- 1State Key Laboratory of Marine Geology, Tongji University, Shanghai, China
- 2College of Marine Sciences, Shanghai Ocean University, Shanghai, China
- 3Key Laboratory of Sustainable Exploitation of Oceanic Fisheries Resources, Ministry of Education, Shanghai Ocean University, Shanghai, China
- 4National Engineering Research Center for Oceanic Fisheries, Shanghai Ocean University, Shanghai, China
- 5Key Laboratory of Ocean Fisheries Exploitation, Ministry of Agriculture and Rural Affairs, Shanghai, China
- 6Scientific Observing and Experimental Station of Oceanic Fishery Resources, Ministry of Agriculture and Rural Affairs, Shanghai, China
- 7Environmental Defense Fund, Boston, MA, United States
Jumbo squid (Dosidicus gigas) plays an important role in food webs throughout the eastern Pacific; however, its trophic ecology is complex, because it is a highly migratory and opportunistic predator. In this study, δ13C and δ15N values were measured along the lateral wall of lower beak to investigate the ontogenetic and spatiotemporal feeding strategy shifts of jumbo squid, respectively. Both year and area had significant influences on δ13C (p < 0.01), while sex and stage had no significant influence on δ13C (p > 0.05). All explanatory variables (sex, stage, year, and area) had significant influences on δ15N (p < 0.05). The differences of niche among life stages indicated that the habitat and feeding habit were likely changed with the growth of individuals. Compared with normal years, the habitat of squids might change during the El Niño year. However, during the strong El Niño year, habitat location changed and niche size decreased compared to normal years. The trophic niche of D. gigas differed between different areas. Stable isotopes in early life stages were most effective for distinguishing different geographic populations. Females and males were likely to inhabit similar geographic areas throughout ontogeny. Unexpectedly, we found evidence to suggest females may have larger habitats and wider trophic niche than males. This study revealed the ontogenetic and spatiotemporal plasticity of habitat and feeding strategy of D. gigas.
Introduction
Jumbo squid is widely distributed from California to Chile in the eastern Pacific (Nigmatullin et al., 2001; Taipe et al., 2001; Field et al., 2007). It is a main prey item of various fishes, marine birds, and mammals (Nigmatullin et al., 2001; Ruiz-Cooley et al., 2006; Espinoza et al., 2017), and is also an active predator of numerous prey species such as copepods, shrimps, crabs, and fishes (Rodhouse and Nigmatullin, 1996; Markaida, 2006; Field et al., 2007). It grows fast and is the largest and one of the most abundant species of the family Ommastrephidae (Nigmatullin et al., 2001; Arkhipkin et al., 2014). It is an important commercial target species off the coasts of western South and Central America and the Gulf of California (Gong et al., 2018a). It is an opportunistic voracious predator with a high capability to adapt to environmental variability (Keyl et al., 2008; Ruiz-Cooley et al., 2013). Furthermore, distribution and habitat for jumbo squid have been shown to change with changes in marine environmental factors (Yu et al., 2016; Trasviña-Carrillo et al., 2018; Yu and Chen, 2018; Hu et al., 2019). Range expansion of D. gigas occurred during a strong 1997–1998 El Niño event, which was suggested to be correlated with wider distribution for spawning eggs and higher success in egg development during anomalously high-temperature events, coupled with migration of smaller squid (Ruiz-Cooley et al., 2013). Yu and Chen (2018) found that ocean warming might shrink the habitat of D. gigas and correspond with a southeastward range-shift in the Southeast Pacific Ocean. Coincident with the variability of the prey items in new habitats, the feeding strategies and diets of D. gigas may shift (Ruiz-Cooley et al., 2006; Ruiz-Cooley et al., 2010; Lorrain et al., 2011). Ruiz-Cooley et al. (2010) analyzed stable isotopic signatures along the proostracum of the gladius, and revealed the ontogenetic and geographic variability of foraging for D. gigas. In this study, we attempted to investigate the shift of feeding strategies of D. gigas by analyzing the ontogenetic and spatiotemporal changes in isotopic niche using the beak lateral wall.
Stable isotope analysis (mainly of δ13C and δ15N) is a useful tool for investigating the trophic ecology of marine animals, including fishes (Trujillo-Olvera et al., 2018; Varela et al., 2018), marine birds (Castillo et al., 2019), cephalopods (Cherel and Hobson, 2005; Ruiz-Cooley et al., 2006; Ruiz-Cooley et al., 2010; Ruiz-Cooley et al., 2013; Merten et al., 2017; Gong et al., 2018b; Hu et al., 2019; Gong et al., 2020), and mammals (Mendes et al., 2007; Ruiz-Cooley et al., 2012). δ13C is used as an indicator of the primary sources of organic matter in the food chain (Post, 2002; McCutchan et al., 2003; Cherel and Hobson, 2007). High δ13C values correspond to zones of high productivity in low latitude and coastal waters (Rau et al., 1989; Miller et al., 2008). It can be used to trace the animals’ habitats and movements, because δ13C changes little (0–1‰) with trophic level (Post, 2002). δ15N tends to enrich stepwise from prey to predators (2–5‰), providing a method to estimate a species’ trophic level (Post, 2002; Bode et al., 2004). However, the δ15N baseline value may be influenced by the oceanographic and biogeochemical processes, which can propagate differently into individual tissues (Ruiz-Cooley and Gerrodette, 2012). The direction and variability of trophic niche among different life stages can reveal shifts of ecological niche. For some species, tissues that grow continuously can record the information of trophic ecology over an individual’s whole life span (Mendes et al., 2007; Hunsicker et al., 2010; Kernaléguen et al., 2016).
Cephalopod beaks are hard structures composed of chitin–protein complexes (Miserez et al., 2007; Miserez et al., 2010). This chemical composition makes them resistant to digestion. Beaks encountered in predator stomachs can be used for identification down to species level (Lu and Ickeringill, 2002; Hu et al., 2018). Though the gladius was demonstrated to be a good tissue to investigate the trophic ecology of squid (Ruiz-Cooley et al., 2010; Lorrain et al., 2011, Gong et al., 2018b), the beak has greater potential because it could be collected from both squids and predator stomachs (Ruiz-Cooley et al., 2006; Hu et al., 2019). Moreover, the beak grows continuously throughout the cephalopod’s lifetime by an incremental accretion of non-reactive biological material (Guerra et al., 2010; Canali et al., 2011; Queirós et al., 2018). Due to these characteristics, beak can be a useful material to investigate cephalopod ontogenetic niche shifts. However, in most previous studies, only whole beak isotopic values have been analyzed, making it difficult to deduce an individual’s trophic shift and/or movement (Ruiz-Cooley et al., 2006; Cherel et al., 2009). The stable isotope signatures along the upper beak hood were used to reveal the ontogenetic shift in diet and migration (Guerra et al., 2010). However, there is no evidence that the increments deposited along the beak hood. Daily deposition of increments on the lateral wall of beaks from the tip of rostrum to the edge of lateral wall has been validated (Perales-Raya et al., 2010). The isotopic values along the lateral wall of beaks could therefore reveal the information spanning the whole lifetime of squid. Queirós et al. (2018) analyzed the isotopic values of different sections on the lateral wall of beaks of Kondakovia longimana, and found that habitat of squid spanned a wide latitudinal range, and trophic level increases throughout its lifetime.
Several literatures suggested that equatorial undercurrent became more intense and pushed warm equatorial surface waters closer to the coast during El Niño events in the southeastern Pacific, and narrowing the suitable habitat of D. gigas (Keyl et al., 2008; Yu and Chen, 2018). Therefore, we hypothesized that the trophic niche of the squid would become smaller and the competition between females and males would become greater during El Niño events. In this study, the samples were collected from both normal years (2013 and 2014) and El Niño years (2015 and 2016). We attempted to analyze the variability of trophic niche between normal years and El Niño years by both life stage and sex.
In this study, we quantified stable isotope values along the lower beak lateral wall of D. gigas, collected from the southeastern Pacific, to (1) explore the feasibility to investigate the trophic ecology of D. gigas using lower beaks lateral wall, and to (2) evaluate ontogenetic and spatiotemporal variability of habitat and feeding strategies for D. gigas. This study improves the knowledge of ecological niche shifts of D. gigas in four perspectives (sex, ontogeny, annual, and geography).
Materials and Methods
Sampling
All jumbo squid were randomly sampled from the catch of Chinese commercial jigging vessels in the Southeastern Pacific. A total of 137 (95 females and 42 males) samples were collected off Peruvian EEZ (exclusive economic zone) from June to September from 2013 to 2016 (Table 1, Figure 1). Forty females were sampled off Chilean EEZ from October to November 2015. In this study, 2013 and 2014 were normal years, followed by El Niño events during 2015 and 2016. The El Niño event in 2016 was much stronger than that in 2015 (Jacox et al., 2016).
