- 1ANSES (French Agency for Food, Environmental and Ocupational Health and Safety, Maisons-Alfort, France
- 2French Research Institute for Exploitation of the Sea (IFREMER), Biodivenv, Le Robert, France
- 3Poison Control Centre, Toulouse University Hospital, Toulouse, France
- 4Chemical, Biological, Radiological and Nuclear (CBRN) Defence, Analytical Chemistry Department, French Defense Procurement Agency (DGA), Vert-le-Petit, France
- 5Laboratory for Food Safety, ANSES (French Agency for Food, Environmental and Occupational Health and Safety), Maisons-Alfort, France
- 6INSERM UMR 1214, Toulouse NeuroImaging Center, Toulouse, France
Toxic algae and their toxins represent an emerging public health issue, particularly due to global warming. The toxicological mechanisms of neurotoxic phycotoxins and their human health effects have been widely described (paralytic, neurological, amnesic, and ciguateric toxins). Food poisoning by bivalve shellfish (mussels, oysters…) can cause serious or even fatal neurological disorders, as bivalves accumulate toxins by feeding on harmful algae. However, retrospective study of human shellfish poisoning highlighted the difficulties in identifying cases related to neurotoxic phycotoxins by gathering clinical and biological diagnostic confirmations on the one hand, as well as environmental contaminations on the other. To improve the collection of shellfish poisoning data, the French Agency for Food, Environmental and Occupational Health and Safety (ANSES), French Poison Control Centers (PCCs), the French Research Institute for Exploitation of the Sea (Ifremer) and the French Defense Procurement Agency (DGA) have developed a prospective surveillance of neurotoxins from algal origin that combines monitoring of poisoning cases (clinical monitoring), of harmful algae and their toxins in shellfish production areas (environmental monitoring), as well as of toxins levels in seafood leftovers and in biological samples from poisoned subjects. This original clinical and environmental monitoring will allow us to have a more complete view of phycotoxin human exposures that are underreported, and to implement measures to protect consumers.
1. Introduction
Marine biotoxins include phycotoxins produced by several species of toxigenic micro-algae that frequently form harmful algal blooms. Several types of marine biotoxins causing neurological signs are described in the literature. Human exposure to these marine biotoxins, most often following the ingestion of contaminated seafood, may be the cause of characteristic clinical manifestations for which the association defined a syndrome. These biotoxins, produced by harmful algae are known to accumulate in tissues of many marine species such as bivalve molluscs (mussels, oysters, scallops, cockles, clams, almonds, razor clams, etc.), fish or other marine organisms (gastropods, crustaceans, corals…).
2. Shellfish poisoning
2.1. Paralytic shellfish poisoning
This intoxication was detected and described for the first time in the 17th century. Since 1689, more than 200 cases of fatal poisoning were reported all over the world (Halstead, 1965; Young et al., 2020; Temple and Hughes, 2022). Cases of poisoning have been described regularly throughout the world since the beginning of the 20th century, including several fatal cases (Canada, Japan, Asia, South America, Europe, North Africa, North America, Australia and New Zealand) (Etheridge, 2010). Many species of dinoflagellates of the genus Alexandrium, Gymnodinium catenatum, as well as cyanobacteria produce the toxins responsible for this syndrome (Anderson et al., 1990; Costa et al., 2015; Belin et al., 2021). Saxitoxin and its derivatives are responsible for this poisoning (Arnich and Thébault, 2018). Saxitoxin is listed as one of the most toxic poisons ever known. It is also classified as a “biological weapon” in France and its possession is subject to declaration and authorization (Chemical Weapons Convention – table 1 - 1993/01/13). The symptoms associated with this poisoning are of several types depending on the degree of severity and the doses of toxins ingested, and essentially correspond to digestive or neurological signs. Thus, at low doses, the signs are generally benign and of types such as headache, nausea, vomiting, oral paresthesia sometimes extending to the neck, face and to the ends of the fingers and toes. The first symptoms appear quickly 5 to 30 minutes after consumption of contaminated shellfish. At a higher dose, the signs are much clearer with paresthesia extending to the limbs, speech disorders, motor incoordination and breathing difficulties (Akaeda et al., 1998; Knaack et al., 2016). In the ultimate cases, at very high doses, respiratory paralysis is observed which can lead to coma and death (Lehane, 2001). There is no antidote. Only rapid respiratory medical assistance (putting on an artificial respirator) is effective. In France, saxitoxin and its derivatives have been described since 1988 (Brittany and Mediterranean coast) and can be found in bivalves and gastropods (Belin et al., 2021).
2.2. Amnesic shellfish poisoning
This syndrome was demonstrated for the first time in Canada in 1987 in Prince Edward Island (Bates et al., 1989). This group poisoning, almost the only one of this magnitude to have been listed in the world, affected 145 people, 22 of whom had to be hospitalized for serious symptoms. Four of them died. The domoic acid produced by diatoms of the genus Pseudo-nitzschia, was identified as the causative toxin. The first signs of poisoning appear 24 hours after ingesting the contaminated products in the form of digestive signs, such as vomiting, nausea and abdominal pain. After 48 hours, the first neurological symptoms appear such as loss of short-term memory, mental confusion, disorientation, dizziness, headaches, drowsiness, even an agitated coma with convulsions (Jeffery et al., 2004). Late effects may result in short-term memory loss. Depending on the degree of intoxication, irremediable brain damage may occur. Domoic acid is particularly present in shellfish collected in European coastal waters, especially in Brittany, the Atlantic coast and the English Channel (Belin et al., 2021). It can therefore potentially be the cause of poisoning in France.
