- 1Dipartimento di Scienze della Terra dell’Ambiente e della Vita (DISTAV), Università di Genova, Genova, Italy
- 2Consorzio Nazionale Interuniversitario per le Scienze del Mare, Roma, Italy
- 3Stazione Zoologica Anton Dohrn, Napoli, Italy
- 4Dipartimento di Scienze della Vita e dell’Ambiente, Università di Cagliari, Cagliari, Italy
- 5Area per la tutela della biodiversità, degli habitat e specie marine protette, Istituto Superiore per la Protezione e Ricerca Ambientale (ISPRA), Roma, Italy
The precious coral Corallium rubrum (Linnaeus, 1758) is a charismatic Mediterranean species. A recent large-scale investigation along the Italian coast highlighted its widespread occurrence at mesophotic and upper bathyal depths, especially on coralligenous and bathyal vertical hardgrounds. The lack of morphometric data limited the considerations on the structure and health status of the populations, fundamental to identify the most vulnerable sites and the correct management actions. For this reason, a ROV dataset, consisting of 624 dives carried out between 40 m and 1825 m in the Ligurian Sea, Tyrrhenian Sea, and Sicily Channel, was analysed to extrapolate quantitative data to describe the populations. Ten random frames were obtained from each of the 170 sites hosting red coral. Density, height, and entanglement were evaluated for about 15700 colonies counted in the frames. The densest populations were mainly found between 40 m and 80 m, with a clear latitudinal density decrease. The mesophotic populations were characterized by both scattered and densely aggregated colonies, while the bathyal ones were dominated by sparse colonies. This study identified 17 major coral areas based on the geographic proximity of the sites hosting red coral and their topographic and oceanographic affinity. The size-frequency distribution of the heights was skewed towards the smaller classes in almost all populations, with a modal class between 2 cm and 4 cm. This study depicted a stress situation of the populations throughout the entire study area, which could be correlated also to the long-term harvesting pressure carried out in the basins. Two additional sources of direct mortality were pointed out in this study. Entanglement to artisanal and recreational fishing gear interested about 18% of the recorded colonies, mainly at mesophotic depths. Almost all populations suffered from mechanical entanglement, with the highest percentages in the Ligurian Sea and Sicilian areas. This study also highlighted a massive occurrence of recent deep mortality events, mainly along the eastern and southern coast of Sardinia and in the Campanian Archipelago. Thirty sites with extensive patches of dead colonies still in place were reported from 70 m to around 200 m, but their formation remains unclear.
Introduction
The precious coral Corallium rubrum (Linnaeus, 1758) is a charismatic Mediterranean species that suffered extensive exploitation for centuries due to its commercial value in the jewellery industry (Tsounis et al., 2006; Bussoletti et al., 2010; Cattaneo-Vietti and Bavestrello, 2010; Tsounis et al., 2010; Cattaneo-Vietti et al., 2017; Cannas et al., 2019). Harvesting has been traditionally exercised with non-selective and highly destructive trawling gear, while, after their ban in 1994, it has been carried out selectively by professional SCUBA divers; this long-term pressure resulted in a serious over-fishing that put this resource into jeopardy (Cattaneo-Vietti et al., 2017). Currently, this species is included in a number of international conventions supporting its controlled harvesting (SPA/BD Protocol Annex III, Bern Convention Annex III, Habitat Directive Annex V and GFCM Recommendations), and in 2016 it was assessed as Endangered in the IUCN Anthozoans Red List for the Mediterranean Sea (IUCN, 2016), recognizing its important ecological role as habitat-former (Garrabou and Harmelin, 2002; Rossi et al., 2017) and its decline over time. Taking into consideration the IUCN assessment, the inclusion of red coral in Appendix II of the Convention on International Trade in Endangered Species of Wild Fauna and Flora (CITES) should be re-evaluated in the near future (Bruckner, 2009; Bussoletti et al., 2010; Bruckner, 2014).
In the past decades, in order to evaluate the distribution and status of the different populations living in the Mediterranean Sea, numerous research studies have been conducted, mainly on littoral banks, often showing an evident state of suffering (FAO, 1988; Cicogna and Cattaneo-Vietti, 1993; Cicogna et al., 1999; Garrabou and Harmelin, 2002; Santangelo et al., 2007; Santangelo et al., 2009; Santangelo and Bramanti, 2010; Tsounis et al., 2010; Bramanti et al., 2014; Montero-Serra et al., 2015). Dramatic size and structural shifts in shallow-water populations have been recognized (Bruckner, 2009), with “forest-like” populations, dominated by large, branched colonies of 30-50 cm height (10-30 mm in diameter) turning into “grass plain-like” populations, dominated by small colonies of 3-5 cm height (5-7 mm in diameter) (Garrabou and Harmelin, 2002; Tsounis et al., 2006; Santangelo et al., 2007; Tsounis et al., 2007; Bramanti et al., 2014). The size shift due to harvesting is not only related to the obvious reason that divers tend to select colonies of larger size, but also to the freeing up of space due to heavy harvesting enhancing larval settling events (Cerrano et al., 1997). The “grass plain-like” condition may persist for a long span of time, because of intra-specific competition (Tsounis et al., 2006), and only in a few Marine Protected Areas has a reversion been recognized after a long-term protection (Bavestrello et al., 2015; Richaume et al., 2021). Moreover, besides harvesting, other factors are known to threaten red coral populations, both direct (e.g., fishing activities and mass mortalities putatively linked to episodic and highly energetic events, like heat waves and volcanic eruptions) and indirect (e.g., climate change-driven events) (e.g., Cerrano et al., 2013; Bavestrello et al., 2014; Lodolo et al., 2017; Cau et al., 2018; Galgani et al., 2018; Enrichetti et al., 2019a).
From a conservation point of view, morphometric data are essential to estimate the health status of the populations, which, in turn, are fundamental to identify the most vulnerable sites and the correct management actions. Although several pieces of evidence are available for shallow-water populations, very little data have been recorded about the morphometric structure of deep populations (Rossi et al., 2008; Priori et al., 2013; Angiolillo et al., 2016; Cau et al., 2016; Carugati et al., 2022). These studies, mainly conducted on a local or regional scale, showed that deep-dwelling populations are generally less harvested than the shallow-water ones, even if high percentages of the colonies are still above the commercial size (≥ 7 mm of basal diameter). However, authors do not agree if there is a size-density relationship in red coral populations with increasing depth, presenting contrasting results.
A recent large-scale investigation along the Italian coast (Toma et al., 2022), taking advantage of an extensive ROV archive, demonstrated that, at basin scale, red coral still shows a wide occurrence, particularly in a large region of the central Tyrrhenian Sea including Tuscan Archipelago, Pontine Archipelago, Gulf of Naples, and the Sardinian coasts. This study also demonstrated that the species occurrence peaks in the mesophotic zone, between 75 m and 125 m, showing a marked preference for biogenic habitats dominated by Crustose Coralline Algae (CCA) and for vertical substrata. However, the lack of morphometric data limited the considerations on the structure and health status of the populations.
The objectives of this study, therefore, were to i) conduct a large-scale morphometric survey on mesophotic and deep populations in the Ligurian Sea, Tyrrhenian Sea, and Sicily Channel, and highlight latitudinal and bathymetrical patterns in colony average size and density, ii) identify the size-frequency patterns in the most representative forests to depict the putative influence of harvesting pressure, and, ultimately, iii) identify other sources of direct mortality which may affect the health status of those populations.
Materials and methods
A large ROV archive was analysed to extrapolate quantitative data with the aim of describing the red coral populations present along the Italian coast. The dataset consisted of 624 ROV dives (each corresponding to a distinct geographical site) carried out between 2006 and 2021 in the Ligurian Sea, Tyrrhenian Sea, and Sicily Channel, between 40 m and 1825 m. Sites were grouped in localities based on their geographic proximity (maximum distance about 5 km), then localities were grouped in areas based on their topographic and oceanographic affinity (e.g., promontory, gulf, shoal), and such areas fell into different macro-areas (4 coastal ones, LIG Ligurian Sea, NCT North-central Tyrrhenian Sea, ST South Tyrrhenian Sea, and SC Sicily Channel, and 1 offshore, SEAM Seamounts) (Table 1).
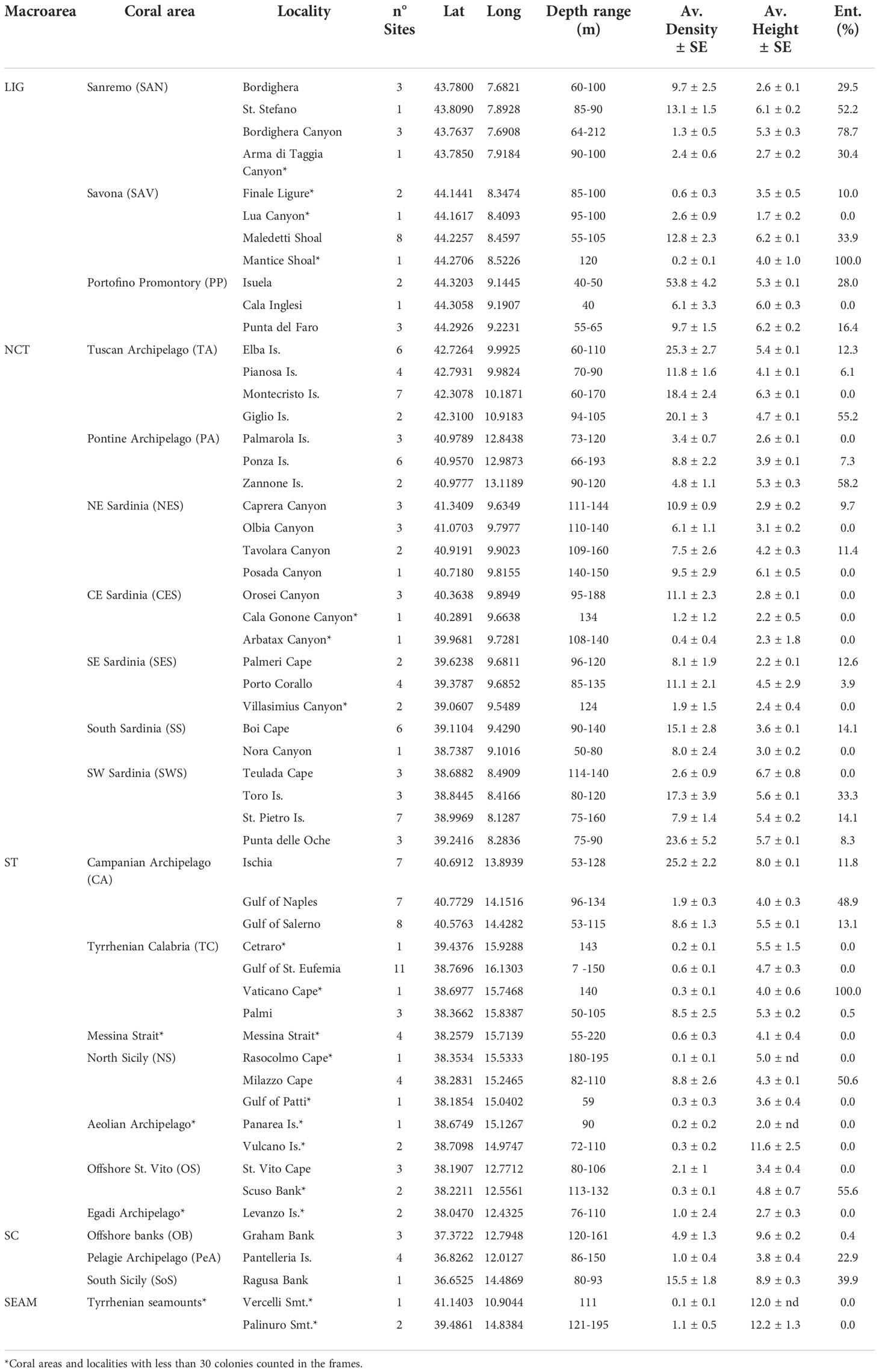
Table 1 Presence of Corallium rubrum along the Italian coast according to macro-area, coral areas (code in brackets), locality, number of sites per locality, latitude, and longitude of the centroid of the locality, depth range, average density and height, and percentage of entanglement of red coral populations in the locality.
