- Guangdong Provincial Key Laboratory of Aquatic Product Processing and Safety, Guangdong Provincial Engineering Technology Research Center of Seafood, Guangdong Province Engineering Laboratory for Marine Biological Products, Guangdong Provincial Modern Agricultural Science and Technology Innovation Center for Subtropical Fruit and Vegetable Processing, College of Food Science and Technology, Guangdong Ocean University, Zhanjiang, China
Introduction: Sargassum fusiform is considered a traditional Chinese medicinal herb to treat many diseases, Sargassum fusiform polysaccharides (SFPS) were found to display many important biological activities. The purpose of this study was to investigate the in vitro antioxidant activity of crude polysaccharide from Sargassum fusiforme and its protective effect against H2O2-induced injury in LO2 cells.
Methods: SFPS was extracted from Sargassum fusiforme using water extraction and alcohol precipitation. High-performance liquid chromatography (HPLC) was used to measure its monosaccharide composition. The in vitro antioxidant activity of SFPS was evaluated using scavenging 1,1-diphenyl-2-picrylhydrazyl (DPPH) and hydroxyl radical assays. Moreover, its hepatoprotective activity was examined by establishing H2O2-induced LO2 cells injury model.
Results and discussion: The results showed that the SFPS was composed of Glc, Ara, Fuc, Gal, Man, Rha and Xyl. Meanwhile, SFPS exhibited strong antioxidant activity and potential hepatoprotective effect. Further studies found that SFPS could alleviate LO2 cells injury induced by H2O2 via decreasing malondialdehyde (MDA) and lactate dehydrogenase (LDH) levels, increasing superoxide dismutase (SOD) and catalase (CAT) activities and inhibiting intracellular reactive oxygen species (ROS) production. These results revealed that SFPS had the ability to protect the liver cells from H2O2-induced liver injury through its antioxidant activity.
1 Introduction
Currently, liver disease has become a global health problem that leads to death accounting for approximately 2 million worldwide each year (Asrani et al., 2019; Neshat et al., 2021). The liver is the essential organ of people and plays a vital role in metabolism, detoxification, and protein production (Cheng et al., 2021). However, liver homeostasis can be disrupted by environmental pollutants, heavy metals, viral infections, drug, alcohol-induced liver injury and autoimmunity. Many studies have shown that liver disease is closely related to oxidative stress. ROS is usually produced as a by-product of oxidative stress, causing apoptosis and damage to lipids, proteins and DNA. Studies have shown that the antioxidant pathway can protect the body from excessive ROS damage. Therefore, inhibition of free radical-induced injury may provide a feasible way to prevent liver disease (Borrelli et al., 2018; Chen et al., 2020; Li et al., 2020).
In recent years, natural food products with elevated antioxidant activity and other health-promoting effects have gained increasing attention for the treatment of liver of diseases. Seaweed-derived polysaccharides have been found with potential in treatment or prevention of liver diseases (Bocanegra et al., 2006; Tsai et al., 2021; Zhao et al., 2021). Sargassum fusiforme is a kind of the seaweed and is mainly distributed in the northwest the of Pacific Ocean. Dietary intake of Sargassum fusiforme was found to be associated with the treatment of several diseases, including neural diseases (Bogie et al., 2019), endocrine diseases (Wu et al., 2022) and gastrointestinal diseases (Zhang et al., 2021), etc. In addition, increasing evidence showed that sargassum fusiforme possessed various bioactivities, such as antitumor activity (Dai et al., 2019), antioxidant activity (An et al., 2022) and immunoenhancement activity (Chen et al., 2017). Meanwhile, sargassum fusiforme could delay the ageing (Zhang et al., 2019). Overall, SFPS exhibits strong antioxidant capacity such as free radical scavenging activity, suggesting that SFPS may have potential therapeutic effects on oxidation-related diseases.
Exogenous H2O2 can cause oxidative stress and induce apoptosis. Increasing evidence has proved that inducing human hepatocyte (LO2) injury has been widely used as a mature model for testing the biological activity of hepatocyte protective compounds in vitro (Fang et al., 2019; Yuan et al., 2020; Wang et al., 2021). However, there are limited reports on the antioxidant effect of SFPS based on the cultured cell model system, especially the comprehensive report on the regulatory effect of SFPS mediated antioxidant pathway on H2O2-induced liver cell injury have not been well investigated (Chen et al., 2016). The protective effect of SFPS on H2O2-induced damage in LO2 cells were demonstrated in this study. Specifically, the antioxidation activity of SFPS as well as its effect on cell viability and apoptosis in H2O2-treated LO2 cells were investigated. And the ROS, malondialdehyde (MDA), lactate dehydrogenase (LDH), superoxide dismutase (SOD) and catalase (CAT) were evaluated. In this experiment, SFPS was used for the first time to screen the in vitro activity of H2O2-induced LO2 cytoprotective effect, providing a theoretical basis for the study of its hepatoprotective effect.
2 Materials and methods
2.1 Chemicals and materials
The LO2 cells used in this study were purchased from Fuheng Biological Company. DEME culture medium, fetal bovine serum (FBS), trypsin digesting solution, phosphate buffer (PBS), penicillin, streptomycin, and dimethyl sulfoxide (DMSO) were all purchased from GIBCO Company. The cell apoptosis kit by ANNEXINV-FITC/PI was obtained from Yeasen Biology Company. MDA, CAT, SOD, and ROS activity test kits were purchased from the Nanjing Institute of Biological Engineering.
Dried sargassum fusiforme was purchased from Xiapu county (Fujian Province). All the raw materials were kept in a dry and dark state.
2.2 SFPS preparation
SFPS was extracted by the hot water extraction method (Ji and You, 2017). The dried leaves of sargassum fusiforme was crushed into powder. The sargassum fusiforme power was pre-extracted by reflux with 95% ethanol to remove pigments. After that, the residue was extracted with boiling water (1:50, v/v) for 4 h and then cooled to room temperature. The water-extracts were filtered, concentrated, and precipitated with 4 vol. of 95% ethanol at 4°C for overnight. After centrifugation, the precipitate was deprotonated by Sevag method (Sevag et al., 1938). Ultimately, the deproteinated supernatant was lyophilized to obtain SFPS.
2.3 General analysis of SFPS
The total carbohydrate content in the SFPS was quantified using the phenol-sulfuric method with fucoidan as the standard (DuBois et al., 2002). The protein contents of SFPS fractions were quantified according to the Bradford method (Bradford, 1976) using bovine serum albumin as the standard. The sulfate content was quantified by Barium sulfate turbidimetry method (Dodgson and Price, 1962).
The monosaccharide composition was analyzed by PMP-HPLC analysis using Agilent 1200 system. Briefly, SFPS was hydrolyzed with 2 M TFA (1mL) at 120 °C for 6 h. After removal of TFA by rotary evaporation, the full hydrolysate was reacted with 500 μL 2 M PMP in methanol and 400 μL aqueous sodium hydroxide (0.3 M) at 70 °C for 1 h. The reaction products were dried and then extracted three times after addition of 0.2 mL of hydrochloric acid (0.3 M), water and chloroform, and filtered for HPLC analysis. The monosaccharide mixture solution was obtained by the same method as above. The mixtures were separated on an ZORBAX Eclipse XDB-C18 (5 μm, 4.6 i.d.× 250 mm) and eluted with PBS: Acetonitrile = 83:17 (%:%) at a flow rate of 1 mL/min.
