- 1MARUM, Center for Marine Environmental Sciences, University of Bremen, Bremen, Germany
- 2Marine Geochemistry, Alfred-Wegener-Institut, Helmholtz Zentrum für Polar- und Meeresforschung, Bremerhaven, Germany
- 3Department of Physics and Earth Sciences, Jacobs University Bremen, Bremen, Germany
- 4Geosciences Department, University of Bremen, Bremen, Germany
To gain more insight into the effect of early diagenetic processes in different redox environments on the molecular characteristics of degradation resistant particulate organic matter, the molecular cyst wall characteristics of the closely related dinoflagellates Impagidinium aculeatum and I. patulum from oxic and anoxic sedimentary environments in the Madeira Abyssal Plain F-turbidite and in the surface sediments of the eastern Mediterranean Sea have been analysed with micro-FTIR spectroscopy. Both species have cysts that are representatives of particulate organic matter being extremely resistant against degradation in natural environments. Their walls primarily consist of a carbohydrate-based polymer. Spectral differences could not be observed between cysts from oxic and anoxic environments, neither from different areas nor from different geological times. However, we identify consistent compositional differences between both species. Compared to I. patulum, cyst walls of I. aculeatum contain more C-O probably of secondary alcohols that might imply a slightly higher resistance to decay of I. patulum. Comparison with cyst species that represent particular organic matter being extremely sensitive and slightly resistant against aerobic degradation in natural settings reveals that cysts walls of the most vulnerable species show C=N, N-H, N-O, C-N bending/stretching, as well as the presence of C=O and C-O bounds. Cyst species that are somewhat less sensitive have a strong indication of the presence of nitrogen in their macromolecules. More resistant species lack nitrogen whereas the most resistant cyst species I. aculeatum and I. patulum show low amounts of C=O.
Introduction
The quality of organic carbon being exported from the ocean surface as well as the depositional redox environment form key elements in the process of carbon sequestration by the biological carbon pump (e.g., Zonneveld et al., 2010; Weber and Bianchi, 2020). Organic carbon included in particulate organic matter (POM) is sequestered in the ocean as a result of a complex mixture of biological oxidation and diagenetic change (e.g., Arndt et al., 2013; Landry et al., 2017). These processes are selective, leading to preferential degradation of the more labile components (e.g., Middelburg, 2018). As a result, organic matter becomes less reactive with water depth such that a considerable part of the POM reaching the deep-sea sediments is relatively recalcitrant (Middelburg, 2019 and references therein). At the sediment-water interface and in the upper sediments, degradation times increase by orders of magnitude compared to those within the water column so that even of relatively degradation-resistant material, only a small fraction escapes mineralization and becomes buried in the accumulating sediment. Oxygen is an important electron acceptor required in the process of mineralization of organic carbon. Bottom water and upper sediment oxygen concentrations are of major importance steering the composition of the microbial and benthic degrader communities. However, oxygen is not the only electron acceptor in sediments but redox processes with a lower energy yield (e.g., nitrate reduction, manganese reduction, iron reduction and sulphate reduction) also accept electrons from organic matter (OM) and take part in its selective degradation (e.g., Kasten et al., 2003; Canfield and Thamdrup, 2009). Although much progress has been made in investigating the OM composition change due to early diagenetic biological, chemical and physical processes (e.g., Hedges and Prahl, 1993; Wakeham et al., 1997; Nierop et al., 2017; Zonneveld et al., 2019), information on the change in molecular characteristics is still limited, especially with respect to the recalcitrant sedimentary organic matter (kerogen). To obtain more insight into these changes, it is important to investigate the effect of early diagenetic processes of different redox environments on the molecular characteristics of such particles. A way to do this is by studying organic microfossils with a characteristic morphology of which the biological affinities are closely constrained; such is the case in dinoflagellate cysts. In accordance with organic matter degradation in general, dinoflagellate cyst degradation appears highly selective (e.g., Zonneveld et al., 1997; Gray et al., 2017). The cysts are structurally diverse with some species being highly labile, whereas others are among the most recalcitrant OM components or show intermediate lability (Versteegh and Zonneveld, 2002; Zonneveld et al., 2019). Many phototrophic species consist of a cellulose-like glucan and are very resistant to early diagenetic decay. The cysts of heterotrophic species consist of a nitrogen-rich glycan which is much more sensitive (Bogus et al., 2014). But there are also clear differences within these groups (e.g., Bogus et al., 2012; Bogus et al., 2014; Gurdebeke et al., 2018). This large species specific variability in sensitivity, combined with their narrowly defined origin, make dinoflagellate cysts very suitable as model POM components with different degradability. In order to shed more light on the influence of the redox environment on the dinoflagellate cyst chemical structure, we analyzed two dinoflagellate cysts with large morphological similarity from different redox sedimentary environments in the Madeira Abyssal Plain F-turbidite and in the surface sediments of the eastern Mediterranean Sea Discovery Basin with Attenuation Total Reflection (ATR) micro-Fourier transform infrared spectroscopy (micro-FTIR).
Both the Madeira Abyssal Plain F-turbidite and sediments from the eastern Mediterranean Discovery Basin provide unique ‘laboratories’ to investigate the long-term effects of in-situ post-depositional anaerobic and aerobic degradation. Within these environments, the effect of different redox conditions can be studied without other factors influencing POM degradation such as the POM flux and composition, sedimentation rates, temperature, rates of encapsulation and sorption of the organic matter. The MAP F-turbidite consists of the distal part of a turbidite that originated at the African shelf and slope and was deposited in the central Atlantic Basin at 127 ka ago (Weaver et al., 1992; Figure 1). As a result of the small particle size and the mineral characteristics as well as the considerable water depth of 5420 m in the region, its sediment is nearly perfectly mixed (ungraded mud; McCave and Jones, 1988). After deposition, the turbidite surfaces became exposed to well-ventilated bottom waters and oxygen penetrated into the sediments resulting in post-depositional aerobic OM degradation (de Lange et al., 1987). Over time, an active oxidation front penetrated downwards into the sediments whereas, in the meantime, pelagic deposition covered the turbidite surface. This process stopped after a few thousand years when the next turbidite blocked oxygen delivery, leaving a so-called ‘palaeo-oxidation’ front behind. Both aerobic and anaerobic processes as well as bioturbation left clearly recognisable signals in the sediments (van Os et al., 1993; Thomson et al., 1998). This allows to study in detail the effects of redox-front-related early diagenetic processes (Zonneveld et al., 2019).
