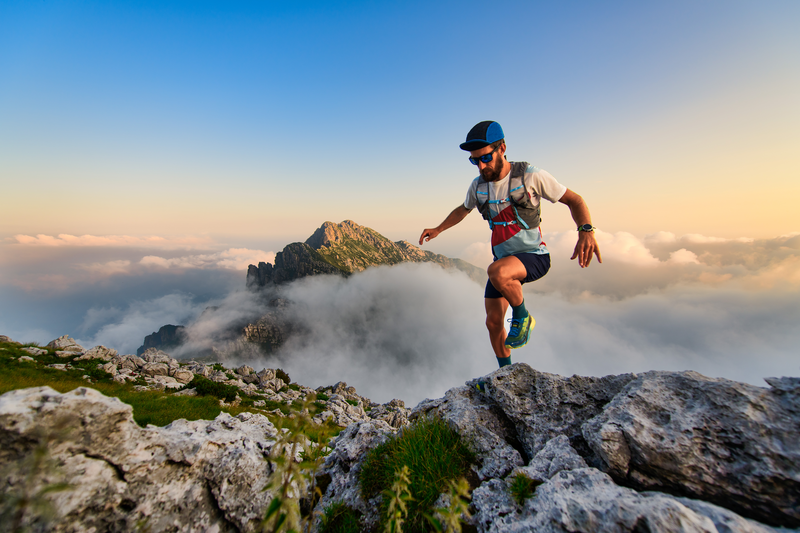
94% of researchers rate our articles as excellent or good
Learn more about the work of our research integrity team to safeguard the quality of each article we publish.
Find out more
ORIGINAL RESEARCH article
Front. Mar. Sci. , 15 September 2022
Sec. Marine Fisheries, Aquaculture and Living Resources
Volume 9 - 2022 | https://doi.org/10.3389/fmars.2022.844158
Assessing the effects of fishing activity and environmental variability on fishing species might help to understand their fluctuations and to manage them accordingly. However, despite the increasing number of studies linking fisheries and environmental data, few had a multi-species approach considering flatfish species and different age groups. Therefore, this study aims to determine the effects of environmental and fishing related variables on commercial flatfish species across different regions of the Portuguese coast. For that, sea surface temperature, North Atlantic Oscillation, upwelling index, wind components, river discharges and fishing effort were related to landings per unit effort (LPUE) of five commercial flatfish species (Pleuronectes platessa, Dicologlossa cuneata, Microchirus spp., Solea lascaris, and Solea solea) fished by the artisanal fleet along different regions of Portuguese coast (Northwestern coast, Southwestern coast, and Southern coast), using a multi-model statistical approach from 1989 to 2009. The fishing effort had the same effect along the life cycle and was negatively related with LPUE trends for most of the species. Regarding to environmental variability, during early phases (larval to juvenile – age-group-0) the factors that affected the recruitment, in addition to being related to the physiological needs (e.g., sea surface temperature) of individuals that will trigger growth and survival, were related to larval transport and dispersion from offshore spawning areas to coastal nursery habitats beyond (e.g., wind components and upwelling). For juvenile and young-adult phases (age-groups I and II), the effects of environmental variability appeared to be linked to physiological needs important for growth and maturation (e.g., temperature). Concluding, different effects were observed depending on the region, species, and life-cycle phase. Therefore, these environmental-fishing relationships should be used when managing local fishing resources.
The variability of fishing stocks and their abundance is one of the main topics of research in fisheries oceanography (e.g., Hjort, 1914; Lehodey et al., 2006). There are several hypotheses that attempt to explain environmental-fisheries recruitment relationships. The classic “critical period” hypothesis suggested by Hjort (1914) pointed out that changes in stock size depend on the strength of the species year-class, determined during early life stages (eggs, larvae, and juveniles) by environmental conditions and food availability. The “match-mismatch” hypothesis proposed by Cushing (1990), indicated that medium-term influences of environmental mechanisms affect larval survival and thereby, inter-annual variability in fish recruitment success. This author highlighted the importance of the matching time of the spawning period and the start of the spring production cycle in coastal areas. Bakun’s triad hypothesis (Bakun, 1996) is an attempt to classify the three main mechanisms favouring early life stages recruitment: i) enrichment (e.g., upwelling events and mixing of waters layers); ii) concentration (e.g., convergence and fronts) and iii) processes favouring the retention within (or toward) an appropriate habitat might improve the chances of larvae stages to reduce their natural mortality and successfully recruit in the fishing stock. In summary, the vulnerability at early life stages crucially determines the recruitment success and consequently, size and structure of fishing stocks.
Currently, in addition to fishing exploitation (Pauly et al., 2002), it is largely recognized that environmental variability can play an important role in fish stock abundance. Climatic and oceanographic variability induces changes on fishing stocks in different ways, at different life-phases (Lloret et al., 2001; Lehodey et al., 2006; Ullah et al., 2012; Leitão et al., 2014; Teixeira et al., 2015) and differently along wide geographic scales (Ravier and Fromentin, 2001). Temperature, salinity, upwelling, winds, tidal currents and oceanic circulation, precipitation and river runoff and nutrients affect productivity, abundance, and distribution of fishing stocks (e.g., Santos et al., 2001; Borges et al., 2003; Erzini, 2005; Lehodey et al., 2006; Brander, 2007; Brander, 2010; Baptista and Leitão, 2014; Baptista et al., 2016; Bueno-Pardo et al., 2020). The interaction of fishing activity and climatic variability can act on abundance and distribution of exploited species (direct effects), and affect the productivity, structure and composition of marine ecosystems (indirect effects) (Lehodey et al., 2006; Brander, 2007; Brander, 2010). Thus, the environmental changes may reduce, or improve, the productivity of fisheries stocks (Brander, 2007; Brander, 2010).
Coastal fishing activity occurs along the Portuguese coast, a transition area dividing cooler temperate waters in the north Europe and warmer subtropical waters in the South. Thus, the Portuguese coast presents distinct oceanographic and climatic regimes (Cunha, 2001; Bettencourt et al., 2004) due to its irregular coastal geography (Mason et al., 2005). Moreover, fishing activity can highly vary from one region to another because of the habitat distribution of commercial fishing stocks and dissimilarities in the technological resources (Sousa et al., 2005; Duarte et al., 2009; Bueno-Pardo et al., 2017). Also, a variation in species distribution has been observed in this region due to climatic changes, with the probability of sub-tropical species increasing in the Iberian Peninsula in the next coming decades (Vinagre et al., 2009; Teixeira et al., 2014), namely in South of Portugal (Leitão et al., 2018; Encarnação et al., 2019). In this context, the evaluation of combined/synchronic fisheries and environmental effects at a local scale (Lloret et al., 2001; Ullah et al., 2012) can result in a more reliable assessment of the specific processes affecting resources. Several authors suggested that regional analyses rather than meta-analyses (e.g., Teixeira and Cabral, 2009; Ullah et al., 2012; Baptista et al., 2014; Leitão et al., 2014) can improve fisheries stock assessment for management purposes (Leitão et al., 2014). Studies on Portuguese fisheries time-series data have linked the environmental and fishing effects on commercial fishing stocks during larval development and recruitment such as those including pelagic species (Santos et al., 2001; Ullah et al., 2012; Leitão et al., 2014), bivalves (Baptista and Leitão, 2014; Baptista et al., 2014), seabreams (Baptista et al., 2016; Leitão et al., 2016), crustaceans (Herraiz et al., 2009) and cephalopods (Ullah et al., 2012), but few of them had a multi-species approach (e.g. Erzini, 2005; Teixeira and Cabral, 2009; Bueno-Pardo et al., 2020).
The polyvalent fleet is one of the largest fishing segments in the Portuguese mainland fisheries, accounting for more than 40% of the total fish landed and employing more than 70% of the fisher’s population (INE, 2013). Characterized by a wide range of passive gears (gill and trammel nets, lines and hooks, traps, and pots), the polyvalent fleet harvests on a diverse range of coastal species (mixed species), switching between fishing techniques depending on the depth, habitat, and season requirements (Teixeira et al., 2011). This multi-gear fleet is responsible for most of the flatfish landings, mainly by means of passive fishing gears such as gill nets and trammel nets. Although in minor quantity, bottom trawling also exploits this group of species. Flatfish commercial species comprises a relatively small proportion of total landings (less than 4%) but due to their high market value, they represent more than 11% of the landings’ economic value (Teixeira and Cabral, 2009).
The flatfish species (Pleuronectiformes order) are widely distributed over the Portuguese coast (Cabral, 2002), inhabiting marine, estuarine, and freshwater environments (Pauly, 1994; Gibson et al., 2014). Flatfishes perform a life-history strategy in which the larvae adopt a pelagic lifestyle, and the adults are benthonic (Ramos et al., 2010). The “migration triangle” hypothesis, from Harden Jones (1968), describes the general life-history strategy of these species: i) spawning occurs in offshore marine waters; ii) in young early stages, flatfishes are transported towards the coast where they settle in shallow nursery habitats such as estuaries or coastal lagoons; iii) then, as juvenile mature they move to the adult habitat (Gibson, 1997; Ramos et al., 2009). Migration patterns vary among flatfish species depending on specific ontogenetic and biological features such as age and size at metamorphosis, spawning period, larval behaviour, and environmental conditions (Minami and Tanaka, 1992; Sogard et al., 2001; Bailey et al., 2005). Therefore, recruitment strength in the fishing stocks has been recognized to be widely determined by the migration, settlement, and survival of larvae on an appropriate nursery habitat (Bell et al., 2001; Warlen et al., 2002; Garcia et al., 2003; Ramos et al., 2010). In flatfish species, the recruitment strength has been linked to the hydrodynamic conditions found during pelagic larval phase (Nielsen et al., 1998), freshwater inputs in estuarine nursery grounds (Le Pape et al., 2003), and density-dependent mechanisms affecting food supply and predation pressure (Cowan et al., 2000; Nash and Geffen, 2000), all of them known to vary among geographical areas (van der Veer et al., 2000).
Considering all these premises, the goal of this study is to determine the effects of environmental and fishing variables on some of the most important commercial flatfish species across different regions of the Portuguese coast (Northwestern coast- IXaCN, Southwestern coast- IXaCS, and Southern coast- IXaS) and along larval (age-group 0) and juvenile/young-adults (age-groups I and II) phases. Understanding the temporal variability in catch rates can provide important information to improve species ecological knowledge and local fisheries management.
The influences of environmental and fishing variables on the Portuguese artisanal flatfish fishery were evaluated in three regions along the Portuguese coast. In its extension of ca. 800 km on the west coast and ca. 200 km on the south coast, the Portuguese coast includes regions with distinct oceanographic, climatic and environmental regimes, such as the Iberian Poleward Current, the Western Iberia Buoyant Plume (river effects), bathymetry, wind regimes and upwelling filaments (Cunha, 2001; Bettencourt et al., 2004; Santos et al., 2007). Also, the Portuguese coast is located in a biogeographical transition zone between temperate and subtropical waters: the Northwestern and the Southwestern coasts represent the subtropical Eastern boundaries of the North Atlantic Ocean (Santos et al., 2001) and the Southern coast which shares characteristics of the Atlantic Ocean and the more saline and warm waters of the Mediterranean Sea (Cunha, 2001). These differences oceanographic environmental and climatic regimes contribute to different fishing regimes across regions (Duarte et al., 2009). According to the International Council of the Exploration of the Sea (ICES), the Portuguese mainland coastal zone is inside the IXa subdivision area, and thus, these areas can be designated as Northwestern coast (IXaCN), Southwestern coast (IXaCS) and Southern coast (IXaS) (Figure 1).
Figure 1 Location of the main fishing ports and freshwater inputs of Portuguese coast divided according to the ICES (International Council of the Exploration of the Sea) subdivisions: Northwest (IXaCN- Central North), Southwest (IXaCS- Central South) and South (IXaS- South).
