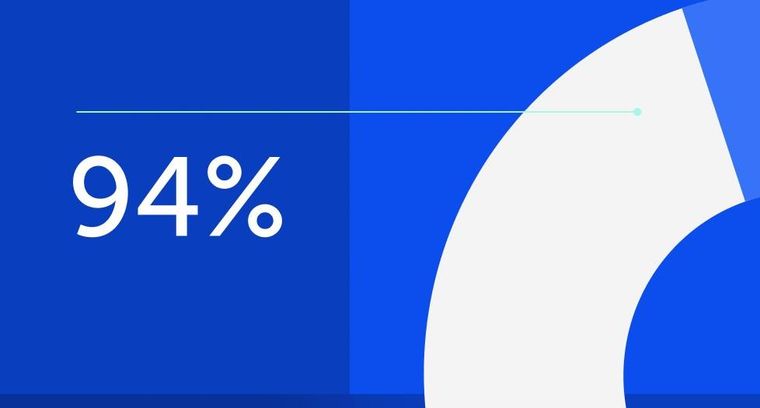
94% of researchers rate our articles as excellent or good
Learn more about the work of our research integrity team to safeguard the quality of each article we publish.
Find out more
ORIGINAL RESEARCH article
Front. Mar. Sci., 28 November 2022
Sec. Marine Biology
Volume 9 - 2022 | https://doi.org/10.3389/fmars.2022.1076929
This article is part of the Research TopicBiological Rhythms of Aquatic Organisms in a Changing ClimateView all 9 articles
A 51-day experiment was conducted to investigate the effects of diurnal temperature fluctuations (DTF) on growth performance, energy metabolism, stress response and gut microbiota of juvenile mud crab Scylla paramamosain. One control and three fluctuation groups were set up, i.e., constant (28 ± 0°C) (CT), slight (SF) (28 ± 2°C), medium (MF) (28 ± 4°C), and large (LF) (28 ± 6°C) DTF. The survival rate tended to decrease with the intensification of DTF, the survival of rate of CT, SF, MF and LF were 80.6 ± 3.9%, 75.0 ± 6.8%, 33.3 ± 6.8%, and 30.6 ± 10.4% respectively. The crab in SF and MF had a shorter, but LF had a longer molt interval compared with the crab in the CT group. Cortisol, blood glucose (GLU), total cholesterol (T-CHO) and triglyceride (TG) levels peaked in the LF group. The exacerbation of DTF caused a dramatic increase in reactive oxygen species (ROS) levels and impacted the antioxidant capacity of juvenile crabs. The relative expression of AMP-activated protein kinase (ampk), heat shock protein 70 (hsp70) and heat shock protein 90 (hsp90) genes was significantly increased in MF group. The expression of Ribosomal protein S6 kinase (s6k) and Mechanistic target of rapamycin (tor) genes was significantly up-regulated in the SF group (P< 0.05) but large DTF caused a decrease in the relative expression of a large number of functional genes. DTF affected the structure and function of gut microbes. The bacterial community changed with the intensification of DTF and alpha diversity continued to rise. Five biomarkers were identified, where Rhodobacterales and Rhodobacterac were significantly more abundant in the CT group, Campylobacterales,Vibrionales and erysipelotrichales were more abundant in the SF, MF and LF groups. In addition, SF also enhanced gut microbes interactions compared with other treatments. These results suggest that drastic environmental DTF reduced the growth and survival of young mud crabs, and the effect was mediated by energy metabolism, antioxidant pathways and gut microbes.
Temperature is one of the most dominant cyclical factors in the natural environment (Whiteley et al., 1997). As the global climate warms, the magnitude of diurnal temperature fluctuations (DTF) increases (Verheyen and Stoks, 2019). For aquatic organisms, the environment is never static. However, it is worth noting that most studies have focused on the effects of rising average temperatures on organisms, which remains a major limitation to understanding the impact of ambient temperature on organisms (Walther et al., 2002; Gunderson et al., 2016). DTF may expose marine organisms to extreme temperatures and have a more profound impact on the physiological processes of aquatic organisms than the effects of average temperatures (Wang and Dillon, 2014). In addition, the importance of DTF in aquaculture cannot be overlooked. Numerous studies have shown the impact of DTF not only on the growth of aquatic animals but also on their survival (Tian and Dong, 2006; Dong et al., 2008a). Interestingly, different studies have found that DTF does not have the same effect on aquatic organisms. Some researchers reckoned that regular temperature fluctuations allow organisms to adapt to their endogenous biorhythms and thus optimize performance (Žák and Reichard, 2020). For example, (Tian and Dong, 2006) found that the growth rates of Chinese shrimp (Fenneropenaeus chinensis) at 25 ± 2°C, 25 ± 3°C, 28 ± 2°C and 31 ± 1°C were significantly higher than their growth rates at constant temperatures of 25°C, 28°C, and 31°C (P< 0.05), respectively. In studies on sea cucumber (Apostichopus japonicus), it was found that growth was higher in both the slight and medium DTF groups (18 ± 2°C, 18 ± 4°C) than in the constant temperature group (18°C), while too large a DTF would put the ginseng in sub-optimal conditions and harm survival and growth (Dong et al., 2008a). Other researchers think that there is no difference in the growth rate of some aquatic organisms at constant temperatures between DTF and mean DTF, e.g. Tahoe sucker (Catostomus tahoensis) (Vondracek et al., 1989).
The mud crab (Scylla paramamosain) is an economically important eurythermic crab widely distributed in China and Southeast Asian countries (Le Vay, 2001). The crab live in the estuary, intertidal zone of the inner bay, quagmire, and beach of high tidal range (Li et al., 2018), experiencing considerable DTF due to the rise and fall of the tide (Newell, 1969). Thus, DTF inevitably strongly affected the living state of mud crab.
Physiological processes in aquatic metazoans are strongly influenced by temperature (Kang et al., 2019). Molting is a crucial physiological process in crustacean growth (Zhou et al., 2017). Temperature is one of the most critical environmental factors affecting the molting of crustaceans (Yuan et al., 2018; Azra et al., 2019; Ren et al., 2021). Ecdysone is an important hormone that controls molt of crustaceans. Molting is influenced by mutually antagonistic ecdysone secreted by the Y-organ and ecdysone-inhibiting hormone (MIH) secreted by the X-organ sinus gland complex in the eyestalk (Mykles, 2011; Hyde et al., 2019). Peripheral tissues convert ecdysteroids into active ecdysone, 20-hydroxy ecdysone (20-HE) (Mykles, 2001). Ecdysone receptor (EcR) forms a heterodimer with the retinoid X receptor (RXR) (Yao et al., 1993). The complex formed by 20-HE combined with the ECR-RXR heterodimer activates the transcription of genes encoding transcription factors such as e75 and ultimately affects the activity of different enzymes that control the degradation of the old cuticle and the formation of the new cuticle, thus influencing molting (Priya et al., 2010).
Molting is an energy consumption course. Metabolites in hemolymph reflect their morphological and physiological adaptation to the environment (Pascual et al., 2003; Hu et al., 2008). Blood glucose (GLU), triglycerides (TG) and total cholesterol (T-CHO) are closely related to their energy metabolism (Ciaramella et al., 2014). Mechanistic target of the rapamycin (mTOR) signaling pathway plays an essential role in energy metabolism (Wullschleger et al., 2006; Saxton and Sabatini, 2017). mTOR can bind to proteins such as Rheb (Ras homolog enriched in brain) to form mTOR Complex 1 (mTORC1) (Saxton and Sabatini, 2017). Ribosomal protein S6 kinase (S6K) is a downstream target of mTORC1 and is an essential activator of synthetic proteins (Saxton and Sabatini, 2017). AMP-activated protein kinase (AMPK) is able to inhibit Rheb, thereby negatively regulating mTOR (Wataya-Kaneda, 2015).
