- 1Molecular Genetics Unit, Okinawa Institute of Science and Technology Graduate University, Okinawa, Japan
- 2Graduate School of Integrated Science for Life, Hiroshima University, Hiroshima, Japan
- 3Centre for Life’s Origins and Evolution, Department of Genetics, Evolution and Environment, University College London, London, United Kingdom
- 4Department of Molecular and Cell Biology, University of California, Berkeley, Berkeley, CA, United States
- 5Chan-Zuckerberg BioHub, San Francisco, CA, United States
Cephalopod research remains limited by the inability to culture species under laboratory conditions for multiple generations to provide continuous access to animals at all stages of the life cycle. Here, we describe a multi-generational laboratory culture system for two emerging cephalopod models: the hummingbird or Berry’s bobtail squid, Euprymna berryi Sasaki, 1929, and Morse’s bobtail squid, Euprymna morsei Verrill, 1881, which are primarily found off mainland Japan. E. berryi wild adults were spawned and raised to the third filial generation, and E. morsei wild adults were spawned and raised to the second filial generation in a closed system at 20°C. We report growth and survivorship data for a cohort of 30 individuals across the first generation raised in captivity. E. berryi and E. morsei grew exponentially during the first 90 and 60 days post-hatching, respectively. Survivorship at the first spawning event for E. berryi and E. morsei was 90% and 77%. E. berryi and E. morsei females spawned after days 112 and 71 days post-hatching, respectively. We describe the life history of each species and how to distinguish sexes. We discuss the challenges of cephalopod culture and how culturing these species address those problems.
Introduction
Cephalopods are widely recognized as the most behaviorally complex invertebrates, attracting attention in the fields of neuroscience, development, and evolution (O’Brien et al., 2018). They have unique characteristics, including adaptive camouflage (Chiao et al., 2015), efficient motor control relative to other mollusks (Levy et al., 2017), and high levels of RNA editing (Liscovitch-Brauer et al., 2017) and transposon activity (Albertin et al., 2015). Furthermore, their capacity to perform complex tasks resembling that of some vertebrate species promoted their inclusion in European Union legislation for animal experimentation and welfare under Directive 2010/63/EU at the same level as vertebrate organisms (European Parliament and Council of the European Union, 2010; Sykes et al., 2012; Smith et al., 2013; Fiorito et al., 2015).
The study of cephalopod development and evolution is a growing area of research that has led to increasing demand for embryos and animals at all stages of their life cycle (Lee et al., 2003; Peyer et al., 2014; Koenig et al., 2016; Navet et al., 2017; Tarazona et al., 2019). While for many purposes wild-caught animals can be studied, and hatchings raised to juvenile or later stages in the laboratory, multigenerational cultures have only been initiated for some cephalopods including octopus (Iglesias et al., 2004; Rosas et al., 2014; Vidal et al., 2014; Maldonado et al., 2019; Grearson et al., 2021), sepioids (Minton et al., 2001; Walsh et al., 2002; Nabhitabhata, 2014), sepiolids (Boletzky et al., 1971; Nabhitabhata et al., 2005; Jones and Richardson, 2010; Nabhitabhata and Nishiguchi, 2014; Sanchez et al., 2019), and the myopsid squid Sepioteuthis lessoniana (Forsythe et al., 1994). Large-scale multigenerational cephalopod culture systems are not only a necessity for forward genetics but is also desirable for targeted approaches like CRISPR-Cas genome editing (Jinek et al., 2012; Doudna and Charpentier, 2014). In cephalopods, gene knockouts by genome editing have been accomplished in the progeny of wild-caught Doryteuthis pealeii (Crawford et al., 2020). Multigenerational cultures will thus help to move forward the field of development and evolution on cephalopods.
In general, each cephalopod species has unique biological characteristics, morphology, and lifestyle that determine which phenomena can be readily studied, as well as disadvantages in terms of difficulty of culture conditions and difficulty of maintenance (Figure 1). For example, cephalopods generally have a high metabolism and food conversion rate but limited fat reserves, requiring frequent feeding (Iglesias et al., 2014; Vidal et al., 2014). Physically larger species such as Sepia officinalis, Sepioteuthis lessoniana, and Octopus vulgaris therefore require correspondingly large aquaria and amounts of food which rapidly become impractical for many laboratory budgets without dedicated marine facilities. Furthermore, most cephalopods are active visual hunters that prefer live prey, which can be costly and labor intensive to provide (Villanueva et al., 2017). Moreover, many cephalopods have evolved different ranges of sociality, with most of the octopus species being solitary and many squids performing group-like behaviors (Sugimoto et al., 2013; Iglesias et al., 2014). Some species may even practice cannibalism (Ibáñez and Keyl, 2010), preventing the culture of more than single animals per tank. Cephalopods that are relatively small at maturity, avoid cannibalism, and are not entirely solitary therefore have advantages for small-scale laboratory culture.
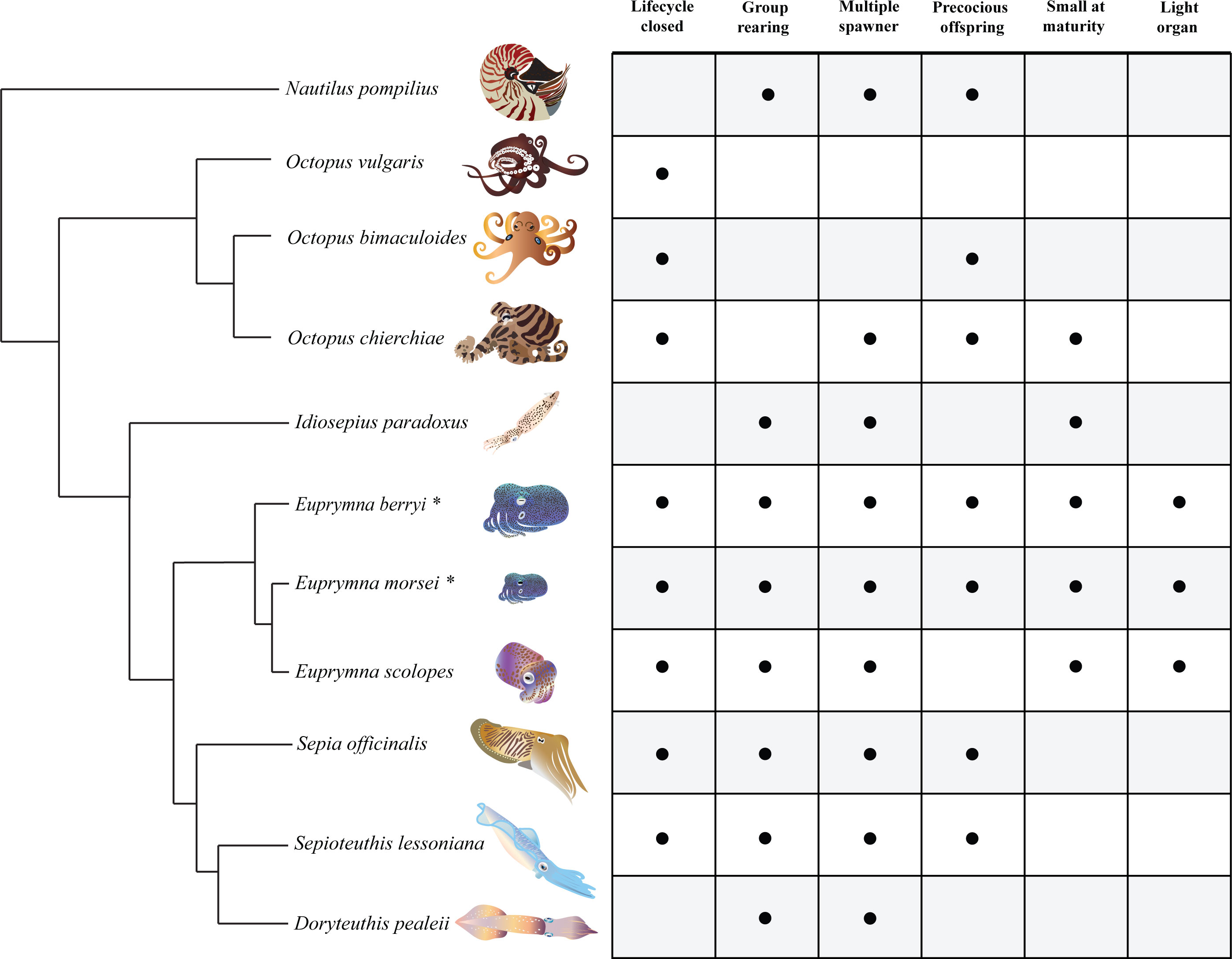
Figure 1 Advantageous Culture Traits of Several Cephalopod Models. Comparison of cephalopod species previously used in laboratory experiments. “Lifecycle closed” refers to a species being cultured across at least one generation. An animal is considered capable of group rearing if minimal aggression and cannibalism is observed, and the stress of group rearing prevents successful culturing efforts. “Multiple spawner” indicates normal multiple spawning events completed by one female. “Precocious offspring” refers to hatchling behaviors similar to adults (including predation). “Small at maturity” refers to an animal with a dorsal mantle length less than 6 cm. Some cephalopod species have evolved a light organ that is bioluminescent. The tree is based on results published by Anderson and Lindgren (2020).
Reproductive (Rocha et al., 2001) and life history traits, including early mode of life (Boyle, 2005; Villanueva et al., 2016), vary among cephalopod species. For most cephalopods, the diet during their early life in their natural habitats is still unknown, limiting the selection of suitable prey to raise them. Some cephalopods lay only very few eggs, e.g., Eumandya parva (Sanchez et al., 2019), and others can produce hundreds or thousands of immature planktonic paralarvae with high mortality, e.g., Octopus vulgaris (Villanueva, 1995), and whose size is too tiny to feed with standard prey in laboratory settings.
Bobtail squid from the subfamily Sepiolinae, i.e., sepiolida clade (Anderson and Lindgren, 2020), are a group of nocturnal cephalopods with relatively small size, correspondingly limited nutritional requirements, short life span, benthopelagic early mode of life, and ability to live at high densities without cannibalism. Female bobtail squid can also mate several times with different males and store spermatangia for around two months for future spawning (Squires et al., 2013; Drerup et al., 2020). These characteristics make them suitable for laboratory culture and a potential model organism for developmental, physiological, behavioral, and genetic assays. Their small size is also ideal for advanced imaging (Kerbl et al., 2013).
