- 1Oceanography Department and Geochemical and Environmental Research Group. Texas A&M University, College Station, TX, United States
- 2Unidad de Química en Sisal, Facultad de Química, Universidad Nacional Autónoma de México, Puerto de Abrigo S/N, Sisal, Yucatán, Mexico
- 3Departamento de Recursos del Mar, Laboratorio de Geoquímica Marina. Centro de Investigacion y de Estudios Avanzados del Instituto Politecnico Nacional (CINVESTAV)-Mérida, Merida, Yucatán, Mexico
Oil extraction and transport activities in the Gulf of Mexico (GoM), along with major marine oil spills and riverine inputs, are exerting environmental pressure on this system by increasing the concentration of oil-related pollutants such as polycyclic aromatic hydrocarbons (PAHs). To fully identify these changes related to oil activities, current PAH levels should be established. Here, we present the PAH concentration and the low molecular weight/high molecular weight (LMW/HMW) ratios obtained in the Perdido Fold Belt area in surface and bottom water at four cruises from May 2016 to September 2017. The Perdido 1 (P1) cruise was conducted in May 2016, the Perdido 2 (P2) cruise in September–October 2016, the Perdido 3 (P3) cruise in June 2017, and the Perdido 4 (P4) cruise in September 2017. Samples were taken during each cruise at up to 3,500-m depth, the deepest ever recorded for the GoM. Results show that the highest concentrations of PAH, LMW PAHs, and HMW PAHs were found in the P4 cruise (1.15, 1.05, and 0.10 µg/L, respectively), well below the 300 µg/L guideline for acute exposure. LMW/HMW ratios show that only the P1 cruise indicates pyrogenic hydrocarbons, while P2, P3, and P4 were petrogenic. The spatial distribution of total PAH, LMW, and HMW showed higher values in the southern and northeastern areas, except for P4, which showed high values related to riverine inputs. The complex hydrodynamic in the region was found to have a significant effect on PAH seasonal changes, river contributions, eddy circulation, and fronts to promote their dispersion.
Introduction
The Gulf of Mexico (GoM) is one of the largest marine ecosystems in the world (Yáñez-Arancibia and Day 2004a; Toledo Ocampo, 2005), supporting important ecosystems as well as economic activities such as tourism, fisheries, and the extraction of hydrocarbons. Specifically, in the Western GoM, the Perdido Fold Belt (PFB) is located in the deep waters of the exclusive economic zones of the USA and Mexico, which has the structural capacity to contain oil and gas reservoirs (Patiño Ruiz et al., 2003). Population increase creates further stress on the environment, particularly on the coast of the Gulf of Mexico. The two closest Mexican states to our study zone are Tamaulipas, with a population in 2020 of 3,527,735 inhabitants, and Veracruz, with a 2020 population of 8,062,579 inhabitants (Instituto Nacional de Estadistica e Informatica [INEGI], 2020).
The presence of oil in the southern GoM is common since there are natural oil seeps (locally known in Mexico as “chapopoteras”), mainly located in the southwestern part of the GoM, where also lies the main Mexican oil extraction industry (Miranda et al., 2004; Murawski et al., 2018). Mitchel et al. (1999); National Research Council Committee on Oil int the Sea (2003), and MacDonald et al. (2015) mentioned that the natural oil input to the GoM ranges between 250,000 and 1.4 million barrels per year. Also, large-scale exploration, transport (Wankhede, 2019), and refining of hydrocarbons are present in its coasts and deep waters (Yáñez-Arancibia and Day 2004a and Yáñez-Arancibia and Day 2004b), since the Burgos basin is the main oil province producing non-associated gas in Mexico (PEMEX, 2013). In addition, extractive activities, transport activities, and also pollutant input by rivers significantly contribute to hydrocarbon concentrations in the GoM (Gracia et al., 2014; Gracia et al., 2016a; Gracia et al., 2016b). Also, two of the main marine oil spills have occurred in the GoM: Ixtoc 1 in 1979–1980 and the Deepwater Horizon in 2010 (Gracia et al., 2016a). Interest in oil pollution in the GoM increased after the Deepwater Horizon accident in 2010, leading to the creation of the Gulf of Mexico Research Initiative (GoMRI), which channeled $300 million to research the spill. The Mexican federal government also funded three series of oceanographic cruises, covering all the GoM once per year in 2010, 2011, and 2012.
Extraction activities exert significant pressure on marine and coastal areas in addition to being a source of different pollutants, whose impact on the natural environment (Salcedo et al., 2017; Soto et al., 2017) is evidenced by the decline of coastal and marine water quality (Botello et al., 2015). Specifically, regarding crude oil, the most toxic compounds that constitute it are the polycyclic aromatic hydrocarbons (PAHs), which are present in 3% to 7% of the crude oil, but they are recognized as carcinogenic and toxic to the environment (ATSDR (Agency for Toxic Substances and Disease Registry), 2005). These PAHs, once released to the water column, could be transported (dispersed or accumulated); in the area, there is a tendency to dispersion related to a constant and rapid forcing of surface water and eddy circulation (Luo et al., 2016; Enríquez et al., 2017; Meza-Padilla et al., 2019). Therefore, transportation to and from the coastal zone is expected, but there is also the presence of eddies and fronts caused by vertical mixing, seasonal changes, and river input. Therefore, the present research aimed to determine the presence and space and time changes of dissolved/dispersed PAHs in the Perdido Fold Belt area in two consecutive years and seasons to evaluate the current pollution status and the processes related to it. In particular, concentrations of dissolved/dispersed PAHs are reported for the first time in the deep areas (over 1,000-m depth) of the GoM.
