- 1National and Local Joint Engineering Research Center of Ecological Treatment Technology for Urban Water Pollution, Wenzhou University, Wenzhou, China
- 2College of Life and Environmental Science, Wenzhou University, Wenzhou, China
- 3Zhejiang Provincial Key Lab for Subtropical Water Environment and Marine Biological Resources Protection, Wenzhou University, Wenzhou, China
During the cultivation of Sargassum fusiforme, sexual reproduction of superior strains can hinder the stable inheritance of their desirable traits and reduce their economic benefits. However, vegetative reproduction of S. fusiforme holdfasts has high potential for subculture. In this study, we investigated the effects of different concentrations of α-naphthaleneacetic acid (NAA), gibberellin (GA3), and 6-benzyladenine (BAP) on the growth and regeneration of S. fusiforme holdfasts. Exogenous application of 1.5, 2 mg·L-1NAA, 1.5 mg·L-1 GA3 or 2, 3 mg·L-1BAP significantly promoted photosynthetic activity and the absorption and utilization of nitrate nitrogen in S. fusiforme holdfasts, thus improving the relative growth rate (RGR) and regeneration rate (RR) and shortening the time for the regeneration of juveniles from S. fusiforme holdfasts to around the 6th day. Among these, the RGR and RR at the end of the culture period with 1.5 mg·L-1 of NAA increased by 118.9% and 67.4%, respectively, compared with those of the control group. However, treatment with 1.5 mg·L-1 GA3 increased the RR of S. fusiforme holdfasts by 58.8% compared with that of the control group. Under BAP treatment at 3 mg·L-1, the RR of S. fusiforme holdfasts increased by 23.4% compared with that of the control group; its promoting effect was thus weaker than that of NAA and GA3. When the concentration of GA3 or BAP was too high (3 mg·L-1; 5 mg·L-1), the RR of the holdfasts decreased by 46.5% and 42.8%, respectively compared with that of the control group. Therefore, exogenous application of NAA at 1.5 mg·L-1, GA3 at 1.5 mg·L-1, and BAP at 3 mg·L-1 can be used to induce regeneration of S. fusiforme holdfasts, shorten the culture time of regenerated seedlings, and obtain more regenerative seedlings, thereby improving economic efficiency.
1 Introduction
Sargassum fusiforme (Harvey) Okamura (Sargassaceae, Phaeophyta) is a perennial macroalga endemic to the nearshore waters of the northwestern Pacific Ocean. Studies have shown that fucoidan, an extract of S. fusiforme, can improve metabolic disorders related to obesity and reduce visceral fat accumulation; further, it is rich in amino acids and trace elements, which have high nutritional and medicinal value (Liu et al., 2021; Zuo et al., 2022). S. fusiforme is a dioecious plant that can reproduce sexually or asexually. Sexual reproduction occurs through the release and union of male and female gametes from male and female plants, respectively with the conspecifics then growing and developing into S. fusiforme juveniles (Lin et al., 2021). In contrast to sexual reproduction, asexual reproduction generally occurs during the late stage of growth. When the seawater temperature rises, the leaves gradually fall off, leaving only the holdfast; when the seawater temperature drops, the holdfast regenerates juveniles (Yoshida and Shimabukuro, 2017; Xu et al., 2022a). Moreover, it has been reported that several Sargassum species, including Sargassum fusiforme, regenerate juveniles through holdfast. These studies also showed that S. fusiforme holdfast had higher tolerance to high temperature and low nutrient conditions during summer (Ito et al., 2009; Yatsuya et al., 2012; Loffler et al., 2018; Endo et al., 2021). Although superior traits of juveniles can be stably maintained through asexual propagation via holdfasts, the number of seedlings produced by holdfasts is currently limited. Therefore, a method to increase the regeneration rate of holdfasts is urgently needed in S. fusiforme culture and is important for increasing the source of S. fusiforme seedlings, especially for stable multiplication of the germplasm of screened desirable cultured strains.
Phytohormones are organic signaling molecules produced by plants through their metabolism and can produce significant physiological effects at low concentrations. The main widely reported plant hormones include auxins, cytokinins, gibberellin, abscisic acid, ethylene (Xiong et al., 2009; Iqbal et al., 2014; Miyakawa and Tanokura, 2017; Waadt, 2020). Numerous studies have shown that although seaweeds have a simple morphology, they share many similarities with vascular plants. Phytohormones control most of the growth and developmental processes in vascular plants; they are also present in seaweeds and may have similar functions in seaweeds (Stirk and Van Staden, 2014). Plant growth regulators (PGRs), are organic compounds that are synthetic (or naturally derived from microorganisms) and have growth and developmental regulatory effects similar to those of natural plant hormones (Rademacher, 2015). Plant hormones such as auxin, gibberellin, and cytokinin can be exogenously applied as PGRs to regulate the growth and reproduction of plants and seaweeds (Duran-Medina et al., 2017; Miyakawa and Tanokura, 2017; Song et al., 2020; Hernandez-Garcia et al., 2021; Nedved et al., 2021; Jiksing et al., 2022). Chen et al. (2022) reported that exogenous application of auxin can promote biomass production and branching in Gracilariopsis lemaneiformis. Further, exogenous addition of PGRs can direct regeneration and callus induction in Sargassum polycystum C. Agardh (Muhamad et al., 2018). Exogenous addition of PGRs may also have a corresponding effect during the germination of S. fusiforme holdfasts.
α-Naphthaleneacetic acid (NAA), gibberellin (GA3), and 6-benzyladenine (BAP) have been reported to promote the growth of macro-green seaweed (Wei et al., 2020). However, the optimal concentrations of the above mentioned PGRs to promote the regeneration of S. fusiforme holdfasts remain unclear. Therefore, it is crucial to determine the optimal concentrations of NAA, BAP, and GA3 for promoting the regeneration of S. fusiforme holdfasts. In this study, we exogenously administered PGRs to investigate their effects on the physiological properties of S. fusiforme holdfasts and the regenerated juveniles. This study has practical significance for the breeding of holdfast seedlings of the economic seaweed S. fusiforme and for the establishment of a stable genetic culture method for superior strains.
2 Materials and methods
2.1 Sample collection and experimental design
In this study, the residual holdfasts from the mature harvest of S. fusiforme culture rafts in Dongtou District, Wenzhou City, Zhejiang Province, were taken and brought back to the laboratory at a low temperature. All S. fusiforme holdfasts were collected from the cultured rafts for superior strains. After washing the epiphytes and impurities on the holdfast surface using seawater, it was soaked for 2 min in 0.38% NaClO and 0.5% KI (Kerrison et al., 2015; Xu et al., 2022a). The soaked holdfast was washed thrice with sterilized seawater, followed by adaptive cultivation for 3 h. Holdfasts under similar conditions were selected for cultivation in groups. Except for the control group, the exogenously applied hormone concentrations during the culture were as follows: α-naphthaleneacetic acid (NAA): 0.5, 1, 1.5, 2.0, and 3.0 mg·L-1; gibberellin (GA3): 0.5, 1, 1.5, 2.0, and 3.0 mg·L-1; and 6-benzyladenine (BAP): 1, 2, 3, and 5 mg·L-1 (Wei et al., 2020). Each group had three replicates, and each replicated had three S. fusiforme holdfasts.
2.2 Determinations of the relative growth rate and regeneration rate
The medium (29‰ seawater, 200 μmol·L-1 NaNO3, 20 μmol·L-1 NaH2PO4) was changed every three days (Zhang et al., 2020); the holdfast was weighed, photographed, and recorded for regeneration. The relative growth rate (RGR) and regeneration rate (RR) were calculated as follows:
where W0 is the initial weight of the holdfast, and Wt is the weight after t days of incubation.
2.3 Determination of photosynthetic activity
Chlorophyll fluorescence kinetics in S. fusiforme holdfasts were determined using Junior-Pam (Walz, Effeltrich, Germany) (Hong et al., 2021). After dark adaptation of the samples for 30 min, the electron transfer rate (rETR) was measured at eight preset incremental light intensities in the range of 0–820 µmol·m-2·s-1 in the area near the tip of the filamentous holdfasts of S. fusiforme, and a fast light curve (RLC) was fitted according to Platt’s formula (Platt et al., 1980):
where rETR is the relative electron transfer rate, rETRm is the maximum relative electron transfer rate, α is the initial slope, reflecting the efficiency of light energy utilization,and β is the photoinhibition parameter.
2.4 Determinations of the maximum net photosynthetic rate and dark respiration rate
After weighing and recording, the holdfast was added to 200 mL of incubated seawater and rotated at a constant speed using a magnetic stirrer at a constant temperature in a water bath (20°C). The light intensity was adjusted to saturation light intensity (400 µmol·m-2·s-1) using LED light, the samples were light-adapted for 3–5 min, and the oxygen content was measured every 30 s using an optical fiber dissolved oxygen meter (Pyro Science FireSting O2, Germany). The above steps were repeated, and maximum net photosynthetic rate (Pm) was obtained after calculation.
