- 1School of Natural Sciences, Macquarie University, Sydney, NSW, Australia
- 2Pepperell Research and Consulting Pty Ltd., Noosaville, QLD, Australia
- 3School of Environmental and Life Sciences, University of Newcastle, Ourimbah, NSW, Australia
- 4Sydney Institute of Marine Science, Mosman, NSW, Australia
Understanding the unique feeding behaviours of oceanic fish, such as marlin, is key to their effective management. Marlin are notoriously difficult to study, however, and the limited research on marlin feeding shows that diet can vary greatly between species and geographic regions. One region where marlin feeding behaviours are particularly poorly understood are temperate eastern Australian waters. This study collected marlin tissue from game fishing tournaments between latitudes 32°43′06.5″S/152°08′50.1″E to 34°40′12.9″S/150°51′34.3″E between 2010 and 2021, and used stable isotope analysis (SIA) to assess the trophic ecology of the three species of marlin occurring in the region: black (Istiompax indica), blue (Makaira nigricans), and striped (Kajikia audax) marlin. All species had similar δ13C values, but δ15N differed between species, with higher variability observed in blue marlin than in the other two species. Sulphur isotopes were key in identifying the relative contribution of coastal or benthic influences on marlin diet, with δ34S suggesting that blue marlin had less coastal/benthic dietary influence than black or striped marlin. Incorporation of δ34S into SIA for marlin is thus recommended for future studies. Some differences in isotope values across locations and dates were found, however, the uneven sample sizes due to the opportunistic sampling limited the ability to understand spatial or seasonal differences. These findings show that marlin followed similar dietary trends to conspecifics in other regions despite temperate eastern Australian waters being one of the few with three marlin species commonly co-occuring. This suggests that interspecies resource competition is not a major force driving the demography of these species in eastern Australian waters. This research highlights a need for specific management strategies at a species level, particularly for blue marlin. Future research incorporating prey isoscapes and baselines assessed over a wider range of marlin sizes is suggested to further improve our knowledge and capacity to manage the marlin of eastern Australian waters.
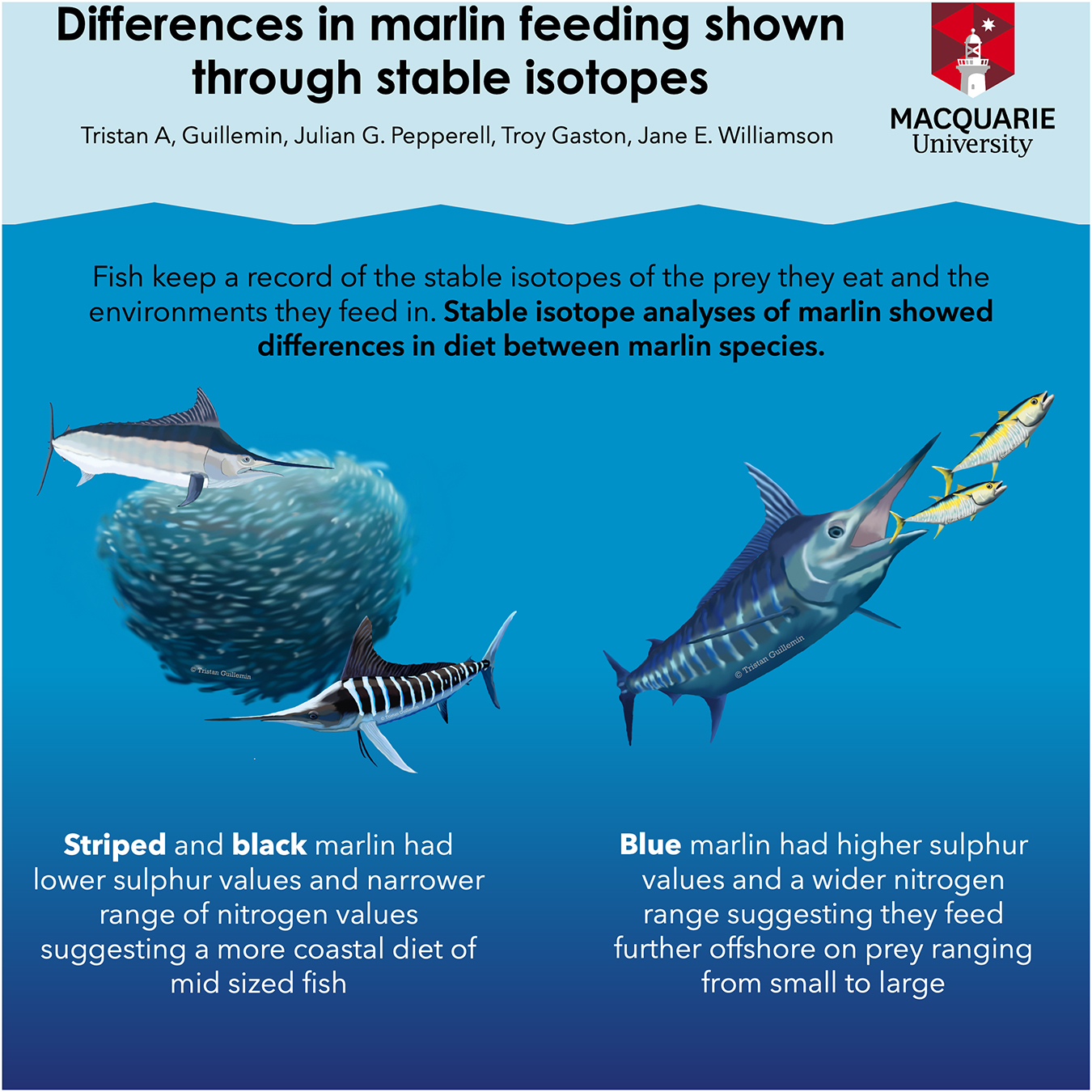
Graphical Abstract. Summarizing some of the key differences revealed by stable isotope analysis of three marlin species (black, blue and striped marlin) in this study. Graphical abstract drawn by TGu, Macquarie University.
Introduction
The role that apex predators play in ecosystem dynamics has long been recognised as pivotal (Estes et al., 2011; Ripple et al., 2014). Due to this perceived importance, apex predators are often the target of conservation and management efforts (Sergio et al., 2008). In ecosystems where multiple predatory species are present, competition for food and trophic partitioning can influence diet and therefore the role that individual species play (Raoult et al., 2015; Curnick et al., 2019). In some instances, high diversity of predators can even lead to functional redundancy in which multiple species play similar trophic roles (Frisch et al., 2016). A lack of understanding of this overlap in trophic ecology can lead to mismanagement of already limited conservation resources (Sergio et al., 2008). Therefore, understanding these inter-predatory interactions is crucial to the efficient management of predatory function in ecosystems.
Marlin are large, oceanic fish considered keystone predators in their environment (Hinman, 1998; Kitchell et al., 2006; Chang et al., 2019). Marlin are also one of the most iconic and targetted fishes by recreational fishers (Ditton and Stoll, 2000; Ward et al., 2012) and are important to commercial fisheries, both as targetted species and bycatch (Restrepo et al., 2003; Langley et al., 2006). Our understanding of their biology and behaviours, however, is limited by the difficulties associated with researching these species and a lack of rigorous data collection on recreational or game fisheries (Holland, 2003; Griffiths, 2012). Managing marlin is particularly challenging as their geographic distributions are large and their often extensive oceanic movements mean that individuals regularly cross multiple jurisdictional boundaries (Hillary et al., 2015). Knowledge of marlin behaviours, including movement and species-specific and regionally specific prey choice are not well understood (Chang et al., 2019). Such information is important when identifying spatial “hotspots” and setting sustainable catch rates and management strategies for recreational and commercial target species such as marlin (Hillary et al., 2015; Lynch et al., 2019).