The number of sites in which Corallium rubrum was present was counted and reported as a percentage of occurrence in the whole dataset. For the quantitative analyses regarding coral areas, only sites (hence localities and areas) with more than 30 colonies were considered.
The depth ranges at which red coral was recorded were noted and ten random frames were obtained for each site targeting the densest coral patches, for a total of 1700 analysed frames. For each picture, density was calculated considering the hardground surface, evaluated using the ROV laser beams, and the exact number of colonies, counted using ImageJ (15730 total counted colonies). The resulting average density of the sites was then defined according to three categories: (1) scattered colonies (< 5 colonies m-2), (2) densely aggregated colonies (5-20 colonies m-2) or (3) very densely aggregated colonies (> 20 colonies m-2). Density values attributed to each category were based on the entire available dataset.
The relationship between density and depth was presented with two different approaches. The first considered the average densities of the sites in four distinct depth ranges (40-80 m, 80-150 m, 150-200 m, and > 200 m). The second analysis considered the percentage distribution of the density categories in the same depth ranges. These latter corresponded to the deepest part of the continental shelf, the shelf break, the upper bathyal range of the continental slope and seamounts, and the bathyal, respectively. The putative effect of latitude on the population average density per macro-area was also investigated by means of a linear regression with r = Pearson’s correlation coefficient.
The height of the colonies was measured in each frame with ImageJ using the laser beams as a reference, to evaluate the morphometry of the populations in the selected coral areas. The relationship between height and depth was investigated by considering the mean height of the populations in the four depth ranges. The putative effect of latitude on the average height was also investigated in the macro-areas by means of a linear regression with r = Pearson’s correlation coefficient. Finally, size-frequency graphs were created for the considered geographic areas, with size classes of 2 cm.
Entanglement to lost fishing gear was evaluated by noting the number of impacted colonies in each frame. Data were presented as the percentage of entangled colonies on the total, as well as the percentage of entangled colonies in each coral area. To investigate the correlation between entanglement and depth, the percentage of entanglement for each area and depth ranges were considered. The putative effect of latitude on the percentage of entanglement of the populations was also investigated for the macro-areas by means of a linear regression with r = Pearson’s correlation coefficient.
Finally, in the sites where dead in-place colonies deprived of living coenenchyme were observed, ten random frames were collected and data of density, height, entanglement, substrate type (rocks, R, and rocks encrusted by CCA, C), and slope of the hardground where C. rubrum was attached were noted to characterize the mortality patches. In the sites where both living and dead populations were present, density values and the average height of the colonies were compared to evaluate the entity and timing of the mass mortality events.
Significant differences in terms of average densities and average heights in the selected coral areas were tested through a Kruskal-Wallis test (data not normally distributed, square root transformed, n=10 - 220, and n=35 - 2913, respectively, for 17 coral areas). Significant differences between average densities, average heights, and average entanglement in the four considered depth ranges were tested through a Kruskal-Wallis test (data not normally distributed, square root transformed, n=3 - 170, for the four depth ranges). All statistical analyses were performed using PAST Software ver. 4.03 (Hammer et al., 2001).
Results
Occurrence and structure of the red coral forests
Red coral populations were present in 170 of the 624 sites considered in this work, corresponding to 27.2% of the total. The sites in which less than 30 colonies were counted were excluded from the quantitative analysis regarding the identification of coral areas, including the seamounts populations, represented by a limited number of colonies (Table 1 and Figure 1). Based on the geographic proximity of the remaining sites, 37 localities with red coral were identified. These were grouped into 17 distinct coral areas based on their topographic and oceanographic affinity, all belonging to the four identified coastal macro-areas. These areas were represented by 84.1% of the investigated sites and included about 99% of the counted colonies.
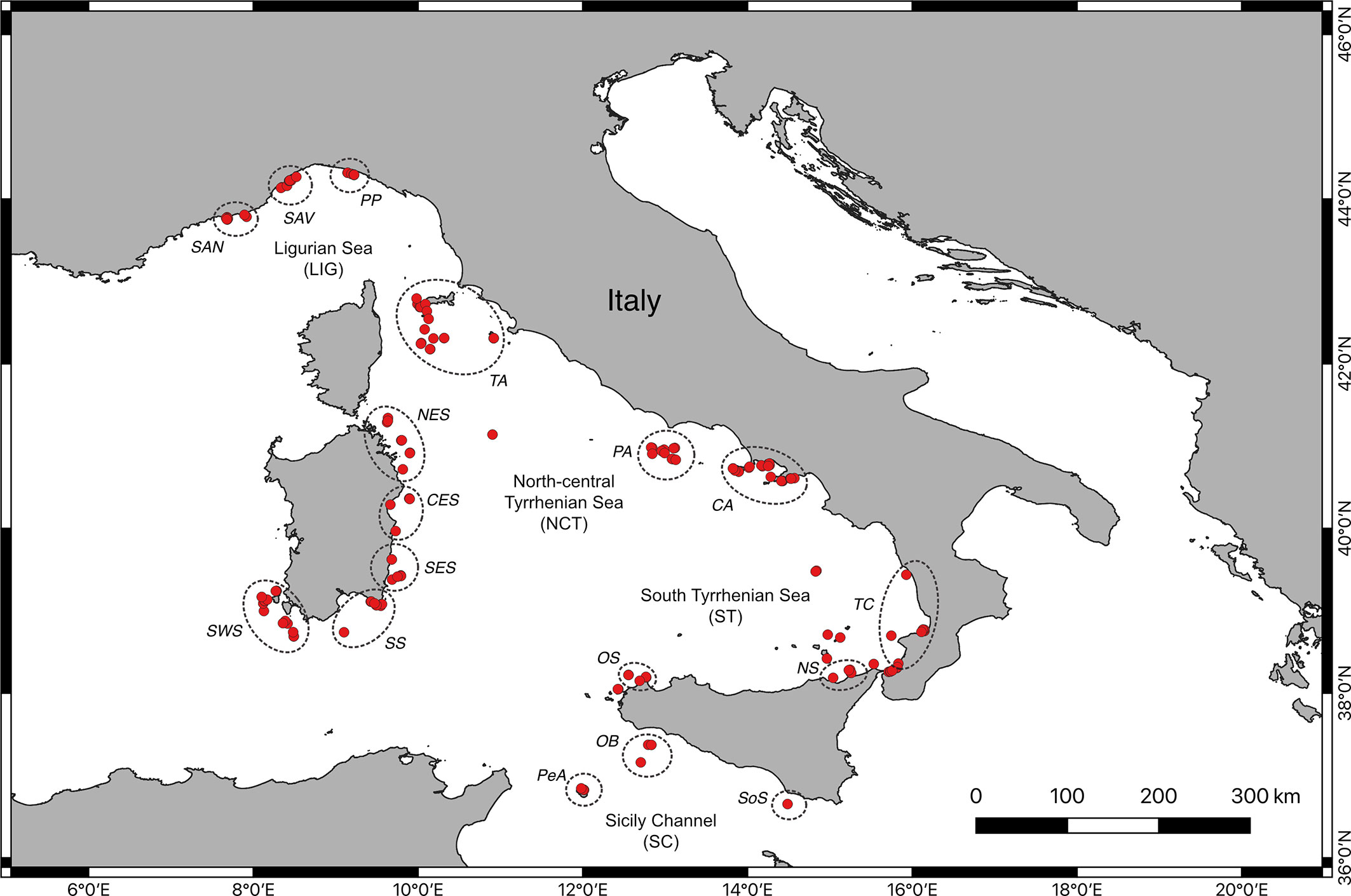
Figure 1 Map of the study area with location of the sites with red coral (red dots) grouped per coral area (dashed circles). For coral areas’ codes, refer to Table 1.
The average density between the 17 coastal coral areas varied between 1.0 ± 0.4 colonies m-2 in the Pelagie Archipelago (namely Pantelleria Island) and 23.8 ± 3.2 colonies m-2 on the Portofino Promontory (Table 1 and Figure 2A). Significant differences were observed among densities in the different coral areas (Kruskal-Wallis, p < 0.0001, Hc = 311.1) (Figures 3A–C). In the Ligurian Sea, average densities were relatively low in the western sector, ranging between 6.6 ± 1.2 colonies m-2 in the Sanremo area and 12.8 ± 2.3 colonies m-2 in the Savona area, in comparison to the eastern one (Figure 2A). Nevertheless, in the Savona area were recorded the maximum values of density of the entire dataset, namely 122 colonies m-2 on the Maledetti Shoal. In the North-central Tyrrhenian Sea, the Tuscan Archipelago and South Sardinia showed significantly higher average densities (19.4 ± 1.4 colonies m-2 and 14.1 ± 2.5 colonies m-2, respectively) (Figures 2A, 3B, C). The localities showing the highest average densities were Elba Island for the Tuscan Archipelago, Ponza Island for the Pontine one, and Caprera Canyon, Orosei Canyon and Porto Corallo for the eastern Sardinian coast (Table 1 and Figure 3D). Along the South and South-West coast of Sardinia, instead, maximal values were recorded in Boi Cape and Punta delle Oche (Figure 3C), reaching densities comparable to the one of the Portofino Promontory (Table 1).
