- 1Centro de Investigación en Ciencias del Mar y Limnología, Universidad de Costa Rica, San José, Costa Rica
- 2Center for Marine Biodiversity and Conservation, Scripps Institution of Oceanography, University of California San Diego, San Diego, CA, United States
- 3Department of Biology, Temple University, Philadelphia, PA, United States
- 4Occidental College, Los Angeles, CA, United States
Deep-sea chemosynthesis-based ecosystems support unique biological communities, but human impacts are an increasing threat. Understanding the life-history traits of species from deep-sea chemosynthesis-based ecosystems can help to develop adequate management strategies, as these can have impacts on ecological responses to changes in the environment. Here we examined the occurrence of sexual dimorphism in the yeti crab Kiwa puravida, an endemic species from the Costa Rican Pacific margin that aggregates at active methane seeps and depends on chemosynthetic bacteria for nutrition. The two morphological features examined included the claws, suspected to be under sexual selection and used for defense, and the carpus of the second pereopod not suspected to be under sexual selection. A total of 258 specimens, 161 males, 81 females, 16 juveniles, were collected from Mound 12 at 1,000-1,040 m depth in 2017 and 2018 and analyzed. We found that males have larger and wider claws than females, while there were no differences in carpus length. These results suggest that claw weaponry is under sexual selection in K. puravida, which is probably related to the mating system of this deep-sea species. This is the first attempt to study the reproductive biology of K. puravida, and additional observations will be necessary to shed more light on this matter.
Introduction
Deep-sea chemosynthesis-based ecosystems support large biomass, and unique and high diversity, mainly through symbiotic relationships of invertebrates with chemosynthetic microorganisms in an otherwise food-limited environment (Rogers et al., 2012; Marsh et al., 2015; Goffredi et al., 2020; Sogin et al., 2020). Since first discovered (Corliss et al., 1979), hydrothermal vents and methane seeps have prompted researchers to study the diversity, ecology, physiology, biogeography, and evolutionary processes of these novel environments (Martin et al., 2008; Moalic et al., 2012; Rogers et al., 2012; Roterman et al., 2018). Methane seeps, in particular, provide an array of ecosystem services such as climate regulation and carbon sequestration (Boetius and Wenzhöfer, 2013; Marlow et al., 2014), supporting habitats for commercially important species (Grupe et al., 2015; Seabrook et al., 2019; Turner et al., 2020), and cultural services (Levin et al., 2016). Considering the relevance of such deep-sea communities, it is important we understand the ecological interactions and life-history traits of deep-sea species (Mengerink et al., 2014; Pereira et al., 2021).
The diversity of species in all corners of our planet is driven by evolutionary forces, and among these, sexual selection plays a key role for many of the most striking shapes and adaptations found in nature (Darwin, 1871; West-Eberhard, 1983; Eberhard et al., 2018). Shuker and Kvarnemo (2021) define sexual selection as “any selection that arises from fitness differences associated with nonrandom success in the competition for access to gametes for fertilization”. Sexual selection mechanisms include competition (i.e., male-male competition) and mate choice (i.e., female choice) (Searcy and Andersson, 1986; Andersson and Simmons, 2006; Jones and Ratterman, 2009). Different intensities of sexual selection between males and females in a particular morphological characteristic can lead to morphological differences between the sexes in body parts other than sexual organs, that is sexual dimorphism (West-Eberhard, 1979; Jones and Ratterman, 2009). In species where males fight against other males to access females, sexual dimorphism is commonly found on the weaponry (McCullough et al., 2016; Rico-Guevara and Hurme, 2019). In many crustaceans, however, weaponry can occur in both males and females, and in some groups the claws represent the only mechanism of aggression and defense (Mariappan et al., 2000).
The yeti crabs (Chirostyloidea: Kiwaidae) are a group of episymbiont-bearing anomuran squat lobsters represented by the genus Kiwa (Schnabel and Ahyong, 2011), with four described species (Kiwa araonae SH Lee et al, 2016, Kiwa hirsuta Macpherson et al., 2005, Kiwa puravida Thurber et al, 2011, and Kiwa tyleri Thatje, Marsh, Roterman, Mavrogortdato and Linse, 2015), and two new, undescribed species (Roterman et al., 2018). All six species have been found exclusively in deep-sea chemosynthesis-based ecosystems, either methane seeps or hydrothermal vents (Roterman et al., 2013; Roterman et al., 2018). A recent phylogeny of the group suggests a split between the clades “bristly” and “plumose”, places the origin of the group in the Eastern Pacific, and proposes a vent origin for Kiwaidae, rather than a seep-to-vent progression as previously suggested (Roterman et al., 2013; Roterman et al., 2018). The kiwaid nutrition is dependent primarily on the chemosynthetic filamentous bacteria that grow among their setae (Goffredi, 2010; Thurber et al., 2011; Thatje et al., 2015a; Zwirglmaier et al., 2015). The only yeti crab known to inhabit methane seeps is K. puravida, an endemic species that occurs at a few methane seep sites on the Costa Rican Pacific margin. Kiwa puravida displays a “dancing” behavior suggested to increase the productivity of its bacterial epibionts (Thurber et al., 2011). The importance of water flow for increasing chemosynthetic production from the bacterial epibionts was affirmed in the vent galatheid crab Shinkaia crosnieri (Watsuji et al., 2017). Differences in bacterial composition among body parts and ontogenetic changes in bacterial communities have been observed in K. puravida (Goffredi et al., 2014). Despite the various studies carried out on yeti crabs, our knowledge of their life-history traits remains poorly understood, limiting our understanding of the biology and vulnerability of this deep-sea group. Our study aimed to examine the occurrence of sexual dimorphism in the claws of K. puravida to further advance our understanding of this species and yeti crab biology. Here we test the hypothesis that the claws of K. puravida are sexually dimorphic, where males have larger claws than females, and discuss the possible implications for the mating system of this deep-sea crustacean.
Material and methods
Sampling
Specimens of the Costa Rican yeti crab Kiwa puravida were collected at Mound 12, in the Costa Rican Pacific margin (8°55.80 N, 84°18.70 W) at depths of 1000–1040 m (Figure 1). Mound 12 is a carbonate mound composed primarily of authigenic carbonates (Klaucke et al., 2008), with ~1-1.6 km in diameter and seepage activity mainly located to the southwest of its summit (Mau et al., 2006). Evidence of variation in seepage activity levels is supported by the presence of slope sediments intercalated with mudflows (Niemann et al., 2013), and measurements revealed annual variation of methane release (Mau et al., 2007). The first studies on the biology of this area reported dominance of tubeworms (Siboglinidae), clam aggregations (Vesicomyidae), mussel beds (Bathymodiolinae), and bacterial mats (Sahling et al., 2008). Levin et al. (2015) found that Mound 12 has some of the highest density (~200 to more than 600 individuals per 200 cm2, the average size of a rock) and diversity (~26 species per rock) of deep-sea fauna on rocks from tubeworm bushes and mussel beds under active seepage among several sites in the Costa Rican Pacific margin. The faunal ensemble composition at Mound 12 shows interannual variability due to natural changes in seepage activity (Pereira et al., 2021).
