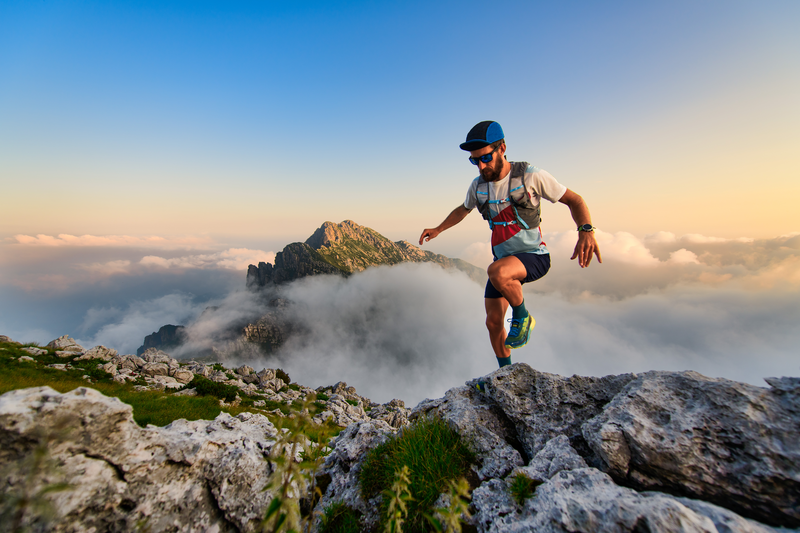
95% of researchers rate our articles as excellent or good
Learn more about the work of our research integrity team to safeguard the quality of each article we publish.
Find out more
ORIGINAL RESEARCH article
Front. Mar. Sci. , 04 January 2023
Sec. Coastal Ocean Processes
Volume 9 - 2022 | https://doi.org/10.3389/fmars.2022.1059353
This article is part of the Research Topic Coastal Ecotones under Anthropogenic Influences: Conservation, Restoration and Realignment View all 14 articles
The functional groups and the ecological health of a constructed oyster reef were investigated during April 2016 to October 2017 in the Yangtze estuary. The data of the long-term monitoring program (14 years) confirmed the feasibility of creating an artificial oyster reef in Yangtze estuary. One or two functional groups were absent from moderate salinity conditions (5.17% - 8.73%), while macrobenthos functional group species richness, abundance, and biomass were greatest under high salinity conditions. The feeding evenness index (jFD) showed that 14 years after construction, the ecosystem health of the artificial oyster reefs were good or high, and was better under high salinity conditions. Ecological health was lower under moderate levels of salinity, possibly due to the absence of some functional groups. Redundancy analysis indicated ecosystem health was associated with changes in salinity and substrate factors. Our study confirmed artificial oyster reef construction is feasible and has a positive effect on estuarine ecosystem health.
Estuaries are highly productive, yet threatened ecological systems that deliver a range of ecosystem services and benefits to human society. However, the health of many estuaries is under threat from high levels of anthropogenic activities and pollution associated with the dense human populations that occur in these regions (Adger et al., 2005). For example, sediment and organic material deposited by rivers in estuaries include nutrients, heavy metals, microplastics, and other contaminants (Chen et al., 2012; Han et al., 2017; Xu et al., 2018) that have led to biodiversity loss and reduced ecological resilience in estuarine environments (Lotze et al., 2006; Liu et al., 2018a).
The recognition of the essential role of estuaries for humans and other organisms has led to the initiation of ecological restoration programs (Lotze et al., 2006), where rehabilitation or construction oyster reefs represent an effective estuarine restoration technique. The biological structure formed by a large number of oysters is called an oyster reef, which is an important coastal habitat, because they provide many ecological services and bring multiple ecological benefits (Grabowski et al., 2022), such as water purification through water filtration and the removal of phytoplankton and heavy metals, and habitat provisioning through creation of three-dimensional habitats for macrobenthos and fish (Breitburg et al., 2000; Coen and Luckenbach, 2000; Grabowski and Peterson, 2007; Thomsen et al., 2007; La Peyre et al., 2014; Liu et al., 2018b). Thus, construction of oyster reefs has been increasingly used for shoreline protection and ecological restoration (La Peyre et al., 2014; Chakraborty, 2016; Walles et al., 2016).
The Yangtze Estuary is the largest in Asia and is one of the most globally important ecotones (Chen et al., 2012) that provides vital ecological services, including breeding grounds for aquatic organisms and stopover and overwintering sites for millions of migratory birds (Xu and Zhao, 2005). However, it is affected by rising sea-level and anthropogenic pressures that have culminated over centuries of overexploitation, reclamation, and pollution (He et al., 2011; Kirwan and Megonigal, 2013; Liu et al., 2016b; Yu et al., 2018); consequently, eutrophication and reduction in biodiversity have led to a deterioration in the ecological health of the Yangtze Estuary (Chai et al., 2006; Chen et al., 2012; Huang et al., 2021; Huang et al., 2022). In response, an oyster reef restoration project was initiated in the region (Quan et al., 2009), based on previous studies that showed the construction of artificial oyster reefs in the Yangtze River estuary was feasible and effective in the restoration of the ecosystem (Quan et al., 2009; Quan et al., 2012; Lv et al., 2016a; Liu et al., 2018b).
