- 1Graduate Program in Veterinary Hygiene (PPGHV), Faculty of Veterinary Medicine, Fluminense Federal University (UFF), Niterói, Brazil
- 2Center for Food Analysis (NAL), Technological Development Support Laboratory (LADETEC), Federal University of Rio de Janeiro (UFRJ), Rio de Janeiro, Brazil
- 3Laboratory of Advanced Analysis in Biochemistry and Molecular Biology (LAABBM), Department of Biochemistry, Federal University of Rio de Janeiro (UFRJ), Rio de Janeiro, Brazil
- 4Agrarian Sciences Center, Department of Zootechnics, Federal University of Paraiba, Areia, Brazil
- 5Laboratório de Avaliação e Promoção da Saúde Ambiental, Instituto Oswaldo Cruz, Fundação Oswaldo Cruz (Fiocruz), Rio de Janeiro, Brazil
- 6Laboratory of Theoretical and Applied Ichthyology, Institute of Biosciences, Federal University of the State of Rio de Janeiro, Rio de Janeiro, Brazil
- 7National Institute of Health Quality Control, Fundação Oswaldo Cruz, Rio de Janeiro, Brazil
- 8Graduate Program in Sanitary Surveillance (PPGVS), National Institute of Health Quality Control (INCQS), Oswaldo Cruz Foundation (FIOCRUZ), Rio de Janeiro, Brazil
- 9Graduate Program in Food Science (PPGCAL), Institute of Chemistry (IQ), Federal University of Rio de Janeiro (UFRJ), Rio de Janeiro, Brazil
- 10Graduate Program in Chemistry (PGQu), Institute of Chemistry (IQ), Federal University of Rio de Janeiro (UFRJ), Rio de Janeiro, Brazil
Mercury (Hg) contamination has increased in the last decades, resulting in human consumption concerns mainly in developing countries. In this context, this study aimed to carry out a health risk assessment regarding the consumption of swimming crabs, shrimp and squid species caught in different regions of the Guanabara Bay, Rio de Janeiro, Brazil. For this purpose, we used calculations that indicated the Estimated Monthly Intake (EMI), Maximum Monthly Intake Rate (IRmm) and Hazard Quotient (HQ). As the target population, the mean weight corresponding to men and women children aged 12 years, young people aged 24 years, and middle-aged people (adults) aged 54 years were used, taking into account the female and male gender. In the studied seafood, the EMI (0.0001 to 0.0006 mg.kg.month-1) was below the monthly intake limit and IRmm (10.3 to 34.8 kg month-1) indicates that large quantities of seafood can be consumed by the population studied, unless the safe limit of monthly intake of the contaminant is reached. The hazard quotient (0.4 to 1.4) indicate potential risks health of children eating swimming crabs and squid and young women eating swimming crabs. Our study also highlights the importance of risk assessments, as even when seafood contains Hg concentrations below established limits, consumption variables must be taken into account, so as not to underestimate the potential health risks.
Introduction
Mercury (Hg) contamination in aquatic environments is a serious concern, especially due to the deleterious effects of this metal on the health of seafood consumers, because of its accumulation and toxic effects (Sadhu et al., 2015; Dias et al., 2016; Harayashiki et al., 2018; Rodrigues et al., 2019a; Rodrigues et al., 2019b; Rodrigues et al., 2020). Mercury is a non-essential metal found both naturally in the environment due to volcanic emanations and atmospheric degassing, and anthropogenically, due to pollution especially caused by mining, sewage activity by industrial sectors like chlor-alkalis and metallurgy plants, fossil fuel burning, and domestic sewage (Baptista Neto et al., 2016; Soares-Gomes et al., 2016; Condini et al., 2017; Harayashiki et al., 2018). In Brazil, the Guanabara Bay estuary, located in the state of Rio de Janeiro, is considered an important Hg contamination area, mainly due to the discharges of industrial and domestic sewage. Despite this, it is a prominent artisanal fishing area in the state, due to a very high diversity of species displaying economic value, found even in polluted waters (Baptista Neto et al., 2016; Rodrigues et al., 2019b; Rodrigues et al., 2020).
Mercury is a highly toxic metal, especially in its organic species, methylmercury (MeHg), where it is more bioavailable for absorption and is excreted more slowly (Arcagni et al., 2018). However, regardless of the chemical species, Hg has the ability to bioaccumulate and biomagnify along the entire food chain, being responsible for several deleterious effects on animal and human health (Arcagni et al., 2018; Chen et al., 2018; Mallory et al., 2018; Taylor and Calabrese, 2018).
In humans, Hg effects are noted mainly after chronic exposures, with the dietary route as the main exposure pathway. Among food items, seafood stands out as the main Hg source for humans, due to the wide distribution of this element in the aquatic environment. In this case, both its inorganic form (displaying an absorption rate between 7 to 15%) and organic form (with an absorption rate of approximately 100% of ingested contents) are responsible for intoxication cases (Hong et al., 2012; Rodrigues et al., 2019a). Acute intoxication cases are also linked to elementary mercury exposure, eliminated in mining industries and activities, which is highly volatile (Tchounwou et al., 2012; Jan et al., 2015). In these cases, symptoms are mainly related to somatosensorial and psychiatric disorders that can progress to death (Ekino et al., 2007). Due to the high liposolubility of Hg, especially in the MeHg form, this element easily crosses the blood-brain and placental barriers, leading to neurological clinical conditions varying in severity, also affecting fetal development. In addition, immunological, cardiovascular and reproductive impairment are also observed (Díez, 2009; Crowe et al., 2017; Gutiérrez-Mosquera et al., 2017; Yin et al., 2017). Chronic intoxication symptoms include cerebellar ataxia, paresthesia of the extremities, somatosensorial disorders, neurological deficits in adults and in children exposed during the prenatal period (Ekino et al., 2007; Bjørklund et al., 2017; Lackner et al., 2018; Rodrigues et al., 2019a). Furthermore, recent studies suggest that Hg exposure may increase an individual’s chance of developing Alzheimer’s disease (Chakraborty, 2017; Pigatto et al., 2018; Yang et al., 2018; Bjørklund et al., 2019).
As mentioned previously, seafood is the main source of Hg exposure to humans. Many studies have evaluated the role of fish consumption regarding health risks due to Hg exposure (Bi et al., 2018; García-Hernández et al., 2018; Kelly et al., 2018), although others animals such as crustaceans (e.g., swimming crabs, crabs and shrimps) and molluscs (e.g., squid) are very popular in global cuisine and also represent an important Hg exposure risk (Koenig et al., 2013; Liu et al., 2019). This is mainly due to the trophic niche position and habitats of these animals. Crabs and shrimp belong to the lower trophic level, comprising benthic organisms that live in close contact with the sediment, a Hg sink, which can favor high contamination in these animals. Squids are pelagic and animals belonging to higher trophic levels, in some cases, at the same level as fish, as they are predators, and in some cases, due to the effect of biomagnification, can host large amounts of Hg in their bodies (Andrade et al., 2014; Taylor and Calabrese, 2018; Das et al., 2019; Liu et al., 2019). Thus, the assessment of potential health risks due to the consumption of these animals should be further explored.
Risks, however, may be underestimated when evaluating only metal concentrations in seafood. Therefore, several factors, such as the consumption frequency of a certain food item, as well as the ingested amounts and exposure period, must also be taken into account. In this regard, the Hazard Quotient (HQ) is noteworthy as an important tool, more accurately representing human health risks, precisely because it considers different factors that interfere in risk occurrence (Copat et al., 2014; Barone et al., 2015; García-Hernández et al., 2018).
Hence, the aim of this study was to carry out a health risk assessment in relation to the consumption of different species of swimming crabs, shrimps and squids captured in different locations in Guanabara Bay, Rio de Janeiro, Brazil. For this purpose, calculations were carried out to assess whether the Hg concentration present in the samples would exceed the tolerable monthly limits, what maximum permitted amount of consumption for the species studied that would not exceed the monthly limit, and the hazard quotient. In this assessment, we simulated the health risk of three populations, children aged 12 years, young people aged 24 years and adults aged between 45 and 54 years, considering men and women separately.
