- 1Key Laboratory of Aquacultural Biotechnology Ministry of Education, School of Marine Sciences, Ningbo University, Ningbo, China
- 2Marine Economic Research Center, Dong Hai Strategic Research Institute, Ningbo University, Ningbo, China
- 3Collaborative Innovation Center for Zhejiang Marine High-efficiency and Healthy Aquaculture, School of Marine Science, Ningbo University, Ningbo, China
A three-month culture experiment was conducted to investigate the effects of the feeding regime on liver health, non-specific immunity, and apoptosis of juvenile rainbow trout under constant light conditions. A total of six experimental groups contained two photoperiods [LL (24L:0D) and LD (12L:12D)] and three feeding regimes [R (random feeding), D (mid-dark stage feeding), L (mid-light stage feeding)], defined as R-LL, D-LL, L-LL, R-LD, D-LD, L-LD. The experiment results revealed a significantly higher alanine aminotransferase (ALT) level in the nocturnal feeding group (D-LD) and significantly higher aspartate aminotransferase (AST) in the R-LL and D-LL groups, indicating possible liver damage in these groups. In addition, high serum levels of immunoglobulins M (IgM), complement 3 (C3), and complement 4 (C4) were observed in the LL (compared to LD), R (LL conditions), and D (LD conditions) groups, suggesting that stress may be present in these groups. Meanwhile, under LL, high cytokine genes (tnf-α, il-1β, il-6, and il-8) expression were observed in the liver and intestine of the L group, possibly reflecting a stronger immune response. In the liver, high malondialdehyde (MDA) content was observed in the LL (compared to LD), R (LD conditions), and D (LL conditions) groups, suggesting that these groups were subjected to oxidative damage. Further, higher apoptosis genes (cytc and bcl-2) expression in the liver was detected in the R and D-LD groups. The highest level of hepatic apoptotic cells was also observed in the D-LD group. Taken together, long-term exposure to LL, random feeding, and nocturnal feeding can cause oxidative damage to juvenile rainbow trout, leading to hepatocyte apoptosis, while scheduled diurnal feeding can alleviate the oxidative damage caused by LL.
Introduction
Rainbow trout (Oncorhynchus mykiss Walbaum, 1792) is an economically important fish and occupies an essential position in global aquaculture. According to the latest Food and Agriculture Organization of the United Nations (FAO, 2022) statistics, global rainbow trout aquaculture production reached 959,600 tons in 2020 (including inland aquaculture of 739,500 tons, marine and coastal aquaculture of 220,100 tons). Rainbow trout has a wide consumer market in China as a high-grade aquatic product and has been farmed since the 1960s. However, as a cold-water fish, the suitable growth temperature for rainbow trout is 12-18°C. Due to the lack of cold-water resources, land-based rainbow trout culture development in China has reached a bottleneck (Huang et al., 2021). In recent years, some scholars tried to aquaculture rainbow trout in the cold-water masses of the Yellow Sea in China (Dong, 2019; Chu et al., 2020). Rainbow trout’s seawater culture is viable and has faster growth rates and higher disease resistance than freshwater (Landless, 1976; Yada et al., 2001; Shepherd et al., 2005). Currently, farming rainbow trout in the cold-water masses of the Yellow Sea is still preliminary, and there are still many problems to be improved.
The first challenge of farming rainbow trout in the Yellow Sea cold-water mass is the water temperature fluctuation, especially in the summer high-temperature period. Currently, submerged offshore fish cages (such as “Shenlan 1”) are used to escape the summer heat, and the fish cages are sunk to the suitable water temperature layer to meet the demand for cultured fish during the hot period (Dong, 2019; Chu et al., 2020). However, the photoperiod is deprived underwater and, as an important zeitgeber, is closely linked to immune function in fish (Esteban et al., 2006). Many studies have reported the impact on the immune system and health status of fish in the absence of photoperiodic environments, affecting the welfare of farmed fish. For example, in largemouth bass (Micropterus salmoides), continuous light (LL) was found to cause a significant increase in thiobarbituric acid reactive substances (TBARS) in the liver, brain, and gills compared to LD (12L:12D), indicating the presence of oxidative damage (Malinovskyi et al., 2022). In Atlantic salmon (Salmo salar L.), LL inhibits vertebral mineralization, leading to elevated deformities (Fjelldal et al., 2005; Wargelius et al., 2009; Fjelldal et al., 2012). Studies in rainbow trout have also found that LL has a stressful impact on the non-specific immune system (Valenzuela et al., 2022). The immune system responds rapidly to stress in fish, as reflected by a rapid increase in blood levels of IgM, C3, and C4 (Demers and Bayne, 2020; Abdollahpour et al., 2021). In European Sea Bass (Dicentrachus labrax L.), both LL and DD (continuous darkness) inhibited growth and caused oxidative stress (Li et al., 2021). In Nile tilapia (Oreochromis niloticus) and African catfish (Clarias gariepinus), both LL and DD were found to cause damage to both fish species, affecting swimming activity, behavior, coloration, and growth, which is a challenge to fish welfare (Mustapha et al., 2014). On the other hand, the liver is the central organ of metabolism, and metabolic disorders caused by the absence of photoperiod are often accompanied by liver damage. Under the healthy condition, there is abundant acid phosphatase (ACP), alkaline phosphatase (AKP), alanine aminotransferase (ALT), aspartate aminotransferase (AST), and lactate dehydrogenase (LDH) in the liver, and once the liver is injured, these enzymes will be released into the blood, so the activity of these enzymes in the serum are widely used as indicators of liver damage (Molayemraftar et al., 2022; Rahman et al., 2022).
Besides photoperiod, food is another important environmental cue able to synchronize organisms’ circadian rhythms (Stephan, 2002; López-Olmeda, 2017). Immune function in many fish exhibits significant circadian rhythms. For example, in zebrafish (Danio rerio), the inflammatory response is regulated by the core biological clock genes period1b and period 2 (Ren et al., 2018). In rainbow trout, significant circadian rhythms were also found for serum bactericidal activity and immune defense factors (Lazado et al., 2018) and in the number of leukocytes and myeloid cells (Montero et al., 2020). Further, the effect of feeding time on fish immune function was also reported in a zebrafish study where fish in the scheduled feeding group exhibited higher levels of lysozyme, β-defensin, and hepcidin transcripts (Lazado et al., 2019). Notably, some studies in mammals have shown that scheduled feeding alleviates biological clock dysregulation caused by photoperiod deficiency (Yamamuro et al., 2020) and prevents the development of metabolic diseases in models of biological clock deficiency (Chaix et al., 2019).
Thus, is it possible to mitigate the impact of photoperiod deficiency on fish immune function by scheduled feeding? This study aimed to investigate the effects of feeding regimes on oxidative stress, liver health, and non-specific immunity in juvenile rainbow trout (Oncorhynchus mykiss) in the absence of a photoperiod. It is expected to provide theoretical support for managing rainbow trout culture in offshore fish cages.
Materials and methods
Ethical issues
All experimental operations involving animals in this study were performed under the approval and supervision of the Animal Care and Use Committee of Ningbo University.
Experimental animals
The juvenile rainbow trout used in the experiments were purchased from a commercial nursery in Shandong province and delivered to the School of Marine Sciences, Ningbo University by live fish transporting vehicle. All juvenile fish were rapidly transferred to a recirculating aquaculture system (RAS, HISHING, Qingdao, China) for one month to acclimatize to the laboratory environment. During the acclimation period, commercial feed (crude protein ≥ 42.0% and crude lipid ≥ 10%, Tech-Bank, Ningbo, China) was randomly provided at about 2% of body weight per day; photoperiod was 12L:12D (lights-on at 6:00, the light intensity on the water surface is 100-200 lx); dissolved oxygen in the culture water was kept saturated; water temperature was at 16.5 ± 1°C; ammonia nitrogen was below 0.05 mg/L; the water circulation rate about 600L/h.
Experimental treatments
This study was a two-factor experiment including two independent variables of photoperiod: 24L:0D (LL) and 12L:12D (LD) and feeding regime: random feeding (R, The feeding schedule was provided by random number generator software [RAND function of Microsoft Excel) according to Nisembaum et al. (2012)], mid-dark stage feeding (D, feeding at 24:00 daily), and mid-light stage feeding (L, feeding at 12:00 daily), for a total of 6 experimental groups (R-LL, D-LL, L-LL, R-LD, D-LD, L-LD), each including 7 culture tanks. At the end of acclimation, a total of 840 juvenile rainbow trout of uniform size (18.98 ± 1.69 g/fish) were randomly placed in 42 culture tanks with 20 fish per tank. The formal culture experiment lasted 3 months, and the culture environment was consistent with the acclimation phase.
Sample collection and treatment
At the end of the culture experiment, fish in all treatment groups were deprived of food for 24 h. Subsequently, 12 fish were randomly retrieved from each tank (there was no fish mortality during the experiment, and a total of 20 fish remained in each tank), anesthetized with MS-222, and immediately weighed and measured body length (growth performance was placed in a separate unpublished manuscript). Afterward, about 2 ml of blood was drawn from the tail vein of each fish using a disposable syringe and stored in two 1.5 mL eppendorf (EP) tubes (4°C) of 1 mL each. One of the EP tubes contained EDTA-K2 to prevent clotting and was used for total blood cell count. Another tube of blood was left overnight at 4°C and then centrifuged in a high-speed freezing centrifuge (3-18K, Sigma, Landkreis Osterode, Germany) at 2500 rpm for 10 min at 4°C. The supernatant (serum) was immediately stored at -80°C until analysis. The fish were dissected immediately after blood sampling, and the liver (one part was fixed directly in 10% neutral formalin), intestine, and dorsal muscle were isolated, quickly frozen in liquid nitrogen, and stored at -80°C until analysis.
The tissue homogenate was prepared according to the following steps: liver, intestine, and muscle tissues were homogenized using pre-cooled saline (4°C, 0.9% NaCl) at a ratio of 1:9 (V: W), and the homogenate was centrifuged at 2500 rpm for 10 min at 4°C in a high-speed freezing centrifuge (Fresco17, Thermo Scientific, Waltham, USA) to obtain the supernatant.
Serum immunological indicators
The serum acid phosphatase (ACP, A060-1-1), alkaline phosphatase (AKP, A059-1-1), alanine aminotransferase (ALT, C009-1-1), aspartate aminotransferase (AST, C010-1-1), albumin (ALB, A028-1-1), and lactate dehydrogenase (LDH, A020-1-2) were measured by commercial kits (Nanjing Jiancheng Bioengineering Institute, Nanjing, China) according to the instructions and the absorbance values were read at 532 nm (ACP, U/100 mL, 100 mL serum interacting with the substrate at 37°C for 15 minutes produced 1 mg of phenol is one unit; AKP, King unit/100 mL, 100 mL serum interacting with the substrate at 37°C for 15 minutes produced 1 mg of phenol is one King unit), 505 nm (ALT, U/L, the pyruvate produced within 1 min of 1 ml serum causes the oxidation of NADH to NAD+ and causes a decrease in absorbance of 0.001 is one unit; AST, U/L, same as ALT), 628 nm (ALB, g/L), and 440 nm (LDH, U/L, 1 L of serum interacting with the substrate at 37°C for 15 minutes produced 1 µmol of pyruvate is one unit) in a microplate reader (Multiskan Go, Thermo Scientific, Waltham, USA), respectively, and the results were calculated according to the formula provided in the instructions.
The serum complement 3 (C3, μg/mL), complement 4 (C4, μg/mL), and immunoglobulins M (IgM, μg/mL) were measured using commercial enzyme-linked immunosorbent assay (ELISA) kits (Shanghai Qiaodu Biotechnology Co., Ltd., Shanghai, China) according to the instructions and the absorbance values were read at 450 nm in a microplate reader (Multiskan Go, Thermo Scientific, Waltham, USA), and the results were calculated according to the formula provided in the instructions.
Total blood cell counts
Total blood cell counts (TBC) were performed using a hemocytometer plate under a light microscope (DN-800M, NOVEL, Nanjing, China), according to Pan et al. (2010).
Antioxidant indicators
The serum, liver, intestine, and dorsal muscle malondialdehyde (MDA, A003-1-2), total antioxidant capacity (T-AOC, A015-1-2), total superoxide dismutase (SOD, A001-1-2), and catalase (CAT, A007-1-1) were measured by commercial kits (Nanjing Jiancheng Bioengineering Institute). Serum and tissue homogenates were analyzed according to the kit instructions, and the absorbance values were read at 532 nm (MDA, nmol/mL for serum; nmol/mgprot for tissue), 520 nm (T-AOC, U/mL for serum; U/mgprot for tissue, each 0.01 increase in absorbance value of the reaction system per minute per milliliter of serum (or per milligram of tissue protein) is one unit), 550 nm (SOD, U/mL for serum; U/mgprot for tissue, the amount of enzyme corresponding to 50% inhibition of SOD in this reaction system is one unit), and 405 nm (CAT, U/mL for serum; U/mgprot for tissue, decomposition of 1 µmol of H2O2 per second per milliliter of serum (or per milligram of tissue protein) is one unit) in a microplate reader (Multiskan Go, Thermo Scientific, Waltham, USA), respectively, and the results were calculated according to the formula provided in the instructions. Meanwhile, the total protein (TP, A045-2-2, g/L) content in the tissue homogenates was measured by the Coomassie brilliant blue method at 595 nm to normalize the antioxidant index in the tissues.
