- 1Department of Chemistry, City University of Hong Kong, Hong Kong, Hong Kong SAR, China
- 2State Key Laboratory of Marine Pollution, City University of Hong Kong, Hong Kong, Hong Kong SAR, China
- 3Department of Applied Biology and Chemical Technology, The Hong Kong Polytechnic University, Hong Kong, Hong Kong SAR, China
- 4Research Institute for Future Food, The Hong Kong Polytechnic University, Hong Kong, Hong Kong SAR, China
Microplastic (MP) pollution has been an emerging problem in the marine environment over the last few decades. Considering wide-ranging negative impacts of MPs on marine organisms, monitoring MP pollution in marine ecosystems is essential and urgent for developing effective management of the problem. An exposure experiment was conducted to assess the potential of the striped barnacle Amphibalanus amphitrite as a bioindicator of MPs. The barnacles were exposed to either polypropylene fibers or fragments for 8 days. A full factorial experiment was conducted, with the shape (fragment, fiber), exposure concentration (10, 100, and 1000 items L-1) and exposure duration (2, 4, and 8 days) as independent factors. The highest number of MPs in A. amphitrite was 21.04 ± 15.22 fragments g-1 and 17.60 ± 13.8 fibers g-1 wet weight recorded after 4 days of exposure, and the MP concentration in the barnacles was positively correlated with the exposure concentration, regardless of the form of MPs. A regression equation relating the MP concentration in the barnacles and that in the water was computed to estimate the MP concentration in the coastal waters of Hong Kong. The predicted values were close to the published data, indicating the potential of A. amphitrite as a bioindicator of MP pollution.
1 Introduction
Since invented in the 1950s, plastics have replaced many traditional materials and join the ranks of the world’s most commonly used materials, reaching a global production of 367 million tons in 2020, and an estimated 4.8 - 12.7 million tons were discharged into the ocean (Jambeck et al., 2015). Microplastics (MPs) are commonly defined as plastic particles smaller than 5 mm in diameter. They are either manufactured as micro-sized plastic particles or originated from the fragmentation of macro-plastic debris. Since MPs and prey of many marine organisms have a similar size range, accidental ingestion of MPs by marine organisms is not uncommon. MPs are found in the digestive tracts of more than 100 species, including planktons (Aytan et al., 2022), bivalves (Santana et al., 2016; Rios-fuster et al., 2022), barnacles (Zhang et al., 2022), crabs (Horn et al., 2019; Zhang et al., 2021), gastropods (Courtene-Jones et al., 2019; Doyle et al., 2019), fish (Collard et al., 2019; Ferreira et al., 2019), and marine mammals (Nelms et al., 2018; Zantis et al., 2021).
The ingested MPs could elicit various health effects. These include reduction of feeding capacity and energy intake (Xu et al., 2017), reduction of feeding rate and fecundity (Yu et al., 2020), growth inhibition (Xia et al., 2020; Silva et al., 2021), oxidative stress (Tlili et al., 2020), immunotoxicity (Revel et al., 2020; Tang et al., 2020), neurotoxicity (Barboza et al., 2020; Kim et al., 2020), and transcriptional changes (Limonta et al., 2019).
Considering the ubiquitous distribution of MPs in the marine environment and the negative impacts of MPs on marine organisms, effective marine monitoring programs for MPs are urgently needed (Gallo et al., 2018).Barnacles are commonly used as biomonitors for pollutants, such as trace metals (Rainbow and Blackmore, 2001) and polybrominated diphenyl ethers (PBDEs) (Chen et al., 2015). Barnacles are filter feeders which have cirri carrying a variety of setae (Chan et al., 2008) to capture plankton in the water column ranging from several millimeters to a few microns (Crisp and Southward, 1961). Since food particles of barnacles and MPs fall into a similar size range, there is a potential for barnacles to be bioindicators of MPs. Several studies have reported on the plastic debris or MPs in the field-collected barnacles. Goldstein and Goodwin (2013) found plastics in the gastrointestinal tract of 33.5% (or 385 individuals) of the gooseneck barnacles (Lepas spp.) obtained from the North Pacific Subtropical Gyre. Thushari et al. (2017) reported 0.23–0.43 particles g−1 tissue in Balanus amphitrite (=Amphibalanus amphitrite) at three study sites in Thailand. Zhang et al. (2022) examined four species of barnacles on the coast of the Yellow Sea, China. The highest MP abundance was found in Balanus albicostatus (ecotype A), with a concentration of 21.59 ± 27.26 items g-1. A similar abundance (10.26 ± 9.76 items g−1) was reported in A. amphitrite in Luk Keng, Hong Kong (Xu et al., 2020). They also found a strong correlation between the MP abundance in A. amphitrite and that in the sediment and concluded that A. amphitrite could be a potential bioindicator of MP pollution.
The tolerance of A. amphitrite was evaluated in laboratory exposure experiments. After A. amphitrite was exposed to MPs of environmentally relevant concentrations, the ingestion of MPs did not cause any lethal or sublethal response (Yu and Chan, 2020a). The tolerance of A. amphitrite to MPs enables this species to become a biomonitors for MP pollution. However, the ingestion of MPs could cause higher mortality of offspring, which may be attributed to lower energy available for gonad development and offspring production (Yu and Chan, 2020b). If there is an impact from MPs on the population sustainability, the competency of A. amphitrite as a long-term bioindicator of MP pollutions has to be considered with caution.
According to Gerhardt (2002), a bioindicator is “a species or group of species that readily reflects the abiotic or biotic state of an environment, represents the impact of environmental change on a habitat, community or ecosystem, or is indicative of the diversity of a subset of taxa or the whole diversity within an area”. According to the applications, bioindicators can be classified as environmental, ecological, or biodiversity indicators. Environmental indicators should respond predictably to environmental disturbance or change (e.g., sentinels, detectors, exploiters, accumulators, bioassay organisms) and are used for diagnosing the state of the environment. Ecological indicators are species sensitive to pollution, habitat fragmentation, or other stresses, and their responses are representative of the community. Biodiversity indicators refer to the use of species richness of an indicator taxon to reflect the species richness of a community (Gerhardt, 2002).
This study was a laboratory exposure experiment, trying to quantify the relationship between MP abundance in the water and that accumulated by A. amphitrite and assess the potential of A. amphitrite to be a bioindicator in predicting environmental concentrations of MPs using regression equations calculated from the exposure experiment. A similar approach was used by Berg and Steinnes (1997) in predicting the absolute deposition rates of heavy metals using concentrations determined in the ground-growing mosses Hylocomium splendens and Pleurozium schreberi. The experiment included two forms of MPs (fiber and fragment), and three concentrations, including one realistic environmental concentration (10 items L−1) (Mak et al., 2020) and two higher concentrations (100 and 1000 items L−1), were prepared for each form of MPs. The accumulation of MPs in A. amphitrite was examined after 2, 4, and 8 days of exposure. Fibers and fragments were chosen for the study because they were the most common forms of MPs found in field-collected barnacles (Xu et al., 2020; Zhang et al., 2022).
2 Materials and methods
2.1 Collection and maintenance of experiment organisms
We collected individuals of the acorn barnacle A. amphitrite from a pebble beach near the Wu Kai Sha pier (114.23°N, 22.42°E). As barnacles might die when separated from the substrate, they were transferred back to the laboratory with the pebbles, which were scrubbed to remove epifauna. The barnacles were kept in 50 L glass aquaria (35 × 50 × 45 cm), with about 40 pebbles per tank equipped with a filtration system and a protein skimmer. Filtered natural seawater (30 psu) was constantly aerated, and 70% of the seawater was renewed every week to maintain the water quality. Light and dark conditions followed cycles of 12 h. The barnacles were fed daily with the alga Skeletonema costatum at 104 - 106 cells L-1. Seawater used in this exposure experiment was collected from the eastern waters of Hong Kong and filtered through 5 µm filter papers before use.
