- 1Chinese Academy of Sciences (CAS) and Shandong Province Key Laboratory of Experimental Marine Biology, Center for Ocean Mega-Science, Institute of Oceanology, Chinese Academy of Sciences, Qingdao, China
- 2Laboratory for Marine Biology and Biotechnology, Qingdao National Laboratory for Marine Science and Technology, Qingdao, China
- 3University of Chinese Academy of Sciences, Beijing, China
- 4School of Marine Science and Engineering, Qingdao Agricultural University, Qingdao, China
- 5College of Fisheries and Life Science, Shanghai Ocean University, Shanghai, China
Cartilaginous fishes, as an important group of jawed vertebrates, hold great promise for improving our understanding of vertebrate evolution. Previous studies have established a good staging scheme for shark embryos. However, to date, little attention has been paid to the development of skate embryos. In this study, we established a fine developmental timeline for the Kong skate. We hatched eggs of the Kong skate (Okamejei kenojei) at fixed temperatures (16.5°C ± 0.5°C) while observing three samples every day and recorded the complete embryonic development. The embryo weight and development time were found to have a quadratic relationship. The weight-at-day curve was fitted by y = 0.0005x2 - 0.0109x + 0.0882 (R² = 0.9406). In addition, complete embryonic development from gastrula to skate larvae took 92 ± 5 days. Here, we divided the entire development process into 39 stages. This shows that the jaw of O. kenojei is developed from the first pair of pharyngeal arches, and its body disc is formed by the fusion of the pectoral fin and head. The eyespots began to form from stage 36 and then became thicker and bigger until the young skate broke out of the egg. Notably, we found that males and females develop differently from stage 31; the end of the male pelvic fins expands inward, which develop into copulatory organ-claspers, whereas in the females, they do not expand. To the best of our knowledge, this is the first study to record the embryonic development of eyes, body discs, eyespots, and other organs of O. kenojei in detail. We hope that our work will provide valuable information for researchers to identify specific time points for developing skate embryos.
Introduction
Skates and their cartilaginous fish relatives shed light on the evolution of vertebrates (Ebert and Sulikowski, 2007; Criswell et al., 2017; Criswell and Gillis, 2020; Sleight and Gillis, 2020). They also offer a great opportunity for biological research, such as the development of cartilage, the landing of vertebrates, and the application of bionics (Venkatesh et al., 2014; Enault et al., 2015; Jung et al., 2018; Johanson et al., 2019; Marconi et al., 2020; Pears et al., 2020; Wang et al., 2021). Furthermore, they have also been investigated as potential model organisms for fish body plans (Freitas et al., 2006; Swenson et al., 2018; Onimaru et al., 2018). Approximately half of the cartilaginous fishes (Batoids, Chondrichthyes: Batoidea; e.g. rays, skates, and guitarfish) have dorsoventrally flattened bodies (Swenson et al., 2018; Bezerra et al., 2019), the most striking of many traits in these groups, with derived pectoral fins morphologies (Dahn et al., 2007; Nakamura et al., 2015; Barry and Crow, 2017). Therefore, it is interesting to question how Batoid got its flat body. As a trait with unique modifications, it is key for understanding the evolution of phenotypic diversification (Tanaka et al., 2002; Enault et al., 2016; Nakamura et al., 2016). At present, studies such as that conducted by Barry (2017) and Nakamura (2015) have shown that the anteriorly expanded pectoral fins of skate fuse to the head, resulting in the flattened body. Nevertheless, the details on the morphological development of skate flat body during the whole embryonic development process are not so much.
Additionally, changes in anatomy come about through changes in development, and developmental changes help us understand the evolution of organisms (Gillis et al., 2009; Baker et al., 2013; Gillis et al., 2013). The embryonic development process of cartilaginous fishes like slow-motion movies of fish development, which offers a great chance for understanding the evolution of vertebrates (Gillis and Hall, 2016; Turner et al., 2019). Nowadays, the developmental timeline of sharks is well established in a variety of studies (Ballard et al., 1993; Xie et al., 2009; Musa et al., 2018; Onimaru et al., 2018). However, many previous embryonic development studies of skates only described several periods of the entire series of embryonic development or were conducted in an environment with a wide temperature range (Clark, 1927; Wang, 1982; Luer et al., 2007; Maxwell et al., 2008; Hoff, 2009; Suriano and Bodznick, 2018), which make it difficult to compare the details of development events among developing cartilaginous fishes (Debiais-Thibaud et al., 2015; Enault et al., 2016; Enault et al., 2018; Criswell et al., 2021; Hirschberger et al., 2021).
These gaps indicate a need to establish a developmental timeline of skates with more details. The O. kenojei is a common species in shallow coastal waters of the western North Pacific, feed on shrimp, fishes, and crabs. The age at maturity of O.kenojei is about 3 years (Ishihara, 1987; Baeck et al., 2011; Misawa et al., 2019). In this research, adult Kong skates were kept under artificial breeding conditions where they laid eggs year around. We collected and hatched the eggs at a relatively fixed temperature, and recorded the development of several organs daily, including the eyes, mouth, and pectoral fins. To the best of our knowledge, this is the first fine developmental timeline of O. kenojei. The main aim of this study was to set a series of normal stages for the development of skates, which were identified by the key features of each stage. This work is an important addition to the body of research on skates.
Materials and Methods
Breeding
The adult Kong skates, O.kenojei were collected from the nearshore waters of the Yellow China Sea, located in the east of China. Then they were kept in pools, measuring 4 m × 4 m × 1 m, with a temperature maintained at 16.5 ± 0.5°C of Haihe Aquatic Breeding Co., Ltd. (Weihai, China). These adult skates were domesticated with iced fish for about six months after which they were fed twice (8:00 AM and 4:00 PM) daily (Figure 1). The animal study was reviewed and approved by the Institutional Animal Care and Use Committee, Institute of Oceanology, Chinese Academy of Sciences.
Sampling
Eggs of the Kong skate, O.kenojei were obtained from these domesticated skates. To reduce the impact on the skate population, we collected eggs only in April, 2021. Eggs laid on the same day were placed in one basket, and then the baskets were labeled by the date of egg-lying. After collecting the eggs for one day, the basket was transferred to an incubation pool with light intensity 70-120 lex, which is same as the breeding pool. Similarly, all baskets were set in one pool (Figure 1). During hatching, we checked the eggs daily, and we calculated the development time (dph) according to the labels of baskets, after which three fresh eggs were randomly selected from each basket according to the development time (dph). We repeated this procedure daily until the juvenile fish escaped the egg case.
Histology Fixation
After selecting the eggs, we used scissors to cut off one side of the egg case and removed the egg jelly between the egg case and embryo with a knife. Thus, the embryo was exposed. Then they were weighed and transferred to the fixative. Notably, before stage 29, the embryo was small so we used straws to transfer the embryos to 4% paraformaldehyde (Boster Biological Technology Co., Wuhan, China). Here, to avoid excessive dehydration and deformation of the embryos, we did not use any fixatives containing ethanol and methanol, as soaking in methanol makes the fine structure of embryos very fragile (Figure 1). However, after stage 30, the yolk tube was clearly visible, and after cutting the tube, we moved the embryos into 4% paraformaldehyde with tweezers. All samples were stored at 4°C overnight. They were then transferred to the key laboratory of experimental marine biology laboratory (IOCAS) by ice box cooler filled with ice for observation.
Observation
The embryos were washed with phosphate buffered saline (Boster Biological Technology Co., Wuhan, China) several times prior to their examination and recording of the stages, after which the embryos were observed and recorded under a ZEISS Stemi 2000-C stereo microscope. The descriptive terminology of embryo morphology was based on the standard established by previous studies (Ballard et al., 1993; Onimaru et al., 2018). After checking each embryo, the representative embryo characteristics of each day were recorded by using the ZEISS Stemi 2000-C stereo microscope at 20-25°C. Based on the characteristics of head, mouth, eyes, external gills, fins and skin pigment patterns, we divided the embryonic development of O. kenojei into different stages, by placing the embryos with similar characteristics in one stage. Its developmental timeline was illustrated using Adobe Photoshop CS6 (Figure 1).
