- 1Cape Research Centre, South African National Parks, Tokai, South Africa
- 2South African Institute for Aquatic Biodiversity (SAIAB), Grahamstown, South Africa
- 3Department of Biological Sciences, Institute for Communities and Wildlife in Africa, University of Cape Town, Cape Town, South Africa
- 4Institute for Coastal and Marine Research, Nelson Mandela University, Gqeberha, South Africa
- 5 Oceanographic Research Institute, Durban, South Africa
- 6Department of Statistics, Nelson Mandela University, Gqeberha, South Africa
- 7Centre for African Conservation Ecology, Zoology Department, Nelson Mandela University, Gqeberha, South Africa
- 8Department of Forestry, Fisheries and the Environment, Oceans and Coasts, Pretoria, South Africa
- 9Blue Wilderness Shark Research Unit, Scottbrough, South Africa
- 10OCEARCH, Park City, UT, United States
- 11Western Cape Department of Agriculture, Veterinary Services, Elsenburg, South Africa
- 12Statistics in Ecology, Environment and Conservation, Department of Statistical Sciences, University of Cape Town, Rondebosch, South Africa
- 13Cape Research and Diver Development, Simon’s Town, South Africa
- 14Oceans Research Institute, Mossel Bay, South Africa
- 15Department of Ichthyology and Fisheries Science, Rhodes University, Grahamstown, South Africa
- 16Dyer Island Conservation Trust, Kleinbaai, South Africa
- 17School of Biological Sciences, University of Western Australia, Perth, WA, Australia
- 18Department of Forestry, Fisheries and the Environment, Fisheries Research and Development, Pretoria, South Africa
- 19KwaZulu-Natal Sharks Board, Umhlanga Rocks, South Africa
- 20Department of Development Studies, School of Economics, Development and Tourism, Nelson Mandela University, Gqeberha, South Africa
- 21Department of Zoology and Institute for Coastal and Marine Research, Nelson Mandela University, Gqeberha, South Africa
- 22Centre for Research into Ecological and Environmental Modelling, School of Mathematics and Statistics, University of St Andrews, St Andrews, United Kingdom
Human activities in the oceans increase the extinction risk of marine megafauna. Interventions require an understanding of movement patterns and the spatiotemporal overlap with threats. We analysed the movement patterns of 33 white sharks (Carcharodon carcharias) satellite-tagged in South Africa between 2012 and 2014 to investigate the influence of size, sex and season on movement patterns and the spatial and temporal overlap with longline and gillnet fisheries and marine protected areas (MPAs). We used a hidden Markov model to identify ‘resident’ and ‘transient’ movement states and investigate the effect of covariates on the transition probabilities between states. A model with sex, total length and season had the most support. Tagged sharks were more likely to be in a resident state near the coast and a transient state away from the coast, while the probability of finding a shark in the transient state increased with size. White sharks moved across vast areas of the southwest Indian Ocean, emphasising the need for a regional management plan. White sharks overlapped with longline and gillnet fisheries within 25% of South Africa’s Exclusive Economic Zone and spent 15% of their time exposed to these fisheries during the study period. The demersal shark longline fishery had the highest relative spatial and temporal overlap, followed by the pelagic longline fishery and the KwaZulu-Natal (KZN) shark nets and drumlines. However, the KZN shark nets and drumlines reported the highest white shark catches, emphasising the need to combine shark movement and fishing effort with reliable catch records to assess risks to shark populations accurately. White shark exposure to shark nets and drumlines, by movement state, sex and maturity status, corresponded with the catch composition of the fishery, providing support for a meaningful exposure risk estimate. White sharks spent significantly more time in MPAs than expected by chance, likely due to increased prey abundance or less disturbance, suggesting that MPAs can benefit large, mobile marine megafauna. Conservation of white sharks in Southern Africa can be improved by implementing non-lethal solutions to beach safety, increasing the observer coverage in fisheries, and continued monitoring of movement patterns and existing and emerging threats.
Introduction
Human activities in the oceans increase the extinction risk of marine megafauna populations (Lewison et al., 2004; Dulvy et al., 2014; McCauley et al., 2015; Rogers et al., 2020). These iconic species are essential for maintaining the structure and function of marine ecosystems, have high cultural and societal value and contribute significantly to the global economy, primarily through tourism (Heithaus et al., 2008; Cisneros-Montemayor et al., 2010; Estes et al., 2011; Pimiento et al., 2020). However, one-third of these species are at risk of extinction (Pimiento et al., 2020). Megafauna species often have broad distributions and are highly migratory, underpinning the need for collaborative international conservation and management interventions (Barkley et al., 2019; Temple et al., 2019). Unfortunately, protecting mobile threatened species across their range is impractical given the limited resources available for surveillance and enforcement over such vast distances, especially in developing countries (Maxwell et al., 2011; Temple et al., 2019). Therefore, directing conservation and management attention to focus areas is the most promising solution.
White sharks (Carcharodon carcharias) are the largest predatory fish and have a circumglobal distribution, but populations are geographically distinct between the northern and southern hemispheres (Bruce and Bradford, 2012; O’Leary et al., 2015; Andreotti et al., 2016). In the global context, the South African population is a local metapopulation with low genetic diversity and needs targeted conservation measures to avoid extinction (Gubili et al., 2012; O’Leary et al., 2015; Andreotti et al., 2016). White sharks are one of the most protected shark species through policy (Rigby et al., 2019), but their populations are inherently vulnerable to human-induced mortality owing to slow growth and low fecundity (Bruce and Bradford, 2012; Hamady et al., 2014; Braccini et al., 2017; Bowlby and Gibson, 2020). They are protected from all consumptive activities and formally protected in several countries, including South Africa, Namibia (Rigby et al., 2019), and recently, Mozambique. The species may benefit further by protecting areas where they spend significantly more time, potentially indicating important areas for feeding or breeding (Huveneers et al., 2018; Rigby et al., 2019).
Sex and size-specific movement patterns may increase the vulnerability of white sharks at different life-history stages (Mucientes et al., 2009; Kanive et al., 2019). Therefore, it is important to analyse these patterns to protect this species better using spatial and fisheries management measures. In the US, Mexico and Australia, white shark regional movements are influenced by sex or size (Jorgensen et al., 2010; Domeier and Nasby-Lucas, 2013; Hoyos-Padilla et al., 2016; Bradford et al., 2020). However, due to limited satellite tracking data from Southern Africa, size-specific movement patterns have not yet been identified until now, and sex-specific patterns have been identified only at individual aggregation sites (Kock et al., 2013; Jewell et al., 2014; Towner et al., 2016). Tracking data on white sharks of both sexes and various sizes across the southwest Indian Ocean would inform a comprehensive regional management plan.
Although there is scientific debate on the South African white shark population’s size, all population estimates are consistently low, ranging from 500 to 2000 individuals (Towner et al., 2013; Andreotti et al., 2016; Andreotti et al., 2017; Irion et al., 2017). In South Africa, white sharks are captured in shark nets and on drumlines along the KwaZulu-Natal (KZN) coast as part of a bather protection program (Dudley and Simpfendorfer, 2006; Rigby et al., 2019). More broadly, in the southwest Indian Ocean, a mean spatial overlap of 64% of white shark movement and pelagic longline fisheries highlights a potential risk of being caught as bycatch in these fisheries (Queiroz et al., 2019), but capture and mortality rates are unknown. Little is known about the potential threat from other fisheries within South Africa’s Exclusive Economic Zone (EEZ), although capture reports in various commercial fisheries are few (Petersen et al., 2009; Attwood et al., 2011; Reed et al., 2020). Identifying potential overlap of threats and the distribution of white sharks across their range, from fisheries capable of catching this species, will help focus and refine management interventions.
Given that fisheries management measures and spatial zonation may be required to reduce the extinction risk of a species, we analysed the movement patterns of white sharks satellite-tagged in South Africa. The overall aim was to identify areas where sharks spend significantly more time (and may thus benefit from spatial zonation) or overlap with fisheries and associated risk (and may thus benefit from additional fisheries management interventions). Consistent with the South African National Biodiversity Assessment for the marine realm (Sink et al., 2019), we refer to these areas as focus areas. These focus areas may, in turn, inform marine protected area (MPA) expansion programmes, Ecologically or Biologically Significant Marine Area(EBSA) delineations, marine spatial planning initiatives (Reed et al., 2020) or fisheries management measures such as effective monitoring and bycatch reduction (Daly et al., 2018; Hays et al., 2019; Heerah et al., 2019). The specific objectives were to: (1) characterise white shark distribution by size and sex; (2) assess the potential relative risk of capture by quantifying the overlap between white shark distribution and longline and gillnet fisheries; and (3) evaluate whether South Africa’s MPA network provides benefit to white sharks and to identify focus areas which may benefit from additional spatial protection.
Methods
Shark Tagging
In March, April and May of 2012, we deployed 34 SPOT5 transmitters (SPOT-257, SPOT-258; Wildlife Computers) on white sharks at South African coastal aggregation sites, namely False Bay (34° 08′ S, 18° 35′ E), Gansbaai (34° 40′ S, 19° 25′ E), Struisbaai (34° 30′ S, 20° 37′ E), Mossel Bay (34° 06′ S, 22° 10′ E) and Algoa Bay (34° 52′ S, 25° 39′ E) (Figure 1). Sharks were caught from a tender vessel using buoyed hand lines with baited circle hooks and towed to a larger research vessel (M/V OCEARCH). Sharks were then temporarily removed from the water using a hydraulic lift system for transmitter attachment on the research vessel (Domeier and Nasby-Lucas, 2013). A qualified veterinarian on-board supervised all sampling and tagging procedures. Once the shark was out of the water, a wet towel was gently placed over its eyes to reduce stress, and using a hose and pump, seawater saturated with oxygen was directed onto the buccal cavity over the gills to produce a mild narcotic effect. Several team members worked on the shark simultaneously to reduce the overall duration out of the water. All sharks were tagged and sampled within 12 minutes. The veterinarian administered appropriate drugs to reduce shark capture myopathy and antibiotics to reduce the risk of infection at the hooking and tagging sites (see Supplementary Material for a list of drugs and dosages). We measured the sharks’ total length (TL) and determined whether they were male or female by the presence or absence of claspers. For analyses we categorised sharks as juveniles (male and female: 175-300 cm TL), sub-adults (male: >300-360 cm TL; females: >300-480 cm TL) and adults (male: >360 cm TL; female: >480 cm TL) (Malcolm et al., 2001). Transmitters were attached to the first dorsal fin using methods described by Bonfil et al. (2005). We coated the tags with Propspeed (Oceanmax Manufacturing, www.propspeed.com), an antifouling product, to reduce the effect of biofouling on the transmitter casings (i.e. Jewell et al., 2011).
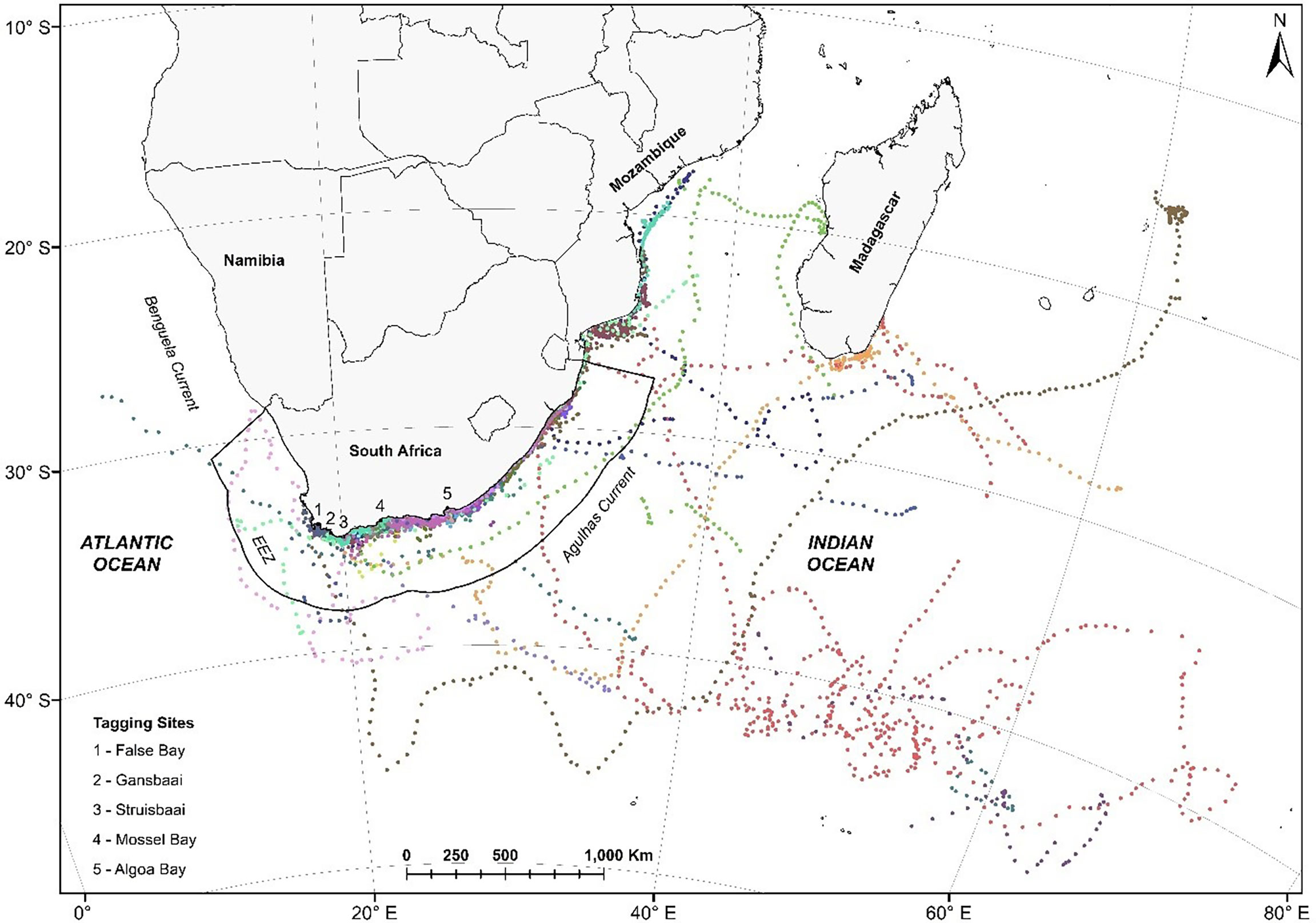
Figure 1 Satellite tracks of 33 white sharks Carcharodon carcharias tagged at five locations along the South African coast in 2012. Each colour represents an individual shark’s track between 2012 and 2014. These are time-regular predicted locations (one location every 12 hours) from a correlated random walk model fitted to the original data, accounting for the estimated CLS Argos location error, using the foieGras package in R (Jonsen and Patterson, 2020).
Track Processing
A total of 20,141 locations were obtained via ARGOS CLS (Argos, 2016) from 34 white sharks between March 2012 and April 2014. We removed the Z class locations (invalid locations) and the duplicated time stamps (ARGOS, 2016). Then, we applied a speed filter to remove unrealistic locations (maximum realistic speed 3 m/sec, following Queiroz et al., 2019), leaving 17,317 locations for analysis. The raw tracks of ARGOS locations from individual sharks were made up of time-irregular locations, with time intervals between consecutive locations of up to several days. To avoid reconstructing individual movement trajectories across these large gaps, we split tracks into segments if the time interval between locations exceeded three days. This time interval was arrived at empirically as a practical balance between having time gaps that meant the animal could be anywhere between observed locations and having too many very short track segments, which meant that estimation of locations (described below) failed. As a result, the track of each shark comprised several segments. Segments shorter than 25 locations in length led to non-convergence of the model used to account for location error and reconstruct the movement path (described below), so we excluded these segments from the analysis. This meant that tracks from shark 5 (ID 117468) were excluded from further analysis. The final dataset used to reconstruct the movement paths comprises 13,370 locations from 171 track segments and 33 individual sharks. The mean time difference between observations was 5.86 hours (standard deviation 9.79 hours).
We used a continuous-time state-space model implemented in Template Model Builder (TMB) within the foieGras R package (Jonsen and Patterson, 2020) to obtain improved estimates of the ARGOS satellite locations and to regularise the locations along the individual trajectories (Jonsen and Patterson, 2020). We chose to reconstruct the trajectories at a temporal resolution of one location every 12 hours using a correlated random walk model. Previous studies (e.g., Queiroz et al., 2019) have used a lower temporal resolution of one location every 24 hours. However, owing to the proximity of some locations to land and our interest in behaviour near the coast, we chose a slightly higher temporal resolution of two locations per day. We did not exclude or correct estimated locations that overlapped with land to avoid making changes that could not be applied systematically to the whole dataset. Locations overlapping land are evident in their error. However, the same degree of error is present throughout the dataset in ways not identifiable away from hard barriers, such as the coast. The final dataset used in the next stage of the analysis consisted of 6,680 time-regular shark locations.