2.2 Preparation of microplastics
Blue PP fragments (63-125 µm) were prepared from stalks for marker pens by cryogenically ground at –196°C using a cryomilling machine ( CryoMill, Retsch, Haan, Germany). Fragments with a size of between 63 and 125 µm were obtained after sieving. For PP fibers, small bundles of fibers separated from orange ropes made from PP were cut by dissecting scissors under a stereomicroscope. Fibers were sieved to obtain the required length (63-250 µm). The MPs remained on the sieve were transferred on filter papers for size measurement and quantification. Micro-Fourier transform infrared spectroscopy (µ-FTIR, Thermo fisher Nicolet iN5, USA) was used to confirm the chemical composition of fragments and fibers.
For size measurement of MPs, photos of the prepared MPs were taken under a stereomicroscope (Leica 165MC) and the perimeter as well as 2D surface area of MPs were measured using ImageJ. The 2D surface area of fragments and fibers were similar (fragments: 6408 ± 605 µm2; fibers: 6246 ± 535 µm2). The shape factor of the MPs was calculated by the following formula (Zhao et al., 2018):
where A represents the 2D surface area (mm2) and P, the perimeter of MPs.
The shape factor of fragments and fibers was 0.31± 0.02 and 0.70 ± 0.02, respectively.
Quantification of MPs was achieved by counting the MPs in a sub-area (1/16) of the filter papers. To remove counting errors caused by uneven distribution of MPs on the filter papers, the suspension of MPs inside the filtering funnel was stirred before turning on the vacuum pump. This suspending, stirring and filtering procedure might repeat several times by adding more DI water into the filtering funnel until the MPs were distributed evenly. Due to the high concentration of MPs in the suspension, the prepared MPs were usually filtered onto several filter papers, to avoid serious overlapping of MPs and to increase the number of replicates for quantification, both of which could further reduce the counting errors.
After quantification of MPs, stock solutions of MPs (10, 100, and 1000 items mL-1) were prepared by transferring the MPs on the filter papers into 50 ml centrifuge tubes with a calculated amount of DI water. To achieve the final exposure concentration (10, 100, and 1000 items L-1), a calculated amount of stock solution was added into the exposure aquarium.
2.3 Bioaccumulation of MPs in barnacles
An 8-day exposure experiment was conducted to investigate the bioaccumulation of MPs in A. amphitrite. A full factorial experiment was conducted, with the shape (fragment, fiber), exposure concentration (10, 100, and 1000 items L-1) and exposure duration (2, 4, and 8 days) as independent factors. This study included 18 treatment groups in total, each containing ten replicates. In addition, ten replicates of the procedural blank were prepared without microplastics in the seawater.
The rostro carinal diameter of barnacles was measured before the exposure, and similar-sized individuals were selected. As a pooled replicate, five barnacles were placed in a 500 mL glass bottle with constant aeration to keep the MPs in suspension and create water flow, which stimulated the barnacles to extend their cirri and filter particles in the water. When more than 5 barnacles were attached to one pebble, which was very common, extra barnacles were removed carefully. The bottles were emptied and washed with filtered seawater during the exposure experiment every 24 h. Then the water was renewed with the subsequent addition of MPs. The barnacles were fed daily with a 5 mL algal solution of S. costatum at 107 cells L-1, immediately after adding MPs.
To determine the MP ingestion, the barnacles were sampled on Day 2, 4, and 8, with their rostro carinal diameter measured. Chemical digestion was used to extract MPs in the body tissue of barnacles, according to Karami et al. (2017). The soft tissue of five barnacles in each pooled replicate was dissected, and the wet weight was measured separately for each barnacle. The tissue was placed in a 100 mL beaker containing 60 mL of 10% potassium hydroxide (KOH). The digestion procedure (~48-96h) was conducted at 40°C in an incubator, with shaking at 200 rpm by an orbital shaker until complete digestion. After filtering the digested solution onto cellulose nitrate filter papers (Membrane Solution, pore size = 5 µm), the filter papers were checked under a stereomicroscope (Leica M165C) at a magnification of 40× - 120×, to identify and quantify the MPs. The MP abundance in the barnacles was estimated as the number of MPs divided by the tissue mass of five barnacles.
2.4 Quality assurance/quality control
The seawater was pre-filtered with 5 µm filter papers and all the glassware and tools were pre-rinsed with DI water for three times. The background contamination was also checked, by conducting control experiments (n = 10), which shared the same procedure except for the addition of MPs, together with the exposure experiments. None of the control groups was contaminated by MPs.
Procedural controls (n=3) for the KOH digestion were conducted daily but without animals. As the digesting procedure might cause loss of MPs, the recovery rate of MPs after the KOH digestion was also evaluated by spiking 10 fragments or fibers into the body of the barnacles and counting the number of recovered MPs after the barnacles going through the KOH digestion.
2.5 Statistical analysis
Even after data transformation, the data failed the normality test (Shapira-Wilk method). Scheirer-Ray-Hare test was conducted for both the fragment and fiber exposure experiments, with the MP concentration and exposure duration being independent factors. To compare the abundance of different forms of MPs in barnacles, Mann Whitney tests were conducted between pairs of the fragment and fiber under the same exposure durations and concentrations. The relationship between the MP abundance in the barnacles and that in the water was analyzed by the linear regression following Berg and Steinnes (1997) and Kono et al. (2012). Berg and Steinnes (1997) used the original data for the linear regression analysis, whereas Kono et al. (2012) used log-transformed data. In this study, both original and log-transformed data were used in the linear regression analysis and the best fit model was reported. Linear regression equations were used to predict the MP concentration in Hong Kong waters based on the MP abundance in field-collected barnacles reported in a previous study (Xu et al., 2020). The Scheirer-Ray-Hare test was conducted by RStudio, and the linear regression analysis by Prism.
3 Results
3.1 MP abundance in barnacles
The highest number of MPs in A. amphitrite was 21.04 ± 15.22 fragments g-1 and 17.60 ± 13.8 fibers g-1 after being exposed to a concentration of 1000 items L-1 for 4 days (Figure 1). The MP abundance in barnacles increased with the MP concentration for both fiber and fragment. The abundance of fragments in the barnacles exposed to 1000 items L−1 was significantly higher than those exposed to 100 items L−1 and 10 items L−1, but there was no difference between 10 items L−1 and 100 items L−1. Differences in the abundance of fibers among all the concentrations were statistically significant (Table 1). The abundance of fragments in the barnacles did not vary with the duration of exposure, but that of fibers was significantly higher after 4 days of exposure than on Day 2 and Day 8 (Table 1). There was no significant difference between the abundance of two forms of MPs for all the exposure durations and concentrations (Table 2).
