- 1Faculty of Fisheries and Protection of Waters, South Bohemian Research Center of Aquaculture and Biodiversity of Hydrocenoses, Research Institute of Fish Culture and Hydrobiology, University of South Bohemia in České Budìjovice, České Budìjovice, Czechia
- 2Biology Centre CAS, Institute of Hydrobiology, České Budìjovice, Czechia
- 3Faculty of Science, University of South Bohemia, České Budìjovice, Czechia
The present study aimed to evaluate cryo-injury during the cryopreservation process in sterlet (Acipenser ruthenus) sperm, focusing on ultrastructural characteristics. Post-thaw sperm quality parameters, including total motility rate, curvilinear velocity (VCL), linearity (LIN), plasma membrane integrity, antioxidant status, DNA damage, and fine ultrastructure were examined on fresh and cryopreserved sperm with/without addition of a single optimal dose of AFPI (10 μg/mL). A lower motility rate, VCL and plasma membrane integrity, and increased DNA damage (p < 0.05) were observed in frozen-thawed spermatozoa with/without AFPI compared to fresh spermatozoa. The morphology and ultrastructure of spermatozoa were affected during the cryopreservation process with/without supplementation of AFPI. Morphological abnormalities were observed in mitochondria (49–54%) and flagellum (55–57%) of cryopreserved spermatozoa with/without AFPI compared to fresh spermatozoa. In conclusion, the morphology and ultrastructure of spermatozoa were slightly changed after cryopreservation of sterlet spermatozoa with/without 10 μg/mL AFPI.
Introduction
Sturgeons (Chondrostei, Acipenseriformes)—members of the ancient Acipenseridae family—have been categorized as a critically endangered species and included in biodiversity conservation programs for population restoration. The deteriorating sturgeon population is the result of growing market demand for caviar and boneless meat (Bronzi et al., 2011; Mims et al., 2011; Golpour et al., 2016a; Niksirat et al., 2017).
Sperm cryopreservation is a powerful approach in aquaculture and can potentially be used for numerous applications in hatcheries and biodiversity programs for endangered species. Synchronization of artificial reproduction, an alternative to decreased broodstock males, selective breeding, and gene banking of endangered species (Lahnsteiner et al., 2002; Billard et al., 2004; Sarvi et al., 2006; Kopeika et al., 2007; Cabrita et al., 2010) is among the most important applications of sperm cryopreservation. However, a deleterious effect of cryopreservation on sperm viability has been reported in many fish species (Glogowski et al., 2002; Asturiano et al., 2007). Cryopreservation may deteriorate sperm quality parameters, such as sperm motility and fertilization ability (Beirão et al., 2012; Zilli et al., 2014). One possible mechanism of cryo-damage is excessive production of reactive oxygen species (ROS) in spermatozoa, which causes oxidative stress, lipid peroxidation, damage to plasma membranes and DNA fragmentation during cryopreservation (Wang et al., 1997; Baumber et al., 2003; Li et al., 2007, 2010; Thomson et al., 2009; Figueroa et al., 2016, 2020). The relatively weak endogenous antioxidant protection of fish sperm may be the cause of this susceptibility (Koppers et al., 2010; Gazo et al., 2013). Moreover, during cryopreservation, spermatozoa are affected by various physical and chemical factors during slow freezing (due to high solute concentration) or rapid freezing (due to intracellular ice formation) (Mazur et al., 1972).
Motility parameters such as percentage of motile spermatozoa, velocity and linearity are commonly used as quality indices for both fresh and cryopreserved spermatozoa and correlate strongly with fertilization ability (Lahnsteiner et al., 2002; Rurangwa et al., 2004; Xin et al., 2020). In addition, plasma membrane and DNA integrity have been considered key factors in assessing the damage caused by sperm freezing and thawing in different species (Labbe et al., 2001; Zilli et al., 2003; Riesco et al., 2012; Figueroa et al., 2016; Xin et al., 2020; Hossen et al., 2021).
Other approaches, such as ultrastructural study using electron microscopy, can provide more specific information, such as damage to the plasma membrane and fragmentation of the flagella. The mentioned approach can provide important information about the morphology and ultrastructural changes in frozen-thawed spermatozoa (Golpour et al., 2017; Balamurugan et al., 2019). Cryo-injury can cause a major challenge for practical application of sperm cryopreservation in aquaculture and fish conservation programs. This technique can lead to lethal and sublethal changes in compartments of the spermatozoa such as acrosome, nucleus, midpiece, flagella, plasma membrane, and cytosol, resulting in impairment of cell structure (Xin et al., 2020).
To protect spermatozoa from cryo-injury, numerous studies have suggested the addition of various proteins, non-enzymatic and enzymatic antioxidants, and antifreeze proteins (AFPs) to cryopreservation protocols (Cabrita et al., 2011; Mogielnicka-Brzozowska and Kordan, 2011; Shaliutina-Kolešová et al., 2014; Figueroa et al., 2017, 2018). Despite the growing success of cryopreservation of fish sperm and the availability of various optimized protocols for cryopreservation of sturgeon spermatozoa, this technique is believed to cause extensive lethal and sublethal cryo-damage to cells (Ciereszko et al., 1996; Urbányi et al., 2004; Boryshpolets et al., 2011). Therefore, the present study aimed to evaluate sperm quality parameters with special attention to ultrastructural damage after applying an established cryopreservation protocol with addition a previously optimized concentration of AFPI in sterlet, Acipenser ruthenus: a widely used model fish from the sturgeon family to study artificial reproduction.
Materials and Methods
Ethics Statement and Animals
This study was performed in accordance with valid legislative regulations in Czech Republic (law No. 166/1996 and no. 246/1992); the permit was issued to No. 2293/2015-MZE-17214 and No. 55187/2016-MZE-17214 in project NAZV QK1710310. All the samplings were carried out with the relevant permission from the Departmental Expert Committee for Authorization of Experimental Projects of the Ministry of Education, Youth and Sports of the Czech Republic (permit No. MSMT 4394/2017-2).
Fish and Sperm Collection
Semen was collected during the natural spawning season (February–April) from six apparently healthy and mature sterlet males (6–7 years, 2–3 kg). The fish were kept in 4 m3 plastic tanks with pond water at a constant flow of 20 l/min (8–10°C) in the hatchery of the Research Institute of Fish Culture and Hydrobiology, Vodnany, Czech Republic. Prior to the experiment, the water temperature was gradually increased to 14–15°C over the course of 24 h. The fish were kept at the above temperature for another 4 days until the beginning of the experiment. Spermiation was induced by intramuscular injection of carp pituitary powder dissolved in 0.9% NaCl solution at a dose of 4 mg/kg body weight. After 24 h, semen was collected at the uro-genital sinus by aspiration with a 4 mm plastic catheter attached to a plastic syringe. The samples were stored on ice before and during the experiments.
Sperm Cryopreservation
Six freshly collected sperm samples with motility greater than 90% (sperm concentration: 0.55–0.75 × 109 spermatozoa mL–1) were diluted separately (1:1) in an extender composed of 23.4 mM sucrose, 0.25 mM KCl, 30 mM Tris-HCl, pH 8.0, 10% methanol (Glogowski et al., 2002; modified by Xin et al., 2018a,b) with/without 10 μg/mL AFPI (optimized protocol according to Xin et al., 2018a). The diluted sperm from each male were immediately loaded into 0.5 mL plastic straws (CRYO-VET, France) and placed on a 3 cm thick polystyrene raft on ice for 10 min of equilibration. The polystyrene raft containing the straws was then transferred and frozen in liquid nitrogen vapor in a styrofoam box. After 10 min, the straws were directly immersed in liquid nitrogen and stored in plastic goblets. Finally, the samples were thawed in a water bath at 40°C for 6 s and then immediately used for further assessments and sample processing.
Spermatozoa Motility and Concentration Assessment
Fresh, cryopreserved, and cryopreserved sperm with an addition of 10 μg/mL AFPI (A/F Protein Inc., Waltham, MA, United States) (cryopreserved + AFP) were activated in an activation medium (AM) consisting of 10 mmol/L Tris-HCl buffer, pH 8.0, 2 mmol/L CaCl2, and 0.125% pluronic acid. For this purpose, the sperm to AM ratio was maintained at approximately 1:50. The sperm was mixed with AM on a 24 × 32 mm microscopic glass slide (ISO 8037/I, Thermo Fisher Scientific, Waltham, MA, United States) at room temperature (21°C). Spermatozoa motility parameters were recorded on the top and bottom of the AM using a CCD video camera (Sony, SSCDC50AP, Tokyo, Japan) operating in 25 frame per second (fps) mode, mounted on an inverted microscope (Olympus IX83, United Kingdom) with a 20 × NIC contrast objective lens (Dadras et al., 2019a). Analysis of recorded videos for curvilinear velocity (VCL; time-averaged velocity of a sperm head along its actual curvilinear path, μm/s), linearity [LIN; defined as straight-line velocity (VSL)/curvilinear velocity (VCL);%] and total motility rate (%) were performed for approximately 100–150 spermatozoa per microscopic field in triplicate (three fields/sample) using ISAS software (PROISER, Paterna, Spain) 15 s after activation. Spermatozoa concentration was determined according to Xin et al. (2018b).
Assessment of Plasma Membrane Integrity
Flow cytometry was used to investigate the integrity of the plasma membrane of fresh and cryopreserved spermatozoa with/without AFPI. 10,000 cells were analyzed for each sample by flow cytometry. Two fluorescent dyes were used to distinguish spermatozoa with intact and impaired membrane integrity. SYBR Green binds to spermatozoa (live and dead), but only dead spermatozoa were labeled with propidium iodide (PI). A total of 2 μL of fresh or cryopreserved sperm samples with/without AFPI were prediluted in 2 mL of phosphate-buffered saline (PBS) (PH 7.4). Then 5 μL of 1:500 diluted SYBR Green (Sigma-Aldrich, St. Louis, MO, United States) was added to the diluted sperm samples. After 5 min, 5 μL of propidium iodide (PI) (Sigma-Aldrich, St. Louis, MO, United States) was added to the sperm diluted with PBS and SYBR Green. After incubating the sperm samples with both fluorescent dyes for 30 min at room temperature, flow cytometry was performed using a fluorescence-activated cell sorter (FACS) Canto II flow cytometer (BD Biosciences). FACS Diva software, v. 5.0 (BD Biosciences) was then used for data analysis. The number of SYBR Green positive events in the FITC channel and the number PI positive events in the PE -Texas Red channel were used to determine dead sperm. Intact plasma membranes (live spermatozoa) were determined as SYBR Green positive and PI negative events. Each analysis was performed in triplicate.
Lipid Peroxidation and Antioxidant Enzyme Activity
Sperm samples (fresh; sperm concentration: 0.55–0.75 × 109 spermatozoa mL–1 and cryopreserved; sperm concentration: 0.27–0.37 × 109 spermatozoa mL–1) sperm with/without AFPI] were centrifuged at 500 × g at 4°C for 20 min to obtain seminal fluid (SF) and a spermatozoa pellet. The pellet was suspended in 50 mM potassium phosphate (KPi) buffer, pH 7.0, containing 0.5 mM EDTA and homogenized in an ice bath using a Sonopuls HD 2070 ultrasonicator (Bandelin Electronic, Berlin, Germany). The homogenate pellet was centrifuged at 10,000 × g at 4°C for 10 min to obtain supernatant. The obtained supernatant (containing spermatozoa) and SF were used to measure the thiobarbituric acid reactive substances (TBARS) and the activities of superoxide dismutase (SOD) and catalase (CAT). The protein concentration of the samples was measured using a BCA Protein Assay Kit (Thermo Fisher Scientific, Waltham, MA, United States).
The CAT activity was determined spectrophotometrically at 240 nm according to the method described by Marklund et al. (1981). The reaction medium consisted of 10 mM K + phosphate buffer containing 0.1 mM EDTA, pH 7.4, and 15 mM H2O2 was used. The activity of CAT was calculated from the H2O2 decomposition rate using the molar extinction coefficient 43.6 M–1 cm–1, and the specific activity was expressed as micromole/min × mg protein.
