Expression of Concern: Novel bioactive compounds from marine sources as a tool for functional food development
- 1Department of Food Technology and Biochemical Engineering, Jadavpur University, Kolkata, India
- 2Department of Food Processing Technology, Malda Polytechnic, West Bengal State Council of Technical Education, Govt. of West Bengal, Malda, India
- 3NatNov Bioscience Private Limited, Balasore, India
- 4Skills Innovation and Academic Network, Institute-ABC, Balasore, India
- 5Faculty of Agro Based Industry, Universiti Malaysia Kelantan, Jeli, Malaysia
- 6School of Health Sciences, Health Campus, Universiti Sains Malaysia, Kubang Kerian, Malaysia
The marine environment is a relatively unexplored source of functional ingredients that can be used in food processing, storage, and fortification in a variety of ways. Marine microorganisms are a possible source of novel bioactive chemicals with potential human utility. Some of these microbes can live in the harsh marine environments, resulting in complex compounds with unique biological properties that can be used in several industrial and biotechnological applications. So far, several marine microorganisms (fungi, myxomycetes, bacteria, and microalgae) have been isolated that produce antioxidant, antibacterial, apoptotic, antitumoral, and antiviral chemicals. Furthermore, it emphasizes the enormous potential for marine microbes to produce very important bioactive chemicals. The main goal of this review is to provide a concise overview of several constituents of marine bioactives. Anticoagulant, anticancer, and hypocholesterolemic effects have been demonstrated for bioactive peptides extracted from fish protein hydrolysates, as well as algal fucans, galactans, and alginates. Furthermore, omega-3 fatty acids are abundant in fish oils and marine microorganisms, while potent antioxidants such as carotenoids and phenolic compounds can be found in crustaceans and seaweeds. This review focuses on the potential use of marine-derived chemicals as functional food ingredients for health maintenance and chronic disease prevention, based on their bioactive qualities.
Introduction
The marine ecosystem continues to be a goldmine of bioactives with great potential as the source of food components for the creation of new functional foods. Many kinds of researches have been done utilizing biotechnologies to discover and synthesize novel compounds to improve the chemical diversity and availability of useful marine compounds (Freitas et al., 2012; Barbosa et al., 2014). The marine environment, one of the most underutilized biological reservoirs, has a diverse range of creatures with distinct biological features. In the literature, algae and microalgae have been mentioned as an excellent resource of bioactive chemicals for application in functional foods (Hayes, 2012). Compounds formed from marine organisms are particularly interesting because of the features of the marine environment, which include varying degrees of salt, temperature, and illumination. More research is being done to find and synthesize marine compounds using biotechnological methods to improve the availability and chemical variety of marine functional components (Freitas et al., 2012; Gallego et al., 2019). Natural compounds derived from marine invertebrates and algae have made significant contributions to contemporary medicine, and there is space for more diversification (Sigwart et al., 2021). Natural organic substances including peptides, proteins, polyether, fatty acids, polysaccharides, and enzymes are among the many specialized and potent bioactive chemicals produced by marine bio-resources. Proteins of marine sources exhibit potential as functional components in foods due to their multiple significant and distinctive qualities, including antibacterial function, foaming ability, and gel-forming capacity (Khora, 2013). Phenolic compounds belong to a group of secondary metabolites with well-established biological functions, causing them to appeal to biotechnological applications. Microalgae are one of the most promising sources for developing eco-sustainable production of natural bioactive metabolites. Photosynthetic organisms that dwell in aquatic environments are known as microalgae and produce fatty acids and carotenoids. They have a broader range of biological and functional diversity. They are also high in other beneficial chemical groups, such as phenolic compounds (Centella et al., 2017; Del Mondo et al., 2021).
Because of its tremendous richness, marine nutrition is a gold mine of innovative healthy food fixes and naturally dynamic combinations such as bioactive peptides, fish oils, and microalgae, macroalgae, and fish proteins. Despite their numerous medical advantages, marine nutraceuticals have been misused for food uses. Marine foods are regarded as an incredible source of proteins of excellent quality, as well as lipids rich in unsaturated fatty acids (Ahmed et al., 2014; Bharat Helkar and Sahoo, 2016). The marine environment, one of the most underutilized biological reservoirs, has a diverse range of creatures with distinct biological features. In the literature, algae and microalgae have been mentioned as an excellent resource of bioactive chemicals for application in functional foods (Hayes, 2012). Compounds formed from marine organisms are particularly interesting because of the features of the marine environment, which include varying degrees of salt, temperature, and illumination. More research is being done to find and synthesize marine compounds using biotechnological methods to improve the availability and chemical variety of marine functional components (Freitas et al., 2012; Gallego et al., 2019). Marine plants survive and thrive in a highly hostile environment by living close to others. Aquatic plants have evolved various physiological changes, such as the production of bioactive chemicals that provide them with protection against grazers. As a response to ecological pressures, they create complex secondary metabolites. Some secondary substances produced by aquatic plants could be effective in preventing pathogenic germs from growing. Photochemical substances are generated from aquatic plants, and their partially purified or refined components (Pooja et al., 2020). Marine-based nutraceuticals can come from several sources, such as marine plants, sponges, and microorganisms; each has its unique set of biomolecules that allow it to thrive in its environment. Because of their polyphenol and carotenoid levels, seaweeds are now being explored as an excellent source of antioxidants. Products derived from post-harvest processing of fish and seafood are also a promising source of marine-based food components. Fish oil (primarily omega-3 polyunsaturated fatty acids, PUFA), vitamins (A, D, and E), shark liver oil, peptides, chitin, chitosan, enzymes, seaweed, and protein hydrolysates (Shahidi and Ambigaipalan, 2015). Marine microorganisms can produce novel glucosidase inhibitors that could be used as anti-diabetic medication options (Trang et al., 2021). The rich biodiversity and abundance of marine and estuarine mollusks, as well as their diverse range of uses as food and nutraceutical resources, have piqued the interest of food technologists and dieticians in recent years. Cephalopoda, Bivalvia, and Gastropoda were the phyla mollusca with the highest nutraceutical applications and bioactive characteristics (Chakraborty and Joy, 2020).
At present, as the consequence of increased awareness of their health advantages and a positive perception of seafood, aquatic food consumption has expanded globally. In comparison to terrestrial sources, marine organisms have developed distinct characteristics and bioactive chemicals due to the diversity of their living conditions. The value of functional food ingredients in terms of health promotion and reduction of disease risk has long been acknowledged (Shahidi and Ambigaipalan, 2015).
The major aim of this review is to highlight a concise illumination of various aspects of marine bioactives that include-
• A precise overview of the sources, activities, and importance of bioactive substances derived from various types of marine organisms.
• Sustainable green processing methods to extract biomolecules from seafood waste
• Application of the marine bioactives in food and nutraceutical industries
This review displays the emerging significance of marine bioactive components, beginning with the sources, classification, potential health benefits, green extraction technologies (Traditional and Emerging techniques), biomedical uses, and their potential food applications.
Significance of Functional Food in Today’s World
Functional food products are described as those that provide a health-promoting activity as well as the traditional nutritional value. In the case purchasing of functional foods, the primary motivation for consumers is their utilization to help in the prevention of chronic illnesses like osteoporosis, Alzheimer’s disease, and cardiovascular disease, as well as to improve health by boosting the immune system and inducing feelings of well-being. At present, functional foods have become a chief focus of the development of novel food products, as they represent one of the primary food classes in the global health spectrum. The development of functional food products is a complicated technique with different success variables than regular new food product development (Khan et al., 2013).
Foods are now designed to not only satisfy hunger and offer essential nutritional constituents for people but also to prevent diseases caused by poor nutrition and to improve human physical and emotional well-being. These foods are termed “functional foods.” The concept of functional foods, which denotes a new way to achieve optimal health by supporting a state of wellness and reducing illness recurrence, arose largely as a result of discoveries in understanding the relationship between nutrition and health. Functional foods can be found in almost every food category, although goods are not evenly distributed across the many segments of the market (Siró et al., 2008).
Importance of Marine Food Sources for Sustainable Development
The Sustainable Development Goals (SDGs) are a worldwide agenda that attempts to encourage measures that promote economic, environmental, and social sustainability. The worldwide marine industry, as one of the primary stakeholders, contributes significantly to global sustainability (Wang X. et al., 2020; Merlo et al., 2021). In tropical and extra-tropical countries, tropical fisheries play a critical function in ensuring food security (Duda and Sherman, 2002; Lam et al., 2020). Microbes come in a wide variety of shapes and sizes, and they perform a critical and often inimitable function in the operation and long-term sustainability of the ecosystem. In the oceanic ecology, both prokaryotic domains are abundant. Aside from them, viruses were 10 times more prevalent in the marine environment than all other microorganisms (Malone et al., 2014). Microorganisms exhibit a vital functionin maintaining the vibrant balance and uprightness of the environment, which is critical because life depends on the continual, microbially mediated change of chemicals in marine habitats and the majority of biogeochemical activities in the land (Pinnaka et al., 2019).
Metabolites From Marine Organisms
Sea microorganisms are considered to be a source of structurally and physiologically unique chemicals with numerous biotechnological uses. Rare functional groups including dichloroimine, isocyanate, isonitrile, and halogenated functional groups as well as distinctive physiochemical features of the marine environment (temperature, osmolality, pressure, and pH) are frequently discovered in marine metabolites. As a result of these facts, bioactive components with properties distinct from those observed in terrestrial habitats have been developed (Rocha-Martin et al., 2014). In recent decades, consumption of aquatic foods has expanded significantly as a consequence of increased consumer awareness of their health-promoting activities and favorable perception of seafood. Marine organisms have derived specific features and bioactive constituents in comparison to terrestrial resources owing to the diversity of their living environments (Shahidi and Ambigaipalan, 2015).
Marine Bacteria
Archaea and eubacteria are two forms of marine bacteria that are increasingly being investigated as potential sources of novel chemicals. Archaea is a fascinating bacterial group because many of them are extremophiles or creatures that live in harsh environments such as the deep oceans, low temperatures, or chemically demanding environments (salinity, pH). The stabilizing element in archaean cell membranes is glycerol tetraethers, as opposed to a catenoid or hopanoid in eubacteria. Extremophiles are considered an excellent source of stable enzymes for industrial uses as well as therapeutic applications. Gram-positive actinomycetes and bacteria make up marine eubacteria (Kiuru et al., 2014).
Actinobacteria create thousands of physiologically active natural chemicals with a wide range of functions. More than half of these bioactive chemicals were discovered in members of the actinobacteria family. Rare actinobacteria found in a variety of environments, including extreme elevations, volcanic locations, and the sea, have recently gained interest. Physiological or metabolic forces under such extreme environmental conditions have been hypothesized to contribute to the synthesis of a diverse range of natural chemicals (Dhakal et al., 2017).
Proteobacteria
Many bioactive chemicals (Bryostatins, Pentabromo-pseudilin, Ectoine, Vibriobactin, Solonamide, Violacein, Thiomarinol, Bromoaltero-chromide) including antibiotics, have been identified from Gram-positive Actinobacteria and filamentous fungus, whereas Gram-negative bacteria have received less attention (Carbonell et al., 2000; Speitling et al., 2007; Buijs et al., 2019; Jia et al., 2022). However, genome mining has recently revealed that Gram-negative bacteria belonging to the phylum Proteobacteria include biosynthetic gene clusters that are likely to encode beneficial chemicals. Many well-known pathogens from the Vibrionaceae, Enterobacteriaceae, and Pseudomonadaceae families are found in the phylum Proteobacteria. Although Proteobacteria is the most prevalent phylum in pelagic marine environments, just a few bioactive chemicals have been identified from this group of organisms (Desriac et al., 2013; Buijs et al., 2019).
Cyanobacteria
Cyanophyta is the only bacterial phylum that derives its energy from photosynthesis and is the only photosynthetic prokaryotes group capable of producing oxygen and absorbing CO2. Although some Cyanobacteria are difficult to cultivate, this group of microorganisms is garnering attention in basic research due to its quick generation period. Cyanobacteria, both unicellular and filamentous, have been gathered over the years, identified on morphological and molecular grounds, and cultivated in axenic cultures using conventional procedures (Stincone and Brandelli, 2020). Screening efforts using target organisms unrelated to those for whom the metabolites developed have revealed several bioactive compounds generated by cyanobacteria and algae. Many of these compounds have greater biological activities and chemical structures that influence a variety of biochemical processes in cells (mainly directed against the photosynthetic process). These compounds are thought to be involved in the management and succession of algal and bacterial populations, and they could be used as natural herbicides or bio-control agents. Many cyanobacteria create secondary metabolites, which are substances that aren’t required for the organism’s main metabolism or growth and are only found in a few taxonomic groupings. Microcystis, Anabaena, Nostoc, and Oscillatoria are cyanobacteria that create a wide range of secondary metabolites. Dolastatin, cryptophycins, and curacin A, among other major marine cyanobacterial compounds identified, were either under preclinical or clinical trials as anticancer medicines (Kiuru et al., 2014).
Microalgae
Sea algae are benthic marine plants that are unicellular or multicellular, macroscopic, and unicellular. The three pigmentation classes of marine algae are Rhodophyceae (red algae), Chlorophyceae (green algae), and Phaeophyceae (blue algae). Pigments, including fucoxanthin for brown, phycobilins for red, and chlorophyll for green algae are responsible for their color. Fucoxanthin, chlorophyll a, and chlorophyll c are the major pigments of brown algae, and they give the algae their greenish-brown hue. Brown algae can reach lengths of up to 45 m. Brown algae are found all over the world and number between 1,500 and 2,000 species (Freile-Pelegrín and Robledo, 2013; Kadam et al., 2013). Microalgae have gotten a lot of attention as an excellent source for the long-term production of a variety of bioactive chemicals. Fatty acids, phycobiliproteins, chlorophylls, carotenoids, and vitamins are just a few examples of compounds that can be found in food additives and constituents. Microalgae bioactive compounds are intriguing as research subjects, and their potential medicinal capabilities suggest that they may have tremendous economic potential soon. Antioxidant, antiviral, antibacterial, antifungal, anti-inflammatory, anticancer, and antimalarial activities are among these qualities (Fu et al., 2017). Because of their photosynthetic activity, microalgae have a critical role in ecosystems as primary producers (de Vera et al., 2018). Microalgae are fascinating natural sources of bioactive elements that include carotenoids, vitamins, enzymes, vital amino acids, PUFAs, minerals, and fiber. Microalgae have emerged as one of the most exciting and inventive sources of novel functional foods due to their potential. Furthermore, microalgae are utilized as functional components to boost the nutritional value of foods and, as a result, have a promising result on human health by boosting human health as well as reducing the occurrence of disease and illness (Matos and Cardoso, 2017). Novel solid-liquid and liquid-liquid extraction methods (high pressure, enzymatic, supercritical, microwave, and ultrasonic) are simple, profitable, and long-term recovery procedures (Menaa et al., 2021). Minerals contained in commercial forms of microalgal biomass could play a key role in functional foods. Oil derivatives fish with beneficial benefits on human health, such as antioxidant, antiviral, antibacterial, and anti-inflammatory properties Gastric ulcers, constipation, anemia, diabetes, and hypertension are all things that can be avoided with this supplement (Camacho et al., 2019). Microalgae have some unique characteristics, including (1) a large number of different strains and species that can be screened for interesting bioactive components that can then be used in commercial products; (2) the ability to isolate them from marine environments or grow biomass in bioreactors; and (3) the use of minimal arable land for their production (Gallego et al., 2019). Because of their simple growth methods, microalgae are seen as a possible alternative feedstock. Microalgae can live in adverse environments such as wastewater, high salinity water, and cold or hot springs, which are unsuitable for conventional agriculture (Khoo et al., 2020).
Macroalgae and Seaweed
In the food industry, microalgae, often known as seaweed, are a relatively untapped source of novel compounds, such as peptides and carbohydrates, which can be used as nutraceuticals. Numerous algae-derived bioactive components have demonstrated several important biological actions, such as antihypertensive and antioxidant, both in vitro and in vivo, which are tightly linked to the chemical structure of the peptides or carbohydrates. Seaweed, particularly bioactive peptides and carbohydrates, are relatively unexplored and intriguing sources of new compounds for application as functional foods and nutraceuticals. Several bioactive chemicals produced from seaweed have demonstrated a great spectrum of biological activity in vitro and in vivo (Zhao et al., 2018; Jesumani et al., 2019). Antihypertensive, antioxidant, anticoagulant, antitumor, anti-inflammatory, and immuno-stimulatory actions are among the most promising biological activity identified by bioactive peptides and polysaccharides. These biological activities have piqued the interest of scientists in producing functional components and researching the link between chemical structure and biological activity (Lafarga et al., 2020). Macroalgae have evolved sophisticated and distinct metabolic processes to survive in harsh marine settings. As a result, natural biologically active components have been mined from these organisms. Many proteinaceous bioactive substances have been discovered through the study of marine creatures. Proteins, amino acids, linear peptides, and amino acid-like components are all examples of these. Furthermore, several macroalgae species have been found to contain considerable amounts of protein (Harnedy and Fitzgerald, 2011). Marine macroalgae are quickly gaining popularity as a source of useful chemicals that can help people stay healthy and avoid sickness. Phlorotannins, a type of polyphenol found only in marine macroalgae, exhibited anti-hyperglycemic and anti-hyperlipidaemic properties in the in vitro studies, and preliminary human trials. Because of their potential to mediate hyperglycemia and hyperlipidemia, phlorotannins are promising components for the creation of functional food items to lower the occurrence of cardiovascular disease and type 2 diabetes (Thangavel and Sridevi, 2015; Rani and Yadav, 2018). A revived interest in seaweeds has resulted from a global desire for the synthesis of key metabolites having nutraceutical value in a sustainable feedstock. Seaweeds have been used in Asian locations since ancient times, and they have recently been shown to offer a variety of medicinal properties. Seaweeds are high in a variety of nutritious components as well as metabolites with medicinal capabilities. As a result, seaweed proteins are a viable source for the food industry. Overall, seaweeds constitute a nutrient-dense food (Tanna and Mishra, 2018).