Material and Methods
Study Area
All animals were collected by bottom trawls at the Guanabara Bay estuary (22° 24′ – 22° 57′ S, 42° 33′ – 43° 19′ W), located in the state of Rio de Janeiro, southeastern Brazil (Figure 1). Seven collection points were chosen, representing the estuarine extension, as follows: Cagarras Island (P1), Copacabana Beach (P2), Urca Beach (P3), Flamengo Beach (P4), Rio de Janeiro seaport (P5), Engenho (P6) and Paquetá Island (P7). A total of 14 bottom trawls (two collections at each point) were carried out at sampling site using a single-port net (25 m net mouth and 6 m high, 25 mm mesh between adjacent nodes). Points were also selected according to the following criteria: presence of port activity and vessel flow (Seaport region), areas of irregular effluent disposal (present in all sampling points, mainly Engenho and Paquetá), leisure areas and tourist attractions (Cagarras Island, Copacabana beach, from Urca, Flamengo and Paquetá), and local fishing activities (present at all sampling points, especially Copacabana, Urca and the Seaport).
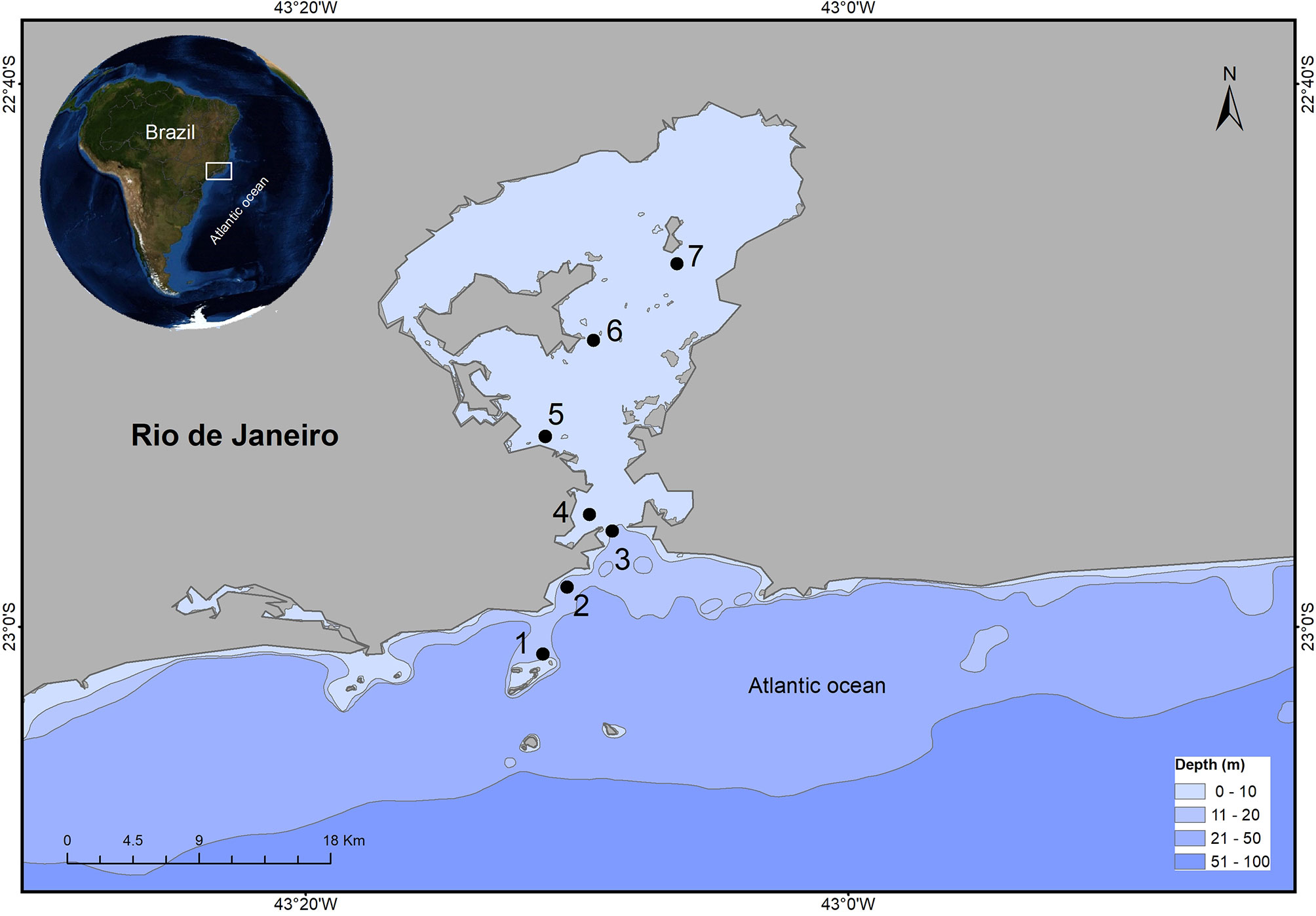
Figure 1 Guanabara Bay shrimp, swimming crabs and squid sampling points: P1, Cagarras Island (23°03’24.86’’S; 43°05’55.95’’W); P2, Copacabana Beach (22°59’24.29’’S; 43°09’05.57’’W); P3, Urca Beach (22°54’10.12’’S; 43°09’05.57’’W); P4, Flamengo Beach (22°55’27.10’’S; 43°09’02.43’’W); P5, Seaport of Rio de Janeiro (22°53’23.73’’S; 43°11’03.45’’W); P6, Engenho-São Gonçalo (22°50’15.76’’S; 43°08’09.97’’W); and P7, Paquetá Island (22°47’18.87’S; 43°07’02.23’’W).
Animals Collected
A total of 125 animals were collected, 48 of which were swimming crabs, 58 shrimps and 19 squids. At each points, we find the following number of animals: 4 at P1 (2 crabs and 2 squids), 17 at P2 (2 crabs, 12 shrimps and 3 squids), 34 at P3 (10 crabs, 21 shrimps and 3 squids), 15 at P4 (6 crabs, 4 shrimps and 5 squids), 28 at P5 (10 crabs and 18 shrimps), 13 at P6 (10 crabs and 3 shrimps) and 14 at P7 (8 crabs and 6 squids) (Table 1). After sampling, the animals were taken to the laboratory, identified up to the genus and species, using specialized literature (Crab Database, 2016; FAO, 2018; WRMS, 2019), and dissection of the musculature was performed for Hg quantification. The musculature was chosen as the target tissue, since it is the main tissue destined for food in the three groups of animals.
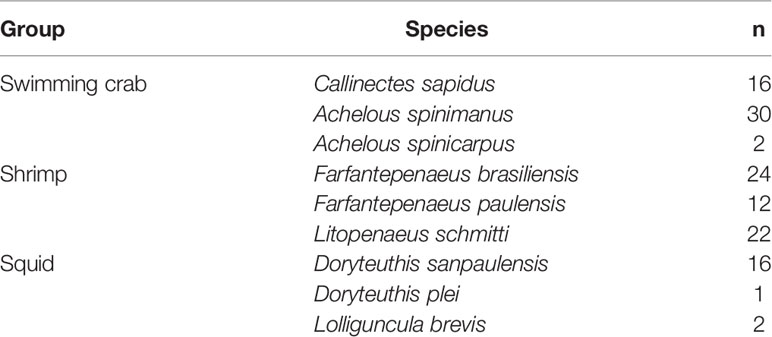
Table 1 Swimming crab, shrimp, and squid species sampled from Guanabara Bay, Rio de Janeiro, Brazil.
Mercury Quantification
Before each sample analysis, blank values were verified to be always less than 0.001 Hg (ng). The boats that received the samples were previously dried in a muffle furnace (650°C at 5 minutes) to remove the possible presence of residues from the previous samples. Total Hg in the animals was determined by the atomic absorption spectrometry method at Direct Mercury Analyzer (DMA-80), following the manufacturer’s recommendations (DMA-80, Milestone, Bergamo, Italy). The equipment was previously calibrated using a standardized Hg solution (Sigma-Aldrich, São Paulo, Brazil). Was prepared a calibration curve from a 1000 mg L−1 Hg stock, used to build the ten-point calibration curve ranging from 0, 0.5, 1, 2, 3, 5, 10, 20, 50, 100 ng g−1 (0–100 ng g−1; y = 22.085 × x − 0.3217; r 2 = 0.9992). Analytical parameter optimization was performed by limit of quantification (LOQ) and limit of detection (LOD). The LOQ and LOD were determined as recommended by 2011/836/EU Regulation, and the methodology validation was based on Torres et al. (2012).