Flatfish (Pleuronectiformes order) fishing data (fishing effort and landings) from the artisanal multi-gear fleet in Portugal were obtained from Direção Geral de Recursos Naturais, Segurança e Serviços Marítimos (DGRM) for the period 1989-2009. A total of five taxa were studied considering their commercial importance: European plaice Pleuronectes platessa Linnaeus, 1785, wedge sole Dicologlossa cuneata (Moreau, 1881), bastard sole Microchirus spp. (Bonaparte, 1833), sand sole Solea lascaris (Risso, 1810), and common sole Solea solea (Linnaeus, 1785). Yearly Landings per Unit Effort (LPUE) were estimated by dividing the annual landings (in kg) by Fishing Effort (FE: fishing days by boat). Due to their commercial value, flatfishes are not considered as discard in these multispecies fisheries and thus, in this study, we considered that landings of flatfish are directly proportional to catches.
In the present study, environmental variable included oceanic, hydrologic and climatic indices. Oceanographic variables included annual and seasonal sea surface temperature (SST; °C), annual and seasonal upwelling index (UPW), and annual and seasonal geostrophic wind data (“u” and “v” wind components). Sea surface temperature data was obtained from Modis-Aqua 4 km satellite imagery available from the NASA Ocean Colour Giovanni website (http://gdata1.sci.gsfc.nasa.gov). The monthly upwelling index was obtained from the Pacific Fisheries and Environmental Laboratory website (www.pfeg.noaa.gov) using port position as input coordinate for data acquisition. Then upwelling port data were averaged to estimate each fishing area average upwelling intensity. Geostrophic wind data (“u” and “v” wind components) was obtained from NASA Jet Propulsion Laboratory California Institute of Technology that comprises high-resolution (0.25 degree) gridded datasets – Physical Oceanography Distributed Active Archive Center – PODAAC (http://podaac.jpl.nasa.gov/dataset/CCMP_MEASURES_ATLAS_L4_OW_L3_5A_MONTHLY_WIND_VECTORS_FLK?ids=&values=) (Atlas et al., 2011). The “u” component (UWIND; m.s-1) represents the east-west winds whereas the “v” component (VWIND; m.s-1) represents the north-south winds. Wind magnitude (WMAG; m s-1) was calculated using “u” and “v” wind components (Baptista et al., 2014):
The annual and seasonal river discharges (RD; dam3) were used as hydrological variables to test the influence of freshwater inputs on flatfish LPUE. Monthly river discharge data were obtained for the period from January to December, for the main Portuguese rivers or basins (Figure 1) including: (i) Cávado, Lima, Douro, Vouga and Mondego for the IXaCN; (ii) Tejo, Sado and Mira for the IXaCS; and (iii) rivers Guadiana, Seco, Rio Arado, Ribeira de Quarteira, Alportel and Almargem for the IXaS. River discharge data were cumulatively grouped in seasons, and the yearly value was estimated by the sum volume contribution of the set of seasons. Monthly flow measurement data from hydrological stations located in the immediacy of the mouth of these rivers were obtained from the Agência Nacional do Ambiente (APA online database, SNIRH: http://snirh.pt/).
All the oceanographic and hydrological variables (SST, UPW, UWIND, VWIND and WMAG) were averaged for each geographical area of the Portuguese coast (Northwest- IXaCN, Southwest- IXaCS, and South- IXaS; Figure 1). For the oceanography raster datasets, the procedure to get tabular data for analyses was the following. The inland port position was extended 12 miles offshore to match with the territorial sea area where most fleet activity is conducted. Then a buffer of 16km were made in each offshore port position. Geospatial operations tools allowed to extract pixel data from raster monthly data sets for each port area vector (area shapefile format). The zonal means values of each oceanographic variable by port were posteriorly averaged by fishing area. Northwest area ranges from Minho at 42° N to Cabo Carvoeiro at 39°21′32″ N, Southwest area from Cabo Carvoeiro to Sagres at 36°59′35″ and South from Sagres at 8°56′54″ W to Vila Real de Santo António at 6° W.
Finally, the yearly and winter North Atlantic Oscillation (NAO) index was also used as a climatic variable (http://www.cgd.ucar.edu/jhurrell/nao.html, last accessed 2010; Hurrell, 1995). Since NAO appears to be particularly active during the winter (Rodwell et al., 1999), NAO winter was also considered for the time series analysis. This index represents the differences in atmospheric pressures between the Azores high-pressure zone (placed at middle latitudes) and Iceland low-pressure zone (located at higher latitudes) (Hurrell, 1995; Hurrell et al., 2003). The NAO index influence precipitation, temperature and wind regimes over most of Northwester Europe (Hurrell, 1995; Witbaard et al., 2005).
In the present study, we aim evaluate the role of regional and seasonal environmental conditions on flatfish species survival rates along their life cycles. Therefore, regional and seasonal models were considered. Seasons were grouped as follows: winter (January to March), spring (April to June), summer (July to September) and autumn (October to December). Thereby, time-series analyses were carried out for each of the species in each of the regions and seasons independently.
For statistical purposes, LPUE was used as the response variable whereas fishing effort (FE) and environmental variables were used as the explanatory variables. LPUE were used as a proxy for yearly fish biomass production and hence, it was the considered the response variable in the models fitted. The variability of fishing recruitment was assumed to be largely determined by survival during the larval and juvenile stages. Consequently, the survival during its early stages was considered to be influenced beyond starvation and predation (Garrido et al., 2015) by environmental and fishing conditions, and thus a critical period determining the state of the stock in the subsequent years (Lloret et al., 2001; Baptista et al., 2016; Leitão et al., 2016). For this reason, the environmental explanatory variables were lagged depending on the time until species reach the gear recruitment age, ensuring that environmental variability was affecting flatfish during their larval (two years for D. cuneata, and three years for Microchirus spp., P. platessa, S. lascaris and S. solea; Table 1), juveniles and young adult stages (one year for D. cuneata, and two and one years for Microchirus spp., P. platessa, S. lascaris and S. solea). Fishing effort can also affect spawning biomass (adults) and recruitment in subsequent years. Thus, to accommodate this effect, FE was also lagged, one, two and three years as environmental variables, to allow reflecting each species LPUE temporal change.
Table 1 Biological and ecological information review of the flatfish species (Pleuronectiformes Order) used in the present study.
A multi-model approach including different time series analyses techniques was applied to find relationships between explanatory variables and the response variable (LPUE): Dynamic Factor Analysis (DFA), Min/Max Autocorrelation Factor Analysis (MAFA) and Generalised Least Squares (GLS). Since different analyses can provide different results, a selection criterion was implemented to identify the explanatory variables with higher probability to explain variability in flatfish LPUE. The probability was defined according to the number of time series techniques that identified the same significant relationship between explanatory variable and LPUE. This procedure allows classification of variables with either a high (the variable is highlighted in two or more models) or low probability to relate with fisheries (Baptista et al., 2016; Leitão et al., 2016).
Before the analyses, annual and seasonal data (response and exploratory variables) were tested for normality (quantile-quantile plots- QQ-plots) and collinearity (pair-plots) (Zuur et al., 2010). After QQ-plots analyses, no transformation was applied to yearly LPUE and explanatory variables. Since the results of the exploratory analysis over explanatory variables (pair-plots) revealed collinearity between yearly northerly wind (VWIND) and wind magnitude (WMAG), these variables were not taken into account together in the same models. As correlations between variables can be nonlinear the spearman correlation coefficient was used. A coefficient of correlation (R- coefficient correlation) of 80% was used as cut off criteria of collinear variables. Regardless of the time series technique used simple models were firstly tested for significance of the relationship without accounting for more than one variable. In situations where more than one variable was selected, the significance of the relationship was tested by accounting for combined statistically significant variables using surrogate testing. Both response and explanatory variables were standardized to convert the data into the same dimensional scale (Zuur et al., 2003b; Zuur et al., 2003a). The standardisation method used was the normalisation which consists in centring the data on zero; (Xi = (Yi - Ŷ))/∂y), where Ŷ is the sample mean, Yi the value of the ith sample and ∂y the sample standard deviation. Statistical analyses were performed using Brodgar software package using R version 3.0.1 (http://www.brodgar.com).
Min/Max Autocorrelation Factor Analysis (MAFA) is a type of principal component analysis (PCA) that can be used for extracting the main trends from time series and for smoothing in short time series. A set of orthogonal linear combinations of the original time series (MAFs) of decreasing smoothness measured by lag-one autocorrelation are constructed (Solow, 1994). The MAFA axes represent a measure of autocorrelation with lag 1. The first axis represents the main trend in the time series, and the others represents less important trends (Solow, 1994). Cross-correlations between MAFA axes and explanatory variables can also be estimated, to allow the identification of significant relationships between trends and explanatory variables (Erzini, 2005; Zuur et al., 2007). The present MAFA analyses considered only a single MAFA trend (autocorrelation time lag 1) for each species and region. Absolute values above the significance cut off level of correlation provided by MAFA were considered to have a significant association with the response variable.
Dynamic Factor Analysis (DFA) is a multivariate, dimension reduction technique that can be used to analyse short and non-stationary time series in terms of common patterns, evaluate the interactions between response variables (LPUE) and determine the effects of explanatory variables (environmental and fishing) on response variables (Zuur et al., 2003b; Zuur et al., 2007; Zuur et al., 2003a). Time series were modelled as a function of a linear combination of common trends, a constant level parameter, one or more explanatory variables and noise (Zuur and Pierce, 2004). The simplest model (1-time series = trend + noise) was used to establish the cut-value based on the Akaike Information Criteria (AIC) to determine significant relations between explanatory variables and the response variable. A single model with an explanatory variable as a parameter (1-time series = trend + explanatory variable + noise) was used for each species within each region, to account for the different environmental conditions among regions (Baptista and Leitão, 2014; Baptista et al., 2016; Leitão et al., 2016). Models were fitted with a diagonal covariance matrix and the AIC was used to compare models. The t-values obtained from the estimation of regression parameters were considered as an indicator of relationship (positive or negative) and its strength between explanatory and response variables (t-values with the absolute value greater than 3 indicate a strong relationship among variables) (Zuur et al., 2003a).
Generalised Least Square (GLS) is an extended linear mixed-effect model in which errors can be correlated and/or have unequal variance (Pinheiro and Bates, 2000; Lloret et al., 2001). Several GLS models were used without autocorrelation and with an autoregressive process required on the error components to allow errors with unequal variance (Zuur et al., 2007). Errors were specified following an autoregressive process of degree 1 which was determined using the partial autocorrelation function and the goodness of fit of an auto-regressive moving average (ARMA) model. This approach assumes that the autocorrelation is highest between consecutive years. Generalised Least Square models an autoregressive process of order 1 and without any autoregressive process were tested and compared (only better models are presented) using the lowest AIC coefficient as decision criterion. The simpler model was selected when the difference in AIC coefficients of two models was smaller than 2 (Zuur et al., 2007). Models were considered significant at the 95% level of confidence. The relationship between the explanatory variables and the response variable was evaluated by the sign (positive or negative) of the slope value.
Seasonal variability was recorded for all the variables tested (Table 2). Sea surface temperature (SST) was higher in the Southern region than in the Northwestern and Southwester regions (Table 2). The SST had similar yearly trends in the three regions with the higher peaks registered in 1989-1990, 1995, 1997 and 2006 (Figure 2A). During the study period, the North Atlantic Oscillation (NAO) Index had positive values until 1995 when a prominent decrease occurred, remaining in a steady state (fluctuating around the mean) thereafter (Figure 2B).
Table 2 Yearly and seasonal means and standard deviations (SD) for oceanographic, hydrological and climatic variables used in the time series analysis for the period from 1989 to 2009 along the Portuguese coast: Northwest (IXaCN), Southwest (IXaCS) and South (IXaS).
