- 1Department of Biological and Environmental Sciences and Technologies, University of Salento, Lecce, Italy
- 2CoNISMa, Consorzio Nazionale Interuniversitario Per le Scienze del Mare, Rome, Italy
- 3Department of Biology, University of Rome “Tor Vergata,” Rome, Italy
- 4Department of Biology, University of Bari Aldo Moro, Bari, Italy
The biodiversity of macrobenthic invertebrates of two artificial hard substrates close to a mariculture plant was assessed in order to understand the effect on the fouling community of an innovative Integrated Multi-Trophic Aquaculture (IMTA) system. The examined hard substrates were (i) vertical bare collectors that are placed around the cages as new colonizable substrates, which were investigated from the early colonization and (ii) artificial hard substrates already present under the cages analyzed over time to observe changes due to the action on the water column by filter feeder organisms colonizing the above vertical collectors. Overall, 186 taxa were collected (both the substrates), of which 99 as sessile habitat-former or structuring macroinvertebrates and 87 as associated fauna, mostly vagile forms. On the vertical collectors 121 taxa were collected, among which 44 sessile structuring species and 77 vagile-associated taxa; on the artificial hard substrates under the cages, 124 taxa were identified, 95 belong to the first category and 29 as associated taxa. The two analyzed substrates shared 43% of sessile species (40) and 22% of associated species (19). At the end of the first year of experimentation, the study revealed Sabella spallanzanii and mussels as the most abundant taxa. Lastly, the communities under the cages showed an increase in biodiversity after the placement of collectors. The changes were attributed to the decrease in particulate matter originating as wastes from the breeding cage, which was intercepted by the filter feeder community developed on the vertical collectors.
Introduction
The exploitation of ocean’s resources to produce feed for the growing global human population needs to identify more sustainable methods to mitigate resulting impacts (Lozano et al., 2010). Indeed, although mariculture is one of the most environmentally efficient ways of producing animal protein, it can cause localized environmental alterations (Kalantzi and Karakassis, 2006; Tomassetti et al., 2009; Grigorakis and Rigos, 2011). By contrast, if carefully designed, mariculture may be an effective strategy to achieve greater positive impact, with the challenge of improving habitat restoration and conservation of marine ecosystems, generating positive ecological, economic, and social impacts (Grabowski and Peterson, 2007; Matthew, 2016; Theuerkauf et al., 2019; Giangrande et al., 2021a).
Relevant examples in sustainable mariculture come from bivalves and seaweed productions, which are activities with a near-zero energy balance, do not require food supply, and produce minimal greenhouse gases and wastes (Aubin et al., 2018); besides, providing new substrates suitable for marine organisms, such activities increase the local biodiversity, enhance the ecosystem functioning, and improve the water quality (Grabowski and Peterson, 2007; Watson et al., 2020). Due to the photosynthetic uptake of carbon dioxide, seaweed aquaculture can also mitigate local effects of ocean acidification by increasing the aragonite saturation state (Rabiei et al., 2014; Mongin et al., 2016). Similarly, bivalves have a positive effect on the water quality, as they feed on both phytoplankton and particulate organic matter and reduce the water organic load (Higgins et al., 2011).
Restoration coupled with production is the current challenge in the sustainable management of marine coastal ecosystems. Oyster reef restoration is a pertinent example: loss of the oyster beds was one of the most dramatic examples of negative human actions on coastal marine ecosystems, with the loss of the ability to immobilizing huge amounts of carbon (Jaris et al., 2019; Rodriguez-Perez et al., 2019) due to the severe impoverishment of the habitat (Thurstan et al., 2017). The restoration of such depleted habitats promised multiple benefits, for this reason, significant efforts were made in restoring them also through market-based solutions to ensure the sustainability of the sector (Grabowski and Peterson, 2007; Tallman and Forrester, 2007; Bayraktarov et al., 2016; Matthew, 2016).
The Integrated Multi-Trophic Aquaculture (IMTA) is another relevant example of the activity inserted within this framework: in fact, the benefits of the integrated approach include minimizing impacts through waste recycling and the production of valuable biomass as a by-product and, at the same time, rising fishes in an optimal environment and thus, improving their performances (Chopin, 2012, 2013, 2020; Reid et al., 2013). Unfortunately, literature-concerning studies about the impacts of the integrated systems on the surrounding environment and the possible sea bottom restoration are still very poor (Carvalho et al., 2006; Borja et al., 2009; Martinez-Garcia et al., 2013).
The present paper is a part of the monitoring program of the ecological status assessment of the marine environment that surrounds an aquaculture plant located in the Mar Grande of Taranto (Ionian Sea), where an innovative IMTA, hosting a new set of bioremediator organisms, such as polychaetes, sponges, bivalves, and macroalgae, has been realized within the EU Remedia Life Project (LIFE16 ENV/IT/000343). One of the major novelties of this project was the experimentation of artificial vertical collectors placed in the water column for enhancing the natural settlement of extractive sessile macroinvertebrates and so evaluating their action as effective bioremediators in improving the environmental quality (Giangrande et al., 2020a). In general, these fouling communities are considered a threat as their settlement on cage nets can limit water exchanges, reduce oxygen levels, and favor the waste accumulation in the surrounding environment, with an impact on fish health and welfare (de Nys and Guenther, 2009; Fitridge et al., 2012). Be that as, the presence of these organisms on dedicated artificial substrates (e.g., biofouling collectors) placed near aquaculture facilities may positively contribute to reducing environmental impacts (Montalto et al., 2020).
The aims of the present study include i) the description of the colonization pattern of benthic community on vertical collectors that consist of bare coconut fiber ropes; ii) the biodiversity assessment on the artificial hard substrates in the mariculture plant area; iii) the influence of vertical collectors on the hard bottom community under the cages; and iv) the proposal of the promising employment of IMTA method for coupling fish production with habitat restoration.
Materials and Methods
Study Area
The study area is located on the south-west coast of the Mar Grande of Taranto (40°25′56″ N; 17°14′19″ E) (Northern Ionian Sea), which, together with the Mar Piccolo, is part of one of the most important coastal marine ecosystems along the Apulian coast (Figure 1A). The Mar Grande of Taranto is a semi-enclosed basin that is connected to the Gulf of Taranto through three artificial dams. The temperature shows seasonal variations typical of the coastal Ionian regions with an average annual value of about 18°C, while the salinity is about 38‰ and is almost uniform over the year. The area is affected by intense anthropogenic activities: a commercial port in the northern portion, a military harbor in the southern portion, four tourist harbors at northeast and southern, the built-up of Taranto at the east, and a lot of mussel farming facilities.
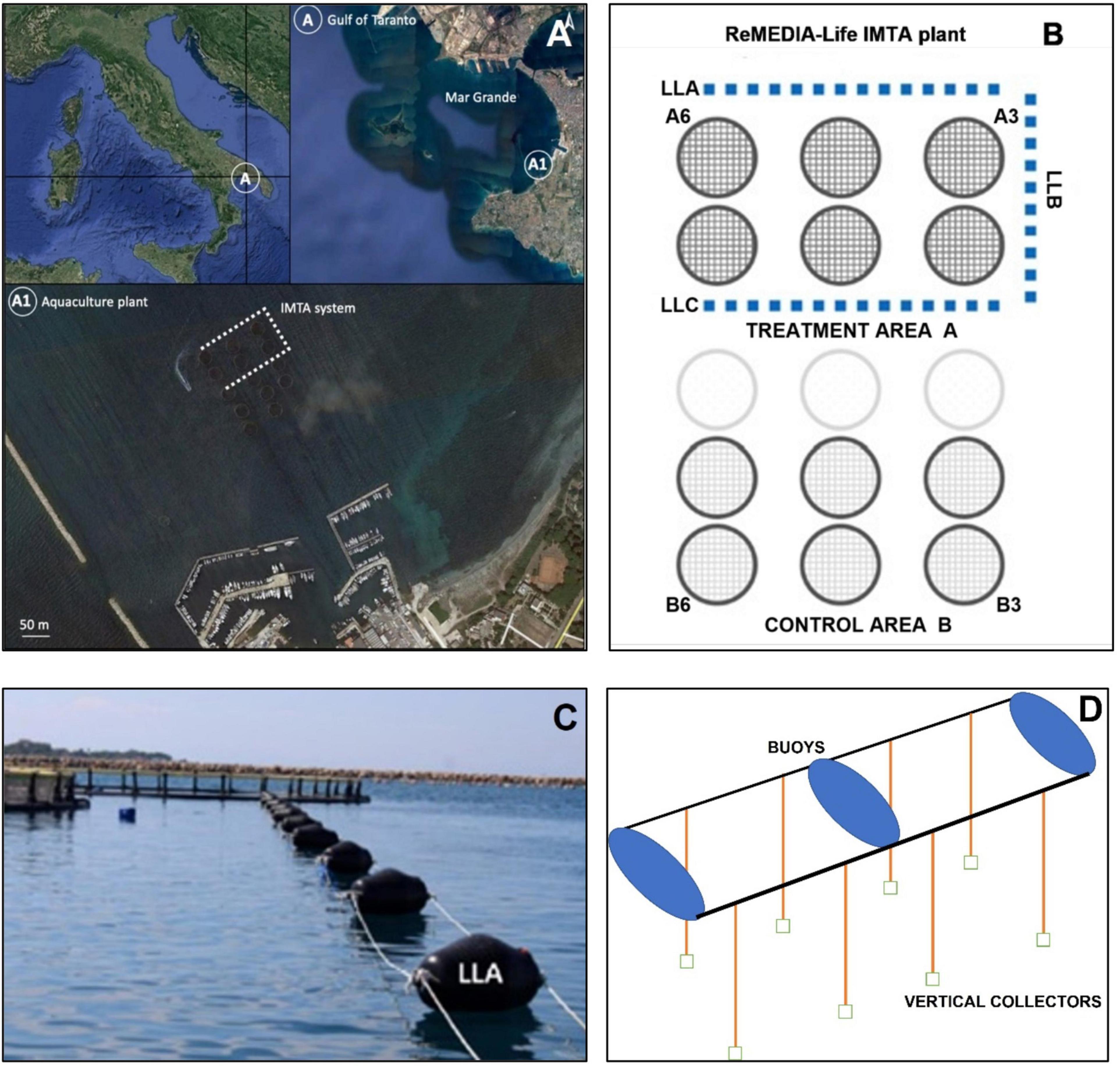
Figure 1. (A) Map of the study site; (B) disposition of the long lines, the blue points represent the three long lines in the area A (treatment area), and the A3, A6, B3, and B6 are indicative of the sampling points of the hard substrate benthic communities; (C) a photograph of the external long line; (D) scheme showing the vertical collectors in two chambers.
Hard bottom communities on both natural and artificial substrates have been widely investigated mostly in the Mar Piccolo (Parenzan, 1969; Gherardi, 1973; Gherardi and Lepore, 1974; Tursi et al., 1974, 1977, 1978, 1979; Scalera-Liaci et al., 1976; Matarrese et al., 1980, 2004; Gherardi et al., 1982, 1993; Montanaro and Tursi, 1983; Scalera-Liaci and Corriero, 1993; Longo et al., 2004, 2007; Pierri et al., 2010, 2019; Lezzi and Giangrande, 2018), where phytobenthic communities, mainly seaweeds, have also been studied (Pierpaoli, 1923; Petrocelli et al., 2019).
The present investigation was performed in the “Maricoltura del Mar Grande” plant, which is a partner of the Remedia Life Project and houses the IMTA experimentation. The aquaculture plant produces about 100 tons of fish a year on a surface of 0.06 Km2 and is located about 600 m from the coast in a semi-confined area of the Mar Grande. It consists of 15 floating cages (Ø 22 m) for breading European seabass Dicentrarchus labrax (Linnaeus, 1758) and sea bream Sparus aurata (Linnaeus, 1758) that go up to a depth ranging from 7 to 12 m (Figure 1B).
