- 1Cross-Research in Environmental Technologies (CRETUS), Department of Applied Economics, University of Santiago de Compostela, A Coruña, Spain
- 2Faculty of Business and Management, University of Santiago de Compostela, A Coruña, Spain
- 3Centro de Investigación Mariña (CIM) and Departamento de Ecoloxía e Bioloxía Animal, Facultade de Ciencias do Mar, Universidade de Vigo, Vigo, Spain
- 4Red Sea Research Center (RSRC) and Computational Bioscience Research Center (CBRC), King Abdullah University of Science and Technology (KAUST), Thuwal, Saudi Arabia
Seagrass ecosystems support human well-being by delivering a wide range of ecosystem services. Particularly important is the significant role they play in food provisioning by supporting fisheries worldwide. Despite their socio-economic importance, it is only recently when they have been considered as important social-ecological systems worldwide. Research focused on the interactions between seagrasses and human activities have been understudied and never addressed from a global approach, even though this knowledge is essential to create relevant policy and management plans, and to promote governance systems, which consider fishers’ needs and rights. Thus, we carried out a global systematic review on trade-offs and/or synergies between seagrasses and fishing activities, aiming to analyse the current state of the art on these interactions, to identify potential gaps in knowledge, and to pinpoint key research priorities. We found a total of 94 publications assessing the relationship between seagrass ecosystems and fisheries, which have tripled between 2001-2021, being most of them empirical studies (90%) with ecological scope (a total of 68). Socioeconomic studies (3%) on the subject were identified as a knowledge gap. Most studies (72%) were carried out in northern hemisphere countries, with an underrepresentation of studies in tropical regions. The studies reporting trade-off (a total of 69) interactions almost tripled those reporting synergies (a total of 31) between seagrass ecosystems and fisheries. Mechanical damage to seagrasses by fishing gear is the main source (51%) of trade-offs, followed by overfishing (28%), while aquaculture cages’ emissions (20%) are also a relevant source of trade-offs. Seafood market demand and conflicts of use were the main drivers for trade-offs. When assessing synergistic interactions, most studies (27 out of 31) reported a larger abundance or recruitment of species with commercial interest mediated by seagrass habitat provision. Globally, seagrass ecosystems are mainly affected by industrial and small-scale fisheries, including aquaculture and shellfisheries, and to a lesser extent, by recreational fisheries. Fisheries management system is not specified in more than half (53) of the reviewed publications, which represents a key knowledge gap with implications for sustainable management. Nevertheless, we document a fast increase in studies covering fishery-seagrass interactions, which, if accompanied by better reporting of the nature of the interactions and the socio-economic context of the fishery, would help improve the sustainable management of both systems.
Introduction
Seagrass are marine flowering plants that form extensive meadows from intertidal to subtidal and can extend down to 60 m worldwide, except Antarctica. Seagrass habitats are a critical component of coastal systems that deliver a broad range of ecosystem services and functions, playing a significant role in supporting human well-being (De la Torre-Castro, 2006; Nordlund et al., 2016; Ruiz-Frau et al., 2017; De los Santos et al., 2019). In particular, seagrass meadows contribute to coastal protection of shorelines (Boudouresque et al., 2016) by reducing wave energy and trapping sediments (Ondiviela et al., 2014). They also improve water quality by filtering excess of nutrients and contaminants (Serrano et al., 2013), control diseases by removing pathogens from the water (Lamb et al., 2017), play a significant role in food security, supporting economies and livelihoods through fisheries (Unsworth et al., 2018), and sequester and store carbon thus mitigating impacts of climate change (Duarte et al., 2013). In fact, the reduction of seagrass capacity to sequester and store carbon because of its decline is also of high international concern, since seagrass loss can lead to significant increase of CO2 into the atmosphere (Arias-Ortiz et al., 2018). Seagrass meadows also provide non-material benefits to human societies, such as recreation, aesthetic, cultural heritage and education (Cullen-Unsworth et al., 2014; Garcia Rodrigues et al., 2017).
They are also of fundamental importance to world fisheries production in different ways since seagrasses are nursery and foraging habitats for commercially important invertebrates and fish (e.g. Unsworth and Cullen, 2010; Lilley and Unsworth, 2014; Nordlund et al., 2016; rev. Unsworth et al., 2018). Seagrass meadows are estimated to provide nursery habitat for at least 20% of the world’s 25 larger-scale fisheries (LSF), including the Alaska (walleye) pollock, the most landed species on the planet (Lilley and Unsworth, 2014; Unsworth et al., 2018). In addition, almost 80% of the 10 most caught species by small-scale fisheries (SSF) are associated with seagrass in tropical and subtropical regions (Unsworth et al., 2018). Seagrasses also support fisheries by providing trophic subsidy to contiguous habitats with adjacent fisheries, and, in the case of SSF, they also provide fishing grounds (Unsworth et al., 2018).
In spite of the critical role of seagrasses, they have shown a worldwide declining trend throughout the 20th century due to cumulative pressures on coastal areas (Waycott et al., 2009; Pendleton et al., 2012; Ruiz-Frau et al., 2017; McKenzie et al., 2020), such as commercial fisheries (trawling), shellfishing (dredging), building of coastal structures, and sewage and nutrient inputs, among others (Cullen-Unsworth and Unsworth, 2013; De los Santos et al., 2019). Nevertheless, encouragingly, increasing awareness about conserving and restoring seagrass meadows have led to a reversal trend involving seagrass recovery across many Australian, Asian, European and North American coastal ecosystems, particularly fast-growing species after restoration programs (De los Santos et al., 2019; Orth et al., 2020; Sinclair et al., 2021).
Although it is evident that fishery activities, including shellfish harvesting, interact with seagrass meadows (Cabaço et al., 2005; Park et al., 2011; Pitanga et al., 2012; Garmendia et al., 2017), the magnitude and impact (positive or negative) of such interactions remain unclear, as some studies report significant decline in seagrass population performance due to shellfishing (e.g. Cabaço et al., 2005), while others not (e.g. Park et al., 2011); even more, results are not always comparable as they might report different species performances. Nonetheless, Nordlund et al. (2018) used experts’ knowledge on 36 global study cases and found that seagrass-based fisheries are of high importance, concluding that wherever seagrass are present, there is most certainly fishing, with recreational, subsistence and commercial value. In addition, (Gagnon et al. 2020) investigated plant-bivalve interactions in coastal habitats (salt marshes, mangroves and seagrass), and reported that most interactions were positive and reciprocal, since plants promoted bivalve survival and abundance by providing substrate and shelter, while bivalves promoted plant growth and survival by stabilizing and fertilizing the sediment and reducing water turbidity. At the same time, (Fales et al. 2020) also carried out a global meta-analysis on ecological variables (e.g. density, size, growth, survival), reporting reciprocal interactions between seagrass and bivalve molluscs, and suggested a variety of outcomes for these interactions, as there are multiple specific mechanisms and spatiotemporal scales enabling the seagrass-bivalves coexistence. However, there is still limited information regarding the interactions between seagrass ecosystems and fisheries as a coupled socio-ecological system.
In this study, we carried out a global systematic literature review of peer-reviewed scientific studies on trade-offs and/or synergies between seagrasses and fishing and aquaculture activities. A trade-off is defined as a situation where the use of one ecosystem service by human activities directly decreases the benefits supplied by another service (e.g. the impact of the management of a fishery on the abundance of seagrasses) (Rodríguez et al., 2006; Bennett et al., 2009). A synergy occurs where the use of one ecosystem service directly increases the benefits supplied by another service (e.g. effectively managing seagrasses helps to improve fish nurseries) (Gilby et al., 2018). Thus, the aims of this paper were (a) to analyse the current state of knowledge on these interactions, in the context of increasing climate change impacts, (b) to identify knowledge gaps, and (c) to pinpoint key research priorities and the way forward beyond the current state of the art.
Methods
Literature Search
Search for published literature in English was performed in Scopus using the following terms in a single string: “seagrass” OR “eelgrass” AND “small-scale fisheries” OR “shellfisheries” OR “fisheries” OR “artisanal” OR “marine” OR “coastal” AND “climate change” OR “global warming”. The terms “subsistence fisheries”, “invertebrate gleaning”, “invertebrate fisheries” and “aquaculture” were included within the string search as a test, and the number of publications was the same as without these terms. Only ‘Articles’ and ‘Reviews’ were included as a type of document, because they have been subjected to a peer-reviewed process. Search results included articles published from 1992 (the first article found about this topic was published in 1992) to March 2022. In addition, despite not appearing in the Scopus searches, additional peer-reviewed articles were also included for being part of the references of publications that did appear in the searches. When a study was identified in a citation, it was scrutinized, and was only included if it met our inclusion criteria. These articles are included as they were directly related to our research objectives.