Thanks to the pioneering efforts of McFall-Ngai and Ruby (Boettcher and Ruby, 1990; Montgomery and McFall-Ngai, 1994; Boettcher et al., 1996; Ruby, 1996; Ruby and Lee, 1998; McFall-Ngai, 1999), the Hawaiian bobtail squid Euprymna scolopes Berry 1913 has been widely adopted as a model for bacterial-metazoan symbiosis in which luminescent Allivibrio fischeri colonize the light organ of these and related species (Boettcher et al., 1996; McFall-Ngai, 1999; McFall-Ngai, 2014). These efforts fostered studies of bobtail squid diversity (Jones et al., 2006) and development (Lee et al., 2003) both morphologically (Lee et al., 2009b) and at the molecular level (Callaerts et al., 2002; Hartmann et al., 2003; Lee et al., 2003; Sanchez et al., 2021). The genome of E. scolopes (Belcaid et al., 2019) has become a reference to study other species in the Euprymna clade (Heath-Heckman and Nishiguchi, 2021; Schmidbaur et al., 2022). Many bobtail squid have also been investigated in studies of associative learning, behavior, and the heritability of personality and fitness traits (Steer et al., 2004; Sinn and Moltschaniwskyj, 2005; Sinn et al., 2006; Sinn et al., 2008; Zepeda et al., 2017). Despite these advantages, a disadvantage of E. scolopes for multigenerational culture is the high mortality of its larval stage (Lee et al., 2009b).
Two species of bobtail squid that are abundant in mainland Japanese waters have the potential to become laboratory models: the hummingbird or Berry’s bobtail Euprymna berryi Sasaki, 1929, and Morse’s bobtail Euprymna morsei Verrill, 1881 (Figures 2A–G). The distribution of these sympatric species extends from Japanese waters southward along the coast of China and westward into the Indian Ocean (Raj and Kalyani, 1971; Okutani and Horita, 1987; Reid and Jereb, 2005; Sundaram and Sreeram, 2008). The spawning season for E. berryi is late April to July in Aichi, Japan (Choe, 1966a), and March and December in Taiwan (Huang, 2006). Adults of E. berryi have been found from April to June in the southern and the Pacific Ocean side of mainland Japan swimming near the water surface at night, while adults have been found as deep as 60 m on the Pacific side of mainland Japan. In a trawl survey off Nobeoka Bay in Miyazaki prefecture, Toriyama et al. found a relatively similar amount of E. morsei across the year with the highest catch from April to June and the lowest from January to March (Toriyama et al., 1970). However, some trawl surveys could have confused both species due to their very similar morphology. Although most fishermen do not discriminate between the two species, they are distinguished by several morphological differences as well as molecular markers (Sanchez et al., 2019). E. morsei (mantle length ≤ 4 cm) is considerably smaller than E. berryi (mantle length ≤ 5cm) (Reid and Jereb, 2005). E. morsei males have enlarged suckers on the ventral sucker rows of arms II, III, and IV, whereas E. berryi have enlarged suckers on both dorsal and ventral sucker rows of arms II and IV (Okutani and Horita, 1987; Norman and Lu, 1997). E. morsei have chromatophores on the dorsal surface of the fins, while E. berryi have chromatophores on both dorsal and ventral surfaces (Okutani and Horita, 1987). Finally, the tentacular suckers in E. morsei have a cylindrical shape but in E. berryi resemble a smoking pipe (Okutani and Horita, 1987; Reid and Jereb, 2005; Huang, 2006).
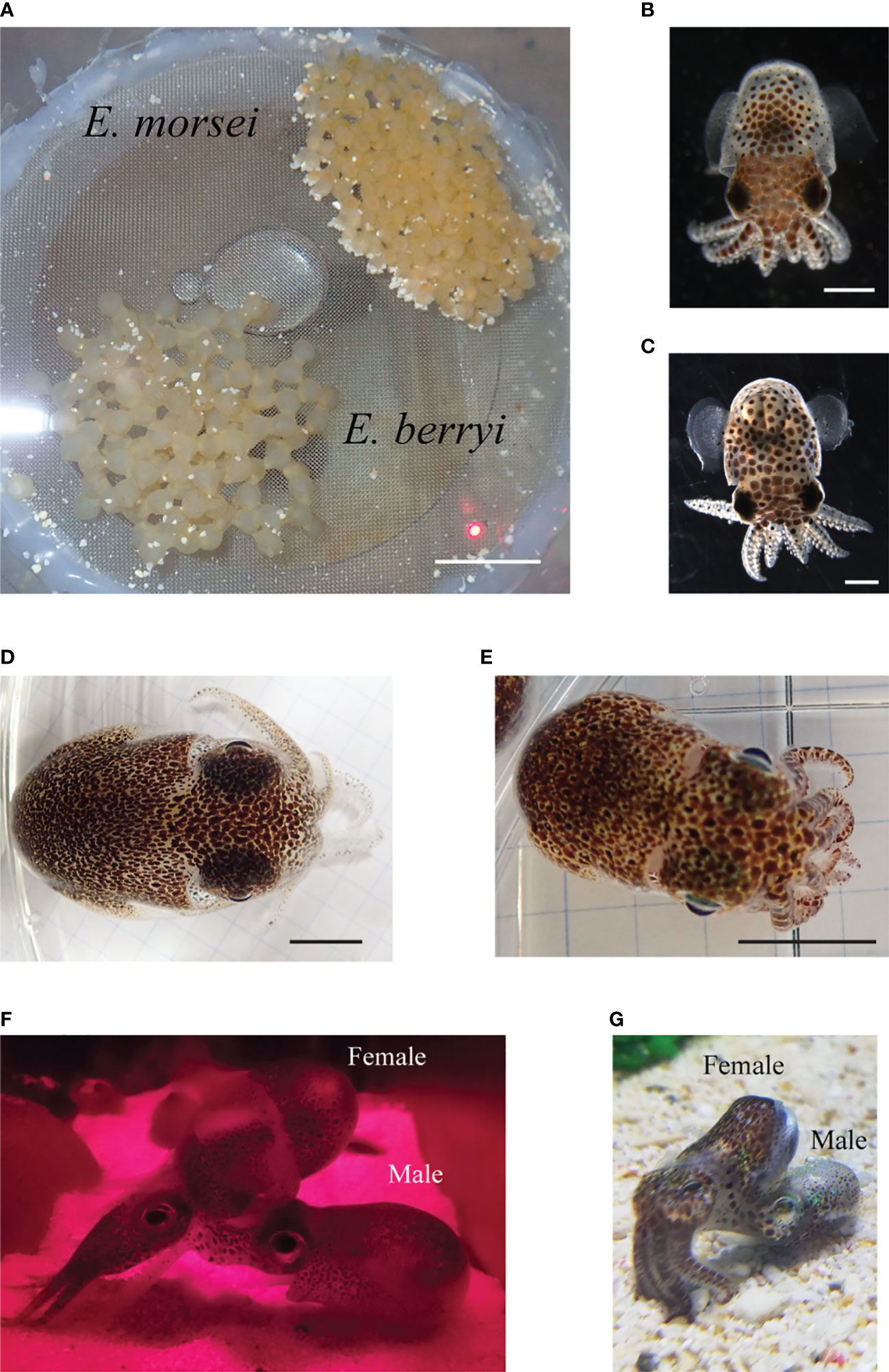
Figure 2 E berryi and E morsei at different life stages. (A) Egg clutches of E berryi (left) and E morsei (right). Scale bar is 5 cm. (B) E berryi at one day post- hatching (dph). Scale bar is 1 mm. (C) E morsei at one dph. Scale bar is 1mm. (D) Mature E berryi at 130 dph. Scale bar is 1 cm. (E) Mature E morsei at 70 dph. Scale bar is 1 cm. (F) E berryi mating. The male (on the right) grasps the female from the ventral side to engage mating. (G) E morsei mating. The smaller male on the right grasps the female from the ventral side to engage mating.
Several previous studies described culturing attempts of E. berryi, E. morsei, and other members of the genus Euprymna (Supplementary Table 1). E. berryi was reared for two months (Choe, 1966a), and E. morsei was raised to reproductive maturity (Ikeda et al., 2003). Euprymna scolopes was successfully raised to the second generation (Hanlon et al., 1997). Several species of Sepiola and Euprymna have been cultured to the second generation (Boletzky et al., 1971; Jones and Richardson, 2010; Sanchez et al., 2019). Further, Euprymna tasmanica and Euprymna hyllebergi have been cultured to the third generation (Nabhitabhata et al., 2005; Nabhitabhata and Nishiguchi, 2014).
Here we report our efforts to develop multigenerational cultures of E. berryi and E. morsei. We closed the lifecycle of both species in a recirculating aquaculture system and measured growth and survivorship for thirty individuals of each species under the same aquarium conditions. This work, along with the development of genomic resources for E. berryi (Gavriouchkina et al., 2022), provides a foundation for the future development of E. berryi and E. morsei as laboratory model organisms.
Materials and methods
Broodstock collection
Both E. berryi and E. morsei were obtained from vendors or from wild collections in southern mainland Japan. Adults are available from vendors seasonally. Adults survived long-distance shipping with commercial couriers using oxygen-saturated seawater and styrofoam-insulated packaging. Adult females of E. berryi and E. morsei were collected from Mie prefecture, Japan from February to June with a set net, and transported using overnight shipping services. Animals were individually packed in 15 L round-bottom transparent 3 mm plastic bags containing 5-7 L of oxygen saturated filtered seawater with excess volume filled with pure oxygen and shipped in expanded polystyrene foam boxes similar to E. scolopes (Hanlon et al., 1997; Cecere and Miyashiro, 2022). Transit time until arrival in the lab was less than 48 h. Upon arriving in Okinawa, animals were acclimated to the temperature (20°C or 23°C) and salinity (~35 gL -1 i.e., parts per thousand) of our culture system. Adults from each species were housed separately. Upon spawning, eggs were removed to two-liter tanks like previous methods (Sanchez et al., 2019). Recently spawned eggs – within the first ten days post spawning - were shipped internationally within 72 h using similar methods to shipping adults apart from using 5 L bags containing 1.5-2 L oxygen saturated filtered seawater. To prevent widespread fouling, eggs were monitored on a daily basis and nonviable eggs removed. Hatchlings were housed in two liter tanks for approximately the first month after hatching.
