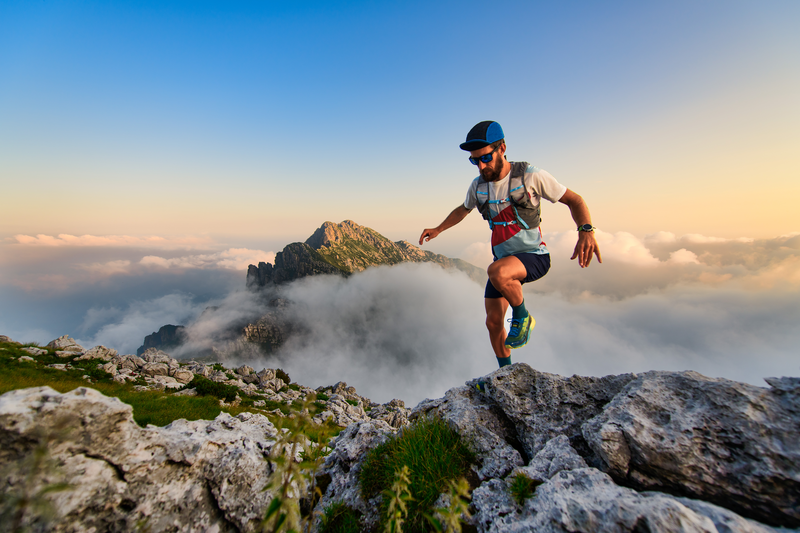
95% of researchers rate our articles as excellent or good
Learn more about the work of our research integrity team to safeguard the quality of each article we publish.
Find out more
ORIGINAL RESEARCH article
Front. Mar. Sci. , 10 November 2022
Sec. Coastal Ocean Processes
Volume 9 - 2022 | https://doi.org/10.3389/fmars.2022.1040308
This article is part of the Research Topic Linking Hydrological and Biological Connectivity to the Restoration and Management of Coastal Ecosystems View all 7 articles
Crab burrows play an important role in saltmarsh wetlands and are a useful indicator of wetland condition. The spatiotemporal distribution of crab burrows varies considerably in tidal wetlands. However, the reasons for these variations are poorly understood, in part, due to the limited availability of comprehensive field data. Based on a two-year continuous observation at a tidal wetland in the northern Jiangsu Coast, China, this study explored the relationship between crab burrow density and environmental variables, including median grain size, water content, organic matter content, soil salinity, and elevation. Our results show that the distribution of crab burrows was unimodal across the shore in winter and spring (Nov-Apr) when air temperature was relatively low, while bimodal in summer and autumn (May-Oct) when temperature was relatively high. The density of crab burrows was larger at areas with higher water content, higher organic matter content, and lower soil salinity, while it was lower with stronger hydrodynamics and lower suspended sediment concentration. Crab burrows were more abundant in vegetated areas than in un-vegetated areas. A backward stepwise model selection was performed based on R-square and Akaike information criterion (AIC) to distinguish the main driving factors that determine crab burrow distribution. Results suggested that the principal driving factors were organic matter content and soil salinity in all the seasons, with the addition of water content in warm seasons. Overall, this study provides a comprehensive field dataset for a more in-depth understanding of crab burrow distribution and a scientific basis for sustainable management of tidal wetlands.
Located at the boundary between the land and the sea, salt marshes are affected by both marine and terrestrial forces at the same time (Zhou et al., 2016; Mcowen et al., 2017). Salt marshes provide important ecosystem services for humanity (Barbier et al., 2011), such as carbon sequestration (Andreo-Martínez et al., 2021; Xiao et al., 2021) and wave energy dissipation (Moller et al., 2001; Pan et al., 2022). Salt marshes also accommodate many types of marine invertebrates such as crabs, flatfishes, and clams (Li et al., 2018). Among these invertebrates, crabs are of special interest because of their high abundance and profound ecological functions. As ecosystem engineers, crabs alter the environment by creating, maintaining, rebuilding, modifying, or destroying habitats (Lawton and Jones, 1995). The biological processes of crabs affect sediment properties (Wilson et al., 2012), vegetation health (Bertness et al., 2009), surface roughness (Widdows and Brinsley, 2002; Meng et al., 2012), water circulation (Carol et al., 2011; Zhou et al., 2020), suspended sediment concentration (Wang et al., 2017). Some crab behavior, e.g., creating and maintaining the burrows by increasing surface turbulence, changing water circulation, binding of sediment grains, and feeding on roots, can reduce the critical erosion threshold of sediment and hence enhance erosion (Mccall and Tevesz, 1982; Wotton and Malmqvist, 2001; Kristensen and Alongi, 2006; Xin et al., 2009; Grabowski et al., 2011; Xin et al., 2012). Meanwhile, crabs are generally an important link in the food chain, feeding on fungi, debris, and cordgrass and providing food for fishes and birds (Weilhoefer, 2011; Chen et al., 2016b; Angelini et al., 2018; Gao and Lee, 2022). Acting as predators and deposit-feeders, crabs play a role in the top-down control of tidal flat ecosystems (Alexander et al., 2016; Souza et al., 2021). Therefore, crabs are often used as indicators of marsh conditions in tidal wetlands (Spivak et al., 1994; Cardoni et al., 2007; Griffiths et al., 2007; Weilhoefer, 2011). Crab burrows are easy to measure and more often used for estimating the density of crabs, and hence have been widely adopted as indicators (Rosa and Borzone, 2008; Weilhoefer, 2011; Schlacher et al., 2016; Stelling-Wood et al., 2016).
An increasing focus in current research of tidal wetlands is how environmental factors regulate the distribution pattern of crab burrows (Vestbo et al., 2018; Hyman et al., 2021; Souza et al., 2021). Previous articles have shown that crab burrow distribution patterns are strongly regulated by abiotic factors (Li et al., 2018). Salinity and substratum preference are, reportedly, the most important factors determining crab burrow distribution (Jones and Simons, 1981; He and Cui, 2015). The majority of previous studies have focused on the distribution of crab burrows among different habitats, at the scale of the specific habitat (Flores et al., 2005; Hamasaki et al., 2011; Li et al., 2018). The different habitats were defined based on the cover of vegetation (Flores et al., 2005; Hamasaki et al., 2011). However, the large-scale distribution patterns of crab burrows across the shore and the longshore variation among the same habitat remain unclear (Turra et al., 2005; Souza et al., 2021). At small scales, the distribution of crab burrows among different zones of the same marsh tussock remains poorly studied. Previous studies showed that it is difficult for crabs to burrow in hard substrates, with complicated root systems (Flores et al., 2005; Bertness et al., 2014). The same marsh tussock has different degrees of root complexity in different zones. Therefore, it is worthwhile to unravel the small-scale detailed spatial preference of crab burrows among different zones of a marsh tussock. Moreover, field observations were mostly conducted in warm seasons, when crabs are more active (Checon and Costa, 2017; Tatsuya et al., 2017; De Grande et al., 2018; Li et al., 2018). However, crabs may display different behaviors in cold seasons (Rosa and Borzone, 2008; Beheshti et al., 2021). Existing studies suggest that crabs prefer to inhabit in vegetated areas as refuge from thermal stress and predation pressure in hot and dry conditions (Coverdale et al., 2012; Bertness et al., 2014). It is therefore necessary to carry out seasonal field observations to explore the temporal distribution of crab burrows.
This study aims to gain insight into the spatiotemporal distribution of crab burrows in a saltmarsh wetland on the Jiangsu Coast, China. Specific questions that we aim to address include: (1) What is the spatiotemporal distribution of crab burrows? (2) How do abiotic factors affect the distribution of crab burrows? To answer these questions, a two-year programme of field observations, at seasonal intervals, was conducted to explore the spatiotemporal distribution of crab burrows. The findings of this study provide a comprehensive field dataset to facilitate a more in-depth understanding of crab burrow distribution, and hence inform the scientific management of tidal wetlands.