Non-specific immunity-related gene expression
The reagents and methods used to extract total RNA from the liver and intestine were consistent with Xu et al. (2022).
A real-time PCR system (QuantStudio™ 6 Flex, Life Technologies, Carlsbad, USA) was used to analyze the relative expression of non-specific immunity-related genes [Cytochrome c(cytc), Caspases 3 (casp3), Caspases 6 (casp6), Caspases 8 (casp8), Bcl2-associated X (bax), B-cell lymphoma-2 (bcl-2), Tumor suppressor p53 (p53), Interleukin-1β (il-1β), Interleukin-6 (il-6), Interleukin-8 (il-8), Tumor necrosis factor-α (tnf-α), Glutathione peroxidase (gpx), Cytosolic Cu/Zn superoxide dismutase (sod1), Mitochondrial Mn superoxide dismutase (sod2), Catalase (cat)] in the liver and intestine. The reaction system and program were consistent with Xu et al. (2022). The specific primers used in this study are shown in Table 1 and synthesized by a commercial company (Youkang Biological Technology Co., Ltd, Hangzhou, China). The relative expression level of target genes is normalized by elongation factor-1α (ef1α) and calculated by the comparative CT method (2-ΔΔCT method) (Livak and Schmittgen, 2001).
Histology and apoptosis analysis
The fixed liver tissue (for 24 h) was flushed with running water overnight, followed by dehydration in gradient alcohol, transparency in alcohol, and xylene solution, and finally liver tissues were embedded in paraffin wax. The liver tissue was subsequently sectioned on a microtome (thickness 5 μm, HM325, Thermo Scientific, Waltham, USA). Liver sections were spread in warm water at 48°C and later dried at 45°C, followed by dewaxing and rehydration in xylene and gradient alcohol, and finally stained using a hematoxylin and eosin (H&E) staining kit (G1120, Solarbio, Beijing, China) following the steps of the instructions. Observations were performed under a light microscope (magnification 200×, CKX53, OLYMPUS, Tokyo, Japan).
The above obtained liver sections were stained with TUNEL using Colorimetric TUNEL Apoptosis Assay Kit (C1098, Beyotime, Shanghai, China) according to the steps of the instructions and then observed under a light microscope (magnification 200×, CKX53, OLYMPUS, Tokyo, Japan).
Statistical analysis
First, all data were checked for homogeneity and normal distribution through Levene’s and Kolmogorov-Smirnov tests, respectively. Then a two-way ANOVA was performed with photoperiod and feeding regime. Meanwhile, t-tests were performed for photoperiod, and one-way ANOVA followed by Duncan’s multiple range test was performed for the feeding regime. P < 0.05 and P < 0.01 were considered significant differences and extremely significant differences, respectively. All statistical analyses were performed on SPSS 22.0 and R 4.1.2 software. Data are presented as box plots (minimum, median, maximum, outliers) or heatmaps (mean).
Results
Liver health indicators
Serum ACP, AKP, and LDH activities were not affected by experimental treatments. In group D, ALT activity was significantly higher in group LD than in group LL. AST activity was lower in group L than in groups R and D. The ALB was significantly lower in group R than in D and L groups (Figure 1).
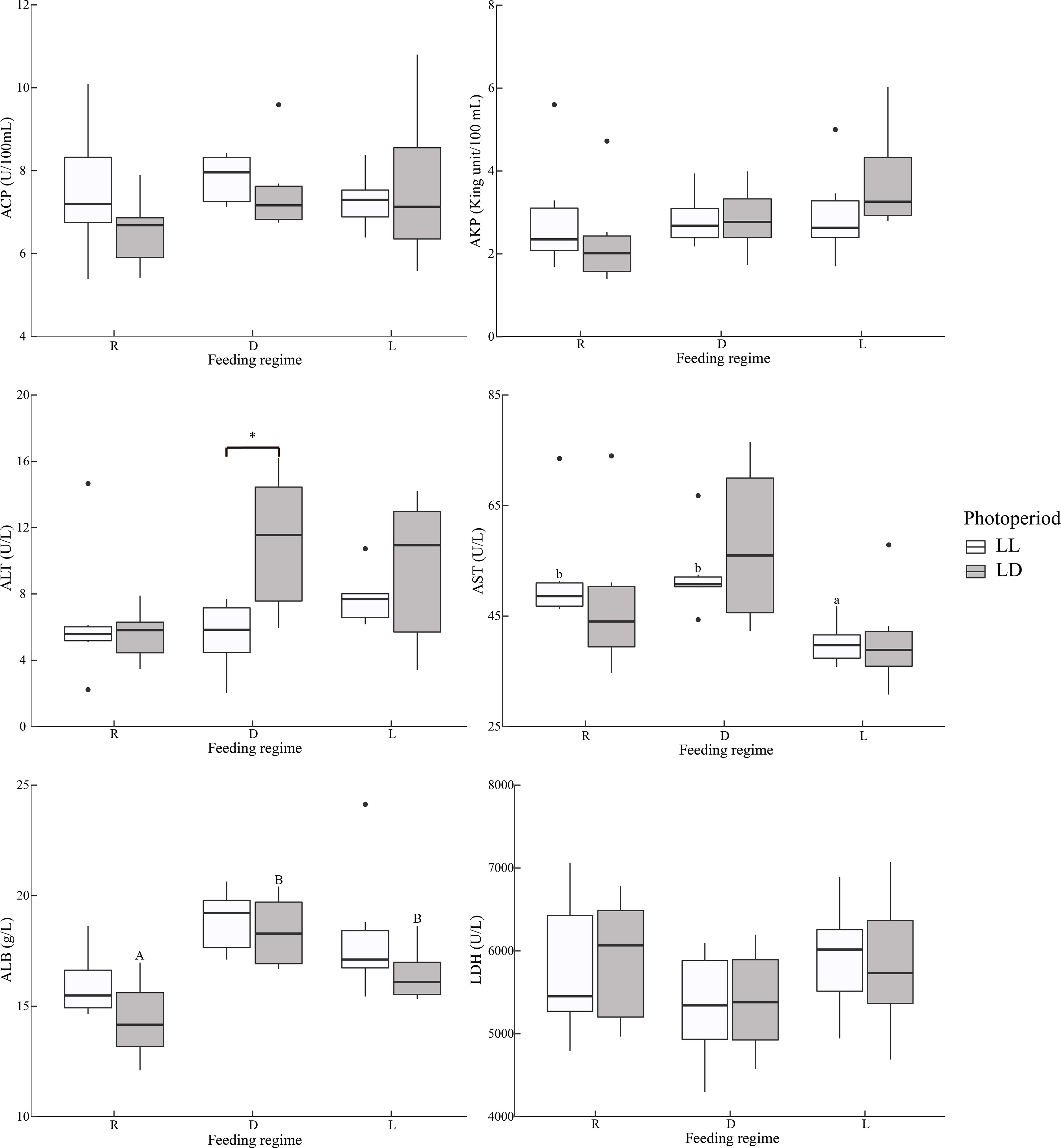
Figure 1 Liver health indicators of juvenile Oncorhynchus mykiss under different experimental treatments (n=6). ACP, Acid phosphatase; AKP, Alkaline phosphatase; ALT, Alanine aminotransferase; AST, Aspartate aminotransferase; ALB, Albumin; LDH, Lactate dehydrogenase; R, random feeding; D, mid-dark stage feeding; L, mid-light stage feeding; LL: 24L: 0D, LD: 12L: 12D. Different lowercase letters and capital letters indicate significant difference among different feeding regimes at the LL (24L: 0D) and LD (12L: 12D, lights-on at 6:00), respectively (P < 0.05). Asterisks denote significant differences between photoperiods at the same feeding regime (*P < 0.05). Points outside the box plot are outliers.
Serum immunological indicators
The scheduled feeding group had higher TBC (Figure 2).
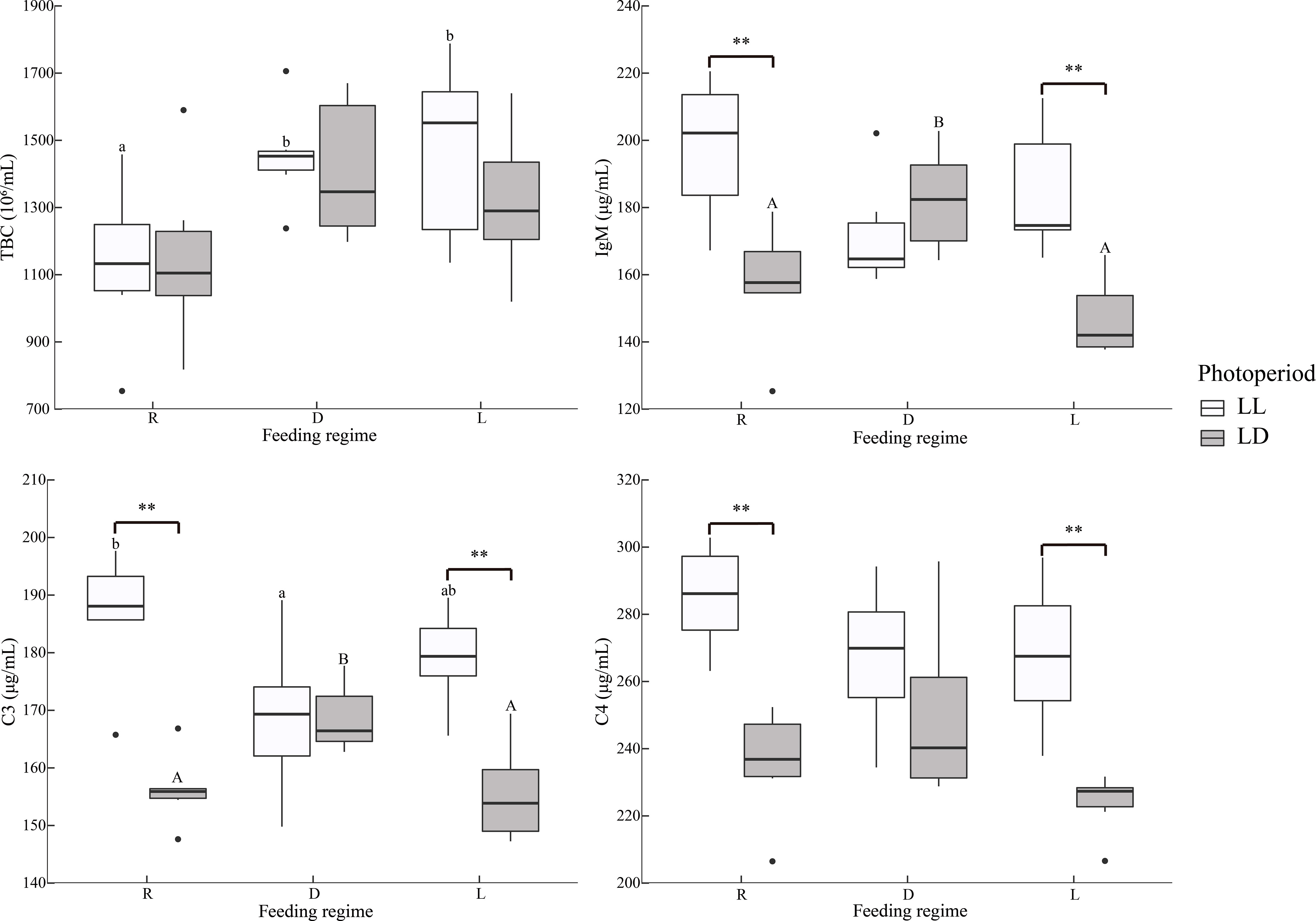
Figure 2 Serum immunological indicators of juvenile Oncorhynchus mykiss under different experimental treatments (n=6). TBC: Total blood cell counts, IgM: Immunoglobulins M, C3, Complement 3; C4, Complement 4; R, random feeding; D, mid-dark stage feeding; L, mid-light stage feeding; LL: 24L: 0D, LD: 12L: 12D. Different lowercase letters and capital letters indicate significant difference among different feeding regimes at the LL (24L: 0D) and LD (12L: 12D, lights-on at 6:00), respectively (P < 0.05). Asterisks denote significant differences between photoperiods at the same feeding regime (**P < 0.01). Points outside the box plot are outliers.