Culture system
The tank system assembled for culturing bobtail squids is a closed tank system that consists of a 200 L filter tank, five 70 L tanks (60 cm x 35 cm x 35 cm), five 2 L tanks (20 cm x 13 cm x 13 cm), two protein skimmers, an ultraviolet sterilizer, and contains filtered natural seawater from the OIST Seragaki Marine Science Station (Supplementary Figure 1). Flow rate for larger tanks is 4.5 to 5 L min-1, and 160 to 180 ml min-1 for smaller tanks. Cleaning and partial exchange of 10% seawater are performed daily, and larger 50% water changes are performed biweekly. Water temperature from 2017 to February 2018 was maintained at 23°C with a chiller and heater and thereafter maintained at 20°C. All measurements were taken from animals kept at 20°C. The following water parameters were maintained between the ranges and monitored daily: salinity - 33 to 37 gL-1, pH - 8.2 to 8.4. The following parameters were measured at least weekly nitrate - 0 to 20 mg L-1, i.e., parts per million (ppm), nitrite - 0 to 0.5 mg L-1 (ppm), ammonia (NH3/NH4) - 0 to 0.25 mg L-1 (ppm).
Artificial plants, coral rubble, and PVC pieces were added to tanks to provide egg laying substrate and refuge. Beach sand collected from nearby beaches was added to aquarias. Sand was autoclaved and rinsed in reverse osmosis-treated water before introducing into aquaria. Enough substrate was given to allow the animals to bury completely. Without any burying substrate, skin lesions can form on the ventral side of the animal from friction with the aquarium floor. Aquaria were spot cleaned daily with siphons. The use of coarse sand collected from beaches or from vendors is adequate to allow the animal refuge while remaining easy to siphon.
Blue light-emitting diodes (450 nm wavelength) were used to create twelve hour light-twelve hour dark diurnal cycles in the laboratory. The photoperiod was shifted similarly to previous methods (Franklin et al., 2014) so “night” begins at noon local time to facilitate feeding and experimentation. We used red light-emitting diodes (665 nm) to observe and feed animals during “night” when they were most active.
Feeding and maintenance conditions
Animals were fed ad libitum, with new shrimp added once daily. From hatching to 40 dph (days post-hatching), both species were fed mysids (Neomysis spp.). Mysids were maintained in a separate tank and fed Artemia sp. nauplii once a day prior to being fed to hatchlings. After 40 dph, both species were fed glass shrimp (Palamonetes spp.), and the freshwater marsh shrimp-Caridina spp.
Both species were also trained to consume cut frozen shrimp (e.g., black tiger prawn) upon reaching maturity.
Frozen shrimp were thawed and cut before presenting to a squid with forceps and moved to simulate living prey. Afterward, contact was made between frozen food and the inner portion of the squid’s arms until the squid either showed signs of stress or voluntarily grasped the frozen food (Supplementary Video 1). After approximately one week of continuous training, squids could attack falling frozen food spontaneously.
In ongoing culture we provide adequate space, refugia, and burying substrate to minimize stress. Adults are kept in a ratio at or greater than 1:1 males to females. Our heuristic for determining appropriate space is to ensure each animal has at least two mantle lengths distance between animals. For our tank dimensions (approximately 70 L, 60 cm x 35 cm x 35 cm and water height of 31 cm), we do not exceed eight fully mature E. berryi individuals per tank (eight squids per 2,100 cm2 floor area, 65,100 cm3 water volume). Assuming a maximum potential mantle length of 6 cm at maturity (Okutani and Horita, 1987), each animal is therefore given a cube of water that is 12 cm on each side, equivalent to 1700 cm3. E. morsei reaches a smaller size at maturity, with a dorsal mantle length (DML) less than 4 cm (Okutani and Horita, 1987; Reid and Jereb, 2005), and therefore can be kept at higher densities than E. berryi. To avoid reproductive attempts and aggression from males, females can be separated from males after mating. We have not observed any overt changes in behavior when adults of either species are isolated.
Survivorship and growth rate
Thirty F1 hatchlings of E. berryi and E. morsei were isolated on the first day after hatching and reared to monitor their survivorship and growth rate. To measure growth rate, wet weight (WW, g) and DML (mm) were measured in five randomly selected individuals approximately every 10 d to maturity to prevent additional stress due to handling. We measured only five individuals each to minimize handling stress on the cohorts. Squid were placed in a transparent reservoir containing aquarium water atop graph paper and imaged using an Olympus TG-5 camera. The FIJI variant of ImageJ (Schindelin et al., 2012) was used for image calibration and DML measurement. All data is expressed as Mean ± SD. Survivorship was calculated (Leverich and Levin, 1979) as the percentage of surviving individuals, I(t) by:
Where Ns(t) is the number of survivors at time t and No is the initial cohort size.
At 115 dph, eleven E. berryi were removed from the study due to lack of space and prey items, and Nowas then adjusted from 30 to 19 for E. berryi. All 27 surviving E. berryi were first removed from the aquaria, then eight of each males and females were selected and placed back in the aquaria. The animals selected were the first animals to be collected. The other 11 E. berryi were removed and euthanized via overdose to the anesthetic ethanol (Abbo et al., 2021). Animals were immersed in a bath of 1% ethanol in filtered seawater. Over a period of thirty minutes, ethanol was gradually introduced until reaching a final concentration of 5% followed by mechanical destruction of the brain (Fiorito et al., 2015; Abbo et al., 2021).
Observations
Behavioral observations of E. berryi were made visually from March 2017 to May 2020. E. morsei observations were from March 2018 to May 2020. Both species were cultured during that time for other experiments.
Results
Culture
We cultured E. berryi and E. morsei under conditions similar to those used for other bobtail squid (Hanlon et al., 1997; Nabhitabhata et al., 2005; Jones and Richardson, 2010; Sanchez et al., 2019) (Methods). We spawned wild-caught adults of both species and cultured E. berryi to the third filial generation and E. morsei to the second filial generation. Growth rate and survivorship were tracked for the first filial generation (Figure 3).
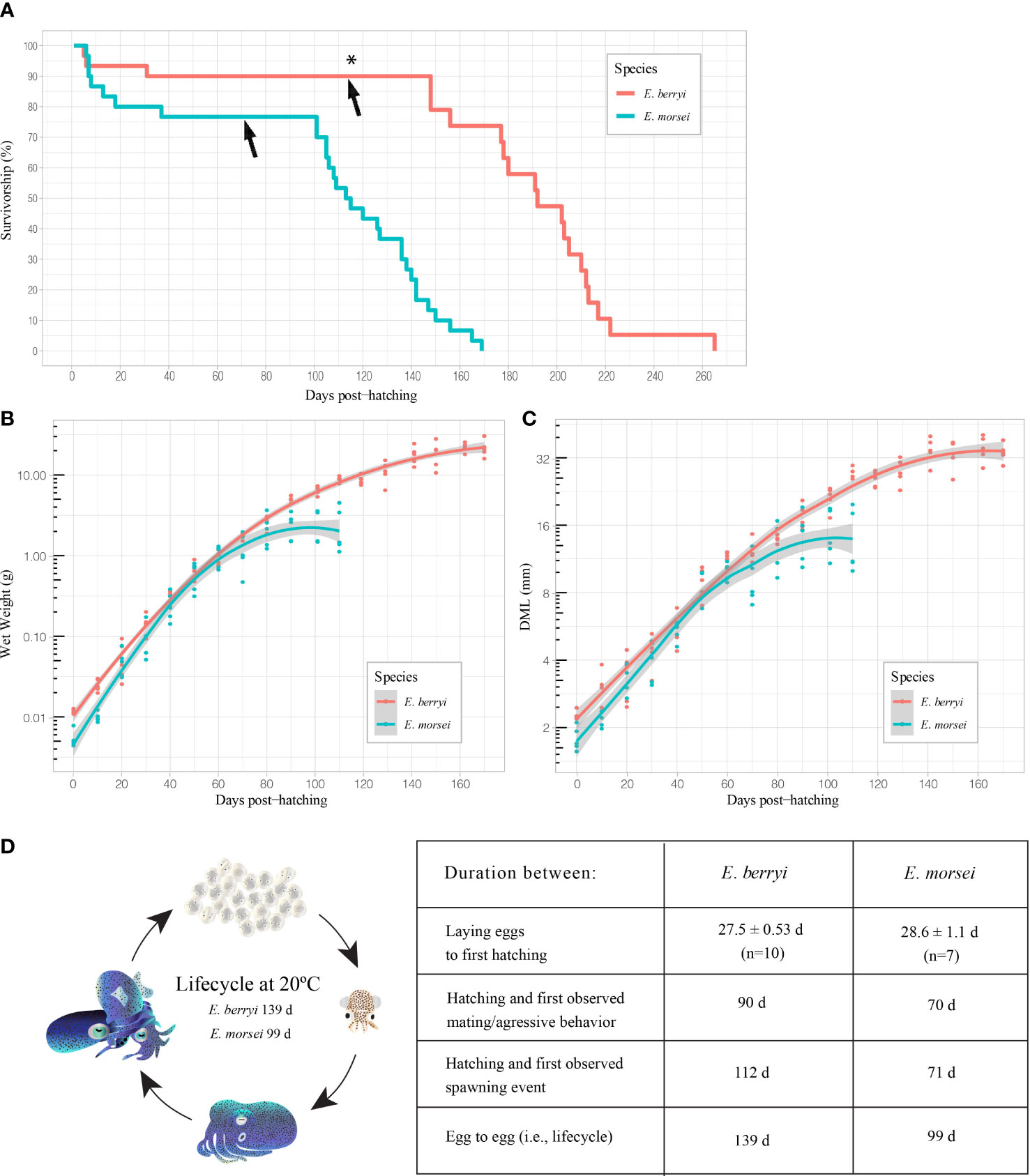
Figure 3 Survivorship, growth rate, and developmental timelines for E berryi and E morsei. (A) Survivorship for each species. For each species, initial population size was 30 individuals. The asterisk (*) represents an artificial reduction in total population size for E berryi from 30 to 19 individuals due to limited tank space. Arrows indicate the first spawning event for each species. (B) Growth rate comparing wet weight (g) to dph on semi-log scale. (C) Growth rate comparing dorsal mantle length (mm) to age on semi-log scale. (D) Comparison of the lifecycle and time between developmental landmarks of both E berryi and E morsei.