Results
Growth Curve of the Kong Skate Embryo
During the complete embryonic development, the mean wet weight of the embryo was positively related to the development time. We observed that the quadratic relationship of embryo weight and days had a correlation of 0.94. The growth curve was fitted using y = 0.0005x2 - 0.0109x + 0.0882 (Figure 2). Before 30 days, the variation in embryo weight was small and the speed of development was slow. After 30 days, the embryos showed rapid growth. The speed of growth increased, and before hatching, the maximum speed was obtained.
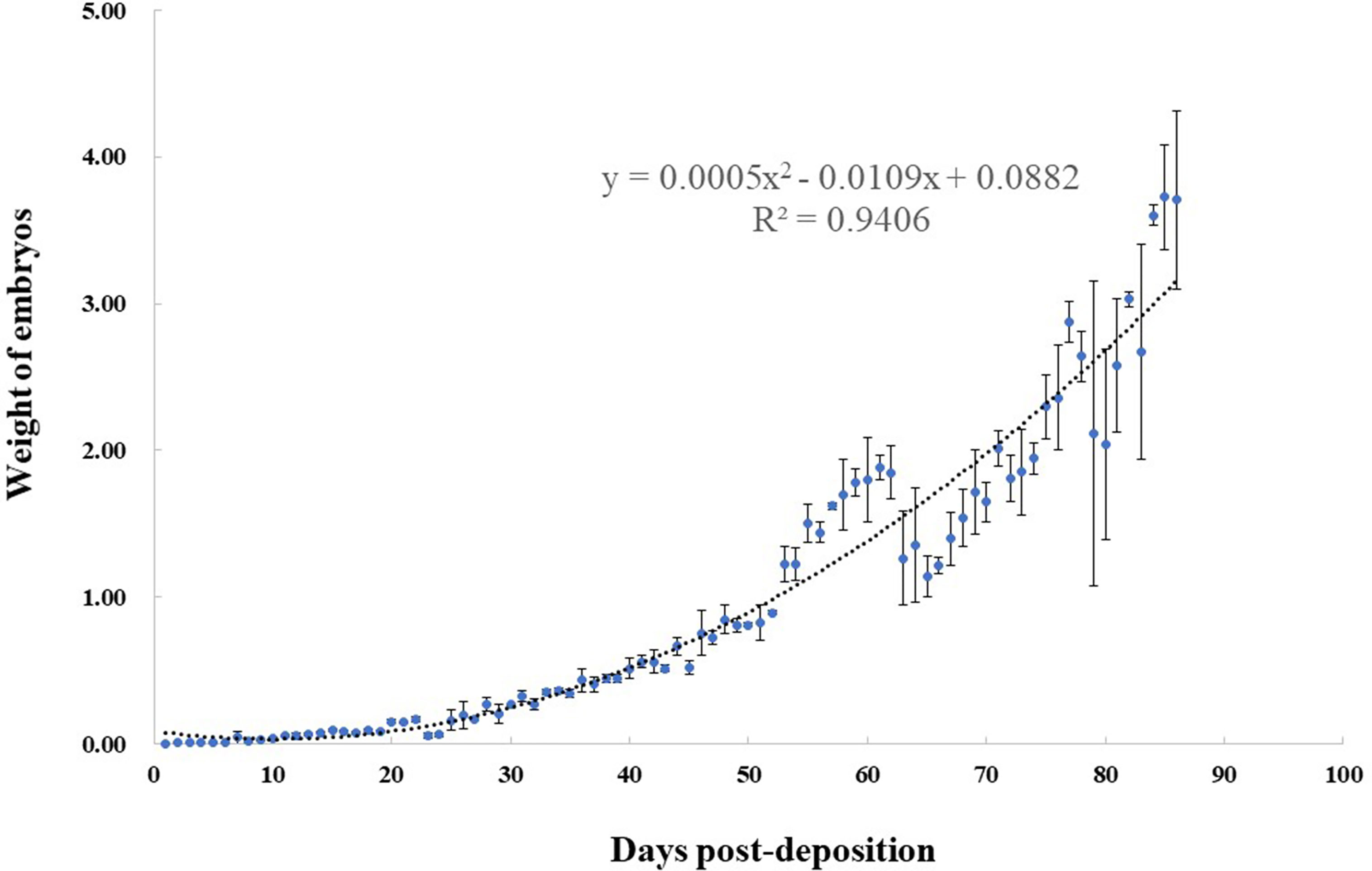
Figure 2 Relationship between weight (g) and days post-deposition (dpd) for Okamejei kenojei embryos. The curve was fitted by y = 0.0005x2 - 0.0109x + 0.0882, (R² = 0.9406).
Fine Staging Scheme for the Kong Skate
Stages 1–8: At these stages, the embryos develop from oosperm to gastrula in the female genital tracts. These stages span approximately 2 days at 16.5 ± 0.5°C before egg-laying. To determine a fine staging scheme before egg-laying, it would be necessary to dissect a large number of pregnant females. However, as the samples are limited and rare, this report focused on the stages after egg-laying.
Stage 9 (0–1 days post-deposition (dpd)): At this stage, most embryos are in the gastrula stage. The blastodisc has a ring-like shape, with a notch in the middle, expanding around (Figure 3).
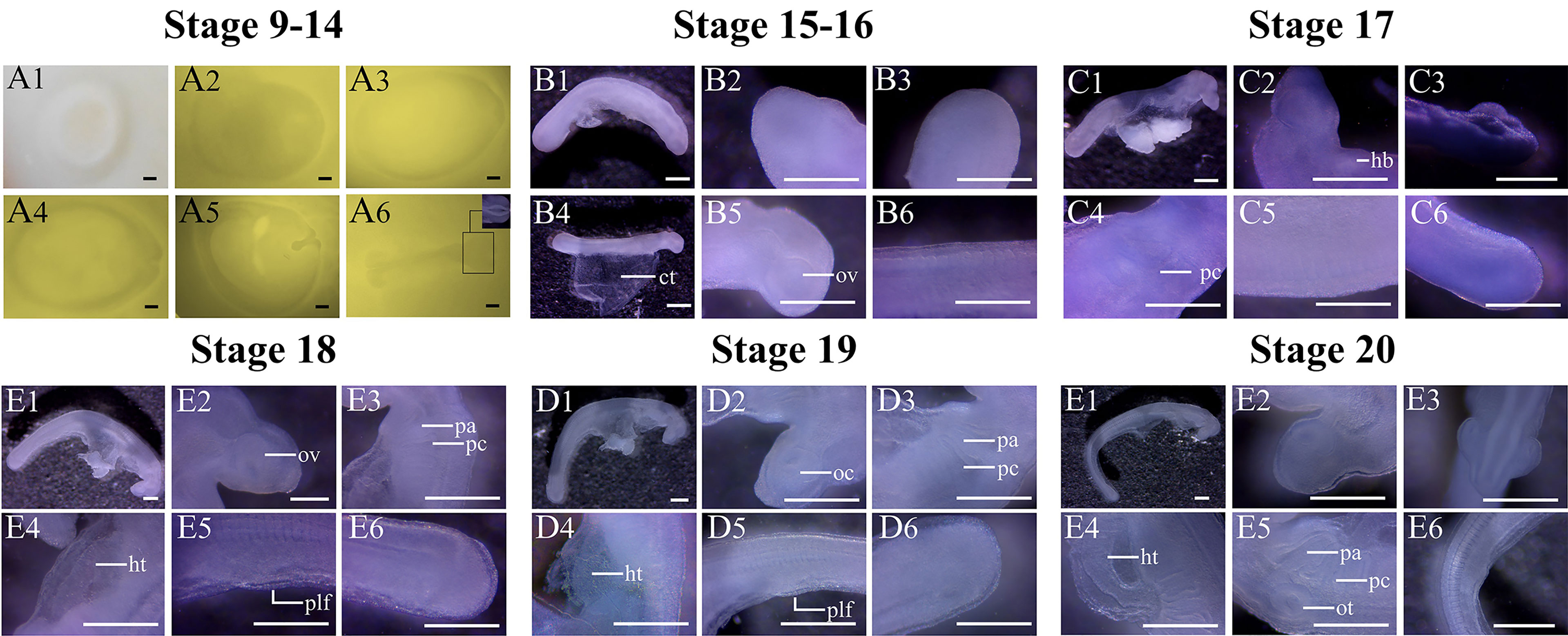
Figure 3 Key characteristics of O. kenojei embryos in developmental stages 9–20. Bar = 0.05 cm. ct, connective tissue; hb, heart bulge; oc, optic cup; ov, optic vesicle; ot, otic placode; pa, pharyngeal arch; pc, pharyngeal cleft; plf, pelvic fin.