It is likely that artificial and restored oyster reefs may be affected by human activities and pollution, such as over-fishing of the nursery beds and oil contamination, therefore, monitoring of such restoration schemes is crucial for their success. Macrobenthos represents a vital component of oyster reefs, and are ideal indicators for use in ecosystem health monitoring schemes due to their limited mobility, ease of collection, and high sensitivity (Borja et al., 2000; Borja et al., 2003; Gamito and Furtado, 2009; Liu et al., 2019); however, studies of oyster reefs have tended to focus on taxonomic macrobenthos community structure, abundance, biomass, diversity, and ecosystem health (Quan et al., 2009; Quan et al., 2012; Lv et al., 2016a; Liu et al., 2018b) that may be more appropriate in the evaluation of biodiversity rather than ecosystem health (Cummins et al., 2005; Peng et al., 2013). Macrobenthos functional groups represent taxa that have similar ecological characteristics, so assessment of their responses to environmental change is more meaningful than that of individuals or taxonomic families, because it provides a more accurate indication of ecosystem-level responses to ecological perturbations (Yang et al., 2017).
Ecological restoration may trigger the emergence of novel functional groups and turnover of existing functional groups (Lv et al., 2018). Previous studies of the Yangtze Estuary oyster reef have quantified biological resources, including macrobenthos and nektons, macrobenthic taxonomic diversity and ecological health (Quan et al., 2007; Quan et al., 2009; Quan et al., 2012; Lv et al., 2016a; Liu et al., 2018b); however, assessment of macrobenthos functional groups remains lacking. Here, we identified macrobenthos functional groups to assess the ecological status of the artificial oyster reef in the Yangtze estuary. Specifically, the objectives were to (1) assess the composition and abundance of macrobenthos functional groups; (2) measure long-term variation in macrobenthos functional group composition; (3) test the relationship between macrobenthos functional groups and environmental variables; and, (4) use macrobenthos functional groups to assess ecosystem health.
The Yangtze estuary (29°30′N–32°00′N, 121°00′E–124°00′E) artificial intertidal oyster reef (c. 260 ha) was constructed in 2004 in the north passage of the southern Yangtze River channel (Figures 1A, B), and comprises two dikes (south and north) with 19 groins (S1-S9 in the south dike and N1-N10 in the north dike). The north dike (total length: 48 km) is located along the southern margin of Hengsha Island, and the south dike (total length: 49.2 km) is located along the northern edge of Jiuduansha National Nature Reserve. The artificial reef is vaulted in cross-section (Figure 1C).
Figure 1 Study site locations. (A) Yangtze River estuary, China; (B) Sampling sites on the artificial intertidal oyster reef; (C) Schematic drawing of the sampling transect and cross-section of the artificial reef; (D) No organisms were recorded from the low-salinity sites (S2 and N2).
Macrobenthos were sampled in April and September 2016, and in August and October 2017 from three paired sites (N = 6) at the two dikes along an increasing salinity gradient, where salinity at sites S2 and N2 was 0.43 ± 0.03 and 0.43 ± 0.04, respectively, S5 and N6 was 6.55 ± 1.38 and 7.86 ± 0.87, respectively, and S8 and N9 was 16.43 ± 0.34 and 15.50 ± 1.09, respectively. At low spring tide from three site (high, mid, and low tidal zones), four quantitative samples of macrobenthos (25 × 25 cm) were collected from each site (Quan et al., 2009), where the high tidal zone was located on the crest of the reef, the mid zone was on the flank, and the low zone was at the reef base. In addition, as many qualitative samples as possible were collected from an area of 25.0 m2 from the sampling center. Samples were immediately sieved (1.0-mm mesh) and the collected macrobenthos were preserved in 75% alcohol prior to subsequent identification at the laboratory to the lowest possible taxonomic level; individuals were counted and weighed to the nearest 0.01g wet weight. No organisms were recorded from both quantitative and qualitative investigations from the low salinity sites (S2 and N2) (Figure 1D).
Water temperature (WT), salinity (SAL), pH, and dissolved oxygen (DO) at the sampling sites were measured using a HQ 40D multi-parameter water quality meter (Hach, Loveland, Colorado, USA). The abundance of oyster (OA) and barnacles (BA) were defined as the substrate variables of the oyster reef.
Including the 2016 and 2017 data collected, changes over a 14-year period in the macrobenthic functional groups were integrated in an analysis of dynamics from 2004 to 2017. Historical data points (2004–2014) were obtained from the literature, namely for September 2004, August 2005, August and November 2007, April and July 2008, May and September 2009, April and November 2011, July and October 2012, August and October 2013, August and October 2014 (Quan et al., 2009; Quan et al., 2012; Lv et al., 2016a).