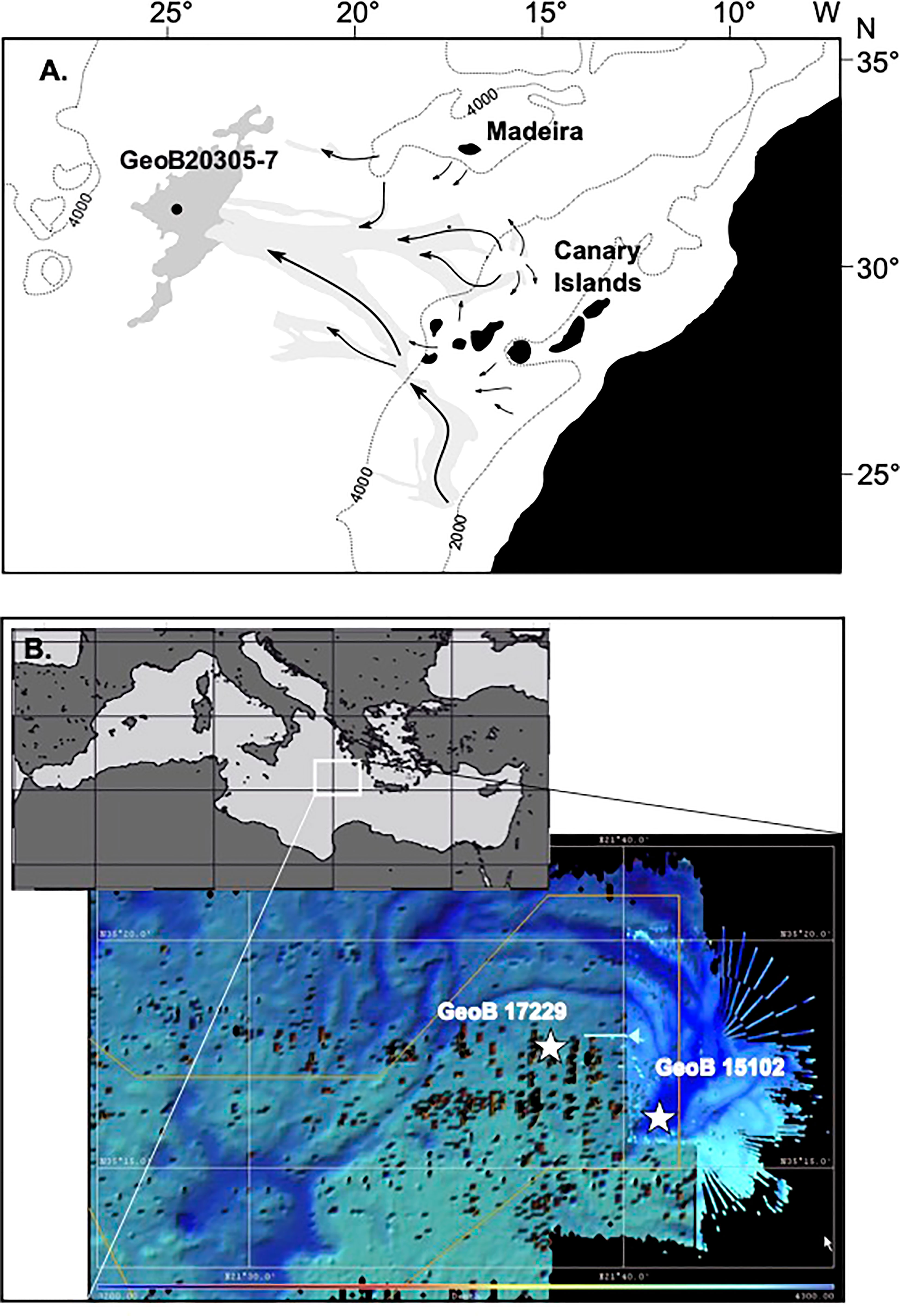
Figure 1 Maps of the core positions on (A) the equatorial Atlantic Ocean off NW Africa depicting the 4000 m and 2000 m depth contours, the position of the Madeira Abyssal Plain (dark grey), major turbidity flow paths (light gray and arrows) and core position. (redrawn from Zonneveld et al., 2019) (B) Map of the Mediterranean Sea with location of the Discovery Basin. Depth contour map with positions of the core locations.
The eastern Mediterranean Discovery Basin is located in the central eastern Mediterranean Sea. The underwater basin is filled by high salinity, oxygen-depleted sulfidic brine waters at a depth below 3500 m (Polymenakou et al., 2007; Bernhard et al., 2015). These brine waters are overlain by well-ventilated eastern Mediterranean deep waters. The region is characterized by low sedimentation rates and oligotrophic upper waters with a low export production. Both anoxic brine sediments and sediment from the direct surroundings obtain highly similar sediment rain but experience different depositional redox environments.
In this study, we analysed thecyst species Impagidinium aculeatum and Impagidinium patulum by means of micro-FTIR. This technique is very efficient to determine species specific molecular characteristics of dinoflagellate cysts (e.g., Versteegh et al., 2007; Bogus et al., 2012; Versteegh et al., 2012; Mertens et al., 2015a; Mertens et al., 2017b; Gurdebeke et al., 2018; Gurdebeke et al., 2020). Morover, it has been useful to determine characteristic differences in the most labile and resistant dinoflagellate cyst species (Bogus et al., 2014). However, interpretation of the micro-FTIR spectra has not always been straightforward due to analytical difficulties such as Mie scattering (e.g., Blümel et al., 2018) and different methodological approaches (e.g., Meyvisch et al., 2022). Nevertheless, the use of attenuation total reflection (ATR) rather than the transmission or reflection modi circumvents most problems of Mie scattering and the method has successfully been used to determine alteration in molecular characteristics as a result of early diagenetic processes in different redox environments (Versteegh et al., 2020). Here we use the method to investigate to what extent the molecular characteristics of both aforementioned resistant dinoflagellate cysts species vary in oxic, and anoxic depositional environments from the Madeira Abyssal Plain f-turbidite and surface sediments of the eastern Mediterranean Sea Discovery Basin and its surroundings.
Material and methods
Material
Gravity core 15102-5 from the Discovery Basin, eastern Mediterranean (35°16’43”N, 21°41’50”E, 3624 m water depth) was collected during research cruise Meteor 84/1 on 12 Feb. 2011 (Zabel et al., 2012; Figure 1A and Table 1). This deep-sea basin is filled with brine water and is anoxic. Sediments were analysed from the uppermost cm that are estimated to represent sub-recent deposits.
Multicore 17229-2 (35°16’98” N 21°37’98”E) was recovered just south of the Discovery Basin from a water depth of 3544 m during FS Poseidon research cruise POS448 CAPRICCIO (Table 1). The upper 29 cm consist of oxic pelagic sediments. Below this depth, the anoxic sediments of the S1 sapropel are present (Zonneveld et al., 2013). Cysts were isolated from the upper cm of this core representing recent/subrecent deposition.
Gravity core GeoB 20305-7 from the Madeira Abyssal Plain F-turbidite and overlying pelagic sediments were obtained in November of 2015 by the FS Maria S. Merian during research cruise MSM48 ADOMIS (Zonneveld et al., 2016; Figure 1B and Table 1) from a water depth of 5421 m at 31°22’333”N, 24°45’211”W. On board, the core was described, sampled and then stored at −20°C to prevent diagenetic processes from altering the geochemical and palynological signal. Samples were taken with steel utensils, wrapped in aluminium foil stored at −80°C on board and kept frozen until analysis in Bremen.
Samples were recovered from section 3 (569-668.5 cm core depth) and section 2 (668.5 - 768.5 cm core depth) with an estimated deposition age between 115-127 ka ago (pelagic deposition) and 127 ka ago (F-turbidite). In these sections, the following sedimentary deposits could be observed from top to bottom (Figure 2): 569-658 cm, unoxidised sediments representing the bottom of the “closing off” E1 turbidite, 658-669 cm, Pelagic bioturbated oxidized sediments (red-colours), 669-684 cm, oxidized bioturbated F-turbidite (pale greyish blue with burrows filled with red pelagic sediments), 684-691 cm, oxidised non-bioturbated turbidite (pale greyish blue), 691-696.2 cm, Purple layer, oxidised layer were several chemical species became reduced after oxygen supply stopped due to deposition of the next turbidite and denitrification/Mn reduction additionally affected OM degradation (purple with dark band on top); 696 cm, visible oxidation front (transition of pale purple (Fe3+) to olive green sediments with Fe2+; below 696 cm, anoxic turbiditic sediments (olive green). Samples 659 and 664 are recovered from pelagic oxidized sediments. Samples 688, 689 and 699 are recovered from the F-turbidite where samples 688 and 689 are derived from post-depositionally oxidized sediments with estimated oxygen exposure time of ca. 10.53 ka (Zonneveld et al., 2019). Sample 699 is recovered from the anoxic part of the turbidite.