Under LL, the R group had higher C3 levels than the D group; under LD, the D group had higher C3 and IgM levels than R and L groups. In the R and L groups, the LL group had significantly higher C3, C4, and IgM (Figure 2).
Antioxidant indicators
Overall, MDA in the liver was significantly higher in the LL group than in the LD group, independent of the feeding regime (Table 2 and Figure 3). Specifically, under LL, the D group was significantly higher than the R group; under LD, the R group was significantly higher than the D and L groups. In group D, LL was significantly higher than that LD. In contrast, SOD activity was significantly lower in the D group than in the R group under LL; in the D group, the LD group was significantly higher than in the LL group; CAT activity was significantly higher in the LD group than in the LL group under all three feeding regimes.
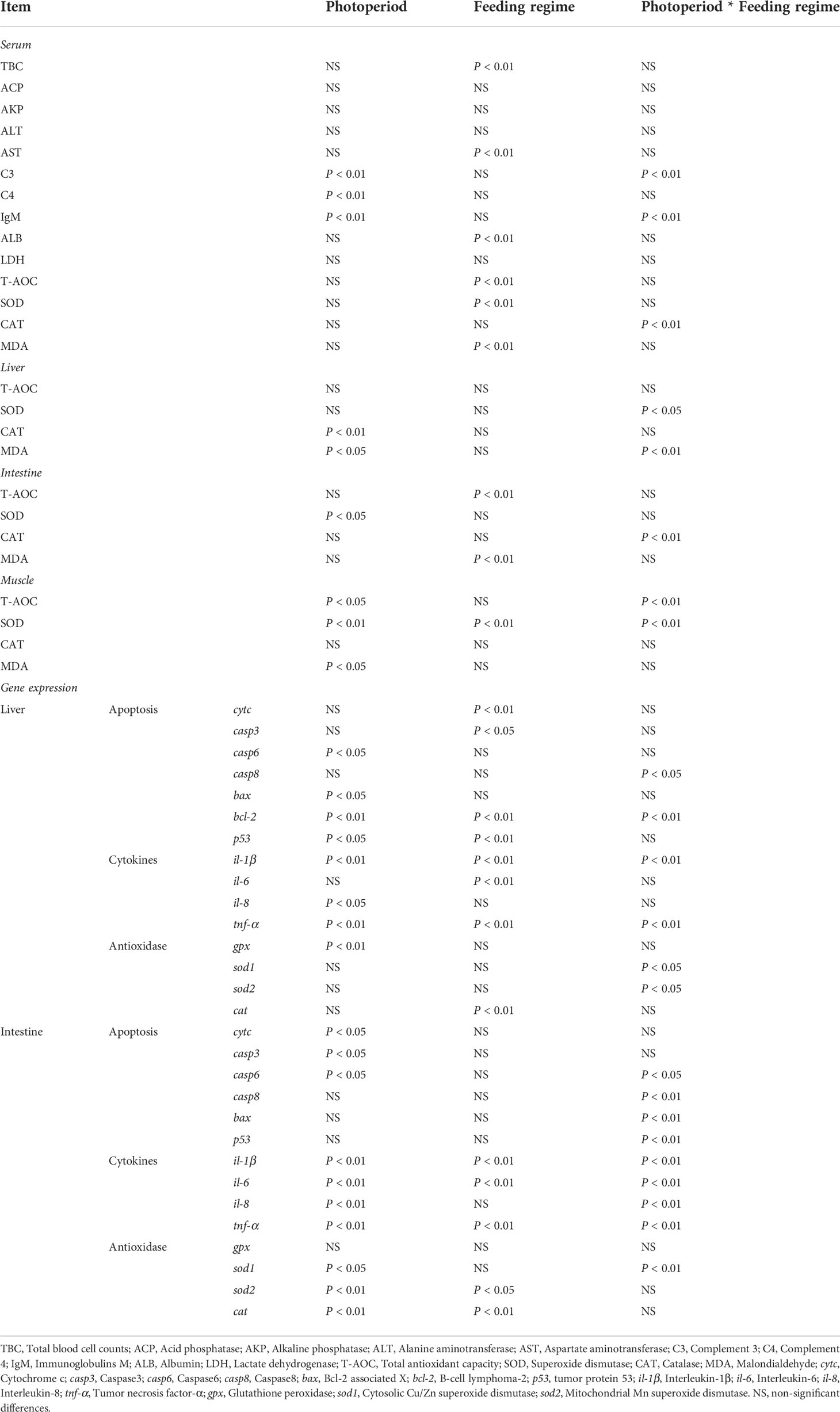
Table 2 Summary of two-way ANOVA: Immunity index and gene expression of juvenile Oncorhynchus mykiss under different experimental treatment.
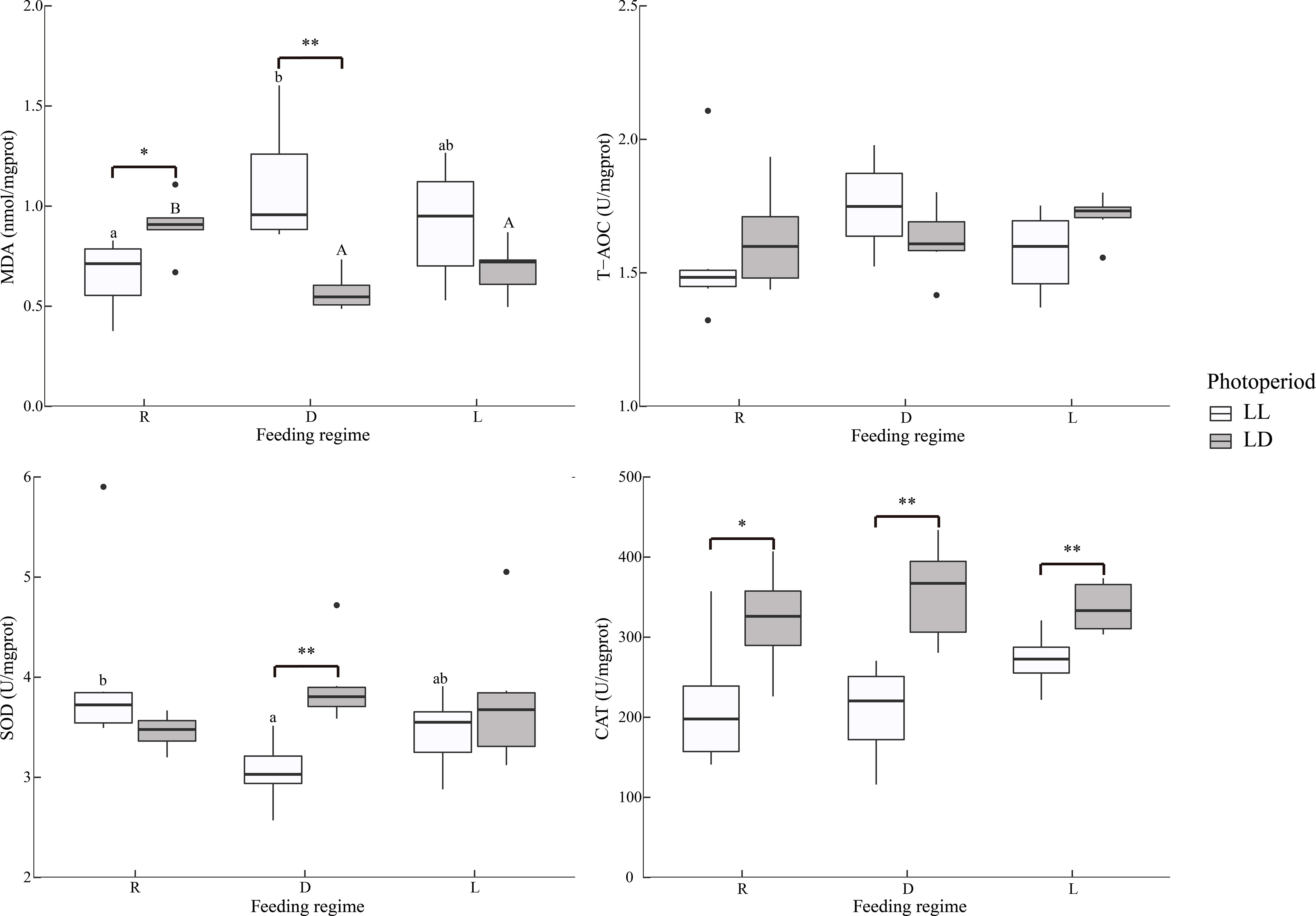
Figure 3 Liver antioxidant indicators of juvenile Oncorhynchus mykiss under different experimental treatments (n=6). MDA: Malondialdehyde, T-AOC: Total antioxidant capacity, SOD: Superoxide dismutase, CAT, Catalase; R, random feeding; D, mid-dark stage feeding; L, mid-light stage feeding; LL: 24L: 0D, LD: 12L: 12D. Different lowercase letters and capital letters indicate significant difference among different feeding regimes at the LL (24L: 0D) and LD (12L: 12D, lights-on at 6:00), respectively (P < 0.05). Asterisks denote significant differences between photoperiods at the same feeding regime (*P < 0.05; **P < 0.01). Points outside the box plot are outliers.
Overall, the MDA in the intestine was significantly lower in the L group than R and D groups, independent of the photoperiod (Table 2 and Figure 4). In contrast, T-AOC was significantly higher in the L group than R and D groups.
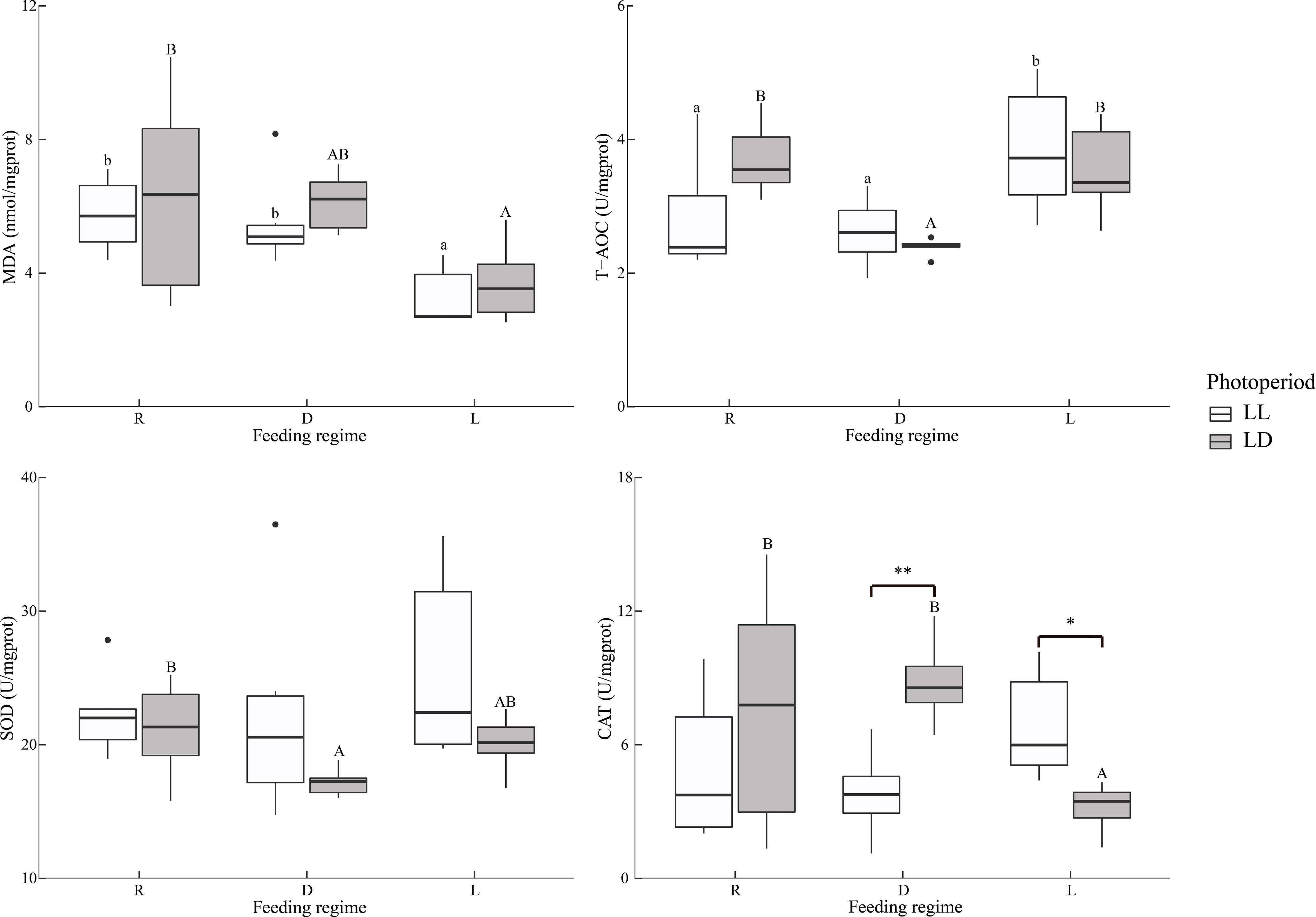
Figure 4 Intestine antioxidant indicators of juvenile Oncorhynchus mykiss under different experimental treatments (n=6). MDA: Malondialdehyde, T-AOC: Total antioxidant capacity, SOD: Superoxide dismutase, CAT, Catalase; R, random feeding; D, mid-dark stage feeding; L, mid-light stage feeding; LL: 24L: 0D, LD: 12L: 12D. Different lowercase letters and capital letters indicate significant difference among different feeding regimes at the LL (24L: 0D) and LD (12L: 12D, lights-on at 6:00), respectively (P < 0.05). Asterisks denote significant differences between photoperiods at the same feeding regime (*P < 0.05; **P < 0.01). Points outside the box plot are outliers.
