- 1School of Life Sciences, La Trobe University, Melbourne, VIC, Australia
- 2Fathom Pacific Pty Ltd., Mordialloc, VIC, Australia
- 3Total Hydrographic Pty Ltd., EndeavourSunshine West, VIC, Australia
Using multibeam echosounding (R2Sonic, Austin, Texas) combined with underwater observation and imagery surveys, this study sought to estimate the size and describe the typology of newly discovered biogenic bryozoan reefs in Western Port (VIC, Australia). The bryozoan species forming the biogenic structure are Triphyllozoon moniliferum, T. munitum (both fenestrate forms and dominant on the reef) and Celleporaria (non-fenestrate form and sub-dominant). The bryozoan biogenic reefs occupy a total area of 1.74 km2. Their distribution appears to be geomorphically and depth-controlled, which might limit their maximum possible extent. The Western Port bryozoan reefs are unique and globally significant because they: (1) occur in shallow water (typically 5–10 m), (2) are dominated by delicate fenestrate colonies of T. moniliferum and T. munitum, (3) have two distinct morphologies (linear and patch-like), (4) form continuous rows of reef interspersed with fine sediment, and (5) the size and vertical relief (up to approximately 1.5 m) are among the largest recorded in the world. In Western Port, these bryozoan reefs are habitat-forming and may represent sites of enhanced biodiversity, which is currently being investigated in another separate study and are likely important habitat utilised by commercially and recreationally fished species. The bryozoan biogenic reefs are a previously unrecognised feature of conservation significance in this Ramsar site. The reefs are potentially vulnerable to physical damage from recreational fishing practices, sediment smothering and scouring, algal encroachment and hydrological alteration. Recommendations for monitoring and potential management of this unique biotope are made.
Introduction
Bryozoans are a diverse phylum of colonial, non-photosythetic filter-feeding aquatic invertebrates, with ∼5700 extant (Horowitz and Pachut, 1994) and ∼15,000 fossil species (Amini et al., 2004). They are commonly referred to as “lace corals”, despite being quite unrelated to corals (phylum: Cnidaria). Marine bryozoan colonies (Class Stenolaemata) produce complex rigid calcareous skeletons (termed “hard” bryozoans), which creates structural microhabitat for a myriad of fauna (Wood and Probert, 2013), such as crustaceans, molluscs, fishes and worms and this ecological function and service is enhanced when colonies combine to form bryozoan biogenic reefs “BBR” (Bradstock and Gordon, 1983). In contrast, “soft” marine bryozoan colonies (i.e., those that do not form a calcium carbonate matrix), can also occur locally in high abundance in the lower strata of kelp-dominated rocky reefs, however, these apparently do not form biogenic reefs. Consequently, complex habitats such as BBR are often biodiversity “hotspots” (Morgado and Tanaka, 2001; Wood et al., 2012; Wilson, 2021) compared to the surrounding habitats and provide food, attachment substrate for sessile organisms, shelter from strong currents as well as concealment from predators for both adult and larval stage organisms. Currently, there are only 54 high-density bryozoan sites known globally (Wood et al., 2012) with only three occurring in Australian waters prior to this study. Virtually nothing is known about the ecological role, biology, extent, and characteristics of the BBR of Western Port (a temperate Australian embayment).
It appears that modern BBR are confined to temperate zones, although bryozoan biogenic habitat has been noted through a latitudinal band between 13°N and 23°S (Wood et al., 2012). Bryozoan biogenic habitat tends to be confined to the lower limits of the photic zone or in shallow turbid waters where they are not out-competed by light-dependent algae, potentially allowing for larger colony sizes to be attained and diverse epifaunal communities to form (Bock et al., 2018). This light-based exclusion/competition scenario is analogous to tropical scleractinian corals and algae (McCook et al., 2001). Favourable growth conditions for bryozoans are thought to require adequate nutrients and an abundant supply of unarmoured phytoplankton that are their primary food source (James et al., 2008).
Several reports of bryozoan “reefs” actually relate to quasi bryozoan-encrusted rock formations [e.g., Abrolhos Shelf, Brazil (Bastos et al., 2018) and bryozoan-rich fossil bioherms (Cuffey et al., 1977; Ernst and Königshof, 2008) and sediments (e.g., Tasmanian continental shelf, James et al., 2008)]. So-called bryozoan “sands” are a feature of the southern Australian shelf sediments that are cited as Holocene in origin (James et al., 2008). Fenestrate bryozoan (i.e., net-like or reticulated skeletal structure) forms typically dominate these Holocene sands although fine branching forms can be locally abundant in deeper water (generally <80 m) where wave energy is substantially dissipated.
The bryozoan reef “thickets” on the Otago Shelf, New Zealand, are true extant bryozoan reefs, forming emergent mounds of various species (see Table 1 for this and other notable extant sites). Wood et al. (2012) describes 50 mm as the size at which heavily calcified bryozoans become habitat-forming and the authors reported growth rates of 0.8 to 5 mm per year, indicating that the habitat-forming BBR likely establish on the scale of decades. As reported herein, epibenthic colonies in the Western Port (WP) bryozoan reefs attain sizes of some 2,000 mm in radius and 500 to 1,200 mm in vertical relief, suggesting century-scale age.
Bryozoa occur throughout most of WP as individual colonies or “clumps” (i.e., non-reef forming), often associated with circalittoral reef (i.e., below the zone of light penetration that supports algal growth) and among seagrasses, Caulerpa spp. beds and growing on jetty pylons. The first indication of the potential presence of BBR comes from the historical records of the mud oyster (Ostrea angasi) dredge fishery that was prevalent between 1820s through to the 1920s. Historical records indicate that fishers interacted with abundant bryozoan reefs:
“Lumps of live coral were often brought up, so big that they blocked the dredge mouth. These lumps were broken up and thrown back. The coral was live and full of sealife, worms, octopus etc.” (Hannan and Bennett, 2010).
Indeed, the interaction between oysters and bryozoans is intriguing given the observations made in the present study of oysters occurring on BBR and associated biodiversity studies indicating the presence of a dense shell layer ∼10–20 cm under the sediment surface in neighbouring habitats.
The general Western Port bryozoan reef area became known to commercial and recreational fishers as “The Corals”, probably named for the fragments of bryozoan skeleton that resemble coral, which is sometimes present upon the retrieval of anchors and fishing equipment. Today there is no commercial fishing in WP, but it is known as a productive recreational fishing area. Furthermore, this area has typically poor visibility, is far from shore and has strong currents making it unfavourable for diving and snorkelling activities, meaning the BBR have avoided attention until now.
The first scientific report of the presence of bryozoan structures in the area came from Blake et al. (2013) who employed towed camera transects and noted “isolated colonies”, comprising “patches of low and high profile broken, and solid reef colonised by dense bryozoans and sparse sponges”. The significance of that discovery was not appreciated until the same towed video footage was reviewed in 2016 during a biotope classification project by the Victorian Department of Environment, Land, Water and Planning “DELWP” (Department of Environment, Land, Water and Planning [DELWP], 2017). Biotope mapping in 2016 made use of multibeam bathymetry collected in 2009 which showed, at a coarse resolution, characteristic seabed textures that instigated the current study. This study sought to characterise the typology and quantify the extent of BBR, establish conservation values and provide critical information for management strategies. This was achieved by utilising high resolution multibeam echosounding (MBES) in conjunction with various ground-truthing surveys.
Materials and Methods
Study Area and Ground-Truthing
The bryozoan biogenic reef (BBR) area is situated in the Eastern Arm of Western Port (WP), VIC, Australia, in an area termed the “Rhyll Segment” (Harris et al., 1979; Jenkins et al., 2013) between French Island, Corinella and Rhyll (Figure 1). Potential reef areas were initially identified based on the seabed textures in a 2009 bay-wide multibeam bathymetry product (2.5 m horizontal resolution) generated by the Port of Hastings Development Authority. Image-based groundtruthing was achieved by inspection of linear seabed texture features detected in the multibeam bathymetry using a BlueROV2 Remote Operated Vehicle (ROV) recording oblique high-definition video (Figure 2). Approximately 1.5 h of ROV footage was acquired in the northern cell. In addition, a drop-camera platform was used to collect imagery while station-keeping or slow drifting over seafloor features of interest and surrounding habitats. The drop camera platform comprised a Splashcam® high-definition video camera recording in an oblique orientation and a GoPro Hero4 with separate lighting collecting still images in planar orientation (see Figure 2). Further, in situ observations supported by underwater photography were made over 10 SCUBA dives in the BBR area (see Figure 2) during various sample collection events between December 2017 and February 2020. Collectively, the results of these groundtruthing efforts were used to injunction with the low-resolution bay-wide multibeam bathymetry to define the survey extents for high-resolution multibeam echosounding (MBES). BBRs were found to be comprised of three species on species and representative samples were collected for taxonomic confirmation by a bryozoan taxonomy expert at the National Museum of Victoria (Australia). Still images and video footage were also shared with bryozoan taxonomy experts at CSIRO (Australia) and National Institute of Water and Atmospheric Research (New Zealand) to confirm species identifications. For this study, the imagery was used to make qualitative assessments of the presence of BBR, its composition and characteristics and there was no quantitative analysis. In a partner study, epifauna species co-inhabiting the reefs were scored from the imagery and these data will be reported in future publication.
