- Laboratório de Ecologia Bentônica, CIENAM and Instituto de Biologia and INCT Estudos Interdisciplinares e Transdisciplinares em Ecologia e Evolução, Universidade Federal da Bahia, Salvador, Brazil
Function in ecology can be understood as the role that each component plays in the surrounding environment. It can be studied through the functional traits of organisms and depends on variations of abundance in time and space. Nevertheless, traits should be clearly associated with functions. The functions performed by estuarine macrofauna along estuarine gradients and its variation in time are scarcely studied. We expected that the functional structure (i.e., the set of functions in a site) would not change significantly over estuarine gradients, even with changes in taxonomic composition, since different taxa may have similar traits, allowing the performance of the same functions. We used polychaete assemblages along three tropical estuaries sampled four different times, to test for differences in functional intensity between estuarine salinity zones (Venice system). From a literature search we selected the most frequent ecological functions performed by estuarine benthic assemblages and we explicitly established which polychaete functional traits, or combinations of traits, were directly related to these functions. Nutrient cycling, bioturbation and fragmentation of organic matter were the most frequent functions. We discovered that the last two were present throughout the entire salinity gradient (i.e., along different salinity zones) but with different intensities. The intensity of functions may also show significant variability in time. Nutrient cycling and fragmentation of organic matter showed strong variation among estuarine zones. Using traits explicitly associated with ecological functions is necessary to investigate function and function intensity. Future studies should investigate how precisely traits may alter specific environmental characteristics and ecosystem properties.
Introduction
Function in ecology can be understood as the role that each component plays in the surrounding environment (i.e., control the fluxes of energy, nutrients, and organic matter) (Calow, 1987; Jax, 2005; Bellwood et al., 2019). But, in the ecological literature, the term is used in different ways depending on the scale (local or regional) and/or the object (individual, population, or ecosystem) (Nunes-Neto et al., 2013; Mlambo, 2014; Queirós et al., 2015; Clare et al., 2016; Luiza-Andrade et al., 2017; Drylie et al., 2020). At the individual level, function is the role that an organism plays in the environment, and at the ecosystem level it refers to the combined effects of all natural processes that sustain an ecosystem and can result in global processes and ecosystem services (Paterson et al., 2012; Snelgrove et al., 2014; Degen et al., 2018).
Determining the roles that organisms play is important to identify key species and understand ecosystem functioning (Bremner, 2008). One way to access the ecological functions performed by species is to classify them into functional groups according to a set of characteristics, i.e., traits, that respond to certain environmental conditions (Díaz and Cabido, 2001). These functional traits, for instance, may describe the way of life, feeding mode and reproduction strategies, which are associated with intrinsic biological characteristics as well as with activities that organisms perform in the ecosystem (Violle et al., 2007; de Bello et al., 2010).
The distribution of functional traits in a community can be a better indicator of ecosystem functioning than assemblage structure, including species abundance, richness and other metrics (e.g., Bolam et al., 2002). For instance, different species can have similar traits, thus performing similar functions. Therefore, functional redundancy may occur when in the absence of one taxon, another (with a similar set of traits) performs the same function (Walker, 1995; Magalhães and Barros, 2011). On the other hand, species with different traits will perform different functions resulting in complementarity and in an increase of functional diversity (Loreau et al., 2001; Hillebrand and Matthiessen, 2009).
It is well known that benthic macroinvertebrates are very important for estuarine and marine habitats and that polychaetes are frequently an abundant and diverse group (Hutchings, 1998; Magalhães and Barros, 2011; Barros et al., 2012). Due to their large morphological variability, they have an array of traits, including different feeding modes, movement capabilities and reproduction types. Through different trait combinations, polychaetes can perform several functions such as sediment stabilization, sediment oxygenation and transport of dissolved material from water to sediment (Braeckman et al., 2010; Wong and Dowd, 2015; Wrede et al., 2018; Bhowmik and Mandal, 2021). For example, the ability to burrow the sediment and construct tubes facilitates the penetration of water and oxygen, therefore increasing oxygenation (i.e., aeration function) of the sediment (Aller, 1988; Shull et al., 2009; Laverock et al., 2011; Kristensen et al., 2012; Queirós et al., 2015; Murphy and Reidenbach, 2016; Wrede et al., 2017). However, how these functions are distributed in space and time depends in part on how species, and thus, their functional traits, respond to environmental variations.
It is well known that estuaries are strong environmental gradients that are subject to great variability in their environmental conditions (e.g., salinity, grain size, and organic matter content) due to the mixing of freshwater and seawater (McLusky and Elliott, 2004). Different taxa interact with the physical and chemical characteristics of the environment, thus environmental variables can act as filters (e.g., Egres et al., 2019), selecting taxa with sets of traits that will occupy different estuarine regions. From this perspective, the environment is a selective force and filter, excluding species that are unable to tolerate certain conditions at a particular location (Kraft et al., 2015). Therefore, environmental variability plays an important role structuring estuarine benthic macroinvertebrate communities (Alves et al., 2020) and selecting traits (e.g., Morais et al., 2019).
The spatial patterns of benthic assemblages in estuaries has been described by several studies. For instance, lower estuarine regions have a greater number of taxa (Ysebaert et al., 2003; Fujii, 2007; Barros et al., 2012, 2014; Alves et al., 2020) in part due to the fact that most species found in estuaries have a marine origin. Thus, sites closer to the marine waters are richer than sites closer to freshwater, as fewer estuarine species can arrive and/or survive in lower salinity (Attrill and Rundle, 2002). It has been suggested that changes of species along the salinity gradient can result in modifications in ecosystem functioning (Oug et al., 2012; van der Linden et al., 2016). However, most functional studies are focused on the effects of environmental impacts on traits (Bremner et al., 2003, 2006a,b; Paganelli et al., 2012) and do not explore how these changes explicitly translate into different functions, or function intensities. Thus, the knowledge on how functions of the benthic community are distributed along estuaries and how it varies in time (e.g., weeks, months, seasons, or years) is still limited (Darr et al., 2014; van der Linden et al., 2016, 2017).
There are a variety of ways to access the ecological function (Petchey and Gaston, 2002; Podani and Schmera, 2006; Villéger et al., 2008; Pla et al., 2012). However, they often consider ecological function as an emerging property of the community, and the information on which taxa or trait play an important role for a given function is frequently overlooked (Teal et al., 2009, 2010; Sturdivant et al., 2012; Queirós et al., 2013, 2015). Many studies use species traits that are not linked to any function (Mlambo, 2014) and this can lead to wrong conclusions about the functioning and the fate of a given estuarine area. For example, the selection of excessive redundant traits can overestimate functional diversity. On the other hand, if an essential trait for a given function is not considered, this can lead to an underestimation of this function.
The aim of the present study was to investigate the distribution pattern of ecological functions over space and time in estuarine environments, through the use of combinations of specific functional traits. For this, we first: (i) defined combinations of functional traits explicitly related to ecological functions in estuaries; (ii) established the intensity of the functions performed by polychaetes; and (iii) tested for potential differences in the intensity of ecological functions in different salinity zones (following the Venice System). Our expectation was that the functional structure would not significantly change along the estuarine gradient (i.e., salinity zones). This expectation assumes that some subsets of taxa are spread along the estuarine gradients (Alves et al., 2020) and will contribute to the function maintenance.
Materials and Methods
Description of the Ecological Functions and Functional Traits Associated
First, we reviewed the literature to identify which were the most important ecological functions for estuarine ecosystems and the sets of functional traits associated. The search was made in ISI Web of Knowledge database using the string: [(ecosyst* OR ecolog* OR estuar*) AND (func* OR trait*)] AND (estuar* OR environm* OR marine OR salinity OR granulometry OR sediment) AND (gradient OR variation) AND (benth*macro* OR macrobenth* OR macrozoobenth* OR macroinvert*). The search, in January of 2019, resulted in 535 articles. From this total, 99 were selected after screening according to the inclusion (studies that used benthic macroinvertebrates including polychaetes or molluscs or crustaceans and marine or estuarine ecosystems) and exclusion criteria (studies carried out in polar regions, lakes, including zooplankton, phytoplankton and studies that did not investigate ecological functions and did not use traits) (for more details see Supplementary Appendix A; Table A.1). We focused on polychaetes due to their abundance, functional importance in estuaries and also due to the availability of information on their traits.
We observed that five functions performed by estuarine benthic assemblages, were extensively reported by the literature: bioturbation, secondary production, sediment stabilization, nutrient cycling and organic matter fragmentation (Table 1). To soundly establish which functional traits of polychaetes have an effect on the estuarine environment, we carefully described, based on our review, how each function is linked to each specific trait, and also how the trait affected the estuarine environment (Table 1 and see Appendix A.1 for reference listed). Through the revised literature we assume that each trait, or combination of traits, does not perform the function with the same intensity. For example, a polychaete that is tubicolous, very motile and large performs more bioturbation than a polychaete that is tubicolous, sedentary and small. Therefore, we assigned scores based on the affinity of each trait, or subsets of traits, with a given function, and a maximum score was attributed to the combination which best performed each function (see details in Table 2). For instance, a polychaete that is tubicolous, motile, with a large body, that feeds subsurface deposit and is infaunal received a higher score, while a minimum score was given to polychaetes that were only tubicolous (Table 2).
