- 1Resource Management Working Group, Leibniz Centre for Tropical Marine Research, Bremen, Germany
- 2Center for Ocean and Society, Christian-Albrechts-University, Kiel, Germany
- 3Laboratorio Costero de Paita, Instituto del Mar del Perú, Piura, Peru
The coast of Peru lies within the tropics under the influence of the cold, nutrient-rich waters of the Humboldt Current and the interannual onslaught of the El Niño phenomenon. The Peruvian upwelling system is exceptionally productive and is comprised of subsystems at different scales along the coast. We aimed to understand the differences between two shallow coastal systems along a latitudinal gradient: Sechura Bay in the north (at the convergence of Humboldt and tropical waters) and Independencia Bay in the central-south (under typical upwelling conditions). We compared their biodiversity, trophic dynamics, community energetics, resource use and underlying abiotic conditions. Our analysis revealed that over the past two decades, Sechura has shown a warming trend, while Independencia has maintained its cold water conditions. Chlorophyll concentrations have risen significantly in both systems, higher values in Sechura suggesting there is an increase in local pressures that could lead to eutrophication. Trophic models of the La Niña 07/08 period revealed that both systems are bottom-up driven with high biomass and production at the lower trophic levels, though top-down controls were also shown, particularly in Sechura. While primary productivity was similar in both systems, differences were found in the structure and size of energy flows. More cycling and higher transfer efficiency were found in Independencia, where phytoplankton-based food chains played the main role in the overall dynamic. In contrast, the detritus food chain appears to be more relevant for energy flow in Sechura. Differences in biota and flow structure relate to the systems’ environmental conditions, i.e., more diverse warm-water species in the north and mostly cold water adapted species (mainly invertebrate filter-feeders and their predators) in the central-south. Catches in both systems were dominated by the diving fisheries and comprised mostly scallops (bottom-cultured), snails and fish in Sechura, and mussels, clams, crabs and fish in Independencia. Overall, system indicators suggest that Sechura is a comparatively less developed system. Independencia shall likely maintain its general highly productive system features, whereas Sechura will continue to be more frequently disturbed by El Niño and ongoing human-driven activities, reducing its overall stability and functionality. In the context of climate change, acknowledging these differences is essential for future adaptive management regimes.
Introduction
Coastal marine areas typically host highly productive ecosystems that support artisanal and industrial fisheries, often vital for local, regional and global economies. In the tropics, despite the year-round high light intensity, productivity is generally lower, though this will also depend on local physical and biogeochemical processes, such as upwelling conditions, light penetration or nutrient input from land (e.g., Forrest et al., 2007). The Peruvian coast is peculiar in that it lies within the tropical region of western South America (∼3.4–18.3° South) under the influence of the cold nutrient-rich waters of the Northern Humboldt Current Upwelling System (NHCUS); one of the four highly productive eastern boundary currents and the most important in terms of catches (Bakun, 1996; Chavez et al., 2008; Heileman et al., 2009; Gutiérrez et al., 2016). The upwelling off Peru is particularly strong because the system has several distinct characteristics, such as the almost constant blow of equatorward winds and its closeness to the equator (Bakun and Weeks, 2008; Chavez et al., 2008). These characteristics generate apt conditions for sustained production of phyto- and zooplankton that favor Small pelagic fish but also support higher trophic species of coastal and oceanic food webs (Taylor and Wolff, 2007; Bakun and Weeks, 2008; Chavez et al., 2008; Belmadani et al., 2012; Echevin et al., 2014). The northern boundary of the NHCUS adjoins the warmer and less productive waters of the Pacific Central-American Large Marine Ecosystem (Heileman, 2009; Heileman et al., 2009), creating a transition area that roughly extends from 3–6°S under normal conditions (Tarazona and Arntz, 2001; Graco et al., 2007; Heileman et al., 2009). The high levels of primary production generated within the NHCUS lead to the decay and precipitation of organic matter down to a poorly ventilated and highly shallow Oxygen Minimum Zone (OMZ). This layer acts as a barrier that concentrates many pelagic and benthic species toward the surface and shallow areas (Taylor and Wolff, 2007; Chavez et al., 2008; Guevara-Carrasco and Bertrand(eds), 2017). The Peruvian upwelling system is substantially affected by the El Niño Southern Oscillation (ENSO) at interannual timescales, especially during its warm El Niño (EN) phase. It generates dramatic oceanographic and atmospheric changes that result in heavy rains in the north coast and the “tropicalization” of typical upwelling areas off central and southern Peru (Graco et al., 2007; Heileman et al., 2009; Espinoza-Morriberón et al., 2019), leading to latitudinal changes in species composition due to mortality and migration (Tarazona and Arntz, 2001; Taylor and Wolff, 2007). Besides supporting one of the largest single-species industrial fisheries worldwide, i.e., anchovy Engraulis ringens, the NHCUS also sustains several other coastal and littoral fish and shellfish of economic importance. In particular, shallow enclosed locations, such as uneven sandy and rocky shores or larger bay systems, can serve as refuge, feeding or nursing grounds, offering appropriate conditions for species to establish and fisheries-dependent social-ecological systems (SES) to develop. In subtidal and intertidal systems, particularly above the OMZ, several fishes, crustaceans, molluscs and algae thrive on mainly sandy and rocky bottoms, sustaining several local multi-species and multi-gear artisanal fisheries (Tarazona and Arntz, 2001; Guevara-Carrasco and Bertrand(eds), 2017). Toward northern Peru, in the transition of Humboldt into tropical waters, higher temperatures and a deeper OMZ lead to differences in species composition and higher biodiversity (Guevara-Carrasco and Bertrand(eds), 2017).
Certain areas along the Peruvian shore may be more productive because of their bathymetry, topography and closeness to a prominent upwelling plume (Tarazona and Arntz, 2001). Two such areas, focus of this study, are Sechura Bay in the north (Sechura Province, within the ecotone of Humboldt and tropical waters) and Independencia Bay in the central-south (Pisco Province, under typical upwelling conditions) (Figure 1). Artisanal fisheries are essential for these highly productive bays and while several species are present in both systems, the main target groups differ between the areas. Additionally, both bays sustain significant stocks of Argopecten purpuratus, a highly profitable bay scallop typically harvested through bottom culture and enhanced-fishery systems. Pisco and Sechura have been the focal points of many ENSO-induced (mainly scallop driven) human migrations over the years (Badjeck, 2008; Mendo et al., 2008; Kluger et al., 2018). Although they share many features, these bays differ in their physical and ecological makeups, as well as in their social-ecological responses to environmental and anthropogenic stressors (Taylor et al., 2008a,b; Badjeck et al., 2009; Guevara-Carrasco and Bertrand(eds), 2017; Kluger et al., 2018). The goal of this study was to enable ecological inter-bay comparison and update current knowledge of both systems’ trophic structure and dynamics. Given their distinct geographic locations along the Peruvian upwelling ecosystem and their peculiar social-ecological characteristics and connectivity, we aim to understand how the two bays differ in biodiversity, resource use, community energetics, and underlying abiotic conditions. The differences and similarities thus recognized will shed some light on the systems’ functioning, energy throughput dynamics, developmental state and resilience. We hypothesize that Independencia is the more productive system as it is located adjacent to the main upwelling center off Peru and is not usually subject to frequent, constant environmental disturbances as Sechura, situated in the northern transition zone. We thus expect Independencia to be dominated by planktivorous species and to have a more efficient energy transfer through these plankton-based food chains. In contrast, due to a higher load of suspended particles from river input and the expansion of scallops aquaculture, Sechura is expected to support a higher relative biomass of detritivores and a more considerable diversity of second-level consumers.
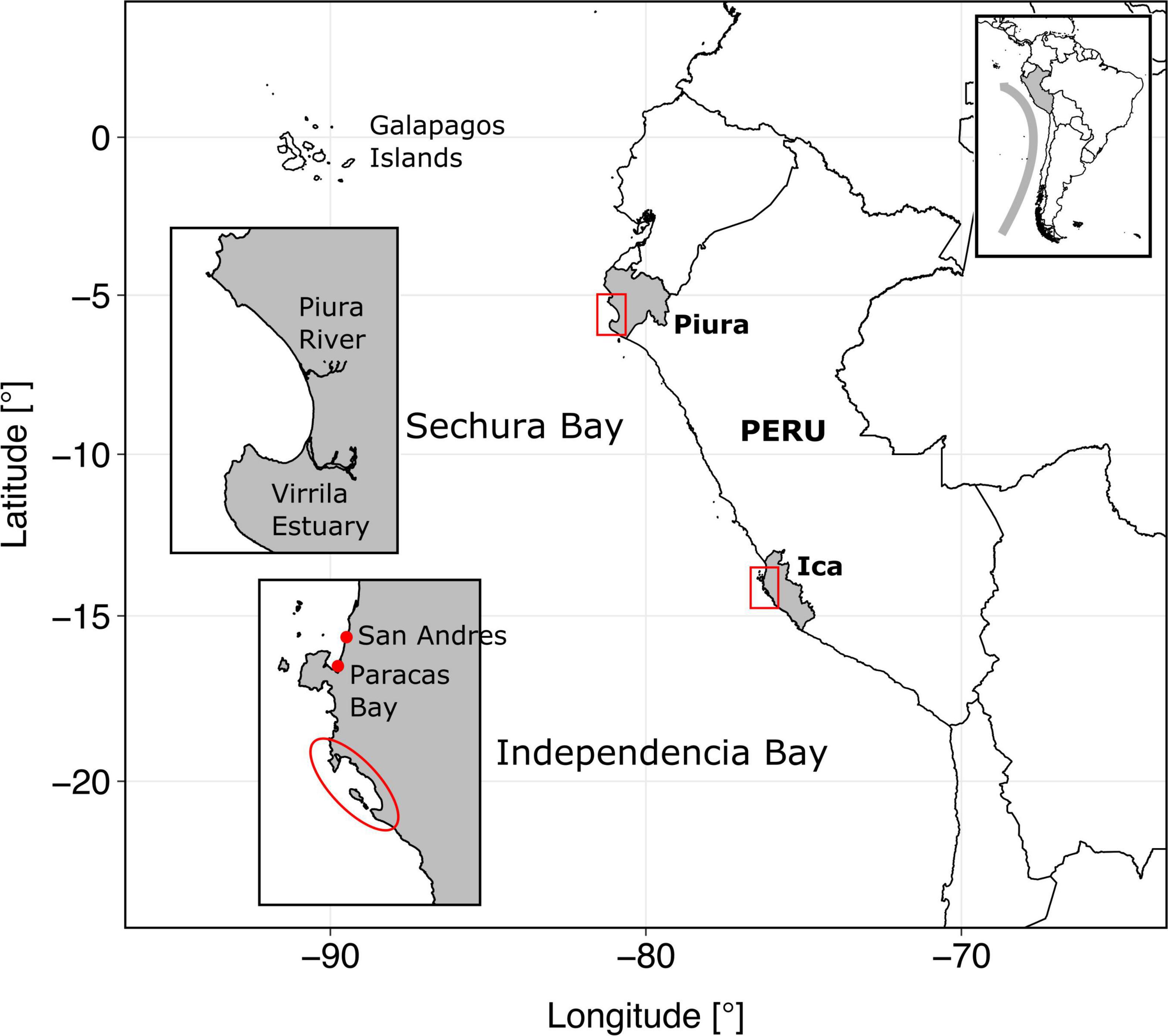
Figure 1. Map of study sites, located in Peru, western coast of South America. The gray curved arrow in the box at the upper right corner represents the Humboldt Current.
The objectives of this study are to (i) use food web analysis to compare the two systems, (ii) analyze their trophic structure, energy flows and resilience, and (iii) discuss the role of fisheries, aquaculture and the environmental envelope. For this purpose, we assembled the available ecological and fisheries data and modeled both systems applying the Ecopath with Ecosim mass balance approach to address such differences in trophic structure and energy flows (Christensen and Pauly, 1992; Christensen and Walters, 2004; Heymans et al., 2016). The Ecopath models built for both systems are intended to serve as the basis for future work on assessing ecological tipping points related to environmental and resource use drivers, emphasizing the dynamics of fisheries and aquaculture.
Materials and Methods
The Study Areas and Their Environmental Envelopes
The Peruvian coastline covers an extension of about 3,000 km of mostly rocky and sandy beaches with several capes and bays. The continental shelf off Peru, defined by the 200 m isobaths, is broader in central Peru (∼65 nautical miles) compared to the northern and southern shores (∼3–25 nautical miles). The two study areas, Sechura and Independencia bays, are located about 1,000 km (∼580 nautical miles) from each other within these narrower portions of the shelf (Figure 1).
Sechura Bay is located in the Piura Region in northern Peru, covering ∼1,000 km2 roughly between 5°10′ and 6°00′ South (at the convergence of Humboldt and tropical waters). The bay can be split into an inner, shallower region (inner bay: about 30 m max depth) in the first 6–10 km from the shore, and a deeper area (outer bay: 60–90 m depth) outward to 35 km distance from the beach. The bay is surrounded by several villages and towns that use, directly or indirectly, the ecosystem services it provides. Among the main economic activities in and around Sechura are fishing, aquaculture, agriculture, mining and tourism. According to the Peruvian Sea Institute (IMARPE, for its Spanish acronym), Sechura Bay has three state-owned landing sites (DPA, in Spanish, which include some infrastructure) and one private pier used for artisanal fleets, plus an industrial (anchovy) landing site in the south tip of Bayovar. It is also known that landings occur directly on the beach of some settlements around the Bay (for example in the fishing village of Constante). Sechura Bay has the capacity to produce, harvest, process and store fish and shellfish under exportation standards.
Independencia Bay lies between 14°10″ and 14°11″ South, adjacent to the southern upwelling center of Peru. It has a smaller area (∼150 km2) than Sechura and is partially enclosed by La Vieja Island. The 30 m isobath lies very close to the shore, fringing at about 1 km. Between this point and the bay’s mouth (∼7 km from the beach), depth varies down to ∼90 m. This bay has no river influx and is surrounded by desertic land with no agricultural systems or human settlements nearby. Economic activities in the bay are fisheries, scallop aquaculture and tourism. It has two main landing sites (according to IMARPE’s data collection) that are only suitable for small-scale activities. The closest important town (and landing site) is Pisco (San Andrés) (Figure 1).
For general environmental comparisons between the systems, we looked into their main oceanographic and atmospheric conditions at different scales (offshore versus shallow bays at different latitudes).
This environmental envelope was mainly assessed using measurements of sea surface temperature (SST) and chlorophyll concentration (Chl-a),which we used as a proxy for primary production through phytoplankton biomass. Both were obtained through available remote sensing data. Time series SST data (2006–2020) were acquired for both bays from the Surface Temperature and Sea Ice Analysis (OSTIA) satellites system (Stark et al., 2007), which records daily average SST (°C) at a high spatial resolution (4 × 4 km). The dataset was supplied by IMARPE and only included values from inside each bay. In the case of Chl-a (mg.m–3), time-series daily average values were provided by IMARPE for the whole Peruvian EEZ (from 1997 to 2019 at 4 km resolution) from the data integration of two satellites: MODIS-Aqua and SeaWifs (NASA). This allowed to filter and compare averaged data from three areas in front of each study site’s approximate latitudinal range: going from inside the bays toward the offshore and beyond the continental shelf.