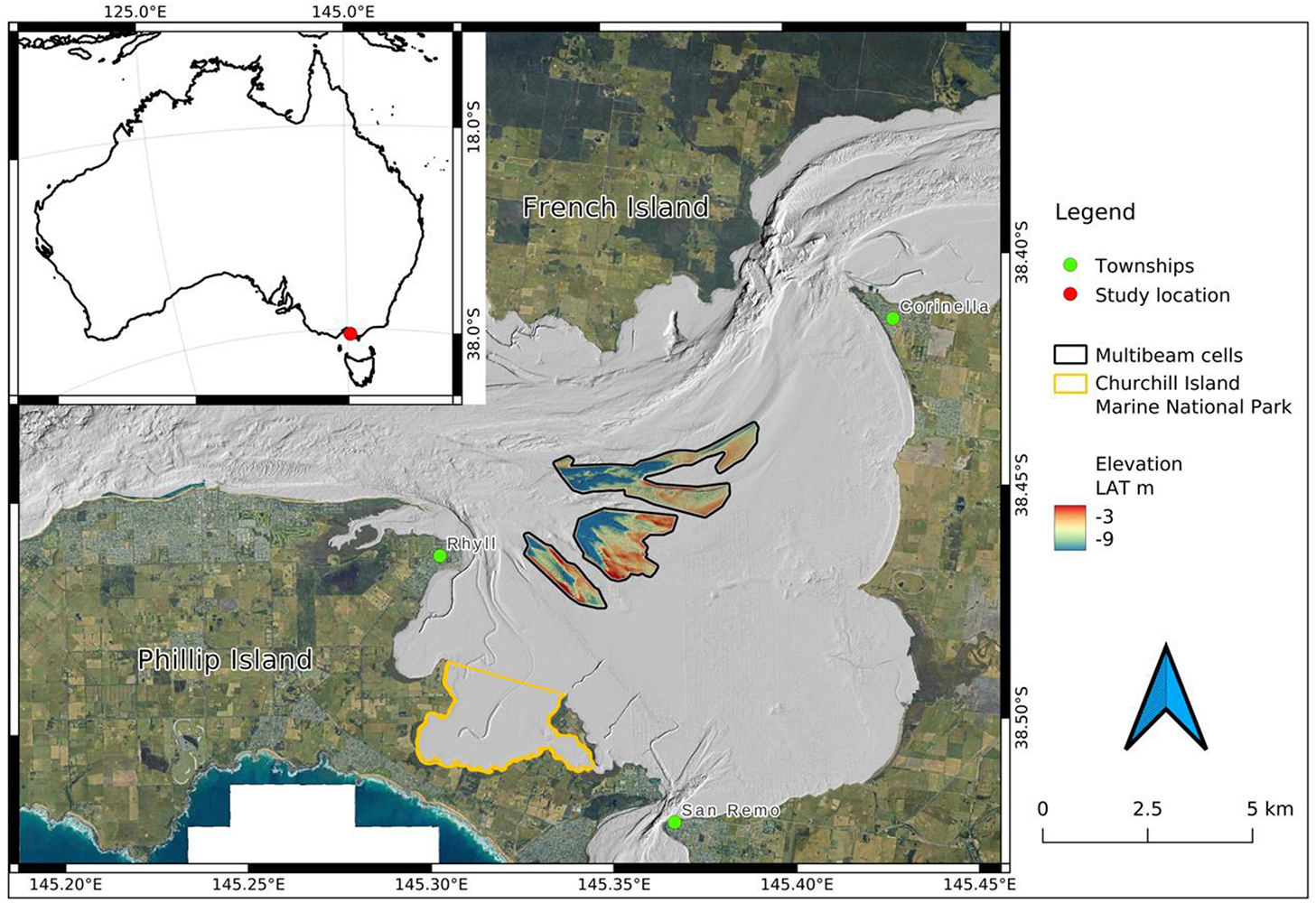
Figure 1. The study site (inset, red dot) and location of the bryozoan biogenic reefs in the East Arm of Western Port. This area is known as the “Rhyll Segment” and is situated between the township of Rhyll and Corinella and north east of the Churchill Island Marine National Park (outlined in orange). Multi-beam bathymetry of the study area (three coloured polygons outlined in black) is highlighted from the multi-beam bathymetry of surrounding waters (light grey). Scale bar applicable for satellite image only. Arrow depicts north.
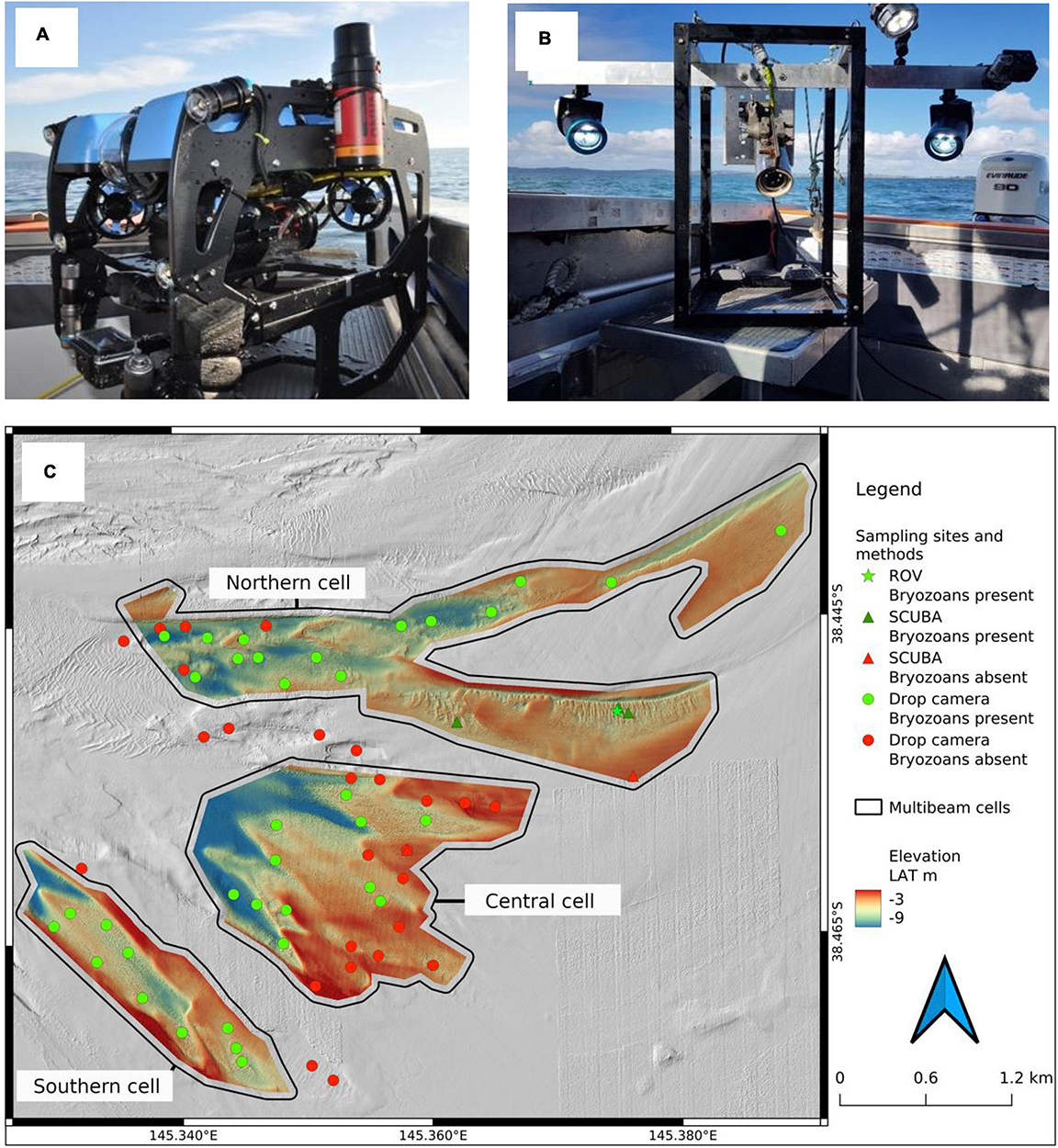
Figure 2. Remote operated vehicle (A) and drop/drift camera (B) used for ground-truthing surveys. Imaging ground-truthing sites (C) showing the presence (green dots) or absence (red dots) of bryozoans in the Northern, Central and Southern cells. Linear BBR are present at the ROV (green star) and SCUBA dive (green triangle) locations. Arrow indicates north.
