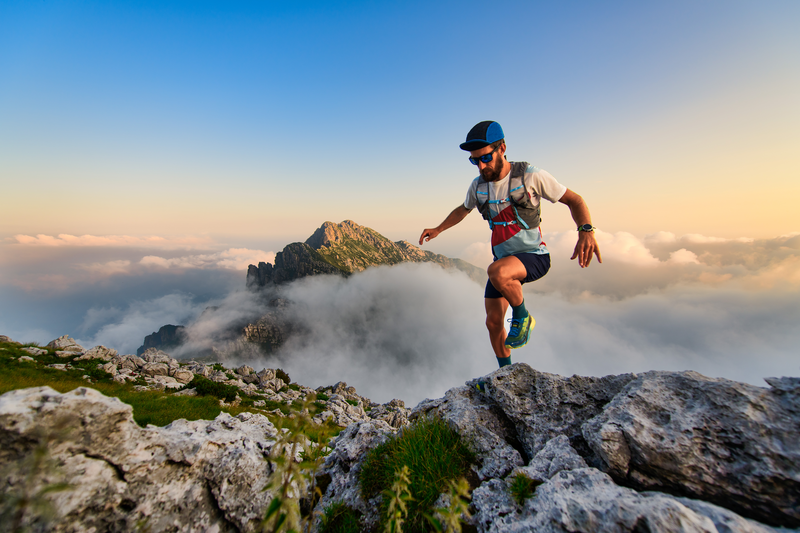
95% of researchers rate our articles as excellent or good
Learn more about the work of our research integrity team to safeguard the quality of each article we publish.
Find out more
ORIGINAL RESEARCH article
Front. Mar. Sci. , 25 April 2022
Sec. Marine Fisheries, Aquaculture and Living Resources
Volume 9 - 2022 | https://doi.org/10.3389/fmars.2022.788339
This article is part of the Research Topic How Overfishing Handicaps Resilience of Marine Resources Under Climate Change View all 10 articles
International objectives for sustainable development and biodiversity conservation require restoring fish populations to healthy levels and reducing fishing impacts on marine ecosystems. At the same time, governments, retailers, and consumers are increasingly motivated to reduce the carbon footprint of food. These concerns are reflected in measures of the EU Common Fisheries Policy (CFP) and the CFP Reform Regulation, which highlighted a need to move from traditional single-stock management toward an ecosystem approach to fisheries management (EAF). Using publicly available landings and effort data combined with estimates of adult population biomass, we develop methods to explore the potential for lowering emissions intensity and impacts on organic carbon stocks through ending overfishing and rebuilding stocks. We use the recent recovery of European hake (Merluccius merluccius) stocks in the Northeast Atlantic as a case study. With a focus on the hake fisheries of France, Spain, and the United Kingdom, we compare 2008 and 2016 fishing years. We make an initial estimate of the influence of changing stock status on greenhouse gas emissions during the fishery phase from fuel use and investigate the potential disturbance of organic carbon in the ecosystem, specifically via identification of bottom trawling overlap with organic-rich muddy sediments, and directly on storage in hake biomass. Our findings indicate that recovery of the hake stock was associated with reductions in overall emissions intensity from fuel and proportional impact on hake populations, however, total emissions from both fuel and landings increased, as did likely disturbance of sedimentary organic carbon in surface sediments due to benthic trawling. Ultimately, the aims of this analysis are to further explore the climate impacts of fisheries and overfishing, and to inform development of EAF in the EU.
Unsustainable resource extraction, biodiversity loss, and climate change threaten the health and longevity of marine ecosystems and fish populations worldwide (IPBES, 2019). Reducing greenhouse gas emissions (GHGs) is essential for reducing the ecological and social impacts of climate change and remains an international priority (United Nations General Assembly resolution [UNFCCC], 2015). Simultaneously, restoring fish populations and marine ecosystems to achieve healthy oceans are cited as international objectives for sustainable development (United Nations General Assembly resolution [UNFCCC], 2015) and conservation of biodiversity (Convention on Biological Diversity, 2021). In the European Union (EU), these objectives are reflected in measures outlined by the Common Fisheries Policy (CFP) (European Union [EU], 2013). The latter reform of the CFP proposed a new framework to manage European fisheries, and amongst several new initiatives, it highlighted a need to move from traditional single-stock management towards an ecosystem approach to fisheries management (EAF) (Prellezo and Curtin, 2015). The CFP Reform Regulation defines the EAF as “… an integrated approach to managing fisheries within ecologically meaningful boundaries which seeks to manage the use of natural resources, taking account of fishing and other human activities, while preserving both the biological wealth and the biological processes necessary to safeguard the composition, structure and functioning of the habitats of the ecosystem affected, by taking into account the knowledge and uncertainties regarding biotic, abiotic and human components of ecosystems” (European Union [EU], 2013).
Ecosystem approach to fisheries management prioritises the wellbeing of ecosystems over economic and social objectives since ecosystem wellbeing is considered a prerequisite for the latter two objectives (Murawski et al., 2008; Baudron et al., 2019). While the current CFP advocates for the implementation of some form of EAF, it remains largely unclear how to include conservation objectives within fisheries management measures in practice. The CFP aims to fish at levels consistent with achieving Maximum Sustainable Yield (MSY) for all exploited stocks (European Union [EU], 2013), an approach which does not necessarily account for impacts on the ecosystem (Ulrich et al., 2017). In Northern European waters, these fishing levels are proposed by the EU’s International Council for the Exploration of the Sea (ICES) which delivers scientific advice for the management of northern European fish stocks. This advice provides biological reference points for commercial stocks, including the level of fishing mortality (F) needed to achieve MSY (FMSY). According to the latest Scientific, Technical and Economic Committee for Fisheries (STECF) CFP monitoring report, stock status of the NE Atlantic (both EU and non-EU waters) has significantly improved since 2003, although many stocks remain overexploited (STECF, 2020a). Among the stocks which are fully assessed, the proportion of those that are overexploited decreased from around 75% to close to 40% over the last ten years. This trend is promising, however, in 2019, the proportion of overexploited stocks (i.e., F > FMSY) for which there are data increased slightly (STECF, 2020a).
Regarding the climate impacts of EU fisheries, many national governments, retailers, and consumers are increasingly motivated to reduce greenhouse gas emissions (GHGs) associated with food production, including fisheries, as a form of climate change mitigation (Iribarren et al., 2010). When considering direct emissions, such as fuel use, trawl fisheries are known to produce, on average, more GHGs per kilogram of catch than virtually any other fishing gear (Tilman and Clark, 2014). The carbon footprint of seafood landed using trawl nets may be similar to, and in some cases exceeds, the footprint of terrestrial staples such as poultry or pork (Tilman and Clark, 2014; Hilborn et al., 2018). Trawl fisheries are also known to have negative effects on the marine environment via significant alterations to abiotic and biogenic structure (Chuenpagdee et al., 2003). Evidence from vessel Automatic Information Systems (AIS) data suggests that the continental shelf surrounding the EU is among the world’s most trawled, although the Celtic and Greater North Seas are slightly less impacted than the waters west of Iberia and within the Mediterranean (Amoroso et al., 2018a).
In addition to direct emissions, fishing activity can affect organic carbon (OC) stocks in the marine environment, with implications for both climate and ecosystem function. One such stock is held in marine sediments, which store substantial amounts of carbon (Atwood et al., 2020; Legge et al., 2020), the active burial of which provides a climate regulation service over thousand-year timescales (Berner, 2003). Where trawling gear interacts with marine sediments, it can introduce oxygen into sub-surface sediment layers, increasing the potential loss of buried carbon through aerobic remineralisation (Aller, 1994; Hulthe et al., 1998). Sediment type is an important factor in the storage of sedimentary OC (Diesing et al., 2017; Smeaton et al., 2021), where OC is largely controlled by the proportion of clay or mud (Hedges and Keil, 1995). Trawling has a larger physical impact on muddy-sediment environments in terms of resuspension, causing significantly higher volumes of OC to be resuspended compared to sandy-bottom environments (O’Neill and Summerbell, 2011), with implications for both carbon flux and the resilience of local biodiversity (Pusceddu et al., 2014; Paradis et al., 2017). There is currently a lack of comprehensive evidence to fully demonstrate the effects of trawling on sedimentary OC stocks, but one likely outcome of regular trawling is prevention of sediment settling processes and, in turn, localised carbon sequestration (Epstein et al., 2022 PREPRINT; Oberle et al., 2016). Furthermore, using the definition outlined in Scheffold and Hense (2020), fish populations are living pools containing stocks of OC. In addition to affecting OC stocks in sediments, by removing fish biomass, fishing activity may prevent OC from sinking in the form of fish carcasses and facilitate conversion of OC in biomass to atmospheric CO2 (Mariani et al., 2020).