Marine Fungi
In terrestrial ecosystems, fungi grow in symbiotic relationships with plants and animals and play an important part in nutrient cycling. Fungi are predicted to grow anywhere in the terrestrial biosphere moisture and a carbon source coexist. At several trophic levels, fungi act as parasites. The role of chytrid fungi in parasitism of marine phytoplankton is becoming more widely recognized, and fungal parasites have been discovered in crustaceans, fish, and even top predators like seals and dolphins that are susceptible to cryptococcosis. Fish fungal parasites are poorly understood, although the most well-known types are the internal parasites known as microsporidia. In the oceans, fungi also serve as important saprotrophs, converting detritus and algae into fungal biomass, which, together with bacterial heterotrophs, is ingested by zooplankton, forming a microbial loop that connects refractory polymers produced from primary productivities (Gladfelter et al., 2019). Owing to their chemical variety and high biological roles, marine fungi were an important source of microbial beginnings. Only a small portion of the worldwide fungal population has been sampled, particularly marine fungi. Marine fungus with diverse genetic origins and extensive metagenomes are a major source of metagenomic information (Wang C. et al., 2021).
Marine Invertebrates
Edible marine invertebrates such as crabs, mollusks, and echinodermata can be used as organic-based biopolymers for a variety of purposes. These biopolymers are composed up of polysaccharides and protein and have been used by humans for decades as food and nutraceutical ingredients to treat various ailments. Biopolymers such as glycosaminoglycans, fucosylated chondroitin sulfate, chitin, and chitosan have recently gained popularity in the biomedical industry for treating a variety of illnesses. These chemicals have anti-cancer, antibacterial, anti-inflammatory, wound healing, and anti-microbial functions, as well as being used in animal feed (Ganesan et al., 2020b). Marine invertebrates are considered as a wide animal kingdom species that contain an abundance of unique functional biopolymers such as proteins, lipids, and polysaccharides with diverse biological functions. These biopolymers have been employed in a variety of applications and as functional food from a health standpoint. Marine invertebrates are a diverse group found throughout the environment, from the intertidal area to the deep ocean. Annelida (marine worms), Porifera (sponges), Cnidaria (corals, jellyfish), mollusks (oysters, prawns, and crayfish), and echinoderms are some of the taxonomic families (starfish, sea cucumbers, and sea urchin). Coastal communities have a long history of employing marine invertebrates in their diets and for medical purposes. Collagen and gelatin are commercially important substances derived from edible marine animals. Collagen makes up about 30% of the total content of all animals’ skins and bones. Collagen generated from marine organisms is a viable replacement for mammalian collagen and is widely used in biomedical applications (Ganesan et al., 2020a).
Sponges have long been known to be high in bioactive compounds. Unusual sterols are abundant in marine sponges, and these sterols exhibit numerous functions in biological membranes. The antimicrobial function has been found in sulfated and alkaloid sterols. Cnidarians are the richest natural producers of prostaglandins, which have just recently been discovered in the soft coral Plexaura hamomala of the Caribbean Sea. Bryozoans have also been used to obtain bioactive compounds. A marine polychaete (phylum Annelida) has been discovered to treat a variety of pathophysiological disorders including arthritis, osteoporosis, and bone cancer, among others. Arenicola marina, a marine annelid, was used to isolate the bioactive chemical. Marine arthropods have also yielded several bioactive chemicals. Limulus Amoebocyte, Lysate and Tachypleus, Amoebocyte Lysate is the most notable bioactive compound derived from marine arthropods (John et al., 2018). It is an aqueous extract of horseshoe crab blood cells (amoebocyte). Shrimp is popular seafood around the world, and its lipids have been investigated for biological activity in both muscle and exoskeleton. This fraction is made up of free fatty acids, triglycerides, carotenoids, and other lipids, and some of these chemicals have been linked to cancer chemoprevention. In both in vivo and in vitro research, carotenoids and PUFAs have been widely examined for their chemopreventive characteristics. Spisulosine, a bioactive molecule obtained from the Hawaian mollusk Elysia rufescens, exhibits antileukemic properties. Synthadotin and Soblidotin are synthetic analogs of Dolstatin, which were obtained from the molluscan species D. auricularia and are currently being tested for therapeutic potential. The mollusk Dolabella auricularia has been shown to produce several anticancer and cytotoxic chemicals known as dolastatins (Papon et al., 2022). Saponins are the most common bioactive chemicals found in Echinoderm metabolites. These can be found in sea urchins, starfish, and sea cucumbers, among other things. Asterosaponins are sterol derivatives, but sea cucumber saponins have been claimed to be terpenoid in origin (Datta et al., 2015).
Marine Fishes
Marine fish have long been considered a healthy food choice, but current advances in the area of nutraceuticals and functional foods have revealed that their value extends beyond nutritive value, as they provide our diet with molecules that represent a vital therapeutic function in the prevention of human diseases. Even by-products from the seafood business have recently been considered as a source of useful constituents including enzymes, peptides, and collagen that could benefit human health or be used in the development of new medications (Šimat, 2021). Fish are the most often consumed marine species and are vital to the global economy. The presence of proteins, minerals (including selenium, calcium, iron, and zinc), unsaturated essential fatty acids, and vitamins, such as Vitamin A, D, E, B3, B6, and B12, contribute to the nutritional benefits of fish consumption. Peptides generated from fermented fish after enzymatic treatment have also been found to be effective therapies for a variety of hypertension, cancer, viral infections, and Alzheimer’s disease (Suleria et al., 2015).
Apart from this, the finfish and crustacean aquaculture business remain heavily reliant on marine capture fisheries for important dietary nutrient inputs such as fish meal and fish oil. This reliance is especially severe in complex aquafeeds for farmed carnivorous finfish and marine shrimp (Kari et al., 2021, 2022).
Marine Actinomycetes
Actinomycetes are gram-positive bacteria that are well recognized for producing a variety of antibiotics. Actinomycetes are primarily free-living creatures in nature; however, some can be harmful. They create symbiotic relationships with a variety of plants and animals. Actinomycetes also have symbiotic relationships with a variety of marine macrofauna, including cone snails, invertebrates, marine sponges (Axinella polypoides, Haliclona etc.), and fora-like insects which are important ecologically (Mahapatra et al., 2020).
Primary Metabolites and Secondary Metabolites From Marine Organisms
The importance of marine bacteria and fungus as potentially promising sources of various biologically active constituents cannot be overstated. Fungi and bacteria from the sea are acrucial source of novel compounds with significant pharmacological and therapeutic promise (Debbab et al., 2010). Omega-3 oils, chitin, chitosan, algal components, carotenoids, and other bioactive constituents are examples of high value-added chemicals having nutraceutical potential that can be used as functional additives. Polysaccharides like chitin and chitosan are gaining popularity. They are thought to be a superb source of lipids with high quantities of unsaturated fatty acids, high-quality protein, and maybe resulting in human health improvement by lowering the dangers of cardiovascular disease (Grienke et al., 2014). Marine bioactive chemicals are a potential source of bio-products with promising applications in biotechnology (Figure 1). Diverse biological activities must be evaluated to discover and identify novel marine natural products (MNPs) (Table 1).
The discovery of novel bioactive chemicals is being accelerated due to the improved sensitivity of omics technologies, particularly next-generation high-throughput sequencing paired with liquid chromatography-mass spectrometry and nuclear magnetic resonance. The most promising MNPs are mycosporine-like amino acids (MAAs), which have been obtained from a variety of marine microorganisms with UV absorption properties (Rosic, 2021).
Bioactive substances and secondary metabolites generated from sponges, crabs, seaweed, algae, fungi, fish, bacteria, and other marine species provide a huge source of healthful meals. The principal functional constituents having biological qualities from marine sources applied as functional foods are pigments, polysaccharides, proteins, lipids, vitamins, minerals, and phenolic compounds. Seaweeds have antioxidant, anticancer, anti-inflammatory, anticoagulant, anti-obesity, and antibacterial functions making them a promising crop. Microalgae and poly-saturated fatty acid-rich fish oils can aid to improve the nutritional profile of food (Prabha et al., 2019).
Algae are photosynthetic polyphyletic organisms that are divided into three families: Rhodophyceae, Phaeophyceae, and Chlorophyceae. In the open ocean, macro-algae have a rapid growth rate, resulting in high biomass production. Macro-algae have higher photosynthetic activity than terrestrial biomass, as well as the benefit of not being reliant on land and water. Chlorophyll and phycobiliproteins are found in red algae, which are multicellular eukaryotic cells. It is the high phycoerythrin concentration that gives them their distinctive red color. Floridian starch, which is similar to amyl, can be metabolized by red seaweed as a food reserve. Brown algae are a type of multicellular algae that can be found in a range of shapes and sizes in the ocean. Some are only a few centimeters long, while others can grow to be 60 m long. Brown algae have high carbohydrate content, with carbohydrates accounting for more than half of the dry biomass. Brown algae are grown in large quantities for food and alginate extraction (Centella et al., 2017; Romano et al., 2017).
Proteins
Proteins are polymers made up of 20 amino acids whose sequence is determined by the genetic code, as well as a variety of additional amino acids. Proteins serve as hormones (insulin), antibodies, and transport proteins (hemoglobin) in biological and food systems, in addition to being nutritionally significant (immunoglobulins). Due to their rich nutritional and medicinal properties, seaweeds have long been utilized as food or herbal medicine to prevent a variety of diseases and ailments. They are also utilized as dietary supplements, herbicides, animal feed, fungicides, and a source of agar, alginate, and carrageenans for numerous industrial and pharmaceutical uses. For several decades, it is commonly acknowledged that seaweeds have been successfully applied as a source of protein (Pooja et al., 2020).
Peptides
Protein hydrolysis for bioactive peptide synthesis, especially peptides obtained from marine proteins, has sparked a lot of interest. These peptides have been isolated from crustaceans, algae, and fish species found in the sea (Table 2). Bioactive peptides can include anywhere from 3 to 20 amino acid residues according to the amino acid content and sequencing. They have biological properties such as antioxidant, antithrombotic, antihypertensive, immunomodulation, anticancer, and antibacterial actions, among others (Hamed et al., 2015). Peptides produced from marine protein sources could be beneficial as vital antihypertensive compounds which can be incorporated incorporation into functional foods (Norris et al., 2013; Cunha and Pintado, 2021).
Amino Acids
The amino acids taurine, Pro, Gln, Ala, Gly, and Arg are abundant in seafood muscles that are water-soluble components. Because water-soluble components are lost during cooking or thermal processing, their beneficial characteristics are expected to be stronger when seafood is lightly prepared. Children’s diets are frequently deficient in amino acids containing sulfur, Lys, and Thr, especially when they are based on grains. Consequently, it is assumed that consuming muscle proteins from fish are high in certain amino acids may improve human nutrition by boosting the nutritional value of foods (Dewapriya and Kim, 2014; Romano et al., 2017).
Lipids and Fatty Acids
Eicosapentaenoic acid (EPA) and docosahexaenoic acid (DHA) are the most common omega-3 PUFA generated from aquatic sources. In human beings, linolenic acid can be extended and desaturated to EPA and DHA to a limited amount. These PUFA can only be obtained via seafood and supplementation. DPA (docosapentaenoic acid) is contained in fish oils in extremely modest concentrations compared to EPA and DHA. In seal blubber oil, however, it is almost as significant as either EPA or DHA. Both unicellular and multicellular marine plants, including phytoplankton and algae, produce EPA and DHA. They make their way up the food chain and end up in the lipids of aquatic animals like fish and marine mammals. W-3 fatty acids have been shown to alter membrane lipid composition, gene expression, cardiovascular health, eicosanoid production, cell signaling cascades, and blood lipid profiles in animal and in vitro investigations. The consumption of fatty acids from natural sources, according to epidemiological research, may impact the initiation and progression of numerous disease conditions, such as cardiovascular disease, autoimmune illness, and cancer (Shahidi and Ambigaipalan, 2015). In seafood, the composition of fatty acids is often characterized by a decreased amount of saturated fatty acids (SFA). Since a link between SFA consumption and the development of cardiovascular disease has been commonly assumed, a reduction in SFA intake is recommended. A present study, however, casts doubt on this idea, with carbohydrates being identified as a possible reason. PUFAs and a large amount of monounsaturated fatty acids (MUFAs) are also found in seafood, both of which are considered healthy if not oxidized (Hoffman et al., 2009).
Carbohydrates and Dietary Fiber
Carbohydrates as a significant substance in marine life, from marine organisms, exhibited antioxidant and immunity enhancement functions, which imparted a vital foundation for anti-aging. The majority of the marine natural carbohydrates are polysaccharides, and a few of them are monosaccharides or oligosaccharides. A disaccharide called trehalose, which was widely present in seaweed and shrimp, with an anti-aging function by controlling Nrf2 and insulin signaling pathway (Wang X. et al., 2021).
Brown algae conatin three main forms of soluble dietary fiber polysaccharides including alginate, fucoidan, and laminaran. Cellulose makes up the majority of the insoluble fiber. Alginate, the major polymer found in brown algae, is made up of polymannuronic acid, polyguluronic acid, and a linear combination of polymannuronic acid and polyguluronic acid. Alginate is available in a variety of forms, including acid and salt (considered to be a vital constituent of the cell wall). Fucoidan, unlike alginate and laminaran, has a very wide and complicated structure. According to the type of brown algae, fucoidan is a sulfated polysaccharide-rich in fucose sugar with other monosaccharides (e.g., low amounts of glucose, glucuronic acid, mannose, xylose, and galactose) (Gurpilhares et al., 2019). Laminaran, fucoidan, and alginate are the three most common kinds of soluble dietary fiber polysaccharides produced by brown algae. Alginate, the major polymer found in brown algae, is made up of polymannuronic acid, polyguluronic acid, and a linear combination of polymannuronic acid and polyguluronic acid (Jin et al., 2015). Brown seaweed polysaccharides have a complex structure that prevents endogenous enzymes in the human intestine from breaking them down. Dietary fiber can be found in alginate, laminaran, and fucoidan. As a result, much work has gone into creating efficient methods for extracting, purifying, and elucidating the structural features of algal prebiotics to increase fiber bioavailability (soluble and insoluble) (Gurpilhares et al., 2019). Chitin and chitosan polymers are natural amino-polysaccharides with multimodal characteristics and distinctive architectural structures. In the last decade, chitin and chitosan derivatives have gotten a lot of attention in the fields of food, biomedical, culinary, biopharmaceutical, and other industrial research, with applications like tissue engineering and wound healing biomaterials (Pati et al., 2021). Chitin can be deacetylated to some extent to create chitosan, chitosan oligosaccharides, and glucosamine, among other things (Pati et al., 2020; Pati et al., 2020). Due to their improved water solubility, these chitosan derivatives are more helpful as nutraceutical components than the completely acetylated, insoluble variety of chitin. Even though the efficiency of chitosan in decreasing fat absorption has been questioned, several chitosan products on the market today are advertised as fat burners and cholesterol reducers. The diversity of biological features of chitosan and its derivatives, such as biodegradability, biocompatibility, and non-toxicity, have sparked commercial interest in chitosan and (Gurpilhares et al., 2019) its derivatives in several fields (Nagaoka et al., 2011; Zhang et al., 2012). Brown algae include three types of soluble dietary fiber polysaccharides: alginate, fucoidan, and laminaran. Cellulose makes up the majority of insoluble fiber. Laminaran is a -glucan composed primarily of 1,3-D-glucopyranose residue that is branched with 1,6-D-glucopyranose and serves as the primary storage carbohydrate of algae (Gurpilhares et al., 2019). Agar, alginate, carrageenan, and fucoidan are marine polysaccharides derived from seaweeds that have a variety of physiological properties and are widely used in food, agriculture, and medicine. However, their poor solubility and low absorption have severely hampered their use. Marine oligosaccharides, as breakdown products of those polysaccharides, have garnered a lot of interest because of their clear biological activity, improved solubility, and superior bioavailability (Zhu et al., 2021).
Pigments
Porphyridium cruentum and A. platensis, marine red algae, can produce up to 8% of phycobiliproteins, which are applied as fluorescent indicators when covalently attached to antibodies, A-protein, biotin, lectins, and hormones. A protein plus phycobilin, a chromophore, makes up phycobiliproteins (connected by covalent bonds). Arthrospira’s phycocyanin and Porphyridium’s phycoerythrin are the most well-known phycobiliproteins. They can be used as non-radioactive markers in fluorescence-based immunology assays, as well as microscopy and DNA assays (De Jesus Raposo et al., 2013).
The presence of carotenoids causes the yellow, orange, and red hues of skin, shells, and exoskeletons o the aquatic species. Algal species have them as well. Carotenoids (especially b-carotene) serve as vitamin A precursors in mammals, which is one of their most important physiological activities. Vitamin A activity has been demonstrated in a-carotene, cryptoxanthin, canthaxanthin, 3,4-dehydro-b-carotene, and b-apo-80-carotenoic acid ethyl ester, and echinenone. In the gastrointestinal lumen, marine carotenoids have been reported to reduce triacylglycerol absorption and inhibit lipase activity. The marine carotenoids fucoxanthin, abundant in brown seaweeds, has been related to cancer (Matsumoto et al., 2010; Manivasagan et al., 2018). Natural astaxanthin is produced by marine microorganisms such as algae (e.g., Chlorella zofingiensis, Chlorococcum, and Haematococcus) and yeast (e.g., Phaffia rhodozyma) (Khoo et al., 2019).
