- 1Osaka Museum of Natural History and C/O Institute for East China Sea, Nagasaki University, Nagasaki, Japan
- 2Department of Biology, Osaka Institute of Technology, Osaka, Japan
- 3Center for Marine Environmental Studies, Ehime University, Ehime, Japan
Stratigraphic cluster analysis using aquatic palynomorphs preserved in the core sediments revealed a rapid eutrophication due to anthropogenic activities from the mid 1960s in Beppu Bay, East Kyushu, Japan. These assemblages were divided into three major units: BP-I, BP-II and BP-III, and also only dinoflagellate cyst assemblages were divided into the following four units in Beppu Bay: BP-A, BP-B, BP-C, and BP-D. Unit boundaries based on aquatic palynomorphs and dinoflagellate cysts were different except in the upper part, BP-III and BP-D, both of which clearly indicated anthropogenic eutrophication in both sea water and bottom sediments. On the other hand, in dinoflagellate cyst assemblages, Unit BP-A was characterized by stable occurrence of Spiniferites bulloideus and Spiniferites hyperacanthus, Lingulodinium machaerophorum of Gonyaulacales, and reduction of heterotrophic Peridinioid Brigantedinium spp. In Unit BP-C there was a clear decrease of L. machaerophorum. Unit BP-B was characterized by decreases of S. bulloideus, S. hyperacanthus, and L. machaerophorum, and little increase of Spniferites bentori. Unit BP-C was characterized by an increase in S. bulloideus and heterotrophic Peridinioid Echinidinium spp. Unit BP-D was subdivided into Subunit BP-D1 where dinoflagellate cysts showed a marked increase in S. bulloideus accompanied by the appearance of L. machaerophorum and Tuberculodinium vancampoae, and Subunit PB-D2 where there was a decrease of total dinoflagellate cysts. From the dinoflagellate cyst assemblages, the marine environment of the period of BP-A Unit was suggested to be warm and stable. However, L. machaerophorum started to decrease in BP-B. The clear decrease of L. machaerophorum suggest that the marine environment became cooler than that of Unit BP-A. Significant increases of S. bulloideus, S. hypearcanthus, L. machaerophorum, T. vancampoae, Brigantedinium spp., and Polykrikos kofoidii were characteristic of Unit BP-D. The increase in total dinoflagellate cyst density and the increase of the ratio of heterotrophic dinoflagellate cysts in Subunit BP-D1 are manifestations of the Oslo fjord Signal and Heterotroph Signal, respectively. In addition, the decrease in microforaminiferal lining that continued from Unit BP-C to Unit BP-D might indicate deterioration of the bottom sediment environment.
Introduction
The remains of organisms that live by aquatic environments are transported and preserved in the sediments in coastal areas. In particular, large amounts of microscopic biological remains (microfossils) are preserved. Among these, microfossils with organic walls are collectively called palynomorphs (Travers, 2007). Among palynomorphs, dinoflagellate cysts appeared in the Triassic period of the Mesozoic era and from then onward and have contributed greatly to the establishment of biostratigraphy and the elucidation of changes in the paleo-ocean environment, as well as diatoms, foraminifera, and coccoliths, which have mineral shells (e.g., Guiot and de Vernal, 2007; Fensome et al., 2009; Zonneveld et al., 2013; Williams et al., 2017; Penaud et al., 2018).
Dinoflagellate cysts are dormant zygotes formed by sexual reproduction with a size of 10–100 μm (Matsuoka and Fukuyo, 2000) and have a cell wall composed of a cellulose-based biopolymer (Kokinos et al., 1998; Versteegh et al., 2012). Since this cell wall is physically and chemically resistant, it is conserved in various fine-grained sediments (Zonneveld et al., 1997), especially in coastal sediments. Due to these characteristics, it has been used to elucidate changes in oceanic climate and biological production on the order of decades to hundreds of years (Thorsen and Dale, 1998; Harland et al., 2013). Focusing on the characteristics of production of dinoflagellates, which are primary producers and also include various heterotrophic species, has also contributed to clarifying changes in lower-order ecosystems associated with changes in nutrient levels (eutrophication) based on changes of dinoflagellate cyst assemblages (Dale et al., 1999; Matsuoka, 1999; Matsuoka et al., 2003; Pospelova et al., 2005).
In recent years, research on palynomorphs other than dinoflagellate cysts, including microforaminiferal linings, crustacean resting eggs, resting cysts and lorica of ciliates, turbellarian egg capsules, testate amoebas, and others has progressed (Belmonte and Rubino, 2019; McCarthy et al., 2021; Mudie et al., 2021; Shumilovskikh et al., 2021). Research on these microfossils is expected to play an important role in studying coastal environmental changes.
The coastal area is the main location for ocean-related human activities, and it responds sensitively to environmental changes in the land and sea areas. Recently, harmful algal blooms have occurred frequently, and their damage to human health and role in decreased biological production are of great concern (IOC1). Marine coastal ecosystems are changing, and it is argued that such changes have been accelerating in recent years (e.g., Clarke et al., 2006; Köster et al., 2007; Maier et al., 2009; Caffrey and Murrell, 2016). Thus, it is necessary to know the changes in ecosystems over time in order to clarify when and to what extent they have progressed. Since the changes in the ecosystem in the coastal areas are extremely regional phenomena, we must clarify them locally.
In order to clarify the beginning of the change in the ecosystem and the actual condition of the change, we investigated the aquatic palynomorphs preserved in the sediment cores collected in Beppu Bay, Western Japan. Beppu Bay is one of most investigated regions for clarifying human-induced environmental changes from the view point of environmental sciences and is regarded as one of candities for GSSP (global boundary stratotype section and point) (Anthropocene Working Group, 2020; Kuwae et al., 2022). However, although there are many related studies of sediment cores from a view point of geochemistry, only stratigraphic changes of the environments using diatoms were investigated at this point in time (Kuwae et al., 2022). In this article, we try to clarify the historical changes of coastal marine environments based on the findings about aquatic palynomorph assemblages and to discuss the primary factors leading to these changes in Beppu Bay for understanding how and when anthropogenic activities affected the low-trophic ecosystems. In addition, important aquatic palynomorphs which are not so much familiar to other than palynologists are briefly introduced for future study.
Materials and Methods
Environmental Setting
Beppu Bay, in the western Seto Inland Sea, northeast Kyushu, Japan (Figure 1) is one of the enclosed bays indirectly affected by the warm Kuroshio Current through Bungo Channel and Hyuga Nada. This bay also is the only basin located within the Japanese coastal zone which exhibits clear autumnal oxygen depletions in bottom waters (0–0.2 mg L–1) (Kameda and Fujiwara, 1995) as well as annual varve sedimentation during the late Holocene in the deepest area (Kuwae et al., 2013). Sediments in the area are continuously deposited with relatively high sedimentation rates (of 0.23–0.30 cm year–1) (Kuwae et al., 2013; Yamada et al., 2016). The high sedimentation rates have allowed for the precise dating of surface sediments using lead-210 (210Pb) (Takahashi et al., 2020) and the reconstruction of excellent proxy records of anthropogenic markers, including increases in concentrations of cesium-137 (137Cs) (Kuwae et al., 2013; Takahashi et al., 2020), polychlorinated biphenyls (PCB; Takahashi et al., 2020), dichloro-diphenyl-trichloroethane (DDT; Nishimuta et al., 2020), brominated fire retardants (Hoang et al., 2021) and microplastics (Masumoto et al., 2018) since year 1950. There are cities in the coastal area around Beppu Bay, Oita City in the south and Beppu City in the west, and an orchard area in the north (Kuwae et al., 2022). The Ohno and Oita Rivers, which drain the Oita Plain, are major rivers discharging to Beppu Bay (Yamada et al., 2016). Thus, this bay is one of most well-investigated sites for understanding anthropogenic activities in the world (Anthropocene Working Group, 2020).
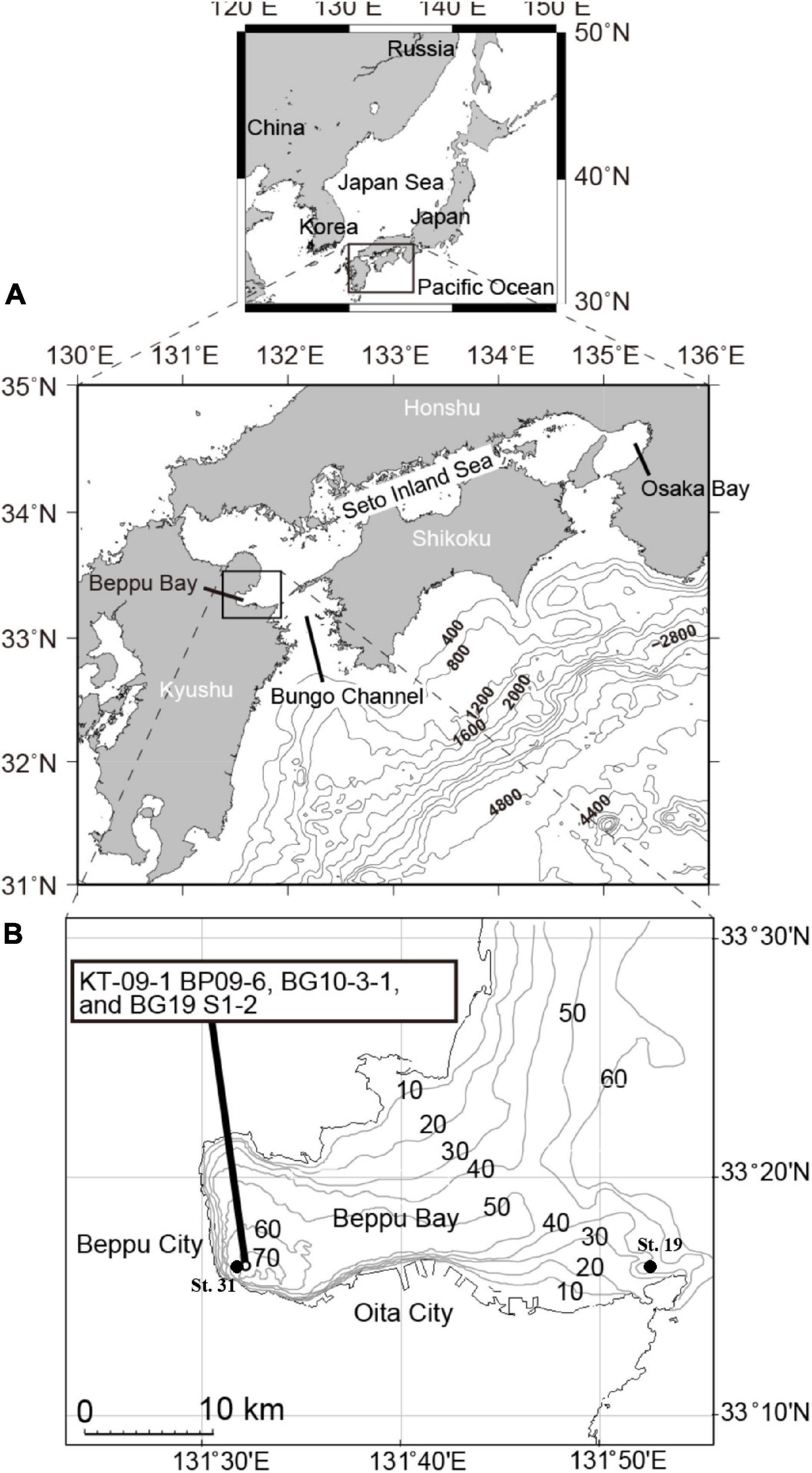
Figure 1. (A,B) Sampling location map and operation points for the shallow sea constant line survey (Senkai Teisen Chosa (STC) in Japanese) carried out by the Oita Prefectural Fisheries Research Department.
Sampling of Sediment Cores
Three cores, namely KT-09-1 BP09-6, BG10-3-1, and BG19 S1-2, collected at the innermost part of Beppu Bay on 2009/3/8, 2010/9/10, 2019/9/10, respectively (Figure 1 and Supplementary Material 1) were obtained for palynomorph analysis. The respective cores were collected using a 10-m-long piston core, a 100-cm-long gravity core (HRL, RIGO Co., Ltd., Saitama, Japan), and a 100-cm-long multiple corer (Ashra, RIGO Co., Ltd., Saitama, Japan).
All of the cores consisted primarily of hemipelagic silty to clayey sediments and a few millimeters or centimeters thickness-event layers with high-density, high-magnetic susceptibility, and coarser grains than those in hemipelagic layers (i.e., turbidites, which were probably formed by flooding and earthquakes). Since the event stratigraphy and chronology at the deepest site in Beppu Bay is well known, these event layers could be correlated between cores at the deepest site in Beppu Bay by lithological features and stratigraphy on visual inspection, CT images, and magnetic susceptibility and each event layer was named as a specific identification code (e.g., event (Ev) 0, 1, 2, 0a, 0b, and so on, in Supplementary Material 2; Kuwae et al., 2013). The ages of these event layers in the sediment (Supplementary Material 3) were determined in previous studies (Kuwae et al., 2013; Takahashi et al., 2020), and were used as time markers and for dating the core samples used in this study.