Literature Review Process
We follow the Preferred Reporting Items for Systematic Reviews and Meta-Analyses (PRISMA) (Moher et al., 2009) for the systematic literature review (Figure 1). Publications were screened by their title and abstracts, which had to mention any type of relationship between seagrass and fisheries-related activities. The number of publications was remarkably reduced from 1135 to 274 after this screening process. After screening their abstracts, full-texts were considered to assess whether they were eligible for the final synthesis. In order to meet the eligibility criteria, publications had to present at least one qualitative or quantitative evaluation addressing trade-offs and/or synergies between seagrass ecosystems and fisheries-related activities. This analysis was carried out on 94 final publications (the detailed list of publications is included in the Supplementary Material).
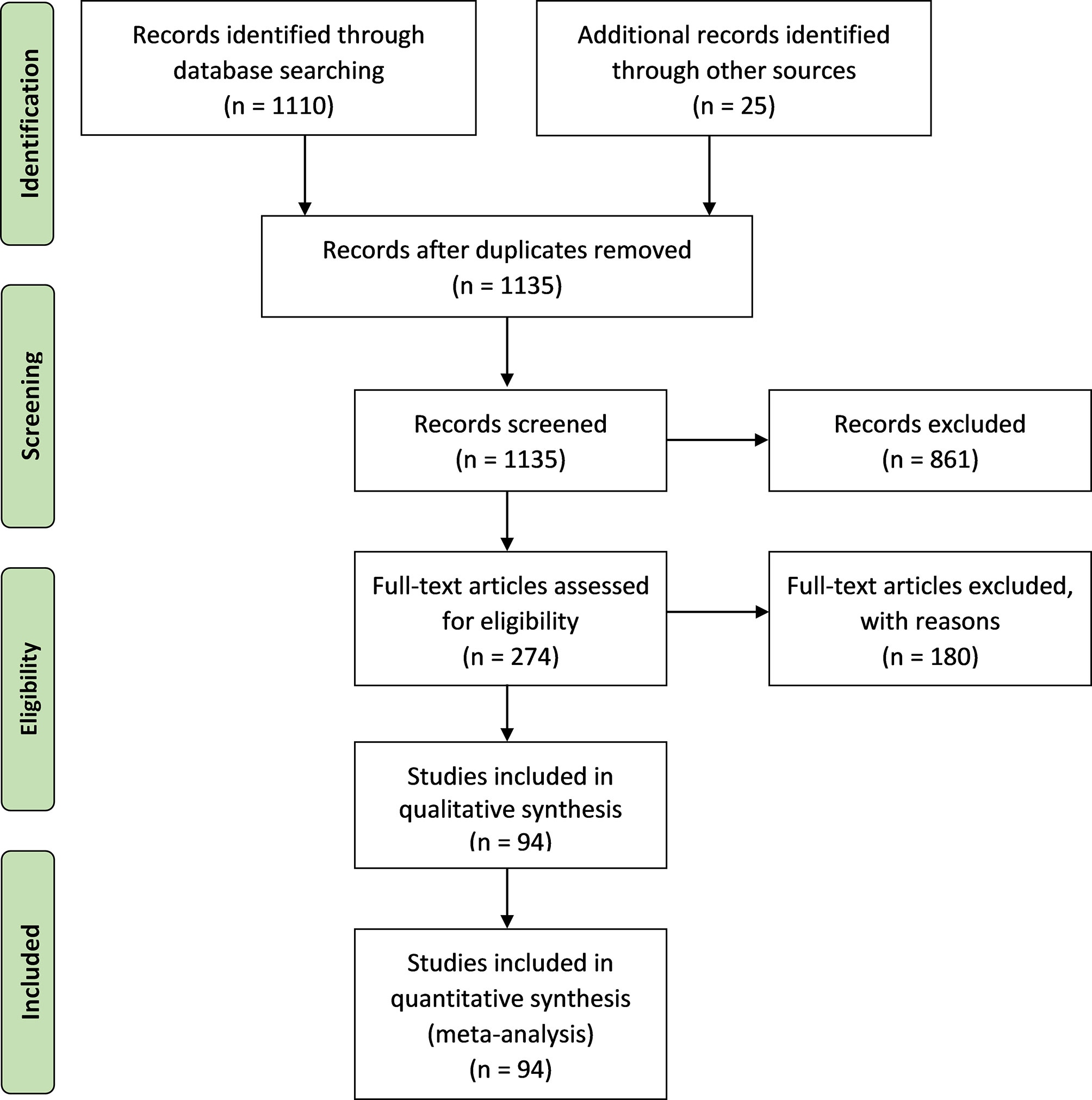
Figure 1 Flow diagram for literature review with the Preferred Reporting Items for Systematic reviews and Meta-Analyses (PRISMA).
To analyse the results of the systematic review, we included 20 variables and their corresponding response categories. Data variables included habitat types, scale of the studies, type of fishing activities, commercial and seagrass species, ecosystem services, fisheries management systems, magnitude of interactions and those associated drivers, methods and data used to assess these interactions (Table 1).
We scored the magnitude of the interactions evaluated in the systematic review, either as trade-off or as synergy. As these scores are not absolute values from the review’s results, the relative quality of the underlying data may affect the robustness of the findings. In order to reflect this uncertainty in our magnitude calculations, we used data quality scores for each interaction in the dataset. For example, if the data suggested the occurrence of an interaction but their effects were not significant or evident by using the studied variables of the publication assessed, the quality score applied was 1 (minimum). We assigned a score 2 (low) when at least one studied variable was significant but did not affect the main outcome of results; score 3 (medium) when there was a significant effect on at least one of the main assessed variables, while a score 4 (high) when the effect was significantly different in most of the analyzed variables and the measure of such effect was at least twofold the reference value. Finally, when an effect was described in a publication, but it was not quantified in any way, the magnitude of the interaction was recorded as not assessed.
Results
Literature Synthesis
The number of publications assessing the relationship between seagrass ecosystems and fisheries has tripled over the last decade, starting from 24 publications in the early 2000s to 70 publications from 2012 to 2021. Prior to 2000, studies assessing the interaction were almost non-existing, at least within the selected criteria. Most of the publications found in the literature synthesis reported empirical studies (85 out of 94) where environmental survey (51 out of 94) was carried out at local or regional scale (73 out of 94) with an ecological scope (68 out of 94), although there was a variety of combinations among the methods and the scope of the assessment, as well as the habitat or the scale at which the studies were conducted Figure 2). A large number of publications (62) contributed within the research field of marine sciences (27), biology (16), ecology (13), environmental sciences (13), and oceanography (4). Seagrass ecosystems research field was represented by three publications, similar to fisheries (3), agriculture (3) and zoology (3). The least represented research fields were global change, geography and tourism (2 each), and veterinary, political sciences and economics (1 each).
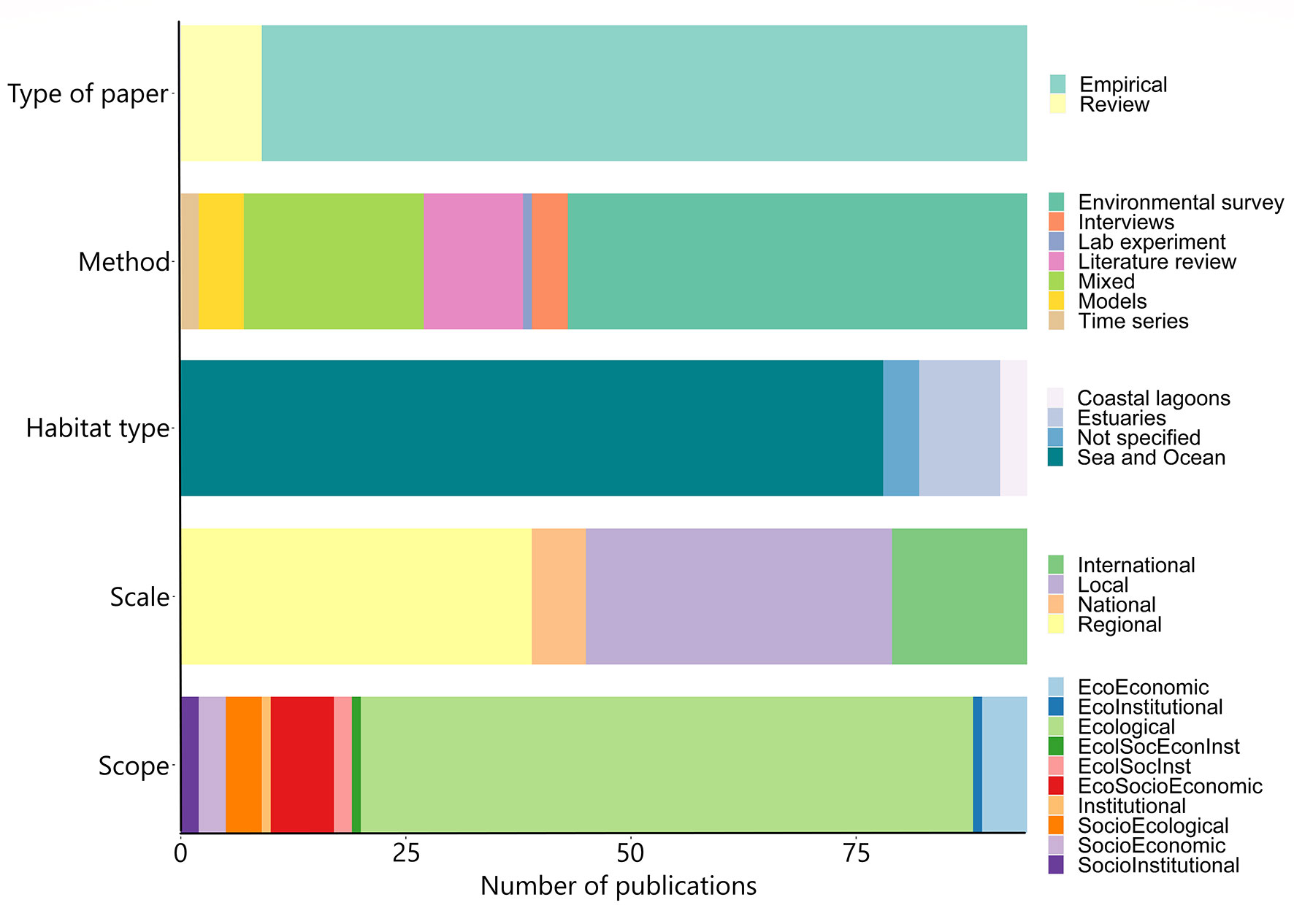
Figure 2 Number of publications considered in each variable category from the literature review (N=94).