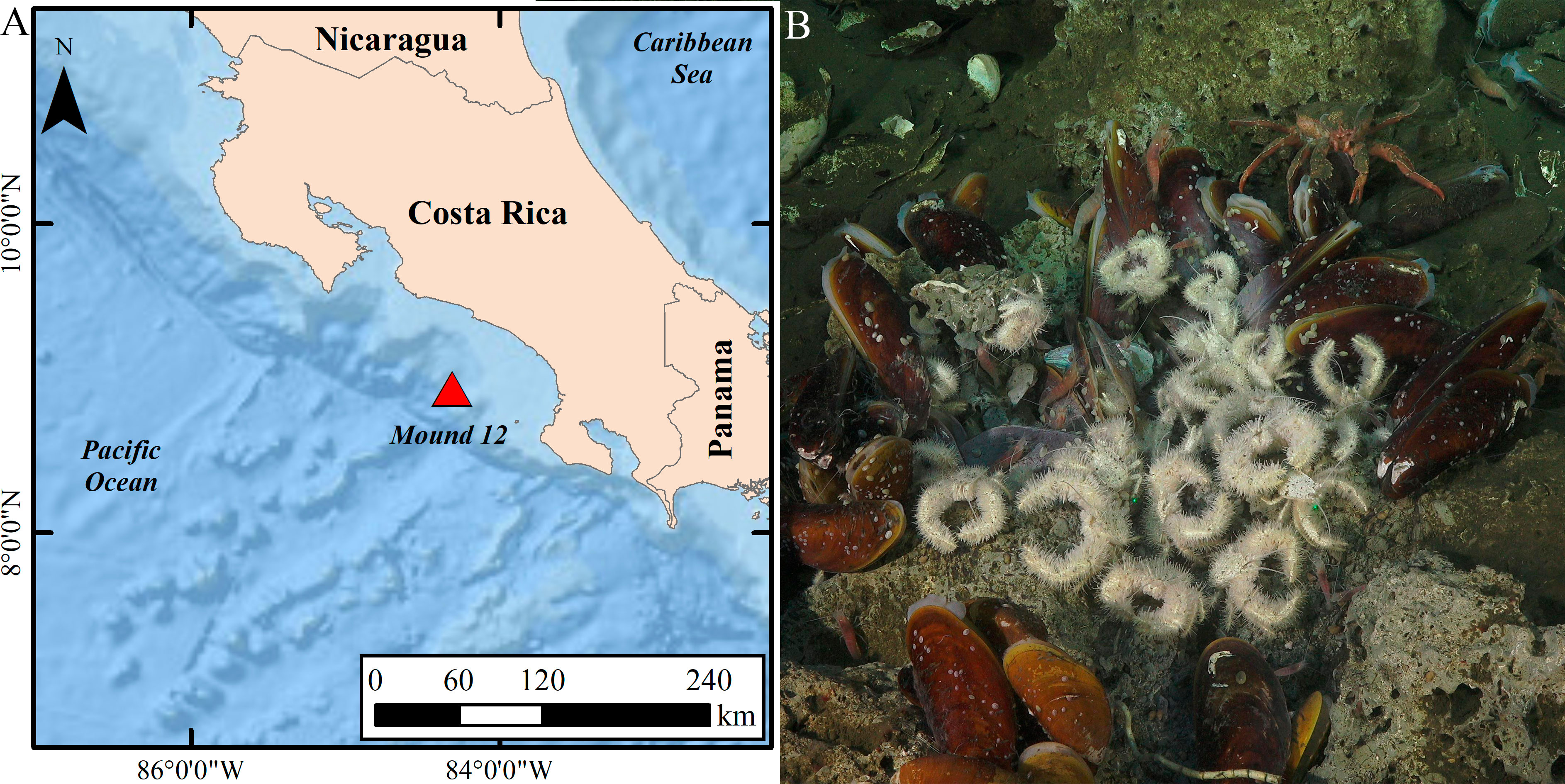
Figure 1 Sampling location (A) and detail of the yeti crabs Kiwa puravida (B) at Mound 12, a methane seep at ~1,000 m deep on the Costa Rican Pacific margin.
Kiwa puravida specimens at Mound 12 were sampled using the HOV Alvin deployed from RV Atlantis, during the expeditions AT 37-13 (Alvin dives: AD4906 and AD4907) and AT 42-03 (Alvin dives: AD4974, AD4975, AD4984, and AD4987), in May-June 2017 and October 2018, respectively. Carbonate rocks, tubeworm bushes, and mussel pots within mussel beds hosting yeti crabs were collected for megafaunal and macrofaunal studies under the collaborative research project “Quantifying the biological, chemical, and physical linkages between chemosynthetic communities and the surrounding deep sea” (ROC Hits, https://www.bco-dmo.org/project/648472). Carbonate rocks were placed into individual compartments, and tubeworm bushes and mussel pots were collected with a specially designed hydraulic net, the Bushmaster (Cordes et al., 2005), that entrapped the tubes. Immediately upon retrieval, samples were kept in the cold room (4°C) as they were processed. Animals, including yeti crabs, were removed from the rocks and bushes, and preserved in 95% ethanol to be sorted, identified, and measured in the laboratories at Scripps Institution of Oceanography (see Pereira et al., 2021) and Temple University. Other yeti crabs were also collected for microbial experiments, and these were measured onboard for morphological measurements and sex determination as described below. All samples were collected with the permission of the Ministerio de Ambiente y Energía of Costa Rica (MINAE): Sistema Nacional de Áreas de Conservación (SINAC: SINAC-CUS-PIR-035-2017, SINAC-CUSBSE-PI-R-032-2018, SINAC-SE-064-2018) and Comisión Nacional para la Gestión de la Biodiversidad (CONAGEBIO: R-070-2018-OT-CONAGEBIO).
Morphological measurements and sex determination
A total of 258 K. puravida specimens (161 males, 81 females, 16 juveniles) were measured. An additional 183 specimens were too small to get adequate measurements (carapace length < 0.3 mm), and 8 others were in bad condition. Measurements of both live specimens onboard and preserved specimens collected from rocks were made with vernier calipers to the nearest 0.01 mm in the laboratory, and measurements of preserved specimens collected from biogenic habitats (tubeworm bushes and mussel beds) were made using ImageJ 1.53r or an Amscope MU1000 calibrated camera attached to a dissecting microscope. The carapace length of K. puravida yeti crabs was measured as the distance from the distal midline of the orbital arch to the posterior dorsal margin of the carapace (Figure 2). We included two measurements of the propodus as a proxy of claw size (chela), which is suspected to present sexual dimorphism: 1) claw length, as the distance from the proximal margin of the propodus of the first pereopod (cheliped) to the distal margin of the fixed finger of the propodus; 2) claw width, as the widest transversal segment of the propodus of the first pereopod (Figure 2). In addition, we measured the length of the carpus of the second pereopod (first walking leg), as the distance from the proximal margin of the carpus to its distal margin (Figure 2). Sex in K. puravida was determined by looking for gonopores in the base of the third pair of pereopods, which are present in females only, and for the modified pair of first pleopods in males (Baba et al., 2008; Baba et al., 2011; Thurber et al., 2011). The specimens were classified as juveniles when the sex could not be determined, lacking gonopores and modified pleopods (these were usually small specimens), and thus were excluded from the analyses. Eggs under the abdomen of ovigerous females were carefully removed and counted. Unfortunately, statistical tests comparing measurements between non-ovigerous females and ovigerous females were not possible due to a small sample size of ovigerous females (n = 3) yielding low statistical power.