Samples for the palynomorph analysis were obtained from the stratigraphic sequence which was dated back to 1000 years using core samples from event (Ev) 8c to Ev 0 for BP09-6, Ev 0 to Ev 0c for BG10-3-1, and from Ev 0c to Ev -1cU.
Palynomorph Analysis
Each sample was divided into portions that were then treated according to the method of Matsuoka and Fukuyo (2000) described below to prepare a concentrated sample for observation of palynomorphs.
About 1 to 2 g of a wet sample was picked up from the divided samples and transferred to a 15-ml chemical-resistant plastic tube. After desalting with distilled water, the sample was treated with hydrochloric acid (37%) and hydrofluoric acid (46%) for 24 h to remove calcareous and siliceous particles, respectively. The sample was fractionated using a sieve with a mesh size of 125 μm and then a mesh size of 10 μm, and distilled water was added to the residue on the sieve with a mesh size of 10 μm to prepare a purified sample for observing palynomorphs. All processes were carried out at the room temperature.
A part of the concentrated sample for observation was taken with a micropipette (GILSON, PIPETMAN, P-1000), dropped onto a slide glass, and palynomorphs were identified and counted using an upright microscope (ASONE Digital Biological Microscope, DN-107T) with 400 to 1000 x magnification.
For calculating water content of the samples, the collected columnar sediment cores were cut in half vertically, and a 1cc cube was pushed into the cross section to collect samples. And then their wet weight was measured. After drying the cube at 50°C for 2 days in dry oven, the dry weight of the cub was measured.
A minimum of 200 palynomorphs were counted per sample to obtain homogeneous data for the statistical analyses.
Age Determination of the Cores
Ages of event layers (Supplementary Material 3) have been determined in previous studies using the constant rate of supply (CRS) model of 210Pb dating (Appleby and Oldfield, 1978) for sediments above Event 0a (R7) (Takahashi et al., 2020) and 14C wiggle match-dating methods (Kuwae et al., 2013) below Event 0a and were used to date the core samples in this study. The ages of events determined by the CRS model were 2005 ± < 1 for Event -1c (R11), 1994 ± < 1 for Event -1b (R9), 1968 ± 4 for Event -1a (R8), and 1925 ± 10 to 30 for Event 0a (R7) (Takahashi et al., 2020). The uncertainty of 14C wiggle match-based age was less than 25 years (Kuwae et al., 2013). The age of each sample was determined by linear-interpolation of the ages and depths of two successive events.
Data of Dissolved Oxygen and Chemical Oxygen Demand
Data of Dissolved Oxygen (DO) and Chemical Oxygen Demand (COD) in the inner and mouth parts of Beppu Bay (St. 31 and St. 19 of the shallow sea constant line survey (Senkai Teisen Chosa; STC) in Japanese) respectively measured at STC carried out by the Oita Prefectural Fisheries Research Department was employed, however, these data were left from year 1972 onward only.
Statistical Analysis
In order to determine temporal changes in coastal aquatic biota of Beppu Bay, stratigraphic changes in aquatic palynomorph assemblages were statistically analyzed using total count numbers of each palynomorphs and total numbers of major palynomorphs including dinoflagellate cysts with software Past 42 under stratigraphic clustering of paired group with Bray-Curtis similarity index. In the case of dinoflagellate cyst assemblages, relative abundances of each species were statistically analyzed in the same way independently. Also, to know temporal change of dissolved oxygen at the bottom layer of sea water, the original data were analyzed with PC-ORD 73.
Results
Aquatic Palynomorph Assemblage
Major aquatic palynomorphs preserved in sediments are summarized in Table 1 and specific species needed further study are introduced in Supplementary Material 4. The main components of aquatic palynomorphs in Beppu Bay were dinoflagellate cysts, microforaminiferal linings, and crustacean resting eggs, which accounted for more than 90% of the total aquatic palynomorph assemblages as shown in Figure 2, Table 2, and Supplementary Material 5. Microforaminiferal linings sometimes accounted for more than 50% of total palynomorphs in the middle and lower depth (for example, year 1395 and 1933), but they decreased rapidly after year 1969. Dinoflagellate cysts were 40 to 50% in the lower middle depth and 60% or more in the upper depth (1979 and 2003). The maximum output of aquatic palynomorphs was 8863 palynomorphs/g in year 1771, the minimum was 2203 palynomorphs/g in year 1960, and the average was 4878 palynomorphs/g. After the maximum output was recorded in year 1771, it decreased significantly after year 1824. After a slight increase from year 1880 to 1933, the minimum output was recorded in year 1960. Furthermore, it increased toward year 1979 and decreased after that. These increases and decreases were mainly due to the production of dinoflagellate cysts and microforaminiferal linings.
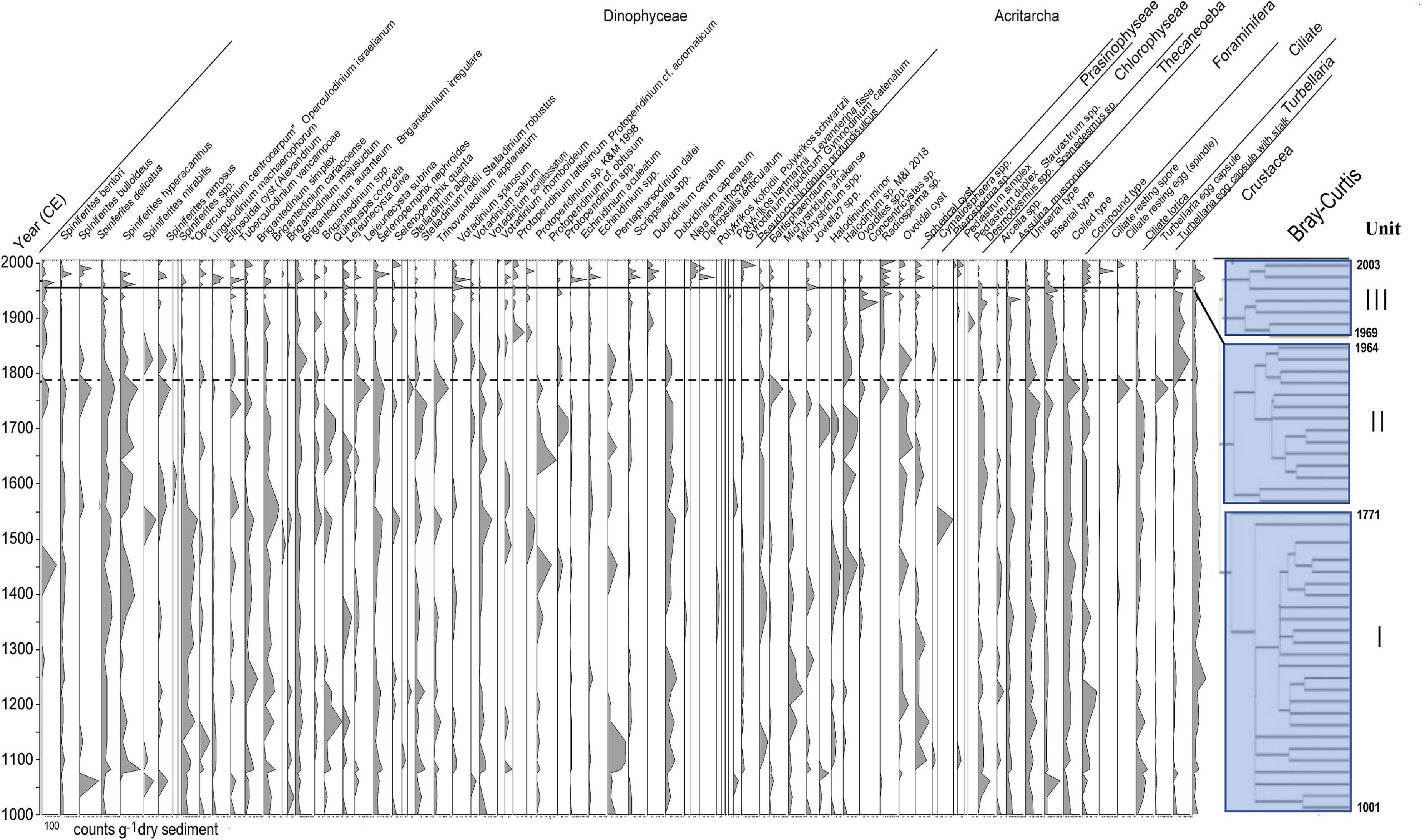
Figure 2. Stratigraphic distribution of all aquatic palynomorphs observed in the core samples of Beppu Bay and Unit division based on stratigraphic cluster analysis using Bray-Curtis method.
The results of the cluster analysis using the aquatic palynomorph assemblage data of Beppu Bay core revealed that these palynomorph assemblages were stratigraphically divided into three major units: BP- I Unit, BP- II Unit, and BP- III Unit. The boundaries were placed at year 1771 between BP- I Unit and BP- II Unit, and year 1964 between BP- II Unit and BP- III Unit (Figure 2).
Dinoflagellate Cysts (Figures 3, 4(1-11), 5, 6))
The average concentration of dinoflagellate cysts appearing throughout all samples was 1954 cysts/g, accounting for about 20% (year 1933) to about 70% (year 2003) of the total aquatic palynomorph assemblage. The maximum number of dinoflagellate cysts was 3546 cysts/g in year 1984, and the minimum number was 661 cysts/g in year 1955. There was a marked decrease in dinoflagellate cysts between year 1854 and 1873, and an increase between year 1960 and 1979.
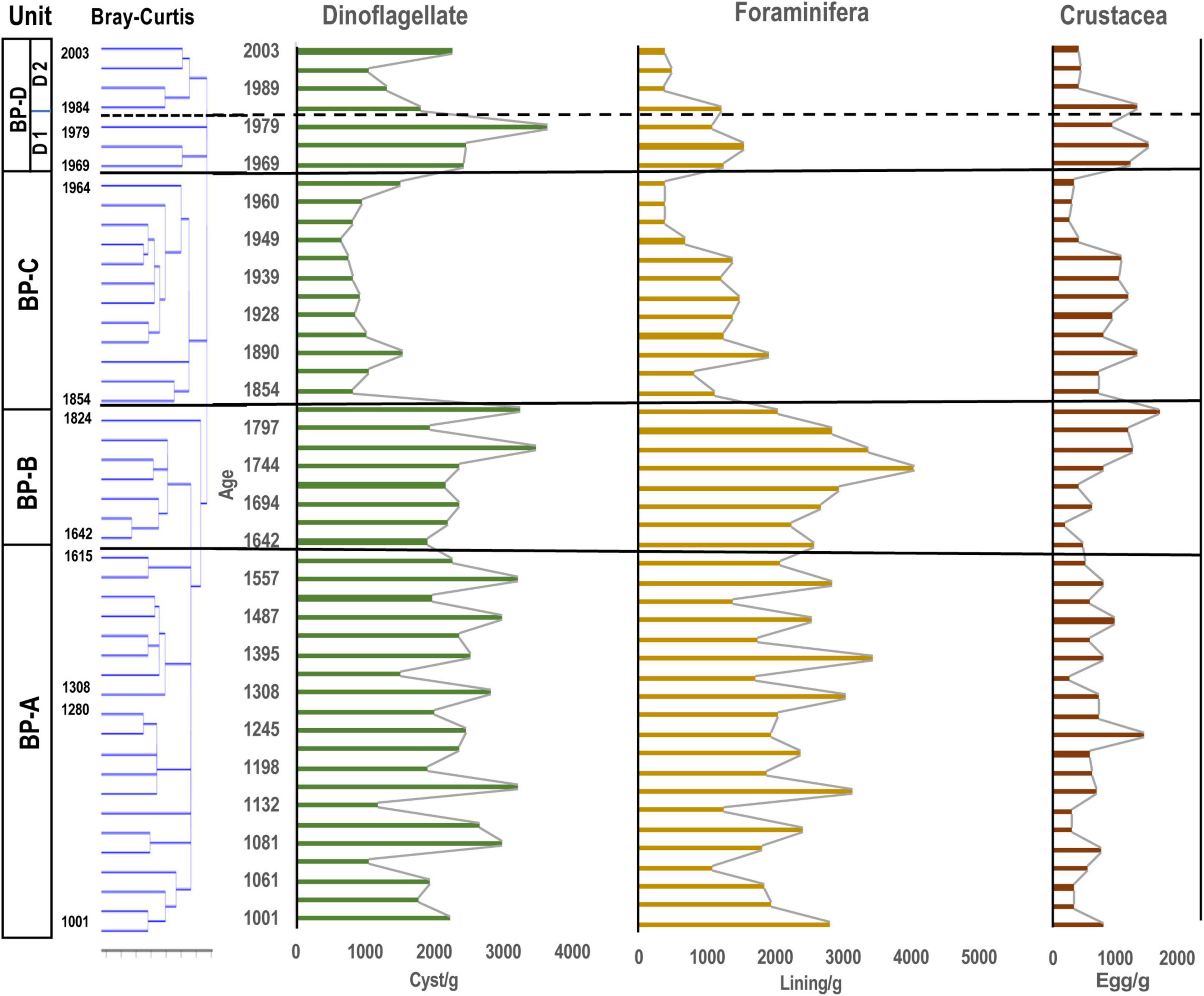
Figure 3. Stratigraphic distribution of major aquatic palynomorphs (dinoflagellate cysts, microforaminiferal linings and crustacean resting eggs) observed in the core samples of Beppu Bay. Unit division in the left column based on the result for dinoflagellate cyst assemblages according to stratigraphic cluster analysis using Bray-Curtis method.