In order to investigate the possible effect of the innovative IMTA system on the surrounding environment, an accurate monitoring survey that includes both biological and physico-chemical parameters in the area before the beginning of the experimentation activities was performed. This ex ante survey aimed to identify the most suitable site to place the experimental modules for IMTA purposes and to assess a reliable reference baseline for assessing the possible environmental changes after the bioremediation activity (Giangrande et al., 2021b). This ex ante analysis revealed an area of the plant, which resulted in more impact and was chosen to place the bioremediation system. Here, a total of three long-lines (LLs) supported by buoys to avoid the sinking, named, LLA, LLB, and LLC, were placed around six cages. This area, i.e., stations A3 and A6, is referred to as the treatment site (Treatment Area A, Figure 1B). The opposite area around other six cages without LLs that include stations B3 and B6 was referred to as the control area (Control Area B, Figure 1B).
In the long lines, the spaces between two consecutive buoys constitute a breeding “chamber” (16 chambers in LLA, 8 in LLB, and 12 in LLC) housing module of bioremediator organisms, such as Polychaeta, sponges, and bivalves, on vertical collectors and macroalgae positioned horizontally on the surface in plastic socks (Giangrande et al., 2020a). In addition, vertical bare collectors consisting of coconut fiber ropes of 2 cm wide and 10 m long were installed in all LLs for supporting the natural settlement of fouling species (Figures 1C,D; see Giangrande et al., 2020a for a better description of the IMTA plant). These latter are the vertical collectors analyzed in the present paper. On such bare collectors, the colonization pattern was investigated in order to detect the success of filter feeder species, especially Sabella spallanzanii (Gmelin, 1791), whose abundant presence in the area is known in the literature (Pierri et al., 2010, 2019; Lezzi and Giangrande, 2018).
Field Work: Sampling and Processing
The Remedia Life Project has a duration of 4 years, and the present study referred to the first 3 years of activity, from 2018 to 2021 that includes two productive cycles (2018–2019 and 2019–2020). The last productive cycle, i.e., 2020–2021, is still in progress. Every year all the collectors were removed and replaced with new ones for the further production cycle. The study involved the analysis of the fouling on the bare vertical collectors and of the community occurring on the artificial hard substrates located under the cages. All the material collected from the vertical structures and hard substrates was sorted and identified in the laboratory at the finest level possible, by specialists of different groups. The nomenclature adopted is reported in the relevant updated check list of the World Register of Marine Species (WoRMS Editorial Board, 2021).
Vertical Structures for Fouling Recruitment
During the first year of the study, in October 2018, a total of 196 bare vertical collectors were placed in the breeding chambers and divided according to LLs length (76 in LLA, 54 in LLB, and 66 in LLC).
The macrofouling colonization of the collectors was monitored in the external (LLA) and internal (LLC) LLs, from December 2018 (S1) to June 2019 (S5) (February, March, and April represents, respectively, S2, S3, and S4) by taking photographs with an underwater digital camera. Fifty-centimeter long portions of collectors were in vivo photographed (3 replicates for each long line) at two different levels (surface 0 m and 10 m depth). Twelve photographs were taken each time for a total of 60 pictures. Images were analyzed using the software ImageJ (Abràmoff et al., 2004) to detect the coverage of the sessile organisms identified to the lowest possible taxonomic level. The total surface area was 100 square centimeters (50 × 2 cm2). A multi-layer coverage was considered, with a potential surface coverage greater than 100%. During this procedure, some organisms were collected for a more accurate identification under stereomicroscope in the laboratory.
The total biodiversity of the vertical collectors was then estimated in December 2019, after the first cycle of experimentation (14 months), when all the organisms were scraped off from collectors considering three replicates of 1 m length for a detailed taxonomic analysis. Both encrusting and interstitial fauna were collected and analyzed after the filtration of the trapped mud with a 0.5 mm mesh sieve. Collected organisms were taken to the laboratories of the Department of Biological and Environmental Sciences and Technologies (Salento University) and of the Department of Biology of the University of Rome “Tor Vergata” for taxonomical studies.
Artificial Hard Substrates Under the Cages
The hard bottom community was studied on permanently immersed artificial hard substrates under the cages, such as iron chains and concrete anchoring blocks, placed on muddy sediment without vegetation cover, at 12 m depth. In order to evaluate the changes in the macrobenthic communities, a survey was conducted each year from 2018 (ex ante analysis) to 2020 in four stations, the two within the treatment area under the fish cages with IMTA system (A3 and A6), and the two within the control area under the fish cages without IMTA system (B3 and B6; Figure 1B). Each year the survey was repeated in two periods of the year, corresponding in the Mediterranean to cold and warm seasons (winter and summer). At each station, photographs were acquired randomly and subsequently analyzed with the ImageJ software annotating the conspicuous fauna species detected and coverage. For a fine identification of the organisms, random samples were collected by scraping off three replicates of 20 cm2 at each station. Samples were taken to the laboratory of the Biology Department (University of Bari Aldo Moro) for sorting and taxonomic analyzes.
Statistical Analysis
Vertical Collectors
Permutation analysis of variance, PERMANOVA (Anderson, 2001), was performed to test for differences in the composition of macrofouling assemblages in relation to three factors: Sampling time (S; five levels: S1, S2, S3, S4, and S5), depth (D; 2 levels: Sh, De), and LL (2 levels: LLA and LLC). When significant interactions were detected (p < 0.05), differences among assemblages across factor’s levels were identified with post hoc pairwise comparison. Prior to the analysis, the coverage data of the detected species were square-root transformed and a triangular similarity matrix was obtained applying the Bray-Curtis index (Bray and Curtis, 1957). Non-metric multidimensional scaling (nMDS) via Bray-Curtis distances on square-root transformed data, combined with clustering analysis, was used to visualize changes in the composition of macrofouling assemblages (nMDS plot). Species that contributed most to similarities among groups of samples were identified using the similarity percentages analysis (SIMPER) and were added as overlay vectors on the nMDS plot. The cutoff criterion for the identification of species responsible for covering collectors was placed at 90%. Mean values of species richness and macrofouling percent coverage were also computed and graphed over time. One-way ANOVA was used to test for differences in species richness and percent coverage across sampling time. Prior, Levene’s test was performed to verify the homogeneity of variances. PERMANOVA, nMDS, and SIMPER analyses were run using the PRIMER v6 + PERMANOVA software (Anderson et al., 2008). One-way ANOVA was performed using STATISTICA 10 software.
Artificial Hard Substrates Under the Cages
Permutation analysis of variance (Anderson, 2001) was performed to test for differences in the composition of macrobenthic assemblages in relation to two factors: Station (St; four levels: A3, A6, B3, and B6) and sampling time (S; three levels: S1, S2, and S3). If necessary, square-root transformed data in a Bray-Curtis similarity matrix with 9,999 permutations were used to perform the analyses. If it was impossible to obtain enough permutations for PERMANOVA analysis, the reference p was obtained using a permutation simulation test (Monte Carlo test). The pairwise test was applied to discover statistically significant differences in each pair of factor levels based on the significant value of PERMANOVA/Monte Carlo tests. nMDS via Bray-Curtis distances on square-root transformed data, combined with clustering analysis, was used to visualize changes over time of the assemblages colonizing the artificial hard bottom under the cages (nMDS plot). To better highlight the changes in the assemblage composition, species characterizing the different sampling periods were added as overlay vectors. The number of taxa identified for each phylum were also computed and graphed in a histogram divided by the station. One-way ANOVA was used to test for differences (treatment vs. control) in the number of taxa across sampling time. Prior, Levene’s test was performed to verify the homogeneity of variances. One-way ANOVA was performed using STATISTICA 10 software. PERMANOVA and nMDS analyses were conducted using PRIMER v6 + PERMANOVA software (Anderson et al., 2008).
Results
Macrobenthos Colonization of Vertical Collectors
After 2 months from the placement of vertical collectors (December 2018, S1), macrofouling has already started to colonize the substrate and was clearly visible: the percent coverage and species richness occurring on the collectors are shown in Figure 2. One-way ANOVA test (Table 1) showed that percent coverage and species richness were significantly affected by sampling time. The first immersion time showed the lowest values of total coverage, which, starting from the second time (February 2019, S2), began to increase progressively, reaching the maximum value (95%) at the end of the period of successional study, after 8 months of colonization (June 2019, S5). Similarly, the lowest value for species richness was observed at the beginning of the experiment; it started to increase from S2 in both the LLs examined, with a slight decrease in S4, reaching the maximum value in the last period of observation.
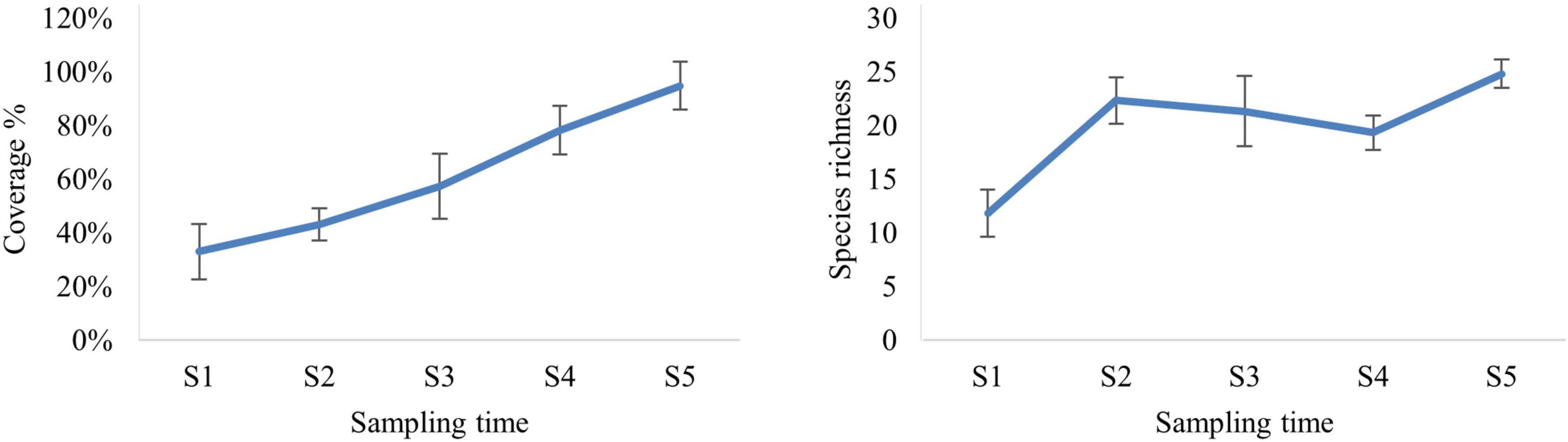
Figure 2. The trend over time of total percent coverage (left) and the number of macrofouling species (right) on vertical collectors.