2.4 UV and IR spectrum analysis
The experimental UV spectrum of polysaccharide fractions (1 mg/mL) were recorded in the region of 400–200 nm using UV–Vis spectrophotometer (TU-1901 Two-beam ultraviolet photometer, China). The extracted polysaccharides (1 mg/mL) were mixed with KBr powder and pressed into disc at the mid, and FT-IR spectrum was recorded in the range 4000-500 cm−1 using a BRUKER TENSOR-27 Fourier infrared spectrometer (Germany).
2.5 Antioxidant activity assays for SFPS
2.5.1 Scavenging activity of DPPH radicals
The antioxidant activity was determined using DPPH as a free radical (Lim et al., 2001). Before measurement, 0.1 mol/L DPPH solution was prepared and dissolved in absolute ethanol and protected from light. Reaction mixture consisting of 0.5 mL SFPS solution and 2 mL DPPH solution was added to each test tube, and then added with 1.5 mL of ultrapure water. After shaken and left to stand for 30 min in the dark, the absorbance of the mixture was determined by spectrophotometer measurement at a wavelength of 517 nm. Vitamin C was used as a positive control. The inhibition percentage is calculated as follows:
where D0 is the absorbance of the blank solution, D1 is the absorbance of the sample group by mixing test sample with DPPH solution and D2 is the absorbance of the solvent control group by mixing test sample without DPPH solution.
2.5.2 Scavenging activity of hydroxyl radical (•OH)
The hydroxyl radical scavenging activity of SFPS was determined with a slight modification of the standard method. The absorbance of SFPS was measured at 510 nm after adding the solution of SFPS with different concentrations into the mixture of 9 mM iron2+ sulfates (anhydrous) and 9 mM salicylic acid and 8 mM hydrogen peroxide at 37°C for 1 hour. In the control, absolute ethanol was used instead of salicylic acid ethanol solution, and in the blank, deionized water was used instead of polysaccharide sample solution. VC was used as a positive control. The removal rate is calculated as follows:
D0 is the absorbance of the blank control, D1 is the absorbance value of the experimental group and D2 is the absorbance value of the control group.
2.6 Hepatoprotective activity assays for SFPS
2.6.1 Cell culture
LO2 cells were grown in DMEM supplemented with 100 U/mL penicillin,10% fetal bovine serum and 100 U/mL streptomycin at 37 °C in 5% CO2. Subculture was carried out when the degree of cell confluence reached to 80%.
2.6.2 Cell viability
The effect of SFPS on the H2O2-induced cytotoxicity was determined, and LO2 cells were plated in 96-well plates at a density of 8×103 cells/mL. The cells were treated with different concentrations of SFPS (0, 0.3, 0.6, 1.25, 2.5 μg/mL) for 12 hours. Then, each well was added with 700 μM H2O2 and cultivated the cells for another 3 hours. MTT assay was utilized to examine cell viability.
2.6.3 Determination of LDH, CAT, SOD activity and MDA level
The assay kits were used to measure the antioxidant index. In brief, the LO2 cells were seeded in each 6-well plate with a density of 1×105 cells/mL. Then cells were cultivated for 24 h. Next, the cells were treated with SFPS for 12 h before the treatment with H2O2 for 3 h. After cells were lysed, the cells supernatant was collected. According to the manufacturer’s instructions, LDH, CAT, SOD and MDA were detected with the corresponding assay kits provided by the Nanjing Jiancheng Bioengineering Institute.
2.6.4 Measurement of ROS production
A cell-permeable probe, DCFH-DA, was used to evaluate the effect of SFPS on ROS production by LO2 cells (Jiao and Zhao, 2002). LO2 cells were prepared into a cell suspension and incubated into a 6-well plate with 1×105 cells/mL. Cells were cultured for 24 h at 37°C in 5% CO2 medium. LO2 cells were exposed to polysaccharides (0.3, 0.6, 1.25, 2.5 μg/mL) for 12 h followed by H2O2 (700 μM) for another 3 h. Next, cells were co-cultured with DCFH-DA for 0.5 h at 37°C in darkness and then washed twice with PBS. The fluorescence production of DCF was observed by a microplate reader (Varioskan Flash, Thermo Scientific, USA).
2.6.5 Cell apoptosis detection
To further assess the protection of SFPS against H2O2-induced cell apoptosis, the annexin V/PI double staining was assessed by Annexin V-FITC/PI apoptosis detection kit (Wang et al., 2017). In brief, LO2 cells were digested with trypsin, centrifuged and collected, and stained with Annexin V-FITC and PI. The cells were determined by commercially available kits according to the manufacturer’s instructions and detected by the flow cytometry (Cytof, Beckman Coulter, USA).
2.7 Statistical analysis
SPSS 20.0 statistical software was used to examine the difference between groups using a t-test.
3 Results
3.1 The physicochemical properties and structural features of SFPS
Firstly, SFPS was prepared by water extraction and alcohol precipitation with a yield of 15.47%. Then, its general composition was measured, and the results were shown in Table 1. The prepared SFPS contained 50.46 ± 0.44% total sugar, 31.30 ± 0.48% sulfate and 1.83 ± 0.04% protein. Further analysis of monosaccharide composition of the SFPS was performed by PMP-HPLC. SFPS were predominantly composed of glucose (Glc), arabinose(Ara), fucose(Fuc), galactose (Gal), mannose (Man), rhamnose (Rha), and xylose (Xyl) with a ratio of 21.06:15.44: 13.33:12.85:12.50:12.43:12.40, indicating that SFPS was a heteropolysaccharide. Moreover, glucose and arabinose were the major monosaccharide of SFPS.
Purity of SFPS was detected by UV spectrophotometer. There was no absorbance at 260 and 280 nm, indicating that the SFPS did not contain nucleic acid and protein (Figure 1A). The results were basically consistent with the chemical composition analysis. However, the protein concentration in SFPS was determined to be 1.88%, which might be attributed to the presence of glycoproteins. The FT-IR spectra of SFPS was shown in Figure 1B. Typical absorption peaks of SFPS was concentrated between 500 cm-1 and 4000 cm-1. The absorption peaks presented at 3248 cm-1, 2758 cm-1 and 1423 cm-1 are O-H stretching vibrations, C-H stretching vibrations, and C-O stretching vibrations within or between the polysaccharide structures. The characteristic peaks between 950-1200 cm-1 correspond to characteristic functional groups on the glycosidic ring, overlapping with the stretching vibration of the C-OH side group and the C-O-C glycosidic band vibration (Chen et al., 2011). Besides, the absorption peak near 845 cm−1 was attributed to the stretching vibration of symmetrical C-O-S (Lajili et al., 2019). The absorption peaks of 1053 cm-1 and 1170 cm-1 indicated that the sugar was a pyranose (Zhao et al., 2005). The characteristic peaks between 840 cm-1 indicated that the polysaccharide was α-pyranose.
3.2 Antioxidant activities of SFPS
The DPPH scavenging activities and hydroxyl radical scavenging activities of SFPS was determined to evaluate its antioxidant property. Different concentrations of SFPS had strong DPPH scavenging efficiency, and the DPPH scavenging rate increased by polysaccharide concentration in a certain concentration range (Figure 2A). Specifically, the DPPH scavenging rate increased smoothly within the polysaccharide concentration between 0-2.0 mg/mL, and a rapid increase of DPPH scavenging rate occurred at the polysaccharide concentration of 2.5 mg/mL, which reached to 80.49 ± 0.21%. Meanwhile, the scavenging effect of SFPS on hydroxyl radicals was obvious at different concentrations (Figure 2B). Within a certain concentration range, the scavenging effect gradually increased by polysaccharide concentration. Specifically, the scavenging effect of SFPS on hydroxyl radical increased rapidly within the polysaccharide concentration between 0.5-1.0mg/mL. After that, the hydroxyl radical scavenging activity grew slowly, and the highest scavenging rate was 67.15 ± 0.50% with SFPS concentration of 2.5 mg/mL. Although the scavenging activity of SFPS was lower than VC, SFPS could dose-dependently scavenge the DPPH and hydroxyl free radical, indicating that SFPS possessed certain antioxidant activity.