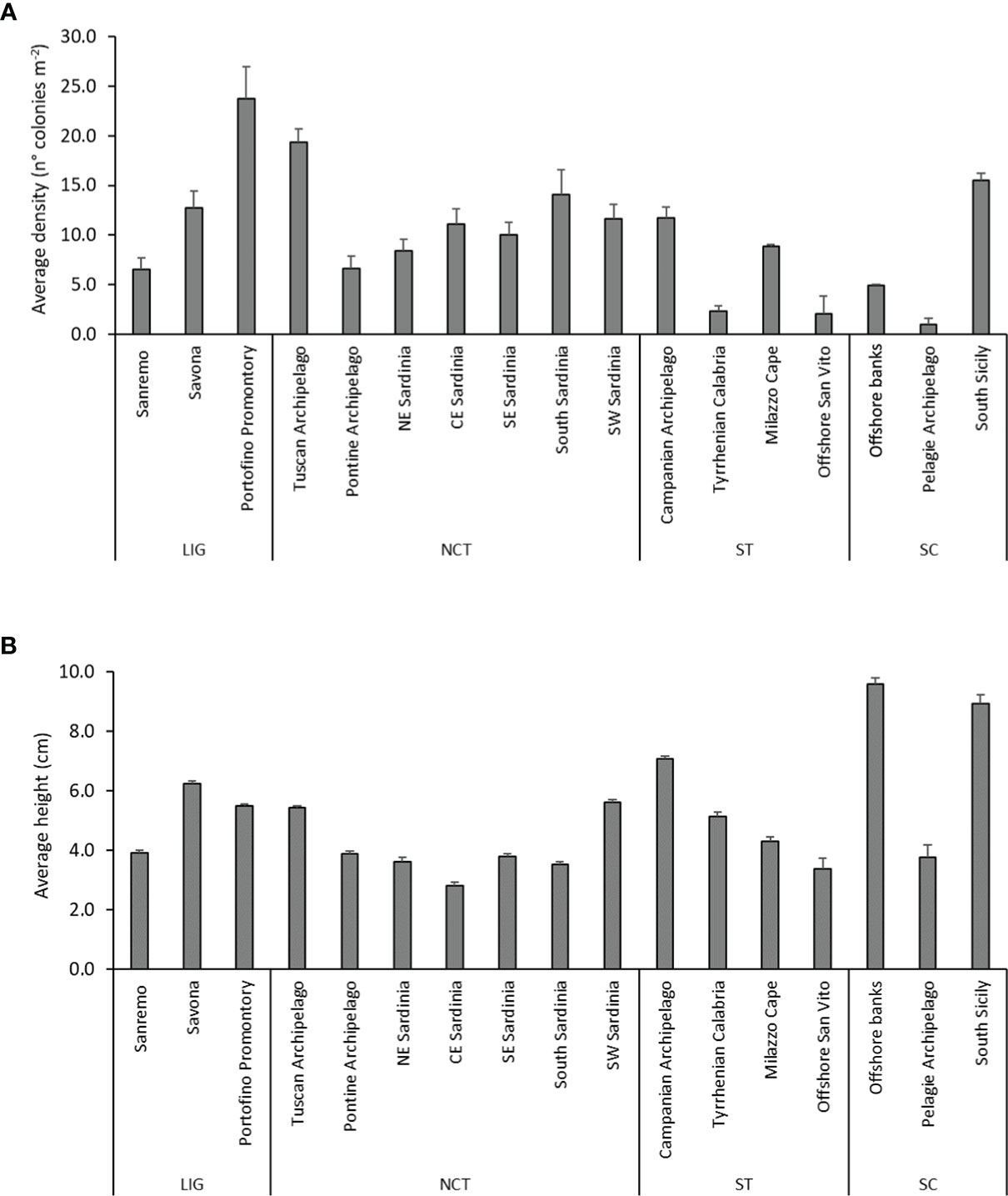
Figure 2 Demographic characteristics of the coral areas. (A) Average density (± SE) and (B) average height (± SE) of the populations in the 17 identified coral areas.
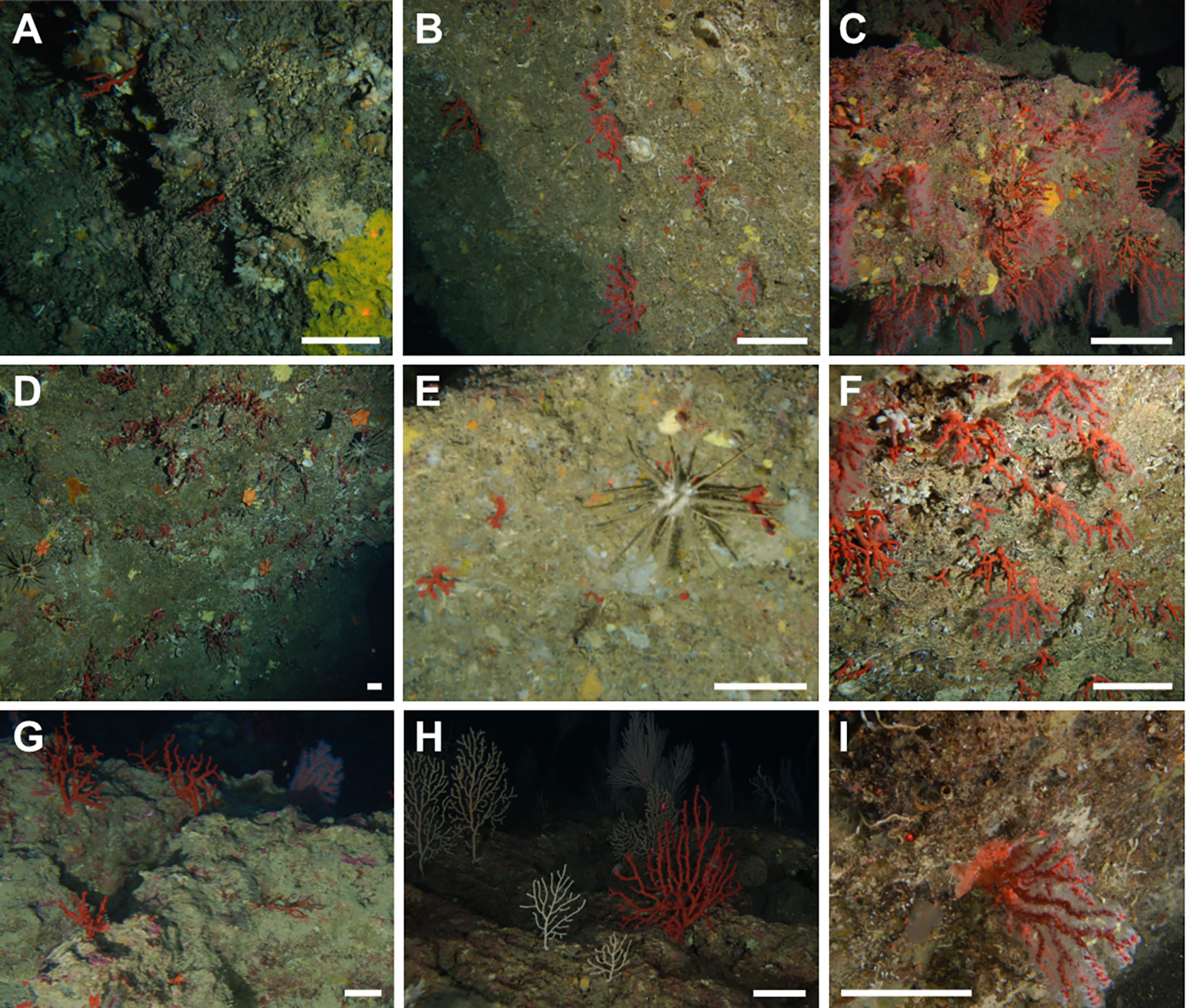
Figure 3 Corallium rubrum aggregations. (A) Scattered colonies on a vertical cliff in Palmi (Tyrrhenian Calabria, 85 m); (B) vertical rocky wall hosting aggregated colonies of C rubrum in Giglio Island (Tuscan Archipelago, 100 m); (C) very dense aggregation of red coral on a sloping CCA-covered rock in Punta delle Oche (SW Sardinia, 85 m); (D) Peltaster placenta (Müller & Troschel, 1842) and Stylocidaris affinis (Philippi, 1845) feeding on a dense aggregation of small living and dead colonies of C rubrum (Porto Corallo, SE Sardinia, 95 m); (E) very small colonies predated by S. affinis (Montecristo Island, Tuscan Archipelago, 84 m); (F) medium-size red coral colonies coexist with the white scleractinian Thalamophyllia gasti (Döderlein, 1913) on the vertical cliffs of Boi Cape (South Sardinia, 108 m); (G) large colonies flourish on the sub-horizontal CCA-covered rocks in the Ragusa Bank (Sicily Channel, 85 m); (H) a large colony surrounded by Eunicella cavolini (Koch, 1887) and Callogorgia verticillata (Pallas, 1766) (Capo Teulada, SW Sardinia, 124 m); (I) a single large colony of the precious coral hanging from a vertical rocky cliff on the Palinuro Seamount (121 m). Scale bar: 10 cm.
In the South Tyrrhenian Sea, the densest populations were observed in the Campanian Archipelago and on Milazzo Cape, with 11.8 ± 1.1 colonies m-2 and 8.8 ± 2.6 colonies m-2, respectively (Figure 2A). In this macro-area, Ischia Island (Campania) and the Milazzo Promontory reached also the highest maximal values (Table 1). The Tyrrhenian Calabrian coast and the sites offshore St. Vito showed very scattered populations (Table 1 and Figures 2A, 3A). Finally, in the Sicily Channel, the South Sicily area (Ragusa Bank) showed the highest average density (15.5 ± 1.8 colonies m-2) with respect to offshore banks (Graham Bank) and Pelagie Archipelago (Pantelleria Island) (Table 1 and Figure 2A). Nonetheless, also this macro-area was overall characterized by scattered populations.
In general, in terms of average height, the investigated coral areas showed a small range of variation, with two significantly higher peaks, both recorded in the Sicily Channel macro-area, namely the offshore banks (9.6 ± 0.2 cm) and South Sicily (8.9 ± 0.3 cm) (Kruskal-Wallis, p < 0.0001, Hc = 2461) (Table 1 and Figures 2B, 3E–I). In the Ligurian Sea, the Maledetti Shoal (Savona) and Punta del Faro (Portofino Promontory) showed the highest average and maximal heights, with colonies reaching up to 20 cm (Table 1). In the North-central Tyrrhenian Sea, the average colony height varied between 2.8 ± 0.1 cm in CE Sardinia and 5.6 ± 0.1 cm in SW Sardinia. Maximal heights were mainly recorded in S and SW Sardinia, up to 25 cm (Boi Cape) (Table 1 and Figures 2B, 3H). In the South Tyrrhenian Sea, a significant peak was recorded in the Campanian Archipelago, where the average height of the colonies was 7.1 ± 0.1 cm (Figure 2B). In this latter coral area, high maximal heights were recorded in all localities (Table 1). No significant correlation was found within the dataset between average height and average density of the colonies (r = 0.2236, graph not shown).
The size-frequency distributions, calculated for the coral areas, were generally skewed towards the small size classes with a long tail of large colonies (Figure 4). The modal classes were generally found in the 2-4 cm size classes for the western Ligurian Sea, Pontine Archipelago, E Sardinia, SW Sardinia and North Sicily. The modal classes were slightly shifted in the 4-6 size classes in the eastern Ligurian Sea, Tuscan Archipelago and Tyrrhenian Calabria. The populations measured in the Campanian Archipelago and in the Sicily Channel showed a more symmetric distribution characterised by a positive kurtosis and a modal class between 6 and 10 cm. In addition, a second mode of larger colonies was found in the western Ligurian Sea (6-10 cm, mainly attributed to the Savona coral area), in the Pontine Archipelago (6-8 cm, mainly attributed to Zannone Island), in the Tyrrhenian Calabria (8-10 cm, mainly attributed to Palmi), and in the Sicily Channel (12-14 cm, mainly attributed to Graham Bank) (Figure 4). Very large colonies were extremely rare: on a total of 15549 measured colonies, only 25 (0.16%) exceeded 20 cm height. These were located in the Ligurian Sea, Tuscan Archipelago, E and SW Sardinia, and in the Campanian Archipelago.
