- 1College of Marine Life Sciences, Ocean University of China, Qingdao, Shandong, China
- 2Key Laboratory of Marine Eco-Environmental Science and Technology, First Institute of Oceanography, Ministry of Natural Resources, Qingdao, Shandong, China
- 3College of Biological and Environmental Engineering, Binzhou University, Binzhou, Shandong, China
- 4Fujian Pingtan Aquatic Product Improved Variety Experiment Co. Ltd., Fuzhou, Fujian, China
The enhancement of harmful ultraviolet-B (UV-B, 280–320 nm wavelength) radiation is a serious problem generally faced by photosynthetic organisms. Previous studies have reported that the currently increasing level of UV-B radiation received on earth can promote the formation of reactive oxygen species (ROS) by altering the antioxidant defense system or by hampering of photosynthesis system of plants. However, the relationship between the UV-B-induced ROS production, antioxidant capacity, and photosynthetic performance in macroalgae remains unclear. In this study, the regulation of the antioxidant system and photosynthetic activity by ROS triggered by UV-B radiation were investigated in an intertidal seaweed, Neoporphyra haitanensis. The contents of hydrogen peroxide and malondialdehyde increased with enhanced UV-B radiation, indicating N. haitanensis experienced oxidative stress. Increased activities of antioxidant enzymes showed a positive response of enzymatic antioxidants to maintain the balance of ROS under low UV-B conditions. Likewise, an accumulation of non-enzymatic antioxidants occurred and glutathione redox status was maintained at a high level, suggesting that the ascorbate–glutathione cycle played an indispensable role in detoxifying ROS. Nevertheless, high UV-B radiation markedly inhibited the efficiency of the enzymatic and non-enzymatic antioxidants, causing oxidative damage and a depression of photosynthesis. The addition of ROS scavengers alleviated the increased oxidation level caused by UV-B radiation, implying that oxidative damage under UV-B condition was ascribed to accumulated ROS. The activation of the antioxidant defense system was also modulated by ROS. Additionally, the decreased photosynthetic activities induced by enhanced UV-B radiation were reversed by ROS scavengers, indicating that ROS-mediated oxidative damage caused by UV-B radiation is one of the important causes of photo-inhibitory damage in N. haitanensis. Overall, these results shed some light on the close relationship between UV-B-induced ROS production, the antioxidant defense system, and photosynthetic performance in macroalgae, which helps to reveal their survival strategies for adapting to highly variable marine intertidal environments.
Introduction
Increased incidence of ultraviolet (UV) radiation reaching the surface of the Earth that has been attributed to the depletion of the ozone layer since the late 1980s is considered a serious environmental problem. Ultraviolet-B (UV-B, 280–320 nm wavelength) is a type of UV light that contains significantly high levels of energy and can potentially to cause major damage to organisms (Qi et al., 2018; Barnes et al., 2019). Alteration in cloud cover, aerosols, and surface albedo caused by climate change exacerbates the difficult challenge posed by enhanced UV-B radiation, especially in low- and mid-latitude regions (Eleftheratos et al., 2020). The UV-B radiation reaching the Earth’s surface is expected to continue to increase in the second half of the 21st century, which will undoubtedly have a continuous and immense impact on living organisms (Dhomse et al., 2019; Meinshausen et al., 2020). In particular, intertidal macroalgae experience unpredictable dynamic environmental changes caused by the tidal cycle, such as changes in radiation, temperature, and rehydration. Enhanced UV-B radiation during low tide will pose a potential threat to their growth, survival, and reproduction.
Fortunately, plants have evolved sophisticated regulatory mechanisms to cope with adverse environmental conditions, among which scavenging pathways of reactive oxygen species (ROS) are ubiquitous (Ahmad et al., 2008). ROS are unavoidable harmful by-products of metabolic processes, and the accumulation of ROS is a common plant response to various environmental stresses. The major members of the ROS family include non-radicals like hydrogen peroxide (H2O2), singlet oxygen (1O2) and free radicals like superoxide anion (O2·–), and hydroxyl radicals (OH·), which differ in their stability, reactivity and transmembrane transport capacity (Mittler, 2017). When plants are exposed to unfavorable conditions, ROS production is exacerbated, activating pathways associated with ROS scavenging (Mishra and Prasad, 2021). Dysregulation in any of these ROS-scavenging processes leads to the generation of excess amounts of ROS, causing extensive damage to protein, DNA and lipids and thereby affecting normal cellular functioning (Narayanaswamy et al., 2016; Juan et al., 2021).
The interplay between ROS-producing and ROS-scavenging pathways determines the tolerance of plants toward adverse environment. High levels of antioxidant enzyme activity and antioxidant content are associated with high stress tolerance (Gururani et al., 2015; Hasanuzzaman et al., 2020). Superoxide dismutase (SOD) is considered to constitute the first defensive barrier against oxidative stress in the antioxidant system owing to its ability to catalyze the highly toxic O2·– to less toxic H2O2 and O2 (del Rio et al., 2003). Then catalase (CAT) and peroxidase (POD) detoxify H2O2 into non-toxic H2O and O2 (Hasanuzzaman et al., 2012). Notably, due to its significantly longer half-life compared to other ROS members, H2O2 can traverse longer distances and cross cell membranes, and is considered to be the most stable and easily disseminated form of oxidative stress (Das and Roychoudhury, 2014; Nadarajah, 2020). Thus, the level of enzyme activity associated with H2O2 production and scavenging is important for plants to resist unfavorable environments. Apart from antioxidant enzymes, non-enzymatic antioxidants are also responsible for maintaining the cellular ROS balance. Ascorbate (AsA) and glutathione (GSH) are the main non-enzymatic antioxidants that are regulated by ascorbate peroxidase (APx), monodehydroascorbate reductase (MDHAR), dehydroascorbate reductase (DHAR), and glutathione reductase (GR). The ascorbate-glutathione (AsA-GSH) cycle has been considered to be the most effective pathway for H2O2 detoxification in plants (Ding et al., 2019). In addition, the defense-related enzyme glutathione peroxidase (GPx) detoxifies H2O2 using GSH as a substrate. The antioxidant defense system composed of multiple antioxidant enzymes and antioxidants is crucial to the survival of macroalgae in the extremely unstable intertidal zone (Yu et al., 2020). Neoporphyra haitanensis L.E. Yang et J. Brodie (Bangiales, Rhodophyta) (redefined from the synonym, Pyropia haitanensis, in May 2020), an intertidal seaweed species with high economic and ecological value, is widely cultured in the southeast of China (Yang et al., 2020; Xu et al., 2020). In nature, N. haitanensis thalli are directly exposed to high levels of UV-B radiation, and their duration of exposure to UV-B radiation is influenced by the tides. The protective role of the antioxidant defense system in scavenging excessive ROS has been found in stress studies of N. haitanensis in response to adverse environmental factors such as high temperature, desiccation, and high salinity (Wang et al., 2019; Wang et al., 2020; Chen et al., 2022). Nevertheless, so far, the response of the antioxidant system in UV-B radiation acclimation of N. haitanensis remains unclear.
In photosynthetic organisms, photosynthesis serves as the foundation for all material and energy metabolism and is capable of responding rapidly to UV radiation (Tokutsu et al., 2021). Ultraviolet-B radiation generally acts directly on the photosynthetic apparatus, with photosystem II (PSII) being the most sensitive and subject to the most severe photo-inhibitory damage (Bornman, 1989). The photoinhibition exhibited by plants under UV-B may be related to an overproduction of ROS. The electron transport chain of the chloroplast is the main site of ROS production (Zhao et al., 2020). When the amount of light energy captured by the photosystems exceeds the energy that is required for photosynthesis, excess excitation energy in the photosynthetic electron transport chain can promote ROS production. In turn, an excessive production of ROS causes oxidative damage to proteins and lipids in the thylakoid membrane, leading to the inactivation of PSII reaction centers. The evidence suggests that photoinhibition is an undesirable consequence of ROS accumulation in plants (Tyystjarvi, 2013). In addition, photoinhibition has been believed to be a controlled protective strategy for dissipating excess energy to prevent further damage, which is accompanied by the passive generation of ROS. An interesting question, therefore, involves determining whether the accumulated ROS are responsible for photoinhibition or rather if photoinhibition promotes the overproduction of ROS.
In this study, the H2O2 content, lipid peroxidation level, antioxidant enzyme activities, and antioxidant content in N. haitanensis were determined under varying UV-B radiation intensities. In addition, ROS scavengers were used to explore the relationship between UV-B-induced ROS and the antioxidant system. This study also attempted to explain the underlying reason for the photoinhibition of macroalgae caused by UV-B radiation by measuring its photosynthetic activity in the presence of ROS scavengers. These results contribute to a better understanding of the antioxidant defense system of intertidal macroalgae under environmental stress, and thus enhance our knowledge related to survival strategies for adapting to the drastic changes occurring in the intertidal zone.
Materials and methods
Sample collection
Initially, N. haitanensis blades were collected from farmed rafts in the vicinity of Tan’nan Bay, China (119.76°E, 25.41°N), and immediately transported to the marine ecology laboratory of the Ocean University of China. Intact and healthy blades were selected; any additional sediments and epiphytic miscellaneous algae were removed. Before the experiment, N. haitanensis thalli were pre-cultured for 3 days in a lighted incubator (GHP-500E, Sanfa, Shanghai, China) at 20°C. Photosynthetically active radiation was set to 50 μmol photons·m−2·s−1 for 12 hours a day. The seawater was supplemented with a sterilized nutrient solution (Provasoli’s enrichment solution medium) and renewed every 2 days.