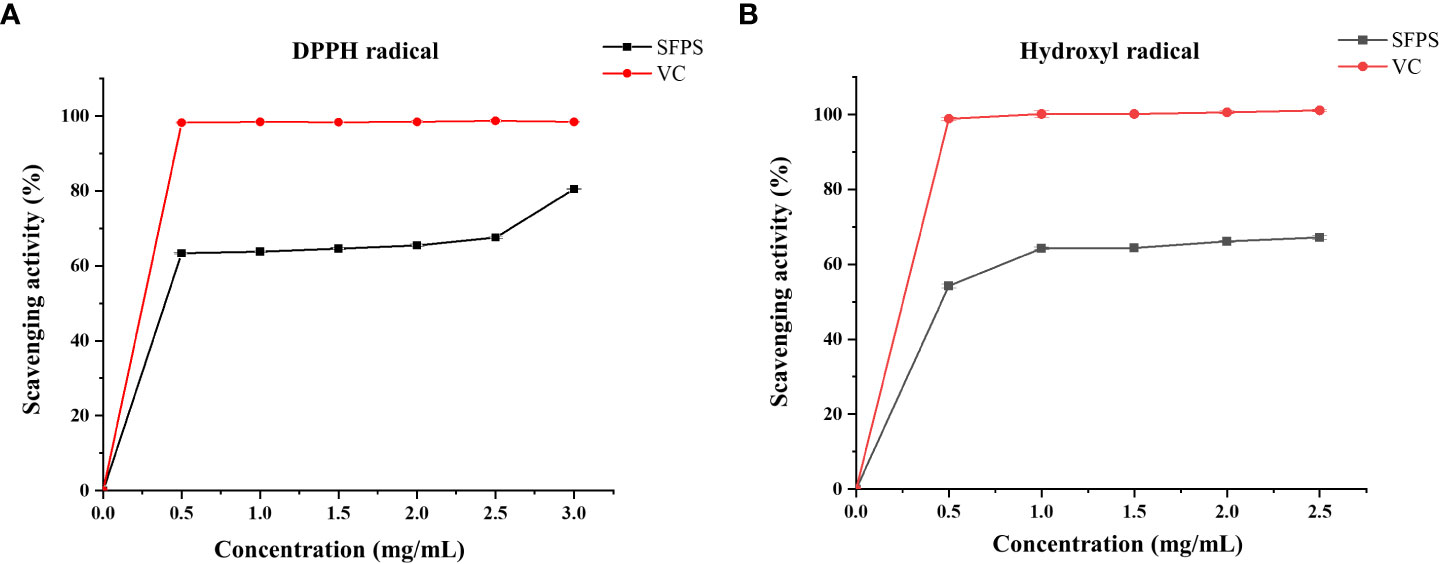
Figure 2 The antioxidant activities of SFPS. (A) DPPH scavenging activity (B) Hydroxyl radical scavenging activity.
3.3 Protective effect of SFPS on H2O2-injured LO2
Our previous research has established a model and found that the best damage model can be obtained by treating at 700 μM H2O2 concentration for 3 h (Chen et al., 2022). Thus, subsequent experiments were conducted based on this model. As shown in Figure 3, compared with the H2O2 group, SFPS could markedly improve the cell viability in a dose-dependent manner. Specifically, the cell viability in SFPS treatment at 0.3, 0.6, 1.25 and 2.5 μg/mL were 56.84 ± 2.92%, 68.40 ± 5.43%, 71.60 ± 1.32% and 74.57 ± 4.31%, respectively. It showed that SFPS might inhibit oxidative stress that induced by H2O2 in LO2 cells.
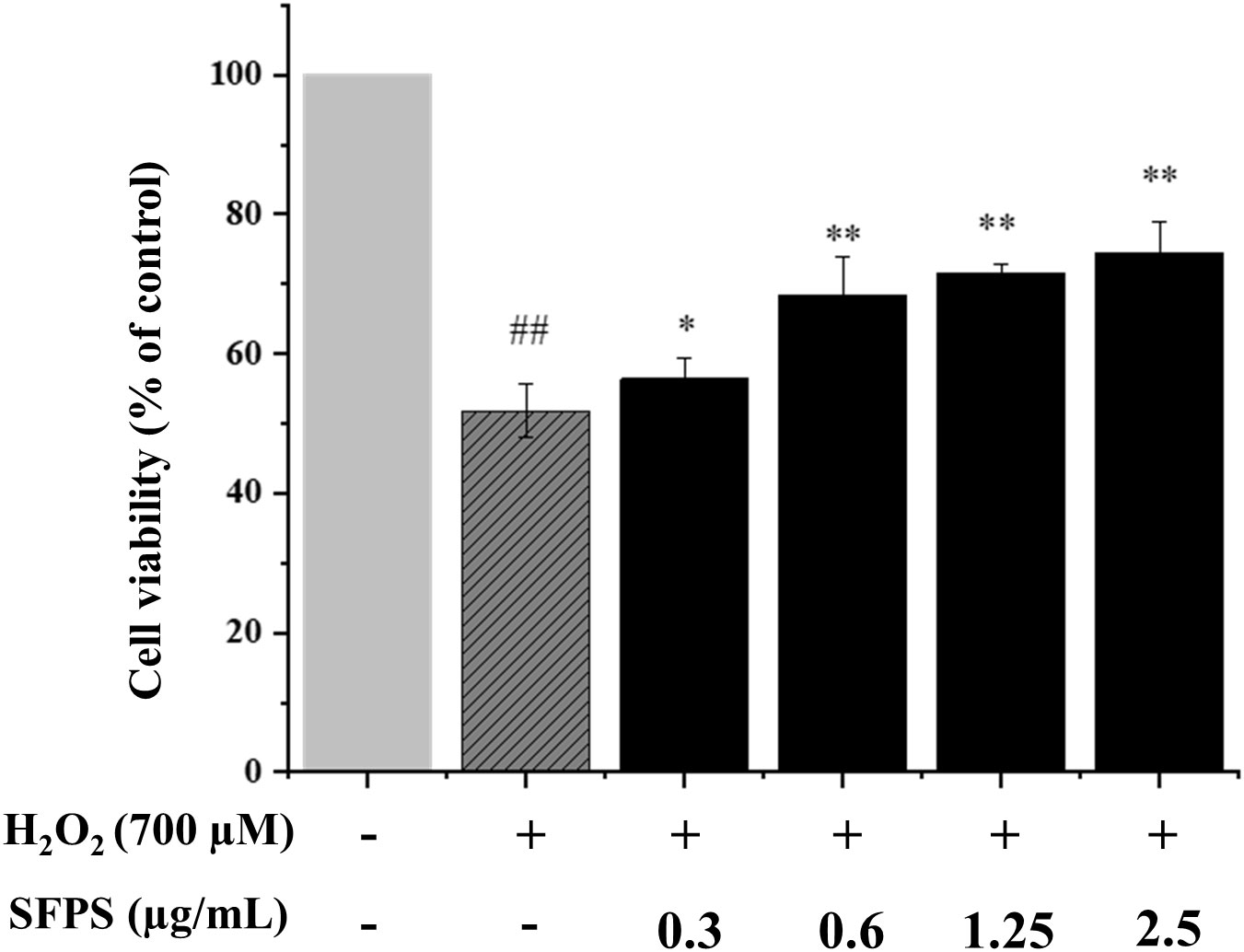
Figure 3 Protective effects of SFPS on H2O2-injured LO2 cells. LO2 cells were precultured with 0.3 to 2.5µg/ml SFPS for 12 h and then treated with 700µM H2O2 for 3 h. Cell viability was measured by MTT assay. p< 0.01 (**) or p< 0.05 (*) indicates that the columns of H2O2 group and SFPS groups are significantly different. p< 0.01 (##) indicates significant difference between the control group and the H2O2 group.