Stage 10 (2 dpd): The blastodisc is oval in shape (Figure 3). One side of the blastodisc becomes thickened, and the embryonic shield begins to form.
Stage 11 (3 dpd): The embryonic shield is clearly visible (Figure 3). The middle part of the embryonic shield, which will develop into a neural fold, is thicker than the border region.
Stage 12 (3.5 dpd): The neural fold is formed in an n-shape. The direction of the ridge of the neural fold is parallel to the long axis of the oval blastodisc (Figure 3).
Stage 13 (4 dpd): The middle part of the neural fold starts to fuse where a constriction can be seen (Figure 3).
Stage 14 (5 dpd): With subsequent embryonic development (Figure 3), the neural fold develops into a neural groove. The anterior part of the neural fold is fusing, which will form the brain.
Stage 15 (6 dpd): The neural groove is fully formed and is tube-like shape (Figure 3). The head of the embryo is clearly visible, which is sharper than that of the posterior part of the embryo.
Stage 16 (7 dpd): The connective tissue between the embryo and the yolk sac develops, and the embryo can be easily separated from the external yolk sac. At this stage, the optic vesicle starts to develop, resulting in a circle-like disc at the head of the embryo (Figure 3). The somites in the middle part of the embryo are clearly visible, but because of the fixative, it is very difficult to count all the somites.
Stage 17 (8 dpd): The head of the embryo becomes sharper. Below the head of the embryo, the pharyngeal clefts are forming (Figure 3). There is a dense region around the pharyngeal clefts, the heart bulge, which will develop into the heart.
Stage 18 (9 dpd): The five pharyngeal clefts are clearly visible, and their size decreases in turn forming six pharyngeal arches (Figure 3). However, the ends of the pharyngeal arches are still adhered to each other, and the whole gill region is still packed in the connective tissue, having no direct contact with the outside. The heart tube has begun to form.
Stage 19 (10 dpd): The pharyngeal clefts grow larger, and their edges are thickened (Figure 3). The heart tube is visible. The optic vesicle develops into the optic cup, with a dip in the middle. The pelvic fins have begun to form, but they are stacked, making it difficult to record their shape.
Stage 20 (11 dpd): The ends of the gill arches are segregated at the end of this stage. Optic cups are protruding from both sides of the head, and the otic placode is clearly visible (Figure 3).
Stage 21 (12 dpd): The six pharyngeal arches can be seen with a wedge shape. The pharyngeal gill arch bends toward the posterior part of the embryo, with an approximately 45° angle to the long axis of the embryo. The mouth is open. The heart tube coils up, resulting in a heart disc in the abdominal region. Pectoral fins have begun to form (Figure 4). The yolk stalk connecting the embryo and yolk sac is formed.
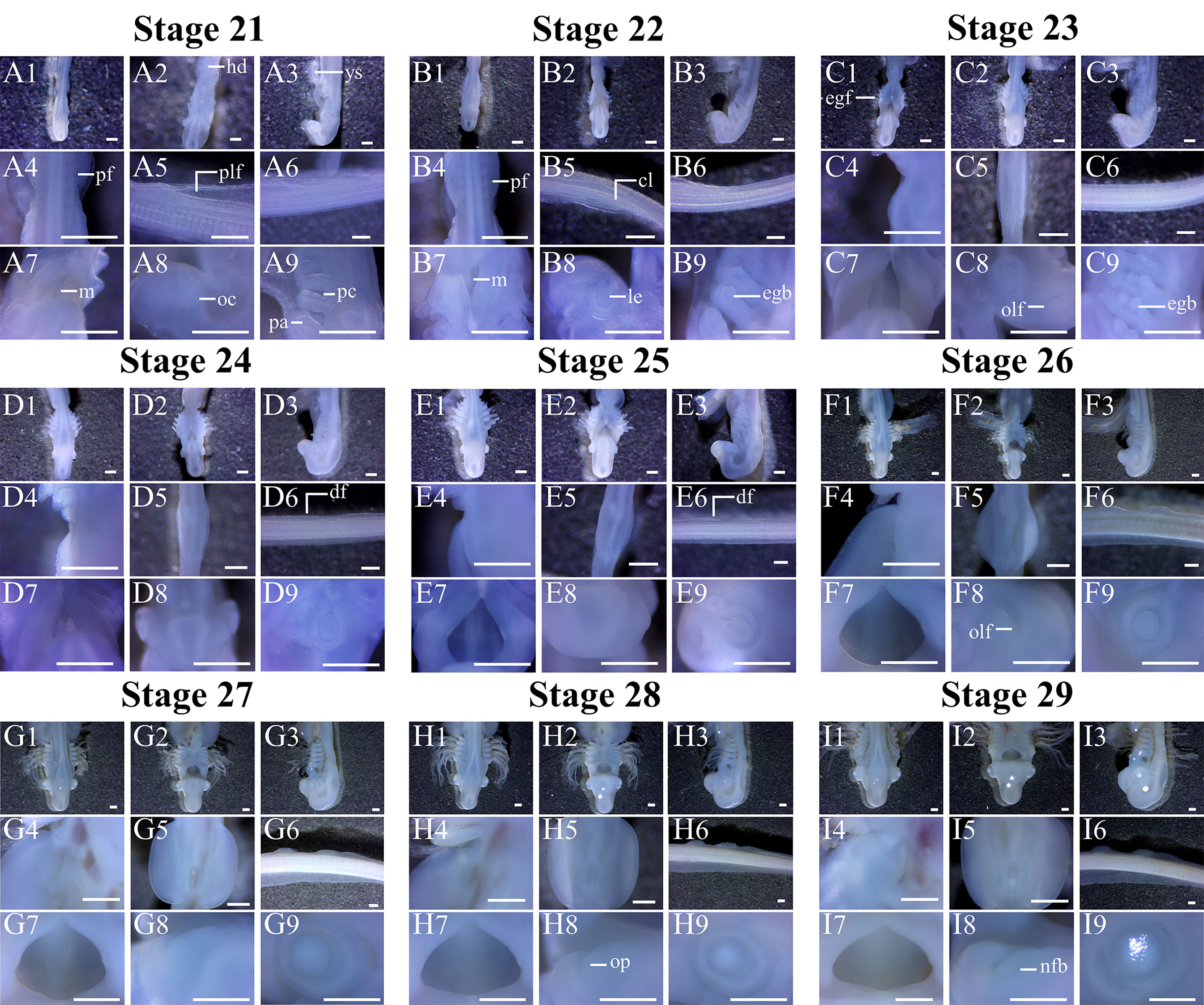
Figure 4 Key characteristics of O. kenojei embryos in developmental stages 21–29. Bar = 0.05 cm. The pictures of each stage labeled with 1, 2, and 3 indicate the changes in the embryo’s head. The pictures labeled with 4, 5, 6, 7, 8, and 9 show the development of the embryo’s pectoral fin, pelvic fin, dorsal fin, mouth, nose, and eye, respectively. cl, cloaca; df, dorsal fin; egb, external gill buds; hd, heart disc; le, lens; m, mouth; nfb, nasal flap buds; oc, optic cup; olf, olfactory placode; op, olfactory pits; pf, pectoral fin; plf, pelvic fin; op, olfactory pits; ys, yolk stalk.
Stage 22 (13 dpd): The pharyngeal arches have elongated. There are three external gill buds on each of the last five pharyngeal arches; the middle external gill bud is the largest among them (Figure 4). The lens in the middle of the eye cups starts to develop; the mouth is water-drop shaped, with a length-width ratio of 2.06:1. The pelvic fins have protruded from both sides of the body, and in the middle of the two pelvic fins, the cloaca can be seen.