Figure 2 Standardized time series of the yearly oceanographic, hydrological and climatic variables for the period from 1989 to 2009 along the Portuguese coast: Northwest (IXaCN), Southwest (IXaCS) and South (IXaS). (A) Sea surface temperature (°C), (B) North Atlantic Oscillation Index, (C) Upwelling Index, (D) Wind magnitude (m.s-1), (E) Easterly wind component (m.s-1), (F) Northerly wind component (m.s-1), and (G) RD – River discharges (dam3).
The intensity of yearly Upwelling Index (UPW) was higher during spring and summer in the Portuguese mainland coastal zone, predominantly in the Northwestern and Southwestern regions (Table 2). Variations over the time showed different trends principally between the Southern and Western regions (IXaCN and IXaCS, Figure 2C). Important upwelling peaks occurred in the Southern region in 1996 and in 2009. In the Northwestern and Southwestern regions noteworthy peaks in upwelling befell in 1992, 1999-2000, 2005 and 2007-2008.
Yearly wind magnitude (WMAG) trends for the three regions shared similar variations over the time (Figure 2D). Remarkably events occurred in 1996 in the Southern region and also in 2008 where all the regions presented the highest values of the time series. Seasons with strong winds were spring and summer, with the strongest winds occurring in the Southwestern region (Table 2). The easterly yearly wind component (UWind) showed different trends among areas until 1998 (Figure 2E). Afterwards the three regions had similar patterns. Positive anomalies (i.e., values above the mean and wind blew from the west) were striking in 1993-1997, 1998-2004 and 2006-2009. Negative anomalies (i.e., values are below the mean and wind blew from the east) were remarkable during the period before 1992 and in 2005. The yearly northerly wind component (VWind) presented similar trends among all the regions varying smoothly along the mean (Figure 2F). The dominance of positive values represents winds coming from the south whereas negative values denote winds blowing from the north. After 2006, an outstanding decrease befell peaking in the lowest values in 2008 regardless of the areas.
Maximum river discharge (RD) volumes occurred during winter and the lowest occurred in summer, for all the regions (Table 2). The discharges were higher in the Northwestern region regardless of season, and significantly lower in the Southern region. The Northwestern and Southwestern regions had similar trends, peaking in 1996, 2001 and 2003 (Figure 2G). The larger volumes discharged in the Southern region occurred in 1989 and in 1996-1997.
Fishing effort and LPUE regional trends showed high variability among areas and for each flatfish species (Figure 3). Landings per unit effort values were higher in IXaCN. The FE of P. platessa showed a decreasing trend in IXaCS (Figure 3A) and was highly variable in IXaCN and IXaS, with a peak in 2004 in IXaCN. The LPUE of P. platessa also revealed decreasing trends in all the regions, with a high isolated peak in 2003 in IXaCN (Figure 3B). The FE of D. cuneata showed an increasing pattern in IXaCN and IXaCS, contrarily to registered in IXaS (Figure 3A). Its LPUE was higher in 1991 and 1993 in IXaCN, in 1991 in IXaCS, and between 1997 and 2003 in IXaS (Figure 3B). Microchirus spp. had highly variable FE and LPUE trends in all the regions, with no clear patterns (Figures 3A, B). The FE of S. lascaris increased along the years in IXaCN and IXaCS and showed more variability in IXaS increasing in 1990 and 2003 and decreasing in 1998 (Figure 3A). Contrarily, its LPUE decreased over the years, with the highest values registered in 1991 and 1993 in IXaCN, and 1995 in IXaCS (Figure 3B). Solea solea FE was concentrated close to the mean, showing an increasing patter from 2005 in all the three regions (Figure 3A). The LPUE of S. solea showed a decreasing pattern, peaking in 1993 in IXaCN, 1995 in all regions, and 2001 and 2005 in IXaCS (Figure 3B).
Figure 3 (A) Standardised yearly fishing effort (number of fishing days by boat, and (B) Landings per unit effort (LPUE: kg per fishing days by boat) for Pleuronectes platessa, Dicologlossa cuneata, Microchirus spp., Solea lascaris, and Solea solea between 1989 and 2009 in Northwestern (IXaCN), Southwestern (IXaCS) and Southern (IXaS) coasts of Portugal.
Explanatory variables selected as significant in more than one model have a high probability to influence flatfish LPUE. Summarized results of explanatory variables with significant relationship with flatfish LPUE for each region are given in Table 3 (age-group 0), Table 4 (age-group I), and Table 5 (age-group II) (for the detailed results of all model approaches see Supplementary Table 1 in Supplementary Material).
Table 3 Summary of results of relationships (significant in two or more time series analysis) between each flatfish species (Landings per unit effort- LPUE) and explanatory variables (fishing effort and environmental factors) using Min/Max Autocorrelation Factor Analysis (MAFA), Dynamic Factor Analysis (DFA) and Generalised Least Squares (GLS) corresponding to the age-group 0 (Lag3 for Pleuronectes platessa, Microchirus spp., Solea lascaris and Solea solea, and Lag2 for Dicologlossa cuneata), along the Portuguese coast: Northwest (IXaCN), Southwest (IXaCS) and South (IXaS).
Table 4 Summary of results of relationships (significant in two or more time series analysis) between each flatfish species (Landings per unit effort- LPUE) and explanatory variables (fishing effort and environmental factors) using Min/Max Autocorrelation Factor Analysis (MAFA), Dynamic Factor Analysis (DFA) and Generalised Least Squares (GLS) corresponding to the age-group I (Lag2 for Pleuronectes platessa, Microchirus spp., Solea lascaris and Solea solea, and Lag1 for Dicologlossa cuneata), along the Portuguese coast: Northwest (IXaCN), Southwest (IXaCS) and South (IXaS).
Table 5 Summary of results of relationships (significant in two or more time series analysis) between each flatfish species (Landings per unit effort- LPUE) and explanatory variables (fishing effort and environmental factors) using Min/Max Autocorrelation Factor Analysis (MAFA), Dynamic Factor Analysis (DFA) and Generalised Least Squares (GLS) corresponding to the age-group II (Lag1 for Pleuronectes platessa, Microchirus spp., Solea lascaris and Solea solea), along the Portuguese coast: Northwest (IXaCN), Southwest (IXaCS) and South (IXaS).
Considering the sign of the effect of explanatory variables on the age-group 0, FE had positive effects on P. platessa in IXaCS, and negative effects on D. cuneta and S. lascaris in IXaCN, S. solea in IXaCS, and on Microchirus spp. in IXaCN and IXaS (Table 3). The SST negatively affected P. platessa during spring (IXaCS), and S. solea in spring (IXaCS and IXaS), summer (IXaCN) and autumn (IXaCS) (Table 3). On the other hand, SST had positive effects on D. cuneata and Microchirus spp. during winter and spring (IXaS). The NAO Index in winter positively affected P. platessa (IXaCN and IXaS), Microchirus spp. and S. lascaris (IXaCN) (Table 3). Upwelling (UPW) Index showed negative effects in P. platessa during summer (IXaCS) and on D. cuneata during spring (IXaS) (Table 3). Upwelling had also positive effects on Microchirus spp. in summer (IXaCN and IXaCS) and autumn (IXaS), and on S. solea during spring and summer (IXaCS). Wind magnitude (WMag) had negative effects on P. platessa during summer (IXaS), Microchirus spp. in spring (IXaCN) and winter (IXaCS), and on S. solea during autumn (IXaCN) (Table 3). However, WMag showed positive effects during summer on Microchirus spp. (IXaS) and S. lascaris (IXaCS). U-wind component (UWind) negatively affected P. platessa during summer (IXaCS and IXaS), D. cuneata and S. solea during spring (IXaCS), and S. lascaris during winter (IXaS). Dicologlossa cuneata was also positively affected by VWind during summer (IXaS), as well as Microchirus spp. during autumn (IXaCN and IXaS) (Table 3). V-wind component (VWind) showed negative effects on D. cuneata during spring (IXaCN and IXaCS), and on S. lascaris and S. solea during summer (IXaCS); and positive effects on Microchirus spp. during winter (IXaS). River discharges positively affected P. platessa during spring (IXaS), D. cuneata in winter (IXaS), and Microchirus spp. during spring (IXaCN and IXaS) and autumn (IXaS); and negatively affected S. solea during spring (IXaCN) (Table 3).
In relation to the effects on age-group I, FE showed positive effects on P. platessa in IXaCS, and negative effects on D. cuneta and S. lascaris in IXaCN, and on Microchirus spp. and S. solea in IXaCS (Table 4). The SST had negative and positive effects on P. platessa in spring (IXaCN) and summer (IXaS), respectively (Table 4). Dicologlossa cuneata was negatively affected by SST in autumn (IXaCS) and positively during winter and spring (IXaS). The SST had also negative effects on Microchirus spp. (IXaS) and S. solea (IXaCN and IXaCS) during spring. The NAO Index in winter had positive effects on D. cuneata (IXaCN), S. lascaris (IXaCS) and S. solea (IXaCN) (Table 4). Dicologlossa cuneata was also negatively affected by NAO winter (IXaS). Upwelling index showed negative effects on P. platessa during winter (IxaCN), spring (IXaS) and summer (IXaCS); and positive effects on Microchirus spp. in winter (IXaCN) (Table 4). Wind magnitude negatively affected P. platessa during summer and autumn (IXaS), Microchirus spp. during spring (IXaS), S. lascaris during winter (IXaCN) and summer (IXaS), and S. solea during autumn (IXaCN) (Table 4). On the other hand, WMag showed positive effects on D. cuneata in summer (IXaCS) and autumn (IXaCS and IXaS), on Microchirus spp. during autumn (IXaCS and IXaS), and on S. solea during winter and spring (IXaCN). U-wind component had negative effects on P. platessa during summer (IXaS), and positive effects on S. lascaris and S. solea during summer (IXaCS) (Table 4). Microchirus spp. was negatively affected by UWind during winter and positively during autumn (IXaCN). V-wind component showed negative effects during summer on P. platessa (IXaS) and D. cuneata (IXaCS), and during autumn on Microchirus spp. (IXaS) (Table 4). Microchirus spp. was also positively affected by VWind during winter (IXaCN), as well as S. solea during summer (IXaS). River discharges had positive effects on P. platessa (IXaCN) and D. cuneata (IXaS) during winter, and on Microchirus spp. during spring (IXaCN); and negative effects on D. cuneata during summer (IXaCN) and autumn (IXaCS) (Table 4).
Concerning to the effects on age-group II, FE showed positive effects on P. platessa in IXaCS and IXaS, and negative effects on S. lascaris in IXaCN and S. solea in IXaCS (Table 5). The SST negatively affected P. platessa during spring (IXaCN and IXaS) and autumn (IXaS), S. lascaris during autumn (IXaCS and IXaS), and S. solea during spring and summer (IXaS) (Table 5). Upwelling index had positive effects on P. platessa during spring (IXaCN) and on Microchirus spp. during summer (IXaS) (Table 5). Wind magnitude had positive effects on P. platessa during spring (IXaS) and summer (IXaCS), and on Microchirus spp. and S. solea during winter (IXaCN); and negative effects on S. lascaris during winter (IXaS) (Table 5). U-wind component positively affected P. platesa during spring (IXaCS) and Microchirus spp. during winter (IXaCN) (Table 5). Pleuronectes platessa was also affected by UWind during summer (IXaCS). V-wind component showed negative effects on P. platessa during summer (IXaCS) and autumn (IXaS), on Microchirus spp. during winter (IXaCN) and autumn (IXaS), and on S. solea during winter (IXaS) and spring (IXaCN and IXaS) (Table 5). Microchirus spp. was also positively affected by VWind during summer (IXaS). Pleuronectes platessa was positively affected by river discharges during summer (IXaCS and IXaS) and negatively during spring (IXaCN) and autumn (IXaS) (Table 5). River discharges had also negative effects on Microchirus spp. (IXaCS), S. lascaris (IXaCS) and S. solea (IXaS) during autumn.
