- 1Department of Aquaculture, National Taiwan Ocean University, Keelung, Taiwan
- 2Center of Excellence for the Oceans, National Taiwan Ocean University, Keelung, Taiwan
- 3Department of Environmental Biology and Fisheries Science, National Taiwan Ocean University, Keelung, Taiwan
The cuttlefish is a commercially important marine species across the world; however, due to the lack of appropriate artificial feed, there is limited future aquaculture development of the cuttlefish. We proposed a novel process comprising (1) feed preference tests and (2) progressive training programs. Through this process, artificial diets can become more suitable for pharaoh cuttlefish (Sepia pharaonis). Feed preference tests (based on vision, touch, and smell) revealed that pharaoh cuttlefish preferred rod-shaped feed to flat rod-shaped or ball-shaped feed, moist feed over dry feed (DF), and DF with agar coating to DF without agar coating. Without olfactory training, some cuttlefish refused to eat artificial feed and eventually died. Through progressive training programs (3- and 6-week programs), a survival rate of >80% was achieved. Although the artificial diets examined in this study resulted in a lower conversion ratio for S. pharaonis than for fresh shrimp, available artificial feed can potentially be adopted for cuttlefish aquaculture through the use of our progressive training program.
Introduction
Cephalopods include octopus, squid, cuttlefish, and nautilus, all of which are found across the world oceans from intertidal areas to deep-sea regions (Boletzky and Villanueva, 2014). They have separate sexes and lack a true larval stage, which means that they develop directly (Boletzky and Villanueva, 2014). Most cephalopods have short life spans (1–2 years) and high rates of growth and feed conversion; all cephalopods are carnivores (Lee et al., 1994; Domingues et al., 2004; Boletzky and Villanueva, 2014; Jiang et al., 2018). The global fishery industry has been increasing its production of cephalopods in the past 20 years and has sustainably met the demand for marine products (Boletzky and Villanueva, 2014; FAO, 2017). Among the various cephalopod species, the cuttlefish is regarded as having considerable potential for aquaculture because of its high nutritional profile and high market value (Gao et al., 2014). The natural distribution of cuttlefish can be divided into two major areas, namely, the Atlantic-Mediterranean and Indo-Pacific seas (Boletzky and Villanueva, 2014). Among the various cuttlefish species, Sepia officinalis is the predominant cuttlefish reared for research in European countries, including Spain, Portugal, and France (Boletzky, 1983; Domingues et al., 2001, 2005; Baeza-Rojano et al., 2010). In East and Southeast Asia (e.g., Thailand, China, Taiwan, and Japan), Sepia pharaonis, Sepia esculenta, and Sepiella japonica are the major species that are cultured (Nabhitabhata, 1978; Hao et al., 2007; Fan et al., 2011; Zheng et al., 2014).
In the early 1960s, Choe and Ohshima (1963) first used live shrimp as feed for rearing cuttlefish and squid and successfully bred Sepioteuthis lessoniana, S. esculenta, Sepia lycidas, and S. japonica. Since then, researchers have mainly used live prey and fresh diets (e.g., shrimp and fish) to rear cuttlefish and squid (Boletzky, 1974; Pascual, 1978; Minton et al., 2001; Villanueva et al., 2017; Jiang et al., 2018). However, the training of non-domesticated animals to accept artificial feed and further development of commercial feed are key factors that influence commercial aquaculture development; thus, minimizing or changing the practice of using live prey is a major challenge in the rearing of cuttlefish (Sorgeloos et al., 2001; Zohar and Mylonas, 2001; Nguyen et al., 2011; Ouraji et al., 2011; Takeuchi and Haga, 2015). Various studies have experimented with the use of artificial feed. Lee et al. (1991) were the first researchers to successfully feed S. officinalis using an artificial diet containing non-marine sources that included hot dogs, chicken puree, and raw chicken. Koueta et al. (2002) and Perrin et al. (2004) used enriched natural and frozen diets to enhance the growth of S. officinalis. Studies have reported positive results after feeding surimi to S. officinalis and highlighted that the protein content of the mantle muscle is a valuable indicator in long-term rearing (Castro et al., 1993; Castro and Lee, 1994). Numerous studies have reported that the higher the percentage of protein (35–50%) in an artificial diet, the better the growth performance of the fed cuttlefish (Domingues et al., 2005, 2008; Ferreira et al., 2010; Gao et al., 2014; Han et al., 2017; Jiang et al., 2021). Some studies have highlighted the crucial role of fatty acids for cuttlefish, particularly eicosapentaenoic acid and docosahexaenoic acid, with cuttlefish requiring high amounts of these nutrients (Perrin et al., 2004; Ferreira et al., 2010; Gao et al., 2014; Wen et al., 2014; Jiang et al., 2018).
Pharaoh cuttlefish (S. pharaonis), which have a unique tiger stripe pattern on their skin, are distributed in the Indo-Pacific sea, the waters north of Japan, and the waters west of the Red Sea (Nabhitabhata and Nilaphat, 1999). The pharaoh cuttlefish is a tropical and large (350–420 mm in length and 4,200–5,000 g in weight) species and a crucial fishery product in Southeast Asia (Nabhitabhata, 1978). Minton et al. (2001) cultured multiple generations of pharaoh cuttlefish under laboratory conditions. Although pharaoh cuttlefish can be reared for multiple generations, its mass production remains difficult. Jiang et al. (2021) proposed an optimum weaning method for pharaoh cuttlefish in mass culture and a specific culture protocol. However, the high cost and unstable quality of the live or fresh diet required for rearing pharaoh cuttlefish limit their mass production (Han et al., 2017; Jiang et al., 2018). Before mass production can be achieved, further information on the nutritional compositions of cuttlefish and their diets are required. Peng et al. (2015) and Jiang et al. (2018) analyzed the crude composition of the muscles of juvenile pharaoh cuttlefish in culture and compared it with the diets that they were fed; their results revealed that cuttlefish muscle and shrimp shared a similar composition. Wen et al. (2014) compared the nutritional quality of wild and cultured pharaoh cuttlefish and reported that the nutritional quality of both wild and cultured cuttlefish was similar to that of the shrimp that were fed to them. Because the nutritional quality of pharaoh cuttlefish is influenced by the prey that they eat, an enriched diet may increase their nutritional quality (Han et al., 2017).
Although the implementation of artificial diets for cuttlefish has been explored, most studies have done so by mixing various fresh diets (e.g., fish and shrimp) to produce moist feed (MF; Han et al., 2017; Jiang et al., 2018). Currently, dry feed (DF) for cuttlefish is unavailable. Our cultivation experience suggests that during the mass culture of cuttlefish, most cuttlefish (wild and artificially reared stocks) only accept bait with complete fish and shrimp shapes, and artificial diets cannot be used unless training is provided. Therefore, we developed a novel process comprising (1) feed preference tests and (2) progressive training programs. Subsequently, we assessed the growth performance achieved with the use of available artificial diets.
Materials and Methods
Cuttlefish
Stocks of pharaoh cuttlefish (S. pharaonis) were obtained from the northeastern region of Taiwan and reared in an outdoor 200,000-L concrete pool in Gongliao Aqua Center, New Taipei City, Taiwan. The exact number of female and male adults could not be determined; their numbers differed for each catch (the cuttlefish were captured in coastal waters). However, the ratio of female to male adults was approximately between 1:3 and 1:5, and a stock of between 100 and 150 adults was maintained in the pool during the breeding season. Pharaoh cuttlefish were fed thawed fish (Scomber spp. and Trichiurus spp.; weight, 100–300 g) three times a day to ensure that each cuttlefish consumed at least one or two fish per day. To maintain the water quality, we removed dead shrimp and shrimp remains once a day. During the breeding season, we maintained a water temperature of between 22 and 26°C, water salinity of 33 ± 1‰, and a photoperiod of approximately 14:10 (light:dark) depending on the weather conditions of a given day. The open-system pool received 24-h fresh flowing seawater (10,000 L/h). After the cuttlefish laid their eggs, these eggs were harvested and placed in indoor 2,500-L fiberglass-reinforced plastic (FRP) tanks for hatching. Hatched seedlings (weight, 0.5 ± 0.06 g; mantle length, 0.8 ± 0.2 cm) were collected in a plastic tank (length, 60 cm; width, 41.5 cm; and water depth, 9.5 cm) with a semicirculating water system (temperature, 26 ± 1°C; salinity, 33 ± 1‰; photoperiod, 12:12 indoor; water change, 100 L/h), and a total of 25,000 seedlings were hatched and collected. We fed live and chilled shrimp (Litopenaeus vannamei; body length, 1.0–2.0 cm) and fresh shrimp (Exopalaemon orientis; body length, 2–5 cm) to the juveniles ate least four times a day, with each individual consuming at least one or two shrimp per meal. The size of a shrimp was approximately between 50 and 150% of the body length of cuttlefish at that stage. Live shrimp were fed to juvenile cuttlefish in the first 2 weeks after they hatched. In the 3rd week, the live shrimp were replaced by chilled shrimp, followed by a combination of chilled and fresh shrimp. The 4-week-old juvenile cuttlefish were then fed fresh oriental shrimp until they were 8 weeks old (weight, 44.3 ± 2.5 g; mantle length, 8.4 ± 2.5 cm). We attempted to maximize the amount of feed consumed by each juvenile and removed shrimp remains and dead shrimp once a day. These 8-week-old cuttlefish were used in the subsequent tests (Figure 1).