During EN events, SST quickly rises in northern Peru (>25°C), leading to tropical-like storms, heavy rainfall and a dramatic freshwater runoff into Sechura Bay, mainly reducing salinity in the system. This water input can also carry particulate matter, nutrients and various pollutants from agricultural land and populated areas nearby, reducing oxygen availability. In Independencia Bay, there can be an SST rise of about 10°C and an increase of oxygen levels in deeper areas, which is very beneficial for many resources, in particular, scallops (Arntz and Tarazona, 1990; Arntz et al., 1991; Mendo and Wolff, 2003; Taylor et al., 2008a). Given the substantial impacts of ENSO, there is constant monitoring of atmospheric and oceanographic conditions along four regions of the equatorial Pacific, using in situ measurements and remote sensing (Takahashi and Dewitte, 2016). For this study, we used the decades-long remote sensing SST anomalies reported by the US National Oceanographic and Atmospheric Agency (NOAA) for the farthest eastern Pacific region, i.e., NIÑO 1 + 2 (Figure 2). These data were used to compare the SST fluctuations and the clearly defined El Niño (EN) and La Niña (LN) periods seen in this broader, general area offshore northern Peru, with the data obtained from the bay systems under study. Based on the information shown in Figure 2, we chose to focus on the cold LN 2007–2008 period for the present study since this period showed the lowest anomalies since 1916 and 1988. The snapshot is also midway between the extraordinary EN 97–98 and the very particular strong EN 2016 (coastal EN). In addition, this year is within a stable period of more than 15 years since many decades, which would have allowed the systems to develop under relatively constant conditions.
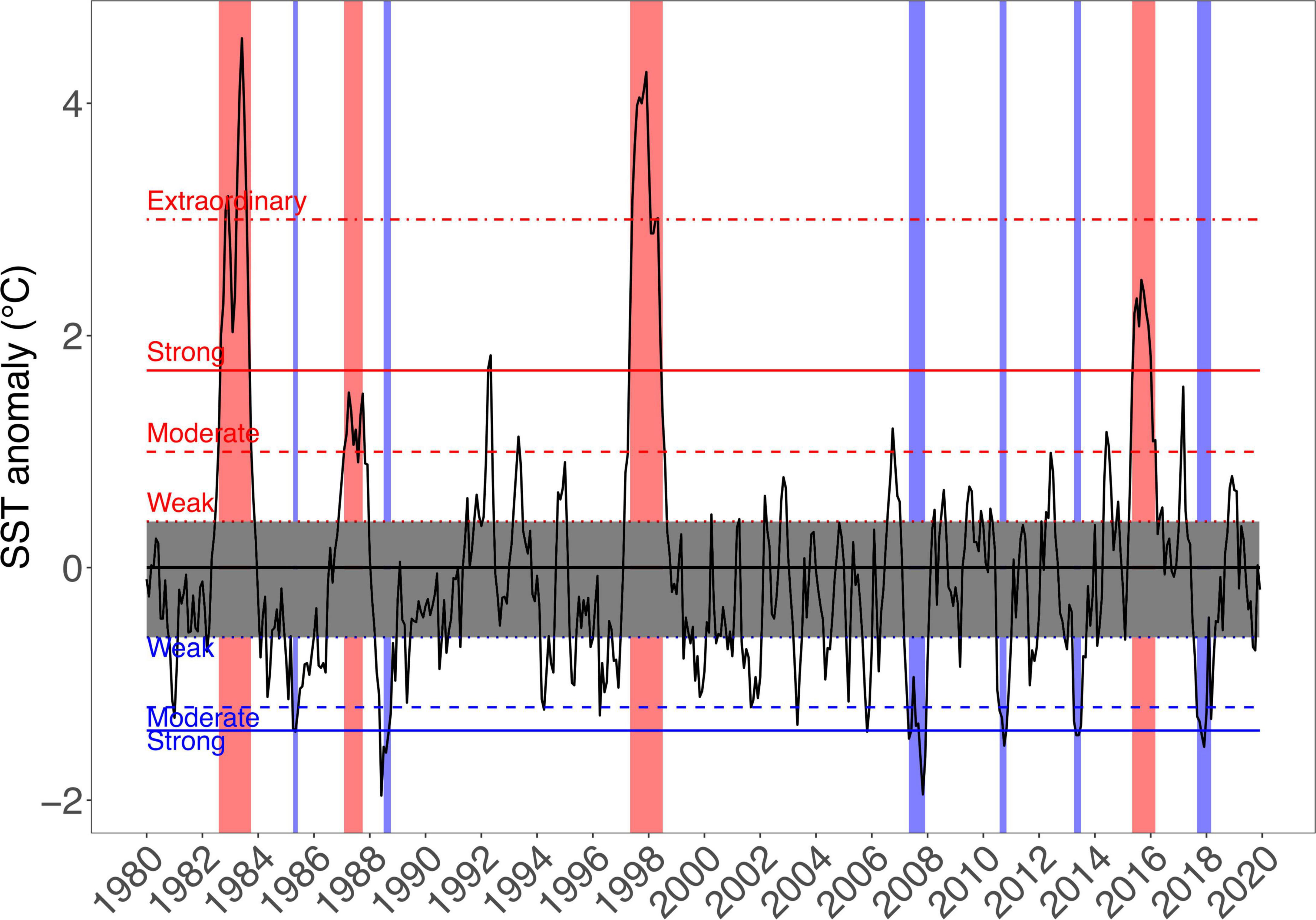
Figure 2. Decades-long data from the US National Oceanographic and Atmospheric Agency (NOAA) website show the SST anomalies since 1980 for the NIÑO 1 + 2 in the eastern Pacific. The periods corresponding to El Niño (EN) and La Niña (LN) events were defined according to the indices used by the National Study of the El Niño Phenomenon (ENFEN, for its Spanish acronym: http://enfen.gob.pe), which is an independent scientific committee from the Peruvian Government. Red and blue bars indicate EN and LN periods, respectively.
Resources and Their Use (Fisheries and Aquaculture)
Given the Peruvian ban on industrial fishing within the first 5 nautical miles offshore, the two systems under study are exploited mainly by small-scale fleets. The Piura Region (to which Sechura Bay belongs) represents approximately 10% of the 3,000 km of Peruvian coastline but hosts ∼30% of all Peruvian fishermen and ∼35% of all artisanal boats and small-scale vessels (Guevara-Carrasco and Bertrand(eds), 2017). In contrast, Ica Region (to which Independencia Bay belongs) has about 14% of the artisanal fishermen and only 6–7% of the boats, with more or less the same length of coastline as Piura (Guevara-Carrasco and Bertrand(eds), 2017).
Artisanal fisheries are critical to both systems, where multiple gears of varying selectivity and fishing power, such as hook and line, purse seine, gillnet, drive and artisanal trawl (Salazar et al., 2020), are used to target numerous benthic and pelagic resources. While several species are present in both bays, the main target groups differ between the two systems. Furthermore, both bays sustain significant stocks of the bay scallop Argopecten purpuratus, a highly profitable species typically harvested through bottom culture and enhanced-fishery systems. Presently, this scallop is one of the national leading export species, having generated more than 1 billion US$ Free On Board (without consider transport costs) between 2001 and 2017 (Bernedo et al., 2017).
The distances between Pisco’s fishing grounds areas are relatively small, so artisanal fishers tend to rotate among the small bays, beaches and islands, namely, Mendieta Beach, Lagunillas Bay, San Gallán Island, Paracas Peninsula, Ballestas Islands and Independencia Bay (Figure 1). In contrast, because of the considerable longer distances between provinces in Piura, nearshore artisanal fishing fleets tend to be site-specific. In Sechura, most landings from these fisheries come from within the bay. However, it is known that a proportion of certain target groups caught in Sechura Bay are landed in other provinces (e.g., squids in Paita), while other species are partially caught elsewhere but landed in Sechura (e.g., shrimps from Talara or octopus from Lobos de Afuera Islands, located about 105 nautical miles to the south).
Reported landings from both bay systems thus also encompass species not necessarily caught inside them, including artisanal and industrial fisheries. For this comparative study, distinguishing between fish caught inside or outside the bays was essential for building the Ecopath models and the subsequent ecological analyses. For this purpose, reported landings (sampled for scientific use) of Sechura Bay and Independencia Bay were obtained from IMARPE through their beach recorders programme from 1996 to 2019. The datasets included the following variables: species, gear, landing site and fishing ground, which allowed us to filter out landings reported exclusively from inside the bays.
Modeling Approach
Mass balance trophic models were built for each bay system using the Ecopath with Ecosim approach (EwE, version 6.5). Ecopath provides a snapshot of the system and helps spot key functional groups and critical energetic pathways; consequently identifying knowledge gaps concerning the functioning of the ecosystem under study (Jarre et al., 1991; Christensen and Pauly, 1992; Jarre-Teichmann, 1998; Christensen and Walters, 2004). Furthermore, these models are useful for fisheries management. All compartments relevant to fish production can be included, allowing for a more fine-tuned assessment of species interactions and their fisheries’ ecological sustainability (Jarre-Teichmann, 1998).
The Ecopath approach achieves mass balance by solving two master linear equations for each functional group (i) in the ecosystem. Consumption (Qi) is split into production (Pi), respiration (Ri) and unassimilated food (UNi, also termed egestion). In turn, the model divides the production (Pi) into predation mortality (M2ij) driven by the biomass of the other predators (Bj) and using the prey-predator diet matrix (DCij); exports from the system, both from fishing activity (Yi) and other exports (Ei); biomass accumulation in the ecosystem (BAi); and baseline mortality or other mortality (1-EEi), where EEi is the ecotrophic efficiency of the group within the system, or the proportion of the production of (i) that is exported out of the ecosystem (i.e., by fishing activity) or consumed by predators within it. The two master equations are summarized as:
To solve these linear equations, Ecopath first requires at least three out of these four parameters for each group: Bi (t.km–2), Pi/Bi (/year), Qi/Bi (/year) and EEi, plus the diet matrix (DCij). It is good practice to let Ecopath estimate the ecotrophic efficiency of all groups, but can also solve for Bi if necessary. Landings per fleet per functional group (t.km–2) can be included and are necessary if Bi is to be estimated by the model.
The models were conceptualized and designed based on previous EwE models of the study areas, which were constructed to study a very warm EN cycle (1996–1998, both bays; Taylor et al., 2008a,b) and to understand aquaculture impacts and dynamics of a neutral year (2010, Sechura; Kluger et al., 2016). The main purpose of this study was to allow for inter-bay system comparison and update current knowledge of their trophic dynamics and ecological responses to environmental and anthropogenic stressors, with emphasis on drivers related to fisheries and aquaculture. The models have been thus designed and built parallel under the main criteria of allowing comparability between the bay systems while using commercially important species relevant to management, and parameterized to represent the annual average situation for the cold 2007–2008 LN period (see section “The Study Areas and Their Environmental Envelopes”).
Model Building, Parameterization and Analysis
Most information of the two study systems regarding species abundance, ecology and fisheries was obtained through IMARPE (see Supplementary Material for a list of species used in the models). The data came from specific surveys of essential resources performed by its coastal laboratories or central office in Lima (e.g., scallop stock surveys at different locations along the coast once or twice a year since the mid-1980s). Some of the information was also obtained directly from previous models (Taylor et al., 2008a,b) and Kluger et al. (2016) and from peer-reviewed literature, which presented and discussed the biology, ecology or modeling of similar ecosystems. The species selected for the different functional groups were defined mainly based on IMARPE’s benthic surveys and artisanal fisheries landings reports (Tables 1, 2).
The spatial boundaries of the two models followed the geographical limits used by Taylor et al. (2008a,b), Kluger et al. (2016), defined as the depth (about 30 m) at which the OMZ begins. Most benthic and demersal species inhabit the region above this point, where IMARPE typically performs its surveys. Following these criteria, the Sechura model area was also restricted to the south portion of the bay, delimited by Piura River in the north (Figure 1).
Input Parameters
Phytoplankton biomasses were calculated from chlorophyll a (Chl-a) (mg.m–3) estimates obtained from remote sensing (see section “The Study Areas and Their Environmental Envelopes”). The transformation of Chl-a into wet weight phytoplankton biomass was done following Taylor et al. (2008b), Kluger et al. (2016). Given the lack of reliable information, the biomass of the zooplankton groups was estimated by Ecopath in both bays, assuming an Ecotrophic Efficiency (EE) of 0.9, following Taylor et al. (2008a,b), Kluger et al. (2016). Jellyfish biomass estimates (t.km–2) were obtained directly through IMARPE’s Research Unit on Superior Predators from cruises between 1996 and 2014. The data was received as tonnes.m–3 and transformed to t.km–2 by multiplying the calculated average values by an average depth of 15 m in both systems.
Biomass values of other benthic invertebrates were estimated from a combination of official IMARPE surveys and published and gray literature, depending on their availability for each system. The primary survey used was the one performed by IMARPE’s General Office of Research in Demersal and Littoral Resources for the bay scallop A. purpuratus, which provided scallop biomass estimates (t.km–2) since 1984 and 1995, for Independencia and Sechura, respectively. These biomass estimates were doubled for both sites for the model period (i.e., 2007–2008), an approximation thought to better reflect reality based on reported underestimation due to aquaculture. This was discussed and validated with experts from both coastal laboratories.
In the case of Sechura, partial information of the biomass of accompanying species could be obtained from some scallop surveys (period 2004–2009). Independencia values were mainly obtained from supplementary material provided by Riascos et al. (2016). Complementary information (in particular for polychaetes and small gastropods and crustaceans) was obtained from the baseline study (Gonzales and Yépez, 2007) for Sechura, and from Gutiérrez (2017) for Independencia. The biomasses of clams in Independencia Bay were obtained from direct surveys conducted by IMARPE (Donayre et al., 2010; Flores et al., 2010; Gutiérrez, 2017).
Furthermore, given that the scallop surveys rely on throwing a metallic square and collecting everything in it, some groups are known to be under sampled, particularly mobile invertebrates or those living in the endobenthos or on rocky substrate. For those groups where reliable information could not be obtained, or under sampling was reported, as well as for those that were not found in the surveys but were part of the landing statistics, their biomass was left for Ecopath to estimate, taking into account EE values obtained from previous models in these areas (Taylor et al., 2008a,b; Kluger et al., 2016). Groups parameterized in this way include, in Sechura: Clams, Misc. filter-feeders, Polychaetes, Sea cucumber and Octopus; and in Independencia: Limpets and chitons, Other grazers, Green sea urchin, Sea cucumber and Octopus.
All values of production and consumption (P/B and Q/B) were estimated from literature, using mainly the FishBase online repository (Froese and Pauly(eds), 2000), published models that use similar species or functional groups under similar conditions, and empirical models from Thomas Brey’s “Population Dynamics in Benthic Invertebrates Virtual Handbook” (Brey, 2001). Some values were guessed estimated based on previous models of the study areas (Taylor and Wolff, 2007; Tam et al., 2008; Taylor et al., 2008a,b; Kluger et al., 2016). Even though IMARPE performs regular stomach content studies for some species along the coast, specific diet composition studies from within the two bays are inexistent. Therefore, the feeding guild and ecology of all species required to fill out the diet matrix for each functional group were mainly obtained and inferred from online repositories (FishBase1) and published literature (Vargas et al., 1999; Chirichigno and Cornejo, 2001; Medina et al., 2004; Tam et al., 2008; Taylor et al., 2008a,b).