Overall, we have different variable relationships between the LPUE of flatfish in Portuguese coast and the explanatory variables considered (FE, SST, NAO, UPW, WMAG, UWIND, VWIND and RD). Also, we have found that these relationships vary between species and regions, and that these relationships are species and region dependent, in agreement with previous works (van der Veer et al., 2000). The environmental variability affects flatfish species in different ways along their life cycle, seasons, and regions along the Portuguese coast. Similarly, FE had opposite effects on the LPUE depending on the region or the species (sometimes positive, sometimes negative). This finding is related to the indirect (sometimes not clear) relationship between LPUE and fish stocks. Hence, positive relationships between LPUE and FE are only expected on healthy stocks, while negative relationships might be found in situations of decreasing stocks or in the case of species that do not form large schools (as is the case of flatfish) (Bueno-Pardo et al., 2020). In the following subsections we discuss the effects of fishing pressure and environmental variability on the age-group 0 (larvae and juveniles), and age-groups I and II (juveniles to young-adults) separately.
In parallel with environmental variables, it has been widely demonstrated that fishing can cause great impacts on fishing stocks (Pauly et al., 2002; Gibson et al., 2014). Our results show that FE is the most relevant variable (more times identified as significant) of the analysed variables, for almost all species and age-groups of flatfish studied. A negative relationship between FE and LPUE was detected for Dicologlossa cuneata and Solea lascaris in the Northwestern region (IXaCN), and for Microchirus spp. and Solea solea in the Southwestern (IXaCS) and Southern (IXaS) regions. As mentioned before, the relationship between LPUE (as for the catch per unit effort, CPUE) has been shown to not be necessarily related to the state of the stock (Harley et al., 2001), but negative relationships between LPUE and FE could only be related to decreasing stocks. The limitation in the management measures of flatfish fisheries in Portugal and the lack of stock assessment have generated some problems in legislation, for example, the minimum landing sizes, local quotas, and total admissible captures (TAC) were regulated only for a couple of species (Teixeira and Cabral, 2009). Consequently, these resources have yet presented evidences of over-exploitation (Rice and Cooper, 2003; Rijnsdorp et al., 2007; Teixeira and Cabral, 2009). Although there are minimum landing sizes defined for flatfish species (e.g., 15 cm for D. cuneata, 18 cm for Microchirus spp., and 24 cm for Solea spp.), undersized flatfishes are being caught, belonging to the age-groups 0 and I (i.e., immature individuals) (Ribeiro et al., 1999; Afonso-Dias et al., 2005). Also, some authors (Teixeira and Cabral, 2009; Andersen et al., 2010; Teixeira et al., 2011) described that flatfish species were caught mainly during the spawning season, a sensitive period for these species, as they concentrate near the coast to spawn and become more vulnerable for fishery. Thus, the fishing pressure exercised on these species could have reduced the spawning stock biomass influencing the recruitment in subsequent years. It is worth to note, however, that the LPUE of Pleuronectes platessa is positively related to FE in the region IXaCS. Here the LPUE time-series presented a decreasing trend, being also the region with lowest effort for this species.
Sea surface temperature (SST) is considered one of the main environmental factors driven biological and ecological changes in the marine environment (Teixeira and Cabral, 2009; Baptista et al., 2018). Larval development in fish species is highly affected by the increase of temperature, accelerating planktonic larval duration and consequently survivorship (Pauly and Pullin, 1988; Pepin, 1991). In the case of flatfish species, SST has been reported to affect growth, maturity, spawning, and development of eggs, larvae, and juveniles (Rijnsdorp and Vingerhoed, 1994; Henderson and Seaby, 2005; Petitgas et al., 2012; Vinagre et al., 2013). In the present study, negative relationships between SST and LPUE of P. platessa were found in the Southwestern region during spring, corresponding to the end of the spawning season and development of early phases (eggs and larvae). These results are in accordance with previous findings reporting negative relationships between P. platessa year-class strength and water temperature in North Atlantic during spawning (van der Veer et al., 2000) and early life periods (van der Veer and Witte, 1999). Teal et al. (2008) also suggested that growth of age-group 0 P. platessa is determined by temperature. LPUE of Solea solea had also a negative relation with SST in Southwestern (spring and summer) and Southern coasts (spring and autumn). Although several studies found positive correlations between SST and Solea spp. in Portuguese estuaries (e.g., Vinagre et al., 2008; Ramos et al., 2009; Gamito et al., 2015), Rijnsdorp et al. (1992) reported that lower SST at the spawning season might enhance the reproductive success of S. solea. Cozzi and Giani (2011) also registered low recruitment rates in years with warm temperatures in Adriatic Sea. Solea solea is a temperate species with a metabolic optimum temperature estimated of 18.8°C (Lefrançois and Claireaux, 2003), possibly suffering thermal stress in Southwestern and Southern regions of Portuguese coast, with temperatures varying between 18.4 and 22.5°C during spring, summer, and autumn (Table 2). During these conditions, the ingestion rates decrease (Yamashita et al., 2001) as well as the growth rates, since the energy is spent to face unfavourable conditions, as previously described in Tagus Estuary (Southwestern coast- IXaCS) (Vinagre and Cabral, 2008; Vinagre et al., 2008). Still, egg mortality in P. platessa and S. solea has also been associated with the increase of pathogens activity caused by warm temperatures (Fonds, 1995; Henderson, 1998).
In addition, time-series analysis also revealed positive relationships between LPUE and SST for D. cuneata and Microchirus spp., beneficiating from winter (IXaS) and spring SST (IXaCS and IXaS), and matching with spawning and larval periods (Andrade, 1990; Santos and Lino, 2010; Table 1). As reported by Lange and Greve (1997), temperature influences the rate of gonadal maturation and spawning time, and consequently recruitment of flatfish species. Désaunay et al. (2006) also reported that winter warming can be favourable for growth and survival of D. cuneata early phases in the Bay of Biscay. Increases in SST may also influence other marine trophic levels such as phytoplankton and zooplankton communities (Colebrook, 1982; Tunberg and Nelson, 1998), important food resources for flatfish larvae. Furthermore, D. cuneata had a subtropical range distribution (Froese and Pauly, 2016) tolerating warmer temperatures than other flatfish species occurring in these waters. In fact, Teixeira et al. (2014) reported the increase of D. cuneata landings in warm years in Portuguese coast. Microchirus spp. LPUE was also positively related with both winter and spring SST, corresponding to their spawning period (February to June; Andrade, 1990; Table 1). Therefore, favourable SST conditions might determine an early spawning and decrease pelagic larval duration (O’Connor et al., 2007) increasing the settlement success through larval retention (Lacroix et al., 2018). Microchirus spp. are a temperate species with a remarkably southern distribution (Quéro et al., 1986) taking advantage of warmer SST conditions. Teixeira and Cabral (2009) reported that temperature was the most important predictor explaining Microchirus azevia landings, by its effects on food resources. Also, Teixeira et al. (2014) and Gamito et al. (2015) demonstrated that Microchirus spp. landings were higher in warm years and lower during cold periods.
Although the same North Atlantic Oscillation (NAO) Index was used for all the regions, LPUE of different flatfish species were associated to it in different regions of the Portuguese coast. The NAO Index was positively related to LPUE of P. platessa (IXaCN and IXaS), Microchirus spp. and S. lascaris (IXaCS) during winter, coinciding with their spawning season and the beginning of the larval period (Andrade, 1990; Félix et al., 2011; Table 1). Thus, high biomass was observed during positive NAO periods, characterized, in the Southern Europe, by a predominance of relatively lower SST (Henriques et al., 2007) and Northern wind conditions favouring upwelling and productivity (Huthnance et al., 2002; Borges et al., 2003; Santos et al., 2004). The correlation between NAO and recruitment of Microchirus spp. and S. solea was previously observed in the Portuguese coast (Gamito et al., 2015). It was related with S. solea growth, due to the possible relation with productivity and food availability (phytoplankton and zooplankton) (Henderson and Seaby, 2005). Also, cold winters could favour the spawning processes of some flatfish species, as previously reported for P. platessa and S. solea (e.g., Rijnsdorp et al., 1992; van der Veer and Witte, 1999), and may also reduce eggs and larval mortality due to lower predator activity or to the match between larval production and their food (Cushing, 1972; Leggett and Deblois, 1994).
Upwelling (UPW) events usually occur along the Portuguese coast during spring and summer (Leitão et al., 2019; Table 2) when equatorward winds occur for consecutive days (Relvas et al., 2007; Vinagre et al., 2007). During upwelling events, the surface water is pushed offshore being replaced by deep, cool, and nutrient enriched waters favouring biological production. Generally, drifting larvae is hampered by these oceanographic events, threatening the chance of flatfish early stages to reach the nursery grounds close to the coast (Vinagre et al., 2008; Vinagre et al., 2007). Thus, upwelling events can have opposite effects on fish larvae and juveniles. Pleuronectes platessa and D. cuneata were negatively correlated with upwelling in summer (Southwest) and spring (South), respectively. These seasons corresponded to the higher upwelling index registered in each region (Table 2), increasing the offshore advection of larvae (Guisande et al., 2001; Landaeta and Castro, 2002) and affecting the recruitment success. Contrarily, Microchirus spp. LPUE was found to be positively related with summer (IXaCN) and autumn (IXaS) upwelling, as well as S. solea with spring and summer upwelling (IXaCS). Although upwelling provokes the offshore advection of the surface water layer, several mechanisms can retain larvae in the nursery grounds near the coast (Gibson, 1994; Moita et al., 2003; Vinagre et al., 2007). It is the case of upwelling shadow zones that may play an important role in the retention of flatfish early stages preventing their offshore advection (Moita et al., 2003; Mason et al., 2005).
Coastal drift currents are affected by wind strength and direction (Moita, 1986; Melo, 1989; Vila-Concejo et al., 2003) influencing the transport and/or the retention of eggs and larvae along the coastline through changes in hydrodynamics (Jager, 2001; Borges et al., 2007; Vinagre et al., 2009; Lacroix et al., 2018), and food supply (Fernández et al., 1993; Santos et al., 2012). Wind magnitude also influences the dispersal of the river plumes into the sea, helping larvae to successfully reach estuarine nursey areas (Tanaka, 1985; Vinagre et al., 2007) by using the chemical cues released by the river discharge and spread by the force of the wind (e.g., Gibson, 1997; Arvelund and Takemura, 2006). Thus, as reported by several authors, the recruitment of flatfish species is related to prevailing wind conditions (e.g., Werner et al., 1997; Nielsen et al., 1998; van der Veer et al., 2000). In the present study, the increase of wind strength negatively affected the LPUE of P. platessa and S. solea during summer (IXaS) and autumn (IXaCN) probably by promoting the larval dispersion from the nursery areas in a way that larval mortality was also enhanced (Vinagre et al., 2009; Lacroix et al., 2018) and by increasing turbidity in shallow waters (Gibson, 1994; Laffargue et al., 2007). On the other hand, S. lascaris larvae have been also found to benefit from the increase of river plumes (Vinagre et al., 2007) and retention in coastal areas (Vinagre et al., 2009) caused by the increase of summer wind magnitude (Southwest). The LPUE of Microchirus spp. was negatively related to wind magnitude during winter (IXaCS) and spring (IXaCN), and positively during summer (IXaS), revealing the importance of regionality and seasonality for recruitment.