In this study, we make a first attempt at combining an established approach for estimating the fuel use of a specific fishery, with novel insights into the additional perturbation of OC stocks caused by fishing. We use landings and effort data associated with the Northern and Southern stocks of European hake (Merluccius merluccius) from 2008 and 2016, which enables a comparison of fishing emissions and OC impacts of the fishery when the Northern stock was depleted (2008) and subsequently rebuilt (2016) (ICES, 2021a). The Northern stock of European hake (hereafter “hake”) encompasses those populations living within the Greater North Sea, Celtic Seas, and the northern Bay of Biscay, while the Southern stock consists of those populations living in the southern Bay of Biscay and Iberian Coast (Figure 1). The combined range for Northern and Southern hake stocks is provided by ICES in terms of the following subareas and divisions: Subarea 4, 6, 7, Divisions 3.a, 8.a-d, 9.a (ICES, 2021a,b).
Figure 1. Map showing the combined ICES Subareas and Divisions where the northern and southern hake stocks are distributed. Shaded areas indicate the approximate depth preference for hake species, 75–400 m [Bathymetry data downloaded from EMODnet Bathymetry Consortium (2020)].
Hake are predatory, demersal fish, usually found at depths of 75–400 m, above muddy or sandy sediments (Lloris et al., 2005; Froese and Pauly, 2021). The Northern and Southern hake stocks may not be two distinct populations biologically but nevertheless are assessed separately for the purpose of fisheries management (Milano et al., 2014). The Northern stock is managed using the MSY approach and as such, the spawning stock biomass (SSB) is estimated during assessment of stock status by ICES. Conversely, the Southern stock is managed under the precautionary principle and SSB is not estimated. The Northern stock was overfished from at least the 1990s to the late 2000s (Murua, 2010; Villasante et al., 2011); on multiple occasions during this time, fishing mortality (F) substantially exceeded the agreed landings target (ICES, 2021a,b). However, in 2004, a recovery plan for hake was introduced which required a 70% reduction in F. Subsequently, the SSB of the Northern stock increased substantially, likely due to the reduced fishing pressure (Baudron and Fernandes, 2014). Between 2008 and 2016, SSB of the Northern stock increased by approximately six-fold, catches more than doubled, and F declined substantially (ICES, 2021a). Overall, catches of hake reported by ICES for 2016 were landed predominantly by long lines (39%), trawls (33%), and gillnets (24%) (ICES, 2017). Each gear type comes with trade-offs from varying levels of ease of use, to efficacy, to selectivity. Trawls, which trap fish as they scrape across the seafloor, are known as “active” gear types as they are continuously dragged by a moving vessel, while long lines and gillnets are considered “passive” gear types as they drift or hang idly in the current. It is worth noting that, despite modifications made to each of these gear types to increase selectivity, all three of these gear types have some issues.
In this paper we focus on the relationship between the carbon impact of the fishery, namely GHGs generated from vessel fuel use, and consider the potential perturbations to the OC stocks in the sediment and hake population, against the rebuilding of the Northern hake stock, from 2008 to 2016. We do not consider emissions from non-fuel inputs associated with fishing, for which estimates vary (for example Parker et al. (2018) assume 25% of total emissions arise from non-fuel sources). The purpose of including a preliminary ecological perturbation analysis in this paper is to introduce the impacts of fishing on OC stocks, in addition to the direct carbon footprint of boats on the water, and to advance information available for the development of ecosystem-based approaches for EU fisheries. Given the nexus between climate change, sustainable resource use, and biodiversity conservation (Sumaila and Tai, 2020), we consider whether the GHG footprint of the hake fishery overall and per tonne of catch were reduced by ending overfishing.
We used publicly available data collected by STECF through the Fisheries Dependent Information (FDI) data call (STECF, 2017) in combination with data we requested from STECF (STECF, 2020b), which included detail on hake landings, fuel use and intensity (litres per tonne of total landings) at the level of individual fishing fleet segments (i.e., vessel size, gear type). The requested data, hereafter referred to as dataset 1, were extracted from the STECF database (STECF, 2020b) for every second year from 2008 to 2018 inclusive, according to the following selection criteria: (i) fleets operating in the North Atlantic Ocean (FAO Area 27 - NAO) and (ii) EU fishing fleet segments in which, in 2018, hake accounted for at least 1% of total landings. France, Spain, and the United Kingdom landed 91% of total hake landings in 2008 (27,416 tonnes), and 78% in 2016 (23,764 tonnes) (STECF, 2020b). Thus, fishing mortality of the hake population within the NEA was dominated by the activities of these three countries, indicating that hake was an important target species for these nations. As such, we used the landings, effort, and fuel data for these three nations to investigate the impacts of the hake fishery in terms of fuel use, sedimentary disturbance, and extraction of OC in biomass. To compare the carbon impacts of the European hake fishery eight years apart, with two vastly different estimates of SSB, we emphasised the relative fuel use and impacts on OC per kg of hake landings, as opposed to focusing on the total emissions and impacts of the fishery. Landings data from STECF (STECF, 2020b) were used in kilograms, and, unless otherwise stated, this is the basic unit of measure we refer to hereafter.
To analyse the GHG footprint associated with fishing activity we focused on the carbon generated by vessels’ fuel use, which includes travel to and from fishing grounds and gear operation. These data were entirely available in dataset 1 (STECF, 2020b). We estimated fuel intensity (FI) (the amount of fuel burned while fishing hake per kilogram of hake landed), and emissions intensity (EI) (the estimated GHGs released while fishing hake per kilogram of hake landed) which is directly related to FI. Estimates for mean FI values were calculated for year and gear type. There were eight types of gear listed in dataset 1 (STECF, 2020b): (1) polyvalent passive gears (PGP), i.e. polyvalent nets, (2) drift/fixed-net gears (DFN), (3) gears with hooks (HOK), (4) demersal trawlers and seiners (DTS), (5) pots and traps (FPO), (6) vessels using active and passive gears (PMP), (7) vessels using “other” active gears (MGO), and (8) pelagic trawlers (TM). For this analysis, we grouped polyvalent passive gears (PGP) and drift/fixed-net gears (DFN) together as “DNGN”. In addition to reporting the cumulative FI value for hake fished by all gear types in 2008 and 2016, we also report on FI values for the “DNGN”, “HOK”, and “DTS” gear types only, as these were relatively well sampled in both 2008 and 2016 (n > 2 for both years) and were responsible for the overwhelming majority of hake landed (>95% of total landings) in both years.
To estimate the disturbance of OC in muddy sediments caused by bottom-contacting otter-trawlers, we used swept area (SA), a measure of the seabed surface area that has been in contact with, or altered by, bottom-contacting fishing gear, such as trawls, and report SA per kg of hake landed. SA is determined by the width of the gear and vessel speed (Church et al., 2016). We calculated and mapped the total SA by country using publicly available FDI data (STECF, 2017), hereafter referred to as dataset 2. Although this calculation does not quantify the amount of OC lost or consider the specific mechanisms by which OC may be lost, the areal overlap provides spatial information about where the biggest impacts of the trawl fishery on surficial sedimentary OC stocks are likely to be.