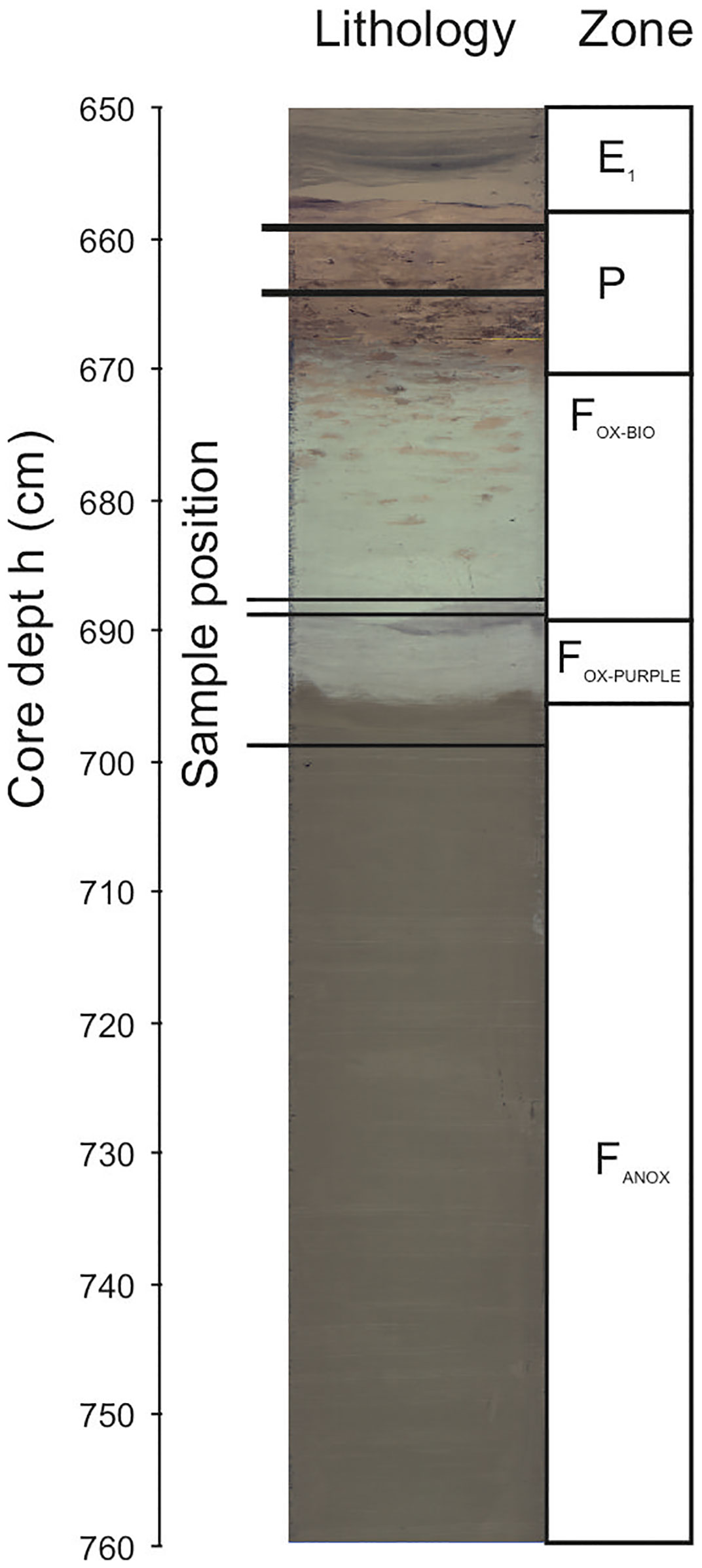
Figure 2 Compiled line scan and schematic drawing of core GeoB 20305-7 sections 2 and 3 depicting sample positions as well as the different redox zones.
Palynomorph isolation
In the laboratory, the samples were treated according to Zonneveld et al. (2019). Shortly thereafter, samples were dried (60°C). Carbonate was removed using HCl (10%), and silicates by adding HF (40%). The remaining sample was sieved using a Stork Veco precision sieve with 20 μm pores. Mild ultrasonic treatment was used to disperse the material and clean the dinoflagellate cysts from material adhering to the cyst walls. The residue was then centrifuged (7 min at 3000 rpm) and concentrated to either 0.5 ml or 1 ml in water. Individual cysts were isolated by placing part of the residue on a microscopic slide and observing this with an inverted microscope. Individual cysts were taken up using hand-drawn micropipettes and placed in a little drop of ultra-clean water. These drops of ultra-clean water were placed on a microscopic slide that was previously rinsed with isopropanol and ultra-clean water. Successively, the cysts were washed until the cysts were optically clean. The cysts were taken up with a little water from the washing droplet using a micropipette and carefully placed on a gold-mirror. Hereafter, excess water is removed from the mirror as much as possible with a thinner micropipette (<20 μm internal diameter). After further airdrying, the infrared spectra of the cysts were determined.
Statistical analyses
The statistical package CANOCO-5 (Smilauer and Leps, 2014) was used for multivariate analyses. Since initial analyses showed that the length of the gradient of maximum variance was less than two standard deviations indicating that linear models such as principal component analyses (PCA) are better suited than nonlinear models (e.g., correspondence analyses, CA) to explain the main trends in the data.
Infrared analyses and data processing
Infrared measurements followed the procedures and equipment described in Versteegh et al. (2020). Shortly, measurements were obtained using a BRUKER Invenio-S spectrometer coupled to a Hyperion 1000 IR microscope equipped with a KBr beam splitter and liquid N2-cooled mercury cadmium telluride (MCT) detector. A total of 256 scans were obtained at 4 cm-1 resolution using a germanium ATR crystal of 100 μm diameter for 31 specimens of I. patulum and 24 specimens of I. aculeatum. The spectra were corrected for atmospheric CO2 and baseline corrected using a concave rubber band correction with 64 baseline points and 2 iterations. Furthermore, we applied an ATR correction algorithm with an average refraction index of 1.5, a 45° angle of incidence and one ATR reflection as parameters (Nunn and Nishikida, 2008). Assignment of characteristic IR group frequencies follows Colthup et al., 1990; Jardine et al. (2017), and published literature. Spectra were standardized on the 1500-1250 cm-1 region (absorption of the y-axis in Figures 3–6 represents a relative value without unit). Standardized spectra were statistically analyzed with the multivariate ordination technique principal component analysis (PCA) using the software package PAST 4.08 (Hammer et al., 2001). Analyses were performed on the whole spectra as well as the fingerprint area between 800-1700 cm-1. The fingerprint area represents that part of the spectra where absorption patterns differ between species (e.g. Meyvisch et al., 2022). For reasons given below, spectra from Impagidinium patulum from sample 664 measured on 28-11-2021 have been excluded from analysis.
Results
Impagidinium patulum
The absorption spectra of I. patulum cysts from both the eastern Mediterranean Discovery Basin and Madeira Abyssal Plain all displayed the same overall characteristics (Figure 3). A major broad absorption of -OH centered at 3380 cm-1 was observed as well as absorptions of CH2 and CH3 symmetric and asymmetric stretching centered at 2930 and 2860 cm-1. Between 1800 and 850 cm-1 three clusters of absorptions were observed. Between 1800-1500 cm-1, a broad region with variable absorptions were observed with strongest absorptions centered around 1650 cm-1 and 1588 cm-1 followed by a minimum at 1483 cm-1. The next cluster from 1483-1250 cm-1 had about equal strength as the former and showed a maximum around 1435 cm-1. A final major cluster of absorptions from 1166-940 cm-1 was generally stronger than both previous ones with strongest absorptions at 1058 cm-1 and 983 cm-1.
Both visual examination of the spectra as well as the PCA analyses displayed no systematic differences between samples from the Eastern Mediterranean and Madeira Abyssal Plain samples of Late Quaternary age. Furthermore, no differences were observed between samples derived from oxic and suboxic to sulfidic depositional environments.