Enzymes, Vitamins, and Minerals
In the food and nutraceutical industries, enzymes can be utilized in the transformation of other molecules into important biotechnological tools. Enzymes, as food ingredients, can affect spoilage, storage, processing, and safety. Lipase, transglutamase, chitinolytic enzymes, polyphenol oxidase, and red algal enzymes utilized in the degradation of starch (such as -1, 4-glucanase) are all supplied from marine sources (Table 3). When compared to their terrestrial equivalents, they have superior catalytic, physical, and chemical capabilities. They can also be deactivated at a moderate temperature (Zhang and Kim, 2012). These enzymes are also utilized as culinary additives. Due to their distinctive activity under abnormal conditions, biomolecules extracted from extremophiles, such as enzymes, can be particularly valuable in the food industry, and extremophiles are usually thought to have a considerable potential to be profitable resources for biotechnological applications. Vitamins and minerals serve several activities in the body, including supplying intracellular transportation and serving as cofactors in metabolic processes. Vitamins and minerals, such as iron, are abundant in seaweeds (Suleria et al., 2015). Xylanases are a family of enzymes that catalyze the release of xylose from hemicellulose. Xylose is a key carbon source for bacteria and is involved in plant-pathogen infection. Algae, bacteria, fungus, protozoa, gastropods, and arthropods are among the creatures that make xylanases. Despite this, microorganisms are favored over plant and animal sources for xylanase synthesis because of their availability, higher volumetric output, structural stability, and simplicity of genetic manipulation. The majority of marine xylanases have been identified from fungus and bacteria, particularly actinomycetes. Microbial sources of marine xylanases have been discovered (Izadpanah et al., 2020). Chitin hydrolysis can be catalyzed by chitinolytic enzymes. Chitinolytic enzymes have gained widespread attention as a result of their medicinal and biotechnological uses. Marine creatures have recently become a new source for commercial enzyme separation and the manufacture of a variety of unique useful chemicals. We could identify microorganisms suited to various extreme situations (halophiles, psicrophiles, thermophiles, and so on) that could be useful in a variety of areas because the sea environment is so diverse (Beygmoradi et al., 2018).
Seaweeds are known for their high mineral (Na, Ca, Mg, P, and K) and trace element (Zn, I, and Mn) content, which is linked to their propensity to retain inorganic sea chemicals because of polysaccharides in the surface of the cell, which can account for up to 36% of dry mass in some species. Many types of seaweed are recommended as food supplements to fulfill daily mineral and trace element demands because they are high in calcium and potassium. Other nutrients that are more prevalent in some shellfish than, say, beef include selenium, iodine, zinc, magnesium, and calcium. Increased selenium consumption in the diet has been related to a reduced risk of cancer. Seaweeds, rather than fortified salt with iodine, are an alternate option to give iodine through diet in particular countries with poor iodine consumption. One of the most promising natural sources of iodine is seaweed.
Probiotics
Gut flora is a collection of microbial species found in the gastrointestinal tract that exceeds 100 trillion bacteria in the human intestine. Eubacterium, Bacteroides, Enterococcus, Prevotella, Clostridium, Staphylococcus, Streptococcus, and Enterobacter, among the most common intestinal microbial species, perform a variety of helpful functions for the host in the utilization of unused sources of energy. In a healthy gut, numerous strains can become pathogenic under particular circumstances. As a result, it has been discovered that limiting the number of hazardous species while fostering a favorable environment. Many approaches to enhancing the positive functions of intestinal microflora have been proposed in this area. Probiotic strains are live microorganisms that are beneficial to gut flora and have the potential to reduce the disease-causing activity of bacteria, and they are one of the most effective ways to promote beneficial microbes in the gut. Prebiotics, eventually, allow for particular changes in the composition and functions of the gastrointestinal microflora, which promotes the overall well-being and health of the host. Probiotics are frequently made up of live Lactobacillus and Bifidobacterium strains. Yogurts, in particular, are fermented dairy products (Dewapriya and Kim, 2014).
Miscellaneous Bioactive Compounds From Marine Organisms
Collagen is abundant in around 30 percent of the trash generated during the preparation of seafood. Marine collagen has the potential to replace mammalian collagen in a variety of applications, including wound dressing. Collagen peptides have also been shown to aid in wound healing and the prevention of age-related bone loss several researchers (Hosseini et al., 2020). Collagen is also a promising substance for the creation of functional biomaterials. Electrospun collagen scaffolds produced from tilapia fish skin have promote wound healing and re-epithelialization in vivo (Ganesan et al., 2020a).
The collagen present in the skin and bones of aquatic creatures is used to make marine gelatin. It is a vital gelling biopolymer that gives flexibility, viscosity, and stability to foods. Fish gelatin is a high-protein raw material made from fish waste, most commonly fish skin and bones. By dry weight, it contains 27–49 percent protein. Gelatin has a monomer composition and a linear structure that allows it to generate high-quality films. Gelatin films have been shown in previous studies to exhibit characteristics of low oxygen permeability and high UV light barrier, allowing them to be employed as packaging or encapsulating materials (Uranga et al., 2016).
Saponins are oligomeric sterol or triterpene glycosides found in a wide range of plant and animal species, including sea cucumbers and starfish. The triterpene form of saponin is found in sea cucumbers, while the steroidal form is found in starfish. In vitro tests have identified sea cucumber saponins as pancreatic lipase inhibitors. The anti-obesity properties of echinoside A and holothurin A, two sulfated triterpene saponins produced from sea cucumber (Pearsonothuria graeffei), were investigated (Wang T. et al., 2018).
Phycobiliproteins (PBPs) are water-soluble pigments present in chloroplast cytoplasm and stroma. Phycoerythrin, a reddish-pink pigment that functions as a primary light-harvesting pigment by transferring light energy to chlorophyll, is found only in red seaweeds. PBPs that emit red and yellow fluorescence such as, phycocyanin (blue color), allophycocyanin (blue color), and phycoerythrin (red hue). Spirulina-derived phycocyanin is one example of how these pigments have been used in culinary dishes as natural colorants (Sudhakar et al., 2019).
Bioactive secondary metabolites found in marine algae have a wide range of therapeutic benefits. Phytosterols are a type of sterol component that is physically and functionally similar to side chains of cholesterol: phytosterols typically have 9 or 10 carbon atoms, whereas side chain of cholesterol has eight carbon atoms. Brown seaweed contains the sterol fucosterol, whereas red seaweed contains cholesterol. The health benefits of phytosterols are primarily due to their ability to lower cholesterol. However, they have been observed to promote various other health-promoting activities, for instance, anti-obesity, antioxidant, anti-diabetic, anti-atherosclerosis, and anti-inflammatory properties (Hannan et al., 2020).
Potential Health Promoting Actions of Marine Bioactives
With longer life expectancies, our nutrition will become increasingly important in maintaining the health status of humans. This is a dilemma for the food sector since consumers want food that is tasty and convenient, as well as healthful and nutritious. In the food industry, marine nutraceuticals are a reasonable and appealing alternative because there are so many functional food constituents that can be created from marine resources (Figure 2). As people grow more concerned about the connection between food and good health, it is also projected that their consumption of fishery products would expand. Furthermore, the concentration of proteins, carbs, lipids, fiber, metabolites, and other compounds in marine algae can vary.
Antioxidant Activity
The marine environment contains a wide variety of habitats from which novel natural product sources can be derived (Table 4). Marine organisms produce various metabolites with unique chemical structures and biologically active properties. Antioxidants are synthetic or natural compounds that can help to prevent or delay cell damage. These compounds shield cells from the harmful effects of free radicals, which are unstable chemicals. Marine sponges, seaweeds, and related microbes create bioactive components that are effective for a boost of health and prevention of illness. These therapeutic actions are due to a complex blend of phytochemicals that contain antioxidants, antibacterial, anticancer, and antiviral properties. These functions are carried out by phenolic compounds, sulfated polysaccharides, and organic acids. Flavonoids, benzoic acid, gallic acid, phlorotannins, and quercy are examples of polyphenolic compounds produced by the marine environment (Domínguez, 2013; Balakrishnan et al., 2014). The sea environment is a rich current resource for bioprospecting, as it covers 70% of the planet and is home to a diverse range of organisms. As research improves, it is becoming clear that there are unknown bacteria that have emerged specific metabolic and genetic pathways for the generation of unusual secondary metabolites. Because they are a rich source of structurally varied bioactive metabolites, fungi play a key role in marine bio-prospecting. Antibiotics, anticancer, antioxidants, and anti-virals are only a few of the bioactive chemicals discovered in marine fungi (Vitale et al., 2020).
Based on their structure, polyphenolic components are antioxidant compounds obtained from micro- and macroalgae. Phenolic components are classified into 10 types. The 10 classes of substances, for instance, phenolic acids, coumarins, xanthones, anthraquinones, flavonoids, stilbenes, and lignins. Polyphenolic components called phlorotannins have been discovered in a variety of brown algae groups and have powerful antioxidant capabilities. Antibacterial, chemopreventive, and UV-protective activities have also been discovered in phlorotannins (Centella et al., 2017). Microalgae and seaweeds produced reactive oxygen species (ROS) as a result of the harsh environmental conditions they are exposed to (such as temperature and intense irradiation) (ROS). Cancer, diabetes, and neurological and inflammatory illnesses have all been related to high levels of ROS. Because ROS reacts with biomolecules like DNA, membrane lipids, and proteins, it causes cell and tissue damage. Seaweeds (macroalgae) use their high cellular concentrations of antioxidant substances, including carotenoids, phycobilins, polyphenols, and vitamins, to deactivate ROS (Cornish and Garbary, 2010). Microalgal-derived carotene is more physiologically active than synthetically generated -carotene, making it a “natural” food additive. Natural carotene contains a variety of carotenoids and other important components not found in synthetic -carotene, and because the tissues of the body regulate its utilization, it can be consumed in greater quantities. Dunaliella salina can also collect considerable levels of xanthophylls under irradiance stress, especially zeaxanthin, which have unique biological features and could be valuable in disease prevention (Lordan et al., 2011).
Anti-obesity Effect
An unbalanced energy intake-to-expend ratio leads to obesity, a chronic metabolic disorder. Accumulation of excessive fat causes high coronary heart disease, blood pressure, high blood cholesterol, type 2 diabetes, and sleep apnea. Obesity has a bad social impact as well. Obese people may be stigmatized and subjected to a variety of prejudices and discrimination as a result of their weight. Obesity has become a global medical issue due to its rising prevalence. Fish oil has been found to help people lose weight. Even if there are few human studies and they are usually conducted over short periods, the results acquired can be useful (Centella et al., 2017). Potential health advantages of marine products are well-known, thanks to their distinct functional components. There are several anti-obesity chemicals generated from microbes, phytoplankton, invertebrates, and vertebrates, among other marine species. So far, the main emphasis of the focused research effort has been on algae investigation. Algae, particularly brown algae, yielded a large number of chemicals that were isolated and investigated for their anti-obesity properties (Hu et al., 2016).
Prevention of Cardiovascular Diseases
Owing to the PUFA present in fatty fish, eating fish has been demonstrated to lower the incidence of CHD. Many researchers have been undertaken to prove the link between eating fish and having a lower risk of CHD. People that eat a lot of seafood, like Alaskans and Japanese, have been demonstrated a lower risk of heart disease. PUFAs like omega-3 aid to prevent breast and prostate cancer (Zheng et al., 2013; Manlusoc et al., 2019). Hyperlipidemia is a condition in which total cholesterol, total triglycerides, and low-density lipoprotein cholesterol levels are high but high-density lipoprotein cholesterol levels are low in the blood (HDL-C). The presence of alginate in the small intestine lumen inhibits fat intake and lowers plasma cholesterol in a variety of diets, according to a study. The most likely cause is an increase in fecal bile and cholesterol excretion. Fucoidan has also been demonstrated to lower blood fat levels by modifying fat absorption, activating lipid metabolic enzymes, and increasing the production of LDL-rmRNA, whereas linolenic acid regulates fat metabolism by accelerating the conversion of LDL to HDL. By reducing LDL levels and increasing HDL levels, atherosclerosis can be avoided if total blood fat levels are reduced (Qin, 2018b).
Effect on Prebiotics and Encouraging Health Benefits
Polysaccharides are observed in all organisms in various forms. Polysaccharides found in macroalgae could potentially be used as prebiotics in the future. Sulfated hetero polysaccharides are abundant in chlorophyta. Brown algae contain alginates, fucans, and laminarin. Red algae are also used to make agar and carrageenans. In vitro and in vivo studies have exhibited the benefits of macroalgal polysaccharides. Laboratory animals were shown to have greater amounts of Bifidobacterium and Lactobacillus, which was encouraging. In the GI tract, pathogenic and good bacteria coexist, but research is focusing on reaching a more positive balance by eliminating potentially dangerous bacteria while encouraging the development of other species with health benefits (increase infection resistance, reduce colon cancer risk, and decrease inflammation) (Al-Sheraji et al., 2013).
Anti-inflammatory Functions
The PUFAs in seafood, particularly omega-3, are thought to have anti-inflammatory characteristics because they can decrease specific inflammation mediators. Inflammation can be reduced by improving the ratio of omega-3 to omega-6 fatty acids in the diet, according to several studies. Omega-6 PUFA-derived eicosanoids are pro-inflammatory and immunoactive, whereas omega-3 PUFA-derived eicosanoids (EPA and DHA) are anti-inflammatory. When compared to omega-3 PUFAs, Western cuisine has a significantly higher ratio of omega-6 PUFAs. Algae have an anti-inflammatory property as well. The anti-inflammatory properties of photosynthetic pigments are well established. Fucoxanthin (Fx) extracted from brown algae, for example, suppresses cyclooxygenase 2 (COX-2) protein expression and lowers prostaglandin E2 (PGE2) production. Pheophytin from Enteromorpha prolifera inhibited the 12-O-tetradecanoylphorbol-13-acetate-induced superoxide radical and inflammatory responses in mice macrophages. Turbinaria ornate polysaccharide is another anti-inflammatory component derived from the brown alga Turbinaria ornate (Wall et al., 2010; Rahman and McFadden, 2011).
Anti-cancer Effect
Cancers are diseases in which cells cease to respond correctly to chemical signals sent by other cells. By entering and manipulating normal tissues, these aberrant cells have the potential to kill. It becomes critical to hunt for chemicals that can cause apoptosis to occur (programmed cells death). Cell deregulation, which occurs when pro-apoptotic signals are lost or anti-apoptotic signals are increased, can result in a range of clinical diseases, including cancer. As a result, promoting apoptosis is a significant anticancer therapeutic target. Several types of researches have been carried out to establish the link between eating fish and avoiding cancer. The hydrolysates were made up of a complicated combination of free amino acids and peptides with molecular weights ranging from 1 to 7 kDa, as well as lipids and NaCl in low concentrations, all of which inhibited cell proliferation (Kim et al., 2013).
Neuroprotective Effects
In the elderly population, one of the primary causes of death is neurodegenerative illnesses. As a result, researchers have been on the lookout for new neuroprotective drugs to prevent apoptosis, neuronal cell damage, dysfunction, and CNS degeneration. Synthetic compounds, despite their neuroprotective properties, can cause anxiety, uneasiness, drowsiness, mouth dryness, and weariness. The consumption of fish and marine algae in East Asia has been related to a low rate of neurodegenerative disorders. Another potential possibility for neuroprotective drugs is marine algae (Suganthy et al., 2010; Pangestuti and Kim, 2011).
Prevention of Arthritis
Arthritis is a disease characterized by joint inflammation that primarily affects the elderly population. The prevention of inflammation, as well as the pain and restricted mobility symptoms that come with it, is a key component of arthritis treatment. Because inflammatory eicosanoids are involved in the occurrence of arthritis, diet can be a possible therapeutic agent; thus, dietary PUFAs have been extensively studied in the treatment of arthritis. After 3–4 months of n-3 PUFA supplementation, positive results were found; including decreased patient-reported a number of bothersome and painful joints, joint pain intensity, and non-steroidal anti-inflammatory medicine use. Supplementing with n-3 PUFAs may even aid with joint pain relief. Clinical studies suggest that ingesting collagen hydrolysates, which can be generated from fish by products, lowers pain in osteoarthritis patients in addition to n-3 PUFAs (Lordan et al., 2011).
Prevention of Asthma
Fish oil or fish with greater than 2% fat has been linked to a lower risk of airway hyper-responsiveness, and kids who consume fresh, oily fish regularly had a lower chances of developing asthma than kids who only consume fish on special occasions. In asthma patients, the benefits of n-3 fatty acid supplementation in the diet have also been confirmed in terms of reduced breathing difficulties and other symptoms, as well as the requirement for lower drug doses (Lordan et al., 2011).
Prevention of Diabetes
To reduce the risk of CVD, diabetic neuropathy, retinopathy, and nephropathy, dietary management for diabetes entails keeping blood glucose and blood lipid levels as close to normal as feasible. The marine environment has a diverse range of physiologically active substances that have yet to be discovered. Exploiting marine resources could yield useful leads with both commercial and scientific implications. Diabetes is a metabolic illness that affects both developed and developing countries and is a leading cause of death and morbidity. There are several anti-diabetics on the market, but their main drawbacks are cost, effectiveness, and side effects (Barde et al., 2015). Several marine extracts have been linked to glycemic control, protection against heart disease and cancer. Consequently, these chemicals could be used as functional food components to help alleviate insulin resistance and diabetes (Lordan et al., 2011).
Prevention of Cognitive Decline and Depression
Among the elder persons, dementia and cognitive impairment are common, and they have an impact on their ability to function independently. The frequency of dementia and cognitive impairment is predicted to rise as the population ages. Several epidemiological researches on the connection between food and cognitive decline recommend that fatty acids may help preserve cognitive function and possibly prevent or delay dementia. Fatty fish and marine n-3 PUFAs, in particular, have been related to a reduced risk. Furthermore, consuming a diet rich in the algae Chlorella reduced oxidative stress. Several epidemiological and nutritional researches have also found that n-3 fatty acid consumption is negatively associated with depression, whereas clinical studies have found that n-3 compounds are beneficial to prevent depressive disorders. Long-chain n-3 PUFA supplementation has been represented in many studies to enhance mood in persons with serious depression (Lordan et al., 2011).
Anti-aging Effect
Because of their unique compounds, marine species have gotten a lot of attention in anti-aging research. The goal of this review is to reveal and debate potential anti-aging compounds found in marine macroalgae, micaroalgae, invertebrates, and vertebrates. To illustrate the action and mechanism of carbohydrate, protein, pigment, flavonoids, fatty acids, and phenols from marine species, anti-aging experimental animals, for instance, Drosophila melanogaster, and Caenorhabditis elegans were used. There were mycosporine-like amino acids in marine macroalgae, microalgae, fungi, and bacteria, which were condensed by cyclohexenone and other types of amino acids and could absorb ultraviolet light (Wang X. et al., 2021).