Briefly, about 0.2700 g ± 0.0030 g of each muscle sample were inserted in a quartz boat and dried under an oxygen stream at 160 °C for 1 min, 650 °C for 2 min, and 650 °C for another 1 min, at 3.1 atm. After drying, the Hg vapor was then desorbed using a gold amalgamation trap and released for reading after heating. The detection is performed at a wavelength of 253.7 nm and the results were expressed as mg kg−1. Analyses were performed in triplicate.
Risk Assessment Calculation
Calculations were first carried out to determine the Estimated Monthly Intake (EMI) (Equation 1) and maximum monthly Ingestion Rate (IRmm) (Equation 2). The EMI calculation was used to verify whether the Hg concentration present in the average amount of seafood consumed will exceed the permitted monthly consumption limit, established by JECFA (FAO, 2011) and called provisional tolerable mouth intake (PTMI) (0.017 mg kg-1 month-1), which was converted from the weekly PTWI value (0.004 mg kg-1). In addition, the IRmm identifies the maximum amount of seafood that can be consumed so as not to exceed the PTMI. Subsequently these data were used to calculate the Hazard Quotient (HQ) (Equation 3). The equations 1 were based on Barone et al. (2015) and equation 3 on Aquino et al. (2017) and followed Environmental Protection Agency guidelines (US-EPA, 1989; US-EPA, 2000). In the HQ equation, values above 1 represent potential damage to consumer health.
EMI: estimated monthly intake; IRmm: maximum monthly Ingestion Rate; IR: ingestion rate (0.093 kg per week and 0.372 per month); C: mercury concentration (mg kg-1); BW: body weight; PTMI: is provisorial tolerable month intake (0.017 mg kg-1 month-1); HQ: hazard quotient; EF: exposure frequency (48 days year-1); ED: exposure duration (12 or 24 or 54 years); RfD: estimate of a safe daily oral exposure (Hg = 0.0001 mg kg-1 day-1; US-EPA, 2000); TA: average exposure time for non-carcinogens (“EF” X “ED”).
In order to obtain a risk assessment for different age groups and taking into account the differences between men and women, we performed calculations based on the average weight of three age groups: children aged 12 years (boys 42 kg and girls 46 kg), young aged 24 years (men 72 kg and women 59 kg) and adults aged 54 years (men 78 kg and women 66 kg). This information was taken from the platform of the Brazilian Institute of Geography and Statistics (IBGE), which contains data from the Family Budget Survey - Population estimates of the median height and weight of children, adolescents and adults, by sex and age, in the state of Rio de Janeiro.
Statistical Analyses
Data normality was checked by the Kolmogorov-Smirnov test. The difference between genera and between species was performed using an ANOVA and Fisher’s Least Significant Difference (LSD) post-hoc test. The Statistica® 12 and OriginPro 2020b Trial software programs were used. A significance level of 0.05 was employed for all assessments.
Results and Discussion
The Hg concentrations detected in the investigated animals were below both Brazilian limits [0.5 mg kg-1 Hg for non-predator and 1.0 for predator species (ANVISA, 2013)] and international guidelines (0.5 mg kg-1 Hg) (ANZFA, 2000; FAO, 2003; DOH, 2004; EU, 2005; CSF, 2018; FSA, 2020). The differences between the concentrations of THg in the species studied are identified in Figure 2, however, we did not identify a statistical difference between species of the same type of animal. Mercury concentrations is only statistically different between the three groups of animals studied. Swimming crabs, showed greater contamination which can be attributed to their benthic habitat, in close contact with contaminated sediment. In addition, the bioturbation movement scarred out by these animals result in the resuspension of contaminated sediment takes place, making previously immobilized Hg bioavailable for absorption by the local biota (Das et al., 2019; Liu et al., 2019). When compared to shrimp, which are categorized as the same trophic level, crabs exhibit higher metal assimilation rates and lower excretion rate, resulting in increased Hg values (Evans et al., 2000). Squid were expected to present higher Hg contents due to their predatory habits. This, however, was not observed herein. This can be attributed to the fact that squid are typically pelagic, remaining, consequently, away from the bottom sediment, where Hg is often absorbed. Additionally, Liu et al. (2019) describe that the biomagnification process is more efficient in benthic chains than pelagic ones, corroborating higher Hg concentrations in crabs compared to squids. In addition, physiological and metabolism differences, as well as related to the varied contamination of the Guanabara Bay collection points may also justify this difference (Das et al., 2019; Liu et al., 2019). Other works presented in a literature review by Rodrigues et al. (2021a) point to the same result found here, indicating that not only for Hg but for other metals, crustaceans, especially crabs and swimming crabs, stand out as important bioaccumulators of these elements, sometimes overcoming the effect of biomagnification (Hosseini et al., 2014; Baki et al., 2018).
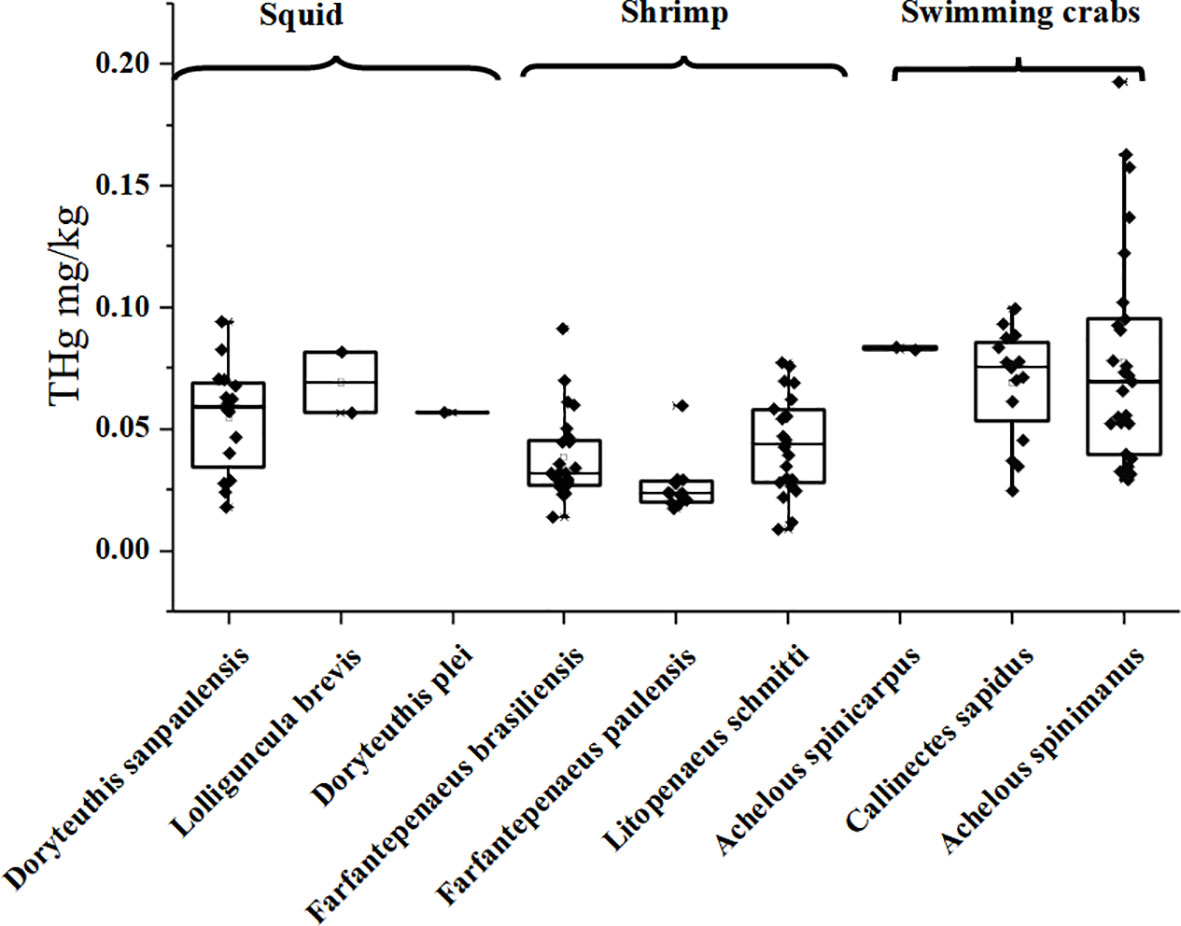
Figure 2 Box-plot representing THg concentrations (mg/kg) in different species of squid, shrimp and swimming crabs collected in Guanabara Bay.
