- 1A.V. Zhirmunsky National Scientific Center of Marine Biology, Russian Academy of Sciences, Vladivostok, Russia
- 2Department of Marine Ecology, Far Eastern Federal University, Vladivostok, Russia
The Kuril Basin and the Kuril-Kamchatka Trench are two interconnected deep-sea ecosystems both located in one of the most highly productive regions of the world’s oceans. The main distinguishing features of these deep-sea ecosystems are the low oxygen concentration in the near-bottom water in the Kuril Basin, and the high hydrostatic pressure in the trench. We investigated the abundance of meio- and macrobenthic nematodes and macrofauna on the Kuril Basin floor (depths of 3,300–3,366 m) and in the Kuril-Kamchatka Trench area (depths of 3,432–9,539 m), as well as the influence of some environmental factors on the quantitative distribution of bottom fauna. This was not studied so far. The study also focused on the species composition and quantitative distribution of Polychaeta and Bivalvia, which were dominant in abundance among macrofaunal samples. The main factors influencing the quantitative distribution of macrofauna and nematodes were depth, oxygen concentration, and structure of bottom sediments. The Kuril Basin bottom communities are characterized by a high abundance of nematodes and macrofauna, a high species richness of polychaetes, and a pronounced dominance of small-sized species of Polychaeta and Bivalvia, which are probably more tolerant to low oxygen concentrations. Compared to the Kuril Basin, the Kuril-Kamchatka Trench area (at depths of 3,432–5,741 m) had a more diverse and abundant macrofauna, and a very high abundance of meio- and macrobenthic nematodes. In the trench (at depths more than 6,000 m), the diversity of macrofauna and the abundance of macrobenthic nematodes decreased, while the abundance of macrofauna increased with increasing depth. On the trench floor, the macrofaunal abundance was highest due to the high density of populations of several bivalve and polychaete species, apparently adapted to the high hydrostatic pressure on the trench floor. Obviously, the high primary production of surface waters supports the diverse and abundant deep-sea bottom fauna in the studied areas of the northwestern Pacific. Furthermore, a large number of animals with chemosynthetic endosymbiotic bacteria were found in the bottom communities of the Kuril Basin and the Kuril-Kamchatka Trench. This suggests a significant contribution of chemosynthetic organic carbon to functioning of these deep-sea ecosystems.
Introduction
The abyssal and hadal zones of the world’s oceans (with depths greater than 3,000 m) occupy more than 50% of Earth’s surface (Smith et al., 2008; Ramirez-Llodra et al., 2010) and include deep basins of seas, vast oceanic plains, canyons, and trenches. These deep-sea regions support ecosystems with unusually high levels of diversity and, in many cases, abundance of bottom animals (Hessler and Sanders, 1967; Belyaev, 1989; Allen and Sanders, 1996; Rex et al., 2005; Danovaro et al., 2014; Jamieson, 2015). Deep, marginal, semi-enclosed seas and ocean trenches differ greatly in their geomorphology, structure of bottom sediments, hydrological and hydrochemical characteristics of the near-bottom water from the vast abyssal plains that occupy a larger part of the ocean floor (Ushakov, 1953; Belyaev, 1989; Ramirez-Llodra et al., 2010; Danovaro et al., 2014; Tyler et al., 2016). The specific environmental conditions in ocean trenches and deep marginal seas largely affect the abundance, species composition, distribution, and adaptation of bottom animals living in deep-sea habitats (Ushakov, 1953; Savilov, 1961; Belyaev, 1989; Danovaro et al., 2010; Jamieson, 2015; Oug et al., 2017; Brandt et al., 2018, 2019; Mironov et al., 2019a). However, the abundance, species diversity and distribution of bottom animals in deep-sea basins of seas and oceanic trenches, as well as the factors responsible for the composition and distribution of their abyssal and hadal communities, are still very poorly understood (Belyaev, 1989; Jamieson et al., 2010; Ramirez-Llodra et al., 2010; Tyler et al., 2016; Howell et al., 2021).
The Sea of Okhotsk, separated from the Pacific Ocean by the Kuril Islands, is one of the largest deep-water marginal seas. The Kuril Basin, with its depths greater than 3,000 m, is located in the deepest, southeasternmost part of the sea (Glukhovsky et al., 1998). The Sea of Okhotsk is characterized by a very high level of primary production in the spring-summer period and has a low oxygen water layer (0.8–1.3 ml l–1, 12–18% saturation) in central part of the sea in depths of 750–1,500 m (Ushakov, 1953; Terziev, 1996). This layer is formed by the oxidation of a significant amount of organic matter produced in the surface layer. Deeper than 1,500 m, the oxygen concentration of water in the Sea of Okhotsk increases due to the influx of more oxygenated deep-sea oceanic waters entering through the deepest Kuril straits. As a result, the oxygen concentration in the water near the floor of the Kuril Basin (at depth more than 3000 m) fluctuates in the range of 1.6–1.9 ml l–1 (22–27% saturation) (Terziev, 1996). Thus, bottom animals of the abyssal zone of the Sea of Okhotsk are exposed to permanent oxygen deficiency in the near-bottom layer of water (Ushakov, 1953; Savilov, 1961; Terziev, 1996), in contrast to the well-oxygenated bottom waters of the oceanic abyssal plains (Smith et al., 2008).
The species composition and the structure of bottom communities in the shelf zone of the Sea of Okhotsk have been sufficiently studied (Ushakov, 1953; Savilov, 1961; Kafanov et al., 2003). However, the analysis of the abundance and quantitative distribution of the abyssal macrofauna of the Kuril Basin was based on only three quantitative samples collected with a Petersen grab (sampling area 0.25 m2) at depths of more than 3,000 m in 1949–1954 (Savilov, 1961). Thus, the composition and abundance of abyssal macrofauna of the Kuril Basin is still poorly studied, and the abundance and quantitative distribution of meiofauna have remained completely unexplored (Ushakov, 1953; Savilov, 1961).
The Kuril-Kamchatka Trench with a maximum depth of 9,600 m is one of the deepest in the world (Vasiliev et al., 1978; Belyaev, 1989; Dreutter et al., 2020; Jamieson and Stewart, 2021). It is separated from the deep-sea basin of the Sea of Okhotsk by the Kuril Ridge, which forms the chain of the Kuril Islands (Glukhovsky et al., 1998). These two deep-sea ecosystems differing in bottom topography, depth, many characteristics of bottom sediments and water masses, are interconnected by numerous straits between the Kuril Islands, with a maximum depth of 2,318 m in the Bussol Strait (Glukhovsky et al., 1998). In contrast to the abyssal fauna of the Kuril Basin, the hadal fauna of the Kuril-Kamchatka Trench has been studied much better (Belyaev, 1989; Shirayama and Kojima, 1994; Kojima et al., 2004; Sasaki et al., 2005; Itoh et al., 2011; Kitahashi et al., 2012, 2013). However, in all studies of the bottom communities in the Kuril-Kamchatka Trench, the major focus was on the morphology and anatomy of new taxa, the species composition of the bottom fauna, the spatial and vertical distribution of species, and faunal relationships with other deep-sea regions of the Pacific Ocean (Zenkevitch et al., 1955; Filatova, 1971; Belyaev, 1989; Sasaki et al., 2005; Okutani et al., 2009). Over the entire period of study, only five quantitative macrofaunal samples were collected from the trench slopes using an Okean grab (sampling area 0.25 m2), of them, four were from depths of 6,000–7,000 m and only one from a depth of 8,355 m (Belyaev, 1989). Thus, the deepest part of the Kuril-Kamchatka Trench (depths over 9,000 m) has remained unexplored in terms of the abundance and quantitative distribution of bottom fauna to date.
Recently, the bottom fauna of the Kuril Basin and the Kuril-Kamchatka Trench were studied by the Russian-German SokhoBio (Sea of Okhotsk Biodiversity Studies) expedition (2015) and the German-Russian KuramBio II (Kurile-Kamchatka Biodiversity Studies II) expedition (2016). The main goal of these expeditions was to study the species composition and distribution patterns of bottom animals, as well as to assess the faunal relationships in the deep-sea ecosystems of the Sea of Okhotsk and the Kuril-Kamchatka Trench separated by such a natural barrier as the Kuril Islands (Malyutina et al., 2018; Brandt et al., 2019). As a result, numerous species from different taxonomic groups of animals were described, the composition of macro- and meiofauna and the patterns of distribution of the main taxonomic groups of animals were studied, the faunal relationship and levels of endemism of these deep-sea areas were estimated (e.g., Adrianov and Maiorova, 2018, 2019; Alalykina, 2018; Brandt et al., 2018, 2019; Chernyshev and Polyakova, 2018, 2019; Fukumori et al., 2018, 2019; Kamenev, 2018a,b, 2019, 2020; Maiorova and Adrianov, 2018a,b; Malyutina and Brandt, 2018, 2020; Mironov et al., 2018, 2019b; Borisanova and Chernyshev, 2019; Brandão et al., 2019; Mordukhovich et al., 2019; Schmidt et al., 2019; Alalykina and Polyakova, 2020; Maiorova and Adrianov, 2020; Saeedi and Brandt, 2020). However, the analysis of the quantitative distribution of macro- and meiofauna in the Kuril Basin has not been completed, and only preliminary results of the macrofauna abundance study have been reported for the Kuril-Kamchatka Trench (Lins and Brandt, 2020).
It should be noted that in the SokhoBio and KuramBio II expeditions a box corer was used for the first time to study the quantitative distribution of macrofauna of the deep-sea ecosystem in the Sea of Okhotsk and the Kuril-Kamchatka Trench. The box corer was shown to be a much more efficient gear for collecting quantitative samples of deep-sea macrofauna compared to the Petersen and Okean grabs (Hessler and Jumars, 1974; Jumars and Hessler, 1976; Filatova, 1982; Belyaev, 1989). In recent years, the composition, abundance, and distribution of meiofauna in the hadal zone of the world’s oceans have been most actively studied on material collected using mainly multicorer (Shirayama and Kojima, 1994; Danovaro et al., 2002; Gambi et al., 2003; Vanhove et al., 2004; Itoh et al., 2011; Kitahashi et al., 2012, 2013; Schmidt and Martínez Arbizu, 2015; Leduc et al., 2016; Leduc and Rowden, 2018; Schmidt et al., 2018, 2019; Wang et al., 2019). Nevertheless, the quantitative distribution of macrofauna in the hadal zone, as before, remains extremely poorly studied due to the major problems associated with collection of quantitative samples using box corer from oceanic trenches with a complex bottom topography, a wide variety of bottom sediments (from very dense with small stones and gravel to “liquid” silts), and great depths (Belyaev, 1989; Jamieson, 2015; Tyler et al., 2016). The estimation of the macrofaunal abundance based on the box-corer samples was carried out only in the Aleutian and Izu-Bonin trenches (one sample each) (Jumars and Hessler, 1976; Shirayama, 1984). Therefore, the study of the abundance of macrofauna and the dominant taxonomic groups of animals in the oceanic trenches still remains one of the most urgent issues in marine biology.
We hypothesize, that the bottom communities of the Kuril Basin and the Kuril-Kamchatka Trench are adapted to the specific habitat conditions in these deep-sea ecosystems. Therefore, the major objectives of the present study were to: (1) study the abundance and quantitative distribution of macrofauna, macro- and meiobenthic nematodes inhabiting the floor of the Kuril Basin and the slopes and floor of the Kuril-Kamchatka Trench; (2) identify environmental factors affecting the taxonomic composition and quantitative distribution of deep-sea macrofauna and nematodes in studied areas; (3) investigate the species composition and abundance of polychaetes and bivalves, as the dominant taxonomic groups of macrofauna in box-corer samples, and compare their species composition and quantitative distribution in the abyssal zone of the Sea of Okhotsk and the abyssal and hadal zones of the adjacent Kuril-Kamchatka Trench area.
Materials and Methods
Sample Collection and Processing
The bottom fauna of the Kuril Basin, the Bussol Strait, and the abyssal zone of the Pacific slope of the Kuril Islands opposite the Bussol Strait was studied during the SokhoBio expedition (RV Akademik M.A. Lavrentyev, cruise no. 71, July 6–August 6, 2015) (Malyutina et al., 2018; Figure 1). The abyssal and hadal faunas in the Kuril-Kamchatka Trench area were studied during the KuramBio II expedition (RV Sonne, cruise no. 250, August 16–September 26, 2016) (Brandt et al., 2020). Detailed descriptions of the geomorphology, hydrological regime and bottom sediments of the Kuril Basin and the Kuril-Kamchatka Trench were given by Kamenev (2018b,2019). In both expeditions, an epibenthic sledge, an Agassiz trawl, and a box corer (with a sampling area of 0.25 m2) were used for sampling macrofauna. In both expeditions, one complete sample suitable for studying the macrofauna abundance was collected using box corer at each station after several attempts. Also, an additional sample was collected with box corer at each station to study sediments and meiofauna in the SokhoBio expedition. To study the meiofauna, three subcores (with a circular surface area of 88.2 cm2) were taken with acryl-glass tubes (with the outer diameter of 110 mm and the inner diameter of 96 mm) from additional box-corer sample at each station. The upper 5 cm of the sediment from a subcore were fixed in 4% buffered formalin.
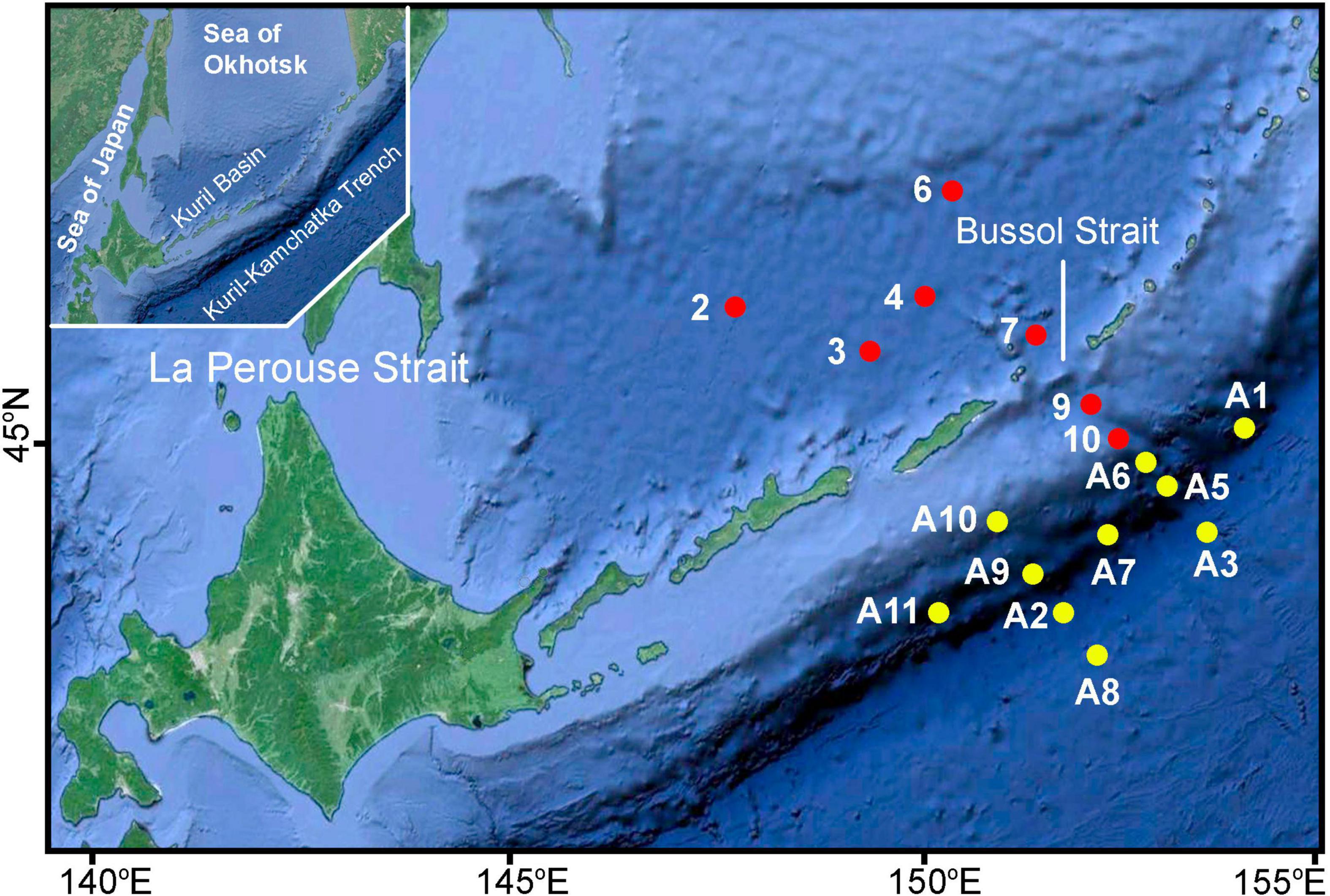
Figure 1. A map of stations sampled in the abyssal zone of the Kuril Basin (Sea of Okhotsk) and abyssal and hadal zones of the Kuril-Kamchatka Trench area by the SokhoBio expedition (2015) (red circles) and KuramBio II expedition (2016) (yellow circles).
The present work is based only on the results of the macrofaunal study of 17 satisfactory box-corer samples. From 2 to 5 box-corer samples were taken from the major topographic environments of the abyssal (depths of 3,000–6,000 m) and hadal (depth more than 6,000 m) zones of the study area of the northwestern Pacific: (1) the floor of the Kuril Basin (St. 2–4, 6, 7; depths of 3,300–3,366 m); (2) the upper part of the oceanic slope of the Kuril Islands opposite the Bussol Strait (St. 9, 10; 3,432 and 4,741 m); (3) the lower part of the oceanic slope of the Kuril Islands and the abyssal plain adjacent to the trench (St. A3, A8, A10; 5,144–5,741 m); (4) the upper part of the slopes of the Kuril-Kamchatka Trench (St. A2, A5, A6; 6,068–7,136 m); (5) the lower part of the trench slopes (St. A1, A9; 8,221 and 8,251 m); (6) the floor of the deepest basins (Vityaz Deep 3 and Sonne Deep) (Dreutter et al., 2020) of the trench (St. A7, A11; 9,492 m and 9,539 m) (Table 1).