Anti-thrombin and Anti-coagulant Functions
Coagulation is a complicated process that aids in the production of clots and is a vital aspect of hemostasis, which is the process of halting blood loss from a damaged vessel after an injury. Anticoagulants are medications that can prevent blood clotting or stop it from forming. They are used to prevent blood clots after surgery and give prompt therapeutic anticoagulation in life-threatening situations such as deep vein thrombosis and pulmonary embolisms. One of the most regularly used anticoagulant substances is heparin, a sulfated polysaccharide present in most mammalian tissues, having been utilized to treat thromboembolic disorders for the past 15 years. Heparin treatment, on the other hand, can cause thrombocytopenia, which is the inability to block thrombin connected to fibrin, as well as ineffectiveness in acquired anti-thrombin deficiencies and undesired bleeding. Compounds such as sulfated polysaccharides and peptides are produced by several marine sources. In marine algae, anti-thrombotic and anti-coagulant effects are observed in sulfated polysaccharides. Anti-thrombotic and anti-coagulant sulfated polysaccharides have been extracted from a variety of marine algae, for instance, red and brown seaweeds. Anti-coagulant fucoidans have been discovered in a variety of brown algae species, with structural and biological activity variations (Suleria et al., 2016).
Anti-allergic Activity
Allergic reactions are the reaction of the immune system to normally harmless environmental chemicals. Mast cells have a crucial role in the pathophysiology of allergy disorders because the allergen-induced IgE436-mediated allergic response activates them. Mast-cell activation causes multiple intracellular activities, including tyrosinase kinase activation; production of reactive oxygen species, which causes a rise in intracellular calcium ion (Ca2+); microtubule polymerization; degranulation; and synthesis of cytokine (Cunha and Pintado, 2021). To combat the problems of food-induced allergies, researchers are currently focusing their efforts on finding anti-allergic compounds from marine sources. The anti-food allergic activity of many plants has been documented in numerous publications, and anti-food allergic components in these plants include flavonoids, terpenes, and other compounds. Recent research has found that marine-derived active substances help to relieve allergies by inhibiting the development of Th2, enhancing Th1 immune function, inhibiting mast cell and basophil degranulation, reducing cytokine production, and regulating intracellular calcium concentration, among other mechanisms (Wang K. et al., 2020).
Anti-microbial Activity
Adaptive immunity is not present in marine invertebrates. Bivalves only have the innate reaction, which means they must create defense systems to adapt to their environment, which is frequently teeming with deadly bacteria and viruses. Their pathogen exposure is increased as a result of their filtering effect. Antibacterial peptides are one of the components involved in defense mechanisms of invertebrates. Antimicrobial peptides (AMPs) are short, cationic, amphipathic peptides with a sequence ranging from 12 to 50 amino acids. They are the first line of defense against infections in animals and plants (Cunha and Pintado, 2021).
Sustainable Green Processing Methods to Extract Biomolecules From Seafood Waste
Seafood processing generates a large number of by-products, which has significant economic and environmental consequences. It is critical to have access to seafood resources to minimize environmental issues and ensure resource sustainability (Ozogul et al., 2021; Šimat et al., 2021).
Green extraction techniques are a potential method for recovering biomolecules from by-products from the seafood industry (Figure 3). These cutting-edge techniques boost extraction yield while minimizing process time and resources. The extraction procedures employed and the operating conditions used are crucial in producing a greater extraction yield and greater target substances quality because of the diversity of seafood by-products, as well as the variances in characteristics of seafood biomolecules. The use of a combination of green approaches may be able to help with the correction of some problems. Furthermore, because laboratory and pilot-scale yields and energy consumption differ, these green procedures must be validated at pilot scale on seafood wastes before being applied in the industry (Bruno et al., 2019).
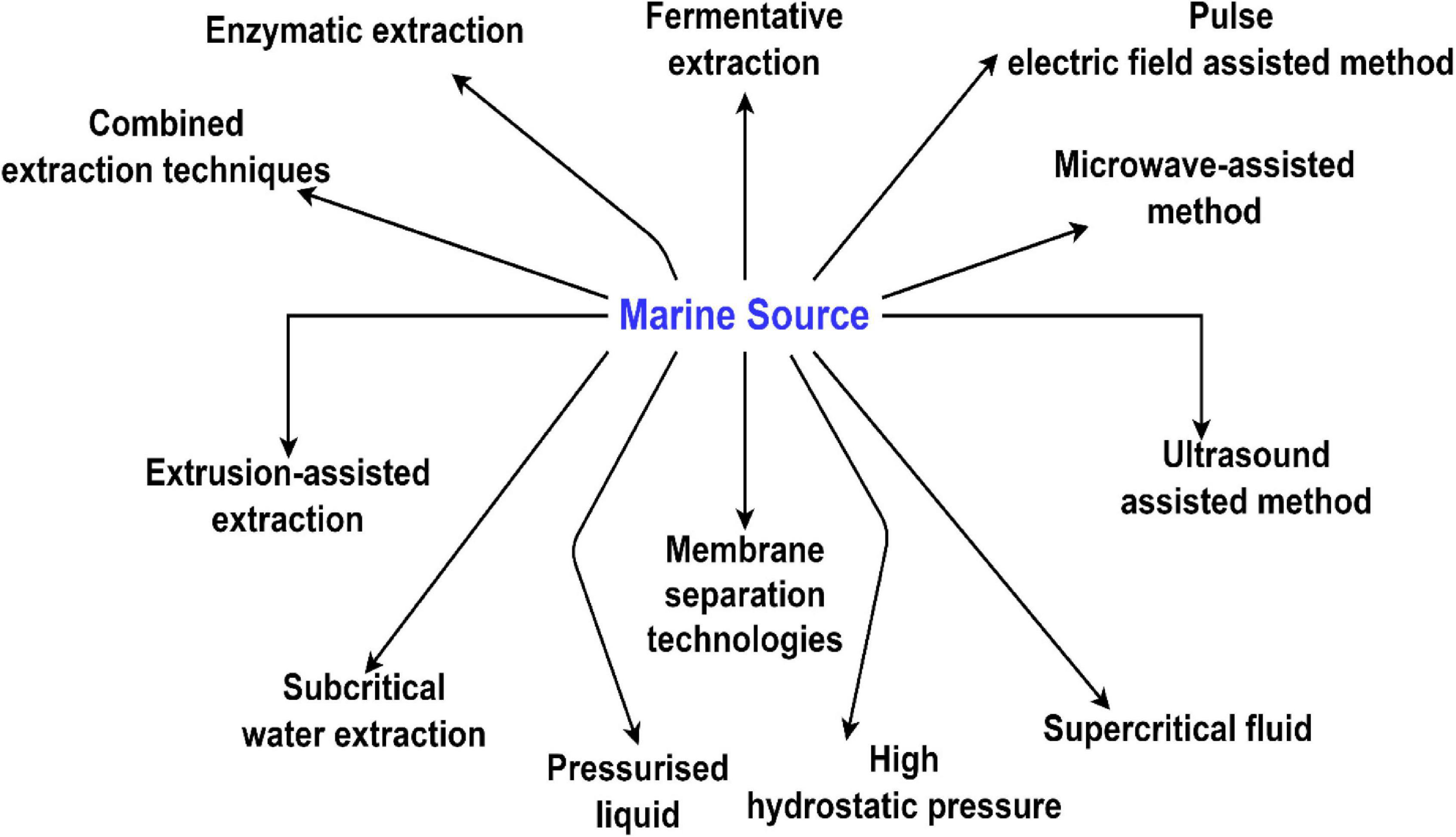
Figure 3. Classification of sustainable green processing methods to extract biomolecules from different marine sources.
Bioactive chemicals found in marine macroalgae can be employed to promote health in several dietary, cosmetic, and medical applications. Bioactivity has been demonstrated for bioactive compounds including polyphenols, polysaccharides, carotenoids, and w-3 fatty acids. These compounds were extracted using modern technologies such as, Supercritical Fluid Extraction, Subcritical Water Extraction, Ultrasound-Assisted Extraction, and Microwave-Assisted Extraction since they have advantages over traditional methods. Process parameters of all techniques must be tuned to get extracts containing the desired bioactive (Ciko et al., 2018). Ionic liquids have developed as an alternate solvent for recovering important proteins from microalgae bioprocessing. The antioxidant properties of the isolated fucoxanthin fraction were satisfactory. In repeated rounds of fucoxanthin extraction, the recyclability of diallylammonium diallylcarbamate was proven. As a result, CO2-based alkyl carbamate ILs could potentially replace traditional organic solvents in the downstream processing of microalgal bioactive chemicals (Khoo et al., 2021).
Traditional Methods
Maceration, percolation, and Soxhlet extraction are common traditional extraction methods. This type of extraction is a common process that may be found throughout the globe. Ethyl ethanoate, ethanol, acetone, water, and methanol, in various combinations, are the most commonly employed solvents, and they are chosen depending on the polarity of the molecules to be extracted. Solvents like hydroalcoholic mixtures are appropriate for this technique since phenolic compounds are hydrophilic. According to certain research, combining a solvent with acids, or instance, citric acid, tartaric acid, or hydrochloric acid can enhance the extraction efficiency of specific substances. Within traditional extraction methods, the Soxhlet extraction produces better results, though it has various demerits, including the degradation of thermolabile substances (such as anthocyanins, hydrolyzable tannins), the use of relatively greater volumes of solvents, and more processing times. To extract lipophilic compounds, the Soxhlet technique is commonly used (Santos-Buelga et al., 2012; Vieira et al., 2018).
Emerging Methods
Pulse Electric Field-Assisted Method
Because it uses less specific energy per processed product, pulsed electric field (PEF) processing is more acceptable due to its environmental and cost-effective benefits. Several food businesses have confirmed the use of PEF-based extraction throughout the previous decade. Many of present procedures of the food industries are less effective and sustainable than PEF-based technology. Different researchers have discovered that when combined with other techniques like osmotic shock and mechanical press, this developing technology is efficient in the extraction process. Furthermore, the combination of PEF and solid/liquid extraction produces fewer food wastes in comparison with existing methods (Arshad et al., 2021). By disrupting cell membranes, PEFs can be used to improve mass transfer techniques. A moderate PEF therapy uses 0.5–1.0 kV/cm field strength with treatment periods of 100–10,000 seconds, or 1–10 kV/cm field strength and treatment times of 5–100 s. Intensity, amplitude, length, amount, and repetition frequency of external electric pulses determine whether reversible or irreversible pores form in membranes. The ability to extract bioactive substances from natural matrices requires the construction of irreversible pores. Treatments using 1–20 kJ/kg specific energy and 0.7–3 kV/cm electric field intensity are commonly used (Grosso et al., 2015).
Microwave-Assisted Method
Microwave radiation is non-ionizing radiation that increases molecular mobility while keeping the structure of the molecule intact. Microwave wavelengths range from 1 to 1 cm, with frequencies ranging from 300 MHz to 300 GHz. MAE is a synergistic combination of two techniques that both work in the same direction, namely energy and mass transfer. During the microwave process, the moisture in the cells evaporates as the temperature rises, resulting in high pressure in the cell. Due to the fracturing of cell walls, this physical alteration in the matrix improves its porosity. The mass transfer is assisted by raising the matrix porosity while also increasing the temperature and pressure. Sample moisture, the microwave power output, processing duration, sample viscosity, frequency, extraction cycles, pressure, sample size, and solvent nature all affect the efficiency of the MAE process (Bruno et al., 2019).
The utilization of microwave potency, which produces alterations in cell structures owing to electromagnetic waves, lies at the heart of this method. Electromagnetic energy is converted into calorific energy by two mechanisms: dipole rotation and ionic conduction. Polyphenols and polysaccharides can be extracted using this method in open (at atmospheric pressure) or closed (at higher pressure) containers. The power and frequency of pressure, microwaves, temperature, the solid-to-solvent ratio, and time are all important parameters to consider while optimizing this form of extraction. It is vital to understand that phenolic compounds can be damaged by high microwave power and high temperatures. When utilizing hydroalcoholic solutions with moderate ethanol concentrations as extraction solvents, these chemicals are easily dissolved. The results of extracting phenolic compounds from brown algae using this extraction method were positive (Kalil et al., 2017; Otero et al., 2019).
Ultrasound Assisted Method
Ultrasonic waves in the 20–1000 kHz range are commonly used to improve extraction efficiency. Ultrasonic waves are mechanical waves that travel through target matrices by compressing and rarefying them. When these waves propagate through a solvent, they create negative pressure, and when sound wave pressure of higher intensities propagates through a solvent, microscopically small holes or bubbles arise. When these spaces or bubbles are filled with a gas or water vapor, the bubbles grow and shrink until they collide, causing cavitation (Donnell et al., 2020).
Ultrasonic waves with a frequency ranging from 20 to 100 kHz are used in this method, which induces bubbles to form due to the pressure difference created. The bubbles then burst and cavitation occurs, triggering particle breakup along with liquid–solid interfaces and the release of bioactive chemicals into the matrix. Low temperatures, short durations, and a small volume of solvent are the key advantages of using Ultrasound Assisted Method (UAE) to extract phenolic chemicals from algae. However, it should be noted that prolonged ultrasonication might raise the temperature, which can jeopardize the stability of phenolic compounds. Frequency, power, and temperature are the parameters to optimize this type of extraction (Heleno et al., 2016). An ultrasonic bath (indirect sonification) or an ultrasonic probe (direct sonification) is two types of ultrasound equipment (direct sonification). These two have different operating conditions and how ultrasonic waves affect the sample. After the sample has been immersed in the ultrasonic bath, the ultrasonic probe is inserted into it. The device is less expensive than other extraction methods, and it can be used with a variety of solvents. UAE operates at low temperatures, allowing for the preservation of thermolabile compounds (Ciko et al., 2018).
Supercritical Fluid Extraction
This is dependent on the supercritical fluid extraction principle, which involves raising temperature and pressure above their critical points while maintaining liquid and gas characteristics (Sánchez-Camargo et al., 2017). The fluid has a density that is similar to liquids and a viscosity that is akin to gasses. Because of its non-toxicity, safety, and low cost, carbon dioxide (CO2) is the most often used solvent for Supercritical Fluid Extraction (SFE). Because of their low viscosity and enhanced diffusion coefficient, supercritical fluids have a significant advantage in terms of mass transfer. Supercritical CO2 can only extract non-polar or low-polarity molecules because it is a non-polar solvent, though it can also extract polar chemicals (Ciko et al., 2018).
High Hydrostatic Pressure
A non-thermal high hydrostatic pressure (HHP) technology combined with polysaccharidases is proposed as a novel method for improving phytochemical extraction from red macroalgae. Palmaria palmata and Solieria chordalis, two macroalgae species, were hydrolyzed with cellulase and hemicellulase under HHP conditions (400 MPa, 20 min). The HHP-assisted enzymatic treatment improves the extraction of certain components like proteins, polyphenols, and polysaccharides; however, the benefits vary greatly depending on the macroalgae species. As a result of the treatment with HHP and hemicellulase, the antioxidant activity of isolated fractions increased by over 2.8 times (Suwal et al., 2019).
High hydrostatic pressure is a non-thermal processing method used to reduce microbial populations and inactivate enzymes in marine food, as well as to treat marine food, dairy, fruits, and vegetables. Under high pressure (100–1000 MPa) and at a temperature of 5–35°C, charged particles were deprotonated, salt bridges were broken down, and weak connections (electrostatic bonds, hydrogen bonds, and hydrophobic bonds) in cell membranes were shattered, resulting in increased cell permeability. Cell permeability increases as pressure rises to the crucial threshold level, improving extracellular fluid flow (Ali et al., 2021).
Pressurized Liquid Extraction
High pressures (10–15 MPa), short processing periods, and temperatures ranging from 50 to 200 °C characterize this form of extraction, which is a green technique because it uses small volumes of non-toxic solvents. Four different solvents (hexane, ethyl acetate, pure ethanol, and 50 percent ethanol) were employed to extract phenolic chemicals from the brown algae Laminaria ochroleuca at 100 bar, with ethanol diluted in water yielding the greatest extraction yield (37 percent for 80°C and 52 percent for 160°C) (Otero et al., 2019). The selectivity and solubility of the different substances in the supercritical fluid are influenced by the extraction conditions, particularly pressure and temperature. Bioactive molecules are preserved and no breakdown occurs because CO2 has a low critical temperature and pressure. Fatty acids, phytosterols, phenolics, carotenoids, and triglycerides are commonly found in SC-CO2 extracts (Duarte et al., 2014; Sánchez-Camargo et al., 2017).
Subcritical Water Extraction
This technique entails keeping water liquid for a short time (5–10 min) at temperatures above its boiling point (100–374°C) while under high pressure (10–60 bar). The change of the dielectric constant with temperature is one of the most vital elements to consider in this type of extraction. Pressure, time, and temperature, as well as choosing the right solvent, are all important aspects to consider and optimize while using this process (Zakaria and Kamal, 2016). SWE uses a small amount of solvent and operates for a short time (5–10 min) at high temperatures (50–200°C) and pressures (50–300 psi). This is the most promising technology for extracting bioactive chemicals, according to published studies. The solvents are held in a liquid state within their critical zone, below their boiling point, with the help of applied temperature and pressure. As the working temperature rises, the viscosity and surface tension of the solvent decrease, improving solubility and mass transfer rate. SWE is an environmentally friendly extraction method since it uses water instead of an organic solvent as a solvent (Duarte et al., 2014).
Extrusion-Assisted Extraction
This is an ancient food processing technique that is used to prepare infant food and ready-to-eat snacks. It has lately been employed for extractive purposes due to its capacity to solubilize particular macromolecules. Heating to high temperatures at high pressure, mechanical mixing, and shearing are all part of the traditional extrusion process. Extrusion-Assisted Extraction (EAE) operates by rupturing cell walls and using a thermo-mechanical action to transform insoluble macromolecules into soluble ones. The walls are strengthened by biomaterial mixing and shearing during EAE, allowing heat to penetrate more freely. Heat, on the other hand, accelerates the cell’s disruption (Huang et al., 2016). The EAE process has several advantages, including a reduction in process time, energy consumption, and solvents required, as well as an increase in extraction yield. Extrusion, on the other hand, is not suggested for thermo-sensitive compounds, and it must be followed by a method to extract the solubilized chemicals. EAE has only been used to extract biomolecules from seafood wastes in a few studies. Extrusion–hydro-extraction (EHE) is a process that involves extruding a by-product solution and extracting the extrudates in water (Bruno et al., 2019).
