- 1Department of Genetics and Cell Biology, Basic Medical College, Qingdao University, Qingdao, China
- 2Biology Institute, Qilu University of Technology, Shandong Academy of Sciences, Jinan, China
- 3Engineering Research Center of Zebrafish Models for Human Diseases and Drug Screening of Shandong Province, Jinan, China
- 4Intensive Care Unit, Qingdao Chengyang District People’s Hospital, Qingdao, China
Background: C-phycocyanin (C-PC), a phycobiliprotein from Spirulina platensis, has been confirmed to be an effective antioxidant. Duing to the large molecular weight protein, C-PC is hardly absorbed in the human gut and easy coagulates into lumps. In light of this, highly pure, easy absorption, and small molecular weight polypeptides has won the attention in the recent past. However, there are few studies on the C-PC bioactive peptides. Thus, the objective of this study was find natural multifunctional antioxidant peptides from C-PC and demonstrate their mechanisms.
Method: In this study, novel antioxidant peptides from pancreatin hydrolysate of C-PC were isolated and purified by using a multi-bioassay-guided method. The DPPH and ABTS radical scavenging in vitro and antioxidant zebrafish model in vivo were used to evaluate the antioxidant activities. Furthermore, the underlying molecular mechanisms of these peptides were investigated by measuring the level of ROS, MDA, SOD, CAT and gene expression in Nrf2 signaling pathway.
Results: Total three novel active peptides, MHLWAAK (Met-His-Leu-Trp-Ala-Ala-Lys), MAQAAEYYR (Met-Ala-Gln-Ala-Ala-Glu-Tyr-Tyr-Arg), and MDYYFEER (Met-ASP-Tyr-Tyr-Phe-Glu-Glu-Arg) were isolated and purified from pancreatin hydrolysate of C-PC. These synthesized peptides displayed high DPPH and ABTS radical scavenging ability. In addition, in a zebrafish model, the three peptides significantly protected zebrafish embryos from H2O2-induced oxidative injury without toxicity by inhibiting ROS generation, preventing MDA formation, and upregulating the activities of SOD and CAT. Further experiments showed that these peptides could significantly regulated oxidative stress via activating the Nrf2 signaling pathway.
Conclusion: This study indicates that the antioxidant peptides from C-PC could serve as powerful antioxidants for preventing and treating various diseases associated with oxidative stress.
Introduction
Reactive oxygen species (ROS) are a class of highly reactive free radicals or molecules containing oxygen, including superoxide free radicals, hydroxyl free radicals, and hydrogen peroxide (Sies and Jones, 2020). At low concentrations, ROS are beneficial to organisms, and have a role of signaling molecules in metabolism (Arulselvan et al., 2016). However, when the body is stimulated by endogenous or exogenous factors, ROS increase and cause oxidative stress, consequently leading to biological damage and occurrence of various diseases (Simon et al., 2000; Bhardwaj and Saraf, 2020). Nuclear factor E2 related factor 2 (Nrf2) as a key regulator of antioxidant signaling regulates ROS production via activating the gene expression of phase II detoxifying/antioxidant enzymes (SOD, CAT, and GSH-Px), playing a pivotal role in antioxidant defense system (Satoh et al., 2006). Antioxidants can transform ROS into more stable radical or non-radical products to prevent the damage of oxidative stress. At present, synthetic antioxidants, such as, butylated hydroxyanisole (BHA), butylated hydroxytoluene (BHT), ethoxyquin, and n-propyl gallate, have been generally applied to clear ROS and free radicals in biological systems. Although they possess a strong antioxidant activity, they have certain potential risks related to health (Hu et al., 2020). Therefore, there is an increasing interest in searching for antioxidants with safe or new from natural sources for treating various diseases.
In recent years, natural active components from marine resources have become the research hotspot due to their limited side effects and potent pharmacological activity (Li et al., 2022). From this perspective, it is very meaningful to discover and develop novel marine drugs. Spirulina attracts great attention for its unique medical value. The mass content (m/m) of C-phycocyanin (C-PC)—a light-harvesting pigment protein composed of α and β subunits—can be up to 60% in Spirulina platensis (Rastogi et al., 2015; Dong et al., 2022). C-PC can be applied in immunological studies as a fluorescent probe or added to food as an artificial pigment (Yang et al., 2022). Moreover, it also has many potential biological functions such as antioxidant, anti-inflammatory, antitumor, antiallergic, and other activities (Romay et al., 2003; Roy et al., 2007; Li et al., 2013; Wu et al., 2016; Piovan et al., 2022). However, as a natural large molecular weight protein, C-PC is hardly absorbed in the human gut and easy coagulates into lumps. In general, during human digestion, approximately one third of proteins are degraded to amino acids and two thirds are broken down to peptides. Therefore, many researchers have shifted their attention to the preparation of highly pure and small polypeptides.
Bioactive peptides are generally composed of between 3 and 20 amino acid residues, and can regulate important body functions through their myriad activities (Chakrabarti et al., 2018). Many reports have revealed that active peptides have been isolated from marine organisms, such as Lizard fish, Pacific cod, Skipjack Tuna Muscle, Ulva lactuca, and Palmaria palmata (Lan et al., 2015; Ngo et al., 2016; Garcia-Vaquero et al., 2019; OC et al., 2020; Zheng et al., 2022). Furthermore, Yu et al. obtained an antioxidant peptide (Pro–Asn–Asn) from enzymatic hydrolysates of Spirulina platensis (Yu et al., 2016). Zeng et al. purified and identified three novel antioxidative peptides from pepsin hydrolysates of Spirulina platensis, and the EYFDALA peptide exhibiting the excellent radical-scavenging activity (Zeng et al., 2020). However, to date, there have been few reports on preparation, identification, and characterization of antioxidant peptides derived from C-PC.
In this work, natural multifunctional antioxidant peptides from C-PC pancreatin hydrolysate were isolated and purified by using gel filtration chromatography (GFC) and reverse-phase high-performance liquid chromatography (RP-HPLC). The amino acid sequences of three peptides were identified by liquid chromatography–tandem mass spectrometry (LC-MS/MS). 2,2’-diphenyl-1-picrylhydrazyl (DPPH), 2,2-azino-bis-3-ethylbenzothiazoline-6-sulfonic acid (ABTS) radical-scavenging activity and H2O2-induced zebrafish were employed to assess the antioxidant properties of the synthetic peptides. Moreover, Quantitative Real-Time PCR (qRT-PCR) was used to detect changes in the expression of Nrf2 signaling pathway related gene to elucidate the peptides protect mechanisms underlying H2O2-induced oxidative stress.
Materials and methods
Materials and chemicals
C-phycocyanin (C-PC) was purchased from Binmei Biotechnology (Taizhou, China) and stored at 4°C for use. Alkaline, compound, papain, pepsin, and pancreatin were obtained from Shanghai Yuanye Bio-Technology Co., Ltd. (Shanghai, China). Sephadex G-100 medium, Sephadex LH-20, 1,1-diphenyl-2-picrylhydrazyl (DPPH), metronidazole, 2,2′-azinobis (3-ethylbenzothiazoline-6-sulfonic acid) diammonium salt (ABTS), and O-phthalaldehyde (OPA) were purchased from Suo Laibao Biotechnology Co., Ltd. (Beijing, China). 2’, 7’-Dichlorofluorescin diacetate (DCFH-DA) was from Sigma-AldrichCo. (St. Louis, USA). All other chemicals and reagents used in this study were of analytical grade.
Zebrafish maintenance
Wild-type AB strain and Transgenic zebrafishes-Tg (krt4:NTR-hKikGR)cy17 zebrafish were used in the study. Male and female zebrafish were housed at 28 ± 0.5°C under a 14 h light/10 h dark cycles. The healthy and sexually mature zebrafish were placed into tank at a male to female ratio of 1:2 overnight, and the embryos were collected at the following morning 10 a.m. Embryos were transferred to E3 culture solution (5 mM NaCl, 0.17 mM KCl, 0.33 mM CaCl2, 0.33 mM MgSO4) after three washes. All experiments were performed on 24 hours post fertilization (hpf) embryos.
Preparation of protein enzymatic hydrolysates of C-PC
Screening of enzymes
Briefly, C-PC was added to deionized water and hydrolyzed by alkaline, compound, papain, pepsin, and pancreatin under optimum conditions of each enzyme for 2 h. The enzymes in the mixture were inactivated in a 95°C water bath for 15 min. After the hydrolysate was centrifuged at 12000 rpm for 15 min, the supernatant was collected and freeze-dried for further use. The DH and DPPH radical-scavenging activity were analyzed as the index to screen the optimal protease.
Single-factor experiments
The effects of four factors, including reaction temperature, enzyme concentration, material-to-liquid ratio, and pH of the buffer, on the DH, DPPH radical-scavenging activity, and comprehensive index were investigated by single-factor experiments.
Optimization of enzymatic hydrolysis conditions
Response surface methodology (RSM) was used to optimize the enzymes hydrolysis condition of C-PC employing the Design Expert 8.0.6.1 version (Stat-Ease, Inc., Minneapolis, USA). Furthermore, according to the single-factor experimental results, a second-order Box-Behnken design (BBD) was used for the 4-factor 29-run BBD.
DH and antioxidant activity
Calculation of DH
DH was calculated as the percentage of α-amino nitrogen (AN) to the total protein nitrogen (TPN) according to Noman et al. with some modifications (Noman et al., 2018) using the following formula:
where C is the concentration of NaOH (mol/L), V1 is NaOH volume (mL), and V2 is the volume of NaOH (mL) in the blank.
The DH was calculated via the following equation:
DPPH radical-scavenging activity
The DPPH radical-scavenging activity of peptides was determined following the approach reported by Wolosiak et al. (Wołosiak et al., 2021). Samples were added to 96-well microliter plates and reacted in the dark for 30 min. The absorbance of the reaction mixtures was measured at 517 nm in a Spectra MR. Deionized water was used as negative control. The IC50 values for DPPH radical activity was assayed.
ABTS radical-scavenging activity
The ABTS radical-scavenging activity of the peptides was determined by the standard protocol (Salar et al., 2017; Rafique et al., 2020). The ABTS radical scavenging of active three peptides were determined by IC50.
Antioxidative activity in zebrafish embryos
Transgenic zebrafishes-Tg (krt4:NTR-hKikGR)cy17 was used to evaluate the antioxidative activity of C-PC peptides in line with the previously reported methods (Zhang et al., 2018; Li et al., 2019). Specifically, 24 hpf zebrafish embryos were first placed on a 24-well plate, and subsequently incubated with 1 mL MTZ (20 mM) and samples of specified concentration for 24 h. Embryos without any treatment were used as blank controls, and embryos treated with MTZ were used as negative controls. The fluorescence spots (FS) on the skin were visualized with a stereological fluorescence microscope (Nikon, Japan). FS counting was done using Image Pro-Plus (IPP).