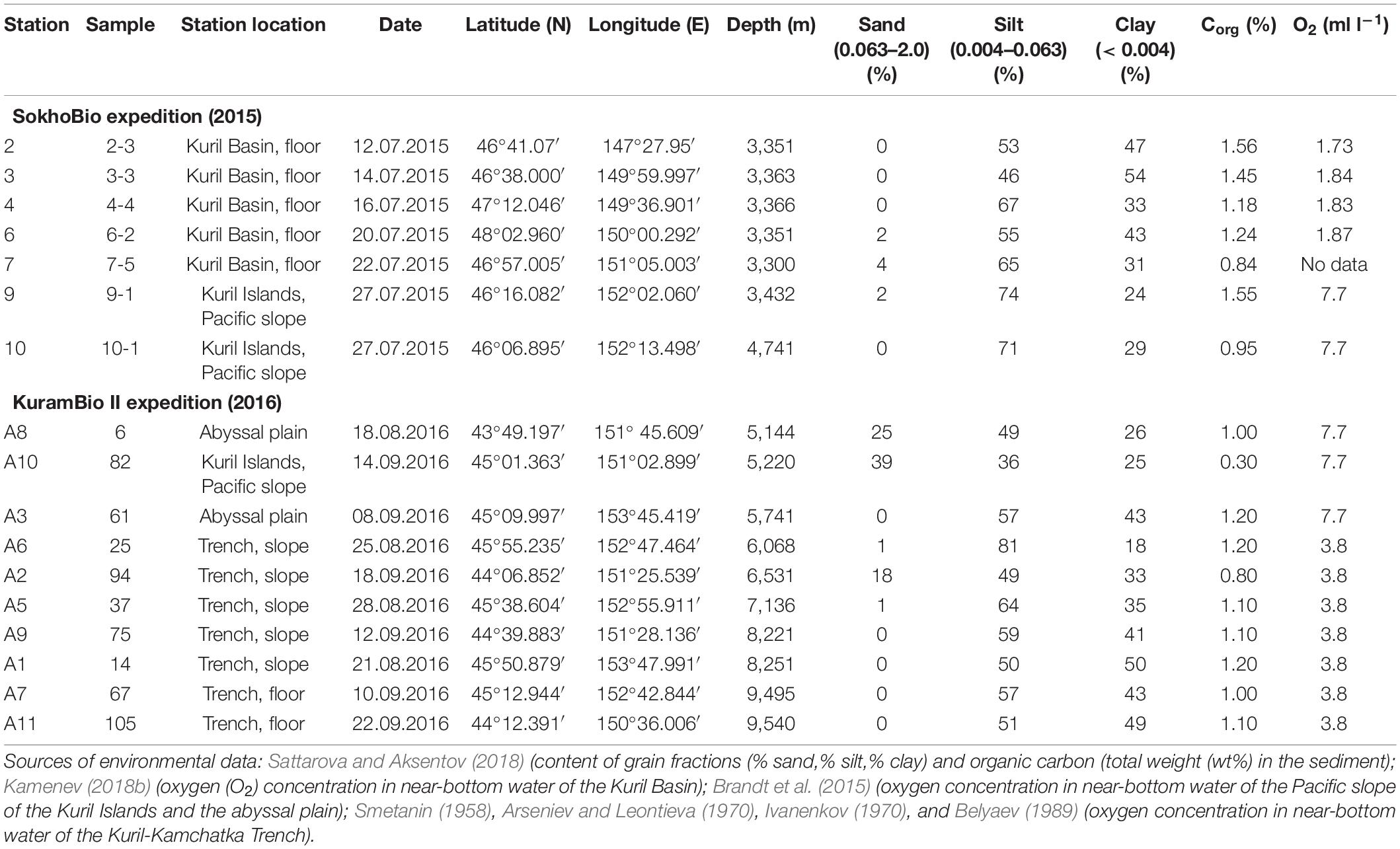
Table 1. Position and depth of box-corer samples and environmental data at the stations of the SokhoBio and KuramBio II expeditions in the Kuril Basin (Sea of Okhotsk) and the Kuril-Kamchatka Trench area (Pacific Ocean).
The surface area of the box corer was entirely preserved for macrofauna analysis. Upon lifting the box corer aboard, the overlying water was siphoned through a 0.3 mm sieve to prevent surface organisms from escaping; then a photograph of the sediment surface was taken. All box-corer samples (upper 20 cm of sediments) collected in the SokhoBio expedition were washed through 1-mm and 500-μm mesh sieves. The KuramBio II expedition used the standardized methods of sample collection and treatment in order to study all size classes of deep-sea bottom fauna. Therefore, in the KuramBio II expedition all box-corer samples were washed through 1-mm, 500-μm, and 300-μm mesh sieves. In the on-board laboratory, all animals were collected from the 1,000- and 500-μm fraction and sorted out under stereomicroscopes into major taxonomic groups of different levels (type, class, order), counted, and fixed in pre-cooled non-denatured 95% ethanol and in a 4% buffered formaldehyde solution. Subsequently, some taxonomic groups of animals were transferred to 70% ethanol for permanent storage. The 300-μm fraction was fixed in pre-cooled non-denatured 95% ethanol. Groups of animals of different taxonomic levels in our study is considered as separate taxa.
In most deep-sea studies, macrofauna consisted of animals retained on a 300-μm sieve. However, it is well known that washing samples through 300-μm mesh sieves provides a large number of animals from meiofaunal taxa (e.g., Nematoda, Harpacticoida, and Ostracoda) (Hessler and Jumars, 1974), whose many species and life stages easily pass through a 300-μm mesh sieve. As a rule, these taxa are overlooked in analyses of macrofauna taxonomic composition and abundance (e.g., Hessler and Jumars, 1974; Jumars and Hessler, 1976; De Smet et al., 2017; Wilson, 2017; Washburn et al., 2021). However, certain meiofaunal taxa are considered as macrofauna in some studies, which hampers comparative analysis of these data (Choi et al., 2004; Yu et al., 2018; Lins and Brandt, 2020). We sequentially washed sediments from the box corer through sieves with mesh sizes of 1,000 μm, 500 μm, and 300 μm and compared these fractions. The 1,000− and 500-μm mesh sieves retained almost all macrofaunal animals. Thus, mainly meiofaunal taxa remained in the 300-μm fraction, with rare exception of a small number of very young specimens of animals from macrofaunal taxa. Therefore, macrofauna in our study is considered as bottom animals retained on 1,000− and 500-μm mesh sieves. Nevertheless, 1,000− and 500-μm mesh sieves retained a large number of meiofaunal animals (nematodes, harpacticoids, ostracods, and kinorhynchs), which we did not take into account in our study (except for nematodes). Large-sized deep-sea nematodes are known to be found in large numbers in the macrofaunal size fraction. Such large nematodes are often considered as one of the macrofauna components (Sharma and Bluhm, 2011; Sharma et al., 2011; Gunton et al., 2017). Therefore, in our work we assessed also the abundance of macrobenthic nematodes from the same box-corer samples (fraction > 500 μm) collected by the SokhoBio and KuramBio II expeditions. Polychaetes and bivalves were identified to species or genus levels, counted, and stored at the A.V. Zhirmunsky National Scientific Center of Marine Biology, Far Eastern Branch, Russian Academy of Sciences. The identified species and morphospecies are referred herein as species.
In the laboratory, meiofauna samples were washed with tap water, screened through a 32-μm sieve, and centrifuged three times (at 4,000 rpm, 6 min) in a solution of colloidal silica (Ludox HS-40). Afterward, meiofauna samples were re-fixed in a formalin solution and stained with Rose Bengal (0.5 g l–1). Macro- and meiobenthic nematodes were sorted out manually under a stereo microscope and counted.
Environmental Data
The following environmental data were used in the analyses of macrofauna samples: depth (m), O2 concentration (ml l–1) in the near-bottom water, percentage of sand (0.063–2.0 mm), silt (0.004–0.063 mm), and clay fractions (< 0.004 mm) in sediments, and total organic carbon (% Corg) (Table 1). Sediment samples for the study of the grain-size structure and organic carbon content were collected with a multicorer at the same stations where box-corer samples had been taken during the same expeditions. Those samples were analyzed by Sattarova and Aksentov (2018). Oxygen concentration of the near-bottom water layer (at a distance of 1 m from the bottom) in the Kuril Basin was measured with a SBE 19plusV2 SEACAT CTD profiler. The oxygen concentration ranged within 1.73-1.87 ml l–1 (Brandt et al., 2018; Kamenev, 2018b). Therefore, in our study we used an average oxygen concentration of 1.8 ml l–1 for the stations sampled in the Sea of Okhotsk. For all stations in the hadal zone of the Kuril-Kamchatka Trench, we used an average oxygen concentration of the near-bottom water layer of 3.6 ml l–1, as inferred from long-term data (Smetanin, 1958; Arseniev and Leontieva, 1970; Ivanenkov, 1970; Belyaev, 1989). For all abyssal stations in the area of the Kuril-Kamchatka Trench, we used an oxygen concentration value of 7.7 ml l–1, which is stable at abyssal depths of 4,865-5,402 m in this area (Brandt et al., 2015).
Statistical Analysis
We have calculated taxon richness (number of identified taxonomic groups per sample), species richness of polychaetes and bivalves (number of species per sample), diversity (Shannon Index, H’) and evenness (Pielou Index, J’) (Magurran, 2012) as well as abundance of macrofaunal taxa, polychaete and bivalve species, macrobenthic nematodes (ind. m–2) and meiobenthic nematodes (ind. 10 cm–2) for each sample. Mean values with standard error (SE) of those indices were calculated for each depth zone. Statistical differences between the depth zones were assessed by one-way analysis of variance (ANOVA), post hoc pairwise comparisons were performed by Tukey’s test. This part of the analysis was performed using a STATISTICA 13 package (StatSoft, 2012).
Cluster and non-metric multidimensional scaling (MDS) analyses were chosen to assess the similarity of species compositions of polychaetes and bivalves found at different stations and to identify areas and depth ranges within the study region of the northwestern Pacific that differ in the species composition of these taxonomic groups. The Bray—Curtis similarity (Bray and Curtis, 1957) was calculated on 4th-root transformation of the bivalve and polychaete species abundance per box corer to define relationships of the faunal composition between all sites (Clarke and Gorley, 2015). The intra-location resemblance was subjected to a group-average linkage cluster analysis. The cluster analysis was performed using a resemblance matrix to produce dendrograms. Clusters were tested using the similarity profile procedure (SIMPROF). The significant branch (SIMPROF, p < 0.05) was used as a prerequisite for defining the faunal complex. MDS was conducted on the resemblance matrix to assess the sample relationship on a 2-dimensional plane. The SIMPER procedure (similarity percentage routine) was used to determine the level of within-group sample dissimilarity (or similarity) and particular species responsible for the differences between groups of samples (Clarke, 1993). Similarities between sample groups were tested using the analysis of similarities (ANOSIM) (Clarke and Warwick, 2001).
The relations between the biological (taxon abundances) and environmental data were investigated using the BIOENV analysis (Clarke and Ainsworth, 1993) and Spearman’s rank correlation (Sokal and Rohlf, 1995). The BIOENV procedure was used to identify the tested environmental variables that best explain the observed patterns in macrofauna distribution (Clarke and Gorley, 2015). The environmental data were logarithm-transformed, normalized (divided by standard deviation), and computed for Euclidean distances. The RELATE test was applied to determine the significance of any relationships between the similarity matrices underlying the biological and environmental data. Multivariate analyses were performed using the PRIMER 7.0 (Clarke and Gorley, 2015).
Results
Abundance and Diversity of Bottom Fauna on the Kuril Basin Floor (Sea of Okhotsk)
A total of 691 specimens of macrofaunal animals belonging to 15 taxa and 1,161 specimens of macrobenthic nematodes were found in box-corer samples (St. 2, 3, 4, 6, and 7) collected from the Kuril Basin floor (Table 2). The total macrofaunal abundance (without nematodes) on the basin floor averaged at 552.8 ± 86.6 ind. m–2. The lowest macrofaunal abundance was recorded at St. 6, which is most remote from the Kuril Islands straits and the La Perouse Strait.
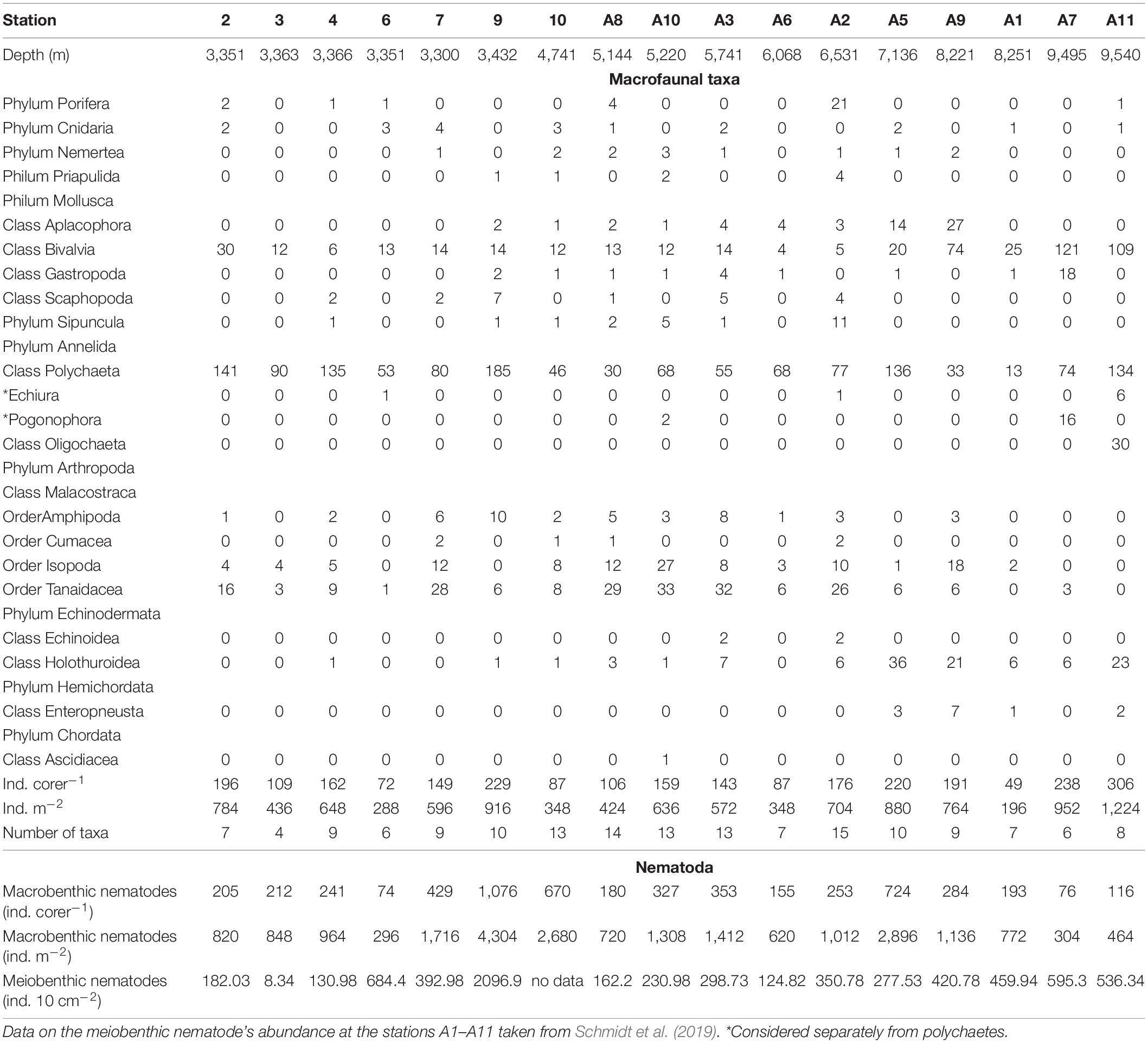
Table 2. Abundance of macrofaunal taxa, macro- and meiobenthic nematodes at the SokhoBio and KuramBio II stations in the Kuril Basin (Sea of Okhotsk) and the Kuril-Kamchatka Trench area (Pacific Ocean).
The abundance of macrobenthic nematodes in all samples, except for St. 7, slightly exceeded the macrofaunal abundance (Tables 2, 3). Their maximum abundance, almost 3-fold higher than the abundance of macrofauna (74.1% of the total abundance of macrofauna and nematodes), was recorded at St. 7, closest to the Bussol Strait (Figure 2). The lowest abundance of macrobenthic nematodes was recorded at St. 6. The abundance of meiobenthic nematodes varied in a very wide range, reaching a maximum value at St. 6.
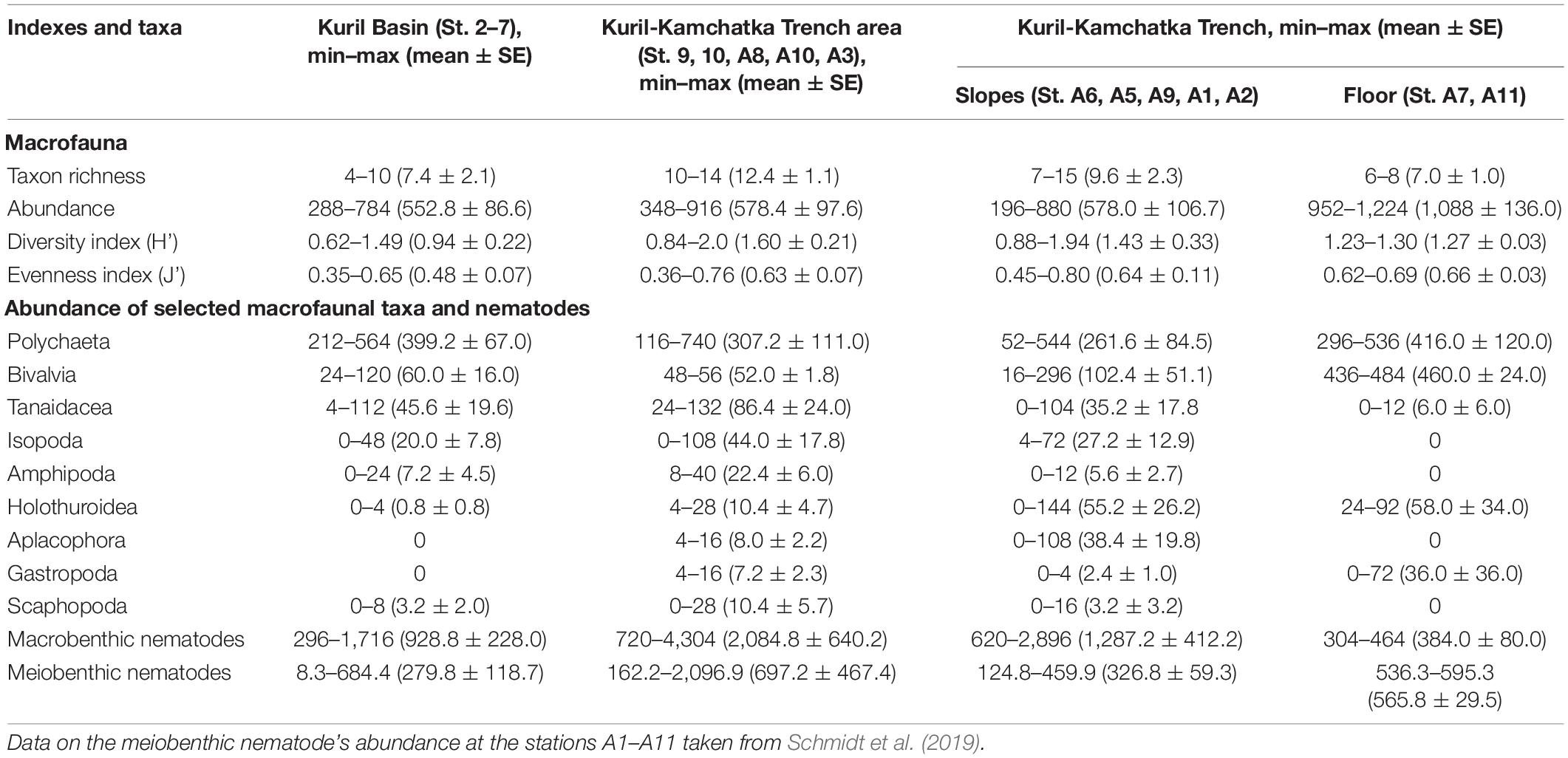
Table 3. Taxon richness (number taxa per sample), abundance (ind. m–2), and diversity indexes of macrofauna and abundance of selected macrofaunal taxa (ind. m–2), macro- (ind. m–2) and meiobenthic (ind. 10 cm–2) nematodes from the quantitative samples collected in the abyssal zone (3,300–3,366 m) of the Kuril Basin (Sea of Okhotsk), abyssal zone (3,432–5,741 m) of the Kuril-Kamchatka Trench area, and hadal zone (6,068–9,539 m) of the Kuril-Kamchatka Trench.