Egg masses
Both E. berryi and E. morsei were collected during the Japanese spring season and we observed mating and spawning of wild-caught adults in the laboratory. We shipped recently spawned eggs internationally and they hatched and were raised without overt abnormalities. Both species laid a large number of eggs per clutch, usually exceeding 200 eggs for E. berryi and 100 eggs for E. morsei (Figure 2A). Wild-caught E. berryi laid an average of 235 ± 75.8 eggs per clutch (n=7), while wild-caught E. morsei laid 153 ± 26.5 eggs per clutch (n=4). Eggs are encapsulated within a jelly coat and laid individually in a clutch. The jelly coat of eggs of both species have an orange tint due to a dye secreted by the maternal accessory nidamental gland. Non-viable eggs have an opaque white appearance.
The period between spawning events for wild E. berryi at 20°C was 6.7 ± 2.7 d (n=18) and was not recorded for E. morsei. Both species demonstrated intermittent terminal spawning and spawned separate clutches continually once reaching sexual maturity (Figure 3A). On one occasion one isolated wild-caught female E berryi laid 9 fertilized clutches over a period of 59 d without any additional mating in the laboratory (presumably using stored spermatangia). E. berryi was observed to live longer in captivity after capture than E. morsei and benefited from more spawning events. E. berryi has higher survivorship and fecundity than E. morsei (Figure 3).
We observed no physical or behavioral abnormalities in sequential generations; however, survivorship of later generations of E. berryi and E. morsei immediately after hatching was noticeably reduced for some clutches. On some occasions, we observed eggs laid outside of the jelly coat and some clutches with many unfertilized eggs.
Growth
Growth of E. berryi and E. morsei, measured by WW or DML, was approximately exponential for the first 90 and 60 dph (Table 1) before reaching species-specific plateaus by 80 and 140 dph, respectively (Figures 3B, C).
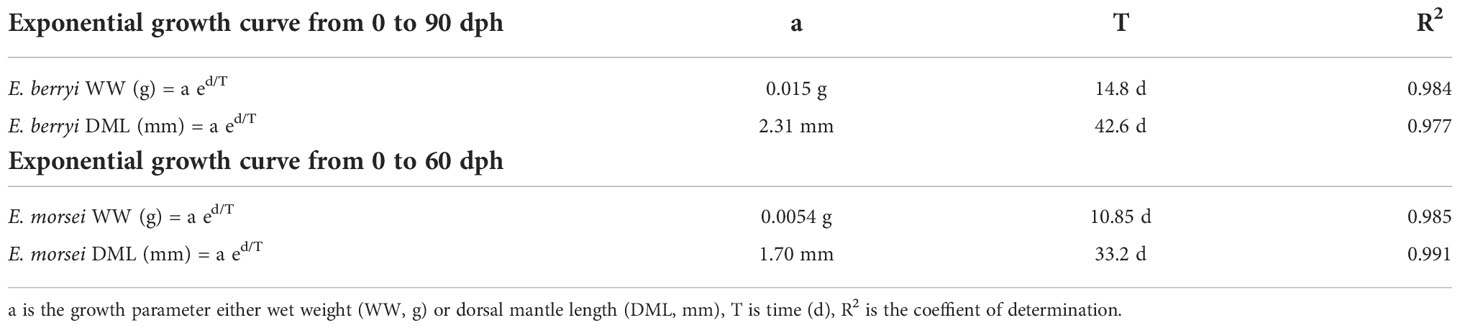
Table 1 Exponential growth curve equations for E. berryi 0-90 days post hatching (dph) and E. morsei 0-60 dph.
We observed a large range of both DML and WW at later stages in both E. berryi and E. morsei (Figures 3B, C). Average E. berryi WW and DML were 17.32 ± 3.82 g and 32.14 ± 4.90 mm for males (n=16), and 23.06 ± 4.90 g and 36.26 ± 3.67 mm for females (n=14). Average E. morsei WW and DML were 1.41 ± 0.15 g and 11.45 ± 1.24 mm for males (n=6), and 3.70 ± 0.42 g and 18.95 ± 2.20 mm for females (n=6). The average female E. berryi weighed 1.33 times larger than males and were 1.13 times longer. The average female E. morsei weighed 2.63 times larger than males and were 1.65 times longer.
Survivorship
Survivorship was 93% for E. berryi and 80% for E. morsei for the first 30 days after hatching (Figure 3A). Survivorship was stable until shortly after spawning began. Thereafter, survivorship declined steadily from ~101 dph in E. morsei and ~148 dph in E. berryi. The oldest E. berryi and E. morsei in our laboratory culture were males and lived 265 dph and 169 dph, respectively. E. berryi outlived E. morsei and took longer to reach spawning age by 42 dph.
Mating and spawning
Sexual maturity was noted when males became aggressive towards conspecifics. No courtship behavior was observed in either species. Aggression appeared similar to mating, i.e., a male would assault, grapple, and possibly bite a conspecific. At 20°C this was first observed at 90 dph in E. berryi and 70 dph in E. morsei. During mating the female is first attacked by the male and the male attempts to grab the ventral head of the female, i.e., the male-to-female neck position. The male maintains control of the female and inserts the hectocotylus holding spermatophores into the mantle of the female (Figures 2F, G).
Spawning events began shortly after the night cycle began. Females spawned on the substrate provided including the tank walls, PVC pipes, rocks, and on imitation plants. As described above, for both species, the female laid each egg individually as part of a large clutch (Supplementary Video 2). Spawning began at night and continued into the day. Females have been observed laying eggs cooperatively on the same substrate simultaneously. Females typically consumed less prey one day before spawning. Females repeatedly laid egg clutches every few days until reaching a late senescent life stage (Figure 3A). Some individuals spawned within three days of shipment. Mature E. berryi females were observed spawning fertilized clutches repeatedly over a period of 100 days at 20°C. No parental care was observed in either species. Egg clutch morphology was different for both species. E. morsei eggs were more densely packed in a clutch, whereas E. berryi eggs were more spaced out (Figure 2A).
Sexual dimorphism
Sexual dimorphism was visually evident at 100 dph for E. berryi and 70 dph for E. morsei. The sex of the animal can be determined by its side profile, size, suckers, and the morphology of the first left-arm (Supplementary Figure 2). Males are smaller than females for both species. The size difference is more pronounced in E. morsei than E. berryi. The side mantle profile in males is sharper in males than in females (Supplementary Figures 2A, B). Fully mature females generally have a bulbous mantle, because of the presence of oocytes in their ovaries, and can be distinguished from males visually in a minimally invasive manner. Males of both species can further be distinguished from females by observing the first arm pair (Supplementary Figure 2C). Males have a modified first left arm known as the hectocotylus which is shorter than the opposing arm and curls slightly outward. Males of both species have large suckers on some rows of certain arms and modified suckers on the hectocotylus (Supplementary Figure 2D), whose patterns can be used to discriminate species of Euprymna (Norman and Lu, 1997). Females have uniform sucker sizes. Female first arms and suckers (Supplementary Figure 2E) are indistinguishable from one another (Norman and Lu, 1997).
Senescence
Males of both species generally outlived females and displayed similar signs of senescence. Characteristics of early senescence include nonfunctional and faded chromatophores, greater susceptibility to infections, and loss of appetite. Signs of later stages of senescence include complete cessation of eating and burying, loss of equilibrium, and continuous labored ventilation.
Discussion
Two promising cephalopod model organisms
The utility and prominence of E. scolopes as a model cephalopod species was discussed by Lee et al. (2009c) who also suggested that, as genomic information becomes available for different cephalopod species, the availability of broodstock and embryos becomes a primary factor in choosing a model system. Here we have explored the culturing of two related Japanese bobtail squid species, E. berryi Sasaki, 1929, and E. morsei Verrill, 1881. We find that E. berryi and E. morsei have comparable life cycles in captivity to E. scolopes (Hanlon et al., 1997), E. hyllebergi (Nabhitabhata et al., 2005), E. tasmanica (Nabhitabhata and Nishiguchi, 2014), E. parva, and E. brenneri (Sanchez et al., 2019) (Table 2). Both E. berryi and E. morsei can be raised in laboratory settings and are intermittent terminal spawners, spawning repeatedly once reaching sexual maturity (Figure 3A). They are therefore well-suited for evo-devo studies, physiological assays, behavioral assays, laboratory culture, and have the potential to be used for gene editing (Crawford et al., 2020). E. berryi has higher survivorship and fecundity than what is reported for other sepiolids including E. morsei, E. scolopes (Hanlon et al., 1997), and S. atlantica (Jones and Richardson, 2010). These characteristics are crucial for establishing genetic lines with mutations that potentially decrease fitness.
Broodstock
Adult wild E. berryi and E. morsei can both be shipped using commercial couriers from their native range in southern mainland, with oxygen-saturated seawater and styrofoam-insulated packaging as described for E. scolopes (Cecere and Miyashiro, 2022), and acclimate well to aquarium conditions. E. berryi and E. morsei are usually caught using a set net round 30m deep, although both species have been collected with dip nets at night near the surface. E. berryi is also caught from the shore by recreational fishermen, and by commercial fishermen by trawling for sale to fish markets. An existing commercial fishery is potentially useful to obtain large numbers of specimens either living, for seeding propagation in captivity or studying behavior, or dead animals for morphological comparisons, isotope analysis, and population genetics. Because E. morsei is a relatively smaller cephalopod, this species is less familiar to the fishing community, which hinders the collection of wild specimens. E. morsei is also similar in size to the adult forms of the sympatric species Lusepiola birostrata and Eumandya parva making identification challenging for nonexperts (Takayama and Okutani, 1992; Bello, 2020). Differences in egg clutch morphology have been used to distinguish sympatric species (Sanchez et al., 2019), and can aid in identifying and collecting eggs in the field.