This study was carried out in the Doulong harbor of Yancheng intertidal flat, in the northern part of the Jiangsu Province, China (Figures 1A, B). The Jiangsu Coast (119°170E–122°200E, 31°330N–35°070N) is located between the Yangtze River Estuary and the abandoned Yellow River Delta, which comprises rich tidal flats sheltered by radial sand ridges (Zhang et al., 2016). The radial sand ridges are characterized by a typical monsoon climate, with an apparent seasonal variation. In general, the most intense monsoon season is from October to March. The prevailing wind is south-east direction in summer and north-west direction in winter. The wave heights which are highly influenced by the monsoon climate in winter are larger than those in summer (Xu et al., 2016). While the influence of a single winter storm is weaker than that of a typhoon, the frequency of winter storms is greater than that of typhoons. Storms, including typhoon and winter storms, could only cause short-term modifications, tidal currents were suggested to be the major hydrodynamic factor. Tidal flow in the study area is characterized by a radial current field governed by the large-scale radial sand ridges (Zhang et al., 1999). On the near-shore coast, tide is semidiurnal and the mean tidal range is 2.5-4.0 m. Due to the influence of rainfall, evaporation, runoff and tide, seawater salinity fluctuates yearly from 21.9 to 33.4 ‰ and the mean seawater salinity is about 27.5 ‰ (Zhang, 2013). The climate in this area is subtropical, with an average annual temperature of 15.3°C and the mean seawater temperature ranges from 5.62 to 31.00°C (Zhang, 2013).
Figure 1 (A) Location of the study area; (B) Sampling site and sections arrangement, the solid lines represent the two sampling sites and the dashed lines represent the repeated sampling sections in each site, green points represent the sampling points in the marsh, red points represent the sampling points in the edge, yellow points represent the sampling points in the bare flat; (C) Photo of Helice tientsinensis and its burrow; (D) Different sizes of burrows in summer and winter; (E) Small-scale zonation of plant tussock.
This study was situated on Doulong harbor from September 2019 to June 2021. Throughout the observational period, seawater temperature ranges were from 3.32 to 30.68°C with an average of 14.8°C. The current velocities fluctuated from 0.02 to 1.50 m/s and were relatively small in cold seasons. The water depth ranged from 0 to 1.52 m in the entire study. The significant wave heights were between 0 and 0.45 m with higher significant wave heights during warm seasons. Therefore, the observational conditions encountered in this research can be regarded as the typical conditions for this site and the observational results can be regarded as the general patterns for crab distribution in this site.
We focus on the distribution of Helice tientsinensis, which tends to distribute the upper and middle part of the intertidal areas and is the most wide-spreading crab species in the Doulong Coast (Huang et al., 2019; Lan et al., 2020). They can remove large amounts of sediment to form a surface mound during daily maintenance and their burrowing activities can generate a concave or convex micro-topography (Figure 1C). The width of the burrows is about 5 cm and almost constant throughout the year, while the depth varies from 20 cm in summer to 50 cm in winter as crabs need to dig deeper to keep warm (Figure 1D). Meanwhile, burrows remain open during high water and may work as passive traps for sediment.
Hydrodynamic parameters were measured using instruments tied to a custom-made frame at both northern and southern sites in September 2020, December 2020, March 2021, and June 2021. In order to maintain the measurement frame stability over the observation period, frame made with two bamboos was pushed into the sediment at least 1.5 m. All instruments were attached to the frame and set in advance. The Acoustic Doppler Current Profiler (ADCP) was mounted vertically onto the frame, and its sensor was located 10 centimeters above the sea bed to measure the tidal parameters. Data of tidal current velocities were measured every 2.5 minutes. The wave recorder (a RBR Company Tide Wave Recorder-2050) was vertically installed 10 centimeters above the bottom to collect time series of water depth and wave parameters. The recorder used pressure sensors to collect burst data every 20 minutes. To investigate the SSC, the Optical Backscatter Sensor (OBS-3A) was used, which was mounted on the same frame in a vertical position 10 centimeters above the sea bed. Data of OBS-3A were collected at 2.5-min intervals. Calibration of OBS-3A is carried out by establishing a fitting curve by relating the on-site sediment concentration of collected water samples and the OBS digital signal (Lewis, 1996; Chen et al., 2020).
The activity of crabs decreases when temperature is either too low or too high. Field observations were carried out in periods when crabs were active, i.e., September 2019 (the mean temperature: 18°C), December 2019 (the mean temperature: 3°C), September 2020 (the mean temperature: 20°C), December 2020 (the mean temperature: 4°C), March 2021 (the mean temperature: 10°C) and June 2021 (the mean temperature: 24°C). For simplicity, spring and winter are referred to as cold seasons while summer and autumn are referred to as warm seasons in this study. Field observations in March and June of 2020 were not conducted, because of travel restrictions for COVID-19.
To investigate the distribution of burrows and environmental variables across the coast, 2 sampling sites, which were referred to as northern site (N-N) and southern site (S-S) respectively, were selected. Each sampling site has five repeated sampling sections, and each sampling section crossed the marshes and bare flats (Figure 1B). The saltmarsh edge of northern site presented a smooth transition and southern site presented a tidal creek in the front of the edge. 8 and 6 sampling points at each sampling section were selected in the vegetated and bare flats, respectively. The distance between two neighboring points was approximately 40 m. Hereafter, the location of each sampling point relative to the marsh edge are abbreviated to “Point ± x”, where “-” indicates a sampling point on bare flat and “+” on vegetated flat, and “x” indicates the distance from the sampling point to marsh edge (in meters). At each point, we randomly took 3 paired samples (1m * 1m) from the upper 5 cm of the sediment using a soil sampler (Ø 5cm) during low tide. The samples were taken back to the laboratory to measure the environmental factors (grain size composition, water content, organic matter content, and soil salinity). The number of crab burrows was counted three times in each sampling point. Elevation and position data were acquired by a Real Time Kinematic (RTK), and was used to calculate the inundation duration.
To explore the distribution of crab burrows among the different parts of marsh tussock, the sampling points were chosen randomly ranging from Point +160 to Point +200 (around the most densely populated zone). Each sampling point had to be a newly born plant tussock with a radius of around 1 m, because the effect of crabs on newly born plants was greater (Coverdale et al., 2012). Based on the small-scale zonation of plant tussocks (Figure 1E), sampling points were divided into three parts: the middle of vegetation (indicated by the circle from the center point to 1 m in diameter), the edge of vegetation (indicated by the ring from 1 to 2 meters in diameter), and the external of vegetation (indicated by the ring from 2 to 3 meters in diameter) (Zhao et al., 2021). The middle and the edge parts were collectively called the vegetated area. Marks were used to ensure that we surveyed the same plant tussock over time. At each part of plant tussock, the number of crab burrows was counted three times and then 3 paired samples from the upper 5 cm of the sediment were collected for the measurement of environmental variables.
The grain size distribution was measured by a laser particle size analyzer (Malvern 3000). Water content was calculated as the ratio of the loss weight (difference between wet and dry weight of the soil) to the dry weight of the soil (oven-dried at 105°C until reaching a constant weight, generally about 6-8h). After that, soil samples were both weighed before and after drying at 600°C for 2h by a muffle furnace to obtain organic matter content. Soil salinity was obtained from the salinity of the soil in solution. The soil solution was prepared using 5-gram of soil dissolved in 100ml of water and salinity was measured using a salinity meter.
Statistical analysis was conducted following several steps. The first step was to compare crab burrow density, the median grain size, water content, organic matter content, soil salinity, and elevation among 14 points at regular (40m) intervals across the marsh zone from land to sea. The second step was to explore the variations in crab burrow density and environmental factors along the shore. The third step was to investigate the spatiotemporal patterns of crab burrow distribution and environmental variables among different zones of plant tussock. The differences between each environmental variable were evaluated by one-way analysis of variance (ANOVA) at the 5% level. The final step was to explore the dominant abiotic drivers in different seasons. Correlations between two factors were examined by the variance inflation factor (VIF) to eliminate highly correlated parameters. A backward stepwise model selection was performed based on R-square and Akaike information criterion (AIC) to explore the main driving factors. In addition, the correlation between crab burrow density and various factors was explored using multiple linear regression. The detailed procedure of the multiple linear regression method carried out can be referred to Appendix A.