Marlin in waters off temperate eastern Australia specifically are difficult to manage due to our poor understanding of their behaviours and pressures they face (Findlay et al., 2003). Three species of marlin commonly occur in these waters: black (Istiompax indica), blue (Makaira nigricans), and striped (Kajikia audax). These marlin typically migrate down from the equator into eastern Australia to feed during the warmer months of the year, though the timings of these migrations vary annually and between species (Ghosn et al., 2015). The average size of each species caught and landed by recreational fishers in this region also varies, with striped marlin averaging 91 kg, black marlin averaging 104 kg, and blue marlin averaging 155 kg (Pepperell, 2018). Tag and release data reveals that the sizes of captured blue and striped marlin are roughly representative of the population. However, some years juvenile black marlin weighing 25–40 kg dominate the size distribution of fish tagged (Ghosn et al., 2015) but are not captured as they fall below the minimum tournament weight cut-off of 60 kg.
Research to date indicates that marlin diet varies between stocks and species and that these patterns are complex (Ortiz et al., 2003; Shimose et al., 2006; Torres Rojas et al., 2013; Chiang et al., 2020). Tagged marlin can display distinct geographical ranges and movement patterns, both within and between species (Ortiz et al., 2003; Domeier et al., 2019). Diets as indicated by stomach content analysis are highly variable both in terms of species consumed and prey size across regions (Ueyanagi and Wares, 1972; Shimose et al., 2006, 2008). Stomach content analyses undertaken in temperate eastern Australia have shown that teleosts were the primary, and squid the secondary prey items for striped marlin in this region (Young et al., 2010). The high variability in diet across regions and limited understanding of diet preferences at different life history stages of these animals constrains cohesive management strategies.
One method of studying the trophic ecology of marlin is through stable isotope analysis (SIA). SIA has been used to examine nutritional origin, trophic relationships, ontogeny and broad spatial movements in a wide range of marine animals (Hesslein et al., 1991; Kiljunen et al., 2006; Rodgers and Wing, 2008; Raoult et al., 2015, 2019; Park et al., 2017; Whitfield, 2017). Typically, this method uses varying isotopic turnover rates of nitrogen (δ15N) and carbon (δ13C) among tissues as tracers of diet source within an organism (Vander Zanden et al., 1997). These two isotopes are commonly used for their ability to discriminate between trophic levels (δ15N) and between foraging location or prey types (δ13C) as well as their low analysis cost (relative to other stable isotopes) (Raoult et al., 2019). Varying isotopic turnover rates of different tissue types allow them to provide different temporal insights into fish isotopes, with muscle tissue generally considered to have turnover rates of 6 months to a year (Madigan et al., 2012; Malpica-Cruz et al., 2012).
The few studies that have assessed SIA in marlin show great variation in δ15N and δ13C isotopic ratios within and between species in a manner similar to gut-content analyses. In blue marlin, for example, δ15N is far more variable than δ13C (Logan and Lutcavage, 2013; Torres Rojas et al., 2013; Chang et al., 2019; Chiang et al., 2020), suggesting this species consistently feeds within similar food webs but consumes prey occupying different trophic levels. Striped marlin show different δ13C and δ15N signatures to blue marlin (Torres Rojas et al., 2013; Acosta-Pachón et al., 2015), suggesting less predictability within this species and a high degree of variability in feeding that could be considered opportunistic. The only study to have evaluated black marlin SIA found high variability in δ13C and δ15N and reported higher δ15N values than in other marlin species (Chiang et al., 2020). Moreover, the studies comparing SIA for two co-occurring marlin species have all documented niche segregation in marlin, occurring between blue and striped marlin off Mexico (Torres Rojas et al., 2013; Ordiano-Flores et al., 2021), and between black and blue marlin off eastern Taiwan (Chiang et al., 2020).
All marlin SIA studies to date have been limited to these two isotopic tracers, however, use of a greater number of tracers allows increased clarity in mixed model analyses of SIA and can result in different and more comprehensive interpretations of trophic ecology (Raoult et al., 2019). Analysis of sulphur (δ34S) as a third natural tracer has been included in some isotope studies due to its’ ability to contrast benthic and pelagic origins, and distinguish terrestrial from oceanic or even anthropogenic sources (Connolly et al., 2004; Croisetiere et al., 2009; Raoult et al., 2019). Black and striped marlin are more commonly caught and encountered inside the continental shelf break whereas blue marlin are caught wide of the shelf break, potentially due to differences in diet between the species (Ghosn et al., 2015). Including δ34S in SIA in addition to the usual δ13C and δ15N would help tease apart trophic ecology for such enigmatic species. A similar research question was asked by Plumlee and Wells (2016), who used δ34S to successfully discriminate between diets of three coastal shark species with perceived differing coastal and pelagic foraging. Other studies have similarly shown that adding δ34S increases certainty when comparing dietary sources between teleost groups with varying degrees of coastal or pelagic influence in their diet (Thomas and Cahoon, 1993; Wells et al., 2008).
This study used nitrogen, carbon and sulphur SIA to assess commonalities and differences in the trophic ecology for three species of marlin occurring off eastern Australian waters. This is the first study to evaluate SIA for marlin in the South Pacific, filling crucial knowledge gaps for the region. This study is also the first to incorporate δ34S in a three tracer approach to evaluate the trophic ecology of marlin species. Two key research questions were posed: (1) do different marlin species in temperate eastern Australian waters share similar isotopic niches, and (2) is the use of δ34S as an environmental tracer in marlin useful for trophic assessments?
Materials and Methods
Sample Collection
White muscle samples (∼1,000 mm3) from black, blue and striped marlin were opportunistically sourced from individuals captured across fourteen game fishing tournaments (a total of 34 tournaments). All tournaments were run by clubs affiliated with the New South Wales (NSW) Game Fishing Association in Australia and occurred between Port Stephens (32°43′06.5″S/152°08′50.1″E) and Kiama (34°40′12.9″S/150°51′34.3″E) between 2018 and 2021 (Table 1). The range of fishing boats from any port was within a 70 km arc. Additional marlin tissue preserved in the same manner as the current study (see below) but from earlier fishing competitions dating back to 2010 were also used. Tissue samples were removed from the ventral surface of each marlin near the anal fin and stored individually and immediately frozen to -30°C until processed. The whole weight of each fish (as weighed by the tournament weighmaster) and the “short length” (tip of the lower jaw to caudal fork; the most commonly used measurement of length in Istiophorid research) were recorded. Sex was determined by making an incision in the underside of the fish and macroscopically inspecting the gonads.
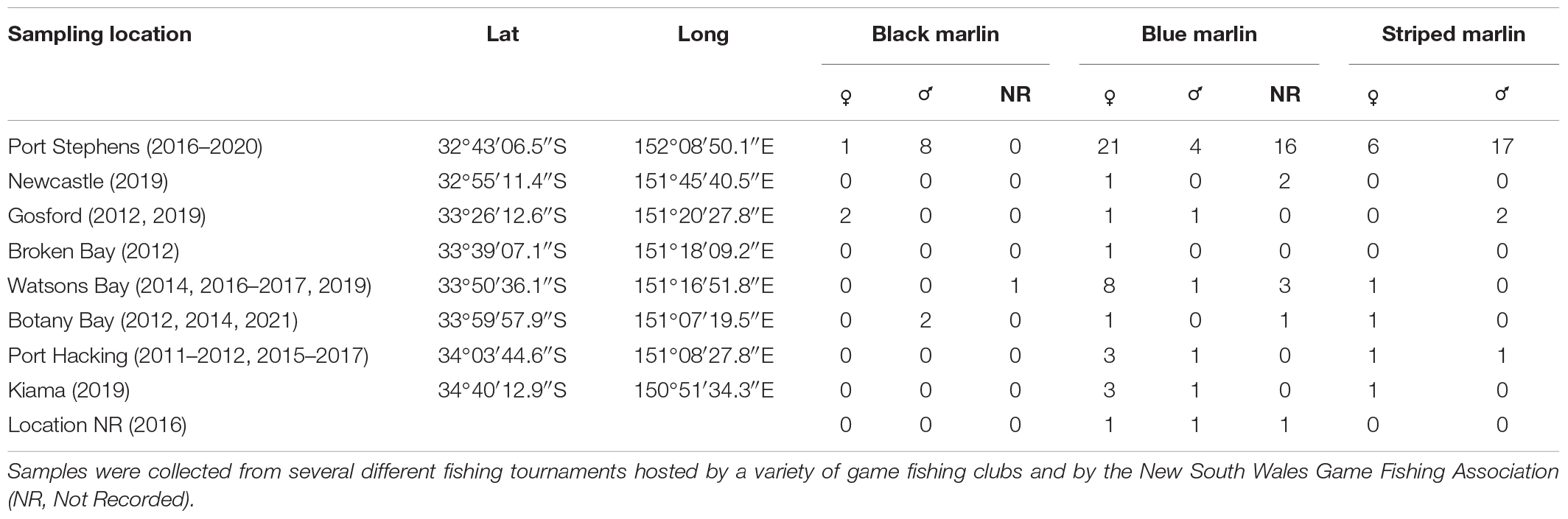
Table 1. Locations from which tissue was collected and the sample size (number of individuals) of tissue collected for three different marlin species: black marlin (Istiompax indica), blue marlin (Makaira nigricans), and striped marlin (Kajikia audax).