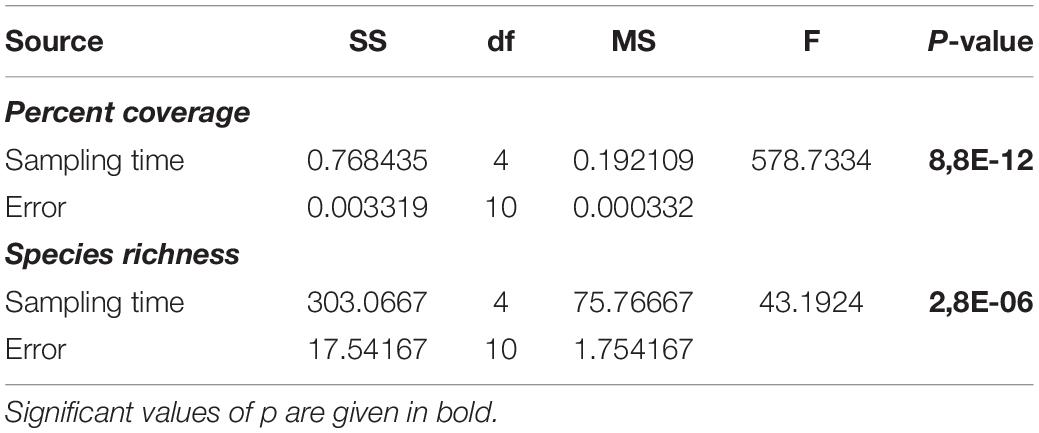
Table 1. Results from one-way ANOVA test on percent coverage and species richness of vertical collectors.
In June 2019, at the end of the successional study, image and laboratory analyses showed that the community had reached a number of 25 sessile species, among which S. spallanzanii and Mytilus galloprovincialis (Lamarck, 1819) were dominant. They showed a similar trend increasing in abundance over time, but at different depth: S. spallanzanii coverage was 1% at the surface and 64% under 4 m up to 10 m depth; by contrast, M. galloprovincialis showed a coverage of 65% at the surface and 2% in depth. Other sabellids, Branchiomma luctuosum (Grube, 1870) and B. boholense (Grube, 1878), were also found less abundant together with S. spallanzanii.
At the endpoint (S5), in the macrofaunal community, both colonial and solitary ascidians were also abundant, especially Phallusia mammillata (Cuvier, 1815) and Styela plicata (Lesueur, 1823): the first more abundant in depth and the second in the shallow samples. Moreover, some remarkable mollusks, such as Ostrea edulis (Linnaeus, 1758) and Limaria hians (Gmelin, 1791), have been found abundantly at various depths along with the collectors.
The multivariate PERMANOVA test (Table 2) showed that the development of the macrofouling assemblage was significantly affected by sampling time, depth, and their interaction (pseudo F = 4.0061; p < 0.05) without any relationship with position (LLx). Post hoc pairwise confirmed that depth across sampling time was responsible for differences in macrofouling assemblage, especially in S5 (Table 3).
The differences in species composition of the macrofaunal assemblages with respect to time and depth are highlighted by the nMDS plot (Figure 3). In the plot, the first sampling time (S1) was characterized mostly by macroalgae, Bugulina calathus (Norman, 1868), Ascidia conchilega (Muller, 1776) and secondarily by Ciona intestinalis (Linnaeus, 1767), Hydroides elegans (Haswell, 1883), and H. dirampha (Mörch, 1863). The temporal samples S2, S3, and S4 were grouped together due to the occurrence of colonial ascidians, i.e., Polyandrocarpa zorritensis (Van Name, 1931), Didemnum coriaceum (Drasche, 1883), Diplosoma listerianum (Milne Edwards, 1841), Botrylloides leachii (Savigny, 1816), and Botryllus schlosseri (Pallas, 1766). Finally, S5 samples were appeared and divided into two sub-groups according to depth with mostly mollusks, i.e., M. galloprovincialis, Pinctada radiata (Leach, 1814), O. edulis in the shallow samples and sabellids, i.e., S. spallanzanii, B. luctuosum, and B. boholense, in the deep samples. SIMPER analysis identified the species mostly responsible for the differences in the macrofaunal community. In June, the last sampling time (S5), M. galloprovincialis and S. spallanzanii mostly contributed to differentiate the shallow samples and the deep ones, respectively (Table 3).
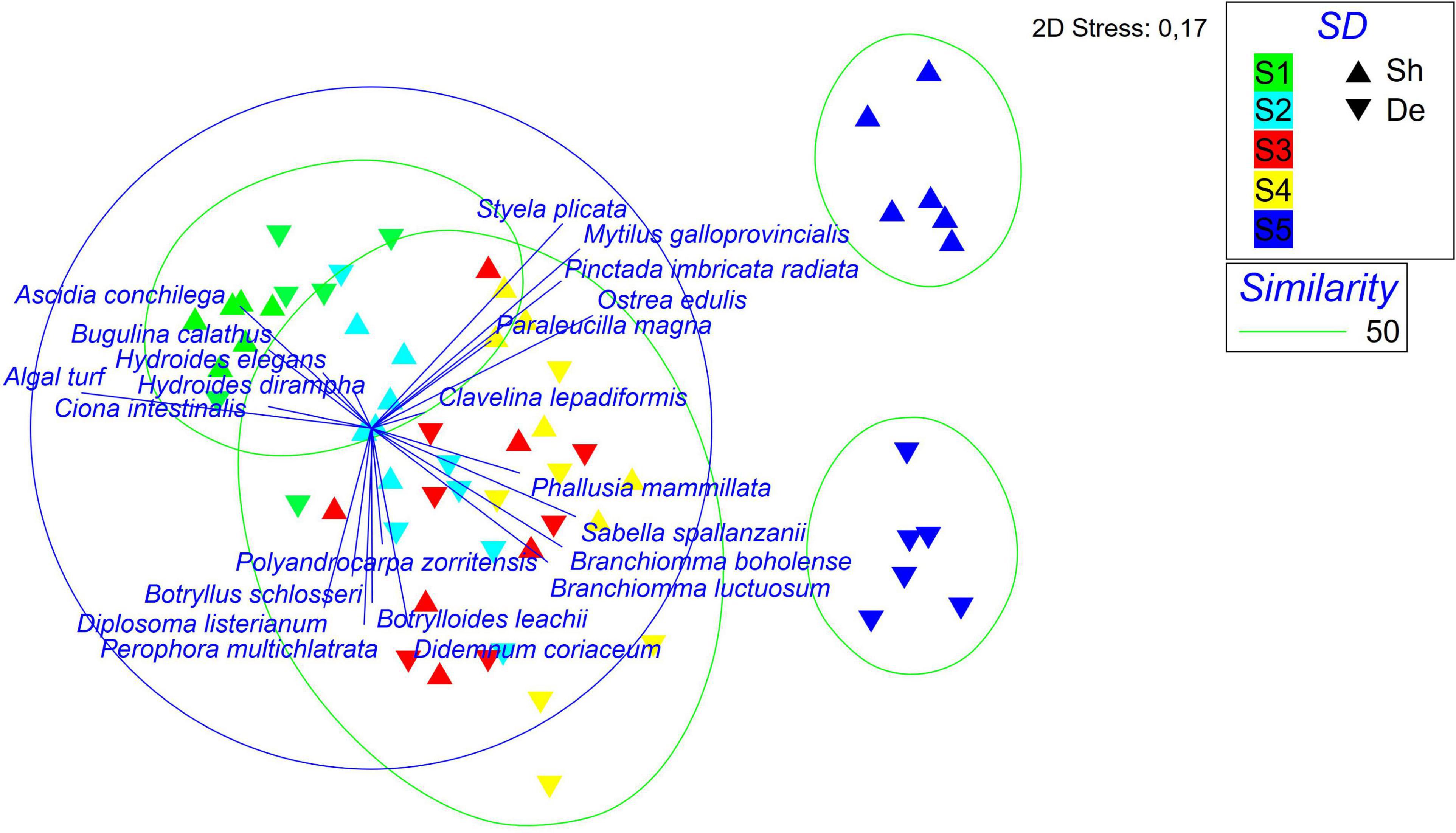
Figure 3. Non-metric multidimensional scaling (nMDS) plot of macrofouling assemblage developed on vertical collectors at different sampling time and depths. S1 = December 2018, S2 = February 2019; S3 = March 2019; S4 = April 2019; S5 = June 2019; Sh = shallow samples, De = deep samples.
Biodiversity of the Artificial Substrates
A total of 186 invertebrate taxa, colonizing the investigated artificial substrates, were identified (Table 4). As regards the vertical collectors, the list includes both the species collected during the successional study from December 2018 to June 2019 and those identified after the sampling of December 2019, when an entire collector was analyzed for a better identification of the taxa. As concerns, the biodiversity was found on artificial hard substrates under the cages, the list refers to the taxa collected during the whole period of study.
Of the 186 taxa identified, 99 species were sessile and can be considered habitat-former or structuring taxa (sensu Barbault, 1991), while 87 were considered associated fauna, and mostly are vagile forms. On the vertical collectors, 121 taxa were collected, among which 44 were sessile structuring species, and 77 were associated fauna; on the artificial hard substrates under the cages, 124 taxa were identified, 95 as sessile structuring species and 29 as associated taxa. Forty sessile species (43%) and 19 associated species (22%) were, respectively, in common between the two analyzed substrates.
Biodiversity on the Vertical Collectors
In December 2019, 14 months after the placement of the experiment, the vertical collectors appeared fully colonized by sessile macroinvertebrates, especially mussels and sabellid polychaetes (Supplementary Figure 1) with a lower contribution of macroalgae. Macroalgae occurred especially during the pioneer phase of colonization as algal turf and disappeared very early, while abundant taxa present in late stages of the fouling community were the red alga Spyridia filamentosa (Wulfen) (Harvey, 1833), seasonally abundant in the superficial area of the collectors, and Dictyota dichotoma (Hudson) (J.V. Lamouroux, 1809) with sparse talus along with all the collectors.
Chordata (Ascidiacea) was the most represented taxon with 19 species, while Porifera was represented by the only alien species Paraleucilla magna (4.5%) (Klautau et al., 2004). The most abundant invertebrate species were M. galloprovincialis, covering more than 50% of the collectors at the surface, and S. spallanzanii becoming dominant deeper, covering 64.2% of the substrate. Other abundant species were the ascidians P. mammillata, S. plicata, and D. coriaceum (9.5, 12.7, and 2.8% of cover, respectively). M. galloprovincialis, S. spallanzanii, and P. mammillata are typical species of the Mediterranean fouling communities; other notable mollusks were O. edulis and L. hians.
Several alien species also contributed to the total biodiversity, e.g., the sabellids B. boholense, B. luctuosum, the hydroids H. elegans and H. dirampha, the calcareous sponge P. magna, the ascidians P. zorritensis (Van Name, 1931), D. bermudensis (Van Name, 1902) and S. plicata, the bryozoan Celleporaria brunnea (Hincks, 1884), the bivalve P. radiata, and the crustaceans Balanus trigonus (Darwin, 1854) and Caprella scaura (Templeton, 1836).
At the end of the first annual cycle, together with the sessile filter-feeder sessile species forming a secondary substrate on the collectors and considered structuring taxa of the community, a large number of vagile species were found on the collector in the trapped mud of the interstice created by encrusting organisms. They were mainly echinoderms and crustaceans collected throughout the entire length of the collector. As regards crustaceans, the anomuran decapod Pisidia bluteli (Risso, 1816) (synonym of P. longimana) and the shrimp Athanas nitescens (Leach, 1814), which are reported to be common on hard artificial reefs and detritic bottoms, especially on oyster and mussel beds (Santelli et al., 2013; Ferreira and Tavares, 2020) was the most abundant species. Amphipods and isopods were also collected in large numbers that include species characteristic of both hard and soft bottom in marine confined areas and lagoons, e.g., Elasmopus rapax (Costa, 1853), Ericthonius punctatus (Spence Bate, 1857), Maera inaequipes (Costa, 1857), Cyathura carinata (Krøyer, 1847), together with hard bottom species associated with ascidians and sponges, e.g., Leucothoe spinicarpa (Abildgaard, 1789), Monocorophium sextonae (Crawford, 1937), and some alien species, e.g., C. scaura and Paracerceis sculpta (Holmes, 1904; Forniz and Sconfietti, 1983; Cantor et al., 2009; Fernandez-Gonzalez and Sanchez-Jerez, 2014, 2017). Remarkable were the records of the echiuran Bonellia viridis and of some gastropod nudibranch species, i.e., Cratena peregrina (Gmelin, 1791), Crimora papillata (Alder and Hancock, 1862), and Doto cervicenigra (Ortea and Bouchet, 1989), which are specialized predators of colonial organisms). Concerning vagile polychaetes, most of the species, e.g., Ceratonereis costae (Grube, 1840), Harmothoe extenuata (Grube, 1840), Lysidice ninetta (Audouin and H Milne Edwards, 1833), Nereis rava (Ehlers, 1868), Platynereis dumerilii (Audouin and Milne Edwards, 1833), Polyophthalmus pictus (Dujardin, 1839), are commonly found on infralittoral rocky bottoms (Mikac et al., 2020), while some species, such as Cirriformia tentaculata (Montagu, 1808) and Naineris setosa (Verrill, 1900), were typical of the soft bottom, the latter is an alien species, recently recorded and spread in muddy sediments of several Mediterranean sites (Khedhri et al., 2014).
