- 1Marine Biodiversity, Exploitation and Conservation, MARBEC, Univ. Montpellier, Ifremer, CNRS, IRD, Montpellier, France
- 2Institut des Sciences de l’Evolution de Montpellier, ISEM, Univ Montpellier, CNRS, IRD, Montpellier, France
Introduction: Natural and synthetic estrogens are pollutants found in aquatic ecosystems at low concentrations reaching ng.L-1 to μg.L-1. At these concentrations, they are able to interfere with the fish endocrine system. When waterborne exposure occurs at early life stages, when blood estrogens concentrations are low, this may have significant consequences for estrogen-sensitive functions such as skeletal development.
Methods: To better understand how (xeno)estrogens may affect early head mineralization, 12 days post-hatch larvae of the European seabass Dicentrarchus labrax were experimentally exposed for 4 days to the natural estrogen estradiol E2 and to the xenoestrogen bisphenol A (BPA), both used at either regulatory concentration of water quality or a 100 times higher concentration. Head mineralization level was assessed using Alizarin red staining, together with the relative quantification of mRNA expression levels of several genes playing key roles in skeletogenesis and estrogen signaling pathways.
Results: We showed that (xeno)estrogen exposure at early larval stage increases the expression of skeleton-associated genes: matrix proteins encoding genes (col1a2, col2a1a, col2a1b, bgp1a, bgp1b, sparc), proteolytic enzyme encoding genes (ctsk) and transcription and signaling factors (sox9a, sox9b, ihha, runx2, rankl). Although transcriptional overexpression of these genes was significant in larvae exposed to 40 ng.L-1 E2 and to 1.6 and 160 μg.L-1 BPA, increased mineralization was detected only in E2-exposed larvae, suggesting a difference in head skeleton development and remodeling in BPA-treated larvae.
Discussion: Our results suggest that these phenotypic differences could be due to the implication of other estrogenic signaling pathways involving both nuclear and membrane-bound estrogen receptors (ERs and GPERs), but also estrogen-related receptors (ERRs). This study brings new insights into the regulatory mechanisms of skeletogenesis by E2 and BPA and into the effects of waterborne exposure to (xeno)estrogens on the early skeletal development of teleost fishes.
Introduction
Aquatic environments are the ultimate reservoirs for many anthropogenic chemicals, including xenohormones, that mimic the functions of natural hormones. One of the currently best-studied groups of endocrine-disrupting chemicals are estrogenic or xenoestrogenic compounds that interact with estrogen receptors. Bisphenol A (BPA), a synthetic polyphenol, represent a group of ubiquitous environmental xenoestrogens that are related in structure, although somewhat different from estrogens. BPA is a monomer by-product of plastic manufacturing or product breakdown and is considered as a concern in aquatic ecosystems and a potential threat to wildlife and public health (Colborn et al., 1994; Flint et al., 2012; Careghini et al., 2015; Kalb et al., 2016; Liu et al., 2021). In seawater, the concentrations of BPA range from several hundred pg.L-1 to several μg.L-1 in polluted areas (Staniszewska et al., 2015) exposing fish to this chemical during critical periods of their development or throughout their entire life cycle. In aquaculture, the use of polycarbonate plastic plumbing and epoxy paints may increase BPA exposure due to potential leaching from aquaculture tanks (Sajiki and Yonekubo, 2003). The additional use of estrogen-enriched diet to increase productivity and the sex ratio in favor of females is of concern (Piferrer, 2001; Gorshkov et al., 2004). If exposure to estrogenic compounds occurs during early developmental stage when blood estrogen concentrations are low or undetectable, such exposure may lead to significant changes and developmental failures (Chin et al., 2018). Given the potential immediate and long-term implications for individual growth, survival, and fitness, identifying the effects and mechanisms of action of environmental (xeno)estrogens in early life stages is essential.
Estrogen signaling is mediated by several types of receptors: nuclear Estrogen Receptors (ERs) generate genomic (direct or tethered) regulation via binding to an Estrogen Response Element (ERE). Membrane-bound estrogen receptors (G protein-coupled estrogen receptors, GPERs) lead to non-genomic regulations and directly trigger cellular signaling events (Xu et al., 2019). The resulting cellular modifications can span time frames from seconds to hours or days (Prossnitz and Barton, 2011; Marino et al., 2012; Zuo and Wan, 2017). Among natural estrogens, 17β-estradiol (E2) is the compound with highest potency toward estrogen receptors (Tremblay and van der Kraak, 1998; Brion et al., 2012; Delfosse et al., 2014; Le Fol et al., 2017). Most vertebrates have two ER-encoding genes: era (or esr1) encoding the ER-alpha protein (ERα), and erb (or esr2) encoding ER-beta protein (ERβ). BPA can mimic the action of the E2 via its binding to ERs and elicit estrogenic action in vivo and in vitro despite a binding affinity to estrogen receptors being about 3 to 4 orders of magnitude lower (Tremblay and van der Kraak, 1998; Björnström and Sjöberg, 2005; Pinto et al., 2014a; Acconcia et al., 2015; Le Fol et al., 2017). BPA is also able to bind GPER (Thomas and Dong, 2006; Périan and Vanacker, 2020). Previous studies have shown that the affinity of estradiol for GPER is some 10-fold lower than it is for ER (Revankar et al., 2007). In contrast, the affinity of BPA for GPER is between 8 and 50 times greater than its affinity for ER, although this represents only a weak binding affinity (Thomas and Dong, 2006).
The genomic effects of the estrogen signaling through ERs are modulated by a family of orphan nuclear receptors: the estrogen-related receptors (ERR). ERRs include ERRα, ERRβ, and ERRγ in mammals, and they show strong sequence similarity to ERα and ERβ in their DNA binding and ligand binding domains, despite their inability to bind estradiol. The ERRs have a stronger affinity to BPA than ERs (5 nM and 0.2 μM respectively for ERα and ERβ; (Gibert et al., 2011)). Studies in human and zebrafish highlighted that BPA may exert ligand-dependent effects disturbing the endogenous estrogen signaling by linking members of the ERRs (Takayanagi et al., 2006; Tohmé et al., 2014), but the putative roles of ERRs in BPA estrogen receptor-independent effects are still poorly addressed.
Though estrogens are commonly referred to as sex hormones, they play many other key physiological roles by acting as signaling molecules in a variety of early functions, including skeleton development and mineral homeostasis (Zuo and Wan, 2017). Estrogen signaling has an effect in all skeletal cell types in bony vertebrates: chondrocytes, osteoblasts, and osteoclasts (Kousteni et al., 2001; Pinto et al., 2014b; Almeida et al., 2017; Zuo and Wan, 2017). Chondrocytes are cells involved in cartilage matrix synthesis and homeostasis. As such, they provide the supporting architecture for bone synthesis during the embryonic and early post-embryonic development. Osteoblasts are involved in the secretion and mineralization of bone, while osteocytes are osteoblasts enclosed in the mineralized matrix. Bone renewal over life is allowed by the degradation activity of osteoclasts (reviewed in Prein and Beier, 2019). According to Hammond and Schulte-Merker (2009), osteoclast activity begins as soon as 12 dpf in zebrafish, synchronously with early head mineralization (Verreijdt et al., 2006; Aceto et al., 2015). Several studies have shown the effect of E2 on chondrogenesis and ossification in the development of the zebrafish craniofacial skeleton (Cohen et al., 2014; Pashay Ahi et al., 2016; Zare Mirakabad et al., 2019) with massive inhibition of cranial cartilage development with high concentrations of E2 (Fushimi et al., 2009; Pashay Ahi et al., 2016). Similarly, embryonic and larval treatment of the estuarine mummichog, Fundulus heteroclitus, impacted skeletal development at high doses of E2 by inhibiting mineralization but not cartilage development (Urushitani et al., 2002).
The European seabass Dicentrarchus labrax is a non-model species of high commercial interest for fisheries and aquaculture where skeletal malformations are a major issue. Although osteoblasts never turn into osteocytes in this species (Kranenbarg et al., 2005; Davesne et al., 2018), mononuclear osteoclasts are still present (Boglione et al., 2013) and the head skeleton development involves cartilage growth and ossification (Darias et al., 2010). In this study, we investigated whether waterborne exposure to (xeno)estrogens impacts early head mineralization in Dicentrarchus labrax. Since reproduction and breeding are managed in aquaculture, experimentation in very early life stages was performed: 12 days post-hatched (dph) larvae were exposed to a four-day treatment with E2 and BPA, both used at a regulatory concentration of water quality and a 100 times more elevated concentration. Mineralization was recorded using Alizarin Red staining and the transcription levels of several genes playing a key role in skeletogenesis and estrogen signaling pathways were measured.
Material and methods
Experimental design
Animals
A first cohort of European seabass Dicentrarchus labrax was used for the developmental longitudinal analysis (6-, 10-, 17-day post-hatch, dph). This cohort was obtained from in vitro fertilization of gametes produced by five unrelated wild native Mediterranean breeders (3 males and 2 females) at the Ifremer marine station of Palavas-les-Flots (France). The eggs were kept in the dark at 12°C and 35 ppt salinity until hatching, then the salinity of the rearing water was gradually decreased from 35 to 25 ppm (5 ppm/day) and larvae were grown in 500 L tank, at 20°C, 16/8 hours light/dark photoperiod. The second cohort for experimental exposure (about 12,000 larvae) was obtained from the aquaculture farm Les Poissons du Soleil (Balaruc les Bains, Hérault, France) from natural reproduction of Mediterranean progenitors. This cohort was transferred directly into McDonald jars at the Ifremer station of Palavas-Les-Flots at the age of 11-dph, when the swim bladder was formed to avoid strong mortality due to manipulation and transfer (10/14 hours light/dark photoperiod, 17°C, 25 ppt salinity, dissolved 02 above 7.5 mg.L-1). Both cohorts were fed twice a day with type AF INVE® live artemia nauplii preys (one prey.mL-1). All the experiments were conducted according to the guidelines of the European Union (directive 86/609) and of the French law (decree 87/848) regulating animal experimentation. The experimental design has been approved by the French legal requirement concerning welfare of experimental animals (APAFIS permit no. 9045-201701068219555).
Experimental exposure and sampling
E2 (17β-œstradiol, ≥98% purity, Sigma-Aldrich) and BPA (bisphenol A, ≥99% purity, Sigma-Aldrich) were dissolved at 40 mg.L-1 and 40 g.L-1 respectively, in ethanol (100%, Fluka). Stock solutions were kept at 4°C in dark conditions. Exposure solutions were prepared by diluting working stocks in filtered 25 ppt seawater at a final vehicle concentration of 0.0008% ethanol in solvent control and all treatment groups.
Exposure was conducted in McDonald jars mainly composed of glass to avoid any additional BPA contamination of the water due to plastic materials. For each condition, 400 larvae of 11-dph were placed in a recirculated closed circuit consisting of two 9-L McDonalds jars connected with a 5-L beaker filled with 25 ppt seawater for a total volume of 22 L per device (see the experimental setup in Figure S1). They were acclimated in this experimental setup for 24 hours, then 12-dph larvae (0.57 ± 0.02 cm standard length, SL) were exposed for 4 days (until 16-dph) to the following nominal concentrations: 0.4 or 40 ng.L-1 E2, 1.6 or 160 μg.L-1 BPA and 0.00008% ethanol for solvent control. The lowest concentration of exposure chosen for E2 and BPA is environmentally realistic and refers to regulatory concentrations applicable to surface water (Water Framework Directive): Environmental Quality Standards (EQS, Directive 2008/105/EC) or PNECaqua when EQS was not available: respectively PNECaqua = 1.6 μg.L-1 for BPA (EC, 2008) and provisional EQS = 0.4 ng.L-1 for E2 (EC, 2018). After this initial water contamination, E2, BPA and ethanol were delivered using a peristaltic pump (8-canals, IPC-N, Ismatec) at a renewal rate of 12 hours, through a BPA-free pharmed tube (diameter 0.51 mm, PharMed® BPT, Saint-Gobain Performance plastics). Half the volume of water was renewed with freshly contaminated water every 48-h, and the working solutions delivered by the peristaltic pump were also freshened. Larvae were fed twice a day during exposure with artemia nauplii from AF INVE® (1 prey.mL-1). The stability of temperature, salinity, dissolved oxygen concentration and ammonia was checked every day.
After 4 days of exposure, 16-dph larvae were collected and euthanized in 100 ppm benzocaine on ice. Twelve larvae of each condition were individually imaged under a binocular stereomicroscope to measure their standard length and to assess possible bladder abnormalities and flash frozen in liquid nitrogen and stored at -80°C for subsequent molecular analysis. The remaining larvae were collected for Alizarin Red staining, used for visualizing the mineralized elements. They were fixed 48-h at 4°C in 4% paraformaldehyde prepared in phosphate buffer saline adjusted at 25 ppt, then dehydrated in ethanol and stored at −20°C.
Biometric and bladder analyses
The mean survival rate between 12 and 16 dph was 29 ± 14%, which is consistent with the survival rates recorded in aquaculture at these early life stages (Villamizar et al., 2011). The mean growth percentages were +18% for solvent control (0.70 ± 0.03 cm) and E2 0.4 ng.L-1 (0.70 ± 0.06 cm), +14% for E2 40 ng.L-1 (0.67 ± 0.04 cm) and + 20% for BPA 1.6 μg.L-1 (0.71 ± 0.04 cm) and BPA 160 μg.L-1 (0.71 ± 0.04 cm) between 12 and 16 dph, without any significant difference in larval size between the different treatments at the end of the exposure. Abnormal swim bladder was observed in 33% of 12-dph individuals at the beginning of the exposure, while it was 37 ± 10% in 16-dph individuals with about ⅔ of over-inflation and ⅓ of under-developed swim bladders. Larvae with abnormal bladder were discarded for subsequent mRNA or Alizarin analyses.