Materials and methods
The PFB area occupies approximately 27,230 km2 in the Western GoM. It is located within the Deep Gulf of Mexico Oil Province (PEMEX, 2013; CNH, 2015) and is home to numerous active systems that produce oil and gas emissions (CNH, 2015).
Water samples were collected in four oceanographic cruises: Perdido 1 (P1), Perdido 2 (P2), Perdido 3 (P3), and Perdido 4 (P4), onboard the B/O Justo Sierra along the Western Gulf of Mexico. Water samples at the surface (5 m) and the bottom were collected at 27 sampling stations during cruise P1 in May 2016, P2 September–October 2016, P3 in June 2017, and P4 in September 2017 (Table 1). Cruises in May or June are considered to be in the dry season, and those in September or October are considered to be in the rainy season.
Water samples from the surface (between 1 and 10 m) and bottom (see Figure 1 for depth reference) were collected in 2-L glass bottles previously cleaned with gas chromatography (GC)-grade hexane (Omnisolv, Sigma-Aldrich Corp., St. Louis, MO, USA), extracted on board (see Herzka et al., 2017 for complete methods), and the extracts were taken to the Marine Geochemistry laboratory (Cinvestav, Merida) for further analysis following the method of Zhendi et al. (1994) (for full methods, please refer to Herguera et al., 2017). Briefly, extracts were separated into fractions in an activated silica gel column. The aromatic fraction was eluted with a 50/50 (vol/vol) mixture of GC-grade dichloromethane and hexane (Omnisolv). Analysis was performed in a Perkin Elmer Clarus 500 GC–mass spectrometry (GC-MS) in selected ion monitoring (SIM) mode. Helium was used as a carrier (1.0 ml/min), injector temperature was 290°C with an initial ramp of 25°C to 160°C and a second ramp of 8°C/min to 290°C with a final time of 15 min. A DB% (30 m × 0.25 mm ID, 0.25 µm film) was used. The PAH standards were from Ultra Scientific (North Kingstown, RI, USA). Internal and surrogate standards (terphenyl-d14, acenaphthene-d10, phenanthrene-d10, chrysene-d12, perylene-d12, and pyrene-d10) as well as external standards were obtained from Ultra Scientific. The limits of detection were between 0.003 and 0.0027 µg/L for individual compounds. Recoveries of surrogate compounds were between 60% and 120%.
Distribution maps were performed with “Ocean Data View” version 5.3 (Schlitzer, 2020). Effect sizes were calculated using the package “sjstats” version 0.18.0 (Lüdecke, 2020) in R version 4.0.2 (R Core Team, 2020).
Results and discussion
The highest mean PAH, LMW PAH, and HMW PAH concentrations were found in the Perdido 4 cruise, whereas the lowest mean concentrations for the three fractions were found in the second cruise (Table 2). The highest concentrations of PAH, LMW PAHs, and HMW PAHs were found in the Perdido 4 cruise (1.15, 1.05, and 0.10 µg/L, respectively). The lowest concentrations of PAH, LMW, and HMW were below the detection limit on cruises Perdido 2 to Perdido 4.
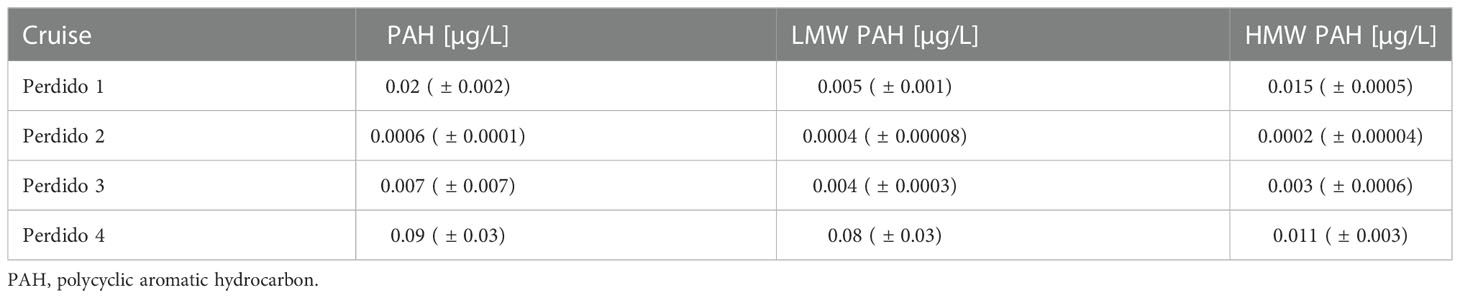
Table 2 Mean total PAH, low molecular weight (LMW), and high molecular weight (HMW) PAH concentrations (in µg/L) ( ± standard error of the mean) of each cruise.
To test for the effect sizes of the two categorical variables (“cruise” and “depth”), F-tests produced low values (lower than 1) for the factor “depth” and the interaction term in a two-way ANOVA, suggesting that temporal differences were more important than depth differences, and thus the focus here is on the F-tests for factor “cruise” only. The ω2 effect size was chosen because ω2 effect sizes indicate the proportion of variance explained by the independent variables and is a less biased effect size measure than η2 (Nakagawa and Cuthill, 2007; Lakens, 2013; Calin-Jageman and Cumming, 2019). Percent variance explained by the factor “cruise” for PAH, LMW PAHs, and HMW PAHs is low in all cases, with 22% for HMW being the highest (Table 3). The 95% confidence interval is also higher for the HMW PAH fraction, which indicates a higher dispersion of values. The 95% confidence interval for ω2 does not include zero for any of the three fractions, which means a “significant” difference in the usual hypothesis testing framework.