Model Analysis
Before the final balancing of the models, the underlying assumptions in relation to biomass ratios, vital rates and total production per trophic level were tested using the pre-balance diagnostics (PREBAL) tool incorporated in Ecopath (Link, 2010; Heymans et al., 2016). A visual assessment of the systems’ structure and flow was done through the basic estimates tables and the Ecopath flow diagrams provided as output in the software. Various indices were then explored and used to describe and compare the two ecosystems. These indices, which are based on thermodynamic concepts, information theory and ecological network analysis (Christensen and Walters, 2004), were extracted from Ecopath via different routines and sorted into these main categories: summary statistics, community energetics, fisheries, energy cycling, biodiversity, system development (network indicators, information) and transfer efficiencies (see Table 3). The relative importance of each functional group within each system was assessed using the keystone indices estimated by Ecopath. They are calculated through the mixed trophic impact (MTI) matrix, which portrays the relative change in biomass of one group based on the infinitesimal increase of another, directly and indirectly, from the composition of their predators and diets (Ulanowicz and Puccia, 1990). Valls et al. (2015) compared keystoneness across 101 EwE models and proposed keystone index #3 (KS3), as an improvement to KS1 (Libralato et al., 2006), which tended to be less balanced and include groups with high biomass as keystone species. By giving more importance to low biomass groups, KS3 corrects this imbalance and focuses on predatory species that disproportionately influence the food-web structure, without bias on abundant or rare species (Valls et al., 2015). Nevertheless, because KS1 gives more weight to each group’s relative trophic impact compared to its biomass, keystoneness via bottom-up and wasp-waist effects can also be reflected. In this study, we used the graphical representation of a classification tree based on the total biomass and relative trophic impact of each group, and focused on the keystoneness rank of KS3, as suggested by Valls et al. (2015) We also used KS1 (Libralato et al., 2006), which is the basis for KS3, to complement and enrich the keystoneness analysis. Ecopath calculates KS1 by estimating two main parameters for each functional group: trophic impact (εi), obtained from the MTI matrix, and a measure of biomass (pi) that corresponds to the contribution of group i to the total biomass in the food web. KS1 is calculated as follows:
The calculation of KS3 uses this formula and modifies the biomass component (1 – pi), exchanging it with a term that uses the biomass ranking of each group i in descending order, named “drank(Bi).” For more detail on these equation and parameters, please refer to Libralato et al. (2006), Valls et al. (2015).
Results
Environmental Envelope
The time series (2006–2020) of monthly averaged SST values from inside each bay suggest relatively stable conditions in both systems during this period (Figure 3A). Both bays showed a similar pattern in seasonal oscillation, though Sechura was about 2.5°C warmer than Independencia throughout the series. This temperature difference was notably higher during the summer months. The largest difference was seen around March 2017 (∼7°C), reflecting the violent increase of SST produced by the Coastal EN in Sechura (Figure 3B).
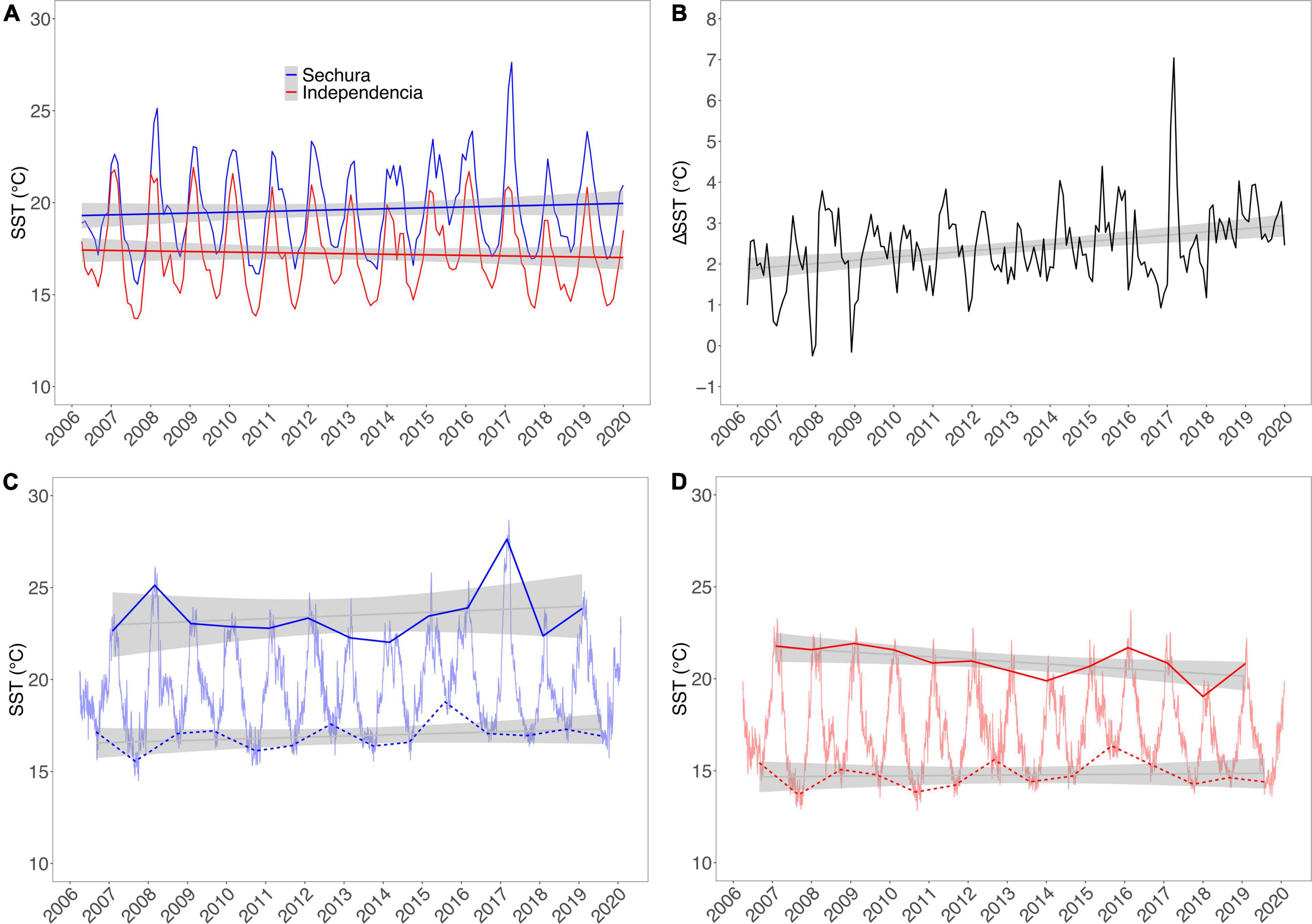
Figure 3. Remotely sensed sea surface temperature (SST°C) inside Sechura Bay (blue) and Independencia Bay (red). Panel (A) Monthly averages with corresponding trendlines. Panel (B) Difference between the monthly average of Sechura minus Independencia. Panels (C,D) Daily averages for Sechura and Independencia, respectively; solid and dotted lines show the average hottest and coldest months, respectively, with their corresponding trendlines. Data source: OSTIA satellites system (as described in main text).
When averaging SST by year, there was an apparent increase in the difference between the average temperature of the two systems (from about 1.5 to 3.0°C), though only Sechura showed increasing values, while Independencia had no clear trend. However, Independencia’s seasonal averages presented opposite directions: summers getting colder and winters warmer (Figure 3D). Sechura maintained a growing SST trend during both seasons, though it could have been skewed slightly by the Coastal EN peak (Figure 3C). Furthermore, the differences in SST between the lowest and highest months of each year showed a decreasing trend in both bays, suggesting that seasonal variability is decreasing at the two sites, disrupted in Sechura only by the Coastal EN SST rise of March 2017 (Figures 3C,D).
The remote sensing of superficial Chl-a (mg.m–3) showed similar yearly average values in the three areas adjacent to Independencia [see Figure 4(right), from inside the bays toward the offshore]. The time series show an increasing trend and similar variability in all three curves, particularly between 1997 and 2005. Beyond this point toward 2019, the trends increased their variability and seemed to separate from each other. In contrast, the Chl-a trends in Sechura are clearly separated, ranging from higher to lower values from inside the bay toward the offshore [Figure 4(left)]. The two offshore curves had no clear tendency, whereas the Chl-a values from inside the bay increased significantly during the whole period (1997–2019). Similarly to the SST trends in Sechura, there is a considerable peak of Chl-a inside the bay around 2016–2017, which could also be associated with the Coastal EN. The average yearly values inside both bays showed that Sechura had a slightly higher Chl-a concentration throughout the series (1997–2019). However, monthly averaged time-series plots showed that the values and variability were very similar in both systems, with a few spikes in Sechura, usually during post-summer months (March–June).
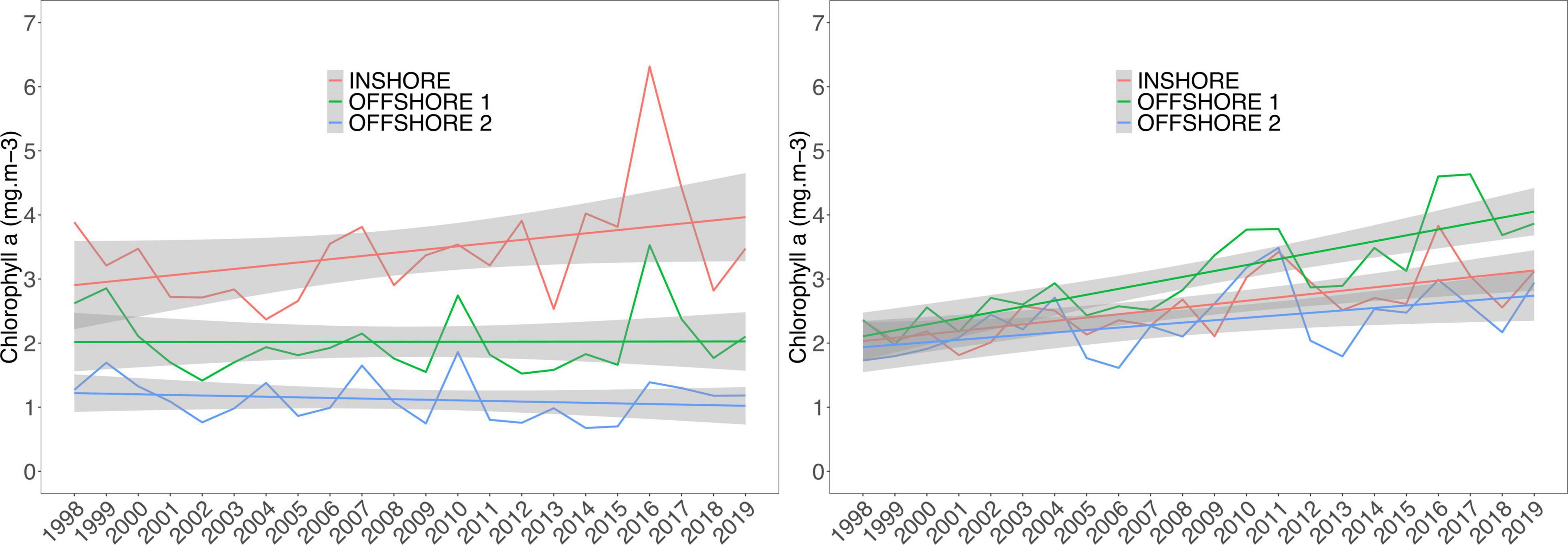
Figure 4. Yearly averaged Chl-a concentration (mg.m–3) for Sechura Bay (left) and Independencia Bay (right), estimated from remote sensing. The values were averaged for three distinct areas per study site: inside each bay (INSHORE), outside the bay area up until the shelf’s slope (OFFSHORE 1), and beyond the platform into the open ocean (OFFSHORE 2). Data source: MODIS-Aqua and SeaWifs satellites (as described in main text).
During the model period (2007–2008), the bays showed similar patterns of seasonal oscillations in both SST and Chl-a trends. On average, Sechura had higher values for both parameters, exceeding Independencia by ∼2°C and ∼0.8 mg.m–3, respectively. Furthermore, Sechura showed distinct peaks of chlorophyll concentration between January and March of each year (2006–2009), a feature not seen in Independencia (Figure 5).
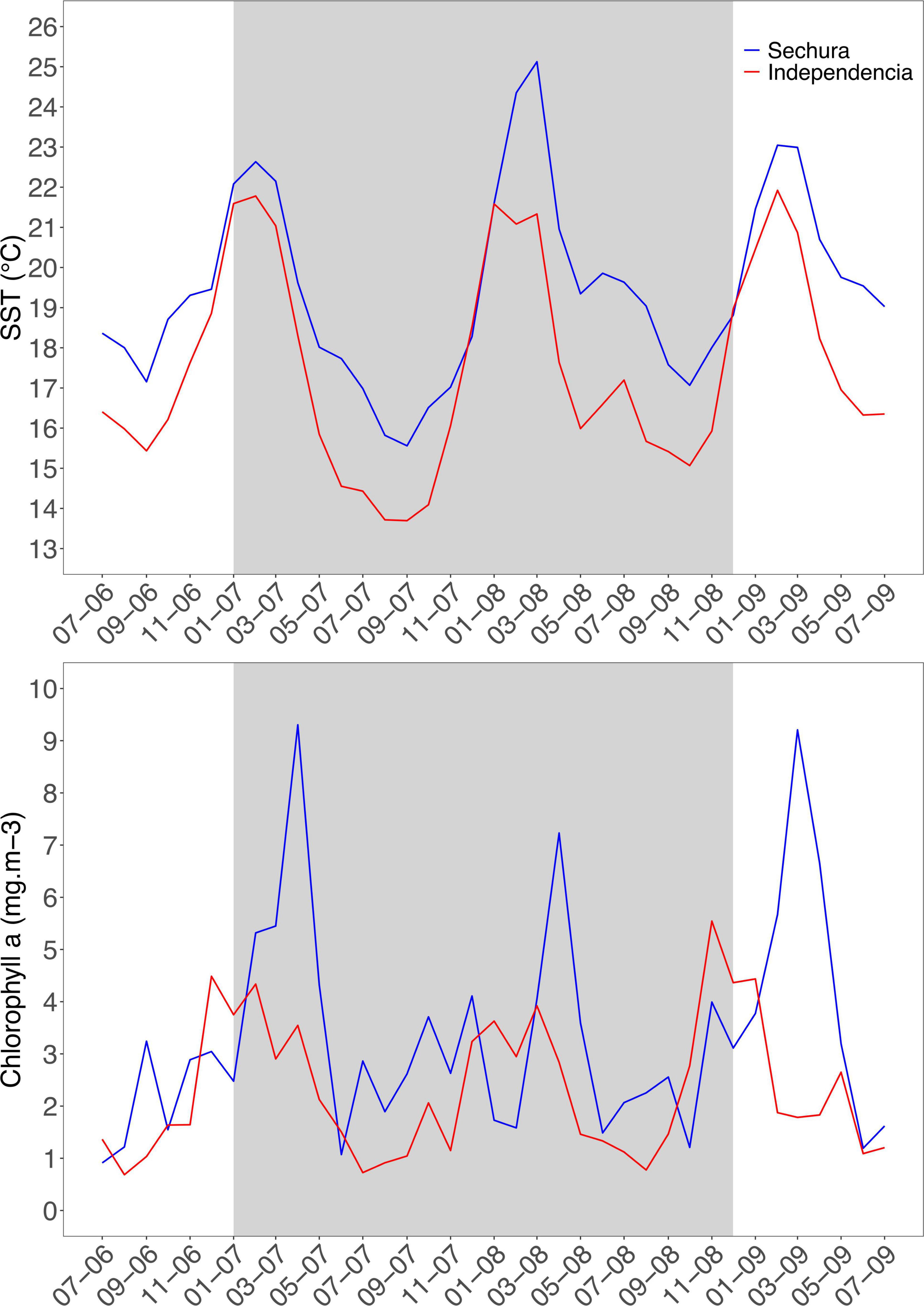
Figure 5. Monthly average (remotely sensed) sea surface temperature (SST°C, top panel) and chlorophyll-a concentration (mg.m–3, bottom panel) inside Sechura Bay (blue) and Independencia Bay (red) between July 2006 and 2009. Gray panel shows the study period (2007–2008). Data sources are the same as Figures 3, 4.
Biodiversity and Resource Use
The two 2007 models differed in their species composition, Sechura exceeding Independencia by 75 species in total (273 vs. 198). When categorized by general taxonomy and feeding guild (11 and 9 groups, respectively), some broad ecological differences can be observed regarding their species abundance distributions (Figure 6, see also list of species in Supplementary Material).