Stable Isotope Analyses
Tissue samples were individually placed in a drying oven (Binder, ED115) and dried for 48 h at 60°C. Desiccated samples were ground into a fine powder using a mortar and pestle, which was rinsed thoroughly with deionised water and dried between each sample. Powdered tissue (∼1 g per sample) was placed into separate 5 mL plastic centrifuge tubes and sent to Griffith University Stable Isotope Laboratory in Queensland, Australia, for analysis. Nitrogen, carbon and sulphur stable isotopes were assessed using a Europa EA GSL element analyser (Europa Scientific Inc., Cincinnati, OH, United States) coupled to a Hydra 20–22 automated Isoprime isotope ratio mass spectrometer (Sercon Ltd., Crewe, Cheshire, United Kingdom). Ratios of 15N:14N (δ15N) were expressed as the relative difference between the sample and a standard of atmospheric nitrogen. Ratios of 13C:12C (δ13C) were expressed as the relative difference between the sample and the PDB (Pee Dee belemnite) standard. Ratios of 34S:32S (δ34S) were expressed as the relative difference between the sample and the Vienna-Canyon Diablo Troilite. Ten standards of bovine liver, glycine NBS127 and glycineLSU 1 delta were run with each tray. The standard deviation for measurements of standards was 0.2–0.4‰ for δ13C, 0.1–0.3‰ for δ15N, and 0.4‰ for δ34S.
Data Analyses
All statistical analyses were undertaken using R (R Development Core Team, 2021). As lipid extractions were not done on the samples, the δ13C values were corrected in individuals with high C:N ratios as per Kiljunen et al. (2006). Linear mixed-effects models were constructed in R using the lme4 package (Bates et al., 2018) with the isotope type included as the response variables in three separate models. Linear models were used to test whether isotope values varied between years and locations or between different sexes and sizes of fish. Significance of each of these main effects were obtained with an ANOVA type III table using the anova function in the lmertest package (Kuznetsova et al., 2017). When comparing isotopic values between marlin species, sex, date (day, month, and year), and weight were included as fixed effects with location included as a random effect in all models. Weight was used as a proxy of size instead of length as length correlated closely with weight, and weight was recorded for a higher proportion of our samples. Residuals from the models met linearity and normality assumptions. Pairwise comparisons among main effects were obtained using the pairwise method and a p-value adjustment equivalent to the Tukey test in the emmeans package (Lenth et al., 2019). Trophic niches for the three marlin species were estimated and compared using the r package nicheROVER (Swanson et al., 2015). Within niche rover, trophic niches were generated using Bayesian analysis of δ13C, δ15N, and δ34S values at 1,000 runs with a probability level of alpha = 0.95. A random 10 niche regions were plotted to create 2-dimensional niche projections, these were plotted alongside biplots and probability plots as part of the nicheROVER package. The size (as individual values and standard deviations) of the trophic niche was calculated based on the parameters μ and Σ in a Bayesian context. This allowed the probability of individual marlin from one species falling within the niche of another species to be calculated.
Results
Tissue was sampled from a total of 13 black marlin (Istiompax indica), 73 blue marlin (Makaira nigricans), and 30 striped marlin (Kajikia audax) (Table 2) and δ13C, δ15N and δ34S were analysed for each fish (Table 3). The majority of samples were obtained during the 2018–2021 seasons, with 1 black marlin, 27 blue marlin, and 6 striped marlin sampled in other seasons (2012–2017). Mixed effects models determined that find weight, sex, date, or sampling locations had no significant effect on any of the three isotopes for black marlin (Supplementary Appendix 1). For blue marlin, sampling location had a significant effect on δ34S values (ANOVA: F(7,36) = 3.7093, p = 0.0040), no other significant effects of weight, sex, date, or sampling location were found (Supplementary Appendix 1). In striped marlin, capture date was found to have significant effects on both δ15N (ANOVA: F(1,21) = 12.6305, p = 0.0019) and δ34S (ANOVA: F(1,21) = 9.1723, p = 0.0064) values, no other significant effects of weight, sex, date, or sampling location were found (Supplementary Appendix 1).
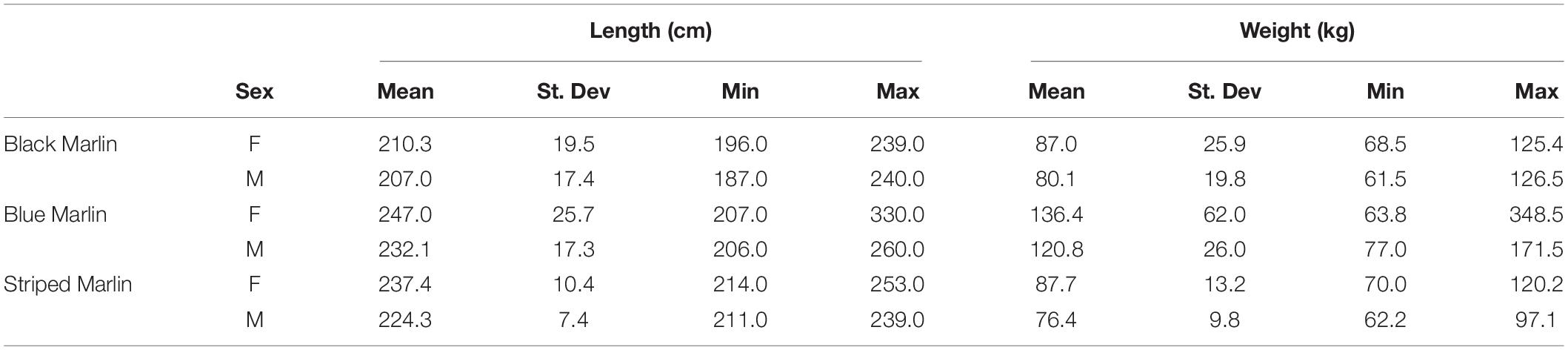
Table 2. Mean size (±1SD) and size range of male and female marlin of each of the three species sampled: black marlin (Istiompax indica), blue marlin (Makaira nigricans), and striped marlin (Kajikia audax).

Table 3. Mean (±1SD), minimum and maximum stable isotope (δ13C, δ15N, and δ34S) values of each of the three species sampled: black marlin (Istiompax indica) blue marlin (Makaira nigricans), and striped marlin (Kajikia audax).
Neither mean δ13C or mean δ15N values differed significantly between any marlin species (Figure 1 and Table 4). Blue marlin had a wider range of both δ13C and δ15N values than black and striped marlin (Figure 2). δ34S also differed significantly between species with blue marlin having significantly higher mean δ34S than both black and striped marlin (Figure 2 and Table 4).