Next, the LED lamp and magnetic stirrer were switched off, the S. fusiforme holdfasts were placed in a light-proof state, and acclimatized to dark conditions for 3–5 min. The magnetic stirrer was turned on; after the reading was stabilized, the readings were recorded using the same method described above, and the dark respiration rate of holdfasts (Rd) were calculated. Both the Pm and Rd were calculated in μmol O2·g-1Fw·h-1. The dissolved oxygen standard was calculated as per the formula shown below (Henley, 1993):
Where Δ is the average value of the difference between the measured values, α is the satiation dissolved oxygen constant at each temperature, and V is the volume of the reaction fluid (mL).
2.5 Determination of the nitrate-nitrogen uptake rate
The nitrate-nitrogen content in the culture medium before absorption and after 24 h was determined in seawater samples collected at the respective time points using the zinc-cadmium reduction method (Sun et al., 2013). The rate of nitrate-nitrogen uptake by S. fusiforme holdfasts in the culture solution was expressed as the rate of reduction of nitrate-nitrogen per hour (μmol NO3-·g-1·h-1). The calculation formula used is as follows:
where N0 is the initial nitrogen concentration of culture solution, Nt is the final nitrogen concentration of culture solution at the time of determination, V is the volume of culture solution (L), M is the wet weight of S. fusiforme holdfasts (g), and t is the incubation time (h).
2.6 Determination of nitrate reductase activity
Nitrate reductase (NR) activity was measured according to Corzo’s method (Corzo and Niell, 1991). Briefly, 0.1 g of S. fusiforme holdfasts was mixed with 10 mL of reaction medium [0.1 M NaH2PO4(buffer, pH 7.5), 0.5 mM EDTA, 0.1% (v/v) propanol, 0.01 mM glucose, 50 mM NaNO3] and aerated with N2 for 10 min, the reaction system was then scaled and incubated for 30 min at 30°C in a dark environment. The supernatant reaction solution (4 mL) was then mixed with 2 mL of 1% sulfonamide and 2 mL of 0.1% N-(1-naphthyl)-ethylenediamine hydrochloride in a test tube and allowed to for 15 min. Absorbance was then measured at 543 nm. The NR activity of S. fusiforme holdfasts was expressed as μmol ·g-1Fw·h-1.
2.7 Determination of glutamine synthetase activity
For determining the glutamine synthetase (GS) activity 0.1 g of S. fusiforme holdfasts was mixed with 5 mL of extraction buffer (pH 8.0) containing 50 mmol·L-1 Tris-HCl, 1 mmol·L-1 EDTA, 10 mmol·L-1 β-mercaptoethanol, 5 mmol·L-1 dithiothreitol (DTT), 10.0 mmol·L-1 MgCl2·7 H2O, 6.6% of polyvinylpolypyrrolidone (PVPP), 0.5 mmol·L-1 phenylmethylsulfonyl (PMSF), and 1 mmol·L-1 cysteine. This was followed by centrifugation at 17000 × g for 20 min at 4°C. The supernatant was collected and the absorbance value was measured at 540 nm. γ-Glutamyl isohydroxamic acid was used to generate the standard curve. One unit of GS activity was defined as the amount of enzyme required to catalyze the production of 1 µmol of γ-glutamyl isohydroxamic acid per minute at 25°C (Setien et al., 2013).
2.8 Determination of glutamate synthase activity
Approximately 0.1 g of S. fusiforme holdfasts was weighed and finely ground with a small amount of quartz sand and 6 mL of enzyme extract [10 mM Tris-HCl (pH 7.6), 1 mM MgCl2, 1 mM EDTA, and 1 mM mercaptoethanol]. The mixture was centrifuged for 30 min (12000 × g, 4°C), 3 mL of the supernatant enzyme reaction solution was collected, and the change in absorbance was measured at 340 nm. One unit of glutamate synthase (GS) activity was defined as consumption of 1 nmol NADH in 1 min by 1 g of tissueand was expressed as nmol-1·min-1·g-1 (Shah et al., 2017).
2.9 Determination of soluble protein content
Soluble proteins in holdfasts were determined using the Coomassie Brilliant Blue G-250 dye binding method (Kochert, 1978). Holdfasts (0.1 g) were added to 5 mL of phosphate buffer (pH 6.8) and ground at low temperature. After centrifugation at 4°C and 4000 rpm for 10 min, 1 mL of supernatant was collected, mixed with 4 mL of Coomassie Brilliant Blue G-250 staining solution, and left for 3 min. The absorbance of the samples was measured at 595 nm. A standard curve (bovine serum albumin standard solution) was used to calculate the soluble protein (SP) content (mg·g-1Fw) of S. fusiforme holdfasts.
2.10 Determination of soluble carbohydrates
Glucose was used to generate the standard curve, and the anthrone method was used for soluble carbohydrates determination (Ershadi et al., 2015). Briefly, holdfasts (0.1 g) were ground thoroughly, mixed well with 9 mL of distilled water and 1 mL of magnesium carbonate suspension, and centrifuged at 4°C for 10 min at 10000 rpm. The supernatant (1 mL) was mixed well with 2 mL of distilled water and 10 mL of anthrone reagent, placed in a boiling water bath at 100°C for 10 min, and cooled to 20°C. The absorbance was measured at 620 nm. The soluble carbohydrate (SC) content (mg·g-1Fw) of the S. fusiforme holdfasts was then calculated.
2.11 Statistical analysis
Statistical analysis was performed using SPSS statistical software. Data are expressed as the mean ± standard deviation (mean ± SD), and differences between the treatment and control groups were analyzed using one-way analysis of variance (ANOVA) and Tukey’s test. Statistical significance was set at p< 0.05.
3 Results
3.1 The regeneration of S. fusiforme holdfasts
Figure 1 shows the morphological changes in S. fusiforme holdfasts under different exogenous hormone treatments. Compared with normally cultured S. fusiforme holdfasts, exogenous addition of NAA at 1.5, 2 mg·L-1, GA3 at 1.5, 2 mg·L-1, or BAP at 2, 3 mg·L-1 could significantly shorten the regeneration time of S. fusiforme holdfasts from around 12 days to about 6 days. However, when the hormone concentration was too low or too high, the rate of juvenile regeneration from holdfasts was not significantly promoted. In fact, high hormone concentrations had a significant inhibitory effect on the regeneration of S. fusiforme holdfasts.
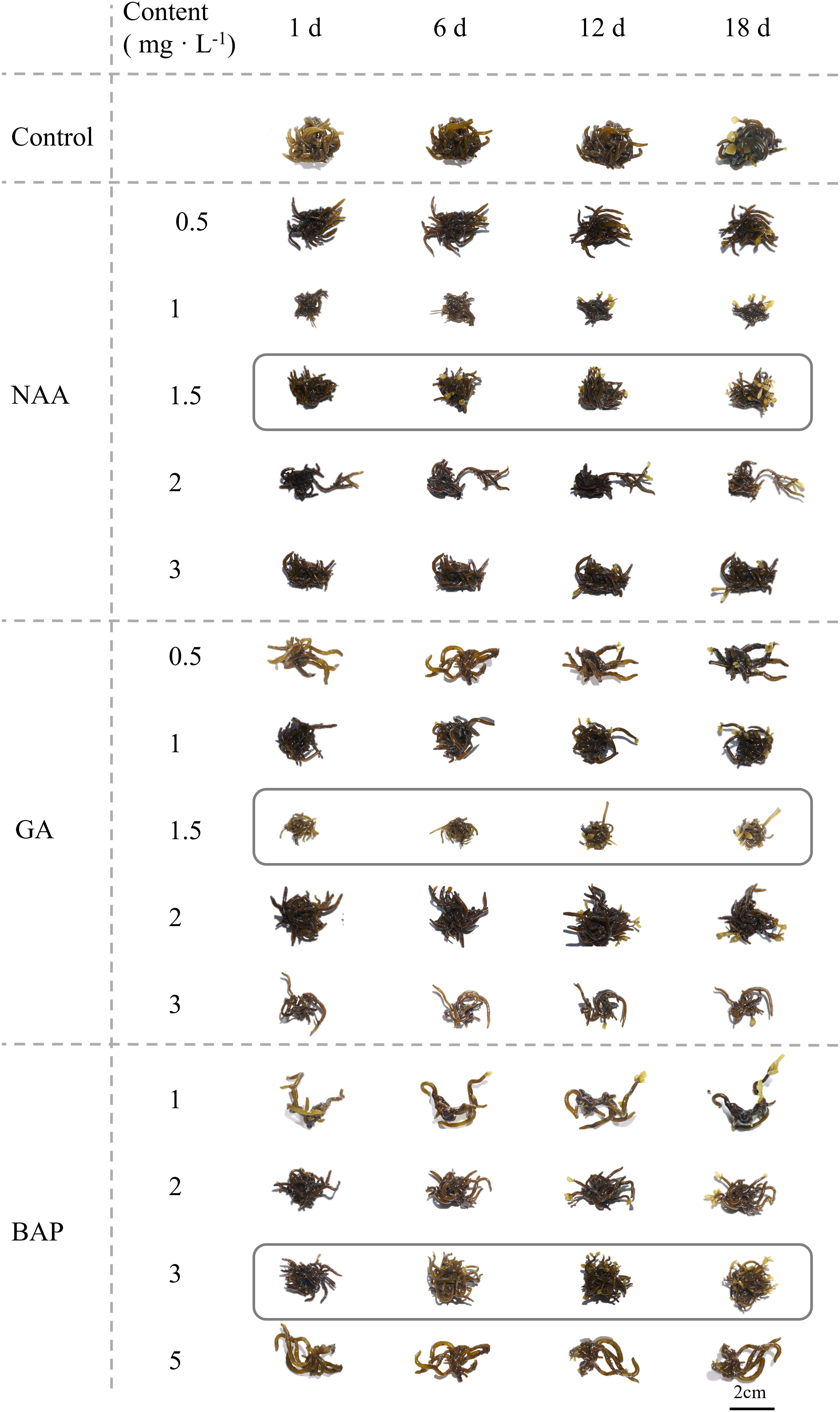
Figure 1 Morphological changes in Sargassum fusiforme holdfasts with different concentrations of three exogenous hormones applied for different incubation periods.