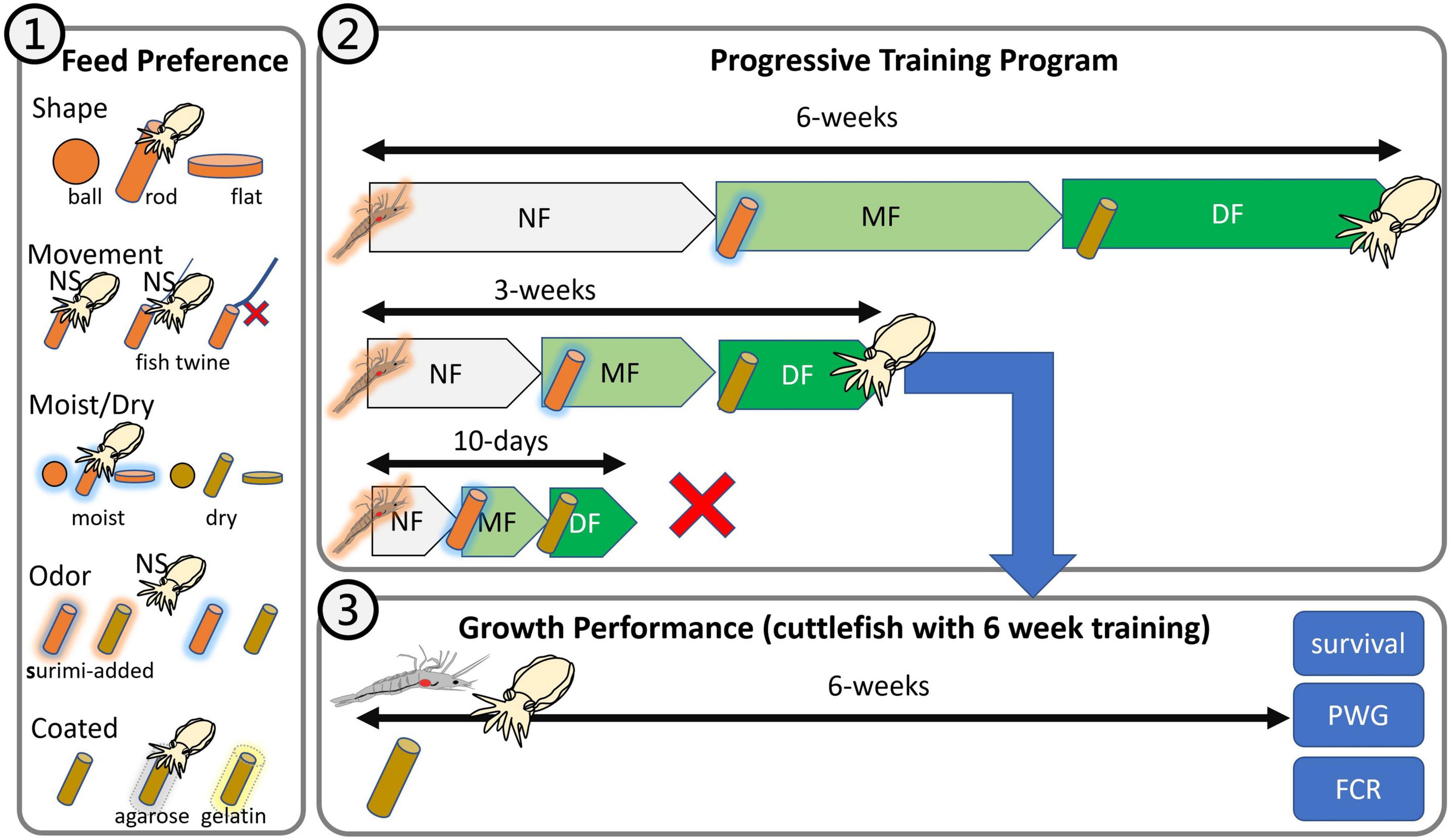
Figure 1. Experimental design of this study. (1) Feed preference test: shape, movement, moistness, odor, and coating; (2) progressive training program: 10-day, 3-week, and 6-week programs; (3) growth performance: feeding of fresh shrimp and dry feed.
Artificial Feed
Before the tests were conducted, the amino acid composition of a single cuttlefish was determined by examining the mantle muscle, viscera, and ovary. The mantle muscle was used as a reference for preparing the artificial feed. The amino acid composition was analyzed in accordance with the method proposed by Alava and Pascual (1987), Table 1. The composition of the prepared artificial feed is listed in Table 2. Fish meal, krill shrimp meal, and poultry meal were crushed and sieved through a sieve with No. 40 mesh (0.38 × 0.38 mm2), and soybean concentrate was crushed and sieved through a sieve with No. 60 mesh (0.25 × 0.25 mm2). Artificial feed was prepared in two forms, namely, MF (feed powder-to-water ratio of 3:1 that was mixed evenly and then shaped per experimental requirements), DF (the procedure used for preparing MF was also applied to DF, but the DF was dried at 40°C for at least 12 h after mixing). Frozen oriental shrimp (E. orientis), the preferred prey of the cuttlefish, was selected as the control treatment because of their size. The crude moisture, protein, fat, and ash contents of the artificial feed (DF) and oriental shrimp were analyzed in accordance with the procedures of the Association of Official Analytical Chemists (AOAC, 2000; Table 3).
Feed Preference Test
The feed preference test was performed to evaluate five components, namely, shape, movement, moistness, odor, and coating (Figure 1). To prevent the training effect from affecting our results, we performed each test using different juveniles. A total of 95 juvenile cuttlefish underwent the feed preference test. All tests were performed in 2,500-L FRP tanks with a semicirculating water system (temperature, 26 ± 1°C; salinity, 33 ± 1‰; photoperiod, 12:12 indoor; water change 2,500 L/h). Cuttlefish were randomly selected from the rearing tank 12 h before the tests were conducted. Five selected cuttlefish formed a group for one trial, and three replications were performed for each treatment. Each group was tested with an interval of at least 12 h between each replication to prevent excessive interference. During the testing process, we randomly gave different feeds from the same trial to five cuttlefish, prevented them from feeding by using inertial selection, and recorded the “latency-to-grab” time that corresponded to each individual cuttlefish. Each feed with a specific state weighed approximately 5 g. Through our long-term observations, we discovered that the cuttlefish would observe the bait for a period of time (usually <60 s) before eating it. Therefore, we discontinued the recording process if a cuttlefish failed to display any foraging behavior within 120 s. That is, we waited for 120 s for each cuttlefish to grab its feed. The time was recorded as 120 s if a cuttlefish abandoned a piece of grabbed feed or if no cuttlefish grabbed any feed during a trial. Each cuttlefish had to be observed eating a piece of grabbed feed for 120 s before the initially observed latency-to-grab time was accepted and recorded.
Shape
We evaluated the feed shape preferred by cuttlefish by shaping MF into rods, balls, and flat rods. To entice cuttlefish to grab a piece of feed, a fishing line (width, 0.2 mm) was inserted and used to move the pieces of feed that were fed to the cuttlefish.
Movement
In this experiment, a rod-shaped MF was used. “Wriggled” feed comprised pieces of feed that were moved using a fishing line (width, 0.2 and 2 mm), and “stationary” feed comprised pieces of feed that were directly fed to cuttlefish without the use of a fishing line.
Moistness
We examined whether the cuttlefish preferred MF or DF. Both MF and DF were shaped into rods, balls, and flat rods.