Features of the Hard Substrate Community Under the Cages
The multivariate PERMANOVA test (Table 5) showed that the development of the macrofouling assemblage was significantly affected by the sampling time, stations, and their interaction (SxSt; pseudo F = 4.7564; p < 0.01) without any relationship with Seasons. The post hoc pairwise test highlighted that all the stations in 2018 had a different composition while in 2019 the differences remained within the stations subjected to the bioremediation treatment (A3 and A6) and within the control stations (B3 and B6) with no differences between the treatment and control stations except in one case [A6, B6, p (perm) = 0.0045]. At the end of the experiment, in 2020, all the stations, both the treatment and the control ones, showed to diverge, without any recorded significance.
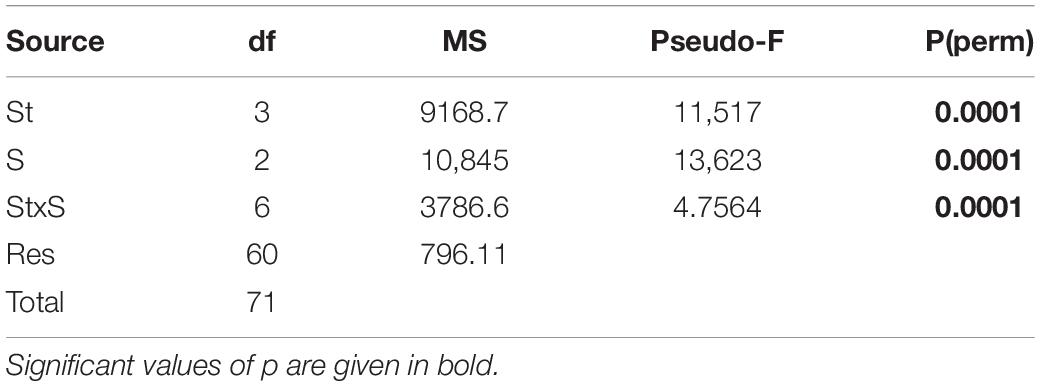
Table 5. Results from permutation analysis of variance (PERMANOVA) analysis of hard substrates under the cages.
As for the general presence of species, at the beginning of the observations (2018), before the positioning of the IMTA system, the artificial hard substrates present under the cages were highly heterogeneous and sparsely populated by macrobenthic organisms with large portions of the substrate covered by sediments. The community was dominated by bivalves, especially M. galloprovincialis and large solitary or colonial ascidians, mainly, Pyura dura (Heller, 1877), S. plicata, Microcosmus spp., D. bermudensis, all of them define together about 80% of the entire macrobenthic community. The algal contribution was practically absent with no difference between the stations investigated (treatment vs. control). One year after the start of the bioremediation experimental plant, the community has undergone an evolution toward greater specific diversity, a reduction in the coverage of the initially dominant species in the treatment area (about 60%), and a shift toward the communities characterizing the control stations. In the control ones, no changes have been registered as shown in Table 6. In particular, tubicolous polychaetes (S. spallanzanii and B. luctuosum) and new ascidians (genera Distaplia, Botrylloides, and Aplidium) were added to the initial community. The alien bivalve P. radiata was added to the mollusks previously found, with a high number of small specimens and much larger and still numerous in the following year (2020). In 2020, a greater complexity and heterogeneity was observed in the samples from the treatment area, detecting a community in which mussels have reduced in number, facilitating the entry of different species of sponges, colonial bryozoans, and colonial ascidians. The control areas have also shown in the last period a constant community structure in terms of the number of species and coverage.
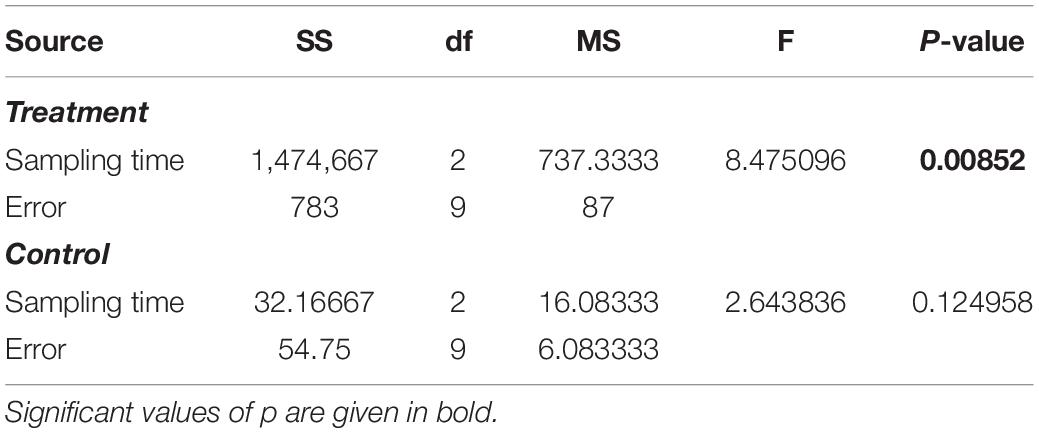
Table 6. Results from one-way ANOVA test (treatment vs. control) on the number of taxa of hard substrates under the cages.
From a taxonomic point of view, overall, a total of 124 taxa were detected on hard substrates under the cages during the studied period. The most represented taxa were Tunicata and Annelida (26 and 24 taxa, respectively) followed by Mollusca and Porifera (20 and 17 taxa, respectively), less represented were Crustacea, Bryozoa, Cnidaria, and Echinodermata (Table 4 and Figure 4).
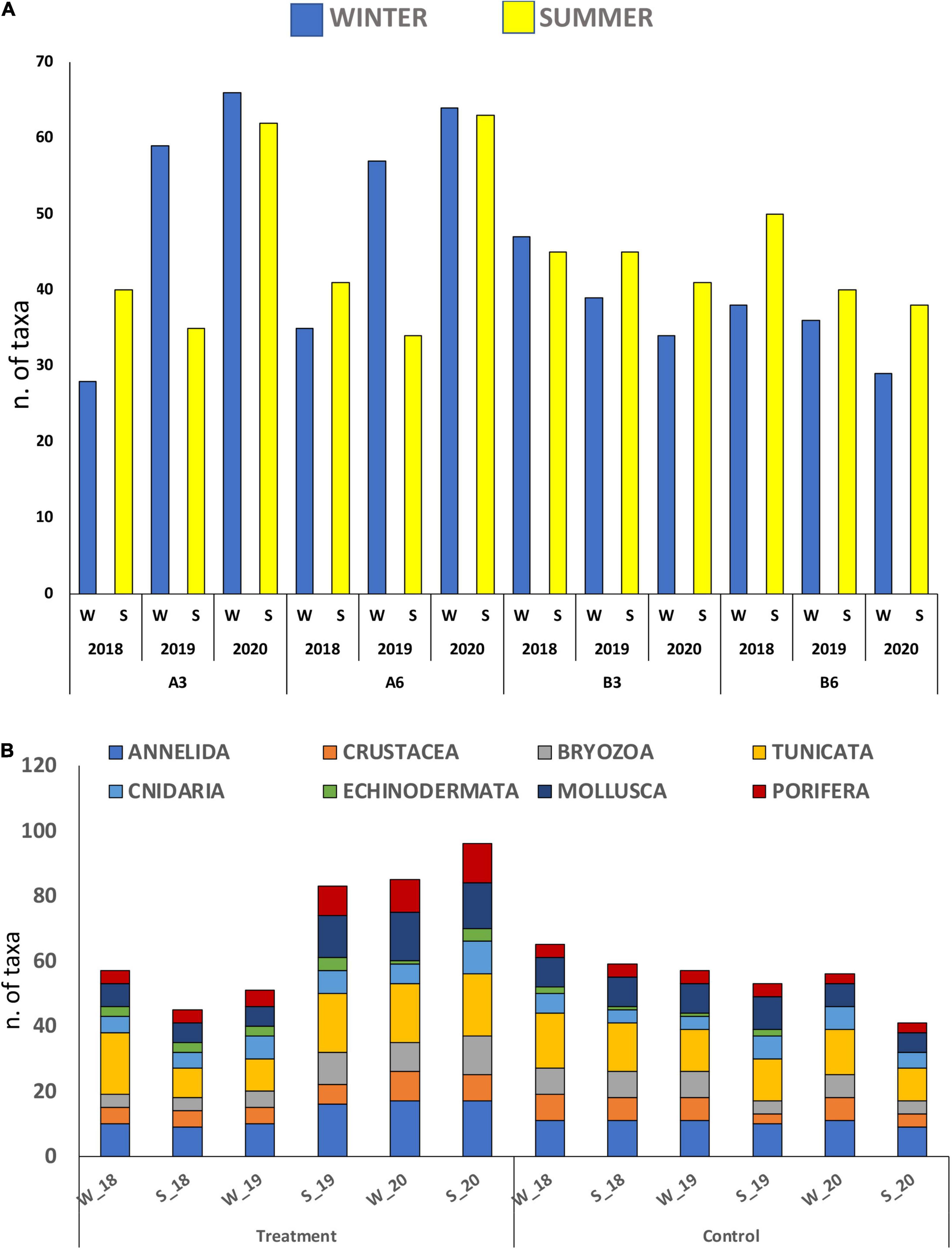
Figure 4. The trend in macrobenthic taxa detected during the 3 years of study on the hard substrates under the fish cages in the treatment (A3 and A6) and control (B3 and B6) sampling points (A) and its composition (B). W = winter; S = summer.
The total number of taxa detected on hard substrates under the cages showed an increase from 2018 to 2020 passing from 86 to 111. Although the overall increase does not seem obvious, the taxa richness showed a continuous increase in the treatment area (A3 and A6 stations), while in the control area (B3 and B6 stations), the number of taxa remains almost constant during the entire period of study (Figures 4A,B). This increase in the treatment area was mainly attributable to species detected in the summer samples where the number of taxa passed from 45 in 2018, to 83 in 2019, up to 96 in 2020, with some taxa, such as annelids, bryozoans, mollusks, poriferans, and ascidians almost doubling the number of species. By contrast in the control area, the number of taxa shows small fluctuations over time with a considerable decrease during the summer of 2020 (Figure 4). It is also worth noting the increase in the number of taxa recorded in the treatment area of the winter samples, which went from 57 in 2018 to 85 in 2020.