The activity of SOD was determined spectrophotometrically at 420 nm as described by Marklund and Marklund (1974). The activity of SOD was measured by the inhibition of autoxidation of pyrogallol by the sample. The addition of the analyzed sample led to the inhibition of autoxidation of 0.2 mM pyrogallol in air-equilibrated 50 mM Tris-HCl buffer containing 1 mM EDTA, pH 8.2. The amount of enzyme inhibiting the reaction (pyrogallol autoxidation) by 50% is considered as one unit of enzyme. The specific activity of SOD was expressed as unit/mg protein.
The TBARS level was determined spectrophotometrically (Asakawa and Matsushita, 1980): briefly, 0.025 mL of a solution of butylated hydroxytoluene (22 mg in 10 mL ethanol), 0.025 mL of a ferric chloride solution (27 mg FeCl3 6H2O in 10 mL water), 0.375 mL of a 0.2 M glycine-hydrochloric acid buffer, pH 3.6, and 0.375 mL of TBA reagent (0.5% TBA and 0.3% sodium dodecyl sulfate) were added to 0.4 mL of the supernatant and heated in a boiling water bath for 15 min. The samples were allowed to cool, then 0.25 mL of glacial acetic acid and 0.5 mL of chloroform were added. After vigorous shaking, the samples were centrifuged at 1,500 × g for 10 min. The absorbance was measured at 535 nm. TBARS content was determined using a molar extinction coefficient of 1.56 105 M–1 cm–1 and expressed as nmol/mg protein sample. All measurements were performed in triplicate for each sample.
Assessment of DNA Damage
DNA integrity of fresh (sperm concentration: 0.57–0.70 × 109 spermatozoa mL–1) and cryopreserved sperm with/without AFPI was assessed using the comet assay. Sperm samples from each male were diluted 1:300 with pre-chilled PBS and stored at 4°C. Agarose gel (0.6% NuSieve GTG Agarose OxiSelect™ with low melting point; Cell Biolabs, Inc., San Diego, United States) was melted at 90°C and cooled to 37–40°C. The agarose gel was mixed with diluted sperm at a ratio of 9:1. Then, 50 μL of the prepared sperm samples were pipetted into each well of the slides (OxiSelect™; Cell Biolabs, INC., United States) and spread over the well. The slides were allowed to solidify at 4°C and then immersed in lysis buffer prepared according to the protocol for the OxiSelect Comet assay with the addition of proteinase K (1 mg/mL of lysis solution). The slides were incubated in the lysis buffer for 5 h at 37°C. After incubation, the lysis buffer was carefully aspirated and replaced with electrophoresis solution (90 mM Tris Base, 90 mM boric acid, 2.5 mM EDTA, pH 8.0). After incubation in the electrophoresis solution for 20 min at room temperature, the slides were carefully transferred to the electrophoresis chamber filled with the electrophoresis solution. Electrophoresis was performed at 35 V and 170 mA for 15 min. The slides were then transferred to a small container of distilled water for 5 min. The slides were then drained well, dehydrated by immersion in 70% ethanol for 5 min, and air-dried. Once the agarose slides were completely dried, they were stained with DAPI (50 μL, 1 μg/mL). The slides were observed under an Olympus Fluoview microscope (Olympus Corp., Tokyo, Ja-pan) using filters with an excitation wavelength of 450–480 nm and recorded with a SONY DXC -9100D video camera (SONY, Tokyo, Japan). Spermatozoa DNA damage (Tail DNA, %) was assessed in one hundred cells for each sample using CASP software (version 1.2.3., 2001).
Ultrastructural Analysis
For scanning electron microscopic (SEM) observations, sperm samples (fresh and cryopreserved sperm with/without AFPI) were fixed in freshly prepared 2.5% glutaraldehyde in 0.1 M phosphate buffer (pH 7.4) at 0–4°C for 3 h, rinsed in the same buffer, and immersed for 2 h in 1.0% osmium tetroxide in 0.1 M phosphate buffer (pH 7.4) at room temperature for 2 h. After rinsing in the buffer, samples were dehydrated in a graded acetone series (30, 50, 70, 80, and 100% for 10 min each). Then, the samples were dried using a critical point dryer (XD -1, Eiko) and coated with gold using a coater (IB -3, Eiko). Finally, the samples were examined and photographed using a scanning electron microscope (Hitachi-S570, Tokyo, Japan and JSM 6300, JEOL Ltd., Akishima, Tokyo, Japan).
For transmission electron microscopy (TEM), the sperm samples were centrifuged at 200 × g for 30 min, and the supernatant was discarded. The samples were fixed with the same solutions used for SEM. Then the samples were dehydrated in a graded acetone series and embedded in Epon812. The solidified blocks were sectioned at 50–70 nm and the sections mounted on copper grids. Post-staining with 2% uranyl acetate in 50% ethanol was performed, followed by staining with Reynolds’ lead citrate (Golpour et al., 2016a,b; Dadras et al., 2019b). The prepared grids were examined and photographed by transmission electron microscopy (JEM -1230, Tokyo, Japan and JEOL 1010, JEOL Ltd., Tokyo, Japan). For TEM, specimens were processed separately for each treatment and then approximately 400 electron micrographs were taken for each treatment. For SEM, about 100 electron micrographs were taken. The electron micrographs were examined for changes in the structure of the spermatozoa. Finally, the best images showing normal structure in the control treatment or altered spermatozoa structure in both treatments (with/without addition of AFP1) were selected.
For quantitative analysis, thirty TEM micrographs of each group studied were evaluated to determine the morphological abnormalities. Quantification of the abnormalities in the micrographs was performed using ImageJ v1 software (Wayne Rasband, United States). The analyses were performed in triplicate.
Statistical Analysis
Experimental values for each studied parameter were obtained by averaging the results of six male fish with three replicates for each male. The mean values of the males were calculated and used for statistical analyses. The data distribution characteristics and homogeneity of dispersion were evaluated using Shapiro-Wilk and Levene’s tests, respectively. The normally distributed data with similar dispersion values were analyzed using one-way ANOVA followed by Tukey’s honest significant difference test. Non-homogeneous data (Tail DNA) were analyzed using a non-parametric Kruskal-Wallis ANOVA followed by multiple comparison of mean ranks for all groups. Statistical significance was accepted at p < 0.05. Data are presented as box plots and bar graphs (mean ± SD). All analyses and plots were performed using STATISTICA v. 12 (Statsoft Inc.)1 and GraphPad Prism v. 8.
Results
Spermatozoa Motility
After dilution with AM, the fresh spermatozoa exhibited 93.3 ± 5%, 182.2 ± 17.8 μm/s and 62 ± 8% for motility rate, VCL and LIN, respectively (Figures 1A–C). A significant decrease in motility rate was observed in cryopreserved spermatozoa (with 10 μg/mL AFPI: 80 ± 6%; without 10 μg/mL AFPI: 78.3 ± 7%) compared with fresh spermatozoa (Figure 1A). The curvilinear velocity (VCL) showed a significant difference between fresh spermatozoa (182.2 ± 17.8 μm/s) and cryopreserved spermatozoa with/without 10 μg/mL AFPI (with AFPI: 141.5 ± 11.4 μm/s; without AFPI: 155.8 ± 8.3 μm/s) (Figure 1B). No significant differences were observed in LIN between fresh and cryopreserved spermatozoa with/without the addition of the antifreeze protein (Figure 1C).
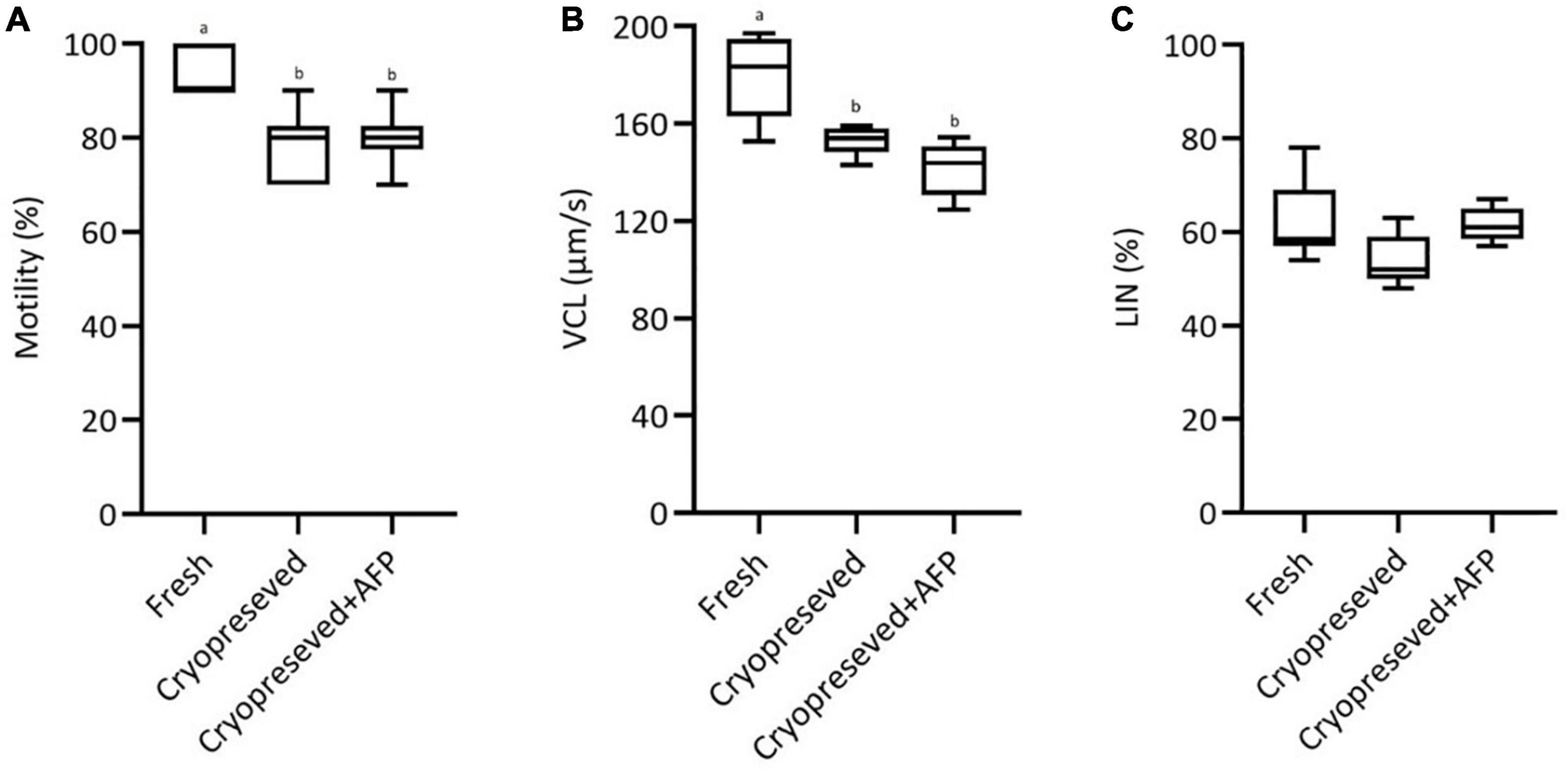
Figure 1. Effect of cryopreservation on motility parameters at 15 s post-activation in fresh, cryopreserved, and cryopreserved spermatozoa with supplementation of 10 μg/mL AFPI (cryopreserved + AFP) in sterlet: (A) motility; (B) curvilinear velocity (VCL); (C) linearity (LIN). Values with different lower-case letters are significantly different [p < 0.05, one-way analysis of variance (ANOVA) followed by Tukey’s honest significant difference test].
Plasma Membrane Integrity
The integrity of the plasma membrane of fresh spermatozoa was 96 ± 2.2%. The spermatozoa with intact plasma membranes were significantly decreased in cryopreserved sperm with (70.4 ± 2.4%) and without AFPI (70.3 ± 3.6%) (Figure 2). No significant difference in spermatozoa membrane integrity was observed between cryopreserved sperm with and without the addition of AFPI (Figure 2).