The nearshore region of the studied tidal flat was affected by both tidal currents and waves. In this study, tidal current velocity, water depth, significant wave height, and SSC were measured (Figure 2). Data in some subplots were missing because of issues in observational apparatus or external disruption (e.g., due to Covid) During the entire field measurement, the variability of tidal current generally followed the same trend. The lowest current velocities were recorded around high tides (minimum current velocities in the northern site and southern site were 0.16 m/s and 0.02 m/s, respectively). Higher current velocities occurred during early flood and late ebb stages (maximum current velocities in the northern and southern site were 1.50 m/s and 1.43 m/s, respectively). The velocity and duration of the flood were similar with those of the ebb. Water depth was similar in the northern site (0.75 ± 0.44 m on average) and the southern site (0.72 ± 0.43 m on average). Significant wave height was higher around high tides. With water depth decreasing, significant wave height reduced. In the northern site, the significant wave height ranged from 0 to 0.45 m, with an average of 0.17 m. In the southern site, lower values were recorded, ranging from 0 to 0.38 m and 0.14 m on average. The value of SSC reflects the combined influence of tide and wave forcing. It should be noted that SSC in the southern site (389 ± 49 g/m3 on average) with lower hydrodynamic strength showed higher value than that in the northern site (318 ± 42 g/m3 on average). This can be attributed to the coarser bottom depositions in the northern site which require larger current velocity to be suspended.
Figure 2 Seasonal hydrodynamic conditions in different sites. (A) September 2020, (B) December 2020, (C) March 2021, (D) June 2021. Vc represents current velocity. D represents water depth. Hs represents the significant wave height. SSC represents suspended sediment concentration.
Different spatial patterns of crab burrow were found in different seasons (Figure 3). The distribution of crab burrows across the shore was unimodal in winter-spring and bimodal in summer-autumn (Figures 3A, B). The onshore peak value of 12 ± 4 int/m2 was found some 160 m landward of the marsh edge and the offshore peak value of 8 ± 2 int/m2 was found about 80 m seaward of the marsh edge. Compared to the other observation times, the overall crab distribution in June 2021 advanced approximately 50 m seaward when the edge of the marsh (characterized by the patches of Spartina alterniflora) moved seaward by a similar distance, with the substrate becoming wet and soft.
Figure 3 The spatial distribution of crab burrow and environmental variables across the shore in different seasons. (A) The density of crab burrows in northern site; (B) The density of crab burrows in southern site; (C) The median grain size in northern site; (D) The median grain size in southern site; (E) The water content in northern site; (F) The water content in southern site; (G) The organic content in northern site; (H) The organic content in southern site; (I) The soil salinity in northern site; (J) The soil salinity in southern site; (K) The elevation in northern site; (L) The elevation in southern site; Data is shown as means ± SE (n=15). The origin of coordinates means the edge of salt marshes. X-axis means the distance from the edge with positive values indicating seaward and negative representing landward. Point +20~+280m (the background color: green) are in the salt marsh. Point -20m~20m (the background color: yellow) are around the edge. Point -20m~-240m (the background color: white) are on the bare flat.
As for the corresponding environmental variables, spatial and temporal variations were also observed. In the cross-shore direction, the median grain size (D) on the bare flat was more than twice that of the salt marsh area and this applied for both sites in different seasons (Figures 3C, D). Water content (W) within the salt marsh was 70-80% higher than on the bare flat (Figures 3E, F). Organic matter content (O) varied among different seasons across the shore. The value of the organic matter content across the shore in winter and spring showed a decreasing trend ranging from 8.7 ± 2.4% to 4.2 ± 1.2%. In summer and autumn, however, it decreased and reached the lowest level around the edge of the marsh (minimum value of 9.5 ± 1.5% was found at Point 0m), and then increased to some extent before gradually decreasing offshore (Figures 3G, H). Soil salinity (S) generally decreased across the shore from the land to the sea, ranging from 9.6 ± 1.3‰ to 5.2 ± 1.2‰ (Figures 3I, J). Sea bed elevation at the salt marsh area was roughly 10% higher than that on the bare flat (Figures 3K, L). Sea bed elevation was also similar around the edge where a slight drop was found except September 2020 in the southern site. In September 2020, a tidal creek meandered to the southern site. Meanwhile, it was worth noting that the elevation in both sites of June 2021 on the bare flat was slightly higher than other seasons.
To better illustrate the temporal distribution patterns at the two sites, mean values were obtained by taking the average of each site (Figure 4). In warm seasons (May-Oct), crab burrow density was double or even triple that in cold seasons (Figure 4A). Median grain size was 10%-30% larger in cold seasons (Nov-Apr) (Figure 4B). Water content in warm seasons was over 24% greater than that in cold seasons (Figure 4C). Organic matter content in warm seasons was 100~200% larger than that in cold seasons (Figure 4D). Soil salinity showed similar values in the different seasons (Figure 4E). Elevation remained relatively stable among different seasons, except the southern site in September 2020 (Figure 4F).
Figure 4 Variation of crab burrow distribution and environmental variables between the different sites in different seasons. (A) The density of crab burrows; (B) The median grain size; (C) The water content; (D) The organic matter content; (E) The soil salinity; (F) The elevation. Data is shown as means of the whole site ± SE. All ANOVA tests are significant (P<0.05 in each case). The white background showed the warm seasons. The blue background showed the cold seasons. Different letters denoted significant differences between treatments.
The distribution of crab burrows and environmental variables across the shore in the same season was similar between southern and northern sites. However, the onshore peak in the southern site was closer to the sea, while the offshore peak in both sites were similar. Meanwhile, there was some slight difference in the absolute values of crab burrows and environmental variables between the two sites (Table 1). Crab burrow density in the southern site (3.22 ± 0.97 int/m2 on average) was obviously higher than that in northern site (2.1 ± 0.75 int/m2 on average) (Figure 4A). Median grain size in the northern site was larger (19.05 ± 3.15 μm on average), compared with the southern site (15.08 ± 3.12 μm on average) (Figure 4B). Water content, organic matter content and soil salinity in both sites showed similar values (Figures 4C–E). Elevation in the northern site was more stable than that in the southern site (Figure 4F). This may be attributed to the meandering of tidal creek in the southern site.
The mean values of current velocity, water depth, significant wave height, and suspended sediment concentration were obtained by taking the average at each site (Table 2). The current velocities in warm seasons were about twice that of cold seasons (Figure 5A). This is probably affected by the combined effect of wind-driven current and ocean currents. As a whole, the current velocities at the northern site (0.88 ± 0.32 m/s on average) were slightly larger than at the southern site (0.76 ± 0.31 m/s on average) (Figure 5A). The water depth in warm seasons was 30%-40% larger than in cold seasons but water depth showed no significant difference between the two sites (p>0.05). Significant wave height in warm seasons was 30%-40% larger than that in cold seasons (Figure 5B). Meanwhile, significant wave heights in the northern site were 20%-30% higher than that in the southern site (Figure 5C). Qualitatively, the suspended sediment concentrations in the southern site were 20%-30% higher than in the northern site in all seasons (Figure 5D). There was similar value in suspended sediment concentrations between September 2020 and December 2020. Noticeably, the suspended sediment concentrations in June 2021 overall increased about 20%. This may have resulted in more deposition on the bare flat in June 2021.
Figure 5 Mean and standard deviation values by different seasons and different sites for (A) The current velocity, (B) The wave depth, (C) The significant wave height, and (D) The suspended sediment concentration. Different letters denote significant difference (p<0.05) between different treatment. The white background showed the warm seasons. The blue background showed the cold seasons. Different letters denoted significant differences between treatments.
