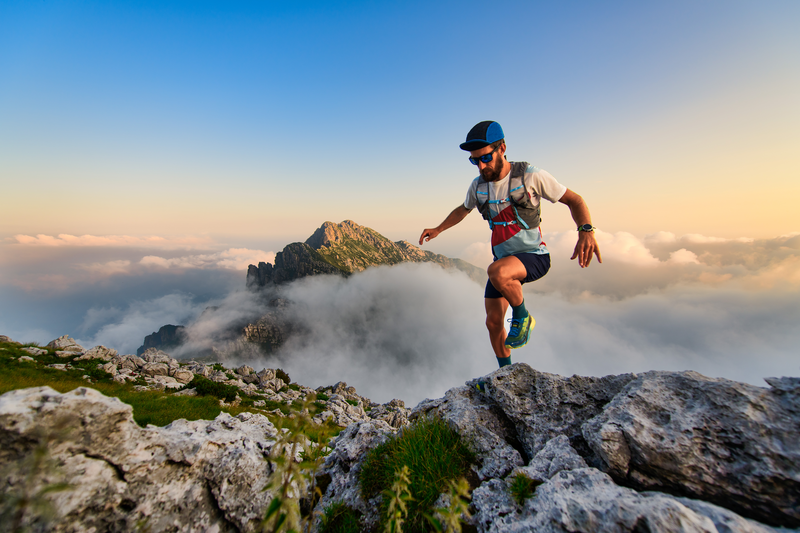
94% of researchers rate our articles as excellent or good
Learn more about the work of our research integrity team to safeguard the quality of each article we publish.
Find out more
ORIGINAL RESEARCH article
Front. Mar. Sci. , 04 January 2023
Sec. Marine Pollution
Volume 9 - 2022 | https://doi.org/10.3389/fmars.2022.1044232
This article is part of the Research Topic Impact of Marine Debris on Marine Ecosystems and Organisms View all 6 articles
The accumulation of seafloor litter changes the habitats of benthic organisms and thus affects their spatial distribution. Previous studies suggested that the accumulation of seafloor litter might contribute to the bloom of Metridium senile fimbriatum, given that seafloor litter could serve as “vectors” for M. senile dispersal and provide with “natural habitat” for their settlement. As a common fouler and invader, M. senile bloom will inevitably affect the benthic ecosystems. The cascading effects of seafloor litter on benthic ecosystems can be explored by studying the interactions between this litter-associated anemone and other co-occurred species. Therefore, this study uses stable isotope analysis to study the food composition of M. senile attached to the seafloor litter and the niche relationship with co-occurred species. First, by comparing the stable isotope ratios of the pedal disc and tentacle of M. senile, it was found that the δ15N of the foot disc is more stable than that of the tentacle, so the pedal disc was chosen as the sampling tissue. The mean δ13C value of M. senile was -19.34 ± 0.86‰, which ranged from -22.30‰ to -18.17‰. For δ15N, the mean value was 12.02 ± 0.95‰, which ranged from 9.22‰ to 13.31‰. Then, we quantified the contribution of the potential food resources of M. senile using the SIMMR model. M. senile has the highest feeding proportion to crustaceans, followed by juvenile fish, zooplankton and other macrobenthos. Meanwhile, M. senile has high uncertainty in the feeding proportion of crustaceans and juvenile fish. In addition, the trophic niche overlap between different functional groups was calculated by the SIBER model, and the results showed that the isotopic niche of M. senile was overlapped with different functional groups, ranging from 34.29 to 35.25%. Therefore, this study suggests that M. senile, as litter-associated carnivorous anemone, interacts with other components in the ecosystems through predation and competition, which also reveals the cascade effect of seafloor litter on the benthic ecosystems of the northern Yellow Sea.
Marine litter pollution is an important factor affecting regional or global marine ecosystems health (UNEP, 2005). It has become a hot issue in the research field of marine environments and has been widely studied by all countries in the world (Borrelle et al., 2020). The entanglement and ingestion of marine litter is one of the important causes threatening marine biodiversity (Rochman et al., 2013). The secretariat of the Convention on Biological Diversity reported that all turtle species, 45% of marine mammal species and 21% of seabird species are likely to be harmed by marine debris (CBD-GEF, 2012). In addition, macrolitter can degrade into microplastics, which always interact with toxic pollutants in marine life and cause them to transport through the food web (Peng et al., 2020).