Recent ecological studies have also shown that the oxidation state can effectively respond to the environmental conditions faced by organisms (Beaulieu and Costantini, 2014). DTF is a source of stress that cannot be ignored. Excessive DTF may lead to thermal stress in aquatic organisms, causing a wide range of behavioral and physiological responses, generating reactive oxygen species (ROS) (Lutterschmidt and Hutchison, 1997; Helmuth and Hofmann, 2001; Carveth et al., 2007; Madeira et al., 2013). In response to oxidative damage from ROS, superoxide dismutase (SOD), catalase (CAT) and glutathione peroxidase (GPX) play a key role (Kong et al., 2012; Cheng et al., 2017). The level of malondialdehyde (MDA) in tissues is an effective indicator of lipid peroxidation (Viarengo et al., 1990). In response to the stress response caused by DTF, heat shock proteins (HSPs) in eukaryotes function as molecular chaperones to repair proteins denatured by heat (Liu et al., 2018). Although this process is energy-intensive, it reduces the high cost of subsequent protein re-synthesis by protecting the existing protein pool (Dong et al., 2008b). One of the most abundant families is the HSP70 family, which is one of the biochemical indicators of crustacean response to heat stress (Yang et al., 2013; Liu et al., 2018).
Furthermore, the gut microbes of aquatic organisms are also affected by temperature (Feng et al., 2021; Han et al., 2022). Differences in the composition and structure of gut microbes can affect a variety of vital physiological activities, such as host growth and development, energy metabolism, and immune competence and have received widespread attention (Zuo et al., 2019; Butt and Volkoff, 2019; Wang et al., 2020). However, to date, few studies have focused on the effects of DTF on growth performance, energy metabolism, stress response, and gut microbes of juvenile mud crab.
Therefore, the aim of this study was to examine the growth performance, energy metabolism, stress response and gut microbes of juvenile mud crabs exposed to DTF for prolonged periods, as a means of understanding the adaptive capacity of juvenile mud crabs to DTF. This study will provide new ideas for the aquaculture of mud crab, as well as a better understanding of adapting the mechanism of marine organisms to DTF.
The crabs used in the present study were purchased from the Xinxing nursery (Xiangshan County, Ningbo City, Zhejiang Province) and transported to the Meishan Campus of Ningbo University in a foam box with seawater-soaked sponge mats. Before starting the experiment, the crabs were kept in a 500L PVC tank at a water temperature of 28°C for 30 days. Commercial feed (40% protein and 6% lipid, Ningbo Tech-Bank Feed Co., Ltd., Ningbo, China) was fed daily at 18:00, and any unconsumed residual feed was siphoned out with a siphon tube at 8:00 the following day.
The experiment had four groups, i.e., constant temperature group (CT, 28°C) and slight (SF, 28 ± 2°C), medium (MF, 28 ± 4°C), and large (LF, 28 ± 6°C) DTF groups respectively, distributed to four mutually independent recirculating aquaculture systems (Dong et al., 2008a; Liu et al., 2022). Each system is equipped with a cotton filter and biofilter and comprises six square containers (0.5 × 0.3 × 0.2 m) with six separate compartments (0.1 × 0.1 × 0.13 m) in each container. A total of 144 juvenile mud crabs (0.146 ± 0.015 g) with complete appendages and similar weight conditions were randomly placed in each compartment, with six replicates (6 crabs per replicate) per group. A computerized thermostat (WK-SM3) was used to heat the water to Tmax (28 + t°C) from 9:00 to 12:00 each day, maintaining Tmax until 21:00. Afterwards, the water temperature was reduced to Tmin (28 – t°C) using a chiller (AO LING HENG YE, LA-160, China) from 21:00 to 24:00 and Tmin was maintained constant until 9:00 the following day. During the experiment, the light cycle was synchronized to warm up and cool down by switching the light source from 9:00 to 21:00 and switching it off from 21:00 to 9:00 the following day. During the experiment, seawater salinity was 25‰ and dissolved oxygen (>6.0 mg L-1), excess commercial feed was fed at 18:00 each day, and the residual feed was sucked out using a siphon tube at 7:00 the following day.
Sampling was conducted at the end of the 51-day culture experiment. After 24 hours of starvation, samples were collected at 12:00 the next day, and the sampling lasted for half an hour. One juvenile mud crab was randomly selected from each replicate in each treatment group, and the juvenile crabs in each group were weighed after wiping off the surface with paper towels. The crab was placed on an ice pack, and the hemolymph was extracted from the pericardial sinus. The samples were centrifuged at 3500r/min at 4°C for 15 min, and the supernatant was collected. The eyestalk, gut, and hepatopancreas were rapidly collected, frozen in liquid nitrogen, and stored at -80°C (Xu et al., 2023).
Two hemolymph samples were randomly selected from each replicate to be mixed, with a total of six samples in each treatment. The assays were performed with crab’s specific ecdysone ELISA kit (Shanghai Huyu Biological Technology Co., Ltd, Shanghai, China). Two samples of eyestalks and hepatopancreas were randomly selected from each replicate. A total of 6 samples per tissue were collected for each treatment. The tissue was added to the mortar after being well pre-chilled with liquid nitrogen. Quickly ground and added to an EP tube containing 1 ml of Trizol reagent (Invitrogen, CA, USA) to extract total RNA. After testing the purity and integrity of RNA using a spectrophotometer and 1% gel electrophoresis, the RNA was reverse transcribed into cDNA using the reverse transcription kit HiFiScript cDNA (CW Biotech. Co. Lid., Shanghai, China). Real-time PCR (LightCycler480II) was performed in a 20 μL reaction system. The primers used for qPCR are listed in Table 1. The relative expression of the ecdysone inhibitory hormone gene(mih), ecdysone receptor gene (ecr), retinoid X receptor gene (rxr), ecdysone inducible gene (e75) in the eyestalk was calculated using the 2-ΔΔCT method with β-actin as the internal reference gene and the CT group as the control group in relative expression (Chen et al., 2022). The relative expression of the heat shock protein 70 gene (hsp70), heat shock protein 90 gene (hsp90), mechanistic target of rapamycin gene (tor), AMP kinase gene (ampk) and S6 kinase gene (s6k) were determined in the hepatopancreas in the same way.
Two hemolymph samples were randomly selected from each replicate to be mixed, with a total of six samples in each treatment. The assays were performed with crab’s specific cortisol ELISA kit (Shanghai Huyu Biological Technology Co., Ltd, Shanghai, China). GLU (A154-1-1), T-CHO (A111-1-1) and TG (A110-1-1) content were determined by commercial kits (Nanjing Jiancheng Bioengineering Institute, Nanjing, China) (Zhu et al., 2021).
Two hepatopancreas samples were randomly selected from each replicate to be mixed, with a total of six samples in each treatment. Before the assay, tissue homogenization was performed by adding four times the weight of the hepatopancreas to the centrifuge tube with PBS. The supernatant was collected after centrifugation at 4°C and 3500 r/min for 15 min. The assays were performed with crab’s specific ROS ELISA kit (Shanghai Huyu Biological Technology Co.,Ltd, Shanghai, China). MDA(A003-1-2), SOD (A001-3-2), GSH (A006-2-1), CAT (A007-1-1) and T-AOC (A015-2-1) content were determined by commercial kits (Nanjing Jiancheng Bioengineering Institute, Nanjing, China) (Chen et al., 2021).
Two gut samples were randomly selected from each replicate of each treatment for genomic DNA extraction using the FastDNA Spin kit (MP Biomedicals,USA), with a total of six samples per treatment. After quality checks, the primers 338F: 5’-ACTCCTACGGGAGGCAGCAG-3’ upstream and 806R: 5’-GGACTACHVGGGTWTCTAAT-3’ downstream were used were amplified by polymerase chain reaction (PCR) (Fan et al., 2019). The V3-V4 region of the 16S rRNA gene was sequenced by Illumina MiSeq through MAGIGENE (Guangdong, China). Raw sequence data are available in the NCBI Sequence Read Archive under BigProject PRJNA898305.
All data were statistically analyzed using SPSS 22.0 (IBM, USA) software. All data in this study are expressed as mean ± SD (n=3). Before analysis, the Kolmogorov - Smirnov and Levene tests were used to test the distribution normality and homogeneity of variance of the original data. One-way ANOVA was used for homogeneous variance data, and Tukey multiple comparisons were used for the significance test. P< 0.05 was considered significant. When the variances were not homogeneous, the nonparametric Kruskal-Wallis test was used for analysis (Liu et al., 2022).
