- 1Nofima AS, The Norwegian Institute of Food, Fisheries and Aquaculture Research, Tromsø, Norway
- 2FiiZK AkvaFresh AS, Trondheim, Norway
Ultrafiltration (UF) membranes are used to successfully remove waterborne virus and bacteria from wastewater and drinking water. However, UF membrane application in aquaculture water treatment is limited. In this study we evaluate the performance of a capillary polyethersulfone UF membrane to remove two benchmark waterborne fish pathogens: i) the infectious pancreatic necrosis virus - IPNV, which is an unenveloped icosahedral virus, and ii) the bacterium Aeromonas salmonicida, which is a Gram-negative, facultative anaerobic bacilli. Moreover, the UF membrane bench-scale unit was tested at two temperatures according to salmonid aquaculture: low (4 - 7°C) and high (16 - 19°C). Sterilised natural seawater was spiked with laboratory cultured pathogens, the water was filtrated, and the membrane permeate collected. Both pathogen solution and permeate were evaluated using a cell culture method to estimate the colony-forming units (CFU/ml) for bacteria presence, a median tissue culture infectious dose (TCID50/ml) assay for virus presence, and real-time quantitative polymerase chain reaction (RT-qPCR) for both bacteria and virus presence. The membrane permeate was negative for both virus and bacteria for all analysis and for both low and high temperatures. The results from this bench-scale study are encouraging for the application of UF membrane technology in aquaculture water treatment to prevent virus and bacteria outbreaks. Further studies should validate this UF membrane technology results in commercial aquaculture conditions.
Introduction
Membrane filtration is typically a pressure-driven process to force water particles through a membrane and retain them. The water that passes through the membrane is known as filtrate or permeate. Microfiltration, ultrafiltration, nanofiltration and reverse osmosis are the main membrane processes differentiated by the pore size. Ultrafiltration (UF) membrane is an emerging technology being applied in a wide range of industries from drinking water production to wastewater treatment to remove fine solids and microorganisms (Goswami and Pugazhenthi, 2020). However, UF membrane technology to treat aquaculture water is limited (Chiam and Sarbatly, 2011).
Currently UF membrane in aquaculture applications is used as support of ultraviolet (UV) irradiation and it was shown to eliminate waterborne microorganisms, small particles, and humic acids (M. Jakobsen personal communication, July 2022). A promising use of UF membrane technology in aquaculture is a stand-alone unit to treat production facilities intake water by removing waterborne viruses and bacteria. Despite the high biosecurity features in aquaculture facilities (Mota et al., 2022), pathogen outbreaks occurred account for several mass mortality events (Murray et al., 2014; Wiik-Nielsen et al., 2017). UF filters pore sizes range from 20 to 100 nm and are composed of non-cellulosic synthetics (such as polyvinylidene fluoride, polyacrylonitrile, or polyethersulfone) that are resistant to heat and chemical attacks, possess a low protein-binding characteristic, and are reusable (Winona et al., 2001; Olszewski et al., 2005). A recent review on membrane filtration usage to remove bacteria and virus from water showed the promising use of this technology for water purification (Goswami and Pugazhenthi, 2020). A particular UF membrane technology, a capillary fiber membrane made of polyethersulfone, was reported to be able to process large water volumes with extreme durability both in a drinking water treatment plant (Ming Chew et al., 2015) and in a textile wastewater treatment plant (Sahinkaya et al., 2019). The few applications of UF membrane technology in aquaculture are combined with ultraviolet (UV) irradiation. A stand-alone application of UF membrane technology can also be relevant for aquaculture operations. However, before an application of this disinfection barrier in aquaculture is suitable, it is necessary to evaluate its removal efficiency of relevant fish viruses and bacteria in aquaculture water quality.
In this study we evaluated the performance of a capillary polyethersulfone UF membrane to remove two benchmark waterborne salmonids pathogens: i) the infectious pancreatic necrosis virus (IPNV), which is an unenveloped icosahedral virus, and ii) the bacterium Aeromonas salmonicida, which is a Gram-negative, facultative anaerobic bacilli. Moreover, the removal efficiency was evaluated at low (4 - 7°C) and high (16 - 19°C) water temperatures for salmonids.