There are many types of marine litter, including but not limited to plastic, glass, fabric, metal, etc. Due to the limitations of observation means and research methods, researches on marine litter mainly focuses on floating litter. In contrast, the investigation and research on seafloor litter, which accounts for more than 50% of the total marine litter, is not in-depth and seriously affects the comprehensive management of marine litter and the health of marine ecosystemss (UNEP, 2005; Madricardo et al., 2020). Seafloor litter is diverse, relatively stable and hard, and is found in most of the world’s oceans (Madricardo et al., 2020). Its wide distribution not only provides a “habitat” and “vector” for the spread of sessile and attached organisms, but also enables many regional organisms to overcome long-standing natural barriers and introduce into other sea areas (Barnes, 2002; Carlton et al., 2017). In addition, seafloor litter increases the population connectivity of benthic organisms between different water layers, which is especially beneficial for the shift in different life history stages of those species. In particular, it can provide shelter for the larval stages of benthic organisms, such as the free-swimming planula larvae stage of jellyfish, leading to jellyfish blooms (Song et al., 2021). On the account of the distinction in roughness, chemical properties and biofilms between artificial and natural substrates, artificial substrates are more beneficial to the proliferation and immigration of Aurelia spp. polyps in the laboratory (Feng et al., 2017a). Similar to jellyfish, sea anemones belong to the phylum Cnidaria. They are also fast-growing, fertile cnidarians that can reproduce both sexually and asexually and have strong regenerative ability in some species (Bocharova, 2016). Given their complex life-history strategies and ecological tolerance, sea anemones are considered to be able to successfully introduce, spread and even invade other ecosystemss in the context of the proliferation of artificial substrates (Glon et al., 2020). Based on field observations and model analysis, Teng et al. (2021a) showed that the extensive distribution of seafloor litter in the northern Yellow Sea provides nature habitat for the distribution of Metridium senile fimbriatum, which promotes the proliferation and diffusion of the M. senile, contributing to sea anemone blooms. As the most abundant and widespread anemone species in the world, M. senile is mainly distributed in the intertidal zone and at a water depth of 100 m (Li and Xu, 2020). In recent years, with the proliferation of artificial substrates, including marine litter, M. senile has not only shown a trend of outbreak in local areas, but also significantly expanded its distribution in the global scope (Xu et al., 2009; Glon et al., 2019; Teng et al., 2021a).
The northern Yellow Sea is a semi-enclosed epicontinental sea surrounded by the Shandong Peninsula, the Liaodong Peninsula and the Korean Peninsula which serve as the spawning grounds, feeding grounds, overwintering grounds and migration channels for a great deal of fishery species (Liu, 1990). As a hotspot area of seafloor litter, the density of seafloor litter in the northern Yellow Sea is the highest in the Bohai Sea, the Yellow Sea and the northern East China Sea (Teng et al., 2021b). Mediated by seafloor litter, the benthic community of the northern Yellow Sea has been gradually dominated by M. senile (Li and Xu, 2020; Teng et al., 2021a). As a common fouler and invader, M. senile blooms will inevitably influence the structure and development of the community, and then affect the energy flow of the benthic ecosystems (Nelson and Craig, 2011; Martin et al., 2015). Therefore, by studying the trophic interaction between litter-associated M. senile and benthic organisms, it can reflect the cascade effect of seafloor litter on the benthic ecosystems of the northern Yellow Sea. The aims of this study were: (1) to determine the trophic characteristics of the M. senile attached to the seafloor litter; (2) to study the feeding effects of M. senile on potential food resources and the niche overlap of M. senile with other functional groups; and (3) to explore the cascade effects of seafloor litter on the benthic ecosystems of the northern Yellow Sea.
The samples were collected from a bottom trawl survey carried out by R/V Bei Dou in the Yellow Sea in October and November 2019. The trawl net was sunk down to the seafloor at each station, which enabled an effective collection of sea anemones, benthic organisms and seafloor litter. The net mouth width is 217 m, and the trawl is carried at approximately 3 knots. Therefore, the swept area of the bottom trawl reached 0.12 km2 per hour, which was enough to obtain most species of fishery resources and macrobenthos. More detailed characteristics of vessels and gears used in the surveys were described by Teng et al. (2021b). To better characterize the impact of M. senile on the benthic ecosystems, we selected sampling sites in the main anemone bloom area of the northern Yellow Sea (Teng et al., 2021a) (Figure 1). By trawling, M. senile and their co-occurred species were collected, including fish, cephalopods, shrimp, crabs, bivalves and other macrobenthos. Detailed information on the collected samples is shown in Table 1. M. senile caught by bottom trawl were stripped from the surface of seafloor litter and their biological characteristics were recorded. All macrobenthic samples were cryopreserved and brought back to the laboratory for species identification and biological determination, and the digestive tract and stable isotope samples were collected. Zooplankton samples were obtained by vertical towing with mesh sizes of plankton nets of 505 μm. Zooplankton were retained in filtered seawater overnight to evacuate gut contents prior to isotopic analysis.
