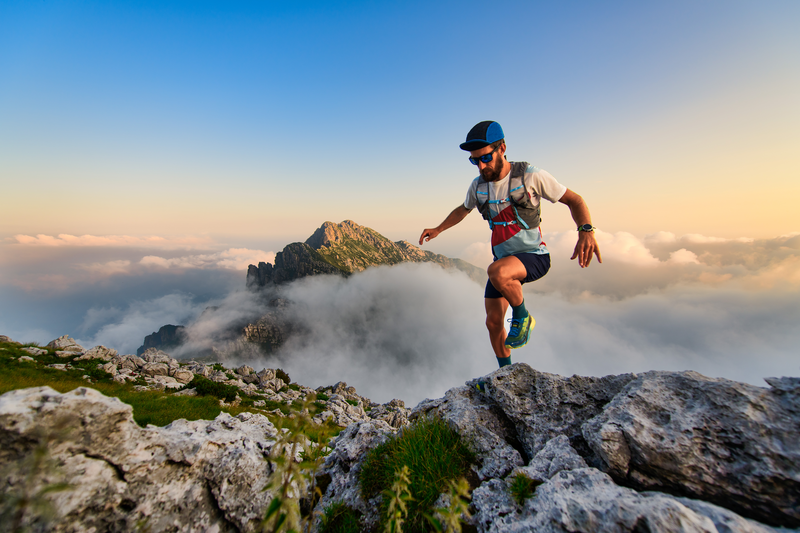
94% of researchers rate our articles as excellent or good
Learn more about the work of our research integrity team to safeguard the quality of each article we publish.
Find out more
ORIGINAL RESEARCH article
Front. Mar. Sci. , 19 May 2022
Sec. Marine Ecosystem Ecology
Volume 9 - 2022 | https://doi.org/10.3389/fmars.2022.753325
This article is part of the Research Topic Toward an Integrated Understanding of Ecological Responses to Variable Climate Changes and Marine Environments View all 9 articles
Tropical cyclones can cause severe destruction of coral reefs with ecological consequences for reef fish communities. Ocean warming is predicted to shorten the return interval for strong tropical cyclones. Understanding the consequences of cyclone impacts on coral reefs is critical to inform local-scale management to support reef resilience and the livelihood security of small-scale fishing communities. Here, we present the first analysis of a tropical cyclone disturbance on coral reefs in Madagascar. We investigate the impact of Cyclone Haruna (category 3 Saffir-Simpson scale) in February 2013 on coral communities, both adults and recruits, and explore the relationship between the severity of cyclone impact with cyclone parameters (wind speed, duration of storm impact and distance from cyclone track) and environmental variables (reef type and reef depth). We use survey data collected as part of a long-term citizen science monitoring programme at 21 coral reef sites between 2012 and 2015 in the Velondriake Locally Managed Marine Area along Madagascar’s southwest coast. Coral cover declined at 19 sites, however damage was spatially heterogeneous ranging from a decrease in coral cover of 1.4% to 45.8%. We found the severity of cyclone damage related to: distance from the cyclone track, duration of cyclone impact and reef depth. The taxonomic and morphological composition of coral communities was significantly different after the cyclone. Notably, there was a decrease in the dominance of branching morphologies, and an increase in the relative abundance of encrusting and massive morphologies. Two years after Cyclone Haruna, mean coral cover had increased and the density of coral recruits increased to above pre-cyclone levels indicating the potential recovery of coral populations. However, recovery to pre-disturbance community composition will likely be hindered by the increasing occurrence of acute and chronic disturbance events.
Coral reefs provide habitat for a third of marine species (Pandolfi et al., 2011) and supply ecosystem services for millions of people worldwide (Woodhead et al., 2019; Eddy et al., 2021) including food provision (Cohen et al., 2019), tourism and recreation (Spalding et al., 2017), and coastal protection (Ferrario et al., 2014). Tropical cyclones (also known as typhoons and hurricanes) are a major natural disturbance to coral reefs and can impact the structure and functioning of reefs at different spatial and temporal scales (Cheal et al., 2017). Ocean warming is predicted to shorten the return interval for strong tropical cyclones (Knutson et al., 2020), increasing their potential to have severe ecological impacts on coral reef ecosystems (Puotinen et al., 2020).
Direct impacts of cyclones on reefs are caused by large waves and currents exerting strong mechanical forces (Madin et al., 2014; Perry et al., 2014). Mechanical reef damage is highly variable and can range from minor fragmentation of coral branches to the dislodgement of entire colonies (Bozec et al., 2015). Indirect impacts of cyclones include intense rainfall and high river loads, which can increase turbidity and decrease salinity on reefs leading to a stress response and mortality of coral organisms (Haapkylä et al., 2013; Perry et al., 2014). High river loads and coastal run off can also lead to the deposition of litter, potentially increasing the likelihood of coral disease (Lamb et al., 2018). Furthermore, increased nutrient availability from land run-off or strong winds bringing up nutrient rich water from depth can cause macroalgal blooms that inhibit coral recruitment (Kuffner et al., 2006; Doropoulos et al., 2014).
The spatial distribution of cyclone damage to coral communities is characteristically patchy (Harmelin-Vivien, 1994). Factors which influence the spatial heterogeneity of cyclone damage include the intensity of waves and their duration near a particular reef; the location, topography and depth of a reef; and the composition and state of the coral community (Mumby, 1999; Fabricius et al., 2008; Beeden et al., 2015; Price et al., 2021). Several studies have aimed to model predictors of cyclone damage (e.g. Puotinen, 2005; Fabricius et al., 2008; Puotinen et al., 2016; Puotinen et al., 2020). The simplest models use distance from the cyclone track as a predictor of damage severity (Edwards et al., 2011; Ban et al., 2015). However, using distance thresholds alone can be ineffective, as evidenced in storms where severe damage has occurred up to 800 km from the cyclone track (Puotinen et al., 2020). An alternative approach is to include the cyclone parameters, wind speed and duration of cyclone winds (Puotinen, 2007; Woolsey et al., 2012; Price et al., 2021). These can be used as proxy for the intensity of cyclone-generated waves (Puotinen, 2005) and can help delineate the region in which cyclone damage would be likely (Puotinen, 2007). All studies agree that any model of cyclone impact using only cyclone parameters is not likely to capture the heterogeneity of damage. This is because at a regional scale, bathymetric and topographic features such as reefs and islands will interact with wave intensity (Young and Hardy, 1993). Within reefs, factors including depth and reef profile cause variability in reef exposure (Woesik et al., 1991). Finally, the vulnerability of coral colonies will differ according to their size and shape. Large colonies and colonies with fragile morphologies are generally more vulnerable to wave damage than smaller colonies with sturdier growth forms (Muko et al., 2013; Madin et al., 2014).
Cyclones can change the supply of ecological goods and services provided by the reef ecosystem (Micheli et al., 2014). Direct impacts of cyclones may change the movement and behaviour pattern of fish species (Kawabata et al., 2010), influencing their availability to fishers (Tobin et al., 2010). Cyclone-associated reduction in the three-dimensional structure of the reef, hard coral cover and coral diversity can decrease the number and variety of habitats available for reef fish (Graham and Nash, 2013; Komyakova et al., 2013). Where major structural changes occur, it will likely have long-term effects on the abundance and diversity of reef-associated species (Bozec et al., 2015; Darling et al., 2017; Pratchett et al., 2018). In coastal communities with strong livelihood dependency on reef fisheries, unpredictability of catch may impact food and income security (Hicks et al., 2021). Madagascar has been identified as a country highly vulnerable to the degradation of coral reefs owing to its significant economic and social reliance on reef resources (Cinner et al., 2012). This is particularly relevant along the southwest coast, where aridity and poor soils limit opportunities for agriculture (Hanisch, 2015), and where there are few other economic or subsistence alternatives to fishing (Barnes-Mauthe et al., 2013). The southwest coast of Madagascar is home to the sea-faring Vezo people (Marikandia, 2001). Vezo are highly reliant on the reef-associated fisheries for their income and food security (Barnes-Mauthe et al., 2013), and the cultural identity of the Vezo is also entwined with the ocean; you are not born Vezo, you become Vezo as you learn skills to master and live off the sea (Astuti, 1995). Preserving coral reefs in this region is vital for the preservation of Vezo livelihoods, food security, culture and identity (Astuti, 1995; Barnes-Mauthe et al., 2013)
The documentation of cyclone impacts on coral reefs is an important precursor to on-going climate adaptation efforts and locally tailored conservation planning, particularly where reliance on coral reefs for food security and livelihoods is high. However, studies assessing the impacts of cyclones on coral reefs are spatially biased. In particular, few studies are available on the impact of cyclones in the Western Indian Ocean (WIO; but see Letourneur et al., 1993; Naim et al., 2000; Scopélitis et al., 2009) and no studies have been found for Madagascar. Here, we present the first published study on the impact of a tropical cyclone on coral reefs in Madagascar. On 22nd February 2013, Cyclone Haruna, a category 3 storm on the Saffir-Simpson scale - with maximum sustained wind speeds of 150 kmh-1 and gusts of up to 185 kmh-1 - made landfall on the southwest coast of Madagascar, in the Velondriake Locally Managed Marine Area (LMMA). We use existing data (2011 – 2015) from a long-term, citizen science reef monitoring programme to investigate the impact of Cyclone Haruna on the coral community at a total of 21 reef sites in Velondriake LMMA. The objectives of this research were to: (a) quantify changes in benthic cover and benthic composition following Cyclone Haruna and (b) examine the influence of cyclone parameters (duration of cyclone impact, wind speed and minimum distance from cyclone track) and environmental variables (reef type and reef depth) on the severity of cyclone impact. This study aims to increase our knowledge of reef system vulnerability to severe storm impacts in the WIO and therefore help improve understanding of climate change-associated threats on the livelihoods of coastal communities.