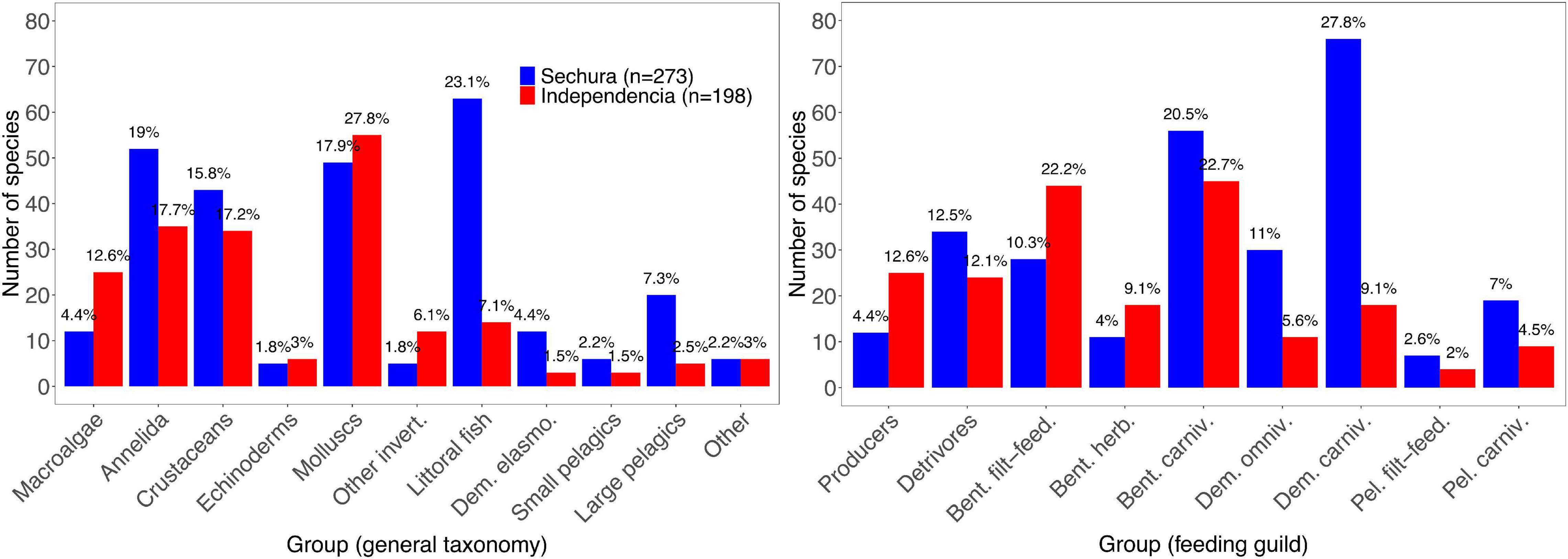
Figure 6. Number of species used in the 2007 Ecopath models of Sechura Bay (blue; n = 273) and Independencia Bay (red; n = 198); grouped in categories (x-axis) based on general taxonomy (left) and feeding guild (right). The total number of species per group is shown in the y-axis, while the data labels represent their respective proportion within each system. Full list of species per model is provided as Supplementary Material.
In Sechura, most species were grouped into Littoral fish (23.1%, 63 species of sea basses, groupers, drums and grunts, among others), Annelida (19%), Molluscs (17.9%) and Crustaceans (15.8%). A quarter (24.9%) of all species in Sechura were categorized as Demersal carnivores (i.e., littoral fish, sharks, rays and Octopus mimus). Benthic carnivores (20.5%) and Detrivores (12.5%) mainly represent species of polychaetes, molluscs, crustaceans and echinoderms.
Conversely, in Independencia, the highest number of species corresponded to the group Molluscs (27.8%), followed by Annelida (17.7%) and Crustaceans (17.2%). Regarding feeding guild, 22.7% of all species used in the model corresponded to Benthic carnivores and 22.2% to Benthic filter-feeders, which is consistent with the high abundance of Molluscs species, primarily snails and bivalves.
Ecopath estimates the trophic level of every functional group using the diet matrix of each system, and calculates the mean trophic level (MTL) as a biomass-based weighted average of all groups (including detritus). MTL was around 2.0 in both systems. It was slightly lower in Sechura, mainly because of its more significant proportion of phytoplankton and macroalgae biomass (∼30%) compared to Independencia (∼18%). The MTL was still relatively low at about 2.4 in the two bays when considering only consumers. The dominance of low TL species can also be perceived when looking at the biomass proportions of primary producers and benthic, demersal and pelagic consumers within each bay (shown as ratios in the Biodiversity section of Table 3).
The largest proportion of the total biomass in each model corresponds to the benthic communities (64% in Sechura; 75% in Independencia), where filter-feeding organisms are the majority. After macroalgae and polychaetes, the most significant biomass values corresponded to Scallops in Sechura and Ribbed mussels in Independencia. Both systems share the bay scallop and several species of clams and other miscellaneous filter-feeders and benthic grazers. However, the group Ribbed mussels, mainly represented by Aulacomya ater, was not found in Sechura. It is worth noting that some fish species also contributed to lowering the trophic levels through omnivory and filter-feeding, such as the demersal omnivore Anisotremus scapularis and the pelagic filter-feeder Sardinops sagax, found in both bays as part of the Miscellaneous littoral fish and Small pelagic fish functional groups, respectively (see Supplementary Material). However, just like with the number of species, they constitute a low proportion of the total biomass in each model compared to their demersal and pelagic carnivorous counterparts (Sechura in particular). According to output from Ecopath, both systems contain ∼75% of their biomass within the first two TLs, while roughly 20% is found in TL 3.
The fisheries indicators of both systems are relatively similar, dominated by diving fisheries [Figure 7 (left)]. Sechura has higher flows of total exports, production and biomass of commercial species in the system, but it is only slightly larger than Independencia in total catch (73.7 vs. 71.2 t.km–2 year). The MTL of the catch (MTLC) is around 2.3 in both systems, relying on the considerably higher proportion of biomass found at lower TLs. The MTLC is higher in Independencia, albeit by a tiny margin, despite having a high proportion of commercially important low-TL benthic biomass (mainly macroalgae, scallops, clams, mussels). Despite having relatively high catches and discards of predatory snails (high TL), the MTLC goes down in Sechura because of the significantly higher availability of scallops per unit of area (Figures 7, 8).
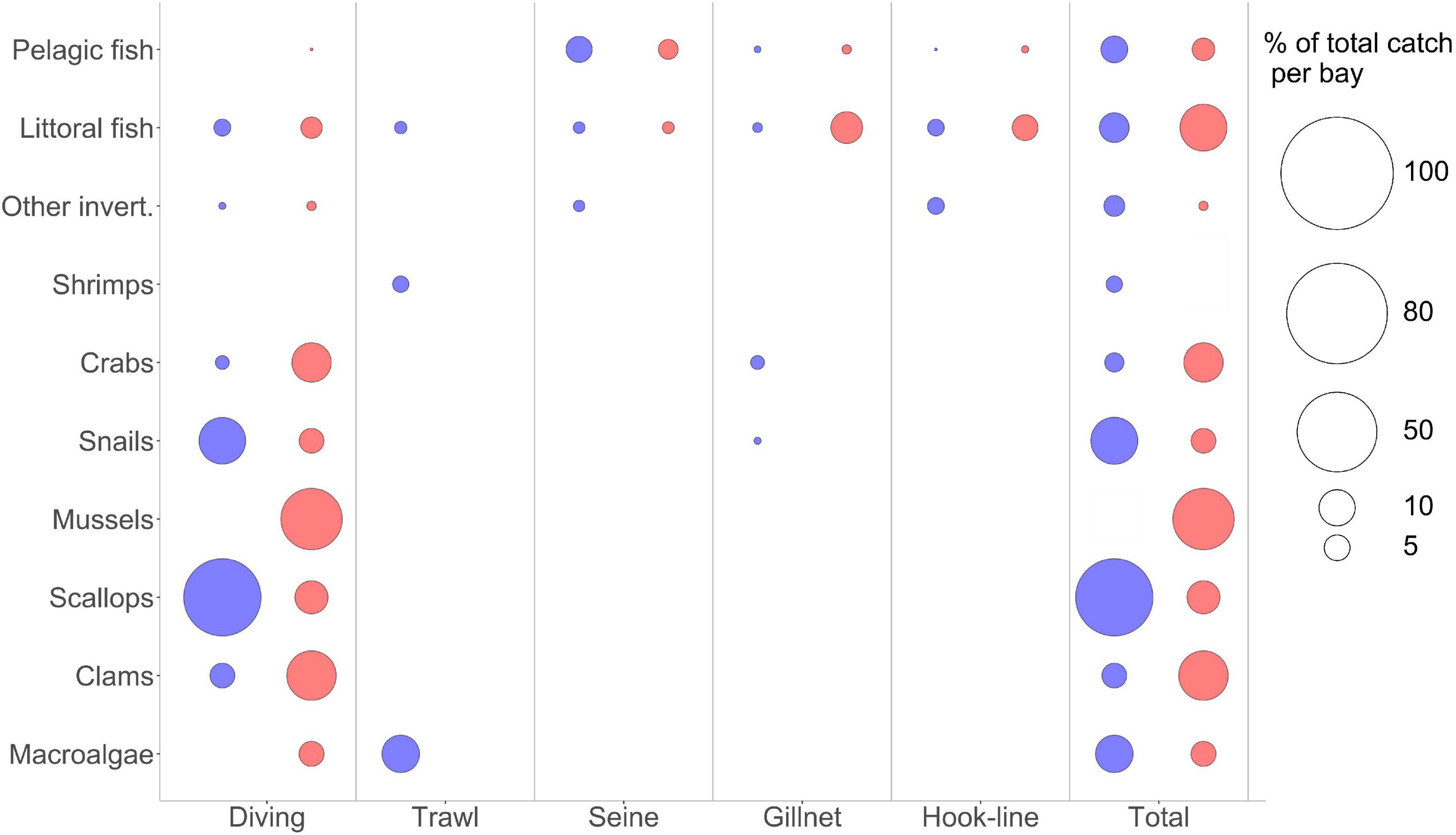
Figure 7. Bubble plots showing the percentage of total catches in the 2007 Ecopath models (Sechura in blue, Independencia in red), categorized by the gear used (x axis) and a broad taxonomical grouping for the target species (y axis). The size of the bubble shows the percentage over each system’s total catch (t km−2 year). Total catches are:Sechura Bay, 73.7 t km−2 year; Independencia Bay, 71.2 t km−2 year.
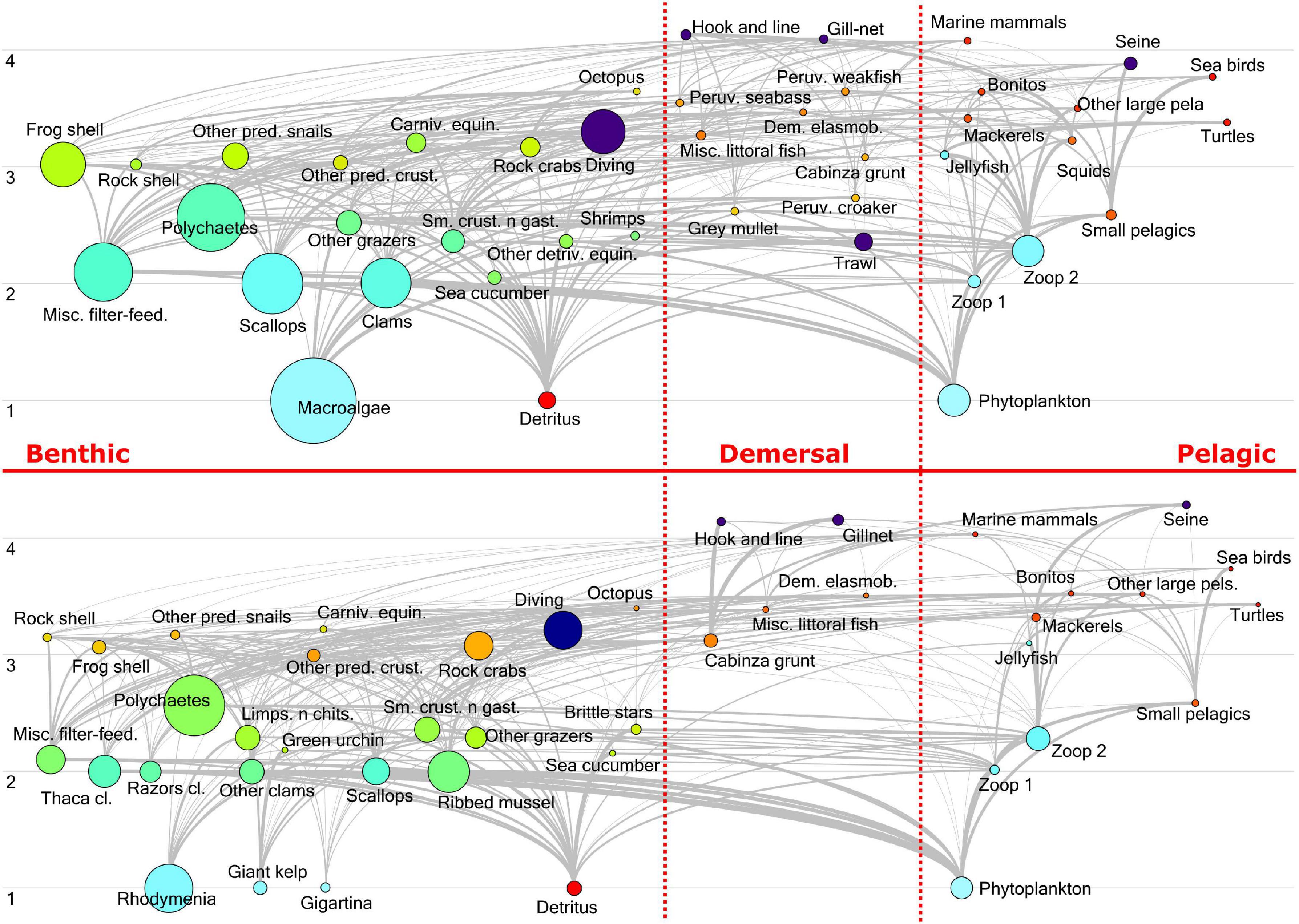
Figure 8. Ecopath flow diagram of Sechura Bay (SB, top) and Independencia Bay (IB, bottom). Bubble sizes represent the biomass (t km–2) of each functional group and the y-axis shows their Trophic Level position. Groups are horizontally organized into Benthic, Demersal and Pelagic.
Also relevant is that there is no macroalgae fishery in Sechura, but 8 t.km–2 per year (more than 10% of total catches) are extracted incidentally through shrimp trawling [Figure 7 (left)]. Independencia fishers target gigartina and giant kelp but extracted only 3.4 t.km–2 per year during 2007–2008. Even when ∼21 t.km–2 of mussels were caught per year in Independencia during that period, the comparatively low catches of scallops and other low-TL groups, as well as the relatively high catches of Rock crabs and Cabinza grunt (20.1 t.km–2 year, ∼28% of total catches), helped increase the MTLC value.
Quite significant was that the gross efficiency (catch/net PP) was almost twice as large in Independencia compared to Sechura, partly reflecting the importance of the Ribbed mussels group in Independencia during the model period (2007–2008). This group represents a tenth of all biomass in this system, but only about 1% of the total production. However, a third of the total landings reported in Independencia for this cold period corresponds to this filter-feeding bivalve.