Alizarin staining and mineralization level determination
Fixed larvae were gradually rehydrated in Ethanol/KOH 0.5% (v/v) then in KOH 0.5%. They were depigmented by incubation in a 0.3% hydrogen peroxide in KOH 0.5% solution for 30 minutes in the dark, washed in KOH 0.5%, incubated overnight in a solution of 0.01% Alizarin red in KOH 0.5%, and washed in KOH 0.5%. Stained larvae were transferred in graded series of KOH 0.5%/glycerol and then stored and imaged in 100% glycerol. Staining was carried out simultaneously for all treatments to avoid technical variability of the staining.
For each pictured individual after Alizarin staining (ventral and lateral views), head mineralized structures were identified and counted. Based on the mineralization level of each cranial unit studied (0: no mineralization, 0.25: 1 quarter mineralized, 0.5: half mineralized, 0.75: 3 quarter mineralized, 1: fully mineralized), an overall mineralization score was assigned to each larva corresponding to the sum of the cranial unit mineralization scores (see Supplementary data Figure S2 as an example of mineralization level quantification).
mRNA expression analysis
Selection of target genes
Sequences of selected genes were retrieved from gene models derived from the seabass genome draft assembly (dicLab v1.0c) (Tine et al., 2014). Orthology of each candidate gene with other vertebrate genes was tested by phylogenetic reconstruction (data not shown). Several classes of genes were selected, including cartilage and bone structural matrix proteins: the type I collagen encoding gene (col1a2), known to be expressed in the perichondrium and osteoblast cells in the zebrafish (Li et al., 2009; Eames et al., 2012), and two homologs of the type II collagen encoding genes col2a1a and col2a1b, known in zebrafish to be divergent in their function, respectively expressed in chondrocytes and perichondrium/osteoblast cells (Dale and Topczewski, 2011; Eames et al., 2012). We further identified calcium-interacting matrix protein encoding genes: a single sparc gene, expressed in osteoblasts of Oreochromis mossambicus (Weigele et al., 2015) and Takifugu rubripes (Kaneko et al., 2016); both bgp1a and bgp1b paralogs present in most teleost fishes including in seabass (Leurs et al., 2021) while the bgp1a gene is known to be expressed in zebrafish osteoblasts (Topczewska et al., 2016). We also selected the tissue nonspecific alkaline phosphatase (alp) gene for its critical role in the mineralization mechanisms (according to Yang et al., 2012), and both paralogs encoding for Indian hedgehog factors (ihha and ihhb), that are expressed by chondrocytes in the zebrafish (Avaron et al., 2006) and ihha has been shown to be a chondrocyte-secreted signaling factor that activates osteoblasts (Paul et al., 2016). We identified three transcription factors that are classically associated with the differentiation of chondrocytes (sox9) and osteoblasts (runx2 and sp7) (Yan et al., 2002; Li et al., 2009; Topczewska et al., 2016). In the zebrafish, both runx2a and runx2b are expressed in osteoblasts and chondrocytes, although with higher levels in osteoblasts (Eames et al., 2012). In the medaka, both sox9a and sox9b are involved in chondrogenic differentiation (Wang et al., 2020). We selected three genes known to be expressed along with osteoclast activity: the rankl gene, an osteoclast activator synthesized by osteoblasts (Imangali et al., 2021), and ctsk and acp5 genes that are two classical markers of osteoclast remodeling activity (To et al., 2015). Note that ctsk is also expressed at early stages of skeletal development in chondrocytes and perichondrium/osteoblast cells in the zebrafish (Petrey et al., 2012; Sharif et al., 2014). Regarding estrogen signaling, we used both duplicates of the estrogen receptor beta erb1 and erb2 (due to the teleost-specific whole genome duplication) and the only known copy for the estrogen alpha receptor era that all have previously been identified in Dicentrarchus labrax (Halm et al., 2004), both genes of membrane G protein-coupled estrogen receptor gpera and gperb identified in Dicentrarchus labrax (Pinto et al., 2018) and the five estrogen receptor-related receptors (erra, errb1 and errb2, errga and errgb) isolated from Dicentrarchus labrax databases.
RNA extraction and reverse transcription
RNA extraction was performed on whole larvae, using the total RNA extraction kit which includes a DNase step (Nucleospin® RNA, Macherey-Nagel, Germany). Quantity and purity of extraction products were verified using a UV spectrophotometer (NanoDrop™ One/OneC Spectrophotometer, Thermo Scientific, Waltham, MA, USA). RNA quality was checked using Bioanalyzer 2100 and RIN levels were all above 8. Reverse transcription was performed using one microgram of RNA using the qScript™ cDNA SuperMix (Quanta Biosciences™) providing all necessary components for first-strand synthesis: buffer, oligo(dT) primers, random primers and qScript reverse transcriptase.
Quantitative real-time polymerase chain reaction
Real time q-PCR conditions were as follows: 2 min denaturation at 95°C followed by 35 cycles (95°C for 30 s, 61°C for 45 s and 72°C for 1 min) followed by a final elongation step at 72°C for 4 min. All measurements were run in triplicates, and no-template control (water) Ct was above 40. The reference genes 18S, EFa and L13 were tested according to previous studies performed in seabass (Mitter et al., 2009). According to Normfinder stability analysis (Andersen et al., 2004), L13 and EFa did not fit the stability criteria and 18S was the most stable reference gene. Therefore, relative mRNA levels were normalized to 18S rRNA levels and expressed as ΔΔCt (Ct, threshold cycle number) as described by (Pfaffl, 2001) using solvent control as a reference for treatment effect comparison, or 6-dph condition as a reference for longitudinal analysis of mineralization-related gene expression. Primer sequences and efficiencies are given in Table 1. Gene expressions with Ct greater than 32 were considered under the limit of quantification, as was the case for era, gpera and errgb genes in both the 6-10-17 dph longitudinal analysis and the analysis of E2 and BPA treatment effects (data not shown). These genes are not further discussed.
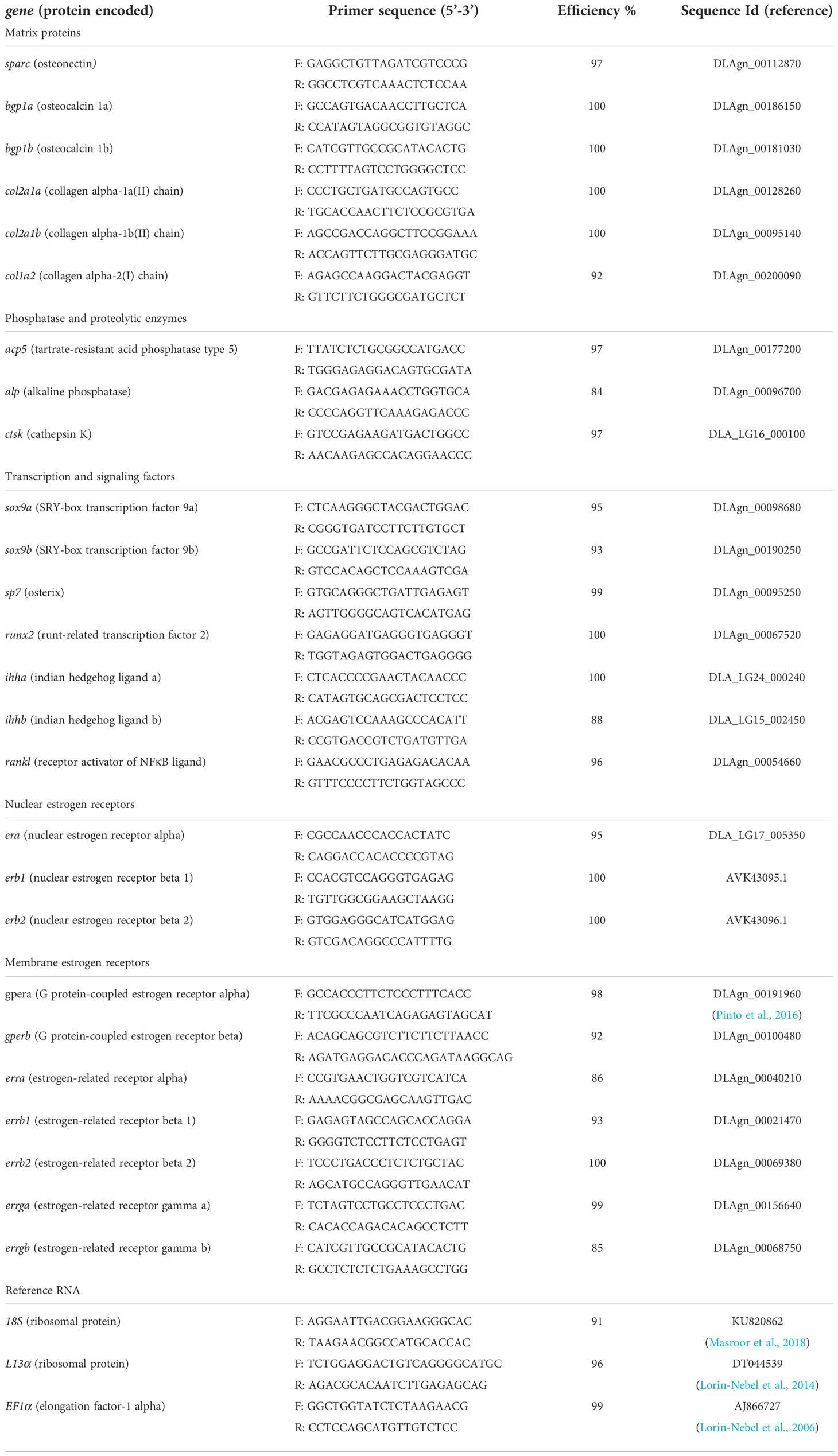
Table 1 Primers used for the analysis of gene expression by quantitative RT-PCR and their efficiency.
Estrogen response element identification
The putative presence of Estrogen Response Elements (EREs) in the non-coding regions surrounding candidate genes was assessed by automatic identification of the ERE consensus motifs (Bourdeau et al., 2004): 5’ AGGTCA nnn TGACCT 3’. On each locus, non-coding regions (10kb before and 5kb after the transcription start) were extracted from the seabass genome and given as input to EREfinder (Anderson and Jones, 2019), which scans the sequences for perfect or close to perfect EREs. EREfinder was used with a 15b-wide sliding window, sliding one base at a time to calculate a dissociation constant (Kd) specific to the estrogen receptor β (rat ERβ). EREfinder reports the inverse of Kd, so that larger values represent stronger binding. For each locus, only the EREs with 1/Kd values higher than 0.08 were kept. These EREs correspond to those with one perfect half-site and only one substitution (AT - CG) on the other half.
Statistics
Statistical analyses were performed on GraphPad Prism (version 6, GraphPad Software Incorporated, La Jolla, CA 268, USA). Since data did not fit normality or homoscedasticity assumptions, differences between groups were tested using the nonparametric Kruskal-Wallis test followed by Dunn’s multiple comparison test. Multiplicity adjusted p-value are given, accounting for familywise error rate due to multiple pairwise comparisons. The strength and direction of the linear relationship between fish standard length and head mineralization level was tested using the nonparametric Spearman correlation test (p<0.05). To compare the effect of treatment on mineralization levels, nonparametric ANCOVA was performed to test differences between predicted non-linear regression curves using the r package fANCOVA (0.6-1). Three pairwise comparisons were made for each data set. Therefore, the significance threshold was adjusted to p <0.017 according to the Bonferroni correction.
Results
Morphological and molecular aspects of mineralization in control rearing conditions
Only the otoliths, cleithrum and jaws are mineralized at 12 dph in control conditions, similarly to the previous description of (Darias et al., 2010) (Figure 1A). In addition to these structures, the parasphenoid, branchial arches and basioccipital bones are mineralized at 16 dph (Figure 1C). At these stages, the vertebrae are not visible as they are not yet mineralized under our rearing conditions (Figure 1).
