- 1Institute of Marine Biology, National Taiwan Ocean University, Keelung, Taiwan
- 2Travel and Tourism Program, Ming Chuan University, Taoyuan, Taiwan
- 3National Museum of Marine Science and Technology, Keelung, Taiwan
- 4Kagoshima City Aquarium, Kagoshima, Japan
- 5Center of Excellence for the Oceans, National Taiwan Ocean University, Keelung, Taiwan
Sea slug (Subclass Heterobranchia) biodiversity is an important element of marine coral reef communities. However, due to their small size and cryptic behavior, few studies have examined their spatiotemporal variation. Taiwan is in the middle of East Asia Island Arcs, where it is one of the hotspots of sea slug diversity. Meanwhile, the northeast coast of this island lies within the subtropical monsoon region, where the climate shifts from a warm southwest monsoon (SW: May to Sep) to a cold northeast monsoon (NE: Oct to Apr). Between the monsoons, distinct temperatures and currents result in different community structures. To understand how those environmental factors (i.e., monsoon season, diel period and water depth) influence sea slug species richness and assemblages, we surveyed these invertebrates monthly at both night and day by diving in a marine protection area (CJ) and at a nearby non-protected site (MR) from May 2020 to Apr 2021. We showed that the species composition in our sampling area was highly consistent with what was found in Okinawa and Pescadores island, but less similar to those in southern Taiwan or Hong Kong. On the northeast coast of Taiwan, sea slug number and abundance were both higher in CJ than MR, but biodiversity (H’) and the species evenness index J’ was lower in CJ than MR. Additionally, cluster analysis indicated that the sea slug assemblages differed between the two sites. Monsoon season significantly influenced both species richness and species assemblages, with species richness during SW being significantly higher than in the NE monsoon and species assemblages also being distinct between these two seasons. Moreover, species richness increased with water depth (within the surveyed range of 0-25 m). Diel period did not exert a strong impact on sea slug biodiversity, except for species richness at CJ, where it was higher during the day than at night. Our study suggested that changing currents between the monsoon seasons could transport individuals originating from either the north or south of these island arcs. Furthermore, temperature and food sources may differ between seasons and depths, thereby influencing sea slug assemblages in this region.
Introduction
Sea slugs, formerly known as the Opisthobranchia, are a polyphyletic group of marine invertebrates in Subclass Heterobranchia, which includes the notable orders Cephalaspidea, Nudibranchia, Pleurobranchida, and Sacoglossa, among others. Those animals are characterized by their gills located posteriorly to the heart unlike most of the other gastropods. Some groups in basal-shelled sea slugs have full shell at maturity, while nudibranchs had complete shell loss at maturity (Gosliner et al., 2018). Most groups among sea slugs have trended towards shell loss in maturity and evolved defense mechanisms, for example, chemical defense, aposematic coloration and camouflage (Gosliner, 2001; Gosliner et al., 2018). In addition, most of them, especially nudibranchs, are hermaphrodites and reproduce by exchanging sperm and laying eggs through copulation. Due to their great morphological diversity and colorful patterning, sea slugs are flagship species for recreational divers. Their considerable species richness contributes significantly to marine biodiversity (Urra et al., 2013; Artüz et al., 2018; Kaligis et al., 2018). Sea slugs exhibit strong niche partitioning, displaying specialized feeding habits (reviewed in Larkin et al., 2018), varied diel activity patterns (Larkin et al., 2018), and microhabitat adaptations (Hussain et al., 2022; Richards Donà et al., 2022). Most sea slugs are carnivorous, and their species-specific food items include sponges, tunicates, bryozoans, crustaceans, hydrozoans, cnidarians and other sea slugs (or their eggs) (review in Behrens, 2005). Some of the nudibranchs were corallivorous which feed on scleractinian corals, and several new species were recorded and described in recent years (Hu et al., 2020a; Hu et al., 2020b). The coral-feeding nudibranch, Phestilla sibogae can quickly consume all living tissue on a coral colony and cause extensive damage to coral colonies without predators (Gochfeld and Aeby, 1997). Some sacoglossan species feed on alga and retain chloroplasts as kleptoplasts for photosynthesis (review in Pierce et al., 2015; Chihara et al., 2020). Additionally, more than the need for nutrition, the kleptocnida species (Aeolidioidea) that feed on Cnidaria may keep the food-derived nematocysts in their body for defense (review in Togawa, 2021). Thus, sea slug assemblages may reflect the diversity or abundance of food sources (Betti et al., 2017), or other resources. Accordingly, a diverse assemblage of sea slugs could reflect a diversity of trophic structures and thus potentially be deployed as a biological indicator (Lock et al., 2010).
To date, there are roughly over 6,000 known species of sea slugs around the world (Gosliner et al., 2018). Globally, the geographical distribution of heterobranch sea slugs shows that the species richness is higher in the tropical zones than temperate zones (Chang et al., 2013). In addition, some tropical regions (e.g., Coral Triangle) in the Indo-Pacific Ocean have higher diversity than other tropical regions, such as the central Pacific and western Indian Ocean (Gosliner et al., 2018). The species richness of the eastern Australian heterobranch sea slugs decreases towards the higher latitudes, with the diversity from approximately 1,000 species in the northern Great Barrier Reef to less than 400 in the southern region of the Bass Strait (Nimbs & Smith, 2017). The area of Festoon Islands (East Asia Island Arcs) is one of the hotspots for sea slug diversity, where more than 1,400 species are found in Japan (Nakano, 2018), 782 species in Taiwan (Chang, 2012), 1,200 species in the Philippines, and 646 species in Papua New Guinea (Gosliner et al., 2018). However, the species richness is relatively lower in the coastal waters of the mainland. The most recent report indicated that there were 257 species in Hong Kong (Chow et al., 2022), but only 196 species were recorded in South Korea (Koh, 2006).
Taiwan is in the middle of East Asia Island Arcs, where the northeast coast displays a classical subtropical monsoon climate, with the monsoon being driven by warm tropical marine air masses from the southwest during the summer (May to Sep, SW monsoon season) and cold continental air masses from the northeast in the winter (Oct to Apr, NE monsoon season) (reviewed in Chang et al., 2006; Chen et al., 2010). Seasonal changes in currents and wind direction are associated with these two monsoon seasons. During the summer, the Kuroshio Current and South China Sea Warm Current bring a warm water mass and tropical species from southerly areas to this region. However, the China Coastal Current dominates this area during the winter, bringing a low-temperature water mass from the north continental waters through the Taiwan strait (reviewed in Tseng & Shen, 2003) (Figure 1). Along with the northeast wind, the China Coastal Current and Taiwan’s “cold dome” also result in stronger upwelling in winter, and consequently higher primary productivity, than in summer (Chang et al., 2009), eliciting distinct floristic compositions (Lin et al., 2018). Since sea slugs play important roles in marine biodiversity and community structures (Burghardt et al., 2006; Smith & Davis, 2019; Papu et al., 2020) and these environmental factors are clearly different between the monsoon seasons, it is reasonable to hypothesize that the species richness and assemblages of sea slugs in this region also differ throughout the year (Su et al., 2009).
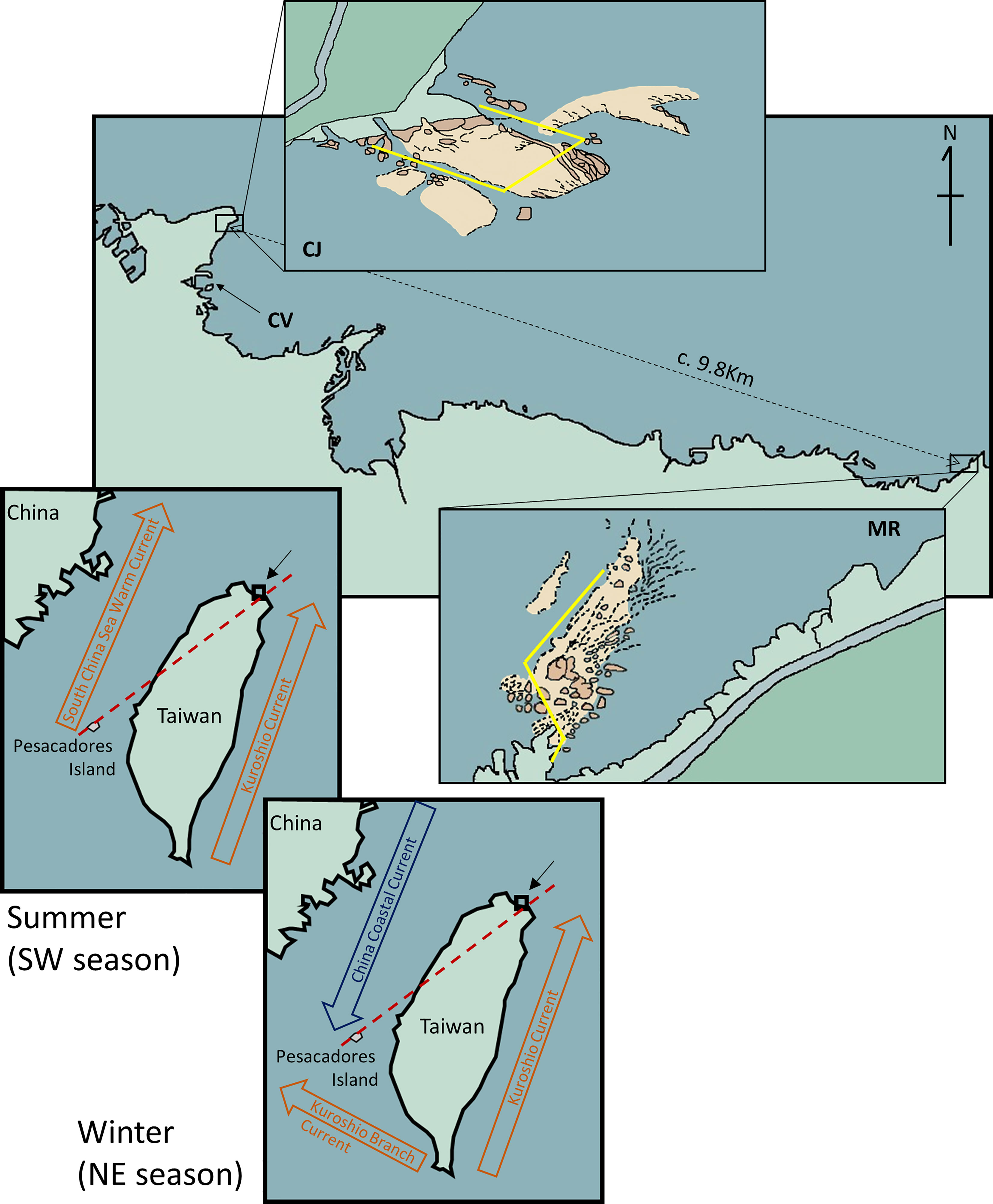
Figure 1 Map of part of the northeastern coast of Taiwan showing the locations of the sites surveyed for this study, CJ and MR. CV is the location where the marine meteorological buoy is deployed at Changtan Village harbor. The inserted figures show the transect line (yellow) for each site. The red dashed line on the map indicates the S-N marine biogeographical line based on Shao et al. (1997), and the current vanes are based on Hu et al. (2000).
The aim of our study was to understand how environmental factors—specifically the monsoon season, diel period, and water depth—influence the richness and species assemblage of sea slugs in a subtropical monsoon region. To this end, we surveyed the sea slugs in marine protected areas on the northeast coast of Taiwan, along with a nearby non-protected area to represent a control site. It may allow us to assess how human activities impact sea slug populations. The surveys were conducted by SCUBA diving monthly and both day and night from May 2020 to Apr 2021. Moreover, we recorded the depth and temperature where each individual was recorded to explore potential sea slug microhabitat preferences.
Material and methods
Study areas
The resource conservation area of Wanghaixiang Chao-Jing Bay (CJ; 25°14481N, 121°80473E, c. 0.15 km2) is located on the northeast coast of Taiwan. It is managed by the government of Keelung City and the National Museum of Marine Science and Technology (NMMST). The control site, Mother Rock Bay (MR; 25°12030N, 121°89971E, location marker 82.5km on the Taiwan Provincial Highway No. 2) lies ~9.8 km east of CJ (Figure 1). The two sites display similar underwater topographies, with maximum depths of ~30 m and an average depth of ~14 m. The substrate of both sites is mainly comprised of rocks and corals at depths<25 m and sandy sediment in deeper areas. All extractive activities are forbidden in CJ, with the principal human impacts being attributable to snorkeling or recreational SCUBA diving. In contrast, commercial fishing, including seashore fishing and SCUBA-based hunting, is allowed at MR.
We also obtained from the Central Weather Bureau of Taiwan a consecutive log of water temperatures from a marine meteorological buoy in the nearby harbor of Changtan Village (25°14053N, 121°80288E) (Figure 1). Since water temperatures clearly differed according to monsoon season as earlier reports (reviewed in Chang et al., 2006; Chen et al., 2010), we focused on comparing the southwest monsoon season (SW, May – Sep) to the northeast monsoon season (NE, Oct-Apr).