The two seamounts where C. rubrum was present (Vercelli and Palinuro) showed very low average density values (0.1 ± 0.1 colonies m-2 and 1.1 ± 0.5 colonies m-2, respectively). The few colonies encountered on the Palinuro Seamount (Figure 3I) measured up to 20 cm (Table 1).
The influence of depth and latitude on the density and size of red coral forests
Considering clustered data according to depth ranges, the populations average density resulted inversely related to depth (Figure 5A), ranging from an average value of 18.6 ± 2.0 colonies m-2 in the first depth range to 0.4 ± 0.1 colonies m-2 in the deepest one (Kruskal-Wallis, p < 0.0001, Hc = 31.18). On the deepest part of the continental shelf (40-80 m), the three density categories were all largely represented (32.7-34.7%), while, with increasing depth, populations were constituted by a higher percentage of populations dominated by scattered colonies, which became the only represented category at bathyal depths (> 200 m) (Figure 5B). No clear depth-related pattern was observed for the colony average size between the clustered depth ranges, varying between a minimum of 4.2 ± 0.1 cm at 80-150 m and a maximum of 6.0 ± 0.1 cm below 200 m. Differences among average height values tested significant only for the 80-150 m depth range (Kruskal-Wallis, p < 0.001, Hc = 15.79, graph not shown).
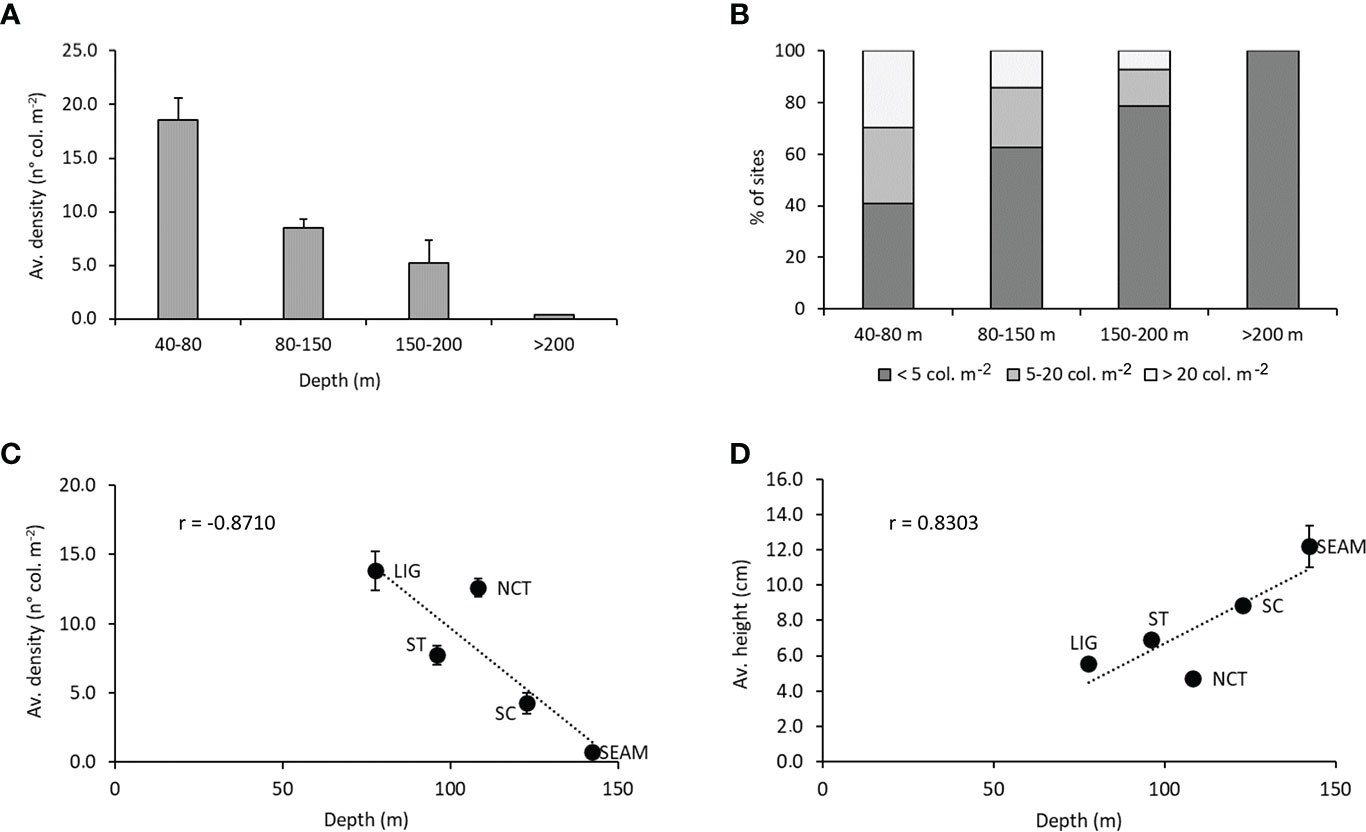
Figure 5 Bathymetric and latitudinal effect. (A) Relationship between average density (± SE) of the populations and depth in the four considered depth ranges. (B) Percentage of sites with scattered populations (dark grey), dense populations (grey), and very dense populations (light grey) in the four depth ranges. (C) Correlation between average density (± SE) of the populations grouped per macro-area and depth. (D) Correlation between average height (± SE) of the populations grouped per macro-area and depth.
The average density of the red coral populations clustered per macro-area showed a strongly significant decreasing pattern with depth, with a maximal value registered in the Ligurian Sea (13.8 ± 1.4 colonies m-2) (Figure 5C). The minimal values were, for the coastal macro-areas, 4.3 ± 0.8 colonies m-2 in the Sicily Channel, and 0.8 ± 0.3 colonies m-2 on the offshore seamounts. On the contrary, a strongly significant correlation was recorded between average colony height and depth in the considered macro-areas, with a peak in the Sicily Channel (8.9 ± 0.2 cm) for coastal macro-areas, and on offshore seamounts (12.2 ± 1.2 cm) (Figure 5D).
Fishing impact
In total, 17.5% of the counted colonies considered in the site selection were entangled in abandoned, lost or otherwise discarded fishing gear (ALDFG), including nets and longlines (Figures 6A–D). Considering the macro-areas, the Ligurian Sea was the one showing the overall highest percentage of entanglement, varying between 24.7% on the Portofino Promontory and 40.8% in the Sanremo area, with a peak in the Bordighera Canyon (78.7%) (Table 1 and Figure 7A). The southern macro-areas followed with single coral areas showing even higher peaks of percentage entanglement than those identified in the Ligurian Sea, such as Milazzo Cape (North Sicily, 50.6%), Ragusa Bank (South Sicily, 39.9%), and Pantelleria Island (Pelagie Archipelago, 22.9%). The North-central Tyrrhenian macro-area overall showed the lowest percentages, ranging from 6.4% (NE Sardinia) to 17.7% (SW Sardinia). Few coral areas showed a very negligible percentage of entanglement (below 0.5%) (Table 1 and Figure 7A).
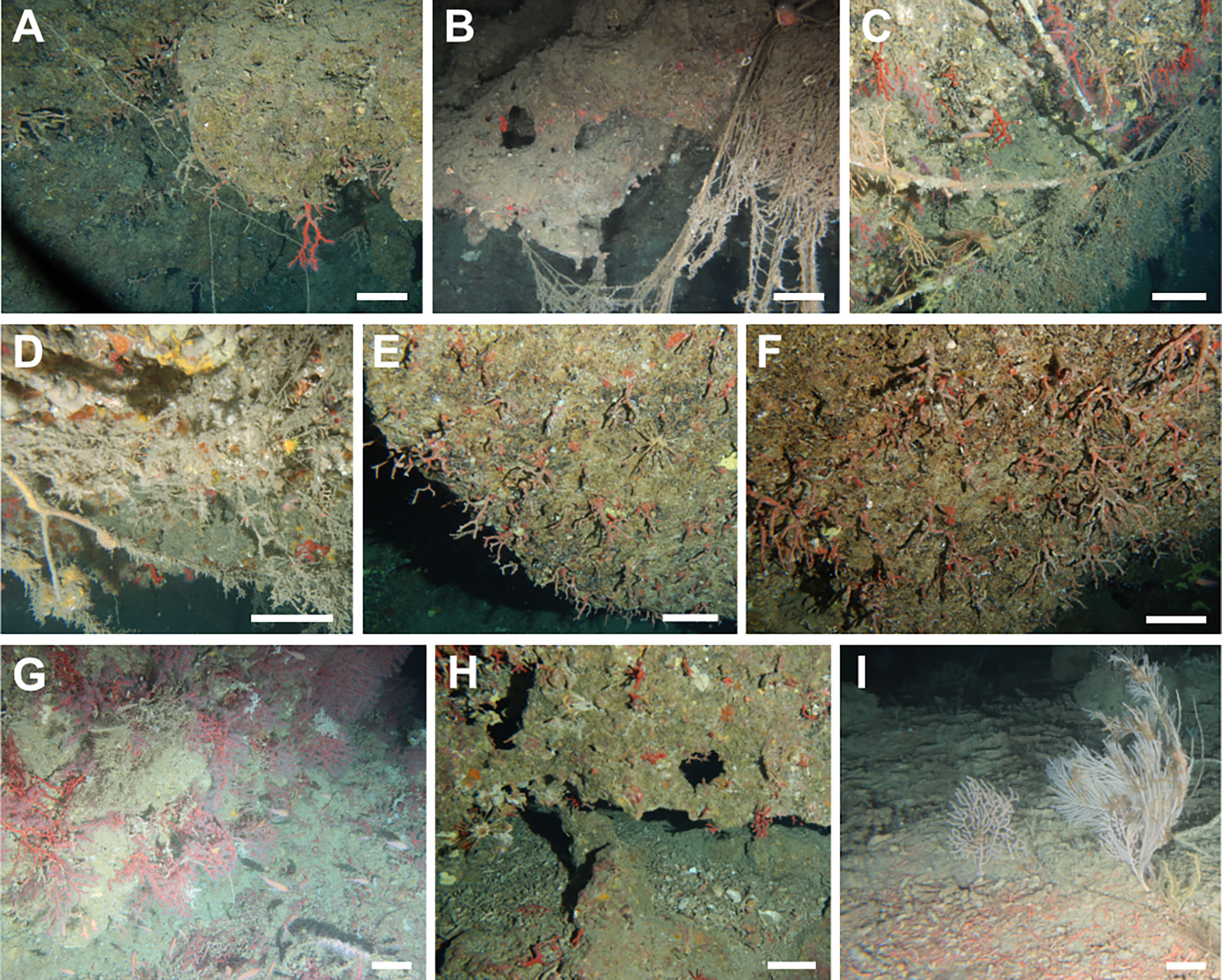
Figure 6 Fishing impact and mass mortalities. (A) Living and dead colonies entangled with a longline (Gulf of Salerno, 98 m); (B–D) nets impacting on Corallium rubrum (Milazzo Cape, 110 m; Ischia Island, 70 m; Bordighera Canyon, 70 m, respectively); (E, F) extensive mass mortality on the vertical rocky cliffs in Boi Cape (South Sardinia, 118 m); (G) large patch of coral fragments beneath flourishing living colonies (Graham Bank, Sicily Channel, 147 m); (H) coral fragments at the base of a rocky boulder hosting living and dead colonies (Porto Corallo, SE Sardinia, 87 m); (I) dense patch of C. rubrum fragments in the detritus (Graham Bank, Sicily Channel, 80 m). Scale bar: 10 cm.
