- 1Akvaplan-niva AS, Fram Centre, Tromsø, Norway
- 2NOFIMA AS, Tromsø, Norway
- 3K-To-9 AS, Hammerfest, Norway
- 4University Centre in Svalbard, Longyearbyen, Norway
The red king crab (RKC, Paralithodes camtschaticus) was introduced to the southern Barents Sea in the 1960s with the aim to develop a new, commercially attractive stock of the species. In the subsequent decades, the stock has indeed become abundant and widespread, but the species’ presence also implies intense predation on benthic biota and thereby severe degradation of benthic ecosystems. Our capacity to monitor and harvest the species efficiently is therefore imperative. Yet, fishermen report highly variable catches despite little variation in the timing and location of fishing, possibly induced by the species’ migratory behavior, which makes the search for crab aggregations time consuming and expensive. Previous studies have shown that the RKC is capable of conducting long-distance migrations, and suggest that the species conduct seasonal migrations between shallow (winter- and springtime) and deep waters (summer and autumn). Here, we applied telemetry to investigate the migratory behavior and habitat utilization of 37 adult individuals of the RKC in a relatively shallow fjord in northern Norway from late May until early November. Approximately half of the crabs (n = 16) left the study area early during the study period, but some individuals (n = 3) were recaptured between 53 and 147 km away from the study area, confirming that the RKC may conduct long-distance migrations. In contrast to expectations, most of the remaining individuals of RKC (n = 16) stayed for a prolonged summer and autumn period and used a limited portion of the fjord. These crabs responded quickly to changes in ambient water temperature, seeking deeper and colder waters masses when the temperature in shallower waters increased. Several individuals showed nearly identical spatio-temporal distributions, which supports earlier observations of crab aggregations. Our data indicates that the area utilization of the RKC is affected by trade-offs between biotic and abiotic factors, in which sub-optimal water temperatures may be tolerated provided appropriate access to food. Thus, our findings suggest that the current knowledge of RKC seasonal migration patterns may need to be revised, which in turn will have implications for managers and the fishing industry. This study demonstrates the high potential of telemetry studies to yield new, high-resolution data and knowledge of species’ ecology.
Introduction
The red king crab (RKC, Paralithodes camtschaticus) is indigenous to the northern Pacific Ocean, but was introduced at the Murman coast (Russia) of the Barents Sea in the 1960s (Orlov and Ivanov, 1978), and has since increased in abundance and expanded its spatial distribution. Dense occurrences of the RKC are currently found from Kolguyev Island (eastern Barents Sea, Russia) in the east to the city of Tromsø, Troms and Finnmark County (Norway) in the west, and the crabs are most numerous in coastal areas (Britayev et al., 2010; Windsland et al., 2014). In Norway, the RKC has high commercial value (first-hand value of catches in Norwegian waters in 2019: ∼30,000,000 €).1 In Norwegian waters, the RKC is managed according to two different approaches. In the areas east of 26°E (North Cape; Figure 1A), the fishery follows a conventional management regime in which both males and females above the minimum landing size of 130 CL can be exploited throughout the year (e.g., Anon, 2019). West of this border an eradication fishery is implemented (Anon, 2007). Accurate monitoring, management and effective harvesting rely on detailed knowledge of the species’ distribution, population structure, and habitat use, features that are likely driven by trade-offs between crab density, food availability, and water temperature (Christiansen et al., 2015). Yet, these features are challenging to assess due to the species’ migratory behavior.
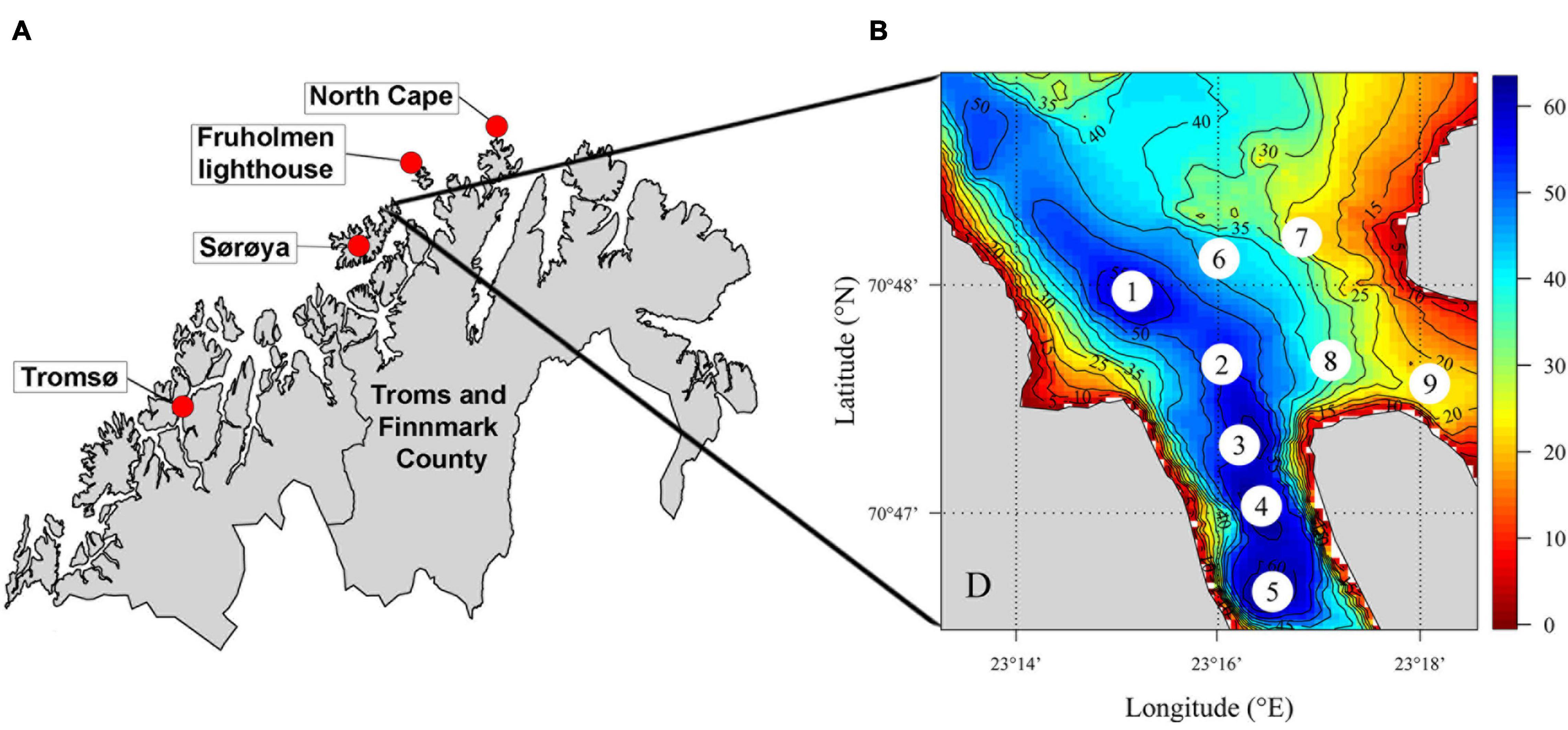
Figure 1. (A) Map of Troms and Finnmark County in northern Norway. The migratory behavior of RKC was investigated in in Gamvikfjorden at Sørøya island in 2016. (B) Bottom topography (water depths in meters) in the study area, with the locations of selected grab sample sites.
The RKC is highly mobile and exhibits both seasonal habitat shifts and considerable long-distance migration. In Northern Pacific waters, individuals of both genders were shown to move > 10 km day–1 (Marukawa, 1933), whereas in Alaska, an average walking distance of nearly 1 km day–1 was maintained for 200 days (Hayes and Montgomery, 1963). Similarly, tag-recapture investigations in Norwegian waters confirm that the RKC is capable of long-range (>100 km) migrations (Windsland et al., 2014). However, most investigations indicate that the RKC largely operates at small spatial scales and avoids extensive migrations (Hayes and Montgomery, 1963; Stone et al., 1992; Windsland et al., 2014), typically residing in fjords and coastal waters (Pinchukov and Sundet, 2011). Within these local home ranges, the RKC is assumed to conduct seasonal migrations between shallow mating areas (during winter- and springtime) and deeper feeding areas (summer and autumn; Sundet and Hjelset, 2010). In another fjord in northern Norway, where the seasonal variation in the depth distribution of RKC was investigated using traps, catches of adult males were made in shallow waters (50 m) in the period between November to May (Sundet and Hjelset, 2010). On the other hand, the catches of adult females in shallow waters mainly occurred during the months January–March (Sundet and Hjelset, 2010). Furthermore, Sundet and Hjelset (2010) speculated that the poor catches of females in April and May were not due to an absence of individuals, but rather due to a reduced tendency to enter traps caused by a low feeding activity during reproductive activities. From June through September (males) or November (females), adult RKC were almost exclusively caught in deeper waters (≥ 150 m; Sundet and Hjelset, 2010). Furthermore, although the RKC tolerates water temperatures of > 15°C (Rodin, 1989), laboratory studies indicate that the RKC prefers water temperatures between 2.5 and 3.5°C and avoid water temperatures > 4°C (Christiansen et al., 2015). As such, seasonal variation in ambient water temperatures plausibly promote migrations between shallow and deep waters. Nevertheless, divers frequently report observations of adult RKC in shallow waters during summertime (Aune, pers. obs.), which suggest that exceptions from the seasonal migration pattern are common. Observations from the Russian part of the Barents Sea suggest that the RKC prefers sandy and muddy sediments and topographies characterized by underwater slopes (Anisimova and Lubin, 2008). However, most data on RKC distributions have been obtained by means of trap fisheries (commercial and scientific) and are therefore biased by variation in the species’ catchability. Indeed, low catchability of the RKC may occur due to life history events such as reproduction and molting (e.g., Sundet and Hjelset, 2010). Moreover, a combination of factors including aggregative behavior, high mobility and seasonal variation in habitat utilization suggest that it is challenging to predict the species’ actual distributions in time and space. Consequently, fishermen report highly variable catches despite low variability in the timing and location of the fishery. Indeed, high-resolution data describing RKC habitat use and migratory and aggregative behavior are largely lacking, and the current knowledge of the species’ general migrations is therefore uncertain.
