- Third Institute of Oceanography, Ministry of Natural Resources, Xiamen, China
Taxonomic information is important to fisheries management and conservation. In this study, we collected 95 specimens of larval fish from Hawaiian waters and a total of 92 mitochondrial COI barcode sequences were obtained, representing 20 species, 18 genera and 12 families. The determined average Kimura-2-parameter distances within species, genera, families, and orders were 0.72%, 25.99%, 26.30%, and 27.50%, respectively, and the mean interspecific distance was found to be 36-fold higher than the mean intraspecific distance. Phylogenetic tree analysis showed that individuals belonging to the same species were clustered together and could be clearly distinguished. The findings of this study can make a valuable contribution to our knowledge of the diversity and dispersal of larval fish in Hawaiian waters.
Introduction
The larval stage of fish is defined as the life stage prior to attaining the full complement of fin ray elements and the complete development of scales. Given that they represent a key trophic link between plankton and higher predators, the larvae of fish play an important role in the efficient functioning of marine ecosystems (Heimeier et al., 2010; Ardura et al., 2016; Mazaheri Kouhanestani et al., 2020). Moreover, larval fish are the basis for the replenishment and sustainable use of fish stocks. Consequently, a thorough understanding of the biology of the early life history of fish is essential for effective fisheries management (Mazaheri Kouhanestani et al., 2020). Specifically, the knowledge of larval ecology provides fundamental information on the reproductive biology of fish, such as the timing and sites of reproduction, migration routes, and success of population recruitment (Wibowo et al., 2017; Wibowo et al., 2018), which are important with respect to monitoring fish ecology, analyzing environmental impacts, developing management and conservation plans, establishing fishing management strategies, and contributing to the conservation of vulnerable and threatened species (Reynalte-Tataje et al., 2011; Wibowo et al., 2018).
The classification and identification of fish larvae are not only of importance concerning taxonomic studies but also key prerequisites from the perspective of fishery management. Ecological studies on the diversity and distribution of the larvae of marine fish require accurate taxonomic identification, which can, however, represent a considerable challenge (Heimeier et al., 2010; Valdez-Moreno et al., 2010). Traditionally, larval identification has been based primarily on the examination of morphological characters such as body shape, pigmentation, meristic count, and trait measurements (Azmir et al., 2017). However, a notable difficulty associated with morphological identification is that larval fish frequently bear little resemblance to adults. Moreover, the larvae of different species often exhibit the same or duplicated characteristics (Victor et al., 2009; Ko et al., 2013; Kwun, 2018). In particular, closely related taxa, such as congeneric and cryptic species, can be notably difficult to identify based on morphology (Matarese et al., 2011; Kwun, 2018). Furthermore, different levels of expertise and capabilities among larval fish taxonomists have made such experience a dependent variable in the morphological identification of larvae (Azmir et al., 2017). Consequently, larval fish identification using traditional morphological characters should be more conservative, and it is better to identify only to the family or genus level, and not to the species level, in order to avoid identification errors (Ko et al., 2013). Such limitations inherent in morphology-based identification systems and the declining number of experienced taxonomists accordingly highlight the necessity of a molecular approach which is a complementary tool to morphological identification (Zhang and Hanner, 2011; Xing et al., 2018). DNA barcoding, the sequencing of an approximately 650-base pair (bp) region of the cytochrome c oxidase I (COI) gene, has gained widespread acceptance in the scientific literature as an accurate, sensitive, rapid, and standard method for the identification of a diverse range of animal lineages, including fish species (Hebert et al., 2003; Kenchington et al., 2017; Xing et al., 2018; Xing et al., 2020a; Xing et al., 2020b). It has been successfully applied in the identification of coral reef ichthyoplankton (Hubert et al., 2015), larval fish in the Eastern Atlantic Ocean (Ardura et al., 2016), the larval fish community in the central Red Sea (Patterson et al., 2017), drifting fish eggs in the Yangtze River (Liu et al., 2018), and larval lantern fish in the Gulf of Mexico (Batta-Lona et al., 2019).
To this end, in the present study, we evaluated the utility of DNA barcoding in establishing the taxonomy of larval fish collected from Hawaiian waters. We believe the findings of this study will significantly enhance our understanding of the species diversity and distribution of larval fish and the reproductive activity of fishes in Hawaiian waters, and we anticipate that our work will make a valuable contribution to documenting and conserving the biodiversity in this region.