Field methods
Between May 2020 and Apr 2021, we conducted 12 monthly diel SCUBA surveys according to a line transect method at the two study sites, amounting to a total of 50 surveys. For safety reasons, SCUBA surveys were only undertaken in slack water when the swell height was<2.5 m and visibility was >2 m. Depending on tidal characteristics, daytime surveys were performed between 10:00 and 16:00 (at least 1 h before sunset or after sunrise), whereas nighttime surveys were conducted between 19:00 and 24:00 (at least 1 h before sunrise or after sunset) to avoid potential crossover of diurnally and nocturnally active species. All SCUBA surveys were performed by at least two divers in each period following the safety regulations issued by National Taiwan Ocean University (NTOU). Nighttime surveys in CJ were approved by the government of Keelung City.
In CJ, two daytime and one nighttime survey were conducted each month during the SW season, whereas one daytime and one nighttime survey per month were performed during the NE season. In MR, two daytime surveys during the SW season and one daytime survey during the NE season were performed each month. One nighttime survey was done each month during the SW season in MR but, due to safety concerns, only one was conducted every 3 months during NE at this site.
At each site, a transect line (~250 m in length) was set at a depth of between 0 and 25 m (Figure 1). Each transect survey took c. 45-50 min to complete. All sea slugs observed within a 1 m belt along the 250-m transect were photographed using a digital camera (Olympus TG-5, within housing) with a flash (D-200, Inon). For both daytime and nighttime surveys, a diving wide-beam torch (LF3100EW, Inon) was used to search for sea slugs, especially those hidden in the crevices of the reef. A diving watch (Mission One, Atoms) continuously recorded depth and temperature at scales of ±0.1 m and ±0.1°C, respectively. After the survey, the data log of depth and temperature was synchronized to the sea slug observational records according to the timeline (Figure 2). All surveys were conducted by the first author (HYC), with buddy divers assisting in guiding him along the transect line and providing photographic support. Species were identified using field guides (Nakano, 2018; Gosliner et al., 2018; Ono and Kato, 2020). Any uncertainties with species identification were resolved by the authors KN and YWC.
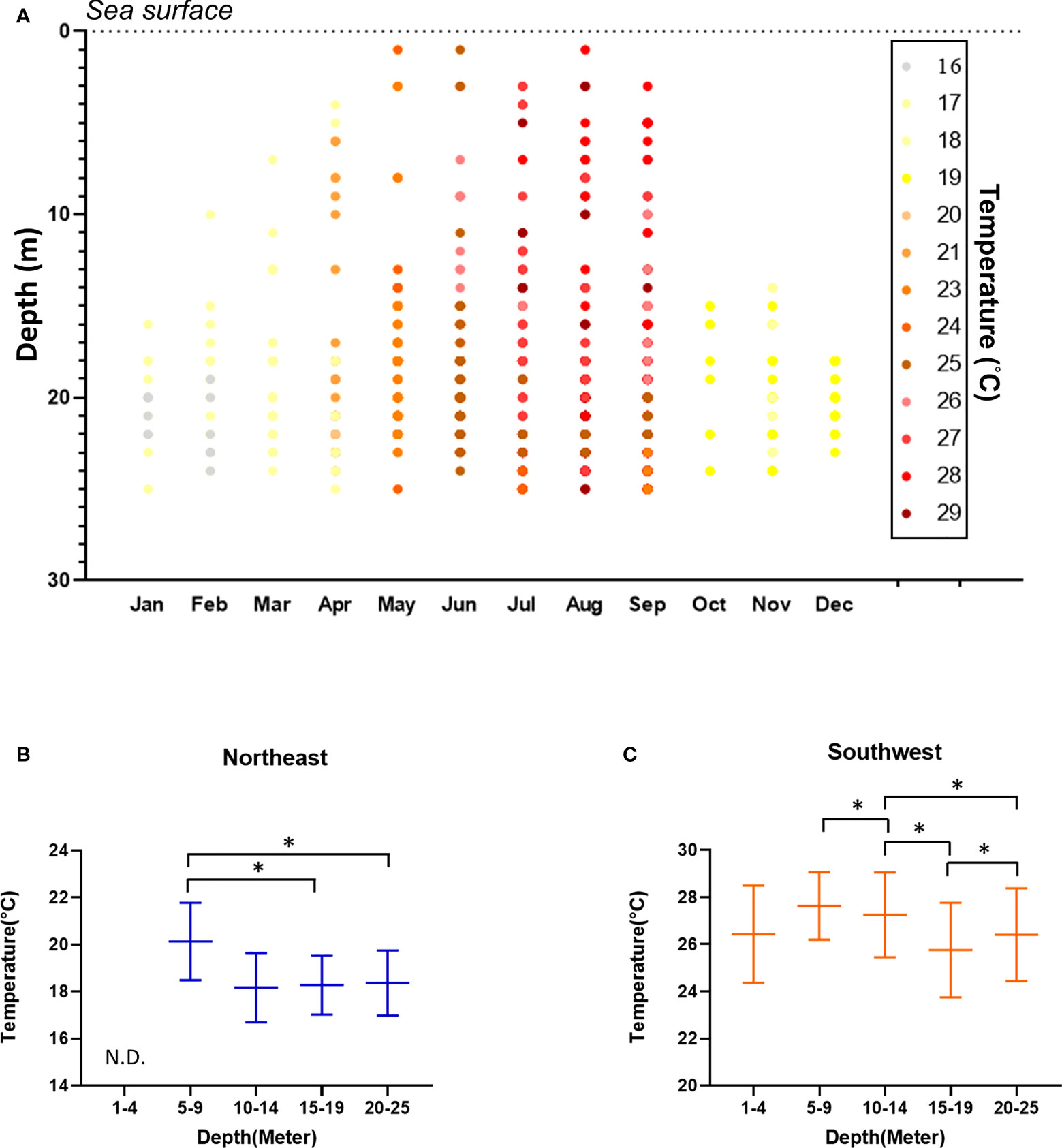
Figure 2 Water temperature, as recorded during the surveys. (A) Heatmap of temperature across depth and months. Water temperature according to depth for the NE season (B) and SW season (C). Mean ± SE; * p< 0.05. N.D. No sea slugs were recorded at a depth of 0-4 m during the NE season, hence there is no temperature data for this depth profile.
A small number of rare or previously undescribed species were collected during the survey after 27 May 2020 with permission from the government of Keelung City for DNA barcoding (data not shown), but no other sea slugs were removed or relocated. Moreover, no underwater objects were moved during any of the surveys. Although current experimental animal protocols do not apply to molluscs, or indeed most invertebrates, any contact in our experiments strictly adhered to 3R ethical principles (replacement, reduction, and refinement) (Russell and Burch, 1959), and followed the guidelines on animal experiments of the Institutional Animal Care and Use Committee of NTOU.
Data analysis
We adopted a descriptive-quantitative approach to analyze our dataset. Species richness is represented as the number of species or individuals recorded in each survey. Since survey effort was not equal between sites, diel periods or seasons, we normalized species richness and abundance against the number of surveys for each category (species/individual counts per survey) (Farrell and Saloner, 1985).
The Shannon-Wiener diversity index (H’) and Pielou’s evenness index (J’) (Heip et al., 1998) were calculated in PRIMER v.6.1.5 (Clarke and Gorley, 2006, licensed to user 3429) to measure dominance and diversity of species in each group:
in which S is the total number of species and pi is the ratio of species i in total abundance.
Using SPSS v.19, species richness and abundance of two groups were compared by performing a Shapiro-Wilk normality test (p > 0.05), followed by a two-tailed Student’s t-test. Multiple group comparisons were performed by two-way ANOVA, followed by post hoc analysis with Tukey’s test. For the data on temperature, relative abundance, biodiversity (H’) and evenness (J’), some of the data were not normally distributed after a Shapiro-Wilk normality test (p< 0.05), so both parametric one-way ANOVA test and non-parametric statistical analyses were deployed, i.e., a one-way Kruskal-Wallis test followed by a post-hoc Dunn test (Błażewicz et al., 2019).
Variation in sea slug assemblages for each group was investigated using a multivariate analysis in PRIMER v.6.1.5. Abundance data was standardized through Log(X+1) transformation and then a Bray–Curtis similarity matrix was generated to calculate similarity among groups. A dendrogram was constructed in CLUSTER with the group average as mode, and then NMDS (non-metric multidimensional scaling) plots were generated to examine the ordination pattern of samples between groups. When distinctive clustering was detected, ANOSIM (analysis of similarities) was used to test for significant differences among pairs of groups (Clarke and Warwick, 2001). This test creates a global R-statistic that ranges from 0 to 1 to represent relative significance levels between groups (Clarke, 1993). Furthermore, for the comparisons that did not form distinctly clustered ordinations by the Bray-Curtis method, the similarity of the data was also analyzed by an additional Jaccard qualitative similarity index (Béguinot, 2019; Inoue et al., 2021).
Results
Overview
According to the log of water temperatures, average sea surface temperatures during May – Sep 2020 (27.29 ± 2.07°C, Mean ± SD) were significantly higher than during Oct 2020 – Apr 2021 (20.10 ± 2.31°C) (Student’s t-test p< 0.001) (Supplementary Data S1). However, due to a cold dome, water temperatures could occasionally drop to as low as 16°C for a short timeframe, as occurred in January 2021.
We identified 121 species from 53 genera and 25 families in a total of 1,300 sea slug observations, which included 3 species of Cephalaspidea, 110 species of Nudibranchia, 2 species of Pleurobranchida, and 6 species of Sacoglossa (Supplementary Data S2). Goniobranchus setoensis (Chromodorididae) and Polycera risbeci (Polyceridae) represented new records for Taiwan, and we recorded another nine species that have never before been reported along the northeast coast of the island (Supplementary Data S2). Among those 121 species, 24 species are previously undescribed, but 15 possess morphological characters consistent with unnamed species in earlier publications (Figure 3). However, the remaining 9 unidentified sea slugs display unique characteristics, i.e., Coryphellina sp.1, Hypselodoris sp., Nembrotha sp.1, Nembrotha sp.2, Platydoris sp., Polycera sp., Sakuraeolis sp.1, Sakuraeolis sp.2, and Thecacera sp.1 (Figure 4).
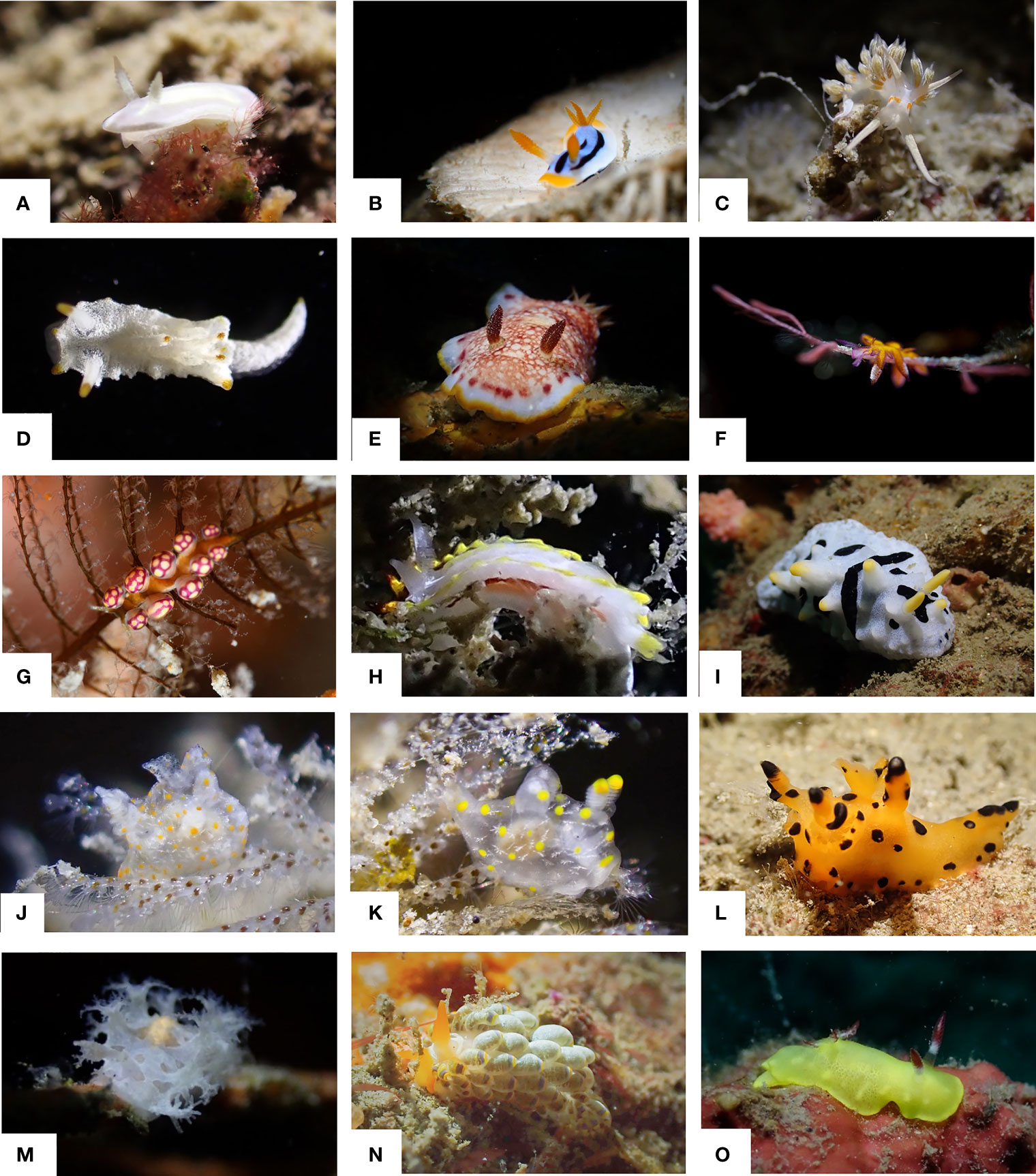
Figure 3 The unnamed species recorded at the two study sites. (A) Ceratosoma sp. [Ceratosoma sp.1 in Nakano (2018)], (B) Chromodoris sp. (Chang, 2012), (C) Cratena sp. [Cratena sp.3 in Nakano (2018)], (D) Goniodoridella sp. [Goniodoridella sp.8 in Ono & Kato (2020)], (E) Goniobranchus sp. [Goniobranchus cf. alderi in Nakano (2018)]. (F) Hermaea sp. [Hermaea sp2. in Gosliner et al. (2018)], (G) Kabeiro sp. [Kabeiro sp.3 in Gosliner et al. (2018)], (H) Okenia sp. [Okenia sp.11 in Nakano (2018)], (I) Phyllidia sp. [Phyllidia sp.8 in Gosliner et al. (2018)], (J) Thecacera sp.2 [Thecacera sp.3 in Nakano (2018)], (K) Thecacera sp.3 [Thecacera sp.14 in Gosliner et al. (2018)], (L) Thecacera sp.4 [Thecacera sp1. in Nakano (2018) and Thecacera sp.8 in Gosliner et al. (2018)], (M) Tritonia sp. [Tritonia sp1 in Nakano (2018) and Tritonia sp3. in Ono & Kato (2020)], (N) Trinchesia sp. [Trinchesia sp.33 in Ono & Kato (2020)] and (O) Verconia sp. [Verconia sp.5 in Gosliner et al. (2018)].