Membrane Separation Technologies
Membrane Separation Technologies (MST) is a technique to distinguish tiny particles from dissolved compounds. In a membrane separation technique, the molecules are isolated depending on their molecular size since the membrane acts as a selective and semi-permeable barrier. Molecules and particles with a diameter less than the membrane pores pass through, while those with a diameter larger than the membrane pores are repelled. On the other hand, at the molecular level, microporous membranes can segregate particles (nanofiltration and reverse osmosis). The molecule is segregated mostly depending on its chemical composition in this case (Nędzarek et al., 2017).
The bulk of membranes currently on the market are made comprised of inorganic materials (ceramic membranes made of zirconium, aluminum, titanium, and silicium oxides) and organic polymers (polyamides and polysulfones). The solvent permeability and separation selectivity of the membrane are the most important qualities. Microfiltration, ultrafiltration, nanofiltration, and reverse osmosis are the most common membrane technologies utilized in the seafood business, and they range greatly in structure and function. MST is primarily utilized in the seafood processing industry to recover and separate biomolecules from by the byproducts (Bruno et al., 2019).
Fermentative Extraction
It has been found that fermenting crustacean waste yields a higher extraction yield than chemical extraction. Fermentation, on the other hand, has been used extensively in the case of shrimp waste. During bacterial fermentation operations, the proteins and minerals in the shrimp shells are successfully eliminated, increasing the extraction efficiency of carotenoids without harming the quality. Although astaxanthin is greatly unstable, it can be stabilized through lactic acid fermentation, potentially boosting manufacturing yield by lowering the byproduct losses. The shrimp waste is both perishable and seasonal, as the fermenting method creates carotenoid-rich liquor that can be stored for a long time (75 days) under normal storage conditions, which is impossible to achieve with typical extraction methods. Shrimp waste fermentation takes a long period and necessitates a constant temperature to be successful. Regular pH monitoring is also necessary for optimal carotenoid production. Fermentation, rather than ensilaging, is a useful approach for demineralization, deproteination, and proteolysis (Routray et al., 2019).
Enzymatic Extraction
Enzymatic extraction saves energy and time by eliminating chemical contamination caused by leftover solvents and it is another ecologically acceptable method that is quickly being researched in the case of nutraceutical application of extracted value-added compounds. To extract astaxanthin, enzymes (proteases and lipases) from several sources are used, including microbial, bovine, porcine, and cod sources. Enzymes are affected by temperature, acidity, and other environmental factors, and they can be used at different stages of the process to create a wide range of products and by-products (Armenta and Guerrero-Legarreta, 2009). After fermentation and/or other digestion processes, enzymatic digestion is utilized as a final step in the processing of crustacean detritus. Astaxanthin is extracted in the form of carotenoprotein, which is a mixture of carotenoid and lipoprotein that provides both carotenoids and protein. Astaxanthin is extracted in a form called carotenoprotein, which is a mixture of carotenoid and lipoprotein that provides both carotenoids and protein. Carotenoprotein is a bluish-brown stable complex form that degrades to its natural reddish-orange color when exposed to heat. Separation of astaxanthin from carotenoproteins is frequently accomplished via enzyme hydrolysis. Protein hydrolysate and astaxanthin can be isolated from enzyme-digested materials (Routray et al., 2019).
Supercritical CO2 Extraction
Numerous temperature and pressure combinations are possible with this method. Furthermore, as a supercritical fluid, CO2, a non-toxic gas, is utilized, causing the fluid to behave both like a liquid and a gas at the same time, making extraction easier. Using co-solvents like ethanol, the polarity of CO2 can be altered, and polar components can be removed as a result. The thermal destruction of phytochemicals is prevented by using low temperatures and pressure (Jimenez-Lopez et al., 2021). CO2 is used as a supercritical fluid in this approach.
This fluid is frequently used due to its non-toxicity, non-flammability, cost-effectiveness, and widespread availability with a high purity grade. Supercritical CO2 Extraction (SC-CO2) extraction can be employed with several pressure-temperature combinations, making it the method of choice for a wide range of end products. Because it allows for the use of low pressure (7.386 MPa) and low temperature, SC-CO2 is a popular method in lab-scale facilities (31.60°C, the critical point of CO2). A combination of low temperatures and pressure ensures the retention of thermolabile phytochemicals (Alara et al., 2021).
Pressurized Hot Water Extraction
Pressurized Hot Water Extraction (PHWE) is a type of PLE that extracts using water as the solvent. It is also known as superheated water extraction (SHWE), subcritical water extraction, or pressurized low polarity water (PLPW) extraction. Water has numerous advantages in terms of versatility and environmental effect, and it can be utilized to extract usable molecules from a variety of source materials, including plants and food waste. The idea behind PHWE is to use water at temperatures higher than its atmospheric boiling point while keeping it as a liquid by applying pressure; physical and chemical properties of water change dramatically under these conditions. Furthermore, as the temperature rises, the viscosity and surface tension decrease, while diffusivity rises, improving the extraction method in terms of efficiency and speed. Furthermore, temperature affects the solubility of water, favoring the solubility of various types of compounds and altering its selectivity. Water is also the most environmentally friendly solvent available, perfectly adhering to the Green Chemistry rules. As with PLE, using solvents above their boiling temperatures allows for faster extractions; The PHWE, on the other hand, is a more environmentally friendly extraction technology because it uses water instead of organic solvents. This type of extraction optimization, on the other hand, is frequently empirical, failing to take into account experimental discoveries from related domains or theoretical connections. Although PHWE is a promising technology, additional research in the marine field is required; there are currently just a few applications dealing with bioactive extraction from marine sources (Hayes, 2012).
Combined Extraction Techniques
In some cases, a combination of extraction methods may be required to improve phytochemical extraction. This is the case when a single extraction procedure is insufficient to extract all of the desired phytochemicals from the source material. As a result, using various extraction methods to extract such phytochemicals more efficiently may be a viable choice (Alara et al., 2021). Green technologies have a lot of benefits, but they also have certain drawbacks. These limitations can be overcome by employing a combination of green approaches, which increases extraction efficiency while also improving the quality of target compounds. The protein hydrolysate product rejection coefficient was in the range of 90% after scaling up the enzymatic hydrolysis and coupling the reactor with an ultrafiltration machine to concentrate the hydrolysates (Tonon et al., 2016).
Marine By-Products
Bioactive peptides can be mined in large quantities from seafood products and by-products. Marine proteins contain new bio-functional peptides in their main structure, which can be exploited in food and medicinal applications due to their structural variety (Sila and Bougatef, 2016). By-products from the processing of seafood often account for 20–60% of the original raw material. Offal, head, and tail (27 percent of the fish) gathered by eviscerating, cutting, and fileting operations account for the majority of residues in pelagic fish including tuna, cod, mackerel, anchovy, and herring. The second significant residues gathered during skinning and cutting processes (25 percent of the fish) include skin, bones, blood, and frames. The majority of these by-products are currently transferred to fish meal facilities, which generate fish meal and fish oil. By far the most lucrative non-edible product made from marine wastes is fish meal (Ferraro et al., 2010; Ngo et al., 2011; Table 5).
Owing to their extraordinarily nutritious and value-added substances, modern food processing companies generate marine goods and by-products, and they play an important role in the regular function and enhancement of human health. By-products of industrial marine processing are currently produced in large quantities, and these by-products can be ingested by people. Skulls, viscera, bones, scales, skin, blood, and shells are among the by-products. Depending on the species and processing parameters, marine by-products account for 50–70% of all harvested marine animals, and they are typically discarded as wastes. The by-products of marine processing, on the other hand, contain significant amounts of extremely nutritional substances. Bioactive compounds can be found in proteins, minerals, vitamins, and PUFAs such as EPA and DHA. Proteins, enzymes, hydroxyapatite, oil, collagen, and gelatine are examples of high-value-added chemicals applied in the pharmaceutical and nutraceutical sectors (Ali et al., 2021).
Biomedical Uses of Bioactive Compounds
A diverse spectrum of marine-derived bioactive compounds is available for a variety of biotechnological and medical uses. Natural materials can be converted into high-value-added goods of interest after careful consideration. Potential candidates include marine-derived bio-products, which contain a large number of pharmacologically active elements with a wide chemical and structural variety. As a result, they have a lot of potentials to create high-value therapeutics (Centella et al., 2017).
Application as Functional Food Ingredients
Carotenoids, chlorophyll, DHA, EPA, and astaxanthin are only a few of the functional components found in algae. These components provide several advantages for value-added food applications and are in high demand in today’s market. Because the cost of producing these nutraceutical products is so expensive, researchers are exploring ways to increase yield using nanotechnology (Koyande et al., 2021).
Edible marine invertebrates such as crabs, mollusks, and echinodermata can be used as organic-based biopolymers for a variety of purposes. These biopolymers are composed up of polysaccharides and proteins, and have been used by humans for decades as food and nutraceutical ingredients to treat various ailments (Hosseini et al., 2020). Biopolymers such as glycosaminoglycans, fucosylated chondroitin sulfate, chitin, and chitosan have recently gained popularity in the biomedical industry for treating a variety of illnesses. These chemicals have antibacterial, anti-inflammatory, anti-cancer, wound healing, hypoglycemia, and anti-microbial functions, as well as being used in animal feed (Qin, 2018a; Ganesan et al., 2020b). While being generally unaffected by harmful anthropogenic activities, the marine environment represents a massive source of bioactive chemicals. Many chemicals present in seafood, including pigments, enzymes, proteins, polysaccharides, peptides, PUFAs, phenolics, and other secondary metabolites, have broader applications in a variety of industries such as the food industry (Figure 4). These compounds are found in prokaryotes, microalgae, and macroalgae, as well as crabs, sponges, and other invertebrates, as well as a variety of vertebrates (Karunarathne et al., 2020).
Due to its cellulose structure, the cell wall of microalgae is usually tough to digest, inhibiting protein absorption when consumed. Microalgae protein hydrolysates have enhanced digestibility and peptide and amino acid availability, making them more valuable in food applications. To enhance their application in food items, the importance of marine biopeptides in human nutrition should be emphasized, including:
• they are easier to absorb by the gastrointestinal tract in comparison with intact protein;
• they have numerous bioactivities described that can improve human health;
• Because maritime biopeptides are generated from marine species, they can contribute to long-term development;
• they can contribute to sustainable.
Food Colorant
Color is believed to be the primary element seen by the senses, and it has played an important part in the acceptance of foods for millennia to improve their actual appearance and quality (Ghosh et al., 2021, 2022).
The microalgal pigment is a natural food coloring component with commercial use. Carotene is found in large concentrations in some microalgae (besides beta carotene). Other sorts of colors can also be seen in microalgae. Beta carotene is used as a food pigment (most notably to give margarine its yellow color), as a food additive to enhance the color of fish flesh and egg yolks, and to enhance the health and fertility of grain-fed cattle. Natural Beta Carotene has physical features that distinguish it from synthetic Beta Carotene (Aman et al., 2021). Natural Beta Carotene is a far superior anti-carcinogen and anti-heart disease agent because it is fat-soluble. The role of microalgae as a food coloring source is limited, however, because algal-derived food coloring is not photostable and bleaches with cooking. Despite this constraint, the market for microalgae-derived food coloring has enormous potential. Dunaliella salina is cultivated to produce beta-carotene, a photosynthetic pigment. Beta-carotene is an orange color and a vitamin C supplement (Zeid and Dhar, 2012). Lutein is a high-value bioactive molecule that has greater industrial applications in aquaculture, poultry farming, food processing, and health care (Low et al., 2020).
Other Food Application
Marine proteins are employed in food owing to their unique features, including the ability to film foam and gel form. Marine gelatin is used as a food additive after the partial hydrolysis of collagen due to its gel-forming capacity, enhancement of texture, water-holding ability, and food product longevity. Marine polysaccharides come from numerous creatures and have several qualities that make them excellent for use in food. Marine polysaccharides, for example, can bind greater amounts of water and disperse it in meals. Agar can be utilized in the confectionery business due to its high sugar content, bland taste, and lack of flavor in jellies, jams, fruit desserts, puddings, and custards. By modifying their water binding, foaming, and emulsifying capabilities, carrageenans are utilized to vary the texture of a range of meals. Because of their gel-forming capacity, other marine polysaccharides including, alginate, chitosan, and fucoidan are also good raw materials for edible, biodegradable films. Antimicrobial substances, edible films, additives, nutraceuticals (e.g., enhancing dietary fiber, decreasing fat absorption), and water purifiers are just a few of the uses for chitin, chitosan, and its derivatives in food. Other marine polysaccharides, for instance, alginate, chitosan, and fucoidan, have a gel-forming potential that makes them ideal raw ingredients for edible, biodegradable films (Suleria et al., 2016).
Conclusion
In the era of modern world biotechnology, research on marine-derived bioactive chemicals is gaining traction. Natural marine-derived bioactive substances are favored over chemical-based manufactured formulations because they are more accessible and have fewer adverse effects. This study looks at the biotechnological and medical use of marine-derived bioactive substances. Bioactives and nutraceuticals can be found in abundance in marine resources. Due to the numerous potential health benefits, food products, supplements, and natural health products containing marine bioactives are predicted to command a large market. The addition of three fatty acids in foods has been at the forefront of research and development since they have proven to be the most beneficial in treating a variety of health concerns. Many recent studies have focused on marine algae and their nutraceutical contents, with potential health advantages linked to the three fatty acids, antioxidants, and other bioactives found in these organisms. Marine microorganisms have gotten a lot of attention in bio-discoveries because of the unique physicochemical traits that they have developed as a result of surviving in the harsh circumstances of the sea. Marine organisms, particularly sponges, and their symbiotic fungus and bacteria, have produced a plethora of bioactive natural compounds with unique structures, according to a broad prospectus. Although the use of PLE and SFE for bioactives extraction is now considered a mature field, new advancements that can greatly boost the interest in these approaches are still needed. Methods such as SFE, UAE, SWE, and MAE can be used to isolate certain bioactive chemicals, according to the findings. It is possible to optimize their process parameters, desired extraction yield, and chemical makeup of the extracts.
We believe that the use of novel metabolites produced from marine microorganisms in current nutraceuticals and functional foods with advanced health claims is not far off.
Future research should give emphasize on-
• Further research and development of marine functional foods should be pursued in the hopes that, in the future, their regular inclusion in the human diet would result in a reduction in the incidence and severity of numerous ailments.
• Despite the wide range of applications for marine bioactives in food, an additional multidisciplinary study is required in all areas, including chemical composition, biotechnology, extraction, bioactivity, and toxicity.
• Furthermore, new fermentation technology approaches are required for the cost-effective commercial synthesis of marine microbial metabolites.
• To ensure the future success of marine natural health at specialty products as new and novel therapeutic entities that can make a significant contribution to the treatment of human ailments as well as disease risk reduction and health care cost reduction, new technologies and efficient collaborations between academia and industry will be required.
Author Contributions
SG and TS: conceived and designed the experiments. SG, TS, SP, RC, and HE: writing—original draft preparation. SG, TS, SP, RC, ZK, and HE: formatting, editing according journal guidelines, and writing—review and editing. All authors: read and agreed to the published version of the manuscript.
Funding
This study was supported by National Conservation Trust Fund for Natural Resources from Ministry of Energy and Natural Resources Malaysia (Grant No: 304/PPSK/6150219).
Conflict of Interest
SP is employed by NatNov Bioscience Pvt. Ltd.
The remaining authors declare that the research was conducted in the absence of any commercial or financial relationships that could be construed as a potential conflict of interest.
The reviewer AF declared a shared affiliation, though no other collaboration, with one of the author ZK to the handling editor.
Publisher’s Note
All claims expressed in this article are solely those of the authors and do not necessarily represent those of their affiliated organizations, or those of the publisher, the editors and the reviewers. Any product that may be evaluated in this article, or claim that may be made by its manufacturer, is not guaranteed or endorsed by the publisher.
References
Afifi, A. H., El-Desoky, A. H., Kato, H., Mangindaan, R. E. P., De Voogd, N. J., Ammar, N. M., et al. (2016). Carteritins A and B, cyclic heptapeptides from the marine sponge Stylissa carteri. Tetrahedron Lett. 57, 1285–1288.
Ahmed, A. B. A., Adel, M., Karimi, P., and Peidayesh, M. (2014). Pharmaceutical, Cosmeceutical, and Traditional Applications of Marine Carbohydrates, 1st Edn. Amsterdam: Elsevier.
Al Khawli, F., Martí-Quijal, F. J., Ferrer, E., Ruiz, M. J., Berrada, H., Gavahian, M., et al. (2020). Aquaculture and its by-products as a source of nutrients and bioactive compounds. Adv. Food Nutr. Res. 92, 1–33. doi: 10.1016/bs.afnr.2020.01.001
Alara, O. R., Abdurahman, N. H., and Ukaegbu, C. I. (2021). Extraction of phenolic compounds: a review. Curr. Res. Food Sci. 4, 200–214.
Ali, A., Wei, S., Liu, Z., Fan, X., Sun, Q., Xia, Q., et al. (2021). Non-thermal processing technologies for the recovery of bioactive compounds from marine by-products. Lwt 147:111549.
Al-Sheraji, S. H., Ismail, A., Manap, M. Y., Mustafa, S., Yusof, R. M., and Hassan, F. A. (2013). Prebiotics as functional foods: a review. J. Funct. Foods 5, 1542–1553.