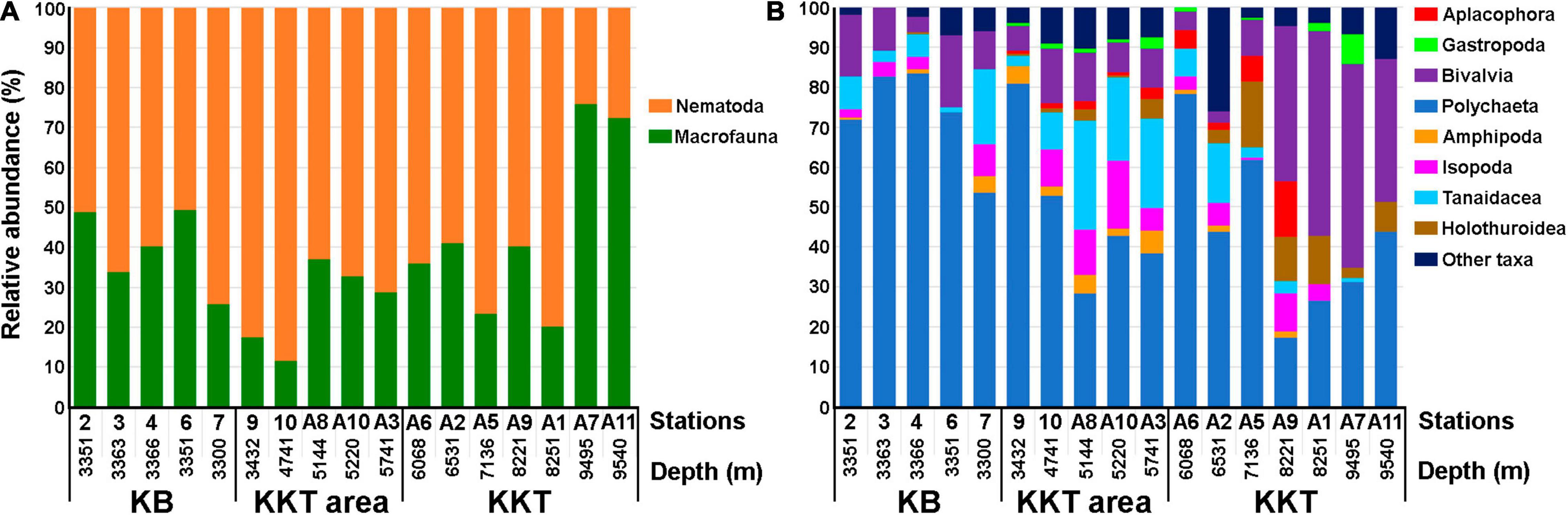
Figure 2. Bar plots indicating the relative abundance of macrofauna and macrobenthic nematodes (A) and macrofaunal taxa (B) in the box-corer samples taken from the different depths of the studied areas of the northwestern Pacific. KB – Kuril Basin (Sea of Okhotsk); KKT – Kuril-Kamchatka Trench.
The number of taxonomic groups of animals in the samples varied from 4 (St. 3) to 9 (St. 4 and 7) (Table 3). Polychaetes (72.8 ± 8.1% of the total abundance of macrofauna), crustaceans (13.8 ± 5.0%) and bivalves (11.5 ± 4.2%) were present in all samples and dominated in abundance, except for St. 7, contributed nearly 86.0–93.6% of the total macrofaunal abundance (Figure 2). At St. 7, which is closest to the Bussol Strait, the largest abundance of crustaceans (32.0%) was recorded. Among them, tanaids (9.1 ± 4.6% of the total macrofaunal abundance) and isopods (3.3 ± 2.0%) were the most abundant.
The average values of the Shannon’s diversity and Pielou’s evenness indices calculated for macrofauna were low, reflecting the pronounced dominance of polychaetes and bivalves in most samples. The exception was St. 7, where these indices had high values (H′ = 1,45; J′ = 0,66) in accordance with the relatively high abundance of many taxonomic groups of the macrofauna.
Abundance and Diversity of Bottom Fauna in the Abyssal Zone of the Kuril-Kamchatka Trench Area (Pacific Ocean)
In the samples collected from the abyssal zone of the Kuril-Kamchatka Trench area (St. 9, 10, A8, A10, A3; depths of 3,432–5,741 m), a total of 723 specimens of macrofaunal animals belonging to 20 taxonomic groups and 2,606 specimens of macrobenthic nematodes were found (Table 2). The macrofaunal abundance at these abyssal depths averaged at 578.4 ± 97.6 ind. m–2 (Table 3). The maximum macrofauna abundance (916 ind. m–2) was recorded from the oceanic slope of the Kuril Islands opposite the Bussol Strait (St. 9). At depths greater than 5,000 m, the macrofaunal abundance varied within 432–572 ind. m–2.
The macrobenthic nematode abundance varied widely, with an average of 2,084.8 ± 1,125.8 ind. m–2. Their proportion in the total number of macrofaunal animals was 62.5–88.5% (Figure 2). At St. 9 and 10, the macrobenthic nematode abundance was highest (reaching more than 4,000 ind./m2) and 4–7-fold higher than the macrofaunal abundance (Table 3). At depths greater than 5,000 m, the nematode abundance was lower than 1,500 ind. m–2. The meiobenthic nematode abundance was also highest at St. 9 and almost 10-fold higher than the abundance of meiobenthic nematodes at depths greater than 5,000 m.
The number of taxonomic groups of macrofauna varied from 10 to 14 (Table 3). Nine of them were present in almost all samples (polychaetes, crustaceans (amphipods, isopods and tanaids), bivalves, gastropods, aplacophorans, sipunculans, and holothurians). Polychaetes (48.7 ± 9.0%), crustaceans (29.3 ± 6.7%), and bivalves (9.9 ± 1.4%) dominated in abundance (Figure 2). The maximum proportion of polychaetes (80.8%) was recorded at the shallowest station 9. At depths more than 5,000 m, the proportion of polychaetes in the macrofauna was below 43%. Among crustaceans, tanaids and isopods were the most abundant taxa in the samples. The proportion of tanaids and isopods reached maximum values at depths more than 5,000 m, where their total proportion exceeded 38%.
The values of the Shannon’s diversity and Pielou’s evenness indices calculated for macrofauna were significantly higher (ANOVA, p < 0.05) compared to those for the Sea of Okhotsk, reaching maximum values (H′ = 2.0; J′ = 0.76) at depths greater than 5,000 m, where several taxonomic groups of animals were found in all samples in relatively large numbers and the dominance of polychaetes was less pronounced. In the upper part of the abyssal zone at depths of 3,432 m (St. 9), where the proportion of polychaetes in the abundance of macrofauna was maximum, the diversity of macrofauna was, correspondingly, minimum (H′ = 0.84; J′ = 0.36).
Abundance and Diversity of Bottom Fauna in the Hadal Zone of the Kuril-Kamchatka Trench
In the samples collected from the hadal zone of the Kuril-Kamchatka Trench, a total of 1,268 specimens of macrofauna belonging to 21 taxonomic groups and 1,801 specimens of macrobenthic nematodes were found (Table 2). The abundance of macrofauna varied from 196 to 1,224 ind. m–2. The lowest abundance of macrofauna was observed in samples collected from the lower part of the trench slope (St. A1) and from the floor of the canyon that cuts through the Kuril Islands slope from the Bussol Strait to the Kuril-Kamchatka Trench (St. A6). The maximum macrofaunal abundance was found in samples collected from the floor of the deepest basins of the trench (St. A7, A11).
The abundance of macrobenthic nematodes on the trench slopes varied from 620 ind. m–2 (St. A6) to 2,896 ind. m–2 (St. A5), which was 1.5–3-fold higher than the macrofaunal abundance. The lowest abundance of macrobenthic nematodes was recorded from the trench floor (St. A7, A11), where their density was 3–fold lower than that of macrofauna (24.2–27.5% of the total abundance) (Figure 2).
In general, with increasing depth, the number of considered taxonomic groups of animals in the samples from the trench decreased. The maximum number of taxa (15) was recorded from the upper part of the slope (St. A2), and the minimum number (6) found from the greatest depth of the trench (St. A7) (Table 3). The most numerous in the samples were polychaetes (43.2 ± 8.0%), bivalves (27.5 ± 8.1%), crustaceans (8.2 ± 3.2%), holothurians (7.6 ± 2.2%), and aplacophorans (3.8 ± 1.9%) (Figure 2). Only polychaetes and bivalves were present in all samples and dominated in abundance. The proportion of polychaetes was the highest (43.8–78.2%) in the samples collected from the upper part of the trench slopes at depths of 6,068–7,136 m (St. A6, A2, A5). In the lower part of the slopes and on the trench floor (depth more than 8,000 m), the polychaete proportion in the macrofauna was much smaller (17.3–43.8%), although its abundance remained high, reaching 536 ind. m–2 on the trench floor (St. A11). The proportion of bivalves varied within a wide range (2.8–51.0%). In the upper part of the trench slopes (St. A6, A2, A5), the bivalve proportion was up to 9.1%. However, at depths more than 8,000 m, the bivalve proportion in the macrofaunal abundance increased to 38.7-51.0%, with maximum abundance (484 ind. m–2) on the trench floor. In addition to polychaetes and bivalves, samples from the depths of 6,531–8,221 m (St. A2, A5, A9) contained holothurians, crustaceans (mostly tanaids and isopods), and aplacophorans in large numbers (26.0–39.2% of the total macrofaunal abundance). Among them, holothurians were the most abundant. Holothurians were the third dominant in abundance group of animals after polychaetes and bivalves in the sample collected from the maximum depth of the trench (St. A11). Besides, oligochaetes, gastropods, and pogonophorans were also found in large numbers in the samples collected from a depth more than 9,000 m.
The Shannon’s diversity and Pielou’s evenness indices calculated for samples from the trench were similar (ANOVA, p > 0.05) to those from the oceanic abyssal zone, since in trench samples with lower taxonomic richness were many abundant taxa.
Effect of Environmental Factors on Quantitative Distribution of Bottom Fauna
BIOENV analyses of abundance data showed that a large amount of biological variation (with the correlation coefficient ranging from 0.357 to 0.440) can be explained by three environmental variables (water depth, clay content, and oxygen concentration); additional variables did not increase the correlation coefficient. These relationships are statistically significant (p < 0.01 for each of the 4 models using the RELATE procedure) (Table 4). The best combination of environmental variables explaining the variation in the abundance of macrofauna is depth and clay content (correlation coefficient, 0.440).
In general, depth is a complex factor since many environmental factors are changed with depth. According to our data, the abundances of holothurians, enteropneustans, and bivalves positively correlated with depth (p < 0.05) (Table 4). The abundance of amphipods, gastropods, aplacophorans, as well as sipunculans and priapulids, showed positive relationships with the oxygen concentration, while the abundance of bivalves and aplacophorans was greatly influenced by the clay content of sediments (p < 0.05). In addition, the clay content had a negative effect on the abundance of amphipods, tanaids, sipunculans, and priapulids. The correlation of the polychaete and nematode abundances with all environmental variables considered in the analysis was not significant.
Species Richness, Diversity, Abundance, and Faunal Assemblages of Polychaetes and Bivalves
Polychaetes were the richest group of animals in terms of number of species in the samples. A total of 97 species of polychaetes belonging to 37 families (excluding echiurans and pogonophorans) were found in the box-corer samples (Supplementary Table 1). The largest number of species belonged to the families Ampharetidae (10 species) and Paraonidae (9 species). The rest of the families were represented by no more than 5 species. The number of species in the samples varied from 6 to 29. The richest samples in the number of polychaete species were collected on the floor of the Kuril Basin, where the species number per sample varied from 17 to 29 (23.8 ± 2.4). The smallest number of species was found at St. 6 most remotely located from the Kuril Islands straits.
In the Pacific Ocean, the largest number of polychaete species was found in samples collected in the abyssal zone (14–26 species per sample, with an average of 17.8 ± 2.5), where the maximum number of species was observed at the shallowest-water station 9, while at the rest of the stations the species number was smaller than 20. In the hadal zone, the number of polychaete species in the samples decreased with depth (to an average of 12.0 ± 2.1), although the polychaete species richness in the upper part of the trench slope at depths between 6,068–7,136 m (St. A2, A5, and A6) still remained high (17–19 species per sample). The smallest number of species (6) was recorded from the samples collected on the trench floor (St. A7 and A11).
Bivalves were most frequently recorded as the second dominant group of animals after polychaetes. In the Kuril-Kamchatka Trench at depths greater than 8,000 m, they dominated the macrofauna in abundance. A total of 25 bivalve species belonging to 10 families were identified in the samples. More than half of the species (13) belonged to the family Thyasiridae, and 3 species belonged to the family Vesicomyidae. The rest of the families were represented by 1–2 species each. The number of species in the samples varied from 1 to 7. The samples from the floor of the Sea of Okhotsk were richer in number of species compared to those from the other study areas. Here, from 3 to 7 species were found per sample (average, 5.0 ± 0.7). In the abyssal zone of the Pacific Ocean, the number of species per sample varied from 2 to 5 (average, 4.0 ± 0.6). In the hadal zone, the number of species varied widely (1–7) and reached 4 even at the maximum depth of 9,495 m (St. A7).
Cluster and MDS analyses (Figure 3) of quantitative data on polychaete and bivalve species showed that all the analyzed stations formed the following five groups (with a similarity level of more than 35%), which differed significantly (ANOSIM; global R = 0.903; significance level, 0.1%): (A) all stations conducted on the floor of the Kuril Basin (depths of 3,207–3,366 m); (B) the shallowest-water station 9 located in the upper part of the abyssal zone of the Pacific Ocean (depth of 3,432 m); (C) stations carried out in the lower part of the oceanic abyssal zone and in the hadal zone of the upper slopes of the Kuril-Kamchatka Trench (depth of 4,741–7,136 m); (D) stations conducted in the lower part of the trench slopes (depths of 8,221 and 8,251 m); (E) stations performed on the floor of the deepest basins of the trench (depths of 9,495 and 9,540 m).
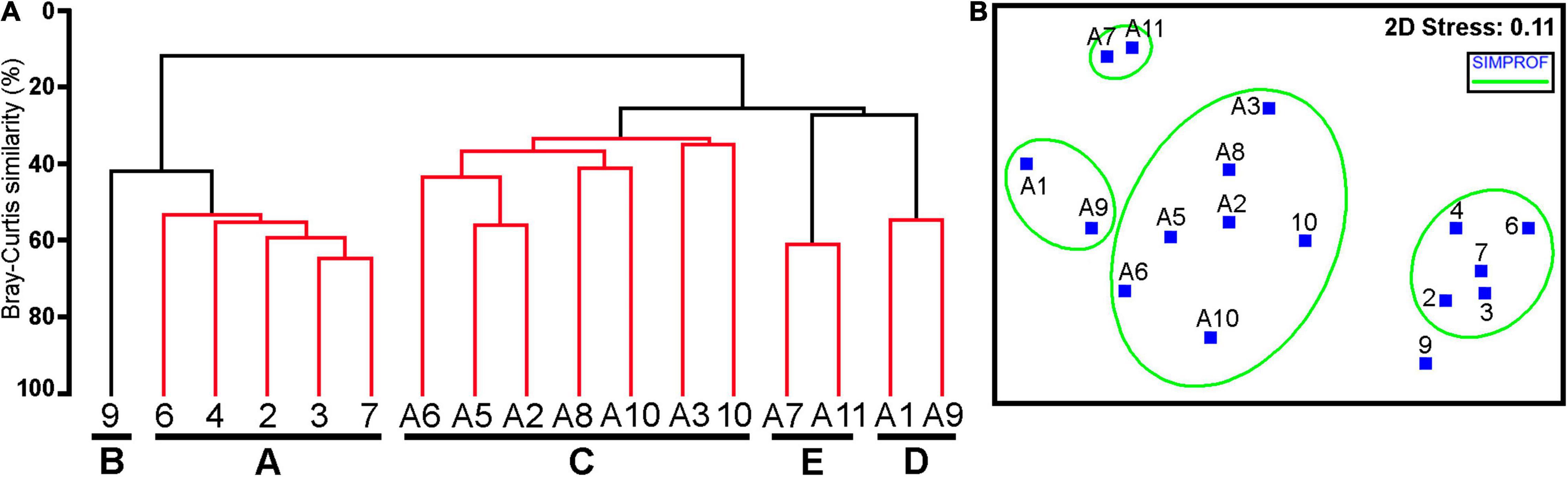
Figure 3. Cluster (A) and MDS (B) based on quantitative similarities (fourth root transformed data) among the bivalve and polychaete assemblages of box-corer samples taken at 17 stations by the SokhoBio and KuramBio II expeditions. (Red lines indicate the samples that cannot be significantly differentiated by SIMPROF).