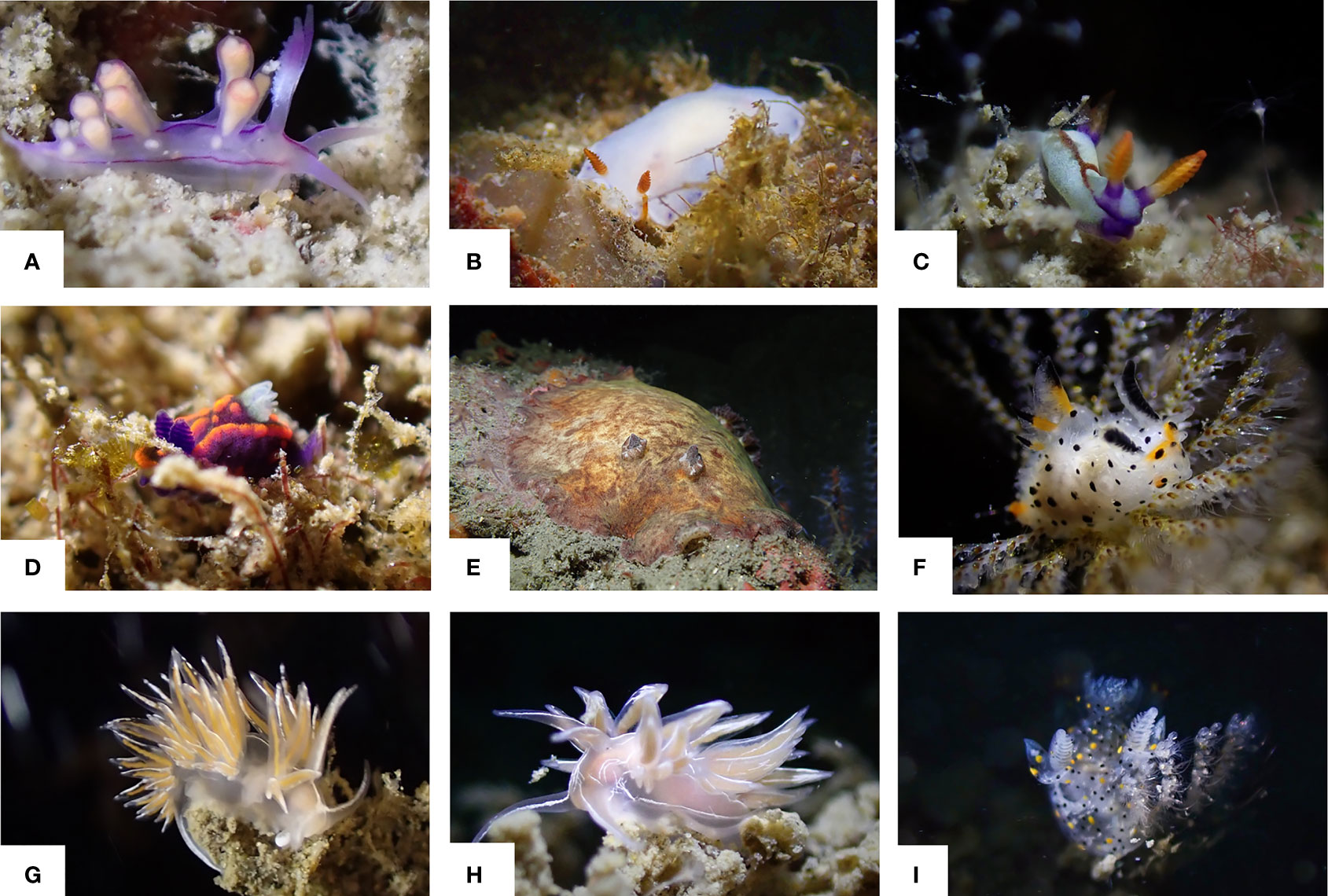
Figure 4 Undescribed types of sea slugs found at the two sites. (A) Coryphellina sp.1, (B) Hypselodoris sp., (C) Nembrotha sp.1, (D) Nembrotha sp.2, (E) Platydoris sp., (F) Polycera sp., (G) Sakuraeolis sp.1, (H) Sakuraeolis sp.2, and (I) Thecacera sp.1.
We observed 100 species of sea slugs in CJ and 86 at MR (Table 1), with 33 species specific to CJ and another 67 species only occurring in MR. Species from all four orders were present in CJ, but Pleurobranchida was absent from MR. Although the total number of species was higher in CJ than MR, values for the biodiversity and evenness indexes were both lower for CJ than for MR (Table 1).
Underwater temperature was significantly higher in SW than NE at all depths (one-way Kruskal-Wallis test df = 1, p< 0.001; ANOVA, F = 3348.508, p< 0.001), and water temperature declined according to increasing water depth for both seasons (one-way Kruskal-Wallis test/ANOVA df = 4, NE: p = 0.047/p< 0.001 (F = 468.633); SW: p< 0.001/p< 0.001 (F = 1800.559)) (Figure 2). We detected more sea slug species during the SW season (114 species, 52 genera and 24 families) compared to the NE season (46 species, 23 genera and 13 families). Moreover, values for the biodiversity and evenness indexes were both higher in SW than in NE (one-way Kruskal-Wallis test/ANOVA; df = 1, p< 0.005/p< 0.001 (H’: F = 38.827; J’: F = 1.129), for both indexes). We recorded 75 species of sea slug only during SW, and another 7 only in the NE season. Additionally, 47 species were exclusively diurnal and a further 10 were nocturnal, with the remaining 64 species being recorded during both nighttime and daytime surveys (Table 1).
Species richness and evenness
Standardized numbers of species and individuals recorded per survey in the SW season were significantly higher than for the NE season (Figure 5) (Table 2). The effect of monsoon season on species richness and species abundance was similar across sites (Figure 6A). However, the interaction between these two factors was not statistically significant (Table 2).
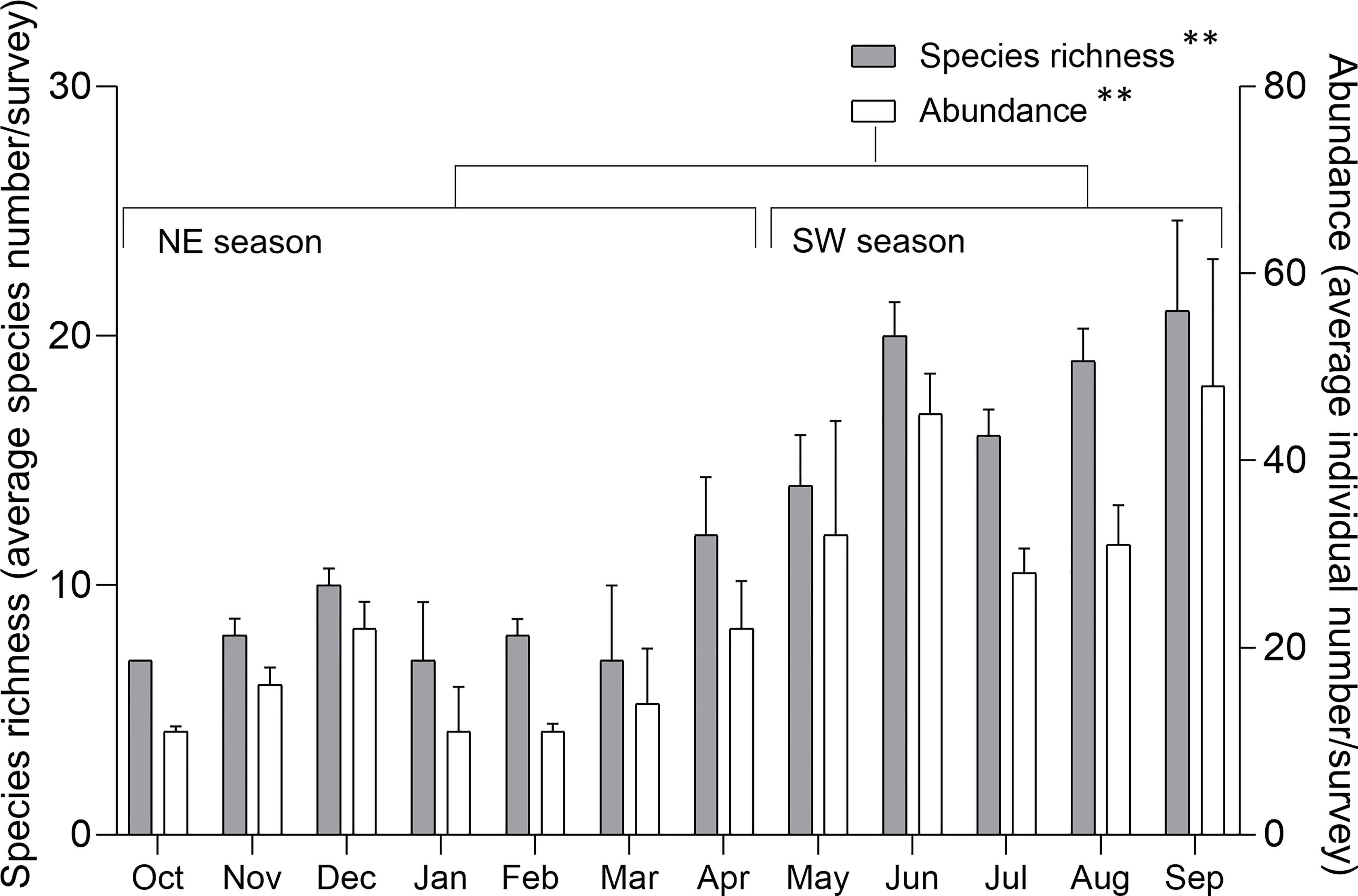
Figure 5 Species richness (left scale) and abundance (right scale) of sea slugs across the year. Species richness and abundance are represented by the standardized species number and records per survey, respectively. Mean ± SE; ** p< 0.001 (ANOVA test).