Stage 23 (14–15 dpd): The pelvic fins grow on both sides. The olfactory placode is clearly visible at the front of the head (Figure 4). One side of the eye cup is growing vigorously, resulting in a water-drop shape. The aspect ratio of the mouth is 1.91:1. The external gill filaments have begun to grow.
Stage 24 (16 dpd): Two dorsal fin buds can be seen. The anterior portion of the pectoral fins begins to grow. The external gills are approximately 0.5 mm long. The length-width ratio of the mouth is 1.78:1.
Stage 25 (17 dpd): The anterior end of the pectoral fins is close to the sixth gill arch. The pelvic fins expand outside. The aspect ratio of the mouth is 1.20:1.
Stage 26 (18–20 dpd): The front end of the pectoral fins is close to the sixth gill arch. The two dorsal fin buds are clearly visible. The mouth is changing its shape to a diamond-shape, with a length-width ratio of 0.92:1, and the globular lens can be seen in the circular optic cups. At this stage, the width of the pelvic fins is approximately 0.4 mm (Figure 4). The external gill filaments continue to elongate.
Stage 27 (21–23 dpd): Three dorsal fin buds can be seen. The eyes do not have pigmentation (Figure 4). The aspect ratio of the mouth is 0.90:1.
Stage 28 (24–25 dpd): The maxilla and mandible gradually close, converting the mouth from being diamond-shaped to bell-shaped. The length-width ratio of the mouth is 0.76:1, and olfactory pits can be seen (Figure 4).
Stage 29 (25–27 dpd): The front ends of the pectoral fins sharpen and start to bend inward. The lens are C-shaped and pigments starts to accumulate on both of its sides. The structure of external gill filaments is clearly visible, which is a blood capillary loop with some red blood cells in it. Olfactory pits elongate, resulting in two nasal-flap buds. In this stage, the length-width ratio of the mouth is 0.65:1. The width of the pelvic fins is approximately 0.5 mm (Figure 4).
Stage 30 (28–31 dpd): The bent pectoral fins mark this stage, and the shape of the mouth becomes oval, with a length-width ratio of 0.51:1. The pigment in the eye is thicker than it was at the Stage 29. The pigment is now U-shaped (Figure 5).
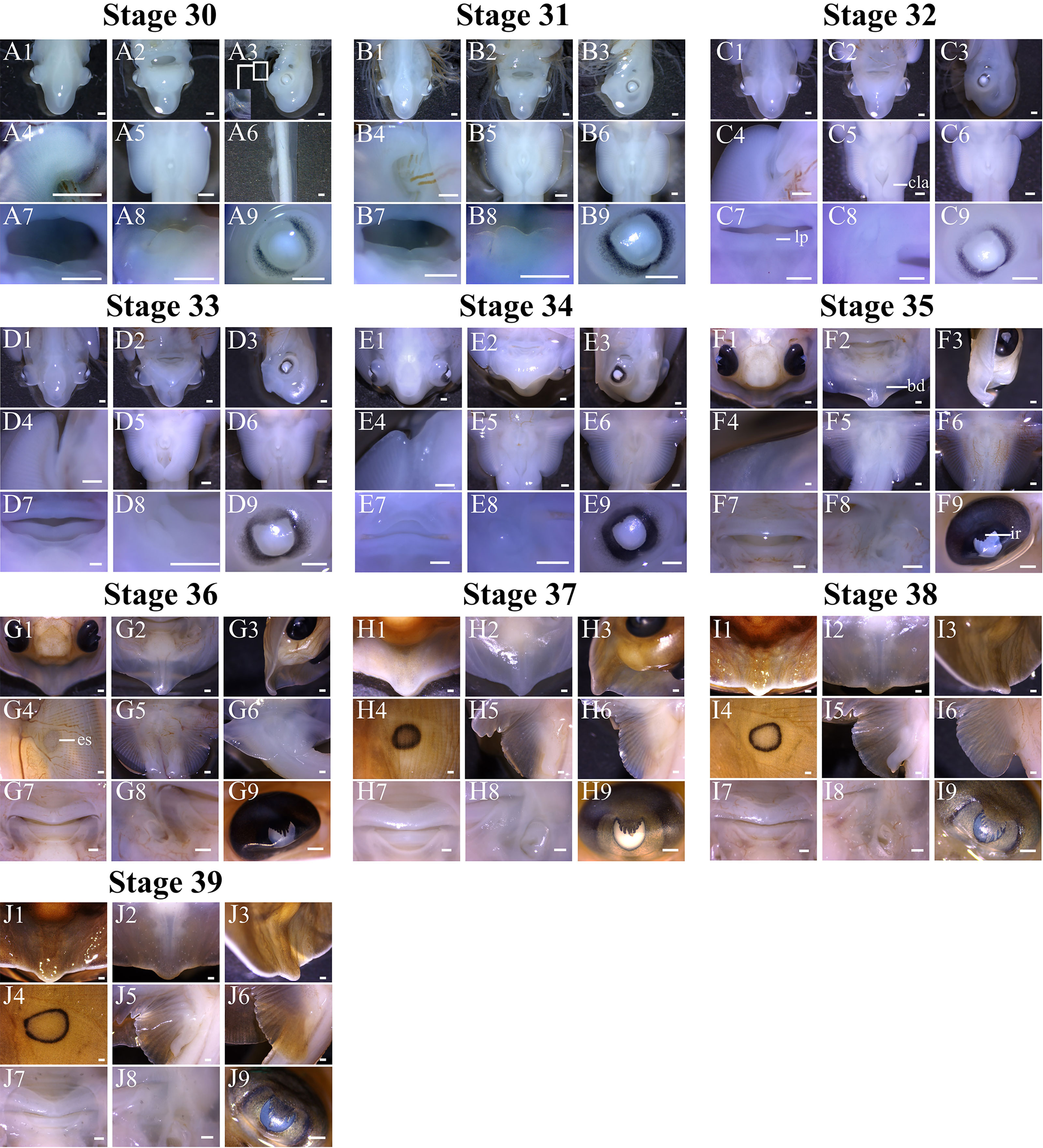
Figure 5 Key characteristics of O. kenojei embryos in developmental stages 30–39. Bar = 0.05 cm. The pictures of each stage labeled with 1, 2, and 3 show the changes in the embryo’s head. The labels (A4, B4, C4, D4, E4, F4) indicate the pectoral fin. (G4, H4, I4, J4) show the eyespot. (A5, B5, C5, D5, E5, F5, G5, H5, I5, J5) indicate the development of male pelvic fin. (A6) shows the dorsal fin. (B6, C6, D6, E6, F6, G6, H6, I6, J6) show the development of the female pelvic fin. The pictures of each stage labeled with 7, 8, and 9 show the development of the embryo’s mouth, nose, and eyes, respectively. cla, clasper; lp, lip; bd, body disc; ir, irises; es, eyespot.
Stage 31 (31–33 dpd): The most striking feature at this stage is the differentiation of the pelvic fins. At this stage the males and females begin to develop differently. In males, the end of the pelvic fins expands inward, which will develop into claspers, whereas in the females, they do not expand. Meanwhile, the front part of the pectoral fins is hook-shaped. The aspect ratio of the mouth is 0.34:1 (Figure 5).
Stage 32 (34–41 dpd): Around arches, the lips can be seen. In this stage, because of the further closure of the maxilla and mandible, mouth shows a slit-like shape with an aspect ratio of 0.11:1. The inner-curved edges of the front part of the pectoral fins start to straighten. Pelvic fins are butterfly-shaped, and the pelvic fin girdle begins to form.
Stage 33 (41–45 dpd): The pigment accumulates around the lens with a circular shape. The snout is shown in front of the head, fusing with the pectoral fins. The aspect ratio of the mouth is 0.01:1 (Figure 5).
Stage 34 (46–50 dpd): The mouth can fully close. The pectoral fins expand forward, starting to form the body disc. However, there are still two gaps on both sides of the head. The pigment shows a palm-like shape above the lens, elongating downward. The male pelvic fins form a lobe-like structure at their posterior end. The skin of the embryo shows pigmentation (Figure 5).
Stage 35 (51–58 dpd): The body disc forms fully, and the anterior part of the body disc curls up. The whole eyes are black, in addition to the pupils. Palm-like irises are clearly visible. The male pelvic fins are transforming into the shape of a club (Figure 5).