2.3. Neurotoxic shellfish poisoning
This intoxication currently concerns only a few regions of the world, the Gulf of Mexico, Florida, Texas, North Carolina in the northern hemisphere and New Zealand in the southern hemisphere. This poisoning has been described for a very long time as early as 1844. There is no poisoning described in Europe. This intoxication is caused by the brevetoxins produced by, among other things, Karenia brevis (Gymnodinium breve) (Pierce et al., 2003; Pierce et al., 2005; Watkins et al., 2008; Wolny et al., 2015). As with other poisonings, these toxins impact humans consuming marine animals ranging from invertebrates to marine mammals at the top of the food chain (Flewelling et al., 2005; Naar et al., 2007; Hinton and Ramsdell, 2008; Fire et al., 2015). Populations become poisoned by ingesting seafood, but also by inhaling aerosols containing toxins (Pierce et al., 2003; Pierce et al., 2005). Symptoms related to this poisoning depend on the route of contact with the toxin. By ingestion, neurological and gastrointestinal syndromes appear one to three hours after consumption. There are no reported fatal cases. Recovery is complete 24 to 48 hours after ingestion. Exposure to aerosols containing brevetoxins can be responsible for eye irritation, dry cough and asthma-like respiratory discomfort (Arnich et al., 2021; Abdullah et al., 2022). Brevetoxins were detected for the first time in France in mussels from the Diana lagoon in Corsica in 2018 (Guillou et al., 2002; Amzil et al., 2021). The species of microalgae producing these toxins have not yet been identified.
2.4. Tetrodotoxin poisoning
This poisoning is mainly reported in Asia following the consumption of puffer fish (or fugu) or gastropods, and is the cause of several deaths each year, mainly in Japan, Hong Kong and Bangladesh (Mahmud et al., 2000; Ahmed, 2006; Jen et al., 2007; Guardone et al., 2020; Cheung and Chan, 2022). To date, no case of poisoning by tetrodotoxins has been described following the consumption of shellfish. The symptoms are those that may be associated with a neurotoxin and occur from a few minutes after consumption and up to 4 hours after the meal. Observable symptoms include oral and perioral paresthesias, accompanied by nausea, vomiting and sometimes with diarrhea and abdominal pain, dizziness, pallor, malaise, changes in deep sensation, throat and larynx are observed at the origin of dysphagia or even complete aphagia, as well as dysphonia, bilateral mydriasis, bradycardia, hypotension, hypersialorrhoea, hypothermia, hypersudation, asthenia, cyanosis of the extremities and lips, petechial haemorrhages on the body (You et al., 2015). Death occurs by cardio-respiratory arrest in the most serious cases. Tetrodotoxins have been detected in Europe (Italy (Bordin et al., 2021) and Holland). They are also present in France (shellfish and gastropods), but in small amounts (Hort et al., 2020).
2.5. Pinnatoxin poisoning
Pinnatoxins are cyclic imines produced by dinoflagellates of the genus Vulcanodinium (Nézan and Chomérat, 2011). No cases of human poisoning by ingestion by pinnatoxins have been reported to date. In 2013, Vulcanodinium rugosum was identified in the Persian Gulf (Al Muftah et al., 2016) and in 2015 in Cuba in Cienfuegos Bay. Vulcanodinium rugosum is suspected to be the cause of skin lesions of bathers by contact in Cuba (Moreira-González et al., 2021). Based on pharmacological and acute toxicity data in mice, an extrapolation to humans of the effects observed after acute exposure in mice has been suggested (Delcourt et al., 2021). The following symptoms, after consuming shellfish or contact with contaminated sea water, can be attributed to pinnatoxins: neuromuscular signs (myasthenic syndrome associated with in particular hypotonia, dysphagia, ptosis), neurological signs (pyramidal syndrome, seizures convulsions), anticholinergic syndrome (dryness of the mucous membranes, constipation, mydriasis, accommodation disorders, painful exophthalmia, sinus tachycardia, acute urinary retention), respiratory signs (dyspnea which can go as far as an acute respiratory distress syndrome in the most serious), and cardiovascular signs (arterial hypotension, tachycardia). In France, V. rugosum was identified by Ifremer in 2011, from water samples from the Ingril lagoon on the Mediterranean coast in Occitanie in water samples taken in 2009 (Hess et al., 2013; Abadie et al., 2016). Since that date and up until 2020, elevated pinnatoxin concentrations were measured in Ingril mussels every year for several months. Vic and Prévost lagoons (near Ingril lagoon), the Diana lagoon in Corsica and the Scoré areas in Brittany, are also affected by the presence of pinnatoxins, but to a lesser extent than Ingril (Arnich 2021). Professional and recreational fishing, as well as the farming of all shellfish groups, has been prohibited in the Ingril lagoon by prefectural decree as of January 1, 2020.
2.6. Regulations
In European Union, the presence of certain phycotoxins, including amnesic (ASP) and paralytic (PSP) toxins, is regulated in shellfish under the “Hygiene Package” (Regulation (EC) No. 854/2004 of April 29, 2004) (Nicolas et al., 2017). The “Hygiene Package” is a set of European regulations directly applicable in all Member States. It applies to the entire agri-food sector from primary, animal and plant production to distribution to the end consumer, including the agri-food industry, catering trades and transport. This legislation relating to hygiene entered into force on January 1, 2006. The maximum regulatory levels in shellfish are listed in Regulation (EC) No. 853/2004 of April 29, 2004 (Annex III, section VII, chapter V). Regulatory thresholds are thresholds above which shellfish are considered unfit for consumption and withdrawn from the market. Concerning the toxins causing neurotoxic syndromes, the regulatory threshold for saxitoxins in shellfish is 800 µg of saxitoxin equivalent/kg of total flesh, and that of domoic acid is 20 mg/kg of total flesh (Nicolas et al., 2017).
2.7. Poisoning by phycotoxins at the origin of neurological disorders in France
We recently conducted a retrospective study of poisoning cases by bivalve shellfish (oysters, mussels and scallops) recorded by the French Poison Control Centers (PCCs) from 2012 to 2019, looking for a link with environmental monitoring data when possible, specifically in order to identify those cases presenting neurological signs compatible with neurotoxic phycotoxins (Sinno-Tellier et al., 2022). Among the 619 shellfish poisoning cases recorded by the PCCs between 2012 and 2019, 22% (n = 134) had reported at least one neurological symptom (headache, dizziness or paraesthesia). The review of medical records for the 134 patients led to suspicion of 14 cases of PSP and one case of ASP.