Differences in isotope richness and assimilation efficiency in various tissues often lead to bias in the analysis of food resources or trophic levels of predators (Liu et al., 2019). Therefore, we first compared the carbon and nitrogen stable isotope varations in the foot disc and tentacle of M. senile, and then selected appropriate tissues to characterize the feeding ecology of M. senile. For potential food resources of M. senile, white muscle of fish was collected near the first dorsal fin, and abdominal muscle was collected from crustaceans (Zhao et al., 2022). Carcass and wrist muscles were taken in cephalopods. The shell and stomach were removed, and the internal tissues of shellfish were used for stable isotope analysis. For other smaller invertebrates, if there was not enough white muscle tissue, the entire body was used for stable isotope analysis. All samples were freeze-dried and homogenized using a mortar and pestle, and ground tissue samples were loaded into a tin boat for stable isotope analyses.
All isotope samples were measured with an elemental analyzer and an isotope ratio mass spectrometer (Iso Prime 100, Iso Prime Corporation, Cheadle, UK) at Yellow Sea Fisheries Research Institute (YSFRI), Chinese Academy of Fishery Sciences (CAFS). Stable isotope ratios are expressed in the standard δ-unit notation (δ13C and δ15N) and are defined by the following equation:
where X represents 13C or 15N and R represents 13C/12C or 15N/14N. Rsample was measured for biota samples and Rstandard is an international standard (Vienna Pee Dee belemnite limestone carbonate for carbon and atmospheric air for nitrogen). The analytical precision of δ13C and δ15N was better at 0.084‰ and 0.066‰, respectively.
Paired-Samples t-test was used to test whether there were significant differences in δ13C and δ15N between the tentacles and foot discs of M. senile. Additionally, to examine whether ontogenetic shifts had an impact on feeding habits, simple linear regressions were conducted to determine the relationship between body weight and δ13C and δ15N.
Bayesian mixing models were adopted to quantify the proportional contributions of a potential food resource to the diet of each species using the Stable Isotope Mixing Model in R (simmr) (Parnell et al., 2010; Parnell et al., 2013). The simmr model allows for uncertainties associated with isotopic signatures and diet-to-tissue discrimination factors, which were set to 1 ± 0.3‰ and 3 ± 0.3‰ for δ13C and δ15N, respectively (Parnell et al., 2013; Phillips et al., 2014). Potential prey items used in the model were derived from coelenteron content analysis, as well as a prior knowledge about their feeding preferences (Tsurpalo and Kostina, 2003; Liu et al., 2020). To quantify the proportion of different food resources, prey items with similar taxonomic status were grouped as a potential food resource, and the mean ± SD of their δ13C and δ15N values was calculated. Different food resources were grouped into four categories in total, namely zooplankton, crustaceans, juvenile fish and macrobenthos. Among them, juvenile fish are identified as those less than 1 year old, which can be easily judged according to their body length (Chen, 1991).
To order to compare the niche relationships among different feeding functional groups, Stable Isotope Bayesian Ellipses in R (SIBER) was used to calculate the trophic niche breadth of consumers and niche overlap between them (Jackson et al., 2011). We divided the benthic community into three functional groups: 1) M. senile; 2) crustaceans and young fish (main food resources of M. senile, shown in part 3.2); and 3) adult fish and cephalopods (potential competitors of M. senile). The trophic niche breadth was measured by corrected Standard Ellipse Area (SEAc), which was estimated as a quantification of the isotopic δ-space, to avoid bias owing to small sample sizes (Jackson et al., 2011). All calculations of trophic niche metrics were completed using the Stable Isotope Mixing Model in R with the “simmr” package (Parnell et al., 2013) and Stable Isotope Bayesian Ellipses in R with the “SIBER” package (Jackson et al., 2011).
