- 1Departamento de Biología Animal, Edafología y Geología, Universidad de La Laguna, Santa Cruz de Tenerife, Spain
- 2Instituto Universitario de Enfermedades Tropicales y Salud Pública de Canarias, Universidad de La Laguna, Santa Cruz de Tenerife, Spain
- 3Departamento de Obstetricia y Ginecología, Pediatría, Medicina Preventiva y Salud Pública, Toxicología, Medicina Legal y Forense y Parasitología, Universidad de La Laguna, San Cristóbal de La Laguna, Spain
- 4Red de Investigación Cooperativa en Enfermedades Tropicales (RICET), Madrid, Spain
- 5CIBER de Enfermedades Infecciosas (CIBERINFEC), Instituto de Salud Carlos III, Madrid, Spain
In recent years, recurrent sea urchin mass mortalities in the Canary Islands have been registered. These mortality-related events have decimated 93% of the eastern Atlantic populations of the barren-forming sea urchin Diadema africanum. Two severe episodes of rough southeastern seas led to winter storms in February 2010 (Xynthia) and February 2018 (Emma) and preceded the last mass mortality event. We hypothesized that these events are related to the mass mortalities registered during the February in those years. Previous studies identified Neoparamoeba branchiphila as the causal agent of the disease, possibly acting in synergy with Vibrio alginolyticus and/or other pathogens. To determine the link between winter storms and the sea urchin pathogen community, we monitored the marine pathogen assemblage before and after the winter storm Filomena (February 2020) on Tenerife Island, on different habitats (sea water, sediment and algae) and in four species of sea urchin hosts (D. africanum, Arbacia lixula, Paracentrotus lividus and Sphaerechinus granularis). A total of six pathogens, including N. branchiphila, Vexillifera minutissima, Acanthamoeba sp., Vahlkampfia sp., V. alginolyticus and green colonies of Vibrio spp., were identified. Only small amoebas were found in sea urchins, while Vibrio species were more common in seawater, sediment and algae substrates. V. alginolyticus was occasionally detected in three sea urchins specimens, while N. branchiphila was found in the coelom of all four sea urchin studied. As previously hypothesized, a significant pathogen increment in seawater and in the sea urchin species D. africanum and P. lividus, was found after Filomena. Our results confirmed the relationship between the winter storms and marine pathogen dynamics. However, further studies are needed to demonstrate the direct relationship between these pathogen increases and the sea urchin mass mortalities.
Introduction
Over the last several years, the frequency and severity of disease spread in marine animals is increasing (Harvell et al., 1999; Lafferty et al., 2004; Feehan et al., 2012; Nowak and Archibald, 2018), a process that is related to an unbalanced ecosystem caused by human activities. It is anticipated that this growth will continue in the currently climate change context (Harvell et al., 2002). The echinoderms are one of the taxa having several reports of spread diseases that have been associated with massive mortality events (Lessios et al., 1984; Tajimaa et al., 2007; Hernández et al., 2020; Sweet, 2020). Since 1970, extensive mortality events involving sea urchins caused by pathogenic agents has been reported in almost 30 genera of sea urchins from different parts of the world (Maes and Jangoux, 1984; Tajimaa et al., 2007; Feehan et al., 2012; Wang et al., 2013). However, the most studied cases are the green sea urchin Strongylocentrotus droebachiensis off the coast of Nova Scotia (Scheibling and Stephenson, 1984; Scheibling et al., 2010; Feehan et al., 2012) and D. antillarum in the Caribbean Sea (Lessios et al., 1984); the latest because of the strong effects on the structure and dynamics of the coral reef communities of the Caribbean, which have had a very slow recovery after 30 years and are still far from their initial states (Lessios, 2016). In Nova Scotia, the massive mortalities events are cyclicals and are associated with storm-induced paramoebiasis, better known as the “Killer Storm” (Scheibling and Lauzon-Guay, 2010; Scheibling et al., 2010; Feehan et al., 2012).
The spread of these diseases has been associated with extremes in environmental conditions, such as storms and sea temperature increases, which synergistically facilitate the dispersion of pathogenic agents thus causing an increase in their presence in the ecosystems over the last several years because of the global warming (Tajimaa et al., 2007; Scheibling and Lauzon-Guay, 2010; Scheibling et al., 2010; Feehan et al., 2012). Because sea urchins are key herbivores in the benthic communities, any density-related changes could introduce a risk for the stability and the structure of the benthic ecosystems and generate an impact throughout trophic cascade processes (Fernandez et al., 2006; Clemente et al., 2014; Trowbridge et al., 2019). In the East Atlantic Ocean, two mass mortality events have been reported, one after the storms Xynthia in February 2010 and one after Emma in February 2018 (Hernández et al., 2020) that affected the sea urchin Diadema africanum. The first mortality event was observed to last from October 2009 to April 2010 during which the sea urchin population was reduced by 65%; however, this event occurred without compromising the reproductive success of the species (Clemente et al., 2014). During the second event, the population was reduced by 93% around Tenerife and La Palma Islands (Hernández et al., 2020) and promoted a stable shift toward a macroalgae-dominated system (Sangil and Hernández, 2021). These mortality events also affected the Madeira Archipelago; but in contrast to what happened around La Palma and Tenerife Islands, some recovery of the sea urchin populations after the second mortality event did occur (Gizzi et al., 2020). All mortality cases were attributed to sea urchin bald disease because of the similarities in the lesions observed in the epidermis of echinoids. In the first set of studies, it was suggested that Vibrio algynoliticus was the principal pathogenic agent and during the second event, only Neoparamoeba branchiphila was found in moribund individuals. Nevertheless, in the first event the sea urchin specimens under study were collected in an advanced state of disease or were even dead. Therefore, the possibility of a synergy with other causative pathogens, such as the amoeba N. branchiphila, was not ruled out (Dyková et al., 2011; Clemente et al., 2014). It is relevant to highlight at this point that a similar amoeba (Paramoeba invadens) was identified as the causative agent of the disease in the sea urchins Strongylocentrotus droebachiensis in the west Atlantic. Both periods of mortality were associated with unusually rough seas due to southwest storms in February 2010 and 2018, suggesting that this kind of storm also induces paramoebiasis in the East Atlantic, supporting the hypothesis “Killer Storm” that has been suggested for the West Atlantic shores (Scheibling and Lauzon-Guay, 2010; Scheibling et al., 2010; Feehan et al., 2012). This hypothesis supposes a positive correlation between the intensity of the storm and the propagation of paramoebiasis (Scheibling and Lauzon-Guay, 2010). The East Atlantic mortality events presented completely different average temperatures; hence, no clear relationship with sea surface temperatures can be found (Hernández et al., 2020).
Despite the number of extensive reported mortality events, in many cases, the causal agents had not been studied, and in most of these cases, it was not identified because of the difficulties related to the microbiological studies (Trowbridge et al., 2019; Sweet, 2020). Nonetheless, bacteria, fungus, protozoa and even algae have been considered as infectious agents (Wang et al., 2013), probably potentiated by the interaction of multiple stress drivers such as temperature changes, storms and environmental degradation (Trowbridge et al., 2019). The identification of pathogens in the marine environment is difficult, but this type of study can be harder and bring more challenges when free-living amoebae (FLA) are studied. In the first place, amoebae are diverse, they have a cosmopolitan distribution, and they can be isolated from most environments and infect many different animals. Because they have a two-stage life cycle, morphological studies are based on the identification of trophozoites and cysts (Walochnik, 2018). The observation of FLA requires many hours in front of a microscope, and sometimes it is difficult to focus on an agar medium. The observation of cysts is widely used in amoebae identification; yet, many times, this finding is not supported with molecular data (Walochnik, 2018). In this situation, we found another drawback because DNA extraction of marine amoebae requires high quantity of cells; also, the procurement and maintenance of axenic cultures is complicated because the amoebae usually are in a mixed culture with bacteria (Nowak and Archibald, 2018). In some cases, we must consider a symbiotic relation between the amoeba and bacteria, because intracellular presence of Vibrio sp. has been detected in amoebae (Mac Phail et al., 2020). In addition, the availability of molecular marker data and the isolated strains of invertebrates in collections are low (Dyková et al., 2007, 2011). This drawback makes molecular studies even more difficult.
On January 6–8, the storm, Filomena, arrived at the Canaries after originating inside the United States (US) and moved through the Atlantic until it was positioned in the north of the Canary Islands. This storm produced intense rains and generalized winds of 70 to 80 km/h from the SW and NW, which generated waves of 2 to 3 m (AEMET, 2021). These conditions seemed appropriate for obtaining information on the dynamics of the pathogen community after the passage of the storm and its possible relationship with sea urchin mortalities. Therefore, the presence of this pathogen before and after Filomena was examined in different type of substrates (water, sediment and algae) and sea urchin species (Diadema africanum, Paracentrotus lividus, Sphaerechinus granularis and Arbacia lixula) to understand the mechanisms of the introduction of pathogens and its dynamics to determine how the sea urchin mass extensive mortality events could occur.
Materials and Methods
Sampling from four locations on the island of Tenerife was conducted (28°16′07″North, 16°36′20″West): Boca Cangrejo, Abades, La Caleta de Adeje and Punta de Teno (Figure 1) before the storm season in August 2020 and during the month of January 2021, some days after the Filomena was finished. The storm produced waves of 2 to 3 m from the S and SW (Figure 2). Generally, Tenerife Island is affected by trade winds that generate waves of moderate energy with an annual medium height of 1.4 m. Between October and March, the sea swell intensifies and winds from the North Atlantic approach the island from the north/northwest (NNW) and northwest (NW), producing waves of 2 to 3 m. Due to the orientation of coasts, the eastern and southern shores of the island are more protected from the NW/NNW swells, and the average wave is less than in other orientations (Yanes et al., 2006).