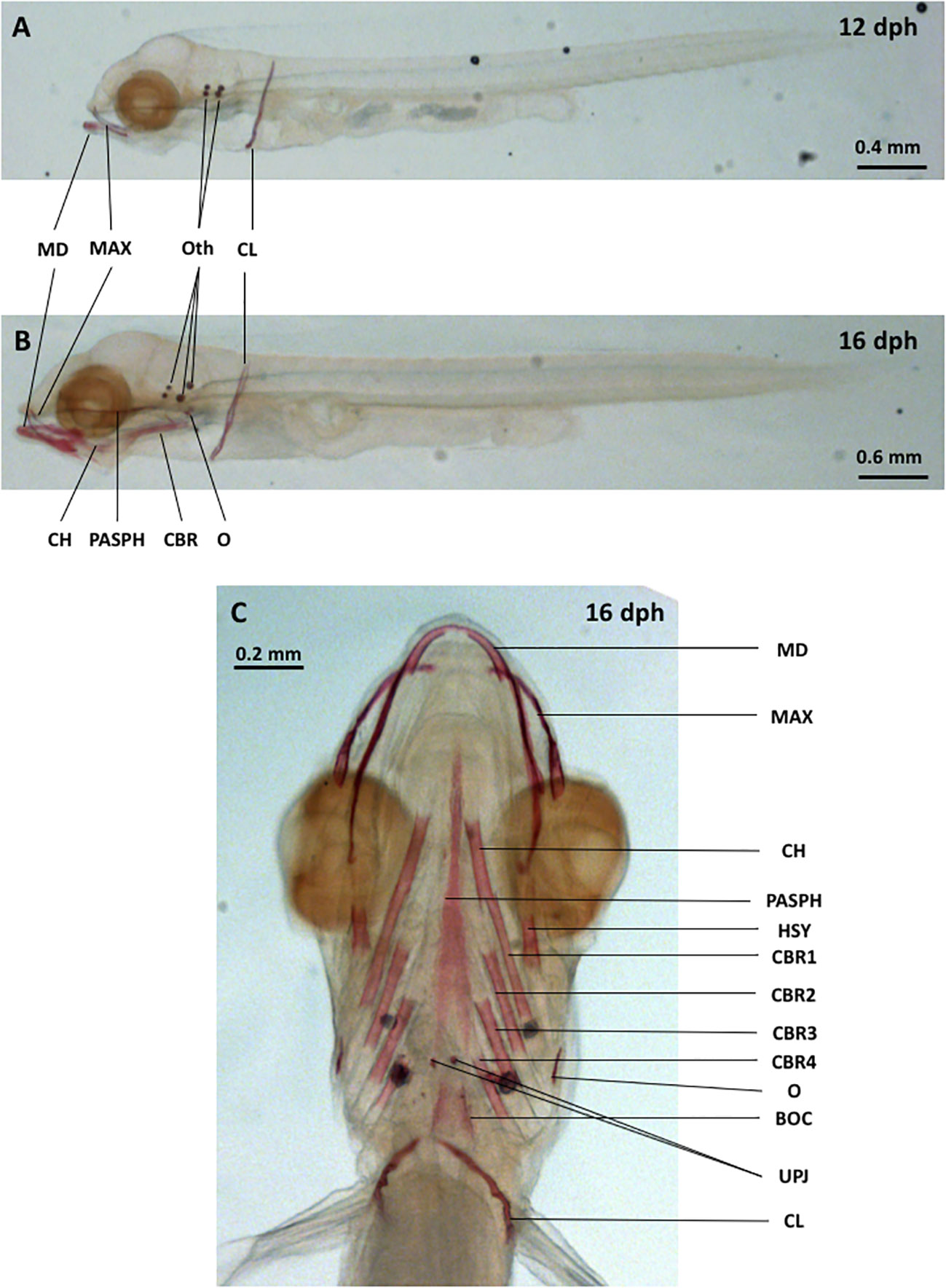
Figure 1 Lateral views of D. labrax larvae at 12 dph [(A) 5.4 mm SL] and 16 dph [(B) 6.2 mm SL]. Ventral view of D. labrax osteocranium at 16 dph [(C) 7.1 mm SL]. BOC, basioccipital; CBR 1-4, ceratobranchial 1-4; CH, ceratohyal; CL, cleithrum; HSY, hyosymplectic; MAX, maxillary; MD, mandibular; O, opercular; Oth, otholiths; PASPH, parasphenoid; UPJ, upper pharyngeal jaw. Bone nomenclature was assigned according to Gluckmann et al., 1999 and Kužir et al., 2004.
Three larval developmental time points were chosen to compare the expression levels of selected genes involved in early skeletal initiation in a first cohort: (i) 6 dph, when only the cleithrum and maxillary have started their mineralization; (ii) 10 dph, when the mandibular has started its mineralization; (iii) 17 dph, when the mineralization of many other cephalic structures is undergoing (Table 2).
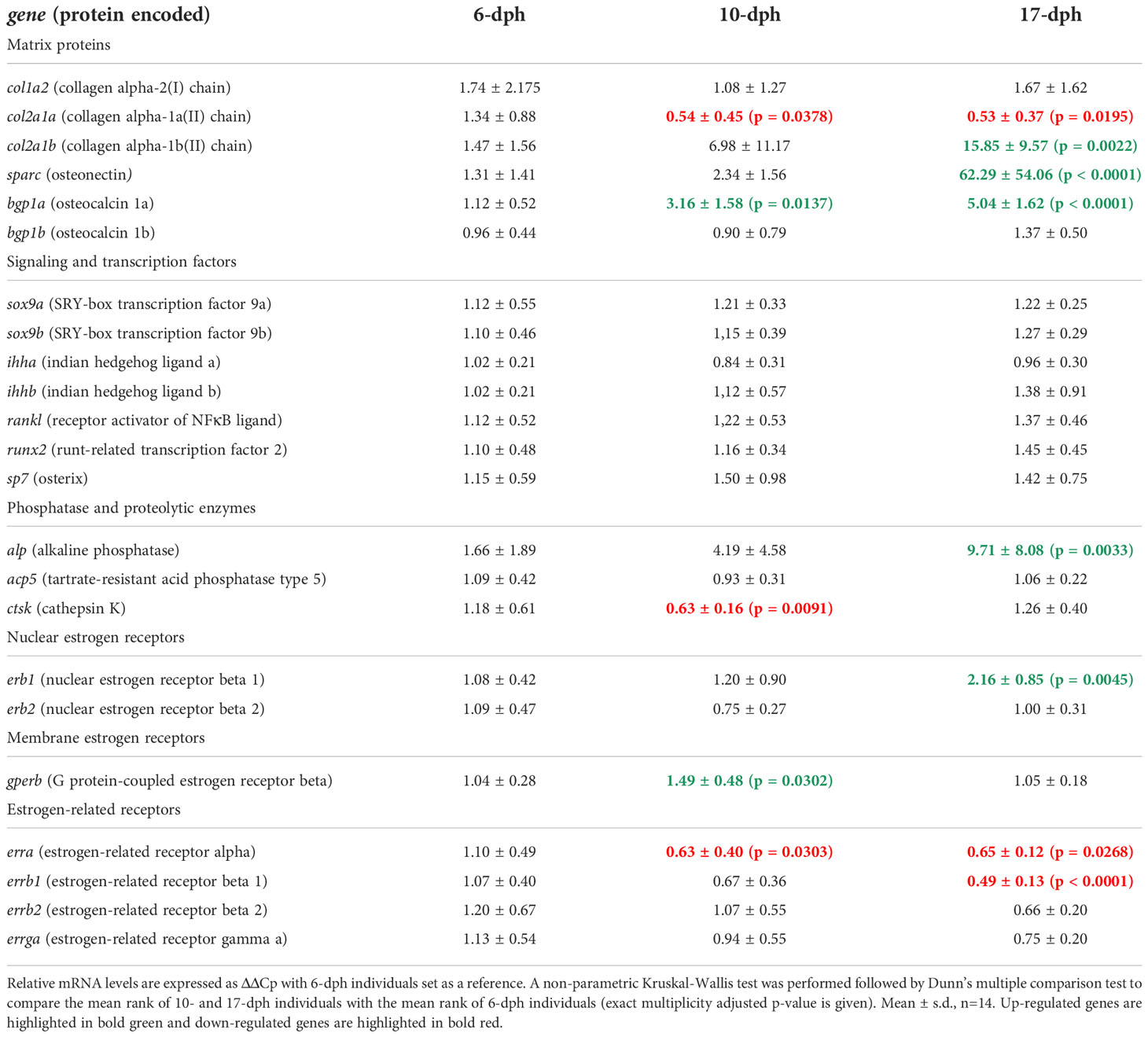
Table 2 Longitudinal analysis of mineralization-related genes and estrogenic endocrine control related genes mRNA expression according to developmental timing in 6-, 10- and 17-dph individuals maintained in control rearing conditions (first cohort).
While col1a2 expression appears constant over time, the chondrocyte-associated col2a1a showed a decrease in expression level between 6 and 10 dph which was maintained at 17dph, while the perichondrium/osteoblast-associated col2a1b increased expression between 6 and 17 dph. These patterns were consistent with the timing of mineralization initiation in the head of the larvae. The three genes sparc, bgp1a and alp exhibited an increase in their expression levels detected at 17 dph, with a start as early as 10 dph for bgp1a (Table 2). Regarding ihha and ihhb, sox9a and sox9b, runx2 and sp7 genes, no significant transcriptional regulation of these signaling and transcription factors was detected according to developmental timing (Table 2). Though a 1.9-fold transient decrease in ctsk mRNA expression was observed at 10 dph, when compared with its expression at 6 dph, the expression of rankl and acp5 genes did not significantly change in the earliest phase of skeletal mineralization (Table 2).
Regarding estrogenic signaling related genes, we detected an increased expression of erb1 between 10 and 17 dph, but no variation of erb2 in this time window (Table 2). Concerning the gper family, only gperb was detected and its mRNA expression increased between 6 and 10 dph. Among the five estrogen-related receptors, errb2 and errga expression is constant over time, erra and errb1 expression decreases at 10 dph and 17 dph, respectively.
Comparisons between gene expression levels show that extracellular matrix genes have the highest levels of expression, i.e. the three structural collagen genes (col1a2, col2a1a, col2a1b) and sparc are 10 to 100 times more represented than the others (Figure 2), which highlights the intense secretory activity of skeletal cells (chondrocytes and osteoblasts) over this period. In addition, the estrogen-related receptors erra, errb1 and errga are about one order of magnitude more expressed than the nuclear estrogen receptors erb1 and erb2 (Figure 2) suggesting their potential functioning in ER signaling modulation at these developmental stages, despite not identifying in which cell types they are expressed.
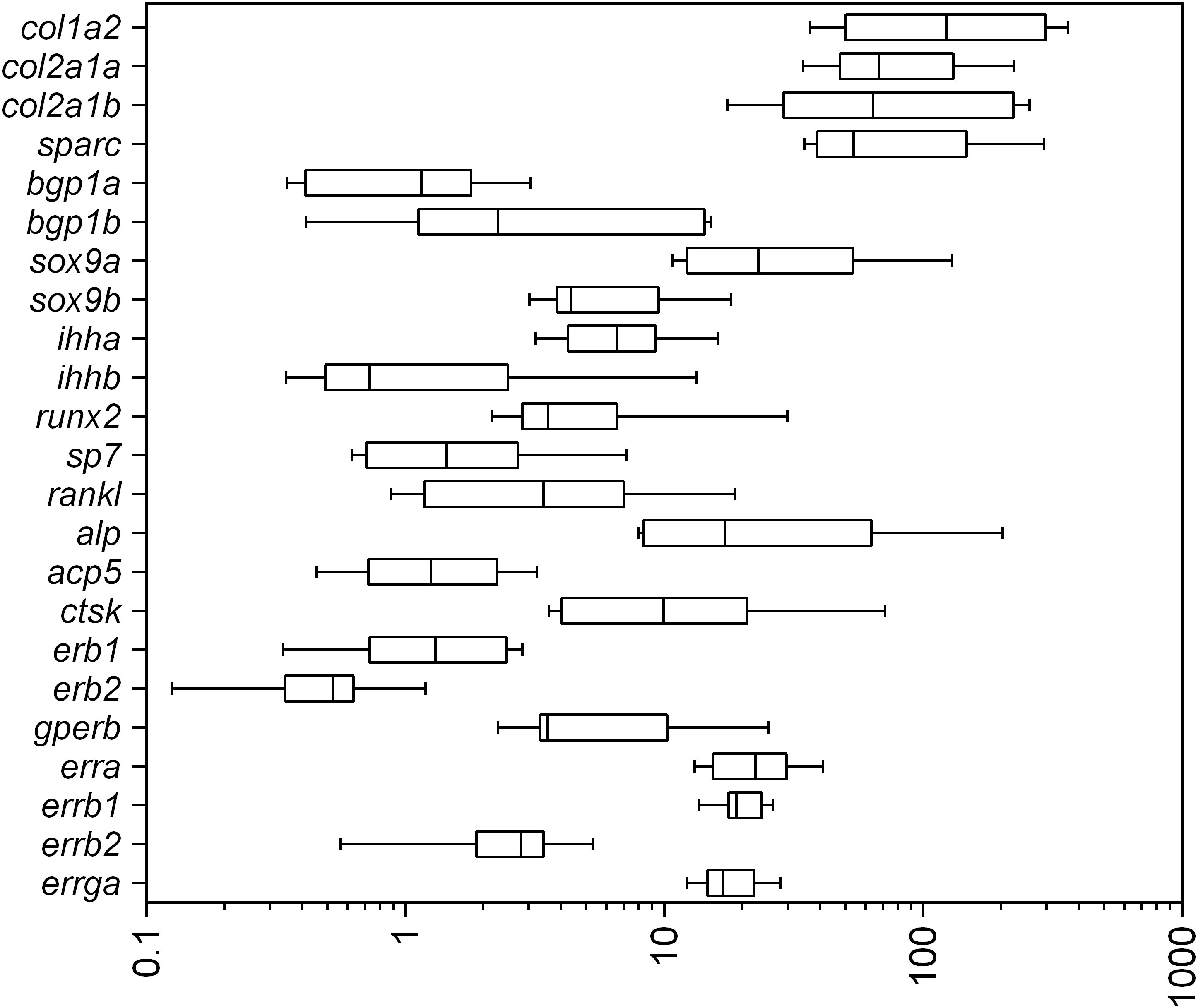
Figure 2 Relative mRNA levels of mineralization-related genes and estrogen-related endocrine control in 16-dph (n=8-10) individuals maintained in control rearing conditions (second cohort). Relative mRNA levels are expressed as ΔΔCp with the bgp1a gene used as a reference. In boxplots, hinges indicate first and third quartiles; whiskers indicate the min and max values, and horizontal lines indicate the median.