Time Series Analysis of Movement Characteristics
We fitted a hidden Markov model (Zucchini et al., 2016) to step lengths and turning angles between consecutive time-regular shark locations. We used the momentuHMM R package (McClintock and Michelot, 2018), which implements computationally efficient model fitting routines using maximum likelihood. We identified two movement states which we interpreted as “resident” and “non-resident” (hereafter referred to as ‘transient’) and investigated the effect of individual and temporal covariates on the transition probabilities between states. Owing to the lack of information on white shark movement ecology at the scale of ocean basins, the time resolution of the data, and in the interest of biological interpretability (Pohle et al., 2017), we did not explore the possibility of more than two movement states. The candidate covariates included sex (male, female), life stage (juvenile, subadult, adult), TL (cm), day of the year and season. We also explored the effect of the day of the year on the shape of the state variable distributions to check whether the states’ characteristics changed with seasonality, for example, if the mean step length in the transient state increased or decreased at certain times of the year. We compared the candidate models using the AIC weights and used the Viterbi algorithm to calculate the most likely state sequence (global decoding) (Zucchini et al., 2016). We also calculated the probability of the transient movement state at each location (local decoding of state probability), in addition to the most likely state sequence provided by the Viterbi algorithm. State probabilities give an idea of uncertainty in state assignments along each track segment. Details on the candidate models, model fitting and model assessment are included in the Supplementary Material.
Spatial Analyses
We carried out two spatial analyses of white shark movement based on the results of the HMM. First, to quantify the exposure of white sharks to the risk of incidental capture in fisheries, we examined the overlap between white shark locations and the spatial distribution of fishing effort in fisheries that could potentially catch them. Second, to quantify the use of MPAs by white sharks, we examined the time spent and distance covered by sharks inside and outside MPAs.
We aggregated the 6,680 time-regular shark location data into 0.1 degree planning units, which we used in spatial analyses. These units corresponded to the approximate error ( ± 12 km) associated with the poorest location class (B) from the SPOT satellite tags (Patterson et al., 2010). Following Queiroz et al. (2019), data were aggregated within larger planning units (0.25 degrees). However, spatial scales larger than 0.1 degrees were too inaccurate for this study because they often incorporated coastal habitat within pelagic fishing zones, which inflated fisheries overlap, and exceeded the size of the smaller MPAs, making it impossible to test for an effect. Of all white shark locations recorded in this study, most (75%) occurred within the South African EEZ, so we limited our analyses to planning units within the continental EEZ. One of the 33 tagged sharks was excluded from the analyses because no tracks were left inside the EEZ after initial processing (as described under the section “Track Processing” above), leaving data from 32 sharks to be used in this part of the analysis.
The analytical approach used for fisheries and MPAs was different because the fisheries data consisted of counts of hooks or nets within each planning unit. In contrast, the MPA data were binary, either inside or outside an MPA.
White Shark Movement and Fishing Effort Overlap
Due to the high proportion of shark locations within the South African EEZ, we only considered the overlap between tagged white sharks and longline and gillnet fisheries within the EEZ, for which fishing effort data were available for the same period over which sharks were tracked (2012-2014) and used fishing gear capable of potentially catching white sharks. These included the pelagic longline fishery, which targets swordfish, tunas, and large sharks like mako sharks (Isurus oxyrinchus) and blue sharks (Prionace glauca) and the demersal shark longline fishery that targets soupfin sharks (Galeorhinus galeus), smoothhound sharks (Mustelus mustelus) and bronze whaler sharks (Carcharhinus brachyurus) (Da Silva et al., 2015; Taylor et al., 2016). Additionally, a combination of gillnets and drumlines are deployed along the KwaZulu-Natal coast between Port Edward and Richards Bay to capture large, potentially dangerous sharks for bather safety. Species targeted in this program are white sharks, bull sharks (Carcharhinus leucas) and tiger sharks (Galeocerdo cuvier) (Dudley and Simpfendorfer, 2006). Beyond the spatial and temporal overlap of white sharks with fisheries, we also obtained white shark catch reports per fishery where possible. We converted precaudal length to total length for reporting purposes, according to Casey and Pratt (1985). In addition to commercial catch data, we obtained catch data from a scientific demersal shark longline survey operated by the Department of Forestry, Fisheries and the Environment under their National Research Surveys.
Total position counts per planning unit were normalised by dividing by the number of individual sharks occupying that planning unit (Walli et al., 2009) to reduce tagging location bias and varying shark track lengths. We refer to this as the normalised shark density. We calculated an exposure index representing sharks’ spatial exposure to fisheries by multiplying the total fishing effort (total number of hooks or nets) by the normalised shark density. We restricted the temporal overlap of the analysis to periods when both the respective fishery was actively fishing, and white sharks were present. Following Taylor and Small (2009), we divided each year into quarters: Q1 (Jan-Mar), Q2 (Apr-Jun), Q3 (Jul-Sep), Q4 (Oct-Dec) and calculated the average number of days the sharks overlapped per quarter with the demersal shark longline and pelagic longline fishing sectors. Shark net and drumline effort in KZN are similar all year (except in June and July when some gear is removed due to the annual sardine run). The proportion of spatial overlap with fisheries for sharks in a ‘transient or ‘resident’ state, ‘male’ or ‘female’ and ‘sub-adult’ or ‘juvenile’ were also calculated. We combined adults and sub-adults into the sub-adult class because of the small sample of tagged adult animals.
White Shark Movement and Marine Protected Area Overlap
MPAs established before and after tagging were deemed to contribute to the MPA estate, referred to as “current MPAs” in the results. However, to compare results, we repeated the analysis using only those MPAs that existed during the tracking period (we refer to these as “existing MPAs”). Most of South Africa’s MPAs were proclaimed in 2019, after the tracking period. Thus “current MPAs” refer to those that existed prior to 2019, combined with those proclaimed in 2019.
Using the 6,680 time-regular white shark locations, we created track lines joining consecutive points of an individual and maintained the transient/resident state information. Track lines were overlaid with the planning units (grid cell used for spatial planning) to calculate the length of the track and the time spent of each individual in each planning unit. For these calculations, we assumed a straight-line distance (d) between shark locations and used the 12-hour time difference (t) between consecutive points to allow calculations of velocity (v) (where v = d/t). We used the velocity to determine the proportion of time spent in each planning unit. We coded each planning unit as inside or outside an MPA to calculate the proportion of time that individual sharks spent in MPAs.
Since MPA and planning unit boundaries do not coincide, we allocated planning units to MPAs only if >10% of their area fell within an MPA. A larger cut off was avoided to prevent the exclusion of many smaller MPAs along the coastline from the MPA estate. We then calculated the hours spent by each individual in each MPA (and outside MPAs) when in transient or resident states. We analysed data in ArcGIS (version 10.6) software.
We statistically tested four hypotheses based on a visual assessment of the shark movement data in relation to existing MPAs (i.e. those proclaimed prior to 2019) and current MPAs (i.e. existing and 2019 MPAs combined). The first hypothesis was that white sharks spent more time or covered greater distances in the existing (hypothesis 1a) and current (1b) MPA estate than expected. We defined the planning domain as only those planning units visited by any shark (total area = 235,873 km2). Of this area, 34,896 km2 fell in current MPAs (14.8%), and 8,541 km2 fell in existing MPAs (3.6%). We used this proportion as a proxy for the proportion of time one would expect the sharks to spend within MPAs if they randomly utilise their planning domain. We used a single sample t-test to compare the mean proportion of time (of all individuals combined) inside MPAs to the expected proportion of time spent. A boxplot confirmed that the data followed a normal distribution. We performed the same analysis for track length instead of time spent which also met the assumption of normality.
The second and third hypotheses were that the mean proportion of time that white sharks spend in MPAs (relative to the planning domain) differs between males and females or between subadult sharks versus juveniles, respectively. We combined adults and sub-adults into the sub-adult class because of the small sample of tagged adult animals and used a Welch two-sample t-test to avoid the assumption of equal variances between the groups. A boxplot confirmed that both variables followed a normal distribution. We performed the same analysis for track length instead of time spent.
The fourth hypothesis was that white sharks spend more time in individual MPAs than would be expected. We defined the planning domain as only those planning units visited by any sharks that visited that specific MPA for each MPA-specific analysis. We used the proportion of these planning units within the MPA as a proxy for the proportion one would expect the sample of sharks to spend within that MPA if they randomly utilise their planning domain. Sharks visited 29 MPAs, but four of these were visited by only single sharks, and we excluded them from the analyses. Therefore, we ran analyses using 25 MPAs, of which ten were existing MPAs and 15 were proclaimed in 2019. We used a single sample t-test to compare the mean proportion of the track length or time inside an individual MPA to the expected proportion of time spent. The significance level was adjusted for multiple testing using the Bonferroni adjustment. A boxplot confirmed that the data followed a normal distribution. The same analysis was performed for track length instead of time spent.
We then used point density estimation to investigate the areas of utilisation. This analysis aimed to identify focus areas utilised by sharks that do not fall within MPAs, which could then be considered for future protection. We ran all statistical analyses in R Core Team (2020) and the point density estimation in ArcGIS.
Results
Geographic Movements
Argos satellite tracks were obtained for 33 white sharks ranging in size from 251 – 420 cm TL (316 ± 15; mean ± SE, n = 13) for males and 228 – 505 cm TL (369 cm ± 16.4, mean ± SE, n = 20) for females (Table 1). These represented three adults (2 males; 1 female), 18 sub-adults (5 males; 12 females) and 13 juveniles (6 males; 7 females) (Table 1). The SPOT5 tags’ transmission duration ranged from 36 – 1155 days (374.5 ± 277.28 days, median ± SD). White sharks tagged in South Africa moved across a vast area of the southwest Indian Ocean, encompassing coastal and pelagic zones of South Africa, Mozambique, Madagascar and the high seas (Figure 1). Nine out of 33 sharks (27%) visited Mozambique at least once, and six out of 33 sharks (18%) visited the Madagascar area at least once. Apart from coastal areas of South Africa, southern Mozambique and southern Madagascar were areas frequented by large (>3 m) female white sharks (Figure 1). Only three (8%) of the tagged white sharks swam extensively westwards up the west coast of South Africa.
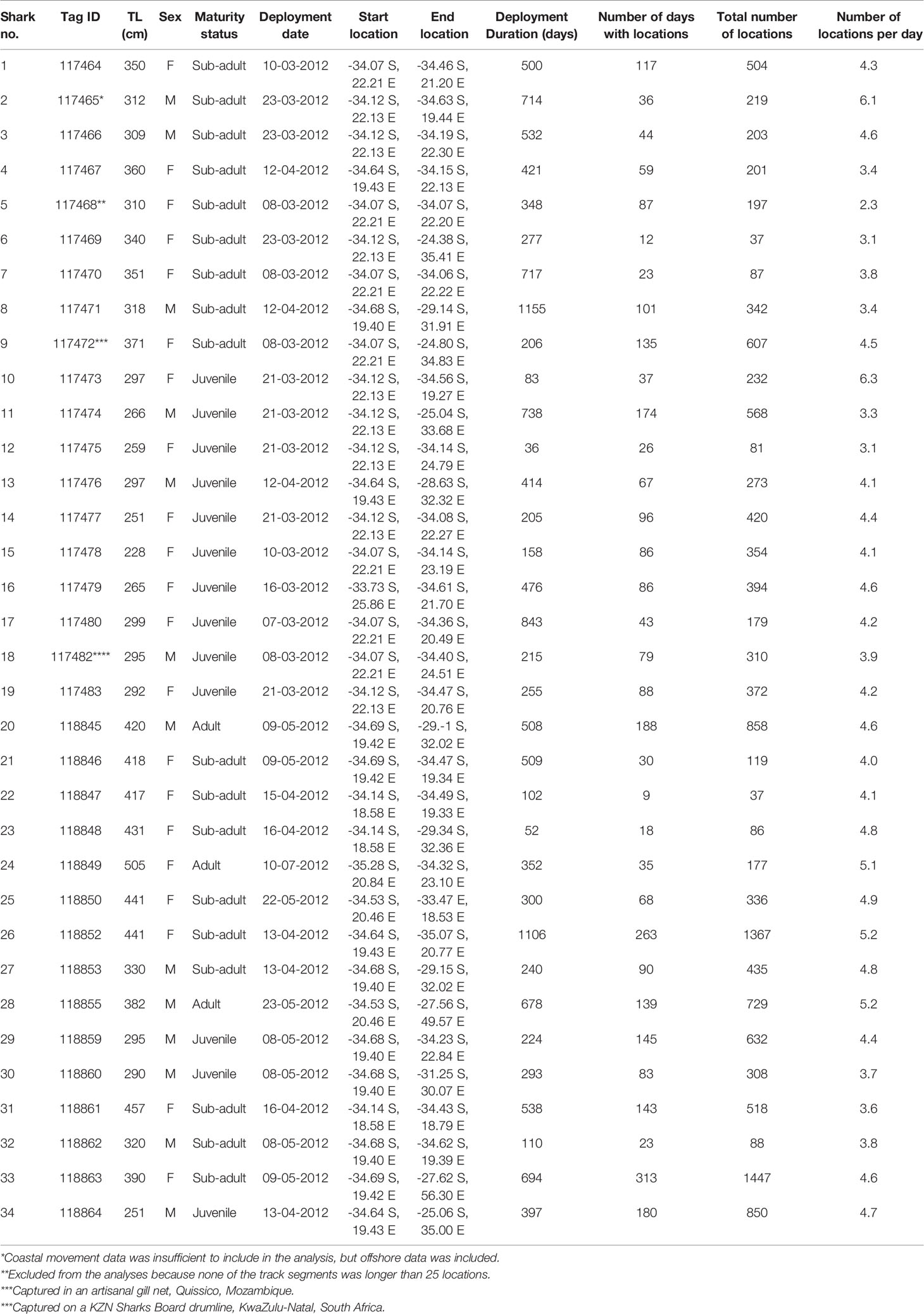
Table 1 SPOT5 transmitter (Wildlife Computers) summary information for white sharks Carcharodon carcharias tagged in South Africa in 2012.
There was no systematic difference between female and male sharks in the quality or quantity of tracking data (Table 1). This includes the total number of locations (Welch two-sample t-test, t = -0.62, df = 31.00, p-value = 0.54), the number of days with locations (Welch two-sample t-test, t = -0.81, df = 30.79, p-value = 0.42) and the number of locations per day (Welch two-sample t-test, t = -0.16, df = 25.04, p-value = 0.88).
Time Series Analysis of Movement Characteristics
According to the AIC weights, we found that a model with sex, TL and the sine and cosine of the day of the year as covariates on the transition probabilities had the most support (70%). The only other model with any support was one with life stage (adult, subadult, and juvenile) instead of TL (29%). Since life stage is determined using TL, we consider that both models capture the same patterns in the data. Using the model with the most support, we estimated the state-dependent distributions of step length and turning angle. We found that one state is characterised by slow, undirected movement (mean ~11 km per 12 hours, SD 10 km – just under 1 km per hour), and the other fast, directed movement (mean ~52 km per 12 hours, SD 28 km – just over 4 km per hour). We refer to the two states as ‘resident’ and ‘transient’ movement patterns. The state-dependent distributions are shown in Figure 2 and have been weighted by the proportion of locations assigned to each state by the Viterbi algorithm (Zucchini et al., 2016).