The RKC is omnivorous, and ∼100 prey species have been recorded in their stomachs, mostly including polychaetes, mollusks, crustaceans, echinoderms, fish, and algae (Britayev et al., 2010; Falk-Petersen et al., 2011; Fuhrmann et al., 2017). The diet in the southern Barents Sea is comparable to that in their home range in the northern Pacific Ocean (Falk-Petersen et al., 2011). Although the king crab is a generalist, it seems to prefer certain types of prey, such as polychaetes and bivalves (e.g., the scallop, Chlamys islandica; Jørgensen and Primicerio, 2007; Britayev et al., 2010). The RKC has been shown to effect the ecosystem structure and function in areas where it is abundant, causing bioturbation and abundance declines of taxa common in the northeast Atlantic including non-motile polychaetes, bivalves, and echinoderms (Falk-Petersen et al., 2011; Oug et al., 2011, 2018). This fact, and the recognition that it is an introduced alien species, requires authorities to enact policies to limit the species’ footprint. To facilitate such efforts, detailed knowledge of the species’ seasonal variation in migratory behavior and habitat utilization is required.
In this study, we applied acoustic telemetry to investigate the summer and autumn migratory behavior of the RKC in Gamvikfjorden, a fjord in northern Norway recently invaded by the species. As a consequence of the high but strongly variable catches of the RKC in the fjord and adjacent areas during recent years, the fishermen spent considerable amounts of time and fuel searching for crab aggregations. This issue motivated the implementation of a field study aiming to shed light on drivers of the species’ spatio-temporal distribution patterns. Focusing on a period of > 5 months (the 24th of May to the 1st of November 2016), we integrated triangulated telemetry data from 37 adult (CL = 15.5–20.5 cm) RKC individuals with high-resolution data on bathymetry and water temperature. Our overall aim for the study was to obtain detailed data and knowledge on RKC environmental preferences, migratory behavior, and activity level (e.g., walking speed), to facilitate effective monitoring, management and harvesting of this introduced species.
Aiming to assess how telemetry data correspond with our current understanding of RKC seasonal migrations, we hypothesized that
1. A proportion of the tagged RKC individuals would reside in the study area for a prolonged period.
2. The crabs would show seasonal variation in behavior, including area utilization and habitat choice (e.g., bottom depth and water temperature).
Materials and Methods
Study Area
Gamvikfjorden is located at the north-western side of the island Sørøya in northern Norway (Figures 1A,B). The study area extended across most of the fjord (Figure 1), with direct access to the climatic and oceanographic conditions of the Barents Sea to the north. The fjord is deeper (maximum depth is ∼63 m) than the area in the 5 km radius outside the fjord mouth (20–50 m), and the fjord bathymetry and topography is steep on the western side and in the inner parts, whereas flatter and partially sandy on the eastern side. The study area included the inner and central parts of the fjord (Figure 1), with water depths ranging between 1 and 63 m (mean depth of study area ∼35 m).
Investigations of Seafloor Topography, Sediments, and Benthic Fauna
High-resolution seabed topography data were obtained from the Norwegian Mapping Authority and aggregated to a raster grid with a spatial resolution of 35 m × 35 m (Figure 1B).
Sediment sampling using a grab (0.1 m2 van Veen grab equipped with 2 × 5 kg lead weights) was conducted at 9 locations within the study area to collect data on sediment quality (texture, chemistry) as well as composition and density of benthic fauna (macrofauna and infauna known to be consumed by RKC). The sediment sampling was conducted on the 12th of October 2019. The sediment sample sites were located along gradients of bottom depth and distance from the fjord mouth (Figure 1B and Supplementary Table 1). Sediments from the upper 5 cm were selected from one sediment sample per location for common chemical and sediment analyses [total organic carbon (TOC), total nitrogen (TN), and the TOC/TN (C/N) ratio]. The coarseness of the sediments was defined as the percentage of the sediment grains being < 0.063 mm (i.e.,% pelite), where a high pelite percentage indicates fine sediments. The remaining sediments in the grab were sieved through 1 mm sieve, and the composition of the benthos assemblage was identified to major taxonomic groups and counted on site (Supplementary Table 2).
Tagging, Tracking, and Positioning
Baited square pots were used to catch 37 adult RKC individuals (24 males and 13 females) in Gamvikfjorden on the 24th of May 2016 (Table 1). The crabs were in the body weight (BW) range 1.2–3.5 kg, carapace width (CW) range 13–20 cm and carapace length (CL) range 15.5–20.5 cm. The CL values implies that all crabs were sexually mature (Hjelset et al., 2009). Each crab was tagged with an acoustic transmitter (tag types ATID-MP-13 or ADT-MP-13, transmission rate: 240–360 s random interval, mass with attachment in water ∼8.5 g, Thelma Biotel AS, Norway)2 attached to the fourth pereopod, and the crabs were then carefully released back into the water at the same location where they were captured. Tag to body weight ratio was < 0.8%, i.e., well below the general rule of thumb of 2% of the study object (e.g., Cooke et al., 2004).
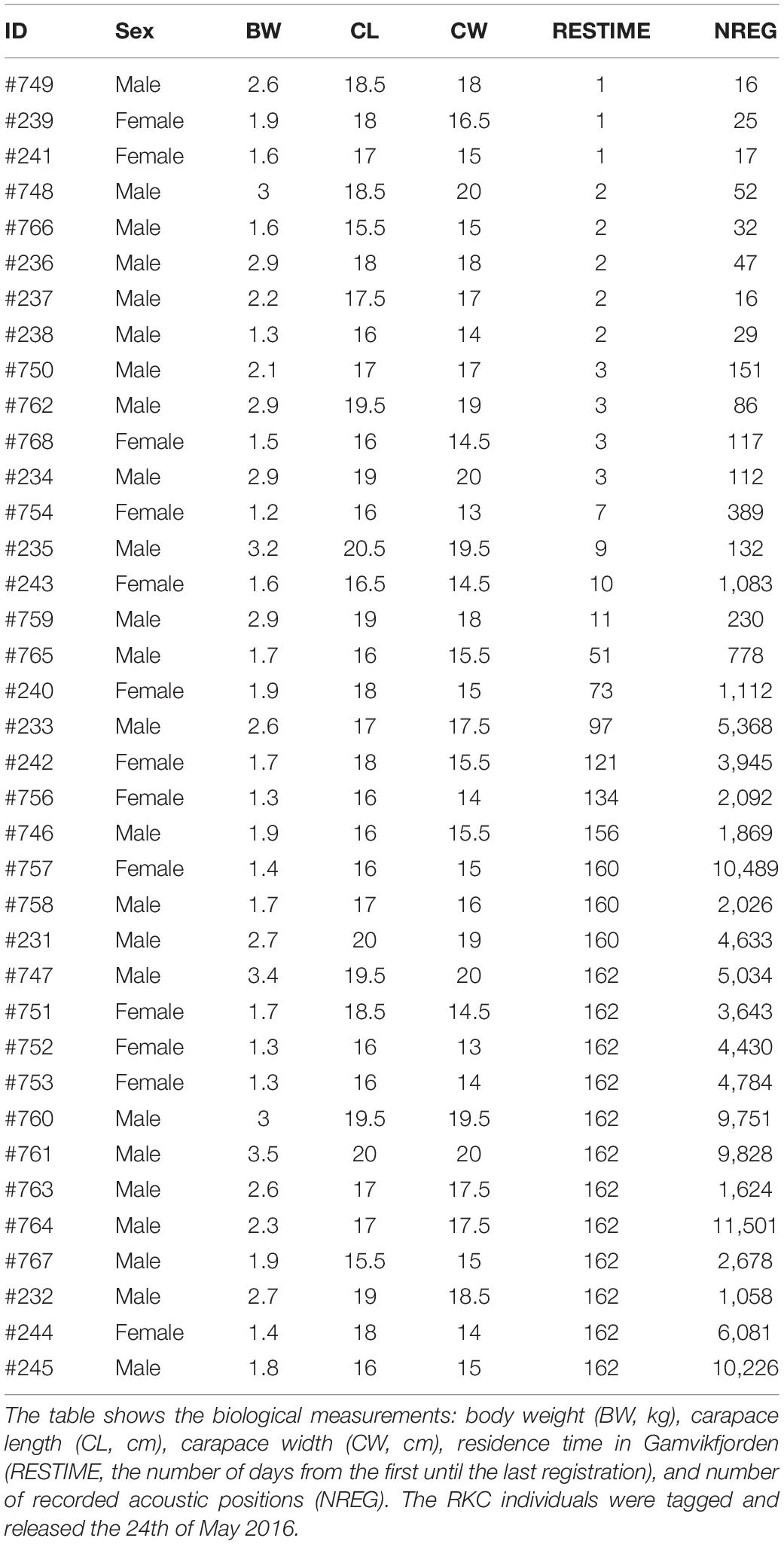
Table 1. Overview of acoustically tagged RKC included in a telemetry study in Gamvikfjorden, northern Norway, in 2016.
Acoustic receivers (n = 54) equipped with temperature sensors (type TBR-700, Thelma Biotel AS) were deployed close to the seabed throughout the inner part of Gamvikfjorden on the 25th of April 2016 (Figure 2). These receivers were located according to a pre-defined grid system, which allowed for detailed triangulation of the crabs’ positions during the period from the 24th of May until the 1st of November 2016. Harsh weather conditions caused the loss of 16 receivers, and the acoustic coverage was therefore limited in some parts of the fjord (in particular in the inner part of the study area; Figure 2). Data obtained by the 38 remaining receivers (Supplementary Table 3) resulted in a position dataset consisting of 105,484 pairs of accurate spatial coordinates and associated time indications, describing the seasonal movements of the 37 tagged crabs within the study area. These recordings constituted the basis for further numerical analyses. An exhaustive description of the methodology is provided in Aune et al. (2022).