We defined five macrobenthos functional groups according to feeding strategy (Zhu and Lu, 2003; Peng et al., 2013; Yang et al., 2017) that comprised planktivore (Pl) herbivore (He); predator (Pr); omnivore (O); and, detritivore (D). From these, we defined four trophic levels (Figure 2), where the first level comprised primary producers (we did not survey this group), the second level comprised primary consumers (Pl, He, and D), the third level comprised omnivore secondary consumers of levels I and II, while the fourth level comprised predatory (Pr) secondary consumers of levels II and III.
Figure 2 Trophic levels and food web of the microbenthic community, redrawn from Li et al. (2007).
We used CLUSTER (hierarchical clustering) and NMDS (two-dimensional nonmetric multidimensional scaling) in PRIMER v5.0 to test the degree of similarity of the macrobenthic functional groups (Clarke and Gorley, 2001). Macrobenthos functional group data were fourth-root transformed to calculate the community similarity according to the Bray-Curtis similarity index, and similarity analysis (ANOSIM 2-way) was used to test for tidal zone and site differences in the abundance of macrobenthos functional groups.
Detrended correspondence analysis determined linear redundancy analysis (RDA) was more appropriate than a unimodal analysis (maximum gradient length of the axes<3) to describe the effects of environmental variables on macrobenthos functional group abundance. With the exception of pH, environmental data were log10(x+1) transformed prior to analysis.
A feeding evenness index (jFD) was used to assess the ecological health status of the macrobenthos trophic levels, following the methodology reported by (Gamito and Furtado, 2009):
where H’ is the Shannon–Wiener index and n is the number of trophic groups. Ecosystem health was determined as low (jFD =<0.20), poor (jFD = 0.20-0.40), moderate (jFD = 0.40-0.60), good (jFD = 0.60-0.80), and high (jFD = >0.80) (Gamito et al., 2012).
Two-way ANOVA, with post-hoc Turkey test, was used to test the effects of site and tidal zone on macrobenthos species richness and abundance, and biomass of macrobenthos functional group at P< 0.05. When assumptions of homogeneity of variances were not met, data were fourth-root transformed; if variances remained non-homogenous, we applied the Scheirer-Ray-Hare extension of the Kruskal-Wallis test. Associations between environmental variables and macrobenthos were tested using correlation analysis at P< 0.05. Analyses were performed using SPSS statistics 19.0 and presented data are means ± SEM.
The species composition of the macrobenthos functional groups varied between 2004 and 2017, where total number of species increased immediately after reef creation, before it declined in 2008, and then peaked in 2011 (36 species); species richness remained stable from 2012 to 2017 (Figure 3). The number of functional groups increased with restoration time, from three in 2004 (O, He, and Pl) to five from 2009 onwards (Figure 3).
Figure 3 Long-term variation in macrobenthos functional group species richness. Pr, predator; D, detritivore; O, omnivore; He, herbivore; and Pl, planktivore.
We identified three and four macrobenthos functional groups at the moderate salinity sites N6 and S5, respectively (Figure 4 and Table 1), and five groups at both high salinity sites (S8 and N9) during 2016-2017. O and He groups comprised higher numbers of species at the high salinity sites (S8 and N9) than at the moderate-salinity (S5 and N6) sites (P< 0.05, Table 2). There were more species in the O group in the low intertidal zone, but fewer in the mid zone; species richness varied among the three tidal zones (P< 0.05, Table 2).
Figure 4 Average functional group species richness. Pr, predator; D, detritivore; O, omnivore; He, herbivore; and Pl, planktivore.
Table 2 Study site and tidal zone effects on macrobenthic functional group species richness during 2016-2017.
Abundance of C, O, and He and biomass of C were higher in high-salinity (N9 and S8) sites than in the moderate salinity sites (N5 and S6) (P<0.05, Tables 3–6), and there was no effect of tidal zone on abundance and biomass of the five functional groups (Tables 5, 6).
Table 3 Abundance of macrobenthos functional groups in the constructed intertidal oyster reef in Yangtze River Estuary during 2016-2017.
Table 4 Biomass of macrobenthic functional groups in the constructed intertidal oyster reef in Yangtze River Estuary during 2016-2017.
Table 5 Effect of study site and tidal zone on macrobenthos functional group abundance in the constructed intertidal oyster reef in Yangtze River Estuary during 2016-2017.
Table 6 Effect of study site and tidal zone on macrobenthos functional group biomass in the constructed intertidal oyster reef in Yangtze River Estuary during 2016-2017.
Cluster analysis revealed similarities and differences in macrobenthos functional groups among sites and tidal zones, where there were three clusters comprising S8-L, S8-M, N9-L, S5-M, S8-H, N9-H, and N9-M; S5-H; and, N6-H, N6-M, and N6-L (Figure 5A). The nMDS analysis results were consistent with the cluster analysis, with a stress value = 0.08 indicating the results were reliable (Figure 5B). There were site differences in macrobenthos functional groups (ANOSIM 2 Global R = 0.719; P = 0.029); however, there were no tidal zone differences (Global R = 0.167; P = 0.34).
Figure 5 (A) Cluster and (B) non-metric multidimensional scaling (NMDS) ordinations of the macrobenthos functional groups.
We found that jFD values for the macrobenthos functional groups were<0.8 in the moderate salinity sites (S5 and N6), indicating ecological health was good, and >0.8 in the high salinity sites (S8 and N9), indicating high quality ecological health (Figure 6).