In this work we used samples for quantification of Hg, the musculature of the animals. This is because this is the main tissue intended for consumption by aquatic organisms in general (Bisi et al., 2012; Ruus et al., 2017; Arcagni et al., 2018). However, this tissue is not the one with the greatest tropism for the accumulation of metals such as Hg. Rodrigues et al. (2021a) present in their review work a table that points out that, in crustaceans, the main bioaccumulating organ is the hepatopancreas, and secondly the gills, followed by the musculature. In other aquatic organisms, the liver is the organ that performs the function of the hepatopancreas of crustaceans. For this reason, it is also the main accumulator of xenobiotics in fish and cephalopods, such as squid (Azevedo et al., 2016; Mallory et al., 2018; Murillo-Cisneros et al., 2018). This organ stands out for its role in the detoxification process of contaminants, especially for the presence and activity of the metallothionein protein responsible for this mechanism (Azevedo et al., 2012; Hosseni et al., 2013). It has a high percentage of amino, nitrogen and sulfur groups used to sequester metals (Hosseni et al., 2013). On the other hand, despite not being the main site of Hg accumulation, the musculature also plays an important role as a long-term reservoir (Azevedo et al., 2012). This is because when compared to the liver/hepatopancreas, the musculature has a low potential for metal clearance. Even with low concentrations of metallothionein, Hg reaches the muscle through amino acid thiol ligands that transport it to that tissue (Onsanit and Wang, 2011; Murillo-Cisneros et al., 2018). Thus, based on the importance of muscle consumption, its evaluation is essential, even though it is not the main reservoir of Hg.
Still, on the aspects that can influence the concentration of the contaminant, in addition to the biotic part, there are also abiotic factors, such as water temperature, salinity, oxygenation and pH. These factors were not evaluated in this study; however, based on other previously published studies, it is possible to indicate that such factors are responsible for influencing the bioavailability, speciation, and toxicity of Hg in the Guanabara Bay region (Rodrigues et al., 2019b; Rodrigues et al., 2020; Rodrigues et al., 2021b). The salinity of BG varies between 31 and 35 g.L-1, being closer to the salinity of the marine environment, while the pH is around 8, indicating an alkaline environment (Rodrigues et al., 2021b). Environments with such a salinity rate have a lower concentration of Hg when compared to freshwater habitats. This is because the sulfide is present in saline water complexes with Hg, making it less available for absorption by the biota. As for pH, the bioavailability of Hg is increased in environments where it tends to be acidity (Wang et al., 2010; Dong et al., 2016; Reinhart et al., 2018; Costa et al., 2019). As for BG temperature, varies between 17 and 25°C, and oxygenation between 3 and 6 mg.L-1 (Rodrigues et al., 2021b). Temperature influences animal metabolism, with higher temperatures responsible for promoting greater excretion of metals such as Hg (Ando et al., 2010). Regarding oxygenation, lower concentrations of oxygen can favor a greater uptake of the same and consequently a greater gill absorption of the contaminants, in addition to favoring the process of methylation of Hg, due to the better activity in this environment of the sulfate-reducing bacteria responsible for the process (Sadhu et al., 2015). However, most of the characteristics of BG end up disfavoring the acquisition of Hg by the biota due to its low availability for absorption. In addition, a large amount of local organic matter complexes with Hg also reduces its bioavailability (Kehrig et al., 2007). Thus, regardless of the risk factor for consumption, the low concentrations of Hg in BG can be justified by unfavorable abiotic conditions, such as an intensely polluted environment.
The maximum amounts of each seafood species that can be consumed in order not to exceed the monthly intake limit (IRmm) and the EMI values, indicating the concentration of THg consumed monthly through the ingestion of the studied seafood, are shown in Table 2. The EMI results indicate that for all species the THg concentration consumed (93 g per week, 372 g per month) from seafood is very low and below the PTMI limit (0.017 mg.kg.month-1). Besides, the results on the IRmm calculation, shown in Table 2, indicate that the estimated amount of consumption allowed to not exceed the provisional tolerable monthly intake for Hg is very high, reaching 34.8 kg for adults and 10.3 for children, demonstrating consumption security, since the quantity that is possibly consumed in the region is very low. General data obtained through statistics available at the Brazilian Institute of Geography and Statistics (IBGE, 2020), indicate that the average per capita consumption of seafood, except fish, in Brazil is 0.2 g day-1 representing 0.3% of total consumption of these food items. Due to the lack of information in the literature regarding the consumption amount and frequency of the groups of animals investigated herein for the state of Rio de Janeiro, the EMI and HQ calculations were performed using an amount of 93 g month-1 of seafood. This value was based on the work by Costa et al. (2021), who indicated the weekly consumption of 93g shrimp by the Brazilian population, a value that we extrapolated to the other animals studied.
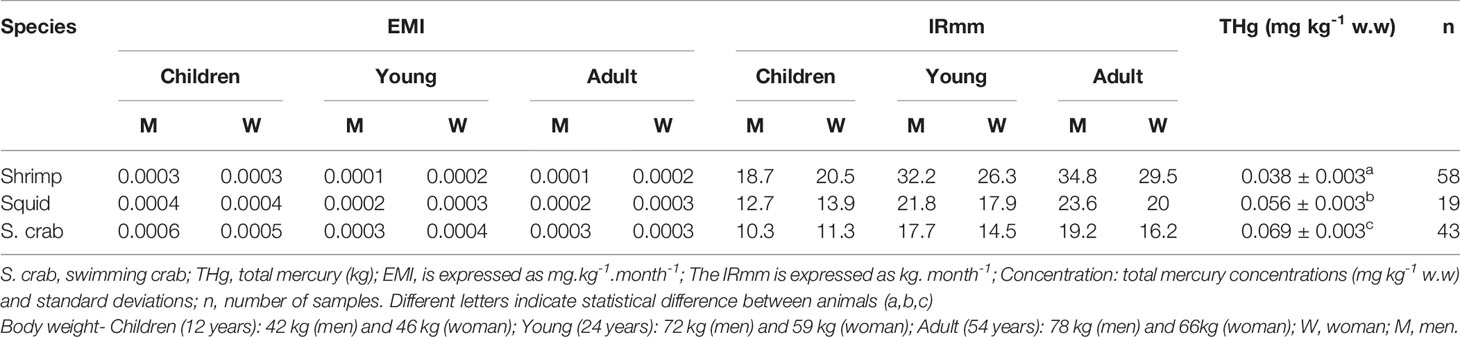
Table 2 Maximum consumption rates for squid, shrimp, and swimming crabs sampled from Guanabara Bay, Rio de Janeiro, southeastern Brazil, per month (IRmm in kg month-1) and EMI (mg kg-1 month-1).
Our expectation was that the results obtained in these studies were in line with other results in the literature that demonstrate that, although the concentrations of Hg in these seafood species are below the limit, when considering the amount consumed and the frequency, the calculation would indicate the existence of health risk (HQ>1). In this sense, according to Table 3 we obtained this result when we evaluated the child population of both sexes and women consumers of swimming crabs and squids and young people (24 years old), consumers of swimming crabs. For example, Okati and Esmaili-sari (2017) in their study of fish and shrimp collected in three cities (Bandar Abbas, Bushehr and Mahshahr) located on the coast of the Persian Gulf reported an amount of monthly shrimp consumption (Paeneus semisulcatus and Metapenaeus affinis) (IRmm g month-1) of 450 g for children and 990 g for adults, which would result in HQ values of 1.32 and 0.82, respectively. Copat et al. (2014) also identified that, although Hg concentrations in some seafood items from the Gulf of Catania (Ionian Sea) [species: Donax trunculu (crustaceans), Arnoglossus laterna, Mullus barbatu, Engraulis encrasicolus, Trachurus trachurus, Scomber scombrus (all fish)] were within the limit, the intake calculations applying the size of the meals exceeded the doses recommended by JECFA and the HQ values for Hg were greater than 1 in some cases.

Table 3 Results of the Hazard Quotient evaluation in three age groups of the consumer population of squid, shrimp, and swimming crabs sampled from Guanabara Bay, Rio de Janeiro, southeastern Brazil.
