- 1School of Environmental Science and Engineering, Southern Marine Science and Engineering Guangdong Laboratory (Zhuhai), Sun Yat-sen University, Guangzhou, China
- 2Guangdong Provincial Key Laboratory of Environmental Pollution Control and Remediation Technology, Sun Yat-sen University, Guangzhou, China
- 3School of Marine Science, Guangdong Provincial Key Laboratory of Marine Resources and Coastal Engineering, Sun Yat-sen University, Guangzhou, China
- 4Institute of Urban Environment, Chinese Academy of Sciences, Xiamen, China
- 5South China Institute of Environmental Sciences, Ministry of Ecology and Environment, Guangzhou, China
- 6Simon F. S. Li Marine Science Laboratory, School of Life Sciences and Earth System Science Programme, The Chinese University of Hong Kong, Shatin, Hong Kong SAR, China
Mangroves around the world settle along coasts and estuaries, where they usually encounter aquaculture effluent that causes eutrophication and regulates the nitrogen cycle in their ecosystems. Moreover, the physical–chemical features of sediment in mangrove habitats might be altered by coastal wind, waves, and currents more frequently under the condition of increasing extreme climatic events. These events affect the process of colonization/regeneration of mangroves. To study how exogenous nitrogen input and sediment texture affect root system establishment of mangrove propagules and the early growth of seedlings, in turn, we set up a mesocosm experiment to explore the morphological and physiological responses of the root system of two mangroves, i.e., Kandelia obovata and Sonneratia apetala. We found that the root morphological features of mangrove seedlings mostly showed significant differences across the sediment texture treatment; K. obovata seedlings were more intensively influenced than S. apetala seedlings. It could be ascribed that the roots of K. obovata seedlings were more sensitive to sediment texture than S. apetala seedlings. Conversely, the treatment of exogenous nitrogen input showed a slighter even non-significant influence on the root features of mangrove seedlings. These results suggest that the seedlings of S. apetala and K. obovata performed similarly. In estuaries and coasts featuring eutrophication and various sediment textures, the conservation and restoration of mangroves should consider these conditions to ensure the sustainable development of mangroves.
Introduction
Mangroves mainly occur throughout tropical and sub-tropical coastal areas, usually in sheltered estuaries and bays with lower wind and waves energy (Romañach et al., 2018; Friess et al., 2019). These forests provide numerous ecosystem functions and benefits, such as carbon sequestration, storm and wave damping, and marine and coastal biodiversity conservation (Alongi, 2008; Lee et al., 2014). Despite the ecological importance of mangrove forests, the global area of mangrove forests has presented a continuously decreasing trend. It has been estimated that an annual deforestation rate of 0.16–0.39% of mangrove forests has occurred since the 2000s (Valiela et al., 2001; He et al., 2018). Mangrove forests have undergone degradation and loss by 1646 km2 globally from 2000 to 2012 (Hamilton and Casey, 2016). Despite continuous mangrove recession throughout the world, over the last two decades, China has observed a resilient trend in which the mangrove area had increased to 34472.14 ha in 2013, achieving a 56.69% increment from 2001 (Fan and Wang, 2017). In general, the worldwide loss of mangroves might be attributed to population growth and social development in the coastal zone (Thomas et al., 2017; Hargan et al., 2020). Recognized for their tremendous and irreplaceable ecological functionalities and services, the restoration of mangroves is currently an issue of considerable interest for ecology and conservation currently (Barbier, 2016).
For the natural reproduction process, mangroves are embellished by their unique viviparous and water-disposal features. Stranded propagules could anchor and grow rapidly under appropriate conditions after ripening and falling from the branches (Rabinowitz, 1978). Mangrove propagules can have a long-distance dispersal through the tide and current. Hence, the early seedling establishment and development of mangroves are regulated by many internal and external factors. The seedling may be affected by drag forces of fluid motion or buried or excavated when transported (Balke et al., 2011, 2014). Different mangrove species may exhibit distinct regenerating and colonizing strategies in accordance with their reproductive traits, which is closely related to their roles and niches in the successional stages of natural mangroves. The anchorage and establishment of seedlings are essentially vital to natural mangrove colonization or artificial restoration on the dynamic and unstable sediments. Mangroves are typical bio-geomorphic ecosystems in which their occurrence is strongly affected by coastal sedimentation dynamics (Kamal et al., 2017). Although mangrove forests usually occur in sheltered coastlines with more tranquil environments, physical conditions, such as sediment deposition, sediment erosion, and the physical components of sediment, can still affect the seedling establishment and even the floral composition of mangrove forests. The early establishment and development of seedling roots are sensitive to the physical composition of sediment in mangroves (Jiang et al., 2008).
In southern China, Kandelia obovata is one of the dominant native mangrove species (Sheue et al., 2003). The viviparous propagules of K. obovata grow rapidly once they drop into the soft and muddy substrate. Due to the great convenience of collecting and planting propagules directly on the mudflat, K. obovata has been highly preferred for many mangrove restoration programs in the past three decades. Another mangrove species usually recommended for restoration is Sonneratia apetala Buch.-Ham., which was introduced from Bangladesh to China in 1985 (Ren et al., 2009). Unlike the native and slow-growing K. obovata, S. apetala is non-viviparous and establishes seedlings on tidal flats via seeds released from the ripened fruit (Liao et al., 2004). As an introduced species with high adaptability and fast-growing characteristics, S. apetala had been widely planted in southern China for mangrove afforestation programs, and its plantation has expanded to 20.8 km2 in the last two decades (Yang et al., 2018).
Many studies had previously shed light on the impacts of sediment dynamics, i.e., erosion or accretion of sediment, on the survival and growth of mangrove seedlings (Ellison, 1999; Balke et al., 2013). Therefore, sediment dynamics are crucial when considering the natural dispersal and colonization of mangrove seedlings (Balke et al., 2011). The allocation of root biomass was significantly different, exhibiting resistance to erosion between different sediment types in a mesocosm experiment with two saltmarsh species (Schwarz et al., 2015). The sediment texture may also cause root traits to respond to the inundation of trees (Wang et al., 2015). However, less is known about how sediment texture affects the development of mangrove root systems during the early growth stage.
Three major anthropogenic sources are responsible for the additional nutrient input to mangrove forests, including domestic sewage, agricultural drainage, and coastal aquaculture effluent. Lee (2016) estimated the scale of the C and N influx surrounding the South China Sea and compared it to the C and N input contributed by mangroves. Notably, the nutrients exported by artificial sources are more likely used by decomposers, e.g., microbes and algae, compared with those from mangrove forests, indicating that eutrophication will occur more frequently on the coast, especially where mangrove forests retreat (Lee, 2016). Sun et al. (2020) also verified that persistent aquaculture effluent input would substantially enhance eutrophication levels in mangrove forests. Most nutrients were eventually deposited and accumulated in the mangrove sediment, and the soil total organic carbon (TOC), total nitrogen (TN), and total phosphorus (TP) content increased in mangroves compared to those of unvegetated tidal flats (Sun et al., 2020). Continuous overloading of terrigenous nutrients may pose irreversible impacts on coastal ecosystems, such as temperate saltmarshes and tropical mangroves, resulting in the degradation and loss of existing wetland ecosystems (Ruiz et al., 2001; Lefcheck et al., 2018). Usually, mangrove propagules spread at the margin of the forest and along the tidal creek. The seedlings develop and colonize in the tidal creeks, which are frequently affected by the aquaculture effluent in southern China. The morphological and physiological features of seedling root systems may be sensitive to the additional nutrient input. However, the influence of persistent nutrient input on mangroves during their early growth stage remains vague and challenging. The influence of nutrient input on modifying the patterns of colonization and competition of native/exotic mangroves is still unclear.