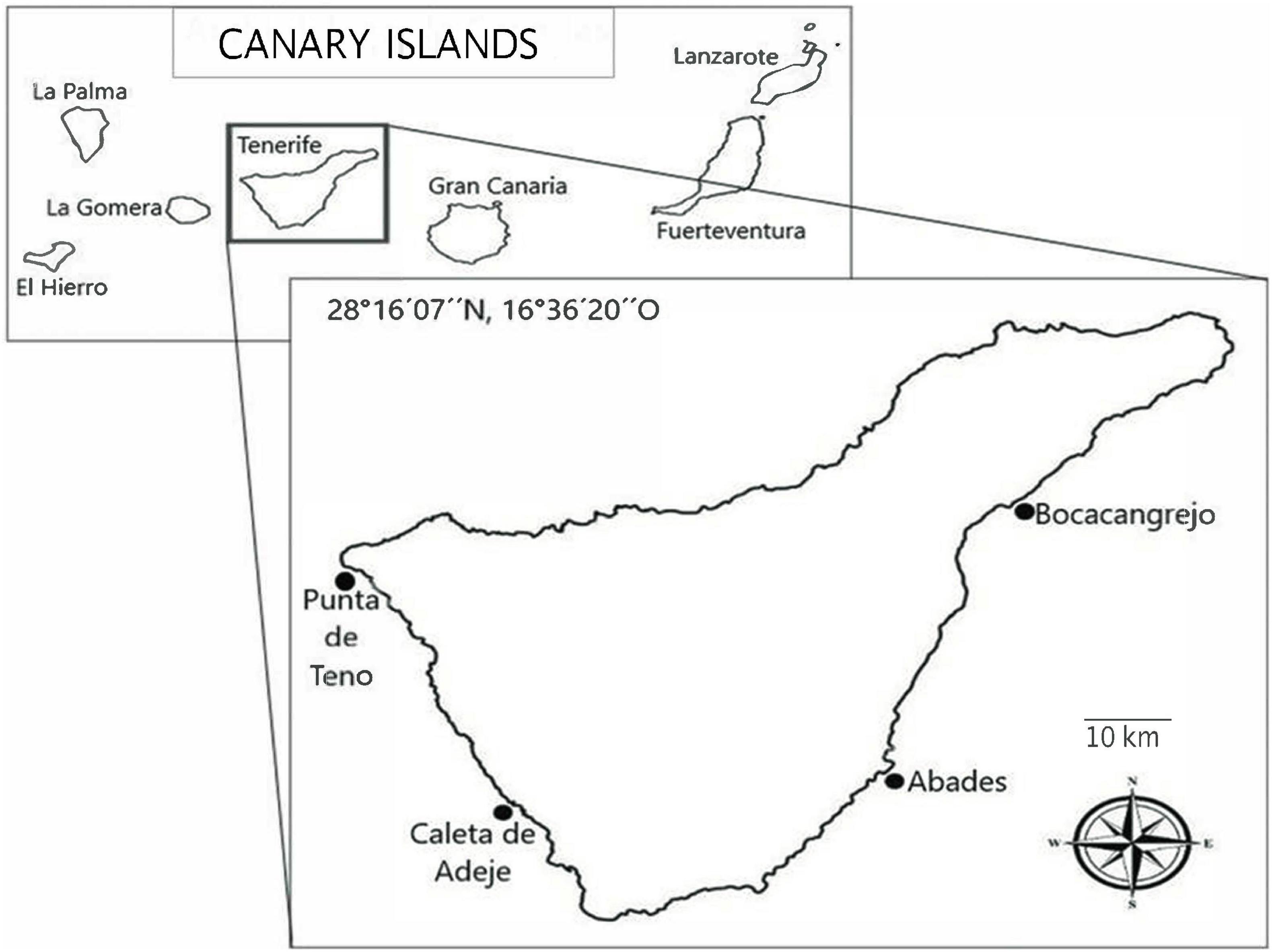
Figure 1. Map showing the sampling localities visited in the present work off Tenerife Island (Canary Archipelago, Spain).
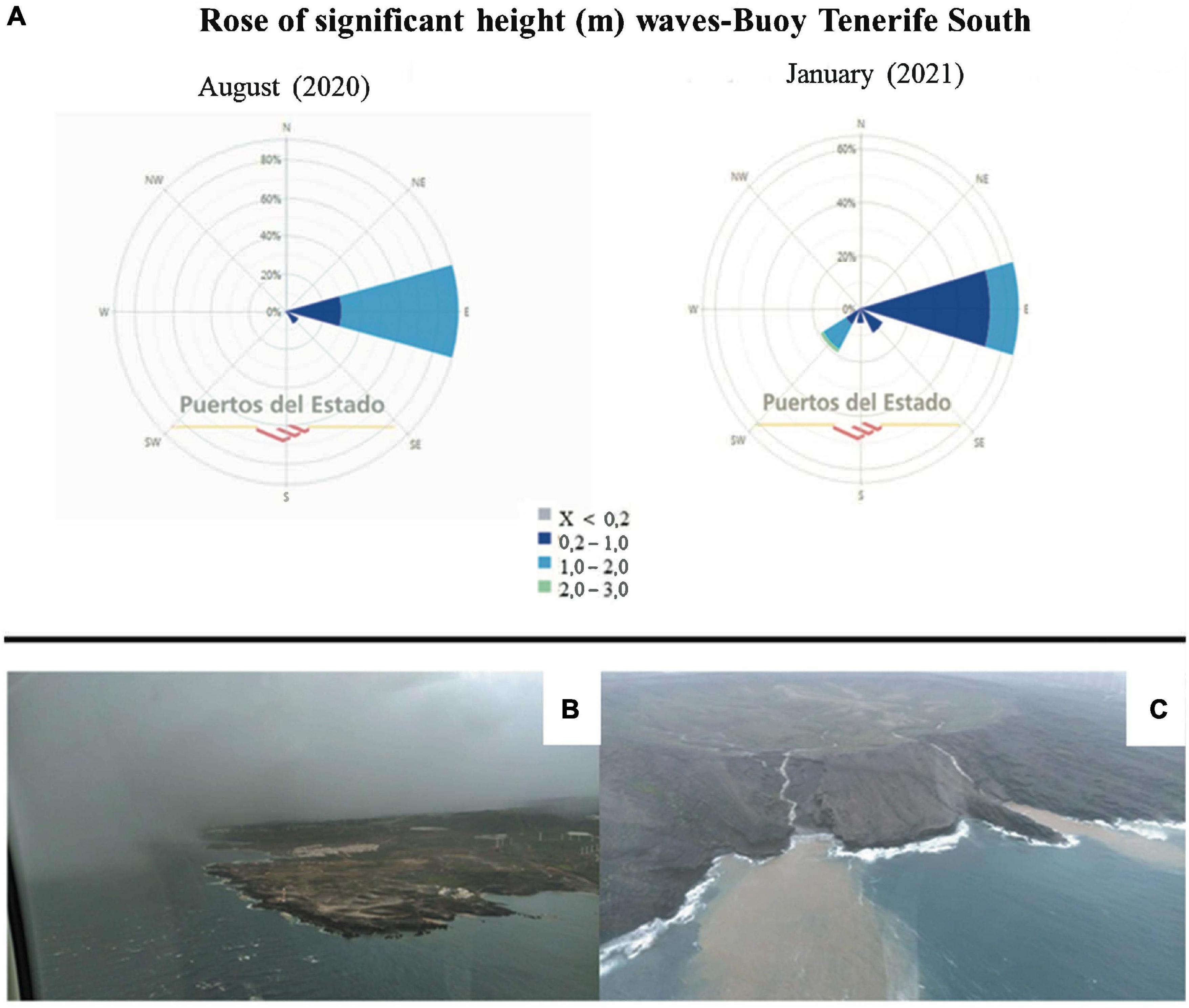
Figure 2. (A) Significant wave height Rose (m) for August 2020 (pre-storm) and January 2021 (post-storm) from the buoy of Tenerife South (del Estado, 2021). (B) Image of Pta. Rasca (south tip of Tenerife), where the westerly weather is clearly visible during the period of Filomena storm; (C) Image of Montaña Pelada (Tenerife), where the flow of the ravines in the post-storm period is clearly visible. Aerial photographs by Ignacio Herranz Duarte.
To locate and characterize the presence of pathogens, we selected three types of substrates: (1) sea water, (2) sediment and (3) algae; we also evaluated the possibility that some of the common sea urchin species (D. africanum, A. lixula, P. lividus, and S. granularis) could act as pathogen hosts. For each locality, we collected by free diving three replicates of sediment using 50 ml cores, 25 cm2 of macroalgae community and 1.5 L of water; each replicate was collected at about 1–2 m depth and spaced about 3 m apart. Additionally, depending on the availability, three individuals for each sea urchins were collected by visual searching between 1 and 3 m depth for 45 min (Table 1). All samples were transported in the refrigerator to the laboratory facilities.
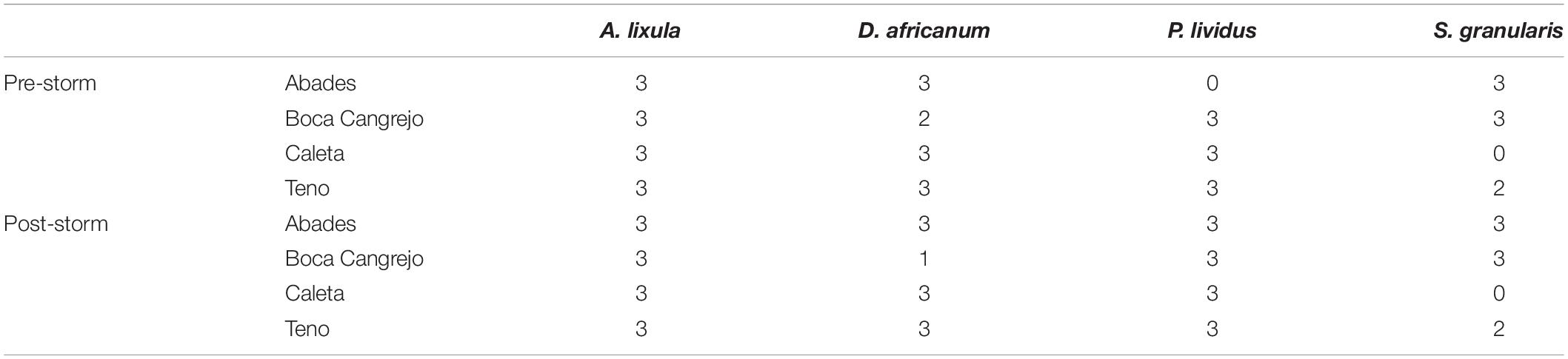
Table 1. Number of sea urchin specimens of each species collected before and after the storm Filomena.