Kuril Basin (Sea of Okhotsk)
The stations (group A) performed on the floor of the Kuril Basin with a relatively high intra-group similarity (SIMPER; average similarity, 56.16%) were the least similar in species composition and quantitative abundance of polychaetes and bivalves to the groups of stations C–E conducted in the abyssal and hadal zones of the Pacific Ocean (SIMPER, average dissimilarity of groups A and C–E > 87%). A total of 53 polychaete species belonging to 30 families were found on the Kuril Basin floor (Supplementary Table 1). More than half of the species (31 species, 58.5%) from the Sea of Okhotsk were also found in the samples collected from the Pacific Ocean. The families richest in number of species were Ampharetidae (8 species), Paraonidae (5 species), and Trichobranchidae (4 species). Only 7 polychaete species (13.2%) were recorded from all samples. Almost all of them were the most abundant species. About a third of the species (17 species, 32.1%) were found in more than 50% of the samples, and most (19 species, 35.8%) were found in only one sample. The most abundant species were Prionospio sp. 2, Anobothrus sonne, Chaetozone sp. 1, Levinsenia gracilis, Tanseimaruana vestis, and Labioleanira okhotica (Table 5). The total abundance of these species in all samples exceeded 50% of the total abundance of polychaetes. The average value of the Shannon’s diversity indices was the highest in the group of stations located in the Kuril Basin, in comparison with other groups, reaching maximum values at St. 7, where the high abundance of the largest number of polychaete species was recorded. The average Pielou’s evenness index was also high, reflecting a large number of polychaete species with a relatively high abundance inhabiting the floor of the Kuril Basin.
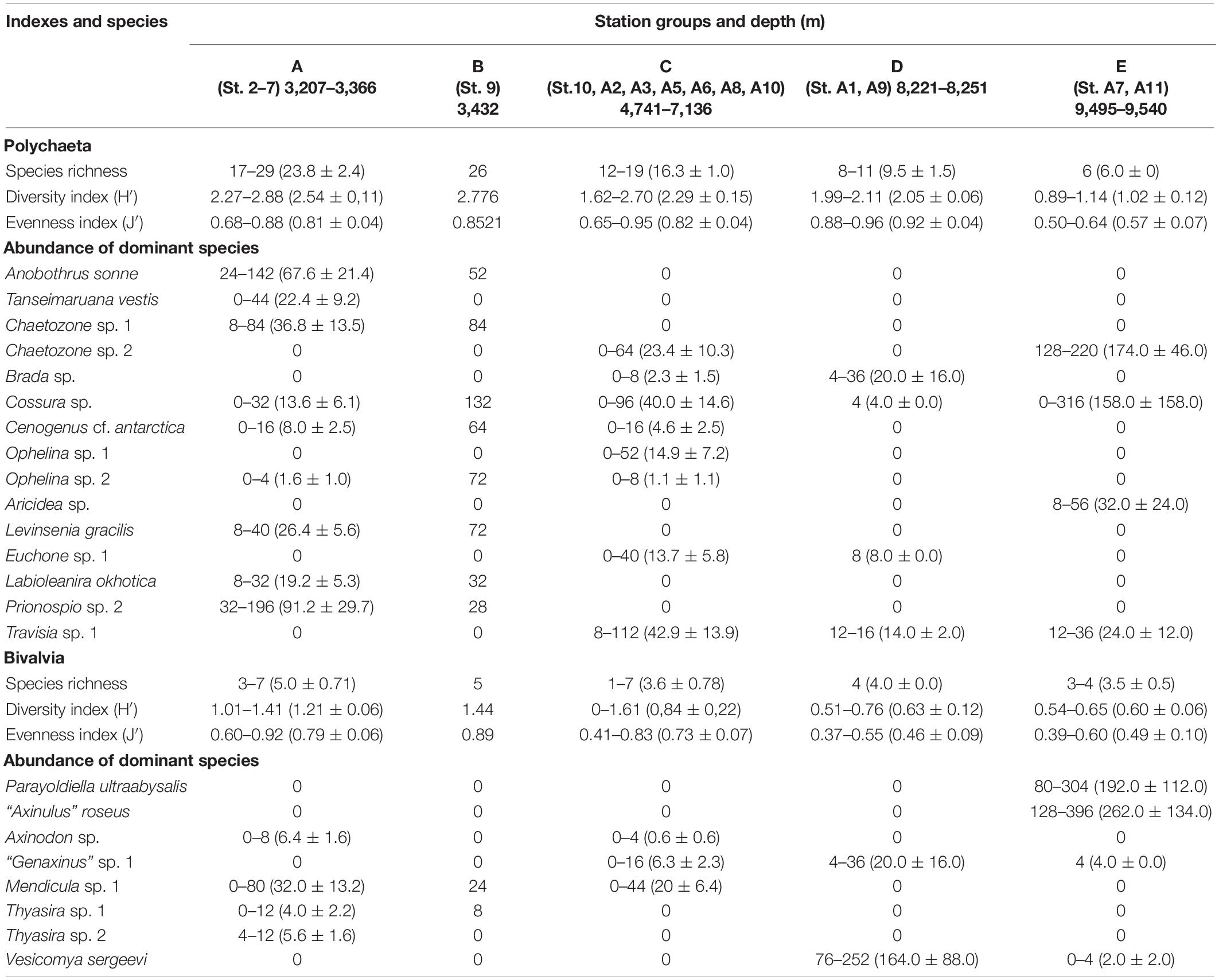
Table 5. Species richness (number of species per sample), diversity indexes, and abundance (min—max, mean ± SE, ind. m–2) of dominant species of polychaete and bivalve assemblages in five station groups (A–E), identified using Cluster and MDS analyzes of quantitative data (Figure 3).
In the Sea of Okhotsk, 9 species of bivalves belonging to 5 families were found in the box-corer samples, of which 3 species were also recorded from the Pacific Ocean (Supplementary Table 1). Most of the species (5 species) belonged to the family Thyasiridae. Thyasirids were present in all samples and provided the major contribution to the abundance of bivalves on the Kuril Basin floor. Their proportion in the total abundance of bivalves exceeded 83% in each sample. The species of the rest of the families were found at one or two stations, in most cases, one specimen per sample. The most abundant species was the small thyasirid Mendicula sp. 1 (Table 5). The values of the Shannon’s diversity and Pielou’s evenness indices calculated for the bivalve assemblage from this group of stations were maximal.
Pacific Ocean
In the samples collected in the Pacific Ocean, 76 species of polychaetes belonging to 34 families and 20 species of bivalves from 9 families were identified. The faunal assemblage of polychaetes and bivalves at the shallowest-water oceanic station 9 (“group” B) was most similar to that in the Kuril Basin (SIMPER, average dissimilarity of groups A and B = 58.16%). This station was characterized not only by the most abundant, but also by the most diverse and rich polychaete fauna in terms of number of species compared to other deeper-water oceanic stations (Table 5). The species composition of polychaetes was most similar to the abyssal fauna of the Sea of Okhotsk (80.8% of species were common with those from the Sea of Okhotsk and 23.1% are common with those from the oceanic stations). At this station, almost all the species most abundant on the Kuril Basin floor also dominated in abundance. In addition, Cossura sp., Ophelina sp. 2, and Ñenogenus cf. antarctica, recorded from most samples collected in the Kuril Basin, were also found here in large numbers.
Of the 5 species of bivalves found at this station, two species were also recorded from the Sea of Okhotsk. The most abundant species, as in the Sea of Okhotsk, were thyasirids with their proportion being 78.6% of the total bivalve abundance.
Compared to the group of stations A performed on the Kuril Basin floor, group C included a larger number of stations covering a wide range of depths (4,741–7,136 m) in the abyssal and hadal zones with diverse biotopes. Nevertheless, the polychaete assemblage of this group had the same species richness (53 species belonging to 30 families) and similar diversity (ANOVA, p > 0.05) (Table 5). The families Paraonidae (5 species) and Spionidae (4 species) proved to be the richest in terms of number of species. Only one species, Travisia sp. 1, was found in all samples. Most species (23 species, 43.4%) were recorded from only one sample. The most abundant and frequently encountered species (found in more than half of the samples of this group of stations) were Travisia sp. 1, Notomastus latericeus, Cossura sp., Chaetozone sp. 2, Ophelina sp. 1, and Euchone sp. 1.
The faunal assemblage of bivalves from stations of group C included 14 species from 6 families. More than half of the species (8 species, 57.1%) belonged to the family Thyasiridae. The contribution of thyasirids to the total abundance of bivalves in all samples, except for St. A5 (7,136 m), was higher than 80%, sometimes reaching 100%. Only two species from this family (“Genaxinus” sp. 1 and Mendicula sp. 1) were found in more than half of the samples; of them, Mendicula sp. 1 was the most abundant species, as in the Sea of Okhotsk.
The deepest-water groups of stations D and E were the least similar to the rest of the oceanic stations (SIMPER; average dissimilarity of groups D, E and B, C > 66%). Polychaete and bivalve assemblages of group D were characterized by less diversity and species richness compared to the assemblages of shallower-water groups of stations. The abundance of polychaetes at these depths also reduced sharply (Table 2). The most abundant species here was Brada sp. (Table 5). Unlike polychaetes, bivalves had a greatly increased abundance mainly due to the formation of very abundant populations of Vesicomya sergeevi at these depths, which was found only in the hadal zone of the trench.
The samples (group E), collected from the maximum depth of the trench, were characterized by the least diversity and a low species richness of polychaete and bivalve assemblages, as well as a very high abundance of these animals. Among polychaetes, the dominant species were Chaetozone sp. and Cossura sp., which showed a wide range of distribution depths (more than 5,000 m) and were recorded from all the studied depths. The dominant bivalve species were “Axinulus” roseus and Parayoldiella ultraabysalis. Unlike the dominant polychaetes, these species were found only in the deepest basins of the trench.
Discussion
Kuril Basin (Sea of Okhotsk)
As shown earlier, the bottom macrofauna on the Kuril Basin floor is generally rich and is not inferior in taxonomic diversity to the abyssal macrofauna of the Kuril-Kamchatka Trench region (Brandt et al., 2015, 2018). However, the species richness of most taxonomic groups of macrofauna dominating in abundance at the bottom of the Kuril Basin is lower than that in the abyssal zone of the Pacific Ocean off the Kuril Islands (Fukumori et al., 2018, 2019; Kamenev, 2018b,2019; Malyutina and Brandt, 2018; Mironov et al., 2018, 2019b; Golovan et al., 2019). This can be caused by many factors, including differences in the amount of material collected and the area of the regions covered by the study, a narrower range of abyssal depths, less habitat diversity and a lower oxygen concentration in the bottom layer of the Kuril Basin, a greater isolation of the Sea of Okhotsk from the Pacific Ocean (Ushakov, 1953; Savilov, 1961; Fukumori et al., 2018; Kamenev, 2018b; Mironov et al., 2018). Only polychaete fauna was proved to be richer in terms of number of species on the Kuril Basin floor compared to the abyssal zone of the studied regions of the Northwest Pacific (Alalykina, 2015, 2018). Also, the number of considered macrofaunal taxa in the box-corer samples collected from the Kuril Basin floor was 1.5–2-fold smaller than in the samples collected from abyssal zone of the Pacific region adjacent to the Sea of Okhotsk (Tables 3, 6). The relatively small number of macrofaunal taxa in the box-corer samples is probably due to fact that many species of various taxonomic groups form sparser populations on the Kuril Basin floor, as compared to the macrofauna in the oceanic abyssal zone. The low density of populations of many species on the Kuril Basin floor can be associated with both a wide distribution of homogeneous, very fine-grained sediments and a low oxygen content of the near-bottom layer of water. The abundance of many taxonomic groups showed a negative correlation with the finest fraction (clay content) of sediments and a positive correlation with the oxygen concentration of the near-bottom water (Table 4). However, low oxygen concentration may be the main factor affecting the species composition and quantitative distribution of bottom animals at the maximum depths in the Sea of Okhotsk.
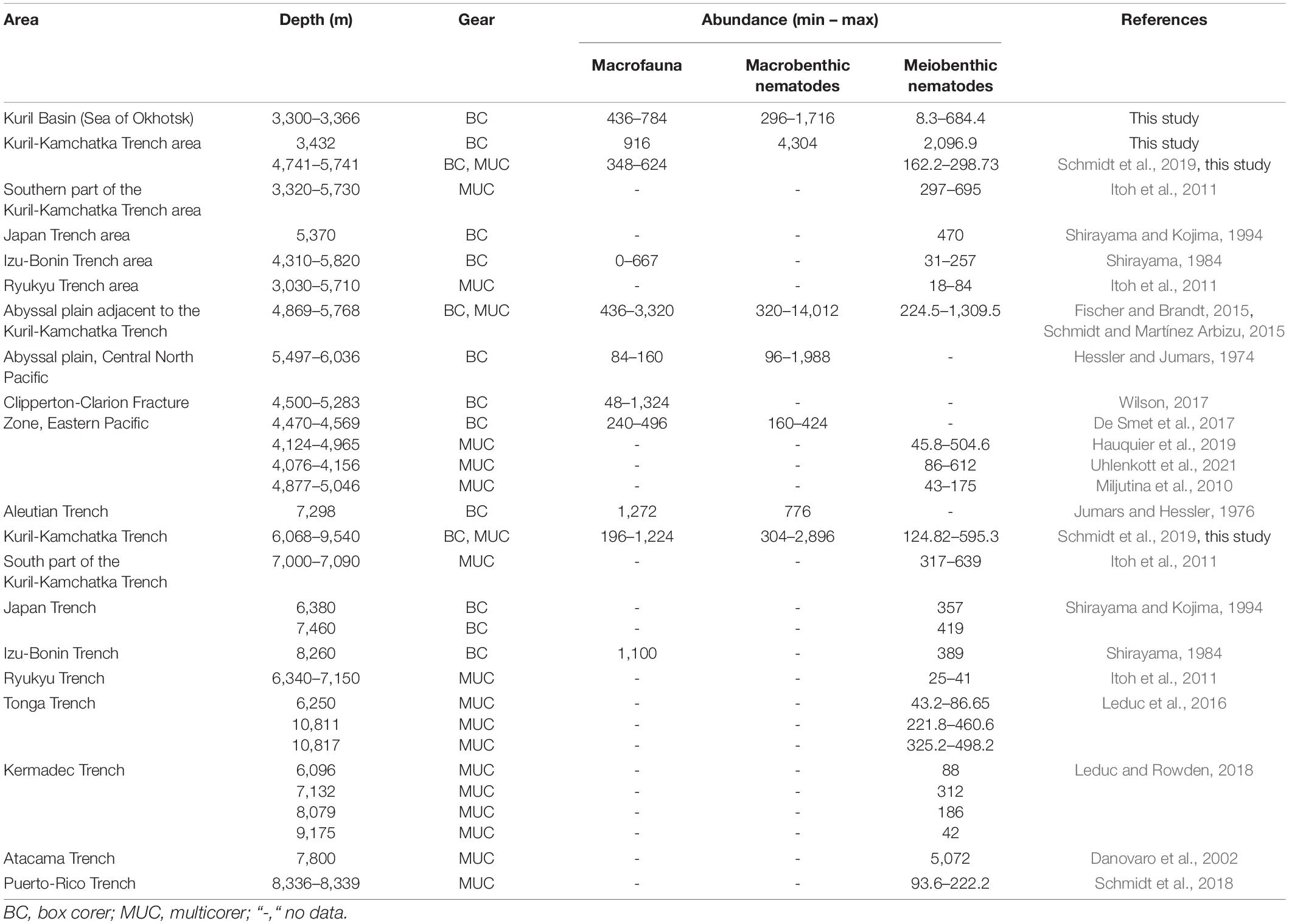
Table 6. Abundance of macrofauna (ind. m–2), macro- (ind. m–2) and meiobenthic (ind. 10 cm–2) nematodes from different abyssal areas (depths more than 3,000 m) of the North Pacific and trenches.
As also reported previously, the bottom fauna on the Kuril Basin floor is characterized by a low biomass (about 0.3 g m–2) and sparse populations of many mega- and macrofauna species (Ushakov, 1953; Savilov, 1961). An assumption was made that one of the major factors negatively affecting the distribution and abundance of macrofauna in the abyssal zone of the Sea of Okhotsk is the low oxygen concentration of the near-bottom layer of water. Long-term (more than half a century) investigations of the hydrochemical regime of water masses in the Sea of Okhotsk have shown the constancy of oxygen concentration in the water near the floor of the Kuril Basin within 1.6–1.9 ml l–1, and the absence of seasonal and annual fluctuations (Terziev, 1996). Thus, the bottom fauna on the Kuril Basin floor is exposed for a long time to chronic oxygen deficiency.
As numerous studies have shown, bottom fauna is generally tolerant to oxygen concentration decreases to 2.0 ml l–1 for a relatively short time. However, a critical oxygen concentration in water for many invertebrates is about 2.0 ml l–1; below this level, a response of benthic organisms can be observed (Diaz and Rosenberg, 1995; Karlson et al., 2002; Vaquer-Sunyer and Duarte, 2008; Zeppilli et al., 2018). Diaz and Rosenberg (1995) provide a list of hypoxic systems across the world and the effects of low oxygen concentrations on benthic communities. Unfortunately, the Sea of Okhotsk was not included in this extensive list of regions of the world’s oceans with oxygen deficiency. According to Diaz and Rosenberg (1995), the deep-water part (depths more than 750 m) of the Sea of Okhotsk with a concentration of oxygen in the water 0.8–1.9 ml l–1 can be categorized as a water system with a moderate level (with oxygen decline to about 0.5 ml l–1) of persistent hypoxia (year-round hypoxia).
The response of benthic communities to hypoxia can be very different, depending on the oxygen concentration, the duration of hypoxia exposure (temporary or periodic), and many associated environmental factors (water temperature and salinity, organic matter content and grain-size structure of sediments, etc.) (Gooday et al., 2009). A decrease in the oxygen concentration below 2 ml l–1 causes a reduction in the taxonomic diversity and species richness of macro- and meiofauna, a decrease in the abundance of macrofauna, a pronounced dominance of certain taxonomic groups and species of bottom animals resistant to hypoxia, a change in the size structure of animal populations, etc. (Diaz and Rosenberg, 1995; Karlson et al., 2002; Levin et al., 2009). In quantitative samples collected from the floor of the Kuril Basin, polychaetes and bivalves dominated in abundance and frequency of occurrence. As is known, these of animals, along with isopods and echinoderms, dominate in abyssal bottom communities of various regions of the world’s oceans in number of species and abundance (Hessler and Jumars, 1974; Glover et al., 2002; Brandt et al., 2005, 2007, 2019; Alalykina, 2013, 2015, 2018; Kamenev, 2013, 2015, 2018b,2019; Bonifácio et al., 2020). However, in the abyssal zone of the Sea of Okhotsk, the degree of dominance of these groups is markedly higher than, e.g., in abyssal macrofaunal communities of the northwestern Pacific Ocean (Figure 2; Fischer and Brandt, 2015). Polychaetes and bivalves have been reported as the groups of animals most resistant to hypoxia (Diaz and Rosenberg, 1995; Levin, 2003; Levin et al., 2009). Therefore, in waters with low oxygen concentrations, these animals often dominate communities of macrofauna in abundance (Levin, 2003). It is likely that the pronounced dominance of polychaetes and bivalves and the high species richness of polychaetes on the Kuril Basin floor are also due to the relatively low oxygen concentrations in the near-bottom water layer, which negatively affect other taxonomic groups of macrofauna. Unlike most taxonomic groups, whose abundance positively correlated with the oxygen level in the near-bottom water layer, the quantitative distribution of polychaetes in the study regions showed the highest negative correlation with oxygen concentrations (Table 4).