Table 3 F-test, effect size (ω2), minimum, maximum, and 95% confidence interval for the “cruise” factor in a two-way ANOVA for the three PAH fractions.
Based on differences in their physical characteristics and environmental behavior, some PAH indices could suggest their origin. One such index is the ratio of low-molecular-weight (LMW PAHs with 2 or 3 benzene rings) to high-molecular-weight compounds (HMW, four or more benzene rings). A ratio higher than 1 indicates a petrogenic origin (PAH originates from petroleum), and a ratio lower than 1 indicates a pyrogenic origin (from incomplete combustion of organic matter) (Soclo et al., 2000; Magi et al., 2002; Freitas da Silva et al., 2007). LMW/HMW ratios were below 1 for the P1 cruise (Table 4), and the ratio was higher than 1 for the other three cruises, indicating that these compounds are from petroleum inputs.
Percent variance explained by the factor “cruise” for the LMW/HMW ratio, as indicated by ω2, is low at 8% (Table 5). The 95% confidence interval for ω2 does not include zero for any of the three fractions, which means a “significant” difference in the usual hypothesis testing framework.
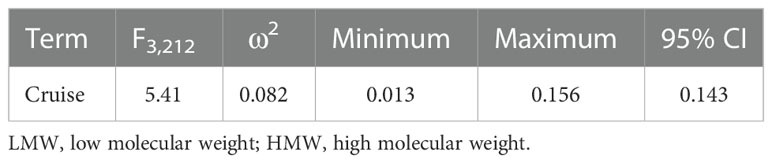
Table 5 F-test, effect size (ω2), minimum, maximum, and 95% confidence interval for the “cruise” factor in a one-way ANOVA for the LMW/HMW ratio.
The spatial distribution of total PAH, LMW, and HMW (Figure 2) shows a consistent spatial trend for the surface and bottom samples, with higher values in the southern and northeastern parts of the study zone, except for the P4 cruise, which shows