. 13, 510–515. doi: 10.1002/ieam.1913
Kaposi, K. L., Mos, B., Kelaher, B. P., and Dworjanyn, S. A. (2014). Ingestion of microplastic has limited impact on a marine larva. Environ. Sci. Technol. 48, 1638–1645. doi: 10.1021/es404295e
Karuppasamy, P. K., Ravi, A., Vasudevan, L., Elangovan, M. P., Mary, P. D., Vincent, S. G., et al. (2020). Baseline survey of micro and mesoplastics in the gastro- intestinal tract of commercial fish from Southeast coast of the Bay of Bengal. Mar. Pollut. Bull. 153:110974. doi: 10.1016/j.marpolbul.2020.110974
Kasamesiri, P., and Thaimuangphol, W. (2020). Microplastics ingestion by freshwater fish in the Chi river, Thailand. Intern. J. Geomate 18, 114–119. doi: 10.21660/2020.67.9110
Kim, D., Chae, Y., and An, Y.-J. (2017). Mixture toxicity of nickel and microplastics with different functional groups on Daphnia magna. Environ. Sci. Technol. 51, 12852–12858. doi: 10.1021/acs.est.7b03732
Kim, J.-S., Lee, H.-J., Kim, S.-K., and Kim, H.-J. (2018). Global pattern of microplastics (MPs) in commercial food-grade salts: sea salt as an indicator of seawater MP pollution. Environ. Sci. Technol. 52, 12819–12828. doi: 10.1021/acs.est.8b04180
Koelmans, A. A. (2015). “Modeling the role of microplastics in bioaccumulation of organic chemicals to marine aquatic organisms. a critical review,” in Marine Anthropogenic Litter, eds M. Bergmann, L. Gutow, and M. Klages (Berlin: Springer), 309–324.
Koelmans, A. A., Besseling, E., Foekema, E., Kooi, M., Mintenig, S., Ossendorp, B. C., et al. (2017). Risks of plastic debris: unravelling fact, opinion, perception, and belief. Environ. Sci. Technol. 51, 11513–11519.
Koelmans, A. A., Diepens, N. J., and Mohamed Nor, N. H. (2022). “Weight of evidence for the microplastic vector effect in the context of chemical risk assessment,” in Microplastic in the Environment: Pattern and Process. Environmental Contamination Remediation & Management, ed. M. S. Bank (Cham: Springer), doi: 10.1007/978-3-030-78627-4_6
Kong, Q., Li, Y., Xu, X., Cheng, J., Sun, Y., Zhang, L., et al. (2020). Microplastics interfere with mixotrophic Ochromonas eliminating toxic Microcystis. Chemosphere 265:129030. doi: 10.1016/j.chemosphere.2020.129030
Krause, S., Baranov, V., Nel, H. A., Drummond, J. D., Kukkola, A., Hoellein, T., et al. (2021). Gathering at the top? Environmental controls of microplastic uptake and biomagnification in freshwater food webs. Environ. Pollut. 268:115750. doi: 10.1016/j.envpol.2020.115750
Lambert, S., and Wagner, M. (2018). “Microplastics are contaminants of emerging concern in freshwater environments: an overview,” in Freshwater Microplastics, eds M. Wagner and S. Lambert (Cham: Springer), 1–23. doi: 10.1016/j.scitotenv.2021.151989
Latchere, O., Audroin, T., Hétier, J., Métais, I., and Châtel, A. (2021). The need to investigate continuums of plastic particle diversity, brackish environments and trophic transfer to assess the risk of micro and nanoplastics on aquatic organisms. Environ. Pollut. 273:116449. doi: 10.1016/j.envpol.2021.116449
Lebreton, L., and Andrady, A. (2019). Future scenarios of global plastic waste generation and disposal. Palgrave Commun. 5, 1–11. doi: 10.1057/s41599-018-0212-7
Lei, L., Wu, S., Lu, S., Liu, M., Song, Y., Fu, Z., et al. (2018). Microplastic particles cause intestinal damage and other adverse effects in zebrafish Danio rerio and nematode Caenorhabditis elegans. Sci. Total Environ. 619–620, 1–8. doi: 10.1016/j.scitotenv.2017.11.103
LeMoine, C. M., Kelleher, B. M., Lagarde, R., Northam, C., Elebute, O. O., and Cassone, B. J. (2018). Transcriptional effects of polyethylene microplastics ingestion in developing zebrafish (Danio rerio). Environ. Pollut. 243, 591–600. doi: 10.1016/j.envpol.2018.08.084
Lenaker, P. L., Baldwin, A. K., Corsi, S. R., Mason, S. A., Reneau, P. C., and Scott, J. W. (2019). Vertical distribution of microplastics in the water column and surficial sediment from the Milwaukee river basin to Lake Michigan. Environ. Sci. Technol. 53, 12227–12237. doi: 10.1021/acs.est.9b03850
Leslie, H., Brandsma, S., Van Velzen, M., and Vethaak, A. (2017). Microplastics en route: field measurements in the Dutch river delta and Amsterdam canals, wastewater treatment plants, North Sea sediments and biota. Environ. Int. 101, 133–142. doi: 10.1016/j.envint.2017.01.018