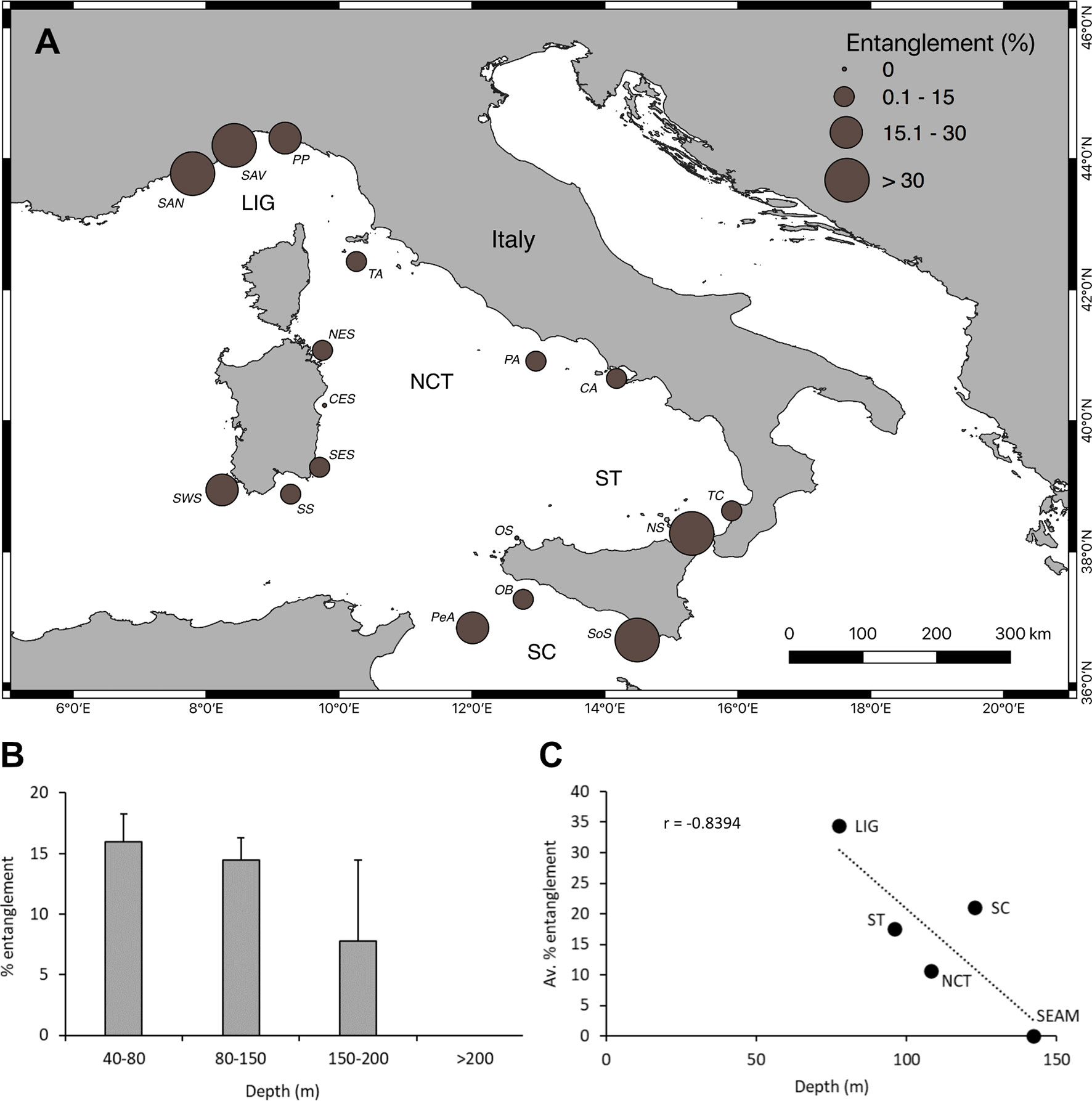
Figure 7 Fishing impact. (A) Map of the coral areas showing evident traces of impact (entanglement) by nets and longlines. The size of the dots refers to the average percentage of entanglement. (B) Relationship between the average percentage of entangled colonies and depth in the four depth ranges. (C) Correlation between the average percentage entanglement per macro-area and depth.
An inverse relationship between the average percentage of entangled colonies and depth was found, with the deepest populations being the less impacted (Kruskal-Wallis, p = ns, Hc = 5.34) (Figure 7B). The average percentage entanglement ranged from 16.0 ± 2.3% for the populations found along the deepest part of the continental shelf (40-80 m) to virtually absent for those at bathyal depths (>200 m).
The average percentage entanglement of the red coral colonies clustered per macro-area showed a strongly significant decreasing pattern with depth, with offshore seamount populations free from fishing impact (Figure 7C).
Deep mass mortalities
In 30 of the explored sites (17.6%), large patches of dead colonies still in place, ascribed to mass mortality events, were observed at mesophotic and upper bathyal depths (70-198 m, with the maximum recorded in the Caprera Canyon, NE Sardinia) (Table 2). 25 of these sites presented both living and dead populations, while 5 presented exclusively dead colonies. In addition, 8 sites showed no dead colonies in place on the hardgrounds, but detectable large patches of coral rubble on the nearby detritic seafloor. These latter sites were found between 80 m and 160 m along the southern Sardinian coast and nearby the Graham Bank and were often located nearby living populations (Table 2 and Figures 6G–I).
Mass mortality events were mainly recorded in Sardinia Island (57% of the sites with dead colonies), particularly in Boi Cape (S Sardinia, 5 sites, between 90 m and 140 m), and in Porto Corallo (SE Sardinia, 4 sites, between 85 m and 135 m) (Table 2 and Figures 6E, F). Another coral area particularly impacted by these events was the Campanian one: dead populations were recorded in the Gulf of Naples (96-134 m) and in the Salerno Gulf (70-115 m). Large dead patches of red coral were found also in the Pontine Archipelago (110-193 m) and along the North Sicilian coast, including the Aeolian Archipelago (107-128 m) (Table 2). Dead in-place patches were absent in the Ligurian Sea, in the Sicily Channel and on seamounts. Considering both living and dead colonies in the 9 involved coral areas, it was possible to report an incidence of mortality of less than 50% of the colonies in one-third of the populations, between 50 and 75% in about one-fifth of the populations and between 75 and 100% in the remaining 44% of the populations. Mass mortality events interested populations settled on vertical hardgrounds, including outcropping rocks (19 sites) and rocks covered by CCA (11 sites) (Figures 6E–I).
The overall density and height comparison between living and dead colonies, when co-occurring, showed no differences (an average density of about 9 colonies m-2 and an average height of about 4 cm). Nevertheless, locally, some differences were recorded in terms of density (Figure 8). In particular, 14 sites, corresponding to 57% of the sites where both living and dead colonies were simultaneously present, showed a higher density of dead colonies with respect to living ones, with the maximum average density (55.6 ± 9.6 colonies m-2) recorded in Boi Cape (Figure 8).
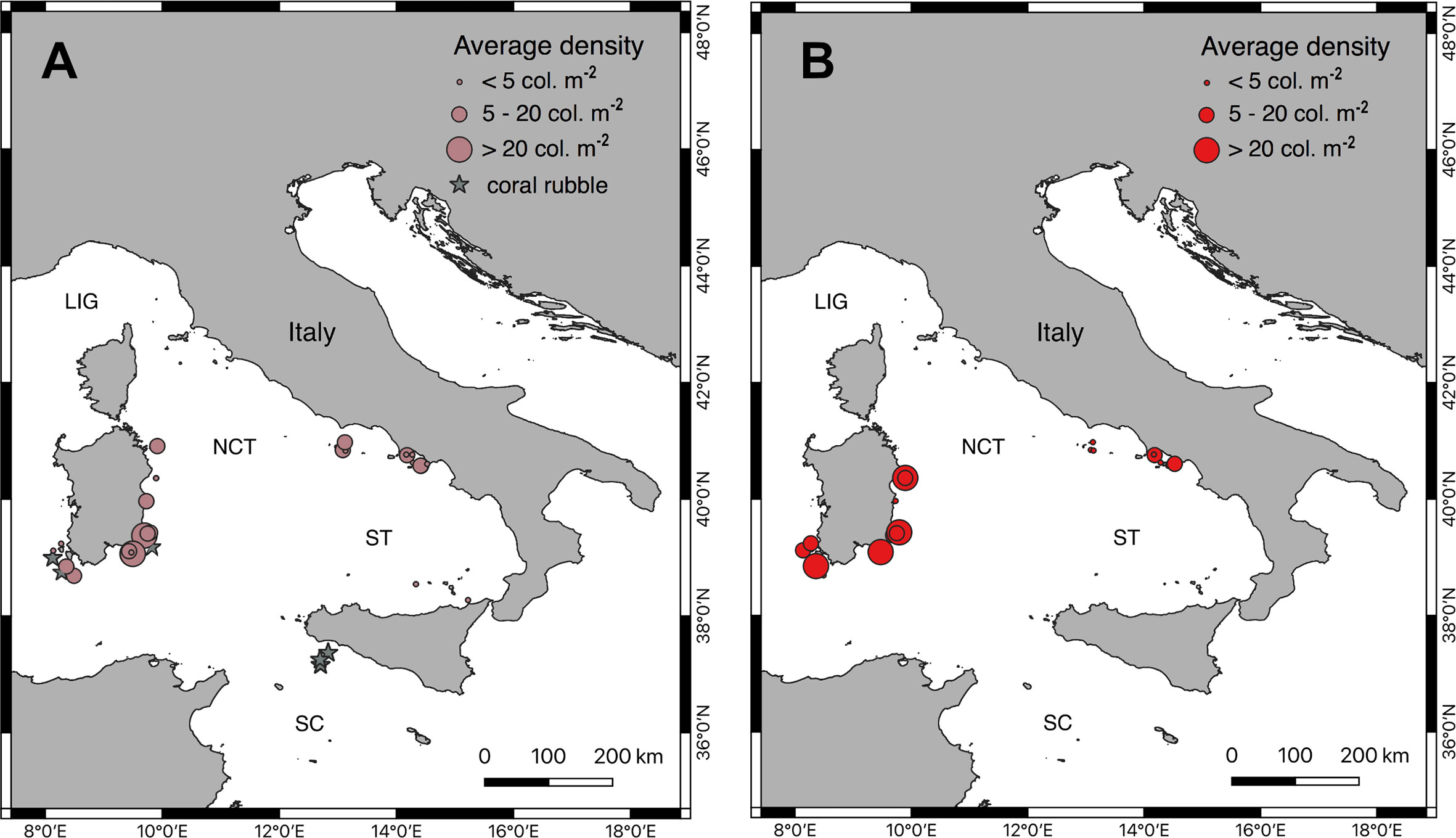
Figure 8 Deep mass mortalities. Comparative maps of the sites where dead (A) and living (B) colonies were observed. The size of the dots refers to the average density of the populations. Grey stars indicate the sites where patches of coral rubble were visible in the sediment.
Dead colonies occasionally suffered from a secondary direct impact from lost fishing gear. Traces of entanglements, visible on up to 52% of the colonies (Teulada Cape, SW Sardinia), were found in 33% of the sites with mortality events (Table 2).