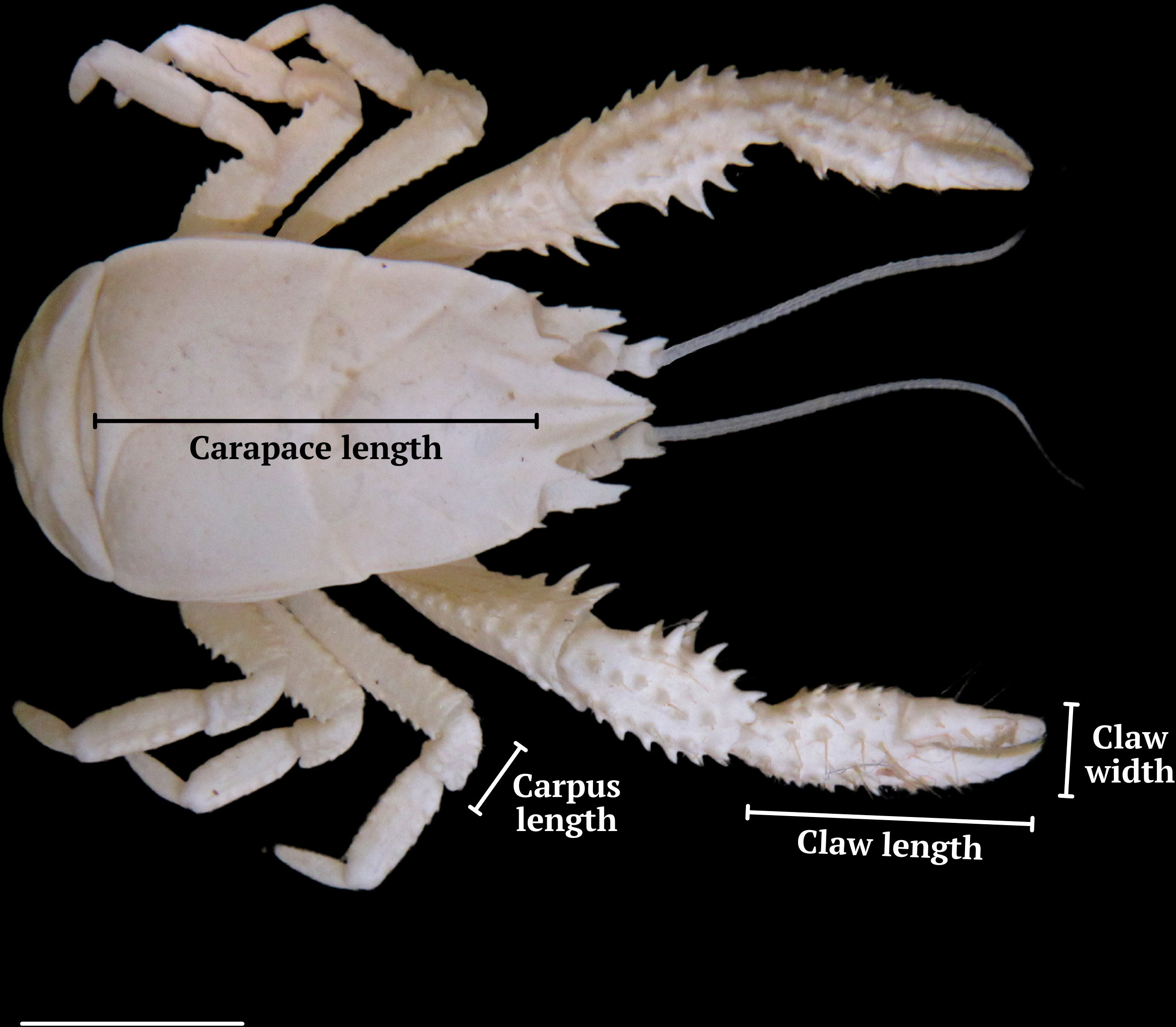
Figure 2 Morphological measurements of carapace length, claw length, claw width, and carpus length of the second pereopod in specimens of Kiwa puravida from Mound 12, Costa Rican Pacific margin. Scale bar: 0.5mm.
Statistical analyses
Normal distribution and homogeneity of variance were confirmed through quantile-quantile plots and fitted values-residuals plots. An ANOVA was conducted to check for sexual dimorphism in carapace length, and a chi-squared test was conducted to examine whether there was a significant deviation from a 1:1 sex ratio within the sample. We tested our hypothesis that males would have proportionally larger claws than females using one-way Analysis of Covariance (ANCOVA). ANCOVAs are useful to analyze the influence of a categorical predictor on a dependent variable while controlling a continuous predictor covariable, and to compare the slopes of the regressions of the dependent variable with the continuous predictor covariable by the categorical predictor (Rutherford, 2011). ANCOVAs have been successfully used in other sexual dimorphism studies with anomuran species (Hendrickx and Papiol, 2019; Palaoro et al., 2020). We considered claw length and claw width, both suspected to present sexual dimorphism as the dependent variables, as well as the length of the carpus of the second pereopod, a structure that is not suspected to present sexual dimorphism. The ANCOVAs for each dependent variable were fitted with sex as the categorical predictor and carapace length as the continuous predictor covariable, including the interaction between both predictor variables. Data of juvenile yeti crabs were excluded from the ANCOVAs. All statistical analyses were performed using RStudio (RStudio Team, 2022).
Results
We measured 161 (62%) males, 81 (31%) females, and 16 (6%) juveniles, showing an overall male bias with a sex ratio of 1:0.5 in the sampled population (X2 = 38.168, p < 0.0001, d.f. = 1). The largest and smallest males measured were 29.38 mm and 0.40 mm (carapace length), and the largest and smallest females measured were 18.46 mm and 0.46 mm (carapace length). There was no difference in average size (carapace length) between males and females (ANOVA: F1,240 = 3.88, p = 0.14). A summary of the mean and standard deviation (SD) for carapace length, claw length and width (propodus of the first pereopod), and carpus length of the second pereopod is in Table 1. We found three ovigerous females, with carapace length ranging between 15.66 mm and 18.22 mm, and number of eggs ranging from 38 to 159.

Table 1 Number of specimens of Kiwa puravida from Mound 12, Costa Rican Pacific margin, mean (± standard deviation) carapace length (mm), claw length and width (propodus of the first pereopod, mm), and carpus length of the second pereopod (mm) of all specimens and by sex (males, females, and juveniles).
Males had longer claws than females (ANCOVA: F1,202 = 9.07, p = 0.003; Figure 3A), and the slope of the relationship between claw length and carapace length was steeper in males than in females (F = 11.93, p = 0.0006). There were no differences in the claw width between males and females (ANCOVA: F1,201 = 2.98, p = 0.086; Figure 3B), but the slope of the relationship between claw width and carapace length in males was steeper than in females (F = 10.04, p = 0.002). As expected, there were no differences in the length of carpus of the second pereopod between sexes (ANCOVA: F1,206 = 2.95, p = 0.087; Figure 3C) and in the slope between carpus length and carapace length (F = 4.542, p = 0.13426). All the ANCOVA outcomes are summarized in Table 2.
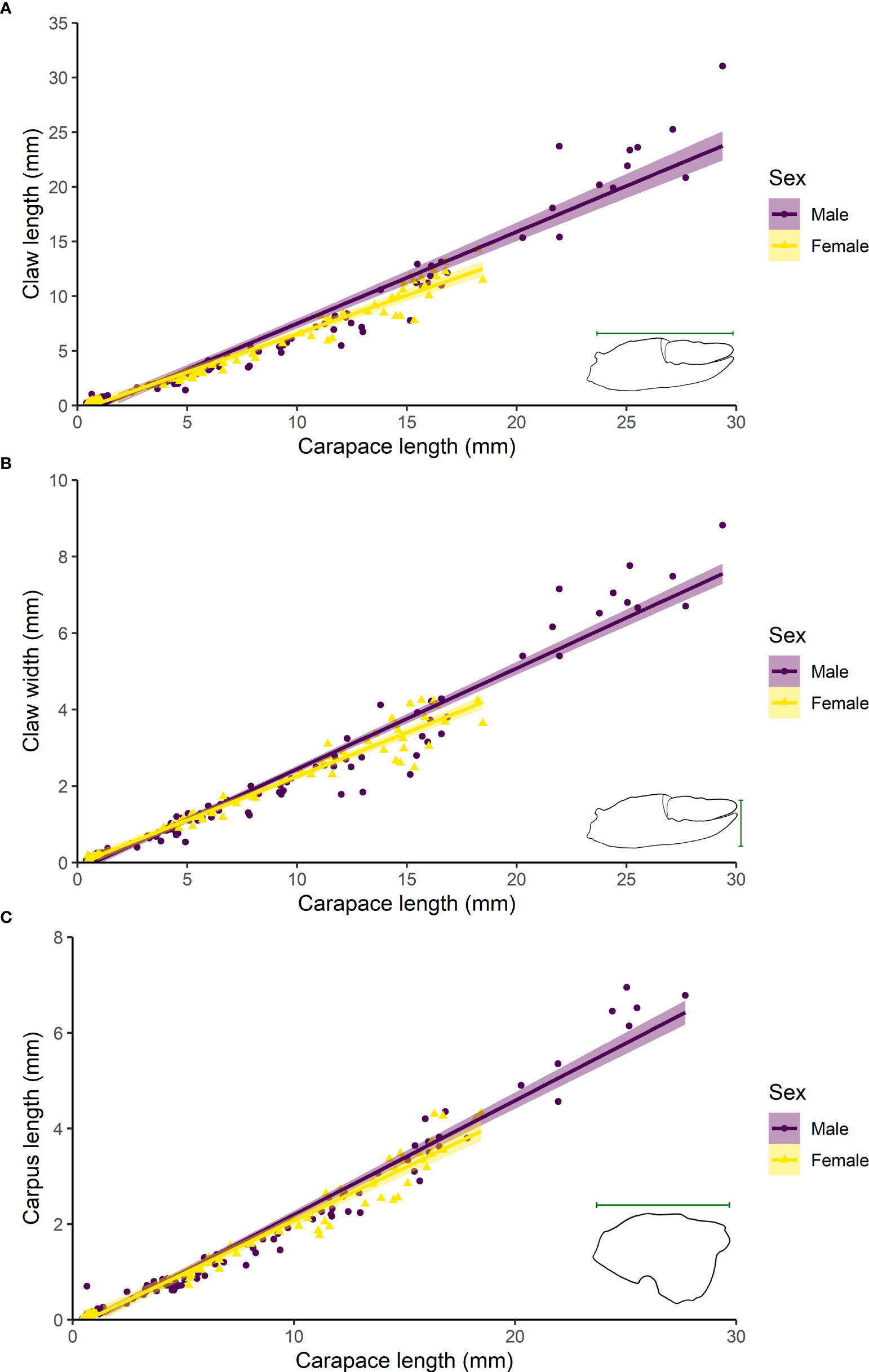
Figure 3 Comparison of the relationship of the claw length (A), claw width (B), and carpus length of the second pereopod (C) with the carapace length by sex (adult males and females) of Kiwa puravida, 2011 from Mound 12, Costa Rican Pacific margin. Data were fitted in linear regression models, shading represents 95% confidence interval around the model fit.