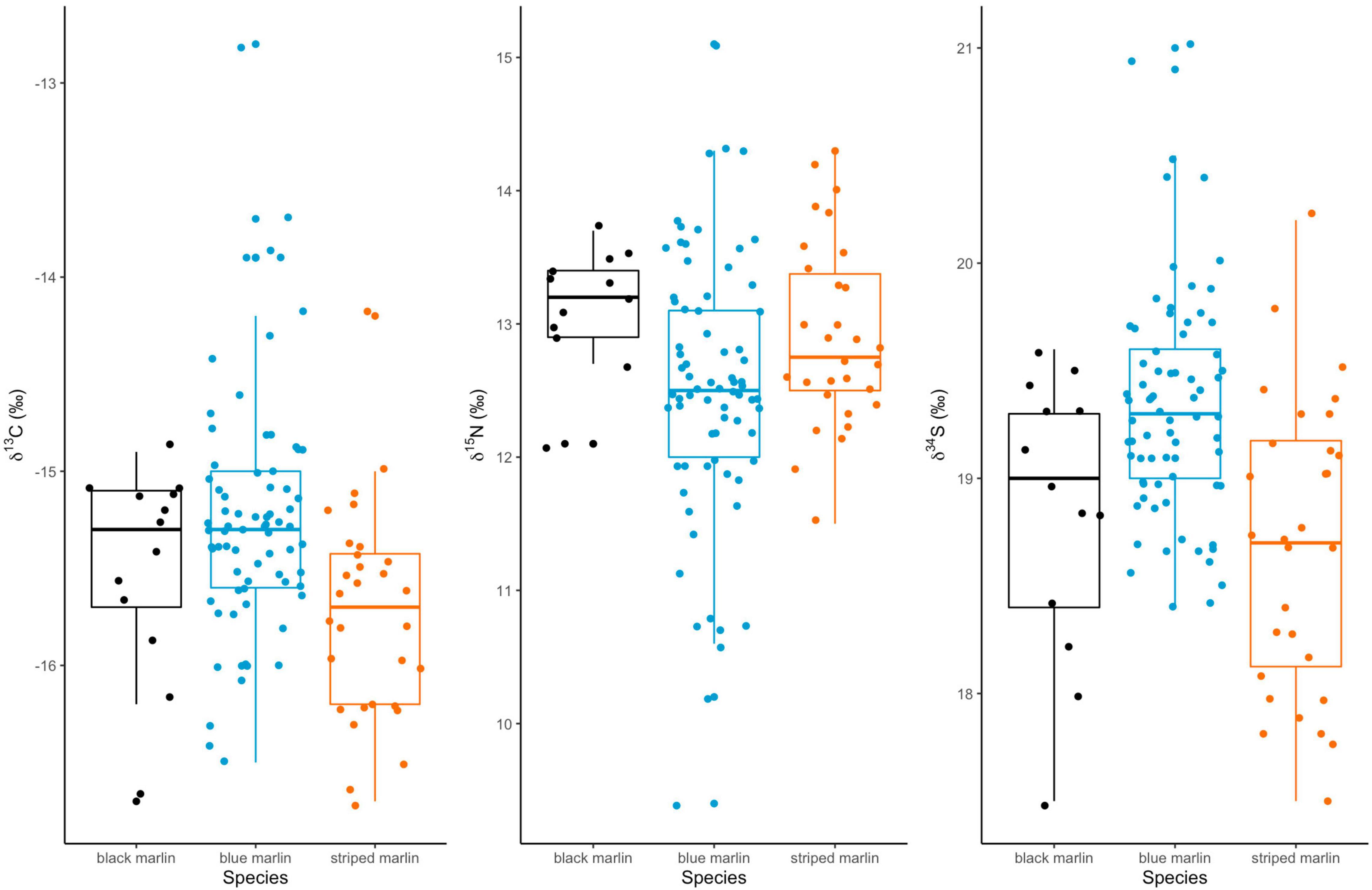
Figure 1. Boxplots of mean isotope (δ13C, δ15N, and δ34S) values for three marlin species: black marlin (Istiompax indica), blue marlin (Makaira nigricans), and striped marlin (Kajikia audax).

Table 4. Results of ANOVA tests run on linear mixed effects models comparing isotope (δ13C, δ15N and δ34S) values between three marlin species: black marlin (Istiompax indica) blue marlin (Makaira nigricans), and striped marlin (Kajikia audax).
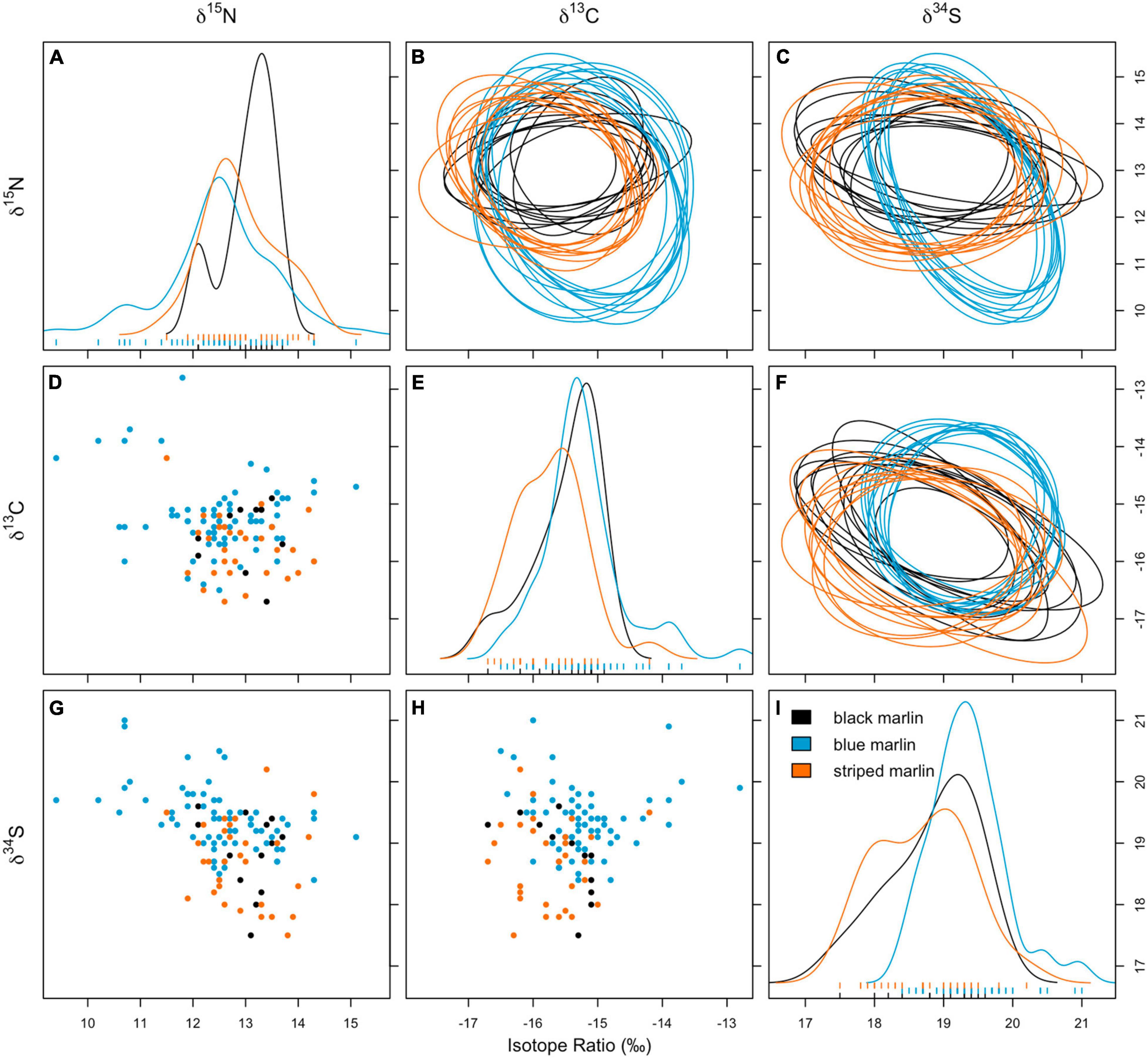
Figure 2. Biplots for δ13C vs. δ15N (D), δ15N vs. δ34S (G), and δ13C vs. δ34S (H); density plots showing distribution of isotope values of δ15N (A), δ13C (E), and δ34S (I); and bayesian ellipse areas (10 runs plotted) using posterior estimates of ellipses for δ13C vs. δ15N (B), δ15N vs. δ34S (C), and δ13C vs. δ34S (F) for three marlin species: black marlin (Istiompax indica), blue marlin (Makaira nigricans), and striped marlin (Kajikia audax).