Materials and Methods
Sample Collection
Sampling was conducted at four stations in Hawaiian waters in October 2019, the locations of which are shown in Figure 1. Larval fish were sampled using a bongo net with a mouth opening of 80 cm diameter and mesh size of 500 μm. Whole specimens were preserved in 95% ethanol during sampling and subsequently transported to the laboratory. The collected larvae were initially separated from the remaining zooplankton under a dissection microscope, photographed, and thereafter sorted into different morphotypes according to their basic morphological characters. The voucher specimens were deposited in the Marine Biological sample Museum at the Third Institute of Oceanography, Ministry of Natural Resources.
DNA Extraction, PCR Analysis, and Sequencing
Total DNA was extracted from individual larvae using a DNeasy Tissue Kit (QIAGEN), with the quality and quantity of the extracted DNA is determined using a N50-Touch spectrophotometer (NanoPhotometer, Germany). Sequences of approximately 655 bp within the 5’ region of the mitochondrial COI gene were amplified using the upstream primers jgLCO1490 TITCIACIAAYCAYAARGAYATTGG and jgHCO2198 TAIACYTCIGGRTGICCRAARAAYCA (Geller et al., 2013). The 25-µL reaction mixtures contained 16.0 of µL ultrapure water, 2.5 µL of 10× PCR buffer, 2.0 µL of MgCl2 (25 mM), 0.5 µL of each dNTP (10 mM), 0.5 µL of each primer (100 nmol), 0.5 µL of Taq DNA polymerase (5 U/μL), and 1 µL of DNA template. The thermal cycling conditions consisted of an initial denaturation step of 2 min at 95°C, followed by 37 cycles of denaturation (94°C, 45 s), annealing (48°C, 30 s), and extension (72°C, 1 min), with a final extension at 72°C for 8 min, after which the reaction mixtures were held at 4°C. The samples were subsequently run on 1.0% agarose gels for analysis of the amplification products, the sequencing in both directions of which was performed by Sangon Biotech (Shanghai, China).
Data Analysis
The SeqMan package of DNAStar software was used to splice the forward and reverse sequences determined for each sample. Specimens were identified by comparing the COI sequences thus obtained with those in the NCBI database using dedicated statistical tools, and we adopted a sequence similarity of at least 98% as a threshold to determine the validity of species identification (Armani et al., 2015; Xing et al., 2020b). Subsequently, the sequences were arranged using ClustalW in MEGA6.0 software. Pairwise genetic distances were calculated using the Kimura-2-parameter (K2P) distance model (Kimura, 1980).
The best-fit evolutionary models were selected based on Bayesian Information Criterion (BIC) by using ModelFinder (Kalyaanamoorthy et al., 2017). Bayesian Inference phylogenies were inferred using MrBayes 3.2.6 (Ronquist et al., 2012) (2 parallel runs, 2000000 generations), in which the initial 25% of sampled data were discarded as burn-in. Maximum likelihood (ML) phylogenies were inferred using IQ-TREE (Nguyen et al., 2015) for 2000 standard bootstraps, as well as the Shimodaira–Hasegawa–like approximate likelihood-ratio test (Guindon et al., 2010). The phylogenetic trees were viewed and edited using iTOL (available at https://itol.embl.de/) (Letunic and Bork, 2021).
Results
A total of 95 larvae, representing a range of different fish families and ontogenetic stages, were collected for molecular identification, among which, three samples failed to yield an amplified PCR product despite amplification using different combinations of primers and annealing temperatures. The remaining 92 samples were successfully barcoded and categorized into 12 families, 18 genera, and 20 species (GenBank accession numbers and taxonomic data are listed in Table 1), based on BLAST searches of the NCBI database. Among the 20 species, 3 (52 individuals) could not be matched to corresponding species in the database and could only be assigned to the genus at the lowest taxonomic level. Among 92 samples 35 samples were collected at the B4 station, which has more samples than the other three stationsB1(16), B2(22) and B3(19). Three kinds of scombrid fishes were found at B2 and B3 stations, albacore (Thunnus alalunga), blue marlin (Makaira nigricans) and skipjack tuna (Katsuwonus pelamis), comprising 6.5% of the total sample. Only Parupeneus sp. were found at four stations, dominating the collections at frequencies of 46 and 50%, respectively. Myctophidae with 8 species was the family that has the most species.