Our retrospective study highlighted the difficulties in identifying cases related to neurotoxic phycotoxins by gathering clinical and biological diagnostic confirmations on the one hand, as well as environmental contaminations on the other. Moreover, a recent systematic analysis reported that few formal epidemiological studies, except for ciguatera poisoning, were related to cases of intoxication by neurotoxic phycotoxins in the world (Young et al., 2020).
2.8. Prospective surveillance of shellfish poisonings by neurotoxins
To improve the collection of shellfish poisoning data, the French Agency for Food, Environmental and Occupational Health and Safety (ANSES), French Poison Control Centers (PCCs), the French Research Institute for Exploitation of the Sea (Ifremer) and the French Defense Procurement Agency (DGA) have developed a prospective surveillance of PSP that combines monitoring of poisoning cases (clinical monitoring), of harmful algae and their toxins in shellfish production areas (environmental monitoring), as well as of toxins levels in seafood leftovers and in biological samples from poisoned subjects. First, we have developed a questionnaire to collect all relevant data when neurological signs related to shellfish consumption are reported during the first call to a PCC: clinical signs associated with marine neurotoxins, quantity of shellfish ingested, type and location of purchase, and shellfish production area. The patients should keep and freeze any meal leftovers for subsequent analyses. Measurement of toxin concentrations in unconsumed shellfish are key, especially when poisoning is associated with shellfish harvested from uncontrolled areas. The suspected cases should be referred to the emergency department of a hospital, for collection of biological samples that may be frozen (in particular for a posteriori testing for marine toxins, even those not yet regulated in European countries such as pinnatoxins and brevetoxins). These samples will help to confirm the poisoning. Finally, ANSES has set up daily automatic monitoring of cases of shellfish poisoning recorded in the PCC national database to detect any suspected case the day following the call to the PCC. The aims are to launch appropriate investigations, alert the competent authorities, and inform the population as soon as possible. Therefore, any healthcare professional suspecting this type of poisoning should immediately contact a PCC (Figure 1).
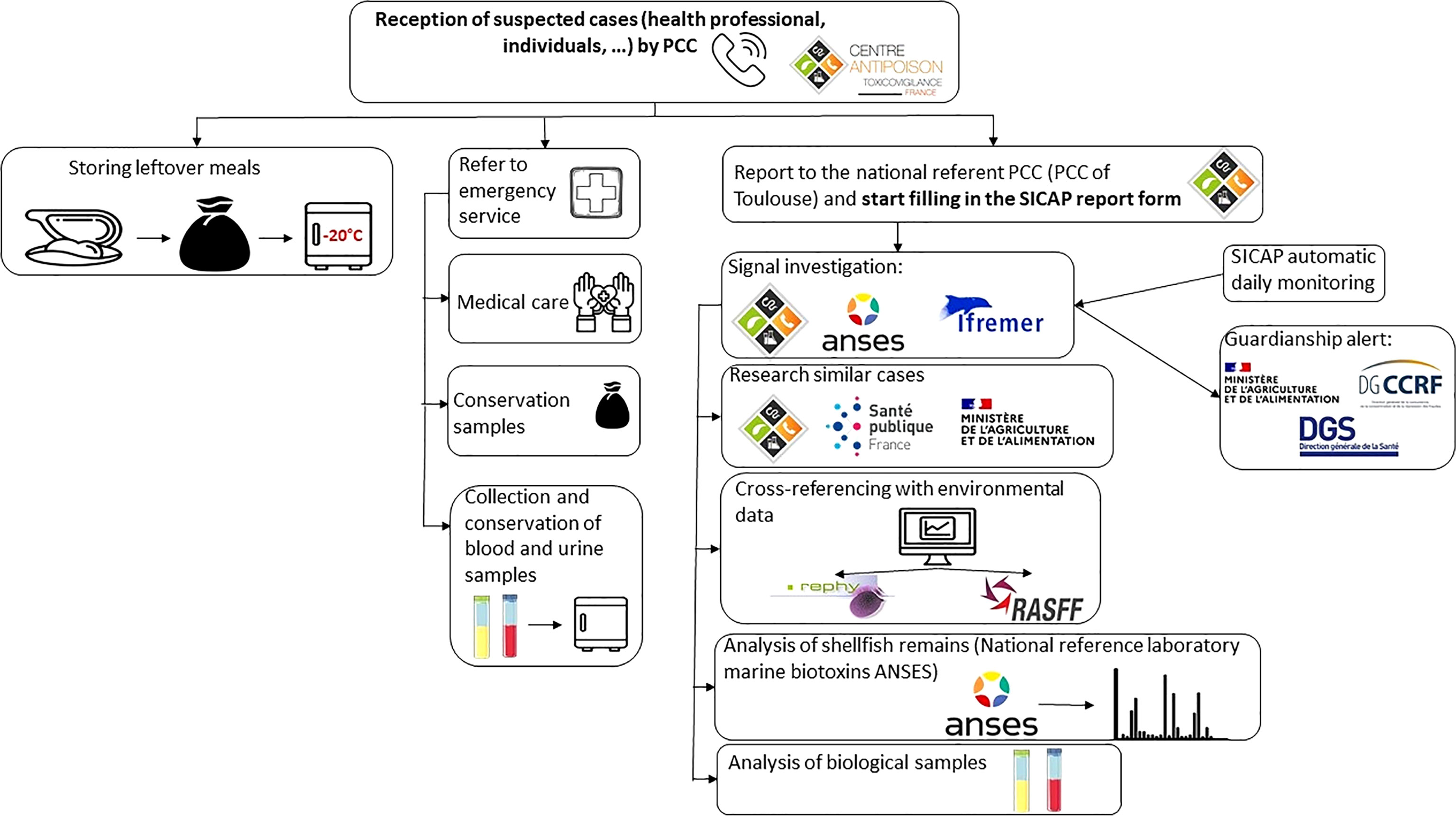
Figure 1 Scheme for the management of a suspected case of shellfish poisonings following the developed prospective surveillance in France.