Monte Carlo tests for the first and all Redundancy analysis (RDA) canonical axes were significant (P = 0.004), indicating these parameters may be important in explaining the composition of the macrobenthos functional groups. The first axis explained 62.4% of total variation in the functional group data and all canonical axes accounted for 72.3% of the variance in the functional group abundance-environment relationship. Functional groups were correlated with environmental variables: groups O, Pl, D, and He were positively correlated with barnacle abundance, oyster abundance, salinity, and temperature, and negatively correlated with pH, whereas group C was negatively correlated with dissolved oxygen (Figure 7).
Figure 7 Redundancy analysis ordination of the macrobenthos functional groups and environmental variables (S5,
S8,
N6, and
N9). DO, dissolved oxygen; WT, water temperature; SAL, water salinity; BA, barnacle abundance; and OA, oyster abundance.
Restored and artificial oyster reefs play a vital role in improving the ecological health status of estuarine environments, because they improve water quality and provide a three-dimensional structure that may be colonized by macrobenthos and fish (Breitburg et al., 2000; Thomsen et al., 2007). In the first year (2004) since its creation, the artificial oyster reef in the Yangtze estuary was found to have become host to three functional groups (comprising five species) of macrobenthos; the species richness of the functional groups rapidly increased with time, with the exception of 2008, before it peaked in 2011 and remained stable from 2012. This increase in functional group species richness occurred after oyster abundance had stabilized (2011) (Liu et al., 2018b) and prior to the increase in macrobenthos taxonomic diversity that occurred from 2012 to 2016-2017 (Liu et al., 2018b). These results support previous studies that have indicated the oyster reefs in the Yangtze Estuary be develop into self-sustaining systems (Walles et al., 2016; Liu et al., 2018b).
Water salinity is a key factor that affects the distribution of macrobenthos in estuarine habitats (Holland et al., 1987; Liu et al., 2018a), where species richness, abundance, and biomass increase with increase in salinity (Yuan et al., 2002). In this study, the increase in salinity (from S5 and N6 to S8 and N9) resulted in an increase in species richness, abundance, and biomass of macrobenthic functional group (P< 0.05; Figure 7), indicating salinity affects the distribution of macrobenthos functional groups in the artificial Yangtze Estuary oyster reefs. Adaptation to salinity varies among species (Yuan et al., 2002); for example, Phascolosoma esculenta, which was only collected from the high salinity sites (S8 and N9), belongs to the D functional group, and has a survival salinity of 5-30 ‰, with a salinity range for embryo development of 17.10-30.15 ‰ (Jin et al., 2011). We found that functional group D, whose main species comprise polychaetes and Sipuncula that are sensitive to fluctuations in environmental conditions (Dauvin and Ruellet, 2007; Cai, 2015), was absent from the moderate salinity study sites (S5 and N6). There are marked seasonal changes in natural environmental variables, such as salinity and dissolved oxygen, and tidal conditions in the area where study sites S5 and N6 were located: it is possible that mud trapped at the bottom of the oyster reef is removed by tidal action, resulting in a lack of food source required by functional group D.
Salinity not only influences estuarine macrobenthos distribution patterns, but also affects growth and survival of oysters (Hulathduwa and Brown, 2006; Liu et al., 2018b). Effects of salinity on oyster size and abundance vary with region, therefore oyster substrate of the artificial reefs is likely to similarly vary with region, and may lead to diverse distribution patterns of macrobenthos functional groups. Our RDA confirmed that salinity, barnacle abundance, and oyster abundance (substrate parameters) were the main factors that influenced the distribution of macrobenthos functional groups in the Yangtze River estuary artificial oyster reefs (Figure 7). Although there were differences in growth and abundance of oysters between regions and at different tidal zones within a region, there was no difference in macrobenthos functional group species richness, abundance or biomass, with the exception of species richness of group D among the tidal zones. These results indicate that while there are differences in substrate habitat with salinity and among tidal zones within a salinity range, the distribution pattern of macrobenthos functional groups varies with salinity, but not with different tidal zone, such that tidal zone and associated effects on substrate elicit little effect on the artificial oyster reef macrobenthic functional groups in the Yangtze Estuary. This finding is inconsistent with those of other local natural habitats, such as Jiuduansha and Quanzhou Bay (Zhu and Lu, 2003; Zhuo et al., 2014), possibly because the habitat of artificially constructed oyster reef lacks diversity. We suggest enrichment and diversification of artificial oyster reef habitats may improve the recruitment of functional group types to increase biodiversity and ecosystem stability.