The experimental treatments did not affect MDA levels in muscles (Figure 5).
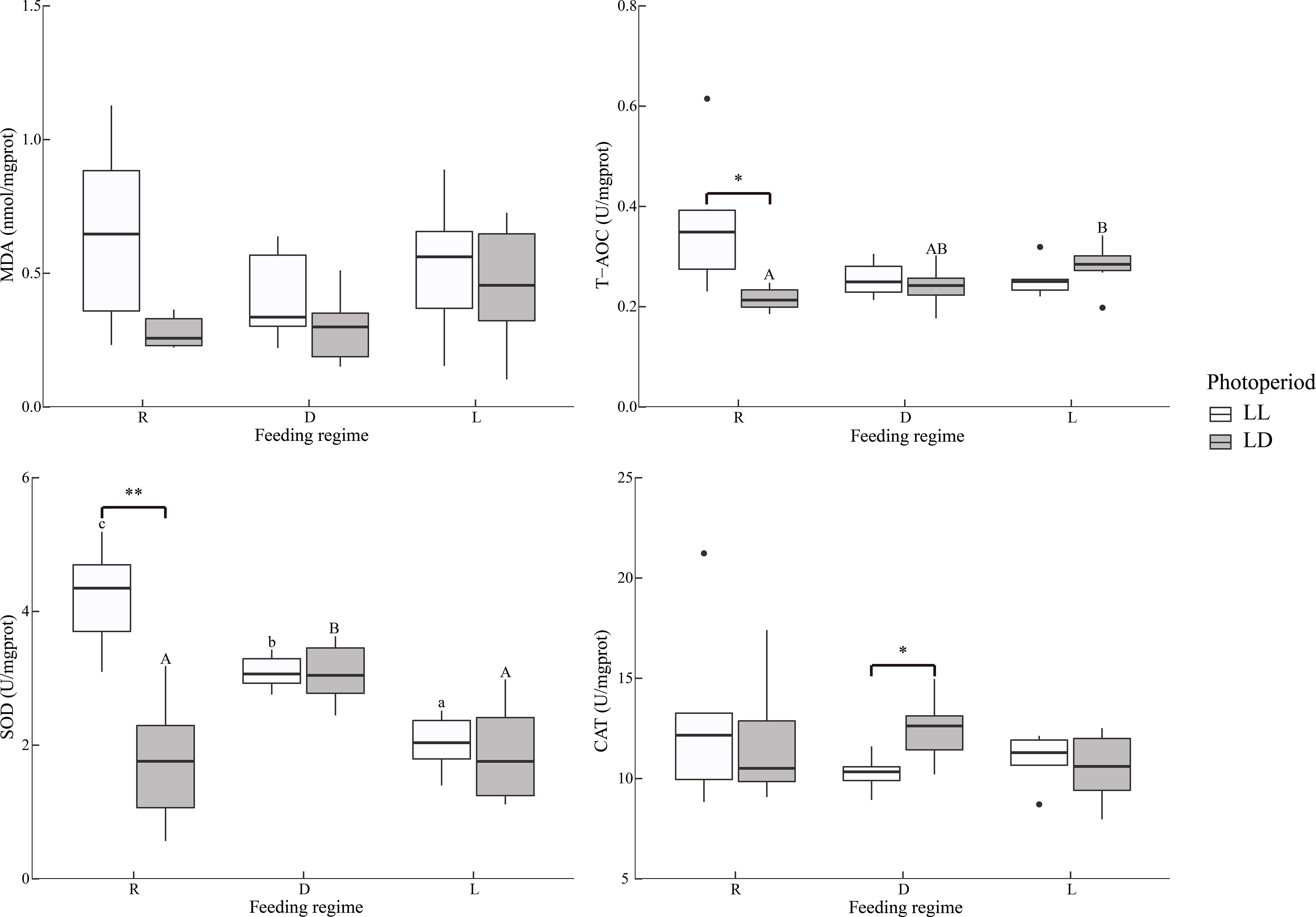
Figure 5 Dorsal muscle antioxidant indicators of juvenile Oncorhynchus mykiss under different experimental treatments (n=6). MDA, Malondialdehyde; T-AOC, Total antioxidant capacity; SOD, Superoxide dismutase; CAT, Catalase; R, random feeding; D, mid-dark stage feeding; L, mid-light stage feeding; LL: 24L: 0D, LD: 12L: 12D. Different lowercase letters and capital letters indicate significant difference among different feeding regimes at the LL (24L: 0D) and LD (12L: 12D, lights-on at 6:00), respectively (P < 0.05). Asterisks denote significant differences between photoperiods at the same feeding regime (*P < 0.05; **P < 0.01). Points outside the box plot are outliers.
In serum, MDA levels were significantly lower in the R group than in the D and L groups, independent of photoperiod (Table 2 and Figure 6). Similarly, T-AOC and SOD activities were significantly lower in the R group than in the D and L groups.
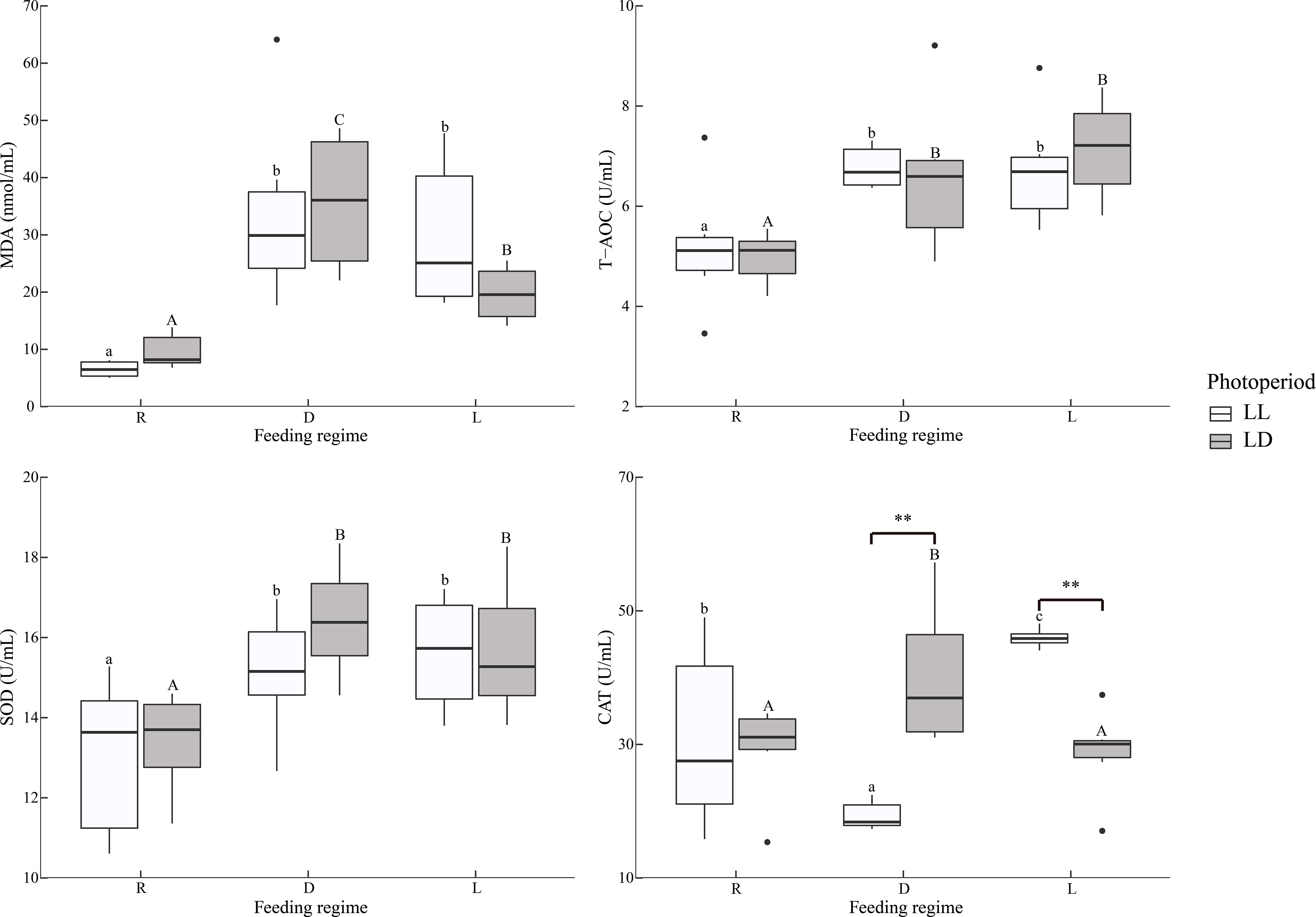
Figure 6 Serum antioxidant indicators of juvenile Oncorhynchus mykiss under different experimental treatments (n=6). MDA, Malondialdehyde; T-AOC, Total antioxidant capacity; SOD, Superoxide dismutase; CAT, Catalase; R, random feeding; D, mid-dark stage feeding; L, mid-light stage feeding; LL: 24L: 0D, LD: 12L: 12D. Different lowercase letters and capital letters indicate significant difference among different feeding regimes at the LL (24L: 0D) and LD (12L: 12D, lights-on at 6:00), respectively (P < 0.05). Asterisks denote significant differences between photoperiods at the same feeding regime (**P < 0.01). Points outside the box plot are outliers.
Liver and intestinal gene expression
Apoptosis, cytokine, and antioxidant gene expression in the liver and intestine were detected in this experiment (Figure 7 and Supplementary Figure 1).