Multibeam Echosounding Surveys
The vessel-based MBES surveys were completed in the northern, central and southern cells, for a total area of 6.4 km2 at a resolution of 0.5 × 0.5 m (see Figure 2). MBES surveys were completed over two periods: 9–10 October 2019 and 11–14 February 2020. Transect direction was dictated by the shape and position of the survey area extents but was principally in east-west direction. The MBES was completed using an R2Sonic® Dual Head 2020 multibeam system (340, 400 kHz frequency). Positioning was achieved with an Applanix® POS MV Wavemaster system with the real-time kinematic (RTK) satellite position validated against the Victorian Permanent Survey Mark “Bittern PM 259”. The MBES were corrected to Lowest Astronomical Tide (LAT). Validation of the vertical data was achieved by deploying a cage to the seafloor at the survey site which had a RTK Global Navigation Satellite System (GNSS) receiver target installed at a known distance above the seabed. The MBES system captured data over the subsea target, resulting in a Total Horizontal Uncertainty (THU) of ± 0.1 m and Total Vertical Uncertainty (TVU) of ± 0.05 m. Data processing used the Hypack® 2020 system and digital elevation models for the survey areas were produced at a horizontal resolution of 0.5 m × 0.5 m.
Reef Area Calculation
Bryozoan reefs were visible in the digital elevation models (DEMs) in each of the three cells. However, fully automated terrain analysis and habitat modelling routines failed to reliably distinguish BBR due to interference patterns introduced by seams in the DEM and textures associated with Zostera nigricaulis and seasonal Caulerpa cactoides beds. Therefore, a supervised approach that relied on the circularity of reef structures was applied as follows:
1. Apply slope calculation on bathymetry raster.
2. Bryozoan biogenic reefs were detected as circular features with slope ≥7 degrees in the northern cell and 5 degrees in the central and southern cells. Extract rasters of those slope values and convert to a binary raster that was a proxy for “reef” and “non-reef” pixels (Figure 3). As will be discussed below, in the central and southern cell, there was a depth control of bryozoans and an additional rule of >4 m water depth was applied to the slope-based detection.
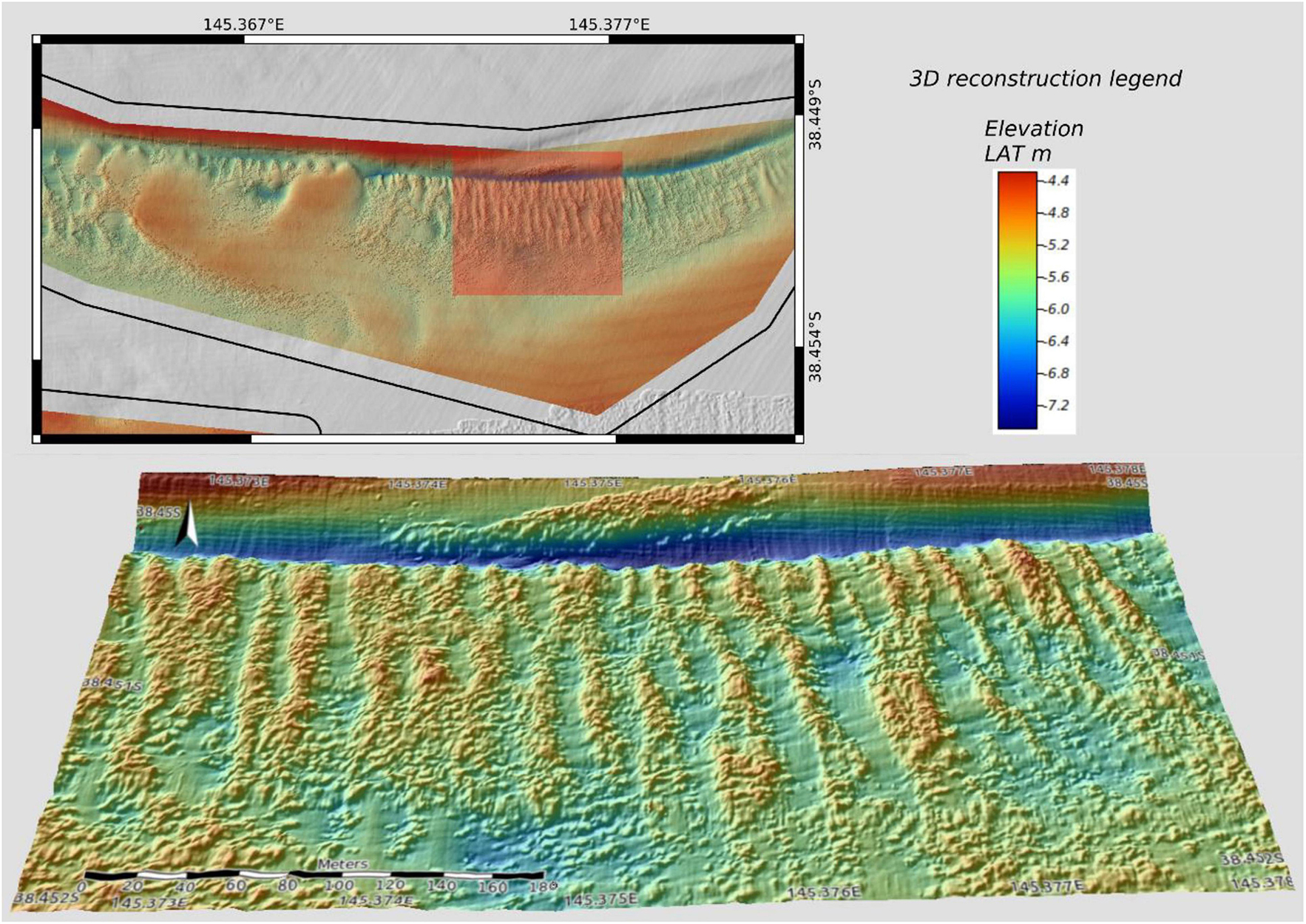
Figure 3. 3D reconstruction of Linear Bryozoan Reef (LBR) formations in part of the northern cell. Colours represent depth range. The northern boundary is associated with a deeper gully feature (dark blue horizontal feature). Arrow indicates north. Scale bar (black and white horizontal line) in the bottom panel equals 180 m.
3. Grow the resultant slope raster to omit fragmented cells using the Geographic Resources Analysis Support System (GRASS) tools:
a. r. grow function –1 m cell size.
b. r. neighbours’ function – neighbourhood window of five cells.
4. Convert raster to polygon.
5. Use “delete holes” tool to fill annulus features using a minimum cell area of 1 m2.
6. Use Fix Geometries tool to correct polygon spatial inconsistencies.
7. Visual check and manual polygon clean-up of obvious errors by overlaying on vertically exaggerated hill-shaded bathymetry.
Detections were checked by overlapping resultant polygons on a vertically exaggerated DEM and confirming against actual ground-truthing locations.
Results
Biogenic bryozoan reefs (BBR) cover a total area of 1.74 km2 (Figure 4A). The total area of BBR is comprised of 0.78 km2, 0.91 km2, and 0.05 km2 for the northern, central and southern cells, respectively (Figures 2, 4A). Taxonomic collections confirmed that the linear form of BBR is composed exclusively of three bryozoan species: Triphyllozoon moniliferum, Triphyllozoon munitum and Celleporaria foliata (Figures 5, 6). The Triphyllozoon species are fenestrate, mounded colonies with intricate laminal folding, while C. foliata is a non-fenestrate species forming plate-like colonies that can be mounded, spreading or encrusting. Qualitative in situ observations suggest that the two Triphyllozoon species are present in approximately equal proportions and that these two species are dominant on the BBRs, with the cover of C. foliata being relatively low.