Both the mean δ15N and δ13C of tentacle of M. senile were higher than those of the foot disc, but there was no significant difference between them (p>0.05 for both cases) (Figure 2). The maximum and minimum values of δ15N are 19.20‰ and 6.56‰, respectively, which both appear in the tentacle samples (Figure 2A). This indicates that the δ15N value of tentacles is unstable and beyond the reasonable range. For the δ13C value, the tentacle and foot disc are relatively stable and within a reasonable range (Figure 2B). Therefore, the foot disc was finally chosen as the tissue for carbon and nitrogen stable isotope determination to represent the trophic characteristics of M. senile.
Figure 2 Differences in δ15N (A) and δ13C (B) among different tissues (foot disc and tentacles) of M. senile.
For all samples, the mean δ13C value of M. senile was -19.34 ± 0.86‰, which ranged from -22.30‰ to -18.17‰. For δ15N, the mean value was 12.02 ± 0.95‰, which ranged from 9.22‰ to 13.31‰. As shown in Figure 3, the values of δ15N and δ13C increased with the increasing of the body weight of M. senile. However, Pearson correlation analysis showed that there was no significant correlation between body weight and δ15N or δ13C. (p > 0.05).
The simmr results revealed that crustacean and juvenile fish were two principal food resources for M. senile, which also showed different degrees of dependence on zooplankton and macrobenthos (Figure 4). Crustaceans and juvenile fish accounted for 46.8% and 36.9% of the food resources of M. senile, respectively (Figure 4). There is a negative correlation between juvenile fish and crustaceans (Figure 5), which also leads to the uncertainty of the contribution of these two food resources to the feeding composition of M. senile (Figure 4). In contrast, density distributions of zooplankton and macrobenthos were concentrated, which accounted for 6.8% and 9.5% of the food resources of M. senile, respectively (Figures 4, 5).
Figure 5 Density distribution of the feeding proportion of different food resources of M. senile and the correlation between them (on the right side of the diagonal in the figure, contour lines indicate the proportion relationship between different food resources; on the left side of the diagonal, is the correlation coefficient between different food resources).
The SEAc value of M. senile was 2.77, which was higher than that of the other groups (Table 2). As shown in Figure 6B, the mean value of stable isotopes (δ13C and δ15N) of M. senile is different from its principal food resources (juvenile fish and crustaceans) and potential competitors (adult fish and cephalopod). However, trophic niche overlap analysis showed that the maximum niche overlap was 35.25% between M.senile with adult fish and cephalopod (Figure 6A; Table 3).
Figure 6 Isotopic niche width/overlap among different functional groups in the benthic ecosystem of the northern Yellow Sea. (Ellipse in (A) is the standard ellipses area (SEA), representing the isotopic niche of different functional groups; dotted lines are the total area (TA) of the convex hulls representing the total niche width. The small ellipse in (B) represents 95% confidence intervals for the means of δ13C and δ15N; purple represents M. senile; yellow represents adult fish and cephalopod; The blue represents crustaceans and juvenile fish).
Table 3 Area of overlap between small sample size-corrected SIBER ellipses of different functional group.
García-Gómez et al. (2021) systematically described the vector effect of marine litter and found that a wide variety of organisms can colonize plastic litter, including both microorganisms and macrofauna species. Many sessile and attached organisms have a preference for marine litter, which leads to the dominance on the surface of litter (Richardson et al., 2009; Purcell, 2012; Janßen et al., 2013). Meanwhile, changes in species abundance and community composition on the surface of marine litter may affect the interactions among ecosystems components. Through in situ experiments, Feng et al. (2017b) found that fouling organisms on marine litter selectively suppress on polyps of different scyphozoan species by competition and predation. M. senile is ubiquitous in cold temperate fouling communities, and the population dynamics of M. senile determine the direction of community succession at different temporal scales (Nelson and Craig, 2011). In recent years, the distribution of M. senile is expending worldwide (GBIF, 2022). Similarly, M. senile has become the dominant species in the benthic ecosystems of northern Yellow Sea, and its occurrence frequency has increased significantly since 1959 (Chen, 1987; Li, 2003; Xu et al., 2009). As a direct indicator of trophic level, the δ15N of M. senile indicates that it has a high trophic level, which is consistent with previous research (Liu et al., 2020). Paralyzed by the anemone’s filaments, the prey is captured by the tentacles and transported to the mouth (Tsurpalo and Kostina, 2003). With the increase in body weight, the δ15N of M. senile showed an increasing trend, which is consistent with many marine organisms (Cushing, 1975; Caddy et al., 1998). It is worth noting that small anemones also had a large niche width in this study, indicating that small individuals also had relatively strong feeding ability. This is mainly because the anemone is an opportunistic predator and its active hunting ability is poor. Therefore, the species passing through or near the anemone’s habitat have a more significant impact on the anemone’s feeding than its body size (Anthony, 1997). This also explains the diverse diet of M. senile, including zooplankton, mollusks, crustaceans and other invertebrates, and even fish (Sebens and Koehl, 1984; Tsurpalo and Kostina, 2003).