In this study, we set up a mesocosm experiment to explore the growth and physiological responses of the propagule root system of two mangrove species, i.e., K. obovata and S. apetala, which are typically used in mangrove restoration and afforestation programs in southern China. Both the mangrove species had naturalized and dispersed extensively along the coasts. However, whether to plant the fast-growing exotic mangroves or slow-growing native ones, e.g., S. apetala vs. K. obovata in mangrove restoration programs is still controversial and inconclusive (Ren et al., 2009). A comparison of their performance in various restoration sites featured by different sediment and nutrient input conditions may give valuable reference for the selection of mangrove species when conducting a restoration program in the future. The effects of sediment physical properties and additional N input on the growth and development of mangrove roots had been examined during their early growth period. It was hypothesized that the exotic S. apetala could be more adaptive to sediment texture and N input conditions than K. obovata and that the root system of different mangrove species may respond differently to sediment physical properties and N input in accordance with their morphological and physiological traits.
Materials and Methods
Collection and Cultivation of Mangrove Propagules
In April 2020, the ripened propagules of K. obovata with similar individual lengths and weights were collected from the Pearl River Estuary, southern China. Later in July 2020, the fruits of S. apetala were collected in the same place, and seeds were removed from the ripened and softened fruits within 2 weeks. The propagules and seeds were cultivated for 15 days by placing them in nursing trays for acclimation before being planted for the mesocosm experiment. Simulated semidiurnal tides with a daily inundation period of 8 h were also applied to the seedlings according to the nursing process of mangroves described previously by Tam and Wong (1997).
Mesocosm Experiments of Seedling Cultivation
After propagules or seeds were acclimated for 15 days, the seedlings of K. obovata and S. apetala were then transplanted in three categories of sediment treatments of sand (SA), mud (MU), and a mixture of mud and sand by the volumetric ratio of 1:1 (MI) to test the influence of sediment texture on the growth and root development of mangrove seedlings. The bulk density and sediment mean value of grain size were measured as 1.104 ± 0.253 g⋅cm–3 and 131.73 ± 12.49 μm, 0.823 ± 0.374 g⋅cm–3 and 55.37 ± 8.68 μm, and 0.644 ± 0.208 g⋅cm–3 and 11.54 ± 2.86 μm for SA, MI, and MU, respectively, prior to the experiment. «According to the Report on the State of the Ecology and Environment in China» in the past 5 years,1 one of the major pollutions in the nearshore sea areas, the major pollution indicator in key spawning grounds, feeding grounds, migration channels as well as in nature reserve areas for key fishery resources were inorganic nitrogen in China. Moreover, the main inorganic nitrogen pollutants in marine aquaculture wastewater was NH4+ (Yang et al., 2019; Ding et al., 2020; Liu et al., 2020). Therefore, to evaluate the impact of additional N input on the growth of mangrove seedlings, N as NH4Cl was added to the seawater of the mesocosm at concentrations of 0 (CK), 10 (N10), and 50 (N50) mg N⋅L–1. The set N content in seawater referred to the upper limit (10 mg N⋅L–1, N10) and five times (50 mg N⋅L–1, N50) the aquaculture effluent discharge in the coastal water of southern China (Li et al., 2013). Salinity in the mesocosm was set to 10 PSU according to the pore water salinity detected randomly in situ to meet the conditions of the mangrove in Pearl River Estuary, where the propagules were collected. There were nine tanks for each species in this experiment and divided into three groups for the 20th-, 40th-, and 60th-day cultivation experiment. Each group of tanks was for the three N input treatments, respectively. In each of the three tanks in one group contained seedlings planted in three different texture sediments. To avoid loss of seedlings’ death during the experiment, five seedlings were planted in each sediment type. In total, 18 water tanks were used for mesocosm compartments, including two mangrove species, three categories of sediment treatment, and three concentrations of additional N input. Before the cultivation experiment, the seedlings were placed into a pot of 10 cm in height and 10 cm in diameter with different sediment treatments and then placed into the tank containing seawater with different concentrations of additional N input. The mean height of seedlings of K. obovata and S. apetala were 23.1 and 6.2 cm, respectively. The daily inundation period was unified to 8 h by pumping seawater between the mesocosm tanks and storage tanks with an automatic water pump.
Measurement of Morphological and Physiological Parameters of Mangrove Seedlings’ Root
On the 20th, 40th, and 60th day of the cultivation experiment, the seedlings of both mangrove species were randomly chosen from each tank for the data collection process of morphological and physiological traits. Three replications were set up for the sediment and N input treatments. The measured and analyzed parameters of root samples were root respiratory rate, root length, root surface area, root volume, root branch number, root carbon content (TOC), root nitrogen content (TN), porosity (POR), nitrate reductase activity (NR), and moisture content. The specific methods of measurement and analysis were as follows:
Root Morphology Measurement
The WinRHIZO root analyzer equipped with an image capture system and a professional version of WinRHIZOpro 2005b analysis software (Fan Sheng Technology Co., Ltd., Shijiazhuang, China) was used to scan the root surface. When the scanning process was completed, the WinRHIZO root image analyzer was applied to obtain the morphological parameters of the fine roots scanned. The number, diameter, volume, and absorption surface area of fine roots at all levels were directly obtained through the image analysis process. After scanning, the weight of each level of the root system was measured.
Root Physiology Measurement
The in vitro root system method was used to measure the fine root respiratory rate. Since there are different levels of fine roots based on the root position in the branching hierarchy of the root system and may have different functions and compositions (Zhang et al., 2021). Therefore, root samples were selected from the live fine roots at various levels as much as possible and were oven-dried to constant weight at 65°C. Root samples with a dry weight of 0.05–0.40 g were selected from the live fine roots at various levels and smeared on the fine root section with Vaseline to prevent traumatic breathing. Each fine root was wrapped with soaked filter paper, placed in an aluminum container, and connected to the AZG-300 portable soil/water greenhouse gas monitor (Ao Zuo Ecological Instrument Co., Ltd., Beijing, China) to measure the fine root respiratory rate. The aluminum container was immersed in dark and room temperature water to maintain a temperature similar to that of the soil (Lovelock et al., 2006b). Because the filter paper absorbs and removes CO2 when measuring the fine root respiratory rate, the filter paper was measured separately as a blank and subtracted from the value of the fine root respiratory rate.
To determine the organic carbon and total nitrogen content of roots, the samples of roots were oven-dried and ground. Then, the organic carbon content and total nitrogen content of roots were measured by a Vario EL CHN autoanalyzer (Elementar Inc., Germany).
The porosity of the root was detected via the pycnometer method (Luxmoore et al., 1972; Mei et al., 2009), and the equation for calculation is as follows:
where r refers to the weight of the fresh roots, p refers to the weight of the pycnometer filled with water, Pr refers to the total weight of the fresh roots in the pycnometer filled with water, and Pvr refers to the total weight of the fresh roots and the pycnometer filled with water after vacuuming.