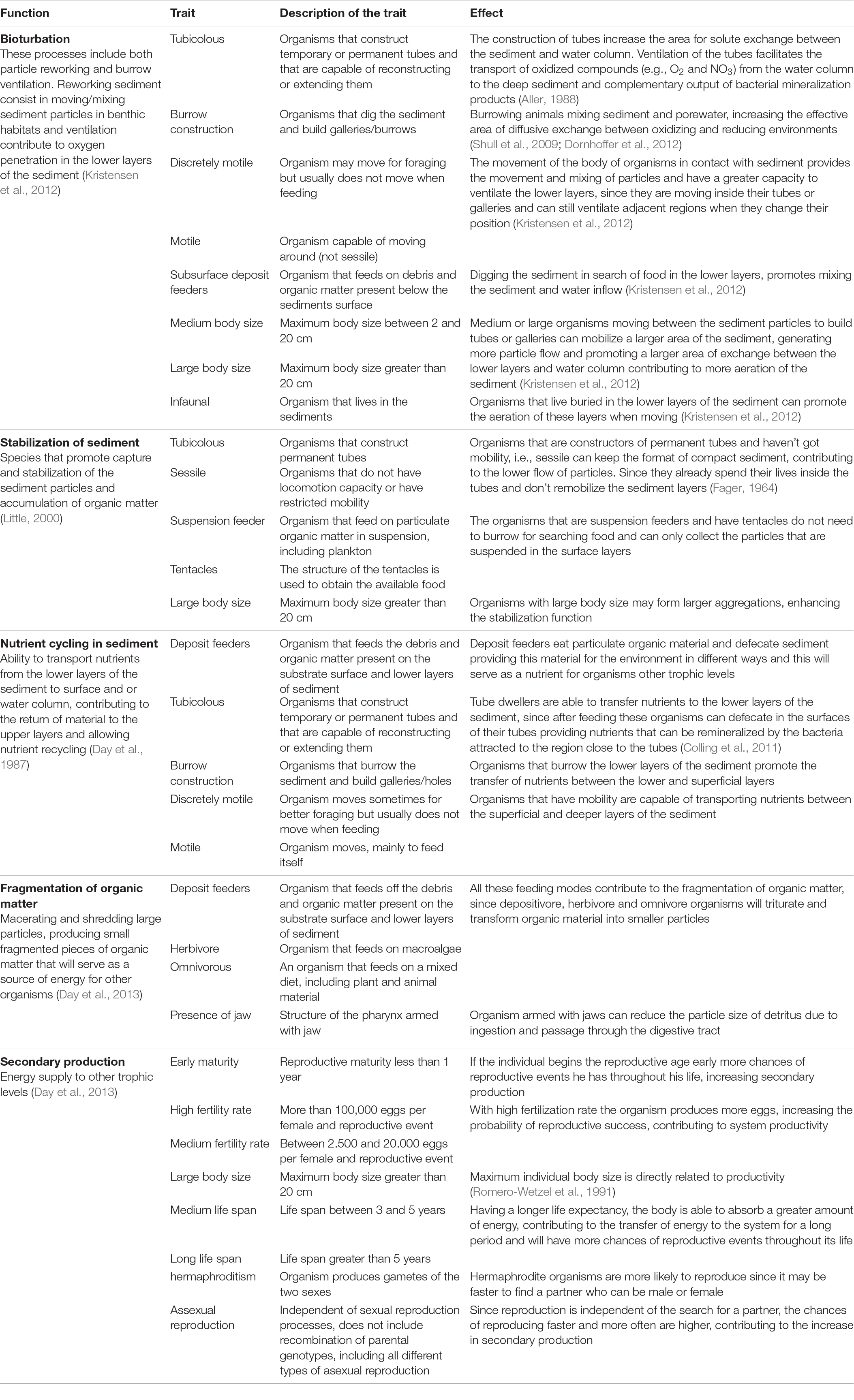
Table 1. Description of each ecological function used in this study, linked with specific polychaetes functional traits, and description of its effect on the estuarine ecosystem.
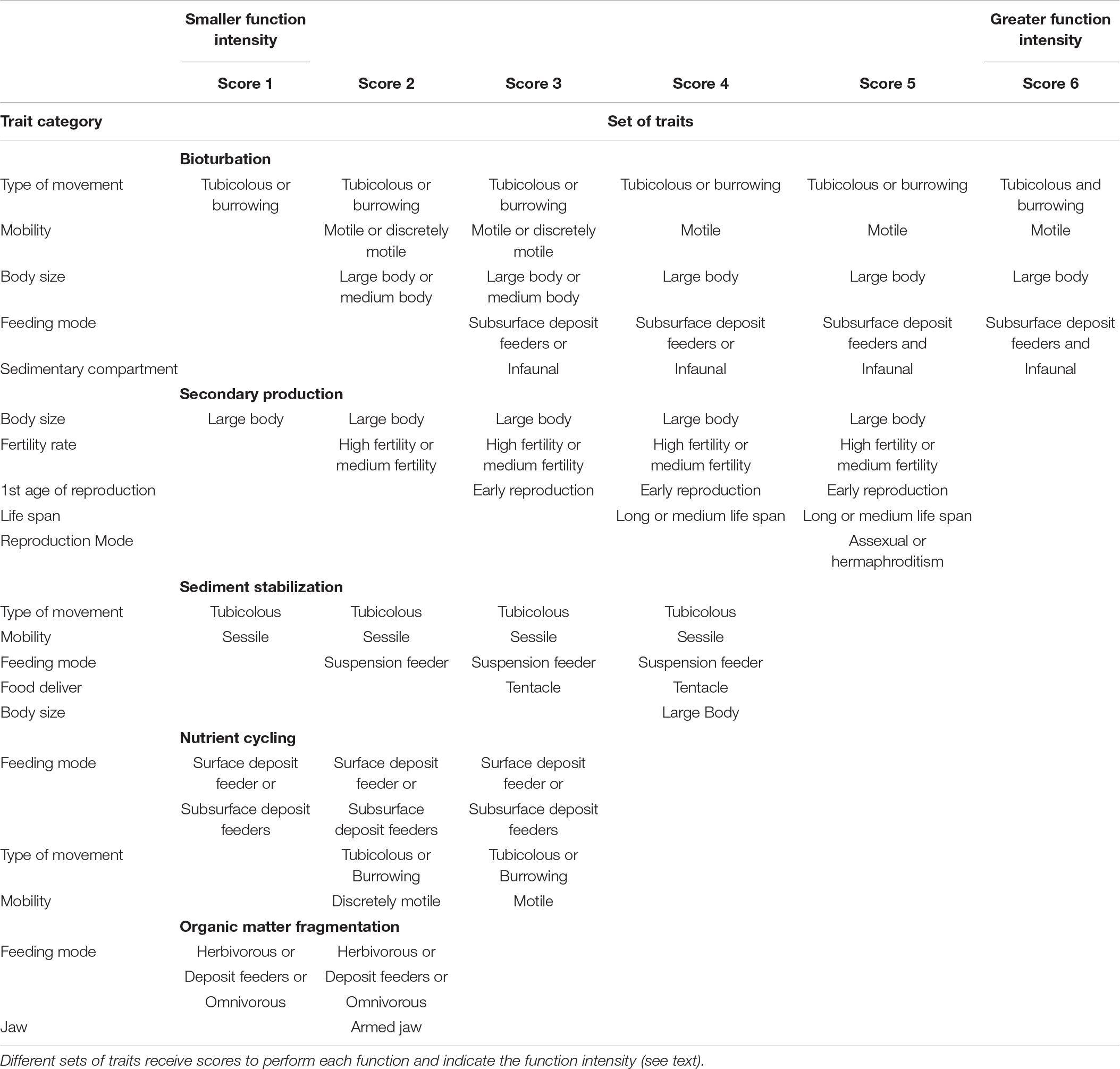
Table 2. Sets of traits that polychaetes must have to perform important ecological functions in estuaries.
We built a matrix of polychaete family traits and a script in R (R Development Core Team, 2011; Supplementary Appendix B) that analyzed each line of this matrix, selected the families that had the traits of interest for each specific ecological function (as in Table 1), and assigned the informed score based on the combinations of traits (as in Table 2). The routine also assigned to every family the maximum possible score for each ecological function (see the detailed explanation in Appendix B). Finally, the scores of all functions were standardized to a range from 0 to 1 (Supplementary Appendix A; Table A.2) since some functions presented six classes of possible trait combinations (i.e., bioturbation, Table 2) while others showed only two (i.e., fragmentation, Table 2). Thenceforth, the density (number of individuals per m2) of each taxa on each station was multiplied by the scores of ecological functions at this same station. Consequently, it was possible to obtain values representing the intensity of each ecological function along the estuaries (Supplementary Appendix A; Table A.3).
Distribution of Ecological Functions
Data Acquisition
In order to access the distribution of benthic ecological functions throughout the estuarine ecosystem, the benthic assemblage fauna dataset, obtained from several research projects developed by Tropical Marine Ecology group,1 was used. This data set was partially published previously (e.g., Barros et al., 2014, 2021; Alves et al., 2020).
We used the density (number of individuals per m2) of polychaete families along the salinity gradient of the estuaries of the Jaguaripe River (sampled on 05/2006, 08/2007, 07/2010, and 08/2014), Paraguaçu River (05/2005, 12/2005, 06/2011, and 08/2014) and Subaé River (06/2004, 03/2006, 04/2011, and 03/2013) (Figure 1 and Supplementary Appendix A; Table A.4). Each estuary was sampled along 10 (Paraguaçu and Jaguaripe) or 11 stations (Subaé) every single time. At each station, two sites 20–50 m apart, were sampled. At each site, either three replicates were taken using a van Veen grab (0.054 m2) (in Paraguaçu) or four replicates using cores (0.0078 m2) (in Subaé and Jaguaripe). Samples were sieved in the field using a 0.5 mm mesh, preserved with ethanol and transported to the lab. In the lab, samples were sorted and the invertebrates were mostly identified to family level. This taxonomic level has already been used to show precise ecological patterns (Souza and Barros, 2015) and previous works also used polychaete family for the analysis of biological traits (Otegui et al., 2016). The detailed sampling and processing methodology was published elsewhere (Hatje et al., 2006; Barros et al., 2008, 2012; Magalhães and Barros, 2011; see Appendix A.7 to complementary environmental data for each sampling station such granulometry and salinity). Furthermore, to investigate if there were differences in the functional intensity (i.e., the product of polychaetes density and polychaete function score) between the estuarine salinity zones, we adopted the Venice system to classify each sampling station in the salinity zone as euhaline (30–40), polyhaline (18–30), mesohaline (5–18), and oligohaline (0.5–5) (Venice System, 1958). The salinity data was obtained between 2004 and 2013, in different sampling occasions, using a calibrated water quality analyzer (Hydrolab DataSonde 4A, Loveland, Colorado, United States) and an optical refractometer (Instrutherm, model RTS-101 ATC, Loveland, Colorado, United States). In order to group the sampling stations of the different estuaries according to the Venice system, a cluster analysis was used based on Ward’s criterion using untransformed salinity data based on the similarity matrix with Euclidean distance (see Krull et al., 2014).