Material and methods
Experimental design
Two pathogens (IPNV and A. salmonicida) at two water temperatures (low: 4 - 7 °C and high: 16 - 19 °C) were used to test the removal performance of an UF membrane. Each condition was replicated three times in a total of 12 tests. Each test consisted in spiking sterile seawater (10 L) with a laboratory grown pathogen (virus or bacteria) and pumping the water through an UF membrane bench-scale unit. Water samples were collected from the seawater pathogen solution and from the membrane permeate to assess the presence and quantity of pathogens.
Water matrices and chemical analysis
The water used in this study was collected from surface seawater (0 – 1 m depth) at Håjafjorden 69°80'42.8áN 19°01'58.8áE (Tromsø, Norway) during May and June 2022. After collection, the water was sterilised using a high-pressure steam steriliser (SX-700E, TOMY Digital Biology, Japan) and stored refrigerated until testing; at 4°C for low temperature test and 16°C for high temperature test. The sterilised seawater was analysed for the presence of IPNV and A. salmonicida, and it was negative for both microorganisms. Further, the water was characterised for salinity and pH (Tetra con 925 and Sentix 940 sensors, Multi 3630 IDS, WTW, Germany), turbidity (ORION AQ4500, Thermo Scientific®, Thermo Fisher Scientific, USA), and ultra-violet transmittance (UVT %), an indirect measure of turbidity (Table 1). The water absorbance at λ=254 nm (uniSPEC 2 Spectrophotometer, Lab logistics group, Germany) was measured and the UVT was calculated as follows:
where:
UVT = ultraviolet transmittance (%)
a = absorbance at 254 nm wavelength
Ultrafiltration membrane bench-scale unit
The UF membrane was installed as a bench-scale unit (FiiZK Aqua, Trondheim, Norway) and the experimental trials were carried out in two refrigerated rooms at Nofima research facilities (Tromsø, Norway). The UF membrane bench-scale unit is illustrated in Figure 1A. Briefly, it consisted of a cylindrical tank number 1 to add the pathogen solution, a magnet gear pump (MDG-M4S6B220, Iwaki CO, Japan), a water flow meter, a temperature sensor, a water pressure sensor, a UF membrane (Figure 1B), and a cylindrical tank number. 2 to collect the membrane permeate. The capillary membrane consisted of 63 fibres, each fibre had 7 capillaries with an inner diameter of 0.9 mm (dizzer® modules with Multibore® 0.9 membrane, inge GmbH, Germany) and was 50 cm in length measuring a total area of 0.5 m2. The membrane material was polyethersulfone and the pore size was appr. 20 nm. The membrane was operated as inside-out filtration, i.e., pressured water and particles were forced into the membrane fibres capillaries where water and particles smaller than 20 nm escape forming the membrane permeate which flowed to a cylindrical tank number 2. Standard commercial operation settings were used in the tests (Table 1), such as pressure< 1 bar and flux ~50 L/h/m2.