E2 and BPA exposures disrupt morphological and molecular aspects of larval mineralization
Variation of head mineralization levels in 16-dph larvae is represented in Figure 3. Head mineralization score is positively correlated to the standard length for every treatment with Spearman correlation coefficient values ranging between 0.68 and 0.88 (p<0.001). Larvae exposed to E2 40 ng.L-1 for 4 days exhibit significantly higher head mineralization levels compared to the solvent control, along the full range of observed sizes (from 0.55 to 0.75 cm; nonparametric ANCOVA, p<0.01, Figure 3A). Conversely, no significant difference in head mineralization level was statistically detected between larvae exposed to E2 0.4 ng.L-1, BPA 1.6 and 160 μg.L-1 compared to the solvent control.
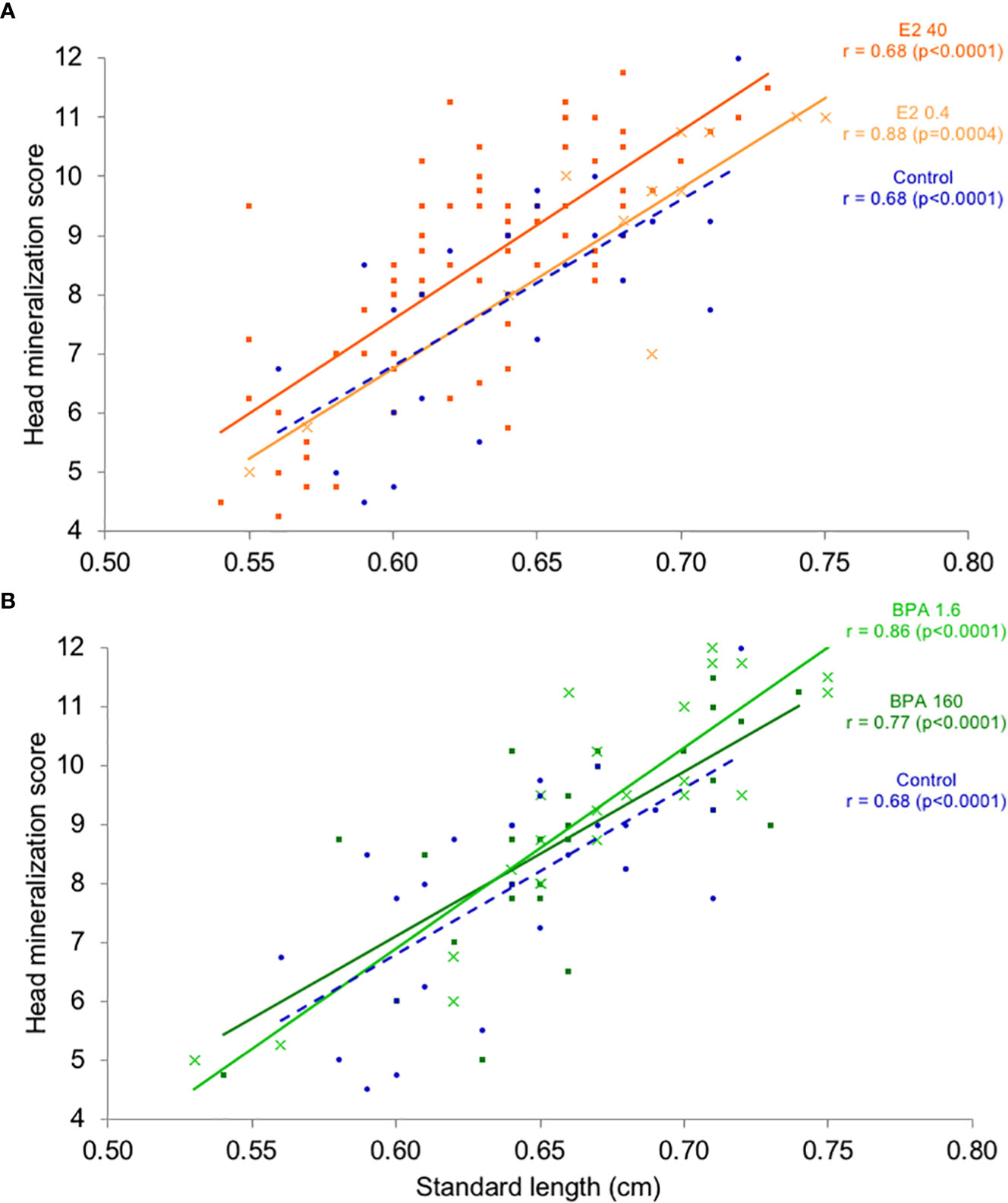
Figure 3 Distribution of head mineralization level as a function of the fish standard length (cm) in 16-dph individuals exposed for 4 days at nominal concentrations of E2 0.4 and 40 ng.L-1 (A), BPA 1.6 and 160 μg.L-1 (B) and ethanol 0.0008% (v/v) as a solvent control (A, B). Linear regression curves are shown to illustrate the linear relationship. Since data does not fit normality assumption, a non-parametric Spearman’s correlation coefficient r was calculated to evaluate the strength and the direction of the monotonic relationship. A nonparametric analysis of covariance was used to test the difference between two treatments. The p-value is given in the table (ns, not significant according to the Bonferroni adjusted p<0.017 threshold).
No significant effect of E2 0.4 ng.L-1 treatment could be detected on the expression of any of the selected skeletogenesis-related genes. Conversely, the E2 40 ng.L-1 induced a significant increase in expression of 9 out of 16 genes (Figure 4), four of them are expressed by osteoblasts (col1a2, col2a1b, sparc and runx2), one (ihha) is expressed by hypertrophic chondrocytes to activate osteoblast differentiation, both sox9a and sox9b are expressed during chondrogenesis and two genes (rankl, ctsk) are expected to be expressed by osteoclasts. The expression of skeletal genes is significantly modified only with high concentrations of E2 and seems to act mostly on bone secreting (osteoblasts) and degrading (osteoclasts) cells.
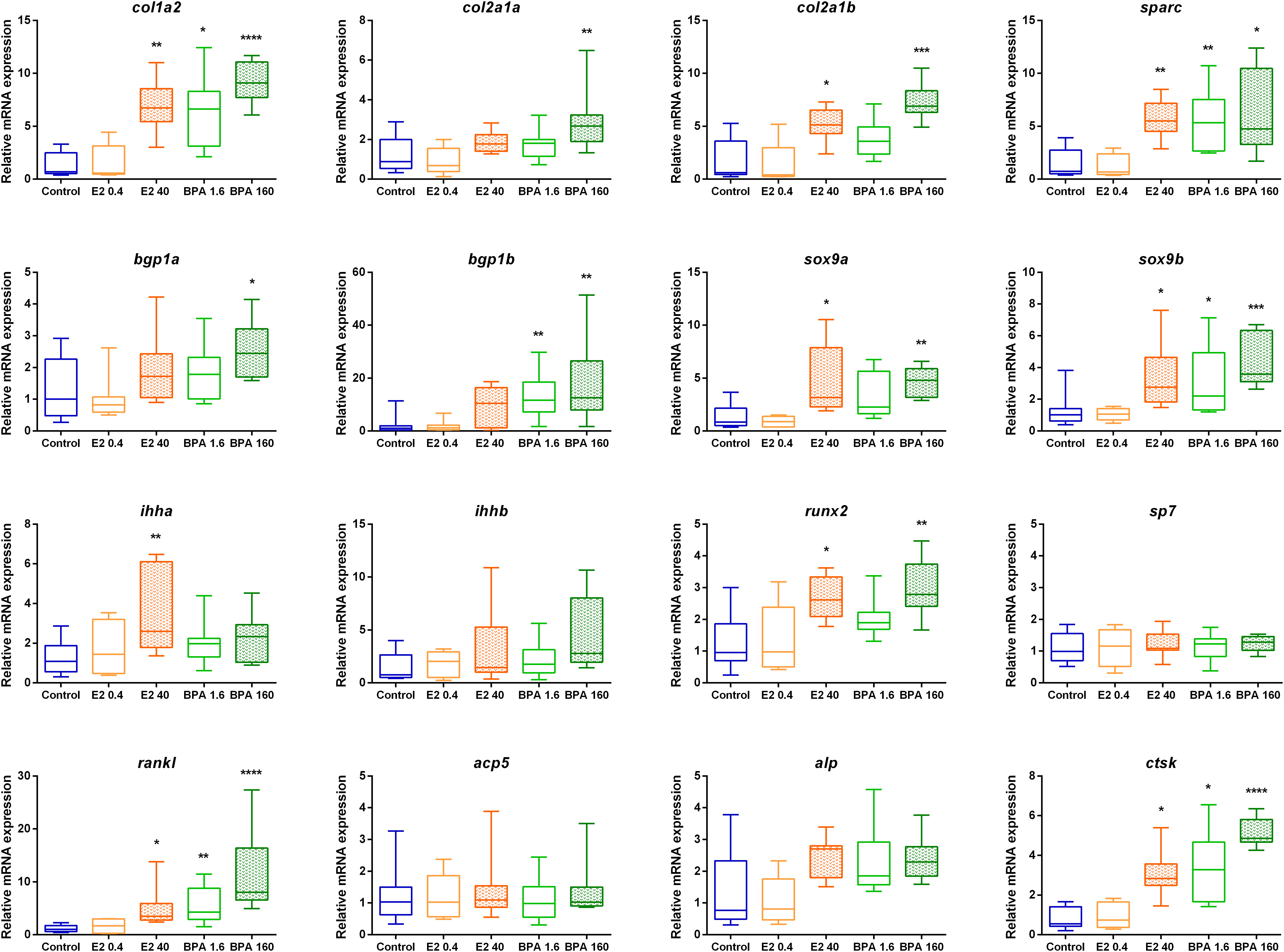
Figure 4 Relative mRNA expression of mineralization-related genes in 16-dph individuals exposed for 4 days at nominal concentrations of E2 0.4 and 40 ng.L-1, BPA 1.6 and 160 μg.L-1, and ethanol 0.0008% (v/v) as a solvent control. Relative mRNA levels are expressed as ΔΔCp with solvent control as a reference (n=8-10 individuals). In boxplots, hinges indicate first and third quartiles; whiskers indicate the min and max values, and horizontal lines indicate the median. Difference between treatments was tested using the non-parametric multiple comparison test Kruskal-Wallis, followed by Dunn’s post-hoc test. Black stars indicate significant differences compared to the solvent control (multiplicity adjusted p-value: *p < 0.05; **p < 0.01, ***p < 0.001, ****p < 0.0001).
In contrast, we observed a general tendency towards a dose-dependent up-regulation of most tested genes in both BPA exposure experiments (Figure 4). Exposure to BPA 1.6 μg.L-1 induced a significant over-expression of 6 of the 16 studied genes: three extracellular matrix markers (col1a2, bgp1b and sparc), the transcription factor sox9b and the osteoclast markers rankl and ctsk. This effect is quite similar to the observed results of E2 40 ng.L-1 treatment. In contrast, the exposure of BPA 160 μg.L-1 has a significant effect for 11 of the 16 genes studied, and often with a stronger overexpression than the values obtained after E2 40 ng.L-1 exposure (Figure 4; Table 3). The BPA treatment with high concentration therefore has a stronger impact on gene expression for all skeletal cells, both quantitatively and qualitatively.