Trophic Structure and Ecosystem Functioning
The two systems had similar functional groups in their makeup (Tables 1, 2), with variations based on certain species that were considerably more relevant in the official surveys and landings reports, as well as on their commercial value. When comparing the models’ general statistics, Sechura shows considerably larger flows of mass compared to Independencia. Besides having higher total biomass (t.km–2), consumption and respiratory flows (t.km–2 year), the sum of all exports in Sechura more than doubles that of Independencia (Table 3), while the sums of all production and total system throughput (TST) are about 1.5 times higher. The ratios of primary production over respiration (PP/R) and biomass (PP/B) were also considerably higher in Sechura. However, the total biomass supported per unit of throughput (B/TST) was higher in Independencia by about 10%. Indicators also showed higher cycling of TST (and more paths for it to cycle through) in Independencia than Sechura, notably when excluding detritus.
Both MTI plots share regions of similar positive and negative effects (Figure 9). Besides predator-prey impacts, negative effects can be see on certain regions along the diagonal, evidencing mostly competition between groups of similar trophic level and habitat, such as the benthic Rock crabs and Predatory snails, or the pelagic Bonitos and Mackerels. However, there seem to be slightly more negative pairwise interactions in Independencia than in Sechura.
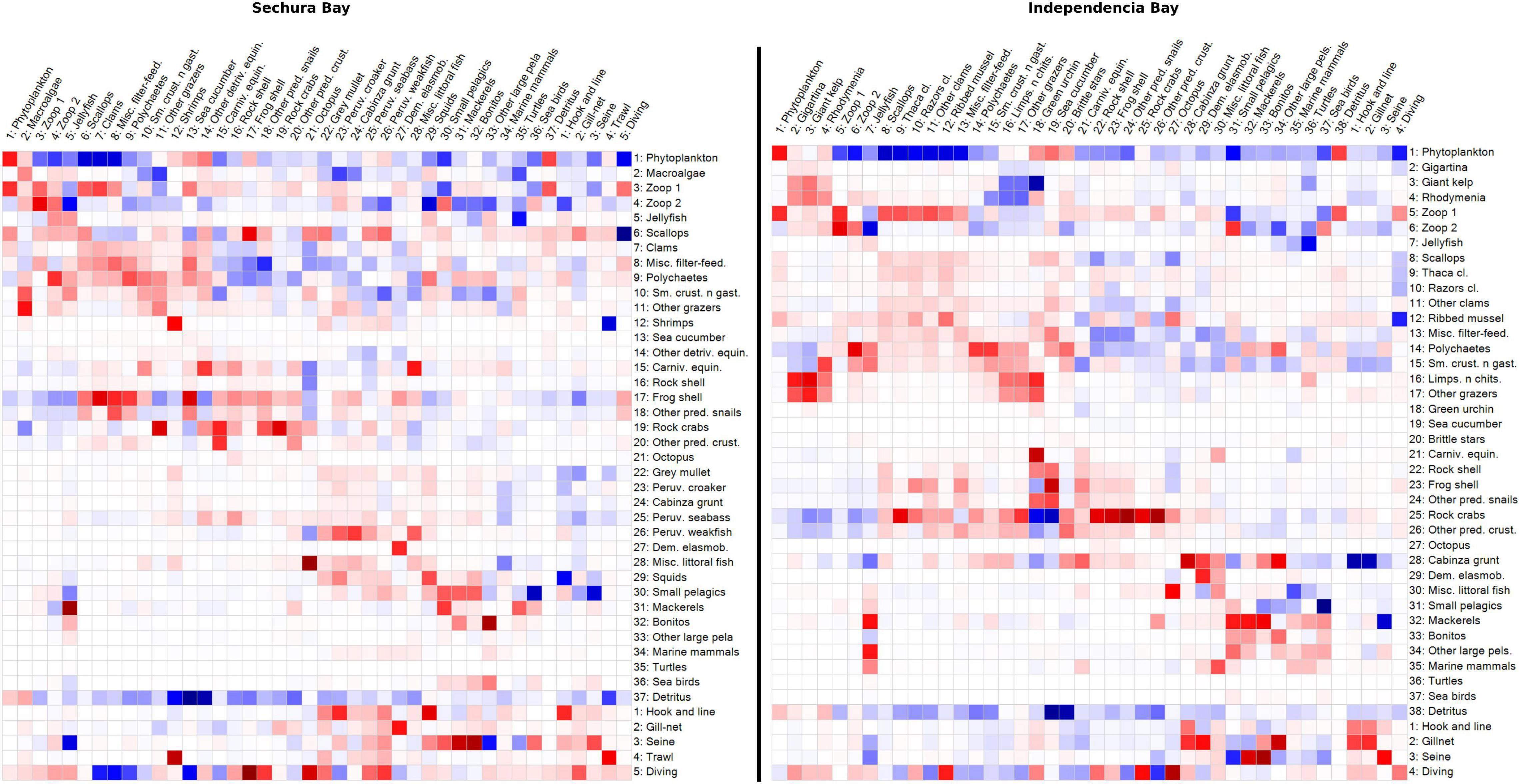
Figure 9. Mixed trophic impact (MTI) grid plots of Sechura Bay (left panel) and Independencia Bay (right panel) ecosystem models. The color in each square indicates the proportional impact (blue = positive; red = negative) that one functional group (rows) has on another (columns) within each ecosystem. The groups include fleets.
As expected, the most positive impacts occurred on fleets: Scallops on Diving, Shrimps on Trawl, and Small pelagics on Seine net in Sechura; Cabinza grunt on Hook and line and Gill-net, and Mackerels on Seine net. The positive impact of Detritus on detritivores was also quite salient in both systems, compared to most of the impact relations, suggesting its essential role in them. Consumption of primary production (phytoplankton and macroalgae) was also very significant, though it appears to be slightly more relevant in Independencia. In particular, the herbivore Green urchin is heavily impacted by Giant kelp. More than a quarter of the total biomass in Sechura is from the group Macroalgae. However, this group does not appear to greatly impact directly other groups of the system, even when it represented ∼10% of total catches (t.km–2year), which came from discards of the trawl fishery targeting mainly shrimps. This agrees with the fact that macroalgae are very little consumed in coastal systems directly, since most of its biomass ends up as detritus in the system (Walton et al., 2022). Despite the high proportion of Polychaetes biomass in both systems (∼15% in Sechura and ∼25% in Independencia), and in spite of having a broad feeding guild, there does not seem to be a highly impactful interaction (positive or negative) between these organisms and the rest of the groups.
The scatter plot used to classify the different functional groups based on their trophic impact and biomass showed that not one group was identified as keystone in either bay system (Figure 10). Only one group barely fell into the keystone category: Bonitos in Sechura. Relatively close to this keystone box were Mackerels, Peruvian weakfish, Misc. littoral fish and Small pelagics. Consequently, these groups were top five in the KS3 rank positions shown in Table 3 and Supplementary Material. KS1 however shows Phytoplankton, Zooplankton 2 and the carnivorous Frog shell as the top three ranked keystone groups, consistent with the high value assigned by this index to the trophic impact values.
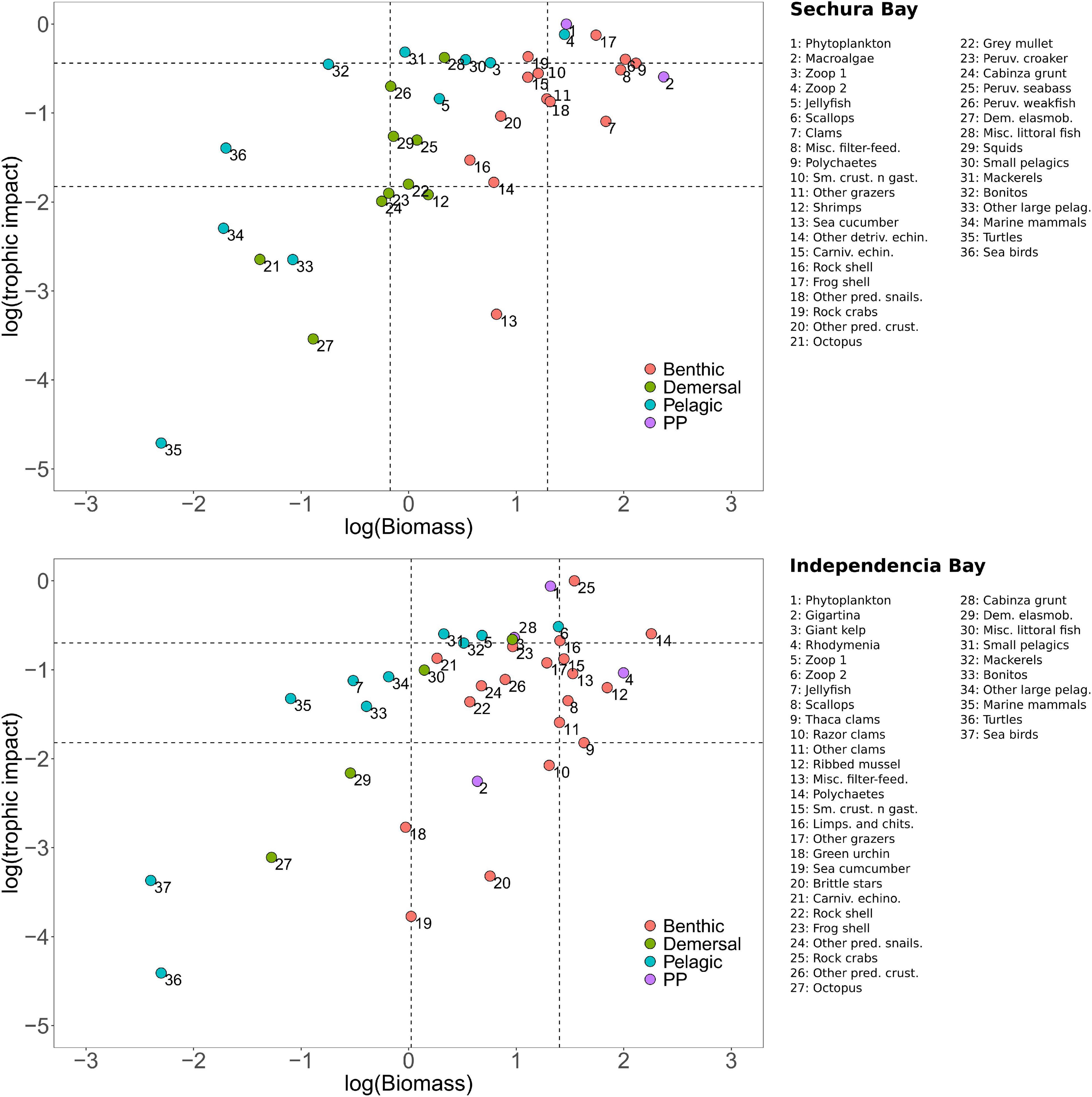
Figure 10. Scatterplot of log-transformed relative trophic impact (squared values) versus log-transformed biomass of each functional group (Bi, t.km–2) for each model: Sechura Bay (A) and Independencia Bay (B), following Valls et al. (2015). The plot is divided into four sub-panels based on a classification tree that uses the minimum, maximum and quartile (Q1 and Q3) values: (i) keystone groups (upper-left box), (ii) high-impact–high-biomass groups (upper-right box), (iii) low-impact–low-biomass groups (lower-left box), and (iv) low-impact–high-biomass groups (lower-right box). Intermediate groups are spread on the rest of the plotting area.
Independencia did not show any keystone groups but had Small pelagics, Mackerels and Carnivorous echinoderms near the upper-left keystone box (Figure 10). These groups were also found within the top five keystone species according to KS3, also including Phytoplankton and Zooplankton 1. On the other hand, consistent with the scatter plot, KS1 placed Rock crabs and Phytoplankton as the two groups with the highest keystone value, given their considerably higher trophic impact (see Supplementary Material), followed by Zooplankton 2, Small pelagics and Zooplankton 1 (Table 2).
Discussion
This paper compares two bay systems of the Peruvian Humboldt Current separated latitudinally by about 1,000 km of coastline. In the following segment, the system features analyzed are synthesized and discussed, looking at three aspects: environmental envelope, biodiversity and resource use, and trophic structure and ecosystem functioning.
Environmental Envelope
Over the past 15 years, Sechura SST has been about 2.5°C higher than Independencia on average, and even though they show similar seasonal variation, the difference between their average yearly SST seems to be increasing. Chlorophyll-a levels inside the bays have also been higher in Sechura, showing an increasing trend in both systems since 1997. During the study period (2007–2008), Sechura had in average more chlorophyll concentration (∼0.8 mg.m–3) than Independencia, and showed distinct peaks during the summer.
In contrast with global trends, historical time series of yearly averaged SST (reconstructed from in situ data, paleoceanographic proxies and model simulations) show a significant steady cooling of main upwelling areas off Peru, particularly during the second half of the 20th century (Wolff, 2010; Gutiérrez et al., 2011; Espinoza-Morriberón, 2018). This cooling has also been associated with intensifying alongshore winds that drive upwelling and increase marine productivity (Gutiérrez et al., 2011), which is supported by records and models of chlorophyll (Chl-a) that show a positive trend (mg.m–3/decade) during the same period (Espinoza-Morriberón, 2018). The increased productivity also partly explains a deoxygenation process of the NHCUS and the subsequent shoaling of its OMZ upper limit over the last few decades (Bertrand et al., 2010; Gutiérrez et al., 2011, 2016; Espinoza-Morriberón, 2018), even when some site-specific oxygenation has been shown in recent decades (Callao, 12°02′S, 77°29′W; Graco et al., 2017).
These trends along the Peruvian coast, usually estimated and averaged within a large coastal band (particularly south of 6°S and 100 km offshore), have typically been used to address large-scale and long-term variability questions commonly associated with ENSO. For the most part, these trends apply to the whole NHCUS. Still, specific latitudinal differences exist, particularly in main upwelling areas and near the transition zone of Humboldt and Equatorial waters. However, it has been suggested that the remote forcing of equatorial source waters is the primary driver of these trends compared to local forcing mechanisms, such as nearshore winds (Espinoza-Morriberón, 2018). Thus, the intrusion of oceanic water masses is associated with long-term variability at a large scale (in the NHCUS). They are commonly assumed to dominate the nearshore and bay systems dynamics.
In situ time-series measurements show a general trend of higher SST with decreasing latitude. However, some site-specific differences exist. For example, values recorded in Pisco (∼14°S) are usually about 1°C warmer than in Paita (∼5°S) (Wolff, 2010; Gutiérrez et al., 2011). For the present study, remote sensing was used to determine SST and Chl-a inside both bays, mainly because in situ measurements were not standardized between both bays (e.g., from stations not representing the whole area or from different depths not always clarified). Satellite information allowed us to analyze the two systems based on surface water information that was standardized and comparable.
These SST time series (2006–2020), yearly averaged for inside each bay, showed an increasing trend in Sechura Bay compared to a slightly descending trend in Independencia. Both curves drift apart from an average difference of ∼1.5°C in 2006 to ∼3°C in 2020. However, it can be argued that this fifteen-year span is not long enough to assess SST long-term trends inside the bays with certainty. In Independencia, water temperature has been recorded by IMARPE at the La Vieja Island station since 1983, though published data stops in 2005 (Wolff et al., 2007). Even though it has been published as SST, these values seem too low compared to the SST satellite data from 2006–2020. IMARPE has not fully clarified this, but it appears that either the 1983–2005 dataset is from bottom water measurements, or the sampling time was always in the morning when the water temperature is usually lower, or both. In contrast, satellite information gave us a daily average of SST for each bay area. In any case, the La Vieja time series does not show a general trend, despite the observed peaks associated with EN. Both time series together suggest that the average temperature in Independencia has had a relatively small variation since 1983, with a slight tendency toward cooling in recent years. A longer reliable SST time series has not been found for Sechura.