The study was conducted in southwest Madagascar within the Velondriake LMMA (43°13`30 E, 22°04`22 S), 150km north of the regional capital of Toliara (Figure 1). The Velondriake LMMA stretches along 40km of coastline, incorporating 35 coastal villages with a combined population of approximately 8000 people (see Gardner et al., 2020 for further information on Velondriake). For those living in the LMMA, seafood provides 99% of protein, and 87% of the adult population derive their livelihoods from small-scale fishing (Barnes-Mauthe et al., 2013).
Figure 1 (A) The position of study region in relation to the path of Cyclone Haruna (solid red line) on the 22nd Feb 2013. Dotted line is boundary for the Velondriake Locally Managed Marine Area. (B) The location of reef sites in relation to the path of Cyclone Haruna (solid red line) on satellite image. Reef depth is indicated by the size of the orange circles.
The marine area of the Velondriake LMMA covers approximately 600 km2. The reef complex in the LMMA is characterised by a fringing and barrier reef system with several patch reefs situated in a 5km wide channel. The region has relatively high annual variation in sea surface temperature (McClanahan et al., 2009) with a range of up to 11°C recorded (22-33°C in 2015; Blue Ventures, unpublished data) and the presence of large, anticyclonic eddies, caused by the interaction of the south equatorial current with the Madagascar landmass (Quartly and Srokosz, 2004). The distinctive oceanographic climate has resulted in a biogeographically unique reef assemblage that includes corals endemic to Madagascar such as Pocillopora fungiformis and Stylophora madagascarensis (Veron, 2002), and 430 species of reef fish (Nadon et al., 2008).
Coral surveys were undertaken between 2011 and 2015 as part of a long-term citizen science reef monitoring programme run by the NGO Blue Ventures (www.blueventures.org) in the Velondriake LMMA. The survey programme was designed to monitor the health of coral reef ecosystems over time across the LMMA as a whole. Surveys took place on reef sites comprising patch, fringing and barrier reefs of varying depths, spread across the north, central and southern parts of Velondriake. As part of our data preparation, we selected a subset of the Blue Ventures dataset, including the sites that had data available up to two years before and after Cyclone Haruna. This resulted in the inclusion of 21 sites in total (11 patch, 5 barrier and 5 fringing reefs) with a depth range of 3 -13m (Figure 1). Sites were surveyed between one and three times per year. Benthic data was not available at every site, each year. Sites included in each analysis are outlined in the Supplementary Information (Tables S1, S2). The following outlines the survey methodologies used by the Blue Ventures teams.
Point Intersect Transects (PITs) were used to survey hard coral cover (HCC) and the morphology of adult corals (>10cm in diameter). Transects were placed randomly at each site running east to west for all patch reefs and west-facing sites and, north to south for south facing sites. The number of transects undertaken ranged between 6 and 30 and was determined by the size of the site. One observer per transect made fifty observations at 20 cm intervals along each 9.8 m transect: the first being at 0.0 m and the fiftieth at 9.8 m. The type of benthic organism or substrate at each point was identified. Hard corals were recorded by their morphology (branching, digitate, tabular, foliose, encrusting, massive, solitary or columnar). In addition, quadrat surveys were used to assess the community assemblage of adult corals following the methods in (McClanahan, 2004). Seven randomly positioned 4 m2 quadrats were completed at each site. The quadrats were haphazardly placed by the observer who randomly selected a direction of travel and a random amount of fin kicks (between 5 and 20) to determine the quadrat location. All colonies with diameter >10 cm were counted in each quadrat. Colonies were identified to genus level except for Porites which was classified as either ‘branching’ or ‘massive’. The sub-genus Synarea was included within Porites.
Coral recruits and juvenile corals ≤ 10 cm diameter were surveyed using 20 randomly placed 0.1 m2 quadrats. Quadrat surveys were undertaken by two observers per quadrat. The size of each colony was measured to the nearest 0.5 cm and recorded to genus level.
PITs have been identified as a time efficient survey method of monitoring disturbance over large reefs areas with non-specialist surveyors (Facon et al., 2016). To ensure data accuracy, before undertaking PITs, the Blue Ventures citizen scientists underwent training in survey and identification of corals and other benthic life forms. To be eligible to undertake surveys, participants were required to pass a computer test and an in-water identification tests with an accuracy rate of 90%. Following completion of these tests, participants undertook a PIT with a member of trained staff. On the PIT test, the participant’s data had to be in 100% agreement with the data collected by the staff member when identifying major groups (e.g., between coral, algae, substrate), and citizen scientists had to correctly identify organisms within these major groups to at least 80% accuracy (e.g., branching or massive coral growth forms, calcareous or turf algae). Quadrat surveys of the adult coral community assemblage and the recruit community assemblage (where identifying to genus level was required) were undertaken only by trained field staff.
We investigated the severity of cyclone damage in relation to three continuous cyclone parameters: (i) wind speed (average maximum wind speed when the cyclone was within 70km of the reef site in km/h), (ii) duration (the number of hours the cyclone was within 70km of the reef site) and (iii) distance (the minimum distance from centre of the cyclone to the reef site in km). Wind speed and track data for Cyclone Haruna and historic cyclones in the region were extracted from the International Best Track Archive for Climate Stewardship (Knapp et al., 2010). Seventy kilometres was selected as the distance threshold as it is within the range observed for severe damage following cyclone events of similar intensity to Cyclone Haruna (Manzello et al., 2007; Puotinen, 2007).
Generalized linear mixed model (GLMM) analysis was used to assess variation of cyclone impact on (i) hard coral cover (ii) taxonomic richness (number of coral genera per site) and (iii) and recruit density (number of colonies <10cm diameter per m2). We divided the survey data into four time periods related to the date of the cyclone. We refer to the two years before the cyclone as year -2 (22nd February 2011 - 22nd February 2012) and year -1 (23rd February 2012 - 22nd February 2013) and the two years after the cyclone as year 1 (23rd February 2013 - 22nd February 2014) and year 2 (23rd February 2013 - 22nd February 2014). GLMM analysis of hard coral cover and recruit density included survey data two years before and two years after the cyclone. For taxonomic richness, data was available for only one year before and two years after the cyclone. GLMM analysis was selected to allow for the inclusion of fixed and random effects. Fixed effects included cyclone parameters wind speed, duration and distance and environmental variables and reef type and reef depth (Table S1). We also included a fixed effect (before/after) for whether a survey was conducted before or after the cyclone occurred to quantify the overall impact of the cyclone on coral cover, taxonomic richness and coral recruits. The before/after term was allowed to interact with cyclone parameters to try and understand how exactly cyclones impact on coral communities (Laird and Ware, 1982). We also included reef type and reef depth in interaction with the before/after term to determine if different reef environments were impacted differently by the cyclone. We examined Pearson correlation coefficients and Variance Inflation Factors (VIFs) to assess potential multi-collinearity between fixed effect variables. Wind and duration metrics were found to be strongly negatively correlated (r = -0.83 to - 0.85). We therefore did not include both wind and duration in the same model, and calculated VIF for the two potential subsets of predictors. The highest VIF for any fixed effect in all subsets of models was 1.15. In each model, site was included as a random effect. Observer identity was included as a random effect in models investigating taxonomic richness and recruit density.
We assessed whether model assumptions were followed using graphical procedures, evaluating homogeneity of variance by plotting residuals against each fixed effect and against fitted values. All numerical explanatory variables were scaled by standardisation to the same mean and variance in order to compare their relative effects on response variables. Taxonomic richness was modelled as a poisson distribution with a log link function. Hard coral cover and recruit density were modelled as a negative binomial distribution with a log link function (poisson models for these variables were overdispersed). All models were fit using Laplace Approximation. For each of the GLMM analyses, null models (with no fixed effects), a model including only before/after as a fixed effect and all possible combinations of fixed effects in interaction with the before/after term were tested. Model performance was compared using Akaike’s Information Criterion, corrected for small sample sizes (AICc; Akaike, 1974). The model with the smallest AICc value was considered the ‘best’ model and competing models were considered equivalent if ΔAICc < 2. In addition to AIC, we used Likelihood Ratio Tests to compare the goodness of fit and test the significance of individual fixed effects when dropped from the best model.