We calculated SA per kg of hake landed for France, Spain and the UK using the landings data in dataset 1 (STECF, 2020b), which included hake landings, hake landings as a percentage of total landings, and effort by fishing segment (STECF, 2020b), but did not contain spatial data. Instead, dataset 2 (STECF, 2017), which included fishing effort available per ICES rectangle, was downloaded for annexes IIA, IIB, BOB, and CEL1 and years 2008 and 2016 (see Table 1). The data were filtered for otter trawling gears only (OT) and for the following countries: France, Spain, and the United Kingdom (data were available for England, Northern Ireland, and Scotland). Dataset 2, which provided effort, landings, and spatial data (STECF, 2017), did not contain hake landings for otter trawlers in France or Spain in 2008, or hake landings as a percentage of total landings. As such, all hake landings data for both 2008 and 2016 for France, Spain, and the United Kingdom were used from dataset 1 (STECF, 2020b). Dataset 1 were filtered by gear type “DTS”, which includes demersal trawlers and demersal seiners, on the assumption that these were mostly otter trawls, since, in European waters, effort by otter trawlers is an order of magnitude greater than that of demersal seiners (Eigaard et al., 2016; Supplementary Table 1). To see clearer differences in SA between depleted and recovered stocks it would be best to compare fleets that landed a high percentage of hake, as detailed in dataset 1, however, we were unable to disaggregate the effort of those fleets in dataset 2, which contained the spatial data. Therefore, for the purpose of making a comparison between two years with different stock statuses, the use of OT spatial data from dataset 2 with the total landings for France, Spain and the United Kingdom from the DTS segment in dataset 1 was the best possible approach given the limitations of the data.
Table 1. International Council for the Exploration of the Sea (ICES) Annexes and the fishing areas they encompass.
Finally, to estimate the fishery’s impacts on the OC stock of the hake population, we first calculated the carbon removed by each country and by the different fleet segments using the landings reporting in dataset 1 for 2008 and 2016. We again grouped polyvalent passive gears (PGP) and drift/fixed-net gears (DFN) together as “DNGN,” and report OC removal values for the “DNGN,” “HOK,” and “DTS” fishing segments for 2008 and 2016. We also estimated the OC stock in the adult hake population for 2008 and 2016 and the proportion of OC in hake stock removed by total landings by France, Spain, and the United Kingdom cumulatively using dataset 1 and SSB estimates for the northern stock only (ICES, 2021a). This analysis was limited to using SSB estimates for the Northern stock as a proxy for the adult hake population of both stocks as SSB estimates were not available for the Southern stock, and the landings data in dataset 1 could not be disaggregated spatially to ascertain whether landings were from the Northern or Southern stocks. We reasoned that this approach was appropriate for our purpose of comparison between the two years given that total landings estimated by ICES for the Northern stock were much higher at 47,822 tonnes in 2008, and 107,530 tonnes in 2016 (ICES, 2021a), than for the Southern stock, at 16,773 tonnes for 2008 and 12,443 tonnes for 2016 (ICES, 2021b). We note that our results are therefore underestimates of the OC stock in the adult hake population of the NEA for 2008 and 2016, and overestimates of the OC removed by landings as a proportion of the adult hake population in those two years.
Hake are caught as part of a mixed-species fishery with several other target species, which makes it challenging to partition and confidently assign impacts to hake versus other species that end up in the same net. Nonetheless, we provide estimates for FI, emissions intensity (EI), and SA for hake landed by all trawlers, noting that the figures reported herein are useful for comparing the fishery impacts between a depleted and recovered stock, and do not represent precise point estimates for hake’s total carbon footprint. Estimates and statistical analyses were calculated in R version 4.0.3 (R Core Team, 2020), ArcGIS version 10.1, and Microsoft Excel.
To estimate the amount of fuel consumed in the pursuit of hake, we assumed that the fuel used to land hake was directly proportional to the ratio of hake landings to total landings for that fishing segment. That is, for each fishing segment reported in dataset 1 (STECF, 2020b), we assumed:
Where F is fuel and L is landings. Thus, we assumed that the quotient of total fuel consumption over total catch (i.e., the FI for total catch) was a reasonable proxy for the FI of hake. On this basis, we estimated FI using the following equation:
Where Fhake is estimated as:
Mean FI values for the three gear types were calculated by first summing the estimated values for Fhake, G (in litres) and then the reported values for Lhake, G (in kg), and subsequently dividing these as follows:
Where G is gear type and Y is year.
To convert FI to emission intensity (EI), we assumed that most of the fishing vessels in the EU operate using marine diesel (Borrello et al., 2013), which has a published density of 890 kg/m3 (ExxonMobil, 2021), equivalent to 890 g/L. The fuel-to-emissions conversion factor for marine diesel (MD), as published by the International Maritime Organisation (IMO, 2015), is 3.206 g of CO2/g of MD. After converting between units, we arrived at the conversion factor of 2.853 kg CO2/L of fuel. Note that this number does not account for the carbon-dioxide equivalent of: (i) other GHGs released during combustion, e.g., methane and nitrous oxide; (ii) upstream emissions associated with fuel production, e.g., drilling and refining; nor (iii) downstream emissions borne from processing, e.g., freezing, packaging, and distribution. In this respect, our estimates for GHGs released by fuel consumption are likely quite conservative.
Emission intensity estimates (reported as kg CO2 per kg hake) were derived by multiplying FI values by a factor of 2.853 kg of CO2/L of fuel, using the following equation:
Swept area was calculated using standardised values for door width (km) and trawl speed (knots) for the Otter trawl class BENTHIS métier code: “OT_MIX_DMF_PEL,” which is used to target hake (Eigaard et al., 2016). Following recommendations in the OSPAR CEMP guidelines (OSPAR Commission, 2017) (Agreement 2017-09) and Gerritsen et al. (2013), we used the equation:
Where Effort (fishing hours) from dataset 2 (STECF, 2017) was provided with spatial data (standardised ICES rectangles), 0.07621 km is the reported average door width and 3.4 knots is average trawl speed (ICES, 2016; OSPAR Commission, 2017); and 1.852 is the conversion factor for knots to km/hr. The final SA estimates between the countries and two fishing years are compared on a relative basis. Only the average door width and vessel speed were reported by ICES (2016) and OSPAR Commission (2017), yet the width of the trawl doors is a function of the vessel size and length of the sweeps, which can vary between 25 and 250 m, and the towing speed can vary from 2 to 4 knots (Eigaard et al., 2016). Thus, there is a degree of uncertainty within the estimated SA results.
Resulting SA estimates were spatially mapped for individual countries and as combined totals for 2008 and 2016, using a Natural Breaks Classification scheme (Holt, 2008). Swept Area Ratios (SAR), i.e., the proportion of each grid cell area that is trawled, were not deemed a suitable metric for this study due to the large area of the ICES rectangles (at 0.5° × 1°, these are approximately 4,000 km2) in combination with the low spatial resolution of dataset 2 (STECF, 2017), as it was not possible to determine where within the ICES square the reported activity occurred (Amoroso et al., 2018b). As outlined above, SA per kg of hake was calculated using total annual estimated SA (km2) and hake landings (kg) data for each country as reported in dataset 1 (STECF, 2020b).
Shapefiles for seabed substrate classified to the simplified Folk 5 classification were downloaded from EMODnet Geology (EMODnet Geology, 2016; Supplementary Figure 1). The total SA polygons for 2008 and 2016 were overlaid with the classified sediment layers and a spatial analysis was performed that extracted the areas of ‘mud to muddy sands’ (having the highest relative OC contents; e.g., Smeaton et al., 2021) directly swept by the otter trawls, and therefore considered to be impacted. We calculated the total area of the sediments considered to be impacted for both years.