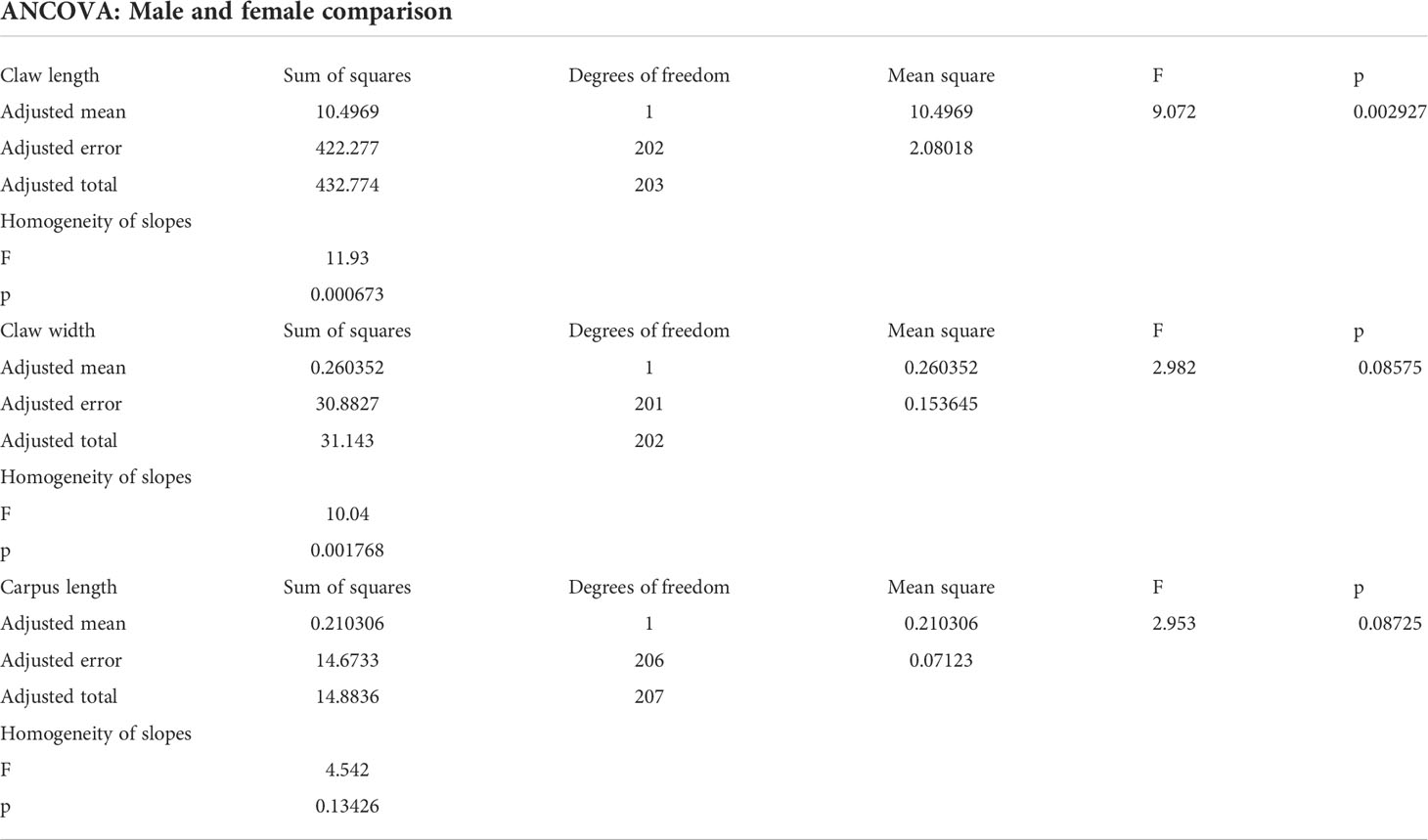
Table 2 ANCOVA outcomes: Sum of squares, degrees of freedom, mean squares, F values, p values, and homogeneity of slopes (F value and p value) for the relationship of claw length, claw width, and carpus length (second pereopod) with carapace length by sex in (adult males and females) of Kiwa puravida from Mound 12, Costa Rican Pacific margin.
Discussion
Our initial hypotheses that the yeti crab Kiwa puravida exhibits sexual dimorphism with males having larger claws than females was supported. Results suggest that the claws of male yeti crabs are larger and therefore under sexual selection, perhaps representing an advantage for reproduction (Andersson and Simmons, 2006; Jones and Ratterman, 2009; Shuker and Kvarnemo, 2021). Similar patterns of claw sexual dimorphism have been reported for species of Chrisostyloidea squat lobsters such as Uroptychus alcocki and Uroptychus scambus (Schnabel, 2009), and other deep-sea anomurans including Cervimunida johni (Lezcano et al., 2015), Munida intermedia (Mori et al., 2004), Munida iris (Williams and Brown, 1972), Munida microphtalma (Kassuga et al., 2008), Munida rugosa (Claverie and Smith, 2007; Claverie and Smith, 2010), Munidopsis erinacea (Tavares and Campinho, 1998), Munidopsis kaiyoae (Schnabel and Bruce, 2006), Munidopsis scobina (Creasey et al., 2000), Munidopsis sigsbei (Tavares and Campinho, 1998), Munidopsis papanui (Schnabel and Bruce, 2006), Pleuroncodes monodon (Lezcano et al., 2015), and Shinkaia crosnieri (Tsuchida et al., 2003). Although this is the first time that sexual dimorphism in weaponry is tested for yeti crabs in the family Kiwaidae, studies conducted on Kiwa tyleri from the Southern Ocean hydrothermal vent fields found that males have larger carapace length than females, and also reported that sexual dimorphism of the claw is apparent in this species, but this was not tested (Marsh et al., 2015; Thatje et al., 2015a). In contrast, we observed no difference in carapace length between sexes of K. puravida.