high values in front of the Mexican Laguna Madre. There is no obvious oceanographic feature to explain this (Figure 3), although Luo et al. (2016); Enríquez et al. (2017); Meza-Padilla et al. (2019), and Arcega-Cabrera et al. (2021) mentioned that the transport to and from the coastal zone is expected to be related to river input, vertical mixing, and seasonal changes. There is a secondary maximum for the P1 cruise across the Mexican Laguna Madre for total and low-molecular-weight PAH, which coincides with lower salinity values (Figure 3), suggesting a terrestrial input. This agrees with the mean LMW/HMW ratio for this cruise (0.313, Table 3), which indicates a pyrogenic origin for PAH in this cruise. This same pattern was shown by Arcega-Cabrera and Dótor-Almazán (2021), using the fluoranthene/fluoranthene+pyrene index, finding that for surface and near the bottom water, PAHs from oil source (petrogenic) and from wood, plants, and mineral carbon (pyrogenic) were corroborated, agreeing with our results.
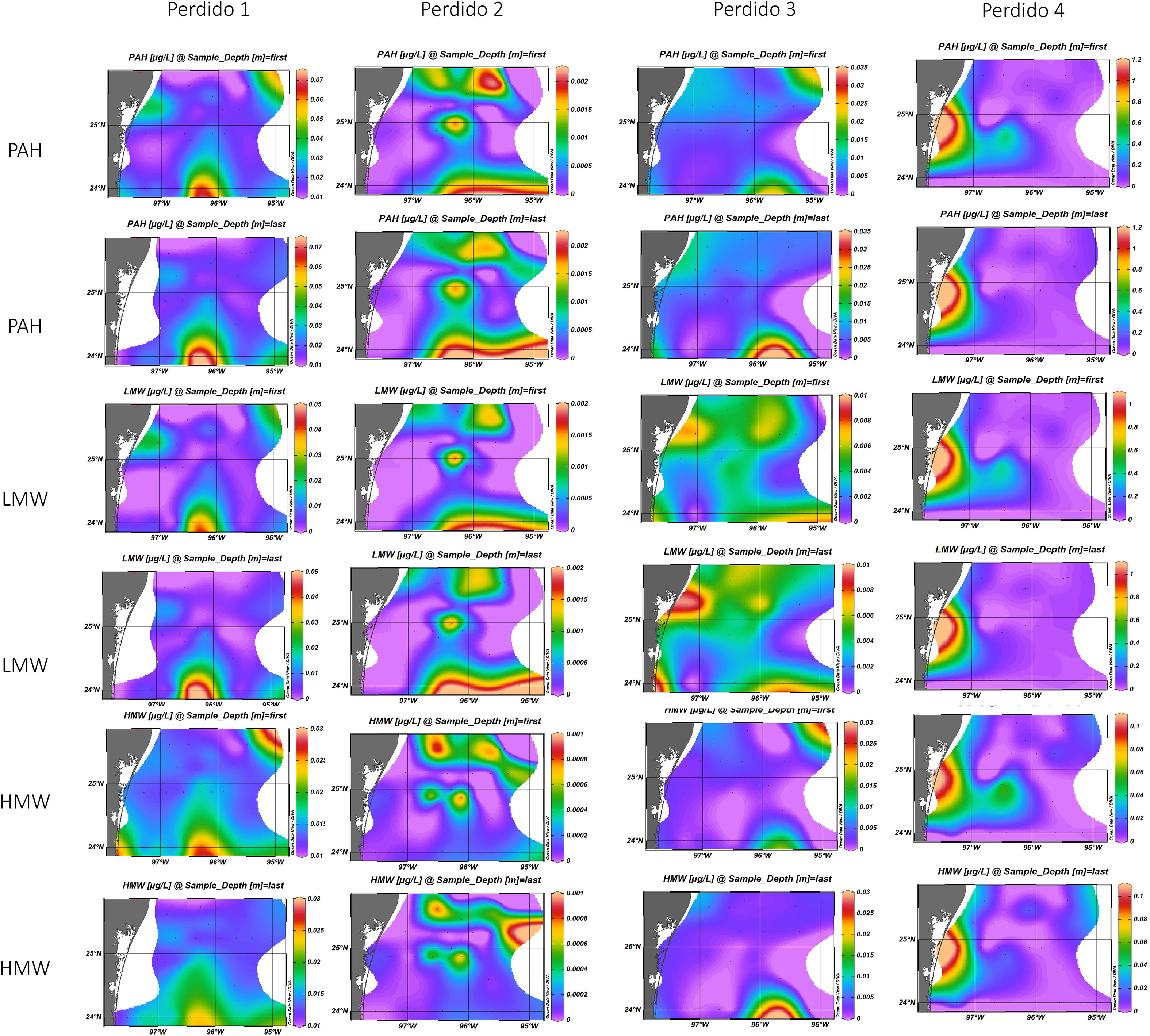
Figure 2 Spatial distribution of total polycyclic aromatic hydrocarbons (PAHs), LMW (low molecular weight), and HMW (high molecular weight) for the Perdido 1, 2, 3, and 4 cruises. First = surface sample (ca. 1–10 m); Last = bottom sample (please refer to Figure 1 for approximated depth).
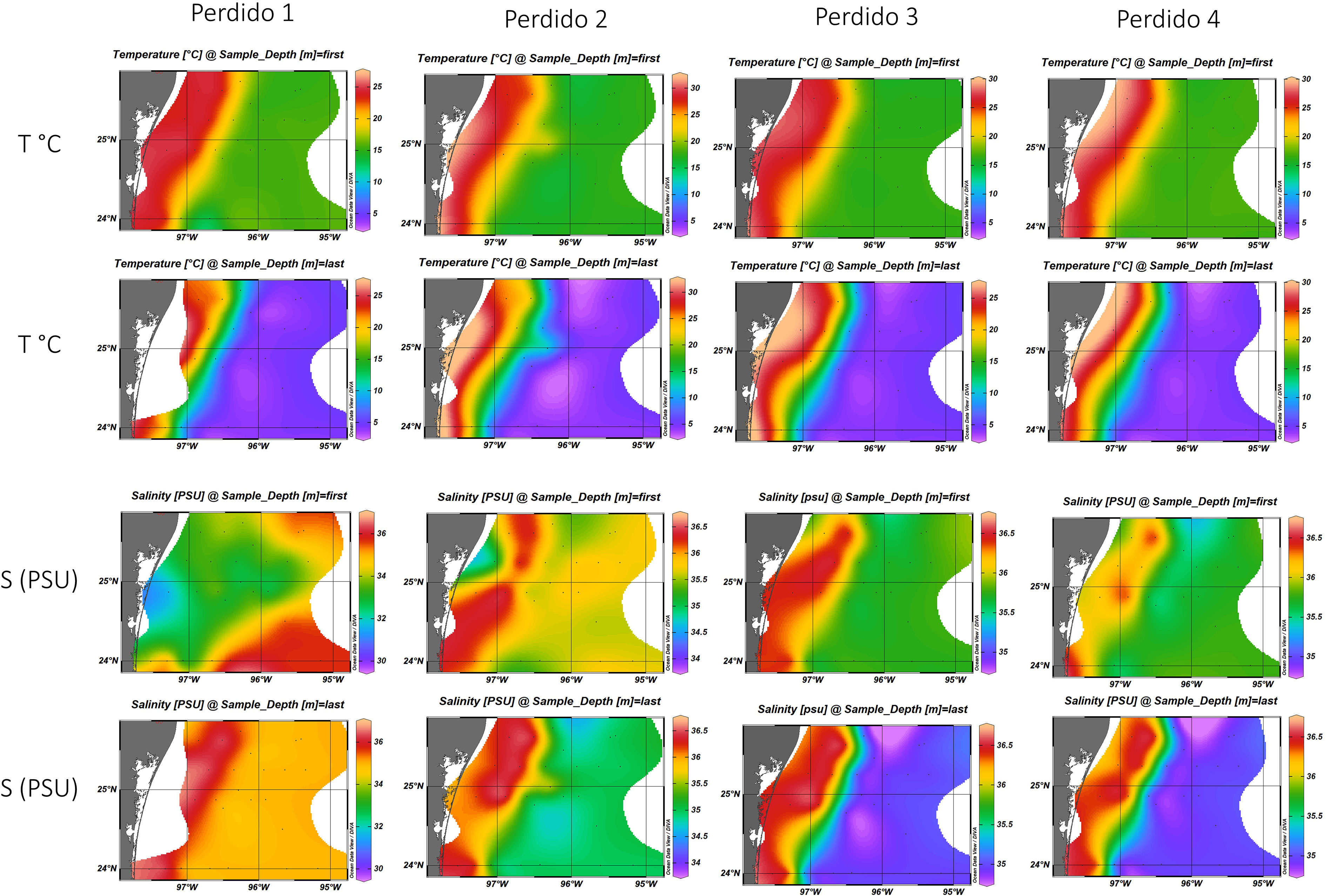
Figure 3 Spatial temperature and salinity variations for the Perdido 1, 2, 3, and 4 cruises. First = surface sample (ca. 1–10 m); Last = bottom sample (please refer to Figure 1 for approximated depth).