3.2 Growth and regeneration rates of S. fusiforme holdfasts
All NAA concentrations in the range of 1.5, 2 mg·L-1 significantly increased the RGR of S. fusiforme holdfasts (p< 0.05); further, 1.5 mg·L-1 of NAA increased the RGR of holdfasts by 118.9% at the end of the culture. In contrast, low concentrations (0.5, 1 mg·L-1) of NAA did not have a significant effect on RGR by the ninth day of incubation (p< 0.05; Figure 2A). As shown in Figure 2B, the RGR of holdfasts was not significant (p< 0.05) when incubated at or above 2 mg·L-1 GA3. Relative to the other GA3 concentrations, the highest RGR was observed in holdfasts grown at 1.5 mg·L-1, with an increase of 54.2% over the control group (p< 0.05). BAP promoted the growth of holdfasts when its concentration did not exceed 3 mg·L-1, and its promotion effect was the most significant (p< 0.05) at 3 mg·L-1, with a 103.8% increase in RGR. However, a high BAP concentration (5 mg·L-1) inhibited the growth of S. fusiforme holdfasts after 12 days of incubation (Figure 2C).
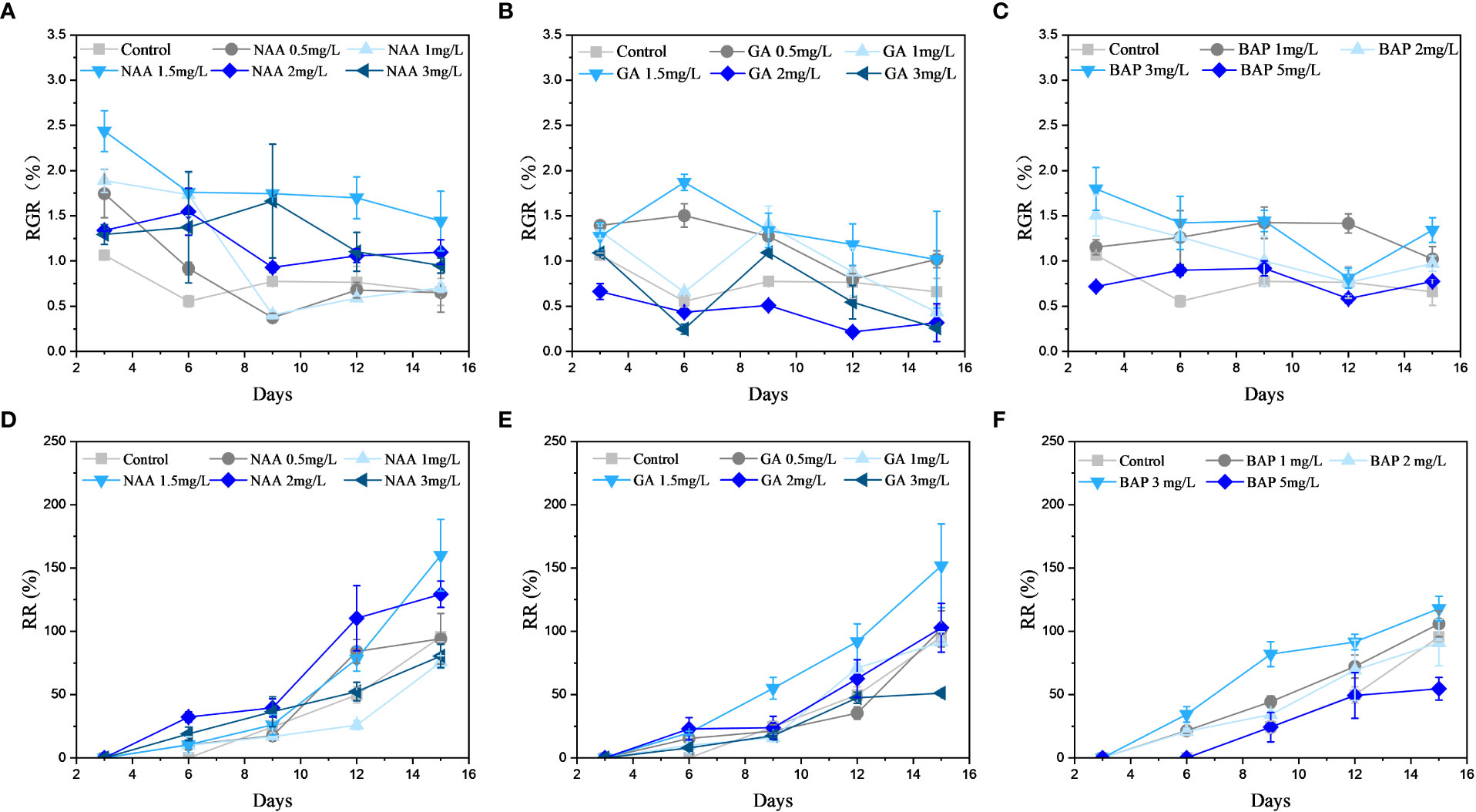
Figure 2 Effects of three exogenous hormones on the relative growth rate (RGR) and regeneration rate (RR) of Sargassum fusiforme holdfasts. (A–C): relative growth rate; (D–F): regeneration rate.
NAA at a concentration of 1.5 mg·L-1 had the most significant (p< 0.05) promotion effect on the RR of S. fusiforme holdfasts, which was 167.4% compared to that of the control (Figure 2D). At a GA3 concentration of 1.5 mg·L-1, the RR of the holdfasts was 158.8% higher than that of the control (p< 0.05; Figure 2E). In contrast, BAP at a concentration of 3 mg·L-1 had the most significant promotion effect on the RR of holdfasts, increasing the RR by 123.4% relative to the control (p< 0.05; Figure 2F). However, high concentrations of GA3 and BAP inhibited the regeneration of S. fusiforme holdfasts.
3.3 Effects of different concentrations of exogenous hormone treatments on the photosynthesis of S. fusiforme holdfasts
Exogenous addition of NAA significantly promoted the rETR of holdfasts compared to that of the control, but the difference in the promotion effect between the different concentrations of NAA was not significant (p< 0.05; Figure 3A); however, the increase in rETRm values of S. fusiforme holdfasts was greater at NAA concentrations of 1.5 and 2 mg·L-1 at 26.1% and 26.3% (Figure 3B). As shown in Figures 3C, D, the most significant promotion of photosynthesis in holdfasts was observed when the concentration of GA3 was 1.5 mg·L-1; and the rETRm values of S. fusiforme holdfasts at this concentration increased by 40.6% relative to that of the control (p< 0.05). Further, BAP at 2 mg·L-1 significantly promoted rETR in holdfasts, and the growth rate of rETRm values of S. fusiforme holdfasts at this concentration was 50.1% (p< 0.05; Figures 3E, F).