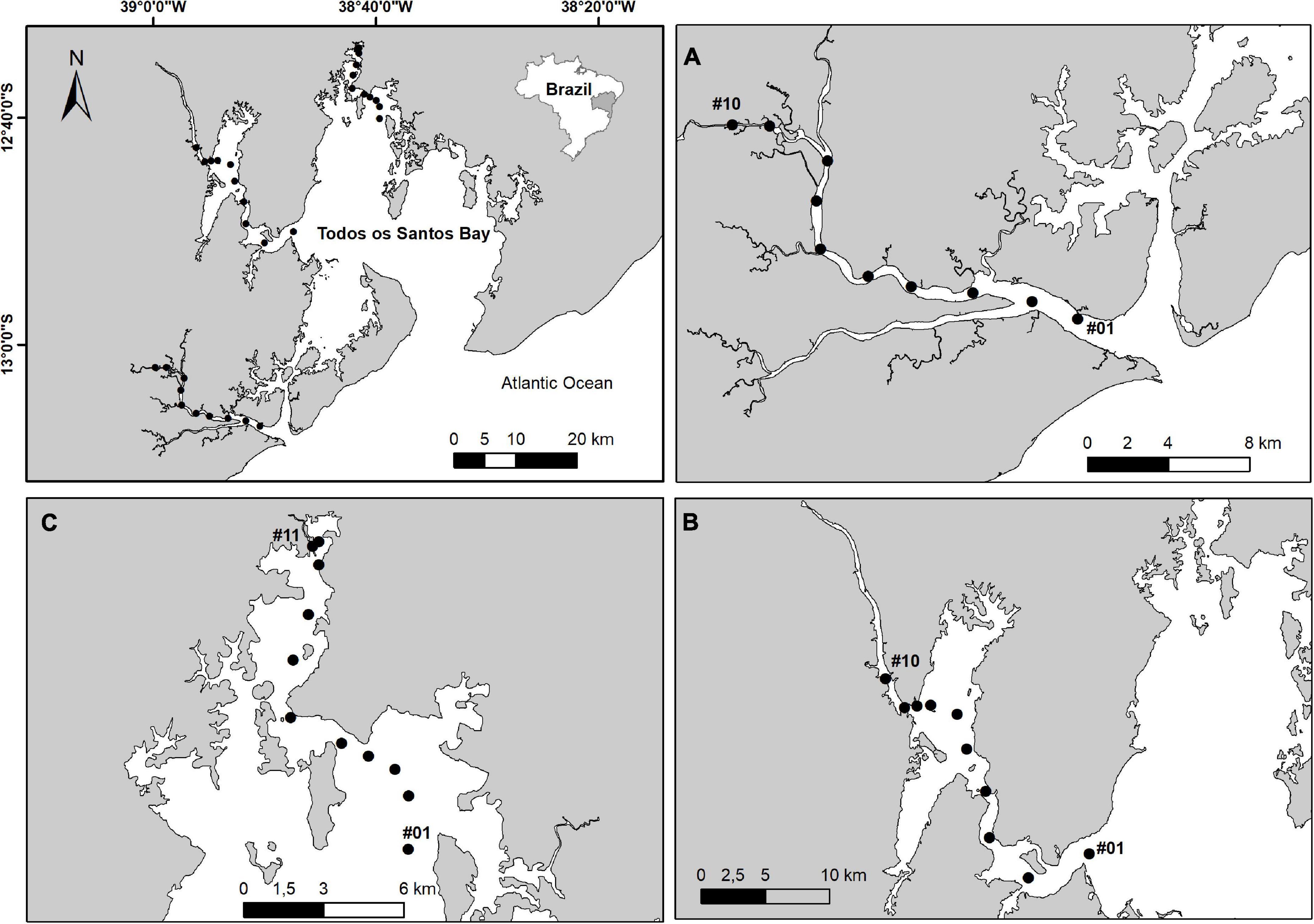
Figure 1. Distribution of the sampling stations in Jaguaripe (A), Paraguaçu (B), and Subaé (C) estuaries in Todos os Santos Bay, northeast of Brazil.
Data Selection
A matrix was constructed with the taxonomic classification of the polychaetes families and the presence or absence of selected functional traits (Supplementary Appendix A; Table A.5). The elected functional traits of each taxa were assessed through literature search and online databases. The categories of traits such as feeding mode, food delivered, mobility, type of movement, sedimentary compartment and body size of each family were consulted mainly through the work of Jumars et al. (2015). Traits associated to reproduction such as reproduction mode, egg size, age of first reproduction, life span, fecundity rate, and the trait material used for burrow construction were searched through the Polytraits database (Faulwetter et al., 2014), the Biological Traits Information Catalog - BIOTIC (Marine Life Information Network, 2006) and also through Rouse and Pleijel (2001).
Statistical Analysis
From the matrix of distributions of functions intensity (i.e., number of individuals per m2 of each taxa on each station multiplied by the scores of ecological functions at this same station, see section “Data Acquisition”), a Bray-Curtis similarity matrix between stations at each estuary was created. Based on this matrix, in order to test for potential differences on function intensity at different zones and times within each estuary, a two-factor permutational multivariate analysis of variance (PERMANOVA) was performed using PRIMER v.6 software (Clarke and Warwick, 2001). Factors in these analyses were salinity zone (fixed with four levels: oligohaline, polyhaline, mesohaline and euhaline) and sampling dates (random with four levels, see section “Data Acquisition” for the specific sampling dates at each estuary). Additional PERMANOVA pair-wise tests (Anderson et al., 2008) were performed whenever necessary.
Results
Description of the Ecological Functions and Functional Traits Associated
The most important ecological functions performed by estuarine polychaetes were bioturbation of sediment, fragmentation of organic matter, stabilization of sediment, nutrient cycling and secondary production. A total of 11 categories of traits were used to describe these functions (type of movement, mobility, body size, feeding mode, food deliver, jaw, sedimentary compartment, fertility rate, first age of reproduction, life span, and reproduction mode; Table 2). Different traits and sets of traits were considered necessary for the organism to perform each ecological function with different intensities (i.e., scores in Table 2). For instance, to perform bioturbation the organism must be tubicolous or burrower (see Table 2 Bioturbation, score 1) but, we observed that the function was greater, i.e., more intense, when the traits mobile or discretely mobile and medium or large body size were also present, since those features increase the amount of sediment being bioturbated (Table 2). The maximum score indicates high function intensity. In this sense, in the bioturbation case (Score 6) the highest intensity performance was achieved by a polychaete that is (i) tubicolous and burrower, (ii) motile, (iii) large, (iv) subsurface deposit feeder, and (v) infaunal (Table 2 Bioturbation, score 6). The same framework was used to determine the intensity of the other functions.
Secondary production function was accessed through the combination of traits related to reproduction and body size. Large body size was considered an important trait, since the maximum size of an individual is directly related to productivity, and when associated with other traits (high or medium fertility rate, long or medium life span, asexual reproduction or hermaphroditism and early age of first reproduction) lead to an increase in intensity of this function (Table 2).
Distribution of Ecological Functions
The distribution of functions varied across estuarine systems, zones and sampling dates (Figure 2). Bioturbation and fragmentation of organic matter were present along the entire estuarine gradients showing similar patterns of variation within zones. Their intensities generally decreased in the middle estuary, in the polyhaline and/or mesohaline zones, and increased in the upper estuary (i.e., oligohaline zone). Nutrient cycling function decreased toward regions with lower salinities, especially in the Jaguaripe estuary (Figure 2A). The intensity of sediment stabilization was generally very low and, when observed (i.e., 08/2007 and 07/2010 in Jaguaripe, 06/2004 in Subaé and all dates in Paraguaçu estuary), was mainly found in lower and middle estuary (Figure 2). The intensity of secondary production appeared to increase in lower salinity regions in the upper estuary. This function showed null or low intensity in mesohaline zones (Figure 2). In a few stations, due to the absence of polychaetes, no ecological function was registered. This was mainly observed at some points of the Subaé estuary (Figure 2C), but in the first station of Paraguaçu (Figure 2B, 08/2014) and in the last station of Jaguaripe (Figure 2A, 05/2006).
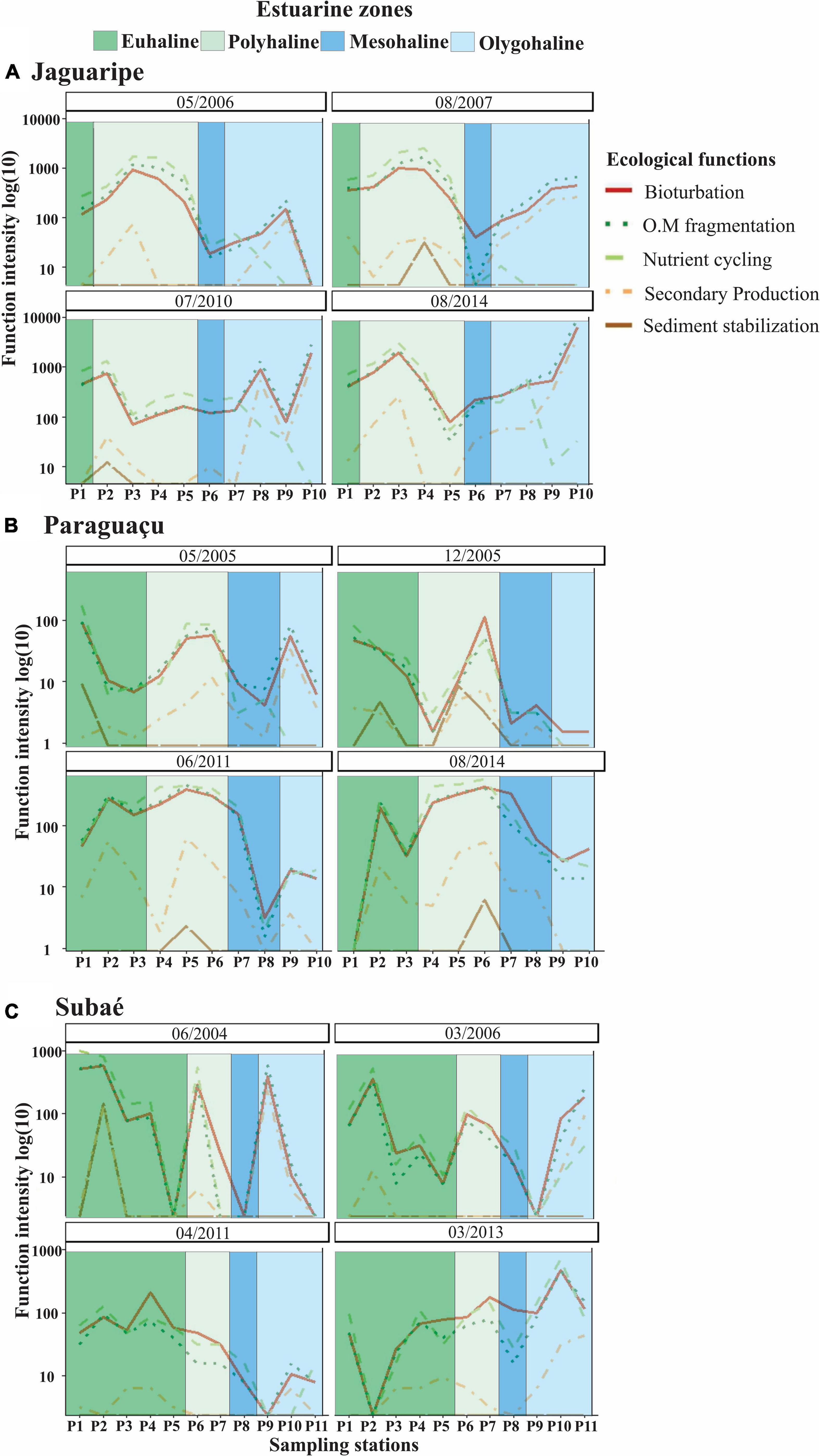
Figure 2. Function intensities performed by polychaetes along the estuaries of Jaguaripe estuary (A), Paraguaçu (B), and Subaé (C) at Todos os Santos Bay, Brazil, at different sampled occasions.