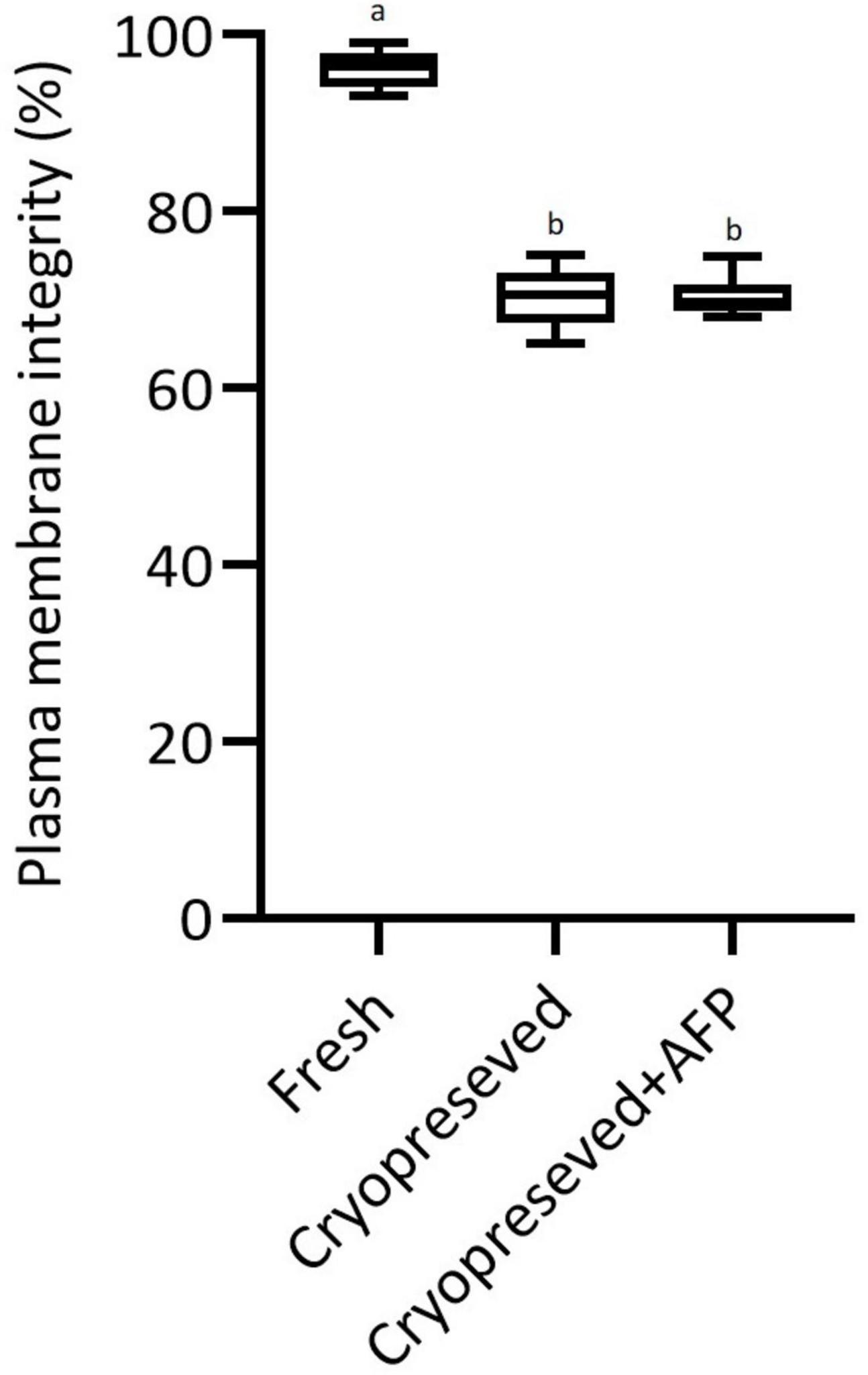
Figure 2. Effect of cryopreservation on plasma membrane integrity in fresh, cryopreserved, and cryopreserved spermatozoa with supplementation of 10 μg/mL AFPI (cryopreserved + AFP) in sterlet. Values with different lower-case letters are significantly different [p < 0.05, one-way analysis of variance (ANOVA) followed by Tukey’s honest significant difference test].
Lipid Peroxidation and Antioxidant Enzyme Activity
The protein concentrations of seminal fluid and spermatozoa were in the range of 0.17–0.8 and 0.58–1.71 mg/mL, respectively. The CAT and SOD activities in the spermatozoa and seminal fluid of each group are shown in Figures 3A,B, respectively. No significant difference was observed among the studied groups with regard to the enzymatic antioxidant activities (SOD and CAT). The TBARS value also showed no difference among the groups (Figure 3C).
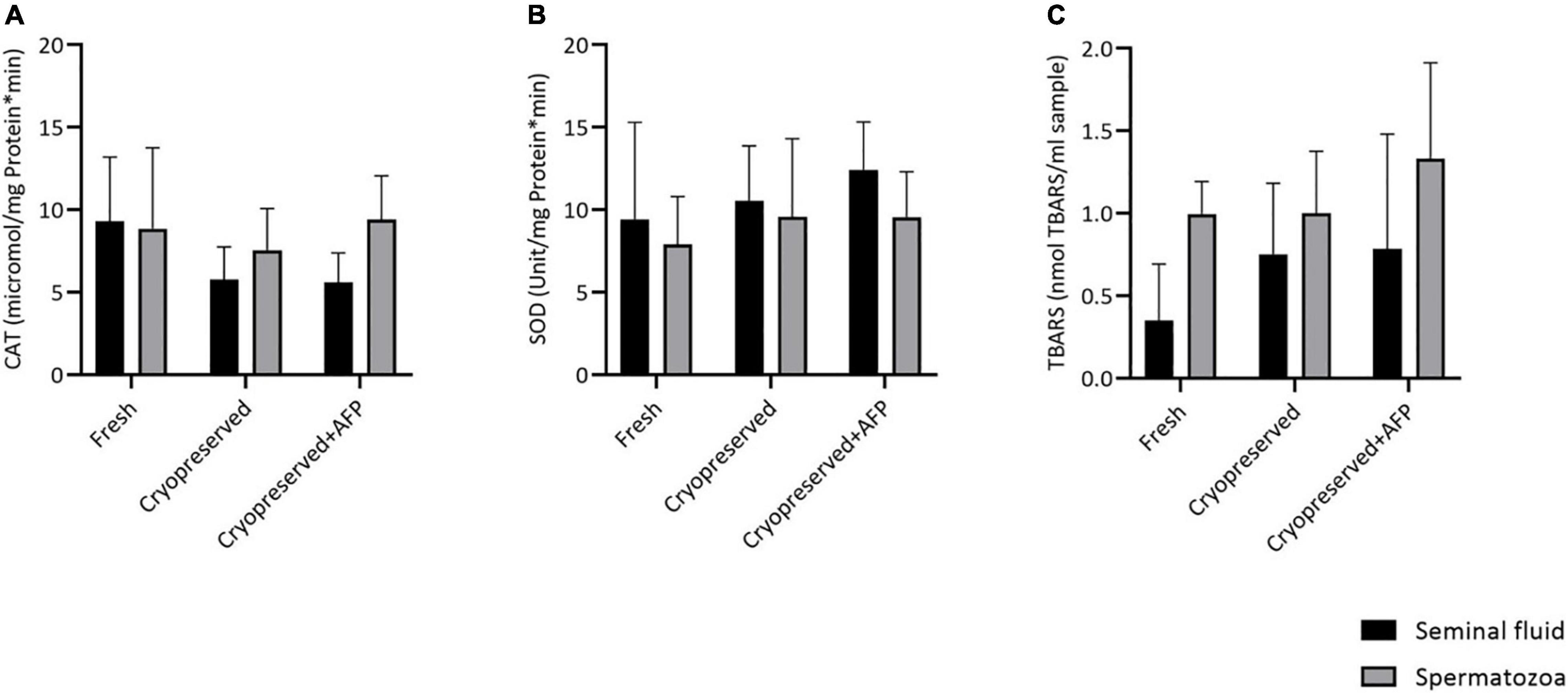
Figure 3. Effect of cryopreservation on lipid peroxidation and antioxidant enzyme activities in fresh, cryopreserved, and cryopreserved sperm with supplementation of 10 μg/mL AFPI (cryopreserved + AFP) in sterlet spermatozoa and seminal fluid (mean ± SD): (A) catalase activity (CAT); (B) superoxide dismutase activity (SOD); (C) thiobarbituric acid-reactive substances (TBARS) [p < 0.05, one-way analysis of variance (ANOVA) followed by Tukey’s honest significant difference test].
DNA Damage
An illustration of the assay used to assess DNA damage for all groups is shown in Figures 4A–C. The evaluation of tail DNA%, which is representative of DNA damage, showed that the level of DNA fragmentation was significantly higher in cryopreserved spermatozoa with (4.8%) and without (4.9%) AFPI than in fresh spermatozoa (2.2%) (Figure 5). The cryopreserved spermatozoa supplemented with 10 μg/mL AFPI showed no difference in tail DNA% compared to those without AFPI (Figure 5).
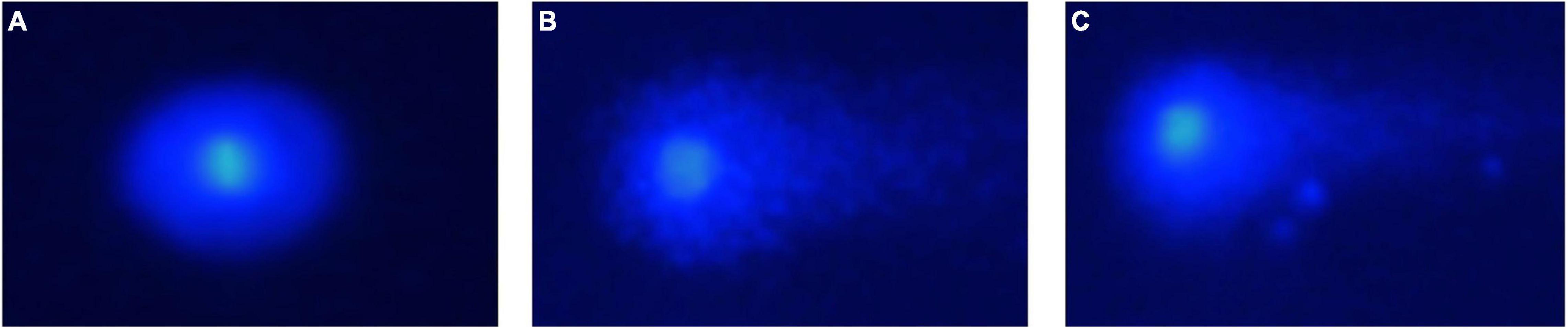
Figure 4. Photomicrograph of comets with different levels of DNA damage in sterlet spermatozoa post-cryopreservation: (A) fresh spermatozoa; (B) cryopreserved spermatozoa; (C) cryopreserved spermatozoa with supplementation of 10 μg/mL AFPI.