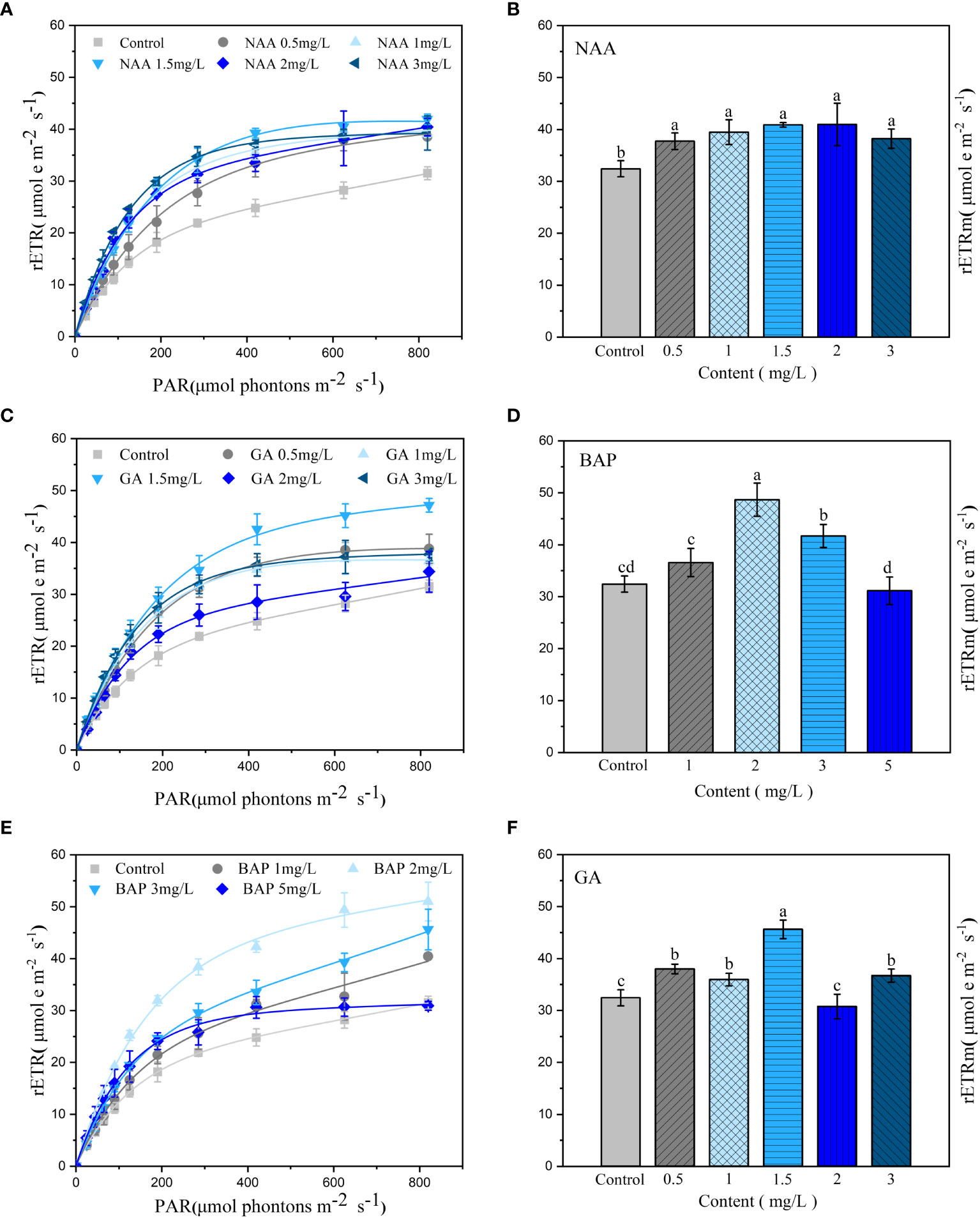
Figure 3 Effects of three exogenous hormones on photosynthetic activities in Sargassum fusiforme holdfasts. (A, C, E): relative electron transfer rate (rETR); (B, D, F): the maximum relative electron transfer rate (rETRm). Values are the mean ± standard deviation (n = 3). Different letters indicate significant differences between different culture conditions (p < 0.05).
3.4 Photosynthesis and respiration of S. fusiforme holdfasts
Exogenous application of NAA significantly promoted the Pm of holdfasts but had a lower effect on Rd; further, the promotion effect was not significantly different among concentrations (p< 0.05; Figure 4A). The promotion of the saturated irradiance oxygen evolution was significant when the GA3 concentration was 0.5, 1.5 mg·L-1, with the maximum promotion effect observed at 1.5 mg·L-1. In contrast, the dark respiration rate of holdfasts was significantly enhanced at GA3 concentrations of 0.5 mg·L-1 and 1.5 mg·L-1, with the strongest respiration at 0.5 mg·L-1 (p< 0.05; Figure 4B). The Pm of holdfasts significantly increased when the BAP concentration was 2, 3 mg·L-1; the maximum increase was observed at a concentration of 3 mg·L-1. The effect of exogenous BAP application on dark respiration was not significant compared to that in the control and was only slightly higher at 1 and 5 mg·L-1 compared to the other treatment groups (p< 0.05; Figure 4C).
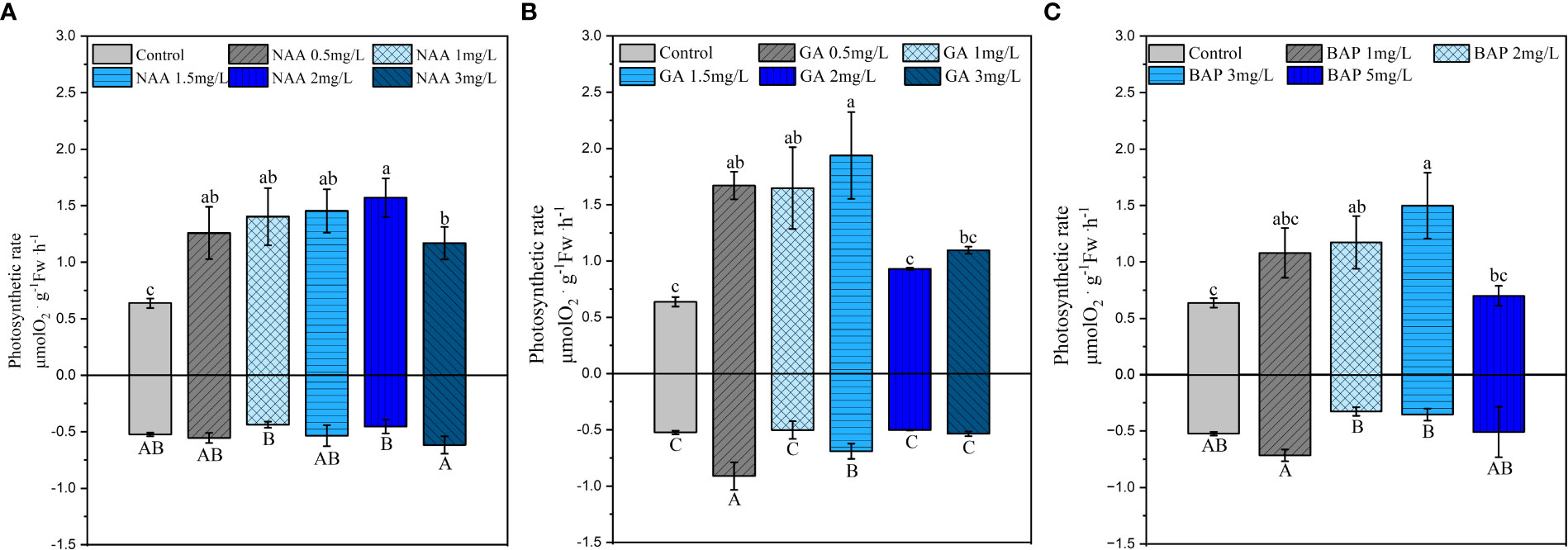
Figure 4 Effects of three exogenous hormones on the maximum net photosynthetic rate (Pm) and dark respiration rate (Rd) in Sargassum fusiforme holdfasts (A, B). Values are the mean ± standard deviation (n = 3). Different letters indicate significant differences between different culture conditions (p < 0.05).
3.5 The uptake of nitrate-nitrogen by S. fusiforme holdfasts
The ability of holdfasts to take up nitrate-N was significantly enhanced when the NAA concentration was 0.5, 2 mg·L-1, and the highest rate of nitrate-N uptake was observed at 1.5 mg·L-1 (p< 0.05; Figure 5A). Compared to that of the control, NR activity was significantly higher when the NAA concentration was at 1.5 mg·L-1 (p< 0.05; Figure 5D). The promotion of N uptake was not significant for low or high GA3 (0.5, 1 mg·L-1, 3 mg·L-1) treatments and was the most significant only at 1.5 mg·L-1, which showed an increase by 54.3% in the rate of uptake (p< 0.05; Figure 5B). As shown in Figure 5E, the NR activity in S. fusiforme holdfasts did not change significantly at 0.5 mg·L-1 of GA3; when the GA3 concentration reached 1, 3 mg·L-1 NR activity was significantly enhanced in holdfasts (p< 0.05). Exogenous application of BAP significantly promoted the rate of nitrate-nitrogen uptake by holdfasts, with the greatest uptake with the 2 mg·L-1 BAP treatment, showing an increase by 57.3% compared to that of the control (p< 0.05; Figure 5C). BAP treatment at 1, 3 mg·L-1 significantly enhanced the S. fusiforme holdfasts and the highest NR activity was observed when the BAP concentration was 3 mg·L-1 (p< 0.05) (Figure 5F).