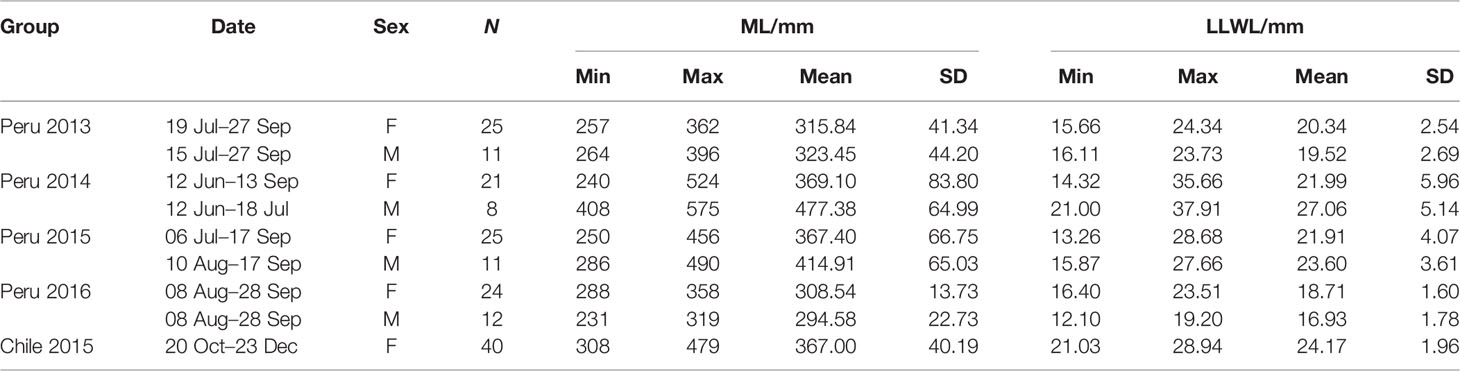
Table 1 Sample information of D. gigas in southeastern Pacific (ML corresponds to the mantle length; LLWL corresponds to the lower beak lateral wall length; “Peru 2013” represents samples caught off Peruvian EEZ in 2013, “Chile 2015” represents samples caught off Chilean EEZ in 2015, and so on).
All specimens were immediately frozen on board. After defrosting the samples in the laboratory, the mantle length and body weight were measured. Meanwhile, sex was identified. The beaks of squids were extracted and stored in 70% alcohol, which does not significantly affect the isotopic composition of beaks (Ruiz-Cooley et al., 2011). Morphometric measurements were conducted for beaks using digital calipers.
Beak Processing and Stable Isotope Analysis
Five sections (the tip of the rostrum, 5.0 mm, 10.0 mm, and 15.0 mm along the lateral wall, and the edge of the lateral wall) were cut along the lower lateral wall from the tip to edge following the “V” shape of the growth lines, which represent five different stages of the squid (Figure 2). Each section’s width is 1.0 mm. All sections were cleaned in ultrasonic cleaner for 10 min and rinsed with distilled water. They were dried and homogenized into fine power for stable isotope analysis.
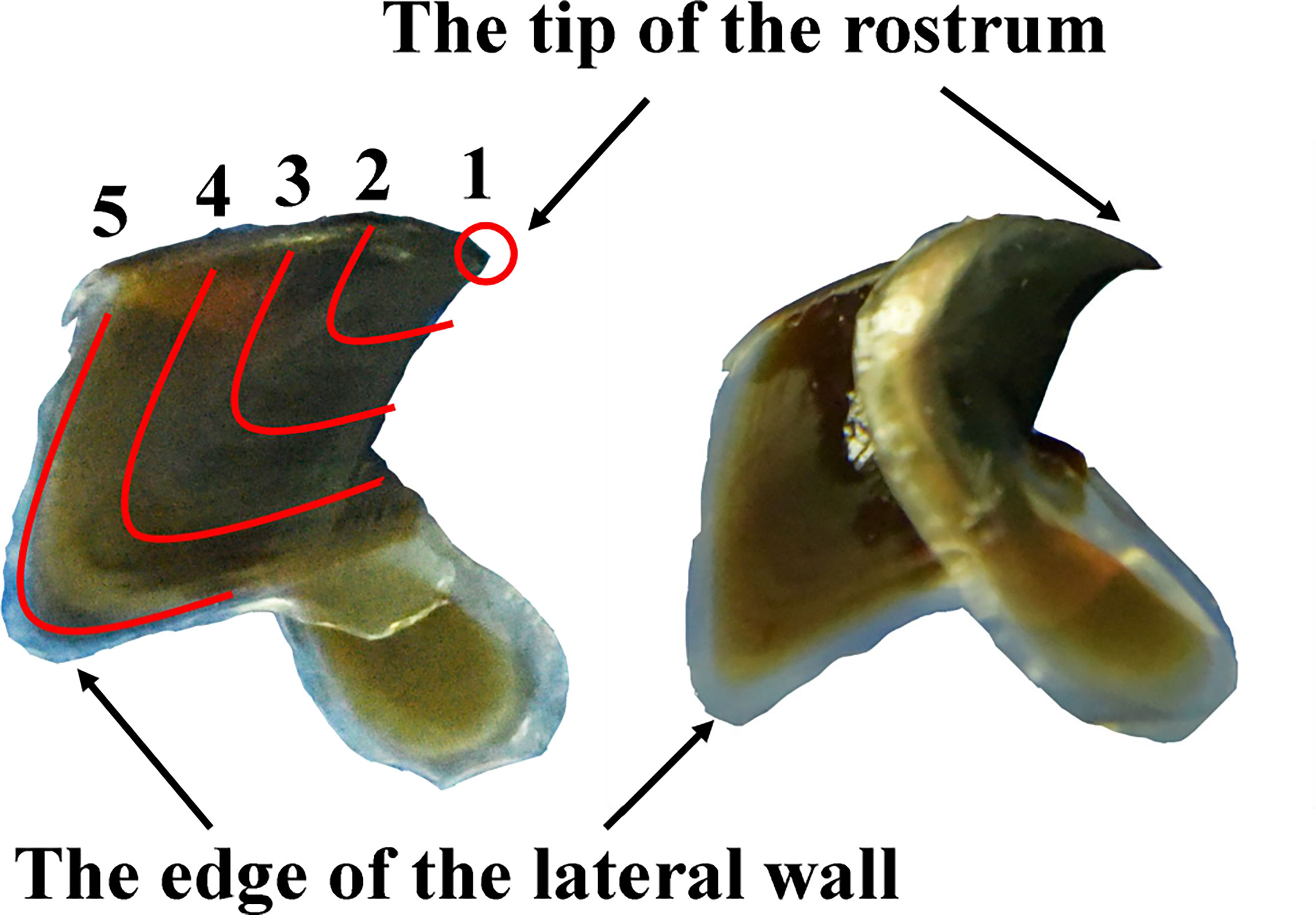
Figure 2 Lateral view of the lateral wall for lower beak (1–5 represent the tip of the rostrum, 5.0 mm, 10.0 mm, and 15.0 mm along the lateral wall, and the edge of the lateral wall, respectively).
A total of 876 subsamples were prepared for SIA. One-milligram powder of each sample was pooled in tin capsule. The SIA was conducted using an ISOPRIME 100 isotope ratio mass spectrometer (Isoprime Corporation, Cheadle, UK) and a vario ISOTOPE cube elemental analyzer (Elementar Analysensyteme GmbH, Hanau, Germany) at Shanghai Ocean University. To calibrate the system and compensate for drift, the international reference materials [USGS 24 (−16.049‰ v PDB), USGS 26 (53.7‰ v N2) and a laboratory reference (protein, −26.98‰ v PDB and 5.96‰ v N2)] were used for calibration every ten samples. The measurement errors of δ13C and δ15N were less than 0.1‰ for both δ13C and δ15N.
where X is 13C or 15N, and Rsample and Rstandard are the ratios of 13C/12C or 15N/14N of the sample and the standard, respectively.
Generalized Linear Model
Generalized linear model (GLM) is a mathematical extension of linear models that allow for non-linearity and non-constant variance structures. These traits enable GLMs to be commonly used in ecological studies (Guisan et al., 2002; Kim et al., 2012; Kato et al., 2016). To investigate the effects of sex, ontogeny, year, and area on the stable isotopes in beaks, GLMs were built as follows:
where SI is the measured isotopic ratio (δ13C or δ15N) in the beak; Stage is the life stage of squid; Year is the year of sampling; Area is the sampling area of squid; Sex is the sex of squid; and ε is the error of the model. Statistical analyses were carried out using the glm2 package in R 3.5.1.
Variation in Isotopic Values
The gender variability in isotopic values was analyzed using a two-tailed t-test for a given area, year, and life stage. The ontogenetic variability in isotopic values was determined using ANOVA for a given area, year, and sex, and the pairwise comparisons among five life stages were evaluated using Tukey HSD (honest significant difference) test. For interannual variability, the same procedure was followed, except a Tukey HSD test considered pairwise comparisons between years.
We analyzed the geographic variability between Peru and Chile in isotopic values using a two-tailed t-test for a given sex and life stage. Statistical analyses were performed using SPSS 19.0.
Comparison of Isotopic Niches
The standard ellipse corrected area (SEAc) was used to determine the isotopic niche width and overlap between genders, life stages, years, and areas. This approach is unbiased with sample size and allows robust comparison among data sets (Jackson et al., 2011). Variation in SEAc size was statistically evaluated by comparing the posterior distributions of standard ellipse area using the SIBER package in R. Statistical analyses were performed using R 3.5.1.