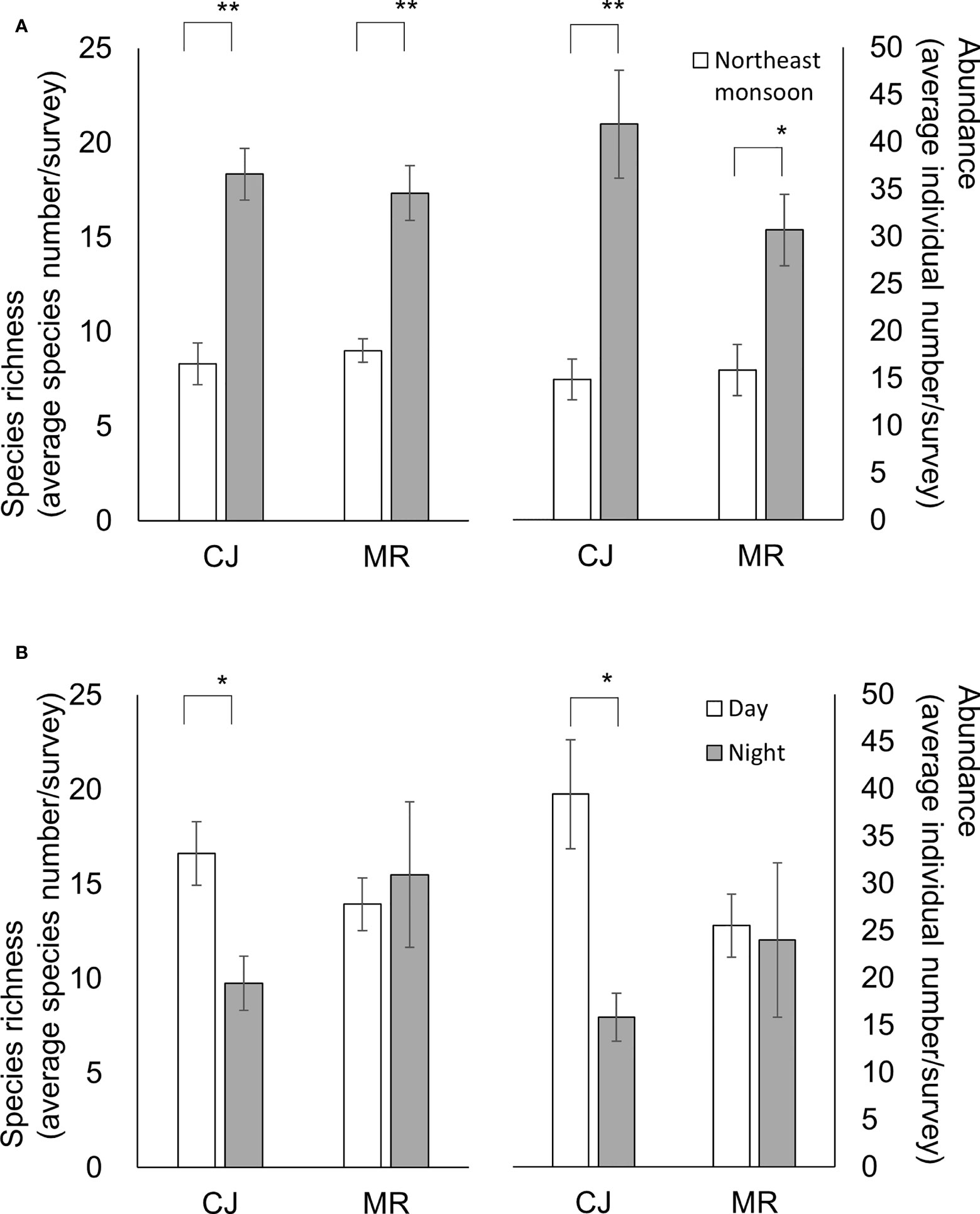
Figure 6 Species richness (left scale) and abundance (right scale) for the two sites according to (A) monsoon season or (B) diel period. Species richness and abundance are represented by the standardized species number and records per survey, respectively. Mean ± SE; ** p< 0.001, * p< 0.05 (ANOVA test).
Species richness and the diversity index increased in line with depth (Figure 7). Interestingly, in general, more species and/or individuals were found during SW relative to NE for various depth profiles: 5-9 m (Student t-test, p = 0.064 or 0.045, respectively); 15-19 m (Student t-test, p = 0.005 and 0.024, respectively); and 20-25 m (Student t-test, p< 0.001 in both comparisons). Thus, monsoon season and depth exert a significant co-dependent influence on both the species richness and diversity (Table 2). However, the survey site had no impact on this pattern (Table 2).
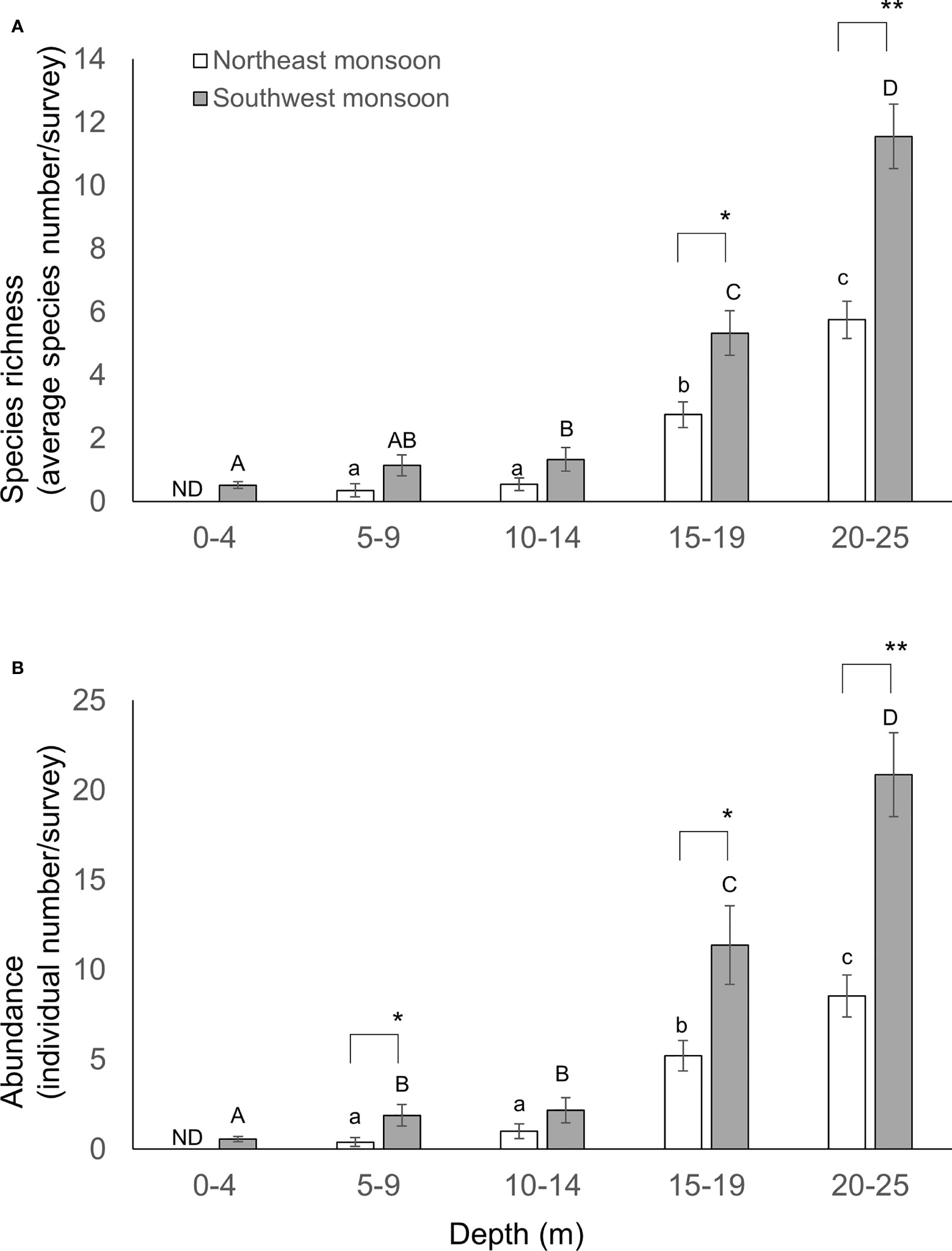
Figure 7 Species richness (A) and abundance (B) of sea slugs across water depth. Species richness and abundance are represented by the standardized species number and records per survey, respectively. Different letters indicate statistical significance (ANOVA test, p< 0.05). Mean ± SE; ** p< 0.001, * p< 0.05 (ANOVA test).
Our results revealed that for daytime and nighttime surveys, there were higher species richness (Student t-test, p< 0.001 for both comparisons) and abundance (Student t-test, p< 0.001 and = 0.003, respectively) during the SW than the NE season (Figure 8). However, an influence of diel period was only detected for species abundance during the NE season (Student t-test, p = 0.034) (Figure 8). There was a clear trend for species richness and abundance in CJ to be higher in the daytime than nighttime (Student t-test, p = 0.006 and 0.002, respectively), but that was not the case for MR (Student t-test, p = 0.642 and 0.843, respectively) (Figure 6B). Furthermore, although values for the biodiversity index were higher during daytime than nighttime surveys, the opposite was true for evenness index values (Table 1) (one-way Kruskal-Wallis test/ANOVA; df = 1, p = 0.115/p = 0.077 (F = 3.281) and p = 0.67/p = 0.42 (F = 0.663), respectively). Overall, diel period did not appear to be a significant factor influencing the number or diversity of sea slugs in this region, with no obvious interaction with factors other than the survey site (Table 2).
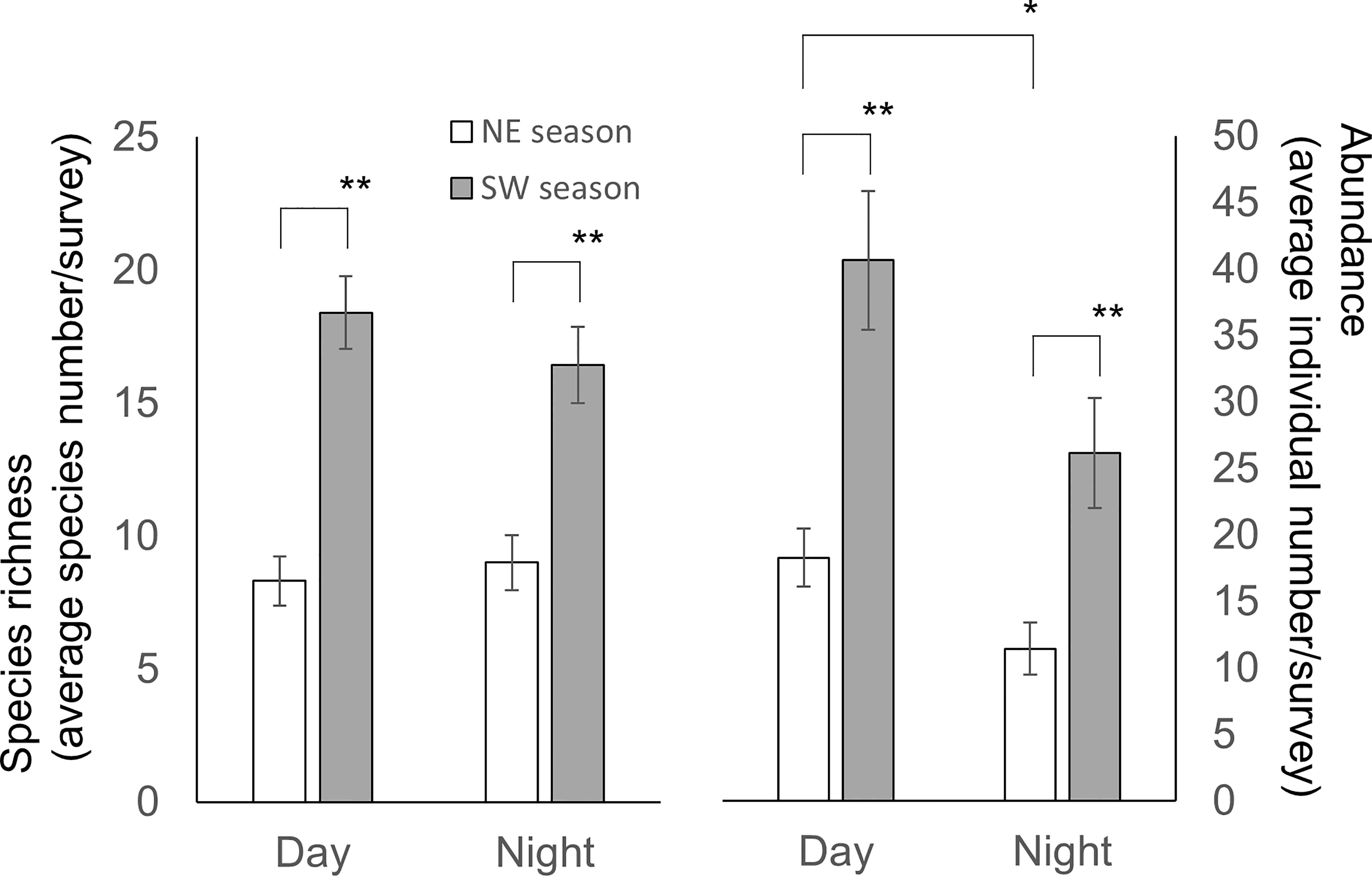
Figure 8 Species richness (left scale) and biodiversity index (H’) (right scale) according to monsoon season and diel period. Species richness is represented by the standardized species number per survey. Mean ± SE; * p< 0.05, ** p< 0.001 (Species richness: Student t-test; H’: Kruskal-Wallis test).
Species assemblages
The most abundant species in our dataset was Phyllidiella pustulosa, representing ~18% of all our records. P. pustulosa also proved to be the most dominant species in CJ throughout the year, but densities were higher during NE than in SW (Figure 9) (one-way Kruskal-Wallis test/ANOVA, df = 1, p = 0.004/p = 0.002 (F = 12.191)). Proportions of this species were lower in MR than CJ in both seasons (one-way Kruskal-Wallis test/ANOVA, df = 1, NE: p = 0.031/p = 0.001 (F = 16.296) and SW: p = 0.004/p = 0.002 (F = 11.699), respectively) (Figure 9A). In contrast, Dermatobranchus primus (a species absent from CJ) was the dominant species at the MR site in both seasons (one-way Kruskal-Wallis test/ANOVA df = 1, p = 0.337/p = 0.101 (F = 3.003)) (Figure 9B).