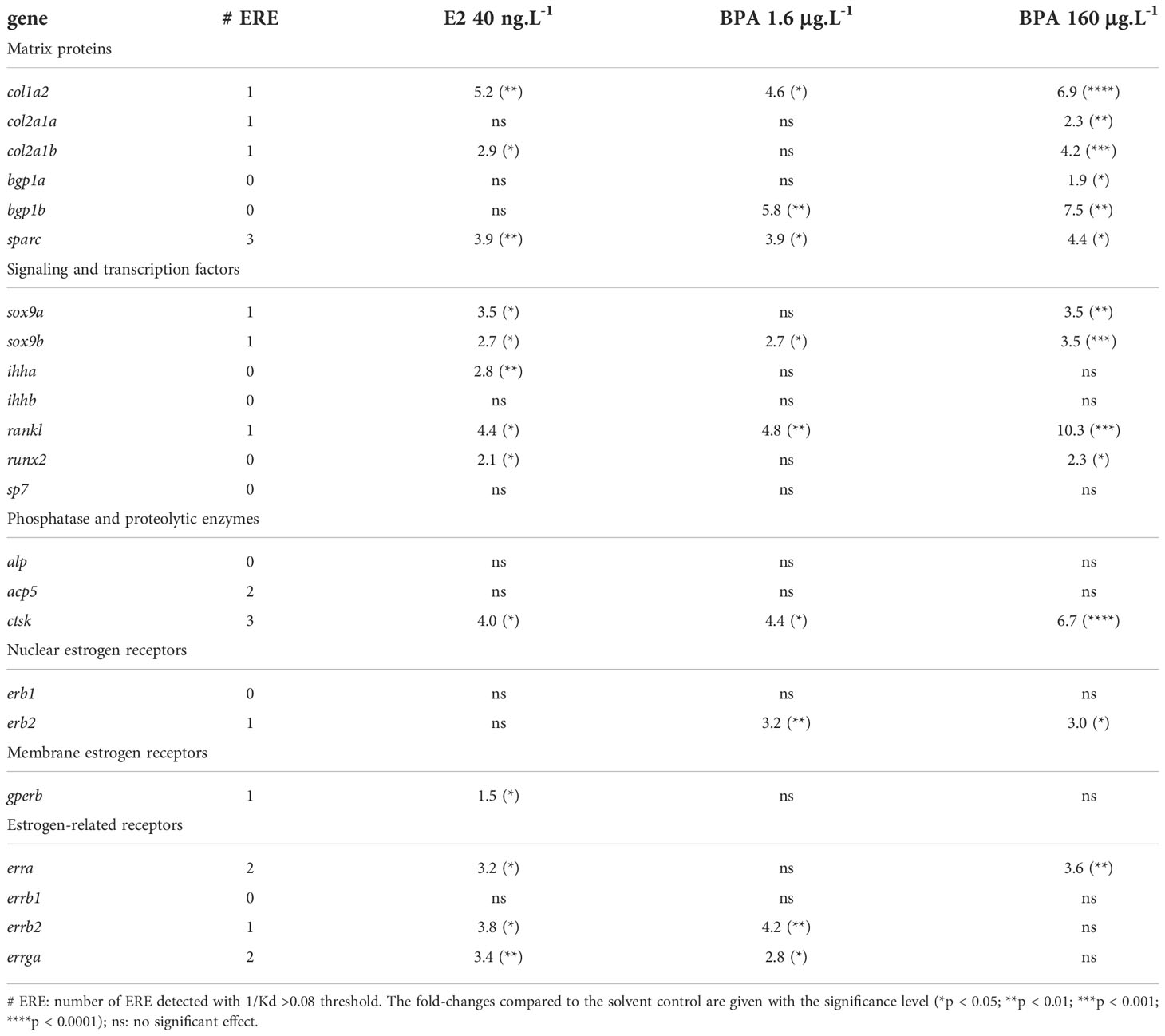
Table 3 ERE consensus motif detection and summary of the significant effects of E2 and BPA treatments on the expression of the selected genes.
E2 and BPA exposures impact differentially the expression of er, gper and err gene families
No significant effect of the E2 0.4 ng.L-1 treatment could be detected on the expression of any of the ER or ERR encoding genes (Figure 5). Conversely, E2 40 ng.L-1 induced a significant overexpression of gperb, erra, errb2 and errga. This exposure condition also induced a weak (about 2-fold) but non-significant up-regulation of erb1 and erb2 (Table 3). Three estrogen-related receptors were induced in response to BPA: errb2 and errga at BPA 1.6 μg.L-1, and erra at BPA 160 μg.L-1. In contrast to the results obtained with E2 treatments, gperb mRNA expression was not significantly modified by BPA exposure but the expression of erb2 was significantly overexpressed compared to solvent control in response to both tested concentrations of BPA. As a consequence, in both cases, the effects of exposure might be amplified by a putative better availability of estrogen receptors (Gperb with E2, or ERβ with BPA), and of estrogen-related receptors.
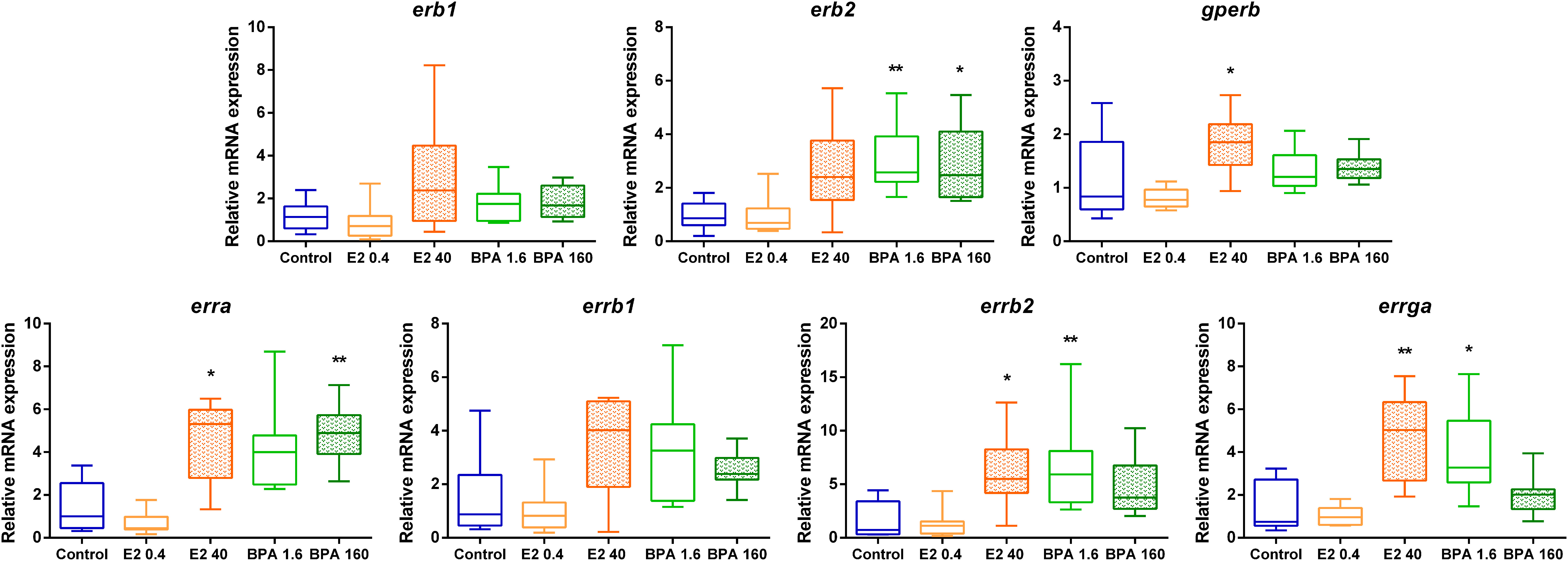
Figure 5 Relative mRNA expression of nuclear and membrane estrogen receptor genes and estrogen-related receptor genes in 16-dph individuals exposed for 4 days at nominal concentrations of E2 0.4 and 40 ng.L-1, BPA 1.6 and 160 μg.L-1, and ethanol 0.0008% (v/v) as a solvent control. Relative mRNA levels are expressed as ΔΔCp with solvent control as a reference (n=8-10 individuals). In boxplots, hinges indicate first and third quartiles; whiskers indicate the min and max values, and horizontal lines indicate the median. Difference between treatments was tested using the non-parametric multiple comparison test Kruskal-Wallis, followed by Dunn’s post-hoc test. Black stars indicate significant differences compared to the solvent control (multiplicity adjusted p-value: *p < 0.05; **p < 0.01).
Putative EREs are found in most of up-regulated skeletal genes
The estrogen-ER genomic signaling pathway involves the binding of ERs to ERE in the promoter of the target genes. ERRs can also bind the ERE and they may act as modulators of this genomic pathway (Acconcia et al., 2015; Tanida, 2022). We tested the presence of putative ERE consensus sequences in the non-coding sequences of all 23 genes, with 1/Kd>0.08 threshold: nine genes displayed one putative ERE (col1a2, col2a1a, col2a1b, sox9a and sox9b, rankl, erb2, gperb, errb2) while acp5, erra and errga had two putative EREs and sparc and ctsk had three in their vicinity (Table 3). In these conditions, no ERE was identified in the 15kb surrounding the promoter region for bgp1a and bgp1b, ihha and ihhb, runx2, sp7, alp, erb1 or errb1. Putative EREs were therefore identified in the vicinity of 14 of the tested genes, of which 13 showed modified expression after E2 and/or BPA exposure. Only acp5 showed no significant change in its expression after E2 or BPA exposure despite the detection of two putative EREs. The expression of col2a1a and erb2 was not altered in the E2 treatments but increased in the BPA treatments. Of the 9 genes without any detected ERE, 5 genes (ihhb, sp7, alp, erb1 and errb1) display no response to E2 and BPA treatments, supporting independence of their transcriptional regulation from a genomic estrogen signaling. Despite the absence of putative ERE, two genes (bgp1a and bgp1b) have their expression induced by the BPA treatments only, the ihha gene had its expression induced by E2 40 ng.L-1, and runx2 had its expression increased at E2 40 ng.L-1 and BPA 160 μg.L-1.
Discussion
Early larval stages of skeletal development are impacted by estrogenic compounds
Since early developmental stages are often more sensitive to xenobiotic exposure, studying the potential precocious deleterious effects of xenobiotics is of great interest in ecotoxicology and aquaculture. The potential physiological disturbance due to estrogenic compounds exposure occurring at early life stages, when blood estrogen concentrations are low or undetectable, have to be addressed, because low concentration of estrogens may potentially lead to significant endocrine disruption or other developmental failures (Chin et al., 2018). In non-model species of ecological interest, early life stages are generally less considered due to rearing or other technical difficulties, yet in the European seabass Dicentrarchus labrax, as in other fish species, critical developmental steps for survival occur (Chatain and Dewavrin, 1989): mouth opening and exotrophy start around 4-5 dph (Barnabé, 1974; Cucchi et al., 2012) and the correct establishment of the swim bladder occurs between 3 and 7 dph (Chatain, 1986). In our study, the rates of swim bladder anomalies observed were in the range of those commonly observed in aquaculture in Dicentrarchus labrax (Chatain and Dewavrin, 1989; Miller, 2009). Its early skeletal development is cephalic and is linked with the function of collecting food and the development of the respiratory function. We detected an acceleration of head mineralization as a result of specifically the E2 40 ng.L-1 treatment (not detected in any BPA treatment, or lower E2 treatment), showing that exposure to waterborne estrogens has an impact on fish skeletal development even at early larval stages.
In the present study, despite the lack of phenotypical effects in most of our 4-day treatments, results obtained after exposure to E2 (40 ng.L-1) and BPA (1.6 and 160 µg.L-1) suggest that both molecules exert a stimulatory effect on skeleton-related genes, in a dose dependent manner. The identification of putative ERE in the promoters of most of the up-regulated skeleton genes supports the hypothesis of genomic regulatory pathways as a result of E2 and BPA exposure. The concentration of E2 40 ng.L-1 induced a significant increase in expression of genes known to be expressed by osteoblasts and hypertrophic chondrocytes, involved in osteoblast differentiation. This transcriptional induction of osteoblast-linked gene expression suggests a stimulation of osteoblast activity, but also of osteoblast differentiation (runx2 and ihha are early cell differentiation markers), having as a physiological consequence more head mineralization (Figures 3A, 4, 6). In addition, the concentration of E2 40 ng.L-1 also induced a significant increase in expression of genes known to be expressed by osteoclasts involved in bone remodeling. A lower concentration of E2 did not induce such changes in gene expression, suggesting that estrogenic signal has to be strong to have an effect at early stages where the level of estrogen receptors, and therefore estrogen sensitivity, is low. Interestingly, ihha and runx2 do not have putative ERE while both their expression was increased upon treatment with E2 40 ng.L-1. This up-regulation could be indirect and mediated by other transcription factors, or could be due to non-genomic regulation pathways (Björnström and Sjöberg, 2005) including, at least in part, Gperb (Pinto et al., 2014b).