The analysis of similarities (ANOSIM) test is a non-parametric test that can be used to assess for significant differences between the composition of different groups. ANOSIM was used to test if there is a significant difference between coral community composition before and after Cyclone Haruna. A one-way, pairwise ANOSIM based on Bray-Curtis distance on a square root transformed compositional matrix (species by survey) was conducted to determine the significance of differences in the (i) taxonomic composition of adult corals (ii) morphological composition of adult corals and the (iii) taxonomic composition of coral recruits, one year before and one year after Cyclone Haruna. Sites included in the analysis are listed in Table S2. The identification of which taxa and morphologies were most important at driving the differences pre and post cyclone were conducted using similarity percentage (SIMPER) analysis. Wilcoxon signed rank tests were used to assess if there was a significant difference in mean coral recruit size before and after the cyclone. Mean relative cover of coral genera and coral morphologies and the relative frequency of recruits size classes were calculated for each year as the number of colonies of each coral genera, morphology or recruit size class per site divided by the total number of observations at that site.
Analysis was conducted using the ‘lme4’ package (Bates et al., 2007) and the ‘vegan’ package (Oksanen et al., 2007) in R Version 1.0.143 (R Core Team, 2020).
Mean coral cover in the study region was 37.8% ( ± 1.2 SE) in year –2 and 36.9% ( ± 1.1 SE) in year -1. During the first year after the cyclone (year 1), mean coral cover declined to 21.1% ( ± 0.9). Coral cover declined across all surveyed sites, but the extent of coral decline varied among locations from -1.4% to -45.8%. Two years after the cyclone (year 2) mean coral cover increased slightly to 23.0% ( ± 1.1 SE; Figure 2). However, recovery between year 1 and 2 was not observed at all sites and changes in coral cover ranged from – 11% to + 21.9%.
Figure 2 (A) Hard coral cover and (B) mean taxonomic richness (C) mean relative cover of coral morphologies and (D) mean relative cover of coral genera at reef sites in the Velondriake Locally Managed Marine Area before and after Cyclone Haruna (22nd February 2013). Values are for adult coral colonies with diameter >10cm. (A, B) The solid line in the box represents the median; the top and bottom of the boxes represent the 25th and 75th percentiles; the whiskers above and below the box extend no further than 1.5*the interquartile range; the dots represent outliers and (n) is number of sites. N is number of sites. (C, D) Values are for surveys within one year before and one year after Cyclone Haruna and bars are standard error.
The most parsimonious models based on AICc values (Table 1) and Likelihood Ratio Tests (Table S3) contained the cyclone parameters duration and distance and the environmental characteristic reef depth, all in interaction with the before/after term (Table S4). The model results indicate that cyclone duration had a significant negative effect, with sites in the path of the cyclone for longer showing a greater decrease in coral cover. Distance and reef depth were also found to have negative effects, with sites located furthest from the cyclone track and sites at a greater depth having greater losses in coral cover after the cyclone. The difference between the marginal R2 (0.21) and the conditional R2 (0.56) of the best model indicates unexplained site-to-site variation in hard coral cover. Including wind speed and reef type in the models did not improve the model fit. Our best model slightly under-predicted hard coral cover where coral cover was highest, but overall we found a largely linear relationship between observed hard coral cover and that predicted by the model (Figures S1, S2). When we removed those surveys with the highest coral cover from the model, the same results are obtained with respect to which fixed effects are included in the best model and the directionality of their influence on hard coral cover. An additional analysis assessing proportional change in coral cover rather than raw coral cover values gave highly similar results with respect to duration, depth and distance [see Supplementary Material (Tables S5, S6)].
Table 1 Hard coral cover (HCC), taxonomic richness and recruit density were modelled as a function of three cyclone parameters (i) wind (average maximum wind speed when the cyclone was within 70km of the reef site in m/s), (ii) duration (number of hours the cyclone was within 70km of the reef site) (iii) distance (minimum distance from centre of the cyclone to the reef site in km) and two reef characteristics (i) reef type and (ii) reef depth.
A total of 53 coral genera were identified across all the surveys. Mean taxonomic richness was 18 (SE ±2.12) one year before (year -1) and 16 (SE ± 1.50) one year after the cyclone (year 1; Figure 2). The most parsimonious models (ΔAIC < 2) were the null model and one with the before/after term (Table 1). The before/after term was not significant, suggesting the cyclone did not consistently change taxonomic richness up or down. Including cyclone parameters (duration, distance and wind speed) and reef type and depth did not significantly improve the model.
The taxonomic and morphological composition showed a significant change when comparing one year before (year-1) and one year after the cyclone (year 1; Genera: ANOSIM, R = 0.07, P < 0.05; Morphology: ANOSIM; R = 0.12, P <0.05). This dissimilarity was driven by changes in the abundance of the dominant coral taxa Acropora, Pocillopora, Galexea and Stylophora (SIMPER: 18.0%, 9.3%, 5.5% and 4.9%, respectively) and branching, encrusting and massive morphologies (SIMPER: 34.4%, 20.4%, 8.1%, respectively). From surveys conducted before the cyclone, the relative cover of Acropora ranged from 10% to 55% between sites. The mean relative cover of Acropora across the region decreased slightly in the year following the cyclone. Other branching genera, Pocillopora and Stylophora also decreased in relative cover following the cyclone. Massive Porites had the greatest increase in relative cover (Figure 2). In the year following the cyclone, the relative cover of branching corals decreased from 34.2% (± 3.05) to 24.34% (± 3.65) and encrusting and massive corals became the dominant morphologies [relative cover of 32.21% ( ± 3.00) and 28.34% (± 2.78) respectively; Figure 2].
The greatest decrease in density of coral recruits was observed between the two years before the cyclone from 31.8 (± 2.08) recruits/m2 in year -2 to 24.5 (± 1.93) recruits/m2 in year -1 (Figure 3). In year 1 after Cyclone Haruna, the density of recruits continued to decrease to a mean of 20.5 (± 1.21) recruits/m2. In the second-year post-cyclone, recruit density increased to above pre-cyclone levels [28.9 (± 2.22) recruits per m-2]. Using a negative binomial GLMM, several models were statistically equivalent (ΔAIC<2), and had limited predictive power (Table 1). Likelihood ratio tests revealed adding cyclone parameters to the model did not improve the model accuracy (p > 0.05) and therefore the null model was assumed the best model.
Figure 3 (A) Recruit density (B) relative frequency of recruit colony sizes and (C) relative cover of coral genera, for coral recruits and juvenile corals (colony diameter < 10cm) before and after Cyclone Haruna (22nd February 2013). (A) The solid line in the box represents the median; the top and bottom of the boxes represent the 25th and 75th percentiles; the whiskers above and below the box extend no further than 1.5 times the interquartile range; the dots represent outliers and (n) is number of sites. (B, C) Values are for surveys within one year before and one year after Cyclone Haruna and bars are standard error.
The composition of coral recruits differed significantly one year before and after the cyclone (ANOSIM, R = 0.11, P < 0.05). Acropora, Pocillopora and Favia contributed most towards the dissimilarity (SIMPER; 9.2%, 8.3% and 7.0% respectively). Acropora was the dominant genus of recruits and after the cyclone, increased in mean relative abundance across the study region from 15.1% (± 1.5) to 17.0% ( ± 2.23). Pocillopora and Favia became less abundant and there was an increase in the relative count of massive Porites, Psammacora and Seriatopora (Figure 3). No significant change was observed in the mean size class of recruits following the cyclone (Wilcoxon signed-rank; V=54, p > 0.05: Figure 3).
The present study found that Cyclone Haruna had a spatially heterogeneous impact on coral cover and the community composition of coral reefs located in the Velondriake LMMA in southwest Madagascar. Coral loss was greatest at sites with the longest duration of cyclone impact and at the deepest sites and sites furthest from cyclone track. There was a significant difference in the taxonomic and morphological composition of the coral assemblage before and after the cyclone. Two years after the cyclone, hard coral cover increased, and the density of coral recruits increased to above pre-cyclone levels indicating potential for recovery of the coral population.
Patterns of taxonomic richness and recruit density were found not to be related to cyclone parameters and reef type was found not to have a significant influence on the impact of the cyclone. In addition, the high conditional R2 relative to marginal, indicates that there is site-to-site variation in hard coral cover which are not explained by the variables in the model. It is likely environmental variables not captured in our investigation, such as the orientation of reefs and the influence of localised topography on wave exposure, will have had some influence on the pattern of cyclone impact on the coral community (Woodley et al., 1981; Harmelin-Vivien, 1994; Mumby, 1999). Furthermore, when compared to other reports on cyclone impact on corals (e.g Fabricius et al., 2008; Beeden et al., 2015; Price et al., 2021), this study covers a small region with relatively low variation in wind speed, duration of cyclone impact and distance from the cyclone track. Low variation in cyclone parameters could be a contributing reason as to why we did not find further significant relationships between the cyclone parameters and responses in the coral population.