The density of macrofauna, with its low biomass recorded from the Kuril Basin floor, was generally as high as in the abyssal zone of the Kuril-Kamchatka Trench area, primarily, due to the high abundance of several small-sized species of polychaetes (up to 10 mm long) and bivalves (with shells smaller than 5 mm) in samples, which are probably more resistant to hypoxia. The predominance of small species in the macrofauna was also observed in other areas with low oxygen concentrations in the water (Levin, 2003). The high organic matter content of bottom sediments in the Sea of Okhotsk probably supports the high abundance of polychaetes and bivalves. It is known that abundance and availability of food can partially compensate for the adverse environmental conditions associated with low oxygen concentrations in the water (Diaz and Rosenberg, 1995; Levin, 2003; Hanz et al., 2019).
On the Kuril Basin floor, the proportion of animals containing endosymbiotic chemosynthetic bacteria in the macrofauna composition also increases. The most characteristic species in macrofaunal communities on the Kuril Basin floor is the pogonophoran Lamellisabella zachsi, which forms extensive, although sparse, populations there (Ushakov, 1953; Savilov, 1961; Karaseva et al., 2019). Pogonophorans are known to rely on endosymbiotic chemoautotrophic bacteria for nutrition, and their anatomy and physiology are adapted to their need to obtain both oxygen and reduced sulfur compounds (Southward, 1982; Naganuma et al., 2005; Southward et al., 2005). Among bivalves, thyasirids were the dominant group in terms of species richness and abundance in box-corer samples; of them, many also contained endosymbiotic chemosynthetic bacteria (Southward, 1986; Dufour, 2005; Oliver, 2014). Thyasirids made up more than one third (36.4%) of the species richness of the abyssal bivalve fauna in the Sea of Okhotsk and 76.3% of the total number of live bivalve specimens in all samples collected with various sampling gear types from the Kuril Basin floor during the SokhoBio expedition (Kamenev, 2018b). The increase in the proportion of animals containing symbiotic chemosynthetic bacteria in the bottom fauna is a characteristic feature of bottom communities in hypoxia areas (Levin, 2003).
Stable isotope and fatty acid analyses have also shown that organic matter derived from chemosynthetic bacteria is one of the carbon sources for macrobenthic nematodes in the Kuril Basin (Mordukhovich et al., 2018). It is probable that chemosynthetic bacteria inhabit sediments with high organic matter content on the Kuril Basin floor in large abundances. Dense mats of sulfur-oxidizing bacteria comprising the diet of multicellular animals have been found in areas with a low oxygen concentration and a high organic content (Levin, 2003). Furthermore, extensive areas with an increased concentration of methane in bottom sediments (more than 5 × 103 ml kg–1) have been found on the Kuril Basin floor, and there are probably numerous cold methane seeps widespread in the Sea of Okhotsk to a depth of 2,200 m (Lüdmann and Wong, 2003; Jin et al., 2011; Obzhirov et al., 2018; Derkachev et al., 2021). In the Sea of Okhotsk, extensive bacterial mats and aggregations of various macrofaunal animal species containing chemosynthetic endosymbiotic bacteria are formed in areas with an increased concentration of methane in sediments and methane seeps (Kuznetsov et al., 1989; Kamenev et al., 2001; Kamenev, 2009; Kharlamenko et al., 2016, 2019; Karaseva et al., 2019). Thus, the primary production created by chemosynthetic bacteria is, apparently, also an important source of organic matter in the diet of deep-sea bottom animals inhabiting the Sea of Okhotsk.
The abundance of macro- and meiobenthic nematodes on the Kuril Basin floor varied greatly, being, on average, lower than in the abyssal zone of the Pacific Ocean region adjacent to the Kuril Islands (Table 3). Nematodes are known to dominate the meiofauna of deep-sea ecosystems in abundance (Rosli et al., 2018; Zeppilli et al., 2018), and are a metazoan meiofaunal group most tolerant to low oxygen (Cook et al., 2000; Neira et al., 2001; Levin, 2003). Also, their abundance, as well as the abundance of many macrofaunal animals, depends on the organic matter content and the structure of sediments. Many researchers reported that nematodes tend to fine sediments with a predominance of silt (e.g., Gutzmann et al., 2004; Netto et al., 2005; Schmidt and Martínez Arbizu, 2015). In our study, the abundance of macrobenthic nematodes had a relatively high but not significant positive correlation with the silt content (r = 0.38, p > 0.05) and a negative correlation with the clay content (r = −0.38, p > 0.05). It is probably that the high clay content of bottom sediments on the Kuril Basin floor negatively affects the quantitative distribution of nematodes. It is also likely that specific local environmental conditions are formed in the water layer near the very bottom and in the upper sediment layer of the Kuril Basin, which significantly affects the composition and distribution of the bottom fauna. A characteristic peculiarity is that the lowest abundance of macrofauna and macrobenthic nematodes and the total proportion of polychaetes and bivalves of 91.7% were recorded at St. 6, which is the most remote station from the Kuril Straits and the La Perouse Strait and, accordingly, is less influenced by oceanic and marine waters entering through these straits. The maximum abundance of meiobenthic nematodes was also observed at this station (Table 2). At St. 7, the closest to the Bussol Strait, we recorded, on the contrary, the maximum taxonomic diversity of macrofauna and abundance of macrobenthic nematodes, and also the minimum proportion of polychaetes and bivalves in the total abundance of macrofauna. It cannot be ruled out that the oxygen concentration at the very bottom varies markedly and differs from the oxygen concentration measured in hydrochemical studies of water at a distance of 1 m from the bottom. It is known that a strong gradient in oxygen concentrations often occurs close to the sediment surface (Diaz and Rosenberg, 1995). Therefore, the actual oxygen concentration having an effect on the bottom fauna may have been markedly lower than that measured at 1 m from the bottom and varied between different areas of the Kuril Basin floor depending on local conditions.
A comparative analysis of the composition and quantitative distribution of the bottom fauna using studies of previous years (Ushakov, 1953; Savilov, 1961) showed the relative stability of the taxonomic diversity of macrofauna, the composition of the dominant species, and the specifics of the quantitative distribution of bottom communities on the Kuril Basin floor. The abyssal bottom fauna of the Sea of Okhotsk has been exposed for a long time to hypoxic conditions and has probably adapted to living in conditions of chronic oxygen deficiency. The relatively high taxonomic diversity, species richness, and abundance of bottom fauna on the basin floor are probably due to the favorable trophic conditions for the bottom animals. The Kuril Basin is characterized by very high rates of sedimentation, large amounts of suspended detritus in the near-bottom water layer (Savilov, 1961), and high content of organic matter in the sediments (0.84–1.92%) (Sattarova and Aksentov, 2018). The high content of organic matter in the sediments may be related to very high level of phytoplankton primary production of the Sea of Okhotsk (260–350 gC m–2 per year) (Shuntov, 2001). It has been shown that in various regions of the world’s oceans, seafloor oxygen minimum zones tend to have stable hypoxia, and are frequently associated with the production of large quantities of high-quality organic matter which contributes to the development of stable fauna with a relatively low diversity but high abundance (Levin, 2003; Levin et al., 2009). This combination of hypoxia and high organic matter content creates a unique habitat in marine ecosystems that supports a community with high energy fluxes. In offshore areas with permanent oxygen deficiency, the benthic communities have apparently adapted to lower oxygen concentrations (Levin, 2003). Thus, the adaptation to permanent hypoxia on the Kuril Basin floor, as well as the abundance of organic matter reaching the floor of the basin, probably contributes to the formation of a relatively diverse and abundant abyssal bottom fauna in the Sea of Okhotsk, which is connected with the oceanic abyssal fauna through the deep-sea straits between the Kuril Islands. Many deep-sea eurybathic macrofaunal species from the upper abyssal zone of the Pacific Ocean could enter the Sea of Okhotsk through the deep-sea straits between the Kuril Islands and adapt to living on the Kuril Basin floor in low-oxygen conditions (Kamenev, 2018b; Malyutina and Brandt, 2018; Mironov et al., 2018).
Abyssal Zone of the Kuril-Kamchatka Trench Area (Pacific Ocean)
In the abyssal zone of the Kuril-Kamchatka Trench area, we recorded a generally high abundance of macrofauna and nematodes which was higher than at similar depths of some regions in the North Pacific (Table 6). However, it was markedly lower than in the abyssal plain adjacent to the Kuril-Kamchatka Trench (Fischer and Brandt, 2015; Schmidt and Martínez Arbizu, 2015). The northwestern Pacific Ocean region adjacent to the Kuril Islands, with its high level of primary production and a large terrigenous runoff of organic matter from the continent and the islands, is one of the most productive regions of the world’s oceans, characterized by a high organic matter content of bottom sediments (Sokolova, 1976, 1981; Itoh et al., 2011; Kitahashi et al., 2012, 2013; Stewart and Jamieson, 2018; Du et al., 2021). The abundance of food material provides favorable habitat conditions for a diverse and abundant bottom fauna (Filatova, 1960; Belyaev, 1989; Brandt et al., 2015; Fischer and Brandt, 2015; Schmidt and Martínez Arbizu, 2015), especially on the abyssal plain, where more organic matter is accumulated in bottom sediments than on the oceanic slopes of the Kuril Islands (Sattarova and Aksentov, 2018). Also, the higher density of macrobenthic nematodes on the oceanic abyssal plain near the Kuril-Kamchatka Trench may be explained in part by the differences in the methods used to study of the box-corer samples. In our study, unlike Fischer and Brandt (2015), we did not count macrobenthic nematodes on a 300 μm sieve.
The density of macrofauna and macrobenthic nematodes at the studied abyssal depths varied quite significantly and probably depended on the structure of bottom sediments, the dynamics of near-bottom waters, the bottom topography and other local habitat conditions, which are more diverse than on the floor of the Kuril Basin, or on the abyssal plain adjacent to the trench. For example, bottom sediments at St. 10 with the lowest density of macrofauna (Table 2) were represented by a very thin surface layer of silt with a high organic matter content covering a very dense and thick layer of coarse-grained, black volcanic sand with an admixture of clay. At this station, all macrofauna was found only on the sediment surface and in the thin surface layer, while the infauna was dominated by small macrofaunal species and, primarily, macrobenthic nematodes, whose proportion in the total macrofauna composition was 88.51%. The species richness of the major taxonomic groups of macrofauna at this station was also low, as evidenced by the results of processing of samples collected with a trawl and an epibenthic sledge (Alalykina, 2018; Fukumori et al., 2018; Kamenev, 2018b; Malyutina and Brandt, 2018; Mironov et al., 2018). On the other hand, at St. 9, which had the most abundant and rich in the number of species macrofauna and the highest abundance of nematodes (Table 2), sediments were mainly represented by loose silty sand and a relatively thick surface layer of silt with a high content of organic matter (Table 1). The combination of these factors contributed to the formation of abundant infauna here, mainly polychaetes and nematodes. The active outflow of Sea of Okhotsk waters enriched in organic matter and nutrients occurs through the Bussol Strait characterized by extremely strong currents (Moroz and Bogdanov, 2007; Zakharkov et al., 2007). In this area, where the station was performed, very favorable feeding conditions for the bottom fauna have apparently been formed on the canyon floor. Such favorable habitat conditions for the deep-sea benthic fauna are probably not a unique phenomenon for the slopes of the Kuril Islands. On the oceanic slope of the northern Kuril Islands, even more abundant aggregations of macrofauna (2,555–3,520 ind. m–2) and macrobenthic nematodes (5,096–8,992 ind. m–2) were found at a depth of 4,977–4,980 m (Fischer and Brandt, 2015).
The Hadal Zone of the Kuril-Kamchatka Trench
The taxonomic diversity and species richness of the fauna in the oceanic trenches are reduced with increasing depth. Also, the abundance of bottom macrofauna increases in eutrophic trenches with depth (Belyaev, 1989; Jamieson, 2015). Changes in the taxonomic composition and quantitative distribution of macrofauna in the trenches are most significant at depths over 8,000 m. It has been shown that, apparently, the major factor responsible for the depletion of the taxonomic and species richness of the bottom fauna in trenches is the hydrostatic pressure, which increases with depth (Belyaev, 1989; Jamieson, 2015; Swan et al., 2021). In the lower part of the hadal zone of almost all the examined trenches, holothurians and bivalves dominated in abundance. Polychaetes, isopods, and amphipods were also found in large numbers there (Filatova, 1960, 1971; Belyaev and Mironov, 1977; Belyaev, 1989; Jamieson, 2015). These patterns of quantitative distribution of macrofauna are also characteristic of the Kuril-Kamchatka Trench, where, with increasing depths (especially at depths of more than 8,000 m), the taxonomic diversity decreased and the macrofauna abundance increased compared to those in the abyssal zone. Depth was the main factor having an effect on the taxonomic macrofauna composition and the quantitative proportions of the major taxonomic groups in the box-corer samples (Table 5). At the maximum depths of the Kuril-Kamchatka Trench, despite a low number of taxonomic groups of animals, the abundance of macrofauna appeared to be maximum in the box-corer samples, mainly due to the high density of populations of two bivalve species, “Axinulus” roseus and Parayoldiella ultraabysalis, and two polychaete species, Chaetozone sp. 2 and Cossura sp.
In the upper part of the trench slopes (6,000–7,150 m), the taxonomic diversity and macrofaunal abundance in the samples varied greatly, probably, depending on the local habitat conditions. The slopes of the Kuril-Kamchatka Trench, like those of all oceanic trenches, are characterized by complex bottom topography and mosaic pattern of bottom sediments (Belyaev, 1989; Jamieson, 2015; Jamieson and Stewart, 2021). Landslides and turbidity flows often occur on steep slopes as a result of seismic activity and water currents. The complex geomorphology of the trench slopes, increased water dynamics, heterogeneity of substrates, and instability of bottom sediments have a great effect on the composition and abundance of bottom fauna, as well as on sampling of bottom animals from trenches (Belyaev, 1989; Jamieson, 2015; Kamenev, 2019). For example, stations A1 and A9 were conducted on the slopes of the trench at similar depths (8,251 and 8,221 m, respectively) and were characterized by approximately the same grain-size structures and organic matter contents of sediments. However, the abundance of macrofauna at St. A9 was more than 4-fold higher. These differences in macrofaunal abundance are presumably related to the structure and density of bottom sediments at these stations. At St. A1 with a minimum abundance of macrofauna, the bottom sediments in the box-corer sample were represented by very dense compressed silty clay covered by a thin (1–2 cm) loose surface layer of silt. At St. A9 the thickness of the loose surface layer of sediments in the sample was 10 cm, with relatively soft silty clay beneath. Accordingly, the loose and thick surface layer of bottom sediments at this station provided more favorable habitat for the abundant macrofauna.
The relatively high taxonomic diversity of macrofauna and the species richness of polychaetes and bivalves in the box-corer samples collected from the upper part of the trench slopes are caused by many factors, of which the major ones are, probably, the heterogeneity of habitats, the abundance of organic matter in bottom sediments, and the patterns of vertical distribution of many benthic animal species. It has been reported that the heterogeneity of habitats and the abundance of organic matter in trenches leads to an increase in the species richness and diversity of bottom fauna (Belyaev, 1989; Jamieson et al., 2010; Jamieson, 2015; Stewart and Jamieson, 2018; Brandt et al., 2019; Kamenev, 2019). Furthermore, depths within 6,000–7,000 m are a transitional zone between the abyssal and hadal faunas, where the species richness of macrofauna is higher (Belyaev, 1989; Jamieson, 2015) compared to the adjacent abyssal areas due to the simultaneous presence of mainly abyssal and hadal species. The example of the vertical distribution of bivalves in the Kuril-Kamchatka Trench shows that for many mainly abyssal and hadal species, these depths are, respectively, the lower and upper limits of their vertical distribution (Kamenev, 2019).
On the floor of the deepest basins of the Kuril–Kamchatka Trench, the macrofaunal abundance was maximum and much higher than previously assumed. In the deepest quantitative sample collected earlier with an Okean grab from a depth of 8,355 m in the Kuril-Kamchatka Trench, the density of macrofauna (except nematodes) was 228 ind. m–2 (the density of polychaetes and bivalves was 80 ind. m–2 for each group) (Filatova, 1971; Belyaev, 1989). The much higher values of macrofaunal abundance in the Kuril-Kamchatka Trench that we obtained are related, to a greater extent, with the use of a box corer for sampling, which is a more advanced gear for quantitative assessment of macrofauna at great depths and is devoid of many shortcomings typical of Okean grab (Filatova, 1982; Belyaev, 1989). The abundance of macrofauna on the floor of the Kuril-Kamchatka Trench is comparable to that recorded from the floors of the Aleutian and Izu-Bonin trenches (Table 6). The high density of macrofauna in the deepest basins of the Kuril-Kamchatka Trench was confirmed by trawl samples, where the number of collected animals reached 20,000 (Belyaev, 1989).
The high abundance of animals on the Kuril-Kamchatka Trench floor, as in the Aleutian and Izu-Bonin trenches, is probably explained by the high organic matter content of bottom sediments and the abundance of food available for bottom animals (Belyaev, 1989). Recent studies have shown that the organic matter flux to the floors of oceanic trenches comes from a variety of sources such as terrigenous runoff, vertical sinking of particulate organic matter from the ocean surface, lateral transport from slopes, large amounts of sediment moving down the trench slopes to the floor as a result of landslides and tectonic activity, remains of dead large animals, and production of chemosynthetic communities (Belyaev, 1989; Ichino et al., 2015; Jamieson, 2015; Liu et al., 2018; Xu et al., 2018). Therefore, the organic matter content of sediments on the trench floor (especially in depressions or basins of a relatively flat bottom) is, as a rule, higher than in sediments on the trench slopes. The ocean trenches located in highly productive zones are much richer in species diversity and abundance of bottom fauna compared to the trenches in oligotrophic and low-productive waters of the tropical latitudes of the world’s oceans (Belyaev and Mironov, 1977; Belyaev, 1989; Jamieson, 2015).