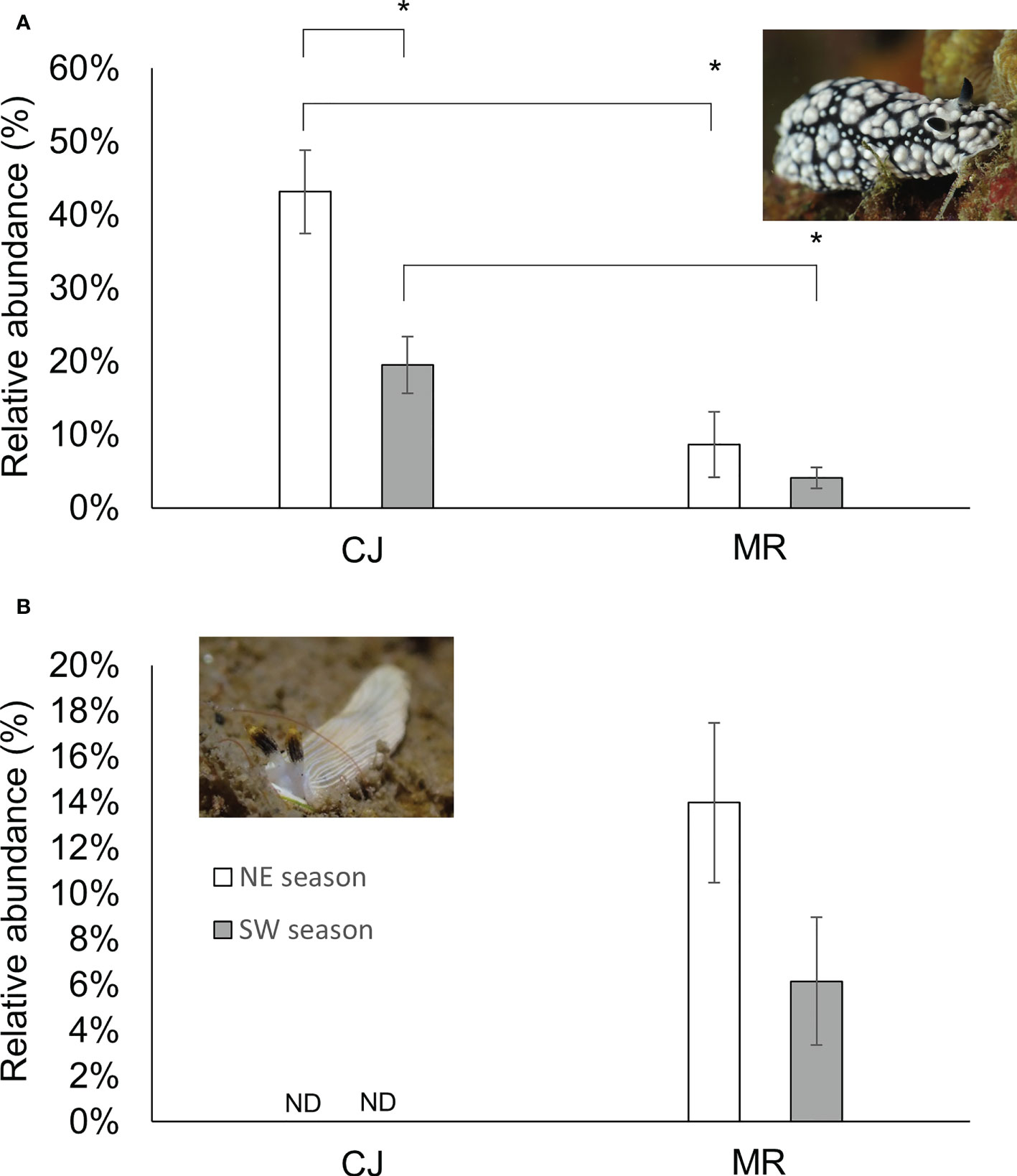
Figure 9 Proportions of abundance for Phyllidiella pustulosa (A) or Dermatobranchus primus (B) at the two sites. Mean ± SE; * p< 0.05 (Kruskal-Wallis test). ND No individual was found.
CLUSTER analysis of monthly sea slug datasets revealed two main groups at a Bray-Curtis similarity level of 40% (Figure 10A), consistent with the two monsoon seasons in this region (SW: May – Sep and NE: Oct – Apr) (Figure 11A) (ANOSIM, R = 0.358; p = 0.001). Both of these main groups could be further subdivided into two clades; NE comprised two clades covering Oct – Jan and Feb – Apr, and SW comprised May – Jun and Jul – Sep clades (Figure 11A). CLUSTER analysis of our sea slug observations identified three groups at a similarity level of 30% according to water depth (ANOSIM, R = 0.494; p = 0.001) (Figure 10B). The sea slug assemblage at 0 – 4 m proved quite distinctive, with CLUSTER further subdividing the remaining two depth-related groups into 15 – 19 m and 20 – 25 m or 5 – 9 m and 10 – 14 m clades, respectively (Figure 11B). In addition, sea slug assemblages formed distinct clades at a similarity level of 50% according to water temperature, with those occurring at high temperatures (22-29°C) being quite different to those at low temperatures (16-19°C) (Figures 10C, 11C).
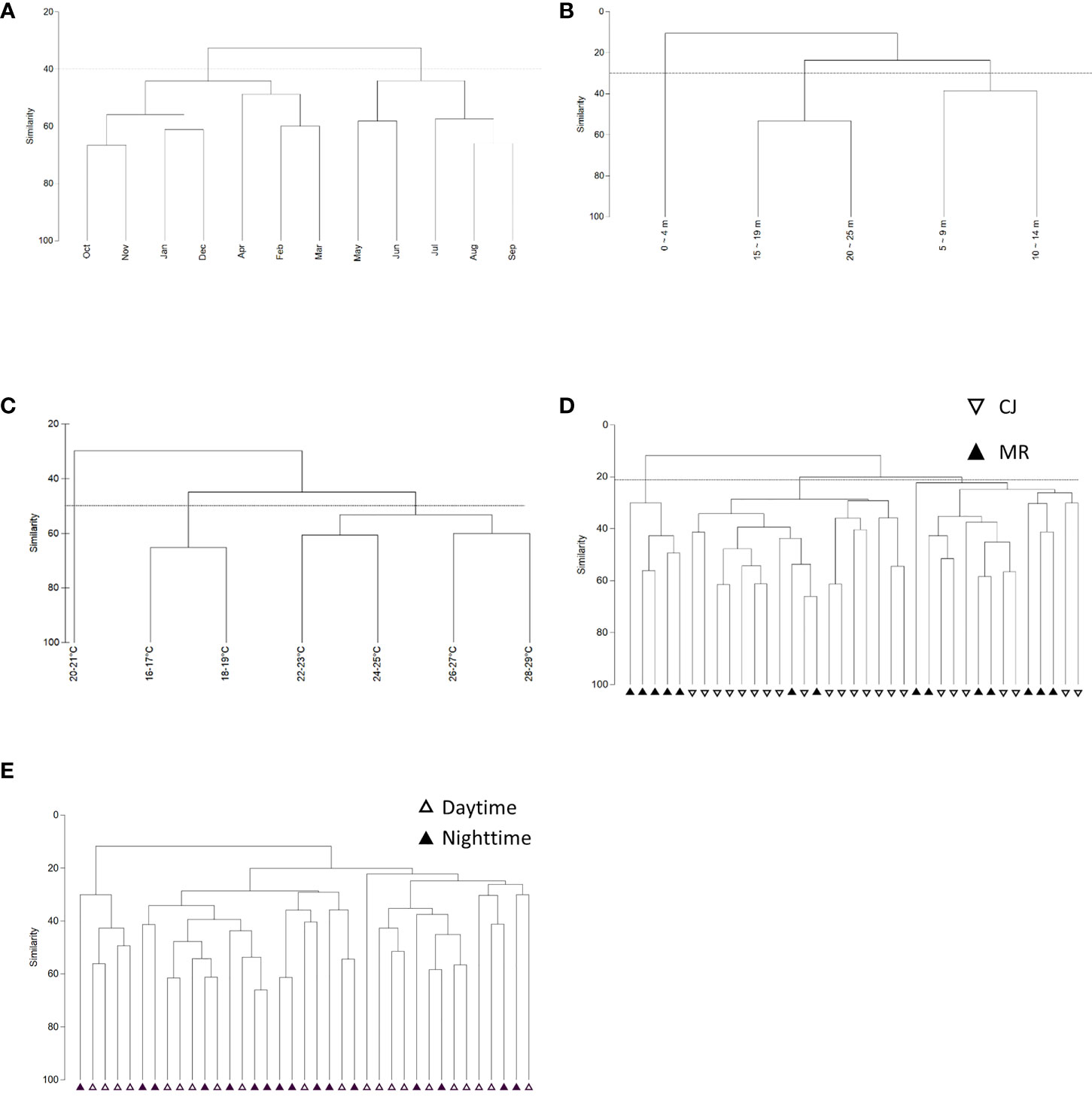
Figure 10 Dendrogram of sea slug assemblages according to (A) month, (B) depth profile, (C) temperature profile, (D) study site, and (E) diel period.
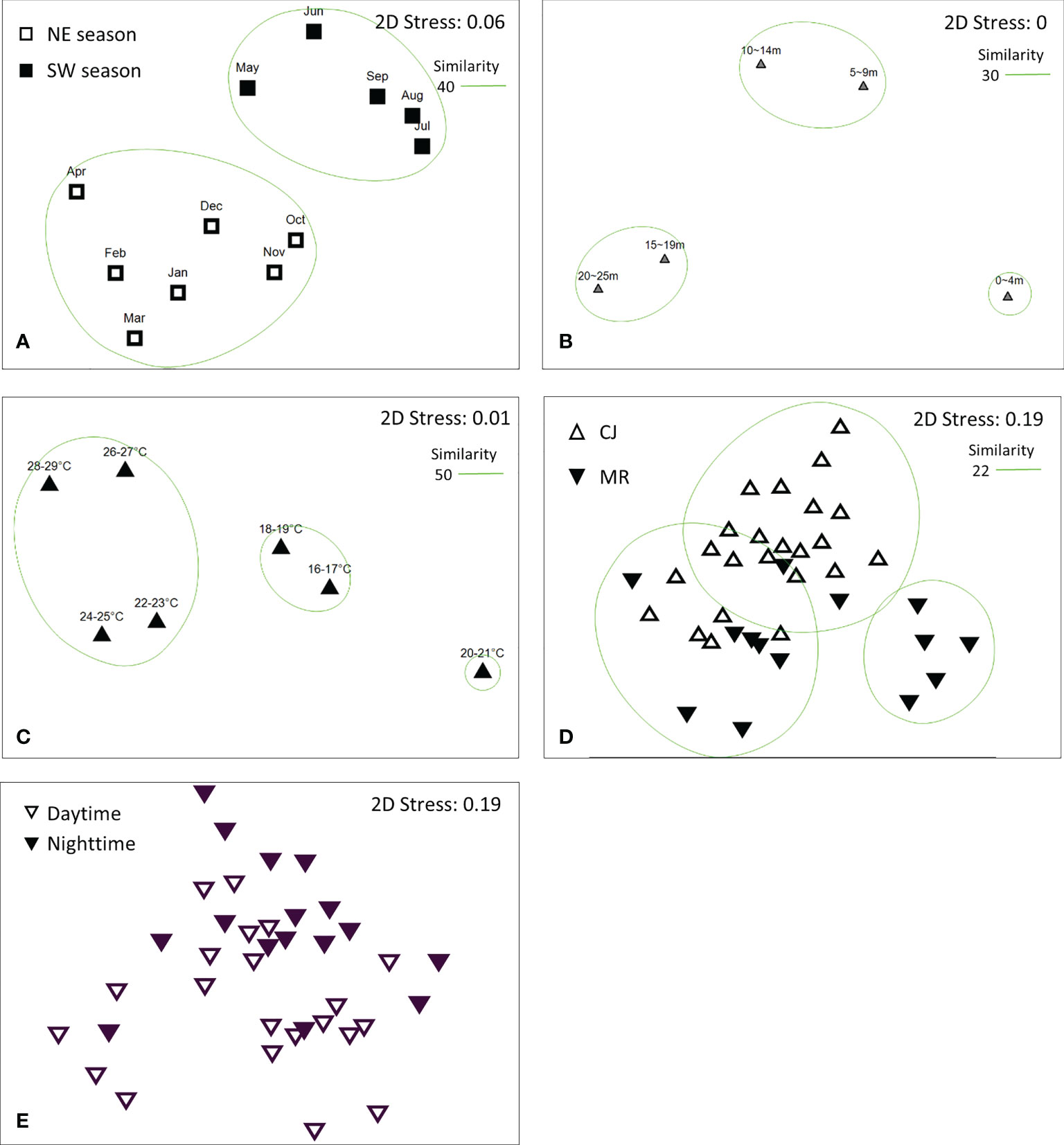
Figure 11 Non-metric multidimensional scaling (NMDS) analysis of sea slug assemblages according to (A) month, (B) depth profile, (C) temperature profile, (D) study site, and (E) diel period.