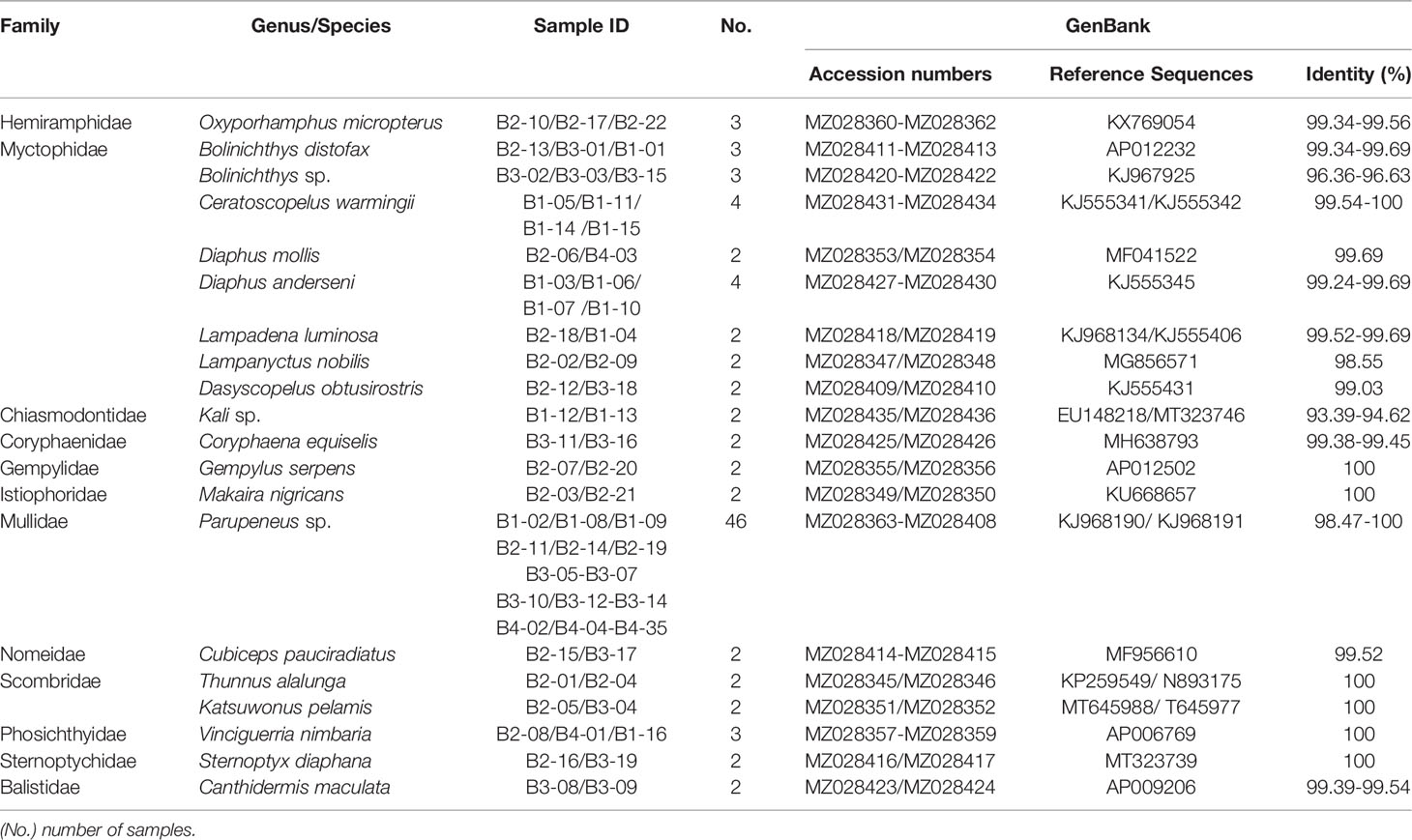
Table 1 Species composition and diversity of fish larvae collected from four sampling stations of Hawaiian waters.
After editing, we obtained a consensus length of 655 bp for all barcode sequences, none of which was found to contain stop codons, insertions, or deletions. The overall mean nucleotide base frequencies observed in these COI sequences were as follows: T (28.30%), C (29.30%), A (22.90%), and G (19.50%). K2P model analysis of the intraspecific, interspecific, and intergeneric genetic divergences of the samples revealed that intraspecific distances among larval sequences ranged from 0% to 1.61%, with an average distance of 0.72%, and with the largest distance of 1.61% being found in Diaphus mollis (Table 2). Genetic distances between species ranged from 9.79% to 36.38%, with an average of 25.99%, which is 36-fold higher than the average genetic distance within species. The genetic distance between genera ranged between 11.40% and 39.60%, with an average of 26.30%, whereas that between families varied between 19.70% and 39.60%, with an average of 27.50% (the minimum genetic distance of genera considers only sequences of different species, the minimum genetic distance for families considers only sequences of different genera, and so on).