Aman, M., Hossein, M., Saeed, A., Seyede, M., and Hosseini, M. (2021). Microbial pigments as an alternative to synthetic dyes and food additives: a brief review of recent studies. Bioprocess Biosyst. Eng. 45, 1–12. doi: 10.1007/s00449-021-02621-8
Ameen, F., AlNadhari, S., and Al-Homaidan, A. A. (2021). Marine microorganisms as an untapped source of bioactive compounds. Saudi J. Biol. Sci. 28, 224–231. doi: 10.1016/j.sjbs.2020.09.052
Armenta, R. E., and Guerrero-Legarreta, I. (2009). Amino acid profile and enhancement of the enzymatic hydrolysis of fermented shrimp carotenoproteins. Food Chem. 112, 310–315.
Arshad, R. N., Abdul-Malek, Z., Roobab, U., Qureshi, M. I., Khan, N., Ahmad, M. H., et al. (2021). Effective valorization of food wastes and by-products through pulsed electric field: a systematic review. J. Food Process Eng. 44:e13629.
Balakrishnan, D., Kandasamy, D., and Nithyanand, P. (2014). A review on antioxidant activity of marine organisms. Int. J. Chemtech Res. 6, 3431–3436.
Barbosa, M., Valentão, P., and Andrade, P. B. (2014). Bioactive compounds from macroalgae in the new millennium: implications for neurodegenerative diseases. Mar. Drugs 12, 4934–4972. doi: 10.3390/md12094934
Barde, S. R., Sakhare, R. S., Kanthale, S. B., Chandak, P. G., and Jamkhande, P. G. (2015). Marine bioactive agents: a short review on new marine antidiabetic compounds. Asian Pac. J. Trop. Dis. 5, S209–S213.
Beygmoradi, A., Homaei, A., Hemmati, R., Santos-Moriano, P., Hormigo, D., and Fernández-Lucas, J. (2018). Marine chitinolytic enzymes, a biotechnological treasure hidden in the ocean? Appl. Microbiol. Biotechnol. 102, 9937–9948. doi: 10.1007/s00253-018-9385-7
Bharat Helkar, P., and Sahoo, A. (2016). Review: food industry by-products used as a functional food ingredients. Int. J. Waste Resour. 6:3.
Borba, D., De, Paes, L., Kato, N., Pessoa, A., and Durães, L. (2019). Marine prebiotics: polysaccharides and oligosaccharides obtained by using microbial enzymes. Food Chem. 280, 175–186. doi: 10.1016/j.foodchem.2018.12.023
Bruno, S. F., Ekorong, F. J. A. A., Karkal, S. S., Cathrine, M. S. B., and Kudre, T. G. (2019). Green and innovative techniques for recovery of valuable compounds from seafood by-products and discards: a review. Trends Food Sci. Technol. 85, 10–22.
Buijs, Y., Bech, P. K., Vazquez-Albacete, D., Bentzon-Tilia, M., Sonnenschein, E. C., Gram, L., et al. (2019). Marine Proteobacteria as a source of natural products: advances in molecular tools and strategies. Nat. Prod. Rep. 36, 1333–1350. doi: 10.1039/c9np00020h
Camacho, F., Macedo, A., and Malcata, F. (2019). Potential industrial applications and commercialization of microalgae in the functional food and feed industries: a short review. Mar. Drugs 17:312. doi: 10.3390/md17060312
Carbonell, G. V., Della Colleta, H. H., Yano, T., Darini, A. L., Levy, C. E., and Fonseca, B. A. (2000). Clinical relevance and virulence factors of pigmented Serratia marcescens. FEMS Immunol. Med. Microbiol. 28, 143–149. doi: 10.1111/j.1574-695X.2000.tb01469.x
Centella, M. H., Arévalo-Gallegos, A., Parra-Saldivar, R., and Iqbal, H. M. N. (2017). Marine-derived bioactive compounds for value-added applications in bio- and non-bio sectors. J. Clean. Prod. 168, 1559–1565. doi: 10.1016/j.jclepro.2017.05.086
Chakraborty, K., and Joy, M. (2020). High-value compounds from the molluscs of marine and estuarine ecosystems as prospective functional food ingredients: an overview. Food Res. Int. 137:109637. doi: 10.1016/j.foodres.2020.109637
Chan-Higuera, J. E., Santacruz-Ortega, H. D. C., Carbonell-Barrachina, A. A., Burgos-Hernández, A., Robles-Sánchez, R. M., Cruz-Ramírez, S. G., et al. (2019). Xanthommatin is behind the antioxidant activity of the skin of dosidicus gigas. Molecules 24:3420. doi: 10.3390/molecules24193420
Cheng, C., Othman, E. M., Reimer, A., Grüne, M., Kozjak-Pavlovic, V., Stopper, H., et al. (2016). Ageloline a, new antioxidant and antichlamydial quinolone from the marine sponge-derived bacterium Streptomyces sp. SBT345. Tetrahedron Lett. 57, 2786–2789. doi: 10.1016/j.tetlet.2016.05.042
Cheng, M. M., Tang, X. L., Sun, Y. T., Song, D. Y., Cheng, Y. J., Liu, H., et al. (2020). Biological and chemical diversity of marine sponge-derived microorganisms over the last two decades from 1998 to 2017. Molecules 25:853. doi: 10.3390/molecules25040853
Chi, C. F., Wang, B., Hu, F. Y., Wang, Y. M., Zhang, B., Deng, S. G., et al. (2015). Purification and identification of three novel antioxidant peptides from protein hydrolysate of bluefin leatherjacket (Navodon septentrionalis) skin. Food Res. Int. 73, 124–129. doi: 10.1016/j.foodres.2014.08.038
Ciko, A. M., Jokić, S., Šubarić, D., and Jerković, I. (2018). Overview on the application of modern methods for the extraction of bioactive compounds from marine macroalgae. Mar. Drugs 16, 348. doi: 10.3390/md16100348
Cornish, M. L., and Garbary, D. J. (2010). Antioxidants from macroalgae: potential applications in human health and nutrition. Algae 25, 155–171. doi: 10.4490/algae.2010.25.4.155
Cunha, S. A., and Pintado, M. E. (2021). Bioactive peptides derived from marine sources: biological and functional properties. Trends Food Sci. Technol. 119, 348–370. doi: 10.1016/j.tifs.2021.08.017
Datta, D., Nath Talapatra, S., and Swarnakar, S. (2015). Bioactive compounds from marine invertebrates for potential medicines – an overview. Int. Lett. Nat. Sci. 34, 42–61. doi: 10.18052/www.scipress.com/ilns.34.42
De Jesus Raposo, M. F., De Morais, R. M. S. C., and De Morais, A. M. M. B. (2013). Health applications of bioactive compounds from marine microalgae. Life Sci. 93, 479–486. doi: 10.1016/j.lfs.2013.08.002
de Vera, C. R., Crespín, G. D., Daranas, A. H., Looga, S. M., Lillsunde, K. E., Tammela, P., et al. (2018). Marine microalgae: promising source for new bioactive compounds. Mar. Drugs 16, 1–12. doi: 10.3390/md16090317
Debashish, G., Malay, S., Barindra, S., and Joydeep, M. (2005). Marine enzymes. Adv. Biochem. Eng. Biotechnol. 96, 189–218.
Debbab, A., Aly, A. H., Lin, W. H., and Proksch, P. (2010). Bioactive compounds from marine bacteria and fungi: minireview. Microb. Biotechnol. 3, 544–563. doi: 10.1111/j.1751-7915.2010.00179.x
Del Mondo, A., Smerilli, A., Ambrosino, L., Albini, A., Noonan, D. M., Sansone, C., et al. (2021). Insights into phenolic compounds from microalgae: structural variety and complex beneficial activities from health to nutraceutics. Crit. Rev. Biotechnol. 41, 155–171. doi: 10.1080/07388551.2021.1874284
Desriac, F., Jégou, C., Balnois, E., Brillet, B., Le Chevalier, P., and Fleury, Y. (2013). Antimicrobial peptides from marine proteobacteria. Mar. Drugs 11, 3632–3660. doi: 10.3390/md11103632
Dewapriya, P., and Kim, S. K. (2014). Marine microorganisms: an emerging avenue in modern nutraceuticals and functional foods. Food Res. Int. 56, 115–125. doi: 10.1016/j.foodres.2013.12.022
Dhakal, D., Pokhrel, A. R., Shrestha, B., and Sohng, J. K. (2017). Marine rare actinobacteria: isolation, characterization, and strategies for harnessing bioactive compounds. Front. Microbiol. 8:1106. doi: 10.3389/fmicb.2017.01106
Domínguez, H. (2013). “Algae as a source of biologically active ingredients for the formulation of functional foods and nutraceuticals,” in Functional Ingredients from Algae Foods Nutraceuticals, ed. H. Dominguez (Amsterdam: Elsevier). doi: 10.1016/bs.afnr.2021.02.007
Donnell, C. O., Tiwari, B. K., and Ojha, K. S. (2020). Trends in Analytical Chemistry Ultrasound Technology for the Extraction of Biologically Active Molecules from Plant, Animal and Marine Sources. Amsterdam: Elsevier.
Duarte, K., Justino, C. I. L., Gomes, A. M., Rocha-Santos, T., and Duarte, A. C. (2014). Green Analytical Methodologies for Preparation of Extracts and Analysis of Bioactive Compounds. Amsterdam: Elsevier.
Duda, A. M., and Sherman, K. (2002). A new imperative for improving management of large marine ecosystems. Ocean Coast. Manag. 45, 797–833. doi: 10.1016/s0964-5691(02)00107-2
Ferraro, V., Cruz, I. B., Jorge, R. F., Malcata, F. X., Pintado, M. E., and Castro, P. M. L. (2010). Valorisation of natural extracts from marine source focused on marine by-products: a review. Food Res. Int. 43, 2221–2233. doi: 10.1016/j.foodres.2010.07.034
Fitton, J. H. (2011). Therapies from fucoidan; multifunctional marine polymers. Mar. Drugs 9, 1731–1760. doi: 10.3390/md9101731
Freile-Pelegrín, Y., and Robledo, D. (2013). “Bioactive phenolic compounds from algae,” in Bioactive Compounds from Marine Foods, eds B. Hernández-Ledesma and M. Herrero (Chichester: John Wiley & Sons, Inc). doi: 10.1002/9781118412893.ch6
Freitas, A. C., Rodrigues, D., Rocha-Santos, T. A. P., Gomes, A. M. P., and Duarte, A. C. (2012). Marine biotechnology advances towards applications in new functional foods. Biotechnol. Adv. 30, 1506–1515. doi: 10.1016/j.biotechadv.2012.03.006
Fu, W., Nelson, D. R., Yi, Z., Xu, M., Khraiwesh, B., Jijakli, K., et al. (2017). Bioactive compounds from microalgae: current development and prospects. Stud. Nat. Prod. Chem. 54, 199–225. doi: 10.3390/molecules26040943
Gallego, R., Bueno, M., and Herrero, M. (2019). Sub- and supercritical fluid extraction of bioactive compounds from plants, food-by-products, seaweeds and microalgae – an update. TrAC-Trends Anal. Chem. 116, 198–213. doi: 10.1016/j.trac.2019.04.030
Ganesan, A. R., Saravana Guru, M., Balasubramanian, B., Mohan, K., Chao Liu, W., Valan Arasu, M., et al. (2020b). Biopolymer from edible marine invertebrates: a potential functional food. J. King Saud Univ. Sci. 32, 1772–1777. doi: 10.1016/j.jksus.2020.01.015
Ganesan, A. R., Mohanram, M. S. G., Balasubramanian, B., Ho Kim, I., Seedevi, P., Mohan, K., et al. (2020a). Marine invertebrates’ proteins: a recent update on functional property. J. King Saud Univ. Sci. 32, 1496–1502. doi: 10.1016/j.jksus.2019.12.003
Ghanbarzadeh, M., Golmoradizadeh, A., and Homaei, A. (2018). Carrageenans and carrageenases: versatile polysaccharides and promising marine enzymes. Phytochem. Rev. 17, 535–571. doi: 10.1007/s11101-018-9548-2
Ghosh, S., Sarkar, T., Das, A., and Chakraborty, R. (2021). Micro and nanoencapsulation of natural colors: a holistic view. Appl. Biochem. Biotechnol. 193, 3787–3811. doi: 10.1007/s12010-021-03631-8
Ghosh, S., Sarkar, T., Das, A., and Chakraborty, R. (2022). Natural colorants from plant pigments and their encapsulation: an emerging window for the food industry. LWT 153:112527. doi: 10.1016/j.lwt.2021.112527
Gogineni, V., and Hamann, M. T. (2018). Marine natural product peptides with therapeutic potential: chemistry, biosynthesis, and pharmacology. Biochim. Biophys. Acta Gen. Subj. 1862, 81–196. doi: 10.1016/j.bbagen.2017.08.014
Gogineni, V., Schinazi, R. F., and Hamann, M. T. (2015). Role of marine natural products in the genesis of antiviral agents. Chem. Rev. 115, 9655–9706. doi: 10.1021/cr4006318
Grienke, U., Silke, J., and Tasdemir, D. (2014). Bioactive compounds from marine mussels and their effects on human health. Food Chem. 142, 48–60. doi: 10.1016/j.foodchem.2013.07.027
Grosso, C., Valentão, P., Ferreres, F., and Andrade, P. B. (2015). Alternative and efficient extraction methods for marine-derived compounds. Mar. Drugs 13, 3182–3230. doi: 10.3390/md13053182
Gurpilhares, D. D. B., Cinelli, L. P., Simas, N. K., Pessoa, A., and Sette, L. D. (2019). Marine prebiotics: polysaccharides and oligosaccharides obtained by using microbial enzymes. Food Chem. 280, 175–186.
Hamed, I., Özogul, F., Özogul, Y., and Regenstein, J. M. (2015). Marine bioactive compounds and their health benefits: a review. Compr. Rev. Food Sci. Food Saf. 14, 446–465.
Hamzeh, A., Rezaei, M., Khodabandeh, S., Motamedzadegan, A., and Noruzinia, M. (2018). Antiproliferative and antioxidative activities of cuttlefish (Sepia pharaonis) protein hydrolysates as affected by degree of hydrolysis. J. Food Meas. Charact. 12, 721–727. doi: 10.1007/s11694-017-9685-0
Hannan, A., Al, A., Sohag, M., Dash, R., and Haque, N. (2020). Phytomedicine Phytosterols of marine algae: Insights into the potential health benefits and molecular pharmacology. Phytomedicine 69:153201. doi: 10.1016/j.phymed.2020.153201
Haoujar, I., Cacciola, F., Abrini, J., Mangraviti, D., Giu, D., Oulad, Y., et al. (2019). The contribution of carotenoids, phenolic compounds, and flavonoids to the antioxidative properties of marine microalgae isolated from mediterranean morocco imane. Molecules 24, 1–17. doi: 10.3390/molecules24224037
Harnedy, P. A., and Fitzgerald, R. J. (2011). Bioactive proteins, peptides, and amino acids from macroalgae. J. Phycol. 47, 218–232. doi: 10.1111/j.1529-8817.2011.00969.x
Hayes, M. (2012). Marine Bioactive Compounds: Sources, Characterization and Applications. Berlin: Springer.
Heleno, S. A., Diz, P., Prieto, M. A., Barros, L., Rodrigues, A., Barreiro, M. F., et al. (2016). Optimization of ultrasound-assisted extraction to obtain mycosterols from Agaricus bisporus L. by response surface methodology and comparison with conventional Soxhlet extraction. Food Chem. 197, 1054–1063. doi: 10.1016/j.foodchem.2015.11.108
Hemker, A. K., Nguyen, L. T., Karwe, M., and Salvi, D. (2020). Effects of pressure-assisted enzymatic hydrolysis on functional and bioactive properties of tilapia (Oreochromis niloticus) by-product protein hydrolysates. Lwt 122:109003. doi: 10.1016/j.lwt.2019.109003
Hoffman, D. R., Boettcher, J. A., and Diersen-Schade, D. A. (2009). Toward optimizing vision and cognition in term infants by dietary docosahexaenoic and arachidonic acid supplementation: a review of randomized controlled trials. Prostaglandins Leukot. Essent. Fat. Acids 81, 151–158. doi: 10.1016/j.plefa.2009.05.003
Hosseini, S. F., Rezaei, M., and Mcclements, D. J. (2020). Bioactive functional ingredients from aquatic origin: a review of recent progress in marine- derived nutraceuticals. Crit. Rev. Food Sci. Nutr. doi: 10.1080/10408398.2020.1839855 [Epub ahead of print].