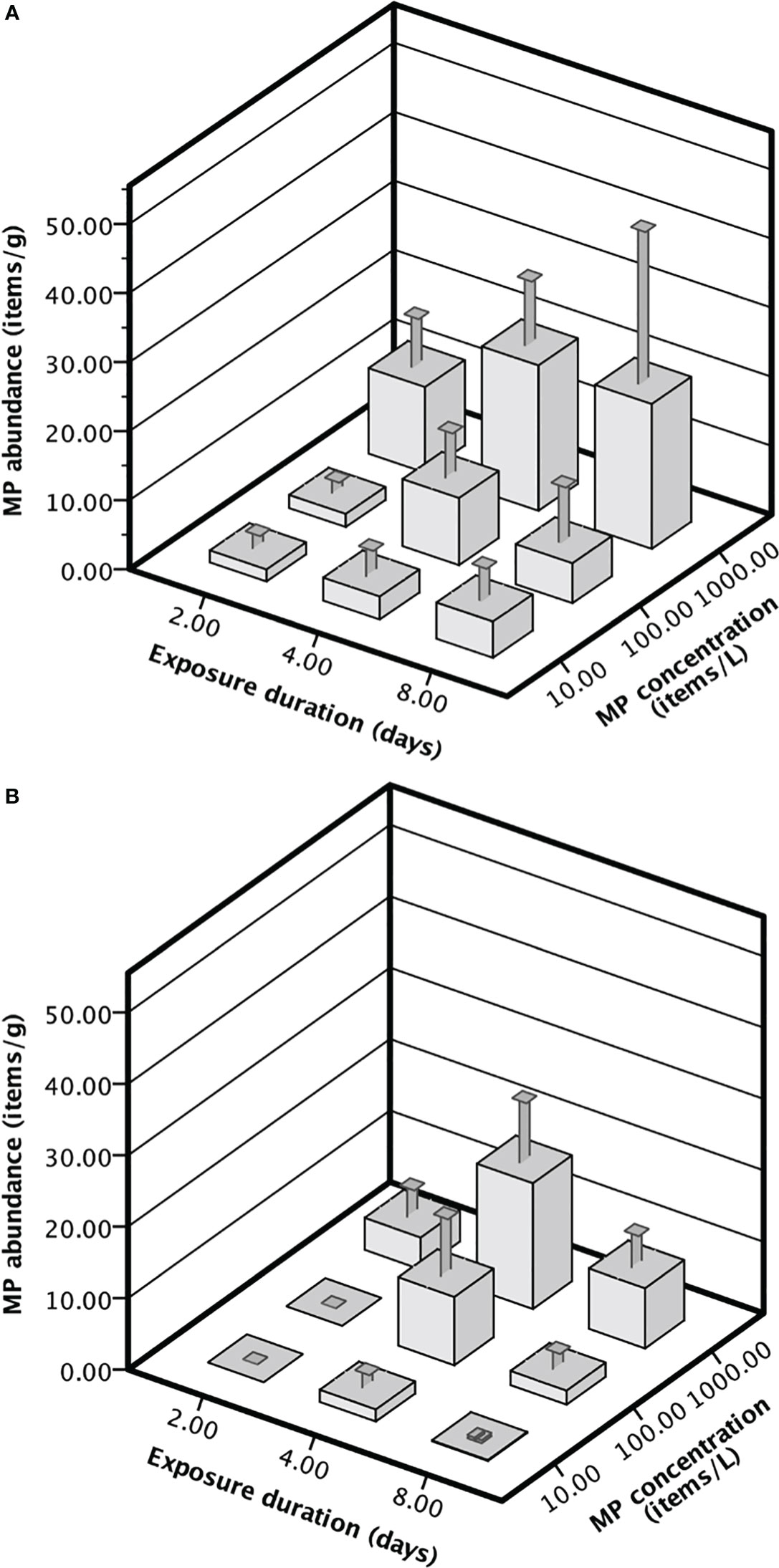
Figure 1 The MP abundance (mean ± SE, n = 10) in A amphitrite exposed to different concentrations of (A) fragment, or (B) fiber for 2, 4, or 8 days.
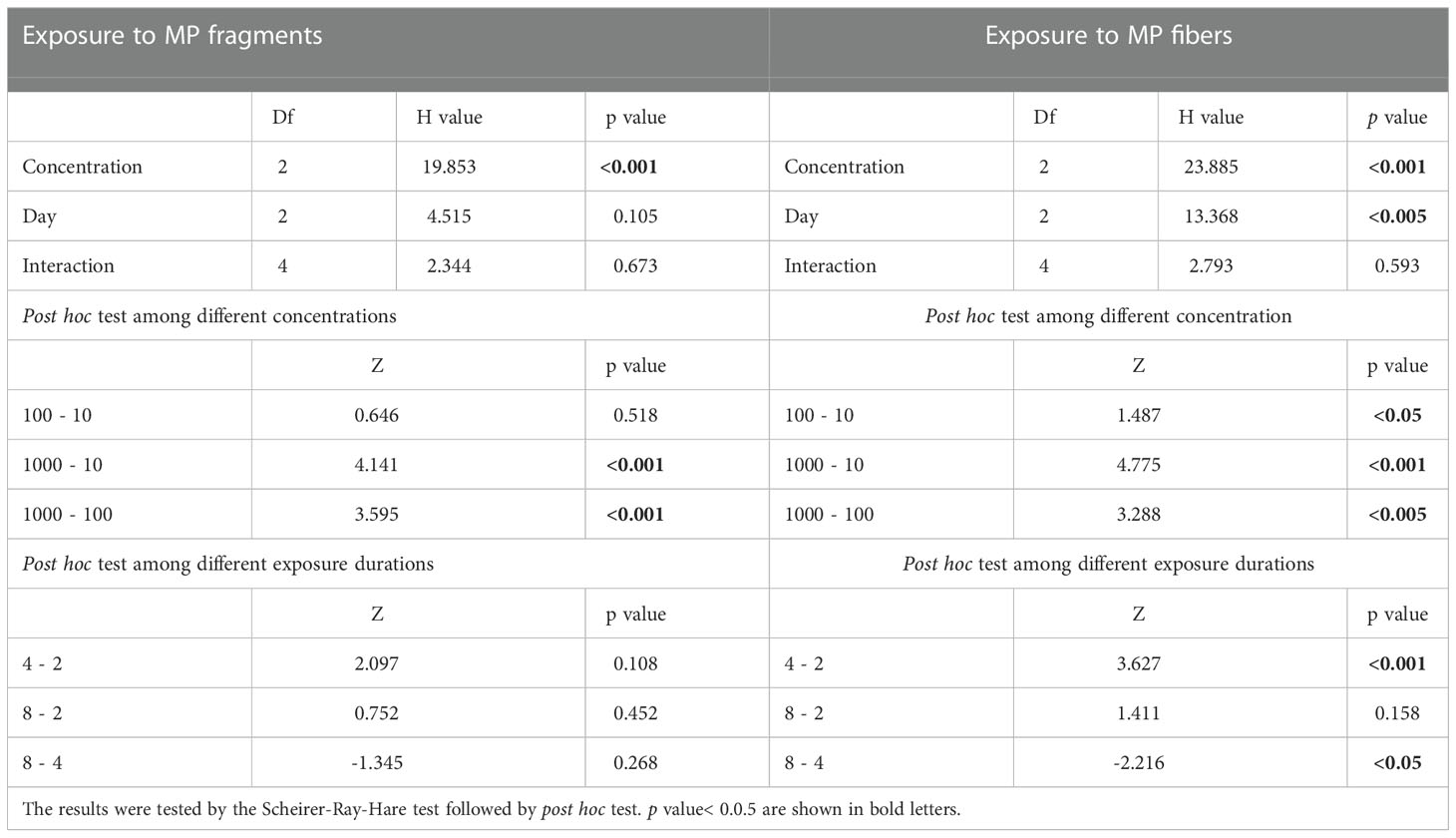
Table 1 The effect of exposure duration and MP concentration on the abundance of fragments and fibers in A. amphitrite.