Sample Process and Experimental Design
Each sample was grown in two different media: (1) petri plate (60 mm × 15 mm) of thiosulphate-citrate-bile-sucrose Agar (TCBS) Merck™, a selective medium for Vibrio isolation, composed of bile salts, thiosulphate, citrate and alkaline pH of 8.6. These components inhibit the growth of other bacteria and the saline characteristics of the medium promote the growth of Vibrio spp. The differentiation process is based on fermentation of as sucrose detected by indicators (thymol blue and bromothymol blue), which change color to yellow via a sucrose reaction. Strains undergoing sucrose fermentation produce yellow colonies, while the others show green colonies. The sizes of the colonies can be different, but generally, they have a diameter of 0.5 mm minimum (Corry et al., 1995) and (2) the other medium used was a petri plate (100 mm × 20 mm) of nutritious granulated agar (ANN) DIFCO™ 2%, a solid medium for amoebae isolation but does not guarantee exclusive growth of amoebae. Both culture mediums were prepared following the instructions of the manufacturer using Milli-Q® water.
Sea water samples were prepared using a vacuum ramp PALL No 1540 and nitrocellulose membranes with pores of 0.45 μm diameter. The first 100 ml from each bottle was filtered. Using a Bunsen burner and tongs, the membrane was separated from the filter and was grown on the TCBS plate after which the remaining water was filtered, and the membrane was placed face down in the Petri plates with ANN to facilitate transfer from the membrane to the culture medium.
For the algae assemblages samples, with the help of tweezers, a small piece of algae turf of 2 cm3 were seeded directly onto both growing media. An individual of each algae species that composed the turf was left for later taxonomical identification (Table 2).
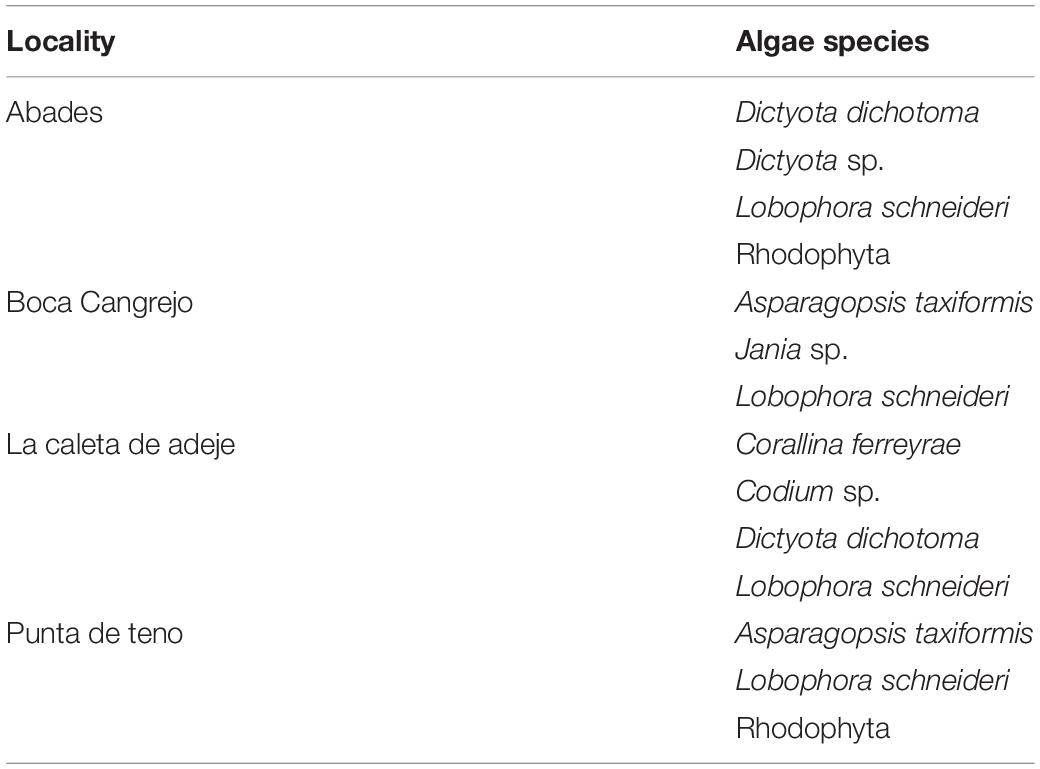
Table 2. Algae species community that composed the turfs used for cultivation on growing media at each locality.
For the sediment samples, a cotton swab was introduced for the obtaining a small sample, which was later added directly to both growing media.
For the sea urchin coelom samples, the sea urchin was positioned on its back and using a 29 Gx 1.27 cm insulin syringe, 1 ml of coelom from each sea urchin was extracted. The syringe was introduced in the peristomal membrane at an angle that avoided contact with the Aristotle’s lantern (Dyková et al., 2011), and the coelomic fluid was cultured in each selective medium.
Vibrio sp. Isolation
A total of 76 samples from pre-storm and 78 from post-storm were cultured and incubated at 37°C for 24 h in a temperature controlled room. After which time the presence of Vibrio spp. colonies was identified using API (Analytical Profile Index) 20E test.
Amoebae Isolation
A total of 76 cultures from pre-storm and 78 from post-storm were incubated at 20°C in the laboratory and observed weekly using an inverted microscope Leica DMIL at 10 and 20 X to identify the presence of amoebae (Pussard and Pons, 1977). We took pictures using the microscope EVOS Floid Imaging System. Additionally, 30 replications were made to isolate amoebas and keep the cultures free of bacteria and fungi.
Identification of the Amoeba Species Present in the Cultures
Identification was made using morphological characters such as the shape of trophozoites, the pseudocysts for the genus Neoparamoeba (Lima et al., 2017) and the double-walled cysts shape unique in Acanthoamoeba. For the DNA extraction, two procedures were used. For the first procedure, 2 ml of the cold Page’s amoeba saline solution (PAS) was used in the Petri plate and amoebae were separated with a staff and the liquid was pipetted into an Eppendorf tube for centrifugation for 20 min at 1500 rpm. The supernatant was collected and the DNA was extracted in an automatized system Maxwell 16®. In the case that the amoebae were found in just one area of the plate and in a very small proportion, that particular piece of agar was cut and put directly into a cartridge for the extraction of DNA in the Maxwell 16®. In both cases, after 40 min, the DNA was collected from the elution buffer cell and stored at −20°C until PCR was performed.
The amplification of DNA was carry out with a Termocycler Arktik™ (Thermo Scientific™), using 18S rRNA specific primers for free living amoebae (FLA) and for Valkampfidae Amoebae (VAHL), following the protocol described in Reyes-Batlle et al. (2021). The primers were P-FLA F 5′-CGCGGTAATTCCAGCTCCAATAGC-3′/P-FLA R 5′-CAGGTTAAGGTCTCGTTCGTTAAC-3′ (Tsvetkova et al., 2004) and VAHL-1 5′-GTCTTCGTAGGTGAACCTGC-3′/VAHL-2 3′-CCGCTTACTGATATGCTTAA-5′ (De Jonckheere and Brown, 2005). The expected amplicon was between 500 and 550 pb long. For the mixture, 5 μl buffer, 1 μl dNTPs, 0.5 μl forward and 0.5 μl reverse primers, 0.25 μl Taq, and 32.75 μl water were used. For each sample, two sets were prepared, the first one with 10 μl of DNA, and the second one with 4 μl of DNA and 6 μl of water. For the positive control of FLA 6 μl Acanthoamoeba DNA + 4 μl MIC, for the positive control of VAHL 6 μl Naegleria fowleri DNA + 4 μl MIC and for the negative control in both cases 10 μl MIC were used. Once the samples were amplified, the electrophoresis was performed using a 2% agarose gel with 1% TAE buffer. A charged buffer was used to weight the fragments and the marker PCRBIO Ladder IV, which works from 100 to 1500 pb, was used to calculate the molecular weights of the DNA samples. P. brachiphila sequence have been uploaded to ZENODO and are free accessible (Lorenzo-Morales and Hernández, 2020).
Statistical Analyses
We used the SPSS 19 (IBM) and PRIMER7 + PERMANOVA (Anderson et al., 2008; Clarke et al., 2014) statistical packages to perform all analyses. Chi2 test was applied specifically for the most frequently detected pathogen species (Vibrio algynoliticus, Neoparamoeba branchiphila and Vexillifera minutissima) (Figure 3) to determine significant variations on their presence/absence proportion after the passage of the storm. For the pathogen assemblage analysis the presence/absence of pathogen data was used for calculating the similarity matrix using Bray–Curtis index. The triangular matrix of similarities was then used to obtain a cluster grouping to determine the principal groups of pathogens. Using the SIMPROF procedure, significant differences between groups were determined. A non-metric MDS was used for better visualize the obtained results. The effects of time (pre-/post-storm periods) and the substrate/host (sea water, sediment, algae and sea urchin species) on the pathogen community were tested using a two-way factor permutational multivariate analysis of variance (PERMANOVA) in which both factors were treated as fixed factors with two and seven levels, respectively.