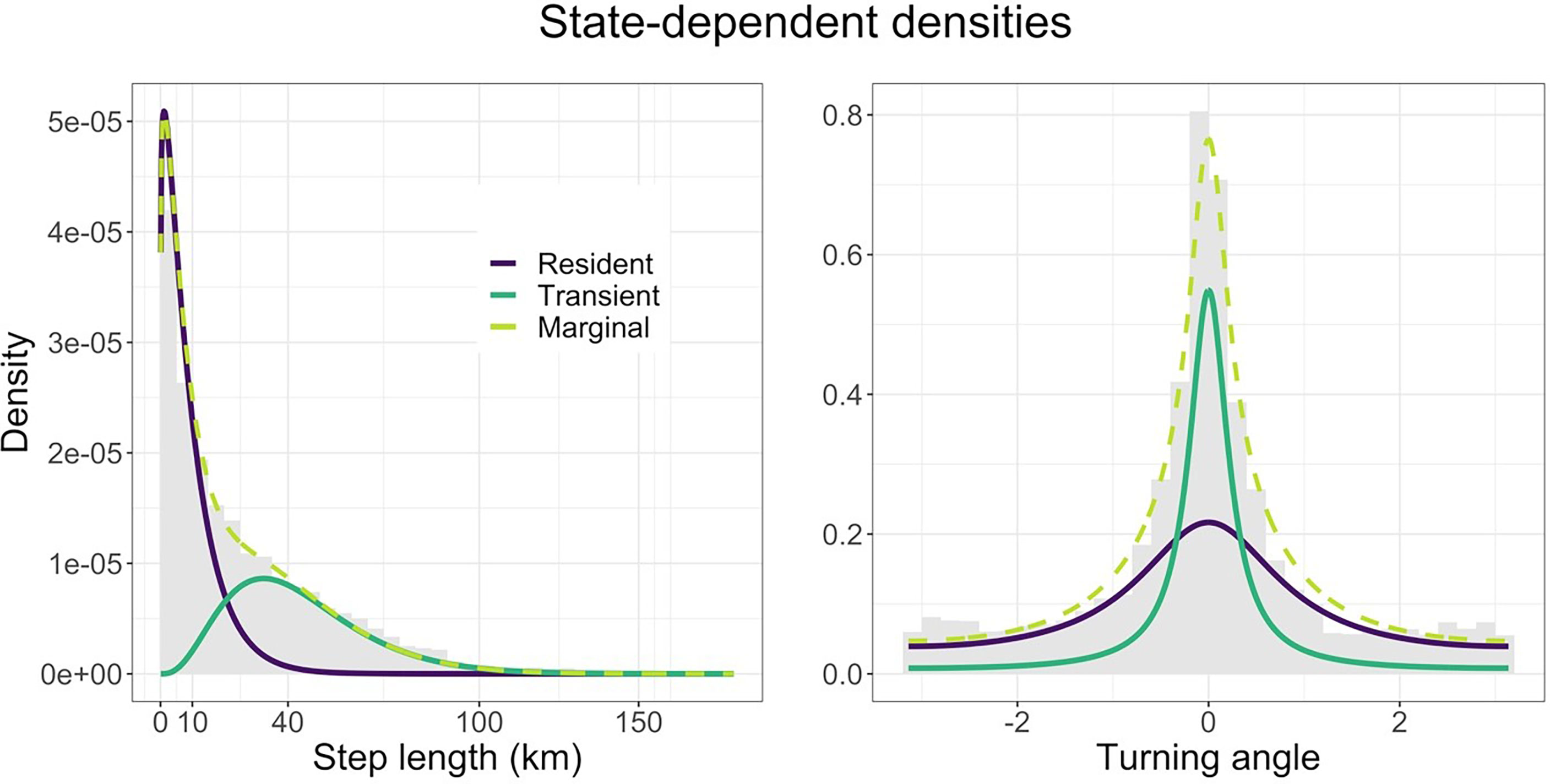
Figure 2 Estimated state-dependent distribution of step length (km) and turning angle (radians). The resident state (purple) is made up of smaller steps and bigger turns (slow, undirected movement), while the transient state (sea green) is made up of bigger steps and smaller turns (fast, directed movement). The marginal distribution (lime) shows the sum of the two densities, while the data appear as a grey histogram.
Males and females were more likely to adopt resident movement behaviour near the coast (Figure 3). In contrast, sub-adult and adult females were the only ones to travel extensively away from the coast over the tagging period (Figure 4).
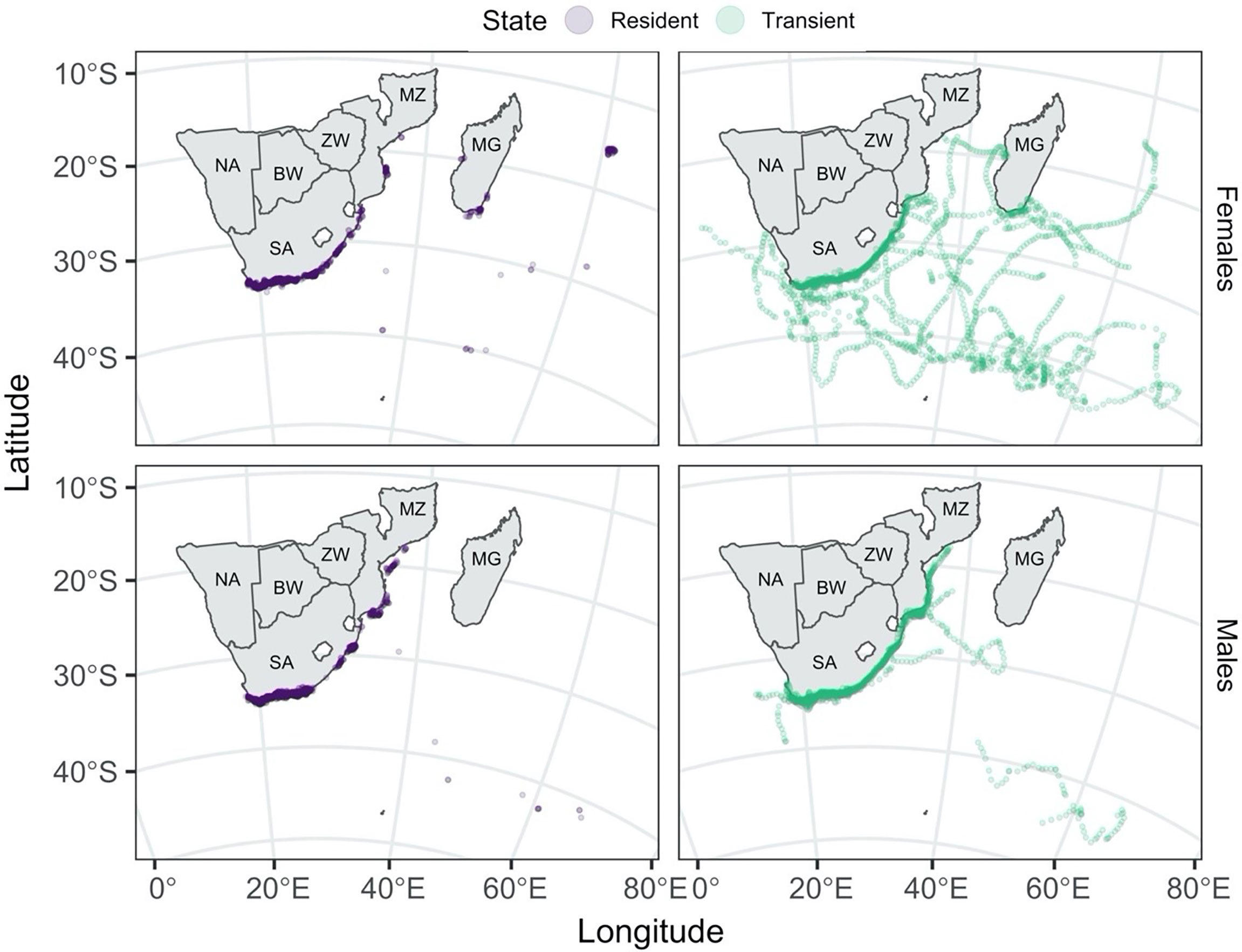
Figure 3 Predicted shark locations coloured by Viterbi-decoded states. The tracks are separated by state (resident in purple: left column, transient in sea green: right column) and sex (females: top row, males: bottom row). Country codes are shown on the map: SA, South Africa; NA, Namibia; BW, Botswana; ZW, Zimbabwe; MZ, Mozambique; MG, Madagascar.
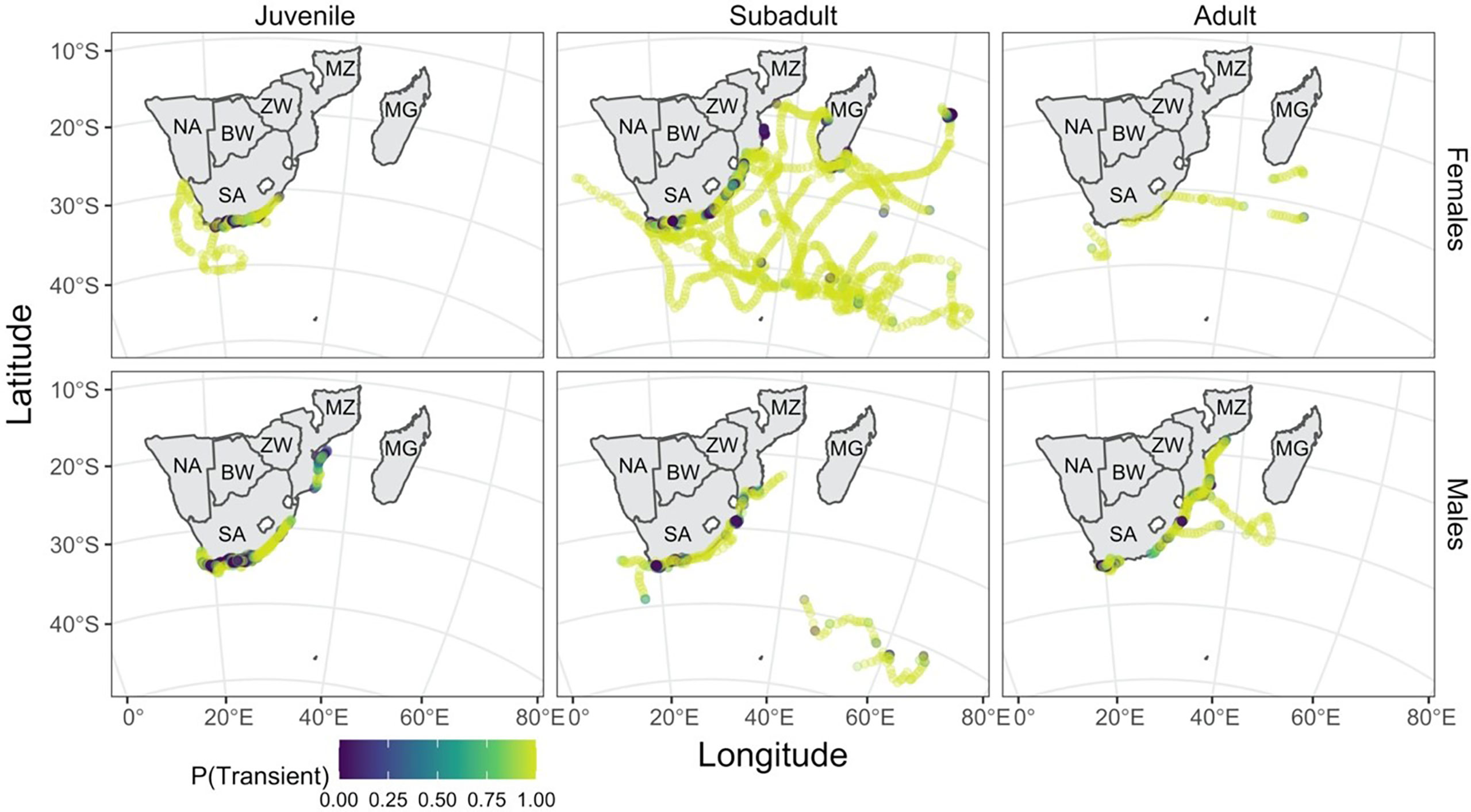
Figure 4 Predicted shark locations are coloured by the probability of a given location belonging to the transient state, where lighter locations indicate a high probability of being transient. This illustrates the level of uncertainty in state assignment; the closer the colour to either end of the colour scale, the greater the certainty in state assignment. The tracks are separated here by life stage based on size (juvenile: left column, subadult: middle column, adult: right column) and by sex (females: top row, males: bottom row). Country codes are shown on the map: SA, South Africa; NA, Namibia; BW, Botswana; ZW, Zimbabwe; MZ, Mozambique; MG, Madagascar.
The effect of time of the year on the probability of finding a shark in a given state differed for males and females and by life stage within sex. Adult and subadult females were consistently more likely to be in a transient state throughout the year, with a high probability (Figure 5), while adult males were only more likely to be transient during the summer months. Subadult male and juvenile female sharks were only more likely to be in a resident state in the winter months (between days 120 and 300), by a narrow margin. Juvenile males were the only group more likely to be in a resident state throughout the year.
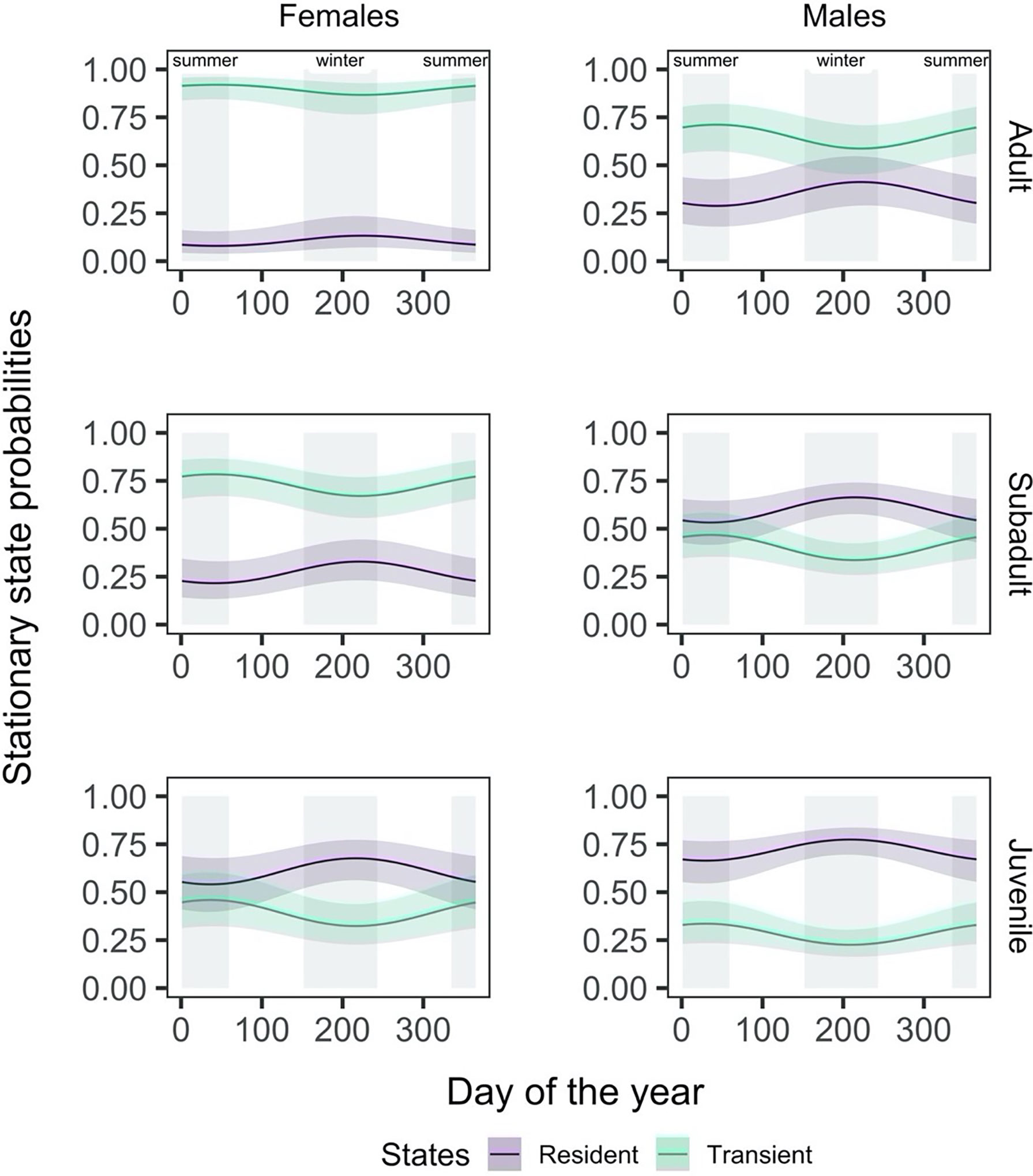
Figure 5 The effect of day of the year on the probability of finding a shark in a given state, shown here by sex (females: left column, males: right column) and life stage (adult: top row, subadult: middle row, juvenile: bottom row). The shading around the state line represents the 95% confidence interval around the mean effect.
We found strong evidence that the probability of finding a female shark in the transient movement state increased with size and was consistently high from about 350 cm (Figure 6). There was weak evidence that the smallest female sharks (200 - 250 cm) were more likely to be in a resident state in winter, and there was uncertainty in the most likely state for this group during summer. Male sharks showed a similar pattern, with an increase in the probability of being transient with size, but there was strong evidence for an increased probability of finding small males (up to about 300 cm) in a resident state in both winter and summer. This suggests a change in behaviour at a larger size than females (approximately 280 cm for females, compared to 350 cm for males). There were fewer small and large males than females in the dataset, seen in the relationship’s limits (Figure 6, right column).