3.4 Effect of SFPS on CAT, SOD, MDA and LDH levels
In H2O2 treatment group, CAT activity and SOD levels were 3.23 ± 0.28 U/mL and 1.25 ± 0.02 U/mL, which were significantly decreased compared with the control group. However, CAT activity and SOD levels were significantly higher in SFPS pretreatment group than the H2O2-treatment group, and the two indicators were increased by SFPS concentration (p<0.05). Specifically, CAT activity and SOD levels were 5.42 ± 0.03 U/mL, 5.99 ± 0.02 U/mL, 7.24 ± 0.03 U/mL, 7.98 ± 0.09 U/mL (Figure 4A), 1.29 ± 0.02 U/mL, 1.40 ± 0.03 U/mL, 1.44 ± 0.06 U/mL and 1.62 ± 0.06 U/mL at SFPS of 0.3, 0.6, 1.25 and 2.5 μg/mL (Figure 4B).
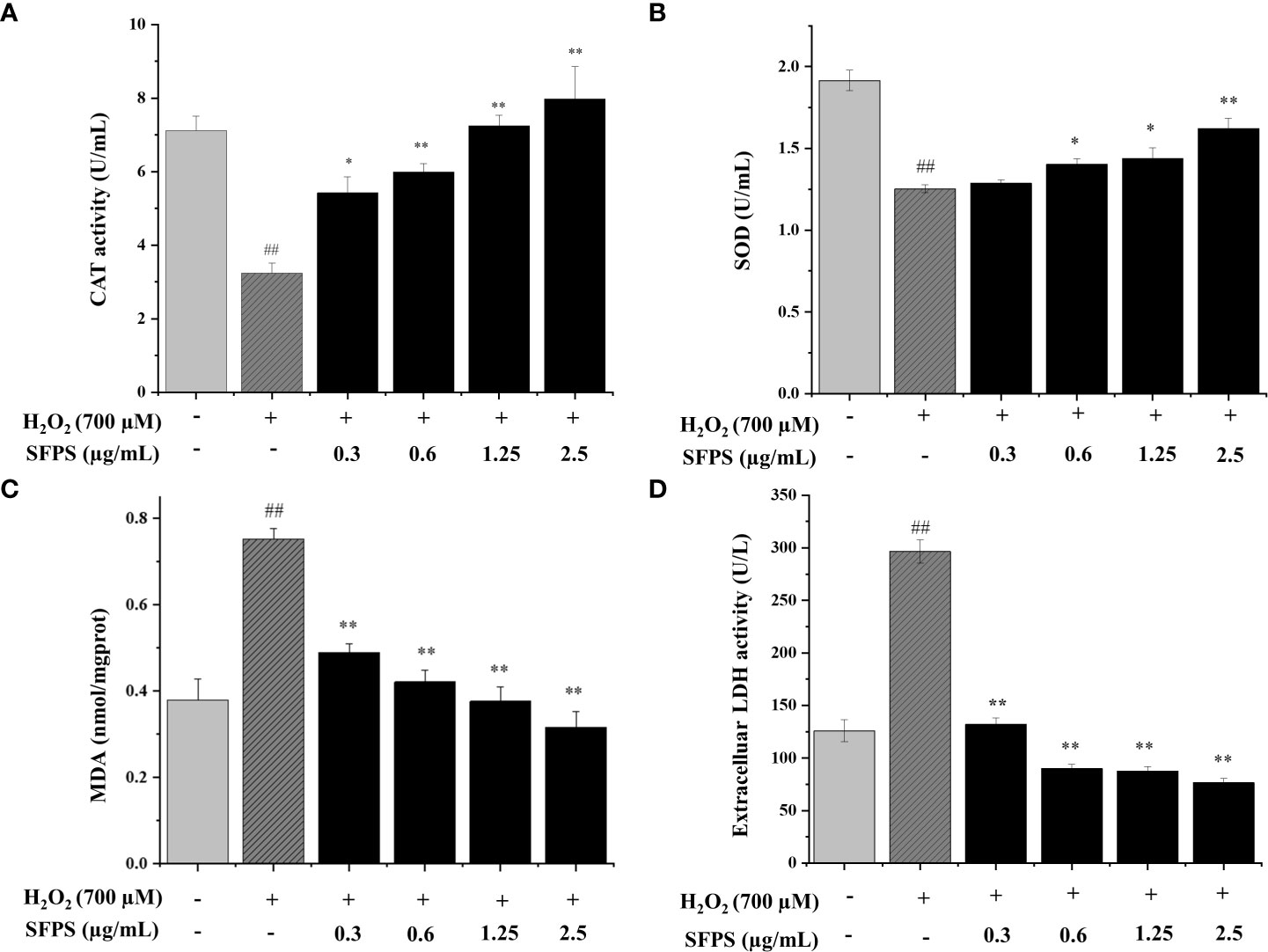
Figure 4 Effects of SFPS on CAT (A), SOD (B), MDA (C) and LDH leakage (D) in H2O2-treated LO2 cells. p< 0.01 (**) or p< 0.05 (*) means that columns between H2O2 group and SFPS groups are significantly different. p< 0.01 (##) means that columns between the control group and the H2O2 group are significantly different.
The MDA level in H2O2 group was the highest, indicating that H2O2 successfully induced lipid peroxidation (Figure 4C). Meanwhile, the intracellular MDA levels in SFPS groups were significantly lower than H2O2 group, suggesting that the addition of SFPS greatly declined oxidative injuries in LO2 cells. At the concentration of 0.3, 0.6, 1.25, 2.5 μg/mL, the intracellular MDA levels were dramatically decreased to 0.49 ± 0.02, 0.42 ± 0.02, 0.38 ± 0.03, 0.32 ± 0.04 mmol/mgprot, respectively.
The LDH levels in cultured supernatant were determined to analyze the effect of SFPS on the extent of oxidative injury in LO2 cells. As shown in Figure 4D, LDH levels were significantly increased in H2O2-treatment group. Pretreatment with SFPS decreased LDH level in dose-dependent manner. Notably, LDH were only 76.68± 3.76 U/mL with the pretreatment of SFPS at 2.5 μg/mL, which was much better than the H2O2 treatment group (296.48± 11.13 U/mL). These above results suggested that SFPS could reverse the reduction of antioxidant enzyme (SOD and CAT) levels and the increase in lipid peroxidation (MDA) and LDH levels in the H2O2 treatment group.
3.5 Influence of SFPS on H2O2-induced LO2 cell apoptosis
The effect of SFPS on cell apoptosis in H2O2-treated LO2 was measured by detection of Annexin V/PI double staining and PI staining. The results were shown in Figure 5. H2O2 caused significant apoptosis in LO2 cells. The apoptosis rate increased from 15.58 ± 1.77% to 52.22 ± 0.68% when cells were incubated with H2O2 (p<0.01). However, treatment with SFPS at different concentrations could attenuate the apoptosis significantly, and cell apoptosis decreased slightly with the increasing concentration of SFPS. These results indicated that SFPS inhibited apoptosis and cell death.