Stage 36 (59–62 dpd): The body disc is clearly visible. The edge of the snout at the anterior of the body disc is visible. The eyespots first appear on the dorsal side of the embryo, with a ring-like shape. Golden pigment starts accumulating around the pupils. At this stage, the teeth are visible. Nasal flaps form a serrated edge (Figure 5).
Stage 37 (63–71 dpd): The eyespots are clearly visible, and the crura is fully formed. The golden pigment forms a ring structure around the pupils, spreading all around the eyes (Figure 5), except for the area above the palm-like irises, which is brown.
Stage 38 (72–90 dpd): The eyespots become bigger and thicker. At this stage, the whole eyes are golden, except for the pupils, which are covered by the palm-like irises (Figure 5). The area above the palm-like irises is still brown.
Stage 39 (91 dpd until hatching): The external yolk is almost completely absorbed. The external yolk sac is button-shaped and empty. The stomach of the embryo is completely filled with yolk. The egg case has opened (Figure 5). At this stage, the embryo is like a miniature adult but with a whip-like tail and is ready to hatch. After the baby skate comes out of the egg case, if there is no external energy intake, the internal yolk sac will gradually disappear, within 2 weeks (Figure 6).
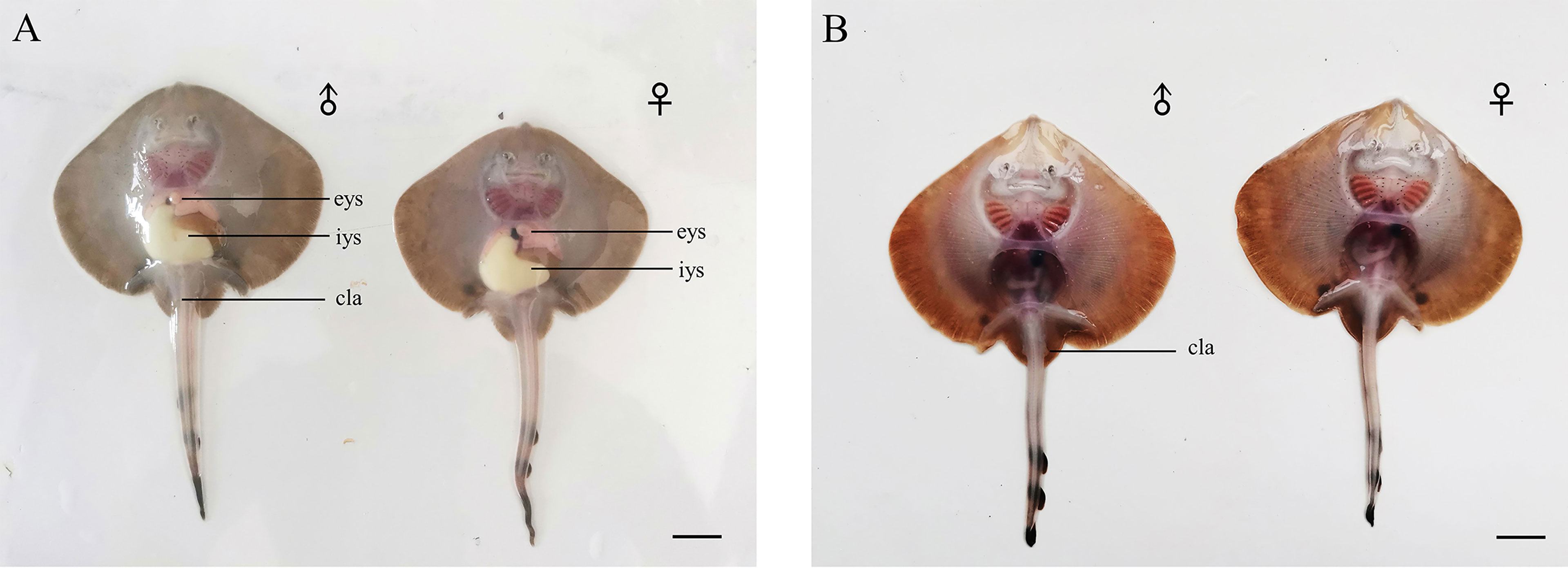
Figure 6 The Kong skate larvae. (A) Newly hatched larvae possess a large internal yolk sac. (B) Two weeks after hatching, the internal yolk sac of larvae disappears. Bar=1cm; eys, external yolk sac; iys, internal yolk sac; cla, clasper.
Summary of the Embryonic Development of the Kong Skate
To visualize and compare these development events at each stage, we illustrated the embryonic development timeline of the Kong skate using 10 days as the period to identify the main changes in each organ, i.e., the identification was done every 10 days. This helps us to compare different developmental events along the timeline (Figure 7). The results showed that the development of the mouth and eyes began in the early stage of embryonic development, and the accumulation of eye pigment and the development of the pectoral fin essentially began at the same time. At approximately one-third of the way into the embryonic development process, the male and female pelvic fins develop differently, and the male copulatory organs begin to develop. Eyespots appear at approximately two-third of the way into the embryonic development process, expanding gradually until hatching.
Comparative Embryonic Development in Skates and Sharks
By comparing the staging schemes of sharks and skates, we found that although the staging standards and limitations of samples vary in different species, which lead to the variation in the quantity of stages and the unknown in some development processes, the main developmental events in the embryonic development timeline of sharks and skates are conservative (Figure 8). Similarly, the timeline of O. kenojei in this study is paralleled to others.
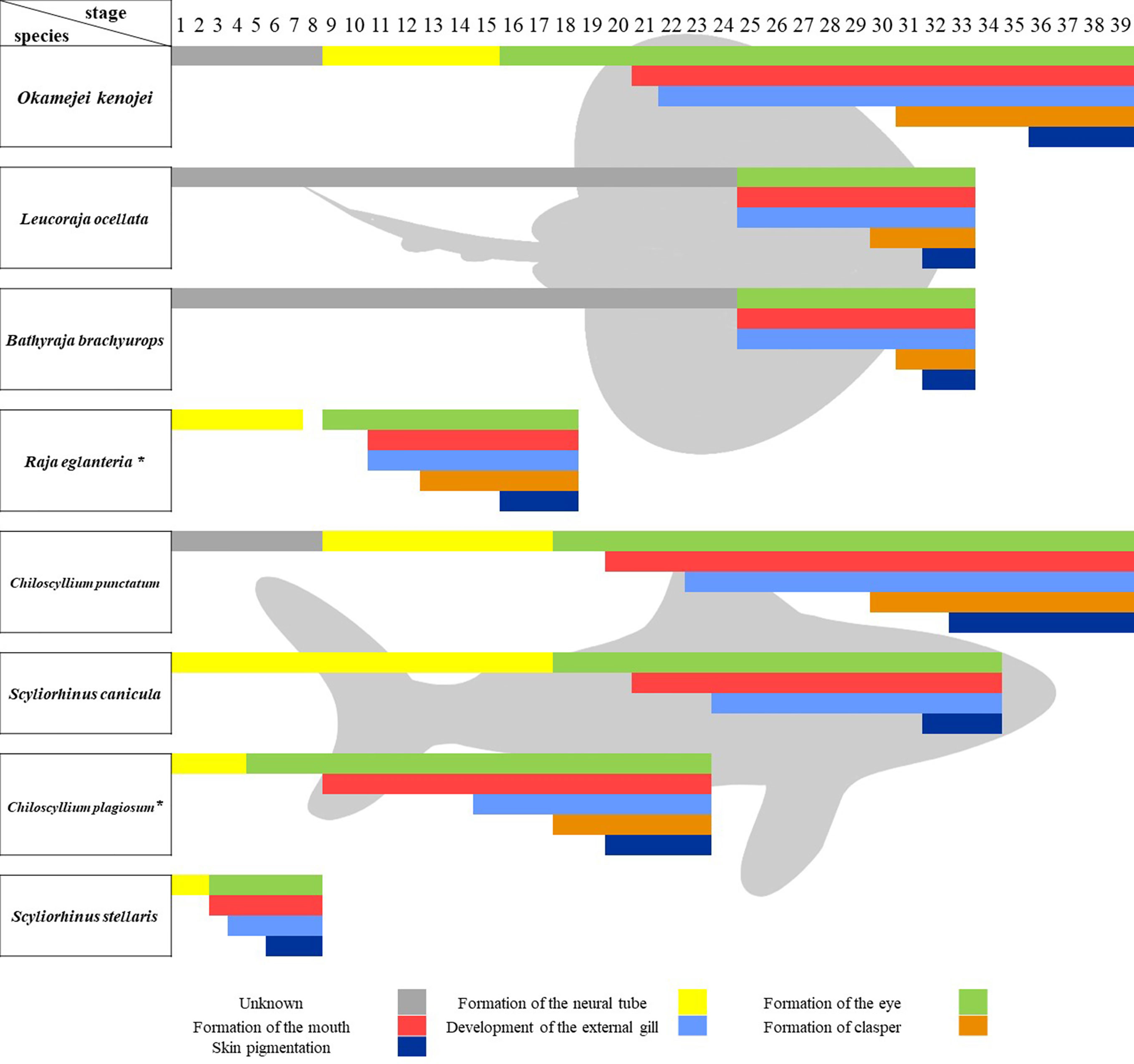
Figure 8 Comparison of embryonic development timeline in sharks and skates. Comparison of the main developmental events in developing sharks and skates. *stages are divided according to the development time and pictures in the research.