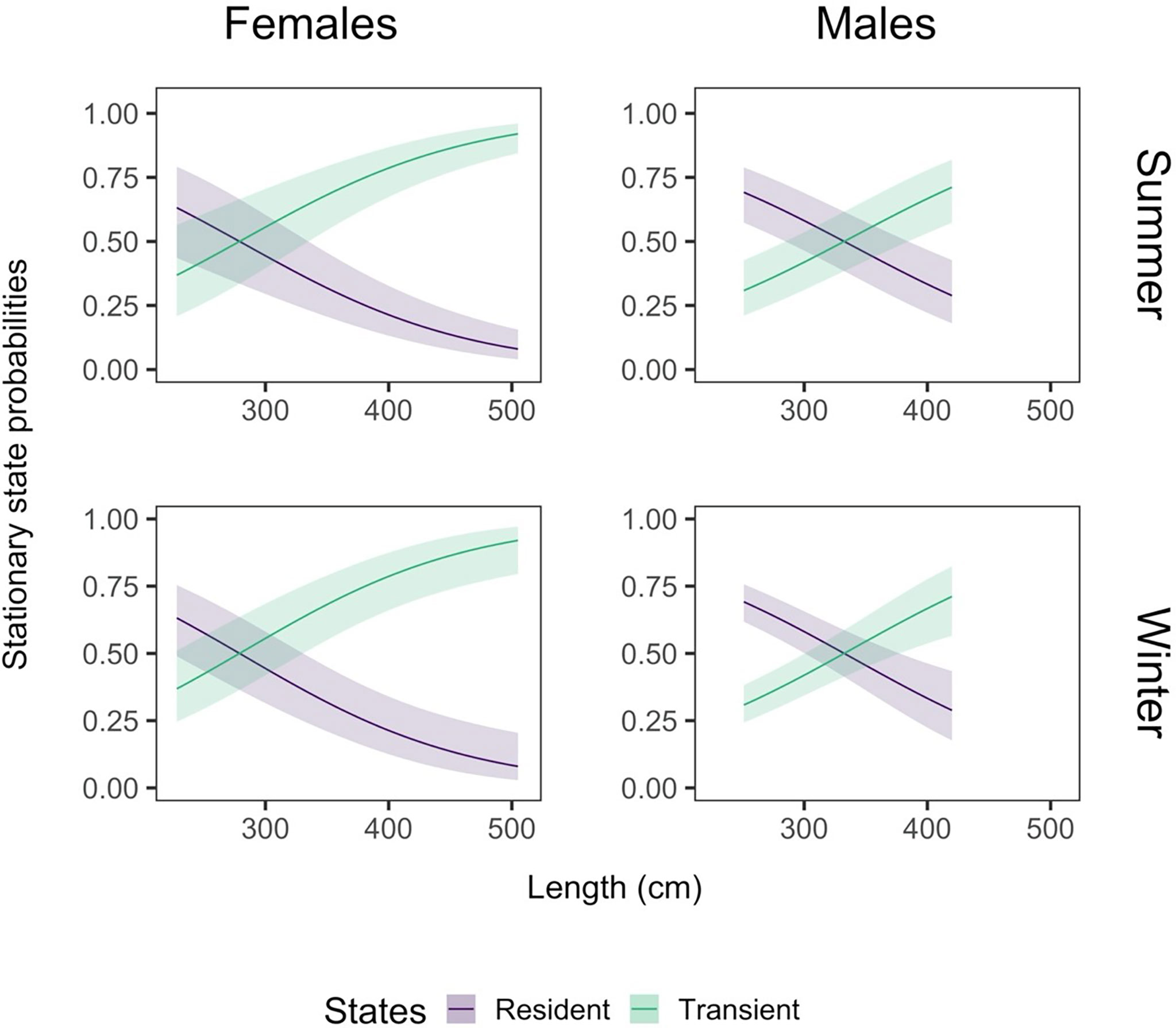
Figure 6 The effect of total length on the probability of finding a shark in a given state. The effect of length is shown here by sex (females: left column, males: right column) and season (summer: top row, winter: bottom row). We define seasonality according to the peak of mature transient movement and the trough of immature resident movement, as seen in Figure 5. Therefore, we define summer as day 40 of the year (9 February) and winter as day 220 (8 August). The shading around the state line represents the 95% confidence interval around the mean effect.
White Shark Movement and Fishing Effort Overlap
Over the study period, one white shark died in a gillnet set in Southern Mozambique and another on a KZN Sharks Board drumline (Table 1). White shark tracks and fishing effort varied over the study period (see Supplementary Material), but overall white sharks overlapped with longline and gillnet fisheries within 25% of the total area occupied in the South African EEZ and spent 15% of their time exposed to these fisheries during the study period. Spatial and temporal overlap was highest, 14% and 10%, respectively, between white sharks and the demersal shark longline sector, followed by 11% and 4% within the pelagic longline sector, and 1% and 1% within the KZN shark nets and drumlines (Table 2).

Table 2 The spatial and temporal overlap between white sharks (Carcharodon carcharias) and longline and gillnet fishing effort in the South African Exclusive Economic Zone.
Sharks most exposed to the demersal longlining were those that were resident (71%), male (59%) and juvenile (82%). The fishery with the second-highest overlap was the pelagic longline sector, with those sharks that were transient (81%), female (67%) and sub-adult/adult (51%) most exposed. The fishery with the third-highest overlap was shark nets and drumlines with those sharks that were transient (62%), female (69%) and juvenile (69%) most exposed (Table 2).
Areas of highest fishing exposure included mainly inshore areas within the vicinity of Algoa Bay, Jeffrey’s Bay, Sedgefield, Stillbaai and Gansbaai (demersal shark longline), where resident immature sharks were most exposed, as well as central to southern KZN (shark nets and drumlines) where transient immature, female sharks were most exposed (Figure 7). The overlap with the pelagic longline sector was relatively widespread and sporadic and overlapped most with transient female sharks (Figure 7).
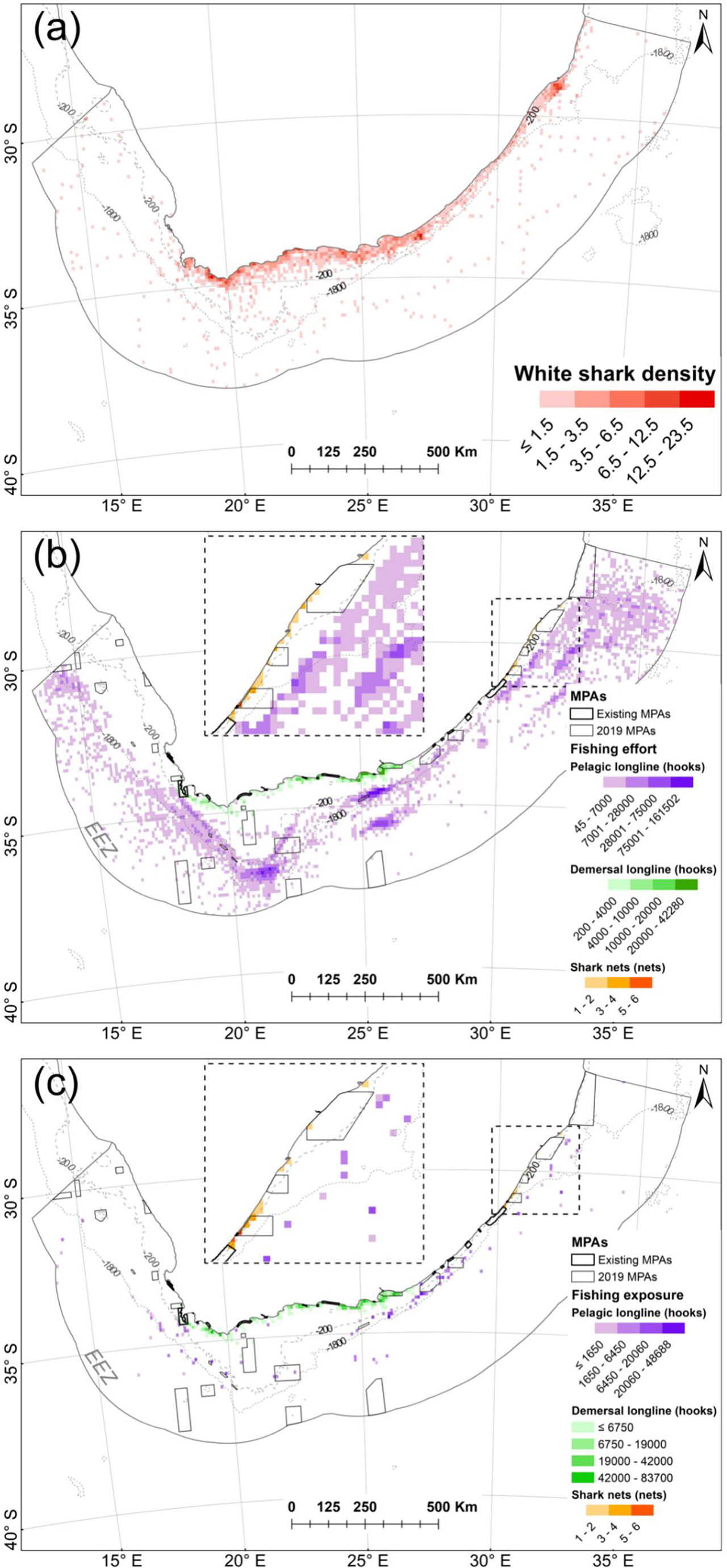
Figure 7 (A) Normalized shark density in the South African EEZ between 2012 and 2014. (B) Total fishing effort within the EEZ by fishing sector between 2012 and 2014. (C) White shark fishing exposure within the EEZ represented by fishery sector. MPAs in existence during tracking are referred to as “existing” MPAs; others were proclaimed in 2019.
White shark catch records were hard to obtain as there is no central database of white shark incidental catches, and statistics are kept in different databases according to fishery type. We could find no white shark reports in the pelagic shark longline fishery, and only two white sharks (unknown size and sex; one released alive) were reported in the demersal shark longline fishery (DFFE, unpublished data). In the scientific national demersal shark longline survey, 106 demersal shark longlines (11933 hooks) were set between False Bay and Port Alfred between 2008 and 2016. In total, two juvenile white sharks (both female), 200 and 280 cm TL, respectively, were caught in Algoa Bay during one summer survey. One died, and one was released alive. No other white shark interactions were reported. In contrast, 1317 white sharks were captured in the KwaZulu-Natal shark nets and drumlines between 1978 and 2018 (mean 32 sharks/year). A total of 209 (16%) sharks were released alive. More females were caught in the shark nets (527 males, 666 females, 47 unknown) and drumlines (30 males, 43 females, 4 unknown). Most white sharks caught were juveniles of both sexes (Table 3). The average size of white sharks caught in the shark nets is 265 cm TL and in the drumlines is 275 cm TL.
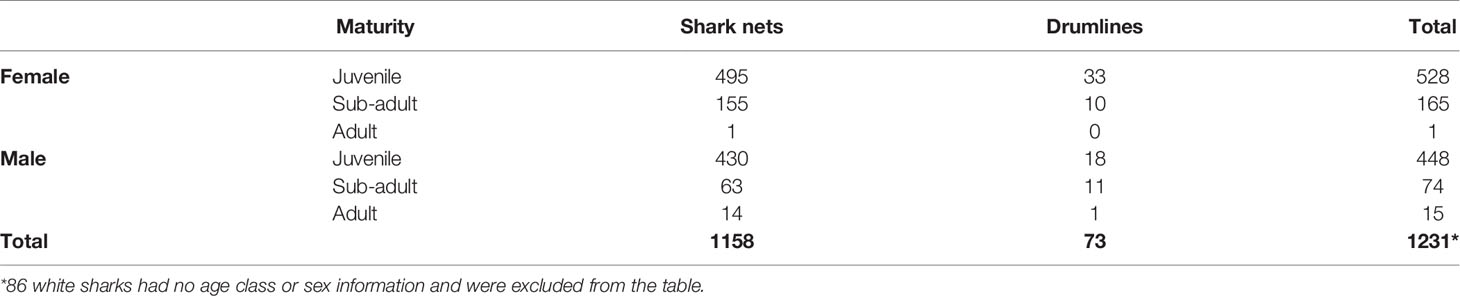
Table 3 Catches of white sharks (Carcharodon carcharias) in the KwaZulu-Natal beach safety program from 1978 – 2018 (shark nets) and 2007 – 2018 (drumlines).
White Shark Movement and Marine Protected Area Overlap
Inside the South African EEZ, the 32 individual white sharks spent 50,260 hours and covered 68,878 km (73% of the time and 41% of the distance in a resident state). The percent of time spent and distances covered within the current MPA estate were 23% and 21%. Figure 8 shows the relationship between hours spent and the current MPA estate.
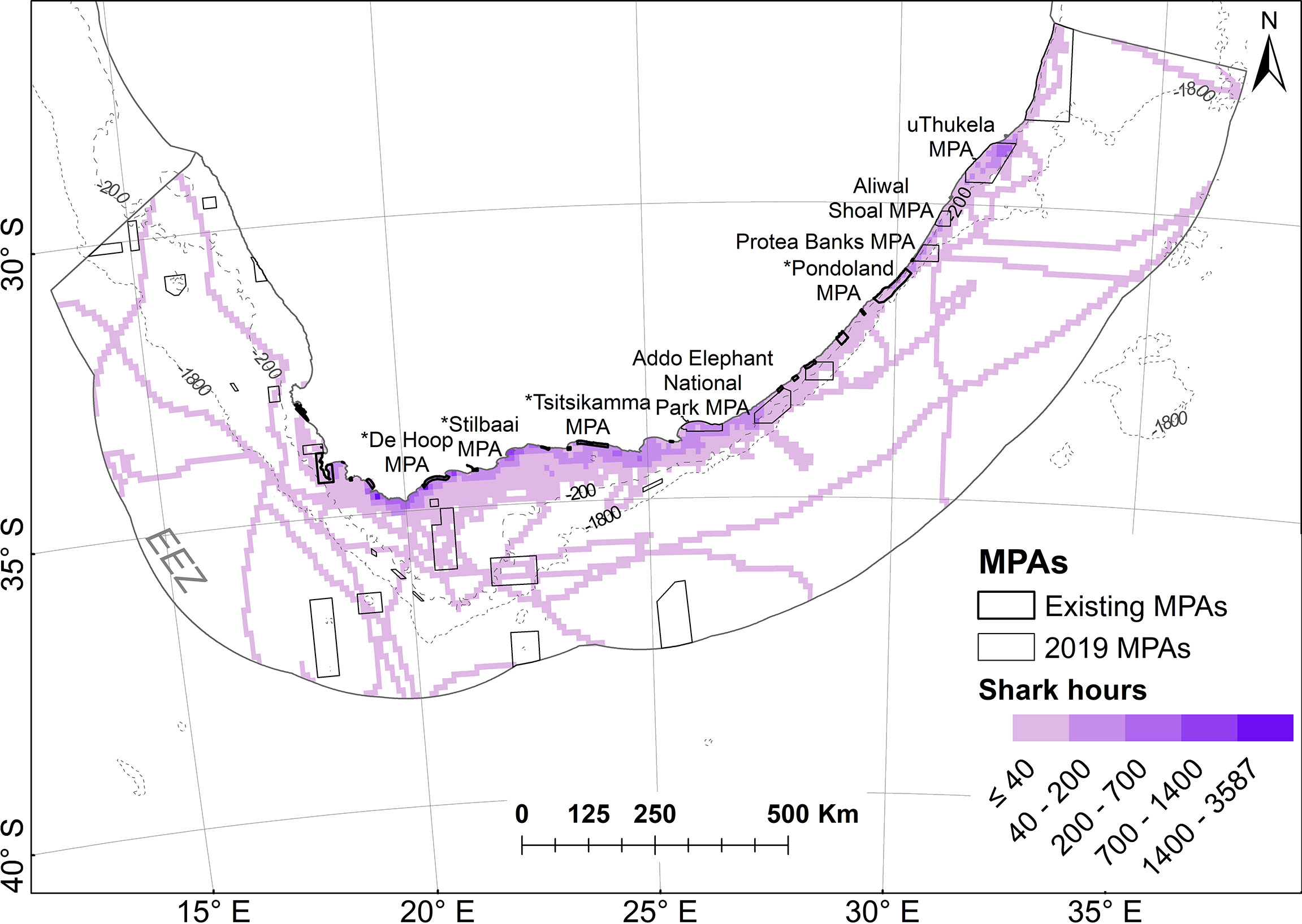
Figure 8 Time spent (in hours) of all white sharks in planning units within South Africa’s EEZ in relation to marine protected area (MPA) boundaries. Shark hours are shaded on natural breaks in the data. MPAs in existence during tracking are referred to as “existing” MPAs and are indicated with a * in Figure 10; others were proclaimed in 2019.