Results
Variability in Isotopic Values
The results of GLMs suggested that both year and area had significant influences on δ13C (p < 0.001), while sex and stage had no significant influence on δ13C (p > 0.05) (Table 2). All explanatory variables (sex, stage, year, and area) had significant influences on δ15N (p < 0.05) (Table 2).
For the sexual variability off Peru, δ13C significantly differed between genders for stage 3 and 5 in 2013 (t-test, p < 0.05), while no significant difference was found for other groups (t-test, p > 0.05) (Table 3 and Figure 3). δ15N significantly differed between genders for stage 2, 3, and 4 in 2014 (t-test, p < 0.05), and no significant difference was found for other groups (t-test, p > 0.05) (Table 3 and Figure 3).
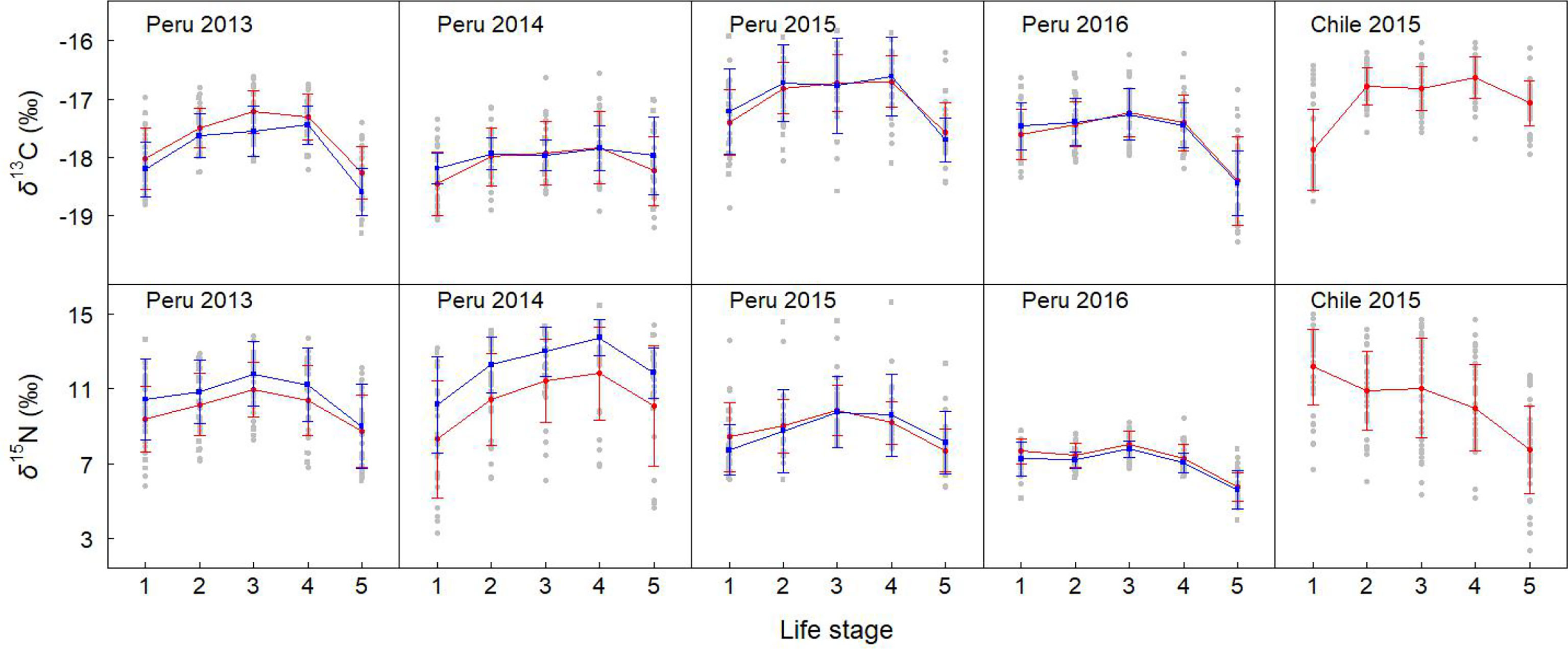
Figure 3 Mean ± standard deviation isotopic values in lower beaks of females (red solid circle) and males (blue solid square) in each life stage. “Peru 2013” represents the samples collected off Peru during 2013, and so on.
For the ontogenetic variability, We found significant differences in δ13C among five stages for Peru 2016 and Chile 2015 (ANOVA, p < 0.001). No significant difference was found for Peru 2013, 2014, and 2015 (ANOVA, p > 0.05) (Table 3 and Figure 3). δ15N differed among five stages for Peru 2014 and 2016 and Chile 2015 (ANOVA, p < 0.001), and no significant difference was found for Peru 2013 and 2015 (ANOVA, p > 0.05) (Table 3 and Figure 3).
For the annual variability off Peru, δ13C significantly differed among 4 years for stage 1 and 2 of females and for stage 1, 2, and 3 of males (ANOVA, p < 0.05) (Table 3 and Figure 3). δ15N significantly differed among 4 years for all stages of both females and males (ANOVA, p < 0.01) (Table 3 and Figure 3).
For the geographic variability, δ13C had significant differences between Peru and Chile in 2015 for stage 1 and 5 of females (t-test, p < 0.01) (Table 3 and Figure 3). δ15N had significant differences between Peru and Chile in 2015 for stage 1, 2, and 3 of females (t-test, p < 0.05) (Table 3 and Figure 3).
Ontogenetic Variation in Isotopic Niche
Off Peru, the niche overlaps between stage 1 and stages 3 and 4 in 2013, 2014, and 2015 ranged from 0 to 19% for females and from 0 to 23% for males, while overlaps for the same life stages in 2016 ranged from 33% to 59% for females and from 55% to 92% for males (Tables 4, 5 and Figure 4). The niche overlaps between stages 1 and 5 for Peru 2013, 2014, and 2015 ranged from 62% to 66% for females and from 29% to 57% for males, while there was no overlap for those in 2016 (Tables 4, 5 and Figure 4). Expect for 2014, there was no overlap between stage 5 and stages 2, 3, and 4 for squids off Peru (Tables 4, 5 and Figure 4).
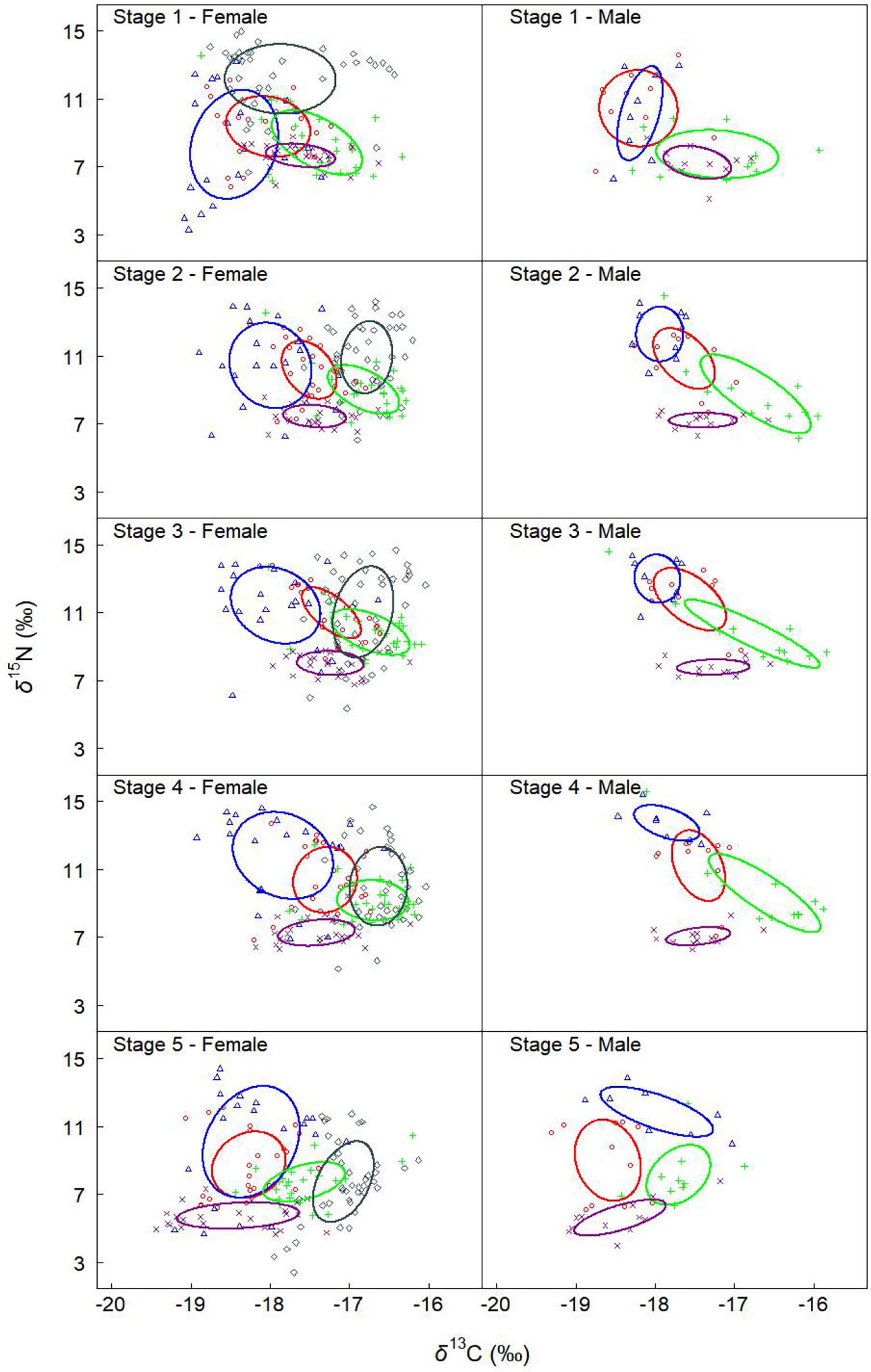
Figure 4 Ontogenetic variation in the isotopic niche of females and males for different groups. Solid lines enclose the standard ellipse areas (SEAc), showing the isotopic niche of D. gigas from different areas and years (red, blue, green, dark slate gray, and dark magenta represent Peru 2013, Peru 2014, Peru 2015, Chile 2015, and Peru 2016, respectively). “Peru 2013” represents the samples collected off Peru during 2013, and so on.