The dissolved/dispersed PAH concentrations obtained in this study are higher than those reported by Botello et al. (2015) for the coast of Tamaulipas on two cruises made in 2010 and 2011, right after the Deepwater Horizon spill. Botello et al. (2015) reported that all water samples they collected had total PAH concentrations below their detection limit (around 0.03 µg/L), while in this study, they were in an approximate range from undetected to 1.15 µg/L. For the Northern Gulf of Mexico, Wade et al. (2016) analyzed the results of more than 20,000 water samples collected before and after the Macondo well spill. They reported that 84% of the samples analyzed had results lower than 1 µg/L and consider this to be the “natural” or “background” concentration for the Gulf of Mexico. Wade et al. (2016) also reported that 79% of the samples analyzed had concentrations of less than 0.056 µg/L, while in this study, they were in an approximate range from undetected to 1.15 µg/L. To assess possible risk to marine organisms, the concentrations found in this study for total, LMW, and HMW PAHs were compared to the guidelines suggested in the Screening Quick Reference Tables (Buchman, 2008). Only the acute exposure level is reported for marine waters, and it is the same for all three fractions, 300 µg/L. This value is higher than the maximum concentrations reported here, and thus, the risk to aquatic organisms is very low.
Differences for the previous and actual concentrations of hydrocarbons, and in particular PAH, can be caused by several factors, from differences in analytical methods (although analytical quality controls assure the quality of results diminishing the significance of this factor) or sampling times up to real regional differences for the concentrations of these compounds. Hydrocarbon analyses in any of its fractions in the GoM are complicated by the presence of many natural seeps, known in Mexico as “chapopoteras”. MacDonald et al. (2015) reported the presence of 914 natural seeps in the Gulf of Mexico, which in total discharge to the surface 2.5 to 9.4 × 104 m3 year−1, while they calculated that the Macondo spill in 2010 discharged 22.6 × 103 m3 to the surface. The high input of hydrocarbons from natural sources to the Gulf of Mexico produces a high background concentration, which makes it more difficult to assess human impacts.
Also, in the Perdido Fold Belt area, there are contributions of agricultural and industrial activities, as well as wastewater inputs through local river systems (Arcega-Cabrera et al., 2021), and according to Reilly et al. (1991); Le Blanc (1994), and Murawski et al. (2020), there are significant hydrocarbon and metal inputs from the discharge of 140 × 10 6 m3 of production wastewater from approximately 3,000 platforms in the GoM. Also, Vidal-Martinez (2021) showed that some of the sampled fish in the area had a medium-to-high metabolite concentration related to exposure and probable damage from PAH that could be stressful for the organisms.
In addition to this, an intriguing possibility is that spatial and temporal differences observed in hydrocarbons and other pollutant concentrations in the Western Gulf of Mexico may be due, at least in part, to east-to-west transport by eddies that promote a rapid and constant forcing of surface water (Luo et al., 2016; Enríquez et al., 2017 and Meza-Padilla et al., 2019; Guerrero et al., 2020). Therefore, transport to and from the coastal zone is common in this area caused by the vertical mixing and seasonal changes in contributions from river discharges as reported by Arcega-Cabrera et al. (2021) for metals. In addition, a continental water influence, including contributions from the continental shelf and southern part of the GoM, has also been reported by Enríquez et al. (2017) and Meza-Padilla et al. (2019).
Transport in the deep gulf by eddies has been inferred from modeling studies and observational campaigns (Morey et al., 2020). During the time of the Perdido cruises, there were two active eddies in the Western Gulf of Mexico, the Olympus (active from June 2015 to June 2016) and Poseidon (active from April 2016 to April 2017) eddies (Woods Hole Group, 2020).
All of the abovementioned factors could be promoting the PAH observed seasonal changes, causing dispersion of the PAH by being forced from river contributions and also eddy circulation and fronts.
Conclusions
PAH concentration showed significant differences between cruises (seasons), and some of the samples showed a higher concentration than the ones previously reported. These seasonal changes could be related to the presence of natural seeps, riverine input, and oil extraction and transport activities. Therefore, these factors could be promoting changes in the system that could turn out to be stressful for the system at the GoM.
The source of polycyclic aromatic hydrocarbons was mainly petrogenic with exception of the first cruise, suggesting that inputs are mainly from the oil industry in the area, although riverine input is also present and varies according to hydrological season.
The complex hydrodynamic and diverse sources inputs in the Perdido area are directing the seasonal changes of PAH. Transport from and to the coast, eddies, and fronts are working together to disperse the PAH from riverine input and oil extraction and transport activities.