Li, C., Busquets, R., and Campos, L. C. (2020). Assessment of microplastics in freshwater systems: a review. Sci. Total Environ. 707:135578. doi: 10.1016/j.scitotenv.2019.135578
Li, D., Deng, Y., Wang, S., Du, H., Xiao, G., and Wang, D. (2020a). Assessment of nanopolystyrene toxicity under fungal infection condition in Caenorhabditis elegans. Ecotoxicol. Environ. Saf. 197:110625. doi: 10.1016/j.ecoenv.2020.110625
Li, D., Ji, J., Yuan, Y., and Wang, D. (2020b). Toxicity comparison of nanopolystyrene with three metal oxide nanoparticles in nematode Caenorhabditis elegans. Chemosphere 245:125625. doi: 10.1016/j.chemosphere.2019.125625
Li, J., Liu, F., and Chen, J. P. (2018). Microplastics in freshwater systems: a review on occurrence, environmental effects, and methods for microplastics. Water Res. 137, 362–374. doi: 10.1016/j.watres.2017.12.056
Li, W., Chen, X., Li, M., Cai, Z., Gong, H., and Yan, M. (2022). Microplastics as an aquatic pollutant affect gut microbiota within aquatic animals. J. Hazard. Mater. 423(Part B):127094. doi: 10.1016/j.jhazmat.2021.127094
Li, W. C., Tse, H. F., and Fok, L. (2016). Plastic waste in the marine environment: a review of sources, occurrence and effects. Sci. Total Environ. 566, 333–349. doi: 10.1016/j.scitotenv.2016.05.084
Lima, A. R. A., Costa, M. F., and Barletta, M. (2014). Distribution patterns of microplastics within the plankton of a tropical estuary. Environ. Res. 132, 146–155. doi: 10.1016/j.envres.2014.03.031
Limonta, G., Mancia, A., Benkhalqui, A., Bertolucci, C., Abelli, L., Fossi, M. C., et al. (2019). Microplastics induce transcriptional changes, immune response and behavioral alterations in adult zebrafish. Sci. Rep. 9:15775. doi: 10.1038/s41598-019-52292-5
Lin, W., Jiang, R., Xiao, X., Wu, J., Wei, S., Liu, Y., et al. (2020). Joint effect of nanoplastics and humic acid on the uptake of PAHs for Daphnia magna: a model study. J. Hazard. Mater. 391:122195. doi: 10.1016/j.jhazmat.2020.122195
Liu, J., Xu, D., Chen, Y., Zhao, C., Liu, L., Gu, Y., et al. (2022). Adverse effects of dietary virgin (nano)microplastics on growth performance, immune response, and resistance to ammonia stress and pathogen challenge in juvenile sea cucumber Apostichopus japonicus (Selenka). J. Hazard. Mater. 423(Part A):127038. doi: 10.1016/j.jhazmat.2021.127038
Liu, Z., Cai, M., Wu, D., Yu, P., Jiao, Y., Jiang, Q., et al. (2020). Effects of nanoplastics at predicted environmental concentration on Daphnia pulex after exposure through multiple generations. Environ. Pollut. 256:113506. doi: 10.1016/j.envpol.2019.113506
Liu, Z., Yu, P., Cai, M., Wu, D., Zhang, M., Huang, Y., et al. (2019). Polystyrene nanoplastic exposure induces immobilization, reproduction, and stress defense in the freshwater cladoceran Daphnia pulex. Chemosphere 215, 74–81. doi: 10.1016/j.chemosphere.2018.09.176
Long, M., Moriceau, B., Gallinari, M., Lambert, C., Huvet, A., Raffray, J., et al. (2015). Interactions between microplastics and phytoplankton aggregates: impact on their respective fates. Mar. Chem. 175, 39–46. doi: 10.1016/j.marchem.2015.04.003
Lu, Y. F., Zhang, Y., Deng, Y. F., Jiang, W., Zhao, Y. P., Geng, J. J., et al. (2016). Uptake and accumulation of polystyrene microplastics in zebrafish (Danio rerio) and toxic effects in liver. Environ. Sci. Technol. 50, 4054–4060. doi: 10.1021/acs.est.6b00183
Luan, L., Wang, X., Zheng, H., Liu, L., Luo, X., and Li, F. (2019). Differential toxicity of functionalized polystyrene microplastics to clams (Meretrix meretrix) at three key development stages of life history. Mar. Pollut. Bull. 139, 346–354. doi: 10.1016/j.marpolbul.2019.01.003
Ma, Y.-F., and You, X.-Y. (2021). Modelling the accumulation of microplastics through food webs with the example Baiyangdian Lake, China. Sci. Total Environ. 762:144110. doi: 10.1016/j.scitotenv.2020.144110
Macfadyen, G., Huntington, T., and Cappell, R. (2009). Abandoned, Lost or Otherwise Discarded Fishing Gear. UNEP Regional Seas Reports and Studies No.185; FAO Fisheries and Aquaculture Technical Paper, No. 523. Rome: FAO, 115.