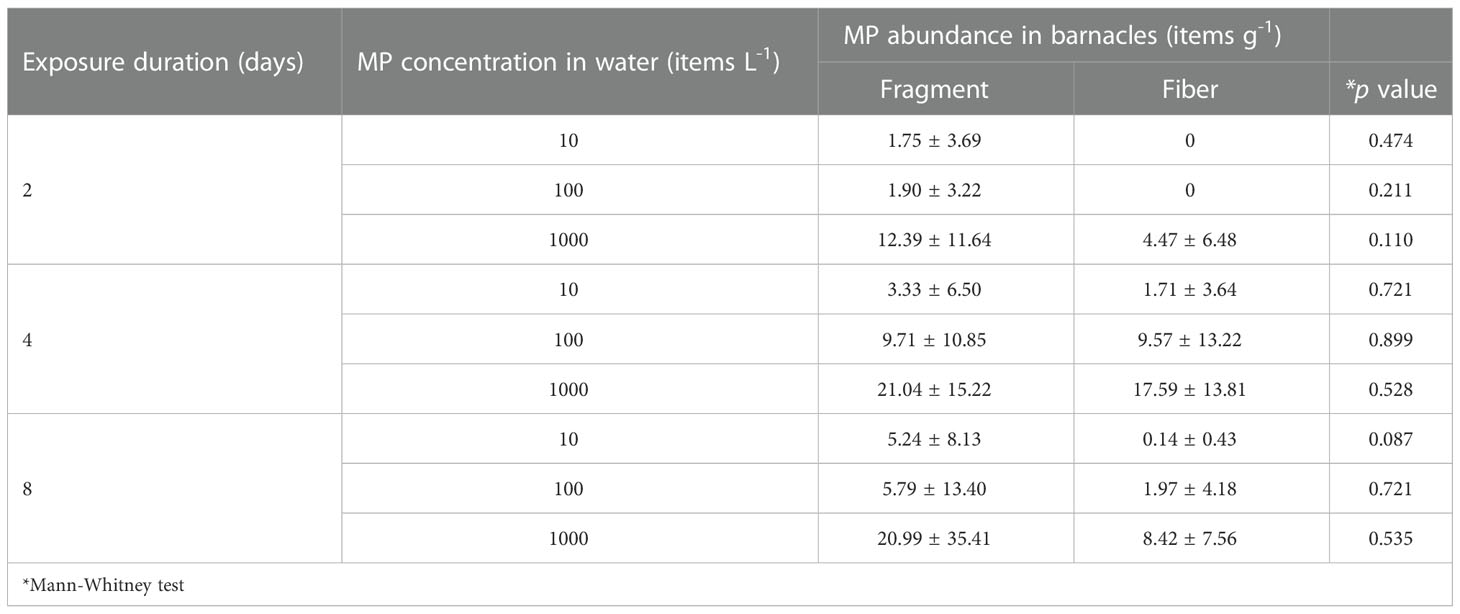
Table 2 Comparisons between the concentration of fragments and fibers in barnacles under the same exposure conditions.
3.2 Regression between MP abundance and exposure concentration
To validate A. amphitrite as a bioindicator of MPs, linear regression equations were computed to relate the mean MP abundance in the barnacles and that in the water for both the 4-day and 8-day exposure groups. Since the relationships were not linear for the 4-day exposure groups (Figure 2), the exposure concentrations in the water were log-transformed. Two regression lines are computed (Figure 2), and the equations are as follows:
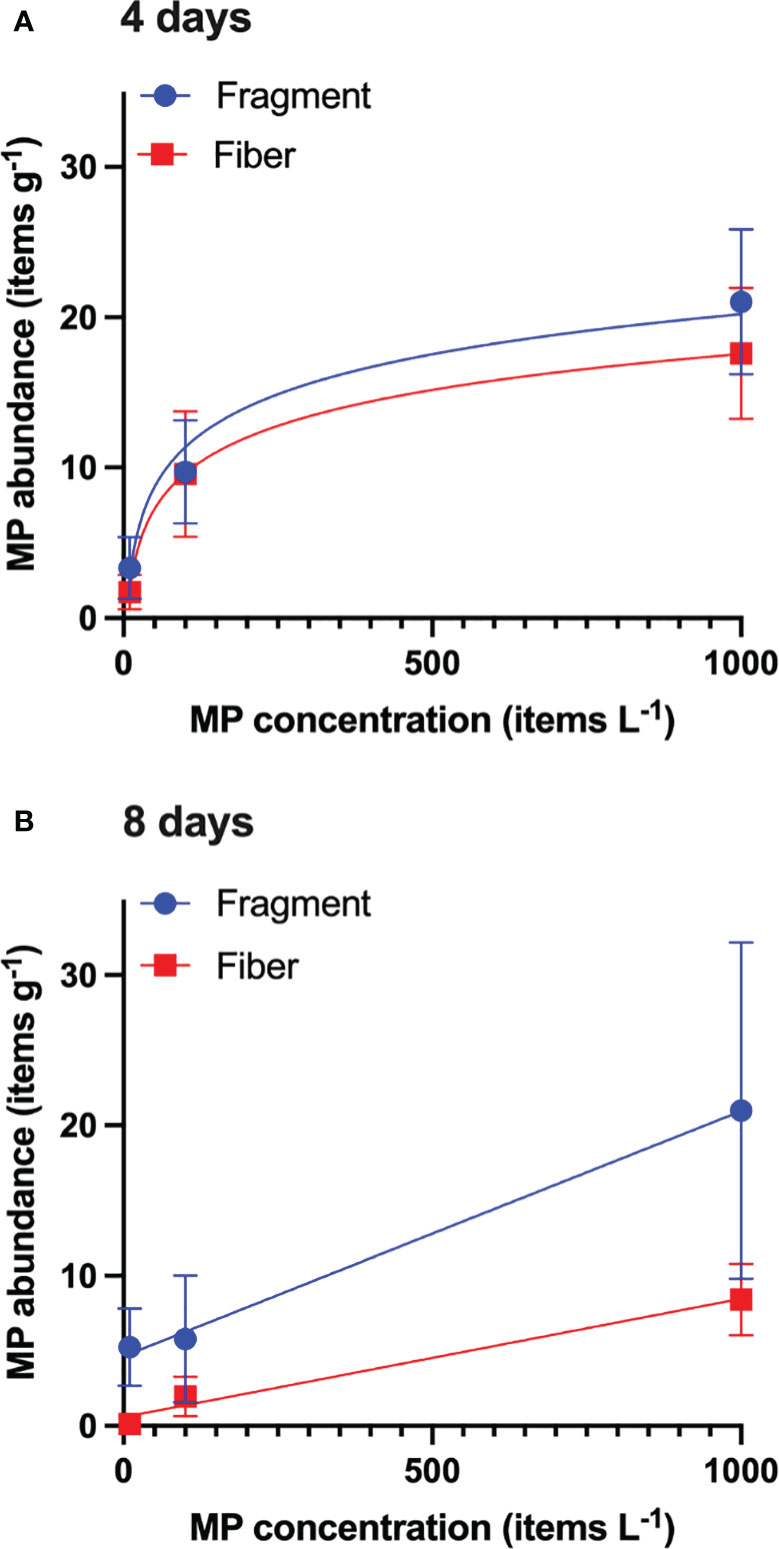
Figure 2 MP abundance in barnacles exposed for (A) 4 days and (B) 8 days. Trends based on lines of best fit (regression) are provided to show the relationships. Data are means ± standard error of the mean; n = 10.
(Equation 1)
(Equation 2)
where
MPbarnacle is the MP abundance in barnacles (items g-1), and
MPwater is the exposure concentration in the water (items L-1)
Regression equations relating MPs (fragment and fiber) in the barnacles and that in the water were also computed for the 8-day exposure group (Figure 2):
(Equation 3)
(Equation 4)
Only the regression equations computed using 4-day exposure data for fibers and 8-day exposure for fragments were significant. Since the fiber is the most dominant form of microplastics in the field-collected barnacles, the MP concentration in the water of each study site in Xu et al. (2020) was estimated by converting Equation 2, which becomes:
(Equation 5)
where
MPenvironment is the MP concentration in the water at each study site (items L-1), and MPbarnacle is the MP concentration in field-collected barnacles reported upon by Xu et al. (2020).