UV-B radiation treatments
Each sample was transferred to a square plastic dish (13 cm ×13 cm) containing 300 mL cultured seawater for UV-B radiation treatments. The intensities of UV-B radiation were set to three levels: control (0 W·m−2), low (0.5 W·m−2), and high (1 W·m−2), according to our previous experiments (Xue et al., 2022). Ultraviolet-B radiation was provided by UV-B lamp tubes (TL-K 40 W/10R, Philips, Poland). The UV-B intensity was determined using a radiometer equipped with a UV-B sensor (UV-B 297, Beijing Normal University, Beijing, China). The UV-B radiation system was continuously irradiated for more than 12 h prior to use to allow for stabilization of the UV output. Except for UV-B intensity, other experimental conditions were the same as culture conditions.
H2O2 and lipid peroxidation content
The H2O2 content of each sample was measured according to the method of Contreras-Porcia et al. (2011). After samples weighed to 0.1 g and 1 mL of lysis buffer was added, tissue homogenates were prepared by an automatic tissue grinding machine (JXFSTPRP-48L, Jingxin, Shanghai, China). The supernatant was transferred after centrifugation at 12,000 g at 4°C for 10 min. The H2O2 content was determined by hydrogen peroxide content detection kit (Solarbio, Beijing, China) and determined with an enzyme-labeled instrument (ELX808IU, BioTek Instruments, Inc., Winooski, VT, USA). The produced intracellular ROS was tagged by 2′,7′-dichlorofluorescin diacetate (DCFH-DA, Sigma, San Diego, CA, USA) fluorescent staining. The samples were incubated with 40 mL of seawater containing 10 μM DCFH-DA at room temperature, and kept away from light during the entire process. After incubation, the thalli were rinsed three times in filtered seawater, and the H2O2 was immediately observed in situ using a fluorescence microscope (RVL-100-G, Echo, Boston, MA, USA).
The lipid peroxidation levels were measured by a degradation product of lipid peroxidation, malondialdehyde (MDA). Samples weighing 0.1 g was ground in liquid nitrogen and MDA content was determined by a micro malondialdehyde assay kit (Solarbio).
Antioxidant enzyme activity and antioxidant content
Fresh samples were ground with liquid nitrogen and tissue homogenates were prepared for further enzyme activity analysis. The activities of SOD, CAT, POD APx, DHAR, MDHAR, GR, and GPx were estimated using commercially available assay kits (Solarbio). The concentrations of DHA, AsA, GSSG, GSH, and T-GSH content were measured using assay kits obtained from Nanjing Jiancheng Bioengineering Institute (Nanjing, China). The protein concentration was determined using a BCA protein kit (Solarbio).
ROS scavenger treatments
To reveal the involvement of H2O2 in the UV-B-induced changes in the antioxidant metabolism and photosynthetic performance, thalli were treated with different ROS scavengers. Sodium benzoate (SB) is considered as a general free radical scavenger (Nagalakshmi and Prasad, 1998; Dey et al., 2021), 1,2-dihydroxy-benzene-3,5-disulphonic acid (Tiron) is a specific O2·– scavenger (Wise, 1995; Xing et al., 2016), and dimethylthiourea (DMTU) is a specific H2O2 scavenger (Levine et al., 1994; Liu et al., 2020). The concentrations of ROS scavengers were modified from the method of Shiu and Lee (2005). The thalli were incubated in 300 mL sterile seawater containing 10 mM SB, 10 mM Trion or 20 mM DMTU, for 1 h in darkness, and then treated with UV-B radiation.
Photosynthetic performance
After dark adaptation for 20 min, the samples were transferred into a chlorophyll fluorescence imaging system (Imaging-PAM; Heinz Walz, Effeltrich, Germany) to measure photosynthetic performance of N. haitanensis. Parameters indicating photosynthetic activity, such as maximal PSII quantum yield (Fv/Fm), the effective PSII quantum yield (Y(II)), the non-photochemical quenching (NPQ), the photochemical quenching coefficient (qP), the electron transport rate (ETR), and the non-regulated non-photochemical quantum yield (Y(NO)), were calculated and determined by the manufacturer’s ImagingWinGigE software.
Statistical analysis
All data in this study were showed as mean ± standard deviation (SD) for three replicates. Statistical analysis was conducted using IBM SPSS 25.0 software. The differences between groups were identified using one-way analysis of variance (ANOVA) with the significance level was set as P < 0.05. The least significant difference (LSD) test was used for pairwise comparisons.
Results
Changes of H2O2 and MDA contents under UV-B radiation
Ultraviolet-B radiation activated H2O2 production in N. haitanensis (Figures 1A, B). The H2O2 contents in N. haitanensis at UV-B radiation appeared to be a dose-dependent response with the extension of exposure time. In situ fluorescence staining intuitively demonstrated the effect of UV-B radiation promoting ROS production. The cells showed light green fluorescence under low UV-B radiation, while the algal cells showed bright green fluorescence with unclear cell boundaries after exposure to high UV-B radiation for 5 h. These observed results were consistent with the changes of H2O2 content.
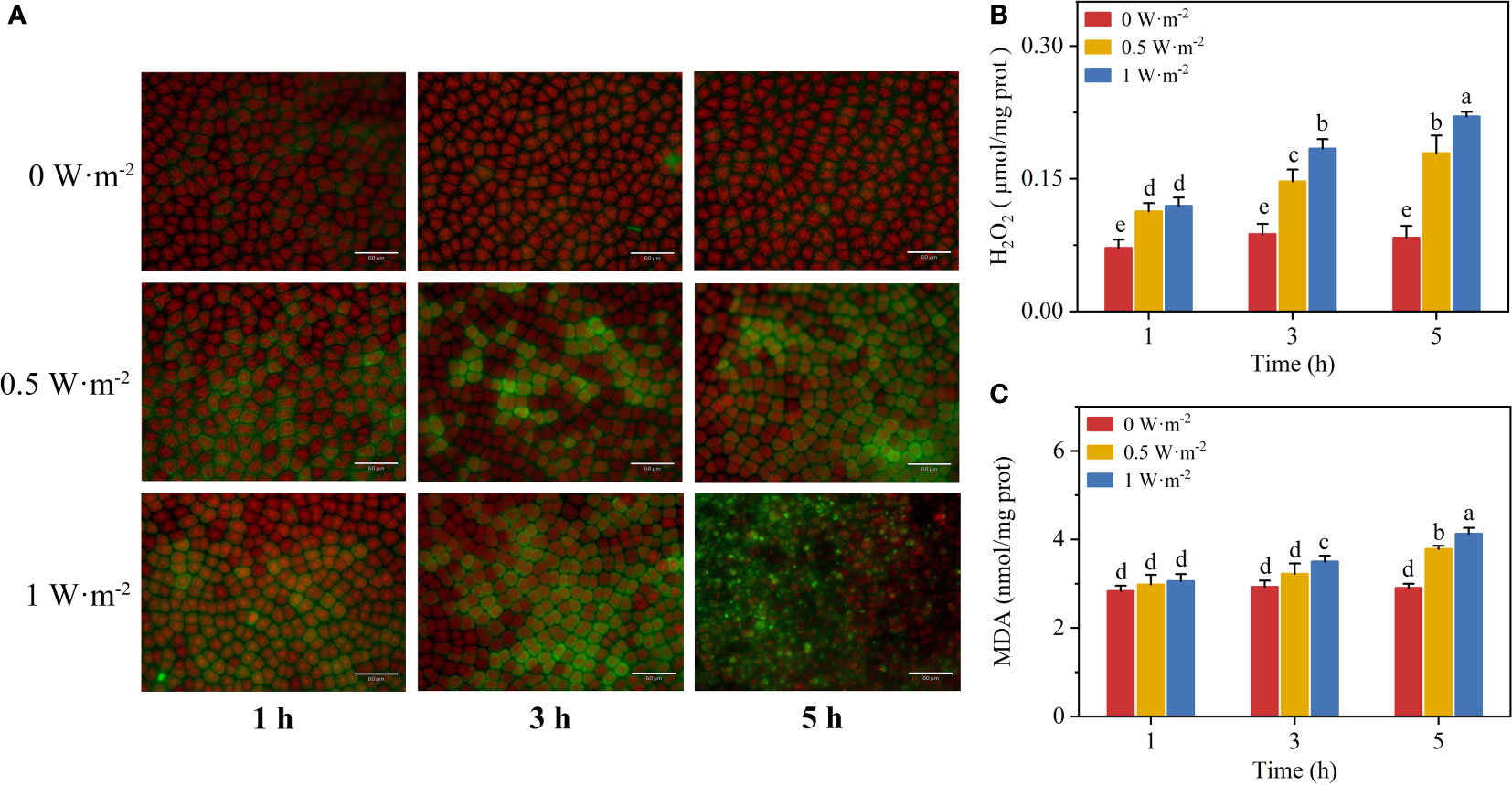
Figure 1 Effects of ultraviolet-B (UV-B) radiation on hydrogen peroxide (H2O2) and malondialdehyde (MDA) content of Neoporphyra haitanensis: (A) fluorescent staining of H2O2 with 2,7-dichlorofluorescein diacetate after UV-B radiation exposure for 1 h, 3 h, and 5 h; (B) variations in H2O2 content; (C) variations in MDA content. Values are represented as means ± SD (n = 3). Different lowercase letters indicate a significant difference among groups according to ANOVA and the LSD test (P < 0.05); the same lowercase letters indicate no significant difference between the groups (P > 0.05).
Enhanced UV-B radiation aggravated membrane lipid peroxidation in N. haitanensis (Figure 1C). No significant change in MDA content (ANOVA, P > 0.05) was observed under low UV-B radiation for 3 h. The MDA content of algae in the high UV-B group significantly increased with the extension of exposure time (ANOVA, P < 0.05).