Odor
We also examined the olfactory effect of feed. Shrimp surimi (made from oriental shrimp) was mixed with artificial feed powder at a 1:1 ratio to prepare surimi-supplemented dry feed (SDF) and surimi-supplemented moist feed (SMF). Subsequently, the DF, MD, SDF, and SMF were all shaped into rods.
Coated
To imitate the texture of fresh shrimp, agarose or gelatin was coated on rod-shaped DF. DF was soaked in agarose or gelatin liquid (60°C) to prepare coated feed (CDF). After the coating process was completed, the CDF was dugout, and the excess coating was removed to retain only a thin layer of coating over the feed. The weight of the feed before and after coating was approximately 5 and 5.5 g, respectively. The non-coated DF was used in the control treatment for this trial.
Progressive Training Program
This test was performed in 500-L plastic tanks with an open water system (temperature, 24–26°C; salinity, 30–35‰, photoperiod 12:12 indoor), and the water exchange rate was 100% every 2 h. We trained cuttlefish to eat artificial feed by applying three steps as follows: (1) soaking oriental shrimp into artificial feed liquid (powder-to-water ratio of 1:2) for 30 min at 4°C and then feeding the shrimp to cuttlefish, (2) feeding rod-shaped MF to cuttlefish, and (3) feeding rod-shaped DF to cuttlefish. The 10-day, 3-week, and 6-week training programs were implemented in triplicate, and the training period for each of the three steps was 3 days, 1, and 2 weeks for the 10-day, 3-, and 6-weeks training programs, respectively. The cuttlefish received three meals per day, and the shrimp remains and dead cuttlefish were removed daily. In this test, each group consisted of five cuttlefish, and we recorded their survival rate for each period (according to training time). A step was considered a failure if <80% (n < 4) of the cuttlefish survived, but the program was continued and only stopped when complete mortality was reached. The outcomes provided an indication of whether the training program was suitable for cuttlefish.
Growth Experiment
In the growth experiment, 8-week-old cuttlefish (n = 20) underwent a 3-week training program that was identical to that described in the “Progressive training program” section. Each group comprised five individual cuttlefish, and three replicated groups were established; an additional group was established as the control group. Since the 8-week-old cuttlefish exhibited individual differences in their body size, cuttlefish in a given group were similarly sized (determined visually) but may have differed in terms of weight. Each cuttlefish in the experimental and control groups received approximately 5 g of artificial feed (rod-shaped DF) and oriental shrimp, respectively. The feeding frequency was two meals a day (11:00 and 21:00), which was determined on the basis of our rearing experience. Remains (feed and shrimp) were removed daily. The test was performed in 500-L plastic tanks with an open water system (temperature, 24–26°C; salinity, 30–35‰), and the water exchange rate was 100% every 2 h. After the growth test was completed, the following indicators were calculated: percentage weight gain (PWG) = (final body weight – initial body weight)/initial body weight × 100%; feed conversion ratio (FCR) = feed intake/(final body weight – initial body weight).
Statistical Analysis
A completely randomized design was used in this study, and the results are presented in terms of means ± standard deviations. Latency-to-grab time (refer to the “Shape,” “Movement,” “Odor,” and “Coated” sections), PWG, and FCR data were subjected to one-way analyses of variance (ANOVAs), and latency-to-grab time data relating to moistness (refer to the “Moistness” section) were subjected to the two-way ANOVA; Tukey’s honestly significant difference test was used to evaluate the differences between treatments. Statistical analyses were conducted using SPSS version 19 (IBM, Armonk, Westchester County, NY, United States.).
Results
Feed Preference Test
Shape
In the test evaluating the preference of cuttlefish with respect to feed shape, the recorded latency-to-grab time was considerably shorter for the rod- and flat rod-shaped feed than for the ball-shaped feed (Figure 2). The shortest time was recorded for rod-shaped feed (15.60 ± 8.99 s), followed by flat rod-shaped feed (25.48 ± 6.21 s) and ball-shaped feed (120 s; the ball-shaped feed was not grabbed by cuttlefish).
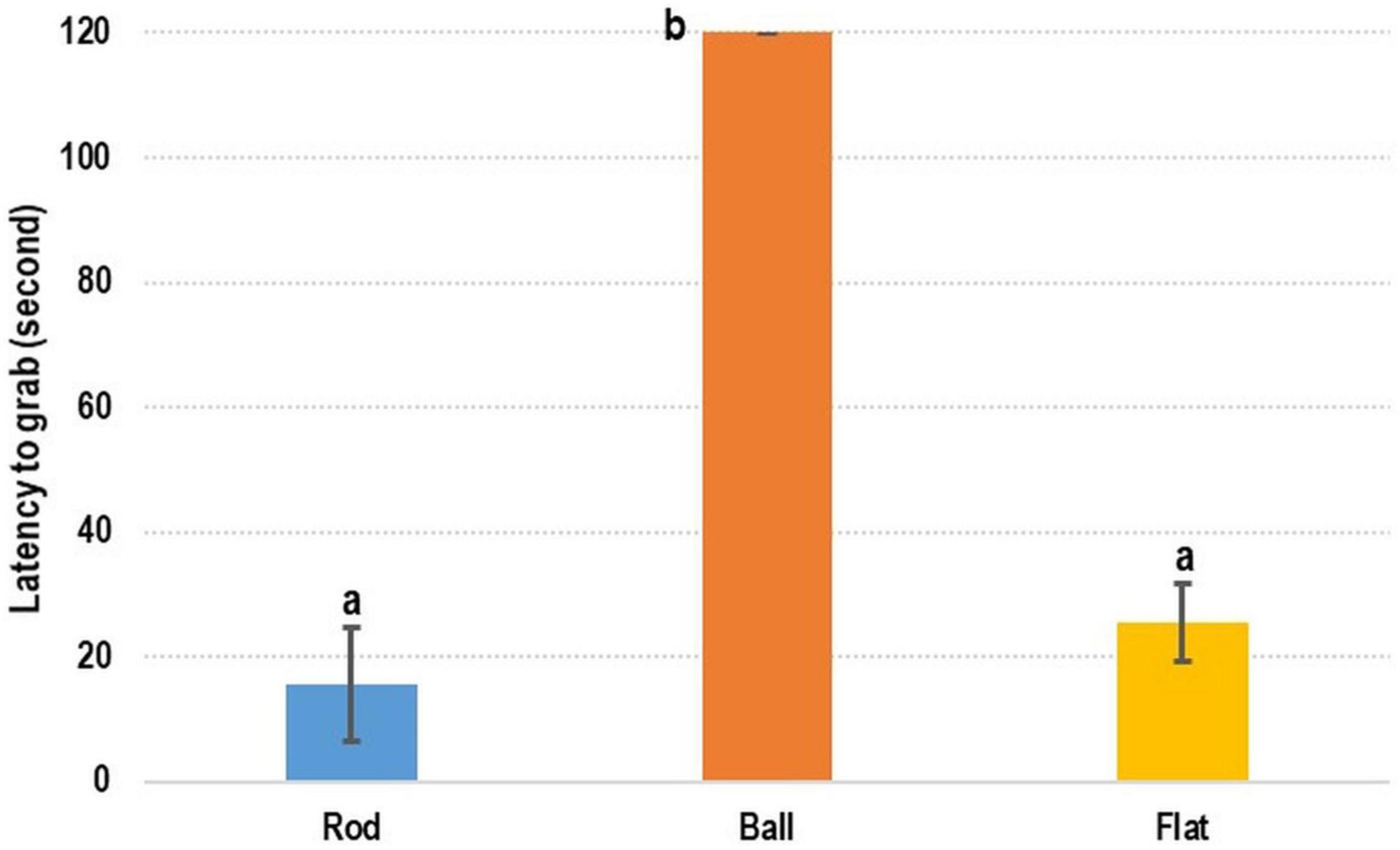
Figure 2. Testing of feed shape preference of cuttlefish. Feed shape affected the latency-to-grab time of cuttlefish. Rod- and flat rod-shaped feed were more attractive to cuttlefish. The latency-to-grab time was significantly shorter for rod- and flat rod-shaped feed than for ball-shaped feed (p < 0.05).
Movement
No statistical difference was observed in the movement experiments (Figure 3). The latency-to-grab times were 14.60 ± 7.61 s, 8.02 ± 2.38 s, and 7.66 ± 2.84 s for the experiments in which a 2-mm fishing line was used, a 0.2-mm fishing line was used, and no fishing line was used, respectively. The time required when a 2-mm fishing line was used was longer than that required when a 0.2-mm fishing line or no fishing line was used.