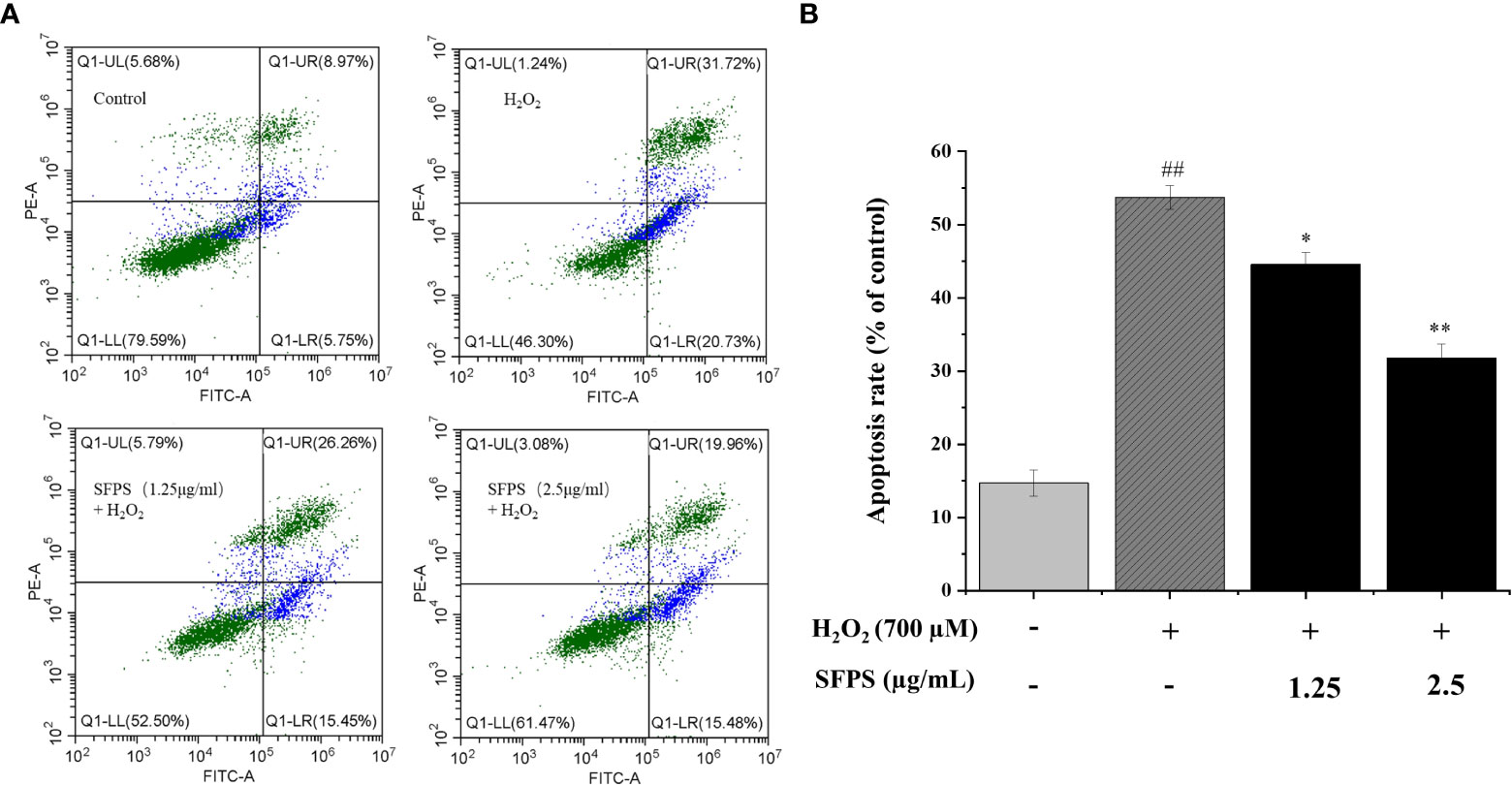
Figure 5 Influence of SFPS on H2O2-induced LO2 cell apoptosis. (A) LO2 cells were preincubated with SFPS (1.25 and 5 µg/mL) for 12 h before the treatment with H2O2 for 3 h. Then, LO2 cells were collected and co-stained with Annexin V and PI for 10 min. The upper right quadrants and lower right quadrants represent the ratios of late apoptotic cells early apoptotic cells, respectively. (B) Effect of SFPS on the apoptosis rate of H2O2-treated LO2 cells. p< 0.01 (**) or p< 0.05 (*) means that columns between H2O2 group and SFPS groups are significantly different. p< 0.01 (##) means that columns between the control group and the H2O2 group are significantly different.
3.6 Effect of SFPS on ROS production induced by H2O2
ROS generation was monitored by the measurement of fluorescence intensity to assess the mechanisms of SFPS for decreasing H2O2-induced cytotoxicity and apoptosis. The results were shown in Figure 6. Compared to the blank control group, the green fluorescence in LO2 cells treated with hydrogen peroxide was significantly enhanced, which the relative amount of ROS in the damaged group was 298.52± 5.18% (p<0.01). Treatment of cells with SFPS dramatically downregulated the levels of H2O2-induced ROS formation in LO2 cells. Specifically, the relative amount of ROS in the SFPS-treated group declined in a concentration-dependent manner, which were 159.58 ± 11.31%, 138.72 ± 6.90%, 115.47 ± 6.27%, and 100.38 ± 7.62%, respectively. These results indicate that SFPS exerts protective effects against oxidative stress induced by H2O2 in cells.
