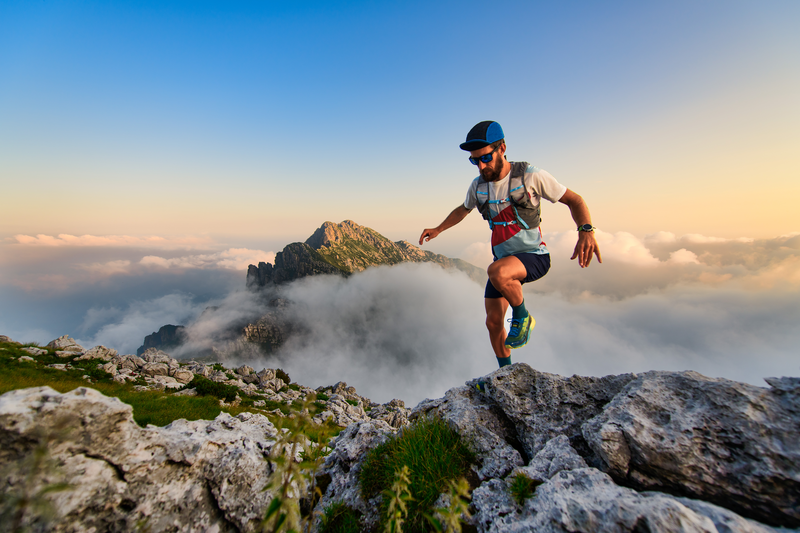
95% of researchers rate our articles as excellent or good
Learn more about the work of our research integrity team to safeguard the quality of each article we publish.
Find out more
ORIGINAL RESEARCH article
Front. Mar. Sci. , 24 November 2022
Sec. Marine Biotechnology and Bioproducts
Volume 9 - 2022 | https://doi.org/10.3389/fmars.2022.1043123
This article is part of the Research Topic Boosting the Potential of Algae for Biomass Production, Valorisation, and Bioremediation View all 9 articles
The objective of this work was to test the growth of the freshwater microalga Chlamydopodium fusiforme MACC-430 in diluted piggery wastewater (PWW) for biomass production which is a potential producer of biostimulating and antimicrobial compounds. The productivity and effectiveness in removing nitrogen and phosphorus by the cultures were tested outdoors in open ponds by comparing the mineral medium BG-11 medium (control) and piggery wastewater (PWW). Daily biomass yields were found 14.7 ± 4.6g m-2 d-1 in BG-11 and 17.6 ± 6.7g m-2 d-1 in 10-fold diluted wastewater (PWW10) with deionized water. Further increase in the biomass productivity yield (33.1 ± 5.6g m-2 d-1) was achieved with more concentrated PWW (5-fold dilution – PWW5). Nitrogen removal rate (N-NH4+) reached 21.4 ± 2.9mg L-1 d-1 in cultures grown in PWW10 and increased to 38.4±16.3mg L-1 d-1 using the PWW5. The use of undiluted PWW strongly increased the bacterial load, which caused the loss of the culture. The photosynthetic performance measured as the maximum fluorescence yield Fv/Fm ratio in both the PWW10 and BG-11 cultures showed a cyclic behavior, as it decreased during the day and recovered at night. However, in the cultures grown in diluted PWW the Fv/Fm ratio slightly increased throughout the cultivation. Between PWW10 and BG-11, no significant differences were observed in photosynthetic oxygen evolution; on the contrary, PWW5 showed a 30% reduction compared to PWW10 and BG-11. Significantly lower chlorophyll and carotenoid contents were found in the cultures grown in PWW compared to BG-11. Biochemical composition showed a slightly higher protein content in biomass grown in PWW10 (53.4%) compared to BG-11 (52.7%). The protein content further increased (61.3%) when the more concentrated PWW5 was used. The biomass, particularly that produced on PWW, revealed antimicrobial activity against plant pathogens, including fungi, and oomycete, while rather weak biostimulant activity was determined for the C. fusiforme biomass independently of the cultivation medium. Results demonstrated that C. fusiforme is a robust strain suitable to be grown in diluted PWW outdoors with a high nutrient removal rate.
Nowadays, the world is facing several challenges: population growth, depletion of fossil raw materials, climate change, and many associated environmental problems, such as drought affecting large areas of the planet. To this end the concept of circular economy has been introduced, that is, a production and consumption model which involves, among others, the recycling of waste products as thoroughly as possible, allowing an extension of their life cycle (Barros et al., 2020). One of the waste nutrient sources is piggery wastewater (PWW) which production in Europe accounts for hundreds of millions of cubic meters containing high concentrations of organic matter and nutrients (de Godos et al., 2009).
Microalgae are being increasingly recognized as a potential source of food and feed supplements and biomaterials. They have been considered good candidates for the production of biofuels, biodiesel, biogas, and biohydrogen (Chew et al., 2017; Singh and Das, 2018; Siebert and Torzillo, 2018; Touloupakis et al., 2021). Some microalgae strains can also produce compounds that can be used as biostimulants such as auxins and cytokinins, and biopesticides (Stirk et al., 2013; Chojnacka et al., 2015; Ranglová, 2018; Carneiro et al., 2021; Grivalský et al., 2022; Morillas-España et al., 2022). To expand the microalgal industry, there is a need to test new species suitable for the production of renewable biological resources and the conversion of these into high-value products, such as food, feed, bio-based products, and bioenergy to meet societal needs (Koller et al., 2014). Recently Chlamydopodium has been tested to produce proteins (Touloupakis et al., 2020) and for wastewater treatment to produce phytostimulants (Morillas-España et al., 2022).
Microalgal species, particularly Chlamydomonas, Scenedesmus, and Chlorella, have been investigated for piggery wastewater treatment (de Godos et al., 2009; Wang et al., 2010; Min et al., 2011; Kao et al., 2012; Wang et al., 2015; Ayre et al., 2017; Qi et al., 2017). Chlorella sp. can remove nitrogen, and phosphorus and reduce chemical oxygen demand in raw centrate (Li et al., 2011) while substantial removal of nitrogen, phosphorus, and inorganic carbon from piggery wastewater has been documented in Chlamydomonas mexicana (Abou-Shanab et al., 2013). The use of wastewater with high contents of nitrogen and phosphorus to cultivate microalgae can help reduce production costs, which is a mandatory condition to scale up the process at an industrial level. It has been calculated that nutrient supply in large-scale cultivation of microalgae accounts for 26% of variable costs (Norsker et al., 2011).
Cultivation of microalgae fits well within the circular economy since one of its possible utilizations is to recover nutrients (carbon, nitrogen, and phosphorus) from wastewaters to produce biomass, with lower costs and energy demands than those associated with conventional treatment processes. The process was proposed many years ago by Oswald and Golueke (1960). In recent years, many studies have demonstrated the feasibility of cultivating microalgae in various types of wastewaters for nutrient removal and biomass production (Li et al., 2011; Abou-Shanab et al., 2013; Shen, 2014; Ge and Champagne, 2016; Luo et al., 2016). Digestate from pig farms contains nutrients such as nitrogen and phosphorus, which can cause eutrophication in natural water bodies (Cai et al., 2013; Ayre et al., 2017) and can be utilized for microalgal cultivation instead (Wang et al., 2015; Ayre et al., 2017).
In this work, we compared the productivity and nutrient removal by Chlamydopodium fusiforme cultivated in a synthetic medium (BG-11) and diluted piggery wastewater (PWW) in fully controlled mini-ponds outdoors. To gain more insight into the physiology of the organism outdoors, we coupled photosynthesis and fluorescence analysis to growth measurements. Understanding the growth physiology of this organism is a prerequisite for its scale-up to an industrial level. A preliminary investigation of the bioactivity of biomass produced on both synthetic medium and PWW was also performed.
The freshwater microalga Chlamydopodium fusiforme (Chlorophyceae) (hereafter C. fusiforme) was obtained from Prof. Vince Ördög, Szechényi István University in Mosonmagyárovár, Hungary as strain designated MACC-430 (Figure 1). This microalga is fast-growing green strain that was selected due to its potential biostimulant and antimicrobial activities (Takács et al., 2019; Kumar et al., 2021). The inoculum was prepared in the laboratory using vertical glass columns (5-cm light path, 400mL working volume) in the mineral medium BG-11 (Rippka et al., 1979) under a continuous light intensity of 150μmol photons m-2 s-1 (provided by cool white lamps Dulux L, 55W/840, Osram, Italy), at 28°C and mixed by bubbling a mixture of air/CO2 (98:2v/v).
Figure 1 Light microscope photograph of C. fusiforme cells (By dr. Claudio Sili, CNR-IBE). Bar = 5μm.