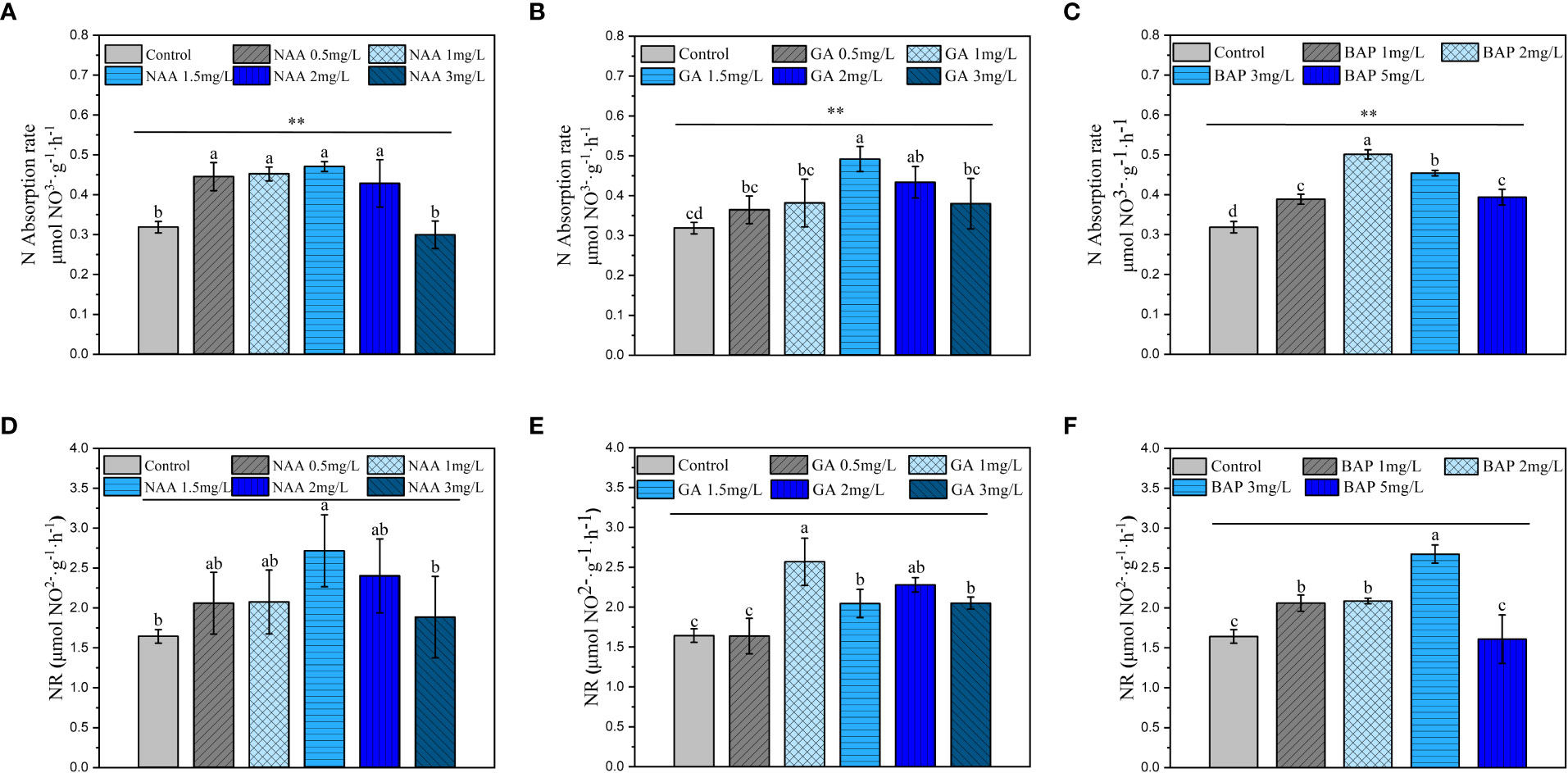
Figure 5 Effects of three exogenous hormones on the uptake and reduction of nitrate nitrogen by Sargassum fusiforme holdfasts. (A–C): uptake rate of nitrate nitrogen by holdfasts under different culture conditions; (D–F): nitrate reductase activity in holdfasts under different culture conditions. Values are the mean ± standard deviation (n = 3). Different letters indicate significant differences between different culture conditions (p < 0.05). **indicates intergroup variability between different concentrations of exogenous hormones (**p < 0.01).
3.6 The conversion of nitrogen by S. fusiforme holdfasts
Exogenously applied NAA treatment significantly enhanced GS activity in S. fusiforme holdfasts compared to that of the control, with the strongest GS activity observed in holdfasts treated with 1.5 mg·L-1 NAA (p< 0.05; Figure 6A). GOGAT activity in the holdfasts was significantly enhanced when the NAA concentration was 1, 1.5 mg·L-1 and 3 mg·L-1; the most significant promotion of GOGAT was observed at 1.5 mg·L-1 (p< 0.05; Figure 6D). Different concentrations of GA3 showed significant enhancement of GS activity, with the strongest enhancement observed at 1.5 mg·L-1 (p< 0.05; Figure 6B). GA3 significantly enhanced GOGAT activity at 1.5 mg·L-1 and 2 mg·L-1, and the most significant effect was observed at 1.5 mg·L-1 (p< 0.05; Figure 6E). The GS activity was significantly increased when the BAP concentrations ranged from 1 to 3 mg·L-1, and the strongest effect was observed at 2 mg·L-1 (p< 0.05; Figure 6C). As shown in Figure 6F, GOGAT activity was significantly enhanced by different concentrations of BAP, with the most significant (p< 0.05) promotion effect observed at a BAP concentration was 3 mg·L-1.
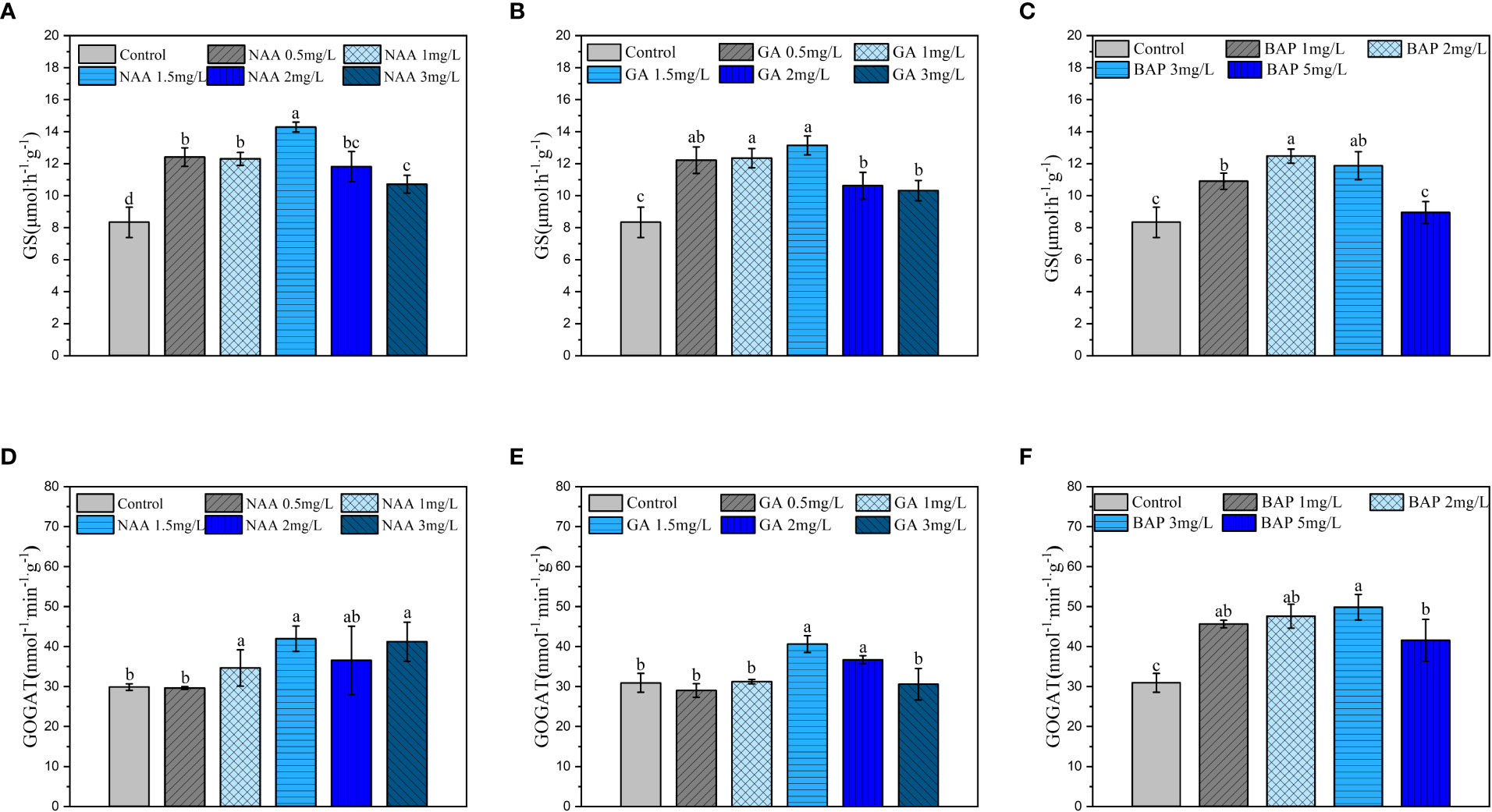
Figure 6 Effects of three exogenous hormones on nitrogen assimilation by Sargassum fusiforme holdfasts. (A–C): glutamine synthetase (GS) activity in holdfasts under different culture conditions; (D–F): glutamate synthase (GOGAT) activity in holdfasts under different culture conditions. Values are the mean ± standard deviation (n = 3). Different letters indicate significant differences between different culture conditions (p < 0.05).