Crab burrow distribution and environmental variables varied across the different zones of marsh tussock in different seasons (Table 3). In the vegetated area, crab burrow density was double that found in un-vegetated areas (Figure 6A). The difference between seasons was mainly characterized by the preference for the middle or the edge of the marsh tussock. In winter and spring, the density of crab burrows in the middle and the edge of the tussock showed no significant difference (p<0.05), while in summer and autumn the density of crab burrows in the middle of the tussock was about half of that in the edge. This may be attributed to the complexity of plant roots. Median grain size in the tussock (8.84 ± 2.5 μm on average) was similar with that in un-vegetated areas (9.35 ± 0.9 μm on average) (Figure 6B). Nevertheless, median grain size in vegetated areas fluctuated more widely than in un-vegetated areas. Water content differed among different seasons. Namely water content in the middle and edge areas of the tussock (55.75 ± 5.7% on average) was higher than the external areas (48.1 ± 1.9% on average) in warm seasons and showed similar values (57.2 ± 4.6% on average) in cold seasons (Figure 6C). The overall organic matter content in summer and autumn was twice that measured for winter and spring (Figure 6D). Simultaneously, organic matter content was 20% smaller for un-vegetated areas than in the tussock. The soil salinity in winter and spring was 10% higher than that in summer and autumn (Figure 6E). The largest values of soil salinity were in the external areas of the tussock (8.55 ± 0.7‰ on average). This may be affected by gradients in the evaporation and drainage. Elevation in the middle and edge of the tussock was 5% higher than that in the external areas of the tussock, regardless of season (Figure 6F).
Figure 6 Box plots of crab burrow distribution and environmental variables among different plant zones and in different seasons. (A) The density of crab burrows; (B) The median grain size; (C) The water content; (D) The organic matter content; (E) The soil salinity; (F) The elevation. Data is shown as means ± SE (n=10). All ANOVA tests are significant (P<0.05 in each case). A box plot is a chart that shows data from a five-number summary including minimum, Q1 (First Quartile), median, Q3 (third Quartile), and maximum (from top to bottom). Different letters denoted significant differences between treatments.
All environmental variables were involved in the best predictive model (Table 4). In our initial multiple regression model (IM), we checked Variance Inflation Factor (VIF) to eliminate inappropriate variables out of the model. Then a backward stepwise regression is used to determine which parameter was more predictive. Model selection was based on R2 and AIC. The error greater than 1% was defined as a significantly worse fit model.
Table 4 Summary outputs of the multiple linear regressions. A backward stepwise model selection was performed based on R2 and AIC.
The dominant abiotic factors driving crab burrow distribution were as follows: organic matter content and soil salinity in cold seasons; water content, organic matter content, and soil salinity in warm seasons. The other abiotic factors did not seem to correlate well with crab burrow distribution.
The spatiotemporal distribution of crab burrow exhibited similar patterns across the shore. To explore the temporal heterogeneity, six field observations were carried out. The crab burrow distributed from Point +280 (salt marsh) to Point -240 (bare flat) in summer and autumn, while across-shore crab burrow distribution was from Point +280 (salt marsh) to Point 0 (marsh edge) in winter and spring. The distribution of crab burrows in warm seasons were spread out over larger areas and with greater density (Rosa and Borzone, 2008; Beheshti et al., 2021; Bopp et al., 2021; Souza et al., 2021). The result showed that the bare flat in front of the marsh edge reached a larger organic matter content in warm seasons, which made the soil humid and crumby to engage crab settlement. Meanwhile, in warm seasons crabs are often more active and widespread. The onshore peak in four seasons was always found in the middle part of the marsh tussock, with appropriate water content, organic matter content, and soil salinity conditions. Previous studies showed that these three abiotic factors are particularly important, because (1) water content relieves desiccation stress, (2) organic matter is a key food resource for crabs and (3) inappropriate soil salinity limits the distribution of crabs (Reinsel and Dan, 1995; Thrush et al., 2003; Colpo and Negreiros-Fransozo, 2013; Li et al., 2018; Peer et al., 2018). Meanwhile, the plant biomass in the middle of salt marsh tussock was moderate. The offshore peak only in summer and autumn always appeared in the front of the edge, where soil substrate was wetter and softer. Moreover, biofilm and fungi were abundant in warm seasons which provided nutrition for crabs on the bare flat. This was also in consistent with that organic content on the bare flat was obviously higher in warm season than in cold season. Therefore, crabs showed up on bare flat, and abandoned their burrows in cold season. Particularly, in September 2020, the tidal creek, which was moist, approached the northern cross-sections. A previous study of Li et al. (2018) reported the same phenomenon that crabs preferred habitats with softer and wetter edaphic conditions with enough food resources. It was worth noting that the distribution of crab burrows in June 2021 tended to prograde seaward by about 50m. At the same time, the edge of marshes and the patches of Spartina alterniflora were observed to advance seaward by the similar distance. It was accompanied by the substrate in front of the marsh edge turning wet and soft. Plants can provide shade and reduce the evaporation to provide a comfortable shelter for crabs. In addition, plants can prevent crabs from thermal stress and predator attack. Previous studies have shown that crabs prefer habitats in vegetated areas because there is less thermal stress and predation pressure (Coverdale et al., 2012; Bertness et al., 2014). Around Point +280 and Point -240 crab burrows disappeared suggesting a spatial limit for the Helice tientsinensis distribution in Jiangsu Coast. The median grain size was largest offshore (Zhou et al., 2016), and therefore limited the crab burrow distribution (Chartosia et al., 2006). In the marshes further shoreward, soil salinity was usually high due to poor drainage and strong evaporation, which resulted in large bare salt patches and limited the crab burrow distribution (Qiang et al., 2009; Li et al., 2016). Meanwhile, the plants close to the landside salt marsh were too dense for crabs to burrow in. Crab burrow density reached a lower level around the marsh edge. The hydrodynamics were more dynamic in front of the marsh edge, where plants could induce complex flow structures with intense wave breaking. Meanwhile, the bed elevation profile indicated that there was a slight cliff around the marsh edge, again as a result of wave-plant interactions. Previous studies reported that the bathymetry changed dramatically around the marsh edge, which might prejudice crab survival (Zhao, 2020).
The mean crab burrow density in warm seasons was larger than that in cold seasons. Meanwhile, the range of crab burrows distribution in warm seasons was wider than in cold seasons, since crabs in warm seasons behave more actively, e.g., feeding, courtship and reproduction (Rosa and Borzone, 2008; Beheshti et al., 2021; Souza et al., 2021). The median grain size in winter and spring was larger than that in summer and autumn, consistent which have identified a seasonal shift in the median grain size from coarser sediments in cold seasons to finer sediments in warm seasons (Herman et al., 2001; Van Wesenbeeck et al., 2007; van der Wal et al., 2008; Coverdale et al., 2012). The water content and the soil salinity in warm seasons were higher than that in cold seasons, because crab burrows enhance drainage and evaporation (Xin et al., 2016; Xiao et al., 2019; Zhou et al., 2020). Meanwhile, the organic matter content increased in warm seasons as the decomposition of microorganisms mainly occurs in the thermophilic and mesophilic stages during composting (Chen et al., 2020).
The spatial distribution patterns of crab burrows showed similar tendencies and certain quantity differences along the shore. The mean crab burrow density in the northern site was lower than that in the southern site. Meanwhile, the mean median grain size in the northern site was larger than that in the southern site. No significant differences were found in the other four parameters in the two sites. To a large extent, the median grain size was affected by hydrodynamics. The current velocities and significant wave heights in the northern site were generally higher than that in the southern site, namely stronger hydrodynamics in the northern site. Existing studies have shown that the interior of the burrow tunnel and surface around the burrow entrance can be affected by tidal currents during the flood tide, and can be destroyed during storms (Botto and Iribarne, 2000). Our results showed that the mean crab burrow density was overall lower in the northern site, suggesting that there might be a link between hydrodynamic conditions and the density of crabs. Noticeably, the hydrodynamics in the northern site were slightly stronger, while the suspended sediment concentrations in the northern site were lower, and this can be attributed to the larger median grain size in northern site. Shear at mixed layer base caused by wave-current interaction was too low to resuspend surface sediment. Previous studies reported that of the presence of crabs can reduce the critical erosion threshold and enhance erosion to some degree. Crabs directly affect the erosion threshold by binding and carrying the sediment to create and maintain burrows and indirectly influence the erosion resistance by feeding on the plant roots to cause plant death and reduce the soil integrity (Mccall and Tevesz, 1982; Bortolus and Iribarne, 1999; Wotton and Malmqvist, 2001; Escapa et al., 2007; Grabowski et al., 2011; Hu et al., 2021). There was stronger hydrodynamics in the northern site, while there was lower SSC in the northern site. This is not only attributed to the coarser bottom depositions in the northern site which require larger current velocity to be suspended, but also owing to higher crab burrow densities in southern site which stirring up sediment to transport. This study may provide new evidence that crabs stir up sediment to enhance sediment transport. In particular, the suspended sediment concentrations in June 2021 generally increased, which might have contributed to the deposition in front of the marsh edge. Hence, the hydrodynamics would seem to have a direct impact on crab distribution but also an indirect impact by altering the sediment properties.