Off Chile, no overlap was found between stage 1 and stages 2, 3, 4, and 5 for females in 2015, while overlaps ranged from 17% to 99% between other stages (Tables 4, 5 and Figure 4).
Annual and Geographic Variation in Isotopic Niche
For the annual variation of females off Peru, there were relatively large overlaps between 2013 and 2014 throughout the life stages, and the maximum overlap (98%) was observed during stage 5 (Table 6, Figure 4). The overlap was 62% between 2015 and 2016 during stage 1, then no overlap was found from stage 2 to 5. The overlap between 2015 and 2013 and 2014 ranged from 6% to 37% and 0 to 5% throughout the life stage. There was no overlap between 2016 and 2013, 2014, and 2015 after stage 1. Except for stages 3 and 5, SEAcs in 2016 were significantly smaller than those in 2013 (p < 0.01). SEAcs in 2016 were significantly smaller than those in 2014 throughout the life stage (p < 0.001).
For the annual variation of males off Peru, largest overlaps occurred during stage 1 between 2013 and 2014 (86%), and 2015 and 2016 (95%) (Table 7, Figure 4). The isotopic niches in 2015 and 2016 were separated after stage 1. There were overlaps between 2013 and 2015 during stages 2, 3, and 4, while no overlap was observed between 2016 and 2013 and 2014 throughout the life stage. All SEAcs in 2016 were smaller than those in 2014 and significantly less than those in 2013 throughout the life stage (p < 0.05).
For the geographic variation of females in 2015, only 1% of niche overlapped between Peru and Chile during stage 1, while overlaps ranged from 29% to 81% after stage 1 (Table 6, Figure 4). Expect for stage 2, SEAcs off Chile were significantly greater than those off Peru (p < 0.05).
Sexual Variation in Isotopic Niche
Off Peru, niche overlaps between females and males ranged from 55% to 76%, 68% to 79%, 67% to 100%, and 78% to 99% in 2013, 2014, 2015, and 2016, respectively (Table 8 and Figures 5, 6). In 2013 and 2016, no significant difference was found for SEAc between females and males. In 2014, SEAcs of females were significantly greater than those of males (p < 0.05). In 2015, SEAcs of females were significantly less than those of males during stages 3 and 4 (p < 0.01), while there was no significant difference between females and males during stages 1, 2, and 5.
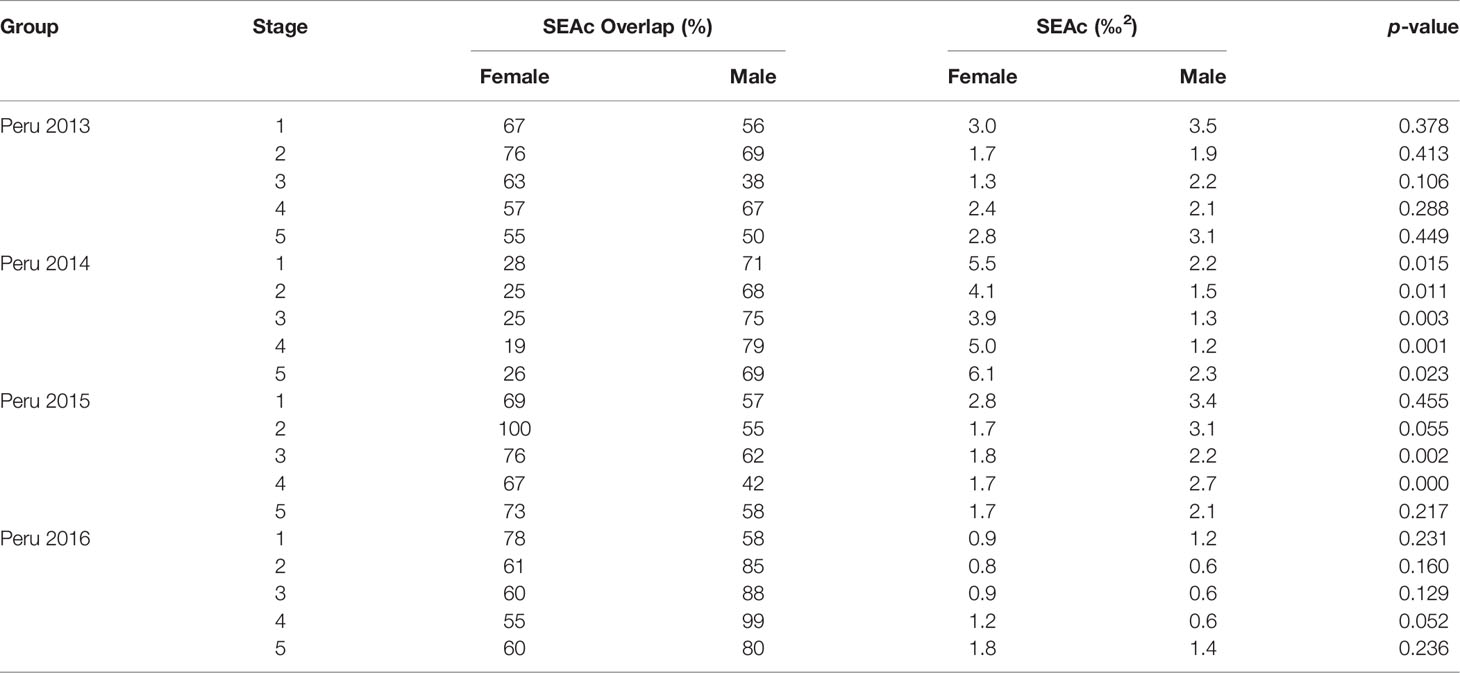
Table 8 Sexual variation in isotopic niche off Peru in different years (“Peru 2013” represents the samples collected off Peru during 2013, and so on).
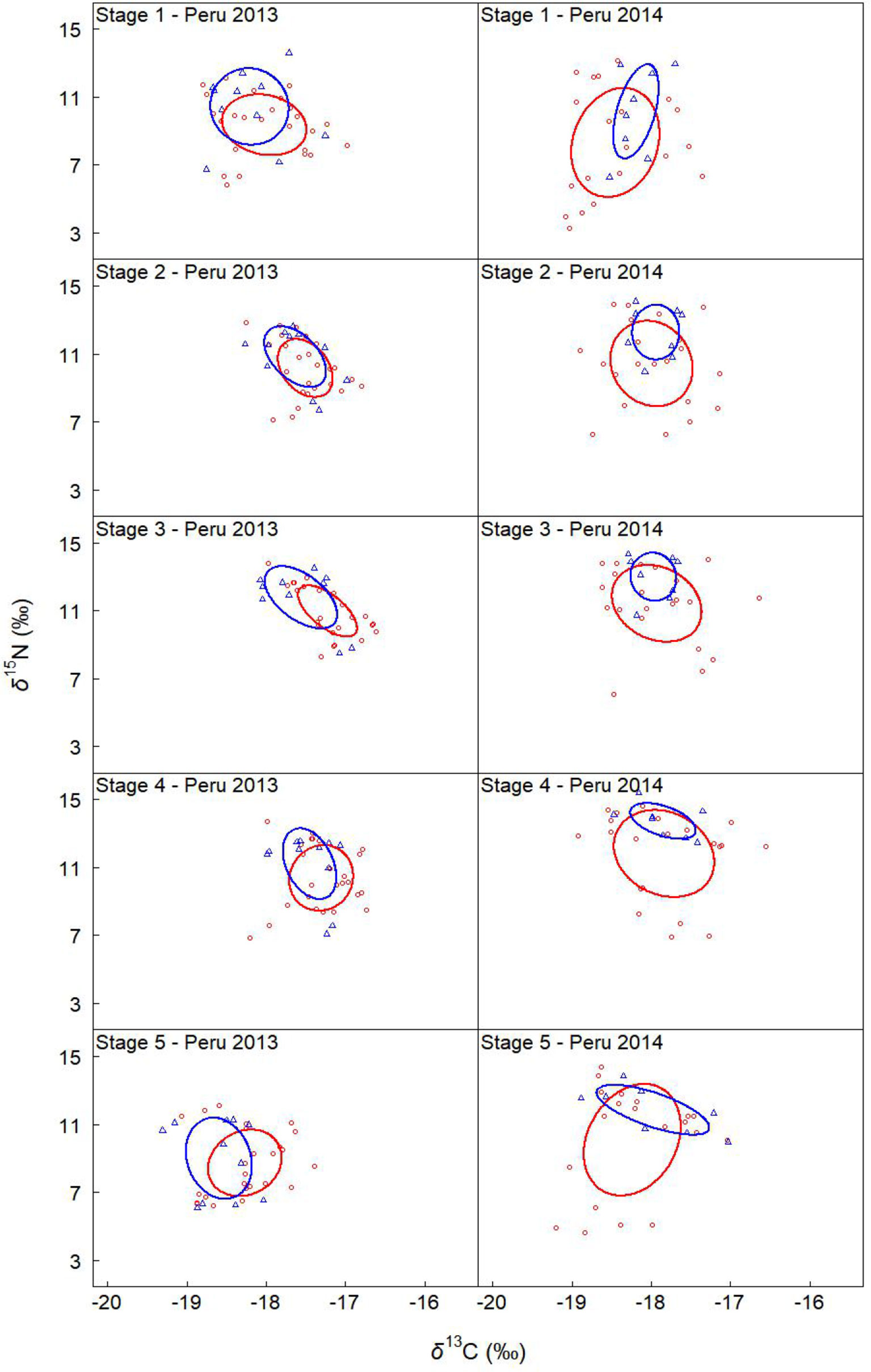
Figure 5 Sexual variation in the isotopic niche off Peru in 2013 and 2014. Solid lines enclose the standard ellipse areas (SEAc), showing the isotopic niche of females and males (red and blue represent female and male, respectively). “Peru 2013” represents the samples collected off Peru during 2013, and so on.