Proper care of sepiolid eggs is necessary to prevent fouling and maintain high hatching rates (Lee et al., 2009a). Females of E. scolopes host bacterial consortium in their accessory nidamental gland that is secreted to eggs during spawning to protect them from predation (Kerwin et al., 2019). We indirectly observed the same feature of E. berryi and E. morsei; specifically, we noted orange-dyed accessory nidamental glands, whose pigments are generated from carotenoids produced by symbiotic bacterial communities (Pichon et al., 2005). Eggs can be kept in incubating tanks with constant water flow in dark conditions to further inhibit microbial growth (Choe, 1966a). Our eggs were maintained at constant conditions with minimal disturbance as failure to do so can cause premature hatching and decreased survivorship (Hanlon et al., 1997).
The stocking density and male:female ratio are important to consider in cephalopod culture. Crowding has been shown to induce stress and decrease fecundity in Sepia officinalis (Forsythe et al., 2002), Sepioteuthis lessoniana (LaRoe, 1971; Boal and Gonzalez, 1998) and Euprymna scolopes (Hanlon et al., 1997). As most bobtail squid adopt benthic lifestyles quickly (Table 2), the ratio of animals to floor area is also relevant. A low male:female ratio can reduce stress from mating events and prevent forced copulation. We achieved mating and spawning with a low (1:1-1:2) male to female ratio though it is preferable to separate males from females as males become aggressive, similar to E. tasmanica (Nabhitabhata and Nishiguchi, 2014).
Few studies exist on the effects of inbreeding depression on cephalopod culture. Sepia officinalis grown for seven consecutive generations developed decreased fertility in later generations, and the seventh generation failed to produce viable offspring (Forsythe et al., 1994). There are also accounts of a decreased size at maturity for cephalopods cultured to multiple generations (Iglesias et al., 2014). Thus, maintaining the genetic diversity of a colony, by careful interbreeding of separate subpopulations, or the introduction of new alleles by the steady addition of wild animals to the culture, may be necessary to support healthy laboratory colonies. Euprymna hyllebergi and Euprymna tasmanica were cultured for three generations without the introduction of wild-caught specimens. Growth rates were similar across generations and no obvious abnormalities relating to inbreeding were observed (Nabhitabhata and Nishiguchi, 2014). No physical or behavioral abnormalities were observed in both species in sequentially cultured generations; however, sometimes survivorship immediately after hatching was noticeably reduced for some clutches due to some unknown phenomenon similarly reported in E. scolopes (Hanlon et al., 1997). Additionally, rare clutches contained many unfertilized eggs and aberrant jelly coats, and more work should be done to understand and improve these traits. Based on our findings, it should be feasible to maintain a culture of both E. berryi and E. morsei for several generations. Genetic diversity can be maintained by introducing wild caught individuals seasonally (February to June) when vendors in Japan are able to supply additional animals.
Prey and hunting
Activity patterns were similar to what is described for other Euprymna species (Hanlon et al., 1997; Nabhitabhata et al., 2005; Nabhitabhata and Nishiguchi, 2014; Sanchez et al., 2019; Drerup et al., 2020) and animals became active at “night” - emerging from the substrate and discarding the sand coat for hunting and mating. During the “day”, animals bury themselves under the sandy substrate for shelter and to avoid predators (Hanlon et al., 1997; Rodrigues et al., 2010; Drerup et al., 2020). An alternating 12 hour light-dark cycle is sufficient to mimic natural diurnal cycles (Franklin et al., 2014). As for other Euprymna, E. berryi and E. morsei cover their body, head, and arms with sand but leave their eyes exposed (Nabhitabhata et al., 2005; Hanlon and Messenger, 2018; Sanchez et al., 2019; Drerup et al., 2020).
While adults can be fed frozen shrimp, hatchlings and juveniles require live food, similar to other Euprymna spp. (Choe, 1966b; Hanlon et al., 1997; Ikeda et al., 2003; Nabhitabhata and Nishiguchi, 2014; Sanchez et al., 2019). To feed hatchlings of either species, mysids can be collected from inshore locations and reared on a diet of Artemia spp. (Lussier et al., 1988). Hatchlings of both species attacked adult mysids often larger than themselves similar to other species of Euprymna (Okutani and Horita, 1987; Hanlon et al., 1997; Nabhitabhata et al., 2005; Sanchez et al., 2019). While adults were taught to spontaneously grasp frozen prey, they would sometimes ignore non-moving prey. Fully mature adult females consumed more food relative to adult males possibly due to ongoing egg production.
Hatchling behavior
For both E. berryi and E.morsei, hatching from an egg clutch occurs over a period of several days. E. berryi settled and established a benthic lifestyle shortly after hatching in agreement with (Choe, 1966a) and similar to Euprymna tasmanica (Nabhitabhata and Nishiguchi, 2014) and Eumandya parva (Sanchez et al., 2019). E. berryi and E. morsei exhibited a brief nektobenthic paralarval stage similar to what is described for Euprymna hyllebergi (Nabhitabhata et al., 2005) and unlike hatchling behavior of Euprymna scolopes, Eumandya pardalota, and Euprymna brenneri (Hanlon et al., 1997; Sanchez et al., 2019) which displayed surface swimming phototaxic paralarval stages the first month after hatching (Table 2). In our culture, E. berryi and E. morsei could consume prey within 24 hours after hatching, two days earlier than previously reported for E. berryi (Choe, 1966a).
Growth and sexual dimorphism
E. berryi and E. morsei followed similar growth patterns to other Euprymna spp., including early growth stages of E. scolopes (Hanlon et al., 1997) and E. hyllebergi (Nabhitabhata et al., 2005) (Table 2). For comparison, E. scolopes raised at 23°C experienced exponential growth from hatchling to 83 dph (Hanlon et al., 1997). E. hyllebergi demonstrated an exponential growth phase the first 30 dph when raised at 28°C (Nabhitabhata et al., 2005). E. tasmanica raised at 20°C experienced exponential growth from 7 to 44 dph was followed by approximately linear growth from 58 to 140 dph (Moltschaniwskyj and Carter, 2010). As with other bobtail squid, adult males of E. berryi and E. morsei can be definitively distinguished from females by their characteristically modified first left arm, the hectocotylus, which is shorter than the opposing arm and curls outward; female left and right first arms are indistinguishable (Okutani and Horita, 1987; Norman and Lu, 1997). Sexual dimorphism becomes visually evident ~90-100 dph for E. berryi and ~70 days for E. morsei, concurrent with aggressive behavior in males.
Survivorship
Both E. berryi and E. morsei recorded higher survivorship in the first 30 dph (93% and 80%) compared to the 73% survivorship reported for E. scolopes (Hanlon et al., 1997). Neither species exhibited long-lived pelagic paralarval stages after hatching, which could contribute to higher survivorship in captivity relative to E. scolopes. E. morsei was previously reared at 22.5°C and survived for 97 to 128 dph (Ikeda et al., 2003). Our E. morsei grew more slowly and lived longer, possibly due to being cultured at a lower temperature (20°C) and thus having a lower metabolic rate (Iglesias et al., 2014). E. berryi recorded the longest lifespan of any cultured Euprymna spp. (Table 2).
Reproductive maturity and mating behavior
Mating behavior is similar to what was observed in other Euprymna spp. without any obvious courtship behavior (Moynihan, 1983; Hanlon et al., 1997; Nabhitabhata et al., 2005; Squires et al., 2013; Sanchez et al., 2019; Drerup et al., 2020). Females stored spermatangia deposited during matings similarly to other Euprymna spp. (Hanlon et al., 1997; Squires et al., 2013). As females were observed laying eggs cooperatively on the same substrate simultaneously; it is necessary to separate females to track parental lineage without genotyping. Male squids were sometimes aggressive towards conspecifics; therefore, crowding should be avoided especially as squids reach sexual maturity.
Spawning
Both E. berryi and E. morsei are multiple spawners similar to other members of the genera Euprymna and Sepiola (Huang, 2006; Rodrigues et al., 2011; Squires et al., 2013). Adult females of both species laid egg clutches every few days until reaching a late senescent life stage similar to other Euprymna species (Hanlon et al., 1997; Nabhitabhata et al., 2005; Squires et al., 2013). Spawning events were observed within three days of shipment of wild animals, possibly stimulated by the stress of transport (Cecere and Miyashiro, 2022). Mature E. berryi were observed spawning fertilized clutches repeatedly over a period of 100 days at 20°C. Egg clutch morphology differs across sepiolids, and may be used to differentiate sympatric species (Sanchez et al., 2019). Similarly, we found eggs more densely packed in E. morsei clutches than in E. berryi (Figure 2A).
Concluding remarks
Protocols established for E. scolopes are readily adapted for E. berry and E. morsei. Some existing protocols, including in situ hybridization (Lee et al., 2009e), micro-CT (Kerbl et al., 2013), immunohistochemistry (Lee et al., 2009d), and hemocyte collection (Collins and Nyholm, 2010) have already been described in E. berryi (Gavriouchkina et al., 2022), and protocols for infection with symbiotic bacteria (Naughton and Mandel, 2012), behavioral assays, injury treatment, and electrophysiology (Howard et al., 2019) are expected to be transferable from E. scolopes other bobtail species.
Established cultures of E. berryi and E. morsei will allow for comparative studies among bobtail squids in the genus Euprymna. Genomic and transcriptomic data are publicly available for both E. berryi and E. morsei (Sanchez et al., 2019) and other related species (Sanchez et al., 2021), and a genome sequence of E. berryi has recently been reported (Gavriouchkina et al., 2022). The widespread distribution of E. morsei and E. berryi in conjunction with the ability to ship adults and recently spawned eggs should allow more researchers access to these model bobtail squids, and also offers opportunities to find adaptations acquired by different populations.
Data availability statement
The datasets presented in this study can be found in online repositories. The names of the repository/repositories and accession number(s) can be found below: https://figshare.com/search?q=10.6084%2Fm9.figshare.21063211.
Ethics statement
Japan has no specific regulations regarding cephalopods used for research purposes and cephalopods do not fall under the Japanese legislation ‘Act on Humane Treament and Management of Animals (Ogden et al., 2016). All procedures were approved by the OIST Animal Care and Use Committee (approval ID: 2018-204). Procedures and animal cultural protocols followed the guidelines set by Directive 2010/63/EU for cephalopods (Fiorito et al., 2015) and animal welfare guidelines set by OIST Animal Care and Use Committee. Efforts were made to provide the highest quality care and reduce the suffering of animals.