The increase in the abundance of meiobenthic nematodes (which was maximum on the bottom of the deepest basins of the Kuril-Kamchatka Trench) with depth is probably also associated with the increased organic matter content of bottom sediments of the trench (Schmidt et al., 2019). The increase in the abundance of meiofauna with increasing depth have been repeatedly reported in other studies on eutrophic trenches (George and Higgins, 1979; Shirayama and Kojima, 1994; Danovaro et al., 2002; Leduc et al., 2016; Schmidt et al., 2018; Wang et al., 2019). However, the abundance of large macrobenthic nematodes, unlike meiobenthic ones, on the Kuril-Kamchatka Trench floor was minimum and generally lower than in the abyssal zone of the eutrophic regions of the North Pacific. The phenomenon of meiofaunal miniaturization was reported earlier for both oligotrophic and eutrophic deep-sea waters of the world’s oceans (Shirayama, 1984; Schewe and Soltwedel, 1999; Danovaro et al., 2002; Wang et al., 2019). After studying the meiofauna of the eutrophic Atacama Trench, Danovaro et al. (2002) showed that meiofaunal miniaturization cannot be related to the reduced food availability or to oligotrophic conditions, as previously suggested by Schewe and Soltwedel (1999). Danovaro et al. (2002) assumed that most likely other factors are responsible for the body size reduction in meiobenthic animals at hadal depths. The decrease in the sizes of nematodes at the maximum depth of the eutrophic Kuril-Kamchatka Trench with the high organic matter content of bottom sediments, as in the Atacama Trench, is apparently not associated with low food inputs to the trench floor. It is possible that the reduction in body size is one of nematodes’ adaptations to extreme habitat conditions on the trench floor exposed to very high hydrostatic pressure (Zeppilli et al., 2018).
One of the key factors also having an effect on the structure of bottom communities in the trenches is the environmental instability of, in particular, bottom sediments. Oceanic trenches are located in zones of seismic activity which often cause landslides and turbidity flows in areas of steep slopes of trenches, which negatively affects bottom communities (Jumars and Hessler, 1976; Belyaev, 1989; Oguri et al., 2013; Bigham et al., 2021). In one of the samples from maximum depths of the Kuril-Kamchatka Trench, we found a small amount of silt characteristic of trench floor sediments and a rolled lump of very dense clay of approximately 0.5 m in diameter, which occupied almost the entire volume of the box corer. It was obvious that this lump had rolled down from the trench slope to its floor as a result of a landslide. Landslides on trench slopes are not a rare phenomenon, since aggregations of large boulders consisting of compressed clay with a diameter of up to 1 m are frequently found in fine silts on trench floors (Belyaev, 1989). Landslides and turbidity flows can have catastrophic consequences for bottom communities, up to mass mortality of animals on large areas of the trench floor (Belyaev, 1989). Moreover, landslides and turbidity flows cause habitat heterogeneity in trenches and canyons, which affects the diversity and abundance of bottom macrofauna (Belyaev, 1989; Bigham et al., 2021). It is very likely that the differences in the taxonomic composition and abundance of macrofauna at the deepest stations A11 and A7 are associated with the differences in the local habitat conditions for animals living on the trench floor.
It is worth mentioning that in the samples collected from depths more than 8,000 m in the trench, animals with endosymbiotic bacteria or previously recorded from areas of hydrothermal vents and cold seeps were found in large numbers. At a depth greater than 8,000 m (St. A1 and A9), the samples were dominated by the bivalve Vesicomya sergeevi, which is an endemic of the Kuril-Kamchatka Trench distributed down to maximum depths and forming the most abundant populations in the lower part of slopes (Filatova, 1971; Belyaev, 1989; Krylova et al., 2015; Kamenev, 2019). Krylova et al. (2000) found an abundance of endosymbiotic bacteria of two phyla, presumably chemoautotrophic, in gill tissues of this bivalve species. Furthermore, a large number of pogonophorans and gastropods from the family Neomphalidae and the genus Sahlingia were found at St. A7 at a depth of 9,495 m (Fukumori et al., 2019). Pogonophorans are widely distributed over the hadal zone of the Kuril-Kamchatka Trench, where they form sometimes abundant populations at depths greater than 9,000 m. In one of the trawl samples from a depth of 9,000 m, the number of pogonophoran specimens was almost 1,500, which proved to be the second most abundant group after holothurians in this sample (Belyaev, 1989). Also, all previously described gastropod species from the family Neomphalidae and the genus Sahlingia were found only in areas of various gas hydrothermal vents and cold seeps (Warén and Bouchet, 2001). A large number of oligochaetes recorded from St. A11 (depth 9,540 m) were previously found only to a depth of 7,298 m (Aleutian Trench) (Erséus, 1979). Many of oligochete species also have endosymbiotic chemosynthetic bacteria (Dubilier et al., 1995, 2005; Blazejak et al., 2005). The abundance of animals containing endosymbiotic chemosynthetic bacteria in the trench may be due to the cold seeps on the slopes and floor of the Kuril-Kamchatka Trench (Fukumori et al., 2019). For example, hadal cold seep communities in the Japan Trench were found to a depth of 7,326 m (Fujikura et al., 1999; Okutani et al., 1999; Sasaki et al., 2005). Also, Li et al. (2021) suggest that the chemoautotrophic activity in the oceanic crust could be an additional source of organic carbon in trench sedimentary environments. Thus, our study confirms that abundant bottom communities, some of which supposedly utilize nutrients produced by chemosynthetic bacteria, are formed within the abyssal and hadal zones in the area of the Kuril-Kamchatka Trench, located in the eutrophic region of the Pacific Ocean.
Conclusion
(1) This study has demonstrated that the abyssal communities of the Kuril Basin (Sea of Okhotsk) are quite stable and adapted to constant oxygen deficiency at depths of more than 3000 m. The high content of organic matter in the sediments of the Kuril Basin probably partially compensates for the negative effect of oxygen deficiency on bottom fauna. However, local conditions on the basin floor, particularly the oxygen concentration at the surface of the sediment, can significantly affect the composition, distribution and abundance of bottom fauna, which requires more detailed studies of this deep-sea ecosystem. The exchange of deep-sea bottom faunas between the Sea of Okhotsk and the Pacific Ocean occurs via the deep-water straits between the Kuril Islands. It is possible that part of the populations of bottom animals in the Sea of Okhotsk are constantly replenished by Pacific populations living under more favorable conditions. Further studies of the bottom fauna will allow a more accurate assessment of the faunal relationships of the deep-sea ecosystems of the Sea of Okhotsk and the Pacific Ocean.
(2) The high primary production of surface waters and the inflow of Okhotsk Sea waters rich in organic matter through the straits of the Kuril Islands support a diverse and abundant bottom fauna in the abyssal and hadal zones of the Kuril-Kamchatka Trench region. The most favorable conditions for the bottom communities exist in the upper abyssal zone of the oceanic slope of the Kuril Islands at depths of less than 4000 m. Probably, the bathyal bottom fauna of the oceanic slope of these islands at depths of less than 3000 m is no less diverse and abundant. However, this assumption can be substantiated by extensive additional studies of the bathyal bottom fauna of the Kuril Islands and its relationships with the abyssal fauna.
(3) We have shown that the abundance of macrofauna at the bottom of the eutrophic Kuril-Kamchatka Trench is much higher than previously reported. High hydrostatic pressure is probably the main limiting factor affecting the vertical distribution of animals in the hadal zone of the trench. Also, the distribution of bottom animals in the trench is greatly influenced by the substrate heterogeneity and instability on its slopes. A small number of macrofaunal species that were able to adapt to bottom habitats of the trench form more abundant populations, compared to those in the abyssal zone of the studied northwestern Pacific region. The dominant species of bivalves on the trench floor are endemic to the hadal zone and were only recorded at depths greater than 6000 m. However, the dominant species of polychaetes had a wide vertical distribution (more than 5000 m) and were found outside the trench. A number of taxonomic groups and species of macrofauna that form abundant populations on the trench floor harbor chemosynthetic endosymbiotic bacteria. This indicates the importance of chemosynthetic organic carbon for sustaining bottom communities at the maximum depths of the trench. Further studies of the bottom communities of oceanic trenches will allow us to assess in detail the contribution of chemosynthetic organic carbon to the functioning of the deepest ecosystems of the World Ocean.
Data Availability Statement
The original contributions presented in the study are included in the article/Supplementary Material, further inquiries can be directed to the corresponding author/s.
Author Contributions
GK identified the bivalve species, performed the data analyses, and wrote the first draft of the manuscript. VM collected, sorted nematodes, and provided the nematode data. IA identified the polychaete species. AC identified the nemertine species and main macrofaunal taxa in the samples. AM identified the echiurans, priapulids, and sipunculans species. All authors carried out the sampling and the subsequent sample processing on board, contributed to manuscript revision, read, and approved the submitted version.
Funding
This research was supported by the Ministry of Science and Higher Education of the Russian Federation (Grant No. 13.1902.21.0012, Agreement No. 075-15-2020-796).
Conflict of Interest
The authors declare that the research was conducted in the absence of any commercial or financial relationships that could be construed as a potential conflict of interest.
Publisher’s Note
All claims expressed in this article are solely those of the authors and do not necessarily represent those of their affiliated organizations, or those of the publisher, the editors and the reviewers. Any product that may be evaluated in this article, or claim that may be made by its manufacturer, is not guaranteed or endorsed by the publisher.
Acknowledgments
We are very grateful to A. Brandt (Senckenberg Research Institute and Natural History Museum, and Goethe University Frankfurt, Frankfurt), chief scientist of the KuramBio II expeditions, and to M.V. Malyutina (A.V. Zhirmunsky National Scientific Center of Marine Biology, Far Eastern Branch, Russian Academy of Sciences, Vladivostok), coordinator of the Russian team of the KuramBio and KuramBio II expeditions, for invitation to join the deep-sea KuramBio and KuramBio II expeditions (RV Sonne, 2012, 2016), and to the scientific staff of the expeditions and the ship crews for their assistance during the expeditions; to E.P. Shvetsov (A.V. Zhirmunsky National Scientific Center of Marine Biology, Far Eastern Branch, Russian Academy of Sciences, Vladivostok) for help with the translation of the manuscript into English; to the Associate Editor P. Bhadury and two reviewers for comments on the manuscript.
Supplementary Material
The Supplementary Material for this article can be found online at: https://www.frontiersin.org/articles/10.3389/fmars.2022.812464/full#supplementary-material
References
Adrianov, A. V., and Maiorova, A. S. (2018). Meristoderes okhotensis sp. nov. – the first deepwater representative of kinorhynchs in the Sea of Okhotsk (Kinorhyncha: Cyclorhagida). Deep. Res. II Top. Stud. Oceanogr. 154, 99–105. doi: 10.1016/j.dsr2.2017.10.011
Adrianov, A. V., and Maiorova, A. S. (2019). Echinoderes ultraabyssalis sp. nov. from the Kuril-Kamchatka Trench – the first hadal representative of the Kinorhyncha (Kinorhyncha: Cyclorhagida). Prog. Oceanogr. 178:102142. doi: 10.1016/j.pocean.2019.102142
Alalykina, I. L. (2013). Preliminary data on the composition and distribution of polychaetes in the deep-water areas of the north-western part of the Sea of Japan. Deep. Res. II Top. Stud. Oceanogr. 8, 164–171. doi: 10.1016/j.dsr2.2012.07.038
Alalykina, I. L. (2015). Polychaete composition from the abyssal plain adjacent to the Kuril-Kamchatka Trench with the description of a new species of Sphaerephesia (Polychaeta: Sphaerodoridae). Deep. Res. II Top. Stud. Oceanogr. 111, 166–174. doi: 10.1016/j.dsr2.2014.09.006
Alalykina, I. L. (2018). Composition of deep-sea polychaetes from the SokhoBio expedition with a description of a new species of Labioleanira (Annelida: Sigalionidae) from the Sea of Okhotsk. Deep. Res. II Top. Stud. Oceanogr. 154, 140–158. doi: 10.1016/j.dsr2.2018.04.004
Alalykina, I. L., and Polyakova, N. E. (2020). New deep-sea species of Anobothrus (Annelida: Ampharetidae) from the Kuril-Kamchatka Trench and adjacent abyssal regions. Prog. Oceanogr. 182:102237. doi: 10.1016/j.pocean.2019.102237
Allen, J. A., and Sanders, H. L. (1996). The zoogeography, diversity and origin of the deep-sea protobranch bivalves of the Atlantic: the epilogue. Prog. Oceanogr. 38, 95–153. doi: 10.1016/S0079-6611(96)00011-0
Arseniev, V. S., and Leontieva, V. V. (1970). Water Masses of Southern Part of the Kuril-Kamchatka trench in the summer of 1966. Tr. Instituta Okeanol. Akad. Nauk SSSR 86, 10–20.
Belyaev, G. M., and Mironov, A. N. (1977). Bottom fauna of the West Pacific deep-sea trenches. Trudy Instituta Okeanologii AN SSSR 108, 7–24.
Bigham, K. T., Rowden, A. A., Leduc, D., and Bowden, D. A. (2021). Review and syntheses: impacts of turbidity flows on deep-sea benthic communities. Biogeosciences 18, 1893–1908. doi: 10.5194/bg-18-1893-2021
Blazejak, A., Erse’us, C., Amann, R., and Dubilier, N. (2005). Coexistence of bacterial sulfide oxidizers, sulfate reducers, and spirochetes in a gutless worm (Oligochaeta) from the Peru Margin. Appl. Environ. Microbiol. 71, 1553–1561. doi: 10.1128/AEM.71.3.1553-1561.2005
Bonifácio, P., Martínez Arbizu, P., and Menot, L. (2020). Alpha and beta diversity patterns of polychaete assemblages across the nodule province of the eastern Clarion-Clipperton Fracture Zone (equatorial Pacific). Biogeosciences 17, 865–886. doi: 10.5194/bg-17-865-2020
Borisanova, A. O., and Chernyshev, A. V. (2019). A new loxosomatid species from the Kuril-Kamchatka trench area: Loxosomella marcusorum sp. n., the first record of hadal Entoprocta. Prog. Oceanogr. 178:102146. doi: 10.1016/j.pocean.2019.102146
Brandão, S. N., Hoppema, M., Kamenev, G. M., Karanovic, I., Riehl, T., Tanaka, H., et al. (2019). Review of Ostracoda (Crustacea) living below the Carbonate Compensation Depth and the deepest record of a calcified ostracod. Prog. Oceanogr. 178:102144. doi: 10.1016/j.pocean.2019.102144
Brandt, A., Alalykina, I. L., Brix, S., Brenke, N., Błażewicz, M., Golovan, O. A., et al. (2019). Depth zonation of Northwest Pacific deep-sea macrofauna. Prog. Oceanogr. 176:102131. doi: 10.1016/j.pocean.2019.102131
Brandt, A., Brenke, N., Andres, H. G., Brix, S., Guerrero-Kommritz, J., Mühlenhardt-Siegel, U., et al. (2005). Diversity of peracarid crustaceans (Malacostraca) from the abyssal plain of the Angola Basin. Org. Divers. Evol. 5, 105–112. doi: 10.1016/j.ode.2004.10.007
Brandt, A., Brix, S., Brökeland, W., Choudhury, M., Kaiser, S., and Malyutina, M. (2007). Deep-sea isopod biodiversity, abundance, and endemism in the Atlantic sector of the Southern Ocean-Results from the ANDEEP I-III expeditions. Deep. Res. Part II Top. Stud. Oceanogr. 54, 1760–1775. doi: 10.1016/j.dsr2.2007.07.015
Brandt, A., Brix, S., Riehl, T., and Malyutina, M. (2020). Biodiversity and biogeography of the abyssal and hadal Kuril-Kamchatka trench and adjacent NW Pacific deep-sea regions. Prog. Oceanogr. 181:102232. doi: 10.1016/j.pocean.2019.102232
Brandt, A., Elsner, N. O., Malyutina, M. V., Brenke, N., Golovan, O. A., Lavrenteva, A. V., et al. (2015). Abyssal macrofauna of the Kuril-Kamchatka Trench area (Northwest Pacific) collected by means of a camera–epibenthic sledge. Deep. Res. II Top. Stud. Oceanogr. 111, 175–187. doi: 10.1016/j.dsr2.2014.11.002
Brandt, A., Frutos, I., Bober, S., Brix, S., Brenke, N., Guggolz, T., et al. (2018). Composition of abyssal macrofauna along the Vema Fracture Zone and the hadal Puerto Rico Trench, northern tropical Atlantic. Deep. Res. II Top. Stud. Oceanogr. 148, 35–44. doi: 10.1016/j.dsr2.2017.07.014
Bray, J. R., and Curtis, J. T. (1957). An ordination of the upland forest communities of southern wisconsin. Ecol. Monogr. 27, 325–349.
Chernyshev, A. V., and Polyakova, N. E. (2018). Nemerteans from deep-sea expedition SokhoBio with description of Uniporus alisae sp. nov. (Hoplonemertea: Reptantia s.l.) from the Sea of Okhotsk. Deep. Res. II Top. Stud. Oceanogr. 154, 121–139. doi: 10.1016/j.dsr2.2017.09.022
Chernyshev, A. V., and Polyakova, N. E. (2019). Nemerteans from the deep-sea expedition KuramBio II with descriptions of three new hoplonemerteans from the Kuril-Kamchatka Trench. Prog. Oceanogr. 178:102148. doi: 10.1016/j.pocean.2019.102148
Choi, J. W., Kim, D. S., Hyun, J. H., and Lee, C. H. (2004). Macrozoobenthic communities of the deep sea sediments in the Northeastern Pacific Ocean. Ocean Polar Res. 26, 367–376. doi: 10.4217/OPR.2004.26.2.367
Clarke, K. R. (1993). Non-parametric multivariate analyses of changes in community structure. Aust. J. Ecol. 18, 117–143. doi: 10.1111/j.1442-9993.1993.tb00438.x
Clarke, K. R., and Ainsworth, M. (1993). A method of linking multivariate community structure to environmental variables. Mar. Ecol. Prog. Ser. 92, 205–219.
Clarke, K. R., and Warwick, R. M. (2001). Change in Marine Communities: An Approach to Statistical Analysis and Interpretation, 2nd Edn. Plymouth: PRIMER-E Ltd.