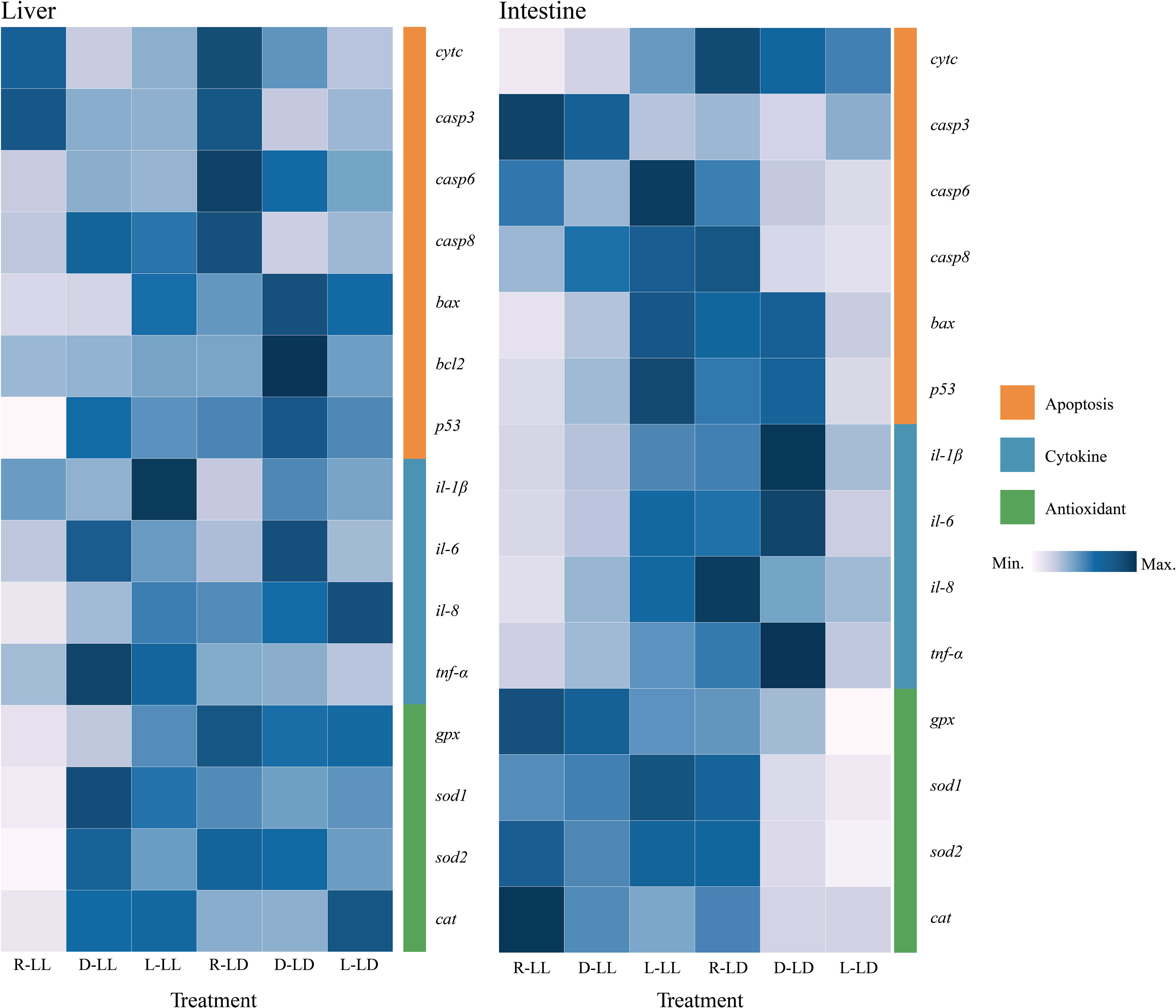
Figure 7 Liver and intestinal apoptosis, cytokine, and antioxidant-related gene expression of juvenile Oncorhynchus mykiss under different experimental treatments (n=6). R: random feeding, D: mid-dark stage feeding, L: mid-light stage feeding, LL: 24L: 0D, LD: 12L: 12D. cytc: Cytochrome c, casp3: Caspases 3, casp6: Caspases 6, casp8: Caspases 8, bax: Bcl2-associated X, bcl-2: B-cell lymphoma-2, p53: Tumor suppressor p53, il-1β: Interleukin-1β, il-6: Interleukin-6, il-8: Interleukin-8, tnf-α: Tumor necrosis factor-α, gpx: Glutathione peroxidase, sod1: Cytosolic Cu/Zn superoxide dismutase, sod2: Mitochondrial Mn superoxide dismutase, cat: Catalase.
In the liver, cytc gene expression was significantly higher in the R group under both photoperiods; in the D group, the LD was significantly higher than the LL. The p53 gene was significantly lower in the R group under LL. In the R group, the LD was significantly higher than LL. The bax gene was significantly higher in the L group under LL. The bcl-2 gene was significantly higher in the D group under LD; in the D group, the LD was significantly higher than the LL. The tnf-α gene was significantly lower in the R group under LL. In the D and L groups, the LL was significantly higher than LD. The il-1β gene was significantly higher in the L group under LL and significantly lower in the R group under LD. In the R and L groups, LL was significantly higher than LD. in the D group, LD was significantly higher than LL. The il-6 gene was significantly higher in group D under LL; the D group was significantly higher than the R and L groups under LD. The gpx gene was significantly higher in the L group under LL. The LL was significantly lower than LD in the R and D groups. The sod1 and sod2 genes were significantly lower in the R group under LL. In the R group, LL was significantly lower than LD. The cat genes were significantly lower in the R group under LL; under LD, the L group was significantly higher than the R and D groups. In the R group, LL was significantly lower than LD.
In the R group, the cytc gene in the intestine was significantly lower in LL than in LD. The p53 and bax genes were significantly higher in the L group under LL and significantly lower in the L group under LD. In the R group, LL was significantly lower than LD; in the L group, LL was significantly higher than LD. The casp6 and casp8 genes were significantly higher in the R group under LD. The tnf-α, il-1b, il-6, and il-8 genes exhibited significantly higher in the L group under LL. The L group was lower than the R and D groups under LD. In the R and D groups, LL was significantly lower than LD. The expression trends of antioxidant enzyme genes were also consistent, with the expression of sod1, sod2, and cat genes significantly higher in the R group under LD. The sod1 and sod2 genes were higher in LL than in LD under both D and L groups. The cat gene expression was highest in the R group under LL; in the R and L groups, LL was higher than LD.
Liver histology and apoptosis
The observation of liver paraffin sections with H&E staining revealed pathological changes between the six treatment groups (Figure 8). The liver parenchyma was looser in the R group, and some cellular vacuolation (black triangles) was present between the different treatment groups. The R and D groups observed significant capillary dilatation (black circles). Erythrocyte sludge in the blood sinusoids (black circles and green arrows) was observed in the R-LL, D-LL, R-LD, and D-LD groups.
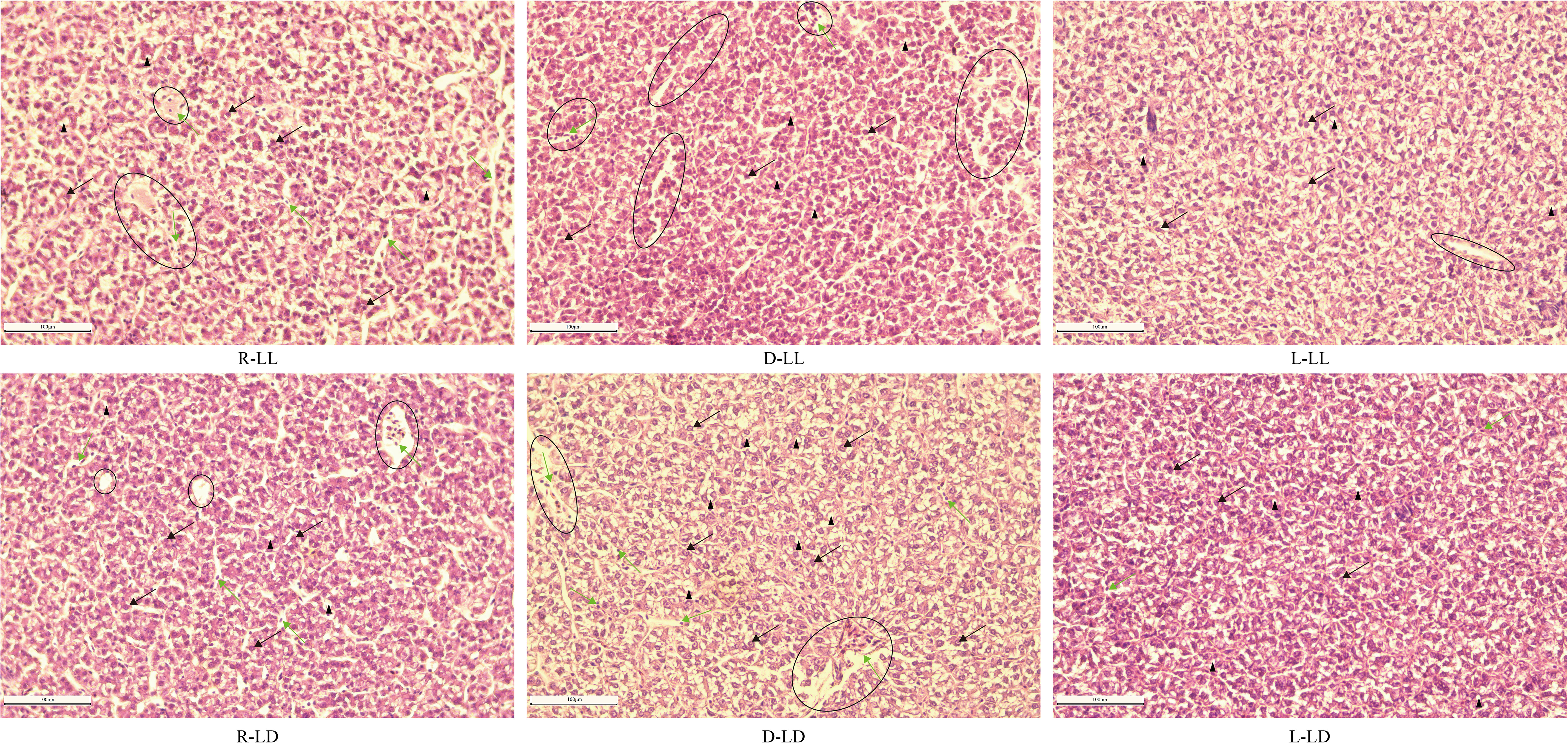
Figure 8 The histology of the liver in juvenile Oncorhynchus mykiss under different experimental treatments (200 × magnification, scale bars = 100 μm, slice thickness = 7 μm). R: random feeding, D: mid-dark stage feeding, L: mid-light stage feeding, LL: 24L: 0D, LD: 12L: 12D. The black arrow points to hepatocyte; the green arrow points to blood sinusoid including erythrocyte; the black triangle points to cavitation; the black circle points to vein including erythrocyte.
TUNEL staining of liver tissue sections revealed that there were almost no apoptotic cells in the L group under both photoperiods, and apoptosis was most severe in the D-LD group (Figure 9).
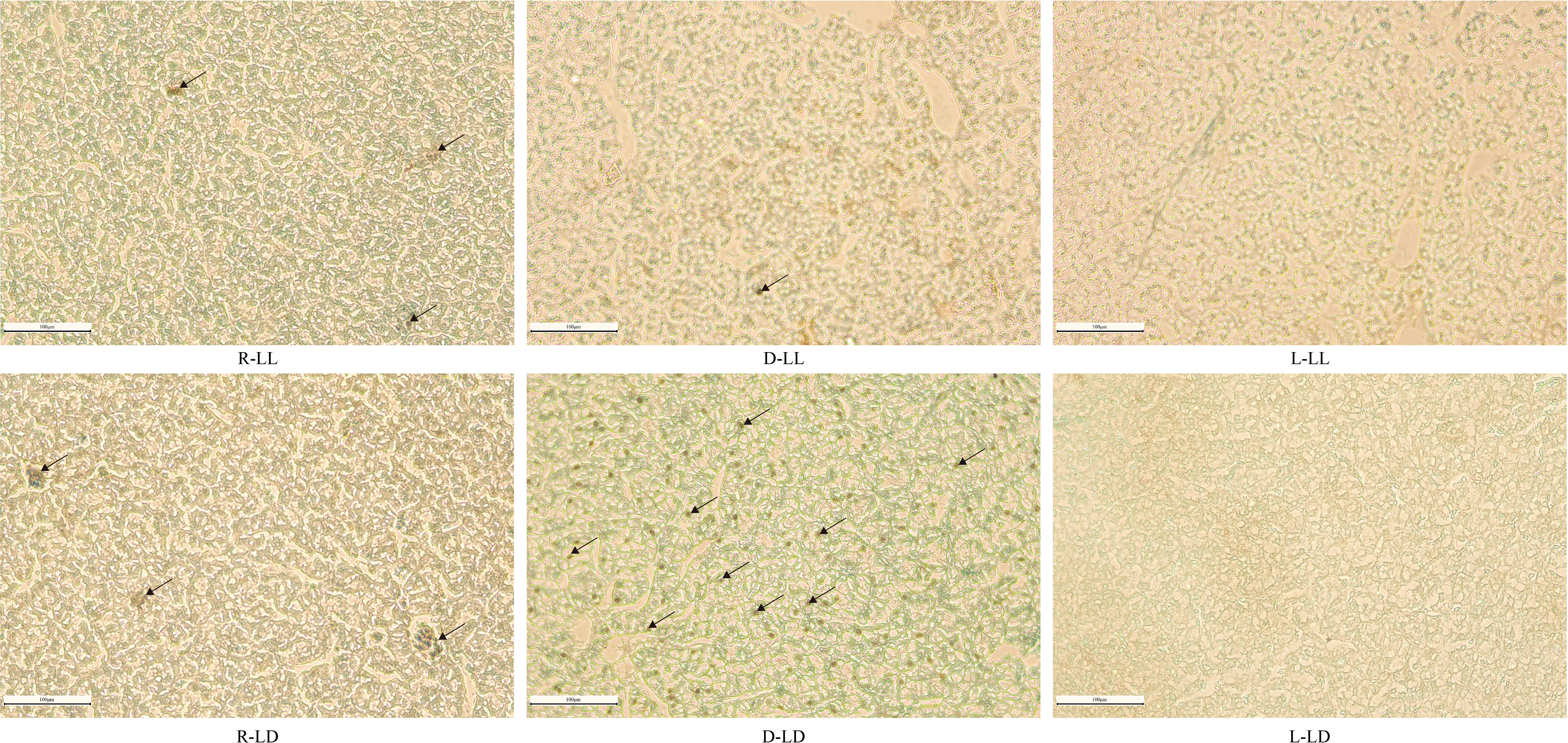
Figure 9 TUNEL staining analysis of liver in juvenile Oncorhynchus mykiss under different experimental treatments (200 × magnification, scale bars = 100 μm, slice thickness = 7 μm). R: random feeding, D: mid-dark stage feeding, L: mid-light stage feeding, LL: 24L: 0D, LD: 12L: 12D. The black arrows point to apoptotic cells.