Different methods were used in the papers, although most of them (69) were focused on obtaining data related to biological (e.g. variables such as abundance, reproductive effort, seagrass or fish biomass, size, growth, survival, coverage) or environmental parameters (e.g. sediment composition, organic matter, granulometry, water quality, oceanographic data), which enabled comparisons between disturbed and undisturbed seagrass meadows.
In a smaller proportion of studies (22), several socioeconomic variables were taken into account along with biological or environmental ones: twelve publications related the presence or abundance of seagrass or seafood to socioeconomic variables like harvesting experience, income, human development index, landings or subsistence on harvesting; six publications related seagrass conditions to social variables like stakeholders engagement, perceptions on governance or well-being, boaters’ profile, likelihood to use buoys or willingness to pay for their use; while four studies used economic variables like monetary value of seagrass, annual production of commercial species, or income, in relation to seagrass abundance. Only three publications were exclusively focused on the use of socioeconomic variables like number of licensed harvesters, monetary value of seafood, awareness of seagrass ecosystem services, frequency and purpose of collecting seafood, frequency of recreation in seagrass meadows, perception of seagrass condition, or perceived threats to seagrass.
Most of the studies used quantitative methods (74), while others used a combination of qualitative and quantitative methods (20). In most cases (55), multivariate analysis was the most used study method, followed by mapping (16), ecological modelling (10), interviews or workshops (7) and socioeconomic evaluations (3).
Many publications (N=40; 48%) considered the four types of ecosystem services categories delivered by seagrasses [provisioning, regulating, supporting and cultural services; based on Millennium Ecosystem Assessment (MEA), 2005], followed by 16 publications relating only three of these services in different combinations. Different ecosystem services bundles were also identified. For example, the combination of provisioning and supporting services were observed in 16 publications; regulating and supporting services were analysed in only 2, while the combined analysis of provisioning and cultural services were only assessed in 1 study. For those studies focusing on a single delivered service, the provisioning service was the most analysed (14 out of 16).
Most publications (N=74; 80%) included in the literature synthesis did not assess climate change. However, several studies (20) did consider climate change by modelling future scenarios (8), evaluating time series of data (6), measuring temperature response (3) and carbon sequestration (2), or carrying out ecosystem valuation (1). Carbon sequestration and storage by seagrass ecosystems dominated the focus of recent publications, when the words “climate change” or “global warming” were included in the search; however, they were not included in the synthesis as they did not directly assess seagrass-fisheries interactions.
Geographic Distribution
Most studies (N=68; 72%) were carried out in northern hemisphere countries Figure 3), mainly in the West and East coast of the USA and Canada, in the Atlantic coast of Western Europe and Mediterranean Sea (namely Spain). Only ten studies were developed in the Pacific Ocean, mostly in Australia and Indonesia (N=5; 6% each), while there was a considerable lack of studies in East Africa (N=7; 8%) (no studies were found in West Africa) and the Caribbean (N=1; 1%), despite the fact that seagrass-based fisheries are likely to be important in these areas (e.g. De la Torre-Castro et al., 2014).
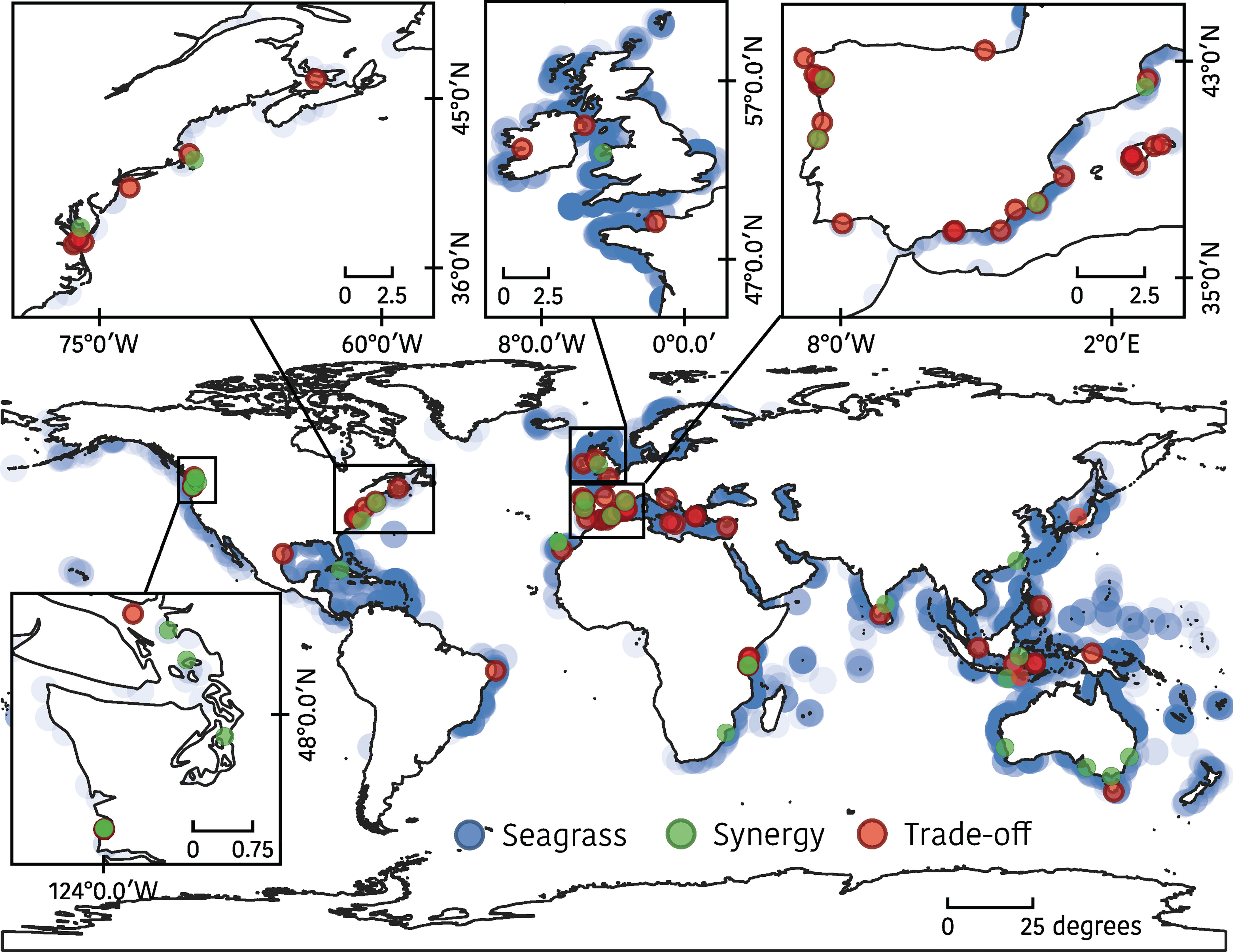
Figure 3 Geographical distribution of publication’ study sites. Trade-offs are shown in red and synergies in green (N=89). Global seagrass distribution is shown in blue (UNEP-WCMC and Short, 2021). Global reviews are not included to avoid duplications.
Our results also highlight that historical research efforts conducted to study trade-offs and/or synergies between seagrasses and fishing activities were mainly developed in those areas of the world where seagrasses are abundant, namely North America, Europe and Oceania Figure 3) (Short et al., 2007). However, the results also suggest that the research done in these developed regions is still limited, except in Europe where we found the largest number of relevant publications, mainly addressing trade-offs; although, naturally, fewer studies do not imply less research quality. As a result, most relevant studies were developed in marine ecosystems with temperate seagrass species, such as Zostera marina (30 publications), Posidonia oceanica (19), Z. noltei (13) and Cymodocea nodosa (8). Other species with more tropical distribution (e.g. species of the genera Thalassia, Halodule and Halophila) were poorly represented in the studies assessed (Figure 4).