Results for hypotheses 1 to 4 are presented in Table 4. White sharks spent significantly more time (and covered significantly longer distances) in MPAs than can be expected based on the size of MPAs relative to the planning domain used by sharks. This was the case for existing and current MPAs. Males and females, and age classes, did not use MPAs differently. At a finer scale in current MPAs, sharks spent significantly more time in De Hoop (existing) (p = 0.012), Tsitsikamma (existing) (p = 0.022) and Addo Elephant National Park (proclaimed in 2019) (p = 0.006) MPAs than can be expected based on the size of these MPAs relative to the planning domain used by the sharks that visit these MPAs. Sharks cover significantly longer distances in De Hoop (p = 0.011), Stillbaai (existing) (p = 0.007), Tsitsikamma (p = 0.012), Addo Elephant National Park (p = 0.003), Pondoland (existing) (p = 0.018), Protea Banks (proclaimed in 2019) (p = 0.015) and Aliwal Shoal (proclaimed in 2019) (p = 0.014) MPAs than can be expected. Aliwal Shoal existed before 2019 as a small MPA (125 km2) and was expanded to a much larger MPA in 2019 (678 km2); we thus do not treat it as an existing MPA.
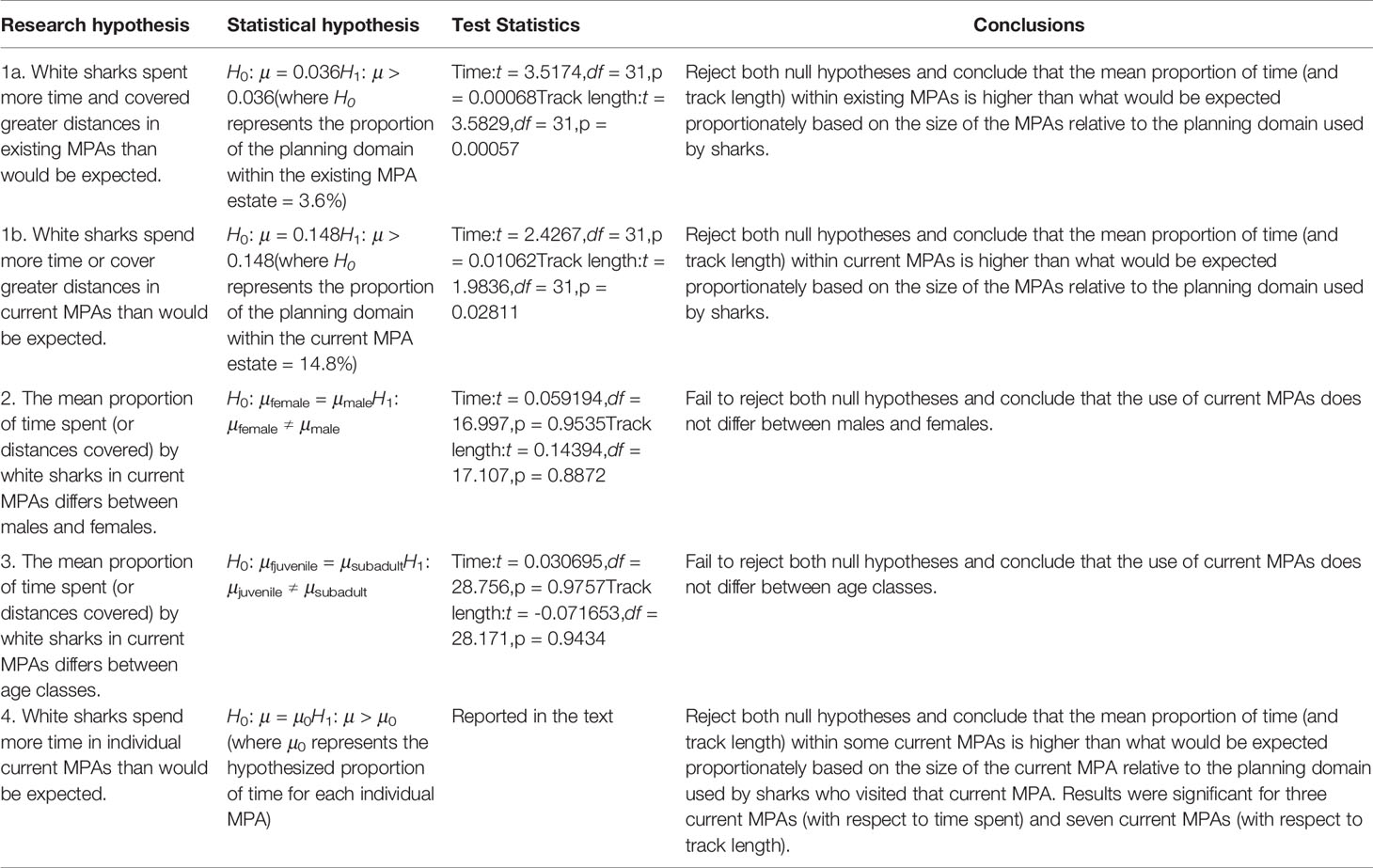
Table 4 Research hypotheses and results of white shark Carcharodon carcharias use of marine protected areas (MPAs) using time spent and track length.
Results of the point density analysis show important areas used by white sharks on the Cape south coast (adjacent to the tagging locations of Gansbaai, Struisbaai and Mossel Bay, as can be expected), with a previously unknown cluster in the northern restricted zone of the uThukela MPA (newly proclaimed), which is >1150 km away from the closest tagging site (Figure 9).
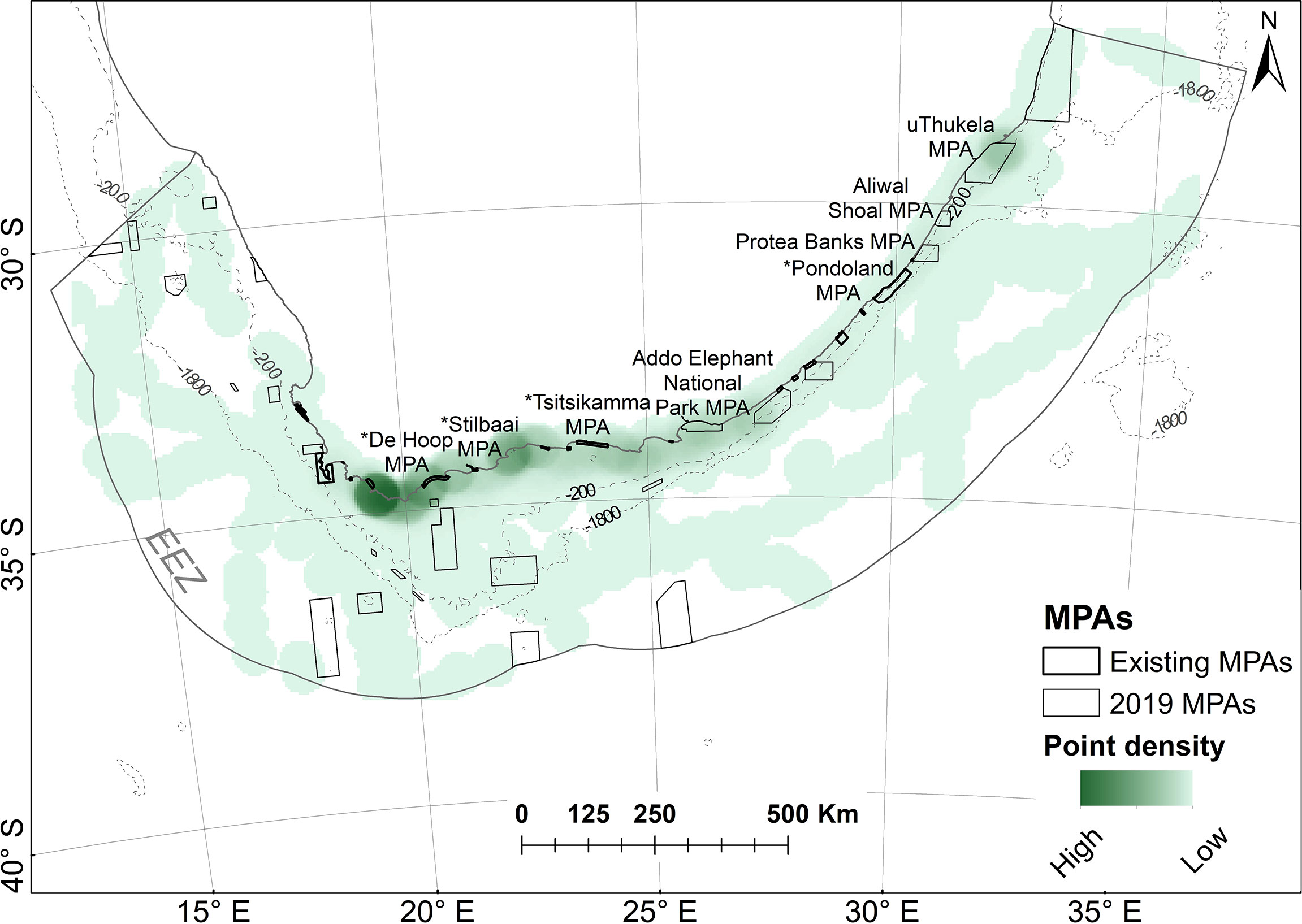
Figure 9 Point densities of white sharks in relation to marine protected areas (MPAs). MPAs in existence during tracking are referred to as “existing” MPAs and are indicated with a * in Figure 10; others were proclaimed in 2019.
Figure 10 shows the percentage of time spent by sharks in individual MPAs when all tracks are combined (all) or if only resident or transient state tracks are used. The cluster noted in Figure 9 in the uThukela MPA is also supported here.
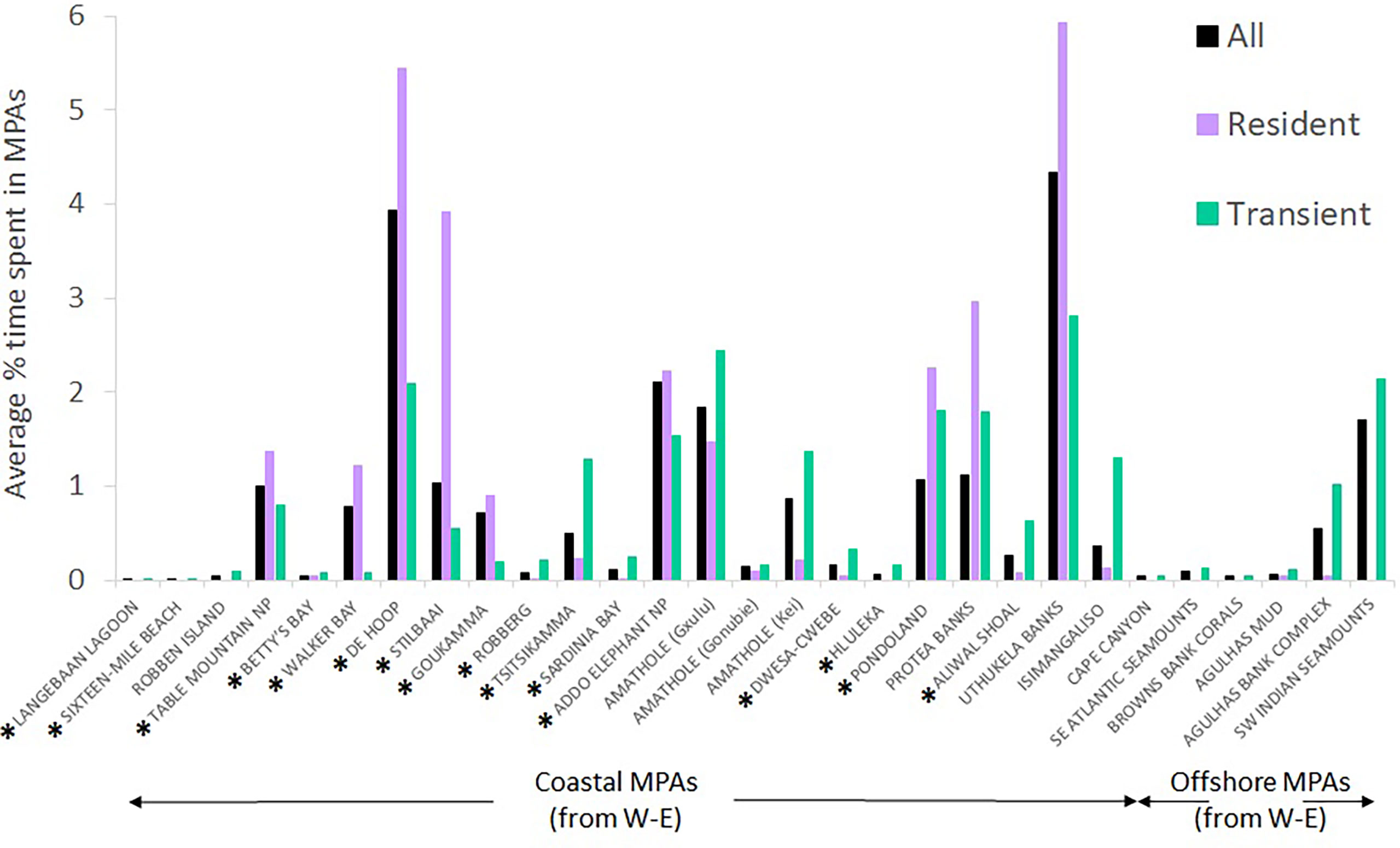
Figure 10 The average percentage of time spent by white sharks Carcharodon carcharias in the 29 marine protected areas they visited, depending on whether data were combined (all), or divided into resident versus transient states. MPAs in existence during tracking are indicated with a *; others were proclaimed in 2019.
Discussion
Satellite tracking improves our understanding of species movement across their range. It is also a powerful tool for identifying the overlap of pressures and threats with species’ spatial and temporal distribution and opportunities to focus our conservation and management interventions. In this study, we used statistical modelling of satellite tracking data to describe the spatiotemporal movement patterns of white sharks in the southwest Indian Ocean, estimate their exposure to capture risk and their relative use of protected areas. By comparing sex-specific, size-specific and seasonal movement with relevant fishing effort and South Africa’s MPA network, we have identified opportunities for improved conservation, spatial planning actions and management interventions.
White sharks tagged in this study moved extensively along the coastal areas of South Africa, crossing borders into Mozambique and even Madagascar. These sharks thus crossed several countries’ respective EEZs and spent time in Areas Beyond National Jurisdictions (high seas). The extent of their movements was similar to that reported by Bonfil et al. (2005), which identified temporary residency to coastal bays and long-distance coastal migrations along the Southern African coast. These diverse movement strategies have been identified for white sharks in Australia and the United States (Jorgensen et al., 2010; Bradford et al., 2020) and highlight this species’ adaptability and their role in connecting a diverse range of habitats and ecosystems. However, unlike Bonfil et al. (2005), we recorded no transboundary movement between South Africa and Australia. Our results, coupled with previously published movement and genetic results (Pardini et al., 2000; Bonfil et al., 2005; Andreotti et al., 2016), suggest that transboundary movement between South Africa and Australia is rare.
Notably, only three of the 34 tagged sharks (and none of the 32 white sharks tagged in Bonfil et al. (2005)) moved extensively westwards of Cape Point. Cape Point is a transition zone between the warmer Agulhas Shelf ecoregion and the colder Southern Benguela Shelf ecoregion (Sink et al., 2019) and acts as an oceanographic barrier for many marine species from invertebrates to teleosts, elasmobranchs and marine mammals (Elwen et al., 2010; Griffiths et al., 2010; Scott et al., 2012). As regional endotherms, white sharks have high metabolic demands (Ezcurra et al., 2012) and high energy requirements (Carey et al., 1982; Watanabe et al., 2019). We propose that the cold, upwelled, and often oxygen-depleted waters along the west coast of South Africa (Jarre et al., 2015) may not provide suitable conditions for white sharks, especially for extended periods (see also Hammerschlag et al., 2017). Therefore, even though the west coast is highly productive with abundant prey, including several extensive Cape fur seal colonies, it might be too energetically expensive for white sharks to forage or breed in this region.