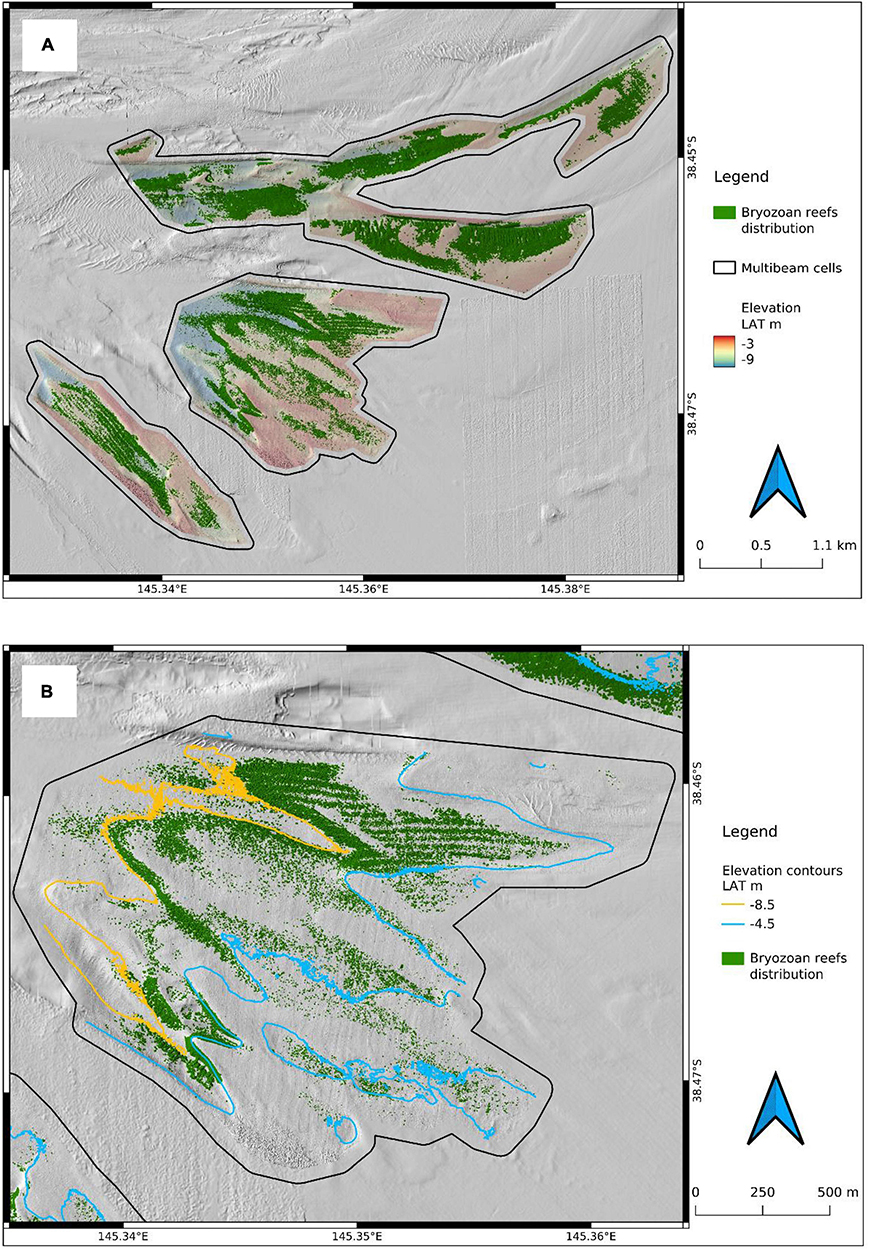
Figure 4. Total extent of detected bryozoan reef (A) indicated in green in and the surveyed area (black polygons) of bryozoan reefs in the central cell (green, B) in relation to depth contours (orange = 8.5 m, blue = 4.5 m). Blue arrows denote north.
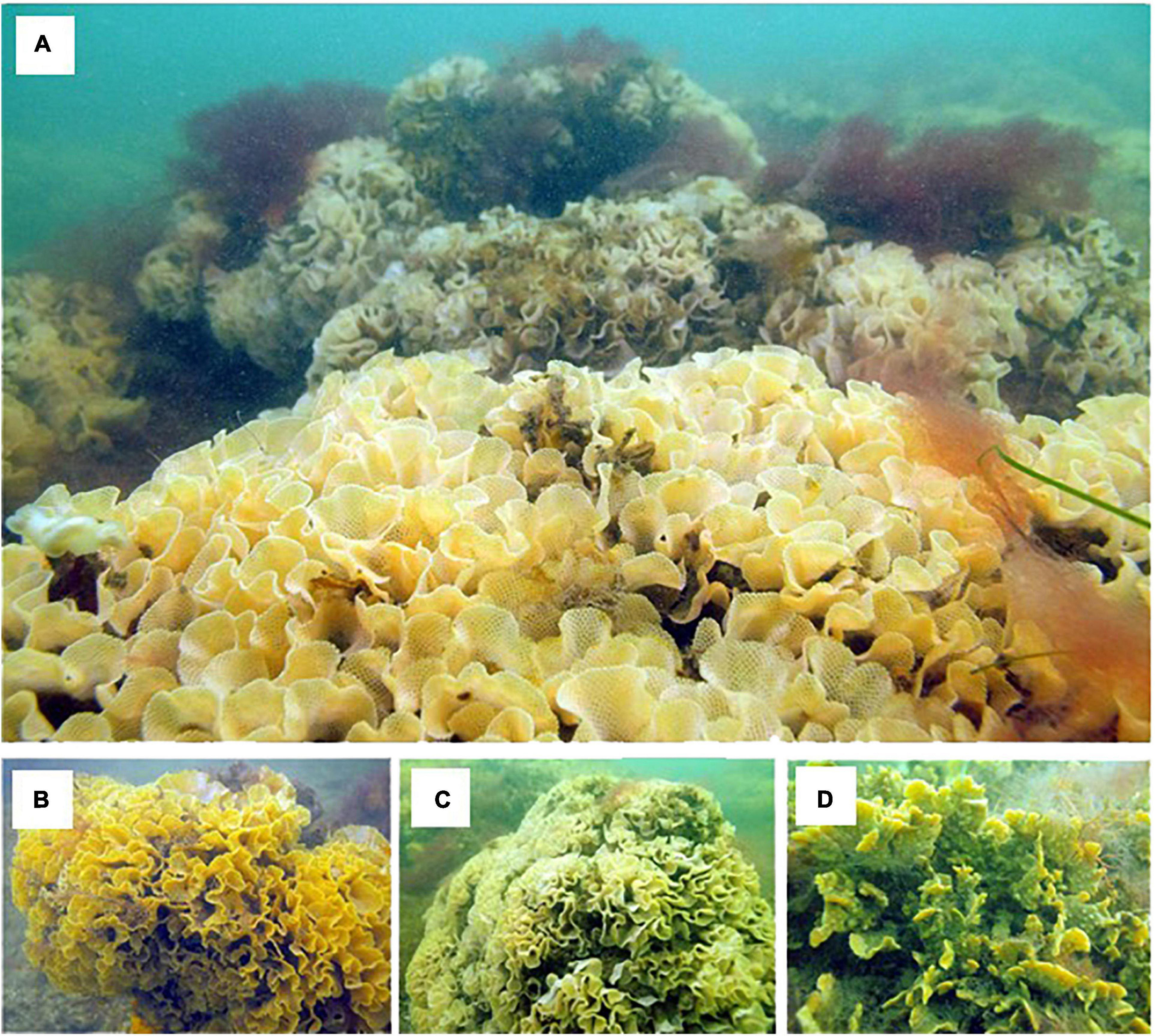
Figure 5. Bryozoan biogenic reef (“BBR”, A) and the three dominant species of which they are comprised. Triphyllozoon munitum (B), Triphyllozoon moniliferum (C) and Celleporaria foliata (D).
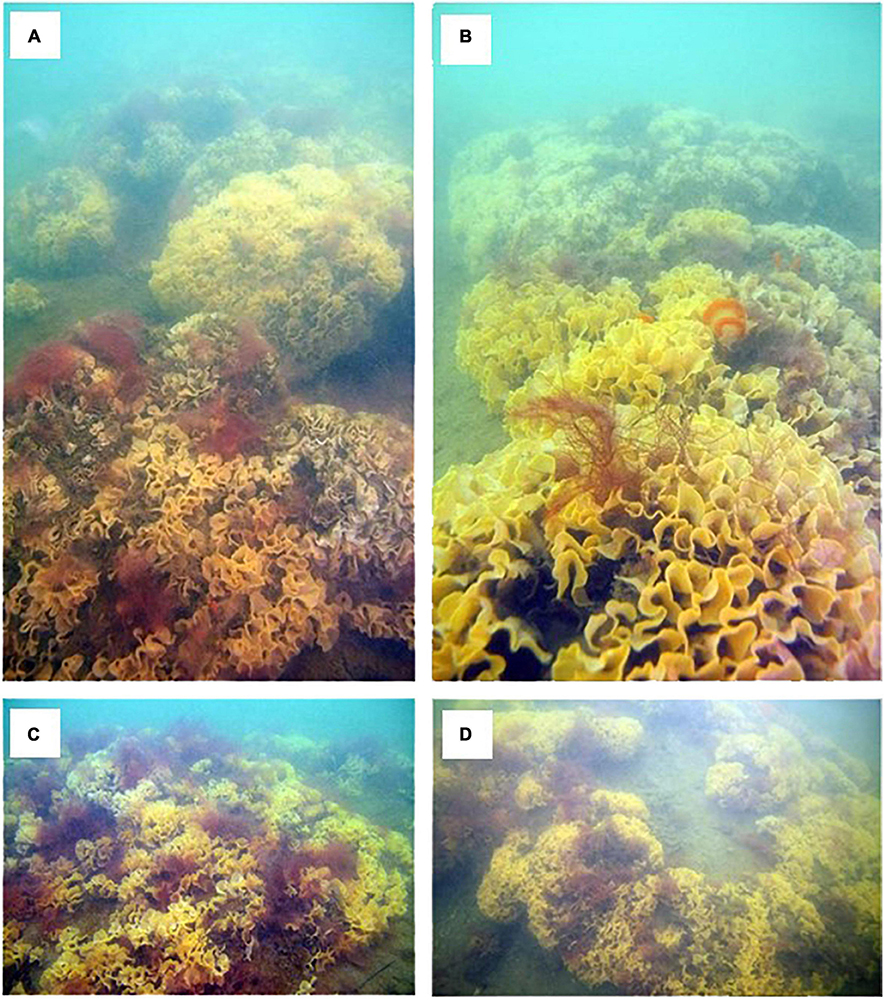
Figure 6. Images from the linear reef – northern cell, (A,B). Note presence of tufted fine red algae (potentially seasonal) that may be competition with bryozoans (C). The presence of cavities and gaps in some large colonies may reflect damage (D).