The cultures of C. fusiforme that were tested in these trials using PWW as a source of nutrients, were cultivated outdoors in cylindrical bioreactors (mini-ponds) 14cm depth, with a 10-L working volume and an illuminated surface area of 0.07m2. The mini ponds were placed in a greenhouse to protect the culture from the rain (Figure 2). Cultures in BG-11 (control) were compared with cultures grown in 10-fold diluted (hereafter PWW10), 5-fold diluted PWW (hereafter PWW5), and undiluted PWW. Since only two fully controlled mini ponds were available, each comparison between BG-11 and PWW was carried out independently. Each experiment lasted for 2 weeks. A semi-continuous harvesting regimen was adopted, by daily removing 25% of the culture. In the experiment with undiluted PWW, the daily dilution rate was reduced to 10% to reduce the toxic effect of ammonia. A stainless steel coil connected to a cooling system was placed in the ponds to keep the maximum culture temperature within 28°C. Culture pH was maintained at optimal value of 7.5 by the automatic addition of CO2. Trials were carried out in summer under the climatic conditions of Sesto Fiorentino (Florence, Italy), latitude 43° 49’ 54 N longitude 11° 11′ 57″ E.
Figure 2 General view of the cultivation set-up used for the experiment with C. fusiforme grown in piggery wastewater and a mineral medium BG-11. In-situ Chlorophyll fluorescence measurements were recorded in two identical cylindrical bioreactors at 5-min intervals during the day (08:30-18:00h) using a modulated fluorimeter (Junior-PAM, Walz GmbH, Effeltrich, Germany) controlled by PC via USB interface.
The PWW used in these trials was taken from a private farm near Florence (Italy) and was obtained after anaerobic digestion in a biogas plant (digestate). The original piggery digestate was first centrifuged (drum speed) using a centrifuge separator (mod. SE05.1SMX-ALG-Q0P0, Seital, Vicenza, Italy) to remove most of the insoluble/coarse solid particles. The recovered supernatant was mixed, and then analyzed for major nutrient content and frozen to preserve its composition and prevent the increase of the bacterial load (Figure S1). The average composition of the piggery wastewater used in this study is summarized in Table 1. The chemical oxygen demand (COD) was 10,160mg L-1, TN of about 1000mg L-1 (mostly N-NH4+), TP of 224mg L-1, and pH 8.2. Solid particle concentration in the PWW was 226mg L-1.
The determination of biomass dry weight (DW) was performed in triplicate. Samples (10mL) were filtered using pre-weighed glass microfiber filters (Whatman GF/F, Maidstone, England), washed twice with deionized water, oven-dried at 105°C for 3h, and weighed after equilibration to room temperature (10min) in a desiccator. Lyophilized biomass used for analyses was obtained from frozen samples (−20°C) using a 5PASCAL LIO10P instrument (24h at 0.1mbar).
Pigments were extracted in 90% acetone in water and determined spectrophotometrically. Chlorophyll a (Chl a) and b (Chl b) concentrations were calculated according to Jeffrey and Humphrey (1975), while carotenoids were measured according to Parsons and Strickland (1963).
The concentration of individual carotenoids was assessed in 90% acetone extracts by Varian Multisolvent pump ProStar 210, coupled with a photodiode array detector Varian ProStar 335, using a column Luna, C8 (Phenomenex, USA), according to Van Heukelem and Thomas (2001). For the analysis, all reagents were HPLC grade, except the tetra-butyl ammonium acetate salt solution, pH 6.5, which was prepared with distilled water and sterilized by filtration. Eluent solutions were: solvent A, 80:20 (v/v) methanol, 28mM tetra-butyl ammonium acetate; solvent B, 100% methanol. For the elution, with a flow rate of 0.8 mL min-1, a linear gradient was used as follows: starting with 100% solvent A, 100% of solvent B was reached in 25 minutes, maintained for 2 minutes, then 100% of solvent A was reached in 3 minutes.
Total biomass carbohydrate content was assessed using the phenol-sulfuric acid method with D+ glucose as standard (Dubois et al., 1956). The carbon and nitrogen content of the biomass was measured using a CHNOS Analyzer (Flash EA, 1112 Series (Thermo Electron Corporation, USA). The nitrogen content was used to determine the crude protein content using 6.25 as the nitrogen-to-protein conversion factor (Benedict, 1987).
Culture samples were withdrawn from the mini ponds daily in the early morning (before and after dilution) and the evening. After centrifugation, the supernatant was analyzed for ammonium, nitrate and phosphate concentrations and COD using a C99 Multiparameter, Bench Photometer (Hanna Instruments Inc. RI, USA). All sample analyses were carried out in triplicate. Briefly, phosphorus was measured with the ascorbic acid method, ammonium using the Nessler reactive method while the nitrates were quantified with the cadmium reduction method according to the Standard Methods for the Examination of Water and Wastewater (1989). All the analytical reagents were provided by Hanna Instruments Inc. (USA). The nutrient removal efficiency (RE) was calculated as follows:
where:Nin = total nutrient concentration measured in the morning after diluting the culture with PWW
Nout = total nutrient concentration measured the following morning before culture dilution with PWW
Microbiological analysis was performed to quantify the microbial load in the cultures. Anaerobic bacteria were determined by plate count using Nutritive Agar. The samples were diluted to the 10-7 decimal scale and each dilution was spread in triplicate on sterile and disposable Petri dishes. Incubation was carried out over 48h at 30°C. Results were expressed as CFU mL-1.
The photon flux density (PFD) at the culture mini-pond surface was measured by a LI-190SB cosine quantum sensor connected to a LI-250A quantum radiometer/photometer (Li-Cor, Inc., Lincoln, USA). The daily global solar radiation values were obtained from the LaMMA Agrometeorological Station (http://www.lamma.rete.toscana.it, CNR-IBE, Florence, Italy).
For the measurement of light attenuation, different PWW dilutions were prepared using deionized water. The measurements were carried out in 5-cm vertical transparent Pyrex columns exposed to solar light, in the afternoon of a summer sunny day when the direct solar was perpendicular to the column surface and using a Li-Cor 250A quantum radiometer equipped with a LI-193 Spherical Underwater Quantum Sensor which was positioned in the center of the column at about half the height of the column (about 20cm from the horizontal plane).
Chlorophyll fluorescence measurements were carried out with a pulse amplitude-modulation fluorometer (PAM-2100, H. Walz, Effeltrich, Germany) operated using PamWin software (version 2.00f). The ratio between variable and maximum fluorescence, Fv/Fm, was used to determine the maximum photochemical quantum yield of photosystem II (PSII); it indicates the capacity of the system to absorb light through the reaction centers and the light-harvesting complex and expresses the maximum quantum efficiency of primary photochemistry (Strasser et al., 2004). For this purpose, culture samples were incubated in the dark for 15min to relax any energy-dependent quenching. In addition, just before sending a flash for the Fm determination, the sample was illuminated with a far-red light pulse for 10s (above 700nm, 10W m−2) to fully oxidize the plastoquinone pool (PQ).
Rapid light-response curves (LRCs) of diluted, dark-adapted (0.2 to 0.3g DW L−1; 5-10min) microalgal samples taken from the cultures were measured off-situ in a light-protected measuring chamber with mixing (3mL glass cuvette, light path of 10mm) using a pulse-amplitude-modulation fluorimeter (PAM-2500, H. Walz, Germany). The minimum and maximum fluorescence levels (F0, Fm) were determined using a weak modulated light (<0.15µmol photons m–2 s–1, frequency of 0.5–1kHz) in the dark-adapted samples (actinic irradiance = 0; first step of LRC).
In-situ chlorophyll fluorescence measurements were recorded at 5-min intervals during the day (08:00-18:00h) using a modulated fluorimeter (Junior-PAM, Walz GmbH, Effeltrich, Germany) controlled by a PC via USB interface. A plastic fibreoptics (150cm, 1.5mm diameter) and a spherical PAR sensor (US-SQS, Walz GmbH, Effeltrich, Germany) were submerged – side by side – directly into the microalgae culture at a depth of 10mm to record fluorescence and irradiance simultaneously. Blue light-emitting diodes (LED, 460nm) were used to apply the saturating pulses, measuring and actinic lights. WinControl-3 software was used for data acquisition and recording. Using the measurement setup detailed above, the incident photosynthetically active irradiance (EPAR) and the actual quantum yield in the light, ΔF/Fm′ [=(Fm′−F′)/Fm′] where F′ is the steady state fluorescence in the light and Fm′ is the maximum fluorescence after the application of the saturation pulse measured. The relative electron transport rate through PSII (rETR; r.u.) was calculated as rETR (= ΔF/Fm′ × EPAR).
In principle, non-photochemical quenching (NPQ) [= (Fm – Fm’)/Fm’ = Y(NPQ)/Y(NO)] is a measure of futile (not used for photochemistry) energy dissipation as heat. It is inversely related to photochemistry (ΔF/Fm′) and is considered a safety valve protecting PSII reaction centers from damage by excess irradiance.