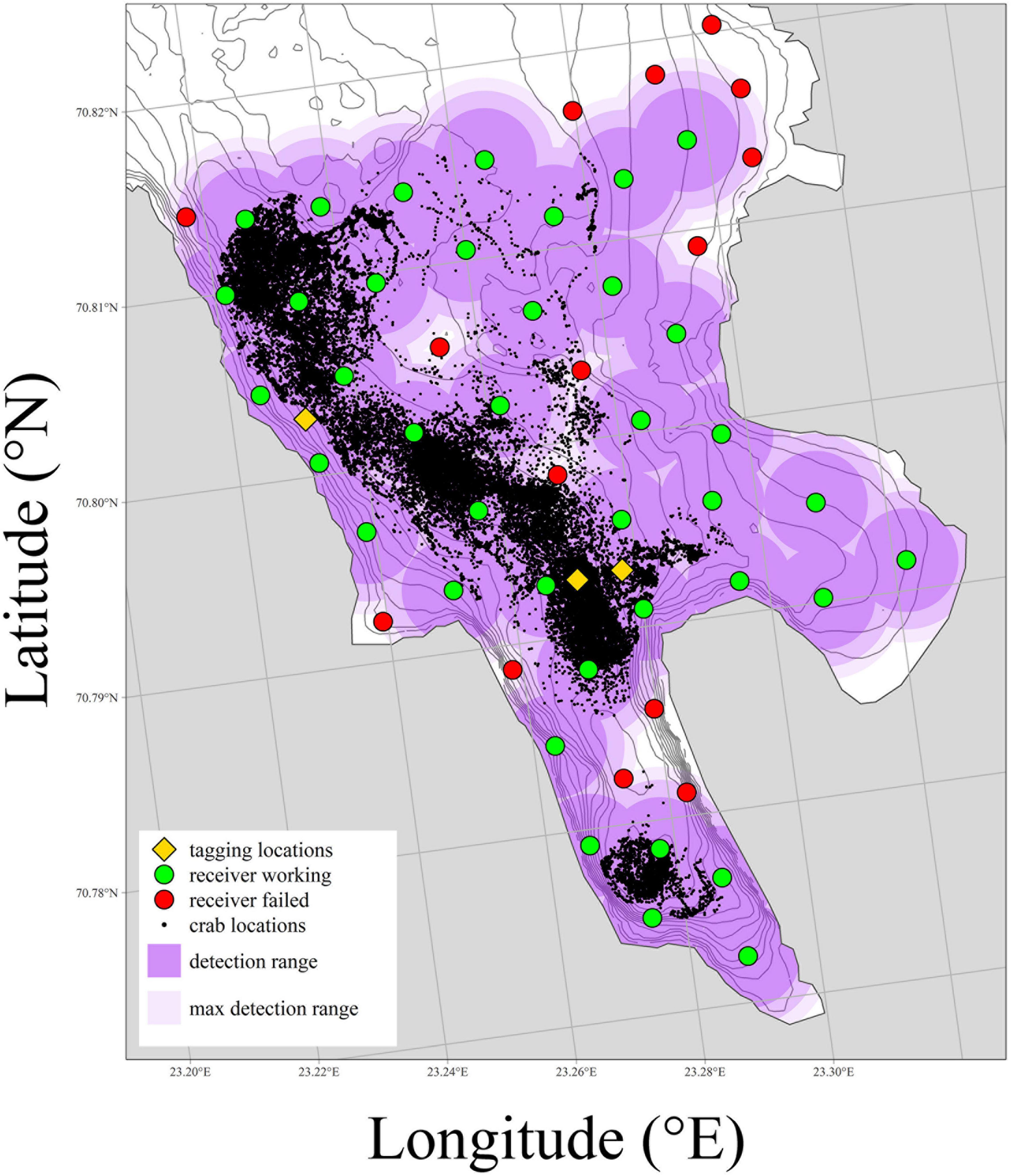
Figure 2. Overview map showing the study fjord, locations of receivers (both working and failed), locations where tagged individuals were released and all estimated locations of tagged red king crab (Paralithodes camtschaticus). Additionally, estimated detection ranges for each working receiver have been overlayed in three levels. These correspond to—in decreasing color intensity—minimum, mean and maximum distance all crab locations had to their nearest working receiver. See Supplementary Table 3 for positions of acoustic receivers.
Numerical Analyses
To assess the seasonal variability of water temperature in the study area, the temperature recordings from the acoustic receivers were assessed as a function of time for each receiver position. Also, to explicitly assess daily range and difference in temperature within the study area, we calculated daily mean temperatures at each receiver position.
Fjord oceanography is known to be affected by meteorological conditions such as strong winds (Inall and Gillibrand, 2010). In order to investigate whether the seasonal variation in water temperature could be related to wind conditions, we obtained data on daily maximum wind speed recorded at the closest weather station, located at Fruholmen lighthouse, located 42 km northeast of Gamvikfjorden (Figure 1A). These data were freely available from https://seklima.met.no/observations.
To assess the relationships between benthic faunal composition and associated covariates (data on bottom depths and sediment properties), a canonical correspondence analysis (CCA; ter Braak, 1986) was conducted using the R package vegan (Oksanen et al., 2020).
Sex-specific seasonal variation in migratory behavior and habitat utilization was assessed by means of the latitude and water depth that the crabs used, as well as the water temperature they experienced during the study period. Water depths associated with crab locations were obtained from the gridded bottom topography data. As the study area lies approximately along a north-south gradient, crab movements in and out of the fjord were assessed by means of the latitude of each recording. For each individual, estimates of the minimum walking speed were calculated as the distance between two successive recordings divided by the time difference between these two recordings, and the daily averages of these estimates were calculated. The seasonal progression of these daily minimum walking speed estimates was assessed by means of a linear regression model.
For each recorded crab location, the temperature experienced by the crab was obtained from the closest acoustic receiver. Monthly individual space use for each crab was estimated as the central location occupied by each individual (not taking into account depth). Total monthly space use by all tagged crabs in turn was calculated using minimum convex polygons (MCP) encompassing all recorded locations each month. Individual home ranges were estimated as 100% MCP of all relocations recorded by each respective crab. This analysis was only conducted for individuals with at least 9 days of data. All analyses were carried out in R 4.0.0 (R Core Team, 2020).
Results
Seafloor Samples
Grab sample data from selected locations along gradients of latitude and water depth indicate spatial variation in sediment properties and benthic community structure (both composition and abundance) (Supplementary Tables 1, 2). In the CCA, axis 1 accounted for 81.0% of the variation, characterized by a gradient in depth and bottom substrate (Figure 3). Indeed, pelite, TOC and TN values increased with increasing water depth, and showed the highest values at > 45 m water depth (Supplementary Tables 1, 2). Polychaetes constituted the most abundant benthos group at most of the grab sample stations. Bivalves were only present in the stations shallower than 45 m. Crustaceans and the echinoderms were also generally more abundant at shallow than deeper stations.
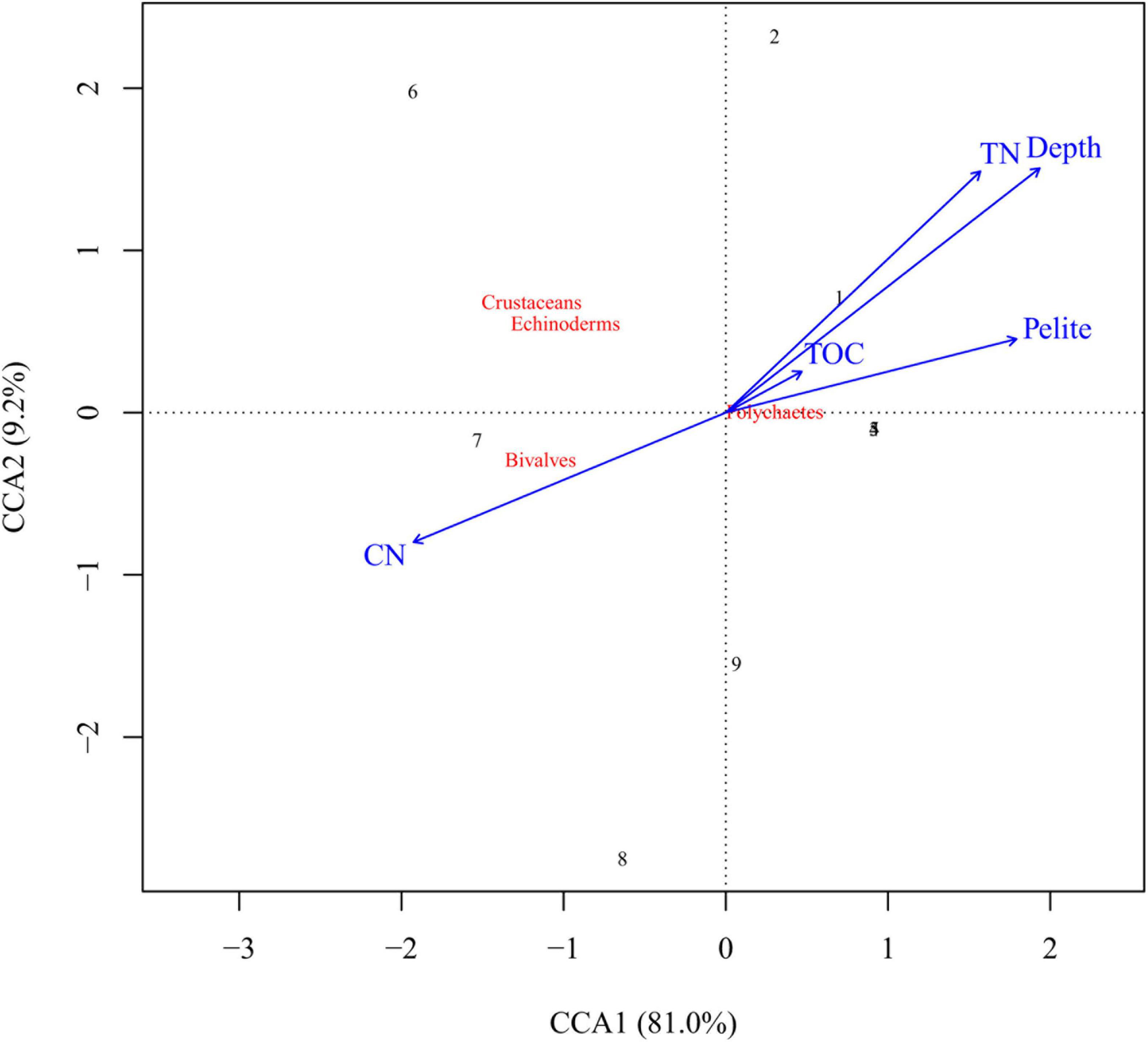
Figure 3. CCA plot of benthic taxa composition as function of selected environmental variables, based on grab samples collected from the seabed in Gamvikfjorden the 12th of October 2019. TOC, total organic carbon; TN, total nitrogen; Pelite = fine fraction of the sediment (% < 0.063 mm); CN, the C:N ration (i.e., TOC:TN). The numbers refer to the grab sample sites (Supplementary Table 1).