Preparation of C-PC peptides
Separation of peptide by Sephadex G-100
C-phycocyanin hydrolysate (C-PCH) was first separated by Sephadex column according to the MWs. The dried powder of Sephadex was soaked in distilled water for at least 24 h or longer. Before sample injection, the column was equilibrated with at least 3–5 column volumes. Lyophilized C-PCH sample (500 mg) was dissolved in 5 mL of deionized water and loaded on Sephadex column (2.0 × 40 cm dimension). The eluent was deionized water, and the flow rate was 0.5 mL/min. The fractions were combined and concentrated according to the TLC analysis results. Finally, their antioxidant activities were measured by assaying DPPH and ABTS radicals-scavenging activity.
Separation of peptide by Sephadex LH-20
The fraction with the highest antioxidant property was further separated by Sephadex LH-20 gel filtration column (2 × 100 cm dimension) according to polarity. The Sephadex LH-20 was macerated in 60% to 70% ethanol overnight. Moreover, the mixture was adequately stirred in order to remove any residuals. A total of 500 mg of the sample was dissolved in a small amount of methanol and loaded on the Sephadex column, followed by elution with 50% methanol–H2O at a flow rate of 0.75 mL/min. In all cases, the samples were merged by silica gel TLC plate, and concentrated, dried, and stored at −80°C until use. Similarly, the DPPH radical and ABTS radical-scavenging activities were measured as described above.
Purification of peptide by RP-HPLC
The antioxidant active peptides of C-PC were purified with a ZORBAX, 300 Extent-C18 columns (4.6×250 mm, 5 μm) in an HPLC Hitachi L-2000 system (Hitachi, Japan). The column temperature was 20°C, flow rate was 0.8 mL/min, sample concentration was 1 mg/mL, detection wavelength was 214 nm, and injection volume was 50 μL. The mixed gradient elution conditions were 3%–50% acetonitrile elution for 30 min. The main peak was collected and lyophilized for the antioxidant assay.
Identification and synthesis of C-PC peptide
Identification of de novo peptide sequences of C-PC was done via EASY-Nlc1000 chromatography system coupled to an LTQ Orbitrap Velos Pro mass spectrometer (Thermo Finnigan, Bremen, Germany) in accordance with the previously published methods (Lassoued et al., 2015; Agrawal et al., 2016). The identified peptides were synthesized via an Fmoc-protected solid-phase procedure by Cellmano Biotech Co., Ltd. (Hefei, China).
Safety assessment of active peptides
Healthy zebrafish embryos at 24 hpf were selected and treated with different concentrations of peptides (5, 10, 20, 40, 80, 160, 320 μg/mL) in 24-well cell culture plates (10 eggs per well, with 2 mL of bathing medium) for continuous development until 48 hpf. The morphological development of zebrafish was observed and photographed using a dissecting microscope (Olympus, Tokyo, Japan).
Measurement of heartbeat and survival rate
The heart-beat rate of both atrium and ventricle of zebrafish were measured at 48 hpf after induction of H2O2 under a stereomicroscope. Counting and recording number of heartbeats in 1 min in eight of each group (Kang et al., 2013). The survival rate was calculated at 48 hpf after induction of H2O2 by zebrafish embryos mortality.
Measurement of ROS generation
At 48 hpf, the ROS level in the zebrafish larvae was determined using a 2,7-dichlorofluoresceindiacetate (DCF-DA) probe. The larvae were placed in 24-well plates supplemented with DCF-DA (10 mg/mL) and purified water to a final concentration of 10 μg/mL, and incubated at 28°C in the dark for 1 h. After that, each group was washed with phosphate buffer solution (PBS) and photographed on a fluorescence microscope (Olympus, Tokyo, Japan). The fluorescence intensity was quantified by Image Pro-Plus (IPP).
Determination of oxidative stress indicators
At 48 hpf, 40 zebrafish of each group were collected, ultrasonically broken in ice bath, and then centrifuged (10000 g, 10 min, 4°C). Lastly, the supernatant was removed for the following experiments. Malondialdehyde (MDA), Micro Catalase (CAT), and superoxide dismutase (SOD) activity in the zebrafish were detected in accordance with the instruction manual (Suo Laibao Biotechnology Co., Ltd, Beijing, China).
Analysis of gene expression by qRT-PCR
Total RNA was extracted using a FastPure® Cell/Tissue Total RNA Isolation Kit V2 (Vazyme, Nanjing, China) and then reverse transcribed into cDNA using a HiScript® III Reverse Transcriptase (Vazyme, Nanjing, China) following the manufacturer’s protocol. Quantitative RT-PCR was performed using AceQ® qPCR SYBR Green Master Mix on the LightCycler® 96 (Roche, Switzerland) system. rps18 was used as an internal reference gene for normalization of mRNA levels. The primers sequences for genes of zebrafish are provided in Table 1.
Molecular docking
Molecular docking study was performed to examine the interactions between peptide and receptor protein, and -CDocker was used to predict the affinity of peptide binding to oxidative stress–related target proteins in our study. In the experiment, Keap1 (4ZY3.pdb) was chosen as receptor. The X-ray crystal structure of receptors were obtained from RCSB Protein Data Bank (http://www.rcsb.org). The 3D structure of peptides was drawn, and energy was minimized with the Chem3D 2020 installer (CambridgeSoft Co., MA, USA). From the crystal structure of these receptors, we removed water molecules and added hydrogen molecules with Discovery Studio 2019 installer (Accelrys Software, Inc., San Diego, CA, USA). The simulations were performed using cavity detection at the coordinates (x, y, z) of −43.8784, −0.498661, and −6.9621 (4ZY3). All the binding pockets were chosen at a radius of 30 Å. The result of molecular docking was expressed in terms of values of -CDocker energy and -CDocker interaction energy (kcal/mol).
Statistical analysis
All data are presented as mean ± standard deviation. Statistical analysis was performed using GraphPad Prism 9 for Windows (GraphPad Prism Software, San Diego, CA). One-way ANOVA was used to evaluate differences among multiple groups. p< 0.05 was treated as statistically significant.
Results and discussion
Preparation of protein enzymatic hydrolysates of C-PC
In our study, five different proteases (alkaline, compound, papain, pepsin, and pancreatin) were used to hydrolyze C-PC under the same conditions. The antioxidant activities of different enzymatic hydrolysates were determined by both degree of hydrolysis (DH) assay and DPPH radical-scavenging activity. As shown in Figures 1A, B, pancreatin was demonstrated to have the highest radical-scavenging activity and DH, and both were more than 50%. Pancreatin is one of the major proteolytic enzymes in enzymatic hydrolysis experiments, and is readily available and relatively cheap. Besides, it can also activate zymogens such as carboxypeptidase and phospholipase. Therefore, we selected pancreatin as a candidate for further C-PC hydrolysis to produce active peptides.
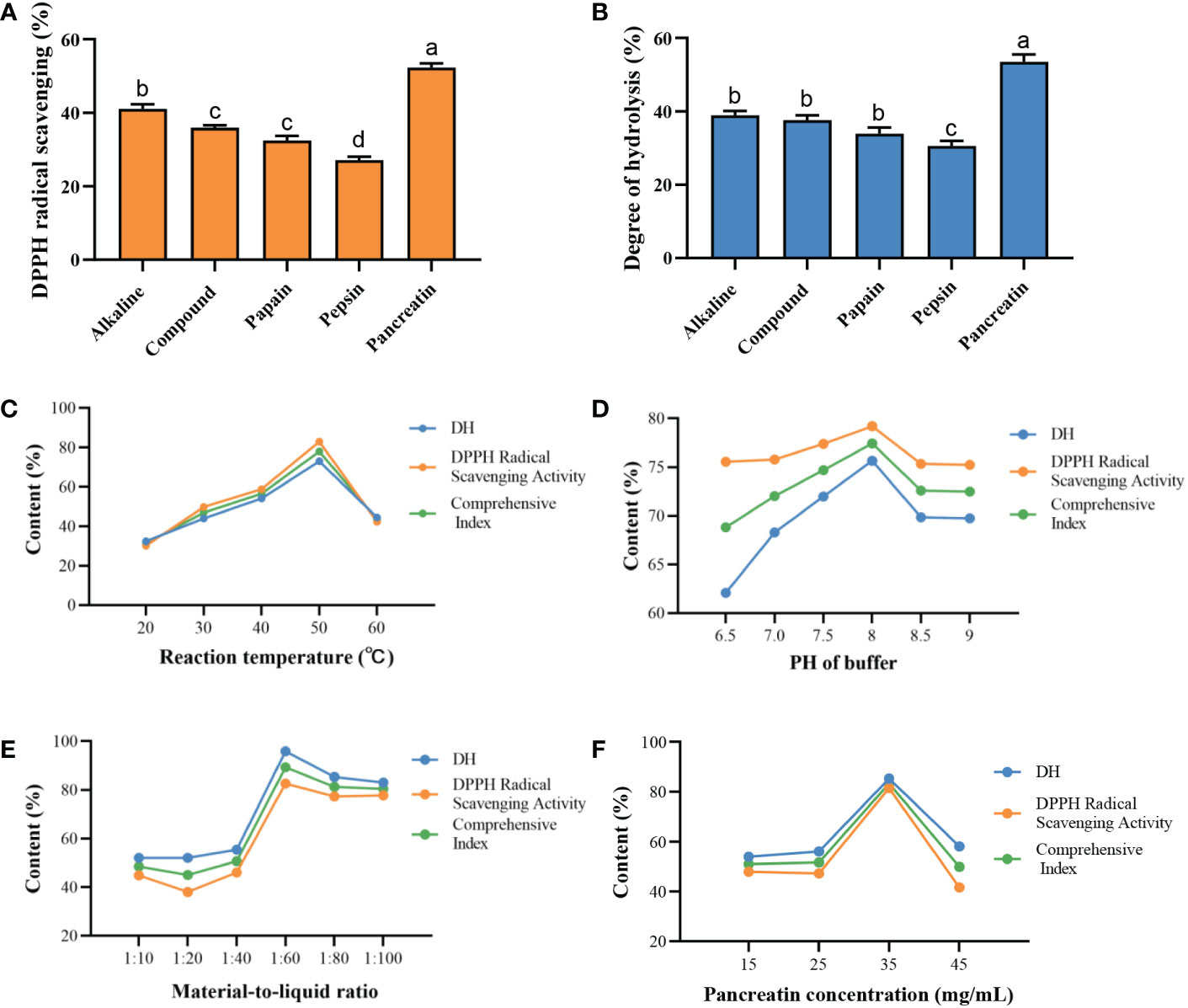
Figure 1 Preparation of protein enzymatic hydrolysates of C-PC. Effects of different proteases on the (A) DPPH radical scavenging activity. (B) Degree of hydrolysis (DH). DH and DPPH radical scavenging activity vary with effects of (C) Reaction temperature. (D) PH of buffer. (E) Material-to-liquid ratio. (F) Pancreatin concentration. a-dValues with the different letters indicate a significant difference (p < 0.05).