Discussion
This work considered the widest demographic dataset ever produced on Corallium rubrum, with a specific focus on the mesophotic and upper bathyal depths of the Italian coast. This species confirms to be widespread in the basin, with seventeen coral areas identified based on their geographic proximity and topographic and oceanographic affinity. Among them, some showed high density and considerable size of the colonies (such as those of the Portofino Promontory, Tuscan Archipelago, South and South-western Sardinia, Campanian Archipelago and South Sicily), representing important larval stock for the species, and these may be the target of specific management actions as required by the most recent species and habitat regional assessments (Otero et al., 2017; Montefalcone et al., 2021).
In general, the investigated populations consistently showed a size-frequency distribution skewed towards small-size colonies, with low variability among the areas and among depth ranges. Although it is recognized the possibility of a limited bias in the measures obtained through bidimensional ROV footage with respect to the ones derived by three-dimensional techniques (Lagudi et al., 2022), the overall patterns appear clear. The size of colonial organisms such as C. rubrum is known to be potentially locally constrained by numerous environmental parameters (e.g., food and substrate availability, temperature, currents, depth, slope) (e.g., Cau et al., 2016; Galli et al., 2016) as well as by anthropic pressures, mainly harvesting and fishing (e.g., Tsounis et al., 2006; Rossi et al., 2008; Bruckner, 2009; Cattaneo-Vietti et al., 2016). Over the spatial scale here considered, these factors may be synergically responsible for the recorded variations, especially when considering the occurrence, in certain coral areas (western Ligurian Sea, Pontine Archipelago, Tyrrhenian Calabria, Sicily Channel), of multiple cohorts of colonies. However, overall, it seems that, among all factors, depth does not influence the size of the colonies. This is in accordance with Carugati et al. (2022), reporting no significant variation of colony height with depth in the North-western Sardinian populations (50-290 m), but contrasts with the findings of other authors sustaining the major size (as well as diameter and branching) of deep-dwelling colonies, a trend mainly attributed to a lower harvesting pressure (e.g., Tsounis et al., 2007; Rossi et al., 2008; Priori et al., 2013; Angiolillo et al., 2016; Cau et al., 2016). Moreover, the same authors provided evidence of a size/density relationship within C. rubrum populations, with larger, but more scattered colonies in deeper waters, that did not result from the present dataset.
Together with indeed ecological considerations, the homogeneous reduced size of the red coral populations in the entire study area could be related also to a stressful situation due to the long-term harvesting pressure carried out in all the considered basins (Cattaneo-Vietti et al., 2016). Already Bruckner (2009, 2014), based on data derived from the landed harvested material, supported the use of size reduction in time as the main indicator of the harvesting impact on the demography of the populations. Similar conclusions were also derived from in-situ local studies, carried out both on shallow and deep populations. Tsounis et al. (2006); Tsounis et al. (2007) and Rossi et al. (2008) clearly demonstrated that the harvesting pressure is the main constraint driving population structure at Cap de Creus (Spain, NW Mediterranean Sea), reporting a colony size in shallow overexploited populations noticeably smaller than that of deeper, less harvested populations (average colony size: 3.0 ± 1.7 cm, and 7.8 ± 3.3 cm, respectively, in shallow and deep populations). Significant differences in terms of basal diameter and maximum height emerged from the comparison between harvested and non-harvested populations around Marseilles (France) (Garrabou and Harmelin, 2002). Finally, larger colony sizes have been observed also in populations located inside protected areas, where harvesting is forbidden, as in the Medes Islands Marine Reserve (Spain) and on Ischia Island (Regno di Nettuno MPA, Campania, Italy), suggesting that the harvesting pressure has a deleterious effect on the population structure of the precious coral (Linares et al., 2010; Angiolillo et al., 2016). Our data, coming from a wide sampling effort, provide additional support to this hypothesis.
Differently from height, density is strongly driven by depth, with a linear decrease from shallow to deep sites, confirming the preliminary qualitative results obtained by Toma et al. (2022) and in accordance with known literature (e.g., Rossi et al., 2008; Carugati et al., 2022). In addition, on the continental shelf and shelf break, higher variability in terms of density was observed, with dense aggregations and populations made of sparse colonies equally present, while at bathyal depth, populations of scatted colonies were dominant. A similar pattern was already reported in Cap de Creus (North-western Mediterranean Sea) at 50-230 m (Rossi et al., 2008). The present work enhances the idea that the self-thinning process (i.e., the intra-specific competition regulating population density and structure, based on space limitations, namely hardgrounds availability) (Cau et al., 2016), is a valid explanation for the density-depth pattern observed on a large scale for C. rubrum, but this trend is not necessarily associated to an increase of the colonies’ size with depth. It has to be considered that this latter trend of size with depth emerged from studies targeting single populations located in restricted geographic and depth ranges, and often deeply harvested since historical times in shallow waters. The present study, instead, considers a much larger area (about 680,000 m2), and a wider depth range (from the deep circalittoral to the deep bathyal), including coastal and offshore sites subjected to a larger variety of environmental constraints and human pressures. This allowed obtaining a larger picture of the population structure of red coral, damping local influences. Interesting large-scale patterns, both in terms of density and height, emerged from the latitudinal point of view, probably related to unknown gradients of environmental (e.g., currents, temperature, silting, nutrients) and biological (e.g., competition with other co-occurring components of the assemblages) characteristics. In fact, populations showing higher densities and smaller colony heights characterize the mesophotic hardgrounds of the northern areas, with respect to the southern ones.
In terms of coastal-offshore gradient, seamounts, despite hosting only a few sparse red coral colonies generally of large size, offered some insights into the demographic trends of this species. The distance of these structures from the coast seems to act as a barrier for the larval dispersion of the species, which is generally considered on a short-medium range (Costantini et al., 2007; Martínez-Quintana et al., 2015; Costantini and Abbiati, 2016; Guizien et al., 2020). High densities of C. rubrum observed on other Mediterranean seamounts may be related to their closer proximity to the coast (de la Torriente et al., 2018), as well as to more favourable trophic conditions than those found on the offshore reliefs investigated here (30-70 NM from the nearest coastal populations). However, once settled, offshore red coral populations may potentially be subjected to lower levels of anthropic impact since these structures have never been interested by coral harvesting (Cattaneo-Vietti et al., 2016) and are known to have a modest fishing pressure (Bo et al., 2014), hence supporting larger sizes.
The remoteness of a site, influencing its anthropic accessibility, both in terms of depth and distance, has been considered a factor driving the demography of the red coral populations (Rossi et al., 2008). In this study, demersal artisanal and recreational fishing activities emerged as serious and widespread threats to the habitats including red coral at almost all coastal sites and depths. Colonies entangled in fishing gear were visible in almost 90% of the coral areas, with those in the Ligurian Sea and Sicily Channel being among the most impacted, and those in the Tuscan Archipelago and eastern Sardinian coast among the least. This pattern has been highlighted in numerous studies conducted on the deep megabenthic assemblages of these regions (Bo et al., 2014; Angiolillo et al., 2015; Cattaneo-Vietti et al., 2017; Cau et al., 2017). Highly impacted benthic communities have been documented along the Ligurian coast, especially in the western sector (Enrichetti et al., 2019a; Enrichetti et al., 2019b). On the contrary, the Portofino Promontory resulted among the less impacted Ligurian coral areas. This could be due to the proximity of the studied mesophotic populations to the coastal Marine Protected Area. Here, in fact, a reversion in the size-structure shift was observed by Bavestrello et al. (2015), reporting flourishing dense populations of large colonies at shallow depths after almost two decades of protection. The positive effects of restrictions on red coral populations have been reported also in North-West Sardinia, where large colonies (modal size classes 10-15 cm and 15-20 cm) were registered by Onboard Scientific Observes in catches from areas subjected to stricter harvesting measures compared to other countries within the GFCM competence area (Follesa et al., 2013; Carugati et al., 2020). This specific area within the Sardinian Sea was already reported as a hotspot of occurrence of C. rubrum (Toma et al., 2022). These works support the importance of MPAs and other protection measures in the conservation and management of red coral. This is particularly true if we consider the high frequency of the indirect damages of fishing activities occurring in the deepest part of the continental shelf and along the shelf break, where the densest and more diversly aggregated populations were observed.
The present study also highlighted how, besides fishing and harvesting pressures, mass mortality events, registered down to 200 m, may represent significant stresses for mesophotic and upper bathyal red coral populations. Our data enlarge the current knowledge about red coral mass mortality events identifying a Tyrrhenian and Sicilian belt of dead red coral zones. The causes of these catastrophic events remain, however, uncertain. Similar phenomena in coastal ecosystems (0-40 m), including also red coral, have been linked to modifications in environmental conditions triggered by global changes (e.g., thermal anomalies) (Harvell et al., 1999; Cerrano et al., 2000; Pérez et al., 2000; Garrabou et al., 2001; Harley et al., 2006; Garrabou et al., 2009; Cerrano et al., 2013). However, in the deep regions where red coral mortalities have been reported, far from the influence of the seasonal thermocline, other putative causes should be addressed. For example, the red coral mass mortality event reported along the coast of Provence in 1987 (Rivoire, 1991; Arnoux et al., 1992), between 80 and 160 m, was attributed to polluted waters driven by the dominant currents in that area. The deep circulation of warm waters in proximity to volcanic areas (Bavestrello et al., 2014) combined with the seafloor movements in seismic areas (Lodolo et al., 2017; Bavestrello et al., 2021) may explain the extensive mortality events observed in the Pontine Archipelago, Campanian Archipelago and the offshore banks of the Sicily Channel. For the Sardinian sites, however, there is no clear explanation of the putative factors triggering the coral death, neither for the population along the North-eastern sector (Carugati et al., 2022) nor for those in the southern one. Most of the mortalities were recorded in submarine canyons, which may support the fact that the particular environmental conditions (e.g., turbidity currents) occurring in these systems very close to the shorelines, may have caused the events (Moccia et al., 2021). However, the co-occurrence, in the same sites, of different structuring filter-feeding anthozoans (e.g., Antipathella subpinnata (Ellis and Solander, 1786), Eunicella cavolini (Koch, 1887)) in apparently good health status would exclude a wide clogging event triggered by a massive deposition of silt (Moccia et al., 2022). Certainly, mass mortality events, both in shallow and deep waters, should be considered as additional factors significantly shaping the demography of red coral populations in the short term.
This work provides valuable information on the distribution and population structure of a precious overexploited species from a large-scale perspective. Deep coral areas, with particularly high density and size, were identified, as well as the areas most affected by fishing impact and mass mortality events. All this information is fundamental to have a comprehensive picture of the demography of an endangered species and to depict the best conservation actions.
Data availability statement
The raw data supporting the conclusions of this article will be made available by the authors, without undue reservation.
Author contributions
Conceptualization, MT, GB, MB. methodology, validation, formal analysis, MT, GB, MB. investigation, resources, data curation, writing-original draft preparation, MT, MB, GB, SC, AC, MA. writing-review and editing, MT, MB, GB, SC, AC, MA. visualization, supervision, project administration, GB, MB, SG, FA, SC. All authors contributed to the article and approved the submitted version.