The Jaguaripe estuary showed differences in functional structure among salinity zones (p = 0.0117), and the pairwise comparisons indicated significant differences between euhaline and oligohaline zones (p = 0.0012; Table 3A and Figure 2A). The euhaline zone presented higher intensity of bioturbation, fragmentation, nutrient cycling, and lower secondary production (Figure 2A). On the other hand, in the oligohaline zone an increase in secondary production intensity and a decrease in nutrient cycling was observed. Although variations of some functions have been observed along the Jaguaripe estuary, the only function that individually showed a significant difference along the salinity zones was nutrient cycling (p = 0.0004). Pairwise tests showed that nutrient cycling in euhaline and polyhaline zones was significantly larger than in the oligohaline zone (p = 0.0043 and p = 0.0014, respectively; Figure 3, Supplementary Appendix A; Table A.6 I. Jaguaripe).
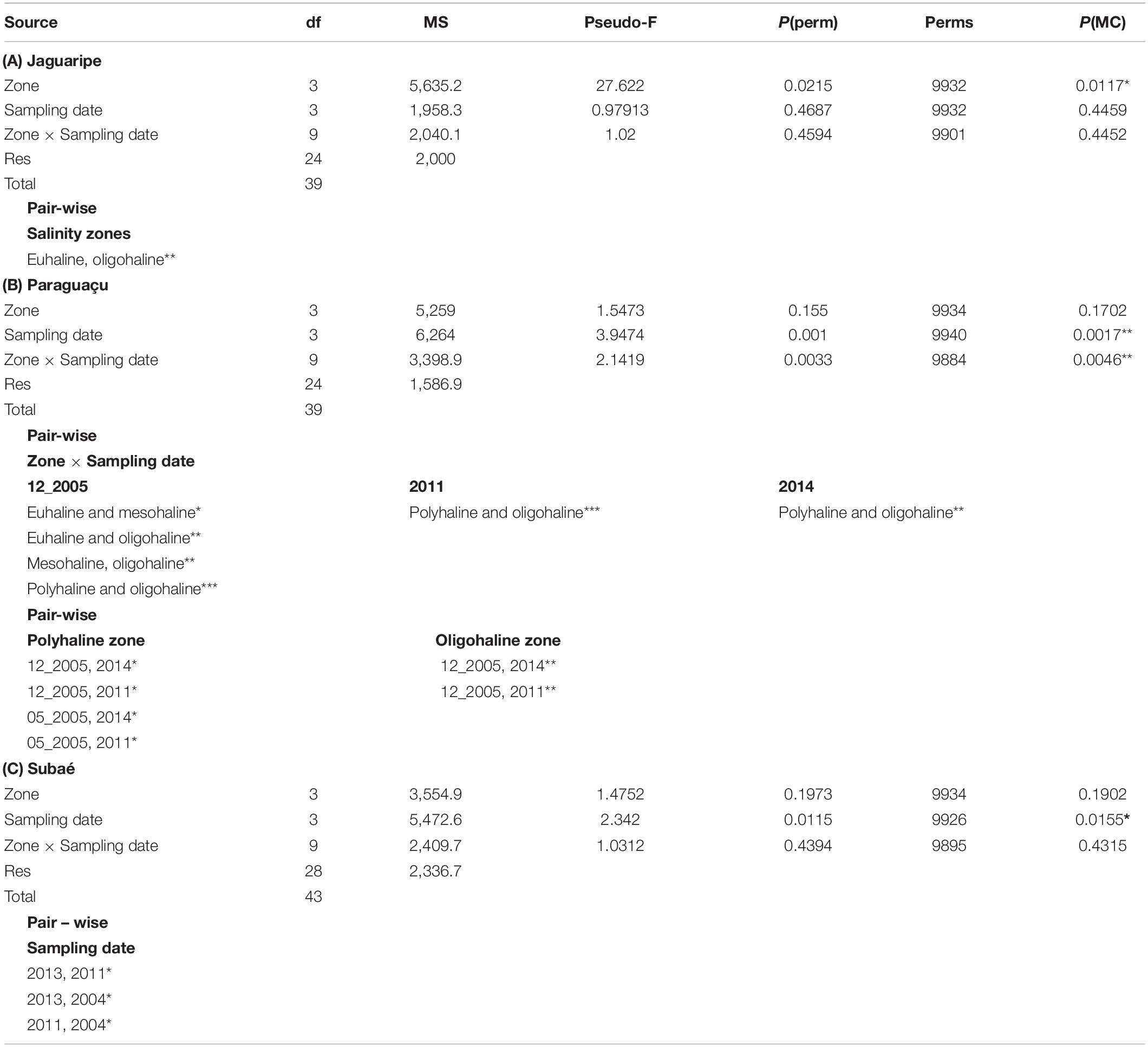
Table 3. Permanova test and pairwise comparisons testing differences in functional structure of polychaetes assemblages in (A) Jaguaripe, (B) Paraguaçu, and (C) Subaé estuaries (*p < 0.05, **p < 0.01; ***p < 0.001).
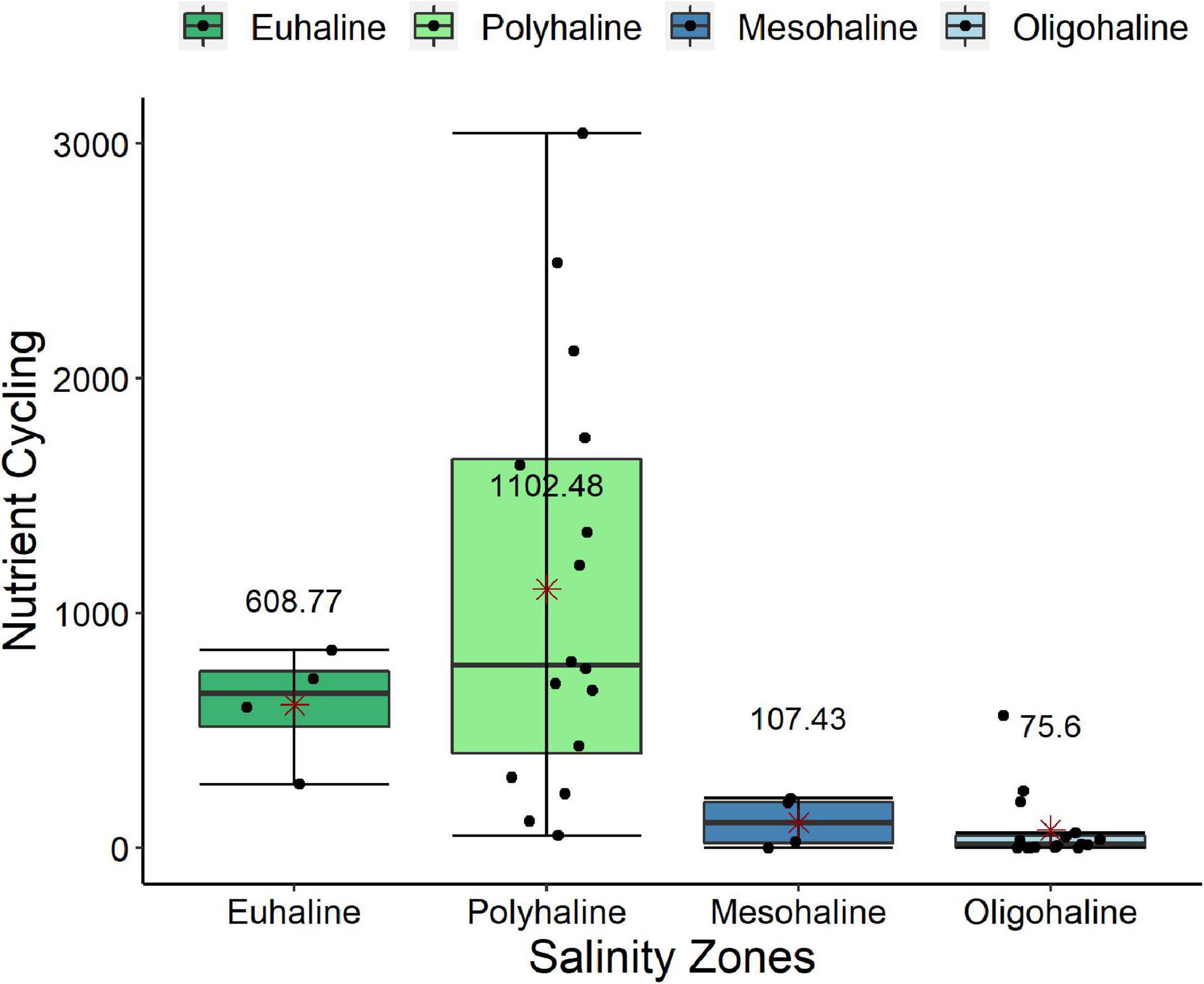
Figure 3. Boxplot of Nutrient Cycling potential performed by polychaetes in different salinity zones along Jaguaripe estuary in all sampled occasions. Points represent the samples. The red asterisk represents the mean value and is shown by the numbers on the boxplots. The medians are represented by the dash in the middle of the boxplots and the error bar is represented by the vertical line.
Paraguaçu estuary showed a significant interaction between salinity zones and sampling dates (p = 0.0046; Table 3B). Fewer polychaete functions were performed in the oligohaline zone than in the euhaline zone in one occasion (12/2005; p = 0.008) and in the polyhaline zone in other two occasions (2011, p = 0.0007; 2014, p = 0.0015; Table 3B and Figure 2B). The polyhaline zone in Paraguaçu presented all the functions (three out of four times) but these functions decreased in the oligohaline zone along with the loss of sediment stabilization and a remarkable reduction in the secondary production. Nutrient cycling and fragmentation of organic matter showed significant differences for interaction between zone and sampling dates (p = 0.0007; p = 0.0026, respectively; see Supplementary Appendix A; Table A.6 II. Paraguaçu). For these two functions, pairwise tests for the polyhaline zone showed a significant difference in the two dates of 2005 in relation to 2011 and 2014 (Supplementary Appendix A; Table A.6 II. Paraguaçu). The intensity of nutrient cycling and fragmentation of organic matter in the polyhaline zone was higher on the two most recent sampled occasions (Figure 4). In these two sampling occasions, we also observed an increase in function intensity of the euhaline zone in direction to the polyhaline zone but a decline in mesohaline and oligohaline zones (Figure 4). The bioturbation function showed a significant difference only for sampling dates (p = 0.0013; Supplementary Appendix A; Table A.6 II. Paraguaçu).