Blue marlin had the largest isotopic niche (25.85 ± 1SD 4.25) followed by striped marlin (22.49 ± 1SD 3.74) and then black marlin (11.95 ± 1SD 3.29). Black marlin showed the highest probability to be found in niche regions of other marlin with an 85.98% probability for blue marlin and 89.60% striped marlin (Figure 3). Blue marlin had the lowest probability to be found in the niche region of other species with 50.95% chance to occur within the black marlin niche and 66.73% chance for the striped marlin niche (Figure 3). Lastly, the chances for striped marlin to fall within niche of black marlin and blue marlin were 63.68 and 70.61%, respectively (Figure 3).
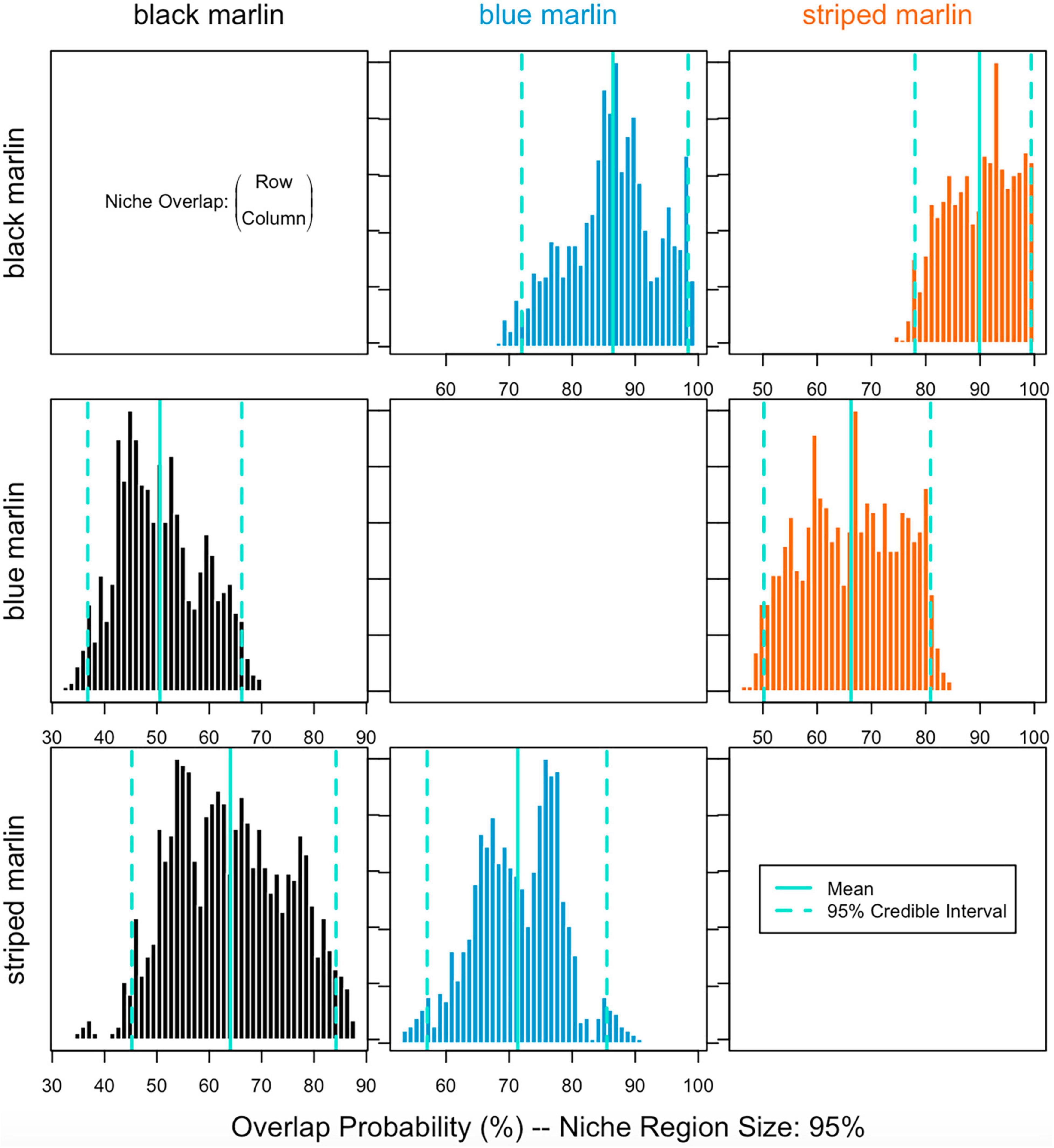
Figure 3. Probability of individual marlin isotope values of placing it within the isotopic niche of another marlin species (1,000 runs with probability level of alpha = 95%) for three marlin species: black marlin (Istiompax indica), blue marlin (Makaira nigricans), and striped marlin (Kajikia audax).
Discussion
Overall isotopic values and ranges were similar between marlin, but there were some differences which suggest some disparities in their diets and/or movements in temperate eastern Australian waters. In particular, the isotopic niche that blue marlin occupied differed to that occupied by black and striped marlin. The similar mean δ13C and δ15N values across marlin species suggest all three feed on similar types and sizes of prey sources and likely within similar environments. However, wider range of both δ13C and δ15N values in blue marlin than in the other two species suggests greater dietary variability in prey size/trophic level and prey type for this species. δ34S was useful in differentiating between blue marlin and the other two species. Specifically, the significantly higher mean and narrower range of δ34S values of blue marlin are indicative of a less benthic or coastal influence in their prey. These results suggest a slight disparity between the ecological roles played by different marlin species off eastern Australia. Statistical analyses showed blue marlin δ34S differed significantly between locations and that striped marlin δ15N and δ34S differed significantly between sampling dates. The locations and dates in which marlin isotopes differed significantly had sample sizes of 4 or less. The effect of location and date was noted and included in our models, however, it is unclear whether these results are ecologically relevant or whether our opportunistic sampling limited the ability to build a robust dataset. Samples from a greater latitude and range of dates are needed to determine the ecological significance of these effects.
Stable isotope analysis of marlin in our study typically supported what is known from other isotope studies in other areas of the world for these species. The only other study to have analysed black marlin isotopes, undertaken in Taiwan, recorded remarkably similar means and ranges for both δ15N and δ13C (Chiang et al., 2020) possibly suggesting similarities in feeding ecology between the two regions for this species. While specific values differed, blue marlin in our study demonstrated similarly higher variability in δ15N compared to δ13C observed in other regions (Logan and Lutcavage, 2013; Torres Rojas et al., 2013; Chang et al., 2019; Chiang et al., 2020). The two studies that have analysed striped marlin isotopes (both undertaken in the Gulf of California) report higher variability in δ15N and δ13C values than found here (Torres Rojas et al., 2013; Acosta-Pachón et al., 2015). Every region has its own isotopic baselines and behaviors limiting cross regional comparison (Cherel and Hobson, 2007), however, there were similarities between regions which may help uncover big-picture marlin isotope ecology with future isoscape information.
Mean δ13C values suggested little overall difference between the prey sources or food webs utilised by all three species of marlin on the east coast of Australia, except for potentially higher prey variability in blue marlin. However, stomach content analyses undertaken on marlin in other regions consistently show both inter- and intra- specific differences and variability in prey species (Ueyanagi and Wares, 1972; Abitia-Cardenas et al., 1999; Shimose et al., 2006, 2008). While the similarities we observed in δ13C values may reflect similar prey use in Australian marlin, they may also reflect the limitations of using δ13C in discriminating diets in oceanic fish. Specifically, oceanic δ13C variability is largely driven by latitudinal gradients in phytoplankton carbon (Raoult et al., 2020), δ13C may therefore not be as effective in discriminating between prey sources within a relatively narrow latitude of open ocean. Future studies using δ13C isotopes in oceanic predators may benefit from collecting prey isoscapes or from using additional trophic ecology methods such as stomach content or fatty acid analysis (Young et al., 2018).