In general, healthy environments contain most types of feeding guild, whereas degraded environments tend to be dominated by a few trophic groups, with others absent (Gamito and Furtado, 2009; Peng et al., 2013). In this study, we found one or two groups were absent from the moderate salinity study sites (S5 and N6), where they included functional groups that play an important role in estuarine food web nutrient cycling and energy flow. For example, Pl is the main source of nutrition for predators and omnivores, and plays an important role in controlling the growth of plankton, such as cyanobacteria (Figure 2). The absence of this functional group from the moderate salinity study sites indicates compromised ecological health, as supported by our results for jFD, and previous results for AMBI, M-AMBI, and ABC curve (Liu et al., 2018b). Our ANOSIM 2 analysis showed site differences in ecological health, where it was better in the high salinity study sites. These results indicate that 14 years after construction, the habitat status of the artificial oyster reefs in the Yangtze Estuary was good or high, and was better under high salinity conditions (S8 and N9) than under moderate salinity (S5 and N6). Long-term artificial oyster reef monitoring confirms the feasibility of artificial oyster reef construction in the Yangtze estuary, and can be applied to estuarine areas or tidal flats within the same salinity range, such as east shoal of Nanhui, which are mainly affected by reclamation (Liu et al., 2018a). Coupled with the complex habitat of natural intertidal zone, it is inevitably more conducive to the ecological restoration of artificial oyster reef in these areas.
We found that macrobenthos functional group structure was more heterogeneous under high salinity conditions (S8 and N9) than under moderate levels of salinity (S5 and N6). Substrate parameters and environmental variables, such as dissolved oxygen, pH, temperature, and salinity, were similar between S5 and N6, and S8 and N9. However, functional group species richness, abundance, and biomass, and jFD of the southern dike (S5 and S8) was higher than in the north dike (N6 and N9), possibly due to site conditions. The southern region of the oyster reef in the Yangtze Estuary is proximate to the ecologically protected Jiuduansha Nature Reserve (Zhang et al., 2018) that may affect the structure and ecological environment of macrobenthos (Lv et al., 2016b). In contrast, the lack of such protection measures in the north dike may render the oyster and macrobenthos in this area susceptible to human activities, such as overfishing. Therefore, we suggest the establishment of a nature reserve to protect this important oyster reef habitat in the Yangtze estuary.
In summary, artificial oyster reef was feasibility and has positive effect on the ecological health in the Yangtze estuary. Further, the ecological health was worse in medium salinity than high salinity, may due to the absence of functional groups. The release of species of absent functional group can be taken in the medium salinity region. The artificial oyster reef may be disturbed by human activities, thus a nature reserve should be established to protect this important habitat in the Yangtze estuary.
The raw data supporting the conclusions of this article will be made available by the authors, without undue reservation.
YNZ: Conceptualization, Methodology, Resources, Formal analysis, Investigation, Data curation, Visualization, Writing - original draft, Writing - review and editing. HW: Conceptualization, Methodology, Resources, Visualization, Data curation, Writing - review and editing. YLZ: Conceptualization, Methodology, Resources, Visualization, Funding acquisition, Writing - review and editing. JD: Writing - original draft, Writing - review and editing. ZZ: Investigation, Data curation, Writing - review and editing. HZ: Conceptualization, Methodology, Writing - review and editing. ZL: Conceptualization, Methodology, Resources, Visualization, Supervision, Writing - review and editing, Project administration, Funding acquisition. All authors contributed to the article and approved the submitted version.
This study was sponsored by the Natural Science Foundation of Zhejiang Province of China (LQ22C030003), the special fund of State Environmental Protection Key Laboratory of Environmental Health Impact Assessment of Emerging Contaminants (SEPKL-EHIAEC-202201), the National Natural Science Foundation of China (42207323, 52009023) and the Scientific Reuter Foundation for Scholars of Hangzhou Normal University (2021QDL063).
We thank Elixigen for English language editing. We thank the editor and reviewers for their constructive and insightful feedback that improved the manuscript.
The authors declare that the research was conducted in the absence of any commercial or financial relationships that could be construed as a potential conflict of interest.
All claims expressed in this article are solely those of the authors and do not necessarily represent those of their affiliated organizations, or those of the publisher, the editors and the reviewers. Any product that may be evaluated in this article, or claim that may be made by its manufacturer, is not guaranteed or endorsed by the publisher.
Adger W. N., Hughes T. P., Folke C., Carpenter S. R., Rockström J. (2005). Social-ecological resilience to coastal disasters. Science 309, 1036–1039. doi: 10.1126/science.1112122
Bao Y., Hu Z., Li H., Ge B., Cheng H. (2008). Seasonal variation and functional groups of macrobenthic communities at diked and natural tidal flat China. Acta Entomol. Sin. 51, 1099–1128. doi: : 10.17520/biods.2015264
Borja A., Franco J., Pérez V. (2000). A marine biotic index to establish the ecological quality of soft-bottom benthos within European estuarine and coastal environments. Mar. Pollut. Bull. 40, 1100–1114. doi: 10.1016/S0025-326X(00)00061-8
Borja A., Muxika I., Franco J. (2003). The application of a marine biotic index to different impact sources affecting soft-bottom benthic communities along European coasts. Mar. Pollut. Bull. 46, 835–845. doi: 10.1016/S0025-326X(03)00090-0
Breitburg D. L., Coen L. D., Luckenbach M. W., Mann R., Posey M., Wesson J. A. (2000). Oyster reef restoration: Convergence of harvest and conservation strategies. J. Shellfish. Res. 19, 371–377. doi: 10.1093/plankt/22.6.1213
Chai C., Yu Z., Song X., Cao X. (2006). The status and characteristics of eutrophication in the Yangtze river (Changjiang) estuary and the adjacent East China Sea China. Hydrobiologia 563, 313–328. doi: 10.1007/s10750-006-0021-7
Chakraborty P. (2016). Oyster reef restoration in controlling coastal pollution around India: A viewpoint. Mar. Pollut. Bull. 115, 190–193. doi: 10.1016/j.marpolbul.2016.11.059
Chen J. (2001). Primary study on chrouosome of corophium sinense. J. Fujian. Fisher. 1, 011. doi: 10.14012/j.cnki.fjsc.2001.01.011
Chen Y., Liu R., Sun C., Zhang P., Feng C., Shen Z. (2012). Spatial and temporal variations in nitrogen and phosphorous nutrients in the Yangtze river estuary. Mar. Pollut. Bull. 64, 2083–2089. doi: 10.1016/j.marpolbul.2012.07.020
Clarke K., Gorley R. N. (2001). PRIMER (Plymouth routines in multivariate ecological research) v5: User manual/tutorial (Plymouth: Primer-E Ltd), 1–91.