Using Equation 5 and the MP abundance in the field-collected barnacles (Xu et al., 2020), the mean and medium predicted MP concentration in the coastal waters was 31.26 items L-1, and 14.10 items L-1, respectively (Table 3, Figure 3).
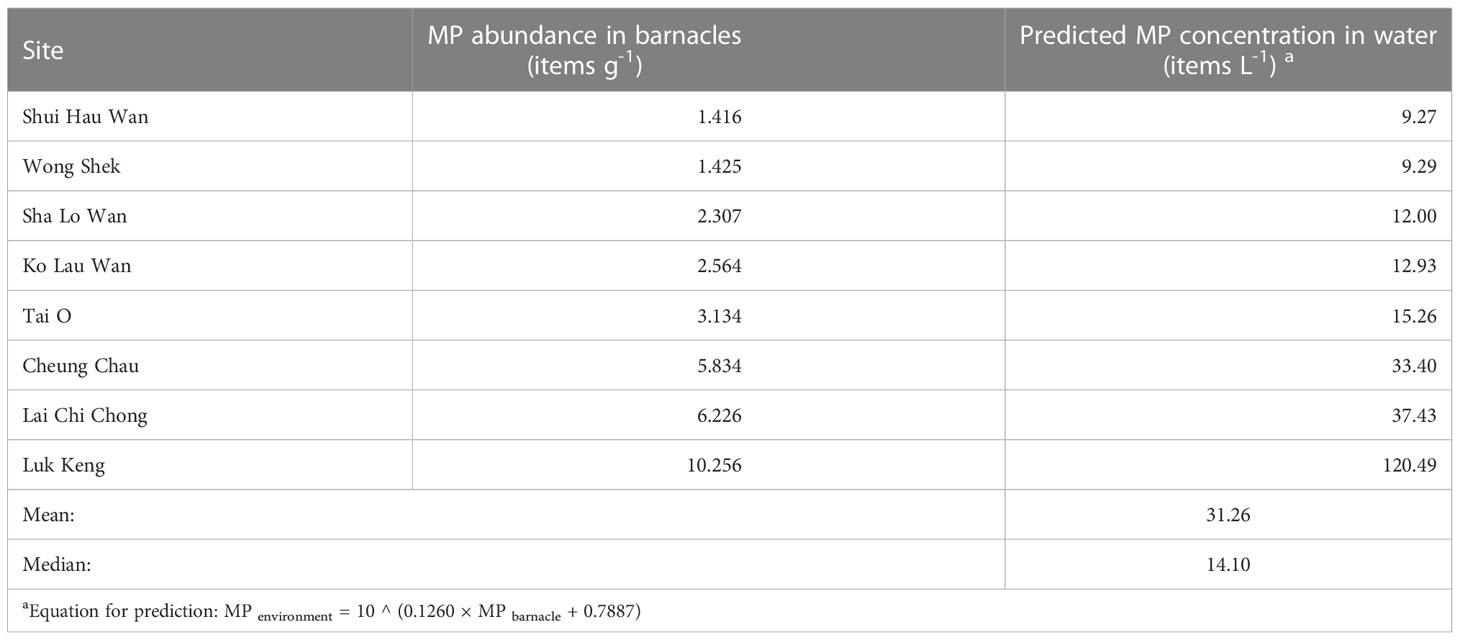
Table 3 MP concentrations in the water predicted from the measured MP abundance in barnacles in our previous study (Xu et al., 2020), using the equation computed for the 4-day exposure group.
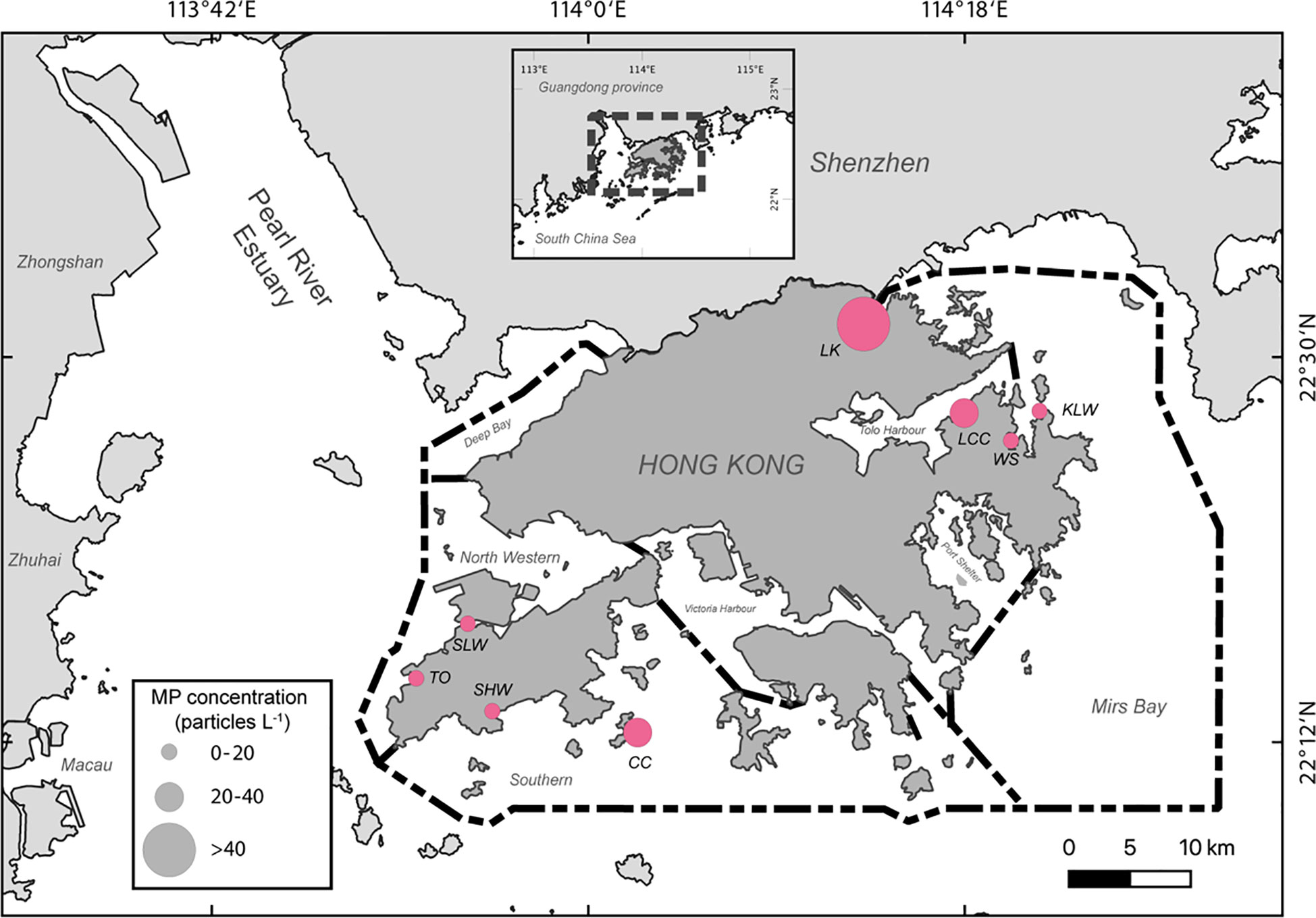
Figure 3 Predicted MP concentration in the water from different sites around Hong Kong. There are four sites in the eastern waters: Luk Keng (LK), Lai Chi Chong (LCC), Ko Lau Wan (KLW), and Wong Shek (WS); four sites in the western waters: Sha Lo Wan (SLW), Tai O (TO), Shui Hau Wan (SHW) and Cheung Chau (CC).