Water Temperature Regime
Strong spatial and seasonal variation in bottom water temperature was observed, and the seasonal water temperature variability largely depended on the water depth (Figure 4A and Supplementary Figure 1). When the acoustic receivers were deployed (i.e., 25th of April), relatively homogeneous water temperatures were recorded, ranging from 3.8 to 4.2°C (not shown in the figures). One month later, when the tagged crabs were released into the study area (i.e., 24th of May), the water masses had started to heat up, but with a stronger temperature increase in shallow than in deeper water masses, resulting in an increased temperature range (4.8–6.5°C). The largest difference in water temperature within the study area was observed on the 14th of July (range: 7.3–10.8°C), after which the water temperature at the shallowest stations started to decrease. In the beginning of October, the water temperatures at the deepest stations started to decline from a maximum of 9.0°C, after which the temperature difference between shallow and deep parts of the fjord was never greater than 0.9°C. At the last day of the study period (the 1st of November), the water temperatures ranged from 7.7 to 8.4°C.
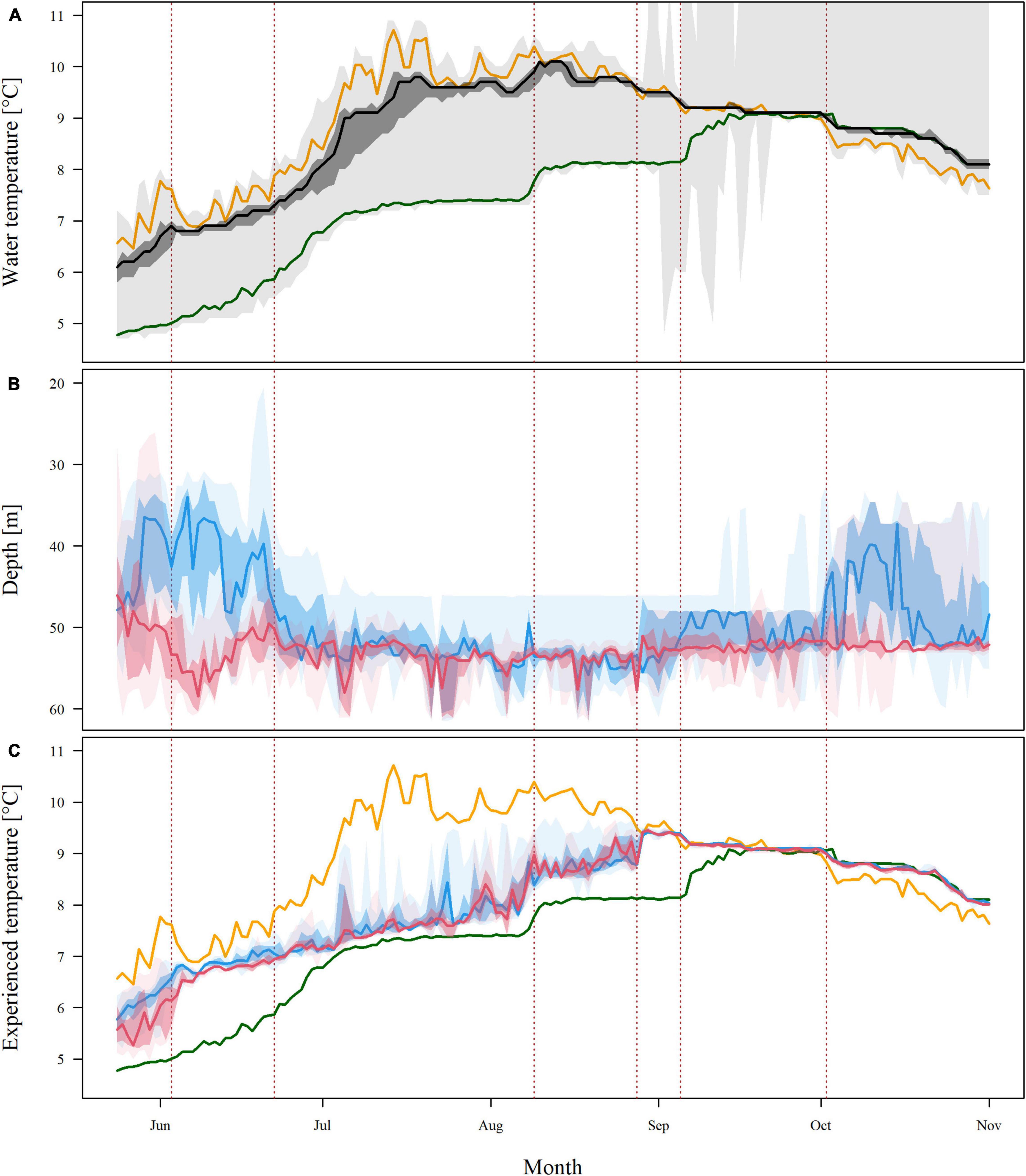
Figure 4. Seasonal data on water temperature and habitat utilization of 37 acoustically tagged individuals the red king crab (RKC, Paralithodes camtschaticus) in Gamvikfjorden (northern Norway) from the 24th of May until the 1st of November 2016. Solid lines show the overall running median values, the dark shaded areas show the data between the 25th and the 75th percentiles, and the lightly shaded areas show the first and third quartiles. Dotted red lines indicate dates of water mass exchanges. See Supplementary Table 3 for positions of temperature loggers. (A) Seasonal variation in water temperature. The orange line shows the water temperature at logger 121 (highest average temperature), the green line shows the water temperature at logger 100 (lowest average temperature), the black line show the overall running temperature median value. (B) Seasonal variation in daily mean depth utilization of the RKC individuals, separated into males (blue) and females (red). (C) Seasonal variation in daily mean experienced water temperature of the RKC individuals, separated into males (blue) and females (red). The orange line shows the water temperature at logger 121 (highest average temperature), the green line shows the water temperature at logger 100 (lowest average temperature).
With one exception (i.e., at acoustic receiver #124, located at a water depth of 46 m), the average bottom water temperature over the entire study period was lower in areas deeper than 45 m as compared to shallower areas. Shallow parts of the fjord (< 45 m water depth) showed a relatively continuous increase in water temperature, whereas temperature increases in deeper parts of the fjord (> 45 m water depth) largely depended on episodic water exchange with the surrounding oceanic water masses. We identified six prominent water exchange events, occurring on the 4th of June, 23rd of June, 8th of August, 29th of August, 6th of September, 3rd of October and 18th of October. An examination of historic weather data revealed that the important water exchange events coincided with distinct events of strong winds (> 19 ms–1; Supplementary Figure 2). Specifically, on average, the six episodes of water exchange identified were associated with maximum daily wind speeds of 22.4 ± 1.9 ms–1. In contrast, the average of the daily maximum wind speed over the entire study period was 13.6 ± 4.4 ms–1.
General Migration Behavior
The detection range estimated as the maximum distance of each crab location to the nearest working receiver was on average 400 m up to a maximum of 476 m (Figure 2). The residence time of the crabs (i.e., the number of days from the first until the last registration) varied from 1 to 162 days (mean: 80 days). Whereas 16 crabs left the study area during the first 11 days, 5 crabs stayed in the study area for between 51 and 134 days, and the remaining 16 crabs stayed in the study area for at least 156 days. Harsh weather conditions caused the loss of several acoustic receivers during the study period, and therefore some parts of study area were poorly covered, which in turn made individual crabs “disappear” from the study area during certain days (Supplementary Figures 3, 4). The average number of crabs recorded per day was 12.5 ± 3.8 crabs. Depending on the residence time and the time spent in poorly covered parts of the fjord, the number of records per crab during the entire study period varied from 16 to 11,501 (mean number of records per crab: 2,851).
Position data revealed that the crabs mostly resided in the central, deep trench and largely avoided the shallower parts of the fjord (examples given in Figure 5). The crabs that left the study area shortly after the release typically followed remarkably straight lines toward the fjord mouth (Figures 5A,B). On the other hand, those crabs that stayed in the study area for an extended period of time typically made several movements between the northern and southern parts of the fjord, largely (but not always) following the central deep trench (Figures 5C,D).