Magni, S., Della Torre, C., Garrone, G., D’Amato, A., Parenti, C., and Binelli, A. (2019). First evidence of protein modulation by polystyrene microplastics in a freshwater biological model. Environ. Pollut. 250, 407–415. doi: 10.1016/j.envpol.2019.04.088
Magri, D., Sanchez-Moreno, P., Caputo, G., Gatto, F., Veronesi, M., Bardi, G., et al. (2018). Laser ablation as a versatile tool to mimic polyethylene terephthalate nanoplastic pollutants: char- acterization and toxicology assessment. ACS Nano 12, 7690–7700. doi: 10.1021/acsnano.8b01331
Malafaia, G., de Souza, A. M., Pereira, A. C., Gonçalves, S., da Costa Araújo, A. P., Ribeiro, R. X., et al. (2020). Developmental toxicity in zebrafish exposed to polyethylene microplastics under static and semi-static aquatic systems. Sc. Total Environ. 700:134867. doi: 10.1016/j.scitotenv.2019.134867
Mao, Y., Ai, H., Chen, Y., Zhang, Z., Zeng, P., Kang, L., et al. (2018). Phytoplankton response to polystyrene microplastics: perspective from an entire growth period. Chemosphere 208, 59–68. doi: 10.1016/j.chemosphere.2018.05.170
Maršić-Lučić, J., Lušić, J., Tutman, P., Bojanić Varezić, D., Šiljić, J., and Pribudić, J. (2018). Levels of trace metals on microplastic particles in beach sediments of the island of Vis, Adriatic Sea, Croatia. Mar. Pollut. Bull. 137, 231–236. doi: 10.1016/j.marpolbul.2018.10.027
Mataji, A., Taleshi, M. S., and Balimoghaddas, E. (2020). Distribution and characterization of microplastics in surface waters and the Southern Caspian sea coasts sediments. Arch. Environ. Contam. Toxicol. 78, 86–93. doi: 10.1007/s00244-019-00700-2
Mattsson, K., Ekvall, M. T., Hansson, L. A., Linse, S., Malmendal, A., and Cedervall, T. (2014). Altered behavior, physiology, and metabolism in fish exposed to polystyrene nanoparticles. Environ. Sci. Technol. 49, 553–561. doi: 10.1021/es5053655
Meng, Y., Kelly, F. J., and Wright, S. L. (2020). Advances and challenges of microplastic pollution in freshwater ecosystems: a UK perspective. Environ. Pollut. 256:113445. doi: 10.1016/j.envpol.2019.113445
Miandad, R., Kumar, R., Barakat, M. A., Basheer, C., Aburiazaiza, A. S., Nizami, A. S., et al. (2018). Untapped conversion of plastic waste char into carbon-metal LDOs for the adsorption of Congo red. J. Colloid Interface Sci. 511, 402–410. doi: 10.1016/j.jcis.2017.10.029
Mintenig, S. M., Int-Veen, I., Loder, M. G. J., Primpke, S., and Gerdts, G. (2017). Identification of microplastic in effluents of waste water treatment plants using focal plane array-based micro-Fourier-transform infrared imaging. Water Res. 108, 365–372. doi: 10.1016/j.watres.2016.11.015
Moore, C. J. (2008). Synthetic polymers in the marine environment: a rapidly increasing, long-term threat. Environ. Res. 108, 131–139. doi: 10.1016/j.envres.2008.07.025
Morgana, S., Ghigliotti, L., Estévez-Calvar, N., Stifanese, R., Wieckzorek, A., Doyle, T., et al. (2018). Microplastics in the Arctic: a case study with sub-surface water and fish samples off Northeast Greenland. Environ. Pollut. 275B, 1078–1086. doi: 10.1016/j.envpol.2018.08.001
Müller, A., Österlund, H., Marsalek, J., and Viklander, M. (2020). The pollution conveyed by urban runoff: a review of sources. Sci. Total Environ. 709:136125. doi: 10.1016/j.scitotenv.2019.136125
Naidoo, T., and Glassom, D. (2019). Decreased growth and survival in small juvenile fish, after chronic exposure to environmentally relevant concentrations of microplastic. Mar. Pollut. Bull. 145, 254–259. doi: 10.1016/j.marpolbul.2019.02.037
Nakano, T. (2010). Synthesis, structure, and function of [pi]-stacked polymers. Polym. J. 42, 103–123.
National Geographic Society (2021). Food Web | National Geographic Society. Available online at: https://www.nationalgeographic.org/encyclopedia/food-web/ (accessed on February 16, 2021)
Nel, H. A., Dalu, T., and Wasserman, R. J. (2018). Sinks and sources: assessing microplastic abundance in river sediment and deposit feeders in an austral temperate urban river system. Sci. Total Environ. 612, 950–956. doi: 10.1016/j.scitotenv.2017.08.298
Nelms, S. E., Duncan, E. M., Broderick, A. C., Galloway, T. S., Godfrey, M. H., Hamann, M., et al. (2016). Plastic and marine turtles: a review and call for research. ICES J. Mar. Sci. 73, 165–181. doi: 10.1093/icesjms/fsv165
Nelms, S. E., Galloway, T. S., Godley, B. J., Jarvis, D. S., and Lindeque, P. K. (2018). Investigating microplastic trophic transfer in marine top predators. Environ. Pollut. 238, 999–1007. doi: 10.1016/j.envpol.2018.02.016
Nematollahi, M. J., Moore, F., Keshavarzi, B., Vogt, R. D., Nasrollahzadeh Saravi, H., and Busquets, R. (2020). Microplastic particles in sediments and waters, south of Caspian Sea: frequency, distribution, characteristics, and chemical composition. Ecotoxicol. Environ. Saf. 206:111137. doi: 10.1016/j.ecoenv.2020.111137
Oberbeckmann, S., Löder, M. G. J., and Labrenz, M. (2015). Marine microplastic-associated biofilms; a review. Environ. Chem. 12, 551–562.