Differences in the Alpha Diversity Index were tested using the Kruskal-Wallis test in SPSS 22.0. Principal coordinate analysis (PCoA) based on Bray-Curtis distances was used to visualize the beta diversity of the samples and ANOSIM to test for significance. Linear discriminant analysis effect size (LEfSe) was used to identify and characterize dominant species with significantly different abundance in bacterial communities between groups (Segata et al., 2011). The KEGG (Kyoto encyclopedia of genes and genomes) metabolic pathway was predicted by PICRUSt2 (Langille et al., 2013). Gephi.0.9.2 was used to visualize the molecular ecological network and calculate the network topology, including network nodes, edges, average path length, average degree, and clustering coefficient (Du et al., 2020).
Survival rates for the CT, SF, MF, and LF groups were 80.6 ± 3.9%, 75.0 ± 6.8%, 33.3 ± 6.8, and 30.6 ± 10.4%, respectively (Figure 1A). Constant temperature and slight DTF had no significant effect on the survival of juvenile crabs (P > 0.05). However, moderate and excessive DTF significantly reduced the survival rate of juvenile crabs (P< 0.05). In addition, DTF also had a significant effect on the growth of juvenile crabs (P< 0.05). Slight DTF had a significant growth-promoting effect on juvenile crabs, with the SF group SGR (4.62 ± 0.12% day-1) being significantly higher than the CT (3.87 ± 0.19% day-1), MT (4.17 ± 0.17% day-1) and LT (3.13 ± 0.13% day-1) groups (P< 0.05) (Figure 1B). The molt interval was significantly shorter in the SF (11.92 ± 1.43 days) and MF (12.60 ± 0.54 days) groups compared to the CT (14.81 ± 0.55 days) group (P< 0.05). The molt interval was significantly higher in the LF group (19.78 ± 1.13 days) compared to the other three groups (P< 0.05) (Figure 1C).
Figure 1 Effects of different levels of DTF (28°C, 28 ± 2°C, 28 ± 4°C, 28 ± 6°C) on juvenile crab survival (A), specific growth rate (B) and inter-molt period (C). Values are expressed as mean ± SD (n=3). Different superscripts indicate significant differences between treatments (P< 0.05).
The levels of ecdysone were significantly higher in the SF and MF groups than in the CT and LF groups (P< 0.05) (Figure 2A). The expression of mih gene was significantly higher in the CT group than in all other groups (P< 0.05) (Figure 2B). The relative expression of ecr and e75 genes was significantly higher in the MF group than in all other groups (P< 0.05) (Figures 2C, E). There was no significant difference in the relative expression of rxr gene between SF and MF groups (P > 0.05), and LF group was significantly lower than the other three groups (P > 0.05) (Figure 2D).
Figure 2 Ecdysone levels in haemolymph (A). Relative expression of mih (B), ecr (C), rxr (D) and e75 (E) gene of the eyestalk. Values are expressed as mean ± SD (n=3). Different superscripts indicate significant differences between treatments (P< 0.05).
The level of cortisol in hemolymph tended to increase significantly with the exacerbation of DTF (P< 0.05) (Figure 3A). There was no significant difference in GLU levels between the MF (8.02 ± 1.24 mmol L-1) and LF (7.29 ± 0.76 mmol L-1) groups (P > 0.05), but both were significantly higher than the CT (4.52 ± 0.47 mmol L-1) and SF groups (3.95 ± 0.20 mmol L-1) (P< 0.05) (Figure 3B). T-CHO and TG levels showed a significant increase in both MF and LF compared to the CT and SF groups (P< 0.05) (Figures 3C, D).
Figure 3 Effects of different levels of DTF (28°C, 28 ± 2°C, 28 ± 4°C, 28 ± 6°C) on cortisol (A), GLU (B), TG (C) and T-CHO (D) in juvenile crabs. Values are expressed as mean ± SD (n=3). Different superscripts indicate significant differences between treatments (P< 0.05).
Moderate DTF caused a significant upregulation (P< 0.05) of the relative expression of the ampk gene in the hepatopancreas of juvenile crabs, but there were no significant differences between the CT, SF and LF groups (P > 0.05) (Figure 4A). The relative expression of the tor gene was significantly higher in the SF group than in the other three groups, and significantly lower in the LF group than in the CT and MF groups (P< 0.05) (Figure 4B). s6k gene expression was similar to that of the tor gene (Figure 4C). hsp70 gene expression was significantly higher in the MF group than in the other three groups (P< 0.05) (Figure 4D). The relative expression of the hsp90 gene was not significantly different between the MF and SF groups (P > 0.05), but was significantly higher in the MF group than in the CT and LF groups (P< 0.05) (Figure 4E).
Figure 4 Relative expression of ampk (A), tor (B), s6k (C), hsp70 (D) and hsp90 (E) in the hepatopancreas of juvenile crabs. Values are expressed as mean ± SD (n = 3). Different superscripts indicate significant differences between treatments (P< 0.05).
DTF had a significant effect on ROS and antioxidant capacity (P< 0.05). The ROS concentration in juvenile crabs’ hepatopancreas increased significantly with the increased diurnal temperature simultaneously (P< 0.05) (Figure 5A). T-AOC, SOD and CAT activities were significantly higher in the SF group than in the other three groups (P< 0.05) (Figures 5B, C, F). GSH in SF and LH groups was significantly higher than that in CT and MF groups (P< 0.05) (Figure 5D). The MDA content was significantly higher in the MF (575.62 ± 109.71 nmol ml-1) and LF (598.77 ± 26.55 nmol ml-1) groups than in the CT (160.49 ± 38.05 nmol ml-1) and SF (212.96 ± 34.65 nmol ml-1) groups (P< 0.05) (Figure 5E).
Figure 5 Effects of different levels of DTF (28°C, 28 ± 2°C, 28 ± 4°C, 28 ± 6°C) on ROS (A), T-AOC (B), SOD (C), GSH (D), MDA (E) and CAT (F) in juvenile crabs. Values are expressed as mean ± SD (n = 3). Different superscripts indicate significant differences between treatments (P< 0.05).
Regression analysis of the alpha-diversity indices found that Richness, Shannon index, Pielous’s eveness and Phylogentic diversity showed an increasing trend with the drastic degree of DTF (Figure 6). A comparison of the Principal Coordinate Analysis (PCoA) method based on the Bray_curtis distance algorithm revealed that CT, SF, MF and LF showed significant clustering (Figure 7). The ANOSIM analysis showed significant differences in bacterial community structure between the four groups (R=0.327, P=0.001) (Table 2). There were no significant differences (P > 0.05) between the CT and SF groups and between the CT and LF groups. The rest of the groups were significantly different from each other (P< 0.05). Relative community abundance at the phylum level (Figure 8A) indicates that nine known phyla were identified, and sequences from any known phylum that could not be classified were classified as “other”. Of these, Firmicutes and Proteobacteria predominate in the gut of juvenile crab. Campilobacterota accounted for a significantly higher proportion in the SF group (8.35 ± 4.22%) than in CT (0.11 ± 0.06%), MF (0.62 ± 0.43%) and LF (0.70 ± 0.60%) (P< 0.05). The first nine dominant genera were selected to compare the abundance of communities between groups at the genus level (Figure 8B), and sequences of any known genera that could not be classified were classified as “other”. The main genera in the CT, SF, MF and LF groups were Vibrio (25.76 ± 11.05%, 36.05 ± 12.32%, 52.79 ± 6.63%, 21.77 ± 5.41%), Streptococcus (0.57 ± 0.51%, 0.54 ± 0.52%, 0.94 ± 0.78%, 2.36 ± 1.39%), Pseudoalteromonas (6.43 ± 5.12%, 3.43 ± 1.25%, 2.04 ± 0.21%, 4.12 ± 1.94%), Mycoplasmataceae_uncultured (34.60 ± 8.24%, 21.46 ± 2.70%, 16.25 ± 10.40%, 27.75 ± 12.86%), Lactococcus (1.32 ± 1.73%, 0.63 ± 0.81%, 0.63 ± 0.56%, 2.06 ± 1.86%), Fusibacter (4.45 ± 4.59%, 0.27 ± 0.13%, 0.07 ± 0.04%, 0.54 ± 0.63%), Exiguobacterium (2.03 ± 2.21%, 5.80 ± 7.77%, 5.07 ± 4.93%, 7.87 ± 3.22%), Carnobacterium (3.15 ± 2.17%, 1.46 ± 0.98%, 2.22 ± 1.34%, 3.60 ± 3.03%) found that the abundance of Vibrio tended to increase and then decrease with the intensification of DTF. According to the set screening criteria (LDA > 4), LEfSe showed that five biomarkers were significantly more abundant in relative abundance in each of the CT, SF, MF, and LF groups (Figure 9). Among them, Rhodobacterales and Rhodobacterac were significantly more abundant in the CT group, Campylobacterales in the SF group, while Vibrionales in the MF group and erysipelotrichales in the LF group. Metabolic functions were compared between all groups in a 2-level KEGG analysis by using clustered heat map analysis. Pathways related to amino acid metabolism, carbohydrate metabolism, metabolism of terpenoids and polyketides, metabolism of other amino acids, biosynthesis of other secondary metabolites, nucleotide metabolism, lipid metabolism, xenobiotics biodegradation and metabolism, glycan biosynthesis and metabolism, metabolism of cofactors and vitamins, and energy metabolism were relatively abundant in the MF group (Figure 10). The microbial network structure of each group was different (Figure 11). The intestinal microbiota network of juvenile crabs in the SF group consisted of 155 nodes and 1179 edges and had the highest microbial network complexity. Compared with the other groups, the SF group had a higher average degree, average path length, and clustering coefficient (Table 3).