The results of this research constitute an invaluable reference for PAH behavior at the GoM given the spatial, time, and economic effort made with the extensive sampling; this allows us to achieve a set of robust data, although further research should be performed to better understand the processes affecting the concentration and transport of hydrocarbons in the complex and environmentally relevant system of the GoM.
Data availability statement
The raw data supporting the conclusions of this article will be made available by the authors, without undue reservation.
Author contributions
GG-B designed the sampling scheme; GG-B and FA-C did most of data analysis; all authors contributed to writing the manuscript; VC-M did most of sample analysis. All authors contributed to the article and approved the submitted version.
Funding
The authors wish to acknowledge funding for research provided by Consejo Nacional de Ciencia y Tecnologia (CONACYT) and Secretaria de Energia-Fondo Sectorial CONACYT-Secretaria de Energia-Hidrocarburos project #201441.
Acknowledgments
This is a contribution of the Consorcio de Investigacion del Golfo de Mexico (CIGoM). We thank M.C. Ismael Oceguera Vargas and M.C. Elizabeth Lamas Cosío for laboratory work and data analysis.
Conflict of interest
The authors declare that the research was conducted in the absence of any commercial or financial relationships that could be construed as a potential conflict of interest.
Publisher’s note
All claims expressed in this article are solely those of the authors and do not necessarily represent those of their affiliated organizations, or those of the publisher, the editors and the reviewers. Any product that may be evaluated in this article, or claim that may be made by its manufacturer, is not guaranteed or endorsed by the publisher.
References
Arcega-Cabrera F., Dótor-Almazán A. (2021). Hidrocarburos (Tomo IV) in “Atlas de línea base ambiental del golfo de méxico” (Coordinators) Herzka S., Zaragoza Álvarez R. A., Peters E. M., Hernández Cárdenas G. CICESE Carretera Ensenada-Tijuana No. 3918, Zona Playitas, CP. 22860, Ensenada, B.C. México. Available at: https://www.cicese.edu.mx https://atlascigom.cicese.mx ISBN: 978-607-8811-04-5. Available at: https://atlascigom.cicese.mx/map_data/T004/ATLAS-TOMO-04.pdf.
Arcega-Cabrera F., Gold−Bouchot G., Lamas−Cosío E., Dótor−Almazán A., Ceja−Moreno V., Zapata−Pérez O., et al. (2021). “Vanadium and cadmium in water from the perdido area, Northwest of the gulf of Mexico: 2 years’ monitoring and current status,” in Bulletin of environmental contamination and toxicology. doi: 10.1007/s00128-021-03212-9
ATSDR (Agency for Toxic Substances and Disease Registry) (2005). Toxicology profile of polyaromatic hydrocarbons (Boca Ratón, Florida: CRC Press).
Botello A. V., Soto L. A., Ponce-Velez G., Villanueva S. (2015). Baseline for PAHs and metals in NW gulf of Mexico related to the deepwater horizon oil spill. Est. Coast. Shelf Sci. 156, 124–133. doi: 10.1016/j.ecss.2014.11.010
Buchman M. F. (2008). “Screening quick reference tables,” in NOAA OR and r report 08-1 (Seattle WA: Office of Response and Restoration Division, National Oceanic and Atmospheric Administration), 34.
Calin-Jageman R. J., Cumming G. (2019). “The new statistics for better science: Ask how much, how uncertain, and what else is known,” in Statistical inference in the 21st century, vol. 73. (Milton Park, in Oxfordshire, UK: The American Statistician), S1, 271–280. doi: 10.1080/00031305.2018.1518266
CNH (2015) Golfo de méxico-aguas profundas norte. cinturón plegado perdido, cinturón subsalino, cordilleras mexicanas. síntesis geológico-petrolera. Available at: www.cnh.gob.mx.
Enríquez C., Mariño-Tapia I., Meza-Padilla R., Ruiz Angulo A., Appendini C. (2017). “Condiciones termohalinas y de circulación en el polígono perdido,” in Resumen de la reunion anual de la unión geofísica mexicana (Puerto Vallarta: Ciudad de México, México. Unión Geofísica Mexicana).
Freitas da Silva T., de Almeida Azevedo D., Radler de Aquino Neto F. (2007). Distribution of polycyclic aromatic hydrocarbons in surface sediments and waters from guanabara bay, Rio de Janeiro, Brazil. J. Braz. Chem. Soc. 18 (3), 628–637. doi: 10.1590/S0103-50532007000300021
Gracia A., Alexander-Valdés H. M., Ortega-Tenorio P. L., y Frausto Castillo J. A. (2016a). “Source and distribution of polycyclic aromatic hydrocarbon in the IXTOC I spill area,” in Gulf of Mexico oil spill & ecosystem science conference(Tampa, Florida: EUA).
Gracia A., Alexander-Valdés H. M., Ortega-Tenorio P. L., Frausto-Castillo J. A. (2016b). “Distribución de hidrocarburos en columna de agua y sedimentos del sur del golfo de méxico,” in Reunión anual 2016 de la unión geofísica mexicana.
Gracia A., F. Vázquez G., Enciso Sánchez G., y Alexander Valdés H. M. (2014). “Composición y volumen de contaminantes de las descargas costeras al golfo de méxico,” in Golfo de méxico. contaminación e impacto ambiental: diagnóstico y tendencias. Eds. Botello A. V., Rendón von Osten J., Benítez y G. Gold-Boucht J. (Coastal & Estuarine Research Federation: Seattle, WA 98133 | United States), 787–816.