Coen L. D., Luckenbach M. W. (2000). Developing success criteria and goals for evaluating oyster reef restoration: Ecological function or resource exploitation? Ecol. Eng. 15, 323–343. doi: 10.1016/S0925-8574(00)00084-7
Cummins K. W., Merritt R. W., Andrade P. C. (2005). The use of invertebrate functional groups to characterize ecosystem attributes in selected streams and rivers in south Brazil. Stud. Neotrop. Fauna. Environ. 40, 69–89. doi: 10.1080/01650520400025720
Dauvin J., Ruellet T. (2007). Polychaete/amphipod ratio revisited. Mar. Pollut. Bull. 55, 215–224. doi: 10.1016/j.marpolbul.2006.08.045
Gamito S., Furtado R. (2009). Feeding diversity in macroinvertebrate communities: A contribution to estimate the ecological status in shallow waters. Ecol. Indic. 9, 1009–1019. doi: 10.1016/j.ecolind.2008.11.012
Gamito S., Patrício J., Neto J. M., Teixeira H., Marques J. C. (2012). Feeding diversity index as complementary information in the assessment of ecological quality status. Ecol. Indic. 19, 73–78. doi: 10.1016/j.ecolind.2011.08.003
Grabowski J. H., Baillie C. J., Baukus A., Carlyle R., Fodrie F. J., Gittman R. K., et al. (2022). Fish and invertebrate use of restored vs. natural oyster reefs in a shallow temperate latitude estuary. Ecosphere 13, e4035. doi: 10.13140/RG.2.2.24829.51681
Grabowski J. H., Peterson C. H. (2007). Restoring oyster reefs to recover ecosystem services. Ecosyst. engineers.: Plants to. protists. 4, 281–298. doi: 10.1016/S1875-306X(07)80017-7
Han D., Cheng J., Hu X., Jiang Z., Mo L., Xu H., et al. (2017). Spatial distribution risk assessment and source identification of heavy metals in sediments of the Yangtze river estuary China. Mar. Pollut. Bull. 115, 141–148. doi: 10.1016/j.marpolbul.2016.11.062
He W. P., Li Y. X., Liu M., Radhakrishnan K., Li Z. J., Murphy B., et al. (2011). Reproductive biology of coilia mystus (Linnaeus) from the Yangtze estuary China: Responses to overexploitation. J. Appl. Ichthyol. 27, 1197–1202. doi: 10.1111/j.1439-0426.2011.01767.x
Holland A., Shaughnessy A. T., Hiegel M. H. (1987). Long-term variation in mesohaline Chesapeake bay macrobenthos: Spatial and temporal patterns. Estuaries 10, 227–245. doi: 10.2307/1351851
Huang Y., Huang Y., Du X., Li Y., Tian J., Chen Q., et al. (2022). Assessment of macrobenthic community function and ecological quality after reclamation in the changjiang (Yangtze) river estuary wetland. Acta Oceanolog. Sin. 41, 96. doi: 10.1007/s13131-022-2046-9
Huang Y., Li Y., Chen Q., Huang Y., Tian J., Cai M., et al. (2021). Effects of reclamation methods and habitats on macrobenthic communities and ecological health in estuarine coastal wetlands. Mar. Pollut. Bull. 168, 112420. doi: 10.1016/j.marpolbul.2021.112420
Hulathduwa Y. D., Brown K. M. (2006). Relative importance of hydrocarbon pollutants salinity and tidal height in colonization of oyster reefs. Mar. Environ. Res. 62, 301–314. doi: 10.1016/j.marenvres.2006.04.069
Jin C.-H., Zhu J.-Q., Xu S.-J., Wang W. (2011). Study on the embryonic and larval development of phascolosoma esculenta. Oceanolog. Limnolog. Sin. 1, 014. doi: 10.1007/s13131-011-0129-0
Kirwan M. L., Megonigal J. P. (2013). Tidal wetland stability in the face of human impacts and sea-level rise. Nature 504, 53. doi: 10.1038/nature12856
La Peyre M., Furlong J., Brown L. A., Piazza B. P., Brown K. (2014). Oyster reef restoration in the northern gulf of Mexico: Extent methods and outcomes. Ocean. Coast. Manage. 89, 20–28. doi: 10.1016/j.ocecoaman.2013.12.002
Li H., Bao Y., Hu Z., Ge B. (2007). Seasonal dynamics of macrobenthic functional groups and trophic levels in the bridge construction zone at the south bank of hangzhou bay China. J. Zool. 6, 1011–1023. doi: 10.1016/S1005-8885(07)60162-9