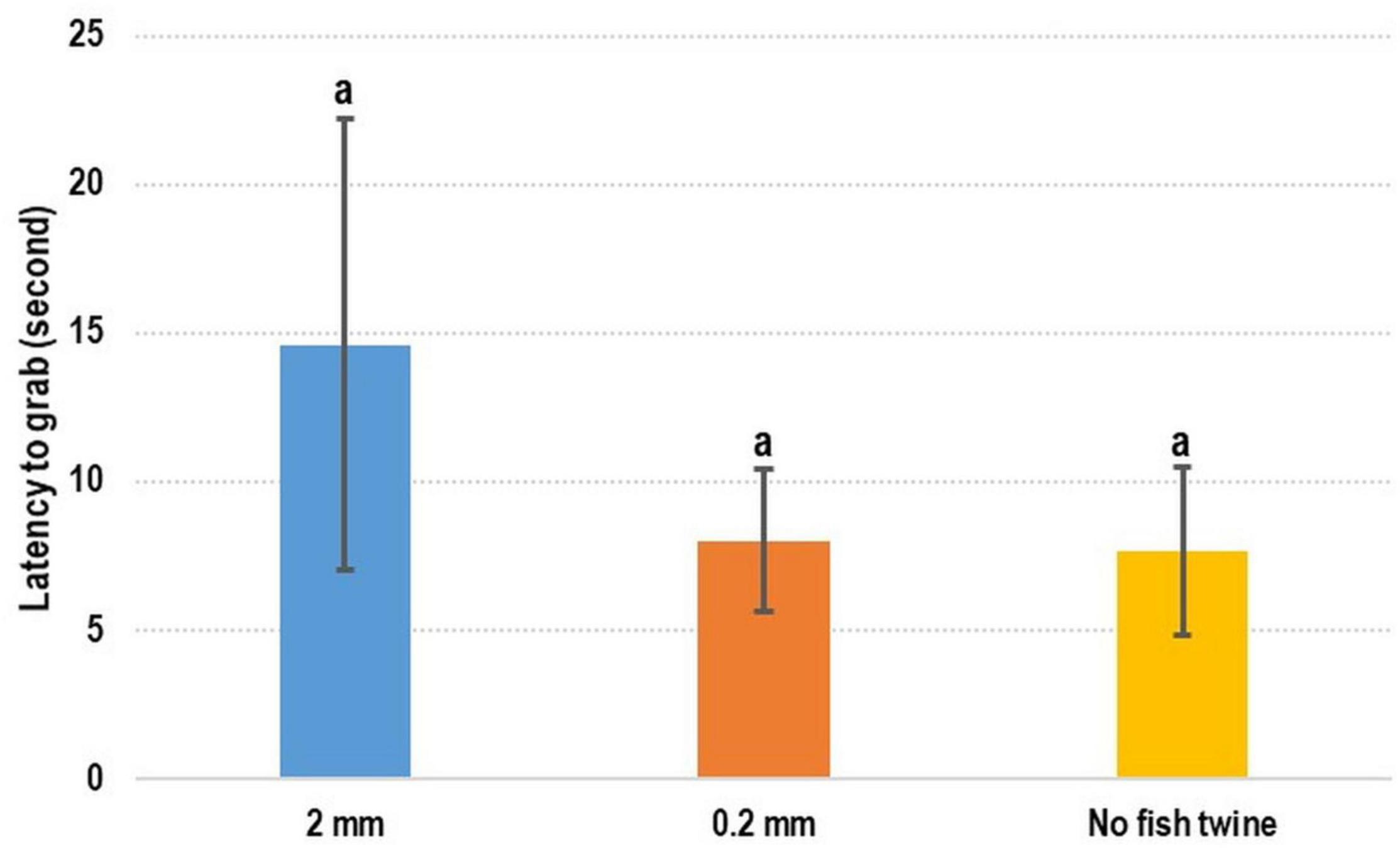
Figure 3. Test for evaluating the use of fishing line in training programs. No significant difference between these three treatments was observed. The use of a fishing line to drag and wriggle feed did not attract cuttlefish to eat the feed.
Moistness
In terms of the preference of cuttlefish for DF or MF, the rod- and flat rod-shaped MF exhibited the shortest latency-to-grab times of 17.38 ± 7.16 s and 23.64 ± 2.34 s, respectively (Figure 3). The latency-to-grab times for the ball-shaped MF (70.24 ± 27.38 s), rod-shaped DF (87.08 ± 30.28 s), and flat rod-shaped DF (91.96 ± 25.31) were similar. No cuttlefish grabbed the ball-shaped DF (120 s). Relative to the various types of MF, the latency-to-grab times of the various types of DF were significantly longer (p < 0.05).
Odor
The addition of shrimp surimi did not enhance the attractiveness of the artificial feed to cuttlefish (Figure 4). No significant difference was observed between the treatments. The latency-to-grab time was slightly shorter for dry SF than for the other types of feed; however, the longest latency-to-grab time was recorded for moist SF. Specifically, the latency-to-grab times of SMF, SDF, MF, and DF were 29.92 ± 16.02 s, 13.28 ± 3.18 s, 23.46 ± 9.30 s, and 18.72 ± 7.00 s, respectively.
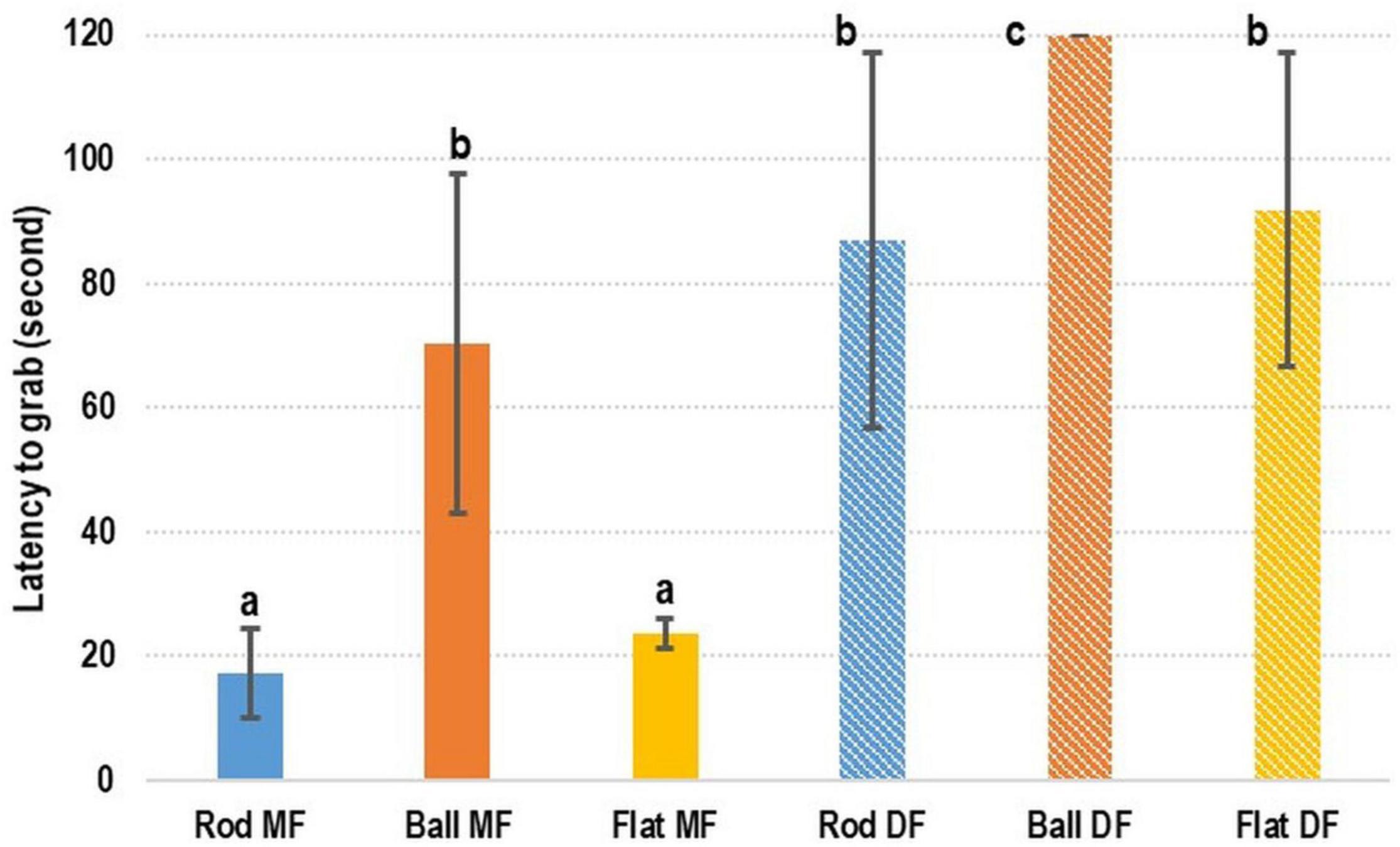
Figure 4. Testing of feed type preference of cuttlefish. Cuttlefish preferred moist feed (MF) over dry feed (DF) regardless of the shape. Lowercase letters indicate significant differences (Tukey’s honestly significant difference test; p < 0.05) in examined variables between diets.