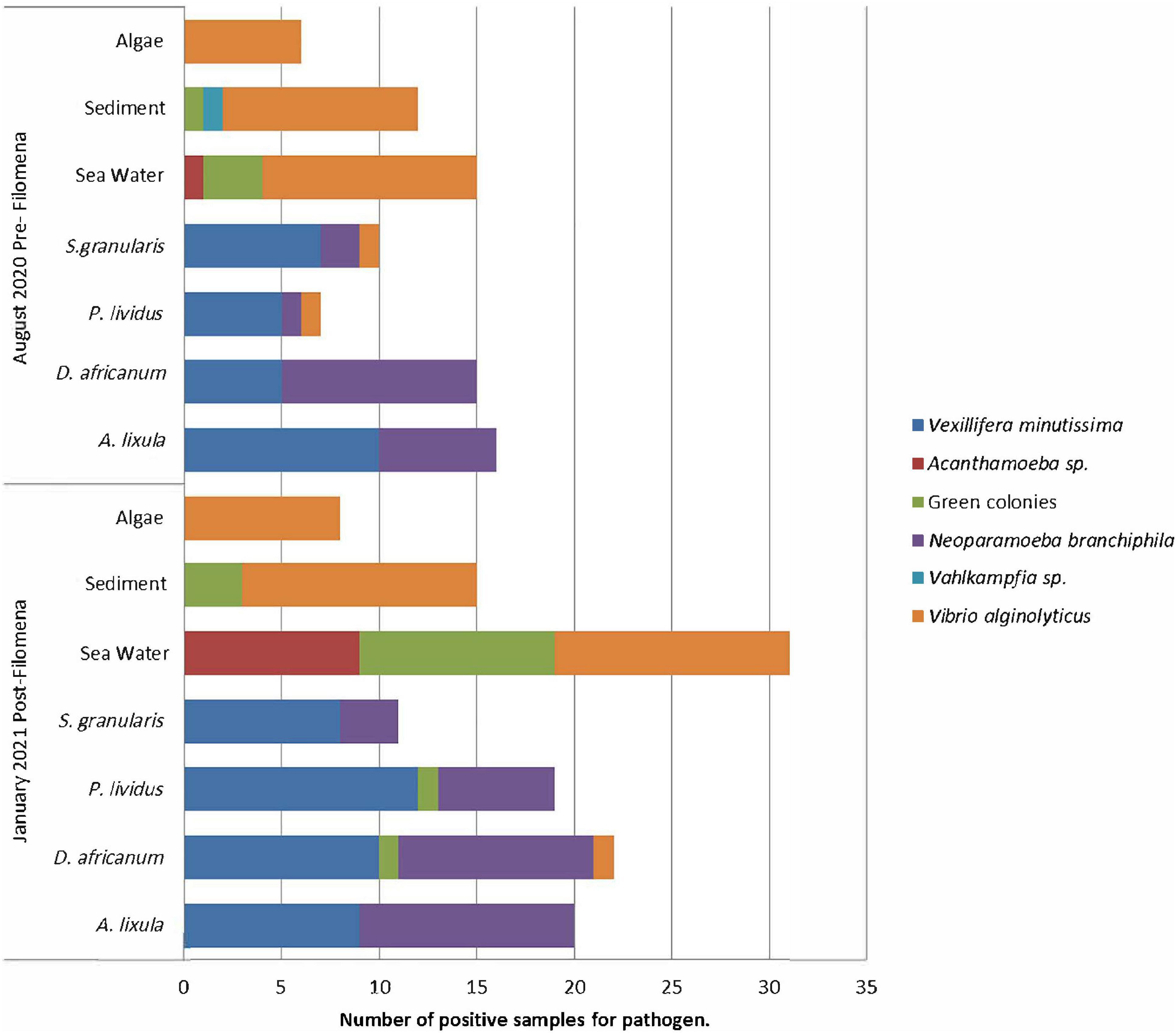
Figure 3. Bar chart of the number of positive samples for the different pathogens grown on the different substrates/host collected before (pre-) and after (post-) the Filomena storm.
Results
A total of 156 cultures from the August 2020 sampling were examined, 78 in ANN culture media and 78 in TCBS culture media. In January 2021, 152 cultures were examined, 76 in ANN and 76 in TCBS. Six different pathogens were found: (1) yellow colonies of the bacteria Vibrio alginolyticus, (2) green colonies of bacteria Vibrio sp., and (3) the amoeba Acanthamoeba sp., (4) the amoeba Vahlkampfia sp., (5) the amoeba Neoparamoeba branchiphila and (6) the amoeba Vexillifera minutissima. In January 2021 (post-Filomena storm) a higher number of positive per sample were obtained for all pathogens. Particularly, in the seawater samples, highlighted by the large number of positive for three pathogens: (1) green colonies of Vibrio sp., (2) V. alginolyticus and (3) Acanthamoeba sp. (Figure 3).
Vibrio spp. Isolation
In 62 analyzed samples, 29 before the storm and 33 after, yellow colonies identified as V. alginolyticus were observed on the three substrates (Figures 4A–C,E,F) and in three sea urchin’s species, before storm in the case of P. lividus and S. granularis; and after in the case of D. africanum (Figures 4A,D). Additionally, in the sea water sample from Teno, collected in August 2020 (pre-storm), it was found Vibrio harveyi (Figure 4G). Also, the growth of green colonies, which increased by 13%, was observed, specifically in sea water samples, sediment and two species of sea urchins (Figures 3, 4D).
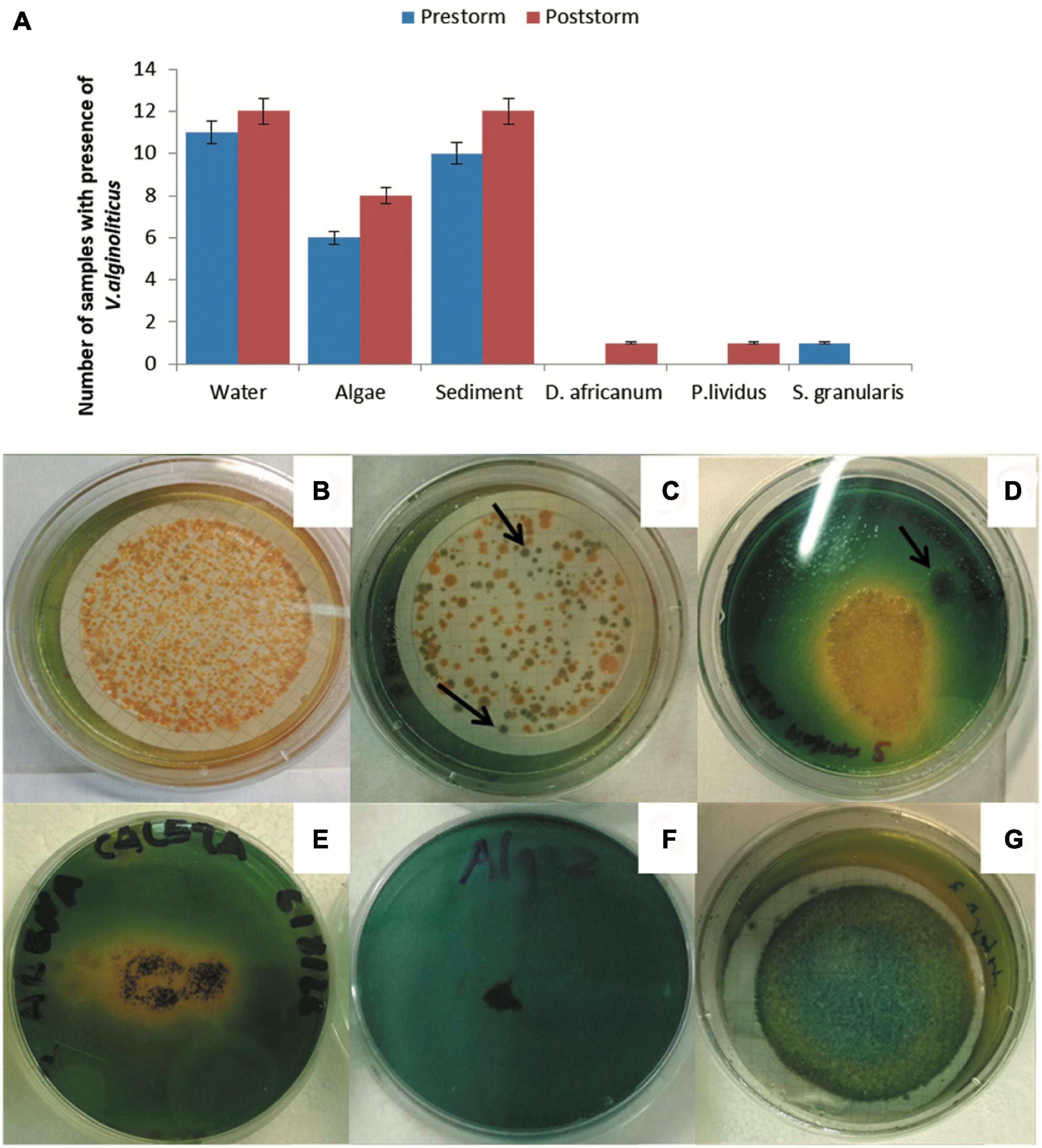
Figure 4. Isolation of Vibrio species: (A) the bar graph shows the presence of V. alginolyticus on substrates/hosts before and after of Filomena’s storm (5% error bars). Cultures photographs: (B) positive growth of yellow colonies of V. alginolyticus post-storm in water sample from Teno; (C) positive growth of V. alginolyticus post-storm in water sample from Abades; (D) Positive growth of V. alginolyticus and green colonies (see arrow) post-storm in coelomic liquid of Diadema africanum from La Caleta de Adeje; (E) positive growth of V. alginolyticus pre-storm in sediment collected in La Caleta de Adeje; (F) negative growth of algae sample from Boca Cangrejo; (G) positive growth of V. harveyi in water sample from Teno.
Amoebas Isolation
A total of 156 ANN plates were followed. A cyst and trophozoites of Acanthamoeba sp. in a sample of sea water from La Caleta de Adeje (Figure 5A) and a cyst of Vahlkampfia sp. (Figure 5B) in a sediment sample from Abades were found in samples taken before the storm. N. branchiphila was observed on 19 plates containing sea urchin coelomic fluid (Figure 5C) and V. minutissima was found in 27 plates of sea urchins (Figure 5F). Most of the post-Filomena substrate samples presented Acanthamoeba sp. The sequencing of one of the strains allowed the identification of genotype T4. Even though the positive control did not run adequately, the molecular marker allowed us to deduce that the amplified fragment was around 500 pb, and it was Acanthamoeba. The other amoeba found in January 2021 was N. branchiphila, identified by the trophozoites and the pseudocyst (Figures 5D,F). When compared with samples from August 2020, this amoeba showed a 13% increase in amount (Figure 5A) while V. minutissima had an increase of 15% (Figure 5F).