Movement Influenced by Sex, Size and Season
We found that sex and size influence the spatiotemporal movement of white sharks in Southern Africa, consistent with behaviour described from Australia and the United States (Jorgensen et al., 2010; Domeier and Nasby-Lucas, 2012; Hoyos-Padilla et al., 2016; Franks et al., 2021; Lee et al., 2021). Subadult and adult females ranged across 45 degrees of longitude in the southwest Indian Ocean and regularly occurred offshore. On the other hand, juvenile and subadult males stayed almost exclusively in the coastal region. This is similar to white sharks’ behaviour in southwestern Australia (Bradford et al., 2020) and western North Atlantic (Franks et al., 2021), where large females covered broader longitudinal ranges and spent more time in offshore waters, while males mainly stayed on the continental shelf. Our results support Bonfil et al. (2005) conclusion that connectivity between white shark populations is facilitated by females rather than males, as Pardini et al. (2001) proposed. However, we rarely record adult white sharks in South Africa, especially females (Ryklief et al., 2014; Hewitt et al., 2018), with only three tagged in our study and Bonfil et al. (2005). In this study, large white sharks are more likely to be in a transient state near the coast, possibly resulting in fewer opportunities to be recorded or tagged, and this may also partially explain why we see fewer adults at these coastal aggregation sites. However, very large and sometimes pregnant female white sharks have been caught in gillnet fisheries eastwards in Kenya, Tanzania and Madagascar and are suspected to originate from South Africa (Cliff et al., 2000; Zuffa et al., 2002). The current information suggests that South Africa is an important area for juveniles and subadults and that the waters further east and offshore are important when they reach maturity. Focus areas for adult white sharks in Southern Africa remain unknown and present a significant knowledge gap in need of attention.
Feeding is considered an important driver of shark occurrence at coastal aggregation sites as these encompass pinniped colonies and other productive and biodiverse coastal areas (Martin et al., 2005; Dicken and Booth, 2013; Jewell et al., 2013; Kock et al., 2013; Towner et al., 2016; Kock et al., 2018). The dominance of resident movement near the coast by the mostly sexually immature sharks that make up our dataset, and the increase in the probability of transient movement with shark size, suggest a change in needs and constraints with maturity. Therefore, we propose that the resident movement state most likely represents feeding, patrolling or resting at or near important feeding areas, analogous to area-restricted search behaviour (Towner et al., 2016; Bailey et al., 2019), while the transient state likely represents migratory or travelling behaviour, but without precluding feeding. Muscle and blood samples were also collected for stable isotope analysis from the sharks tagged in this study, which, upon later analysis, may provide further insight into the relationship between movement strategies and trophic ecology. The coast may provide more predictable, concentrated foraging opportunities but more competition with conspecifics or other top predators than the open ocean, where sharks may have to travel longer, farther and deeper to find prey. Larger, maturing sharks can expand their foraging range offshore to take advantage of open ocean resources and avoid competition with conspecifics and other large predators. Mature sharks may also need to travel wider and further to find suitable mates.
The seasonal occurrence of white sharks is pronounced at all major aggregation sites worldwide (Klimley et al., 2001; Martin et al., 2005; Anderson et al., 2011; Dicken and Booth, 2013; Kock et al., 2013; Bruce and Bradford, 2015; Skomal et al., 2017). In our study, the season also influenced the behavioural state of white sharks, with juvenile females and subadult males more likely to be in a resident state during the austral winter months. This coincides with the predictable feeding opportunities and highest predation rates at Cape fur seals colonies (Martin et al., 2005; Dicken and Booth, 2013; Towner et al., 2016). Overall, juvenile female and subadult male white sharks are the most frequently recorded age classes at South African aggregation sites (Ryklief et al., 2014; Hewitt et al., 2018). Juvenile males are more likely to be in a resident state all year round, while the opposite is true for subadult and adult females and adult males. This may explain why they are recorded at South African aggregation sites less frequently than other demographic categories (Ryklief et al., 2014; Hewitt et al., 2018), which can help improve population modelling.
White Shark Movement and Fishing Effort Overlap
White sharks overlapped with longline and gillnet fisheries within 25% of South Africa’s Exclusive Economic Zone and spent 15% of their time exposed to these fisheries during the study period. The spatial and temporal overlap was highest for the demersal shark longline fishery, followed by the pelagic longline fishery and the shark nets and drumlines. Sharks most exposed to the demersal shark longline fishery were disproportionately resident, immature male sharks that spend the most time inshore in areas of the highest fishing effort. However, there have only been two reported white shark captures in this fishery (DFFE, unpublished data). Non-reporting of threatened and protected species is a concern in commercial fisheries (Campana, 2016) and needs to be considered when interpreting catch reports. However, the catches from the demersal shark longline fishery’s scientific equivalent (presented in this paper) support the low number of white shark captures, with only two white sharks caught over eight years and >11 000 set hooks. Furthermore, in Australia and the United States, white sharks are rarely caught on the demersal longline fishing gear component of demersal shark fisheries (gill nets capture most white sharks), providing further support for low captures in this fishery (McAuley and Simpfendorfer, 2003; Morgan et al., 2009; Dewar et al., 2013; Curtis et al., 2014; Braccini and Waltrick, 2019). The demersal shark longline fishery targets soupfin and smoothhound sharks and generally uses relatively small baited hooks with nylon traces (no steel) that most white sharks could bite through, although entanglement in longlines is possible.
The exposure risk of white sharks to pelagic longline fisheries within the South African EEZ was lower than for the demersal shark longline fishery and occurred over a larger area and in offshore areas. The exposure risk was disproportionately high for subadult and adult female sharks in a transient movement state. Pelagic longline fisheries use larger hooks than the demersal shark longline fishery. However, no steel traces are permitted, similar to the demersal shark fishery (DFFE permit conditions), although entanglements are also possible. No white shark captures have been reported in this fishery by the industry or independent observers (DFFE, unpublished data; Petersen et al., 2009; Attwood et al., 2011; Reed et al., 2020), but as with other commercial fisheries, species misidentification (possibly with mako sharks) and non-reporting of threatened and protected species remain concerns. However, like with the demersal shark longline fishery, it is unlikely that substantial white sharks are caught in this fishery and likely present a relatively low risk to white sharks.
The shark nets and drumlines set along the KwaZulu-Natal coast had much lower spatial and temporal overlap (1%) than the demersal shark and pelagic longline fisheries but caught a substantial number of white sharks (on average 32 sharks/year). The exposure risk was highest for juvenile female sharks in a transient state. Indeed, the catch composition from the shark net and drumline programme confirms that juvenile female sharks had the highest catch rates, providing support for this being a meaningful exposure risk estimate. The biggest difference between the shark nets and drumlines and the longline fisheries is that the KZN bather protection program targets large sharks and uses fishing gear capable of catching white sharks such as gillnets and large, baited hooks with steel traces (chain) to prevent sharks’ biting through the traces. Our results emphasise the need to combine spatiotemporal shark movement and fisheries overlap with reliable catch reports to assess risks to shark populations accurately. Our results show that the KZN shark nets and drumlines pose the largest relative risk to white sharks out of the fisheries assessed in this study, even though it had the lowest overlap with white shark movement.
One of the fisheries not assessed here, but where we know white sharks are caught, is the recreational sport and trophy angling. Given the high coastal occurrence of juvenile sharks of both sexes in a resident movement state, combined with a fishery that uses equipment to target large sharks, captures are likely sizeable. The Oceanographic Research Cooperative Fish Tagging Project’s catch records support this, with 510 records of white sharks caught over the past 35 years (1984-2020), most before protecting the species in 1991 (Jordaan and Mann, 2020). Furthermore, in one of the identified focus areas, Algoa Bay, a total of 58 white shark catches (1.5-2.5 m total length) were recorded from interviews with shore anglers between July 2009 and December 2011 (Dicken and Booth, 2013). White sharks are caught and released in most cases, but post-survival is unknown, with some records of white sharks washing ashore dead after capture and release (DFFE, unpublished data). The increasing use of technology, i.e. drones and heavy tackle, to target large sharks for sport (Gallagher et al., 2017; Winkler et al., 2022) means that this fishery could be an unknown source of mortality, warranting further investigation.
In addition to the direct risk of capture, fisheries may also have indirect consequences for predator populations in the form of altered trophic interactions or resource competition (Stevens et al., 2000; Piroddi et al., 2011; Grémillet et al., 2018). Negative impacts on marine species from fisheries have mainly been demonstrated for specialist predators or central place foragers that feed on forage fish, e.g. African penguins (Pichegru et al., 2009). However, no published studies so far have demonstrated similar effects on shark populations to the best of our knowledge. Unlike specialist predators, white sharks are generalist predators feeding on a variety of invertebrates, teleosts, chondrichthyans and marine mammals and predate on prey that is most abundant and easy to catch (Cliff et al., 1989; Martin et al., 2005; Kerr et al., 2006; Hussey et al., 2012; Kim et al., 2012; Tamburin et al., 2020). Fishing pressure on prey populations may influence food availability, which could influence shark movement and distribution, but the extent is unknown and needs further investigation. Whether or not fishing impacts white shark survival through resource competition is currently unknown and untested. However, white sharks’ generalist diet and diverse movement strategies across several ecosystems, coupled with large, stable Cape fur seal colonies and a range of prey options, increase their resilience to impacts from fisheries. In addition to implementing an ecosystem approach to fisheries management that considers predators’ needs (Shannon et al., 2010; Parker et al., 2020), an additional way to mitigate any potential risks from the indirect effect of fisheries is by establishing and effectively managing MPAs.
White Sharks Spend More Time in Marine Protected Areas
Given that white sharks are protected in South Africa, we assume that sharks visiting MPAs benefit from diverse, healthy ecosystems that potentially increase prey availability and have less disturbance. White sharks of both sexes and all age classes spent significantly more time and travelled greater distances inside South African MPAs than can be expected by chance, suggesting a preference for protected areas. This included existing MPAs at the time of tracking and those proclaimed in 2019. In the case of sharks visiting areas that later became MPAs, it is relevant to note that these newer MPAs were designed to expand existing MPAs or represent highly productive areas that contribute to biodiversity conservation goals with the least impact on competing industries (primarily fishing and mining). Significant use of areas that later became the new MPAs is thus not unexpected, given that their design criteria included known areas of diversity and productivity. There is much scientific debate about the effectiveness of MPAs to conserve large, wide-ranging predators (Breen et al., 2015) like white sharks. However, studies have shown that if positioned in essential breeding or feeding habitats, they can benefit megafauna (Williams et al., 2009; Boerder et al., 2017; Daly et al., 2018). Benefits will depend on the MPA’s size, level of protection, the amount of time spent inside MPAs, and the life-history stage protected (Hays et al., 2019; Peñaherrera-Palma et al., 2020). In this study, given that most white sharks were immature, it is likely that these MPAs are important for feeding due to increased prey availability, or for resting, due to less disturbance from anthropogenic activities. Our results contribute to the growing body of evidence that well designed MPAs can benefit large, mobile marine megafauna.
Several coastal MPAs were used by white sharks significantly more than others, namely, the De Hoop, Stillbaai, Tsitsikamma, Addo Elephant, Pondoland, Protea Banks, Aliwal Shoal and uThukela MPAs. We also identified several areas significantly used by white sharks in a resident state that are not yet part of the MPA network. These areas are clear focus areas for marine spatial planning, located in or adjacent to the primary tagging locations of Gansbaai, Struisbaai and Mossel Bay, and not included in MPAs. They may indicate high biodiversity or prey availability, which can be used in MPA expansion, delineating Ecological or Biological Significant Areas (EBSAs) or Critical Biodiversity Areas (CBAs), and in marine area plans as required by South Africa’s marine spatial planning legislation. However, some focus areas may not have been identified in this study, e.g. False Bay is a well-known aggregation site year-round (Hewitt et al., 2018; Kock et al., 2013), but only three of the sharks in this study were tagged at this site, and thus it may be under-represented.
Management and Policy Recommendations
South Africa should lead a regional conservation and management plan, given that white sharks move extensively between several Southern African countries. Encouragingly, Mozambique has recently formally protected white sharks from all fisheries alongside other large megafauna (Maritime Fisheries Regulation (REPMAR) — Decree 89/2020). National Plans of Action for Sharks and Shark Biodiversity Plans could be the vehicles for such a plan. Regional marine spatial plans can also facilitate cooperation amongst countries, e.g. the regional marine spatial planning strategy is currently being developed for the Western Indian Ocean by the Nairobi Convention Secretariat of the United Nations Environment Programme. As the fishery reporting the highest catches of white sharks, the KZN bather protection program should continue its ongoing efforts to reduce the number of nets and drumlines, where possible (Guyomard et al., 2019). In the long-term, lethal methods should be replaced with non-lethal methods (McPhee et al., 2021) and coupled with an education program around shark risk as used in other regions such as Cape Town (Shark Spotters, Kock et al., 2012) or Western Australia (Shark Smart, McAuley et al., 2017). This would have a positive impact on threatened species, including white sharks. Given that the demersal shark longline fishery overlaps with white shark movement, mainly in the resident state, and uses fishing gear that may catch small juveniles, good observer coverage is needed to monitor catches. In the absence of physical observers, a newly established electronic monitoring pilot programme in the demersal shark longline sector should be extended within the sector and to the pelagic longline sector to shed more light on fisheries interactions between these fleets and white sharks. A centralised national database of white shark fishing bycatch risk and mortality across fisheries is also needed to improve population modelling and projections. Lastly, MPAs with a high white shark presence should include white sharks in their biodiversity monitoring programs. Enforcement of current MPAs to safeguard high biodiversity and future MPA expansion efforts may mediate the direct (i.e. capture) and indirect (e.g. resource competition and disturbance) impacts on the Southern African white shark population. Since this study, white sharks have abruptly disappeared from the False Bay and Gansbaai (Hammerschlag et al., 2019, Towner et al. submitted) aggregation sites with sporadic sightings. The reasons for the disappearance are under investigation but emphasise the need for continued monitoring of movement patterns related to environmental and biological drivers and existing and emerging threats. However, the main results from this study and consequently the management recommendations remain relevant.
Conclusions
By combining animal movement modelling and spatiotemporal analyses of risk exposure from fisheries and protection from MPAs, we have provided policy-relevant results that can be used to inform management interventions. We determined demographic differences in risk exposure, identified the fisheries with the greatest impact relative to spatial or temporal shark exposure and provided a behavioural context for that impact. Our inferences can further refine place-based conservation to protect the most vulnerable demographic groups and ultimately contribute to safeguarding this species from extinction in the southwest Indian Ocean.
Data Availability Statement
The datasets presented in this study can be found in online repositories. The names of the repository/repositories and accession number(s) can be found below: 10.5281/zenodo.5575189.
Ethics Statement
We collected data according to the South African Department of Environmental Affairs: Oceans and Coasts (now the Department of Forestry, Fisheries and the Environment) protocols and adhered to the legal requirements of South Africa. All research methods were approved and conducted under the South African Department Forestry, Fisheries and the Environment: Oceans and Coasts permitting authority (Permit #RES2012/OCEARCH/umbrella-project).
Author Contributions
AAK, TP, ATL, and RD conceptualized the study and the design of the methodology. MM, RJ, CF, AK, PK, EG, AT, OJ, CS, MLD, DI, and MJS collected or contributed data. TP, RD, ATL, VG, and AK analysed and interpreted the data; AK led the writing of the manuscript. All authors contributed critically to the drafts and gave final approval for publication.
Funding
The study was made possible through generous funding by Fischer Productions for fieldwork and equipment costs. TP was supported by a postdoctoral fellowship funded by the Nelson Mandela University Research Career Development Office (2016-2018) and funding from the South African Research Chairs Initiative awarded to Prof AT Lombard by the National Research Foundation, and by a Royal Society Newton International Fellowship (2018-2020, NF170682).
Conflict of Interest
The authors declare that the research was conducted in the absence of any commercial or financial relationships that could be construed as a potential conflict of interest.
Publisher’s Note
All claims expressed in this article are solely those of the authors and do not necessarily represent those of their affiliated organizations, or those of the publisher, the editors and the reviewers. Any product that may be evaluated in this article, or claim that may be made by its manufacturer, is not guaranteed or endorsed by the publisher.
Acknowledgments
We would like to thank A Boyd, H Oosthuizen and D Anders from the Department of Forestry, Fisheries and the Environmental: Oceans and Coasts Branch for operational support and permits to conduct this work in South Africa. A Hewitt, formerly with Shark Spotters; G Oelofse, H Gold and S Liell-Cock from the City of Cape Town; S Waries from Shark Spotters; G Cliff, and S Dudley formerly from the KwaZulu Natal Sharks Board; M Wcisel and W Chivell from the Dyer Island Conservation Trust; the entire crew and support team of the M/V OCEARCH, especially Captain B McBride, and white shark cage diving operators, are thanked for their operational support and assistance in the field. Thank you to T. Wolf for assistance with the figures, and Dr R Hueter, Chief Scientist, OCEARCH, for your valuable comments on the draft manuscript.