Using carbon measurements from six hake, 15–20 cm long and 25–35 g in wet mass, as recorded by Czamanski et al. (2011), where dry weight was 42.9 ± 6%, we calculated that OC was approximately 11.33% of hake wet weight. Dataset 1 was used to estimate the OC removed from the hake population (combined Northern and Southern stocks) in landings by country for France, Spain and the UK (STECF, 2020b), using the equation:
Where Landings are in kg, C is country, and Y is year. Using a 94% conversion rate of landed OC to CO2 emissions described by Mariani et al. (2020), CO2 emissions from total hake landings for gear type (DNGN, HOK, and DTS), for all three countries cumulatively, for 2008 and 2016 were calculated using the following equation:
Where G is gear type and Y is year. To calculate the OC stock in the adult hake population for 2008 and 2016, estimates of SSB were used for Northern stock only (ICES, 2021a), since ICES does not estimate the SSB for the southern stock, using the equation:
Where Y is year. The range of OC in SSB was calculated using the high, mean, and low estimates of SSB reported by ICES (2021a). Finally, to make a meaningful comparison between 2008, when the stock was considered overfished, and 2016, when it was considered rebuilt, the proportion of OC removed in total hake landings for the three countries was calculated as a percentage of the total OC stock in the hake population, again using the SSB for Northern stock only and the years 2008 and 2016. For this, we used the equation:
Again, the range was calculated using the high, mean, and low estimates of SSB.
Between 2008 and 2016, the total fuel consumption for all fishing segments that landed hake for France, Spain, and the United Kingdom increased by 29% (from ∼49M litres to ∼63M litres), relative to hake landings more than doubling (from ∼45M kg to ∼95.5Mkg). Simultaneously, the FI (and by extension, EI) for hake landed by the gear types DNGN (polyvalent passive gears and drift/fixed-net gears), HOK (gears with hooks), and DTS (demersal trawlers and seiners) decreased by 11–54% (Figure 2 and Table 2). Additional analyses on these differences are included in the Supplementary Information. The sample size was extremely small for those fishing segments that landed primarily hake (i.e., where hake accounted for ≥50% of the total landings), and therefore not particularly viable for statistical analyses. However, there was a declining trend in FI over time (Supplementary Figure 2) and, as FI is directly related to EI, the same can very likely be said for emissions per kg of hake.
Figure 2. Boxplot of individual Fuel Intensity (FI) estimates for 2008 and 2016, grouped by gear type, where each data point represents an FI estimate for a particular fishing fleet segment, calculated according to Equation (2). Sample sizes for each gear type (i.e., the total number of fishing segments using that gear), in either year, are all equal to or greater than n = 8, and are listed in Table 2. DNGN, polyvalent-, drift-, or fixed-net gear types; DTS, demersal trawlers and seiners; HOK, vessels using hooks.
Table 2. The reported total landings and estimated total fuel, mean Fuel Intensity (FI), and mean Emission Intensity (EI) for hake landed by vessels equipped with particular gear types (DNGN, HOK, DTS, or all gear types) in years 2008 and 2016.
The CO2 produced in pursuit of hake (and, realistically, other species associated with the fishery) was influenced by the type of gear used to target them. For example, in 2016, hake that was landed using demersal trawlers or seiners (DTS) was approximately 37% more carbon intensive than the mean FI for the entire fishery and approximately 65% more carbon intensive than hake landed by vessels using polyvalent-, drift-, or fixed-net gears (DNGN) (Figure 2 and Table 2).
An increase in effort by vessels using otter trawls, and subsequently total SA, occurred for all three nations from 2008 and 2016, although the hake landings by otter trawl decreased overall (Figure 3). Vessels that landed hake for France, Spain, or the United Kingdom using otter trawls swept a cumulative total area of 230,103 and 520,856 km2 in 2008 and 2016 respectively. Conversely, the total reported landings of hake by otter trawls were reduced from approximately 27,416 tonnes in 2008, to 23,764 tonnes in 2016. The total SA values for the two annual snapshots are presented spatially in Supplementary Figure 3. In terms of SA per kg of landed hake, when comparing the depleted stock of 2008 to the rebuilt stock of 2016, only the United Kingdom exhibited a lower result (33% decrease), while SA per kg of landed hake increased for France by 40% and Spain by 8-fold (Figure 3). For France, this is a result of a combined increase in effort and landings; however, for Spain, while there is a large increase in effort in 2016, reported hake landings were greatly reduced: less than 50% of 2008’s catch.
Figure 3. (Top left) Swept area by otter trawls by country for 2008 and 2016 (STECF, 2017). (Top right) Hake landings by “DTS” by country, with percentage of total hake landings in parenthesis, for 2008 and 2016 (STECF, 2020b). (Bottom left) Swept area per kg of hake by country for 2008 and 2016.
As we have emphasised throughout the text, it is important to note that this is a mixed fishery and that hake forms a percentage of the overall landings, thus the SA is not attributable to the hake fishery alone. However, irrespective of which species were caught, the area of sediment swept represents the disturbance caused in relation to the hake landed. The spatial distribution of SA per individual nation for each year is shown in Supplementary Figure 4. Overall, the total spatial distribution of effort is quite similar for both years. The most intense activity occurred within the Bay of Biscay, following the western coastline of France, and within the Moray Firth region of the Greater North Sea. The English Channel sees increased effort in 2016, albeit consistent in distribution. In 2008, there was activity within the southern North Sea and along the northern and western coastline of Spain which is not seen in 2016; instead, in 2016, a larger distribution of activity in the Celtic seas to the south-west of Ireland occurred. Using a somewhat crude area calculation provided by the spatial resolution of the ICES rectangles, we calculate an increase in the area of specifically muddy sediments disturbed by otter trawls from 5.72 km2 in 2008 to 31.82 km2 in 2016 (Figure 4). In 2008, disturbance of muddy sediments occurred off the west coast of France; northern coastline of Spain and west coast of Portugal; within the northern North Sea; and in the Celtic Sea to the west of Ireland. In 2016, there was less disturbance, and less activity generally, around the coasts of Spain and Portugal and the North Sea, and relatively consistent disturbance on the west coast of France. However, disturbance of a larger area of muddy sediments occurred in the Celtic Sea to the west of Ireland.
Figure 4. Swept area by otter trawlers that landed hake for Spain, France, and the United Kingdom in 2008 and 2016 overlaid with muddy sediment types, with impacted muddy sediments highlighted (EMODnet Geology, 2016).
The total OC in hake landings increased between 2008 and 2016 for all three countries, with an additional 5,728 tonnes of OC landed in 2016 cumulatively compared to 2008 (Table 3). Subsequently, total CO2 emissions from OC in landed hake increased from 4.8M kg in 2008, to 10.2M kg in 2016, including increases in CO2 emissions from hake landed using polyvalent passive gears, drift/fixed-net gears, and gears with hooks (Figure 5). However, a decrease of 0.4M kg CO2 was observed for hake landed using demersal trawlers and seiners, as fewer hake overall were landed using that gear type in 2016. Despite total OC removal and emissions from OC in hake landings increasing between the two years, the proportion of the OC stock in the hake pool removed by landings in 2016 was much lower (31%) than that in 2008 (96%) (Table 3), and the OC stock in the adult hake population was approximately 6.5 times larger in 2016 than in 2008 (Figure 5).
Figure 5. Conceptual diagram of emissions and OC impacts by segment (demersal trawlers and/or demersal seiners; drift and fixed net gear types, including polyvalent gears; and vessels using hooks), and for total (all gear types) for 2008 and 2016.
Despite the limitations of the data, comparisons between the relative impacts of the fishery when the stock was overfished, as in 2008, and rebuilt, as in 2016, were possible. We have demonstrated improvements in efficiency and proportional OC storage in living stocks in 2016 compared to 2008. However, increased fishing in 2016 also resulted in increased total emissions from both fuel and landed hake, and increased ecosystem disturbance. First, we found that all gears were more fuel efficient in 2016 compared to 2008, suggesting an increase in catch per unit effort, where the unit of effort is fuel. Second, in 2016, when stocks had been rebuilt, more OC remained in the living pool of hake compared to when stocks were depleted in 2008, both in terms of total biomass and proportionally. Third, increased fishing effort in 2016 using bottom trawling resulted in higher disturbance of sedimentary OC, however, the hake catch from trawling was lower in 2016, suggesting this increase may have been due to trawls targeting other species than hake. Finally, as may be expected with increased landings, higher total fuel emissions and CO2 release from hake landings in the Northeast Atlantic occurred once stocks were rebuilt. However, this analysis does not consider the displacement of effort and catch that may occur when a stock in one geographic location is depleted, while demand for that seafood remains constant. For instance, in 2008 frozen hake imported to Spain from outside the EU was higher than imports reported for the five subsequent years (EUMOFA, 2015), which suggests that the full emissions and OC impacts borne from the depleted stock may be higher, perhaps substantially so, from a global perspective. Likewise, we did not consider post-processing differences in our analysis, which may undergo changes when fish are imported rather than landed and marketed fresh. While our aim was to provide insights on the impacts of fisheries that will ultimately be useful for the development of EAF, such additional assessments would also improve the information available to the consumer at the point of purchase.