The ML tree and Bayesian based on an analysis of the COI sequences of collected larvae and reference sequences available in the public domain, the reliability of which was confirmed by bootstrap values (Table 1 and Figures 2, 3). The ML tree construction was performed using the TIM2+I+G4+F model, meanwhile, the best fit models of Bayesian was GTR+I+G+F. Both of the trees showed that individuals of the same species clustered along the same tree branch and could be clearly distinguished from those of other species. This clustering was found to correspond to the genetic distance values obtained, thereby indicating that the species could be effectively distinguished. Moreover, we detected no taxonomic deviation at the species level and accordingly established that a majority of the examined species could be authenticated based on analyses using the barcoding approach (Table 2).
Discussion
Accurate identification of larval fish at the species level is important to the distribution, abundance, ecology, and behavior of the early stages of those species inhabiting Hawaiian waters. In the present study, we employed DNA barcoding to identify the taxonomy of larval fish inhabiting waters surrounding the Hawaiian Islands, successfully barcoding 92 of the 95 collected samples that were assigned to fish in 12 families, 18 genera, and 20 species. Among 20 species, 17 species were identified at the species level, based on a similarity threshold greater than 98% in comparisons with database sequences. These results accordingly confirm that DNA barcoding can be successfully applied to identify a majority of the sequenced samples of larval fish at the species level, and thereby contribute significantly to our current understanding of the diversity of larval fish communities within Hawaiian waters. However, given the lack of an accurate, large, and robust database with reliable reference sequences, we were unable to classify the remaining three at the species level, which could only be assigned identity at the genus level, as Bolinichthys sp., Kali sp., and Parupeneus sp., respectively. Incomplete databases represent a substantial bottleneck with respect to species identification (Ko et al., 2013; Wang et al., 2019), as has been reported by Ardura et al. (2016) for Eastern Atlantic Ocean fish larvae and Leis (2015) for Indo-Pacific fish larvae. These findings serve to highlight that the success of DNA barcoding for species identification is particularly dependent on the availability of high-quality reference sequences in public sequence libraries and taxonomists will play a vital role in completing such a global database. Hopefully, additional sampling of adult fish will solve these taxonomic questions.
The efficacy of species identification based on DNA barcoding is also dependent on both sufficient inter-and intraspecific divergence (Xing et al., 2020b), with larger barcode-related intra- and interspecific differences in genetic distance contributing to the more successful identification of species (Dhar and Ghosh, 2017). In the present study, we used the minimum interspecific and maximum intraspecific divergence to define the barcode gap and found this more efficient than using the means, with both maximum and mean intraspecific distance values (1.61% and 0.72%, respectively) being inferior to interspecific distances (9.79–36.38%, with a mean value of 25.99%), with no overlap. The mean interspecific distance (25.99%) was found to be 36-fold higher than the mean intraspecific distance (0.72%), which is higher than the 29-fold difference reported for the DNA barcoding of Taiwan Strait fishes and the 25-fold difference obtained for Australian marine fishes (Ward et al., 2005; Xing et al., 2018). These results accordingly confirmed that DNA barcoding can be effectively applied in identifying the larval fish in Hawaiian waters. In the present study, we constructed Bayesian and ML trees based on COI sequences, the reliability of which was confirmed by bootstrap evaluation (Figures 2, 3). The trees revealed the same topology for the clustering of closely related or well-identified species, which were grouped in the same clade or sister clades. All individuals of larval fish samples were grouped into the same specific level compared with the identified target species, the genera and families were well clustered. The two phylogenetic trees indicated that DNA barcoding has high efficiency in species identification and showed the phylogenetic relationships of the species.