Supplementary Material
The Supplementary Material for this article can be found online at: https://www.frontiersin.org/articles/10.3389/fmars.2022.811985/full#supplementary-material
References
Anderson S. D., Chapple T. K., Jorgensen S. J., Klimley A. P., Block B. A. (2011). Long-Term Individual Identification and Site Fidelity of White Sharks, Carcharodon Carcharias, Off California Using Dorsal Fins. Marine Biol. 158, 1233–1237. doi: 10.1007/s00227-011-1643-5
Andreotti S., Von Der Heyden S., Henriques R., Rutzen M., Meÿer M., Matthee C. A. (2017). Erring on the Side of Caution: Reply to Irion Et Al.(2017). Marine Ecol. Prog. Ser. 577, 257–262. doi: 10.3354/meps12284
Andreotti S., Von Der Heyden S., Henriques R., Rutzen M., Meÿer M., Oosthuizen H., et al. (2016). New Insights Into the Evolutionary History of White Sharks, Carcharodon Carcharias. J. Biogeography 43, 328–339. doi: 10.1111/jbi.12641
Argos. (2016). Argos User’s Manual: Worldwide Tracking and Environmental Monitoring by Satellite (New York: CLS America).
Attwood C. G., Petersen S. L., Kerwath S. E. (2011). Bycatch in South Africa’s Inshore Trawl Fishery as Determined From Observer Records. ICES J. Marine Sci. 68, 2163–2174. doi: 10.1093/icesjms/fsr162
Bailey H., Lyubchich V., Wingfield J., Fandel A., Garrod A., Rice A. (2019). Empirical Evidence That Large Marine Predator Foraging Behavior is Consistent With Area-Restricted Search Theory. Ecology 100, e02743. doi: 10.1002/ecy.2743
Barkley A. N., Gollock M., Samoilys M., Llewellyn F., Shivji M., Wetherbee B., et al. (2019). Complex Transboundary Movements of Marine Megafauna in the Western Indian Ocean. Anim. Conserv. 22, 420–431. doi: 10.1111/acv.12493
Boerder K., Bryndum-Buchholz A., Worm B. (2017). Interactions of Tuna Fisheries With the Galápagos Marine Reserve. Marine Ecol. Prog. Ser. 585, 1–15. doi: 10.3354/meps12399
Bonfil R., Meÿer M., Scholl M. C., Johnson R., O’brien S., Oosthuizen H., et al. (2005). Transoceanic Migration, Spatial Dynamics, and Population Linkages of White Sharks. Science 310, 100–103. doi: 10.1126/science.1114898
Bowlby H. D., Gibson A. J. F. (2020). Implications of Life History Uncertainty When Evaluating Status in the Northwest Atlantic Population of White Shark (Carcharodon Carcharias). Ecol. Evol. 10, 4990–5000. doi: 10.1002/ece3.6252
Braccini M., Rensing K., Langlois T., Mcauley R. (2017). Acoustic Monitoring Reveals the Broad-Scale Movements of Commercially Important Sharks. Marine Ecol. Prog. Ser. 577, 121–129. doi: 10.3354/meps12251
Braccini J. M., Waltrick D. (2019). Species-Specific at-Vessel Mortality of Sharks and Rays Captured by Demersal Longlines. Mar. Policy 99, 94–98. doi: 10.1016/j.marpol.2018.10.033
Bradford R., Patterson T. A., Rogers P. J., Mcauley R., Mountford S., Huveneers C., et al. (2020). Evidence of Diverse Movement Strategies and Habitat Use by White Sharks, Carcharodon Carcharias, Off Southern Australia. Marine Biol. 167, 1–12. doi: 10.1007/s00227-020-03712-y
Breen P., Posen P., Righton D. (2015). Temperate Marine Protected Areas and Highly Mobile Fish: A Review. Ocean Coastal Manage. 105, 75–83. doi: 10.1016/j.ocecoaman.2014.12.021
Bruce B. D., Bradford R. W. (2012). “Habitat Use and Spatial Dynamics of Juvenile White Sharks, Carcharodon carcharias Eastern Australia Global”, In: Global Perspectives on the Biology and Life History of the White Shark (Perspect. Biol. Boca Raton: CRC Press), 225-253.
Bruce B. D., Bradford R. (2015). Segregation or Aggregation? Sex-Specific Patterns in the Seasonal Occurrence of White Sharks Carcharodon Carcharias at the Neptune Islands, South Australia. J. Fish Biol. 87, 1355–1370. doi: 10.1111/jfb.12827
Campana S. E. (2016). Transboundary Movements, Unmonitored Fishing Mortality, and Ineffective International Fisheries Management Pose Risks for Pelagic Sharks in the Northwest Atlantic. Can. J. Fisheries Aquat. Sci. 73, 1599–1607. doi: 10.1139/cjfas-2015-0502
Carey F. G., Kanwisher J. W., Brazier O., Gabrielson G., Casey J. G., Pratt H. L. (1982). Temperature and Activities of a White Shark, Carcharodon Carcharias. Copeia 1982, 254–260. doi: 10.2307/1444603
Casey J. G., Pratt J. H. L. (1985). Distribution of the White Shark, Carcharodon Carcharias, in the Western North Atlantic. Memoirs South. California Acad. Sci. 9, 2–14. doi: 10.1139/f85-121
Cisneros-Montemayor A. M., Sumaila U. R., Kaschner K., Pauly D. (2010). The Global Potential for Whale Watching. Mar. Policy 34, 1273–1278. doi: 10.1016/j.marpol.2010.05.005
Cliff G., Compagno L. J. V., Smale M. J., van der Elst R. P., Wintner S. P. (2000). First Records of White Sharks, Carcharodon Carcharias, From Mauritius,· Zanzibar, Madagascar and Kenya. South Afr. J. Sci. 96, 365–367. doi: 10.10520/AJA00382353_8942
Cliff G., Dudley S., Davis B. (1989). Sharks Caught in the Protective Gill Nets Off Natal, South Africa. 2. The Great White Shark Carcharodon Carcharias (Linnaeus). South Afr. J. Marine Sci. 8, 131–144. doi: 10.2989/02577618909504556
Curtis T. H., Mccandless C. T., Carlson J. K., Skomal G. B., Kohler N. E., Natanson L. J., et al. (2014). And Pratt, H.L., JrSeasonal Distribution and Historic Trends in Abundance of White Sharks, Carcharodon Carcharias, in the Western North Atlantic Ocean. PloS One 9, e99240. doi: 10.1371/journal.pone.0099240
Daly R., Smale M. J., Singh S., Anders D., Shivji M., K. Daly C. A., et al. (2018). Refuges and Risks: Evaluating the Benefits of an Expanded MPA Network for Mobile Apex Predators. Divers. Distrib. 24, 1217–1230. doi: 10.1111/ddi.12758
Da Silva C., Booth A., Dudley S., Kerwath S., Lamberth S., Leslie R., et al. (2015). The Current Status and Management of South Africa’s Chondrichthyan Fisheries. Afr. J. Marine Sci. 37, 233–248. doi: 10.2989/1814232X.2015.1044471
Dewar H., Eguchi T., Hyde J., Kinzey D. H., Kohin S., Moore J., et al. (2013). Status Review of the Northeastern Pacific Population of White Sharks (Carcharodon Carcharias) Under the Endangered Species Act. (California: National Oceanic and Atmospheric Administration (NOAA)). Available at: https://repository.library.noaa.gov/view/noaa/17705.
Dicken M. L., Booth A. J. (2013). Surveys of White Sharks (Carcharodon Carcharias) Off Bathing Beaches in Algoa Bay, South Africa. Marine Freshwater Res. 64, 530–539. doi: 10.1071/MF12336
Domeier M. L., Nasby-Lucas N. (2012). “Sex-Specific Migration Patterns and Sexual Segregation of Adult White Sharks,” in Carcharodon Carcharias, in the Northeastern Pacific. Global Perspectives on the Biology and Life History of the White Shark (Boca Raton: CRC Press), 133–146.
Domeier M. L., Nasby-Lucas N. (2013). Two-Year Migration of Adult Female White Sharks (Carcharodon Carcharias) Reveals Widely Separated Nursery Areas and Conservation Concerns. Anim. Biotelemetry 1, 1–10. doi: 10.1186/2050-3385-1-2
Dudley S. F., Simpfendorfer C. A. (2006). Population Status of 14 Shark Species Caught in the Protective Gillnets Off KwaZulu–Natal Beaches, South Africa 1978–2003. Marine Freshwater Res. 57, 225–240. doi: 10.1071/MF05156
Dulvy N. K., Fowler S. L., Musick J. A., Cavanagh R. D., Kyne P. M., Harrison L. R., et al. (2014). Extinction Risk and Conservation of the World’s Sharks and Rays. eLife 3, e00590. doi: 10.7554/eLife.00590
Elwen S. H., Thornton M., Reeb D., Best P. B. (2010). Near-Shore Distribution of Heaviside’s (Cephalorhynchus Heavisidii) and Dusky Dolphins (Lagenorhynchus Obscurus) at the Southern Limit of Their Range in South Africa. Afr. Zool. 45, 78–91. doi: 10.1080/15627020.2010.11657256
Estes J. A., Terborgh J., Brashares J. S., Power M. E., Berger J., Bond W. J., et al. (2011). Trophic Downgrading of Planet Earth. Science 333, 301–306. doi: 10.1126/science.1205106
Ezcurra J. M., Lowe C. G., Mollet H. F., Ferry L. A., O’Sullivan J. B. (2012). Captive Feeding and Growth of Young-of-the-Year White Sharks, Carcharodon carcharias, at the Monterey Bay Aquarium. Global Perspectives on the Biology and Life History of the White Shark. (CRC Press). 3–15.
Franks B. R., Tyminski J. P., Hussey N. E., Braun C. D., Newton A. L., Thorrold S. R., et al. (2021). Spatio-Temporal Variability in White Shark (Carcharodon Carcharias) Movement Ecology During Residency and Migration Phases in the Western North Atlantic. Front. Marine Sci. 8, 744202. doi: 10.3389/fmars.2021.744202
Gallagher A. J., Hammerschlag N., Danylchuk A. J., Cooke S. J. (2017). Shark Recreational Fisheries: Status, Challenges, and Research Needs. Ambio 46, 385–398. doi: 10.1007/s13280-016-0856-8
Grémillet D., Ponchon A., Paleczny M., Palomares M.-L. D., Karpouzi V., Pauly D. (2018). Persisting Worldwide Seabird-Fishery Competition Despite Seabird Community Decline. Curr. Biol. 28, 4009–4013. doi: 10.1016/j.cub.2018.10.051
Griffiths C. L., Robinson T. B., Lange L., Mead A. (2010). Marine Biodiversity in South Africa: An Evaluation of Current States of Knowledge. PloS One 5, e12008. doi: 10.1371/journal.pone.0012008
Gubili C., Duffy C. A., Cliff G., Wintner S. P., Shivji M., Chapman D., et al. (2012). Application of Molecular Genetics for Conservation of the White Shark, Carcharodon Carcharias, L. 24, 1758. Global Perspect. Biol. Life History White Shark 357–380. doi: 10.1201/b11532-28
Guyomard D., Perry C., Tournoux P. U., Cliff G., Peddemors V., Jaquemet S. (2019). An Innovative Fishing Gear to Enhance the Release of non-Target Species in Coastal Shark-Control Programs: The SMART (Shark Management Alert in Real-Time) Drumline. Fisheries Res. 216, 6–17. doi: 10.1016/j.fishres.2019.03.011
Hamady L. L., Natanson L. J., Skomal G. B., Thorrold S. R. (2014). Vertebral Bomb Radiocarbon Suggests Extreme Longevity in White Sharks. PloS One 9, e84006. doi: 10.1371/journal.pone.0084006
Hammerschlag N., Meÿer M., Seakamela S. M., Kirkman S., Fallows C., Creel S. (2017). Physiological Stress Responses to Natural Variation in Predation Risk: Evidence From White Sharks and Seals. Ecology 98, 3199–3210. doi: 10.1002/ecy.2049
Hammerschlag N., Williams L., Fallows M., Fallows C. (2019). Disappearance of White Sharks Leads to the Novel Emergence of an Allopatric Apex Predator, the Sevengill Shark. Sci. Rep. 9, 1–6. doi: 10.1038/s41598-018-37576-6
Hays G. C., Bailey H., Bograd S. J., Bowen W. D., Campagna C., Carmichael R. H., et al. (2019). Translating Marine Animal Tracking Data Into Conservation Policy and Management. Trends Ecol. Evol. 34, 459–473. doi: 10.1016/j.tree.2019.01.009
Heerah K., Dias M., Delord K., Oppel S., Barbraud C., Weimerskirch H., et al. (2019). Important Areas and Conservation Sites for a Community of Globally Threatened Marine Predators of the Southern Indian Ocean. Biol. Conserv. 234, 192–201. doi: 10.1016/j.biocon.2019.03.037
Heithaus M. R., Frid A., Wirsing A. J., Worm B. (2008). Predicting Ecological Consequences of Marine Top Predator Declines. Trends Ecol. Evol. 23, 202–210. doi: 10.1016/j.tree.2008.01.003
Hewitt A. M., Kock A. A., Booth A. J., Griffiths C. L. (2018). Trends in Sightings and Population Structure of White Sharks, Carcharodon Carcharias, at Seal Island, False Bay, South Africa, and the Emigration of Subadult Female Sharks Approaching Maturity. Environ. Biol. Fishes. 101, 39–54. doi: 10.1007/s10641-017-0679-x
Hoyos-Padilla E. M., Klimley A. P., Galván-Magaña F., Antoniou A. (2016). Contrasts in the Movements and Habitat Use of Juvenile and Adult White Sharks (Carcharodon Carcharias) at Guadalupe Island, Mexico. Anim. Biotelemetry 4, 14. doi: 10.1186/s40317-016-0106-7
Hussey N. E., Mccan H. M., Cliff G., Dudley S. F. J., Wintner S. P., Fisk A. T. (2012). Size-Based Analysis of Diet and Trophic Position of the White Shark, Carcharodon Carcharias. Global Perspect. Biol. Life History White Shark. 3, 27–49. doi: 10.1201/b11532-5
Huveneers C., Apps K., Becerril-García E. E., Bruce B., Butcher P. A., Carlisle A. B., et al. (2018). Future Research Directions on the “Elusive” White Shark. Front. Marine Sci. 5, 455. doi: 10.3389/fmars.2018.00455
Irion D. T., Noble L. R., Kock A. A., Gennari E., Dicken M. L., Hewitt A. M., et al. (2017). Pessimistic Assessment of White Shark Population Status in South Africa: Comment on Andreotti Et Al.(2016). Marine Ecol. Prog. Ser. 577, 251–255. doi: 10.3354/meps12283
Jarre A., Hutchings L., Crichton M., Wieland K., Lamont T., Blamey L., et al. (2015). Oxygen-Depleted Bottom Waters Along the West Coast of S Outh A Fric–2011. Fisheries Oceanography 24, 56–73. doi: 10.1111/fog.12076
Jewell O. J. D., Johnson R. L., Gennari E., Bester M. N. (2013). Fine Scale Movements and Activity Areas of White Sharks (Carcharodon Carcharias) in Mossel Bay, South Africa. Environ. Biol. Fishes 96, 881–894. doi: 10.1007/s10641-012-0084-4
Jewell O. J. D., Wcisel M. A., Gennari E., Towner A. V., Bester M. N., Johnson R. L., et al. (2011). Effects of Smart Position Only (SPOT) Tag Deployment on White Sharks Carcharodon Carcharias in South Africa. PloS One 6, e27242. doi: 10.1371/journal.pone.0027242
Jewell O. J. D., Wcisel M. A., Towner A. V., Chivell W., van der Merwe L., Bester M. N. (2014). Core Habitat Use of an Apex Predator in a Complex Marine Landscape. Marine Ecol. Prog. Ser. 506, 231–242. doi: 10.3354/meps10814
Jonsen I., Patterson T. (2020). Foiegras: Fit Continuous-Time State-Space and Latent Variable Models for Filtering Argos Satellite (and Other) Telemetry Data and Estimating Movement Behaviour. 2019. R Package Version 0.4.0 (Sydney: Macquarie University).
Jordaan G., Mann B. (2020). ORI-Cooperative Fish Tagging Project: Summary of the Tag and Recapture Data for the Great White Shark (Carcharodon Carcharias) Caught Along the Southern African Coastline: 1984-2020. Data Report Oceanographic Res. Institute Durban 2020_08, 5.