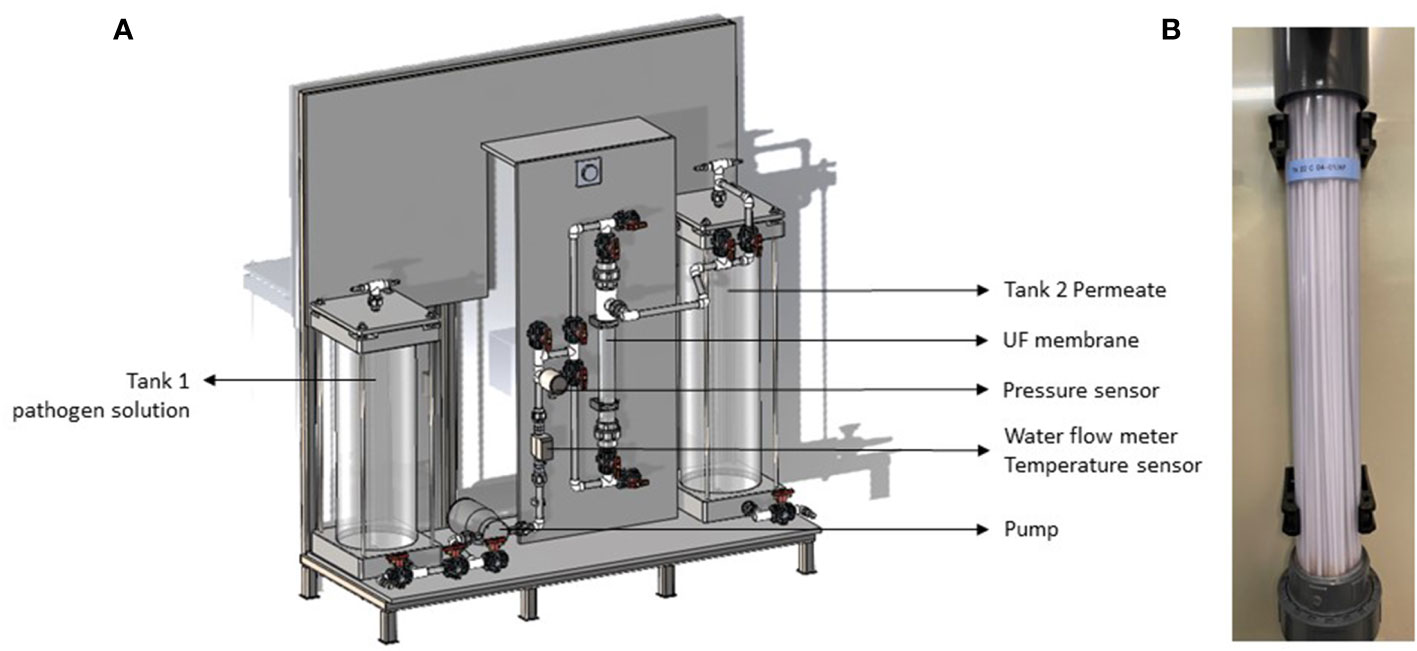
Figure 1 Sketch of the ultrafiltration membrane bench-scale unit (A) and picture of the membrane tested (B).
Disinfection of bench-scale unit
The bench-scale unit was disinfected between each test using a sodium hypochlorite solution. The disinfection protocol consisted in filtering 10 L of ultrapure water (18.2 MΩ/cm, PURELAB Chorus, ELGA Lab water, Veolia, USA) mixed with a sodium hypochlorite solution of 5% active chlorine (Thermo scientific, Belgium) at a concentration 5 ml/L (v/v), followed by rinsing the unit with 10 L of ultrapure water to remove any traces of the disinfection solution. The disinfection efficiency was assessed twice, one for IPNV and one for A. salmonicida, by collecting water samples for microorganism detection analysis and was negative both times. At the end of each test, the membrane permeate was treated with the above sodium hypochlorite solution at a concentration 20 ml/L (v/v) for 48h - 72h before the water was disposed into the domestic wastewater system.
Virus and bacterium preparation
IPN virus was isolated from the pylorus of an infected Atlantic salmon (Salmo salar) and preserved in a minimum essential media (MEM; Ref. 21090-022, Gibco, USA) with 2% fetal bovine serum (FBS, product number F7524, Sigma Aldrich) at -80 °C. The virus titration was estimated 1 x 104.1 TCID50/ml and 1 x 107.8 TCID50/ml. At the day of each trial IPNV serum was defrosted and 18.4 ml of virus stock solution was added to the 10 L refrigerated sterile seawater.
Aeromonas salmonicida subsp. salmonicida bacteria was isolated from the headkidney of Atlantic salmon and preserved in a glycerol and liquid heart infusion (BHI) solution at -80 °C. Prior to each test, bacteria isolates were defrosted and grown on blood agar standard Petri plates with 2% NaCl for 5 days. After, 1 colony forming unit (CFU) was transferred to 20 ml of BHI broth 4 days prior to testing for further bacteria growth. Two days before testing, 20 ml BHI culture was transferred to 400 ml of BHI liquid culture. This bacteria stock solution was 105 CFU/ml and 100 ml was added to the 10 L refrigerated sterile seawater.
Water sampling and filtration
IPNV water samples from both unit tanks were collected in triplicate (100 -200 ml) per test and filtered under vacuum through an electropositive charge filter disc (Zeta Plus 1MDS, Lenntech, The Netherlands) using a filtration pump (Milliflex Oasis, Millipore, Molsheim, France). The filter was stored in 100 µl nuclease-free water (Life technologies, USA) at -80 after filtration. For virus RNA extraction the whole filter was placed in a lysis buffer (RNAdvance Tissue kit, A32646, Beckman Coulter) and incubated for 30 min. at 37 °C. Thereafter, the solution (300 uL) was transferred to tubes for further extraction in a BiomekI5 automated workstation (Beckman Coulter). Extracted RNA (9 uL) was used to make cDNA, using High-Capacity RNA-to-cDNA™ Kit (4387406, Applied Biosystems).