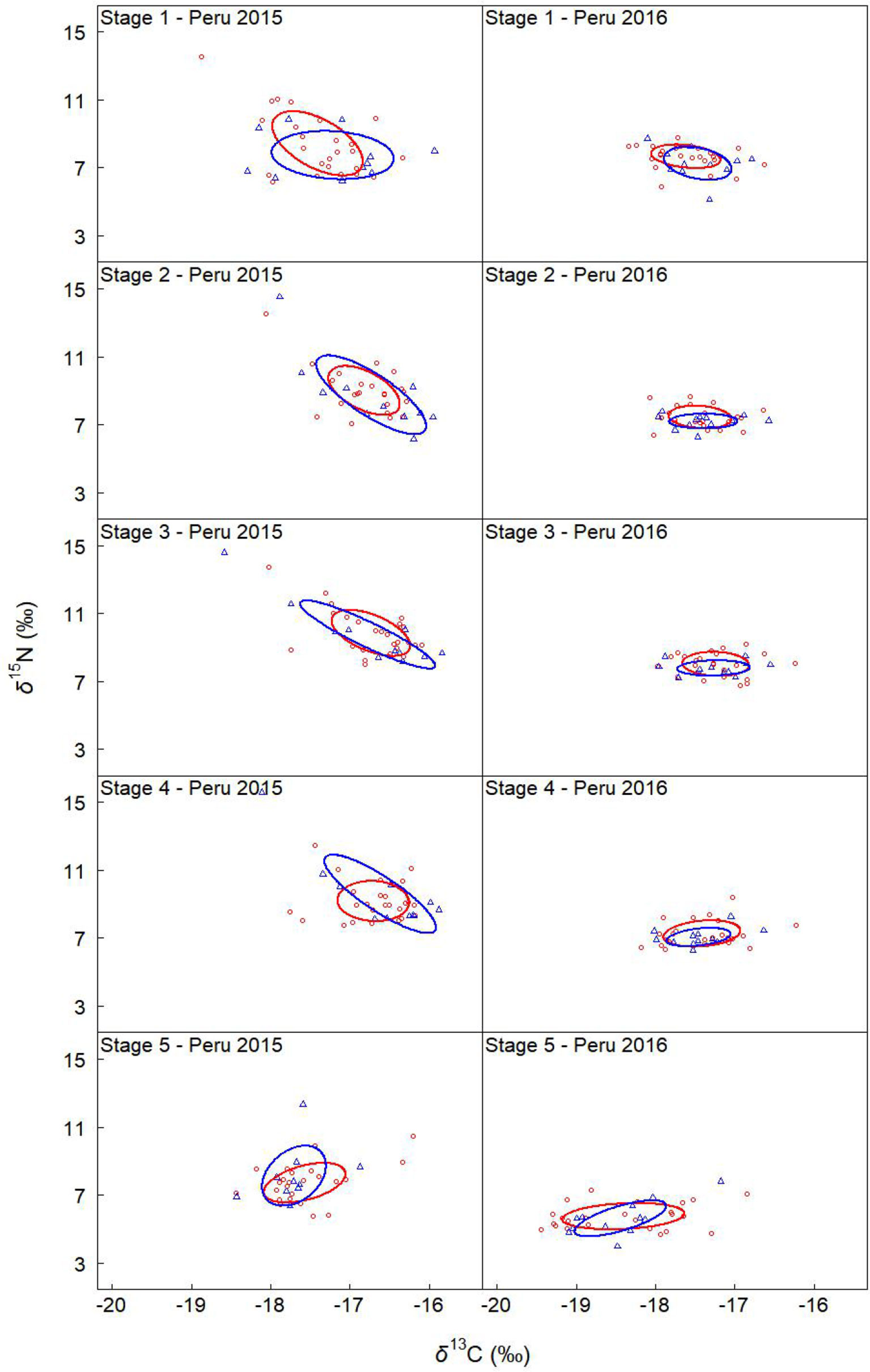
Figure 6 Sexual variation in the isotopic niche off Peru in 2015 and 2016. Solid lines enclose the standard ellipse areas (SEAc), showing the isotopic niche of females and males (red and blue represents female and male, respectively). “Peru 2013” represents the samples collected off Peru during 2013, and so on.
Discussion
Ontogenetic Variation in Isotopic Niche
We found that life stage had no significant influence on δ13C, which might be because D. gigas did not migrate over large geographic areas (Ruiz-Cooley et al., 2010). However, life stage had strong significant influences on δ15N. δ15N fluctuated with stage off Peru from 2014 to 2016 and decreased off Chile in 2015, which contrasted to some previous studies that δ15N was positively related with the growth of squids (Ruiz-Cooley et al., 2006; Ruiz-Cooley et al., 2010; Espinoza et al., 2017; Trasviña-Carrillo et al., 2018). D. gigas is an opportunistic predator and a highly migratory species (Nigmatullin et al., 2001; Ruiz-Cooley and Gerrodette, 2012). δ15N in beaks could be influenced not only by the available food but also by the baseline δ15N value (Lorrain et al., 2011; Li et al., 2017; Liu et al., 2018). Moreover, δ15N was observed to fluctuate and decreased with growth of individual either in previous studies (Lorrain et al., 2011; Li et al., 2017), indicating high diversity of prey items and different migratory routes D. gigas (Ruiz-Cooley et al., 2013). The stomach content analysis showed that the food of D. gigas off Peru was dominated by fish (in particular Vinciguerria sp., Nomeidae) and cephalopods (D. gigas and other species), but crustaceans such as euphausiids can also make a large contribution to the diet (up to 45% by mass) (Lorrain et al., 2011). Pardo-Gandarillas et al. (2014) found that the main prey species in the stomachs of D. gigas from coastal and oceanic waters of Chile were Macruronus novaezelandiae and Trachurus murphyi, respectively, which contributed 36.9% and 84.5% by mass to the diet, respectively.
Off Peru, niche overlaps between stage 1 and stages 3 and 4 in 2013, 2014, and 2015 were very small, while overlaps between stage 1 and stages 2 and 5 were large (Tables 4, 5 and Figure 4). We suggested that squids likely lived in a similar habitat during stages 1 and 2, then moved to a different habitat during stages 3 and 4. Previous studies suggested that majority of paralarvaes are carried northward in the Humboldt Current, and then juveniles grow in the Humboldt Current and will eventually be carried to the west in the South Equatorial Current (Anderson and Rodhouse, 2001; Keyl et al., 2008). Then, adult squid migrate southward and come back to the spawning grounds. In 2016, overlaps between stage 1 and stages 2, 3, and 4 were large, while no overlap was observed between stages 1 and 5. The overlaps between life stages in 2016 indicated that squids inhabited and foraged in a similar area from paralarval to adult phase and experienced a short horizontal migration, which was probably caused by the strong El Niño event. The temperature of sea water has a strong influence on the abundance and distribution of squids (Waluda and Rodhouse, 2006; Waluda et al., 2006; Yu et al., 2016; Yu and Chen, 2018). During the strong El Niño event in 2016, the equatorial undercurrent became more intense and pushed warm equatorial surface waters closer to the coast, which weakened the coastal upwelling current and narrowed the suitable habitat for squid (Keyl et al., 2008; Yu and Chen, 2018). Yu and Chen (2018) evaluated the effects of ocean warming to the habitat quality and spatial distribution of D. gigas off Peru, and found that the potential habitat of D. gigas tended to shrink and move southeastward under ocean warming.