Next, the effects of the reaction temperature, pH of buffer, material-to-liquid ratio, and enzyme concentration on the antioxidant activity of enzymatic hydrolysates were investigated by single factor experiment. As shown in Figure 1C, the DH and DPPH radical-scavenging activity of C-PCH gradually increased as the temperature raised, and the peak level of the antioxidant capacity occurred at 50°C. The DH and radical-scavenging activity increased when pH of the buffer was increased from 6.5 to 9, and the highest comprehensive index was obtained at pH 8 (Figure 1D). The antioxidant capacity varied with the difference in the material-to-liquid ratio but peaked at 1:60 (Figure 1E). In Figure 1F, the enzyme concentration of 35 mg/mL exhibited the strongest antioxidant activity. Therefore, we determined the optimal enzymatic hydrolysis conditions for the following surface methodology (RSM) experiments as follows: reaction temperature of 50°C, pH of 8, material-to-liquid ratio of 1:60, and enzyme concentration of 35 mg/mL.
Based on the results of the single-factor experiments, the conditions of RSM and antioxidant activity by Box–Behnken and Design (BBD) were obtained. The results of the statistical analysis of the quadratic model of DH and DPPH were significant (p< 0.05). To further explore the influence of the four variables on the DH and DPPH radical-scavenging activity, response surface plots were created (Figure S1). Finally, the optimum conditions were predicted as follows: reaction temperature (49°C), pH of the buffer (8.0), material-to-liquid ratio (1:60.6), and enzyme concentration (55.18 mg/mL). Under these conditions, the maximum DH and DPPH radical-scavenging activity ratio were predicted to be 88.36% and 87.60%, respectively.
Purification of antioxidant peptides from C-PC hydrolysates
Gel filtration chromatography (GFC) is a functional and effective separation approach based on different molecular weight (MW) (Ó'Fágáin et al., 2017; Qiao et al., 2022). It has been constantly used to isolate peptides with different MWs from marine protein hydrolysates (Han et al., 2020; Sridhar et al., 2021). As shown in Figure 2A, the C-PC hydrolysates (C-PCH) were fractionated into C-PCH1, C-PCH2, C-PCH3, C-PCH4, and C-PCH5 by a Sephadex G-100 chromatographic column. Among them, the C-PCH3 had the highest antioxidant activity with DPPH radical-scavenging activity of 31.71% ± 0.90% and ABTS radical-scavenging activity of 27.45% ± 2.67% at the concentration of 10 mg/mL (Figure 2B). In our experiment, the C-PCH3 did not have the lowest MW compared with other parts of the enzymatic hydrolysates, but its free radical–scavenging activity was significantly stronger (p< 0.05), suggesting that the antioxidant activity of C-PC was not only influenced by MW but also by the amino acid composition and sequence (Wang et al., 2021; Qiao et al., 2022). Herein, the C-PCH3 was further separated by Sephadex LH-20.
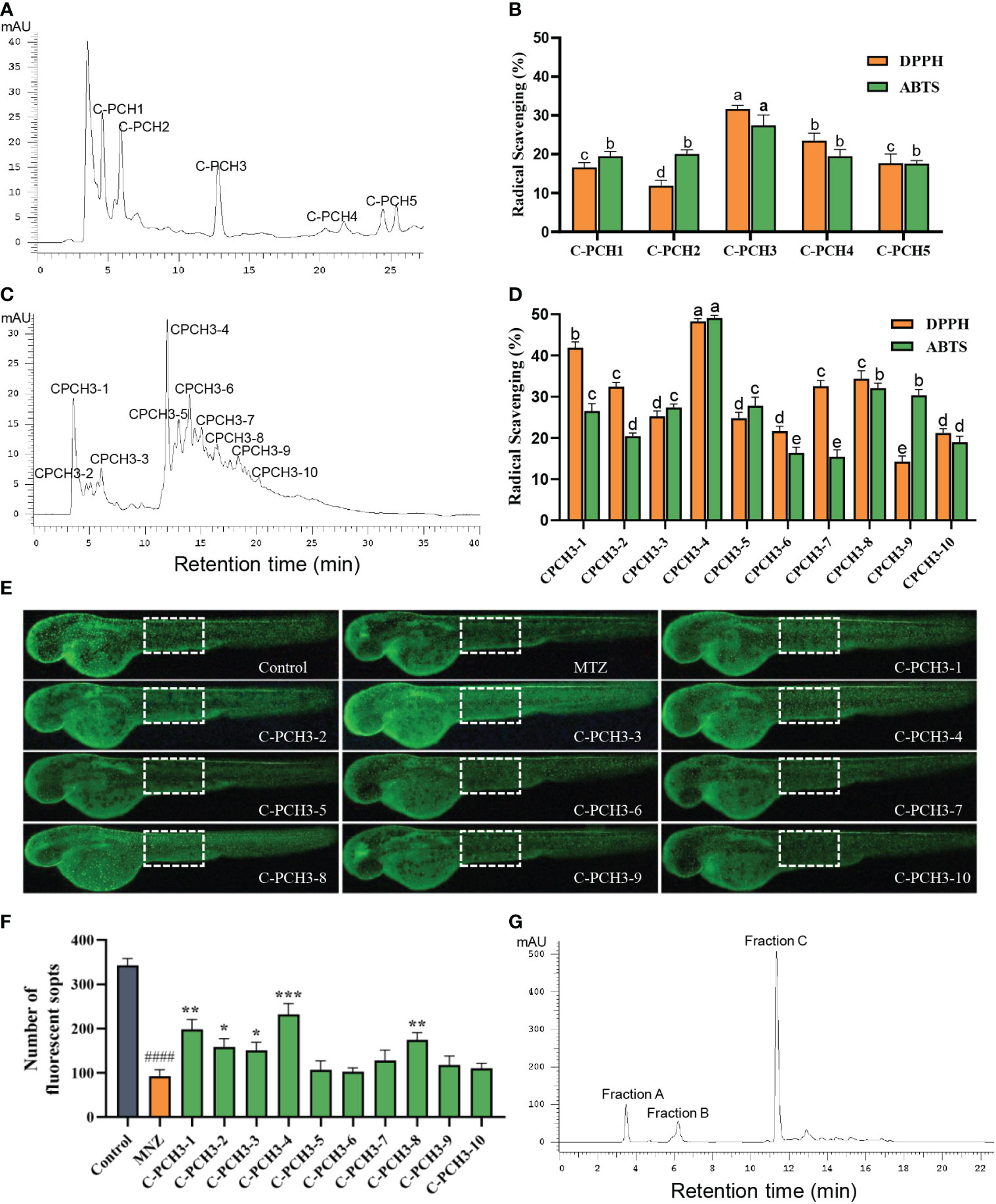
Figure 2 Purification of antioxidant peptides from C-PC hydrolysates (C-PCH). (A) Separation of C-phycocyanin hydrolysate (C-PCH) by Sephadex G-100 gel filtration chromatography. (B) Radical scavenging activities of corresponding subfractions. (C) Further separation of C-PCH3 by Sephadex LH-20 gel filtration chromatography. (D) Radical scavenging activities of corresponding subfractions. a-eValues with the different letters indicate a significant difference (p < 0.05). All data were expressed as mean ± standard deviation of triplicate experiments. (E) In vivo antioxidant activity of transgenic fluorescent zebrafish Tg(krt4:NTR-hKikGR)cy17 treated with MTZ (10 mM) and C-PCH3-1 to C-PCH3-10 (50 μg/mL). (F) FS number statistic results of all groups. Data are represented as the mean ± SEM (n=15). *p < 0.05, **p < 0.01, ***p < 0.001 compared with the MTZ group. ####p < 0.0001 compared with the control group. Scale bar, 200 μm. (G) Purification of C-PCH3-4 by RP-HPLC on a 300 Extent-C18 column.
Sephadex LH-20 is an alkylated cross-linked dextran that is widely used because it is convenient, rapid, and efficient (Bartley et al., 1972). As indicated in Figure 2C, the C-PCH3 was then divided into 10 fractions (C-PCH3-1 to C-PCH3-10) by using Sephadex LH-20 separation. In addition, as shown in Figure 2D, C-PCH3-4 showed the best DPPH and ABTS radical-scavenging activity at the concentration of 10 mg/mL compared with all other fractions (48.28% ± 0.64% and 48.44% ± 1.25%). These results proved that the radical-scavenging activity of C-PCH3-4 was significantly improved after Sephadex LH-20 separation. Furthermore, the antioxidant activities of the samples were further proven by a transgenic zebrafish line of Tg (krt4:NTR-hKikGR)cy17. Metronidazole (MTZ) can induce oxidative damage on the skin of zebrafish, ROS overproduction; thus, the number of fluorescent spots (FS) can be used to evaluate antioxidant activity of peptides (Kulkarni et al., 2018). Sample with antioxidant activity can remove ROS from transgenic zebrafish and prevent the apoptosis of skin cells. Hence, an increased number of FS are observed (Li et al., 2019). As depicted in Figure 2E, the number of FS was significantly reduced in the skin of zebrafish treated with MTZ compared with the control group, meaning that the MTZ-induced model was successful. Compared with the model group, the treatment with MTZ at a concentration of 50 μg/mL significantly increased the number of FS on the skin of zebrafish. Among them, the C-PCH3-4 had more FS than the other nine groups (Figure 2F). Thus, in order to identify the most active peptides, C-PCH3-4 was further purified by RP-HPLC.
RP-HPLC is an effective chromatographic method used to purify crude peptides and separate small molecules below 1000 Da (Chi et al., 2015; Agrawal et al., 2016). From Figure 2G, it is evident that the C-PCH3-4 was purified into three different fractions (Fraction A, Fraction B, Fraction C) based on hydrophobicity and elution times by RP-HPLC on a C18 column. The more hydrophobic fractions have a longer adsorption time on this column and vice versa. Among them, Fraction C, which is the main peak and has strong hydrophobicity, was collected at an elution time of 11 min. Therefore, Fraction C was finally analyzed for sequencing of the peptides.
Identification of antioxidant peptides
According to Figure 3, three different peptides composed of 7, 9, and 8 amino acid residues in Fraction C were identified by UPLC-MS/MS system according to the amino acid sequence by comparing with database (mascot and NCBI), and they were named P1, P2, and P3, respectively. The sequences were identified as MHLWAAK (P1), MAQAAEYYR (P2), and MDYYFEER (P3), respectively. As shown in Table 2, The molecular masses of the three peptides were 856.06, 1101.4913, and 1151.4594 Da, and their pI were 9.71, 7.00, and 6.58, respectively. P1 had higher content of hydrophobic amino acids and lower MW than P2 and P3. Next, we evaluated the antioxidant activity of the identified peptides using in vitro chemical assays and in vivo antioxidant zebrafish. The results suggest that all three peptides showed high free radical scavenging activity, which showed concentration-dependent increase from 2 to 10 mg/mL. The IC50 values of P1 and P2 on DPPH and ABTS were lower than phycocyanin, but P3 higher than phycocyanin (Figures S3A, B). In addition, the fluorescent spots (FS) number on zebrafish skin cells of P1 and P2 at a low concentration showed significant difference (p< 0.01) compared with model group. There was no significant difference in the number of FS between the P3 at a low, medium concentration and negative control. No difference between phycocyanin and model group was found in low, medium, and high concentration (Figures S2, S3). Overall, these results indicate that compared with P3 and phycocyanin, P1 and P2 showed higher antioxidant capacity. Literature reports have also indicated that the ABTS IC50 of peptide (LSPGEL) from Allophycocyanin was 5.35 mg/mL, higher than that of our antioxidant peptides (Hu et al., 2022). In fact, the peptides of the in vivo antioxidant experiment revealed excellent antioxidant activity compared with the DPPH and ABTS radical-scavenging experiments. There were two possible reasons for this difference (Sies and Jones, 2020): the peptides were absorbed and degraded in the zebrafish larvae to generate secondary metabolites with a stronger antioxidant activity (Arulselvan et al., 2016); the peptides could act on reactive oxygen free radicals caused by oxidative stress in the body. Previous studies have demonstrated that peptides with low MW (500–1500 Da) and hydrophobic amino acids could play a more important role in antioxidant systems (Ma et al., 2018; Islam et al., 2021). Moosmann and Behl reported that oxidant–antioxidant systems are more accessible to low molecular peptides than to large peptides and proteins (Moosmann and Behl, 2002). Peptides of Urechis unicinctus Visceral have been reported to show a relatively high antioxidant activity due to the abundance of hydrophobic amino acids (Li et al., 2022).