A. salmonicida water samples from both tanks were collected in triplicate (100 ml) per test and filtered under vacuum through a cellulose ester MCE 0.45 µm sterilised filter (Millipore, Molsheim, France) using a filtration pump (Milliflex Oasis, Millipore, Molsheim, France). Filters were transferred to 2 ml tubes containing 200 µl of MilliQ water and heated for 10 min at 95°C to disrupt the bacteria cells. Bacteria lysate was used as a template in the real time quantitative polymerase chain reaction (RT-qPCR) assay.
Real-time quantitative polymerase chain reaction assay
QuantStudio™ Real-Time PCR System (Applied Biosystems, United States) was used to perform RT-qPCR. The RT-qPCR assays were performed in a final volume of 20 µl reaction mixture containing 2 µL of DNA template, 5.6 µL of nuclease-free water, 10 µl of 2X PowerUp™ SYBR® Green Master Mix (Thermo Fisher Scientific, USA) and 1.2 µl of primer. (Table 2). All samples were run in duplicate. The thermocycling parameters were as follows: 20 s of preincubation at 95°C, amplification with 40 cycles at 95°C for 1 s and 60°C for 20 s. A dissociation stage for 1 s followed at 95°C, 20 s at 60°C, and 1 s at 95°C. A five-step standard curve of 2-fold dilution series was prepared from pooled cDNA to determine the amplification efficiencies.
Median tissue culture infectious dose (TCID50) assay
Prior to virus quantification, chinook salmon embryo cells (CHSE-214, LOT 00/F/031, ECACC, England) were grown in culture flasks with 9 mL of MEM and 8% FBS for one week at 20°C with 5% CO2. Two days prior to virus quantification CHSE culture was moved to the 96-well microtiter plates (200 μL/well).
To quantify the virus, samples were added in 200 µl volumes to six wells per dilution (10-fold serial dilution, 2% FBS and MEM) of 96-well microtiter plates containing CHSE. The plates were incubated at 20°C with 5% CO2 for 7 days. After the incubation period, the 96-well microtiter plates were examined for infection by visible cytopathic effect (CPE) using an inverted phase contrast microscope (TMS-F, Nikon, Japan). TCID50 was calculated according to the method of Kärber (1931).
Bacteria plating and counting of colony-forming units
A. salmonicida water samples from both tanks were collected in duplicate (100 ml) per test and filtered under vacuum through a cellulose ester MCE filter as described above. Filters were immediately placed in blood agar 2% NaCl standard Petri plates and incubated at 12°C for 3-5 days. After the incubation period, bacteria colonies were counted, and the results calculated as colony forming units per ml of sample (CFU/mL). Only plates with CFU between 25 and 250 were considered.
Results and discussion
Two benchmark waterborne salmonid pathogens were selected to represent a typical pathogen in these fish species’ aquaculture operations. The infectious pancreatic necrosis virus (IPNV), which is an unenveloped icosahedral virus with an average size of around 55 – 70 nm is responsible for abnormal erratic corkscrew swimming and anorexia in fish (Dobos, 1995; Dopazo, 2020). The A. salmonicida is a Gram-negative bacterium with an average size 500 – 2000 nm, and is the causative agent of furunculosis, a bacterial septicaemia of salmonid fish (Pickup et al., 1996; Dallaire-Dufresne et al., 2014). The removal efficiency of water microorganisms was previously shown to be membrane and water quality specific (Goswami and Pugazhenthi, 2020). Microfiltration and ultrafiltration are the main membrane sizes used for water disinfection and their pore size range from 10 – 5000 nm (Jacangelo et al., 1997). For example, a suspension of poliovirus, one of the smallest known viruses that ranges between 28 - 30 nm, was found to pass through a microfiltration membrane but to be retained in a UF membrane (Madaeni et al., 1995). The UF membrane used in the current study has an approximate pore size of 20 nm (according to the manufacturer). The removal efficiency of IPNV and A. salmonicida by the UF membrane used in this study was 100%, i.e., the membrane permeate was found to be virus and bacteria free in all the replicated tests. Through consideration of the sizes of the target organisms and the membrane pore size, it was apparent that removal of these organisms was due to the membrane acting as a physical barrier that retained the microorganisms’ particles.