When comparing groups comprising different animals, Zhang et al. (2018) identified that fish from major markets in Beijing exhibited higher HQ values than other types of seafood, such as squid and shrimp, which presented HQ values below 1.0. Okati and Esmaili-sari (2017), when comparing fish and shrimp species from different locations within the study region of three cities (Bandar Abbas, Bushehr and Mahshahr) located on the Persian Gulf coast, reported only one HQ value greater than 1.0, regarding shrimp consumption in a population of children and women from fishing communities. Barone et al. (2015) in their study with different species of cephalopods, fish, and crustaceans, identified that none of the groups had values greater than 1.0 in the HQ calculation, and shrimp and squid species from Italy were those that obtained the lowest values. Mishra et al. (2007) also identified HQ<1 values for crabs, praws and bivalves species. These results are in part in agreement with our study, since we did not find values greater than 1 associated with the risk of shrimp consumption and we only identified risk for children consuming squid. In our study, the main risk was associated with the consumption of swimming crabs, since these animals had the highest concentration of Hg when compared to other groups of animals.
Another fundamental aspect to be debated is the difference between the sex and age of the population studied. It is clear that the child population is more likely to develop health problems associated with the ingestion of this contaminant via seafood consumption, since they have a lower body mass, in addition to being with the organism in the development phase (Zamora-Arellano et al., 2017; García-Hernández et al., 2018). These weaknesses may favor the greatest risk to this population. Thus, Zhang et al. (2018) also identified a higher health risk in the 2 to 7-year-old age group with regard to the consumption of certain species of fish, shrimp and squid found in the Beijing market contaminated with Hg. Therefore, the body weight item is an important influencing factor in the risk assessment, since in the evaluated population, the highest HQ values found are in groups with lower body mass, such as children and young and adult women.
Women, mainly in the 20-29 age group, are considered to be of reproductive age. These women, whether pregnant or looking to become pregnant, are sometimes recommended not to consume fish, precisely because it is an important source of metals with genotoxic, carcinogenic and mutagen potential, such as Hg, which has the ability to cross the blood-brain and placental barrier (Ekino et al., 2007; Bjørklund et al., 2017; Lackner et al., 2018). Thus, García-Hernández et al. (2018) in their study identified that women from a fishing community in Kino Bay, Sonora, Mexico were at high risk in relation to MeHg, especially in the age group between 40-49 years, while women between 20-29 years were at lower risk. The study then relates this result to a lower frequency of consumption of seafood in the age group that comprises women of reproductive age, due to this recommendation and a tendency to reduce consumption due to reproduction. Okati and Esmaili-sari (2017) also identified a higher risk for the population of children and women of reproductive age. In this case, such a result was seen in individuals belonging to fishing communities, where the consumption of this type of food is higher than when compared to the non-fishing population.
Another issue that has not been evaluated and of worth highlighting is the issue of heat treatment to which the seafood is subjected before being consumed. In general, treatments that subject food to heating can lead to direct losses of Hg through volatilization or indirect losses, changing the speciation of this metal to a more volatile form (for example, CH3Hg-CH3) (Schmitd et al., 2018; Liao et al., 2019). Heat can also lead to changes in solubility and induce metal complexation with other biological components, such as proteins, amino acids or insoluble compounds, thus precipitating during cooking (Maulvault et al., 2011; Schmitd et al., 2018). In addition, several studies indicate that heat treatment can lead to reduced digestibility through changes in conformation or structural losses of the protein associated with Hg. Consequently, these changes can reduce the susceptibility to the action of the protease, decreasing the bioaccessibility of the metal during the gastrointestinal digestion process (Maulvault et al., 2011; Cano-Sancho et al., 2015; Liao et al., 2019). Thus, considering the heat treatment to reduce the Hg concentration in the food and to reduce the bioaccessibility of the metal at the time of digestion, it is possible to indicate that the Hg concentrations that are already low in the seafood studied here, will be lower when subjected to heat treatment, such as cooking, so the health risk may be even lower than the values provided here.
Surprisingly, the Hg risk from consuming seafood was low. It is noteworthy, considering that Guanabara Bay and nearby beaches have water unsuitable for bathing, and several locations in the Bay have intense tailings odors all year round. In this way, contamination by domestic sewage might be being released into this bay in greater quantities, and consequently causing much impacts, when compared to industrial waste.
Conclusions
Mercury contamination in crabs, squid, and shrimp from Guanabara Bay is below the limit proposed by Brazilian and international legislation. However, when we consider the average weight of the population, frequency, and quantity consumed, we reveal the risk to the people of infantile and young women consumers of the species with the highest concentration of Hg. Our study reinforces the importance of studying these indicators, not underestimating the risks of Hg consumption when only contamination in seafood is determined. The present study is notable for addressing commercially important but understudied seafood species concerning Hg risk assessments. We also reinforce that, as it is an element that bioaccumulates in the body throughout life, it is not certain that there is no harm to the health of the adult population related to mercury contamination and that the existence of public policies to control contamination by Hg and other pollutants is fundamental in the ecosystem of Guanabara Bay.
Data Availability Statement
The raw data supporting the conclusions of this article will be made available by the authors, without undue reservation.
Author Contributions
PR: Investigation, Formal analysis, Data curation, Writing – original draft, Writing – review and editing. RF: Investigation, Formal analysis, Data curation, Writing – original draft, Writing – review and editing. DR: Formal analysis, Data curation, Writing – review and editing. RH-D: Investigation, Formal analysis, Supervision, Conceptualization, Resources, Data curation, Writing – original draft, Writing – review and editing. LS: Supervision, Conceptualization, Resources. CC-J: Supervision, Conceptualization, Resources, Data curation, Writing – original draft, Writing – review and editing. All authors contributed to the article and approved the submitted version.
Funding
The authors are thankful for the financial support provided by the Fundação de Amparo à Pesquisa do Estado do Rio de Janeiro (FAPERJ) Brazil — grant number [E‐26/203.049/2017, E26/201.167/2020 and E-26/201.947/2020]; the Conselho Nacional de Desenvolvimento Científico e Tecnológico (CNPq) Brazil – [process no. 313119/2020-1; PELD Baía de Guanabara] and the Coordenação de Aperfeiçoamento de Pessoal de Nível Superior (CAPES) Brazil — Finance Code 001.
Conflict of Interest
The authors declare that the research was conducted in the absence of any commercial or financial relationships that could be construed as a potential conflict of interest.
The reviewer CCA declared a shared affiliation with the author RAHD to the handling editor at the time of review.
Publisher’s Note
All claims expressed in this article are solely those of the authors and do not necessarily represent those of their affiliated organizations, or those of the publisher, the editors and the reviewers. Any product that may be evaluated in this article, or claim that may be made by its manufacturer, is not guaranteed or endorsed by the publisher.
References
Ando M., Seoka M., Mukai Y., Jye M. W., Miyashita S., Tsukamasa Y. (2010). Effect of Water Temperature on the Feeding Activity and the Resultant Mercury Levels in the Muscle of Cultured Bluefin Tuna Thunnus Orientalis (Temminck and Schlegel). Aquac. Res. 42 (4), 516–524. doi: 10.1111/j.1365-2109.2010.02647.x
Andrade L. S., Frameschi I. F., Costa R. C., Castilho A. L., Fransozo A. (2014). The Assemblage Composition and Structure of Swimming Crabs (Portunoidea) in Continental Shelf Waters of Southeastern Brazil. Cont. Shelf. Res. 94, 8–16. doi: 10.1016/j.csr.2014.12.005
ANVISA (2013). “Agência Nacional De Vigilância Sanitária (ANVISA), Ministério Da Saúde. RDC N° 42 De 29 De Agosto De 2013,” in Dispõe Sobre O Regulamento Técnico MERCOSUL Sobre Limites Máximos De Contaminantes Inorgânicos Em Alimentos (Brasília, DF, 29 ago. 2020: Diário Oficial [da] República Federativa do Brasil).