Extreme winds and waves caused by cyclones dislodge and damage coral colonies causing a decline in coral cover (McLeod et al., 2019). Cyclone Haruna caused coral cover to decline in the Velondriake LMMA by a mean 15.8% in the study region, but the spatial distribution damage was substantially patchy. A mosaic of impact severity is typical of cyclone damage (Harmelin-Vivien, 1994; Guillemot et al., 2010; Perry et al., 2014; Cooke and Marx, 2015), as coral vulnerability is influenced by factors from local (Madin et al., 2012; Madin et al., 2014) to regional scales (Young and Hardy, 1993). No reports of cyclone impact of similar strength events are available for comparison in the WIO. Cyclone damage varies substantially between cyclone events and reef regions. However, the decline observed at the present study site is comparable to the severity of damage caused by category 3 Cyclone Rona (1999) on the Great Barrier Reef (16% decline in average HCC; Cheal et al., 2002) and the average reduction of coral cover caused by hurricanes in the Caribbean (17% decline on average HCC; Gardner et al., 2005).
Previous studies have found sustained wind speed, storm duration and distance from the storm track as effective predictors of coral cover loss (Puotinen, 2007; Fabricius et al., 2008). For Cyclone Haruna, the duration of cyclone impact, the distance from the cyclone path and depth of the reef were the most effective predictors of coral cover decline. Across the study region, reef sites were within 70km of Cyclone Haruna for between 18 to 24 hours. Reef sites within the threshold distance for a longer duration, experienced more severe coral loss. The duration of cyclone impact at a reef site will impact the severity of coral loss as short exposure to high energy waves will only break or dislodge fragile colonies, while persistent exposure to high energy waves will have time to weaken and remove stronger and larger areas of reef framework (Puotinen et al., 2016). Reef depth and distance from the cyclone track modulated the effect of the cyclone on coral cover, with coral loss greater at deeper reef sites and reef sites located further away from the cyclone track. The maximum surveyed reef depth was 13 m, well within the range of which direct mechanical damage to a reef from a tropical cyclone has been observed (refs in Harmelin-Vivien, 1994). For this study, it is possible that more severe coral decline occurred on deeper reefs as they were generally located offshore, exposed to cyclone waves arriving from the open ocean. On the other hand, shallower reef sites located behind the offshore barrier may have been more sheltered as wave energy was dissipated by the barrier reef (Done, 1992; Fabricius et al., 2008). The destruction potential of waves that were generated from the cyclone as it crossed the inshore area are likely to have been limited by shallower depth and shorter fetch (Young and Hardy, 1993). Cyclone damage related to distance from cyclone path is often unpredictable as evidenced by corals located in the direct path of the cyclone remaining unchanged (Massel and Done, 1993), yet coral up to 800km away experiencing severe decline (Puotinen et al., 2020). All reef sites in the study region were located close enough to the cyclone track (between 8.7 km and 39.3 km) in which severe damage would be expected (Fabricius et al., 2008). It is likely that the pattern of coral decline was strongly influenced by localised topography producing refraction of storm waves (Harmelin-Vivien, 1994). The rate of damage would be higher at sites struck directly by cyclone-induced waves as opposed to those sheltered by an adjacent reef. As Cyclone Haruna crossed directly from offshore to inshore in the study region, the reefs were impacted by cyclone-induced waves from multiple directions, which may have produced complex patterns of damage that could not be captured in our model.
Cyclone Haruna did not significantly impact the taxonomic richness of the adult coral community in the study region. The relative difference of the cyclone impact on coral cover compared to taxonomic richness can be partially attributed to the fact that dominant branching morphologies reduced in cover but did not disappear (Rogers, 1993; Fabricius et al., 2008). Following the cyclone, the dominant coral morphology changed from branching to encrusting and massive. A loss of the more fragile coral morphologies (branching and tabular) can be expected from cyclone-induced damage, as they are more vulnerable to fragmentation or dislodgement from extreme waves (Baldock et al., 2014). At some sites, we detected an increase in the relative cover of Acropora in the year following the cyclone. This increase may be caused by fragmentation of existing Acropora colonies concurrent with the mortality of other genera. Overtime, fragments can attach and fuse with underlying coral colonies or substrate and survive (Linares et al., 2011), but due to tissue damage or partial mortality, are also more at risk of mortality from secondary impacts such as bioerosion or fouling from macroalgae (Knowlton et al., 1981). In other cyclone events, mortality from secondary impacts has caused severe mortality rates of Acropora to be recorded from 5 months to over a year after a cyclone event (Knowlton et al., 1981; Muko et al., 2013), however, this does not seem to be the case within the Velondriake LMMA following Cyclone Haruna.
Following declines in coral cover after a disturbance, the survivorship of coral recruits will play a key role in the structuring of the future adult coral assemblage (Coles and Brown, 2007). We observed the greatest decrease in recruit density in the two years before Cyclone Haruna. Interannual variability in recruit density relative to adult coral cover may occur as small individuals can be dislodged or killed outright by biotic (Doropoulos et al., 2014) or abiotic factors (Edmunds et al., 2010) that cause larger individuals only partial or no mortality (Babcock, 1991). Recruit density continued to decrease at some sites in the year following Cyclone Haruna, although we found no significant impact of the cyclone across the region. From our data, it is not possible to extrapolate the influence of Cyclone Haruna compared to other biotic and abiotic pressures on recruit density. Compared to adult coral cover, the relationship between cyclone parameters (wind speed, distance from cyclone track and duration of cyclone impact) and coral recruits may be less pronounced as small colonies will have significantly less drag than their adult counterparts (Harmelin-Vivien, 1994). It is also likely that parameters not captured in our model, such as local scale topography and reef rugosity provided localised shelter for smaller colonies, creating a mosaic of impact severity at an intra-reef scale.
Following the cyclone, observations indicate an increase in macroalgae cover (Blue Ventures, unpublished data). In other regions, algal blooms caused by severe storms have been linked to the failure of coral recruitment and survivorship, as recruits can be outcompeted by the algae for suitable settlement substrate (Baldock et al., 2014; Doropoulos et al., 2014). However, following Cyclone Haruna we found no significant difference in the relative abundance of recruit size classes (1-10cm) and two years after the cyclone recruit density increased to above pre-cyclone levels. This suggests macroalgae did not inhibit the survivorship of recruits which had settled before the cyclone or the settlement of new arrivals.
The taxonomic composition of coral recruits was significantly different following Cyclone Haruna. There was an increase in the relative abundance of sturdier genera including Porites massive, Psammacora, Montipora. This increase can likely be attributed to sturdier morphologies being less fragile to wave action during the cyclone (Baldock et al., 2014) and self- recruitment from surviving adult colonies (Graham et al., 2011). These genera may also have a competitive advantage to secondary cyclone impacts as they are relatively more tolerant to stress (Darling et al., 2013). The genus Acropora is the most abundant genus in the study region, so its recovery is crucial for the maintenance of the coral ecosystem. The ability of Acropora to rapidly colonise space following a disturbance through fragmentation or recruitment may explain the increase in the relative abundance of Acropora recruits after Cyclone Haruna (Pratchett et al., 2020). This is consistent with previous studies where Acropora has rapidly recovered in the aftermath of disturbance events, attributed to fast growth and high levels of recruitment (Sheppard et al., 2002; Linares et al., 2011).
The persistence of the coral community assemblage is dependent on the return interval and intensity of disturbance events (Pratchett et al., 2020). The reef community in southwest Madagascar has evolved with relatively frequent cyclones (Puotinen et al., 2020). Since 1913, there have been 15 cyclones recorded in the region, 13 of these have been category 1 on the Saffir-Simpson scale (Knapp et al., 2010). The remaining two cyclones were Cyclone Earnest (category 3) in 2005 and Cyclone Haruna. No other reports regarding the impact of cyclones on coral reefs in southwest Madagascar are available. However, we can assume the severity of damage caused by category 1 cyclones would be, in general, less than observed following Cyclone Haruna (Fabricius et al., 2008). It is predicted anthropogenic climate change will cause the global average intensity of tropical cyclones to increase (Knutson et al., 2020) and therefore the return interval between damaging cyclones to shorten (Puotinen et al., 2020). Predictions of changes to the size, frequency and spatial distribution of cyclones are more uncertain (Knutson et al., 2020) however, evidence suggests the poleward migration of the latitude at which cyclones reach maximum intensity is already occurring (Kossin et al., 2014). If this trend continues, tropical cyclones may become more prevalent in southwest Madagascar.
The high rates of replenishment of Acropora may be sufficient for the reef ecosystem to recover between cyclone impacts (Thompson and Dolman, 2010). However, mass coral bleaching and mortality caused by marine heatwaves (MHWs) will likely be the leading control on the composition of reef communities (Loya et al., 2001) and lead to an increase in the relative abundance of thermally tolerant species (Woesik et al., 2011). Since the late 20th century, MHWs have increased in frequency, intensity and duration in the Mozambique Channel and southwest Madagascar has experienced the longest and most extreme MHWs in the region (Mawren et al., 2021). Under a high emission scenario (RCP 8.5), MHWs are predicted to become more intense and prolonged in the WIO (Jacobs et al., 2021). When the impact of tropical cyclones and MHWs are combined in a disturbance system of threats that also includes Acanthaster spp invasions (Bigot and Quod, 2000), coral diseases (Sheridan et al., 2014) and anthropogenic pressures, such as overfishing (Brenier et al., 2011; Gilchrist et al., 2020; Gough et al., 2020), it is likely the reef composition will change to be dominated by stress tolerant coral taxa (Loya et al., 2001; Woesik et al., 2011).