UF membrane pore size was previously reported to be influenced by temperature (8.9 - 28.5 °C), where the pore size reduces at lower temperatures (Ma et al., 2017). In the current study, the UF performance was evaluated at a low (4 - 7°C) and high (16 - 19°C) temperature ranges for salmonid aquaculture, and both resulted in a 100% virus and bacteria removal. However, the pressure (bar) before the membrane was 11% higher at low temperature when compared to higher temperature, which may be a result on the effect of temperature on pore size as described by Ma et al. (2017).
Bacteria and virus removal were evaluated according to two different methods: TCDI50/CFU platting and RT-qPCR. Table 3 shows RT-qPCR threshold cycle (Ct) values, estimated titres as TCID50/ml and colony forming units as CFU/ml for both pathogen solution and membrane permeate. Virus titres and bacteria plating returned similar results as RT-qPCR. However, further membrane validation in commercial conditions should consider RT-qPCR method, as it generates faster results, and the primers can be designed specifically for the microorganism of interest.
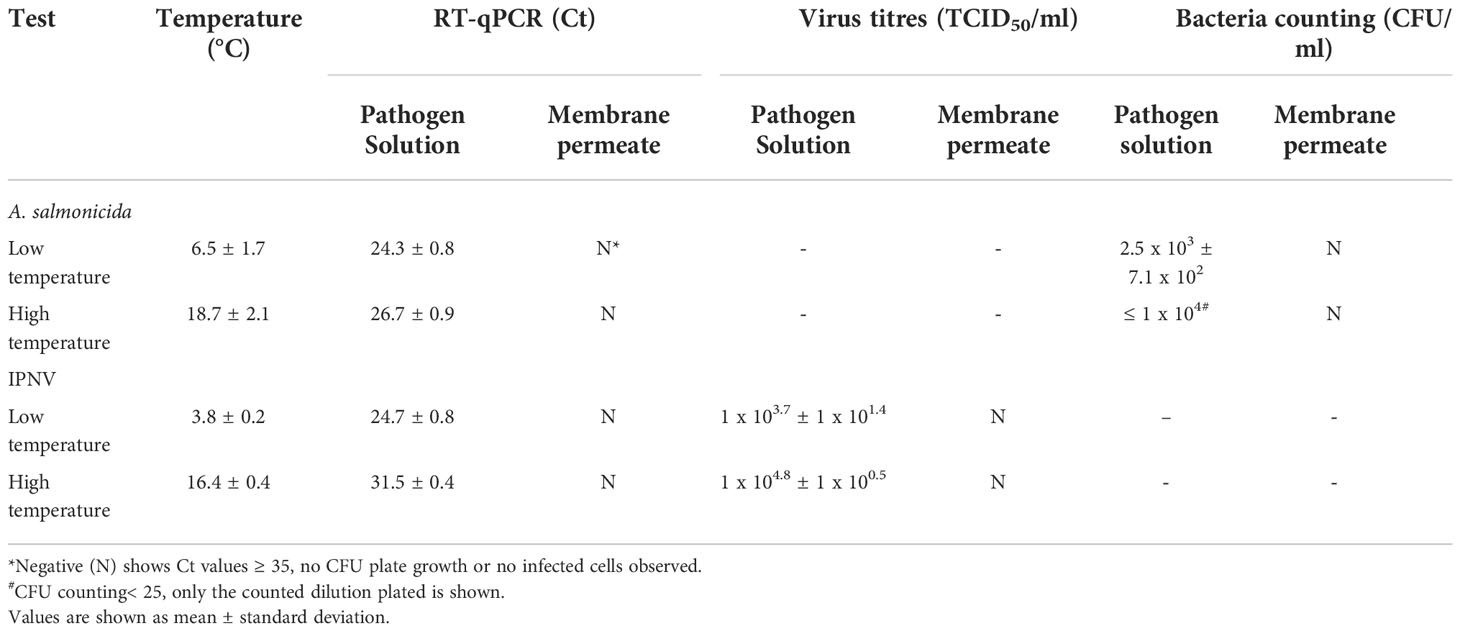
Table 3 Water temperature, RT-qPCR cycle threshold (Ct) values, estimated virus titres (TCID50/ml) and bacteria colony forming units (CFU/ml).