Sessile structuring species under the cages can be mainly ascribed to a typical fouling community of confined environments as observed on the collectors as well, with the addition of species typical of the hard bottom of less transient communities, such as poriferans (Table 4). This community was mainly characterized by a high number of exclusive bryozoans, namely, C. brunnea, Cradoscrupocellaria bertholletii (Audouin, 1826), Crisia denticulata (Lamarck, 1816), Crisia fistulosa (Heller, 1867), Crisularia aperta (Hincks, 1886), and 14 exclusive species of poriferans not found on vertical collectors. Lastly, hydroids and serpulidae were also well represented.
As shown in Figure 4B, at the end of the observations, in the treatment area, annelids, mollusks, and bryozoans were the main groups showing a noticeable increase in the number of species. As regards sessile structuring organisms, its increasing was especially due to B. calathus, Scrupocellaria scruposa (Linnaeus, 1758), and Savignyella lafontii (Audouin, 1826) as regards bryozoans; Lissoclinum weigelei (Lafargue, 1968) and D. bermudensis as regards ascidians, this last becoming very abundant at present; again Eudendrium racemosum (Cavolini, 1785), Halecium petrosum (Stechow, 1919), H. pusillum (M. Sars, 1857) as regards hydroids; and lastly to several sponges, such as Clathrina coriacea (Montagu, 1814), Crambe (Schmidt, 1862), Haliclona mediterranea (Griessinger, 1971), Sarcotragus spinosulus (Schmidt, 1862), and Sycon ciliatum (Fabricius, 1780). The increase in species richness is, however, also due to associated fauna.
Vagile fauna showed on the contrary a taxonomic pattern poorer in species than the vertical collectors even if partially overlapped. As already mentioned, excluding some cases, such as N. setosa, the vagile fauna found here is not typical of confined environments.
The multivariate analysis conducted on species diversity among samples is well evident in Figure 5 where the points concerning the control and treatment area appeared clearly separated at the beginning of the investigation (2018 and part of 2019) and resulted again separated in 2020. Indeed, the analysis showed three clusters: A first one containing the station-points of the treatment area (of the year 2018 and winter 2019), which appeared separate on the left of the plane; the second cluster located in the middle that includes the stations of the control area for the years 2018 and 2019 (winter); and the third more conspicuous cluster embracing all the stations relative to the year 2020 and the summer of 2019. The MDS plot confirmed the species most responsible for the distinction of clusters, among which most of the Porifera species for the third cluster.
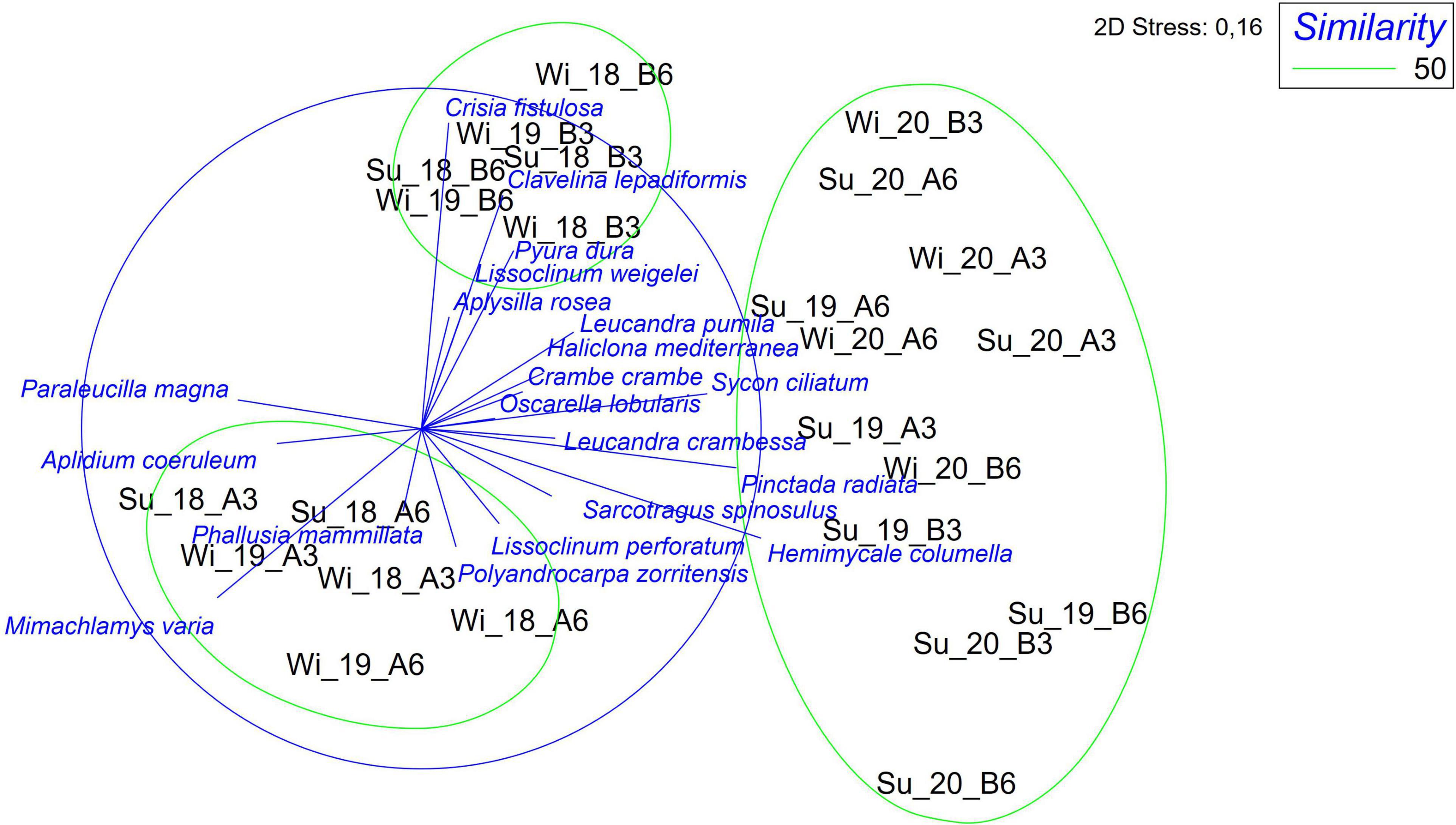
Figure 5. Non-metric multidimensional scaling (nMDS) plots showing the hard substrate with species characterizing the different sampling periods as overlay vectors. Wi = winter; Su = summer.
The changes that occurred in the environment after the placement of the bioremediating collectors were highlighted from some photographs, which show as the particulate was higher in 2018 (Supplementary Figure 2).
Discussion
The analysis of biodiversity in the IMTA plant area of the Mar Grande of Taranto showed a high number of species for both the two examined artificial substrates, vertical collectors hanging from buoys, and artificial hard substrates on the bottom under the cages. Although major differences have been highlighted between the two communities, in terms of the number and composition of species, also relevant similarities have been revealed due to a high number of ascidian species and the high abundance of S. spallanzanii and M. galloprovincialis. Artificial hard substrates on the bottom showed a higher number of sessile species, while vagile forms, mostly amphipods inhabiting the amount of mud trapped in the interstices among sessile species, were more abundant on vertical collectors. Such vagile faunal composition corresponds to that of typical fouling and/or infralittoral hard bottom assemblages affected by mud deposition (Giangrande et al., 2020a; Mikac et al., 2020).
Differences in the composition of sessile fauna between the two substrates can probably be attributed to the different degree of maturity of the communities under the cages when compared to that on the vertical collectors. In the first case, the high number of bryozoans and especially of poriferans, the latter uncommon in the fouling communities of Mar Grande of Taranto (Giangrande et al., 2021c), attested to the advanced stage of the assemblage on the artificial hard substrates under the cages; while the only sponge P. magna, together with the typical fouling elements C. coriacea and S. ciliatum (Longo et al., 2004; Longo and Pronzato, 2011), highlighted the early stage of developing community on the vertical collectors. Indeed, the immersion time of vertical collectors placed during the activity of the Remedia Life is certainly shorter than that of the substrates present under the cages. Although not easily estimated, the latter have been present in the area for at least 10 years and in any case much older than the collectors.
The study of collector colonization indeed suggested the residence time as one of the main drivers in the observed patterns, promoting both the coverage and the number of species increasing in time, as highlighted by other studies on fouling communities (Anderson and Underwood, 1994; Underwood and Chapman, 2006; Antoniadou et al., 2010, 2011; Pierri et al., 2010).
Multivariate analysis showed that the immersion duration and depth had a significant effect on the fouling community development of the collectors. In the beginning, few pioneer species, e.g., the bryozoan B. calathus and the ascidian A. conchilega growing on the considerable algal felt, settled on the collectors at all depths and in all long-lines, contributing to the similarity among samples. Later in the colonization stage, the benthic assemblages differentiated mostly according to depth. By contrast, the LL factor did not affect species composition and abundance, except for the one difference observed in LLA, where the highest macroalgal bloom occurred in April probably affected the settlement of benthic species. Major differences in the LL assemblages were recorded during the first and intermediate stage of colonization, as those between the LLC and LLA LLs, due to the abundance of colonial ascidians, mainly D. coriaceum, D. listerianum, and B. leachii, which probably recruited in springtime. These species acted as early successional species and rapidly covered bare substrate, but only for a short time due to the weak competitive capacity, as already observed by Worcester (1994) and Stachowicz et al. (2002). At an advanced stage, the benthic assemblage on the long-lines converged to a “stable state,” which is dominated by the late-successional species, i.e., M. galloprovincialis and S. spallanzanii.
Such a “stable point” was reached after a period of 9 months when the community was characterized by an assemblage typical of the shallow depth, 0–3 m, dominated by M. galloprovincialis, and a deeper assemblage (3–10 m depth) dominated almost exclusively by sabellid worms. This community pattern remained unchanged until the collector removal in December 2019 and so it was considered the final stage of the fouling community on the collectors.
It is worth noting that an identical pattern of succession has been observed during the following years, 2020 and 2021, throughout the study period. The observed pattern of community development agreed with previous studies, which highlighted the relevant role of the availability of propagule over time and in depth in influencing the fouling community and this latter in turn in influencing the subsequent community phases (Lezzi and Giangrande, 2018). Besides the final assemblages, clearly agreed to the succession endpoints observed in the same period and in the same area by Lezzi and Giangrande (2018). It is however noteworthy that while Lezzi and Giangrande (2018) reported the alien sabellid Branchiomma boholense plays a dominant role in the colonization process, in this study, the native sabellid Sabella spallanzanii remained the dominant species throughout the present investigation, with other sabellids act as associated species. Further studies showed the late-successional stages to be dominated by species particularly efficient in the use of available energy (Anderson and Underwood, 1994; Antoniadou et al., 2010, 2011; Cifuentes et al., 2010).
Overall, after 1 year of immersion, each vertical collector was revealed to be centralizers of biodiversity, being capable of attracting propagules of a large number of both sessile and vagile species. Hard bottom communities under the cages indicate as the macroinvertebrates settled on above vertical collectors can enhance local biodiversity. They are active in the extraction of the particulate matter from the water column and therefore favor the enhancement of the most suitable environment for the benthic organisms on the sea bottom. According to this pattern of increasing efficiency, the biodiversity of the artificial hard bottom under the cages showed a remarkable increase from the year 2018 (before placement of bioremediators) and 2019–2020 (after the placement of bioremediators) (Table 6). Last but not least, the appearance of several species of Porifera that are not part of confined nutrient-rich waters further confirms this scenario, showing a shift from a fouling community to a hard substrate community more typical of natural wild environments.