A shift in the community composition to an assemblage dominated by massive and robust stress-tolerant genera has already been observed areas of southwest Madagascar (Harris et al., 2010) and in other reef regions (Hughes, 1994; Fine et al., 2019). Dominance of less complex morphologies will alter the habitat availability and likely lead to a decline in the biodiversity and abundance of reef associated organisms (Pratchett et al., 2008). Small-bodied and juvenile reef fish are particularly vulnerable to a decreased reef complexity (Richardson et al., 2017; Fontoura et al., 2020) they rely on the coral for refuge and nutrition (Gratwicke and Speight, 2005).
In Vezo small-scale fisheries, reef fish represent 30% to 50% of target fish species (Blue Ventures, unpublished data). Therefore, a change to reef fish populations may have implications for the food security and livelihoods of Vezo communities. While it is not possible to remove the threat of cyclone damage to corals, marine management measures can help reduce the likelihood of catastrophic regime shifts. For example, protecting a diversity of coral habitats from destructive fishing gear will provide opportunity for a diversity in ecosystem responses (Nyström et al., 2008) and provide an insurance policy against losing ecosystem functions (van Nes and Scheffer, 2005). Where herbivorous fish are heavily exploited, removing fishing pressure can support the existence of diverse functional groups of herbivores and reduce the risk of a shift from coral to algal dominance after a disturbance event (Graham et al., 2015). Within the Velondriake LMMA, no-take zones permanently closed to extractive activities have been found to have significantly higher fish biomass than fished sites (786 ± 247 kg/ha vs 247 ± 33 kg/ha; Gilchrist et al., 2020), indicating their potential role in coral recovery.
In the present study, we used long term monitoring data collected by trained field staff (nationals and international) and international citizen science volunteers to assess the impact of Cyclone Haruna. This programme was not designed to monitor disturbance-related responses in coral health, and therefore variability in the spatial distribution and sample replication of surveys inhibited deeper investigation on the impact of Cyclone Haruna on reefs. However, despite challenges and limitations in data analysis, in remote and data-scarce regions, using the data that is available is critical to support local reef health and strengthen regional knowledge of ecological and societal outcomes for coral reefs and dependent communities. Engaging local stakeholders in reef monitoring is critical to foster public support for coral reef conservation and local ownership of management efforts (Aswani et al., 2015). Monitoring programmes must be accompanied by skills training and sustainable financing (Hattam et al., 2020). In locations with economic constraints, citizen science provides an opportunity to support robust large scale and long-term monitoring (Chandler et al., 2017). Monitoring approaches should be designed to address localised threats to coral health and social-ecological dynamics, yet guided by global best practices (e.g. Global Coral Reef Monitoring Network; Hill and Wilkinson, 2004) to allow for the aggregation of data at a global level (Freiwald et al., 2018). As disturbance regimes increase on coral reefs, monitoring coral reef status and trends will provide critical data which can be used to inform local marine management and national and international obligations to support reef health (Obura et al., 2019).
This paper presents the first published record of the impact of a tropical cyclone on coral reefs in Madagascar. Disturbances, such as cyclones, have major impacts on the structure and functioning of coral reef communities. The frequency of severe disturbances to coral communities is predicted to increase as climate change progresses, with the potential for severe consequences for those that rely on reef-associated marine resources for their livelihoods. Cyclone Haruna had an immediate impact on the hard coral cover and community assemblage on the coral reefs located in the Velondriake LMMA. Following the cyclone, the morphological composition shifted from being dominated by branching genera to massive and encrusting genera. Two years after the cyclone, hard coral cover increased, and the density of coral recruits increased to above pre-cyclone levels indicating the potential for recovery of the coral population. Our results contribute to extending the geographic evidence of cyclone impacts on reefs and increase knowledge of cyclone impacts on reefs in the WIO. Understanding coral community responses to disturbances is important to inform and support effective reef and resource management actions.
For the Velondriake LMMA, we recommend ensuring that no take zones are in place, and that these areas protect a range of habitats with distinctive coral assemblages. Velondriake currently has a network of five coral reef no take zones, so we recommend further research exploring whether these NTZs protect a range of coral assemblages.
As climate-driven impacts on reefs increase in frequency and severity, a more geographically equitable picture of these impacts are needed. Often, areas that are data deficient are also places where coastal communities have a high dependence on seafood and coral reefs for nutrition, and where lives are most closely entangled with the sea. Without a detailed picture of cyclone impacts and recovery, we cannot tailor management recommendations to account for local, or even regional ecology and oceanography, ultimately further threatening both unique coral ecosystems, and the lives and livelihoods of the people who depend on them.
The original contributions presented in the study are included in the article/Supplementary Material. Further inquiries can be directed to the corresponding author. Data is available here: https://figshare.com/projects/southwest_Madagascar_coral_data/128954.
AC and HG and conceived and wrote the paper. HG and CGa were involved in data collection. AC and KD undertook data analysis. KD, CGa, CGo, SR, and AW contributed sections of the manuscript and/or reviewed and edited manuscript text. All authors contributed to the article and approved the submitted version.
Funding for this research was provided by NERC through an E4 DTP studentship (NE/S007407/1).
The authors declare that the research was conducted in the absence of any commercial or financial relationships that could be construed as a potential conflict of interest.
All claims expressed in this article are solely those of the authors and do not necessarily represent those of their affiliated organizations, or those of the publisher, the editors and the reviewers. Any product that may be evaluated in this article, or claim that may be made by its manufacturer, is not guaranteed or endorsed by the publisher.
We are grateful to the many field scientists and volunteers who aided in the collection of data for this study in addition to all the staff involved in the Blue Ventures Expeditions programme. Thank you to the boat captains who ensured our colleagues could safely conduct reef surveys and the Velondriake LMMA Management Association for allowing us to survey within the LMMA. Thank you to Prof. Sandy Tudhope for his helpful comments and review of the manuscript in its final stages and thank you to the anonymous reviewers who provided valuable comments to the earlier version of this manuscript.
The Supplementary Material for this article can be found online at: https://www.frontiersin.org/articles/10.3389/fmars.2022.753325/full#supplementary-material
Akaike H. (1974). A New Look at the Statistical Model Identification. IEEE Trans. Auto. Contr. 19, 716–723. doi: 10.1109/TAC.1974.1100705
Astuti R. (1995). “The Vezo are Not a Kind of People”: Identity, Difference, and “Ethnicity” Among a Fishing People of Western Madagascar. Am. Ethnolog. 22, 464–482. doi: 10.1525/ae.1995.22.3.02a00010
Aswani S., Mumby P. J., Baker A. C., Christie P., McCook L. J., Steneck R. S., et al. (2015). Scientific Frontiers in the Management of Coral Reefs. Front. Mar. Sci. 0. doi: 10.3389/fmars.2015.00050
Babcock R. C. (1991). Comparative Demography of Three Species of Scleractinian Corals Using Age- and Size-Dependent Classifications. Ecol. Monogr. 61, 225–244. doi: 10.2307/2937107
Baldock T. E., Karampour H., Sleep R., Vyltla A., Albermani F., Golshani A., et al. (2014). Resilience of Branching and Massive Corals to Wave Loading Under Sea Level Rise–A Coupled Computational Fluid Dynamics-Structural Analysis. Mar. Poll. Bull. 86, 91–101. doi: 10.1016/j.marpolbul.2014.07.038
Ban S. S., Pressey R. L., Graham N. A. J. (2015). Assessing the Effectiveness of Local Management of Coral Reefs Using Expert Opinion and Spatial Bayesian Modeling. PloS One 10, e0135465. doi: 10.1371/journal.pone.0135465
Barnes-Mauthe M., Oleson K. L. L., Zafindrasilivonona B. (2013). The Total Economic Value of Small-Scale Fisheries With a Characterization of Post-Landing Trends: An Application in Madagascar With Global Relevance. Fish. Res. 147, 175–185. doi: 10.1016/j.fishres.2013.05.011
Bates D., Mächler D., Bolker B., Walker S. (2007). Fitting Linear Mixed-Effects Models Using lme4. J. Stat. Softw. 67(1), 1–48. doi: 10.18637/jss.v067.i01
Beeden R., Maynard J., Puotinen M., Marshall P., Dryden J., Goldberg J., et al. (2015). Impacts and Recovery From Severe Tropical Cyclone Yasi on the Great Barrier Reef. PloS One 10, e0121272. doi: 10.1371/journal.pone.0121272
Bigot L., Quod J. (2000). Coral Bleaching in the Indian Ocean Islands: Ecological Consequences and Recovery in Madagascar, Comoros, Mayotte and Reunion. CORDIO/SAREC Mar. Sci. Progr 108–113.