3.7 Soluble protein and carbohydrate accumulation in S. fusiforme holdfasts
When the NAA concentration was greater than 0.5 mg·L-1, the SP content increased significantly in holdfasts, and it further increased with increasing NAA concentration (p< 0.05; Figure 7A). Exogenous application of NAA also significantly increased the content of SC in S. fusiforme holdfasts compared to that in the control, but the difference between different concentrations was not significant (p< 0.05; Figure 7D). Different concentrations of GA3 significantly increased the content of SP and SC in holdfasts, with the highest SP and SC contents observed at the 1.5 mg·L-1 GA3 treatment (p< 0.05; Figures 7B, E). BAP treatment significantly increased the SP content in S. fusiforme holdfasts in a dose-dependent manner (p< 0.05; Figure 7C). The SC content in holdfasts was significantly increased at BAP concentrations of 2, 3 mg·L-1, with the highest SC content observed at 3 mg·L-1 BAP (p< 0.05; Figure 7F).
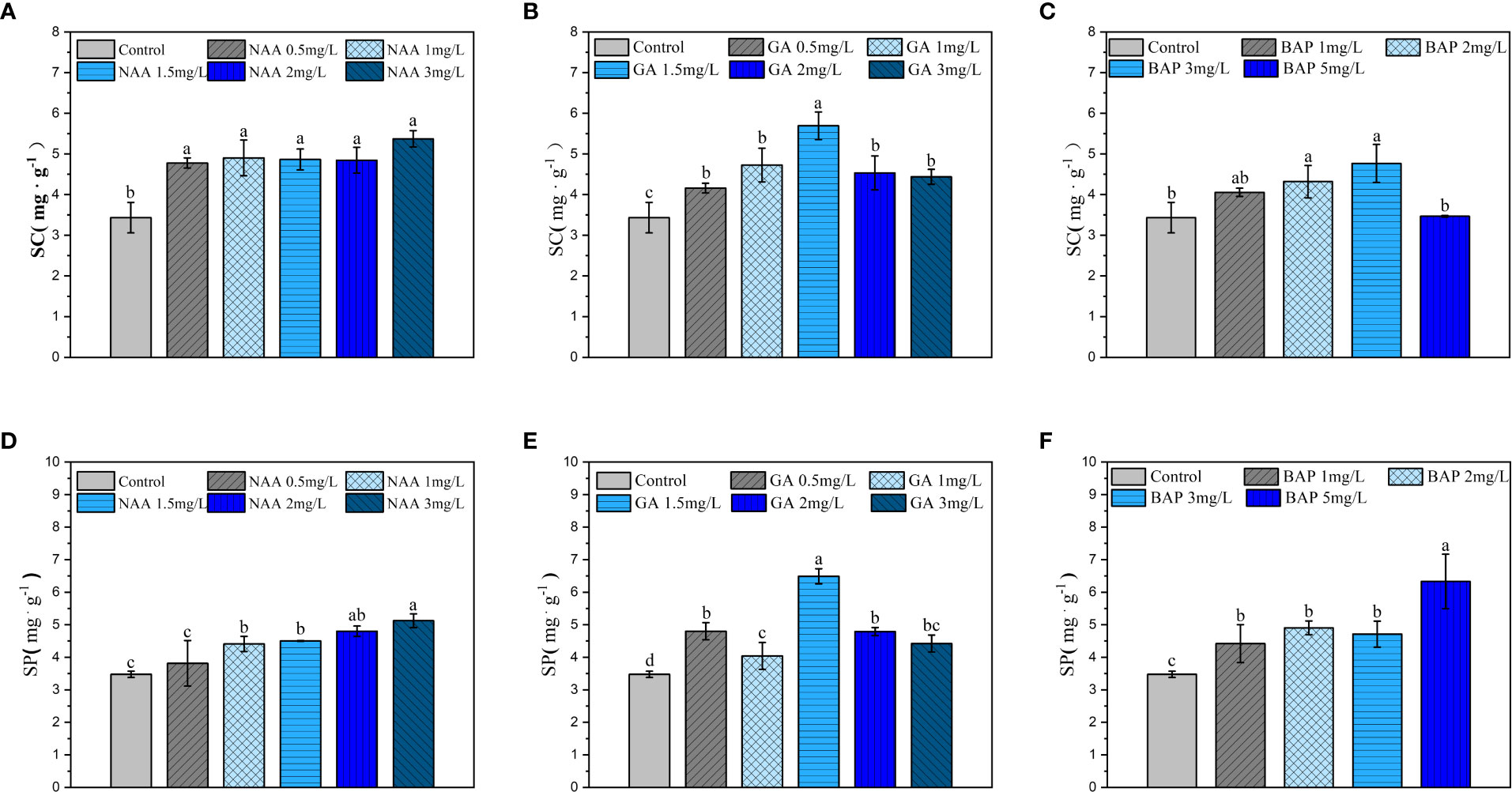
Figure 7 Effects of three exogenous hormones on the accumulations of soluble protein and carbohydrate in Sargassum fusiforme holdfasts. (A–C): soluble protein contents in the holdfasts under different culture conditions; (D–F): soluble carbohydrate contents in holdfasts under different culture conditions. Values are mean ± standard deviation (n = 3). Different letters indicate significant differences between different culture conditions (p < 0.05).
4 Discussion
The type and content of PGRs remarkably affect the photosynthetic rate and pigment content of seaweeds, and the appropriate concentrations of phytohormones promote the photosynthetic rate, carbonic anhydrase activity, and C/N ratio of seaweeds (Wei et al., 2020). Light and growth factors regulate each other to maintain a balance for normal plant growth (Xu et al., 2018). In the present study, exogenous application of NAA significantly enhanced photosynthesis in holdfasts, but the difference between different concentrations examined in this study was not significant.
After - N is absorbed by algae, it continues to be reduced to - N by NR reduction and then enters the GS/GOGAT cycle to synthesize nitrogenous compounds, such as proteins and nucleic acids (Balotf et al., 2016; Liu et al., 2022). In this study, exogenous addition of NAA significantly promoted the nitrogen uptake and transformation capacity of S. fusiforme holdfasts with the maximum promoting effect observed at 1.5 mg·L-1. Compared with that of GOGAT, NAA had a more significant enhancing effect on GS activity in holdfasts. This indicates that NAA treatment at 0.5, 2 mg·L-1 significantly promoted the rate of nitrate nitrogen uptake and the ability to convert it to amino acids in S. fusiforme holdfasts, with significant differences in the effects between concentrations only observed in the results of GS activity assays. and NAA help increase the uptake of N, P, K, and Ca2+ in some higher plants, and exogenous application of NAA during tissue culture is known to increase the activities of key enzymes in the nitrogen assimilation pathway, including NR, GS, and GOGAT (Srivastava and Dwivedi, 2003; Tavakoli Hasanaklou et al., 2021). These conclusions are highly consistent with our experimental results.
In this study, exogenous addition of GA3 promoted photosynthesis in S. fusiforme holdfasts with maximum promotion at a concentration of 1.5 mg·L-1. GA is involved in light signal perception, such as photosensitive pigment pathways during seed regeneration, photomorphogenesis during yellowing and stem elongation, and photoperiodic regulation during development (Wu et al., 2019; Hu et al., 2022). During S. fusiforme holdfast regeneration, GA3 may achieve photosynthesis-promoting effects by regulating the photoperiod and light sensitivity. In a previous study, addition of 20 μM GA3 to the in vitro medium of Strelitzia reginae conidia embryos increase nitrate reductase activity (Figueiredo et al., 2021). In this study, exogenous application of 1.5 GA3 mg·L-1 significantly promoted the uptake and transformation of nitrate-nitrogen by S. fusiforme holdfasts.
Exogenous addition of different BAP concentrations increased the relative electron transfer rate of photosystem II in S. fusiforme holdfasts. The photosynthesis of holdfasts was sensitive to BAP concentration during the regulated process, and its effect decreased with increasing or decreasing concentrations, showing the most significant promotion effect at 2 mg·L-1. Exogenous addition of cytokinin has been shown to modulate the inhibitory effect of physical stress on the PSII operating efficiency in maize leaves, thus improving gas exchange; further, BAP-treated plants have higher ΦPSII and ETR than untreated plants under stress conditions (Acidri et al., 2020; Wang et al., 2022). Moreover, adenine-type cytokinins have been shown to enhance the activities of carbon-metabolizing and nitrogen-assimilating enzymes in Chlorella vulgaris (Piotrowska and Czerpak, 2009). In the present study, exogenous application of 1–3 mg·L-1 BAP significantly enhanced the activity of nitrogen-assimilating enzymes in S. fusiforme holdfasts, suggesting that cytokinins may have similar regulatory mechanisms in macroalgae and microalgae.