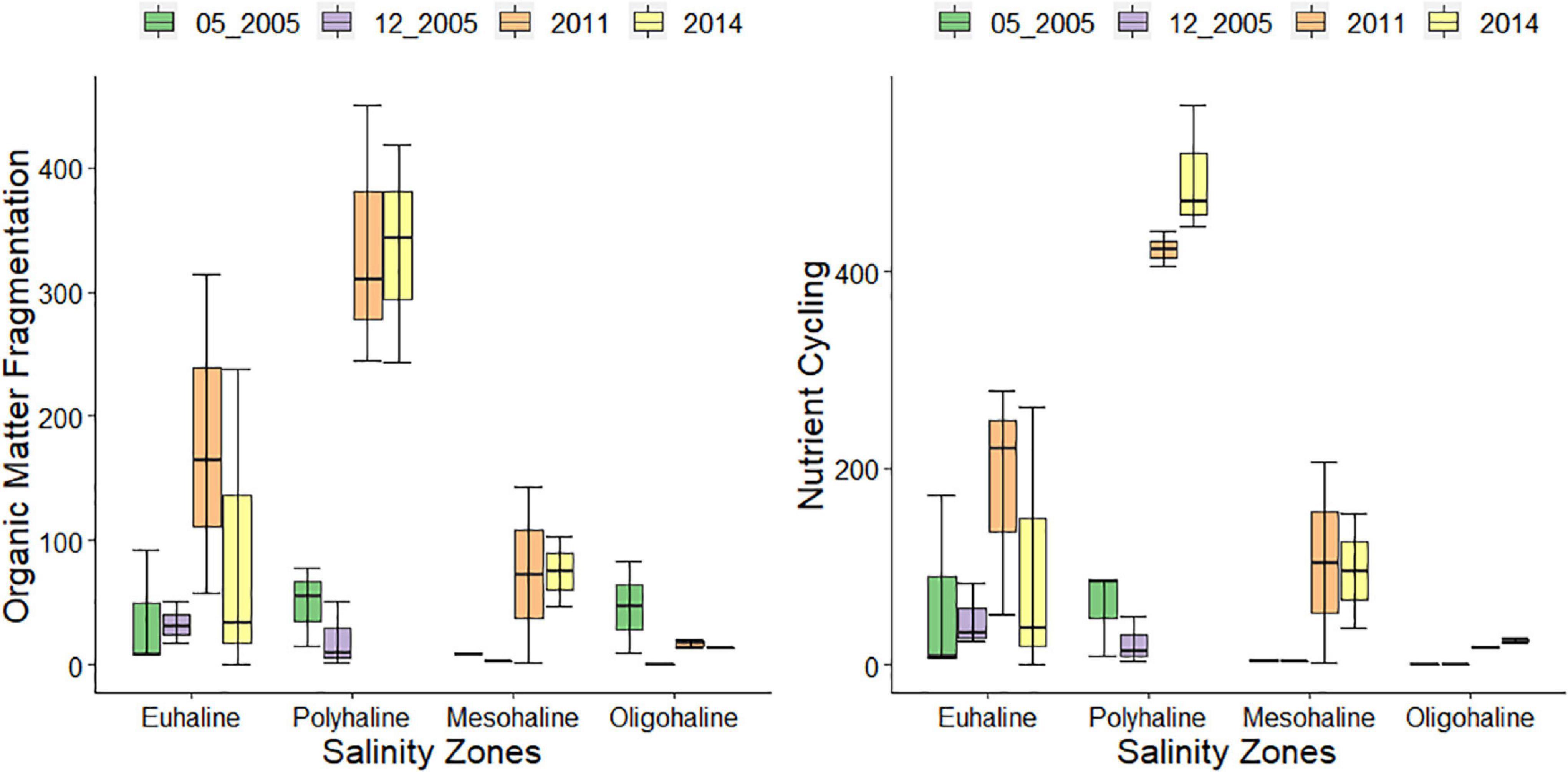
Figure 4. Boxplot of organic matter fragmentation and Nutrient Cycling estimated functions performed by polychaetes at different salinity zones and sampling dates along Paraguaçu estuary. The medians are represented by the dash in the middle of the boxplots and the error bar is represented by the vertical line.
Subaé estuary showed significant temporal differences in functional structure (p = 0.0155; Table 3C and Figure 2C). The sampling date 2004 depicted a high variability in functional structure contrasting to the results from 2011 and 2013 (Table 3C and Figure 2C). In addition, 2013 showed increases in the intensity of ecological functions, generally toward the upper estuary, except in point 2, thus differing from 2011 when there was a decrease in overall functions (p = 0.015; Table 3C and Figure 2C).
The intensity of bioturbation, nutrient cycling and secondary production performed by polychaetes in the Subaé estuary showed significant variability among sampling dates (respectively, p = 0.0349, p = 0.001, and p = 0.0206; Supplementary Appendix A; Table A.6 III.Subaé). While secondary production showed significant differences between the salinity zones (p = 0.0371) amidst the mesohaline with euhaline zones (p = 0.0142) and oligohaline zone (p = 0.0036), in general the oligohaline zone showed higher intensity for secondary production than the other zones (Figure 5, Supplementary Appendix A; Table A.6 III.Subaé).
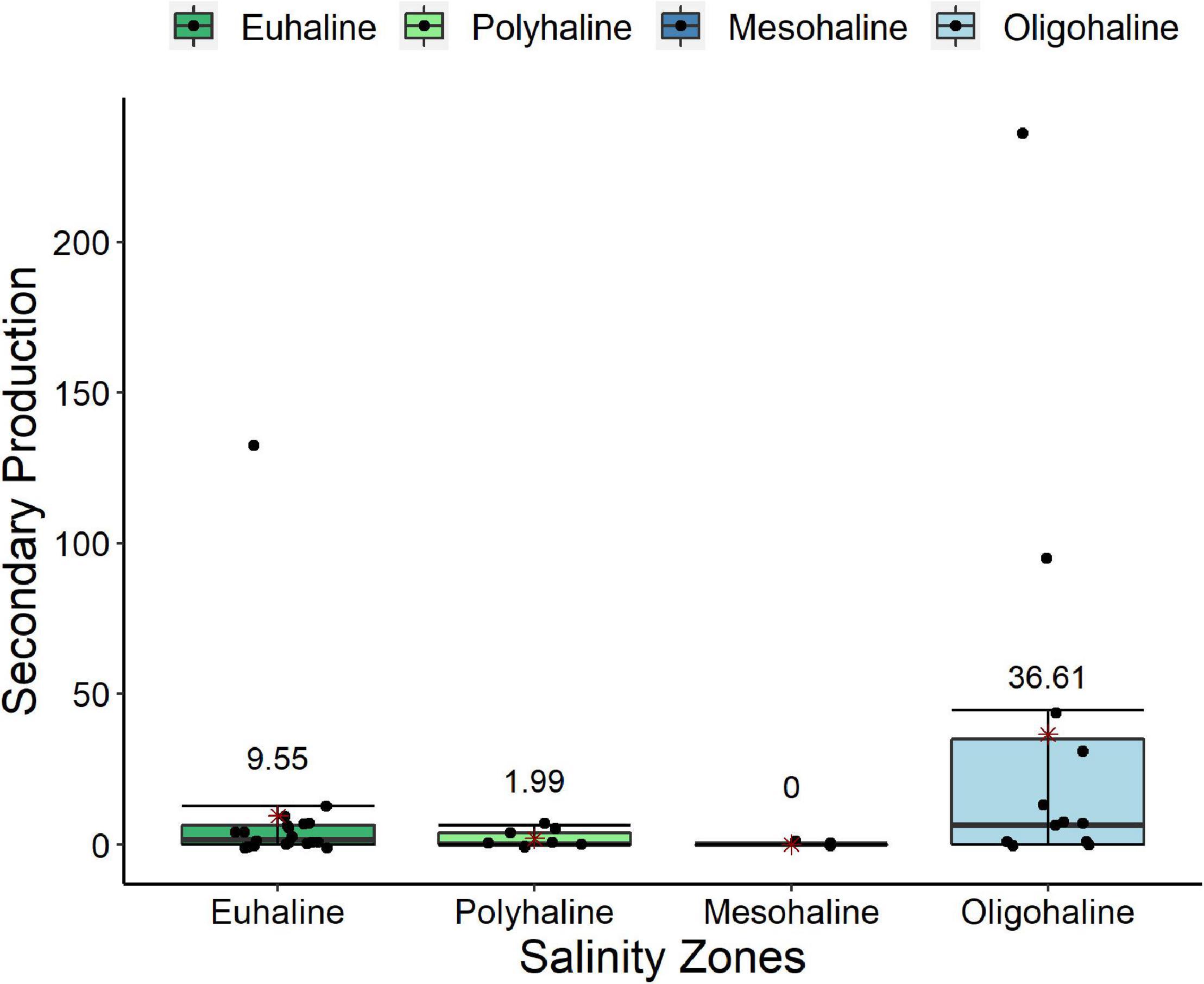
Figure 5. Boxplot of Secondary production potential performed by polychaetes along salinity zones in Subaé estuary. Points represent the samples. The red asterisk represents the mean value and is shown by the numbers on the boxplots. The medians are represented by the dash in the middle of the boxplots and the error bar is represented by the vertical line.
Discussion
Bioturbation, organic matter fragmentation and nutrient cycling were the most frequent functions performed by polychaetes along the estuarine systems, remaining present throughout the gradient but with variable intensities. Although bioturbation is frequently perceived as a small-scale phenomenon, this function has important roles in estuarine systems. For example, it causes topographic variation, increases in aeration and oxygenation of deep layers of sediment, directly affecting community metabolism, changes in organic content, reworking of sediments, reduction of sediment compaction and influences the fate of pollutants (metals, organic pollutants) (e.g., Little, 2000; Gray and Elliott, 2009; Day et al., 2013). Bioturbation is even more relevant in areas with muddy sediments, with low permeability, low concentrations of oxygen and a greater accumulation of contaminants (i.e., reduced water exchanges between sediments and water column) (Hatje et al., 2006; Mermillod-Blondin, 2011). In the three estuaries investigated, the highest percentage of fine sediments was found mainly in high salinity zones (Barros et al., 2012). Virtually all taxa identified in the three estuaries were classified as bioturbators, with the exception of two less frequent and abundant taxa (Sphaerodoridae and Sigalionidae). Trichobranchidae showed the highest score for bioturbation, being a subsurface deposit feeder, mobile, with a large body and tubicolous. These polychaetes can burrow the sediment in search of food in the lower layers and promote mixing of the sediment and water inflow (Kristensen et al., 2012; Jumars et al., 2015).