Changes of antioxidant enzyme activities under UV-B radiation
Ultraviolet-B radiation significantly affected the activities of antioxidant enzymes involved in ROS scavenging (Figure 2). The SOD activity increased gradually with an increasing exposure time under low and high UV-B radiation. Both POD and CAT activities were significantly induced by low UV-B radiation (ANOVA, P < 0.05). Although high UV-B radiation increased the activity of CAT and POD within 3 h, it was followed by a decrease as time increased. The APx activity increased rapidly under low and high UV-B radiation with a peak occurring at the 3 h and then decreased substantially. The change of DHAR activity was similar to the changes in CAT activity. Compared with DHAR, MDHAR seemed to be more easily activated at high UV-B radiation. The GR activity only significantly increased under low UV-B radiation. The GPx activity increased with increasing UV-B radiation, while it experienced inhibition by high UV-B radiation after exposure for 5 h.
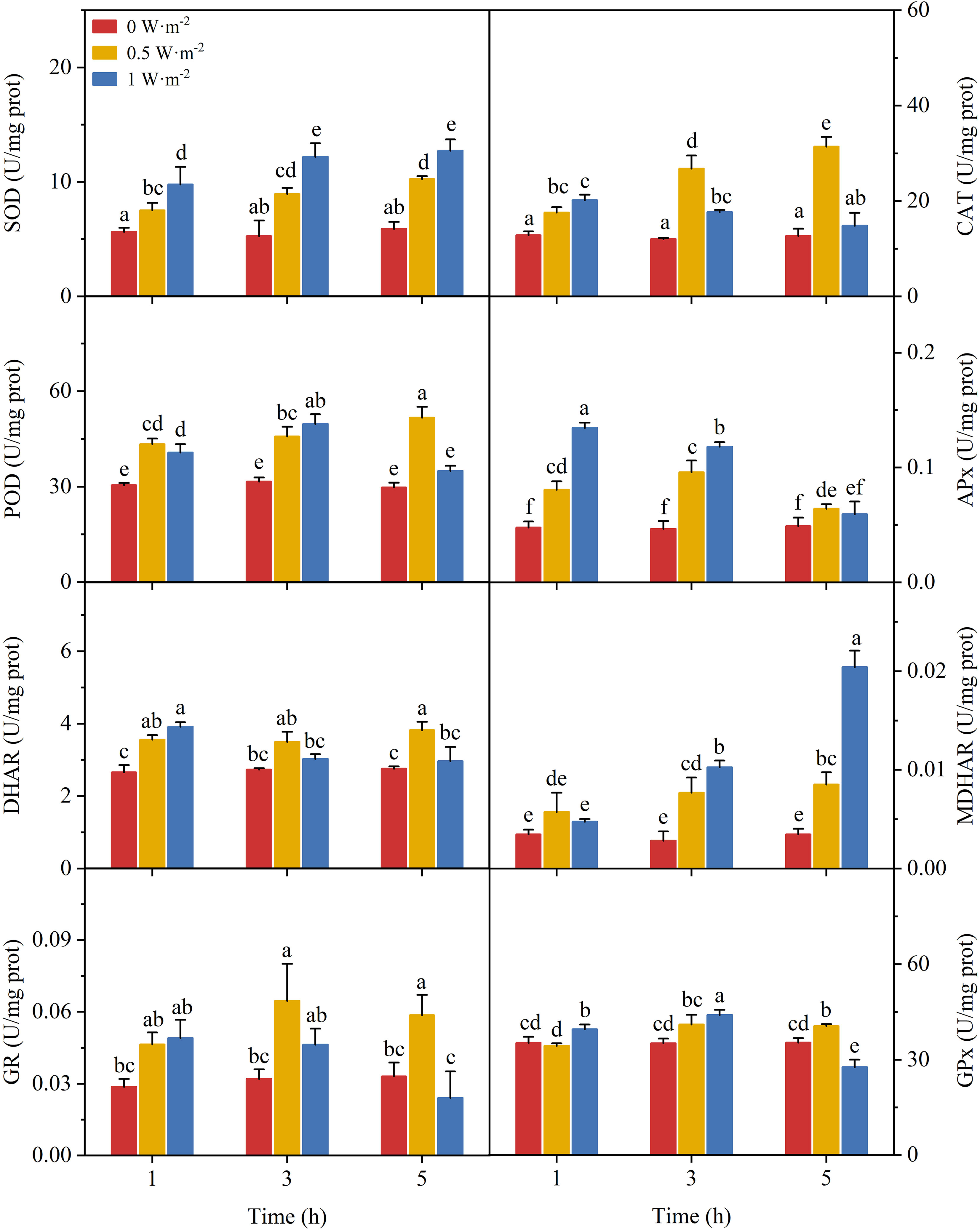
Figure 2 Effects of ultraviolet-B radiation on antioxidant enzyme activity of Neoporphyra haitanensis. Values are represented as means ± SD (n = 3). Different lowercase letters indicate a significant difference among groups according to ANOVA and the LSD test (P < 0.05); others are significantly different (P > 0.05). SOD, superoxide dismutase; CAT, catalase; POD, peroxidase; APx, ascorbate peroxidase; DHAR, dehydroascorbate reductase; MDHAR, monodehydroascorbate reductase; GR, glutathione reductase; GPx, glutathione peroxidase.
Changes of AsA and GSH contents under UV-B radiation
The activity of the AsA-GSH cycle varied with increasing intensity and duration of UV-B radiation (Figure 3). The ratios of AsA/DHA and GSH/GSSG were calculated separately to determine the regeneration rates of AsA and GSH. The reduction/oxidation ratio also reflects the cellular redox state and ROS-mediated cytotoxicity. The levels of T-GSH, GSH, and GSSG showed an increase under the low UV-B exposure. The GSH/GSSG ratio increased significantly after exposure to low UV-B radiation for 1 h. The continuous high UV-B radiation resulted in a low GSH/GSSG ratio in N. haitanensis. The AsA content increased when stimulated by UV-B radiation. The change in DHA content was similar to the change in AsA content. However, the AsA/DHA value decreased after low UV-B exposure for 3 h, but increased significantly after 5 h-irradiation. High UV-B radiation significantly decreased the AsA/DHA ratio.
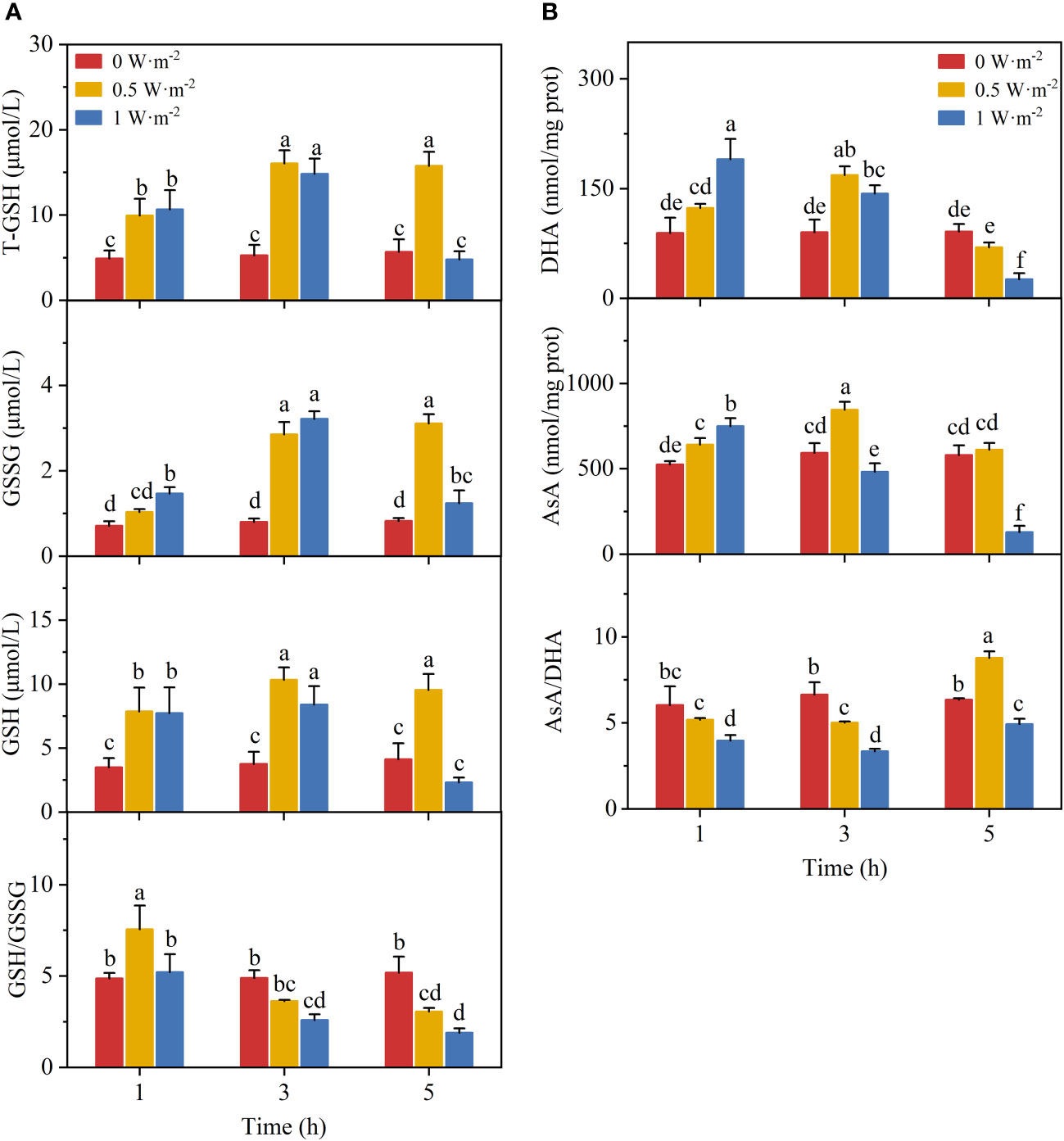
Figure 3 The changes of ascorbate-glutathione cycle in Neoporphyra haitanensis in response to increasing ultraviolet-B radiation: (A) variations in total glutathione (T-GSH), glutathione disulfide (GSSG), glutathione (GSH), and the ratio of GSSG/GSH; (B) variations in dehydroascorbate (DHA), ascorbate (AsA), and the ratio of AsA/DHA. Values are represented as means ± SD (n = 3). Different lowercase letters indicate a significant difference among groups according to ANOVA and the LSD test (P < 0.05); the same lowercase letters indicate no significant difference between the groups (P > 0.05).