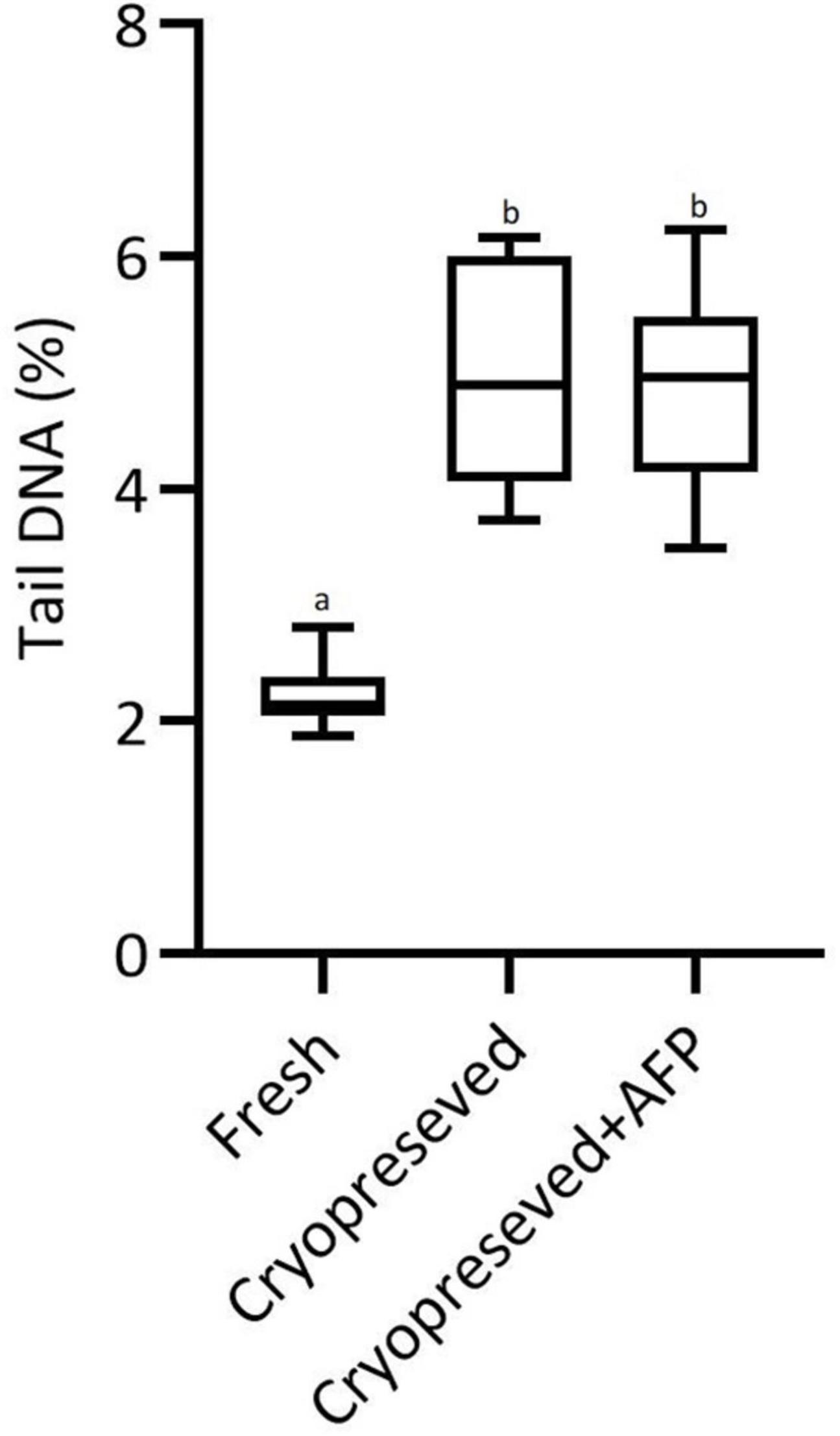
Figure 5. The percent of DNA in the tail as measured in the comet assay before (fresh) and after cryopreservation of sterlet spermatozoa without (cryopreserved) and with supplementation of 10 μg/mL AFPI (cryopreserved + AFP). Values with different lower-case letters are significantly different (p < 0.05, non-parametric Kruskal–Wallis ANOVA followed by multiple comparison of mean ranks for all groups).
Ultrastructural Changes
Sterlet spermatozoa consist of an elongated head with an acrosome, a cylindrical midpiece, and a flagellar region surrounded by the plasma membrane (Figures 6A, 7A, 8A, 9A). The SEM micrographs show no damage to the fresh spermatozoa, while morphological damages such as swelling of midpiece and detachment of the plasma membrane of flagellum were clearly observed in cryopreserved spermatozoa with/without AFP (Figures 6A–C).
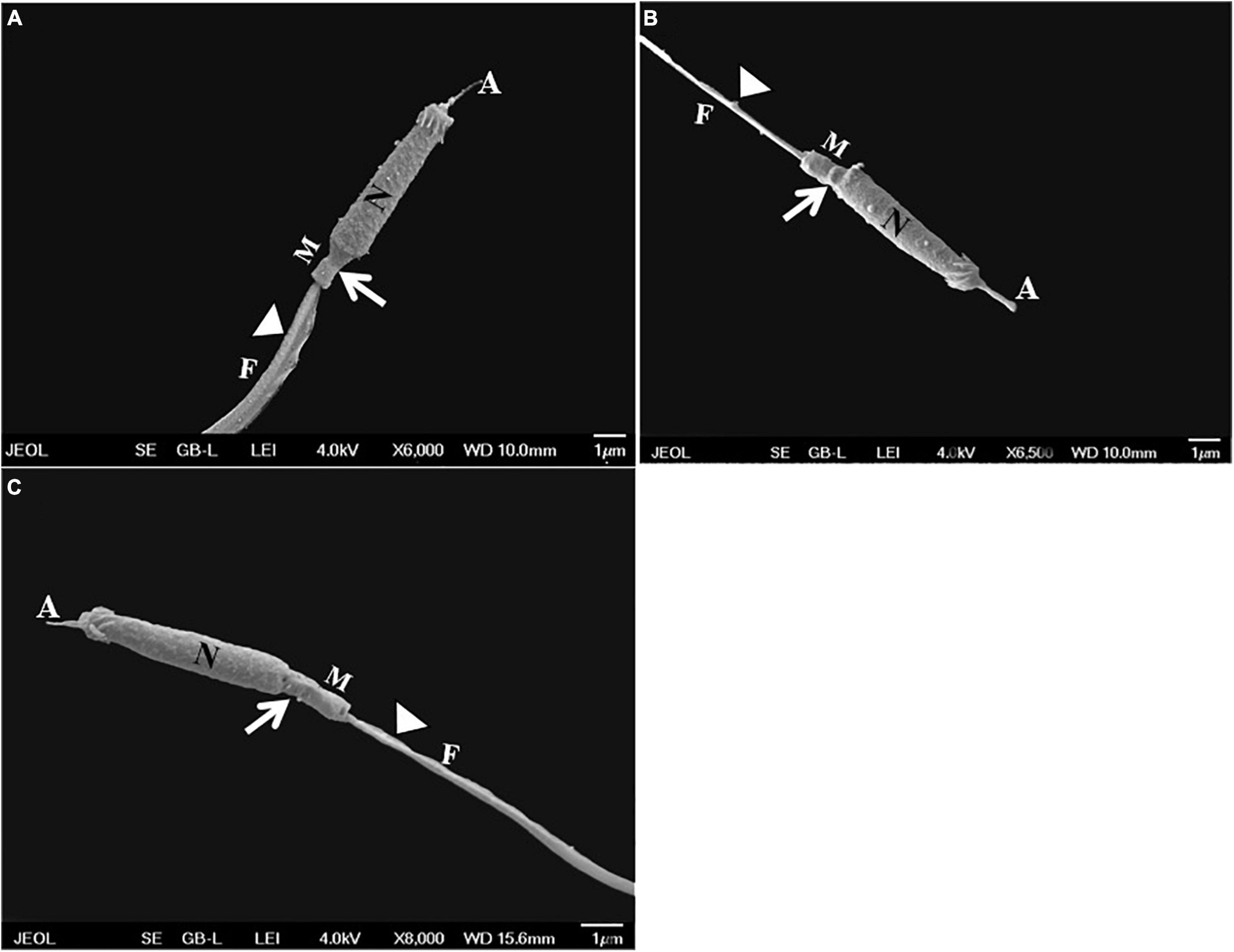
Figure 6. Scanning electron micrographs showing the ultrastructure of sterlet spermatozoa in: (A) fresh spermatozoa without damage in the ultrastructure; (B) cryopreserved spermatozoa without supplementation of 10 μg/mL AFPI showing swollen midpiece (white arrows), and ruptured and detached flagellum (white arrowheads); and (C) cryopreserved spermatozoa with supplementation of 10 μg/mL AFPI showing swollen midpiece (white arrows) and detached flagellum (white arrowheads). A, acrosome; N, nucleus; M, mitochondria; F, flagellum.
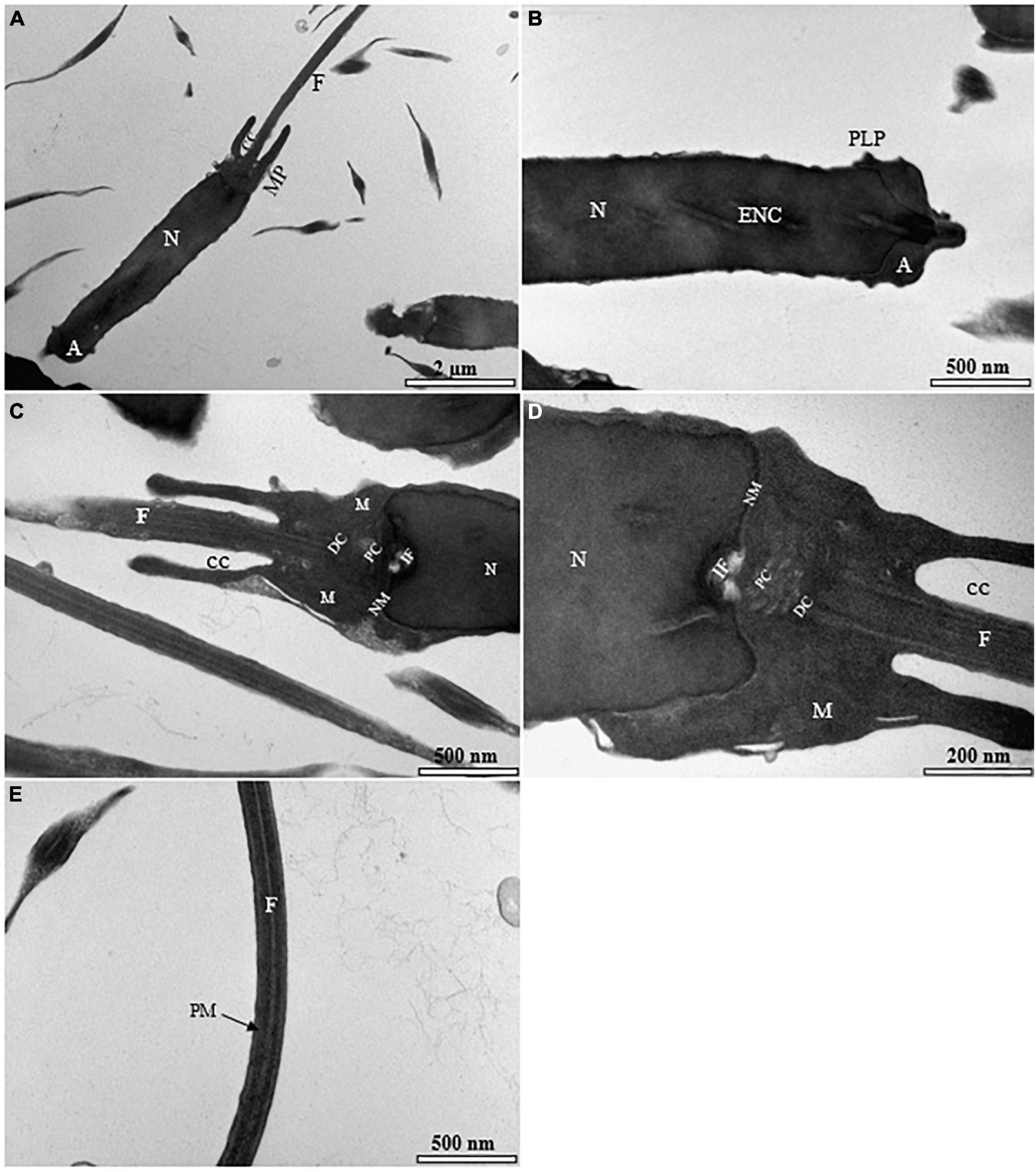
Figure 7. Transmission electron micrographs of the fresh spermatozoon in sterlet. (A) Longitudinal sagittal section of the spermatozoon showing different parts of the cell; (B) higher magnification longitudinal section of the truncate of spermatozoon exhibiting acrosome (A), endonuclear canals (ENC), nucleus (N), and posterolateral projections (PLP); (C) ultrastructure of the midpiece showing nuclear membrane (NM), flagellum (F) separated by cytoplasmic channel (CC), mitochondrion (M), distal centriole (DC), proximal centriole (PC), and implantation fossa (IF); (D) higher magnification of spermatozoon midpiece region displaying various normal and unchanged sections of the spermatozoon; (E) higher magnification of spermatozoon flagellum showing cytoplasmic canal without abnormality in the plasma membrane (PM).