4 Discussion
4.1 Accumulation and ejection of MPs by barnacles
The concentration of both the fragment and fiber in the barnacles increased from Day 2 to Day 4. No further increase, however, was observed from Day 4 to Day 8 for the fragment, while a considerable decrease was found for the fiber. The MP concentration in an organism is a balance between the rates of MP ingestion and removal. Since the accumulation of MPs was the highest on Day 4, this indicates the efficiency of MP removal was improved in the latter part of the experiment. Crisp and Southward (1961) reported that compared with those feeding on animal food, barnacles feeding on dinoflagellate with a thick cell wall produced larger numbers of fecal pellets containing undamaged plant cells. Since MPs are undigestible, they should be treated the same as dinoflagellates, and were eliminated by the barnacles through fecal production. When more and more MPs were accumulated in the intestine, this may trigger a faster removal of MPs, as shown in the latter part of the experiment. When the amount of inorganic particulates in the water increases, marine mussels reduce the gut retention time of ingested particles. This strategy allows the mussels to remove the non-nutritious particles efficiently (Galimany et al., 2013). Laboratory experiments that monitor the gut retention time of barnacles upon exposure to different MP concentrations can clarify whether barnacles possess a similar adaptation for handling MPs.
Fragments and fibers used in this experiment have a similar surface area, but the former has a smaller shape factor. Although there was no significant difference between the abundance of fibers and fragments in the barnacles for all the exposure concentrations and durations, the removal of fibers was more efficient than fragments after 8 days of exposure, resulting in an insignificant difference in the fiber concentration between Day 2 and Day 8. The results agree with Zhao et al. (2018), who reported that plastics in the feces of mussels had larger shape factors, while plastics with smaller shape factors tend to retain in digestive glands/guts. The irregular shape of fragments could also lengthen their retention time in the digestive system of barnacles and might induce physical damage to the gut by their sharp edges, as demonstrated in other marine organisms (Wright et al., 2013).
4.2 Evaluation of A. amphitrite as a bioindicator for MP pollution
Using marine organisms, such as barnacles, as bioindicators of pollutants have pros and cons compared with direct water sampling. MPs could accumulate in barnacles during a period. Therefore, the concentration of MPs represents time-averaged pollution. By contrast, direct water sampling only reflects the MP concentration at the time of sampling, resulting in large temporal variations in the MP concentration in water due to fast water movement in the sea. The daily tidal movement further complicates the distribution of MPs in the coastal waters (Malli et al., 2022). Although the disadvantage of direct water sampling could be overcome by repeated sampling, this inevitably increases both the labor and time cost of the monitoring program. On the other hand, the MP abundance in barnacles could be several orders of magnitude smaller than the corresponding MP concentration in the water column, and the rapid removal of MPs aggravates the problem through fecal production.
The present study has demonstrated a positive correlation between the MP concentration in barnacles and the exposure concentration, regardless of the form of MPs and the exposure duration. The results indicate that A. amphitrite could be a potential bioindicator of MP pollution. To validate the accuracy of the predicted MP concentrations in the coastal waters, results were compared with published data. In a study of MPs from the effluents of sewage treatment works and stormwater discharging into Victoria Harbor, Hong Kong, the average concentration of MPs ranged from 0.59 to 10.816 items L-1, with the highest concentration recorded as 11.927 items L-1 (Mak et al., 2020). Sewage and water samples were collected from sewage treatment works and stormwater drains, respectively, in Hong Kong in 2021, and the MP abundance ranged from 0.07 to 91.9 items L-1 in the sewage samples and from 0.4 to 36.48 items L-1 in the water samples (Zhang et al., 2022). The MP concentration in the surface water of small-scale estuaries in Shanghai, China, ranged from 13.53 to 44.94 items L-1 (Zhang et al., 2019), the highest MP concentration recorded in China (Wang et al., 2022). The predicted concentrations of MPs in the coastal waters of Hong Kong using 4-day exposure data (predicted mean: 31.26 items L-1, medium: 14.10 items L-1), therefore, were close to the published data but on the high side.
Since the accumulation of MPs was the highest on Day 4, using the 4-day exposure data to estimate the environmental concentrations of MPs in the coastal waters could improve the prediction accuracy, mainly because the MP concentration in the barnacles is generally very low. However, the removal of MPs by A. amphitrite was more efficient after 8 days of exposure, which probably better reflects the long-term accumulation of MPs in the barnacles. The predictive power of the model can be improved by increasing the frequency of sampling during the exposure duration. The simplification of the exposure conditions in the experiment further limits the use of the experimental results in estimating the environmental concentration of MPs in the coastal waters (Herricks and Schaeffer, 1985). In the field environment, the accumulation of pollutants can be influenced by multiple uncontrollable factors, such as disease, weather, and other environmental stresses (Su et al., 2019). For example, the seasonal variability in metal and metalloid burdens was reported for mussels (Knopf et al., 2020). In the ocean quahog (Arctica islandica), copper, zinc, and cadmium concentrations were generally lower in winter when the lead concentration was the highest (Swaileh, 1996). These variations were attributed to the seasonality of the reproductive cycle and food availability, resulting in changes in body weight and composition (Sheehan and Power, 1999). In contrast to a relatively stable laboratory experiment, field populations of barnacles are constrained by various environmental stresses. For example, barnacles are protected by the closure of opercular plates and stop feeding during low tides when they are exposed to air. Seasonal fluctuations in temperature also influence the feeding behavior of barnacles. Nishizaki and Carrington (2014) reported that Balanus glandula showed a clear thermal optimum between 10 and 15°C, and feeding was reduced at high (25°C) and low (5°C) temperatures. A better understanding of the seasonal variations in the physiology of barnacles is essential to the development of more realistic models in predicting the environmental concentrations of MPs.
The gut retention time and egestion of MPs could influence the accumulation of MPs and further influence the prediction accuracy. According to the results from an exposure experiment with barnacle larvae, the retention time of MPs was inversely proportional to the size of MPs. Larger MPs were expelled in a shorter time, while MPs with a smaller size were retained for a longer time (Yu et al., 2021). If adult barnacles could also expel larger MPs quickly and retain smaller ones, the absence of larger MPs in barnacles could introduce bias to the estimation of MPs concentration in the environment. In addition, the water quality could also influence the depuration of MPs by barnacles. Barnacle species in the estuarine area can depurate MPs faster than species in pristine waters (Yu et al., 2021). Aging and biofouling could also influence the accumulation of MPs in organisms (Vroom et al., 2017). To further explore the potential of barnacles as bioindicators, aged MPs or MPs with biofilms could be used. All in all, factors that can influence the accumulation of MPs in barnacles could be included in the prediction equation, based on further experiments with more independent variables.
The accuracy of the prediction can further be improved by increasing the number of MP concentrations in the exposure experiment and incorporating more environmental factors, e.g., seasonal change in temperature, in the experimental design.
Data availability statement
The raw data supporting the conclusions of this article will be made available by the authors, without undue reservation.
Author contributions
S-GC - Supervision, Funding acquisition, Resources, Methodology, Writing - review and editing XX - Methodology, Data curation, Software, Writing - original draft C-YW - Funding acquisition, Resources, Methodology JF - Funding acquisition, Methodology, Resources. All authors contributed to the article and approved the submitted version.
Funding
This study was supported by the Marine Ecology Enhancement Fund, Hong Kong (MEEF2018011) and the research project 9220116 of the City University of Hong Kong.
Conflict of interest
The authors declare that the research was conducted in the absence of any commercial or financial relationships that could be construed as a potential conflict of interest.