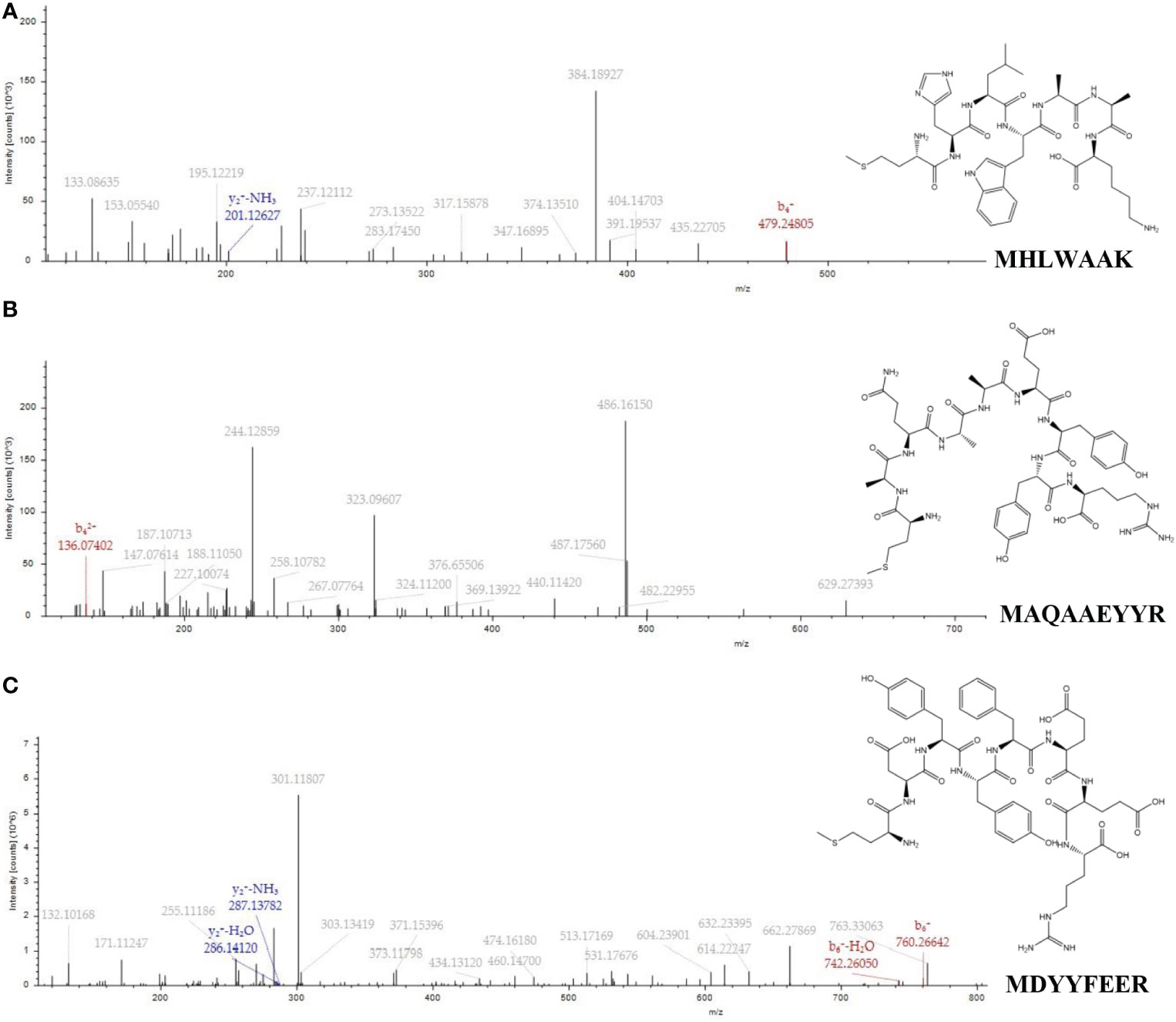
Figure 3 MS/MS spectra and structures of the three peptides from C-Phycocyanin (C-PC). (A) MHLWAAK. (B) MAQAAEYYR. (C) MDYYFEER.
Protective effect of peptides in H2O2-induced oxidative damaged model of zebrafish
In the present study, the effects of the three identified peptides on the survival rate and morphology of zebrafish embryos were investigated. Zebrafish larvae at 24 hpf were treated with different concentrations of active peptides (5, 10, 20, 40, 80, 160, and 320 μg/mL) for 24 h, and no malformation or death occurred below 160 μg/mL (Figures 4A, B). Thus, these peptides were safe and effective at concentrations below 160 μg/mL. H2O2, as a stable molecule, which could easily induce cell death via oxidative signaling, is commonly used to induce oxidative stress in zebrafish embryos. As shown in Figure 4C, survival rate of embryos at 48 hpf with different treatments are calculated. Survival rate of the H2O2 treated group were significantly decreased compared with the control group. All three peptides treatment at 20 µg/mL significantly reduced the mortality. The heart beating rate detected in the H2O2-treated zebrafish embryos was 148 ± 4.31/min, and higher than that in not un-treated control embryos whose rate was 129 ± 6.86/min (Figure 4D). In addition, the heart beating rate in P1, P2, and P3 were 134 ± 4.43/min, 133 ± 5.24/min, and 139 ± 2.12/min at the highest concentration, respectively, which were significantly lower than that of the model group (p< 0.05). Therefore, these results showed that P1, P2, and P3 could significantly protect zebrafish larvae from H2O2-induced oxidative damage.
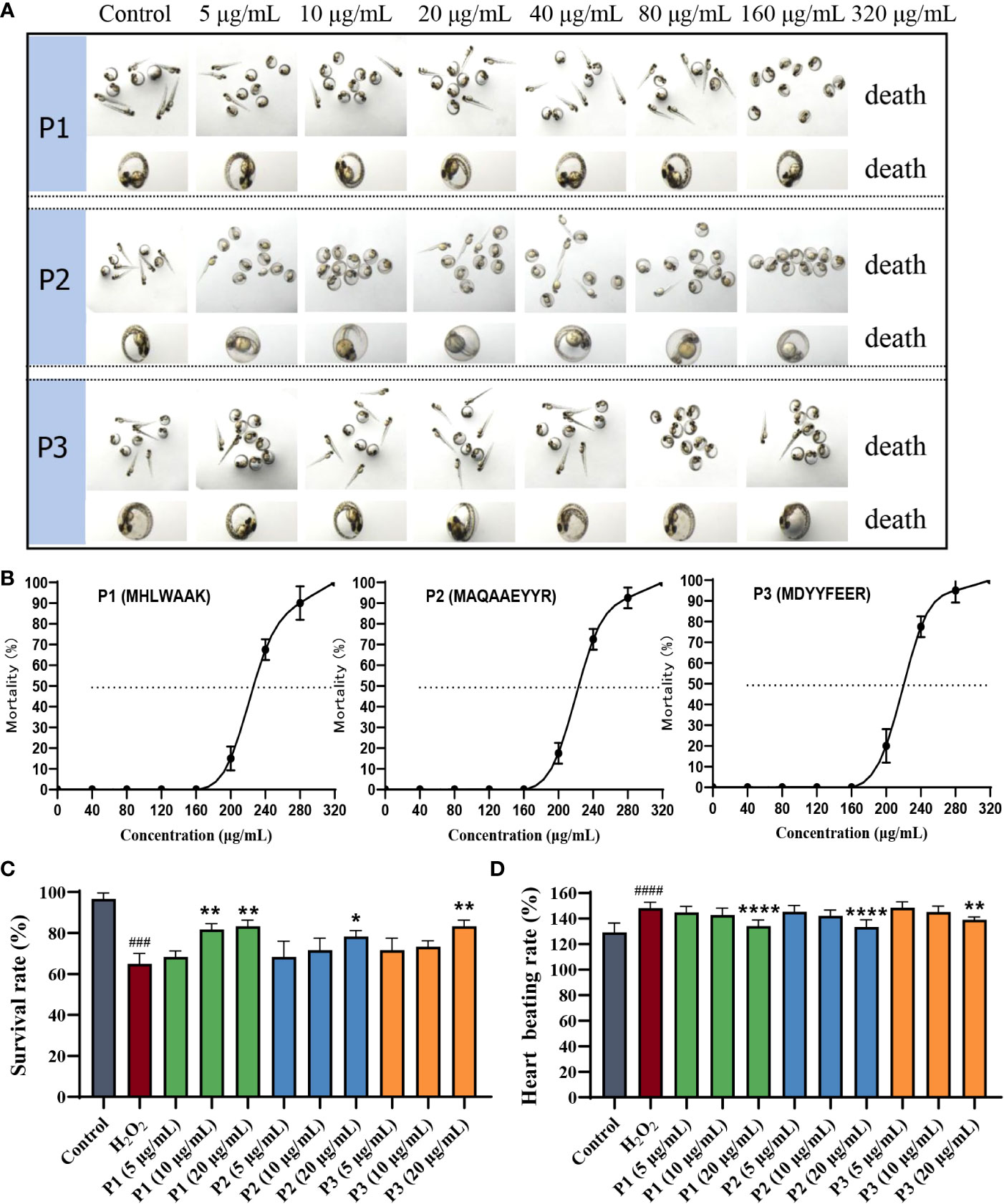
Figure 4 Protective effect of antioxidant peptides on H2O2-induced oxidative damaged in zebrafish embryos. (A) The morphology of zebrafish larvae at 48 hpf. (B) The mortality of zebrafish larvae at 48 hpf. (C) Survival rates. (D) Heart beating rates. *p < 0.05, **p< 0.01, ****p < 0.0001 vs. the H2O2 group. ###p < 0.001, ####p < 0.0001 vs. the blank control group.
Effects of peptides on the levels of ROS, MDA, SOD, and CAT in H2O2-induced oxidative damaged model of zebrafish
In order to evaluate whether the enzymatic antioxidative system play an important role in zebrafish larvae, the ROS, MDA content and SOD, CAT activity were measured.