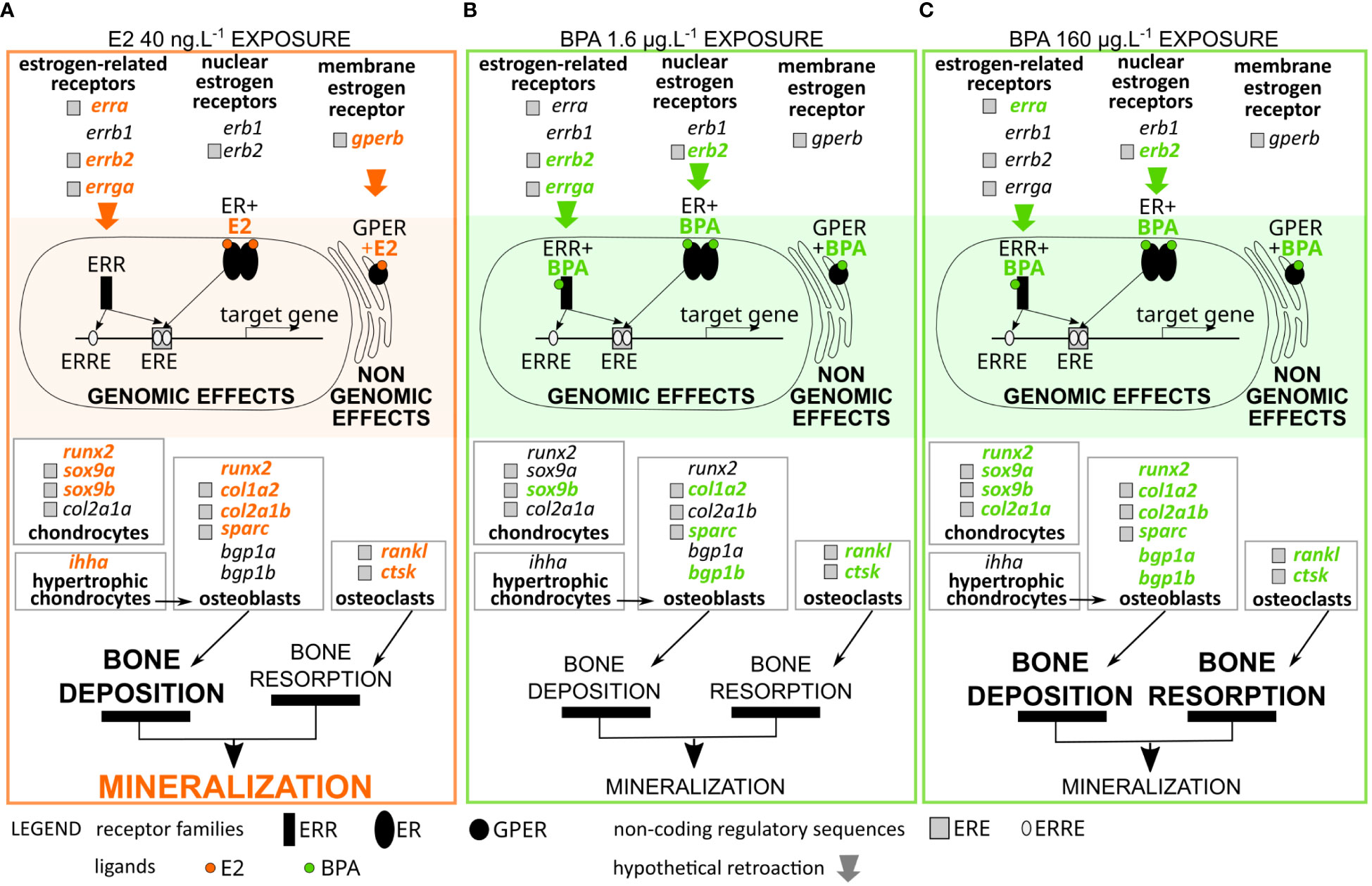
Figure 6 Transcriptional and physiological effects of E2 exposure (orange color, (A) 40 ng.L-1), and BPA exposure (green color, (B) 1.6 µg.L-1, (C) 160 µg.L-1). Bold and colored gene names in each panel represent transcriptional upregulation in this condition. Grey squares with gene names represent the putative presence of ERE. Colored arrows represent putative retroaction of the exposure on the signaling pathway, on either its genomic or non-genomic aspects. Bone deposition and resorption, and mineralization, are sized following according to the putative effects of each exposure onto these physiological processes (see discussion for details).
Compared to E2, BPA treatment exerted an overlapping but wider positive effect on the transcriptional expression of skeletal genes. Notably, the matrix proteins encoding genes col2a1a, bgp1a and bgp1b were upregulated only by BPA and not by E2 (Figures 4, 6). These genes are considered to be chondrocytes and osteoblasts derived (Dale and Topczewski, 2011; Eames et al., 2012; reviewed in Leurs et al., 2021) though in situ hybridization techniques would help to localize mRNA expression to specific cell types in the seabass. However, the significant stimulatory effect of BPA was not correlated to any significant change in head mineralization (Figure 3B). The lack of significant induction of major precocious signaling and transcription factors (namely sox9a, ihha, ihhb, runx2) may explain that the head of larvae exposed to BPA 1.6 μg.L-1 was not more mineralized than in controls. In contrast, BPA 160 μg.L-1 exposure resulted in the same or stronger transcriptional activation of mineralization-related genes, as compared to E2 40 ng.L-1 (Table 3). However, a stronger activation of osteoclast-related genes was detected in BPA 160 μg.L-1 exposed larvae, as shown by higher levels of transcription of rankl (10.3-fold changes versus 4.4-fold in E2 40 ng.L-1) and ctsk (6.7-fold changes versus 4.0-fold in E2 40 ng.L-1) (Table 3). This suggests that the balance between bone mineralization (due to chondrocytes and osteoblast markers activation) and bone degradation (due to osteoclast markers activation) may be differentially regulated by E2 and BPA at elevated concentrations (Figure 6). To confirm this hypothesis, osteoclast activity should be validated by TRAP/ACP5 staining in larval tissues. Overall, our results suggest that E2 exerts a positive physiological effect on head mineralization and that BPA does not strictly mimic this positive effect at skeletal level, though BPA can transcriptionally induce the same genes as E2 (Figure 6).
BPA versus E2 effects
The genomic effect of both E2 and BPA may act through the ERβ1 endogenously up-regulated in larvae during the studied developmental stages (Table 2). In both BPA treatments, this genomic effect may be also amplified through ERβ2 whose expression was induced (Figure 5). However, E2 40 ng.L-1 exposure may activate or amplify a non-genomic activity as the expression level of gperb is increased (Figure 5). In mammals, GPER is expressed in osteocytes, osteoclasts, osteoblasts and chondrocytes and is involved in estrogen-induced bone growth and development (Urushitani et al., 2002; Heino et al., 2008). GPERs are known to be involved in scale mineralization in seabass (Pinto et al., 2016). It may be hypothesized that the difference between E2 and BPA effects is an over-activation of the non-genomic pathway by E2, as opposed to an over-activation of the genomic pathway by BPA. This may explain the different outcome in mineralization. Despite this difference, both molecules similarly impact erra, errb2 and errga expression levels. During bone development, ERRs were previously shown to be involved in the differentiation and functional activation of osteoblasts and osteoclasts (Bonnelye and Aubin, 2013). The fact that EREs were found in the erb2, gperb, erra, errb2 and errga promoters supports the hypothesis for a potential crosstalk between nuclear ERs and orphan ERRs in the European seabass. Indeed, the ERRs have been postulated to modulate E2 signaling by either synergizing or competing with ERs in regulating multiple shared transcriptional targets (Horard and Vanacker, 2003; Cheung et al., 2013). In contrast to ERs, ERRs assume an active state without a ligand being bound to the ligand binding domain (LBD) (Kallen et al., 2004) which makes them constitutive transcription factors when linked to their ERR-response Element (ERRE). However, BPA was suggested to modify ERRs genomic activity through its binding, making BPA also a modulator of ERR activity (Tohmé et al., 2014). In addition, the presence of ERREs was identified in human bgp (Wang and Wang, 2013). As a consequence, some observed variations between E2 and BPA exposure effects on the transcriptional gene activation, such as those of bgp1a and bgp1b, might be coming from this effect of BPA on ERRs. In mice, in silico analysis found putative ERRE sites in the promoter regions of a number of skeletal development genes, including ihh and runx2 (Cardelli et al., 2013). This could explain observed upregulations of genes without an identified ERE (ihha and runx2) in our E2 and/or BPA treatments. Our data highlight the importance of including ERRs in future research on the effects of (xeno)estrogens on physiology, given their putative interaction with estrogen signaling pathways, especially in teleost where their role is probably very underestimated.
The action of E2 and BPA also do not seem to be strictly similar on ERRs: in our experiment, the expression of erra was activated by high concentrations of both E2 and BPA, whereas errb2 and errga were activated only following E2 40 ng.L-1 and BPA 1.6 μg.L-1 exposure (Figure 5). In mouse, ERRα is highly expressed throughout the osteoblast developmental sequence and plays a physiological role in bone formation in vitro (Bonnelye et al., 2001) and has been shown to regulate the expression of sox9a/b and the downstream genes necessary for cartilage development during zebrafish embryogenesis (Kim et al., 2015). As a consequence, our variation of sox9a and sox9b expression might also result from disruption of the endogenous ERR effects. In mice, ERRγ negatively regulates chondrocyte proliferation and positively regulates matrix synthesis to coordinate growth plate organization (Cardelli et al., 2013), and its activity is thought to be dependent on Runx2 (Cardelli and Aubin, 2014). ERRγ was also previously shown to negatively regulate osteoblast differentiation and bone formation (Jeong et al., 2009). These variations effects of ERRγ on skeletal cells may also explain why E2 treatment result in more calcium deposition, while BPA treatment does not lead to this morphological effect.
This study brings new insights into the regulatory mechanisms of skeletogenesis by E2 and into the effects of waterborne exposure to BPA on early skeletal development of a marine teleost fish. More detailed insights into their effects on cell activity would require functional and cell-centered studies, in particular in situ hybridization of genes associated with mineralization for their specific cell type localization, and activity assays such as TRAP/ACP5 or ALP. Regarding the regulation of estrogenic signals, complex ligand-dependent and ligand-independent pathways could be at stake, involving the different subfamilies of estrogen receptors, with potential cross-talks. Our results suggest that the role of ERR and the interaction between estrogen-linked signaling pathways are probably very underestimated especially in teleost. Last, beside the membrane-bound and estrogen(-related) receptors, emerging data from in vitro and in silico models show that BPA binds with a significant number of hormone receptors, including androgen receptors, as well as the thyroid hormone receptor, glucocorticoid receptor, and PPARγ (Delfosse et al., 2012; MacKay and Abizaid, 2018; Cimmino et al., 2020), all of them playing a role in the development and maintenance of cartilage and bone (Zuo and Wan, 2017; Gouveia et al., 2018; Chou et al., 2021). This wider range of signalization for BPA might be another aspect explaining the differences observed between E2 and BPA treated larvae.
Data availability statement
The original contributions presented in this study are included in the article and Supplementary Material, further inquiries can be directed to the corresponding authors.
Ethics statement
The animal study was reviewed and approved by the french Comité d’éthique en expérimentation animale Languedoc Roussillon CEEA-036 (APAFIS permit no. 9045-201701068219555).
Author contributions
EF, MD-T and CM-M conceived the project, performed the experiments, analyzed the data, and wrote the manuscript. EG, GD, SL contributed to conception and design of the study, management of animal exposure. EF, CM-M, EP and CB contributed to qPCR and Alizarin red staining analyses. NL contributed to the phylogeny analyses and ERE finding. All authors contributed to read and approved the submitted version.
Funding
The project was supported by the Centre Méditerranéen de l’Environnement et de la Biodiversité (ANR- 10-LABX-0004 Skelestro project).
Acknowledgments
Data used in this study were produced using the High-Debit PCR, Génotypage-Séquençage (Gen-Seq) and Montpellier Bioinformatics Biodiversity (MBB) platforms supported by the LabEx CeMEB, an ANR “Investissements d’avenir” program (ANR- 10-LABX-04-01).
Conflict of interest
The authors declare that the research was conducted in the absence of any commercial or financial relationships that could be construed as a potential conflict of interest.
Publisher’s note
All claims expressed in this article are solely those of the authors and do not necessarily represent those of their affiliated organizations, or those of the publisher, the editors and the reviewers. Any product that may be evaluated in this article, or claim that may be made by its manufacturer, is not guaranteed or endorsed by the publisher.
Supplementary material
The Supplementary Material for this article can be found online at: https://www.frontiersin.org/articles/10.3389/fmars.2022.1062334/full#supplementary-material
Supplementary Figure 1 | Experimental setup for treatments: two Mac Donald jars were connected to allow for an optimal larval density per jar. The 5-L glass beaker serves as a transitional container that collects the overflow from both jars but also as a receiver of the continuous recontamination provided by the peristaltic pump. A recirculation pump returns the contaminated water to the 2 jars. The flow rate of the whole system is regulated by quarter-turn valves.
Supplementary Figure 2 | Quantification of the level of head mineralization from alizarin red staining: comparison between a TS larva versus an E2 40-treated larva of the same size. For each larva, the picture of its stained head and the corresponding mineralization score table are given as an example, to illustrate the method.