Trade-Offs and/or Synergies Between Seagrasses and Fishing Activities
The publications reporting trade-off interactions almost tripled those reporting synergies between seagrass ecosystems and fisheries (Table 2). Mechanical damage to seagrasses by fishing gear was the main source of trade-offs identified in the analysed literature, where most publications (N=35; 51% of trade-off origin) described, from small to large magnitude effects, caused by trawling, boat propellers or anchoring, digging, dredging and deployment of structures such as fish fences. Overfishing was the second most observed source of trade-offs (N=19; 28%), with most of the publications indicating a medium negative effect. Aquaculture cages’ emissions were also a relevant source of trade-offs (N=14; 20%), and at least one publication reported episodes of invasive species associated with aquaculture. Trade-offs were almost evenly driven by seafood market demand (according to 46 publications) and conflicts of use (33 publications).
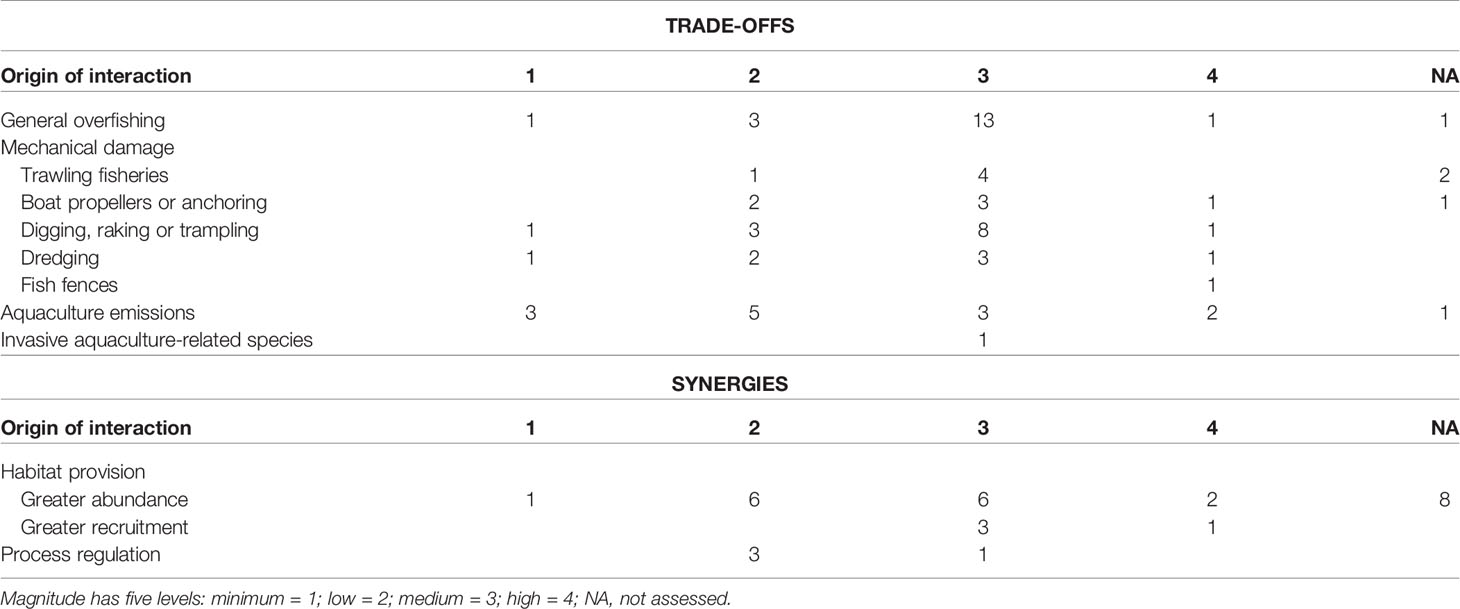
Table 2 Summary of origin and magnitude of observed interactions in the literature synthesis (number of publications where a particular interaction and magnitude was observed).
Regarding synergistic interactions, most studies reported larger abundance (N=23; 74% of synergies) or recruitment (N=4; 15%) of species with commercial interest mediated by seagrass habitat provision and by the regulation of beneficial processes for performance of populations (N=4; 13%), such as water quality improvement or sediment stabilization.
Relationships Between Drivers of Changes, Seagrasses and Fishing Activities
Globally, in most of the locations where the studies were reported, seagrass habitats were subjected to a variety of fishing activities (36% mixed activities, Figure 5), as industrial and small scale commercial fisheries, including shellfisheries, or finfish aquaculture and recreational fisheries, in different combinations and proportions of targeted species groups (e.g. fish, molluscs and crustaceans). Commercial fisheries alone represented more than 20% of the activities analysed within the interactions with seagrasses. Although about half of the publications did not specify what type of commercial fishery was involved in the study, SSF were the most observed fishery (37%), followed by LSF (11%) and then a small proportion of both types. Aquaculture had a similar presence (16%) to commercial fisheries within the analysed publications (mainly through shellfish - namely oyster culture- and finfish aquaculture), where the main cultivated species were the Pacific oyster Crassostrea gigas, and the gilthead seabream, Sparus aurata and European seabass, Dicentrarchus labrax, respectively. The publications that described shellfisheries as the main activity (14%), mostly mentioned molluscs (without specification) as the target group, while recreational fishery (exclusively assessed in almost 5% of the publications) targeted both fish (25%) and molluscs (25%), with a large proportion of unspecific target species (50%). Similarly, near 10% of the analysed publications did not specify the type of fishery activity performed and were encompassed within other anthropogenic activities.
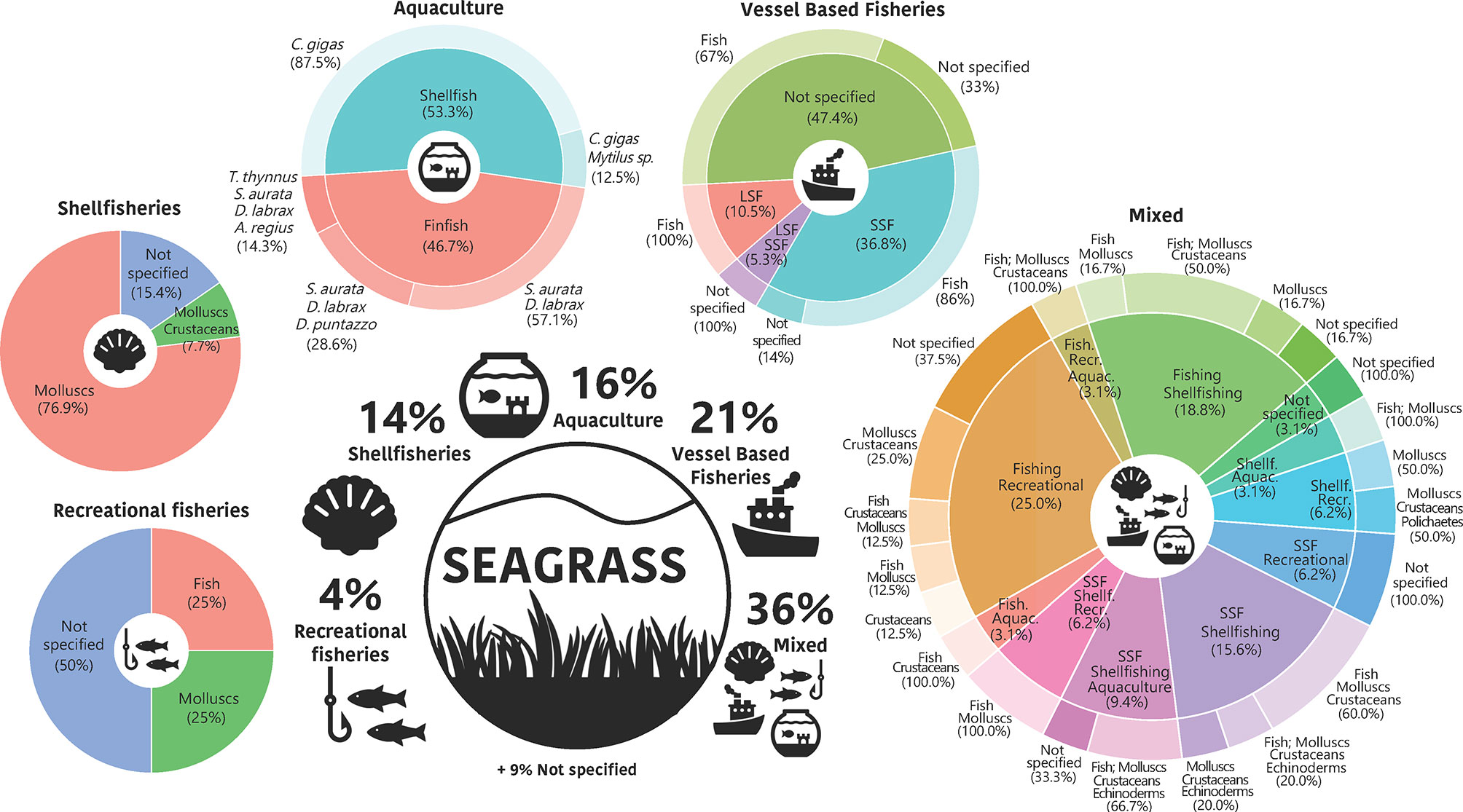
Figure 5 Main fisheries-related activities carried out on seagrass areas according to the literature synthesis. Fish, Vessel Based Fisheries; LSF, large-scale fisheries; SSF, small-scale fisheries; Aquac., Aquaculture; Shellf., Shellfisheries; Recr., Recreational fisheries; C. gigas, Crassostrea gigas (Thunberg, 1793); S. aurata, Sparus aurata (Linnaeus, 1758), D. labrax, Dicentrarchus labrax (Linnaeus, 1758); T. thynnus, Thunnus thynnus (Linnaeus, 1758); A. regius, Argyrosomus regius (Asso, 1801); D. puntazzo, Diplodus puntazzo (Walbaum, 1792).