Many squat lobsters are commonly found living in large groups (Lovrich and Thiel, 2011), and sometimes in high densities (60-4,000 individuals per m2) such as K. tyleri (Marsh et al., 2015; Thatje et al., 2015a). In addition, a few species might congregate near resources, especially species whose nutrition relies on chemosynthetic episymbionts (Baeza, 2011; Marsh et al., 2015). According to the review of Thiel and Lovrich (2011), high-density aggregations of squat lobsters might prompt competition or antagonistic interactions for food, territory, or potential mating partners, however, most evidence suggests that these animals rarely have aggressive intraspecific interactions, and retreating is more common. Some documented agonistic interactions over resources or mates might include spreading the chelipeds, raising the body from the bottom using the walking legs, approaching the opponent, and grabbing the chelipeds of the opponent in the most aggressive interactions (Berril, 1970; Parzefall and Wilkens, 1975; Antonsen and Paul, 1997; Tulipani and Boudrias, 2006). Kiwa puravida is known to occur in dense groups (Thurber et al., 2011), thus it is expected to observe some level of antagonistic interactions in this species.
Antagonistic behavior has previously been observed in yeti crabs. Kiwa tyleri has been shown fighting (Marsh et al., 2015), while K. puravida has been shown displaying a conspicuous interaction, although it was unclear whether it was courtship or competitive display (Thurber et al., 2011). During this research, we observed yeti crabs waving their claws apparently to fend off other individuals (Supplementary Material Video). We also observed similar behavior directed at other species that got close, such as Alvinocaris shrimps (Supplementary Material Video). The occurrence of sexual dimorphism in claws and the observation of agonistic interactions could indicate that the claws of K. puravida males are under sexual selection derived from male-male competition for potential mating partners. In addition, we observed one individual using its fourth pereopod to fend off an Alvinocaris shrimp (Supplementary Material Video), suggesting that further observations and behavioral studies will help to better understand antagonistic behavior in K. puravida and conduct future morphological studies in other structures.
Sexual selection can also arise from mate choice, for example females choosing males for mating (Searcy and Andersson, 1986; Andersson and Simmons, 2006; Jones and Ratterman, 2009). In squat lobsters, mating behavior is usually commenced by the male approaching the female, and, in some species this behavior might include grasping and keeping the female in a precopula position using the chelipeds or pereopods (Thiel and Lovrich, 2011). Such courtship displays and extended precopulatory positions have been reported in Galathea strigosa (Brandes, 1897; Heitler et al., 1983), Pleuroncodes monodon (M Thiel unpublished in Thiel and Lovrich, 2011), and Pleuroncodes planipes (Serrano-Padilla and Aurioles-Gamboa, 1995). Our understanding about yeti crab reproductive behaviors is very limited, except for the different life-history trait adaptations among sexes in K. tyleri (Marsh et al., 2015) and the footage of a potential courtship display of K. puravida, as mentioned earlier.
Competition for territory has also been discussed for squat lobsters living in aggregations (Thiel and Lovrich, 2011), thus it could also play a significant role as a mechanism for the occurrence of sexual dimorphism of claws in K. puravida. Large densities of K. puravida have been reported from carbonate rocks with up to 40 individuals/200 cm2 on a single rock (Pereira et al., 2021; Pereira et al., 2022). They are called “dancing yeti crabs” due to their rhythmic arm movements; they wave their claws in seeping waters (Thurber et al., 2011) to presumably supply oxygen and sulfide to the episymbiotic bacteria (Goffredi et al., 2014). These movements might increase the area occupied by each specimen and create even more conflict among individuals (see Supplementary Material Video). If territory represents an advantage for males to access potential mating partners, then competition for territory could underpin in the mechanisms causing sexual selection of the claws in males of K. puravida.
In K. puravida, the claws provide a surface for episymbiotic chemosynthetic bacterial farming (Thurber et al., 2011). Thus, bigger claws might prove advantageous for feeding by providing larger surface area for bacterial farming. Our specimens were collected mostly from active seep areas, where chemosynthetic bacterial growth is the highest compared to lower seepage activity areas (Case et al., 2015). The male-biased sex ratio of 1:0.5 observed in our sampled population of K. puravida mainly from active seep areas could indicate different diet adaptations and spatial distribution between sexes, and these could be associated with males having bigger claws, allowing for a larger area for bacteria farming. In addition, K. puravida may periodically feed on detritus as needed (Thurber et al., 2011). Their epibiotic bacterial community changes between early and later life-cycle stages potentially due to environmental factors (Goffredi et al., 2014). Differences in the epibiont community composition have also been observed between males and females of K. tyleri (identified previously as Kiwa sp. nov. ESR) from hydrothermal vents in the East Scotia Ridge, leading to clear differences in isotopic niches reflecting the different food sources that were assimilated among sexes (Zwirglmaier et al., 2015). Males of K. tyleri are predominant in assemblages close to active venting areas in high densities, while females were observed further away from the active areas, and egg-bearing females need to leave to colder surrounding areas to protect the embryos that have a protracted and lecithotrophic development; also, differences in life-history trait adaptations between sexes have been reported (Marsh et al., 2015; Thatje et al., 2015b; Zwirglmaier et al., 2015). These observations suggest that environmental factors could be influencing behavioral and morphological characteristics in kiwaids. However, this seems unlikely in K. puravida since they comb bacteria from all parts of their body, including abdomen and legs, not just claws (see Goffredi et al., 2014), and if farming is increased in males, we would expect males to be larger than females due to increased nutrition, which we did not observe. It is also important to note that our sampled population included specimens from different assemblages (or yeti parties, as we called them due to their ‘dance moves’), and more research is needed to better understand whether K. puravida males and females have different life-history traits that could contribute to the difference in claw size, for example, incorporating spatial distribution and stable isotope analyses (Reid et al., 2013; Zwirglmaier et al., 2015).
There is an extensive body of work reviewing mating systems in Decapoda, factors influencing it, and its consequences for other aspects of life-history traits (see Asakura, 2016). The occurrence of sexual dimorphism and the observation of antagonistic interactions, which can potentially be related to competition for resources (i.e., food, territory, mating partners) could influence the mating system of K. puravida. According to Asakura (2016), the most common types of mating systems in free-living decapods are 1) “short courtship”, with short copulatory events and low levels of aggression in males; and 2) “precopulatory guarding”, with longer precopulatory and copulatory interactions and higher levels of aggression in males. Evidence suggests that both duration and aggressiveness are not found in discrete categories, but display a gradient from short to long or no-aggressive to highly aggressive, respectively, in this group. For example, in two deep-water squat lobsters that exhibit precopulatory guarding, the guarding duration was shorter in P. monodon than in C. johni, and the authors proposed that these differences can be related to species-specific life-history traits such as mobility and intraspecific aggression, both expected to be higher in C. johni (Espinoza-Fuenzalida et al., 2012). Furthermore, the cheliped shape in these two species show a relation to the mating system and possibly to male aggressiveness, where C. johni large males have more arched chelipeds to inflict puncture wounds on opponents, while in P. monodon the chelipeds were straighter (Lezcano et al., 2015). In K. puravida, we found that males have larger claws that may be used as weaponry in male-male competition for access to females, and some antagonistic interactions have been observed (Thurber et al., 2011; Supplementary Material Video). Considering this, we propose that K. puravida might present a mating system that resembles the precopulatory guarding type. Further observations on the mating and reproductive behavior of this yeti crab will be necessary to determine its mating system.
Research on reproductive biology and other life-history traits of these deep-sea species will enhance our capacity to develop adequate management strategies (Mengerink et al., 2014; Pereira et al., 2021), necessary under the current scenario of increasing pressures on exploration and exploitation of resources in the deep sea (Ramírez-Llodra et al., 2011; Norse et al., 2012; Heffernan, 2019). Knowledge about the reproductive behavior of squat lobsters is limited (Thiel and Lovrich, 2011), and this is especially true for yeti crabs. The biology of yeti crabs is obscure due to the complexity of studying these species, which are only found in deep-sea chemosynthetic habitats, and individual species have highly restricted distributions. Seven specimens of K. puravida survived during seven months in captivity at the aquarium facilities of Centro de Investigación en Ciencias del Mar y Limnología (CIMAR), in tanks with 8°C water temperature and with a few sulfide chips provided weekly, but not through multiple generations (personal observation). Further direct and detailed observations and analyses of specimen morphology and behavior from collections or videos from deep submersible vehicles (HOVs, ROVs) will be essential to enhance our knowledge of these crustaceans and their life-history strategies.