Sabella spallanzanii, the dominant species on the bare collectors (Giangrande et al., 2020b), is one of the most efficient species in removing particulate organic matter from the water column (Giangrande et al., 2005; Licciano et al., 2005; Cavallo et al., 2007; Stabili et al., 2010, 2014). The collector immersion in the month of October, which corresponds to the spawning period of S. spallanzanii, drove the success of this species in substrate colonization. The full coverage of the collectors by sabellids resulted in a very efficient tool for bioremediation purposes since the worms located in depth can also intercept the wastes produced by mussels and so well addressed one of the main targets of the Remedia Life Project. Besides, also the role of Porifera was relevant in cleaning the water around the cages, particularly, removing the bacterial component (Stabili et al., 2006, 2008; Longo et al., 2010, 2016).
Major effects of bivalve culture on benthic macrofauna (Fabi et al., 2009; McKindsey et al., 2012; Drouin et al., 2015) and on the water column are well known (Prins et al., 1997). Variations in benthic communities among locations are largely due to two main mechanisms: the addition of artificial substrates linked to the farming and the increasing organic load. Similarly, to the mussel farms, the mariculture facilities add considerable physical structure, such as ropes and anchor blocks, which result in available substrate for sessile hard bottom organisms. When fouled, such structures promote an increase in the abundance and diversity of fauna on-farm sites when compared to non-farm sites (Dumbauld et al., 2009; Forrest et al., 2009; McKindsey et al., 2011; Drouin et al., 2015).
More artificial structures mimicking natural reefs could be added under the cages, providing new habitats suitable for a rich benthic assemblage development. The restoration associated with aquaculture involving the use of artificial structures is an extension of the pioneer concept of the artificial reefs (Relini et al., 2007, 2012) and represents a new application of the artificial structure philosophy (Giangrande et al., 2021c). The original purposes of the artificial reefs were the enhancement of fishery and the improvement of coastal management, as the protection of the seabed from illegal fishing and the increase of wild fish populations (Bombace et al., 1994; Relini et al., 2012). The artificial structures applied to the sustainable aquaculture have the additional functions of nursery areas, protection of biodiversity, and habitat for recreational activities. Benthic artificial structures were, however, rarely associated to IMTA that demonstrating as organisms are able to successfully exist in high enrichment areas as long as suitable habitat and environmental conditions can be maintained (Robinson et al., 2011). Some interesting experiments carried out in the central Adriatic Sea where submerged structures were coupled with off-shore mussel farming increasing mussel productivity (Fabi and Spagnola, 2001).
In our experimental system, the marked changes in species composition that have occurred over time on the artificial hard bottom structures under the cages in the treatment site have been demonstrated by the multivariate analysis results. These highlighted the clear distinction of the ex ante samples of the treatment area from the control samples, while a high similarity resulted between the treatment samples and control samples at the end of the study. Moreover, we demonstrated, on the one hand, that the biodiversity of the site was increased by the addition of the artificial substrate and, on the other hand, that the action on the water column due to the filtering activity of the fouled structures increases the biodiversity also on the artificial structures on the sea bottom. Confirming this, our further data, still under investigation (Borghese et al., in prep.1), suggested that a noticeable improvement in the quality of both the water column and soft bottom communities under the cages is due to the filtration by the fouler organisms. Indeed, the particulate matter from the aquaculture activity has been demonstrated to be intercepted by the fouler on vertical collectors and transformed into their biomass, with an estimated production of about 6 Q of polychaete worms and 400 L of sponges at each annual cycle. In addition, the LL structures also acted as a mechanical filter capable of trapping about 600 kg of suspended particulate matter per year (Giangrande et al., 2020a).
We also proved that the best bioremediation performance of the ideated IMTA system was reached by the addition of 172 vertical collectors for Porifera rearing to the 196 bare collectors for sabellids, mollusks, and ascidians here investigated (Giangrande et al., 2020a). We supposed that the combined actions of all these filter feeder invertebrates resulted in a very efficient bioremediation rate, with a clarification of the water column, that allows the amelioration of the bottom benthic assemblage observed on the artificial substrates under the cages. Furthermore, the very abundant muddy-tube builder amphipods found on the collectors may also have an active role in removing the suspended sediment and the organic particles from the water column and so might be efficient contributors to bioremediate the seawater (Gonzalez-Silvera et al., 2015; Fernandez-Gonzalez et al., 2018; Giangrande et al., 2020b).
Lastly, despite the fouling development has always been considered a negative factor for the mariculture production (Fitridge et al., 2012), our study proved that the fouling also has a positive effect on the ecosystem quality and thus supports the mariculture activities.
Conclusion
The ideated IMTA system in the area of the Mar Grande of Taranto provides promising clues that combined with aquaculture activities can improve the environment. Indeed, our study suggested that in the Mediterranean semi-enclosed areas, the IMTA can effectively couple production with restoration purposes. The vertical collectors placed around the cages as support for bioremediators can increase the biodiversity, with positive effects also on the fauna of the hard substrates on the bottom. The filtration activity of the foulers settled on the collectors reduces the supply of nutrients sinking toward the bottom, reducing the surplus of organic load and creating better conditions for the benthic organisms settled under the cages. When artificial structures are also placed under the cages, mimicking natural reefs, they act as restorative structures, providing new habitats suitable for a rich benthic assemblage development, obviously characteristic of the surrounding habitat. These “underwater gardens” play manifold roles: from the supply of larvae for the fauna of the vertical collectors to the creation of submerged structures, i.e., artificial reefs, capable to attract fishes and underwater tourism. At the same time, the fouling settled on the vertical structures placed around the fish cages increase the production of the area, as the fouling organisms consume the wastes from the in-shore mariculture plants for their food and so transforming the wastes into the biomass of economic value. Despite the great effort required for collector management, this technology opens new perspectives on the challenges related to the use of the by-product biomass.
Data Availability Statement
The original contributions presented in the study are included in the article/Supplementary Material, further inquiries can be directed to the corresponding author/s.
Author Contributions
DA, AG, CL, and CP conceived of the presented idea and wrote the first draft of the manuscript. AG, CL, and CP conceived and planned the experiments. DA, JB, RT, and CP carried out the experiments and with MG, AG, and CL analyzed the samples. All authors contributed to the interpretation of the results, to the implementation of the manuscript, and approved the submitted version.
Funding
This research was funded by the EUROPEAN COMMUNITY, Remedia Life Project LIFE16 ENV/IT/000343.
Conflict of Interest
The authors declare that the research was conducted in the absence of any commercial or financial relationships that could be construed as a potential conflict of interest.
Publisher’s Note
All claims expressed in this article are solely those of the authors and do not necessarily represent those of their affiliated organizations, or those of the publisher, the editors and the reviewers. Any product that may be evaluated in this article, or claim that may be made by its manufacturer, is not guaranteed or endorsed by the publisher.
Supplementary Material
The Supplementary Material for this article can be found online at: https://www.frontiersin.org/articles/10.3389/fmars.2022.842616/full#supplementary-material
Footnotes
- ^ Borghese, J., Arduini, D., Del Pasqua, M., Gravina, M. F., Musco, L., and Giangrande, A. (in prep). Evaluating the Bioremediation Effect of an Innovative IMTA System in the Mar Grande of Taranto (Mediterranean Sea, Italy).
References
Abràmoff, M. D., Magalhães, P. J., and Ram, S. J. (2004). Image processing with ImageJ. Biophotonics Int. 11, 36–42.
Anderson, M. J. (2001). A new method for non-parametric multivariate analysis of variance. Austral Ecol. 26, 32–46. doi: 10.1046/j.1442-9993.2001.01070.x
Anderson, M. J., and Underwood, A. J. (1994). Effects of substratum on the recruitment and development of an intertidal estuarine fouling assemblage. J. Exp. Mar. Bio. Ecol. 184, 217–236. doi: 10.1016/0022-0981(94)90006-x
Anderson, M. J., Gorley, R. N., and Clarke, K. R. (2008). PERMANOVA+ for PRIMER: Guide to Software and Statistical Methods. Plumoth: PRIMER-E Ltd.
Antoniadou, C., Voultsiadou, E., and Chintiroglou, C. (2010). Benthic colonization and succession on temperate sublittoral rocky cliffs. J. Exp. Mar. Bio. Ecol. 382, 145–153. doi: 10.1016/j.jembe.2009.11.004
Antoniadou, C., Voultsiadou, E., and Chintiroglou, C. (2011). Seasonal patterns of colonization and early succession on sublittoral rocky cliffs. J. Exp. Mar. Bio. Ecol. 403, 21–30. doi: 10.1016/j.jembe.2011.04.001
Aubin, J., Fontaine, C., Callier, M., and Roque d’orbcastel, E. (2018). Blue mussel (Mytilus edulis) Bouchot culture in Mont-St Michel Bay: potential mitigation effects on climate change and eutrophication. Int. J. Life Cycle Assess. 23, 1030–1041. doi: 10.1007/s11367-017-1403-y
Barbault, R. (1991). Ecological constraints and community dynamics: linking community patterns to organismal ecology. The case of tropical herpetofaunas. Acta Oecol. 12, 139–163.
Bayraktarov, E., Saunders, M. I., Abdullah, S., Mills, M., Beher, J., Possingham, H. P., et al. (2016). The cost and feasibility of marine coastal restoration. Ecol. Appl. 16, 1055–1074. doi: 10.1890/15-1077
Bombace, G., Fabi, G., Fiorentini, L., and Speranza, S. (1994). Analysis of the efficacy of artificial reefs located in five different areas of the Adriatic Sea. Bull. Mar. Sci. 55, 559–580.
Borja, Á., Rodríguez, J. G., Black, K., Bodoy, A., Emblow, C., Fernandes, T. F., et al. (2009). Assessing the suitability of a range of benthic indices in the evaluation of environmental impact of fin and shellfish aquaculture located in sites across Europe. Aquaculture 293, 231–240. doi: 10.1016/j.aquaculture.2009.04.037
Bray, J. R., and Curtis, J. T. (1957). An ordination of the upland forest communities of southern Wisconsin. Ecol. Monogr. 27, 326–349.
Cantor, M., Leite Siqueira, S. G., Cruvinel, G. T., and Pedini Leite, F. P. (2009). Occurrence of the amphipod Leucothoe spinicarpa (Abildgaard, 1789) (Amphipoda) in the ascidian Phallusia nigra (Urochordata, Ascidiacea) in Southeastern Brazil. Nauplius J. Brazilian Crustac. Soc. 17, 13–17.
Carvalho, S., Barata, M., Pereira, F., Gaspar, M. B., da Fonseca, L. C., and Pousao-Ferreira, P. (2006). Distribution patterns of macrobenthic species in relation to organic enrichment within aquaculture earthen ponds. Mar. Pollut. Bull. 52, 1573–1584. doi: 10.1016/j.marpolbul.2006.09.005
Cavallo, D., Pusceddu, A., Danovaro, R., and Giangrande, A. (2007). Particulate organic matter uptake rates of two benthic filter-feeders (Sabella spallanzanii and Branchiomma luctuosum) candidates for the clarification of aquaculture wastewaters. Mar. Pollut. Bull. 54, 622–625. doi: 10.1016/j.marpolbul.2006.11.024
Chopin, T. (2012). Seaweed aquaculture provides diversified products, key ecosystem functions. Part I. Lesser-known species group tops mariculture output. Glob. Aqua. Advocate 15, 24–27.
Chopin, T. (2013). Integrated multi-trophic aquaculture–ancient, adaptable concept focuses on ecological integration. Glob. Aqua. Advocate 16, 16–19.
Chopin, T. (2020). Reducing the carbon footprint of ocean-derived food production (fisheries and aquaculture) and shifting diets: another opportunities to make the ocean part of the solution to climate change. Aquaculture 23, 12–13.