Aquino L. F. M. C., Ribeiro R. O. R., Simoes J. S., Mano S. B., Mársico E. T., Conte-Junior C. A. (2017). Mercury Content in Whey Protein and Potencial Risk for Human Health. J. Food Compos. Anal. 59, 141–144. doi: 10.1016/j.jfca.2017.02.014
Arcagni M., Juncos R., Rizzo A., Pavlin M., Fajon V., Arribére M. A., et al. (2018). Species- and Habitat-Specific Bioaccumulation of Total Mercury and Methylmercury in the Food Web of a Deep Oligotrophic Lake. Sci. Tot. Environ. 612, 1311–1319. doi: 10.1016/j.scitotenv.2017.08.260
Australia and New Zealand Food Authority (ANZFA). (2000). Provisional Regulation Limitation of Contamination in Food, Specifications and Standards for Foods, Food Additives, etc. Under The Food Sanitation Laws, April 2004, Standard 1.4.1, Contamination and Natural Toxicants, Maximum Level of Metal Contaminates in Food, Australia and New Zealand Food Authority Amendment No.53 to Food Standard Code, Commonwealth of Australia.
(2016). Crab Database, Taxon Tree of Crabs. Available at: https://www.crabdatabase.info/en/crabs (Accessed 12 December 2021).
Azevedo L. S., Almeida M. G., Bastos W. R., Suzuki M. S., Recktenvald M. C. N. N., Bastos M. T. S., et al. (2016). Organotropism of Methylmercury in Fish of the Southeastern of Brazil. Chemosphere 185, 746–753. doi: 10.1016/j.chemosphere.2017.07.081
Azevedo J., Souza S., Oliveira T., Ulrich J. (2012). Tissue-Specific Mercury Concentrations in Two Catfish Species From the Brazilian Coast. Braz. J. Ocean. 60 (2), 211–219. doi: 10.1590/S1679-87592012000200011
Baki M. A., Hossain M. M., Akter J., Quraishi S. B., Haque Shojib M. F., Atique Ullah A. K. M., et al. (2018). Concentration of Heavy Metals in Seafood (Fishes, Shrimp, Lobster and Crabs) and Humanhealth Assessment in Saint Martin Island, Bangladesh. Ecotoxicol. Environ. Saf. 159, 153–163. doi: 10.1016/j.ecoenv.2018.04.035
Baptista Neto J. A., Barreto C. F., Vilela C. G., Fonseca E. M., Melo G. V., Barth O. M. (2016). Environmental Change in Guanabara Bay, SE Brazil, Based in Microfaunal, Pollen and Geochemical Proxies in Sedimentary Cores. Ocean. Coast. Manag. 143, 4–15. doi: 10.1016/j.ocecoaman.2016.04.010
Barone G., Storelli A., Garofalo R., Busco V. P., Quaglia N. C., Centrone G., et al. (2015). Assessment of Mercury and Cadmium via Seafood Consumption in Italy: Estimated Dietary Intake (EWI) and Target Hazard Quotient (THQ). Food Addit. Contam.: Part A. 32 (8), 1277–1286. doi: 10.1080/19440049.2015.1055594
Bi B., Liu X., Guo X., Lu S. (2018). Occurrence and Risk Assessment of Heavy Metals in Water, Sediment, and Fish From Dongting Lake, China. Environ. Sci. Pollut. Res. 25 (34), 34076–34090. doi: 10.1007/s11356-018-3329-8
Bisi T. L., Lepoint G., Azevedo A. D. F., Dorneles P. R., Flach L., Das K., et al. (2012). Trophic Relationships and Mercury Biomagnification in Brazilian Tropical Coastal Food Webs. Ecol. Indic. 18, 291–302. doi: 10.1016/j.ecolind.2011.11.015
Bjørklund G., Dadar M., Mutter J., Aaseth J. (2017). The Toxicology of Mercury: Current Research and Emerging Trends. Environ. Res. 159, 545–554. doi: 10.1016/j.envres.2017.08.051
Bjørklund G., Tinkov A. A., Dadar M., Rahman M. M., Chirumbolo S., Skalny A. V., et al. (2019). Insights Into the Potential Role of Mercury in Alzheimer’s Disease. J. Mol. Neurosci. 67 (4), 511–533. doi: 10.1007/s12031-019-01274-3
Cano-Sancho G., Perello G., Maulvault A. L., Marques A., Nadal M., Domingo J. L. (2015). Oral Bioaccessibility of Arsenic, Mercury and Methylmercury in Marine Species Commercialized in Catalonia (Spain) and Health Risks for the Consumers. Food Chem. Toxicol. 85, 34–40. doi: 10.1016/j.fct.2015.09.012
Center of Food Safety (CSF) Hong Kong. (2018). “Food Legislation/Guidelines, Part V (Food and Drugs) of the Public Health and Municipal Services Ordinance (Cap. 132),” in Food Adulteration (MetallicContaminatio) Regulations. Hong Kong: Health and Municipal Services Ordinance. Available at: https://www.cfs.gov.hk/english/food_leg/food_leg_mc.html.
Chakraborty P. (2017). Mercury Exposure and Alzheimer’s Disease in India—an Imminent Threat? Sci. Tot. Environ. 589, 232–235. doi: 10.1016/j.scitotenv.2017.02.168
Chen M. M., Lopez L., Bhavsar S. P., Sharma S. (2018). What's Hot About Mercury? Examining the Influence of Climate on Mercury Levels in Ontario Top Predator Fishes. Environ. Res. 162, 63–73. doi: 10.1016/j.envres.2017.12.018
Condini M. V., Hoeinghaus D. J., Roberts A. P., Soulen B. K., Garcia A. M. (2017). Mercury Concentrations in Dusky Grouper Epinephelus Marginatus in Littoral and Neritichabitats Along the Southern Brazilian Coast. Mar. Pollut. Bull. 115 (1–2), 266–272. doi: 10.1016/j.marpolbul.2016.12.006
Copat C., Vicenti M., D'Agati M. G., Arena G., Mauceri V., Grasso A., et al. (2014). Mercury and Selenium Intake by Seafood From the Ionian Sea: A Risk Evaluation. Ecotoxicol. Environ. Saf. 100, 87–92. doi: 10.1016/j.ecoenv.2013.11.009
Costa B. N. S., Almeida H. P., Silva B. C. P., Figueiredo L. G., Oliveira A. M., Lima M. O. (2019). Macrobrachium Amazonicum (Crustacea, Decapoda) Used to Biomonitor Mercury Contamination in Rivers. Arch. Environ. Contam. Toxicol. 78, 245–253. doi: 10.1007/s00244-019-00683-0
Costa B. S., Custódio F. B., Deus V. L., Hoyos D. C. M., Gloria M. B. A. (2021). Mercury in Raw and Cooked Shrimp and Mussels and Dietary Brazilian Exposure. Food Control 121, 107669. doi: 10.1016/j.foodcont.2020.107669
Crowe W., Allsopp P. J., Watson G. E., Magee P. J., Strain J. J., Armstrong D. J., et al. (2017). Mercury as an Environmental Stimulus in the Development of Autoimmunity- a Systematic Review. Autoimmun. Rev. 16 (1), 72–80. doi: 10.1016/j.autrev.2016.09.020
Das S., Tseng L. C., Chou C., Wang L., Souisssi S., Hwang J. S. (2019). Effects of Cadmium Exposure on Antioxidant Enzymes and Histological Changes in the Mud Shrimp Austinogebia Edulis (Crustacea: Decapoda). Environ. Sci. Pollut. Res. 26 (8), 7752–7762. doi: 10.1007/s11356-018-04113-x
Dias D., Bessa J., Guimarães S., Soares M. E., Bastos M., Texeira H. M. (2016). Inorganic Mercury Intoxication: A Case Report. Forensic Sci. Int. 259, e20–e24. doi: 10.1016/j.forsciint.2015.12.021
Díez S. (2009). Human Health Effects of Methylmercury Exposure. Rev. Environ. Contam. Toxicol. 198, 111–132. doi: 10.1007/978-0-387-09647-6_3
Dong W., Liu J., Wei L., Jingfeng Y., Chernick M., Hinton D. E. (2016). Developmental Toxicity From Exposure to Various Forms of Mercury Compounds in Medaka Fish (Oryzias Latipes) Embryos. Peer. J. 4, e2282. doi: 10.7717/peerj.2282
Ekino S., Susa M., Ninomiya T., Imamura K., Kitamura T. (2007). Minamata Disease Revisited: An Update on the Acute and Chronic Manifestations of Methyl Mercury Poisoning. J. Neurol. Sci. 262 (1–2), 131–144. doi: 10.1016/j.jns.2007.06.036
European Commission. (2005). Commission Regulation (EC) No 78/ 2005 of 19 January 2005 Amending Regulation (EC) No 466/2001 as Regards Heavy Metals. Available at: http://eur-lex.europa.eu/LexUriServ/LexUriServ.do?uri=OJ:L:2005:016:0043:0045:EN:PDF (Accessed 26 Feb 2020).