ROS serve an important role in cell signaling and in maintaining the homeostasis. Oxidative stress occurs when the ROS levels exceed antioxidant defense systems. ROS generation in H2O2-induced oxidative stress was measured in zebrafish using fluorescent probe DCF-DA. As shown in Figure 5A, the fluorescence in the H2O2-induced group was significantly brighter and stronger than that in the other groups, and the ROS level was higher. These results proved that the model was successfully established. In addition, compared to that in the H2O2 group, the ROS levels in the zebrafish treated with a high concentration of P1, P2, and P3 was significantly lower (P< 0.05). No significant difference was observed between the low, medium concentration groups of three peptides and model group (P > 0.05) (Figure 5B). These results demonstrated that P1, P2, and P3 played a protective role at high concentration on H2O2-induced zebrafish oxidative damage by reducing ROS.
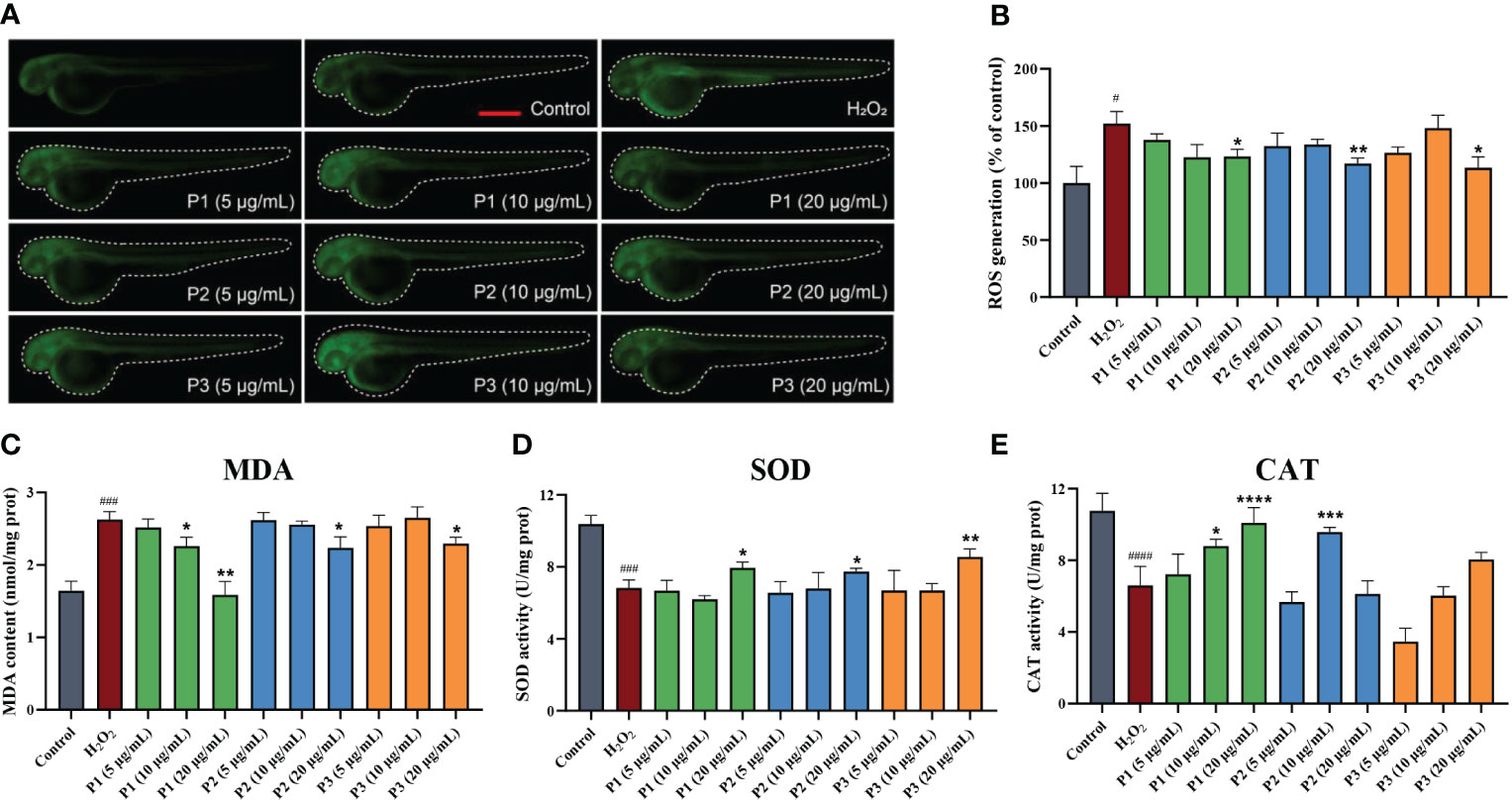
Figure 5 Three novel antioxidant peptides inhibited H2O2-induced oxidative stress in zebrafish. (A) The ROS fluorescent images. (B) The levels of ROS production. (C) MDA level. (D) SOD activity. (E) CAT activity. *p < 0.05, **p < 0.01, ***p < 0.001, ****p < 0.0001 vs. the model group. #p < 0.05, ###p < 0.001, ####p < 0.0001 vs. the control group.
MDA is a metabolic product of a large numbers of oxygen free radicals, which indirectly reflects the degree of tissue peroxidation (Moosmann and Behl, 2002). Figure 5C shows the effects of peptides on the MDA content in H2O2 oxidative damaged zebrafish. After treatment with H2O2 for 24 h, MDA content in zebrafish larvae was significantly increased (2.62 ± 0.09 nmol/mg prot) (P< 0.05) compared to that control group, proving that H2O2 increased oxidative stress in zebrafish. Pretreated with P1, P2, and P3 at the concentration of 5, 10, and 20 μg/mL, the MDA level observed at high and medium concentrations of P1 and high concentration of P2 and P3, were significantly lower (P< 0.05) than that observed in the H2O2 group. It could be seen that he antioxidant activity of P1 was stronger than that P2 and P3.
SOD is an endogenous antioxidant enzyme that converts superoxide anion (O2−) into hydrogen peroxide (H2O2) and oxygen (O2) (Zhang et al., 2014). It can indirectly reflect the ability to remove or quench oxygen free radicals. As shown in Figure 5D, SOD activity was decreased in the H2O2-induced group compared with the normal control group. However, compared with the model group (6.83 ± 0.36 U/mg prot), the SOD activity in high-dose groups of P1 (7.94 ± 0.26 U/mg prot), P2 (7.74 ± 0.15 U/mg prot), and P3 (8.55 ± 0.36 U/mg prot) was significantly elevated (p< 0.05), while there was no significant difference in the low-dose and medium-dose group of peptides. In summary, the three peptides could protect the zebrafish larvae from oxidative damage induced by H2O2 only at higher concentrations.
The effects of the peptides on CAT activity in zebrafish are shown in Figure 5E. Compared to the control group, H2O2 treatment significantly inhibited CAT activity (P< 0.05) by 63.17%, while the CAT activity of peptides treatment group was significantly enhanced. Specifically, compared to the H2O2-induced group (6.60 ± 0.87 U/mg prot), the activities of CAT in the P1 treatment group were increased by 33.44% and 52.95% at medium and high concentrations (10 and 20 μg/mL), respectively. The CAT activities were increased by 45.23% at 10 μg/mL of P2, compared to that observed in the model group. In addition, there was no significant difference in the CAT should be modified to CAT activities (P > 0.05) of P3 treatment group (3.47 ± 0.61 U/mg prot, 6.03 ± 0.41 U/mg prot, and 8.05 ± 0.32 U/mg prot) at different concentrations compared to the model group. These results showed that P1 and P2 showed better antioxidant capacities than P3. It was noted that the MDA content and CAT activity of P3 was significantly worse than that of P1, while the activities of SOD of P3 group were better than that of P1, suggesting that there were other antioxidant enzyme systems and small molecules playing a key role in exerting antioxidant effects in zebrafish larvae (Sun et al., 2022).
The effect of peptides on gene expression in H2O2-induced oxidative damaged of zebrafish
The Keap1/Nrf2 pathway is one of the most important defense and survival pathways to reduce external oxidative damage, and is also a key regulator of cellular stress response caused by ROS (Yi et al., 2020). It can regulate the expression of antioxidant enzymes such as HO-1, NQO1, GCLC, GCLM, SOD, CAT, and GSH, which is essential for maintaining the redox balance of the body. Above we have demonstrated the three peptides showed antioxidant abilities by regulating the endogenous antioxidant enzyme activities. Therefore, in order to explore whether they inhibited H2O2-induced oxidative damage via the Nrf2 signaling pathway, we selected genes associated with oxidative stress and assessed their expression by qRT-PCR.
As shown in Figures 6A–C, compared to the control group, the relative mRNA levels of SOD, CAT, and GSH-Px in H2O2-induced group decreased by 13.77%, 13.54%, and 31.64%, respectively. The mRNA levels of SOD in the zebrafish larvae treated with different concentrations of P1, P2, and P3 were increased by 0.14 – 0.32, 0.09 – 0.29, 0.15 – 0.20, respectively. P1, P2, and P3 treatment at different concentrations upregulated the mRNA levels of CAT by 0.00 – 0.13, 0.00 – 0.06, and 0.08 – 0.16, respectively. Similarly, Different concentrations treatment of P1, P2, and P3, the mRNA levels of GSH-Px were elevated by 0.04 – 0.35, 0.15 – 0.26, and 0.00 – 0.17 folds, respectively. Notably, compared to P2 and P3, P1 showed higher gene expression levels of SOD, CAT, and GSH-Px, which was agreement with the previous study conducted measuring the activities of antioxidant enzymes.