Cook, A. A., John, P., Hawkins, L. E., Mitchell, N., and Levin, L. A. (2000). Nematode abundance at the oxygen minimum zone in the Arabian Sea. Deep. Res. II Top. Stud. Oceanogr. 47, 75–85. doi: 10.1016/S0967-0645(99)00097-1
Danovaro, R., Company, J. B., Corinaldesi, C., D’Onghia, G., Galil, B., Gambi, C., et al. (2010). Deep-sea biodiversity in the Mediterranean Sea: the known, the unknown, and the unknowable. PLoS One 5:e11832. doi: 10.1371/journal.pone.0011832
Danovaro, R., Gambi, C., and Della Croce, N. (2002). Meiofauna hotspot in the Atacama Trench, eastern South Pacific Ocean. Deep. Res. I Oceanogr. Res. Pap. 49, 843–857. doi: 10.1016/S0967-0637(01)00084-X
Danovaro, R., Snelgrove, P. V. R., and Tyler, P. (2014). Challenging the paradigms of deep-sea ecology. Trends Ecol. Evol. 29, 465–475. doi: 10.1016/j.tree.2014.06.002
De Smet, B., Pape, E., Riehl, T., Bonifácio, P., Colson, L., and Vanreusel, A. (2017). The community structure of deep-sea macrofauna associated with polymetallic nodules in the eastern part of the Clarion-Clipperton Fracture Zone. Front. Mar. Sci. 4:103. doi: 10.3389/fmars.2017.00103
Derkachev, A. N., Nikolaeva, N. A., Tsoy, I. B., Baranov, B. V., Barinov, N. N., Mozherovskiy, A. V., et al. (2021). A long-lived center of gas-fluid emanations on the western slope of the Kuril Basin (Sea of Okhotsk). Lithol. Miner. Resour. 59, 309–332.
Diaz, R. J., and Rosenberg, R. (1995). Marine benthic hypoxia: a review of its ecological effects and the behavioral responses of benthic macrofauna. Oceanogr. Mar. Biol. An Annu. Rev. 33, 245–303.
Dreutter, S., Steffen, M., Martínez Arbizu, P., and Brandt, A. (2020). Will the “top five” deepest trenches lose one of their members? Prog. Oceanogr. 181:102258. doi: 10.1016/j.pocean.2019.102258
Du, M., Peng, X., Zhang, H., Ye, C., Dasgupta, S., Li, J., et al. (2021). Geology, environment, and life in the deepest part of the world’s oceans. Innovation 2:100109. doi: 10.1016/j.xinn.2021.100109
Dubilier, N., Blazejak, A., and Rühland, C. (2005). Symbioses between bacteria and gutless marine oligochaetes. Prog. Mol. Subcell. Biol. 41, 251–275. doi: 10.1007/3-540-28221-1_12
Dubilier, N., Giere, O., Distel, D. L., and Cavanaugh, C. M. (1995). Characterization of chemoautotrophic bacterial symbionts in a gutless marine worm Oligochaeta, Annelida) by phylogenetic 16S rRNA sequence analysis and in situ hybridization. Appl. Environ. Microbiol. 61, 2346–2350. doi: 10.1128/aem.61.6.2346-2350.1995
Dufour, S. C. (2005). Gill anatomy and the evolution of symbiosis in the bivalve family Thyasiridae. Biol. Bull. 208, 200–212. doi: 10.2307/3593152
Erséus, C. (1979). Taxonomic revision of the marine genera Bathydrilus Cook and Macroseta Erséus (Oligochaeta. Tubificidae), with descriptions of six new species and subspecies. Zool. Scr. 8, 139–151. doi: 10.1111/j.1463-6409.1979.tb00625.x
Filatova, Z. A. (1960). On the question of the quantitative distribution of benthic fauna in the central part of the Pacific Ocean. Tr. Instituta Okeanol. Akad. Nauk SSSR 41, 85–97.
Filatova, Z. A. (1971). On some mass species of bivalve mollusks from the ultra-abyssal zone of the Kuril-Kamchatka Trench. Tr. Instituta Okeanol. Akad. Nauk SSSR 92, 46–60.
Filatova, Z. A. (1982). On some problems of the quantitative investigations of the deep-sea bottom fauna. Tr. Instituta Okeanol. Akad. Nauk SSSR 117, 5–18.
Fischer, V., and Brandt, A. (2015). Composition of the abyssal infauna of the Kuril-Kamchatka area (NW Pacific) collected with a box corer. Deep. Res. II Top. Stud. Oceanogr. 111, 26–33. doi: 10.1016/j.dsr2.2014.10.002
Fujikura, K., Kojima, S., Tamaki, K., Maki, Y., Hunt, J., and Okutani, T. (1999). The deepest chemosynthesis-based community yet discovered from the hadal zone, 7326 m deep, in the Japan Trench. Mar. Ecol. Prog. Ser. 190, 17–26. doi: 10.3354/meps190017
Fukumori, H., Hasegawa, K., and Kano, Y. (2018). Abyssal gastropods in the Sea of Okhotsk (Vetigastropoda and Caenogastropoda). Deep. Res. II Top. Stud. Oceanogr. 154, 187–196. doi: 10.1016/j.dsr2.2017.09.013
Fukumori, H., Takano, T., Hasegawa, K., and Kano, Y. (2019). Deepest known gastropod fauna: species composition and distribution in the Kuril-Kamchatka Trench. Prog. Oceanogr. 178:102176. doi: 10.1016/j.pocean.2019.102176
Gambi, C., Vanreusel, A., and Danovaro, R. (2003). Biodiversity of nematode assemblages from deep-sea sediments of the Atacama Slope and Trench (South Pacific Ocean). Deep. Res. I Oceanogr. Res. Pap. 50, 103–117. doi: 10.1016/S0967-0637(02)00143-7
George, R. Y., and Higgins, R. P. (1979). Eutrophic hadal benthic community in the Puerto Rico Trench. Ambio Spec. Rep. 6, 51–58.
Glover, A. G., Smith, C. R., Paterson, G. L. J., Wilson, G. D. F., Hawkins, L., and Sheader, M. (2002). Polychaete species diversity in the central Pacific abyss: local and regional patterns, and relationships with productivity. Mar. Ecol. Prog. Ser. 240, 157–170. doi: 10.3354/meps240157
Glukhovsky, B. H., Goptareva, N. P., and Terziyeva, F. S. (1998). Hydrometeorology and Hydrochemistry. Vol. IX. Sea of Okhotsk. Issue 1. Hydrometeorological Conditions. St.-Petersburg: Gidrometeoizdat Press.
Golovan, O. A., Błażewicz, M., Brandt, A., Jażdż;ewska, A. Ì, Jóźwiak, P., Lavrenteva, A. V., et al. (2019). Diversity and distribution of peracarid crustaceans (Malacostraca) from the abyss adjacent to the Kuril-Kamchatka Trench. Mar. Biodivers. 49, 1343–1360. doi: 10.1007/s12526-018-0908-3
Gooday, A. J., Levin, L. A., Aranda da Silva, A., Bett, B. J., Cowie, G. L., Dissard, D., et al. (2009). Faunal responses to oxygen gradients on the Pakistan margin: a comparison of foraminiferans, macrofauna and megafauna. Deep Sea Res. II Top. Stud. Oceanogr. 56, 488–502. doi: 10.1016/j.dsr2.2008.10.003
Gunton, L. M., Bett, B. J., Gooday, A. J., Glover, A. G., and Vanreusel, A. (2017). Macrofaunal nematodes of the deep Whittard Canyon (NE Atlantic): assemblage characteristics and comparison with polychaetes. Mar. Ecol. 38:e12408. doi: 10.1111/maec.12408
Gutzmann, E., Martínez Arbizu, P., Rose, A., and Veit-Köhler, G. (2004). Meiofauna communities along an abyssal depth gradient in the Drake Passage. Deep Sea Res. II Top. Stud. Oceanogr 51, 1617–1628. doi: 10.1016/j.dsr2.2004.06.026
Hanz, U., Wienberg, C., Hebbeln, D., Duineveld, G., Lavaleye, M., Juva, K., et al. (2019). Environmental factors influencing benthic communities in the oxygen minimum zones on the Angolan and Namibian margins. Biogeosciences 16, 4337–4356. doi: 10.5194/bg-16-4337-2019
Hauquier, F., Macheriotou, L., Bezerra, T., Egho, G., Martínez Arbizu, P., and Vanreusel, A. (2019). Distribution of free-living marine nematodes in the clarion-clipperton zone: implications for future deep-sea mining scenarios. Biogeosciences 16, 3475–3489. doi: 10.5194/bg-16-3475-2019
Hessler, R. R., and Jumars, P. A. (1974). Abyssal community analysis from replicate box cores in the central North Pacific. Deep Sea Res. 21, 185–209.
Hessler, R. R., and Sanders, L. H. (1967). Faunal diversity in the deep-sea. Deep Sea Res. Oceanogr. Abstr. 14, 65–78. doi: 10.1016/0011-7471(67)90029-0
Howell, K. L., Hilário, A., Allcock, A. L., Bailey, D., Baker, M., Clark, M. R., et al. (2021). A decade to study deep-sea life. Nat. Ecol. Evol. 5, 265–267. doi: 10.1038/s41559-020-01352-5
Ichino, M. C., Clark, M. R., Drazen, J. C., Jamieson, A. J., Jones, D. O. B., Martin, A. P., et al. (2015). The distribution of benthic biomass in hadal trenches: a modelling approach to investigate the effect of vertical and lateral organic matter transport to the seafloor. Deep Sea Res. I Oceanogr. Res. Pap. 100: 21–33. doi: 10.1016/j.dsr.2015.01.010
Itoh, M., Kawamura, K., Kitahashi, T., Kojima, S., Katagiri, H., and Shimanaga, M. (2011). Bathymetric patterns of meiofaunal abundance and biomass associated with the Kuril and Ryukyu trenches, western North Pacific Ocean. Deep Sea Res. I Oceanogr. Res. Pap. 58, 86–97. doi: 10.1016/j.dsr.2010.12.004
Ivanenkov, V. N. (1970). Chemical properties of the deep waters of the Kuril-Kamchatka Trench. Tr. Instituta Okeanol. Akad. Nauk SSSR 86, 21–29.
Jamieson, A. J. (2015). The Hadal Zone: Life in the Deepest Oceans. Cambridge: Cambridge University Press.
Jamieson, A. J., and Stewart, H. A. (2021). Hadal zones of the Northwest Pacific Ocean. Prog. Oceanogr. 190:102477. doi: 10.1016/j.pocean.2020.102477
Jamieson, A. J., Fujii, T., Mayor, D. J., Solan, M., and Priede, I. G. (2010). Hadal trenches: the ecology of the deepest places on Earth. Trends Ecol. Evol. 25, 190–197. doi: 10.1016/j.tree.2009.09.009
Jin, Y. K., Kim, Y. G., Baranov, B., Shoji, H., and Obzhirov, A. (2011). Distribution and expression of gas seeps in a gas hydrate province of the northeastern Sakhalin continental slope, Sea of Okhotsk. Mar. Pet. Geol. 28, 1844–1855. doi: 10.1016/j.marpetgeo.2011.03.007
Jumars, P. A., and Hessler, R. R. (1976). Hadal community structure: implications from the Aleutian Trench. J. Mar. Res. 547–560.
Kafanov, A. I., Labay, V. S., and Pecheneva, N. V. (2003). Biota and Macrobenthic Communities of the Northeast Sakhalin Lagoons. Yuzhno-Sakhalinsk: Sakhalin Research Institute of Fisheries & Oceanography Press.
Kamenev, G. M. (2009). North Pacific species of the genus Solemya Lamarck, 1818 (Bivalvia: Solemyidae), with notes on Acharax jonhsoni (Dall, 1891). Malacologia 51, 233–261.
Kamenev, G. M. (2013). Species composition and distribution of bivalves in bathyal and abyssal depths of the Sea of Japan. Deep Sea Res. II Top. Stud. Oceanogr 86–87, 124–139. doi: 10.1016/j.dsr2.2012.08.004
Kamenev, G. M. (2015). Composition and distribution of bivalves of the abyssal plain adjacent to the Kuril-Kamchatka Trench (Pacific Ocean). Deep Sea Res. II Top. Stud. Oceanogr. 111, 188–197. doi: 10.1016/j.dsr2.2014.08.005
Kamenev, G. M. (2018a). Three new species of the genus Hyalopecten (Bivalvia: Pectinidae) from the abyssal and hadal zones of the North-western Pacific Ocean. J. Mar. Biol. Assoc. U. K. 98, 357–374. doi: 10.1017/S0025315416001387
Kamenev, G. M. (2018b). Bivalve molluscs of the abyssal zone of the Sea of Okhotsk: species composition, taxonomic remarks, and comparison with the abyssal fauna of the Pacific Ocean. Deep Sea Res. II Top. Stud. Oceanogr. 154, 230–248. doi: 10.1016/j.dsr2.2017.10.006
Kamenev, G. M. (2019). Bivalve mollusks of the Kuril-Kamchatka Trench, Northwest Pacific Ocean: species composition, distribution and taxonomic remarks. Prog. Oceanogr. 176:102127. doi: 10.1016/j.pocean.2019.102127
Kamenev, G. M. (2020). Three new deep-sea species of Thyasiridae (Mollusca: Bivalvia) from the abyssal plain of the northwestern Pacific Ocean and hadal depths of the Kuril-Kamchatka Trench. PeerJ 8:e10405. doi: 10.7717/peerj.10405
Kamenev, G. M., Nadtochy, V. A., and Kuznetsov, A. P. (2001). Conchocele bisecta (Conrad, 1849) (Bivalvia: Thyasiridae) from cold-water methane-rich areas of the Sea of Okhotsk. Veliger 44, 84–94.
Karaseva, N., Gantsevich, M., Obzhirov, A. I., Shakirov, R. B., Starovoytov, A., Smirnov, R., et al. (2019). Siboglinids (Annelida. Siboglinidae) as possible indicators of carbohydrates on the case of the Sea of Okhotsk. Dokl. Akad. Nauk. 486, 127–130. doi: 10.31857/S0869-56524861127-130
Karlson, K., Rosenberg, R., and Bonsdorff, E. (2002). Temporal and spatial large-scale effects of eutrophication and oxygen deficiency on benthic fauna in Scandinavian and Baltic waters, a review. Oceanogr. Mar. Biol. An Annu. Rev. 4, 427–489. doi: 10.1201/9780203180594.ch8
Kharlamenko, V. I., Kamenev, G. M., Kalachev, A. V., Kiyashko, S. I., and Ivin, V. V. (2016). Thyasirid bivalves from the methane seep community off Paramushir Island (Sea of Okhotsk) and their nutrition. J. Mol. Stud. 82, 391–402. doi: 10.1093/mollus/eyw004
Kharlamenko, V. I., Kiyashko, S. I., Sharina, S. N., Ivin, V. V., and Krylova, E. M. (2019). An ecological study of two species of chemosymbiotrophic bivalve molluscs (Bivalvia: Vesicomyidae: Pliocardiinae) from the Deryugin Basin of the Sea of Okhotsk using analyses of the stable isotope ratios and fatty acid compositions. Deep. Res. I Oceanogr. Res. Pap. 150:103058. doi: 10.1016/j.dsr.2019.06.004
Kitahashi, T., Kawamura, K., Kojima, S., and Shimanaga, M. (2013). Assemblages gradually change from bathyal to hadal depth: a case study on harpacticoid copepods around the Kuril Trench (north-west Pacific Ocean). Deep. Res. I Oceanogr. Res. Pap. 74, 39–47. doi: 10.1016/j.dsr.2012.12.010
Kitahashi, T., Kawamura, K., Veit-Köhler, G., Danovaro, R., Tietjen, J., Kojima, S., et al. (2012). Assemblages of Harpacticoida (Crustacea: Copepoda) from the Ryukyu and Kuril Trenches, north-west Pacific Ocean. J. Mar. Biol. Assoc. U. K. 92, 275–286. doi: 10.1017/S0025315411001536
Kojima, S., Fujikura, K., and Okutani, T. (2004). Multiple trans-Pacific migrations of deep-sea vent/seep-endemic bivalves in the family Vesicomyidae. Mol. Phylogenet. Evol. 32, 396–406. doi: 10.1016/j.ympev.2004.02.016
Krylova, E. M., Drozdov, A. L., and Mironov, A. N. (2000). Presence of bacteria in gills of hadal bivalve “Vesicomya” sergeevi Filatova, 1971. Ruthenica 10, 76–79.
Krylova, E. M., Kamenev, G. M., Vladychenskaya, I. P., and Petrov, N. B. (2015). Vesicomyinae (Bivalvia: Vesicomyidae) of the Kuril-Kamchatka Trench and adjacent abyssal regions. Deep. Res. II Top. Stud. Oceanogr. 111, 198–209. doi: 10.1016/j.dsr2.2014.10.004
Kuznetsov, A. P., Rass, T. S., and Galkin, S. V. (1989). Benthic community of Paramushir gas hydrated source. Zool. J. 58, 5–14.
Leduc, D., and Rowden, A. A. (2018). Nematode communities in sediments of the Kermadec Trench. Southwest Pacific Ocean. Deep. Res. I Oceanogr. Res. Pap. 134, 23–31. doi: 10.1016/j.dsr.2018.03.003
Leduc, D., Rowden, A. A., Glud, R. N., Wenzhöfer, F., Kitazato, H., and Clark, M. R. (2016). Comparison between infaunal communities of the deep floor and edge of the tonga trench: possible effects of differences in organic matter supply. Deep. Res. I Oceanogr. Res. Pap. 116, 264–275. doi: 10.1016/j.dsr.2015.11.003
Levin, L. A. (2003). Oxygen minimum zone benthos: adaptation and community response to hypoxia. Oceanogr. Mar. Biol. An Annu. Rev. 41, 1–45.
Levin, L. A., Ekau, W., Gooday, A. J., Jorissen, F., Middelburg, J. J., Naqvi, S. W. A., et al. (2009). Effects of natural and human-induced hypoxia on coastal benthos. Biogeosciences 6, 2063–2098.