Hu, X., Tao, N., Wang, X., Xiao, J., and Wang, M. (2016). Marine-derived bioactive compounds with anti-obesity effect: a review. J. Funct. Foods 21, 372–387. doi: 10.2174/0929867324666170602082620
Huang, C. Y., Kuo, J. M., Wu, S. J., and Tsai, H. T. (2016). Isolation and characterization of fish scale collagen from tilapia (Oreochromis sp.) by a novel extrusion-hydro-extraction process. Food Chem. 190, 997–1006. doi: 10.1016/j.foodchem.2015.06.066
Izadpanah, F., Homaei, A., Fernandes, P., and Javadpour, S. (2018). Marine microbial L-asparaginase: biochemistry, molecular approaches and applications in tumor therapy and in food industry. Microbiol. Res. 208, 99–112. doi: 10.1016/j.micres.2018.01.011
Izadpanah, F., Homaei, A., Fernandes, P., Hemmati, R., Dijkstra, B. W., and Khajeh, K. (2020). BBA - proteins and proteomics Xylanases from marine microorganisms: a brief overview on scope, sources, features and potential applications. BBA Proteins Proteomics 1868:140312. doi: 10.1016/j.bbapap.2019.140312
Jesumani, V., Du, H., Aslam, M., Pei, P., and Huang, N. (2019). Potential use of seaweed bioactive compounds in skincare—a review. Mar. Drugs 17:688. doi: 10.3390/md17120688
Jia, X., Yang, J., Wang, Z., Liu, R., and Xie, R. (2014). Polysaccharides from Laminaria japonica show hypoglycemic and hypolipidemic activities in mice with experimentally induced diabetes. Exp. Biol. Med. 239, 1663–1670. doi: 10.1177/1535370214537751
Jia, Z. J., Xiaoyu, T., Michelle, Z., Darlene, N., and Moore, B. S. (2022). Broad-host-range expression reveals native and host regulatory elements that influence heterologous antibiotic production in gram-negative bacteria. MBio 8:e1291-17. doi: 10.1128/mBio.01291-17
Jimenez-Lopez, C., Pereira, A. G., Lourenço-Lopes, C., Garcia-Oliveira, P., Cassani, L., Fraga-Corral, M., et al. (2021). Main bioactive phenolic compounds in marine algae and their mechanisms of action supporting potential health benefits. Food Chem. 341:128262. doi: 10.1016/j.foodchem.2020.128262
Jin, W., Zhang, W., Liang, H., and Zhang, Q. (2015). The structure-activity relationship between marine algae polysaccharides and anti-complement activity. Mar. Drugs 3, 1–15. doi: 10.3390/md14010003
John, B. A., Nelson, B. R., Sheikh, H. I., Cheung, S. G., Wardiatno, Y., Dash, B. P., et al. (2018). A review on fisheries and conservation status of Asian horseshoe crabs. Biodivers. Conserv. 27, 3573–3598. doi: 10.1007/s10531-018-1633-8
Jung, H. A., Islam, M. N., Lee, C. M., Oh, S. H., Lee, S., Jung, J. H., et al. (2013). Kinetics and molecular docking studies of an anti-diabetic complication inhibitor fucosterol from edible brown algae Eisenia bicyclis and Ecklonia stolonifera. Chem. Biol. Interact. 206, 55–62. doi: 10.1016/j.cbi.2013.08.013
Kadam, S. U., Tiwari, B. K., and O’Donnell, C. P. (2013). Application of novel extraction technologies for bioactives from marine algae. J. Agric. Food Chem. 61, 4667–4675. doi: 10.1021/jf400819p
Kalil, S. J., Moraes, C. C., Sala, L., and Burkert, C. A. V. (2017). Bioproduct Extraction From Microbial Cells by Conventional and Nonconventional Techniques. Amsterdam: Elsevier.
Kari, Z. A., Kabir, M. A., Dawood, M. A., Razab, M. K. A. A., Ariff, N. S. N. A., Sarkar, T., et al. (2022). Effect of fish meal substitution with fermented soy pulp on growth performance, digestive enzyme, amino acid profile, and immune-related gene expression of African catfish (Clarias gariepinus). Aquaculture 546:737418. doi: 10.1016/j.aquaculture.2021.737418
Kari, Z. A., Kabir, M. A., Mat, K., Rusli, N. D., Razab, M. K. A. A., Ariff, N. S. N. A., et al. (2021). The possibility of replacing fish meal with fermented soy pulp on the growth performance, blood biochemistry, liver, and intestinal morphology of African catfish (Clarias gariepinus). Aquac. Rep. 21:100815. doi: 10.1016/j.aqrep.2021.100815
Karunarathne, S., Mendis, E., and Kim, S. (2020). The potential of developing additives from marine bioresources for the food industry the functions and roles of food additives. Encylopedia Mar. Biotechnol. 3085–3107. doi: 10.1002/9781119143802.ch137
Khan, R. S., Grigor, J., Winger, R., and Win, A. (2013). Functional food product development - opportunities and challenges for food manufacturers. Trends Food Sci. Technol. 30, 27–37. doi: 10.1016/j.tifs.2012.11.004
Khoo, K. S., Chew, K. W., Yew, G. Y., Leong, W. H., Chai, Y. H., Show, P. L., et al. (2020). Recent advances in downstream processing of microalgae lipid recovery for biofuel production. Bioresour. Technol. 304:122996. doi: 10.1016/j.biortech.2020.122996
Khoo, K. S., Lee, S. Y., Ooi, C. W., Fu, X., Miao, X., Ling, T. C., et al. (2019). Recent advances in biorefinery of astaxanthin from Haematococcus pluvialis. Bioresour. Technol. 288:121606. doi: 10.1016/j.biortech.2019.121606
Khoo, K. S., Ooi, C. W., Chew, K. W., Foo, S. C., and Show, P. L. (2021). Bioprocessing of Chaetoceros calcitrans for the recovery of fucoxanthin using CO2-based alkyl carbamate ionic liquids. Bioresour. Technol. 322:124520. doi: 10.1016/j.biortech.2020.124520
Khora, S. S. (2013). Marine fish-derived bioactive peptides and proteins for human therapeutics. Int. J. Pharm. Pharm. Sci. 65, 249–260.
Kim, E. K., Kim, Y. S., Hwang, J. W., Lee, J. S., Moon, S. H., Jeon, B. T., et al. (2013). Purification and characterization of a novel anticancer peptide derived from Ruditapes philippinarum. Process Biochem. 48, 1086–1090. doi: 10.1016/j.procbio.2013.05.004
Kim, S. K., and Dewapriya, P. (2014). “Enzymes from fish processing waste materials and their commercial applications,” in Seafood Processing By-Products, ed. S. K. Kim (New York, NY: Springer), 183–196. doi: 10.1007/978-1-4614-9590-1_10
Kiuru, P., Valeria D’Auria, M., Muller, C. D., Tammela, P., Vuorela, H., and Yli-Kauhaluoma, J. (2014). Exploring marine resources for bioactive compounds. Planta Med. 80, 1234–1246. doi: 10.1055/s-0034-1383001
Koyande, A. K., Chew, K. W., Manickam, S., Chang, J. S., and Show, P. L. (2021). Emerging algal nanotechnology for high-value compounds: a direction to future food production. Trends Food Sci. Technol. 116, 290–302. doi: 10.1016/j.tifs.2021.07.026
Lafarga, T., Acién-Fernández, F. G., and Garcia-Vaquero, M. (2020). Bioactive peptides and carbohydrates from seaweed for food applications: natural occurrence, isolation, purification, and identification. Algal Res. 48:101909. doi: 10.1016/j.algal.2020.101909
Lam, V. W. Y., Allison, E. H., Bell, J. D., Blythe, J., Cheung, W. W. L., Frölicher, T. L., et al. (2020). Climate change, tropical fisheries and prospects for sustainable development. Nat. Rev. Earth Environ. 1, 440–454.
Lapeña, D., Vuoristo, K. S., Kosa, G., Horn, S. J., and Eijsink, V. G. H. (2018). Comparative assessment of enzymatic hydrolysis for valorization of different protein-rich industrial byproducts. J. Agric. Food Chem. 66, 9738–9749. doi: 10.1021/acs.jafc.8b02444
Lordan, S., Ross, R. P., and Stanton, C. (2011). Marine bioactives as functional food ingredients: potential to reduce the incidence of chronic diseases. Mar. Drugs 9, 1056–1100. doi: 10.3390/md9061056
Low, K. L., Idris, A., and Yusof, N. M. (2020). Novel protocol optimized for microalgae lutein used as food additives. Food Chem. J. 307:125631. doi: 10.1016/j.foodchem.2019.125631
Mahapatra, G. P., Raman, S., Nayak, S., Gouda, S., Das, G., and Patra, J. K. (2020). Metagenomics approaches in discovery and development of new bioactive compounds from marine actinomycetes. Curr. Microbiol. 77, 645–656. doi: 10.1007/s00284-019-01698-5
Malone, T. C., DiGiacomo, P. M., Gonçalves, E., Knap, A. H., Talaue-McManus, L., and de Mora, S. (2014). A global ocean observing system framework for sustainable development. Mar. Policy 43, 262–272. doi: 10.1016/j.marpol.2013.06.008
Manivasagan, P., Bharathiraja, S., Moorthy, S., Mondal, S., Seo, H., Lee, K. D., et al. (2018). Critical reviews in biotechnology marine natural pigments as potential sources for therapeutic applications. Crit. Rev. Biotechnol. 38, 745–761. doi: 10.1080/07388551.2017.1398713
Manlusoc, J. K. T., Hsieh, C. L., Hsieh, C. Y., Salac, E. S. N., Lee, Y. T., and Tsai, P. W. (2019). Pharmacologic application potentials of sulfated polysaccharide from marine algae. Polymers 11:1163. doi: 10.3390/polym11071163
Martins, D. A., Custódio, L., Barreira, L., Pereira, H., Ben-Hamadou, R., Varela, J., et al. (2013). Alternative sources of n-3 long-chain polyunsaturated fatty acids in marine microalgae. Mar. Drugs 11, 2259–2281. doi: 10.3390/md11072259
Mateos, R., Pérez-Correa, J. R., and Domínguez, H. (2020). Bioactive properties of marine phenolics. Mar. Drugs 18, 1–65. doi: 10.3390/md18100501
Matos, J., and Cardoso, C. (2017). Function microalgae as healthy ingredients for functional food: a review. Food Funct. 8, 2672–2685. doi: 10.1039/c7fo00409e
Matsumoto, M., Hosokawa, M., Matsukawa, N., Hagio, M., Shinoki, A., Nishimukai, M., et al. (2010). Suppressive effects of the marine carotenoids, fucoxanthin and fucoxanthinol on triglyceride absorption in lymph duct-cannulated rats. Eur. J. Nutr. 49, 243–249. doi: 10.1007/s00394-009-0078-y
Mayakrishnan, V., Kannappan, P., Abdullah, N., and Ahmed, A. B. A. (2013). Cardioprotective activity of polysaccharides derived from marine algae: an overview. Trends Food Sci. Technol. 30, 98–104. doi: 10.1016/j.tifs.2013.01.007
Melgosa, R., Trigueros, E., Sanz, M. T., Cardeira, M., Rodrigues, L., Fernández, N., et al. (2020). Supercritical CO2 and subcritical water technologies for the production of bioactive extracts from sardine (Sardina pilchardus) waste. J. Supercrit. Fluids 164:104943. doi: 10.1016/j.supflu.2020.104943
Menaa, F., Wijesinghe, U., Thiripuranathar, G., Althobaiti, N. A., Albalawi, A. E., Khan, B. A., et al. (2021). Marine algae-derived bioactive compounds: a new wave of nanodrugs? Mar. Drugs 19:484. doi: 10.3390/md19090484
Merlo, S., Gabarrell Durany, X., Pedroso Tonon, A., and Rossi, S. (2021). Marine microalgae contribution to sustainable development. Water 13:1373. doi: 10.3390/w13101373
Moi, I. M., Leow, A. T. C., Ali, M. S. M., Rahman, R. N. Z. R. A., Salleh, A. B., and Sabri, S. (2018). Polyunsaturated fatty acids in marine bacteria and strategies to enhance their production. Appl. Microbiol. Biotechnol. 102, 5811–5826. doi: 10.1007/s00253-018-9063-9
Nagaoka, I., Igarashi, M., Hua, J., Ju, Y., Yomogida, S., and Sakamoto, K. (2011). Recent aspects of the anti-inflammatory actions of glucosamine. Carbohydr. Polym. 84, 825–830. doi: 10.1016/j.carbpol.2010.04.007
Nakashima, Y., Egami, Y., Kimura, M., Wakimoto, T., and Abe, I. (2016). Metagenomic analysis of the sponge Discodermia reveals the production of the cyanobacterial natural product kasumigamide by “entotheonella. PLoS One 11:e0164468. doi: 10.1371/journal.pone.0164468
Nam, P. V., Van Hoa, N., Anh, T. T. L., and Trung, T. S. (2020). Towards zero-waste recovery of bioactive compounds from catfish (Pangasius hypophthalmus) by-products using an enzymatic method. Waste Biomass Valorization 11, 4195–4206. doi: 10.1007/s12649-019-00758-y
Nędzarek, A., Drost, A., Tórz, A., and Bogusławska-Wąs, E. (2017). The use of a micro- and ultrafiltration cascade system for the recovery of protein, fat, and purified marinating brine from brine used for herring marination. Food Bioprod. Process. 106, 82–90. doi: 10.1016/j.fbp.2017.09.001
Ngo, D. H., Wijesekara, I., Vo, T. S., Van Ta, Q., and Kim, S. K. (2011). Marine food-derived functional ingredients as potential antioxidants in the food industry: an overview. Food Res. Int. 44, 523–529. doi: 10.1016/j.foodres.2010.12.030
Nikoo, M., Benjakul, S., Yasemi, M., Ahmadi Gavlighi, H., and Xu, X. (2019). Hydrolysates from rainbow trout (Oncorhynchus mykiss) processing by-product with different pretreatments: antioxidant activity and their effect on lipid and protein oxidation of raw fish emulsion. Lwt 108, 120–128. doi: 10.1016/j.lwt.2019.03.049
Norris, R., Harnedy, P. A., and FitzGerald, R. J. (2013). Antihypertensive peptides from marine sources. Bioact. Compd. Mar. Foods Plant Anim. Sources 228, 506–517. doi: 10.1016/j.foodchem.2017.02.039
Otero, P., López-Martínez, M. I., and García-Risco, M. R. (2019). Application of pressurized liquid extraction (PLE) to obtain bioactive fatty acids and phenols from Laminaria ochroleuca collected in Galicia (NW Spain). J. Pharm. Biomed. Anal. 164, 86–92. doi: 10.1016/j.jpba.2018.09.057
Ozogul, F., Cagalj, M., Šimat, V., Ozogul, Y., Tkaczewska, J., Hassoun, A., et al. (2021). Recent developments in valorisation of bioactive ingredients in discard/seafood processing by-products. Trends Food Sci. Technol. 116, 559–582. doi: 10.1016/j.tifs.2021.08.007
Pangestuti, R., and Kim, S. K. (2011). Neuroprotective effects of marine algae. Mar. Drugs 9, 803–818. doi: 10.3390/md9050803
Papon, N., Copp, B. R., and Courdavault, V. (2022). Marine drugs: biology, pipelines, current and future prospects for production. Biotechnol. Adv. 54:107871. doi: 10.1016/j.biotechadv.2021.107871
Pati, S., Chatterji, A., Dash, B. P., Nelson, B. R., Sarkar, T., Shahimi, S., et al. (2020). Structural characterization and antioxidant potential of chitosan by γ-irradiation from the carapace of horseshoe crab. Polymers 12:2361. doi: 10.3390/polym12102361
Pati, S., Sarkar, T., Sheikh, H. I., Bharadwaj, K. K., Mohapatra, P. K., Chatterji, A., et al. (2021). γ-Irradiated chitosan from Carcinoscorpius rotundicauda (Latreille, 1802) improves the shelf life of refrigerated aquatic products. Front. Mar. Sci. 8:498.
Patra, S., Praharaj, P. P., Panigrahi, D. P., Panda, B., Bhol, C. S., Mahapatra, K. K., et al. (2020). Bioactive compounds from marine invertebrates as potent anticancer drugs: the possible pharmacophores modulating cell death pathways. Mol. Biol. Rep. 47, 7209–7228. doi: 10.1007/s11033-020-05709-8
Pelay-Gimeno, M., Meli, A., Tulla-Puche, J., and Albericio, F. (2013b). Rescuing biological activity from synthetic phakellistatin 19. J. Med. Chem. 56, 9780–9788. doi: 10.1021/jm401520x
Pelay-Gimeno, M., García-Ramos, Y., Jesús Martin, M., Spengler, J., Molina-Guijarro, J. M., Munt, S., et al. (2013a). The first total synthesis of the cyclodepsipeptide pipecolidepsin a. Nat. Commun. 4:2352. doi: 10.1038/ncomms3352
Pinnaka, A. K., Radha, N., and Tanuku, S. (2019). Microbial Diversity in Ecosystem Sustainability and Biotechnological Applications. Berlin: Springer.
Pooja, K., Rani, S., Rana, V., and Pal, G. K. (2020). Aquatic Plants as a Natural Source of Antimicrobial and Functional Ingredients. Amsterdam: Elsevier.
Prabha, S. P., Nagappan, S., Rathna, R., Viveka, R., and Nakkeeran, E. (2019). Blue Biotechnology: a Vision for Future Marine Biorefineries. Amsterdam: Elsevier.
Prabhu, R. H., Bhise, K. S., and Patravale, V. B. (2017). Marine Enzymes in Cancer: a New Paradigm, 1st Edn. Amsterdam: Elsevier.
Qin, Y. (2018a). 6 - Applications of Bioactive Seaweed Substances in Functional Food Products. Cambridge, MA: Academic Press.
Rahman, M. M., and McFadden, G. (2011). Modulation of NF-κB signalling by microbial pathogens. Nat. Rev. Microbiol. 9, 291–306. doi: 10.1038/nrmicro2539
Rocha-Martin, J., Harrington, C., Dobson, A. D. W., and O’Gara, F. (2014). Emerging strategies and integrated systems microbiology technologies for biodiscovery of marine bioactive compounds. Mar. Drugs 12, 3516–3559. doi: 10.3390/md12063516
Rodgers, M. L., Takeshita, R., and Griffitt, R. J. (2018). Deepwater Horizon oil alone and in conjunction with Vibrio anguillarum exposure modulates immune response and growth in red snapper (Lutjanus campechanus). Aquat. Toxicol. 204, 91–99. doi: 10.1016/j.aquatox.2018.09.002
Romano, G., Costantini, M., Sansone, C., Lauritano, C., Ruocco, N., and Ianora, A. (2017). Marine microorganisms as a promising and sustainable source of bioactive molecules. Mar. Environ. Res. 128, 58–69. doi: 10.1016/j.marenvres.2016.05.002
Rosic, N. N. (2021). Recent advances in the discovery of novel marine natural products and mycosporine-like amino acid UV-absorbing compounds. Appl. Microbiol. Biotechnol. 105, 7053–7067. doi: 10.1007/s00253-021-11467-9
Routray, W., Dave, D., Cheema, S. K., Ramakrishnan, V. V., and Pohling, J. (2019). Biorefinery approach and environment-friendly extraction for sustainable production of astaxanthin from marine wastes. Crit. Rev. Biotechnol. 39, 469–488. doi: 10.1080/07388551.2019.1573798
Ruiz-Torres, V., Encinar, J. A., Herranz-López, M., Pérez-Sánchez, A., Galiano, V., Barrajón-Catalán, E., et al. (2017). An updated review on marine anticancer compounds: the use of virtual screening for the discovery of small-molecule cancer drugs. Molecules 22:1037. doi: 10.3390/molecules22071037
Sagar, S., Kaur, M., and Minneman, K. P. (2010). Antiviral lead compounds from marine sponges. Mar. Drugs 8, 2619–2638. doi: 10.3390/md8102619
Saide, A., Martínez, K. A., Ianora, A., and Lauritano, C. (2021). Unlocking the health potential of microalgae as sustainable sources of bioactive compounds. Int. J. Mol. Sci. 22:4383. doi: 10.3390/ijms22094383
Saidi, S., Deratani, A., Belleville, M. P., Amar, R., and Ben. (2014). Production and fractionation of tuna by-product protein hydrolysate by ultrafiltration and nanofiltration: impact on interesting peptides fractions and nutritional properties. Food Res. Int. 65, 453–461. doi: 10.1016/j.foodres.2014.04.026
Salamone, M., National, I., Cuttitta, A., National, I., Seidita, G., and Rigogliuso, S. (2011). Proteolytic marine enzymes and their applications. Mar. Res. 1–12.