2.9. Clinical monitoring
The monitoring of poisoning cases is carried out by the eight French PCCs. French PCCs provide 24/7 teleconsultations during which physicians or pharmacists with expertise in toxicology conduct a telephone interview with the patient or the healthcare professional who calls for urgent medical advice and/or toxicological expertise. All PCC cases, corresponding to “patients”, are collected in the French PCC national database (SICAP) in medical records, corresponding to “events”, each event containing either a single case or collective cases related to the same exposure. Each record is related to one or several exposure agents registered in the French National Product and Formulation Database (FNPFD) of the SICAP. When necessary, cases are followed up by the PCC and new information is recorded in the SICAP. Cases are defined as symptomatic human poisoning in relation to bivalve mollusc consumption in France, regardless of symptomatology, registered by the PCCs. Cases associated with agents referenced in the “Bivalve shellfish” class of the FNPFD during the study period are extracted from the SICAP. A keyword search in the free-text sections of the medical record supplemented the identification of the cases: %mussel%, %oyster%, %scallops%, %clam%. All medical records are reviewed by the toxicologist investigators working on the study to determine whether or not the exposure agent was a bivalve and if so, which one. For cases that could be linked with neurotoxic phycotoxins, the clinical severity is assessed using the poisoning severity score (PSS), composed of five levels ranging from PSS0 (no symptoms) to PSS4 (death). The overall severity of a case is the severity level of its most severe symptom. The probability of a causal link between exposure to an agent and the onset of a symptom, syndrome, or disease, was established according to the toxicovigilance method (https://tv.antipoison.fr/v7.6/Calcul_imputabilite.html), using five levels (no causal link, not excluded/doubtful, possible, probable, and very probable). The causal link is assessed according to six independent factors: exposure, symptoms, chronology, metrology or biological dosages, differential diagnosis and bibliography. For each factor, a degree of certainty is coded (0, 1, 2).
In addition, ANSES and the PCCs have set up automatic daily monitoring of cases of shellfish poisoning recorded in the SICAP. Also, any health professional who is aware of a patient reporting neurological signs after consuming shellfish must immediately contact a PCC who can guide the action to be taken, make the link with the search for toxins in shellfish and alert the authorities, national and regional health services in order to take management and prevention measures, depending on the elements of the investigation. A specific questionnaire (Supplementary Figure 1) was developed to collect all the necessary data. This mainly involves looking for the clinical signs described in syndromes linked to marine neurotoxins, documenting their origin, place of purchase and production area, and estimating the quantity of shellfish ingested. An important point is then to ask intoxicated people to keep leftover meals and freeze them at home for analysis. The competent health authorities may be responsible for recovering them if additional investigations are carried out. Finally, the course of action provides for the person to be sent to the emergency room in order to take biological blood and urine samples to keep (in particular to search for saxitoxins in the urine a posteriori), which will be useful to confirm the intoxication.
2.10. Environmental monitoring
There is a national system for monitoring regulated marine biotoxins (Belin et al., 2021). This monitoring is carried out on one hand at the level of the marine production areas (REPHY for phytoplankton and REPHYTOX for regulated phycotoxins and EMERGETOX for non-regulated emerging phycotoxins) and on the other hand at the stage of placing shellfish on the market in the framework of monitoring plans and control plans set up each year by the Directorate General for Food (DGAL).
The French Research Institute for the Exploitation of the Sea (Ifremer) is responsible for providing the State and other legal persons governed by public law with its support for the exercise of their responsibilities, in particular for the control of the quality of products from the sea and the marine environment (decree of 5 June 1984 amended). As such, Ifremer performs a regulatory mission and a public service activity delegated by the Ministry of Agriculture through the DGAL. The implementation of monitoring of phytoplankton and phycotoxins since 1984 responds to this mission. The monitoring of phycotoxins in shellfish is the subject of a subsidy for public service charges and an annual agreement with the DGAL. Since 2016, the monitoring of phytoplankton and phycotoxins has been reorganised into two separate networks that consider the environmental (REPHY) and health (REPHYTOX) components. These two components were associated prior to 2016.
REPHY, for the environmental component, is entitled: “Network for the Observation and Monitoring of Phytoplankton and Hydrology in Coastal Waters”. The monitoring of phytoplankton species within the framework of the REPHY, including that of harmful and toxic species, contributes to the implementation of monitoring of phycotoxins. According to the procedures specific to each REPHY monitoring site, the appearance in the environment of toxic species triggers alerts considered in REPHYTOX.
REPHYTOX, for the health component, is entitled: “Monitoring network for phycotoxins in marine organisms”. It has been managed by the decentralised State services since 2018. Ifremer is associated with this network as “Project Management Assistance”. REPHYTOX is responsible for researching and monitoring regulated toxins likely to accumulate in consumer marine products, in particular bivalve molluscs, present in production areas or in professionally exploited natural deposits. The surveillance carried out by REPHYTOX applies to shellfish in their natural environment, i.e. in production areas (parks, channels, bouchots, etc.) or in professional fishing areas. REPHYTOX is made up of several hundred shellfish sampling locations, sufficient to provide the necessary information to the administrations responsible for managing the health risk and responsible for taking official decisions to ban fishing and the marketing of contaminated shellfish.
The EMERGTOX system, monitoring the emergence of marine biotoxins in shellfish, is implemented in addition to national monitoring systems for regulated toxins, in particular REPHYTOX. Coordinated by the DGAL, this monitoring system has several objectives. First, the identification of known regulated and unregulated toxins, listed in France or which may be introduced into France via ballast water or trade between countries and which are likely to represent a danger through the consumption of shellfish. Secondly, the acquisition of data on the main groups of lipophilic and hydrophilic toxins listed at international level, to contribute to the assessment of the risks linked to the presence of new or emerging toxins in shellfish, such as pinnatoxins and tetrodotoxins. Thirdly, during the detection of toxins not yet listed in France, the network must enable the identification of emerging toxic micro-algae, via retrospective expertise of the results of analyses of water samples associated with EMERGTOX points, carried out in the REPHY framework. The EMERGTOX system concerns eleven bivalve mollusc production areas spread over the entire French metropolitan coast and is implemented by the Ifremer laboratories and the marine biotoxins laboratory from ANSES.