Conclusion
The filtration of IPNV and A. salmonicida suspensions using a 20 nm capillary polyethersulfone membrane completely removed the microorganism. Water temperature did not affect membrane removal efficiency in the tested range (4 - 19 °C), though lower temperatures resulted in higher membrane water pressure. Moreover, both classical microorganism detection techniques, i.e., virus titres and bacteria plating, and the advanced detection technique RT-qPCR returned the same findings. Further studies should validate this UF membrane technology in commercial aquaculture conditions.
Data availability statement
The original contributions presented in the study are included in the article/Supplementary Material. Further inquiries can be directed to the corresponding author.
Author contributions
VM: Conceptualization, Project administration, Investigation, Formal analysis, Visualization, Writing – original draft, Writing – review & editing. HB: Conceptualization, Methodology, Formal analysis, Writing – review & editing. MK: Conceptualization Methodology, Resources. KM: Conceptualization. MJ: Conceptualization, Visualization, Writing – review & editing. All authors contributed to the article and approved the submitted version.
Funding
This project is part of CtrlAQUA SFI, Center for research-based innovation, and funded by the Research Council of Norway (grant number 237856/O30) and the partners of the center.
Acknowledgments
The authors are thankful for Are Nylund and Heidrun Nylund (University of Bergen, Norway) for kindly providing the electropositive charge filter discs and Lill-Heidi Johansen (Nofima, Norway) for helping with IPNV protocols. The authors would also like to acknowledge the dedication to construction of the UF unit by the technical staff in FiiZK AkvaFresh AS.
Conflict of interest
The authors VM and HB declare that they have no known competing financial interests or personal relationships that could have appeared to influence the work reported in this paper. The authors MK, KM and MJ declare the following financial interests or personal relationships which may be considered as potential competing interests: professional relationship with a commercial company selling the ultrafiltration membrane tested.
Publisher’s note
All claims expressed in this article are solely those of the authors and do not necessarily represent those of their affiliated organizations, or those of the publisher, the editors and the reviewers. Any product that may be evaluated in this article, or claim that may be made by its manufacturer, is not guaranteed or endorsed by the publisher.
Supplementary material
The Supplementary material for this article can be found online at: https://www.frontiersin.org/articles/10.3389/fmars.2022.1037017/full#supplementary-material
References
Balcazar J. L., Vendrell D., De Blas I., Ruiz-Zarzuela I., Girones O., Muzquiz J. L. (2007). Quantitative detection of Aeromonas salmonicida in fish tissue by real-time PCR using self-quenched, fluorogenic primers. J. Med. Microbiol. 56, 323–328. doi: 10.1099/jmm.0.46647-0
Chiam C. K., Sarbatly R. (2011). Purification of aquacultural water: Conventional and new membrane-based techniques. Separation Purification Rev. 40, 126–160. doi: 10.1080/15422119.2010.549766
Dallaire-Dufresne S., Tanaka K. H., Trudel M. V., Lafaille A., Charette S. J. (2014). Virulence, genomic features, and plasticity of Aeromonas salmonicida subsp. salmonicida, the causative agent of fish furunculosis. Veterinary Microbiol. 169, 1–7. doi: 10.1016/j.vetmic.2013.06.025
Dobos P. (1995). The molecular biology of infectious pancreatic necrosis virus (IPNV). Annu. Rev. Fish. Dis. 5, 25–54. doi: 10.1016/0959-8030(95)00003-8
Dopazo C. P. (2020). The infectious pancreatic necrosis virus (IPNV) and its virulence determinants: What is known and what should be known. Pathogens 9, 94. doi: 10.3390/pathogens9020094
Goswami K. P., Pugazhenthi G. (2020). Credibility of polymeric and ceramic membrane filtration in the removal of bacteria and virus from water: A review. J. Environ. Manage. 268, 110583. doi: 10.1016/j.jenvman.2020.110583