Fast chlorophyll fluorescence induction kinetics (OJIP curves) were measured off-situ by a portable fluorimeter (AquaPen AP-100, P.S.I. Ltd. Brno, Czech Republic). The chlorophyll fluorescence induction kinetic (OJIP test) was recorded in diluted (0.2 to 0.3g DW L−1) samples, dark-adapted for 5-10min. Samples were transferred to a 3 mL measuring cuvette (light path of 10mm) which was placed in a light-protected holder of the fluorimeter in front of the detector (adjustable measuring light pulses, ~2.5µs) while red LEDs served as high-intensity actinic light from both sides of the cuvette (up to 3,000µmol photons m-2 s-1), perpendicular to the detector. The OJIP curve was measured in the time range between 50µs to 1s when the signal rises rapidly from the origin (O) to the highest peak (P) via two infections – J and I (Strasser et al., 1995). The O point (50µs) of the fluorescence induction curve represents a minimum value (designated as constant fluorescence yield F0) when PQ electron acceptors (QA and QB) of the PSII complex are oxidized. The inflexion J occurs after ~2-3ms of illumination and reflects the dynamic equilibrium (quasi-steady-state) between QA and QA-. The J–I phase (at 30-50ms) is due to the closure of the remaining centers, and the I–P (ends at about 300-500ms) corresponds to a full reduction of the plastoquinone pool (equivalent to the maximum fluorescence level Fm) (Strasser et al., 2004; Goltsev et al., 2016). From the fluorescence levels at the J and I points, the variables Vj and Vi were calculated as follows: Vj = (F2ms-F0)/(Fm-F0) and Vi = (F30ms-F0)/(Fm-F0).
Oxygen evolution measurements were carried out in triplicate on 2mL culture samples (chlorophyll content 4mg L-1), using a Liquid-Phase Oxygen Electrode Chamber (3, Hansatech Instruments, UK) at 28°C and equipped with an oxygen control electrode unit (Oxy-lab+, Hansatech Instruments, UK). The light was supplied via a red LED light source (LH36/2R, Hansatech Instruments, UK) at 637nm wavelength providing 600μmol photons m-2 s-1 PFD. The dissolved O2 concentration in the sample was continuously monitored at an acquisition rate of 0.2reads s-1. Dark respiration rates were measured after the photosynthesis rates had been measured.
The bioactivity of freeze-dried C. fusiforme biomass was determined according to the procedures described previously (Suárez-Estrella et al., 2014; Carneiro et al., 2021; Ranglová et al., 2021). The antimicrobial activity of freeze-dried C. fusiforme biomass was evaluated by antagonism bioassay. The activity of all extracts was tested against two fungi – Fusarium oxysporum f.sp. melonis and Rhizoctonia solani (further abbreviated as F. oxysporum and R. solani), and one oomycete – Phytophthora capsici (further abbreviated as P. capsici). All strains were provided by the Spanish Type Culture Collection (CECT) at the Science Park of the University of Valencia. The growth inhibition of individual phytopathogens was calculated. The biostimulant activity was detected by seed germination bioassay on cress seeds (Lepidium sativum) using water extracts at two different concentrations 0.5 and 2.0mg DW mL-1, respectively. The percentage of seed germination was calculated while distilled water was used as the control.
Descriptive statistics (means, standard deviation) of measured parameters were calculated using GraphPad Prism software (version 5.1 and 9.3 for Windows, GraphPad Software, La Jolla, California, USA). Three replicates were employed for every treatment and/or condition. One-way ANOVA was applied to detect a statistical difference between conditions (BG-11; PWW10; PWW5). The Tukey HDS (Honestly Significant Difference) posthoc test was used to compare means when ANOVA results were statistically significant (P < 0.05). ANOVA analyses were performed using a calculator prepared by CC 2016 Navendu Vasavada (http://astatsa.com/OneWay-Anova) with TukeyHSD/).
The dark brown color of PWW and its high content of ammonia (≈1,000mg L-1) hampered its direct usability in phototrophic cultivation. Therefore, PWW was diluted 5 and 10-folds with deionized water and tested the feasibility of the growth and photosynthesis performance of C. fusiforme in comparison with BG-11 medium which was used as a control. Figure 3 shows the relationship between the PWW dilution factor and light attenuation. To achieve a light transmission of 50% it was necessary to dilute the PWW almost 10 times (PWW10). Cultures grown in PWW5 received one-fourth (400µmol photons m-2 s-1) of the incident light intensity at noon time in summer, resulting in less light available for the algal cells especially in the morning and in the afternoon hours, which may have limited the growth (Figure S2). In Figure S3, the daily changes in biomass concentrations are shown in the C. fusiforme cultures grown with different dilutions of PWW and BG-11. As can be seen, a quasi-steady state was reached after about 5 days in all the cultures.
The mean values of gross and net areal and volumetric productivity obtained during two weeks in BG-11 medium and 10 and 5-fold diluted PWW are presented in Figure 4. To facilitate the comparison of the night losses recorded in the different trials, data are also summarized in Table 2. The culture grown in PWW10 attained 30% higher gross yields compared to the control (BG-11), however, when net productivities are compared, the differences in productivity are not significant. This is explained by the higher night biomass loss recorded in the culture grown in PWW10 (Table 2). Compared to both BG-11 and PWW10, significantly higher productivities were obtained using the more concentrated PWW5. The net daily biomass yields were 14.7g m-2 d-1 in BG11, 17.6g m-2 d-1 in PWW10 and 33.1g m-2 d-1 in PWW5 (Table 2). The higher productivities obtained using PWW5 (5-fold diluted) could be related to the larger amount of nutrients available to this culture. The total viable count of associated bacteria (aerobic microbiota) in the culture grown in PWW was slightly higher compared to BG-11 culture (on average 650×106 and 4 ×106CFU mL-1, respectively) and not likely to affect the filtration procedure used to assess growth and productivity.
Figure 4 Box-plot and results of the Tukey HDS post-hoc test showing the difference in gross and net areal productivity obtained by C. fusiforme grown outdoors in BG-11 medium and in differently diluted PWW. The boundaries of the rectangles indicate the 25th and 75th percentiles, the horizontal bars indicate the median and the x symbol is the mean value. The vertical bars indicate upper and lower distribution limits. Boxplots sharing common letters (a–c; A–C) are not significantly different (p < 0.05). Solar irradiance measured on the horizontal surface was also reported for each cultivation period.
Table 2 Gross and net volumetric and areal productivity of C. fusiforme cultivated outdoors in BG-11 and PWW.
Experiments were also carried out with undiluted PWW, which culminated with the loss of the cultures within a few days. Moreover, bacterial load in undiluted PWW was very high which hampered the separation of the biomass from the culture medium. In undiluted PWW, cells of C. fusiforme formed aggregates with bacteria resulting in the flocculation of the culture (Figure S4). Compared to the culture grown in PWW5, that cultivated in undiluted PWW did not reach steady state conditions. This culture started with very high amounts of ammonia, phosphate, and COD and since it was subjected to a daily dilution rate of 25%, they increased by 230, 56, and 2500mg L-1 each day, respectively, which resulted in toxicity to the cells. To reduce the toxic ammonia effect of the undiluted PWW addition the daily dilution rate was reduced from 25% to 10%. However, even in this case, growth evaluation by DW increase was not feasible, and even though a chlorophyll productivity of about 3.5 mg L-1 d-1 (data not shown) was attained in both undiluted PWW cultures (25% and 10% dilution rate), steady state of the cultures was never reached, and over time, contamination (by bacteria, fungi, and protozoa) became increasingly higher limiting culture growth, especially when the dilution rate of 25% was adopted.
Analyses of major nutrients (N-NH4+, N-NO3-, P-PO43-, and COD) were performed every day in cultures grown in diluted PWW, in the morning before and after dilution, and at sunset. The N-NH4+, P-PO43-, and COD supplied daily through culture dilution were 23, 5.6, and 254mg L-1 using PWW10, hence they were two times greater with PWW5. Nutrient removal rates (RR) in the cultures grown in PWW10 and PWW5 are reported in Table 3. N-NH4+ removal in cultures grown on PWW10 and PWW5 was on average 93% and 83%, respectively. The removal capacity of COD was 41% using PWW10 and increased to 73% in PWW5. Since no growth of C. fusiforme occurred at night, it is reasonable to conclude that the COD decrease was due to bacterial growth.
The trend of nitrogen concentration over time in the semi-continuous cultures of C. fusiforme grown in PWW10 and PWW5 is shown in Figure S5. In the morning, after the culture dilution with PWW10, the N-NH4+ reached its maximum value of about 40mg L-1 which dropped to about 20mg L-1 at the end of the day as a result of microalgal growth. In the culture on PWW5, N-NH4+ showed a similar trend but with higher values, between 100 and 70mg L-1 in the morning and evening, respectively (Figure S5). However, in the experiments, the trend of the concentration of N-NH4+ both in the morning and evening was decreasing. In Table 4 the conversion of N-NH4+ from the mini-pond cultures into biomass is also reported. In general, around 50% of the total N-NH4+ removed daily was fixed in the biomass, the remaining being lost by outgassing or by bacterial uptake. Nitrogen starvation can be excluded in the cultures on PWW as ammoniacal N was always present at the end of the light period and also at the end of the dark period, although in a lower concentration (Figure S5). P-PO43- was in excess and could sustain even higher growth than that achieved. The amount of iron supplied with PWW was indeed significantly lower compared with the BG-11 medium, and this could account for the decrease in pigment content observed (see below).