2.11. Measurement of toxin level in food
Measurement of toxin concentrations in unconsumed shellfish represents a key point in making the diagnosis of a human shellfish poisoning. As mentioned in the questionnaire, patients are encouraged to keep and freeze any meal leftovers for subsequent analyses.
Toxin levels are measured using a method based on Turner et al. (Turner et al., 2015). In brief, an ultrahigh-performance liquid chromatography utilising hydrophilic interaction with tandem mass spectrometry method (HILIC-MS/MS) is implemented for the quantitative determination of the paralytic shellfish poisoning toxins (PSTs) in shellfish. PSTs are extracted from 5 g of shellfish tissue by heating with 5 mL of 1% acetic acid. After centrifugation, 5 µL of ammonia solution is added to 1 ml of the supernatant, which is then purified through a desalting solid-phase extraction clean-up (SPE) using a graphitised carbon cartridge, diluted with acetonitrile and analysed by HILIC-MS/MS with a gradient elution. PST analogues determined are N-sulfocarbamoyl gonyautoxin-2 (C1), N-sulfocarbamoyl gonyautoxin-3 (C2), N-sulfocarbamoyl gonyautoxin-1 (C3) and N-sulfocarbamoyl gonyautoxin-4 (C4), decarbamoyl gonyautoxin-1 (dcGTX1), decarbamoyl gonyautoxin-2 (dcGTX2), decarbamoyl gonyautoxin-3 (dcGTX3), decarbamoyl gonyautoxin-4 (dcGTX4), gonyautoxin-1 (GTX1), gonyautoxin-2 (GTX2), gonyautoxin-3 (GTX3), gonyautoxin-4 (GTX4), gonyautoxin-5 (GTX5), gonyautoxin-6 (GTX6), deoxydecarbamoyl saxitoxin (doSTX), decarbamoyl saxitoxin (dcSTX), decarbamoyl neosaxitoxin (dcNEO), saxitoxin (STX), neosaxitoxin (NEO). Total toxicity is calculated as saxitoxin di-hydrochloride equivalents by applying the Toxicity Equivalence Factor (TEF) for each congener.
The method for the determination of ASP in shellfish is a Reverse-Phase High Performance Liquid Chromatography with Ultra Violet detection (RP HPLC-UV). The method is based on the EU-Harmonised Standard Operating Procedure, which builds on a method developed by Quilliam (Quilliam, 1995). Domoic acid (DA) and epidomoic acid (epi-AD) are extracted by 50% aqueous methanol from a homogenised shellfish tissue. After centrifugation, the supernatant is filtered through a filter membrane and analysed by RP-HPLC in isocratic elution with UV detection. The DA content, expressed as the sum of domoic acid and epidomoic acid, is calculated using an external standard.
2.12. Measurement of toxin level in human matrices
As phycotoxins are not currently measured in a medical biology laboratory, our protocol is based on a collaboration with a French military laboratory where protocols for marine toxin measurement were used in the context of Chemical, Biological, Radiological and Nuclear (CBRN) risk. Patients should be referred to the emergency department of a hospital, for collection of biological samples that may be frozen. These samples will help to confirm the poisoning.
Saxitoxins and gonyautoxins will be analysed by liquid chromatography (LC) coupled to mass spectrometry (MS) either by high resolution (HR) on a quadrupole time of flight (QTOF) or by low resolution (LR) tandem mass spectrometry (MS/MS) on a triple quadrupole (TQ) in multiple reaction monitoring (MRM) mode. Saxitoxin and its analogues are excreted under their intact form in human urine and can be detected and distinguished after solid phase extraction (SPE) by LC-MS/MS at ppb level in order to demonstrate the intoxication by these toxins. Saxitoxin and its analogues can also be searched for forensic intoxication purposes in human plasma samples, in the same way as the urine matrix, after a sample treatment to minimise matrix effects and obtain an enrichment factor in order to reach a ppb level sensitivity. Two types of LC-MS/MS experiments would be explored: one with HILIC separation of the intact saxitoxin and its analogues and the other with a reversed phase chromatography on C18 column after off-line oxidation by H2O2. The estimated quantitation of each identified toxin would be performed by comparison of the peak areas of references of saxitoxin and its analogues spiked in treated biological matrices at known concentrations, and the analysed samples.
3. Discussion
Toxic algae and their toxins represent an emerging public health issue, particularly due to global warming (Berdalet et al., 2015; Brandenburg et al., 2019; Trainer et al., 2020). However, even though the toxic mechanisms and the effects on human health have been described for a certain number of them (paralytic, neurotoxic, amnesic, ciguateric toxins, etc.), very little published data make it possible to know the extent of poisoning in humans both in terms of the number of poisonings, sequelae following acute exposure, and effects during chronic exposure. A recent bibliographic meta-analysis reports that the number of epidemiological studies reporting cases of phycotoxin poisoning is very low (Young et al., 2020). This study highlighted shortcomings in surveillance systems and epidemiological studies, as well as a very limited use of toxin measurements in humans and the scarcity of studies on chronic exposure. A recent study, based on a retrospective analysis (2012-2019) of cases of shellfish poisoning causing neurological signs recorded by French PCC, has shed light on the difficulties of clinical and biological diagnostic confirmation of on the one hand, as well as environmental contamination on the other hand, from these poisonings.