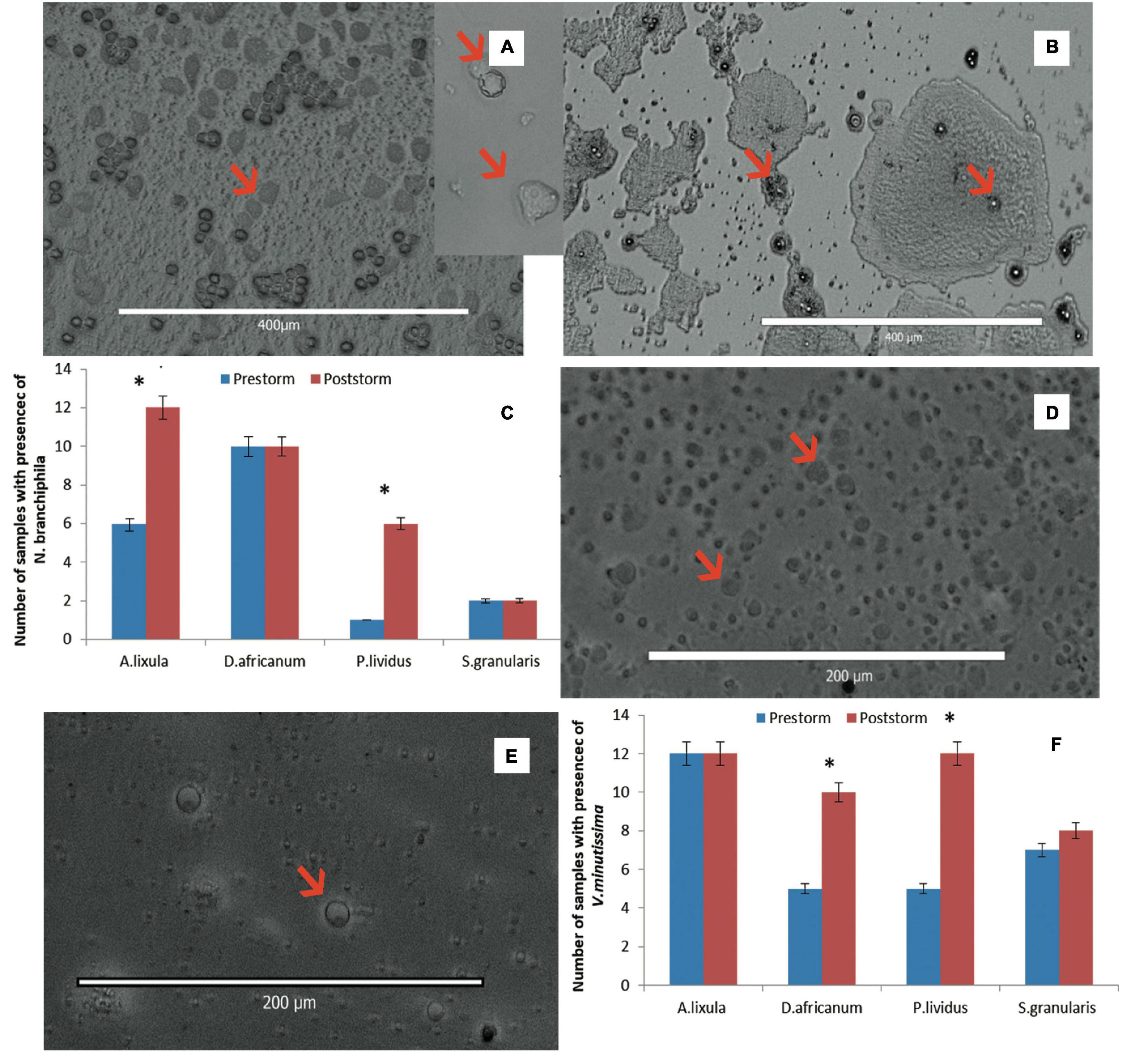
Figure 5. (A) Trophozoites and cyst of Acanthamoeba genotype T4, the arrows shows the double-walled cysts and the shape of trophozoite; (B) cysts of Vahlkampfia sp. in a sediment sample from Abades; (C) bar graph that shows the presence of N. branchiphila in sea urchins before (pre-) and (post-) Filomena’s storm (5% error bars), asterisks shows significant differences of P < 0,005; (D) pseudocysts of N. branchiphila (E) typical pseudocysts of N. branchiphila; (F) bar graph that shows the presence of Vexillifera minutissima in sea urchins before (pre-) and (post-) Filomena’s storm (5% error bars), asterisks shows significant differences of P < 0,005.
Statistical Analysis
The Chi2 test found no differences on the presence/absence of V. alginolyticus on samples proportion after the passage of the Filomena storm (Figure 4A). However, a significant increase of samples with N. brachiphila was found for the sea urchins A. lixula and P. lividus (Figure 5C); parallel to an increase of samples with V. minutissima presence in the case of D. africanum and P. lividus (Figure 5F).
The groups obtained by SIMPROF (Figure 6), better visualized with the MDS plot (Figure 7) were classified by different pathogen communities. In general, we just observed V. minutissima and N. branchiphila in the sea urchin species, while in the sea water, sediment, and algae turf samples, the most observed colonies were Vibrio algynoliticus. Additionally, we found atypical observations that included Acanthamoeba in sea water samples, Vahlkampfia in sediment samples, and Vibrio spp. (green colonies) in four sea urchin samples. The PERMANOVA analysis (Table 3) showed a significant interaction between the factor “pre- and post-storm” and the factor “substrate/hosts” on the presence of pathogens. In other words, Filomena produced a significant change in the presence of pathogens that depend on the substrate/hosts studied. A significant increase in the presence of pathogens was found in sea water samples and the hosts A. lixula, D. africanum and P. lividus. No effect was found in the other substrates/hosts (Figure 3).
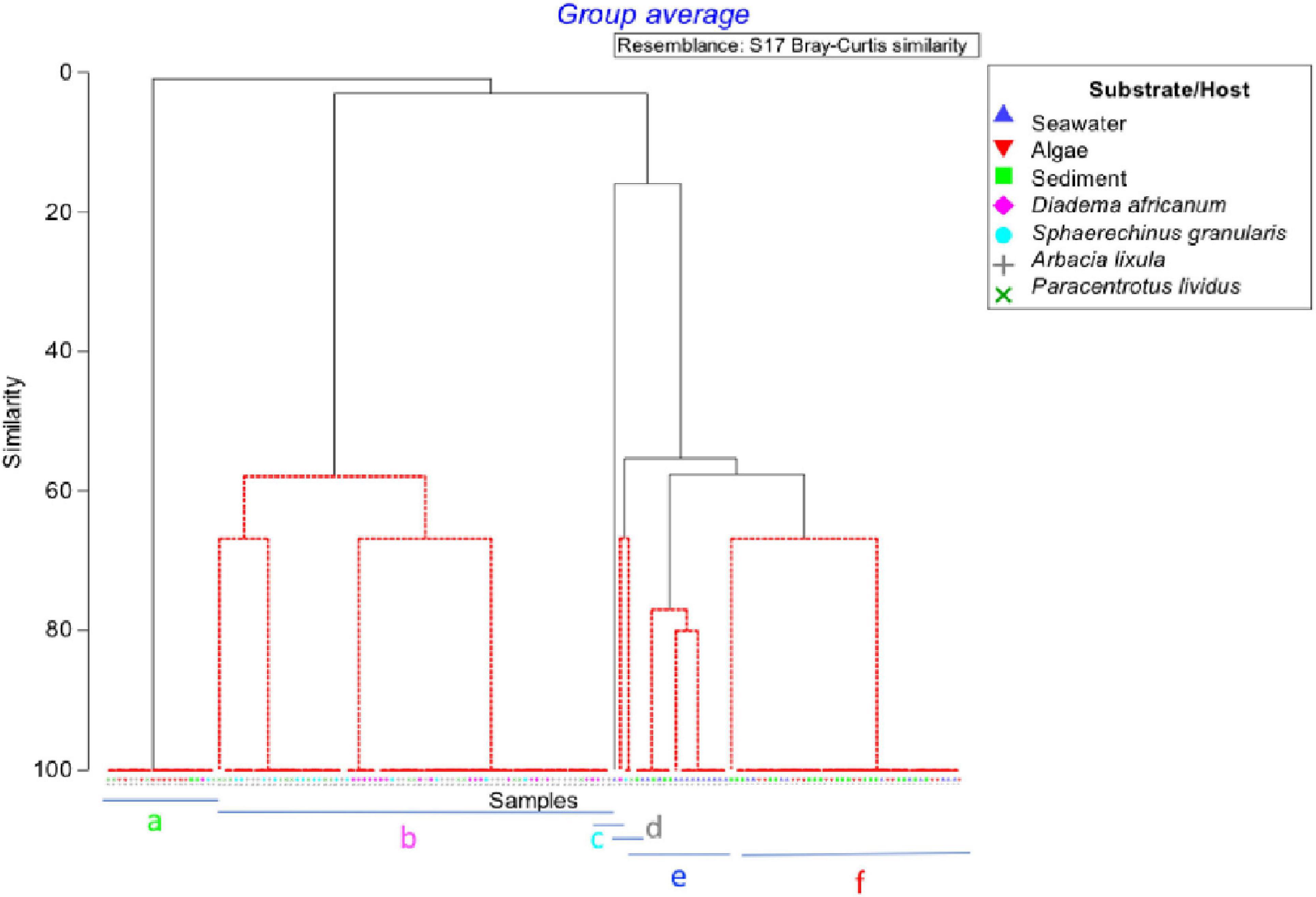
Figure 6. SIMPROF dendrogram showing the significant six clusters of samples formed in red. Each group has a letter from a to f.