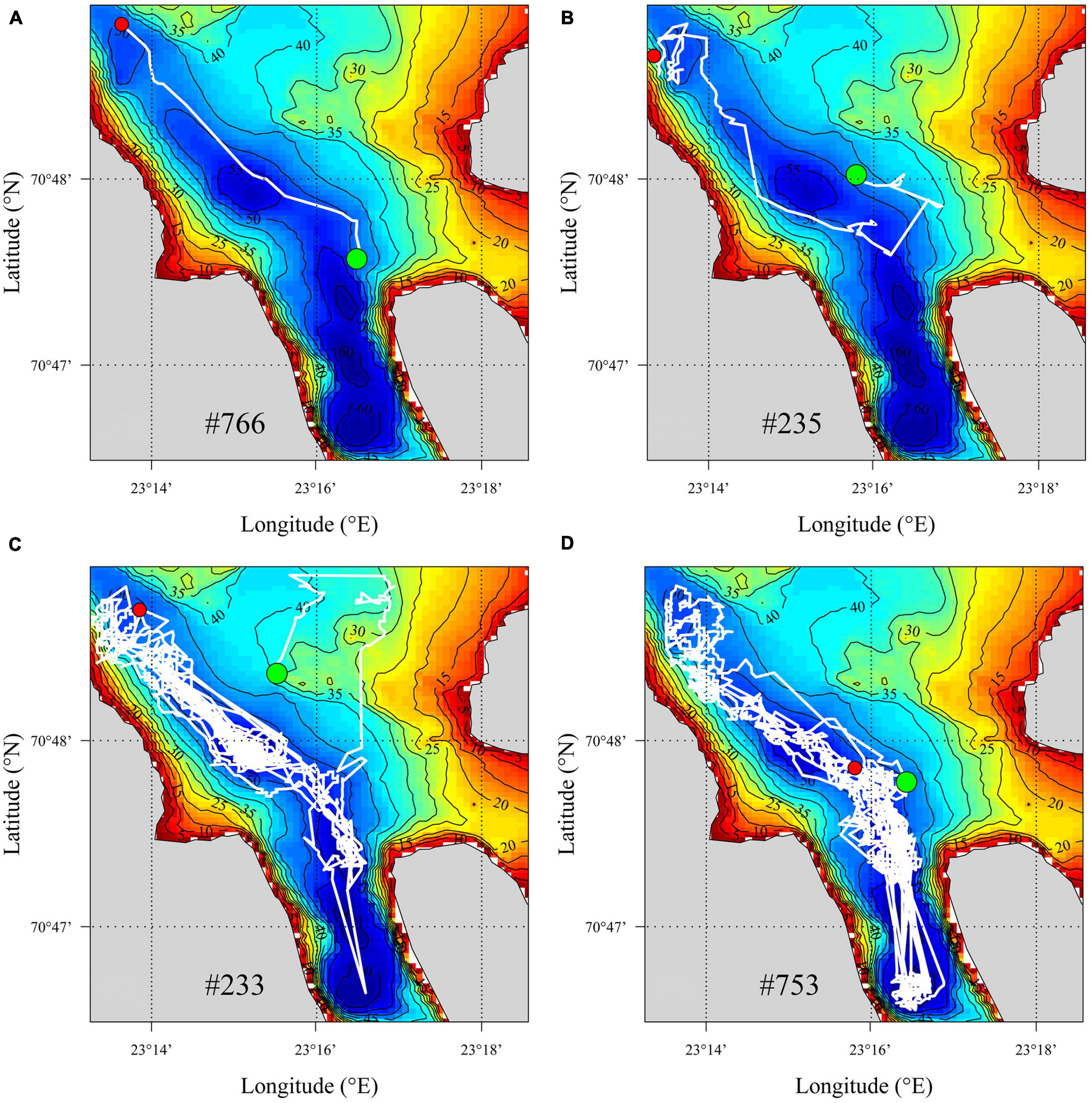
Figure 5. Illustration of movement patterns of four selected individuals of acoustically tagged red king crab (Paralithodes camtschaticus) released in Gamvikfjorden, northern Norway, from the 24th of May 2016. White lines indicate the shortest distance between successive recordings and the green and red dots indicate spatial positions of the first and last recordings, respectively. (A) Crab #766, which left the study area after 15 h; (B) crab #235, which left the study area after 9 days; (C) crab #233, which left the study area after 97 days; (D) crab #753, which stayed in the study area throughout the study period (i.e., for 162 days until the 1st of November 2016).
Seasonal Variation in Depth, Latitude, and Experienced Temperature
The crabs displayed strong seasonal variation in water depth, latitude, and experienced temperature (Figures 4B,C and Supplementary Figure 5). From the end of June until the beginning of October, the crabs were largely found in > 50 m water depth, whereas also shallower waters (∼30–50 m) were utilized by male crabs earlier and later than this period (Figure 4B). With one exception (i.e., 8th of August, a date when only three crabs were registered because they moved into areas with limited acoustic coverage due to the loss of acoustic receivers; Figure 2 and Supplementary Figures 3, 4), the crabs were found significantly deeper during the period 23rd of June to the 7th of September (both dates associated with water mass exchange) compared to at the beginning of the study period (p < 0.05). Males showed a tendency to utilize shallower parts of the fjord more than the females, and displayed a stronger seasonal variation in depth “preference.”
From the beginning of the study period until the middle of July, the crabs were largely found in the central and northern part of the study area (north of 70.79°N; Figure 6 and Supplementary Figure 5). In July, some crabs moved into the southernmost (innermost) parts of the fjord, whereas others stayed in the outer part of the fjord, resulting in the most widespread crab distribution observed during the study period. From mid-August onward, the crabs were mostly recorded in the mid-latitudes of the study area (70.79–70.80°N).
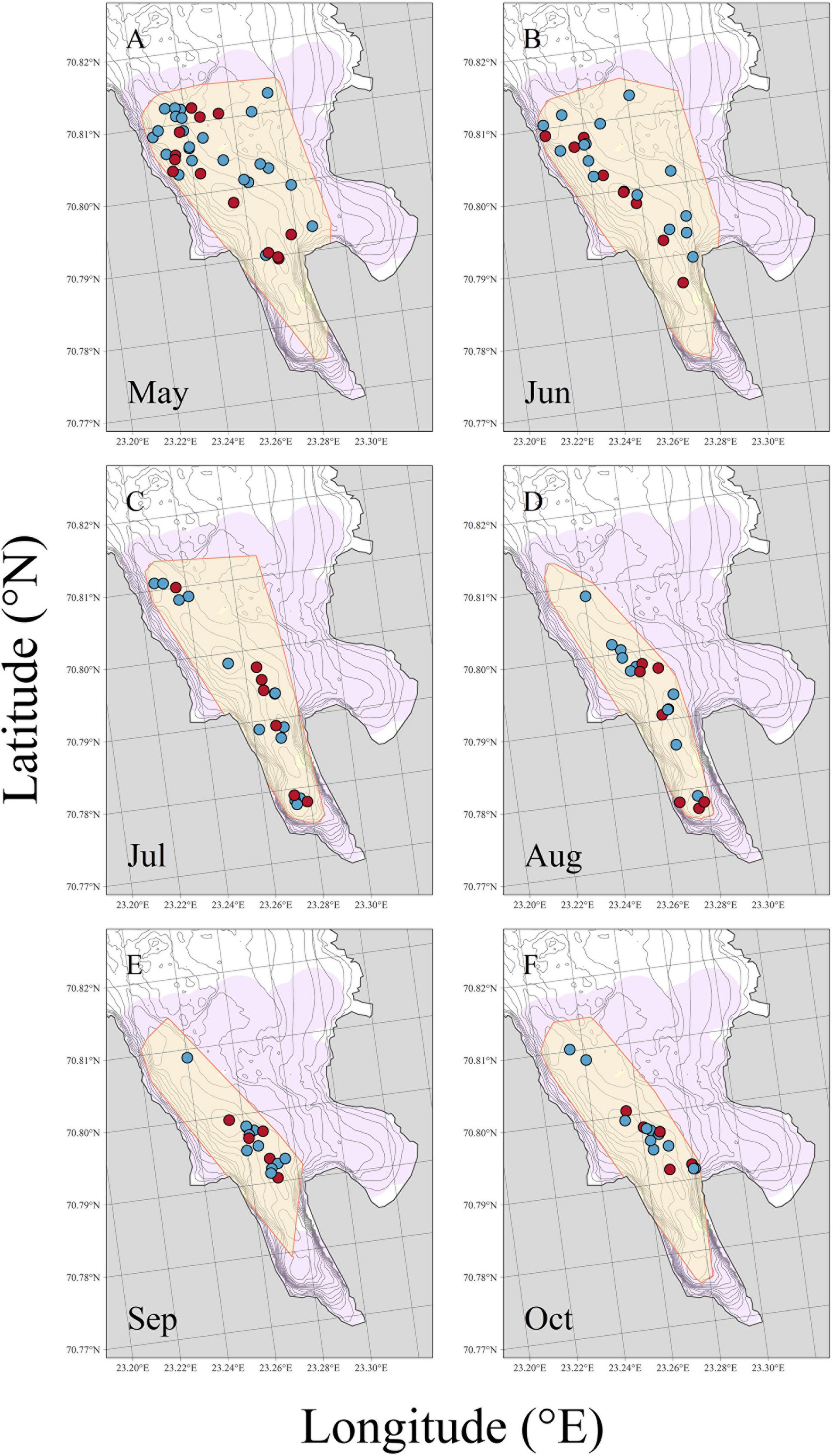
Figure 6. Monthly (May–October) sex-specific space use of each tagged individuals of the red king crab (Paralithodes camtschaticus) released in Gamvikfjorden, northern Norway, the 24th of May 2016. Each symbol corresponds to monthly individual space use for each crab estimated as the central location occupied by each individual in given month. Males and females are displayed in blue and red, respectively. Yellow shapes in each panel illustrate the entire area occupied by both sexes in a given month (as 100% MCP). The purple shape in each panel delimits the area where crab locations could be detected (details in “Materials and Methods” section). (A) May, (B) June, (C) July, (D) August, (E) September, and (F) October.
The crabs experienced water temperatures that were slowly increasing from ∼5.5 to 6.5°C in the beginning of the study period to ∼8°C in the beginning of August (Figure 4C). On the 8th of August, the experienced temperature rose quickly from ∼8.0 to 8.5°C, followed by 3 weeks of slow increase and a new rapid increase to maximum values of > 9°C on the 29th of August. From this date and to the end of the study period, there was minimal variation among crabs with respect to the water temperature that they experienced, which declined to ∼8°C in the end of the study period. Several of the tagged crabs (i.e., crab #245, #757, and #760) showed nearly identical profiles of depth, latitude and experienced temperature.
From May until late August, the crabs appeared to counteract the effect of increasing ambient water temperatures on their experienced temperatures by gradually moving deeper and further into the fjord. Consequently, the area that they utilized contracted as the water masses got warmer. Rapid movements were mostly associated with accelerated increases in ambient water temperature due to water mass exchange events (i.e., quickly rising water temperatures at the locations where they were found just before the movement; see vertical green lines in Figure 6 for dates of important water exchange events). As such, a change in temperature in shallow waters did not necessarily affect the crabs, which were mostly found in deeper waters. Although the lowest water temperatures were found in the innermost part of the fjord, the crabs preferred the area where the second coldest water masses were found (i.e., in the central deep trench; Figures 4C, 6). The median distance from the tagging location was 1,200 m across individual crabs (1st–3rd quartile, 90–1,775 m). Among those individuals that left the study area early in the study period (n = 16), the maximum distance from the tagging location was 1,482 m (1st–3rd quartile, 1,313–2,697 m). Median home ranges used by male and female RKC during the study period were 230 m2 (range: 28–507 m2) and 221 m2 (range: 45–385 m2), respectively.