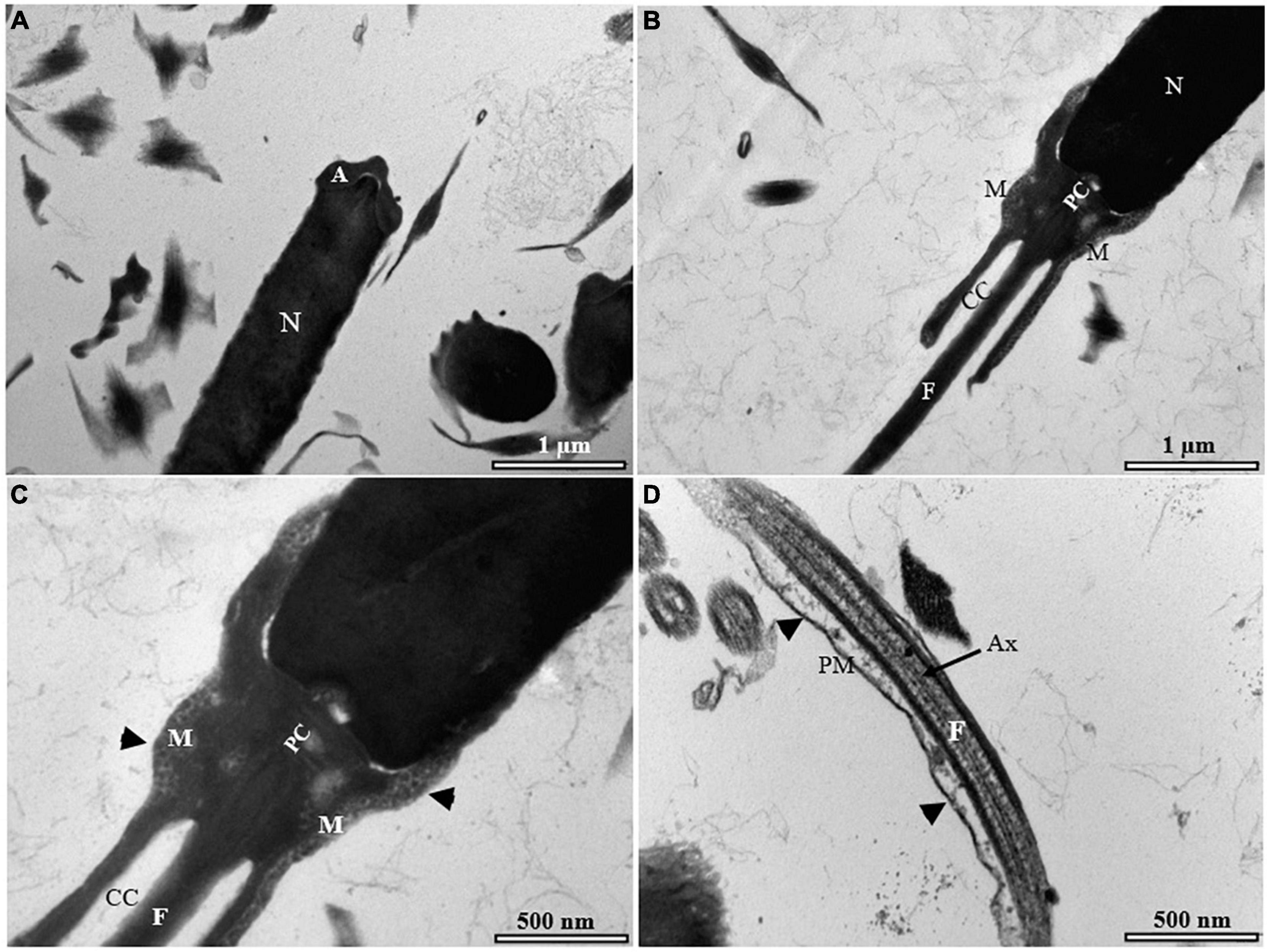
Figure 8. Transmission electron micrographs of cryopreserved spermatozoon in sterlet. (A) Longitudinal sagittal section of cryopreserved spermatozoon showing different parts of the cell; (B) longitudinal section of cryopreserved spermatozoon with some abnormalities in the midpiece; (C) higher magnification of spermatozoon midpiece showing weakening of the midpiece, and particularly, loss of the mitochondria envelope (black arrowheads indicate the mitochondrial crests); (D) higher magnification of spermatozoon flagellum showing detachment and rupture of the plasma membrane of the flagellum (black arrowheads). A, acrosome; Ax, axenome; CC, cytoplasmic channel; F, flagellum; M, mitochondrion; N, nucleus; PM, plasma membrane.
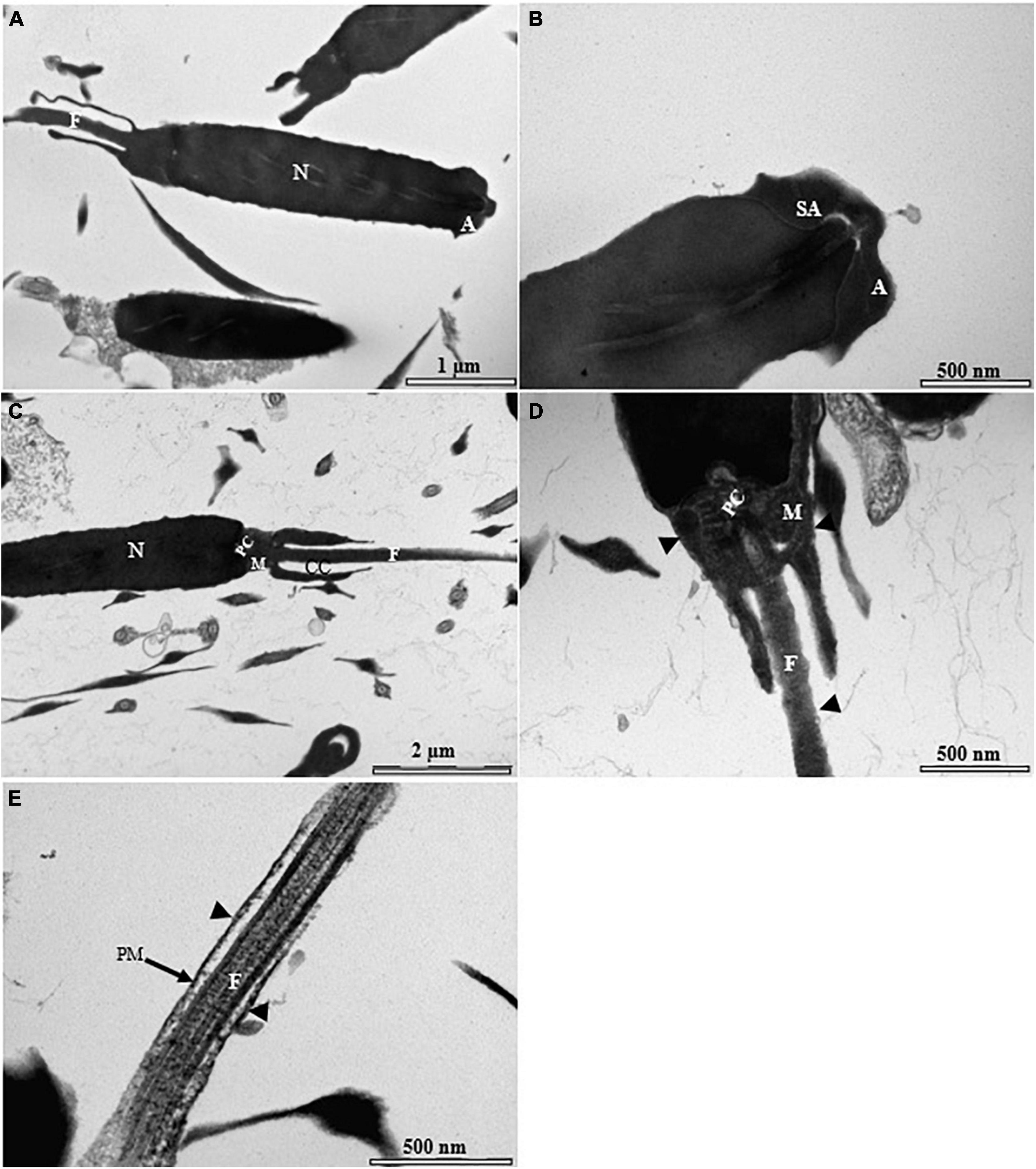
Figure 9. Transmission electron micrographs of cryopreserved spermatozoon with supplementation of antifreeze protein type I (AFPI) in sterlet. (A) Longitudinal sagittal section of cryopreserved spermatozoon showing different parts of the cell; (B) higher magnification of cryopreserved spermatozoon head region showing the unchanged acrosom, sub-acrosome area, and nucleus; (C) longitudinal sagittal section of midpiece exhibiting damage to the midpiece spermatozoon; (D) higher magnification of spermatozoon midpiece region showing visible damage in the mitochondria, midpiece envelope, and plasma membrane of the flagellum (black arrowheads); (E) higher magnification of spermatozoon flagellum showing rupture of the plasma membrane of the flagellum (black arrowheads). A, acrosome; Ax, axenome; CC, cytoplasmic channel; F, flagellum; M, mitochondrion; N, nucleus; PM, plasma membrane.
Most of the visualized fresh spermatozoa showed normal morphology and ultrastructure (Figure 7A). The homogeneous nucleus was surrounded by the nuclear membrane (Figure 7B), and the mitochondria were irregularly dispersed in the cytoplasm with smooth matrix structures (Figures 7C,D). The flagellum was separated by the cytoplasmic channel as in other sturgeon spermatozoa (Figure 7E). The ultrastructure of the spermatozoa was partially affected by the standard cryopreservation procedure with/without AFPI. In both cases, visible changes were observed in the structure of the spermatozoa (Figures 8B–D, 9B–E). Partial damage to the structure of the midpiece and flagellum was visible (Figures 8C,D, 9D,E). Abnormalities of varying severity were observed, such as changes in the structure of the midpiece and detachment of the plasma membrane in the flagellum (Figures 8B,C, 9C,D). In addition, changes in the position of the mitochondrion and, in some cases, the absence of this organelle were observed (Figure 9D). Moreover, partial rupture of the plasma membrane in the flagellum of spermatozoa was observed after the standard cryopreservation procedure with/without AFPI (Figures 8D, 9E).
Partial morphological abnormalities of the mitochondria were observed in a small number of fresh spermatozoa (15 ± 15.07%) (Figure 10A). Significant mitochondrial damage (p < 0.05) was observed in cryopreserved spermatozoa with (49.16 ± 18%) and without AFPI (54.28 ± 15.11%) (Figure 10A). Moreover, the percentage of fresh spermatozoa with morphological changes in the flagellum was 9.16 ± 9.96% (Figure 10B). However, increased morphological abnormalities of the flagellum were observed in cryopreserved spermatozoa with (55 ± 13.22%) and without AFP (57.14 ± 12.53%) (Figure 10B).