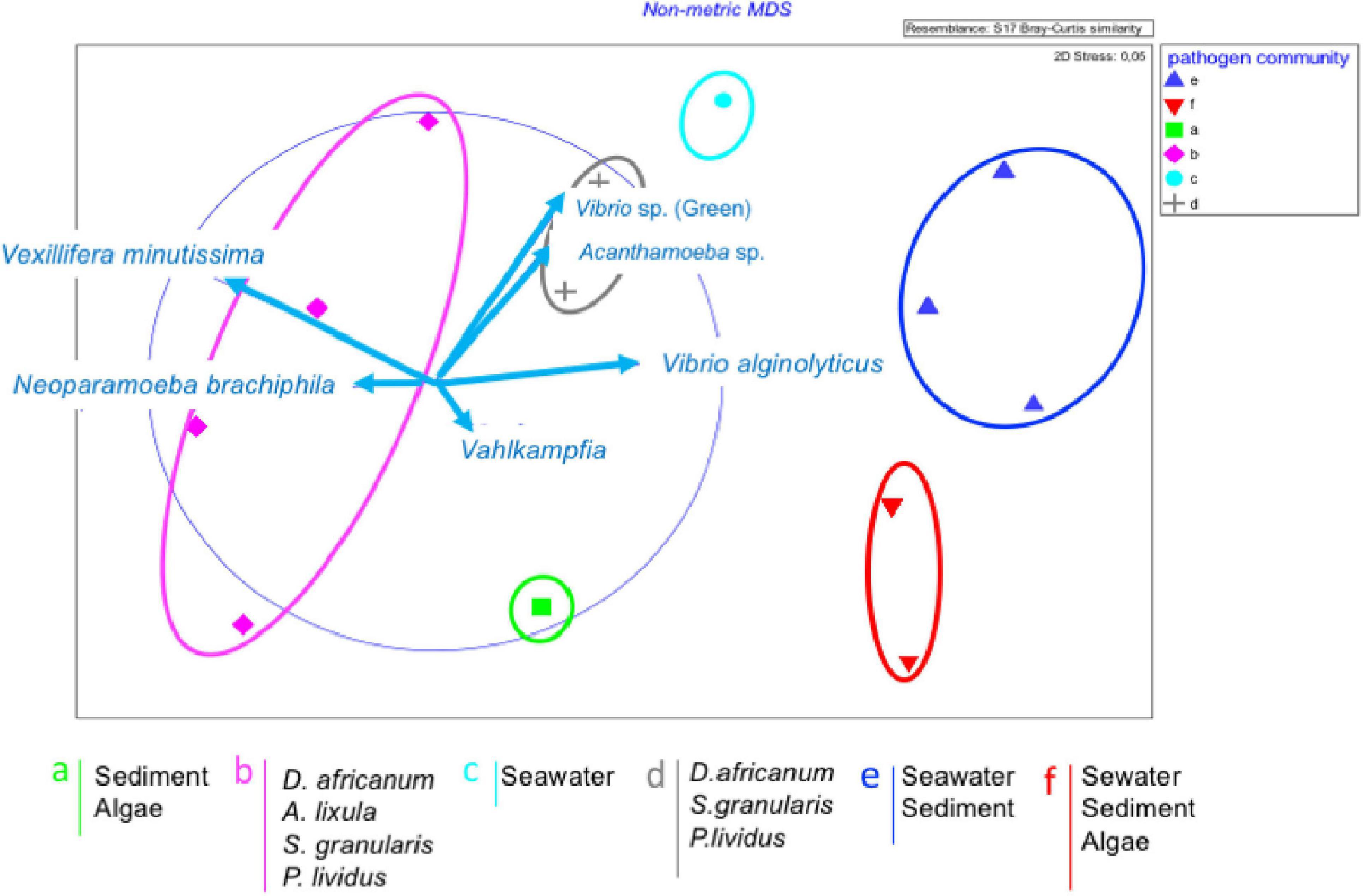
Figure 7. Non-metric multidimensional scaling (nMDS) plots of the dominant pathogen clusters and assemblages. Dominant pathogens which highlight the differences are shown as vector.
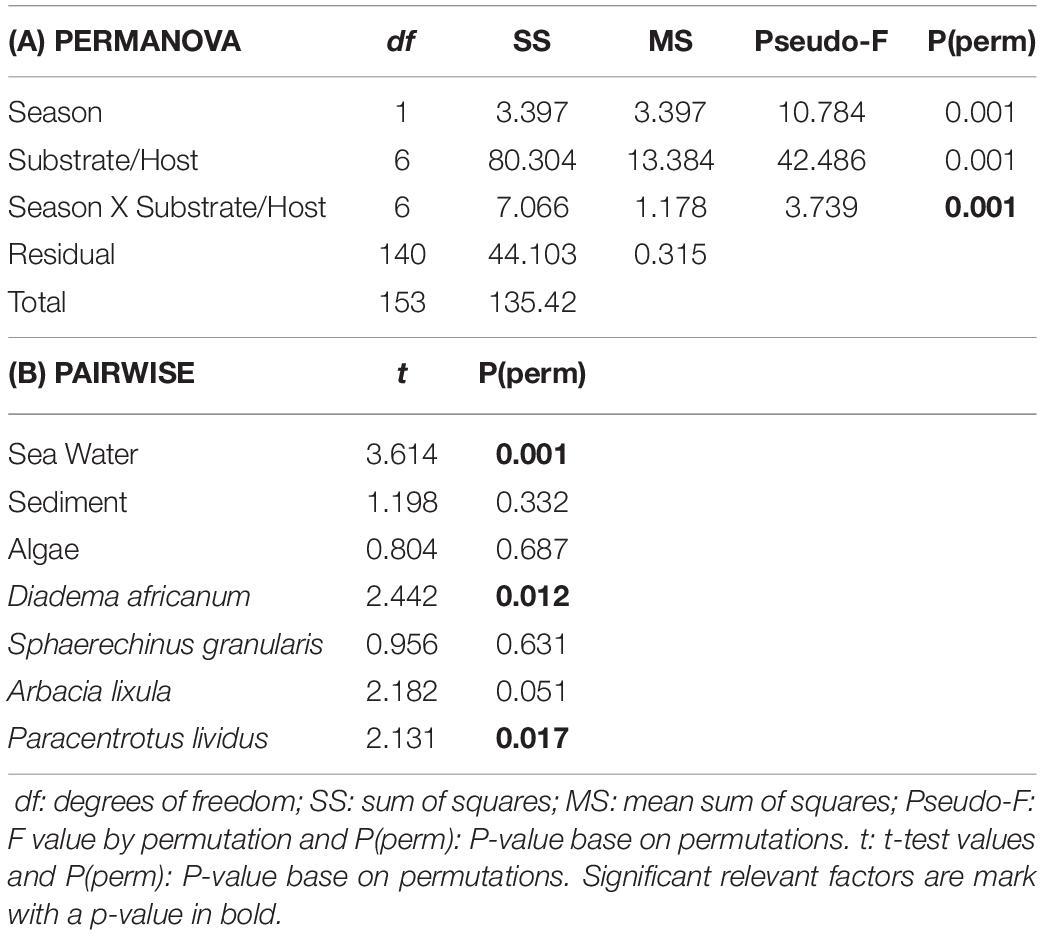
Table 3. (A) PERMANOVA analysis of the pathogen assemblages for the factors “Season” (Pre-/Post- storm), “Substrate/Host” (Sea Water, Sediment, Algae, Diadema africanum, Sphaerechinus granularis, Arbacia lixula, Paracentrotus lividus) and their interactions. (B) PAIWISE analysis of the interaction term. Season (Pre-/Post- storm) is compared across the levels of “Substrate/Host” term.
Discussion
We found six different pathogens in the studied samples: (1) Vibrio alginolyticus, (2) green colonies of Vibrio sp., (3) Acanthamoeba sp., (4) Vahlkampfia sp., (5) Neoparamoeba branchiphila and (6) V. minutissima. The pathogen community depended on the studied substrate/host, for example N. branchiphila and V. minutissima were found only in sea urchins, while Vibrio sp. was present in sea water, sediment and algae samples. The presence of N. branchiphila was exclusively on the coelom of the four studied species of sea urchin. Filomena storm produced significant changes in the pathogen communities of seawater and the hosts, A. lixula, D. africanum and P. lividus. The main effect was an increase in their presence in that substrate and hosts. However, no extensive sea urchin mortality event was reported that year, perhaps because Filomena was a mild storm especially with respect to the intensity of winds and waves from the S and SW since the values did not reach the intensity during Emma and Xynthia as reported by Hernández et al. (2020).
Vibrio sp. was always found in sea urchins and V. alginolyticus in most of the seawater, sediment and algae samples. This result can be interpreted as a stable pathogen community of the sea urchins. In this sense bacteria of the genus Vibrio sp. have been previously associated with the development of skin lesions in different species of echinoids (Becker et al., 2008; Sweet, 2020). Specifically, V. alginolyticus was previously isolated from moribund and dead individuals during the sea urchin mortality event of 2009–2010 that occurred in the East Atlantic (Clemente et al., 2014). Also, it is important to mention that V. harveyi, a marine bacterium characterized by bioluminescence that is associated with vertebrate and invertebrate diseases (Austin and Zhang, 2006), was found in one of the seawater samples. Vibrio has also been associated with poor water quality of the environment (Martony et al., 2018). In relation to the algae substrate, we detected Vibrio sp. but no amoebae were found; this finding could be a result of the secondary metabolites of the selected algae substrate, which can have antimicrobial, antioxidant and cytotoxic activities (Gamal, 2010; García-Davis et al., 2018; Carroll et al., 2019).
We only found V. minutissima, which is a marine amoeba previously isolated from sediments in the Baltic Sea (Garstecki and Arndt, 2000), and N. branchiphila, which has previously been related to the extensive mortality of D. africanum in the East Atlantic (Dyková et al., 2011; Hernández et al., 2020), in the sea urchin samples. Nonetheless, N. branchiphila has only been observed in moribund individuals of D. africanum (Dyková et al., 2011) and in healthy individuals of P. lividus and Heliocidaris erythrogramma (Dyková et al., 2007). In this case, any of the studied sea urchins presented symptoms of the disease. The genus Neoparamoeba has also been observed in conjunction with Vexillifera sp. confirming a pathogen community; which is also present in the gills of the Atlantic salmon (Bermingham and Mulcahy, 2007). N. branchiphila was not observed in any of the seawater, sediment or algae samples in our study, suggesting that the amoeba does not have a stable population in these substrates or that the storms did not have the magnitude to promote vertical mixing in the studied localities. Acanthamoeba sp. was only found in the seawater samples; this amoeba is an opportunistic free-living parasite widely distribute worldwide and can be found in several environments, such as sweet water bodies, seawater, air and different kinds of soil (Lorenzo-Morales et al., 2005a). On the Canary Islands, this amoeba has been previously isolated from many seawater samples (Lorenzo-Morales et al., 2005b). Is interesting to highlight here that one of the isolated strains of Acanthamoeba sp., which was identified by the irregular shape of the trophozoites and cysts with double wall with hexagonal or star shape (Lorenzo-Morales et al., 2005b), was sequenced and identified as genotype T4. This genotype is widely distributed in environments shared with humans and is also associated with some human pathologies even in some animals (Martinez, 1991; Macivera et al., 2013). Even though no studies associating Acanthamoeba sp. as a sea urchin pathogen (Tajimaa et al., 2007; Becker et al., 2008; Wang et al., 2013), Acanthamoeba sp. is part of the pathogen community of seawater with Vibrio sp. and both presented increases with the passage of the storm. This result shows that the storm had an influence on the pathogen’s propagation (Gizzi et al., 2020). We also found cysts of Vahlkampfia genus in the sediments; this species has been associated with human infections and can be localized to several aquatic and terrestrial environments (De Jonckheere, 2006; Reyes-Batlle et al., 2021).