Figure 6 α-diversity index in gut microbes of juvenile crabs. Values are expressed as mean ± SD (n = 3). Different superscripts indicate significant differences between treatments (P< 0.05). R2 and P values after linear regression analysis showed the correlation between α-diversity indices and DTF.
Figure 7 Principal coordinate analysis (PCoA) plots of the gut microbial community of juvenile crabs at different levels of DTF (28°C, 28 ± 2°C, 28 ± 4°C, 28 ± 6°C).
Table 2 ANOMIS results for the community structure of the gut microbiota (OTUs) of juvenile crabs at different levels of DTF (28°C, 28 ± 2°C, 28 ± 4°C, 28 ± 6°C) based on Bray-Curtis distances.
Figure 8 Relative abundance of bacterial communities at the gut microbiota phylum (A) and genus (B) level in juvenile crabs at different levels of DTF (28 °C, 28 ± 2°C, 28 ± 4°C, 28 ± 6°C).
Figure 9 Linear discriminant analysis (LDA) effect sizes (LEfSe) were used to identify potentially unique biomarkers for each group. The circles represent the phylogenetic level from the phylum to the genus. The diameter of each circle is proportional to the abundance of the taxonomic unit. Differences are indicated by the colour of the most abundant class.
Figure 10 Cluster heat map showing Pearson correlations between pathways associated with different degrees of DTF (28°C, 28 ± 2°C, 28 ± 4°C, 28 ± 6°C) and gut microbial metabolism in juvenile crabs, with red representing positive correlations and cyan representing negative correlations.
Figure 11 A network of relationships between gut microbial taxa of juvenile crabs at different levels of DTF (28°C, 28 ± 2°C, 28 ± 4°C, 28 ± 6°C). The size of the nodes indicates the relative abundance of OTUs, and the nodes are coloured by different modules.
Table 3 Topological characteristics of the gut microbial network of juvenile crabs at different levels of DTF (28°C, 28 ± 2°C, 28 ± 4°C, 28 ± 6°C).
The results of this study showed that DTF had a significant effect on the survival and growth of juvenile crabs. However, increased DTF resulted in a substantial decrease in juvenile crab survival and SGR. Moderate levels of DTF have been found to promote the growth of aquatic organisms such as Daphnia pulex (Van As et al., 1980), mud crab (Panopeus herbstii) (Dame and Vernberg, 1978), fiddler crab (Uca pugilator) (Dame and Vernberg, 1978) and Chinese shrimp (Penaeus chinensis) (Miao and Tu, 1996). The ability of DTF to promote aquatic growth is mainly attributed to a decrease in metabolic rate (Dame and Vernberg, 1978), an increase in feeding rate (Diana, 1984; Tian and Dong, 2006), and an increase in feed conversion efficiency (Tian and Dong, 2006). In contrast, Prakoso et al. (2021) concluded that DTF inhibited the growth of giant gourami (Osphronemus goramy) larvae without affecting their survival. Surprisingly, however, the growth performance of juvenile crab, a broadly temperate species, decreased when DTF exceeded ±2°C. The optimum DTF for juvenile crabs in this study was ±2°C. Similar results have been found in studies of other crustaceans. For example, Tian and Dong, 2006 concluded that the optimum DTF for Chinese shrimp (Fenneropenaeus chinensis) was 1 to 3°C. (Miao and Tu, 1996) concluded that the optimum DTF for Chinese shrimp (Penaeus chinensis) was 2.39°C. Some studies have shown that intertidal shellfish, such as the Pacific Oyster (Crassostrea gigas), can withstand high DTF (Zhu et al., 2016). The difference could be mainly attributed to the fact that crab is more agile and could avoid exposure to extreme DTF.
Previous studies have found that periodic fluctuations in environmental factors such as salinity (Su et al., 2010), light color (Guo et al., 2012) and light intensity (Guo et al., 2013) have a facilitating effect on molting in crustaceans. However, the relationship between molt and DTF has not been thoroughly investigated. In the present study, both ±2°C and ±4°C DTF resulted in a significantly shorter inter-molt period in juvenile crabs, while the inter-molt period was significantly longer when DTF reached ±6°C. This is closely related to molting hormone levels and the expression of molt-related genes (Chen et al., 2021). The results showed that DTF at ±2°C and ±4°C inhibited the expression of mih genes in the eyestalks of juvenile crabs and increased the relative expression of ecr, rxr and e75 genes as well as the level of molting hormone, thus promoting molting. In addition, the environmental stress caused by DTF in the LF group inhibited the Y-organ’s secretion of molting hormone through up-regulation of mih gene expression, which contributed to the significant increase in molting interval in the LF group.
Cortisol has been used in numerous studies as an indicator of stress in animals (Yong et al., 2020). As a catabolic hormone, an increase in its level is often accompanied by an increase in energy expenditure (Christiansen et al., 2007). In the present study, DTF increased cortisol levels; however, GLU, TG, and T-CHO levels in the SF group did not increase in parallel. On the other hand, GLU, TG, and T-CHO also increased when juvenile crabs were exposed to higher DTF, suggesting the metabolic energy requirements are increased under fluctuating ambient temperature conditions, as previously observed in Pacific white shrimp (Litopenaeus vannamei) (Wang et al., 2019). These results indicate slighter DTF did not increase energy expenditure, and a moderate increase in cortisol was found to stimulate feeding and growth (Bernier et al., 2004). In addition, this study revealed that the mTOR signaling pathway plays a crucial role in the energy metabolism of juvenile crabs after exposure to DTF. In this study, the expression of ampk gene was found to be up-regulated and tor and s6k gene expression was down-regulated in the presence of DTF at ±4°C. This suggests that the heat stress caused by DTF in the MF group increases the AMP: ATP ratio in juvenile crab cells, thereby activating AMPK (Hardie et al., 2006). AMPK negatively regulates mTOR and S6K downstream by inhibiting Rheb, directing the energy used for protein synthesis and growth in response to DTF (Anttila et al., 2013; Han et al., 2013).
The high metabolic energy demand is often accompanied by the production of ROS (Yang and Lian, 2020). Aquatic organisms resist ROS production due to DTF by activating endogenous antioxidant enzymes such as SOD and CAT (Sachdev et al., 2021). In the present study, juvenile crabs in the LF group showed higher levels of ROS and MDA in the hepatopancreas, while the activities of T-AOC, SOD and CAT were inhibited. These results suggest that DTF may have caused oxidative stress in the hepatopancreas of juvenile crabs. The high levels of MDA in the MF and LF groups further confirmed the hypothesis.
Several studies on the genes of aquatic HSPs have reported that changes in environmental temperature induce upregulation of HSP-related gene expression (Rungrassamee et al., 2010; Dong et al., 2011). The increased hsp70 and hsp90 gene expression when juvenile crabs were exposed to DTF was similar to the results of the above studies. Since the upregulation of HSPs genes can be considered a form of cellular defense (Ueda and Boettcher, 2009), it suggests that prolonged DTF puts juvenile crabs in a state of heat stress when DTF reaches ±4°C. Notably, the lack of sufficient HSPs response in juvenile crabs when DTF reached ±6°C may contribute to the mortality in the LF group.