Intriguingly, sea slug assemblages were not dichotomous for the two survey sites in neither Bray–Curtis (ANOSIM, R = 0.352; p = 0.001) nor Jaccard similarity indexes (ANOSIM, R = 0.225; p = 0.002; Supplementary Data S4). At a similarity level of 22%, Bray–Curtis CLUSTER subdivided the combined sea slug dataset from MR and CJ into three clades, with only some of the MR records from the winter season (Jan – Apr) forming a distinct cluster and site-based ordinations for the other two clades overlapping (Figures 10D; 11D). Moreover, although both species richness and biodiversity differed significantly between daytime and nighttime surveys, the sea slug assemblages did not form distinctly clustered ordinations according to diel period by Bray–Curtis (ANOSIM, R = 0.093; p = 0.032) (Figures 10E, 11E) or Jaccard similarity indexes (ANOSIM, R = 0.077; p = 0.052; Supplementary Data S4).
Discussion
Sea slugs of the northeast Taiwan coast
To date, the species of sea slugs that have been reported from Taiwanese waters represent ~14% of known species worldwide. More species are known to occur in Kenting National Park (at the tropical southern tip of the island) (587 species; Chang, 2012), but at least 291 species have been recorded along the northeastern coast (Chang, 2012). Among the 121 species we recorded in this study, 93 species (c. 76.9%) have been reported previously in this area (Chang, 2012). Additionally, 101 of the species (c. 83.4%) were also found around nearby Okinawa Island, Japan according to the checklists of Ono, 1999; Ono, 2004) and records by a local diving team (Diving team Snack Sunfkin, 2015). However, the consistent species number has been found far lower in the regions close to the mainland. For instance, the species found both in this study and in South Korea (Koh, 2006) or Hong Kong (Chow et al., 2022) represent the ratios of 23.1% and 34.7% (Korea: 28/121; Hong Kong: 42/121) (Supplementary Data S1).
In Taiwan, a marine biogeographical line divides subtropical species from tropical species, which is oriented S to N and is largely defined by ocean currents and water temperature rather than latitude (Figure 1) (Shao et al., 1997). Our sampling sites lie almost directly on this boundary line. We found that the sea slug assemblage of our study site was more similar (based on the Bray-Curtis index) to that of subtropical Taiwan, e.g., the Pescadores Islands (Penghu) (47.5%), than a tropical area, e.g., Kenting National Park (37.7%). Moreover, apart from species such as Phyllidiella pustulosa and Hexabranchus sanguineus that are widely distributed throughout Taiwanese coastal waters, we recorded many species only found around the Pescadores Islands and along the northeast coast, e.g., Tambja pulcherrima, Ceratosoma gracillimum, Ceratosoma trilobatum, Chromodoris orientalis, Chromodoris rubrocornuta, Hypselodoris festiva, Diversidoris flava, Verconia hongkongiensi, Carminodoris estrelyado and Phyllidiopsis loricata (Chang, 2012).
We found that season appeared to be the most important factor influencing species richness and the assemblage of sea slugs at our study sites. Seasonal shifts in species richness have been reported previously for the Mediterranean Sea, where temperature variation was postulated as being the main factor modulating sea slug diversity (Betti et al., 2017). However, similar investigations of subtidal Northern California (Nybakken, 1978) or intertidal Penghu Island, Taiwan (Su et al., 2009) found no evidence of significant seasonality in sea slug species numbers.
We detected a few species only in the NE season, e.g., Hypselodoris tryoni, Bornella anguilla, Siphopteron brunneomarginatum and Sakuraeolis sp.2. Nevertheless, we observed far more sea slug species during the SW season (Figure 12A). Monsoon wind patterns certainly create distinct temperature profiles in this region (Chang et al., 2009), which are important drivers of biogeographical distributions. For instance, it is well known that the monsoon influences the assemblages of coral reef communities, such as fishes (reviewed in Harborne, 2013), benthic crustacea (Kramer et al., 2014), molluscs (Zuschin & Stachowitsch, 2007), and corals (reviewed in Hoey et al., 2016). This may also shift the food items of the area and influence the population of the species with specialized feeding habits in turn (Tanamura & Hirose, 2016). Similarly, the temperature has been identified as the primary factor responsible for seasonal changes in the species richness of sea slugs in the Mediterranean (Betti et al., 2017), and it may also determine their dispersal range. For example, due to climate change, elevated seawater temperatures may extend the range of some tropical or subtropical sea slug species to higher latitudes (Nimbs et al., 2015; Nimbs & Smith, 2016; Nimbs and Smith, 2018; Smith & Nimbs, 2022).
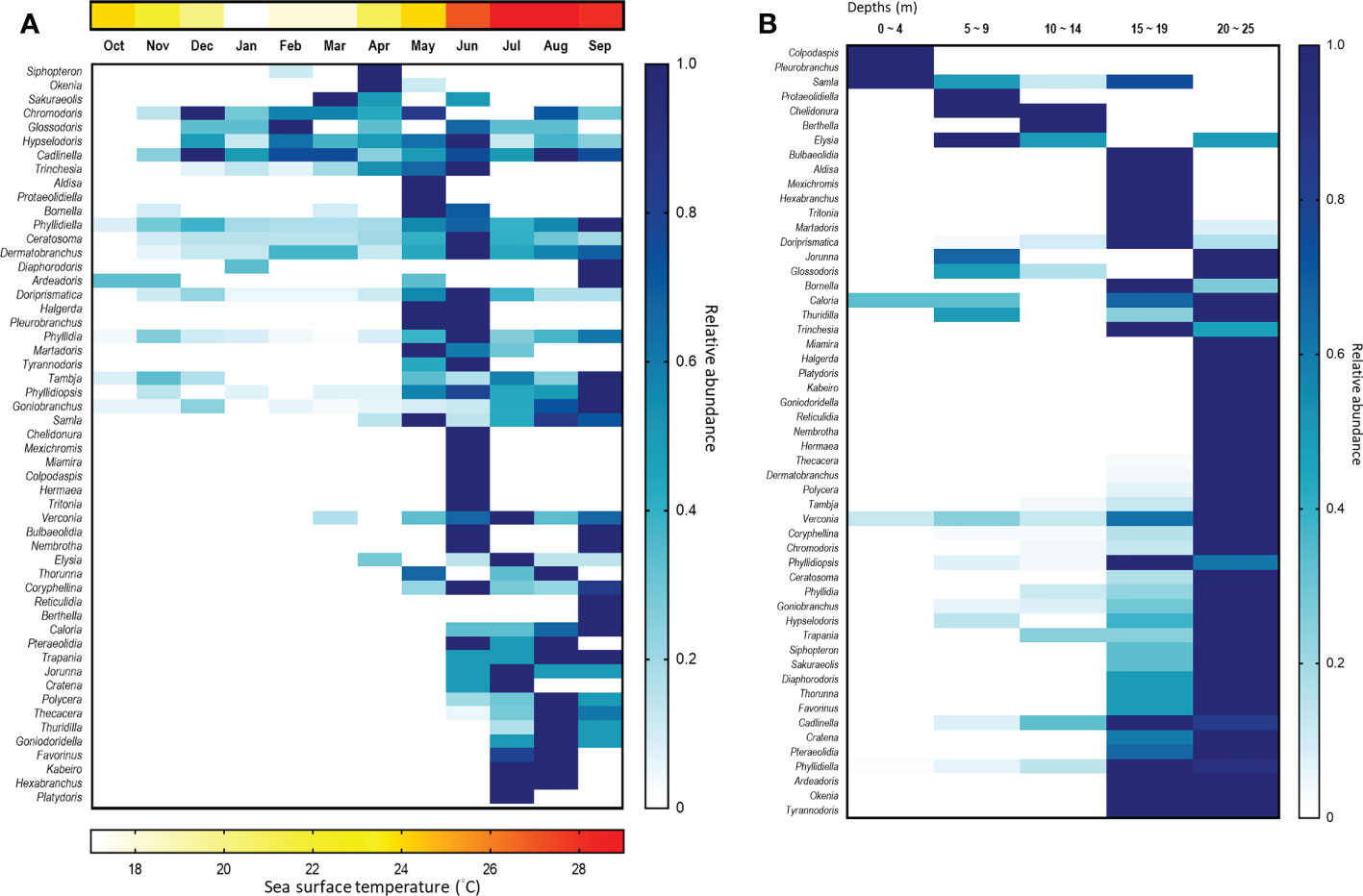
Figure 12 Heatmap of sea slug genera found according to (A) month and (B) depth profile. The blue scale represents the relative density of each genus, which has been normalized against the month with the greatest abundance. The red scale represents the average temperature of each month.
Shifting water masses may also contribute to altered species assemblages between monsoon seasons. In Taiwanese waters, plankton assemblages are strongly defined by the water masses that shift seasonally (Tseng and Shen, 2003; Huang et al., 2004; Chou et al., 2012). For largely sedentary species with a planktonic larval stage, such as coral reef fishes, previous studies have indicated that changing current directions may alter larval recruitment (Chiu & Chen, 1998; Su et al., 2011; Hsieh et al., 2011; Rabbaniha et al., 2013). Significantly, the planktotrophic larval stage is important in most nudibranchs (Clark, 1975), as well as in some sacoglossids (Collin, 2012), for dispersal and exploitation of new habitats (Scheltema, 1986). The changing currents between the monsoon seasons could transport individuals originating from either the north or south, with contributions from currents in both directions potentially giving rise to similar species compositions between the northeastern Taiwanese coast and nearby areas. Nevertheless, the duration of the planktotrophic stage and the settlement mechanism, e.g. habitat preference (Yiu and Qiu, 2022), are also likely critical to sea slug dispersal (reviewed in Todd & Doyle, 1981), but those details remain to be elucidated for many species.
In contrast to our finding of a seasonal pattern of changing sea slug assemblages, there was no clear diurnal variation in species richness or assemblages. A previous study of Green Island (southeastern Taiwan) also revealed no significant impact of diel period on species richness (Chang et al., 2013). Nevertheless, that study found that the total number of individuals was two-fold greater at night than during the day, with cluster analysis showing distinct species compositions between day and night, particularly during the summer. Phyllidiella pustulosa was the dominant species during the daytime at Green Island, but Tritonia sp. was the most frequently recorded species at night (Chang et al., 2013). Similar diel variation was also uncovered by a study of Port Stephens (southeastern coast of Australia), in which more sea slug individuals were observed at night than during the day (Larkin et al., 2018). That same study reported daily variation in the activity of the dominant species, Bulla sp., which was only recorded at night.
Our results also showed that sea slug species richness and assemblages vary across the water depth we sampled. Most species were found at depths >15 m, and sea slugs appear to be rare at 0 – 4 m in our study sites. Accordingly, the species assemblages varied across depths (Figure 12B). Intriguingly, these water depth preferences did not appear consistent within a genus. For example, Samla bicolor was the dominant species at 0 – 4 m, representing >57% of the records from that depth profile, but it was not found deeper than 10 m. In contrast, Samla rubropurpurata only inhabited depths of >15 m. Our field observations indicate that both these Samla species feed on hydroids, but may target different species to enable resource partitioning.
Water temperature is lower at greater depth (Figure 2) (Supplementary Data S3). However, compared to seasonal temperature variation, temperature seems to vary less as depth from the surface increases, especially in subtropical regions (de Boyer Montégut et al., 2004). Notably, light penetration in ocean water is greatly influenced by depth, as water molecules scatter and absorb sunlight, altering spectral bandwidth and reducing light intensity (Pope and Fry, 1997). Optical properties are known to influence diverse biogeochemical processes that determine the primary productivity of an ecosystem and also impact the activities of marine organisms (Mascarenhas & Keck, 2018), potentially contributing to vertical species stratification. In addition, we found that few sea slugs were present at 0 – 4 m during the NE season (Figure 7). Shallow water habitats are at higher risk of exposure to wave-mediated mechanical disturbances compared to those in deep water (reviewed in Smith et al., 2019). During the NE season, onshore winds may exacerbate this physical disturbance in the low tidal zone (Huang et al., 2004), which may further reduce sea slug abundance in shallow water.
We found that the non-protected area MR, had lower species richness than CJ, but exhibited higher species evenness and overall biodiversity due to the high abundance of dominant species than in the MPA site. Marine protected areas (MPA) are essential to conserving oceanic biodiversity and maintaining local biological resources (reviewed in Kelleher, 1999). When managed effectively, MPAs promote both biodiversity and living biomass within them (reviewed in Topor et al., 2019; Zhao et al., 2020). CJ has been designated a no-take protected area since 2016, in which the strict regulations do help to recover the reef community in this area, e.g. fish abundance, (TEIA, 2018; Huang, 2020). However, the low hunting pressure may also benefit the growth of dominant species population. On the other hand, human impacts are still non-negligible in CJ. According to records taken by an onshore surveillance camera (Keelung City Smart Control Platform: https://keelung.leotekiot.com/), 70440 visitors went diving at CJ across the diving season (SW) in 2020. Such a high density of human activity undoubtedly exerts pressure on the local ecosystem. Previous studies of other MPAs have indicated that SCUBA diving is a potential stressor that may impact coral reefs and sediment, independently of the level of training or experience of the diver (Di Franco et al., 2009; Luna et al., 2009). Our study did not find firm evidence of a particular influence of human activities on the sea slug community at CJ, but it should still be considered in any management plan for the MPA.