Together with wind magnitude, the variability of the wind direction also played an important role on larval transport (Lacroix et al., 2018). In fact, in our study, D. cuneata and Microchirus spp. LPUE were positively related with Westerly winds (UWIND) in Southern coast (IXaS) during spring and autumn, respectively, meaning that recruitment was higher when the wind blew from the west. These winds are responsible for upwelling conditions in the Southern coast of Portugal (Fiúza, 1983; Baptista et al., 2016), but with less intensity than on the Western coast (IXaCN and IXaCS) due to the oceanographic characteristics. Therefore, it is more likely that these winds might influence the surface coastal drift current retaining eggs and larvae in the coastal areas and preventing them to run away to the open sea. On the contrary, negative relationships were found in P. platessa (IXaCS and IXaS), S. lascaris (IXaS), D. cuneata and S. solea (IXaCS). In these cases, recruitment enhanced when the winds bellow from the East, corresponding to downwelling conditions in Southern coast of Portugal (Fiúza, 1983; Baptista et al., 2016). Therefore, winds blowing from the east may enhance the retention of flatfish eggs and larvae in coastal areas (Moita, 1986; Vinagre et al., 2009; Baptista et al., 2016). Furthermore, specific characteristics, such as temperature, salinity, and nutrients of the water transported by the Easterly winds (e.g., from the Mediterranean Sea in Southern regions) might have a positive effect on the survivorship of flatfish larvae, improving the recruitment success and consequently, the landings on the following years. In relation to the Northerly wind component (VWIND), winds from South increased the recruitment of Microchirus spp. during winter in Southern region. These winds seem to transport and retain larvae in coastal nursery areas (Vinagre et al., 2009). Contrarily, in Western coast, winds from North (negative values) improved the recruitment of D. cuneata during spring (IXaCN and IXaCS), and S. lascaris and S. solae during summer (IXaCS). In Western coast of Portugal, these winds are responsible for upwelling events (Relvas et al., 2007), increasing biological production (Vinagre et al., 2007) and recruitment of flatfish species.
Variability in river discharges has important consequences for recruitment of fish species, leading to changes in salinity, organic matter input, food abundance and currents (Vinagre et al., 2007). Pleuronectes platessa and Microchirus spp. LPUE were positively related with spring river discharge, and D. cuneata with winter river discharge, that matches with the seasons where these species spawn and larval development occurs (Andrade, 1990; Santos and Lino, 2010; Félix et al., 2011; Table 1). Microchirus spp. LPUE also were positively related to river discharges during autumn, corresponding to a post-metamorphosis and juvenile phase. In fact, larvae living on the coast take advantage of the increase of food abundance driven by the inputs of organic matter from the river discharges (Cushing, 1996; Lloret et al., 2001). The positive relationship between river discharge and the landings of marine fish in Portugal has been recently described using multiple DFA models (Bueno-Pardo et al., 2020), resulting to be especially relevant in the South region during winter. Larvae of various organisms can follow cues released by river plumes such as odour, temperature, salinity and turbidity (e.g., Gibson, 1997; Arvelund and Takemura, 2006) which cloud orient them to the coastal nursery areas where food resources and refuge are available (Tanaka, 1985; Tamburri et al., 1996). Thus, high river discharge increases the river plumes to a wider area, increasing the possibility of being detected by flatfish larvae spawned on the coast (Vinagre et al., 2007). On the other hand, river discharges can also have negative impacts on flatfish LPUE, such as S. solae during spring at the Northwestern region. Despite being described that S. solea larvae benefit from the increase of river discharges to ingress into Portuguese estuaries (Martinho et al., 2010; Vinagre et al., 2007; Martinho et al., 2009), Ramos et al. (2009) reported that this species is not able to migrate into Lima estuary (Northwest) due to the increase of river flow enhancing turbidity. Turbidity, an indirect consequence of the river runoff, can affect the passive tidal migration of flatfish larvae (Ramos et al., 2009). Thus, years with high river discharges during spring, when larvae ingress into nursery habitats, could compromise their estuarine migration, and consequently the recruitment of S. solea.
After spending the first months (at least six months) in coastal nursery grounds to complete their development, many juveniles of flatfish species migrate into deeper coastal waters (Dorel et al., 1991; Gibson, 1997), where they will grow and reach sexual maturity, returning or not to inshore habitats during their life cycle (Gibson, 1997). Thus, as shown by present results, the effects of environmental variability on age-groups I and II of flatfish species seems to be related with growth and maturation. In fact, Fuiman (1997) described the juvenile phase as a period dominated by growth, and the adulthood a period dominated by reproduction. As previously reported, the SST could affect metabolic and physiological rates (Brander et al., 2003) and food availability (Cushing, 1990) which in turn affect growth of flatfish juveniles (Yamashita et al., 2001; Teal et al., 2008), since the energy expended for growth will be diverted to face adverse temperatures (Vinagre et al., 2008). In fact, despite flatfishes are quite sedentary, they can perform small migrations to avoid unfavourable conditions, like temperature (Marchand, 1991). Temperature could also affect the duration of maturation of flatfish species by its influence on gonadal maturation (Lange and Greve, 1997). Beyond the thermal conditions, hydrographic conditions could also have an impact on distribution and abundance of juvenile and young-adult flatfishes (Yeung and Yang, 2017), such as coastal currents affected by wind strength and direction. Yet, juveniles and adults living on the coast could also take advantage of the increase of food abundance driven by the inputs of organic matter from the river discharges (Cushing, 1996; Lloret et al., 2001).
It should be noted that in most cases where the environmental effect was repeated from one year to the next, this effect occurred in the following year, that is, if the effect occurred in the age-group 0, it was repeated in the age-group I and not in the age-group II. In other words, it appears that the effects of environmental variability over the life cycle of flatfish species have a gradual transition over the life cycle phases. Also, although there are some effects of environmental variability during the juvenile-young-adults’ phases (age-groups I and II), these effects are stronger during the larval-juvenile phase (age-group 0), as previously described. For example, it was reported that the year-class abundance is determined by environmental factors experienced early in life in many temperate flatfish species, like plaice (P. platessa) (e.g., van der Veer et al., 1990), sole (S. solea) (Rijnsdorp et al., 1992; Henderson and Seaby, 1994), flounder (Platichthys flesus) (van der Veer et al., 1991; Henderson and Seaby, 1994), and dad (Limanda limanda) (Henderson and Seaby, 1994). Thus, the year-class strength of flatfish species is fixed by the end of the first year (Henderson and Seaby, 1994).
In conclusion, LPUE of P. platessa age-group 0 (recruitment) is favored by an increase in NAO index and river discharge, and a decrease in SST, UPW, WMag and UWind, for age-group I by an increase in river discharge, and a decrease in UPW, WMag, UWind and VWind, and for age-group II by an increase in UPW and WMag, and a decrease in SST and VWind; LPUE for D. cuneata age-group 0 in enhanced by an increase in SST and river discharge, and a decrease in UPW and VWind, and for age-group I by an increase in SST and WMag, and a decrease in VWind and river discharge; LPUE of Microchirus spp. age-group 0 is favored by an increase in SST, NAO index, UPW, UWind, VWind and river discharge, and a decrease in WMag, for age-group I by an increase in UPW, WMag and river discharge, and a decrease in SST, and for age-group II by an increase in UPW, WMag and UWind, and a decrease in VWind and river discharge; LPUE for S. lascaris age-group 0 in enhanced by an increase in NAO index and WMag, and a decrease in UWind and VWind, for age-group I by an increase in NAO, UWind and VWind, and a decrease in WMag, and for age-group II by a decrease in SST, WMag and river discharge; LPUE for S. solea age-group 0 in enhanced by an increase in UPW, and a decrease in SST, WMag, UWind, VWind and river discharge, for age-group I by an increase in NAO, WMag and UWind, and a decrease in SST, and for age-group II by an increase in WMag, and a decrease in SST, VWind and river discharge. From the point of view of management, we have evidenced that an increase in fishing effort is negative for all the species and age-groups evaluated except for P. platessa.
In this multi-species study, it has been found that relationships between explanatory variables (environmental and fishing) and response variables (LPUE) vary among species, regions, and life cycle phase. Intra-specific biological differences within the Pleuronectiformes order and species ecological distribution range result in different patterns among flatfishes regarding environmental-fisheries associations. Furthermore, the heterogenic geography of the Portuguese coast and the different climatic and oceanographic conditions that occur along it, could explain the variability found between regions. Environmental variables tend to function in reactional cascades frequently depending on the pattern of another one (e.g., changes in sea surface temperature might be in part the result of upwelling events enhanced by an increase in the wind magnitude in a certain region). Thus, it can be observed that significant results are usually linked to this “domino effect” between environmental variables. Flatfish early (age-group 0) phases are enhanced when they can successfully reach the nursery grounds. Consequently, the retention or transport of eggs and larvae toward the coast is an important factor being reflected in the LPUE of subsequent years. Food availability is also an essential aspect influencing larval recruitment. Food resources can increase in response to several environmental conditions such as upwelling, sea surface temperature or winds. Therefore, matching the events of high productivity with the recruitment of flatfish early stages could make the difference between a good fishing year and a bad one (high LPUE vs low LPUE). On the other hand, during juveniles to young-adult phase (age-groups I and II), the increase of LPUE in the next years seems to be related to environmental variables that affect growth and maturation. Thus, SST – a variable consistently identified in this study across regions and species as fundamental for understanding LPUE – plays a fundamental role in the maturation processes of the organisms, determining the time that the species spends in the plankton before reaching the nursery areas to conduct metamorphoses into the next life phase and influencing the growth and maturation of juveniles and young-adults.
LPUE are key for fisheries management as it is directly related to the revenues obtained by fishers. This parameter has a different meaning than the total landings or captures, with which LPUE are not necessarily related. Managers of marine fisheries should consider both perspectives and understand the links between them, the environmental variables and fishing effort for a correct decision making. In this work we have identified the main potential drivers of LPUE of flatfish in Portugal, providing a better understanding of their effects and the different age-groups of the species. The strong importance of fishing effort determining LPUE, and the region-species specific influence of the environmental variables, in a scenario of climate change, makes necessary to adopt recurrent monitoring of the stocks and environmental variables to manage fishing effort in the most sustainable way.
Publicly available datasets were analyzed in this study. This data can be found here: this data was requested to Portuguese STAT fisheries office and can only be used for scientific proposes.
Ethical review and approval was not required for the animal study because N/A - this is fisheries time series statistical data.
Conceptualization – VB and FL; Investigation – VB and IP; Methodology – VB and IP; Resources – MT and FL; Supervision – FL; Writing, original draft – VB, IP, and JB-P; Writing, review & editing – VB, IP, JB-P, MT, and FL. All authors contributed to the article and approved the submitted version.
This study received Portuguese national funds from FCT – Foundation for Science and Technology through project UID/Multi/04326/2020, CLIMFISH project – A framework for assess vulnerability of coastal fisheries to climate change in Portuguese coast founded by Portugal 2020, n2/SAICT/2017 – SAICT (Projetos de IC&DT), and from operational program MAR2020-FEAMP through project CLIMA-PESCA – Vulnerabilidade do Setor das Pescas às alterações climáticas: medidas de adaptação (MAR-01.03.02-FEAMP-0052). VB was funded through project LuandaWaterFront – “Luanda Bay Ecological Assessment: A waterfront based approach to reduce environmental risks and increase quality of life” (333191101) supported by Aga Khan, Network for Development and the Portuguese Foundation for Science and Technology (FCT), and by Portuguese Foundation for Science and Technology (FCT) under the 4th Edition of Individual Call to Scientific Employment Stimulus (2021.00956.CEECIND). JB-P was funded by EU H2020 (FutureMARES, contract no. 869300). FL was funded by FCT – Foundation for Science and Technology in the ambit of the contract program DL57/2016/CP1361/CT0008.
The authors declare that the research was conducted in the absence of any commercial or financial relationships that could be construed as a potential conflict of interest.