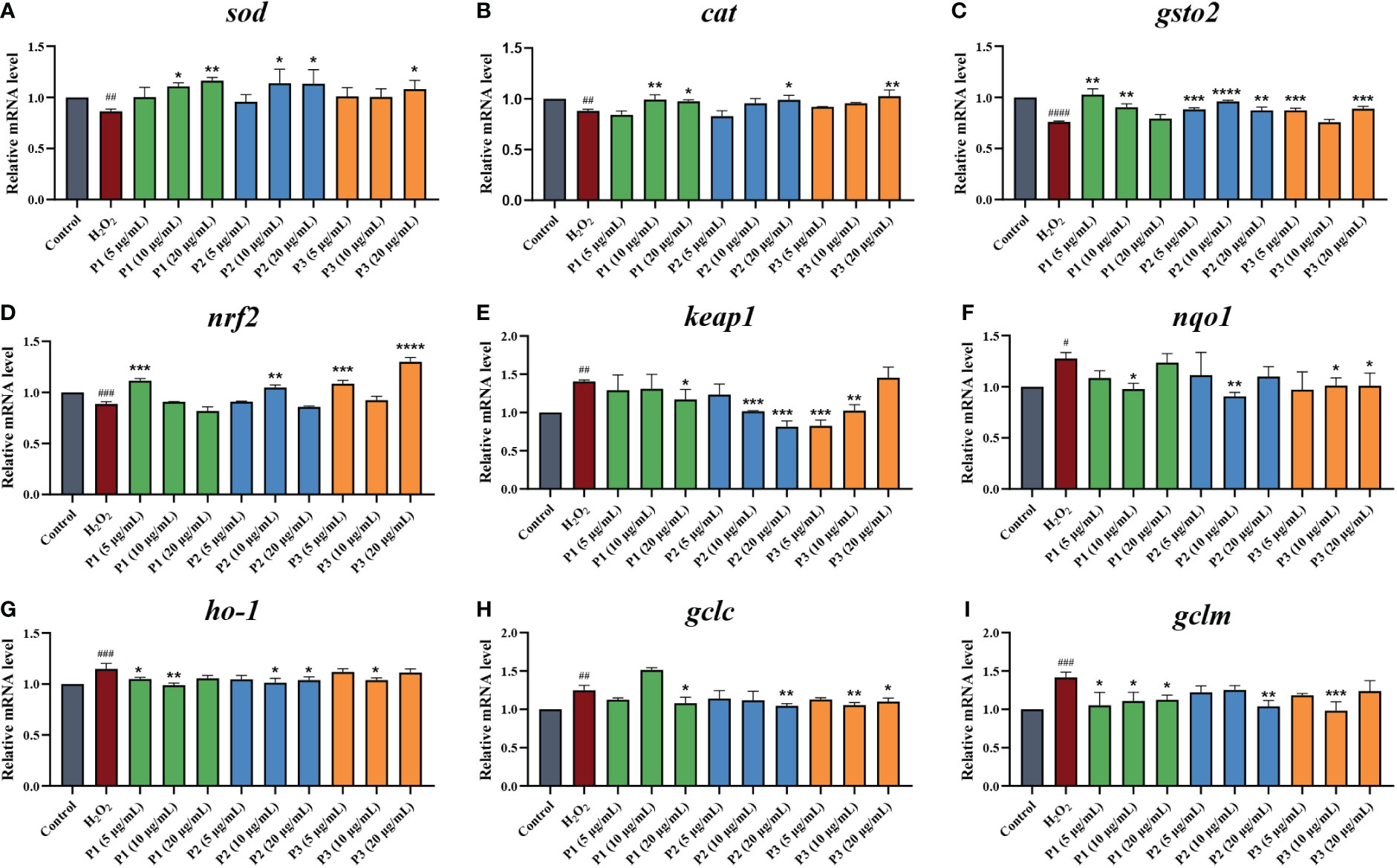
Figure 6 Effect of antioxidant peptides on the relative mRNA expression levels of (A) SOD. (B) CAT. (C) GSH-Px. (D) NRF2. (E) KEAP1. (F) NQO1. (G) HO-1. (H) GCLC. (I) GCLM. *p < 0.05, **p < 0.01, ***p < 0.001, ****p < 0.0001 vs. the H2O2 group. #p < 0.05, ##p < 0.01, ###p < 0.001, ####p < 0.0001 vs. the blank control group.
We next investigated the mRNA expression levels of NRF2 and KEAP1 (Figures 6D, E). The lowest NRF2 gene level (0.258 ± 0.020) and the highest KEAP1 gene level (7.54 ± 0.25) were observed in the H2O2 treatment group without the peptide. The gene expression levels of NRF2 were increased by 2.32% (P1, 10 μg/mL) to 46.43% (P3, 20 μg/mL) in comparison with H2O2 treatment group. In addition, a range of concentrations of peptides treatment could significantly downregulated the gene expressions of KEAP1, from 0.07-fold by P1 (10 μg/mL) to 0.70-fold by P3 (5 μg/mL). This result suggested that these 3 peptides isolated and purified from C-PC promote dissociation of the Nrf2/Keap1 complex, leading to stabilization and activation of NRF2.
Furthermore, we also evaluated the expression of downstream antioxidant genes, including haem oxygenase 1 (ho-1), nad(p)h quinone dehydrogenase 1 (nqo1), and the glutamate-cysteine ligase modification subunits gclm and gclc (Figures 6F–I). It was found that H2O2 treatment along significantly increased mRNA levels of Nrf2 downstream factors. Compared to the model group, HO-1, NQO1, GCLM, and GCLC expression in zebrafish larvae were significantly down-regulated in 3 peptides at different concentrations treatment groups.
Molecular docking simulation
Molecular docking is an effective way to predict the interactions between the receptor protein and small molecules (ligands) by calculating the binding sites, the interaction energies, and other interaction information (Hu et al., 2022). In this study, CDocker was applied to further analyze the interactions between the active peptides and the Keap1 protein. All three peptides displayed a stable docking structure with the Keap1 protein according to values of -CDocker energy and -CDocker interaction energy (Table 3). The results of the molecular docking analysis of the three peptides and Keap1 protein are shown in Figure 7, in which the left side is the lowest docking energy between Keap1 protein and the peptides, and the right side presents the theoretical binding modes. The results indicate that the three peptides were successfully docked against Keap1, and combined with Keap1 residues through conventional hydrogen bonds, carbon hydrogen bonds, and pi-alkyl. It was found that MHLWAAK could form three conventional hydrogen bonds with residues of Val418, Val420, and Val608, and nine carbon hydrogen bonds and π donor hydrogen bonds with residues of Gly367, Arg415, Ile416, Gly462, Val463, Val465, Val512, Ala556, and Val606. MAQAAEYYR formed five conventional hydrogen bonds with Keap1 residues Val369, Arg415, Ala510, Val514, and Ser602, and four carbon hydrogen bonds with residues of Gly364, Val465, Val467, and Cys513. MDYYFEER formed 10 conventional hydrogen bonds with Val369, Arg415, Val418, Val420, Val465, Val467, Arg483, Ser508, Val512, and Val608. Consequently, our study demonstrated the involvement of the Keap1/Nrf2 signaling pathway in peptides-exerted antioxidant action, which may aid to thoroughly understand the underlying molecular mechanism. According to our research, the possible molecular mechanisms for the protective effects of three novel antioxidant peptides against H2O2-induced oxidative damaged are presented in Figure 8.
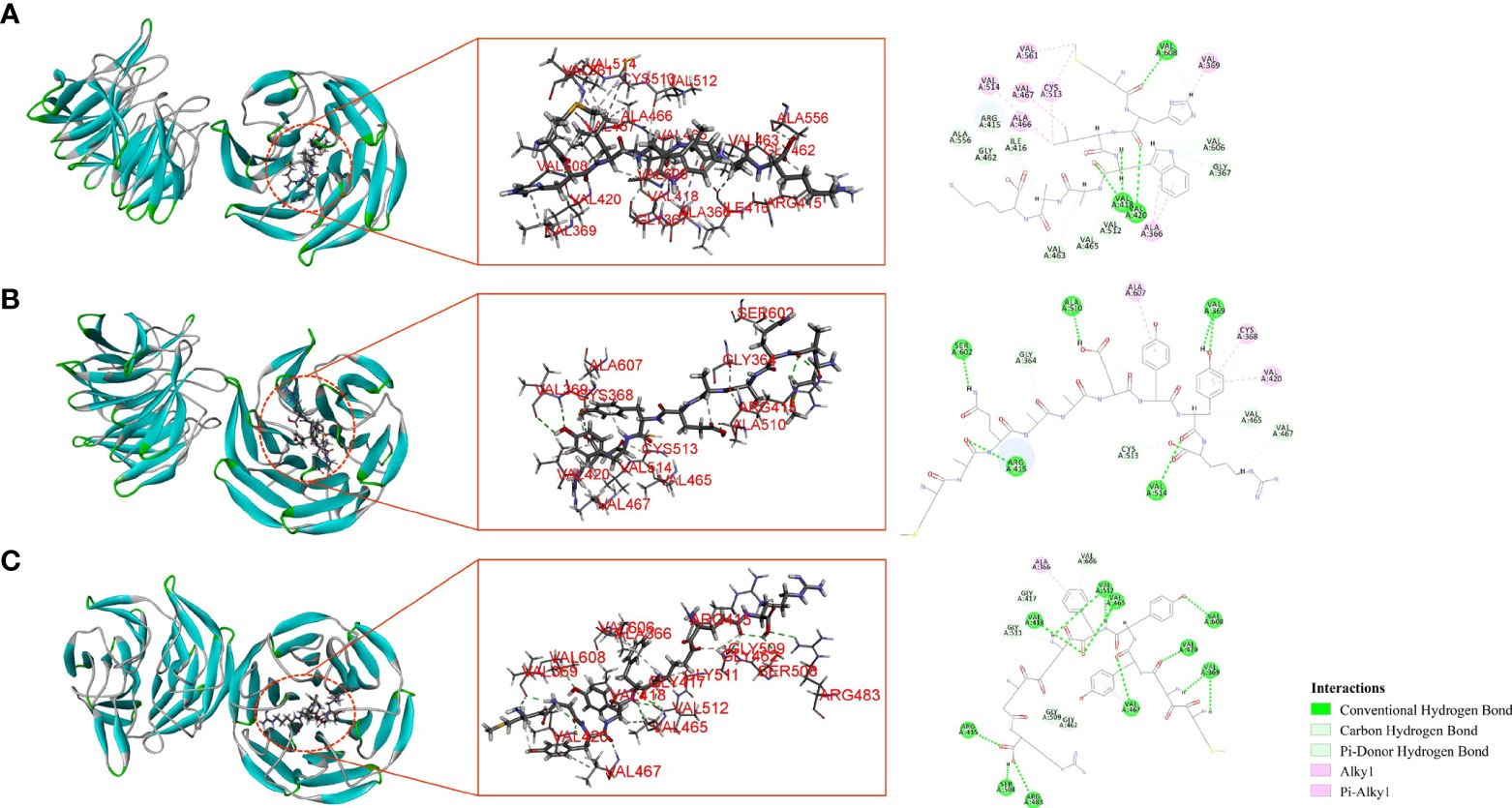
Figure 7 Molecular interactions between Keap1 and antioxidant peptides. MHLWAAK (A), MAQAAEYYR (B), and MDYYFEER (C).