In summary, our study showed that the sea slug composition on the northeast coast of Taiwan was higher in similarity to the species recorded in the adjacent sites of East Asia Island Arcs than what was found in the coastal waters of the mainland. Furthermore, on a smaller geographic scale, we found that the species assemblage in the study area was more consistent with the sea slug assemblage in the subtropical Pescadores Islands than in the tropical Kenting National Park. A seasonal shift of currents which results in the differences in water temperature may involve the dispersal pattern of sea slugs by transporting pelagic larvae. In this study, sea slug communities displayed high diversity, exhibiting distinctive assemblages according to monsoon season. Water depth and site represented two important factors contributing to the spatial variation of sea slugs. Monsoon season and water depth may drive differences in temperature and food sources that, in turn, impact community structure (Figure 13). We hypothesized that divergent levels of physical disturbance and human activities among depths and/or sites may influence species abundance and richness further. Our study highlights that surveying across seasons is necessary to describe the actual diversity and abundance of heterobranch sea slugs in subtropical monsoon regions. Moreover, the depth profile should also be considered in assessments of sea slug biodiversity. Additionally, long-term monitoring is required to illustrate how MPAs contribute to sea slug biodiversity and that of other cryptic taxa.
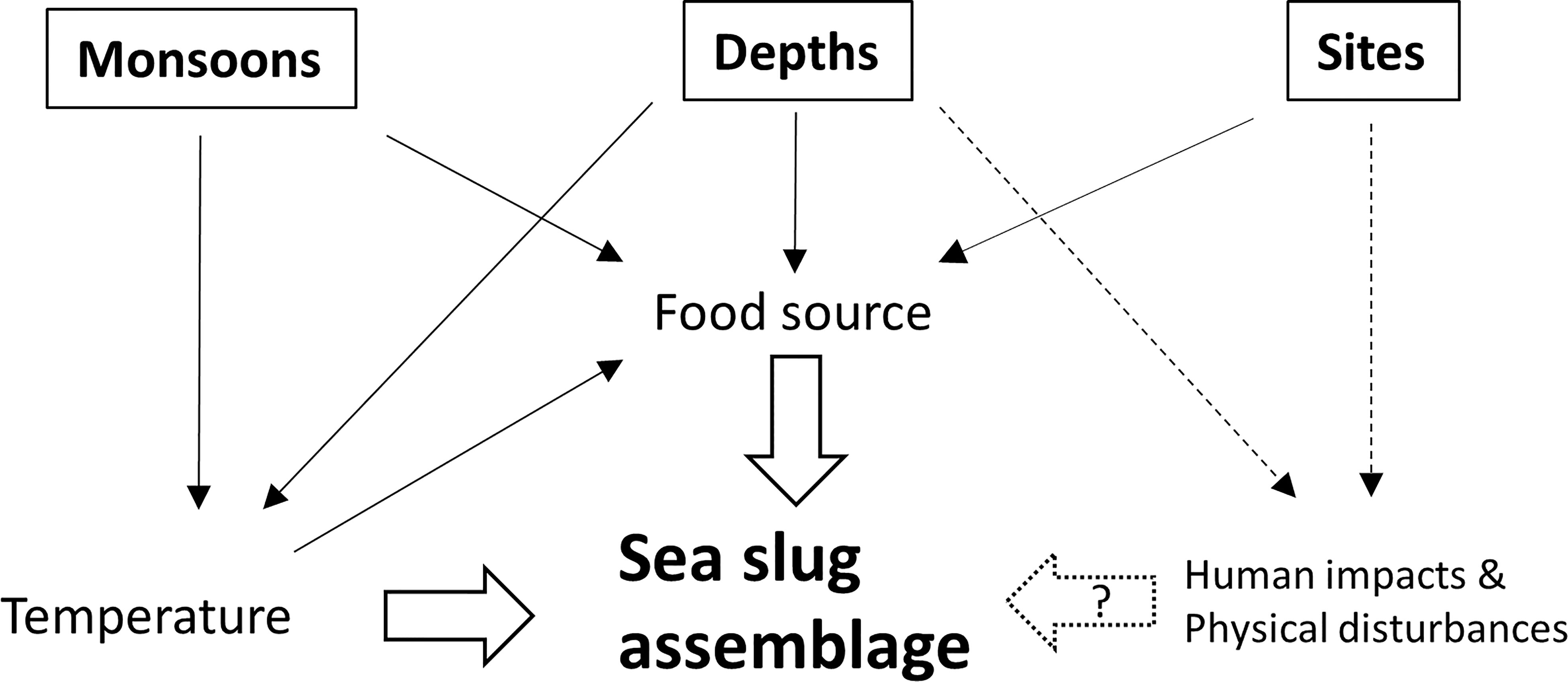
Figure 13 Schematic diagram of the potential effects of temperature, food sources, human impacts and physical disturbances on sea slug assemblages across different seasons, depths and sites. The dash lines indicate the hypothesized effects.
Data availability statement
The original contributions presented in the study are included in the article/Supplementary Material. Further inquiries can be directed to the corresponding author.
Author contributions
YS, HC, L-SC, and Y-WC conceived and designed the experiments. YS, HC, Y-WC and KN performed the experiments. HC and YS analyzed the data. YS, HC, KN and Y-WC wrote the manuscript. All authors contributed to the article and approved the submitted version.
Funding
This study was supported by grants from the Ministry of Science and Technology, Taiwan (108-2621-M-019-002-MY3 and 109-2621-M-019-001) to YS, (108-2637-B-346-001) to Y-WC.
Acknowledgments
We very much appreciate the support from the Center of Excellence for the Oceans and Intelligent Maritime Research Center, National Taiwan Ocean University, from its Featured Areas Research Center Program within the framework of the Higher Education Sprout Project by the Ministry of Education (MOE) in Taiwan. We would like to thank the government of Keelung City, National Museum of Marine Science and Technology, and the Changtan Village Inspection Office of the Coast Guard Administration for assistance. We appreciate the Central Weather Bureau of Taiwan for kindly providing temperature data. The article was improved by comments from three reviewers and the manuscript was edited for language usage by Dr. John O’Brien.
Conflict of interest
The authors declare that the research was conducted in the absence of any commercial or financial relationships that could be construed as a potential conflict of interest.
Publisher’s note
All claims expressed in this article are solely those of the authors and do not necessarily represent those of their affiliated organizations, or those of the publisher, the editors and the reviewers. Any product that may be evaluated in this article, or claim that may be made by its manufacturer, is not guaranteed or endorsed by the publisher.
Supplementary material
The Supplementary Material for this article can be found online at: https://www.frontiersin.org/articles/10.3389/fmars.2022.1042961/full#supplementary-material
Supplementary Data Sheet 1 | Sea surface temperature data log.
Supplementary Data Sheet 2 | Checklist of the species recorded in this study.
Supplementary Data Sheet 3 | Data log of depths and underwater temperature.
Supplementary Data Sheet 4 | Dendrogram and Non-metric multidimensional scaling (NMDS) analysis of sea slug assemblages according to (A) study site and (B) diel period, using Jaccard qualitative similarity index.
Supplementary Data Sheet 5 | Sea slug records across seasons.
Supplementary Data Sheet 6 | Sea slug records across depth profiles.
Supplementary Data Sheet 7 | Sea slug records for the two sites.
References
Artüz M. L., Tunçer S., Poursanidis D. (2018). Contributions to the biodiversity of sea slugs and shelled relatives of the Sea of marmara (Mollusca: Gastropoda). Cahiers Biologie Mar. 59, 267–276. doi: 10.21411/CBM.A.88763B1A
Béguinot J. (2019). Influence of environmental heterogeneity on the species composition, species richness and species abundances unevenness in reef-associated conus communities (Neogastropoda) from Papua new-Guinea. Asian J. Environ. Ecol. 10 (3), 1–21. doi: 10.9734/ajee/2019/v10i330116
Behrens D. W. (2005). Nudibranch behaviour (Jacksonville, FL., U.S.A: New World Publications, Inc.).
Betti F., Bava S., Cattaneo-Vietti R. (2017). Composition and seasonality of a heterobranch assemblage in a sublittoral, unconsolidated, wave-disturbed community in the Mediterranean Sea. J. Molluscan Stud. 83 (3), 325–332. doi: 10.1093/mollus/eyx019
Błażewicz M., Jóźwiak P., Menot L., Pabis K. (2019). High species richness and unique composition of the tanaidacean communities associated with five areas in the pacific polymetallic nodule fields. Prog. Oceanog. 176, 102141. doi: 10.1016/j.pocean.2019.102141
Burghardt I., Carvalho R., Eheberg D., Gerung G., Kaligis F., Mamangkey G., et al. (2006). Molluscan diversity at bunaken national park, sulawesi. Linn. Wallacea 2, 29–43.
Chang Y. W. (2012). “Study on the biodiversity of opisthobranchs in Taiwan and adjacent islands,” in Master thesis (Kaohsiung, Taiwan: National Sun Yat-sen University).
Chang Y. W., Chen T. C., Willan R. C., Mok H. K., Yu M. H. (2013). Diel variation affects estimates of biodiversity and abundance of nudibranch (Gastropoda) faunas. Nautilus 127 (1), 19–28.
Chang Y., Shimada T., Lee M. A., Lu H. J., Sakaida F., Kawamura H. (2006). Wintertime sea surface temperature fronts in the Taiwan strait. Geophys. Res. Lett. 33 (23), L23603. doi: 10.1029/2006GL027415
Chang Y. L., Wu C. R., Oey L. Y. (2009). Bimodal behavior of the seasonal upwelling off the northeastern coast of Taiwan. J. Geophys. Res.: Ocean. 114, C03027. doi: 10.102008JC005131
Chen J. M., Li T., Shih C. F. (2010). Tropical cyclone–and monsoon-induced rainfall variability in Taiwan. J. Climate 23 (15), 4107–4120. doi: 10.1175/2010JCLI3355.1
Chihara S., Nakamura T., Hirose E. (2020). Seasonality and longevity of the functional chloroplasts retained by the sacoglossan sea slug Plakobranchus ocellatus van hasselt 1824 inhabiting a subtropical back reef off Okinawa-jima island, Japan. Zool. Stud. 59, 65. doi: 10.6620/ZS.2020.59-65
Chiu T. S., Chen C. S. (1998). A study on the status of ichthyoplankton in the waters around Taiwan. J. Fish. Soc. Taiwan 25 (3), 161–170. doi: 10.29822/JFST.199809.0001
Chou C., Tseng L. C., Ou C. H., Chen Q. C., Hwang J. S. (2012). Seasonal succession of planktonic copepods in bight environments of northeastern Taiwan. Zool Stud. 51 (8), 1380–1396.
Chow L. H., Yu V. P. F., Kho Z. Y., See G. C. L., Wang A., Baker D. M., et al. (2022). An updated checklist of sea slugs (Gastropoda, heterobranchia) from Hong Kong supported by citizen science. Zool. Stud. 61, 52. doi: 10.3897/zookeys.1042.64474
Clark K. B. (1975). Nudibranch life cycles in the Northwest Atlantic and their relationship to the ecology of fouling communities. Helgoländer Wissenschaftliche Meeresuntersuchungen Plymouth Marine Laboratory 27 (1), 28–69. doi: 10.1007/BF01611686
Clarke K. R. (1993). Non-parametric multivariate analyses of changes in community structure. Aust. J. Ecol. 18 (1), 117–143. doi: 10.1111/j.1442-9993.1993.tb00438.x
Clarke K. R., Gorley R. N. (2006). PRIMER V6: User Manual/Tutorial. 192, PRIMER-e (Plymouth, US: Plymouth Marine Laboratory).
Clarke K. R., Warwick R. M. (2001). Change in marine communities. An approach to statistical analysis and interpretation (Plymouth, U.K: Natural Environment Research Council) 2, p. 1–68.
Collin R. (2012). Nontraditional life-history choices: what can “intermediates” tell us about evolutionary transitions between modes of invertebrate development? Integr. Comp. Biol. 52 (1), 128–137. doi: 10.1093/icb/ics065
de Boyer Montégut C., Madec G., Fischer A. S., Lazar A., Iudicone D. (2004). Mixed layer depth over the global ocean: An examination of profile data and a profile-based climatology. J. Geophys. Res.: Ocean. 109, C12003. doi: 10.1029/2004JC002378
Di Franco A., Milazzo M., Baiata P., Tomasello A., Chemello R. (2009). Scuba diver behaviour and its effects on the biota of a Mediterranean marine protected area. Environ. Conserv. 36 (1), 32–40. doi: 10.1017/S0376892909005426
Diving team Snack Sunfkin (2015). Marine species of oura bay. 123 (Kagoshima, Japan: Nanpou Shinsya Kagoshima).
Farrell J., Saloner G. (1985). Standardization, compatibility, and innovation. RAND J. Econ. 16, 70–83. doi: 10.2307/2555589
Gochfeld D., Aeby G. (1997). Control of populations of the coral-feeding nudibranch Phestilla sibogae by fish and crustacean predators. Mar. Biol. 130, 63–69. doi: 10.1007/s002270050225
Gosliner T. M. (2001). Aposematic coloration and mimicry in opisthobranch mollusks: new phylogenetic and experimental data. Bollettino malacologico 37, 163–170.