Funding
The large ROV dataset employed in the present study was built including observations conducted along the Italian coast during several ROV campaigns. These campaigns were financed by: Ministero dell’Ambiente e della Tutela del Territorio e del Mare (Project 2010, Red Coral); Ministero delle Politiche Agricole, Alimentari e Forestali (Project 2012, “Use of ROV in the management of deep Corallium rubrum populations”; L.R. 7 Agosto 2007, no. 7. “Struttura spaziale, di popolazione e genetica dei banchi di Corallium rubrum del Mediterraneo centro occidentale”); Istituto Superiore per la Ricerca e la Protezione Ambientale (ISPRA) and Calabrian Regional Council for Environment (“Monitoraggio della Biodiversità Marina in Calabria”, grant no. 327 MoBioMarCal); Ministry of Instruction, University and Research (MIUR) (grant no. 2010Z8HJ5M_011 PRIN 2010–2011); Autonomous Region of Sardinia (RAS); Agenzia Regionale per la Protezione dell’Ambiente Ligure (grant no. 127/2015, 109/2016, 110/2017, within the Marine Strategy Framework Monitoring Program, ARPAL n. 177/2014); EU-ENPI CBC MED 2007–2013, “Ecosystem conservation and sustainable artisanal fisheries in the Mediterranean basin” (ECOSAFIMED) (grant no. II-B/2.1/1073); Greenpeace-ISPRA, 2012 - I tesori sommersi del Canale di Sicilia; D.R.A. Assessorato Territorio Ambiente Regione Siciliana-Asse 3 Linea di intervento 3.2.1.2 of POR FESR Sicilia 2007-2013, aim to assess the marine biodiversity in Sicily; Local Management Plan of the Unit Management between Calavà Cape and Milazzo Cape - P0044509. ISPRA REGIONE SICILIA; SIA-SIC Study of the impact on fishing from war and merchant ships wrecks in the Strait of Sicily and in other Sicilian seas. - 2010-2012 - ISPRA/Regione Sicilia; PRIN (Progetti di Rilevante Interesse Nazionale) project “Tyrrhenian Seamounts ecosystems: an Integrated Study (TySEc)” financed by the Italian Ministry of Research and Instruction and by the Global Census of Marine Life on Seamounts (CenSeam, New Zealand); SIR-MIUR_BIOMOUNT Project (Biodiversity Patterns of the Tyrrhenian Seamounts) (grant no. RBSI14HC9O); Curiosity Driven 2020-2021 (UniGe) - “Lost coral reefs: ancient deep-sea bioherms in a modern world” (100022-2020-MB-CURIOSITY_Bo). This research was supported by a National Biodiversity Future Center Grant (CN_00000033). The funders had no role in study design, data collection and analysis, decision to publish, or preparation of the manuscript.
Acknowledgments
The authors would like to thank the members of all the crews for their help, dedication, experience, and technical expertise during the field surveys.
Conflict of interest
The authors declare that the research was conducted in the absence of any commercial or financial relationships that could be construed as a potential conflict of interest.
Publisher’s note
All claims expressed in this article are solely those of the authors and do not necessarily represent those of their affiliated organizations, or those of the publisher, the editors and the reviewers. Any product that may be evaluated in this article, or claim that may be made by its manufacturer, is not guaranteed or endorsed by the publisher.
References
Angiolillo M., di Lorenzo B., Farcomeni A., Bo M., Bavestrello G., Santangelo G., et al. (2015). Distribution and assessment of marine debris in the deep tyrrhenian Sea (NW Mediterranean Sea, Italy). Mar. pollut. Bull. 92 (1-2), 149–159. doi: 10.1016/j.marpolbul.2014.12.044
Angiolillo M., Gori A., Canese S., Bo M., Priori C., Bavestrello G., et al. (2016). Distribution and population structure of deep-dwelling red coral in the Northwest Mediterranean. Mar. Ecol. 37 (2), 294–310. doi: 10.1111/maec.12274
Arnoux A., Harmelin J. G., Monod J. L., Romaña L. A., Zibrowius H. (1992). Altérations des peuplements benthiques de roches profondes en méditerranée nord-occidentale: quelques aspects biologiques et molysmologiques. C R Acad. Sci. Ser. III Sci. Vie 314, 219–225.
Bavestrello G., Bo M., Bertolino M., Betti F., Cattaneo-Vietti R. (2015). Long-term comparison of structure and dynamics of the red coral metapopulation of the portofino promontory (Ligurian sea): A case-study for a marine protected area in the mediterra- nean Sea. Mar. Ecol. 36, 1354–1363. doi: 10.1111/maec.12235
Bavestrello G., Bo M., Calcagnile L., Canessa M., D’Elia M., Quarta G., et al. (2021). The sub-fossil red coral of sciacca (Sicily channel, Mediterranean sea): colony size and age estimates. Facies 67 (2), 1–10. doi: 10.1007/s10347-020-00620-x
Bavestrello G., Bo M., Canese S., Sandulli R., Cattaneo-Vietti R. (2014). The red coral populations of the gulfs of Naples and Salerno: human impact and deep mass mortalities. Ital. J. Zoology 81 (4), 552–563. doi: 10.1080/11250003.2014.950349
Bo M., Bava S., Canese S., Angiolillo M., Cattaneo-Vietti R., Bavestrello G. (2014). Fishing impact on deep Mediterranean rocky habitats as revealed by ROV investigation. Biol. Conserv. 171, 167–176. doi: 10.1016/j.biocon.2014.01.011
Bramanti L., Vielmini I., Rossi S., Tsounis G., Iannelli M., Cattaneo-Vietti R., et al. (2014). Demographic parameters of two populations of red coral (Corallium rubrum l. 1758) in the north Western Mediterranean. Mar. Biol. 161 (5), 1015–1026. doi: 10.1007/s00227-013-2383-5
Bruckner A. W. (2009). Rate and extent of decline in corallium (pink and red coral) populations: existing data meet the requirements for a CITES appendix II listing. Mar. Ecol. Prog. Ser. 397, 319–332. doi: 10.3354/meps08110
Bruckner A. W. (2014). Advances in management of precious corals in the family corallidae: Are new measures adequate? Curr. Opin. Environ. Sustainability 7, 1–8. doi: 10.1016/j.cosust.2013.11.024
Bussoletti E., Cottingham D., Bruckner A., Roberts G., Sandulli R. (2010). Proceedings of the international workshop on red coral science, management, and trade: Lesson from the Mediterranean (Naples, Italy), 2009 (Silver Spring, MD: NOAA Technical Memorandum CRCP-13). 1–233.
Cannas R., Follesa M. C., Cau Al., Cau A., Friedman K. (2019). Global report on the biology, fishery and trade of precious corals. FAO Fisheries Aquacult. Circular 1184, 1–254. Available at: https://www.proquest.com/scholarly-journals/global-report-on-biology-fishery-trade-precious/docview/2330015194/se-2?accountid=15964.
Carugati L., Cau A., Follesa M. C., Melis R., Moccia D., Porcu C, et al. (2020). On board scientific observers provide a realistic picture of harvesting and management priorities for the precious red coral (Corallium rubrum l.). Front. Mar. Sci. 7, 1–12. doi: 10.3389/fmars.2020.00482
Carugati L., Moccia D., Bramanti L., Cannas R., Follesa M. C., Salvadori S., et al. (2022). Deep-dwelling populations of Mediterranean Corallium rubrum and Eunicella cavolini: Distribution, demography, and Co-occurrence. Biology 11 (2), 333. doi: 10.3390/biology11020333
Cattaneo-Vietti R., Bavestrello G. (2010). “Sustainable use and conservation of precious corals in the Mediterranean,” in Biohistory of precious corals. Ed. Iwasaki N. (Tokyo: Tokai University Press), 1–364.
Cattaneo-Vietti R., Bavestrello G., Bo M., Canese S., Vigo A., Andaloro F. (2017). Illegal ingegno fishery and conservation of deep red coral banks in the Sicily channel (Mediterranean Sea). Aquat. Conservation: Mar. Freshw. Ecosyst. 27 (3), 604–616. doi: 10.1002/aqc.2731
Cattaneo-Vietti R., Bo M., Cannas R., Cau Al., Follesa C., Meliadò E. (2016). An Italian overexploited treasure: past and present distribution and exploitation of the precious red coral Corallium rubrum (L. 1758) (Cnidaria, anthozoa). Ital. J. Zoology 83, 443–455. doi: 10.1080/11250003.2016.1255788
Cau A., Alvito A., Moccia D., Canese S., Pusceddu A., Rita C., et al. (2017). Submarine canyons along the upper sardinian slope (Central Western Mediterranean) as repositories for derelict fishing gears. Mar. pollut. Bull. 123 (1-2), 357–364. doi: 10.1016/j.marpolbul.2017.09.010
Cau A., Bramanti L., Cannas R., Follesa M. C., Angiolillo M., Canese S., et al. (2016). Habitat constraints and self-thinning shape Mediterranean red coral deep population structure: implications for conservation practice. Sci. Rep. 6 (1), 1–10. doi: 10.1038/srep23322
Cau A., Bramanti L., Cannas R., Moccia D., Padedda B. M., Porcu C., et al. (2018). Differential response to thermal stress of shallow and deep dwelling colonies of Mediterranean red coral (Corallium rubrum l. 1758). Adv. Oceanogr. Limnol. 9, 13–18. doi: 10.4081/aiol.2018.7275
Cerrano C., Bavestrello G., Bianchi C. N., Cattaneo-Vietti R., Bava S., Morganti C., et al. (2000). A catastrophic mass-mortality episode of gorgonians and other organisms in the ligurian Sea (North-western Mediterranean), summer 1999. Ecol. Lett. 3 (4), 284–293. doi: 10.1046/j.1461-0248.2000.00152.x
Cerrano C., Bavestrello G., Cattaneo-Vietti R. (1997). Light influence on planulae emission and settlement in Eudendrium glomerafum cnidaria, hydrozoa. Biol. Marina Mediterr. 4 (1), 30–33.
Cerrano C., Cardini U., Bianchelli S., Corinaldesi C., Pusceddu A., Danovaro R. (2013). Red coral extinction risk enhanced by ocean acidification. Sci. Rep. 3 (1), 1–7. doi: 10.1038/srep01457
Cicogna F., Bavestrello G., Cattaneo-Vietti R. (1999). Red coral and other Mediterranean octocorals: Biology and protection (Roma: Ministero per le Politiche Agricole), 1–238.
Cicogna F., Cattaneo-Vietti R. (1993). Red coral in the Mediterranean Sea, art, history and science (Roma: Ministero delle Risorse Agricole, Alimentari e Forestali), 1–263.