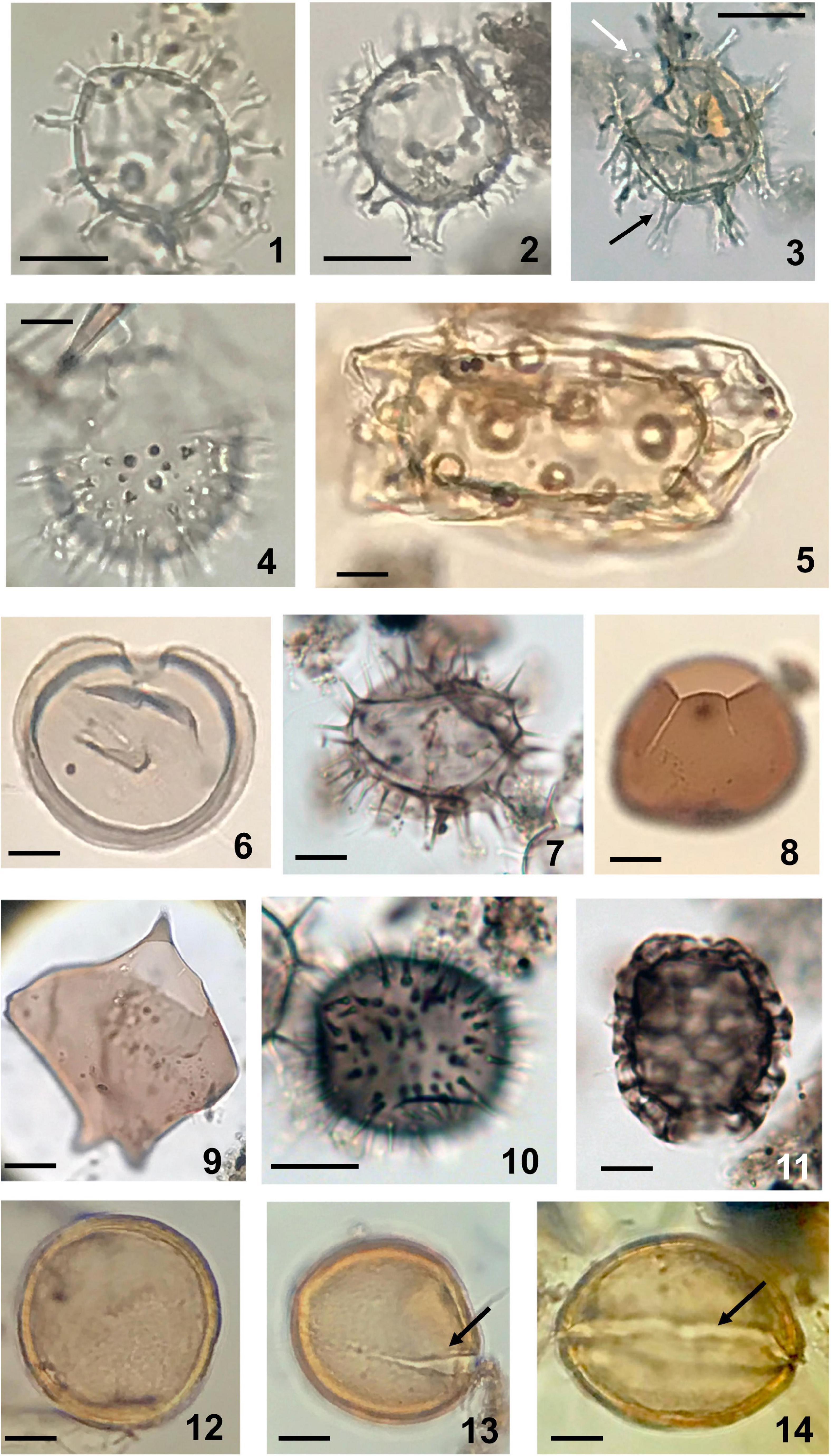
Figure 4. Dinoflagellate cysts (1-11) and acritarch (112-14) observed in sediments of Beppu Bay. (1) Spiniferites bulloideus (Deflandre and Cookson) Sarjeant; photosynthetic. Sample BG19 St 1 2 10-11. (2) Spiniferites delicatus Reid; photosynthetic. Sample BG19 St 1-2 10-11. (3) Hiddenocysta matsuokae P. Gurdebeke, V. Pospelova, K.N. Mertens, and S. Louwye; probably photosynthetic, black arrow indicating fenestrate process, white arrow indicating larger precingular archeopyle. In statistical analysis this species is included in Spiniferites spp. Sample BG19 St 1-2 14-15. (4) Lingulodinium machaerophorum (Deflandre and Cookson) Wall; photosynthetic. Sample BG19 St 1-2 14-15. (5) Tuberculodinium vancampoae Rossignol; photosynthetic. Sample BG19 St 1-2 28-29. (6) Selenopemphix nephroides Benedeck; heterotrophic. Sample BG19 St 1-2 10-11. (7) Selenopemphix quanta (M.R. Bradford) Matsuoka; heterotrophic. Sample BG19 St 1-2 14-15. (8) Votadinium calvum Reid; heterotrophic. Sample BG19 St 1-2 64-65. (9) Cyst of Protoperidinium latissimum* (Kofoid) Balech; heterotrophic. Sample BP09-6 3-22. (10) Cyst of Niea acanthocysta* (H. Kawami, M. Iwataki, and K. Matsuoka) T. Liu, K.N. Mertens, and H. Gu; heterotrophic. Sample BG19 St 1-2 14-15. (11) Cyst of Polykrikos kofoidii* Chatton; heterotrophic. Sample BG 19 St1-2 14-15. (12-14) Acritarch, Joviella? sp.; 12; Sample BP 09 4-53, 13; Sample BP 09 4-60, 14; Sample BP 09 4-81. Scale bars; 20 μm.
The photosynthetic group mainly includes species belonging to Gonyaulacales, Peridiniales such as Scrippsiella* and Gymnodiniales such as Levanderina fissa*, Pseudocochlodinium profundisulcus* and Gymnodinium catenatum*. The relative contribution of all dinoflagellate cysts was 61% (year 1979) to 12% (year 1890) of the total palynomorphs observed. In the heterotrophic group, other Peridiniales (Protoperidinioid, Diplopsalid) and Polykrikos* of Gymnodiniales were the main components, and they accounted for more than 50% of all dinoflagellate cysts except for a few samples with lower counts.
In Gonyaulacales, Spiniferites (mainly S. bulloideus and S. hyperacanthus) were dominant, accounting for more than 90% of Gonyaulacales in year 1979. In addition, S. bentori constituted nearly 20% from year 1964 to 1984. Lingulodinium machaerophorum was abundant before year 1824, accounting for more than 40% of Gonyaulacales in year 1222 and 1557. Tuberculodinium vancampoae was also observed in almost all samples, although its densities were relatively low. The appearance of Operculodinium centrocarpum sensu Wall and Dale was very low.
In the Peridiniales, Brigantedinium spp. which accounted for about 70% of Peridiniales throughout this taxon, occurred in all samples. In Brigantedinium, species that could be identified based on the archeopyle morphology and cyst size were B. simplex, B. cariacoense, B. majusculum, and B. irregulare. In other Peridiniales, various cysts belonging to the family Protoperidiniaceae occurred. These were Lejeunecysta subrina, Quinquecuspis concreta, Selenopemphix nephroides, S. quanta, Stelladinium abei, S. robustus, Trinovantedinium applanatum, Votadinium spinosum, V. rhomboideum, Echinidinium aculeatum, Echinidinium spp., and others. They constituted around 10% of dinoflagellate cyst assemblages and did not become dominant. In addition, Dubridinium cavatum, Niea acanthocysta*, and others frequently appeared in the Diplopsalid group, although they constituted only a few percent.
In the Gymnodiniales, a small amount of Polykrikos hartmannii* appeared in year 1395 and year 1452, but after year 1955, it occurred in a few percent of all dinoflagellate cyst assemblages. There were many heterotrophic Polykrikos kofoidii* (Figure 5).
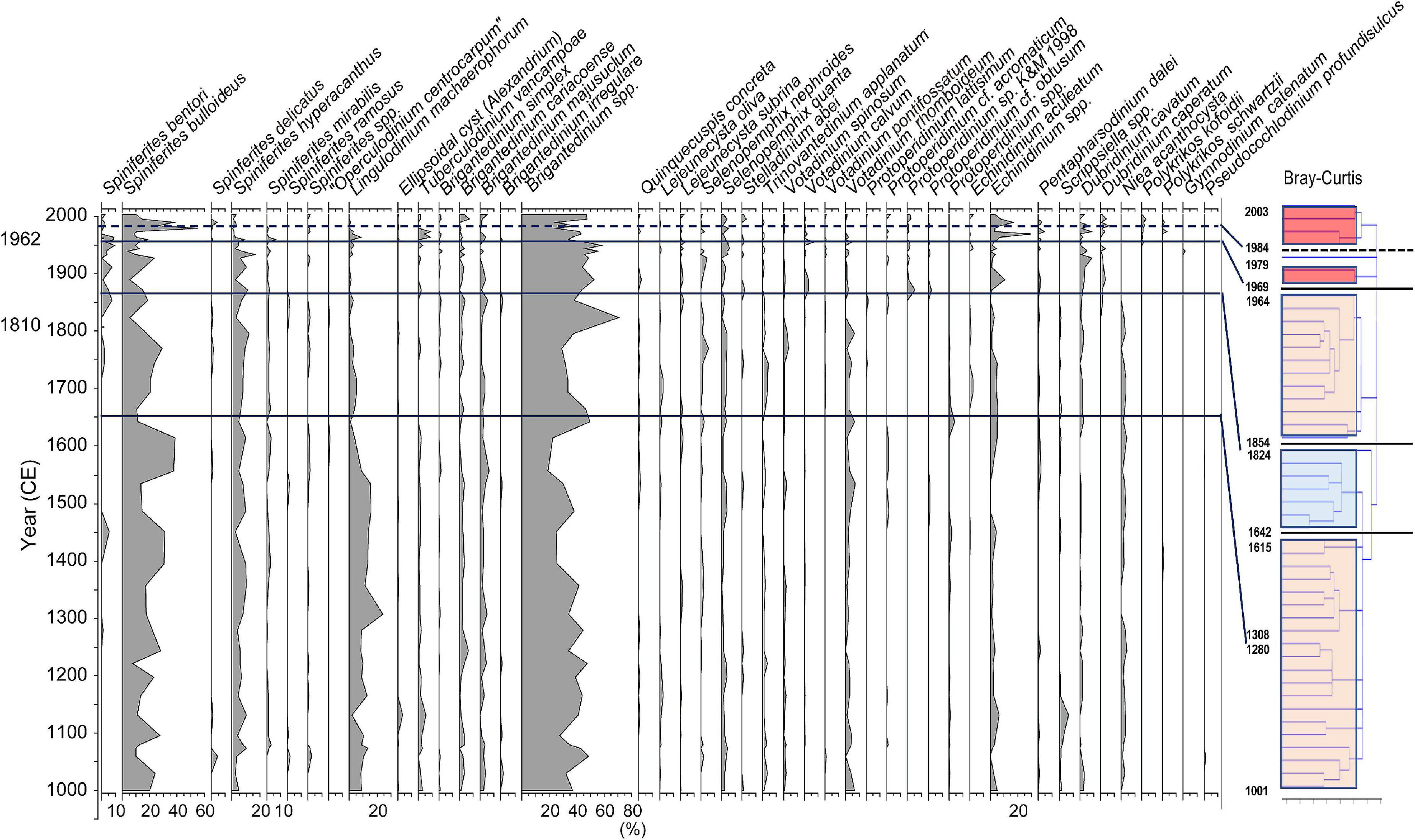
Figure 5. Stratigraphic distribution of major groups of dinoflagellate cysts and Unit division based on stratigraphic cluster analysis using Bray-Curtis method.