Discussion
Blood indicators closely relate to fish’s physiological, metabolic, and health status. When external environmental factors impact fish, they are usually reflected in blood indicators (Casanovas et al., 2021; Witeska et al., 2022). Some serum enzymes and metabolites are widely used as a reflection of liver functional statuses in fish, such as alkaline phosphatase (AKP, Casillas and Ames, 1985), acid phosphatase (ACP, Molayemraftar et al., 2022), alanine aminotransferase (ALT, Kim and Kang, 2016; Zhang et al., 2016), aspartate aminotransferase (AST, Kim and Kang, 2016; Zhang et al., 2016), lactate dehydrogenase (LDH, Rahman et al., 2022) and albumin (ALB, Mukherjee et al., 2022). In the present study, ALT was only significantly higher in the D-LD group than in the L-LD group. High levels of ALT in serum are considered a marker of fish liver damage (Hong et al., 2022). Therefore, this study showed that nocturnal feeding led to liver damage in juvenile rainbow trout under an LD environment. In addition, under LL, significantly low levels of AST were observed in the L group. Like ALT, high levels of AST in serum also reflect liver damage (Hong et al., 2022). This suggests that under LL conditions, scheduled diurnal feeding can alleviate liver damage in juvenile rainbow trout. In addition, significantly low ALB was detected in the random feeding group, suggesting that random feeding may cause stress to juvenile rainbow trout. Similarly, under chronic high salt stress, African catfish (Clarias gariepinus) serum levels of ALT and AST were increased, and ALB levels were decreased (Dawood et al., 2022).
Like mammals, fish’s immune system includes both cellular and humoral immunity. A significantly higher total blood cell count (TBC) was observed in the scheduled feeding group, especially in the LL environment. Blood cells are an important component of the immune system in fish, and they play an irreplaceable role in innate and adaptive immunity (Kordon et al., 2018; Zhu and Su, 2022). High levels of TBC respond to a stronger immune function. Fish humoral immunity consists mainly of immunoglobulin and complements (Abdollahpour et al., 2021). Immunoglobulin M (IgM) is the dominant immunoglobulin in fish serum and is the first line of defense in the adaptive immune response (Xia et al., 2014; Mashoof and Criscitiello, 2016). The complement system is an important component of innate immunity in teleost and plays a role in adaptive immunity as a bridge between innate and adaptive immunity (Bavia et al., 2022). IgM triggers the classical pathway of complement activation, and the immune complex of antigen, IgM, and activated complement 3 (C3) significantly enhances the B-cell antibody response (Boes, 2000). In the complement system, C3 is the central component that plays an important role (Meng et al., 2019). Also, complement 4 (C4) plays an important role in complement activation and humoral defense (Holland and Lambris, 2002). Previous studies have shown a rapid increase in serum C3 in rainbow trout exposed to acute stress (Demers and Bayne, 2020). When grass carp (Ctenopharyngodon idella) are infested with Aeromonas hydrophila, the level of C3 increases to help repair damaged tissues (Meng et al., 2019). On the other hand, chronic stress also resulted in increased IgM and C3 levels in northern snakehead (Channa argus) serum (Li et al., 2019a; Li et al., 2019b) and common carp (Cyprinus carpio) liver (Qiu et al., 2018). Similarly, chronic high concentrations of thyroxine treatment induced stress in the sterlet sturgeon (Acipenser ruthenus) (significant high levels of serum cortisol, glucose, and lactate) while causing an increase in serum IgM, C3, and C4 levels (Abdollahpour et al., 2021). The present study observed high serum IgM, C3, and C4 levels in the LL, R, and D groups. The above results suggested that long-term exposure to LL and random/nocturnal feeding may be a stressor for juvenile rainbow trout and affect the immune system.
Cytokines are small molecular glycoproteins secreted by immune cells and play an important role in the innate immune response (Sakai et al., 2021). In northern snakehead (Channa argus), long-term exposure to selenium resulted in the upregulation of il-1β, il-8, and tnf-α genes expression (Li et al., 2019a; Li et al., 2019b). When pufferfish (Takifugu rubripes) were treated with lipopolysaccharide or polyinosinic-polycytidylic acid, the expression of il-1β, il-6, and tnf-α genes showed a significant increase (Sakai et al., 2021). Similarly, Chronic exposure to the ionic liquid [C8mim] Br resulted in increased levels of il-1β, il-6, and tnf-α in silver carp (Hypophthalmichthys molitrix) spleen (Ma et al., 2019). The above results suggest that the cytokine gene expression would be upregulated in some fish under stress. In this study, LL upregulated the expression of cytokine genes in the liver of juvenile rainbow trout. In addition, cytokine gene expression in the liver and intestine was significantly upregulated in the D-LD group. This result further indicated that LL and nocturnal feeding might cause stress to juvenile rainbow trout.
Photoperiodic changes are considered a chronic stressor in fish (Valenzuela et al., 2022). In the present study, the malondialdehyde (MDA) content in the liver of the LL group was significantly higher than that of the LD, while the catalase (CAT) activity was significantly lower than that of the LD. Similar results were reported for European Sea Bass (Dicentrachus labrax L.) (Li et al., 2021) and largemouth bass (Micropterus salmoides) (Malinovskyi et al., 2022). In addition, the feeding regime also affected the liver MDA content. Under LL, the D group obtained significantly higher MDA and lowest superoxide dismutase (SOD) activity, while under LD, the R group obtained significantly higher MDA. A significantly lower MDA was observed in the intestine under both photoperiods in the L group. Feeding that does not match its feeding rhythm has been found to negatively impact animal health and welfare (Oishi and Hashimoto, 2018), and rainbow trout are typically diurnal feeders (Iigo and Tabata, 1997; Bolliet et al., 2001). In addition, random feeding has been widely reported as a stressor in fish (Sánchez et al., 2009; Saiz et al., 2021). An unsuitable environment is a challenge and a stressor for fish. Anti-stress is a highly energy-consuming process (Chen et al., 2021), and fish need to satisfy additional energy requirements by increasing their energy metabolic rate (De Boeck et al., 2000; Lu et al., 2018). As a natural sub-product of cellular respiration, reactive oxygen species (ROS) production in mitochondria is also elevated under stress conditions (Luo et al., 2006; Mailloux, 2020). ROS is a highly reactive molecule; under normal conditions, organisms have a complete antioxidant system, including SOD and CAT, that can effectively scavenge ROS (Hoseinifar et al., 2020). MDA is a product of lipid peroxidation and is widely used to reflect the degree of oxidative damage in fish (Mendes et al., 2009; Sun et al., 2022). The above results suggest that long-term exposure to LL and random/nocturnal feeding can cause oxidative damage to juvenile rainbow trout.
However, the opposite result was observed in the serum, with significantly lower MDA levels in the random feeding group than in the scheduled feeding group (D and L). One possible reason relates to the unsaturation of fatty acids in the serum, with more unsaturated fatty acids being more susceptible to oxidation (Lynn and Brown, 1959; Zhang et al., 2007), and we detected a higher ratio of monounsaturated and polyunsaturated fatty acids in the serum of the scheduled feeding group (data in a separate unpublished article). Additional evidence was found for significantly higher serum total antioxidant capacity (T-AOC) and SOD activities in the scheduled feeding group, suggesting that the antioxidant capacity was not limited in the scheduled feeding group.
Severe oxidative stress often causes cellular damage and apoptosis (Chandra et al., 2000). Two main pathways initiate apoptosis, the endogenous pathway (mitochondrial pathway) and the exogenous pathway (death receptor pathway). The endogenous apoptotic process begins with the release of cytochrome C (Cytc) from the mitochondria into the cytoplasm induced by cell death signals, which activates caspase and initiates the apoptotic program (Cheng et al., 2015). The exogenous pathway is activated by tumor necrosis factor (TNF) family receptors, which receive extracellular death signals and activate downstream caspase to initiate apoptosis (Gao et al., 2013). In addition, BCL-2 and BAX regulate apoptosis by affecting the release of mitochondrial Cytc during apoptosis (Cory and Adams, 2002). BAX is regulated by upstream p53 (Liu et al., 2005). p53 repairs cell DNA damage or induces cancer cell apoptosis (Polyak et al., 1997; Fridman and Lowe, 2003). In addition, p53 affects the transcription of gpx and mnsod genes in response to oxidative stress (Hussain et al., 2004). In this study, liver cytc gene expression was upregulated in the R group, and bcl2 gene expression was upregulated in the D-LD group. Under LL, the expression of p53 and bax genes was significantly higher in the L group than in the R group. This result indicated that the livers of the R and D-LD groups were subjected to oxidative stress and initiated the process of apoptosis. And the higher expression of the p53 gene may reflect the repair of damaged cells. A similar expression trend to p53 was also reflected in the expression of antioxidant enzyme genes gpx, sod1, sod2, and cat, which further supported that the scheduled feeding (especially the L group) mitigated oxidative damage by increasing the expression of antioxidant enzyme genes, thereby reducing apoptosis.
Under LL, p53 and bax gene expression in the intestine was significantly higher in the L group than in the R group. However, the opposite phenomenon appeared under LD, with the lowest p53 expression level in the L group. The antioxidant genes sod1 and sod2 showed a similar trend under LD. The lowest MDA levels were detected in the L-LD group, which indicated that the rainbow trout in this group were subjected to minimal oxidative stress and therefore, low levels of p53, sod1, and sod2 gene expression also indicated that the fish were in a low-stress state. Similarly, the expression levels of casp6 and casp8 genes were significantly lower in the L-LD group.
Further H&E staining of liver paraffin sections revealed the presence of different degrees of pathological changes between the different groups. The R and D groups had significant capillary dilatation and erythrocyte sludge. This result suggested that random and nocturnal feeding could damage the liver of juvenile rainbow trout. On the other hand, TUNEL staining was used to detect apoptosis in liver cells (Liu et al., 2022), and it was found that a large number of apoptotic cells were observed in the D-LD group, and almost no apoptotic cells were observed in L-LL and L-LD. Overall, the histological observations of liver sections demonstrated that random and nocturnal feeding caused damage to the liver of rainbow trout, resulting in apoptosis. In contrast, there was no liver damage or apoptosis in the diurnal feeding group.
Conclusions
In this study, chronic exposure to LL caused oxidative stress in juvenile rainbow trout and affected liver health and immune system function. On the other hand, random and nocturnal feeding was also a stressor for juvenile rainbow trout, causing oxidative damage and apoptosis. The oxidative damage of LL was mitigated by scheduled diurnal feeding. Therefore, scheduled diurnal feeding could mitigate oxidative damage to rainbow trout from the lack of a photoperiodic environment. Further studies are needed to elucidate the biological clock mechanisms underlying the effects of photoperiod and feeding time on the health and welfare of juvenile rainbow trout.
Data availability statement
The raw data supporting the conclusions of this article will be made available by the authors, without undue reservation.
Ethics statement
The animal study was reviewed and approved by Animal Care and Use Committee of Ningbo University.
Author contributions
HX: methodology, data curation, visualization, and writing - original draft; CS: conceptualization and review and editing; YY, CW, CS, and CM: resources, review and editing, and supervision. All authors contributed to the article and approved the submitted version.
Funding
This study was supported by the National Key Research and Development Program of China (Project No. 2019YFD0901000), The Province Key Research and Development Program of Zhejiang (Project No. 2021C02047), and K.C. Wong Magna Fund in Ningbo University.
Acknowledgments
The authors thank Professor Herve Migaud for his constructive comments on this research.
Conflict of interest
The authors declare that the research was conducted in the absence of any commercial or financial relationships that could be construed as a potential conflict of interest.
Publisher’s note
All claims expressed in this article are solely those of the authors and do not necessarily represent those of their affiliated organizations, or those of the publisher, the editors and the reviewers. Any product that may be evaluated in this article, or claim that may be made by its manufacturer, is not guaranteed or endorsed by the publisher.