Fragmentation of organic matter also plays relevant roles such as reducing the particle size of detritus due to ingestion and passage through the digestive tract, increasing the susceptibility to microbial attack, enhancing rates of decomposition of detritus favoring active bacteria and mobilizing nutrients (Day et al., 2013). Nereididae was one of the most frequent and abundant taxa and, since they are omnivorous and have jaws, these organisms can reduce the particle size of detritus (Jumars et al., 2015). This taxon contributed to the maintenance of fragmentation intensity along the estuarine gradient. Bioturbation and fragmentation were performed more intensively in euhaline zones, decreasing in mesohaline but increasing in oligohaline zones. In this zone many polychaetes do not tolerate the low salinity conditions, therefore Nereididae can be found and are functionally crucial for increase the bioturbation and fragmentation in the upper estuary.
The variation of intensity in nutrient cycling along estuaries was similar to fragmentation, but frequently decreased in the upper estuary (i.e., oligohaline zone). Nutrient cycling increases nutrient fluxes, mobilization and transferring of nutrients between different sediment compartments (Day et al., 1987). Many taxa showed traits that allowed them to perform this function, contributing to the maintenance of nutrient cycling along the estuary. For example, Cirratulidae and Orbiniidae, which were quite frequent and more abundant in the lower estuary (e.g., euhaline and polyhaline zone), contributed to an increase in nutrient cycling in these zones. Additionally, Capitellidae (also abundant in these zones) were also found in the upper estuary (e.g., Oligohaline zones). Therefore, cirattulids and capitellids, being depositivorous, mobile, burrowing and infaunal, are especially important for cycling the nutrients in estuaries.
Stabilization of sediment, although less observed, was more frequent in the polyhaline zone and sometimes in the euhaline zone (i.e., Paraguaçu and Subaé estuaries). It was performed by traits found in Sabellidae, Serpullidae, and Terebellidae. These taxa occurred in the lower Subaé and Paraguaçu estuaries at one occasion and in the middle of Jaguaripe and Paraguaçu estuaries. Sabellidae and Serpulidae share several traits, such as tentacular crown and the capacity for building mucous and sediment tubes. These taxa are sessile and suspension feeders like Terebellidae and largely dependent upon bottom currents to bring particles within range of their collecting systems (Jumars et al., 2015). In many large Sabellidae and Terebellidae, the tube is occupied permanently while serpulids can secrete calcareous tubes. Stabilization promotes resistance to erosion by water flow and may facilitate recruitment of some taxa (Little, 2000). We did not observe this function in the oligohaline zones, which are usually composed of coarser sediments (Barros et al., 2012) a result of strong currents which may not allow the construction of semi-permanent tubes.
Benthic secondary production in general is high in the estuarine system when compared with other aquatic ecosystems (Day et al., 2013). It controls the energy flow through the food web, supporting a high diversity of organisms in the sediments and water column (e.g., larger invertebrates, demersal nekton and wading and diving birds) (Day et al., 2013). This function may be affected by stresses of the estuarine environment, such as salinity variation (Day et al., 2013). Polychaete secondary production was remarkably smaller than bioturbation and fragmentation but increased in oligohaline zones, reaching similar proportions to those other functions in this region. Information about the reproductive traits of polychaetes is not always accessible and, since we did not use biomass but body size as a proxy, proper formal testes are needed for evaluating if secondary production in estuarine sediments is really more intense in the upper estuary.
There are a few studies on ecological function along estuarine gradients (Oug et al., 2012; Otegui et al., 2016; van der Linden et al., 2016) but they are mostly based in the variability of all biological traits (Bremner et al., 2006a,b; Paganelli et al., 2012; van der Linden et al., 2012, 2017; Veríssimo et al., 2012; Darr et al., 2014; Egres et al., 2019). It has been suggested that the use of any functional traits can result in a redundant trait or in traits not necessarily related to the functions (Mlambo, 2014; Beauchard et al., 2017; Luiza-Andrade et al., 2017). Therefore, functions are often not explicitly addressed, neither are traits precisely associated with specific functions. Some studies use indexes that gather all traits information and calculate functional diversity in the environment (Lefcheck et al., 2015; van der Linden et al., 2016), but the interpretation of which function is varying might be complex.
The understanding of ecosystem functioning improves when we define how combinations of functional traits are related to specific functions and the mechanism by which these traits perform their roles (de Bello et al., 2010; Beauchard et al., 2017). In the present study, traits were clearly associated with function allowing the evaluation of function intensity along an ecological gradient.
Synthesizing information from the literature on which traits are associated with specific ecological functions is the first step for experiments to be performed and to actually test the effect of specific functional traits on sedimentary processes and ecosystem properties (de Bello et al., 2010; Mlambo, 2014). Much experimental work is needed to evaluate who (i.e., trait) does what (i.e., function) and at which intensity. For instance, experiments measuring how the movement of an organism, when building galleries and feeding on deposits, will affect the fragmentation of organic matter, are necessary.
This study showed that the distribution of ecological functions is dynamic, and varies in time and space. Variability was observed in the patterns of functions at different times, especially in the estuaries of Paraguaçu and Subaé. where the intensity of some polychaetes functions increased in time. In the Paraguaçu estuary, the change in sediment stabilization in the different sampling dates along the salinity zones was marked. This may be caused by differences in hydrodynamical regimes which shows the demand for research investigating the drivers of variation in function intensity under different situations (e.g., low to extreme river flows).
Biological assemblages and their distribution of functional traits determine the roles that will be performed at each environment and make possible to predict how the environment might recover from different disturbances (e.g., dredging event, dam construction) (de Bello et al., 2010; Bolam, 2014). For instance, functional redundancy allows the function to continue to be performed (Walker et al., 2004; Olsgard et al., 2008; Magalhães and Barros, 2011). That is, even in the absence of a taxon, due to some disturbance, there may be other species with similar traits capable of keeping the function (i.e., functional compensation; Walker, 1995; Naeem, 1998). Thus, ecological functions are indicative of ecosystem health and resilience and are intrinsically related to various ecosystem services that benefit society (Millennium Ecosystem Assessment, 2005; Strong et al., 2015).
It is important to investigate how each set of environmental characteristics (e.g., salinity, particle size, and organic matter) and how human impacts can affect the distribution of ecological functions. Using traits associated with specific ecological functions, rather than all available traits, allows one to observe patterns of function variation in space and time. Future studies may investigate how each function responds to different variables and also empirically test how traits alter environmental characteristics (sediment oxygenation, nutrient content) and ecosystem properties (resilience).
Data Availability Statement
The original contributions presented in the study are included in the article/Supplementary Material, further inquiries can be directed to the corresponding author.
Author Contributions
AM: conceptualization, formal analysis, data curation, methodology, and writing – original draft. FB: supervision, conceptualization, formal analysis, data curation, methodology, and writing – review and editing. Both authors contributed to the article and approved the submitted version.
Funding
The data were generated with different research projects funded by CNPq (Grants 302642/2008-0; 306332/2014-0; 304907/2017-0; 478265/2008-5; 405793/2016-2; and 441264/2017-4; INCT IN-TREE) and by FAPESB (PET 0035). FB was supported by CNPq (PQ 306332/2014-0; 304907/2017-0).
Conflict of Interest
The authors declare that the research was conducted in the absence of any commercial or financial relationships that could be construed as a potential conflict of interest.
Publisher’s Note
All claims expressed in this article are solely those of the authors and do not necessarily represent those of their affiliated organizations, or those of the publisher, the editors and the reviewers. Any product that may be evaluated in this article, or claim that may be made by its manufacturer, is not guaranteed or endorsed by the publisher.
Acknowledgments
We thank the Instituto Kirimurê Baía de Todos os Santos for their support and facilities. ATA is thankful to Coordenação de Aperfeiçoamento de Pessoal de Nível Superior (CAPES). We gratefully acknowledge João Bosco Leite Gusmão Junior and Igor Cristino Silva Cruz for helping with suggestions on an early version of this manuscript and Amanda Campos for helping with the data analysis. We also thank the Laboratório de Ecologia Bentônica team involved in the fieldwork and sample processing which generated the data used in this manuscript.
Supplementary Material
The Supplementary Material for this article can be found online at: https://www.frontiersin.org/articles/10.3389/fmars.2022.780318/full#supplementary-material
Footnotes
References
Aller, R. C. (1988). “Benthic fauna and biogeochemical processes in marine sediments: the role of burrow structures,” in Nitrogen Cycling in Coastal Marine Environments, eds T. H. Blackburn and J. Sørensen (Chichester: Wiley), 301–338.
Alves, A. T., Petsch, D. K., and Barros, F. (2020). Drivers of benthic metacommunity structure along tropical estuaries. Sci. Res. 10:1739. doi: 10.1038/s41598-020-58631-1
Anderson, M. J., Gorley, R. N., and Clarke, K. R. (2008). PERMANOVA + for PRIMER: Guide to Software and Statistical Methods. Auckland: University of Auckland and PRIMER-E, 214.