Publisher’s note
All claims expressed in this article are solely those of the authors and do not necessarily represent those of their affiliated organizations, or those of the publisher, the editors and the reviewers. Any product that may be evaluated in this article, or claim that may be made by its manufacturer, is not guaranteed or endorsed by the publisher.
References
Aytan U., Esensoy F. B., Senturk Y. (2022). Microplastic ingestion and egestion by copepods in the black Sea. Sci. Total Environ. 806, 150921. doi: 10.1016/j.scitotenv.2021.150921
Barboza L. G. A., Lopes C., Oliveira P., Bessa F., Otero V., Henriques B., et al. (2020). Microplastic in wild fish from north East Atlantic ocean and its potential for causing neurotoxic effects, lipid oxidative damage, and human health risks associated with ingestion exposure. Sci. Total Environ. 717, 134625. doi: 10.1016/j.scitotenv.2019.134625
Berg T., Steinnes E. (1997). Use of mosses (Hylocomium splendens and pleurozium schreberi) as biomonitors of heavy metal deposition: From relative to absolute deposition values. Environ. Pollut. 98, 61–71. doi: 10.1016/S0269-7491(97)00103-6
Chan B. K. K., Garm A., Høeg J. T. (2008). Setal morphology and cirral setation of thoracican barnacle cirri: Adaptations and implications for thoracican evolution. J. Zool. 275, 294–306. doi: 10.1111/j.1469-7998.2008.00441.x
Chen L., Lam J. C. W., Zhang X., Pan K., Guo C., Lam P. K. S., et al. (2015). Relationship between metal and polybrominated diphenyl ether (PBDE) body burden and health risks in the barnacle balanus amphitrite. Mar. Pollut. Bull. 100, 383–392. doi: 10.1016/J.MARPOLBUL.2015.08.020
Collard F., Gasperi J., Gabrielsen G. W., Tassin B. (2019). Plastic particle ingestion by wild freshwater fish: A critical review. Environ. Sci. Technol 53, 12974–12988. doi: 10.1021/acs.est.9b03083
Courtene-Jones W., Quinn B., Ewins C., Gary S. F., Narayanaswamy B. E. (2019). Consistent microplastic ingestion by deep-sea invertebrates over the last four decade–2015), a study from the north East Atlantic. Environ. Pollut. 244, 503–512. doi: 10.1016/j.envpol.2018.10.090
Crisp D. J., Southward A. J. (1961). Different types of cirral activity of barnacles. Philos. Trans. R. Soc Lond. B. Biol. Sci. 243, 271–307. doi: 10.1098/rstb.1961.0003
Doyle D., Gammell M., Frias J., Griffin G., Nash R. (2019). Low levels of microplastics recorded from the common periwinkle, littorina littorea on the west coast of Ireland. Mar. Pollut. Bull. 149, 110645. doi: 10.1016/j.marpolbul.2019.110645
Ferreira G. V. B., Barletta M., Lima A. R. A. (2019). Ecological patterns of estuarine ecocline resources use and microplastics contamination of top predator fishes. Sci. Total Environ. 655, 292–304. doi: 10.1016/J.SCITOTENV.2018.11.229
Gallo F., Fossi C., Weber R., Santillo D., Sousa J., Ingram I., et al. (2018). Marine litter plastics and microplastics and their toxic chemicals components: the need for urgent preventive measures. Environ. Sci. Eur. 30, 13. doi: 10.1186/s12302-018-0139-z
Galimany E., Rose J. M., Dixon M. S., Wikfors G. H.. (2013). Quantifying feeding behavior of ribbed mussels (Geukensia demissa) in two urban sites (Long Island Sound, USA) with different seston characteristics. Estuaries Coast 36, 1265–1273.
Goldstein M. C., Goodwin D. S. (2013). Gooseneck barnacles ( lepas spp.) ingest microplastic debris in the north pacific subtropical gyre. PeerJ 1, e184. doi: 10.7717/peerj.184
Herricks E. E., Schaeffer D. J. (1985). Can we optimize biomonitoring? Environ. Manage. 9, 487–492. doi: 10.1007/BF01867323
Horn D., Miller M., Anderson S., Steele C. (2019). Microplastics are ubiquitous on California beaches and enter the coastal food web through consumption by pacific mole crabs. Mar. Pollut. Bull. 139, 231–237. doi: 10.1016/J.MARPOLBUL.2018.12.039
Jambeck J. R., Geyer R., Wilcox C., Siegler T. R., Perryman M., Andrady A., et al. (2015). Plastic waste inputs from land into the ocean. Science 80. doi: 10.1126/science.1260352
Karami A., Golieskardi A., Choo C. K., Romano N., Ho Y., Salamatinia B. (2017). A high-performance protocol for extraction of microplastics in fish. Sci. Total Environ. 578, 485–494. doi: 10.1016/j.scitotenv.2016.10.213
Kim J. H., Yu Y., Choi J. H. (2021). Toxic effects on bioaccumulation, hematological parameters, oxidative stress, immune responses and neurotoxicity in fish exposed to microplastics: A review. J. Hazard. Mater. 413, 125423. doi: 10.1016/j.jhazmat.2021.125423
Knopf B., Fliedner A., Radermacher G., Rüdel H., Paulus M., Pirntke U., et al. (2020). Seasonal variability in metal and metalloid burdens of mussels: using data from the German environmental specimen bank to evaluate implications for long-term mussel monitoring programs. Environ. Sci. Eur. 32, 1–13. doi: 10.1186/s12302-020-0289-7
Kono Y., Rahajoe J. S., Hidayati N., Kodamatani H., Tomiyasu T. (2012). Using native epiphytic ferns to estimate the atmospheric mercury levels in a small-scale gold mining area of West Java, Indonesia. Chemosphere 89, 241–248. doi: 10.1016/j.chemosphere.2012.04.027
Limonta G., Mancia A., Benkhalqui A., Bertolucci C., Abelli L., Fossi M. C., et al. (2019). Microplastics induce transcriptional changes, immune response and behavioral alterations in adult zebrafish. Sci. Rep. 9. doi: 10.1038/s41598-019-52292-5
Mak C. W., Tsang Y. Y., Leung M. M. L., Fang J. K. H., Chan K. M. (2020). Microplastics from effluents of sewage treatment works and stormwater discharging into the Victoria harbor, Hong Kong. Mar. Pollut. Bull. 157, 111181. doi: 10.1016/j.marpolbul.2020.111181
Malli A., Corella-Puertas E., Hajjar C., Boulay A. M. (2022). Transport mechanisms and fate of microplastics in estuarine compartments: A review. Mar. Pollut. Bull 177, 113553. doi: 10.1016/j.marpolbul.2022.113553
Nelms S. E., Galloway T. S., Godley B. J., Jarvis D. S., Lindeque P. K. (2018). Investigating microplastic trophic transfer in marine top predators. Environ. Pollut. 238, 999–1007. doi: 10.1016/j.envpol.2018.02.016
Nishizaki M. T., Carrington E. (2014). Temperature and water flow influence feeding behavior and success in the barnacle balanus glandula. Mar. Ecol. Prog. Ser. 507, 207–218. doi: 10.3354/meps10848
Rainbow P. S., Blackmore G. (2001). Barnacles as biomonitors of trace metal availabilities in Hong Kong coastal waters: Changes in space and time. Mar. Environ. Res. 51, 441–463. doi: 10.1016/S0141-1136(00)00254-3
Revel M., Yakovenko N., Caley T., Guillet C., Châtel A., Mouneyrac C. (2020). Accumulation and immunotoxicity of microplastics in the estuarine worm hediste diversicolor in environmentally relevant conditions of exposure. Environ. Sci. Pollut. Res. 27, 3574–3583. doi: 10.1007/s11356-018-3497-6