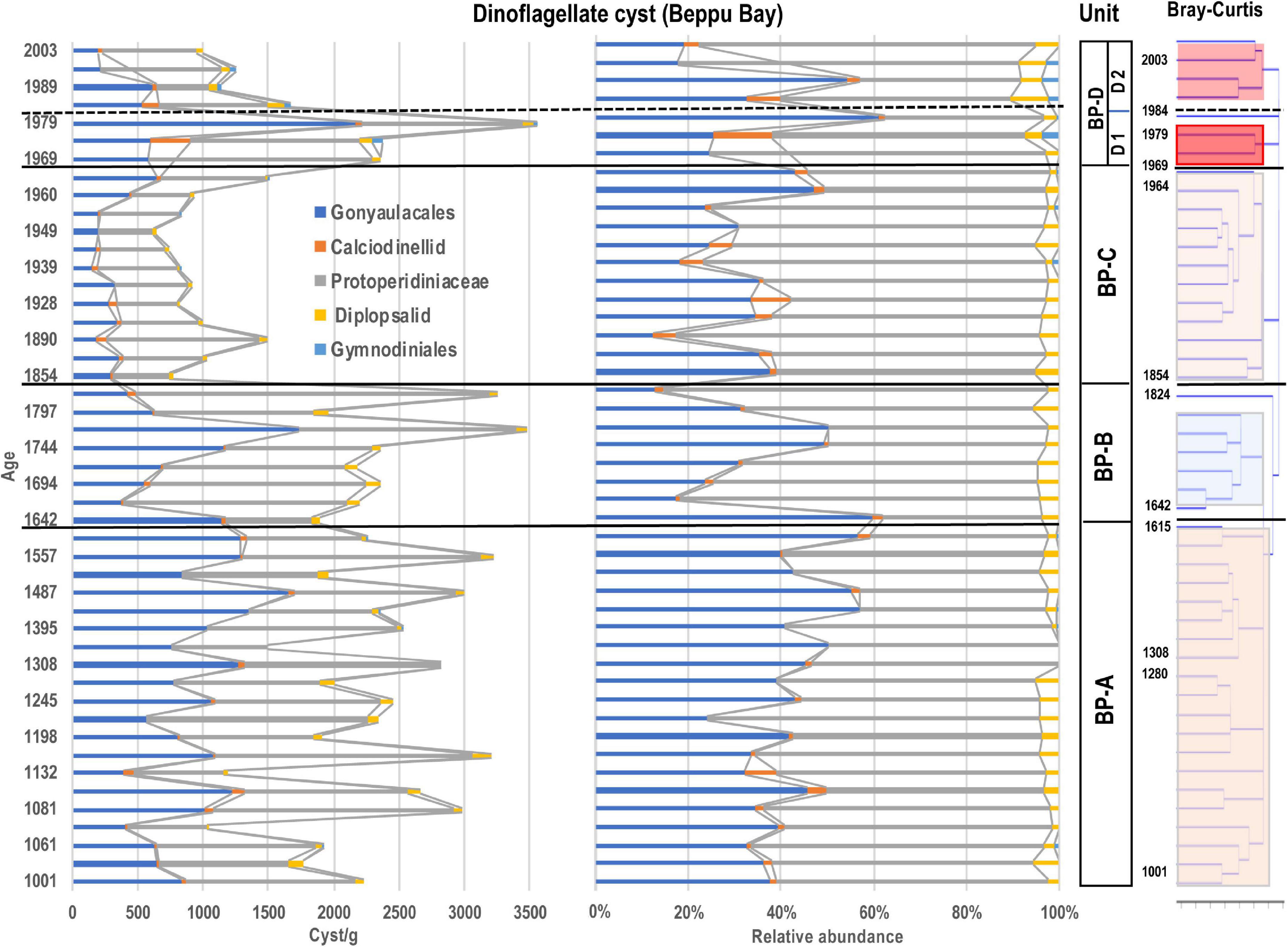
Figure 6. Stratigraphic abundances of dinoflagellate cysts and Unit division based on stratigraphic cluster analysis using Bray-Curtis method.
The ratio between the photosynthetic group (auto-mixotroph) mainly composed of Gonyaulacales and calciodinellid and the heterotrophic group mainly composed of Protoperidinid and Diplopsalid was ranged between 80% (year 1824) to 40% (year 1989). Except for year 1960 to 1979, when the photosynthetic group increased to 50% or more, the heterotroph group was dominant throughout the cores.
Microforaminiferal Linings (Figures 3, 7(2-4))
Microforaminiferal linings were the next most abundant after dinoflagellate cysts with an average of 1802 linings/g in all samples, and the highest number being 4055 linings/g in year 1744, and the minimum number being 366 linings/g in year 1989 (Figure 8). After a rapid decrease after year 1744, the number increased slightly from year 1854 to 1955, except in year 1933, but was nearly constant (1158 linings/g), and then after year 1960 it increased slightly until year 1984 (929 linings/g). However, it decreased (412 linings/g) almost continuously from then until year 2003.
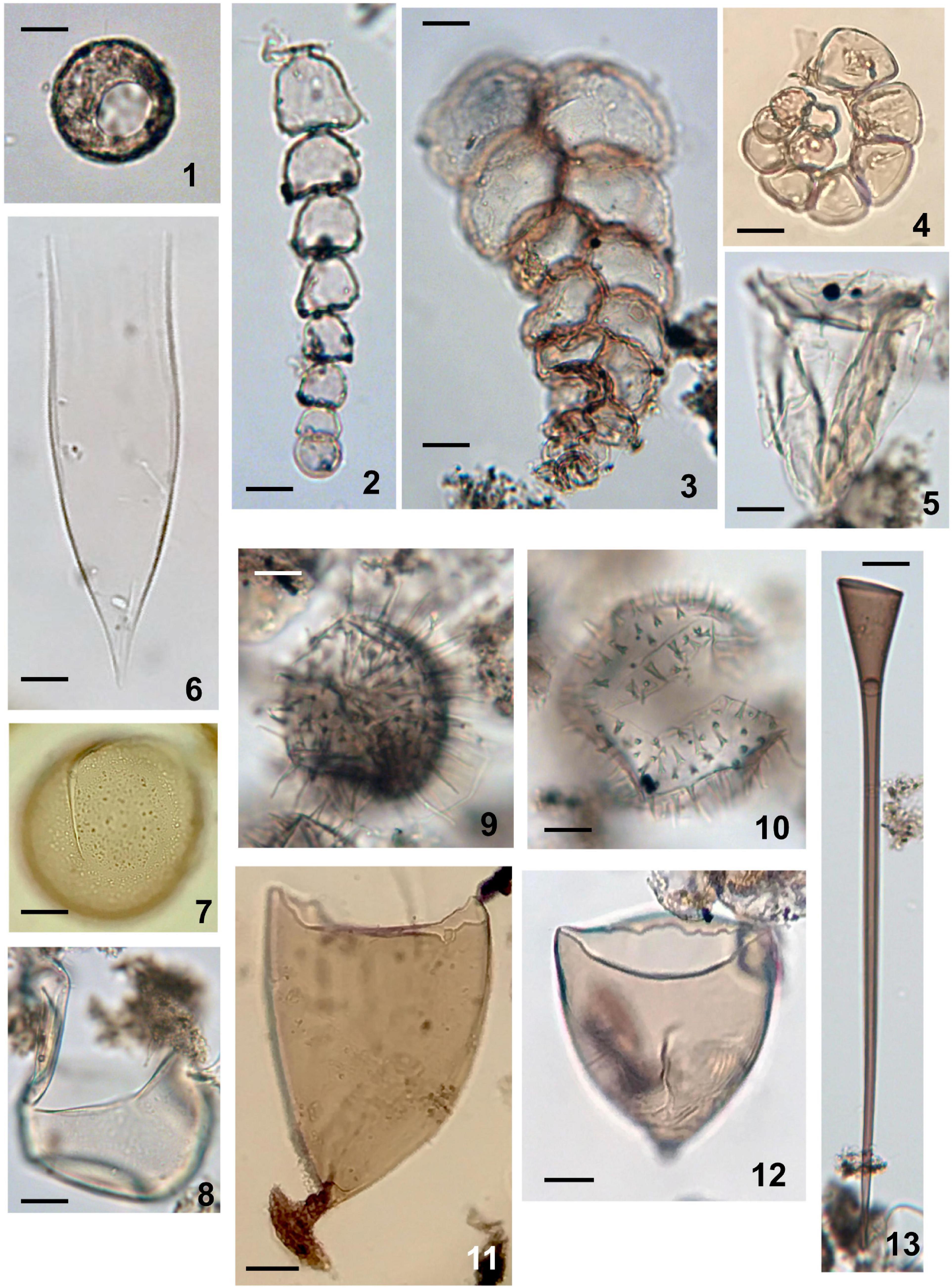
Figure 7. Aquatic palynomorphs except for dinoflagellate cysts and acritarchs observed in sediments of Beppu Bay. (1) Testate amoeba; Arcella sp. Sample BG19 St 1-2 14-15. (2) Microforaminiferal lining uniserial type (Reophax sp.). Sample BG 19 St 1-2 14-15. (3) Microforaminiferal lining biserial type (Textularia cf. tenuissima). Sample BG 19 St 1-2 14-15. (4) Microforaminiferal lining coiled type (Buccella sp.). Sample BG 19 St 1-2 10-11. (5) Ciliate; Favella sp. Sample BG 19 St 1-2 14-15. (6) Ciliate; damaged lorica of Dadayiella sp. Sample BG 19 St 1-2 14-15. (7) Crustacean resting egg with finely granular surface. Arrow indicating rupture for hatching. Sample BG 19 St 1-2 14-15. (8) Crustacean resting egg with smooth surface. Arrow indicating rupture for hatching. Sample BG 19 St 1-2 14-15. (9) Crustacean resting egg ornamented with long flexuous spines. Sample BG 19 St 1-2 14-15. (10) Crustacean resting egg ornamented with short and solid spines. Arrow indicating rupture for hatching. Sample BG 19 St 1-2 14-15. (11) Turbellarian egg capsule with short and stout stalk. Arrow indicating basal attachment. Sample BP 09-6 3-89. (12) Turbellarian egg capsule with very short stalk. Sample BP 09-6 5-45. (13) Turbellarian egg capsule with very long stalk. Sample BG 19 St 1-2. (14-15) Scale bars; 20 μm.
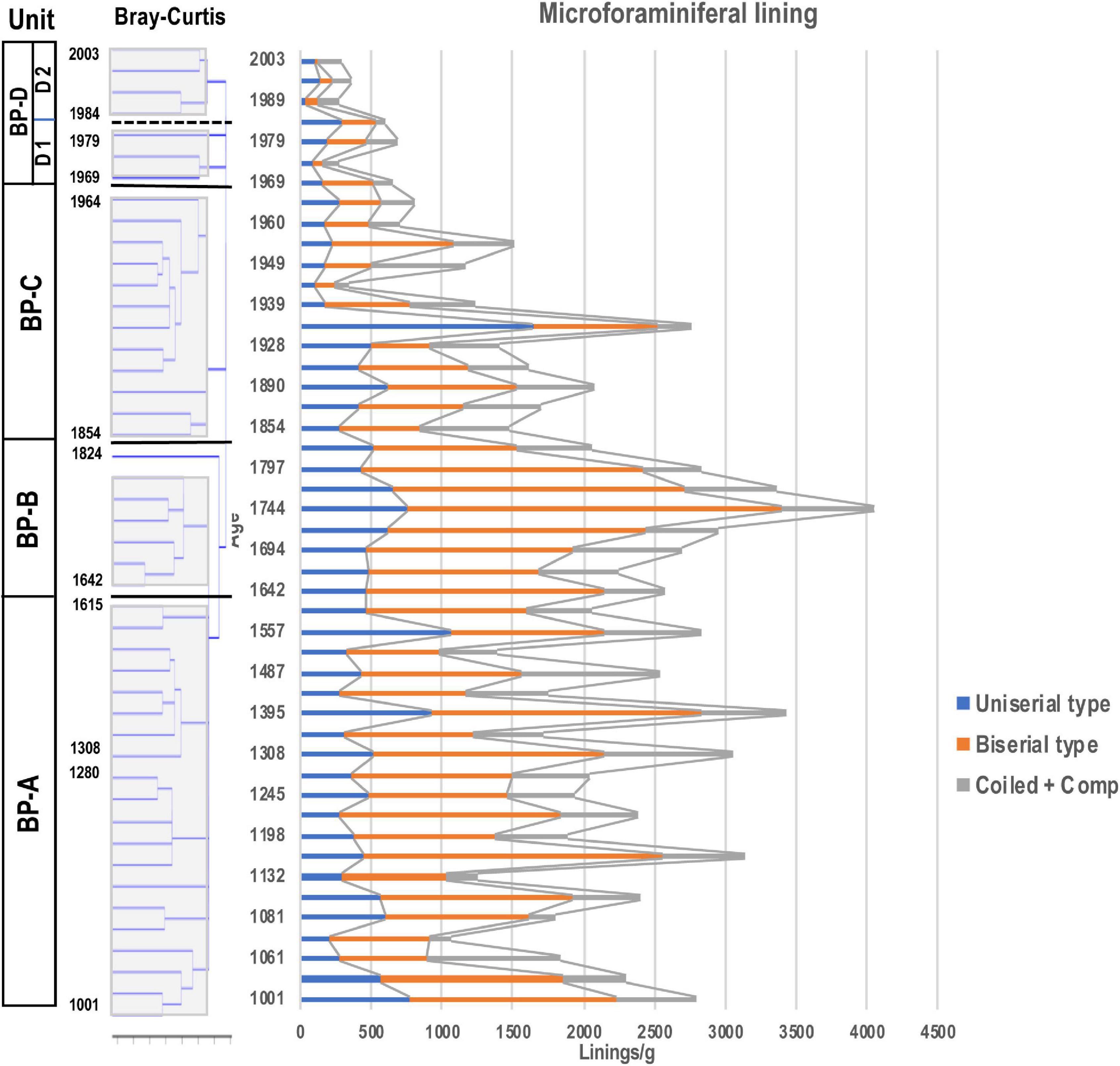
Figure 8. Stratigraphic distribution of microforaminiferal linings. Unit division in the left column based on the result for dinoflagellate cyst assemblages resulting from stratigraphic cluster analysis using Bray-Curtis method.