The average value of chlorophyll content in C. fusiforme grown in BG-11 medium was 3.8 ± 0.4% of DW and decreased significantly to 2.3 ± 0.4% and 2.8 ± 0.2% in the cultures grown in PWW10 and PWW5, respectively. Despite the lower chlorophyll content, the culture in PWW5 exhibited the highest rate of chlorophyll increase (7mg L-1 d-1) because of the significantly greater biomass yield, while in undiluted PWW cultures a progressive decline of the chlorophyll content was observed (Figure 5, insert). A similar trend was observed in total carotenoid content, with a 36% reduction in the culture both in PWW10 and PWW5, compared to the BG-11 culture (from 0.87 ± 0.17% of DW in BG-11 to about 0.56 ± 0.17% of DW in PWW) (Figure 5). However, the ratio between carotenoids and chlorophyll a was approximately constant in all the cultures (0.29 in BG-11 and 0.29-0.31 in PWW), despite a slightly, but significantly higher value observed in the culture on PWW10 as compared to the BG-11 culture.
Figure 5 Pigment content (total Chl and total Carotenoids) of C. fusiforme grown outdoors in BG-11, 10-fold diluted PWW (PWW10), 5-fold diluted PWW (PWW5) and undiluted PWW. The ratio between total carotenoid and Chla is also reported. Data are means ± SD (n =10) except for undiluted PWW. Bars designated by the same letter are not significantly different (p < 0.05). Insert graph shows the progressive decline of total Chlorophyll content in culture cultivated on undiluted PWW.
A comparison of carotenoid composition between cultures grown in PWW10 and BG-11 is reported in Table S1. In all cultures, a low induction of the xanthophyll cycle pigments was observed. Zeaxanthin could not be detected either in BG-11 or in PWW10. However, some differences were found in the antheraxanthin content. Overall, during the daylight period, the cultures in BG-11 exhibited a more consistent increase of antheraxanthin, compared to those grown in PWW10 (81% and 24%, respectively) and this trend was confirmed at the end of the experiment, when in BG-11 this carotenoid was more than doubled, while in PWW10 the cultures saw an increase by 23%. The violaxanthin content did not show strong changes during the experiment period, except for a decrease of 12% recorded in cultures grown in PWW10 at the end of the trial. These changes were reflected in the xanthophyll pool content with the highest increase of 20% and the values of the de-epoxidation state (DES), with a maximum increase of 50%, both of which were observed in the cultures grown on BG-11 (Table S1). Concerning the other carotenoids, β-carotene content followed the same trend in all cultures, lutein decreased in all cultures, and the highest decline of 17% was found in cultures grown on PWW10, at the start (Table S1). For neoxanthin and loraxanthin, the most evident differences were detected on August 1st in the culture grown in PWW10, with a 28% and 11% increase respectively, and in the culture on BG-11 that saw a decline of 33% of loraxanthin.
Biochemical composition showed a higher protein content in the biomass grown in PWW compared to BG-11 (53%). N content and protein content further increased (up to 61.2%) when the more concentrated PWW5 was used (Table 5).
Table 5 Elemental composition, total protein, and carbohydrate contents of C. fusiforme biomass produced outdoors in BG-11 and PWW.
Changes in relative electron transport rate (rETR) were recorded in-situ/online in the cultures during the day using a portable fluorimeter (Figure 6). The results showed that the values of rETR were generally higher (42 ± 8.9) in the cultures grown in PWW10 as compared to the BG-11-grown cultures (28 ± 4.4). These data suggest a better photosynthetic ability to use absorbed light energy, correlated with biomass productivity (Table 2).
Figure 6 Diurnal changes of relative electron transport rate (rETR) measured in situ in C. fusiforme cultures in outdoor reactors on Days 1, 3, 5, 7, and 8. (A) Culture grown in BG-11 medium; (B) Culture grown in 10 times diluted pig manure PWW10.
Measurements of maximum quantum yield of PSII photochemistry (Fv/Fm), photosynthetic oxygen evolution, and respiration rate were performed on culture samples taken at certain time intervals during the day (8:00 and 18:00). In Figure 7A, the effect of daylight on the Fv/Fm ratio of the different cultures is shown. In all cultures, the maximum quantum yield of PSII photochemistry (Fv/Fm) decreased during the daylight hours and was restored during the night. The Fv/Fm ratio of the PWW10 culture was higher than that of the control culture (BG-11) (Figure 7A). Moreover, in BG-11 cultures both the declines in the Fv/Fm ratio recorded during the day and the following recovery phases at the night, were larger than that recorded in PWW cultures. However, after 4 days (BG-11) and 7 days of cultivation in PWW, the Fv/Fm stabilized to about 0.72 and 0.78, respectively. In the culture grown in PWW5, Fv/Fm remained more constant (average value 0.7) with lower variations between morning and evening (data not shown),
Figure 7 Changes in the maximum quantum yield of PSII photochemistry (Fv/Fm) (A) and rate of photosynthetic oxygen evolution (B) of C. fusiforme cultures grown outdoors in BG-11 medium and PWW10 (10-fold dilution), with light (white bars) and dark (grey bars) periods evidenced.
Net photosynthetic oxygen evolution measurements revealed no significant difference between PWW10 and the control (BG-11) (Figure 7B); on the contrary, PWW5 showed a 30% lower oxygen evolution than both PWW10 and the control (data not shown). Respiration rates were up to 3 times higher in PWW10 compared to BG-11 (Figure 8). However, when gross photosynthesis was compared, that is, adding respiration to net photosynthesis rates, then the cultures in PWW10 resulted in higher activity than those grown in BG-11. The contribution of bacteria to respiration rate in PWW still cannot be ruled out
Figure 8 Changes in the respiration rate of C. fusiforme cultures grown outdoors in BG-11 medium and PWW10 (10-fold dilution), with light (white bars) and dark (grey bars) periods evidence.
Rapid light-response curves were measured every day off situ using samples taken from outdoor reactors at certain day periods. As an example, LRCs measured at 14:00h (highest electron transport activity due to the peak irradiance) in the control and the culture grown in PWW10 were compared (Figure 9). It is shown that the maximum rETR was higher in the PWW cultures values as compared to those grown in BG-11.
Figure 9 Changes in the maximum electron transport rate rETRmax (calculated from RLCs) in C. fusiforme were measured on Days 1, 3, 5, 7, and 8 in the samples taken from outdoor reactors. (A) Cultures grown in BG-11 media and (B) Cultures grown in PWW(10-fold diluted).
Fast fluorescence induction kinetics, also called OJIP curves, indicating electron transport steps through the PSII complex show the two-inflection points J and I. These are represented by the Vj and Vi variables which indicate the redox status of the acceptor side of the PSII complex (down-regulation/overreduction of photosynthetic electron transport). If increased, Vj and Vi reflect a slow-down of electron transport in the PSII complex due to the overreduction of the plastoquinone pool. The trends of the Vj and Vi variables measured at 14:00h indicated that the electron transport rate in the BG-11 was slightly lower during the last three days of the trial as compared to that in the PWW10 culture (Figure 10). These days the values of Vj in BG-11 media were 13% higher and, in the case of Vi variables, almost 18% higher in the control compared with PWW.
Figure 10 Changes in the (A) Vj and (B) Vi variables (calculated from the curves of fast fluorescence induction kinetics) in the samples of C. fusiforme cultures grown in BG-11 (A) and in 10-fold diluted PWW (PWW10) (B). Measurements were performed at 14:00h during a one-week outdoor trial.
Results of the dual culture bioassay are summarized in Table S2. In general, higher activity against fungi and the oomycete P.capsici was determined for the C. fusiforme biomass grown in PWW compared to the BG-11 medium. The highest antifungal activity of 42.7% was found when biomass was grown in the ten-times diluted piggery wastewater (PWW10). The weak biostimulant activity of 101.0%, determined by seed germination, was found only in the biomass obtained from the cultivation in PWW10 (Table S3). Practically, rather weak biostimulant activity was determined for the C. fusiforme biomass independently of the cultivation medium.
The growth of the C. fusiforme cultures outdoors using diluted PWW as a sole source of nutrients was compared with the mineral medium BG-11. To prevent excessive rise of pH resulting in growth reduction and excessive release of ammonia in the atmosphere, culture pH was controlled by automatic addition of CO2 (Silva-Benavides and Torzillo, 2012; Sutherland et al., 2015). Indeed, the concern with the increasing amounts of greenhouse gases in the atmosphere may hamper ammonia stripping achieved mainly by increased pH to maintain microalgal photosynthesis, as a way of reducing its content in the residual water. In the environment, ammonia is converted into N2O, which, over 100 years, has 298 times more impact per unit weight compared to CO2. For these reasons, considerable recycling of N in the biomass is desirable (Park et al., 2010) and the additional cost of supplying CO2 needs to be compensated by a higher biomass yield which could lead to an increase of extracted valuable products for agricultural use such as biofertilisers and biostimulants (Morillas-España et al., 2022).