The waters surrounding the Hawaiian Islands are important spawning grounds, because of their diverse complex physical and chemical environments. The number of larvae suggests many species of fish spawning in the water off Hawaiian, such as scombrid, goatfish, and lanternfishes. Lobel and Robinson (1986) reviewed the current patterns in the vicinity of the Hawaiian Islands and concluded that mesoscale current and eddy systems are important to the larval fish. They can entrap larval fishes and retain them offshore for long enough periods for them to complete the planktonic phase of their life history and these eddy currents can account for some passive return to the vicinity where the eggs originated. Scombrid fishes are important worldwide for their economic and ecological value. The composition of scombrid larvae around Hawaii has been described as being dominated by yellowfin tuna, skipjack tuna, and bullet tunas (Strasburg et al., 1960; Boehlert and Mundy, 1994). In the present study, all scombrid larvae were successfully identified to species using the COI marker. Three species of scombrid fishes T. alalunga, M. nigricans and K. pelamis were found to comprise 6.5% of the total sample, similar to the result found by Paine et al. (2008) and more than 2.5% by Boehlert and Mundy (1994). The possible reason for this was that Boehlert and Mundy didn’t use the molecular method, lots of scombrid larval fishes couldn’t identify to genus or species. Previous works on scombrid larva have been limited by reliance on morphological identification. These types of analyses typically have many larvae that can not be identified to species level because they are too small to have developed distinguishing morphological characteristics or are too disfigured (Strasburg et al.,1960; Beckley and Leis, 2000).
Parupeneus sp. belonging to the goatfish family Mullidae, which are one of the most valuable reef fishes in Hawaii. sp. In this study, Parupeneus sp. was the dominant species, 46 individuals comprised 50% of the total samples. It was collected at all four stations, especially the B4 station which has 32 individuals. The main reasons for the distribution were physical and biological factors. It is widely accepted that nearly all fish species that live on coral reefs have mobile life-history stages, spending either the egg or larval stages or both as members of the plankton which drift passively at the mercy of oceanic currents (Smith et al., 1987). Parupeneus sp. belongs to the family Mullidae which are reef species with pelagic eggs. Those coral reef fishes like to select the spawning sites where eddies or gyres tend to keep the eggs in the vicinity of the reefs (Johannes, 1978; Smith et al., 1987). The distance of spawning site to the island is also very important to coral reef fishes because the retention of larvae within a radius enabling return to the spawning site is vital for population maintenance.
Conclusions
In conclusion, the results of this study provide convincing evidence that combining morphological knowledge and DNA barcoding can be served as an effective tool for an accurate species identification of larval fish in Hawaiian waters, and could thereby make a valuable contribution to species diversity surveys. Among the 95 larval fish samples collected, 92 were successfully barcoded and identified as belonging to 12 families, 18 genera, and 20 species. Our findings indicate that many species of fish spawning in the waters off Hawaiian, such as scombrid, goatfish and lanternfishes, and also emphasize the need to obtain a larger number of reference COI barcodes for fish species to enhance the potential utility of barcoding concerning gaining estimates of larval fish community diversity. The findings of this study enhance our current knowledge regarding larval diversity and dispersal, a stage of which is considered an essential facet of fisheries management.
Data Availability Statement
The datasets presented in this study can be found in online repositories. The names of the repository/repositories and accession number(s) can be found in the article/supplementary material.
Ethics Statement
Ethical review and approval was not required for the animal study because this research is about the fish larvae, no protected species.
Author Contributions
Conceptualization: BX and CW; Funding acquisition: CW, BX, and RS; Investigation: ZZ, XC, and QW; Methodology: YW and CW; Project administration: CW and XC; Writing original draft: BX; Writing review and editing: BX and PX. All authors contributed to the article and approved the submitted version.
Funding
The Project of Monitoring and Protection of Ecological Environment in the East Pacific Ocean Sponsored by COMRA (No. DY135-E2-5-03), Scientific Research Foundation of Third Institute of Oceanography, MNR [grant numbers 2017009, 2019015], Global Change and Air-sea Interaction II under contract (No. GASI-01-NPAC-Stsum), National Natural Science Foundation of China (No. 41406216), and The Marine Biological Sample Museum Upgrade And Expansion (No.GASI-01-02-04).
Conflict of Interest
The authors declare that the research was conducted in the absence of any commercial or financial relationships that could be construed as a potential conflict of interest.
Publisher’s Note
All claims expressed in this article are solely those of the authors and do not necessarily represent those of their affiliated organizations, or those of the publisher, the editors and the reviewers. Any product that may be evaluated in this article, or claim that may be made by its manufacturer, is not guaranteed or endorsed by the publisher.
Acknowledgments
We would like to thank the Pacific Remote Islands Marine National Monument/U.S. Fish and Wildlife Service/Department of the Interior for their support, as well as the National Oceanic and Atmospheric Administration.