Overall, the increase in available hake biomass once the stock was rebuilt correlates with increased efficiency across passive gear types and proportional reduction of fishing impacts on the living pool of OC. We found that the volume of CO2 emissions produced by vessels landing hake was influenced by the type of gear used, with the passive gillnets, hook and line, and polyvalent gears generally outperforming trawls in terms of emissions and EI. With regard to sediment disturbance, the rebuilt fishery (2016) performed better than the depleted fishery (2008) in instances where hake landings produced by trawlers were more-or-less consistent between the two years (in France and the United Kingdom for example), compared to instances where the landings from trawlers between 2008 and 2016 were substantially reduced (as in the case of Spain). These observations are likely linked to the improved stock status of hake in 2016 compared to 2008, however, the hake fishery is a mixed fishery, thus the changes in EI and impacts of the benthic trawl gear on sediment cannot be attributed to hake stock status and hake landings alone. A greater proportion of the overall disturbance due to trawling effort in 2016 is likely to be attributable to other species, given the decline in hake landings by trawlers observed in 2016, particularly for the Spanish fleet. Unfortunately, we were unable to explore this observation further due to the limitations of the datasets. Nevertheless, the SA analysis provides useful insights into the potential disruptions to sedimentary OC posed by trawlers that land hake. Notably, hake habitat typically encompasses muddy sediments, which generally hold more OC (Diesing et al., 2017; Smeaton et al., 2021) and are resuspended for longer periods of time relative to other sediment types (O’Neill and Summerbell, 2011), implying a greater potential for OC loss via remineralisation or lateral transport offshore (Paradis et al., 2019; Epstein et al., 2022). This is not to suggest, however, that other types of seabed sediments are impervious to the impacts of trawling, both in terms of carbon and biodiversity—impacts relevant to EAF. For example, while both muddy and gravelly sediments experience relatively high benthic community depletion rates following a trawl, recovery rates for infaunal benthic communities are higher in mud than gravel due to the presence of faster-growing species (Pitcher et al., 2022).
As an early attempt to consider fishery impacts on OC in sediments and biomass using current publicly available data, there are limitations to what we could surmise. Firstly, we recognise the limitations of using two datasets which cannot be consistently filtered either by gear type or by landings with spatial data and, as such, note that the final estimates for SA per kg of hake are unreliable. Furthermore, our estimates of SA are likely to be over-estimated, not least because of the overlap of ICES rectangles with land area along coastlines (Figure 3). This is a function of the spatial scale of available data. We carried out a comparative analysis using a recent, much higher resolution dataset to illustrate this point (i.e., 0.05° × 0.05° as opposed to 0.5° × 1), however, we note that the SA data themselves are not comparable due to differences in the way that STECF (2017) and ICES (2021c) aggregate data. For instance, while landings data for hake are available at a country level by year from STECF (2020b), the data are aggregated into a broader gear type, for example “demersal trawls and seines” are categorised simply as “DTS.” Conversely, while the ICES data are disaggregated into specific designs of otter trawl (seven spatial layers exist for otter trawls within the high-resolution ICES dataset), the data comprise all landed species and for all countries per year.
To illustrate the advantages of high-resolution spatial data, we compared our 2016 SA calculated using dataset 2 (STECF, 2017) with the ICES (2021c) dataset of the 2016 spatial layer for the BENTHIS métier code “OT_DMF,” which refers to otter trawling of demersal fisheries. This BENTHIS métier code includes hake as a target species according to Eigaard et al. (2016) although hake is not specifically mentioned for this gear type by ICES (2021c). We compared the spatial distribution of SA for the area of high-intensity SA values off the west coast of France and north coast of Spain using these two datasets (Supplementary Figure 5). The higher resolution ICES dataset visibly reduced the estimated footprint and provided a much-improved spatial analysis of areas that are heavily impacted by trawling. An analysis of this nature might inform highly targeted management interventions, if required. The second analysis relates to estimates of the overall area of muddy sediment impacted using data of different scales/resolutions (Supplementary Figure 6). When we extracted the areas of muddy sediment that overlap with the SA data from dataset 2 and the ICES data (ICES, 2021c), we did not see a large difference in the disturbed area estimated by the two different resolutions. Overall, despite the data limitations described above, it is evident that the results of any spatial analysis strongly depend on the spatial scale of the dataset (Amoroso et al., 2018b) and highlights the importance of data resolution when estimating and considering fishing impacts. Therefore, with finer spatial resolution of effort data, combined with sediment maps and OC content estimates, quantification of disturbance to sediment OC are likely to improve.
Our analysis of fishing impacts on the living OC stock is focused on hake biomass only, due to the data available. Including dynamics between the target species, competitors, predators, and prey would improve the analysis and better inform management of living OC stocks under different fishing conditions. Consideration of the fishing impacts on bycatch and, when assessing a mixed fishery such as hake, other target species would be necessary, thus reporting catch composition and bycatch by segment would enable a more thorough analysis of fishing impacts on living OC stocks. Furthermore, better understanding and incorporation of a target species’ natural history and trophic dynamics into the analysis could shed further light on the impact of fisheries on OC in living stocks. For instance, consideration of the age at which a species is fished may provide insights on the longevity of the OC stock in that population. The recorded maximum longevity for hake ranges from 20 to 25 years (Vitale et al., 2016); if individuals live for 20 years, they can effectively store OC for two decades. Hake occupies different trophic levels as their diet changes throughout their life stages, thus the prey controlled by juvenile and adult hake are different. Adult hake over 30cm in length, which are the target of hake fisheries, prey on demersal fishes such as blue whiting and, where adult and juvenile hake distributions overlap, they show a preference for cannibalism (Mahe et al., 2007). Removal of large hake may therefore release smaller hake from predation, increasing the chances that juveniles will reach adulthood and recruit to the fishery, while a reduction in fishing pressure on larger hake could have the opposite effect (Mahe et al., 2007). At the same time, larger hake females may facilitate faster rebuilding where stocks are depleted, as they have a higher fecundity than smaller hake females and produce more eggs (Cerviño et al., 2013). Overfishing of predators can cause widespread changes in the ecosystem as prey are released from predation, with implications extending beyond the biomass of the target population, to food webs, habitats, and ecosystem services (Östman et al., 2016; Norderhaug et al., 2021). While they are not considered a deep-sea species, hake can be found at depths down to 1000m (Morales-Muñiz et al., 2018), thus may provide a link between shallower and deep sea habitats, which play a key role in global ecological and biogeochemical processes (Danovaro et al., 2008).
The objectives of this study were to explore how ending overfishing of hake and stock recovery affected (i) the carbon emissions from fuel use during fishing activity; (ii) disturbance of OC in sediments by trawling; and (iii) impacts on the OC stocks in the population of the target species. Earlier analysis of emissions from the hake fishery in Spain considered the entire life cycle of the fishery, from extraction to processing and consumption (Vázquez-Rowe et al., 2011). However, our approach was to consider the fishery from the perspective of EAF, and thus, the only fuel emissions we considered were those caused by fishing activity directly. Our study was also limited to a snapshot view of fisheries impacts when the hake stock was depleted, in 2008, and rebuilt, in 2016. As such, we did not seek to establish the reasons for stock depletion and recovery, thus, the effects we report are not necessarily a product of ending overfishing. However, it is likely that reduced fishing pressure, required by the recovery plan introduced in 2004, contributed to the increase in hake biomass of the Northern stock (Baudron and Fernandes, 2014).