The BBR fall into two distinct structural categories; “linear” bryozoan reef (LBR) and “patch” bryozoan reef (PBR). The LBR are characterised by continuous bed coverage of large bryozoan colonies orientated in a north-south pattern whereas the PBR are characterised conglomerate colonies that can individually large, but not continuous bed coverage and with no regular orientation.
Linear Bryozoan Reefs
The LBRs morphology was observed only in the northern cell (see 3D reconstruction, Figure 3) and consisted of continuous reef mounds that are linearly aligned in approximately north-south orientated rows (see Figure 7). The reef rows ranged from ∼ 3 m to some 30 m in width and the vertical relief of LBR rows ranges from ∼ 50–120 cm above the surrounding sediment. Water depths in the LBR zones ranges from approximately 4.8–6 m. The sediment shoal separating the linear reef zones has a depth of approximately 4 m.
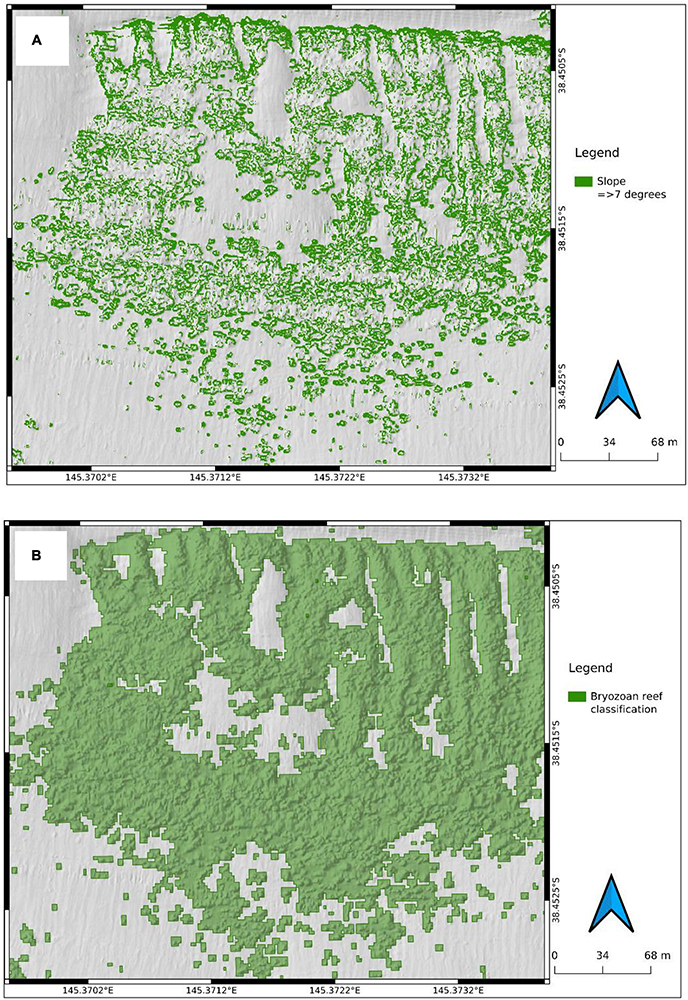
Figure 7. Example of the slope raster (A) and the polygonised “linear” and “patch” reef generated by the reef detection workflow (B) in the northern cell. Top part of panel (B) clearly displays top-to-bottom orientated continuous linear reef whereas the middle of the panel shows patch reef configuration.
In the northern cell there were three discrete areas of LBR, interrupted by a sediment shoal (see Figure 2). This sediment shoal was present in the 2009 bathymetry in addition to this new bathymetry and so does not appear to be a recent feature or to have changed significantly since 2009. The northern edge of the contiguous reef rows terminates with a distinctive gully feature and a steep slope leading to a raised bank (see Figure 3).
In the northern cell, linear reef bands were separated by tracts of very fine silty muds with depauperate infauna. We hypothesise that the baffled colony structure and morphology of the reefs running perpendicular to the prevailing current flow has the effect of slowing currents causing the settlement and trapping of fine particles that are otherwise transported in tidal flows. The multibeam bathymetry showed signs of sediment processes, with potential sediment accretion on the eastern sides of reef rows. Due to the high density of habitat-forming bryozoans in the linear reefs, these are speculated to harbour the highest matrix-associated biodiversity of co-occurring fauna.
Patch Bryozoan Reef
The PBR were observed in the northern, central and southern cells. Patch reefs occurred in 4.5–8.5 m water depth (Figure 4B). In some areas, the PBR were locally dense but these patches were of a different form to the LBRs. Ground-truthing of PBR has not been as thorough as that for the strikingly unusual LBR and field visual observations have been hampered by poor visibility. However, field observations suggest that individual reef patches represent sites of enhanced localised biodiversity of matrix-associated fauna compared to the surrounding sediment beds.
The discovery of PBR in the southern cell significantly expanded the initial expected distribution of bryozoan reefs. Furthermore, discovery of PBR in the far north and east portions of the northern cell represent new findings. Some PBR in the northern cell are associated with apparent remnant, or newly forming, LBR features, which requires future investigation.
Discussion
Ecophysical Controls
The Western Port BBRs occur in seafloor depressions at the terminus of sub-channels. Harris et al. (1979) noted that due to the proximity of the Rhyll Segment to the Eastern Entrance of to bay, currents in this area do not display the directional symmetry that is typical of other sites but rather are generally counter-clockwise circulation pattern. During our surveys, underwater and surface observations were that tidal currents in the BBR areas are lower than those in the neighbouring main channels and there is significant eddying. This reduced current flow is likely to be required for the establishment and colonies with fine fenestrate lamellae that may be prohibited by the stronger currents in the main axis of nearby channels.
Linear bryozoan “swards” in Foveaux Strait, New Zealand are orientated parallel to the peak tidal current direction (Cranfield et al., 2003). The approximately north-south alignment of the LBR may be associated with the pattern of northerly movement of flood tide currents from eastern entrance, along the eastern coast toward Corinella reported by Harris et al. (1979). The Western Port LBRs may further baffle current flow and eddying owing to their perpendicular orientation to the current flow in the area, potentially creating conditions that are conducive to additional density-dependent growth and recruitment (Cranfield et al., 2003; James et al., 2008).
Subtle depth controls on bryozoan distribution were observed and these are exemplified in the central cell. Bryozoan reefs in the central cell were generally constrained to the depth range ∼4.5–8.5 m. Bryozoan reefs generally did not encroach onto the banks to the east and south of the central cell shallower than 4.5 m where Zostera nigricaulis seagrass and Caulerpa cactoides algae beds occur. Few bryozoans were detected deeper than 8.5 m in the central cell. Interestingly, this ecophysical model of bryozoan reefs bordering the offshore extent seagrass beds is reflected in the historical accounts from the oyster dredging fishery, in which fishers reported highest oyster catches at the offshore extent of seagrass (Hannan and Bennett, 2010).
On the Lacepede Shelf, South Australia, Hageman et al. (1995) identified factors of nutrients, temperature, water chemistry, environmental stability, substrate, competition, and secondary factors of water depth and currents as the potential abiotic drivers of bryozoan distributions. Additionally, on the Tasmanian continental shelf, Amini et al. (2004) noted bryozoan morphotype associations with water depth, energy regimes, sedimentation rate and substrate type, with fenestrate and foliose forms occupying deeper water where energy and sedimentation rates were low and where hard substrate is more abundant. The presence of shallow, dense growth of fenestrate bryozoan forms in the WP reefs is therefore unique and may be related to the relatively sheltered conditions, low wave action and current energy at this location. Across the northern, central, and southern cells in our study area, nutrient supply, water chemistry, temperature and water circulation patterns are presumed to be constant. Therefore, the depth-related patterns observed may be related to competition with seagrass and Caulerpa cactoides at the shallow end of their distribution. Alternatively, historic differential parent substrate types at the time of reef formation may explain today’s distribution. Limitations to distribution at the deep end of the distribution may be related to increased current exposure and substrate differences. Furthermore, given the generally turbid nature of this embayment, UV penetration would be typically reduced and hence closely mimic deeper habitats in which bryozoan reefs are more commonly found. Furthermore, the turbid conditions in the BBR area may be limiting competition with algae.