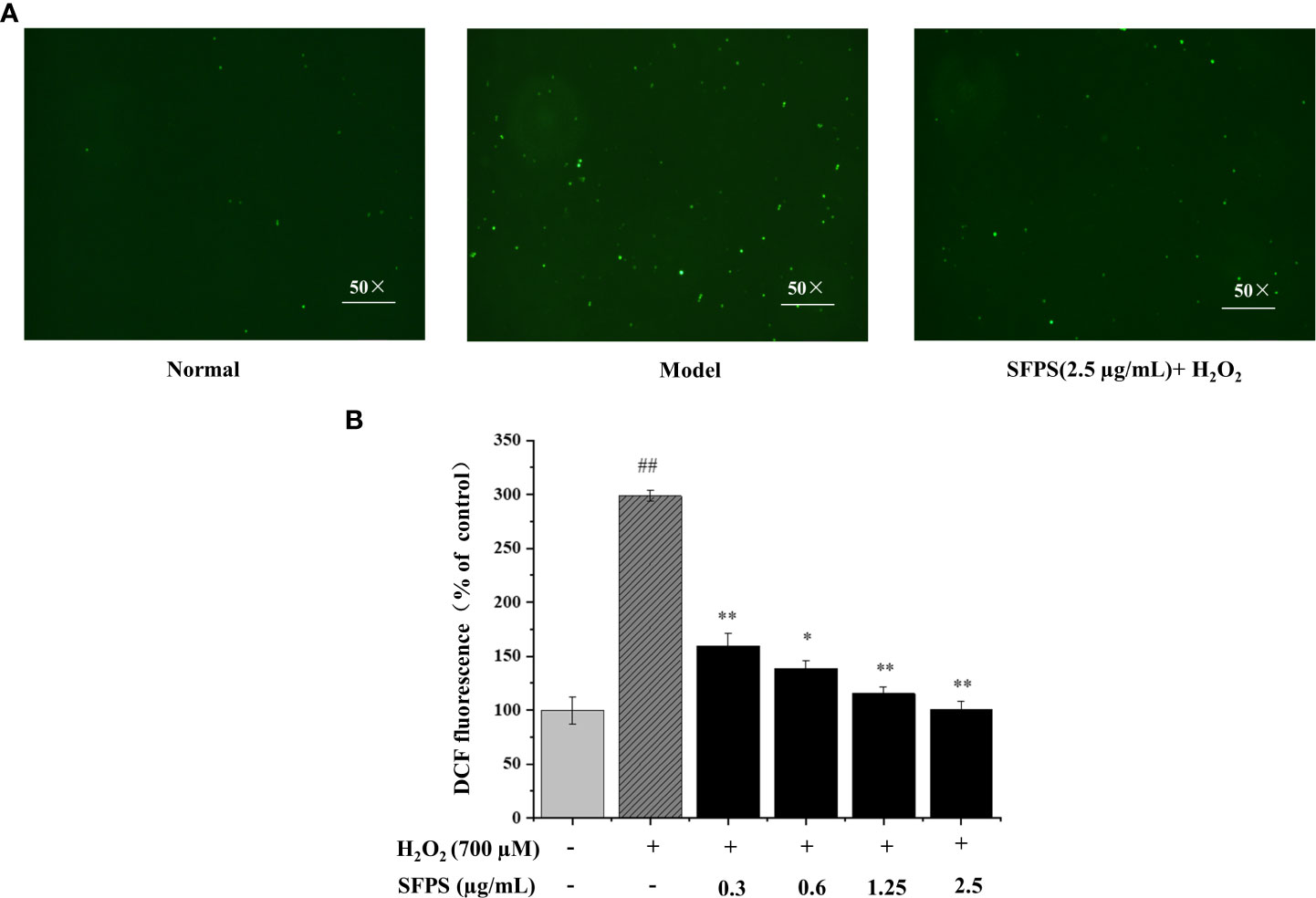
Figure 6 Effect of SFPS pretreatment on intracellular reactive oxygen species (ROS) in H2O2-induced LO2 cells. (A) The fluorescence intensity of DCF image of cells after treatments as examined by fluorescence microscopy (magnification 50×). LO2 cells were incubated with 2.5 µg/mL SFPS for 12 h and then treated with 700 µM H2O2 for 3 h. (B) Effect of SFPS on DCF fluorescent density of H2O2-treated LO2 cells. DCF fluorescent density was observed by the ROS assay kit. p< 0.01 (**) or p< 0.05 (*) indicates that the columns of H2O2 group and SFPS groups are significantly different. p< 0.01 (##) indicates significant difference between the control group and the H2O2 group.
4 Discussion
Sargassum fusiform, a kind of edible brown algae, has strong pharmacological and biological activities. Sargassum fusiform has been used as a therapeutic medicine 4000 years ago (Huang et al., 2006). Polysaccharides are the main constituents of Sargassum fusiform, and Sargassum fusiform polysaccharides has been shown to possess the effect of promoting hepatocyte activity in vitro (Chen et al., 2016). In this study, the polysaccharide SFPS was successfully isolated from Sargassum fusiform by hot water extraction, ethanol precipitation and Sevage deproteinization. Then, the content of carbohydrate, sulfate and protein in SFPS, the structure of SFPS as well as its antioxidant activity were determined. The chemical composition analysis showed that SFPS was mainly composed of fucose with some sulfate heterosaccharides. Further, the analysis of infrared spectroscopy indicated that SFPS was sulfate polysaccharides. The sulfate content of SFPS was higher than that of most of the published raw polysaccharides of Sargassum fusiform (Meinita et al., 2021). Various studies have shown that sulfated polysaccharides exhibit different or stronger biological activities, such as antioxidant (Kim et al., 2016). Among them, the antioxidant activity of sulfate polysaccharide is positively correlated with the number of sulfate groups. Moreover, fucose and galactose have been reported to associate with antioxidant activity (Wang L. et al., 2018). In this study, we also found that the strong antioxidant activity of SFPS could be attributed to their high sulfation content and higher amount of fucose and galactose, which was consistent with the previous report (Seedevi et al., 2017; Jridi et al., 2019).
The antioxidant activity of polysaccharide is often characterized by cell damage model in vitro. The method of H2O2 had been widely used to establish oxidative stress model in vitro. Oxidative stress has been linked to many diseases (Khatri et al., 2018; Daenen et al., 2019; Sadasivam et al., 2022). The liver is the most important organ in the metabolism of xenobiotic, which is particularly vulnerable to oxidative stress affects. Many studies have shown that antioxidant therapy is effective in treating these diseases. Antioxidants of natural origin have the advantage of non-toxicity and good biocompatibility to delay or reduce substrate oxidation and protect the body from oxidative damage caused by excess free radicals (Zhu et al., 2020). Maintaining an appropriate ROS level is necessary for normal physiological function. Activation of reactive oxygen species (ROS) leads to lipid peroxidation of cell membranes, and the products of peroxidation bind to biomolecules, leading to disease production (Yuan et al., 2015). CAT and SOD are the professional enzymes dedicated to preventing enzymes dedicated to preventing the build-up of intracellular H2O2 (Wang Y. et al., 2018; Raza et al., 2021). Therefore, the enhancement of CAT and SOD activity indicates the enhancement of ROS scavenging activity. LDH enzyme is a typical marker of the plasma damage membrane. MDA is a biomarker of oxidative (Chen et al., 2016). Our current study showed that SFPS could attenuate H2O2-induced oxidative stress in LO2 cells. The data showed that SFPS pretreatment significantly increased cell viability as measured by the MTT assay. SFPS pretreatment reduced MDA and LDH levels and increased SOD and CAT enzyme activities. In addition, SFPS pretreatment also significantly inhibited the production of reactive oxygen species.
There is growing evidence that cellular stress and cell death triggered by different causes underlie most acute and chronic liver diseases. Currently, the phenotypic form of cell death in liver disease is apoptosis20. In the present study, the apoptosis of LO2 cells was significantly reduced after SFPS treatment, and the effect of the SFPS-treated group was better than that of the H2O2-treated group, indicating that SFPS could effectively protect against H2O2-induced oxidative damage of LO2. These results suggest that SFPS can reduce ROS production and attenuate oxidative stress-induced LO2 cell injury and apoptosis, thereby protecting LO2 from hydrogen peroxide oxidative stress damage.
However, the relationship between the bioactivity of SFPS and their structure remains unclear. The effectiveness and mechanism of SFPS in vivo need to be further verified. In addition, it is important to explore the possible synergistic mechanism between the polysaccharide and other components. To better utilize this kind of resource, further work on purification and structure analysis of SFPS is necessary in the future research.
5 Conclusion
In this study, the in vitro antioxidant activity of SFPS and its vitro protective activity against LO2 injury in human hepatocytes were investigated. Our results showed that SFPS not only exhibited strong antioxidant activity in vitro, but also improved the viability of oxidatively damaged cells. Moreover, SFPS protected cells from H2O2-induced oxidative stress injury by attenuating the production and accumulation of ROS, reducing MDA levels and intracellular LDH leakage, increasing the activity of antioxidant enzymes such as SOD and CAT, and reducing apoptosis. In conclusion, SFPS possesses well antioxidant ability and exhibits protective effects against H2O2-induced oxidative stress in LO2 cells.
Data availability statement
The original contributions presented in the study are included in the article/supplementary material. Further inquiries can be directed to the corresponding authors.
Author contributions
JL and ZW did field observations and proposed the idea for this study. JL, ZW, SZ, and JC designed the research. SZ and JC acquired the funding. JL performed the research, analyzed the data, and prepared the original manuscript. ZW, JC, BL, XC, RL, JG, XL, BS, and SZ provided logistical support, and revised the manuscript. All authors contributed to the article and approved the submitted version.