Fishing Activities Management
Fisheries management system was not specified in most of the publications (54), while a few studies mentioned some undetailed type of management or regulation plan (12). Marine Protected Areas (MPAs) were the management system observed in 12 publications; Territorial Users Rights for Fishing (TURFs) was the management system described in 9 studies; the requirement of aquaculture license was indicated in 3 studies; Total Allowable Catches (TACs) were mentioned in 2 studies, and also two publications pointed out to a system management based on closed seasons. In the same way, many publications did not mention stakeholders (38) or only included some references to management effort (6), while government or administrations were mentioned in 38 studies, followed by scientists, fisheries sector, local communities and NGOs, with 11, 10, 9 and 6 mentions, respectively.
Discussion
Our systematic review shows a rapid growth of studies evaluating the relationships between seagrass ecosystems and fishing activities over the last decade. Trade-off interactions tripled the synergistic ones, driven by seafood market demand and conflicts of use in most cases. Globally, seagrass ecosystems are mainly affected by commercial fisheries of large and small scale, aquaculture and shellfisheries, and to a lesser extent, by recreational fisheries. These activities are enhanced as a result of the increased abundance and recruitment of commercially important species in this type of habitat.
Our results are consistent with the literature indicating that scientific interest in seagrasses has increased in the last decades (Fulton et al., 2020). It is likely that initiatives such as the Millennium Ecosystem Assessment published in 2005 have contributed to increase in awareness and interest on these habitats, as well as theoretical frameworks to better understand the dynamics of multiple ecosystem services (Bennett et al., 2009). Despite the benefits of seagrass ecosystems for human well-being, they had received less attention, in terms of media and scientific reports, than other coastal ecosystems extensively studied, such as coral reefs, salt marshes and mangrove forests (Duarte et al., 2008b; De la Torre-Castro et al., 2014). Seagrasses provide numerous valuable ecosystem services (Costanza et al., 1997) but, as in many other marine habitats, several studies have indicated high rates of global loss for seagrass ecosystems (Duarte, 2002; Orth et al., 2006; Duarte et al., 2008a; Waycott et al., 2009; Pendleton et al., 2012; De los Santos et al., 2019), exceeding those of tropical forests (Duarte et al., 2008b).
Our research indicates that in a relevant proportion, multiple fisheries activities occur on the same seagrass ecosystem (Figure 5, mixed activities), which is also consistent with Nordlund et al. (2018) results, who observed high similarity in seagrass fishery activity across several locations. Therefore, it seems that seagrass meadows tend to be exposed to several threats derived from fisheries and to other multiple anthropogenic threats, such as deterioration of water quality or coastal development (Duarte et al., 2008a; De los Santos et al., 2019). Overfishing is an important source of impact for seagrass, according to our analysis, which is in line with other studies stating that overfishing is linked to the main changes suffered in the structure of seagrass ecosystems (Baden et al., 2012; Alati et al., 2020). Increasing demand for seafood products supporting much of the 60% increase in per capita consumption of animal protein over the last decades (Béné et al., 2015), likely drives the impacts of overfishing in seagrass ecosystems.
Aquaculture alone represented 16% of the activities carried out in the areas where seagrass and fisheries interact, according to our literature synthesis. This aquaculture proportion does not take into account the cases where aquaculture occurred simultaneously with other activities such as recreational fishing, shellfishing or SSF, which would increase such proportion (Figure 5, mixed activities). From our literature review results, all the studies reported a negative impact of aquaculture practices, mainly emissions from fish cages but also by harvesting practices (e.g. Ruiz et al., 2001; Díaz-Almela et al., 2008; Kletou et al., 2018 Pérez et al., 2008), that ultimately led to degradation of seagrass meadows. Aquaculture has been considered as a potential sustainable solution for feeding the world’s population with products of marine origin. Currently, aquaculture generates around half (52%) of all fishery product production for human consumption (FAO, 2020). Through aquaculture it is possible to reduce not only the dependence of wild fisheries, but also the impacts of overfishing on marine ecosystems, however, aquaculture must be carried out sustainably in order to fulfill these expectations of beneficial effects (FAO, 2018). Suspended animal aquaculture usually generates organic enrichment via biodeposition (Dumbauld et al., 2009), transforming the sedimentary and biological structure of benthic communities into less diverse and poorer environments, a pattern that is shared with diverse anthropogenic sources (Pearson and Rosenberg, 1978). Generally, biodeposition from finfish farms and other anthropogenic sources is greater than those produced by shellfish farms (Pohle et al., 2001; Crawford et al., 2003), although benthic communities response will depend on the species cultured, the environment setting or the stock density (Dumbauld et al., 2009).
Our results suggest that after commercial fisheries and aquaculture, shellfisheries alone represent around 15% of the activities interacting with seagrass ecosystems. Several authors have described negative effects of clam harvesting (and its associated trampling) on seagrass mostly by changes in the species population structure through the reduction of shoot density and total seagrass biomass (Cabaço et al., 2005; Dumbauld et al., 2009; Barañano et al., 2017; Garmendia et al., 2017; De los Santos et al., 2019; Ferris et al., 2019). Mechanical damages by bivalves harvesting (namely clams) and other fisheries were among the main causes of trade-off interaction in our analysis. Other studies have also observed the same pattern regarding mechanical practices and stated that the harvest method and intensity cannot only influence the initial impact but also the recovery time of the meadow (Ferris et al., 2019). Manual methods of harvest are suggested as a source of lower disturbances, since they usually imply smaller harvested areas and result in faster recovery times (e.g. Cabaço et al., 2005).
The global research efforts of seagrass-fishery studies is biased toward temperate zones of the Northern hemisphere, mainly USA and Europe, despite the largest abundance and diversity of seagrass species found in tropical zones, especially in the Indo-Pacific region (Short et al., 2007). We found a mismatch between the research efforts and the hot-spot habitat distribution that most likely can be explained by the socioeconomic differences among regions (Nordlund et al., 2018). This is also supported by the fact that species of tropical distribution (Figure 4) were underrepresented in the analysed publications, where species from more developed temperate regions predominated (Short et al., 2007). Such an imbalance of research efforts usually translates into reduced funding priorities, conservation awareness, capacity building and management support in developing regions, where seagrass-based fisheries are likely to be of most importance. For example, we found that most of the synergies described in the scientific literature belong to studies developed through the coasts of Australia and the USA, where governments adopted spatial management strategies aimed at the protection of seagrass habitats. In fact, seagrass ecosystems are considered a priority for conservation in the USA and Europe and their protection is enforced (De los Santos et al., 2019; Muething et al., 2020).
Conservation of seagrass ecosystems should be a priority worldwide, as the multiple ecosystem services they offer are threatened under the increasing impacts and threats from climate change (Duarte et al., 2018), most likely through the loss of suitable habitats (Dolch and Reise, 2010; Dolch et al., 2013; Short et al., 2016; Ondiviela et al., 2020). Seagrasses are valuable ecosystems for mitigation of climate change given their capacity for carbon sequestration. In fact, their organic carbon stored per unit area is similar to that of forests (Fourqurean et al., 2012), and their ability to serve as carbon sinks has been estimated to account for up to $250 million USD in large seagrass ecosystems like the ones in Southeast Asia (Stankovic et al., 2021). However, the evidence suggests that such capacity diminishes with anthropogenic impacts (Duarte et al., 2013), which was a concern observed in our literature search when the terms “climate change” or “global warming” were included, as the vast majority of publications were related to the carbon sink function delivered by seagrasses. Even more, climate change will likely affect the magnitude and character of the interactions between seagrasses and anthropogenic activities like fisheries. For instance, in the West Coast of Australia, the heatwave caused by El Niño during the 2010-2011 summer, resulted in a massive collapse of a ~1300 km2 seagrass ecosystem. This caused not only the increase of carbon stock emissions during the six consecutive years, but also had a large impact on marine food webs, including fish communities, invertebrates, birds and marine mammals (Serrano et al., 2021). Additional anthropogenic pressure with physical disturbance on the sedimentary carbon stocks of seagrasses (like the one caused by fishery-related activities) can cause a decrease of up to 85% in the seagrass carbon stocks (Román et al., 2022). Therefore, the precautionary approach should be considered when designing management plans aimed at the use of the space where seagrasses and human activities overlap.