References
Ardura A., Morote E., Kochzius M., Garcia-Vazquez E. (2016). Diversity of Planktonic Fish Larvae Along a Latitudinal Gradient in the Eastern Atlantic Ocean Estimated Through DNA Barcodes. Peerj 4, e2438. doi: 10.7717/peerj.2438
Armani A., Guardone L., Castigliego L., D’Amico P., Messina A., Malandra R., et al. (2015). DNA and Mini-DNA Barcoding for the Identification of Porgies Species (Family Sparidae) of Commercial Interest on the International Market. Food Control 50, 589–596. doi: 10.1016/j.foodcont.2014.09.025
Azmir I., Esa Y S. M. N. A., Md Yasin I., Md Yusof F. (2017). Identification of Larval Fish in Mangrove Areas of Peninsular Malaysia Using Morphology and DNA Barcoding Methods. J. Appl. ICHTHYOL 00, 1–9. doi: 10.1111/jai.13425
Batta-Lona P. G., Galindo-Sánchez C. E., Arteaga M. C., Robles-Flores J., Jiménez-Rosenberg S.P.A. (2019). DNA Barcoding and Morphological Taxonomy: Identification of Lanternfish (Myctophidae) Larvae in the Gulf of Mexico. Mitochondrial DNA A. 30 (20), 375–383. doi: 10.1080/24701394.2018.1538364
Beckley L. E., Leis J. M. (2000). Occurrence of Tuna and Mackerel Larvae (Family: Scombridae) Off the East Coast of South Africa. Mar. Freshwater Res. 51 (8), 777–782. doi: 10.1071/MF00044
Boehlert G. W., Mundy B. C. (1994). Vertical and Onshore-Offshore Distributional Patterns of Tuna Larvae in Relation to Physical Habitat Features. Mar. Ecol. Prog. Ser. 107, 1–13. doi: 10.3354/meps107001
Dhar B., Ghosh S. K. (2017). Mini-DNA Barcode in Identification of the Ornamental Fish: A Case Study From Northeast India. Gene 627, 248–254. doi: 10.1016/j.gene.2017.06.043
Geller J., Meyer C., Parker M., Hawk H. (2013). Redesign of PCR Primers for Mitochondrial Cytochrome C Oxidase Subunit I for Marine Invertebrates and Application in All-Taxa Biotic Surveys. Mol. Ecol. Resour. 13 (5), 851–861. doi: 10.1111/1755-0998.12138
Guindon S., Dufayard J. F., Lefort V., Anisimova M., Hordijk W., Gascuel O. (2010) New Algorithms and Methods to Estimate Maximum-Likelihood Phylogenies: Assessing the Performance of PhyML 3.0. Syst. Biol. 59 (3), 307–321. doi: 10.1093/sysbio/syq010
Hebert P. D., Cywinska A., Ball S. L., deWaard J. R. (2003). Biological Identifications Through DNA Barcodes. Proc. Biol. Sci. 270 (1512), 313–321. doi: 10.1098/rspb.2002.2218
Heimeier D., Lavery S., Sewell M. A. (2010). Using DNA Barcoding and Phylogenetics to Identify Antarctic Invertebrate Larvae Lessons From a Large Scale Study. Mar. Genomics 3 (3-4), 165–177. doi: 10.1016/j.margen.2010.09.004
Hubert N., Espiau B., Meyer C., Planes S. (2015). Identifying the Ichthyoplankton of a Coral Reef Using DNA Barcodes. Mol. Ecol. Resour. 15 (1), 57–67. doi: 10.1111/1755-0998.12293
Johannes R. E. (1978). Reproductive Strategies of Coastal Marine Fishes in the Tropics. Environ. Biol. Fish 3 (1), 65–84. doi: 10.1007/BF00006309
Kalyaanamoorthy S., Minh B. Q., Wong T. K., Von Haeseler A., Jermiin L. S. (2017) ModelFinder: Fast Model Selection for Accurate Phylogenetic Estimates. Nature Methods 14 (6), 587–589. doi: 10.1038/nmeth.4285
Kenchington E. L., Baillie S. M., Kenchington T. J., Bentzen P. (2017). Barcoding Atlantic Canada’s Mesopelagic and Upper Bathypelagic Marine Fishes. PloS One 12 (9), e0185173. doi: 10.1371/journal.pone.0185173