To determine the root nitrate reductase activity, the collected root tissue was rinsed in cold tap water and cold deionized water and refrigerated for further use. The tissue was milled with 0.1 M tris buffer (pH 7.5) and 1 mM cysteine. A buffer-tissue ratio of 6:1 was applied. The filtrate was centrifuged at 20,000 g for 20 min, and the supernatant solution was mixed with 1.0 ml of 0.1 M potassium phosphate buffer, 0.2 ml of 0.1 M KNO3, and 0.5 ml of 1.36 × 10–3 M DPNH. After incubation at 27°C for 20 min, the solutions were diluted with 4 ml of deionized water, and N-1-naphthyl-ethylene diamine hydrochloride reagent was added to the tubes and intensively mixed. Then, the optical density was measured at 540 nm with a spectrophotometer (UV-755B, Shanghai Precision Scientific Instrument Co., Ltd., Shanghai, China; Hageman and Flesher, 1960).
The root samples were oven-dried at 65°C to a constant weight. The ratio between the weight loss of water and the weight of the root sample was calculated as water content.
Statistical Analyses
The data obtained in the experiment were analyzed using IBM SPSS statistic 22.0 software (SPSS Inc., United States). All data were tested for normality and log-transformed if necessary. The differences in growth and physiological parameters of seedlings across different mangrove species, sediment texture, and N input treatments were explored with a three-way analysis of variance (ANOVA). Significant differences among multiple means were determined by the Student–Newman–Keuls (SNK) test at the α = 0.05 level. The association of parameters was analyzed by the Pearson correlation test using R software (R Core Team, 2019). Origin (version 8.0) was applied to export graphs. Since the interval of seedlings’ sampling was 20 days, the morphological and physiological features of mangrove seedlings did not change obviously between different sampling times in their early growth stage. Hence, the factor of “sampling time” was excluded in the statistical analysis followed.
Results
Responses of Mangrove Seedling Roots to Sediment Texture and N Input
The root morphological features of mangrove seedlings exhibited distinct patterns across different sediment treatments in the mesocosm experiment (Figure 1 and Supplementary Table 1). Seedlings of K. obovata and S. apetala cultivated in the SA had significantly lower root length, root surface area, root volume, and amounts of roots than those cultivated in MU and MI. Seedlings of K. obovata cultivated in SA (6.62%) had a significantly higher root porosity, indicating a more aerial condition in the sediment compared to those cultivated in MI (5.48%), while such pattern was not applied to those of S. apetala. The root growth of seedlings cultivated in MU and MI was not hindered compared to seedlings cultivated in SA. However, in terms of physiological features, no significant difference was detected among all sediment treatments except the seedlings of S. apetala cultivated in MU exhibited significantly lower root NR activity than those cultivated in SA and MI (Figure 1Supplementary Table 1).
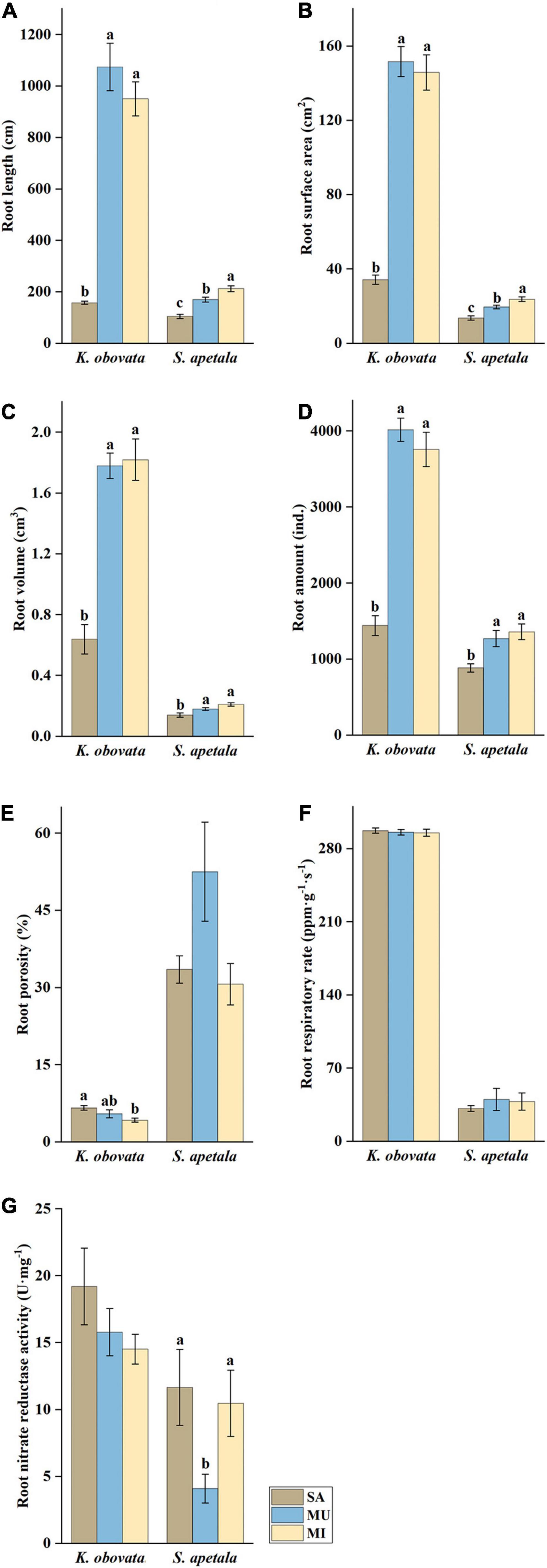
Figure 1. Physiological and morphological root parameters (A) root length, (B) root surface area, (C) root volume, (D) amount of roots, (E) root porosity, (F) root respiratory rate, and (G) nitrate reductase activity of mangrove seedlings roots treated with different sediment texture for 60 days in a mesocosm experiment (means ± SE). Different letters indicate significant differences among different sediment textures in one species (p < 0.05).
Apart from the patterns of different sediment treatments, the treatments of additional N input had no significant influence on either the morphological or physiological parameters of mangrove seedling roots for S. apetala. The same pattern of morphological parameters was also applied to the seedlings of K. obovata, while obviously higher root respiratory rate and root NR activity were detected from its seedlings in treatments of CK and N50, respectively (Figure 2 and Supplementary Table 1).
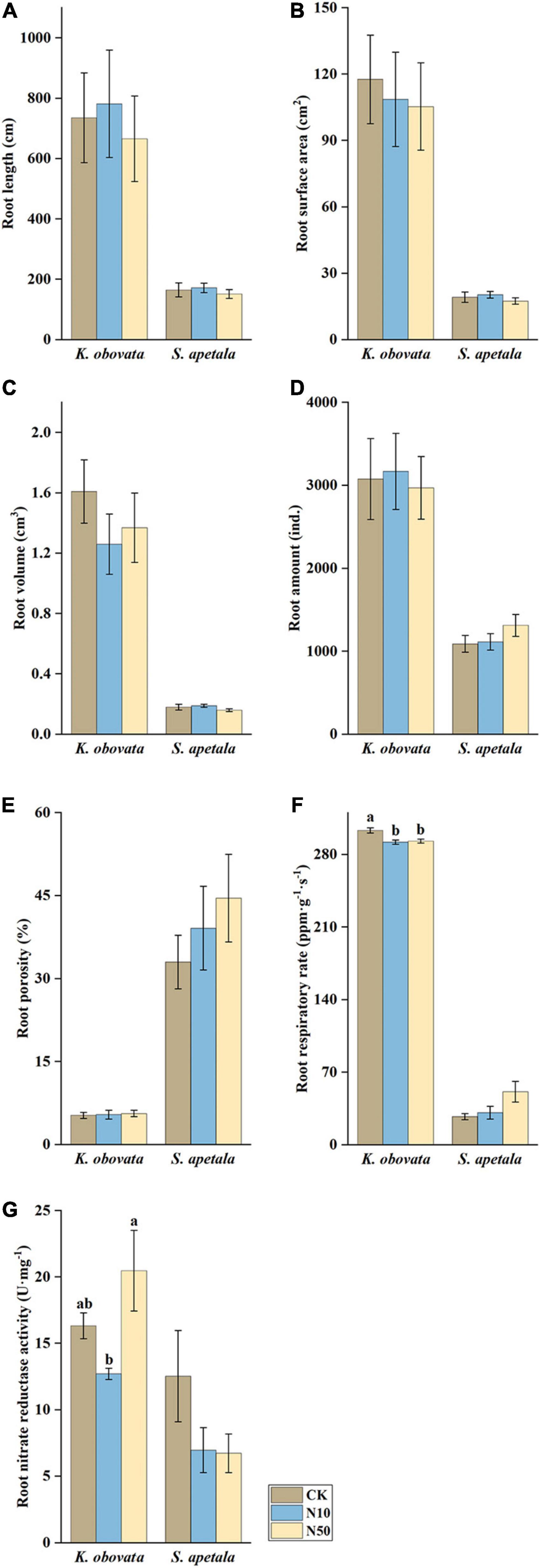
Figure 2. Physiological and morphological root parameters (A) root length, (B) root surface area, (C) root volume, (D) amount of roots, (E) root porosity, (F) root respiratory rate, and (G) nitrate reductase activity of mangrove seedlings roots treated with different nitrogen concentrations for 60 days in a mesocosm experiment (means ± SE). Different letters indicate significant differences among different nitrogen concentration treatments in one species (p < 0.05).