Oliveira, M., Ribeiro, A., Hylland, K., and Guilhermino, L. (2013). Single and combined effects of microplastics and pyrene on juveniles (0+ group) of the common goby Pomatoschistus microps (Teleostei, Gobiidae). Ecol. Indic. 34, 641–647.
Ory, N. C., Sobral, P., Ferreira, J. L., and Thiel, M. (2017). Amberstripe scad Decapterus muroadsi (Carangidae) fish ingest blue microplastics resembling their copepod prey along the coast of Rapa Nui (Easter Island) in the South Pacific subtropical gyre. Sci. Total Environ. 586, 430–437. doi: 10.1016/j.scitotenv.2017.01.175
Padervand, M., Lichtfouse, E., Robert, D., and Wang, C. (2020). Removal of microplastics from the environment, a review. Environ. Chem. Lett. 18, 807–828.
Pannetier, P., Morin, B., Le Bihanic, F., Dubreil, L., Clérandeau, C., Chouvellon, F., et al. (2020). Environmental samples of microplastics induce significant toxic effects in fish larvae. Environ. Int. 134:105047. doi: 10.1016/j.envint.2019.105047
Parga, M. L. (2012). Hooks and sea turtles: a veterinarian’s perspective. Bull. Mar. Sci. 88, 731–741.
Paul-Pont, I., Lacroix, C., González Fernández, C., Hégaret, H., Lambert, C., Le Goïc, N., et al. (2016). Exposure of marine mussels Mytilus spp. to polystyrene microplastics: toxicity and influence on fluoranthene bioaccumulation. Environ. Pollut. 216, 724–737. doi: 10.1016/j.envpol.2016.06.039
Pellini, G., Gomiero, A., Fortibuoni, T., Ferr‘a, C., Grati, F., Tassetti, N., et al. (2018). Characterization of microplastic litter in the gastrointestinal tract of Solea solea from the Adriatic Sea. Environ. Pollut. 234, 943–952. doi: 10.1016/j.envpol.2017.12.038
Pico, Y., Alvarez-Ruiz, R., Alfarhan, A. H., El-Sheikh, M. A., Alshahrani, H. O., and Barcelo, D. (2020). Pharmaceuticals, pesticides, personal care products and microplastics contamination assessment of Al-Hassa irrigation network (Saudi Arabia) and its shallow lakes. Sci. Total Environ. 701:135021.
Pitt, J. A., Kozal, J. S., Jayasundara, N., Massarsky, A., Trevisan, R., Geitner, N., et al. (2018a). Uptake, tissue distribution, and toxicity of polystyrene nanoparticles in developing zebrafish (Danio rerio). Aquat. Toxicol. 194, 185–194. doi: 10.1016/j.aquatox.2017.11.017
Pitt, J. A., Trevisan, R., Massarsky, A., Kozal, J. S., Levin, E. D., and Di Giulio, R. T. (2018b). Maternal transfer of nanoplastics to offspring in zebrafish (Danio rerio): a case study with nanopolystyrene. Sci. Total Environ. 643, 324–334. doi: 10.1016/j.scitotenv.2018.06.186
Pittura, L., Avio, C. G., Giuliani, M. E., d’Errico, G., Keiter, S. H., Cormier, B., et al. (2018). Microplastics as vehicles of environmental PAHs to marine organisms: combined chemical and physical hazards to the Mediterranean mussels’, Mytilus galloprovincialis. Front. Mar. Sci. 5:103. doi: 10.3389/fmars.2018.00103
Porter, A., Lyons, B. P., Galloway, T. S., and Lewis, C. (2018). Role of marine snows in microplastic fate and bioavailability. Environ. Sci. Technol. 52, 7111–7119. doi: 10.1021/acs.est.8b01000
Pozo, K., Gomez, V., Torres, M., Vera, L., Nuñez, D., Oyarzún, P., et al. (2019). Presence and characterization of microplastics in fish of commercial importance from the Biobío region in Central Chile. Mar. Pollut. Bull. 140, 315–319. doi: 10.1016/j.marpolbul.2019.01.025
Prata, J. C., Lavorante, B. R. B. O., Montenegro, M. D. B. S. M., and Guilhermino, L. (2018). Influence of microplastics on the toxicity of the pharmaceuticals procainamide and doxycycline on the marine microalgae Tetraselmis chuii. Aquat. Toxicol. 197, 143–152. doi: 10.1016/j.aquatox.2018.02.015
Prokić, M. D., Radovanović, T. B., Gavrić, J. P., and Faggio, C. (2019). Ecotoxicological effects of microplastics: examination of biomarkers, current state and future perspectives. Trends Anal. Chem. 111, 37–46. doi: 10.1016/j.trac.2018.12.001
Qiao, R., Lu, K., Deng, Y., Ren, H., and Zhang, Y. (2019a). Combined effects of polystyrene microplastics and natural organic matter on the accumulation and toxicity of copper in zebrafish. Sci. Total Environ. 682, 128–137. doi: 10.1016/j.scitotenv.2019.05.163
Qiao, R., Deng, Y., Zhang, S., Wolosker, M. B., Zhu, Q., Ren, H., et al. (2019b). Accumulation of different shapes of microplastics initiates intestinal injury and gut microbiota dysbiosis in the gut of zebrafish. Chemosphere 236:124334. doi: 10.1016/j.chemosphere.2019.07.065