The nitrogen (N-NH4+) concentration in the original digestate (>1,000mg L-1) exceeded that measured in BG-11 medium (250mg L−1 in the form of N-NO3-.) Regarding phosphorus (P-PO43-), the BG-11 medium contains 7mg L−1, significantly less than the 224mg L−1 measured in the PWW digestate. It also contained organic matter, with a COD of about 10,000mg L−1. Important to note that the digestate can contain lower concentrations of relevant compounds such as sulfate, potassium, calcium, magnesium, manganese, zinc, and copper, all of which are necessary for the development of microalgal cells (Morales et al., 2015). Due to the high dilution of the digestate, the nutrients for microalgal growth decreased significantly, with N still at values of 100 and 200mg L−1, i.e., close to the values which have been reported as toxic for microalgae (Collos and Harrison, 2014) and with some other micro- and trace elements in limiting amount (Table S4).
The composition of the piggery digestate effluent used in this study was similar to that reported by Li et al. (2019) and Sánchez-Zurano et al. (2021). The N/P ratio for the culture media varied between 3 and 30. Using the BG-11 medium in these trials, the N/P ratio was around 35, whereas in the digestate the value was 4.3 (considering N-NH4+/P-PO43-). According to these data, PWW digestate is rich in phosphorous (concerning that requested by the nitrogen content). The N/P ratio in microalgal biomass ranges from 4/1 to nearly 40/1, and a range of approximately 6.8–10 has been considered an optimal value for freshwater microalgae growth and high nutrient removal efficiency (Li et al., 2019). The significantly higher productivity attained by diluted PWW as compared to BG-11 can be related to the better N/P ratio.
Changes in the Fv/Fm ratio of both the PWW10 and BG-11 cultures showed logical cycling due to irradiance intensity, i.e., it decreased during the day and recovered at night (Figure 7A). The amplitude of the night/day oscillations in Fv/Fm were higher in the BG-11 cultures indicating greater light stress compared to the cultures grown in PWW10. The better performance of the latter cultures was illustrated by the fluorescence measurement performed in-situ. These measurements were carried out online during the daylight period so that they give a clear picture of the physiological status of the cultures. A similar trend was also observed with the daily measurements of the rapid light-response curves (RLC) using samples taken from the outdoor ponds at certain day periods. The maximum rETR estimated from the measurements of RLC confirmed the better performance of cultures of C. fusiforme grown in PWW10 (Figure 9). A similar behavior was also recorded with cultures grown with PWW5 (results not shown). Analysis of VJ and Vi calculated from the OJIP curves also indicated higher constraints experienced by cultures grown in BG-11. Indeed, the Vi and Vj variables were lower in the PWW cultures meaning that the PQ acceptors were less reduced and electrons flow was not slowed down in the PSII complex (Figure 10). As expected, the better performance of the cultures shown by chlorophyll fluorescence measurements was confirmed by the higher productivity of the cultures grown in PWW. Most likely, the higher light availability in the BG-11 grown cells, due to the higher medium transparency as compared to PWW, may cause downregulation of photosynthesis due to excess light. Another positive effect of PWW can be the presence of bacterial populations which may produce metabolites stimulating the growth of microalgae (Quijano et al., 2017; Sánchez Zurano et al., 2020).
The chlorophyll content was unexpectedly lower in the cultures grown in PWW and particularly in those grown with PWW10. Indeed, the higher biomass concentration in these cultures and the lower transparency of the culture medium should have enhanced the photolimitation and thus promoted the accumulation of chlorophyll. However, since the availability of nitrogen was decreasing from BG-11 to PWW10 it is conceivable that lower nitrogen content may have affected the chlorophyll synthesis by cells (Ferreira et al., 2015). Indeed, the nitrogen content in chlorophyll is relevant reaching 6.26%. The chlorophyll a/b ratio which is frequently used as an index of photoacclimation of cells to the light usually increases with irradiance (Givinish, 1988). In these trials, it resulted in a slightly higher value in the PWW cultures, particularly in the one grown with PWW5, probably as a result reduction in light-harvesting complex accessory pigments in wastewater (Chuka-ogwude et al., 2020).
The results of carotenoid composition indicated that PWW cultures were less effective in inducing antioxidant carotenoid synthesis. This is mainly represented by a lower induction of the xanthophyll cycle in these cultures, compared to the ones grown on BG-11. In addition to the highest antheraxanthin content, associated with the highest de-epoxidation state (DES), the lowest lutein content could indicate that the reductive pressure on the photosynthetic apparatus, was lower in the PWW cultures than in the BG-11 ones. These findings are in line with what was observed in the cultures grown in PWW, which exhibited values of VJ lower than the ones found on BG-11, as already discussed in paragraph 4.1. In particular, the VJ parameter reflects the level of reduction of the plastoquinone electron acceptors, and its increase may reveal the occurrence of oxidative stress activating defensive strategies to counteract the effect of ROS generation (Carol and Kuntz, 2001; Xi et al., 2021). In photosynthetic microorganisms, carotenoid synthesis is one of the main processes involved in these defense mechanisms, and it may imply the increase of both specific antioxidant carotenoids and total carotenoids content (Masojidek et al., 1999; Masojidek et al., 2004; Shi et al., 2020). Although significant photo-oxidative stress could not be detected in the cultures, these grown in PWW showed a better photosynthetic performance than the ones grown on BG-11, revealing the higher rETR. Hence, low reductive pressure on the photosynthetic apparatus may explain the lowest carotenoid content in these cultures, both in terms of the total amount and specific antioxidant carotenoids.
The turbid nature of PWW and the high ammonia content (>1,000mg L-1) are two limiting factors to its usability, especially for growing phototrophic organisms (Sánchez-Zurano et al., 2021). In PWW5 the color strongly reduced (by 75%) the penetration of light into the cultures. While this strong reduction in light may result beneficial in the middle of the day when light intensity may cause photoinhibition, it may be limiting the growth in the morning and afternoon hours (Torzillo and Vonshak, 2013). Nevertheless, cultures grown in PWW5 were more productive than those grown in PWW10. Very likely, cultures grown in PWW10 were nutrient-limited, which prevented full exploitation of light. On the other hand, it is conceivable that cultures grown in PWW5 could have further increased their productivity if the light limitation due to digestate turbidity was less severe. Therefore, dilution of the PWW, while improving light penetration, also reduces the amount of nutrients for growth thus hampering culture productivity. In microalgae cultivation, one has to be aware of two important constraints: ammonia toxicity, and dark color. Surprisingly, the cultures grown in PWW performed much better than those grown in the synthetic medium, indicating that PWW successfully meets all the nutrient requirements for the growth of C. fusiforme. We may speculate that PWW contains compounds and microorganisms which can stimulate growth (Kang et al., 2021; Grivalsky et al., 2022).
Using secondary-treated urban wastewater supplemented with centrate, three strains of Chlorella and one of Chlamydopodium fusiforme were cultivated at the small-scale level in a laboratory to evaluate their biostimulant properties. The highest biomass productivity was found for C. fusiforme, with a value of 0.38g L-1 d-1 (Morillas-España et al., 2022). De Godos and co-workers evaluated the performance of the indigenous Chlorella vulgaris strain in two 464-L outdoor raceway ponds (HRAPs) using 20- and 10-fold diluted piggery manure under Meditteranean climatic conditions in Spain (de Godos et al., 2009). Both HRAPs showed average COD and TKN removal efficiency of 76% and 88%, respectively, and biomass productivity ranging from 21 to 28g m-2 d-1.
The use of undiluted digestate would be desirable, particularly in dry regions due to the scarce availability of water, a problem which has become dramatic recently as a result of global warming, with increasing drought periods in large areas of the Earth. Unfortunately, the culturing of microalgae in pure digestate is strongly limited by an excess of ammonia nitrogen, which can be only marginally alleviated by the pH control (Ayre et al., 2017). At high pH, above 9, ammonia is the dominant chemical species and toxicity is mainly caused by its diffusion through the cell membrane resulting in detrimental ammonia accumulation in the cells (Boussiba and Gibson, 1991). Ammonia toxicity causes damage to the manganese (Mn) cluster of the oxygen-evolving complex of the PSII complex, causing considerable sensitivity of PSII to photodamage (Drath et al., 2008).
An interesting strategy based on the use of PWW diluted with seawater allowed the cultivation of the marine N. gaditana in thin-layer cascade reactors operated in semi-continuous mode at two dilution rates of 0.30 and 0.40d-1 (Jiménez Veuthey et al., 2022). The biomass productivity ranged between 11 and 16g m-2 d-1 in autumn and summer, respectively. Moreover, the use of seawater to dilute digestate (and save freshwater) can provide some important micro- and trace elements for growth. However, this strategy is limited to the growth of marine species, while freshwater strains may require preliminary bioprospecting and testing of strains under higher salinity (Chuka-Ogwude et al., 2020).
Piggery slurry was also used as the sole nutrient source to produce biomass of Scenedesmus almeriensis using laboratory-scale bubble column photobioreactors. The optimal dilution of the slurry to enable the processing of the largest amount of piggery slurry per liter was 1:20 which resulted in biomass productivity of 0.68g L-1 d-1, comparable to the standard growth medium (Ciardi et al., 2022).