With respect to the morphological and physiological response of two mangrove seedlings, K. obovata and S. apetala, significant differences were observed between the mangrove species and the interaction of sediment texture and mangrove species (Supplementary Table 1). The mangrove species differed significantly in all parameters. Therefore, we analyzed the responses of two mangrove species, K. obovata and S. apetala, in the treatment of sediment texture and nitrogen input for further results (Supplementary Tables 2, 3). In terms of sediment texture, the morphological traits as root length, the amount of roots, root surface area, and root volume were lower in SA in both mangrove species (Figure 1). These parameters were higher in K. obovata than in S. apetala. The viviparous propagules of K. obovata were characterized by their larger size compared to those of S. apetala, which could have resulted in their higher morphological parameters to meet the demands of early anchorage and colonization after falling onto the dynamic tidal flats. About the physiological features, the root porosity of K. obovata seedlings in SA was higher than those in MI, but for the root respiratory rate and root NR activity, they presented no significant difference among the sediment treatments. The root NR activity of S. apetala seedlings had the different profile for root porosity and root respiratory rate that root NR activity was higher in SA and MI than in MU. The root respiratory rate of S. apetala seedlings showed a similar pattern to K. obovata seedlings. In contrast to the root NR activity, the root porosity of K. obovata was far lower than that of S. apetala, especially in MU sediment, indicating that S. apetala seedlings had more physiological adaptability toward the MU treatment than K. obovata seedlings. For nitrogen input, except root porosity, both the morphological and physiological parameters of K. obovata were higher than those of S. apetala. However, the features of S. apetala presented no significant difference among nitrogen input treatments, while the root respiratory rate and root NR activity of K. obovata showed a significant difference among nitrogen input treatments. The highest root respiratory rate of K. obovata was found in the control (CK), which showed a significant difference with other treatments. The lowest NR of K. obovata was found in N50.
Regarding the interaction of nitrogen input and sediment texture, no significant interaction between the two treatments for K. obovata was detected in all parameters, while there was a significant interaction between the two treatments for root length in S. apetala. Although the root length of S. apetala did not show a significant difference in different nitrogen inputs, the interaction showed a significant difference, which means that the combination of the two treatments might influence the stability of S. apetala seedling roots. Sediment texture and species had a significant interaction on most of the parameters, except root respiratory rate and NR, which indicated different responses for these parameters between the two mangrove species. For root length, the interaction of nitrogen input and sediment texture was only detected in S. apetala. The root respiratory rate was affected by nitrogen input only in K. obovata (Supplementary Tables 1–3).
Correlation Across the Morphological and Physiological Traits of Mangrove Seedlings
A bivariate correlation analysis was applied to explore the common response of morphological and physiological traits to the sediment texture treatment. For K. obovata, the root respiratory rate had a significant negative correlation with root length and amount of roots and a significant positive correlation with NR in all sediment treatments. The root respiratory rate was significantly negatively correlated with root surface area in MI and MU but significantly positively correlated with root volume in SA. For the morphological traits of K. obovata seedlings, many features were interdependent among treatments. Root length had a significantly positive correlation with root surface area and amount of roots, while root surface area was significantly positively correlated with root volume in the three sediment treatments. However, root volume was negatively correlated with the amount of roots in SA and positively correlated with MU. A significant positive correlation of root surface area with the amount of roots was found in MU and MI but not SA. The root porosity rate had a significant negative correlation with root length and root surface area only in MU. In MU and MI, NR was negatively correlated with the amount of roots, and it was also significantly negatively correlated with root length and root surface area in MI. However, there was no significant correlation of NR with other traits in SA, except for root respiratory rate.
For S. apetala, root respiratory rate was not significantly correlated with other traits among sediment treatments. To morphological traits, root length was significantly positively correlated with root surface area, root volume, and amount of roots among sediment treatments. Root surface area had a significant positive correlation with root volume in the three sediment treatments. A significant positive correlation of root porosity with NR was observed in the SA and MI, while the amount of roots had a significant positive correlation with root surface area in SA and MU.
Compared with K. obovata, the traits of S. apetala seedlings had less significant correlations across all sediment treatments, and most of the correlations were observed for morphological rather than physiological traits. The only significant correlation involved in the physiological traits of S. apetala was that NR had a significant positive correlation with root porosity in MI and SA. However, such a correlation was not observed in K. obovata. For K. obovata, many morphological traits significantly interacted with physiological traits. The seedlings of K. obovata exhibited a more sensitive response toward sediment treatments than S. apetala (Figure 3).