First of all, certain neurological symptoms, which may be part of the clinical picture of intoxication by neurotoxins, are not very specific (dizziness, headache, etc.) when they are isolated. They alone do not make it possible to make a diagnosis of intoxication by neurotoxins in the absence of other information in the file. The review of the files generally does not make it possible to distinguish whether questions on the symptoms that could be associated with marine neurotoxins had been systematically asked at the time of the call or not. A second major difficulty comes from the lack of confirmation of the diagnosis by the detection and/or quantitation of neurotoxins in human matrices (plasma, urine), which is not currently carried out routinely by city or hospital biology laboratories. It is thus noted that the diagnosis of PSP or ASP does not appear in the SICAP medical file nor in the hospital reports, and was only mentioned by the expert editors after proofreading and analysis of the files, and correlation with environmental data. Thus, intoxications by marine neurotoxins seem to be underestimated, due both to the difficulties of diagnosis resulting from a certain ignorance of these toxins by the medical profession, as well as to the great difficulty of confirming the diagnosis by a dosage of these toxins in human matrices (plasma, urine).
The origin of the shellfish is essential in order to be able to seek information on possible levels of toxins detected from REPHYTOX or RASFF (Rapid Alert System for Food and Feed) examiners if the shellfish have been imported. This search for information greatly contributes to the diagnosis. This information needs to be collected for each suspected case situation. Indeed, a third limitation stems from the difficulty of linking clinical data and environmental data, which are not accessible to emergency physicians or PCC toxicologists at the time of the call. Since the observation of the first episodes of PSP contamination in shellfish production areas in 1988, it has always been very difficult in France to link the consumption of shellfish to human poisoning. It is difficult to show that the poisonings described in the data recorded by the PCC can be linked to episodes of contamination of the marine environment where the shellfish are raised and caught. In addition, the severity of the poisoning remains dependent on the level of contamination of the shellfish consumed. The higher the dose of toxin ingested, the greater the symptoms expressed. Even if the regulations already incorporate this principle, and the thresholds set try to take the dose effect into account, the sensitivity of the analysis techniques and the absence of toxicological data can limit consumer protection. Thus, in the case of paralysing toxins (associated with the PSP syndrome), the routine use of a bioassay made it impossible to go below a threshold of around 350 µg STX eq/kg of shellfish meat (threshold of the bioassay method). In the June 2019 alert, the concentrations of saxitoxins measured in the mussels of the incriminated batch (313 µg/kg of mussel flesh) were below the regulatory threshold (800 µg/kg of shellfish flesh). The link between the symptoms and exposure to paralytic toxins was established thanks to the reporting to RASFF by the distributor of the product, which was not mandatory given the concentrations measured below the regulatory limit. The two probable cases of Italian mussel poisoning (PSP) identified as a result of prospective surveillance seem to confirm that the threshold of 800 µg eq STX/kg is not protective enough (Delcourt et al., 2021).
Moreover, it is often not possible to recover leftover meals (forgetting to ask, lack of leftover meals or thrown in the trash by the consumer, etc.), in order to look for the presence of these toxins in the shellfish consumed. It is therefore important that this request be made as soon as possible, as soon as the call is made to the PCC. The dosages of toxins in the remains of shells are indeed essential during poisoning by shells collected by amateur shore fishermen, in uncontrolled areas. Similarly, the collection of plasma and urine samples from patients will allow both confirmation of intoxication, but will also make it possible to enrich knowledge of toxicokinetics and the dose-response effects of these phycotoxins.
Together, this original clinical, analytical and environmental monitoring will allow us to have a more complete view of phycotoxin human exposures that are underreported, and to implement measures to protect consumers.
Data availability statement
The original contributions presented in the study are included in the article/Supplementary Material. Further inquiries can be directed to the corresponding author.
Author contributions
ND, SS-T and EA conceptualized the manuscript. All authors contributed to the article and approved the submitted version. ND supervised the project.
Conflict of interest
The authors declare that the research was conducted in the absence of any commercial or financial relationships that could be construed as a potential conflict of interest.
Publisher’s note
All claims expressed in this article are solely those of the authors and do not necessarily represent those of their affiliated organizations, or those of the publisher, the editors and the reviewers. Any product that may be evaluated in this article, or claim that may be made by its manufacturer, is not guaranteed or endorsed by the publisher.
Supplementary material
The Supplementary Material for this article can be found online at: https://www.frontiersin.org/articles/10.3389/fmars.2022.1089585/full#supplementary-material
References
Abadie E., Muguet A., Berteaux T., Chomérat N., Hess P., Roque D’OrbCastel E., et al. (2016). Toxin and growth responses of the neurotoxic dinoflagellate Vulcanodinium rugosum to varying temperature and salinity. Toxins (Basel) 8, E136. doi: 10.3390/toxins8050136
Abdullah L., Ferguson S., Niedospial D., Patterson D., Oberlin S., Nkiliza A., et al. (2022). Exposure-response relationship between K. brevis blooms and reporting of upper respiratory and neurotoxin-associated symptoms. Harmful Algae 117, 102286. doi: 10.1016/j.hal.2022.102286
Ahmed S. (2006). Puffer fish tragedy in Bangladesh: an incident of Takifugu oblongus poisoning in degholia, khulna. Afr. J. Mar. Sci. 28, 457–458. doi: 10.2989/18142320609504197
Akaeda H., Takatani T., Anami A., Noguchi T. (1998). Mass outbreak of paralytic shellfish poisoning due to ingestion of oysters at tamano-ura, goto islands, Nagasaki, Japan. J. Food Hygienic Soc. Japan (Japan) 39, 272–274.