Funding
This work was funded by the Guangdong Basic and Applied Basic Research Foundation (2020A1515010860 and 2021A1515012455), Guangdong Ocean University Innovation Program (230419100), Nanhai Youth Scholar Project of Guangdong Ocean University (002029002009), the Project of Science and Technology of Zhanjiang City (2020A01034 and 2022A01045), the Scientific Research Foundation of Guangdong Ocean University (no. R17034), and the Innovative Team Program of High Education of Guangdong Province (2021KCXTD021), Excellent Graduate Thesis Cultivation Program (040505042203 and 040505042204).
Conflict of interest
The authors declare that the research was conducted in the absence of any commercial or financial relationships that could be construed as a potential conflict of interest.
Publisher’s note
All claims expressed in this article are solely those of the authors and do not necessarily represent those of their affiliated organizations, or those of the publisher, the editors and the reviewers. Any product that may be evaluated in this article, or claim that may be made by its manufacturer, is not guaranteed or endorsed by the publisher.
References
An Y., Liu H., Li X., Liu J., Chen L., Jin X., et al. (2022). Carboxymethylation modification, characterization, antioxidant activity and anti-UVC ability of sargassum fusiforme polysaccharide. Carbohydr. Res. 515, 108555. doi: 10.1016/j.carres.2022.108555
Asrani S. K., Devarbhavi H., Eaton J., Kamath P. S. (2019). Burden of liver diseases in the world. J. Hepatol. 70, 151–171. doi: 10.1016/j.jhep.2018.09.014
Bocanegra A., Benedí J., Sánchez-Muniz F. J. (2006). Differential effects of konbu and nori seaweed dietary supplementation on liver glutathione status in normo- and hypercholesterolaemic growing rats. Br. J. Nutr. 95, 696–702. doi: 10.1079/bjn20051682
Bogie J., Hoeks C., Schepers M., Tiane A., Cuypers A., Leijten F., et al. (2019). Dietary sargassum fusiforme improves memory and reduces amyloid plaque load in an alzheimer’s disease mouse model. Sci. Rep. 9, 4908. doi: 10.1038/s41598-019-41399-4
Borrelli A., Bonelli P., Tuccillo F. M., Goldfine I. D., Evans J. L., Buonaguro F. M., et al. (2018). Role of gut microbiota and oxidative stress in the progression of non-alcoholic fatty liver disease to hepatocarcinoma: Current and innovative therapeutic approaches. Redox Biol. 15, 467–479. doi: 10.1016/j.redox.2018.01.009
Bradford M. M. (1976). A rapid and sensitive method for the quantitation of microgram quantities of protein utilizing the principle of protein-dye binding. Anal. Biochem. 72, 248–254. doi: 10.1016/0003-2697(76)90527-3
Cheng M. L., Nakib D., Perciani C. T., MacParland S. A. (2021). The immune niche of the liver. Clin. Sci. Lond. Engl. 135, 2445–2466. doi: 10.1042/CS20190654
Chen P., He D., Zhang Y., Yang S., Chen L., Wang S., et al. (2016). Sargassum fusiforme polysaccharides activate antioxidant defense by promoting Nrf2-dependent cytoprotection and ameliorate stress insult during aging. Food Funct. 7, 4576–4588. doi: 10.1039/C6FO00628K
Chen X., Jin J., Tang J., Wang Z., Wang J., Jin L., et al. (2011). Extraction, purification, characterization and hypoglycemic activity of a polysaccharide isolated from the root of ophiopogon japonicus. Carbohydr. Polym. 83, 749–754. doi: 10.1016/j.carbpol.2010.08.050
Chen J., Li J., Fan T., Zhong S., Qin X., Li R., et al. (2022). Protective effects of curcumin/cyclodextrin polymer inclusion complex against hydrogen peroxide-induced LO2 cells damage. Food Sci. Nutr. 10, 1649–1656. doi: 10.1002/fsn3.2787
Chen Z., Tian R., She Z., Cai J., Li H. (2020). Role of oxidative stress in the pathogenesis of nonalcoholic fatty liver disease. Free Radic. Biol. Med. 152, 116–141. doi: 10.1016/j.freeradbiomed.2020.02.025
Chen H., Zhang L., Long X., Li P., Chen S., Kuang W., et al. (2017). Sargassum fusiforme polysaccharides inhibit VEGF-a-related angiogenesis and proliferation of lung cancer in vitro and in vivo. biomed. Pharmacother. 85, 22–27. doi: 10.1016/j.biopha.2016.11.131
Daenen K., Andries A., Mekahli D., Van Schepdael A., Jouret F., Bammens B. (2019). Oxidative stress in chronic kidney disease. Pediatr. Nephrol. Berl. Ger. 34, 975–991. doi: 10.1007/s00467-018-4005-4
Dai Y.-L., Jiang Y.-F., Lee H. G., Jeon Y.-J., Kang M.-C. (2019). Characterization and screening of anti-tumor activity of fucoidan from acid-processed hijiki (Hizikia fusiforme). Int. J. Biol. Macromol. 139, 170–180. doi: 10.1016/j.ijbiomac.2019.07.119
Dodgson K., Price R. (1962). A note on the determination of the ester sulphate content of sulphated polysaccharides. Biochem. J. 84, 106–110. doi: 10.1042/bj0840106
DuBois M., Gilles K. A., Hamilton J. K., Rebers P. A., Smith F. (2002). Colorimetric method for determination of sugars and related substances. ACS Publ 28(350–356). doi: 10.1021/ac60111a017
Fang K., Wu F., Chen G., Dong H., Li J., Zhao Y., et al. (2019). Diosgenin ameliorates palmitic acid-induced lipid accumulation via AMPK/ACC/CPT-1A and SREBP-1c/FAS signaling pathways in LO2 cells. BMC Complement. Altern. Med. 19, 255. doi: 10.1186/s12906-019-2671-9
Huang X., Zhou H., Zhang H. (2006). The effect of sargassum fusiforme polysaccharide extracts on vibriosis resistance and immune activity of the shrimp, fenneropenaeus chinensis. Fish Shellfish Immunol. 20, 750–757. doi: 10.1016/j.fsi.2005.09.008
Jiao H., Zhao B. (2002). Cytotoxic effect of peroxisome proliferator fenofibrate on human HepG2 hepatoma cell line and relevant mechanisms. Toxicol. Appl. Pharmacol. 185, 172–179. doi: 10.1006/taap.2002.9538
Jridi M., Nasri R., Marzougui Z., Abdelhedi O., Hamdi M., Nasri M. (2019). Characterization and assessment of antioxidant and antibacterial activities of sulfated polysaccharides extracted from cuttlefish skin and muscle. Int. J. Biol. Macromol. 123, 1221–1228. doi: 10.1016/j.ijbiomac.2018.11.170
Khatri N., Thakur M., Pareek V., Kumar S., Sharma S., Datusalia A. K. (2018). Oxidative stress: Major threat in traumatic brain injury. CNS Neurol. Disord. Drug Targets 17, 689–695. doi: 10.2174/1871527317666180627120501
Kim S.-Y., Kim E.-A., Kim Y.-S., Yu S.-K., Choi C., Lee J.-S., et al. (2016). Protective effects of polysaccharides from psidium guajava leaves against oxidative stresses. Int. J. Biol. Macromol. 91, 804–811. doi: 10.1016/j.ijbiomac.2016.05.111