The nutrient removal rates of C. fusiforme grown outdoors in the bioreactors were comparable with most of the data reported in the literature both indoors and outdoors. For example, with N. gaditana, nitrogen removal from the piggery slurry was total (Jiménez Veuthey et al., 2022). In contrast, when using fertilizers as the nutrient source, the nitrogen was in the form of N-NO3-, and the removal capacity was 63-73%. It is conceivable that the better removal capacity found in cultures grown with digestate was increased by ammonia outgassing. A similar trend for ammonium removal was published by Sánchez-Zurano et al. (2020). The authors found that using a thin-layer cascade reactor, Scenedesmus sp. can entirely uptake the N-NH4+ present in municipal wastewater. The N-NH4+ consumption ranged from 0.75 to 1.53g m-2 d-1) (a depuration efficiency greater than 90%) whereas the nitrate consumption was lower than the ammonium consumption, ranging from 0 to 0.11g m-2 d-1. Phosphorus removal, on the other hand, showed an opposite pattern to that observed for nitrogen (Sánchez-Zurano et al., 2020)
Several laboratory studies carried out under artificial light have focused on the use of microalgae for nitrogen and phosphorus removal from the food industry and agro-industrial wastewater (Esteves et al., 2021). In particular, on piggery manure (anaerobically digested) grown Chlorella vulgaris showed an N-NH4+ and phosphorus RE of 84% and 22%, respectively; on piggery wastewaters (anaerobically digested), Chlorella sorokiniana and activated sludge native bacteria obtained a nitrogen RE and phosphorus RE of 94-100% and 70-90%, and Chlamydomonas mexicana was capable to remove 60-65% of both N and P; on the agro-industrial mixture (anaerobically digested), Parachlorella kessleri removed more than 98% of N and 80-90% of P. Finally, Scenedesmus obliquus (FACHB-31) cultivation in diluted the liquid anaerobic digestate of piggery slurry was investigated by Xu et al. (2015). Total nitrogen and total phosphorous were removed in amounts of 58–75% and 70–89%, respectively. Chlorella zofingiensis cultivated on piggery manure digestate resulted in total nitrogen removal efficiency of 69–83% (Zhao et al., 2013).
Concerning evaluation of antimicrobial activity of C. fusiforme biomass, an antifungal activity was observed mainly when cultures were grown in diluted piggery wastewater (PWW5 and PWW10), while no activity against fungi, such as R. solani and F. oxysporum, was determined when biomass was grown in the BG-11 medium. This interesting behaviour may be explained by the fact that PWW may stimulate synthesis of antimicrobial substances by microalgae to cope with microbial contaminants usually found in wastewater. Microalgae, can produce among the others, unsaturated lattones, glycosides, sulphur containing compouds, phenols ans phenol glycosides, saponins and phytoalexin, fatty acdis, acrylic acid, terpenes and phenols showing antibacterial and antifungal activity (Skulberg, 2007). A similar antifungal activity of C. fusiforme biomass against the oomycete P. capsici was determined for C. vulgaris (Ranglova et al., 2021) and Tetradesmus. acutus biomass (Grivalský et al., 2022), respectively, when centrate from municipal wastewater treatment plant was used for cultivation Finally, a rather weak biostimulant activity was determined for the C. fusiforme biomass independently of the cultivation medium.
The present study demonstrates that the microalga C. fusiforme is a robust organims suitable to be cultivated in diluted piggery manure digestate. Its productivity significantly surpassed that attainable with synthetic medium BG-11 rich in nutrients, particularly nitrate, and specifically studied for the optimal growth of algae. Antifungal activity resulted influenced by the culture medium used for cultivation of C. fusiforme. Indeed, out of 3 species utilized for biopesticide tests, two resulted in negatives for biomass grown in BG-11. This fact together to the better growth attained in PWW indicate that PWW performs much better than synthetic medium and thus it is an optimal substrate to be used in microalgal biotechnology. The comparison made in fully controlled mini-ponds, outdoors, allowed us to get more accurate information on the performance of C. fusiforme grown on piggery digestate. Biomass from these experiments was found to be richer in proteins. These results are relevant for the scale-up of this species at an industrial level utilizing wastewater, thus widening the spectrum of microalgae potentially suitable to be used for both nutrient recycling and the production of bioactive substances.
The raw data supporting the conclusions of this article will be made available by the authors, without undue reservation.
GZ, Conceptualization, methodology, investigation, result summary, draft writing.; AMSB design of experiments, investigation, result summary; TS, Experimental work: KR, Conceptualization, methodology, investigation; JM, fund acquisition, conceptualization, writing and editing., CF, HPLC analysis, writing., BC, Experimental work, and English editing. ET, Investigation, data summary, writing, and editing. GT, fund acquisition, conceptualization, draft writing, and revising. All authors contributed to the article and approved the submitted version.
The work has been supported by the H2020 project “SABANA” funded by the European Union’s Horizon 2020 research and innovation program under grant agreement No 727874.
We thank Mr A. Dodero for his technical assistance with the CHNOS elemental analysis and dr. Francisca Suárez Estrella for bioactivity assays.
The authors declare that the research was conducted in the absence of any commercial or financial relationships that could be construed as a potential conflict of interest.
All claims expressed in this article are solely those of the authors and do not necessarily represent those of their affiliated organizations, or those of the publisher, the editors and the reviewers. Any product that may be evaluated in this article, or claim that may be made by its manufacturer, is not guaranteed or endorsed by the publisher.
The Supplementary Material for this article can be found onlineat: https://www.frontiersin.org/articles/10.3389/fmars.2022.1043123/full#supplementary-material
Figure S1 | Piggery wastewater pre-treatment (top) and the 10L mini-pond with C. fusiforme MACC-430 culture (bottom).
Figure S2 | Light attenuation measured in different PWW dilutions. Circle = Ambient PFD; diamond, PWW10, triangles, PWW5. The light attenuations with medium prepared with PWW10 and PWW5 were deducted from data presented in Figure 3. Time of the day refers to summer Central European Standard Time (CEST).
Figure S3 | Growth curve of C. fusiforme MACC-430 during the experimental periods. Growth is expressed as dry biomass concentration; vertical grey bars indicate the dark period.
Figure S4 | Macroscopic and microscopic evidence of bacterial load in undiluted PWW culture.
Figure S5 | N-NH4+ concentration (mg L-1) of C. fusiforme MACC-430 cultures grown outdoors in PWW10 (black symbol) and PWW5 (red symbol) during the experimental periods. Vertical grey bars indicate the dark period.