The spatiotemporal patterns of crab burrow distribution and abiotic drivers varied among different parts of the same marsh tussock. Crab burrow density was larger in vegetated areas than in un-vegetated areas. Shading by plants could reduce stress of predation and dehydration (He et al., 2015; Chen et al., 2016a). Meanwhile, plants provide a food resource and reduce the physical stresses on crabs. Our results showed that water content and organic matter content were higher in vegetated areas than in un-vegetated areas, while soil salinity was lower in vegetated areas than in un-vegetated areas. Comparing warm and cold seasons, crab burrow density in the middle of the marsh tussock decreased and more crabs were attracted to the marsh edge. The main reason for this was that the substrate became too hard to burrow into, due to the growth of middle vegetation (Bertness et al., 2014; Wang et al., 2014). Meanwhile, crab burrow density in the vegetated areas during the warm seasons was higher than in cold seasons. With temperature rising, crabs move to vegetated areas to decrease the risk of exposure to high thermal and predation stresses rises (Stone and O'Clair, 2001; Morris, 2005; Walther et al., 2009; Li et al., 2020; Andreo-Martínez et al., 2021). The mean value of median grain size in the marsh area was similar to the mean value in un-vegetated area. Nevertheless, the fluctuation of the median grain size in the vegetated area was larger than in the un-vegetated area. More crab disturbance was attributed to the wider range of median sediment grain size (Chen et al., 2016a). Vital activities for crabs, such as ingestion, excretion, and movement, can bind sediment grains and disturb the soil structure. The elevation in the vegetated area was higher because plants can retard surface runoff, trap sediment, and promote siltation (Moller et al., 2001). Meanwhile, elevation reflects inundation duration and overlong inundation affects the activity of crabs (Li et al., 2018).
The field data were used to explore and predict the spatiotemporal distribution of crab burrows on tidal flat-marsh systems, adopting a response model approach. Response models have been applied successfully to study other macro-benthos in soft sediments (Ysebaert et al., 2002; Thrush et al., 2003; Thrush et al., 2005; Ellis et al., 2006). Response models represent a top-down correlation between crabs and environmental variables (Li et al., 2018, Noor and Asmat, 2019, and Qiu et al., 2021). The correlation reflects a relationship similar to cause and effect. Previous response models have explored the relationship between crab burrows and sediment grain sizes (Ysebaert et al., 2002; Thrush et al., 2003). However, other abiotic factors may also be important and have not been systematically considered in previous response models. Significant difference existed in different seasons, and therefore the analysis examined the four seasons, as well as the response over all seasons. In this study, the response models were best explained using a subset of the environmental variables, drawn from median grain size, water content, organic matter content, soil salinity, and bed elevation. The result showed that the main influences on crab burrow distribution in cold seasons were organic matter content and soil salinity; while in warm seasons water content also played a significant role. Organic material, like leaf litter, debris, and fungi were fed by crabs (He et al., 2015; Chen et al., 2016b). Detailed analysis results (Appendix A) showed that organic content showed positive relationship with crab burrow density. These results were generally consistent with the preference to habitats with softer and wetter edaphic conditions and adequate food resources (Li et al., 2018). Our result showed that burrow densities were higher in vegetated areas than in un-vegetated areas. Plants provided leaf litter and debris for crabs. In warm season, biofilm and fungi were abundant which provided nutrition for crabs on the bare flat. This is consistent with our result that the distribution of crab burrows in warm season expanded into the bare flat. Soil salinity was related positively with crab burrow density in winter and spring, while negatively in summer and autumn. Crabs also exhibited subtly different preferences in the different seasons in response to variations in soil salinity (Horn and Tolley, 2008). The result showed that water content was also governing factor in warm seasons. Desiccation was a fundamental factor in the survival of crabs (Bortolus et al., 2002). When crabs abandoned their burrows, they would die quickly during high temperature (Beigt et al., 2003). Our study found that crabs preferred bare flat which was frequently flooded. Escapa et al. (2007) also showed that crabs preferred sites such as tidal creek heads and drainage basins, where water continues flowing even during low tides and accumulates after rainy days (Yuan et al., 2022). Compared with salt marsh edge, the frequency of fully flooding internal salt marshes was less. The internal salt marshes studied is fully flooded only about 40 times per year (Minkoff et al., 2006). The burrows in internal salt marshes had greater depths and volumes allow more water retention during low tides.
Crabs can be used as an indicator for the health of salt marsh ecosystems (Spivak et al., 1994; Cardoni et al., 2007; Griffiths et al., 2007; Weilhoefer, 2011). The response models provide a tool to predict the distribution of crab burrow density. In this study, a model using five environmental variables had the largest explanatory power. Correlations between crab burrow distribution and environmental variables may also be affected by other variables that were not included in this analysis. Future work should therefore consider other environmental variables and sites in different environmental settings, to both test the findings presented here and further improve the response model. More detailed relationship between crab burrow density and environmental variables could also be explored in laboratory experiments.
Spatiotemporal distribution across the shore of crab burrows showed seasonal patterns in Doulong Harbor. Our results showed that patterns of crab burrow distribution were more strongly affected by organic matter content and soil salinity in cold season, while water content played an important role in warm season. With temperature increasing and plants growing, crabs preferred to settle around the edge of plant tussocks. This study provides a basis for exploring the spatiotemporal patterns of the dominant crab species Helice Tientsinensis in the Northern Jiangsu Coast. More detailed relationships between crab burrow density and environmental variables could potentially be explored in the more controlled environment provided by laboratory experiments.
The raw data supporting the conclusions of this article will be made available by the authors, without undue reservation.
XC and ZZ did field observations and proposed the idea for this study. XC, ZZ, and HZ designed the research. ZZ, ZG, and CZ acquired the funding. XC performed the research, analysed the data, and prepared the original manuscript. ZZ, QH, HZ, TB, ZG, IT, and CZ provided logistical support, and revised the manuscript. All authors contributed to the article and approved the submitted version.
This study is supported by the Natural Science Foundation of Jiangsu Province (Grant No. BK20200077), the National Natural Science Foundation of China (NSFC, Grant Nos. 41976156, 51925905), the Nantong Science and Technology Bureau (Grant No. MS12021083), the Marine Science and Technology Innovation Project of Jiangsu Province (Grant No. JSZRHYKJ202105), and the Fundamental Research Funds for the Central Universities (Grant No. B210204022).
The authors declare that the research was conducted in the absence of any commercial or financial relationships that could be construed as a potential conflict of interest.
All claims expressed in this article are solely those of the authors and do not necessarily represent those of their affiliated organizations, or those of the publisher, the editors and the reviewers. Any product that may be evaluated in this article, or claim that may be made by its manufacturer, is not guaranteed or endorsed by the publisher.