Quinn, B., Murphy, F., and Ewins, C. (2017). Analytical methods validation of density separation for the rapid recovery of microplastics from sediment. Anal. Methods 9, 1491–1498. doi: 10.1039/c6ay02542k
Rehse, S., Kloas, W., and Zarfl, C. (2016). Short-term exposure with high concentrations of pristine microplastic particles leads to immobilisation of Daphnia magna. Chemosphere 153, 91–99. doi: 10.1016/j.chemosphere.2016.02.133
Renzi, M., Blavskovi ìc, A., Bernardi, G., and Russo, G. F. (2018). Plastic litter transfer from sediments towards marine trophic webs: a case study on holothurians. Mar. Pollut. Bull. 135, 376–385. doi: 10.1016/j.marpolbul.2018.07.038
Revel, M., Lagarde, F., Perrein-Ettajani, H., Bruneau, M., Akcha, F., Sussarellu, R., et al. (2019). Tissue-specific biomarker responses in the blue mussel Mytilus spp. exposed to a mixture of microplastics at environmentally relevant concentrations. Front. Environ. Sci. 7:33. doi: 10.3389/fenvs.2019.00033
Ribeiro, F., O’Brien, J. W., Galloway, T., and Thomas, K. V. (2019). Accumulation and fate of nano- and micro-plastics and associated contaminants in organisms. Trends Anal. Chem. 111, 139–147. doi: 10.1016/j.chemosphere.2020.127344
Ribeiro, F., Garcia, A. R., Pereira, B. P., Fonseca, M., Mestre, N. C., Fonseca, T. G., et al. (2017). Microplastics effects in Scrobicularia plana. Mar. Pollut. Bull. 122, 379–391. doi: 10.1016/j.marpolbul.2017.06.078
Rist, S. E., Assidqi, K., Zamani, N. P., Appel, D., Perschke, M., Huhn, M., et al. (2016). Suspended micro-sized PVC particles impair the performance and decrease survival in the Asian green mussel Perna viridis. Mar. Pollut. Bull. 111, 213–220. doi: 10.1016/j.marpolbul.2016.07.006
Robin, R. S., Karthik, R., Purvaja, R., Ganguly, D., Anandavelu, I., Mugilarasan, M., et al. (2020). Holistic assessment of microplastics in various coastal environmental matrices, southwest coast of India. Sci. Total Environ. 703, 134–147. doi: 10.1016/j.scitotenv.2019.134947
Rochman, C. M., Brookson, C., Bikker, J., Djuric, N., Earn, A., Bucci, K., et al. (2019). Rethinking microplastics as a diverse contaminant suite. Environ. Toxicol. Chem. 38, 703–711. doi: 10.1002/etc.4371
Rochman, C. M., Kurobe, T., Flores, I., and Teh, S. J. (2014). Early warning signs of endocrine disruption in adult fish from the ingestion of polyethylene with and without sorbed chemical pollutants from the marine environment. Sci. Total Environ. 493, 656–661. doi: 10.1016/j.scitotenv.2014.06.051
Rochman, C. M., Hoh, E., Kurobe, T., and Teh, S. J. (2013). Ingested plastic transfers hazardous chemicals to fish and induces hepatic stress. Sci. Rep. 3:3263. doi: 10.1038/srep03263
Rodrigues, J. P., Duarte, A. C., Santos-Echeandía, J., and Rocha-Santos, T. (2019). Significance of interactions between microplastics and POPs in the marine environment: a critical overview. Trends Anal. Chem. 111, 252–260. doi: 10.1016/j.trac.2018.11.038
Rodrigues, M. O., Abrantes, N., Gonçalves, F. J. M., Nogueira, H., Marques, J. C., and Gonçalves, A. M. M. (2018). Spatial and temporal distribution of microplastics in water and sediments of a freshwater system (Antuã River, Portugal). Sci. Total Environ. 633, 1549–1559. doi: 10.1016/j.scitotenv.2018.03.233
Rubio, L., Marcos, R., and Hernandez, A. (2020). Potential adverse health effects of ingested micro- and nanoplastics on humans. lessons learned from in vivo and in vitro mammalian models. J. Toxicol. Environ. Health B Crit. Rev. 23, 51–68. doi: 10.1080/10937404.2019.1700598
Sadri, S. S., and Thompson, R. C. (2014). On the quantity and composition of floating plastic debris entering and leaving the Tamar Estuary, Southwest England. Mar. Pollut. Bull. 81, 55–60. doi: 10.1016/j.marpolbul.2014.02.020
Saeed, T., Al-Jandal, N., Al-Mutairi, A., and Taqi, H. (2020). Microplastics in Kuwait marine environment: results of first survey. Mar. Pollut. Bull. 152:110880. doi: 10.1016/j.marpolbul.2019.110880
Sanchez, W., Bender, C., and Porcher, J. M. (2014). Wild gudgeons (Gobio gobio) from French rivers are contaminated by microplastics: preliminary study and first evidence. Environ. Res. 128, 98–100. doi: 10.1016/j.envres.2013.11.004
Savoca, S., Capillo, G., Mancuso, M., Bottari, T., Crupi, R., Branca, C., et al. (2019). Microplastics occurrence in the Tyrrhenian waters and in the gastrointestinal tract of two congener species of seabreams. Environ. Toxicol. Pharmacol. 67, 35–41. doi: 10.1016/j.etap.2019.01.011