The δ15N results in our research largely aligned with what is known about marlin feeding through dietary studies. The similar δ15N range between black and striped marlin suggested a wider trophic niche or they fed on comparable trophic levels, while the wider range of blue marlin suggested far more variability in prey size. Black and striped marlin are known to feed largely on mid-sized schooling teleosts, though black marlin can also feed on larger pelagic and mesopelagic prey (Ueyanagi and Wares, 1972; Shimose et al., 2008; Young et al., 2010; Chiang et al., 2020; Ordiano-Flores et al., 2021). Blue marlin are known to preferentially feed on scombrids and squid of highly varying size (Shimose et al., 2006, 2007; Rudershausen et al., 2010). Again, the SIA of prey species or a multi-method approach (Young et al., 2018) would allow a more specific identification of the trophic levels at which marlin species feed and is recommended for future studies.
Use of δ34S as an environmental tracer proved constructive in assessment of marlin trophic ecology and differentiating between species. It is known that bacteria in sediments discriminating against specific sulphur isotopes leads to differences in isotope ratios between the coast/benthos and the pelagic environment (Jorgensen, 1979; Connolly et al., 2004). There is a need for more evidence of δ34S differences in oceanic species to establish its use and limits in trophic assessments. As is known through local recreational fisher catches, black and striped marlin typically occur far closer to coast than blue marlin in eastern Australian waters (Ghosn et al., 2015). This created the perfect scenario for testing the effectiveness of δ34S in discriminating coastal dietary influences in large oceanic predators. Consistent with the local knowledge, we found lower δ34S in black and striped marlin and higher δ34S in blue. While more research using δ34S is needed to truly understand the sensitivity of sulphur to benthic influences in diet of mostly pelagic fish, this study provides one of the first examples of δ34S pathways in apex pelagic predatory fish. Interestingly, other studies have recorded diving behaviours in marlin that have been attributed to feeding on more benthic prey (Domeier, 2006; Shimose et al., 2006). As δ34S is typically lower in benthic fish than in pelagic fish (Connolly et al., 2004), the high δ34S values observed in our study were not indicative of any such diving behaviours in marlin. Such differences in results, however, may just reflect limits in our ability to conclude whether this diving behaviour is present or not off eastern Australia due to the small number of studies assessing δ34S and the lack of any prey isoscapes.
The distinct feeding behaviours found in eastern Australian marlin have key implications for effective management strategies. Prey stock management is necessary to maintain populations of predatory fish (Barnett et al., 2010). In having different prey preferences, anthropogenic or natural changes to prey abundance may affect blue marlin differently to black or striped marlin (Layman et al., 2007). This supports the need for species-specific management for marlin off eastern Australia (Hillary et al., 2015). In being the first to use δ34S in marlin research, our findings also have key implications for δ34S use in oceanic environments. This study adds to the growing amount of research showing the effectiveness of δ34S in differentiating feeding patterns in marine species and supports the use of more than two isotopic tracers in stable isotope research.
The opportunistic sampling undertaken and the lack of ability to collect any prey or baseline items greatly limited our capacity to comprehensively understand isotope variation in marlin in this region. While useful in its lack of invasiveness on marlin stocks (i.e., no marlin were collected specifically for this research), a lack of size ranges and sexes for each species makes comprehensive evaluation difficult. Fish smaller than 60 kg, predominantly black marlin, are often caught during tournaments, however, as these fall below the minimum weight allowed at tournaments, our study lacked representation of these smaller marlin. Our samples predominantly came from a single location (Port Stephens) and a narrow range of dates, as such, it limited our ability to determine whether the differences observed in location and date were ecologically relevant. While the size and sex of marlin had no significant effects on the isotope ratios of all three species in our research, this may be an artefact of our sample range. Other studies found mixed results regarding the significance of size, sex and date of capture on marlin isotope ratios (Wells et al., 2010; Logan and Lutcavage, 2013; Torres Rojas et al., 2013; Acosta-Pachón et al., 2015; Chang et al., 2019). Similarly, research from dietary studies directly assessing gut contents found that juvenile blue marlin consumed smaller and more diverse prey than their larger counterparts, and that juvenile blue marlin consume a higher quantity of benthic fishes than adults (Ueyanagi and Wares, 1972; Shimose et al., 2006). SIA of prey samples can be key in not only identifying the most likely prey species consumed, but also in interpreting consumer isotope ratios (Raoult et al., 2019). The lack of prey or baselines largely limited the ability to identify the causes of the observed variability for all three isotopes in marlin. While we are confident in our results for adults in this area, caution should be taken before extrapolating our research beyond the parameters sampled in our study.
This study documented some similarities and some differences in the stable isotope ratios between marlin species and found δ34S to be a key isotope in understanding marlin feeding ecology. Our findings suggest black and striped marlin feed on similar trophic levels relatively near to the coast while blue marlin have higher variability in their trophic level and feed further from the coast. Prey isoscapes and baselines would further our understanding of their trophic ecology and explain some of the differences observed in this study. Nonetheless, our findings are the first to show isotopic values of marlin in the South Pacific and contribute to the global understanding of how marlin feed across oceans and how they may adapt their trophic niches regionally. Further research is needed on the movement, ontogeny and seasonality of marlin in eastern Australian waters. While our findings have revealed trophic differences that have important implications for the conservation and management of these species, more research is needed to effectively manage and protect these keystone predators.
Data Availability Statement
The original contributions presented in the study are included in the article/Supplementary Material, further inquiries can be directed to the corresponding author.
Ethics Statement
Ethical review and approval was not required for the animal study because samples were opportunistically collected from animals already captured and deceased at fishing tournaments. No live animals were handled or killed for the purpose of this study.
Author Contributions
TGu, JW, and JP designed the study. TGu undertook field and laboratory work, analyzed the data and wrote up the research. TGa assisted with analysis of isotope results and write up. JP advised on the research and assisted in sourcing marlin samples. JW led the project and assisted with sample collection, experimental design, analyses and wrote up. All authors contributed to the article and approved the submitted version.
Funding
This research was funded primarily by the New South Wales Department of Primary Industries Recreational Fishing Trust (Grant SS060) and in part by the Department of Biological Sciences at Macquarie University. Tristan Guillemin was supported by a Macquarie University RTP Scholarship. The study was conducted according to the Australian code for the care and use of animals for scientific purposes.
Conflict of Interest
JP was employed by Pepperell Research and Consulting Pty Ltd.
The remaining authors declare that the research was conducted in the absence of any commercial or financial relationships that could be construed as a potential conflict of interest.
Publisher’s Note
All claims expressed in this article are solely those of the authors and do not necessarily represent those of their affiliated organizations, or those of the publisher, the editors and the reviewers. Any product that may be evaluated in this article, or claim that may be made by its manufacturer, is not guaranteed or endorsed by the publisher.
Acknowledgments
We thank the New South Wales Game Fishing Association and the many individual game fishing club officials and anglers who were welcoming, helpful and went out of their way to ensure all researchers at game fishing tournaments were able to access the samples needed. Thanks to all the researchers who volunteered their time to collect samples alongside us. We thank Drew Allen, Louise Tosetto and Vincent Raoult for advice and help with statistical analyses.
Supplementary Material
The Supplementary Material for this article can be found online at: https://www.frontiersin.org/articles/10.3389/fmars.2022.795436/full#supplementary-material
References
Abitia-Cardenas, L. A., Galvan-Magaña, F., Gutierrez-Sanchez, F. J., Rodriguez-Romero, J., Aguilar-Palomino, B., and Moehl-Hitz, A. (1999). Diet of blue marlin Makaira mazara off the coast of Cabo San Lucas, Baja California Sur, Mexico. Fish. Res. 44, 95–100. doi: 10.1016/s0165-7836(99)00053-3
Acosta-Pachón, T. A., Ortega-García, S., and Graham, B. (2015). Stable carbon and nitrogen isotope values of dorsal spine age rings indicate temporal variation in the diet of striped marlin (Kajikia audax) in waters around Cabo San Lucas, Mexico. Rapid Commun. Mass Spectrom. 29, 1676–1686. doi: 10.1002/rcm.7271
Barnett, A., Abrantes, K., Stevens, J. D., Yick, J. L., Frusher, S. D., and Semmens, J. M. (2010). Predator–prey relationships and foraging ecology of a marine apex predator with a wide temperate distribution. Mar. Ecol. Prog. Ser. 416, 189–200.