Author contributions
JJ led the project and all aspects of husbandry. JJ, YH, LZ, RK, GS, and CS were involved in husbandry efforts. YH and CS were involved in obtaining wild specimens. JJ, GS, and YH identified species. JJ and CS took growth measurements. DG, FM, GS, and DR provided guidance. JJ, GS, and DR wrote the manuscript. All authors contributed to the article and approved the submitted version.
Funding
This project was funded by the Okinawa Institute of Science and Technology (OIST) to the Molecular Genetics Unit. DR is also grateful for the generous support of the Marthella Foskett-Brown Chair in Biology at the University of California, Berkeley, and from the Chan-Zuckerberg BioHub. GS is grateful for the support of the 22K15085 Grant-in-Aid for Early-Career Scientists (KAKENHI). FM was supported by a JSPS Kakenhi grant (#19K06620). CS was supported by JSPS KAKENHI Grant-in-Aid for Early-Career Scientists, Grant Number 19K15901.
Acknowledgments
We thank Oleg Simakov and Eric Edsinger for helping to initiate bobtail squid studies at OIST, Bret Grasse, Taylor Sakmar, Spencer Nyholm, Bethany Rader, and Robyn Crook for guidance regarding bobtail squid culturing, Keishu Asada for assisting with culturing efforts, Michael Kuba and Tamar Gutnik for guidance on general cephalopod culturing, and Chika Azama for providing logistical support. We appreciate Aki Masunaga’s beautiful illustrations created for this manuscript. We thank the local fishermen in Hiroshima and Mie prefectures for providing information on the seasonal abundance of bobtail squid and for providing animals for this study. Finally we thank the reviewers whose suggestions improved this manuscript.
Conflict of interest
The authors declare that the research was conducted in the absence of any commercial or financial relationships that could be construed as a potential conflict of interest.
Publisher’s note
All claims expressed in this article are solely those of the authors and do not necessarily represent those of their affiliated organizations, or those of the publisher, the editors and the reviewers. Any product that may be evaluated in this article, or claim that may be made by its manufacturer, is not guaranteed or endorsed by the publisher.
Supplementary material
The Supplementary Material for this article can be found online at: https://www.frontiersin.org/articles/10.3389/fmars.2022.1039775/full#supplementary-material
References
Abbo L. A., Himebaugh N. E., DeMelo L. M., Hanlon R. T., Crook R. J. (2021). Anesthetic efficacy of magnesium chloride and ethyl alcohol in temperate octopus and cuttlefish species. J. Am. Assoc. Lab. Anim. Sci. 60, 556–567. doi: 10.30802/AALAS-JAALAS-20-000076
Albertin C. B., Simakov O., Mitros T., Wang Z. Y., Pungor J. R., Edsinger-Gonzales E., et al. (2015). The octopus genome and the evolution of cephalopod neural and morphological novelties. Nature 524, 220–224. doi: 10.1038/nature14668
Anderson F. E., Lindgren A. R. (2020). Phylogenomic analyses recover a clade of large-bodied decapodiform cephalopods. Mol. Phylogenet. Evol. 156, 107038. doi: 10.1016/j.ympev.2020.107038
Belcaid M., Casaburi G., McAnulty S. J., Schmidbaur H., Suria A. M., Moriano-Gutierrez S., et al. (2019). Symbiotic organs shaped by distinct modes of genome evolution in cephalopods. Proc. Natl. Acad. Sci. U. S. A. 116, 3030–3035. doi: 10.1073/pnas.1817322116
Bello G. (2020). Evolution of the hectocotylus in sepiolinae (Cephalopoda: Sepiolidae) and description of four new genera. Eur. J. Taxon. 1–53. doi: 10.5852/ejt.2020.655
Boal J. G., Gonzalez S. A. (1998). Social behavior of individual oval squids (Cephalopoda, teuthoidea, loliginidae, Sepioteuthis lessoniana) within a captive school. Ethology 104, 161–78. doi: 10.1111/j.1439-0310.1998.tb00059.x
Boettcher K. J., Ruby E. G. (1990). Depressed light emission by symbiotic Vibrio fischeri of the sepiolid squid Euprymna scolopes. J. Bacteriol. 172, 3701–3706. doi: 10.1128/jb.172.7.3701-3706.1990
Boettcher K. J., Ruby E. G., McFall-Ngai M. J. (1996). Bioluminescence in the symbiotic squid Euprymna scolopes is controlled by a daily biological rhythm. J. Comp. Physiol. A 179, 65–73. doi: 10.1007/BF00193435
Boletzky S. V. (1975) The reproductive cycle of sepiolidae (Mollusca, cephalopoda). pubblicazioni della stazione zoologica, napoli (Italy) 39. Available at: http://agris.fao.org/agris-search/search.do?recordID=XE7600708.
Boletzky S. V., Boletzky M. V., Frösch D., Gätzi V. (1971). Laboratory rearing of sepiolinae (Mollusca: Cephalopoda). Mar. Biol. 8, 82–87. doi: 10.1007/BF00349349
Boyle P. R. (2005). Cephalopods: ecology and fisheries (Oxford, UK: Ames, Iowa: Blackwell Pub). Available at: https://openlibrary.org/books/OL17173099M/Cephalopods.
Callaerts P., Lee P. N., Hartmann B., Farfan C., Choy D. W. Y., Ikeo K., et al. (2002). HOX genes in the sepiolid squid Euprymna scolopes: implications for the evolution of complex body plans. Proc. Natl. Acad. Sci. U. S. A. 99, 2088–2093. doi: 10.1073/pnas.042683899
Cecere A. G., Miyashiro T. I. (2022). Impact of transit time on the reproductive capacity of Euprymna scolopes as a laboratory animal. Lab. Anim. Res. 38, 25. doi: 10.1186/s42826-022-00135-2
Chiao C.-C., Chubb C., Hanlon R. T. (2015). A review of visual perception mechanisms that regulate rapid adaptive camouflage in cuttlefish. J. Comp. Physiol. A Neuroethol. Sens. Neural Behav. Physiol. 201, 933–945. doi: 10.1007/s00359-015-0988-5
Choe S. (1966a). On the eggs, rearing, habits of the fry, and growth of some Cephalopoda. Bull. Mar. Sci. 16, 330–348.
Choe S. (1966b). On the growth, feeding rates and the efficiency of food conversion for cuttlefishes and squids. Korean J. Syst. Zool. 9, 12–20.
Collins A. J., Nyholm S. V. (2010). Obtaining hemocytes from the Hawaiian bobtail squid Euprymna scolopes and observing their adherence to symbiotic and non-symbiotic bacteria. J. Vis. Exp. 36, 1–3. doi: 10.3791/1714
Crawford K., Diaz Quiroz J. F., Koenig K. M., Ahuja N., Albertin C. B., Rosenthal J. J. C. (2020). Highly efficient knockout of a squid pigmentation gene. Curr. Biol 30 (17), 3484–3490. doi: 10.1016/j.cub.2020.06.099
Doudna J. A., Charpentier E. (2014). Genome editing. the new frontier of genome engineering with CRISPR-Cas9. Science 346, 1258096. doi: 10.1126/science.1258096
Drerup C., Sykes A. V., Cooke G. M. (2020). Behavioural aspects of the spotty bobtail squid Euprymna parva (Cephalopoda: Sepiolidae). J. Exp. Mar. Bio. Ecol. 530-531, 151442. doi: 10.1016/j.jembe.2020.151442
European Parliament and Council of the European Union. (2010). Directive 2010/63/EU of the European parliament and of the council of 22 September 2010 on the protection of animals used for scientific purposes. Off. J. Eur. Union 53, 33–79.
Fiorito G., Affuso A., Basil J., Cole A., de Girolamo P., D’Angelo L., et al. (2015). Guidelines for the care and welfare of cephalopods in research -a consensus based on an initiative by CephRes, FELASA and the Boyd group. Lab. Anim. 49, 1–90. doi: 10.1177/0023677215580006
Forsythe J. W., DeRusha R. H., Hanlon R. T. (1994). Growth, reproduction and life span of Sepia officinalis (Cephalopoda: Mollusca) cultured through seven consecutive generations. J. Zool. 233, 175–192. doi: 10.1111/j.1469-7998.1994.tb08582.x
Forsythe J., Lee P., Walsh L., Clark T. (2002). The effects of crowding on growth of the European cuttlefish, Sepia officinalis linnaeuReared at two temperatures. J. Exp. Mar. Bio. Ecol. 269, 173–185. doi: 10.1016/S0022-0981(02)00006-0
Franklin A. M., Squires Z. E., Stuart-Fox D. (2014). Does predation risk affect mating behavior? an experimental test in dumpling squid (Euprymna tasmanica). PloS One 9, e115027. doi: 10.1371/journal.pone.0115027
Gavriouchkina D., Tan Y., Ziadi-Künzli F., Hasegawa Y., Piovani L., Zhang L., et al. (2022). A single-cell atlas of bobtail squid visual and nervous system highlights molecular principles of convergent evolution. bioRxiv 2022, 5.26.490366. doi: 10.1101/2022.05.26.490366
Grearson A. G., Dugan A., Sakmar T., Sivitilli D. M., Gire D. H., Caldwell R. L., et al. (2021). The lesser pacific striped octopus, Octopus chierchiae: An emerging laboratory model. Front. Mar. Sci. 8. doi: 10.3389/fmars.2021.753483
Hanlon R. T., Claes M. F., Ashcraft S. E., Dunlap P. V. (1997). Laboratory culture of the sepiolid squid Euprymna scolopes: A model system for bacteria-animal symbiosis. Biol. Bull. 192, 364–374. doi: 10.2307/1542746
Hanlon R. T., Messenger J. B. (2018). Cephalopod behaviour 2nd edn. (Cambridge: Cambridge University Press). doi: 10.1017/9780511843600
Hartmann B., Lee P. N., Kang Y. Y., Tomarev S., de Couet H. G., Callaerts P. (2003). Pax6 in the sepiolid squid Euprymna scolopes: evidence for a role in eye, sensory organ and brain development. Mech. Dev. 120, 177–183. doi: 10.1016/s0925-4773(02)00456-2
Heath-Heckman E., Nishiguchi M. K. (2021). Leveraging short-read sequencing to explore the genomics of sepiolid squid. Integr. Comp. Biol. 61, 1753–1761. doi: 10.1093/icb/icab152
Howard R. B., Lopes L. N., Lardie C. R., Perez P. P., Crook R. J. (2019). Early-life injury produces lifelong neural hyperexcitability, cognitive deficit and altered defensive behaviour in the squid Euprymna scolopes. Philos. Trans. R. Soc Lond. B Biol. Sci. 374, 20190281. doi: 10.1098/rstb.2019.0281
Huang C. P. (2006). Species diversity of the genus Euprymna Steenstrup, 1887 in Taiwan and reproductive cycle of Euprymna berryi Sasaki, 1929. Master's thesis (Taichung, Taiwan: National Chung Hsing University), 1929.