Unexpectedly, in the LF group, the low relative expression of molting genes was accompanied by HSPs-related genes as well as mTOR-related genes. This phenomenon could result from oxidative damage to DNA caused by overmuch ROS that cannot even be scavenged (Lesser, 2006). Many functional genes in juvenile crabs cannot complete their normal transcriptional processes, resulting in mass mortality in the LF group. The possibility of such a scenario is supported by numerous studies that have found that DNA damage occurs when aquatic organisms are exposed to extreme temperatures (Qiu et al., 2011; Yao and Somero, 2012; Cheng et al., 2018).
Environmental temperature is one of the key factors shaping the gut microbial community of aquatic organisms. The effects of their gut microbes on host physiology have been shown in previous studies (Dai et al., 2022; Liu et al., 2022). However, less research has been conducted on the microbial community in the more complex environment of DTF. This study showed that the gut microbe was mainly composed of Proteobacteria, Firmicutes and Bacteroidetes, which confirmed the results of a previous study (Wei et al., 2019). In this study, the relative abundance of Rhodobacterales was found to be significantly higher when the temperature was maintained at a constant level. The bacterial polyhydroxybutyric (PHB) produced by Rhodobacter can become an energy source for aquatic organisms, which is conducive to the growth of aquatic organisms (Zheng et al., 2021). One of these bacteria, Ruegeria, is a member of the Rhodobacterales and is considered a probiotic (Barreto-Curiel et al., 2018). One study found that in Atlantic cod larvae, Ruegeria produced isolates of antibacterial compounds against V. anguillarum, thereby increasing the survival of larvae (Lazado and Caipang, 2014). Thus, one of the reasons for the better survival and growth of juvenile crabs in the CT group.
Conditionally pathogenic bacteria are widely present in the crustacean gut and are usually harmless. However, under certain conditions, Conditionally pathogenic bacteria as Vibrio can cause disease (Zheng et al., 2021). Our study found that varying degrees of DTF influenced the relative abundance of Vibrio. The significantly higher abundance of Vibrio in the MF and Erysipelotrichaceae in the LF may be related to host metabolic disorders or energy metabolism (Chen et al., 2012), contributing to the lower survival rate.
The metabolic activity of gut microbes is critical for the homeostasis and phenotype of the host (Visconti et al., 2019). Functional predictions in juvenile crabs showed that the MF group was significantly higher in all relevant metabolism-related pathways. In contrast, the gut microbial metabolism-related pathways were suppressed in the LF group, confirmed by the down-regulated express a large number of functional genes in the host. Due to intense DTF and increased conditioned pathogenic bacteria, crabs in the MF group require more energy, reducing their growth potential (Zhou et al., 2019). In the LF group, large DTF may impair the function of gut microbes and reduce the host’s ability to cope with DTF, leading to the death of juvenile crabs. Drastic temperature changes disrupt the symbiotic relationship between the host and its symbiotic microbes, causing disease (Zhu et al., 2019) and compromising growth performance. The interactions between gut microbial species were more complex and better connected in juvenile crabs at slight DTF, as evidenced by increased clustering coefficients, averages, and mean path lengths. Unlike the alpha diversity index, microbial interactions did not increase parallel with DTF. A possible reason is that the increasing microbial diversity may disrupt interactions between gut microbes. However, the enhanced microbial interactions in the gut of juvenile crabs may have contributed to the faster growth of juvenile crabs. Similarly, recent results from the analysis of the gut network of Litopenaeus Vannamei showed that the gut microbes of overgrown shrimp had a higher density of correlated microbes compared to normally grown shrimp, promoting digestibility and thus affecting growth (Xiong et al., 2017).
This study investigated the effects of environmental DTF on the growth performance, energy metabolism, stress response, and gut microbes of juvenile mud crabs. The results showed that the higher DTF ( ± 6°C) resulted in reduced growth and survival of young crabs, mediated by the expression of relevant functional genes, energy metabolism, antioxidant pathways, and gut microbes. The optimal DTF was ±2°C. These findings provide a reference to the temperature regulation of mu crab indoor aquaculture and give clues to the adaptation of crustaceans to environmental DTF.
The sequencing data has been deposited into a publicly accessible NCBI repository (accession: PRJNA898305).
JL, SC and ZR: Investigation, Data curation, Visualization, Writing - original draft CM, CW, YY and QW: Review & editing, Funding acquisition. CS: Conceptualization, Writing - review & editing, Project administration, Funding acquisition. All authors contributed to the article and approved the submitted version.
The work was supported by the National Natural Science Foundation of China (Grant Nos. 32172994 and 31972783), The Province Key Research and Development Program of Zhejiang (2021C02047), Key Scientific and Technological Grant of Zhejiang for Breeding New Agricultural Varieties (2021C02069-6), Collaborative Promotion Program of Zhejiang Provincial Agricultural Technology of China (No. 2020XTTGSC03), SanNongLiuFang Zhejiang Agricultural Science and Technology Cooperation Project (2021SNLF029), 2025 Technological Innovation for Ningbo (No. 2019B10010), China Agriculture Research System of MOF and MARA (No. CARS48), the Special research funding from the Marine Biotechnology and Marine Engineering Discipline Group in Ningbo University (No. 422004582), and K. C. Wong Magna Fund in Ningbo University.
The authors declare that the research was conducted in the absence of any commercial or financial relationships that could be construed as a potential conflict of interest.
All claims expressed in this article are solely those of the authors and do not necessarily represent those of their affiliated organizations, or those of the publisher, the editors and the reviewers. Any product that may be evaluated in this article, or claim that may be made by its manufacturer, is not guaranteed or endorsed by the publisher.