All claims expressed in this article are solely those of the authors and do not necessarily represent those of their affiliated organizations, or those of the publisher, the editors and the reviewers. Any product that may be evaluated in this article, or claim that may be made by its manufacturer, is not guaranteed or endorsed by the publisher.
The Supplementary Material for this article can be found online at: https://www.frontiersin.org/articles/10.3389/fmars.2022.844158/full#supplementary-material
Afonso-Dias I., Reis C., Andrade J. P. (2005). Reproductive aspects of Microchirus azevia (Risso 1810) (Pisces: Soleidae) from the south coast of Portugal. Sci. Mar. 69 (2), 275–283. doi: 10.3989/scimar.2005.69n2275
Andersen B. S., Vermard Y., Ulrich C., Hutton T., Poos J. J. (2010). Challenges in integrating short-term behaviour in a mixed-fishery management strategies evaluation frame: A case study of the north Sea flatfish fishery. Fish. Res. 102, 26–40. doi: 10.1016/j.fishres.2009.10.004
Andrade J. P. (1990). A importância da ria Formosa no ciclo biológico de solea senegalensis (Kaup 1858), solea vulgaris (Quensel 1806), solea lascaris (Risso 1810) e microchirus azevia (Capello 1868) (Faro: Universidade do Algarve).
Arvelund M., Takemura A. (2006). The importance of chemical environmental cues for juvenile Lethrinus nebulosus forsskål (Lethrinidae, teleostei) when settling into their first benthic habitat. J. Exp. Mar. Bio. Ecol. 338, 112–122. doi: 10.1016/j.jembe.2006.07.001
Atlas R., Hoffman R. N., Ardizzone J., Leidner S. M., Jusem J. C., Smith D. K., et al. (2011). A cross-calibrated, multiplatform ocean surface wind velocity product for meteorological and oceanographic applications. Bull. Am. Meteorol. Soc 92, 157–174. doi: 10.1175/2010BAMS2946.1
Bailey K. M., Nakata H., van der Veer H. W. (2005). “The planktonic stages of flatfishes: physical and biological interactions in transport process,” in Flatfishes, biology and exploitation. Ed. Gibson R. N. (Oxford: Blackwell), 94–119.
Bakun A. (1996). Patterns in the ocean: Ocean processes and marine population dynamics (La Paz: California Sea Grant College System, La Jolla).
Baptista V., Campos C. J. A., Leitão F. (2016). The influence of environmental factors and fishing pressure on catch rates of Diplodus vulgaris. Estuar. Coasts 39 (1), 258–272. doi: 10.1007/s12237-015-9990-y
Baptista V., Leitão F. (2014). Commercial catch rates of the clam Spisula solida reflect local environmental coastal conditions. J. Mar. Syst. 130, 79–89. doi: 10.1016/j.jmarsys.2013.07.010
Baptista V., Silva P. L., Relvas P., Teodósio M. A., Leitão F. (2018). Sea Surface temperature variability along the Portuguese coast since 1950. Int. J. Climatol. 38 (3), 1145–1160. doi: 10.1002/joc.5231
Baptista V., Ullah H., Teixeira C. M., Range P., Erzini K., Leitão F. (2014). Influence of environmental variables and fishing pressure on bivalve fisheries in an inshore lagoon and adjacent nearshore coastal area. Estuar. Coast. 37, 191–205. doi: 10.1007/s12237-013-9658-4
Bell K., Cowley P., Whitfield A. (2001). Seasonality in frequency of marine access to an intermittently open estuary: Implications for recruitment strategies. Estuar. Coast. Shelf Sci. 52, 327–337. doi: 10.1006/ecss.2000.0709
Bettencourt A., Bricker S. B., Ferreira J. G., Franco A., Marques J. C., Melo J. J., et al. (2004). Typology and reference conditions for Portuguese transitional and coastal waters development of guidelines for the application of the European union water framework directive (Lisbon: Instituto da Agua (INAG)—Institute of Marine Science (IMAR).
Borges T. C. (2010). Biodiversidade nas pescas do Algarve (Sul de Portugal) (Faro: Universidade do Algarve).
Borges R., Ben-Hamadou R., Chícharo M. A., Ré P., Gonçalves E. J. (2007). Horizontal spatial and temporal distribution patterns of nearshore larval fish assemblages at a temperate rocky shore. Estuar. Coast. Shelf. S. 71, 412–428. doi: 10.1016/j.ecss.2006.08.020
Borges M. F., Santos A. M. P., Crato N., Mendes H., Mota B. (2003). Sardine regime shifts off Portugal: A time series analysis of catches and wind conditions. Sci. Mari. 67 (1), 235–244. doi: 10.3989/scimar.2003.67s1235
Brander K. M. (2007). Global fish production and climate change. Proc. Natl. Acad. Sci. U.S.A. 104 (50), 19709–19714. doi: 10.1073/pnas.0702059104
Brander K. M. (2010). Impacts of climate change on fisheries. J. Mar. Syst. 79, 389–402. doi: 10.1016/j.jmarsys.2008.12.015
Brander K. M., Blom G., Borges M. F., Erzini K., Henderson G., MacKenzie B. R., et al. (2003). Changes in fish distribution in the eastern north Atlantic: Are we seeing a coherent response to changing temperature? ICES Mar. Sci. Symp. 219, 261–270.
Bueno-Pardo J., Pierce G. J., Cabecinha E., Grilo C., Assis J., Valavanis V., et al. (2020). Trends and drivers of marine fish landings in Portugal since its entrance in the European union. ICES J. Mar. Sci. 77, 988–1001. doi: 10.1093/icesjms/fsaa010
Bueno-Pardo J., Ramalho S. P., García-Alegre A., Morgado M., Vieira R. P., Cunha M. R., et al. (2017). Deep-sea crustacean trawling fisheries in Portugal: quantification of effort and assessment of landings per unit effort using a vessel monitoring system (VMS). Sci. Rep. 7, 40795. doi: 10.1038/srep40795
Cabral H. N. (2002). On the occurrence of some rare flatfish species in south – European Atlantic waters. Thalassa 18, 31–38.
Colebrook J. M. (1982). Continuous plankton records: phytoplankton, zooplankton and environment, north-East Atlantic and north Sea 1958–1980. Oceanol. Acta 5, 473–480.
Cowan J. H., Rose K. A., de Vries D. R. (2000). Is density-dependent growth in young of the year fishes a question of critical weight? Rev. Fish Biol. Fish. 10, 61–89. doi: 10.1023/A:1008932401381
Cozzi S., Giani M. (2011). River water and nutrient discharges in the northern Adriatic Sea: current importance and long term changes. Cont. Shelf Res. 31, 1881–1893. doi: 10.1016/j.csr.2011.08.010
Cunha M. E. (2001). Physical control of biological processes in a coastal upwelling system: comparison of the effects of coastal topography, river run-off and physical oceanography in the northern and southern parts of western Portuguese coastal waters (Lisbon: Faculdade de Ciência da Universidade de Lisboa).
Cushing D. H. (1972). “The production cycle and the numbers of marine fish,” in Conservation and productivity of natural waters. Eds. Edwards R. W., Garrod D. J. (Oxford: Pergamon Press), 213–232.
Cushing D. H. (1990). Plankton production and year-class strength in fish populations: an update of the match/mismatch hypothesis. Adv. Mar. Biol. 26, 249–293. doi: 10.1016/S0065-2881(08)60202-3
Cushing D. H. (1996). Towards a science of recruitment in fish populations (Oldendorf: Ecology Institute).
Deniel C. (1990). Comparative study of growth of flatfishes on the west coast of Brittany. J. Fish Biol. 37 (1), 149–166. doi: 10.1111/j.1095-8649.1990.tb05936.x
Désaunay Y., Guérault D., Le Pape O., Poulard J. C. (2006). Changes in occurrence and abundance of northern/southern flatfishes over a 20-year period in a coastal nursery area (Bay of vilaine) and on the eastern continental shelf of the bay of Biscay. Sci. Mar. 70 (1), 193–200.
Dinis M. T. (1986). Quatre soleidae de l’estuaire du tage. reproduction etcroissance. essai d’elevage de solea senegalensis kaup (Brest: Université de Bretagne Occidentale).
Dorel D., Koutsikopoulos C., Desaunay Y., Marchand J. (1991). Seasonal distribution of young sole (Solea solea (L.)) in the nursery ground of the bay of vilaine (northern bay of Biscay). Neth. J. Sea Res. 27, 297–306. doi: 10.1016/0077-7579(91)90032-V
Duarte R., Azevedo M., Afonso-Dias M. (2009). Segmentation and fishery characteristics of the mixed-species multi-gear Portuguese fleet. ICES Mar. Sci. Symp. 66 (3), 594–606. doi: 10.1093/icesjms/fsp019
Encarnação J., Morais P., Baptista V., Cruz J., Teodósio M. A. (2019). New evidence of marine fauna tropicalization off the southwestern Iberian peninsula (Southwest Europe). Diversity 11 (4), 48. doi: 10.3390/d11040048
Erzini K. (2005). Trends in NE Atlantic landings (southern portugal): identifying the relative importance of fisheries and environmental variables. Fish. Oceanogr. 14 (3), 195–209. doi: 10.1111/j.1365-2419.2005.00332.x
Félix P. M., Vinagre C., Cabral H. N. (2011). Life-history traits of flatfish in the northeast Atlantic and Mediterranean Sea. J. Appl. Ichthyol. 27 (1), 100–111. doi: 10.1111/j.1439-0426.2010.01623.x
Fernández E., Cabal J., Acuña J. L., Bode A., Botas A., García-Soto C. (1993). Plankton distribution across a slope current-induced front in the southern bay of Biscay. J. Plankton Res. 15, 619–641. doi: 10.1016/j.csr.2006.08.010
Fiúza A. F. G. (1983). “Upwelling patterns off Portugal,” in Coastal upwelling: its sediment record. Eds. Suess E., Thiede J. (New York: Plenum Publishing), 85–98.
Fonds M. (1995). Effect of winter temperature on the brood success of flatfish. annual report 1995 (Texel: Netherlands Institute for Sea Research).
Froese R., Pauly D. (2016). FishBase (Stockholm: World Wide Web electronic publication). Available at: www.fishbase.org.
Fuiman L. A. (1997). What can flatfish ontogenies tell us about pelagic and benthic lifestyles? J. Sea Res. 37 (3-4), 257–267. doi: 10.1016/S1385-1101(97)00013-0
Gamito R., Teixeira C. M., Costa M. J., Cabral H. N. (2015). Are regional fisheries’ catches changing with climate? Fish. Res. 161, 207–216. doi: 10.1016/j.fishres.2014.07.014
Garcia A., Cortes D., Ramirez T., Giraldez A., Carpena A. (2003). Contribution of larval growth rate variability to the recruitment of the bay of malaga anchovy (SW Mediterranean) during the 2000-2001 spawning seasons. Sci. Mar. 67, 477–490. doi: 10.3989/scimar.2003.67n4477
Garrido S., Ben-Hamadou R., Santos A. M. P., Ferreira S., Teodósio M. A., Cotano U., et al. (2015). Born small, die young: Intrinsic, size-selective mortality in marine larval fish. Sci. Rep. 5, 17065. doi: 10.1038/srep17065
Gibson R. N. (1994). Impact of habitat quality and quantity on the recruitment of juvenile flatfishes. Neth. J. Sea Res. 32, 191–206. doi: 10.1016/0077-7579(94)90040-X
Gibson R. N. (1997). Behaviour and the distribution of flatfishes. J. Sea Res. 37 (3-4), 241–256. doi: 10.1016/S1385-1101(97)00019-1
Gibson R. N., Nash R. D., Geffen A. J., van der Veer H. W. (2014). Flatfishes: Biology and exploitation (New Jersey: John Wiley & Sons).