Bozec Y., Alvarez-Filip L., Mumby P. J. (2015). The Dynamics of Architectural Complexity on Coral Reefs Under Climate Change. Global Change Biol. 21, 223–235. doi: 10.1111/gcb.12698
Brenier A., Ferraris J., Mahafina J. (2011). Participatory Assessment of the Toliara Bay Reef Fishery, Southwest Madagascar. Madagasc. Conserv. Dev. 6, 60–67.doi: 10.4314/mcd.v6i2.4
Chandler M., See L., Copas K., Bonde A. M. Z., López B. C., Danielsen F., et al. (2017). Contribution of Citizen Science Towards International Biodiversity Monitoring. Biol. Conserv. 213, 280–294. doi: 10.1016/j.biocon.2016.09.004
Cheal A., Coleman G., Delean S., Miller I., Osborne K., Sweatman H. (2002). Responses of Coral and Fish Assemblages to a Severe But Short-Lived Tropical Cyclone on the Great Barrier Reef, Australia. Coral. Reef. 21, 131–142. doi: 10.1007/s00338-002-0227-8
Cheal A. J., MacNeil M. A., Emslie M. J., Sweatman H. (2017). The Threat to Coral Reefs From More Intense Cyclones Under Climate Change. Global Change Biol. 23, 1511–1524. doi: 10.1111/gcb.13593
Cinner J. E., McClanahan T. R., Graham N. A. J., Daw T. M., Maina J., Stead S. M., et al. (2012). Vulnerability of Coastal Communities to Key Impacts of Climate Change on Coral Reef Fisheries. Global Environ. Change 22, 12–20. doi: 10.1016/j.gloenvcha.2011.09.018
Cohen P. J., Allison E. H., Andrew N. L., Cinner J., Evans L. S., Fabinyi M., et al. (2019). Securing a Just Space for Small-Scale Fisheries in the Blue Economy. Front. Mar. Sci. 6. doi: 10.3389/fmars.2019.00171
Coles S. L., Brown E. K. (2007). Twenty-Five Years of Change in Coral Coverage on a Hurricane Impacted Reef in Hawai’i: The Importance of Recruitment. Coral. Reef. 26, 705–717. doi: 10.1007/s00338-007-0257-3
Cooke C. A., Marx D. J. E. (2015). Assessment of the Impact of Super Storm Sandy on Coral Reefs of Guantánamo Bay, Cuba (United States: Space and Naval Warfare Systems Central Pacific San Diego).
Darling E. S., Graham N. A. J., Januchowski-Hartley F. A., Nash K. L., Pratchett M. S., Wilson S. K. (2017). Relationships Between Structural Complexity, Coral Traits, and Reef Fish Assemblages. Coral. Reef. 36, 561–575. doi: 10.1007/s00338-017-1539-z
Darling E. S., McClanahan T. R., Côté I. M. (2013). Life Histories Predict Coral Community Disassembly Under Multiple Stressors. Global Change Biol. 19, 1930–1940. doi: 10.1111/gcb.12191
Done T. (1992). Effects of Tropical Cyclone Waves on Ecological and Geomorphological Structures on the Great Barrier Reef. Continent. Shelf. Res. 12, 859–872. doi: 10.1016/0278-4343(92)90048-O
Doropoulos C., Roff G., Zupan M., Nestor V., Isechal A. L., Mumby P. J. (2014). Reef-Scale Failure of Coral Settlement Following Typhoon Disturbance and Macroalgal Bloom in Palau, Western Pacific. Coral. Reef. 33, 613–623. doi: 10.1007/s00338-014-1149-y
Eddy T. D., Lam V. W. Y., Reygondeau G., Cisneros-Montemayor A. M., Greer K., Palomares M. L. D., et al. (2021). Global Decline in Capacity of Coral Reefs to Provide Ecosystem Services. One Earth 4, 1278–1285. doi: 10.1016/j.oneear.2021.08.016
Edmunds P. J., Leichter J. J., Adjeroud M. (2010). Landscape-Scale Variation in Coral Recruitment in Moorea, French Polynesia. Mar. Ecol. Prog. Ser. 414, 75–89. doi: 10.3354/meps08728
Edwards H. J., Elliott I. A., Eakin C. M., Irikawa A., Madin J. S., Mcfield M., et al. (2011). How Much Time can Herbivore Protection Buy for Coral Reefs Under Realistic Regimes of Hurricanes and Coral Bleaching? Global Change Biol. 17, 2033–2048. doi: 10.1111/j.1365-2486.2010.02366.x
Fabricius K. E., De’ath G., Puotinen M. L., Done T., Cooper T. F., Burgess S. C. (2008). Disturbance Gradients on Inshore and Offshore Coral Reefs Caused by a Severe Tropical Cyclone. Limnol. Oceanogr. 53, 690–704. doi: 10.4319/lo.2008.53.2.0690
Facon M., Pinault M., Obura D., Pioch S., Pothin K., Bigot L., et al. (2016). A Comparative Study of the Accuracy and Effectiveness of Line and Point Intercept Transect Methods for Coral Reef Monitoring in the Southwestern Indian Ocean Islands. Ecol. Indic. 60, 1045–1055. doi: 10.1016/j.ecolind.2015.09.005
Ferrario F., Beck M. W., Storlazzi C. D., Micheli F., Shepard C. C., Airoldi L. (2014). The Effectiveness of Coral Reefs for Coastal Hazard Risk Reduction and Adaptation. Nat. Commun. 5, 3794. doi: 10.1038/ncomms4794
Fine M., Hoegh-Guldberg O., Meroz-Fine E., Dove S. (2019). Ecological Changes Over 90 Years at Low Isles on the Great Barrier Reef. Nat. Commun. 10, 4409. doi: 10.1038/s41467-019-12431-y
Fontoura L., Zawada K. J. A., D’agata S., Álvarez-Noriega M., Baird A. H., Boutros N., et al. (2020). Climate-Driven Shift in Coral Morphological Structure Predicts Decline of Juvenile Reef Fishes. Global Change Biol. 26, 557–567. doi: 10.1111/gcb.14911
Freiwald J., Meyer R., Caselle J. E., Blanchette C. A., Hovel K., Neilson D., et al. (2018). Citizen Science Monitoring of Marine Protected Areas: Case Studies and Recommendations for Integration Into Monitoring Programs. Mar. Ecol. 39, e12470. doi: 10.1111/maec.12470
Gardner T. A., Côté I. M., Gill J. A., Grant A., Watkinson A. R. (2005). Hurricanes and Caribbean Coral Reefs: Impacts, Recovery Patterns, and Role in Long-Term Decline. Ecology 86, 174–184. doi: 10.1890/04-0141
Gardner C. J., Cripps G., Prémesnil Day L., Dewar K., Gough C., Peabody S., et al. (2020). A Decade and a Half of Learning From Madagascar’s First Locally Managed Marine Area. Conserv. Sci. Pract. 2 (12), e298. doi: 10.1111/csp2.298
Gilchrist H., Rocliffe S., Anderson L. G., Gough C. L. (2020). Reef Fish Biomass Recovery Within Community-Managed No Take Zones. Ocean. Coast. Manage. 192, 105210. doi: 10.1016/j.ocecoaman.2020.105210Get
Gough C. L., Dewar K. M., Godley B. J., Zafindranosy E., Broderick A. C. (2020). Evidence of Overfishing in Small-Scale Fisheries in Madagascar. Front. Mar. Sci. 7. doi: 10.3389/fmars.2020.00317
Graham N. A. J., Jennings S., MacNeil M. A., Mouillot D., Wilson S. K. (2015). Predicting Climate-Driven Regime Shifts Versus Rebound Potential in Coral Reefs. Nature 518, 94–97. doi: 10.1038/nature14140
Graham N. A. J., Nash K. L. (2013). The Importance of Structural Complexity in Coral Reef Ecosystems. Coral. Reef. 32, 315–326. doi: 10.1007/s00338-012-0984-y
Graham N. A. J., Nash K. L., Kool J. T. (2011). Coral Reef Recovery Dynamics in a Changing World. Coral. Reef. 30, 283–294. doi: 10.1007/s00338-010-0717-z
Gratwicke B., Speight M. R. (2005). The Relationship Between Fish Species Richness, Abundance and Habitat Complexity in a Range of Shallow Tropical Marine Habitats. J. Fish. Biol. 66, 650–667. doi: 10.1111/j.0022-1112.2005.00629.x
Guillemot N., Chabanet P., Le Pape O. (2010). Cyclone Effects on Coral Reef Habitats in New Caledonia (South Pacific). Coral. Reef. 29, 445–453. doi: 10.1007/s00338-010-0587-4
Haapkylä J., Melbourne-Thomas J., Flavell M., Willis B. (2013). Disease Outbreaks, Bleaching and a Cyclone Drive Changes in Coral Assemblages on an Inshore Reef of the Great Barrier Reef. Coral. Reef. 32, 815–824. doi: 10.1007/s00338-013-1029-x
Hanisch S. (2015). Improving Cropping Systems of Semi-Arid South-Western Madagascar Under Multiple Ecological and Socio-Economic Constraints. Kassel, Germany, 199 pp.