Li, J., Chen, Z., Li, X., Chen, S., Xu, H., Ta, K., et al. (2021). The sources of organic carbon in the deepest ocean: implication from bacterial membrane lipids in the mariana trench zone. Front. Earth Sci. 9:1–12. doi: 10.3389/feart.2021.653742
Lins, L., and Brandt, A. (2020). Comparability between box-corer and epibenthic-sledge data on higher taxon level: a case study based on deep-sea samples from the NW Pacific. Prog. Oceanogr. 182:102273. doi: 10.1016/j.pocean.2020.102273
Liu, R., Wang, L., Wei, Y., and Fang, J. (2018). The hadal biosphere: recent insights and new directions. Deep. Res. II Top. Stud. Oceanogr. 155, 11–18. doi: 10.1016/j.dsr2.2017.04.015
Lüdmann, T., and Wong, H. K. (2003). Characteristics of gas hydrate occurrences associated with mud diapirism and gas escape structures in the northwestern Sea of Okhotsk. Mar. Geol. 201, 269–286. doi: 10.1016/S0025-3227(03)00224-X
Magurran, A. E. (2012). “Biodiversity in the context of ecosystem function,” in Marine Biodiversity and Ecosystem Functioning: Frameworks, Methodologies, and Integration, eds M. Solan, R. J. Aspden, and D. M. Paterson (Oxford: Oxford University Press), 16–23. doi: 10.1093/acprof:oso/9780199642250.003.0002
Maiorova, A. S., and Adrianov, A. V. (2018a). Deep-sea sipunculans from the Kuril Basin of the Sea of Okhotsk and the adjacent slope of the Kuril-Kamchatka Trench. Deep. Res. II Top. Stud. Oceanogr. 154, 167–176. doi: 10.1016/j.dsr2.2018.06.004
Maiorova, A. S., and Adrianov, A. V. (2018b). Deep-sea spoon worms (Echiura) from the Sea of Okhotsk and the adjacent slope of the Kuril-Kamchatka Trench. Deep. Res. II Top. Stud. Oceanogr. 154, 177–186. doi: 10.1016/j.dsr2.2018.07.010
Maiorova, A. S., and Adrianov, A. V. (2020). Biodiversity of echiurans (Echiura) of the Kuril-Kamchatka Trench area. Prog. Oceanogr. 180:102216. doi: 10.1016/j.pocean.2019.102216
Malyutina, M. V., and Brandt, A. (2018). First records of deep-sea Munnopsidae (Isopoda: Asellota) from the Kuril Basin of the Sea of Okhotsk, with description of Gurjanopsis kurilensis sp. nov. Deep. Res. II Top. Stud. Oceanogr. 154, 275–291. doi: 10.1016/j.dsr2.2017.12.006
Malyutina, M. V., and Brandt, A. (2020). Munnopsidae (Crustacea. Isopoda, Asellota) from the Kuril–Kamchatka Trench with a regional and inter-ocean comparison of their biogeographic and richness patterns. Prog. Oceanogr. 183:102289. doi: 10.1016/j.pocean.2020.102289
Malyutina, M. V., Chernyshev, A. V., and Brandt, A. (2018). Introduction to the SokhoBio (Sea of Okhotsk Biodiversity Studies) expedition 2015. Deep. Res. II Top. Stud. Oceanogr. 154, 1–9. doi: 10.1016/j.dsr2.2018.08.012
Miljutina, M. A., Miljutin, D. M., Mahatma, R., and Galéron, J. (2010). Deep-sea nematode assemblages of the Clarion-Clipperton Nodule Province (Tropical North-Eastern Pacific). Mar. Biodivers. 40, 1–15. doi: 10.1007/s12526-009-0029-0
Mironov, A. N., Dilman, A. B., Minin, K. V., and Malyutina, M. V. (2019a). Submergence of the sublittoral–bathyal species into the abyssal zone of the Sea of Japan. Oceanology 59, 920–930. doi: 10.1134/S0001437019060110
Mironov, A. N., Dilman, A. B., Gebruk, A. V., Kremenetskaia, A. V., Minin, K. V., and Smirnov, I. S. (2019b). Echinoderms of the Kuril-Kamchatka Trench. Prog. Oceanogr. 179:102217. doi: 10.1016/j.pocean.2019.102217
Mironov, A. N., Minin, K. V., Dilman, A. B., and Smirnov, I. S. (2018). Deep-sea echinoderms of the Sea of Okhotsk. Deep. Res. II Top. Stud. Oceanogr. 154, 342–357. doi: 10.1016/j.dsr2.2017.10.003
Mordukhovich, V. V., Kiyashko, S. I., Kharlamenko, V. I., and Fadeeva, N. P. (2018). Determination of food sources for nematodes in the Kuril Basin and eastern slope of the Kuril Islands by stable isotope and fatty acid analyses. Deep. Res. II Top. Stud. Oceanogr. 154, 365–373. doi: 10.1016/j.dsr2.2018.01.003
Mordukhovich, V. V., Semenchenko, A. A., Fadeeva, N. P., and Zograf, J. K. (2019). One new genus and two new free-living deep-sea nematode species with discussion of phylogeny of the family Leptosomatidae Filipjev, 1916. Prog. Oceanogr. 178:102160. doi: 10.1016/j.pocean.2019.102160
Moroz, V. V., and Bogdanov, K. T. (2007). ““Variability of hydrophysical fields in the area of islands arcs of the Far Eastern seas,” in Far Eastern Seas of Russia. Book 1. Oceanologic Research, eds V. B. Lobanov and V. A. Luchin (Moscow: Nauka Press), 387–404.
Naganuma, T., Elsaied, H. E., Hoshii, D., and Kimura, H. (2005). Bacterial endosymbioses of gutless tube-dwelling worms in nonhydrothermal vent habitats. Mar. Biotechnol. 7, 416–428. doi: 10.1007/s10126-004-5089-3
Neira, C., Sellanes, J., Levin, L. A., and Arntz, W. E. (2001). Meiofaunal distributions on the Peru margin: relationship to oxygen and organic matter availability. Deep. Res. I Oceanogr. Res. Pap. 48, 2453–2472. doi: 10.1016/S0967-0637(01)00018-8
Netto, S. A., Gallucci, F., and Fonseca, G. F. C. (2005). Meiofauna communities of continental slope and deep-sea sites off SE Brazil. Deep. Res. I Oceanogr. Res. Pap. 52, 845–859. doi: 10.1016/j.dsr.2004.11.009
Obzhirov, A. I., Baranov, B. V., Shakirov, R. B., Prokudin, V. G., and Mal’tseva, E. V. (2018). Landslide processes on the South-West slope of the Kuril basin of Okhotsk Sea. Geosist. Pereh. Zones 2, 92–98. doi: 10.30730/2541-8912.2018.2.2.092-098
Oguri, K., Kawamura, K., Sakaguchi, A., Toyofuku, T., Kasaya, T., Murayama, M., et al. (2013). Hadal disturbance in the Japan Trench induced by the 2011 Tohoku-Oki earthquake. Sci. Rep. 3, 1–6. doi: 10.1038/srep01915
Okutani, T., Fujikura, K., and Kojima, S. (1999). Two new hadal bivalves of the family Thyasiridae from the plate convergent area of the Japan Trench. Venus 58, 49–54.
Okutani, T. Koshi-ishi, T., Sato, T., Imai, T., and Kato, C. (2009). Vesicomyid fauna in the Chishima (Kurile) Trench: occurrences of a new taxon and Calyptogena extenta. Venus 68, 15–25. doi: 10.18941/venus.68.1-2_15
Oliver, P. G. (2014). TUBULAR GILLS” Extreme gill modification in the thyasiroidea with the description of Ochetoctena tomasi gen. et sp. nov. (Bivalvia: Thyasiroidea). Zoosyst. Evol. 90, 121–132. doi: 10.3897/zse.90.8323
Oug, E., Bakken, T., Kongsrud, J. A., and Alvestad, T. (2017). Polychaetous annelids in the deep Nordic Seas: strong bathymetric gradients, low diversity and underdeveloped taxonomy. Deep. Res. II Top. Stud. Oceanogr. 137, 102–112. doi: 10.1016/j.dsr2.2016.06.016
Ramirez-Llodra, E., Brandt, A., Danovaro, R., De Mol, B., Escobar, E., German, C. R., et al. (2010). Deep, diverse and definitely different: unique attributes of the world’s largest ecosystem. Biogeosciences 7, 2851–2899. doi: 10.5194/bg-7-2851-2010
Rex, M. A., McClain, C. R., Johnson, N. A., Etter, R. J., Allen, J. A., Bouchet, P., et al. (2005). A source-sink hypothesis for abyssal biodiversity. Am. Nat. 165, 163–178. doi: 10.1086/427226
Rosli, N., Leduc, D., Rowden, A. A., and Probert, P. K. (2018). Review of recent trends in ecological studies of deep-sea meiofauna, with focus on patterns and processes at small to regional spatial scales. Mar. Biodivers. 48, 13–34. doi: 10.1007/s12526-017-0801-5
Saeedi, H., and Brandt, A. (2020). Biogeographic Atlas of the Deep NW Pacific Fauna. Sofia: Pensoft Publishers. doi: 10.3897/ab.e51315
Sasaki, T., Okutani, T., and Fujikura, K. (2005). Molluscs from hydrothermal vents and cold seeps in Japan: a review of taxa recorded in twenty recent years (1984-2004). Venus 64, 87–133. doi: 10.18941/venus.64.3-4_87
Sattarova, V. V., and Aksentov, K. I. (2018). Geochemistry of mercury in surface sediments of the Kuril Basin of the Sea of Okhotsk. Kuril-Kamchatka trench and adjacent abyssal plain and northwest part of the Bering Sea. Deep. Res. II Top. Stud. Oceanogr. 154, 24–31. doi: 10.1016/j.dsr2.2017.09.002
Savilov, A. I. (1961). Ecologic characteristics of the bottom communities of invertebrates in the Okhotsk Sea. Tr. Instituta Okeanol. Akad. Nauk SSSR 46, 3–84.
Schewe, I., and Soltwedel, T. (1999). Deep-sea meiobenthos of the central Arctic Ocean: distribution patterns and size-structure under extreme oligotrophic conditions. Vie Milieu 49, 79–92.
Schmidt, C., and Martínez Arbizu, P. (2015). Unexpectedly higher metazoan meiofauna abundances in the Kuril-Kamchatka Trench compared to the adjacent abyssal plains. Deep. Res. II Top. Stud. Oceanogr. 111, 60–75. doi: 10.1016/j.dsr2.2014.08.019
Schmidt, C., Sattarova, V. V., Katrynski, L., and Martínez Arbizu, P. (2019). New insights from the deep: Meiofauna in the Kuril-Kamchatka Trench and adjacent abyssal plain. Prog. Oceanogr. 173, 192–207. doi: 10.1016/j.pocean.2019.02.010
Schmidt, C., Wolf, K. E., Lins, L., Martínez Arbizu, P., and Brandt, A. (2018). Meiofauna abundance and community patterns along a transatlantic transect in the Vema Fracture Zone and in the hadal zone of the Puerto Rico trench. Deep Sea Res. II Top. Stud. Oceanogr. 148, 223–235. doi: 10.1016/j.dsr2.2017.12.021
Sharma, J., and Bluhm, B. A. (2011). Diversity of larger free-living nematodes from macrobenthos (>250 μm) in the Arctic deep-sea Canada Basin. Mar. Biodivers. 41, 455–465. doi: 10.1007/s12526-010-0060-1
Sharma, J., Baguley, J., Bluhm, B. A., and Rowe, G. (2011). Do meio- and macrobenthic nematodes differ in community composition and body weight trends with Depth? PLoS One 6:1–8. doi: 10.1371/journal.pone.0014491
Shirayama, Y. (1984). The abundance of deep sea meiobenthos in the Western Pacific in relation to environmental factors. Oceanol. Acta 7, 113–121.
Shirayama, Y., and Kojima, S. (1994). Abundance of deep-sea meiobenthos off Sanriku. Northeastern Japan. J. Oceanogr. 50, 109–117. doi: 10.1007/bf02233860
Shuntov, V. P. (2001). Biology of the Far-eastern seas of Russia. Vol. 1. Vladivostok: Pacific Fisheries Research Center (TINRO-Center) Press.
Smetanin, D. A. (1958). Hydrochemistry of the Kuril-Kamchatka deep-sea basin. Tr. Instituta Okeanol. Akad. Nauk SSSR 27, 22–54.
Smith, C. R., De Leo, F. C., Bernardino, A. F., Sweetman, A. K., and Arbizu, P. (2008). Abyssal food limitation, ecosystem structure and climate change. Trends Ecol. Evol. 23, 518–528. doi: 10.1016/j.tree.2008.05.002
Sokal, R. R., and Rohlf, F. J. (1995). Biometry: the Principles and Practice of Statistics in Biological Research, 3rd Edn. New York, NY: W.H. Freeman.
Sokolova, M. N. (1976). Large-scale division of the World Ocean by trophic structure of deep-sea macrobenthos. Tr. Instituta Okeanol. Akad. Nauk SSSR 99, 20–30.
Sokolova, M. N. (1981). On characteristic features of the deep-sea benthic eutrophic regions of the World Ocean. Tr. Instituta Okeanol. Akad. Nauk SSSR 115, 5–13.
Southward, E. C. (1982). Bacterial symbionts in Pogonophora. J. Mar. Biol. Assoc. UK 62, 889–906. doi: 10.4161/cib.1.2.7108
Southward, E. C. (1986). Gill symbionts in thyasirids and other bivalve molluscs. J. Mar. Biol. Assoc. UK 66, 889–914. doi: 10.1017/s0025315400048517
Southward, E. C., Schulze, A., and Gardiner, S. L. (2005). Pogonophora (Annelida): form and function. Hydrobiologia 53, 227–251. doi: 10.1007/s10750-004-4401-6
Stewart, H. A., and Jamieson, A. J. (2018). Habitat heterogeneity of hadal trenches: considerations and implications for future studies. Prog. Oceanogr. 161, 47–65. doi: 10.1016/j.pocean.2018.01.007
Swan, J. A., Jamieson, A. J., Linley, T. D., and Yancey, P. H. (2021). Worldwide distribution and depth limits of decapod crustaceans (Penaeoidea, Oplophoroidea) across the abyssal-hadal transition zone of eleven subduction trenches and five additional deep-sea features. J. Crust. Biol. 41:ruaa102. doi: 10.1093/jcbiol/ruaa102
Terziev, F. S. (1996). Hydrometeorology and Hydrochemistry of the USSR Seas. Volume 9. Sea of Okhotsk. Issue 2. Hydrochemical Conditions and Oceanological Foundations of the Formation of Biological Productivity. Sankt-Petersburg: Hydrometeoizdat Press.
Tyler, P. A., Baker, M., and Ramirez-Llodra, E. (2016). “Deep-sea benthic habitats,” in Biological Sampling in the Deep Sea, eds M. R. Clark, M. Consalvey, and A. A. Rowden (Chichester: John Wiley & Sons), 1–15. doi: 10.1002/9781118332535.ch1
Uhlenkott, K., Vink, A., Kuhn, T., Gillard, B., and Arbizu, P. M. (2021). Meiofauna in a potential deep-sea mining area—influence of temporal and spatial variability on small-scale abundance models. Diversity 13, 1–16. doi: 10.3390/d13010003
Ushakov, P. V. (1953). The Fauna of the Sea of Okhotsk and Conditions of Life in it. Leningrad: Academy of Sciences of USSR Press.
Vanhove, S., Vermeeren, H., and Vanreusel, A. (2004). Meiofauna towards the South Sandwich Trench (750-6300 m), focus on nematodes. Deep. Res. II Top. Stud. Oceanogr. 51, 1665–1687. doi: 10.1016/j.dsr2.2004.06.029
Vaquer-Sunyer, R., and Duarte, C. M. (2008). Thresholds of hypoxia for marine biodiversity. Proc. Natl. Acad. Sci. U. S. A. 105, 15452–15457. doi: 10.1073/pnas.0803833105
Vasiliev, B. I., Ziltsov, E. G., and Suvorov, A. A. (1978). The structure of the paraxial zone of the southwestern part of the Kuril-Kamchatka Trench. Geotektonika 4, 70–78.
Wang, X., Liu, X., and Xu, J. (2019). Distribution patterns of meiofauna assemblages and their relationship with environmental factors of deep sea adjacent to the yap trench Western Pacific Ocean. Front. Mar. Sci. 6, 1–12. doi: 10.3389/fmars.2019.00735
Warén, A., and Bouchet, P. (2001). Gastropoda and Monoplacophora from hydrotermal vents and seeps New taxa and records. Veliger 44, 116–131.
Washburn, T. W., Menot, L., Bonifácio, P., Pape, E., Błażewicz, M., Bribiesca-Contreras, G., et al. (2021). Patterns of macrofaunal biodiversity across the clarion-clipperton zone: an area targeted for seabed mining. Front. Mar. Sci. 8:1–22. doi: 10.3389/fmars.2021.626571
Wilson, G. D. F. (2017). Macrofauna abundance, species diversity and turnover at three sites in the Clipperton-Clarion Fracture Zone. Mar. Biodivers. 47, 323–347. doi: 10.1007/s12526-016-0609-8
Xu, Y., Ge, H., and Fang, J. (2018). Biogeochemistry of hadal trenches: recent developments and future perspectives. Deep. Res. II Top. Stud. Oceanogr. 155, 19–26. doi: 10.1016/j.dsr2.2018.10.006
Yu, O. H., Lee, H.-G., Kim, D., Wi, J. H., Kim, K.-H., and Yoo, C. (2018). Characterization of deep-sea macrofauna in the korean exploration claim area in the Clarion-Clipperton fracture zone Northeastern Pacific Ocean. Ocean Sci. J. 53, 301–314. doi: 10.1007/s12601-018-0029-8
Zakharkov, S., Shtraikhert, E. A., Gordeichuk, T. N., and Vanin, N. S. (2007). “Primary production in the western part of the Sea of Okhotsk during the spring period,” in Far Eastern Seas of Russia. Book 2. Research of Marine Ecosystems and Bioresources, ed. V. P. Chelomin (Nauka Press), 463–480.
Zenkevitch, L. A., Birstein, Y. A., and Belyaev, G. M. (1955). Study of the bottom fauna of the Kuril-Kamchatka basin. Tr. Instituta Okeanol. Akad. Nauk SSSR 12, 345–381.
Keywords: abundance, macrofauna, nematodes, polychaetes, bivalves, abyssal, hadal
Citation: Kamenev GM, Mordukhovich VV, Alalykina IL, Chernyshev AV and Maiorova AS (2022) Macrofauna and Nematode Abundance in the Abyssal and Hadal Zones of Interconnected Deep-Sea Ecosystems in the Kuril Basin (Sea of Okhotsk) and the Kuril-Kamchatka Trench (Pacific Ocean). Front. Mar. Sci. 9:812464. doi: 10.3389/fmars.2022.812464
Received: 10 November 2021; Accepted: 10 January 2022;
Published: 10 February 2022.
Edited by:
Punyasloke Bhadury, Indian Institute of Science Education and Research Kolkata, IndiaReviewed by:
Er Hua, Ocean University of China, ChinaS. Bijoy Nandan, Cochin University of Science and Technology, India
Copyright © 2022 Kamenev, Mordukhovich, Alalykina, Chernyshev and Maiorova. This is an open-access article distributed under the terms of the Creative Commons Attribution License (CC BY). The use, distribution or reproduction in other forums is permitted, provided the original author(s) and the copyright owner(s) are credited and that the original publication in this journal is cited, in accordance with accepted academic practice. No use, distribution or reproduction is permitted which does not comply with these terms.
*Correspondence: Gennady M. Kamenev, Z2VubmFkeS5rYW1lbmV2QG1haWwucnU=