Seagrass ecosystems can remain functional as providers of fundamental ecosystem services in spite of a changing climate if other cumulative impacts do not interfere with their resilience capacity, which can only be achieved by effective fisheries management and conservation measures (Unsworth et al., 2015; Wallner-Hahn et al., 2016). However, the provision of some services may require determining a minimum threshold of habitat area below which the ecosystem fails to effectively deliver the service (De Groot et al., 2012). Effective communication from the scientific community is also essential to increase the awareness of the society and public administrations in order to ensure seagrass conservation (Duarte et al., 2008b).
It is important to highlight that socioeconomic studies were a minority in our literature review, which represents a critical knowledge gap in this research field. However, the scientific literature suggests that it is crucial to understand the relevance of seagrass in supporting the well-being of people, as impacts on seagrass ecosystems go beyond a resource decline, they can affect directly or indirectly human communities depending on coastal systems. Beyond the more obvious loss of fisheries support if a seagrass meadow is lost, a clear example of this connection can be made with tourism. For instance, in Green Island, Australia, tourists are attracted by the high density of turtles, which depend on seagrass for feeding; however, tourists do not necessarily establish the connection between healthy seagrass meadows and the presence of turtles (Cullen-Unsworth et al., 2014).
Insufficient reporting in some of the reviewed literature (ca. 10% of the studies), made it impossible to gather information on some of the variables we considered, such as the cause of the interaction and the type of fishery or the species involved; most likely because those aspects were not within the scope of the analysed studies. Nevertheless, based on the results of our literature review, we advocate for developing studies on this field to improve their reporting on the interactions between seagrass meadows and fisheries. More detailed information about the activities carried out in these habitats will improve our understanding to sustainably manage these interactions (De la Torre-Castro and Lindström, 2010; Wallner-Hahn et al., 2016; Ferris et al., 2019; Riera et al., 2020). In contrast to the well documented significance of coral reefs for fisheries, that of seagrass remains poorly understood. However, we document a fast increase in studies reporting fishery-seagrass interactions, which, if accompanied by improved reporting of the nature of the interactions and the socio-economic context of the fishery, would help improve the sustainable management of seagrass-associated fisheries.
Conclusions
The interest and awareness regarding the importance of seagrass ecosystems for human well-being appears to have rapidly increased in the past two decades. However, seagrasses are globally subjected to multiple threats and pressures from fisheries worldwide, as the studies reporting trade-off interactions almost tripled those reporting synergies between seagrass ecosystems and fisheries. The seafood market demand and the conflicts of use generated in coastal areas are the main drivers for such trade-offs. Results indicate that seagrass ecosystems are mainly affected by industrial and small-scale fisheries, but also by aquaculture and shellfisheries, and to a lesser extent, by recreational fisheries. In most cases, these activities have negative effects on seagrass ecosystems as they cause mechanical damage by their fishing gears or boat propellers, by overfishing itself, or by aquaculture cages’ emissions. Nonetheless, the scientific knowledge on synergistic interactions is improving, as most studies focused on this subject reported a larger abundance or recruitment of species with commercial interest mediated by seagrass habitat provision.
Most of the research effort has been allocated to ecological studies in developed countries with an underrepresentation of studies in tropical regions, which represents a mismatch and a knowledge gap precisely in the regions where seagrass ecosystems are more abundant. Similarly, another relevant gap identified in our review is the lack of studies with an in-depth socioeconomic scope, since even when socioeconomic variables were considered in a study, the ecological approach was dominant. A third gap was identified as the fisheries management system was not specified in many of the reviewed publications, which represents a key knowledge gap with implications for sustainable management. Therefore, we advocate for an improvement in the reporting of future studies regarding fishery-seagrass interactions, as key interdisciplinary studies would help improve the sustainable management of both seagrasses and fisheries.
Data Availability Statement
The original contributions presented in the study are included in the article/Supplementary Material. Further inquiries can be directed to the corresponding author.
Author Contributions
All authors equally contributed to the manuscript.
Funding
This research was supported by the Spanish Ministerio de Ciencia e Innovación/Agencia Estatal de Investigación (10.13039/501100011033) for the ZEUS project (grant RTI2018-095583-B-I00) and by the Autonomous government Xunta de Galicia-FEDER (project ED431C 2021/42): “ERDF A way of making Europe”. SV thanks the Consellería de Educación da Xunta de Galicia (Galicia, Spain) for funding support Grupo de Referencia Competitiva GI-2060 AEMI, under grant ED431C2019/11.
Conflict of Interest
The authors declare that the research was conducted in the absence of any commercial or financial relationships that could be construed as a potential conflict of interest.
Publisher’s Note
All claims expressed in this article are solely those of the authors and do not necessarily represent those of their affiliated organizations, or those of the publisher, the editors and the reviewers. Any product that may be evaluated in this article, or claim that may be made by its manufacturer, is not guaranteed or endorsed by the publisher.
Supplementary Material
The Supplementary Material for this article can be found online at: https://www.frontiersin.org/articles/10.3389/fmars.2022.781713/full#supplementary-material
References
Alati V. M., Olunga J., Olendo M., Daudi L. N., Osuka K., Odoli C., et al. (2020). Mollusc Shell Fisheries in Coastal Kenya: Local Ecological Knowledge Reveals Overfishing. Ocean Coast. Manag 195, 105285. doi: 10.1016/j.ocecoaman.2020.105285
Arias-Ortiz A., Serrano O., Masqué P., Lavery P. S., Mueller U., Kendrick G. A., et al. (2018). A Marine Heatwave Drives Massive Losses From the World’s Largest Seagrass Carbon Stocks. Nat. Clim. Change 8 (4), 338–344. doi: 10.1038/s41558-018-0096-y
Baden S., Emanuelsson A., Pihl L., Svensson C.-J., Åberg P. (2012). Shift in Seagrass Food Web Structure Over Decades is Linked to Overfishing. Mar. Ecol. Prog. Ser. 451, 61–73. doi: 10.3354/meps09585
Barañano C., Fernández E., Méndez G., Troncoso J. S. (2017). Resilience of Zostera Marina Habitats and Response of the Macroinvertebrate Community to Physical Disturbance Caused by Clam Harvesting. Mar. Biol. Res. 13, 955–966. doi: 10.1080/17451000.2017.1307989
Béné C., Barange M., Subasinghe R., Pinstrup-Andersen P., Merino G., Hemre G. I., et al. (2015). Feeding 9 Billion by 2050 – Putting Fish Back on the Menu. Food Secur. 7, 261–274. doi: 10.1007/s12571-015-0427-z
Bennett E. M., Peterson G. D., Gordon L. J. (2009). Understanding Relationships Among Multiple Ecosystem Services. Ecol. Lett. 12, 1394–1404. doi: 10.1111/j.1461-0248.2009.01387.x
Boudouresque C. F., Pergent G., Pergent-Martini C., Ruitton S., Thibaut T., Verlaque M. (2016). The Necromass of the Posidonia Oceanica Seagrass Meadow: Fate, Role, Ecosystem Services and Vulnerability. Hydrobiologia 781, 25–42. doi: 10.1007/s10750-015-2333-y
Cabaço S., Alexandre A., Santos R. (2005). Population-Level Effects of Clam Harvesting on the Seagrass Zostera Noltii. Mar. Ecol. Prog. Ser. 298, 123–129. doi: 10.3354/meps298123
Costanza R., d’Arge R., de Groot R., Farber S., Grasso M., Hannon B., et al. (1997). The Value of the World’s Ecosystem Services and Natural Capital. Nature 387, 253–260. doi: 10.1038/387253a0
Crawford C. M., Macleod C. K. A., Mitchell I. M. (2003). Effects of Shellfish Farming on the Benthic Environment. Aquaculture 224, 117–140. doi: 10.1016/S0044-8486(03)00210-2
Cullen-Unsworth L. C., Nordlund L. M., Paddock J., Baker S., McKenzie L. J., Unsworth R. K. F. (2014). Seagrass Meadows Globally as a Coupled Social–Ecological System: Implications for Human Wellbeing. Mar. Pollut. Bull. 83, 387–397. doi: 10.1016/j.marpolbul.2013.06.001
Cullen-Unsworth L. C., Unsworth R. K. F. (2013). Seagrass Meadows, Ecosystem Services, and Sustainability. Environ. Sci. Policy Sustain. Dev. 55, 14–28. doi: 10.1080/00139157.2013.785864
De Groot R., Brander L., van der Ploeg S., Costanza R., Bernard F., Braat L., et al. (2012). Global Estimates of the Value of Ecosystems and Their Services in Monetary Units. Ecosyst. Serv. 1, 50–61. doi: 10.1016/j.ecoser.2012.07.005
De la Torre-Castro M. (2006). Humans and Seagrasses in East Africa – A Social-Ecological Systems Approach (Stockholm, Sweden: Department of Systems Ecology, Stockholm University). Doctoral dissertation.