In general, accumulation of soluble proteins in plants is strongly influenced by nitrogen uptake and utilization (Chen et al., 2017a; Chen et al., 2018; Tian et al., 2022). When the RR of S. fusiforme holdfasts was higher, the synthesized SP was used more for the growth of regenerated seedlings, despite the fuller uptake and utilization of nitrogen (Xu et al., 2022b). In the present study, the SP content in holdfasts was related to enzymatic activity during nitrogen conversion and utilization, as well as to the RR (juvenile growth) of S. fusiforme holdfasts under these conditions. The SC content was related to plant photosynthesis and respiration (Chen et al., 2017b; Chen et al., 2019; Zhang et al., 2020). In this study, 5 mg·L-1 of BAP enhanced the dark respiration of holdfasts, leading to the depletion of carbohydrates accumulated by photosynthesis. In contrast, the promotion of photosynthesis by other hormones and concentrations in the holdfasts was more consistent with the changes that increased the SC content.
Overall, appropriate concentrations of NAA, GA3, and BAP could promote faster and greater regeneration of juveniles from S. fusiforme holdfasts. Combined with other relevant physiological indicators, the most suitable concentrations of NAA, GA3, and BAP for holdfast regeneration were 1.5 mg·L-1, 1.5 mg·L-1, and 3 mg·L-1, respectively. Therefore, the above concentrations of exogenous hormones can be added in practice in the early stages of culture to shorten the culture time of juveniles’ regeneration and to obtain more regenerated juveniles for improve the economic efficiency. Moreover, although the high concentrations of GA3 and BAP inhibited the regeneration from S. fusiforme holdfasts during the culture process, they did not significantly inhibit the physiological activities of holdfasts by the end of the culture. This property provides a new perspective for regulating the propagation of S. fusiforme holdfasts.
Data availability statement
The original contributions presented in the study are included in the article/supplementary materials. Further inquiries can be directed to the corresponding authors.
Author contributions
LL and BC contributed to complete the experiments, investigate and analyze the data, and wrote original draft of the paper. XZ, LG, GP contributed to sample and process the experiments. ZM contributed to revise the paper. MW and BC contributed to conceptualize, supervise, review and edit the paper. All authors contributed to the article and approved the submitted version.
Funding
This project was funded by the National Key R&D Program of China (No. 2018YFD0901503), the National Natural Science Foundation of China (No.41706147 and 41876124), and the Science and Technology Plan Project of Wenzhou (N20220005).
Acknowledgments
The authors also would like to appreciate all staffs for their helpful assistance during the materials collection.
Conflict of interest
The authors declare that the research was conducted in the absence of any commercial or financial relationships that could be construed as a potential conflict of interest.
Publisher’s note
All claims expressed in this article are solely those of the authors and do not necessarily represent those of their affiliated organizations, or those of the publisher, the editors and the reviewers. Any product that may be evaluated in this article, or claim that may be made by its manufacturer, is not guaranteed or endorsed by the publisher.
References
Acidri R., Sawai Y., Sugimoto Y., Handa T., Sasagawa D., Masunaga T., et al. (2020). Exogenous kinetin promotes the nonenzymatic antioxidant system and photosynthetic activity of coffee (Coffea arabica l.) plants under cold stress conditions. Plants (Basel) 9, 281–301. doi: 10.3390/plants9020281
Balotf S., Kavoosi G., Kholdebarin B. (2016). Nitrate reductase, nitrite reductase, glutamine synthetase, and glutamate synthase expression and activity in response to different nitrogen sources in nitrogen-starved wheat seedlings. Biotechnol. Appl. Biochem. 63, 220–229. doi: 10.1002/bab.1362
Chen B., Lin L., Ma Z., Zhang T., Chen W., Zou D. (2019). Carbon and nitrogen accumulation and interspecific competition in two algae species, pyropia haitanensis and ulva lactuca, under ocean acidification conditions. Aquaculture Int. 27, 721–733. doi: 10.1007/s10499-019-00360-y
Chen X., Tang Y., Sun X., Zhang X., Xu N. (2022). Comparative transcriptome analysis reveals the promoting effects of IAA on biomass production and branching of gracilariopsis lemaneiformis. Aquaculture 548. doi: 10.1016/j.aquaculture.2021.737678
Chen B., Zou D., Du H., Ji Z. (2018). Carbon and nitrogen accumulation in the economic seaweed gracilaria lemaneiformis affected by ocean acidification and increasing temperature. Aquaculture 482, 176–182. doi: 10.1016/j.aquaculture.2017.09.042
Chen B., Zou D., Yang Y. (2017a). Increased iron availability resulting from increased CO2 enhances carbon and nitrogen metabolism in the economical marine red macroalga pyropia haitanensis (Rhodophyta). Chemosphere 173, 444–451. doi: 10.1016/j.chemosphere.2017.01.073
Chen B., Zou D., Zhu M. (2017b). Growth and photosynthetic responses of ulva lactuca (Ulvales, chlorophyta) germlings to different pH levels. Mar. Biol. Res. 13, 351–357. doi: 10.1080/17451000.2016.1267367
Corzo A., Niell F. X. (1991). Determination of nitrate reductase activity in ulva rigida c. agardh by the in situ method. J. Exp. Mar. Biol. Ecol. 146, 181–191. doi: 10.1016/0022-0981(91)90024-q
Duran-Medina Y., Diaz-Ramirez D., Marsch-Martinez N. (2017). Cytokinins on the move. Front. Plant Sci. 8. doi: 10.3389/fpls.2017.00146
Endo H., Sugie T., Yonemori Y., Nishikido Y., Moriyama H., Ito R., et al. (2021). Vegetative reproduction is more advantageous than sexual reproduction in a canopy-forming clonal macroalga under ocean warming accompanied by oligotrophication and intensive herbivory. Plants (Basel) 10. doi: 10.3390/plants10081522
Ershadi A., Karimi R., Mahdei K. N. (2015). Freezing tolerance and its relationship with soluble carbohydrates, proline and water content in 12 grapevine cultivars. Acta Physiologiae Plantarum 38, 2. doi: 10.1007/s11738-015-2021-6
Figueiredo J. R. M., Paiva P., Silva D., Paiva R., Souza R. R., Reis M. (2021). Temperature and GA(3) on ROS and cytogenetic stability during in vitro cultivation of strelitzia zygotic embryos. Ciencia E Agrotecnologia 45. doi: 10.1590/1413-7054202145020220
Henley W. J. (1993). Measurement and interpretation of photosynthetic light-response curves in algae in the context of photoinhibition and diel changes. J. Phycology 29, 729–739. doi: 10.1111/j.0022-3646.1993.00729.x
Hernandez-Garcia J., Briones-Moreno A., Blazquez M. A. (2021). Origin and evolution of gibberellin signaling and metabolism in plants. Semin. Cell Dev. Biol. 109, 46–54. doi: 10.1016/j.semcdb.2020.04.009
Hong M. H., Ma Z. L., Wang X. Y, Shen Y. W, Mo Z. Y., Wu M. J, et al. (2021). Effects of light intensity and ammonium stress on photosynthesis in sargassum fusiforme seedlings. Chemosphere 273. doi: 10.1016/j.chemosphere.2020.128605
Hu D., Li X., Yang Z., Liu S., Hao D., Chao M., et al. (2022). Downregulation of a gibberellin 3beta-hydroxylase enhances photosynthesis and increases seed yield in soybean. New Phytol. 235, 502–517. doi: 10.1111/nph.18153
Iqbal N., Umar S., Khan N. A., Khan M. I. R. (2014). A new perspective of phytohormones in salinity tolerance: Regulation of proline metabolism. Environ. Exp. Bot. 100, 34–42. doi: 10.1016/j.envexpbot.2013.12.006
Ito R., Terawaki T., Satuito C. G., Kitamura H. (2009). Storage, cutting and culture conditions of filamentous roots of hiziki sargassum fusiforme. Aquaculture Sci. 57, 579–585. doi: 10.11233/aquaculturesci.57.579
Jiksing C., Ongkudon M. M., Thien V. Y., Rodrigues K. F., Yong W. T. L. (2022). Recent advances in seaweed seedling production: a review of eucheumatoids and other valuable seaweeds. Algae 37, 105–121. doi: 10.4490/algae.2022.37.5.11
Kerrison P. D., Le H. N., Hughes A. D. (2015). Hatchery decontamination of sargassum muticum juveniles and adults using a combination of sodium hypochlorite and potassium iodide. J. Appl. Phycology 28, 1169–1180. doi: 10.1007/s10811-015-0672-8
Kochert G. (1978). Protein determination by dye binding. Handbook of Phycological Methods: Physiological and Biochemical Methods 91–93. doi: 10.2216/i0031-8884-18-4-439b.1
Lin L., Ma Z., Chen B., Wu M. (2021). Analysis of physiological and ecological functions to mature sporophyte of cultivation sargassum fusiforme based on its organ morphological structure. Oceanologia Et Limnologia Sin. 52, 1047–1057. doi: 10.11964/jfc.20190111639
Liu X., Hu B., Chu C. (2022). Nitrogen assimilation in plants: current status and future prospects. J. Genet. Genomics 49, 394–404. doi: 10.1016/j.jgg.2021.12.006
Liu J., Wu S., Cheng Y., Liu Q., Su L., Yang Y., et al. (2021). Sargassum fusiforme alginate relieves hyperglycemia and modulates intestinal microbiota and metabolites in type 2 diabetic mice. Nutrients 13, 2887. doi: 10.3390/nu13082887
Loffler Z., Graba-Landry A., Kidgell J. T., McClure E. C., Pratchett M. S., Hoey A. S. (2018). Holdfasts of sargassum swartzii are resistant to herbivory and resilient to damage. Coral Reefs 37, 1075–1084. doi: 10.1007/s00338-018-01745-w
Miyakawa T., Tanokura M. (2017). Structural basis for the regulation of phytohormone receptors. Biosci. Biotechnol. Biochem. 81, 1261–1273. doi: 10.1080/09168451.2017.1313696
Muhamad S. N. S., Ling A. P.-K., Wong C.-L. (2018). Effect of plant growth regulators on direct regeneration and callus induction from sargassum polycystum c. Agardh. J. Appl. Phycology 30, 3299–3310. doi: 10.1007/s10811-018-1649-1
Nedved D., Hosek P., Klima P., Hoyerova K. (2021). Differential subcellular distribution of cytokinins: How does membrane transport fit into the big picture? Int. J. Mol. Sci. 22, 3428. doi: 10.3390/ijms22073428
Piotrowska A., Czerpak R. (2009). Cellular response of light/dark-grown green alga chlorella vulgaris beijerinck (Chlorophyceae) to exogenous adenine- and phenylurea-type cytokinins. Acta Physiologiae Plantarum 31, 573–585. doi: 10.1007/s11738-008-0267-y
Platt T., Gallegos C. L., Harrison W. G. (1980). Photoinhibition of photosynthesis in natural assemblages of marine phytoplankton. J.mar.res 38, 341–345.