Bates, D., Maechler, M., Bolker, B., Walker, S., Christensen, R. H. B., Singmann, H., et al. (2018). Package ‘lme4’. Version 1, 437.
Chang, C. T., Chiang, W. C., Chang, Y. C., Musyl, M. K., Sun, C. L., Madigan, D. J., et al. (2019). Stable isotope analysis reveals ontogenetic feeding shifts in Pacific blue marlin (Makaira nigricans) off eastern Taiwan. J. Fish Biol. 94, 958–965. doi: 10.1111/jfb.13902
Cherel, Y, and Hobson, K (2007). Geographical variation in carbon stable isotope signatures of marine predators: a tool to investigate their foraging areas in the Southern Ocean. Mar Ecol Prog Ser 329:281–287
Chiang, W.-C., Chang, C.-T., Madigan, D. J., Carlisle, A. B., Musyl, M. K., Chang, Y.-C., et al. (2020). Stable isotope analysis reveals feeding ecology and trophic position of black marlin off eastern Taiwan. Deep Sea Res. Part II Top. Stud. Oceanogr. 175:104821.
Connolly, R. M., Guest, M. A., Melville, A. J., and Oakes, J. M. (2004). Sulfur stable isotopes separate producers in marine food-web analysis. Oecologia 138, 161–167. doi: 10.1007/s00442-003-1415-0
Croisetiere, L., Hare, L., Tessier, A., and Cabana, G. (2009). Sulphur stable isotopes can distinguish trophic dependence on sediments and plankton in boreal lakes. Freshw. Biol. 54, 1006–1015.
Curnick, D. J., Carlisle, A. B., Gollock, M. J., Schallert, R. J., and Hussey, N. E. (2019). Evidence for dynamic resource partitioning between two sympatric reef shark species within the British Indian Ocean Territory. J. Fish Biol. 94, 680–685. doi: 10.1111/jfb.13938
Ditton, R. B., and Stoll, J. R. (2000). A socio-economic review of recreational billfish fisheries. Mar. Freshw. Res. 54, 545–554. doi: 10.1071/mf01279
Domeier, M. L. (2006). An analysis of Pacific striped marlin (Tetrapturus audax) horizontal movement patterns using pop-up satellite archival tags. Bull. Mar. Sci. 79, 811–825.
Domeier, M. L., Ortega-Garcia, S., Nasby-Lucas, N., and Offield, P. (2019). First marlin archival tagging study suggests new direction for research. Mar. Freshw. Res. 70, 603–608. doi: 10.1071/mf18160
Estes, J. A., Terborgh, J., Brashares, J. S., Power, M. E., Berger, J., Bond, W. J., et al. (2011). Trophic downgrading of planet Earth. Science 333, 301–306. doi: 10.1126/science.1205106
Findlay, J. D., Cross, C. M., and Bodsworth, A. G. (2003). Marlin fisheries management in Australia. Mar. Freshw. Res. 54, 535–543.
Frisch, A. J., Ireland, M., Rizzari, J. R., Lönnstedt, O. M., Magnenat, K. A., Mirbach, C. E., et al. (2016). Reassessing the trophic role of reef sharks as apex predators on coral reefs. Coral Reefs 35, 459–472. doi: 10.1111/cobi.12385
Ghosn, D. L., Collins, D. P., and Gould, A. P. (2015). The NSW Game Fish Tournament Monitoring Program 1994–2013: data summary and assessment of program role and design. Nelson Bay, NSW: NSW Department of Primary Industries
Griffiths, S. P. (2012). Recreational catch composition, catch rates, effort and expenditure in a specialised land-based pelagic game fish fishery. Fish. Res. 127, 40–44.
Hesslein, R., Capel, M., Fox, D., and Hallard, K. (1991). Stable isotopes of sulfur, carbon, and nitrogen as indicators of trophic level and fish migration in the lower Mackenzie River basin, Canada. Can. J. Fish. Aquat. Sci. 48, 2258–2265. doi: 10.1139/f91-265
Hillary, R., Preece, A., Kolody, D., Evans, K., and Davies, C. (2015). Development of an approach to harvest strategy management of internationally managed multi-species fisheries. Fish. Res. Dev. Corporation 203, 1–161.
Hinman, K. (1998). Ocean roulette: conserving swordfish, sharks and other threatened pelagic fish in longline-infested waters. Southeast Leesburg: National Coalition for Marine Conservation
Holland, K. N. (2003). A perspective on billfish biological research and recommendations for the future. Mar. Freshw. Res. 54, 343–347.
Jorgensen, B. B. (1979). A theoretical model of the stable sulfur isotope distribution in marine sediments. Geochim. Cosmochim. Acta 43, 363–374. doi: 10.1016/j.scitotenv.2004.01.011
Kiljunen, M., Grey, J., Sinisalo, T., Harrod, C., Immonen, H., and Jones, R. I. (2006). A revised model for lipid-normalizing δ13C values from aquatic organisms, with implications for isotope mixing models. J. Appl. Ecol. 43, 1213–1222.
Kitchell, J. F., Martell, S. J., Walters, C. J., Jensen, O. P., Kaplan, I. C., Watters, J., et al. (2006). Billfishes in an ecosystem context. Bull. Mar. Sci. 79, 669–682.
Kuznetsova, A., Brockhoff, P. B., and Christensen, R. H. (2017). lmerTest package: tests in linear mixed effects models. J. Stat. Softw. 82, 1–26.
Langley, A., Molony, B., Bromhead, D., Yokawa, K., and Wise, B. (2006). Stock assessment of striped marlin (Tetrapturus audax) in the southwest Pacific Ocean. WCPFC SC2 SA WP-6. Manila, Philippines: Western and Central Pacific Fisheries Commission Scientific Committee, 7–18.
Layman, C. A., Quattrochi, J. P., Peyer, C. M., and Allgeier, J. E. (2007). Niche width collapse in a resilient top predator following ecosystem fragmentation. Ecol. Lett. 10, 937–944. doi: 10.1111/j.1461-0248.2007.01087.x
Lenth, R., Singmann, H., Love, J., Buerkner, P., and Herve, M. (2019). Package ‘emmeans’: Estimated Marginal Means, aka Least-Squares Means. Available online at: https://github.com/rvlenth/emmeans
Logan, J. M., and Lutcavage, M. E. (2013). Assessment of trophic dynamics of cephalopods and large pelagic fishes in the central North Atlantic Ocean using stable isotope analysis. Deep Sea Res. Part II Top. Stud. Oceanogr. 95, 63–73. doi: 10.1016/j.dsr2.2012.07.013
Lynch, T., Smallwood, C., Ochwada-Doyle, F., Lyle, J., Williams, J., Ryan, K., et al. (2019). A cross continental scale comparison of Australian offshore recreational fisheries research and its applications to Marine Park and fisheries management. ICES J. Mar. Sci. 78, 1190–1205. doi: 10.1093/icesjms/fsz092
Madigan, D. J., Litvin, S. Y., Popp, B. N., Carlisle, A. B., Farwell, C. J., and Block, B. A. (2012). Tissue turnover rates and isotopic trophic discrimination factors in the endothermic teleost, Pacific bluefin tuna (Thunnus orientalis). PLoS One 7:e49220. doi: 10.1371/journal.pone.0049220
Malpica-Cruz, L., Herzka, S. Z., Sosa-Nishizaki, O., and Lazo, J. P. (2012). Tissue-specific isotope trophic discrimination factors and turnover rates in a marine elasmobranch: empirical and modeling results. Can. J. Fish. Aquat. Sci. 69, 551–564.
Ordiano-Flores, A., Galván-Magaña, F., Sánchez-González, A., Soto-Jiménez, M. F., and Páez-Osuna, F. (2021). Mercury, selenium, and stable carbon and nitrogen isotopes in the striped marlin Kajikia audax and blue marlin Makaira nigricans food web from the Gulf of California. Mar. Pollut. Bull. 170:112657. doi: 10.1016/j.marpolbul.2021.112657
Ortiz, M., Prince, E. D., Serafy, J. E., Holts, D. B., Davy, K. B., Pepperell, J. G., et al. (2003). Global overview of the major constituent-based billfish tagging programs and their results since 1954. Mar. Freshw. Res. 54, 489–507. doi: 10.1071/mf02028
Park, J. M., Gaston, T. F., and Williamson, J. E. (2017). Resource partitioning in gurnard species using trophic analyses: the importance of temporal resolution. Fish. Res. 186, 301–310. doi: 10.1016/j.fishres.2016.10.005
Pepperell, J. (2018). NSW DPI Game Fish Tagging Program Report 2017-2018. New South Wales, Australia: Pepperell Research, 29.