Among microforaminiferal linings, the biserial type was dominant, followed by the uniserial type. Before year 1744, the average number of the biserial type (Figure 7(7)) was 1281 linings/g, but after then it dropped sharply to 866 linings/g until year 1955 and 195 linings/g until year 2003. The uniserial type (Figure 7(2)) showed an average of 495 linings/g before year 1744, but thereafter their occurrence did not change (465 linings/g until year 1955), but it decreased sharply to 159 linings/g until year 2003. The number of the coiled type linings was smaller than that of uniserial and biserial types, and although they did not dominate in all samples, they also decreased after year 1960.
Ciliates (Figures 7(5-6))
Ciliophora were present in very low percentages throughout all samples, but 711 cells/g were recorded in year 1984 and 147 cells/g in year 1995. This was due to the abundance of loricae of Dadayiella*. In other samples, resting cysts of Favella* and Cyrtostrombidium* sometimes occurred.
Crustaceans (Figures 3, 7(7-10))
Crustacean resting eggs were the most abundant after dinoflagellate cysts and microforaminiferal linings. The average number of resting eggs was 751 eggs/g in all samples; the highest was 1709 eggs/g (year 1709), and the lowest was 284 eggs/g. However, their occurrence patterns were different from those of dinoflagellate cysts and microforaminiferal linings. Until year 1665, the output was almost constant (615 eggs/g on average), but after that, it increased (1005 eggs/g) until year 1944, and then decreased sharply until year 1960 (336 eggs/g). After that, it started to increase again (913 eggs/g).
Other Aquatic Palynomorphs
Testate amoebae (Figure 7(1)) were also obtained from almost all samples, but did not dominate. Their occurrence was a little higher in the sample before year 1771.
Turbellarian egg capsules (Figures 7(11-13)) occurred in almost all samples with various morphologies, consisting of less than a few percent of all palynomorphs, but were relatively abundant in the samples before year 1771 (263 capsules/g; highest value) and decreased thereafter.
Acritarchs (Figures 4(12-14) Is Joviella? sp.)
Acritarchs were also obtained from all samples, but always constituted less than 10% of all palynomorphs and never dominated. After year 1771, they decreased from about 200 to 146 cells/g.
Stratigraphic Divisions of Dinoflagellate Cyst Assemblage
Since the eco-physiological characteristics of palynomorphs except dinoflagellate cysts have not been well investigated so far, dinoflagellate cyst assemblages were statistically analyzed using a stratigraphic and Bray-Curtis method. As the results of the cluster analysis for the dinoflagellate cyst assemblage data dominated in the Beppu Bay core, the dinoflagellate cyst assemblages were divided into four major units.; BP-A Unit (year 1001–1615) from the bottom, BP-B Unit (year 1642–1824), BP-C Unit (year 1854–1964), and BP-D Unit (year 1969–2003). BP-D Unit was further subdivided into BP-D 1 Subunit (year 1969–1979) and BP-D 2 Subunit (year 1984–2003) (Figure 5).
BP- A Unit
Palynomorphs ranged from 2957 to 7583 (5440 on average) palynomorphs/g. Dinoflagellates dominated by Spiniferites hyperacanthus, S, mirabilis, Lingulodinium machaerophorum and Brigantedinium spp. were present at 2268 cysts/g on average, and constituted ca. 40% of the total palynomorphs. The foraminiferal linings were also dominant at 2165 linings/g on average, and constituted more than 40% of total palynomorphs. Crustacean resting eggs were present as 642 eggs/g on average and constituted ca. 10% of total palynomorphs.
BP- B Unit
Palynomorphs slightly increased to within the range of 4968–8863 (6556 on average) palynomorphs/g. The density of dinoflagellate cysts was approximately the same as the density of BP- A Unit, being 2458 cysts/g on average, however, L. machaerophorum remarkably decreased in this unit. The microforaminiferal linings slightly increased in concentration, with 2845 linings/g on average, and represented 40–50% of total palynomorphs; however, toward the upper parts they decreased.
BP- C Unit
Palynomorphs clearly decreased to within the range of 2957–7583 (3380 on average) palynomorphs/g. Dinoflagellate cysts also decreased to 986 cysts/g on average, but their relative proportion among total palynomorphs was over 50% in the upper strata, and 1517 cysts/g were recorded in year 1964. The microforaminiferal linings were present at 1492 linings/g, however, they showed a clear decrease from 20% to 40% of the total palynomorphs in the upper layer. The crustacean resting eggs were present at 777 palynomorphs/g on average and increased their relative proportion to 40% (2070 palynomorphs/g) of total palynomorphs at year 1890.
BP- D Unit
Palynomorphs were present as 2325–5546 palynomorphs/g(3751 on average). Dinoflagellate cysts remarkably increased to 2141cysts/g on average and constituted ca. 40% of total palynomorphs. Spiniferites bulloideus was dominant in this unit. The microforaminiferal linings constituted 40–50% of total palynomorphs; however, both uniserial and biserial types continuously decreased to 449 linings/g on average. The crustacean resting eggs slightly increased to 913 palynomorphs/g.
Dissolved Oxygen and Chemical Oxygen Dissolved After Year 1972
The DO at the bottom layer of the inner part of the bay (St. 31) near the core sampling point was the highest in year 1973 (4.2 mg/L). After this, it once dropped to around 2 mg/L, but increased to nearly 3 mg/L around year 2010. The COD at 0 m decreased from the maximum value of 1.5 mg/L in year 1974 to 0.5 to 1 mg/L. At St. 19 of the mouth of the bay, the DO of the bottom layer was 5 mg/L, but it increased slightly after year 2000, and the COD at 0 m reached a maximum of 1.8 mg/L in year 1974. It decreased to 0.5 mg/L in year 1978, but slightly increased 0.7 mg/L until around year 2000, and has remained at 0.5 mg/L since then 1 (Figure 9).
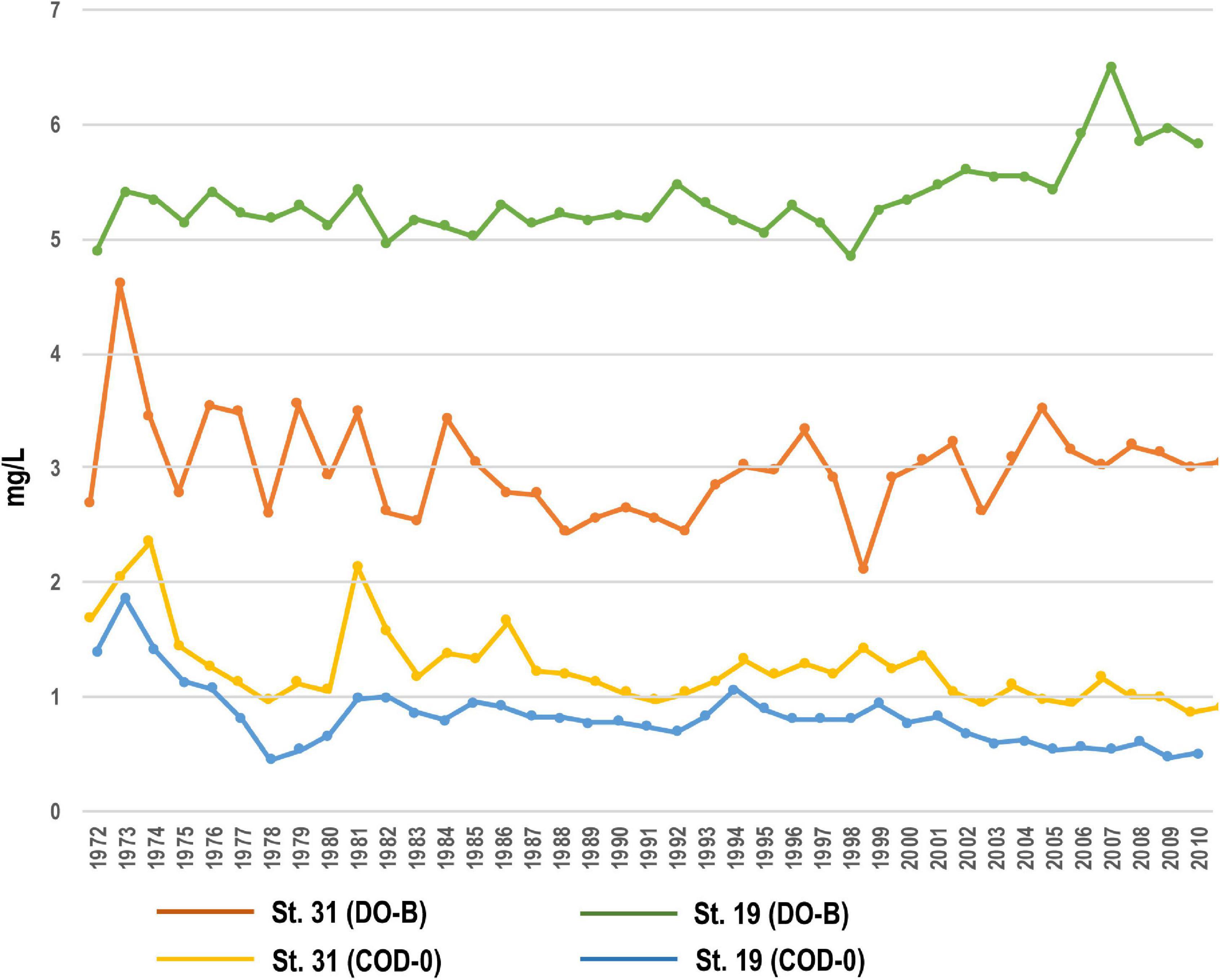
Figure 9. Temporal changes of DO and COD at the inner (St. 31) and mouth (St. 19) parts of Beppu Bay.
Discussion
Marine Environmental Changes Recorded in Dinoflagellate Cysts and Other Palynomorph Assemblages
Although the palynomorph assemblages do not represent all organisms that inhabit in and around Beppu Bay, the changes of these assemblages seemed to reflect the change of ecosystem (biota) in Beppu Bay. The unit boundaries were concordant in BP- III Unit of all palynomorphs and Unit BP-D of dinoflagellate cysts only, although others were slightly different. This means that the change of aquatic biota in this period seems to be larger than that in other periods. As discussed later, these changes were induced by eutrophication due to human activities. Except in the upper part of the Unit, the boundaries suggested by all aquatic palynomorphs and dinoflagellate cysts were different. This might be due to the use of different data sets for statistical analysis. As shown in Table 1, all aquatic palynomorphs included the remains of organisms inhabiting freshwater, brackish water and marine environments, as well as water column and bottom sediments. For example, dinoflagellate cysts originated from planktonic dinoflagellates whereas microforaminiferal linings were from benthic foraminifers. Therefore, the unit divisions resulting from total aquatic palynomorphs might be proxies for wider biota of Beppu Bay (Supplementary Material 6).
In the cluster analysis, BP-D Unit had a lower similarity between each sample than those of other Units, and it is specific that the year 1979 sample did not belong to any cluster. This was only due to extremely dominant Spiniferites bulloideus in the year 1979 sample, but this tendency did not continue. In addition, the cysts of heterotrophic species were common to each sample, but their relative ratios were not constant. The production of such unstable occurrence of dinoflagellate cysts suggested that the coastal marine environment became unstable after year 1964.