Rademacher W. (2015). Plant growth regulators: Backgrounds and uses in plant production. J. Plant Growth Regul. 34, 845–872. doi: 10.1007/s00344-015-9541-6
Setien I., Fuertes-Mendizabal T., Gonzalez A., Aparicio-Tejo P. M., Gonzalez-Murua C., Gonzalez-Moro M. B., et al. (2013). High irradiance improves ammonium tolerance in wheat plants by increasing n assimilation. J. Plant Physiol. 170, 758–771. doi: 10.1016/j.jplph.2012.12.015
Shah J. M., Bukhari S. A. H., Zeng J. B., Quan X. Y., Ali E., Muhammad N., et al. (2017). Nitrogen (N) metabolism related enzyme activities, cell ultrastructure and nutrient contents as affected by n level and barley genotype. J. Integr. Agric. 16, 190–198. doi: 10.1016/S2095-3119(15)61308-9
Song S., Liu J., Tang C., Zhang W., Xu H., Zhang Q., et al. (2020). Metabolism and signaling of auxins and their roles in regulating seed dormancy and germination. Chin. Sci. Bulletin-Chinese 65, 3924–3943. doi: 10.1360/Tb-2020-0509
Srivastava S., Dwivedi U. N. (2003). Modulation of key nitrogen assimilating enzymes by NAA and in vitro culture in cuscuta reflexa. Plant Physiol. Biochem. (Paris) 41, 65–71. doi: 10.1016/s0981-9428(02)00010-4
Stirk W. A., Van Staden J. (2014). Plant growth regulators in seaweeds: Occurrence, regulation and functions. In Bourgougnon N. (ed.) Advances in Botanical Research. Academic Press 71, 125–159. doi: 10.1016/B978-0-12-408062-1.00005-6
Sun H. F., Wang H. W., Yuan C. Y. (2013). Optimization of zinc-cadmium reduction method for determination of nitrate in seawater. Advanced Materials Res. 864-867, 1004–1007. doi: 10.4028/www.scientific.net/AMR.864-867.1004
Tavakoli Hasanaklou H., Ebadi Khazineh Ghadim A., Moradi F., Jahanbakhsh Ghodehkahriz S., Gholipouri A. (2021). The effects of NH4+ and NO3– and plant growth regulators on the accumulation of nutrients, carbohydrates and secondary metabolites of stevia rebaudiana bertoni. Sugar Tech 23, 65–76. doi: 10.1007/s12355-020-00875-2
Tian S., Zheng T., Wu M., Cao C., Xu L., Gu Z., et al. (2022). Differences of photosynthesis and nutrient utilization in sargassum fusiforme and its main epiphyte, ulva lactuca. Aquaculture Res. 53, 3176–3187. doi: 10.1111/are.15830
Waadt R. (2020). Phytohormone signaling mechanisms and genetic methods for their modulation and detection. Curr. Opin. Plant Biol. 57, 31–40. doi: 10.1016/j.pbi.2020.05.011
Wang J., Wang Y. L., Wang D. Y., Huang J. X., Liu Y. B., Zhu M., et al. (2022). Mitigative effect of 6-benzyladenine on photosynthetic capacity and leaf ultrastructure of maize seedlings under waterlogging stress. Photosynthetica 60 (3), 389–399. doi: 10.32615/ps.2022.027
Wei Z., Long C., Yang F., Long L., Mo J., Hu Q., et al. (2020). Effects of plant growth regulators on physiological performances of three calcifying green macroalgae halimeda species (Bryopsidales, chlorophyta). Aquat. Bot. 161. doi: 10.1016/j.aquabot.2019.103186
Wu Y. S., Gong W. Z., Wang Y. M., Yang W. Y. (2019). Shading of mature leaves systemically regulates photosynthesis and leaf area of new developing leaves via hormones. Photosynthetica 57, 303–310. doi: 10.32615/ps.2019.021
Xiong G., Li J., Wang Y. (2009). Advances in the regulation and crosstalks of phytohormones. Chin. Sci. Bull. 54, 4069–4082. doi: 10.1007/s11434-009-0629-x
Xu F., He S., Zhang J., Mao Z., Wang W., Li T., et al. (2018). Photoactivated CRY1 and phyB interact directly with AUX/IAA proteins to inhibit auxin signaling in arabidopsis. Mol. Plant 11, 523–541. doi: 10.1016/j.molp.2017.12.003
Xu L., Lin L., Luo L., Zuo X., Cao C., Jin X., et al. (2022b). Organic acid treatment for removal of epiphytic ulva l. attached to sargassum fusiforme seedlings. Aquaculture 547. doi: 10.1016/j.aquaculture.2021.737533
Xu L., Luo L., Zuo X., Cao C., Lin L., Zheng H., et al. (2022a). Effects of temperature and irradiance on the regeneration of juveniles from the holdfasts of sargassum fusiforme, a commercial seaweed. Aquaculture 557. doi: 10.1016/j.aquaculture.2022.738317
Yatsuya K., Kiyomoto S., Yoshida G., Yoshimura T. (2012). Regeneration of the holdfast in 13 species of the genus sargassum grown along the western coast of Kyushu, south-western Japan. Jpn. J. Phycol 60, 41–45.
Yoshida G., Shimabukuro H. (2017). Seasonal population dynamics of sargassum fusiforme (Fucales, phaeophyta), suo-oshima is., seto inland Sea, Japan-development processes of a stand characterized by high density and productivity. J. Appl. Phycol 29, 639–648. doi: 10.1007/s10811-016-0951-z
Zhang T., Hong M., Wu M., Chen B., Ma Z. (2020). Oxidative stress responses to cadmium in the seedlings of a commercial seaweed sargassum fusiforme. Acta Oceanologica Sin. 39, 147–154. doi: 10.1007/s13131-020-1630-0
Keywords: Sargassum fusiforme, holdfast regeneration, seaweed hormones, breeding, plant growth regulators
Citation: Luo L, Zuo X, Guo L, Pang G, Ma Z, Wu M and Chen B (2023) Effects of exogenous hormones on the regeneration of juveniles from Sargassum fusiforme holdfasts. Front. Mar. Sci. 9:1072391. doi: 10.3389/fmars.2022.1072391
Received: 18 October 2022; Accepted: 15 December 2022;
Published: 04 January 2023.
Edited by:
Rafael R. Robaina, University of Las Palmas de Gran Canaria, SpainReviewed by:
Dong Xu, Yellow Sea Fisheries Research Institute (CAFS), ChinaDu Hong, Shantou University, China
Copyright © 2023 Luo, Zuo, Guo, Pang, Ma, Wu and Chen. This is an open-access article distributed under the terms of the Creative Commons Attribution License (CC BY). The use, distribution or reproduction in other forums is permitted, provided the original author(s) and the copyright owner(s) are credited and that the original publication in this journal is cited, in accordance with accepted academic practice. No use, distribution or reproduction is permitted which does not comply with these terms.
*Correspondence: Mingjiang Wu, wmj@wzu.edu.cn; Binbin Chen, binbch@163.com