Effects of ROS scavengers on UV-B-induced oxidative stress, enzyme activities, and antioxidant contents
Three ROS scavengers, SB, Tiron, and DMTU, used in the experiment had no adverse effect on the oxidation and antioxidant system of the algae (Figure 4). The exposure to the low UV-B radiation for 5 h increased both the contents of H2O2 and MDA and the activities of antioxidant enzymes, including SOD, CAT, POD, APx, DHAR, MDHAR, GR, and GPx. The increased H2O2 and MDA contents caused by UV-B radiation were inhibited by SB, Tiron, and DMTU. Correspondingly, the activities of antioxidant enzymes were significantly decreased in the presence of ROS scavengers compared to the enzyme activity levels after UV-B radiation.
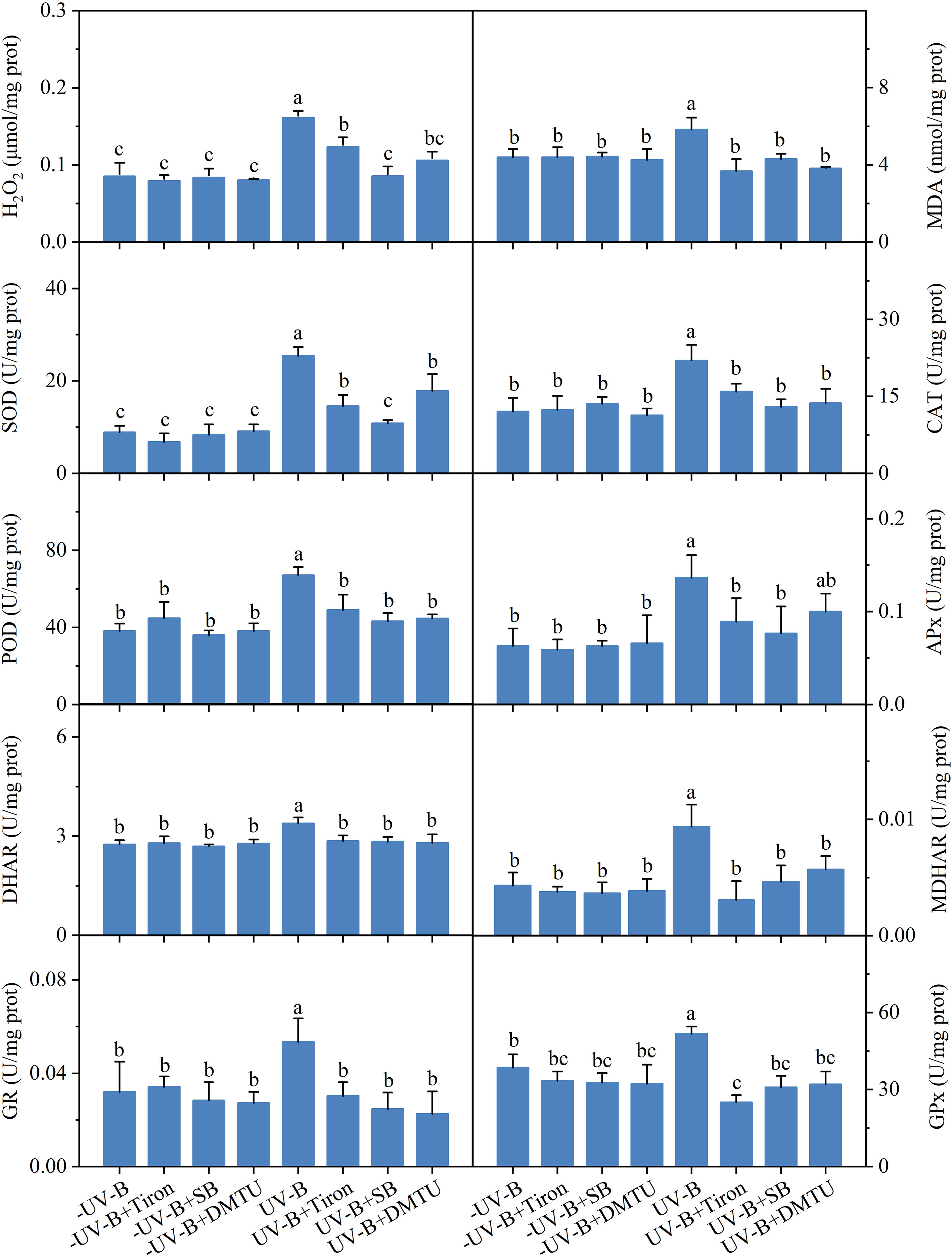
Figure 4 Effects of 10 mM sodium benzoate (SB), 100 μM 1,2-dihydroxy-benzene-3,5-disulphonic acid (Tiron), or 100 μM dimethylthiourea (DMTU) on oxidation and antioxidant system in Neoporphyra haitanensis on exposure to 0.5 W·m−2 ultraviolet-B (UV-B) radiation for 5 h. Values are represented as means ± SD (n = 3). Different lowercase letters indicate a significant difference among groups according to ANOVA and the LSD test (P < 0.05); The same lowercase letters indicate no significant difference between the groups (P > 0.05).
Increased activity of the AsA-GSH cycle upon UV-B exposure was also affected by SB, Tiron, and DMTU (Figure 5). The UV-B-induced increase in the contents of T-GSH, GSSG, and GSH were inhibited by ROS scavengers. After exposed to low UV-B radiation for 5 h, the ratio of GSH/GSSG decreased by 21%. After the addition of ROS scavengers, the GSH/GSSG ratio substantially recovered to almost the original level. The decreased DHA content was reversed by SB, Tiron, and DMTU. The UV-B-induced increase in AsA content and AsA/DHA ratio was inhibited by three ROS scavengers.
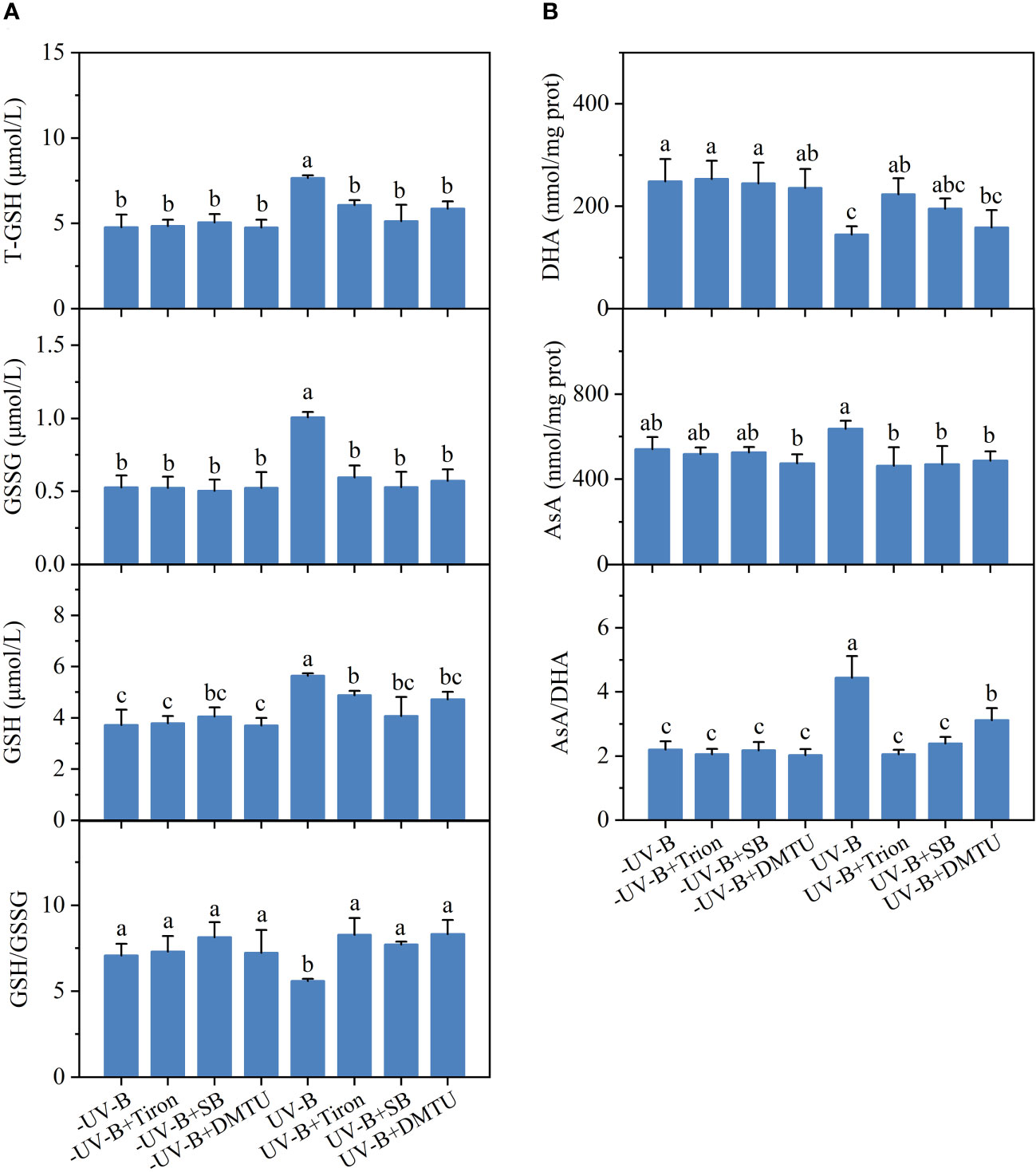
Figure 5 Effects of 10 mM sodium benzoate (SB), 100 μM 1,2-dihydroxy-benzene-3,5-disulphonic acid (Tiron), or 100 μM dimethylthiourea (DMTU) on the ascorbate-glutathione cycle in Neoporphyra haitanensis on exposure to 0.5 W·m−2 ultraviolet-B (UV-B) radiation for 5 h. (A) T-GSH, GSSG, GSH and the ratio of GSSG/GSH. (B) DHA, AsA, and the ratio of AsA/DHA. Values are represented as means ± SD (n = 3). Different lowercase letters indicate a significant difference among groups according to ANOVA and the LSD test (P < 0.05); the same lowercase letters indicate no significant difference between the groups (P > 0.05).