Discussion
In this study, we established a detailed developmental timeline for the Kong skate, hatching eggs of the Kong skate (Okamejei kenojei) at fixed temperatures (16.5°C ± 0.5°C). It was found that the complete embryonic development from gastrula to skate larvae took 92 ± 5 days. The descriptive terminology and staging standard of developing skate morphology were mostly based on the standard of sharks established by Onimaru and Ballard (Ballard et al., 1993; Onimaru et al., 2018) and our result is likely to set a series of normal stages for the development of skates by comparing with sharks, and is applicable cross-species. It is well known that skates are different from sharks, as is the development of their embryos. However, it is complex to compare the differences between sharks and skates, as the staging standard and conditions of incubation varies in different research (Figure 7). Here, we used some developmental events as bars in the timeline of developing embryos to compare cross-species. Ballard’s research is a systematic study of the shark’s embryonic development with 34 stages (Ballard et al., 1993), but it did not provide more details about the development of clasper. The research of Chiloscyllium punctatum conducted by Onimaru raises the highest stage number to 39, describing more details after stage 31 and the key stage of clasper development (Onimaru et al., 2018). Our results are closely paralleled to the record of developing C. punctatum. Especially the development of the mouth, external gill and clasper. However, there are two more stages we used to describe the eye development process, as we found that the eye development in skate is not only a process of pigmentation, but also changes of iris and the color of pigment types, which haven’t been reported before. In addition, in our staging scheme, the skin pigmentation starts from stage 36, while its starts from stage 33 in C. punctatum. To best of our knowledge, this study is the first detailed staging scheme in skates. Luer’s research set a representative timeline of developing skate, but the macroscopic observation of these embryos ignored many details of the development process (Luer et al., 2007), like the development of pelvic fins. Research conducted by Maxwell and Vazquez have recorded some key development stages of pelvic fins and pectoral fins in skate (Maxwell et al., 2008; Vazquez et al., 2020), but the other stages are unknown. Here, we have recorded the complete embryonic development from gastrula to skate larvae with more details, although it kept unknown before stage 9, where the embryos develop in female oviducts. Moreover, we found that although the staging standard varies in different research, the main developmental process in the embryonic timeline was conservative, like the onset time of clasper development was between the onset time of external gill development and skin pigmentation. This result could help researchers optimize the staging scheme of different species by adding some necessary stages between developmental events.
One of the interesting embryonic development events in O. kenojei is the formation of the iris. To the best of our knowledge, this is the first study to record the eye development of skates in detail. At stage 29, the pigment starts accumulating on both sides of the crystalline lens, with a C-shape. At stage 34, the pigment shows a palm-like shape above the lens, elongating downward. Ultimately, a palm-like golden iris can be seen, resulting in the irregular shape of the pupil. Some sharks living in turbid water also have special tapetal reflectance, which is different from the population that lives in transparent water (Litherland et al., 2009). Therefore, we conjectured that the pigment on the iris of elasmobranch fishes might help them to effectively reflect the diffuse light in the water so that the images projected onto the retina of the eye have clear edges that are easy to detect. Traits of organisms are adapted to the environment, and the differences in traits come about through changes in development. In some species, like bluespotted ray Neotrygon kuhlii and eastern shovelnose ray Aptychotrema rostrate, the shape and structure of iris can help them adapt to the environment (Douglas, 2018). The palm-like iris of Kong skate may also have the same function. However, very little attention has been paid to the development of iris in elasmobranchs. It still remained unanswered whether the development of iris is similar among elasmobranchs living in the same environment (Youn et al., 2019).
Claspers of chondrichthyans are one of the complex copulatory organs among vertebrates. Research on copulatory organs may shed light on the evolution of sex and copulation (Ahlberg et al., 2009; Long et al., 2014; Trinajstic et al., 2015). However, very little attention has been paid to the development of claspers. Here, we describe the development of male skate claspers that appear to form at stage 31. Due to the lack of sex-specific molecular markers, this study could not identify the sex of skate embryos, especially the embryos at the early stage of clasper development. However, we found that this trait is dimorphic among different individuals at the same stage; therefore, it may have a strong correlation with sex. Significantly, research on Leucoraja erinacea showed that androgen plays an important role in the development of male skate claspers at early stages (O'Shaughnessy et al., 2015). However, at that time, more evidence was needed to determine if Leydig cells, a type of cell that secretes androgen, in the testis had completed differentiation.
To the best of our knowledge, we were the first to record the yespot development of O. kenojei. After stage 36, the pigment of the eyespots appears on the dorsal side of the embryo. With the development of the embryo, the eyespots become larger and the pigment becomes thicker. Before hatching, an oval pigment pattern, with a diameter of approximately 5 mm, was clearly visible. Other skates also have a similar pigment pattern (Janez and Sueiro, 2007). Similarly, it is common that with the development of the larvae, the pigment pattern of some elasmobranchs become thinner or disappear, such as bamboo sharks, Chiloscyllium punctatum (Harahush et al., 2007; Onimaru et al., 2018; Kuraku, 2021), Alaska skate, Bathyraja parmifera (Hoff, 2009). Juvenile bamboo sharks have pigmentation bands on their skin, but it is hard to identify the edges of each pigmentation band in adult skin. Generally, pigmentation bands are most commonly found in juvenile sharks, while eyespots are normally present in juvenile batoids. Pigment patterns vary considerably between these groups. It is interesting to question how elasmobranch fishes got these different colored patterns and do these patterns play the same ecological role.
Conclusion
In this study, we provide the first detailed record of the embryonic development of O. kenojei. The entire embryonic development process was divided into 39 stages. A breakdown of these stages shows that the difference between males and females appeared after stage 31, and the jaw of O. kenojei developed from the first pair of pharyngeal arches. The body disc of O. kenojei is formed at stage 35 by fusing the pectoral fins and head. Moreover, the eyespots began to form from stage 36, becoming thicker and bigger until the young skate broke out of the egg. To our knowledge, this is the first study to record the embryonic development of O. kenojei in detail.
Data Availability Statement
The original contributions presented in the study are included in the article/supplementary material. Further inquiries can be directed to the corresponding authors.
Ethics Statement
The animal study was reviewed and approved by Institutional Animal Care and Use Committee (IACUC) at the Institute of Oceanology, Chinese Academy of Sciences (IOCAS).
Author Contributions
GG was responsible for the whole experiment design and operation. XZ was responsible for providing the overall idea, and others assisted in sampling. All authors contributed to the article and approved the submitted version.
Funding
This work was supported by National Key Research and Development Program (2018YFD0901204), Key Special Project for Introduced Talents Team of Southern Marine Science and Engineering Guangdong Laboratory (Guangzhou) (GML2019ZD0402), Major Agricultural Application Technology Innovation Project of Shandong Province (SD2019YY011), Qingdao National Laboratory for Marine Science and Technology (2018SDKJ0502-2), China Agriculture Research System (CARS-47), Major Science and Technology for Scientific and Technological Innovation Projects (Shandong) (2019JZZY020710), and STS Project (KFZD-SW-106, ZSSD-019, 2017T3017, 2019T3022).