Guisande C., Cabanas J. M., Vergara A. R., Riveiro I. (2001). Effect of climate on recruitment success in Atlantic Iberian sardine Sardina pilchardus. mar. Ecol. Prog. Ser. 223, 243–250. doi: 10.3354/meps223243
Harley S. J., Myers R. A., Dunn A. (2001). Is catch-per-unit-effort proportional to abundance? Can. J. Fish. Aquat. 58, 1760–1772. doi: 10.1139/f01-112
Henderson P. (1998). On the variation in dab Limanda limanda recruitment: a zoogeographic study. J. Sea Res. 40, 131–142. doi: 10.1016/S1385-1101(98)00020-3
Henderson P. A., Seaby R. M. (1994). On the factors influencing juvenile flatfish abundance in the lower Severn estuary, england. neth. J. Sea Res. 32 (3-4), 321–330. doi: 10.1016/0077-7579(94)90009-4
Henderson P. A., Seaby R. M. (2005). The role of climate in determining the temporal variation in abundance, recruitment and growth of sole Solea solea in the Bristol channel. J. Mar. Biol. Assoc. U.K. 85, 197–204. doi: 10.1017/S0025315405011069h
Henriques M., Gonçalves E. J., Almada V. C. (2007). Rapid shifts in a marine fish assemblage follow fluctuations in winter sea conditions. Mar. Ecol. Prog. Ser. 340, 259–270. doi: 10.3354/meps340259
Herraiz I. G., Torres M. A., Farina A. C., Freirec J., Cancelo J. R. (2009). The NAO index and the long-term variability of Nephrops norvegicus population and fishery off West of Ireland. Fish. Res. 98, 1–7. doi: 10.1016/j.fishres.2009.03.006
Hjort J. (1914). Fluctuations in the great fisheries of northern Europe viewed in the light of biological research. Rapp. P.-v Réun. Cons. Int. Explor. Mer 20, 1–228.
Hurrell J. W. (1995). Decadal trends in the north Atlantic oscillation: regional temperatures and precipitation. Science 269 (5224), 676–679. doi: 10.1126/science.269.5224.676
Hurrell J. W., Kushnir Y., Ottersen G. (2003). An overview of the north Atlantic oscillation, the north Atlantic oscillation. Geophysical Monograph. 134, 1–35. doi: 10.1029/GM134
Huthnance J. M., Aken H. M. V., White M., Barton E. D. (2002). Ocean margin exchange – water flux estimates. J. Mar. Syst. 32, 107–137. doi: 10.1016/S0924-7963(02)00034-9
Jager Z. (2001). Transport and retention of flounder larvae (Platichthys flesus l.) in the dollard nursery (Ems estuary). J. Sea Res. 45, 153–171. doi: 10.1016/S1385-1101(01)00043-0
Lacroix G., Barbut L., Volckaert F. A. (2018). Complex effect of projected sea temperature and wind change on flatfish dispersal. Glob. Change Biol. 24 (1), 85–100. doi: 10.1111/gcb.13915
Laffargue P., Lagardère F., Rijnsdorp A. D., Fillon A., Amara R. (2007). Growth performances of juvenile sole Solea solea under environmental constraints of embayed nursery areas. Aquat. Living Resour. 20 (3), 213–221. doi: 10.1051/alr:2007034
Landaeta M. F., Castro L. R. (2002). Spring spawning and early nursery zone of the mesopelagic fish Maurolicus parvipinnis at the coastal upwelling zone off talcahuano, central Chile. Mar. Ecol. Prog. Ser. 226, 179–191. doi: 10.3354/meps226179
Lange U., Greve W. (1997). Does temperature influence the spawning time, recruitment and distribution of flatfish via its influence on the rate of gonadal maturation? Dtsch. Hydrogr. Z. 49 (2-3), 251–263. doi: 10.1007/BF02764037
Lefrançois C., Claireaux G. (2003). Influence of ambient oxygenation and temperature on metabolic scope and scope for heart rate in the common sole, Solea solea. mar. Ecol. Prog. Ser. 259, 273–284. doi: 10.3354/meps259273
Leggett W. C., Deblois E. (1994). Recruitment in marine fishes: is it regulated by starvation and predation in the egg and larval stages? Neth. J. Sea Res. 32, 119–134. doi: 10.1016/0077-7579(94)90036-1
Lehodey P., Alheit J., Barange M., Baumgartner T., Beaugrand G., Drinkwater, et al. (2006). Climate variability, fish and fisheries. J. Climate 19, 5009–5030. doi: 10.1175/JCLI3898.1
Leitão F., Alms V., Erzini K. (2014). A multi-model approach to evaluate the role of environmental variability and fishing pressure in sardine fisheries. J. Mar. Syst. 139, 128–138. doi: 10.1016/j.jmarsys.2014.05.013
Leitão F., Baptista V., Teodósio M. A., Hughes S. J., Vieira V., Chícharo L. (2016). The role of environmental and fisheries multi-controls in white seabream (Diplodus sargus) artisanal fisheries in Portuguese coast. Reg. Environ. Change 16, 163–176. doi: 10.1007/s10113-014-0726-5
Leitão F., Baptista V., Vieira V., Laginha Silva P., Relvas P., Teodósio M. A. (2019). A 60-year time series analyses of the upwelling along the Portuguese coast. Water 11 (6), 1285. doi: 10.3390/w11061285
Leitão F., Maharaj R. R., Vieira V. M., Teodósio A., Cheung W. W. (2018). The effect of regional sea surface temperature rise on fisheries along the Portuguese Iberian Atlantic coast. Aquat. Conserv.: Mar. Freshw. Ecosyst. 28 (6), 1351–1359. doi: 10.1002/aqc.2947
Le Pape O., Désaunay Y., Guérault D. (2003). Relationship between fluvial discharge and sole (Solea solea l.) recruitment in the bay of Biscay (France). ICES Mar. Sci. Symp. 219, 241–248.
Lloret J., Lleonart J., Solé I., Fromentin J. M. (2001). Fluctuations of landings and environment conditions in the north-western Mediterranean Sea. Fish. Oceanogr. 10 (1), 33–50. doi: 10.1046/j.1365-2419.2001.00151.x
Marchand J. (1991). The influence of environmental conditions on settlement, distribution and growth of 0-group sole (Solea solea (L.)) in a macrotidal estuary (Vilaine, France). Neth. J. Sea Res. 27 (3-4), 307–316. doi: 10.1016/0077-7579(91)90033-W
Martinho F., Dolbeth M., Viegas I., Baptista J., Cabral H. N., Pardal M. A. (2010). Does the flatfish community of the mondego estuary (Portugal) reflect environmental changes? J. Appl. Ichthyol. 26 (6), 843–852. doi: 10.1111/j.1439-0426.2010.01486.x
Martinho F., Dolbeth M., Viegas I., Cabral H. N., Pardal M. A. (2009). Environmental effects on the recruitment variability in nursery species. Estuar. Coast. Shelf. Sci. 83, 460–468. doi: 10.1016/j.ecss.2009.04.024
Mason E., Coombs S., Oliveira P. B. (2005). An overview of the literature concerning the oceanography of the eastern north Atlantic region. Relatório Científico-Técnico do Instituto Investigação das Pescas e do Mar 33, 58.
Melo J. L. B. S. (1989). Caracterização hidro-oceanográfica da ria Formosa. An. Inst. Hid. 10, 107–123.
Minami T., Tanaka M. (1992). Life history cycles in flatfish from the northwestern pacific, with particular reference to their early life histories. Neth. J. Sea Res. 29, 35–48. doi: 10.1016/0077-7579(92)90006-Z
Moita I. (1986). Plataforma continental. carta dos sedimentos superficiais. notícia explicativa da folha SED Vol. 7 (Lisbon: Instituto Hidrográfico).
Moita T., Oliveira P. B., Mendes J. C., Palma A. S. (2003). Distribution of chlorophyll-a and gymnodinium catenatum associated with coastal upwelling plumes off central Portugal. Acta Oecol. 24, 125–132. doi: 10.1016/S1146-609X(03)00011-0
Nash R. D. M., Geffen A. J. (2000). The influence of nursery ground processes in the determination of year-class strength in juvenile plaice Pleuronectes platessa l. in port Erin bay, Irish Sea. J. Sea Res. 44, 101–110. doi: 10.1016/S1385-1101(00)00044-7
Nielsen E., Bagge O., MacKenzie B. R. (1998). Wind-induced transport of plaice (Pleuronectes platessa): early life-history stages in the skagerrak-kattegat. J. Sea Res. 39, 11–28. doi: 10.1016/S1385-1101(97)00014-2
O’Connor M., Bruno J. F., Gaines S. D., Halpern B. S., Lester S. E., Kinlan B. P., et al. (2007). Temperature control of larval dispersal and the implications for marine ecology, evolution, and conservation. Proc. Natl. Acad. Sci. U. S. A. 104, 1266–1271. doi: 10.1073/pnas.0603422104
Pauly D. (1994). A framework for latitudinal comparisons of flatfish recruitment. Neth. J. Sea Res. 32, 107–118. doi: 10.1016/0077-7579(94)90035-3
Pauly D., Christensen V., Guénette S., Pitcher T. J., Sumaila U. R., Walters C. J. (2002). Towards sustainability in world fisheries. Nature 418, 689–695. doi: 10.1038/nature01017
Pauly D., Pullin R. S. V. (1988). Hatching time and spherical, pelagic, marine fish eggs in response to temperature and egg size. Environ. Biol. Fish. 22, 261–271. doi: 10.1007/BF00004892
Pepin P. (1991). Effect of temperature and size on development, mortality, and survival rates of the pelagic early life history stages of marine fish. Can. J. Fish. Aquat. Sci. 4, 503–518. doi: 10.1139/f91-065
Petitgas P., Rijnsdorp A. D., Dickey-Collas M., Engelhard G. H., Peck M. A., Pinnegar J. K., et al. (2012). Impacts of climate change on the complex life cycles of fish. Fish. Oceanogr. 22, 121–139. doi: 10.1111/fog.12010
Quéro J. C., Desoutter M., Lagardère F., UNESCO (1986). “Soleidae,” in Fishes of the north-eastern Atlantic and the Mediterranean. Eds. Whithead P. J. P., Bauchot M. L., Hureau J. C., Nielsen J., Tortonese E. (Paris: UNESCO), 1308–1324.
Ramos S., Ré P., Bordalo A. A. (2009). Environmental control on early life stages of flatfishes in the Lima estuary (NW Portugal). Estuar. Coast. Shelf Sci. 83 (2), 252–264. doi: 10.1016/j.ecss.2009.03.031
Ramos S., Ré P., Bordalo A. A. (2010). Recruitment of flatfish species to an estuarine nursery habitat (Lima estuary, NW Iberian peninsula). J. Sea Res. 64 (4), 473–486. doi: 10.1016/j.seares.2010.01.010
Ravier C., Fromentin J. M. (2001). Long-term fluctuations in the eastern Atlantic and Mediterranean bluefin tuna population. ICES Mar. Sci. Symp. 58 (6), 1299–1317. doi: 10.1006/jmsc.2001.1119
Relvas P., Barton E. D., Dubert J., Oliveira P. B., Peliz Á., da Silva J. C. B., et al. (2007). Physical oceanography of the western Iberia ecosystem: latest views and challenges. Prog. Oceanogr. 74, 149–173. doi: 10.1016/j.pocean.2007.04.021
Ribeiro F., Ramos S., Santos P. (1999). Evaluation of the amount of undersized sand sole solea lascaris (Risso 1810) caught in the Portuguese fisheries (Stockholm: Council Meeting of the International Council for the Exploration of the Sea).