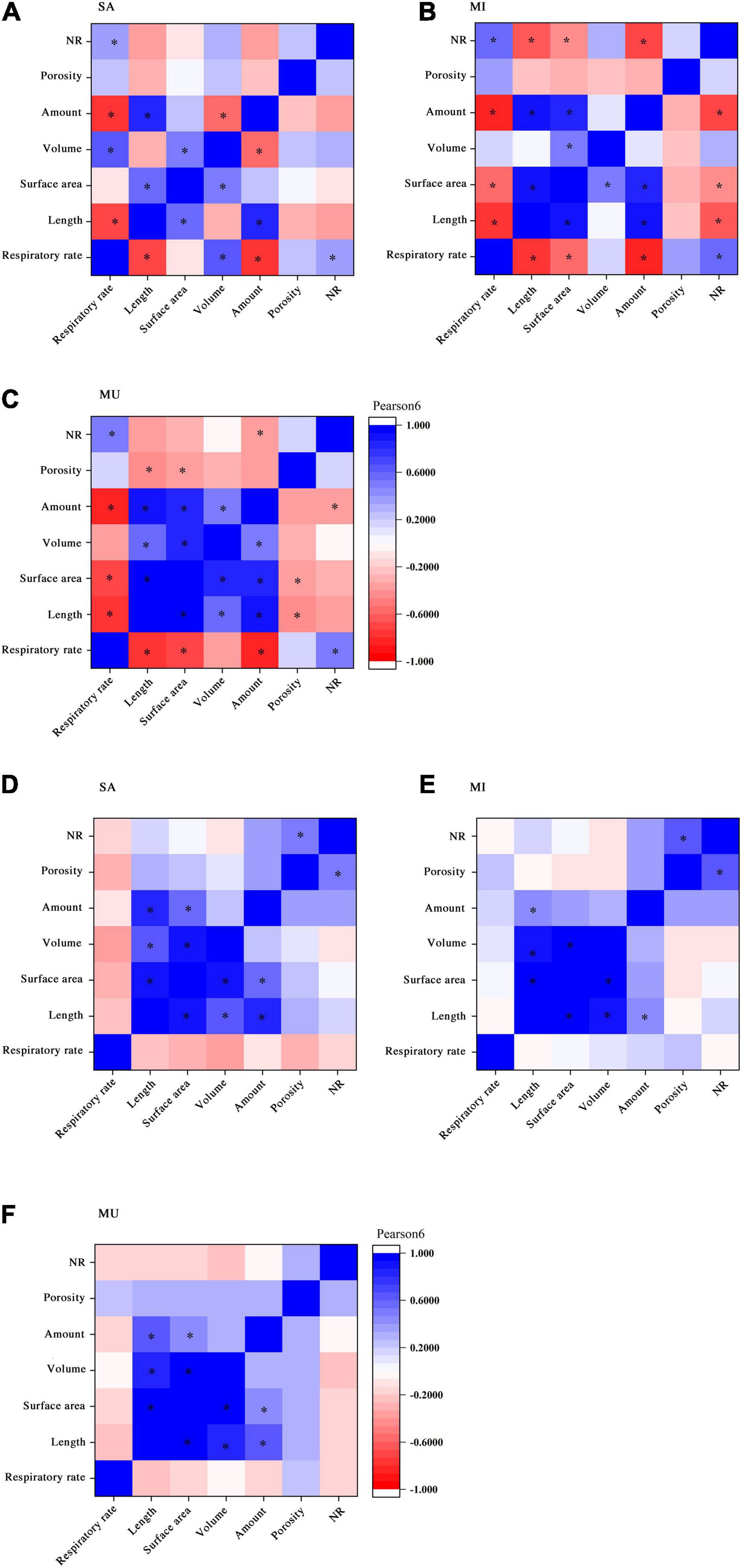
Figure 3. Correlation between parameters of root morphology and physiology in sediment types [sand (SA), mud (MU), and mixture of mud and sand by volumetric ratio of 1:1 (MI)]. Graphs panels (A–C) represent the correlation of parameters in K. obovata, and graphs panels (D–F) represent the correlation of parameters in S. apetala. *Indicates a significant difference (p < 0.05) among the sediment treatments.
Discussion
Different Responses of Mangrove Seedling Roots to the Treatments of Sediment Texture and N Input Concentration
Root morphology is one of the fundamental indicators for describing the development and growth of plants (Lynch, 1995), and the sediment physical characteristics are key factors that affect root morphology. In this study, root length, root surface area, root volume, and the amount of roots were considered to briefly describe the mangrove seedlings’ root morphology in treatments of sediment texture and nitrogen input. The results of root morphological features treated with different sediment textures showed a significant difference. The four morphological features had a less active response in SA treatment than in MU and MI treatments, except for root porosity. There were no significant differences between all sediment textures. Most of the morphological features in SA were less than half of those in MU and MI. The sediment physical characteristics may influence the morphological features of mangrove seedlings’ roots, thus modifying the nutrient uptake of plants by affecting their root growth. For instance, the rooted submerged macrophyte Vallisneria natans develops thinner and longer roots to facilitate the absorption of nutrients in sediment with more sand (Xie et al., 2005), which is not consistent with our findings. Opposing patterns of root morphological features were observed in another submerged macrophyte, Myriophyllum spicatum; the root length decreased in sediment containing more sand (Wang et al., 2009; Bai et al., 2015). It can be inferred that the response of root morphological features to different sediment textures is species dependent. Furthermore, anoxia in sediment may play another key role in root growth and development. Increased sediment anoxic conditions might hamper the growth of roots and cause mortality of shoots in seagrass (Terrados et al., 1999). For mangroves extending along the coastline, mangrove plants are always flooded by the periodical tide, which results in the anoxia of sediment captured and settled by their massive aboveground root systems (Furukawa et al., 1997; Reef et al., 2010). Therefore, anoxic conditions played an important role in root morphology. Plants responded positively along the gradient in redox potential, showing that the emerged marsh plants had morphological responses that affected roots and biomass to meet their oxygen demand under anaerobic conditions (White and Ganf, 2001). For clay loam, similar to MU in our mesocosm experiment, more severe oxygen deficiency was observed compared to SA treatment. Thus, the morphological features of mangroves planted in MU were promoted more than those planted in SA.
In general, due to the high denitrification rate of vigorous microbial activities in anaerobic sediment (Flores-Mireles et al., 2007; Zhang et al., 2020), nitrogen limitation has been manifested to be ubiquitous in most mangroves (Lovelock et al., 2006a). Consequently, mangrove plants are susceptible to external nutrient input. The growth of the root system could be accelerated by moderate nutrient input (Wang et al., 2009; Chen et al., 2020). However, in our study, no significant difference in root features was detected among various levels of nitrogen input. The root development of both mangrove species was not affected by the simulated additional N input at the early growth stage after colonization along tidal creeks, which are frequently impacted by massive aquaculture effluent. Moreover, for the physiological features, including root respiratory rate and NR, there was no significant difference across the treatments of neither sediment texture nor nitrogen input concentration. The fine root respiratory rate obviously correlated to the nitrogen concentration that had been previously attested in terrestrial forests (Burton et al., 2002). Additional fertilization with nitrogen could not markedly enhance the fine root respiratory rate of mangroves. Such physiological responses in mangrove roots were attributed to the availability of physiologically active compounds of nitrogen and the phosphate limitation in soil, which may influence the root respiratory rate (Lovelock et al., 2006b). This interpretation was in line with our findings in the mesocosm experiment. Although mangrove root physiological activities were hindered in sediment with lesser aerated conditions (Lee et al., 1996; Ellison, 1999), the root respiratory rate did not vary across different sediment textures in our experiment. For aquatic macrophytes, root respiratory rates were closely correlated with root porosity, indicating that these plants can actively cope with the anaerobic stress (Lemoine et al., 2012). However, such variation was not observed in seedlings of K. obovata and S. apetala during the early growth stage.
Adaptation to Sediment Texture of Different Mangrove Seedlings Was Linked to Their Biological Traits
Sediment texture constructs environmental conditions, such as nutrient availability and oxygen content, affecting the development of roots and colonization by seedlings (Lenssen et al., 1999; Krauss et al., 2014). In our experiment, all analyzed features showed a significant difference between mangrove species, and a species-specific adaptive mechanism of mangroves was at play. On this basis, how mangrove seedlings build up their early root structure in different sediment textures has been investigated. Notably, the sediment texture and mangrove species had a significant interaction with root length, root surface area, root volume, amount of roots, and root porosity.
For root morphological traits of two mangrove species, those of K. obovata were collectively higher than those of S. apetala. This is highly pertinent to the strategy of colonization presented by their propagules. As K. obovata is typically viviparous, characterized by its larger and longer propagules, namely the hypocotyl, a more extensive and robust root system is required in favor of its anchorage on the soft and dynamic tidal flats. In contrast, S. apetala is non-viviparous and reproduces numerous tiny and lightweight seeds with smaller and thinner roots. Despite the different root systems of the two mangrove species, they responded similarly to different sediment treatments. A more proliferating root system was found in the muddy sediment with a lower bulk density and finer particle size. The growth of littoral marsh plants might be hampered when cultivated with the soil of a higher bulk density. Plants have to deploy more biomass in proportion to the underground part to ensure the successful colonization of seedlings (Lenssen et al., 1999). Similarly, this finding also applies to the seedlings of mangrove plants in our study.