Link Between Bivalves and Bryozoans
There appears to be an association between the mud oysters (Ostrea angasi) and BBRs. Field surveys identified mud oyster clumps intermingled with bryozoan colonies, particularly Celleporaria foliata. This association is supported by the fact that the BBR area was an important mud oyster fishery between 1850–1900 (Hannan and Bennett, 2010). Our field surveys which involved diver hand-cores, also identified the presence of a dense subsurface bivalve shell layer consisting of O. angasi and Anadara trapezia shell. It is speculated that these observations are indicative of previously bivalve beds that may have served as a settlement substrate for bryozoans and that is markedly different to the surface sediments today. Biogenic bivalve reefs have been severely impacted in Victoria (Ford and Hamer, 2016) and are now the subject of significant restoration investment. The apparent BBR-mud oyster association further adds to the uniqueness and ecological significance of the Western Port BBRs.
Potential Threats
The Victorian Government is actively seeking to increase reactional fishing activity (Target 1 Million initiative), which may increase the pressure associated with physical damage from anchors and fishing equipment (potentially visible in Figure 6D). GPS coordinates for the site, known to fishers as “The Corals”, are widely available. Recreational fishing activity should be monitored in terms of spatial intensity (has commenced as part of the Western Port Bryozoan Reefs Project and as per recommendation #4 in Ford and Gilmour, 2013) and linked to a program to monitor colony damage. Responsible anchoring practices and the use of new technological advancements in trawling motors, for example, could be investigated to minimise physical impacts. Precedence for this exists for the Port Phillip Bay shellfish reef restoration project (Gillies et al., 2018), where fishers were educated about techniques to use current and wind conditions to maximise fishing on-reef structures while placing anchors in appropriate off-reef positions.
In WP, bryozoan reefs are potentially threatened by sedimentation and scouring. Sediment transport models have shown that the BBR site is exposed to southerly transport of sediment particles via ebb tidal water circulation from the north-eastern sector of the bay (north of French Island), through the tidal divide and into the Rhyll segment of East Arm. Once deposited, these fine sediment particles can become resuspended by wind-driven wave energy. No data are available on sedimentation specific to the BBRs, however, previous studies identified the Rhyll segment of East Arm as an area of very fine sediments (Marsden et al., 1979) and depositional processes (Hancock et al., 2001). Our field studies identified the presence of thick deposits of fine silt between the rows of LBRs that is differentiated from the muddy sands further away from the LBRs, suggesting localised sorting and trapping of fine particles. Initiatives currently underway to decrease sediment inputs include rehabilitation of mangrove and saltmarsh habitat and replanting of intertidal seagrasses and riparian vegetation (Department of Environment, Land, Water and Planning [DELWP], 2017; Melbourne Water, 2018). While these initiatives are predominantly focussed on protecting seagrass meadows, they will also benefit BBRs.
To date, very limited marine pest incursions or outbreaks have been documented in the WP region. The algae wakame, Undaria pinnatifida, now prevalent throughout neighbouring Port Phillip Bay, presents a potential risk to BBRs as they present a large area of hard substrate that may be colonised by the algae. The Northern Pacific seastar, Asterias amurensis, also prevalent throughout Port Phillip Bay, presents a risk to the abundant bivalve community associated with the BBR and a potentially large risk to bryozoans directly. Other Asteroidea, such as Patiriella regularis and Coscinasterias calamaria have are known to consume bryozoan zooids (Gordon, 1972), thus is it reasonable to speculate that Northern Pacific seastar could do likewise.
Increasing industrialisation and growing needs for larger and more frequent commercial and passenger shipping operations within WP, in addition to increasing recreational vessel activity, increases the risk of potential marine pest introductions. Monitoring efforts should expand in step with this increasing risk, both at port locations and at highly sensitive locations such as the BBR complex.
Fortunately, the BBR of WP have, until now, have largely escaped anthropogenic destruction and so conservation efforts can focus on preservation rather than later restoration. In stark contrast, there are several examples in New Zealand whereby, despite many years of protection, various bryozoan populations have failed to sufficiently recover from past destructive fishing practises (Cranfield et al., 2004; Rowden et al., 2004; Wood et al., 2012, 2013; Wood and Probert, 2013; Mello et al., 2021).
Further Research and Recommendations for Management
The Western Port Bryozoan Reef Project is engaged in concurrent studies of; reef macrofauna biodiversity, bryozoan colony age and growth, bryozoan reef fish biomass and citizen science acoustic monitoring methods. These studies are occurring at multiple spatial scales from the sub-meter scale to investigate biological interactions, to area-based management scales. The Victorian Government is embarking on a process of identifying indicators of Good Environmental Status (GES), adopting the general framework of the European Union’s Marine Strategy Framework Directive (European Commission [EC], 2008). Good Environmental Status is a quantitative or qualitative description of the condition/character of the environment that managers wish to maintain or drive toward improvement (see Table 2). The GES is linked to ecosystem models and indicators for monitoring and establishing monitoring objectives. GES indicators that could be applied to BBR are listed in Table 3. Non-destructive techniques are required and data to inform these indicators can be derived from acoustics, imagery, physicochemical studies. Poor underwater visibility at the BBR location put a focus on acoustic methods of monitoring which has strongly informed related studies of the project.
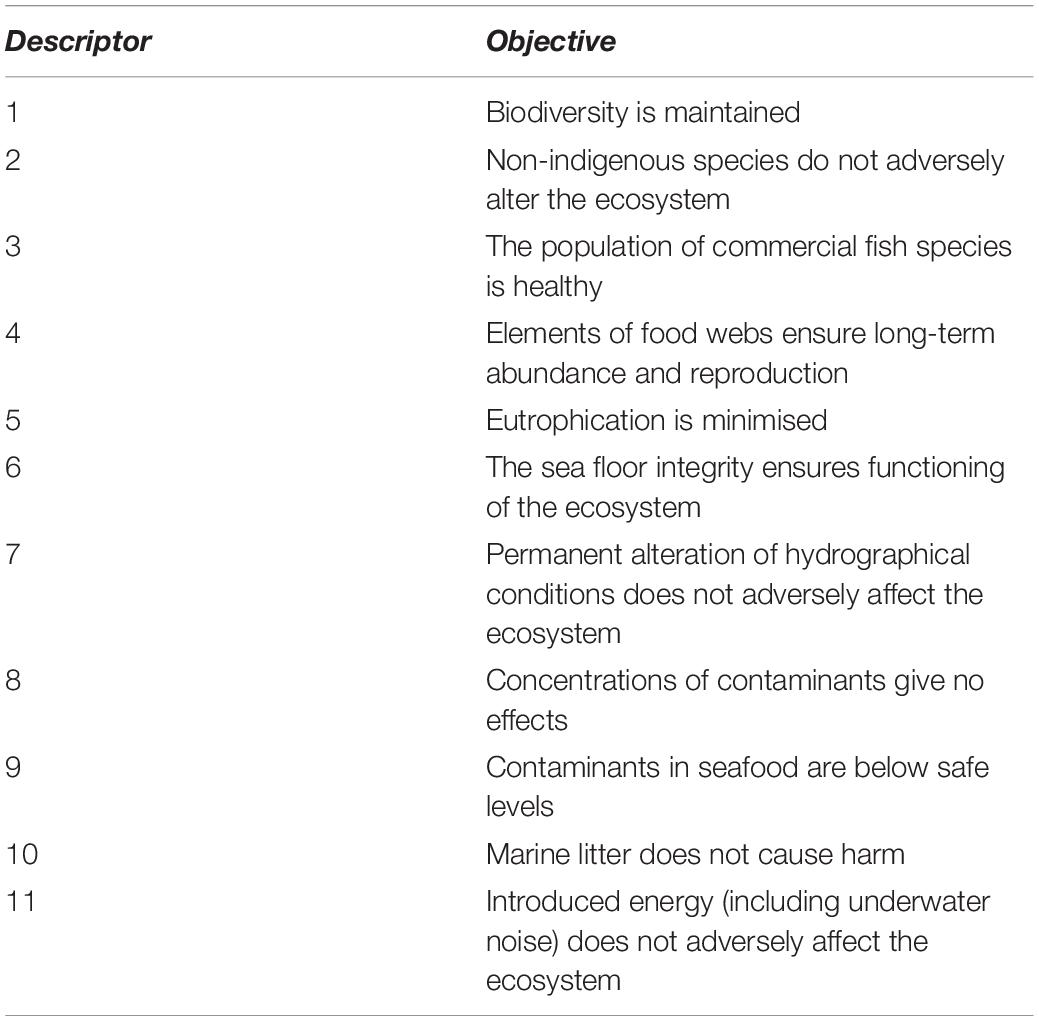
Table 2. Eleven descriptors of Good Environmental Status (GES). These 11 descriptors are relevant for the BBRs of WP and based from European Commission [EC], 2008.