Li X., Cai L., Zhuo Y., Guo T., Rao Y., Yan L., et al. (2014). Functional groups of macrofauna in xunpu intertidal zone quanzhou bay. Mar. Sci. Bull. 33, 497–504. doi: 10.1007/s11434-013-0074-8
Li S., Liu Y., Li F., Zhang Y., Xu Z., Lü Z., et al. (2013). Macrobenthic functional groups in laizhou bay East China. Chin. J. Ecol. 32, 380–388. doi: 10.13292/j.1000-4890.2013.0143
Liu Z., Chen M., Li Y., Huang Y., Fan B., Lv W., et al. (2018a). Different effects of reclamation methods on macrobenthos community structure in the Yangtze estuary China. Mar. Pollut. Bull. 127, 429–436. doi: 10.1016/j.marpolbul.2017.12.038
Liu Z., Fan B., Huang Y., Yu P., Li Y., Chen M., et al. (2019). Assessing the ecological health of the chongming dongtan nature reserve China using different benthic biotic indices. Mar. Pollut. Bull. 146, 76–84. doi: 10.1016/j.marpolbul.2019.06.006
Liu K., Lin H., He X., Huang Y., Lin J., Mu J., et al. (2016a). Functional feeding groups of macrozoobenthos and their relationships to environmental factors in xiamen coastal waters. Haiyang. Xuebao. 38, 95–105. doi: : 10.3969/j.issn.0253-4193.2016.12.010
Liu Z., Lv W., Huang Y., Fan B., Li Y., Zhao Y. (2016b). Effects of cadmium on lipid metabolism in female estuarine crab chiromantes dehaani. Comp. Biochem. Physiol. Part C.: Toxicol. Pharmacol. 188, 9–16. doi: 10.1016/j.cbpc.2016.06.002
Liu Z., Yu P., Chen M., Cai M., Fan B., Lv W., et al. (2018b). Macrobenthic community characteristics and ecological health of a constructed intertidal oyster reef in the Yangtze estuary China. Mar. Pollut. Bull. 135, 95–104. doi: 10.1016/j.marpolbul.2018.07.019
Lotze H. K., Lenihan H. S., Bourque B. J., Bradbury R. H., Cooke R. G., Kay M. C., et al. (2006). Depletion degradation and recovery potential of estuaries and coastal seas. Science 312, 1806–1809. doi: 10.1126/science.1128035
Lv W., Huang Y., Liu Z., Yang Y., Fan B., Zhao Y. (2016a). Application of macrobenthic diversity to estimate ecological health of artificial oyster reef in Yangtze estuary China. Mar. Pollut. Bull. 103, 137–143. doi: 10.1016/j.marpolbul.2015.12.029
Lv W., Liu Z., Yang Y., Huang Y., Fan B., Jiang Q., et al. (2016b). Loss and self-restoration of macrobenthic diversity in reclamation habitats of estuarine islands in Yangtze estuary China. Mar. Pollut. Bull. 103, 128–136. doi: 10.1016/j.marpolbul.2015.12.030
Lv W., Ma C., Yu J., Tian W., Yuan X., zhao Y. (2013). Macrobenthic functional groups at the reclamation and natural tidal flats of hengsha East shoal the estuary of changjiang river. Acta Ecolog. Sin. 33, 6825–6833. doi: 10.5846/stxb201207100969
Lv W., Zhou W., Zhao Y. (2018). Macrobenthos functional groups as indicators of ecological restoration in reclaimed intertidal wetlands of china’s Yangtze estuary. Regional. Stud. Mar. Sci. 22, 93–100. doi: 10.1016/j.rsma.2018.06.003
Peng S., Zhou R., Qin X., Shi H., Ding D. (2013). Application of macrobenthos functional groups to estimate the ecosystem health in a semi-enclosed bay. Mar. Pollut. Bull. 74, 302–310. doi: 10.1016/j.marpolbul.2013.06.037
Quan W., Humphries A. T., Shen X., Chen Y. (2012). Oyster and associated benthic macrofaunal development on a created intertidal oyster (Crassostrea ariakensis) reef in the Yangtze river estuary China. J. Shellfish. Res. 31, 599–610. doi: 10.2983/035.031.0302
Quan W.-M., Zhang J.-P., Ping X.-Y., Shi L.-Y., Li P.-J., Chen Y.-Q. (2007). Purification function and ecological services value of crassostrea sp. in Yangtze river estuary. Ying. yong. sheng. tai. xue. bao. = J. Appl. Ecol. 18, 871–876.