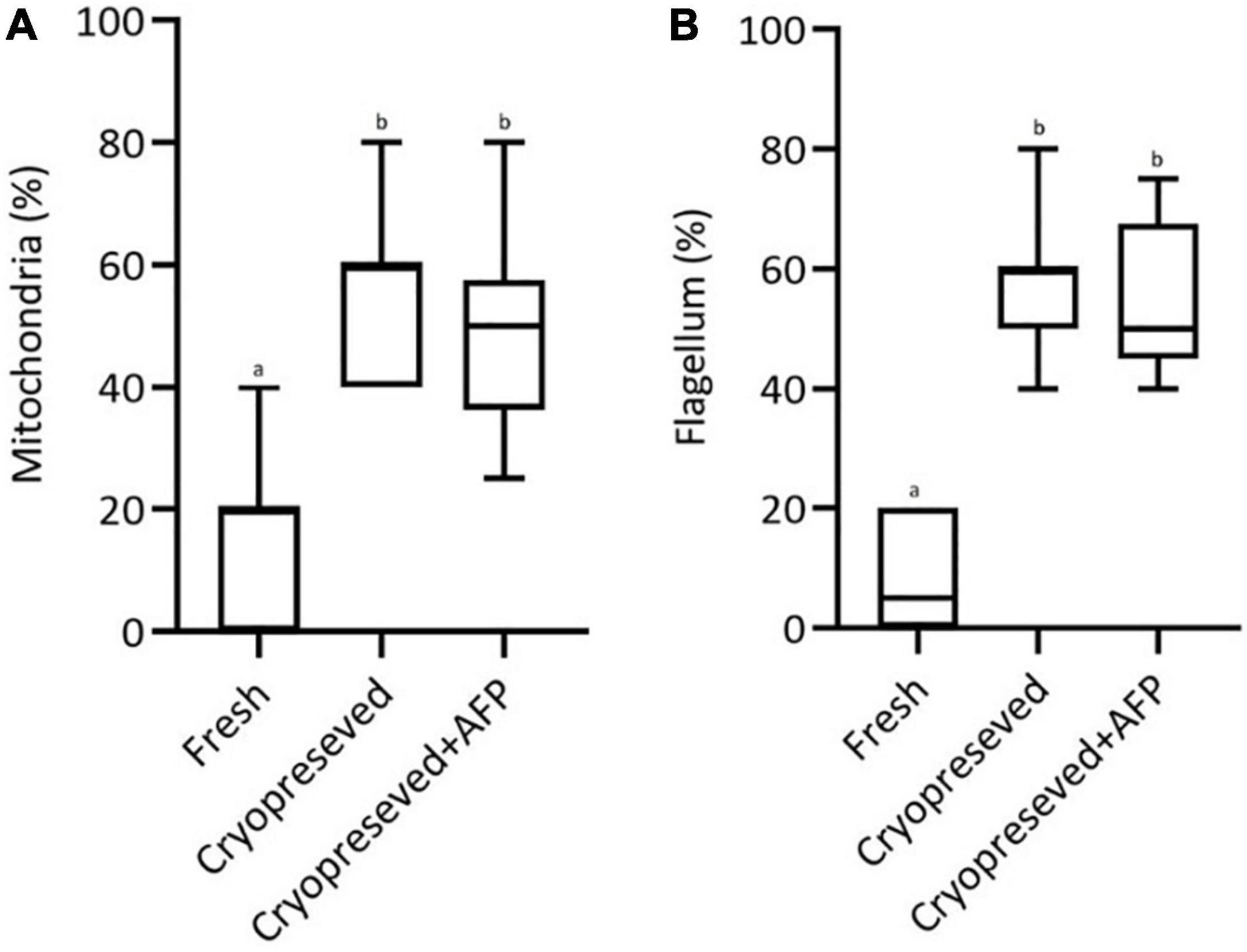
Figure 10. Quantification of morphological alterations in (A) mitochondria and (B) flagellum, comparing fresh, cryopreserved, and cryopreserved spermatozoa with supplementation of 10 μg/mL AFPI (cryopreserved + AFP). Values with different lower-case letters are significantly different [p < 0.05, one-way analysis of variance (ANOVA) followed by Tukey’s honest significant difference test].
Discussion
Despite the practical application of sperm cryopreservation in more than 200 freshwater and marine fish species (Tsai and Lin, 2012), cryo-injuries in various cell compartments, damage to the plasma membrane (Lahnsteiner et al., 1996), changes in DNA integrity (Zilli et al., 2003), and impaired spermatozoa motility and velocity (Linhart et al., 2000) are blamed for lower fertilization and hatching rates in most fish species when frozen sperm are used (Suquet et al., 1998; Li et al., 2006).
A significant decrease in motility of sterlet cryopreserved spermatozoa has been reported (Boryshpolets et al., 2011; Xin et al., 2018b). In the present study, a significant decrease in motility rate and VCL was observed in cryopreserved spermatozoa with/without supplementation of 10 μg AFPI (an optimized concentration of AFPI perceived in a previous study) compared to fresh spermatozoa. However, a previous study on supplementation of different concentrations of AFPI showed improvement in sperm motility rate after the use of AFPI (Xin et al., 2018a). The post-thaw motility of cryopreserved spermatozoa of Pacific Abalone, Haliotis discus hannai supplemented with 10 μg/mL AFPIII in combination with 8% DMSO, 8% ethylene glycol (EG), 6% propylene glycol (PG), or 2% glycerol (GLY) was significantly improved compared to spermatozoa cryopreserved without AFPIII, though this rate was significantly lower than that of fresh spermatozoa (Hossen et al., 2021). No significant differences in velocity were observed between fresh and cryopreserved spermatozoa with/without the addition of AFPI/AFPIII in sea bream (Zilli et al., 2014) and sterlet (Xin et al., 2018a,b).
The structural and functional integrity of the plasma membrane is considered one of the most important indicators of sperm quality after cryopreservation (Muller et al., 2008; Ahmad et al., 2014; Cuevas-Uribe et al., 2015). In the present study, a significant decrease was observed in the plasma membrane integrity of cryopreserved spermatozoa with/without supplementation of 10 μg AFPI compared to fresh spermatozoa. The decreased plasma membrane integrity may be due to chemical toxicity and osmotic damage (Dzuba and Kopeika, 2002). These findings are supported by the relative change in the lipid composition of the plasma membrane after spermatozoa freezing (Holt and North, 1984; Beirão et al., 2012).
In the present study, we did not observe significant changes in lipid peroxidation (TBARS) and antioxidant enzymes (CAT and SOD) activities in sterlet spermatozoa and seminal fluid. These results are in agreement with those reported by Shaliutina-Kolešová et al. (2015) in sterlet cryopreserved spermatozoa with 10% dimethylacetamide (DMA) as cryoprotectant. Moreover, studies on European sea bass, Dicentrarchus labrax and salmonids (Lahnsteiner et al., 2011; Martínez-Páramo et al., 2012) did not demonstrate any significant change in lipid peroxidation levels before or after freezing. On the other hand, the induction of oxidative stress in frozen-thawed sperm was reported in some other studies on common carp, Cyprinus carpio (Li et al., 2010) and Asian sea bass, Lates calcarifer (Klaiwattana et al., 2016). The difference between studies could be due to differences in freezing procedures or the type of cryoprotectant used (Sandoval-Vargas et al., 2021).
Spermatozoa DNA quality assessment is a classic quality biomarker of fresh and cryopreserved spermatozoa in many fish species (Zilli et al., 2003; Riesco et al., 2012; Figueroa et al., 2018, 2020). DNA fragmentation in frozen-thawed fish spermatozoa has been reported in the presence and absence of a cryoprotectant (Xin et al., 2020). In the present study, a low level of DNA damage (with AFPI, 4.87%; without AFPI, 4.96%) was observed in cryopreserved spermatozoa according to the criteria published by Linhartova et al. (2015). In agreement with the present study, slight DNA damage was observed in cryopreserved spermatozoa of rainbow trout, Oncorhynchus mykiss (Labbe et al., 2001). It is suggested that ice formation or osmotic stress could break the DNA through mechanical pressure or apoptotic events that may occur during freezing/thawing (Medeiros et al., 2002). Significant levels of DNA fragmentation were detected in the post-thawed spermatozoa of rainbow trout, brown trout Salmo trutta caspius, common carp, European sea bass and sterlet with different cryoprotectants (Billard, 1983; Kornilova et al., 1997; Zilli et al., 2003; Shaliutina-Kolešová et al., 2015). In contrast, 10 μg/mL AFPIII combined with 8% DMSO improved DNA integrity in cryopreserved spermatozoa of Pacific abalone (Hossen et al., 2021). In gray mullet, Mugil cephalus, freezing-thawing process had no effect on DNA integrity (Balamurugan et al., 2019). The low DNA damage observed in the present study might be due to the reduced susceptibility of sperm chromatin due to high degree of chromatin packaging (Singh et al., 2003).
In the present study, the morphology and ultrastructure of sterlet spermatozoa were slightly changed after applying the established protocol of cryopreservation with/without addition of the optimal concentration of AFPI. This is the first report of ultrastructural and morphological changes in frozen-thawed spermatozoa supplemented with/without AFPI in sturgeons. The morphological changes in the present study, such as weakening of the midpiece and rupture of the plasma membrane of the flagellum were visible after thawing of the cryopreserved spermatozoa. Similar effects were observed in the ultrastructure of Atlantic salmon, Salmo salar spermatozoa, in addition to the detachment of the mitochondrion after cryopreservation (Díaz et al., 2019).
Various abnormalities, such as swelling or rupture of the plasma membrane in the flagellum and midpiece, swelling of mitochondria, change of the position of the mitochondria, loss of mitochondrial crests, vacuolization and rupture of axonemes were observed in cryopreserved spermatozoa of Atlantic croaker Micropogonias undulatus (Gwo and Arnold, 1992), grayling Thymallus thymallus (Lahnsteiner et al., 1992), rainbow trout (Lahnsteiner et al., 1996), flounder Paralichthys olivaceus (Zhang et al., 2003), striped bass Morone saxatilis (He and Woods, 2004), pejerrey Odontesthes bonariensis (Gárriz and Miranda, 2013), and Atlantic salmon (Figueroa et al., 2019). The ruptured plasma membrane of the flagellum and deformed region of the midpiece may be caused by an osmotic regulation deficiency or by leakage of the plasmalemma due to changes in its molecular structure mediated by osmotic shock and/or intracellular ice crystals (Grout and Morris, 1987).
Our ultrastructural results showed no additional protection of plasma membrane or mitochondria by cryopreservation with optimal dose of AFPI in sterlet spermatozoa. Despite several advantages of sperm cryopreservation, this approach is associated with extreme freezing and thawing temperatures that can lead to lethal and sublethal changes in the plasma membrane, flagella and mid-piece/mitochondria of spermatozoa (Lahnsteiner et al., 1992; Billard et al., 2004). The occurrence of membrane destabilization after cryopreservation is the result of physical damage caused by the formation of ice crystals within the cell and in the external environment, as well as osmotic stress (Watson and Morris, 1987; Martínez-Páramo et al., 2012). Certain cellular and physiological processes in spermatozoa, such as ion transport, water balance, receptors for fertilization signals, and regulation of fluidity and permeability can be affected by the above changes (Lahnsteiner et al., 2009; Berrios et al., 2010). These changes, including swelling or rupture of the tail, and certain lesions on other organelles can lead to displacement of mitochondria or loss of their function (Taddei et al., 2001). In addition, damage to spermatozoa membrane may also result from cryoprotectant toxicity, duration of exposure, and interactions with phospholipids in the membrane (Gárriz and Miranda, 2013). Changes in head shape and chromatin condensation of vacuoles and tail region have been demonstrated as a result of structural damage in cryopreserved carp spermatozoa (Drokin et al., 2003). Moreover, cryopreservation of Atlantic salmon spermatozoa revealed some membrane damages (morphological abnormalities) in the head (64.7%), mitochondria (62%) and flagellum (66.4%) (Díaz et al., 2019). In the present study, similar morphological abnormalities were observed in the ultrastructure of mitochondria (49–54%) and flagellum (55–57%) of spermatozoa after cryopreservation with/without AFPI. However, it is worth noting that thawed sperm with damaged flagella still have the potential to move, as has been reported in the Siberian sturgeon, Acipenser baerii (Tsvetkova et al., 1996). According to the present results, the observed damage to cryopreserved spermatozoa was not due to oxidative stress. This suggests that physical damage could occur due to the formation of ice crystals within the cell and in the external environment, as well as osmotic stress (Mazur et al., 1972; Grout and Morris, 1987; Cabrita et al., 2010).
Conclusion
In conclusion, the present study shows that cryopreservation of sterlet spermatozoa with/without addition of a single optimal dose of AFPI (10 μg/ml) results in partial changes in the ultrastructural compartments, such as weakening of the midpiece and rupture of the plasma membrane of the flagellum. The present results do not support a role for oxidative stress in spermatozoa cryo-damage.
Data Availability Statement
The raw data supporting the conclusions of this article will be made available by the authors, without undue reservation.
Author Contributions
HD and AG: conceptualization, investigation, and writing—original draft preparation. HD, AG, JL, VD, and IG: methodology. HD: sample collection. HD, AG, and DR: formal analysis and data curation. AG: re-sources. AG, DR, VD, IG, and TP: writing—review and editing. TP: project administration and supervision. All authors have read and agreed to the published version of the manuscript.
Funding
This study was financially supported by the Ministry of Agriculture of the Czech Republic, project NAZV no. QK22020144, and by the Ministry of Education, Youth and Sports of the Czech Republic, project Biodiversity no. CZ.02.1.01./0.0/0.0/16_025/0007370.
Conflict of Interest
The authors declare that the research was conducted in the absence of any commercial or financial relationships that could be construed as a potential conflict of interest.
Publisher’s Note
All claims expressed in this article are solely those of the authors and do not necessarily represent those of their affiliated organizations, or those of the publisher, the editors and the reviewers. Any product that may be evaluated in this article, or claim that may be made by its manufacturer, is not guaranteed or endorsed by the publisher.