Rios-fuster B., Alomar C., Gonz G. P., Lucy D., Rojas S., Fern P., et al. (2022). Assessing microplastic ingestion and occurrence of bisphenols and phthalates in bivalves, fish and holothurians from a Mediterranean marine protected area. Environ. Res. 214, 114034. doi: 10.1016/j.envres.2022.114034
Santana M. F. M., Ascer L. G., Custódio M. R., Moreira F. T., Turra A. (2016). Microplastic contamination in natural mussel beds from a Brazilian urbanized coastal region: Rapid evaluation through bioassessment. Mar. Pollut. Bull. 106, 183–189. doi: 10.1016/j.marpolbul.2016.02.074
Sheehan D., Power A. (1999). Effects of seasonality on xenobiotic and antioxidant defence mechanisms of bivalve molluscs. Comp. Biochem. Physiol. - C Pharmacol. Toxicol. Endocrinol. 123, 193–199. doi: 10.1016/S0742-8413(99)00033-X
Silva C. J. M., Patrício Silva A. L., Campos D., Machado A. L., Pestana J. L. T., Gravato C. (2021). Oxidative damage and decreased aerobic energy production due to ingestion of polyethylene microplastics by chironomus riparius (Diptera) larvae. J. Hazard. Mater. 402, 123775. doi: 10.1016/J.JHAZMAT.2020.123775
Su L., Nan B., Hassell K. L., Craig N. J., Pettigrove V. (2019). Microplastics biomonitoring in Australian urban wetlands using a common noxious fish (Gambusia holbrooki). Chemosphere 228, 65–74. doi: 10.1016/j.chemosphere.2019.04.114
Swaileh K. M. (1996). Seasonal variations in the concentrations of Cu, cd, Pb and zn in arctica islandica l. (Mollusca: Bivalvia) from Kiel bay, Western, Baltic Sea. Mar. Pollut. Bull. 32, 631–635. doi: 10.1016/0025-326X(96)00026-4
Tang Y., Zhou W., Sun S., Du X., Han Y., Shi W., et al. (2020). Immunotoxicity and neurotoxicity of bisphenol a and microplastics alone or in combination to a bivalve species, tegillarca granosa. Environ. Pollut. 265, 115115. doi: 10.1016/j.envpol.2020.115115
Thushari G. G. N., Senevirathna J. D. M., Yakupitiyage A., Chavanich S. (2017). Effects of microplastics on sessile invertebrates in the eastern coast of Thailand: An approach to coastal zone conservation. Mar. Pollut. Bull. 124, 349–355. doi: 10.1016/j.marpolbul.2017.06.010
Tlili S., Jemai D., Brinis S., Regaya I. (2020). Microplastics mixture exposure at environmentally relevant conditions induce oxidative stress and neurotoxicity in the wedge clam donax trunculus. Chemosphere 258, 127344. doi: 10.1016/j.chemosphere.2020.127344
Vroom R. J. E., Koelmans A. A., Besseling E., Halsband C. (2017). Aging of microplastics promotes their ingestion by marine zooplankton. Environ. Pollut. 231, 987–996. doi: 10.1016/j.envpol.2017.08.088
Wang Q., Guan C., Han J., Chai M., Li R. (2022). Microplastics in China Sea: Analysis, status, source, and fate. Sci. Total Environ. 803, 149887. doi: 10.1016/j.scitotenv.2021.149887
Wright S. L., Thompson R. C., Galloway T. S. (2013). The physical impacts of microplastics on marine organisms: A review. Environ. Pollut. 178, 483–492. doi: 10.1016/j.envpol.2013.02.031
Xia X., Sun M., Zhou M., Chang Z., Li L. (2020). Polyvinyl chloride microplastics induce growth inhibition and oxidative stress in cyprinus carpio var. larvae. Sci. Total Environ. 716, 136479. doi: 10.1016/J.SCITOTENV.2019.136479
Xu X. Y., Lee W. T., Chan A. K. Y., Lo H. S., Shin P. K. S., Cheung S. G. (2017). Microplastic ingestion reduces energy intake in the clam atactodea striata. Mar. Pollut. Bull. 124, 798–802. doi: 10.1016/j.marpolbul.2016.12.027
Xu X. Y., Wong C. Y., Tam N. F. Y., Liu H. M., Cheung S. G. (2020). Barnacles as potential bioindicator of microplastic Pollution in Hong Kong. Mar. Pollut. Bull. 154, 111081. doi: 10.1016/j.marpolbul.2020.111081
Yu S. P., Chan B. K. K. (2020a). Effects of polystyrene microplastics on larval development, settlement, and metamorphosis of the intertidal barnacle amphibalanus amphitrite. Ecotoxicol. Environ. Saf. 194, 110362. doi: 10.1016/J.ECOENV.2020.110362
Yu S. P., Chan B. K. K. (2020b). Intergenerational microplastics impact the intertidal barnacle amphibalanus amphitrite during the planktonic larval and benthic adult stages. Environ. Pollut. 267, 115560. doi: 10.1016/J.ENVPOL.2020.115560
Yu S. P., Cole M., Chan B. K. K. (2020). Review: Effects of microplastic on zooplankton survival and sublethal responses. Oceanogr. Mar. Biol. 58, 351–394. doi: 10.1201/9780429351495-7
Yu S. P., Nakaoka M., Chan B. K. K. (2021). The gut retention time of microplastics in barnacle naupliar larvae from different climatic zones and marine habitats. Environ. Pollut. 268, 115865. doi: 10.1016/j.envpol.2020.115865
Zantis L. J., Carroll E. L., Nelms S. E., Bosker T. (2021). Marine mammals and microplastics: A systematic review and call for standardisation. Environ. Pollut. 269, 116142. doi: 10.1016/j.envpol.2020.116142
Zhang T., Song K., Meng L., Tang R., Song T., Huang W., et al. (2022). Distribution and characteristics of microplastics in barnacles and wild bivalves on the coast of the yellow Sea, China. Front. Mar. Sci. 8. doi: 10.3389/fmars.2021.789615
Zhang T., Sun Y., Song K., Du W., Huang W., Gu Z., et al. (2021). Microplastics in different tissues of wild crabs at three important fishing grounds in China. Chemosphere 271, 129479. doi: 10.1016/j.chemosphere.2020.129479
Zhang J., Zhang C., Deng Y., Wang R., Ma E., Wang J., et al. (2019). Microplastics in the surface water of small-scale estuaries in shanghai. Mar. Pollut. Bull. 149, 110569. doi: 10.1016/J.MARPOLBUL.2019.110569
Keywords: microplastics, polypropylene, barnacles, fragments and fibers, bioindicator
Citation: Xu X, Fang JK-H, Wong C-Y and Cheung S-G (2023) Experimental accumulation of microplastics in acorn barnacle Amphibalanus amphitrite and its use in estimating microplastic concentration in coastal waters. Front. Mar. Sci. 9:1081329. doi: 10.3389/fmars.2022.1081329
Received: 27 October 2022; Accepted: 27 December 2022;
Published: 12 January 2023.
Edited by:
Cristina Panti, University of Siena, ItalyReviewed by:
Benny K. K. Chan, Academia Sinica, TaiwanImran Hashmi, Namibia University of Science and Technology, Namibia
Copyright © 2023 Xu, Fang, Wong and Cheung. This is an open-access article distributed under the terms of the Creative Commons Attribution License (CC BY). The use, distribution or reproduction in other forums is permitted, provided the original author(s) and the copyright owner(s) are credited and that the original publication in this journal is cited, in accordance with accepted academic practice. No use, distribution or reproduction is permitted which does not comply with these terms.
*Correspondence: Siu-Gin Cheung, bhsgche@cityu.edu.hk