Data availability statement
The original contributions presented in the study are included in the article/Supplementary Material. Further inquiries can be directed to the corresponding author.
Author contributions
JCAS and OP designed the study. EEC, LL, SG, and JC designed the logistics of the expeditions, and all authors participated on the expeditions as part of the science party and collected the samples. OP and EJC performed the morphometric measurements. JCAS performed the statistical analyses. JCAS and OP wrote the manuscript, with significant contributions from EJC, EEC, LL, SG, and JC. JCAS and OP contributed equally to the manuscript and share first authorship. All authors contributed to the article and approved the submitted version.
Funding
This research was funded by NSF OCE 1635219 awarded to EEC and NSF OCE 1634172 awarded to LL. OP received a Scholarship award from the Association of Women in Science San Diego (2018) and was supported by NSF OCE 2048720 during manuscript preparation.
Acknowledgments
We are grateful to the efforts of many aboard RV Atlantis II expeditions AT 37-13 and AT 42-03, including the captains, crew, pilots, and technicians of HOV Alvin, and science parties. Thanks to O Breedy, B Naranjo, J Lunden, A Stabbins, M Betters, O Ashford, GW Rouse, and V Orphan for their help with sampling on board. We thank these Costa Rican institutions for granting the collection permits: Ministerio de Ambiente y Energía (MINAE), the Sistema Nacional de Áreas de Conservación (SINAC), and the Comisión Nacional para la Gestión de la Biodiversidad (CONAGEBIO). Support for this research was provided by NSF award OCE 1635219. We highly appreciate the valuable comments from two reviewers which enhanced our contribution.
Conflict of interest
The authors declare that the research was conducted in the absence of any commercial or financial relationships that could be construed as a potential conflict of interest.
Publisher’s note
All claims expressed in this article are solely those of the authors and do not necessarily represent those of their affiliated organizations, or those of the publisher, the editors and the reviewers. Any product that may be evaluated in this article, or claim that may be made by its manufacturer, is not guaranteed or endorsed by the publisher.
Supplementary material
The Supplementary Material for this article can be found online at: https://www.frontiersin.org/articles/10.3389/fmars.2022.1051590/full#supplementary-material
References
Andersson M., Simmons L. W. (2006). Sexual selection and mate choice. Trends Ecol. Evol. 21 (6), 296–302. doi: 10.1016/j.tree.2006.03.015
Antonsen B. L., Paul D. H. (1997). Serotonin and octopamine elicit stereotypical agonistic behaviors in the squat lobster munida quadrispina (Anomura, galatheidae). J. Comp. Physiol. A 181 (5), 501–510. doi: 10.1007/s003590050134
Asakura A. (2016). “The evolution of mating systems in decapod crustaceans,” in Decapod crustacean phylogenetics. Eds. Martin J. W., Crandall K. A., Felder D. L. (Florida: CRC Press), 133–194.
Baba K., Ahyong S. T., Macpherson E., Poore G. C. B. (2011). “Morphology of marine squat lobsters,” in The biology of squat lobsters. Eds. Poore G. C. B., Ahyong S. T., Taylor J. (Boca Raton: CSIRO Publishing, Melbourne, and CRC Press), 1–37.
Baba K., Macpherson E., Poore G. C., Ahyong S. T., Bermudez A., Cabezas P., et al. (2008). Catalogue of squat lobsters of the world (Crustacea decapoda: Anomura families chirostylidae, galatheidae and kiwaidae). Zootaxa 1905 (1), 1–220. doi: 10.11646/zootaxa.1905.1.1
Baeza J. A. (2011). “Squat lobsters as symbionts and in chemo-autotrophic environments,” in The biology of squat lobsters. Eds. Poore G. C. B., Ahyong S. T., Taylor J. (Boca Raton: CSIRO Publishing, Melbourne, and CRC Press), 249–270.
Berril M. (1970). The aggressive behavior of Munida sarsi (Crustacea: Galatheidae). Sarsia 43 (1), 1–12.
Boetius A., Wenzhöfer F. (2013). Seafloor oxygen consumption fuelled by methane from cold seeps. Nat. Geosci. 6 (9), 725–734. doi: 10.1038/ngeo1926
Case D. H., Pasulka A. L., Marlow J. J., Grupe B. M., Levin L. A., Orphan V. J. (2015). Methane seep carbonates host distinct, diverse, and dynamic microbial assemblages. mBio 6 (6), e0134. doi: 10.1128/mBio.01348-15
Claverie T., Smith I. P. (2007). Functional significance of an unusual chela dimorphism in a marine decapod: Specialization as a weapon? Proc. R. Soc B 274 (1628), 3033–3038. doi: 10.1098/rspb.2007.1223
Claverie T., Smith I. P. (2010). Allometry and sexual dimorphism in the chela shape in the squat lobster Munida rugosa. Aquat Biol. 8 (2), 179–187. doi: 10.3354/ab00233
Cordes E. E., Hourdez S., Predmore B. L., Redding M. L., Fisher C. R. (2005). Succession of hydrocarbon seep communities associated with the long-lived foundation species Lamellibrachia luymesi. Mar. Ecol. Prog. Ser. 305, 17–29. doi: 10.3354/meps305017
Corliss J. B., Dymond J., Gordon L. I., Edmond J. M., von Herzen R. P., Ballard R. D., et al. (1979). Submarine thermal springs on the Galapagos rift. Science 203 (4385), 1073–1083. doi: 10.1126/science.203.4385.1073
Creasey S., Rogers A., Tyler P., Gage J., Jollivet D. (2000). Genetic and morphometric comparisons of squat lobster, munidopsis scobina (Decapoda: Anomura: Galatheidae) populations, with notes on the phylogeny of the genus Munidopsis. Deep Sea Res. Part II 47, 87–118. doi: 10.1016/S0967-0645(99)00098-3
Eberhard W. G., Rodríguez R. L., Huber B. A., Speck B., Miller H., Buzatto B. A., et al. (2018). Sexual selection and static allometry: The importance of function. Q. Rev. Biol. 93 (3), 207–250. doi: 10.1086/699410
Espinoza-Fuenzalida N. L., Acuña E., Hinojosa I. A., Thiel M. (2012). Reproductive biology of two species of squat lobsters-female receptivity and interbrood intervals. J. Crust Biol. 32 (4), 565–574. doi: 10.1163/193724012X626601
Goffredi S. K. (2010). Indigenous ectosymbiotic bacteria associated with diverse hydrothermal vent invertebrates. Environ. Microbiol. Rep. 2 (4), 479–488. doi: 10.1111/j.1758-2229.2010.00136.x
Goffredi S. K., Gregory A., Jones W. J., Morella N. M., Sakamoto R. I. (2014). Ontogenetic variation in epibiont community structure in the deep-sea yeti crab, Kiwa puravida: convergence among crustaceans. Mol. Ecol. 23 (6), 1457–1472. doi: 10.1111/mec.12439
Goffredi S. K., Tilic E., Mullin S. W., Dawson K. S., Keller A., Lee R. W., et al. (2020). Methanotrophic bacterial symbionts fuel dense populations of deep-sea feather duster worms (Sabellida, Annelida) and extend the spatial influence of methane seepage. Sci. Adv. 6 (14), eaay8562. doi: 10.1126/sciadv.aay8562
Grupe B. M., Krach M. L., Pasulka A. L., Maloney J. M., Levin L. A., Frieder C. A. (2015). Methane seep ecosystem functions and services from a recently discovered southern California seep. Mar. Ecol. 36, 91–108. doi: 10.1111/maec.12243
Heitler W. J., Myers C. M., Maitland D. P. (1983). An intersegmental reflex between the copulatory swimmerets of the male squat lobster, Galathea strigosa. Mar. Behav. Physiol. 10 (2), 103–120. doi: 10.1080/10236248309378611
Hendrickx M. E., Papiol V. (2019). Distribution, biology, and ecology of the deep-water squat lobster Galacantha diomedeae (Crustacea: Decapoda: Galatheoidea: Munidopsidae) in the Mexican pacific. Invertebr Biol. 138 (2), e12248. doi: 10.1111/ivb.12248
Jones A. G., Ratterman N. L. (2009). Mate choice and sexual selection: what have we learned since Darwin? Proc. Natl. Acad. Sci. U.S.A. 106 (Suppl 1), 10001–10008. doi: 10.1073/pnas.0901129106
Kassuga A. D., Silva-Ferreira T. C. G., Ostrovski M. C., Costa T. M. M. (2008). Population structure of Munida microphthalma leach (Crustacea: Decapoda: Galatheidae) from the northern coast of Rio de Janeiro state, Brazil. PanamJAS 3 (2), 116–121.