Schymanski, D., Goldbeck, C., Humpf, H.-U., and Fürst, P. (2018). Analysis of microplastics in water by micro-Raman spectroscopy: release of plastic particles from different packaging into mineral water. Water Res. 129, 154–162. doi: 10.1016/j.watres.2017.11.011
Schuyler, Q., Hardesty, B. D., Wilcox, C., and Townsend, K. (2014). Global analysis of anthropogenic debris ingestion by sea turtles. Conserv. Biol. 28, 129–139. doi: 10.1111/cobi.12126
Selvam, S., Manisha, A., Venkatramanan, S., Chung, S. Y., Paramasivam, C. R., and Singaraja, C. (2020). Microplastic presence in commercial marine sea salts: a baseline study along Tuticorin Coastal salt pan stations, Gulf of Mannar, South India. Mar. Pollut. Bull. 150:110675. doi: 10.1016/j.marpolbul.2019.110675
Sendra, M., Saco, A., Yeste, M. P., Romero, A., Novoa, B., and Figueras, A. (2020). Nanoplastics: from tissue accumulation to cell translocation into Mytilus galloprovincialis hemocytes. Resilience of immune cells exposed to nanoplastics and nanoplastics plus Vibrio splendidus combination. J. Hazard. Mater. 388:121788. doi: 10.1016/j.jhazmat.2019.121788
Setälä, O., Fleming-Lehtinen, V., and Lehtiniemi, M. (2014). Ingestion and transfer of microplastics in the planktonic food web. Environ. Pollut. 185, 77–83. doi: 10.1016/j.envpol.2013.10.013
Setälä, O., Magnusson, K., Lehtiniemi, M., and Norén, F. (2016). Distribution and abundance of surface water microlitter in the Baltic Sea: a comparison of two sampling methods. Mar. Pollut. Bull. 110, 177–183. doi: 10.1016/j.marpolbul.2016.06.065
Scherer, C., Weber, A., and Lambert, S. (2018). “Interactions of microplastics with freshwater biota,” in Freshwater Microplastics. The Handbook of Environmental Chemistry, eds M. Wagner and S. Lambert (Cham: Springer), doi: 10.1007/978-3-319-61615-5_13
Sharma, S., and Chatterjee, S. (2017). Microplastic pollution, a threat to marine ecosystem and human health: a short review. Environ. Sci. Pollut. Res. Int. 27, 21530–21547. doi: 10.1007/s11356-017-9910-8
Shaw, D. G., and Day, R. H. (1994). Colour- and form-dependent loss of plastic micro-debris from the North Pacific Ocean. Mar. Pollut. Bull. 28, 39–43. doi: 10.1016/0025-326X(94)90184-8
Shi, J., Sanganyado, E., Wang, L., Li, P., Li, X., and Liu, W. (2020). Organic pollutants in sedimentary microplastics from eastern Guangdong: spatial distribution and source identification. Ecotoxicol. Environ. Saf. 193:110356. doi: 10.1016/j.ecoenv.2020.110356
Sökmen, T. O., Sulukan, E., Türkoğlu, M., Baran, A., Özkaraca, M., and Ceyhun, S. B. (2020). Polystyrene nanoplastics (20 nm) are able to bioaccumulate and cause oxidative DNA damages in the brain tissue of zebrafish embryo (Danio rerio). Neurotoxicology 77, 51–59. doi: 10.1016/j.neuro.2019.12.010
Steer, M., Cole, M., Thompson, R. C., and Lindeque, P. K. (2017). Microplastic ingestion in fish larvae in the western English Channel. Environ. Pollut. 226, 250–259. doi: 10.1016/j.envpol.2017.03.062
Stelfox, M., Balson, D., and Hudgins, J. (2014). Olive ridley project: actively fighting ghost nets in the Indian Ocean. Hum. Organ. 69, 375–386.
Suman, T. Y., Jia, P.-P., Li, W.-G., Junaid, M., Xina, G.-Y., Wang, Y., et al. (2020). Acute and chronic effects of polystyrene microplastics on brine shrimp: first evidence highlighting the molecular mechanism through transcriptome analysis. J. Hazard. Mater. 400:123220. doi: 10.1016/j.jhazmat.2020.123220
Sussarellu, R., Suquet, M., Thomas, Y., Lambert, C., Fabioux, C., Pernet, M. E. J., et al. (2016). Oyster reproduction is affected by exposure to polystyrene microplastics. Proc. Natl. Acad. Sci. U.S.A. 113, 2430–2435. doi: 10.1073/pnas.1519019113
Sweeney, M. F., Hasan, N., Soto, A. M., and Sonnenschein, C. (2015). Environmental endocrine disruptors: effects on the human male reproductive system. Rev. Endocr. Metab. Disord. 16, 341–357. doi: 10.1007/s11154-016-9337-4
Tan, X., Yu, X., Cai, L., Wang, J., and Peng, J. (2019). Microplastics and associated PAHs in surface water from the Feilaixia Reservoir in the Beijiang River, China. Chemosphere 221, 834–840. doi: 10.1016/j.chemosphere.2019.01.022
Tanaka, K., Takada, H., Yamashita, R., Mizukawa, K., Fukuwaka, M. A., Watanuki, Y., et al. (2013). Accumulation of plastic-derived chemicals in tissues of seabirds ingesting marine plastics. Mar. Pollut. Bull. 69, 219–222. doi: 10.1016/j.marpolbul.2012.12.010
Thouvenot, A., Richardot, M., Debroas, D., and Devaux, J. (1999). Bacterivory of metazooplankton, ciliates and flagellates in a newly flooded reservoir. J. Plankton Res. 21, 1659–1679.