Quan W.-m., Zhu J.-x., Ni Y., Shi L.-y., Chen Y.-q. (2009). Faunal utilization of constructed intertidal oyster (Crassostrea rivularis) reef in the Yangtze river estuary China. Ecol. Eng. 35, 1466–1475. doi: 10.1016/j.ecoleng.2009.06.001
Thomsen M. S., Silliman B. R., McGlathery K. J. (2007). Spatial variation in recruitment of native and invasive sessile species onto oyster reefs in a temperate soft-bottom lagoon. Estuar. Coast. Shelf. Sci. 72, 89–101. doi: 10.1016/j.ecss.2006.10.004
Walles B., Troost K., van den Ende D., Nieuwhof S., Smaal A. C., Ysebaert T. (2016). From artificial structures to self-sustaining oyster reefs. J. Sea. Res. 108, 1–9. doi: 10.1016/j.seares.2015.11.007
Xu Z. (2015). Preliminary study of functional groups and ecological study of macrofauna in jiaozhou bay (Ocean University of China).
Xu P., Peng G., Su L., Gao Y., Gao L., Li D. (2018). Microplastic risk assessment in surface waters: A case study in the changjiang estuary China. Mar. Pollut. Bull. 133, 647–654. doi: 10.1016/j.marpolbul.2018.06.020
Xu H., Zhao Y. (2005). Scientific survey on chongming dongtan migratory birds nature reserve of shanghai (Beijing: China Forestry Publishing House), 2–24.
Yang W., Li X., Sun T., Pei J., Li M. (2017). Macrobenthos functional groups as indicators of ecological restoration in the northern part of china’s yellow river delta wetlands. Ecol. Indic. 82, 381–391. doi: 10.1016/j.ecolind.2017.06.057
Ye J.-s. (2010). Biological characteristics of neanthes japonica and their application in shrimp aquaculture. J. Anhui. Agric. Sci. 15, 069. doi: 10.13989/j.cnki.0517-6611.2010.15.164
Yuan X., Lu J., Liu H. (2002). Distribution pattern and variation in the functional groups of zoobenthos in the changjiang estuary. Acta Ecolog. Sin. 22, 2054–2062. doi: 10.5846/stxb201607011353
Yu P., Liu Z., Wu D., Chen M., Lv W., Zhao Y. (2018). Accumulation of polystyrene microplastics in juvenile eriocheir sinensis and oxidative stress effects in the liver. Aquat. Toxicol. 200, 28–36. doi: 10.1016/j.aquatox.2018.04.015
Zhang S., He X., Wang J., Lin H., Huang Y., Lin J., et al. (2017). Characteristics of the benthic crustacean community structures and functional groups around the luoyang estuary in fujian. Acta Ecolog. Sin. 37, 5961–5972.
Zhang T., Tian B., Bo S., Yuan L., Zhao Y., Yuan X. (2018). Mapping the conservation priority of migratory shorebird habitat on a dynamic deltaic coast. Estuar. Coast. Shelf. Sci. 212, 219–232. doi: 10.1016/j.ecss.2018.07.010
Zheng G., Fan B. (1986). Ecological studees on perinereis aibuhitensis grube in ma yi isjand of zhoushan archipelago. J. Zhejiang. Ocean. Univ. 1, 009.
Zhu X., Lu J. (2003). Functional groups of zoobenthos in the intertidal zone of jiuduansha the Yangtze river estuary. Zool. Res. 24, 355–361.
Keywords: functional group, macrobenthos, Yangtze estuary, oyster reef, ecological restoration
Citation: Zhang Y, Wan H, Zhao Y, Ding J, Zhu Z, Zhang H and Liu Z (2023) Macrobenthic functional group analysis of ecological health of the intertidal artificial oyster reefs in the Yangtze Estuary, China. Front. Mar. Sci. 9:1059353. doi: 10.3389/fmars.2022.1059353
Received: 01 October 2022; Accepted: 12 December 2022;
Published: 04 January 2023.
Edited by:
Guanqiong Ye, Zhejiang University, ChinaReviewed by:
Huang Honghui, South China Sea Fisheries Research Institute (CAFS), ChinaCopyright © 2023 Zhang, Wan, Zhao, Ding, Zhu, Zhang and Liu. This is an open-access article distributed under the terms of the Creative Commons Attribution License (CC BY). The use, distribution or reproduction in other forums is permitted, provided the original author(s) and the copyright owner(s) are credited and that the original publication in this journal is cited, in accordance with accepted academic practice. No use, distribution or reproduction is permitted which does not comply with these terms.
*Correspondence: Zhiquan Liu, bGl1emhpcXVhbjEwMjRAMTYzLmNvbQ==
†These authors have contributed equally to this work
Disclaimer: All claims expressed in this article are solely those of the authors and do not necessarily represent those of their affiliated organizations, or those of the publisher, the editors and the reviewers. Any product that may be evaluated in this article or claim that may be made by its manufacturer is not guaranteed or endorsed by the publisher.
Research integrity at Frontiers
Learn more about the work of our research integrity team to safeguard the quality of each article we publish.