Hiney M., Dawson M., Heery D., Smith P., Gannon F., Powell R. (1992). DNA Probe for Aeromonas salmonicida. Appl. Environ. Microbiol. 58, 1039–1042. doi: 10.1128/aem.58.3.1039-1042.19
Jacangelo J. G., Trussell R. R., Watson M. (1997). Role of membrane technology in drinking water treatment in the united states. Desalination 113, 119–127. doi: 10.1016/S0011-9164(97)00120-3
Julin K., Johansen L.-H., Sommer A.-I. (2009). Reference genes evaluated for use in infectious pancreatic necrosis virus real-time RT-qPCR assay applied during different stages of an infection. J. Virol. Methods 162, 30–39. doi: 10.1016/j.jviromet.2009.07.003
Kärber G. (1931). Beitrag zur kollektiven behandlung pharmakologischer reihenversuche. Naunyn-Schmiedebergs Archiv. Für. Experimentelle Pathol. Und Pharmakol. 162, 480–483. doi: 10.1007/BF01863914
Madaeni S., Fane A., Grohmann G. (1995). Virus removal from water and wastewater using membranes. J. Membrane Sci. 102, 65–75. doi: 10.1016/0376-7388(94)00252-T
Ma B., Wang X., Liu R., Qi Z., Jefferson W. A., Lan H., et al. (2017). Enhanced antimony (V) removal using synergistic effects of fe hydrolytic flocs and ultrafiltration membrane with sludge discharge evaluation. Water Res. 121, 171–177. doi: 10.1016/j.watres.2017.05.025
Ming Chew C., David Ng K., Richard Ooi H., Ismail W. (2015). Malaysia's largest river bank filtration and ultrafiltration systems for municipal drinking water production. Water Pract. Technol. 10, 59–65. doi: 10.2166/wpt.2015.008
Mota V. C., Striberny A., Verstege G. C., Difford G. F., Lazado C. C. (2022). Evaluation of a recirculating aquaculture system research facility designed to address current knowledge needs in Atlantic salmon production. Front. Anim. Sci. 26. doi: 10.3389/fanim.2022.876504
Murray F., Bostock J., Fletcher D. (2014). Review of recirculation aquaculture system technologies and their commercial application (Stirling, U.K: University of Stirling Aquaculture). H.a.I. Enterprise.
Olszewski J., Winona L., Oshima K. H. (2005). Comparison of 2 ultrafiltration systems for the concentration of seeded viruses from environmental waters. Can. J. Microbiol. 51, 295–303. doi: 10.1139/w05-011
Pickup R., Rhodes G., Cobban R., Clarke K. (1996). The postponement of non-culturability in Aeromonas salmonicida. J. Fish. Dis. 19, 65–74. doi: 10.1111/j.1365-2761.1996.tb00121.x
Sahinkaya E., Tuncman S., Koc I., Guner A. R., Ciftci S., Aygun A., et al. (2019). Performance of a pilot-scale reverse osmosis process for water recovery from biologically-treated textile wastewater. J. Environ. Manage. 249, 109382. doi: 10.1016/j.jenvman.2019.109382
Wiik-Nielsen J., Gjessing M., Solheim H., Litlab A., Gjevre A. G., Kristoffersen A., et al. (2017). Ca. Branchiomonas cysticola, ca. Piscichlamydia salmonis and salmon gill pox virus transmit horizontally in Atlantic salmon held in fresh water. J. Fish Dis. 40, 1387–1394. doi: 10.1111/jfd.12613
Keywords: Aeromonas salmonicida, infectious pancreatic necrosis virus - IPNV, Atlantic salmon pathogens, polyether sulfone membrane, ultrafiltration (UF), aquaculture water treatment
Citation: Mota VC, Brenne H, Kojen M, Marhaug KR and Jakobsen ME (2022) Evaluation of an ultrafiltration membrane for the removal of fish viruses and bacteria in aquaculture water. Front. Mar. Sci. 9:1037017. doi: 10.3389/fmars.2022.1037017
Received: 05 September 2022; Accepted: 23 November 2022;
Published: 08 December 2022.
Edited by:
Zhangying Ye, Zhejiang University, ChinaReviewed by:
Saurav Kumar, Central Institute of Fisheries Education (ICAR), IndiaNajiah Musa, University of Malaysia Terengganu, Malaysia
Copyright © 2022 Mota, Brenne, Kojen, Marhaug and Jakobsen. This is an open-access article distributed under the terms of the Creative Commons Attribution License (CC BY). The use, distribution or reproduction in other forums is permitted, provided the original author(s) and the copyright owner(s) are credited and that the original publication in this journal is cited, in accordance with accepted academic practice. No use, distribution or reproduction is permitted which does not comply with these terms.
*Correspondence: Vasco C. Mota, dmFzY28ubW90YUBub2ZpbWEubm8=