Coating
Regarding the preference of cuttlefish for CDF, contradicting results were obtained. The shortest latency-to-grab time was achieved with agarose-coated feed (29.56 ± 8.51 s), the longest latency-to-grab time (120 s) was recorded for gelatin-coated feed, and the latency-to-grab time of the control (DF) was 64.08 ± 11.44 s (Figure 5). The latency-to-grab time was significantly shorter for the agarose-coated feed than for the control and gelatin-coated feed. However, the latency-to-grab time was considerably longer for the gelatin-coated feed than for the control and agarose-coated feed.
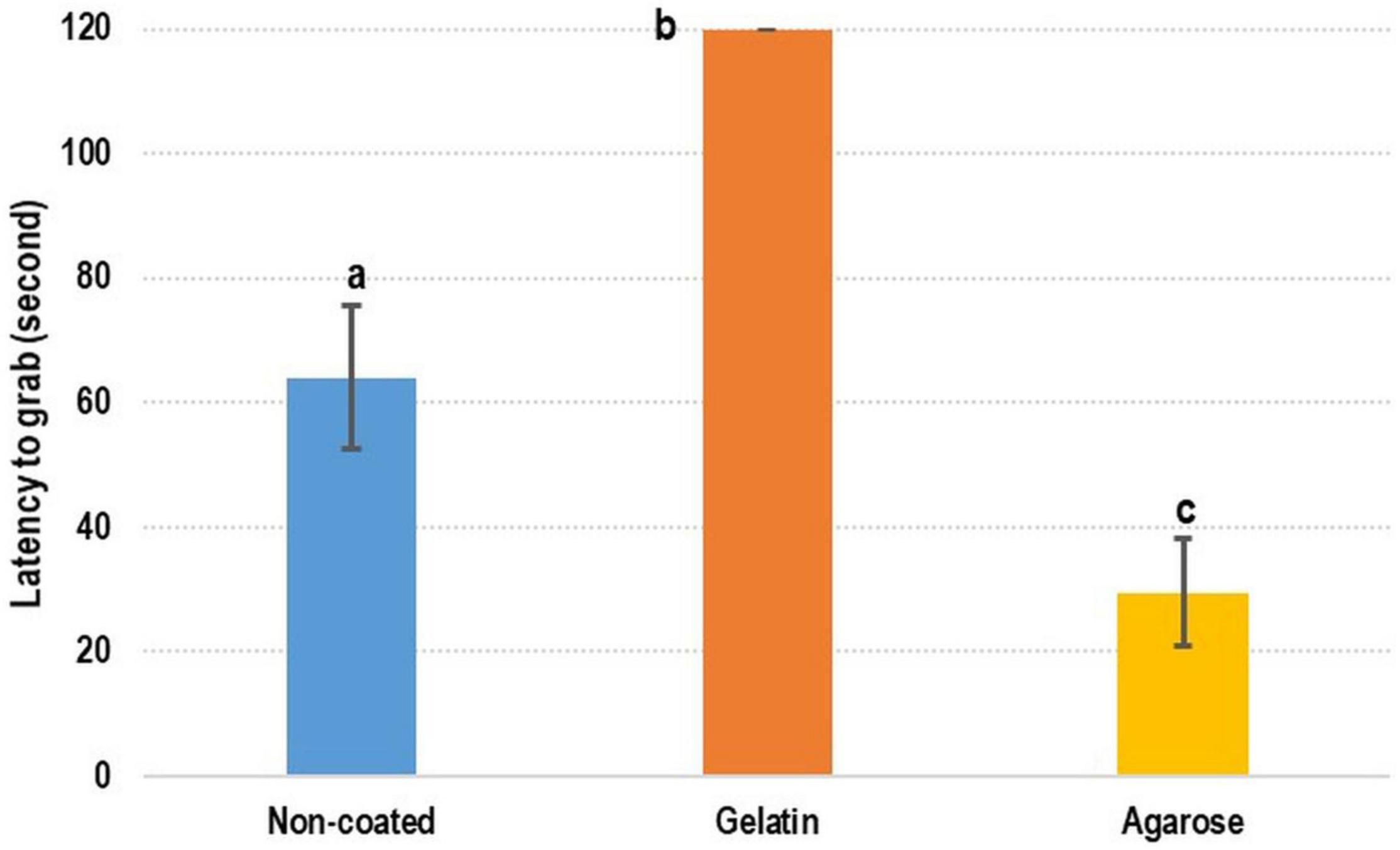
Figure 5. Test of coated feed for cuttlefish. For cuttlefish, the agarose-coated feed was the most preferred feed, followed by non-coated feed and gelatin-coated feed. Lowercase letters indicate significant differences (Tukey’s honestly significant difference test; p < 0.05) in examined variables between diets.
Progressive Training Programs
Only the 6- and 3-week trials were successfully completed (Table 4). The longer the training time, the higher the acceptance and survival rates. Notably, failures in steps 2 and 3 were reported for the 3-week training program, although the final component of the program was nevertheless completed successfully. Given the time that was spent and the final accepted results that was achieved, the 3-week training program was regarded to be more efficient than the conservative 6-week program.
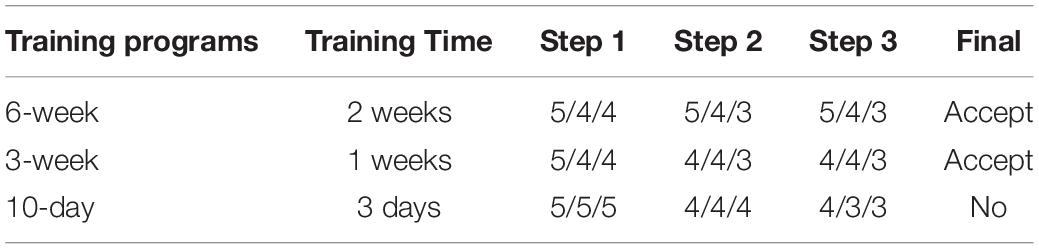
Table 4. Survival status of the five cuttlefish used in each training program for each step and replication.
Growth Performance
The results indicated that experimental groups (artificial feed) did not perform as well as the control group (oriental shrimp; Table 5). No significant difference in initial weight was observed between the experimental and control groups. However, the control group achieved a significantly higher final weight, PWG, and FCR than the artificial feed groups. Better results were achieved with fresh shrimp than with artificial feed for a given feeding weight. This was because the artificial feed was not completed consumed by cuttlefish. In contrast, fresh shrimp was always completely consumed by cuttlefish, although this did not occur immediately (i.e., within 30 min). Nevertheless, the 100% survival rate that was achieved for both groups indicates that artificial feed can be fed to cuttlefish.
Discussion
Cephalopods have complex brains and central nervous systems that provide them with excellent learning and memorization abilities (Cole and Adamo, 2005; Correia et al., 2005; Cartron et al., 2013; Yang and Chiao, 2016; Zepeda et al., 2017; Shinzato et al., 2018; Yasumuro and Ikeda, 2018). As a member of the cephalopod family, the pharaoh cuttlefish has been proven to have number sense and excellent learning ability (Yang and Chiao, 2016; Yasumuro and Ikeda, 2018; Huang et al., 2019). Studies have verified several methods of pharaoh cuttlefish weaning, providing us with reliable message training methods that enable cuttlefish to become accustomed to eating artificial feed (Jiang et al., 2018, 2020, 2021). Our cultural experience (we weaned more than 5000 juveniles per year between 2015 and 2021) suggests that the key challenge is not the weaning process but the reduction of rearing cost and preparation of nutritional diets. Thus, training cuttlefish to eat an artificial diet is crucial to future commercial culture and enhances its efficiency.