Off Chile, the δ13C value in this study was similar to the results of Gong et al. (2018a) using muscle tissue but the δ15N value was much lower. In this study, both δ13C and δ15N significantly differed among ontogenetic stages. δ13C obviously increased from stage 1 to 2, and became stable from stage 2 to 4, then apparently decreased from stage 4 to 5, indicating that the squids lived in a similar area during stages 2–4. δ15N decreased from stage 3 to 4, which was interpreted as a decrease in the trophic position of squids. Besides, there was no overlap for trophic niche between stage 1 and stages 2, 3, 4, and 5 of the D. gigas, indicating that the habitat of the squids changed after paralarval phase. Squids off Chile were suggested to carry out both a south-northward movement within coastal waters and an east-westward movement between oceanic and coastal waters (Nigmatullin et al., 2001; Liu et al., 2016).
Annual Variation in Isotopic Niche
GLMs showed that year had significant influences on both δ13C and δ15N, which contrasted a previous study (Argüelles et al., 2012). We suggested that it is probably because the El Niño event occurred during 2015 and 2016 (Li et al., 2017; Hu et al., 2019). For the annual variability off Peru, δ13C significantly differed among years during stages 1, 2, and 3, while δ15N significantly differed among years during all stages. Therefore, compared with δ13C, year effects on δ15N tended to be greater. Li et al. (2017) also found that δ13C and δ15N had significant differences among years. The variance in δ13C indicated differences of habitat squids experienced among years (Ruiz-Cooley et al., 2010; Lorrain et al., 2011), while the annual variability in δ15N was likely caused by both the variance of habitat and trophic position in different years (Ruiz-Cooley and Gerrodette, 2012; Liu et al., 2018). Except for stage 1 females in 2014, the average δ15N in 2013 and 2014 was greater than those in 2015 and 2016 during every stage (Tables 4, 5 and Figure 4). We suspect that the El Niño event during 2015 and 2016 changed the migration route and reduced the trophic position of squids.
For the annual variation off Peru, relatively large overlaps were observed between 2013 and 2014 (normal years) throughout the life stage. Trophic niches were separated between normal years (2013 and 2014) and El Niño years (2015 and 2016) with the growth of squids. Moreover, we found that there was no overlap between 2015 and 2016 after the paralarvae phase. Therefore, we suggested that the habitat of squids changed during El Niño years and differed between 2015 and 2016. Other studies have noted that regional differences in environmental variables were different between 2015 and 2016 (Jacox et al., 2016). Oceanographic changes during El Niño events may have shortened and changed the horizontal migration routes and reduced the habitat of squids (Keyl et al., 2008; Yu and Chen, 2018). We found that isotopic niche width in 2016 was generally less than this in 2013 and 2014 throughout the life stage. Therefore, for squids, not only habitat changed but also niche size decreased in 2016. The impacts of El Niño event on squids in 2016 were greater than 2015 (only habitat of squids changed in 2015, while both habitat and niche size changed in 2016). The El Niño event was much stronger during the periods squids experienced in 2016 than that in 2015 (Jacox et al., 2016).
Geographic Variation in Isotopic Niche
In this study, GLMs showed that area had significant influences on both δ13C and δ15N, which was in agreement with previous studies (Argüelles et al., 2012; Ruiz-Cooley and Gerrodette, 2012). Gong et al. (2018a) analyzed the stable isotopes of muscle tissue of D. gigas in the offshore waters of the central eastern Pacific and off Peru and Chile, and found that the δ13C and δ15N values were different among origins.
These differences were probably due to the variability in food composition and baseline values across different geographic areas (Ruiz-Cooley and Gerrodette, 2012; Gong et al., 2018a). Latitudinal gradients in δ13C and δ15N values have been recognized in the eastern Pacific and can be propagated up into the squid tissues through the diet (Argüelles et al., 2012; Ruiz-Cooley and Gerrodette, 2012). Differences of nearly 4.0‰ in δ13C values and 8.0‰ in δ15N values were investigated in D. gigas muscle tissues along a 15° latitudinal gradient in the Humboldt Current Ecosystem (Argüelles et al., 2012). δ15N not only provides information of the trophic position but also can be used to discriminate squids among areas (Gong et al., 2018a). The variations of δ15N among life stages off Chile were greater than those off Peru, which was probably caused by differences of assimilated diet and biochemical cycle between different areas (Ruiz-Cooley et al., 2010; Gong et al., 2020). Only 1% overlap was observed during stage 1 between Peru and Chile in 2015. Compared with other stages, stable isotopes during early life history stage best distinguishes different areas. Several studies also noted that stable isotope can be used for distinguishing geographic populations of D. gigas (Ruiz-Cooley et al., 2010; Gong et al., 2018a; Gong et al., 2020).
Sexual Variation in Isotopic Niche
Sex had no significant influence on δ13C. Females and males probably live and forage together in the similar habitat (Post, 2002), which was consistent with a previous study of gladius stable isotopes (Gong et al., 2018b). Moreover, niche overlaps between females and males were large off Peru from 2013 to 2016, further indicating that females and males shared a similar habitat. Gong et al., (2018b) investigated the sexual variability of niche using gladius stable isotope, and also found that the niche overlaps between females and males were large from paralarval stage to 210 days. The isotopic niches between genders only differed during 211–230 days. Results of GLMs showed sex-specific differences in δ15N, and t-test showed that differences in δ15N between females and males only occurred in 2014, which indicated that trophic level of prey items was probably different between females and males (Cherel and Hobson, 2005; Lorrain et al., 2011; Kernaléguen et al., 2016; Trasviña-Carrillo et al., 2018). In 2014, niche width of females was significantly greater than that of males during all life stages. Compared with males, we supposed that females likely had larger habitats and more available food in 2014. Compared with normal years (2013 and 2014), the niche overlaps were generally greater between females and males during El Niño years (2015 and 2016). This might be due to the smaller suitable habitats during El Niño events (Keyl et al., 2008; Yu and Chen, 2018), resulting in greater competition between females and males.
In conclusion, we investigated the ontogenetic and spatiotemporal effects on δ13C and δ15N values in lower beaks of D. gigas, and compared the trophic niches by sex, life stage, year, and area. δ15N was significantly influenced by all explanatory variables, while δ13C was only influenced by year and area. The habitat and feeding habit of squids likely changed due to the niche differentiation. In a weak El Niño year (2015), the habitat of squids changed. However, during a strong El Niño year (2016), habitat changed and niche size decreased. Stable isotope in early life stages was most effective for distinguishing different geographic populations. Females and males probably inhabited a similar area. However, compared with males, females might have larger habitats and more available food. This study improves the understanding of habitat and feeding strategies of D. gigas.
Data Availability Statement
The original contributions presented in the study are included in the article/supplementary material, further inquiries can be directed to the corresponding author/s.
Ethics Statement
The animal study was reviewed and approved by Institutional animal care and use Committee of Shanghai Ocean University.
Author Contributions
GH, RB, and XC conceived and designed the experiments. XC and JL provided the tissue samples. GH performed the experiments and analyzed the data with the help of ZZ and JL. GH wrote the manuscript with the advice of RB and XC. All authors contributed to the manuscript revision and approved the submitted version.
Funding
This work was supported by the National Key Research and Development Program (2019YFD0901404), the Postdoctoral Innovative Talent Support Program (BX20200238), the National Natural Science Foundation of China (NSFC41876141), and the Shanghai Science and Technology Innovation Action Plan (10DZ1207500).
Conflict of Interest
The authors declare that the research was conducted in the absence of any commercial or financial relationships that could be construed as a potential conflict of interest.
Publisher’s Note
All claims expressed in this article are solely those of the authors and do not necessarily represent those of their affiliated organizations, or those of the publisher, the editors and the reviewers. Any product that may be evaluated in this article, or claim that may be made by its manufacturer, is not guaranteed or endorsed by the publisher.
Acknowledgments
We thank all our colleagues at SHOU for their assistance with sample collection and to Chunxia Gao and Jiahong Guo for stable isotope preparation and analysis. We thank Lei Xing for advice on the figures.