Exploration of the OC impacts of fisheries is timely given that the interaction between trawl fishing activity and sediments is increasingly recognised as an important impact for consideration in EAF (De Borger et al., 2021); recent research has highlighted the roles of fish in the carbon cycle and flux (Bianchi et al., 2021; Saba et al., 2021); and there is significant overlap between fishing grounds and areas of high productivity (Cavan and Hill, 2021). Furthermore, EU targets to end overfishing by 2020 have not been met, yet overfishing is known to affect the resilience of fish stocks to climate change and other stressors (Sumaila and Tai, 2020). During this study, we used only publicly available fisheries data and, as such, found various limitations which have resulted in unreliable estimates, particularly for SA and OC in hake stock. Improving the reliability and accuracy of estimates is necessary. Ensuring that data can be consistently disaggregated across public databases, such as by country, fishery segment, BENTHIS métier, effort, and landings, would go a long way toward improving the accuracy of estimates of the impacts of fishing on landed populations and the environment. In addition, spatial data at finer scale resolutions than ICES rectangles are especially important for generating estimates of impacts on benthic environments.
The need for EAF which can lead to better outcomes for climate, biodiversity, and people is widely recognised but not easily operationalised (Link et al., 2020). Climate-based fisheries management must go beyond a target of MSY to include climate-based objectives in EAF. This would, presumably, call for management decisions based on consideration of emissions from fuel, disturbance to OC in sediments and living pools, and the carbon functions of the ecosystem. Reference levels for what constitutes sustainable fishing and desirable stock size could then be decided across priorities, such as carbon sequestration, harvest, ecosystem health, and social and cultural facets. This analysis represents a first step towards ensuring that relevant information from emerging fields of research can be included in the development of EAF approaches and has illuminated some of the complexity involved when using publicly available data to assess fisheries impacts on ecosystems.
Publicly available datasets were analyzed in this study and are referenced. The data provided by STECF (2020b) upon our request can be found here: https://github.com/angela-m/Hake-fishery-recovery-and-climate.
AM, EF, and SV contributed to the initial discussion. AM and EF led the writing of the manuscript. AM, EF, CH, and KB designed the figures and compiled the tables. All authors contributed to the literature survey, drafted and reviewed the manuscript.
EF was supported by the NSF GRFP under Grant No. DGE-1650112. The authors declare that this study received funding from OurFish (to AM). The funder was not involved in the study design, collection, analysis, interpretation of data, the writing of this article, or the decision to submit it for publication.
The authors declare that the research was conducted in the absence of any commercial or financial relationships that could be construed as a potential conflict of interest.
All claims expressed in this article are solely those of the authors and do not necessarily represent those of their affiliated organizations, or those of the publisher, the editors and the reviewers. Any product that may be evaluated in this article, or claim that may be made by its manufacturer, is not guaranteed or endorsed by the publisher.
We would like to thank Bec Hubbard and Mike Walker for their organization of a workshop in March 2020 and symposium in March 2021, attended by AM, EF, and SV, which helped inform the discussions and direction of the manuscript. AM, EF, and SV would like to thank John Driscoll for insightful discussions during the early stages of manuscript development. Data provided by ICES were used unchanged under creative commons license: https://creativecommons.org/licenses/by/4.0/. Information used in Figures 1, 4 and Supplementary Figure 1 was made available by the EMODnet Bathymetry and Geology projects, https://doi.org/10.12770/bb6a87dd-e579-4036-abe1-e649cea9881a, https://www.emodnet-geology.eu/ funded by the European Commission Directorate General for Maritime Affairs and Fisheries. The data layers used for this work were: “EMODnet Bathymetry Consortium (2020): EMODnet Digital Bathymetry (DTM)” and “EMODnet Geology (2016): Seabed Substrate Multiscale FOLK5.”
The Supplementary Material for this article can be found online at: https://www.frontiersin.org/articles/10.3389/fmars.2022.788339/full#supplementary-material
Aller, R. C. (1994). Bioturbation and remineralization of sedimentary organic matter: effects of redox oscillation. Chem. Geol. 114, 331–345. doi: 10.1016/0009-2541(94)90062-0
Amoroso, R. O., Pitcher, C. R., Rijnsdorp, A. D., McConnaughey, R. A., Parma, A. M., and Suuronen, P. (2018a). Bottom trawl fishing footprints on the world’s continental shelves. Proc. Natl. Acad. Sci. U.S.A. 115, E10275–E10282. doi: 10.1073/pnas.1802379115
Amoroso, R. O., Parma, A. M., Pitcher, C. R., McConnaughey, R. A., and Jennings, S. (2018b). Comment on “Tracking the global footprint of fisheries. Science 361, 2016–2018. doi: 10.1126/science.aat6713
Atwood, T. B., Witt, A., Mayorga, J., Hammill, E., and Sala, E. (2020). Global Patterns in Marine Sediment Carbon Stocks. Front. Mar. Sci. 7:165. doi: 10.3389/fmars.2020.00165
Baudron, A. R., and Fernandes, P. G. (2014). ‘Adverse Consequences of Stock Recovery: European Hake, a New “Choke” Species under a Discard Ban?’. Fish Fisher. 16, 563–575. doi: 10.1111/faf.12079
Baudron, A. R., Pecl, G., Gardner, C., Fernandes, P. G., and Audzijonyte, A. (2019). Ontogenetic deepening of Northeast Atlantic fish stocks is not driven by fishing exploitation. Proc. Natl. Acad. Sci. U.S.A. 116, 2390–2392. doi: 10.1073/pnas.1817295116
Berner, R. A. (2003). The long-term carbon cycle, fossil fuels and atmospheric composition. Nature 426, 323–326. doi: 10.1038/nature02131
Bianchi, D., Carozza, D. A., Galbraith, E. D., Guiet, J., and DeVries, T. (2021). Estimating global biomass and biogeochemical cycling of marine fish with and without fishing. Sci. Adv. 7:eabd7554. doi: 10.1126/sciadv.abd7554
Borrello, A., Motova, A., and Dentes, and de Carvalho, N. (2013). Fuel subsidies in the EU fisheries sector (No. IP/B/PECH/IC/2013-114. PE 513.963). France: European Parliament.
Cavan, E. L., and Hill, S. L. (2021). Commercial fishery disturbance of the global ocean biological carbon sink. GCB 28, 1212–1221. doi: 10.1111/gcb.16019
Cerviño, S., Domínguez-Petit, R., Jardim, E., Mehault, S., Piñeiro, C., and Saborido-Rey, F. (2013). Impact of egg production and stock structure on MSY reference points and its management implications for southern hake (Merluccius merluccius). Fisher. Res. 138, 168–178. doi: 10.1016/j.fishres.2012.07.016
Chuenpagdee, R., Morgan, L. E., Maxwell, S. M., Norse, E. A., and Pauly, D. (2003). Shifting gears: assessing collateral impacts of fishing methods in US waters. Front. Ecol. Environ. 1, 517–524. doi: 10.1890/1540-92952003001[0517:SGACIO]2.0.CO;2
Church, N. J., Carter, A. J., Tobin, D., Edwards, D., Eassom, A., Cameron, A., et al. (2016). JNCC Pressure Mapping Methodology. Physical Damage (Reversible Change) - Penetration and/or disturbance of the substrate below the surface of the seabed, including abrasion (No. JNCC Report No. 515). Peterborough: JNCC.
Convention on Biological Diversity (2021). First draft of the post-2020 global biodiversity framework CBD/WG2020/3/3. Brazil: Convention on Biological Diversity.