The Supplementary Material for this article can be found online at: https://www.frontiersin.org/articles/10.3389/fmars.2022.1040308/full#supplementary-material
Alexander T., Bell S. S., Mccann K. S., Kristina M., Armitage A. R. (2016). Predator diet and trophic position modified with altered habitat morphology. PloS One 11, e0147759. doi: 10.1371/journal.pone.0147759
Andreo-Martínez P., Ortiz-Martínez V. M., Muoz A., Menchón-Sánchez P., Quesada-Medina J. (2021). A web application to estimate the carbon footprint of constructed wetlands. Environ Modell Software 135, 104898. doi: 10.1016/j.envsoft.2020.104898
Angelini C., van Montfrans S. G., Hensel M., He Q., Silliman B. R. (2018). The importance of an underestimated grazer under climate change: How crab density, consumer competition, and physical stress affect salt marsh resilience. Oecologia 187, 205–217. doi: 10.1007/s00442-018-4112-8
Barbier E. B., Hacker S. D., Kennedy C., Koch E. W., Stier A. C., Silliman B. R. (2011). The value of estuarine and co-astal ecosystem services. Ecol. Monogr. 81, 169–193. doi: 10.1890/10-1510.1
Beheshti K. M., Endris C., Goodwin P., Pavlak A., Wasson K. (2021). Burrowing crabs and physical factors hasten marsh recovery at panne edges. bioRxiv 17, 435836. doi: 10.1101/2021.03.17.435836
Beigt D., Píccolo M. C., Perillo G. M. E. (2003). Soil heat exchange in Puerto cuatreros tidal flats, Argentina. Cienc. Mar. 29, 595–602. doi: 10.7773/cm.v34i1.1194
Bertness M. D., Brisson C. P., Bevil M. C., Crotty S. M. (2014). Herbivory drives the spread of salt marsh die-off. PloS One 9, e92916. doi: 10.1371/journal.pone.0092916
Bertness M. D., Holdredge C., Altieri A. H. (2009). Substrate mediates consumer control of salt marsh cordgrass on cape cod, New England. Ecology 90, 2108–2117. doi: 10.1890/08-1396.1
Bopp J. J., Sclafani M., Frisk M. G., McKown K., Ziegler-Fede C., Smith D. R., et al. (2021). Telemetry reveals migratory drivers and disparate space use across seasons and age-groups in American horseshoe crabs. Ecosphere 12 (10), e03811. doi: 10.1002/ecs2.3811
Bortolus A., Iribarne O. (1999). Effects of the SW Atlantic burrowing crab Chasmagnathus granulata on a spartina salt marsh. Mar. Ecol. Prog. Ser. 178, 79–88. doi: 10.3354/meps178079
Bortolus A., Schwindt E., Iribarne O. O. (2002). Positive plant–animal interactions in the high marsh of an Argentinean coastal lagoon. Ecology 83, 733–742. doi:10.1890/0012-9658(2002)083[0733:PPAIIT]2.0.CO;2.
Botto F., Iribarne O. (2000). Contrasting effects of two burrowing crabs (Chasmagnathus granulata and Uca uruguayensis) on sediment composition and transport in estuarine environments, estuar. Coast. Shelf S 51, 141–151. doi: 10.1006/ecss.2000.0642
Cardoni D. A., Isacch J. P., Iribarne O. O. (2007). Indirect effects of the intertidal burrowing crab Chasmagnathus granulatus in the habitat use of argentina's south West Atlantic salt marsh birds. Estuar. Coast. 30, 382–389. doi: 10.1007/BF02819385
Carol E. S., Kruse E. E., Pousa J. L. (2011). Influence of the geologic and geomorphologic characteristics and of crab burrows on the interrelation between surface water and groundwater in an estuarine coastal wetland. J. Hydrol 403, 234–241. doi: 10.1016/j.jhydrol.2011.04.007
Chartosia N., Koukouras A., Mavidis M., Kitsos M. S. (2006). Preliminary estimation of the factors influencing the distribution of the midlittoral crab Portumnus lysianassa (Herbs). Hydrobiologia 557, 97–106. doi: 10.1007/s10750-005-1312-0
Checon H. H., Costa T. M. (2017). Fiddler crab (Crustacea: Ocypodidae) distribution and the relationship between habitat occupancy and mouth appendages. Mar. Biol. Res. 13, 618–629. doi: 10.1080/17451000.2016.1273530
Chen H., Hagerty S., Crotty S. M., Bertness M. D. (2016a). Direct and indirect trophic effects of predator depletion on basal trophic levels. Ecology 97, 338–346. doi: 10.1890/15-0900.1
Chen G. C., Lu C., Rong L., Chen B., Hu Q., Yong Y. (2016b). Effects of foraging leaf litter of Aegiceras corniculatum (Ericales, myrsinaceae) by Parasesarma plicatum (Brachyura, sesarmidae) crabs on properties of mangrove sediment: a laboratory experiment. Hydrobiologia 763, 125–133. doi: 10.1007/s10750-015-2367-1
Chen Q., Wang J., Zhang H., Shi H., Liu G., Che J., et al. (2020). Microbial community and function in nitrogen transformation of ectopic fermentation bed system for pig manure composting. Bioresource Technol. 319, 124155. doi: 10.1016/j.biortech.2020.124155
Colpo K. D., Negreiros-Fransozo M. L. (2013). Morphological diversity of setae on the second maxilliped of fiddler crabs (Decapoda: Ocypodidae) from the southwestern Atlantic coast. Invertebr Biol. 132, 38–45. doi: 10.1111/ivb.12004
Coverdale T. C., Altieri A. H., Bertness M. D. (2012). Belowground herbivory increases vulnerability of New England salt marshes to die-off. Ecology 93, 2085–2094. doi: 10.1890/12-0010.1
De Grande F. R., Granado P., Sanches F. H. C., Costa T. M. (2018). Organic matter affects fiddler crab distribution? Results from field and laboratorial trials. Estuar. Coast. Shelf S 212, 138–145. doi: 10.1016/j.ecss.2018.07.005
Ellis J., Ysebaert T., Hume T., Norkko A., Bult T., Herman P., et al. (2006). Predicting macrofaunal species distribution in estuarine gradients using logistic regression and classification systems. Mar. Ecol. Prog. Ser. 316, 69–83. doi: 10.3354/meps316069
Escapa M., Darío R., Perillo G. M. E., Iribarne O. (2007). Direct and indirect effects of burrowing crab Chasmagnathus granulatus activities on erosion of southwest Atlantic sarcocornia-dominated marshes. Limnol Oceanogr 52, 2340–2349. doi: 10.4319/lo.2007.52.6.2340
Flores A. A. V., Abrantes K. G., Paula J. (2005). Estimating abundance and spatial distribution patterns of the bubble crab Dotilla fenestrata (Crustacea: Brachyura). Austral Ecol. 30, 14–23. doi: 10.1111/j.1442-9993.2005.01409.x
Gao X., Lee S. Y. (2022). Feeding strategies of mangrove leaf-eating crabs for meeting their nitrogen needs on a low-nutrient diet. Front. Mar. Sci. 9. doi: 10.3389/fmars.2022.872272
Grabowski R. C., Droppo I. G., Wharton G. (2011). Erodibility of cohesive sediment: The importance of sediment properties. Earth-Sci Rev. 105, 101–120. doi: 10.1016/j.earscirev.2011.01.008
Griffiths M. E., Mohammad B. A., Vega A. (2007). Dry season distribution of land crabs, Gecarcinus quadratus (Crustacea: Gecarcinidae), in corcovado national park. Costa Rica Rev. Biol. Trop. 55, 219–224. doi: 10.15517/rbt.v55i1.6073
Hamasaki K., Matsui N., Nogami M. (2011). Size at sexual maturity and body size composition of mud crabs Scylla spp. caught in don sak, Bandon Bay, Gulf of Thailand. Fisheries Sci. 77, 49–57. doi: 10.1007/s12562-010-0307-6
He Q., Altieri A. H., Cui B. (2015). Herbivory drives zonation of stress-tolerant marsh plants. Ecology 96, 1318–1328. doi: 10.1890/14-0937.1
He Q., Cui B. (2015). Multiple mechanisms sustain a plant-animal facilitation on a coastal ecotone. Sci. Rep. 5, 8612. doi: 10.1038/srep08612
Herman P., Middelburg J. J., Heip C. (2001). Benthic community structure and sediment processes on an intertidal flat: results from the ECOFLAT project. Cont Shelf Res. 21, 2055–2071. doi: 10.1016/S0278-4343(01)00042-5