Conflict of Interest
The authors declare that the research was conducted in the absence of any commercial or financial relationships that could be construed as a potential conflict of interest.
Publisher’s Note
All claims expressed in this article are solely those of the authors and do not necessarily represent those of their affiliated organizations, or those of the publisher, the editors and the reviewers. Any product that may be evaluated in this article, or claim that may be made by its manufacturer, is not guaranteed or endorsed by the publisher.
References
Ahlberg P., Trinajstic K., Johanson Z., Long J. (2009). Pelvic Claspers Confirm Chondrichthyan-Like Internal Fertilization in Arthrodires. Nature 460, 888–889. doi: 10.1038/nature08176
Baeck G. W., Park C. I., Choi H. C., Huh S. H., Park J. M. (2011). Feeding Habits of Ocellate Spot Skate, Okamejei Kenojei (Müller & Henle 1841), in Coastal Waters of Taean, Korea. J. Appl. Ichthyol. 27, 1079–1085. doi: 10.1111/j.1439-0426.2011.01751.x
Baker C. V.H., Modrell M. S., Gillis J.A. (2013). The Evolution and Development of Vertebrate Lateral Line Electroreceptors. J. Exp. Biol. 216, 2515–2522. doi: 10.1242/jeb.082362
Ballard W. W., Mellinger J., Lechenault H. (1993). A Series of Normal Stages for Development of Scyliorhinus Canicula, the Lesser Spotted Dogfish (Chondrichthyes: Scyliorhinidae). J. Exp. Zool. 267, 318–336. doi: 10.1002/jez.1402670309
Barry S. N., Crow K. D. (2017). The Role of HoxA11 and HoxA13 in the Evolution of Novel Fin Morphologies in a Representative Batoid (Leucoraja Erinacea). EVODEVO 8, 24. doi: 10.1186/s13227-017-0088-4
Bezerra M. F., Lacerda L. D., Lai C. T. (2019). Trace Metals and Persistent Organic Pollutants Contamination in Batoids (Chondrichthyes: Batoidea): A Systematic Review. Environ. Pollut. 248, 684–695. doi: 10.1016/j.envpol.2019.02.070
Clark R. S. (1927). Rays and Skates. No. 2-Description of Embryos. J. Mar. Biol. Assoc. U.K. 14, 661–683. doi: 10.1017/S0025315400058409
Criswell K. E., Coates M. I., Gillis J. A. (2017). Embryonic Development of the Axial Column in the Little Skate, Leucoraja Erinacea. J. Morphol. 278 (3), 300–320. doi: 10.1002/jmor.20637
Criswell K. E., Gillis J.A. (2020). Resegmentation is an Ancestral Feature of the Gnathostome Vertebral Skeleton. Elife 9, e51696. doi: 10.7554/elife.51696
Criswell K. E., Roberts L. E., Koo E. T., Head J. J., Gillis J.A. (2021). Hox Gene Expression Predicts Tetrapod-Like Axial Regionalization in the Skate, Leucoraja Erinacea. Proc. Natl. Acad. Sci. U. S. A. 118, e2114563118. doi: 10.1073/pnas.2114563118
Dahn R. D., Davis M. C., Pappano W. N., Shubin N. H. (2007). Sonic hedgehog function in chondrichthyan fins and the evolution of appendage patterning. Nature 445, 311–314. doi: 10.1038/nature05436
Debiais-Thibaud M., Chiori R., Enault S., Oulion S., Germon I., Martinand-Mari C., et al (2015). Tooth and Scale Morphogenesis in Shark: An Alternative Process to the Mammalian Enamel Knot System. BMC Evol. Biol. 15, 292. doi: 10.1186/s12862-015-0557-0
Douglas R. H. (2018). The Pupillary Light Responses of Animals; A Review of Their Distribution, Dynamics, Mechanisms and Functions. Prog. Retin. Eye Res. 66, 17–48. doi: 10.1016/j.preteyeres.2018.04.005
Ebert D. A., Sulikowski J. A. (2007). Preface: Biology of Skates. Environ. Biol. Fishes 80, 107–110. doi: 10.1007/s10641-007-9244-3
Enault S., Auclair C., Adnet S., Debiais-Thibaud M. (2016). A Complete Protocol for the Preparation of Chondrichthyan Skeletal Specimens. J. Appl. Ichthyol. 32, 409–415. doi: 10.1111/jai.13050
Enault S., Muñoz D. N., Silva W. T. A. F., Borday-Birraux V., Bonade M., Oulion S., et al (2015). Molecular Footprinting of Skeletal Tissues in the Catshark Scyliorhinus Canicula and the Clawed Frog Xenopus Tropicalis Identifies Conserved and Derived Features of Vertebrate Calcification. Front. Genet. 6. doi: 10.3389/fgene.2015.00283
Enault S., Muñoz D., Simion P., Ventéo S., Sire J.-Y., Marcellini S., et al (2018). Evolution of Dental Tissue Mineralization: An Analysis of the Jawed Vertebrate SPARC and SPARC-L Families. BMC Evol. Biol. 18, 127. doi: 10.1186/s12862-018-1241-y
Freitas R., Zhang G., Cohn M. J. (2006). Evidence That Mechanisms of Fin Development Evolved in the Midline of Early Vertebrates. Nature 442, 1033–1037. doi: 10.1038/nature04984
Gillis J.A., Dahn R. D., Shubin N. H. (2009). Shared Developmental Mechanisms Pattern the Vertebrate Gill Arch and Paired Fin Skeletons. Proc. Natl. Acad. Sci. U. S. A. 106, 5720–5724. doi: 10.1073/pnas.0810959106
Gillis J.A., Hall B. K. (2016). A Shared Role for Sonic Hedgehog Signalling in Patterning Chondrichthyan Gill Arch Appendages and Tetrapod Limbs. Development 143, 1313–1317. doi: 10.1242/dev.133884
Gillis J.A., Modrell M. S., Baker C. V.H. (2013). Developmental Evidence for Serial Homology of the Vertebrate Jaw and Gill Arch Skeleton. Nat. Commun. 4, 1436. doi: 10.1038/ncomms2429
Harahush B. K., Fischer A. B. P., Collin S. P. (2007). Captive Breeding and Embryonic Development of Chiloscyllium Punctatum Muller & Henle 1838 (Elasmobranchii: Hemiscyllidae). J. Fish Biol. 71, 1007–1022. doi: 10.1111/j.1095-8649.2007.01569.x
Hirschberger C., Sleight V. A., Criswell K. E., Clark S. J., Gillis J.A. (2021). Conserved and Unique Transcriptional Features of Pharyngeal Arches in the Skate (Leucoraja Erinacea) and Evolution of the Jaw. Mol. Biol. Evol. 38, 4187–4204. doi: 10.1093/molbev/msab123
Hoff G. R. (2009). Embryo Developmental Events and the Egg Case of the Aleutian Skate Bathyraja Aleutica (Gilbert) and the Alaska Skate Bathyraja Parmifera (Bean). J. Fish Biol. 74 (3), 483–501. doi: 10.1111/j.1095-8649.2008.02138.x
Ishihara H. (1987). Revision of the Western North Pacific Species of the Genus Raja. Jpn. J. Ichthyol. 34, 241–285. doi: 10.1007/BF03380117
Janez J. A., Sueiro M. C. (2007). Size at Hatching and Incubation Period of Sympterygia Bonapartii (Müller & Henle 1841) (Chondrichthyes, Rajidae) Bred in Captivity at the Temaiken Aquarium. J. Fish Biol. 70, 648–650. doi: 10.1111/j.1095-8649.2007.01332.x
Johanson Z., Martin K., Fraser G., James K. (2019). The Synarcual of the Little Skate, Leucoraja Erinacea: Novel Development Among the Vertebrates. Front. Ecol. Evol. 5. doi: 10.3389/fevo.2019.00012
Jung H., Baek M., D'Elia K. P., Boisvert C., Currie P. D., Tay B.-H., et al (2018). The Ancient Origins of Neural Substrates for Land Walking. Cell 172, 667–682. doi: 10.1016/j.cell.2018.01.013