Jorgensen S. J., Reeb C. A., Chapple T. K., Anderson S., Perle C., Van Sommeran S. R., et al. (2010). Philopatry and Migration of Pacific White Sharks. Proc. R. Soc. London B: Biol. Sci. 277, 679–688. doi: 10.1098/rspb.2009.1155
Kanive P. E., Rotella J. J., Jorgensen S. J., Chapple T. K., Hines J. E., Anderson S. D., et al. (2019). Size-Specific Apparent Survival Rate Estimates of White Sharks Using Mark–Recapture Models. Can. J. Fisheries Aquat. Sci. 76, 2027–2034. doi: 10.1139/cjfas-2018-0142
Kerr L. A., Andrews A. H., Cailliet G. M., Brown T. A., Coale K. H. (2006). Investigations of Δ 14c, δ 13C, and δ 15N in Vertebrae of White Shark (Carcharodon Carcharias) From the Eastern North Pacific Ocean. Environ. Biol. Fishes 77, 337–353. doi: 10.1007/s10641-006-9125-1
Kim S. L., Tinker M. T., Estes J. A., Koch P. L. (2012). Ontogenetic and Among-Individual Variation in Foraging Strategies of Northeast Pacific White Sharks Based on Stable Isotope Analysis. PloS One 7, e45068. doi: 10.1371/journal.pone.0045068
Klimley A. P., Le Boeuf B. J., Cantara K. M., Richert J. E., Davis S. F., Van Sommeran S., et al. (2001). The Hunting Strategy of White Sharks (Carcharodon Carcharias) Near a Seal Colony. Marine Biol. 138, 617–636. doi: 10.1007/s002270000489
Kock A. A., O’riain M. J., Mauff K., Meÿer M., Kotze D., Griffiths C. (2013). Residency, Habitat Use and Sexual Segregation of White Sharks, Carcharodon Carcharias in False Bay, South Africa. PloS One 8, e55048. doi: 10.1371/journal.pone.0055048
Kock A. A., Photopoulou T., Durbach I., Mauff K., Meÿer M., Kotze D., et al. (2018). Summer at the Beach: Spatio-Temporal Patterns of White Shark Occurrence Along the Inshore Areas of False Bay, South Africa. Movement Ecol. 6, 7. doi: 10.1186/s40462-018-0125-5
Kock A. A., Titley S., Petersen W., Sikweyiya M., Tsotsobe S., Colenbrander D., et al. (2012). Shark Spotters: A Pioneering Shark Safety Program in Cape Town, South Africa. Global Perspect. Biol. Life History White Shark 29, 447–466.
Lee K., Butcher P., Harcourt R., Patterson T., Peddemors V., Roughan M., et al. (2021). Oceanographic Conditions Associated With White Shark (Carcharodon Carcharias) Habitat Use Along Eastern Australia. Marine Ecol. Prog. Ser. 659, 143–159. doi: 10.3354/meps13572
Lewison R. L., Crowder L. B., Read A. J., Freeman S. A. (2004). Understanding Impacts of Fisheries Bycatch on Marine Megafauna. Trends Ecol. Evol. 19, 598–604. doi: 10.1016/j.tree.2004.09.004
Martin R. A., Hammerschlag N., Collier R. S., Fallows C. (2005). Predatory Behaviour of White Sharks (Carcharodon Carcharias) at Seal Island, South Africa. JMBA-Journal Marine Biol. Assoc. United Kingdom 85, 1121–1136. doi: 10.1017/S002531540501218X
Malcolm H., Bruce B. D., Stevens J. D. (2001). A Review of the Biology and Status of White Sharks in Australian Waters (CSIRO Div. of Marine Research). doi: 10.4225/08/585eb916877d8
Maxwell S. M., Breed G. A., Nickel B. A., Makanga-Bahouna J., Pemo-Makaya E., Parnell R. J., et al. (2011). Using Satellite Tracking to Optimize Protection of Long-Lived Marine Species: Olive Ridley Sea Turtle Conservation in Central Africa. PloS One 6, e19905. doi: 10.1371/journal.pone.0019905
McAuley R., Bruce B., Keay I., Mountford S., Pinnell T., Whoriskey F. (2017). Broad-Scale Coastal Movements of White Sharks Off Western Australia Described by Passive Acoustic Telemetry Data. Marine Freshwater Res. 68, 1518–1531. doi: 10.1071/MF16222
McAuley R., Simpfendorfer C. A. (2003). “Catch Composition of the Western Australian Temperate Demersal Gillnet and Demersal Longline Fisherie 1994 to 1999”, in Department Fisheries Government Western Australia (North Beach: Department of Fisheries).
McCauley D. J., Pinsky M. L., Palumbi S. R., Estes J. A., Joyce F. H., Warner R. R. (2015). Marine Defaunation: Animal Loss in the Global Ocean. Science 347, 355. doi: 10.1126/science.1255641
McClintock B. T., Michelot T. (2018). momentuHMM: R Package for Generalized Hidden Markov Models of Animal Movement. Methods Ecol. Evol. 9, 1518–1530. doi: 10.1111/2041-210X.12995
McPhee D. P., Blount C., Smith M. P. L., Peddemors V. M. (2021). A Comparison of Alternative Systems to Catch and Kill for Mitigating Unprovoked Shark Bite on Bathers or Surfers at Ocean Beaches. Ocean Coastal Manage. 201, 105492. doi: 10.1016/j.ocecoaman.2020.105492
Morgan A., Cooper P. W., Curtis T., Burgess G. H. (2009). Overview of the US East Coast Bottom Longline Shark Fisher 1994–2003. Marine Fisheries Rev. 71, 23–38.
Mucientes G. R., Queiroz N., Sousa L. L., Tarroso P., Sims D. W. (2009). Sexual Segregation of Pelagic Sharks and the Potential Threat From Fisheries. Biol. Lett. 5, 156–159. doi: 10.1098/rsbl.2008.0761
O’Leary S. J., Feldheim K. A., Fields A. T., Natanson L. J., Wintner S., Hussey N., et al. (2015). Genetic Diversity of White Sharks, Carcharodon Carcharias, in the Northwest Atlantic and Southern Africa. J. Heredity 106, 258–265. doi: 10.1093/jhered/esv001
Pardini A. T., Jones C. S., Noble L. R., Kreiser B., Malcolm H., Bruce B. D., et al. (2001). Sex-Biased Dispersal of Great White Sharks. Nature 412 (6843), 139–140. doi: 10.1038/35084125
Pardini A. T., Jones C. S., Scholl M. C., Noble L. R. (2000). Isolation and Characterization of Dinucleotide Microsatellite Loci in the Great White Shark, Carcharodon Carcharias. Mol. Ecol. 9, 1176–1178. doi: 10.1046/j.1365-294x.2000.00954-4.x
Parker D., Coetzee J. D., Winker H., van der Lingen C. D. (2020). Accounting for Linefish Dependency in the Management of South African Small Pelagic Fisheries. Afr. J. Mar. Sci. 42 (3), 283–294. doi: 10.2989/1814232X.2020.1788160
Patterson T. A., Mcconnell B. J., Fedak M. A., Bravington M. V., Hindell M. A. (2010). Using GPS Data to Evaluate the Accuracy of State–Space Methods for Correction of Argos Satellite Telemetry Error. Ecology 91, 273–285. doi: 10.1890/08-1480.1
Peñaherrera-Palma C., Hobday A., Hearn A., Espinoza E., Shillinger G., Ketchum J., et al. (2020). Assessing the Efficacy of a Marine Reserve to Protect Sharks With Differential Habitat Use. Res. Sq. doi: 10.21203/rs.3.rs-124555/v1
Petersen S., Honig M., Ryan P., Underhill L., Compagno L. J. (2009). Pelagic Shark Bycatch in the Tuna-and Swordfish-Directed Longline Fishery Off Southern Africa. Afr. J. Marine Sci. 31, 215–225. doi: 10.2989/AJMS.2009.31.2.9.881
Pichegru L., Ryan P., Le Bohec C., van der Lingen C., Navarro R., Petersen S., et al. (2009). Overlap Between Vulnerable Top Predators and Fisheries in the Benguela Upwelling System: Implications for Marine Protected Areas. Marine Ecol. Prog. Ser. 391, 199–208. doi: 10.3354/meps08283
Pimiento C., Leprieur F., Silvestro D., Lefcheck J., Albouy C., Rasher D., et al. (2020). Functional Diversity of Marine Megafauna in the Anthropocene. Sci. Adv. 6, eaay7650. doi: 10.1126/sciadv.aay7650
Piroddi C., Bearzi G., Gonzalvo J., Christensen V. (2011). From Common to Rare: The Case of the Mediterranean Common Dolphin. Biol. Conserv. 144, 2490–2498. doi: 10.1016/j.biocon.2011.07.003
Pohle J., Langrock R., Van Beest F. M., Schmidt N. M. (2017). Selecting the Number of States in Hidden Markov Models: Pragmatic Solutions Illustrated Using Animal Movement. J. Agricultural Biol. Environ. Stat 22, 270–293. doi: 10.1007/s13253-017-0283-8
Queiroz N., Humphries N. E., Couto A., Vedor M., Sequeira A., Mucientes G., et al. (2019). Global Spatial Risk Assessment of Sharks Under the Footprint of Fisheries. Nature 572, 461–466. doi: 10.1038/s41586-019-1444-4
Reed J. R., Lombard A. T., Sink K. J. (2020). A Diversity of Spatial Management Instruments can Support Integration of Fisheries Management and Marine Spatial Planning. Mar. Policy 119, 104089. doi: 10.1016/j.marpol.2020.104089
Rigby C., Barreto R., Carlson J., Fernando D., Fordham S., Francis M., et al. (2019). “White Shark (Carcharodon carcharias),” in IUCN Red List of Threatened Species E.T3855A2878674. doi: 10.2305/IUCN.UK.2019-3.RLTS.T3855A2878674.en
Rogers A., Aburto-Oropeza O., Appeltans W., Assis J., Ballance L. T., Cury P., et al. (2020). “Critical Habitats and Biodiversity: Inventory, Thresholds and Governance”, in Nova Southeastern University Marine Environ. Sciecnes Faculty Rep (Fort Lauderdale: Nova Southeastern University (NSU)).
Ryklief R., Pistorius P. A., Johnson R. (2014). Spatial and Seasonal Patterns in Sighting Rate and Life-History Composition of the White Shark Carcharodon Carcharias at Mossel Bay, South Africa. Afr. J. Marine Sci. 36, 449–453. doi: 10.2989/1814232X.2014.967296
Scott R., Griffiths C., Robinson T. (2012). Patterns of Endemicity and Range Restriction Among Southern African Coastal Marine Invertebrates. Afr. J. Marine Sci. 34, 341–347. doi: 10.2989/1814232X.2012.725284
Shannon L. J., Jarre A. C., Petersen S. L. (2010). Developing a Science Base for Implementation of the Ecosystem Approach to Fisheries in South Africa. Prog. Oceanogr. 87 (1–4), 289–303. doi: 10.1016/j.pocean.2010.08.005.
Sink K., van der Bank M., Majiedt P., Harris L., Atkinson L., Kirkman S., et al. (2019). South African National Biodiversity Assessment 2018 Technical Report Volume 4: Marine Realm (Pretoria: South African National Biodiversity Institute (SANBI)).
Skomal G. B., Braun C. D., Chisholm J. H., Thorrold S. R. (2017). Movements of the White Shark Carcharodon Carcharias in the North Atlantic Ocean. Marine Ecol. Prog. Ser. 580, 1–16. doi: 10.3354/meps12306
Stevens J., Bonfil R., Dulvy N. K., Walker P. (2000). The Effects of Fishing on Sharks, Rays, and Chimaeras (Chondrichthyans), and the Implications for Marine Ecosystems. ICES J. Marine Sci. 57, 476–494. doi: 10.1006/jmsc.2000.0724
Tamburin E., Elorriaga-Verplancken F. R., Estupiñan-Montaño C., Madigan D. J., Sánchez-González A., Padilla M. H., et al. (2020). New Insights Into the Trophic Ecology of Young White Sharks (Carcharodon Carcharias) in Waters Off the Baja California Peninsula, Mexico. Marine Biol. 167, 55. doi: 10.1007/s00227-020-3660-8
Taylor S., Braccini J. M., Mcauley R., Fletcher R. (2016). “Review of Potential Fisheries and Marine Management Impacts on the South-Western Australian White Shark Population”, in Department Fisheries Government Western Australia (North Beach: Department of Fisheries).
Taylor F., Small C. (2009). “Spatial and Temporal Overlap Between Seabird Distribution in the Atlantic Ocean and ICCAT Longline Fishing Effort,” in Paper Submitted to the 2008 Inter-Sessional Meeting of the ICCAT Sub-Committee on Ecosystems, vol. 64. (Madrid: Collect. Vol. Sci. Pap. ICCAT), 2383–2397.
Temple A. J., Wambiji N., Poonian C. N., Jiddawi N., Stead S. M., Kiszka J. J., et al. (2019). Marine Megafauna Catch in Southwestern Indian Ocean Small-Scale Fisheries From Landings Data. Biol. Conserv. 230, 113–121. doi: 10.1016/j.biocon.2018.12.024
Towner A. V., Leos-Barajas V., Langrock R., Schick R. S., Smale M. J., Kaschke T., et al. (2016). Sex-Specific and Individual Preferences for Hunting Strategies in White Sharks. Funct. Ecol. 30, 1397–1407. doi: 10.1111/1365-2435.12613
Towner A. V., Wcisel M. A., Reisinger R. R., Edwards D., Jewell O. J. D. (2013). Gauging the Threat: The First Population Estimate for White Sharks in South Africa Using Photo Identification and Automated Software. PloS One 8, e66035. doi: 10.1371/annotation/bb25e7cb-12f7-42e4-9d26-07b9bda87ecc
Walli A., Teo S. L., Boustany A., Farwell C. J., Williams T., Dewar H., et al. (2009). Seasonal Movements, Aggregations and Diving Behavior of Atlantic Bluefin Tuna (Thunnus Thynnus) Revealed With Archival Tags. PloS One 4, e6151. doi: 10.1371/journal.pone.0006151
Watanabe Y. Y., Payne N. L., Semmens J. M., Fox A., Huveneers C. (2019). Swimming Strategies and Energetics of Endothermic White Sharks During Foraging. J. Exp. Biol. 222, jeb185603. doi: 10.1242/jeb.185603
Williams R., Lusseau D., Hammond P. S. (2009). The Role of Social Aggregations and Protected Areas in Killer Whale Conservation: The Mixed Blessing of Critical Habitat. Biol. Conserv. 142, 709–719. doi: 10.1016/j.biocon.2008.12.004
Winkler A. C., Butler E. C., Attwood C. G., Mann B. Q., Potts W. M. (2022). The Emergence of Marine Recreational Drone Fishing: Regional Trends and Emerging Concerns. Ambio. 51, 638–651. doi: 10.1007/s13280-021-01578-y
Zucchini W., Macdonald I. L., Langrock R. (2016). Hidden Markov Models for Time Series: An Introduction Using R, Second Edition (Boca Raton: Chapman and Hall/CRC).
Keywords: conservation, satellite tagging, movement states, marine protected area (MPA), Carcharodon carcharias
Citation: Kock AA, Lombard AT, Daly R, Goodall V, Meÿer M, Johnson R, Fischer C, Koen P, Irion D, Gennari E, Towner A, Jewell OJD, da Silva C, Dicken ML, Smale MJ and Photopoulou T (2022) Sex and Size Influence the Spatiotemporal Distribution of White Sharks, With Implications for Interactions With Fisheries and Spatial Management in the Southwest Indian Ocean. Front. Mar. Sci. 9:811985. doi: 10.3389/fmars.2022.811985
Received: 09 November 2021; Accepted: 10 March 2022;
Published: 07 April 2022.
Edited by:
Yan Ropert-Coudert, UMR7372 Centre d’études biologiques de Chizé (CEBC), FranceReviewed by:
Nuno Queiroz, Centro de Investigacao em Biodiversidade e Recursos Geneticos (CIBIO-InBIO), PortugalRussell Bradford, Commonwealth Scientific and Industrial Research Organisation (CSIRO), Australia
Copyright © 2022 Kock, Lombard, Daly, Goodall, Meÿer, Johnson, Fischer, Koen, Irion, Gennari, Towner, Jewell, da Silva, Dicken, Smale and Photopoulou. This is an open-access article distributed under the terms of the Creative Commons Attribution License (CC BY). The use, distribution or reproduction in other forums is permitted, provided the original author(s) and the copyright owner(s) are credited and that the original publication in this journal is cited, in accordance with accepted academic practice. No use, distribution or reproduction is permitted which does not comply with these terms.
*Correspondence: Alison A. Kock, YWxpc29uLmtvY2tAc2FucGFya3Mub3Jn