Horn J. V., Tolley S. G. (2008). Patterns of distribution along a salinity gradient in the flatback mud crab Eurypanopeus depressus. Gulf Mex Sci. 26, 57–63. doi: 10.18785/goms.2601.06
Hu Z., Borsje B. W., van Belzen J., Willemsen P. W. J. M., Wang H., Peng Y. S., et al. (2021). Mechanistic modeling of marsh seedling establishment provides a positive outlook for coastal wetland restoration under global climate change. Geophys. Res. Lett. 48, e2021GL095596. doi: 10.1029/2021GL095596
Huang Q., Li M., Wang F., Li C. (2019). The parasitic dinoflagellate Hematodinium perezi infecting mudflat crabs, Helice tientsinensis, in polyculture system in China. J. Invertebr Pathol. 166, 107229. doi: 10.1016/j.jip.2019.107229
Hyman A. C., Chiu G. S., Fabrizio M. C., Lipcius R. N. (2021). Spatiotemporal modeling of nursery habitat using bayesian inference: Environmental drivers of juvenile blue crab abundance. Front. Mar. Sci. 9. doi: 10.3389/fmars.2022.834990
Jones M. B., Simons M. J. (1981). Habitat preferences of two estuarine burrowing crabs Helice crassa dana (Grapsidae) and Macrophthalmus hirtipes (Jacquinot) (Ocypodidae). J. Exp. Mar. Biol. Ecol. 56, 49–62. doi: 10.1016/0022-0981(81)90007-1
Kristensen E., Alongi D. M. (2006). Control by fiddler crabs (Uca vocans) and plant roots (Avicennia marina) on carbon, iron, and sulfur biogeochemistry in mangrove sediment. Limnol Oceanogr 51, 1557–1571. doi: 10.4319/lo.2006.51.4.1557
Lan S. Q., Zhang L. W., Yi H. P., Xu C. L., Han G. X. (2020). Food source and feeding habit of Helice tientsinensis from the common reed vegetation in high marsh of yellow river delta, China. J. Appl. Ecol. 31, 319–325. doi: 10.13287/j.1001-9332.202001.034
Lawton J. H., Jones C. G. (1995). Linking species and ecosystems: Organisms as ecosystem engineers (Boston, MA: Springer). doi: 10.1007/978-1-4615-1773-3_14
Lewis J. (1996). Turbidity-controlled suspended sediment sampling for runoff-event load estimation. Water Resour. Res. 32, 2299–2310. doi: 10.1029/96WR00991
Li S., Cui B., Xie T., Bai J., Wang Q., Shi W. (2018). What drives the distribution of crab burrows in different habitats of intertidal salt marshes, yellow river delta, China. Ecol. Indic 92, 99–106. doi: 10.1016/j.ecolind.2017.11.003
Li S., Cui B., Xie T., Xian Zhang M. (2016). Consequences and implications of anthropogenic desalination of salt marshes on macrobenthos. Clean-Soil Air Water 44, 8–15. doi: 10.1002/clen.201400787
Li D., Zhang J., Chen L., Lloyd H., Zhang Z. (2020). Burrow ambient temperature influences helice crab activity and availability for migratory red-crowned cranes grus japonensis. Ecol. Evol. 10, 11523–11534. doi: 10.1002/ece3.6788
Mccall P. L., Tevesz M. (1982). Animal-sediment relations the biogenic alteration of sediments. Sediment Geol. 42, 305–306. doi: 10.1016/0037-0738(85)90049-1
Mcowen C. J., Weatherdon L. V., Bochove J. V., Sullivan E., Blyth S., Zockler C., et al. (2017). A global map of saltmarshes. Biodivers. Data J. 21, e11764. doi: 10.3897/BDJ.5.e11764
Meng X., Jia Y., Shan H., Yang Z., Zheng J. (2012). An experimental study on erodibility of intertidal sediments in the yellow river delta. Int. J. Sediment Res. 27, 240–249. doi: 10.1016/S1001-6279(12)60032-8
Minkoff D. R., Escapa M., Ferramola F. E., Maraschín S. D., Pierini J. O., Perillo G. M. E., et al. (2006). Effects of crab–halophytic plant interactions on creek growth in a S.W. Atlantic salt marsh: A cellular automata model. Estuar. Coast. Shelf Sci. 69, 403–413. doi: 10.1016/j.ecss.2006.05.008
Moller I., Spencer B. A., French J. R., Leggett D. J., Dixon M. (2001). The Sea-defence value of salt marshes: Field evidence from north Norfolk. Water Environ. J. 15, 109–116. doi: 10.1111/j.1747-6593.2001.tb00315.x
Morris S. (2005). Respiratory and acid–base responses during migration and to exercise by the terrestrial crab discoplax (Cardisoma) hirtipes, with regard to season, humidity and behaviour. J. Exp. Biol. 208 (22), 4333–4343. doi: 10.1242/jeb.01918
Noor U. S., Asmat S. S. (2019). Occurrence of heavy metals in sediment and their bioaccumulation in sentinel crab (Macrophthalmus depressus) from highly impacted coastal zone. Chemosphere 221, 89–98. doi: 10.1016/j.chemosphere.2019.01.008
Pan Y., Yin S., Chen Y. P., Yang Y. B., Xu C. Y., Xu Z. S. (2022). An experimental study on the evolution of a submerged berm under the effects of regular waves in low-energy conditions. Coast. Eng. 176, 104169. doi: 10.1016/j.coastaleng.2022.104169.
Peer N., Rishworth G. M., Miranda N. A. F., Perissinotto R. (2018). Biophysical drivers of fiddler crab species distribution at a latitudinal limit. Estuar. Coast. Shelf S 208, 131–139. doi: 10.1016/j.ecss.2018.05.001
Qiang H., Cui B., Cai Y., Deng J., Sun T., Yang Z. (2009). What confines an annual plant to two separate zones along coastal topographic gradients? Hydrobiologia 630, 327–340. doi: 10.1007/s10750-009-9825-6
Qiu D., Cui B., Ma X., Yan J., Cai Y., Xie T., et al. (2021). Reciprocal facilitation between annual plants and burrowing crabs: Implications for the restoration of degraded saltmarshes. J. Ecol. 109, 1828–1841. doi: 10.1111/1365-2745.13608
Reinsel K. A., Dan R. (1995). Environmental regulation of foraging in the sand fiddler crab Uca pugilator (Bosc 1802). J. Exp. Mar. Biol. Ecol. 187, 269–287. doi: 10.1016/0022-0981(94)00192-G
Rosa L. C. D., Borzone C. A. (2008). Spatial distribution of the ocypode quadrata (Crustacea: Ocypodidae) along estuarine environments in the Paranaguá bay complex, Southern Brazil. Rev. Bras. Zool. 25, 383–388. doi: 10.1590/S0101-81752008000300001
Schlacher T. A., Lucrezi S., Connolly R. M., Peterson C. H., Gilby B. L., Maslo B., et al. (2016). Human threats to sandy beaches: A meta-analysis of ghost crabs illustrates global anthropogenic impacts. Estuar. Coast. Shelf S 169, 56–73. doi: 10.1016/j.ecss.2015.11.025
Souza D. G. C., Petraccoa M., Danin A. P. F., Pombo M. (2021). Population structure of and use of space by ghost crabs (Brachyura: Ocypodidae) on an equatorial, macrotidal sandy beach. Estuar. Coast. Shelf S 5 (258), 107376. doi: 10.1016/j.ecss.2021.107376
Spivak E., Anger K., Luppi T., Bas C., Ismael D. (1994). Distribution and habitat preferences of two grapsid crab species in mar chiquita lagoon (Province of Buenos Aires, Argentina). Helgoland Mar. Res. 48, 59–78. doi: 10.1007/bf02366202
Stelling-Wood T. P., Clark G. F., Poore A. G. B. (2016). Responses of ghost crabs to habitat modification of urban sandy beaches. Mar. Environ. Res. 116, 32–40. doi: 10.1016/j.marenvres.2016.02.009
Stone R. P., O'Clair C. E. (2001). Seasonal movements and distribution of dungeness crabs cancer magister in a glacial southeastern Alaska estuary. Mar. Ecol. Prog. Ser. 214, 167–176. doi: 10.3354/meps214167
Tatsuya U., Masaki H., Ryo S., Hisami H., Shiho, Kobayashi (2017). Higher species richness and abundance of fish and benthic invertebrates around submarine groundwater discharge in Obama Bay, Japan. J. Hydrol Regional Stud. 11, 139–146. doi: 10.1016/j.ejrh.2015.11.012
Thrush S. F., Hewitt J. E., Herman P., Ysebaert T. (2005). Multi-scale analysis of species-environment relationships. Mar. Ecol. Prog. Ser. 302, 13–26. doi: 10.3354/MEPS302013