From the perspective of niche competition, the trophic niche of M. senile overlaps with other functional groups, such as crustaceans and fishes. M. senile mainly lives in the Yellow Sea Cold Water Mass, and there are many economic cold-temperate species in this area, such as Cleisthenes herzensteini, Gadus macrocephalus, Sebastes schlegelii, and so on (Wu et al., 2019). Sea anemone blooms may affect the survival of these economic species at early life history stages while potentially squeezing their ecological niche, creating competitive pressures. In addition, most benthic marine invertebrates have a pelagic larval phase, after which they settle preferentially on or near conspecific adults, forming aggregations (Toonen and Pawlik, 1994). Aggregation has often been viewed as an evolutionarily advantageous state in which individuals derive the benefits of protection and reproduction (Danchin and Wagner, 2000; He et al., 2019). As a common fouling organism, M. senile can quickly colonize and aggregate in a new habitat by pedal laceration. The aggregation effect may be more beneficial to rounding up by M. senile and its dominance in niche competition with other functional groups. Therefore, trophic interactions of this carnivorous anemone associated with seafloor litter may not only feed on the early larvae of fishery resources, but also cause niche competition for other species, thus affecting the energy flow of benthic ecosystems in the northern Yellow Sea. However, there are still limited studies on the life-history characteristics and feeding ecology of M. senile. Future research will focus on these two aspects, which will be conducive to studying the impact of seafloor litter on the regional diffusion of sea anemones and the cascading effect on the benthic ecosystems.
As a diploblastic organism, sea anemone does not have a specialized stomach like vertebrates. The retention time of undigested food residue in sea anemone is very short, so traditional dietary analysis methods, such as coelenteron content analysis, have certain limitations (Shick, 1991). Compared with the traditional method, the stable isotope method more accurately reflects the assimilated food resources and the contribution of various food resources to the dietary habits of consumers (Deniro and Epstein, 1978; Post, 2002). Nevertheless, it is worth noting that the selection of food resources is the key to affecting the accuracy of stable isotope analysis. Phillips et al. (2014) suggested that more caution should be exercised in using stable isotope mixing models for species with fewer studies on dietary preferences. In addition, coelenteron content analysis for samples in this study showed that the coelenteron cavity of M. senile was usually empty and only a few samples were dissected with inclusions. Therefore, it should be to strengthen the observation of feeding organisms of M. senile by combining DNA barcoding with analysis of coelenteric inclusions. In addition, aquaculture experiments or field observation experiments can be carried out to verify the feeding capacity of anemones for fish, crustaceans and macrobenthos. After accurately determining the dietary composition of M. senile, the number of food resource samples should be increased to reduce the uncertainty of the model results, so as to better explain the effect of litter-induced anemone blooms on benthic ecosystemss.
Marine litter pollution is becoming increasingly serious and affects the health of marine ecosystems. Most marine litter ends up on the seafloor, changing the habitat and community structure of benthic organisms. The accumulation of seafloor litter in the northern Yellow Sea provides vectors and habitats for the diffusion and distribution of M.senile, which leads to its regional expansion. In this study, the interactions between sea anemones and those co-occurred species in the northern Yellow Sea reflected the cascading effects of seafloor litter on the benthic ecosystems. The results of stable isotope analysis showed that M.senile had a high trophic level, and the benthic crustaceans and juvenile fish were the main food resources of M.senile. In addition, M.senile had different ratios of niche overlap with different functional groups, ranging from 34.29 to 35.25%. Therefore, this study showed that M.senile regulated the energy flow of the benthic ecosystems in the northern Yellow Sea through predation and niche competition. This study provides a new perspective for understanding the ecological effects of seafloor litter and the comprehensive management of marine litter.
The original contributions presented in the study are included in the article/supplementary material. Further inquiries can be directed to the corresponding author.