Attrill, M., and Rundle, S. (2002). Ecotone or ecocline: ecological boundaries in estuaries. Estuar. Coast. Shelf Sci. 55, 929–936. doi: 10.1006/ecss.2002.1036
Barros, F., Blanchet, H., Hammerstrom, K., Sauriau, P., and Oliver, J. (2014). Estuarine, coastal and shelf science a framework for investigating general patterns of benthic b -diversity along estuaries. Estuar. Coast. Shelf Sci. 149, 223–231. doi: 10.1016/j.ecss.2014.08.025
Barros, F., Costa, Y., Reis, A., Martins, A., and Magalhães, W. F. (2021). Monitoramento da Macrofauna Bentônica dos Estuários da Baía de Todos os Santos (BA). v1. Laboratório de Ecologia Bentônica UFBA. Dataset/Occurrence [WWW Document]. Available online at: https://ipt.sibbr.gov.br/sibbr/resource?r=ufba_leb_01&v=1.0
Barros, F., de Carvalho, G. C., Costa, Y., and Hatje, V. (2012). Subtidal benthic macroinfaunal assemblages in tropical estuaries: generality amongst highly variable gradients. Mar. Environ. Res. 81, 43–52. doi: 10.1016/j.marenvres.2012.08.006
Barros, F., Hatje, V., Figueiredo, M. B., Magalhães, W. F., Dórea, H. S., and Emídio, E. S. (2008). The structure of the benthic macrofaunal assemblages and sediments characteristics of the Paraguaçu estuarine system, NE, Brazil. Estuarine. Coast. Shelf Sci. 78, 753–762. doi: 10.1016/j.ecss.2008.02.016
Beauchard, O., Veríssimo, H., Queirós, A. M., and Herman, P. M. J. (2017). The use of multiple biological traits in marine community ecology and its potential in ecological indicator development. Ecol. Ind. 76, 81–96. doi: 10.1016/j.ecolind.2017.01.011
Bellwood, D. R., Streit, R. P., Brandl, S. J., and Tebbett, S. B. (2019). The meaning of the term ‘function’ in ecology: a coral reef perspective. Funct. Ecol. 33, 948–961. doi: 10.1111/1365-2435.13265
Bhowmik, M., and Mandal, S. (2021). Do seasonal dynamics influence traits and composition of macrobenthic assemblages of Sundarbans Estuarine System, India? Oceanologia 63, 80–98. doi: 10.1016/j.oceano.2020.10.002
Bolam, A. S. G. (2014). Macrofaunal recovery following the intertidal recharge of dredged material: a comparison of structural and functional approaches. Mar. Environ. Res. 97, 15–29. doi: 10.1016/j.marenvres.2014.01.008
Bolam, A. S. G., Fernandes, T. F., and Huxham, M. (2002). Diversity, biomass, and ecosystem processes in the marine benthos. Ecol. Monogr. 72, 599–615.
Braeckman, U., Provoost, P., Gribsholt, B., Van Gansbeke, D., Middelburg, J. J., Soetaert, K., et al. (2010). Role of macrofauna functional traits and density in biogeochemical fluxes and bioturbation. Mar. Ecol. Prog. Ser. 399, 173–186. doi: 10.3354/meps08336
Bremner, J. (2008). Species’ traits and ecological functioning in marine conservation and management. J. Exp. Mar. Biol. Ecol. 366, 37–47. doi: 10.1016/j.jembe.2008.07.007
Bremner, J., Rogers, S. I., and Frid, C. L. J. (2003). Assessing functional diversity in marine benthic ecosystems: a comparison of approaches. Mar. Ecol. Prog. Ser. 254, 11–25. doi: 10.3354/meps254011
Bremner, J., Rogers, S. I., and Frid, C. L. J. (2006a). Methods for describing ecological functioning of marine benthic assemblages using biological traits analysis (BTA). Ecol. Ind. 6, 609–622. doi: 10.1016/j.ecolind.2005.08.026
Bremner, J., Rogers, S. I., and Frid, C. L. J. (2006b). Matching biological traits to environmental conditions in marine benthic ecosystems. J. Mar. Syst. 60, 302–316. doi: 10.1016/j.jmarsys.2006.02.004
Calow, P. (1987). Towards a definition of functional ecology. Funct. Ecol. 1, 57–61. doi: 10.2307/2389358
Clare, D. S., Spencer, M., Robinson, L. A., and Frid, C. L. J. (2016). Species densities, biological interactions and benthic ecosystem functioning: an in situ experiment. Mar. Ecol. Prog. Ser. 547, 149–161. doi: 10.3354/meps11650
Clarke, K. R., and Warwick, R. M. (2001). Change in Marine Communities: an Approach to Statistical Analysis and Interpretation, 2nd Edn. Auckland: PRIMER-E Plymouth.
Colling, L. A., and Bemvenuti, C. E., and Organismos bentônicos (2011). in Estudos Oceanográficos: Do Instrumental ao prático, ed. D. Calazans Pelotas, RS, Brazil, 276–281.
Darr, A., Gogina, M., and Zettler, M. L. (2014). Functional changes in benthic communities along a salinity gradient- a western Baltic case study. J. Sea Res. 85, 315–324. doi: 10.1016/j.seares.2013.06.003
Day, J. W. Jr., Hall, C. A. S., Kemp, W. M., and Yanez-Arancibia, A. (1987). Estuarine Ecology. New York, NY: John Wiley and Sons.
Day, J. W., Kemp, W. M., Yanez-Arancibia, A., and Crump, B. C. (2013). Estuarine Ecology, 2nd Edn. Hoboken, NJ: Wiley-Blackwell.
de Bello, F., Lavorel, S., Díaz, S., Harrington, R., Storkey, J., Sandin, L., et al. (2010). Towards an assessment of multiple ecosystem processes and services via functional traits. Biodivers. Conserv. 19, 2873–2893. doi: 10.1007/s10531-010-9850-9
Degen, R., Aune, M., Bluhm, B. A., Cassidy, C., Kedra, M., Kraan, C., et al. (2018). Trait-based approaches in rapidly changing ecosystems: a roadmap to the future polar oceans. Ecol. Ind. 91, 722–736. doi: 10.1016/j.ecolind.2018.04.050
Díaz, S., and Cabido, M. (2001). Vive la différence: plant functional diversity matters to ecosystem processes. Trends Ecol. Evol. 16, 646–655. doi: 10.1016/S0169-5347(01)02283-2
Dornhoffer, T., Waldbusser, G. G., and Meile, C. (2012). Burrow patchiness and oxygen fluxes in bioirrigated sediments. J. Exp. Mar. Biol. Ecol. 412, 81–86. doi: 10.1016/j.jembe.2011.11.004
Drylie, T. P., Lohrer, A. M., Needham, H. R., and Pilditch, C. A. (2020). Taxonomic and functional response of estuarine benthic communities to experimental organic enrichment: consequences for ecosystem function. J. Exp. Mar.Biol. Ecol. 532:151455. doi: 10.1016/j.jembe.2020.151455
Egres, A. G., Hatje, V., Miranda, D. A., Gallucci, F., and Barros, F. (2019). Functional response of tropical estuarine benthic assemblages to perturbation by polycyclic aromatic hydrocarbons. Ecol. Ind. 96, 229–240. doi: 10.1016/j.ecolind.2018.08.062
Fager, E. W. (1964). Marine sediments: effects of a tube building polychaete. Science 143, 356–359. doi: 10.1126/science.143.3604.356
Faulwetter, S., Markantonatou, V., Pavloudi, C., Papageorgiou, N., Keklikoglou, K., Chatzinikolaou, E., et al. (2014). Polytraits: a database on biological traits of marine polychaetes. Biodivers. Data J. 2:e1024. doi: 10.3897/BDJ.2.e1024
Fujii, T. (2007). Spatial patterns of benthic macrofauna in relation to environmental variables in an intertidal habitat in the Humber estuary, UK: developing a tool for estuarine shoreline management. Estuar. Coast. Shelf Sci. 75, 101–119. doi: 10.1016/j.ecss.2007.02.027
Gray, J. S., and Elliott, M. (2009). Ecology of Marine Sediments: Science to Management. Oxford: Oxford University Press, Oxford.
Hatje, V., Barros, F., Figueiredo, D. G., Santos, V. L. C. S., and Peso–Aguiar, M. C. (2006). Trace metal contamination and benthic assemblages in Subaé estuarine system, Brazil. Mar. Pollut. Bull. 52, 982–987. doi: 10.1016/j.marpolbul.2006.04.016
Hillebrand, H., and Matthiessen, B. (2009). Biodiversity in a complex world: consolidation and progress in functional biodiversity research. Ecol. Lett. 12, 1405–1419. doi: 10.1111/j.1461-0248.2009.01388.x
Hutchings, P. (1998). Biodiversity and functioning of polychaetes in benthic sediments. Biodivers. Conserv. 7, 1133–1145. doi: 10.1023/A:1008871430178
Jax, K. (2005). Function and “functioning” in ecology: what does it mean? Oikos 111, 641–648. doi: 10.1111/j.1600-0706.2005.13851.x
Jumars, P. A., Dorgan, K. M., and Lindsay, S. M. (2015). Diet of worms emended: an update of polychaete feeding guilds. Annu. Rev.Mar. Sci. 7, 497–520. doi: 10.1146/annurev-marine-010814-020007
Kraft, N. J. B., Adler, P. B., Godoy, O., James, E. C., Fuller, S., and Levine, J. M. (2015). Community assembly, coexistence and the environmental filtering metaphor. Funct. Ecol. 29, 592–599. doi: 10.1111/1365-2435.12345
Kristensen, E., Penha-Lopes, G., Delefosse, M., Valdemarsen, T., Quintana, C. O., and Banta, G. T. (2012). What is bioturbation? Need for a precise definition for fauna in aquatic sciences. Mar. Ecol. Prog. Ser. 446, 285–302. doi: 10.3354/meps09506
Krull, M., Abessa, D. M. S., Hatje, V., and Barros, F. (2014). Integrated assessment of metal contamination in sediments from two tropical estuaries. Ecotoxicol. Environ. Saf. 106, 195–203. doi: 10.1016/j.ecoenv.2014.04.038
Laverock, B., Gilbert, J. A., Tait, K., Osborn, A. M., and Widdicombe, S. (2011). Bioturbation: impact on the marine nitrogen cycle. Biochem. Soc. Trans. 39, 315–320. doi: 10.1042/BST0390315
Lefcheck, J. S., Bastazini, V. A. G., and Griffin, J. N. (2015). Choosing and using multiple traits in functional diversity research. Environ. Conser. 42, 104–110. doi: 10.1017/S0376892914000307
Little, C. (2000). The Biology of Soft Shores and Estuaries, 1st Edn. Oxford: Oxford University Press, 252.
Loreau, M., Naeem, S., Inchausti, P., Bengtsson, J., Grime, J. P., Hector, A., et al. (2001). Ecology: biodiversity and ecosystem functioning: current knowledge and future challenges. Science 294, 804–808. doi: 10.1126/science.1064088
Luiza-Andrade, A., Montag, L. F., de, A., and Juen, L. (2017). Functional diversity in studies of aquatic macroinvertebrates community. Scientometrics 111, 1643–1656. doi: 10.1007/s11192-017-2315-0
Magalhães, W. F., and Barros, F. (2011). Structural and functional approaches to describe polychaete assemblages: ecological implications for estuarine ecosystems. Mar. Freshw. Res. 62, 918–926. doi: 10.1071/MF10277
Marine Life Information Network (2006). BIOTIC - Biological Traits Information Catalogue. Citadel Hill: Marine Biological Association.
McLusky, D. S., and Elliott, M. (2004). The Estuarine Ecosystem: Ecology, Threats and Management, 3rd edit Edn. Oxford: Oxford University Press.