Studies have reported that for the purpose of training cuttlefish to eat an artificial diet, their feed should be shaped in a form that is similar to the shape of their natural prey. Lee et al. (1991) used various artificial baits as feed for cultured S. officinalis and reported that artificial pellets had the longest latency-to-grab time, whereas rod-shaped artificial feed (e.g., hot dog-shaped feed) exhibited a shorter latency-to-grab time. Castro et al. (1993) and Shinzato et al. (2018) reported that cuttlefish were more attracted to shrimp-shaped bait. Thus, artificial feed should be shaped like a rod or a flat rod. In our tests, juvenile cuttlefish also tended to grab rod- and flat rod-shaped artificial feed (Figure 2). However, the feed-movement test did not reveal any significant difference; that is, pharaoh cuttlefish did not exhibit a preference in this regard (Figure 3). This result is inconsistent with that of Cole and Adamo (2005), who reported that the feed given to S. officinalis must be slowly lowered. Although the fishing lines used in our tests were not used to control the sinking speed of bait, we came to the same conclusion regarding the necessity of allowing feed to sink.
While they are hunting, cuttlefish search, detect, select, grab, handle, and kill their prey, and these activities require experience and learning (Cole and Adamo, 2005; Villanueva et al., 2017). Changes in the movement of a bait may confuse a cuttlefish or cause it to ignore the bait. Our results revealed that pharaoh cuttlefish preferred MF to DF in general, even when they had the same shape (Figure 4). This could be because the sinking speed of MF is higher than that of DF. Moreover, cuttlefish do not necessarily consume the feed that they have detected, selected, grabbed, and handled. We discovered that they may discard a piece of feed and refrain from grabbing it again. We speculate that the similarity of the texture of MF to that of real prey (i.e., soft surface) was the reason why pharaoh cuttlefish preferred MF to DF. As was highlighted in an earlier section, we believe that the texture of artificial feed influences the selection behavior of cuttlefish; this inference is also supported by Lee et al. (1991), who reported that feed texture is essential for cuttlefish weaning. Our results indicate that MF and agarose-coated feed are more suitable feed options for cuttlefish (Figures 4, 5). The latency-to-grab time was shorter for agarose-coated feed and longer for gelatin-coated feed. We believe that these two types of coated feed achieved different results because of their texture. The texture of gelatin CDF was softer than that of agarose CDF. We speculate that the gelatin coating was too “liquid-like,” such that our cultured pharaoh cuttlefish could not identify it as food and refused to eat it as a result. This study is the first to employ coated feed for cuttlefish, and the experiment revealed that coating has a considerable effect on cuttlefish. However, the suitability of various types of coating warrants further research.
The effect of feed odor on cuttlefish was difficult to evaluate. The flavor of surimi-supplemented feed was more similar to that of fresh shrimp, but the latency-to-grab times of surimi-supplemented and normal feed did not differ significantly (Figure 6). This means that cuttlefish rely more on their vision than their sense of smell to locate prey (Correia et al., 2005). However, olfactory effects still merit discussion. Guibé et al. (2010) reported that the visual prey preference of cuttlefish can be changed by odor experiences, and Lee et al. (2020) highlighted that pharaoh cuttlefish embryo can detect environmental cues and change their behavior accordingly after hatching. In our preliminary test, we added a large amount of shrimp surimi to our artificial feed, and some cuttlefish refused to eat such feed and consequently died. Therefore, we refrained from training cuttlefish to accept artificial feed directly. Instead, we changed the odor of fresh shrimp. Accordingly, the first step of the progressive training program involved the soaking of oriental shrimp into artificial feed liquid to change the odor of fresh shrimp. This enabled us to train our cuttlefish to accept artificial feed; some groups even maintained a 100% survival rate throughout the tests (Table 4). These results indicate that the role of olfactory stimulation in training should be considered, even though vision is the primary factor. The combined results of the odor test and training programs revealed that visual training yielded short-term results, whereas olfactory training can be beneficial in the long term. Olfactory training is necessary to enable cuttlefish to eat artificial feed in the long term; this position is supported by Guibé et al. (2010) and Lee et al. (2020).
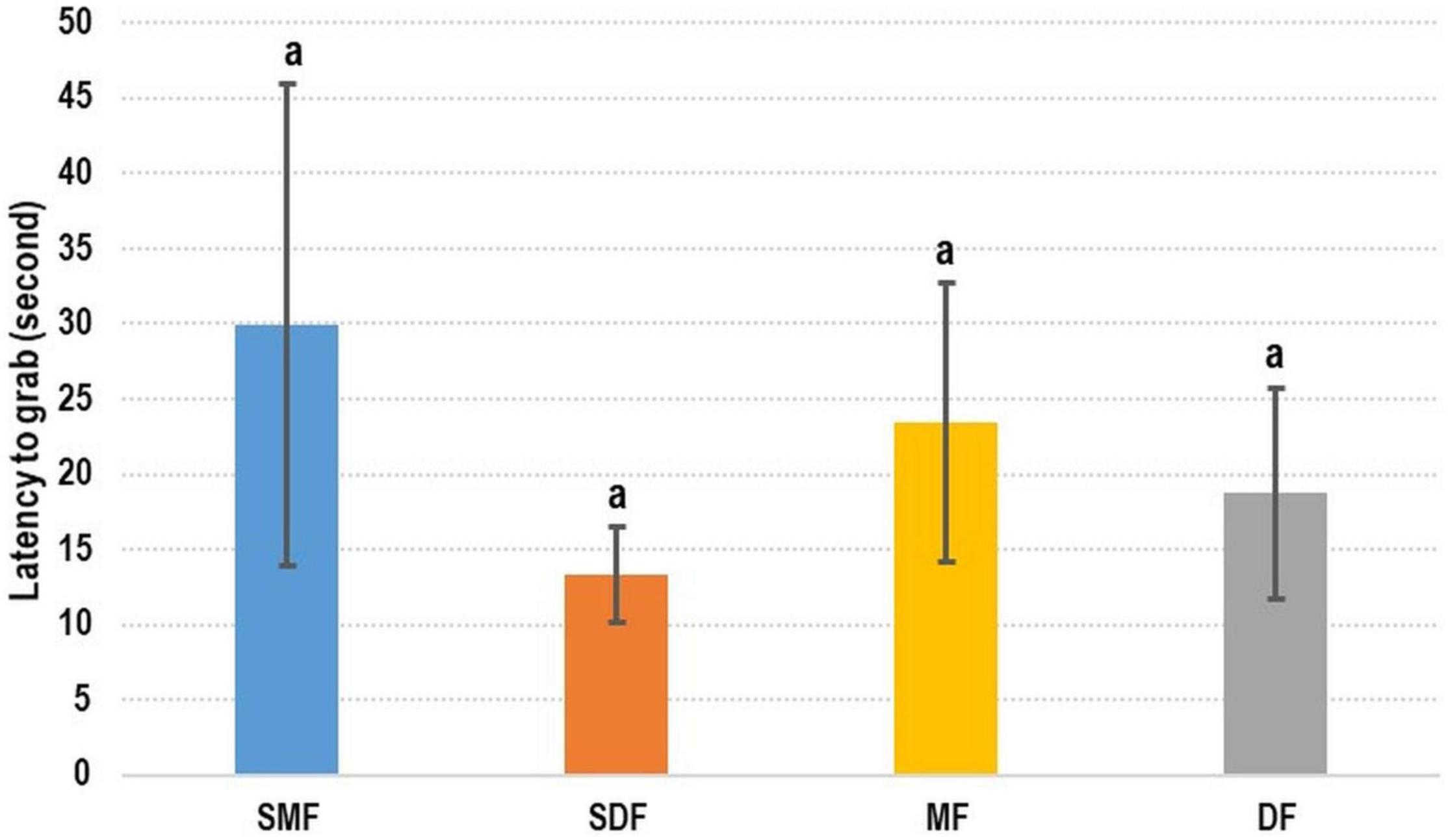
Figure 6. Test of olfactory effect on cuttlefish. No significant difference was observed between feed with and without shrimp surimi. SMF, shrimp surimi-supplemented moist feed; SDF, shrimp surimi-supplemented dry feed; MF, moist feed; DF, dry feed.