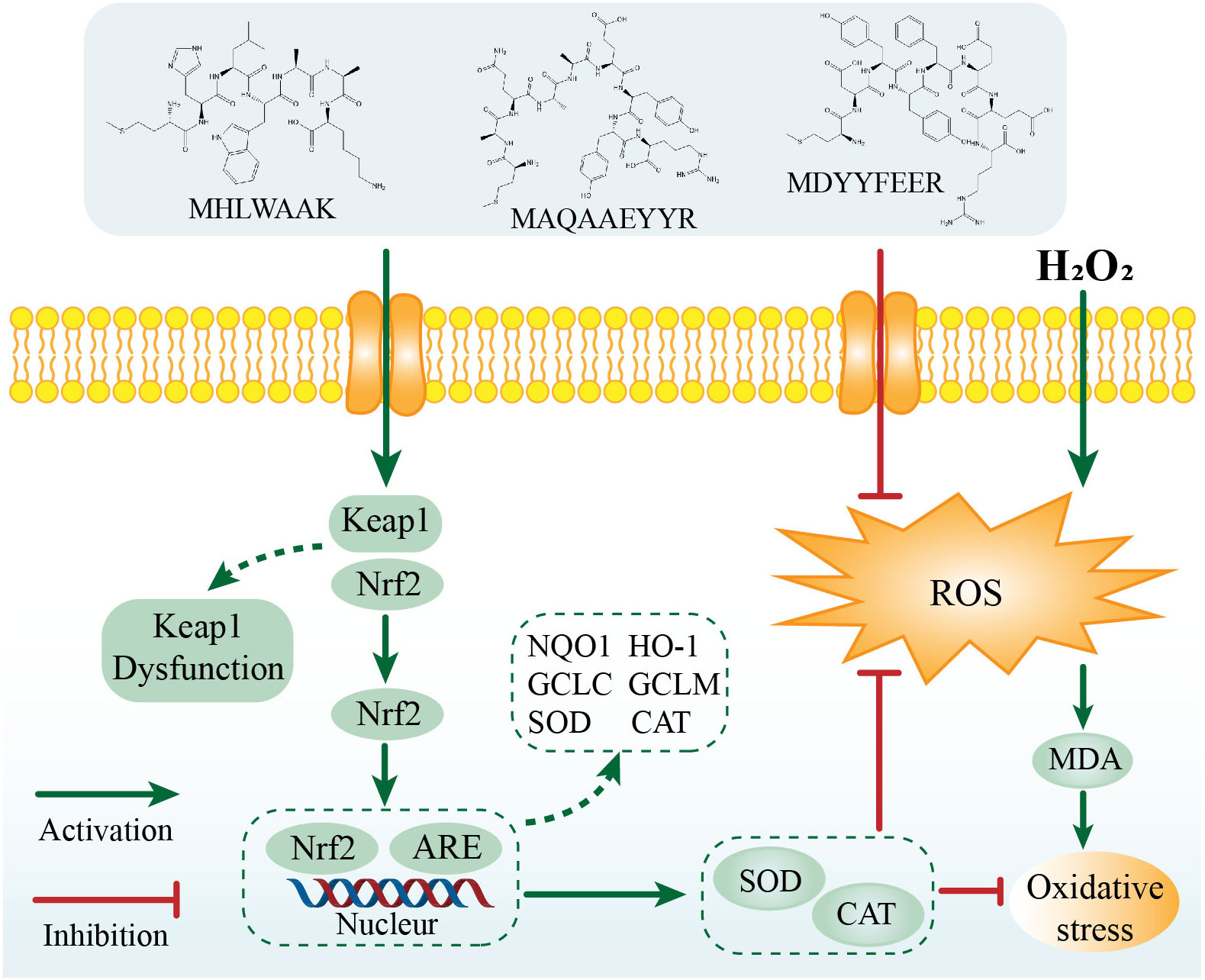
Figure 8 The possible molecular mechanism for the protective effects of three novel antioxidant peptides against H2O2-induced oxidative damaged.
Conclusion
In this study, three novel antioxidant active peptides—MHLWAAK (P1), MAQAAEYYR (P2), and MDYYFEER (P3)—were separated, purified, and characterized by gel filtration chromatography, RP-HPLC, HILIC, and LC-MS/MS. All three peptides exhibited strong antioxidant activities in DPPH and ABTS assays, along with effective ROS-scavenging capacity, increased SOD, CAT activity, and decreased MDA content, and protected tissues against oxidative damage in a zebrafish model. Further experiments demonstrated that the three peptides exerted antioxidant effects by activating the Nrf2 signaling pathway. These findings suggested that these peptides could be potential candidates for treating diseases related to oxidative stress. In addition, as novel antioxidants from natural origins, the C-PC peptides have promising potentials in functional food.
Data availability statement
The original contributions presented in the study are included in the article/Supplementary Material. Further inquiries can be directed to the corresponding authors.
Ethics statement
The animal study was reviewed and approved by Biology Institute, Qilu University of Technology of Animal Ethics Committee. Written informed consent was obtained from the owners for the participation of their animals in this study.
Author contributions
Conceptualization, FX, SZ, and BL; Data curation, FX, YZ, YQ, FY, and GL; Formal analysis, XD, GC, CC, and QZ; Funding acquisition, SZ and BL; Supervision, SZ and BL; Writing—original draft, FX; Writing—review and editing, SZ and BL. All authors contributed to the article and approved the submitted version.
Funding
This work was financially supported by grants from the National Natural Science Foundation of China (No. 81871231, 81471546 and 81001346), Youth Innovation and Technology Plan of Shandong Province (2019KJK016), Science, Education, and Industry Integration Major Innovation Project of Qilu University of Technology (Shandong Academy of Sciences) (2022JBZ01-06), the Key R&D Major Scientific and Technological Innovation Project of Shandong Province (2021CXGC010507) and the Foundation (No. ZZ20190413) of State Key Laboratory of Biobased Material and Green Papermaking, Qilu University of Technology, Shandong Academy of Sciences. Jinan Talent Project for Universities (Nos. 2020GXRC031).
Acknowledgments
We thank letpub (https://www.letpub.com/) for the language editing during the manuscript preparation. We thank Novogene. Co., Ltd. for help with the amino acid sequence analysis.
Conflict of interest
The authors declare that the research was conducted in the absence of any commercial or financial relationships that could be construed as a potential conflict of interest.
Publisher’s note
All claims expressed in this article are solely those of the authors and do not necessarily represent those of their affiliated organizations, or those of the publisher, the editors and the reviewers. Any product that may be evaluated in this article, or claim that may be made by its manufacturer, is not guaranteed or endorsed by the publisher.
Supplementary material
The Supplementary Material for this article can be found online at: https://www.frontiersin.org/articles/10.3389/fmars.2022.1098091/full#supplementary-material
Supplementary Figure 1 | Response surface plots depicting the effects of reaction temperature, pH of buffer, material-to-liquid ratio and enzyme concentration on antioxidant activity of C-PC hydrolysates prepared (A–F) DH; (G–L) DPPH radical scavenging activity.
Supplementary Figure 2 | In vivo antioxidant activity of three isolated P1, P2 and P3. (n = 10, mean ± standard deviation). (A) Transgenic fluorescent zebrafish treated with MTZ (10 mM) and different concentrations of three peptides. (B) Fluorescent spots number statistic results of all groups. *p< 0.05, **p< 0.01, ***p< 0.001 compared with the MTZ group. ####p< 0.0001 compared with the control group. Scale bar, 200 μm.
Supplementary Figure 3 | Bioactivities of C-Phycocyanin (C-PC). (A) DPPH radical scavenging activity. (B) ABTS radical scavenging activity. (C) In vivo visualization of zebrafish skin fluorescence treatment with vehicle. (D) FS number statistic results of all groups. *p< 0.05, vs. the MTZ group. ####p< 0.0001 vs. the blank control group. Scale bar, 200 μm.
References
Agrawal H., Joshi R., Gupta M. (2016). Isolation, purification and characterization of antioxidative peptide of pearl millet (Pennisetum glaucum) protein hydrolysate. Food Chem. 204, 365–372. doi: 10.1016/j.foodchem.2016.02.127
Arulselvan P., Fard M. T., Tan W. S., Gothai S., Fakurazi S., Norhaizan M. E., et al. (2016). Role of antioxidants and natural products in inflammation. Oxid. Med. Cell Longev. 2016, 5276130. doi: 10.1155/2016/5276130
Bartley I. M., Hodgson B., Walker J. S., Holme G. (1972). The use of acid alumina and sephadex LH-20 for the separation and characterization of ethanol-soluble peptides produced by bacillus brevis. Biochem. J. 127 (3), 489–502. doi: 10.1042/bj1270489
Bhardwaj J. K., Saraf P. (2020). N-acetyl-l-cysteine mediated regulation of DNA fragmentation, an apoptotic event, against methoxychlor toxicity in the granulosa cells of ovarian antral follicles. Mutat. Res. Genet. Toxicol. Environ. Mutagen 858-860, 503222. doi: 10.1016/j.mrgentox.2020.503222
Chakrabarti S., Guha S., Majumder K. (2018). Food-derived bioactive peptides in human health: Challenges and opportunities. Nutrients 10 (11), 1738. doi: 10.3390/nu10111738
Chi C.-F., Wang B., Wang Y.-M., Zhang B., Deng S.-G. (2015). Isolation and characterization of three antioxidant peptides from protein hydrolysate of bluefin leatherjacket (Navodon septentrionalis) heads. J. Funct. Foods 12, 1–10. doi: 10.1016/j.jff.2014.10.027
Dong X., Yang F., Xu X., Zhu F., Liu G., Xu F., et al. (2022). Protective effect of c-phycocyanin and apo-phycocyanin subunit on programmed necrosis of GC-1 spg cells induced by H(2) O(2). Environ. Toxicol. 37 (6), 1275–1287. doi: 10.1002/tox.23482
Garcia-Vaquero M., Mora L., Hayes M. (2019). In vitro and in silico approaches to generating and identifying angiotensin-converting enzyme I inhibitory peptides from green macroalga ulva lactuca. Mar. Drugs 17 (4), 204. doi: 10.3390/md17040204
Han J., Huang Z., Tang S., Lu C., Wan H., Zhou J., et al. (2020). The novel peptides ICRD and LCGEC screened from tuna roe show antioxidative activity via Keap1/Nrf2-ARE pathway regulation and gut microbiota modulation. Food Chem. 327, 127094. doi: 10.1016/j.foodchem.2020.127094
Hu X., Dai Z., Jin R. (2022). Purification and identification of a novel angiotensin converting enzyme inhibitory peptide from the enzymatic hydrolysate of lepidotrigla microptera. Foods 11 (13), 1889. doi: 10.3390/foods11131889
Hu X., Liu J., Li J., Song Y., Chen S., Zhou S., et al. (2022). Preparation, purification, and identification of novel antioxidant peptides derived from gracilariopsis lemaneiformis protein hydrolysates. Front. Nutr. 9, 971419. doi: 10.3389/fnut.2022.971419
Hu X. M., Wang Y. M., Zhao Y. Q., Chi C. F., Wang B. (2020). Antioxidant peptides from the protein hydrolysate of monkfish (Lophius litulon) muscle: Purification, identification, and cytoprotective function on HepG2 cells damage by H(2)O(2). Mar. Drugs 18 (3), 153. doi: 10.3390/md18030153
Islam M. S., Hongxin W., Admassu H., Noman A., Ma C., An Wei F. (2021). Degree of hydrolysis, functional and antioxidant properties of protein hydrolysates from grass turtle (Chinemys reevesii) as influenced by enzymatic hydrolysis conditions. Food Sci. Nutr. 9 (8), 4031–4047. doi: 10.1002/fsn3.1903
Kang M. C., Cha S. H., Wijesinghe W. A., Kang S. M., Lee S. H., Kim E. A., et al. (2013). Protective effect of marine algae phlorotannins against AAPH-induced oxidative stress in zebrafish embryo. Food Chem. 138 (2-3), 950–955. doi: 10.1016/j.foodchem.2012.11.005
Kulkarni A. A., Conteh A. M., Sorrell C. A., Mirmira A., Tersey S. A., Mirmira R. G., et al. (2018). An In vivo zebrafish model for interrogating ROS-mediated pancreatic β-cell injury, response, and prevention. Oxid. Med. Cell Longev 2018, 1324739. doi: 10.1155/2018/1324739
Lan X., Liao D., Wu S., Wang F., Sun J., Tong Z. (2015). Rapid purification and characterization of angiotensin converting enzyme inhibitory peptides from lizard fish protein hydrolysates with magnetic affinity separation. Food Chem. 182, 136–142. doi: 10.1016/j.foodchem.2015.02.004
Lassoued I., Mora L., Barkia A., Aristoy M. C., Nasri M., Toldrá F. (2015). Bioactive peptides identified in thornback ray skin's gelatin hydrolysates by proteases from bacillus subtilis and bacillus amyloliquefaciens. J. Proteomics 128, 8–17. doi: 10.1016/j.jprot.2015.06.016