Guerrero L., Sheinbaum J., Mariño-Tapia I., González-Rejón J. J., Perez-Brunius P. (2020). Influence of mesoscale eddies on cross-shelf exchange in the western gulf of Mexico. Continental Shelf Res. 209, 104243. doi: 10.1016/j.csr.2020.104243
Herguera J. C., Hernández-Ayón J.M., Camacho-Ibar V., Delgadillo-Hinojosa F., Huerta M.A., Herrera-Silveira J., et al. (2017). “Manual de procedimientos de muestreos durante los cruceros oceanográficos y resultados de procesos de intercalibración y control de calidad de los laboratorios analíticos,” in Metodología analísitca y procesos de intercalibración y control de calidad de los laboratorios analíticos, vol. 2. (Consorcio de investigación del Golfo de México). Available at: https://goo.gl/GzNnFs.
Herzka S. Z., Aguilar-Trujillo A., Alexander H, Balta P., Arteaga M.C., Bazan C., et al. (2017). “Manual de protocolos de muestreo y procesamiento de muestras durante cruceros oceanográficos; línea de acción 2: Línea base y monitoreo ambiental,” in Resultados del censo nacional de población y vivienda (Consorcio de nvestigación del Golfo de México). Available at: https://cuentame.inegi.org.mx/monografias/informacion/tam/poblacion/default.aspx?tema. https://goo.gl/ju5YdNINEGI.
Lakens D. (2013). Calculating and reporting effect sizes to facilitate cumulative science: a practical primer for t-tests and ANOVAs. Front. Psychol. 4, 863. doi: 10.3389/fpsyg.2013.00863
Le Blanc L. (1994). Industry marches into deepwater: The offshore years, (1954–1994), Offshore, April 36–56.
Lüdecke D. (2020). ‘sjstats’: Statistical functions for regression models (Version 0.18.0). doi: 10.5281/zenodo.1284472
Luo H., Bracc A., Cardona Y., McWilliams J. C. (2016). Submesoscale circulation in the northern gulf of Mexico: Surface processes and the impact of the fresh water river input. OceanModelling 101, 68–82. doi: 10.1016/j.ocemod.2016.03.003
MacDonald I. R., Garcia‐Pineda O., Beet A., Daneshgar Asl S., Feng L., Graettinger G., et al. (2015). Natral and unnatural oil slicks in the gulf of Mexico. J. Geophys. Res. Oceans 120, 8364–8380. doi: 10.1002/2015JC011062
Magi E., Bianco R., Ianni C., Di Carro M. (2002). Distribution of polycyclic aromatic hydrocarbons in the sediments of the Adriatic Sea. Environm. Poll 119, 91–98. doi: 10.1016/S0269-7491(01)00321-9
Meza-Padilla R., Enríquez C., Yonggang L., Appendini C. (2019). Ocean circulation in the Western gulf of Mexico using Self‐Organizing maps. J. Geophysical Reseach (Oceans) 124 (6), 4152–4167. doi: 10.1029/2018JC014377
Miranda F. P., Marmol A. M. Q., Pedroso E. C., Beisl C. H., Welgan P. y, Morales L. (2004). Analysis of RADARSAT-1 data for offshore monitoring activities in the cantarell complex, gulf of Mexico, using the unsupervised semivariogram textural classifier (USTC). Can. J. Remote Sens 30 (3), 424–436. doi: 10.5589/m04-019
Mitchel R., MacDonald I. R., y Kvenvolden K. (1999). Estimates of total hydrocarbon seepage into the gulf of Mexico based on satellite remote sensing images. EOS Suppl. 80 (49), OS 242.
Morey S. L., Gopalakrishnan G., Pallaz Sanz E., AZEVEDO CORREIA DE SOUZA J. M., Donohue K., PÉREZ-BRUNIUS P. (2020). Assessment of numerical simulations of deep circulation and variability in the gulf of Mexico using recent observations. J. Phys. Oceanogr 50, 1045–1064. doi: 10.1175/JPO-D-19-0137.1
Murawski S. A., Hollander D. J., Gilbert S., Gracia A. (2020). Deepwater oil and gas productuion in the Gulf of Mexico and related global trends. In: Murawski S., Ainsworth C., Gilbert S., Hollander D., Paris C., Schlüter M., et al (eds) Scenarios and responses to future deep oils spills. Springer, New York.
Murawski S. A., Peebles E. B., Gracia A., Tunnell J. W. Jr., y Armenteros M. (2018). Comparative abundance, species composition and demographics of continental shelf fish assemblages throughout the gulf of Mexico. Mar. Coast. Fisheries Dynamics: Manage. Ecosystem Sci. 10, 325–346. doi: 10.1002/mcf2.10033
Nakagawa S., Cuthill I. C. (2007). Effect size, confidence interval and statistical significance: a practical guide for biologists. Biol. Rev. 82, 591–605. doi: 10.1111/j.1469-185X.2007.00027.x
National Research Council Committee on Oil int the Sea (2003). Oil in the Sea III: Inputs (Washington, D.C: The National Academies Press).
Patiño Ruiz J., Rodríguez Uribe M. A., Hernández Flores E. R., Lara Rodríguez J., Gómez González A. R. (2003). “El Cinturón plegado mexicano,” in Estructura y potencial petrolero (Boletín de la Asociación Mexicana de Geólogos Petroleros (AMGP), 4–20.