Significant changes in the pathogen community were found after the storm in the sea urchin species A. lixula, P. lividus and D. africanum and in seawater samples, which had an increase of 51% after the storm, maybe because of the vertical mixing of sediments caused by the waves in the case of Vibrio species. The continuous presence of N. branchiphilain in the sea urchin species during both seasons may indicate that these sea urchins may acts as a reservoir for the amoeba. In Nova Scotia, for example, it was also observed that Paramoeba invadens remained in the sea urchin S. droebachiensis that had recovered from the disease. In that case, it was also observed that those sea urchins only developed the diseases when the temperature increased above the infection threshold (Scheibling and Stephenson, 1984; Feehan et al., 2012). This finding could mean that the amoebae remained in the sea urchins for some time after the infection occurred (Jones, 1985; Jones and Scheibling, 1985; Feehan et al., 2013). The presence of N. branchiphila in the sea urchins also could be related to recurrent episodes that had introduced the pathogen to Tenerife’s coast, in a manner like that described by Feehan et al. (2016) for the coasts of Nova Scotia. It appears that the individuals that survived were resistant to the infection (Scheibling et al., 2010). This idea has also been hypothesized for the survivors D. antillarum in the Caribbean Sea (Beck et al., 2014; Lessios, 2016). It may be due to loss of virulence that can happen in the species of genus Neoparamoeba sp. (Dyková et al., 2000), which are maintained in sea urchins but do not induce the symptoms of the disease. In our case, lack of a clear correlation with the sea surface temperature and the mortality events was found (Hernández et al., 2020).
The study of causative agents of diseases that are spread in the marine environment is often complicated due to difficulties in detecting potential pathogens in host tissues that are surrounded by water (Feehan et al., 2013); the presence of just one pathogen does not imply disease development or the identification; therefore, histological studies are needed and also the verification of Koch’s postulates (Martony et al., 2018). Perhaps viewing diseases in the marine environment as the interaction of various agents allows a better understanding of the disease (Sweet, 2020). With respect to the four samples of sea urchin coelom, two in August and two in January, we observed the growing of V. alginolyticus colonies and in only one sample the amoeba was present; however, individuals presenting symptoms of the disease at the moment of capture were seen in some cases (Clemente et al., 2014). Assessing the influence of pathogens on the disease is a difficult task. The presence of bacteria in the coelomic fluid could be the result of a poor-quality environment (Martony et al., 2018), such as observed in the water samples when the pathogen community showed a significant increase after Filomena. Similarly, the presence of N. branchiphila in healthy individuals has been observed and could means that N. branchiphila is not the only trigger of the disease as other agents may be involved in the development of this (Dyková et al., 2011; Clemente et al., 2014; Hernández et al., 2020). The next studies must also verify the pathogenic activity of V. minutissima because the genus has been found in some fish and has shown the capability of colonizing gills in addition to pathogenic activity (Bermingham and Mulcahy, 2007). It is also necessary to evaluate the disease using the Koch’s ecological postulates (Sweet, 2020) for evaluating the dysbiosis and existence of an intermediate host.
Finally, the fact that the winter storms, including Filomena, are related to an increase on the presence of pathogens proves that the environmental changes associated with global change could benefit the presence of pathogens of sea urchins, which are key species in structuring the biodiversity composition of the rocky bottoms in the Atlantic (Hernández et al., 2008). Faced with this situation, continuous monitoring of pathogens in the this region is necessary and must be done in parallel with the monitoring of climatic events as stormy periods, temperature increases, and others that could benefit the presence of the pathogens. In the climate change context, it is important to understand the disease dynamics in marine organisms and the role as inducers of ecosystems have on development management policies.
Data Availability Statement
The original contributions presented in the study are included in the article/supplementary material, further inquiries can be directed to the corresponding author.
Author Contributions
JCH and JL-M conceived of the presented idea. JCH, CES-F, and SG-D contributed the field work. CES-F and MR-B contributed the lab work. JL-M verified the analytical methods. JCH supervised the findings of this work. All authors discussed the results and contributed to the final manuscript.
Conflict of Interest
The authors declare that the research was conducted in the absence of any commercial or financial relationships that could be construed as a potential conflict of interest.
Publisher’s Note
All claims expressed in this article are solely those of the authors and do not necessarily represent those of their affiliated organizations, or those of the publisher, the editors and the reviewers. Any product that may be evaluated in this article, or claim that may be made by its manufacturer, is not guaranteed or endorsed by the publisher.
Acknowledgments
We thank Estefanía González and María Camila Medina for their help during field work. Thanks to Stella Forero and Helman Salazar for their unconditional support. This work has been possible thanks to the Fundación Carolina fellowship award that provide support for CES-F, to the funding of Ministerio de Ciencia e Innovación though the ADAPTIVE-PGC2018-100735-B-I00 (MCIU/AEI/FEDER. UE), by the RICET (project no. RD16/0027/0001 of the programme of Redes Temáticas de Investigación Cooperativa, FIS), CIBER de Enfermedades Infecciosas (CIBERINFEC), Instituto de Salud Carlos III (CB21/13/00100) and Cabildo de Tenerife 21/0587 cofunded by FDCAN and MEDI (Tenerife Innova Programme).
References
AEMET (2021). Informe Sobre el Episodio Meteorológico de Fuertes Nevadas y Precipitaciones Ocasionadas por Borrasca Philomena y Posterior Ola de Frío. [Online]. Available online at: http://www.aemet.es/documentos/es/conocermas/recursos_en_linea/publicaciones_y_estudios/estudios/Informe_episodio_Philomena.pdf (accessed March 9, 2021).
Anderson, M. J., Gorley, R. N., and Clarke, K. R. (2008). PERMANOVA+ for PRIMER: Guide to Software and Statistical Methods. Plymouth: PRIMER-E.
Austin, B., and Zhang, X. H. (2006). Vibrio harveyi: a signicant pathogen of marinevertebrates and invertebrates. Lett. Appl. Microbiol. 43, 119–124.
Beck, G., Miller, R., and Ebersole, J. (2014). Mass mortality and slow recovery of Diadema antillarum: could compromised immunity be a factor? Mar. Biol. 161, 1001–1013.
Becker, P. T., Egea, E., and Eeckhaut, I. (2008). Characterization of bacterial communities associated with the bald sea urchin disease of the echinoid Paracentrotus lividus. J. Invertebr. Pathol. 98, 136–147. doi: 10.1016/j.jip.2007.12.002
Bermingham, M. L., and Mulcahy, M. F. (2007). Neoparamoeba sp. and other protozoans on the gills of Atlantic salmon Salmo salar smolts in seawater. Dis. Aquat. Org. 76, 231–240. doi: 10.3354/dao076231
Carroll, A., Copp, B. R., Davis, R. A., Keyzers, R. A., and Prinsep, M. R. (2019). Marine natural products. Nat. Prod. Rep. 36, 122–173.
Clarke, K. R., Gorley, R. N., Somerfield, P. J., and Warwick, R. M. (2014). Change in Marine Communities: An Approach to Statistical Analysis and Interpretation, 3rd Edn. Plymouth: PRIMER-E.
Clemente, S., Lorenzo-Morales, J., Mendoza, J. C., López, C., Sangil, C., Alves, F., et al. (2014). Sea urchin Diadema africanum mass mortality in the subtropical eastern Atlantic: role of waterbone bacteria in a warming ocean. Mar. Ecol. Prog. Ser. 506, 1–14. doi: 10.3354/meps10829
Corry, J. E. L., Curtis, G. W., and Baird, R. M. (eds) (1995). “Thiosulphate citrate Bile-salt sucrose (TCBS) agar,” in Progress in Industrial Microbiology. Amsterdam: Elseivier, 450–452. doi: 10.1016/s0079-6352(05)80086-4
De Jonckheere, J. F. (2006). Isolation and molecular identification of vahlkampfiid amoebae from an island (Tenerife. Spain). Acta Protozool. 45, 91–96. doi: 10.1016/j.exppara.2017.09.016
De Jonckheere, J. F., and Brown, S. (2005). The identification of vahlkampfiid amoebae by ITS sequencing. Protist 156, 89–96. doi: 10.1016/j.protis.2004.11.001
del Estado, P. (2021). Available online at: http://www.puertos.es/es-es/oceanografia/Paginas/portus.aspx (accessed March 3, 2021).
Dyková, I., Figueras, A., and Peric, Z. (2000). Neoparamoeba page, 1987: light and electron microscopic observations on six strains of different origin. Dis. Aquat. Org. 43, 217–223. doi: 10.3354/dao043217
Dyková, I., Lorenzo-Morales, J., Kostka, M., Valladares, B., and Pecková, H. (2011). Neoparamoeba branchiphila infections in moribund sea urchins Diadema aff. antillarum in Tenerife, Canary Islands, Spain. Dis. Aquat. Org. 95, 225–231. doi: 10.3354/dao02361
Dyková, I., Nowak, B., Pecková, H., Fiala, I., Crosbie, P., and Dvoráková, H. (2007). Phylogeny of Neoparamoeba strains isolated from marine fish and invertebrates as inferred from SSU rDNA sequences. Dis. Aquat. Org. 74, 57–65. doi: 10.3354/dao074057
Feehan, C., Johnson-Mackinnon, J., Scheibling, R. E., Lauzon-Guay, J.-S., and Simpson, A. G. B. (2013). Validating the identuty of Paramoeba invadens, the causative agent of recurrent mass mortality of sea urchins in Nova Scotia, Canada. Dis. Aquat. Org. 103, 209–227. doi: 10.3354/dao02577
Feehan, C., Scheibling, R., and Lauzon-Guay, J.-S. (2012). An outbreak of sea urchin disease associated with a recent hurricane: support for the “killer storm hypothesis” on a local scale. J. Exp. Mar. Biol. Ecol. 413, 159–168. doi: 10.1016/j.jembe.2011.12.003
Feehan, C. J., Scheibling, R. E., Brown, M. S., and Thompson, K. R. (2016). Marine epizootics linked to storms: mechanisms of pathogen introduction and persistence inferred from coupled physical and biological time-series. Limnol. Oceanogr. 61, 316–329.