An exception were spectra obtained from cysts of I. patulum from oxic sample 664 measured on 28.10.2021. (Figure 4 upper panel). These spectra showed a clear absorption centered at 738 cm-1 which was absent in all other spectra of I. patulum. Furthermore, they displayed larger variability notably at 1588 cm-1 and 1058 cm-1 and an absorption maximum at 1402 cm-1 rather than 1435 cm-1. Renewal of the solvent by opening a new bottle of ultrapure water for washing the cysts prior to transfer to the gold coated mirror on 02.11.2021 resulted in the disappearance of the adsorption at 738 cm-1, a reduction of variance and a movement of the maximal adsorption at 1402 cm-1 towards 1435 cm-1 which was consistent with spectra of I. patulum derived from the other samples (Figure 3).
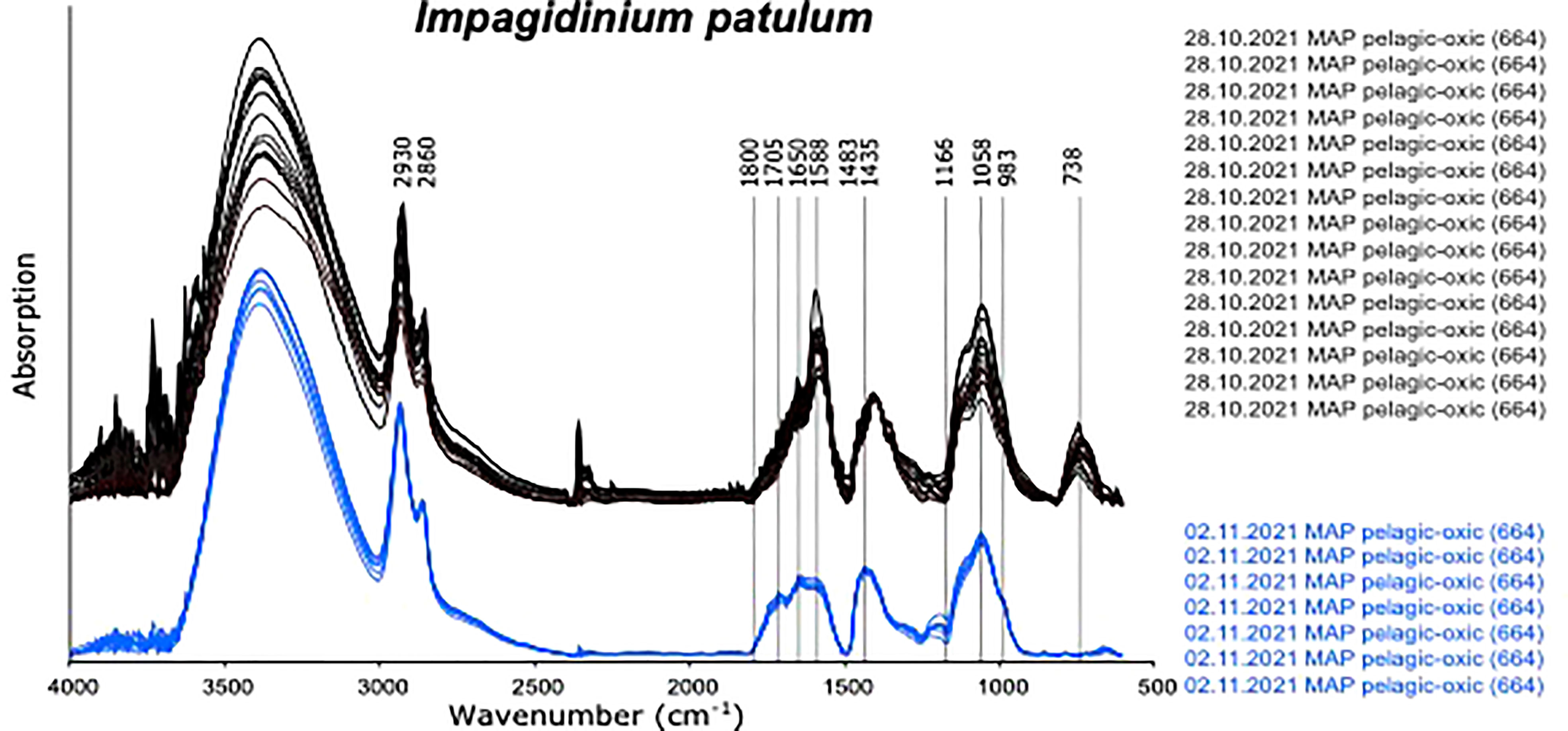
Figure 4 Micro-FTIR spectra of Impagidinium patulum measured on 28.10.2021 and 02.11.2022 displaying the effects of different cleaning procedures.
Impagidinium aculeatum
Spectra of I. aculeatum displayed more variations compared to those of I. patulum. Especially in the 1200-900 cm-1 region and at 1600 cm-1 much variability was observed (Figure 5). Similar to I. patulum, we did not observe differences in spectra between cysts recovered from the different regions, sediments of different age and redox depositional environments.
Similar to I. patulum, a major broad absorption of -OH centered at 3380 cm-1, was observed as well as absorptions of CH2 and CH3 symmetric and asymmetric stretching centered at 2930 and 2860 cm-1. However, normalisation of all spectra on the 1500-1250 cm-1 region showed that for I. patulum, the ratio of the absorption bands 3700-3000 cm-1/1200-900 cm-1 was considerably higher than for I. aculeatum (Figure 6).
Between 850 and 1800 cm-1, three clusters of absorptions were also observed in spectra of I. aculeatum (Figure 5). In the region between 1800-1500 cm-1, strongest absorptions were observed around 1594 cm-1. Absorption maxima were absent at about 1705 cm-1 and 1650 cm-1. The next cluster from 1483-1250 cm-1 had less strength than the former and showed a maximum at 1435 cm-1. A final major cluster of absorptions from 1210-940 cm-1 was stronger than the previous one with strongest absorptions at 1209 cm-1, 1166 cm-1, 1058 cm-1 and 983 cm-1. In contrast to I. patulum, I. aculeatum showed a clear absorption at 1209-1166 cm-1, with higher absorbance at 1594 cm-1 but the spectra lacked a shoulder at 1705 cm-1 and 1650 cm-1 (Figure 6).
Statistical analyses
The Principal Component Analyses clearly separated the spectra of I. patulum and I. aculeatum both when including the complete spectral range between 650-4000 cm-1 as well as focussing on the fingerprint area from 840 - 1900 cm-1 (Figure 7).