Lajili S., Ammar H. H., Mzoughi Z., Amor H. B. H., Muller C. D., Majdoub H., et al. (2019). Characterization of sulfated polysaccharide from laurencia obtusa and its apoptotic, gastroprotective and antioxidant activities. Int. J. Biol. Macromol. 126, 326–336. doi: 10.1016/j.ijbiomac.2018.12.089
Li S., Li H., Xu X., Saw P. E., Zhang L. (2020). Nanocarrier-mediated antioxidant delivery for liver diseases. Theranostics 10, 1262–1280. doi: 10.7150/thno.38834
Lim K.-T., Hu C., Kitts D. D. (2001). Antioxidant activity of a rhus verniciflua stokes ethanol extract. Food Chem. Toxicol. 39, 229–237. doi: 10.1016/S0278-6915(00)00135-6
Meinita M. D. N., Harwanto D., Sohn J.-H., Kim J.-S., Choi J.-S. (2021). Hizikia fusiformis: Pharmacological and nutritional properties. Foods 10, 1660. doi: 10.3390/foods10071660
Neshat S. Y., Quiroz V. M., Wang Y., Tamayo S., Doloff J. C. (2021). Liver disease: Induction, progression, immunological mechanisms, and therapeutic interventions. Int. J. Mol. Sci. 22, 6777. doi: 10.3390/ijms22136777
Raza A., Su W., Gao A., Mehmood S. S., Hussain M. A., Nie W., et al. (2021). Catalase (CAT) gene family in rapeseed (Brassica napus l.): Genome-wide analysis, identification, and expression pattern in response to multiple hormones and abiotic stress conditions. Int. J. Mol. Sci. 22, 4281. doi: 10.3390/ijms22084281
Sadasivam N., Kim Y.-J., Radhakrishnan K., Kim D.-K. (2022). Oxidative stress, genomic integrity, and liver diseases. Molecules 27, 3159. doi: 10.3390/molecules27103159
Seedevi P., Moovendhan M., Vairamani S., Shanmugam A. (2017). Evaluation of antioxidant activities and chemical analysis of sulfated chitosan from sepia prashadi. Int. J. Biol. Macromol. 99, 519–529. doi: 10.1016/j.ijbiomac.2017.03.012
Sevag M. G., Lackman D. B., Smolens J. (1938). THE ISOLATION OF THE COMPONENTS OF STREPTOCOCCAL NUCLEOPROTEINS IN SEROLOGICALLY ACTIVE FORM. J. Biol. Chem. 124, 425–436. doi: 10.1016/S0021-9258(18)74048-9
Tsai M.-Y., Yang W.-C., Lin C.-F., Wang C.-M., Liu H.-Y., Lin C.-S., et al. (2021). The ameliorative effects of fucoidan in thioacetaide-induced liver injury in mice. Mol. Basel Switz. 26, 1937. doi: 10.3390/molecules26071937
Wang Y., Branicky R., Noë A., Hekimi S. (2018). Superoxide dismutases: Dual roles in controlling ROS damage and regulating ROS signaling. J. Cell Biol. 217, 1915–1928. doi: 10.1083/jcb.201708007
Wang W., Deng Z., Feng Y., Liao F., Zhou F., Feng S., et al. (2017). PM2.5 induced apoptosis in endothelial cell through the activation of the p53-bax-caspase pathway. Chemosphere 177, 135–143. doi: 10.1016/j.chemosphere.2017.02.144
Wang C., Liu T., Tong Y. M., Cui R. X. (2021). Ulinastatin protects against acetaminophen-induced liver injury by alleviating ferroptosis via the SIRT1/NRF2/HO-1 pathway. Am. J. Transl. Res. 13 (6), 6031–6042.
Wang L., Oh J. Y., Kim H. S., Lee W., Cui Y., Lee H. G., et al. (2018). Protective effect of polysaccharides from celluclast-assisted extract of hizikia fusiforme against hydrogen peroxide-induced oxidative stress in vitro in vero cells and in vivo in zebrafish. Int. J. Biol. Macromol. 112, 483–489. doi: 10.1016/j.ijbiomac.2018.01.212
Wu J., Jia R.-B., Luo D., Li Z.-R., Lin L., Zheng Q., et al. (2022). Sargassum fusiforme polysaccharide is a potential auxiliary substance for metformin in the management of diabetes. Food Funct. 13, 3023–3035. doi: 10.1039/d1fo02165f
Yuan H., Duan S., Guan T., Yuan X., Lin J., Hou S., et al. (2020). Vitexin protects against ethanol-induced liver injury through Sirt1/p53 signaling pathway. Eur. J. Pharmacol. 873, 173007. doi: 10.1016/j.ejphar.2020.173007
Yuan C., Li Z., Peng F., Xiao F., Ren D., Xue H., et al. (2015). Combination of selenium-enriched green tea polysaccharides and huo-ji polysaccharides synergistically enhances antioxidant and immune activity in mice: Se- GPT with HJP enhances antioxidant and immune activity. J. Sci. Food Agric. 95, 3211–3217. doi: 10.1002/jsfa.7287
Zhang Y., Liu J., Mao G., Zuo J., Li S., Yang Y., et al. (2021). Sargassum fusiforme fucoidan alleviates diet-induced insulin resistance by inhibiting colon-derived ceramide biosynthesis. Food Funct. 12, 8440–8453. doi: 10.1039/d1fo01272j
Zhang Y., Xu M., Hu C., Liu A., Chen J., Gu C., et al. (2019). Sargassum fusiforme fucoidan SP2 extends the lifespan of drosophila melanogaster by upregulating the Nrf2-mediated antioxidant signaling pathway. Oxid. Med. Cell. Longev. 2019, 8918914. doi: 10.1155/2019/8918914
Zhao G., Kan J., Li Z., Chen Z. (2005). Structural features and immunological activity of a polysaccharide from dioscorea opposita thunb roots. Carbohydr. Polym. 61, 125–131. doi: 10.1016/j.carbpol.2005.04.020
Zhao H., Liu S., Zhao H., Liu Y., Xue M., Zhang H., et al. (2021). Protective effects of fucoidan against ethanol-induced liver injury through maintaining mitochondrial function and mitophagy balance in rats. Food Funct. 12, 3842–3854. doi: 10.1039/d0fo03220d
Keywords: Sargassum fusiforme, polysaccharides, H2O2, LO2 cell injury, protective effects
Citation: Li J, Wang Z, Chen J, Luo B, Chen X, Li R, Gao J, Liu X, Song B and Zhong S (2023) Protective effect of Sargassum fusiforme polysaccharides on H2O2-induced injury in LO2 cells. Front. Mar. Sci. 9:1098758. doi: 10.3389/fmars.2022.1098758
Received: 15 November 2022; Accepted: 19 December 2022;
Published: 06 January 2023.
Edited by:
Zedong Jiang, Jimei University, ChinaReviewed by:
Xingwei Xiang, Zhejiang University of Technology, ChinaShuang Song, Dalian Polytechnic University, China
Copyright © 2023 Li, Wang, Chen, Luo, Chen, Li, Gao, Liu, Song and Zhong. This is an open-access article distributed under the terms of the Creative Commons Attribution License (CC BY). The use, distribution or reproduction in other forums is permitted, provided the original author(s) and the copyright owner(s) are credited and that the original publication in this journal is cited, in accordance with accepted academic practice. No use, distribution or reproduction is permitted which does not comply with these terms.
*Correspondence: Jianping Chen, Y2pwNTE2NTU1OTg5QGdkb3UuZWR1LmNu; Saiyi Zhong, enN5bHhjQDEyNi5jb20=
†These authors have contributed equally to this work