Cifuentes, M., Krueger, I., Dumont, C. P., Lenz, M., and Thiel, M. (2010). Does primary colonization or community structure determine the succession of fouling communities? J. Exp. Mar. Bio. Ecol. 395, 10–20. doi: 10.1016/j.jembe.2010.08.019
de Nys, R., and Guenther, J. (2009). “The impact and control of biofouling in marine finfish aquaculture,” in Advances in Marine Antifouling Coatings and Technologies, eds C. Hellio and D. Yebra (Cambridge: Woodhead Publishing), 177–221. doi: 10.1533/9781845696313.1.177
Drouin, A., Archambault, P., Clynick, B. G., Richer, K., and McKindsey, C. W. (2015). Influence of mussel aquaculture on the distribution of vagile benthic macrofauna in les de la Madeleine, eastern Canada. Aquacult. Env. Interac. 6, 175–183. doi: 10.3354/aei00123
Dumbauld, B. R., Ruesink, J. L., and Rumrill, S. S. (2009). The ecological role of bivalve shellfishaquaculture in the estuarine environment: a review with application to oyster and clam culture in West Coast (USA) estuaries. Aquaculture 290, 196–223. doi: 10.1016/j.aquaculture.2009.02.033
Fabi, G., and Spagnola, A. (2001). “Artificial reefs and mariculture,” in Modern Aquaculture in the Coastal Zone, ed. J. Coimbra (Amsterdam: IOS Press), 91–98.
Fabi, G., Manoukian, S., and Spagnolo, A. (2009). Impact of an open-sea suspended mussel culture on macrobenthic community (Western Adriatic Sea). Aquaculture 289, 54–63. doi: 10.1016/j.aquaculture.2008.12.026
Fernandez-Gonzalez, V., and Sanchez-Jerez, P. (2014). First occurrence of Caprella scaura Templeton, 1836 (Crustacea: Amphipoda) on off-coast fish farm cages in the Mediterranean Sea. Helgol. Mar. Res. 68, 187–191. doi: 10.1007/s10152-013-0375-y
Fernandez-Gonzalez, V., and Sanchez-Jerez, P. (2017). Fouling assemblages associated with off-coast aquaculture facilities: an overall assessment of the Mediterranean Sea. Mediterr. Mar. Sci. 18, 87–96. doi: 10.12681/mms.1806
Fernandez-Gonzalez, V., Toledo-Guedes, K., Valero-Rodriguez, J. M., Agraso, M., del, M., and Sanchez-Jerez, P. (2018). Harvesting amphipods applying the integrated multitrophic aquaculture (IMTA) concept in off-shore areas. Aquaculture 489, 62–69. doi: 10.1016/j.aquaculture.2018.02.008
Ferreira, L. A. A., and Tavares, M. (2020). Pisidia longimana (Risso, 1816), a junior synonym of P. bluteli (Risso, 1816) (Crustacea: Decapoda: Anomura: Porcellanidae) and a species distinct from P. longicornis (Linnaeus, 1767). Pap. Avulsos Zool. 60:e20206036. doi: 10.11606/1807-0205/2020.60.36
Fitridge, I., Dempster, T., Guenther, J., and De Nys, R. (2012). The impact and control of biofouling in marine aquaculture: a review. Biofouling 28, 649–669. doi: 10.1080/08927014.2012.700478
Forniz, A., and Sconfietti, R. (1983). Ritrovamento di Paracerceis sculpta (Holmes, 1904) (Isopoda, Flabellifera, Sphaeromatidae) nella laguna di Venezia. Boll. Mus. Civ. St. Nat. 34, 197–203.
Forrest, B. M., Keeley, N. B., Hopkins, G. A., Webb, S. C., and Clement, D. M. (2009). Bivalve aquaculture in estuaries: review and synthesis of oyster cultivation effects. Aquaculture 298, 1–15. doi: 10.1016/j.aquaculture.2009.09.032
Gherardi, M. (1973). “Ricerche sulle comunità fouling del Mar Piccolo di Taranto,” in Atti III Simposio Nazionale Sulla Conservazione Della Natura, ed. Cacucci (Bari: Università di Bari), 55–73.
Gherardi, M., and Lepore, E. (1974). “Insediamenti stagionali delle popolazioni fouling del mar Piccolo di Taranto,” in Atti IV Simposio Nazionale Sulla Conservazione Della Natura, (Bari: Università di Bari), 235–258.
Gherardi, M., Lepore, E., and Sciscioli, M. (1982). Variazioni qualitative e quantitative di un popolamento a policheti del Mar Piccolo di Taranto. Mem. Biol. Mar. Ocean 12, 217–231.
Gherardi, M., Lepore, E., and Sciscioli, M. (1993). Distribution of the Polychaetous Annelids in the Ionian and lower Adriatic Sea: descriptive analysis. Oebalia 19, 27–45.
Giangrande, A., Cavallo, A., Licciano, M., Mola, E., Pierri, C., and Trianni, L. (2005). Utilization of the filter feeder polychaete Sabella. Aquac. Int. 13, 129–136.
Giangrande, A., Gravina, M. F., Rossi, S., Longo, C., and Pierri, C. (2021a). Aquaculture and restoration: perspectives from Mediterranean Sea experiences. Water 13:991. doi: 10.3390/w13070991
Giangrande, A., Licciano, M., Arduini, D., Borghese, J., Pierri, C., Trani, R., et al. (2021b). “Integrated study for the assessment of an inshore mariculture plant impact on the sourronding environment (Ionian Sea),” in 2021 International Workshop on Metrology for the Sea; Learning to Measure Sea Health Parameters (MetroSea) (Piscataway: IEEE), 349–353.
Giangrande, A., Arduini, D., Borghese, J., Del Pasqua, M., Lezzi, M., Petrocelli, A., et al. (2021c). Macrobenthic success of non-indigenous species related to substrate features in the Mar Grande of Taranto, Italy (Mediterranean Sea). BioInvasions Rec. 10, 238–256. doi: 10.3391/bir.2021.10.2.02
Giangrande, A., Pierri, C., Arduini, D., Borghese, J., Licciano, M., Trani, R., et al. (2020a). An innovative IMTA system: polychaetes, sponges and macroalgae co-cultured in a Southern Italian in-shore mariculture plant (Ionian Sea). J. Mar. Sci. Eng. 8:733. doi: 10.3390/JMSE8100733
Giangrande, A., Lezzi, M., Del Pasqua, M., Pierri, C., Longo, C., and Gravina, M. F. (2020b). Two cases study of fouling colonization patterns in the Mediterranean Sea in the perspective of integrated aquaculture systems. Aquac. Rep. 18:100455. doi: 10.1016/j.aqrep.2020.100455
Gonzalez-Silvera, D., Izquierdo-Gomez, D., Fernandez-Gonzalez, V., Martínez-López, F. J., López-Jiménez, J. A., and Sanchez-Jerez, P. (2015). Mediterranean fouling communities assimilate the organic matter derived from coastal fish farms as a new trophic resource. Mar. Pollut. Bull. 91, 45–53. doi: 10.1016/j.marpolbul.2014.12.029
Grabowski, J. H., and Peterson, C. H. (2007). Restoring oyster reefs to recover ecosystem services. Theor. Ecol. Ser. 4, 281–298. doi: 10.1016/S1875-306X(07)80017-7
Grigorakis, K., and Rigos, G. (2011). Aquaculture effects on environmental and public welfare - The case of Mediterranean Mariculture. Chemosphere 85, 899–919. doi: 10.1016/j.chemosphere.2011.07.015
Higgins, C. B., Stephenson, K., and Brown, B. L. (2011). Nutrient bioassimilation capacity of aquacultured oysters: quantification of an ecosystem service. J. Environ. Qual. 40, 271–277. doi: 10.2134/jeq2010.0203
Jaris, H., Brown, D. S., and Proestou, D. A. (2019). Assessing the contribution of aquaculture and restoration to wild oyster populations in a Rhode Island coastal lagoon. Conserv. Genet. 20, 503–516. doi: 10.1007/s10592-019-01153-9
Kalantzi, I., and Karakassis, I. (2006). Benthic impacts of fish farming: meta-analysis of community and geochemical data. Mar. Pollut. Bull. 52, 484–493. doi: 10.1016/j.marpolbul.2005.09.034
Khedhri, I., Lavesque, N., Bonifácio, P., Djabou, H., and Afli, A. (2014). First record of Naineris setosa (Verrill, 1900)(Annelida: Polychaeta: Orbiniidae) in the Western Mediterranean Sea. Bioinvasions Rec. 3, 83–88. doi: 10.3391/bir.2014.3.2.05
Lezzi, M., and Giangrande, A. (2018). Seasonal and bathymetric effects on macrofouling invertebrates’ primary succession in a mediterranean non-indigenous species hotspot area. Mediterr. Mar. Sci. 19, 572–588. doi: 10.12681/mms.14786
Licciano, M., Stabili, L., and Giangrande, A. (2005). Clearance rates of Sabella spallanzanii and Branchiomma luctuosum (Annelida: Polychaeta) on a pure culture of Vibrio alginolyticus. Water Res. 39, 4375–4384. doi: 10.1016/j.watres.2005.09.003
Longo, C., and Pronzato, R. (2011). Fauna d’Italia. Porifera I. Calcarea, Demospongiae (Partim), Hexactinellida, Homoscleromorpha. Milano: Calderini.
Longo, C., Cardone, F., Corriero, G., Licciano, M., Pierri, C., and Stabili, L. (2016). The co-occurrence of the demosponge Hymeniacidon perlevis and the edible mussel Mytilus galloprovincialis as a new tool for bacterial load mitigation in aquaculture. Environ. Sci. Pollut. Res. 23, 3736–3746. doi: 10.1007/s11356-015-5587-z
Longo, C., Corriero, G., Licciano, M., and Stabili, L. (2010). Bacterial accumulation by the Demospongiae Hymeniacidon perlevis: a tool for the bioremediation of polluted seawater. Mar. Pollut. Bull. 60, 1182–1187. doi: 10.1016/j.marpolbul.2010.03.035
Longo, C., Mastrototaro, F., and Corriero, G. (2007). Occurrence of Paraleucilla magna (Porifera: Calcarea) in the Mediterranean Sea. J. Mar. Biol. Assoc. U. K. 87, 1749–1755. doi: 10.1017/S0025315407057748
Longo, C., Scalera Liaci, L., Manuel, M., and Corriero, G. (2004). Notes on the sponge fauna from Mar Grande and Mar Piccolo of Taranto (Apulian, Ionian Sea). Biol. Mar. Mediterr. 11, 444–447.
Lozano, S., Iribarren, D., Moreira, M. T., and Feijoo, G. (2010). Environmental impact efficiency in mussel cultivation. Resour. Conserv. Recycl. 54, 1269–1277. doi: 10.1016/j.resconrec.2010.04.004
Martinez-Garcia, E., Sanchez-Jerez, P., Aguado-Giménez, F., Ávila, P., Guerrero, A., Sánchez-Lizaso, J. L., et al. (2013). A meta-analysis approach to the effects of fish farming on soft bottom polychaeta assemblages in temperate regions. Mar. Pollut. Bull. 69, 165–171. doi: 10.1016/j.marpolbul.2013.01.032
Matarrese, A., Mastrototaro, F., D’onghia, G., Maiorano, P., and Tursi, A. (2004). Mapping of the benthic communities in the Taranto seas using side-scan sonar and an underwater video camera. Chem. Ecol. 20, 377–386. doi: 10.1080/02757540410001727981
Matarrese, A., Tursi, A., Scalera Liaci, L., Montanaro, C., and Cecere, E. (1980). Variazioni di biomassa nell’Ascidiofauna presente sui substrati duri del Mar Piccolo di Taranto. Oebalia 5, 63–79.