Footnotes
References
Ahmad, M., Nasrullah, R., Riaz, H., Sattar, A., and Ahmad, N. (2014). Changes in motility, morphology, plasma membrane and acrosome integrity during stages of cryopreservation of buck sperm. J. S. Afr. Vet. Assoc. 85:972. doi: 10.4102/jsava.v85i1.972
Asakawa, T., and Matsushita, S. (1980). Coloring conditions of thiobarbituric acid test for detecting lipid hydroperoxides. Lipids 15, 137–140. doi: 10.1007/BF02540959
Asturiano, J. F., Marco-Jiménez, F., Peñaranda, D. S., Garzón, D. L., Pérez, L., Vicente, J. S., et al. (2007). Effect of sperm cryopreservation on the European eel sperm viability and spermatozoa morphology. Reprod Domest Anim. 42, 162–166. doi: 10.1111/j.1439-0531.2006.00746.x
Balamurugan, R., Prapaporn, W., and Munuswamy, N. (2019). Sperm activation and effects of cryopreservation on motility, ultrastructure and DNA integrity in Grey mullet Mugil cephalus. Aquac. Rep. 14:100204. doi: 10.1016/j.aqrep.2019.100204
Baumber, J., Ball, B. A., Linfor, J. J., and Meyers, S. A. (2003). Reactive oxygen species and cryopreservation promote DNA fragmentation in equine spermatozoa. J. Androl. 24, 621–628. doi: 10.1002/j.1939-4640.2003.tb02714.x
Beirão, J., Zilli, L., Vilella, S., Cabrita, E., Schiavone, R., and Herráez, M. P. (2012). Improving sperm cryopreservation with antifreeze proteins: effect on gilthead seabream (Sparus aurata) plasma membrane lipids. Biol. Reprod. 86, 1–9. doi: 10.1095/biolreprod.111.093401
Berrios, O., Valdebenito, I., Treulén, F., and Ubilla, A. (2010). Cold storage of sperm of rainbow trout (Oncorhynchus mykiss): effect on motility, intracellular superoxide, plasma membrane integrity and mitochondrial membrane potential. Arch. Med. Vet. 42, 179–186. doi: 10.4067/S0301-732X2010000300009
Billard, R. (1983). Ultrastructure of trout spermatozoa: changes after dilution and deep-freezing. Cell Tissue Res. 228, 205–218. doi: 10.1007/BF00204873
Billard, R., Cosson, J., Noveiri, S. B., and Pourkazemi, M. (2004). Cryopreservation and short-term storage of sturgeon sperm, a review. Aquaculture 236, 1–9. doi: 10.1016/j.aquaculture.2003.10.029
Boryshpolets, S., Dzyuba, B., Rodina, M., Alavi, S. M. H., Gela, D., and Linhart, O. (2011). Cryopreservation of sterlet (Acipenser ruthenus) spermatozoa using different cryoprotectants. J. Appl. Ichthyol. 27, 1147–1149. doi: 10.1111/j.1439-0426.2011.01866.x
Bronzi, P., Rosenthal, H., and Gessner, J. (2011). Global sturgeon aquaculture production: an overview. J. Appl. Ichthyol. 27, 169–175. doi: 10.1111/j.1439-0426.2011.01757.x
Cabrita, E., Ma, S., Diogo, P., Martınez-Paramo, S., Sarasquete, C., and Dinis, M. T. (2011). The influence of certain aminoacids and vitamins on post-thaw fish sperm motility, viability and DNA fragmentation. Anim. Reprod. Sci. 125, 189–195. doi: 10.1016/j.anireprosci.2011.03.003
Cabrita, E., Sarasquete, C., Martínez-Páramo, S., Robles, V., Beirão, J., Pérez-Cerezales, S., et al. (2010). Cryopreservation of fish sperm: applications and perspectives. J. Appl. Ichthyol. 26, 623–635. doi: 10.1111/j.1439-0426.2010.01556.x
Ciereszko, A., Toth, G. P., Christ, S. A., and Dabrowski, K. (1996). Effect of cryopreservation and theophylline on motility characteristics of lake sturgeon (Acipenser fulvescens) spermatozoa. Theriogenology 45, 665–672. doi: 10.1016/0093-691X(95)00412-412
Cuevas-Uribe, R., Chesney, E. J., Daly, J., and Tiersch, T. R. (2015). Vitrification of sperm from marine fishes: effect on motility and membrane integrity. Aquac. Res. 46, 1770–1784. doi: 10.1111/are.12337
Dadras, H., Boryshpolets, S., Golpour, A., Policar, T., Blecha, M., and Dzyuba, B. (2019a). Effects of temperature on sperm motility of burbot Lota lota: spontaneous activation and calcium dependency. J. Fish Biol. 95, 1137–1144. doi: 10.1111/jfb.14110
Dadras, H., Golpour, A., Dzyuba, B., Kristan, J., and Policar, T. (2019b). Ultrastructural feature of spermatogenic cells and spermatozoon in cultured burbot Lota lota. Tissue Cell 61, 1–7. doi: 10.1016/j.tice.2019.08.005
Díaz, R., Lee-Estevez, M., Quiñones, J., Dumorné, K., Short, S., Ulloa-Rodríguez, P., et al. (2019). Changes in Atlantic salmon (Salmo salar) sperm morphology and membrane lipid composition related to cold storage and cryopreservation. Anim. Reprod. Sci. 204, 50–59. doi: 10.1016/j.anireprosci.2019.03.004
Drokin, S. I., Stein, H., and Govorukha, T. P. (2003). Ultrastructure of carp Cyprinus carpio spermatozoa after cooling, dilution and freeze-thawing. CryoLetters 24, 49–55.
Dzuba, B. B., and Kopeika, E. F. (2002). Relationship between the changes in cellular volume of fish spermatozoa and their cryoresistance. Cryoletters 23353–360.
Figueroa, E., Farias, J. G., Lee-Estevez, M., Valdebenito, I., Risopatrón, J., Magnotti, C., et al. (2018). Sperm cryopreservation with supplementation of α-tocopherol and ascorbic acid in freezing media increase sperm function and fertility rate in Atlantic salmon (Salmo salar). Aquaculture 493, 1–8. doi: 10.1016/j.aquaculture.2018.04.046
Figueroa, E., Lee-Estévez, M., Valdebenito, I., Farías, J. G., and Romero, J. (2020). Potential biomarkers of DNA quality in cryopreserved fish sperm: impact on gene expression and embryonic development. Rev. Aquacult. 12, 382–391. doi: 10.1111/raq.12323
Figueroa, E., Lee-Estevez, M., Valdebenito, I., Watanabe, I., Oliveira, R. P. S., Romero, J., et al. (2019). Effects of cryopreservation on mitochondrial function and sperm quality in fish. Aquaculture 511:634190. doi: 10.1016/j.aquaculture.2019.06.004
Figueroa, E., Valdebenito, I., Merino, O., Ubilla, A., Risopatron, J., and Farias, J. G. (2016). Cryopreservation of Atlantic salmon Salmo salar sperm: effects on sperm physiology. J. Fish Biol. 89, 1537–1550. doi: 10.1111/jfb.13052
Figueroa, E., Valdebenito, I., Zepeda, A. B., Figueroa, C. A., Dumorné, K., Castillo, R. L., et al. (2017). Effects of cryopreservation on mitochondria of fish spermatozoa. Rev. Aquac. 9, 76–87. doi: 10.1111/raq.12105
Gárriz, Á, and Miranda, L. A. (2013). Ultrastructure of fresh and post thawed sperm of pejerrey Odontesthes bonariensis (Atheriniformes). Neotrop. Ichthyol. 11, 831–836. doi: 10.1590/S1679-62252013000400011
Gazo, I., Linhartova, P., Shaliutina, A., and Hulak, M. (2013). Influence of environmentally relevant concentrations of vinclozolin on sperm quality, DNA integrity, and antioxidant responses in sterlet Acipenser ruthenus spermatozoa. Chem. Biol. Interact. 203, 377–385. doi: 10.1016/j.cbi.2013.01.004
Glogowski, J., Kolman, R., Szczepkowski, M., Horvath, A., Urbanyi, B., Sieczynski, P., et al. (2002). Fertilization rate of Siberian sturgeon (Acipenser baeri, Brandt) milt cryopreserved with methanol. Aquaculture 211, 367–373. doi: 10.1016/S0044-8486(02)00003-0
Golpour, A., Pšenièka, M., and Niksirat, H. (2016a). Ultrastructural localization of intracellular calcium during spermatogenesis of sterlet (Acipenser ruthenus). Microsc. Microanal. 22, 1155–1161. doi: 10.1017/S1431927616011958
Golpour, A., Pšenička, M., and Niksirat, H. (2016b). Subcellular localization of calcium deposits during zebrafish (Danio rerio) oogenesis. Micron 80, 6–13. doi: 10.1016/j.micron.2015.09.004
Golpour, A., Pšenièka, M., and Niksirat, H. (2017). Subcellular distribution of calcium during spermatogenesis of zebrafish, Danio rerio. J. Morphol. 278, 1149–1159. doi: 10.1002/jmor.20701
Grout, B. W. W., and Morris, G. J. (1987). “Freezing and cellular organization,” in The Effects of Low Temperatures on Biological Systems, eds B. W. W. Grout and G. J. Morris (London: Edward Arnold), 147–174.
Gwo, J. C., and Arnold, C. R. (1992). Cryopreservation of Atlantic croaker spermatozoa: evaluation of morphological changes. J. Exp. Zool. 264, 444–453. doi: 10.1002/jez.1402640410
He, S., and Woods, L. C. I. I. I. (2004). Effects of dimethyl sulfoxide and glycine on cryopreservation induced damage of plasma membranes and mitochondria to striped bass (Morone saxatilis) sperm. Cryobiology 48, 254–262. doi: 10.1016/j.cryobiol.2004.01.009
Holt, W. V., and North, R. D. (1984). Partially irreversible cold-induced lipid phase transitions in mammalian sperm plasma membrane domains: freeze-fracture study. J. Exp. Zool. 230, 473–483. doi: 10.1002/jez.1402300316
Hossen, S., Sharker, M. R., Cho, Y., Sukhan, Z. P., and Kho, K. H. (2021). Effects of antifreeze protein III on sperm cryopreservation of pacific abalone, Haliotis discus hannai. Int. J. Mol. Sci. 22:3917. doi: 10.3390/ijms22083917
Klaiwattana, P., Srisook, K., Srisook, E., Vuthiphandchai, V., and Neumvonk, J. (2016). Effect of cryopreservation on lipid composition and antioxidant enzyme activity of sea bass (Lates calcarifer) sperm. Iran. J. Fish. Sci. 15, 157–169.
Kopeika, E., Kopeika, J., and Zhang, T. (2007). “Cryopreservation of fish sperm,” in Cryopreservation and Freeze-drying Protocols Second, eds J. G. Day and G. N. Stacey (Totowa, NJ: Humana Press), 203–219.
Koppers, A. J., Garg, M. L., and Aitken, R. J. (2010). Stimulation of mitochondrial reactive oxygen species production by unesterified, unsaturated fatty acids in defective human spermatozoa. Free Radic. Biol. Med. 48, 112–119. doi: 10.1016/j.freeradbiomed.2009.10.033
Kornilova, S. V., Leontev, V. S., Grigorev, D. N., and Blagoi, I. P. (1997). Effect of low temperatures on molecular parameters of DNA. BioWzika 42, 99–104.