Harmelin-Vivien M. L. (1994). The Effects of Storms and Cyclones on Coral Reefs: A Review. J. Coast. Res., 211–231.
Harris A., Manahira G., Sheppard A., Gouch C., Sheppard C. (2010). Demise of Madagascar’s Once Great Barrier Reef: Changes in Coral Reef Conditions Over 40 Years. Atoll. Res. Bull. 574, 1–16. doi: 10.5479/si.00775630.574.16
Hattam C., Evans L., Morrissey K., Hooper T., Young K., Khalid F., et al. (2020). Building Resilience in Practice to Support Coral Communities in the Western Indian Ocean. Environ. Sci. Policy 106, 182–190. doi: 10.1016/j.envsci.2020.02.006
Hicks C. C., Graham N. A. J., Maire E., Robinson J. P. W. (2021). Secure Local Aquatic Food Systems in the Face of Declining Coral Reefs. One Earth 4, 1214–1216. doi: 10.1016/j.oneear.2021.08.023
Hill J., Wilkinson C. (2004). Methods for Ecological Monitoring of Coral Reefs. Aust. Insti. Mar. Sci. Townsv. 117, 1–116.
Hughes T. P. (1994). Catastrophes, Phase Shifts, and Large-Scale Degradation of a Caribbean Coral Reef. Science 265, 1547–1551. doi: 10.1126/science.265.5178.1547
Jacobs Z. L., Yool A., Jebri F., Srokosz M., van Gennip S., Kelly S. J., et al. (2021). Key Climate Change Stressors of Marine Ecosystems Along the Path of the East African Coastal Current. Ocean. Coast. Manage. 208, 105627. doi: 10.1016/j.ocecoaman.2021.105627
Kawabata Y., Okuyama J., Asami K., Okuzawa K., Yoseda K., Arai N. (2010). Effects of a Tropical Cyclone on the Distribution of Hatchery-Reared Black-Spot Tuskfish Choerodon Schoenleinii Determined by Acoustic Telemetry. J. Fish. Biol. 77, 627–642. doi: 10.1111/j.1095-8649.2010.02702.x
Knapp K. R., Kruk M. C., Levinson D. H., Diamond H. J., Neumann C. J. (2010). The International Best Track Archive for Climate Stewardship (IBTrACS) Unifying Tropical Cyclone Data. Bull. Am. Meteorolog. Soc. 91, 363–376. doi: 10.1175/2009BAMS2755.1
Knowlton N., Lang J. C., Christine Rooney M., Clifford P. (1981). Evidence for Delayed Mortality in Hurricane-Damaged Jamaican Staghorn Corals. Nature 294, 251–252. doi: 10.1038/294251a0
Knutson T., Camargo S. J., Chan J. C. L., Emanuel K., Ho C.-H., Kossin J., et al. (2020). Tropical Cyclones and Climate Change Assessment: Part II: Projected Response to Anthropogenic Warming. Bull. Am. Meteorolog. Soc. 101, E303–E322. doi: 10.1175/BAMS-D-18-0194.1
Komyakova V., Munday P. L., Jones G. P. (2013). Relative Importance of Coral Cover, Habitat Complexity and Diversity in Determining the Structure of Reef Fish Communities. PloS One 8, e83178. doi: 10.1371/journal.pone.0083178
Kossin J. P., Emanuel K. A., Vecchi G. A. (2014). The Poleward Migration of the Location of Tropical Cyclone Maximum Intensity. Nature 509, 349–352. doi: 10.1038/nature13278
Kuffner I. B., Walters L. J., Becerro M. A., Paul V. J., Ritson-Williams R., Beach K. S. (2006). Inhibition of Coral Recruitment by Macroalgae and Cyanobacteria. Mar. Ecol. Prog. Ser. 323, 107–117. doi: 10.3354/meps323107
Laird N. M., Ware J. H. (1982). Random-Effects Models for Longitudinal Data. Biometrics 38, 963–974. doi: 10.2307/2529876
Lamb J. B., Willis B. L., Fiorenza E. A., Couch C. S., Howard R., Rader D. N., et al. (2018). Plastic Waste Associated With Disease on Coral Reefs. Science 359, 460–462. doi: 10.1126/science.aar3320
Letourneur Y., Harmelin-Vivien M., Galzin R. (1993). Impact of Hurricane Firinga on Fish Community Structure on Fringing Reefs of Reunion Island, SW Indian Ocean. Environ. Biol. Fish. 37, 109–120. doi: 10.1007/BF00000586
Linares C., Pratchett M. S., Coker D. J. (2011). Recolonisation of Acropora Hyacinthus Following Climate-Induced Coral Bleaching on the Great Barrier Reef. Mar. Ecol. Prog. Ser. 438, 97–104. doi: 10.3354/meps09272
Loya Y., Sakai K., Yamazato K., Nakano Y., Sambali H., van Woesik R. (2001). Coral Bleaching: The Winners and the Losers. Ecol. Lett. 4, 122–131. doi: 10.1046/j.1461-0248.2001.00203.x
Madin J. S., Baird A. H., Dornelas M., Connolly S. R. (2014). Mechanical Vulnerability Explains Size-Dependent Mortality of Reef Corals. Ecol. Lett. 17, 1008–1015. doi: 10.1111/ele.12306
Madin J. S., Hughes T. P., Connolly S. R. (2012). Calcification, Storm Damage and Population Resilience of Tabular Corals Under Climate Change. PloS One 7, e46637. doi: 10.1371/journal.pone.0046637
Manzello D. P., Brandt M., Smith T. B., Lirman D., Hendee J. C., Nemeth R. S. (2007). Hurricanes Benefit Bleached Corals. Proc. Natl. Acad. Sci. 104, 12035–12039. doi: 10.1073/pnas.0701194104
Marikandia M. (2001). The Vezo of the Fiherena Coast, Southwest Madagascar: Yesterday and Today. Ethnohistory 48, 157–170. doi: 10.1215/00141801-48-1-2-157
Massel S., Done T. (1993). Effects of Cyclone Waves on Massive Coral Assemblages on the Great Barrier Reef: Meteorology, Hydrodynamics and Demography. Coral. Reef. 12, 153–166. doi: 10.1016/0278-4343(92)90048-O
Mawren D., Hermes J., Reason C. (2021). Marine Heatwaves in the Mozambique Channel. Clim. Dynam. 1–23, 305–327. doi: 10.1007/s00382-021-05909-3
McClanahan T. (2004). The Relationship Between Bleaching and Mortality of Common Corals. Mar. Biol. 144, 1239–1245. doi: 10.1007/s00227-003-1271-9
McClanahan T. R., Castilla J. C., White A. T., Defeo O. (2009). Healing Small-Scale Fisheries by Facilitating Complex Socio-Ecological Systems. Rev. Fish. Biol. Fish. 19, 33–47. doi: 10.1007/s11160-008-9088-8
McLeod I. M., Williamson D. H., Taylor S., Srinivasan M., Read M., Boxer C., et al. (2019). Bommies Away! Logistics and Early Effects of Repositioning 400 Tonnes of Displaced Coral Colonies Following Cyclone Impacts on the Great Barrier Reef. Ecol. Manage. Restor. 20, 262–265. doi: 10.1111/emr.12381
Micheli F., Mumby P. J., Brumbaugh D. R., Broad K., Dahlgren C. P., Harborne A. R., et al. (2014). High Vulnerability of Ecosystem Function and Services to Diversity Loss in Caribbean Coral Reefs. Biol. Conserv. 171, 186–194. doi: 10.1016/j.biocon.2013.12.029
Muko S., Arakaki S., Nagao M., Sakai K. (2013). Growth Form-Dependent Response to Physical Disturbance and Thermal Stress in Acropora Corals. Coral Reefs 32, 269–280 doi: 10.1007/s00338-012-0967-z
Mumby P. J. (1999). Bleaching and Hurricane Disturbances to Populations of Coral Recruits in Belize. Mar. Ecol. Prog. Ser. 190, 27–35. doi: 10.3354/meps190027
Nadon M., Griffiths D., Doherty E., Harris A. (2008). The Status of Coral Reefs in the Remote Region of Andavadoaka, Southwest Madagascar. West. Indian Ocean. J. Mar. Sci. 6, 207–218. doi: 10.4314/wiojms.v6i2.48244
Naim O., Chabanet P., Done T., Tourrand C., Letourneur Y. (2000). Regeneration of a Reef Flat Ten Years After the Impact of the Cyclone Firinga. In 9th International Coral Reef Symposium (Reunion, SW Indian Ocean). Vol. 1, 547–554.