Anttila K., Casselman M. T., Schulte P. M., Farrell A. P. (2013). Optimum temperature in juvenile salmonids: connecting subcellular indicators to tissue function and whole-organism thermal optimum. Physiol. Biochem. Zool. 86 (2), 245–256. doi: 10.1086/669265
Azra M. N., Chen J. C., Hsu T. H., Ikhwanuddin M., Abol-Munafi A. B. (2019). Growth, molting duration and carapace hardness of blue swimming crab, Portunus pelagicus, instars at different water temperatures. Aquacult. Rep. 15, 100226. doi: 10.1016/j.aqrep.2019.100226
Barreto-Curiel F., Ramirez-Puebla S. T., Ringø E., Escobar-Zepeda A., Godoy-Lozano E., Vazquez-Duhalt R., et al. (2018). Effects of extruded aquafeed on growth performance and gut microbiome of juvenile Totoaba macdonaldi. Anim. Feed Sci. Technol. 245, 91–103. doi: 10.1016/j.anifeedsci.2018.09.002
Beaulieu M., Costantini D. (2014). Biomarkers of oxidative status: missing tools in conservation physiology. Conserv. Physiol. 2 (1), 1–16. doi: 10.1093/conphys/cou014
Bernier N. J., Bedard N., Peter R. E. (2004). Effects of cortisol on food intake, growth, and forebrain neuropeptide y and corticotropin-releasing factor gene expression in goldfish. Gen. Comp. Endocrinol. 135 (2), 230–240. doi: 10.1016/j.ygcen.2003.09.016
Butt R. L., Volkoff H. (2019). Gut microbiota and energy homeostasis in fish. Front. Endocrinol. 10, 9. doi: 10.3389/fendo.2019.00009
Carveth C. J., Widmer A. M., Bonar S. A., Simms J. R. (2007). An examination of the effects of chronic static and fluctuating temperature on the growth and survival of spikedace, Meda fulgida, with implications for management. J. Thermal. Biol. 32 (2), 102–108. doi: 10.1016/j.jtherbio.2006.11.002
Cheng C. H., Guo Z. X., Luo S. W., Wang A. L. (2018). Effects of high temperature on biochemical parameters, oxidative stress, DNA damage and apoptosis of pufferfish (Takifugu obscurus). Ecotoxicol. Environ. Saf. 150, 190–198. doi: 10.1016/j.ecoenv.2017.12.045
Cheng C. H., Ye C. X., Guo Z. X., Wang A. L. (2017). Immune and physiological responses of pufferfish (Takifugu obscurus) under cold stress. Fish. Shellfish. Immunol. 64, 137–145. doi: 10.1016/j.fsi.2017.03.003
Chen W., Liu F., Ling Z., Tong X., Xiang C. (2012). Human intestinal lumen and mucosa-associated microbiota in patients with colorectal cancer. PLoS One 7 (6), e39743. doi: 10.1371/journal.pone.0039743
Chen S., Migaud H., Shi C., Song C., Wang C., Ye Y., et al. (2021). Light intensity impacts on growth, molting and oxidative stress of juvenile mud crab Scylla paramamosain. Aquaculture 545, 737159. doi: 10.1016/j.aquaculture.2021.737159
Chen S., Shi C., Migaud H., Song C., Mu C., Ye Y., et al. (2022). Light spectrum impacts on growth, molting, and oxidative stress response of the mud crab Scylla paramamosain. Front. Mar. Sci. 9. doi: 10.3389/fmars.2022.840353
Christiansen J. J., Djurhuus C. B., Gravholt C. H., Iversen P., Christiansen J. S., Schmitz O., et al. (2007). Effects of cortisol on carbohydrate, lipid, and protein metabolism: studies of acute cortisol withdrawal in adrenocortical failure. J. Clin. Endocrinol. Metab. 92 (9), 3553–3559. doi: 10.1210/jc.2007-0445
Ciaramella M. A., Battison A. L., Horney B. (2014). Measurement of tissue lipid reserves in the American lobster (Homarus americanus): hemolymph metabolites as potential biomarkers of nutritional status. J. Crustacean. Biol. 34 (5), 629–638. doi: 10.1163/1937240X-00002262
Dai W., Dong Y., Ye J., Xue Q., Lin Z. (2022). Gut microbiome composition likely affects the growth of razor clam Sinonovacula constricta. Aquaculture 550, 737847. doi: 10.1016/j.aquaculture.2021.737847
Dame R. F., Vernberg F. J. (1978). The influence of constant and cyclic acclimation temperatures on the metabolic rates of Panopeus herbstii and Uca pugilator. Biol. Bull. 154 (2), 188–197. doi: 10.2307/1541121
Diana J. S. (1984). The growth of largemouth bass, Micropterus salmoides (Lacepede), under constant and fluctuating temperatures. J. Fish. Biol. 24 (2), 165–172. doi: 10.1111/j.1095-8649.1984.tb04787.x
Dong Y., Dong S., Ji T. (2008a). Effect of different thermal regimes on growth and physiological performance of the sea cucumber Apostichopus japonicus selenka. Aquaculture 275 (1-4), 329–334. doi: 10.1016/j.aquaculture.2007.12.006
Dong Y., Dong S., Meng X. (2008b). Effects of thermal and osmotic stress on growth, osmoregulation and Hsp70 in sea cucumber (Apostichopus japonicus selenka). Aquaculture 276 (1-4), 179–186. doi: 10.1016/j.aquaculture.2008.01.028
Dong Y. W., Yu S. S., Wang Q. L., Dong S. L. (2011). Physiological responses in a variable environment: relationships between metabolism, hsp and thermotolerance in an intertidal-subtidal species. PLoS One 6 (10), e26446. doi: 10.1371/journal.pone.0026446
Du X., Li S., F K., H Q., W Z., W Y., et al. (2020). Vertical distribution features of microbial quantity, diversity and interactions along soil profiles in an agropasture grassland. Microbiol. China 47 (9), 2789–2806.
Fan L., Wang Z., Chen M., Qu Y., Li J., Zhou A., et al. (2019). Microbiota comparison of pacific white shrimp intestine and sediment at freshwater and marine cultured environment. Sci. Total. Environ. 657, 1194–1204. doi: 10.1016/j.scitotenv.2018.12.069
Feng J., Zhang L., Tang X., Xia X., Hu W., Zhou P. (2021). Season and geography induced variation in sea cucumber (Stichopus japonicus) nutritional composition and gut microbiota. J. Food Composition. Anal. 101, 103838. doi: 10.1016/j.jfca.2021.103838
Gunderson A. R., Armstrong E. J., Stillman J. H. (2016). Multiple stressors in a changing world: the need for an improved perspective on physiological responses to the dynamic marine environment. Annu. Rev. Mar. Sci. 8, 357–378. doi: 10.1146/annurev-marine-122414-033953
Guo B., Mu Y., Wang F., Dong S. (2012). Effect of periodic light color change on the molting frequency and growth of Litopenaeus vannamei. Aquaculture 362, 67–71. doi: 10.1016/j.aquaculture.2012.07.034
Guo B., Wang F., Li Y., Dong S. (2013). Effect of periodic light intensity change on the molting frequency and growth of Litopenaeus vannamei. Aquaculture 396, 66–70. doi: 10.1016/j.aquaculture.2013.02.033
Han M., Gao T., Liu G., Zhu C., Zhang T., Sun M., et al. (2022). The effect of a polystyrene nanoplastic on the intestinal microbes and oxidative stress defense of the freshwater crayfish, Procambarus clarkii. Sci. Total. Environ. 833, 155722. doi: 10.1016/j.scitotenv.2022.155722
Han G. D., Zhang S., Marshall D. J., Ke C. H., Dong Y. W. (2013). Metabolic energy sensors (AMPK and SIRT1), protein carbonylation and cardiac failure as biomarkers of thermal stress in an intertidal limpet: linking energetic allocation with environmental temperature during aerial emersion. J. Exp. Biol. 216 (17), 3273–3282. doi: 10.1242/jeb.084269
Hardie D. G., Hawley S. A., Scott J. W. (2006). AMP-activated protein kinase–development of the energy sensor concept. J. Physiol. 574 (1), 7–15. doi: 10.1113/jphysiol.2006.108944
Helmuth B. S., Hofmann G. E. (2001). Microhabitats, thermal heterogeneity, and patterns of physiological stress in the rocky intertidal zone. Biol. Bull. 201 (3), 374–384. doi: 10.2307/1543615
Huang X., Feng B., Huang H., Ye H. (2017). In vitro stimulation of vitellogenin expression by insulin in the mud crab, Scylla paramamosain, mediated through PI3K/Akt/TOR pathway. Gen. Comp. Endocrinol. 250, 175–180. doi: 10.1016/j.ygcen.2017.06.013
Huang C., Yu K., Huang H., Ye H. (2016). Adenosine monophosphate-activated protein kinase from the mud crab, Scylla paramamosain: cDNA cloning and profiles under cold stress. J. Genet. 95 (4), 923–932. doi: 10.1007/s12041-016-0717-z