Walking Speed
The daily mean walking speed of the crabs ranged from 0.35 to 3.08 m min–1 within the study area (mean 1.14 m min–1; Supplementary Figure 6). There was a weak, yet significant (r2 = 0.12, p < 0.001) decline in daily mean walking speed throughout the study period.
Fishermen recaptured three of the tagged crabs (#234, #237, and #238), all of which left the study area early in the study period. Crab #234 was recaptured close to Vannøya, ∼147 km southwest of Gamvikfjorden in the beginning of April 2017, which accounts for a minimum walking distance of 490 m day–1. Crab #237 was recaptured ∼25 km northwest of Gamvikfjorden, but since the date of recapture of this crab is unknown, it was not feasible to estimate its daily walking speed. The exoskeleton of Crab #238 was recovered in a fishing net deployed east of Rolfsøy, ∼53 km northeast of Gamvikfjorden on the 5th of February. This implies a minimum walking distance of 208 m day–1, followed by molting after being caught in a fishing pot.
Discussion
This study successfully combined acoustic telemetry on RKC migratory behavior with environmental recordings and benthic investigations to reveal the species’ movements in relation to its surroundings. The findings reveal the RKC as a aggregative, highly opportunistic species capable of long-distance migrations, that is capable of withstanding large temperature fluctuations. Furthermore, our results indicate that the RKC can reside far outside its assumed temperature preference (e.g., Christiansen et al., 2015).
What Does the Atlantic Coastal Red King Crab Habitat Look Like?
The grab sample data from Gamvikfjorden revealed several interesting patterns that are probably typical for many Norwegian fjords newly invaded by RKC. For instance, shallow locations (<45 m water depth) showed relatively coarse sediments (low pelite values) and low values of total nitrogen (TN), whereas progressively finer sediments coupled with increasing values of TN were observed at deeper locations. As anticipated, the spatial variation in sediment texture within the study area was reflected in benthic community composition. Polychaetes dominated at most stations and showed the highest numbers in the deep trench, whereas bivalves, echinoderms and crustaceans were almost exclusively found in the shallower parts of the fjord. These findings may indicate that the seabed in the study area can be divided into two distinct categories: “shallow” and “deep,” characterized by different benthic faunas which are both appropriate as food for the RKC (Britayev et al., 2010; Falk-Petersen et al., 2011; Fuhrmann et al., 2017).
The environmental conditions within fjords varies greatly and are dependent on numerous parameters such as thresholds, depth, water exchange and freshwater runoff. In Gamvikfjorden, the deep, inner basin had the highest TOC values, similar to e.g., fjords at Svalbard. This is likely explained by a regular supply of terrestrial, organic material due to e.g., river runoff (Koziorowska et al., 2016). As the inner basin of Gamvikfjorden also showed the highest C/N ratio, a metric suggestive of organic material of terrestrial origin (Koziorowska et al., 2016), we argue that the supply of organic carbon from land (i.e., Sørøya) may have contributed to the high TOC values in the area. Indeed, field notes revealed that the grab sample taken from the inner, deep basin contained substantial amounts of organic material such as small branches. Similarly, a recent study of a river-impacted fjord in northern Norway showed the highest C/N ratio in the innermost part of the fjord (i.e., closest to the river outlet) and the highest TOC further out in the deeper parts of the fjord (McGovern et al., 2020). A few smaller creeks flow into the inner part of the study area, and the location close to the open ocean probably causes transportation of terrestrial material by wind. Our bottom-water temperature data suggest that the bottom water masses of the deep, inner basin are seldomly exchanged during the summer season, and this part of the fjord may thus function as a sedimentation basin for terrestrial organic material. The infrequent water exchange in the deep, inner basin may also cause O2 limitation close to the seabed in this part of the fjord, which is supported by the field team registering the smell of sulfur at the two innermost grab sample locations. Lack of oxygen points toward less favorable conditions for benthos. Indeed, we observed few macrofauna in this part of the fjord. However, early during the study period (July), the deep, inner basin was relatively frequently visited by tagged crabs. It is therefore also possible that predation from RKC contributed to the impoverishment of the benthos community in the small, inner fjord basin, as observed locally in adjacent fjords in northern Norway (Oug et al., 2011, 2018). Despite low water temperatures, generally poor food availability apparently made the deep, inner basin of Gamvikfjorden unfavorable as habitat for the RKC.
Movement Patterns
In contrast to the tagged individuals remaining in the study area for a prolonged period, which typically resided within ∼1.2 km from the tagging locations, recaptures of three tagged crabs (i.e., ∼8% of the studied crabs) up to 147 km from the study area confirm that the RKC is also capable of long-distance migrations. Such migrations have previously been observed in the Barents Sea and in the species’ native areas in the Pacific Ocean (e.g., Marukawa, 1933; Hayes and Montgomery, 1963; Windsland et al., 2014). We cannot demonstrate that additional individuals among the early emigrating crabs made subsequent, long-distance migrations beyond the maximum distance of ∼1,500 m from the tagging locations. Nevertheless, provided the low likelihood of recaptures, these numbers suggest that such migrations are a common feature of the red king crab. The crabs presently studied by use of acoustic telemetry typically displayed walking speeds of up to 3 m min–1 between successive registrations (therefore capable of > 4,000 m d–1), which largely agrees with previous studies (e.g., Jørgensen et al., 2007). Several of the crabs in our study left the fjord along straight paths, yet others moved in seemingly random patterns (as observed in Jørgensen et al., 2007), presumably searching for food. Thus, we observed two modes of movement, likely corresponding with long-distance migration and foraging behaviors, where at least the latter may imply seasonal migrations between shallow and deep waters. It is unclear, however, what prompts an individual crab to choose one mode at any time, or how often and under what circumstances a crab may shift modes. Further telemetry and mark-recapture studies can be correlated with potential food resources and environmental characteristics in future studies. With regard to the recaptured crabs, we therefore assume that the actual walking distances between the study area and the sites of recapture, and consequently the walking speeds, were greater than initially estimated.
Although more than half of the tagged crabs stayed in the study area for several months, 16 of the crabs left the study area within the first 11 days (i.e., before the 4th of June), after which no crabs left the study area for another 40 days. This departure from the shallow study area was not surprising given the general expectation that the RKC move to deeper water masses before June (e.g., Sundet and Hjelset, 2010). Most of the early emigrating crabs followed a consistent and a directed migratory path: following the central, deep trench northwards toward the outer part of the fjord. This suggest that the RKC display a spatial understanding, perhaps prompted by cues from the water mass, and that they may follow specific migratory routes, e.g., along topographic features such as slopes. Indeed, the RKC is often caught in slope areas (Anisimova and Lubin, 2008). Moreover, evidence from Alaskan waters suggest that the RKC conduct long-distance migrations as groups of individuals (Stone et al., 1993), which suggest a means of aggregative behavior. Indeed, several of the tagged crabs showed nearly identical profiles of depth, latitude and, consequently, experienced temperature, and it can certainly not be ruled out that these three individuals co-occurred in time and space with individuals that were not acoustically monitored. Although the formation of dense aggregations is thought to be most common among juvenile RKC (i.e., “podding”), the behavior has also been observed among adult individuals (Jewett and Powell, 1981; Stone et al., 1993). In sum, various observations suggest that RKC individuals co-occur and move collectively, which would facilitate the identification of potential migratory routes. Detailed knowledge of such migratory routes would, if they exist, support effective management and exploitation of this RKC stock.
Interestingly, we note that the 16 early emigrating specimens left the study area before the first major water exchange, which occurred from the 4th of June. A movement northward from the study area into deeper offshore areas implies that the crabs must transit a broad area of 35–50 m water depth. A closer examination of the temperature data from this depth interval revealed water temperatures of ∼5.9–6.6°C the 1st of June (i.e., before the first major water exchange), whereas higher and less variable water temperatures (∼6.6–6.8°C) were observed 5 days later (i.e., after the water exchange event). Although Christiansen et al. (2015) showed that mature RKC prefers temperatures lower than 4°C, they also utilized the 4–6°C temperature range and to a lesser degree water masses warmer than 6°C. We therefore hypothesize that the shift to warmer water masses in the shallower areas north of the study area, largely caused by discrete water exchange events, may have imposed a thermal barrier of ∼6°C that trapped the remaining crabs inside the deeper parts of the study area, preventing migrations to colder offshore areas. Alternatively, our data questions our current knowledge of seasonal migrations between deep and shallow waters. We expected crabs to be present in Gamvikfjorden in May, when the tagging was conducted. Yet the proportion of tagged individuals remaining in the study area for several months was surprisingly high given the assumption that they would reside in deeper waters from June onward (e.g., Sundet and Hjelset, 2010). This may indicate that the crabs remaining in Gamvikfjorden were not hindered from conducting a feeding migration to deeper waters, but on the contrary stayed in the fjord despite sub-optimal water temperatures, possibly due to favorable feeding conditions there. Likewise, it is assumed that mature males that have recently molted may skip spawning and stay in deeper waters throughout the spawning season (Powell et al., 1973; Sundet and Hjelset, 2010). These observations combined point toward a seasonal migration regime that is more flexible or complex than previously thought, which may point toward a means of dispersive migration pattern (e.g., Fayet et al., 2016). Nevertheless, the ectothermic RKC is sensitive to changes in water temperature, as its food conversion efficiency as well as its ability to bind oxygen (i.e., the hemocyanin oxygenation) severely decreases in response to increased water temperature (Decker et al., 2007; Siikavuopio and James, 2015). Thus, a general avoidance of high (> 4°C) temperature waters is expected (Christiansen et al., 2015). However, in a previous study, no relationship between quick, synchronous movements of the RKC and water temperature could be detected (Stone et al., 1992). Contrary, our combined data on crab movements and water temperature variability in the current study area show a tendency for crabs to respond quickly to changes in ambient water temperature. For instance, water exchange events occurring in springtime (as shown by quick changes in water temperature) apparently caused the presently studied crabs to move quickly into deeper, colder water masses. In turn, these water exchange events were largely associated with strong winds at the surface. Surface wind conditions were also shown to be decisive with regard to the dispersal and subsequent settlement of pelagic RKC larvae (Pedersen et al., 2006). Conditions above the sea surface may thus affect more than one life stage of the RKC, and consequently, behavioral responses affecting the spatial distribution of adults may facilitate larval dispersal. As such, a direct link between meteorological conditions, dispersal and distributions in coastal waters seems plausible.