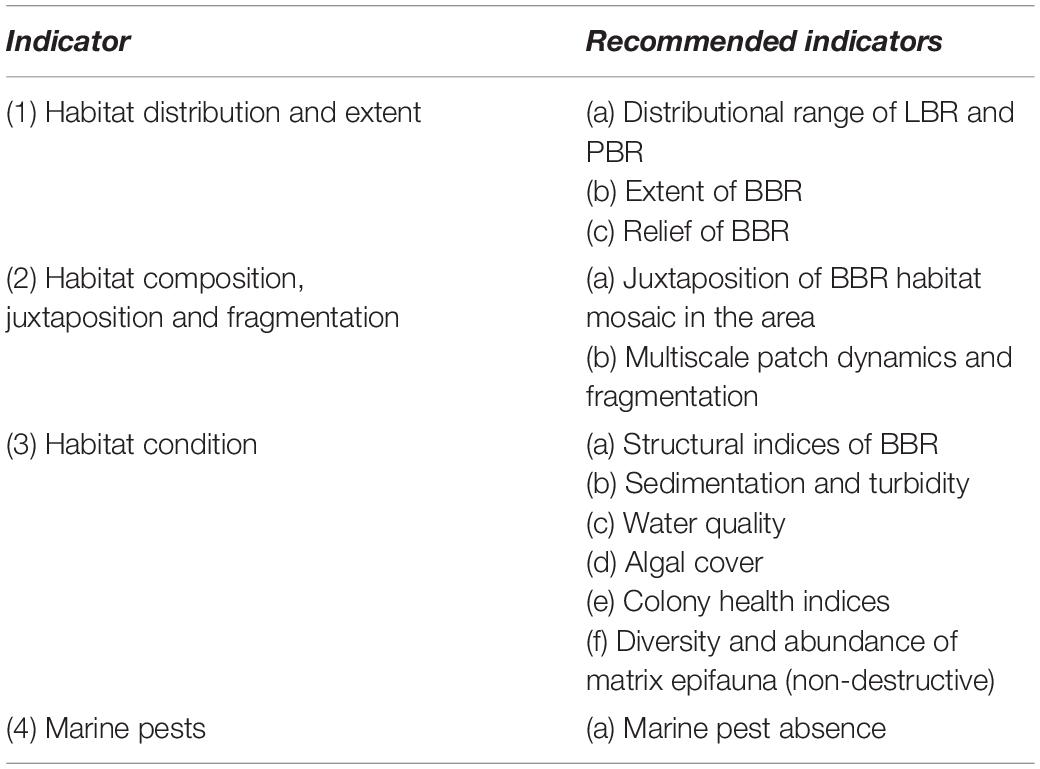
Table 3. Recommended GES indicators for monitoring the WP bryozoan reef complex. These relevant recommendations are based on European Commission [EC] (2008) and tailored to the BBRs of WP.
These unique BBR complexes fall outside of any marine protected areas and are not afforded any legislated protection. The Western Port Bryozoan Reefs Project has nominated the bryozoan reefs for listing under the Victorian Flora and Fauna Guarantee Act 1998 as a community that is prone to a variety of future potential threats that may lead to extinction. Other formal legislative conservation options available include listing the community on the Environment Protection and Biodiversity Conservation Act 1999 (EPBC Act) List of Threatened Ecological Communities.
Conclusion
Whilst there are other kinds of extant BBR known around the world and extinct BBR from the fossil record, there are striking differences that make the WP biotope globally unique. To our knowledge the Western Port BBRs are unique because they are dominated by delicate fenestrate colonies of Triphyllozoon species, occur in shallow water, and can form continuous bed structures of a size and vertical relief (up to 1.5 m) that are among the largest recorded in the world. Other studies of the Western Port Bryozoan Reef Project have shown that the BBR support diverse and abundant invertebrate fauna. Studies into growth rates of the bryozoan species, fish biomass and cost-effective monitoring methods are underway. Increases in biodiversity and abundance of various co-occurring matrix fauna relative to neighbouring habitats have been reported (Wilson, 2021). The Western Port BBRs further contributes to the ecological character of the WP Ramsar site. Much more fundamental research is required to fully understand the ecology of BBRs and the findings of this study can act as a baseline to track future changes in the BBR complex.
Data Availability Statement
The raw data supporting the conclusions of this article will be made available by the authors, without undue reservation.
Author Contributions
AFl and TD were the project organizers, conceived and designed the study, and prepared the manuscript. DD, TW, and AFe collected data, analysed data, and prepared figures. All authors contributed to the article and approved the submitted version.
Funding
This study received funding from AGL Wholesales Pty Ltd., Port Phillip and Westernport Catchment Management Authority. The funder was not involved in the study design, collection, analysis, interpretation of data, the writing of this article or the decision to submit it for publication.
Conflict of Interest
AFl, AFe, and DD are employed by Fathom Pacific Pty Ltd. TW was employed by Total Hydrographic Pty Ltd.
The remaining author declares that the research was conducted in the absence of any commercial or financial relationships that could be construed as a potential conflict of interest.
Publisher’s Note
All claims expressed in this article are solely those of the authors and do not necessarily represent those of their affiliated organizations, or those of the publisher, the editors and the reviewers. Any product that may be evaluated in this article, or claim that may be made by its manufacturer, is not guaranteed or endorsed by the publisher.
Acknowledgments
The project has benefitted greatly from the collaborations with Port of Hastings Development Authority, Victorian Fisheries Authority and the Western Port Biosphere, and Western Port Seagrass Partnership. The Port of Hastings Development Authority and Department of Environment, Land, Water and Planning allowed access to the original 2009 multibeam data.
References
Amini, Z. Z., Adabi, M. H., Burrett, C. F., and Quilty, P. G. (2004). Bryozoan distribution and growth form associations as a tool in environmental interpretation, Tasmania, Australia. Sediment. Geol. 167, 1–15. doi: 10.1016/j.sedgeo.2004.01.010
Barrett, N., Edgar, G., Lawler, M., and Halley, V. (2007). A Quantitative Video Baseline Survey of Reef Biota and Survey of Marine Habitats Within Bathurst Channel, Southwest Tasmania 2002. Tasmania Aquaculture and Fisheries Institute Report, Tasmania, No. 26. Hobart TAS: Tasmanian Aquaculture and Fisheries Institute, University of Tasmania, 55.
Bastos, A. C., Moura, R. L., Moraes, F. C., Vieira, L. C., Braga, J. C., Ramalho, L. V., et al. (2018). Bryozoans are major modern builders of South Atlantic oddly shaped reefs. Sci. Rep. 8:9638. doi: 10.1038/s41598-018-27961-6
Batson, P. B. (2000). The Otago Shelf Bryozoan Thickets: Aspects of Their Distribution, Ecology, and Sedimentology. M.Sc. thesis. Dunedin: University of Otago.
Batson, P. B., and Probert, P. K. (2000). Bryozoan Thickets Off Otago Peninsula. New Zealand Fisheries Assessment Report 2000/46. Wellington: Ministry of Fisheries, 31.
Blake, S., Ball, D., Coots, A., and Smith, T. (2013). Marine Video Survey of Western Port Report. Melbourne, VIC: Fisheries Victoria, Department of Primary Industries, 62.
Bock, P. E., Cook, P. L., and Gordon, D. P. (2018). “Bryozoans in the marine benthos,” in Australian Bryozoa: Biology, Ecology and Natural History, Vol. Volume 1, eds P. L. Cook, P. E. Bock, D. P. Gordon, and H. J. Weaver (Melbourne, VIC: CSIRO Publishing), 157.
Bone, Y., and James, N. P. (1993). Bryozoans as carbonate sediment producers on the cool-water Lacepede Shelf, Southern Australia. Sediment. Geol. 86, 247–271. doi: 10.1016/0037-0738(93)90025-Z
Bone, Y., and Wass, R. (1990). Sub-Recent bryozoan-serpulid buildups in the Coorong lagoon, South Australia. Aust. J. Earth Sci. 37, 207–214.
Bradstock, M., and Gordon, D. (1983). Coral-like bryozoan growths in Tasman Bay, and their protection to conserve commercial fish stocks. N. Z. J. Mar. Freshw. Res. 17, 159–163.
Cocito, S., Sgorbini, S., and Bianchi, C. N. (1998). Aspects of the biology of the bryozoan Pentapora fascialis in the northwestern Mediterranean. Mar. Biol. 131, 73–82. doi: 10.1007/s002270050298
Cranfield, H. J., Manighetti, B., Michael, K. P., and Hill, A. (2003). Effects of oyster dredging on the distribution of bryozoan biogenic reefs and associated sediments in Foveaux Strait, southern New Zealand. Cont. Shelf Res. 23, 1337–1357.