Gosliner T. M., Valdés Á., Behrens D. W. (2018). Nudibranch & sea slug identification: Indo-pacific (Jacksonville, Florida, US: New World Publications).
Harborne A. R. (2013). The ecology, behaviour and physiology of fishes on coral reef flats, and the potential impacts of climate change. J. Fish Biol. 83 (3), 417–447. doi: 10.1111/jfb.12203
Heip C. H., Herman P. M., Soetaert K. (1998). Indices of diversity and evenness. Oceanis 24 (4), 61–88.
Hoey A. S., Howells E., Johansen J. L., Hobbs J. P. A., Messmer V., McCowan D. M., et al. (2016). Recent advances in understanding the effects of climate change on coral reefs. Diversity 8 (2), 12. doi: 10.3390/d8020012
Hsieh H. Y., Lo W. T., Wu L. J., Liu D. C., Su W. C. (2011). Comparison of distribution patterns of larval fish assemblages in the Taiwan strait between the northeasterly and southwesterly monsoons. Zool. Stud. 50 (4), 491–505.
Huang C. Y. (2020). Reef check result of chaojin in 2020 (Taipei, Taiwan: Taiwan Environmental Information Association). Available at: https://teia.tw/ocean_lastestpost/2020-潮境公園珊瑚.
Huang W. P., Kung C. S., Yim J. Z., Chou C. R. (2004). Wave climate study of the northeast taiwan. in the sixth ISOPE Pacific/Asia offshore mechanics symposium. OnePetro. ISOPE-P-04-027.
Hu J., Kawamura H., Hong H., Qi Y. (2000). A review on the currents in the south China Sea: seasonal circulation, south China Sea warm current and kuroshio intrusion. J. Oceanog. 56 (6), 607–624. doi: 10.1023/A:1011117531252
Hussain A., Bhave V., Zari A., Apte D., Ingole B., Nanajkar M. (2022). Records of Sea slug fauna (Gastropoda: Heterobranchia) from the shores of goa, Eastern Arabian Sea. Thalassas: Int. J. Mar. Sci., 1–15. doi: 10.1007/s41208-022-00430-8
Hu J., Zhang Y., Xie J. Y., Qiu J. W. (2020a). A new species of predatory nudibranch (Gastropoda: Trinchesiidae) of the coral pavona decussata. Zool. Stud. 59, 30. doi: 10.6620/ZS.2020.59-30
Hu J., Zhang Y., Yiu S. K. F., Xie J. Y., Qiu J. W. (2020b). A new species of predatory nudibranch (Gastropoda: Trinchesiidae) of the scleractinian coral goniopora. Zool. Stud. 59, 62. doi: 10.6620/ZS.2020.59-62
Inoue T., Yaida Y. A., Uehara Y., Katsuhara K. R., Kawai J., Takashima K., et al. (2021). The effects of temporal continuities of grasslands on the diversity and species composition of plants. Ecol. Res. 36 (1), 24–31. doi: 10.1111/1440-1703.12169
Kaligis F., Eisenbarth J. H., Schillo D., Dialao J., Schäberle T. F., Böhringer N., et al. (2018). Second survey of heterobranch sea slugs (Mollusca, Gastropoda, heterobranchia) from bunaken national park, north sulawesi, Indonesia-how much do we know after 12 years? Mar. Biodiversity Records 11 (1), 1–20. doi: 10.1186/s41200-018-0136-3
Kelleher G. (1999). Guidelines for marine protected areas. 1-53 (Switzerland and Cambridge, UK: IUCN, Gland).
Kramer M. J., Bellwood D. R., Bellwood O. (2014). Benthic Crustacea on coral reefs: a quantitative survey. Mar. Ecol. Prog. Ser. 511, 105–116. doi: 10.3354/meps10953
Larkin M. F., Smith S. D. A., Willan R. C., Davis T. R. (2018). Diel and seasonal variation in heterobranch sea slug assemblages within an embayment in temperate eastern Australia. Mar. Biodiversity 48, 1541–1550. doi: 10.1007/s12526-017-0700-9
Lin S. M., Tseng L. C., Ang P. O., Bolton J., Liu L. C. (2018). Long-term study on seasonal changes in floristic composition and structure of marine macroalgal communities along the coast of northern Taiwan, southern East China Sea. Mar. Biol. 165 (5), 1–17. doi: 10.1007/s00227-018-3344-9
Lock K., Newman P., Burton M. (2010). “Skomer marine nature reserve nudibranch diversity survey 2010,” in CCW regional report(Countryside Council for Wales, UK: Plymouth Marine Laboratory).
Luna B., Pérez C. V., Sánchez-Lizaso J. L. (2009). Benthic impacts of recreational divers in a Mediterranean marine protected area. ICES J. Mar. Sci. 66 (3), 517–523. doi: 10.1093/icesjms/fsp020
Mascarenhas V., Keck T. (2018). “Marine optics and ocean color remote sensing,” in YOUMARES 8–oceans across boundaries: Learning from each other, vol. 41. (Verlag, Cham: Springer).
Nakano R. (2018). Field guide to sea slugs and nudibranchs of Japan (Tokyo. Japan: Bun-ichi Co., Ltd.).
Nimbs M. J., Smith S. D. (2016). Welcome strangers: Southern range extensions for seven heterobranch sea slugs (Mollusca: Gastropoda) on the subtropical east Australian coast, a climate change hot spot. Region. Stud. Mar. Sci. 8, 27–32. doi: 10.1016/j.rsma.2016.08.008
Nimbs M. J., Smith S. D. (2017). An illustrated inventory of the sea slugs of new south Wales, Australia, (Gastropoda: Heterobranchia). Proc. R. Soc. Victoria 128 (2), 44–113. doi: 10.1071/RS16011
Nimbs M. J., Smith S. D. (2018). Beyond capricornia: tropical sea slugs (Gastropoda, heterobranchia) extend their distributions into the Tasman Sea. Diversity 10 (3), 99. doi: 10.3390/d10030099
Nimbs M. J., Willan R. C., Smith S. D. (2015). Range extensions for heterobranch sea slugs (formerly opisthobranch) belonging to the families diaphanidae, plakobranchidae and facelinidae on the eastern coast of Australia Vol. 8 (Plymouth, UK: Marine Biodiversity Records).
Nybakken J. (1978). Abundance, diversity and temporal variability in a California intertidal nudibranch assemblage. Mar. Biol. 45, 129–146. doi: 10.1007/BF00390549
Ono A., Kato S. (2020). Nudibranchs & Sea slug illustrated (Toyko, Japan: Seibundo Shinkosha Publishing).
Papu A., Undap N., Martinez N. A., Segre M. R., Datang I. G., Kuada R. R., et al. (2020). First study on marine heterobranchia (Gastropoda, Mollusca) in bangka archipelago, north sulawesi, Indonesia. Diversity 12, 2, 52. doi: 10.3390/d12020052
Pierce S. K., Curtis N. E., Middlebrooks M. L. (2015). Sacoglossan sea slugs make routine use of photosynthesis by a variety of species-specific adaptations. Invertebrate Biol. 134 (2), 103–115. doi: 10.1111/ivb.12082
Pope R. M., Fry E. S. (1997). Absorption spectrum (380–700 nm) of pure water. II. Integrating cavity measurements. Appl. optics 36 (33), 8710–8723. doi: 10.1364/ao.36.008710
Rabbaniha M., Dehghan S., Seraji F., Norinezhad M., Mohammadkezhad J., Oofi F. (2013). “Review and show distribution pattern of marine fish larvae of Iranian coastal waters Persian gulf by GIS,” in Agricultural research (Tehran, Iran: Education & Extension Organization Iranian Fisheries Research Organization).
Richards Donà A., Evertsen J., Johnsen G. (2022). The role of parapodia and lack of photoacclimation in kleptoplasts of the sacoglossan sea slug plakobranchus ocellatus. Coral Reefs 41 (2), 319–332. doi: 10.1007/s00338-022-02224-z
Russell W. M. S., Burch R. L. (1959). The principles of humane experimental technique (London, UK: Methuen Publishing).
Scheltema R. S. (1986). On dispersal and planktonic larvae of benthic invertebrates: an eclectic overview and summary of problems. Bull. Mar. Sci. 39 (2), 290–322.
Shao K. T., Chen J. P., Wang S. C. (1997). “Biogeography and database of marine fishes in Taiwan waters,” In: Proceedings of the 5th Indo-Pacific Fish Conference, Noumea (Paris: Société française d'ichtyologie). 673–680.
Smith S. D. A., Davis T. R. (2019). Slugging it out for science: Volunteers provide valuable data on the diversity and distribution of heterobranch sea slugs. Molluscan Res. 39, 214–223. doi: 10.1080/13235818.2019.1594600
Smith T. B., Holstein D. M., Ennis R. S. (2019). “Disturbance in mesophotic coral ecosystems and linkages to conservation and management,” in Mesophotic coral ecosystems (Cham: Springer), 911–929.
Smith S. D., Nimbs M. J. (2022). Citizen scientists record significant range extensions for tropical Sea slug species in subtropical Eastern Australia. Diversity 14 (4), 244. doi: 10.3390/d14040244
Su Y., Huang L. J., Chang Y. W., MOK H. K. (2009). Temporal changes in nudibranch composition at a coastal site off penghu (the pescadores) in the Taiwan strait. Zool. Stud. 48, 448–459.
Su W. C., Lo W. T., Liu D. C., Wu L. J., Hsieh H. Y. (2011). Larval fish assemblages in the kuroshio waters east of Taiwan during two distinct monsoon seasons. Bull. Mar. Sci. 87 (1), 13–29. doi: 10.5343/bms.2010.1010
Tanamura D., Hirose E. (2016). Population dynamics of the sea slug Plakobranchus ocellatus (Opisthobranch: Sacoglossa: Elysioidea) on a subtropical coral reef off Okinawa-jima island, Ryukyu archipelago, Japan. Zool. Stud. 55, 42. doi: 10.6620/ZS.2016.55-42
TEIA. (2018). Annual report of reef check results of Taiwan (Taipei, Taiwan: Taiwan Environmental Information Association). Available at: https://www.slideshare.net/reefcheck/2018-146707832.
Todd C. D., Doyle R. W. (1981). Reproductive strategies of marine benthic invertebrates: a settlement-timing hypothesis. Mar. Ecol. Prog. Ser. 4 (7), 75–83. doi: 10.3354/meps004075
Togawa Y. (2021). Studies on cnidophage, specialized cell for kleptocnida, of pteraeolidia semperi (Mollusca: Gastropoda: Nudibranchia) (University of Tsukuba, Ibaraki, Japan: PhD Thesis).
Topor Z. M., Rasher D. B., Duffy J. E., Brandl S. J. (2019). Marine protected areas enhance coral reef functioning by promoting fish biodiversity. Conserv. Lett. 12 (4), e12638. doi: 10.1111/conl.12638
Tseng R. S., Shen Y. T. (2003). Lagrangian Observations of surface flow patterns in the vicinity of Taiwan. Deep Sea Res. Part II: Top. Stud. Oceanog. 50 (6-7), 1107–1115. doi: 10.1016/S0967-0645(03)00012-2
Urra J., Ramírez Á.M., Marina P., Salas C., Gofas S., Rueda J. L. (2013). Highly diverse molluscan assemblages of posidonia oceanica meadows in northwestern alboran Sea (W mediterranean): seasonal dynamics and environmental drivers. Estuar. Coast. Shelf Sci. 117, 136–147. doi: 10.1016/j.ecss.2012.11.005
Yiu S. K. F., Qiu J. W. (2022). Morphology, feeding rate and larval settlement preference of the corallivorous nudibranch Phestilla subodiosa (Nudibranchia: Trinchesiidae) from Hong Kong. Zool. Stud. 61, 59.
Zhao Q., Stephenson F., Lundquist C., Kaschner K., Jayathilake D., Costello M. J. (2020). Where marine protected areas would best represent 30% of ocean biodiversity. Biol. Conserv. 244, 108536. doi: 10.1016/j.biocon.2020.108536
Keywords: sea slug, monsoon, diel period, depth, temperature
Citation: Chan HY, Chang Y-W, Chen L-S, Nishida K and Shao YT (2022) The effect of environmental factors on spatial-temporal variation of heterobranch sea slug community in northern Taiwan. Front. Mar. Sci. 9:1042961. doi: 10.3389/fmars.2022.1042961
Received: 13 September 2022; Accepted: 24 October 2022;
Published: 10 November 2022.
Edited by:
Xiaojun Yan, Zhejiang Ocean University, ChinaReviewed by:
Andrea Oliveira Ribeiro Junqueira, Federal University of Rio de Janeiro, BrazilBenny K. K. Chan, Academia Sinica, Taiwan
Pedro Medina-Rosas, University of Guadalajara, Mexico
Copyright © 2022 Chan, Chang, Chen, Nishida and Shao. This is an open-access article distributed under the terms of the Creative Commons Attribution License (CC BY). The use, distribution or reproduction in other forums is permitted, provided the original author(s) and the copyright owner(s) are credited and that the original publication in this journal is cited, in accordance with accepted academic practice. No use, distribution or reproduction is permitted which does not comply with these terms.
*Correspondence: Yi Ta Shao, aXRzaGFvQG1haWwubnRvdS5lZHUudHc=