Habitat Utilization
Our data from Gamvikfjorden suggest that the habitat choice of the RKC is affected by a trade-off between abiotic factors (e.g., water temperature) and food availability. The king crab is a generalist predator feeding on a wide variety of infauna and epifauna (Fuhrmann et al., 2017), and its movement over the seasonal cycle is likely a response to a combination of food encounter rate, seasonal differences in thermal tolerance, reproduction and trade-offs with e.g., predation risk. Indeed, this is likely behind the general model of seasonal migration patterns observed in some areas, whereby crabs migrate between shallow (winter- and springtime) and deep waters (summer and autumn; Sundet and Hjelset, 2010). Fuhrmann et al. (2015) found 75% lower infaunal biomass in areas of a north Norwegian fjord inhabited by king crabs compared to fjord areas where the crab was absent. Clearly, therefore, movement patterns can at least partially represent a response to food availability, and more detailed investigation of prey fields relative to movement patterns is needed. Tagged crabs were generally active, but typically operated at surprisingly local scales of ∼225 m2 over the entire study period, suggesting factors other than the potential chance of finding food in other areas of the fjord must be acting here.
The crabs that remained in the study area for a prolonged period showed a spatial distribution with clear seasonal variation, moving deeper as the increasing water temperatures made the shallow parts of the fjord progressively less suitable as habitats. These individuals generally utilized a surprisingly small portion of the fjord. Interestingly, a distinct sexual segregation was observed early and late in the study period. Females were predominantly found deeper than 40 m, experiencing lower water temperatures than the males, the latter being commonly found as shallow as 30 m before the first water exchange event (i.e., the 4th of June) and after the deeper parts of the fjord started to cool (i.e., early October). Sexual segregation in RKC has previously been reported (Stiansen et al., 2009; Falk-Petersen et al., 2011), but research data supporting the existence of such behavior is rare (Stone et al., 1993; Taggart et al., 2008; Sundet and Hjelset, 2010). Mating is known to occur in shallow waters during springtime (e.g., Stone et al., 1993; Sundet and Hjelset, 2010). We can therefore assume that the tagged females, all of which were sexually mature, had completed the mating before the tagging and therefore carried eggs throughout the study period. To avoid accelerated egg development and early hatching, the females likely seek cold water masses most of the year. Indeed, RKC in the Barents Sea were found in deep waters (>150 m) from April through December (Sundet and Hjelset, 2010), where the water temperatures were likely low. Possibly constrained to the study area due to the thermal barrier outside Gamvikfjorden, the tagged ovigerous females may have been forced to reside in deep and cold parts of the fjord already from the beginning of the study period. The males, on the other hand, apparently tolerated the slightly higher temperatures found in shallower waters early in the study period and were therefore allowed to feed both in deep and in in relatively shallow waters. As such, females likely had to base their diet on polychaetes while the males could initially benefit from a more diverse diet consisting of polychaetes, bivalves, echinoderms and crustaceans. After the first water exchange event, the males were forced to move progressively into deeper and colder water masses as the ambient water temperature rose. Nevertheless, although the crabs eventually experienced temperatures in the central, deep trench that were substantially higher than they apparently prefer (e.g., Christiansen et al., 2015), they avoided the cold, presumably food-poor inner deep basin. This study was conducted in one fjord only, and the specific hydrography, bathymetric profile, and benthic community structure may have strongly influenced these results, whereas patterns may be different in other fjords. Yet, our findings highlight a trade-off between physical (e.g., water temperature) and biological factors (e.g., access to suitable food) that likely affect distributions of the RKC in coastal waters. This in turn indicates that the current knowledge base of the species’ seasonal migration patterns may need revisions.
Conclusion and Implications
In this paper, we show that the RKC in coastal areas can respond quickly to wind-induced water exchange events, avoiding unfavorable ambient water temperatures. Moreover, our results suggest that the species’ habitat selection likely depends on a trade-off between abiotic and biotic conditions. For instance, provided access to appropriate food, the crabs seem to tolerate temperatures that are much higher than previous studies suggest that they prefer (e.g., Christiansen et al., 2015), which clearly has implications for our understanding of its future distribution patterns in coastal waters. Yet, as the water temperatures in the study area rose, the crabs moved into deeper and colder parts of the fjord, and their walking speed decreased. Indeed, unfavorable temperature conditions have previously been shown to cause reduced activity level of other crab species (e.g., in fiddler crabs, Uca pugilator; Mat et al., 2017). A reduced activity level implies a reduced intensity in the search for food, and as such, high water temperatures may cause a lower likelihood that the crabs are being captured by traps. To assess the degree to which a reduced activity level may affect the access to food, future investigations may integrate data on RKC movement patters and benthic abundance and composition in different environments. This may help estimating the daily amount of potential food covered under differing modes of movement.
About half of the tagged crabs remained in the study area throughout the summer season despite the general expectation that they would move into deeper waters before June (e.g., Sundet and Hjelset, 2010). We propose that the crabs were forced to do so because of a thermal barrier imposed by warm waters in the shallow areas surrounding the study area. Alternatively, other factors (e.g., favorable food conditions or low competition) made the crabs prefer to stay in the area despite sub-optimal water temperatures. In any case, the high proportion of tagged RKC remaining in the study area throughout the summer season, in addition to numerous observations by divers, question the premise that the RKC conduct general seasonal migrations between deep and shallow waters. Indeed, the RKC may occur in shallow, warm waters in summertime, but may remain unaccounted due to a lower catchability during this time of the year. Intuitively, the utilization of a wide depth range (shallow and deep waters) during given periods of the year will affect the density, and consequently the catchability, of the species. To facilitate sound management, future studies should aim to estimate the actual proportion of the RKC stock remaining in shallow fjord areas throughout the year.
Our data also suggest that the crabs may display a means of aggregative behavior, performing migrations as groups of individuals, and that many crabs perform long-distance, high-velocity migrations. The combined effects of aggregative behavior, quick response to environmental changes, and the capability to conduct long and fast migrations, may help explain why many fishermen experience highly variable catches of the RKC despite little variation in the time and geographical location of the fishing. For future studies, we recommend conducting telemetric studies over entire annual cycles, and further investigating whether the RKC follow distinct migration routes along the coast. Furthermore, in recent years, the snow crab (Chionoecetes opilio) has recently appeared in the eastern Barents Sea, rapidly expanding into the areas of the southern Barents Sea where the RKC reside. We, therefore, suggest that telemetry be applied to investigate the interplay between these two species of crabs.
Data Availability Statement
The original contributions presented in the study are publicly available. This data can be found here: https://doi.org/10.5281/zenodo.5823339.
Author Contributions
JJ, GC, and KN conceptualized study. JJ, GC, and MA conducted the fieldwork. MA and BM conducted the numerical analyses. MA wrote first draft of the manuscript. All authors contributed significantly to the manuscript and approved the submitted version.
Funding
We greatly acknowledge funding from Regionale Forskningsfond Nord-Norge (RFFNORD, contract number 257046), with additional financial support from the Norwegian Directorate of Fisheries, and Akvaplan-niva.
Conflict of Interest
MA, JJ, GC, BM, and PR was employed by Akvaplan-niva AS. SS was employed by NOFIMA AS. KN was employed by K-To-9 AS.
Publisher’s Note
All claims expressed in this article are solely those of the authors and do not necessarily represent those of their affiliated organizations, or those of the publisher, the editors and the reviewers. Any product that may be evaluated in this article, or claim that may be made by its manufacturer, is not guaranteed or endorsed by the publisher.
Acknowledgments
We would like to thank the owners of the coastal fishing companies Nergårdfisk AS, T. Hansen Kystfiske AS and Jari Kystfiske AS for initiating and supporting the project Optimal king crab harvesting (“Optimal kongekrabbefangst”; “KONGEFANGST”) with knowledge, field assistance and ship time. We would also like to thank the Norwegian Mapping Authority for providing seabed bathymetry data. We would like to thank Roger Velvin for informative discussions, Mikko Vihtakari for help with R coding, Ann-Cecilie Henriksen and Lars Birkeland Sjetne (Akvaplan-niva) for conducting sediment sampling, and Oda Sofie Bye Wilhelmsen (Akvaplan-niva) for chemical analyses. We are grateful to the three reviewers for their comments and suggestions.
Supplementary Material
The Supplementary Material for this article can be found online at: https://www.frontiersin.org/articles/10.3389/fmars.2022.762087/full#supplementary-material
Footnotes
References
Anisimova, N., and Lubin, P. (2008). The Red King Crab and Benthos Communities. Distribution of the Red King Crab Compared to Benthic Communities. Available online at: https://www.hi.no/resources/publikasjoner/imrpinro/2008/imr-pinro_2008-3_til_web.pdf
Anon (2007). “Stortingsmelding 40,” in Forvaltning av Kongekrabbe [Management of Red King Crab], 144. doi: 10.1108/15285810710739364
Anon (2019). Kongekrabbe i Norsk Sone. Bestandstaksering og Rådgivning 2019. Technical Report. Bergen: Institute of Marine Research.