Rice J., Cooper J. A. (2003). Management of flatfish fisheries – what factors matter? J. Sea Res. 50, 227–243. doi: 10.1016/j.seares.2003.07.001
Rijnsdorp A. D., Daan N., Dekker W., Poos J. J., van Densen W. L. T. (2007). Sustainable use of flatfish resources: Addressing the credibility crisis in mixed fisheries management. J. Sea Res. 57, 114–125. doi: 10.1016/j.seares.2006.09.003
Rijnsdorp A. D., van Beek F. A., Flatman S., Millner R. M., Riley J. D., Giret M., et al. (1992). Recruitment of sole stocks, Solea solea (L.), in the northeast Atlantic. Neth. J. Sea Res. 29 (1-3), 173–192. doi: 10.1016/0077-7579(92)90018-A
Rijnsdorp A. D., Vingerhoed B. (1994). The ecological significance of geographical and seasonal differences in egg size in sole (Solea solea (L.)). Neth. J. Sea Res. 32 (3/4), 255–270. doi: 10.1016/0077-7579(94)90003-5
Rodwell M. J., Rodwell D. P., Folland C. K. (1999). Oceanic forcing of the wintertime north Atlantic oscillation and European climate. Nature 398, 320–323. doi: 10.1038/18648
Santos A. M. P., Chícharo M. A., dos Santos A., Moita T., Oliveira P. B., Peliz A., et al. (2007). Physical–biological interactions in the life history of small pelagic fish in the western Iberia upwelling ecosystem. Prog. Oceanogr. 74 (2-3), 192–209. doi: 10.1016/j.pocean.2007.04.008
Santos M. B., González-Quirós R., Riveiro I., Cabanas J. M., Porteiro C., Pierce G. J. (2012). Cycles, trends, and residual variation in the Iberian sardine (Sardina pilchardus) recruitment series and their relationship with the environment. ICES J. Mar. Sci. 69, 739–750. doi: 10.1093/icesjms/fsr186
Santos M. N., Lino P. G. (2010). Aspects of the fishery, maturation and net selectivity of the wedge sole (Dicologoglossa cuneata) off south-western Iberia: implications for fishery management. J. Mar. Biol. Assoc. U. K. 90 (7), 1465–1474. doi: 10.1017/s0025315409991329
Santos A., Miguel P., Borges M. F., Groom S. (2001). Sardine and horse mackerel recruitment and upwelling off. ICES J. Mar. Sci. 58, 589–596. doi: 10.1006/jmsc.2001.1060
Santos A. M. P., Peliz A. J., Dubert J., Oliveira P. B., Angélico M. M., Ré P. (2004). Impact of a winter upwelling event on the distribution and transport of sardine (Sardina pilchardus) eggs and larvae off western Iberia: a retention mechanism. Cont. Shelf Res. 24, 149–165. doi: 10.1016/j.csr.2003.10.004
Sogard S., Able K., Hagan S. (2001). Long-term assessment of settlement and growth of juvenile winter flounder (Pseudopleuronectes americanus) in new Jersey estuaries. J. Sea Res. 45, 189–204. doi: 10.1016/S1385-1101(01)00048-X
Solow A. R. (1994). Detecting change in the composition of multispecies community. Biometrics 50 (2), 556–565.
Sousa P., Azevedo M., Gomes M. C. (2005). Demersal assemblages off Portugal: mapping, seasonal, and temporal patterns. Fish. Res. 75 (1-3), 120–137. doi: 10.1016/j.fishres.2005.03.012
Tamburri M. N., Finelli C. M., Wethey D. S., Zimmer-Faust R. K. (1996). Chemical induction of larval settlement behavior in flow. Biol. Bull. 191, 367–373. doi: 10.2307/1543009
Tanaka M. (1985). Factors affecting the inshore migration of pelagic larval and demersal juvenile red sea bream Pagrus major to a nursery ground. Trans. Am. Fish. Soc 114, 471–477. doi: 10.1577/1548-8659(1985)114<471:FATIMO>2.0.CO;2
Teal L. R., de Leeuw J. J., van der Veer H. W., Rijnsdorp A. D. (2008). Effects of climate change on growth of 0-group sole and plaice. Mar. Ecol. Prog. Ser. 358, 219–230. doi: 10.3354/meps07367
Teixeira C. M., Batista M. I., Cabral H. N. (2011). Landing profiles and typologies of flatfish fisheries on the Portuguese coast. Aquat. Living Resour. 24 (2), 169–182. doi: 10.1051/alr/2011134
Teixeira C. M., Cabral H. N. (2009). Time series analysis of flatfish landings in the Portuguese coast. Fish. Res. 96 (2-3), 252–258. doi: 10.1016/j.fishres.2008.12.004
Teixeira C. M., Gamito R., Leitão F., Cabral H. N., Erzini K., Costa M. J. (2014). Trends in landings of fish species potentially affected by climate change in Portuguese fisheries. Reg. Environ. Change 14 (2), 657–669. doi: 10.1007/s10113-013-0524-5
Teixeira C. M., Gamito R., Leitão F., Murta A. G., Cabral H. N., Erzini K., et al. (2015). Environmental influence on commercial fishery landings of small pelagic fish in Portugal. Reg. Environ. Change 16, 709–716. doi: 10.1007/s10113-015-0786-1
Tunberg B. G., Nelson W. G. (1998). Do climatic oscillations influence cyclical patterns of soft bottom macrobenthic communities on the Swedish west coast? Mar. Ecol. Prog. Ser. 170, 85–94. doi: 10.3354/meps170085
Ullah H., Leitão F., Baptista V., Chícharo L. (2012). An analysis of the impacts of climatic variability and hydrology on the coastal fisheries, Engraulis encrasicolus and Sepia officinalis, of Portugal. Ecohydrol. Hydrobiol. 12 (4), 337–352. doi: 10.2478/v10104-012-0026-y
van der Veer H. W., Berghahn R., Miller J. M., Rijnsdorp A. D. (2000). Recruitment in flatfish, with special emphasis on north Atlantic species: Progress made by the flatfish symposia. ICES Mar. Sci. Symp. 57, 202–215. doi: 10.1006/jmsc.1999.0523
van der Veer H. W., Bergman M. J. N., Dapper R., Witte J. I. J. (1991). Population dynamics of an intertidal 0-group flounder Platichthys flesus population in the western Dutch wadden Sea. Mar. Ecol. Prog. Ser. 73, 141–148.
van der Veer H. W., Pihl L., Bergman M. J. N. (1990). Recruitment mechanisms in north Sea plaice Pleuronectes platessa. Mar. Ecol. Prog. Ser. 64, 1–12.
van der Veer H. W., Witte J. I. J. (1999). Year-class strength of plaice Pleuronectes platessa in the southern bight of the north Sea: a validation and analysis of the inverse relationship with winter seawater temperature. Mar. Ecol. Prog. Ser. 184, 245–257. doi: 10.3354/meps184245
Vila-Concejo A., Ferreiro Ò., Matias A., Dias J. M. A. (2003). The first two years of an inlet: sedimentary dynamics. Cont. Shelf Res. 23, 1425–1445. doi: 10.1016/S0278-4343(03)00142-0
Vinagre C., Amara R., Maia A., Cabral H. N. (2008). Latitudinal variation in spawning season and growth of 0-group sole, Solea solea (L.). Estuar. Coast. Shelf Sci. 78, 521–528. doi: 10.1016/j.ecss.2008.01.012
Vinagre C., Cabral H. N. (2008). Prey consumption by the juvenile soles, Solea solea and Solea senegalensis, in the tagus estuary, Portugal. Estuar. Coast. Shelf Sci. 78 (1), 45–50. doi: 10.1016/j.ecss.2007.11.009
Vinagre C., Costa M. J., Cabral H. N. (2007). Impact of climate and hydrodynamics on sole larval immigration towards the tagus estuary, Portugal. Estuar. Coast. Shelf Sci. 75 (4), 516–524. doi: 10.1016/j.ecss.2007.05.035
Vinagre C., Maia A., Amara R., Cabral H. N. (2013). Spawning period of Senegal sole, Solea senegalensis, based on juvenile otolith microstructure. J. Sea Res. 76, 89–93. doi: 10.1016/j.seares.2012.11.004
Vinagre C., Santos F. D., Cabral H. N., Costa M. J. (2009). Impact of climate and hydrology on juvenile fish recruitment towards estuarine nursery grounds in the context of climate change. Estuar. Coast. Shelf. Sci. 85 (3), 479–486. doi: 10.1016/j.ecss.2009.09.013
Warlen S. M., Able K. W., Laban E. H. (2002). Recruitment of larval Atlantic menhaden (Brevoortia tyrannus) to north Carolina and new Jersey estuaries: evidence for larval transport northward along the east coast of the united states. Fish. Bull. 100, 609–623.
Werner F. E., Ouinlan J. A., Blanton B. O., Luettich R. A. Jr. (1997). The role of hydrodynamics in explaining variability in fish populations. J. Sea Res. 37, 195–212. doi: 10.1016/S1385-1101(97)00024-5
Witbaard R., Duineveld G. C. A., Amaro T., Bergman M. J. N. (2005). Growth trends in three bivalve species indicate climate forcing on the benthic ecosystem in the southeastern north Sea. Climate Res. 30, 29–38. doi: 10.3354/cr030029
Yamashita Y., Tanaka M., Miller J. M. (2001). Ecophysiology of juvenile flatfish in nursery grounds. J. Sea Res. 45, 205–218. doi: 10.1016/S1385-1101(01)00049-1
Yeung C., Yang M. S. (2017). Habitat quality of the coastal southeastern Bering Sea for juvenile flatfishes from the relationships between diet, body condition and prey availability. J. Sea Res. 119, 17–27. doi: 10.1016/j.seares.2016.10.002
Zuur A. F., Fryer R. F., Jolliffe I. T., Decker R., Beukema J. J. (2003b). Estimating common trends in multivariate time series using dynamic factor analysis. Environmetrics 14 (7), 665–685. doi: 10.1002/env.611
Zuur A. F., Ieno E. N., Elphick C. S. (2010). A protocol for data exploration to avoid common statistical problems. Methods Ecol. Evol. 1, 3–14. doi: 10.1111/j.2041-210X.2009.00001.x
Zuur A. F., Pierce G. J. (2004). Common trends in northeast Atlantic squid time series. J. Sea Res. 52 (1), 57–72. doi: 10.1016/j.seares.2003.08.008
Keywords: Pleuronectiformes, environmental pressure, recruitment, time-series analyses, multi-model approach
Citation: Baptista V, Blasco IP, Bueno-Pardo J, Teodósio MA and Leitão F (2022) Environmental variability and fishing effects on artisanal flatfish fisheries along the Portuguese coast. Front. Mar. Sci. 9:844158. doi: 10.3389/fmars.2022.844158
Received: 27 December 2021; Accepted: 25 August 2022;
Published: 15 September 2022.
Edited by:
Tomaso Fortibuoni, Istituto Superiore per la Protezione e la Ricerca Ambientale (ISPRA), ItalyReviewed by:
Sanja Matic-Skoko, Institute of Oceanography and Fisheries (IZOR), CroatiaCopyright © 2022 Baptista, Blasco, Bueno-Pardo, Teodósio and Leitão. This is an open-access article distributed under the terms of the Creative Commons Attribution License (CC BY). The use, distribution or reproduction in other forums is permitted, provided the original author(s) and the copyright owner(s) are credited and that the original publication in this journal is cited, in accordance with accepted academic practice. No use, distribution or reproduction is permitted which does not comply with these terms.
*Correspondence: Francisco Leitão, ZmxlaXRhb0B1YWxnLnB0
Disclaimer: All claims expressed in this article are solely those of the authors and do not necessarily represent those of their affiliated organizations, or those of the publisher, the editors and the reviewers. Any product that may be evaluated in this article or claim that may be made by its manufacturer is not guaranteed or endorsed by the publisher.
Research integrity at Frontiers
Learn more about the work of our research integrity team to safeguard the quality of each article we publish.