Tongo, I., and Erhunmwunse, N. O. (2022). Effects of ingestion of polyethylene microplastics on survival rate, opercular respiration rate and swimming performance of African catfish (Clarias gariepinus). J. Hazard. Mater. 423(Part B):127237. doi: 10.1016/j.jhazmat.2021.127237
Torres, G. F., Dioses-Salinas, D. C., Pizarro-Ortega, C. I., and De-la-Torre, G. E. (2021). Sorption of chemical contaminants on degradable and non-degradable microplastics: recent progress and research trends. Sci. Total Environ. 757:143875. doi: 10.1016/j.scitotenv.2020.143875
Turan, N. B., Erkan, H. S., and Engin, G. O. (2021). Microplastics in wastewater treatment plants: occurrence, fate and identification. Process Saf. Environ. Protect. 146, 77–84.
Turner, A., and Holmes, L. A. (2015). Adsorption of trace metals by microplastic pellets in fresh water. Environ. Chem. 12, 600–610. doi: 10.1071/EN14143
van Cauwenberghe, L., Claessens, M., Vandegehuchte, M. B., and Janssen, C. R. (2015). Microplastics are taken up by mussels (Mytilus edulis) and lugworms (Arenicola marina) living in natural habitats. Environ. Pollut. 199, 10–17. doi: 10.1016/j.envpol.2015.01.008
van der Hal, N., Yeruham, E., Shukis, D., Rilov, G., Astrahan, P., and Angel, D. L. (2020). Uptake and incorporation of PCBs by eastern Mediterranean rabbitfish that consumed microplastics. Mar. Pollut. Bull. 150:110697. doi: 10.1016/j.marpolbul.2019.110697
van Sebille, E., Wilcox, C., Lebreton, L., Maximenko, N., Hardesty, B. D., Van Franeker, J. A., et al. (2015). A global inventory of small floating plastic debris. Environ. Res. Lett. 10:124006. doi: 10.1088/1748-9326/10/12/124006
Vegter, A., Barletta, M., Beck, C., Borrero, J., Burton, H., Campbell, M., et al. (2014). Global research priorities to mitigate plastic pollution impacts on marine wildlife. Endanger. Species Res. 25, 225–247.
Veneman, W. J., Spaink, H. P., Brun, N. R., Bosker, T., and Vijver, M. G. (2017). Pathway analysis of systemic transcriptome responses to injected polystyrene particles in zebrafish larvae. Aquat. Toxicol. 190, 112–120. doi: 10.1016/j.aquatox.2017.06.014
von Moos, N., Burkhardt-Holm, P., and Köhler, A. (2012). Uptake and effects of microplastics on cells and tissue of the blue mussel Mytilus edulis L. after an experimental exposure. Environ. Sci. Technol. 46, 11327–11335. doi: 10.1021/es302332w
Waller, C. L., Griffiths, H. J., Waluda, C. M., Thorpe, S. E., Loaiza, T., Moreno, B., et al. (2017). Microplastics in the Antarctic marine system: an emerging area of research. Sci. Total Environ. 598, 220–227. doi: 10.1016/j.scitotenv.2017.03.283
Walkinshaw, C., Lindeque, P. K., Thompson, R., Tolhurst, T., and Cole, M. (2020). Microplastics and seafood: lower trophic organisms at highest risk of contamination. Ecotox. Environ. Saf. 190:110066. doi: 10.1016/j.ecoenv.2019.110066
Watts, A. J., Lewis, C., Goodhead, R. M., Beckett, S. J., Moger, J., Tyler, C. R., et al. (2014). Uptake and retention of microplastics by the shore crab Carcinus maenas. Environ. Sci. Technol. 48, 8823–8830. doi: 10.1021/es501090e
Wilcox, C., Heathcote, G., Goldberg, J., Gunn, R., Peel, D., and Hardesty, B. D. (2014). Understanding the sources and effects of abandoned, lost, and discarded fishing gear on marine turtles in northern Australia. Conserv. Biol. 29, 198–206. doi: 10.1111/cobi.12355
Wilson, D. R., Godley, B. J., Haggar, G. L., Santillo, D., and Sheen, K. L. (2021). The influence of depositional environment on the abundance of microplastic pollution on beaches in the Bristol Channel, UK. Mar. Pollut. Bull. 164:111997. doi: 10.1016/j.marpolbul.2021.111997
Wong, S. L., Nyakuma, B. B., Wong, K. Y., Lee, C. T., Lee, T. H., and Lee, C. H. (2020). Microplastics and nanoplastics in global food webs: a bibliometric analysis (2009–2019). Mar. Pollut. Bull. 158:111432. doi: 10.1016/j.marpolbul.2020.111432
Wright, S. L., Thompson, R. C., and Galloway, T. S. (2013a). The physical impacts of microplastics on marine organisms: a review. Environ. Pollut. 178, 483–492. doi: 10.1016/j.envpol.2013.02.031
Wright, S. L., Rowe, D., Thompson, R. C., and Galloway, T. S. (2013b). Microplastic ingestion decreases energy reserves in marine worms. Curr. Biol. 23, R1031–R1033. doi: 10.1016/j.cub.2013.10.068
Wu, P., Huang, J., Zheng, Y., Yang, Y., Zhang, Y., He, F., et al. (2019). Environmental occurrences, fate, and impacts of microplastics. Ecotoxicol. Environ. Saf. 184:109612. doi: 10.1016/j.ecoenv.2019.109612