PEMEX (2013) BDOE (Base de datos integrada de acuerdo al instructivo de oportunidades exploratorias). Available at: https://www.rondasmexico.gob.mx/media/1039/ac1_cpp.pdf.
R Core Team (2020). R: A language and environment for statistical computing (Vienna, Austria: R Foundation for Statistical Computing). Available at: https://www.R-project.org.
Reilly W. K., O’Farrel T., Rubin M. R. (1991). Development document for 1991 proposed effluent limitation guidelines and new source performancestandards for the offshore subcategory of the oil and gas extraction and point source category (Washington, DC: US EPA).
Salcedo D. L., Soto L. A., Estradas-Romero A., Vázquez-Botello A. (2017). Interannual vriability of soft.bottom macrobenthic communities of the NW gulf of Mexico in reationship to the deepwater horizon oil spill. Mar. Pollut. Bull. 114, 987–994. doi: 10.1016/j.marpolbul.2016.11.031
Soclo H. H., Garrigues P., Ewald M. (2000). Origin of polycyclic aromatic hydrocarbons (PAHs) in coastal marine sediments: Case studies in cotonou (Benin) and aquitaine (France) areas. Mar. Poll. Bull. 40 (5), 387–396. doi: 10.1016/S0025-326X(99)00200-3
Soto L. A., Salcedo D. L., Arvizu K., Botello A. V. (2017). Interannual patterns of the large free-living nematode assemblages in the Mexican Exclusive Economic Zone, NW Gulf of Mexico after the Deepwater Horizon oil spill Ecol. Indicators 79, 371–381. doi: 10.1016/j.ecolind.2017.03.058.
Toledo Ocampo A. (2005). “Marco Conceptual: caracterización ambiental del golfo de méxico,” in Golfo de méxico contaminación e impacto ambiental: Diagnóstico y tendencias, 2da edn. Eds. Botello A. V., Rendón-von Osten J., Gold-Bouchot G., Agraz-Hernández C. (México: Univ. Autón. de Campeche, Univ. Nal. Autón. de México, Instituto Nacional de Ecología), pp, 25–pp, 52.
Vidal-Martinez (2021). “Contaminantes, biomarcadores y bioindicadores en organismos,” in Atlas de línea base ambiental del golfo de méxico (Coordinators) Herzka S, Zaragoza Álvarez R. A, Peters E. M., Hernández Cárdenas G. CICESE Carretera Ensenada-Tijuana No. 3918, Zona Playitas, CP. 22860, Ensenada, B.C. México. Available at: https://www.cicese.edu.mx https://atlascigom.cicese.mx ISBN: 978-607-8811-04-5978-607-99048-2-1. Available at: https://atlascigom.cicese.mx/map_data/T005/ATLAS-TOMO-05.pdf.
Wade T. L., Sericano J. L., Sweet S. T., Knap A. H., Guinasso N. L. Jr (2016). Spatial and temporal distribution of water column total polycyclic aromatic hydrocarbons (PAH) and total petroleum hydrocarbons (TPH) from the Deepwater Horizon (Macondo) incident. Mar. Poll. Bull. 103(1-2), 286–293. doi: 10.1016/j.marpolbul.2015.12.002.
Wankhede A. (2019). A comprehensive list of fuel, diesel and lube oil tanks on a ship (Marine Technology, Marine Insight). Available at: https://www.marineinsight.com/tech/a-comprehensive-list-fuel-diesel-and-lube-oil-tanks-on-a-ship/.
Woods Hole Group (2020). Available at: https://www.horizonmarine.com/loop-current-eddies.
Yáñez-Arancibia A., Day J. W. (2004a). The gulf of Mexico: towards an integration of coastal management with large marine ecosystem management. Ocean Coast. Manage. 47, 537–563. doi: 10.1016/j.ocecoaman.2004.12.001
Yáñez-Arancibia A., Day J. W. (2004b). Environmental sub-regions in the gulf of Mexico coastal zone: the ecosystem approach as an integrated management tool. Ocean Coast. Manage. 47, 727–757. doi: 10.1016/j.ocecoaman.2004.12.010
Keywords: perdido fold belt, dissolved PAH, deep gulf, baseline, environmental assessment
Citation: Gold-Bouchot G, Arcega-Cabrera F and Ceja-Moreno V (2023) Dissolved/dispersed polycyclic aromatic hydrocarbon spatial and temporal changes in the Western Gulf of Mexico. Front. Mar. Sci. 9:1069315. doi: 10.3389/fmars.2022.1069315
Received: 13 October 2022; Accepted: 23 December 2022;
Published: 23 January 2023.
Edited by:
Hernando Bacosa, Mindanao State University-Iligan Institute of Technology (MSU-IIT), PhilippinesReviewed by:
Balram Ambade, National Institute of Technology, Jamshedpur, IndiaJohn Jewish Arellano Dominguez, Iwate Biotechnology Research Center, Japan
Copyright © 2023 Gold-Bouchot, Arcega-Cabrera and Ceja-Moreno. This is an open-access article distributed under the terms of the Creative Commons Attribution License (CC BY). The use, distribution or reproduction in other forums is permitted, provided the original author(s) and the copyright owner(s) are credited and that the original publication in this journal is cited, in accordance with accepted academic practice. No use, distribution or reproduction is permitted which does not comply with these terms.
*Correspondence: Gerardo Gold-Bouchot, ggold@tamu.edu