Abou-Shanab R. A. I., Ji M. K., Kim H. C., Paeng K. J., Jeon B. H. (2013). Microalgal species growing on piggery wastewater as a valuable candidate for nutrient removal and biodiesel production. J. Environ. Manage. 115, 257–264. doi: 10.1016/j.jenvman.2012.11.022
Ayre J. M., Moheimani N. R., Borowitzka M. A. (2017). Growth of microalgae on undiluted anaerobic digestate of piggery effluent with high ammonium concentrations. Algal Res. 24, 218–226. doi: 10.1016/j.algal.2017.03.023
Barros M. V., Salvador R., de Francisco A. C., Piekarski C. M. (2020). Mapping of research lines on circular economy practices in agriculture: From waste to energy. Renew. Sustain. Energy Rev. 131, 109958. doi: 10.1016/j.rser.2020.109958
Benedict R. (1987). Determination of nitrogen and protein content of meat and meat products. J. Assoc. Off. Anal. Chem. 70 (1), 69–74. doi: 10.1093/JAOAC%2F70.1.69A
Boussiba S., Gibson G. (1991). Ammonia translocation in cyanobacteria. FEMS Microbiol. Lett. 88 (1), 1–14. doi: 10.1016/0378-1097(91)90692-4
Cai T., Park S. Y., Li Y. (2013). Nutrient recovery from wastewater streams by microalgae: status and prospects. Renew. Sust. Energy Rev. 19, 360–369. doi: 10.1016/j.rser.2012.11.030
Carneiro M., Ranglová K., Lakatos G. E., Camara Manoel J. A., Grivalský T., Kozhan D. M., et al. (2021). Growth and bioactivity of two chlorophyte (Chlorella and Scenedesmus) strains co-cultured outdoors in two different thin-layer units using municipal wastewater as a nutrient source. Algal Res. 56, 102299. doi: 10.1016/j.algal.2021.102299
Carol P., Kuntz M. (2001). A plastid terminal oxidase comes to light: implications for carotenoid biosynthesis and chlororespiration. Trends Plant Sci. 6, 31–36. doi: 10.1016/s1360-1385(00)01811-2
Chew K. W., Yap J. Y., Show P. L., Suan N. H., Juan J. C., Ling T. C., et al. (2017). Microalgae biorefinery: High value products perspectives. Bioresour. Technol. 229, 53–62. doi: 10.1016/j.biortech.2017.01.006
Chojnacka K., Michalak I., Dmytryk A., Gramza M., Słowiński A., Górecki H. (2015). “Algal extracts as plant growth biostimulants,” in Marine algae extracts: Processes, products, and applications. Eds. Kim S. K., Chojnacka K., 189–212 (Weinheim, Germany: Wiley-VCH Verlag GmbH & Co. KGaA). doi: 10.1002/9783527679577.ch11
Chuka-ogwude D., Ogbonna J. C., Borovitska M. A., Moheimani N. R. (2020). Screening, acclimation and ammonia tolerance of microalgae grown in food waste digestate. J. Appl. Phycol. 32, 3775–3785. doi: 10.1007/s10811-020-02276-0
Ciardi M., Gómez-Serrano C., del Mar Morales-Amaral M., Acién-Fernández F. G., Lafarga T., Fernandez-Sevilla J. M. (2022). Optimisation of Scenedesmus almeriensis production using pig slurry as the sole nutrient source. Algal Res. 61, 102580. doi: 10.1016/j.algal.2021.102580
Collos Y., Harrison P. J. (2014). Acclimation and toxicity of high ammonium concentrations to unicellular algae. Mar. pollut. Bull. 80, 8–23. doi: 10.1016/j.marpolbul.2014.01.006
de Godos I., Blanco S., Garcia-Encina P. A., Becares E., Munoz R. (2009). Long-term operation of high rate algal ponds for the bioremediation of piggery wastewaters at high loading rates. Bioresour. Technol. 100 (19), 4332–4339. doi: 10.1016/j.biortech.2009.04.016
Drath M., Kloft N., Batschauer A., Marin K., Novak J., Forchhammer K. (2008). Ammonia triggers photodamage of photosystem II in the cyanobacterium Synechocystis sp. strain PCC 6803. Plant Physiol. 147 (1), 206–215. doi: 10.1104/pp.108.117218
Dubois A., Gilles J. K., Hamilton P. A., Rebers F. S. (1956). Colorimetric method for determination of sugars and related substances. Anal. Chem. 28, 350–356. doi: 10.1021/ac60111a017
Esteves A. F., Pires J. C. M., Gonçalves A. L. (2021). “Current utilization of microalgae in the food industry beyond direct human consumption,” in Cultured microalgae for the food industry - current and potential applications. Eds. Lafarga T., Acién Fernández G. (London, UK: Academic Press), 199–248. doi: 10.1016/B978-0-12-821080-2.00005-8
Ferreira V. S., Pinto R. F., Sant’Anna C. (2015). Low light intensity and nitrogen starvation modulate the chlorophyll content of Scenedesmus dimorphus. J. Appl. Microbiol. 120, 661–670. doi: 10.1111/jam.13007
Ge S., Champagne P. (2016). Nutrient removal, microalgal biomass growth, harvesting and lipid yield in response to centrate wastewater loadings. Water Res. 88, 604–612. doi: 10.1016/j.watres.2015.10.054
Givinish T. J. (1988). Adaptation to sun and shade: a whole-plant perspective. Funct. Plant Biol. 15, 63–92. doi: 10.1071/PP9880063
Goltsev V. N., Kalaji H. M., Paunov M., Bąba W., Horaczek T., Mojski J., et al. (2016). Variable chlorophyll fluorescence and its use for assessing physiological condition of plant photosynthetic apparatus. Russ. J. Plant Physiol. 63, 869–893. doi: 10.1134/S1021443716050058
Grivalský T., Ranglová K., Lakatos G. E., Camara Manoel J. A., Černá T., Barceló-Villalobos M., et al (2022). Bioactivity assessment, micropollutant and nutrient removal ability of Tetradesmus obliquus cultivated outdoors in centrate from urban wastewater. J. Appl. Phycol. doi: 10.1007/s10811-022-02828-6
Jeffrey S. W., Humphrey G. F. (1975). New spectrophotometric equations for determining chlorophylls a, b, c1 and c2 in higher plants algae and natural phytoplankton. Biochem. Physiol. Pflanz. 167, 191–194. doi: 10.1016/S0015-3796(17)30778-3
Jiménez Veuthey M., Morillas-Espana A., Sanchez-Zurano A., Elvira Navarro-Lopez E., Gabriel Acien Fernandez F. G., Lopez-Segura J. G., et al. (2022). Production of the marine microalga Nannochloropsis gaditana in pilot-scale thin-layer cascade photobioreactors using fresh pig slurry diluted with seawater. J. Water Process. Eng. 48, 102869. doi: 10.1016/j.jwpe.2022.102869
Kang Y., Kim M., Shim C., Bae S., Jang S. (2021). Potential of algae-bacteria synergistic effects on vegetable production. Front. Plant Sci. 12. doi: 10.3389/fpls.2021.656662
Kao C. Y., Chiu S. Y., Huang T. T., Dai L., Hsu L.-K., Lin C. S. (2012). Ability of a mutant strain of the microalga Chlorella sp. to capture carbon dioxide for biogas upgrading. Appl. Energy 93, 176–183. doi: 10.1016/j.apenergy.2011.12.082
Koller M., Muhr A., Braunegg G. (2014). Microalgae as versatile cellular factories for valued products. Algal Res. 6 (A), 52–63. doi: 10.1016/j.algal.2014.09.002
Kumar J., Ramla A., Mallick D., Mishra V. (2021). An overview of some biopesticides and their importance in plant protection for commercial. Plants 10 (6), 1185. doi: 10.3390/plants10061185
Li Y., Chen Y. F., Chen P., Min M., Zhou W., Martinez B., et al. (2011). Characterization of a microalga Chlorella sp. well adapted to highly concentrated municipal wastewater for nutrient removal and biodiesel production. Bioresour. Technol. 102 (8), 5138–5144. doi: 10.1016/j.biortech.2011.01.091
Li K., Liu Q., Fang F., Luo R., Lu Q., Zhou W., et al. (2019). Microalgae-based wastewater treatment for nutrients recovery: A review. Bioresour. Technol. 29, 121934. doi: 10.1016/j.biortech.2019.121934
Luo L., He H., Yang C., Wen S., Zeng G., Wu M., et al. (2016). Nutrient removal and lipid production by Coelastrella sp. in anaerobically and aerobically treated swine wastewater. Biores. Technol. 216, 135–141. doi: 10.1016/j.biortech.2016.05.059
Masojídek J., Torzillo G., Koblízek M., Kopecký J., Bernardini P., Sacchi A., et al. (1999). Photoadaptation of two chlorophyta (Scenedesmus and Chlorella) in laboratory and outdoor cultures: changes of chlorophyll fluorescence quenching and the xanthophyll cycle. Planta 209, 126–135. doi: 10.1007/s004250050614
Masojíidek J., Kopeckýy J., Koblížizek M., Torzillo G. (2004). The xanthophyll cycle in green algae (Chlorophyta): Its role in the photosynthetic apparatus. Plant Biol. 6, 342–349. doi: 10.1055/s-2004-820884
Min M., Wang L., Li Y., Mohr M. J., Hu B., Zhou W., et al. (2011). Cultivating Chlorella sp. in a pilot-scale photobioreactor using centrate wastewater for microalgae biomass production and wastewater nutrient removal. Appl. Biochem. Biotechnol. 165, 123–137. doi: 10.1007/s12010-011-9238-7
Morales-Amaral D. M. M., Gómez-Serrano C., F Acién Fernández F. G., José M. Fernández-Sevilla J. M., Molina-Grima E. (2015). Production of microalgae using centrate from anaerobic digestion as the nutrient source. Algal Res. 9, 297–305. doi: 10.1016/j.algal.2015.03.018
Morillas-España A., Ruiz-Nieto Á., Lafarga T., Acién- F. G., Arbib Z., González-López C. V. (2022). Biostimulant capacity of Chlorella and Chlamydopodium species produced using wastewater and centrate. Biology 11, 1086. doi: 10.3390/biology11071086
Norsker N. H., Barbosa M. J., Vermuë M. H., Wijffels R. H. (2011). Microalgal production - a close look at the economics. Biotechnol. Adv. 29, 24–27. doi: 10.1016/j.biotechadv.2010.08.005
Oswald W. J., Golueke G. G. (1960). Biological transformation of solar energy. Adv. Appl. Microbiol. 2, 223–262. doi: 10.1016/s0065-2164(08)70127-8
Park J., Jin H. F., Lim B. R., Park K. Y., Lee K. (2010). Ammonia removal from anaerobic digestion effluent of livestock waste using green alga Scenedesmus sp. Bioresour. Technol. 101, 8649–8657. doi: 10.1016/j.biortech.2010.06.142
Parson T. R., Strickland J. H. D. (1963). Discussion of spectrophotometric determination of marine plant pigments with revised equation ascertaining chlorophyll and carotenoids. J. Mar. Res. 12, 121–130. doi: 10.1016/0011-7471%2865%2990662-5
Qi F., Xu Y., Yu Y., Liang X., Zhang L., Zhao H., et al. (2017). Enhancing growth of Chlamydomonas reinhardtii and nutrient removal in diluted primary piggery wastewater by elevated CO2 supply. Water Sci. Technol. 10, 2281–2290. doi: 10.2166/wst.2017.111
Quijano G., Arcila J. S., Buitrón G. (2017). Microalgal-bacterial aggregates: Applications and perspectives for wastewater treatment. Biotechnol. Adv. 35, 772–781. doi: 10.1016/j.biotechadv.2017.07.003
Ranglová K. (2018). Available at: https://www.alga.cz/UserFiles/lhotsky/files/Ranglova.pdf (Accessed September 5, 2022).