Compared to S. apetala, in K. obovata, the most porous roots occurred in the sediment composed of sand rather than mud, while the root porosity of K. obovata was lower than that of S. apetala. A higher root porosity rate may indicate higher adaptability to submerged or anoxic conditions by enhancing oxygen exchange (Justin and Armstrong, 1987). It can be inferred that S. apetala seedlings can acclimate more seaward in less elevated habitats than K. obovata does, which is coincident with their natural occurrence along the coastline of southern China (Ren et al., 2009). The strategy of plant roots coping with an anoxic environment may involve the balance between maintaining physiological function and decreasing tissue respiration (Purnobasuki and Suzuki, 2004).
For additional nitrogen input, no significant difference was found in S. apetala, but differences in NR and root respiration were detected in K. obovata. Although many studies reported that nitrogen enrichment could enhance the growth of many plants, no such trends existed were found in our experiment for either mangrove species. The simulated nitrogen input did not seem to significantly influence the early growth stage of mangroves newly colonized along tidal creeks. Notably, under more eutrophic conditions, K. obovata improved its efficiency for nitrogen use but lowered the root respiratory rate, which demonstrated the alteration of carbon allocation (Bae et al., 2015). Thus, the growth of saplings of K. obovata may be impacted in a nutrient-enriched habitat over a longer period.
The Effect of Sediment Texture and Nitrogen Input on Mangrove Conservation
Artificial fertilization has been shown to significantly modify the growth and ecological function of mangroves, especially at the sites of higher sedimentation (Lovelock et al., 2007). Therefore, the impacts of nutrient enrichment will be enlarged based on the sediment texture. The soil bulk density was vital to the establishment and development of the root system which was relevant to the biogeochemical process of mangrove ecosystems (Ola et al., 2019). It has been suggested that the sediment should also be considered when conducting mangrove restoration programs. The result of our study inferred that the seedling roots of K. obovata showed more sensitivity toward sediment texture and nutrient enrichment conditions, suggesting that K. obovata needed more suitable conditions for colonization than S. apetala. Currently, mangroves are widely suffering from terrigenous eutrophication on both regional and global scales (Friess et al., 2019). The nutrient-enriched environment will probably shift the existing status of mangrove populations in their assemblages (Luo et al., 2017; Yu et al., 2021).
In a heavily disturbed and eutrophicated estuary surrounded by megacities, such as the Pearl River Estuary, the natural regeneration and colonization of K. obovata and S. apetala is inclined to vary unintentionally. Both of these mangrove species are universally used in many mangrove restoration programs. Regarding the potential influence of sediment texture and nitrogen input on the early growth of seedlings, it is suggested that sediment and nutrient conditions be considered in conservation and restoration programs for mangroves in the future.
Data Availability Statement
The original contributions presented in the study are included in the article/Supplementary Material, further inquiries can be directed to the corresponding author.
Author Contributions
ZH and YP originally planned and designed the research. YP, LY, ZW, and ZH performed the field work and the mesocosm experiments. LY, HH, LZ, and ZW contributed to the laboratory analysis. YP and ZH wrote the first drafts of the manuscript and were further improved by ZH and SL. All authors commented and approved the manuscript.
Funding
This study was supported by Guangdong Basic and Applied Basic Research Foundation (2020A1515110657), National Nature Science Foundation Grant of China (41771095, 42106159, 91951207, and 42006138), project supported by the Southern Marine Science and Engineering Guangdong Laboratory (Zhuhai) (311021004 and SML2020SP004), and Guangdong Forestry Science and Technology Innovation Project (2021KJCX012 and 2022KJCX019).
Conflict of Interest
The authors declare that the research was conducted in the absence of any commercial or financial relationships that could be construed as a potential conflict of interest.
Publisher’s Note
All claims expressed in this article are solely those of the authors and do not necessarily represent those of their affiliated organizations, or those of the publisher, the editors and the reviewers. Any product that may be evaluated in this article, or claim that may be made by its manufacturer, is not guaranteed or endorsed by the publisher.
Acknowledgments
We sincerely thank Huaye Sun and Mengxing Wu for their help with fieldwork and laboratory analysis.
Supplementary Material
The Supplementary Material for this article can be found online at: https://www.frontiersin.org/articles/10.3389/fmars.2022.793818/full#supplementary-material
Footnotes
References
Alongi, D. M. (2008). Mangrove forests: resilience, protection from tsunamis, and responses to global climate change. Estuar. Coast. Shelf S. 76, 1–13. doi: 10.1016/j.ecss.2007.08.024
Bae, K., Fahey, T. J., Yanai, R. D., and Fisk, M. (2015). Soil nitrogen availability affects belowground carbon allocation and soil respiration in northern hardwood forests of New Hampshire. Ecosystems 18, 1179–1191. doi: 10.1007/s10021-015-9892-7
Bai, X., Chen, K., Zhao, H., and Chen, X. (2015). Impact of water depth and sediment type on root morphology of the submerged plant Vallisneria natans. J. Freshwater Ecol. 30, 75–84. doi: 10.1080/02705060.2014.970672
Balke, T., Bouma, T., Herman, P., Horstman, E., Sudtongkong, C., and Webb, E. (2013). Cross-shore gradients of physical disturbance in mangroves: implications for seedling establishment. Biogeosciences 10, 5411–5419. doi: 10.5194/bg-10-5411-2013
Balke, T., Bouma, T. J., Horstman, E. M., Webb, E. L., Erftemeijer, P. L., and Herman, P. M. (2011). Windows of opportunity: thresholds to mangrove seedling establishment on tidal flats. Mar. Ecol-prog. Ser. 440, 1–9. doi: 10.3354/meps09364
Balke, T., Herman, P. M., and Bouma, T. J. (2014). Critical transitions in disturbance-driven ecosystems: identifying windows of opportunity for recovery. J. Ecol. 102, 700–708. doi: 10.1111/1365-2745.12241
Barbier, E. B. (2016). The protective service of mangrove ecosystems: a review of valuation methods. Mar. Pollut. Bull. 109, 676–681. doi: 10.1016/j.marpolbul.2016.01.033
Burton, A., Pregitzer, K., Ruess, R., Hendrick, R., and Allen, M. (2002). Root respiration in North American forests: effects of nitrogen concentration and temperature across biomes. Oecologia 131, 559–568. doi: 10.1007/s00442-002-0931-7
Chen, J., Liu, L., Wang, Z., Zhang, Y., Sun, H., Song, S., et al. (2020). Nitrogen fertilization increases root growth and coordinates the root-shoot relationship in cotton. Front. Plant Sci. 11:880. doi: 10.3389/fpls.2020.00880
Ding, Y., Guo, Z. S., Mei, J. X., Liang, Z. L., Li, Z. P., and Hpi, X. G. (2020). Investigation into the Novel Microalgae Membrane Bioreactor with Internal Circulating Fluidized Bed for Marine Aquaculture Wastewater Treatment. Membranes-Basel. 10:353. doi: 10.3390/membranes10110353
Ellison, J. C. (1999). Impacts of sediment burial on mangroves. Mar. Pollut. Bull. 37, 420–426. doi: 10.1016/S0025-326X(98)00122-2
Fan, H., and Wang, W. (2017). Some thematic issues for mangrove conservation in China (In Chinese). J. Xiamen Univers. Nat. Sci. 56, 323–330.