Ibáñez C. M., Keyl F. (2010). Cannibalism in cephalopods. Rev. Fish Biol. Fish. 20, 123–136. doi: 10.1007/s11160-009-9129-y
Iglesias J., Fuentes L., Villanueva R. (2014). Cephalopod culture (Dordrecht: Springer Netherlands). 1a. doi: 10.1007/978-94-017-8648-5_23
Iglesias J., Otero J. J., Moxica C., Fuentes L., Sánchez F. J. (2004). The completed life cycle of the octopus (Octopus vulgaris, cuvier) under culture conditions: Paralarval rearing using Artemia and zoeae, and first data on juvenile growth up to 8 months of age. Aquac. Int. 12, 481–487. doi: 10.1023/B:AQUI.0000042142.88449.bc
Ikeda Y., Sakurazawa I., Sakurai Y., Matsumoto G. (2003). Initial trials of squid rearing, maintenance and culture at the brain science institute of RIKEN. Aquac. Sci. 51, 391–400. doi: 10.11233/aquaculturesci1953.51.391
Jinek M., Chylinski K., Fonfara I., Hauer M., Doudna J. A., Charpentier E. (2012). A programmable dual-RNA-guided DNA endonuclease in adaptive bacterial immunity. Science 337, 816–821. doi: 10.1126/science.1225829
Jones B. W., Lopez J. E., Huttenburg J., Nishiguchi M. K. (2006). Population structure between environmentally transmitted vibrios and bobtail squids using nested clade analysis. Mol. Ecol. 15, 4317–4329. doi: 10.1111/j.1365-294X.2006.03073.x
Jones N. J. E., Richardson C. A. (2010). Laboratory culture, growth, and the life cycle of the little cuttlefish Sepiola atlantica (Cephalopoda: Sepiolidae). J. Shellfish Res. 29, 241–246. doi: 10.2983/035.029.0121
Kerbl A., Handschuh S., Nödl M.-T., Metscher B., Walzl M., Wanninger A. (2013). Micro-CT in cephalopod research: Investigating the internal anatomy of a sepiolid squid using a non-destructive technique with special focus on the ganglionic system. J. Exp. Mar. Bio. Ecol. 447, 140–148. doi: 10.1016/j.jembe.2013.02.022
Kerwin A. H., Gromek S. M., Suria A. M., Samples R. M., Deoss D. J., O’Donnell K., et al. (2019). Shielding the next generation: Symbiotic bacteria from a reproductive organ protect bobtail squid eggs from fungal fouling. MBio 10, 1–18. doi: 10.1128/mBio.02376-19
Koenig K. M., Sun P., Meyer E., Gross J. M. (2016). Eye development and photoreceptor differentiation in the cephalopod Doryteuthis pealeii. Development 143, 3168–3181. doi: 10.1242/dev.134254
LaRoe E. T. (1971). The culture and maintenance of the loliginid squids Sepioteuthis sepioidea and Doryteuthis plei. Mar. Biol. 9, 9–25. doi: 10.1007/BF00348813
Lee P. N., Callaerts P., de Couet H. G. (2009a). Culture of Hawaiian bobtail squid (Euprymna scolopes) embryos and observation of normal development. Cold Spring Harb. Protoc. 2009, db.prot5323. doi: 10.1101/pdb.prot5323
Lee P. N., Callaerts P., de Couet H. G. (2009b). The embryonic development of the Hawaiian bobtail squid (Euprymna scolopes). Cold Spring Harb. Protoc. 2009, db.ip77. doi: 10.1101/pdb.ip77
Lee P. N., Callaerts P., De Couet H. G., Martindale M. Q. (2003). Cephalopod hox genes and the origin of morphological novelties. Nature 424, 1061–1065. doi: 10.1038/nature01872
Lee P. N., McFall-Ngai M. J., Callaerts P. (2009c). The Hawaiian bobtail squid (Euprymna scolopes): a model to study the molecular basis of eukaryote-prokaryote mutualism and the development and evolution of morphological novelties in cephalopods. Cold Spring Harbor 4 (11), 1–9. doi: 10.1101/pdb.emo135
Lee P. N., McFall-Ngai M. J., Callaerts P., de Couet H. G. (2009d). Confocal immunocytochemistry of embryonic and juvenile Hawaiian bobtail squid (Euprymna scolopes) tissues. Cold Spring Harb. Protoc. 2009, db.prot5320. doi: 10.1101/pdb.prot5320
Lee P. N., McFall-Ngai M. J., Callaerts P., de Couet H. G. (2009e). Whole-mount in situ hybridization of Hawaiian bobtail squid (Euprymna scolopes) embryos with DIG-labeled riboprobes: II. embryo preparation, hybridization, washes, and immunohistochemistry. Cold Spring Harb. Protoc. 2009, db.prot5322. doi: 10.1101/pdb.prot5322
Leverich W. J., Levin D. A. (1979). Age-specific survivorship and reproduction in Phlox drummondii. Am. Nat. 113, 881–903. doi: 10.1086/283443
Levy G., Nesher N., Zullo L., Hochner B. (2017). “Motor Control in Soft-Bodied Animals: The Octopus”, in Ed. The Oxford handbook of invertebrate neurobiology. Byrne Ed J. H. (Oxford: Oxford University Press). doi: 10.1093/oxfordhb/9780190456757.013.36
Liscovitch-Brauer N., Alon S., Porath H. T., Elstein B., Unger R., Ziv T., et al. (2017). Trade-off between transcriptome plasticity and genome evolution in cephalopods. Cell 169, 191–202.e11. doi: 10.1016/j.cell.2017.03.025
Lussier S. M., Kuhn A., Chammas M. J., Sewall J. (1988). Techniques for the laboratory culture of mysidopsis species (Crustacea: Mysidacea). Environ. Toxicol. Chem. 7 (12), 969–977. doi: 10.1002/etc.5620071203
Maldonado E., Rangel-Huerta E., González-Gómez R., Fajardo-Alvarado G., Morillo-Velarde P. S. (2019). Octopus insularis as a new marine model for evolutionary developmental biology. Biol. Open 8, 1–11. doi: 10.1242/bio.046086
McFall-Ngai M. J. (1999). Consequences of evolving with bacterial symbionts: Insights from the squid-vibrio associations. Annu. Rev. Ecol. Syst. 30, 235–256. doi: 10.1146/annurev.ecolsys.30.1.235
McFall-Ngai M. (2014). Divining the essence of symbiosis: insights from the squid-vibrio model. PloS Biol. 12, e1001783. doi: 10.1371/journal.pbio.1001783
Minton J. W., Walsh L. S., Lee P. G., Forsythe J. W. (2001). First multi-generation culture of the tropical cuttlefish Sepia pharaonis Ehrenberg, 1831. Aquac. Int. 9, 379–392. doi: 10.1023/A:1020535609516
Moltschaniwskyj N. A., Carter C. G. (2010). Protein synthesis, degradation, and retention: mechanisms of indeterminate growth in cephalopods. Physiol. Biochem. Zool. 83, 997–1008. doi: 10.1086/656387
Montgomery M. K., McFall-Ngai M. (1994). Bacterial symbionts induce host organ morphogenesis during early postembryonic development of the squid Euprymna scolopes. Development 120, 1719–1729. doi: 10.1242/dev.120.7.1719
Moynihan M. (1983). Notes on the behavior of Euprymna scolopes (Cephalopoda: Sepiolidae). Behaviour 85, 25–41. doi: 10.1163/156853983x00020
Nabhitabhata J. (2014). “Sepiella inermis”, in Cephalopod culture. Eds. Iglesias J., Fuentes L., Villanueva R. (Dordrecht: Springer Netherlands), 225–240. doi: 10.1007/978-94-017-8648-5_13
Nabhitabhata J., Nilaphat P., Promboon P. (2005). Life cycle of cultured bobtail squid, Euprymna hyllebergi Nateewathan. Spec. Publ. Phuket Mar. Biol. Cent 66, 351–365.
Nabhitabhata J., Nishiguchi M. K. (2014). “Euprymna hyllebergi and Euprymna tasmanica,” in Cephalopod culture. Eds. Iglesias J., Fuentes L., Villanueva R. (Dordrecht: Springer Netherlands), 253–269. doi: 10.1007/978-94-017-8648-5_15
Naughton L. M., Mandel M. J. (2012). Colonization of Euprymna scolopes squid by Vibrio fischeri. J. Vis. Exp. 61, e3758. doi: 10.3791/3758
Navet S., Buresi A., Baratte S., Andouche A., Bonnaud-Ponticelli L., Bassaglia Y. (2017). The pax gene family: Highlights from cephalopods. PloS One 12, e0172719. doi: 10.1371/journal.pone.0172719
Norman M. D., Lu C. C. (1997). Redescription of the southern dumpling squid Euprymna tasmanica and a revision of the genus Euprymna (Cephalopoda: Sepiolidae). J. Mar. Biol. Assoc. United Kingdom 77, 1109–1137. doi: 10.1017/s0025315400038662
O’Brien C. E., Roumbedakis K., Winkelmann I. E. (2018). The current state of cephalopod science and perspectives on the most critical challenges ahead from three early-career researchers. Front. Physiol. 9. doi: 10.3389/fphys.2018.00700
Ogden B. E., Pang William W., Agui T., Lee B. H. (2016). Laboratory animal laws, regulations, guidelines and standards in China mainland, Japan, and Korea. ILAR J. 57, 301–311. doi: 10.1093/ilar/ilw018
Okutani T., Horita E. (1987). Identity of Euprymna berryi Sasaki, 1929 (Cephalopoda: Sepiolidae). Venus 46 (2), 91–107. doi: 10.18941/venusjjm.46.2_91
Peyer S. M., Pankey M. S., Oakley T. H., McFall-Ngai M. J. (2014). Eye-specification genes in the bacterial light organ of the bobtail squid Euprymna scolopes, and their expression in response to symbiont cues. Mech. Dev. 131, 111–126. doi: 10.1016/j.mod.2013.09.004
Pichon D., Gaia V., Norman M. D., Boucher-Rodoni R. (2005). Phylogenetic diversity of epibiotic bacteria in the accessory nidamental glands of squids (Cephalopoda: Loliginidae and idiosepiidae). Mar. Biol. 147, 1323–1332. doi: 10.1007/s00227-005-0014-5
Raj P. J. S., Kalyani N. (1971). Euprymna morsei (Verril) (Sepiolidae: Cephalopoda) from the Indian coast. J. Mar. Biol. Assoc. India 131, 135–375.