Czamanski, M., Nugraha, A., Pondaven, P., Lasbleiz, M., Masson, A., Caroff, N., et al. (2011). Carbon, nitrogen and phosphorus elemental stoichiometry in aquacultured and wild-caught fish and consequences for pelagic nutrient dynamics. Mar. Biol. 158, 2847–2862. doi: 10.1007/s00227-011-1783-7
Danovaro, R., Gambi, C., Dell’Anno, A., Corinaldesi, C., Fraschetti, S., Vanreusel, A., et al. (2008). Exponential Decline of Deep-Sea Ecosystem Functioning Linked to Benthic Biodiversity Loss. Curr. Biol. 18, 1–8. doi: 10.1016/j.cub.2007.11.056
De Borger, E., Tiano, J., Braeckman, U., Rijnsdorp, A. D., and Soetaert, K. (2021). Impact of bottom trawling on sediment biogeochemistry: a modelling approach. Biogeosciences 18, 2539–2557. doi: 10.5194/bg-18-2539-2021
Diesing, M., Kröger, S., Parker, R., Jenkins, C., Mason, C., and Weston, K. (2017). Predicting the standing stock of organic carbon in surface sediments of the North–West European continental shelf. Biogeochemistry 135, 183–200. doi: 10.1007/s10533-017-0310-4
Eigaard, O. R., Bastardie, F., Breen, M., Dinesen, G. E., Hintzen, N. T., Laffargue, P., et al. (2016). Estimating seabed pressure from demersal trawls, seines, and dredges based on gear design and dimensions. ICES J. Mar. Sci. 73, i27–i43. doi: 10.1093/icesjms/fsv099
EMODnet Bathymetry Consortium (2020). EMODnet Digital Bathymetry (DTM). Available online at: https://doi.org/10.12770/bb6a87dd-e579-4036-abe1-e649cea9881a (accessed May 26, 2021).
EMODnet Geology (2016). Seabed Substrates. Available online at: https://www.emodnet-geology.eu/data-products/seabed-substrates/ (accessed July 14, 2021).
Epstein, G., Middelburg, J. J., Hawkins, J. P., Norris, C. R., and Roberts, C. M. (2022). The impact of mobile demersal fishing on carbon storage in seabed sediments. Glob. Chang. Biol. 1–20. doi: 10.1101/2021.07.07.450307
EUMOFA (2015). Case study. Price structure in the supply chain for fresh hake in Spain. European Market Observatory for fisheries and aquaculture products. Rome: Food and Agriculture Organization.
European Union [EU] (2013). Regulation (EU) no 1380/2013 of the European Parliament and of the Council on the Common Fisheries Policy, amending Council Regulations (EC) No 1954/2003 and (EC) No 1224/2009 and repealing Council Regulations (EC) No 2371/2002 and (EC) No 639/2004 and Council Decision 2004/585/EC Regulation (EU) No 1380/2013. Maastricht: EU.
ExxonMobil (2021). Specifications [WWW Document]. ExxonMobil Marine Distillate Fuel. Available online at: https://www.exxonmobil.com/en-gq/commercial-fuel/pds/gl-xx-exxonmobil-marine-distillate-fuel (accessed on Sep 1, 2021).
Froese, R., and Pauly, D. (2021). FishBase. Merluccius merluccius (Linnaeus, 1758). Available online at: https://www.fishbase.se/Summary/SpeciesSummary.php?ID=30&AT=hake (accessed on Jan 9, 2021).
Gerritsen, H. D., Minto, C., and Lordan, C. (2013). How much of the seabed is impacted by mobile fishing gear? Absolute estimates from Vessel Monitoring System (VMS) point data. ICES J. Mar. Sci. 70, 523–531. doi: 10.1093/icesjms/fst017
Hedges, J. I., and Keil, R. G. (1995). Sedimentary organic matter preservation: an assessment and speculative synthesis. Mar. Chem. 49, 81–115.
Hilborn, R., Banobi, J., Hall, S. J., Pucylowski, T., and Walsworth, T. E. (2018). The environmental cost of animal source foods. Front. Ecol. Environ. 16:1822. doi: 10.1002/fee.1822
Holt, J. B. (2008). The topography of poverty in the United States: A spatial analysis using county-level data from the community health status indicators project. Prev. Chronic Dis. 5:A111.
Hulthe, G., Hulth, S., and Hall, P. O. G. (1998). Effect of oxygen on degradation rate of refractory and labile organic matter in continental margin sediments. Geochim. Cosmochim. Acta 62, 1319–1328.
ICES (2017). Hake (Merluccius merluccius) in subareas 4, 6, and 7, and in divisions 3.a, 8.a–b, and 8.d, Northern stock (Greater North Sea, Celtic Seas, and the northern Bay of Biscay). France: ICES.
ICES (2021a). Hake (Merluccius merluccius) in subareas 4, 6, and 7, and in divisions 3.a, 8.a–b, and 8.d, Northern stock (Greater North Sea, Celtic Seas, and the northern Bay of Biscay). In Report of the ICES Advisory Committee, 2021. ICES Advice 2021, hke.27.3a46-8abd. France: ICES.
ICES (2021b). Hake (Merluccius merluccius) in divisions 8.c and 9.a, Southern stock (Cantabrian Sea and Atlantic Iberian waters). In Report of the ICES Advisory Committee, 2021. ICES Advice 2021, hke.27.8c9a. France: ICES.
ICES (2021c). OSPAR request on the production of spatial data layers of fishing intensity / pressure, in: Report of the ICES Advisory Committee, ICES Advice 2021, ICES Technical Service, Sr.2021.11. p. 6. France: ICES.
IMO (2015). Third IMO GHG Study 2014 Executive Summary and Final Report. London, UK: International Maritime Organization.
IPBES (2019). Global Assessment Report on Biodiversity and Ecosystem Services of the Intergovernmental Science-Policy Platform on Biodiversity and Ecosystem Services, eds E. S. Brondizio, J. Settele, S. Díaz, and H. T. Ngo (Bonn: IPBES secretariat).
Iribarren, D., Vázquez-Rowe, I., Hospido, A., Moreira, M. T., and Feijoo, G. (2010). Estimation of the carbon footprint of the Galician fishing activity (NW Spain). Sci. Total Environ. 408, 5284–5294. doi: 10.1016/j.scitotenv.2010.07.082
Legge, O., Johnson, M., Hicks, N., Jickells, T., Diesing, M., Aldridge, J., et al. (2020). Carbon on the Northwest European Shelf: Contemporary Budget and Future Influences. Front. Mar. Sci. 7:143. doi: 10.3389/fmars.2020.00143
Link, J. S., Huse, G., Gaichas, S., and Marshak, A. R. (2020). Changing how we approach fisheries: A first attempt at an operational framework for ecosystem approaches to fisheries management. Fish Fish 21, 393–434. doi: 10.1111/faf.12438
Lloris, D., Matallanas, J., and Oliver, P. A. (2005). Hakes of the world (family Merlucciidae): an annotated and illustrated catalogue of hake species known to date, FAO species catalogue for fishery purposes. Rome: Food and Agriculture Organization of the United Nations.
Mahe, K., Amara, R., Bryckaert, T., Kacher, M., and Brylinski, J. M. (2007). Ontogenetic and spatial variation in the diet of hake (Merluccius merluccius) in the Bay of Biscay and the Celtic Sea. ICES J. Mar. Sci. 64, 1210–1219. doi: 10.1093/icesjms/fsm100
Mariani, G., Cheung, W. W. L., Lyet, A., Sala, E., Mayorga, J., Velez, L., et al. (2020). Let more big fish sink: Fisheries prevent blue carbon sequestration—half in unprofitable areas. Sci. Adv. 6:eabb4848. doi: 10.1126/sciadv.abb4848
Milano, I., Babbucci, M., Cariani, A., Atanassova, M., Bekkevold, D., Carvalho, G. R., et al. (2014). Outlier SNP markers reveal fine-scale genetic structuring across European hake populations (Merluccius merluccius). Mol. Ecol. 23, 118–135. doi: 10.1111/mec.12568
Morales-Muñiz, A., González-Gómez de Agüero, E., Fernández-Rodríguez, C., and Saborido Rey, F. (2018). Hindcasting to forecast. An archaeobiological approach to the European hake (Merluccius merluccius, Linnaeus 1758) fishery: Iberia and beyond. Reg. Stud. Mar. Sci. 21, 21–29. doi: 10.1016/j.rsma.2017.11.001
Murawski, S. A., Davidson, C. N., Hart, Z. NOAA, Balgos, M., Wowk, K., et al. (2008). Ecosystem-based Management and Integrated Coastal and Ocean Management and Indicators for Progress. Global Forum on Oceans, Coasts, and Islands: Working Group on Ecosystem-based Management and Integrated Coastal and Ocean Management and Indicators for Progress.