Kimura M. (1980). A Simple Method for Estimating Evolutionary Rates of Base Substitutions Through Comparative Studies of Nucleotide Sequences. J. Mol. Evol. 16 (2), 111–120. doi: 10.1007/BF01731581
Ko H. L., Wang Y. T., Chiu T. S., Lee M. A., Leu M. Y., Chang K. Z., et al. (2013). Evaluating the Accuracy of Morphological Identification of Larval Fishes by Applying DNA Barcoding. PloS One 8, e53451. doi: 10.1371/journal.pone.0053451
Kwun H. J. (2018). Species Identification of Juvenile Fishes of the Genus Pseudoblennius Using Mitochondrial DNA Barcoding. Mitochondrial DNA Part B 3 (1), 405–408. doi: 10.1080/23802359.2018.1456982
Leis J. M. (2015). Taxonomy and Systematics of Larval Indo-Pacific Fishes: A Review of Progress Since 1981. Ichthyological Res. 62, 9–28. doi: 10.1007/s10228-014-0426-7
Letunic I., Bork P. (2021) Interactive Tree Of Life (iTOL) v5: An Online Tool for Phylogenetic Tree Display and Annotation. Nucleic Acids Res. 49 (W1), W293–W296. doi: 10.1093/nar/gkab301
Liu M. D., Wang D. Q., Gao L., Tian H. W., Liu S. P., Chen D. Q., et al. (2018). Species Diversity of Drifting Fish Eggs in the Yangtze River Using Molecular Identification. PeerJ 6, e5807. doi: 10.7717/peerj.5807
Lobel P., Robinson A. (1986). Transport and Entrapment of Fish Larvae by Ocean Mesoscale Eddies and Currents in Hawaiian Waters. Deep Sea Res. Part A. Oceanographic Res. Papers 33 (4), 483–500. doi: 10.1016/0198-0149(86)90127-5
Matarese A. C., Spies I. B., Busby M. S., Orr J. W. (2011). Early Larvae of Zesticelus Profundorum (Family Cottidae) Identified Using DNA Barcoding. Ichthyological Res. 58 (2), 170–174. doi: 10.1007/s10228-010-0198-7
Mazaheri Kouhanestani Z., Ghorbani R., Fujiwara M., Amini K., Mahmoodi S. (2020). Identification of Larval Stages of Fish in Southeastern Coastal Waters of the Caspian Sea-Golestan Province. Iranian J. Fisheries Sci. 19, 325–439. doi: 10.22092/ijfs.2018.119806
Nguyen L. T., Schmidt H. A., Von Haeseler A., Minh B. Q. (2015) IQ-TREE: A Fast and Effective Stochastic Algorithm for Estimating Maximum-Likelihood Phylogenies. Mol. Biol. Evol. 32 (1), 268–274. doi: 10.1093/molbev/msu300
Paine M. A., McDowell J. R., Graves J. E. (2008). Specific Identification Using COI Sequence Analysis of Scombrid Larvae Collected Off the Kona Coast of Hawaii Island Ichthyological Research 55, 1, 7–16. doi: 10.1007/s10228-007-0003-4
Patterson H. M., Isari S., Pearman J. K., Casas L., Michell C. T., Curdia J., et al. (2017). Exploring the Larval Fish Community of the Central Red Sea With an Integrated Morphological and Molecular Approach. PloS One 12 (8), e0182503. doi: 10.1371/journal.pone.0182503
Reynalte-Tataje D. A., Nakatani K., Fernandes R., Agostinho A., Bialetzki A. (2011). Temporal Distribution of Ichthyoplankton in the Ivinhema River (Mato Grosso do Sul State/ Brazil): Influence of Environmental Variables. Neotropical Ichthyol 9, 427–436. doi: 10.1590/S1679-62252011005000017
Ronquist F., Teslenko M., Van Der Mark P., Ayres D. L., Darling A., Höhna S., et al., (2012) MrBayes 3.2: Efficient Bayesian Phylogenetic Inference and Model Choice Across a Large Model Space. Systematic Biol. 61 (3), 539–542. doi: 10.1093/sysbio/sys029
Smith C. L., Tyler J. C., Stillman L. (1987). Inshore Ichthyoplankton: A Distinctive Assemblage? Bull. Mar Sci. 41 (2), 432–440.
Strasburg D. W. (1960) Estimates of LKarval Tuna Abundance in the Central Pacific[M]. US Fish and Wildlife Service.