Al Muftah A., Selwood A. I., Foss A. J., Al-Jabri H. M. S. J., Potts M., Yilmaz M. (2016). Algal toxins and producers in the marine waters of Qatar, Arabian gulf. Toxicon 122, 54–66. doi: 10.1016/j.toxicon.2016.09.016
Amzil Z., Derrien A., Terre Terrillon A., Duval A., Connes C., Marco-Miralles F., et al. (2021). Monitoring the emergence of algal toxins in shellfish: First report on detection of brevetoxins in French Mediterranean mussels. Mar. Drugs 19, 393. doi: 10.3390/md19070393
Anderson D. M., Kulis D. M., Sullivan J. J., Hall S., Lee C. (1990). Dynamics and physiology of saxitoxin production by the dinoflagellates Alexandrium spp. Mar. Biol. 104, 511–524. doi: 10.1007/BF01314358
Arnich N., Abadie E., Amzil Z., Dechraoui Bottein M.-Y., Comte K., Chaix E., et al. (2021). Guidance level for brevetoxins in French shellfish. Mar. Drugs 19, 520. doi: 10.3390/md19090520
Arnich N., Thébault A. (2018). Dose-response modelling of paralytic shellfish poisoning (PSP) in humans. Toxins (Basel) 10, E141. doi: 10.3390/toxins10040141
Bates S. S., Bird C. J., Freitas A. S. W., de Foxall R., Gilgan M., Hanic L. A., et al. (1989). Pennate diatom Nitzschia pungens as the primary source of domoic acid, a toxin in shellfish from Eastern prince Edward island, Canada. Can. J. Fish Aquat Sci. 46, 1203–1215. doi: 10.1139/f89-156
Belin C., Soudant D., Amzil Z. (2021). Three decades of data on phytoplankton and phycotoxins on the French coast: Lessons from REPHY and REPHYTOX. Harmful Algae 102, 101733. doi: 10.1016/j.hal.2019.101733
Berdalet E., Fleming L. E., Gowen R., Davidson K., Hess P., Backer L. C., et al. (2015). Marine harmful algal blooms, human health and wellbeing: challenges and opportunities in the 21st century. J. Mar. Biol. Assoc. U K 2015, 61–91. doi: 10.1017/S0025315415001733
Bordin P., Dall’Ara S., Tartaglione L., Antonelli P., Calfapietra A., Varriale F., et al. (2021). First occurrence of tetrodotoxins in bivalve mollusks from northern Adriatic Sea (Italy). Food Control 120, 107510. doi: 10.1016/j.foodcont.2020.107510
Brandenburg K. M., Velthuis M., Van de Waal D. B. (2019). Meta-analysis reveals enhanced growth of marine harmful algae from temperate regions with warming and elevated CO2 levels. Global Change Biol. 25, 2607–2618. doi: 10.1111/gcb.14678
Cheung K. S., Chan C. K. (2022). A 12-year retrospective review of tetrodotoxin poisoning in Hong Kong. Hong Kong J. Emergency Med. 10249079221106840. doi: 10.1177/10249079221106841
Costa P. R., Robertson A., Quilliam M. A. (2015). Toxin profile of Gymnodinium catenatum (Dinophyceae) from the Portuguese coast, as determined by liquid chromatography tandem mass spectrometry. Mar. Drugs 13, 2046–2062. doi: 10.3390/md13042046
Delcourt N., Arnich N., Sinno-Tellier S., Franchitto N. (2021). Mild paralytic shellfish poisoning (PSP) after ingestion of mussels contaminated below the European regulatory limit. Clin. Toxicol. (Phila) 59, 76–77. doi: 10.1080/15563650.2020.1788055
Etheridge S. M. (2010). Paralytic shellfish poisoning: seafood safety and human health perspectives. Toxicon 56, 108–122. doi: 10.1016/j.toxicon.2009.12.013
Fire S. E., Flewelling L. J., Stolen M., Durden W. N., Wit M., de Spellman A. C., et al. (2015). Brevetoxin-associated mass mortality event of bottlenose dolphins and manatees along the east coast of Florida, USA. Mar. Ecol. Prog. Ser. 526, 241–251. doi: 10.3354/meps11225
Flewelling L. J., Naar J. P., Abbott J. P., Baden D. G., Barros N. B., Bossart G. D., et al. (2005). Brevetoxicosis: red tides and marine mammal mortalities. Nature 435, 755–756. doi: 10.1038/nature435755a
Guardone L., Maneschi A., Meucci V., Gasperetti L., Nucera D., Armani A. (2020). A global retrospective study on human cases of tetrodotoxin (TTX) poisoning after seafood consumption. Food Rev. Int. 36, 645–667. doi: 10.1080/87559129.2019.1669162
Guillou L., Nézan E., Cueff V., Erard-Le Denn E., Cambon-Bonavita M.-A., Gentien P., et al. (2002). Genetic diversity and molecular detection of three toxic dinoflagellate genera (Alexandrium, dinophysis, and karenia) from French coasts. Protist 153, 223–238. doi: 10.1078/1434-4610-00100
Halstead B. W. (1965) Poisonous and venomous marine animals of the world (Washington, D.C: United States Government Printing Office). Available at: https://www.biblio.com/book/poisonous-venomous-marine-animals-world-3/d/246327587 (Accessed November 3, 2022).
Hess P., Abadie E., Hervé F., Berteaux T., Séchet V., Aráoz R., et al. (2013). Pinnatoxin G is responsible for atypical toxicity in mussels (Mytilus galloprovincialis) and clams (Venerupis decussata) from ingril, a French Mediterranean lagoon. Toxicon 75, 16–26. doi: 10.1016/j.toxicon.2013.05.001
Hinton M., Ramsdell J. S. (2008). Brevetoxin in two planktivorous fishes after exposure to Karenia brevis: implications for food-web transfer to bottlenose dolphins. Mar. Ecol. Prog. Ser. 356, 251–258. doi: 10.3354/meps07267
Hort V., Arnich N., Guérin T., Lavison-Bompard G., Nicolas M. (2020). First detection of tetrodotoxin in bivalves and gastropods from the French mainland coasts. Toxins (Basel) 12, E599. doi: 10.3390/toxins12090599
Jeffery B., Barlow T., Moizer K., Paul S., Boyle C. (2004). Amnesic shellfish poison. Food Chem. Toxicol. 42, 545–557. doi: 10.1016/j.fct.2003.11.010
Jen H.-C., Lin S.-J., Lin S.-Y., Huang Y.-W., Liao I.-C., Arakawa O., et al. (2007). Occurrence of tetrodotoxin and paralytic shellfish poisons in a gastropod implicated in food poisoning in southern Taiwan. Food Addit Contam 24, 902–909. doi: 10.1080/02652030701245171
Knaack J. S., Porter K. A., Jacob J. T., Sullivan K., Forester M., Wang R. Y., et al. (2016). Case diagnosis and characterization of suspected paralytic shellfish poisoning in Alaska. Harmful Algae 57, 45–50. doi: 10.1016/j.hal.2016.03.006
Lehane L. (2001). Paralytic shellfish poisoning: a potential public health problem. Med. J. Aust. 175, 29–31. doi: 10.5694/j.1326-5377.2001.tb143508.x
Mahmud Y., Arakawa O., Noguchi T. (2000). An epidemic survey on freshwater puffer poisoning in Bangladesh. J. Nat. Toxins 9, 319–326.