Averaged by month (2006–2020), both bays exhibited similar seasonal variability, consistent with the synchrony seen in the oscillation of SST (and other parameters) between bay systems along the Humboldt Current coastline (e.g., Sechura and Paracas in Peru and Tongoy in Chile); though it has been suggested that the Peruvian bays have greater fluctuations (Cueto-Vega et al., 2021). Regarding the differences between SST averaged by season, Sechura showed a steady increase during both the warm and cold periods, while Independencia displayed opposing trends, with winters getting warmer and summers colder. The two systems also differed from what was reported for the Galapagos Islands (most northern tip of the NHCUS) for the past ∼30 years, where the summer and winter periods were becoming warmer and colder, respectively; while there was no significant change in mean yearly SST over time (Wolff, 2010).
Our comparative analysis of Chl-a trends from inside the bays toward the offshore is very revealing. In particular, the higher values registered outside Independencia suggest that upwelling is becoming stronger at this latitude, compared to a more stable situation outside Sechura. However, local (within the bay) dynamics might be increasing the Chl-a in Sechura for apparently different reasons, probably from eutrophication due to waste disposal of nearby cities and factories carried into the bay by the Piura River (Loaiza Alamo, 2020). It is worth noting that Independencia does not have cities nearby or riverine water input of any sort.
One of the main problems that face nearshore areas along the NHCUS is the presence of a shallow OMZ (Arntz et al., 1991; Bertrand et al., 2010; Graco et al., 2017). This is particularly the case for bay systems that operate close to their carrying capacity and that have significant anthropogenic stressors, such as the scallop bottom culture of Sechura Bay (Tam et al., 2012; Kluger et al., 2016). Environmental surveys have shown that hypoxia is a persistent phenomenon throughout the year in Peruvian shallow coastal waters, with higher intensity and duration during summer and early fall (Cueto-Vega et al., 2021). Even in northern Peru, where the OMZ is located at depths > 50 m (Bertrand et al., 2010), recent surveys in Sechura Bay showed frequent hypoxia and anoxia events at 10 m depth (Aguirre-Velarde, pers. comm.). The low levels of oxygen at such a shallow depth also create the conditions for anaerobic metabolism, particularly under the frequent intrusions of hydrogen sulfide from deeper waters into coastal areas, known as sulphidic or “milky water” events (Arntz et al., 2006). These are recurring phenomena typically occurring in shallow areas, such as Paracas Bay in Pisco, but also in Sechura, usually creating mass mortalities in such areas due to their high toxicity (Gonzales et al., 2012; Schunck et al., 2013).
Also associated to the OMZ in these areas is the presence of the filamentous “spaghetti” bacteria (genus Thioplaca), which increases its abundance under low oxygen conditions and can act as co-stressors leading to scallop mass mortality (Arntz et al., 1991; Cueto-Vega et al., 2021). Between 2006 and 2008 (pre and post LN period), Gutiérrez (2017) recorded low levels of biomass of Thioplaca in Independencia throughout the period. Cueto-Vega et al. (2021) showed that Paracas Bay had a higher DO variability and more frequent low-DO periods, compared to Sechura, where low-DO was associated with low salinity, linking hypoxia to the stratification occurring usually during the summer months. However, Independencia’s conditions differ from Paracas, since the bay is much deeper and does not show such events (at least as frequent). Although still relatively depleted, oxygen levels in Independencia are typically higher than other similar embayments of the HCUS, in particular at shallower depths, considerably increasing during strong EN conditions (Arntz and Tarazona, 1990; Arntz et al., 1991; Taylor et al., 2008a).
There exist different types of ENSO events, which occur at different intensities and are more pronounced at different locations along the Equatorial Pacific Ocean, commonly known as typical (strong), modoki and coastal EN (Takahashi et al., 2011; Takahashi and Dewitte, 2016; Garreaud, 2018; Dewitte and Takahashi, 2019; Takahashi and Martínez, 2019). The typical strong EN has the most significant impacts along the Peruvian coast, having marked effects at southern latitudes, compared to the other more localized types. This means that while Sechura is constantly perturbed by ENSO interannual variability, Independencia is only rattled by strong episodes. Typical events are also important because they can re-oxygenate the coastal systems by disrupting the OMZ and eliminating the summertime stratification (Wolff, 2018; Cueto-Vega et al., 2021). Independencia’s sand-gravel bottom and narrower shallow area allows for more water exchange and higher oxygenation than Sechura, where ENSO-driven river input increases oxygen consumption inside the bay.
This would allow for the ecosystem to thrive without constantly being perturbed, which might suggest that Independencia is more adapted to this conditions and that its resources could have developed accordingly. However, a longer time-series analysis is required to advance this further.
As part of the NHCUS, the environmental conditions of the bay areas are influenced by both oceanic remote forcing (e.g., equatorial currents, Kelvin waves and coastal trapped waves) and by local wind forcing mechanisms within the NHCUS (Espinoza-Morriberón et al., 2019). However, these results strengthen the point of acknowledging local bay dynamics and variability. As shown by the above analysis, the environmental envelopes described for the bays of Sechura and Independencia show distinctive features, which drive differences in the bay’s biota, resources and general flow structure, as further discussed below.
Biodiversity and Resource Use
During the study period, both systems had very similar catch values, though Sechura Bay comprised about 30% more species than Independencia, representing a biomass difference of ∼140 t.km2. Most biomass in both systems consisted of low-TL filter-feeding benthic organisms, though each bay was represented by different species: A. purpuratus in Sechura and A. ater in Independencia.
The most apparent difference between the bay systems was that the total number of species in Sechura was about 35% higher than in Independencia, mainly due to the presence of more littoral fish species. This is consistent with this region having a broader, relatively shallow platform and a deeper OMZ, which in combination with a higher temperature and primary production inside the bay, set the stage for a highly productive tropical setting that sustains a more diverse food web (Bertrand et al., 2010; Guevara-Carrasco and Bertrand(eds), 2017). The number of species and biomass of benthic invertebrates were similar in both bays. Proportionally, Independencia had about 15% more of these resources compared to Sechura meaning that their proportion was about 15% higher in Independencia than Sechura. The species found within each system align with what is expected for such systems under cold upwelling conditions, which is a combination of tropical and temperate organisms, with Sechura having more of the former group, and Independencia of the latter (Wolff et al., 2003; Guevara-Carrasco and Bertrand(eds), 2017).
Both systems share the bay scallop and several species of miscellaneous benthic invertebrates, but there exist some relevant differences in species composition and biomass. For instance, in Sechura, scallops represent 12% of the biomass and 47% of the catches, compared to only 4% and 8% in Independencia, respectively. More filter-feeders and herbivores are found in Independencia, compared to more species of carnivores and detrivores in Sechura. Most notably, ribbed mussels in Independencia and shrimps in Sechura are two commercially important species that only occur at one site, mainly because of environmental conditions. However, in terms of biomass (t.km–2), mussels represent about 10% of the total biomass and 30% of the catches in Independencia, whereas, in Sechura, shrimps are only 0.2% of the total biomass and 2% of the catches. Notably, shrimp trawling in Sechura, which also targets miscellaneous littoral fish, takes out around 14% of all catches (t.km–2), 80% of which is macroalgae bycatch (Mendo et al., 2020). This biomass is not reported as landed or discarded and includes thousands of animal larvae, juveniles and small adults. Independencia fishers target gigartina and giant kelp but extracted only 3.4 t.km–2 per year during 2007–2008. Furthermore, macroalgae may be more important than portrayed through the models, given their potential to provide large amounts of detrital mater, but also to serve as refuge for several larvae and small organisms. This is an aspect that could be explored in further trophic modeling studies.
Despite the models being built before the 2009 scallop boom, after which the national harvest of scallops (from aquaculture and fishery) increased about 200% in one year (Mendo et al., 2016), the differences in scallops biomass and catches (t.km–2) were very large between the systems. This was mainly due to the steady migration of fishers from Pisco since 2001, and the consequent overloading of scallops in Sechura for aquaculture purposes (Mendo et al., 2016). Without accounting for scallops, landings were dominated by small pelagics in Sechura and molluscs in Independencia. The latter were mostly Ribbed mussels, represented by Aulacomya atra, which seem to have an advantage over other species because of their ability to withstand low levels of oxygen, as they are frequently exposed to hypoxia when closing their valves as an adaptation to intertidal conditions (Cueto-Vega et al., 2021). In contrast, even when scallops (A. purpuratus) can efficiently regulate their oxygen uptake in a broad temperature range, their basal metabolism and survival gets affected if exposed over prolonged periods to hypoxic or anoxic conditions (Aguirre-Velarde et al., 2016; Cueto-Vega et al., 2021). So under normal upwelling conditions in Independencia, low temperature and low oxygen levels favor mussels over scallops, whereas under EN (which increases temperature and oxygenizes the system), scallops can thrive (Wolff, 1987; Taylor et al., 2008a).
During extreme EN events, scallop production at Independencia can increase up to more than fifty-fold (Wolff, 1987; Arntz and Tarazona, 1990; Taylor et al., 2008a). Even though the stock-recruitment relationship gets weakened (Wolff et al., 2007), the increase in water temperature favors scallops, while it negatively impacts its main predators and competitors (Taylor et al., 2008a). It has been suggested that during such events, there is an alternation of species but that the functional niches remain, which seems to be correlated with the speed of colonization and longevity of the species that alternate (Wolff et al., 2003). This is an interesting question to explore in future simulations, given the apparent trends in SST and Chl-a and their potential consequence in the survival and distribution of such species in both systems.
The lack of nearby settlements and river influx into Independencia Bay reduce the risks of anthropogenic contamination through sewage or littering. In contrast, EN effects on the Sechura Bay scallop populations are different and rather negative. When EN causes a quick rise in SST in northern Peru, tropical-like storms develop, and heavy rainfall leads to a dramatic increase in river discharge into the bay, which increases particulate organic matter and reduces salinity and oxygen in the system. Although scallops are well adapted to high temperatures, these conditions are not favorable for the scallop stocks of Sechura, which tend to have massive mortalities during this period (Kluger et al., 2018).
The fisheries indicators of both systems are relatively similar. Total catch in Sechura is only slightly larger than in Independencia (73.7 vs. 71.2 t.km–2 year) and the Mean Trophic Level of The Catch (MTLC) is around 2.3 in both systems due to a higher proportion of biomass found at lower TLs. These values are considerably higher than for other bay systems found within the tropics and even within the Humboldt current system, such as Tongoy Bay in Chile (Wolff, 1994). However, compared to previous models developed for both bays, there are some notable differences. In Independencia, Taylor et al. (2008a) found that the total catch of the system (t.km–2) was significantly smaller in 1996 than that reported in this study, increasing in almost 2,000% in 1998, after EN, due to scallop proliferation. Not only scallops landings were higher in the 2007–2008 period, due to increased cultivation areas, but the high landings of mussels and cabinza grunt were not reported in the 1996 model. A look at the time-series of landings in Independencia might shine some light on why these differences were seen: was it due to an increasing fishery of these species over time or some other factor not perceived in the available data. In the case of Sechura, there is also a big landings difference, particularly of small pelagics, being about 15% higher in 1996, compared to 2007. Having had access to the Sechura landings time-series, we attribute this difference to a misrepresentation of the amount of anchovies landed in the Region, given that some reports include catches from outside the systems.
Catch composition differs between the two 2007 models, with high proportions of commercially important low-TL groups in Independencia (mainly mussels, macroalgae, scallops, and clams) and high catches and discards of high TL species such as predatory snails in Sechura Bay. Sechura also yields a considerably higher scallop harvest per unit of area and Independencia has relatively high catches of Rock crabs and Cabinza grunt (20.1 t.km–2 year, ∼28% of total catches), balancing out the low and high TL species in both systems. A significant difference was found in the gross efficiency (catch/net PP) between both bays, which for Independencia was about twice as high. This is mainly due to the importance of the ribbed mussel, which represented a tenth of all biomass and a third of the total landings in Independencia.
The fisheries data obtained from IMARPE (1996–2019) allowed us to filter the data appropriately and make sure that we used the landings from inside the model areas. It was also possible to identify the proportion of certain target groups caught inside the bays but landed in other sites, and those species that are (partially) caught elsewhere but landed in the bays’ landings sites.
There is a historical, socio-economic connection between Pisco and Sechura, which has been the focal point of ENSO-induced (mainly scallop driven) human migrations over the years (Badjeck, 2008; Mendo et al., 2008; Kluger et al., 2018). This two-way migration’s intricate dynamic is critical for understanding potential social-ecological repercussions in each system. Additionally, while recognizing the influence of offshore waters on the bays’ environmental and ecological characteristics, it is crucial to consider the effects of local inshore conditions.
Trophic Structure and Ecosystem Functioning
The overall energy flows of Sechura exceed those of Independencia, though the latter exhibited a higher capacity for energy cycling and transfer efficiency, particularly when this energy flows from primary producers, in spite of Sechura having higher net primary production. This high production in Sechura supported high-TL pelagic fish, that were shown to exert a top-down control in the system, as evidenced in the analysis keystone indices. However, Sechura had a strong reliance on the detritus food chain, whereas phytoplankton was shown to be more relevant as a keystone group in Independencia, where benthic carnivores also played an important role in the energy flows.
Both systems support a large biomass of low-TL benthic organisms, though it is worth noting that some demersal and pelagic fish species also contributed to lowering the trophic levels through omnivory and filter-feeding. Just like with the number of species, these fish constitute a low proportion of the total biomass in each model compared to their carnivorous counterparts (particularly in Sechura). According to the output from Ecopath, both systems contained ∼75% of their biomass within the first two TLs, while roughly 20% was found in TL 3. This situation resembles that of a typical upwelling (bottom-up driven) system, where most biomass is found and harvested at low TLs, also shown by Wolff (1994) for Tongoy Bay in Chile.
In the two models here presented, Sechura Bay exceeded Independencia Bay (by different margins) in all of the most relevant indices of total community energy flows (t.km–2 year), being more than 20% higher in the sum of all exports, sum of all flows into detritus, total system throughput (TST), sum of all production, net system production and net primary production. In both cases, the TST is relatively high and also reflects the influence of the upwelling system. Independencia had a similar value than that reported for Tongoy (Wolff, 1994), whereas Sechura is about 20% higher. This might be counterintuitive as Sechura is closer to the influence of Equatorial warm waters, and would be expected to exhibit lower relative throughput values. In comparison, the Gulf of Nicoya, located also in the Eastern Pacific, at ∼10°N under typical tropical conditions, shows about 75% less throughput (Wolff et al., 1998; Alms and Wolff, 2019). This shows that both study systems presented here are highly influenced by the upwelling system. Sechura seems to have higher values of TST due to a combination of upwelling influence (which drives primary production of plankton and macroalgae), eutrophication of the bay (through additional nutrient input by riverine waters), higher average temperatures (which lead to enhanced community metabolism), and a lower OMZ (which enlarges the vertical expansion of the system). The catches shown above also relate to this, and are much higher than those presented for the Gulf of Nicoya in 1993 and 2013 (Wolff et al., 1998; Alms and Wolff, 2019).
The systems did not have considerable differences between their total respiration and total biomass (t.km–2 year) values. However, the significantly higher value of net primary production (PP) found in Sechura during the 2007–2008 model period, suggests that this bay was less developed than Independencia, as it is assumed that more mature systems should support higher levels of biomass per unit of PP. This was particularly evident when looking at the very high PP value of Sechura in relation to its respiration (R) value, especially against an idealized stage of maturity (PP/R = 1).
Other indicators, such as cycling indices and transfer efficiencies, also pointed toward Independencia being a more developed, resilient and efficient system than Sechura. In particular, it has a significantly higher cycling of predatory and total energy flows when detritus is excluded. When detritus is included, Independencia still has a higher TST being cycled but only by a small margin (0.09% difference, Table 3). Furthermore, when assessing the ratios of detritivory over herbivory, and of total throughput from detritus over primary production (Table 3, section transfer efficiencies), the importance of detritus in the sustainment of the Sechura system becomes evident.