Effects of ROS scavengers on photosynthetic performance under UV-B radiation
The changes in the photosynthetic performance of N. haitanensis under UV-B radiation were measured in the presence of ROS scavengers (Figure 6). When the algae were exposed to the low UV-B radiation for 5 h, they exhibited a decrease in the Fv/Fm, Y(II), and ETR by a range of 50–70% compared with those without irradiation. The value of the qP decreased by 21.39% after UV-B exposure. The value of NPQ and Y(NO) in N. haitanensis significantly increased by 66.06% and 59.80%, respectively, under UV-B conditions. Decreased Fv/Fm, Y(II), and ETR by UV-B exposure were relieved by three ROS scavengers, and the qP recovered to more than 90% of the initial efficiency. The UV-B-irradiated algae underwent a marked decrease in NPQ with the addition of ROS scavengers. Similarly, three ROS scavengers had a positive effect on the recovery of Y(NO) to pre-radiation levels.
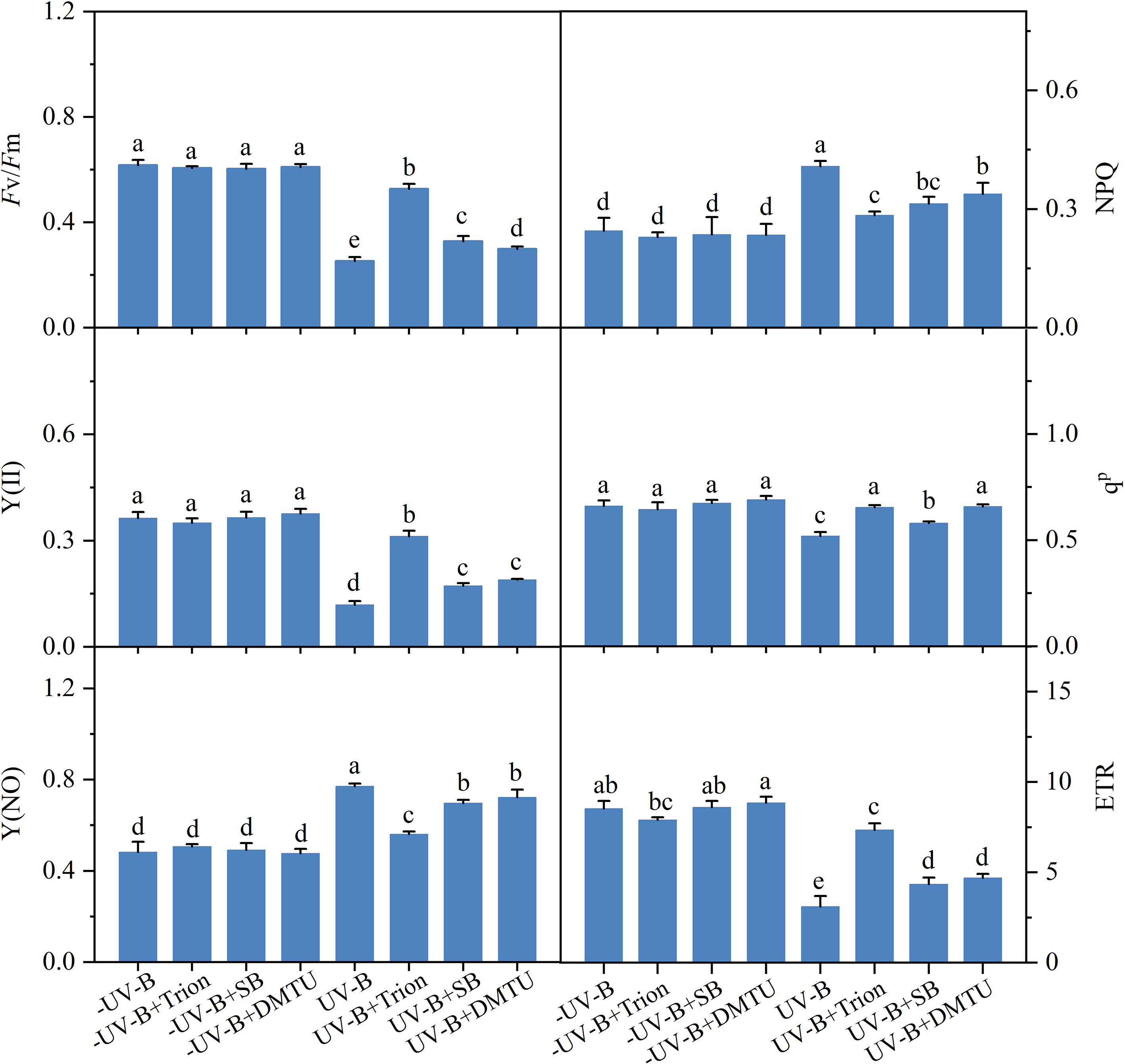
Figure 6 Effects of reactive oxygen species scavengers on the photosynthetic performance in Neoporphyra haitanensis under exposure to 0.5 W·m−2 ultraviolet-B (UV-B) radiation for 5 h. Values are represented as means ± SD (n = 3). All data are the mean values (± SD) from three biological replicates. Different lowercase letters indicate a significant difference among groups according to ANOVA and the LSD test (P < 0.05); the same lowercase letters indicate no significant difference between the groups (P > 0.05). Fv/Fm, the maximal photosystem II quantum yield; Y(II), the effective photosystem II quantum yield; qP, photochemical quenching coefficient; ETR, electron transport rate; Y(NO), non-regulated non-photochemical quantum yield; NPQ, non-photochemical quenching.
Discussion
UV-B-induced oxidative stress in N. haitanensis
Ultraviolet-B radiation is a variable ambient type of radiation whose flux fluctuations may modulate the level of ROS in plants. This UV-B radiation can act on the chloroplasts and mitochondria of photosynthetic organisms, producing a large amount of ROS (Gill and Tuteja, 2010). The generation and retention of large amounts of ROS is thought to be one of the main reasons for the toxic and damaging effects of UV radiation on marine algae (Malanga and Puntarulo, 1995). Accumulated ROS interacted with lipids, resulting in structural changes and lipid peroxidation (Wang et al., 2010). The increase in H2O2 content is positively correlated with the degree of lipid peroxidation (Dai et al., 1997). In this study, an increase in ROS with no significant change in MDA was observed at both low and high UV-B radiation. These results suggest that UV-B radiation triggers changes in ROS production in N. haitanensis, without oxidative damage. With the enhancement of UV-B radiation, a large amount of ROS accumulated, and the content of MDA increased significantly, resulting in oxidative damage. These results suggested that H2O2 detoxification was not achieved when UV-B radiation continuously increased. In addition to high irradiation, there is substantial evidence describing the effects of other environmental stimuli on ROS production. Typical intertidal environmental stresses, such as dehydration, high salinity, and ocean acidification, could induce the accumulation of ROS, leading to oxidative stress in intertidal macroalgae (Kumar et al., 2017; Wang et al., 2019; Liang et al., 2021). It is worth noting that intertidal seaweeds may constantly suffer from combined stresses due to the particularity of their habitat. Future studies not only need to clarify the responses of macroalgae to single source of stress, but also need to explore the resistance, adaptation, and damage mechanisms of macroalgae under combined stresses.
Regulation of the antioxidant system by ROS under UV-B conditions
Studies have shown that antioxidant systems contribute to the self-maintenance of macroalgae under exposure to UV-B radiation (Tuzun et al., 2020). In the process of removing ROS, the antioxidant enzymes CAT, SOD, and POD serve as the first line of the antioxidant defense system (Czegeny et al., 2016), and become critical in suppressing the toxic levels of ROS in cells (Apel and Hirt, 2004). In the present study, the activities of these enzymes increased in N. haitanensis under low UV-B radiation. Plants may also enhance antioxidant capacity through enhancing the AsA-GSH cycle and the regeneration rates of AsA and GSH. Low levels of UV-B radiation increased the accumulation of GSH and GSSG, revealing a positive response of non-enzymatic antioxidants to UV-B exposure similar to that observed in other species of marine algae (Wang et al., 2007; Roy et al., 2021). Furthermore, the activities of GR, DHAR, and GPx involved in GSH regeneration also increased under low UV-B radiation. The GSH/GSSG ratio is a measure of redox and the detoxification state of cells. Thalli treated with low UV-B radiation maintained a highly reducing redox state as evidenced by the increased GSH/GSSG ratios, which may be beneficial for minimizing ROS generation. The antioxidant function of AsA is manifested as the detoxification of H2O2 by APx and regeneration from DHA through MDHAR and DHAR enzymes with the help of GSH (Srivastava et al., 2012). In the study, both AsA and DHA contents increased when thalli were exposed to UV-B radiation, indicating that AsA actively participated in the ROS scavenging under UV-B radiation. The AsA/DHA ratio initially decreased under low UV-B radiation, indicating that a large amount of AsA reacted with ROS, aided by high levels of APx activity. Subsequently, the regeneration of AsA increased due to the decrease in APx activity and the high levels of DHAR and MDHAR activity. Thus, both enzymatic and non-enzymatic antioxidants were activated by low UV-B radiation and responded positively to detoxify ROS to avoid oxidative damage (Figure 7A). This activation of the antioxidant system by UV-B radiation, we believe, is achieved by ROS. These ROS are supposed to be a signal that triggers the plant antioxidant defense system in response to abiotic and biotic stresses (Carvalho and Silveira, 2020). The activities of antioxidant enzymes are regulated by both genetic regulation and environmental stimuli, based on the need and urgency of scavenging the ROS that have been produced in cells (De Gara et al., 2003). Although H2O2 has been found to induce the expression of antioxidant genes in macroalgae (Sung et al., 2009), there is little direct evidence that alterations in antioxidant metabolism under environmental stress are modulated by ROS. In the present study, ROS scavengers were used to determine the relationship between ROS and the induced antioxidant defense system under UV-B exposure in N. haitanensis. The inhibitory effect of a UV-B-induced increase in enzymatic antioxidant activity and non-enzymatic antioxidant regeneration by the H2O2 scavenger DMTU supported the idea that H2O2 participated in the activation of the antioxidant defense system of N. haitanensis. Changes in antioxidant capacity after applying Tiron and SB further confirmed that the antioxidant defense system under UV-B radiation was regulated by ROS. These results suggest that UV-B radiation causes alterations in antioxidant status that are mediated by ROS. Therefore, UV-B exposure, ROS, and a rapid response of the antioxidant system are closely linked in macroalgae. It may be that UV-B activated the production of H2O2, which triggered the antioxidant defense system of N. haitanensis. It has been suggested that the ROS accumulation caused by adverse environmental stimuli rapidly triggers the antioxidant defense system in a variety of ways, including retrograde signaling, transcriptional and post-transcriptional regulation, phosphorylation, and proteolysis (Dvorak et al., 2020). Regardless of the way in which ROS in macroalgae will regulate the antioxidant system under UV-B radiation, rapid ROS production requires an immediate mobilization of the antioxidant protection system to counteract the resulting environmental stress.