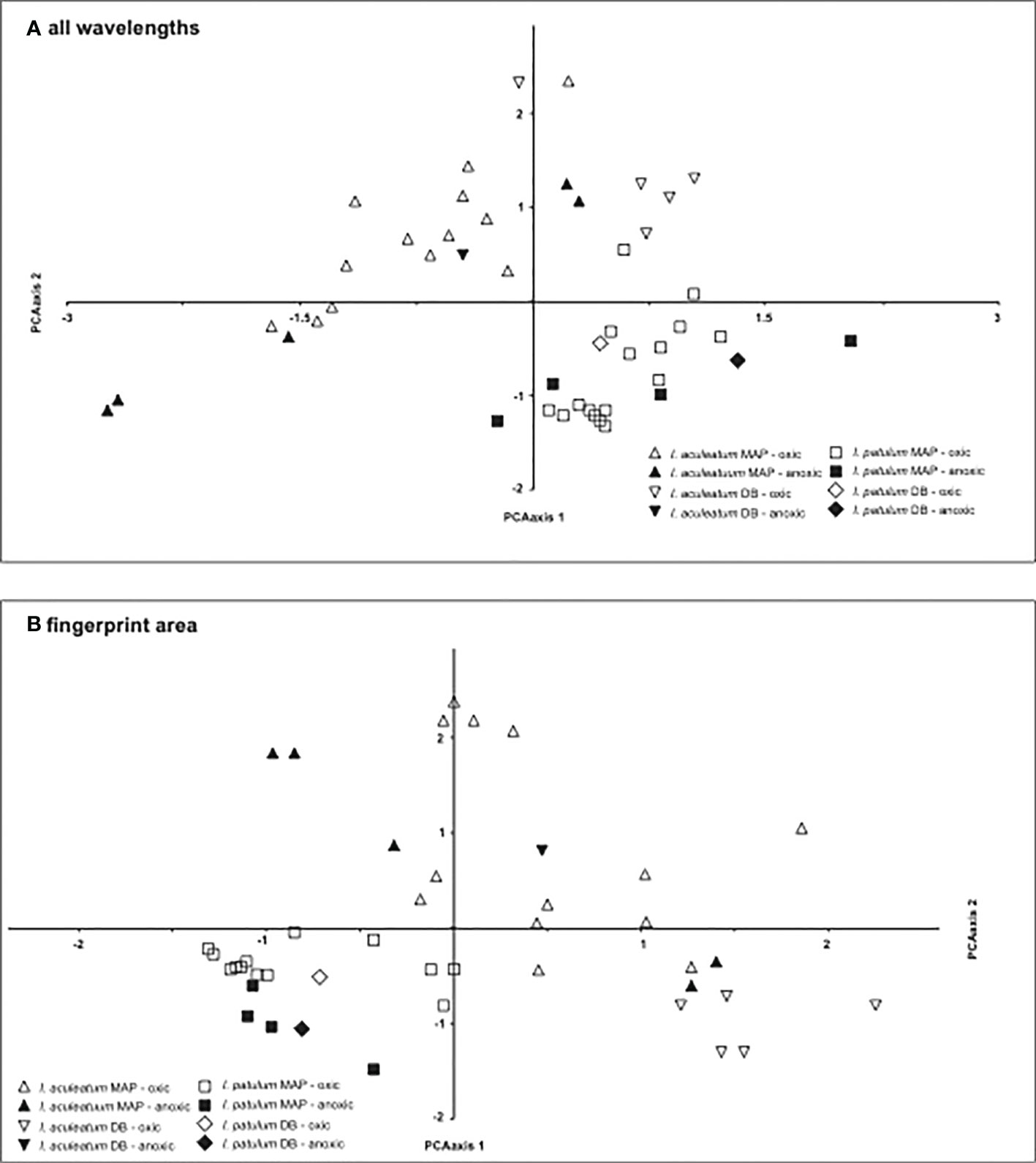
Figure 7 Results of the PCA analysis on (A) complete spectra, (B) fingerprint area between 840-1900 cm-1.
In coherence with the visual results, I. aculeatum showed larger variability than I. patulum and no separation could be made between cysts derived from oxic or anoxic/sulfidic depositional environments.
Discussion
Since the introduction of the technique of micro-FTIR in dinoflagellate research (Kokinos et al., 1998; Versteegh et al., 2004), the technique has increasingly been used to determine the molecular characteristics of dinoflagellate cysts for taxonomic purposes (e.g., Bogus et al., 2012; Versteegh et al., 2012; Mertens et al., 2015a; Mertens et al., 2015b; Mertens et al., 2017a; Mertens et al., 2017b; Gurdebeke et al., 2018; Mertens et al., 2018; Gurdebeke et al., 2020), ecological purposes (Bogus et al., 2014) and the investigation of taphonomic processes (Versteegh et al., 2004; Versteegh et al., 2020). During the last decade, it became evident that although all dinoflagellate cyst species measured so far appear to be primarily consisting of carbohydrate-based polymers, there is large variability in their molecular characteristics that seems to be species specific. Micro-FTIR analyses indicate that differences can be observed in the fingerprint areas between 2700-3600 cm-1 and 800-1700 cm-1. It also became clear that spectra of multiple specimens of the same species might show large variability. Recently, it has been shown that a major part of this variability might be induced by the cyst preparation methods and the way cysts have been measured with transmission or ATR modes (Meyvisch et al., 2022). Meyvisch et al. (2022) clearly showed that measuring cysts with ATR consistently gave a higher repeatability and reproducibility over spectra collected in transmission modes. They found that the use of ATR largely reduced the signal-noise ratio by reducing the number of scattering artefacts. Here we observe that specimens of Impagidinium patulum from the same sample measured at two different days had different spectra with a strong absorption centered at 738 cm-1 and larger scattering which was not observed in earlier measurements. Upon noticing that the water used for cleaning and washing the cysts might have been contaminated by others, we isolated additional cysts which were cleaned by ultra-pure water from a newly opened bottle. Our results showed that despite the fact that we removed additional water with a micropipette with a diameter smaller than the diameter of the dinoflagellate cysts prior to our analyses on 28.10.2021, the contaminated water had left a clear additional signal and induced large scattering of the spectra. Consequently, our results imply that apart from measuring cysts in ATR mode as is shown by Meyvisch et al., 2022, it is of utmost importance to clean the cyst carefully prior to measurement.
Our results show much larger differences between spectra of I. aculeatum compared to I. patulum. I.aculeatum is much smaller than I. patulum, it also has a thinner cell wall and has higher septa relative to its inner body (Plate 1). A smaller size reduces the signal-noise ratio and increases the relative importance of contaminants which would lead to a larger variability in spectra. As such, it makes accessing a good spectrum more challenging for small, thin objects. Moreover, the presence of pronounced septa on an object make it more difficult to achieve good contact between the ATR crystal and the cyst wall, which may be seen in relatively higher CO2 absorptions (see additional information). We also have the impression that in some cases a thinner thickness of the cyst wall increased spectral noise, but more investigation is needed to verify this hypothesis. Our results indicate that the use of micro-FTIR in ATR mode to characterise the species specific molecular characteristics of cysts is more challenging for small, thin-walled cysts compared to larger, more robust cyst species. Furthermore, we assume that obtaining good spectra from cysts with many processes or spines might even be more challenging since cysts with that morphology generally have small organic debris attached which is extremely hard to remove.
Bogus et al. (2014) documented a compiled spectrum of I. patulum based on three cysts collected from a surface sediment sample of the Benguela upwelling region (GeoB 4804, 24°80’60’’S, 12°40’12’’E (Bogus et al., 2014; Figure 3). This spectrum was measured in transmission mode with 265 scans at 8 cm-1 resolution using a Nicolet FTIR spectrometer coupled to a Nicplan microscope. The spectrum also shows a clear absorption minimum at 1166 cm-1, 1483 cm-1 and maxima at 1058 cm-1, 1435 cm-1, and 1650 cm-1. However, in contrast to our study, the cysts measured by Bogus et al. (2014) showed additional absorption at 983 cm-1. Furthermore, the absorption minimum at 1483 cm-1 was much less pronounced as was the absorption between 3650-2700 cm-1. Meyvisch et al. (2022) showed that especially absorptions between 3650-2700 cm-1 can vary largely when measured in transmission mode. Therefore, we assume that the observed differences with our results can be ascribed to the differences in measuring method. With respect to the differences at 983 cm-1 and 1483 cm-1, it is not possible to determine a possible cause since we have no insight in the variability of the spectra obtained from the Benguela upwelling and have no information about eventual contamination.