Aune, M., Jensen, J. L. A., Christensen, G. N., Nilsen, K. T., Merkel, B., and Renaud, P. E. (2022). Telemetry data of red king crab (Paralithodes camtschaticus) migrations in a north Norwegian fjord. Data Brief. 40:107894. doi: 10.1016/j.dib.2022.107894
Britayev, T. A., Rzhavsky, A. V., Pavlova, L. V., and Dvoretskij, A. G. (2010). Studies on impact of the alien Red King Crab (Paralithodes camtschaticus) on the shallow water benthic communities of the Barents Sea. J. Appl. Ichthyol. 26, 66–73. doi: 10.1111/j.1439-0426.2010.01494.x
Christiansen, J. S., Sparboe, M., Saether, B.-S., and Siikavuopio, S. I. (2015). Thermal behaviour and the prospect spread of an invasive benthic top predator onto the Euro-Arctic shelves. Diver. Distrib. 21, 1004–1013. doi: 10.1111/ddi.12321
Cooke, S. J., Hinch, S. G., Wikelski, M., Andrews, R. D., Kuchel, L. J., Wolcott, T. G., et al. (2004). Biotelemetry: a mechanistic approach to ecology. Trends Ecol. Evol. 19, 334–343. doi: 10.1016/j.tree.2004.04.003
Decker, H., Hellmann, N., Jaenicke, E., Lieb, B., Meissner, U., and Markl, J. (2007). Minireview: recent progress in hemocyanin research. Integr. Comp. Biol. 47, 631–644. doi: 10.1093/icb/icm063
Falk-Petersen, J., Renaud, P., and Anisimova, N. (2011). Establishment and ecosystem effects of the alien invasive red king crab (Paralithodes camtschaticus) in the Barents Sea–a review. ICES J. Mar. Sci. 68, 479–488. doi: 10.1093/icesjms/fsq192
Fayet, A. L., Freeman, R., Shoji, A., Boyle, D., Kirk, H. L., Dean, B. J., et al. (2016). Drivers and fitness consequences of dispersive migration in a pelagic seabird. Behav. Ecol. 27, 1061–1072. doi: 10.1093/beheco/arw013
Fuhrmann, M. M., Pedersen, T., and Nilssen, E. M. (2017). Trophic niche of the invasive red king crab Paralithodes camtschaticus in a benthic food web. Mar. Ecol. Prog. Ser. 565, 113–129. doi: 10.3354/meps12023
Fuhrmann, M. M., Pedersen, T., Ramasco, V., and Nilssen, E. M. (2015). Macrobenthic biomass and production in a heterogenic subarctic fjord after invasion by the red king crab. J. Sea Res. 106, 1–13. doi: 10.1016/j.seares.2015.09.003
Hayes, M. L., and Montgomery, D. T. (1963). Movement of king crabs tagged and released in Shumagin Island Area, 1957–1962. U.S Fish and Wildlife Service. Spec. Sci. Rep. Fish. 458:7.
Hjelset, A. M., Sundet, J. H., and Nilssen, E. M. (2009). Size at sexual maturity in the female red king crab (Paralithodes camtschaticus) in a newly settled population in the Barents Sea, Norway. J. Northwest Atl. Fish. Sci. 41, 173–182. doi: 10.2960/j.v41.m633
Inall, M. E., and Gillibrand, P. (2010). “The physics of midlatitude fjords: a review,” in Fjord Systems and Archives, Vol. 344, eds J. A. Howe, W. E. N. Austin, M. Forwick, and M. Paetzel (London: Geological Society), 17–33.
Jewett, S. C., and Powell, G. C. (1981). Nearshore movement of king crab. Alaska Seas Coasts 9, 6–8.
Jørgensen, L. L., and Primicerio, R. (2007). Impact scenario for the invasive red king crab Paralithodes camtschaticus (Tilesius, 1815) (Reptantia, Lithodidae) on Norwegian, native, epibenthic prey. Hydrobiologia 590, 47–54. doi: 10.1007/s10750-007-0756-9
Jørgensen, T., Løkkeborg, S., Fernö, A., and Hufthammer, M. (2007). Walking speed and area utilization of red king crab (Paralithodes camtschaticus) introduced to the Barents Sea coastal ecosystem. Hydrobiologia 582, 17–24. doi: 10.1007/s10750-006-0542-0
Koziorowska, K., Kuliński, K., and Pempkowiak, J. (2016). Sedimentary organic matter in two Spitsbergen fjords: terrestrial and marine contributions based on carbon and nitrogen contents and stable isotopes composition. Cont. Shelf. Res. 113, 38–46. doi: 10.1016/j.csr.2015.11.010
Marukawa, H. (1933). Biological and fishery research on Japanese king crab Paralithodes camtschatica (Tilesius). J. Imper. Fish. Exper. Stat. 4, 37–152.
Mat, A. M., Dunster, G. P., Sbragaglia, V., Aguzzi, J., and de la Iglesia, H. O. (2017). Influence of temperature on daily locomotor activity in the crab Uca pugilator. PLoS One 12:e0175403. doi: 10.1371/journal.pone.0175403
McGovern, M., Poste, A. E., Oug, E., Renaud, P. E., and Trannum, H. C. (2020). Riverine impacts on benthic biodiversity and functional traits: a comparison of two sub-Arctic fjords. Estuar. Coast. Shelf. Sci. 240:106774.
Oksanen, J., Blanchet, F. G., Kindt, R., Legendre, P., O’Hara, R. B., Simpson, G. L., et al. (2020). vegan: Community Ecology Package. In R Package Version 2.5-7.
Orlov, Y. I., and Ivanov, B. G. (1978). On the introduction of the Kamchatka King crab Paralithodes camtschatica (Decapoda: Anomura: Lithodidae) into the Barents Sea. Mar. Biol. 48, 373–375. doi: 10.1007/BF00391642
Oug, E., Cochrane, S. K. J., Sundet, J. H., Norling, K., and Nilsson, H. C. (2011). Effects of the invasive red king crab (Paralithodes camtschaticus) on soft-bottom fauna in Varangerfjorden, northern Norway. Mar. Biodiv. 41, 467–479. doi: 10.1007/s12526-010-0068-6
Oug, E., Sundet, J. H., and Cochrane, S. K. J. (2018). Structural and functional changes of soft-bottom ecosystems in northern fjords invaded by the red king crab (Paralithodes camtschaticus). J. Mar. Syst. 180, 255–264. doi: 10.1016/j.jmarsys.2017.07.005
Pedersen, O. P., Nilssen, E. M., Jørgensen, L. L., and Slagstad, D. (2006). Advection of the Red King Crab larvae on the coast of North Norway—A Lagrangian model study. Fish. Res. 79, 325–336. doi: 10.1016/j.fishres.2006.03.005
Pinchukov, M. A., and Sundet, J. H. (2011). “Red king crab,” in The Barents Sea. Ecosystem, Resources, Management. Half a Century of Russian-Norwegian Cooperation, eds T. Jakobsen and V. K. Ozhigin (Trondheim: Tapir Academic Press), 160–167.
Powell, G. C., Shafford, B., and Jones, M. (1973). Reproductive biology of young adult king crabs Paralithodes camtschatica (Tilesius) at Kodiak, Alaska. Proc. Natl. Shellf. Assoc. 63, 77–87.
R Core Team (2020). R: A Language and Environment for Statistical Computing. Vienna: R Foundation for Statistical Computing.
Rodin, V. E. (1989). “Population biology of the king crab, Paralithodes camtschatica Tilesius, in the North Pacific Ocean,” in Proceedings of the International Symposium on King Tanner Crabs, ed. B. R. Melteff (Anchorage, AK: University of Alaska Sea Grant Program), 133–144
Siikavuopio, S. I., and James, P. (2015). Effects of feed intake, growth and oxygen consumption in adult male king crab Paralithodes camtshaticus held in captivity and fed manufactured diets. Aquac. Res. 46, 602–608. doi: 10.1111/are.12207
Stiansen, J. E., Korneev, O., Titov, O., and Arneberg, P. (2009). Joint Norwegian-Russian Environmental Status 2008. Report on the Barents Sea Ecosystem. Part II–Complete report. IMR/PINRO Joint Report Series, 2009. Bergen: Havforskningsinstituttet, 1–375.
Stone, R. P., O’Clair, C. E., and Shirley, T. C. (1992). Seasonal migration and distribution of female red king crabs in a southeast Alaskan estuary. J. Crust. Biol. 12, 546–560. doi: 10.1163/193724092x00030
Stone, R. P., O’Clair, C. E., and Shirley, T. C. (1993). Aggregating behaviour of ovigerous female red king crab, Paralithodes camtschaticus, in Auke Bay, Alaska. Can. J. Fish. Aquat. Sci. 50, 750–758. doi: 10.1139/f93-086
Sundet, J. H., and Hjelset, A. M. (2010). “Seasonal depth distribution of the red king crab (Paralithodes camtschaticus) in Varangerfjorden, Northern Norway,” in Biology and Management of Exploited Crab Populations under Climate Change, eds G. H. Kruse, G. L. Eckert, R. J. Foy, R. N. Lipcius, B. Sainte-Marie, D. L. Stram, et al. (Fairbanks, AK: Alaska Sea Grant), doi: 10.4027/bmecpcc.2010.20
Taggart, S. J., Mondragon, J., Andrews, A. G., and Nielsen, J. K. (2008). Spatial patterns and movements of red king and Tanner crabs: implications for the design of marine protected areas. Mar. Ecol. Prog. Ser. 365, 151–163. doi: 10.3354/meps07493
ter Braak, C. J. F. (1986). Canonical correspondence analysis: a new eigenvector technique for multivariate direct gradient analysis. Ecology 67, 1167–1179. doi: 10.2307/1938672
Keywords: crustaceans, efficient fisheries, seasonal variation, migration, temperature, trade-offs
Citation: Aune M, Jensen JLA, Siikavuopio SI, Christensen GN, Nilsen KT, Merkel B and Renaud PE (2022) Space and Habitat Utilization of the Red King Crab (Paralithodes camtschaticus) in a Newly Invaded Fjord in Northern Norway. Front. Mar. Sci. 9:762087. doi: 10.3389/fmars.2022.762087
Received: 20 August 2021; Accepted: 17 January 2022;
Published: 04 March 2022.
Edited by:
Jorge M. Pereira, University of Coimbra, PortugalReviewed by:
María Paula Sotelano, CADIC-CONICET, ArgentinaJan Marcin Weslawski, Institute of Oceanology (PAN), Poland
Lucas Krüger, Instituto Antártico Chileno (INACH), Chile
Copyright © 2022 Aune, Jensen, Siikavuopio, Christensen, Nilsen, Merkel and Renaud. This is an open-access article distributed under the terms of the Creative Commons Attribution License (CC BY). The use, distribution or reproduction in other forums is permitted, provided the original author(s) and the copyright owner(s) are credited and that the original publication in this journal is cited, in accordance with accepted academic practice. No use, distribution or reproduction is permitted which does not comply with these terms.
*Correspondence: Magnus Aune, magnus.aune@akvaplan.niva.no