Marine Environmental Changes Recorded in Dinoflagellate Cyst Assemblages at Millennium Scale in Beppu Bay
As previously pointed out, dinoflagellate cysts were most dominant in the aquatic palynomorphs, and their eco-physiologies were well investigated. Therefore, for understanding the environmental changes of Beppu Bay, the temporal changes of dinoflagellate cyst assemblages should be clarified. As results of statistical analysis, dinoflagellate cyst assemblages were divided into four major units as shown previously.
BP-A Unit (Year 1001 to 1615): Warm Period
In BP-A Unit, the occurrence of all palynomorphs fluctuated, but it was within a certain range and nearly stable. The number of aquatic palynomorphs in this unit is 5759 palynomorphs/g, which is abundant in the core sample. Dinoflagellate cysts and microforaminiferal linings account for 80 to 90% of all palynomorph assemblages. In BP-A Unit, dinoflagellate cysts, foraminifera linings, and crustacean resting eggs also fluctuated. The relationship between these fluctuations and the rapid tide of the Kuroshio, which has a relatively short cycle and affects the marine environment of the Bungo Channel, is an important topic for further study.
Harada et al. (1994) presented the nearly 9,000 years’ evolution of the dinoflagellate cyst assemblages in the core samples collected in the inner part of Beppu Bay, which was north of the core samping site in this study. After Harada et al. (1994), the study of dinoflagellates cysts, especially those of heterotrophic species, has greatly progressed, and many cyst species have been described thereafter, so it is difficult to quote the data at that time as they were, but the species of photosynthetic Spiniferites bulloideus and Lingulodinium machaerophorum have been consistent. Therefore, the occurrence patterns of L. machaerophorum in the core sample of Harada et al. (1994) seemed to be rather constant at 100 cysts/ml of wet sediment for the past 2000 years. According to Zonneveld et al. (2013), L. machaerophorum is a warm water species. Based on these data, it appears that the marine environment of BP-A Unit was rather stable and warm judging from the dinoflagellate cyst assemblages. This warm period might correspond to the Medieval Warm Period suggested in Marcott et al. (2013).
BP-B Unit (Year 1642 to 1824): Cool Period
The biserial type of microforaminiferal lining has been decreasing since its highest appearance value was recorded in year 1744. This suggests that the bottom environments of Beppu Bay started to deteriorate in this period. A remarkable decrease of L. machaerophorum characterizing BP-B Unit suggested that the water temperature in Beppu Bay might have become lower than that of BP-A Unit. The heterotrophic Protoperidinium species consisting of Brigantedinium spp., Selenopenphix quanta, Votadinium rhomboideum, and Echinidinium spp. suggested that dominant phytoplankton might shift from photosynthetic dinoflagellates to diatoms which are prey for heterotrophic dinoflagellates, mainly Protoperidinium species (Gaines and Taylor, 1984; Jacobson and Anderson, 1986).
BP-C Unit (Year 1854 to 1964): Warmer Period
In BP- C Unit, the total count of palynomorphs was 3543 palynomorphs/g, which was lower than that in BP-A Unit and BP-B Unit. Among them, dinoflagellate cysts and microforaminiferal linings decreased to about 70% of all palynomorphs, and crustacean resting eggs increased instead. The decrease of Spiniferites spp. and Lingulodinium machaerophorum did not mean that the biological productivity in the inner part of Beppu Bay had decreased, because the increase in crustacean resting eggs indicated that it was possible that there was a change in phytoplankton as primary producers, for example from dinoflagellates to diatoms during this period. In Kuwae et al. (2022), diatom flora also changed around year 1850 in their D2a zone of Beppu Bay core sediments.
Increase of S. bentori, a warm water species discussed in Zonneveld et al. (2013) with sporadic appearance of Tuberclodinium vancampoae suggested a climatic change toward warmer conditions in the upper layer. On the other hand, the decrease in microforaminiferal linings of the uniserial type (e.g., Reophax spp.) and the Biserial type (e.g., Texturalia spp.) inhabiting the surface layer of sediments might indicate degraded bottom environments in Beppu Bay (Names of foraminifera inferred from Sugaya and Nakao, 1983). In year 1854, a tsunami of about 2 m height due to the Ansei Nankai Earthquake struck Beppu Bay4. Since the sediments in the shallow waters along the Sanriku coast were much disturbed when this region was hit by the Chile Tsunami and the Great East Japan Earthquake (e.g., Matsuoka et al., 2018), there is no doubt that Beppu Bay was also affected by the tsunami caused by the Ansei Nankai Earthquake. However, how much the bottom habitats were affected cannot be evaluated at this time. In addition to this, Shimada et al. (2012) suggested that the vegetation around Beppu Bay was changed from a poor to a rich forest condition covered with Pinus (Diploxylon) and Cryptomeria around year 1850, based on pollen analysis. This change of the vegetation on the land might have influenced the transport of sediment into the coasts of Beppu Bay and then changed the coastal environments.
BP-D Unit (Year 1969 to 2003): Anthropogenic Eutrophication
In the dinoflagellate cyst assemblage of BP-D Unit, the photosynthetic species Spiniferites bulloideus prominently proliferated to 2011 cysts/g in year 1979, and Lingulodinium machaerophorum and Tuberclodinium vancampoae also increased to near the level of BP-A Unit. This is a manifestation of the “Oslo fjord signal” of Dale (2009), which suggested an increase in photosynthetic species, indicating cultural eutrophication reflected by the dinoflagellate cyst assemblages. In addition, the increase of heterotrophic dinoflagellate cysts named as “Heterotroph signal” of Matsuoka (1999); Dale (2009) also showed eutrophication. This period coincides with the period of frequent large-scale red tides of a raphidophycean, Chattonella marina, and a dinoflagellate, Karenia mikimotoi (late 1960s to 1970s) in the Seto Inland Sea. Interestingly, Kuwae et al. (2022) showed that the dominant pigment preserved in the core sediments of Beppu Bay changed from fucoxanthin to diatoxanthin around the 1960s. This result suggests that dominant primary producers might change from diatoms to phytoflagellates including dinoflagellates. It has been pointed out that the cause of such a large-scale red tide was the load of nutrients from the land, that is, the progress of artificial eutrophication (Okaichi, 1997).
Therefore, BP-D1 Subunit (year 1964 to 1979) of the dinoflagellate cyst assemblages seemed to reflect the anthropogenic eutrophication caused by the increase of the population around Beppu Bay since the 1950s (Beppu City data5). Furthermore, it is speculated that the total nitrogen and total phosphate supplied from the land to the bay in the 1960s and 1970s reached 800 ton/day and 80 ton/day, respectively, which might have contributed to increasing the primary productivity (Tsugeki et al., 2016). In the subsequent BP-D2 Subunit, dinoflagellate cysts, microforaminiferal linings, and crustacean eggs decreased to the level of the BP-C Unit. This may reflect the reduction in nutrient load due to the improvement of drainage regulation and purification function from the land, as mentioned by Tsugeki et al. (2016).
BP-D1 Unit was characterized by the increase of Gymnodinialean Polykrikos kofoidii* and Polykrikos schwartzii*. These Polykrikos* species are heterotrophic, and use nematocysts to capture prey organisms such as Alexandrium catenella, Gymnodinium catenatum, Karenia mikimotoi, Prorocentrum sigmoides, and other flagellates and engulf them at the ventral area (Cho and Matsuoka, 2000; Matsuoka et al., 2000). This way of feeding is different from that of Protoperidinium species, which use a capture membrane called as the feeding veil, to wrap prey organisms such as diatoms and assimilate their cytoplasm (Gaines and Taylor, 1984; Jacobson and Anderson, 1986, Jeong, 1994). That is, the appearance of Polykrikos* suggests an increase in swimming-powered prey organisms such as flagellar microalgae. In other words, since the increases of P. kofoidii* and P. schwartzii* cysts suggest the emergence of flagellates such as C. marina and K. mikimotoi, which favor the environment of stratified seawater, the primary production in the BP-D1 Subunit might be dependent on flagellates rather than diatoms.
The existence of BP-D1 and -D2 Subunits could be controlled by the eutrophication of seawater due to the reclamation of tidal flats and the increase of urban wastewater by the regulation of the discharge of nutrients by law and the increase of sewage treatment capacity. This strongly suggests that it is difficult to improve the bottom sediment over a wide area.
Observational data are only available after year 1972, but COD around year 1974 suggest that the entire bay was rich in nutrients, which coincides with the frequent red tides in the entire Seto Inland Sea. DO of the bottom layer has remained almost unchanged since year 1972 at the mouth of the bay, but it shows that oxygen consumption was predominant at the inner part of the bay until around year 1990. This reflects the fact that organic matter in the water was deposited on the seafloor and its decomposition promoted oxygen consumption. Summarizing the above, it can be judged that eutrophication was progressing around year 1972 included BP-D1 Unit, and once the water quality improved slightly, but the improvement of the bottom layer environment in the inner part of the bay was not remarkable. The environment of seawater and sediment in the inner part of the bay shown in the observation data is consistent with the environmental change inferred from the aquatic palynomorph community (BP-III Unit and BP-D1 Unit).
Deterioration of Bottom Environments Reflected by Microforaminiferal Linings in Beppu Bay
In Beppu Bay core samples, the biserial type and the uniserial type dominated, and the coiled type was rather few as mentioned above. The foraminiferal community indicated that the bottom sediments of the inner part of Beppu Bay consisted of mud with rich organic substance. Kuwae et al. (2022) suggested that total sulfur in sediments clearly increased after year 1968. This increase induced hypoxia bottom sediments and then decreased microforaminiferal linings.
The remarkable deterioration of the bottom habitats suggested by the decrease of microforaminiferal linings in BP-D1 Subunit, especially the biserial type and uniserial type, continued to BP-D2 Subunit and progressed further in BP-C Unit. This deterioration of sediments may be due to the rapid decrease in tidal flat area due to reclamation on the southern coast of Beppu Bay during the BP-D1 Subunit period (1970s)6, when industrialization along the southern coast of this Bay started. As in Ariake Sound of Western Kyushu discussed below, similar bottom environmental changes occurred in Beppu Bay.
Other Coastal Regions Around Japanese Islands
The analysis of dinoflagellate cyst and other aquatic microfossil assemblages in coastal sediments in Japan has revealed that artificial eutrophication has progressed since the 1960s and that the lower trophic system has changed significantly, including the occurrence of large-scale red tides.
In Tokyo Bay, the dinoflagellate cyst density decreased once in the mid-1940s (The Second World War), but then increased sharply after the 1960s, and the proportion of heterotrophic species cysts, mainly Protoperidinioid cysts and accompanied by considerable amount of Polykrikos* cysts, contributed to that increase. These changes, as the Heterotroph signal in dinoflagellate cyst assemblages, reflected the nutrient enrichment caused by the urbanization around Tokyo Bay (Matsuoka, 1999).
In Ariake Sound located on the opposite side of Kyushu respect to Beppu Bay, changes in the dinoflagellate cyst assemblages in core samples of Isahaya Bay and the inner part of Ariake Sound were investigated. According to Matsuoka (2004), Shin et al. (2010a,b), the density of dinoflagellate cysts increased sharply in Isahaya Bay and the inner part of Ariake Sound after the 1960s, and the proportion of heterotrophic species cysts, mainly Protoperidinium* species, clearly increased in Isahaya Bay (Matsuoka, 2004). The cause was that the disappearance of tidal flats by artificial reclamation reduced the processing capacity of organic matter carried from the land by the organisms inhabiting there, and the construction of vertical embankments by reclamation reduced vertical mixing and enhanced stratification of sea water (Manda and Matsuoka, 2006). The resultant stratification due to a sharp reduction in speed of bottom current, promoted the decomposition of organic matter in the seafloor, resulting in an anoxic condition at the boundary between seawater and sediments (Matsuoka, 2006). The cause of artificial eutrophication in the Ariake Sound was not urbanization but large-scale tidal flat reclamation after the 1960s. As introduced above, similar artificial eutrophication progressed around Beppu Bay after the 1960s.
In Osaka Bay, paleoenvironmental studies were conducted on materials other than dinoflagellate cysts using diatoms (Hirose et al., 2008), ostracods (Yasuhara et al., 2003), and benthic foraminifera (Tsujimoto et al., 2006). Taken together, these results showed that the marine environment recovered once in the latter half of the 1940s after a slight increase in nutrient load in the 1900s, but since the 1960s, the artificial nutrient load has increased sharply until the present. The factors that led to such environmental changes are thought to be the reclamation of tidal flats, population growth, industrialization and urbanization around Osaka Bay.