Samaranayaka, A. G. P., Kitts, D. D., and Li-Chan, E. C. Y. (2010). Antioxidative and angiotensin-I-converting enzyme inhibitory potential of a pacific hake (merlucclus productos) fish protein hydrolysate subjected to simulated gastrointestinal digestion and caco-2 cell permeation. J. Agric. Food Chem. 58, 1535–1542. doi: 10.1021/jf9033199
Sánchez-Camargo, A. D. P., Ibáñez, E., Cifuentes, A., and Herrero, M. (2017). Bioactives obtained from plants, seaweeds, microalgae and food by-products using pressurized liquid extraction and supercritical fluid extraction. Compr. Anal. Chem. 76, 27–51. doi: 10.1016/bs.coac.2017.01.001
Santos-Buelga, C., Gonzalez-Manzano, S., Dueñas, M., and Gonzalez-Paramas, A. M. (2012). Extraction and isolation of phenolic compounds. Methods Mol. Biol. 864, 427–464. doi: 10.1007/978-1-61779-624-1_17
Shaheen, F., Ziaee, M. A., Ali, S. A., Simjee, S. U., Ahmed, A., and Choudhary, M. I. (2016). The first solid-phase synthesis and structural studies on phakellistatin 15. Rec. Nat. Prod. 10, 397–406.
Shahidi, F., and Ambigaipalan, P. (2015). Novel functional food ingredients from marine sources. Curr. Opin. Food Sci. 2, 123–129. doi: 10.1016/j.cofs.2014.12.009
Shin, D., Byun, W. S., Moon, K., Kwon, Y., Bae, M., Um, S., et al. (2018). Coculture of marine Streptomyces sp. with Bacillus sp. produces a new piperazic acid-bearing cyclic peptide. Front. Chem. 6:498. doi: 10.3389/fchem.2018.00498
Siddharth, S., and Vittal, R. R. (2018). Evaluation of antimicrobial, enzyme inhibitory, antioxidant and cytotoxic activities of partially purified volatile metabolites of marine streptomyces sp.s2a. Microorganisms 6:72. doi: 10.3390/microorganisms6030072
Sigwart, J. D., Blasiak, R., Jaspars, M., Jouffray, J. B., and Tasdemir, D. (2021). Unlocking the potential of marine biodiscovery. Nat. Prod. Rep. 38, 1235–1242. doi: 10.1039/d0np00067a
Sila, A., and Bougatef, A. (2016). Antioxidant peptides from marine by-products: Isolation, identification and application in food systems. a review. J. Funct. Foods 21, 10–26. doi: 10.1016/j.jff.2015.11.007
Šimat, V. (2021). Nutraceuticals and pharmaceuticals from marine fish and invertebrates. Mar. Drugs 19:401. doi: 10.3390/md19070401
Šimat, V., Čagalj, M., Skroza, D., Gardini, F., Tabanelli, G., Montanari, C., et al. (2021). Sustainable sources for antioxidant and antimicrobial compounds used in meat and seafood products. Adv. Food Nutr. Res. 97, 55–118. doi: 10.1016/bs.afnr.2021.03.001
Šimat, V., Elabed, N., Kulawik, P., Ceylan, Z., Jamroz, E., Yazgan, H., et al. (2020). Recent advances in marine-based nutraceuticals and their health benefits. Mar. Drugs 18, 1–40. doi: 10.3390/md18120627
Siró, I., Kápolna, E., Kápolna, B., and Lugasi, A. (2008). Functional food. product development, marketing and consumer acceptance-a review. Appetite 51, 456–467. doi: 10.1016/j.appet.2008.05.060
Sorres, J., Ramos, S., Thoison, O., and Al-mourabit, A. (2012). Pipestelides A - C: cyclodepsipeptides from the pacific marine sponge Pipestela candelabra. J. Nat. Prod. 75, 759–763. doi: 10.1021/np200714m
Speitling, M., Smetanina, O. F., Kuznetsova, T. A., and Laatsch, H. (2007). Bromoalterochromides A and A’, unprecedented chromopeptides from a marine pseudoalteromonas maricaloris strain KMM 636T. J. Antibiot. 60, 36–42. doi: 10.1038/ja.2007.5
Stabili, L., Rizzo, L., Caprioli, R., and Leone, A. (2021). Jellyfish bioprospecting in the mediterranean sea: antioxidant and lysozyme-like activities from Aurelia coerulea (Cnidaria, Scyphozoa) Extracts. Mar. Drugs 19:619. doi: 10.3390/md19110619
Stincone, P., and Brandelli, A. (2020). Marine bacteria as source of antimicrobial compounds. Crit. Rev. Biotechnol. 40, 306–319. doi: 10.1080/07388551.2019.1710457
Subin, S. R., Mohan, A., Khiari, Z., Udenigwe, C. C., and Mason, B. (2018). Yield, physicochemical, and antioxidant properties of Atlantic salmon visceral hydrolysate: comparison of lactic acid bacterial fermentation with Flavourzyme proteolysis and formic acid treatment. J. Food Process. Preserv. 42, 1–11. doi: 10.3390/fermentation6010001
Sudhakar, M. P., Kumar, B. R., Mathimani, T., and Arunkumar, K. (2019). A review on bioenergy and bioactive compounds from microalgae and macroalgae-sustainable energy perspective. J. Clean. Prod. 228, 1320–1333. doi: 10.1016/j.jclepro.2019.04.287
Suganthy, N., Karutha Pandian, S., and Pandima Devi, K. (2010). Neuroprotective effect of seaweeds inhabiting South Indian coastal area (Hare Island, Gulf of Mannar Marine Biosphere Reserve): cholinesterase inhibitory effect of Hypnea valentiae and Ulva reticulata. Neurosci. Lett. 468, 216–219. doi: 10.1016/j.neulet.2009.11.001
Suleria, H. A. R., Gobe, G., Masci, P., and Osborne, S. A. (2016). Marine bioactive compounds and health promoting perspectives; innovation pathways for drug discovery. Trends Food Sci. Technol. 50, 44–55. doi: 10.1016/j.tifs.2016.01.019
Suleria, H. A. R., Osborne, S., Masci, P., and Gobe, G. (2015). Marine-based nutraceuticals: an innovative trend in the food and supplement industries. Mar. Drugs 13, 6336–6351. doi: 10.3390/md13106336
Suwal, S., Perreault, V., Marciniak, A., Tamigneaux, É, Deslandes, É, Bazinet, L., et al. (2019). Effects of high hydrostatic pressure and polysaccharidases on the extraction of antioxidant compounds from red macroalgae, Palmaria palmata and Solieria chordalis. J. Food Eng. 252, 53–59. doi: 10.1016/j.jfoodeng.2019.02.014
Tamadoni Jahromi, S., and Barzkar, N. (2018). Marine bacterial chitinase as sources of energy, eco-friendly agent, and industrial biocatalyst. Int. J. Biol. Macromol. 120, 2147–2154. doi: 10.1016/j.ijbiomac.2018.09.083
Tanna, B., and Mishra, A. (2018). Metabolites unravel nutraceutical potential of edible seaweeds: an emerging source of functional food. Compr. Rev. Food Sci. Food Saf. 17, 1613–1624. doi: 10.1111/1541-4337.12396
Thangavel, P., and Sridevi, G. (2015). Environmental Sustainability: Role of Green Technologies. Berlin: Springer.
Tonon, R. V., Dos Santos, B. A., Couto, C. C., Mellinger-Silva, C., Brígida, A. I. S., and Cabral, L. M. C. (2016). Coupling of ultrafiltration and enzymatic hydrolysis aiming at valorizing shrimp wastewater. Food Chem. 198, 20–27. doi: 10.1016/j.foodchem.2015.11.094
Tran, T. D., Pham, N. B., Fechner, G. A., Hooper, J. N. A., and Quinn, R. J. (2014). Potent cytotoxic peptides from the Australian marine sponge Pipestela candelabra. Mar. Drugs 12, 3399–3415. doi: 10.3390/md12063399
Trang, N. T. H., Tang, D. Y. Y., Chew, K. W., Linh, N. T., Hoang, L. T., Cuong, N. T., et al. (2021). Discovery of α-Glucosidase inhibitors from marine microorganisms: optimization of culture conditions and medium composition. Mol. Biotechnol. 63, 1004–1015. doi: 10.1007/s12033-021-00362-3
Uranga, J., Leceta, I., Etxabide, A., Guerrero, P., and Caba, K. D. (2016). Cross-linking of fish gelatins to develop sustainable films with enhanced properties. Eur. Polym. J. 78, 82–90. doi: 10.1016/j.eurpolymj.2016.03.017
Urda, C., Pérez, M., Rodríguez, J., Jiménez, C., Cuevas, C., and Fernández, R. (2016). Pembamide, a N-methylated linear peptide from a sponge Cribrochalina sp. Tetrahedron Lett. 57, 3239–3242.
Varijakzhan, D., Loh, J. Y., Yap, W. S., Yusoff, K., Seboussi, R., Lim, S. H. E., et al. (2021). Bioactive compounds from marine sponges: fundamentals and applications. Mar. Drugs 19:246. doi: 10.3390/md19050246
Vásquez, P., Zapata, J. E., Chamorro, V. C., García Fillería, S. F., and Tironi, V. A. (2021). Antioxidant and angiotensin I-converting enzyme (ACE) inhibitory peptides of rainbow trout (Oncorhynchus mykiss) viscera hydrolysates subjected to simulated gastrointestinal digestion and intestinal absorption. Lwt 154:112834.
Vaz, B. D. S., Moreira, J. B., Morais, M. G. D., and Costa, J. A. V. (2016). Microalgae as a new source of bioactive compounds in food supplements. Curr. Opin. Food Sci. 7, 73–77.
Venugopal Menon, V., and Lele, S. S. (2012). Nutraceuticals and Bioactive Compounds from Seafood Processing Waste. Berlin: Springer.
Vieira, V., Prieto, M. A., Barros, L., Coutinho, J. A. P., Ferreira, I. C. F. R., and Ferreira, O. (2018). Enhanced extraction of phenolic compounds using choline chloride based deep eutectic solvents from Juglans regia L. Ind. Crops Prod. 115, 261–271.
Vitale, G. A., Coppola, D., Esposito, F. P., Buonocore, C., Ausuri, J., Tortorella, E., et al. (2020). Antioxidant molecules from marine fungi: methodologies and perspectives. Antioxidants 9, 1–35. doi: 10.3390/antiox9121183
Wall, R., Ross, R. P., Fitzgerald, G. F., and Stanton, C. (2010). Fatty acids from fish: the anti-inflammatory potential of long-chain omega-3 fatty acids. Nutr. Rev. 68, 280–289. doi: 10.1111/j.1753-4887.2010.00287.x
Wang, C., Fan, Q., Zhang, X., Lu, X., Xu, Y., Zhu, W., et al. (2018). Isolation, characterization, and pharmaceutical applications of an exopolysaccharide from aerococcus uriaeequi. Mar. Drugs 16:337. doi: 10.3390/md16090337
Wang, C., Tang, S., and Cao, S. (2021). Antimicrobial Compounds from Marine Fungi. Netherlands: Springer.
Wang, K., Pramod, S. N., Pavase, T. R., Ahmed, I., Lin, H., Liu, L., et al. (2020). An overview on marine anti-allergic active substances for alleviating food-induced allergy. Crit. Rev. Food Sci. Nutr. 60, 2549–2563. doi: 10.1080/10408398.2019.1650716
Wang, T., Jónsdóttir, R., and Ólafsdóttir, G. (2009). Total phenolic compounds, radical scavenging and metal chelation of extracts from Icelandic seaweeds. Food Chem. 116, 240–248.
Wang, T., Xue, C., Zhang, T., and Wang, Y. (2018). Trends in food science & technology the improvements of functional ingredients from marine foods in lipid metabolism. Trends Food Sci. Technol. 81, 74–89.
Wang, X., Luo, H., Yu, W., Ma, R., You, S., Liu, W., et al. (2016). A thermostable Gloeophyllum trabeum xylanase with potential for the brewing industry. Food Chem. 199, 516–523. doi: 10.1016/j.foodchem.2015.12.028
Wang, X., Yuen, K. F., Wong, Y. D., and Li, K. X. (2020). How can the maritime industry meet sustainable development goals? an analysis of sustainability reports from the social entrepreneurship perspective. Transp. Res. Part D Transp. Environ. 78:102173.
Wang, X., Zhang, Z., Zhang, S., Yang, F., Yang, M., Zhou, J., et al. (2021). Antiaging compounds from marine organisms. Food Res. Int. 143:110313. doi: 10.1016/j.foodres.2021.110313
Xu, H. L., Kitajima, C., Ito, H., Miyazaki, T., Baba, M., Okuyama, T., et al. (2012). Antidiabetic effect of polyphenols from brown alga Ecklonia kurome in genetically diabetic KK-A y mice. Pharm. Biol. 50, 393–400. doi: 10.3109/13880209.2011.601464
Yaghoubzadeh, Z., Peyravii Ghadikolaii, F., Kaboosi, H., Safari, R., and Fattahi, E. (2020). Antioxidant activity and anticancer effect of bioactive peptides from rainbow trout (Oncorhynchus mykiss) skin hydrolysate. Int. J. Pept. Res. Ther. 26, 625–632.
Zakaria, S. M., and Kamal, S. M. M. (2016). Subcritical water extraction of bioactive compounds from plants and algae: applications in pharmaceutical and food ingredients. Food Eng. Rev. 8, 23–34.
Zeid, A., and Dhar, D. (2012). Commercial and industrial applications of micro algae – a review. J. Algal Biomass Utln. 3, 89–100.
Zhan, K. X., Jiao, W. H., Yang, F., Li, J., Wang, S. P., Li, Y. S., et al. (2014). Reniochalistatins A-E, cyclic peptides from the marine sponge Reniochalina stalagmitis. J. Nat. Prod. 77, 2678–2684. doi: 10.1021/np5006778
Zhang, C., and Kim, S. K. (2012). Application of Marine Microbial Enzymes in the Food and Pharmaceutical Industries, 1st Edn. Amsterdam: Elsevier.
Zhang, D., Shu, C., Lian, X., and Zhang, Z. (2018). New antibacterial bagremycins F and G from the marine-derived streptomyces sp. ZZ745. Mar. Drugs 16:330. doi: 10.3390/md16090330
Zhang, H. J., Yi, Y. H., Yang, G. J., Hu, M. Y., Cao, G. D., Yang, F., et al. (2010). Proline-containing cyclopeptides from the marine sponge phakellia fusca. J. Nat. Prod. 73, 650–655. doi: 10.1021/np9008267
Zhang, J., Zhang, W., Mamadouba, B., and Xia, W. (2012). A comparative study on hypolipidemic activities of high and low molecular weight chitosan in rats. Int. J. Biol. Macromol. 51, 504–508. doi: 10.1016/j.ijbiomac.2012.06.018
Zhao, C., Yang, C., Liu, B., Lin, L., Sarker, S. D., Nahar, L., et al. (2018). Bioactive compounds from marine macroalgae and their hypoglycemic benefits. Trends Food Sci. Technol. 72, 1–12.
Zheng, J. S., Hu, X. J., Zhao, Y. M., Yang, J., and Li, D. (2013). Intake of fish and marine n-3 polyunsaturated fatty acids and risk of breast cancer: meta-analysis of data from 21 independent prospective cohort studies. BMJ 347:f3706. doi: 10.1136/bmj.f3706
Zheng, L. H., Wang, Y. J., Sheng, J., Wang, F., Zheng, Y., Lin, X. K., et al. (2011). Antitumor peptides from marine organisms. Mar. Drugs 9, 1840–1859. doi: 10.3390/md9101840
Keywords: food biotechnology, seafood, marine bioactive, future food, bioprospecting
Citation: Ghosh S, Sarkar T, Pati S, Kari ZA, Edinur HA and Chakraborty R (2022) Novel Bioactive Compounds From Marine Sources as a Tool for Functional Food Development. Front. Mar. Sci. 9:832957. doi: 10.3389/fmars.2022.832957
Received: 10 December 2021; Accepted: 17 January 2022;
Published: 10 February 2022.
Edited by:
Jianhua Fan, East China University of Science and Technology, ChinaReviewed by:
Dibyajit Lahiri, University of Engineering & Management, IndiaAnis Fadhlina, Universiti Malaysia Kelantan, Malaysia
Pau Loke Show, University of Nottingham, Malaysia
Copyright © 2022 Ghosh, Sarkar, Pati, Kari, Edinur and Chakraborty. This is an open-access article distributed under the terms of the Creative Commons Attribution License (CC BY). The use, distribution or reproduction in other forums is permitted, provided the original author(s) and the copyright owner(s) are credited and that the original publication in this journal is cited, in accordance with accepted academic practice. No use, distribution or reproduction is permitted which does not comply with these terms.
*Correspondence: Hisham Atan Edinur, ZWRpbnVyQHVzbS5teQ==; Runu Chakraborty, Y3J1bnVAaG90bWFpbC5jb20=
†ORCID: Tanmay Sarkar, orcid.org/0000-0003-3869-1604; Siddhartha Pati, orcid.org/0000-0002-8276-0008; Runu Chakraborty, orcid.org/0000-0003-4026-2542
‡These authors have contributed equally to this work