Hu Y., Tan B., Mai K., Ai Q., Zheng S., Cheng K. (2008). Growth and body composition of juvenile white shrimp, Litopenaeus vannamei, fed different ratios of dietary protein to energy. Aquacult. Nutr. 14, 499–506. doi: 10.1111/j.1365-2095.2007.00555.x
Hyde C. J., Elizur A., Ventura T. (2019). The crustacean ecdysone cassette: A gatekeeper for molt and metamorphosis. J. Steroid Biochem. Mol. Biol. 185, 172–183. doi: 10.1016/j.jsbmb.2018.08.012
Kang H. Y., Lee Y. J., Song W. Y., Kim T. I., Lee W. C., Kim T. Y., et al. (2019). Physiological responses of the abalone Haliotis discus hannai to daily and seasonal temperature variations. Sci. Rep. 9 (1), 1–13. doi: 10.1038/s41598-019-44526-3
Kong X., Wang G., Li S. (2012). Effects of low temperature acclimation on antioxidant defenses and ATPase activities in the muscle of mud crab (Scylla paramamosain). Aquaculture 370, 144–149. doi: 10.1016/j.aquaculture.2012.10.012
Langille M. G., Zaneveld J., Caporaso J. G., McDonald D., Knights D., Reyes J. A., et al. (2013). Predictive functional profiling of microbial communities using 16S rRNA marker gene sequences. Nat. Biotechnol. 31 (9), 814–821. doi: 10.1038/nbt.2676
Lazado C. C., Caipang C. M. A. (2014). Atlantic Cod in the dynamic probiotics research in aquaculture. Aquaculture 424, 53–62. doi: 10.1016/j.aquaculture.2013.12.040
Lesser M. P. (2006). Oxidative stress in marine environments: biochemistry and physiological ecology. Annu. Rev. Physiol. 68, 253–278. doi: 10.1146/annurev.physiol.68.040104.110001
Le Vay L. (2001). Ecology and management of mud crab Scylla spp. Asian Fisher. Sci. 14 (2), 101–112. doi: 10.33997/j.afs.2001.14.2.001
Li Y., Ai C., Liu L. (2018). Mud crab, Scylla paramamosain china’s leading maricultured crab. Aquacult. China: Success Stories Modern Trends 226–233. doi: 10.1002/9781119120759.ch3_4
Liu J., Shi C., Ye Y., Ma Z., Mu C., Ren Z., et al. (2022). Effects of temperature on growth, molting, feed intake, and energy metabolism of individually cultured juvenile mud crab Scylla paramamosain in the recirculating aquaculture system. Water 14 (19), 2988. doi: 10.3390/w14192988
Liu Z. M., Zhu X. L., Lu J., Cai W. J., Ye Y. P., Lv Y. P. (2018). Effect of high temperature stress on heat shock protein expression and antioxidant enzyme activity of two morphs of the mud crab Scylla paramamosain. Comp. Biochem. Physiol. Part A.: Mol. Integr. Physiol. 223, 10–17. doi: 10.1016/j.cbpa.2018.04.016
Lutterschmidt W. I., Hutchison V. H. (1997). The critical thermal maximum: history and critique. Can. J. Zool. 75 (10), 1561–1574. doi: 10.1139/z97-783
Madeira D., Narciso L., Cabral H. N., Vinagre C., Diniz M. S. (2013). Influence of temperature in thermal and oxidative stress responses in estuarine fish. Comp. Biochem. Physiol. Part A.: Mol. Integr. Physiol. 166 (2), 237–243. doi: 10.1016/j.cbpa.2013.06.008
Miao S., Tu S. (1996). Modeling effect of thermic amplitude on growing Chinese shrimp, Penaeus chinensis (Osbeck). Ecol. Model. 88 (1-3), 93–100. doi: 10.1016/0304-3800(95)00072-0
Mykles D. L. (2001). Interactions between limb regeneration and molting in decapod crustaceans. Am. Zool. 41 (3), 399–406. doi: 10.1093/icb/41.3.399
Mykles D. L. (2011). Ecdysteroid metabolism in crustaceans. J. Steroid Biochem. Mol. Biol. 127 (3-5), 196–203. doi: 10.1016/j.jsbmb.2010.09.001
Newell R. C. (1969). Effect of fluctuations in temperature on the metabolism of intertidal invertebrates. Am. Zool. 9 (2), 293–307. doi: 10.1093/icb/9.2.293
Pascual C., Gaxiola G., Rosas C. (2003). Blood metabolites and hemocyanin of the white shrimp, Litopenaeus vannamei: the effect of culture conditions and a comparison with other crustacean species. Mar. Biol. 142, 735–745. doi: 10.1007/s00227-002-0995-2
Prakoso V. A., Pouil S., Cahyanti W., Sundari S., Arifin O. Z., Subagja J., et al. (2021). Fluctuating temperature regime impairs growth in giant gourami (Osphronemus goramy) larvae. Aquaculture 539, 736606. doi: 10.1016/j.aquaculture.2021.736606
Priya T. J., Li F., Zhang J., Yang C., Xiang J. (2010). Molecular characterization of an ecdysone inducible gene E75 of Chinese shrimp Fenneropenaeus chinensis and elucidation of its role in molting by RNA interference. Comp. Biochem. Physiol. Part B.: Biochem. Mol. Biol. 156 (3), 149–157. doi: 10.1016/j.cbpb.2010.02.004
Qiu J., Wang W. N., Wang L. J., Liu Y. F., Wang A. L. (2011). Oxidative stress, DNA damage and osmolality in the pacific white shrimp, Litopenaeus vannamei exposed to acute low temperature stress. Comp. Biochem. Physiol. Part C.: Toxicol. Pharmacol. 154 (1), 36–41. doi: 10.1016/j.cbpc.2011.02.007
Ren X., Wang Q., Shao H., Xu Y., Liu P., Li J. (2021). Effects of low temperature on shrimp and crab physiology, behavior, and growth: A review. Front. Mar. Sci. 8, 746177. doi: 10.3389/fmars.2021.746177
Rungrassamee W., Leelatanawit R., Jiravanichpaisal P., Klinbunga S., Karoonuthaisiri N. (2010). Expression and distribution of three heat shock protein genes under heat shock stress and under exposure to Vibrio harveyi in Penaeus monodon. Dev. Comp. Immunol. 34 (10), 1082–1089. doi: 10.1016/j.dci.2010.05.012
Sachdev S., Ansari S. A., Ansari M. I., Fujita M., Hasanuzzaman M. (2021). Abiotic stress and reactive oxygen species: Generation, signaling, and defense mechanisms. Antioxidants 10 (2), 277. doi: 10.3390/antiox10020277
Saxton R. A., Sabatini D. M. (2017). mTOR signaling in growth, metabolism, and disease. Cell 168 (6), 960–976. doi: 10.1016/j.cell.2017.02.004
Segata N., Izard J., Waldron L., Gevers D., Miropolsky L., Garrett W. S., et al. (2011). Metagenomic biomarker discovery and explanation. Genome Biol. 12 (6), 1–18. doi: 10.1186/gb-2011-12-6-r60
Su Y., Ma S., Feng C. (2010). Effects of salinity fluctuation on the growth and energy budget of juvenile Litopenaeus vannamei at different temperatures. J. Crustacean. Biol. 30 (3), 430–434. doi: 10.1651/09-3269.1
Tian X., Dong S. (2006). The effects of thermal amplitude on the growth of Chinese shrimp Fenneropenaeus chinensis (Osbeck 1765). Aquaculture 251 (2-4), 516–524. doi: 10.1016/j.aquaculture.2005.05.031
Ueda N., Boettcher A. (2009). Differences in heat shock protein 70 expression during larval and early spat development in the Eastern oyster, Crassostrea virginica (Gmelin 1791). Cell Stress Chaperones. 14 (4), 439–443. doi: 10.1007/s12192-008-0096-3
Van As J. G., Comhrinck C., Reinecke A. J. (1980). An experimental evaluation of the influence of temperature on the natural rate of increase of Daphnia pulex de geer. J. Limnol. Soc. South. Afr. 6 (1), 1–4. doi: 10.1080/03779688.1980.9633199
Verheyen J., Stoks R. (2019). Temperature variation makes an ectotherm more sensitive to global warming unless thermal evolution occurs. J. Anim. Ecol. 88 (4), 624–636. doi: 10.1111/1365-2656.12946
Viarengo A., Canesi L., Pertica M., Poli G., Moore M. N., Orunesu M. (1990). Heavy metal effects on lipid peroxidation in the tissues of mytilus gallopro vincialis lam. Comp. Biochem. Physiol. Part C.: Comp. Pharmacol. 97, 37–42. doi: 10.1016/0742-8413(90)90168-9
Visconti A., Le Roy C. I., Rosa F., Rossi N., Martin T. C., Mohney R. P., et al. (2019). Interplay between the human gut microbiome and host metabolism. Nat. Commun. 10 (1), 1–10. doi: 10.1038/s41467-019-12476-z
Vondracek B., Cech J. J., Buddington R. K. (1989). Growth, growth efficiency, and assimilation efficiency of the Tahoe sucker in cyclic and constant temperature. Environ. Biol. Fishes. 24 (2), 151–156. doi: 10.1007/BF00001285
Walther G. R., Post E., Convey P., Menzel A., Parmesan C., Beebee T. J., et al. (2002). Ecological responses to recent climate change. Nature 416 (6879), 389–395. doi: 10.1038/416389a