Xia, X., Sun, M., Zhou, M., Chang, Z., and Li, L. (2020). Polyvinyl chloride microplastics induce growth inhibition and oxidative stress in Cyprinus carpio var. larvae. Sci. Total Environ. 716:136479. doi: 10.1016/j.scitotenv.2019.136479
Xiang, Y., Jiang, L., Zhou, Y., Luo, Z., Zhi, D., Yang, J., et al. (2021). Microplastics and environmental pollutants: key interaction and toxicology in aquatic and soil environments. J. Hazard. Mater. 422:126843. doi: 10.1016/j.jhazmat.2021.126843
Yu, P., Liu, Z., Wu, D., Chen, M., Lv, W., and Zhao, Y. (2018). Accumulation of polystyrene microplastics in juvenile Eriocheir sinensis and oxidative stress effects in the liver. Aquat. Toxicol. 200, 28–36. doi: 10.1016/j.aquatox.2018.04.015
Zazouli, M., Nejati, H., Hashempour, Y., Dehbandi, R., Nam, V. T., and Fakhri, Y. (2022). Occurrence of microplastics (MPs) in the gastrointestinal tract of fishes: a global systematic review and meta-analysis and meta-regression. Sci. Total Environ. 815:152743. doi: 10.1016/j.scitotenv.2021.152743
Zhang, B., Chao, J., Chen, L., Liu, L., Yang, X., and Wang, Q. (2022). Research progress of nanoplastics in freshwater. Sci. Total Environ. 757:143791. doi: 10.1016/j.scitotenv.2020.143791
Zhang, C., Chen, X., Wang, J., and Tan, L. (2017). Toxic effects of microplastics on marine microalgae Skeletonema costatum: interactions between microplastics and algae. Environ. Pollut. 220, 1282–1288. doi: 10.1016/j.envpol.2016.11.005
Zhang, X., Chen, J., and Li, J. (2020a). The removal of microplastics in the wastewater treatment process and their potential impact on anaerobic digestion due to pollutants association. Chemosphere 251:126360. doi: 10.1016/j.chemosphere.2020.126360
Zhang, R., Wang, M., Chen, X., Yang, C., and Wu, L. (2020b). Combined toxicity of microplastics and cadmium on the zebrafish embryos (Danio rerio). Sci. Total Environ. 743:140638. doi: 10.1016/j.scitotenv.2020.140638
Zhang, R., Silic, M. R., Schaber, A., Wasel, O., Freeman, J. L., and Sepúlveda, M. S. (2020c). Exposure route affects the distribution and toxicity of polystyrene nanoplastics in zebrafish. Sci. Total Environ. 724:138065. doi: 10.1016/j.scitotenv.2020.138065
Zheng, Y., Li, J., Cao, W., Liu, X., Jiang, F., Ding, J., et al. (2019). Distribution characteristics of microplastics in the seawater and sediment: a case study in Jiaozhou Bay, China. Sci. Total Environ. 674, 27–35. doi: 10.1016/j.scitotenv.2019.04.008
Zhu, H., Fu, S., Zou, H., Su, Y., and Zhang, Y. (2021). Effects of nanoplastics on microalgae and their trophic transfer along the food chain: recent advances and perspectives. Environ. Sci. Process. Impacts 23, 1873–1883.
Keywords: microplastics, nanoplastics, emerging contaminants, food chain, trophic transfer, ecotoxicological effects
Citation: Benson NU, Agboola OD, Fred-Ahmadu OH, De-la-Torre GE, Oluwalana A and Williams AB (2022) Micro(nano)plastics Prevalence, Food Web Interactions, and Toxicity Assessment in Aquatic Organisms: A Review. Front. Mar. Sci. 9:851281. doi: 10.3389/fmars.2022.851281
Received: 09 January 2022; Accepted: 14 February 2022;
Published: 09 March 2022.
Edited by:
Lingzhan Miao, Hohai University, ChinaCopyright © 2022 Benson, Agboola, Fred-Ahmadu, De-la-Torre, Oluwalana and Williams. This is an open-access article distributed under the terms of the Creative Commons Attribution License (CC BY). The use, distribution or reproduction in other forums is permitted, provided the original author(s) and the copyright owner(s) are credited and that the original publication in this journal is cited, in accordance with accepted academic practice. No use, distribution or reproduction is permitted which does not comply with these terms.
*Correspondence: Nsikak U. Benson, bmJlbnNvbkBjb3ZlbmFudHVuaXZlcnNpdHkuZWR1Lm5n
Disclaimer: All claims expressed in this article are solely those of the authors and do not necessarily represent those of their affiliated organizations, or those of the publisher, the editors and the reviewers. Any product that may be evaluated in this article or claim that may be made by its manufacturer is not guaranteed or endorsed by the publisher.
Research integrity at Frontiers
Learn more about the work of our research integrity team to safeguard the quality of each article we publish.