The animal study was reviewed and approved by Institutional Animal Care and Use Committee of the Yellow Sea Fisheries Research Institute, Chinese Academy of Fishery Sciences.
GT: Conceptualization, Data curation, Investigation, Methodology, Formal analysis, Visualization, Writing - original draft and writing—review & editing. GT and XS: Project administration, Funding acquisition, Writing - review & editing. XJ and XS: Supervision, Resources. All authors contributed to the article and approved the submitted version.
This study was supported by the National Natural Science Foundation of China (42176151), China Postdoctoral Science Foundation (2022M713470) and Key Research and Development Program of Shandong Province (2022CXPT013).
We thank the colleagues from the Division of Fishery Resources and ecosystems, Yellow Sea Fisheries Research Institute, Chinese Academy of Fishery Sciences, for measuring and sampling during the surveys. We also thank Dr. Xiujin Wei and Dr. Yongsong Zhao for her help in stable isotope sample processing and data analysis. Special thanks to two reviewers for their valuable and constructive comments on the manuscript.
The authors declare that the research was conducted in the absence of any commercial or financial relationships that could be construed as a potential conflict of interest.
All claims expressed in this article are solely those of the authors and do not necessarily represent those of their affiliated organizations, or those of the publisher, the editors and the reviewers. Any product that may be evaluated in this article, or claim that may be made by its manufacturer, is not guaranteed or endorsed by the publisher.
Anthony K. R. N. (1997). Prey capture by the sea anemone Metridium senile (L.): Effects of body size, flow regime. Biol. Bull. 192 (1), 73–86. doi: 10.2307/1542577
(2022) GBIF secretariat: GBIF backbone taxonomy. Available at: https://www.gbif.org/species/5183974.
Barnes D. K. A. (2002). Invasions by marine life on plastic debris. Nature 416, 808–809. doi: 10.1038/416808a
Bocharova E. (2016). “Reproduction of Sea anemones and other hexacorals,” in The cnidaria, past, present and future. Eds. Goffredo S., Dubinsky Z. (Springer, Cham). doi: 10.1007/978-3-319-31305-415
Borrelle S. B., Ringma J., Law K. L., Monnahan C. C., Lebreton L., McGivern A., et al. (2020). Predicted growth in plastic waste exceeds efforts to mitigate plastic pollution. Science 369, 1515–1518. doi: 10.1126/scienceaba3656
Caddy J., Csirk J., Garcia S. M., Grainger R. J. R. (1998). How pervasive is “Fishing down marine food webs”. Science 282, 183. doi: 10.1126/science.282.5393.1383a
Carlton J. T., Chapman J. W., Geller J. B., Miller J. A., Carlton D. A., McCuller M. I., et al. (2017). Tsunami-driven rafting: transoceanic species dispersal and implications for marine biogeography. Science 357 (6358), 1402–1406. doi: 10.1126/scienceaao1498
CBD-GEF (Secretariat of the Convention on Biological Diversity and the Scientific and Technical Advisory Panel-GEF). (2012). Impacts of marine debris on biodiversity: current status and potential solutions, montreal, technical series No. 67, 61.
Chen G. X. (1987). Marine fishery environment in China (Zhejiang Science and Technology Press), 210–211.