Matthew, G. (2016). Fifteen years of Rhode Island Oyster Restoration: A Performance Evaluation and Cost-Benefit Analysis. Kingston: University of Rhode Island. Master’s thesis. doi: 10.23860/thesis-griffin-matthew-2016
McKindsey, C. W., Archambault, P., and Simard, N. (2012). Spatial variation of benthic infaunal communities in baie de Gasp (eastern Canada) influence of mussel aquaculture. Aquaculture 357, 48–54. doi: 10.1016/j.aquaculture.2012.05.037
McKindsey, C. W., Archambault, P., Callier, M. D., and Olivier, F. (2011). Influence of suspended and off-bottom mussel culture on the sea bottom and benthic habitats: a review. Can. J. Zool. 89, 622–646. doi: 10.1139/z11-037
Mikac, B., Licciano, M., Jaklin, A., Iveša, L., Giangrande, A., and Musco, L. (2020). Diversity and distribution patterns of hard bottom polychaete assemblages in the North Adriatic Sea (Mediterranean). Diversity 12:408. doi: 10.3390/d12100408
Mongin, M., Baird, M. E., Hadley, S., and Lenton, A. (2016). Optimising reef-scale CO2 removal by seaweed to buffer ocean acidification. Environ. Res. Lett. 11:034023. doi: 10.1088/1748-9326/11/3/034023
Montalto, V., Rinaldi, A., Ape, F., Mangano, M. C., Gristina, M., Sarà, G., et al. (2020). Functional role of biofouling linked to aquaculture facilities in Mediterranean enclosed locations. Aquac. Environ. Interact. 12, 11–22. doi: 10.3354/aei00339
Montanaro, C., and Tursi, A. (1983). Settlement of bryozoans in the Mar Piccolo of Taranto (Southern Italy) from 1972 to 1982. Oebalia 9, 91–103.
Petrocelli, A., Cecere, E., and Rubino, F. (2019). Successions of phytobenthos species in a Mediterranean transitional water system: the importance of long term observations. Nat. Conserv. 34, 217–246. doi: 10.3897/natureconservation.34.30055
Pierpaoli, I. (1923). Prima contribuzione allo studio delle alghe del Golfo di Taranto. Riv. Biol. 5, 1–19.
Pierri, C., Colangelo, P., Del Pasqua, M., Longo, C., and Giangrande, A. (2019). Consequences of the experimental removal of Sabella spallanzanii (Gmelin, 1791) from the fouling assemblage of a Mediterranean harbour. Mediterr. Mar. Sci. 20, 476–486. doi: 10.12681/mms.19732
Pierri, C., Longo, C., and Giangrande, A. (2010). Variability of fouling communities in the Mar Piccolo of Taranto (Northern Ionian Sea, Mediterranean Sea). J. Mar. Biol. Assoc. U. K. 90, 159–167. doi: 10.1017/s0025315409990798
Prins, T. C., Smaal, A. C., and Dame, R. F. (1997). A review of the feedbacks between bivalve grazing and ecosystem processes. Aquat. Ecol. 31, 349–359. doi: 10.1023/A:1009924624259
Rabiei, R., Phang, S. M., Yeong, H. Y., Lim, P. E., Ajdari, D., Zarshenas, G., et al. (2014). Bioremediation efficiency and biochemical composition of Ulva reticulata Forsskål (Chlorophyta) cultivated in shrimp (Penaeus monodon) hatchery effluent. Iran. J. Fish. Sci. 13, 621–639.
Reid, G. K., Chopin, T., Robinson, S. M. C., Azevedo, P., Quinton, M., and Belyea, E. (2013). Weight ratios of the kelps, Alaria esculenta and Saccharina latissima, required to sequester dissolved inorganic nutrients and supply oxygen for Atlantic salmon, Salmo salar, in Integrated Multi-Trophic Aquaculture systems. Aquaculture 408-409, 34–46. doi: 10.1016/j.aquaculture.2013.05.004
Relini, G., Lanteri, L., Franco, A., and Cimmino, C. (2012). “Fishes of artificial reefs in the gulf of Genoa,” in Proceedings of the 47th EMBS, 3–7 September, Arendal, 129.
Relini, G., Relini, M., Palandri, G., Merello, S., and Beccornia, E. (2007). History, ecology and trends for artificial reefs of the Ligurian sea, Italy. Hydrobiologia 580, 193–217. doi: 10.1007/s10750-006-0453-0
Robinson, S. M. C., Martin, J. D., Cooper, J. A., Lander, T. R., Reid, G. K., Powell, F., et al. (2011). The role of three dimensional habitats in the establishment of Integrated Multi-Trophic Aquaculture (IMTA) Systems. Bull. Aquacul. Assoc. Canada 109, 23–29.
Rodriguez-Perez, A., James, M., Donnan, D. W., Henry, T. B., Møller, L. F., and Sanderson, W. G. (2019). Conservation and restoration of a keystone species: understanding the settlement preferences of the European oyster (Ostrea edulis). Mar. Pollut. Bull. 138, 312–321. doi: 10.1016/j.marpolbul.2018.11.032
Santelli, A., Punzo, E., Scarcella, G., Strafella, P., Spagnolo, A., and Fabi, G. (2013). Decapod crustaceans associated with an artificial reef (Adriatic Sea). Mediterr. Mar. Sci. 14, 64–75. doi: 10.12681/mms.682
Scalera-Liaci, L., and Corriero, G. (1993). Distribution of the sponge fauna from the Mar Piccolo and the Mar Grande (Taranto, Ionian Sea). Biol. Mar. Suppl. Not. Soc. Ital. Biol. Mar. 1, 317–318.
Scalera-Liaci, L., Sciscioli, M., and Fiordiponti, F. (1976). Distribuzione dei Poriferi del Mar Piccolo di Taranto. Oebalia 2, 3–19.
Stabili, L., Licciano, M., Giangrande, A., Longo, C., Mercurio, M., Marzano, C. N., et al. (2006). Filtering activity of Spongia officinalis var. adriatica (Schmidt) (Porifera, Demospongiae) on bacterioplankton: implications for bioremediation of polluted seawater. Water Res. 40, 3083–3090. doi: 10.1016/j.watres.2006.06.012
Stabili, L., Licciano, M., Lezzi, M., and Giangrande, A. (2014). Microbiological accumulation by the Mediterranean invasive alien species Branchiomma bairdi (Annelida, Sabellidae): potential tool for bioremediation. Mar. Pollut. Bull. 86, 325–331. doi: 10.1016/j.marpolbul.2014.06.047
Stabili, L., Licciano, M., Longo, C., Corriero, G., and Mercurio, M. (2008). Evaluation of microbiological accumulation capability of the commercial sponge Spongia officinalis var. adriatica (Schmidt) (Porifera, Demospongiae). Water Res. 42, 2499–2506. doi: 10.1016/j.watres.2008.02.008
Stabili, L., Schirosi, R., Licciano, M., Mola, E., and Giangrande, A. (2010). Bioremediation of bacteria in aquaculture waste using the polychaete Sabella spallanzanii. N. Biotechnol. 27, 774–781. doi: 10.1016/j.nbt.2010.06.018
Stachowicz, J. J., Fried, H., Osman, R. W., and Whitlatch, R. B. (2002). Biodiversity, invasion resistance, and marine ecosystem function: reconciling pattern and process. Ecology 83, 2575–2590. doi: 10.1890/0012-9658(2002)083[2575:birame]2.0.co;2
Tallman, J. C., and Forrester, G. E. (2007). Oyster grow-out cages function as artificial reefs for temperate fishes. Trans. Am. Fish. Soc. 136, 790–799. doi: 10.1577/t06-119.1
Theuerkauf, S. J., Morris, J. A., Waters, T. J., Wickliffe, L. C., Alleway, H. K., and Jones, R. C. (2019). A global spatial analysis reveals where marine aquaculture can benefit nature and people. PLoS One 14:e0222282. doi: 10.1371/journal.pone.0222282
Thurstan, R. H., Pandolfi, J. M., and zu Ermgassen, P. S. E. (2017). “Animal forests through time: historical data to understand present changes in marine ecosystems,” in Marine Animal Forests: The Ecology of Benthic Biodiversity Hotspots, eds S. Rossi, L. Bramanti, A. Gori, and O. Covadonga (Cham: Springer International Publishing), 947–963. doi: 10.1007/978-3-319-21012-4_31
Tomassetti, P., Persia, E., Mercatali, I., Vani, D., Marussso, V., and Porrello, S. (2009). Effects of mariculture on macrobenthic assemblages in a western Mediterranean site. Mar. Pollut. Bull. 58, 533–541. doi: 10.1016/j.marpolbul.2008.11.027
Tursi, A., Lepore, E., and Gherardi, M. (1977). “Relazione fra inclinazione del substrato e insediamento degli organismi bentonici,” in Atti IX Congresso SIBM, eds F. Cinelli, E. Fresi, and L. Mazzella (Lacco Ameno d’Ischia: Napoli), 439–450.
Tursi, A., Matarrese, A., Sciscioli, M., Vaccarella, R., and Chieppa, M. (1979). Variazioni di biomassa nel Mar Piccolo di Taranto e loro rapporto con i banchi naturali di Mitili. Oebalia 5, 49–70.
Tursi, A., Pastore, M., and Panetta, P. (1974). “Aspetti ecologici del Mar Piccolo di Taranto: Ascidie, Crostacei Decapodi e Molluschi,” in Atti del IV Simposio Nazionale sulla Conservazione della Natura, (Bari: Università di Bari), 93–117.
Tursi, A., Piscitelli, G., Gherardi, M., and Matarrese, A. (1978). Aspetti ecologici del porto di Taranto (Mar Grande). Oebalia 4, 41–78.
Underwood, A. J., and Chapman, M. G. (2006). Early development of subtidal macrofaunal assemblages: relationships to period and timing of colonization. J. Exp. Mar. Bio. Ecol. 330, 221–233. doi: 10.1016/j.jembe.2005.12.029
Watson, S. C. L., Preston, J., Beaumont, N. J., and Watson, G. J. (2020). Assessing the natural capital value of water quality and climate regulation in temperate marine systems using a EUNIS biotope classification approach. Sci. Total Environ. 744:140688. doi: 10.1016/j.scitotenv.2020.140688
Worcester, S. E. (1994). Adult rafting versus larval swimming: dispersal and recruitment of a botryllid ascidian on eelgrass. Mar. Biol. 121, 309–317. doi: 10.1007/bf00346739
WoRMS Editorial Board (2021). World Register of Marine Species. Available online at: https://www.marinespecies.org (accessed December 15, 2021).
Keywords: biodiversity, artificial hard substrates, mariculture, fouling, macrobenthic invertebrates, Mediterranean Sea
Citation: Arduini D, Borghese J, Gravina MF, Trani R, Longo C, Pierri C and Giangrande A (2022) Biofouling Role in Mariculture Environment Restoration: An Example in the Mar Grande of Taranto (Mediterranean Sea). Front. Mar. Sci. 9:842616. doi: 10.3389/fmars.2022.842616
Received: 23 December 2021; Accepted: 24 February 2022;
Published: 25 March 2022.
Edited by:
Massimo Ponti, University of Bologna, ItalyReviewed by:
Daniel Gonzalez-Silvera, University of Stirling, United KingdomJasmine Ferrario, University of Pavia, Italy
Copyright © 2022 Arduini, Borghese, Gravina, Trani, Longo, Pierri and Giangrande. This is an open-access article distributed under the terms of the Creative Commons Attribution License (CC BY). The use, distribution or reproduction in other forums is permitted, provided the original author(s) and the copyright owner(s) are credited and that the original publication in this journal is cited, in accordance with accepted academic practice. No use, distribution or reproduction is permitted which does not comply with these terms.
*Correspondence: Caterina Longo, Y2F0ZXJpbmEubG9uZ29AdW5pYmEuaXQ=