Flores-Mireles, A. L., Winans, S. C., and Holguin, G. (2007). Molecular characterization of diazotrophic and denitrifying bacteria associated with mangrove roots. Appl. Environ. Microb. 73, 7308–7321. doi: 10.1128/AEM.01892-06
Friess, D. A., Rogers, K., Lovelock, C. E., Krauss, K. W., Hamilton, S. E., Lee, S. Y., et al. (2019). The state of the world’s mangrove forests: past, present, and future. Annu. Rev. Env. Resour. 44, 89–115. doi: 10.1146/annurev-environ-101718-033302
Furukawa, K., Wolanski, E., and Mueller, H. (1997). Currents and sediment transport in mangrove forests. Estuar. Coast. Shelf S. 44, 301–310. doi: 10.1006/ecss.1996.0120
Hageman, R., and Flesher, D. (1960). Nitrate reductase activity in corn seedlings as affected by light and nitrate content of nutrient media. Plant Physio. 35:700. doi: 10.1104/pp.35.5.700
Hamilton, S. E., and Casey, D. (2016). Creation of a high spatio-temporal resolution global database of continuous mangrove forest cover for the 21st century (CGMFC-21). Global Ecol. Biogeogr. 25, 729–738. doi: 10.1111/geb.12449
Hargan, K. E., Williams, B., Nuangsaeng, B., Siriwong, S., Tassawad, P., Chaiharn, C., et al. (2020). Understanding the fate of shrimp aquaculture effluent in a mangrove ecosystem: aiding management for coastal conservation. J. Appl. Ecol. 57, 754–765. doi: 10.1111/1365-2664.13579
He, Z., Peng, Y., Guan, D., Hu, Z., Chen, Y., and Lee, S. Y. (2018). Appearance can be deceptive: shrubby native mangrove species contributes more to soil carbon sequestration than fast-growing exotic species. Plant Soil 432, 425–436. doi: 10.1007/s11104-018-3821-4
Jiang, J., Zhou, C., An, S., Yang, H., Guan, B., and Cai, Y. (2008). Sediment type, population density and their combined effect greatly charge the short-time growth of two common submerged macrophytes. Ecol. Eng. 34, 79–90. doi: 10.1016/j.ecoleng.2008.07.003
Justin, S., and Armstrong, W. (1987). The anatomical characteristics of roots and plant response to soil flooding. New Phytol. 106, 465–495. doi: 10.1111/j.1469-8137.1987.tb00126.x
Kamal, S., Warnken, J., Bakhtiyari, M., and Lee, S. Y. (2017). Sediment distribution in shallow estuaries at fine scale: in situ evidence of the effects of three-dimensional structural complexity of mangrove pneumatophores. Hydrobiologia 803, 121–132. doi: 10.1007/s10750-017-3178-3
Krauss, K. W., McKee, K. L., Lovelock, C. E., Cahoon, D. R., Saintilan, N., Reef, R., et al. (2014). How mangrove forests adjust to rising sea level. New Phytol. 202, 19–34. doi: 10.1111/nph.12605
Lee, S. (2016). From blue to black: anthropogenic forcing of carbon and nitrogen influx to mangrove-lined estuaries in the South China Sea. Mar. Pollut. Bull. 109, 682–690. doi: 10.1016/j.marpolbul.2016.01.008
Lee, S., Tan, W., and Havanond, S. (1996). Regeneration and colonisation of mangrove on clay-filled reclaimed land in Singapore. Hydrobiologia 319, 23–35. doi: 10.1007/BF00020968
Lee, S. Y., Primavera, J. H., Dahdouh-Guebas, F., McKee, K., Bosire, J. O., Cannicci, S., et al. (2014). Ecological role and services of tropical mangrove ecosystems: a reassessment. Global Ecol. Biogeogr. 23, 726–743. doi: 10.1111/geb.12155
Lefcheck, J. S., Orth, R. J., Dennison, W. C., Wilcox, D. J., Murphy, R. R., Keisman, J., et al. (2018). Long-term nutrient reductions lead to the unprecedented recovery of a temperate coastal region. Proc. Natl. Acad. Sci. USA. 115, 3658–3662. doi: 10.1073/pnas.1715798115
Lemoine, D. G., Mermillod-Blondin, F., Barrat-Segretain, M. H., Massé, C., and Malet, E. (2012). The ability of aquatic macrophytes to increase root porosity and radial oxygen loss determines their resistance to sediment anoxia. Aquat. Ecol. 46, 191–200. doi: 10.1007/s10452-012-9391-2
Lenssen, J. P., Menting, F. B., van der Putten, W. H., and Blom, C. W. (1999). Effects of sediment type and water level on biomass production of wetland plant species. Aquat. Bot. 64, 151–165. doi: 10.1016/S0304-3770(99)00012-1
Li, Y. M., Liu, M., Wang, P., and Li, Y. J. (2013). Analysis of the water quality of aquaculture wastewater of Litopenaeus vannamei Boeen in tropics. J. Aquacul. 34, 42–45.
Liao, B. W., Zheng, S. F., Chen, Y. J., Li, M., and Li, Y. D. (2004). Biolofical characteristics and ecological adaptability for non-indigenous manfrove species Sonneratia apetala (In Chinese). Chinese J. Ecol. 23, 10–15.