Nyström M., Graham N., Lokrantz J., Norström A. V. (2008). Capturing the Cornerstones of Coral Reef Resilience: Linking Theory to Practice. Coral. Reef. 27, 795–809. doi: 10.1007/s00338-008-0426-z
Obura D. O., Aeby G., Amornthammarong N., Appeltans W., Bax N., Bishop J., et al. (2019). Coral Reef Monitoring, Reef Assessment Technologies, and Ecosystem-Based Management. Front. Mar. Sci. 0. doi: 10.3389/fmars.2019.00580
Oksanen J., Blanchet F. G., Friendly M., Kindt R., Legendre P., McGlinn D., et al. (2020). Vegan: Community Ecology Package. R Package Version 2.5-7 10, 719. Available at: https://CRAN.R-project.org/package=vega
Pandolfi J. M., Connolly S. R., Marshall D. J., Cohen A. L. (2011). Projecting Coral Reef Futures Under Global Warming and Ocean Acidification. Science 333, 418–422. doi: 10.1126/science.1204794
Perry C. T., Smithers S. G., Kench P. S., Pears B. (2014). Impacts of Cyclone Yasi on Nearshore, Terrigenous Sediment-Dominated Reefs of the Central Great Barrier Reef, Australia. Geomorphology 222, 92–105. doi: 10.1016/j.geomorph.2014.03.012
Pratchett M. S., McWilliam M. J., Riegl B. (2020). Contrasting Shifts in Coral Assemblages With Increasing Disturbances. Coral. Reef. 39, 783–793. doi: 10.1007/s00338-020-01936-4
Pratchett M. S., Munday P. L., Wilson S. K., Graham N. A., Cinner J. E., Bellwood D. R., et al. (2008). “Effects of Climate-Induced Coral Bleaching on Coral-Reef Fishes—Ecological and Economic Consequences,” in Oceanography and Marine Biology (Boca Raton, FL: CRC Press), 257–302.
Pratchett M. S., Thompson C. A., Hoey A. S., Cowman P. F., Wilson S. K. (2018). “Effects of Coral Bleaching and Coral Loss on the Structure and Function of Reef Fish Assemblages,” in Coral Bleaching: Patterns, Processes, Causes and Consequences. Eds. van Oppen M. J. H., Lough J. M.. Boca Raton, FL: CRC Press.(Cham: Springer International Publishing), 265–293. doi: 10.1007/978-3-319-75393-5_11
Price B. A., Harvey E. S., Mangubhai S., Saunders B. J., Puotinen M., Goetze J. S. (2021). Responses of Benthic Habitat and Fish to Severe Tropical Cyclone Winston in Fiji. Coral. Reef. 40, 807–819. doi: 10.1007/s00338-021-02086-x
Puotinen M. L. (2005). An Automated GIS Method for Modeling Relative Wave Exposure Within Complex Reef-Island Systems: A Case Study of the Great Barrier Reef. Zerger A., Argent R. M.MODSIM: Int Congr Modelling and Simulation, Dec 2005, 170–176. Modelling and Simulation Society of Australia and New Zealand
Puotinen M. L. (2007). Modelling the Risk of Cyclone Wave Damage to Coral Reefs Using GIS: A Case Study of the Great Barrier Ree–2003. Int. J. Geograph. Inf. Sci. 21, 97–120. doi: 10.1080/13658810600852230
Puotinen M., Drost E., Lowe R., Depczynski M., Radford B., Heyward A., et al. (2020). Towards Modelling the Future Risk of Cyclone Wave Damage to the World’s Coral Reefs. Global Change Biol. 26, 4302–4315. doi: 10.1111/gcb.15136
Puotinen M., Maynard J. A., Beeden R., Radford B., Williams G. J. (2016). A Robust Operational Model for Predicting Where Tropical Cyclone Waves Damage Coral Reefs. Sci. Rep. 6, 26009. doi: 10.1038/srep26009
Quartly G., Srokosz M. (2004). Eddies in the Southern Mozambique Channel. Deep. Sea. Res. Part II.: Top. Stud. Oceanogr. 51, 69–83. doi: 10.1016/j.dsr2.2003.03.001
R Core Team (2020). R: A Language and Environment for Statistical Computing. R Foundation for Statistical Computing, Vienna, Austria. Available at: https://www.R-project.org/
Richardson L. E., Graham N. A. J., Pratchett M. S., Hoey A. S. (2017). Structural Complexity Mediates Functional Structure of Reef Fish Assemblages Among Coral Habitats. Environ. Biol. Fish. 100, 193–207. doi: 10.1007/s10641-016-0571-0
Rogers C. S. (1993). Hurricanes and Coral Reefs: The Intermediate Disturbance Hypothesis Revisited. Coral. Reef. 12, 127–137. doi: 10.1007/BF00334471
Scopélitis J., Andréfouët S., Phinn S., Chabanet P., Naim O., Tourrand C., et al. (2009). Changes of Coral Communities Over 35 Years: Integrating in Situ and Remote-Sensing Data on Saint-Leu Reef (La Réunion, Indian Ocean). Estua. Coast. Shelf. Sci. 84, 342–352. doi: 10.1016/j.ecss.2009.04.030
Sheppard C. R., Spalding M., Bradshaw C., Wilson S. (2002). Erosion vs. Recovery of Coral Reefs After 1998 El Niño: Chagos Reefs, Indian Ocean. AMBIO: A. J. Hum. Environ. 31, 40–48. doi: 10.1016/j.marpolbul.2010.03.033
Sheridan C., Baele J. M., Kushmaro A., Fréjaville Y., Eeckhaut I. (2014). Terrestrial Runoff Influences White Syndrome Prevalence in SW Madagascar. Mar. Environ. Res. 101, 44–51. doi: 10.1016/j.marenvres.2014.08.003
Spalding M., Burke L., Wood S. A., Ashpole J., Hutchison J., Zu Ermgassen P. (2017). Mapping the Global Value and Distribution of Coral Reef Tourism. Mar. Policy 82, 104–113. doi: 10.1016/j.marpol.2017.05.014
Thompson A. A., Dolman A. M. (2010). Coral Bleaching: One Disturbance Too Many for Near-Shore Reefs of the Great Barrier Reef. Coral. Reef. 29, 637–648. doi: 10.1007/s00338-009-0562-0
Tobin A., Schlaff A., Tobin R., Penny A., Ayling A., Krause B., et al. (2010). Adapting to Change: Minimising Uncertainty About the Effects of Rapidly-Changing Environmental Conditions on the Queensland Coral Reef Fin Fish Fishery (Townsville, Qld, Australia: Fishing and Fisheries Research Centre, James Cook University).
van Nes E. H., Scheffer M. (2005). Implications of Spatial Heterogeneity for Catastrophic Regime Shifts in Ecosystems. Ecology 86, 1797–1807. doi: 10.1890/04-0550
Woesik R. V., Ayling A. M., Mapstone B. (1991). Impact of Tropical Cyclone “Ivor” on the Great Barrier Reef, Australia. J. Coast. Res. 7, 551–557.
Woesik R., van, Sakai K., Ganase A., Loya Y. (2011). Revisiting the Winners and the Losers a Decade After Coral Bleaching. Mar. Ecol. Prog. Ser. 434, 67–76. doi: 10.3354/meps09203
Woodhead A. J., Hicks C. C., Norström A. V., Williams G. J., Graham N. A. (2019). Coral Reef Ecosystem Services in the Anthropocene. Funct. Ecol. 33, 1023–1034. doi: 10.1111/1365-2435.13331
Woodley J. D., Chornesky E. A., Clifford P. A., Jackson J. B. C., Kaufman L. S., Knowlton N., et al. (1981). Hurricane Allen’s Impact on Jamaican Coral Reefs. Science 214, 749–755. doi: 10.1126/science.214.4522.749
Woolsey E., Bainbridge S. J., Kingsford M. J., Byrne M. (2012). Impacts of Cyclone Hamish at One Tree Reef: Integrating Environmental and Benthic Habitat Data. Mar. Biol. 159, 793–803. doi: 10.1007/s00227-011-1855-8
Keywords: coral, tropical cyclone, Madagascar, hurricane, citizen science, reef resilience, Western Indian Ocean (WIO) region, Acropora
Citation: Carter AL, Gilchrist H, Dexter KG, Gardner CJ, Gough C, Rocliffe S and Wilson AMW (2022) Cyclone Impacts on Coral Reef Communities in Southwest Madagascar. Front. Mar. Sci. 9:753325. doi: 10.3389/fmars.2022.753325
Received: 04 August 2021; Accepted: 06 April 2022;
Published: 19 May 2022.
Edited by:
Irene D. Alabia, Hokkaido University, JapanReviewed by:
Wei-Chuan Chiang, Council of Agriculture, TaiwanCopyright © 2022 Carter, Gilchrist, Dexter, Gardner, Gough, Rocliffe and Wilson. This is an open-access article distributed under the terms of the Creative Commons Attribution License (CC BY). The use, distribution or reproduction in other forums is permitted, provided the original author(s) and the copyright owner(s) are credited and that the original publication in this journal is cited, in accordance with accepted academic practice. No use, distribution or reproduction is permitted which does not comply with these terms.
*Correspondence: Amber L. Carter, YW1iZXIuY2FydGVyQGVkLmFjLnVr
Disclaimer: All claims expressed in this article are solely those of the authors and do not necessarily represent those of their affiliated organizations, or those of the publisher, the editors and the reviewers. Any product that may be evaluated in this article or claim that may be made by its manufacturer is not guaranteed or endorsed by the publisher.
Research integrity at Frontiers
Learn more about the work of our research integrity team to safeguard the quality of each article we publish.