Supplementary material
The Supplementary Material for this article can be found online at: https://www.frontiersin.org/articles/10.3389/fmars.2022.1036289/full#supplementary-material
References
Abdollahpour H., Falahatkar B., van der Kraak G. (2021). The effects of long-term thyroxine administration on hematological, biochemical and immunological features in sterlet sturgeon (Acipenser ruthenus). Aquaculture 544, 737065. doi: 10.1016/j.aquaculture.2021.737065
Bavia L., Santiesteban-Lores L. E., Carneiro M. C., Prodocimo M. M. (2022). Advances in the complement system of a teleost fish, Oreochromis niloticus. Fish Shellfish Immunol. 123, 61–74. doi: 10.1016/j.fsi.2022.02.013
Boes M. (2000). Role of natural and immune IgM antibodies in immune responses. Mol. Immunol. 37 (18), 1141–1149. doi: 10.1016/S0161-5890(01)00025-6
Bolliet V., Aranda A., Boujard T. (2001). Demand-feeding rhythm in rainbow trout and European catfish-synchronisation by photoperiod and food availability. Physiol. Behav. 73 (4), 625–633. doi: 10.1016/S0031-9384(01)00505-4
Casanovas P., Walker S. P., Johnston H., Johnston C., Symonds J. E. (2021). Comparative assessment of blood biochemistry and haematology normal ranges between Chinook salmon (Oncorhynchus tshawytscha) from seawater and freshwater farms. Aquaculture 537, 736464. doi: 10.1016/j.aquaculture.2021.736464
Casillas E., Ames W. (1985). Hepatotoxic effects of CCl4 on English sole (Parophrys vetulus): possible indicators of liver dysfunction. Comp. Biochem. Physiol. C Comp. Pharmacol. Toxicol. 84 (2), 397–400. doi: 10.1016/0742-8413(86)90112-X
Chaix A., Lin T., Le H. D., Chang M. W., Panda S. (2019). Time-restricted feeding prevents obesity and metabolic syndrome in mice lacking a circadian clock. Cell Metab. 29 (2), 303–319. doi: 10.1016/j.cmet.2018.08.004
Chandra J., Samali A., Orrenius S. (2000). Triggering and modulation of apoptosis by oxidative stress. Free Radical Biol. Med. 29 (3-4), 323–333. doi: 10.1016/S0891-5849(00)00302-6
Cheng C. H., Yang F. F., Liao S. A., Miao Y. T., Ye C. X., Wang A. L., et al. (2015). High temperature induces apoptosis and oxidative stress in pufferfish (Takifugu obscurus) blood cells. J. Thermal Biol. 53, 172–179. doi: 10.1016/j.jtherbio.2015.08.002
Chen S. J., Migaud H., Shi C., Song C. B., Wang C. L., Ye Y. F., et al. (2021). Light intensity impacts on growth, molting and oxidative stress of juvenile mud crab Scylla paramamosain. Aquaculture 545, 737159. doi: 10.1016/j.aquaculture.2021.737159
Chu Y. I., Wang C. M., Parka J. C., Lader P. F. (2020). Review of cage and containment tank designs for offshore fish farming. Aquaculture 519, 734928. doi: 10.1016/j.aquaculture.2020.734928
Cory S., Adams J. M. (2002). The BCL2 family: Regulators of the cellular life-or-death switch. Nat. Rev. Cancer 2 (9), 647–656. doi: 10.1038/nrc883
Dawood M. A. O., Noreldin A. E., Sewilam H. (2022). Blood biochemical variables, antioxidative status, and histological features of intestinal, gill, and liver tissues of African catfish (Clarias gariepinus) exposed to high salinity and high-temperature stress. Environ. Sci. pollut. Res. 29, 56357–56369. doi: 10.1007/s11356-022-19702-0
De Boeck G., Vlaeminck A., van der Linden A., Blust R. (2000). The energy metabolism of common carp (Cyprinus carpio) when exposed to salt stress: An increase in energy expenditure or effects of starvation? Physiol. Biochem. Zool. 73 (1), 102–111. doi: 10.1086/316717
Demers N., Bayne C. (2020). Immediate increase of plasma protein complement C3 in response to an acute stressor. Fish Shellfish Immunol. 107, 411–413. doi: 10.1016/j.fsi.2020.10.022
Dong S. L. (2019). Researching progresses and prospects in large salmonidae farming in cold water mass of yellow Sea. Periodical Ocean Univ. China 49 (03), 1–6. doi: 10.16441/j.cnki.hdxb.20180303
Esteban M. A., Cuesta A., Rodriguez A., Meseguer J. (2006). Effect of photoperiod on the fish innate immune system: a link between fish pineal gland and the immune system. J. Pineal Res. 41 (3), 261–266. doi: 10.1111/j.1600-079X.2006.00362.x
FAO (2022). The state of world fisheries and aquaculture 2022. towards blue transformation (Rome: FAO). doi: 10.4060/cc0461en
Ferain A., Delbecque E., Neefs I., Dailly H., De Saeyer N., Van Larebeke M., et al. (2021). Interplay between dietary lipids and cadmium exposure in rainbow trout liver: Influence on fatty acid metabolism, metal accumulation and stress response. Aquat. Toxicol. 231, 105676. doi: 10.1016/j.aquatox.2020.105676
Fjelldal P. G., Hansen T., Breck O., Ørnsrud R., Lock E. J., Waagbø R., et al. (2012). Vertebral deformities in farmed Atlantic salmon (Salmo salar l.) - etiology and pathology. J. Appl. Ichthyol. 28 (3), 433–440. doi: 10.1111/j.1439-0426.2012.01980.x
Fjelldal P. G., Nordgarden U., Berg A., Grotmol S., Totland G. K., Wargelius A., et al. (2005). Vertebrae of the trunk and tail display different growth rates in response to photoperiod in Atlantic salmon, Salmo salar l., post-smolts. Aquaculture 250 (1-2), 516–524. doi: 10.1016/j.aquaculture.2005.04.056
Fontagné-Dicharry S., Véron V., Larroquet L., Godin S., Wischhusen P., Aguirre P., et al. (2020). Effect of selenium sources in plant-based diets on antioxidant status and oxidative stress-related parameters in rainbow trout juveniles under chronic stress exposure. Aquaculture 529, 735684. doi: 10.1016/j.aquaculture.2020.735684
Fridman J. S., Lowe S. W. (2003). Control of apoptosis by p53. Oncogene 22, 9030–9040. doi: 10.1038/sj.onc.1207116
Gao D., Xu Z. E., Zhang X. Y., Zhu C. C., Wang Y. N., Min W. P. (2013). Cadmium triggers kidney cell apoptosis of purse red common carp (Cyprinus carpio) without caspase-8 activation. Dev. Comp. Immunol. 41 (4), 728–737. doi: 10.1016/j.dci.2013.08.004
Holland M. C. H., Lambris J. D. (2002). The complement system in teleosts. Fish Shellfish Immunol. 12 (5), 399–420. doi: 10.1006/fsim.2001.0408
Hong H. R., Liu Z., Li S. Q., Wu D., Jiang L. Q., Li P. X., et al. (2022). Zinc oxide nanoparticles (ZnO-NPs) exhibit immune toxicity to crucian carp (Carassius carassius) by neutrophil extracellular traps (NETs) release and oxidative stress. Fish shellfish Immunol. 129, 22–29. doi: 10.1016/j.fsi.2022.07.025
Hoseinifar S. H., Yousefi S., Van Doan H., Ashouri G., Gioacchini G., Maradonna F., et al. (2020). Oxidative stress and antioxidant defense in fish: the implications of probiotic, prebiotic, and synbiotics. Rev. Fisheries Sci. Aquaculture 29 (2), 198–217. doi: 10.1080/23308249.2020.1795616
Huang M., Yang X. G., Zhou Y. G., Ge J., Davis D. A., Dong Y. W., et al. (2021). Growth, serum biochemical parameters, salinity tolerance and antioxidant enzyme activity of rainbow trout (Oncorhynchus mykiss) in response to dietary taurine levels. Mar. Life Sci. Technol. 3 (4), 449–462. doi: 10.1007/s42995-020-00088-2
Hussain S. P., Amstad P., He P. J., Robles A., Lupold S., Kaneko I., et al. (2004). p53-induced up-regulation of MnSOD and GPx but not catalase increases oxidative stress and apoptosis. Cancer Res. 64 (7), 2350–2356. doi: 10.1158/0008-5472.CAN-2287-2
Iigo M., Tabata M. (1997). Circadian rhythms of locomotor activity in the rainbow trout Oncorhynchus mykiss. Fisheries Sci. 63 (1), 77–80. doi: 10.2331/fishsci.63.77
Iturriaga M., Espinoza M. B., Poblete-Morales M., Feijoo C. G., Reyesa A. E., Molina A., et al. (2017). Cytotoxic activity of Flavobacterium psychrophilum in skeletal muscle cells of rainbow trout (Oncorhynchus mykiss). Veterinary Microbiol. 210, 101–106. doi: 10.1016/j.vetmic.2017.09.009
Kim J. H., Kang J. C. (2016). Changes in hematological parameters, plasma cortisol, and acetylcholinesterase of juvenile rockfish, Sebastes schlegelii supplemented with the dietary ascorbic acid. Aquac. Rep. 4, 80–85. doi: 10.1016/j.aqrep.2016.07.001
Kordon A. O., Karsi A., Pinchuk L. (2018). Innate immune responses in fish: Antigen presenting cells and professional phagocytes. Turkish J. Fisheries Aquat. Sci. 18 (9), 1123–1139. doi: 10.4194/1303-2712-v18_9_11
Landless P. J. (1976). Acclimation of rainbow trout to sea water. Aquaculture 7 (2), 173–179. doi: 10.1016/0044-8486(76)90006-5
Lazado C. C., Gesto M., Madsen L., Jokumsen A. (2018). Interplay between daily rhythmic serum-mediated bacterial killing activity and immune defence factors in rainbow trout (Oncorhynchus mykiss). Fish Shellfish Immunol. 72, 418–425. doi: 10.1016/j.fsi.2017.11.025
Lazado C. C., Nayak S., Khozin-Goldberg I., Zilberg D. (2019). The gut mucosal barrier of zebrafish (Danio rerio) responds to the time-restricted delivery of Lobosphaera incisa-enriched diets. Fish Shellfish Immunol. 89, 368–377. doi: 10.1016/j.fsi.2019.04.012
Li M. Y., Guo W. Q., Guo G. L., Zhu X. M., Niu X. T., Shan X. F., et al. (2019b). Effect of sub-chronic exposure to selenium and Allium mongolicum regel flavonoids on Channa argus: Bioaccumulation, oxidative stress, immune responses and immune-related signaling molecules. Fish Shellfish Immunol. 91, 122–129. doi: 10.1016/j.fsi.2019.05.002
Liu Z. H., Lu H. M., Shi H. L., Du Y. C., Yu J., Gu S., et al. (2005). PUMA overexpression induces reactive oxygen species generation and proteasome-mediated stathmin degradation in colorectal cancer cells. Cancer Res. 65, 1647–1654. doi: 10.1158/0008-5472.CAN-04-1754
Liu E. G., Zhao X. Q., Li C. J., Wang Y. F., Li L. L., Zhu H., et al. (2022). Effects of acute heat stress on liver damage, apoptosis and inflammation of pikeperch (Sander lucioperca). J. Thermal Biol. 106, 103251. doi: 10.1016/j.jtherbio.2022.103251
Livak K. J., Schmittgen T. D. (2001). Analysis of relative gene expression data using real-time quantitative PCR and the 2-△△CT method. Methods 25 (4), 402–408. doi: 10.1006/meth.2001.1262
Li X., Wei P. P., Liu S. T., Tian Y., Ma H., Liu Y. (2021). Photoperiods affect growth, food intake and physiological metabolism of juvenile European Sea bass (Dicentrachus labrax l.). Aquaculture Rep. 20, 100656. doi: 10.1016/j.aqrep.2021.100656
Li M. Y., Zhu X. M., Tian J. X., Liu M., Wang G. Q. (2019a). Bioaccumulation, oxidative stress, immune responses and immune-related genes expression in northern snakehead fish, channa argus, exposure to waterborne selenium. Mol. Biol. Rep. 46 (1), 947–955. doi: 10.1007/s11033-018-4550-8
López-Olmeda J. F. (2017). Nonphotic entrainment in fish. Comp. Biochem. Physiol. Part A 203, 133–143. doi: 10.1016/j.cbpa.2016.09.006
Lu Y. L., Nie M. M., Wang L., Xiong Y. H., Wang F., Wang L. J., et al. (2018). Energy response and modulation of AMPK pathway of the olive flounder Paralichthys olivaceus in low-temperature challenged. Aquaculture 484, 205–213. doi: 10.1016/j.aquaculture.2017.11.031
Luo Y., Su Y., Lin R. Z., Shi H. H., Wang X. R. (2006). 2-chlorophenol induced ROS generation in fish carassius auratus based on the EPR method. Chemosphere 65 (6), 1064–1073. doi: 10.1016/j.chemosphere.2006.02.054