Liu, X. G., Shao, Z. Y., Chen, G. F., Lu, S. M., Gu, Z. J., Zhu, H., et al. (2020). Ecological engineering in pond aquaculture: a review from the whole-process perspective in China. Rev. Aquacult. 13, 1060–1076. doi: 10.1111/raq.12512
Lovelock, C. E., Ruess, R. W., and Feller, I. C. (2006b). Fine root respiration in the mangrove Rhizophora mangle over variation in forest stature and nutrient availability. Tree Physiol. 26, 1601–1606. doi: 10.1093/treephys/26.12.1601
Lovelock, C. E., Feller, I. C., Ball, M. C., Engelbrecht, B. M., and Ewe, M. L. (2006a). Differences in plant function in phosphorus-and nitrogen-limited mangrove ecosystems. New Phytol. 172, 514–522. doi: 10.1111/j.1469-8137.2006.01851.x
Lovelock, C. E., Feller, I. C., Ellis, J., Schwarz, A. M., Hancock, N., Nichols, P., et al. (2007). Mangrove growth in New Zealand estuaries: the role of nutrient enrichment at sites with contrasting rates of sedimentation. Oecologia 153, 633–641. doi: 10.1007/s00442-007-0750-y
Luo, L., Meng, H., Wu, R. N., and Gu, J. D. (2017). Impact of nitrogen pollution/deposition on extracellular enzyme activity, microbial abundance and carbon storage in coastal mangrove sediment. Chemosphere 177, 275–283. doi: 10.1016/j.chemosphere.2017.03.027
Luxmoore, R., Sojka, R., and Stolzy, L. (1972). Root Porosity and Growth Responses of Wheat to Aeration and Light Intensity. Soil Sci. 113, 354–357. doi: 10.1097/00010694-197205000-00009
Lynch, J. (1995). Root architecture and plant productivity. Plant Physiol. 109:7–13. doi: 10.1104/pp.109.1.7
Mei, X., Ye, Z., and Wong, M. H. (2009). The relationship of root porosity and radial oxygen loss on arsenic tolerance and uptake in rice grains and straw. Environ. Pollut. 157, 2550–2557. doi: 10.1016/j.envpol.2009.02.037
Ola, A., Gauthier, A. R., Xiong, Y., and Lovelock, C. E. (2019). The roots of blue carbon: responses of mangrove stilt roots to variation in soil bulk density. Biol. Lett. 15:20180866. doi: 10.1098/rsbl.2018.0866
Purnobasuki, H., and Suzuki, M. (2004). Aerenchyma formation and porosity in root of a mangrove plant, Sonneratia alba (Lythraceae). J. Plant Res. 117, 465–472. doi: 10.1007/s10265-004-0181-3
R Core Team (2019). R: a language and environment for statistical computing. Vienna: R foundation for statistical computing. Available online at: https://www.r-project.org
Rabinowitz, D. (1978). Dispersal properties of mangrove propagules. BIotropica 10, 47–57. doi: 10.2307/2388105
Reef, R., Feller, I. C., and Lovelock, C. E. (2010). Nutrition of mangroves. Tree Physiol. 30, 1148–1160. doi: 10.1093/treephys/tpq048
Ren, H., Lu, H., Shen, W., Huang, C., Guo, Q., and Jian, S. (2009). Sonneratia apetala Buch. Ham in the mangrove ecosystems of China: an invasive species or restoration species? Ecol. Eng. 35, 1243–1248. doi: 10.1016/j.ecoleng.2009.05.008
Romañach, S. S., DeAngelis, D. L., Koh, H. L., Li, Y., Teh, S. Y., Barizan, R. S. R., et al. (2018). Conservation and restoration of mangroves: global status, perspectives, and prognosis. Ocean Coast. Manage. 154, 72–82. doi: 10.1016/j.ocecoaman.2018.01.009
Ruiz, J. M., Pérez, M., and Romero, J. (2001). Effects of fish farm loadings on seagrass (Posidonia oceanica) distribution, growth and photosynthesis. Mar. Pollut. Bull. 42, 749–760. doi: 10.1016/S0025-326X(00)00215-0
Schwarz, C., Bouma, T., Zhang, L., Temmerman, S., Ysebaert, T., and Herman, P. (2015). Interactions between plant traits and sediment characteristics influencing species establishment and scale-dependent feedbacks in salt marsh ecosystems. Geomorphology 250, 298–307. doi: 10.1016/j.geomorph.2015.09.013
Sheue, C. R., Liu, H. Y., and Yong, J. W. (2003). Kandelia obovata (Rhizophoraceae), a new mangrove species from Eastern Asia. Taxon 52, 287–294. doi: 10.2307/3647398
Sun, H., He, Z., Zhang, M., Yen, L., Cao, Y., Hu, Z., et al. (2020). Spatial variation of soil properties impacted by aquaculture effluent in a small-scale mangrove. Mar. Pollut. Bull. 160:111511. doi: 10.1016/j.marpolbul.2020.111511
Tam, N. F., and Wong, Y. S. (1997). Accumulation and distribution of heavy metals in a simulated mangrove system treated with sewage. Asia-Pacific Confer. Sci Manage. Coast. Environ. 123, 67–75. doi: 10.1007/978-94-011-5234-1_8
Terrados, J., Duarte, C., Kamp-Nielsen, L., Agawin, N., Gacia, E., Lacap, D., et al. (1999). Are seagrass growth and survival constrained by the reducing conditions of the sediment? Aquat. Bot. 65, 175–197. doi: 10.1016/S0304-3770(99)00039-X
Thomas, N., Lucas, R., Bunting, P., Hardy, A., Rosenqvist, A., and Simard, M. (2017). Distribution and drivers of global mangrove forest change, 1996–2010. PLoS One 12:e0179302. doi: 10.1371/journal.pone.0179302
Valiela, I., Bowen, J., and York, J. K. (2001). Mangrove forests: One of the world’s threatened major tropical environments: at least 35% of the area of mangrove forests has been lost in the past two decades, losses that exceed those for tropical rain forests and coral reefs, two other well-known threatened environments. Bioscience 51, 807–815. doi: 10.1641/0006-3568(2001)051[0807:MFOOTW]2.0.CO;2
Wang, L., Zhao, C., Li, J., Liu, Z., and Wang, J. (2015). Root plasticity of Populus euphratica seedlings in response to different water table depths and contrasting sediment types. PLoS One 10:e0118691. doi: 10.1371/journal.pone.0118691
Wang, S., Jin, X., Jiao, L., and Wu, F. (2009). Response in root morphology and nutrient contents of Myriophyllum spicatum to sediment type. Ecol. Eng. 35, 1264–1270. doi: 10.1016/j.ecoleng.2009.05.012
White, S. D., and Ganf, G. G. (2001). The influence of convective flow and sediment type on root morphology in Typha domingensis. Aquat. Bot. 70, 151–161. doi: 10.1016/S0304-3770(00)00145-5
Xie, Y., An, S., Yao, X., Xiao, K., and Zhang, C. (2005). Short-time response in root morphology of Vallisneria natans to sediment type and water-column nutrient. Aquat. Bot. 81, 85–96. doi: 10.1016/j.aquabot.2004.12.001
Yang, J. Z., Hu, Y. H., Luo, Y., and Luo, C. Q. (2018). Study on the distribution status and dynamic changes of mangroves in Guangdong Province (In Chinese). Forest. Environ. Sci. 34, 28–31.
Yang, P., Yang, H., Lai, D. Y. F., Jin, B. S., and Tong, C. (2019). Production and uptake of dissolved carbon, nitrogen, and phosphorus in overlying water of aquaculture shrimp ponds in subtropical estuaries. China. Environ. Sci. Pollut. R. 26, 21565–21578. doi: 10.1007/s11356-019-05445-y
Yu, H., Liu, X. Y., Chao, Y., Peng, Y. S., Yu, X. L., Gu, H., et al. (2021). Co-symbiosis of arbuscular mycorrhizal fungi (AMF) and diazotrophs promote biological nitrogen fixation in mangrove ecosystems. Soil Biol. Biochem. 161:108382. doi: 10.1016/j.soilbio.2021.108382
Zhang, M., Dai, P., Lin, X., Hetharua, B., Zhang, Y., and Tian, Y. (2020). Nitrogen loss by anaerobic ammonium oxidation in a mangrove wetland of the Zhangjiang Estuary. China. Sci. Total Environ. 698:134291. doi: 10.1016/j.scitotenv.2019.134291
Keywords: mangrove, nitrogen input, sediment type, seedling root establishment, colonization
Citation: He Z, Yen L, Huang H, Wang Z, Zhao L, Chen Z, Lee SY and Peng Y (2022) Linkage Between Mangrove Seedling Colonization, Sediment Traits, and Nitrogen Input. Front. Mar. Sci. 9:793818. doi: 10.3389/fmars.2022.793818
Received: 12 October 2021; Accepted: 17 February 2022;
Published: 16 March 2022.
Edited by:
Zeng Zhou, Hohai University, ChinaReviewed by:
Dongdong Shao, Beijing Normal University, ChinaGuanglong Qiu, Guangxi Mangrove Research Center, Guangxi Academy of Sciences, China
Copyright © 2022 He, Yen, Huang, Wang, Zhao, Chen, Lee and Peng. This is an open-access article distributed under the terms of the Creative Commons Attribution License (CC BY). The use, distribution or reproduction in other forums is permitted, provided the original author(s) and the copyright owner(s) are credited and that the original publication in this journal is cited, in accordance with accepted academic practice. No use, distribution or reproduction is permitted which does not comply with these terms.
*Correspondence: Yisheng Peng, cHlpc2hAbWFpbC5zeXN1LmVkdS5jbg==