For both I. aculeatum and I. patulum, we could not observe consistent spectral differences between cysts recovered from oxic and anoxic environments, between cysts recovered from different areas and between cysts recovered from sediments deposited at different geological times (Figures 3, 5). Early diagenetic effects have been observed on the molecular characteristics of Thalassiphora pelagica cyst walls (Versteegh et al., 2020) whereby cysts from an anoxic sulfidic environment deposited about 40 Ma ago, differed markedly from cysts of the same species being deposited in oxic environments. In the sulfidic environment, anaerobic cross-linking, such as natural vulcanization, resulted in cysts being enriched in CHx and to a lesser extent in C=O (enhanced adsorptions at 3000-2800 cm-1 and 1600-1750 cm-1) and having a relative loss of O-H (reduced adsorptions at 3550-3200 cm-1 and around 1335 cm-1). In our study, we do not see any of these changes in cysts derived from sediments of the Discovery Basin (eastern Mediterranean) where anoxic sulfidic conditions prevail (Bernhard et al., 2015). Consequently, there is no indication that the process of natural vulcanisation is sufficiently fast to affect (sub) recent cysts of the investigated Impagidinium species post-depositionally on these short timescales.
We do observe, consistent differences in micro-FTIR ATR spectra of I. aculeatum and I. patulum (Figures 6, 7). Compared to I. patulum, I. aculeatum shows higher absorbance at 1166 cm-1 and an associated shoulder near 1209 cm-1 whereas I. patulum shows no or very minor absorptions in this region. This might indicate additional C-O vibration that might be associated with a slightly different carbohydratic structure of I. aculeatum. In combination with the structurally lower relative absorption at the 3700-3000 cm-1 region one possible explanation would be that I. aculeatum would contain relatively more secondary alcohols. This may explain why that I. aculeatum seems to be slightly less recalcitrant than I. patulum in the bioturbated and oxidized sediments of the MAP F-turbidite (Zonneveld et al., 2019). However, more studies are required to verify this assumption.
To date, micro-FTIR ATR spectra exist for few dinoflagellate cyst taxa. The majority of micro-FTIR spectra of dinoflagellate cysts have been measured in transmission mode (e.g., Bogus et al., 2012; Bogus et al., 2014; Mertens et al., 2015a; Mertens et al., 2015b; Mertens et al., 2017a; Mertens et al., 2017b; Gurdebeke et al., 2018; Gurdebeke et al., 2020) and often only one cyst has been analysed with only scarce information on the cleaning procedure. Moreover, spectra were often not corrected for Mie scattering or other spectral artefacts and information is mostly absent on the spectral variance of a species. However, detailed correlation is possible with spectra of species given in Meyvisch et al. (2022) and Versteegh et al. (2020). Meyvisch et al. (2020) and Versteegh et al. (2020) provide detailed information on species that are very vulnerable to early diagenetic aerobic degradation in modern sediments: Brigantedinium spp., Trinovantedinium applanatum, Votadinium calvum, Quincesuspis concreta, and more resistant cysts species; Spiniferites bentorii, Lingulodinium polyedrum (= L. machaerophorum). Of the latter species, S. bentorii has been considered to be less resistant than L. polyedrum which in turn is less resistant than I. aculeatum and I. patulum (e.g., Zonneveld et al., 1997; Kodrans-Nsiah et al., 2008; Zonneveld et al., 2008; Gray et al., 2017; Zonneveld et al., 2019). Compared to the more resistant species that show minimal adsorption at 1500 cm-1, spectra of the most vulnerable species all show enhanced absorption between 1200-1650 cm-1 that suggests the presence of C=N, N-H, N-O, C-N bending/stretching (= periodic motion of the atoms of the molecule relative to each other along an angle (bending) or in the length of a bond (stretching) as well as conjugated and possibly also aromatic C=C stretching. We assume that the presence of nitrogen and olefins in the macromolecule would, apart from making the molecules more reactive, also make them more prone to microbial decay. Preference for ‘food’ containing nitrogen of the microbial community would then be an additional explanation for their lower resistance to decay.
Compared to I. patulum and I. aculeatum, micro-FTIR spectra of S. bentorii and L. polyedrum have higher absorptions around 1705 cm-1 and compared to I. patulum, higher absorptions around 1200 cm-1. This suggests that their molecules have more C=O and C-O bounds. In aerobic settings, Spiniferites species and L. polyedrum degrade faster than Impagidinium species with L. polyedrum being less resistant than Spiniferites. The presence of more C=O and C-O bounds in their macromolecule might suggest a higher presence of the comparatively reactive carboxylic acids and ester bonds. Spiniferites bentorii shows additionally higher absorption between 1200-1400 suggesting which is however difficult to interpret.
With respect to an increasing trend in vulnerability, the most sensitive species show high absorption for C=N, N-H, N-O, C-N, C=O and C-O bounds, the somewhat more resistant S. bentorii, shows lower C=N, N-H, N-O, C-N, whereas the even more resistant L. polyedrum shows high absorption for C=O and C-O bounds but lacks nitrogen. The resistant I. aculeatum shows high absorption for C-O whereas the potentially most resistant species, I. patulum, does not show high absorption in any of these regions. Consequently, our results show that with increasing resistance to degradation, the proportion of C-O increases. We thus observe different macromolecular characteristics for cyst species with different sensibility to degradation. We therefore erect the hypothesis that these molecular differences are also responsible for the differences in degradability of the above-mentioned species. To further elucidate this link, more detailed chemical characterisation is needed. Our study therefore shows that carbohydrate-based marine particles produced by closely related biological entities have different molecular characteristics and therefore contribute differently to the carbon sequestration in the ocean floor.
Conclusions
To asses more insight into the effect of early diagenetic processes in different redox environments on the molecular characteristics of degradation resistant particulate organic matter, the molecular cyst wall characteristics of the closely related dinoflagellates Impagidinium aculeatum and I. patulum from oxic and anoxic sedimentary environments in the Madeira Abyssal Plain F-turbidite and in the surface sediments of the eastern Mediterranean Sea have been analysed with micro-FTIR spectroscopy. We show that it is of utmost importance to clean the cyst carefully prior to measurement to reduce the spectral variance and avoid the introduction of additional signals and reduce the spectral variance. We observe a larger scattering in spectra of I. aculeatum compared to I. patulum which is assumed to be caused by the smaller cyst size, thinner cysts and the presences of protrusions in I. aculeatum.
No consistent spectral differences have been observed between cysts recovered from oxic and anoxic environments, cysts recovered from different areas and cysts recovered from sediments deposited at different geological times. Furthermore, no indication of molecular change as a result of natural vulcanisation in the sulfidic environment of the Discovery Basin have been observed.
Compared to I. patulum, cyst walls of I. aculeatum contain more C-O probably related to the presence of higher amounts of secondary alcohols that might imply a slightly higher resistance against decay in I. patulum. Comparison with other species implies that cyst walls of most vulnerable species show C=N, N-H, N-O, C-N bending/stretching, as well as the presence of C=O and C-O bounds. Cyst species that are somewhat less sensitive have lower absorbance at the C=C stretching region, but there is a strong indication of the presence of nitrogen in their macromolecules. More resistant species typically have high relative absorptions in the 1200-950 cm-1 region and they lack nitrogen whereas the even more resistant cyst species I. aculeatum and I. patulum show low amounts of C=O.
Data availability statement
The original contributions presented in the study are included in the article/supplementary material, further inquiries can be directed to the corresponding author/s.
Author contributions
KZ was leading part of the expeditions collecting sample material, GV was responsible for the collection of material during these expeditionsl. Both GV and KZ processed and analysed the majority of material. Together they interpreted and discussed the data and wrote the manuscript. Both had an equal share in this process. All authors contributed to the article and approved the submitted version.
Funding
The financial support in the form of positions of Elinor Tessin and GV was provided by the Deutsche Forschungsgemeinschaft funded Cluster of Excellence “The Ocean Floor – Earth’s Uncharted Interface” Unit: “The ocean floor as RECEIVER”.
Acknowledgments
Elinor Tessin is thanked for analyzing part of the cyst from samples MAP 688, GeoB 1510 and GeoB 17229.
Conflict of interest
The authors declare that the research was conducted in the absence of any commercial or financial relationships that could be construed as a potential conflict of interest.