Li B., Chu X. M., Xu Y. J., Yang F., Lv C. Y., Nie S. M. (2013). CD59 underlines the antiatherosclerotic effects of c-phycocyanin on mice. BioMed. Res. Int. 2013, 729413. doi: 10.1155/2013/729413
Li X., Cui L., Feng G., Yu S., Shao G., He N., et al. (2022). Collagen peptide promotes DSS-induced colitis by disturbing gut microbiota and regulation of macrophage polarization. Front. Nutr. 9, 957391. doi: 10.3389/fnut.2022.957391
Li J., Lu J., Asakiya C., Huang K., Zhou X., Liu Q., et al. (2022). Extraction and identification of three new urechis unicinctus visceral peptides and their antioxidant activity. Mar. Drugs 20 (5), 293. doi: 10.3390/md20050293
Li A. L., Shen T., Wang T., Zhou M. X., Wang B., Song J. T., et al. (2019). Novel diterpenoid-type activators of the Keap1/Nrf2/ARE signaling pathway and their regulation of redox homeostasis. Free Radic. Biol. Med. 141, 21–33. doi: 10.1016/j.freeradbiomed.2019.06.001
Ma Y., Wu Y., Li L. (2018). Relationship between primary structure or spatial conformation and functional activity of antioxidant peptides from pinctada fucata. Food Chem. 264, 108–117. doi: 10.1016/j.foodchem.2018.05.006
Moosmann B., Behl C. (2002). Secretory peptide hormones are biochemical antioxidants: structure-activity relationship. Mol. Pharmacol. 61 (2), 260–268. doi: 10.1124/mol.61.2.260
Ngo D.-H., Vo T.-S., Ryu B., Kim S.-K. (2016). Angiotensin- I- converting enzyme (ACE) inhibitory peptides from pacific cod skin gelatin using ultrafiltration membranes. Process Biochem. 51 (10), 1622–1628. doi: 10.1016/j.procbio.2016.07.006
Noman A., Xu Y., Al-Bukhaiti W. Q., Abed S. M., Ali A. H., Ramadhan A. H., et al. (2018). Influence of enzymatic hydrolysis conditions on the degree of hydrolysis and functional properties of protein hydrolysate obtained from Chinese sturgeon (Acipenser sinensis) by using papain enzyme. Process Biochem. 67, 19–28. doi: 10.1016/j.procbio.2018.01.009
Ó'Fágáin C., Cummins P. M., O'Connor B. F. (2017). Gel-filtration chromatography. Methods Mol. Biol. 1485, 15–25. doi: 10.1007/978-1-4939-6412-3_2
OC J., Meaney S., Williams G. A., Hayes M. (2020). Extraction of protein from four different seaweeds using three different physical pre-treatment strategies. Molecules 25 (8), 2005. doi: 10.3390/molecules25082005
Piovan A., Filippini R., Argentini C., Moro S., Giusti P., Zusso M. (2022). The effect of c-phycocyanin on microglia activation is mediated by toll-like receptor 4. Int. J. Mol. Sci. 23 (3), 1440. doi: 10.3390/ijms23031440
Qiao Q. Q., Luo Q. B., Suo S. K., Zhao Y. Q., Chi C. F., Wang B. (2022). Preparation, characterization, and cytoprotective effects on HUVECs of fourteen novel angiotensin-I-Converting enzyme inhibitory peptides from protein hydrolysate of tuna processing by-products. Front. Nutr. 9, 868681. doi: 10.3389/fnut.2022.868681
Rafique R., Khan K. M., Arshia, Chigurupati S., Wadood A., Rehman A. U., et al. (2020). Synthesis, in vitro α-amylase inhibitory, and radicals (DPPH & ABTS) scavenging potentials of new n-sulfonohydrazide substituted indazoles. Bioorg Chem. 94, 103410. doi: 10.1016/j.bioorg.2019.103410
Rastogi R. P., Sonani R. R., Madamwar D. (2015). Effects of PAR and UV radiation on the structural and functional integrity of phycocyanin, phycoerythrin and allophycocyanin isolated from the marine cyanobacterium lyngbya sp. A09DM. Photochem. Photobiol. 91 (4), 837–844. doi: 10.1111/php.12449
Romay C., González R., Ledón N., Remirez D., Rimbau V. (2003). C-phycocyanin: a biliprotein with antioxidant, anti-inflammatory and neuroprotective effects. Curr. Protein Pept. Sci. 4 (3), 207–216. doi: 10.2174/1389203033487216
Roy K. R., Arunasree K. M., Reddy N. P., Dheeraj B., Reddy G. V., Reddanna P. (2007). Alteration of mitochondrial membrane potential by spirulina platensis c-phycocyanin induces apoptosis in the doxorubicinresistant human hepatocellular-carcinoma cell line HepG2. Biotechnol. Appl. Biochem. 47 (Pt 3), 159–167. doi: 10.1042/BA20060206
Salar U., Khan K. M., Chigurupati S., Taha M., Wadood A., Vijayabalan S., et al. (2017). New hybrid hydrazinyl thiazole substituted chromones: As potential α-amylase inhibitors and radical (DPPH & ABTS) scavengers. Sci. Rep. 7 (1), 16980. doi: 10.1038/s41598-017-17261-w
Satoh T., Okamoto S.-i., Cui J., Watanabe Y., Furuta K., Suzuki M., et al. (2006). Activation of the Keap1/Nrf2 pathway for neuroprotection by electrophillic phase II inducers. Proc. Natl. Acad. Sci. 103 (3), 768–773. doi: 10.1073/pnas.0505723102
Sies H., Jones D. P. (2020). Reactive oxygen species (ROS) as pleiotropic physiological signalling agents. Nat. Rev. Mol. Cell Biol. 21 (7), 363–383. doi: 10.1038/s41580-020-0230-3
Simon H. U., Haj-Yehia A., Levi-Schaffer F. (2000). Role of reactive oxygen species (ROS) in apoptosis induction. Apoptosis 5 (5), 415–418. doi: 10.1023/A:1009616228304
Sridhar K., Inbaraj B. S., Chen B. H. (2021). Recent developments on production, purification and biological activity of marine peptides. Food Res. Int. 147, 110468. doi: 10.1016/j.foodres.2021.110468
Sun J., Zhou C., Cao J., He J., Sun Y., Dang Y., et al. (2022). Purification and characterization of novel antioxidative peptides from duck liver protein hydrolysate as well as their cytoprotection against oxidative stress in HepG2 cells. Front. Nutr. 9, 848289. doi: 10.3389/fnut.2022.848289
Wang J., Wang Y. M., Li L. Y., Chi C. F., Wang B. (2021). Twelve antioxidant peptides from protein hydrolysate of skipjack tuna (Katsuwonus pelamis) roe prepared by flavourzyme: Purification, sequence identification, and activity evaluation. Front. Nutr. 8, 813780. doi: 10.3389/fnut.2021.813780
Wołosiak R., Drużyńska B., Derewiaka D., Piecyk M., Majewska E., Ciecierska M., et al. (2021). Verification of the conditions for determination of antioxidant activity by ABTS and DPPH assays-a practical approach. Molecules 27 (1), 50. doi: 10.3390/molecules27010050
Wu Q., Liu L., Miron A., Klímová B., Wan D., Kuča K. (2016). The antioxidant, immunomodulatory, and anti-inflammatory activities of spirulina: An overview. Arch. Toxicol. 90 (8), 1817–1840. doi: 10.1007/s00204-016-1744-5
Yang F. H., Dong X. L., Liu G. X., Teng L., Wang L., Zhu F., et al. (2022). The protective effect of c-phycocyanin in male mouse reproductive system. Food Funct. 13 (5), 2631–2646. doi: 10.1039/D1FO03741B
Yi G., Din J. U., Zhao F., Liu X. (2020). Effect of soybean peptides against hydrogen peroxide induced oxidative stress in HepG2 cells via Nrf2 signaling. Food Funct. 11 (3), 2725–2737. doi: 10.1039/C9FO01466G
Yu J., Hu Y., Xue M., Dun Y., Li S., Peng N., et al. (2016). Purification and identification of antioxidant peptides from enzymatic hydrolysate of spirulina platensis. J. Microbiol. Biotechnol. 26 (7), 1216–1223. doi: 10.4014/jmb.1601.01033
Zeng Q. H., Wang J. J., Zhang Y. H., Song Y. Q., Liang J. L., Zhang X. W. (2020). Recovery and identification bioactive peptides from protein isolate of spirulina platensis and their in vitro effectiveness against oxidative stress-induced erythrocyte hemolysis. J. Sci. Food Agric. 100 (9), 3776–3782. doi: 10.1002/jsfa.10408
Zhang S., Dong Z., Peng Z., Lu F. (2014). Anti-aging effect of adipose-derived stem cells in a mouse model of skin aging induced by d-galactose. PloS One 9 (5), e97573. doi: 10.1371/journal.pone.0097573
Zhang S. S., Han L. W., Shi Y. P., Li X. B., Zhang X. M., Hou H. R., et al. (2018). Two novel multi-functional peptides from meat and visceral mass of marine snail neptunea arthritica cumingii and their activities in vitro and in vivo. Mar. Drugs 16 (12), 473. doi: 10.3390/md16120473
Keywords: C-phycocyanin, antioxidant peptides, zebrafish, molecular docking, Nrf2 signaling pathway
Citation: Xu F, Zhang Y, Qiu Y, Yang F, Liu G, Dong X, Chen G, Cao C, Zhang Q, Zhang S and Li B (2022) Three novel antioxidant peptides isolated from C-phycocyanin against H2O2-induced oxidative stress in zebrafish via Nrf2 signaling pathway. Front. Mar. Sci. 9:1098091. doi: 10.3389/fmars.2022.1098091
Received: 14 November 2022; Accepted: 02 December 2022;
Published: 23 December 2022.
Edited by:
Bin Wang, Zhejiang Ocean University, ChinaReviewed by:
Zhong-Ji Qian, Guangdong Ocean University, ChinaHuoxi Jin, Zhejiang Ocean University, China
Copyright © 2022 Xu, Zhang, Qiu, Yang, Liu, Dong, Chen, Cao, Zhang, Zhang and Li. This is an open-access article distributed under the terms of the Creative Commons Attribution License (CC BY). The use, distribution or reproduction in other forums is permitted, provided the original author(s) and the copyright owner(s) are credited and that the original publication in this journal is cited, in accordance with accepted academic practice. No use, distribution or reproduction is permitted which does not comply with these terms.
*Correspondence: Shanshan Zhang, cWluZ3NodWliYWlrYWlAMTI2LmNvbQ==; Bing Li, bGliaW5nXzUxNkBxZHUuZWR1LmNu