Kuraku S. (2021). Shark and Ray Genomics for Disentangling Their Morphological Diversity and Vertebrate Evolution. Dev. Biol. 477, 262–272. doi: 10.1016/j.ydbio.2021.06.001
Litherland L., Collin S. P., Fritsches K. A. (2009). Visual Optics and Ecomorphology of the Growing Shark Eye: A Comparison Between Deep and Shallow Water Species. J. Exp. Biol. 212, 3583–3594. doi: 10.1242/jeb.028589
Long J. A., Mark-Kurik E., Johanson Z., Lee M. S.Y., Young G. C., Min Z., et al (2014). Copulation in Antiarch Placoderms and the Origin of Gnathostome Internal Fertilization. Nature 517, 196–199. doi: 10.1038/nature13825
Luer C. A., Walsh C. J., Bodine A. B., Wyffels J. T. (2007). Normal Embryonic Development in the Clearnose Skate, Raja Eglanteria, With Experimental Observations on Artificial Insemination. Environ. Biol. Fishes 80, 239–255. doi: 10.1007/s10641-007-9219-4
Marconi A., Hancock-Ronemus A., Gillis J. A. (2020). Adult Chondrogenesis and Spontaneous Cartilage Repair in the Skate, Leucoraja Erinacea. eLife 9, e53414. doi: 10.7554/eLife.53414
Maxwell E. E., Frobisch N. B., Heppleston A. C. (2008). Variability and Conservation in Late Chondrichthyan Development: Ontogeny of the Winter Skate (Leucoraja Ocellata). Anat. Rec. Adv. Integr. Anat. Evol. Biol. 291, 1079–1087. doi: 10.1002/ar.20719
Misawa R., Narimatsu Y., Endo H., Kai Y. (2019). Population Structure of the Ocellate Spot Skate (Okamejei Kenojei) Inferred From Variations in Mitochondrial DNA (mtDNA) Sequences and From Morphological Characters of Regional Populations. Fish. Bull. 117, 24–36. doi: 10.7755/FB.117.1.4
Musa S. M., Czachur M. V., Shiels H. A. (2018). Oviparous Elasmobranch Development Inside the Egg Case in 7 Key Stages. PloS One 13, e0206984. doi: 10.1371/journal.pone.0206984
Nakamura T., Gehrke A. R., Lemberg J., Szymaszek J., Shubin N. H. (2016). Digits and Fin Rays Share Common Developmental Histories. Nature 537, 225. doi: 10.1038/nature19322
Nakamura T., Klomp J., Pieretti J., Schneider I., Gehrke A. R., Shubin N. H. (2015). Molecular Mechanisms Underlying the Exceptional Adaptations of Batoid Fins. Proc. Natl. Acad. Sci. U. S. A. 112, 15940–15945. doi: 10.1073/pnas.1521818112
O'Shaughnessy K. L., Dahn R. D., Cohn M. J. (2015). Molecular Development of Chondrichthyan Claspers and the Evolution of Copulatory Organs. Nat. Commun. 6, 6698. doi: 10.1038/ncomms7698
Onimaru K., Motone F., Kiyatake I., Nishida K., Kuraku S. (2018). A Staging Table for the Embryonic Development of the Brownbanded Bamboo Shark (Chiloscyllium Punctatum). Dev. Dyn. 247, 712–723. doi: 10.1002/dvdy.24623
Pears J. B., Johanson Z., Trinajstic K., Dean M. N., Boisvert C. A. (2020). Mineralization of the Callorhinchus Vertebral Column (Holocephali; Chondrichthyes). Front. Genet. 11. doi: 10.3389/fgene.2020.571694
Sleight V. A., Gillis J.A. (2020). Embryonic Origin and Serial Homology of Gill Arches and Paired Fins in the Skate, Leucoraja Erinacea. Elife 9, e60635. doi: 10.7554/eLife.60635
Suriano C. M., Bodznick D. (2018). Morphological Development of the Dorsal Hindbrain in an Elasmobranch Fish (Leucoraja Erinacea). Zool. Lett. 4, 28. doi: 10.1186/s40851-018-0111-1
Swenson J. D., Klomp J., Fisher R. A., Crow K. D. (2018). How the Devil Ray Got its Horns: The Evolution and Development of Cephalic Lobes in Myliobatid Stingrays (Batoidea: Myliobatidae). Front. Ecol. Evol. 6. doi: 10.3389/fevo.2018.00181
Tanaka M., Münsterberg A., Anderson W.G., Prescott A. R., Hazon N., Tickle C. (2002). Fin Development in a Cartilaginous Fish and the Origin of Vertebrate Limbs. Nature 416, 527–531. doi: 10.1038/416527a
Trinajstic K., Boisvert C., Long J., Maksimenko A., Johanson Z. (2015). Pelvic and Reproductive Structures in Placoderms (Stem Gnathostomes). Biol. Rev. 90, 467–501. doi: 10.1111/brv.12118
Turner N., Mikalauskaite D., Barone K., Flaherty K., Senevirathne G., Adachi N., et al (2019). The Evolutionary Origins and Diversity of the Neuromuscular System of Paired Appendages in Batoids. Proc. R. Soc B. 286, 20191571. doi: 10.1098/rspb.2019.1571
Vazquez D. M., de Astarloa J. M. D., Gabbanelli V., Mabragana E. (2020). Comparative Embryonic Development Patterns in Three Deep-Water Skates From the Southwest Atlantic. Deep Sea Res. Part I Oceanogr. Res. Pap. 161, 103301. doi: 10.1016/j.dsr.2020.103301
Venkatesh B., Lee A. P., Ravi V., Maurya A. K., Lian M. M., Swann J. B., et al (2014). Elephant Shark Genome Provides Unique Insights Into Gnathostome Evolution. Nature 505, 174–179. doi: 10.1038/nature12826
Wang Z. M. (1982). Preliminary Observation on Embryonic Development of Raja Porosa. J. Fish. China 02, 153–163.
Wang P. B., Al Azad M. A. R., Yang X., Martelli P. R., Cheung K. Y., Shi J. H., et al (2021). Self-Adaptive and Efficient Propulsion of Ray Sperms at Different Viscosities Enabled by Heterogeneous Dual Helixes. Proc. Natl. Acad. Sci. 118, e2024329118. doi: 10.1073/pnas.2024329118
Xie Y. J., Wang J., Su Y. Q., Xu X. J., Fu M. N. (2009). Study on Embryonic Development of White Spotted Bambooshark, Chiloscyllium Plagiosum. J. Fish. China. 33, 742–754.
Yang J. X., Jun W., Yong Q. ,. S., Jin X. X., Meng N. F. (2009). Study on Embryonic Development of White Spotted Bambooshark, Chiloscyllium Plagiosum. J. Fish. China 33, 742–754.
Keywords: skate, batoids, Okamejei kenojei, embryonic stages, development
Citation: Guang G, Zhizhong X, Yuting M, Xiao C, Yongshuang X and Jun L (2022) How the Skate Embryo Develops: A Fine Developmental Timeline of Kong Skate Okamejei kenojei (Müller & Henle, 1841). Front. Mar. Sci. 9:831926. doi: 10.3389/fmars.2022.831926
Received: 09 December 2021; Accepted: 19 April 2022;
Published: 18 May 2022.
Edited by:
Cristina Porcu, University of Cagliari, ItalyReviewed by:
Ernesto Maldonado, National Autonomous University of Mexico, MexicoMarleen Klann, Okinawa Institute of Science and Technology Graduate University, Japan
Xin Qi, Ocean University of China, China
Copyright © 2022 Guang, Zhizhong, Yuting, Xiao, Yongshuang and Jun. This is an open-access article distributed under the terms of the Creative Commons Attribution License (CC BY). The use, distribution or reproduction in other forums is permitted, provided the original author(s) and the copyright owner(s) are credited and that the original publication in this journal is cited, in accordance with accepted academic practice. No use, distribution or reproduction is permitted which does not comply with these terms.
*Correspondence: Xiao Zhizhong, eHp6QHFkaW8uYWMuY24=; Li Jun, anVubGlAcWRpby5hYy5jbg==