Klaucke I., Masson D. G., Petersen C. J., Weinrebe W., Ranero C. R. (2008). Multifrequency geoacoustic imaging of fluid escape structures offshore Costa Rica: Implications for the quantification of seep processes. Geochem Geophys. Geosyst 9, Q04010. doi: 10.1029/2007GC001708
Lee S. H., Lee W. K., Won Y. J. (2016). A new species of yeti crab, genus Kiwa macpherson, Jones and segonza (Decapoda: Anomura: Kiwaidae), from a hydrothermal vent on the Australian-Antarctic ridge. J. Crust Biol. 36 (2), 238–247. doi: 10.1163/1937240X-00002418
Levin L. A., Baco A. R., Bowden D. A., Colaco A., Cordes E. E., Cunha M. R., et al. (2016). Hydrothermal vents and methane seeps: rethinking the sphere of influence. Front. Mar. Sci. 3. doi: 10.3389/fmars.2016.00072
Levin L. A., Mendoza G. F., Grupe B. M., Gonzalez J. P., Jellison B., Rouse G., et al. (2015). Biodiversity on the rocks: macrofauna inhabiting authigenic carbonate at Costa Rica methane seeps. PloS One 10 (7), e0131080. doi: 10.1371/journal.pone.0136129
Lezcano A. H., Penna M. A., Márquez F., Thiel M. (2015). Variation in cheliped form in two species of squat lobsters (Decapoda: Anomura) from Chile. Braz. J. Oceanogr 63, 303–310. doi: 10.1590/S1679-87592015088006303
Lovrich G. A., Thiel M. (2011). “Ecology, physiology, feeding and trophic role of squat lobsters,” in The biology of squat lobsters. Eds. Poore G. C. B., Ahyong S. T., Taylor J. (Boca Raton: CSIRO Publishing, Melbourne, and CRC Press), 183–222.
Macpherson E., Jones W., Segonzac M. (2005). A new squat lobster family of galatheoidea (Crustacea, decapoda, anomura) from the hydrothermal vents of the pacific-Antarctic ridge. Zoosystema 27 (4), 709–723.
Mariappan P., Balasundaram C., Schmitz B. (2000). Decapod crustacean chelipeds: an overview. J. Biosci. 25 (3), 301–313. doi: 10.1007/BF02703939
Marlow J. J., Steele J. A., Ziebis W., Thurber A. R., Levin L. A., Orphan V. J. (2014). Carbonate-hosted methanotrophy represents an unrecognized methane sink in the deep sea. Nat. Commun. 5 (1), 1–12. doi: 10.1038/ncomms6094
Marsh L., Copley J. T., Tyler P. A., Thatje S. (2015). In hot and cold water: differential life-history traits are key to success in contrasting thermal deep-sea environments. J. Anim Ecol. 84 (4), 898–913. doi: 10.1111/1365-2656.12337
Martin W., Baross J., Kelley D., Russell M. J. (2008). Hydrothermal vents and the origin of life. Nat. Rev. Microbiol. 6, 805–814. doi: 10.1038/nrmicro1991
Mau S., Rehder G., Arroyo I. G., Grossler J., Suess E. (2007). Indications of a link between seismotectonics and CH4 release from seeps off Costa Rica. Geochem Geophys. Geosyst 8 (4), Q04003. doi: 10.1029/2006GC001326
Mau S., Sahling H., Rehder G., Suess E., Linke P., Soeding E. (2006). Estimates of methane output from mud extrusions at the erosive convergent margin off Costa Rica. Mar. Geol. 225, 129–144. doi: 10.1016/j.margeo.2005.09.007
McCullough E. L., Miller C. W., Emlen D. J. (2016). Why sexually selected weapons are not ornaments. Trends Ecol. Evol. 31 (10), 742–751. doi: 10.1016/j.tree.2016.07.004
Mengerink K. J., Van Dover C. L., Ardron J., Baker M., Escobar-Briones E., Gjerde K., et al. (2014). A call for deep-ocean stewardship. Science 344 (6185), 696–698. doi: 10.1126/science.1251458
Moalic Y., Desbruyères D., Duarte C. M., Rozenfeld A. F., Bachraty C., Arnaud-Haond S. (2012). Biogeography revisited with network theory: Retracing the history of hydrothermal vent communities. Syst. Biol. 61 (1), 127–137. doi: 10.1093/sysbio/syr088
Mori M., Sbrana M., Sartor P., De Ranieri S. (2004). Aspetti bio-ecologici di Munida intermedia (Crustacea, decapoda, anomura) nell’arcipelago toscano meridionale (Tirreno settentrionale). Atti Soc Toscana Sci. Nat. Mem. B 111, 43–53.
Niemann H., Linke P., Knittel K., MacPherson E., Boetius A., Brückmann W., et al. (2013). Methane-carbon flow into the benthic food web at cold seeps – a case study from the Costa Rica subduction zone. PloS One 8 (10), e74894. doi: 10.1371/journal.pone.0074894
Norse E. A., Brooke S., Cheung W. W., Clark M. R., Ekeland I., Froese R., et al. (2012). Sustainability of deep-sea fisheries. Mar. Pol. 36 (2), 307–320. doi: 10.1016/j.marpol.2011.06.008
Palaoro A. V., Muniz D. G., Santos S. (2020). Harder, better, faster, stronger: Weapon size is more sexually dimorphic than weapon biomechanical components in two freshwater anomuran species. J. Morphol 281 (9), 1098–1109. doi: 10.1002/jmor.21234
Parzefall J., Wilkens H. (1975). Zur ethologie augenreduzierter tiere. untersuchungen an Munidopsis polymorpha koelbel (Anomura, galatheidae). Ann. Speleol 30, 325–335.
Pereira O. S., Gonzalez J., Mendoza G. F., Le J., Coscino C. L., Lee R. W., et al. (2021). The dynamic influence of methane seepage on macrofauna inhabiting authigenic carbonates. Ecosphere 12 (10), e03744. doi: 10.1002/ecs2.3744
Pereira O. S., Gonzalez J., Mendoza G., Le J., McNeill M., Ontiveros J., et al. (2022). Does substrate matter in the deep sea? a comparison of bone, wood, and carbonate rock colonizers. PloS One 17 (7), e0271635. doi: 10.1371/journal.pone.0271635
Ramírez-Llodra E., Tyler P. A., Baker M. C., Bergstad O. A., Clark M. R., Escobar E., et al. (2011). Man and the last great wilderness: human impact on the deep sea. PloS One 6 (8), e22588. doi: 10.1371/journal.pone.0022588
Reid W. D., Sweeting C. J., Wigham B. D., Zwirglmaier K., Hawkes J. A., McGill R. A., et al. (2013). Spatial differences in East Scotia ridge hydrothermal vent food webs: Influences of chemistry, microbiology and predation on trophodynamics. PloS One 8 (6), e65553. doi: 10.1371/journal.pone.0065553
Rico-Guevara A., Hurme K. J. (2019). Intrasexually selected weapons. Biol. Rev. 94 (1), 60–101. doi: 10.1111/brv.12436
Rogers A. D., Tyler P. A., Connelly D. P., Copley J. T., James R., Larter R. D., et al. (2012). The discovery of new deep-sea hydrothermal vent communities in the southern ocean and implications for biogeography. PloS Biol. 10 (1), e1001234. doi: 10.1371/journal.pbio.1001234
Roterman C. N., Copley J. T., Linse K. T., Tyler P. A., Rogers A. D. (2013). The biogeography of the yeti crabs (Kiwaidae) with notes on the phylogeny of the chirostyloidea (Decapoda: Anomura). Proc. R. Soc B 280 (1764), 20130718. doi: 10.1098/rspb.2013.0718
Roterman C. N., Lee W. K., Liu X., Lin R., Li X., Won Y. J. (2018). A new yeti crab phylogeny: Vent origins with indications of regional extinction in the East pacific. PloS One 13 (3), e0194696. doi: 10.1371/journal.pone.0194696
RStudio Team (2022). RStudio: Integrated development environment for r. RStudio (Boston, MA: PBC). Available at: http://www.rstudio.com/.