The growth performance of cuttlefish that were fed artificial feed was less favorable than that of cuttlefish that were fed fresh shrimp (Table 5). Domingues et al. (2008) examined the growth performance of cuttlefish that were fed frozen baits and artificial diets. They reported that despite the addition of fresh fish and squid in the artificial diets, the feeding and growth rates were lower among the cuttlefish that were fed artificial diets than among those that were fed frozen baits. Ferreira et al. (2010) balanced the nutritional composition of frozen baits and artificial diets; however, they observed that the growth rate of cuttlefish that were fed artificial diets was still lower than that of cuttlefish that were fed frozen baits. Most studies have reported unfavorable results for artificial diets (Lee et al., 1991; Castro et al., 1993; Castro and Lee, 1994; Domingues et al., 2005, 2008; Ferreira et al., 2010; Han et al., 2017); however, Domingues et al. (2005) and Han et al. (2017) have suggested that a high crude protein content increases the weight of the cuttlefish. Although our artificial feed contained approximately 53% of crude protein, PWG, and FCR of the cuttlefish that were fed artificial feed were still less than those of the cuttlefish that were fed fresh shrimp. We discovered that the artificial feed was not always consumed completely by the cuttlefish, whereas the fresh shrimp was almost always consumed completely by the cuttlefish. The preference of cuttlefish for fresh shrimp might explain why they disliked the artificial feed and engaged in foraging behavior only when they were starving. Although our study revealed that the growth performance of cuttlefish that were fed artificial feed was less favorable than that of cuttlefish fed with fresh shrimp, no cuttlefish died during our tests. This indicates that the artificial feed is suitable for cuttlefish.
Conclusion
In this study, we observed that pharaoh cuttlefish preferred rod-shaped, moist artificial feed to other types of feed that were shaped differently or dry. We also discovered that the texture of feed should be similar to that of the prey of cuttlefish. Pharaoh cuttlefish can be trained to eat DF through odor stimulation, and this training process should be longer than 3 weeks (for 8-week-old juveniles). The use of compound feed for long-term cuttlefish rearing is possible, but the nutritional composition of the compound feed that is used should be studied further.
Data Availability Statement
The original contributions presented in the study are included in the article/Supplementary Material, further inquiries can be directed to the corresponding author.
Ethics Statement
The animal study was reviewed and approved by Institutional Animal Care and Use Committee of National Taiwan Ocean University (NTOU).
Author Contributions
C-HM, C-HLn, Y-CC, C–HLo, and T-HH contributed to conception and design of the study. C-HM, C-HLn, and Y-CC collected the data. C-HM, Y-CC, and H-TL performed the statistical analysis. C-HM and T-HH wrote the first draft of the manuscript. C-HLn, Y-CC, H-TL, and C-HLo wrote sections of the manuscript. All authors contributed to manuscript revision, read, and approved the submitted version.
Funding
This work was supported by grants from the Center of Excellence for the Oceans (National Taiwan Ocean University), which were financially supported by the Featured Areas Research Center Program within the framework of the Higher Education Sprout Project by the Ministry of Education, ROC (Taiwan). This work was also supported by the 2018–2020 Ministry of Science and Technology, Taiwan (MOST107-2313-B-019-004, MOST108-2313-B-019-001, and MOST109-2313-B-019-004).
Conflict of Interest
The authors declare that the research was conducted in the absence of any commercial or financial relationships that could be construed as a potential conflict of interest.
Publisher’s Note
All claims expressed in this article are solely those of the authors and do not necessarily represent those of their affiliated organizations, or those of the publisher, the editors and the reviewers. Any product that may be evaluated in this article, or claim that may be made by its manufacturer, is not guaranteed or endorsed by the publisher.
Acknowledgments
We would like to thank Chuan-Chin Chiao (NTHU) for his communication and encouragement.
Supplementary Material
The Supplementary Material for this article can be found online at: https://www.frontiersin.org/articles/10.3389/fmars.2022.765272/full#supplementary-material
References
Alava, V. R., and Pascual, F. P. (1987). Carbohydrate requirements of Penaeus monodon (Fabricius) juveniles. Aquaculture 61, 211–217. doi: 10.1016/0044-8486(87)90150-5
AOAC (2000). Official Methods of Analysis, 17th Edn. Gaithersburg, MD: The Association of Official Analytical Chemists Press.
Baeza-Rojano, E., García, S., Garrido, D., Guerra-García, J. M., and Domingues, P. (2010). Use of Amphipods as alternative prey to culture cuttlefish (Sepia officinalis) hatchlings. Aquaculture 300, 243–246. doi: 10.1016/j.aquaculture.2009.12.029
Boletzky, S. V. (1974). Effets de la sous-nutrition prolongée sur le développement de la coquille de Sepia officinalis L. (Mollusca, Cephalopoda). Bull. Soc. Zool. Fr. 99, 667–673.
Boletzky, S. V. (1983). “Sepia officinalis,” in Cephalopod Life Cycles, ed. P. R. Boyle (London: Academic Press), 31–52.
Boletzky, S. V., and Villanueva, R. (2014). “Cephalopod biology,” in Cephalopod Culture, eds J. Iglesias, L. Fuentes, and R. Villanueva (Dordrecht: Springer Press), 3–14. doi: 10.1007/978-94-017-8648-5_1
Cartron, L., Darmaillacq, A. S., and Dickel, L. (2013). The “prawn-in-the-tube” procedure: what do cuttlefish learn and memorize? Behav. Brain Res. 240, 29–32. doi: 10.1016/j.bbr.2012.11.010
Castro, B. G., DiMarco, F. P., DeRusha, R. H., and Lee, P. G. (1993). The effects of surimi and pelleted diets on the laboratory survival, growth, and feeding rate of the cuttlefish Sepia oficinalis L. J. Exp. Mar. Biol. Ecol. 170, 241–252. doi: 10.1016/0022-0981(93)90155-h
Castro, B. G., and Lee, P. G. (1994). The effects of semi-purified diets on growth and condition of Sepia officinalis L. (Mollusca: Cephalopoda). Comp. Biochem. Physiol. Part A Physiol. 109, 1007–1016. doi: 10.1016/0300-9629(94)90250-x
Choe, S., and Ohshima, Y. (1963). Rearing of cuttlefishes and squids. Nature 197, 307–307. doi: 10.1038/197307a0
Cole, P. D., and Adamo, S. A. (2005). Cuttlefish (Sepia officinalis: Cephalopoda) hunting behavior and associative learning. Anim. Cogn. 8, 27–30. doi: 10.1007/s10071-004-0228-9
Correia, M., Domingues, P. M., Sykes, A., and Andrade, J. P. (2005). Effects of culture density on growth and broodstock management of the cuttlefish, Sepia officinalis (Linnaeus, 1758). Aquaculture 245, 163–173. doi: 10.1016/j.aquaculture.2004.12.017
Domingues, P., Ferreira, A., Marquez, L., Andrade, J. P., Lopez, N., and Rosas, C. (2008). Growth, absorption and assimilation efficiency by mature cuttlefish (Sepia officinalis) fed with alternative and artificial diets. Aquac. Int. 16, 215–229. doi: 10.1007/s10499-007-9139-5
Domingues, P., Sykes, A., Sommerfield, A., Almansa, E., Lorenzo, A., and Andrade, J. P. (2004). Growth and survival of cuttlefish (Sepia officinalis) of different ages fed crustaceans and fish. Effects of frozen and live prey. Aquaculture 229, 239–254. doi: 10.1016/s0044-8486(03)00351-x
Domingues, P. M., Dimarco, P. F., Andrade, J. P., and Lee, P. G. (2005). Effect of artificial diets on growth, survival and condition of adult cuttlefish, Sepia officinalis Linnaeus, 1758. Aquac. Int. 13, 423–440. doi: 10.1007/s10499-005-6978-9
Domingues, P. M., Kingston, T., Sykes, A., and Andrade, J. P. (2001). Growth of young cuttlefish, Sepia officinalis (Linnaeus 1758) at the upper end of the biological distribution temperature range. Aquac. Res. 32, 923–930. doi: 10.1046/j.1365-2109.2001.00631.x
Fan, F., Yin, F., Peng, S. M., Wang, J. G., Sun, P., and Shi, Z. H. (2011). Impacts of starvation on biochemical indices and behaviors of Sepiella maindroni juveniles. Chin. J. Ecol. 30, 2262–2268.
Ferreira, A., Marquez, L., Almansa, E., Andrade, J. P., Lorenzo, A., and Domingues, P. M. (2010). The use of alternative diets to culture juvenile cuttlefish, Sepia officinalis: effects on growth and lipid composition. Aquac. Nutr. 16, 262–275.
Gao, X. L., Jiang, X. M., and Le, K. X. (2014). Analysis and evaluation of nutritional components in different tissues of wild Sepia pharaonis. Chin. J. Anim. Nutr. 26, 3858–3867.