Evans D., Kathman R., Walker W. (2000). Trophic Accumulation and Depuration of Mercury by Blue Crabs (Callinectes Sapidus) and Pink Shrimp (Penaeus Duorarum). Mar. Environ. Res. 49 (5), 419–434. doi: 10.1016/S0141-1136(99)00083-5
Food and Agriculture Organization (FAO) (2003). Food and Agriculture Organization (FAO) Heavy Metal Regulations–Faolex. Legal Notice No.66/2003. Eritrea: FAO/FAOLEX. Available at: https://www.informea.org/en/legislation/heavy-metals-regulations-ln-no-66-2003
Food and Agriculture Organization of the United Nations (FAO) (2011). Evaluations of Certain Contaminants in Food: SeventySecond Report of the Joint FAO/WHO Expert Committee on Food Additives (Roma: Food and Agriculture Organization/WHO), 115.
Food and Agriculture Organization of the United Nations. (2018). “FAO FishFinder,” in Species Fact Sheets. Roma: Food and Agriculture Organization/WHO. Available at: http://www.fao.org/fishery/species/2632/en.
Food Standards Agency (FSA) UK (2020). “Chemical Contaminant Monitoring,” in Limit for Chemical Contaminants. United Kingdom: Food Standards Agency. Available at: https://www.food.gov.uk/business-guidance/chemical-contaminant-monitoring.
García-Hernández J., Ortega-Vélez M. I., Contreras Paniagua A. D., Aguilera-Márquez D., Leyva-García G., Torre-Cosío J. (2018). Mercury Concentrations in Seafood and the Associated Risk in Women With High Fish Consumption From Coastal Villages of Sonora, Mexico. Food Chem. Toxicol. 120, 367–377. doi: 10.1016/j.fct.2018.07.029
Gutiérrez-Mosquera H., Sujitha S. B., Jonathan M. P., Sarkar S. K., Medina-Mosquera F., Ayala-Mosquera H., et al. (2017) 68:83–90. Mercury Levels in Human Population From a Mining District in Western Colombia. J. Environ. Sci. 68, 83–90. doi: 10.1016/j.jes.2017.12.007
Harayashiki C. A. Y., Reichelt-Brushett A., Cowden K., Benkendorff K. (2018). Effects of Oral Exposure to Inorganic Mercury on the Feeding Behaviour and Biochemical Markers in Yellowfin Bream (Acanthopagrus Australis). Mar. Environ. Res. 134, 1–15. doi: 10.1016/j.marenvres.2017.12.018
Hong Y. S., Kim Y. M., Lee K. E. (2012). Methylmercury Exposure and Health Effects. J. Prev. Med. Public Health 45 (6), 353–363. doi: 10.3961/jpmph.2012.45.6.353
Hosseini M., Nabavi S. M. B., Parsa Y., Nabavi S. N. (2014). Mercury Accumulation in Food Chain of Fish, Crab and Sea Bird From Arvand River. J. Mar. Sci. Res. 4, 14. doi: 10.4172/2155-9910.1000148
Hosseni M., Nabavi S. M., Parsa Y. (2013). Bioaccumulation of Trace Mercury in Trophic Levels of Benthic, Benthopelagic, Pelagic Fish Species, and Sea Birds From Arvand River, Iran. Biol. Trace Elem. Res. 156 (1–3), 175–180. doi: 10.1007/s12011-013-9841-2
Instituto Brasileiro de Geografía e Estatística (IBGE) (2020). “Pesquisa De Orçamentos Familiares 2017-2018,” in Análise do Consumo Alimentar Pessoal No Brasil / IBGE, Coordenação De Trabalho E Rendimento (Rio de Janeiro: IBGE), 147p.
Jan A. T., Azam M., Siddiqui K., Ali A., Choi I., Haq Q. M. (2015). Heavy Metals and Human Health: Mechanistic Insight Into Toxicity and Counter Defense System of Antioxidants. Int. J. Mol. Sci. 16 (12), 29592–29630. doi: 10.3390/ijms161226183
Kehrig H. A., Costa M., Malm O. (2007). Estudo Da Contaminação Por Metais Pesados Em Peixes E Mexilhões Da Baía De Guanabara- Rio De Janeiro. Trop. Oceanog. 35 (1), 32–50. doi: 10.5914/tropocean.v35i1-2.5081
Kelly B. C., Myo A. N., Pi N., Bayen S., Leakhena P. C., Chou M., et al. (2018). Human Exposure to Trace Elements in Central Cambodia: Influence of Seasonal Hydrology and Food-Chain Bioaccumulation Behaviour. Ecotoxicol. Environ. Saf. 162, 112–120. doi: 10.1016/j.ecoenv.2018.06.071
Koenig S., Solé M., Fernández-Gómez C., Díez S. (2013). New Insights Into Mercury Bioaccumulation in Deep-Sea Organisms From the NW Mediterranean and Their Human Health Implications. Sci. Tot. Environ. 442, 329–335. doi: 10.1016/j.scitotenv.2012.10.036
Lackner J., Weiss M., Muller-Graf C., Greiner M. (2018). Disease Burden of Methylmercury in the German Birth Cohort 2014. PloS One 13 (1), ee0190409. doi: 10.1371/journal.pone.0190409
Liao W., Wang G., Zhao W., Zhang M., Wu Y., Liu X., et al. (2019). Change in Mercury Speciation in Seafood After Cooking and Gastrointestinal Digestion. J. Haz. Mat. 375, 130–137. doi: 10.1016/j.jhazmat.2019.03.093
Liu Q., Xu X., Zeng J., Shi X., Liao Y., Du P., et al. (2019). Heavy Metal Concentrations in Commercial Marine Organisms From Xiangshan Bay, China,and the Potential Health Risks. Mar. Pollut. Bull. 141, 215–226. doi: 10.1016/j.marpolbul.2019.02.058
Mallory M. L., O'Driscoll N. J., Klapstein S., Varela J. L., Ceapa C., Stokesbury M. J. (2018). Methylmercury in Tissues of Atlantic Sturgeon (Acipenser Oxyrhynchus) From the Saint John River, New Brunswick, Canada. Mar. Pollut. Bull. 126, 250–254. doi: 10.1016/j.marpolbul.2017.11.024
Maulvault A. L., Machado R., Afonso C., Lourenço H. M., Nunes M. L., Coelho I., et al. (2011). Bioaccessibility of Hg, Cd and As in Cooked Black Scabbard Fish and Edible Crab. Food Chem. Toxicol. 49, 2808–2815. doi: 10.1016/j.fct.2011.07.059
Mishra S., Bhalke S., Saradhi I. V., Suseela B., Tripathi R. M., Pandit G. G., et al. (2007). Trace Metals and Organometals in Selected Marine Species and Preliminary Risk Assessment to Human Beings in Thane Creek Area, Mumbai. Chemosphere 69 (6), 972–978. doi: 10.1016/j.chemosphere.2007.05.013
Murillo-Cisneros D. A., O'Hara T. M., Castellini J. M., Sánchez- González A., Elorriaga-Verplancken F. R., Marmolejo-Rodríguez A. J., et al. (2018). Mercury Concentrations in Three Ray Species From the Pacific Coast of Baja California Sur, Mexico: Variations by Tissue Type, Sex and Length. Mar. Pollut. Bull. 126, 77–85. doi: 10.1016/j.marpolbul.2017.10.060
Okati N., Esmaili-sari A. (2017). Hair Mercury and Risk Assessment for Consumption of Contaminated Seafood in Residents From the Coast of the Persian Gulf, Iran. Environ. Sci. Pollut. Res. 25 (1), 639–657. doi: 10.1007/s11356-017-0432-1
Onsanit S., Wang W. X. (2011). Sequestration of Total and Methyl Mercury in Different Subcellular Pools in Marine Caged Fish. J. Haz. Mater. 198, 113–122. doi: 10.1016/j.jhazmat.2011.10.020
Pigatto P. D., Costa A., Guzzi G. (2018). Are Mercury and Alzheimer’s Disease Linked? Sci. Tot. Environ. 613, 1579–1580. doi: 10.1016/j.scitotenv.2017.09.036
Reinhart B. L., Kidd K. A., Curry R. A., O'driscoll N. J., Pavey S. A. (2018). Mercury Bioaccumulation in Aquatic Biota Along a Salinity Gradient in the Saint John River Estuary. J. Environ. Sci. 68, 41–54. doi: 10.1016/j.jes.2018.02.024