Publisher’s note
All claims expressed in this article are solely those of the authors and do not necessarily represent those of their affiliated organizations, or those of the publisher, the editors and the reviewers. Any product that may be evaluated in this article, or claim that may be made by its manufacturer, is not guaranteed or endorsed by the publisher.
References
Arndt S., Joergensen B. B., LaRowe D. E., Middelburg J. J., Pancost R. D., Regnier P. (2013). Quantifying the degradation of organic matter in marine sediments: A review and synthesis. Earth-Sci. Rev. 123, 53–86. doi: 10.1016/j.earscirev.2013.02.008
Bernhard J. M., Morrison C. R., Pape E., Beaudoin D. J., Todaro M. A., Pachiadaki M. G., et al. (2015). Metazoans of redoxcline sediments in Mediterranean deep-sea hypersaline anoxic basins. BMC Biol. 13, 1–21. doi: 10.1186/s12915-015-0213-6
Blümel R., Lukacs R., Zimmermann B., Bağcıoğlu M., Kohler A. (2018). Observation of mie ripples in the synchrotron Fourier transform infrared spectra of spheroidal pollen grains. J. Opt. Soc Am. A 35, 1769. doi: 10.1364/JOSAA.35.001769
Bogus K., Harding I. C., King A., Charles A. J., Zonneveld K. A. F., Versteegh G. J. M. (2012). The composition and diversity of dinosporin in species of the apectodinium complex (Dinoflagellata). Rev. Pal. Pal. 183, 21–31. doi: 10.1016/j.revpalbo.2012.07.001
Bogus K., Mertens K. N., Lauwaert J., Harding I. C., Vrielinck H., Zonneveld K. A. F., et al. (2014). Differences in the chemical composition of organic-walled dinoflagellate resting cysts from phototrophic and heterotrophic dinoflagellates. J. Phyc. 50, 254–266. doi: 10.1111/jpy.12170
Canfield D. E., Thamdrup B. (2009). Towards a consistent classification scheme for geochemical environments, or, why we wish the term 'suboxic' would go away. Geobiol 7, 385–392. doi: 10.1111/j.1472-4669.2009.00214.x
Colthup N. B., Daly L. H., Wiberly S. E. (1990). Introduction to infrared and raman spectroscopy. 3rd ed (London: Academic Press Limited), 1–547.
de Lange G. J., Jarvis I., Kuijpers A. (1987). Geochemical characteristics and provenance of late quaternary sediments from the Madeira abyssal plain, n Atlantic. Geol. Soc Lond. Spec. Pub. 31, 147–165. doi: 10.1144/GSL.SP.1987.031.01.12
Gray D., Zonneveld K. A. F., Versteegh G. J. M. (2017). Species-specific sensitivity of dinoflagellate cysts to aerobic degradation: A five-year natural exposure experiment. Rev. Pal. Pal. 247, 175–187. doi: 10.1016/j.revpalbo.2017.09.002
Gurdebeke P. R., Mertens K. N., Bogus K., Marret F., Chomérat N., Vrielinck H., et al. (2018). Taxonomic re-investigation and geochemical characterization of reid’s, (1974) species of Spiniferites from holotype and topotype material. Palyn 42, 93–110. doi: 10.1080/01916122.2018.1465735
Gurdebeke P. R., Mertens K. N., Meyvisch P., Bogus K., Pospelova V., Louwye S. (2020). Hiddenocysta matsuokae gen. et sp. nov. from the Holocene of Vancouver island, British Columbia, Canada. Palyn 43, 13–114. doi: 10.1080/01916122.2020.1750500
Hammer O., Harper D. A. T., Ryan P. D. (2001). Past: Paleontological statistics software package for education and data analysis. Palaeont. Elec. 4, 1–9.
Hedges J. I., Prahl F. G. (1993). “Early diagenesis: consequences for applications of molecular biomarkers,” in Organic geochemistry, principles and applications. Eds. Engel M. H., Macko S. A., Jones D. S. (New York: Plenum Press), 237–253.
Jardine P. E., Abernethy F. J., Lomax B. H., Gosling W. D., Fraser W. T. (2017). Shedding light on sporopollenin chemistry, with reference to UV reconstructions. Rev. Pal. Pal. 238, 1–6. doi: 10.1016/j.revpalbo.2016.11.014
Kasten S., Zabel M., Heuer V., Hensen C. (2003). “Processes and signals of non-steady state diagenesis in deep-sea sediments,” in The south Atlantic in the late quaternary. Eds. Wefer G., Mulitza S., Rathmeyer V. (Berlin: Springer), 431–459.
Kodrans-Nsiah M., de Lange G. J., Zonneveld K. A. F. (2008). A natural exposure experiment on short-term species-selective aerobic degradation of dinoflagellate cysts. Rev. Pal. Pal. 152, 32–39. doi: 10.1016/j.revpalbo.2008.04.002
Kokinos J. P., Eglinton T. I., Goñi M. A., Boon J. J., Martoglio P. A., Anderson D. M. (1998). Characterisation of a highly resistant biomacromolecular material in the cell wall of a marine dinoflagellate resting cyst. Org. Geochem. 28, 265–288. doi: 10.1016/S0146-6380(97)00134-4
Landry Z., Swan B. K., Herndl G. J., Stepanauskas R., Giovannoni S. J. (2017). SAR202 genomes from the dark ocean predict pathways for the oxidation of recalcitrant dissolved organic matter. MBio 8, e00413–e00417. doi: 10.1128/mBio.00413-1
McCave I. N., Jones K. N. (1988). Deposition of ungraded muds from high-density non-turbulent turbidity currents. Nature 333, 250–252.
Mertens K. N., Gu H., Pospelova V., Chomérat N., Nézan E., Gurdebeke P. R., et al. (2017a). First record of resting cysts of the benthic dinoflagellate Prorocentrum leve in a natural reservoir in gujan-mestras, gironde, France. J. Phyco. 53, 1193–1205. doi: 10.1111/jpy.12582
Mertens K. N., Gu H., Takano Y., Price A. M., Pospelova V., Bogus K., et al. (2017b). The cyst-theca relation of Trinovantedinium pallidifulvum, with erection of protoperidinium lousianensis sp. nov. and their phylogenetic position within the conica group. Palyn 41, 183–202. doi: 10.1080/01916122.2016.1147219
Mertens K. N., Nieuwenhove N. V., Gurdebeke P. R., Aydin H., Bogus K., Bringué M., et al. (2018). The dinoflagellate cyst genera Achomosphaera evitt 1963 and spiniferites mantell 1850 in pliocene to modern sediments: A summary of round table discussions. Palyn 10, 10–44. doi: 10.1080/01916122.2018.1465739
Mertens K. N., Takano Y., Yamaguchi A., Gu H., Bogus K., Kremp A., et al. (2015a). The molecular characterization of the enigmatic dinoflagellate Kolkwitziella acuta reveals an affinity to the excentrica section of the genus Protoperidinium. Syst. Biodiv. 13, 509–524. doi: 10.1080/14772000.2015.1078855
Mertens K. N., Wolny J., Carbonell-Moore C., Bogus K., Ellegaard M., Limoges A., et al. (2015b). Corrigendum to taxonomic re-examination of the toxic armoured dinoflagellate Pyrodinium bahamense plate 1906: Can morphology or LSU sequencing separate P. bahamense var. compressum from var. bahamense? Harm. Algae. 42, 71–72. doi: 10.1016/j.hal.2014.12.002
Meyvisch P., Gurdebeke P. R., Vrielinck H., Neil Mertens K., Versteegh G., Louwye S. (2022). Attenuated total reflection (ATR) micro-Fourier transform infrared (Micro-FT-IR) spectroscopy to enhance repeatability and reproducibility of spectra derived from single specimen organic-walled dinoflagellate cysts. Appl. Spectrosc. 76, 235–254. doi: 10.1177/00037028211041172
Middelburg J. J. (2018). Reviews and syntheses: To the bottom of carbon processing at the seafloor. Biogeosc 15, 413–427. doi: 10.5194/bg-15-413-2018
Middelburg J. J. (2019). Marine carbon biogeochemistry. a primer for earth system scientist (Switzerland: SpringerOpen, Springer Nature Switzerland AG, Chem), 118. doi: 10.1007/978-3-030-10822-9
Nierop K., Reichert G., Veld H., Sinnighe-Damsté J. (2017). The influence of oxygen exposure time on the composition of macromolecular organic matter as revealed by surface sediments on the Murray ridge (Arabian Sea). Geochim. Cosmochim. Acta 206, 40–56. doi: 10.1016/j.gca.2017.02.032
Nunn S., Nishikida K. (2008). Advanced ATR correction algorithm, 50581, Thermo Fisher Appl. Notes., Vol. 2008. 1–3.