Guibé, M., Boal, J. G., and Dickel, L. (2010). Early exposure to odors changes later visual prey preferences in cuttlefish. Dev. Psychobiol. 52, 833–837. doi: 10.1002/dev.20470
Han, Q., Wang, Y., Lv, T., Han, Q., and Jiangx, X. (2017). Effects of dietary lipids on the growth performance, survival, and digestive enzymes of juvenile cuttlefish, Sepia lycidas. J. World Aquac. Soc. 48, 963–971.
Hao, Z. L., Zhang, X. M., and Zhang, P. D. (2007). Biological characteristics and mutiplication techniques of Sepia esculenta. Chin. J. Ecol. 26, 601–606.
Huang, Y. H., Lin, H. J., Lin, L. Y., and Chiao, C. C. (2019). Do cuttlefish have fraction number sense? Anim. Cogn. 22, 163–168. doi: 10.1007/s10071-018-01232-3
Jiang, M., Chen, Q., Zhou, S., Han, Q., Peng, R., and Jiang, X. (2021). Optimum weaning method for pharaoh cuttlefish, Sepia pharaonis Ehrenberg, 1831, in small-and large-scale aquaculture. Aquac. Res. 52, 1078–1087. doi: 10.1111/are.14963
Jiang, M., Han, Z., Sheng, P., Peng, R., Han, Q., and Jiang, X. (2020). Effects of different weaning protocols on survival, growth and nutritional composition of pharaoh cuttlefish (Sepia pharaonis) Juvenile. J. Ocean Univ. China 19, 1421–1429. doi: 10.1007/s11802-020-4479-0
Jiang, M. W., Peng, R. B., Wang, S. J., Zhou, S. N., Chen, Q. C., Huang, C., et al. (2018). Growth performance and nutritional composition of Sepia pharaonis under artificial culturing conditions. Aquac. Res. 49, 2788–2798. doi: 10.1111/are.13741
Koueta, N., Boucaud-Camou, E., and Noel, B. (2002). Effect of enriched natural diet on survival and growth of juvenile cuttlefish Sepia officinalis L. Aquaculture 203, 293–310. doi: 10.1016/s0044-8486(01)00640-8
Lee, P. G., Forsythe, J. W., DiMarco, P. F., DeRusha, R. H., and Hanlon, R. T. (1991). Initial palatability and growth trials on pelleted diets for cephalopods. Bull. Mar. Sci. 49, 362–372.
Lee, P. G., Turk, P. E., Yang, W. T., and Hanlon, R. T. (1994). Biological characteristics and biomedical applications of the squid Sepioteuthis lessoniana cultured through multiple generations. Biol. Bull. 186, 328–341. doi: 10.2307/1542279
Lee, Y. C., Darmaillacq, A. S., Dickel, L., and Chiao, C. C. (2020). Effects of embryonic exposure to predators on the postnatal defensive behaviors of cuttlefish. J. Exp. Mar. Biol. Ecol. 524, 151288. doi: 10.3389/fphys.2017.00981
Minton, J. W., Walsh, L. S., Lee, P. G., and Forsythe, J. W. (2001). First multi-generation culture of the tropical cuttlefish Sepia pharaonis Ehrenberg, 1831. Aquac. Int. 9, 375–392.
Nabhitabhata, J. (1978). Rearing Experiment on Economic Cephalopod-II: Cuttlefish Sepia pharaonis Ehrenberg. Bangkok: Department of Fisheries Press.
Nabhitabhata, J., and Nilaphat, P. (1999). Life cycle of cultured pharaoh cuttlefish, Sepia pharaonis Ehrenberg, 1831. Phuket Mar. Biol. Cent. Spec. Publ. 19, 25–40.
Nguyen, H. Q., Reinertsen, H., Wold, P. A., Tran, T. M., and Kjørsvik, E. (2011). Effects of early weaning strategies on growth, survival and digestive enzyme activities in cobia (Rachycentron canadum L.) larvae. Aquac. Int. 19, 63–78. doi: 10.1007/s10499-010-9341-8
Ouraji, H., Khalili, K. J., Ebrahimi, G., and Jafarpour, S. A. (2011). Determination of the optimum transfer time of kutum (Rutilus frisii kutum) larvae from live food to artificial dry feed. Aquac. Int. 19, 683–691.
Pascual, E. (1978). Crecimiento y alimentacíon de tres generaciones de Sepia officinalis en cultivo. Investig. Pesq. 42, 421–442.
Peng, R. B., Le, K. X., Jiang, X. M., Wang, Y., and Han, Q. X. (2015). Effects of different diets on the growth, survival, and nutritional composition of juvenile cuttlefish, Sepia pharaonis. J. World Aquac. Soc. 46, 650–664. doi: 10.1111/jwas.12235
Perrin, A., Le Bihan, E., and Koueta, N. (2004). Experimental study of enriched frozen diet on digestive enzymes and growth of juvenile cuttlefish Sepia officinalis L. (Mollusca Cephalopoda). J. Exp. Mar. Biol. Ecol. 311, 267–285. doi: 10.1016/j.jembe.2004.05.012
Shinzato, S., Yasumuro, H., and Ikeda, Y. (2018). Visual stimuli for the induction of hunting behavior in cuttlefish Sepia pharaonis. Biol. Bull. 234, 106–115. doi: 10.1086/697522
Sorgeloos, P., Dhert, P., and Candreva, P. (2001). Use of the brine shrimp, Artemia spp., in marine fish larviculture. Aquaculture 200, 147–159. doi: 10.1016/s0044-8486(01)00698-6
Takeuchi, T., and Haga, Y. (2015). Development of microparticulate diets with special reference to Pacific bluefin tuna, abalone, and Japanese spiny lobster: a review. Fish. Sci. 81, 591–600. doi: 10.1007/s12562-015-0883-6
Villanueva, R., Perricone, V., and Fiorito, G. (2017). Cephalopods as predators: a short journey among behavioral flexibilities, adaptions, and feeding habits. Front. Physiol. 8:598. doi: 10.3389/fphys.2017.00598
Wen, J., Chen, D. H., and Zeng, L. (2014). Comparison in nutritional quality between wild and cultured cuttlefish Sepia pharaonis. Chin. J. Oceanol. Limnol. 32, 58–64. doi: 10.1007/s00343-014-3083-9
Yang, T. I., and Chiao, C. C. (2016). Number sense and state–dependent valuation in cuttlefish. Proc. Royal Soc. B 283:20161379. doi: 10.1098/rspb.2016.1379
Yasumuro, H., and Ikeda, Y. (2018). Environmental enrichment affects the ontogeny of learning, memory, and depth perception of the pharaoh cuttlefish Sepia pharaonis. Zoology 128, 27–37. doi: 10.1016/j.zool.2018.05.001
Zepeda, E. A., Veline, R. J., and Crook, R. J. (2017). Rapid associative learning and stable long-term memory in the squid Euprymna scolopes. Biol. Bull. 232, 212–218. doi: 10.1086/693461
Zheng, X. D., Lin, X. Z., Liu, Z. S., Wang, Z. K., and Zheng, W. B. (2014). “Sepiella japonica,” in Cephalopod Culture, eds J. Iglesias, L. Fuentes, and R. Villanueva (Dordrecht: Springer Press), 241–252. doi: 10.1007/978-94-017-8648-5_14
Keywords: cephalopods, hatchery, aquaculture, behavior, domestication
Citation: Ma C-H, Lin C-H, Chang Y-C, Lee H-T, Liou C-H and Hsu T-H (2022) Potential Plasticity of Artificial Feed Preference in Juvenile Pharaoh Cuttlefish (Sepia pharaonis) Through Progressive Training Programs. Front. Mar. Sci. 9:765272. doi: 10.3389/fmars.2022.765272
Received: 26 August 2021; Accepted: 19 January 2022;
Published: 07 March 2022.
Edited by:
Michael Phillips, WorldFish (Malaysia), MalaysiaReviewed by:
Bin Xia, Qingdao Agricultural University, ChinaYuzuru Ikeda, University of the Ryukyus, Japan
Copyright © 2022 Ma, Lin, Chang, Lee, Liou and Hsu. This is an open-access article distributed under the terms of the Creative Commons Attribution License (CC BY). The use, distribution or reproduction in other forums is permitted, provided the original author(s) and the copyright owner(s) are credited and that the original publication in this journal is cited, in accordance with accepted academic practice. No use, distribution or reproduction is permitted which does not comply with these terms.
*Correspondence: Te-Hua Hsu, cmVhbGdpZ2lAZ21haWwuY29t