Both keystone indices prioritize groups based on their total relative trophic impact in relation to their biomass. However, KS3 (Valls et al., 2015) gives a higher importance to groups with lower biomass, thus typically identifying keystone species at higher trophic levels. In contrast, KS1 (Libralato et al., 2006) allows species at lower trophic (basal) levels to have high keystoneness if their trophic impact is relatively high.
Even when KS3 has been shown to be more balanced than KS1 (Valls et al., 2015), we decided to look into both indices, given that the two bays in this study are heavily bottom-up driven, as expected for upwelling systems. This way we could see through slightly different filters which functional groups were short-listed as keystone species and enrich the analysis.
The scatterplots of trophic impact versus biomass displaying the different functional groups per system, did not show any of these groups as being keystone, according to the classification boxes. This could be indicative of a high fishing impact, as ecosystems affected by this tend have less prevalence of keystone species (Heymans et al., 2012; Valls et al., 2015). Still, the two keystone indices analyzed show a ranking of high to low keystoneness for all functional groups in each system.
When looking at KS3 in Sechura, pelagic and littoral fish occupied the top five keystone groups, where the predatory Bonitos and Mackerels were the highest. Independencia also had Mackerels within the top five keystone groups, though the highest in the rank was the filter-feeding Small pelagics. However, the group Carnivorous echinoderms also appeared as a keystone species in Independencia, hinting to the importance of both the benthic and the pelagic pathways. Furthermore, given that Independencia also included the basal groups Phytoplankton and Zooplankton 1 within the high ranked groups, these results suggest that this system is more stable with relative importance of both basal and higher trophic level groups.
The apparently higher top-down control of pelagic flows in Sechura, which also exhibited longer energy pathways (as seen in the higher TLs of Bonitos and Mackerels), might suggest that this system is more developed than its southern counterpart. However, Ecopath network analysis revealed that Sechura had a shorter average path length and a reduced capacity for recycling energy within the system, which are general indicators of system immaturity. Still, Sechura’s higher net primary production has been able to sustain these longer and relevant pelagic chains, though it was shown that a large proportion of this production is not being directly consumed in Sechura, having a higher reliance on the detrital pool, compared to Independencia.
As expected, KS1 shows a slightly different story, though it is consistent with the general description of the systems thus far. As stated before, this index tends to give keystone status to groups that have relatively large biomass, based on their high trophic impact. Both bays have Phytoplankton and Zooplankton within the top five highest-ranked keystone groups, showing the importance of these basal groups in such upwelling driven areas. As with KS3, Mackerels and Miscellaneous littoral fish are also shown as relevant groups in Sechura, pointing toward the relevance of these high-TL pelagic food chains, though a large proportion of the latter group’s diet comes from benthic invertebrates. In Independencia, the groups that are repeated in the top five of both indices are Phytoplankton, Small pelagics and Zooplankton 1, emphasizing the role of the planktonic energy route in this system. The benthic carnivores Frog shell and Rock crabs as keystone groups in Sechura and Independencia, respectively, a result also perceived in the MTI analysis This shows that the high-TL benthic community is very relevant in both systems, particularly in Independencia, as shown for other similar coastal upwelling systems of the eastern Pacific (Ortiz and Wolff, 2002; Ortiz et al., 2013; González et al., 2016).
One of the most salient result coming out of the keystone indices however is the role of the Phytoplankton group in each bay. In Independencia, it appears to be so important that both indices place it second in their respective keystone ranking. In Sechura, Phytoplankton appears first in the ranking when using KS1, but is placed tenth in the keystones rank list of KS3, likely due to the fact that its biomass is relatively higher and that Sechura relied more on the detritus energy flows, as shown above. It is worth noting that high PP could also increase the detritus in the system when there is not enough consumption of phytoplankton and macroalgae by herbivores (i.e., grazing and filtering). In some cases, this situation might kickstart a positive feedback loop where oxygen is too depleted, potentially causing mass mortalities and thus much more detrital pools (Peña et al., 2010; Braeckman et al., 2019; Walton et al., 2022).
Synthesis and Considerations for Management
We aimed to understand the latitudinal similarities and differences between shallow-coastal areas along Peru by building and analyzing the trophic models (La Niña 07/08 period) of two important sites: Sechura Bay in the north (at the convergence of Humboldt and tropical waters) and Independencia Bay in the central-south (under typical upwelling conditions). We compared their biodiversity, resource use, community energetics and underlying abiotic conditions and hypothesized that Independencia is the more productive system, as it is located adjacent to the main upwelling center off Peru and has not been subject to constant environmental and anthropogenic disturbances as Sechura; partly because Independencia is part of the Paracas Bay Reserve, which is under government monitoring. On the other hand, Sechura is surrounded by human settlements and is situated in the northern transition zone, where impacts from El Niño are more prominent with drastic environmental changes that perturb the system more often than in Independencia, usually only affected by strong events. Even when Independencia has relatively higher oxygen values than other bays within the HCUS, both systems generally have low oxygen levels, though Sechura is more prone to substantial and frequent hypoxia events.
Our analysis showed significant distinctions in the environmental conditions of both bays, where sea surface temperature differences seem to have amplified in recent years. Sechura has shown a warming trend over the years, while Independencia has maintained its cold water conditions. Chlorophyll concentrations have increased greatly in the bays over the past two decades, surprisingly higher inside Sechura, possibly affected by local environmental and anthropogenic pressures. The models revealed that both systems are bottom-up driven with high biomass and production at the lower trophic levels, though keystone indices revealed the relevance of top-down control, particularly in Sechura. It is the shallowness of the two bays that allows the benthic and demersal assemblages to proliferate under average conditions of great primary production, even with reduced oxygen levels. Furthermore, the reoxygenation process happening during ENSO seem to boost this proliferation, particularly in Independencia.
Productivity was very similar in both systems, with differences in the structure and size of their energy flows. More cycling was done in Independencia, where phytoplankton-based food chains played an essential role in the overall dynamics. In contrast, the detritus food chain appears to be crucial for energy flow in Sechura. Differences in biota and flow structure relate to the systems’ environmental conditions, i.e., more diverse warm-water fish and shellfish (carnivores and detrivores) in the north and mostly invertebrates (filter-feeders and carnivores) adapted to high-circulating cold water in the central-south. Catches in both systems were dominated by the diving fisheries and comprised mostly scallops (bottom-cultured), snails and fish in Sechura, and mussels, clams, crabs and fish in Independencia. Even though Sechura is highly depended on the detrital food chain, the higher amount of primary production (phytoplankton and macroalgae) sustains a pelagic food web, particularly of high trophic level carnivores that appear to exert a top-down control of the system. In contrast, Independencia is more depended on the phytoplankton food chain, which appears to transfer the energy more efficiently through both benthic and pelagic systems.
Overall, system indicators suggest the northern bay is a comparatively less developed system that heavily relies on detrital energy flows to cope with the constant environmental perturbation and anthropogenic pressures. When management regimes and adaptation to climate change are considered, both bays differ. Independencia shall likely maintain its general highly productive system features, strengthening its filter-feeding food chains and increasing resilience over the following years. In contrast, further warming, El Niño and human-driven perturbations may increasingly disrupt Sechura in the near future, reducing its overall stability and functionality. Furthermore, there seems to be increasing pressure to start new aquaculture ventures inside bay systems in Peru, with particular interest in Sechura, which pose interesting scenarios to test the potential social-ecological impacts of future management policies. Further work on trophic-model simulations can help address questions related to social-ecological tipping points linked to environmental and resource use drivers.
Data Availability Statement
The data analyzed in this study is subject to the following licenses/restrictions: The scallop surveys and fisheries data can be partially obtained from published literature (as commented in Materials and Methods of this manuscript). The complete datasets belong to the “Instituto del Mar del Perú (IMARPE)” and was obtained through the Data Agreement of the Humboldt-Tipping Project. The authors are not authorized to publish the original/raw data. Requests to access these datasets should be directed to http://www.imarpe.gob.pe/imarpe/servicios/formulario-atencion.
Author Contributions
AD, GR, LK, and MW contributed to the conception and structure of the study. AD organized and processed all datasets, while CC provided insight on the data collection process by the IMARPE, built and ran the Ecopath models and its analyses, while GR and MW revised and provided in-depth commentary on them, and wrote the first draft of the manuscript. All authors contributed to manuscript revision, read, and approved the submitted version.
Funding
This study was developed as part of the project Humboldt-Tipping (https://humboldt-tipping.org/en), sponsored by the Federal Ministry of Education and Research of Germany.
Conflict of Interest
The authors declare that the research was conducted in the absence of any commercial or financial relationships that could be construed as a potential conflict of interest.
Publisher’s Note
All claims expressed in this article are solely those of the authors and do not necessarily represent those of their affiliated organizations, or those of the publisher, the editors and the reviewers. Any product that may be evaluated in this article, or claim that may be made by its manufacturer, is not guaranteed or endorsed by the publisher.
Acknowledgments
We are grateful for the support given at different hierarchical levels by the “Instituto del Mar del Perú” (IMARPE) in the facilitation, synthesis, processing and discussion of relevant information, from the start of the Humboldt Tipping Project and even through the COVID-19 pandemic.
Supplementary Material
The Supplementary Material for this article can be found online at: https://www.frontiersin.org/articles/10.3389/fmars.2022.829424/full#supplementary-material
Footnotes
References
Aguirre-Velarde, A., Jean, F., Thouzeau, G., and Flye-Sainte-Marie, J. (2016). Effects of progressive hypoxia on oxygen uptake in juveniles of the Peruvian scallop, Argopecten purpuratus (Lamarck, 1819). Aquaculture 451, 385–389. doi: 10.1016/j.aquaculture.2015.07.030
Alms, V., and Wolff, M. (2019). The gulf of nicoya (Costa Rica) fisheries system: two decades of change. Mar. Coast. Fish. 11, 139–161. doi: 10.1002/mcf2.10050
Arntz, W. E., Gallardo, V. A., Gutiérrez, D., Isla, E., Levin, L. A., Mendo, J., et al. (2006). El Nino and similar perturbation effects on the benthos of the Humboldt, California, and Benguela current upwelling ecosystems. Adv. Geosci. 6, 243–265. doi: 10.1144/GSL.SP.1991.058.01.10
Arntz, W. E., and Tarazona, J. (1990). “Global ecological consequences of the 1982-83 El Nino-Southern Oscillation,” in Global Ecological Consequences of the 1982-83 El Nino-Southern Oscillation, ed. P. W. Glynn (Amsterdam: Elsevier), 323–360.
Arntz, W. E., Tarazona, J., Gallardo, V. A., Flores, L. A., and Salzwedel, H. (1991). “Benthos communities in oxygen deficient shelf and upper slope areas of the Peruvian and Chilean Pacific coast, and changes caused by El Niño,” in Modern and Ancient Continental Shelf Anoxia, eds R. V. Tyson and T. H. Pearson (London: Geological Society of London), 131–154.
Badjeck, M. C. (2008). Vulnerability of Coastal Fishing Communities to Climate Variability and Change: Implications for Fisheries Livelihoods and Management in Peru. Bremen: Universität Bremen.
Badjeck, M. C., Mendo, J., Wolff, M., and Lange, H. (2009). Climate variability and the Peruvian scallop fishery: the role of formal institutions in resilience building. Clim. Change 94, 211–232. doi: 10.1007/s10584-009-9545-y
Bakun, A. (1996). Patterns in the Ocean: Ocean Processes and Marine Population Dynamics. San Diego, CA: California Sea Grant.
Bakun, A., and Weeks, S. J. (2008). The marine ecosystem off Peru: what are the secrets of its fishery productivity and what might its future hold? Prog. Oceanogr. 79, 290–299. doi: 10.1016/j.pocean.2008.10.027
Belmadani, A., Echevin, V., Dewitte, B., and Colas, F. (2012). Equatorially forced intraseasonal propagations along the Peru-Chile coast and their relation with the nearshore eddy activity in 1992-2000: a modeling study. J. Geophys. Res. Oceans 117:C04025. doi: 10.1029/2011JC007848
Bernedo, D., Gómez, H., León, H., and Quispe, J. (2017). Anuario Estadístico Pesquero y Acuícola 2017: La Actividad Productiva del Sector en Números. Lima: Ministerio de la Producción.
Bertrand, A., Ballón, M., and Chaigneau, A. (2010). Acoustic observation of living organisms reveals the upper limit of the oxygen minimum zone. PLoS One 5:e10330. doi: 10.1371/journal.pone.0010330
Braeckman, U., Pasotti, F., Vázquez, S., Zacher, K., Hoffmann, R., Elvert, M., et al. (2019). Degradation of macroalgal detritus in shallow coastal Antarctic sediments. Limnol. Oceanogr. 64, 1423–1441. doi: 10.1002/lno.11125
Brey, T. (2001). Population Dynamics in Benthic Invertebrates. A Virtual Handbook. Bremerhaven: Alfred Wegener Institute for Polar and Marine Research.
Chavez, F. P., Bertrand, A., Guevara-Carrasco, R., Soler, P., and Csirke, J. (2008). The northern humboldt current system: brief history, present status and a view towards the future. Prog. Oceanogr. 79, 95–105. doi: 10.1016/j.pocean.2008.10.012
Chirichigno, F. N., and Cornejo, U. R. M. (2001). Catálogo Comentado de Los Peces Marinos del Peru. Callao: Instituto del Mar del Peruì.
Christensen, V., and Pauly, D. (1992). ECOPATH II - a software for balancing steady-state ecosystem models and calculating network characteristics. Ecol. Modell. 61, 169–185. doi: 10.1016/0304-3800(92)90016-8
Christensen, V., and Walters, C. J. (2004). Ecopath with ecosim: methods, capabilities and limitations. Ecol. Modell. 172, 109–139. doi: 10.1016/j.ecolmodel.2003.09.003
Cueto-Vega, R., Flye-Sainte-Marie, J., Aguirre-Velarde, A., Jean, F., Gil-kodaka, P., and Thouzeau, G. (2021). Size-based survival of cultured Argopecten purpuratus (L, 1819) under severe hypoxia. J. World Aquac. Soc. 53, 151–173. doi: 10.1111/jwas.12777
Dewitte, B., and Takahashi, K. (2019). Diversity of moderate El Niño events evolution?: role of air – sea interactions in the eastern tropical Pacific. Clim. Dyn. 52, 7455–7476. doi: 10.1007/s00382-017-4051-9
Donayre, S., Flores, D., and Guardia, A. (2010). Evaluación Poblacional de Gari Solida en Bahía Independencia, Pisco, Vol. 42. Lima: Instituto del Mar del Perú.
Echevin, V., Albert, A., Lévy, M., Graco, M., Aumont, O., Piétri, A., et al. (2014). Intraseasonal variability of nearshore productivity in the Northern humboldt current system: the role of coastal trapped waves. Cont. Shelf Res. 73, 14–30. doi: 10.1016/j.csr.2013.11.015
Espinoza-Morriberón, D. (2018). Interannual and Decadal Variability of the Primary Productivity and Oxygen Minimum Zone in the Peruvian Upwelling System. Paris: Sorbonne Université.
Espinoza-Morriberón, D., Echevin, V., Colas, F., Tam, J., Gutierrez, D., Graco, M., et al. (2019). Oxygen variability during ENSO in the tropical South Eastern Pacific. Front. Mar. Sci. 5:526. doi: 10.3389/fmars.2018.00526
Flores, D., Donayre, S., Zeballos, J., and Sarmiento, H. (2010). Evaluación Poblacional de Ensis Macha en Bahía Independencia, Pisco, Vol. 42. Lima: Instituto del Mar del Perú.