Murua, H. (2010). The Biology and Fisheries of European Hake, Merluccius merluccius, in the North-East Atlantic. Adv. Mar. Biol. 58, 97–154. doi: 10.1016/B978-0-12-381015-1.00002-2
Norderhaug, K. M., Nedreaas, K., Huserbråten, M., and Moland, E. (2021). Depletion of coastal predatory fish sub-stocks coincided with the largest sea urchin grazing event observed in the NE Atlantic. Ambio 50, 163–173. doi: 10.1007/s13280-020-01362-4
Oberle, F. K. J., Storlazzi, C. D., and Hanebuth, T. J. J. (2016). What a drag: Quantifying the global impact of chronic bottom trawling on continental shelf sediment. J. Mar. Syst. 159, 109–119. doi: 10.1016/j.jmarsys.2015.12.007
O’Neill, F. G., and Summerbell, K. (2011). The mobilisation of sediment by demersal otter trawls. Mar. Pollut. Bull. 62, 1088–1097. doi: 10.1016/j.marpolbul.2011.01.038
OSPAR Commission (2017). CEMP Guidelines: Common Indicator Extent of Physical Damage to predominant and special habitats (BH3), OSPAR Agreement 2017-09). London, UK: OSPAR Commission.
Östman, Ö, Eklöf, J., Eriksson, B. K., Olsson, J., Moksnes, P., and Bergström, U. (2016). Top-down control as important as nutrient enrichment for eutrophication effects in North Atlantic coastal ecosystems. J. Appl. Ecol. 53, 1138–1147. doi: 10.1111/1365-2664.12654
Paradis, S., Puig, P., Masqué, P., Juan-Diáz, X., Martín, J., and Palanques, A. (2017). Bottom-trawling along submarine canyons impacts deep sedimentary regimes. Sci. Rep. 7, 1–12. doi: 10.1038/srep43332
Paradis, S., Pusceddu, A., Masqué, P., Puig, P., Moccia, D., Russo, T., et al. (2019). Organic matter contents and degradation in a highly trawled area during fresh particle inputs (Gulf of Castellammare, southwestern Mediterranean). Biogeosciences 16, 4307–4320. doi: 10.5194/bg-16-4307-2019
Parker, R. W. R., Blanchard, J. L., Gardner, C., Green, B. S., Hartmann, K., Tyedmers, P. H., et al. (2018). Fuel use and greenhouse gas emissions of world fisheries. Nat. Clim. Change 8, 333–337. doi: 10.1038/s41558-018-0117-x
Pitcher, C. R., Hiddink, J. G., Jennings, S., Collie, J., Parma, A. M., Amoroso, R., et al. (2022). Trawl impacts on the relative status of biotic communities of seabed sedimentary habitats in 24 regions worldwide. PNAS 119, 1–11. doi: 10.1073/pnas.2109449119/-/DCSupplemental.Published
Prellezo, R., and Curtin, R. (2015). Confronting the implementation of marine ecosystem-based management within the Common Fisheries Policy reform. Ocean Coastal Manag. 117, 43–51. doi: 10.1016/j.ocecoaman.2015.03.005
Pusceddu, A., Bianchelli, S., Martín, J., Puig, P., Palanques, A., Masqué, P., et al. (2014). Chronic and intensive bottom trawling impairs deep-sea biodiversity and ecosystem functioning. PNAS 111, 8861–8866. doi: 10.1073/pnas.1405454111
R Core Team (2020). R: A language and environment for statistical computing. Vienna: R Foundation for Statistical Computing.
Saba, G. K., Burd, A. B., Dunne, J. P., Hernández-León, S., Martin, A. H., Rose, K. A., et al. (2021). Toward a better understanding of fish-based contribution to ocean carbon flux. Limnol. Oceanogr. 66, 1639–1664. doi: 10.1002/lno.11709
Scheffold, M. I. E., and Hense, I. (2020). Quantifying Contemporary Organic Carbon Stocks of the Baltic Sea Ecosystem. Front. Mar. Sci. 7:571956. doi: 10.3389/fmars.2020.571956
Smeaton, C., Hunt, C. A., Turrell, W. R., and Austin, W. E. N. (2021). Marine Sedimentary Carbon Stocks of the United Kingdom’s Exclusive Economic Zone. Front. Earth Sci. 9:593324. doi: 10.3389/feart.2021.593324
STECF (2017). FDI data call 2017: Effort [hours fished] by rectangle & quarter. Avilable online at: https://stecf.jrc.ec.europa.eu/dd/effort/graphs-quarter (accessed on Mar 8, 21).
STECF (2020a). Monitoring the performance of the Common Fisheries Policy (STECF-Adhoc-20-01). Luxembourg: Publications Office of the European Union.
STECF (2020b). The 2020 Annual Economic Report on the EU Fishing Fleet (STECF 20-06) Annex. EUR 28359 EN. Luxembourgh: Publications Office of the European Union.
Sumaila, U. R., and Tai, T. C. (2020). End Overfishing and Increase the Resilience of the Ocean to Climate Change. Front. Mar. Sci. 7:523. doi: 10.3389/fmars.2020.00523
Tilman, D., and Clark, M. (2014). Global diets link environmental sustainability and human health. Nature 515, 518–522. doi: 10.1038/nature13959
Ulrich, C., Vermard, Y., Dolder, P. J., Brunel, T., Jardim, E., Holmes, S. J., et al. (2017). Achieving maximum sustainable yield in mixed fisheries: a management approach for the North Sea demersal fisheries. ICES J. Mar. Sci. 74, 566–575. doi: 10.1093/icesjms/fsw126
United Nations General Assembly resolution [UNFCCC] (2015). Paris Agreement to the United Nations Framework Convention on Climate Change. United Nations General Assembly resolution. 2015. Transforming our world: the 2030 Agenda for Sustainable Development. A/RES/70/1. Brazil: UNFCCC.
Vázquez-Rowe, I., Moreira, M. T., and Feijoo, G. (2011). Life Cycle Assessment of fresh hake fillets captured by the Galician fleet in the Northern Stock. Fisher. Res. 110, 128–135. doi: 10.1016/j.fishres.2011.03.022
Villasante, S., do Carme, García-Negro, M., González-Laxe, F., and Rodríguez, G. R. (2011). Overfishing and the Common Fisheries Policy: (un)successful results from TAC regulation? Fish Fisher. 12, 34–50.
Keywords: fisheries, carbon emissions, hake (Merluccius merluccius), stock recovery, sedimentary organic carbon, sustainable fisheries, ecosystem based management (EBM), ecosystem based approach for fisheries management
Citation: Martin AH, Ferrer EM, Hunt CA, Bleeker K and Villasante S (2022) Exploring Changes in Fishery Emissions and Organic Carbon Impacts Associated With a Recovering Stock. Front. Mar. Sci. 9:788339. doi: 10.3389/fmars.2022.788339
Received: 02 October 2021; Accepted: 15 February 2022;
Published: 25 April 2022.
Edited by:
Rashid Sumaila, The University of British Columbia, CanadaReviewed by:
Natalia Serpetti, Joint Research Centre, ItalyCopyright © 2022 Martin, Ferrer, Hunt, Bleeker and Villasante. This is an open-access article distributed under the terms of the Creative Commons Attribution License (CC BY). The use, distribution or reproduction in other forums is permitted, provided the original author(s) and the copyright owner(s) are credited and that the original publication in this journal is cited, in accordance with accepted academic practice. No use, distribution or reproduction is permitted which does not comply with these terms.
*Correspondence: Angela Helen Martin, YW5nZWxhaGVsZW5tYXJ0aW5AZ21haWwuY29t
Disclaimer: All claims expressed in this article are solely those of the authors and do not necessarily represent those of their affiliated organizations, or those of the publisher, the editors and the reviewers. Any product that may be evaluated in this article or claim that may be made by its manufacturer is not guaranteed or endorsed by the publisher.
Research integrity at Frontiers
Learn more about the work of our research integrity team to safeguard the quality of each article we publish.