Lynn W. S., Brown R. H. (1959). Oxidation and activation of unsaturated fatty acids. Arch. Biochem. Biophys. 81 (2), 353–362. doi: 10.1016/0003-9861(59)90213-9
Ma J. G., Chen X., Xin G. Y., Li X. Y. (2019). Chronic exposure to the ionic liquid [C8 mim] br induces inflammation in silver carp spleen: Involvement of oxidative stress-mediated p38MAPK/NF-kappa b signalling and microRNAs. Fish Shellfish Immunol. 84, 627–638. doi: 10.1016/j.fsi.2018.09.052
Mailloux R. J. (2020). An update on mitochondrial reactive oxygen species production. Antioxidants 9, 472. doi: 10.3390/antiox9060472
Malinovskyi O., Rahimnejad S., Stejskal V., Boňko D., Stará A., Velíšek J., et al. (2022). Effects of different photoperiods on growth performance and health status of largemouth bass (Micropterus salmoides) juveniles. Aquaculture 548, 737631. doi: 10.1016/j.aquaculture.2021.737631
Mashoof S., Criscitiello M. F. (2016). Fish immunoglobulins. Biology 5, 45. doi: 10.3390/biology5040045
Mendes R., Cardoso C., Pestana C. (2009). Measurement of malondialdehyde in fish: A comparison study between HPLC methods and the traditional spectrophotometric test. Food Chem. 112 (4), 1038–1045. doi: 10.1016/j.foodchem.2008.06.052
Meng X. Z., Shen Y. B., Wang S. T., Xu X. Y., Dang Y. F., Zhang M., et al. (2019). Complement component 3 (C3): An important role in grass carp (Ctenopharyngodon idella) experimentally exposed to Aeromonas hydrophila. Fish Shellfish Immunol. 88, 189–197. doi: 10.1016/j.fsi.2019.02.061
Molayemraftar T., Peyghan R., Jalali M. R., Shahriari A. (2022). Single and combined effects of ammonia and nitrite on common carp, Cyprinus carpio: Toxicity, hematological parameters, antioxidant defenses, acetylcholinesterase, and acid phosphatase activities. Aquaculture 548, 737676. doi: 10.1016/j.aquaculture.2021.737676
Montero R., Strzelczyk J. E., Chan J. T. H., Verleih M., Rebl A., Goldammer T., et al. (2020). Dawn to dusk: Diurnal rhythm of the immune response in rainbow trout (Oncorhynchus mykiss). Biology-Basel 9 (1), 8. doi: 10.3390/biology9010008
Mukherjee D., Saha S., Chukwuka A. V., Ghosh B., Dhara K., Saha N. C., et al. (2022). Antioxidant enzyme activity and pathophysiological responses in the freshwater walking catfish, Clarias batrachus Linn under sub-chronic and chronic exposures to the neonicotinoid, thiamethoxam®. Sci. Total Environ. 836, 155716. doi: 10.1016/j.scitotenv.2022.155716
Mustapha M. K., Oladokun O. T., Salman M. M., Adeniyi I. A., Ojo D. (2014). Does light duration (photoperiod) have an effect on the mortality and welfare of cultured Oreochromis niloticus and Clarias gariepinus? Turkish J. Zool. 38 (4), 466–470. doi: 10.3906/zoo-1309-23
Nisembaum L. G., Velarde E., Tinoco A. B., Azpeleta C., de Pedro N., Alonso-Gómez A. L., et al. (2012). Light-dark cycle and feeding time differentially entrains the gut molecular clock of the goldfish (Carassius auratus). Chronobiol. Int. 29, 665–673. doi: 10.3109/07420528.2012.686947
Oishi K., Hashimoto C. (2018). Short-term time-restricted feeding during the resting phase is sufficient to induce leptin resistance that contributes to development of obesity and metabolic disorders in mice. Chronobiol. Int. 35 (11), 1576–1594. doi: 10.1080/07420528.2018.1496927
Panserat S., Plagnes-Juan E., Gazzola E., Palma M., Magnoni L. J., Marandel L., et al. (2020). Hepatic glycerol metabolism-related genes in carnivorous rainbow trout (Oncorhynchus mykiss): insights into molecular characteristics, ontogenesis, and nutritional regulation. Front. Physiol. 11. doi: 10.3389/fphys.2020.00882
Pan L. Q., Xie P., Hu F. W. (2010). Responses of prophenoloxidase system and related defence parameters of Litopenaeus vannamei to low salinity. J. Ocean Univ. China 9 (3), 273–278. doi: 10.1007/s11802-010-1711-3
Polyak K., Xia Y., Zweier J. L., Kinaler K. W., Vogelstein B. (1997). A model for p53-induced apoptosis. Nature 389 (6648), 300–305. doi: 10.1038/38525
Qiu W. H., Zhan H. Y., Tian Y. Q., Zhang T., He X., Luo S. S., et al. (2018). The in vivo action of chronic bisphenol f showing potential immune disturbance in juvenile common carp (Cyprinus carpio). Chemosphere 205, 506–513. doi: 10.1016/j.chemosphere.2018.04.105
Rahman A. N. A., Mohamed A. A., Dahran N., Farag M. F. M., Alqahtani L. S., Nassan M. A., et al. (2022). Appraisal of sub-chronic exposure to lambada-cyhalothrin and/or methomyl on the behavior and hepato-renal functioning in Oreochromis niloticus: Supportive role of taurine-supplemented feed. Aquat. Toxicol. 250, 106257. doi: 10.1016/j.aquatox.2022.106257
Ren Y. C., Men X. H., Yu Y., Li B., Zhou Y. G., Zhao C. Y. (2022). Effects of transportation stress on antioxidation, immunity capacity and hypoxia tolerance of rainbow trout (Oncorhynchus mykiss). Aquaculture Rep. 22, 100940. doi: 10.1016/j.aqrep.2021.100940
Ren D. L., Zhang J. L., Yang L. Q., Wang X. B., Wang Z. Y., Huang D. F., et al. (2018). Circadian genes period1b and period2 differentially regulate inflammatory responses in zebrafish. Fish Shellfish Immunol. 77, 139–146. doi: 10.1016/j.fsi.2018.03.048
Saiz N., Gomez-Boronat M., De Pedro N., Delgado M. J., Isorna E. (2021). The lack of light-dark and feeding-fasting cycles alters temporal events in the goldfish (Carassius auratus) stress axis. Animals 11 (3), 669. doi: 10.3390/ani11030669
Sakai M., Hikima J., Kono T. (2021). Fish cytokines: current research and applications. Fisheries Sci. 87 (1), 1–9. doi: 10.1007/s12562-020-01476-4
Sánchez J. A., López-Olmeda J. F., Blanco-Vives B., Sánchez-Vázquez F. J. (2009). Effects of feeding schedule on locomotor activity rhythms and stress response in sea bream. Physiol. Behav. 98 (1-2), 125–129. doi: 10.1016/j.physbeh.2009.04.020
Shepherd B. S., Drennon K., Johnson J., Nichols J. W., Playle R. C., Singer T. D., et al. (2005). Salinity acclimation affects the somatotropic axis in rainbow trout. Am. J. Physiol.-Regul. Integr. Comp. Physiol. 288 (5), R1385–R1395. doi: 10.1152/ajpregu.00443.2004
Stephan F. K. (2002). The “other” circadian system: food as zeitgeber. J. Biol. Rhythms 17 (4), 284–292. doi: 10.1177/074873002129002591
Sun X. Q., Zhao X., Li L., Gao Q. F., Dong S. L. (2022). Growth, oxidative stress and the non-specific immune response of Oncorhynchus mykiss exposed to different light spectra. Aquaculture Res. 53 (11), 4210–4216. doi: 10.1111/are.15915
Valenzuela A., Rodríguez I., Schulz B., Cortés R., Acosta J., Campos V., et al. (2022). Effects of continuous light (LD24:0) modulate the expression of lysozyme, mucin and peripheral blood cells in rainbow trout. Fishes 7 (1), 28. doi: 10.3390/fishes7010028
Wargelius A., Fjelldal P. G., Nordgarden U., Hansen T. (2009). Continuous light affects mineralization and delays osteoid incorporation in vertebral bone of Atlantic salmon (Salmo salar l.). J. Exp. Biol. 212 (5), 656–661. doi: 10.1242/jeb.024000
Witeska M., Kondera E., Ługowska K., Bojarski B. (2022). Hematological methods in fish - not only for beginners. Aquaculture 547, 737498. doi: 10.1016/j.aquaculture.2021.737498
Xia H., Wu K., Liu W. J., Gul Y., Wang W. M., Zhang X. Z. (2014). Molecular cloning and expression analysis of immunoglobulin m heavy chain gene of blunt snout bream (Megalobrama amblycephala). Fish Shellfish Immunol. 40 (1), 129–135. doi: 10.1016/j.fsi.2014.06.026
Xu H. Y., Dou J., Wu Q. Y., Ye Y. F., Song C. B., Mu C. K., et al. (2022). Investigation of the light intensity effect on growth, molting, hemolymph lipid, and antioxidant capacity of juvenile swimming crab Portunus trituberculatus. Front. Mar. Sci. 9. doi: 10.3389/fmars.2022.922021
Yada T., Azuma T., Takagi Y. (2001). Stimulation of non-specific immune functions in seawater-acclimated rainbow trout, Oncorhynchus mykiss, with reference to the role of growth hormone. Comp. Biochem. Physiol. Part B: Biochem. Mol. Biol. 129, 695–701. doi: 10.1016/S1096-4959(01)00370-0
Yamamuro D., Takahashi M., Nagashima S., Wakabayashi T., Yamazaki H., Takei A., et al. (2020). Peripheral circadian rhythms in the liver and white adipose tissue of mice are attenuated by constant light and restored by time- restricted feeding. PloS One 15 (6), e0234439. doi: 10.1371/journal.pone.0234439
Zante M. D., Borchel A., Brunner R. M., Goldammer T., Rebl A. (2015). Cloning and characterization of the proximal promoter region of rainbow trout (Oncorhynchus mykiss) interleukin-6 gene. Fish Shellfish Immunol. 43 (1), 249–246. doi: 10.1016/j.fsi.2014.12.026
Zeng C., Hou Z. S., Zhao H. K., Xin Y. R., Liu M. Q., Yang X. D., et al. (2021). Identification and characterization of caspases genes in rainbow trout (Oncorhynchus mykiss) and their expression profiles after Aeromonas salmonicida and Vibrio anguillarum infection. Dev. Comp. Immunol. 118, 103987. doi: 10.1016/j.dci.2020.103987
Zhang C. X., Huang F., Li J., Wang L., Song K., Mai K. S. (2016). Interactive effects of dietary magnesium and vitamin e on growth performance, body composition, blood parameters and antioxidant status in Japanese seabass (Lateolabrax japonicus) fed oxidized oil. Aquac. Nutr. 22, 708–722. doi: 10.1111/anu.12393
Zhang W. H., Shi B., Shi J. (2007). A theoretical study on autoxidation of unsaturated fatty acids and antioxidant activity of phenolic compounds. J. Am. Leather Chem. Assoc. 102 (3), 99–105.
Zhou Z. Y., Zhou B., Chen H. M., Tang X. X., Wang Y. (2019). Reactive oxygen species (ROS) and the calcium-(Ca2+) mediated extrinsic and intrinsic pathways underlying BDE-47-induced apoptosis in rainbow trout (Oncorhynchus mykiss) gonadal cells. Sci. Total Environ. 656, 778–788. doi: 10.1016/j.scitotenv.2018.11.306
Keywords: photoperiod, diurnal feeding, oxidative damage, apoptosis, rainbow trout
Citation: Xu H, Shi C, Ye Y, Mu C and Wang C (2022) Effects of different photoperiods and feeding regimes on immune response, oxidative status, and tissue damage in juvenile rainbow trout (Oncorhynchus mykiss). Front. Mar. Sci. 9:1036289. doi: 10.3389/fmars.2022.1036289
Received: 04 September 2022; Accepted: 27 October 2022;
Published: 10 November 2022.
Edited by:
Amalia Pérez-Jiménez, University of Granada, SpainReviewed by:
Ishtiyaq Ahmad, University of Kashmir, IndiaCristina Trenzado, University of Granada, Spain
Patricia Diaz-Rosales, Centro de Investigación en Sanidad Animal, CISA (INIA-CSIC), Spain
Copyright © 2022 Xu, Shi, Ye, Mu and Wang. This is an open-access article distributed under the terms of the Creative Commons Attribution License (CC BY). The use, distribution or reproduction in other forums is permitted, provided the original author(s) and the copyright owner(s) are credited and that the original publication in this journal is cited, in accordance with accepted academic practice. No use, distribution or reproduction is permitted which does not comply with these terms.
*Correspondence: Ce Shi, shice3210@126.com