Reid A., Jereb P. (2005) Family sepiolidae, in cephalopods of the world (Rome: FAO). Available at: http://www.fao.org/3/ac479e/ac479e00.htm (Accessed May 8, 2020).
Rocha F., Guerra A., González A. F. (2001). A review of reproductive strategies in cephalopods. Biol. Rev. Camb. Philos. Soc 76, 291–304. doi: 10.1017/s1464793101005681
Rodrigues M., Garcí M. E., Troncoso J. S., Guerra A. (2010). Burying behaviour in the bobtail squid Sepiola atlantica (Cephalopoda: Sepiolidae). Ital. J. Zool. 77, 247–251. doi: 10.1080/11250000903282899
Rodrigues M., Garcí M. E., Troncoso J. S., Guerra Á. (2011). Spawning strategy in Atlantic bobtail squid Sepiola atlantica (Cephalopoda: Sepiolidae). Helgol. Mar. Res. 65, 43–49. doi: 10.1007/s10152-010-0199-y
Rosas C., Gallardo P., Mascaró M., Caamal-Monsreal C., Pascual C. (2014). “Octopus maya”, in Cephalopod culture. Eds. Iglesias J., Fuentes L., Villanueva R. (Dordrecht: Springer Netherlands), 383–396. doi: 10.1007/978-94-017-8648-5_20
Ruby E. G. (1996). Lessons from a cooperative, bacterial-animal association: the Vibrio fischeri-Euprymna scolopes light organ symbiosis. Annu. Rev. Microbiol. 50, 591–624. doi: 10.1146/annurev.micro.50.1.591
Ruby E. G., Lee K. H. (1998). The Vibrio fischeri-Euprymna scolopes light organ association: Current ecological paradigms. Appl. Environ. Microbiol. 64, 805–812. doi: 10.1128/AEM.64.3.805-812.1998
Sanchez G., Fernández-Álvarez F.Á., Taite M., Sugimoto C., Jolly J., Simakov O., et al. (2021). Phylogenomics illuminates the evolution of bobtail and bottletail squid (order sepiolida). Commun. Biol. 4, 819. doi: 10.1038/s42003-021-02348-y
Sanchez G., Jolly J., Reid A., Sugimoto C., Azama C., Marlétaz F., et al. (2019). New bobtail squid (Sepiolidae: Sepiolinae) from the Ryukyu islands revealed by molecular and morphological analysis. Commun. Biol. 2, 465. doi: 10.1038/s42003-019-0661-6
Schindelin J., Arganda-Carreras I., Frise E., Kaynig V., Longair M., Pietzsch T., et al. (2012). Fiji: an open-source platform for biological-image analysis. Nat. Methods 9, 676–682. doi: 10.1038/nmeth.2019
Schmidbaur H., Kawaguchi A., Clarence T., Fu X., Hoang O. P., Zimmermann B., et al. (2022). Emergence of novel cephalopod gene regulation and expression through large-scale genome reorganization. Nat. Commun. 13, 2172. doi: 10.1038/s41467-022-29694-7
Sinn D. L., Apiolaza L. A., Moltschaniwskyj N. A. (2006). Heritability and fitness-related consequences of squid personality traits. J. Evol. Biol. 19, 1437–1447. doi: 10.1111/j.1420-9101.2006.01136.x
Sinn D. L., Gosling S. D., Moltschaniwskyj N. A. (2008). Development of shy/bold behaviour in squid: context-specific phenotypes associated with developmental plasticity. Anim. Behav. 75, 433–442. doi: 10.1016/j.anbehav.2007.05.008
Sinn D. L., Moltschaniwskyj N. A. (2005). Personality traits in dumpling squid (Euprymna tasmanica): context-specific traits and their correlation with biological characteristics. J. Comp. Psychol. 119, 99–110. doi: 10.1037/0735-7036.119.1.99
Smith J. A., Andrews P. L. R., Hawkins P., Louhimies S., Ponte G., Dickel L. (2013). Cephalopod research and EU directive 2010/63/EU: Requirements, impacts and ethical review. J. Exp. Mar. Bio. Ecol. 447, 31–45. doi: 10.1016/j.jembe.2013.02.009
Squires Z. E., Norman M. D., Stuart-Fox D. (2013). Mating behaviour and general spawning patterns of the southern dumpling squid Euprymna tasmanica (Sepiolidae): A laboratory study. J. Molluscan Stud. 79, 263–269. doi: 10.1093/mollus/eyt025
Steer M. A., Moltschaniwskyj N. A., Nichols D. S., Miller M. (2004). The role of temperature and maternal ration in embryo survival: using the dumpling squid Euprymna tasmanica as a model. J. Exp. Mar. Bio. Ecol. 307, 73–89. doi: 10.1016/j.jembe.2004.01.017
Sugimoto C., Yanagisawa R., Nakajima R., Ikeda Y. (2013). Observations of schooling behaviour in the oval squid Sepioteuthis lessoniana in coastal waters of Okinawa island. Mar. Biodivers. Rec. 6, e34. doi: 10.1017/S1755267213000067
Sundaram S., Sreeram M. P. (2008). First record of sepiolid squid, Euprymna berryi Sasaki, 1929 from the west coast of India. J. Mar. Biol. Assoc. India 50, 221–223.
Sykes A. V., Baptista F. D., Gonçalves R. A., Andrade J. P. (2012). Directive 2010/63/EU on animal welfare: a review on the existing scientific knowledge and implications in cephalopod aquaculture research. Rev. Aquac. 4, 142–162. doi: 10.1111/j.1753-5131.2012.01070.x
Takayama K., Okutani T. (1992). Identity of Sepiola parva Sasaki, 1913 and S. birostrata Sasaki, 1918 in the Northwest Pacific (Cephalopoda: Sepiolidae). Venus 51, 203–214. doi: 10.18941/venusjjm.51.3_203
Tarazona O. A., Lopez D. H., Slota L. A., Cohn M. J. (2019). Evolution of limb development in cephalopod mollusks. Elife 8, 1–19. doi: 10.7554/eLife.43828
Toriyama M., Kudo S., Kuroki T. (1970) On the small - trawl fishery in the nobeoka - wan - II. Available at: http://feis.fra.affrc.go.jp/publi/bull_nansei/bull_nansei0402.pdf.
Vidal E. A. G., Villanueva R., Andrade J. P., Gleadall I. G., Iglesias J., Koueta N., et al. (2014). Cephalopod culture: current status of main biological models and research priorities. Adv. Mar. Biol. 67, 1–98. doi: 10.1016/B978-0-12-800287-2.00001-9
Villanueva R. (1995). Experimental rearing and growth of planktonic Octopus vulgaris from hatching to settlement. Can. J. Fish. Aquat. Sci. 52, 2639–2650. doi: 10.1139/f95-853
Villanueva R., Perricone V., Fiorito G. (2017). Cephalopods as predators: A short journey among behavioral flexibilities, adaptions, and feeding habits. Front. Physiol. 8. doi: 10.3389/fphys.2017.00598
Villanueva R., Vidal E. A. G., Fernández-Álvarez F.Á., Nabhitabhata J. (2016). Early mode of life and hatchling size in cephalopod molluscs: Influence on the species distributional ranges. PloS One 11, e0165334. doi: 10.1371/journal.pone.0165334
Walsh L. S., Turk P. E., Forsythe J. W., Lee P. G. (2002). Mariculture of the loliginid squid Sepioteuthis lessoniana through seven successive generations. Aquaculture 212, 245–262. doi: 10.1016/S0044-8486(02)00126-6
Keywords: Euprymna, Euprymna berryi, Euprymna morsei, cephalopod, bobtail squid, model organism, aquaculture, developmental biology
Citation: Jolly J, Hasegawa Y, Sugimoto C, Zhang L, Kawaura R, Sanchez G, Gavriouchkina D, Marlétaz F and Rokhsar D (2022) Lifecycle, culture, and maintenance of the emerging cephalopod models Euprymna berryi and Euprymna morsei. Front. Mar. Sci. 9:1039775. doi: 10.3389/fmars.2022.1039775
Received: 08 September 2022; Accepted: 11 November 2022;
Published: 02 December 2022.
Edited by:
Roger Villanueva, Institute of Marine Sciences, (CSIC), SpainReviewed by:
Jaruwat Nabhitabhata, Prince of Songkla University, ThailandCatalina Perales-Raya, Centro Oceanográfico de Canarias (IEO-CSIC), Spain
Copyright © 2022 Jolly, Hasegawa, Sugimoto, Zhang, Kawaura, Sanchez, Gavriouchkina, Marlétaz and Rokhsar. This is an open-access article distributed under the terms of the Creative Commons Attribution License (CC BY). The use, distribution or reproduction in other forums is permitted, provided the original author(s) and the copyright owner(s) are credited and that the original publication in this journal is cited, in accordance with accepted academic practice. No use, distribution or reproduction is permitted which does not comply with these terms.
*Correspondence: Jeffrey Jolly, Jeffrey.A.Jolly@gmail.com; Daniel Rokhsar, dsrokhsar@gmail.com
†Present address: Jeffrey Jolly, Marine Climate Change Unit, Okinawa Institute of Science and Technology Graduate University, Okinawa, Japan
Yuko Hasegawa, Brain Functional Dynamics Collaboration Laboratory, Rikagaku Kenkyūjyo (RIKEN) Center for Brain Science, Saitama, Japan
Chikatoshi Sugimoto, Department of Biology, Keio University, Yokohama, Kanagawa, Japan