Danchin E., Wagner R. H. (2000). Benefits of membership. Science 287 (5454), 804–805. doi: 10.1126/science.287.5454.803e
Deniro M. J., Epstein S. (1978). Carbon isotopic evidence for different feeding patterns in two hyrax species occupying the same habitat. Science 201 (4359), 906–908. doi: 10.1126/science.201.4359.906
Feng S., Lin J. N., Sun S., Zhang F. (2017a). Artificial substrates preference for proliferation and immigration in aurelia aurita (s. l.) polyps. Chin. J. Oceanol. Limn. 35 (1), 153–162. doi: 10.1007/s00343-016-5230-y
Feng S., Wang S. W., Zhang G. T., Sun S., Zhang F. (2017b). Selective suppression of in situ proliferation of scyphozoan polyps by biofouling. Mar. pollut. Bull. 114 (2), 1046–1056. doi: 10.1016/j.marpolbul.2016.10.062
García-Gómez J. C., Garrigós M., Garrigós J. (2021). Plastic as a vector of dispersion for marine species with invasive potential. a review. Front. Ecol. Evol. 9. doi: 10.3389/fevo.2021.629756
Glon H., Daly M., Carlton J. T., Flenniken M. M., Currimjee Z. (2020). Mediators of invasions in the sea: life history strategies and dispersal vectors facilitating global sea anemone introductions. Biol. Invasions. 16, 1–28. doi: 10.1007/s10530-020-02321-6
Glon H. E., Haruka Y., Daly M., Nakaoka M. J. H. (2019). Temperature and salinity survival limits of the fluffy sea anemone, Metridium senile (L.), in Japan. Hydrobiologia 830, 303–315. doi: 10.1007/s10750-018-3879-2
He J., Dai Q., Qi Y., Wu Z., Fang Q., Su P., et al. (2019). Aggregation pheromone for an invasive mussel consists of a precise combination of three common purines. iScience 19, 691–702. doi: 10.1016/j.isci.2019.08.022
Jackson A. L., Inger R., Parnell A. C., Bearhop S. (2011). Comparing isotopic niche widths among and within communities: SIBER - stable isotope Bayesian ellipses in r. J. Anim. Ecol. 80 (3), 595–602. doi: 10.1111/j.1365-2656.2011.01806x
Janßen H., Augustin C. B., Hinrichsen H. H., Kube S. (2013). Impact of secondary hard substrate on the distribution and abundance of Aurelia aurita in the western Baltic Sea. Mar. pollut. Bull. 75 (1-2), 224–234. doi: 10.1016/j.marpolbul.2013.07.027
Li R. G. (2003). Macrobenthos from China shelf and adjacent waters Vol. 79 (Beijing: China Ocean Press).
Liu B., Chen L., Li B., Hou X., Feng G., Li X., et al. (2019). Carbon and nitrogen stable isotopes variations in different tissues of macrobenthos in the intertidal zone. HaiYang XueBao 41 (4), 74–82. doi: 10.3969/j.issn.0253-4193.2019.04.007
Liu X. (1990). Investigation and Planning of Fishery Resources in the Yellow Sea and Bohai Sea (China Ocean Press).
Liu X. (1990). Investigation and planning of fishery resources in the yellow sea and bohai sea. (China Ocean Press).
Liu X., Ni D., Zhong X., Zhang Z. (2020). Structure of benthic food web and trophic relationship of macrofauna in the yellow Sea. J. Ocean U. China. 50 (9), 20–33. doi: 10.16441/j.cnki.hdxb.20200093
Li Y., Xu K. D. (2020). Species diversity and faunal characteristics of the order actiniaria cnidaria: Anthozoa in the seas of China. Oceanologia Et Limnologia Sinica. 5103, 434–443. doi: 10.11693/hyhz20191100237
Madricardo F., Ghezzo M., Nesto N., Kiver W. J. M., Faussone G. C., Fiorin R., et al. (2020). How to deal with seafloor marine litter: an overview of the state-of-the-art and future perspectives. Front. Mar. Sci. 7. doi: 10.3389/fmars.2020.505134
Martin J. P., Garese A., Sar A., Acuña F. H. (2015). Fouling community dominated by Metridium senile (Cnidaria: Anthozoa: Actiniaria) in bahía San julián (southern Patagonia, Argentina). Scientia Marina 79 (2), 211–221. doi: 10.3989/scimar.04082.21A
Nelson M. L., Craig S. F. (2011). Role of the sea anemone metridium senile in structuring a developing subtidal fouling community. Mar. Ecol. Prog. Ser. 421, 139–149. doi: 10.3354/meps08838
Parnell A. C., Inger R., Bearhop S., Jackson A. L. (2010). Source partitioning using stable isotopes: coping with too much variation. PloS One 5 (3), e9672. doi: 10.1371/journal.pone.0009672
Parnell A. C., Phillips D. L., Bearhop S., Semmens B. X., Ward E. J., Moore J. W., et al. (2013). Bayesian Stable isotope mixing models. Environmetrics 24 (6), 387–399. doi: 10.1002/env.2221
Peng L., Fu D., Qi H., Lan C. Q., Yu H., Ge C. (2020). Micro- and nano-plastics in marine environment: Source, distribution and threats - a review. Sci. Total Environ. 698, 134254. doi: 10.1016/j.scitotenv.2019.134254