References
Acconcia F., Pallottini V., Marino M. (2015). Molecular mechanisms of action of BPA. Dose-Response 13, 155932581561058. doi: 10.1177/1559325815610582
Aceto J., Nourizadeh-Lillabadi R., Marée R., Dardenne N., Jeanray N., Wehenkel L., et al. (2015). Zebrafish bone and general physiology are differently affected by hormones or changes in gravity. PloS One 10, e0126928. doi: 10.1371/journal.pone.0126928
Almeida M., Laurent M. R., Dubois V., Claessens F., O’Brien C. A., Bouillon R., et al. (2017). Estrogens and androgens in skeletal physiology and pathophysiology. Physiol. Rev. 97, 135–187. doi: 10.1152/physrev.00033.2015
Andersen C. L., Jensen J. L., Ørntoft T. F. (2004). Normalization of real-time quantitative reverse transcription-PCR data: a model-based variance estimation approach to identify genes suited for normalization, applied to bladder and colon cancer data sets. Cancer Res. 64, 5245–5250. doi: 10.1158/0008-5472.CAN-04-0496
Anderson A. P., Jones A. G. (2019). Erefinder: Genome-wide detection of oestrogen response elements. Mol. Ecol. Resour 19, 1366–1373. doi: 10.1111/1755-0998.13046
Avaron F., Hoffman L., Guay D., Akimenko M. A. (2006). Characterization of two new zebrafish members of the hedgehog family: atypical expression of a zebrafish indian hedgehog gene in skeletal elements of both endochondral and dermal origins. Dev. Dyn 235, 478–489. doi: 10.1002/dvdy.20619
Barnabé G. (1974). “Mass rearing of the bass Dicentrarchus labrax l,” in The early life history of fish. Ed. Blaxter J. H. S. (Berlin, Heidelberg: Springer Berlin Heidelberg), 749–753. doi: 10.1007/978-3-642-65852-5_62
Björnström L., Sjöberg M. (2005). Mechanisms of estrogen receptor signaling: convergence of genomic and nongenomic actions on target genes. Mol. Endocrinol. 19, 833–842. doi: 10.1210/me.2004-0486
Boglione C., Gavaia P., Koumoundouros G., Gisbert E., Moren M., Fontagné S., et al. (2013). Skeletal anomalies in reared European fish larvae and juveniles. part 1: normal and anomalous skeletogenic processes. Rev. Aquacult 5, S99–S120. doi: 10.1111/raq.12015
Bonnelye E., Aubin J. E. (2013). An energetic orphan in an endocrine tissue: a revised perspective of the function of estrogen receptor-related receptor alpha in bone and cartilage. J. Bone Miner Res. 28, 225–233. doi: 10.1002/jbmr.1836
Bonnelye E., Merdad L., Kung V., Aubin J. E. (2001). The orphan nuclear estrogen receptor-related receptor alpha (ERRalpha) is expressed throughout osteoblast differentiation and regulates bone formation in vitro. J. Cell Biol. 153, 971–984. doi: 10.1083/jcb.153.5.971
Bourdeau V., Deschênes J., Métivier R., Nagai Y., Nguyen D., Bretschneider N., et al. (2004). Genome-wide identification of high-affinity estrogen response elements in human and mouse. Mol. Endocrinol. 18, 1411–1427. doi: 10.1210/me.2003-0441
Brion F., Le Page Y., Piccini B., Cardoso O., Tong S.-K., Chung B., et al. (2012). Screening estrogenic activities of chemicals or mixtures in vivo using transgenic (cyp19a1b-GFP) zebrafish embryos. PloS One 7, e36069. doi: 10.1371/journal.pone.0036069
Cardelli M., Aubin J. E. (2014). ERRγ is not required for skeletal development but is a RUNX2-dependent negative regulator of postnatal bone formation in male mice. PloS One 9, e109592. doi: 10.1371/journal.pone.0109592
Cardelli M., Zirngibl R. A., Boetto J. F., McKenzie K. P., Troy T.-C., Turksen K., et al. (2013). Cartilage-specific overexpression of ERRγ results in chondrodysplasia and reduced chondrocyte proliferation. PloS One 8, e81511. doi: 10.1371/journal.pone.0081511
Careghini A., Mastorgio A. F., Saponaro S., Sezenna E. (2015). Bisphenol a, nonylphenols, benzophenones, and benzotriazoles in soils, groundwater, surface water, sediments, and food: a review. Environ. Sci. pollut. Res. Int. 22, 5711–5741. doi: 10.1007/s11356-014-3974-5
Chatain B. (1986). La vessie natatoire chez Dicentrarchus labrax et Sparus auratus. Aquaculture 53, 303–311. doi: 10.1016/0044-8486(86)90361-3
Chatain B., Dewavrin G. (1989). Influence des anomalies de développement de la vessie natatoire sur la mortalité de Dicentrarchus labrax au cours du sevrage. Aquaculture 78, 55–61. doi: 10.1016/0044-8486(89)90005-7
Cheung N. K. M., Cheung A. C. K., Ye R. R., Ge W., Giesy J. P., Au D. W. T. (2013). Expression profile of oestrogen receptors and oestrogen-related receptors is organ specific and sex dependent: the Japanese medaka Oryzias latipes model. J. Fish Biol. 83, 295–310. doi: 10.1111/jfb.12164
Chin K.-Y., Pang K.-L., Mark-Lee W. F. (2018). A review on the effects of bisphenol a and its derivatives on skeletal health. Int. J. Med. Sci. 15, 1043–1050. doi: 10.7150/ijms.25634
Chou Y.-S., Chuang S.-C., Chen C.-H., Ho M.-L., Chang J.-K. (2021). G-Protein-Coupled estrogen receptor-1 positively regulates the growth plate chondrocyte proliferation in female pubertal mice. Front. Cell Dev. Biol. 9. doi: 10.3389/fcell.2021.710664
Cimmino I., Fiory F., Perruolo G., Miele C., Beguinot F., Formisano P., et al. (2020). Potential mechanisms of bisphenol a (BPA) contributing to human disease. Int. J. Mol. Sci. 21, E5761. doi: 10.3390/ijms21165761
Cohen S. P., LaChappelle A. R., Walker B. S., Lassiter C. S. (2014). Modulation of estrogen causes disruption of craniofacial chondrogenesis in Danio rerio. Aquat Toxicol. 152, 113–120. doi: 10.1016/j.aquatox.2014.03.028
Colborn T., vom Saal F. S., Soto A. M. (1994). Developmental effects of endocrine-disrupting chemicals in wildlife and humans. Environ. Impact Assess. Rev. 14, 469–489. doi: 10.1016/0195-9255(94)90014-0
Cucchi P., Sucré E., Santos R., Leclère J., Charmantier G., Castille R. (2012). Embryonic development of the sea bass Dicentrarchus labrax. Helgol Mar. Res. 66, 199–209. doi: 10.1007/s10152-011-0262-3
Dale R. M., Topczewski J. (2011). Identification of an evolutionarily conserved regulatory element of the zebrafish col2a1a gene. Dev. Biol. 357, 518–531. doi: 10.1016/j.ydbio.2011.06.020
Darias M. J., Lan Chow Wing O., Cahu C., Zambonino-Infante J. L., Mazurais D. (2010). Double staining protocol for developing European sea bass ( Dicentrarchus labrax ) larvae. J. Appl. Ichthyol 26, 280–285. doi: 10.1111/j.1439-0426.2010.01421.x
Davesne D., Meunier F. J., Schmitt A. D., Friedman M., Otero O., Benson R. B. J. (2018). The phylogenetic origin and evolution of acellular bone in teleost fishes: insights into osteocyte function in bone metabolism. PeerJ Preprints 94 (4), 1338–1363. doi: 10.7287/peerj.preprints.27406v1
Delfosse V., Grimaldi M., Cavaill èsV., Balaguer P., Bourguet W. (2014). Structural and functional profiling of environmental ligands for estrogen receptors. Environ. Health Perspect. 122, 1306–1313. doi: 10.1289/ehp.1408453
Delfosse V., Grimaldi M., Pons J.-L., Boulahtouf A., le Maire A., Cavailles V., et al. (2012). Structural and mechanistic insights into bisphenols action provide guidelines for risk assessment and discovery of bisphenol a substitutes. Proc. Natl. Acad. Sci. U.S.A. 109, 14930–14935. doi: 10.1073/pnas.1203574109
Eames B. F., Amores A., Yan Y.-L., Postlethwait J. H. (2012). Evolution of the osteoblast: skeletogenesis in gar and zebrafish. BMC Evol. Biol. 12, 27. doi: 10.1186/1471-2148-12-27
EC (2018) Commission implementing decision (EU) 2018/840 of 5 June 2018 establishing a watch list of substances for union-wide monitoring in the field of water policy pursuant to directive 2008/105/EC of the European parliament and of the council and repealing commission implementing decision (EU) 2015/495 (notified under document C(2018) 3362). Available at: http://data.europa.eu/eli/dec_impl/2018/840/oj.
Flint S., Markle T., Thompson S., Wallace E. (2012). Bisphenol a exposure, effects, and policy: a wildlife perspective. J. Environ. Manage 104, 19–34. doi: 10.1016/j.jenvman.2012.03.021
Fushimi S., Wada N., Nohno T., Tomita M., Saijoh K., Sunami S., et al. (2009). 17beta-estradiol inhibits chondrogenesis in the skull development of zebrafish embryos. Aquat Toxicol. 95, 292–298. doi: 10.1016/j.aquatox.2009.03.004
Gibert Y., Sassi-Messai S., Fini J.-B., Bernard L., Zalko D., Cravedi J.-P., et al. (2011). Bisphenol a induces otolith malformations during vertebrate embryogenesis. BMC Dev. Biol. 11, 4. doi: 10.1186/1471-213X-11-4
Gluckmann I., Huriaux F., Focant B., Vandewalle P. (1999). Postembryonic development of the cephalic skeleton in Dicentrarchus labrax (Pisces, perciformes, serranidae). Bull. Mar. Sci. 65, 11–36.
Gorshkov S., Gorshkova G., Colorni B., Gordin H. (2004). Effects of natural Estradiol-17b and synthetic 17a-ethynylestradiol on direct feminization of European Sea bass Dicentrarchus labrax. J. World Aquaculture Soc. 35, 167–177. doi: 10.1111/j.1749-7345.2004.tb01072.x
Gouveia C. H. A., Miranda-Rodrigues M., Martins G. M., Neofiti-Papi B. (2018). Thyroid hormone and skeletal development. Vitam Horm. 106, 383–472. doi: 10.1016/bs.vh.2017.06.002
Halm S., Martínez-Rodríguez G., Rodríguez L., Prat F., Mylonas C. C., Carrillo M., et al. (2004). Cloning, characterisation, and expression of three oestrogen receptors (ERalpha, ERbeta1 and ERbeta2) in the European sea bass, Dicentrarchus labrax. Mol. Cell Endocrinol. 223, 63–75. doi: 10.1016/j.mce.2004.05.009
Hammond C. L., Schulte-Merker S. (2009). Two populations of endochondral osteoblasts with differential sensitivity to hedgehog signalling. Development 136, 3991–4000. doi: 10.1242/dev.042150
Heino T. J., Chagin A. S., Sävendahl L. (2008). The novel estrogen receptor G-protein-coupled receptor 30 is expressed in human bone. J. Endocrinol. 197, R1–R6. doi: 10.1677/JOE-07-0629
Horard B., Vanacker J.-M. (2003). Estrogen receptor-related receptors: orphan receptors desperately seeking a ligand. J. Mol. Endocrinol. 31, 349–357. doi: 10.1677/jme.0.0310349
Imangali N., Phan Q. T., Mahady G., Winkler C. (2021). The dietary anthocyanin delphinidin prevents bone resorption by inhibiting rankl-induced differentiation of osteoclasts in a medaka (Oryzias latipes) model of osteoporosis. J. Fish Biol. 98, 1018–1030. doi: 10.1111/jfb.14317
Jeong B.-C., Lee Y.-S., Park Y.-Y., Bae I.-H., Kim D.-K., Koo S.-H., et al. (2009). The orphan nuclear receptor estrogen receptor-related receptor gamma negatively regulates BMP2-induced osteoblast differentiation and bone formation. J. Biol. Chem. 284, 14211–14218. doi: 10.1074/jbc.M808345200
Kalb A. C., Kalb A. L., Cardoso T. F., Fernandes C. G., Corcini C. D., Varela Junior A. S., et al. (2016). Maternal transfer of bisphenol a during nursing causes sperm impairment in Male offspring. Arch. Environ. Contam Toxicol. 70, 793–801. doi: 10.1007/s00244-015-0199-7
Kallen J., Schlaeppi J.-M., Bitsch F., Filipuzzi I., Schilb A., Riou V., et al. (2004). Evidence for ligand-independent transcriptional activation of the human estrogen-related receptor alpha (ERRalpha): crystal structure of ERRalpha ligand binding domain in complex with peroxisome proliferator-activated receptor coactivator-1alpha. J. Biol. Chem. 279, 49330–49337. doi: 10.1074/jbc.M407999200
Kaneko T., Freeha K., Wu X., Mogi M., Uji S., Yokoi H., et al. (2016). Role of notochord cells and sclerotome-derived cells in vertebral column development in fugu, Takifugu rubripes: histological and gene expression analyses. Cell Tissue Res. 366, 37–49. doi: 10.1007/s00441-016-2404-z
Kim Y.-I., No Lee J., Bhandari S., Nam I.-K., Yoo K.-W., Kim S.-J., et al. (2015). Cartilage development requires the function of estrogen-related receptor alpha that directly regulates sox9 expression in zebrafish. Sci. Rep. 5, 18011. doi: 10.1038/srep18011
Kousteni S., Bellido T., Plotkin L. I., O’Brien C. A., Bodenner D. L., Han L., et al. (2001). Nongenotropic, sex-nonspecific signaling through the estrogen or androgen receptors: dissociation from transcriptional activity. Cell 104, 719–730. doi: 10.1016/S0092-8674(02)08100-X
Kranenbarg S., van Cleynenbreugel T., Schipper H., van Leeuwen J. (2005). Adaptive bone formation in acellular vertebrae of sea bass (Dicentrarchus labrax l.). J. Exp. Biol. 208, 3493–3502. doi: 10.1242/jeb.01808
Kužir S., Kozarić Z., Nejedli S. (2004). Development of mandibular arch in European sea bass, Dicentrarchus labrax (Linnaeu) from the “Cenmar” hatchery, Croatia. Veterinarski arhiv 74, 321–330.