Mermillod-Blondin, F. (2011). The functional significance of bioturbation and biodeposition on biogeochemical processes at the water-sediment interface in freshwater and marine ecosystems. J. N. Am. Benthol. Soc. 30, 770–778. doi: 10.1899/10-121.1
Millennium Ecosystem Assessment (2005). Ecosystems and Human Well-Being: Biodiversity Synthesis. Washington, DC: World Resources Institute, 100.
Mlambo, M. C. (2014). Not all traits are “functional”: insights from taxonomy and biodiversity-ecosystem functioning research. Biodivers. Conserv. 23, 781–790. doi: 10.1007/s10531-014-0618-5
Morais, G. C., Gusmao, J. B., Oliveira, V. M., and Lana, P. (2019). Macrobenthic functional trait diversity at multiple scales along a subtropical estuarine gradient. Mar. Ecol. Prog. Ser. 624, 23–37. doi: 10.3354/meps13033
Murphy, E. A. K., and Reidenbach, M. A. (2016). Oxygen transport in periodically ventilated polychaete burrows. Mar. Biol. 163, 14. doi: 10.1007/s00227-016-2983-y
Naeem, S. (1998). Species redundancy and ecosystem reliability. Conserv. Biol. 12, 39–45. doi: 10.1111/j.1523-1739.1998.96379.x
Nunes-Neto, N. F., do Carmo, R. S., and El-Hani, C. N. (2013). The concept of function in contemporary ecology. Rev. Filosofia 25, 43–73. doi: 10.7213/revistadefilosofiaaurora.7765
Olsgard, F., Schaanning, M. T., Widdicombe, S., Kendall, M. A., and Austen, M. C. (2008). Effects of bottom trawling on ecosystem functioning. J. Exp. Mar. Bio. Ecol. 366, 123–133. doi: 10.1016/j.jembe.2008.07.036
Otegui, M. B., Brauko, K. M., and Pagliosa, P. R. (2016). Matching ecological functioning with polychaete morphology: consistency patterns along sedimentary habitats. J. Sea Res. 114, 13–21. doi: 10.1016/j.seares.2016.05.001
Oug, E., Fleddum, A., Rygg, B., and Olsgard, F. (2012). Biological traits analyses in the study of pollution gradients and ecological functioning of marine soft bottom species assemblages in a fjord ecosystem. J. Exp. Mar. Biol. Ecol. 432–433, 94–105. doi: 10.1016/j.jembe.2012.07.019
Paganelli, D., Marchini, A., and Occhipinti-ambrogi, A. (2012). Functional structure of marine benthic assemblages using biological traits analysis (BTA): a study along the emilia-romagna coastline (Italy, North-West Adriatic Sea). Estuar. Coast. Shelf Sci. 96, 245–256. doi: 10.1016/j.ecss.2011.11.014
Paterson, D. M., Defew, E. C., and Jabour, J. (2012). “Ecosystem function and co-evolution of terminology in marine science and management,” in Marine Biodiversity and Ecosystem Functioning, 1st Edn, eds M. Solan, R. J. Aspden, and D. M. Paterson (Oxford: Oxford University Press), 24–33. doi: 10.1093/acprof:oso/9780199642250.003.0003
Petchey, O. L., and Gaston, K. J. (2002). Functional diversity (FD), species richness and community composition. Ecol. Lette. 5, 402–411. doi: 10.1046/j.1461-0248.2002.00339.x
Pla, L., Casanoves, F., and Di Rienzo, J. (2012). “Functional diversity indices,” in SpringerBriefs in Environmental Science Quantifying Functional Biodiversity, eds L. Pla, F. Casanoves, and J. Di Rienzo (Dordrecht: Springer), 27–51. doi: 10.1007/978-94-007-2648-2_3
Podani, J., and Schmera, D. (2006). On dendrogram-based measures of functional diversity. Oikos 115, 179–185. doi: 10.1111/j.2006.0030-1299.15048.x
Queirós, A. M., Birchenough, S. N. R., Bremner, J., Godbold, J. A., Parker, R. E., Romero-Ramirez, A., et al. (2013). A bioturbation classification of European marine infaunal invertebrates. Ecol. Evol. 3, 3958–3985. doi: 10.1002/ece3.769
Queirós, A. M., Stephens, N., Cook, R., Ravaglioli, C., Nunes, J., Dashfield, S., et al. (2015). Can benthic community structure be used to predict the process of bioturbation in real ecosystems? Prog. Oceanog. 137, 559–569. doi: 10.1016/j.pocean.2015.04.027
R Development Core Team (2011). R: A Language and Environment for Statistical Computing. Vienna: R Foundation for Statistical Computing.
Romero-Wetzel, R., and Marina-Beatrice Gerlach, S. A. (1991). Abundance, biomass, size-distribution and bioturbation potential of deep-sea macrobenthos on the Voring Plateau (1200-1500 m Norwegian Sea). Meeresfors 33, 247–265.
Shull, D. H., Benoit, J. M., Wojcik, C., and Senning, J. R. (2009). Infaunal burrow ventilation and pore-water transport in muddy sediments. Estuar. Coast. Shelf Sci. 83, 277–286. doi: 10.1016/j.ecss.2009.04.005
Snelgrove, P. V. R., Thrush, S. F., Wall, D. H., and Norkko, A. (2014). Real world biodiversity-ecosystem functioning: a seafloor perspective. Trends Ecol. Evolut. 29, 398–405. doi: 10.1016/j.tree.2014.05.002
Souza, G. B. G., and Barros, F. (2015). Analysis of sampling methods of estuarine benthic macrofaunal assemblages: sampling gear, mesh size, and taxonomic resolution. Hydrobiologia 743, 157–174. doi: 10.1007/s10750-014-2033-z
Strong, J. A., Andonegi, E., Bizsel, K. C., Danovaro, R., Elliott, M., Franco, A., et al. (2015). Marine biodiversity and ecosystem function relationships: the potential for practical monitoring applications. Estuar. Coast. Shelf Sci. 161, 46–64. doi: 10.1016/j.ecss.2015.04.008
Sturdivant, S. K., Díaz, R. J., and Cutter, G. R. (2012). Bioturbation in a declining oxygen environment, in situ observations from wormcam. PLoS One 7:e34539. doi: 10.1371/journal.pone.0034539
Teal, L., Parker, E., and Solan, M. (2010). Sediment mixed layer as a proxy for benthic ecosystem process and function. Mar. Ecol. Prog. Ser. 414, 27–40. doi: 10.3354/meps08736
Teal, L., Parker, R., Fones, G., and Solan, M. (2009). Simultaneous determination of in situ vertical transitions of color, pore-water metals, and visualization of infaunal activity in marine sediments. Limnol. Oceanogr. 54, 1801–1810.
van der Linden, P., Borja, A., Rodríquez, J. G., Muxika, I., Galparsoro, I., Patrício, J., et al. (2016). Spatial and temporal response of multiple trait-based indices to natural- and anthropogenic seafloor disturbance (effluents). Ecol. Ind. 69, 617–628. doi: 10.1016/j.ecolind.2016.05.020
van der Linden, P., Marchini, A., Smith, C. J., Dolbeth, M., Simone, L. R. L., Marques, J. C., et al. (2017). Functional changes in polychaete and mollusc communities in two tropical estuaries. Estuar. Coast. Shelf Sci. 187, 62–73. doi: 10.1016/j.ecss.2016.12.019
van der Linden, P., Patrício, J., Marchini, A., Cid, N., Neto, J. M., and Marques, J. C. (2012). A biological trait approach to assess the functional composition of subtidal benthic communities in an estuarine ecosystem. Ecol. Ind. 20, 121–133. doi: 10.1016/j.ecolind.2012.02.004
Venice System (1958). Symposium on the classification of brackish waters, venice, April 8-14, 1958. Arch. Ocean. Limn. 11, 1–248.
Veríssimo, H., Bremner, J., Garcia, C., Patríco, J., van der Linden, P., and Marques, J. C. (2012). Assessment of the subtidal macrobenthic community functioning of a temperate estuary following environmental restoration. Ecol. Ind. 23, 312–322. doi: 10.1016/j.ecolind.2012.04.020
Villéger, S., Mason, N. W. H., and Mouillot, D. (2008). New multidimensional functional diversity indices for a multifaceted framework in functional ecology. Ecology 89, 2290–2301. doi: 10.1890/07-1206.1
Violle, C., Navas, M. L., Vile, D., Kazakou, E., Fortunel, C., Hummel, I., et al. (2007). Let the concept of trait be functional! Oikos 116, 882–892. doi: 10.1111/j.2007.0030-1299.15559.x
Walker, B. H. (1995). Conserving biological diversity through ecosystem resilience. Conserv. Biol. 9, 747–752.
Walker, B. H., Holling, C. S., Carpenter, S. R., and Kinzig, A. (2004). Resilience, adaptability and transformability in social–ecological systems. Ecol. Soc. 9:5. doi: 10.5751/ES-00650-090205
Wong, M. C., and Dowd, M. (2015). Patterns in taxonomic and functional diversity of macrobenthic invertebrates across seagrass habitats: a case study in Atlantic Canada. Estuar. Coast. 38, 2323–2336. doi: 10.1007/s12237-015-9967-x
Wrede, A., Beermanna, J., Dannheima, J., Gutowa, L., and Breya, T. (2018). Organism functional traits and ecosystem supporting services – A novel approach to predict bioirrigation. Ecol. Ind. 91, 737–743. doi: 10.1016/j.ecolind.2018.04.026
Wrede, A., Dannheim, J., Gutow, L., and Brey, T. (2017). Who really matters: influence of german bight key bioturbators on biogeochemical cycling and sediment turnover. J. Exp. Mar. Biol. Ecol. 488, 92–101. doi: 10.1016/j.jembe.2017.01.001
Keywords: ecosystem functioning, functional traits, estuarine macroinvertebrates, sediment, salinity gradient
Citation: Martins AD and Barros F (2022) Ecological Functions of Polychaetes Along Estuarine Gradients. Front. Mar. Sci. 9:780318. doi: 10.3389/fmars.2022.780318
Received: 20 September 2021; Accepted: 09 February 2022;
Published: 01 March 2022.
Edited by:
Susana Carvalho, King Abdullah University of Science and Technology, Saudi ArabiaReviewed by:
Nafsika Papageorgiou, University of Crete, GreeceSumit Mandal, Presidency University, India
Copyright © 2022 Martins and Barros. This is an open-access article distributed under the terms of the Creative Commons Attribution License (CC BY). The use, distribution or reproduction in other forums is permitted, provided the original author(s) and the copyright owner(s) are credited and that the original publication in this journal is cited, in accordance with accepted academic practice. No use, distribution or reproduction is permitted which does not comply with these terms.
*Correspondence: Amanda Domingues Martins, martins.adomingues@gmail.com