De la Torre-Castro M., Di Carlo G., Jiddawi N. S. (2014). Seagrass Importance for a Small-Scale Fishery in the Tropics: The Need for Seascape Management. Mar. Pollut. Bull. 83, 398–407. doi: 10.1016/j.marpolbul.2014.03.034
De la Torre-Castro M., Lindström L. (2010). Fishing Institutions: Addressing Regulative, Normative and Cultural-Cognitive Elements to Enhance Fisheries Management. Mar. Policy 34, 77–84. doi: 10.1016/j.marpol.2009.04.012
De los Santos C., Krause-Jensen D., Alcoverro T., Marbà N., Duarte C. M., van Katwijk M. M., et al. (2019). Recent Trend Reversal for Declining European Seagrass Meadows. Nat. Commun. 10, 3356. doi: 10.1038/s41467-019-11340-4
Díaz-Almela E., Marbà N., Álvarez E., Santiago R., Holmer M., Grau A., et al. (2008). Benthic Input Rates Predict Seagrass (Posidonia Oceanica) Fish Farm-Induced Decline. Mar. Pollut. Bull. 56, 1332–1342. doi: 10.1016/j.marpolbul.2008.03.022
Dolch T., Buschbaum C., Reise K. (2013). Persisting Intertidal Seagrass Beds in the Northern Wadden Sea Since the 1930s. J. Sea Res. 82, 134–141. doi: 10.1016/j.seares.2012.04.007
Dolch T., Reise K. (2010). Long-Term Displacement of Intertidal Seagrass and Mussel Beds by Expanding Large Sandy Bedforms in the Northern Wadden Sea. J. Sea Res. 63, 93–101. doi: 10.1016/j.seares.2009.10.004
Duarte C. M. (2002). The Future of Seagrass Meadow. Environ. Conserv. 29, 192–206. doi: 10.1017/S0376892902000127
Duarte C. M., Borum J., Short F. T., Walker D. I. (2008a). Seagrass Ecosystems: Their Global Status and Prospects, In: Aquatic Ecosystems: Trends and Global Prospects, Cambridge University Press 281–294. doi: 10.1017/CBO9780511751790.025
Duarte C. M., Dennison W. C., Orth R. J., Carruthers T. J. B. (2008b). The Charisma of Coastal Ecosystems: Addressing the Imbalance. Estuaries Coasts J. CERF 31, 233–238. doi: 10.1007/s12237-008-9038-7
Duarte C. M., Losada I. J., Hendriks I. E., Mazarrasa I., Marbà N. (2013). The Role of Coastal Plant Communities for Climate Change Mitigation and Adaptation. Nat. Clim. Change 3, 961–968. doi: 10.1038/nclimate1970
Duarte B., Martins I., Rosa R., Matos A. R., Roleda M. Y., Reusch T. B. H., et al. (2018). Climate Change Impacts on Seagrass Meadows and Macroalgal Forests: An Integrative Perspective on Acclimation and Adaptation Potential. Front. Mar. Sci. 5. doi: 10.3389/fmars.2018.00190
Dumbauld B. R., Ruesink J. L., Rumrill S. S. (2009). The Ecological Role of Bivalve Shellfish Aquaculture in the Estuarine Environment: A Review With Application to Oyster and Clam Culture in West Coast (USA) Estuaries. Aquaculture 290, 196–223. doi: 10.1016/j.aquaculture.2009.02.033
Fales R. J., Boardman F. C., Ruesink J. L. (2020). Reciprocal Interactions Between Bivalve Molluscs and Seagrass: A Review and Meta-Analysis. J. Shellfish Res. 39, 547–562. doi: 10.2983/035.039.0305
FAO (2018). “The State of World Fisheries and Aquaculture 2018,” in Meeting the Sustainable Development Goals (Rome: FAO).
FAO (2020). “The State of World Fisheries and Aquaculture 2020,” in Sustainability in Action, (Rome: FAO). doi: 10.4060/ca9229en
Ferris B. E., Conway-Cranos L. L., Sanderson B. L., Hoberecht L. (2019). Bivalve Aquaculture and Eelgrass: A Global Meta-Analysis. Aquaculture 498, 254–262. doi: 10.1016/j.aquaculture.2018.08.046
Fourqurean J. W., Duarte C. M., Kennedy H., Marbà N., Holmer M., Mateo M. A., et al. (2012). Seagrass Ecosystems as a Globally Significant Carbon Stock. Nat. Geosci. 5, 505–509. doi: 10.1038/ngeo1477
Fulton C. J., Berkström C., Wilson S. K., Abesamis R. A., Bradley M., Åkerlund C., et al. (2020). Macroalgal Meadow Habitats Support Fish and Fisheries in Diverse Tropical Seascapes. Fish Fish. 21, 700–717. doi: 10.1111/faf.12455
Gagnon K., Rinde E., Bengil E. G. T., Carugati L., Christianen M. J. A., Danovaro R., et al. (2020). Facilitating Foundation Species: The Potential for Plant–Bivalve Interactions to Improve Habitat Restoration Success. J. Appl. Ecol. 57, 1161–1179. doi: 10.1111/1365-2664.13605
Garcia Rodrigues J., Conides A. J., Rivero Rodriguez S., Raicevich S., Pita P., Kleisner K. M., et al. (2017). Marine and Coastal Cultural Ecosystem Services: Knowledge Gaps and Research Priorities. One Ecosyst. 2, e12290. doi: 10.3897/oneeco.2.e12290
Garmendia J. M., Valle M., Borja A., Chust G., Lee D.-J., Rodríguez J. G., et al. (2017). Effect of Trampling and Digging From Shellfishing on Zostera Noltei (Zosteraceae) Intertidal Seagrass Beds. Sci. Mar. 81, 121–128. doi: 10.3989/scimar.04482.17A
Gilby B. L., Olds A. D., Connolly R. M., Maxwell P. S., Henderson C. J., Schlacher T. A. (2018). Seagrass Meadows Shape Fish Assemblages Across Estuarine Seascapes. Mar. Ecol. Prog. Ser. 588, 179–189. doi: 10.3354/meps12394
Kletou D., Kleitou P., Savva I., Attrill M. J., Antoniou C., Hall-Spencer J. M. (2018). Seagrass Recovery After Fish Farm Relocation in the Eastern Mediterranean. Mar. Environ. Res. 140, 221–233. doi: 10.1016/j.marenvres.2018.06.007
Lamb J. B., van de Water J. A. J. M., Bourne D. G., Altier C., Hein M. Y., Fiorenza E. A., et al. (2017). Seagrass Ecosystems Reduce Exposure to Bacterial Pathogens of Humans, Fishes, and Invertebrates. Science 355, 731–733. doi: 10.1126/science.aal1956
Lilley R. J., Unsworth R. K. F. (2014). Atlantic Cod (Gadus Morhua) Benefits From the Availability of Seagrass (Zostera Marina) Nursery Habitat. Global Ecol. Conserv. 2, 367–377. doi: 10.1016/j.gecco.2014.10.002
McKenzie L. J., Nordlund L. M., Jones B. L., Cullen-Unsworth L. C., Roelfsema C., Unsworth R. K. F. (2020). The Global Distribution of Seagrass Meadows. Environ. Res. Lett. 15, 074041. doi: 10.1088/1748-9326/ab7d06
Millennium Ecosystem Assessment (MEA) (2005). Ecosystems and Human Well-Being: Synthesis (Washington, DC: Island Press).