Valdez-Moreno M., Vásquez-Yeomans L., Elías-Gutiérrez M., Ivanova N. V., Hebert P. D. N. (2010). Using DNA Barcodes to Connect Adults and Early Life Stages of Marine Fishes From the Yucatan Peninsula, Mexico: Potential in Fisheries Management. Mar. Freshwater Res. 61 (6), 665–671. doi: 10.1071/MF09222
Victor B., Hanner R., Shivji M., Hyde J., Caldow C. (2009). Identification of the Larval and Juvenile Stages of the Cubera Snapper, Lutjanus Cyanopterus, Using DNA Barcoding. Zootaxa 2215, 24–36. doi:10.11646/zootaxa.2215.1.2
Wang Y. H., Duan J. N., Shi H. L., Guo J. X., Wang X. Y., Gao T. X., et al. (2019). Species Identification of Small Fish in Xixuan Island Coastal Waters of Zhoushan Using DNA Barcoding. J. Appl. Ichthyol 00, 1–10. doi: 10.1111/jai.13995
Ward R. D., Zemlak T. S., Innes B. H., Last P. R., Hebert P. D. N. (2005). DNA Barcoding Australia’s Fish Species. Philos. Trans. R. Soc. B-Biol Sci. 360 (1462), 1847–1857. doi: 10.1098/rstb.2005.1716
Wibowo A., Wahlberg N., Vasemägi A. (2017). DNA Barcoding of Fish Larvae Reveals Uncharacterised Biodiversity in Tropical Peat Swamps of New Guinea, Indonesia. Mar. Freshwater Res. 68 (6), 1079–1084. doi: 10.1071/MF16078
Wibowo A. P., Zamroni A. S., Priatna A., Yusuf H. N. (2018). Using DNA Barcode to Improve the Identification of Marine Fish Larvae, Case Study Coastal Water Near Jakarta and Banda Sea, Indonesia. Indonesian Fish Res. J. 24 (1), 23–30. doi: 10.15578/ifrj.24.1.2018.37-44
Xing B. P., Chen X. Y., Zhang Z. L., Sun R. X., Xiang P., Lin M., et al. (2020a). Genetic Identification of Ophichthid Fishes Through DNA Barcoding. Mitochondrial DNA Part B 5 (2), 1970–1974. doi: 10.1080/23802359.2020.1756940
Xing B. P., Lin H. S., Zhang Z. L., Wang C. G., Wang Y. G., Wang J. J. (2018). DNA Barcoding for Identification of Fish Species in the Taiwan Strait. PloS One 13, e0198109. doi: 10.1371/journal.pone.0198109
Xing B. P., Zhang Z. P., Sun R. X., Wang Y. G., Lin M., Wang C. G. (2020b). Mini-DNA Barcoding for the Identification of Commercial Fish Sold in the Markets Along the Taiwan Strait. Food Control 112, 107143. doi: 10.1016/j.foodcont.2020.107143
Keywords: molecular identification, fish larvae, DNA barcoding, diversity, Hawaiian waters
Citation: Xing B, Wang C, Wu Q, Wang Y, Chen X, Sun R, Zhang Z and Xiang P (2022) Species Identification of Larval Fish in Hawaiian Waters Using DNA Barcoding. Front. Mar. Sci. 9:825395. doi: 10.3389/fmars.2022.825395
Received: 30 November 2021; Accepted: 31 March 2022;
Published: 28 April 2022.
Edited by:
Liping Liu, Shanghai Ocean University, ChinaReviewed by:
Jose Julian Tavera, University of Valle, ColombiaAnil Mohapatra, Estuarine Biology Regional Centre, India
Copyright © 2022 Xing, Wang, Wu, Wang, Chen, Sun, Zhang and Xiang. This is an open-access article distributed under the terms of the Creative Commons Attribution License (CC BY). The use, distribution or reproduction in other forums is permitted, provided the original author(s) and the copyright owner(s) are credited and that the original publication in this journal is cited, in accordance with accepted academic practice. No use, distribution or reproduction is permitted which does not comply with these terms.
*Correspondence: Peng Xiang, eGlhbmdwZW5nQHRpby5vcmcuY24=
†ORCID: Bingpeng Xing, orcid.org/0000-0002-4963-6574
Yanguo Wang, orcid.org/0000-0002-8995-6468
Xiaoyin Chen, orcid.org/0000-0002-6665-7588
Rouxin Sun, orcid.org/0000-0003-0774-5868
Peng Xing, orcid.org/0000-0002-3193-0775