Fernandez, C., Pasqualini, V., Boudouresque, C.-F., Johnson, M., Ferrat, L., Caltagirone, A., et al. (2006). Effect of an exceptional rainfall event on the sea urchin (Paracentrotus lividus) stock and seagrass distribution in a Mediterranean coastal lagoon. Estuar. Coast. Shelf Sci. 68, 259–270. doi: 10.1016/j.ecss.2006.02.020
Gamal, A. A. E. (2010). Biological importance of marine algae. Saudi Pharm. J. 18, 1–25. doi: 10.1002/9781119977087.ch1
García-Davis, S., Sifaoui, I., Reyes-Batlle, M., Viveros-Valdez, E., Piñero, J. E., Lorenzo-Morales, J., et al. (2018). Anti-Acanthamoeba activity of brominated Sesquiterpenes from Laurencia johnstonii. Mar. Drugs 16:443. doi: 10.3390/md16110443
Garstecki, T., and Arndt, H. (2000). Seasonal abundances and community structure of benthic rhizopods in shallow lagoons of the southern Baltic Sea. Eur. J. Protistol. 36, 103–115.
Gizzi, F., Jiménez, J., Schäfer, S., Castro, N., Costa, S., Lourenço, S., et al. (2020). Before and after a disease outbreak: tracking a keystone species recovery from a mass mortality event. Mar. Environ. Res. 156:104905. doi: 10.1016/j.marenvres.2020.104905
Harvell, C. D., Kim, K., Burkholder, J. M., Colwell, R. R., Epstein, P. R., Grimes, D. J., et al. (1999). Emerging marine diseases climate links and anthropogenic factors. Science 285, 1505–1510. doi: 10.1126/science.285.5433.1505
Harvell, C. D., Mitchell, C. E., Ward, J. R., Altizer, S., Dobson, A. P., Ostfeld, R. S., et al. (2002). Climate warming and disease risks for terrestrial and marine biota. Science 296, 2158–2162. doi: 10.1126/science.1063699
Hernández, J. C., Clemente, S., Sangil, C., and Brito, A. (2008). The key role of the sea urchin Diadema antillarum in controlling macroalgae assemblages throughout the Canary Islands (eastern subtropical Atlantic): a spatio-temporal approach. Mar. Environ. Res. 66:259. doi: 10.1016/j.marenvres.2008.03.002
Hernández, J. C., Sangil, C., and Lorenzo-Morales, J. (2020). Uncommon southwest swells trigger sea urchin disease outbreaks in Eastern Atlantic archipelagos. Ecol. Evol. 10, 7963–7970. doi: 10.1002/ece3.6260
Jones, G. (1985). Paramoeba invadens n.sp. (Amoebida, Paramoebidae); a pathogenic amoeba from the Sea urchin, Strongyloccentrotus droebachiennsis, in Eastern Canada. J. Protozool. 32, 564–569. doi: 10.1111/j.1550-7408.1985.tb03075.x
Jones, G. M., and Scheibling, R. E. (1985). Paramoeba sp. (Amoebida, Paramoebidae) as thepossible causative agent of sea urchin mass mortality in Nova Scotia. J. Parasitol. 71, 559–565.
Lafferty, K. D., Porter, J. W., and Ford, S. (2004). Are diseases increasing in the ocean? Ann. Rev. Ecol. Evol. Syst. 35, 31–54.
Lessios, H. A. (2016). The great Diadema antillarum Die-Off: 30 years later. Ann. Rev. Mar. Sci. 8, 267–283.
Lessios, H. A., Robertson, D. R., and Cubit, J. D. (1984). Spread of diadema mass mortality through the Caribbean. Science 226(4672), 335–337. doi: 10.1126/science.226.4672.335
Lima, P. C., Taylor, R. S., and Cook, M. (2017). Pseudocyst formation in the marine parasitic amoeba Neoparamoeba perurans: a short-term survival strategy to abrupt salinity variation. J. Fish Dis. 40, 1109–1113. doi: 10.1111/jfd.12588
Lorenzo-Morales, J., and Hernández, J. C. (2020). “Paramoeba brachiphila rDNA sequence [Data set],” in Ecology and Evolution (Geneva: Zenodo). doi: 10.5281/zenodo.3716129
Lorenzo-Morales, J., Lindo, J. F., Martinez, E., Calder, D., Figueruelo, E., Valladares, B., et al. (2005a). Pathogenic Acanthamoeba strains from water sources in Jamaica, West Indies. Ann. Trop. Med. Parasitol. 99, 751–758. doi: 10.1179/136485905X65215
Lorenzo-Morales, J., Ortega-Rivas, A., Foronda, P., Martínez, E., and Valladares, B. (2005b). Isolation and identification of pathogenic Acanthamoeba strains in Tenerife, Canary Islands, Spain from water sources. Parasitol. Res. 95, 273–277. doi: 10.1007/s00436-005-1301-2
Mac Phail, D., Koppenstein, R., Maciver, S. K., Paley, R., Longshaw, M., Henriquez, F. L., et al. (2020). Vibrio species are predominantly intracellular within cultures of Neoparamoeba perurans, causative agent of Amoebic Gill Disease(AGD). Aquaculture 532:736083.
Macivera, S. K., Asifa, M., Simmena, M. W., and Lorenzo-Morales, J. (2013). A systematic analysis of Acanthamoeba genotype frequency correlated with source and pathogenicity: T4 is confirmed as a pathogen-rich genotype. Eur. J. Protistol. 49, 217–221. doi: 10.1016/j.ejop.2012.11.004
Maes, P., and Jangoux, M. (1984). The bald-sea-urchin disease: a biopathological approach. Helgolander Meeresunters 37, 217–224. doi: 10.1007/bf01989306
Martinez, A. J. (1991). Infection of the central nervous system due to Acanthamoeba. Rev. Infect. Dis. 13, S399–S402.
Martony, M., Pouder, D., Yanong, R., Kiryu, Y., Landsberg, J. H., Isaza, R., et al. (2018). Establishing a diagnostic technique for coelomocentesis in the long-spined sea urchin Diadema antillarum. J. Aquat. Anim. Health 30, 325–331. doi: 10.1002/aah.10043
Nowak, B. F., and Archibald, J. M. (2018). Opportunistic but lethal: the mystery of Paramoebae. Trends Parasitol. 34, 404–419. doi: 10.1016/j.pt.2018.01.004
Pussard, M., and Pons, R. (1977). Morphologie de la paroi kystique et taxonomie du genre Acanthamoeba. Protistologica 13, 557–598.
Reyes-Batlle, M., Díaz, F. J., Sifaoui, I., Rodríguez-Expósito, R., Rizo-Liendo, A., Piñero, J. E., et al. (2021). Free living amoebae isolation in irrigation waters and soils of an insular arid agroecosystem. Sci. Total Environ. 753:141833. doi: 10.1016/j.scitotenv.2020.141833
Sangil, C., and Hernández, J. C. (2021). Recurrent large-scale sea urchin mass mortalities and the establishment of a long-lasting alternative macroalgae-dominated community state. Limnol. Oceanogr. 1–14. doi: 10.1002/lno.11966
Scheibling, R., Feehan, C., and Lauzon-Guay, J. S. (2010). Disease outbreaks associated with recent hurricanes cause mass mortality of sea urchin in Nova Scotia. Mar. Ecol. Prog. Ser. 408, 109–116.
Scheibling, R., and Lauzon-Guay, J.-S. (2010). Killer storms: North Atlantic hurricanes and disease outbreaks in sea urchins. Limnol. Oceanogr. 55, 2331–2338. doi: 10.4319/lo.2010.55.6.2331
Scheibling, R. E., and Stephenson, R. L. (1984). Mass mortality of Strongylocentrotus droebachiensis (Echinodermata: Echinoidea) off Nova Scotia, Canada. Mar. Biol. 78, 153–164.
Sweet, M. (2020). “Sea urchin diseases: effects from individuals to ecosystems,” in Sea Urchins: Biology and Ecology, ed. J. M. Lawrence (Amsterdam: Elsevier B.V).
Tajimaa, K., Machado Cunha da Silva, J. R., and Lawrence, J. M. (2007). “Disease in Sea urchins,” in Edible Sea Urchins: Biology and Ecology, ed. J. M. Lawrence (Amsterdam: Elsevier Science B.V.), 167–182.
Trowbridge, C. D., Little, C., Plowman, C. Q., Williams, G. A., Pilling, G. M., Morritt, D., et al. (2019). No “Silver bullet”: multiple factors control population dynamics of European purple sea urchins in lough hyne marine reserve, Ireland. Estuar. Coast. Shelf Sci. 226:106271
Tsvetkova, N., Schild, M., Panaiotov, S., Kurdova-Mintcheva, R., Gottstein, B., Walochnik, J., et al. (2004). The identification of free-living environmental isolates of amoebae from Bulgaria. Parasitol. Res. 92, 405–413. doi: 10.1007/s00436-003-1052-x
Walochnik, J. (2018). “Amoebae,” in Parasitic Protozoa of Farm Animals and Pets, eds M. Florin-Christensen and L. Schnittger (Cham: Springer).
Wang, Y. N., Chang, Y. Q., and Lawrence, J. M. (2013). “Disease in Sea urchins,” in Sea Urchins: Biology and Ecology, ed. J. M. Lawrence (Amsterdam: Elsevier B.V), 179–186.
Keywords: Killer Storm, pathogens, sea urchin, mass mortalities, communities
Citation: Salazar-Forero CE, Reyes-Batlle M, González-Delgado S, Lorenzo-Morales J and Hernández JC (2022) Influence of Winter Storms on the Sea Urchin Pathogen Assemblages. Front. Mar. Sci. 9:812931. doi: 10.3389/fmars.2022.812931
Received: 10 November 2021; Accepted: 28 January 2022;
Published: 07 March 2022.
Edited by:
Anett Kristin Larsen, UiT The Arctic University of Norway, NorwayReviewed by:
Cinthya Santos, Federal Fluminense University, BrazilAlejandro H. Buschmann, University of Los Lagos, Chile
Copyright © 2022 Salazar-Forero, Reyes-Batlle, González-Delgado, Lorenzo-Morales and Hernández. This is an open-access article distributed under the terms of the Creative Commons Attribution License (CC BY). The use, distribution or reproduction in other forums is permitted, provided the original author(s) and the copyright owner(s) are credited and that the original publication in this journal is cited, in accordance with accepted academic practice. No use, distribution or reproduction is permitted which does not comply with these terms.
*Correspondence: José Carlos Hernández, am9jYXJoZXJAdWxsLmVz