Moher D., Liberati A., Tetzlaff J., Altman D. G., The Prisma Group (2009). Preferred Reporting Items for Systematic Reviews and Meta-Analyses: The PRISMA Statement. PloS Med. 6, e1000097. doi: 10.1371/journal.pmed.1000097
Muething K. A., Tomas F., Waldbusser G., Dumbauld B. R. (2020). On the Edge: Assessing Fish Habitat Use Across the Boundary Between Pacific Oyster Aquaculture and Eelgrass in Willapa Bay, Washington, USA. Aquac. Environ. Interact. 12, 541–557. doi: 10.3354/aei00381
Nordlund L. M., Koch E. W., Barbier E. B., Creed J. C. (2016). Seagrass Ecosystem Services and Their Variability Across Genera and Geographical Regions. PloS One 12, e0169942. doi: 10.1371/journal.pone.0169942
Nordlund L. M., Unsworth R. K. F., Gullström M., Cullen-Unsworth L. C. (2018). Global Significance of Seagrass Fishery Activity. Fish Fish. 19, 399–412. doi: 10.1111/faf.12259
Ondiviela B., Galván C., Recio M., Jiménez M., Juanes J. A., Puente A., et al. (2020). Vulnerability of Zostera Noltei to Sea Level Rise: The Use of Clustering Techniques in Climate Change Studies. Estuaries Coasts 43, 2063–2075. doi: 10.1007/s12237-020-00742-z
Ondiviela B., Losada I. J., Lara J. L., Maza M., Galván C., Bouma T. J., et al. (2014). The Role of Seagrasses in Coastal Protection in a Changing Climate. Coast. Eng. 87, 158–168. doi: 10.1016/j.coastaleng.2013.11.005
Orth R. J., Carruthers T. J., Dennison W. C., Duarte C. M., Fourqurean J. W., Heck K. L., et al. (2006). A Global Crisis for Seagrass Ecosystems. BioScience 56, 987–996. doi: 10.1641/0006-568(2006)56[987:AGCFSE]2.0.CO;2
Orth R. J., Lefcheck J. S., McGlathery K. S., Aoki L., Luckenbach M. W., Moore K. A., et al. (2020). Restoration of Seagrass Habitat Leads to Rapid Recovery of Coastal Ecosystem Services. Sci. Adv. 6, eabc6434. doi: 10.1126/sciadv.abc6434
Park S. R., Kim Y. K., Kim J. H., Kang C. K., Lee K. S. (2011). Rapid Recovery of the Intertidal Seagrass Zostera Japonica Following Intense Manila Clam (Ruditapes Philippinarum) Harvesting Activity in Korea. J. Exp. Mar. Biol. Ecol. 407, 275–283. doi: 10.1016/j.jembe.2011.06.023
Pearson T. H., Rosenberg R. (1978). Macrobenthic Succession in Relation to Organic Enrichment and Pollution of the Marine Environment. Annu. Rev. Ocean. Mar. Biol. 16, 229–311.
Pendleton L., Donato D. C., Murray B. C., Crooks S., Jenkins W. A., Sifleet S., et al. (2012). Estimating Global “Blue Carbon” Emissions From Conversion and Degradation of Vegetated Coastal Ecosystems. PloS One 7, e43542. doi: 10.1371/journal.pone.0043542
Pérez M., García T., Invers O., Ruiz J. M. (2008). Physiological Responses of the Seagrass Posidonia Oceanica as Indicators of Fish Farm Impact. Mar. Pollut. Bull. 56, 869–879. doi: 10.1016/j.marpolbul.2008.02.001
Pitanga M. E., Montes M. J. F., Magalhães K. M., Reis T. N. V. (2012). Quantification and Classification of the Main Environmental Impacts on a Halodule Wrightii Seagrass Meadow on a Tropical Island in Northeastern Brazil. Ann. Braz. Acad. Sci. 84, 35–42. doi: 10.1590/S0001-37652012000100005
Pohle G., Frost B., Findlay R. (2001). Assessment of Regional Benthic Impact of Salmon Mariculture Within the Letang Inlet, Bay of Fundy. ICES J. Mar. Sci. 58, 417–426. doi: 10.1006/jmsc.2000.1039
Riera R., Delgado J. D., Moro L., Herrera R., Becerro M. A. (2020). Difficulties to Identify Global and Local Key Biodiversity Areas in Diverse and Isolated Marine Jurisdictions. J. Coast. Conserv. 24, 13. doi: 10.1007/s11852-020-00732-0
Rodríguez J. P., Beard T., Bennett E. M., Cumming G. S., Cork S. J., Agard J., et al. (2006). Trade-Offs Across Space, Time, and Ecosystem Services. Ecol. Soc. 11, 28. doi: 10.5751/ES-01667-110128
Román M., de los Santos C., Román S., Santos R., Troncoso J. S., Vázquez E., Olabarria C.. (2022). Loss of Surficial Sedimentary Carbon Stocks in Seagrass Meadows Subjected to Intensive Clam Harvesting. Mar. Environ. Res. 175, 105570. doi: 10.1016/j.marenvres.2022.105570
Ruiz-Frau A., Gelcich S., Hendriks I. E., Duarte C. M., Marbà N. (2017). Current State of Seagrass Ecosystem Services: Research and Policy Integration. Ocean Coast. Manage. 149, 107–115. doi: 10.1016/j.ocecoaman.2017.10.004
Ruiz J. M., Pérez M., Romero J. (2001). Effects of Fish Farm Loadings on Seagrass (Posidonia Oceanica) Distribution, Growth and Photosynthesis. Mar. Pollut. Bull. 42, 749–760. doi: 10.1016/S0025-326X(00)00215-0
Serrano O., Arias-Ortiz A., Duarte C. M., Kendrick G. A., Lavery P. S. (2021). “Impact of Marine Heatwaves on Seagrass Ecosystems,” in Ecosystem Collapse and Climate Change (Cham: Springer), 345–364. doi: 10.1007/978-3-030-71330-0_13
Serrano O., Martínez-Cortizas A., Mateo M. A., Biester H., Bindler R. (2013). Millennial Scale Impact on the Marine Biogeochemical Cycle of Mercury From Early Mining on the Iberian Peninsula. Global Biogeochem. Cycles 27, 21–30. doi: 10.1029/2012GB004296
Short F. T., Carruthers T., Dennison W., Waycott M. (2007). Global Seagrass Distribution and Diversity: A Bioregional Model. J. Exp. Mar. Biol. Ecol. 350, 3–20. doi: 10.1016/j.jembe.2007.06.012
Short F. T., Kosten S., Morgan P. A., Malone S., Moore G. E. (2016). Impacts of Climate Change on Submerged and Emergent Wetland Plants. Aquat. Bot. 135, 3–17. doi: 10.1016/j.aquabot.2016.06.006
Sinclair E. A., Sherman C. D., Statton J., Copeland C., Matthews A., Waycott M., et al. (2021). Advances in Approaches to Seagrass Restoration in Australia. Ecol. Manage. Restor. 22, 10–21. doi: 10.1111/emr.12452
Stankovic M., Ambo-Rappe R., Carly F., Dangan-Galon F., Fortes M. D., Hossain M. S., et al. (2021). Quantification of Blue Carbon in Seagrass Ecosystems of Southeast Asia and Their Potential for Climate Change Mitigation. Sci. Total Environ. 783, 146858. doi: 10.1016/j.scitotenv.2021.146858
UNEP-WCMC, Short F. T. (2021). “Global Distribution of Seagrasses (Version 7.1),” in Seventh Update to the Data Layer Used in Green and Short, (2003) (Cambridge (UK: UN Environment World Conservation Monitoring Centre). doi: 10.34892/x6r3-d211
Unsworth R. K. F., Collier C. J., Waycott M., Mckenzie L. J., Cullen-Unsworth L. C. (2015). A Framework for the Resilience of Seagrass Ecosystems. Mar. Pollut. Bull. 100, 34–46. doi: 10.1016/j.marpolbul.2015.08.016
Unsworth R. K. F., Cullen L. C. (2010). Recognising the Necessity for Indo-Pacific Seagrass Conservation. Conserv. Lett. 3, 63–73. doi: 10.1111/j.1755-263X.2010.00101.x
Unsworth R. K. F., Nordlund L. M., Cullen-Unsworth L. C. (2018). Seagrass Meadows Support Global Fisheries Production. Conserv. Lett. 12, e12566. doi: 10.1111/conl.12566
Wallner-Hahn S., Molander F., Gallardo G., Villasante S., Eklöf J. S., Jiddawi N. S., et al. (2016). Destructive Gear Use in a Tropical Fishery: Institutional Factors Influencing the Willingness-and Capacity to Change. Mar. Policy 72, 199–2010. doi: 10.1016/j.marpol.2016.07.001
Keywords: seagrass, fisheries, fishing, shellfishing, aquaculture, trade-offs, synergies, global review
Citation: Herrera M, Tubío A, Pita P, Vázquez E, Olabarria C, Duarte CM and Villasante S (2022) Trade-Offs and Synergies Between Seagrass Ecosystems and Fishing Activities: A Global Literature Review. Front. Mar. Sci. 9:781713. doi: 10.3389/fmars.2022.781713
Received: 23 September 2021; Accepted: 17 March 2022;
Published: 22 April 2022.
Edited by:
Manuel J. Zetina-Rejón, Instituto Politécnico Nacional (IPN), MexicoReviewed by:
Maricela de la Torre-Castro, Stockholm University, SwedenMichael Njoroge Githaiga, University of Embu, Kenya
Copyright © 2022 Herrera, Tubío, Pita, Vázquez, Olabarria, Duarte and Villasante. This is an open-access article distributed under the terms of the Creative Commons Attribution License (CC BY). The use, distribution or reproduction in other forums is permitted, provided the original author(s) and the copyright owner(s) are credited and that the original publication in this journal is cited, in accordance with accepted academic practice. No use, distribution or reproduction is permitted which does not comply with these terms.
*Correspondence: Mariana Herrera, bWhlcnJlcmEuYmlvbG9nQGdtYWlsLmNvbQ==