Wang G., Dillon M. E. (2014). Recent geographic convergence in diurnal and annual temperature cycling flattens global thermal profiles. Nat. Climate Change 4 (11), 988–992. doi: 10.1038/nclimate2378
Wang Z., Qu Y., Yan M., Li J., Zou J., Fan L. (2019). Physiological responses of pacific white shrimp Litopenaeus vannamei to temperature fluctuation in low-salinity water. Front. Physiol. 10, 1025. doi: 10.3389/fphys.2019.01025
Wang Z., Zhou J., Li J., Zou J., Fan L. (2020). The immune defense response of pacific white shrimp (Litopenaeus vannamei) to temperature fluctuation. Fish. Shellfish Immunol. 103, 103–110. doi: 10.1016/j.fsi.2020.04.053
Wataya-Kaneda M. (2015). Mammalian target of rapamycin and tuberous sclerosis complex. J. Dermatol. Sci. 79 (2), 93–100. doi: 10.1016/j.jdermsci.2015.04.005
Wei H., Wang H., Tang L., Mu C., Ye C., Chen L., et al. (2019). High-throughput sequencing reveals the core gut microbiota of the mud crab (Scylla paramamosain) in different coastal regions of southern China. BMC Genomics 20 (1), 1–12. doi: 10.1186/s12864-019-6219-7
Whiteley N. M., Taylor E. W., El Haj A. J. (1997). Seasonal and latitudinal adaptation to temperature in crustaceans. J. thermal. Biol. 22 (6), 419–427. doi: 10.1016/S0306-4565(97)00061-2
Wullschleger S., Loewith R., Hall M. N. (2006). TOR signaling in growth and metabolism. Cell 124 (3), 471–484. doi: 10.1016/j.cell.2006.01.016
Xiong J., Dai W., Zhu J., Liu K., Dong C., Qiu Q. (2017). The underlying ecological processes of gut microbiota among cohabitating retarded, overgrown and normal shrimp[J]. Microb. Ecol. 73 (4), 988–999. doi: 10.1007/s00248-016-0910-x
Xu H., Liu T., Feng W., He J., Han T., Wang J., et al. (2023). Dietary phosphatidylcholine improved the survival, growth performance, antioxidant, and osmoregulation ability of early juvenile mud crab Scylla paramamosain. Aquaculture 563, 738899. doi: 10.1016/j.aquaculture.2022.738899
Yang S., Lian G. (2020). ROS and diseases: Role in metabolism and energy supply. Mol. Cell. Biochem. 467 (1), 1–12. doi: 10.1007/s11010-019-03667-9
Yang Y. N., Ye H., Huang H., Li S., Liu X., Zeng X., et al. (2013). Expression of Hsp70 in the mud crab, Scylla paramamosain in response to bacterial, osmotic, and thermal stress. Cell Stress Chaperones. 18 (4), 475–482. doi: 10.1007/s12192-013-0402-6
Yao T. P., Forman B. M., Jiang Z., Cherbas L., Chen J., McKeown M., et al. (1993). Functional ecdysone receptor is the product of EcR and ultraspiracle genes. Nature 366 (6454), 476–479. doi: 10.1038/366476a0
Yao C. L., Somero G. N. (2012). The impact of acute temperature stress on hemocytes of invasive and native mussels (Mytilus galloprovincialis and Mytilus californianus): DNA damage, membrane integrity, apoptosis and signaling pathways. J. Exp. Biol. 215 (24), 4267–4277. doi: 10.1242/jeb.073577
Yong A. S. K., Mok W. Y., Tamrin M. L. M., Shapawi R., Kim Y. S. (2020). Effects of dietary nucleotides on growth, survival and metabolic response in whiteleg shrimp, Litopenaeus vannamei against ammonia stress condition. Aquacult. Res. 51 (6), 2252–2260. doi: 10.1111/are.14570
Yuan Q., Qian J., Ren Y., Zhang T., Li Z., Liu J. (2018). Effects of stocking density and water temperature on survival and growth of the juvenile Chinese mitten crab, Eriocheir sinensis, reared under laboratory conditions. Aquaculture 495, 631–636. doi: 10.1016/j.aquaculture.2018.06.029
Žák J., Reichard M. (2020). Fluctuating temperatures extend median lifespan, improve reproduction and reduce growth in turquoise killifish. Exp. Gerontol. 140, 111073. doi: 10.1016/j.exger.2020.111073
Zhao M., Wang W., Zhang F., Ma C., Liu Z., Yang M. H., et al. (2021). A chromosome-level genome of the mud crab (Scylla paramamosain estampador) provides insights into the evolution of chemical and light perception in this crustacean. Mol. Ecol. Resour. 21 (4), 1299–1317. doi: 10.1111/1755-0998.13332
Zheng L., Xie S., Zhuang Z., Liu Y., Tian L., Niu J. (2021). Effects of yeast and yeast extract on growth performance, antioxidant ability and intestinal microbiota of juvenile pacific white shrimp (Litopenaeus vannamei). Aquaculture 530, 735941. doi: 10.1016/j.aquaculture.2020.735941
Zhou L., Chen C., Xie J., Xu C., Zhao Q., Qin J. G., et al. (2019). Intestinal bacterial signatures of the “cotton shrimp-like” disease explain the change of growth performance and immune responses in pacific white shrimp (Litopenaeus vannamei). Fish. shellfish. Immunol. 92, 629–636. doi: 10.1016/j.fsi.2019.06.054
Zhou L., Li S., Wang Z., Li F., Xiang J. (2017). An eclosion hormone-like gene participates in the molting process of palaemonid shrimp Exopalaemon carinicauda. Dev. Genes Evol. 227 (3), 189–199. doi: 10.1007/s00427-017-0580-9
Zhu S., Long X., Turchini G. M., Deng D., Cheng Y., Wu X. (2021). Towards defining optimal dietary protein levels for male and female sub-adult Chinese mitten crab, Eriocheir sinensis reared in earthen ponds: Performances, nutrient composition and metabolism, antioxidant capacity and immunity. Aquaculture 536, 736442. doi: 10.1016/j.aquaculture.2021.736442
Zhu Q., Zhang L., Li L., Que H., Zhang G. (2016). Expression characterization of stress genes under high and low temperature stresses in the pacific oyster, Crassostrea gigas. Mar. Biotechnol. 18 (2), 176–188. doi: 10.1007/s10126-015-9678-0
Zhu L., Liao R., Wu N., Zhu G., Yang C. (2019). Heat stress mediates changes in fecal microbiome and functional pathways of laying hens. Appl. Microbiol. Biotechnol. 103 (1), 461–472. doi: 10.1007/s00253-018-9465-8
Zuo Z. H., Shang B. J., Shao Y. C., Li W. Y., Sun J. S. (2019). Screening of intestinal probiotics and the effects of feeding probiotics on the growth, immune, digestive enzyme activity and intestinal flora of Litopenaeus vannamei. Fish. Shellfish Immunol. 86, 160–168. doi: 10.1016/j.fsi.2018.11.003
Keywords: mud crab, diurnal temperature fluctuations, growth, energy metabolism, gut microbes
Citation: Liu J, Chen S, Ren Z, Ye Y, Wang C, Mu C, Wu Q and Shi C (2022) Effects of diurnal temperature fluctuations on growth performance, energy metabolism, stress response, and gut microbes of juvenile mud crab Scylla paramamosain. Front. Mar. Sci. 9:1076929. doi: 10.3389/fmars.2022.1076929
Received: 22 October 2022; Accepted: 09 November 2022;
Published: 28 November 2022.
Edited by:
Xiaolong Gao, Xiamen University, ChinaReviewed by:
Xianliang Meng, Yellow Sea Fisheries Research Institute (CAFS), ChinaCopyright © 2022 Liu, Chen, Ren, Ye, Wang, Mu, Wu and Shi. This is an open-access article distributed under the terms of the Creative Commons Attribution License (CC BY). The use, distribution or reproduction in other forums is permitted, provided the original author(s) and the copyright owner(s) are credited and that the original publication in this journal is cited, in accordance with accepted academic practice. No use, distribution or reproduction is permitted which does not comply with these terms.
*Correspondence: Zhiming Ren, cmVuemhpbWluZ0BuYnUuZWR1LmNu; Ce Shi, c2hpY2UzMjEwQDEyNi5jb20=
Disclaimer: All claims expressed in this article are solely those of the authors and do not necessarily represent those of their affiliated organizations, or those of the publisher, the editors and the reviewers. Any product that may be evaluated in this article or claim that may be made by its manufacturer is not guaranteed or endorsed by the publisher.
Research integrity at Frontiers
Learn more about the work of our research integrity team to safeguard the quality of each article we publish.