References
Anderson C. I., Rodhouse P. G. (2001). Life Cycles, Oceanography and Variability: Ommastrephid Squid in Variable Oceanographic Environments. Fish. Res. 54 (1), 133–143. doi: 10.1016/S0165-7836(01)00378-2
Argüelles J., Lorrain A., Cherel Y., Graco M., Tafur R., Alegre A., et al. (2012). Tracking Habitat and Resource Use for the Jumbo Squid Dosidicus Gigas: A Stable Isotope Analysis in the Northern Humboldt Current System. Mar. Biol. 159 (9), 2105–2116. doi: 10.1007/s00227-012-1998-2
Arkhipkin A., Argüelles J., Shcherbich Z., Yamashiro C. (2014). Ambient Temperature Influences Adult Size and Life Span in Jumbo Squid (Dosidicus Gigas). Can. J. Fish. Aquat. Sci. 72 (3), 400–409. doi: 10.1139/cjfas-2014-0386
Bode A., Alvarez-Ossorio M. T., Carrera P., Lorenzo J. (2004). Reconstruction of Trophic Pathways Between Plankton and the North Iberian Sardine (Sardina Pilchardus) Using Stable Isotopes. Sci. Mar 68 (1), 165–178. doi: 10.3989/scimar.2004.68n1165
Canali E., Ponte G., Belcari P., Rocha F., Fiorito G. (2011). Evaluating Age in Octopus Vulgaris: Estimation, Validation and Seasonal Differences. Mar. Ecol. Prog. Ser. 441, 141–149. doi: 10.3354/meps09399
Castillo J., Yorio P., Gatto A. (2019). Shared Dietary Niche Between Sexes in Magellanic Penguins. Austral. Ecol. 44 (4), 635–647. doi: 10.1111/aec.12706
Cherel Y., Hobson K. A. (2005). Stable Isotopes, Beaks and Predators: A New Tool to Study the Trophic Ecology of Cephalopods, Including Giant and Colossal Squids. P. R. Soc. Lond. B-Biol. Sci. 272 (1572), 1601–1607. doi: 10.1098/rspb.2005.3115
Cherel Y., Hobson K. A. (2007). Geographical Variation in Carbon Stable Isotope Signatures of Marine Predators: A Tool to Investigate Their Foraging Areas in the Southern Ocean. Mar. Ecol. Prog. Ser. 329, 281–287. doi: 10.3354/meps329281
Cherel Y., Ridoux V., Spitz J., Richard P. (2009). Stable Isotopes Document the Trophic Structure of a Deep-Sea Cephalopod Assemblage Including Giant Octopod and Giant Squid. Biol. Lett. 5, 364–367. doi: 10.1098/rsbl.2009.0024
Espinoza P., Lorrain A., Ménard F., Cherel Y., Tremblay-Boyer L., Argüelles J., et al. (2017). Trophic Structure in the Northern Humboldt Current System: New Perspectives From Stable Isotope Analysis. Mar. Biol. 164 (4), 86. doi: 10.1007/s00227-017-3119-8
Field J. C., Baltz K., Phillips A. J., Walker W. A. (2007). Range Expansion and Trophic Interactions of the Jumbo Squid, Dosidicus Gigas, in the California Current. Cal. Coop. Ocean. Fish Investig. Rep. 48, 131–146.
Gong Y., Li Y., Chen X., Chen L. (2018a). Potential Use of Stable Isotope and Fatty Acid Analyses for Traceability of Geographic Origins of Jumbo Squid (Dosidicus Gigas). Rapid. Commun. Mass. Sp. 32 (7), 583–589. doi: 10.1002/rcm.8071
Gong Y., Li Y. K., Chen X. J., Yu W. (2020). Trophic Niche and Diversity of a Pelagic Squid (Dosidicus Gigas): A Comparative Study Using Stable Isotope, Fatty Acid, and Feeding Apparatuses Morphology. Front. Mar. Sci. 7, 642. doi: 10.3389/fmars.2020.00642
Gong Y., Ruiz-Cooley R. I., Hunsicker M. E., Li Y., Chen X. (2018b). Sexual Dimorphism in Feeding Apparatus and Niche Partitioning in Juvenile Jumbo Squid Dosidicus Gigas. Marine Ecol. Prog. Ser. 607, 99–112. doi: 10.3354/meps12768
Guerra Á., Rodríguez-Navarro A. B., González Á.F., Romanek C. S., Alvarez-Lloret P., Pierce G. J. (2010). Life-History Traits of the Giant Squid Architeuthis Dux Revealed From Stable Isotope Signatures Recorded in Beaks. ICES J. Mar. Sci: J. Con 67 (7), 1425–1431. doi: 10.1093/Icesjms/fsq091
Guisan A., Edwards T. C. Jr., Hastie T. (2002). Generalized Linear and Generalized Additive Models in Studies of Species Distributions: Setting the Scene. Ecol. Model 157 (2-3), 89–100. doi: 10.1016/S0304-3800(02)00204-1
Hu G., Boenish R., Gao C., Li B., Chen X., Chen Y., et al. (2019). Spatio-Temporal Variability in Trophic Ecology of Jumbo Squid (Dosidicus Gigas) in the Southeastern Pacific: Insights From Isotopic Signatures in Beaks. Fish. Res. 212, 56–62. doi: 10.1016/j.fishres.2018.12.009
Hu G., Fang Z., Liu B., Chen X., Staples K., Chen Y. (2018). Using Different Standardized Methods for Species Identification: A Case Study Using Beaks From Three Ommastrephid Species. J. Ocean U. China 17 (2), 355–362. doi: 10.1007/s11802-018-3441-x
Hunsicker M. E., Essington T. E., Aydin K. Y., Ishida B. (2010). Predatory Role of the Commander Squid Berryteuthis Magister in the Eastern Bering Sea: Insights From Stable Isotopes and Food Habits. Mar. Ecol. Prog. Ser. 415, 91–108. doi: 10.3354/meps08750
Jackson A. L., Inger R., Parnell A. C., Bearhop S. (2011). Comparing Isotopic Niche Widths Among and Within Communities: SIBER–Stable Isotope Bayesian Ellipses in R. J. Anim. Ecol. 80 (3), 595–602. doi: 10.1111/j.1365-2656.2011.01806.x
Jacox M. G., Hazen E. L., Zaba K. D., Rudnick D. L., Edwards C. A., Moore A. M., et al. (2016). Impacts of the 2015–2016 El Niño on the California Current System: Early Assessment and Comparison to Past Events. Geophys. Res. Lett. 43 (13), 7072–7080. doi: 10.1002/2016GL069716
Kato Y., Sakai M., Nishikawa H., Igarashi H., Ishikawa Y., Vijai D., et al. (2016). Stable Isotope Analysis of the Gladius to Investigate Migration and Trophic Patterns of the Neon Flying Squid (Ommastrephes Bartramii). Fish. Res. 173, 169–174. doi: 10.1016/j.fishres.2015.09.016
Kernaléguen L., Arnould J., Guinet C., Cazelles B., Richard P., Cherel Y. (2016). Early-Life Sexual Segregation: Ontogeny of Isotopic Niche Differentiation in the Antarctic Fur Seal. Sci. Rep.-UK 6, 33211. doi: 10.1038/srep33211
Keyl F., Argüelles J., Mariátegui L., Tafur R., Wolff M., Yamashiro C. (2008). A Hypothesis on Range Expansion and Spatio-Temporal Shifts in Size-at-Maturity of Jumbo Squid (Dosidicus Gigas) in the Eastern Pacific Ocean. Cal. COFI. Rep. 49, 119–128.
Kim S. L., Tinker M. T., Estes J. A., Koch P. L. (2012). Ontogenetic and Among-Individual Variation in Foraging Strategies of Northeast Pacific White Sharks Based on Stable Isotope Analysis. PloS One 7 (9), e45068. doi: 10.1371/journal.pone.0045068
Li Y., Gong Y., Zhang Y., Chen X. (2017). Inter-Annual Variability in Trophic Patterns of Jumbo Squid (Dosidicus Gigas) Off the Exclusive Economic Zone of Peru, Implications From Stable Isotope Values in Gladius. Fish. Res. 187, 22–30. doi: 10.1016/j.fishres.2016.11.005
Liu B. L., Cao J., Truesdell S. B., Chen Y., Chen X. J., Tian S. Q. (2016). Reconstructing Cephalopod Migration With Statolith Elemental Signatures: A Case Study Using Dosidicus gigas. Fish. Sci. 82 (3), 425–433. doi: 10.1007/s12562-016-0978-8
Liu B. L., Lin J. Y., Chen X. J., Jin Y., Wang J. T. (2018). Inter-And Intra-Regional Patterns of Stable Isotopes in Dosidicus Gigas Beak: Biological, Geographical and Environmental Effects. Mar. Freshwater Res. 69 (3), 464–472. doi: 10.1071/MF17144
Lorrain A., Argüelles J., Alegre A., Bertrand A., Munaron J.-M., Richard P., et al. (2011). Sequential Isotopic Signature Along Gladius Highlights Contrasted Individual Foraging Strategies of Jumbo Squid (Dosidicus Gigas). PloS One 6 (7), e22194. doi: 10.1371/journal.pone.0022194
Lu C., Ickeringill R. (2002). Cephalopod Beak Identification and Biomass Estimation Techniques: Tools for Dietary Studies of Southern Australian Finfishes. Museum Victoria Sci. Rep. 6, 1–65. doi: 10.24199/j.mvsr.2002.06
Markaida U. (2006). Food and Feeding of Jumbo Squid Dosidicus Gigas in the Gulf of California and Adjacent Waters After the 1997–98 El Niño Event. Fish. Res. 79 (1), 16–27. doi: 10.1016/j.fishres.2006.02.016