Le Fol V., Aït-Aïssa S., Sonavane M., Porcher J.-M., Balaguer P., Cravedi J.-P., et al. (2017). In vitro and in vivo estrogenic activity of BPA, BPF and BPS in zebrafish-specific assays. Ecotoxicol Environ. Saf. 142, 150–156. doi: 10.1016/j.ecoenv.2017.04.009
Leurs N., Martinand-Mari C., Ventéo S., Haitina T., Debiais-Thibaud M. (2021). Evolution of matrix gla and bone gla protein genes in jawed vertebrates. Front. Genet. 12. doi: 10.3389/fgene.2021.620659
Li N., Felber K., Elks P., Croucher P., Roehl H. H. (2009). Tracking gene expression during zebrafish osteoblast differentiation. Dev. Dyn 238, 459–466. doi: 10.1002/dvdy.21838
Liu J., Zhang L., Lu G., Jiang R., Yan Z., Li Y. (2021). Occurrence, toxicity and ecological risk of bisphenol a analogues in aquatic environment – a review. Ecotoxicol Environ. Saf. 208, 111481. doi: 10.1016/j.ecoenv.2020.111481
Lorin-Nebel C., Boulo V., Bodinier C., Charmantier G. (2006). The Na+/K+/2Cl- cotransporter in the sea bass Dicentrarchus labrax during ontogeny: involvement in osmoregulation. J. Exp. Biol. 209, 4908–4922. doi: 10.1242/jeb.02591
Lorin-Nebel C., Budzinski H., Le Ménach K., Devier M. H., Charmantier G., Gros R., et al. (2014). 4-nonylphenol disrupts osmoregulation in the European sea-bass Dicentrarchus labrax. J. Xenobiotics 4, 94–96. doi: 10.4081/xeno.2014.4905
MacKay H., Abizaid A. (2018). A plurality of molecular targets: The receptor ecosystem for bisphenol-a (BPA). Horm. Behav. 101, 59–67. doi: 10.1016/j.yhbeh.2017.11.001
Marino M., Pellegrini M., La Rosa P., Acconcia F. (2012). Susceptibility of estrogen receptor rapid responses to xenoestrogens: Physiological outcomes. Steroids 77, 910–917. doi: 10.1016/j.steroids.2012.02.019
Masroor W., Farcy E., Gros R., Lorin-Nebel C. (2018). Effect of combined stress (salinity and temperature) in European sea bass Dicentrarchus labrax osmoregulatory processes. Comp. Biochem. Physiol. Part A: Mol. Integr. Physiol. 215, 45–54. doi: 10.1016/j.cbpa.2017.10.019
Miller B. (2009). Early life history of marine fishes. Ed. Nummela S. (Oakland, CA: University of California Press). doi: 10.1525/california/9780520249721.001.0001
Mitter K., Kotoulas G., Magoulas A., Mulero V., Sepulcre P., Figueras A., et al. (2009). Evaluation of candidate reference genes for QPCR during ontogenesis and of immune-relevant tissues of European seabass (Dicentrarchus labrax). Comp. Biochem. Physiol. B Biochem. Mol. Biol. 153, 340–347. doi: 10.1016/j.cbpb.2009.04.009
Pashay Ahi E., Walker B. S., Lassiter C. S., Jónsson Z. O. (2016). Investigation of the effects of estrogen on skeletal gene expression during zebrafish larval head development. PeerJ 4, e1878. doi: 10.7717/peerj.1878
Paul S., Schindler S., Giovannone D., de Millo Terrazzani A., Mariani F. V., Crump J. G. (2016). Ihha induces hybrid cartilage-bone cells during zebrafish jawbone regeneration. Development 143, 2066–2076. doi: 10.1242/dev.131292
Périan S., Vanacker J.-M. (2020). GPER as a receptor for endocrine-disrupting chemicals (EDCs). Front. Endocrinol. (Lausanne) 11. doi: 10.3389/fendo.2020.00545
Petrey A. C., Flanagan-Steet H., Johnson S., Fan X., de la Rosa M., Haskins M. E., et al. (2012). Excessive activity of cathepsin K is associated with cartilage defects in a zebrafish model of mucolipidosis II. Dis. Model. Mech. 5, 177–190. doi: 10.1242/dmm.008219
Pfaffl M. W. (2001). A new mathematical model for relative quantification in real-time RT-PCR. Nucleic Acids Res. 29, e45. doi: 10.1093/nar/29.9.e45
Piferrer F. (2001). Endocrine sex control strategies for the feminization of teleost fish. Aquaculture 197, 229–281. doi: 10.1016/S0044-8486(01)00589-0
Pinto P. I. S., Andrade A. R., Estêvão M. D., Alvarado M. V., Felip A., Power D. M. (2018). Duplicated membrane estrogen receptors in the European sea bass (Dicentrarchus labrax): Phylogeny, expression and regulation throughout the reproductive cycle. J. Steroid Biochem. Mol. Biol. 178, 234–242. doi: 10.1016/j.jsbmb.2017.12.019
Pinto P. I. S., Estêvão M. D., Andrade A., Santos S., Power D. M. (2016). Tissue responsiveness to estradiol and genistein in the sea bass liver and scale. J. Steroid Biochem. Mol. Biol. 158, 127–137. doi: 10.1016/j.jsbmb.2015.12.023
Pinto P., Estêvão M., Power D. (2014b). Effects of estrogens and estrogenic disrupting compounds on fish mineralized tissues. Mar. Drugs 12, 4474–4494. doi: 10.3390/md12084474
Pinto C., Grimaldi M., Boulahtouf A., Pakdel F., Brion F., Aït-Aïssa S., et al. (2014a). Selectivity of natural, synthetic and environmental estrogens for zebrafish estrogen receptors. Toxicol. Appl. Pharmacol. 280, 60–69. doi: 10.1016/j.taap.2014.07.020
Prein C., Beier F. (2019). ECM signaling in cartilage development and endochondral ossification. Curr. Top. Dev. Biol. 133, 25–47. doi: 10.1016/bs.ctdb.2018.11.003
Prossnitz E. R., Barton M. (2011). The G-protein-coupled estrogen receptor GPER in health and disease. Nat. Rev. Endocrinol. 7, 715–726. doi: 10.1038/nrendo.2011.122
Revankar C. M., Mitchell H. D., Field A. S., Burai R., Corona C., Ramesh C., et al. (2007). Synthetic estrogen derivatives demonstrate the functionality of intracellular GPR30. ACS Chem. Biol. 2, 536–544. doi: 10.1021/cb700072n
Sajiki J., Yonekubo J. (2003). Leaching of bisphenol a (BPA) to seawater from polycarbonate plastic and its degradation by reactive oxygen species. Chemosphere 51, 55–62. doi: 10.1016/S0045-6535(02)00789-0
Sharif F., de Bakker M. A. G., Richardson M. K. (2014). Osteoclast-like cells in early zebrafish embryos. Cell J. 16, 211–224.
Staniszewska M., Koniecko I., Falkowska L., Krzymyk E. (2015). Occurrence and distribution of bisphenol a and alkylphenols in the water of the gulf of Gdansk (Southern Baltic). Mar. pollut. Bull. 91, 372–379. doi: 10.1016/j.marpolbul.2014.11.027
Takayanagi S., Tokunaga T., Liu X., Okada H., Matsushima A., Shimohigashi Y. (2006). Endocrine disruptor bisphenol a strongly binds to human estrogen-related receptor γ (ERRγ) with high constitutive activity. Toxicol. Lett. 167, 95–105. doi: 10.1016/j.toxlet.2006.08.012
Tanida T. (2022). Molecular dynamics of estrogen-related receptors and their regulatory proteins: roles in transcriptional control for endocrine and metabolic signaling. Anat Sci. Int. 97, 15–29. doi: 10.1007/s12565-021-00634-7
Thomas P., Dong J. (2006). Binding and activation of the seven-transmembrane estrogen receptor GPR30 by environmental estrogens: a potential novel mechanism of endocrine disruption. J. Steroid Biochem. Mol. Biol. 102, 175–179. doi: 10.1016/j.jsbmb.2006.09.017
Tine M., Kuhl H., Gagnaire P.-A., Louro B., Desmarais E., Martins R. S. T., et al. (2014). European Sea bass genome and its variation provide insights into adaptation to euryhalinity and speciation. Nat. Commun. 5, 5770. doi: 10.1038/ncomms6770
Tohmé M., Prud’homme S. M., Boulahtouf A., Samarut E., Brunet F., Bernard L., et al. (2014). Estrogen-related receptor γ is an in vivo receptor of bisphenol a. FASEB J. 28, 3124–3133. doi: 10.1096/fj.13-240465
Topczewska J. M., Shoela R. A., Tomaszewski J. P., Mirmira R. B., Gosain A. K. (2016). The morphogenesis of cranial sutures in zebrafish. PloS One 11, e0165775. doi: 10.1371/journal.pone.0165775
To T. T., Witten P. E., Huysseune A., Winkler C. (2015). An adult osteopetrosis model in medaka reveals the importance of osteoclast function for bone remodeling in teleost fish. Comp. Biochem. Physiol. C Toxicol. Pharmacol. 178, 68–75. doi: 10.1016/j.cbpc.2015.08.007
Tremblay L., van der Kraak G. (1998). Use of a series of homologous in vitro and in vivo assays to evaluate the endocrine modulating actions of β-sitosterol in rainbow trout. Aquat. Toxicol. 43, 149–162. doi: 10.1016/S0166-445X(98)00051-4
Urushitani H., Shimizu A., Katsu Y., Iguchi T. (2002). Early estrogen exposure induces abnormal development of Fundulus heteroclitus. J. Exp. Zool 293, 693–702. doi: 10.1002/jez.10161
Verreijdt L., Debiais-Thibaud M., Borday-Birraux V., van der heyden C., Sire J.-Y., Huysseune A. (2006). Expression of the dlx gene family during formation of the cranial bones in the zebrafish (Danio rerio): Differential involvement in the visceral skeleton and braincase. Dev. Dyn. 235, 1371–1389. doi: 10.1002/dvdy.20734
Villamizar N., Blanco-Vives B., Migaud H., Davie A., Carboni S., Sánchez-Vázquez F. J. (2011). Effects of light during early larval development of some aquacultured teleosts: A review. Aquaculture 315, 86–94. doi: 10.1016/j.aquaculture.2010.10.036
Wang H., Chen H., Chernick M., Li D., Ying G.-G., Yang J., et al. (2020). Selenomethionine exposure affects chondrogenic differentiation and bone formation in Japanese medaka (Oryzias latipes). J. Hazard Mater 387, 121720. doi: 10.1016/j.jhazmat.2019.121720
Wang H., Wang J. (2013). Estrogen-related receptor alpha interacts cooperatively with peroxisome proliferator-activated receptor-gamma coactivator-1alpha to regulate osteocalcin gene expression: ERRα regulating osteocalcin gene expression. Cell Biol. Int. doi: 10.1002/cbin.10148
Weigele J., Franz-Odendaal T. A., Hilbig R. (2015). Expression of SPARC and the osteopontin-like protein during skeletal development in the cichlid fish Oreochromis mossambicus. Dev. Dyn 244, 955–972. doi: 10.1002/dvdy.24293
Xu S., Yu S., Dong D., Lee L. T. O. (2019). G Protein-coupled estrogen receptor: A potential therapeutic target in cancer. Front. Endocrinol. 10. doi: 10.3389/fendo.2019.00725
Yang Y., Wandler A. M., Postlethwait J. H., Guillemin K. (2012). Dynamic evolution of the LPS-detoxifying enzyme intestinal alkaline phosphatase in zebrafish and other vertebrates. Front. Immunol. 3. doi: 10.3389/fimmu.2012.00314
Yan Y.-L., Miller C. T., Nissen R. M., Singer A., Liu D., Kirn A., et al. (2002). A zebrafish sox9 gene required for cartilage morphogenesis. Development 129, 5065–5079. doi: 10.1242/dev.129.21.5065
Zare Mirakabad H., Farsi M., Malekzadeh Shafaroudi S., Bagheri A., Iranshahi M., Moshtaghi N. (2019). Comparison the effect of ferutinin and 17β-estradiol on bone mineralization of developing zebrafish (Danio rerio) larvae. Int. J. Mol. Sci. 20, E1507. doi: 10.3390/ijms20061507
Keywords: estradiol, bisphenol A, skeletogenesis, bone mineralization, estrogen signaling, seabass Dicentrarchus labrax
Citation: Farcy E, Potier E, Leurs N, Gasset E, Dutto G, Lallement S, Bourdy C, Debiais-Thibaud M and Martinand-Mari C (2022) Short-term effects of estradiol and bisphenol A on gene expression associated with early head mineralization in the seabass Dicentrarchus labrax. Front. Mar. Sci. 9:1062334. doi: 10.3389/fmars.2022.1062334
Received: 05 October 2022; Accepted: 29 November 2022;
Published: 15 December 2022.
Edited by:
Demetrio Raldua, Spanish National Research Council (CSIC), SpainReviewed by:
Angela Sequeira, Université de Bordeaux, FrancePaulo Gavaia, University of Algarve, Portugal
Copyright © 2022 Farcy, Potier, Leurs, Gasset, Dutto, Lallement, Bourdy, Debiais-Thibaud and Martinand-Mari. This is an open-access article distributed under the terms of the Creative Commons Attribution License (CC BY). The use, distribution or reproduction in other forums is permitted, provided the original author(s) and the copyright owner(s) are credited and that the original publication in this journal is cited, in accordance with accepted academic practice. No use, distribution or reproduction is permitted which does not comply with these terms.
*Correspondence: Emilie Farcy, ZW1pbGllLmZhcmN5QHVtb250cGVsbGllci5mcg==; Camille Martinand-Mari, Y2FtaWxsZS5tYXJ0aW5hbmQtbWFyaUB1bW9udHBlbGxpZXIuZnI=