Rodrigues P. A., Ferrari R. G., dos Santos L. N., Conte-Junior A. C. (2019a). Mercury in Aquatic Fauna Contamination: A Systematic Review on its Dynamics and Potential Health Risks. J. Environ. Sci. 84, 205–218. doi: 10.1016/j.jes.2019.02.018
Rodrigues P. A., Ferrari R. G., Hauser-Davis R. A., dos Santos L. N., Conte-Junior C. A. (2019b). Seasonal Influences on Swimming Crab Mercury Levels in an Eutrophic Estuary Located in Southeastern Brazil. Environ. Sci. Pollut. Res. 27, 3473–3482. doi: 10.1007/s11356-019-07052-3
Rodrigues P. A., Ferrari R. G., Hauser-Davis R. A., Neves dos Santos L., Conte-Junior C. A. (2020). Dredging Activities Carried Out in a Brazilian Estuary Affect Mercury Levels in Swimming Crabs. Int. J. Environ. Res. Public Health 17 (12), 4396. doi: 10.3390/ijerph17124396
Rodrigues P. A., Ferrari R. G., Kato L. S., Hauser-Davis R. A., Conte-Junior C. A. (2021a). A Systematic Review on Metal Dynamics and Marine Toxicity Risk Assessment Using Crustaceans as Bioindicators. Biol. Trace Elem. Res. 200 (2), 881–903. doi: 10.1007/s12011-021-02685-3
Rodrigues P. A., Ferrari R. G., Rosário D. K. A., Hauser-Davis R. A., Lopes A. P., Santos A. F. N. G., et al. (2021b). Interactions Between Mercury and Environmental Factors: A Chemometric Assessment in Seafood From an Eutrophic Estuary in Southeastern Brazil. Aquat. Toxicol. 236, 105844. doi: 10.1016/j.aquatox.2021.105844
Ruus A., Hjermann D. O., Beylich B., Schoyen M., Oxnevad S., Green N. W. (2017). Mercury Concentration Trend as a Possible Result of Changes in Cod Population Demography. Mar. Environ. Res. 130, 85–92. doi: 10.1016/j.marenvres.2017.07.018
Sadhu A. K., Kim J. P., Furrell H., Bostock B. (2015). Methyl Mercury Concentrations in Edible Fish and Shellfish From Dunedin, and Other Regions Around the South Island, New Zealand. Mar. Pollut. Bull. 101 (1), 386–390. doi: 10.1016/j.marpolbul.2015.10.013
Schmitd L., Figueroa J. A. L., Vecchia P. D., Duarte F. A., Mello P. A., Caruso J. A., et al. (2018). Bioavailability of Hg and Se From Seafood After Culinary Treatments. Microchem. J. 139, 363–371. doi: 10.1016/j.microc.2018.03.009
Soares-Gomes A., Da Gama B. A. P., Baptista Neto J. A., Freire D. G., Cordeiro R. C., Machado W., et al. (2016). An Environmental Overview of Guanabara Bay, Rio De Janeiro. Reg. Stud. Mar. Sci. 8 (2), 319–330. doi: 10.1016/j.rsma.2016.01.009
South African Department of Health (DOH) (2004). “Foodstuffs, Cosmetics and Disinfectants Act, (Act No. 54 of 1972),” in Government Gazette No. R. 500. Republic of South Africa: Government Gazette. Available at: http://web.crownfood.co.za/wp-content/uploads/2017/07/Maximum-levels-for-Metals-in-Foodstuffs-R500.pdf.
Taylor D. L., Calabrese N. M. (2018). Mercury Content of Blue Crabs (Callinectes Sapidus) From Southern New England Coastal Habitats: Contamination in an Emergent Fishery and Risks to Human Consumers. Mar. Pollut. Bull. 126, 166–178. doi: 10.1016/j.marpolbul.2017.10.089
Tchounwou P. B., Yedjou C. G., Patlolla A. K., Sutton D. J. (2012). Heavy Metal Toxicity and the Environment. Experientia. Suppl. 101, 133–164. doi: 10.1007/978-3-7643-8340-4_6
Torres D. P., Martins-Teixeira M. B., Silva E. F., Queiroz H. M. (2012). Method Development for the Control Determination of Mercury in Seafood by Solid-Sampling Thermal Decomposition Amalgamation Atomic Absorption Spectrometry (TDA AAS). Food Addit. Contam. Part A 29, 625–632. doi: 10.1080/19440049.2011.642310
United States Environmental Protection Agency, US-EPA (1989). “Risk Assessment Guidance for Superfund Volume I,” in Human Health Evaluation Manual (Part A), Interim Final. EPA 540/1–89/002 (Washington DC: United States Environmental Protection Agency).
United States Environmental Protection Agency, US-EPA (2000). “Guidance for Assessing Chemical Contamination Data for Use in Fish Advisories Volume II,” in Risk Assessment and Fish Consumption Limits EPA/823- B94-004 (Washington DC: United States Environmental Protection Agency).
Wang R., Wong M. H., Wang W. X. (2010). Mercury Exposure in the Freshwater Tilapia Oreochromis Niloticus. Environ. Pollut. 15 (8), 2694–2701. doi: 10.1016/j.envpol.2010.04.019
Word Register of Marine Spieies (WRMS). Available at: http://www.marinespecies.org/aphia.php?p=search (Accessed July, 15, 2021).
Yang Y. W., Liou S. H., Hsueh Y. M., Lyu W. S., Liu C. S., Liu H. J., et al. (2018). Risk of Alzheimer’s Disease With Metal Concentrations in Whole Blood and Urine: A Casecontrol Study Using Propensity Score Matching. Toxicol. Appl. Pharmacol. 356, 8–14. doi: 10.1016/j.taap.2018.07.015
Yin R., Zhang W., Sun G., Feng Z., Hurley J. P., Yang L., et al. (2017). Mercury Risk in Poultry in the Wanshan Mercury Mine, China. Environ. Pollut. 230, 810–816. doi: 10.1016/j.envpol.2017.07.027
Zamora-Arellano N. Y., Ruelas-Inzunza J., García-Hernández J., Arturo-Ilizaliturri C. A., Betancourt-Lozano M. (2017). Linking Fish Consumption Patterns and Health Risk Assessment of Mercury Exposure in a Coastal Community of NW Mexico. Hum. Ecol. Risk Assess. 23 (6), 1505–1521. doi: 10.1080/10807039.2017.1329622
Keywords: crustacean, mollusc, hazard quotient, mercury, Guanabara bay
Citation: Rodrigues PdA, Ferrari RG, Rosário DKAd, Hauser-Davis RA, Santos LNd and Conte-Junior CA (2022) Mercurial Contamination: A Consumer Health Risk Assessment Concerning Seafood From a Eutrophic Estuary in Southeastern Brazil. Front. Mar. Sci. 9:765323. doi: 10.3389/fmars.2022.765323
Received: 26 August 2021; Accepted: 29 April 2022;
Published: 27 May 2022.
Edited by:
Geórgenes Hilário Cavalcante, Federal University of Alagoas, BrazilReviewed by:
Heyue Zhang, Hohai University, ChinaCristine Couto Almeida, Oswaldo Cruz Institute (FIOCRUZ), Brazil
Israel Medina-Gómez, Instituto Politécnico Nacional de México, Mexico
Copyright © 2022 Rodrigues, Ferrari, Rosário, Hauser-Davis, Santos and Conte-Junior. This is an open-access article distributed under the terms of the Creative Commons Attribution License (CC BY). The use, distribution or reproduction in other forums is permitted, provided the original author(s) and the copyright owner(s) are credited and that the original publication in this journal is cited, in accordance with accepted academic practice. No use, distribution or reproduction is permitted which does not comply with these terms.
*Correspondence: Rafaela Gomes Ferrari, cmFmYWVsYS5mZXJyYXJpQGFjYWRlbWljby51ZnBiLmJy