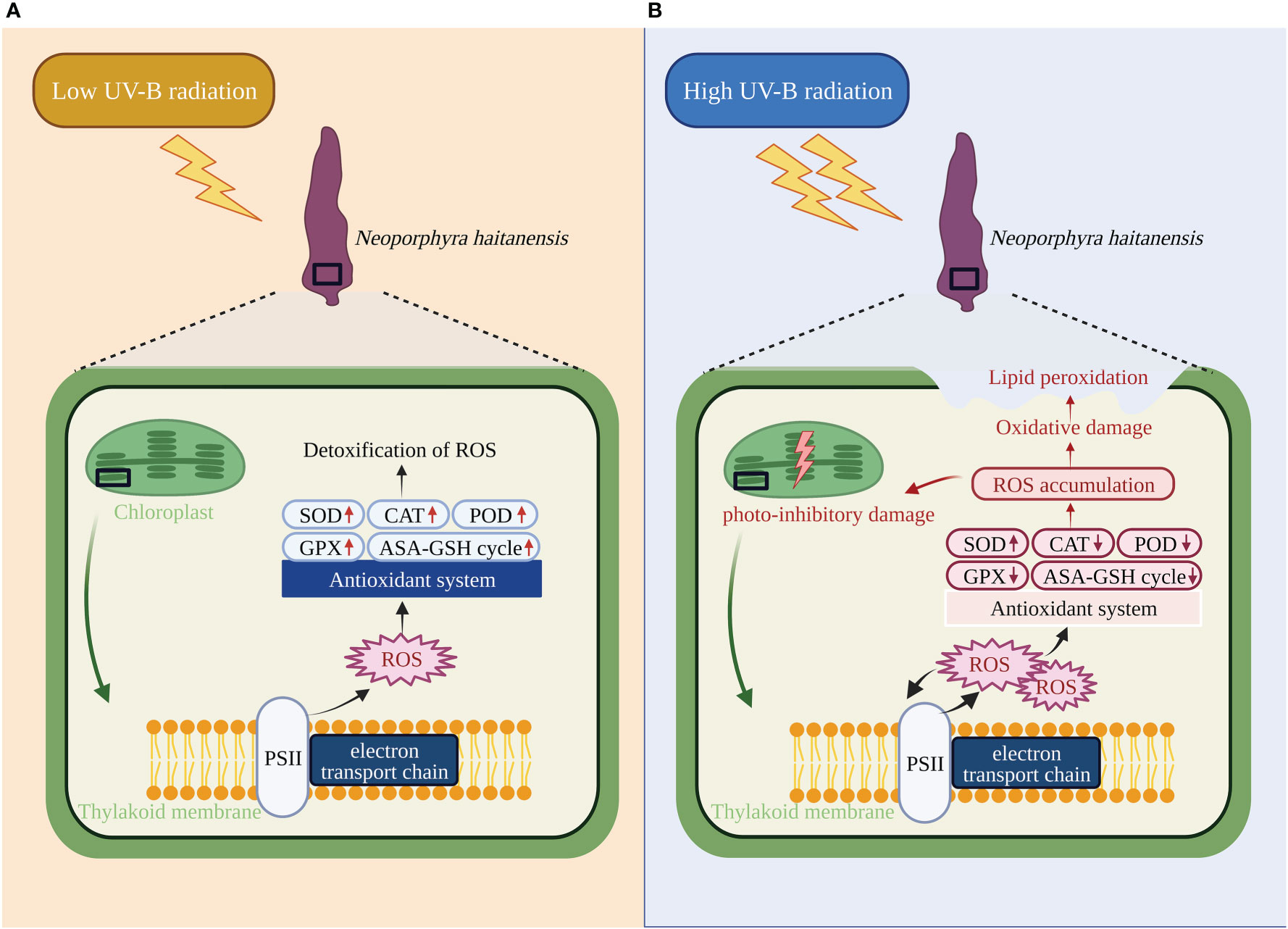
Figure 7 Responses of the antioxidant system in Neoporphyra haitanensis to ultraviolet-B (UV-B) radiation and subsequent photosynthetic system damage. Notations next to the enzymes in red designate changes during: (A) low UV-B radiation for 5 h with respect to the control; (B) high UV-B radiation for 5 h with respect to that of low UV-B radiation.
With the extension of UV-B radiation exposure time, the activities of antioxidant enzymes (including CAT, POD, and GPx) were inhibited and this was accompanied by oxidative damage. The GSH and AsA contents of N. haitanensis decreased under high UV-B stress, which is consistent with the findings in other marine algae (Singh et al., 2012). The low levels of GSH and AsA redox state (decreased ratios of GSH/GSSG and AsA/DHA) in N. haitanensis indicated the existence of elevated cellular toxicity and oxidative stress upon high UV-B exposure. Likewise, the inhibition in APx, DHAR, and GR activities under high UV-B radiation resulted in the insufficiency of the AsA-GSH cycle, subsequently resulting in the excess accumulation of H2O2 in N. haitanensis. Apparently, the deregulation of antioxidant enzymes under continuous UV-B stress affected the efficiency of ROS removal, directly breaking the dynamic balance between ROS and antioxidant defense, leading to a dramatic increase of ROS in cells (Figure 7B). The underlying molecular mechanism by which ROS regulate the antioxidant system under UV-B radiation may be delicate and complex, and further molecular validation is required.
ROS-mediated damage to photosynthetic performance of N. haitanensis under UV-B radiation
Photosynthesis and oxidative stress are intimately related in photosynthetic organisms, as the imbalance between light energy absorption and the effective use of light energy may contribute to the overproduction of ROS (Krieger-Liszkay, 2005). Photoinhibition and ROS accumulation are two major processes with known ubiquitous occurrence in plants under unfavorable conditions; however, the opinions of various researchers on their interrelation are disparate. One view holds that excessive accumulation of ROS in plant cells is the underlying reason causing photoinhibition and photodamage under stress conditions (Triantaphylides et al., 2008; Triantaphylides and Havaux, 2009; Takahashi and Badger, 2011). An alternative view argues that photoinhibition plays a protective role as an active strategy, and it essentiality prevents the overproduction of ROS and the inactivation of downstream components of the photosynthetic electron transport chain (Lima-Melo et al., 2019). In the present study, an improvement of the algal photosynthetic efficiency occurred after the removal of ROS induced by UV-B radiation, accompanied by a reduction in photodamage and a restoration of electron transport, indicating the occurrence of photo-inhibitory damage is closely related to the accumulation of ROS in N. haitanensis. Moreover, ROS scavengers alleviated the lipid peroxidation in N. haitanensis caused by UV-B radiation. We believe that the ROS scavenging system could not remove excess ROS efficiently under enhanced UV-B radiation, which caused irreversible oxidative damage to the photosynthetic apparatus and membrane damage of algal cells (Figure 7B). Therefore, ROS-mediated oxidative damage caused by UV-B radiation is one of the important causes of photo-inhibitory damage in N. haitanensis. In our previous study, we found that N. haitanensis could prevent ROS production by improving NPQ under enhanced UV-B radiation, forming a solid method for algae to avoid an excessive accumulation of ROS (Xue et al., 2022). In the present study, the increased NPQ induced by UV-B radiation was inhibited by ROS scavengers, demonstrating that NPQ and the antioxidant system are collectively responsible for maintaining the ROS balance that allows algae to resist ROS-mediated oxidative damage under low UV-B radiation. Although the photosynthetic performance of UV-irradiated algae increased to varying degrees after the addition of ROS scavengers, the performance was still significantly lower than that of algae without UV-B radiation. Hence, in addition to oxidative damage, UV-B radiation may cause other types of damage to the structure and function of the photosynthetic apparatus of N. haitanensis, such as destroying D1 protein, degrading photopigments, or damaging DNA (Cheng et al., 2016; Yadav et al., 2020), and the underlying direct damage mechanism needs to be further studied.