Costantini F., Abbiati M. (2016). Into the depth of population genetics: pattern of structuring in mesophotic red coral populations. Coral Reefs 35, 39–52. doi: 10.1007/s00338-015-1344-5
Costantini F., Fauvelot C., Abbiati M. (2007). Fine-scale genetic structuring in Corallium rubrum: evidence of inbreeding and limited effective larval dispersal. Mar. Ecol. Prog. Ser. 340, 109–119. doi: 10.3354/meps340109
de la Torriente A., Serrano A., Fernández-Salas L. M., García M., Aguilar R. (2018). Identifying epibenthic habitats on the seco de los olivos seamount: Species assemblages and environmental characteristics. Deep Sea Res. Part I: Oceanographic Res. Papers 135, 9–22. doi: 10.1016/j.dsr.2018.03.015
Enrichetti F., Bava S., Bavestrello G., Betti F., Lanteri L., Bo M. (2019a). Artisanal fishing impact on deep coralligenous animal forests: a Mediterranean case study of marine vulnerability. Ocean Coast. Manage. 177, 112–126. doi: 10.1016/j.ocecoaman.2019.04.021
Enrichetti F., Bo M., Morri C., Montefalcone M., Toma M., Bavestrello G., et al. (2019b). Assessing the environmental status of temperate mesophotic reefs: A new, integrated methodological approach. Ecol. Indic. 102, 218–229. doi: 10.1016/j.ecolind.2019.02.028
FAO (1988). GFCM technical consultation on red coral of the Mediterranean. FAO Fish Report 413:1–159.
Follesa M. C., Cannas R., Cau A., Pedoni C., Pesci P., Cau A. (2013). Deep-water red coral from the island of Sardinia (north-western mediterranean): a local example of sustainable management. Mar. Freshw. Res. 64 (8), 706–715. doi: 10.1071/MF12235
Galgani F., Pham C. K., Claro F., Consoli P. (2018). Marine animal forests as useful indicators of entanglement by marine litter. Mar. pollut. Bull. 135, 735–738. doi: 10.1016/j.marpolbul.2018.08.004
Galli G., Bramanti L., Priori C., Rossi S., Santangelo G., Tsounis G., et al. (2016). Modelling red coral (Corallium rubrum) growth in response to temperature and nutrition. Ecol. Model. 337, 137–148. doi: 10.1016/j.ecolmodel.2016.06.010
Garrabou J., Coma R., Bensoussan N., Bally M., Chevaldonné P., Cigliano M., et al. (2009). Mass mortality in northwestern Mediterranean rocky benthic communities: effects of the 2003 heat wave. Global Change Biol. 15 (5), 1090–1103. doi: 10.1111/j.1365-2486.2008.01823.x
Garrabou J., Harmelin J. G. (2002). A 20-year study on life-history traits of a harvested long-lived temperate coral in the NW Mediterranean: Insights into conservation and management needs. J. Anim. Ecol. 71, 966–978. doi: 10.1046/j.1365-2656.2002.00661.x
Garrabou J., Perez T., Sartoretto S., Harmelin J. G. (2001). Mass mortality event in red coral Corallium rubrum populations in the Provence region (France, NW Mediterranean). Mar. Ecol. Prog. Ser. 217, 263–272. doi: 10.3354/meps217263
Guizien K., Viladrich N., Martínez-Quintana Á., Bramanti L. (2020). Survive or swim: different relationships between migration potential and larval size in three sympatric Mediterranean octocorals. Sci. Rep. 10 (1), 1–12. doi: 10.1038/s41598-020-75099-1
Hammer Ø., Harper D. A., Ryan P. D. (2001). PAST: Paleontological statistics software package for education and data analysis. Palaeontologia electronica 4 (1), 9. doi: 10.1002/9780470750711
Harley C. D., Randall Hughes A., Hultgren K. M., Miner B. G., Sorte C. J., Thornber C. S., et al. (2006). The impacts of climate change in coastal marine systems. Ecol. Lett. 9 (2), 228–241. doi: 10.1111/j.1461-0248.2005.00871.x
Harvell C. D., Kim K., Burkholder J. M., Colwell R. R., Epstein P. R., Grimes D. J., et al. (1999). Emerging marine diseases–climate links and anthropogenic factors. Science 285 (5433), 1505–1510. doi: 10.1126/science.285.5433.1505
IUCN. (2016). Available at: https://www.iucn.org/content/mediterranean-anthozoans.
Lagudi A., Bruno F., Collina M., Rossi L., Rende S. F., Angiolillo M. (2022). A photogrammetric approach to evaluate the ecological status of cold-water corals: first results from the Italian monitoring within the marine streategy framework directive . UNEP/MAP - SPA/RAC, 2022. In Proceedings of the 4th Mediterranean Symposium on the conservation of Coralligenous & other Calcareous Bio-Concretions. (Genova, Italy, 20-21 September 2022). Bouafif C., Ouerghi A., edits, SPA/RAC publi., Tunis, 184 p.
Linares C., Bianchimani O., Torrents O., Marschal C., Drap P., Garrabou J. (2010). Marine protected areas and the conservation of long-lived marine invertebrates: the Mediterranean red coral. Mar. Ecol. Prog. Ser. 402, 69–79. doi: 10.3354/meps08436
Lodolo E., Sanfilippo R., Rajola G., Canese S., Andaloro F., Montagna P., et al. (2017). The red coral deposits of the Graham bank area: constraints on the Holocene volcanic activity of the Sicilian channel. GeoResJ 13, 126–133. doi: 10.1016/j.grj.2017.04.003
Martínez-Quintana A., Bramanti L., Viladrich N., Rossi S., Guizien K. (2015). Quantification of larval traits driving connectivity: the case of Corallium rubrum (L. 1758). Mar. Biol. 162 (2), 309–318. doi: 10.1007/s00227-014-2599-z
Moccia D., Carugati L., Follesa M. C., Cannas R., Carbonara P., Pusceddu A., et al. (2022). Environmental status and geomorphological characterisation of seven black coral forests on the sardinian continental shelf (NW Mediterranean Sea). Biology 11 (5), 732. doi: 10.3390/biology11050732
Moccia D., Cau A., Bramanti L., Carugati L., Canese S., Follesa M. C., et al. (2021). Spatial distribution and habitat characterization of marine animal forest assemblages along nine submarine canyons of Eastern Sardinia (central Mediterranean Sea). Deep Sea Res. Part I: Oceanographic Res. Papers 167, 103422. doi: 10.1016/j.dsr.2020.103422
Montefalcone M., Tunesi L., Ouerghi A. (2021). A review of the classification systems for marine benthic habitats and the new updated Barcelona convention classification for the Mediterranean. Mar. Environ. Res. 169, 105387. doi: 10.1016/j.marenvres.2021.105387
Montero-Serra I., Linares C., García M., Pancaldi F., Frleta-Valić M., Ledoux J. B., et al. (2015). Harvesting effects, recovery mechanisms, and management strategies for a long-lived and structural precious coral. PloS One 10 (2), e0117250. doi: 10.1371/journal.pone.0117250
Otero M. D. M., Numa C., Bo M., Orejas C., Garrabou J., Cerrano C. (2017). Overview of the conservation status of Mediterranean anthozoa (Spain: IUCN. Málaga), 73.
Pérez T., Garrabou J., Sartoretto S., Harmelin J. G., Francour P., Vacelet J. (2000). “Mortalité massive d’invertébrés marins: un événement sans précédent en méditerranée nord-occidentale,” in Comptes rendus de l'Académie des sciences-series III-sciences de la vie, vol. 323. , 853–865.
Priori C., Mastascusa V., Erra F., Angiolillo M., Canese S., Santangelo G. (2013). Demography of deep-dwelling red coral populations: Age and reproductive structure of a highly valued marine species. Estuarine Coast. shelf Sci. 118, 43–49. doi: 10.1016/j.ecss.2012.12.011
Richaume J., Cheminée A., Drap P., Bonhomme P., Cadene F., Ferrari B., et al. (2021). 3D photogrammetry modeling highlights efficient reserve effect apparition after 5 years and stillness after 40 for red coral (Corallium rubrum) conservation in French MPAs. Front. Mar. Sci. 8. doi: 10.3389/FMARS.2021.639334/BIBTEX
Rivoire G. (1991). “Mortalité de corail et de gorgones en profondeur au large des côtes provençales,” in Les Espèces marines à protéger en méditerranée. Eds. Boudouresque C. F., Avon M., Gravez V. (France: GIS Posidonies), 53–59.
Rossi S., Bramanti L., Gori A., Orejas C. (Eds.) (2017). Marine animal forests: the ecology of benthic biodiversity hotspots (Cham: Springer International Publishing), 1–1366.
Rossi S., Tsounis G., Orejas C., Padrón T., Gili J. M., Bramanti L., et al. (2008). Survey of deep-dwelling red coral (Corallium rubrum) populations at cap de creus (NW Mediterranean). Mar. Biol. 154 (3), 533–545. doi: 10.1007/s00227-008-0947-6
Santangelo G., Bramanti L. (2010). Quantifying the decline in Corallium rubrum populations. Mar. Ecol. Prog. Ser. 418, 295–297. doi: 10.3354/meps08897
Santangelo G., Bramanti L., Iannelli M. (2007). Population dynamics and conservation biology of the over-exploited Mediterranean red coral. J. Theor. Biol. 244, 416–423. doi: 10.1016/j.jtbi.2006.08.027
Santangelo G., Bramanti L., Vielmini I., Iannelli M. (2009). “What we have learned about red coral and what we need to learn for its rational management,” in Proceedings of the first international workshop on corallium science, management, and trade (Silver Spring, MD: NOAA Technical Memorandum NMFS-OPR-43 and CRCP-8), 71–86.
Toma M., Bo M., Cattaneo-Vietti R., Canese S., Canessa M., Cannas R., et al. (2022). Basin-scale occurrence and distribution of mesophotic and upper bathyal red coral forests along the Italian coasts. Mediterr. Mar. Sci. 23 (3), 484–498. doi: 10.12681/mms.28052
Tsounis G., Rossi S., Gili J.-M., Arntz W. (2006). Population structure of an exploited benthic cnidarian: The case study of red coral (Corallium rubrum l.). Mar. Biol. 149, 1059–1070. doi: 10.1007/S00227-006-0302-8
Tsounis G., Rossi S., Gili J. M., Arntz W. E. (2007). Red coral fishery at the Costa brava (NW mediterranean): case study of an overharvested precious coral. Ecosystems 10 (6), 975–986. doi: 10.1007/s10021-007-9072-5
Keywords: Corallium rubrum, morphometry, vulnerability, deep mass mortalities, ROV, Mediterranean Sea
Citation: Toma M, Bo M, Giudice D, Canese S, Cau A, Andaloro F, Angiolillo M, Greco S and Bavestrello G (2022) Structure and status of the Italian red coral forests: What can a large-scale study tell? Front. Mar. Sci. 9:1073214. doi: 10.3389/fmars.2022.1073214
Received: 18 October 2022; Accepted: 28 November 2022;
Published: 12 December 2022.
Edited by:
Alexander Ereskovsky, UMR7263 Institut méditerranéen de biodiversité et d’écologie marine et continentale (IMBE), FranceReviewed by:
Maria Flavia Gravina, University of Rome “Tor Vergata”, ItalyDimitris Poursanidis, terrasolutions marine environment research, Greece
Copyright © 2022 Toma, Bo, Giudice, Canese, Cau, Andaloro, Angiolillo, Greco and Bavestrello. This is an open-access article distributed under the terms of the Creative Commons Attribution License (CC BY). The use, distribution or reproduction in other forums is permitted, provided the original author(s) and the copyright owner(s) are credited and that the original publication in this journal is cited, in accordance with accepted academic practice. No use, distribution or reproduction is permitted which does not comply with these terms.
*Correspondence: Margherita Toma, bWFyZ2hlcml0YS50b21hQGVkdS51bmlnZS5pdA==
†ORCID: Margherita Toma, orcid.org/0000-0003-4038-5673