Plumlee, J. D., and Wells, R. D. (2016). Feeding ecology of three coastal shark species in the northwest Gulf of Mexico. Mar. Ecol. Prog. Ser. 550, 163–174.
R Development Core Team (2021). R: a language and environment for statistical computing. Vienna, Austria: R Foundation for Statistical Computing.
Raoult, V., Broadhurst, M. K., Peddemors, V. M., Williamson, J. E., and Gaston, T. F. (2019). Resource use of great hammerhead sharks Sphyrna mokarran off eastern Australia. J. Fish Biol. 95, 1430–1440. doi: 10.1111/jfb.14160
Raoult, V., Gaston, T. F., and Williamson, J. E. (2015). Not all sawsharks are equal: species of co-existing sawsharks show plasticity in trophic consumption both within and between species. Can. J. Fish. Aquat. Sci. 72, 1769–1775. doi: 10.1139/cjfas-2015-0307
Raoult, V., Trueman, C. N., Kingsbury, K. M., Gillanders, B. M., Broadhurst, M. K., Williamson, J. E., et al. (2020). Predicting geographic ranges of marine animal populations using stable isotopes: a case study of great hammerhead sharks in eastern Australia. Front. Mar. Sci. 7:594636. doi: 10.3389/fmars.2020.594636
Restrepo, V., Prince, E. D., Scott, G. P., and Uozumi, Y. (2003). ICCAT stock assessments of Atlantic billfish. Mar. Freshw. Res. 54, 361–367.
Ripple, W. J., Estes, J. A., Beschta, R. L., Wilmers, C. C., Ritchie, E. G., Hebblewhite, M., et al. (2014). Status and ecological effects of the world’s largest carnivores. Science 343:1241484. doi: 10.1126/science.1241484
Rodgers, K. L., and Wing, S. R. (2008). Spatial structure and movement of blue cod Parapercis colias in Doubtful Sound, New Zealand, inferred from δ13C and δ15N. Mar. Ecol. Prog. Ser. 359, 239–248.
Rudershausen, P. J., Buckel, J. A., Edwards, J., Gannon, D. P., Butler, C. M., and Averett, T. W. (2010). Feeding ecology of blue marlins, dolphinfish, yellowfin tuna, and wahoos from the North Atlantic Ocean and comparisons with other oceans. Trans. Am. Fish. Soc. 139, 1335–1359. doi: 10.1577/t09-105.1
Sergio, F., Caro, T., Brown, D., Clucas, B., Hunter, J., Ketchum, J., et al. (2008). Top predators as conservation tools: ecological rationale, assumptions, and efficacy. Annu. Rev. Ecol. Evol. Syst. 39, 1–19. doi: 10.1146/annurev.ecolsys.39.110707.173545
Shimose, T., Shono, H., Yokawa, K., Saito, H., and Tachihara, K. (2006). Food and feeding habits of blue marlin, Makaira nigricans, around Yonaguni Island, southwestern Japan. Bull. Mar. Sci. 79, 761–775.
Shimose, T., Yokawa, K., Saito, H., and Tachihara, K. (2007). Evidence for use of the bill by blue marlin, Makaira nigricans, during feeding. Ichthyol. Res. 54, 420–422.
Shimose, T., Yokawa, K., Saito, H., and Tachihara, K. (2008). Seasonal occurrence and feeding habits of black marlin, Istiompax indica, around Yonaguni Island, southwestern Japan. Ichthyol. Res. 55, 90–94. doi: 10.1007/s10228-007-0004-3
Swanson, H. K., Lysy, M., Power, M., Stasko, A. D., Johnson, J. D., and Reist, J. D. (2015). A new probabilistic method for quantifying n-dimensional ecological niches and niche overlap. Ecology 96, 318–324. doi: 10.1890/14-0235.1
Thomas, C. J., and Cahoon, L. B. (1993). Stable isotope analyses differentiate between different trophic pathways supporting rocky-reef fishes. Mar. Ecol. Prog. Ser. 95, 19–19.
Torres Rojas, Y., Hernandez Herrera, A., Ortega-Garcia, S., and Domeier, M. (2013). Stable isotope differences between blue marlin (Makaira nigricans) and striped marlin (Kajikia audax) in the southern Gulf of California, Mexico. Bull. Mar. Sci. 89, 421–436.
Ueyanagi, S., and Wares, P. (1972). “Synopsis of biological data on striped marlin, Tetrapturus audax (Philippi), 1887,” in Proceedings of the International Billfish Symposium, Kailua–Kona, Hawaii, eds R. S. Shomura and F. Williams (Washington, DC: United States Government Printing Office), 9–12.
Vander Zanden, M. J., Cabana, G., and Rasmussen, J. B. (1997). Comparing trophic position of freshwater fish calculated using stable nitrogen isotope ratios (δ15N) and literature dietary data. Can. J. Fish. Aquat. Sci. 54, 1142–1158.
Ward, P., Mazur, K., Stenekes, N., Kancans, R., Curtotti, R., Summerson, R., et al. (2012). A socioeconomic evaluation of three eastern Australian game-fishing regions. ABARES report to client prepared for the Fisheries Research and Development Corporation. Canberra: Australian Bureau of Agricultural and Resource Economics and Sciences.
Wells, R. D., Cowan, J. H. Jr., and Fry, B. (2008). Feeding ecology of red snapper Lutjanus campechanus in the northern Gulf of Mexico. Mar. Ecol. Prog. Ser. 361, 213–225. doi: 10.1371/journal.pone.0144051
Wells, R. D., Rooker, J. R., and Prince, E. D. (2010). Regional variation in the otolith chemistry of blue marlin (Makaira nigricans) and white marlin (Tetrapturus albidus) from the western North Atlantic Ocean. Fish. Res. 106, 430–435. doi: 10.1016/j.fishres.2010.09.017
Whitfield, A. K. (2017). The role of seagrass meadows, mangrove forests, salt marshes and reed beds as nursery areas and food sources for fishes in estuaries. Rev. Fish Biol. Fish. 27, 75–110.
Young, J. W., Lansdell, M. J., Campbell, R. A., Cooper, S. P., Juanes, F., and Guest, M. A. (2010). Feeding ecology and niche segregation in oceanic top predators off eastern Australia. Mar. Biol. 157, 2347–2368. doi: 10.1007/s00227-010-1500-y
Keywords: marlin, trophic ecology, stable isotope, feeding, gamefish, sulphur
Citation: Guillemin TA, Pepperell JG, Gaston T and Williamson JE (2022) Deciphering the Trophic Ecology of Three Marlin Species Using Stable Isotope Analysis in Temperate Waters Off Southeastern Australia. Front. Mar. Sci. 9:795436. doi: 10.3389/fmars.2022.795436
Received: 15 October 2021; Accepted: 25 January 2022;
Published: 30 March 2022.
Edited by:
Nuno Queiroz, Centro de Investigacao em Biodiversidade e Recursos Geneticos (CIBIO-InBIO), PortugalReviewed by:
Sofia Ortega-Garcia, Instituto Politécnico Nacional (IPN), MexicoTatiana Acosta-Pachon, Universidad Autónoma de Baja California Sur, Mexico
Copyright © 2022 Guillemin, Pepperell, Gaston and Williamson. This is an open-access article distributed under the terms of the Creative Commons Attribution License (CC BY). The use, distribution or reproduction in other forums is permitted, provided the original author(s) and the copyright owner(s) are credited and that the original publication in this journal is cited, in accordance with accepted academic practice. No use, distribution or reproduction is permitted which does not comply with these terms.
*Correspondence: Tristan A. Guillemin, dHJpc3Rhbi5ndWlsbGVtaW5Ac3R1ZGVudHMubXEuZWR1LmF1