Moreira-González A. R., Comas-González A., Valle-Pombrol A., Seisdedo-Losa M., Hernández-Leyva O., Fernandes L. F., et al. (2021). Summer bloom of Vulcanodinium rugosum in cienfuegos bay (Cuba) associated to dermatitis in swimmers. Sci. Total Environ. 757, 143782. doi: 10.1016/j.scitotenv.2020.143782
Naar J. P., Flewelling L. J., Lenzi A., Abbott J. P., Granholm A., Jacocks H. M., et al. (2007). Brevetoxins, like ciguatoxins, are potent ichthyotoxic neurotoxins that accumulate in fish. Toxicon 50, 707–723. doi: 10.1016/j.toxicon.2007.06.005
Nézan E., Chomérat N. (2011). Vulcanodinium rugosum gen. et sp. nov. (Dinophyceae), un nouveau dinoflagellé Marin de la côte méditerranéenne française. Cryptogamie Algologie 32, 3–18. doi: 10.7872/crya.v32.iss1.2011.003
Nicolas M., Belin C., Favre P., Rudloff L. (2017). “Surveillance des phycotoxines dans les coquillages,” in Bulletin epidémiologique, santé animale et alimentation n°77/numéro spécial-surveillance sanitaire des aliments (ANSES) 23–27.
Pierce R. H., Henry M. S., Blum P. C., Hamel S. L., Kirkpatrick B., Cheng Y. S., et al. (2005). Brevetoxin composition in water and marine aerosol along a Florida beach: Assessing potential human exposure to marine biotoxins. Harmful Algae 4, 965–972. doi: 10.1016/j.hal.2004.11.004
Pierce R. H., Henry M. S., Blum P. C., Lyons J., Cheng Y. S., Yazzie D., et al. (2003). Brevetoxin concentrations in marine aerosol: Human exposure levels during a Karenia brevis harmful algal bloom. Bull. Environ. Contam Toxicol. 70, 161–165. doi: 10.1007/s00128-002-0170-y
Quilliam M. A. (1995). Analysis of diarrhetic shellfish poisoning toxins in shellfish tissue by liquid chromatography with fluorometric and mass spectrometric detection. J. AOAC Int. 78, 555–570.
Sinno-Tellier S., Abadie E., de Haro L., Paret N., Langrand J., Le Roux G., et al. (2022). Human poisonings by neurotoxic phycotoxins related to the consumption of shellfish: study of cases registered by the French poison control centres from 2012 to 2019. Clin. Toxicol. (Phila) 60, 759–767. doi: 10.1080/15563650.2022.2034840
Temple C., Hughes A. (2022). A case of fatal paralytic shellfish poisoning in Alaska. Clin. Toxicol. (Phila) 60, 414–415. doi: 10.1080/15563650.2021.1967373
Trainer V. L., Moore S. K., Hallegraeff G., Kudela R. M., Clement A., Mardones J. I., et al. (2020). Pelagic harmful algal blooms and climate change: Lessons from nature’s experiments with extremes. Harmful Algae 91, 101591. doi: 10.1016/j.hal.2019.03.009
Turner A. D., McNabb P. S., Harwood D. T., Selwood A. I., Boundy M. J. (2015). Single-laboratory validation of a multitoxin ultra-performance LC-hydrophilic interaction LC-MS/MS method for quantitation of paralytic shellfish toxins in bivalve shellfish. J. AOAC Int. 98, 609–621. doi: 10.5740/jaoacint.14-275
Watkins S. M., Reich A., Fleming L. E., Hammond R. (2008). Neurotoxic shellfish poisoning. Mar. Drugs 6, 431–455. doi: 10.3390/md20080021
Wolny J. L., Scott P. S., Tustison J., Brooks C. R. (2015). Monitoring the 2007 Florida east coast Karenia brevis (Dinophyceae) red tide and neurotoxic shellfish poisoning (NSP) event. ALGAE 30, 49–58. doi: 10.4490/algae.2015.30.1.049
Young N., Sharpe R. A., Barciela R., Nichols G., Davidson K., Berdalet E., et al. (2020). Marine harmful algal blooms and human health: A systematic scoping review. Harmful Algae 98, 101901. doi: 10.1016/j.hal.2020.101901
Keywords: shellfish poisoning, monitoring program, human exposure, harmful algae, phycotoxin
Citation: Sinno-Tellier S, Abadie E, Guillotin S, Bossée A, Nicolas M and Delcourt N (2023) Human shellfish poisoning: Implementation of a national surveillance program in France. Front. Mar. Sci. 9:1089585. doi: 10.3389/fmars.2022.1089585
Received: 04 November 2022; Accepted: 21 December 2022;
Published: 11 January 2023.
Edited by:
Christine Johanna Band-Schmidt, Centro Interdisciplinario de Ciencias Marinas (IPN), MexicoReviewed by:
Patricia A. Tester, Retired, Beaufort, NC, United StatesSilvio Sosa, University of Trieste, Italy
Ernesto Garcia-Mendoza, Center for Scientific Research and Higher Education in Ensenada (CICESE), Mexico
Copyright © 2023 Sinno-Tellier, Abadie, Guillotin, Bossée, Nicolas and Delcourt. This is an open-access article distributed under the terms of the Creative Commons Attribution License (CC BY). The use, distribution or reproduction in other forums is permitted, provided the original author(s) and the copyright owner(s) are credited and that the original publication in this journal is cited, in accordance with accepted academic practice. No use, distribution or reproduction is permitted which does not comply with these terms.
*Correspondence: Nicolas Delcourt, delcourt.n@chu-toulouse.fr