Ranglová K., Lakatos G. E., Camara Manoel J. A., Grivalský T., Estrella F. S., Fernandez F. G. A., et al. (2021). Growth, biostimulant and biopesticide activity of the MACC-1 Chlorella strain cultivated outdoors in inorganic medium and wastewater. Algal Res. 53, 102136. doi: 10.1016/j.algal.2020.102136
Rippka R., Deruelles J., Waterbury J. B., Herdman M., Stanier R. Y. (1979). Generic assignments, strain histories and properties of pure cultures of cyanobacteria. J. Gen. Microbiol. 111, 1–61. doi: 10.1099/00221287-111-1-1
Sánchez-Zurano A., Ciardi M., Lafarga T., Fernández-Sevilla J. M., Bermejo R., Molina-Grima E. (2021). Role of microalgae in the recovery of nutrients from pig manure. Processes 9, 203. doi: 10.3390/pr9020203
Sánchez-Zurano A., Garrido Cárdenas J. A., Gómez Serrano C., Morales Amaral M., Acién-Fernández F. G., Fernández Sevilla J. M., et al. (2020). Year-long assessment of a pilot-scale thin-layer reactor for microalgae wastewater treatment. variation in the microalgae-bacteria consortium and the impact of environmental conditions. Algal Res. 50, 101983. doi: 10.1016/j.algal.2020.101983
Shen Y. (2014). Carbon dioxide bio-fixation and wastewater treatment via algae photochemical synthesis for biofuels production. RSC Adv. 4, 49672–49722. doi: 10.1039/C4RA06441K
Shi T. Q., Wang L. R., Zhang Z. X., Sun X. M., Huang H. (2020). Stresses as first-line tools for enhancing lipid and carotenoid production in microalgae. Front. Bioeng. Biotechnol. 8. doi: 10.3389/fbioe.2020.00610
Siebert M., Torzillo G. (2018). Microalgal hydrogen production: Achievements and perspectives (The Royal Society of Chemistry: Cambridge, UK Press). doi: 10.1039/9781849737128
Silva-Benavides A. M., Torzillo G. (2012). Nitrogen and phosphorus removal through laboratory batch cultures of Chlorella vulgaris and cyanobacterium Plankthotrix isothrix grown as monoalgal and as co-cultures. J. Appl. Phycol. 24, 26–276. doi: 10.1007/s10811-011-9675-2
Singh H., Das D. (2018). “Biofuels from microalgae: Biohydrogen,” in Energy from microalgae. Eds. Jacob-Lopes E., Queiroz Zepka L., Queiroz M. I. (Switzerland: Springer), 201–228. doi: 10.1016/j.scitotenv.2021.152417
Skulberg O. (2007). “Bioactive chemicals in microalgae”: Handbook of microalgal culture, biotechnology and applied phycology. Ed. Richmond A., Blackwell, Oxford, 485–512. doi: 10.1002/9780470995280.ch30
Stirk W. A., Ördög V., Novák O., Rolčík J., Strnad M., Bálint P., et al. (2013). Auxin and cytokinin relationships in 24 microalgal strains. J. Phycol. 49, 459–467. doi: 10.1111/jpy.12061
Strasser R. J., Srivastava A., Govindjee (1995). Polyphasic chlorophyll a fluorescence transient in plants and cyanobacteria. Photochem. Photobiol. 61, 33–42. doi: 10.1111/j.1751-1097.1995.tb09240.x
Strasser R. J., Tsimili M. M., Srivastava A. (2004). “Analysis of the chlorophyll a fluorescence transient,” in Chlorophyll a fluorescence: a signature of photosynthesis. advances in photosynthesis and respiration. Eds. Papageorgiou G. C., Govindjee (Springer, Dordrecht Press), 321–362. doi: 10.1007/978-1-4020-3218-9_12
Suárez-Estrella F., Ros M., Vargas García M., López M., Moreno J. (2014). Control of Xanthomonas campestris pv. vesicatoria using agroindustrial waste-based compost. J. Plant Pathol. 96, 243–248. doi: 10.4454/JPP.V96I2.028
Sutherland D. L., Howard C., Turnbull M. H., Broady P. A., Craggs R. J. (2015). The effects of CO2 addition along a pH gradient on wastewater microalgal photo-physiology, biomass production and nutrient removal. Water Res. 70, 9–26. doi: 10.1016/j.watres.2014.10.064
Takács G., Gergely I., Molnár Z., Ördög V. (2019). “Plant biostimulating effects of the green alga chlamydopodium fusiforme on winter wheat in field experiments,” in Book of abstract, 9th symposium on microalgae and seaweed products in Plant/Soil systems(Mosonmagyaróvár, Hungary), 34.
Torzillo G., Vonshak A. (2013). “Environmental stress physiology with reference to mass cultures,” in Handbook of microalgal culture: Applied phycology and biotechnology: Second edition. Eds. Richmond A., Hu Q. (New York: John Wiley & Sons), 90–113. doi: 10.1002/9781118567166.ch6
Touloupakis E., Faraloni C., Silva Benavides A. M., Masojídek J., Torzillo G. (2021). Sustained photobiological hydrogen production by Chlorella vulgaris without nutrient starvation. Int. J. Hydrog. Energy. 46, 3684–3694. doi: 10.1016/j.ijhydene.2020.10.257
Touloupakis E., Tartari G., Chini Zittelli G., Torzillo G. (2020). Growth and photosynthetic performance of Chlamydopodium fusiforme cells cultivated in BG11 and Bristol media. J. Appl. Phycol 32, 145–152. doi: 10.1007/s10811-019-01900-y
Van Heukelem L., Thomas C. S. (2001). Computer-assisted high-performance liquid chromatography method development with applications to the isolation and analysis of phytoplankton pigments. J. Chromat. 910 (1), 31–49. doi: 10.1016/s0378-4347(00)00603-4
Wang Y., Guo W., Yen H. W., Ho S. H., Lo Y. C., Cheng C. C., et al. (2015). Cultivation of Chlorella vulgaris JSC-6 with swine wastewater for simultaneous nutrient/COD removal and carbohydrate production. Bioresour. Technol. 198, 619–625. doi: 10.1016/j.biortech.2015.09.067
Wang L., Li Y., Chen P., Min M., Chen Y., Zhu J., et al. (2010). Anaerobic digested dairy manure as a nutrient supplement for cultivation of oil-rich green microalgae Chlorella sp. Bioresour. Technol. 101 (8), 2623–2628. doi: 10.1016/j.biortech.2009.10.062
Xi Y., Kong F., Chi Z. (2021). ROS induce β-carotene biosynthesis caused by changes of photosynthesis efficiency and energy metabolism in Dunaliella salina under stress conditions. Front. Bioeng. Biotechnol. 8. doi: 10.3389/fbioe.2020.613768
Xu J., Zhao Y., Zhao G., Zhang H. (2015). Nutrient removal and biogas upgrading by integrating freshwater algae cultivation with piggery anaerobic digestate liquid treatment. Appl. Microbiol. Biotechnol. 99, 6493–6501. doi: 10.1007/s00253-015-6537-x
Keywords: microalgae cultivation, Chlamydopodium fusiforme, photosynthesis, nutrient removal, productivity, piggery wastewater
Citation: Zittelli GC, Silva Benavides AM, Silovic T, Ranglová K, Masojídek J, Cicchi B, Faraloni C, Touloupakis E and Torzillo G (2022) Productivity and nutrient removal by the microalga Chlamydopodium fusiforme grown outdoors in BG-11 and piggery wastewater. Front. Mar. Sci. 9:1043123. doi: 10.3389/fmars.2022.1043123
Received: 13 September 2022; Accepted: 07 November 2022;
Published: 24 November 2022.
Edited by:
Maja Berden Zrimec, AlgEn, Algal Technology Centre, SloveniaReviewed by:
FGabriel Acien Fernández, University of Almeria, SpainCopyright © 2022 Zittelli, Silva Benavides, Silovic, Ranglová, Masojídek, Cicchi, Faraloni, Touloupakis and Torzillo. This is an open-access article distributed under the terms of the Creative Commons Attribution License (CC BY). The use, distribution or reproduction in other forums is permitted, provided the original author(s) and the copyright owner(s) are credited and that the original publication in this journal is cited, in accordance with accepted academic practice. No use, distribution or reproduction is permitted which does not comply with these terms.
*Correspondence: Giuseppe Torzillo, Z2l1c2VwcGUudG9yemlsbG9AY25yLml0
Disclaimer: All claims expressed in this article are solely those of the authors and do not necessarily represent those of their affiliated organizations, or those of the publisher, the editors and the reviewers. Any product that may be evaluated in this article or claim that may be made by its manufacturer is not guaranteed or endorsed by the publisher.
Research integrity at Frontiers
Learn more about the work of our research integrity team to safeguard the quality of each article we publish.