Sahling H., Masson D. G., Ranero C. R., Hühnerbach V., Weinrebe W., Klaucke I., et al. (2008). Fluid seepage at the continental margin offshore Costa Rica and southern Nicaragua. Geochem. Geophys. Geosyst. 9 (5), Q05S05. doi: 10.1029/2008GC001978
Schnabel K. E. (2009). A review of the new Zealand chirostylidae (Anomura: Galatheoidea) with description of six new species from the kermadec islands. Zool. J. Linn. Soc 155 (3), 542–582. doi: 10.1111/j.1096-3642.2008.00449.x
Schnabel K. E., Ahyong S. (2011). A new classification of the chirostyloidea (Crustacea: Decapoda: Anomura). Zootaxa 2687, 56–64. doi: 10.11646/zootaxa.2687.1.4
Schnabel K. E., Bruce N. L. (2006). New records of Munidopsis (Crustacea: Anomura: Galatheidae) from new Zealand with description of two new species from a seamount and underwater canyon. Zootaxa 1172, 49–67. doi: 10.5281/zenodo.2645699d
Seabrook S., De Leo F. C., Thurber A. R. (2019). Flipping for food: The use of a methane seep by tanner crabs (Chionoecetes tanneri). Front. Mar. Sci. 6. doi: 10.3389/fmars.2019.00043
Searcy W. A., Andersson M. (1986). Sexual selection and the evolution of song. Annu. Rev. Ecol. Syst. 17, 507–533. doi: 10.1146/annurev.es.17.110186.002451
Serrano-Padilla V., Aurioles-Gamboa D. (1995). “Ecología reproductiva de la langostilla,” in La langostilla: Biología, ecología y aprovechamiento. Eds. Aurioles-Gamboa D., Balart E. F. (Mexico: CIBNOR), 109–123.
Shuker D. M., Kvarnemo C. (2021). The definition of sexual selection. Behav. Ecol. 32 (5), 781–794. doi: 10.1093/beheco/arab055
Sogin E. M., Leisch N., Dubilier N. (2020). Chemosynthetic symbioses. Curr. Biol. 30 (19), R1137–R1142. doi: 10.1016/j.cub.2020.07.050
Tavares M., Campinho P. (1998). Three new records of deep-sea squat lobsters of the genus Munidopsis whiteaves from the southwestern Atlantic ocean (Decapoda: Galatheidae). Crust Res. 27, 88–100. doi: 10.18353/crustacea.27.0_88
Thatje S., Marsh L., Roterman C. N., Mavrogordato M. N., Linse K. (2015a). Adaptations to hydrothermal vent life in. Kiwa tyleri New species yeti crab East Scotia Ridge Antarctica PloS One 10 (6), e0127621. doi: 10.1371/journal.pone.0127621
Thatje S., Smith K. E., Marsh L., Tyler P. A. (2015b). Evidence for protracted and lecithotrophic larval development in the yeti crab Kiwa tyleri from hydrothermal vents of the East Scotia ridge, southern ocean. Sexuality Early Dev. Aquat Org. 1 (2), 109–116. doi: 10.3354/sedao00011
Thiel M., Lovrich G. A. (2011). “Agonistic behavior and reproductive biology of squat lobsters,” in The biology of squat lobsters, vol. 20 . Eds. Poore G. C. B., Ahyong S. T., Taylor J. (Boca Raton: CSIRO Publishing, Melbourne, and CRC Press), 223.
Thurber A. R., Jones W. J., Schnabel K. (2011). Dancing for food in the deep sea: bacterial farming by a new species of yeti crab. PloS One 6 (11), e26243. doi: 10.1371/journal.pone.0026243
Tsuchida S., Fujiwara Y., Fujikura K. (2003). Distribution and population structure of the galatheid crab Shinkaia crosnieri (Decapoda: Anomura: Galatheidae) in the southern Okinawa trough. Japan J. Benth 58, 84–88. doi: 10.5178/benthos.58.84
Tulipani D. C., Boudrias M. A. (2006). Behaviors of the pelagic red crab Pleuroncodes planipes (Decapoda: Anomura: Galatheidae) observed in captivity. Crust Res. 6, 67–79. doi: 10.18353/crustacea.Special2006.6_67
Turner P. J., Ball B., Diana Z., Fariñas-Bermejo A., Grace I., McVeigh D., et al. (2020). Methane seeps on the US Atlantic margin and their potential importance to populations of the commercially valuable deep-sea red crab, Chaceon quinquedens. Front. Mar. Sci. 7. doi: 10.3389/fmars.2020.00075
Watsuji T. O., Tsubaki R., Chen C., Nagai Y., Nakagawa S., Yamamoto M., et al. (2017). Cultivation mutualism between a deep-sea vent galatheid crab and its chemosynthetic epibionts. Deep-Sea Res. I: Oceanogr Res. Pap 127, 13–20. doi: 10.1016/j.dsr.2017.04.012
West-Eberhard M. J. (1979). Sexual selection, social competition, and evolution. Proc. Am. Philos. Soc 123, 222–234.
West-Eberhard M. J. (1983). Sexual selection, social competition, and speciation. Q. Rev. Biol. 58, 155–183. doi: 10.1086/413215
Williams A. B., Brown W. S. (1972). Notes on structure and parasitism of Munida iris a. Milne edwards (Decapoda, galatheidae) from north Carolina, USA. Crustaceana 22 (3), 303–308.
Zwirglmaier K., Reid W. D., Heywood J., Sweeting C. J., Wigham B. D., Polunin N. V., et al. (2015). Linking regional variation of epibiotic bacterial diversity and trophic ecology in a new species of kiwaidae (Decapoda, anomura) from East Scotia ridge (Antarctica) hydrothermal vents. Microbiologyopen 4 (1), 136–150. doi: 10.1002/mbo3.227
Keywords: sexual selection, deep sea, methane seep, yeti crab, Eastern Pacific, Anomura, Kiwaidae, squat lobster
Citation: Azofeifa-Solano JC, Pereira OS, Cowell EJ, Cordes EE, Levin LA, Goffredi SK and Cortés J (2022) Sexual dimorphism in the methane seep-dwelling Costa Rican yeti crab Kiwa puravida (Decapoda: Anomura: Kiwaidae). Front. Mar. Sci. 9:1051590. doi: 10.3389/fmars.2022.1051590
Received: 23 September 2022; Accepted: 17 October 2022;
Published: 08 November 2022.
Edited by:
Paris Vasileios Stefanoudis, University of Oxford, United KingdomReviewed by:
Katrin Linse, British Antarctic Survey (BAS), United KingdomCraig M. Young, University of Oregon, United States
Copyright © 2022 Azofeifa-Solano, Pereira, Cowell, Cordes, Levin, Goffredi and Cortés. This is an open-access article distributed under the terms of the Creative Commons Attribution License (CC BY). The use, distribution or reproduction in other forums is permitted, provided the original author(s) and the copyright owner(s) are credited and that the original publication in this journal is cited, in accordance with accepted academic practice. No use, distribution or reproduction is permitted which does not comply with these terms.
*Correspondence: Juan Carlos Azofeifa-Solano, ZWF6b2ZlaWZhMkBnbWFpbC5jb20=
†These authors have contributed equally to this work and share first authorship