Cranfield, H. J., Michael, K. P., and Doonan, I. J. (1999). Changes in the distribution of epifaunal reefs and oysters during 130 years of dredging for oysters in Foveaux Strait, southern New Zealand. Aquat. Conserv. 9, 461–483. doi: 10.1002/(sici)1099-0755(199909/10)9:5<461::aid-aqc353>3.0.co;2-z
Cranfield, H. J., Rowden, A. A., Smith, D. J., Gordon, D. P., and Michael, K. P. (2004). Macrofaunal assemblages of benthic habitat of different complexity and the proposition of a model of biogenic reef habitat regeneration in Foveaux Strait, New Zealand. J. Sea Res. 52, 109–125. doi: 10.1016/j.seares.2003.12.003
Cuffey, R. J., Fonda, S. S., Kosich, D. F., Gebelein, C. D., Bliefnick, D. M., and Soroka, L. G. (1977). “Modern tidal-channel bryozoan reefs at Joulters Cay (Bahamas),” in Proceedings of the 3rd International Coral Reef Symposium, Miami, FL, 339–345.
Department of Environment, Land, Water and Planning [DELWP] (2017). Western Port Ramsar Site Management Plan. East Melbourne, VIC: DELWP, 160.
Ernst, A., and Königshof, P. (2008). The role of bryozoans in fossil reefs - an example from the Middle Devonian of the Western Sahara. Facies 54:613. doi: 10.1007/s10347-10008-10149-10341
European Commission [EC] (2008). Directive 2008/56/EC of the European Parliament and of the Council of 17 June 2008. Establishing a Framework for Community Action in the Field of Marine Environmental Policy (Marine Strategy Framework Directive). Luxembourg: Commission of the European Communities.
Ford, J. R., and Gilmour, P. (2013). The State of Recreational Fishing in Victoria: A Review of Ecological Sustainability and Management Options. A report to the Victorian National Parks Association, Melbourne. Melbourne, VIC: Victorian National Parks Association.
Ford, J. R., and Hamer, P. (2016). The forgotten shellfish reefs of coastal Victoria: documenting the loss of a marine ecosystem over 200 years since European settlement. R. Soc. Vic. 128, 87–105.
Gillies, C. L., McLeod, I. M., Alleway, H. K., Cook, P., Crawford, C., Creighton, C., et al. (2018). Australian shellfish ecosystems: past distribution, current status and future direction. PLoS One 13:e0190914. doi: 10.1371/journal.pone.0190914
Gordon, P. D. (1972). Biological relationships of an intertidal Bryozoan population. J. Nat. Hist. 6, 503–514. doi: 10.1080/00222937200770461
Hageman, S. J., Bone, Y., McGowran, B., and James, N. P. (1995). Modern bryozoan assemblages and distribution on the cool-water Lacepede Shelf, southern Australian margin. Aust. J. Earth Sci. 42, 571–580. doi: 10.1080/08120099508728226
Hancock, G. J., Olley, J. M., and Wallbrink, P. J. (2001). Sediment Transport and Accumulation in Western Port. Canberra, ACT: CSIRO, 54.
Harris, J. E., Hinwood, J. B., Marsden, M. A. H., and Sternberg, R. W. (1979). Water movements, sediment transport and deposition, Western Port, Victoria. Mar. Geol. 30, 131–161. doi: 10.1016/0025-3227(79)90010-0
Horowitz, A. S., and Pachut, J. F. (1994). Lyellian bryozoan percentages and the fossil record of the recent bryozoan fauna. PALAIOS 9, 500–505. doi: 10.2307/3515139
James, N. P., Martindale, R. C., Malcolm, I., Bone, Y., and Marshall, J. (2008). Surficial sediments on the continental shelf of Tasmania, Australia. Sediment. Geol. 211, 33–52. doi: 10.1128/AEM.69.5.2463-2483.2003
James, N., Feary, D., Betzler, C., Bone, Y., Holbourn, A., Machiyama, H., et al. (2004). Origin of Late Pleistocene bryozoan reef mounds Great Australian Bight. J. Sediment. Res. 74, 20–48. doi: 10.1306/062303740020
Jenkins, G., Kenner, T., and Brown, A. (2013). Determining the Specificity of Fish-Habitat Relationships in Western Port. CAPIM Technical Report #26. Parkville VIC: The University of Melbourne, 64.
Jones, E. J. (2006). Bryozoan thickets on Otago shelf, New Zealand: A Quantitative Assessment of the Epibenthos using Underwater Photography. Dunedin: University of Otago.
Madurell, T., Zabala, M., Dominguez-Carrió, C., and Gili, J. M. (2013). Bryozoan faunal composition and community structure from the continental shelf off Cap de Creus (Northwestern Mediterranean). J. Sea Res. 83, 123–136. doi: 10.1016/j.seares.2013.04.013
Marsden, M. A. H., Mallett, C. W., and Donaldson, A. K. (1979). Geological and physical setting, sediments and environments, Western Port, Victoria. Mar. Geol. 30, 11–46. doi: 10.1016/0025-3227(79)90004-5
McCook, L., Jompa, J., and Diaz-Pulido, G. (2001). Competition between corals and algae on coral reefs: a review of evidence and mechanisms. J. Int. Soc. Reef Stud. 19, 400–417. doi: 10.1007/s003380000129
Melbourne Water. (2018). Understanding the Western Port Environment 2018: A Summary of Research Findings from the Western Port Environment Research Program 2011-2017 and Priorities for Future Research. Melbourne, VIC: Melbourne Waters, 112.
Mello, H. L., Gordon, D. P., Ryland, J. S., and Smith, A. M. (2021). Protecting the small: preliminary investigation of bryozoan community change in New Zealand’s oldest marine reserve, New Zealand. J. Mar. Freshw. Res. 1–3. doi: 10.1080/00288330.2021.1990971 [Epub ahead of print].
Morgado, E. H., and Tanaka, M. O. (2001). The macrofauna associated with the bryozoan Schizoporella unicornis in southeastern Brazil. Sci. Mar. 65, 173–181. doi: 10.3989/scimar.2001.65n3173
Probert, P. K., Batham, E. J., and Wilson, J. B. (1979). Epibenthic macrofauna off southeastern New Zealand and mid-shelf bryozoan dominance. N. Z. J. Mar. Freshw. Res. 13, 379–392. doi: 10.1080/00288330.1979.9515814
Rowden, A. A., Warwick, R. M., and Gordon, D. P. (2004). Bryozoan biodiversity in the New Zealand region and implications for marine conservation. Biodivers. Conserv. 13, 2695–2721.
Wilson, N. K. (2021). Biodiversity and Seasonal Fluctuations of Invertebrates Associated with Western Port (Victoria, Australia) Bryozoan Biogenic Reefs. Masters thesis. Bundoora, VIC: La Trobe. doi: 10.26181/6184c825a7da5
Wood, A. C. L., and Probert, P. K. (2013). Bryozoan-dominated benthos of Otago shelf, New Zealand: its associated fauna, environmental setting and anthropogenic threats. J. R. Soc. N. Z. 43, 231–249. doi: 10.1080/03036758.03032012.03756819
Wood, A. C. L., Probert, P. K., Rowden, A. A., and Smith, A. M. (2012). Complex habitat generated by marine bryozoans: a review of its distribution, structure, diversity, threats and conservation. Aquat. Conserv. 22, 547–563.
Keywords: Western Port, biogenic habitat, bryozoan reefs, multibeam bathymetry, Triphyllozoon and Celleporaria
Citation: Dutka TL, Fejer AJ, Williams T, Donnelly DM and Flynn AJ (2022) Extent and Characteristics of a Newly Discovered Unique Bryozoan Biogenic Reef Complex. Front. Mar. Sci. 9:841131. doi: 10.3389/fmars.2022.841131
Received: 22 December 2021; Accepted: 02 February 2022;
Published: 24 February 2022.
Edited by:
Carolyn J. Lundquist, National Institute of Water and Atmospheric Research (NIWA), New ZealandReviewed by:
Mohammad Reza Shokri, Shahid Beheshti University, IranGuillermo Horta-Puga, Universidad Nacional Autónoma de México, Mexico
Copyright © 2022 Dutka, Fejer, Williams, Donnelly and Flynn. This is an open-access article distributed under the terms of the Creative Commons Attribution License (CC BY). The use, distribution or reproduction in other forums is permitted, provided the original author(s) and the copyright owner(s) are credited and that the original publication in this journal is cited, in accordance with accepted academic practice. No use, distribution or reproduction is permitted which does not comply with these terms.
*Correspondence: Travis Luke Dutka, dC5kdXRrYUBsYXRyb2JlLmVkdS5hdQ==