Conclusion
The results demonstrated that ROS were involved in the response of N. haitanensis to UV-B radiation stress and played an important role in the activation of the antioxidant system and the regulation of photosynthesis. Enzymatic and non-enzymatic antioxidants were activated by low UV-B radiation and responded positively to maintain an ROS balance. High UV-B radiation markedly inhibited the activities of antioxidant enzymes as well as the efficiency of the AsA-GSH cycle, causing ROS accumulation and oxidative damage. This study also found that alterations in antioxidant metabolism under UV-B stress were modulated by ROS. In addition, the photo-inhibitory damage of N. haitanensis under UV-B radiation was observed, and the underlying reason may contribute to ROS-mediated oxidative damage caused by UV-B radiation. The results of the study provide evidence for elucidating the adaptive strategy of intertidal macroalgae in highly variable marine environments.
Data availability statement
The original contributions presented in the study are included in the article/supplementary material. Further inquiries can be directed to the corresponding authors.
Author contributions
SX: investigation, methodology, data curation, formal analysis, software, writing of the original draft. YZ: investigation, methodology, formal analysis, conceptualization, funding acquisition, resources, validation, supervision, writing, and review & editing. JC: software, visualization. SS: software, visualization. LG: investigation, methodology. XT: data curation, conceptualization, funding acquisition, project administration, validation, supervision, writing, and review & editing. All authors contributed to the article and approved the submitted version.
Funding
This study was supported by the NSFC-Shandong Joint Fund (Grants No. U1806213), the National Natural Science Foundation of China (Grants No. 42006144), the National Natural Science Foundation of China (Grants No. 42176154), and the National Key R&D Program of China (Grants No. 2019YFD0901204).
Conflict of interest
Author LG was employed by Fujian Pingtan Aquatic Product Improved Variety Experiment Co. Ltd.
The remaining authors declare that the research was conducted in the absence of any commercial or financial relationships that could be construed as potential conflicts of interest.
Publisher’s note
All claims expressed in this article are solely those of the authors and do not necessarily represent those of their affiliated organizations, or those of the publisher, the editors and the reviewers. Any product that may be evaluated in this article, or claim that may be made by its manufacturer, is not guaranteed or endorsed by the publisher.
References
Ahmad P., Sarwat M., Sharma S. (2008). Reactive oxygen species, antioxidants and signaling in plants. J. Plant Biol. 51 (3), 167–173. doi: 10.1007/BF03030694
Apel K., Hirt H. (2004). Reactive oxygen species: metabolism, oxidative stress, and signal transduction. Annu. Rev. Plant Biol. 55, 373–399. doi: 10.1146/annurev.arplant.55.031903.141701
Barnes P. W., Williamson C. E., Lucas R. M., Robinson S. A., Madronich S., Paul N. D., et al. (2019). Ozone depletion, ultraviolet radiation, climate change and prospects for a sustainable future. Nat. Sustain. 2 (7), 569–579. doi: 10.1038/s41893-019-0314-2
Bornman J. F. (1989). New trends in photobiology: Target sites of UV-b radiation in photosynthesis of higher plants. J. Photochem. Photobiol. B. 4 (2), 145–158. doi: 10.1016/1011-1344(89)80001-6
Carvalho F. E. L., Silveira J. A. G. (2020). “H2O2-retrograde signaling as a pivotal mechanism to understand priming and cross stress tolerance in plants,” in Priming-mediated stress and cross-stress tolerance in crop plants. Ed. Hossain M. A. (Pittsburgh: Academic Press), 57–78.
Chen H., Chu J. S.-C., Chen J., Luo Q., Wang H., Lu R., et al. (2022). Insights into the ancient adaptation to intertidal environments by red algae based on a genomic and multiomics investigation of Neoporphyra haitanensis. Mol. Biol. Evol. 39 (1), msab315. doi: 10.1093/molbev/msab315
Cheng D. D., Zhang Z. S., Sun X. B., Zhao M., Sun G. Y., Chow W. S. (2016). Photoinhibition and photoinhibition-like damage to the photosynthetic apparatus in tobacco leaves induced by pseudomonas syringae pv. tabaci under light and dark conditions. BMC Plant Biol. 16, 29–39. doi: 10.1186/s12870-016-0723-6
Contreras-Porcia L., Thomas D., Flores V., Correa J. A. (2011). Tolerance to oxidative stress induced by desiccation in Porphyra columbina (Bangiales, rhodophyta). J. Exp. Bot. 62 (6), 1815–1829. doi: 10.1093/jxb/erq364
Czegeny G., Matai A., Hideg E. (2016). UV-B effects on leaves-oxidative stress and acclimation in controlled environments. Plant Sci. 248, 57–63. doi: 10.1016/j.plantsci.2016.04.013
Dai Q., Yan B., Huang S., Liu X., Peng S., Miranda M. L. L., et al. (1997). Response of oxidative stress defense systems in rice (Oryza sativa) leaves with supplemental UV-b radiation. Physiol. Plantarum 101 (2), 301–308. doi: 10.1111/j.1399-3054.1997.tb01000.x
Das K., Roychoudhury A. (2014). Reactive oxygen species (ROS) and response of antioxidants as ROS-scavengers during environmental stress in plants. Front. Environ. Sci. 2. doi: 10.3389/fenvs.2014.00053
De Gara L., de Pinto M. C., Tommasi F. (2003). The antioxidant systems vis-à-vis reactive oxygen species during plant–pathogen interaction. Plant Physiol. Biochem. 41 (10), 863–870. doi: 10.1016/s0981-9428(03)00135-9
del Rio L. A., Sandalio L. M., Altomare D. A., Zilinskas B. A. (2003). Mitochondrial and peroxisomal manganese superoxide dismutase: differential expression during leaf senescence. J. Exp. Bot. 54 (384), 923–933. doi: 10.1093/jxb/erg091
Dey T., Das S., Majumdar A., Kar R. K. (2021). Apoplastic reactive oxygen species mediated escape growth of root during illumination in Vigna radiata (L.) wilczek seedlings. Acta Physiol. Plant 43 (11), 145–154. doi: 10.1007/s11738-021-03313-2
Dhomse S. S., Feng W., Montzka S. A., Hossaini R., Keeble J., Pyle J. A., et al. (2019). Delay in recovery of the Antarctic ozone hole from unexpected CFC-11 emissions. Nat. Commun. 10 (1), 5781–5792. doi: 10.1038/s41467-019-13717-x
Ding H., Wang T., Zhang P., Lu Y., Yan X. (2019). High-temperature resistance of the thin-blade strain of Pyropia haitanensis and its pilot cultivation in mariculture farm. J. Appl. Phycol. 32 (4), 2261–2270. doi: 10.1007/s10811-019-01885-8
Dvorak P., Krasylenko Y., Zeiner A., Samaj J., Takac T. (2020). Signaling toward reactive oxygen species-scavenging enzymes in plants. Front. Plant Sci. 11. doi: 10.3389/fpls.2020.618835
Eleftheratos K., Kapsomenakis J., Zerefos C. S., Bais A. F., Fountoulakis I., Dameris M., et al. (2020). Possible effects of greenhouse gases to ozone profiles and DNA active UV-b irradiance at ground level. Atmosphere 11 (3), 228–245. doi: 10.3390/atmos11030228
Gill S. S., Tuteja N. (2010). Reactive oxygen species and antioxidant machinery in abiotic stress tolerance in crop plants. Plant Physiol. Biochem. 48 (12), 909–930. doi: 10.1016/j.plaphy.2010.08.016
Gururani M. A., Venkatesh J., Tran L. S. (2015). Regulation of photosynthesis during abiotic stress-induced photoinhibition. Mol. Plant 8 (9), 1304–1320. doi: 10.1016/j.molp.2015.05.005
Hasanuzzaman M., Bhuyan M., Parvin K., Bhuiyan T. F., Anee T. I., Nahar K., et al. (2020). Regulation of ROS metabolism in plants under environmental stress: a review of recent experimental evidence. Int. J. Mol. Sci. 21 (22), 8695–8736. doi: 10.3390/ijms21228695
Hasanuzzaman M., Hossain M. A., da Silva J. A. T., Fujita M. (2012). “Plant response and tolerance to abiotic oxidative stress: antioxidant defense is a key factor,” in Crop stress and its management: Perspectives and strategies. Eds. Venkateswarlu B., Shanker A. K., Shanker C., Maheswari M. (Dordrecht: Springer Netherlands), 261–315.