McCutchan J. H., Lewis W. M., Kendall C., McGrath C. C. (2003). Variation in Trophic Shift for Stable Isotope Ratios of Carbon, Nitrogen, and Sulfur. Oikos 102 (2), 378–390. doi: 10.1034/j.1600-0706.2003.12098.x
Mendes S., Newton J., Reid R. J., Zuur A. F., Pierce G. J. (2007). Stable Carbon and Nitrogen Isotope Ratio Profiling of Sperm Whale Teeth Reveals Ontogenetic Movements and Trophic Ecology. Oecologia 151 (4), 605–615. doi: 10.1007/s00442-006-0612-z
Merten V., Christiansen B., Javidpour J., Piatkowski U., Puebla O., Gasca R., et al. (2017). Diet and Stable Isotope Analyses Reveal the Feeding Ecology of the Orangeback Squid Sthenoteuthis Pteropus (Steenstrup 1855) (Mollusca, Ommastrephidae) in the Eastern Tropical Atlantic. PloS One 12 (12), e0189691. doi: 10.1371/journal.pone.0189691
Miller T. W., Brodeur R. D., Rau G. H. (2008). Carbon Stable Isotopes Reveal Relative Contribution of Shelf-Slope Production to the Northern California Current Pelagic Community. Limnol. Oceanogr. 53 (4), 1493–1503. doi: 10.4319/lo.2008.53.4.1493
Miserez A., Li Y., Waite J. H., Zok F. (2007). Jumbo Squid Beaks: Inspiration for Design of Robust Organic Composites. Acta Biomater. 3 (1), 139–149. doi: 10.1016/j.actbio.2006.09.004
Miserez A., Rubin D., Waite J. H. (2010). Cross-Linking Chemistry of Squid Beak. J. Biol. Chem. 285 (49), 38115–38124. doi: 10.1074/jbc.M110.161174
Nigmatullin C. M., Nesis K., Arkhipkin A. (2001). A Review of the Biology of the Jumbo Squid Dosidicus Gigas (Cephalopoda: Ommastrephidae). Fish. Res. 54 (1), 9–19. doi: 10.1016/S0165-7836(01)00371-X
Pardo-Gandarillas M. C., Lohrmann K. B., George-Nascimento M., Ibáñez C. M. (2014). Diet and Parasites of the Jumbo Squid Dosidicus Gigas in the Humboldt Current System. Molluscan Res. 34 (1), 10–19. doi: 10.1080/13235818.2013.860870
Perales-Raya C., Bartolomé A., García-Santamaría M. T., Pascual-Alayón P., Almansa E. (2010). Age Estimation Obtained From Analysis of Octopus (Octopus Vulgaris Cuvier 1797) Beaks: Improvements and Comparisons. Fish. Res. 106 (2), 171–176. doi: 10.1016/j.fishres.2010.05.003
Post D. M. (2002). Using Stable Isotopes to Estimate Trophic Position: Models, Methods, and Assumptions. Ecology 83 (3), 703–718. doi: 10.1890/0012-9658(2002)083[0703:USITET]2.0.CO;2
Queirós J. P., Cherel Y., Ceia F. R., Hilário A., Roberts J., Xavier J. C. (2018). Ontogenic Changes in Habitat and Trophic Ecology in the Antarctic Squid Kondakovia Longimana Derived From Isotopic Analysis on Beaks. Polar Biol. 41 (12), 2409–2421. doi: 10.1007/s00300-018-2376-4
Rau G. H., Takahashi T., Des Marais D. J. (1989). Latitudinal Variations in Plankton δ13c: Implications for CO2 and Productivity in Past Oceans. Nature 341 (6242), 516. doi: 10.1038/341516a0
Rodhouse P., Nigmatullin C. M. (1996). Role as Consumers. Phil Trans. R Soc. Lond B 351, 1003–1022. doi: 10.1098/rstb.1996.0090
Ruiz-Cooley R. I., Ballance L. T., McCarthy M. D. (2013). Range Expansion of the Jumbo Squid in the NE Pacific: δ 15 N Decrypts Multiple Origins, Migration and Habitat Use. PloS One 8 (3), e59651. doi: 10.1371/journal.pone.0059651
Ruiz-Cooley R. I., Engelhaupt D. T., Ortega-Ortiz J. G. (2012). Contrasting C and N Isotope Ratios From Sperm Whale Skin and Squid Between the Gulf of Mexico and Gulf of California: Effect of Habitat. Mar. Biol. 159 (1), 151–164. doi: 10.1007/s00227-011-1795-3
Ruiz-Cooley R. I., Garcia K. Y., Hetherington E. D. (2011). Effects of Lipid Removal and Preservatives on Carbon and Nitrogen Stable Isotope Ratios of Squid Tissues: Implications for Ecological Studies. J. Exp. Mar. Biol. Ecol. 407 (1), 101–107. doi: 10.1016/j.jembe.2011.07.002
Ruiz-Cooley R. I., Gerrodette T. (2012). Tracking Large-Scale Latitudinal Patterns of δ13c and δ15n Along the E Pacific Using Epi-Mesopelagic Squid as Indicators. Ecosphere 3 (7), 1–17. doi: 10.1890/ES12-00094.1
Ruiz-Cooley R., Markaida U., Gendron D., Aguiniga S. (2006). Stable Isotopes in Jumbo Squid (Dosidicus Gigas) Beaks to Estimate Its Trophic Position: Comparison Between Stomach Contents and Stable Isotopes. Mar. Biol. Associat. UK 86 (2), 437. doi: 10.1017/S0025315406013324
Ruiz-Cooley R., Villa E. C., Gould W. R. (2010). Ontogenetic Variation of δ13c and δ15n Recorded in the Gladius of the Jumbo Squid Dosidicus Gigas: Geographic Differences. Mar. Ecol. Prog. Ser. 399, 187–198. doi: 10.3354/meps08383
Taipe A., Yamashiro C., Mariategui L., Rojas P., Roque C. (2001). Distribution and Concentrations of Jumbo Flying Squid (Dosidicus Gigas) Off the Peruvian Coast Between 1991 and 1999. Fish. Res. 54 (1), 21–32. doi: 10.1016/S0165-7836(01)00377-0
Trasviña-Carrillo L., Hernández-Herrera A., Torres-Rojas Y., Galván-Magaña F., Sánchez-González A., Aguíñiga-García S. (2018). Spatial and Trophic Preferences of Jumbo Squid Dosidicus Gigas (D’Orbigny 1835) in the Central Gulf of California: Ecological Inferences Using Stable Isotopes. Rapid Commun. Mass Spectr. 32 (15), 1225–1236. doi: 10.1002/rcm.8147
Trujillo-Olvera A., Ortega-Garcia S., Tripp-Valdez A., Escobar-Sanchez O., Acosta-Pachon T. A. (2018). Feeding Habits of the Swordfish (Xiphias Gladius Linnaeu) in the Subtropical Northeast Pacific. Hydrobiologia 822 (1), 173–188. doi: 10.1007/s10750-018-3680-2
Varela J. L., Rojo-Nieto E., Sorell J. M., Medina A. (2018). Using Stable Isotope Analysis to Assess Trophic Relationships Between Atlantic Bluefin Tuna (Thunnus Thynnus) and Striped Dolphin (Stenella Coeruleoalba) in the Strait of Gibraltar. Mar. Environ. Res. 139, 57–63. doi: 10.1016/j.marenvres.2018.04.016
Waluda C. M., Rodhouse P. G. (2006). Remotely Sensed Mesoscale Oceanography of the Central Eastern Pacific and Recruitment Variability in Dosidicus Gigas. Mar. Ecol. Prog. 310 (8), 25–32. doi: 10.3354/meps310025
Waluda C. M., Yamashiro C., Rodhouse P. G. (2006). Influence of the ENSO Cycle on the Light-Fishery for Dosidicus Gigas in the Peru Current: An Analysis of Remotely Sensed Data. Fish. Res. 79 (1), 56–63. doi: 10.1016/j.fishres.2006.02.017
Yu W., Chen X. (2018). Ocean Warming-Induced Range-Shifting of Potential Habitat for Jumbo Flying Squid Dosidicus Gigas in the Southeast Pacific Ocean Off Peru. Fish. Res. 204, 137–146. doi: 10.1016/j.fishres.2018.02.016
Keywords: Dosidicus gigas, isotopic niche, stable isotope, ontogeny, spatiotemporal changes, sex, El Niño
Citation: Hu G, Boenish R, Zhao Z, Li J and Chen X (2022) Ontogenetic and Spatiotemporal Changes in Isotopic Niche of Jumbo Squid (Dosidicus gigas) in the Southeastern Pacific. Front. Mar. Sci. 9:806847. doi: 10.3389/fmars.2022.806847
Received: 01 November 2021; Accepted: 10 March 2022;
Published: 11 April 2022.
Edited by:
Enrique Morales-Bojórquez, Centro de Investigación Biológica del Noroeste (CIBNOR), MexicoReviewed by:
Alberto Sánchez-González, Instituto Politécnico Nacional (IPN), MexicoMartin F. Soto-Jimenez, National Autonomous University of Mexico, Mexico
Sergio Aguíñiga, Instituto Politécnico Nacional (IPN), Mexico
Rocio Ruiz-Cooley, Center for Scientific Research and Higher Education in Ensenada (CICESE), Mexico
Copyright © 2022 Hu, Boenish, Zhao, Li and Chen. This is an open-access article distributed under the terms of the Creative Commons Attribution License (CC BY). The use, distribution or reproduction in other forums is permitted, provided the original author(s) and the copyright owner(s) are credited and that the original publication in this journal is cited, in accordance with accepted academic practice. No use, distribution or reproduction is permitted which does not comply with these terms.
*Correspondence: Guanyu Hu, gyhu@shou.edu.cn; Xinjun Chen, xjchen@shou.edu.cn