Thrush S. F., Hewitt J. E., Norkko A., Nicholls P. E., Funnell G. A., Ellis J. I. (2003). Habitat change in estuaries: Predicting broad-scale responses of intertidal macrofauna to sediment mud content. Mar. Ecol. Prog. Ser. 263, 101–112. doi: 10.3354/meps263101
Turra A., Gon Alves M., Denadai M. R. (2005). Spatial distribution of the ghost crab ocypode quadrata in low-energy tide-dominated sandy beaches. J. Nat. Hist 39, 2163–2177. doi: 10.1080/00222930500060165
Van der Wal D., Herman P. M. J., Forster R. M., Ysebaert T., Rossi F., Knaeps E., et al. (2008). Distribution and dynamics of intertidal macrobenthos predicted from remote sensing: Response to microphytobenthos and environment. Mar. Ecol. Prog. Ser. 367, 57–72. doi: 10.3354/meps07535
Van Wesenbeeck B. K., Van De Koppel J., Herman P. M. J., Bakker J. P., Bouma T. J. (2007). Biomechanical warfare in ecology; negative interactions between species by habitat modification. Oikos 116, 742–750. doi: 10.1111/j.0030-1299.2007.15485.x
Vestbo S., Obst M., Fernandez F. J. Q., Intanai I., Funch P. (2018). Present and potential future distributions of asian horseshoe crabs determine areas for conservation. Front. Mar. Sci. 5. doi: 10.3389/fmars.2018.00164
Walther K., Sartoris F. J., Bock C., Pörtner H. O. (2009). Impact of anthropogenic ocean acidification on thermal tolerance of the spider crab hyas araneus. Biogeosciences 6, 2207–2215. doi: 10.5194/bg-6-2207-2009
Wang M., Gao X., Wang W. (2014). Differences in burrow morphology of crabs between Spartina alterniflora marsh and mangrove habitats. Ecol. Eng 69, 213–219. doi: 10.1016/j.ecoleng.2014.03.096
Wang A., Ye X., Du Y., Yin X. (2017). Hydrodynamic and biological mechanisms for variations in near-bed suspended sediment concentrations in a spartina alterniflora marsh-a case study of Luoyuan Bay, China. Estuar. Coast. 40, 1540–1550. doi: 10.1007/s12237-017-0253-y
Weilhoefer C. L. (2011). A review of indicators of estuarine tidal wetland condition. Ecol. Indic 11, 514–525. doi: 10.1016/j.ecolind.2010.07.007
Widdows J., Brinsley M. (2002). Impact of biotic and abiotic processes on sediment dynamics and the consequences to the structure and functioning of the intertidal zone. J. Sea Res. 48 (2), 143–156. doi: 10.1016/S1385-1101(02)00148-X
Wilson C. A., Hughes Z. J., FitzGerald D. M. (2012). The effects of crab bioturbation on mid-Atlantic saltmarsh tidal creek extension: Geotechnical and geochemical changes. Estuar. Coast. Shelf S 106, 33–44. doi: 10.1016/j.ecss.2012.04.019
Wotton R. S., Malmqvist B. (2001). Feces in aquatic ecosystems. Bioscience 51, 537–544. doi: 10.1641/0006-3568(2001)051[0537:FIAE]2.0.CO;2
Xiao K., Wilson A. M., Li H., Ryan C. (2019). Crab burrows as preferential flow conduits for groundwater flow and transport in salt marshes: A modelling study. Adv. Water Resour. 132, 103408. doi: 10.1016/j.advwatres.2019.103408
Xiao K., Wilson A. M., Li H., Santos I. R., Tamborski J., Smith E., et al. (2021). Large CO2 release and tidal flushing in salt marsh crab burrows reduce the potential for blue carbon sequestration. Limnol Oceanogr 66, 14–29. doi: 10.1002/lno.11582
Xin P., Jin G., Ling L., Barry D. A. (2009). Effects of crab burrows on pore water flows in salt marshes. Adv. Water Resour. 32, 439–449. doi: 10.1016/j.advwatres.2008.12.008
Xin P., Kong J., Li L., Barry D. A. (2012). Effects of soil stratigraphy on pore-water flow in a creek-marsh system. J. Hydrol 475, 175–187. doi: 10.1016/j.jhydrol.2012.09.047
Xin P., Yu X., Lu C., Li L. (2016). Effects of macro-pores on water flow in coastal subsurface drainage systems. Adv. Water Resour. 87, 56–67. doi: 10.1016/j.advwatres.2015.11.007
Xu F., Tao J., Zhou Z., Coco G., Zhang C. (2016). Mechanisms underlying the regional morphological differences between the northern and southern radial sand ridges along the Jiangsu Coast, China. Mar. Geol. 371, 1–17. doi: 10.1016/j.margeo.2015.10.019
Ysebaert T., Meire P., Herman P., Verbeek H. (2002). Macrobenthic species response surfaces along estuarine gradients: Prediction by logistic regression. Mar. Ecol. Prog. Ser. 225, 79–95. doi: 10.3354/meps225079
Yuan S. Y., Xu L., Tang H. W., Xiao Y., Carlo G. (2022). The dynamics of river confluences and their effects on the ecology of aquatic environment: A review. J. Hydrodyn. 34, 1–4. doi: 10.1007/s42241-022-0001-z.
Zhang C. (2013). Basic status of offshore marine environmental resources (Jiangsu Province: China Ocean Press).
Zhang Q., Gong Z., Zhang C., Townend I., Jin C., Li H. (2016). Velocity and sediment surge: What do we see at times of very shallow water on intertidal mudflats? Cont Shelf Res. 113, 10–20. doi: 10.1016/j.csr.2015.12.003
Zhang C., Zhang D., Zhang J., Wang Z. (1999). Tidal current-induced formation-storm-induced change-tidal current-induced recovery: Interpretation of depositional dynamics of formation and evolution of radial sand ridges on the yellow Sea seafloor. China Earth Sci. 42, 1–12. doi: 10.1007/BF02878492
Zhao L. (2020). Self-organized spatial patterning in intertidal salt marshes and its ecosystem functioning, Ph.D. thesis (East China Normal University, Shanghai).
Zhao L., Zhang K., Siteur K., Li X., Liu Q., Koppel J. (2021). Fairy circles reveal the resilience of self-organized salt marshes. Sci. Adv. 7 (6), eabe1100. doi: 10.1126/sciadv.abe1100
Zhou T., Xin P., Li L., Barry D. A., Simunek J. (2020). Effects of large macropores on soil evaporation in salt marshes. J. Hydrol 584, 124754. doi: 10.1016/j.jhydrol.2020.124754
Keywords: crab burrow, marine invertebrates, ecosystem engineers, salt marsh, wetland, spatiotemporal distribution
Citation: Chen X, Zhou Z, He Q, Zhang H, Bouma T, Gong Z, Townend I and Zhang C (2022) Role of abiotic drivers on crab burrow distribution in a saltmarsh wetland. Front. Mar. Sci. 9:1040308. doi: 10.3389/fmars.2022.1040308
Received: 09 September 2022; Accepted: 20 October 2022;
Published: 10 November 2022.
Edited by:
Luzhen Chen, Xiamen University, ChinaReviewed by:
Tian Xie, Beijing Normal University, ChinaCopyright © 2022 Chen, Zhou, He, Zhang, Bouma, Gong, Townend and Zhang. This is an open-access article distributed under the terms of the Creative Commons Attribution License (CC BY). The use, distribution or reproduction in other forums is permitted, provided the original author(s) and the copyright owner(s) are credited and that the original publication in this journal is cited, in accordance with accepted academic practice. No use, distribution or reproduction is permitted which does not comply with these terms.
*Correspondence: Zeng Zhou, emVuZy56aG91QGhodS5lZHUuY24=
Disclaimer: All claims expressed in this article are solely those of the authors and do not necessarily represent those of their affiliated organizations, or those of the publisher, the editors and the reviewers. Any product that may be evaluated in this article or claim that may be made by its manufacturer is not guaranteed or endorsed by the publisher.
Research integrity at Frontiers
Learn more about the work of our research integrity team to safeguard the quality of each article we publish.