Froese, R., and Pauly, D. (eds). (2000). FishBase 2000: Concepts, Design and Data Sources. Los Banos, CA: ICLARM.
Forrest, B. M., Gillespie, P. A., Cornelisen, C. D., and Rogers, K. M. (2007). Multiple indicators reveal river plume influence on sediments and benthos in a New Zealand coastal embayment. N. Z. J. Mar. Freshw. Res. 41, 13–24. doi: 10.1080/00288330709509892
Garreaud, R. D. (2018). A plausible atmospheric trigger for the 2017 coastal El Niño. Int. J. Climatol. 38, e1296–e1302. doi: 10.1002/joc.5426
Gonzales, E. I., and Yépez, V. E. (2007). Estudio de Línea Base del Ámbito Marino de la Bahía de Sechura. Lima: Instituto del Mar del Perú.
Gonzales, I., Jacinto, P., Segura, M., and Macalupu, J. (2012). Mortandad de Concha de Abanico Frente a Vichayo, Barrancos y Parachique (Sechura), 16 Febrero 2012. IMARPE Piura, Technical Report. Paita: Instituto del Mar del Perú, IMARPE.
González, J., Ortiz, M., Rodríguez-zaragoza, F., and Ulanowicz, R. E. (2016). Assessment of long-term changes of ecosystem indexes in Tongoy Bay (SE Pacific coast): based on trophic network analysis. Ecol. Indic. 69, 390–399. doi: 10.1016/j.ecolind.2016.04.019
Graco, M. I., Ledesma, J., Flores, G., and Girón, M. (2007). Nutrientes, oxígeno y procesos biogeoquímicos en el sistema de surgencias de la corriente de Humboldt frente a Perú. Rev. Peru. Biol. 14, 117–128.
Graco, M. I., Purca, S., Dewitte, B., Castro, C. G., Morón, O., Ledesma, J., et al. (2017). The OMZ and nutrient features as a signature of interannual and low-frequency variability in the Peruvian upwelling system. Biogeosciences 14, 4601–4617. doi: 10.5194/bg-14-4601-2017
Guevara-Carrasco, R., and Bertrand, A. (eds). (2017). Atlas de la Pesca Artesanal del mar del Perú. Lima: IMARPE-IRD.
Gutiérrez, C. F. (2017). El Macrobentos de Fondo Blando Somero de Bahía Independencia, Pisco, Perú, Asociado al Evento La Nina 2007. Lima: Universidad Nacional Mayor de San Marcos.
Gutiérrez, D., Akester, M., and Naranjo, L. (2016). Productivity and sustainable management of the Humboldt current large marine ecosystem under climate change. Environ. Dev. 17, 126–144. doi: 10.1016/j.envdev.2015.11.004
Gutiérrez, D., Bouloubassi, I., Sifeddine, A., Purca, S., Goubanova, K., Graco, M., et al. (2011). Coastal cooling and increased productivity in the main upwelling zone off Peru since the mid-twentieth century. Geophys. Res. Lett. 38:L07603. doi: 10.1029/2010GL046324
Heileman, S. (2009). “XIV-48 pacific central-American coastal: LME # 11,” in The UNEP Large Marine Ecosystem Report: A Perspective on Changing Conditions in LMEs of the World’s Regional Seas, eds K. Sherman and G. Hempel (Nairobi: United Nations Environment Programme).
Heileman, S., Guevara, R., Chavez, F., Bertrand, A., and Soldi, H. (2009). “XVII-56 humboldt current?: LME # 13,” in The UNEP Large Marine Ecosystem Report: A Perspective on Changing Conditions in LMEs of the World’s Regional Seas, eds K. Sherman and G. Hempel (Nairobi: United Nations Environment Programme).
Heymans, J. J., Coll, M., Libralato, S., and Christensen, V. (2012). “Ecopath theory, modeling, and application to coastal ecosystems,” in Treatise on Estuarine and Coastal Science, eds E. Wolanski and D. McLusky (Waltham, MA: Academic Press), 93–113.
Heymans, J. J., Coll, M., Link, J. S., Mackinson, S., Steenbeek, J., Walters, C., et al. (2016). Best practice in ecopath with ecosim food-web models for ecosystem-based management. Ecol. Modell. 331, 173–184. doi: 10.1016/j.ecolmodel.2015.12.007
Jarre, A., Muck, P., and Pauly, D. (1991). Two approaches for modelling fish stock interactions in the Peruvian upwelling ecosystem. ICES Mar. Sci. Symp. 193, 171–184.
Jarre-Teichmann, A. (1998). The potential role of mass balance models for the management of upwelling ecosystems. Ecol. Appl. 8, 93–103.
Kluger, L. C., Kochalski, S., Aguirre-Velarde, A., Vivar, I., and Wolff, M. (2018). Coping with abrupt environmental change: the impact of the coastal El Niño 2017 on artisanal fisheries and mariculture in North Peru. ICES J. Mar. Sci. 76, 1122–1130. doi: 10.1093/icesjms/fsy171
Kluger, L. C., Taylor, M. H., Mendo, J., Tam, J., and Wolff, M. (2016). Carrying capacity simulations as a tool for ecosystem-based management of a scallop aquaculture system. Ecol. Modell. 331, 44–55. doi: 10.1016/j.ecolmodel.2015.09.002
Libralato, S., Christensen, V., and Pauly, D. (2006). A method for identifying keystone species in food web models. Ecol. Model. 195, 153–171. doi: 10.1016/j.ecolmodel.2005.11.029
Link, J. S. (2010). Adding rigor to ecological network models by evaluating a set of pre-balance diagnostics: a plea for PREBAL. Ecol. Model. 221, 1580–1591. doi: 10.1016/j.ecolmodel.2010.03.012
Loaiza Alamo, I. (2020). Marine Species Associated to Peruvian Scallop Argopecten purpuratus Culture: Trophic Interactions and Contaminant Exposure. Ghent: Ghent University.
Medina, M., Araya, M., and Vega, C. (2004). Alimentación y relaciones tróficas de peces costeros de la zona norte de Chile. Investig. Mar. 32, 33–47. doi: 10.4067/s0717-71782004000100004
Mendo, J., James, M., Gil-kodaka, P., Gozzer, R., Martina, J., Gómez, I., et al. (2020). Desarrollo de un Modelo Dinámico de co-Manejo Para la Protección de la Biodiversidad en una Pesquería de Arrastre de Langostino en el Perú. Lima: Universidad Nacional Agraria La Molina, doi: 10.13140/RG.2.2.26215.37280
Mendo, J., and Wolff, M. (2003). El impacto de el niño sobre la producción de concha de abanico (Argopecten purpuratus) en bahía independencia, Pisco, Perú. Ecol. Apl. 2, 51–57. doi: 10.21704/rea.v2i1-2.247
Mendo, J., Wolff, M., Carbajal, W., Gonzáles, I., and Badjeck, M. (2008). “Manejo y explotación de los principales bancos naturales de concha de abanico (Argopecten purpuratus) en la costa Peruana,” in Estado Actual Del Cultivo y Manejo de Moluscos Bivalvos y Su Proyección Futura: Factores Que Afectan Su Sustentabilidad En América Latina. Taller Técnico Regional de La FAO, eds A. Lovatelli, S. Vannuccini, and D. McLeod (Rome: FAO), 101–114.
Mendo, J., Wolff, M., Mendo, T., and Ysla, L. (2016). Scallop fishery and culture in Peru. Dev. Aquac. Fish. Sci. 40, 1089–1109. doi: 10.1016/B978-0-444-62710-0.00028-6
Ortiz, M., Levins, R., Campos, L., Berrios, F., Campos, F., Jordán, F., et al. (2013). Identifying keystone trophic groups in benthic ecosystems: implications for fisheries management. Ecol. Indic. 25, 133–140. doi: 10.1016/j.ecolind.2012.08.020
Ortiz, M., and Wolff, M. (2002). Trophic models of four benthic communities in Tongoy Bay (Chile): comparative analysis and preliminary assessment of management strategies. J. Exp. Mar. Biol. Ecol. 268, 205–235.
Peña, M. A., Katsev, S., Oguz, T., and Gilbert, D. (2010). Modeling dissolved oxygen dynamics and hypoxia. Biogeosciences 7, 933–957. doi: 10.5194/bg-7-933-2010
Riascos, J. M., Uribe, R. A., Donayre, S., Flores, D., Galindo, O., Quispe, C., et al. (2016). Human footprints on benthic communities in marine reserves: a study case in the most productive upwelling system worldwide. Mar. Ecol. Prog. Ser. 557, 65–75. doi: 10.3354/meps11857
Salazar, C. M., Bandín, R., Castagnino, F., and Monteferri, B. (2020). Artes y Métodos de Pesca del Perú - Serie Ilustrativa. Lima: Sociedad Peruana de Derecho Ambiental, 52.
Schunck, H., Lavik, G., Desai, D. K., Großkopf, T., Kalvelage, T., Löscher, C. R., et al. (2013). Giant hydrogen sulfide plume in the oxygen minimum zone off peru supports chemolithoautotrophy. PLoS One 8:e68661. doi: 10.1371/journal.pone.0068661
Stark, J. D., Donlon, C. J., Martin, M. J., and Mcculloch, M. E. (2007). “OSTIA: an operational, high resolution, real time, global sea surface temperature analysis system,” in Proceedings of the OCEANS 2007 - Europe (Aberdeen: IEEE). doi: 10.1109/OCEANSE.2007.4302251
Takahashi, K., and Dewitte, B. (2016). Strong and moderate nonlinear El Niño regimes. Clim. Dyn. 46, 1627–1645. doi: 10.1007/s00382-015-2665-3
Takahashi, K., and Martínez, A. G. (2019). The very strong coastal El Niño in 1925 in the far-eastern Pacific. Clim. Dyn. 52, 7389–7415. doi: 10.1007/s00382-017-3702-1
Takahashi, K., Montecinos, A., Goubanova, K., and Dewitte, B. (2011). ENSO regimes: reinterpreting the canonical and Modoki El Nio. Geophys. Res. Lett. 38:L10707. doi: 10.1029/2011GL047364
Tam, J., Taylor, M. H., Blaskovic, V., Espinoza, P., Ballón, R. M., Díaz, E., et al. (2008). Progress in oceanography trophic modeling of the Northern humboldt current ecosystem, part i: comparing trophic linkages under La Niña and El Niño conditions. Prog. Oceanogr. 79, 352–365. doi: 10.1016/j.pocean.2008.10.007
Tam, J., Espinoza, D., Oliveros, R., Romero, C., and Ramos, J. (2012). “Modelos de simulación y determinación de la capacidad de carga de la concha de abanico Argopecten purpurats,” in Informe Final–Estudio Bio-Oceanográfico para Determinación de la Capacidad de Carga en la Bahía de Sechura, ed. R. Cisneros Burga (Instituto del Mar del Perú, IMARPE–Dirección General de Investigaciones en Acuicultura), 241–253.
Tarazona, J., and Arntz, W. (2001). “The peruvian coastal upwelling system,” in Coastal Marine Ecosystems of Latin America, eds E. Seeliger and B. Kjerfve (Berlin: Springer), 229–244. doi: 10.1007/978-3-662-04482-7_17
Taylor, M. H., and Wolff, M. (2007). Trophic modeling of eastern boundary current systems: a review and prospectus for solving the “Peruvian Puzzle.” Rev. Peru. Biol. 14, 87–100.
Taylor, M. H., Wolff, M., Mendo, J., and Yamashiro, C. (2008a). Changes in trophic flow structure of independence bay (Peru) over an ENSO cycle. Prog. Oceanogr. 79, 336–351. doi: 10.1016/j.pocean.2008.10.006
Taylor, M. H., Wolff, M., Vadas, F., and Yamashiro, C. (2008b). Trophic and environmental drivers of the sechura bay ecosystem (Peru) over an ENSO cycle. Helgol. Mar. Res. 62, 15–32. doi: 10.1007/s10152-007-0093-4
Valls, A., Coll, M., Christensen, V., and Ellison, A. M. (2015). Keystone species: toward an operational concept for marine biodiversity conservation. Ecol. Monogr. 85, 29–47. doi: 10.1890/14-0306.1
Vargas, M., Fuentes, P., Hernáez, P., Olivares, A., and Rojas, P. (1999). Relaciones tróficas de cinco peces costeros comunes en el área submareal del norte de Chile (20°11′-20°20′8). Rev. Biol. Trop. 47, 601–604. doi: 10.15517/rbt.v47i3.19214
Walton, M. E. M., Browne, R., Griffiths, J. N., Cartwright, D., Robins, P., Malham, S. K., et al. (2022). Kelp detritus: unutilized productivity or an unacknowledged trophic resource? Sci. Total Environ. 820:153191. doi: 10.1016/j.scitotenv.2022.153191
Wolff, M. (1987). Population dynamics of the Peruvian scallop Argopecten purpuratus during the El Nino phenomenon of 1983. Can. J. Fish. Aquat. Sci. 44, 1684–1691. doi: 10.1139/f87-207
Wolff, M. (1994). A trophic model for Tongoy Bay -a system exposed to suspended scallop culture (Northern Chile). J. Exp. Mar. Biol. Ecol. 182, 149–168. doi: 10.1016/0022-0981(94)90048-5
Wolff, M. (2010). Galapagos does not show recent warming but increased seasonality. Galapagos Res. 67, 38–44.
Wolff, M., Koch, V., Chavarría, J. B., and Vargas, J. A. (1998). A trophic flow model of the Golfo de Nicoya, Costa Rica. Rev. Biol. Trop. 46(Suppl. 6), 63–79.
Wolff, M., Wosnitza-Mendo, C., and Mendo, J. (2003). “The Humboldt Current – trends in exploitation, protection and research,” in Large Marine Ecosystems of the World, eds G. Hempel and K. Sherman (Amsterdam: Elsevier), 279–309.
Wolff, M. (2018). “El niño – Curse and blessing for Peru’s upwelling system,” in Scientific Partnership for a Better Future, eds G. Hempel, I. Hempel, and A.-K. Hornidge (Bremen: Edition Falkenberg), 73–76.
Keywords: ecosystem modelling, Peruvian upwelling system, shallow bays, climate variability, latitudinal comparison, resource management, bottom-up energy flow, Ecopath food web model
Citation: Del Solar A, Romagnoni G, Kluger LC, Céspedes CMS and Wolff M (2022) Comparative Food Web Analysis of Two Peruvian Bay Systems Along a Latitudinal Gradient: Resource Use and the Environmental Envelope. Front. Mar. Sci. 9:829424. doi: 10.3389/fmars.2022.829424
Received: 05 December 2021; Accepted: 17 February 2022;
Published: 30 March 2022.
Edited by:
Angel Borja, Technological Center Expert in Marine and Food Innovation (AZTI), SpainReviewed by:
Tomás I. Marina, CONICET Centro Austral de Investigaciones Científicas, ArgentinaPasquale Ricci, University of Bari Aldo Moro, Italy
Copyright © 2022 Del Solar, Romagnoni, Kluger, Céspedes and Wolff. This is an open-access article distributed under the terms of the Creative Commons Attribution License (CC BY). The use, distribution or reproduction in other forums is permitted, provided the original author(s) and the copyright owner(s) are credited and that the original publication in this journal is cited, in accordance with accepted academic practice. No use, distribution or reproduction is permitted which does not comply with these terms.
*Correspondence: Alonso Del Solar, alonso.delsolar@leibniz-zmt.de
†ORCID: Alonso Del Solar, orcid.org/0000-0003-0950-2640; Giovanni Romagnoni, orcid.org/0000-0002-0460-5360; Lotta C. Kluger, orcid.org/0000-0003-1433-4477; Carlos M. Salazar Céspedes, orcid.org/0000-0003-3703-1663; Matthias Wolff, orcid.org/0000-0002-0212-7596