Phillips D. L., Inger R., Bearhop S., Jackson A. L., Moore J. W., Parnell A. C., et al. (2014). Best practices for use of stable isotope mixing models in food-web studies. Can. J. Zool. 92 (10), 823–835. doi: 10.1139/cjz-2014-0127
Post D. M. (2002). Using stable isotopes to estimate trophic position: models, methods, and assumptions. Ecology 83 (3), 703–718. doi: 10.1890/0012-9658(2002)083
Purcell J. E. (2012). Jellyfish and ctenophore blooms coincide with human proliferations and environmental perturbations. Annu. Rev. Mar. Sci. 4 (1), 209–235. doi: 10.1146/annurev-marine-120709-142751
Richardson A. J., Bakun A., Hays G. C., Gibbons M. J. (2009). The jellyfish joyride: causes, consequences and management responses to a more gelatinous future. Trends Ecol. Evol. 24 (6), 312–322. doi: 10.1016/j.tree.2009.01.010
Rochman C. M., Browne M. A., Halpern B. S., Hentschel B. T., Hoh E., Karapanagioti H. K., et al. (2013). Classify plastic waste as hazardous. Nature 494 (7436), 169–171. doi: 10.1038/494169a
Sebens K. P., Koehl M. A. R. (1984). Predation on zooplankton by the benthic anthozoans Alcyonium siderium (Alcyonacea) and Metridium senile (Actiniaria) in the new England subtidal. Mar. Biol. 81, 255–271. doi: 10.1007/BF00393220
Song X., Lyu M., Zhang X., Ruthensteiner B., Ahn I., Pastorino G., et al. (2021). Large Plastic debris dumps: new biodiversity hot spots emerging on the deep-sea floor. Environ. Sci. Technol. Lett. 8 (2), 148–154. doi: 10.1021/acs.estlett.0c00967
Teng G., Jin X., Fu C., Guan L., Jin Y., Chen Y., et al. (2021a). Is seafloor litter contributing to sea anemone blooms? Sci. Total Environ. 759, 143479. doi: 10.1016/j.scitotenv.2020.143479
Teng G., Shan X., Jin X., Yang T. (2021b). Marine litter on the seafloors of the bohai Sea, yellow Sea and northern East China Sea. Mar. pollut. Bull. 169 (11), 112516. doi: 10.1016/j.marpolbul.2021.112516
Toonen R. J., Pawlik J. R. (1994). Foundations of gregariousness. Nature 370, 511–512. doi: 10.1038/370511a0
Tsurpalo A. P., Kostina E. E. (2003). Feeding characteristics of three species of intertidal sea anemones of the south kuril islands. Russ. J. Mar. Biol. 29 (1), 31–40. doi: 10.1023/A:1022823819872
UNEP (2005). “Marine litter: An analytical overview,” in Intergovernmental oceanographic commission of the united nations educational, scientific and cultural organisation (Nairobi: UNEP).
Wu Q., Ying Y. P., Tang Q. S. (2019). Changing states of the food resources in the yellow Sea large marine ecosystems under multiple stressors. Deep-Sea Res. Pt. Ii. 163, 29–32. doi: 10.1016/j.dsr2.2018.08.004
Xu W., Zeng X., Sheng F., Zhang Y., Tang G. (2009). Survey of the macrobenthos by trawling in the northern yellow Sea. J. Ocean U. China. S1, 19–24. doi: 10.7666/dy1501789
Keywords: Metridium senile fimbriatum, trophic interaction, cascade effects, seafloor litter, northern Yellow Sea
Citation: Teng G, Shan X and Jin X (2023) Cascade effects of seafloor litter on benthic ecosystems in the northern Yellow Sea. Front. Mar. Sci. 9:1044232. doi: 10.3389/fmars.2022.1044232
Received: 14 September 2022; Accepted: 07 December 2022;
Published: 04 January 2023.
Edited by:
Nora Fung-Yee Tam, City University of Hong Kong, ChinaCopyright © 2023 Teng, Shan and Jin. This is an open-access article distributed under the terms of the Creative Commons Attribution License (CC BY). The use, distribution or reproduction in other forums is permitted, provided the original author(s) and the copyright owner(s) are credited and that the original publication in this journal is cited, in accordance with accepted academic practice. No use, distribution or reproduction is permitted which does not comply with these terms.
*Correspondence: Xiujuan Shan, c2hhbnhqQHlzZnJpLmFjLmNu
Disclaimer: All claims expressed in this article are solely those of the authors and do not necessarily represent those of their affiliated organizations, or those of the publisher, the editors and the reviewers. Any product that may be evaluated in this article or claim that may be made by its manufacturer is not guaranteed or endorsed by the publisher.
Research integrity at Frontiers
Learn more about the work of our research integrity team to safeguard the quality of each article we publish.