Therefore, it can be concluded that artificial eutrophication around the western Japanese archipelago was caused by the period of high economic growth accompanied with reclamation of tidal flats, urbanization, and industrialization after the 1960s. However, as a result of subsequent restrictions on the influx of nutrients that lead to eutrophication, oligotrophic conditions in coastal areas are progressing now and biological production is declining for example in the Seto Inland Sea (Abo and Yamamoto, 2019).
Consequently, such coastal environmental changes have been well preserved in not only dinoflagellate cyst but also other aquatic palynomorph assemblages. Therefore, further eco-physiological investigations for these aquatic palynomorph groups are needed.
Summary
The sediment cores collected at the innermost part of Beppu Bay included various planktonic and benthic aquatic palynomorphs consisting of dinoflagellate cysts, prasinophycean phycoma, algal cells of chlorophyceae, organic shells of testate ameboae, resting cysts and lorica of ciliates, microforaminiferal linings, crustacean resting eggs and fragments of bodies, turbellarian egg capsules, and acritarchs. Among them, dinoflagellate cysts, microforaminiferal linings, and crustacean resting eggs dominated.
As results of stratigraphic cluster analysis for all aquatic palynomorph assemblages and dinoflagellate cyst assemblages, these palynomorphs and dinoflagellate cysts were divided into several units respectively.
The boundary of both upper units, BP-III Unit for all palynomorphs and BP-D Unit for dinoflagellate cysts, was the same era between year 1964 and 1969. However, other boundaries were different. This might have been caused by their different ecological components, since microforaniniferal linings represent a benthic life form, whereas dinoflagellate cysts and Crustaean resting eggs indicated planktonic life form.
Results from BP-III and BP-D Units suggested that artificial eutrophication started in year 1964 and in 1969, and this change of biota in Beppu Bay was the most drastic in the past 1000 years.
The eutrophication might have been induced by anthropogenic activities such as reclamation of tidal flats, urbanization due to increased population, and industrialization involving the development of larger plants around Beppu Bay.
Since non-dinoflagellate aquatic palynomorphs like microforaminiferal linings, crustacean resting eggs, turbellarian egg capsules and other micro-remains will be useful for reconstructing paleoenvironments, further taxonomical and eco-physiological studies of these palynomorphs are needed.
Data Availability Statement
The raw data supporting the conclusions of this article will be made available by the authors, without undue reservation.
Author Contributions
KM and MK planned the basic design of the project. MK collected the samples and contributed to making an age model. KM and NK analyzed the samples and observed the palynomorphs and revised the earlier draft of the manuscript. KM wrote a draft of the article. All authors approved the submitted version.
Funding
This study was supported financially by Grants-in-Aid for Scientific Research (22340155, 21244073, and 18H01292) from the Japan Society for the Promotion of Science and a research grant from the Mitsui and Co., Ltd., Environment Fund (R09-B022). The cooperative research program (09A043, 10A020, and 19A007) of the Center for Advanced Marine Core Research, Kochi University, also supported this study.
Conflict of Interest
The authors declare that the research was conducted in the absence of any commercial or financial relationships that could be construed as a potential conflict of interest.
Publisher’s Note
All claims expressed in this article are solely those of the authors and do not necessarily represent those of their affiliated organizations, or those of the publisher, the editors and the reviewers. Any product that may be evaluated in this article, or claim that may be made by its manufacturer, is not guaranteed or endorsed by the publisher.
Acknowledgments
We thank Hidejiro Onishi for conducting sampling with E/R/V ISANA of Ehime University and the crew of the R/V Tansei-Maru (cruises KT-09-01) of the Ocean Research Institute, University of Tokyo, for sampling. We also appreciate Wen Liu for his technical support in statistical analysis and Oita Prefectural Fisheries Research Department for kindly providing the data of STC. We also thank three reviewers and Fernando Rubio whose constructive suggestions and comments were very useful for improving the earlier manuscript.
Supplementary Material
The Supplementary Material for this article can be found online at: https://www.frontiersin.org/articles/10.3389/fmars.2022.843824/full#supplementary-material
Footnotes
- ^ http://hab.ioc-unesco.org/
- ^ https://www.nhm.uio.no/english/research/infrastructure/past/
- ^ https://www.wildblueberrymedia.net/pcord
- ^ https://www.pref.oita.jp/soshiki/13550/jishinkiroku.html
- ^ https://www.city.beppu.oita.jp/doc/sisei/kakusyukeikaku/sousei/2ki/vision.pdf
- ^ https://www.biodic.go.jp/reports/4-11/q211.html
References
Abo, K., and Yamamoto, T. (2019). Oligotrophocation and its measures in the Seto Inland Sea. Jpn. Fish. Res. Educ. Agen. 49, 21–26.
Anthropocene Working Group (2020). Report of activities 2020, Newsletter of the Anthropocene Working Group. California: Anthropocene Working Group, 31.
Appleby, P. G., and Oldfield, F. (1978). The Calculation of Lead-210 Dates Assuming a Constant Rate of Supply of Unsupported 210Pb to the Sediment. CATENA 5, 1–8.
Armstrong, H. A., and Brasier, M. D. (2007). Microfossils, 2nd Edn. London: Blackwell publishing Ltd, viii+296.
Belmonte, G., and Rubino, F. (2019). “Resting cysts from coastal marine plankton,” in Oceanography and Marine Biology: Annual Review, Vol. 57, eds S. J. Hawkins, A. L. Allcock, A. E. Bates, L. B. Firth, I. P. Smith, S. E. Swearer, et al. (Boca Raton: CRC Press), 1–186.
Caffrey, J. M., and Murrell, M. C. (2016). “A historical perspective on eutrophication in the Pensacola Bay Estuary, FL, USA,” in Aquat. Microb. Ecol. Biogeochem.: A dual perspective, eds P. M. Gilbert and T. M. Kana (Switzerland: Springer International Publishing), 199–213. doi: 10.1007/978-3-319-30259-1_16
Cho, H.-J., and Matsuoka, K. (2000). Cell lyses of a phagotrophic dinoflagellate, Polykrikos fokoidii feeding on Alexandrium tamarense. Plank Biol. Ecol. 47, 134–136.
Clarke, A. L., Conle, Y. D. J., Anderson, N. J., Adser, F., Andren, E., de Jonge, V. N., et al. (2006). Long-term trends in eutrophication and nutrients in the coastal zone. Limnol. Oceanol. 51, 385–397.
Dale, B. (2009). Eutrophication signals in the sedimentary record of dinoflagellate cysts in coastal waters. J. Sea Res. 61, 103–113. doi: 10.1016/j.scitotenv.2003.08.003
Dale, B., Thorsen, T. A., and Fjellsa, A. (1999). Dinoflagellate cysts as indicators of cultural eutrophication in the Oslofjord, Norway. Est. Coast. Shelf Sci. 48, 371–382.
Dolan, J. R., Montagnes, D. J. S., Agatha, S., Wayne Coats, D., and Diane, K. (2013). “Introduction to tintinnids,” in The Biology and Ecology of Tinntinid Ciliates, eds J. R. Dolan, D. J. S. Montagnes, S. Agatha, W. Coats, and D. K. Stoecker (United Kingdom: Wiley-Blackwell), 1–16.
Downie, C., Evitt, W. R., and Sarjeant, W. A. S. (1963). Dinoflagellates, hystrichospheres, and the classification of the acritarchs. Stanford Univ. Publ. Geosci. 7, 1–16.
Fensome, R. A., Williams, G. L., and MacRae, R. A. (2009). Late Cretaceous and Cenozoic fossil dinoflagellates and other palynomorphs from the Scotian Margin off shore eastern Canada. J. System. Palaeont. 7, 1–79.
Gaines, G., and Taylor, F. J. R. (1984). Extracellular digestion in marine dinoflagellates. J. Plank. Res. 6, 1057–1061.
Guiot, J., and de Vernal, A. (2007). “Chapter Thirteen Transfer Functions: Methods for Quantitative Paleoceanography based on microfossils,” in Developments in Marine Geology, eds C. Hillaire-Marcel and A. Vernal (Amsterdam: Elsevier), 523–563.
Gurdebeke, P. R., Mertens, K. N., Takano, Y., Yamaguchi, A., Bogus, K., Micah Dunthorn, M., et al. (2018). The affiliation of Hexasterias problematica and Halodinium verrucatum sp. nov. to ciliate cysts based on molecular phylogeny and cyst wall composition. Europ. J. Protistol. 66, 115–135. doi: 10.1016/j.ejop.2018.09.002
Hansen, P. J. (1991). Quantitative importance and trophic role of heterotrophic dinoflagellates in a coastal pelagical food web. MEPS 73, 253–261.
Harada, K., Endo, A., and Matsuoka, K. (1994). Uzubennmousou Kaseki wo Mochiita Beppuwann ni okeru Kohyouki no Kaiyoukannkyou Hennsenn no Fukugen (Reconstruction of the post glacial marine environments using dinoflagellate cysts in Beppu Bay). Gekkan Chikyu 16, 709–716.
Harland, R., Asteman, I. P., and Nordberg, K. (2013). A two-millennium dinoflagellate cyst record from Gullmar Fjord, a Swedish Skagerrak sill fjord. Palaeogeogr. Palaeoclimatol. Palaeoecol. 392, 247–260. doi: 10.1016/j.palaeo.2013.09.006
Hirose, K., Yasuhara, M., Tsujimoto, A., Yamazaki, H., and Yoshikawa, S. (2008). The succession of diatom assemblages and anthropogenically-induced environmental changes over the last 120 years, Osaka Bay, Japan. Daiyonki Kenkyu 47, 287–296. doi: 10.4116/jaqua.47.287
Hoang, A. Q., Aono, D., Watanabe, I., Kuwae, M., Kunisue, T., and Takahashi, S. (2021). Contamination levels and temporal trends of legacy and current-use brominated flame retardants in a dated sediment core from Beppu Bay, southwestern Japan. Chemosphere 266:129180. doi: 10.1016/j.chemosphere.2020.129180
Jacobson, D. M., and Anderson, D. M. (1986). Thecate heterotrophic dinoflagellates: feeding behavior and mechanism. J. Phycol. 22, 249–258.
Jeong, H.-J. (1994). Predation by the heterotrophic dinoflagellate Protoperidinium cf. divergens on copepod eggs and early naupliar stages. MEPS 114, 203–208. doi: 10.3354/meps114203
Kameda, T., and Fujiwara, T. (1995). Ventilation time and anoxia of the Benthic cold water in Beppu Bay. Bull. Coast. Oceanogr. 33, 59–68.
Kitazato, H. (1981). Observation of behavior and mode of life of benthic foraminifera in laboratory. Bull. Earth Sci. Shizuoka Univ. 6, 61–71.
Kokinos, J. P., Eglinton, T. I., Goni, M. A., Boon, J. J., Martog, A., and Anderson, D. M. (1998). Characterization of a highly resistant biomacromolecular material in the cell wall of a marine dinoflagellate resting cyst. Org. Geochem. 28, 265–288.
Köster, D., Lichter, J., Lea, P. D., and Nurse, A. (2007). Historical eutrophication in a river-estuary complex in mid-coast Maine. Ecol. Appl. 17, 765–778. doi: 10.1890/06-0815
Kuwae, M., Tsugeki, N. K., Amano, A., Agusa, T., Suzuki, Y., Tsutsumi, J., et al. (2022). Evidence of human-induced marine degradation in anoxic coastal sediments of Beppu Bay, Japan, as an Anthropocene marker in East Asia. Anthropocene 37:100318.
Kuwae, M., Yamamoto, M., Ikehara, K., Irino, T., Takemura, K., Sagawa, T., et al. (2013). Stratigraphy and wiggle-matching-based age-depth model of late Holocene marine sediments in Beppu Bay, southwest Japan. J. Asian Earth Sci. 69, 133–148. doi: 10.1016/j.jseaes.2012.07.002
Maier, G., Nimmo-Smith, J., Glegg, G. A., Tappin, A. D., and Worsfold, P. J. (2009). Estuarine eutrophication in the UK: current incidence and future trends. Mar. Freshw. Ecosyst. 19, 43–56. doi: 10.1002/aqc.982
Manda, A., and Matsuoka, K. (2006). Changes in tidal currents in the Ariake Sound due to reclamation. Estuar. Coast. 29, 645–652. doi: 10.1007/bf02784289
Marcott, S. A., Shakun, J. D., Clark, P. U., and Mix, A. C. (2013). A reconstruction of regional and global temperature for the past 11,300 years. Science 339:1198. 1228026 doi: 10.1126/science
Masumoto, M., Kuwae, M., and Hinata, H. (2018). Flux of sedimentary microplastics in Beppu Bay, Japan. Proc. Jpn. Soc. Civil Engin. B 2, 1321–1326.