Polymenakou P. N., Stephanou E. G., Tselepides A., Bertilsson S. (2007). Organic matter preservation and microbial community accumulations in deep-hypersaline anoxic basins. Geomicrobiol. J. 24, 19–29. doi: 10.1080/01490450601134283
Smilauer P., Leps J. (2014). Multivariate analysis of ecological data using canoco 5 (Cambridge: Cambridge University Press), 1–362.
Thomson J., Jarvis I., Green R. H., Green D. A., Clayton T. (1998). Mobility and immobility of redoc-sensitive elements in deep-sea turbidites during shallow burial. Geochim. Cosmochim. Acta 62, 643–656. doi: 10.1016/S0016-7037(97)00378-5
van Os B., Visser H.-J., Middelburg J. J., de Lange G. J. (1993). Occurrence of thin, metal-rich layers in deep-sea sediments: A geochemical characterization of copper remobilization. Deep Sea Res. I: Oceanogr. Res. Pap. 40, 1713–1730.
Versteegh G., Blokker P., Bogus K., Harding I., Lewis J., Oltmanns S., et al. (2012). Infra-red spectroscopy, flash pyrolysis, thermally assisted hydrolysis and methylation (THM) in the presence of tetramethylammonium hydroxide (TMAH) of cultured and sediment-derived Lingulodinium polyedrum (Dinoflagellata) cyst walls. Org. Geochem. 43, 92–102. doi: 10.1016/j.orggeochem.2011.10.007
Versteegh G. J. M., Blokker P., Marshall C. R., Pross J. (2007). Macromolecular composition of the dinoflagellate cyst Thalassiphora pelagica (Oligocene, SW Germany). Org. Geochem. 38, 1643–1656. doi: 10.1016/j.orggeochem.2007.06.007
Versteegh G. J. M., Blokker P., Wood G., Collinson M. E., Sinninghe Damsté J. S., de Leeuw J. W. (2004). Oxidative polymerization of unsaturated fatty acids as a preservation pathway for microalgal organic matter. Org. Geochem. 35, 1129–1139. doi: 10.1016/j.orggeochem.2004.06.012
Versteegh G. J. M., Houben A. J. P., Zonneveld K. A. F. (2020). Better molecular preservation of organic matter in an oxic than in a sulfidic depositional environment: evidence from Thalassiphora pelagica (Dinoflagellate, Eocene) cysts. Biogeosc 17, 3545–3561. doi: 10.5194/bg-17-3545-2020
Versteegh G. J. M., Zonneveld K. A. F. (2002). Use of selective degradation to separate preservation from productivity. Geol 30, 615–618. doi: 10.1130/0091-7613(2002)030<0615:UOSDTS>2.0.CO;2
Wakeham S. G., Lee C., Hedges J. I., Hernes P. J., Peterson M. L. (1997). Molecular indicators of diagenetic status in marine organic matter. Geochim. Cosmochim. Acta 61, 5363–5369. doi: 10.1016/S0016-7037(97)00312-8
Weaver P. P. E., Rothwell R. G., Ebbing J., Gunn D., Hunter P. M. (1992). Correlation, frequency of emplacement and source directions of megaturbidites on the Madeira abyssal plain. Mar. Geol. 109, 1–20. doi: 10.1016/0025-3227(92)90218-7
Weber T., Bianchi D. (2020). Efficient particle transfer to depth in oxygen minimum zones of the pacific and Indian oceans. Front. Earth. Sci. 8, 376. doi: 10.3389/feart.2020.00376
Zabel M., Aiello I., Becker K., Braun S., Broda N., Dibke C., et al. (2012). Biogeochemistry and methane hydrates of the black sea; oceanography of the mediterranean; shelf sedimentation and cold water carbonates - cruise no. M84/1 - February 09 – February 22, 2011 – Valletta (Malta) – Istanbul (Turkey). 1–36
Zonneveld K., Albert M., Boom L., Donner B., Ebersbach F., Friese C., et al. (2016). Aerobic degradation of particulate organic matter and benthic microbial turnover rates reflecting ocean redox conditions off NW Africa (ADOMIS). maria s. merian MSM 48. November 2 – November 25, 2015 ponta delgada (Portugal) – las palmas (Spain) (Bremen: MARUM-Zentrum für Marine Umweltwissenschaften Bremen). doi: 10.2312/cr_msm48
Zonneveld K., Baumann K.-H., Clotten C., Donner B., Gray D., Iwanczuk N., et al. (2013). Report and preliminary results of R/V POSEIDON cruise POS448 CAPRICCIO. calabrian and Adriatic past river input and carbon conversIon in the eastern Mediterranean Messina – Messina, 6 – 23 march 2013 296, 1–47.
Zonneveld K. A. F., Gray D. D., Kuhn G., Versteegh G. J. M. (2019). Postdepositional aerobic and anaerobic particulate organic matter degradation succession reflected by dinoflagellate cysts: The Madeira abyssal plain revisited. Mar. Geol. 408, 87–109. doi: 10.1016/j.margeo.2018.11.010
Zonneveld K. A. F., Versteegh G. J. M., de Lange G. J. (1997). Preservation of organic walled dinoflagellate cysts in different oxygen regimes: A 10,000 years natural experiment. Mar. Micropal. 29, 393–405. doi: 10.1016/S0377-8398(96)00032-1
Zonneveld K. A. F., Versteegh G. J. M., Kasten S., Eglinton T. I., Emeis K. C., Huguet C., et al. (2010). Selective preservation of organic matter in marine environments; processes and impact on the sedimentary record. Biogeosc 7, 483–511. doi: 10.5194/bg-7-483-2010
Keywords: micro-FTIR (Fourier transform infrared spectroscopy), dinoflagellate cyst, particulate organic matter (POM) preservation, redox environments, molecular characteristics
Citation: Versteegh GJM and Zonneveld KAF (2022) Micro-Fourier transform infrared spectroscopy of degradation-resistant organic microfossils; Influence of preservation environment and phylogeny. Front. Mar. Sci. 9:1040543. doi: 10.3389/fmars.2022.1040543
Received: 09 September 2022; Accepted: 24 October 2022;
Published: 07 December 2022.
Edited by:
Gordon T. Taylor, Stony Brook University, United StatesReviewed by:
Sandy McLachlan, Geological Survey of Canada, CanadaMarianne Ellegaard, University College Copenhagen, Denmark
Copyright © 2022 Versteegh and Zonneveld. This is an open-access article distributed under the terms of the Creative Commons Attribution License (CC BY). The use, distribution or reproduction in other forums is permitted, provided the original author(s) and the copyright owner(s) are credited and that the original publication in this journal is cited, in accordance with accepted academic practice. No use, distribution or reproduction is permitted which does not comply with these terms.
*Correspondence: Karin A. F. Zonneveld, a3pvbm5ldmVsZEBtYXJ1bS5kZQ==