Juan C. A., Perez de la Lastra J. M., Plou F. J., Perez-Lebena E. (2021). The chemistry of reactive oxygen species (ROS) revisited: Outlining their role in biological macromolecules (DNA, lipids and proteins) and induced pathologies. Int. J. Mol. Sci. 22 (9), 4642–4662. doi: 10.3390/ijms22094642
Krieger-Liszkay A. (2005). Singlet oxygen production in photosynthesis. J. Exp. Bot. 56 (411), 337–346. doi: 10.1093/jxb/erh237
Kumar A., AbdElgawad H., Castellano I., Lorenti M., Delledonne M., Beemster G., et al. (2017). Physiological and biochemical analyses shed light on the response of Sargassum vulgare to ocean acidification at different time scales. Front. Plant Sci. 8. doi: 10.3389/fpls.2017.00570
Levine A., Tenhaken R., Dixon R., Lamb C. (1994). H2O2 from the oxidative burst orchestrates the plant hypersensitive disease resistance response. Cell 79 (4), 583–593. doi: 10.1016/0092-8674(94)90544-4
Liang Z. R., Liu F., Wang W., Zhang P., Yuan Y., Yao H., et al. (2021). A reasonable strategy for Caulerpa lentillifera j. agardh (Bryopsidales, chlorophyta) transportation based on the biochemical and photophysiological responses to dehydration stress. Algal Res. 56, 102304. doi: 10.1016/J.ALGAL.2021.102304
Lima-Melo Y., Alencar V., Lobo A. K. M., Sousa R. H. V., Tikkanen M., Aro E. M., et al. (2019). Photoinhibition of photosystem I provides oxidative protection during imbalanced photosynthetic electron transport in Arabidopsis thaliana. Front. Plant Sci. 10. doi: 10.3389/fpls.2019.00916
Liu T., Ye X., Li M., Li J., Qi H., Hu X. (2020). H2O2 and NO are involved in trehalose-regulated oxidative stress tolerance in cold-stressed tomato plants. Environ. Exp. Bot. 171, 103961–103969. doi: 10.1016/j.envexpbot.2019.103961
Malanga G., Puntarulo S. (1995). Oxidative stress and antioxidant content in Chlorella vulgaris after exposure to ultraviolet-b radiation. Physiol. Plantarum 94, 672–679. doi: 10.1111/j.1399-3054.1995.tb00983.x
Meinshausen M., Nicholls Z. R. J., Lewis J., Gidden M. J., Vogel E., Freund M., et al. (2020). The shared socio-economic pathway (SSP) greenhouse gas concentrations and their extensions to 2500. Geosci. Model. Dev. 13 (8), 3571–3605. doi: 10.5194/gmd-13-3571-2020
Mishra P., Prasad S. M. (2021). Low dose UV-b radiation induced mild oxidative stress impact on physiological and nutritional competence of Spirulina (Arthrospira) species. Plant Stress 2, 100039–100048. doi: 10.1016/j.stress.2021.100039
Nadarajah K. K. (2020). ROS homeostasis in abiotic stress tolerance in plants. Int. J. Mol. Sci. 21 (15), 5208. doi: 10.3390/ijms21155208
Nagalakshmi N., Prasad M. N. V. (1998). Copper-induced oxidative stress in Scenedesmus bijugatus: protective role of free radical scavengers. Bull. Environ. Contam. Toxicol. 61, 623–628. doi: 10.1007/S001289900806
Narayanaswamy N., Narra S., Nair R. R., Saini D. K., Kondaiah P., Govindaraju T. (2016). Stimuli-responsive colorimetric and NIR fluorescence combination probe for selective reporting of cellular hydrogen peroxide. Chem. Sci. 7 (4), 2832–2841. doi: 10.1039/c5sc03488d
Qi J., Zhang M., Lu C., Hettenhausen C., Tan Q., Cao G., et al. (2018). Ultraviolet-b enhances the resistance of multiple plant species to lepidopteran insect herbivory through the jasmonic acid pathway. Sci. Rep. 8 (1), 277. doi: 10.1038/s41598-017-18600-7
Roy U. K., Nielsen B. V., Milledge J. J. (2021). Antioxidant production in Dunaliella. Appl. Sci. 11 (9), 3959–3982. doi: 10.3390/app11093959
Shiu C. T., Lee T. M. (2005). Ultraviolet-b-induced oxidative stress and responses of the ascorbate-glutathione cycle in a marine macroalga Ulva fasciata. J. Exp. Bot. 56 (421), 2851–2865. doi: 10.1093/jxb/eri277
Singh V. P., Srivastava P. K., Prasad S. M. (2012). Differential effect of UV-b radiation on growth, oxidative stress and ascorbate-glutathione cycle in two cyanobacteria under copper toxicity. Plant Physiol. Biochem. 61, 61–70. doi: 10.1016/j.plaphy.2012.09.005
Srivastava P. K., Singh V. P., Prasad S. M. (2012). Compatibility of ascorbate-glutathione cycle enzymes in cyanobacteria against low and high UV-b exposures, simultaneously exposed to low and high doses of chlorpyrifos. Ecotoxicol. Environ. Saf. 83, 79–88. doi: 10.1016/j.ecoenv.2012.06.009
Sung M. S., Hsu Y. T., Hsu Y. T., Wu T. M., Lee T. M. (2009). Hypersalinity and hydrogen peroxide upregulation of gene expression of antioxidant enzymes in Ulva fasciata against oxidative stress. Mar. Biotechnol. 11 (2), 199–209. doi: 10.1007/s10126-008-9134-5
Takahashi S., Badger M. R. (2011). Photoprotection in plants: a new light on photosystem II damage. Trends Plant Sci. 16 (1), 53–60. doi: 10.1016/j.tplants.2010.10.001
Tokutsu R., Fujimura-Kamada K., Yamasaki T., Okajima K., Minagawa J. (2021). UV-A/B radiation rapidly activates photoprotective mechanisms in Chlamydomonas reinhardtii. Plant Physiol. 185 (4), 1894–1902. doi: 10.1093/plphys/kiab004
Triantaphylides C., Havaux M. (2009). Singlet oxygen in plants: production, detoxification and signaling. Trends Plant Sci. 14 (4), 219–228. doi: 10.1016/j.tplants.2009.01.008
Triantaphylides C., Krischke M., Hoeberichts F. A., Ksas B., Gresser G., Havaux M., et al. (2008). Singlet oxygen is the major reactive oxygen species involved in photooxidative damage to plants. Plant Physiol. 148 (2), 960–968. doi: 10.1104/pp.108.125690
Tuzun N., Debecker S., Stoks R. (2020). Strong species differences in life history do not predict oxidative stress physiology or sensitivity to an environmental oxidant. J. Anim. Ecol. 89 (7), 1711–1721. doi: 10.1111/1365-2656.13235
Tyystjarvi E. (2013). Photoinhibition of photosystem II. Int. Rev. Cell Mol. Biol. 300, 243–303. doi: 10.1016/B978-0-12-405210-9.00007-2
Wang G., Hao Z., Anken R. H., Lu J., Liu Y. (2010). Effects of UV-b radiation on photosynthesis activity of Wolffia arrhiza as probed by chlorophyll fluorescence transients. Adv. Space Res. 45 (7), 839–845. doi: 10.1016/j.asr.2009.12.004
Wang G., Hu C., Li D., Zhang D., Li X., Chen K., et al. (2007). The response of antioxidant systems in Nostoc sphaeroides against UV-b radiation and the protective effects of exogenous antioxidants. Adv. Space Res. 39 (6), 1034–1042. doi: 10.1016/j.asr.2007.03.022
Wang W., Xu Y., Chen T., Xing L., Xu K., Xu Y., et al. (2019). Regulatory mechanisms underlying the maintenance of homeostasis in Pyropia haitanensis under hypersaline stress conditions. Sci. Total Environ. 662, 168–179. doi: 10.1016/j.scitotenv.2019.01.214
Wang D., You W., Chen N., Cao M., Tang X., Guan X., et al. (2020). Comparative quantitative proteomics reveals the desiccation stress responses of the intertidal seaweed Pyropia haitanensis. J. Phycol. 56 (6), 1664–1675. doi: 10.1111/JPY.13052-19-272
Wise R. R. (1995). Chilling-enhanced photooxidation: The production, action and study of reactive oxygen species produced during chilling in the light. Photosynth. Res. 45, 79–97. doi: 10.1007/BF00032579
Xing X., Zhou Q., Xing H., Jiang H., Wang S. (2016). Early abscisic acid accumulation regulates ascorbate and glutathione metabolism in soybean leaves under progressive water stress. J. Plant Growth Regul. 35 (3), 865–876. doi: 10.1007/s00344-016-9588-z
Xue S., Zang Y., Chen J., Shang S., Tang X. (2022). Effects of enhanced UV-b radiation on photosynthetic performance and non-photochemical quenching process of intertidal red macroalgae Neoporphyra haitanensis. Environ. Exp. Bot. 199, 104888–104898. doi: 10.1016/j.envexpbot.2022.104888
Xu N., Xu K., Wang W., Xu Y., Ji D., Chen C., et al. (2020). Nutrient enrichment improves growth and food quality of two strains of the economic seaweed Pyropia haitanensis. Front. Mar. Sci. 7, 544582–544591. doi: 10.3389/fmars.2020.544582
Yadav A., Singh D., Lingwan M., Yadukrishnan P., Masakapalli S. K., Datta S. (2020). Light signaling and UV-b-mediated plant growth regulation. J. Integr. Plant Biol. 62 (9), 1270–1292. doi: 10.1111/jipb.12932
Yang L. E., Deng Y. Y., Xu G. P., Russell S., Lu Q. Q., Brodie J. (2020). Redefining Pyropia (Bangiales, rhodophyta): Four new genera, resurrection of Porphyrella and description of Calidia pseudolobata sp. nov. from China. J. Phycol. 56 (4), 862–879. doi: 10.1111/jpy.12992
Yu B., Yang J., Niu J., Wang G. (2020). Antioxidant responses to hyperosmolarity stress in the intertidal Pyropia yezoensis (Bangiales, rhodophyta). Algal Res. 48, 101930–101938. doi: 10.1016/j.algal.2020.101930
Keywords: enhanced UV-B radiation, intertidal macroalgae, oxidative damage, photoinhibition, reactive oxygen species scavenging
Citation: Xue S, Zang Y, Chen J, Shang S, Gao L and Tang X (2022) Ultraviolet-B radiation stress triggers reactive oxygen species and regulates the antioxidant defense and photosynthesis systems of intertidal red algae Neoporphyra haitanensis. Front. Mar. Sci. 9:1043462. doi: 10.3389/fmars.2022.1043462
Received: 13 September 2022; Accepted: 17 October 2022;
Published: 02 November 2022.
Edited by:
Yunyan Deng, Institute of Oceanology (CAS), ChinaReviewed by:
Loretto Contreras-Porcia, Andres Bello University, ChileVinod K. Kannaujiya, Banaras Hindu University, India
Copyright © 2022 Xue, Zang, Chen, Shang, Gao and Tang. This is an open-access article distributed under the terms of the Creative Commons Attribution License (CC BY). The use, distribution or reproduction in other forums is permitted, provided the original author(s) and the copyright owner(s) are credited and that the original publication in this journal is cited, in accordance with accepted academic practice. No use, distribution or reproduction is permitted which does not comply with these terms.
*Correspondence: Yu Zang, zangyu@fio.org.cn; Xuexi Tang, tangxx@ouc.edu.cn