Labbe, C., Martoriati, A., Devaux, A., and Maisse, G. (2001). Effect of sperm cryopreservation on sperm DNA stability and progeny development in rainbow trout. Mol. Reprod. Dev. 60, 397–404. doi: 10.1002/mrd.1102
Lahnsteiner, F., Berger, B., Weismann, T., and Patzner, R. (1996). Changes in morphology, physiology, metabolism, and fertilization capacity of rainbow trout semen following cryopreservation. Prog. Fish Cult. 58, 149–159. doi: 10.1577/1548-8640(1996)058<0149:cimpma>2.3.co;2
Lahnsteiner, F., Mansour, N., and Kunz, F. A. (2011). The effect of antioxidants on the quality of cryopreserved semen in two salmonid fish, the brook trout (Salvelinus fontinalis) and the rainbow trout (Oncorhynchus mykiss). Theriogenology 76, 882–890. doi: 10.1016/j.theriogenology.2011.04.019
Lahnsteiner, F., Mansour, N., and Weismann, T. (2002). The cryopreservation of spermatozoa of the burbot, Lota lota (Gadidae, Teleostei). Cryobiology 45, 195–203. doi: 10.1016/S0011-2240(02)00140-142
Lahnsteiner, F., Mansour, N., McNiven, M. A., and Richardson, G. F. (2009). Fatty acids of rainbow trout (Oncorhynchus mykiss) semen: composition and effects on sperm functionality. Aquaculture 298, 118–124. doi: 10.1016/j.aquaculture.2009.08.034
Lahnsteiner, F., Weismann, T., and Patzner, R. A. (1992). Fine structural changes in spermatozoa of the grayling, Thymallus thymallus (Pisces: Teleostei), during routine cryopreservation. Aquaculture 103, 73–84. doi: 10.1016/0044-8486(92)90280-X
Li, J., Liu, Q. H., and Zhang, S. C. (2006). Evaluation of the damage in fish spermatozoa cryopreservation. Chin. J. Oceanol. Limnol. 24, 370–377. doi: 10.1007/BF02842852
Li, P., Li, Z. H., Dzyuba, B., Hulak, M., Rodina, M., and Linhart, O. (2010). Evaluating the impacts of osmotic and oxidative stress on common carp (Cyprinus carpio, L.) sperm caused by cryopreservation techniques. Biol. Reprod. 83, 852–858. doi: 10.1095/biolreprod.110.085852
Li, Z. L., Lin, Q. L., Liu, R. J., Xie, W. Y., and Xiao, W. F. (2007). Reducing oxidative DNA damage by adding antioxidants in human semen samples undergoing cryopreservation procedure. Zhonghua Yi Xue Za Zhi 87, 3174–3177.
Linhart, O., Rodina, M., and Cosson, J. (2000). Cryopreservation of sperm in common carp Cyprinus carpio: sperm motility and hatching success of embryos. Cryobiology 41, 241–250. doi: 10.1006/cryo.2000.2284
Linhartova, P., Gazo, I., Shaliutina-Kolesova, A., Hulak, M., and Kaspar, V. (2015). Effects of tetrabrombisphenol A on DNA integrity, oxidative stress, and sterlet (Acipenser ruthenus) spermatozoa quality variables. Environ. Toxicol. 30, 735–345. doi: 10.1002/tox.21953
Marklund, S., and Marklund, G. (1974). Involvement of the superoxide anion radical in the autoxidation of pyrogallol and a convenient assay for superoxide dismutase. Eur. J. Biochem. 47, 469–474. doi: 10.1111/j.1432-1033.1974.tb03714.x
Marklund, S., Nordensson, I., and Back, O. (1981). Normal CuZn superoxide dismutase, Mn superoxide dismutase, catalase and glutathione peroxidase in Werner’s syndrome. J. Gerontol. 36, 405–409. doi: 10.1093/geronj/36.4.405
Martínez-Páramo, S., Diogo, P., Dinis, M. T., Herráez, M. P., Sarasquete, C., and Cabrita, E. (2012). Incorporation of ascorbic acid and α-tocopherol to the extender media to enhance antioxidant system of cryopreserved sea bass sperm. Theriogenology 77, 1129–1136. doi: 10.1016/j.theriogenology.2011.10.017
Mazur, P., Leibo, S. P., and Chu, E. H. Y. (1972). A two-factor hypothesis of freezing injury: evidence from Chinese hamster tissue-culture cells. Exp. Cell Res. 71, 345–355. doi: 10.1016/0014-4827(72)90303-5
Medeiros, C. M. O., Forell, F., Oliveira, A. T. D., and Rodrigues, J. L. (2002). Current status of sperm cryopreservation: why isn’t it better? Theriogenology 57, 327–344. doi: 10.1016/s0093-691x(01)00674-674
Mims, S. D., Tsvetkova, L. I., Wayman, R. W., Horvath, A., Urbanyi, B., and Gomelskyi, B. (2011). “Cryopreservation of sturgeon and paddlefish sperm,” in Cryopreservation in Aquatic Species, eds T. R. Tiersch and C. C. Green (Baton Rouge, LA: World Aquaculture Society), 366–380. doi: 10.1016/s0305-0491(99)00167-4
Mogielnicka-Brzozowska, M., and Kordan, W. (2011). Characteristics of selected seminal plasma proteins and their application in the improvement of the reproductive processes in mammals. Pol. J. Vet. Sci. 14, 489–499. doi: 10.2478/v10181-011-0074-z
Muller, K., Muller, P., Pincemy, G., Kurz, A., and Labbe, C. (2008). Characterization of sperm plasma membrane properties after cholesterol modification: consequences for cryopreservation of rainbow trout spermatozoa. Biol. Reprod. 78, 390–399. doi: 10.1095/biolreprod.107.064253
Niksirat, H., Andersson, L., Golpour, A., Chupani, L., and James, P. (2017). Quantification of egg proteome changes during fertilization in sterlet Acipenser ruthenus. Biochem. Biophys. Res. Commun. 490, 189–193. doi: 10.1016/j.bbrc.2017.06.019
Riesco, M. F., Martínez-Pastor, F., Chereguini, O., and Robles, V. (2012). Evaluation of Zebrafish (Danio rerio) PGCs viability and DNA damage using different cryopreservation protocols. Theriogenology 77, 122–130. doi: 10.1016/j.theriogenology.2011.07.024
Rurangwa, E., Kime, D. E., Ollevier, F., and Nash, J. P. (2004). The measurement of sperm motility and factors affecting sperm quality in cultured fish. Aquaculture 234, 1–28. doi: 10.1016/j.aquaculture.2003.12.006
Sandoval-Vargas, L., Jiménez, M. S., González, J. R., Villalobos, E. F., Cabrita, E., and Isler, I. V. (2021). Oxidative stress and use of antioxidants in fish semen cryopreservation. Rev. Aquac. 13, 365–387. doi: 10.1111/raq.12479
Sarvi, K., Niksirat, H., Amiri, B. M., Mirtorabi, S. M., Rafiee, G. R., and Bakhtiyari, M. (2006). Cryopreservation of semen from the endangered Caspian brown trout (Salmo trutta caspius). Aquaculture 256, 564–569. doi: 10.1016/j.aquaculture.2006.02.012
Shaliutina-Kolešová, A., Cosson, J., Lebeda, I., Gazo, I., Shaliutina, O., Dzyuba, B., et al. (2015). The influence of cryoprotectants on sturgeon (Acipenser ruthenus) sperm quality, DNA integrity, antioxidant responses, and resistance to oxidative stress. Anim. Reprod. Sci. 159, 66–76. doi: 10.1016/j.anireprosci.2015.05.014
Shaliutina-Kolešová, A., Gazo, I., Cosson, J., and Linhart, O. (2014). Protection of common carp (Cyprinus carpio L.) spermatozoa motility under oxidative stress by antioxidants and seminal plasma. Fish Physiol. Biochem. 40, 1771–1781. doi: 10.1007/s10695-014-9966-z
Singh, N. P., Muller, C. H., and Berger, R. E. (2003). Effects of age on DNA double-strand breaks and apoptosis in human sperm. Fertil. Steril. 80, 1420–1430. doi: 10.1016/S0015-0282(03)02249-2240
Suquet, M. D., Dreanno, C., Petton, B., Normant, Y., Omnes, M. H., and Billard, R. (1998). Long term effects of the cryopreservation of turbot (Psetta maxima) spermatozoa. Aquat. Living Resour. 11, 45–48. doi: 10.1016/S0990-7440(99)80030-80038
Taddei, A. R., Barbato, F., Abelli, L., Canese, S., Moretti, F., Rana, K. J., et al. (2001). Is cryopreservation a homogeneous process? Ultrastructure and motility of untreated, prefreezing, and postthawed spermatozoa of Diplodus puntazzo (Cetti). Cryobiology 42, 244–255. doi: 10.1006/cryo.2001.2328
Thomson, L. K., Fleming, S. D., Aitken, R. J., De Iuliis, G. N., Zieschang, J. A., and Clark, A. M. (2009). Cryopreservation-induced human sperm DNA damage is predominantly mediated by oxidative stress rather than apoptosis. Human Reprod. 24, 2061–2070. doi: 10.1093/humrep/dep214
Tsai, S., and Lin, C. (2012). Advantages and applications of cryopreservation in fisheries science. Braz Arch. Biol. Technol. 55, 425–434. doi: 10.1590/S1516-89132012000300014
Tsvetkova, L. I., Cosson, J., Linhart, O., and Billard, R. (1996). Motility and fertilizing capacity of fresh and frozen-thawed spermatozoa in sturgeons Acipenser baeri and A. ruthenus. J. Appl. Ichthyol. 12, 107–112. doi: 10.1111/j.1439-0426.1996.tb00071.x
Urbányi, B., Horváth, Á, and Kovács, B. (2004). Successful hybridization of Acipenser species using cryopreserved sperm. Aquac. Int. 12, 47–56. doi: 10.1023/B:AQUI.0000017186.59304.6d
Wang, A. W., Zhang, H., Ikemoto, I., Anderson, D. J., and Loughlin, K. R. (1997). Reactive oxygen species generation by seminal cells during cryopreservation. J. Urol. 49, 921–925. doi: 10.1016/s0090-4295(97)00070-8
Watson, P. F., and Morris, G. J. (1987). Cold shock injury in animal cells. Symp. Soc. Exp. Biol. 41, 311–340.
Xin, M., Sterba, J., Shaliutina-Kolesova, A., Dzyuba, B., Lieskovska, J., Boryshpolets, S., et al. (2018a). Protective role of antifreeze proteins on sterlet (Acipenser ruthenus) sperm during cryopreservation. Fish Physiol. Biochem. 44, 1527–1533. doi: 10.1007/s10695-018-0538-535
Xin, M., Tučková, V., Rodina, M., Kholodnyy, V., Dadras, H., Boryshpolets, S., et al. (2018b). Effects of antifreeze proteins on cryopreserved sterlet (Acipenser ruthenus) sperm motility variables and fertilization capacity. Anim. Reprod. Sci. 196, 143–149. doi: 10.1016/j.anireprosci.2018.07.007
Xin, M., Niksirat, H., Shaliutina-Kolešová, A., Siddique, M. A. M., Sterba, J., Boryshpolets, S., et al. (2020). Molecular and subcellular cryoinjury of fish spermatozoa and approaches to improve cryopreservation. Rev. Aquac. 12, 909–924. doi: 10.1111/raq.12355
Zhang, Y. Z., Zhang, S. C., Liu, X. Z., Xu, Y. Y., Wang, C. L., Sawant, M. S., et al. (2003). Cryopreservation of flounder (Paralichthys olivaceus) sperm with a practical methodology. Theriogenology 60, 989–996. doi: 10.1016/s0093-691x(03)00097-99
Zilli, L., Beirão, J., Schiavone, R., Herraez, M. P., Gnoni, A., and Vilella, S. (2014). Comparative proteome analysis of cryopreserved flagella and head plasma membrane proteins from sea bream spermatozoa: effect of antifreeze proteins. PLoS One 9:e99992. doi: 10.1371/journal.pone.0099992
Keywords: DNA damage, antioxidant enzyme, membrane integrity, motility, ultrastructural change
Citation: Dadras H, Golpour A, Rahi D, Lieskovská J, Dzyuba V, Gazo I and Policar T (2022) Cryopreservation of Sterlet, Acipenser ruthenus Spermatozoa: Evaluation of Quality Parameters and Fine Ultrastructure. Front. Mar. Sci. 9:783278. doi: 10.3389/fmars.2022.783278
Received: 25 September 2021; Accepted: 18 February 2022;
Published: 11 March 2022.
Edited by:
Satya Panigrahi, Indira Gandhi Centre for Atomic Research (IGCAR), IndiaReviewed by:
Yusuf Bozkurt, Iskenderun Technical University, TurkeyElias Figueroa, Universidad Católica de Temuco, Chile
Copyright © 2022 Dadras, Golpour, Rahi, Lieskovská, Dzyuba, Gazo and Policar. This is an open-access article distributed under the terms of the Creative Commons Attribution License (CC BY). The use, distribution or reproduction in other forums is permitted, provided the original author(s) and the copyright owner(s) are credited and that the original publication in this journal is cited, in accordance with accepted academic practice. No use, distribution or reproduction is permitted which does not comply with these terms.
*Correspondence: Hadiseh Dadras, ZGFkcmFzQGZyb3YuamN1LmN6, ZmguZGFkcmFzQGdtYWlsLmNvbQ==
†These authors have contributed equally to this work and share first authorship