Matsuoka, K. (1999). Eutrophication recorded in dinoflagellate cyst assemblages - a case of Yokohama Port, Tokyo Bay, Japan. Sci. Total Environ. 231, 17–35. doi: 10.1016/s0048-9697(99)00087-x
Matsuoka, K. (2004). Changes in the Aquatic Environment of Isahaya Bay, Ariake Sound, West Japan: from the view point of dinoflagellate cyst assemblage. Bull. Coast. Oceanog. 42, 55–59.
Matsuoka, K. (2006). Medium- to long-term environmental changes in the inner part of Ariake Sound recorded in the dinoflagellate cyst assemblages. Sci. Tot. Environ. 264, 65–93.
Matsuoka, K., and Ando, T. (2021). Review-Turbellarian egg capsule as one type of aquatic palynomorph; reconsideration of Tintinnomorph. Laguna 28, 15–35. doi: 10.1002/jmor.1051880103
Matsuoka, K., Cho, H.-J., and Jacobson, D. M. (2000). Observations of the feeding behavior and growth rates of the heterotrophic dinoflagellate Polykrikos kofoidii (Polykrikaceae, Dinophyceae). Phycologia 39, 82–86. doi: 10.2216/i0031-8884-39-1-82.1
Matsuoka, K., and Fukuyo, Y. (2000). Technical Guide for modern dinoflagellate cyst study. i+ 29pp, 17 figures, 7 tables, 22 plates. Tokyo: Japan Society for the Promotion of Science.
Matsuoka, K., Ikeda, Y., Kaga, S., Kaga, M., and Ogata, T. (2018). Repercussions of the Great East Japan Earthquake tsunami on ellipsoidal Alexandrium cysts (Dinophyceae) in Ofunato Bay, Japan. Mar. Environ. Res. 135, 123–135. doi: 10.1016/j.marenvres.2018.01.001
Matsuoka, K., Joyce, B. L., Kotani, Y., and Matsuyama, Y. (2003). Modern dinoflagellate cysts in hypertrophic coastal waters of Tokyo, Bay, Japan. J. Plank. Res. 25, 1461–1470.
McCarthy, F. M. G., Pilkington, P. M., Volik, O., Heyde, A., and Cocker, S. L. (2021). “Non-pollen palynomorphs in freshwater sediments and their palaeolimnological potential and selected applications,” in Applications of Non-Pollen Palynomorphs: from Palaeoenvironmental Reconstructions to Biostratigraphy, Vol. 511, eds F. Marret, J. O’Keefe, P. Osterloff, M. Pound, and L. Shumilovskikh (London: Geological Society), doi: 10.1144/SP511-2020-109
McMinn, A., Bolch, C., and Hallegraeff, G. (1992). Cobricosphaeridium Harland and Sarjeant: Dinoflagellate cyst or copepod egg? Micropaleontol. 38, 315–316.
Meisterfeld, R. (2002). “Order Arcellinida Kent, 1880,” in The Illustrated Guide to the Protozoa. Vol. 2, Second edition, eds J. J. Lee, G. F. Leedale, and P. Bradbury (Kansas: Society of Protozoologists), 827–860. doi: 10.1016/j.protis.2005.03.002
Mudie, P. J., Marret, F., Gurdbeke, P. R., Hartman, J. D., and Reid, P. C. (2021). “Marine dinocysts, acritarchs and less well-known NPP: tintinnids, ostracod and foraminiferal linings, copepod and worm remains,” in Applications of Non-Pollen Palynomorphs: from Palaeoenvironmental Reconstructions to Biostratigraphy, eds F. Marret, J. O’Keefe, P. Osterloff, M. Pound, and L. Shumilovskikh (London: Geological Society), 511. doi: 10.1144/SP511-2020-55
Nishimuta, K., Ueno, D., Takahashi, S., Kuwae, M., Kadokami, K., Miyawaki, T., et al. (2020). Use of comprehensive target analysis for determination of contaminants of emerging concern in a sediment core collected from Beppu Bay, Japan. Environ. Pollut. 272:115587. doi: 10.1016/j.envpol.2020.115587
Okaichi, T. (1997). Science of Red Tides (Akashio no Kagaku), 2nd Edn. Tokyo: Koseisha-Koseikaku, 337.
Park, M., Boalch, G. T., Jowett, R., and Harbour, D. S. (1978). The genus Peterosperma (Prasinophyceae): species with a single equatorial ala. J. Mar. Bio. Ass. U.K. 58, 239–276.
Penaud, A., Hardy, W., Lambert, C., Marret, F., Masure, E., Servais, T., et al. (2018). Dinoflagellate fossils: Geological and biological applications. Rev. Micropaléont. 61, 235–254.
Pospelova, V., Chmura, G. L., Boothman, W., and Latimer, J. S. (2005). Spatial distribution of modern dinoflagellate cysts in polluted estuarine sediments from Buzzards Bay (Massachusetts, USA) embayments. MEPS 292, 23–40.
Sherr, E., and Sherr, B. (1988). Role of microbes in pelagic food webs: a revised concept. Limnol. Oceanog. 33, 187–197.
Shimada, M., Takahara, H., Kuwae, M., Yamamoto, M., Ikehara, K., Irino, T., et al. (2012). Late Holocene human impact on vegetation changes around Beppu Bay in northeast Kyushu, southwest Japan based on the influx pollen data dated by a wiggle-matching. Jpn. J. Palynol. 58, 212–213.
Shin, H. H., Matsuoka, K., Yoon, Y. H., and Kim, Y.-O. (2010a). Response of dinoflagellate cyst assemblages to salinity changes in Yeoja Bay, Korea. Mar. Micropaleont. 77, 15–24.
Shin, H. H., Mizushima, K., Oh, S. J., Park, J. S., Noh, I. H., Iwataki, M., et al. (2010b). Reconstruction of historical nutrient levels in Korean and Japanese coastal areas based on dinoflagellate cyst assemblages. Mar. Pol. Bull. 60, 1243–1258. doi: 10.1016/j.marpolbul.2010.03.019
Shumilovskikh, L. S., O’Keefe, J. M. K., and Marret, F. (2021). “An overview of the taxonomic groups of NPPs. In Applications of Non-pollen Palynomorphs: from palaeoenvironmental reconstructions to biostratigraphy,” in Applications of Non-Pollen Palynomorphs: from Palaeoenvironmental Reconstructions to Biostratigraphy, eds F. Marret, J. O’Keefe, P. Osterloff, M. Pound, and L. Shumilovskikh (London: Geological Society), 511.
Shumilovskikh, L. S., Schlütz, F., Lorenz, M., and Tomaselli, B. (2019). Non-pollen palynomorphs notes: 3. Phototrophic loricate euglenoids in palaeoecology and the effect of acetolysis on Trachelomonas loricae. Rev. Palaeobot. Palynol. 270, 1–7.
Stancliffe, R. P. W. (1989). Microforaminiferal linings: Their classification, biostratigraphy and paleoecology, with special reference to specimens from British Oxfordian sediments. Micropaleont. 35, 337–352. doi: 10.2307/1485676
Sugaya, M., and Nakao, S. (1983). Distribution of recent shallow water foraminifera of Bungo Suido. Bull. Geol. Surv. Jpn. 34, 483–496.
Takahashi, S., Anh, H. Q., Watanabe, I., Aono, D., Kuwae, M., and Kunisue, T. (2020). Characterization of mono- to deca-chlorinated biphenyls in a well-preserved sediment core from Beppu Bay, Southwestern Japan: Historical profiles, emission sources, and inventory. Sci. Total Environ. 743:140767. doi: 10.1016/j.scitotenv.2020.140767
Taniguchi, A. (1975). “A role and position of zooplankton in marine ecosystem,” in Marine Science Basic Series 6, ed. S. Motoda (Tokyo: Tokai University Press), 119–235.
Thorsen, T., and Dale, B. (1998). Climatically influenced distribution of Gymnodinium catenatum during the past 2000 years in coastal sediments of southern Norway. Palaeog. Palaeoc. Palaeoecol. 143, 159–177.
Topping, J. N., Murray, J. W., and Pond, D. Z. W. (2006). Sewage effects on the food source and diet of benthic formaninifera living in anoxic sediment: a microcosm experiment. J. Exp. Mar. Biol. Ecol. 329, 239–250.
Tsugeki, N. K., Kuwae, M., Tani, Y., Guo, X.-Y., Omori, K., and Takeoka, H. (2016). Temporal variations in phytoplankton biomass over the past 150 years in the western Seto Inland Sea, Japan. J. Oceanog. 73, 309–320. doi: 10.1007/s10872-016-0404-y
Tsujimoto, A., Nomura, R., Yasuhara, M., Yamazaki, H., and Yoshikawa, S. (2006). Impact of eutrophication on shallow marine benthic foraminifers over the last 150 years in Osaka Bay, Japan. Mar. Micropaleont. 60, 258–268.
Uye, S., and Takamatsu, K. (1990). Feeding interactions between planktonic copepods and red-tide flagellates from Japanese coastal waters. MEPS 59, 97–107. doi: 10.3354/meps059097
van Geel, B. (2001). “non-pollen palynomorphs,” in Tracking Environmental Change Using Lake Sediments. Volumen 3: Terrestrial, Algal and Siliceous Indicators, eds J. P. Smol, H. J. B. Birks, and W. M. Last (Netherlands: Kluwer Academic Publishers), 99–119.
van Waveren, I. M. (1993). Chitinous palynomorphs and palynodebris representing crustacean exoskeleton remains from sediments of the Banda Sea (Indonesia). Geologica Ultraiectina Mededelingen van den Faculteit Aardwetenschappen Universiteit Utrecht 104, 18–51.
Versteegh, G. J. M., Blokker, P., Bogus, K. A., Harding, I. C., Lewis, J., Oltmanns, S., et al. (2012). Infra red spectroscopy, flash pyrolysis, thermally assisted hydrolysis and methylation (THM) in the presence of tetramethylammonium hydroxide (TMAH) of cultured and sediment-derived Lingulodinium polyedrum (Dinoflagellata) cyst walls. Org. Chem. 43, 92–102. doi: 10.1016/j.orggeochem.2011.10.007
Wall, D. (1962). Evidence from Recent plankton regarding the biological affinities of Tasmanites Newton 1875 and Leiosphaeridia Eisenack 1958. Geol. Mag. 99:362.
Wall, D., and Dale, B. (1968). Modern dinoflagellate cysts and the evolution of the Peridiniales. Micropaleont. 14, 265–304.
Williams, G. L., Fensome, R. A., and MacRae, R. A. (2017). DINOFLAJ3. American Association of Stratigraphic Palynologists, Data Series 2. Available online at: http://dinoflaj.smu.ca/dinoflaj3 (accessed January 20, 2022).
Yamada, K., Takemura, K., Kuwae, M., Ikehara, K., and Yamamoto, M. (2016). Basin filling related to the Philippine Sea Plate motion in Beppu Bay, southwest Japan. J. Asian Earth Sci. 117, 13–22. doi: 10.1016/j.jseaes.2015.12.008
Yasuhara, M., Yamazaki, H., Irizuki, T., and Yoshikawa, S. (2003). Temporal changes of ostracode assemblages and anthropogenic pollution during the last 100 years, in sediment cores from Hiroshima Bay, Japan. Holocene 13, 527–536.
Zonneveld, K. A. F., Marret, F., Versteegh, G. J. M., Bonnet, S., Bouimetarhan, I., Crouch, E., et al. (2013). Atlas of modern dinoflagellate cyst distribution based on 2405 datapoints. Rev. Palaeobotan. Palynol. 191, 1–197.
Keywords: aquatic palynomorph, dinoflagellate cyst, microforaminiferal lining, anthropogenic eutrophication, Beppu Bay, Anthropocene
Citation: Matsuoka K, Kojima N and Kuwae M (2022) Marine Environmental Change Induced by Anthropogenic Activities – From a Viewpoint of Aquatic Palynomorph Assemblages Preserved in Sediment Cores of Beppu Bay, West Japan. Front. Mar. Sci. 9:843824. doi: 10.3389/fmars.2022.843824
Received: 27 December 2021; Accepted: 16 February 2022;
Published: 28 March 2022.
Edited by:
Fernando Rubino, Water Research Institute (IRSA) (CNR), ItalyReviewed by:
Haifeng Gu, Third Institute of Oceanography, State Oceanic Administration, ChinaHilal Aydin, Manisa Celal Bayar University, Turkey
Audrey Limoges, University of New Brunswick, Canada
Copyright © 2022 Matsuoka, Kojima and Kuwae. This is an open-access article distributed under the terms of the Creative Commons Attribution License (CC BY). The use, distribution or reproduction in other forums is permitted, provided the original author(s) and the copyright owner(s) are credited and that the original publication in this journal is cited, in accordance with accepted academic practice. No use, distribution or reproduction is permitted which does not comply with these terms.
*Correspondence: Kazumi Matsuoka, a2F6dS1tdGtAbmFnYXNha2ktdS5hYy5qcA==